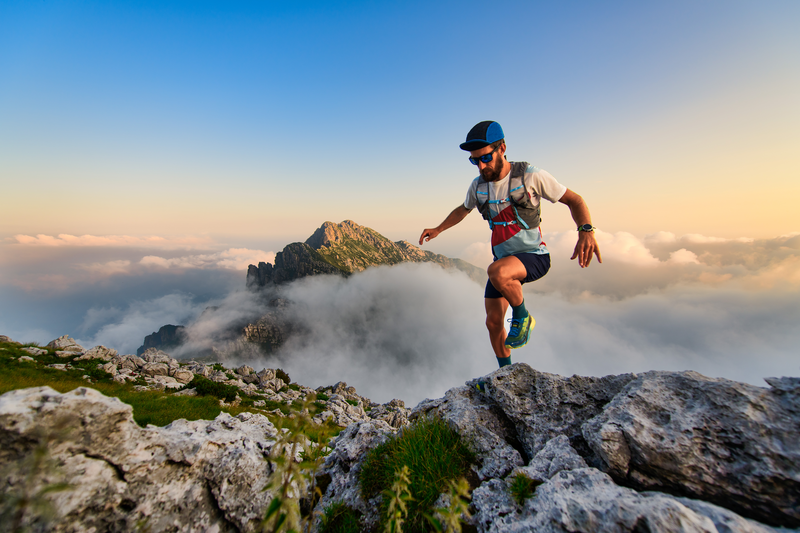
94% of researchers rate our articles as excellent or good
Learn more about the work of our research integrity team to safeguard the quality of each article we publish.
Find out more
ORIGINAL RESEARCH article
Front. Mar. Sci. , 22 July 2022
Sec. Marine Megafauna
Volume 9 - 2022 | https://doi.org/10.3389/fmars.2022.925749
This article is part of the Research Topic Contributions of Zoos and Aquariums to the Advancement of Marine Science View all 14 articles
Fundamental characteristics of the reproductive biology of female sand tiger sharks Carcharias taurus are needed to understand the periodicity, seasonality and environmental factors essential for reproduction in this iconic species. Animals in managed care, such as aquariums, provide the unique opportunity for longitudinal study in contrast to in situ sharks that are examined opportunistically, and at a single point in time. Additionally, comparison of reproductive observations from successfully reproducing in situ sharks and aquarium sharks may help elucidate reasons for lack of reproduction among aquarium sharks and aid the development of assisted reproductive techniques for managed populations. Reproductive status of in situ and aquarium female sharks was assessed using ultrasonography and plasma hormone (17β-estradiol, testosterone, and progesterone) monitoring. The reproductive cycle was divided into eight stages based on ovarian activity and uterine contents. In situ sharks were sampled from Delaware Bay (n = 29), North Carolina (n = 39) and South Carolina (n = 11) during April-November from 2015–2020. Nineteen aquarium females from five aquaria were examined longitudinally for two or more consecutive years. Reproductive regionalization was observed among in situ females with the majority (83%) of North Carolina females in an active state of reproduction and all Delaware females in a resting reproductive state. All aquarium females had a pattern of reproductive cycling that was consistent with alternating years of activity and rest with confirmed biennial (n = 7) or triennial (n = 3) reproductive cycles with spring seasonality. In contrast to in situ females, aquarium females often retained uterine eggs for 9-20 months after ovulation in the absence of a developing embryo(s). Pre-ovulatory aquarium females had significantly higher concentrations of 17β-estradiol, testosterone and progesterone than other reproductive stages. For females in the ovulatory stage, in situ females had higher testosterone than aquarium females. Endocrine differences between successfully reproducing in situ females and aquarium females likely contribute to the limited reproductive success observed for this species in managed care and may be a reflection of diminished seasonal cues and environmental differences.
Sand tiger sharks Carcharias taurus are one of fifteen extant species belonging to the Lamniformes order of sharks. Lamnoid sharks include some of the most ancient and recognizable shark species such as basking sharks Cetorhinus maximus, megamouth sharks Megachasma pelagios, and white sharks Carcharodon carcharias (Gilmore et al., 2005). The reproductive mode for all Lamniformes is oophagy, a matrotrophic aplacental mode of reproduction where developing embryos are supplied with eggs as a supplemental form of nutrition. Detailed information about the reproductive life history of this order of sharks is deficient due to their rarity and/or protected status, with five species classified as ‘Vulnerable’, four species classified as ‘Endangered”, and the sand tiger shark classified as ‘Critically Endangered’ by the International Union for Conservation of Nature’s (IUCN) Red List of Threatened Species (Rigby et al., 2021). Among lamniform species, most is known about the reproductive life history and biology of sand tiger sharks from studies of populations in the western North Atlantic (WNA) (Springer, 1948; Gilmore et al., 1983; Castro, 2009), western South Atlantic (WSA) (Lucifora et al., 2002), Australia (Bansemer and Bennett, 2008; Bansemer and Bennett, 2009) and South Africa (Bass et al., 1975; Dicken et al., 2006; Smale et al., 2007; Smale et al., 2012).
Aquariums have included sand tiger sharks in large mixed-species exhibits for nearly a century (Koob, 2004), but they do not readily reproduce in managed care (Henningsen et al., 2017; Willson and Smith, 2017; Wyffels et al., 2020a). Sharks are observed mating, but young rarely result. Evidence that females cycle in aquariums comes from observations of uterine metritis from retained egg cases and the release of egg cases into the habitat, commonly described as egg shedding, dumping or dropping (George et al., 2017). For sharks in managed care, reproduction may be affected by environmental, social, demographic and/or physiological differences from in situ populations (Henningsen et al., 2004a; Henningsen et al., 2004b; Henningsen et al., 2017). Endocrine dysfunction and poor gamete quality for male sand tiger sharks in managed care has been demonstrated, and likely contributes to limited reproductive success for this species in aquaria (Wyffels et al., 2020b).
Sand tiger shark females mature at 8-10 years (Goldman et al., 2006) with a lifespan of up to 40 years (Passerotti et al., 2014) and have a synchronous and seasonal reproductive cycle (Gilmore et al., 1983; Lucifora et al., 2002; Smale et al., 2007). At the start of the reproductive cycle, the ovary increases in size with maturation of tens of thousands of vitellogenic follicles that are ovulated and subsequently encapsulated by the oviducal (nidamental or shell) gland over a period of about 200 days (Gilmore et al., 2005; Castro, 2009). In addition to oophagy, sand tiger sharks utilize adelphophagy or intrauterine cannibalism, a rare reproductive strategy, early in gestation (Springer, 1948; Gilmore et al., 1983). During late gestation the ovary is no longer vitellogenic and the embryo relies on internally stored yolk from its distended yolk stomach. Gestation is estimated to be 280-300 days and parturition occurs in late winter or early spring depending on location, with more northern locations lagging behind warmer southern locations (Gilmore et al., 2005; Wyffels et al., 2020a).
The periodicity of the reproductive cycle of female sand tiger sharks has been described as annual (Gilmore, 1993), biennial (Branstetter and Musick, 1994; Lucifora et al., 2002; Dicken et al., 2006; Castro, 2009) and triennial (Bansemer and Bennett, 2009). Because gestation is less than a year, biennial and triennial reproductive cycles include one or more resting years after each active reproductive year, a common strategy for many large coastal elasmobranchs. The reproductive status of sand tiger shark females can be described as active or resting, based on ovarian activity and uterine contents. During an active year, females in the WNA undergo vitellogenesis in spring, are gravid during summer, fall and winter, and give birth in late winter and early spring (Gilmore et al., 2005; Wyffels et al., 2020a). Females in a resting year are not gravid and possess an avitellogenic or quiescent ovary during the same seasons. In the wild, reproductively active and resting females have been found to segregate spatially (Branstetter and Musick, 1994; Lucifora et al., 2002; Dicken et al., 2006; Smale et al., 2007), therefore, sampling a large part of their range is required to observe all reproductive stages and to accurately describe periodicity. In the WNA, females in an active reproductive state have been observed from the North Carolina and Florida Atlantic coast but not from more northern parts of their range (Gilmore et al., 2005).
Determining reproductive cycle length is simplified if the same shark can be examined over time, but this is not feasible for in situ populations because recapture of the same individual is uncommon. In contrast, by leveraging non-lethal methods including ultrasonography and endocrine profiling, the reproductive status of sharks in aquariums can be characterized longitudinally, throughout a complete reproductive cycle, and used to confirm patterns observed for in situ sharks where each female is examined at a single point in time during her reproductive cycle. There are few studies of the reproductive endocrinology of sand tiger sharks and none include samples from in situ females throughout their reproductive cycle (Henningsen et al., 2008; Henningsen et al., 2015; Wyffels et al., 2020b). In situ data is valuable as a standard of comparison for reproductive (Mylniczenko et al., 2019; Wyffels et al., 2020b) and general health parameters (Otway, 2015) of managed sharks and rays, especially in cases where the managed population fails to produce young.
Characterizing endocrine patterns associated with reproduction for in situ female sand tiger sharks will provide basic information needed to understand if female endocrine dysfunction contributes to the lack of reproduction for sand tiger sharks in managed care. The objective of this study was to contribute to our understanding of female reproduction of this iconic species by: (1) development of non-lethal methods to assess the reproductive status; (2) investigate reproductive seasonality and periodicity, and (3) compare reproductive characteristics of successfully reproducing in situ females to aquarium females.
In situ sharks were sampled from Delaware (DE) (n = 29) and South Carolina (SC) (n = 11) using longlining methods with 25-100 (16/0) circle hooks with flattened barbs baited with frozen-thawed Atlantic mackerel (Scomber scombrus). Lines were set throughout the day and soaked for 30 minutes to 2 hours before retrieval. In situ sharks sampled from North Carolina (NC) (n = 39) were caught using conventional rod and reel tackle, 25 miles offshore from Morehead City with angling time less than 10 minutes. Sharks (n = 79) were examined once during the study and fishing occurred between April-November 2015–2020. All sharks were tagged with conventional NMFS shark tags before release (Kohler and Turner, 2001). Sand tiger sharks (n = 42) housed at AZA institutions: former Georgia Aquarium affiliate Marineland Dolphin Adventure, Ripley’s Aquarium of the Smokies, Ripley’s Aquarium at Myrtle Beach, Ripley’s Aquarium of Canada and Wildlife Conservation Society’s New York Aquarium were examined 1–4 times annually for 1–5 years with a subset (n = 18) examined opportunistically and less frequently during the study. Aquarium environmental conditions for regularly monitored sharks (n = 24) are given in Table 1. The study was approved by the South-East Zoo Alliance for Reproduction & Conservation Institutional Animal Care and Use Committee (IACUC) and sharks were managed in accordance with AZA-accredited institutional husbandry guidelines.
In situ sand tiger sharks were restrained boat-side or transferred using a stretcher to a water-filled livewell aboard the vessel for examination. Restraint of sharks examined boat-side was accomplished using the fishing leader and a tail rope to secure the shark. Aquarium sand tiger sharks were manually restrained within a nylon cargo net or stretcher. Ventilatory support was provided with a submerged pump directed towards the shark’s mouth, and respiration rate was monitored throughout restraint (Stamper, 2004). Sharks were examined using tonic immobility or they remained upright or canted to either side as necessary for procedures.
Shark examinations were limited to 30 minutes. Blood (15 ml) was collected from the ventral tail vein from a ventral or lateral approach and immediately transferred to lithium heparin coated blood collection tubes without separator gel (BD Vacutainer, Franklin Lakes, NJ). Blood samples collected from in situ sharks were held in a cooler chilled with ice packs until processing at the end of the day. Plasma was collected after centrifugation (22-25°C, 1500 x g, 5 min) and stored frozen (-20°C) for steroid hormone analysis.
For the purposes of this study, sharks < 220 cm TL were considered juveniles, 220-230 cm TL subadults and >230 cm TL adults. Ultrasonography using an Edge II with a rC60xi 5-2 MHz transducer (Fujifilm SonoSite, Inc., Bothell, WA) or Ibex Pro with a Cli3.8 2.5–5 MHz curvilinear transducer (E.I. Medical Imaging, Loveland, CO) was performed to examine the ovary and uteri of females 220cm TL and larger (n = 98). The uterus was remarked to be collapsed or empty (Figure 1A) or to contain fluid, egg cases (Figure 1B) or developing embryos (Figure 1C). The ovary was either small and quiescent or enlarged with vitellogenic follicles (Figures 2A, B). Females with a vitellogenic ovary and/or developing embryos were considered to be in an active reproductive state. Females with an avitellogenic ovary and an empty uterus were considered to be in a resting reproductive state.
Figure 1 Ultrasonography was used to determine contents of the paired uteri. Sonographs (longitudinal) show a uterus (u) with no contents typical of resting females (A), a uterus with egg cases (*) (B), or both uteri, one with egg cases and the other with a developing embryo (dotted outline, arrow indicates snout) and egg cases (*) in early gestation (C). Each egg case (D) contains multiple eggs enclosed by a soft and pliable case secreted by the oviducal gland. Aquarium females without developing embryos were observed to release hundreds of uterine egg cases (E) up to 2 years after ovulation.
Figure 2 Sand tiger shark females develop large vitellogenic ovaries with thousands of follicles (A) during spring of their active reproductive year. Using ultrasonography (B), with the transducer in transverse orientation on midline just caudal to the pectoral girdle, the ovary (Ov) is juxtaposed to liver (L) and extends caudal to the anterior oviduct. A cystic ovary (C) is composed of yellow yolky vitellogenic follicles and fluid filled cysts (*) that appear anechoic in sonograms (D). Scale (cm) is at the right (B) or top and left (D) edge of sonograms.
One or more measures of shark length from the tip of the nose to: the tip of the tail in a natural position as total length (TL), the fork in the tail as fork length (FL), and the precaudal pit as precaudal length (PCL) was collected as examination time and field conditions allowed. Length data for measurements not collected directly were calculated using measured lengths and the previously established relationships between TL, FL and PCL for WNA sand tiger sharks (Goldman et al., 2006). Age was calculated from TL using female specific coefficients for the Von Bertalanffy growth function (Goldman et al., 2006). Age calculations for aquarium sharks were determined from TL at time of collection and known time in managed care. Maturity was assigned to sharks using direct evidence of reproductive cycling from ultrasonography including observation of vitellogenic follicles, uterine egg cases or embryos. Without direct evidence, maturity was assigned from total length using size at maturity determined in this study and previously reported size at maturity for sand tiger sharks in the WNA (Springer, 1948; Gilmore et al., 1983; Castro et al., 1999).
Plasma samples were analyzed using a competitive double antibody enzyme immunoassay (EIA) protocol using antibodies for 17β-estradiol (E2) (1,3,5(10)-estratrien-3, 17β-diol; R0008), testosterone (T) (testosterone-6-carboxymethyl oxime; R156/7), and progesterone (P4) (11α-hemisuccinate progesterone; R4859) and corresponding horseradish peroxidase (HRP) conjugates all sourced from C. Munro, Clinical Endocrinology Lab, University of California, Davis, CA. Antibody cross-reactivity for E2 (Amaral et al., 2013), T (Penfold et al., 2013) and P4 (Graham et al., 2001) have been reported previously. Plasma samples were extracted using diethyl ether before E2 and P4 assays and T was measured from neat plasma. Plasma was double ether-extracted by placing 1 ml into a 16 x 125 glass tube and adding 5 ml of diethyl ether (Fisher Scientific Co., LLC, Pittsburgh, PA) and vortexing for 1 min. The samples were frozen in liquid nitrogen, and the ether supernatant decanted into a second glass tube. The plasma was re-extracted, and the combined ether supernatants were air-dried, reconstituted in 1 ml of methanol (Fisher Scientific), and sonicated for 5 min. The methanol fraction was air-dried and reconstituted in 250 μl of EIA buffer (195 ml stock A (0.23 M NaH2PO4), 305 ml stock B (0.2 M Na2HPO4), 0.15 M NaCl, 1 g/L BSA; all chemicals from Sigma-Aldrich, Inc. St. Louis, MO 68178 USA). Serial dilutions of pooled plasma generated dose response curves parallel to serially-diluted standard. Assay sensitivity was 0.08 ng/ml (E2 and P4) and 0.02 ng/ml (T). Recovery of testosterone spiked plasma yielded 56% whereas recovery of unextracted plasma yielded 96%. Differences in processing techniques have previously been published, demonstrating the importance of validations for each hormone by sex (Graham et al., 2016). Recovery of known amounts of synthetic E2, T and P4 added to pools of plasma was 80% (regression equation: 1.2817x + 4.8721, r2 = 0.967), 96% (regression equation: 1.0252x + 0.003, r2 = 0.998) and 96% (regression equation: 1.0252x + 0.003, r2 = 0.998), respectively. Extracted plasma samples were assayed neat or diluted 1:2 for E2, and neat for P4. Non-extracted plasma was assayed 1:4 for T. Inter-assay coefficients of variation for high and low controls were 4.6% and 3.4% (E2), 10.3% and 6.3% (T) and 10.3% and 6.3% (P4). Mean intra-assay coefficients of variation were 2.73 ± 2.8%, 2.41 ± 0.08% and 3.42 ± 0.14% for E2, T, and P4, respectively. Hormone values were reported as ng/ml.
Antibody reactivity was investigated using reverse-phase high performance liquid chromatography mass spectrometry (HPLC/MS). Pooled serum from in situ samples previously determined in EIA assays to contain a relatively high concentration of one or more hormones (E2, T, and P4) was ether-extracted and dried as previously described. The pooled sample was desalted with a Sep-Pak Vac C-18 solid phase extraction column (WAT020805, Waters, Franklin, MA, 02038-3119 USA) and dissolved in 70% (v/v) methanol. Hormone standards (E2, T, and P4) were prepared singly at 40 ng/ml in 70% methanol and combined into one mixed standard. Separation of steroid hormones was carried out using a Waters HPLC/MS (Autopur) mass-directed preparative purification system with Waters 2767 Sample Manager and SQD2 MS using a Sunfire® Prep C18 OBD Column (19 × 100 mm, 5 μm particle size) eluted with a linear gradient of A [Millipore H2O/HPLC-grade Acetonitrile (ACN) (95:5 v/v + 0.1% formic acid)] and B [ACN (+ 0.1% formic acid)], time = 0 min A:B (95:5 v/v), time = 6 min A:B (0:100 v/v) and flow rate of 20 ml/min. Beginning 1 minute after sample loading (500 μl) and until all standards were eluted based on visual inspection of peaks at hormone specific m/z ESI pos [M+1] (273.16 E2, 289.42 T and 315.36 P4) (~6 minutes), 1ml fractions were collected for subsequent evaluation of immunoreactivity. Fractions from mixed standards and pooled plasma were dried, reconstituted in EIA assay buffer, and analyzed for E2, T, and P4 via EIA as described previously.
Descriptive statistics reported include mean, range and standard deviation (SD). Baseline concentration for each hormone was calculated using samples from all in situ sharks and an iterative algorithm (Brown et al., 1999). The mean concentration of each hormone from all in situ shark samples was calculated, and samples with values greater than 2 SD above the mean were removed before recalculation of the mean. This process was repeated successively until no samples with values greater than the mean + 2 SD remained. The mean of the remaining data was designated as the baseline and hormone concentrations greater than baseline + 2 SD were considered elevated. A Kruskal-Wallis test with pairwise post-hoc Dunn test using Benjamini Hochberg alpha adjustments was used to determine the effect of reproductive stage on steroid hormone concentration for in situ sharks. Linear mixed modeling (LMM) was used to assess the significance of reproductive stage on hormone concentration for aquarium sharks. Mixed models included individual as a random factor to account for unbalanced repeated measures for aquarium sharks with reproductive stage and environment or aquarium as potential factors or explanatory variables. Model fit was compared using likelihood ratio tests (LRT). For models with a significant LRT, explanatory factors were retained if the model Bayesian Information Criteria (BIC) was reduced (Schwarz, 1978). Final models were fit by restricted maximum likelihood (REML) with error degrees of freedom calculated using the Satterthwaite method. Differences for significant factors were explored using post-hoc pairwise comparisons with Tukey adjusted p-values and Kenward-Roger adjusted degrees of freedom. Mixed models were used to compare hormone concentration between in situ and aquarium sharks for common reproductive stages (resting and ovulatory). Models included the reproductive stage, population (aquarium or in situ) and their interaction with individual as a random factor to account for unbalanced repeated measures for aquarium sharks. Kendall’s correlation coefficients between steroid hormone pairs were calculated for aquarium and in situ sharks. Statistical analyses were conducted using R Version 4.0.3 (R Core Team, 2016) with packages lme4 Version 1.1-14 (Bates et al., 2015), lmerTest Version 2.0-33 (Kuznetsova et al., 2017), emmeans Vesion 1.3.1 (Lenth, 2018) and ggplot2 Version 3.3.2 (Wickham, 2009). An alpha of P < 0.05 was considered significant.
Shark length ranged from 145–299 cm TL (107-226 cm PCL) with more immature sharks sampled from DE compared to NC and SC (Table 2). The smallest female confirmed mature by ultrasonography observation of a vitellogenic ovary and uterine egg cases was 231 cm TL (175 cm PCL), and this was used as a threshold for maturity for this study (Table 3). Age calculated from TL for each size cohort indicates juveniles were up to 7.5 years old, subadults 8.2–9.2 years old and adult sharks 9.6 years or older (Table 3). Aquarium subadults were 224 ± 7 cm TL (n = 5, range 217–236 cm) and adults 264 ± 12 cm TL (n = 23, range 242–291 cm). Aquarium juveniles were not included in the study.
During this study, aquarium sharks were examined collectively 206 times without incident. One ovulatory stage female examined at the beginning of this study died within 24 hours of handling due to gut torsion. In an effort to minimize future risk of torsion, examination procedures for sharks were modified and females remained upright with incomplete rotation as required with few exceptions. Samples from 10 females were excluded from analyses because they were collected at the time of necropsy or health complications were discovered during physical examination of the female. Handling for 30 minutes or less did not result in observable negative effects upon release for in situ sharks and aquarium sharks swam normally upon release and resumed eating at their normally scheduled feedings, 24-48 hours post-examination.
The reproductive cycle of mature females was divided into active (pre-ovulatory, ovulatory, early gestation, midgestation, late gestation and postpartum) and resting (resting) stages based on ultrasonography observations of ovarian activity and uterine contents (Table 4). Females sampled from SC were observed in both active (n = 1) and resting (n = 3) reproductive states (Table 2). Females from NC (n = 23) were predominately (83%) in an active reproductive state with 3 resting females sampled, one each for the months of June – August. Females sampled in DE were either immature or in a resting reproductive state (Table 2).
Among sharks sampled in an active reproductive state, only one female sampled during April in SC was in the pre-ovulatory stage (Table 4). All other sharks observed in an active reproductive state were sampled from NC. Ovulatory females with uterine egg cases (Figures 1B, D) were observed in July, August, October and November (Table 2). The majority of mature sand tiger sharks sampled off the NC coast in late summer and early fall were confirmed pregnant (Figure 1C). No samples were collected from late gestation and postpartum stage females.
Aquarium females were examined in all months except January and September and observed in pre-ovulatory, ovulatory and resting reproductive stages. A second resting reproductive stage, designated as retaining (Table 4), describes females with an avitellogenic ovary and uterine egg cases without observable evidence of fertilization or embryonic development. Nearly half of all females were observed in the retaining stage for one or more physical examinations. Six observations of egg release (Figure 1E) were reported. Two females released egg cases in March and April, within a year of ovulation. The remaining 4 observations of egg case release were for egg cases retained in the uteri for more than a year after they were ovulated. Duration for the retaining stage calculated from the first observation of retaining at a physical examination to a reported egg shedding event was 301 ± 55 days. A single postpartum blood sample was collected in April from a female that was successfully mated and became pregnant during the study timeframe (Table 5) (Wyffels et al., 2020a).
A peak in pre-ovulatory stage females was observed April–June followed by ovulatory stage females in June and August (Table 5). Resting stage females were observed throughout the year and in nine of the ten months that females were examined. Retaining stage females were observed in six of the ten months that females were examined including spring, summer and fall. The largest percentage of females in a resting reproductive stage was observed in November with approximately half of females examined in February, March, July and August also in a resting reproductive stage (Table 5).
The minimum duration for pre-ovulatory and ovulatory stages calculated from sequential examinations where the female remained in the same reproductive stage was 70–119 days (n = 6) and 111–209 days (n = 4), respectively. Considering sequential examinations for pre-ovulatory and ovulatory stages together, the ovary contained vitellogenic follicles for 146–280 days (n = 4) during the active year of the reproductive cycle. The two oldest females in the study had a cystic ovary that resolved partially or completely when vitellogenesis commenced (Figures 2C, D).
The resting state (combining retaining and resting stages) for 8 reproductive cycles from 6 aquarium females was 327 ± 24 days. Two females moved from an institution without seasonal changes in temperature to an institution with seasonal changes in temperature had a winter ovulatory cycle before relocation followed by an abbreviated resting period of more than 105 days but less than 216 days before their next cycle in spring. Two females were observed in a resting state for longer than a year, the first for more than 546 days and less than 727 days and the second for more than 472 days and in a resting state as of the completion of the study.
Nineteen females were examined longitudinally for two or more consecutive years to determine reproductive cycle length (Table 6). Females had a pattern of reproductive cycling that was consistent with alternating years of activity and rest with confirmed biennial (n = 7) or triennial (n = 3) reproductive cycles. One of the two triennial females was newly mature (10 years old) but the second female was nearly 20 years old. The time for one complete reproductive cycle was 1084 days for one female with a triennial cycle and 747 and 722 days for 2 females with a biennial cycle. The shortest time for a complete reproductive cycle (572 days) was observed for one of the two relocated females described above with an abbreviated resting stage. No females followed an annual reproductive cycle; an active reproductive year was always followed by, and/or preceded by, a resting reproductive year. Females (n = 9) that were observed first in a resting year and followed for 3 reproductive seasons or fewer could not be confirmed as biennial or triennial before the end of the study.
Table 6 Reproductive cycle length and demographics of longitudinally monitored aquarium sand tiger sharks Carcharias taurus.
Plasma immunoreactivity for HPLC fractions of pooled, ether-extracted plasma occurred in the same fractions as E2, T and P4 standards.
Hormone concentration generally increased with shark size and reproductive activity (Figures 3A, 4A, 5A). Baseline and elevated (baseline + 2 SD) hormone concentration determined from an iterative analysis of samples from all sharks was 0.124 and 0.326 ng/ml for E2, 1.12 and 2.87 ng/ml for T, and 0.173 and 0.303 ng/ml for P4. Kendall’s correlation coefficient indicates weak or no correlation between steroid hormones: P4 and T (τb = 0.003, P = .9745), E2 and T (τb = -0.01, P = 0.92), and E2 and P4 (τb = -0.25, P = 0.009). Among steroid hormones, E2 was measured in the smallest proportion of mature sharks and in the lowest concentrations. E2 was measured in plasma from 47% of immature sharks and 43% of mature sharks (Figure 3A). It was either not present or below the assay detection limit (0.08 ng/ml) for remaining samples. E2 was elevated in plasma from 24% of mature sharks in an active stage of reproduction, not elevated for mature sharks in a resting stage of reproduction and elevated for 3% of immature sharks (Table 7). Reproductive stage had a statistically significant effect on E2 concentration (H(4) = 11, P = 0.0264, n = 54). Post-hoc pairwise comparisons using a Benjamini Hochberg-adjusted alpha were significant between early gestation and midgestation stages (P = 0.0221) (Figure 3B). A trend for elevated E2 concentration for ovulatory females was observed but not significant. The highest E2 measured was from a pre-ovulatory stage female sampled in April (Figure 3C).
Figure 3 Plasma 17β-estradiol concentration by (A) shark length, (B) reproductive stage, and (C) month for in situ sand tiger sharks. Color indicates reproductive stage and the dotted line represents the threshold for elevated hormone concentration. Early gestation and midgestation 17β-estradiol were significantly different (*P = 0.02).
Figure 4 Plasma testosterone concentration by (A) shark length, (B) reproductive stage, and (C) month for in situ sand tiger sharks. Color indicates reproductive stage and the dotted line represents the threshold for elevated hormone concentration. There was a significant difference between immature and resting (*P = 0.0443), ovulatory (**P = 0.00107), early gestation (*P = 0.0161) and midgestation (****P <.0001) stages. Testosterone concentration was highest in the fall, associated with gestational females.
Figure 5 Plasma progesterone concentration by (A) shark length, (B) reproductive stage, and (C) month for in situ sand tiger sharks. Color indicates reproductive stage and the dotted line represents the threshold for elevated hormone concentration.
Table 7 Sand tiger shark Carcharias taurus plasma samples with hormone concentrations below detection or elevated by reproductive stage.
Testosterone was measured in 95% of immature sharks and 100% of mature sharks (Figure 4A). Testosterone was elevated in 95% of mature sharks in an active stage of reproduction compared with 43% of sharks in a resting stage of reproduction, and 10% of immature sharks (Table 7). Testosterone concentration was significantly affected by reproductive stage (H(4) = 39.65, P <.0001, n = 68) with increasing concentration upon maturity and for females progressing through successive stages of reproduction (Figure 4B). There was a significant difference between immature and resting (P = 0.0443), ovulatory (P = 0.00107), early gestation (P = 0.0161) and midgestation (P <.0001) stages (Figure 4B). Testosterone concentration was highest in the fall, associated with gestational females (Figure 4C).
Progesterone was measured in plasma from 97% of immature sharks and 91% of mature sharks (Figure 5A). Progesterone was elevated in plasma from 59% of mature sharks in an active stage of reproduction compared with 17% of sharks in a resting stage of reproduction and 25% of immature sharks (Table 7). Reproductive stage had a statistically significant effect on P4 concentration (H(4) = 10.6, P = 0.0308, n = 54) (Figure 5B). Post-hoc pairwise comparisons of unadjusted alpha values revealed significant differences between resting and ovulatory (P = 0.0395), resting and midgestation (P = 0.0145), ovulatory and early gestation (P = 0.0371) and early and midgestation (P = 0.0184) however, after Benjamini-Hochberg adjustments, all p-values exceeded the threshold for significance (Figure 5B). The highest P4 concentrations among mature females were measured during the ovulatory stage and all midgestation females had elevated P4. Generally, P4 concentrations rose for females in active states of reproduction from spring to summer and fall (Figure 5C).
Steroid hormones from 18 aquarium sharks sampled during their active and resting states revealed that the majority of females have dynamic changes in hormone concentration associated with reproductive stage. However, five females (Females 14 –18) were relatively refractory, with hormone concentrations consistently below or near baseline regardless of reproductive stage (Figure 6). Kendall’s correlation coefficient indicates weak or no correlation between steroid hormone pairs for aquarium sharks: P4 and T (τb = 0.28, P <0.001), E2 and T (τb = 0.23, P <0.001), and E2 and P4 (τb = 0.06, P = 0.32). Plasma E2 was elevated in the majority (68%) of samples from aquarium females in an active reproductive state but only a quarter (26%) of samples from sharks in a resting reproductive state and immature sharks (Table 7). Half (51%) of plasma samples from sharks in a resting reproductive state and a third (35%) of samples from immature sharks had E2 levels below the detection limit (Table 7). Testosterone was detected in nearly all mature sharks and 70% of immature sharks. More than half (54%) of mature females in an active state of reproduction had elevated T compared with 21% of resting state (resting and retaining stages) females (Table 7). Progesterone was elevated for the majority (63%) of sharks in an active stage of reproduction compared with only 10% of resting state sharks (Table 7). Environment (aquarium) was not associated with hormone concentration, but reproductive stage was significant. Pre-ovulatory stage aquarium sharks had significantly elevated E and P4 compared to immature, resting, retaining, and ovulatory stage sharks (Figure 7). Testosterone also was elevated for pre-ovulatory compared to immature, resting and retaining stages but not different from ovulatory stage sharks (Figure 7). The only aquarium female to become pregnant (Female 1) during the study had the highest overall range of hormone concentrations for samples collected during resting and active reproductive states (Wyffels et al., 2020a) (Figure 6). Two females with a cystic ovary (Females 7 and 13) had hormone concentrations within the range observed for aquarium females (Figure 6).
Figure 6 Plasma (A)17β-estradiol (B) testosterone and (C) progesterone concentration for aquarium sharks monitored longitudinally in resting and active reproductive years. Each symbol represents a different timepoint for each female. In situ shark data were combined into gravid and non-gravid categories with each symbol representing a single point in time for different females. The shape and color of each point indicate reproductive stage.
Figure 7 Plasma (A)17β-estradiol (B) testosterone (C) and progesterone concentration (marginal mean and 95% confidence interval) by reproductive stage for aquarium sand tiger sharks. Different letters indicate significant (P < 0.05) differences between stages within each hormone plot.
The proportion of plasma samples with elevated P4 concentrations was comparable for aquarium and in situ sharks (Table 7). In contrast, T concentration for aquarium sharks was persistently lower and E2 concentration was persistently higher compared to in situ shark plasma hormone concentrations (Table 7). Statistical comparison of hormone concentration between in situ and aquarium sharks was limited to reproductive stages that were overlapping between the two populations: resting and ovulatory. Hormone concentrations for immature sharks were not compared between populations because in situ samples were predominantly from juveniles and aquarium samples entirely from subadults. There was no difference in E2, T or P4 between in situ and aquarium sharks for resting females. Similarly, E2 and P4 were not different between populations for ovulatory stage females but T was significantly higher (P < 0.001) for in situ sharks.
Seasonal patterns of ovulation and gestation confirm that the reproductive cycle of female sand tiger sharks is biennial or triennial with a breeding season during spring and early summer for sharks in the WNA. Critical to accomplishing research with threatened and protected species, nonlethal methods, including ultrasonography and endocrine profiling, were developed and shown to be effective for reproductive monitoring. Handling and restraint of aquarium sharks allowed a unique opportunity to assess and refine examination techniques, as post-release swimming patterns and food intake could be easily monitored. Examination procedures were optimized to minimize risk of post-procedure complications observed previously for females with uterine egg cases and fragile, large vitellogenic ovaries susceptible to torsion and rupture (Daly and Jones, 2017; Penfold and Wyffels, 2019). Similar to male sand tiger sharks, endocrine profiles for female sharks under managed care were different than observed for in situ counterparts (Wyffels et al., 2020b).
The biennial or triennial reproductive cycle of female sand tiger sharks is synchronized with seasonal migrations in fall and spring associated with reproduction (Gilmore, 1993). Females in early stages of their active year of reproduction were observed in spring and early summer in the Carolinas (Gilmore et al., 1983; Castro, 2009). Because reproduction is synchronous in this species, if females reproduce annually, all females examined during the same timeframe would be in a similar stage of reproduction. However, during summer, fishing occurred in NC and DE and females were segregated by reproductive condition with active females in NC and resting females in DE. The pattern indicates one or more year of rest between active reproductive cycles as has been proposed for the WNA population (Branstetter and Musick, 1994) as well as the SWA (Lucifora et al., 2002), South African (Dicken et al., 2006) and Australian (Bansemer and Bennett, 2009) sand tiger shark populations.
A reproductive cycle that includes a minimum of one (biennial) and a maximum of two (triennial) resting years was confirmed through longitudinal monitoring of aquarium-housed sharks. Previously hormone profiles supported a biennial cycle for a longitudinally monitored aquarium female (Henningsen et al., 2008) and the addition of ultrasonography in this study corroborates endocrine data and shows unequivocally the cycle length. The majority of females in this study had a biennial cycle, similar to proportions described for a critically endangered population at Wolf Rock, southeast Queensland, Australia (Bansemer and Bennett, 2009). Biennial and triennial cycles were observed for aquarium sharks exposed to various combinations of natural, seasonal or constant photothermal regimes, suggesting the periodicity is not a function of light or temperature. Reproductive age may have a role in periodicity with newly mature and older females adopting longer cycles. The energetic demands of reproduction appear rate-limiting for sand tiger sharks and necessitate a year of rest to recuperate and replenish hepatic reserves and prepare a new cohort of ovarian follicles (Castro et al., 1999). Young are nearly half the size of mature females at parturition and the female must store enough energy to sustain her and the developing embryos during a relatively long gestation. Food consumption patterns from male and female sand tiger sharks show decreased consumption trends during the mating season, and for females, increased consumption in preparation for ovulation and again postpartum has been observed (Townsend and Gilchrist, 2017; Willson and Smith, 2017; Wyffels et al., 2020a). Plasticity in reproductive periodicity may be possible with females initiating or halting a reproductive cycle in response to poor body condition or unfavorable environmental factors (Johnson and Snelson, 1996; Bansemer and Bennett, 2009; Driggers and Hoffmayer, 2009; Furumitsu et al., 2019).
Biennial and triennial cycle plasticity has been described in other species such as the Argentinian lizards, Liolaemus pictus and Liolaemus elongatus (Ibargüengoytía and Cussac, 1996; Ibargüengoytía and Cussac, 1998). Liolaemus pictus exhibit vitellogenesis and pregnancy in alternate years, sometimes skipping a year of reproduction for a biennial to triennial cycle (Ibargüengoytía and Cussac, 1996). Similarly, L. elongatus has an annual or biennial reproductive cycle (Ibargüengoytía and Cussac, 1998). This suggests that the enormous amounts of energy required to produce the large numbers of yolky eggs must be replaced before egg laying can commence again, and so resting periods of one to two years are essential.
The reproductive cycle for seasonally reproducing sharks is annual, biennial or even triennial (Castro, 2009). Two important factors that influence timing of the reproductive cycle for seasonal aquatic species, water temperature and day length (Heupel et al., 1999; Mull et al., 2008; Mull et al., 2010; Dharmadi and White, 2015; Nozu et al., 2018; Elisio et al., 2019), also vary annually. This suggests that changing water temperature and day length may promote reproduction for sharks under managed care (Henningsen et al., 2004a; Henningsen et al., 2017). Aquarium females exposed to seasonal changes in temperature and/or day length cycled predictably and exhibited the same seasonality as their in situ counterparts. Similar to male sand tiger sharks, aquarium females that were maintained in habitats without seasonal changes sometimes cycled unpredictably (Wyffels et al., 2020b). Aquarium females shifted seasonality in response to changes in environment that resulted in predictable cycling in late spring similar to in situ females. This suggests plasticity in cycle length between biennial and triennial for in situ females may be possible, especially if linked to resource availability, which has implications for management of sand tiger sharks. Intraspecific variation in reproductive life-history characteristics within a population and between geographically distinct populations is known for several shark species and environmental factors including temperature have been proposed as potential drivers (Yamaguchi et al., 2000; Driggers and Hoffmayer, 2009; Hoffmayer et al., 2013; Trinnie et al., 2014). No aquarium female in this study cycled annually, highlighting that even with sufficient resources provided in managed care, females are not able to reproduce more frequently than every other year.
Reproductive longevity, time to maturity, and reproductive cycle length are critical parameters for estimating reproductive productivity for any species. Shark age is difficult to assess because of the nonlinear relationship between vertebral and somatic growth and the diminishing rate of growth with age (Natanson et al., 2018). Age estimates from age-length relationships are most accurate for sand tiger sharks 12 years and younger (Passerotti et al., 2014). In this study, half of the aquarium females monitored were wild-sourced when 12 years or younger so their age estimate is relatively reliable compared to the other half, 13-16 years old when wild-sourced, and whose age is likely underestimated. The youngest aquarium females were 8-9 years old at the time of their first reproductive cycle which aligns with previous estimates of age to maturity at 8-10 years based on band deposition in vertebrae (Goldman et al., 2006). In addition, two subadults monitored for 3 years were 8 years old at the end of the study, and one of the two cycled for the first time in the last year of the study.
Size at maturity for females in the WNA has been described as 220–230 cm TL (Springer, 1948; Gilmore et al., 1983; Branstetter and Musick, 1994). However, in this study, the range of lengths for in situ females confirmed mature by observation of uterine eggs or young based on antemortem, non-lethal ultrasonography was 231–299 cm TL. This overlaps with the size reported for pregnant females in the WNA (229- 312 cm TL) (Springer, 1948; Gilmore et al., 1983; Castro, 2009). This suggests that females in the WNA 220- 230 cm TL are predominately maturing subadults and not yet reproducing. In the SWA pregnant females were a similar size (n = 12, 226–252 cm TL) (Sadowsky, 1970) but pregnant females from South Africa are larger, 240-272 cm TL (Bass et al., 1975; Smale, 2002). Sand tiger sharks have a prominent precaudal pit making determination of PCL a more robust option for reporting lengths because it is not subject to differences in tail position (stretch or natural) or significant flux during measurement.
Aquarium females as old as 30 years continued to cycle and four of the oldest females (25-30 years) monitored in the study (n=2) or examined upon necropsy (n=2) had a cystic ovary. Cystic ovaries have been observed in older/larger elasmobranchs of multiple species and may be part of the aging process and reproductive senescence or be associated with disease (Mylniczenko et al., 2019). Ovarian cysts are fluid filled sacs that are distinguished from vitellogenic follicles by their generally larger size and homogeneous dark appearance during ultrasound. The difference in echogenicity between cysts and developing follicles reflects the contents, with yolky vitellogenic follicles appearing heterogeneous and hypoechoic compared to anechoic cysts that contain translucent fluid. Because the two oldest aquarium sharks in the study were older than 12 years when wild-sourced, their actual age is likely underestimated and the true lifespan is likely more than 30 years as was suggested from bomb radiocarbon dating (Passerotti et al., 2014). This suggests females are immature for a third of their lifespan and reproduce for the remaining two-thirds. If a female lives for 30 years and produces 2 young every other year (biennial) or every three years (triennial), she will produce 14-20 young during her lifetime. The low reproductive productivity (Bass et al., 1975; Gilmore et al., 1983; Branstetter and Musick, 1994) and long time to maturity (Goldman et al., 2006; Passerotti et al., 2014) are reasons for the high susceptibility of this species to anthropomorphic threats including fishing pressure (Kilfoil et al., 2017), climate change, and loss of critical habitat. Understanding and using the full range of life history characteristics, especially reproductive parameters, is critical for population assessment, which in turn informs management and conservation plans.
Like all vertebrates, elasmobranch reproduction is regulated by gonadotropin releasing hormones (GnRH) and the hypothalamic-pituitary-gonadal (HPG) axis, with hormones serving as chemical messengers. Among elasmobranchs, endocrine studies are uncommon and few of the more than 1200 species have had the endocrine component of their reproductive cycle characterized. Complicating interpretation of hormone profiles, elasmobranchs utilize a wide range of reproductive modes (Wourms, 1977). Hormone functionality among elasmobranchs may be as equally diverse as reproductive mode and somewhat species-specific, however, functional commonalities for steroid hormones have been described (Awruch, 2013).
Sand tiger sharks are matrotrophic and viviparous reproducing via oophagy and adelphophagy and as a result, have a protracted period of ovulation that overlaps with gestation. Elevated E2 was observed for pre-ovulatory females, linking it to vitellogenesis similar to other studies that have suggested estrogens in elasmobranchs may regulate vitellogenesis, oviducal gland function, and egg case progression in the oviduct (Manire et al., 1995; Tricas et al., 2000; Marina et al., 2008; Elisio et al., 2019). Early gestation showed a trend for higher E2 compared with midgestation. This also supports a link between E2 and vitellogenesis because ovulatory activity tapers during mid-gestation as embryos switch from consumption of newly ovulated egg cases to yolk from their distended yolk stomachs.
For females, testosterone may serve as a precursor for E2 and/or have its own physiological role in reproduction. Among elasmobranchs, T has been reported to stimulate mating behavior, promote sperm storage, and maintain embryonic diapause (Rasmussen and Murru, 1992; Manire et al., 1995; Koob and Callard, 1999; Tricas et al., 2000; Waltrick et al., 2012; Elisio et al., 2019). There is no evidence of sperm storage or diapause in sand tiger sharks, but because T was elevated for pre-ovulatory aquarium sharks it may serve as an E2 precursor and it may also promote pre-copulatory behavior. Testosterone remained elevated for ovulatory aquarium females supporting a link to ovulation or egg case progression in the oviduct. Testosterone was a maturity biomarker and distinguished immature in situ sharks from all reproductive stages of mature in situ sharks. Only one in situ subadult female had elevated T and based on her size (228 cm TL, 196 cm FL, 169 cm PCL) she was either mature in a resting state of reproduction or near maturity. Among in situ sharks, evidence for an association between T and ovulation comes from elevated levels for ovulatory through midgestation sharks. The large variance in T concentration observed during midgestation might be associated with in situ females concluding ovulatory activity. Additionally, although there was only one in situ pre-ovulatory female sampled, she had elevated T and E2 supporting the precursor hypothesis. Alternatively, T may have physiological roles associated with energetics and stressors related to seasonal migrations (Rankin, 1991) for sand tiger sharks as was preliminarily shown for juvenile male tiger sharks (Wosnick et al., 2018) and has been shown for birds (Ramenofsky and Wingfield, 2006; Lymburner et al., 2016) and diadromous fishes (Ueda et al., 1984; Munakata et al., 2000; Munakata et al., 2001; Crossin et al., 2009; Sudo et al., 2011).
Progestogens are thought to serve as maturational hormones (Manire et al., 1995; Snelson et al., 1997; Tricas et al., 2000; Marina et al., 2008; Fujinami and Semba, 2020). Progesterone for aquarium sharks was significantly elevated for pre-ovulatory sharks and a trend for higher concentrations was evident in ovulatory females but not in other stages. For in situ sand tiger sharks, P4 was the least diagnostic steroid hormone for assessing reproduction with concentrations from mature and immature sharks overlapping for most reproductive stages. Gonadal progesterone is secreted by post-ovulatory follicles (corpus luteum), however; progesterone also may be derived from interrenal gland secretions. Observation of elevated P4 for pre-ovulatory and ovulatory sharks supports a maturational role for this hormone but the source of P4 for pre-ovulatory aquarium sharks requires further investigation and consideration. It is possible for elasmobranch pre-ovulatory follicles to undergo atresia and become steroidogenic (Waltrick et al., 2017). Sand tiger sharks females have voluminous uteri and some aquarium females may have commenced ovulation but the few uterine egg cases present elluded detection during examination. In situ sharks afford another hypothesis. The cause for elevated P4 observed for immature females and mature females in their resting year may be due to its more broad functionality in elasmobranch reproduction and physiology through activation of interrenal mineralocorticoid receptors (Wheaton et al., 2018; Baker and Katsu, 2020). Acute physiological stressors, such as restraint during physical examinations and blood draws have been shown to alter circulating hormone concentration within minutes of handling for some species (Cooper et al., 1995; Moe and Bakken, 1996; Krause et al., 2014). The short-term stress associated with fishing and subsequent restraint may have caused ephemeral spikes in interrenal-derived progesterone, confounding potential differences associated with reproductive stage. Increasing the number of sharks sampled and minimizing stress associated with fishing may help elucidate the role of this hormone for sand tiger sharks.
Comparable endocrine data for sand tiger sharks is available for an aquarium female housed at National Aquarium (Henningsen et al., 2008). Over 16 months of monitoring (without ultrasonography), a single peak in E2 and P4 was observed during late winter and early spring, corresponding to when pre-ovulatory vitellogenic females were observed in this study. A single and delayed but temporally overlapping peak in T was observed also (Henningsen et al., 2008). Although the methodology for measuring hormones differed, the patterns observed were similar providing secondary validation to this study.
Similar to reproducing in situ sharks, all plasma samples from the aquarium female that became pregnant during the study had elevated T concentrations. Endocrine dysfunction, especially T, may be associated with the poor reproductive success observed for this species in managed care. This theory is supported by the higher T concentration for ovulating in situ females compared to aquarium females. Other comparisons between in situ and aquarium females were hampered by a lack of samples in comparable stages of reproduction and a low number of samples with high variability in hormone concentration.
Plasma from all reproductive stages except gestation was collected from aquarium females but because aquarium females are not readily reproducing, endocrine patterns may not be representative of normal reproduction for the species (Henningsen et al., 2008). The endocrinology of successfully reproducing in situ sand tiger sharks is important as a biological standard that can be used to understand reproductive dysfunction. Females in aquariums continue to cycle but their ability to become pregnant may be diminished by an improper hormone milieu. It is also possible that pregnancy fails very early in gestation and goes undetected in aquarium females. Reproduction, including gamete quality and hormone concentrations, are often disrupted for fish in managed care as a result of differences from in situ habitat, social dynamics, nutrition and photothermal regimes (Henningsen et al., 2008; Henningsen et al., 2015; Zupa et al., 2017).
Noninvasive reproductive monitoring includes observations of mating and egg release for aquarium females. Egg release is proof of reproductive cycling but egg retention for up to 2 years effectively uncouples ovulation and egg dropping events and makes the timing of reproductive cycles impossible to interpret without routine monitoring. In some instances, retained egg cases can cause egg metritis that can result in animal death that may be prevented through routine reproductive monitoring and treatment (George et al., 2017). Reproductive hormones distinguished mature females in situ and pre-ovulatory females in aquariums. For other reproductive stages, hormones must be interpreted alongside additional reproductive data to be informative. Future studies should include additional steroid hormones that may have a role in reproduction for this species. Physical examination using ultrasonography remains the best non-lethal assessment method to determine reproductive stage for sand tiger sharks. Additional research is needed to understand control of the reproductive cycle and identify alternative biomarkers of reproduction, energetic markers for instance. Understanding the drivers of reproduction for sand tiger sharks has important implications for their conservation. Spatial segregation of in situ females depending on reproductive status may be linked to environmental parameters important for either gestation or postpartum energy recovery. Identification and protection of resources critical for sand tiger shark reproduction such as migration corridors, gestation and parturition grounds, and seasonal prey will support survival and reproduction. Elucidating environmental factors associated with successful reproduction in aquarium sharks and the wild population will help advance our understanding of reproduction for this species and in turn, inform impactful conservation policy.
The raw data supporting the conclusions of this article will be made available by the authors upon reasonable request.
The animal study was reviewed and approved by The South-East Zoo Alliance for Reproduction and Conservation Institutional Animal Care and Use Committee and carried out in accordance with AZA-accredited institutional husbandry guidelines.
JW and LP obtained funding for the research and conceived the study. JW, RG, EC, TC, FL, CB, VL, MH, and AN conducted aquarium shark examinations and RG, EC, and JW conducted in situ shark examinations. JW curated, analyzed and interpreted the data, completed HPLC/MS work, prepared the figures and tables and drafted the manuscript. LP provided project oversight, data interpretation and manuscript revisions. All authors contributed to and approved the final manuscript.
This work was supported in part by a grant from the SeaWorld & Busch Gardens Conservation Fund to JW and LP. HPLC/MS work was supported by the Delaware COBRE program, with a grant from the National Institute of General Medical Sciences – NIGMS (5 P30 GM110758-02) from the National Institutes of Health.
The content is solely the responsibility of the authors and does not necessarily represent the official views of the National Institutes of Health.
The authors declare that the research was conducted in the absence of any commercial or financial relationships that could be construed as a potential conflict of interest.
All claims expressed in this article are solely those of the authors and do not necessarily represent those of their affiliated organizations, or those of the publisher, the editors and the reviewers. Any product that may be evaluated in this article, or claim that may be made by its manufacturer, is not guaranteed or endorsed by the publisher.
This paper is dedicated to Alan Henningsen, a friend and colleague, for his many contributions and enduring role in aquarium-led elasmobranch research. We thank the expert husbandry and veterinary staff at Georgia Aquarium and Marineland Dolphin Adventure (Marineland), Ripley’s Aquarium of the Smokies, Ripley’s Aquarium at Myrtle Beach, Ripley’s Aquarium of Canada and Wildlife Conservation Society’s New York Aquarium. We thank North Carolina Aquariums, Florida Aquarium and Adventure Aquarium for providing opportunistic samples from their aquarium sharks and Cayman Adams for endocrine data and validated EIA assays. Alan Henningsen from the National Aquarium generously contributed sand tiger shark plasma samples for hormone assay validation. Thanks to Papa Nii Asare-Okai, the Director of the Mass Spectrometry Facility at the University of Delaware for expertise and assistance with HPLC/MS. We especially are indebted to Ripley’s Aquariums, North Carolina Aquariums, the National Aquarium, and Cami McCandless and the Cooperative Atlantic States Pupping and Nursery Survey (COASTSPAN) and Cooperative Shark Tagging Program (CSTP) administered by the Apex Predators Program (APP) with Brian Gervelis for collaborative in situ sample collection efforts. This research benefitted from discussions of sand tiger shark reproduction and life history with Grant Gilmore, Dewayne Fox, Alan Henningsen, and Jose Castro. Finally, the authors thank Lara Metrione and reviewers for constructive comments during the peer review process.
Amaral R. S., Rosas F. C. W., da Silva V. M. F., Nichi M., Oliveira C. A. (2013). Endocrine Monitoring of the Ovarian Cycle in Captive Female Amazonian Manatees (Trichechus inunguis). Anim. Reprod. Sci. 142, 84–88. doi: 10.1016/j.anireprosci.2013.09.001
Awruch C. A. (2013). Reproductive Endocrinology in Chondrichthyans: The Present and the Future. Gen. Comp. Endocrinol. 192, 60–70. doi: 10.1016/j.ygcen.2013.05.021
Baker M. E., Katsu Y. (2020). Progesterone: An Enigmatic Ligand for the Mineralocorticoid Receptor. Biochem. Pharmacol. 177, 113976. doi: 10.1016/j.bcp.2020.113976
Bansemer C. S., Bennett M. B. (2008). Multi-Year Validation of Photographic Identification of Grey Nurse Sharks, Carcharias taurus, and Applications for non-Invasive Conservation Research. Mar. Freshw. Res. 59, 322–331. doi: 10.1071/MF07184
Bansemer C., Bennett M. (2009). Reproductive Periodicity, Localised Movements and Behavioural Segregation of Pregnant Carcharias taurus at Wolf Rock, Southeast Queensland, Australia. Mar. Ecol. Prog. Ser. 374, 215–227. doi: 10.3354/meps07741
Bass A. J., D’Aubrey J. D., Kistnasamy N. (1975). Sharks of the East Coast of Southern Africa. IV. The Families Odontaspididae, Scapanorhynchidae, Isuridae, Cetorhinidae, Alopiidae, Orectolobidae and Rhiniodontidae. Investig. Rep. Oceanogr. Res. Inst. 39, 1–102.
Bates D., Mächler M., Bolker B., Walker S. (2015). Fitting Linear Mixed-Effects Models Using lme4. J. Stat. Software 67, 1–48. doi: 10.18637/jss.v067.i01
Branstetter S., Musick J. A. (1994). Age and Growth Estimates for the Sand Tiger in the Northwestern Atlantic Ocean. Trans. Am. Fish. Soc 123, 242–254. doi: 10.1577/1548-8659(1994)123<0242:AAGEFT>2.3.CO;2
Brown J. L., Schmitt D. L., Bellem A., Graham L. H., Lehnhardt J. (1999). Hormone Secretion in the Asian Elephant (Elephas Maximus): Characterization of Ovulatory and Anovulatory Luteinizing Hormone Surges. Biol. Reprod. 61, 1294–1299. doi: 10.1095/biolreprod61.5.1294
Castro J. I. (2009). Observations on the Reproductive Cycles of Some Vivparous North American Sharks. Aqua Int. J. Ichthyol. 15, 205–222.
Castro J. I., Woodley C. M., Brudek R. L. (1999). A Preliminary Evaluation of the Status of Shark Species. FAO Fish. Tech. Pap. 380, 1–72.
Cooper C., Evans A., Cook S., Rawlings N. C. (1995). Cortisol, Progesterone and β-Endorphin Response to Stress in Calves. Can. J. Anim. Sci. 75, 197–201. doi: 10.4141/cjas95-029
Crossin G. T., Hinch S. G., Cooke S. J., Cooperman M. S., Patterson D. A., Welch D. W., et al. (2009). Mechanisms Influencing the Timing and Success of Reproductive Migration in a Capital Breeding Semelparous Fish Species, the Sockeye Salmon. Physiol. Biochem. Zool. 82, 635–652. doi: 10.1086/605878
Daly J. J., Jones R. (2017). “The Use of Reproductive Technologies in Breeding Programs for Elasmobranchs in Aquaria,” in The Elasmobranch Husbandry Manual II: Recent Advances in the Care of Sharks, Rays and Their Relatives. Eds. Smith M., Warmolts D., Thoney D., Hueter R., Murray M., Ezcurra J. (Columbus: Special Publication of the Ohio Biological Survey), 363–374.
Dharmadi F., White W. T. (2015). Species Composition and Aspects of the Biology of Orectolobiformes From Indonesian Waters. J. Fish Biol. 86, 484–492. doi: 10.1111/jfb.12569
Dicken M., Smale M., Booth A. (2006). Spatial and Seasonal Distribution Patterns of the Ragged-Tooth Shark Carcharias taurus Along the Coast of South Africa. Afr. J. Mar. Sci. 28, 603–616. doi: 10.2989/18142320609504210
Driggers W. B., Hoffmayer E. R. (2009). Variability in the Reproductive Cycle of Finetooth Sharks, Carcharhinus isodon, in the Northern Gulf of Mexico. Copeia 2009, 390–393. doi: 10.1643/CE-08-167
Elisio M., Awruch C. A., Massa A. M., Macchi G. J., Somoza G. M. (2019). Effects of Temperature on the Reproductive Physiology of Female Elasmobranchs: The Case of the Narrownose Smooth-Hound Shark (Mustelus schmitti). Gen. Comp. Endocrinol. 284, 113242. doi: 10.1016/j.ygcen.2019.113242
Fujinami Y., Semba Y. (2020). Non-Lethal Assessment of Reproductive Stage for Female Blue Sharks Prionace glauca Using Sex Steroid Hormones. J. Fish Biol.96, 1501–1504. doi: 10.1111/jfb.14312
Furumitsu K., Wyffels J. T., Yamaguchi A. (2019). Reproduction and Embryonic Development of the Red Stingray Hemitrygon akajei From Ariake Bay, Japan. Ichthyol. Res. 66, 419–436. doi: 10.1007/s10228-019-00687-9
George R. H., Haywood P., Steeil J., Aczm D., Box P. O., Baine K., et al. (2017). “Diagnosis and Treatment of Common Reproductive Problems in Elasmobranchs,” in The Elasmobranch Husbandry Manual II: Recent Advances in the Care of Sharks, Rays and Their Relatives. Eds. Smith M., Warmolts D., Thoney D., Hueter R., M M., Ezcurra J. (Columbus, Ohio: Special Publication of the Ohio Biological Survey), 357–362. Available at: https://sites.google.com/site/elasmobranchhusbandry/manual.
Gilmore R. G. (1993). Reproductive Biology of Lamnoid Sharks. Environ. Biol. Fishes 38, 95–114. doi: 10.1007/BF00842907
Gilmore R. G., Dodrill J. W., Linley P. A. (1983). Reproduction and Embryonic Development of the Sand Tiger Shark, Odontaspis taurus (Rafinesque). Fish. Bull. 81, 201–224.
Gilmore R. G., Putz O., Dodrill J. (2005). “Oophagy, Intrauterine Cannibalism and Reproductive Strategy in Lamnoid Sharks,” in Reproductive Biology and Phylogeny of Chondrichthyes: Sharks, Batoids and Chimaeras. Ed. Hamlett W. C. (Enfield, NJ: Science Publishers), 435–462.
Goldman K. J., Branstetter S., Musick J. A. (2006). A Re-Examination of the Age and Growth of Sand Tiger Sharks, Carcharias taurus, in the Western North Atlantic: The Importance of Ageing Protocols and Use of Multiple Back-Calculation Techniques. Environ. Biol. Fishes 77, 241–252. doi: 10.1007/s10641-006-9128-y
Graham K. M., Mylniczenko N. D., Burns C. M., Bettinger T. L., Wheaton C. J. (2016). Examining Factors That may Influence Accurate Measurement of Testosterone in Sea Turtles. J. Vet. Diagn. Invest. 28 (1), 12–19. doi: 10.1177/1040638715618989
Graham L., Schwarzenberger F., Möstl E., Galama W., Savage A. (2001). A Versatile Enzyme Immunoassay for the Determination of Progestogens in Feces and Serum. Zoo Biol. 20, 227–236. doi: 10.1002/zoo.1022
Henningsen A. D., Murru F. L., Rasmussen L. E. L., Whitaker B. R., Violetta G. C. (2008). Serum Levels of Reproductive Steroid Hormones in Captive Sand Tiger Sharks, Carcharias taurus (Rafinesque), and Comments on Their Relation to Sexual Conflicts. Fish Physiol. Biochem. 34, 437–446. doi: 10.1007/s10695-008-9202-9
Henningsen A., Smale M., Garner R., Kinnumen N. (2004b). “Reproduction, Embryonic Development, and Reproductive Physiology of Elasmobranchs,” in The Elasmobranch Husbandry Manual: Captive Care of Sharks, Rays and Their Relatives. Eds. Smith M., Warmolts D., Thoney D., Hueter R. E. (Columbus: Special Publication of the Ohio Biological Society), 227–236.
Henningsen A. D., Smale M. J., Gordon I., Garner R., Marin-Osorno R., Kinnunen N. (2004a). “Captive Breeding and Sexual Conflict in Elasmobranchs,” in The Elasmobranch Husbandry Manual: Captive Care of Sharks, Rays and Their Relatives. Eds. Smith M., Warmolts D., Thoney D., Hueter R. E. (Columbus: Special Publication of the Ohio Biological Society), 239–250.
Henningsen A., Street E. P., Claus E., Street E. P., Littlehale D., Choromanski J., et al. (2017). “Reproduction of the Sand Tiger Sharks, Carcharias taurus, in Aquaria : A Framework for a Managed Breeding Program,” in The Elasmobranch Husbandry Manual II: Recent Advances in the Care of Sharks, Rays and Their Relatives. Eds. Smith M., Warmolts D., Thoney D., Hueter R., M M., Ezcurra J. (Columbus: Special Publication of the Ohio Biological Survey), 375–390.
Henningsen A. D., Whitaker B. R., Kight K., Hess D. L., Hadfield C., Zohar Y. (2015). The Use of a Gonadotropin Releasing Hormone Antagonist in Captive Sand Tiger Sharks, Carcharias taurus, and the Serum Levels of the Antagonist and Reproductive Steroid Hormones. J. Zoo Aquarium Res. 3, 107–115. doi. 10.19227/jzar.v3i3.120
Heupel M. R., Whittier J. M., Bennett M. B. (1999). Plasma Steroid Hormone Profiles and Reproductive Biology of the Epaulette Shark, Hemiscyllium ocellatum. J. Exp. Zool. 284, 586–594. doi: 10.1002/(SICI)1097-010X(19991001)284:5<586::AID-JEZ14>3.0.CO;2-B
Hoffmayer E. R., Driggers W. B., Jones L. M., Hendon J. M., Sulikowski J. A. (2013). Variability in the Reproductive Biology of the Atlantic Sharpnose Shark in the Gulf of Mexico. Mar. Coast. Fish. 5, 139–151. doi: 10.1080/19425120.2013.783518
Ibargüengoytía N. R., Cussac V. E. (1996). Reproductive Biology of the Viviparous Lizard, Liolaemus pictus (Tropiduridae) Biennial Female Reproductive Cycle? Herpetol. Journal1 6, 137–143.
Ibargüengoytía N. R., Cussac V. E. (1998). Reproduction of the Viviparous Lizard Liolaemus elongatus in the Highlands of Southern South America Plastic Cycles in Response to Climate. Herpetol. J. 8, 99–105.
Johnson M. R., Snelson F. F. (1996). Reproductive Life History of the Atlantic Stingray, Dasyatis sabina (Pisces, Dasyatidae), in the Freshwater St. Johns River, Florida. Bull. Mar. Sci. 59, 74–88.
Kilfoil J., Fox D., Wetherbee B., Carlson J. (2017). Targeted Catch-and-Release of Prohibited Sharks: Sand Tigers in Coastal Delaware Waters. Fisheries 42, 281–287. doi: 10.1080/03632415.2017.1306974
Kohler N. E., Turner P. A. (2001). Shark Tagging: A Review Of Conventional Methods and Studies. Environ. Biol. Fishes 60, 191–224. doi: 10.1023/A:1007679303082
Koob T. J. (2004). “Elasmobranchs in the Public Aquarium: 1860 to 1930,” in The Elasmobranch Husbandry Manual: Captive Care of Sharks, Rays and Their Relatives. Eds. Smith M., Warmolts D., Thoney D., Hueter R. E. (Columbus: Special Publication of the Ohio Biological Survey), 1–14.
Koob T. J., Callard I. P. (1999). Reproductive Endocrinology of Female Elasmobranchs: Lessons From the Little Skate (Raja erinacea) and Spiny Dogfish (Squalus acanthias). J. Exp. Zool. 284, 557–574. doi: 10.1002/(SICI)1097-010X(19991001)284:5<557::AID-JEZ12>3.0.CO;2-P
Krause J. S., Dorsa D., Wingfield J. C. (2014). Changes in Plasma Concentrations of Progesterone, Dehydroepiandrosterone and Corticosterone in Response to Acute Stress of Capture, Handling and Restraint in Two Subspecies of White-Crowned Sparrows. Comp. Biochem. Physiol. Part A Mol. Integr. Physiol. 177, 35–40. doi: 10.1016/j.cbpa.2014.07.019
Kuznetsova A., Brockhoff P. B., Christensen R. H. B. (2017). Lmertest Package: Tests in Linear Mixed Effects Models. J. Stat. Software 82, 1–26. doi: 10.18637/jss.v082.i13
Lenth R. (2018) Emmeans: Estimated Marginal Means, Aka Least-Squares Means. Available at: https://cran.r-project.org/package=emmeans.
Lucifora L., Menni R. C., Escalante A. H. (2002). Reproductive Ecology and Abundance of the Sand Tiger Shark, Carcharias taurus, From the Southwestern Atlantic. ICES J. Mar. Sci. 59, 553–561. doi: 10.1006/jmsc.2002.1183
Lymburner A. H., Kelly T. R., Hobson K. A., MacDougall-Shackleton E. A., MacDougall-Shackleton S. A. (2016). Testosterone, Migration Distance, and Migratory Timing in Song Sparrows Melospiza melodia. Horm. Behav. 85, 102–107. doi: 10.1016/j.yhbeh.2016.06.013
Manire C. A., Rasmussen L. E. L., Hess D. L., Hueter R. E. (1995). Serum Steroid Hormones and the Reproductive Cycle of the Female Bonnethead Shark, Sphyrna tiburo. Gen. Comp. Endocrinol. 97, 366–376. doi: 10.1006/gcen.1995.1036
Marina P., Salvatore V., Maria M. D. F., Franca R., Giuseppina D. G., Maurizio R., et al. (2008). Effect of 17β-Estradiol and Progesterone on Vitellogenesis in the Spotted Ray Torpedo marmorata Risso 1810 (Elasmobranchii: Torpediniformes): Studies on Females and on Estrogen-Treated Males. Gen. Comp. Endocrinol. 157, 125–132. doi: 10.1016/j.ygcen.2008.04.011
Moe R. O., Bakken M. (1996). Effect of Repeated Blood Sampling on Plasma Concentrations of Cortisol and Testosterone and on Leucocyte Number in Silver Fox Vixens (Vulpes vulpes). Acta Agriculturae Scandinavica Section A — Anim. Sci. 46, 2, 111–2, 116. doi: 10.1080/09064709609415859
Mull C. G., Lowe C. G., Young K. A. (2008). Photoperiod and Water Temperature Regulation of Seasonal Reproduction in Male Round Stingrays (Urobatis halleri). Comp. Biochem. Physiol. Part A Mol. Integr. Physiol. 151, 717–725. doi: 10.1016/j.cbpa.2008.08.029
Mull C. G., Lowe C. G., Young K. A. (2010). Seasonal Reproduction of Female Round Stingrays (Urobatis halleri): Steroid Hormone Profiles and Assessing Reproductive State. Gen. Comp. Endocrinol. 166, 379–387. doi: 10.1016/j.ygcen.2009.12.009
Munakata A., Amano M., Ikuta K., Kitamura S., Aida K. (2000). Inhibitory Effects of Testosterone on Downstream Migratory Behavior in Masu Salmon, Oncorhynchus masou. Zoolog. Sci. 17, 863–870. doi: 10.2108/zsj.17.863
Munakata A., Amano M., Ikuta K., Kitamura S., Aida K. (2001). The Effects of Testosterone on Upstream Migratory Behavior in Masu Salmon, Oncorhynchus masou. Gen. Comp. Endocrinol. 122, 329–340. doi: 10.1006/gcen.2001.7646
Mylniczenko N. D., Sumigama S., Wyffels J. T., Wheaton C. J., Guttridge T. L., Dirocco S., et al. (2019). Ultrasonographic and Hormonal Characterization of Reproductive Health and Disease in Wild, Semiwild, and Aquarium-Housed Southern Stingrays (Hypanus americanus). Am. J. Vet. Res. 80, 931–942. doi: 10.2460/ajvr.80.10.931
Natanson L. J., Skomal G. B., Hoffmann S. L., Porter M. E., Goldman K. J., Serra D. (2018). Age and Growth of Sharks: Do Vertebral Band Pairs Record Age? Mar. Freshw. Res. 69, 1440. doi: 10.1071/MF17279
Nozu R., Murakumo K., Yano N., Furuyama R., Matsumoto R., Yanagisawa M., et al. (2018). Changes in Sex Steroid Hormone Levels Reflect the Reproductive Status of Captive Female Zebra Sharks (Stegostoma fasciatum). Gen. Comp. Endocrinol. 265, 174–179. doi: 10.1016/j.ygcen.2018.03.006
Otway N. M. (2015). Serum Biochemical Reference Intervals for Free-Living Sand Tiger Sharks ( Carcharias taurus ) From East Australian Waters. Vet. Clin. Pathol. 44, 262–274. doi: 10.1111/vcp.12254
Passerotti M. S., Andrews A. H., Carlson J. K., Wintner S. P., Goldman K. J., Natanson L. J. (2014). Maximum Age and Missing Time in the Vertebrae of Sand Tiger Shark (Carcharias taurus): Validated Lifespan From Bomb Radiocarbon Dating in the Western North Atlantic and Southwestern Indian Oceans. Mar. Freshw. Res. 65, 674–687. doi: 10.1071/MF13214
Penfold L. M., Hallager S., Boylan J., de Wit M., Metrione L. C., Oliva M. (2013). Differences in Fecal Androgen Patterns of Breeding and Nonbreeding Kori Bustards (Ardeotis kori). Zoo Biol. 32, 54–62. doi: 10.1002/zoo.21034
Penfold L. M., Wyffels J. T. (2019). “Reproductive Science in Sharks and Rays,” in Advances in Experimental Medicine and Biology. Eds. Comizzoli P., Brown J., Holt W. (New york, New york: Springer, Cham), 465–488. doi: 10.1007/978-3-030-23633-5_15
Ramenofsky M., Wingfield J. C. (2006). Behavioral and Physiological Conflicts in Migrants: The Transition Between Migration and Breeding. J. Ornithol. 147, 135. doi: 10.1007/s10336-005-0050-4
Rankin M. A. (1991). Endocrine Effects on Migration. Am. Zool. 31, 217–230. doi: 10.1093/icb/31.1.217
Rasmussen L. E. L., Murru F. L. (1992). Long-Term Studies of Serum Concentrations of Reproductively Related Steroid-Hormones in Individual Captive Carcharinids. Aust. J. Mar. Freshw. Res. 43, 273–281. doi: 10.1071/MF9920273
R Core Team (2016). R: A Language and Environment for Statistical Computing (Vienna, Austria: R Foundation for Statistical Computing).
Rigby C. L., Carlson J., Derrick D., Dicken M., Pacoureau N., Simpfendorfer C. (2021) Carcharias taurus. The IUCN Red List of Threatened Species2021: E.T3854A2876505. Available at: https://dx.doi.org/10.2305/IUCN.UK.2021-2.RLTS.T3854A2876505.en (Accessed 04 June 2022).
Sadowsky V. (1970). On the Dentition of the Sand Tiger Shark Odntaspis taurus, From the Vicinity of Caneneia, Brazil. Bolm Inst Ocean. S Paulo 18, 37–44. doi: 10.1590/S0373-55241969000100005
Schwarz G. (1978). Estimating the Dimension of a Model. Ann. Stat. 6, 461–464. doi: 10.1214/aos/1176344136
Smale M. J. (2002). Occurrence of Carcharias taurus in Nursery Areas of Eastern and Western Cape, South Africa. Mar. Freshw. Res. 53, 551–556. doi: 10.1071/MF01129
Smale M., Booth A., Farquhar M., Meÿer M., Rochat L. (2012). Migration and Habitat Use of Formerly Captive and Wild Raggedtooth Sharks (Carcharias taurus) on the Southeast Coast of South Africa. Mar. Biol. Res. 8, 115–128. doi: 10.1080/17451000.2011.617756
Smale M. J. B., Cliff G. C., Dicken M. L. A., Booth A. J. A., Smale M. J. B., Cliff G. C. (2007). Spatial and Seasonal Distribution Patterns of Juvenile and Adult Raggedtooth Sharks (Carcharias taurus) Tagged Off the East Coast of South Africa. Mar. Freshw. Res. 58, 127–134. doi: 10.1071/MF06018
Snelson F. F., Rasmussen L. E., Johnson M. R., Hess D. L. (1997). Serum Concentrations of Steroid Hormones During Reproduction in the Atlantic Stingray, Dasyatis sabina. Gen. Comp. Endocrinol. 108, 67–79. doi: 10.1006/gcen.1997.6949
Springer S. (1948). Oviphagous Embryos of the Sand Shark, Carcharias taurus. Copeia 1948, 153. doi: 10.2307/1438449
Stamper M. A. (2004). “Immobilization of Elasmobranchs,” in The Elasmobranch Husbandry Manual: Captive Care of Sharks, Rays and Their Relatives. Eds. Smith M., Warmolts D., Thoney D., Hueter R. (Columbus: Special Publication of the Ohio Biological Survey, Inc), 281–296.
Sudo R., Suetake H., Suzuki Y., Utoh T., Tanaka S., Aoyama J., et al. (2011). Dynamics of Reproductive Hormones During Downstream Migration in Females of the Japanese Eel, Anguilla japonica. Zoolog. Sci. 28, 180–188. doi: 10.2108/zsj.28.180
Townsend R., Gilchrist S. (2017). “Preliminary Evidence for a Biennial Feeding Strategy Related to Reproduction in Female Sand Tiger Sharks, Carcharias taurus,” in The Elasmobranch Husbandry Manual II: Recent Advances in the Care of Sharks, Rays and Their Relatives. Eds. Smith M., Warmolts D., Thoney D., Hueter R., M M., Ezcurra J. (Columbus: Special Publication of the Ohio Biological Survey), 153–157.
Tricas T. C., Maruska K. P., Rasmussen L. E. (2000). Annual Cycles of Steroid Hormone Production, Gonad Development, and Reproductive Behavior in the Atlantic Stingray. Gen. Comp. Endocrinol. 118, 209–225. doi: 10.1006/gcen.2000.7466
Trinnie F. I., Walker T. I., Jones P. L., Laurenson L. J. (2014). Regional Differences in the Reproductive Parameters of the Sparsely-Spotted Stingaree, Urolophus paucimaculatus, From South-Eastern Australia. Mar. Freshw. Res. 65, 943. doi: 10.1071/MF13275
Ueda H., Hiroi O., Hara A., Yamauchi K., Nagahama Y. (1984). Changes in Serum Concentrations of Steroid Hormones, Thyroxine, and Vitellogenin During Spawning Migration of the Chum Slamon, Oncorhynchus keta. Gen. Comp. Endocrinol. 53, 203–211. doi: 10.1016/0016-6480(84)90243-0
Waltrick D., Awruch C., Simpfendorfer C. (2012). Embryonic Diapause in the Elasmobranchs. Rev. Fish Biol. Fish. 22, 849–859. doi: 10.1007/s11160-012-9267-5
Waltrick D. S., Simpfendorfer C. A., Awruch C. A. (2017). A Review on the Morphology of Ovarian Follicles in Elasmobranchs: A Case Study in Rhizoprionodon taylori. J. Morphol. 278, 486–499. doi: 10.1002/jmor.20644
Wheaton C., Mylniczenko N., Rimoldi J., Gadepalli R., Hart R., O’Hara B., et al. (2018). Challenges, Pitfalls and Surprises: Development and Validation of a Monoclonal Antibody for Enzyme Immunoassay of the Steroid 1α-Hydroxycorticosterone in Elasmobranch Species. Gen. Comp. Endocrinol. 265, 83–89. doi: 10.1016/j.ygcen.2018.01.028
Willson K., Smith M. (2017). “Reproduction of the Sand Tiger Shark, Carcharias taurus (Rafinesque), at UnderWater World SEA LIFE Mooloolaba From 1992 - 2012,” in The Elasmobranch Husbandry Manual II: Recent Advances in the Care of Sharks, Rays and Their Relatives. Eds. Smith M., Warmolts D., Thoney D., Hueter R., Ezcurra J. (Columbus: Special Publication of the Ohio Biological Survey), 391–401.
Wosnick N., Rangel B. D. S., Afonso A. S., Bornatowski H., Hazin F. H. V., Moreira R. G., et al. (2018). Hormones and Migration in Tiger Sharks (Galeocerdo cuvier): Can They be Related? aqua Int. J. Ichthyology. 24 (1), 9–14.
Wourms J. P. (1977). Reproduction and Development in Chondrichthyan Fishes. Am. Zool. 17, 379–410. doi: 10.1093/icb/17.2.379
Wyffels J., Coco C., Schreiber C., Palmer D., Clauss T., Bulman F., et al. (2020a). Natural Environmental Conditions and Collaborative Efforts Provide the Secret to Success for Sand Tiger Shark Carcharias taurus Reproduction in Aquaria. Zoo Biol. 39, 355–363. doi: 10.1002/zoo.21558
Wyffels J. T., George R., Adams L., Adams C., Clauss T., Newton A., et al. (2020b). Testosterone and Semen Seasonality for the Sand Tiger Shark Carcharias taurus†. Biol. Reprod. 102, 876–887. doi: 10.1093/biolre/ioz221
Yamaguchi A., Taniuchi T., Shimizu M. (2000). Geographic Variations in Reproductive Parameters of the Starspotted Dogfish, Mustelus manazo, From Five Localities in Japan and in Taiwan. Environ. Biol. Fishes 57, 221–233. doi: 10.1023/A:1007558324902
Keywords: triennial cycle, biennial cycle, progesterone, testosterone, 17β-estradiol, ultrasonography, egg retention, Grey nurse shark
Citation: Wyffels JT, George R, Christiansen EF, Clauss TM, Newton AL, Hyatt MW, Buckner C, LePage V, Latson FE and Penfold LM (2022) Reproductive Cycle and Periodicity of In Situ and Aquarium Female Sand Tiger Sharks Carcharias taurus from the Western North Atlantic. Front. Mar. Sci. 9:925749. doi: 10.3389/fmars.2022.925749
Received: 21 April 2022; Accepted: 17 June 2022;
Published: 22 July 2022.
Edited by:
Steven T. Kessel, Shedd Aquarium, United StatesReviewed by:
Cynthia Awruch, Consejo Nacional de Investigaciones Científicas y Técnicas (CONICET), ArgentinaCopyright © 2022 Wyffels, George, Christiansen, Clauss, Newton, Hyatt, Buckner, LePage, Latson and Penfold. This is an open-access article distributed under the terms of the Creative Commons Attribution License (CC BY). The use, distribution or reproduction in other forums is permitted, provided the original author(s) and the copyright owner(s) are credited and that the original publication in this journal is cited, in accordance with accepted academic practice. No use, distribution or reproduction is permitted which does not comply with these terms.
*Correspondence: Jennifer T. Wyffels, d3lmZmVsc0B1ZGVsLmVkdQ==
Disclaimer: All claims expressed in this article are solely those of the authors and do not necessarily represent those of their affiliated organizations, or those of the publisher, the editors and the reviewers. Any product that may be evaluated in this article or claim that may be made by its manufacturer is not guaranteed or endorsed by the publisher.
Research integrity at Frontiers
Learn more about the work of our research integrity team to safeguard the quality of each article we publish.