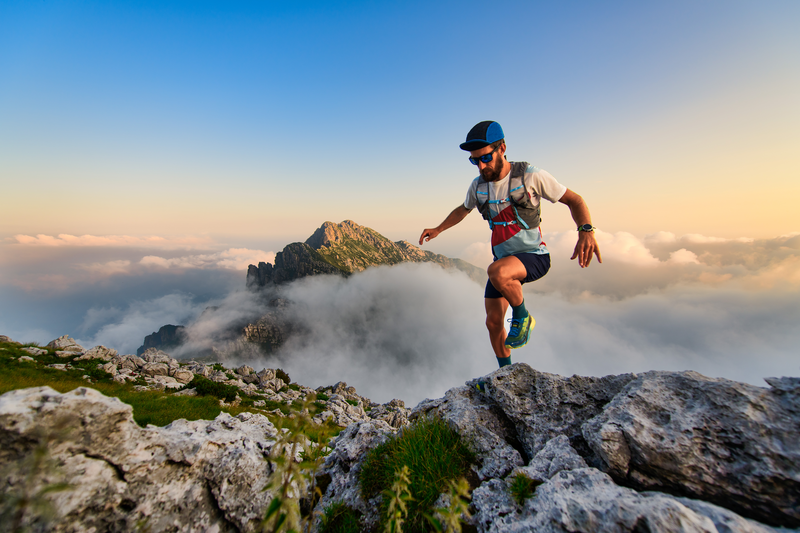
94% of researchers rate our articles as excellent or good
Learn more about the work of our research integrity team to safeguard the quality of each article we publish.
Find out more
ORIGINAL RESEARCH article
Front. Mar. Sci. , 21 June 2022
Sec. Aquatic Physiology
Volume 9 - 2022 | https://doi.org/10.3389/fmars.2022.926106
This article is part of the Research Topic The Relationship Between Nutrition and Stress on Intestinal Health and Reproduction in Aquatic Animals View all 8 articles
The objective was to investigate the improvement of the flesh quality and nutritional value of hybrid grouper (♀ Epinephelus fuscoguttatus × ♂ E. lanceolatu) by Lactobacillus pentosus (BL-15). The two diets were prepared with 9% oxidized fish oil (OFO negative control group) or 9% fresh fish oil (FFO positive control group) as the major lipid source, and the OFO diet were sprayed with L. pentosus at 1.0×107, 1.0×108, 1.0×109 CFU/g, respectively. Five kinds of diets were fed to hybrid grouper with initial weight of 21.36 ± 0.03 g for 60 days. The results showed that (1) consumption of OFO diet significantly increased muscle crude lipid, total cholesterol, and total monounsaturated fatty acids contents and significantly decreased muscle C20:5n3, C22:6n3, ∑PUFA levels of grouper (p < 0.05), whereas the addition of L. pentosus to OFO diet significantly reduced muscle crude lipid, malondialdehyde, reactive oxygen radicals, total cholesterol concentration, increased C20:5n3, C22:6n3, ∑PUFA levels, increased muscle crude protein content, and enhanced muscle hardness, gumminess, and chewiness compared with FFO or OFO group (p < 0.05). (2) In addition, ingestion of OFO diet significantly increased muscle phenylalanine content and significantly decreased aspartic acid content (p < 0.05), while the addition of L. pentosus restored aspartic acid to the level not significantly different from that of FFO group (p > 0.05). The addition of L. pentosus also increased tyrosine, lysine, leucine, phenylalanine, arginine, and alanine content compared to FFO group at the same time (p < 0.05). (3) Furthermore, the intake of OFO diet would lead to deterioration in muscle flavor quality and increase in noxious odors in grouper, and the addition of L. pentosus can mitigate or rectify these detrimental consequences. In further analysis, consumption of OFO group significantly downregulated the mRNA expression of muscle growth factors and caused serious damage to the muscle antioxidant system (p < 0.05), which was protected against by the potent antioxidant properties of L. pentosus. We recommend the supplementation level of L. pentosus to be 1.0×109 CFU/g under the highly oxidized conditions of the present trial fish oil.
Lipid has the essential nutrients for aquaculture animals and is an integral part of cells, providing essential fatty acids, energy supply and storage, and protein conservation (Glencross, 2009). Fish oil with high content of unsaturated fatty acids (HUFAs), specifically docosahexaenoic acid (DHA) and eicosapentaenoic acid (EPA), has been widely consumed in the marine feed industries (Zhu et al., 2012; Yan et al., 2020a; Yan et al., 2020b). Nevertheless, HUFAs are highly sensitive to oxidation and break down into harmful substances such as aldehydes, ketones, and alcohols during the production, shelf-life, and utilization, as they are sensitive to factors such as heat, light, moisture, oxygen, and metals in feed additives. (Frankel, 1980). Eventually, it would have serious implications for the quality and health of the animals. Lipid content in marine aquaculture fish diets is typically 12%–15% (Yoshii et al., 2010), and mariculture objects are normally located in hot and humid subtropical or tropical areas, providing favorable conditions for the oxidation of fish oil in diets. As a result, the oxidation of fish oil in ocean fish diets has become a serious problem for the sustainable development of mariculture.
People demand better seafood with the improvement of the world economy and life standards, and the decline in fish quality and health due to oxidative rancidity of diets is one of the most common challenges in aquaculture today. Recently, more and more attention has been paid to the study of oxidized lipids of diets in relation to oxidative stress (Long et al., 2021; Long et al., 2022a; Long et al., 2022b). Zhang et al. (2017) demonstrated in a study on mudskipper (Misgurnus anguillicaudatus) that oxidized fish oil (OFO) diets can cause liver lipid deposition; growth performance of juvenile hybrid tilapia (Oreochromis niloticus× O. aureus) was reduced, and liver malondialdehyde (MDA) levels were increased by consumption of OFO diets (Huang and Huang, 2004). They mainly focused on the influence of OFO on antioxidant capacity, biochemical parameters, and growth performance (Chen et al., 2011; Yuan et al., 2014). Moreover, OFO also can have a negative impact on the quality of fish meat (fatty acid and amino acid composition, flavor, vitamin E content, shelf life, etc.) in addition to decreasing the nutritional value of the diet itself (Lewis-Mccrea and Lall, 2007; Gao et al., 2012). Earlier works also showed that consumption of OFO diets led to less n-3 polyunsaturated fatty acids (PUFAs) and more MDA in the muscle of largemouth bass (Micropterus salmoides) (Yuan et al., 2014), Atlantic cod (Gadus morhua) (Ying et al., 2008), Japanese flounder (Paralichthys olivaceus) (Gao et al., 2014), and Japanese sea bass (Lateolabrax japonicus) (Han et al., 2012), which has severe impact on the nutritional value and quality of the fish.
High growth rate, disease resistance, high nutritional value, tasty flesh, and adaptability to the conditions have made hybrid grouper (♀ Epinephelus fuscoguttatus × ♂ E. lanceolatu) become a major culture species in Chinese southeast coast (Liu et al., 2018; Liu et al., 2021). As a superior source of protein, grouper production can be up to around 200,000 tons/year. However, our preliminary study found that the consumption of OFO feeds affected the flesh nutritional value and quality of grouper to some extent, which will seriously affect the sales market of grouper. Lactobacillus has a variety of probiotic functions; it not only can regulates the immune system and maintains the balance of intestinal flora, but it also has a certain antioxidant capacity, can remove reactive oxygen molecules, and the reactive oxygen molecules in body can maintain a relatively stable state (Tiiu et al., 2002; Jeongmin et al., 2005; Lin et al., 2020). In addition, Lactobacillus can improve muscle brightness by inhibiting lipid peroxidation and delaying the formation of methemoglobin, while improving muscle tenderness by affecting fatty acid metabolism (Li et al., 2014). Lactobacillus pentosus (BL-15) is an important lactic acid bacterium with a variety of functions including immune enhancement and antioxidant properties. However, its improvement on the deterioration of flesh quality caused by OFO needs to be explored. Hence, the aim of this experiment was to investigate the regulation of flesh quality of hybrid grouper by adding L. pentosus to OFO feeds, and the study was expected to provide a reference for solving the problems of decreased flesh quality of grouper caused by OFO.
Fish oil (fresh fish oil, FFO or OFO) was used as the main lipid source to formulate two groups of iso-nitrogenous (48.92%) and iso-lipid diets (11.96%). OFO was obtained by heating and aeration, the peroxide value (POV) of the OFO was 122.85 ± 1.76 mmol/kg, and the malondialdehyde (MDA) content was 121 mg/kg. All ingredients were well crushed and mixed with 30%–40% distilled water. The diets were manufactured by a twin-screw extruder (F–26, South China University of Technology, Guangdong Province, China) to a diameter of 2.5 mm, then air-dried to a moisture content of about 10% at room temperature, and crushed and sieved to the suitable size and held at −20°C. Table 1 shows the diet formulation and nutritional composition. L. pentosus (BL-15) at concentrations of 1.0×107, 1.0×108, and 1.0×109 CFU/g were added to OFO diet as treatment groups and recorded as OFOM7, OFOM8, and OFOM9. L. pentosus was added on the day of feeding, and the dominant bacterium was isolated and identified from the starfish by Guangdong Ocean University College of Food Science and Technology (Tang, 2020). The bacteria were cultured in MRS medium (Beijing Landbridge Technology Co.) under anaerobic conditions at 37°C for 24 h. The suspensions were centrifuged at 5,000g for 10 min, rinsed twice with sterile saline, and stored in sterile saline at 4°C. The number of bacterial cells in the suspensions was determined by turbidimetric method.
Guangdong Ocean University Institutional Animal Care and Use Committee Ethics Review Committee approved (Zhanjiang, China) the study. Groupers were obtained in local breeding farms in the Donghai Island (Zhanjiang, China) and temporarily cultured to trial size in outdoor concrete pools. A total of 450 hybrid groupers (21.36 ± 0.03 g) were distributed randomly into 15 fiberglass barrels (500 L) after 24 h of fasting. FFO, OFO, OFOM7, OFOM8, and OFOM9 diets were fed twice a day (8:00 and 16:00) on a full stomach, respectively. The experiment was carried out in three replicates and cultured for 60 days. Approximately 70% of the water in the tank was changed daily to keep the water environment. A 20.0-cm diameter × 30.0 cm length of polyvinyl chloride (PVC) pipe per tank served as a shelter for the groupers (Lin et al., 2007). They were kept under natural illumination, pH 7.9 ± 0.1, water temperature of 29 ± 1°C, salinity of 28, and dissolved oxygen concentration maintained at ≥ 5.0 mg/L. Total ammonia and nitrite concentrations were maintained at <0.05 mg/L, which were tested by using a PTF-001B multiparameter water quality detector (WBD Biotechnology Co., Ltd., China).
Sampling was conducted after 24 h of starvation at the end of the test. The skin of the fish was peeled off with bone forceps after anesthesia with eugenol (1:10,000), and the dorsal muscles were separated. The samples used to determine muscle compositions, fatty acids, amino acids, and textural parameters were kept on ice. Samples for the determination of texture parameters were cut into 2 cm × 2 cm × 0.3 cm slices, and the same sampling method was ensured for all samples. Volatile and enzyme activity samples were stored in 2.0 ml lyophilized tubes in liquid nitrogen. Samples of molecules were put into tubes containing RNA later, kept overnight at 4°C, and then moved to a −80°C refrigerator for mRNA expression detection. The samples above were determined immediately after sample collection.
Hardness, springiness, cohesiveness, and other textural parameters of muscle were measured using a textural instrumentation (S-PRO, 175 Food Technology Corporation, Rockville, MD, USA). Chinese standard methods determined the peroxide value (GB 5009.227-2016), malondialdehyde (MDA) (GB/T 28717-2012), and amino acid (GB/T18246-2019) levels. Diets and muscle proximate composition were analyzed using the AOAC prescribed methods (Lee, 1995). Fatty acids were analyzed by a gas chromatograph (7890A, Agilent Technologies Inc., USA) according to Shantha and Ackman (1990). Muscle biochemical indicators include reactive oxygen radicals (ROS), MDA, total protein (TP), triglycerides (TG) and total cholesterol (TC) were analyzed using Shanghai Enzyme-linked Biotechnology Co. Ltd. ELISA kits. The samples were homogenized with saline at a ratio of 1:9, the supernatant was taken after centrifugation, and then the subsequent assay analysis was performed in strict accordance with the kit instructions. Volatiles were identified by headspace solid-phase microextraction (HS-SPME) and gas chromatography-mass spectrometry (Agilent 7890A-5975C). It is based on a computer search of the NIST08 spectral library, supported by manual analysis of the spectra and qualitative analysis in conjunction with published literature. The peak area was normalized to obtain the relative percentages of each volatile constituent by using Excel data handling system. TransGen Biotech (Beijing, China) RNA kit and Accurate Biology Evo M-MLV kit (Hunan, China) were used for RNA extraction and cDNA synthesis, respectively. The quantitative real-time polymerase chain reaction (RT-qPCR) was carried out on 384-well plates in a reaction volume of 10 µl (1 µl cDNA, 0.8 µl primer, 3.2 µl RNse Free dH2O, and 5µl of SYBR® Green Real-Time PCR Master Mix). Primers for the internal reference gene (β-actin) and target genes were designed based on previously reported grouper sequences (Table 2). Calculation of relative expressions using the 2−ΔΔCt method (Livak and Schmittgen, 2001).
The results of all data were analyzed by one-way ANOVA with SPSS version 20.0 (SPSS Inc., USA) after homogeneity of variance. Then, the Tukey test was used to test whether the differences between groups were significant and was considered statistically significant when p < 0.05. All results were given as average ± standard error (SEM).
There was no significant difference in fish oil quality on muscle moisture, crude lipid, and crude protein of grouper (p > 0.05), but muscle moisture, crude protein, and crude lipid of grouper were slightly increased after ingestion of OFO diet (Table 3). The muscle moisture and crude protein content of grouper gradually increased with the increase in L. pentosus concentration in OFO feed, and both reached the maximum value at 1.0 × 109 CFU/g and were significantly higher than those in the FFO group (p < 0.05). Meanwhile, the addition of L. pentosus to OFO feed significantly reduced muscle crude lipid content in grouper (p < 0.05).
Ingestion of OFO feed significantly reduced total protein (TP) content of muscle in grouper (p < 0.05), and the addition of L. pentosus can recover the TP level, which could be recovered to the same level as FFO group when added at a concentration of 1.0 × 109 CFU/g. At the same time, ingestion of OFO feed increased MDA, ROS, TG, and TC levels of grouper muscle to different degrees. However, their contents were reduced by adding L. pentosus to OFO diet, and the lowest values were found in the OFOM9 group, which were significantly lower than those in the OFO group except TG (p < 0.05). Muscle TG content among groups had no significant difference (p > 0.05) (Table 4).
After 60 days, the muscle texture parameters of groupers are shown in Table 5; the quality of fish oil and L. pentosus did not affect the springiness, cohesiveness, and resilience of grouper muscle (p > 0.05). Although hardness, gumminess, and chewiness were not influenced by fish oil quality (p > 0.05), the added 1.0 × 109 CFU/g L. pentosus to OFO diet significantly improved muscle hardness, gumminess, and chewiness (p < 0.05).
The fatty acid composition of grouper muscle is shown in Table 6; compared to FFO group, the consumption of OFO diet significantly increased the content of saturated fatty acids (SAFAs), such as C15:0, C17:0, and C18:0, and total monounsaturated fatty acids (∑MUFA) and C18:1n9 in the muscle, while it significantly decreased the content of C20:5n3, C22:6n3, and total polyunsaturated fatty acids (∑PUFA) (p < 0.05). The fatty acid composition of OFOM7 and OFOM8 groups was similar to that of the OFO group, and the fatty acid composition of the OFOM9 group was similar to that of the FFO group, with no significant differences (except for C18:0) (p > 0.05). Compared to the OFO group, muscle C17:0, C18:1n9, and C22:1n9 levels in the OFOM9 group were significantly reduced, and ∑PUFA levels was significantly increased (p < 0.05).
The amino acid composition of grouper muscle is shown in Table 7; consumption of OFO diet significantly upregulated phenylalanine content and significantly reduced aspartic acid content in grouper muscle (p < 0.05), and the content of other amino acids was not affected by fish oil quality (p > 0.05). Compared with the OFO group, there was no significant effect of L. pentosus addition on amino acid composition of grouper muscle (p > 0.05). In addition, there was also no significant effect of fish oil quality and L. pentosus addition on muscle indispensable amino acid (IAA)/total amino acids (TAAs). However, the content of tyrosine, lysine, leucine, phenylalanine, arginine, IAA, and alanine in grouper muscle was significantly increased by the addition of appropriate amount of L. pentosus to OFO diet compared with FFO group (p < 0.05).
A total of 154 volatile compounds were identified in grouper muscle (Figure 1), including 88 compounds identified in the FFO group, 65 in the OFO group, 82 in the OFOM7 group, 87 in the OFOM8 group, and 83 in the OFOM9 group, of which 37 volatile compounds were identified in all groups. These 154 volatile compounds are mainly composed of alcohols, acids, ketones, amines, aldehydes, alkenes, alkanes, aromatic, esters, etc. (Table 8). Compared to the FFO group, muscle amine and esters were significantly lower after ingestion of OFO diet (p < 0.05) and recovered to the level that was not significantly different from the FFO group after the addition of appropriate amounts L. pentosus to the OFO diet (p > 0.05). The OFO group had significantly higher aromatics than the FFO group (p < 0.05), while there was no significant difference with other groups (p > 0.05). Although aldehydes, ketones, and other (unclassified) compounds did not differ significantly between FFO and OFO groups (p > 0.05), the addition of L. pentosus significantly increased the levels of these substances (p < 0.05). Nevertheless, alcohols, acids, alkanes, and alkenes were not significantly different among groups (p > 0.05). In addition, we performed stacked of volatile compounds with an average of over 1% for all groups (Figure 1). The most abundant volatile compounds identified in grouper muscle included oxime-methoxy-phenyl-, nonanal, 2,4-di-tert-butylphenol, and 1-decene, and the composition of volatile compounds in the muscle is significantly influenced by the ingestion of diet. Compared with the FFO group, ingestion of OFO feed can cause a reduction or even the disappearance of compounds in the muscle such as 1,2-benzenedicarboxylic acid, bis(2-methylpropyl) ester, acetic acid, butyl ester, caprolactam, cedrol, decane, 4-methyl-, dibutyl phthalate, dodecane, 2,6,11-trimethyl-, ethanol, 2-(tetradecyloxy)-, hexadecane, 2,6,10,14-tetramethyl-, hexadecanoic acid, methyl ester, hexanal, hexanedioic acid, bis(2-ethylhexyl) ester, propanoic acid, 2-methyl-, butyl ester, tridecanoic acid, and methyl ester; however, the addition of a certain level of L. pentosus to OFO diet increased the content of partial volatile compounds such as 1,2-benzenedicarboxylic acid, bis(2-methylpropyl) ester, cedrol, decane, 4-methyl-, dibutyl phthalate, dodecane, 2,6,11-trimethyl-, ethanol, 2-(tetradecyloxy)-, hexadecane, 2,6,10,14-tetramethyl-, hexadecanoic acid, methyl ester, hexanal, tridecanoic acid, and methyl ester. In addition, the ingestion of OFO diet increased the content of some volatile compounds such as 11-dodecenol, 1-octanol, 1-octen-3-ol, 2,4-di-tert-butylphenol, 2-tetradecene (E)-, dodecanal, heptanal, nonanal, octadecanal, and tridecane of muscle. The addition of L. pentosus to OFO diet regulates volatile compounds in grouper muscle, particularly reducing levels of 2,4-di-tert-butylphenol, nonanal, octadecanal, tridecane, etc. compared with the OFO group. In addition, L. pentosus also contributed to the appearance of new volatile compounds such as hexadecanoic acid, ethyl ester, and octanal in muscles of groupers.
Figures 2A, B show the relative expression of muscle growth factor mRNA in grouper; myogenin (myog) and myogenic differentiation (myod) were significantly downregulated when ingesting OFO diet compared with the FFO group (p < 0.05), whereas the addition of L. pentosus to OFO diet could upregulate the mRNA expression levels of myog and myod, which were significantly higher than in the OFO and OFOM9 groups (p < 0.05), but still lower than in the FFO group. Although ingestion of OFO diet had no significant effect on the mRNA expression of myofactar5 (mrf5) and myogenic regulatory factor 4 (mrf4) of grouper muscle, the addition of 1.0×109 CFU/g L. pentosus to OFO diet significantly upregulated the mRNA expression levels of mrf5 and mrf4 (p < 0.05). The mRNA expression of myostatin (mstn) was significantly downregulated after ingesting OFO diet (p < 0.05). In addition, ingestion of OFO diet can significantly upregulate the mRNA expression of insulin-like growth factor 1 (igf1) and collagen type I alpha1 (col1a1) in grouper muscle, while the addition of L. pentosus to OFO diet can significantly downregulate their expression (p < 0.05). While the mRNA expression of insulin-like growth factor 2 (igf2) was significantly lower in the OFO group than in the FFO group (p < 0.05), there was no significant difference among L. pentosus-treated groups and FFO group (p > 0.05). The mRNA expression of collagen type I alpha2 (col1a2) was not significantly different between FFO and OFO groups (p > 0.05), but the addition of L. pentosus to the OFO diet significantly downregulated the expression of col1a2 (p < 0.05). Figure 2 showed the relative mRNA expression of muscle antioxidant-related genes; consumption of OFO diet significantly reduced the mRNA expression of muscle gpx, cat, hsp70, and hsp90 in grouper compared to the that in the FFO group (p < 0.05); moreover, the mRNA expression of gpx, cat, hsp70, and hsp90 in the muscle was significantly upregulated when L. pentosus was added to OFO diet, and the expression was highest and significantly higher than those in the OFO group when supplemented at 1.0×109 CFU/g (p < 0.05).
Figure 2 Relative mRNA expression of muscle growth factors (A, B) and antioxidant-related genes (C) of grouper fed different diets. myog, myogenin; myod, myogenic differentiation; myf5, myofactar5; mrf4, myogenic regulatory factor 4; mstn2, myostatin type 2; igf1, insulin-like growth factor 1; igf2, insulin-like growth factor 2; col1a1, collagen type I alpha1; col1a2, collagen type I alpha2; cat, catalase; gpx, glutathione peroxidase; hsp70, heat shock protein 70; hsp90, heat shock protein 90. Different lowercase letters represent significant differences between groups (P < 0.05).
The muscle constitutes the most vital component of fish for human consumption, and its nutritional content is particularly important. The nutritional value is highly dependent on its biochemical composition, including crude protein and crude lipid, which is also considered to be a marker for evaluating the flesh quality (Vijayavel and Balasubramanian, 2006). A previous study (Yu et al., 2022) has demonstrated that consumption of OFO diet reduces muscle crude protein and crude lipid of Amur sturgeon (A. schrenckii), which was consistent with the trend in this study. The addition of 1.0×109 CFU/g L. pentosus to the OFO diet significantly increased the muscle crude protein content and significantly reduced the crude lipid content compared to the FFO group. Similar studies (Amoah et al., 2019) have also shown that probiotics can increase the crude protein content of Pacific white shrimp (Litopenaeus vannamei). Lactobacillus can remove reactive oxygen molecules from cells to reduce lipid peroxidation due to its strong antioxidant capacity, which may be one of the reasons why L. pentosus reduces muscle crude lipid level (Tiiu et al., 2002; Jeongmin et al., 2005).
Muscle biochemical parameters are also important indicators of the quality of fish muscle. MDA is the end product of lipid peroxidation, and its level reflects the extent of oxidative damage to the body by free radicals and reactive oxygen species (Yan et al., 2021) and is also highly biotoxic and can lead to damage to cell structure and function (Ming et al., 2012). Although fish oil quality did not significantly affect muscle MDA and ROS content, the addition of L. pentosus significantly reduced muscle MDA and ROS content, meaning that L. pentosus plays a key regulatory character in the prevention of muscle lipid peroxidation. Whereas consumption of OFO diet significantly increased muscle TC content, the addition of L. pentosus to OFO diet significantly reduced TC content, and these variations were consistent with variations in muscle crude lipid. These variations were attributed to the oxidation of highly unsaturated fatty acids to harmful substances such as alcohols, aldehydes, acids, and ketones, which affected the absorption and conversion of lipids by the organs, resulting in lipid deposition, and the addition of L. pentosus, which has strong antioxidant capacity, to OFO diet can improve the intestinal flora by inhibiting harmful microorganisms and promoting the growth of probiotics (Sun et al., 2015), thus promoting the metabolism of harmful substances and reducing lipid deposition and lipid peroxidation.
Texture is an important indicator of fish quality and can significantly affect the organoleptic properties of fish products. Hardness, springiness, cohesiveness, gumminess, chewiness, and resilience reflected the textural properties of the muscle. To a certain extent, the firmer the muscle, the higher the chewing power and the better the taste (Han et al., 2021). The quality of dietary fish oil consumed in this study did not have a significant effect on the hardness, cohesiveness, gumminess, chewiness, and resilience of grouper, but the addition of 1.0×109 CFU/g L. pentosus to OFO diet significantly increased muscle hardness, gumminess, and chewiness, which implied that L. pentosus rather than fish oil quality can make the muscles more compact and gelatinous to improve hardness and further improve the chewiness of muscles. Furthermore, the addition of L. pentosus significantly increased the crude protein and TP content of muscle, so we suspected that the addition of L. pentosus may have helped to preserve the texture of fish by slowing down the degradation of muscle proteins and maintaining the binding between muscle cells (Zhou et al., 2011).
Previous studies have shown that the fatty acid composition of fish muscle was influenced not only by the type of dietary lipid source consumed (Yan et al., 2020a) but also by the quality of the dietary lipid (Yu et al., 2022). In the present study, consumption of OFO diet significantly increased SUFAs (C15:0, C17:0, C18:0, and C18:1n9) and MUFAs (C18:1n9) content and significantly decreased PUFAs (C20:5n3, C22:6n3) content of grouper muscle. This was primarily because marine fish lacked the ability to synthesize LC-PUFAs themselves (Ghioni et al., 1999; Tocher and Ghioni, 1999), and the oxidation of LC-PUFA in FA to substances such as alcohols, aldehydes, acids, and ketones reduced the amount of LC-PUFA in diet and thereby reducing the amount of deposition in muscle (Chen et al., 2019), which suggested that lipid type plays a critical role in muscle nutritional quality. Previous studies have reported that L. pentosus had antimicrobial (Guerreiro et al., 2014; Zheng et al., 2016), immune enhancing (Izumo et al., 2010; Izumo et al., 2011), and heavy metal detoxifying properties (Osadolor et al., 2013). Furthermore, the addition of 1.0×109 CFU/g of L. pentosus to the OFO diet significantly increased muscle ∑PUFA levels and significantly decreased C17:0, C18:1n9, and C22:1n9 levels, and its fatty acid composition was similar to that of the FFO group. We hypothesize that L. pentosus or its secretions promote the uptake and conversion of oxidation products in OFO by the grouper intestine and may have the potential to restore oxidation products to LC-PUFA, or L. pentosus can synthesize LC-PUFA or improve the intestinal bacterium profile, which can lead to an increase in LC-PUFA production by the bacterium. Of course, these are all speculations, and the exact reasons need to be further verification.
The leucine, lysine, and arginine were the main IAA in the muscles of grouper, and the glutamic acid and aspartic acid were the main dispensable amino acids in this study. This was consistent with existing research findings (Yang et al., 2022). IAA/TAA represents an essential indicator for the nutritional value of aquatic proteins, and the IAA/TAA for high-quality proteins is generally thought to be about 0.4 (Yuan et al., 2020). The IAA/TAA of grouper muscle was about 0.5 in the present study, which indicated that it was a high-quality protein supplier. Phenylalanine was approved by the Food and Agriculture Organization (FAO) and the Joint Committee of Experts of the World Health Organization (WHO) as a sweet amino acid; serine plays a character in the lipid and fatty acids metabolism and growth of muscle, manufacture and processing of cell membranes, and synthesis of muscle tissue and sheaths that surround nerve cells. In this study, the consumption of OFO diet significantly increased the muscle phenylalanine acid content and significantly decreased the aspartic acid content of grouper. Therefore, OFO inhibits the normal growth of grouper muscle cells and the metabolic transport and deposition of fatty acids, which is an important reason for the decrease in muscle LC-PUFA content. While phenylalanine, an aromatic amino acid, may be associated with the odor of OFO, we also identified volatile compounds in the muscle. The addition of L. pentosus to OFO diet had no significant effect on the grouper muscle amino acid profile compared to that in the OFO group but significantly increased the levels of tyrosine, lysine, leucine, phenylalanine, arginine, IAA, and alanine compared to that in the FFO group, which also indicated the plasticity of L. pentosus in amino acid deposition, but the exact mechanism needs to be further investigated.
The sensory characteristics, other than the nutritional quality of the muscle, also decide the level of consumption of aquatic products by consumers, in which an important factor affecting the sensory characteristics is the flavor (Hardy and Lee, 2010). Volatile odor substances in muscles are associated with the odor of fresh fish, which determines consumer acceptability. Volatile compound diversity in grouper muscle fed OFO diet was substantially reduced and restored by the addition of L. pentosus, which suggested a disruptive effect of OFO on muscle flavor of grouper and a promising application of L. pentosus in antioxidation of fish oil. Muscle differential volatile compounds are mainly composed of esters, aldehydes, amines, and aromatic compounds. Sulfur- and nitrogen-containing compounds are considered to be important odor-active components; the amines are generally considered to be related to the freshness and fishy taste of aquatic products, and appropriate amines can improve the freshness of aquatic products. In this study, muscle amines were greatly reduced after consumption of OFO diet and were restored after the addition of L. pentosus, so FFO and L. pentosus can promote the accumulation of freshness in grouper muscle at a certain level. Low-threshold aldehydes have an important function for the formation of flavor substances in aquatic animals, which were produced rapidly in the process of lipid oxidation (Wang et al., 2016). Previous reports have shown that aldehydes are associated with fishy, greasy (Wang and Chen, 2005), and grassy (Wang et al., 2016) flavors of aquatic products and make up the majority of volatile substances. Whereas the OFO group in this study had a large increase in aldehydes, these aldehydes mainly included benzaldehyde, dodecanal, heptanal, and nonanal, which may be related to the oxidation of LC-PUFA to alcoholic aldehydes (Frankel, 1980). Most aldehydes can lead to unpleasant, irritating, earthy, pungent, and fishy odors (Long et al., 2013). The aldehydes of L. pentosus-treated groups were again dramatically reduced, which may be related to the strong antioxidative properties of L. pentosus, and it may facilitate the metabolism of harmful substances by accelerating the breakdown of substances such as aldehydes and ketones in order to reduce damage to host. Most esters bring a fruity or floral taste to food, and they were formed by the esterification of alcohols and carboxylic acids (Yang et al., 2012). There was a significant decrease in total esters in the muscle of OFO group, implying deterioration of muscle flavor. The addition of L. pentosus restores the esters flavor of the muscle, which is also associated with a reduction in aldehydes that reduces the fishy and pungent taste. The most important reason may be attributed to the fact that the metabolites of Lactobacillus lactis are predominantly 1,2-benzenedicarboxylic acid bis(2-methylpropyl) ester and 1,2-benzenedicarboxylic acid dibutyl ester, which were identified in the previous study (Pan et al., 2011). The increase in total aromatics of muscle in the OFO group was mainly due to the increase in the level of 2,4-di-tert-butylphenol, which itself has strong antioxidant properties. The reason for the increase in 2,4-di-tert-butylphenol maybe to alleviate or repair oxidative stress or damage to the body caused by the ingestion of OFO diet, but the long-term or large amount intake of such fish by humans has the risk of causing deformities and cancer (Zhao et al., 2020). Ultimately, the intake of OFO diet can cause a deterioration in muscle flavor quality and an increase in noxious odors in grouper, and the addition of L. pentosus can mitigate or rectify these detrimental consequences.
Muscle growth and quality are under the control of multiple molecular mechanisms (Salem et al., 2013). Muscle growth and catabolism determine the physical nutritional status, and the molecular response of muscle to various diets can be determined by analyzing the expression of genes associated with myogenic signaling pathways (Johansen and Overturf, 2006). Myogenic regulatory factors growth-related factors (myf5, mrf4, myog, myod, mstn 2, col1a-1 and col1a-2, igf1, and igf2) belong to the intrinsic factors controlling the progression, activation, and derivatization of myogenic cells (Watabe, 1999). Myog has essential functions in myogenic cell differentiation, while myod and myfs constitute the majority of myogenic factors (Kassar-Duchossoy et al., 2004). In addition, the muscle modulates the muscle and usually inhibits the growth of the skeletal muscle (McPherron et al., 1997). Igf1 in fish muscle has abundant receptors as an important positive regulatory growth factor. It can promote cell activation, proliferation, and differentiation (Castillo et al., 2006), whereas igf2 is a negative regulator (Snijders et al., 2015). In addition, col1a-1 and col1a-2 regulate the muscle fibers stiffness and diameter (Zhao et al., 2018). Muscle myog, myod, and mstn were all downregulated significantly by consumption of OFO diet, and these changes further demonstrated the detrimental impact of OFO on muscle growth. These adverse effects are fortunately eliminated by the addition of L. pentosus, so we thought that L. pentosus might also have a role in muscle growth and development. Compared with the FFO group, the expression of igf2 was downregulated, and igf1, col1a1 were significantly upregulated. This may be attributed to the increased muscle MDA and TG levels in grouper fed OFO diets. Furthermore, the deposition of lipid and harmful substances had a stimulating impact on muscle growth, which was expressed as a negative feedback regulation. L. pentosus, a probiotic with strong antioxidative properties, was able to eliminate the damage caused by lipid peroxidation products, ultimately showing similar results to FFO group.
A previous study had identified antioxidant-related factors (gpx, cat, hsp70, and hsp90) in vivo and play a critical function in the protection of antioxidant system. GPX helps maintain the structural and functional components of cell membranes by catalyzing the destruction of peroxides (Yan et al., 2021). CAT can reduce oxidative damage by bringing free radical synthesis and elimination into dynamic balance (Li et al., 2007). hsps could prevent protein denaturation, refold damaged proteins, or prevent irreversible degradation of damaged proteins to regulate stress tolerance (Sung et al., 2018). OFO diet ingestion downregulated muscle cat, gpx, hsp70, and hsp90 expression compared to FFO diet ingestion. The results provide further evidence that OFO caused damage to muscle antioxidant system of grouper, which was consistent with the above findings that OFO caused increased muscle TC and MDA contents in grouper (Long et al., 2022c). Furthermore, all of these antioxidant factors genes were significantly upregulated by adding a certain amount of L. pentosus to OFO diet, which was also attributed to the strong antioxidant capacity of L. pentosus to mitigate lipid peroxidation product damage in an organism. The result that L. pentosus improved the mRNA expression of antioxidant factor genes was also confirmed in a previous study (Gao et al., 2018) on abalone (Haliotis discus hannai Ino).
Overall, the peroxidation products of LC-PUFA in fish oil may cause oxidative damage to grouper muscle to increase the deposition of harmful substances and lipids, ultimately leading to deterioration of muscle nutritional value and flavor. L. pentosus, as a powerful antioxidant probiotic, can reduce the damage of lipid peroxidation products of muscle in grouper by preventing oxidative damage, and L. pentosus or its metabolites may have a potential role in the absorption and transformation of fatty acids and lipid peroxidation products; the detailed mechanism needs to be further explored. Therefore, L. pentosus can be used as a probiotic additive to improve the nutritional value, taste, and flavor of aquatic animal muscle, and under the conditions of the present experiment, we recommend the supplementation level of L. pentosus to be 1.0×109 CFU/g.
The original contributions presented in the study are included in the article/supplementary material. Further inquiries can be directed to the corresponding author.
The animal study was reviewed and approved by Guangdong Ocean University Institutional Animal Care and Use Committee Ethics Review Committee.
Design of the study: XY, XD, and BT. Experimental operation: XY, SP, ZL, SL, TL, XS, and YY. Sampling and data analysis: XY, SP, ZL, SL, and TL, XS, and YY. Drafting of the paper: XY. Paper revision: XD. All authors contributed to the article and approved the submitted version.
This study was funded by the National Natural Science Foundation of China (NSFC31972808), the General Program of Natural Science Foundation of Guangdong Province (2021A1515011165), Department of Education of Guangdong Province (2021ZDZX4005), Postgraduate Education Innovation Project of Guangdong Ocean University (202139), Science and Technology Bureau of Zhanjiang (Grant Nos. 2020A03010 and 2020A05003), Research and Demonstration of Precision Functional Compound Feed Technology of Major Cultured Fishes and Shrimps in South China (2021B0202050002), and the China Agriculture Research System of MOF and MARA (CARS-47).
The authors declare that they have no known competing financial interests or personal relationships that could have appeared to influence the work reported in this paper.
All claims expressed in this article are solely those of the authors and do not necessarily represent those of their affiliated organizations, or those of the publisher, the editors and the reviewers. Any product that may be evaluated in this article, or claim that may be made by its manufacturer, is not guaranteed or endorsed by the publisher.
Amoah K., Huang Q., Tan B., Zhang S., Chi S., Yang Q., et al. (2019). Dietary Supplementation of Probiotic Bacillus Coagulans ATCC 7050, Improves the Growth Performance, Intestinal Morphology, Microflora, Immune Response, and Disease Confrontation of Pacific White Shrimp, Litopenaeus Vannamei. Fish. Shellfish. Immunol. 87, 796–808. doi: 10.1016/j.fsi.2019.02.029
Castillo J., Ammendrup-Johnsen I., Codina M., Navarro I., Gutiérrez J. (2006). IGF-I and Insulin Receptor Signal Transduction in Trout Muscle Cells. Am. J. Physiology-Regul. Integr. Comp. Physiol. 290, R1683–R1690. doi: 10.1152/ajpregu.00294.2005
Chen Y., Liu Y., Yang H., Yuan Y., Liu F., Tian L., et al. (2011). Effect of Dietary Oxidized Fish Oil on Growth Performance, Body Composition, Antioxidant Defence Mechanism and Liver Histology of Juvenile Largemouth Bass Micropterus Salmoides. Aquacult. Nutr. 18, 321–331. doi: 10.1111/j.1365-2095.2011.00900.x
Chen S., Zhuang Z., Yin P., Chen X., Zhang Y., Tian L., et al. (2019). Changes in Growth Performance, Haematological Parameters, Hepatopancreas Histopathology and Antioxidant Status of Pacific White Shrimp (Litopenaeus Vannamei) Fed Oxidized Fish Oil: Regulation by Dietary Myo-Inositol. Fish. Shellfish. Immunol. 88, 53–64. doi: 10.1016/j.fsi.2019.02.023
Gao J., Koshio S., Ishikawa M., Yokoyama S., Mamauag R. (2014). Interactive Effects of Vitamin C and E Supplementation on Growth Performance, Fatty Acid Composition and Reduction of Oxidative Stress in Juvenile Japanese Flounder Paralichthys Olivaceus Fed Dietary Oxidized Fish Oil. Aquaculture 422-423, 84–90. doi: 10.1016/j.aquaculture.2013.11.031
Gao J., Koshio S., Ishikawa M., Yokoyama S., Ren T., Komilus C., et al. (2012). Effects of Dietary Palm Oil Supplements With Oxidized and Non-Oxidized Fish Oil on Growth Performances and Fatty Acid Compositions of Juvenile Japanese Sea Bass, Lateolabrax Japonicus. Aquaculture 324, 97–103. doi: 10.1016/j.aquaculture.2011.10.031
Gao X., Zhang M., Li X., Han Y., Wu F., Liu Y. (2018). The Effects of Feeding Lactobacillus Pentosus on Growth, Immunity, and Disease Resistance in Haliotis Discus Hannai Ino. Fish. Shellfish. Immunol. 78, 42–51. doi: 10.1016/j.fsi.2018.04.010
Ghioni C., Tocher D. R., Bell M. V., Dick J. R., Sargent J. R. (1999). Low C18 to C20 Fatty Acid Elongase Activity and Limited Conversion of Stearidonic Acid, 18: 4 (N–3), to Eicosapentaenoic Acid, 20: 5 (N–3), in a Cell Line From the Turbot, Scophthalmus Maximus. Biochim. Biophys. Acta-Mol. Cell Biol. Lipids. 1437, 170–181. doi: 10.1016/S1388-1981(99)00010-4
Glencross B. (2009). Exploring the Nutritional Demand for Essential Fatty Acids by Aquaculture Species. Rev. Aquacult. 1, 71–124. doi: 10.1111/j.1753-5131.2009.01006.x
Guerreiro J., Monteiro V., Franco M., Martinez R. C. R., Todorov S. D., Fernandes P. (2014). Lactobacillus Pentosus B231 Isolated From a Portuguese PDO Cheese: Production and Partial Characterization of its Bacteriocin. Probiot. Antimicrobial. Proteins. 6, 95–104. doi: 10.1007/s12602-014-9157-3
Han Q., Ma X., Huang C. (2021). Analysis on the Nutritional Components and Quality Characteristics of Pelteobagrus Fulvidraco Muscle in Dongting Lake. J. Food Saf. Quality. 12, 9102–9108. doi: 10.19812/j.cnki.jfsq11-5956/ts.2021.23.017
Han Y., Ren T., Jiang Z., Jiang B., Gao J., Koshio S., et al. (2012). Effects of Palm Oil Blended With Oxidized Fish Oil on Growth Performances, Hematology, and Several Immune Parameters in Juvenile Japanese Sea Bass, Lateolabrax Japonicas. Fish. Physiol. Biochem. 38, 1785–1794. doi: 10.1007/s10695-012-9675-4
Hardy R. W., Lee C. (2010). Aquaculture Feed and Seafood Quality. Bull. Fisheries Res. Agency. 31, 43–50.
Huang C., Huang S. (2004). Effect of Dietary Vitamin E on Growth, Tissue Lipid Peroxidation, and Liver Glutathione Level of Juvenile Hybrid Tilapia, Oreochromis Niloticus X O. Aureus, Fed Oxidized Oil. Aquaculture 237, 381–389. doi: 10.1016/j.aquaculture.2004.04.002
Izumo T., Izumi F., Nakagawa I., Kitagawa Y., Shibata H., Kiso Y. (2011). Influence of Lactobacillus Pentosus S-PT84 Ingestion on the Mucosal Immunity of Healthy and Salmonella Typhimurium-Infected Mice. Biosci. Microflora. 30, 27–35. doi: 10.12938/bifidus.30.27
Izumo T., Maekawa T., Ida M., Noguchi A., Kitagawa Y., Shibata H., et al. (2010). Effect of Intranasal Administration of Lactobacillus Pentosus S-PT84 on Influenza Virus Infection in Mice. Int. Immunopharmacol. 10, 1101–1106. doi: 10.1016/j.intimp.2010.06.012
Jeongmin L., Kwon-Tack H., Moon-Soo H., Jun-Haeng L., Kun-Young P. (2005). Resistance of Lactobacillus Plantarum KCTC 3099 From Kimchi to Oxidative Stress. J. Medicinal. Food. 8, 299–304. doi: 10.1089/jmf.2005.8.299
Johansen K. A., Overturf K. (2006). Alterations in Expression of Genes Associated With Muscle Metabolism and Growth During Nutritional Restriction and Refeeding in Rainbow Trout. Comp. Biochem. Physiol. Part B: Biochem. Mol. Biol. 144, 119–127. doi: 10.1016/j.cbpb.2006.02.001
Kassar-Duchossoy L., Gayraud-Morel B., Gomès D., Rocancourt D., Buckingham M., Shinin V., et al. (2004). Mrf4 Determines Skeletal Muscle Identity in Myf5: Myod Double-Mutant Mice. Nature 431, 466–471. doi: 10.1038/nature02876
Lewis-Mccrea L., Lall S. (2007). Effects of Moderately Oxidized Dietary Lipid and the Role of Vitamin E on the Development of Skeletal Abnormalities in Juvenile Atlantic Halibut (Hippoglossus Hippoglossus). Aquaculture 262, 142–155. doi: 10.1016/j.aquaculture.2006.09.024
Lin H. Z., Liu Y. J., He J. G., Zheng W. H., Tian L. X. (2007). Alternative Vegetable Lipid Sources in Diets for Grouper, Epinephelus Coioides (Hamilton): Effects on Growth, and Muscle and Liver Fatty Acid Composition. Aquacult. Res. 38, 1605–1611. doi: 10.1111/j.1365-2109.2007.01811.x
Lin A., Wang W., Chen G., Zhang J., Huang J., Pan C., et al. (2020). Effects of Two Lactic Acid Bacteria on Growth Performance and Activities of Digestive and non-Specific Immune Enzymes of Juvenile Cobia (Rachycentron Canadum). J. Guangdong. Ocean Univer. 40, 1122–1117. doi: 10.3969/j.issn.1673-9159.2020.05.014
Liu H., Li L., Stephen A., Tang Z., Fan W., Tan B., et al. (2021). Effects of Dietary Yeast Culture Supplementation on Growth, Intestinal Morphology, Immunity, and Disease Resistance in Epinephelus Fuscoguttatus♀×Epinephelus Lanceolatu♂. J. Guangdong. Ocean Univer. 41, 1–11. doi: 10.3969/j.issn.1673-9159.2021.03.001
Liu Y., Wang J., Li B., Qiao H., Liu X., Hao T., et al. (2018). Dietary Manganese Requirement of Juvenile Hybrid Grouper, Epinephelus Lanceolatus× E. Fuscoguttatus. Aquacult. Nutr. 24, 215–223. doi: 10.1111/anu.12549
Livak K. J., Schmittgen T. D. (2001). Analysis of Relative Gene Expression Data Using Real-Time Quantitative PCR and the 2– ΔΔCT Method. Methods 25, 402–408. doi: 10.1006/meth.2001.1262
Li T., Zeng D., Ni X., Wang Z., Xin J., Shen X. (2014). Effect of Lactic Acid Bacteria Fermented Feed on Growth Performance, Meat Quality and Serum Antioxidant Activity of Pigs. J. Hunan. Agric. University. 40, 192–195. doi: 10.13331/j.cnki.jhau.2014.02.017
Li K., Zheng T., Tian Y., Xi F., Yuan J., Zhang G., et al. (2007). Beneficial Effects of Bacillus Licheniformis on the Intestinal Microflora and Immunity of the White Shrimp, Litopenaeus Vannamei. Biotechnol. Lett. 29, 525–530. doi: 10.1007/s10529-006-9291-4
Long S., Dong X., Liu H., Yan X., Tan B., Zhang S., et al. (2022a). Effect of Dietary Oxidized Fish Oil on Liver Function in Hybrid Grouper (♀ Epinephelus Fuscoguttatus×♂ Epinephelus Lanceolatus). Aquacult. Rep. 22, 101000. doi: 10.1016/j.aqrep.2021.101000
Long S., Dong X., Tan B., Zhang S., Chi S., Yang Q., et al. (2022c). The Antioxidant Ability, Histology, Proximate, Amino Acid and Fatty Acid Compositions, and Transcriptome Analysis of Muscle in Juvenile Hybrid Grouper (♀ Epinephelus Fuscoguttatus×♂ Epinephelus Lanceolatus) Fed With Oxidized Fish Oil. Aquaculture 547, 737510. doi: 10.1016/j.aquaculture.2021.737510
Long S., Dong X., Tan B., Zhang S., Xie S., Yang Q., et al. (2021). Growth Performance, Antioxidant Ability, Biochemical Index in Serum, Liver Histology and Hepatic Metabolomics Analysis of Juvenile Hybrid Grouper (♀ Epinephelus Fuscoguttatus×♂ Epinephelus Lanceolatus) Fed With Oxidized Fish Oil. Aquaculture 545, 737261. doi: 10.1016/j.aquaculture.2021.737261
Long S., Dong X., Yan X., Liu H., Tan B., Zhang S., et al. (2022b). The Effect of Oxidized Fish Oil on Antioxidant Ability, Histology and Transcriptome in Intestine of the Juvenile Hybrid Grouper (♀ Epinephelus Fuscoguttatus×♂ Epinephelus Lanceolatus). Aquacult. Rep. 22, 100921. doi: 10.1016/j.aqrep.2021.100921
Long B., Wang X., Zhang F., Cao J., Liu Y. (2013). Analysis of Volatile Flavor Compounds of Farmed Silurus Meridionalis Chenmeat by Simultaneous Distillation Extraction and Gas Chrom Atography-Mass Spectrometr. Food Sci. 34, 188–192. doi: 10.7506/spkx1002-6630-201324039
McPherron A. C., Lawler A. M., Lee S.-J. (1997). Regulation of Skeletal Muscle Mass in Mice by a New TGF-P Superfamily Member. Nature 387, 83–90. doi: 10.1038/387083a0
Ming J., Xie J., Xu P., Ge X., Liu W., Ye J. (2012). Effects of Emodin and Vitamin C on Growth Performance, Biochemical Parameters and Two HSP70s mRNA Expression of Wuchang Bream (Megalobrama Amblycephala Yih) Under High Temperature Stress. Fish. Shellfish. Immunol. 32, 651–661. doi: 10.1016/j.fsi.2012.01.008
Osadolor H. B., Igharo O., Okuo R., Anukam K. (2013). Evaluation of Serum Levels of Cadmium and Lead in Occupationally Exposed Painters With Administration of Probiotic (Lactobacillus Pentosus Kca 1) Supplemented Yogurt: A Pilot Study. Med. Biomed. Res. 12, 166–173.
Pan Y., Huang Z., Zhang X., He Y., Shi W., Liu Y. (2011). Identification of Symbiotic or Epiphytic Microbe HSL36 From Mangrove and Analysis of its Main Metabolites by GC-Ms. J. Guangdong. Ocean. University. 31, 73–78. doi: 10.3969/j.issn.1673-9159.2011.06.012
Salem M., Manor M. L., Aussanasuwannakul A., Kenney P. B., Weber G. M., Yao J. (2013). Effect of Sexual Maturation on Muscle Gene Expression of Rainbow Trout: RNA-Seq Approach. Physiol. Rep. 1, 100–120. doi: 10.1002/phy2.120
Shantha N. C., Ackman R. G. (1990). Nervonic Acid Versus Tricosanoic Acid as Internal Standards in Quantitative Gas Chromatographic Analyses of Fish Oil Longerchain N-3 Polyunsaturated Fatty Acid Methyl Esters. J. Chromatogr. B Biomed. Sci. App. 533, 1–10. doi: 10.1016/S0378-4347(00)82182-9
Snijders T., Nederveen J. P., McKay B. R., Joanisse S., Verdijk L. B., van Loon L. J., et al. (2015). Satellite Cells in Human Skeletal Muscle Plasticity. Front. Physiol. 6. doi: 10.3389/fphys.2015.00283
Sung Y. Y., Rahman N. A., Shazili N. A. M., Chen S., Lv A., Sun J., et al. (2018). Non-Lethal Heat Shock Induces Hsp70 Synthesis and Promotes Tolerance Against Heat, Ammonia and Metals in Post-Larvae of the White Leg Shrimp Penaeus Vannamei (Boone 1931). Aquaculture 483, 21–26. doi: 10.1016/j.aquaculture.2017.09.034
Sun M., Zhang L., Liu M., Wang S. (2015). Lactobacillus Pentosus and its Application in the Food Industry. China Brewing. 34, 11–14. doi: 10.11882/j.issn.0254-5071.2015.06.003
Tang H. (2020). Screening and Identification of Symbiotic Microorganisms of Starfish With Antibacterial and Antioxidant Activity and Separation and Identification of Active Substances (Zhanjiang: Guangdong Ocean University).
Tiiu K., Mihkel Z., Marika M., Tiiu V., Heidi A., Ceslava K., et al. (2002). Two Antioxidative Lactobacilli Strains as Promising Probiotics. Int. J. Food Microbiol. 72, 215–224. doi: 10.1016/S0168-1605(01)00674-2
Tocher D. R., Ghioni C. (1999). Fatty Acid Metabolism in Marine Fish: Low Activity of Fatty Acyl Δ5 Desaturation in Gilthead Sea Bream (Sparus Aurata) Cells. Lipids 34, 433–440. doi: 10.1007/s11745-999-0382-8
Vijayavel K., Balasubramanian M. P. (2006). Fluctuations of Biochemical Constituents and Marker Enzymes as a Consequence of Naphthalene Toxicity in the Edible Estuarine Crab Scylla Serrata. Ecotoxicol. Environ. Safety 63, 141–147. doi: 10.1016/j.ecoenv.2005.02.004
Wang X., Chen J. (2005). Analysis of Flavors in Silver Carp Meat by Headspace Solid Phase Microextraction Combined With GC-Ms. J. Shanghai. Fisheries University. 14, 176–180.
Wang S., He Y., Wang Y., Tao N., Wu X., Wang X., et al. (2016). Comparison of Flavour Qualities of Three Sourced Eriocheir Sinensis. Food Chem. 200, 24–31. doi: 10.1016/j.foodchem.2015.12.093
Watabe S. (1999). Myogenic Regulatory Factors and Muscle Differentiation During Ontogeny in Fish. J. Fish. Biol. 55, 1–18. doi: 10.1111/j.1095-8649.1999.tb01042.x
Yan X., Chen Y., Dong X., Tan B., Liu H., Zhang S., et al. (2021). Ammonia Toxicity Induces Oxidative Stress, Inflammatory Response and Apoptosis in Hybrid Grouper (♀ Epinephelus Fuscoguttatus×♂ E. Lanceolatu). Front. Mar. Sci. 8. doi: 10.3389/fmars.2021.667432
Yan X., Dong X., Tan B., Zhang S., Chi S., Yang Q., et al. (2020a). Influence of Different Oil Sources on Growth, Disease Resistance, Immune Response and Immune-Related Gene Expression on the Hybrid Grouper (♀ Epinephelus Fuscoguttatus×♂ E. Lanceolatu), to Vibrio Parahaemolyticus Challenge. Fish. Shellfish. Immunol. 99, 310–321. doi: 10.1016/j.fsi.2020.02.025
Yan X., Dong X., Tan B., Zhang S., Chi S., Yang Q., et al. (2020b). Effects of Alternative Dietary Oils on Lipid Metabolism and Related Gene Expression in Hybrid Grouper (♀Epinephelus Fuscoguttatus × ♂E. Lanceolatu). Aquacult. Nutr. 27, 105–115. doi: 10.1111/anu.13168
Yang Q., Qiu Y., Yu Y., Xiao X., Wu H. (2012). Volatile Component Analysis in Fresh and Defatted Deodorized Pseudosciaena Crocea. Food Sci. 33, 206–210.
Yang X., Zhi X., Song Z., Wang G., Zhao X., Chi S., et al. (2022). Flesh Quality of Hybrid Grouper (Epinephelus Fuscoguttatus ♀ × E. Lanceolatus ♂) Fed With Hydrolyzed Porcine Mucosa Supplemented Low Fishmeal Diet. Anim. Nutr. 8, 114–124. doi: 10.1016/j.aninu.2021.05.011
Ying Z., Lall S., Shahidi F. (2008). Effects of Dietary Oxidized Oil and Vitamin E on the Growth, Blood Parameters and Body Composition of Juvenile Atlantic Cod Gadus Morhua (Linnaeus 1758). Aquacult. Res. 39, 1647–1657. doi: 10.1111/j.1365-2109.2008.02038.x
Yoshii K., Takakuwa F., Nguyen H., Masumoto T., Fukada H. (2010). Effect of Dietary Lipid Level on Growth Performance and Feed Utilization of Juvenile Kelp Grouper Epinephelus Bruneus. Fisheries Sci. 76, 139. doi: 10.1007/s12562-009-0191-0
Yuan Y., Chen Y., Liu Y., Yang H., Liang G., Tian L. (2014). Dietary High Level of Vitamin Premix can Eliminate Oxidized Fish Oil-Induced Oxidative Damage and Loss of Reducing Capacity in Juvenile Largemouth Bass (Micropterus Salmoides). Aquacult. Nutr. 20, 109–117. doi: 10.1111/anu.12057
Yuan Y., Wang X., Jin M., Jiao L., Sun P., Betancor M. B., et al. (2020). Modification of Nutritional Values and Flavor Qualities of Muscle of Swimming Crab ( Portunus Trituberculatus ): Application of a Dietary Lipid Nutrition Strategy. Food Chem. 308, 125607. doi: 10.1016/j.foodchem.2019.125607
Yu H., Ren Y., Wei H., Xing W., Xu G., Li T., et al. (2022). Dietary Oxidized Fish Oil Negatively Affected the Feed Utilization, Health Status and Fillet Quality of Juvenile Amur Sturgeon, A. Schrenckii. Aquaculture 546, 737290. doi: 10.1016/j.aquaculture.2021.737290
Zhang Y., Yang L., Liang X., Cao X., Huang L., Jie Y., et al. (2017). Hepatic Transcriptome Analysis and Identification of Differentially Expressed Genes Response to Dietary Oxidized Fish Oil in Loach Misgurnus Anguillicaudatus. PloS One 12, e0172386. doi: 10.1371/journal.pone.0172386
Zhao F., Wang P., Lucardi R. D., Su Z., Li S. (2020). Natural Sources and Bioactivities of 2,4-Di-Tert-Butylphenol and Its Analogs. Toxins 12, 35. doi: 10.3390/toxins12010035
Zhao H., Xia J., Zhang X., He X., Li L., Tang R., et al. (2018). Diet Affects Muscle Quality and Growth Traits of Grass Carp (Ctenopharyngodon Idellus): A Comparison Between Grass and Artificial Feed. Front. Physiol. 9. doi: 10.3389/fphys.2018.00283
Zheng P.-X., Fang H.-Y., Yang H.-B., Tien N.-Y., Wang M.-C., Wu J.-J. (2016). Lactobacillus Pentosus Strain LPS16 Produces Lactic Acid, Inhibiting Multidrug-Resistant Helicobacter Pylori. J. Microbiol. Immunol. Infect. 49, 168–174. doi: 10.1016/j.jmii.2014.04.014
Zhou R., Liu Y., Xie J., Wang X., Gao Q., Chen Q., et al. (2011). Effects of Electrolyzed Water on Texture and Quality of Obscure Puffer Fish (Takifugu Obscurus) During Cold Storage. Trans. CSAE. 27, 365–369. doi: 10.3969/j.issn.1002-6819.2011.10.064
Keywords: fish oil, flavor, Lactobacillus pentosus, muscle quality, oxidative damage, texture
Citation: Yan X, Pan S, Li Z, Dong X, Tan B, Long S, Li T, Suo X and Yang Y (2022) Amelioration of Flesh Quality in Hybrid Grouper (♀ Epinephelus fuscoguttatus × ♂ E. lanceolatu) Fed With Oxidized Fish Oil Diet by Supplying Lactobacillus pentosus. Front. Mar. Sci. 9:926106. doi: 10.3389/fmars.2022.926106
Received: 22 April 2022; Accepted: 17 May 2022;
Published: 21 June 2022.
Edited by:
Jun Qiang, Freshwater Fisheries Research Center (CAFS), ChinaReviewed by:
Bo Liu, Freshwater Fisheries Research Center (CAFS), ChinaCopyright © 2022 Yan, Pan, Li, Dong, Tan, Long, Li, Suo and Yang. This is an open-access article distributed under the terms of the Creative Commons Attribution License (CC BY). The use, distribution or reproduction in other forums is permitted, provided the original author(s) and the copyright owner(s) are credited and that the original publication in this journal is cited, in accordance with accepted academic practice. No use, distribution or reproduction is permitted which does not comply with these terms.
*Correspondence: Xiaohui Dong, ZG9uZ3hpYW9odWkyMDAzQDE2My5jb20=
Disclaimer: All claims expressed in this article are solely those of the authors and do not necessarily represent those of their affiliated organizations, or those of the publisher, the editors and the reviewers. Any product that may be evaluated in this article or claim that may be made by its manufacturer is not guaranteed or endorsed by the publisher.
Research integrity at Frontiers
Learn more about the work of our research integrity team to safeguard the quality of each article we publish.