- 1Marine Science Group, Department of Biological, Geological and Environmental Sciences, University of Bologna, Bologna, Italy
- 2Fano Marine Center, The Inter-Institute Center for Research on Marine Biodiversity, Resources and Biotechnologies, Fano, Italy
- 3Department of Chemistry “Giacomo Ciamician”, University of Bologna, Bologna, Italy
- 4The Mina and Everard Goodman Faculty of Life Sciences, Bar-Ilan University, Ramat Gan, Israel
Basic information on the reproductive biology of many scleractinian corals species is limited or entirely lacking, particularly from temperate zones, though it is essential for a better understanding of their ecology. This study describes the morphological aspects and the annual cycle of gametogenesis and biometric parameterss of the papillose cup coral Paracyathus pulchellus collected at Palinuro (Italy, Southern Tyrrhenian Sea), filling a knowledge gap about the reproductive biology of a widespread Mediterranean and Northern Atlantic coral. Samples of P. pulchellus were collected by SCUBA diving between 5- and 10-meters depth during 18 monthly collections from June 2010 to December 2011. Sexually active polyps displayed either oocytes or spermaries, indicating that P. pulchellus was gonochoric. The sex ratio of sexually active polyps was 1:1. Gametogenesis began with undifferentiated germ cells arose in the gastrodermis that migrated towards the mesoglea of the mesentery where they completed the development. During spermatogenesis, spermary diameter increased from 25 to 83 µm. Oocyte diameter ranged from 9 to 146 µm and during oogenesis the nucleus/cytoplasm ratio decreased due to the accumulation of yolk. The nucleus migrated to the periphery of the oocyte adhering closely to the cell membrane. No embryo was observed in the coelenteric cavity of the polyps, suggesting an external development of planktotrophic larvae due to the small-sized mature oocytes and a possible broadcast spawning reproductive mode. Gonadal index of both females and males increased significantly from August until November and fertilization took place from November to January. Only sexually inactive individuals were observed from February to April, suggesting a quiescence period in both males and females. Seasonal variations in water temperature and photoperiod may have a key role in regulating gametogenesis. The analysis of the main biometric parameters (polyp width, height, dry skeletal mass, volume, surface/volume ratio and bulk skeletal density) showed a negative correlation between size and skeletal density, and no sexual dimorphism.
Introduction
Reproduction has a fundamental role in the population structure, dynamics, and distribution of benthic animals, which are an important component of marine ecosystems. Studying reproductive traits can provide relevant evolutionary information, which may be crucial for understanding the short- and long-term impacts of climate change that are occurring in the Mediterranean Sea (Airi et al., 2014; Airi et al., 2017; Gizzi et al., 2017; Marchini et al., 2020; Marchini et al., 2021). Within Scleractinian corals, the current global knowledge on reproduction is the result of many decades of research in which studies have identified an exceptional diversity in the reproductive patterns (Harrison and Wallace, 1990). Corals are modular organisms and the variety of reproductive processes and modes they employ is testament to their exceptional regeneration ability, developmental plasticity, and adaptability (Baird et al., 2009; Harrison, 2011). Substantially, four combinations of reproductive patterns can be recognized: propagation mode (asexual or sexual), sexuality (hermaphroditic or gonochoric), reproductive mode (broadcasting or brooding) and embryonic development (coeloblastic or stereoblastic; Harrison, 2011).
Most scleractinians are hermaphrodites (Harrison and Wallace, 1990; Richmond and Hunter, 1990; Harrison, 2011), while gonochorism occurs only in 26% of the species studied (Harrison, 2011). Gonochoric coral species include colonies or individual polyps that produce only male or female gametes over their lifetime (this condition is also referred to as dioecy, separate sexes) (Harrison and Wallace, 1990; Richmond, 1997; Harrison, 2011). Oocytes and spermaries originate from interstitial cells, they grow and develop within the mesenteries, enclosed by mesoglea and gastrodermis (Harrison, 1990).
Fertilization can be either external, when corals release gametes in the water column (broadcast spawning), or internal, when gametes encounter within the celenteric cavity of the polyp (brooding) (Harrison and Wallace, 1990). Broadcast spawning is typical for 82.7% of the 428 coral species for which the mode of development has been documented (Harrison, 2011). Most corals studied so far are hermaphroditic and broadcast spawning, while gonochoric brooding is the least frequent combination (Baird et al., 2009; Harrison, 2011).
The reproductive traits have been reported in more than 450 (Harrison and Wallace, 1990; Richmond and Hunter, 1990; Richmond, 1997; Baird et al., 2009; Harrison, 2011) of the more than 1,500 coral species identified (Harrison, 2011). Most of these studies were carried out on tropical and subtropical scleractinians, while information on the reproductive biology of scleractinians from temperate zones is limited. Although there are 33 species of scleractinians in the Mediterranean Sea (Zibrowius, 1980), data on their reproduction come from old observations (Lacaze-Duthiers, 1873) and from studies on the species Oculina patagonica (Fine et al., 2001), Balanophyllia europaea (Goffredo and Telò, 1998; Goffredo et al., 2002), Leptopsammia pruvoti (Goffredo et al., 2005; Goffredo et al., 2006), Astroides calycularis (Goffredo et al., 2010; Goffredo et al., 2011a), Cladocora caespitosa (Kružić et al., 2008; Kersting et al., 2013; Hadjioannou, 2019), and Caryophyllia inornata (Goffredo et al., 2012; Marchini et al., 2015).
The analysis of biometric parameters has been used in different studies to investigate aspects of coral individual growth patterns (Goffredo and Chadwick-Furman, 2003; Goffredo et al., 2004; Goffredo et al., 2011b) and how these can change in correlation to environmental factors such as solar radiation and seawater temperature (Goffredo et al., 2007; Caroselli et al., 2015) and depth (Özalp et al., 2018). In these studies, polyp width, height, volume, surface/volume ratio and bulk skeletal density are correlated to polyp length. The study of the correlation between width ad length can indicate either an isometric growth, in which the coral has a more circular shape of the calyx, or an allometric growth, where length increases more rapidly than width and which leads to a more oval oral surface (Goffredo and Chadwick-Furman, 2003).
Paracyathus pulchellus (Figure 1A) is a caryophylliid coral widespread in the Western and Eastern basins of the Mediterranean Sea (Fenner et al., 2013). Moreover, this species is found in the Eastern Atlantic between Portugal, the Azores, the Gulf of Guinea (Zibrowius, 1980), and in the Western Atlantic from North Carolina to Brazil (Cairns, 1979; Cairns, 2000). P. pulchellus colonizes hard substrates such as vertical walls, caves, and wrecks (Zibrowius, 1980; Fenner et al., 2013). Its reported depth range varies from 17 to 1260 m (Casado-Amezua et al., 2015; World Register of Marine Species1). The species is classified as Data Deficient by the IUCN and investigation on its occurrence is highly recommended (Casado-Amezua et al., 2015). The purpose of this study was thus to provide the first investigation on the reproductive biology of P. pulchellus, using specimens from a Mediterranean population (Figure 1).
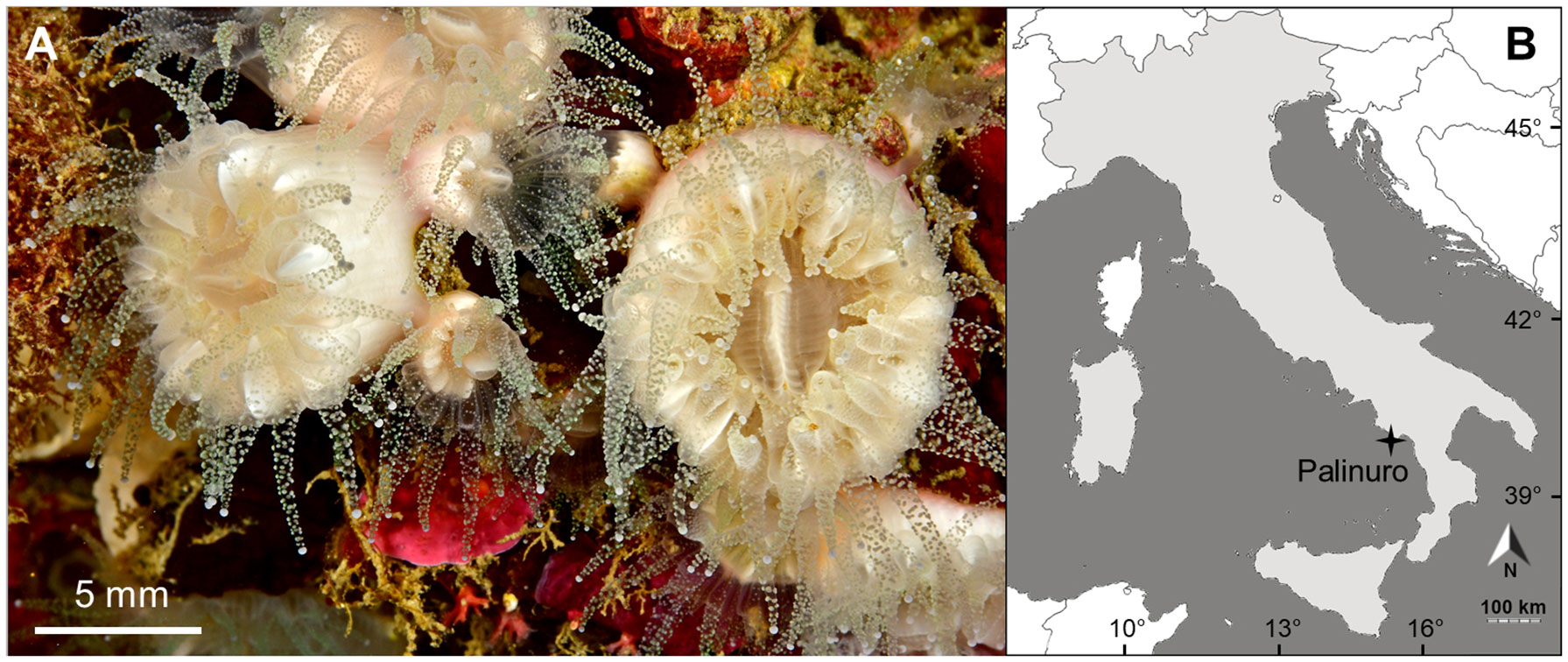
Figure 1 (A) Living specimens of Paracyathus pulchellus photographed by Francesco Sesso; (B) Map of sampling site located at Palinuro (Italy, 40˚02’N and 15˚16’E).
Materials and methods
Sampling
Samples of Paracyathus pulchellus were collected in a cave named Grotta Azzurra (Blue Cave) at Palinuro (Italy, Southern Tyrrhenian Sea: 40˚02’N and 15˚16’E; Figure 1B), during 18 monthly collections from June 2010 to May 2011. Twenty polyps were haphazardly collected each month by SCUBA diving between 5- and 10-meters depth. Polyps were immediately fixed in saturated formalin solution (90% seawater and 10% formaldehyde) and transferred to the lab for biometric and histological analyses.
Environmental parameters
Photoperiod data were obtained from an online database (http://www.eurometeo.com). Depth Thermometer Temperature (DTT; °C) was measured by digital thermometers (I-Button DS1921H, Maxim Integrated Products) placed at the depth and site of collection for the entire sampling period. Sea Surface Temperature data (°C) were recorded from the National Mareographic Network of the Institute for the Environmental Protection and Research (ISPRA). These data were measured by mareographic stations (SM3810 manufactured by the Society for the Environmental and Industrial monitoring; SIAP + MICROS), placed close to the sampling sites. A linear regression was produced between DTT and SST data (Depth Temperature, DT = 1.3818∗SST + 7.243) to estimate temperatures during periods in which sensors were lost due to bad weather conditions. In this study we considered the monthly average Depth Temperature (DT; °C) of one year of sampling (n = 12 monthly temperatures).
Biometric analysis
Biometric analyses were performed on 102 polyps of which we measured the length (l, maximum axis of the oral disc; Figure 2), width (w, minimum axis of the oral disc) and height (h, oral-aboral axis of the polyp) using a caliper. The volume was calculated using the formula v = h × (l/2) × (w/2) × π (Goffredo et al., 2002). Surface/volume (S/V) ratio was calculated by dividing S by V. The surface parameter was obtained as a sum of the surface of the oral disc π * (l/2)* (w/2) and the lateral surface of the coral calculated with the formula (Ramanujan, 1914; Caroselli et al., 2015).
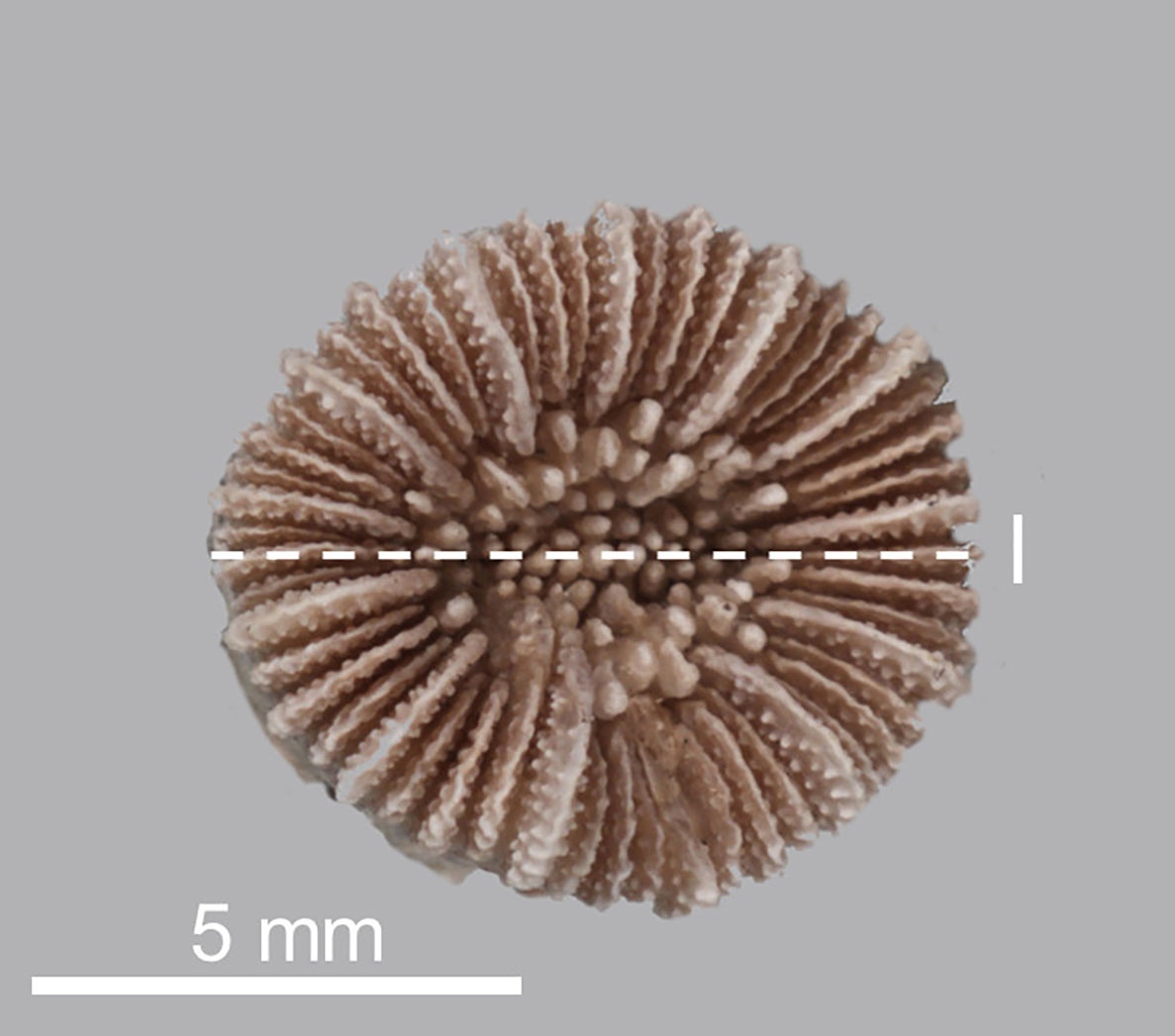
Figure 2 Skeleton of Paracyathus pulchellus. Dotted line indicates polyp length (l, maximum axis of the oral disc).
The wet mass of all 102 corals was measured using a digital precision balance. The wet mass of 10 additional corals spanning the length range 4.4 - 9.65 mm was measured, then they were immersed in a 10% commercial bleach solution for 3 days to remove the soft tissue and then skeletons were dried for 4 days. Each of these 10 corallites was observed under a binocular microscope to remove fragments of substratum and deposits produced by other organisms, then their dry skeletal mass was measured with a digital precision balance. Using the linear regression between the wet and the dry skeletal mass of the 10 selected corallites the dry skeletal mass of all corals was then estimated. Skeletal bulk density (D) was calculated by dividing the dry skeletal mass (M) by the volume (V; Caroselli et al., 2015).
Histological analysis
Histological analysis was performed on 57 polyps (Supporting Information Table S1). After a post fixation in Bouin solution to ensure a better fixation and staining of the tissues, polyps were decalcified using EDTA, dehydrated through a graded alcohol series from 80 to 100%, clarified in histolemon and embedded in paraffin. Serial transverse sections were cut at 7 µm intervals along the oral-aboral axis, from the oral to the aboral pole of the entire polyp, using a microtome. Finally, tissues were stained with Mayer’s hematoxylin and eosin (Goffredo et al., 2002; Marchini et al., 2015).
Gamete size
Histological observations and cytological readings were performed using the light microscope NIKON Eclipse 80i and the image analysis system NIKON NIS-Elements D 3.1. The maximum and minimum diameter of the spermaries and oocytes in nucleated sections were measured and classified into developmental stages in accordance with other studies on gametogenesis in scleractinians (Goffredo et al., 2005; Goffredo et al., 2010). Gamete size was determined as the mean of the two diameters (Goffredo et al., 2005; Goffredo et al., 2010). A gonadal development index was calculated as the percentage of body volume occupied by the germ cells (Hall and Hughes, 1996). The volume of each reproductive element was estimated as described in the subsection “Gonadal index” of Goffredo et al., 2002.
Definitions
The following definitions were used: sexually active polyps, individuals that displayed a gametogenetic activity; male polyps, individuals that displayed spermaries; female polyps, individuals that displayed oocytes; sexually inactive polyps, individuals that did not display a gametogenetic activity.
Statistical analysis
To determine the presence of sexual dimorphism, 12 female and 13 male polyps were used for a comparative analysis of the biometrical parameters (length, width, height, volume, S/V ratio, dry skeletal mass and bulk skeletal density). These comparisons were made by conducting a one-way permutation multivariate analysis of variance (PERMANOVA) using the software Primer v6 – Quest Research Limited (Anderson, 2001). The analysis was conducted with 999 permutations and included the Monte Carlo correction for small sample size. The test was performed using sex as the only fixed effects factor and was based on Euclidean distances. A Student’s t-test was used to compare each biometrical parameter between female and male polyps and to compare length between sexually active and sexually inactive polyps.
Results
The population of P. pulchellus was found and collected from 5 meters depth, shallowing the bathymetric range reported by IUCN for this species that previously started from 17 meters.
Sexual condition and reproductive mode
Paracyathus pulchellus was found to be gonochoric since all specimens analyzed had either spermaries or oocytes, and none showed both types of germ cells. Sexual dimorphism was not observed, neither at level of overall morphology (PERMANOVA, df = 24, p = 0.834) nor according to each biometric parameter (Tables 1, 2; Figure 3). The sex ratio of sexually active polyps was 1:1 (chi-square test, χ 2 = 0.04, df= 1, p > 0.05). In the months in which both males and females showed germ cells (from May to January), 18 sexually inactive polyps were observed (Supporting Information Table S1). The mean size of the sexually inactive polyps was homogeneous to that of the sexually active ones (mean length: sexually inactive = 7.6 mm, 95% CI = 7.1-8.1, N=18, sexually active = 7.8 mm, 95% CI= 7.4-8.2, N=25, Student’s test p > 0.05). No embryo was observed in the coelenteric cavity, indicating an external development and a possible broadcast spawning reproductive mode.

Table 1 General PERMANOVA results of biometric parameters distribution between male and female polyps.
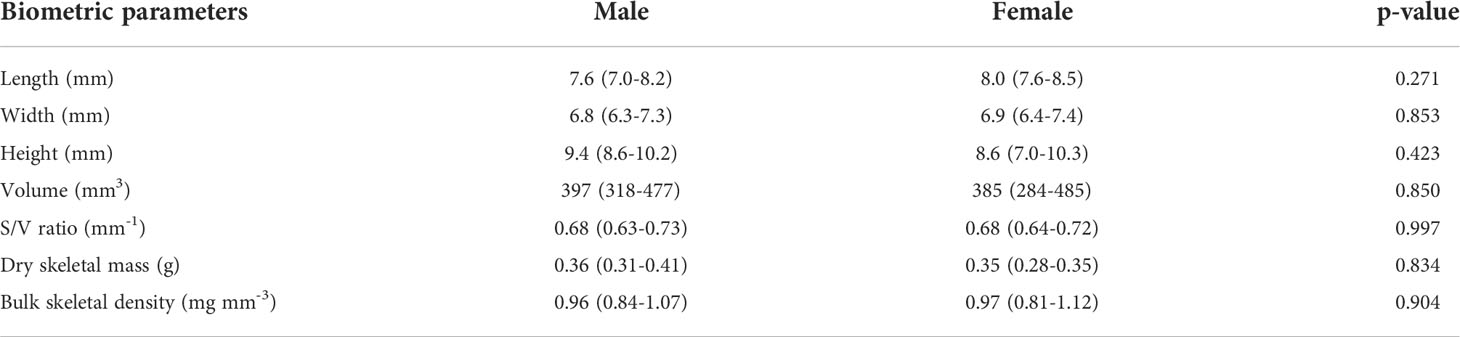
Table 2 Mean biometric parameters, 95% confidence intervals (CI) and t-test p-value of sexually active polyps (males and females).
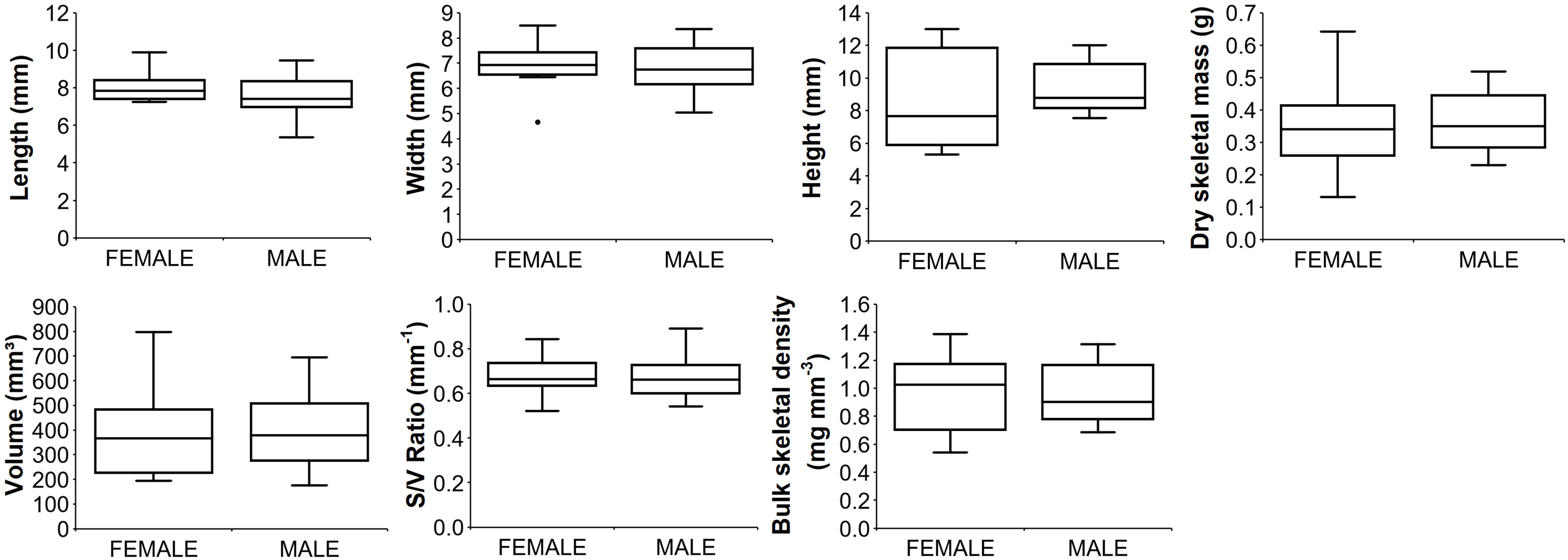
Figure 3 Boxplots of biometric parameters for male and female polyps. Median (solid horizontal line), first and third quartiles (box outline), and outliers (circles). Number of polyps analyzed: 12 for females; 13 for males.
Male gametogenesis
Spermaries were found in the mesentery and were made up of groups of germ cells and delineated by mesogleal envelope (Figure 4). A total of 4,531 spermaries were identified and measured (Supporting Information Table S1). Five stages of maturation were identified:
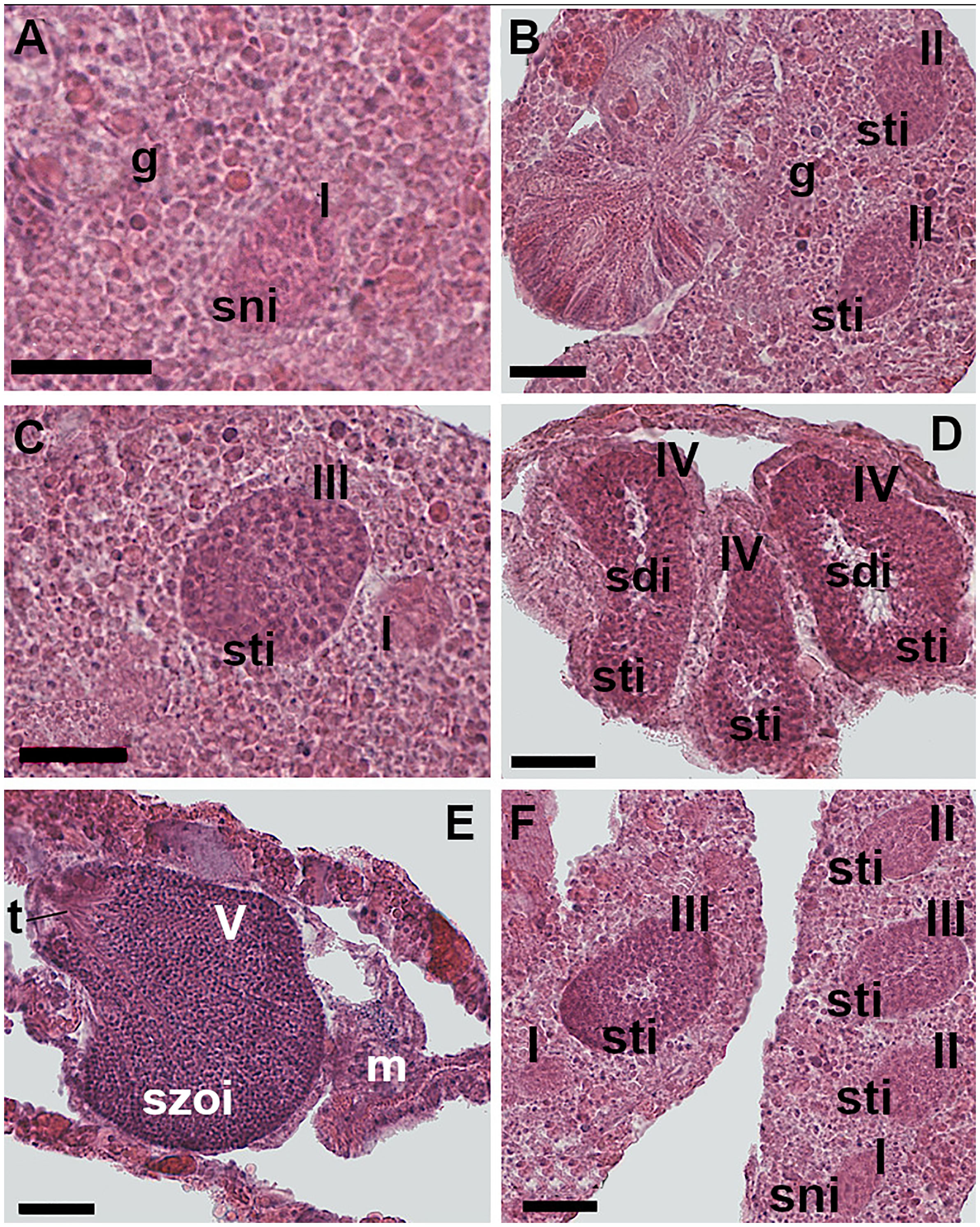
Figure 4 Spermatogenesis. (A) Stage I: undifferentiated germ cells disposed in the gastrodermis layers of the mesentery. The spermaries are made up of a group of spermatogonia. (B) Stage II: the spermaries are made up by spermatocytes involved in the process of meiosis. (C) Stage III: the spermary, containing spermatocytes undergoing meiosis, is delineated by a wall that has arisen from the mesoglea. (D) Stage IV: the spermary presents an external layer of spermatocytes and an internal mass of spermatids, recognizable by the presence of a tail. (E) Stage V: the spermary is made up of a mass of spermatozoa. Shortly before leaving the spermary, mature spermatozoa form a bouquet, with their tails all facing in the same direction. (F) Spermaries of stages I, II, and III located in the same mesentery. [g: gastrodermis; m: mesoglea; sni: spermatogonia; sti: spermatocytes; sdi: spermatids; szoi: spermatozoa; t: spermatozoa tails; I, II, III, IV, V: spermary developmental stage]. Scale bars are 25 μm.
Stage I - undifferentiated germ cells arose in the gastrodermis and then migrated toward the mesoglea of the mesentery where they regrouped forming the spermary. The spermary was made up of an early aggregation of spermatogonia (Figure 4A). Spermaries had a mean diameter of 25 µm, 95% CI = 24-26, N= 213.
Stage II - the spermary was made up of a group of spermatocytes undergoing meiosis. The mesogleal layer enveloping the spermary had not yet formed a wall (Figure 4B). Spermary mean diameter was 38 µm, 95% CI = 37-39, N= 331.
Stage III - the spermary, still made up of a group of spermatocytes undergoing meiosis, was delineated by a clearly differentiated wall formed by the mesoglea (Figure 4C). Spermary mean diameter was 61 µm, 95% CI = 60-62, N= 1,683.
Stage IV - the spermary showed a centripetal maturation gradient: less mature and larger germ cells (spermatocytes) were located at the periphery of the spermary, whereas more mature and smaller ones (spermatids) were located in the center (Figure 4D). Spermary mean diameter was 83 µm, 95% CI = 82-84, N= 1,663.
Stage V - the spermary was made up of a mass of spermatozoa with their tails all facing in the same direction (an arrangement known as a ‘‘bouquet’’; Fadlallah and Pearse, 1982a; Figure 4E). Spermary mean diameter was 83 µm, 95% CI = 81-85, N= 641.
Female gametogenesis
The oocytes were oval-shaped and located in the mesenteries (Figure 5). A total of 25,102 oocytes were identified and measured (Supporting Information Table S1). The diameter of the oocytes ranged from 9 to 146 µm and their mean diameter was 46 µm, 95% CI = 45.7-46.3, N= 25,102. The early stages of oogenesis were visible in the mesentery gastrodermal layers. Early oocytes had a centrally located spherical nucleus and a high ratio of nucleus to cytoplasm (Figure 5A). In the intermediate stages the nucleus/cytoplasm ratio decreased due to the accumulation of yolk (Figure 5B). In the more advanced stages, the ratio further reduced since cell size increased in parallel to the storage of yolk in the oocyte cytoplasm (Figures 5C, D). The nucleus had also migrated to the cell periphery and adhered closely to the cell membrane (Figures 5C, D). During oogenesis, the nucleolus was always positioned on the periphery of the nucleus (Figures 5C, D).
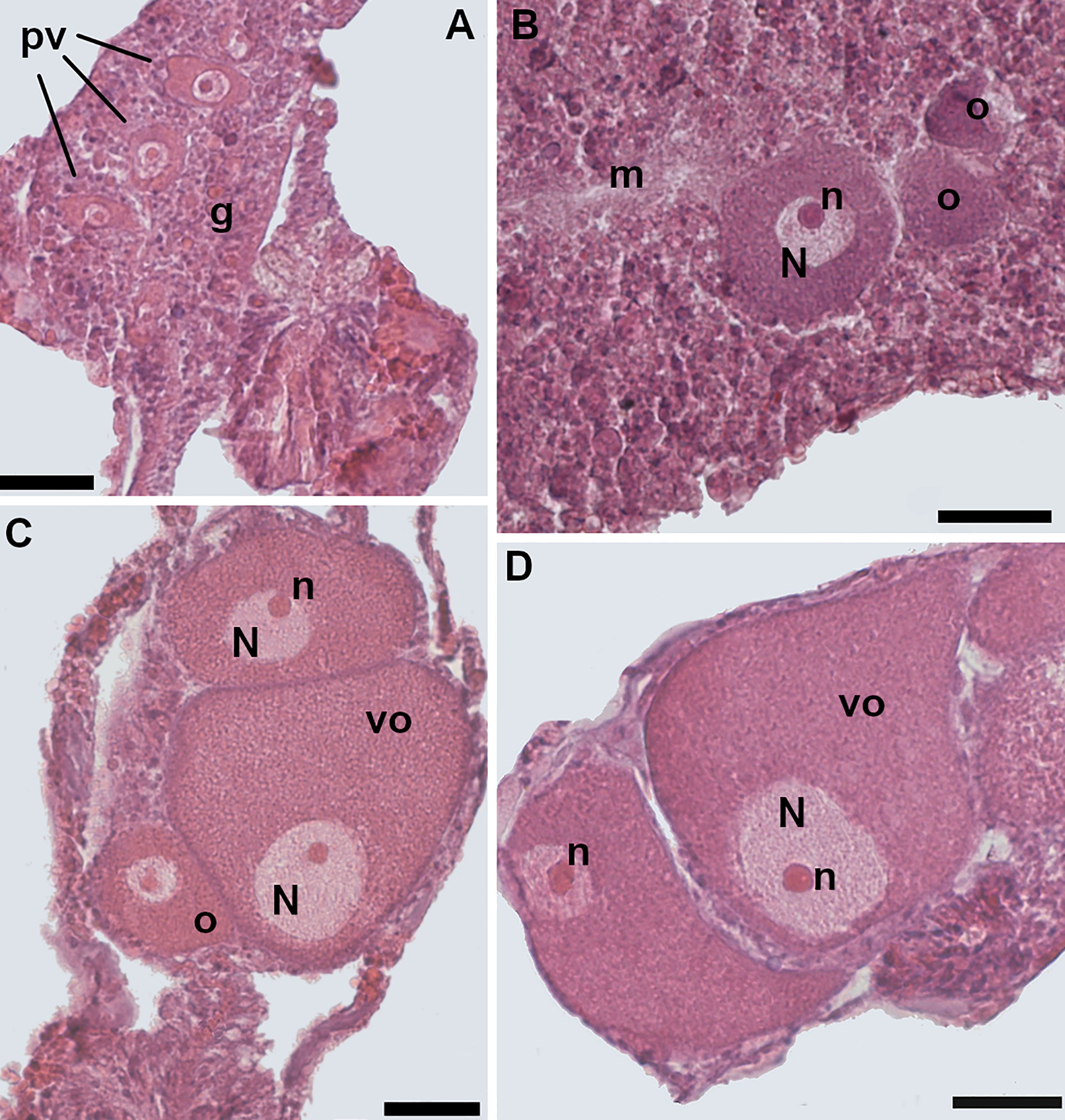
Figure 5 Oogenesis. (A) Early stage: three previtellogenic oocytes in the gastrodermis of the mesentery, characterized by a high nucleus/cytoplasm ratio. (B) Intermediate stage: vitellogenic oocyte located in the mesoglea. The spherical-shaped nucleus is still located in the central portion of the cell. The nucleus/cytoplasm ratio is reduced. (C) Late stage: the nucleus of the oocyte has started to migrate toward the cell periphery. Note two smaller vitellogenic oocytes in the same section. (D) Late stage: two mature oocytes, located in the mesentery; the nucleus/cytoplasm ratio is greatly reduced. [o, oocyte; m, mesoglea; N, nucleus; n, nucleolus; pv, previtellogenic oocyte; vo, vitellogenic oocyte; g, gastrodermis]. Scale bars are 25 μm.
Annual reproductive cycle
Gonadal index of both females and males increased significantly from August until November, the months in which both the photoperiod and water temperature decreased after the maximum of the year (Figures 6A, B). During late autumn and winter, late maturation stages of both oocytes (mean diameter ranged from 67.3 to 93.8 µm) and spermaries (IV and V) were observed (Supporting Information Table S2). After this period, both type of gametes disappeared (Figure 6B and Supporting Information Table S2), suggesting that the fertilization was occurred. Immediately after the fertilization period, we observed the emptying of gametes in both males and females (Figure 6B and Supporting Information Table S2) and the consequent increase in sexually inactive polyps (Figure 6C). In late spring and summer new oocytes (mean diameter ranged from 20.9 to 38.8 µm) and the development of early stages of spermary maturation (I, II, III) were detected (Supporting Information Table S2).
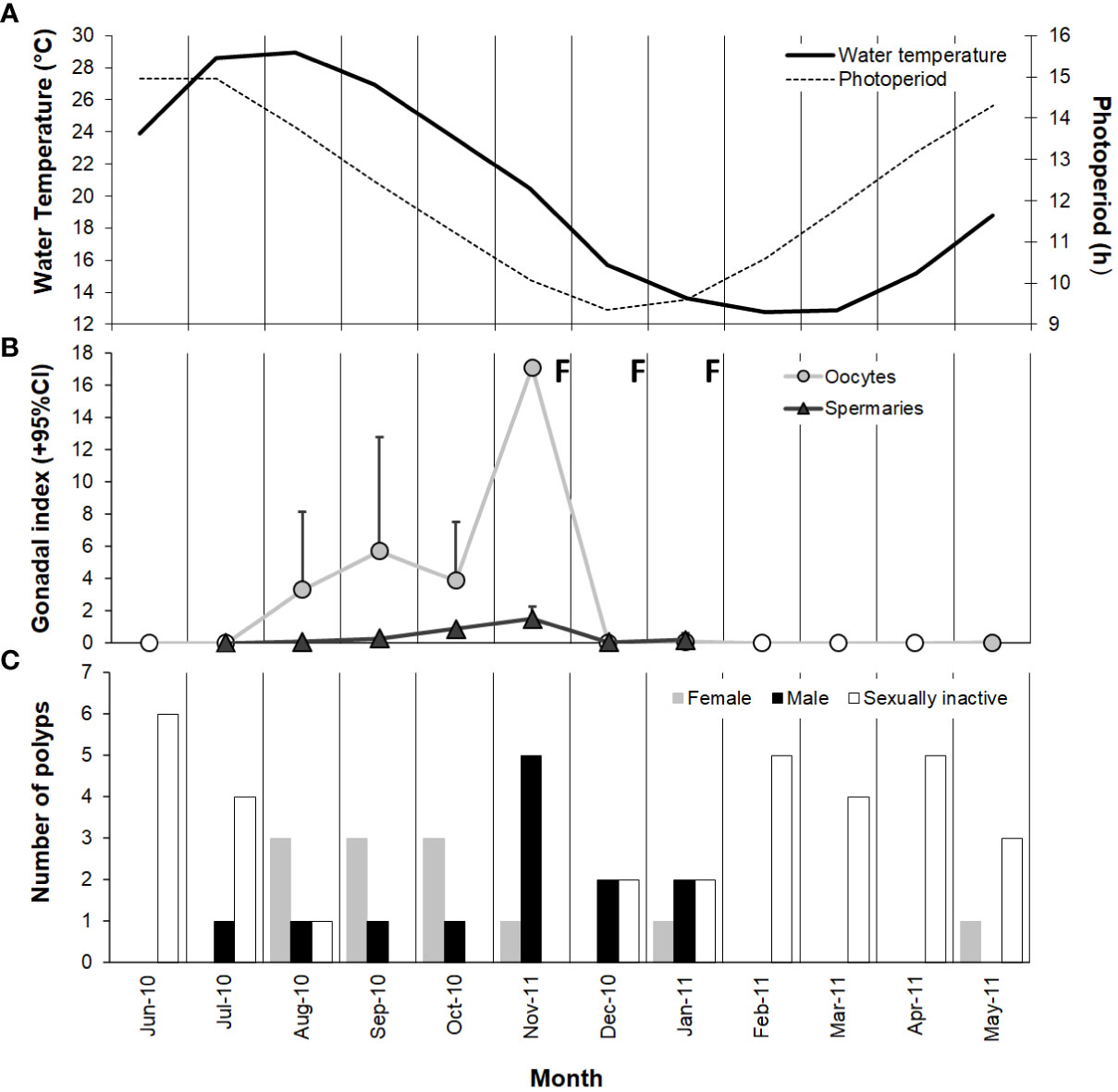
Figure 6 Variation in (A) water temperature and photoperiod, (B) gamete development (monthly mean + 95% confidence interval), and (C) number of female, male, and sexually inactive polyps from June 2010 to May 2011 at Palinuro. Note that the value ranges on the ordinate axes are different. ○ no oocytes detected; Δ no spermary detected; F, fertilization.
Biometry
Polyp length was selected as the main biometric parameter since it was the best indicator of dry skeletal mass and has been used as the measure of size in biometric, reproductive biology, and population dynamic studies of other solitary corals (Bell and Turner, 2000; Goffredo and Chadwick-Furman, 2003; Goffredo et al., 2010; Caroselli et al., 2015). Polyp width, height, dry skeletal mass and volume all correlated positively with polyp length (Figure 7). Surface/volume ratio and bulk skeletal density correlated negatively with polyp length (Figure 7). Since the regression equation exponent was< 1 (95% confidence interval = 0.798-0.959), the increase in polyp width compared with that of polyp length showed an allometric growth, with polyp length increasing more rapidly than width.
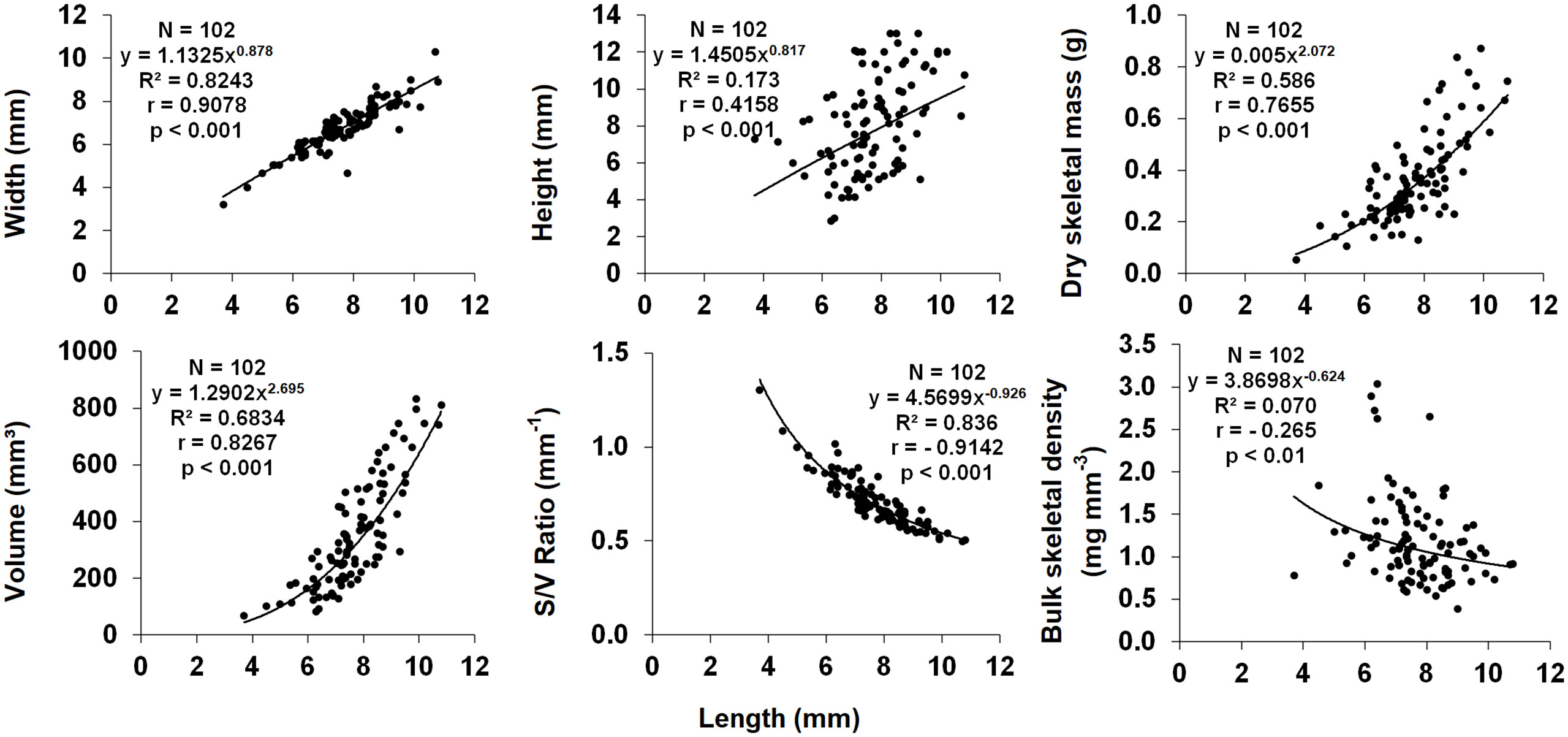
Figure 7 Dependence of biometric parameters on polyp length. N, number of individuals; R2, coefficient of determination; r, Pearson correlation coefficient.
Discussion
Sexual condition and reproductive mode
Each sexually active polyp of Paracyathus pulchellus contained a single type of germ cell, indicating gonochorism. As expected for gonochoric corals, male and female polyps did not show sexual dimorphism, likely excluding protandry or protogynous hermaphroditism (Harrison, 2011). Within the genus Paracyathus, P. stearnsii (Fadlallah and Pearse, 1982b) is another case of gonochorism. The sex ratio in a population with random mating is normally 1:1 (Maynard-Smith, 1978), as it has been observed in this population of P. pulchellus. Similarly, the sex ratio among individuals of P. stearnsii, collected off the coast of California, did not seem to differ from 1:1 (Fadlallah and Pearse, 1982b). Based on the absence of embryos in all the polyps analyzed, it is highly likely that the embryos of P. pulchellus have an external development. Within the Caryophylliidae family, almost all species studied develop the embryos externally, with the exceptions of Caryophyllia cyathus, C. inornata, C. huinayensis, Tethocyathus endesa, and some records of brooding in C. smithii (Table 3). Sexual condition (gonochorism vs. hermaphroditism) in scleractinians tends to be phylogenetically correlated and therefore a constant feature within each species, genus, and family, while reproductive mode (brooding vs. external development) tends to be a plastic trait and more variable (Harrison, 1985; Harrison and Wallace, 1990). However, in an increased number of families the presence of both gonochoric and hermaphroditic species has been reported (Harrison, 2011). Sexuality can vary not only at a genus and family level but also among populations of the same species. For example, Astroides calycularis collected at Palinuro (Southern Tyrrhenian Sea, Italy) and at Punta de la Mona (Alboran Sea, Spain) was described as gonochoric species both at the polyp and colony level (Goffredo et al., 2010; Goffredo et al. 2011a; Casado-Amezua et al. (2013), while Lacaze-Duthiers (1873) described A. calycularis from the Algerian coasts as monoic hermaphroditic, observing hermaphroditic colonies formed by gonochoric polyps with some cases of polyps that exhibited a simultaneous hermaphroditism. Another example is the species Cladocora caespitosa, the only shallow water scleractinian coral that can be considered a reef-building in the Mediterranean basin. Colonies from the Western and Eastern Mediterranean Sea were observed to be gonochoric (Kersting et al., 2013; Hadjioannou, 2019), while Kružić et al. (2008) described this species as hermaphroditic in the Adriatic Sea. Given the wide distribution of P. pulchellus, which spans the entire Mediterranean and Northern Atlantic, investigations on populations from other sites are needed to check if the reproductive traits observed in this study are maintained across the whole species areal.
No significant differences were found in the average size between sexually active and inactive polyps during the reproductive period, thus, the inactive polyps could be quiescent. Since most of the inactive polyps have been observed at the beginning of gametogenesis (May-July; Supporting Information Table S1), it is likely that they do not have yet begun to develop gametes. While the inactive polyps observed at the end of gametogenesis (December-January; Supporting Information Table S1) may have already released their gametes for fertilization.
Male gametogenesis
The morphological aspects of male gametogenesis in Paracyathus pulchellus correspond to those of other scleractinians of this and other families with the same or different reproductive traits. For example, aspects of spermatogenesis observed in P. pulchellus have been described, among Caryophylliidae family, in the gonochoric and broadcasting P. stearnsii (Fadlallah and Pearse, 1982b), Solenosmilia variabilis (Pires et al., 2014), Desmophyllum dianthus (Feehan et al., 2019), and Desmophyllum pertusum (Brooke and Järnegren, 2013; Pires et al., 2014), in the gonochoric and brooding Caryophyllia inornata (Goffredo et al., 2012), and in the hermaphroditic and broadcasting species C. ambrosia, C. sequenzae, and C. cornuformis (Waller et al., 2005). Spermatogenesis with similar morphological traits was also observed in other families: e.g., in the gonochoric and brooding Balanophyllia elegans (Dendrophylliidae; Fadlallah and Pearse, 1982a), Leptopsammia pruvoti (Dendrophylliidae; Goffredo et al., 2005), and Astroides calycularis (Dendrophylliidae; Goffredo et al., 2010), in the hermaphroditic and brooding Balanophyllia europaea (Dendrophylliidae; Goffredo et al., 2002), Madracis mirabilis, M. senaria, M. decactis, M. formosa, M. carmabi, and M. pharensis (Pocilloporidae; Vermeij et al., 2004), in the gonochoric and broadcasting Euphyllia ancora (Euphylliidae; Shikina et al., 2012) , Enallopsammia rostrata (Dendrophylliidae; Pires et al., 2014) and Fungiacyathus marenzelleri (Fungiacyathidae; Waller et al., 2002), in the hermaphroditic and broadcasting Mussismilia hispida (Mussidae; Neves and Pires, 2002), Acropora cervicornis (Acroporidae; Vargas-Angel et al., 2006).
Female gametogenesis
In corals, oocyte development varies according to the reproductive mode. Generally, brooding species produce a relatively small number of large-sized oocytes rich in reserves (Shlesinger et al., 1998), while broadcasting species produce and release a relatively large number of small oocytes (Fadlallah, 1983; Richmond and Hunter, 1990). Paracyathus pulchellus showed many relatively small oocytes that ranged from 9 to 146 µm with a mean diameter of 46 µm, similar to those described for P. stearnsii which produces oocytes ranged from 5 to 175 µm in diameter (Fadlallah and Pearse, 1982b). The morphological aspects of oogenesis observed in P. pulchellus are also similar to those described for some species of the Caryophylliidae family. For example, both Caryophyllia inornata (that broods its embryos but probably of agamic origin with a possible external fertilization) and C. smithii produce oocytes with spherical or oval shape and a diameter respectively ranging from 12 to 382 µm with a mean diameter of 69 µm (Goffredo et al., 2012; Marchini et al., 2015) and from 130-150 µm (Tranter et al., 1982). However, oocyte diameter can vary considerably among individuals of the same species inhabit different environments (Harriott, 1983) or within a genus (Babcock et al., 1986; Richmond and Hunter, 1990). In contrast, the three deep-coral species C. cornuformis, C. sequenzae, and C. ambrosia have oocytes with maximum sizes that are 2-5 times greater ranged from 350 to 700 µm (Waller et al., 2005). It seems that the size of mature oocytes of the genus Caryophyllia increases with an increment in depth of the habitat of the species, as an adaptation to oligotrophic environments, since large oocytes and a consequent development of lecithotrophic larvae should not require an exogenous food supply (Shilling and Manahan, 1994; Waller et al., 2005). Species that produce small oocytes, as for P. pulchellus, which contain less energy reserves, usually generate small planktotrophic larvae (Jaeckle, 1995; Sewell and Manahan, 2001; Prowse et al., 2008) with a long pelagic larval phase, during which they must find food sources in the water (Pechenik, 1990). To enhance growth and larval development, the release of gametes should occur during periods that coincide with the presence of a higher amount of food in the water (Tyler et al., 1982). In P. stearnsii, Fadlallah and Pearse (1982b), through artificial fertilization, observed the formation of swimming planulae, but none were able to settle, probably due to lack of food. Similarly, C. smithii was observed to have planktotrophic planulae which start to feed 48 hours after fertilization when they are totally formed, and that swim and develop for 8 to 10 weeks before starting to settle (Tranter et al., 1982). In P. pulchellus, when the oocytes shifted from immature to mature, their shape started to change from round to elliptical. This pattern was found in other species of the same family but also in corals of different genus and different regions such as Acropora hyacinthus in Northwestern Philippines (Jamodiong et al., 2018), Plesiastrea versipora in Sidney Harbour (Madsen et al., 2014), and Diploria labyrinthiformis in the Colombian Caribbean (Alvarado et al., 2004), and others. As was observed in P. pulchellus, and in other species belonging to the same family, such as Goniocorella dumosa and Solenosmilia variabilis (Burgess and Babcock, 2005), during the final phases of oogenesis in scleractinians and more generally in anthozoans, the nucleus migrates to the cell periphery and adheres to the plasma membrane (Szmant-Froelich et al., 1985). The typical U-shaped phase (in which the nucleus becomes indented assuming a sickle) that is observed in some scleractinians (e.g., Goffredo et al., 2005; Shikina et al., 2012; Park et al., 2020) was not observed in P. pulchellus, as in no other species within the Caryophylliidae family, such as G. dumosa, S. variabilis (Burgess and Babcock, 2005; Pires et al., 2014), Desmophyllum pertusum (Brooke and Järnegren, 2013; Pires et al., 2014), and C. ambrosia, C. sequenzae and C. cornuformis (Waller et al., 2005).
Annual reproductive cycle
Histological observations showed that sexual reproduction of Paracyathus pulchellus follows a single seasonal reproductive cycle. The maturation of gametes required about 9 months (from May to January, though mature males and females predominated from July to January; Figures 6B, C) and occurred simultaneously. Synchronous gametogenesis has also been documented for another solitary coral within the Paracyathus genus, P. stearnsii, in which spermaries and oocytes follow an annual cycle and are closely paralleled (Fadlallah and Pearse, 1982b). The same pattern has been observed for several coral species from Mediterranean Sea such as Balanophyllia europaea, Leptopsammia pruvoti, Astroides calycularis, and Caryophyllia inornata (Goffredo et al., 2002; Goffredo et al., 2006; Goffredo et al., 2011a; Marchini et al., 2015).
Both seawater temperature and photoperiod are often mentioned as important factors that controls gametogenetic cycles in many anthozoans (Babcock et al., 1994; Acosta and Zea, 1997; Colley et al., 2000; Glynn et al., 2008). P. pulchellus showed an acceleration in gamete maturation during the months in which both photoperiod and water temperature decrease, while fertilization took place when photoperiod reaches the minimum of the year. However, studies on other Mediterranean corals showed an increase in gonadal development rate in the months during which both photoperiod and seawater temperature increase (Goffredo et al., 2002; Goffredo et al., 2006; Marchini et al., 2015). In individuals of C. inornata studied at Elba Island, gonadal index increases from March to May, coinciding with an increase of photoperiod and seawater temperature. Fertilization takes place from April until July, when photoperiod reached the maximum of the year (Marchini et al., 2015). The solitary corals L. pruvoti and B. europaea at Calafuria have a significant increase in gonadal development in the months during which photoperiod becomes longer after its annual minimum, and fertilization takes place in the period when both photoperiod and seawater temperature are increasing (Goffredo et al., 2002; Goffredo et al., 2006). Thus, the seasonal variation of seawater temperature and photoperiod seems to be crucial in the gamete development for these temperate corals, even though they can trigger different processes of the reproductive cycle. The dissimilarity observed in the timing of gonadal development of these Mediterranean corals, could be explained by the different larvae development mode. In fact, for the developing larvae also food availability is an important factor (Lessios, 1981). Since Mediterranean Sea is characterized by the highest Chlorophyll-a values in winter (Barale et al., 2008), a gonadal development in this period could be a strategy for brooding corals that need energy to produce large oocytes with subsequent development of lecithotrophic larvae whose feeding depends on the yolk present in the large oocytes. On the other hand, planktotrophic larvae that develop in the water column (as in the case of P. pulchellus), need a constant supply of food throughout the early stages of their lives.
Biometry
All biometric parameters analyzed in this study correlated significantly with polyp length (Figure 7), consistently with previous studies on other solitary coral species (Goffredo et al., 2007; Caroselli et al., 2015). Bulk skeletal decreased with increasing polyp size. The relationship between skeletal density and size can be interpreted in terms of relationship between calcification and linear extension (calcification = skeletal density × linear extension rate; Lough and Barnes, 2000). In Paracyathus pulchellus, as known for other solitary corals (Goffredo et al., 2004; Goffredo et al., 2007; Caroselli et al., 2015), the linear extension rate is expected to decrease as size (age) increases. The decrease of skeletal density with increasing size could be explained as a greater decrease in calcification than in linear extension rate with increasing size, as already suggested for Caryophyllia inornata (Caroselli et al., 2015) and Leptopsammia pruvoti (Goffredo et al., 2007).
The exponent of the w/l relationship for P. pulchellus polyps was< 1, (CI 95% = 0.798-0.959). This indicates an allometric growth of the corallite, in which polyp length increases more rapidly than width, thus producing a more oval-shaped oral disc. This shape of the oral disc has been put in relation to sedimentation stress in some coral species (e.g., Balanophyllia europaea, Goffredo et al., 2007, and C. smithii, Bell and Turner, 2000), as it can facilitate sediment removal compared to circular oral discs (Foster et al., 1988). The width:length relationship has also been studied for other solitary corals. However, also several solitary species living on walls or under overhangs (e.g., with the oral disc facing downwards) such as L. pruvoti (Goffredo et al., 2007) and C. inornata (Caroselli et al., 2015) show an allometric growth, leading to a more circular oral disc even if they are less subject to sedimentation, because sediment removal is mainly carried out by gravity (Goffredo et al., 2007). Other factors such as a better zooplankton capture ability may thus be linked to the shape of the oral disc (Caroselli et al., 2015).
Conclusions
The population of Paracyathus pulchellus from Palinuro shallows the bathymetric range of this species up to 5 m depth. It was gonochoric with a sex-ratio 1:1. No embryo was found in the coelenteric cavity of the polyps, suggesting an external development of the larvae and a possible broadcast spawning reproductive mode. The study of the gametogenesis morphology showed a spermary development similar to other scleractinians (even those not belonging to the same family) and mature small-sized oocytes, such as those found in other caryophyllids living in the same habitat conditions, suggesting a possible planktotrophic larval development. Gametogenesis follows an annual cycle and appears to be influenced by temperature and photoperiod variations. Finally, the study of biometry showed a correlation between the main biometric parameters and polyp length and an allometric growth that leads to a more oval-shaped calyx. This is the first investigation on gametogenesis of P. pulchellus whose data could be useful for future studies assessing the impact of global climate change on reproduction, and thus the survival of these endangered organisms.
Data availability statement
The raw data supporting the conclusions of this article will be made available by the authors, without undue reservation.
Author contributions
GF, ZD, and SG conceived the research; CM, EC, and SG performed the fieldwork; CM, VF, MC, and EC performed the analyses; CM, VF, MC, and EC wrote the manuscript; GF and ZD gave conceptual advice; SG contributed to the reagents, materials, and analysis tools. All authors participated to the scientific discussion and gave final approval for publication.
Funding
The research leading to these results has received funding from the European Research Council under the European Union’s Seventh Framework Programme (FP7/2007–2013)/ERC grant agreement n° [249930-CoralWarm: Corals and global warming: the Mediterranean versus the Red Sea].
Acknowledgments
Thanks to the diving center Pesciolino Sub for their logistical assistance during the sampling collection and the Scientific Diving School (www.sdseducational.org) for technical and logistical support for fieldwork. The research leading to these results has received funding from the European Research Council under the European Union’s Seventh Framework Programme (FP7/2007–2013)/ERC grant agreement n° [249930-CoralWarm: Corals and global warming: the Mediterranean versus the Red Sea]. The experiment complied with current Italian law.
Conflict of interest
The authors declare that the research was conducted in the absence of any commercial or financial relationships that could be construed as a potential conflict of interest.
Publisher’s note
All claims expressed in this article are solely those of the authors and do not necessarily represent those of their affiliated organizations, or those of the publisher, the editors and the reviewers. Any product that may be evaluated in this article, or claim that may be made by its manufacturer, is not guaranteed or endorsed by the publisher.
Supplementary material
The Supplementary Material for this article can be found online at: https://www.frontiersin.org/articles/10.3389/fmars.2022.925586/full#supplementary-material
Footnotes
References
Acosta A., Zea S. (1997). Sexual reproduction of the reef coral Montastrea cavernosa (Scleractinia: Faviidae) in the Santa Marta area, Caribbean coast of Colombia. Mar. Biol. 128, 141–148. doi: 10.1007/s002270050077
Airi V., Gizzi F., Falini G., Levy O., Dubinsky Z., Goffredo S. (2014). Reproductive efficiency of a Mediterranean endemic zooxanthellate coral decreases with increasing temperature along a wide latitudinal gradient. PloS One 9, e91792. doi: 10.1371/journal.pone.0091792
Airi V., Prantoni S., Calegari M., Lisini Baldi V., Gizzi F., Marchini C., et al. (2017). Reproductive output of a non-zooxanthellate temperate coral is unaffected by temperature along an extended latitudinal gradient. PloS One 12, e0171051. doi: 10.1371/journal.pone.0171051
Alvarado C. E.M., García U. R., Acosta A. (2004). Sexual reproduction of the reef-building coral Diploria labyrinthiformis (Scleractinia: Faviidae), in the Colombian Caribbean. Rev. Biol. Trop. 52, 859–868.
Anderson M. J. (2001). A new method for non-parametric multivariate analysis of variance. Austral. Ecol. 26, 32–46. doi: 10.1046/j.1442-9993.2001.01070.x
Babcock R. C., Bull G. D., Harrison P. L., Heyward A. J., Oliver J. K., Wallace C. C., et al. (1986). Synchronous spawning of 105 scleractinian coral species on the great barrier reef. Mar. Biol. 90, 379–394. doi: 10.1007/BF00428562
Babcock R. C., Wills B. L., Simpson C. J. (1994). Mass spawning of corals on high latitude coral reef. Coral. Reefs. 13, 161–169. doi: 10.1007/BF00301193
Baird A. H., Guest J. R., Willis B. L. (2009). Systematic and biogeographical patterns in the reproductive biology of scleractinian corals. Ann. Rev. Ecol. Evol. Syst. 40, 551–571. doi: 10.1146/annurev.ecolsys.110308.120220
Barale V., Jaquet J. M., Ndiaye M. (2008). Algal blooming patterns and anomalies in the Mediterranean Sea as derived from the SeaWiFS data set, (1998–2003). Remote Sens. Environ. 112, 3300–3313. doi: 10.1016/j.rse.2007.10.014
Bell J. J., Turner J. R. (2000). Factors influencing the density and morphometrics of the cup coral Caryophyllia smithii in lough hyne. J. Mar. Biol. Assoc. UK. 80, 437–441. doi: 10.1017/S0025315400002137
Brooke S., Järnegren J. (2013). Reproductive periodicity of the scleractinian coral Lophelia pertusa from the Trondheim fjord, Norway. Mar. Biol. 160, 139–153. doi: 10.1007/s00227-012-2071-x
Burgess S. N., Babcock R. C. (2005). “Reproductive ecology of three reef-forming, deep-sea corals in the new Zealand region”, in Cold-water corals and ecosystems. Eds. Freiwald A., Roberts J. M. (Berlin Heidelberg: Springer-Verlag), 701–713.
Cairns S. D. (1979). The deep-water scleractinia of the Caribbean and adjacent waters. Stud. Fauna. Curaçao. Other. Caribbean. Islands. 57, 1–341.
Cairns S. D. (2000). A revision of the shallow-water azooxanthellate scleractinia of the western Atlantic. Stud. Natural History. Caribbean. Region. 75, 1–231.
Caroselli E., Nanni V., Levy O., Falini G., Dubinsky Z., Goffredo S. (2015). Latitudinal variations in biometry and population density of a Mediterranean solitary coral. Limnol. Oceanogr. 60, 1356–1370. doi: 10.1002/lno.10100
Casado-Amezua P., Aguilar R., Ozalp B., Terrón-Sigler A., Goffredo S., Linares C. L. (2015)Paracyathus pulchellus. In: The IUCN red list of threatened species 2015. Available at: https://www.iucnredlist.org/species/50149125/51215323 (Accessed April 21, 2022).
Casado-Amezua P., Gasparini G., Goffredo S. (2013). Phenological and morphological variations in the Mediterranean orange coral Astroides calycularis between two distant localities. Zoology 116, 159–167. doi: 10.1016/j.zool.2012.10.005
Colley S. B., Feingold J. S., Pena J., Glynn P. W. (2000). Reproductive ecology of Diaseris distorta (Michelin) (Fungiidae) in the Galapagos islands, Ecuador. Proc. 9th. Int. Coral. Reef. Symp. 1, 373–379.
Fadlallah Y. H. (1983). Sexual reproduction, development and larval biology in scleractinian corals. a review. Coral. Reefs. 2, 129–150. doi: 10.1007/BF00336720
Fadlallah Y. H., Pearse J. S. (1982a). Sexual reproduction in solitary corals: Overlapping oogenic and brooding cycles and benthic planulas in Balanophyllia elegans. Mar. Biol. 71, 223–231. doi: 10.1007/BF00397039
Fadlallah Y. H., Pearse J. S. (1982b). Sexual reproduction in solitary corals: synchronous gametogenesis and broadcast spawning in Paracyathus stearnsii. Mar. Biol. 71, 233–239. doi: 10.1007/BF00397040
Feehan K. A., Waller R. G., Häussermann V. (2019). Highly seasonal reproduction in deep-water emergent Desmophyllum dianthus (Scleractinia: Caryophylliidae) from the Northern Patagonian Fjords. Mar. Biol. 166: 52. doi: 10.1007/s00227-019-3495-3
Fenner D., Riolo F., Vittorio M. (2013). New records of scleractinian corals from shallow waters of the Ionian coast of Italy. Mar. Biodivers. Rec. 6, 1–5. doi: 10.1017/S1755267213001073
Fine M., Zibrowius H., Loya Y. (2001). Oculina patagonica: a non-lessepsian scleractinian coral invading the Mediterranean Sea. Mar. Biol. 138, 1195–1203. doi: 10.1007/s002270100539
Fisk D. A. (1981). Studies of two free-living corals and their common sipunculan associate at wistari reef (Great barrier reef). [master’s thesis] ([Brisbane (QLD)]: University of Queensland).
Foster A. B., Johnson K. G., Schultz L. L. (1988). Allometric shape change and heterochrony in the freeliving coral Trachyphyllia bilobata (Duncan). Coral. Reefs. 7, 37–44. doi: 10.1007/BF00301980
Gizzi F., de Mas L., Airi V., Caroselli E., Prada F., Falini G., et al. (2017). Reproduction of an azooxanthellate coral is unaffected by ocean acidification. Sci. Rep. 7, 13049. doi: 10.1038/s41598-017-13393-1
Glynn P. W., Colley S. B., Mate J. L., Corte´s J., Guzman H. M., Bailey R. L. (2008). Reproductive ecology of the azoxanthellate coral Tubastraea coccinea in the equatorial Eastern pacific: Part v. dendrophylliidae. Mar. Biol. 153, 529–544. doi: 10.1007/s00227-007-0827-5
Goffredo S., Telò T. (1998). Hermaphroditism and brooding in the solitary coral Balanophyllia europaea (Cnidaria, anthozoa, scleractinia). Ita. J. Zool. 65, 159–165. doi: 10.1080/11250009809386740
Goffredo S., Arnone S., Zaccanti F. (2002). Sexual reproduction in the Mediterranean solitary coral Balanophyllia europaea (Scleractinia, dendrophylliidae). Mar. Ecol. Prog. Ser. 229, 83–94. doi: 10.3354/meps229083
Goffredo S., Chadwick-Furman N. E. (2003). Comparative demography of mushroom corals (Scleractinia, fungiidae) at eilat, northern red Sea. Mar. Biol. 142, 411–418. doi: 10.1007/s00227-002-0980-9
Goffredo S., Mattioli G., Zaccanti F. (2004). Growth and population dynamics model of the Mediterranean solitary coral Balanophyllia europaea (Scleractinia, dendrophylliidae). Coral. Reefs. 23, 433–443. doi: 10.1007/s00338-004-0395-9
Goffredo S., Radetic J., Airi V., Zaccanti F. (2005). Sexual reproduction of the solitary sunset cup coral Leptopsammia pruvoti (Scleractinia, dendrophylliidae) in the mediterranean. 1. morphological aspects of gametogenesis and ontogenesis. Mar. Biol. 147, 485–495. doi: 10.1007/s00227-005-1567-z
Goffredo S., Airi V., Radetic J., Zaccanti F. (2006). Sexual reproduction of the solitary sunset cup coral Leptopsammia pruvoti (Scleractinia, dendrophylliidae) in the mediterranean. 2. quantitative aspects of the annual reproductive cycle. Mar. Biol. 148, 923–932. doi: 10.1007/s00227-005-0137-8
Goffredo S., Caroselli E., Pignotti E., Mattioli G., Zaccanti F. (2007). Variation in biometry and population density of solitary corals with environmental factors in the Mediterranean Sea. Mar. Biol. 152, 351–361. doi: 10.1007/s00227-007-0695-z
Goffredo S., Gasparini G., Marconi G., Putignano M. T., Pazzini C., Zaccanti F. (2010). Gonochorism and planula brooding in the Mediterranean endemic orange coral Astroides calycularis (Scleractinia: Dendrophylliidae). morphological aspects of gametogenesis and ontogenesis. Mar. Biol. Res. 6, 421–436. doi: 10.1080/17451000903428488
Goffredo S., Gasparini G., Marconi G., Putignano M. T., Pazzini C., Airi V., et al. (2011a). Sexual reproduction in the Mediterranean endemic orange coral, Astroides calycularis (Scleractinia, dendrophylliidae). Bull. Mar. Sci. 87, 589–604. doi: 10.5343/bms.2010.1068
Goffredo S., Caroselli E., Gasparini G., Marconi G., Putignano M. T., Pazzini C., et al. (2011b). Colony and polyp biometry and size structure in the orange coral Astroides calycularis (Scleractinia: Dendrophylliidae). Mar. Biol. Res. 7, 272–280. doi: 10.1080/17451000.2010.492222
Goffredo S., Marchini C., Rocchi M., Airi V., Caroselli E., Falini G., et al. (2012). Unusual pattern of embryogenesis of Caryophyllia inornata (Scleractinia, caryophylliidae) in the Mediterranean Sea: Maybe agamic reproduction? J. Morphol. 273, 943–956. doi: 10.1002/jmor.20039
Hadjioannou L. (2019). Ecological and biological responses to environmental changes, in the endemic scleractinian coral cladocora caespitosa (Linnaeus 1767), in cyprus. [Ph.D. thesis] (Nicosia (CY: University of Cyprus).
Hall V. R., Hughes T. P. (1996). Reproductive strategies of modular organisms: Comparative studies of reef-building corals. Ecology 77:950–963. doi: 10.2307/2265514
Harriott V. J. (1983). Reproductive ecology of four scleractinian species at lizard island, great barrier reef. Coral. Reefs. 2, 9–18. doi: 10.1007/BF00304727
Harrison P. L. (1985). “Sexual characteristics of scleractinian corals: Systematic and evolutionary implications”, in Proceedings of the fifth international coral reef congress Tahiti. Eds. Gabrié C., Salvat B., 337–342.
Harrison P. L. (1990). Sperm morphology and fertilization strategies in scleractinian corals. Adv. Invert. Reprod. 5, 299–304.
Harrison P. L. (2011). “Sexual reproduction of scleractinian corals”, in Coral reefs: An ecosystem in transition. Eds. Dubinsky Z., Stambler N. (Berlin: Springer), 59–85.
Harrison P. L., Wallace C. C. (1990). “Reproduction, dispersal and recruitment of scleractinian corals”, in Ecosystem of the world. coral reefs. Ed. Dubinsky Z. (Amsterdam: Elsevier), 33–207.
Heltzel P. S., Babcock R. C. (2002). Sexual reproduction, larval development and benthic planulae of the solitary coral Monomyces rubrum (Scleractinia: Anthozoa). Mar. Biol. 140, 659–667. doi: 10.1007/s00227-001-0745-x
Heran T., Laudien J., Häussermann V., Försterra G., Hartig A., Richter C. (2018). Early life history of the cold-water coral caryophyllia huinayensis from the Chilean fjord region (Valdivia, Chile: Univ. Chile).
Hiscock K., Howlett R. M. (1977). “The ecology of caryophyllia smithii stokes and broderip on south western coasts of the British isles”, in Underwater research. Eds. Drew E. A., Lythgoe J. N., Woods J. D. (London: Academic Press), 319–344.
Jaeckle W. B. (1995). “Variation in the size, energy content and biochemical composition of invertebrate eggs: Correlates to the mode of larval development”, in Ecology of marine invertebrate larvae. Ed. McEdward L. (Boca Raton: CRC Press), 49–77.
Jamodiong E. A., Maboloc E. A., Villanueva R. D., Cabaitan P. C. (2018). Gametogenesis and inter-annual variability in the spawning pattern of Acropora hyacinthus in northwestern Philippines. Zool. Stud. 57, 46. doi: 10.6620/ZS.2018.57-46
Kersting D. K., Casado C., Lopez-Legentil S., Linares C. (2013). Unexpected patterns in the sexual reproduction of the Mediterranean scleractinian coral Cladocora caespitosa. Mar. Ecol. Progr. Ser. 486, 165–171. doi: 10.3354/meps10356
Kružić P., Žuljević A., Nikolić V. (2008). Spawning of the colonial coral Cladocora caespitosa (Anthozoa, scleractinia) in the southern Adriatic Sea. Coral. Reefs. 27, 337–341. doi: 10.1007/s00338-007-0334-7
Lacaze-Duthiers H. D. (1873). Developpment des coralliaires. Actinaires a polypiers. Arch. Zool. Exp. Gen. 2, 269–348.
Lessios H. A. (1981). Reproductive periodicity of the echinoids diadema and echinometra on the two coasts of Panama. J. Exp. Mar. Biol. Ecol. 50, 47–61. doi: 10.1016/0022-0981(81)90062-9
Lough J. M., Barnes D. J. (2000). Environmental controls on growth of the massive coral porites. J. Exp. Mar. Biol. Ecol. 245, 225–243. doi: 10.1016/S0022-0981(99)00168-9
Madsen A., Madin J. S., Tan C. H., Baird A. H. (2014). The reproductive biology of the scleractinian coral Plesiastrea versipora in Sydney harbour, Australia. Sex Early. Dev. Aquat. Org. 1, 25–33. doi: 10.3354/sedao00004
Marchini C., Airi V., Fontana R., Tortorelli G., Rocchi M., Falini G., et al. (2015). Annual reproductive cycle and unusual embryogenesis of a temperate coral in the Mediterranean Sea. PloS One 10, e0141162. doi: 10.1371/journal.pone.0141162
Marchini C., Gizzi F., Pondrelli T., Moreddu L., Marisaldi L., Montori F., et al. (2021). Decreasing pH impairs sexual reproduction in a Mediterranean coral transplanted at a CO2 vent. Limnol. Oceanogr. 9999, 1–11. doi: 10.1002/lno.11937
Marchini C., Tortorelli G., Guidi E., Airi V., Falini G., Dubinsky Z., et al. (2020). Reproduction of the azooxanthellate coral Caryophyllia inornata is not affected by temperature along an 850 km gradient on the Western Italian coast. Front. Mar. Sci. 6. doi: 10.3389/fmars.2019.00785
Neves E. G., Pires D. O. (2002). Sexual reproduction of Brazilian coral Mussismilia hispida (Verrill 1902). Coral. Reefs. 21, 161–168. doi: 10.1007/s00338-002-0217-x
Özalp H. B., Caroselli E., Raimondi F., Goffredo S. (2018). Skeletal growth, morphology and skeletal parameters of a temperate, solitary and zooxanthellate coral along a depth gradient in the dardanelles (Turkey). Coral. Reefs. 37, 633–646. doi: 10.1007/s00338-018-1687-9
Park J. S., Keshavmurthy S., Subramaniam T., Park S. R., Kang C. K., Choi K. S. (2020). Annual gametogenesis patterns in two high-latitude corals, Alveopora japonica and Oulastrea crispata, from jeju island, south Korea. Estuaries. Coasts. 43, 477–486. doi: 10.1007/s12237-019-00539-9
Pechenik J. A. (1990). Delayed metamorphosis by larvae of benthic marine invertebrates: Does it occur? Is there a price to pay? Ophelia 32, 63–94. doi: 10.1080/00785236.1990.10422025
Pires D. O., Silva J. C., Bastos N. D. (2014). Reproduction of deep-sea reef-building corals from the southwestern Atlantic. Deep-Sea Res. Part II. 99, 51–63. doi: 10.1016/j.dsr2.2013.07.008
Prowse T. A. A., Sewell M. A., Byrne M. (2008). Fuels for development: evolution of maternal provisioning in asterinid sea stars. Mar. Biol. 153, 337–349. doi: 10.1007/s00227-007-0809-7
Richmond R. H. (1997). “Reproduction and recruitment in corals: critical links in the persistence of reefs”, in Life and death of coral reefs. Ed. Birkeland C. (New York: Chapman & Hall), 175–197.
Richmond R. H., Hunter C. L. (1990). Reproduction and recruitment of corals: comparisons among the Caribbean, the tropical pacific, and the red Sea. Mar. Ecol. Progr. Ser. 60, 185–203. doi: 10.3354/meps060185
Sewell M. A., Manahan D. T. (2001). “Echinoderm eggs; biochemistry and larval biology”, in Echinoderms 2000: Proceedings of the 10th international conference, Dunedin (Boca Raton: CRC Press), 55–58.
Shikina S., Chen C. J., Liou J. Y., Shao Z. F., Chung Y. J., Lee Y. H., et al. (2012). Germ cell development in the scleractinian coral Euphyllia ancora (Cnidaria, anthozoa). PloS One 7, e41569. doi: 10.1371/journal.pone.0041569
Shilling F. M., Manahan D. T. (1994). Energy metabolism and amino acid transport during early development of antarctic and temperate echinoderms. Biol. Bull. 187, 398–407. doi: 10.2307/1542296
Shlesinger Y., Goulet T. L., Loya Y. (1998). Reproductive patterns of scleractinian corals in the northern red Sea. Mar. Biol. 132, 691–701. doi: 10.1007/s002270050433
Szmant-Froelich A., Reutter M., Riggs L. (1985). Sexual reproduction of Favia fragum (Esper): Lunar patterns of gametogenesis, embryogenesis and planulation in Puerto Rico. Bull. Mar. Sci. 37, 880–892.
Tranter P. R. G., Nicholson D. N., Kinchington D. (1982). A description of spawning and post-gastrula development of the cool temperate coral Caryophyllia smithii (Stokes and broderip). J. Mar. Biol. Assoc. U K. 62, 845–854. doi: 10.1017/S0025315400044106
Tyler P. A., Pain S. L., Gage J. D. (1982). The reproductive biology of the deep-sea asteroid Bathybiaster vexillifer. J. Mar. Biol. Assoc. U K. 62, 57–69. doi: 10.1017/S0025315400020105
Vargas-Angel B., Colley S. B., Hoke S. M., Thomas J. D. (2006). The reproductive seasonality and gametogenic cycle of Acropora cervicornis off broward county, Florida, USA. Coral. Reefs. 25, 110–122. doi: 10.1007/s00338-005-0070-9
Vermeij M. J. A., Sampayo E., Bröker K., Bak R. P. M. (2004). The reproductive biology of closely related coral species: Gametogenesis in Madracis from the southern Caribbean. Coral. Reefs. 23, 206–214. doi: 10.1007/s00338-004-0368-z
von Koch G. (1897). Entwicklung von Caryophyllia cyathus. mittheilungen aus der zoologischen station zu neapel 12, 755–772.
Waller R. G., Tyler P. A. (2005). The reproductive biology of two deep-water, reef-building scleractinians from the NE Atlantic ocean. Coral. Reefs. 24, 514–522. doi: 10.1007/s00338-005-0501-7
Waller R., Tyler P., Gage J. (2002). Reproductive ecology of the deep-sea scleractinian coral Fungiacyathus marenzelleri (Vaughan 1906) in the northeast Atlantic ocean. Coral. Reefs. 21, 325–331. doi: 10.1007/s00338-002-0252-7
Waller R. G., Tyler P. A., Gage J. D. (2005). Sexual reproduction in three hermaphroditic deep-sea Caryophyllia species (Anthozoa: Scleractinia) from the NE Atlantic ocean. Coral. Reefs. 24, 594–602. doi: 10.1007/s00338-005-0031-3
Keywords: sexuality, reproductive mode, gametogenesis, sex ratio, scleractinia, Mediterranean Sea
Citation: Marchini C, Fossati V, Cerpelloni M, Caroselli E, Falini G, Dubinsky Z and Goffredo S (2022) Sexual reproduction and biometry of the non-zooxanthellate papillose cup coral Paracyathus pulchellus. Front. Mar. Sci. 9:925586. doi: 10.3389/fmars.2022.925586
Received: 21 April 2022; Accepted: 13 September 2022;
Published: 06 October 2022.
Edited by:
Manuel Maldonado, Center for Advanced Studies of Blanes, (CSIC), SpainReviewed by:
Esti Kramarsky-Winter, Ben-Gurion University of the Negev, IsraelBenny K. K. Chan, Academia Sinica, Taiwan
Copyright © 2022 Marchini, Fossati, Cerpelloni, Caroselli, Falini, Dubinsky and Goffredo. This is an open-access article distributed under the terms of the Creative Commons Attribution License (CC BY). The use, distribution or reproduction in other forums is permitted, provided the original author(s) and the copyright owner(s) are credited and that the original publication in this journal is cited, in accordance with accepted academic practice. No use, distribution or reproduction is permitted which does not comply with these terms.
*Correspondence: Erik Caroselli, erik.caroselli@unibo.it; Chiara Marchini, chiara.marchini2@unibo.it