- 1Univ Brest, Ifremer, CNRS, Unité BEEP, Plouzané, France
- 2Univ Brest, Ifremer, CNRS, Unité GEOCEAN, Plouzané, France
- 3Ifremer, Unité RDT, Plouzané, France
To date, two main vent faunal assemblages have been described on active sulfide edifices along the northern Mid-Atlantic Ridge (nMAR): one dominated by bathymodiolin mussels in low temperature areas and the other dominated by alvinocaridid shrimp in warmer habitats. In this study, we describe the ecology of new types of assemblage, dominated by gastropods, that are recurrent in several nMAR vent fields, from ~830 m to 3500 m depth. We assessed and compared the composition, abundance, diversity and trophic niche of these assemblages from three vent fields (Menez Gwen, Lucky Strike and Snake Pit) and characterized their habitats in terms of key environmental conditions. These assemblages, first seen during the Momarsat cruise in 2012 at the Lucky Strike vent field, were investigated during several subsequent cruises. They appear to be widespread along the nMAR, forming two distinct assemblages, one dominated by Lepetodrilus atlanticusat the shallowest vent field Menez Gwen, and the other by Peltospira smaragdina at the other investigated fields. Our data seem to indicate that these gastropods dominate an intermediate habitat at MAR vents and may play an important ecological role in these communities.
Introduction
Located on mid-oceanic ridges and back-arc basins, hydrothermal vent communities rely on chemosynthetic processes to sustain dense, but low diversity faunal assemblages (Tunnicliffe, 1991). Since the discovery of vent faunal assemblages in 1977, several studies have focused on the factors driving their spatial distribution in different regions of the world’s oceans and at different spatial scales. At the edifice scale, active sulfide structures are colonized by large engineer species that often live in association with symbiotic microorganisms (Govenar, 2012). These foundation species provide substratum, refuge and food to various associated macro- and meiofaunal taxa (Van Dover, 2002; Van Dover, 2003; Zekely et al., 2006; Govenar and Fisher, 2007; Sarrazin et al., 2015). They form recurrent faunal assemblages that are distributed along a mixing gradient between the cold, oxygenated surrounding seawater and the hot (up to 400°C), often acidic, hydrothermal fluids (e.g. Gebruk et al., 1997; Sarrazin et al., 1997; Sarradin et al., 1999; Marsh et al., 2012; Sen et al., 2013). Over the years, several studies have demonstrated the influence of environmental conditions on species distribution (Sarrazin et al., 1999; Bates et al., 2005; Van Dover and Doerries, 2005; Cuvelier et al., 2009; Cuvelier et al., 2011a; Sen et al., 2013; Husson et al., 2017) and dynamics (Copley et al., 1997; Cuvelier et al., 2011b; Cuvelier et al., 2014; Lelievre et al., 2017) on these large sulfide edifices. The observed mosaics of faunal assemblages are linked to the patchiness of vent emissions (Sarrazin et al., 1997; Sarrazin et al., 1999; Luther et al., 2001; Gollner et al., 2010; Podowski et al., 2010; Marsh et al., 2012; Nye et al., 2013; Sarrazin et al., 2015; Sarrazin et al., 2020). Particularly important in explaining these small-scale patterns are the available concentrations of reduced chemical compounds (e.g. hydrogen sulfide, methane and hydrogen) that are involved in chemosynthetic pathways (Jannasch, 1985; Matabos et al., 2008; Schmidt et al., 2008; Luther et al., 2012) as well as those of metals and oxygen (Desbruyères et al., 2000; Cuvelier et al., 2011a; Martins et al., 2011;). Although vital for the hydrothermal fauna, these chemicals can also have deleterious effects, depending on their concentrations (Martins et al., 2011). Thus, the spatial distribution of vent species results from a trade-off between their tolerance to the environment and their nutritional needs. On top of environmental conditions, biotic factors such as facilitation, predation or competition also interact and modulate the realized niche of these species (e.g. Mullineaux et al., 2003; Sancho et al., 2005; Podowski et al., 2010; Mullineaux et al., 2012).
The northern Mid-Atlantic Ridge (nMAR) harbors 11 known active vent fields between 12°N and 37°N (Beaulieu and Szafranski, 2020; see Gollner et al., 2021 for a map of these fields). Two faunal assemblages appear to be dominant on active edifices at the studied sites: those dominated by the engineer taxon Bathymodiolus spp. and those dominated by alvinocaridid shrimp (Desbruyères et al., 2000; Sarrazin et al., 2015; Sarrazin et al., 2020; Hernández-Ávila et al., 2022). In 2012, the discovery of a distinct faunal assemblage, dominated by small (~1 cm) gastropods and not described before, raised our interest. Although these small assemblages were sometimes visually difficult to locate from a submersible, we quickly realized that they were widespread not only at Lucky Strike, but also at many other vent fields of the nMAR (Marcon et al., 2013; Wheeler et al., 2013). Following this discovery, we dedicated dive time during six different cruises to gather biological and environmental data aiming to describe their composition, distribution and ecological role.
The objectives of the present study were to describe and compare the structure of these gastropod assemblages in terms of composition, density and diversity at three vent fields (Menez Gwen, Lucky Strike and Snake Pit) located on the nMAR, to describe their habitats and assess their realized ecological niches using stable isotopes. We address the following questions: Are there differences in faunal community structure between the gastropod assemblages at the three vent fields? What are the environmental conditions characterizing their habitats and are they similar among fields?
Materials and Methods
Sampling Sites
Three vent fields, characterized by different geological settings along the nMAR, were investigated for this study: Menez Gwen (MG), Lucky Strike (LS) and Snake Pit (SP) (Figure 1A). They differ in depth, fluid chemistry (Charlou et al., 2000), background seawater temperature and composition of faunal assemblages (Charlou et al., 2000; Desbruyères et al., 2001; Sarrazin et al., 2020). MG (37°50’.47’’N; 31°33’.37’’W, Figure 1B) is the shallowest known vent system of the nMAR, with a mean depth of 850 m. The hydrothermally active sites are concentrated on the southern and eastern flanks of this small 15 km diameter volcano (Charlou et al., 2000) and are partly colonized by dense assemblages of Bathymodiolus azoricus mussels (Desbruyères et al., 2001; Marcon et al., 2013; Sarrazin et al., 2020). Mirocaris fortunata shrimp assemblages are visible close to high-temperature fluids and gastropod patches were previously reported from video observations (Marcon et al., 2013). In contrast to the two other vent fields, environmental conditions at MG include colder end-member fluids (up to 280°C) and higher seawater background temperatures (8.8°C). In addition, the presence of a relatively strong current at a shallow depth near this field – trending southeast to northwest – probably acts as a strong barrier to larval dispersal towards more southern fields (Sarrazin et al., 2020). The 1 km² LS vent field (37°17’.51′’N; 32°16’.66′’W, Figure 1C) has been extensively studied over the past 25 years (Langmuir et al., 1997) and has been host to the EMSO-Azores observatory since 2010 (Cannat et al., 2011). Lying at ca. 1700 m depth, LS is located in the center of three volcanic cones that surround a fossilized lava lake (Ondréas et al., 2009). The magmatic chamber is situated at 3 km below the seafloor, feeding about 20 active sulfide edifices (Singh et al., 2006). B. azoricus mussel assemblages largely dominate the biomass of these edifices, but M. fortunata assemblages are sparse (Desbruyères et al., 2001; Cuvelier et al., 2009; Cuvelier et al., 2011a; Sarrazin et al., 2015; Husson et al., 2017; Sarrazin et al., 2020). The mussel assemblages preferentially colonize low temperature habitats (4.4 – 6.1°C), whereas those of M. fortunata are located in slightly warmer and more variable habitats (5.2 – 9.5°C; Husson et al., 2017). The gastropod assemblages described in this study, present on only a few edifices, have never been described prior to this study. Background temperatures at this field are 4.5°C (Sarrazin et al., 2020). The SP vent field (23°22’.06’’N; 44°56’.99’’W, Figure 1D) is located on the flank of a volcanic cone, 25 km south of the Kane fracture zone (Karson et al., 1987). It harbors several active and inactive sulfide structures that are perched on top of a large talus mound (Fouquet et al., 1993). Dense populations of Rimicaris exoculata shrimp colonize the warmest parts of the edifices (Segonzac, 1993; Methou et al., 2019) along with three other species (Rimicaris chacei, M. fortunata, Alvinocaris markensis) present in lower abundances. Moreover, a few structures are also colonized by Bathymodiolus puteoserpentis mussel assemblages (Maas et al., 1999). Background temperatures at this field are 2.6°C (Hernández-Ávila et al., 2022).
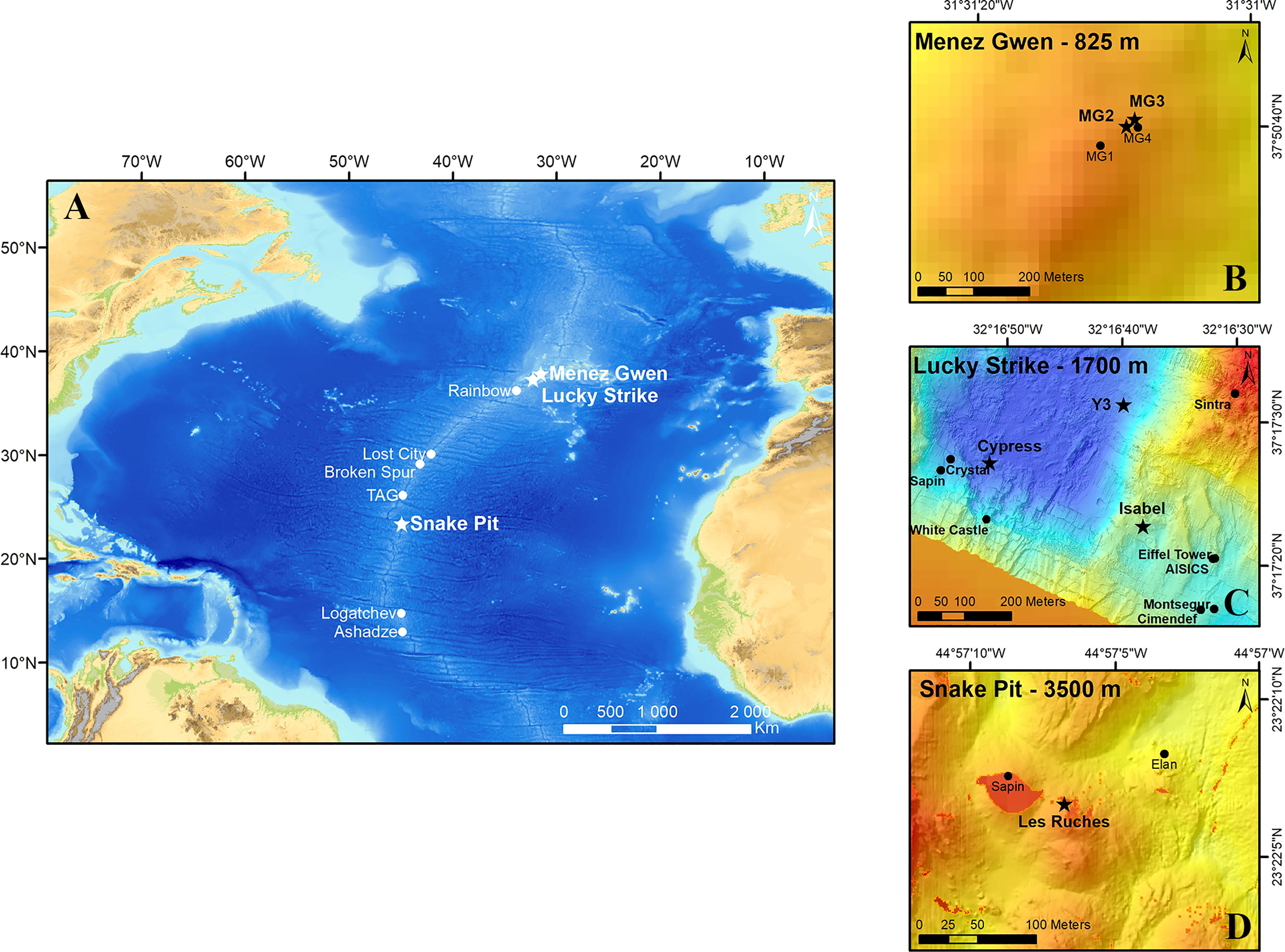
Figure 1 (A) Location of the three vent fields studied along the northern Mid-Atlantic Ridge: Menez Gwen (MG), Lucky Strike (LS) and Snake Pit (SP). (B-D). Locations (stars) of the sampling sites on MG (B), LS (C) and SP (D).
Faunal Sampling and Habitat Characterization
Twelve gastropod assemblages were collected during several cruises and using the suction sampler on the ROV Victor6000 from five sampling locations in the MG (MG3), LS (Y3, Cypress, Isabel) and SP (Les Ruches) vent fields along the nMAR (Table 1 and Figures 1, 2). The habitat chemistry of these assemblages was characterized prior to faunal sampling using the in situ chemical analyzer CHEMINI (Vuillemin et al., 2009), which measures total dissolved sulfide and iron concentrations. In addition, fluid samples within the habitat were collected using the PEPITO water sampler (Sarradin et al., 2009). The availability of these instruments on the submersible depended on the dive/cruise, which explains discrepancies between samples (Table 1). The chemical characterization was also carried out on five additional assemblages in which no faunal samples were taken (see Table 1) and on the two other dominant assemblages of the nMAR (Table S1). In 2014, two thermistor chains were deployed in a gastropod assemblage at the Y3 vent site in LS to capture the longer-term temporal variability of temperature. Each chain was composed of six thermochron i-buttons© enclosed in titanium cases and the thermistors recorded hourly temperature measurements over 5.5 months from 23 July to 31 December 2014, for a total of 3977 data points per probe. The chains were recovered in 2015, but 50% of the thermistors were damaged and could not be read (Table S2).
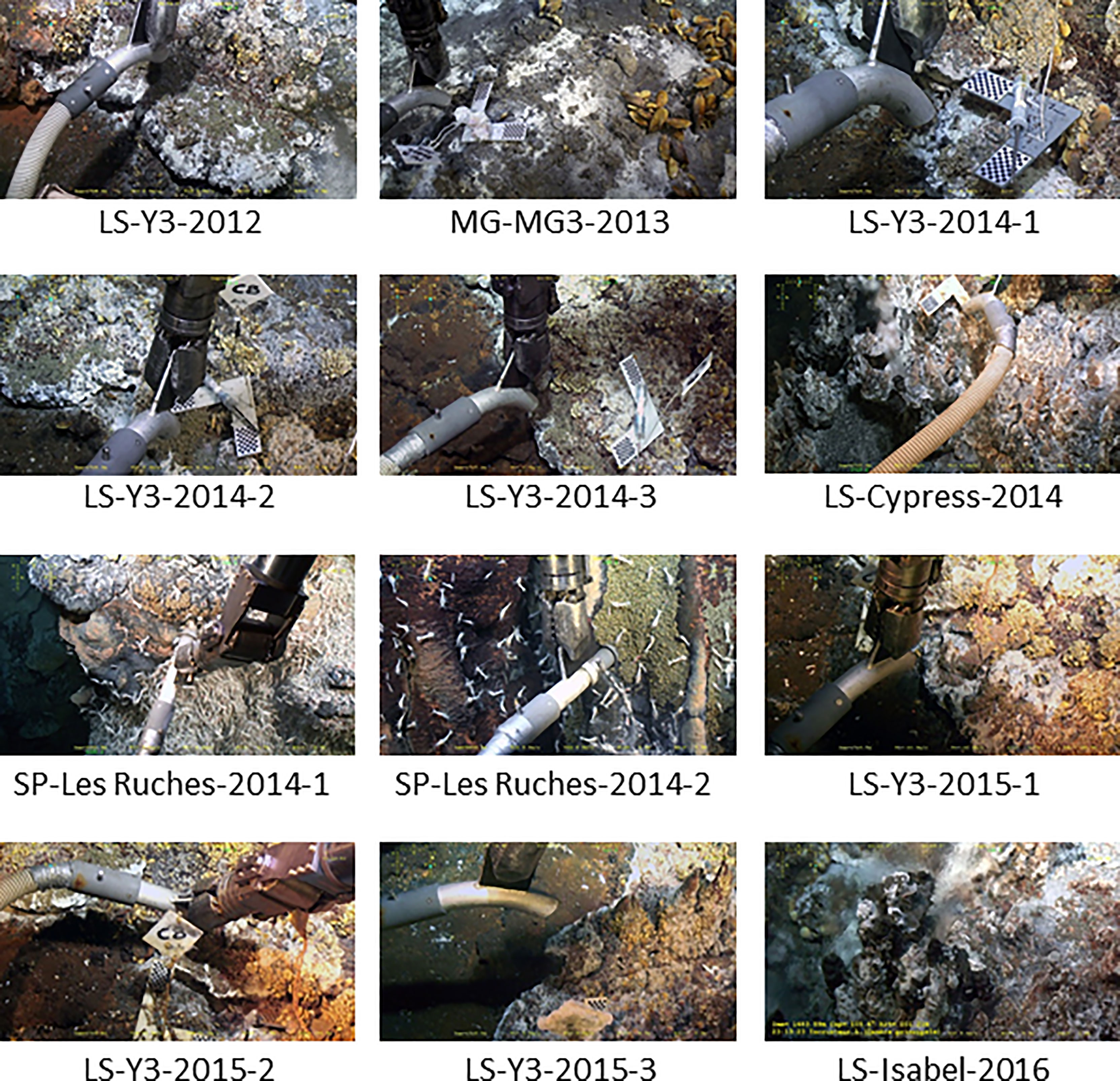
Figure 2 Twelve gastropod assemblages were characterized and sampled during the present study at three vent fields (Menez Gwen, Lucky Strike and Snake Pit) at the northern Mid-Atlantic Ridge. Samples were taken during different cruises in 2012 (Momarsat), 2013 (Biobaz), 2014 (Momarsat for LS, Bicose for SP), 2015 & 2016 (Momarsat). A physico-chemical characterization was carried out on each assemblage prior to faunal sampling. Faunal samples were collected using the ROV suction sampler. A target was placed in the field of the camera and sampling surfaces were estimated from video snapshots using ImageJ software© (see Sarrazin et al., 1997 for protocol).
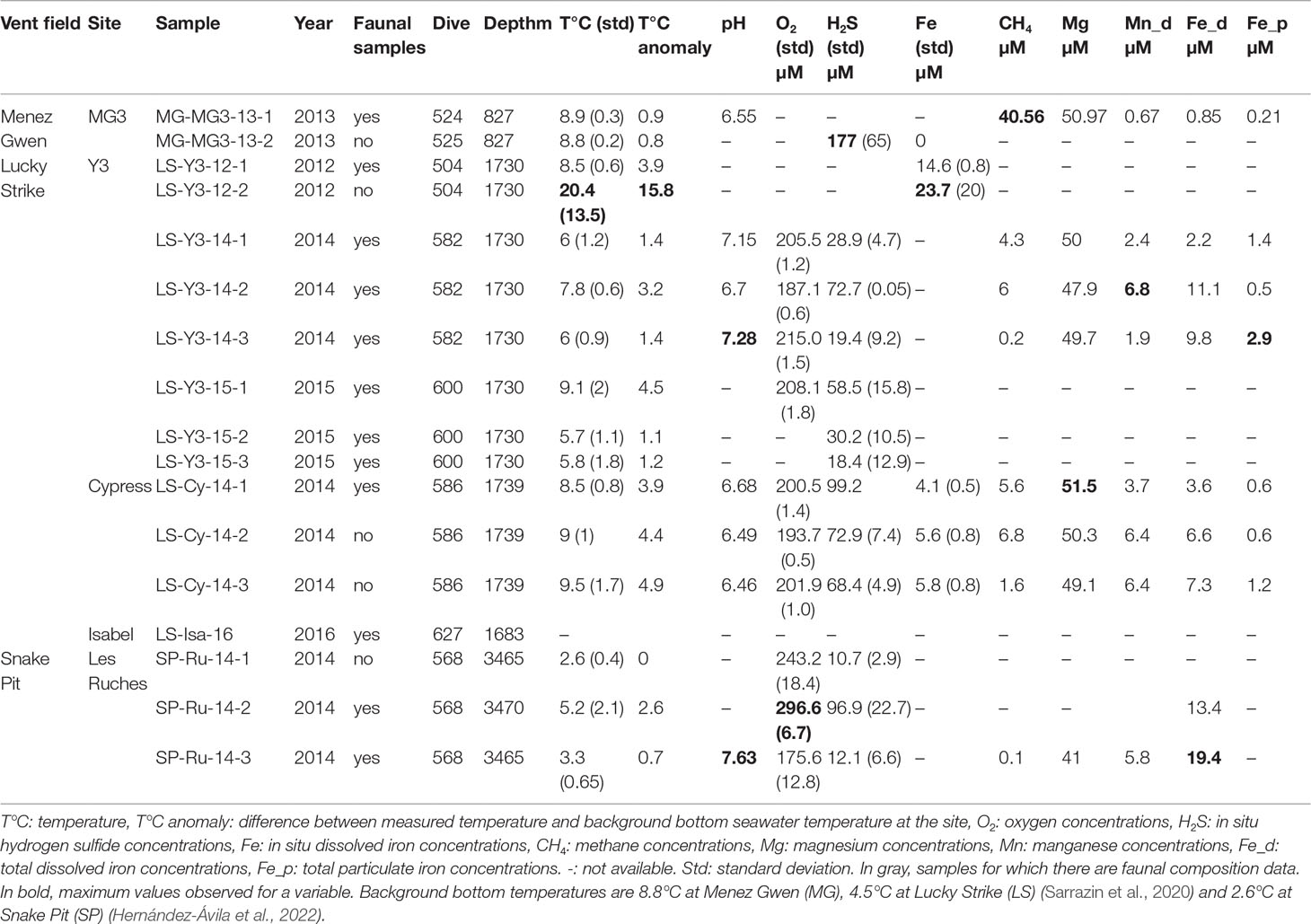
Table 1 Sampling characteristics and mean environmental conditions in the different gastropod assemblages studied.
Faunal Sample Processing
For each sample, the total sampled area was estimated from a video capture using a checkerboard (7 mm squares) as a scale, deployed in the camera field of view (Figure 2). Surface measurements were performed using Image J© (Schneider et al., 2012). When the checkerboard was not available, scale was assessed from the known size of the submersible suction sampler nozzle, visible on video sequences. Each faunal sample was sieved on 300 μm (macrofauna) and 20 μm (meiofauna) meshes. Individuals in the macrofaunal compartment were sorted, counted and identified to the lowest possible taxonomic level, and those of the meiofauna were identified at higher taxonomic levels.
Stable Isotope Analyses
To characterize their trophic ecology, stable isotope ratios of carbon and nitrogen were analyzed in 61 specimens of four gastropod species (Lepetodrilus atlanticus, Protolira valvatoides, Divia briandi and Peltospira smaragdina, Table S3). After sampling, individuals were either directly frozen, or preserved in ethanol or formalin solutions. Whole individuals were analyzed due to their small body sizes. Gastropods were extracted from their shells, freeze-dried for 24 h and ground to a homogeneous powder using a ball mill. Samples were then sent to the Laboratoire d’analyse en écologie aquatique et en sédimentologie at the Université du Québec à Trois-Rivières (CA) or the Littoral Environnement et Sociétés stable isotope facility at La Rochelle Université (FR) for analyses using continuous flow-elemental analysis-isotope ratio mass spectrometry (CF-EA-IRMS). Isotope ratios were expressed using the widespread δ notation (Coplen, 2011) in ‰ and relative to the international references Vienna Pee Dee belemnite (for carbon) and atmospheric air (for nitrogen). Analytical precision based on repeated measurements of the same sample was less than 0.3‰ for both δ13C and δ15N. Given that chemical preservation can alter stable isotope compositions, correction factors experimentally measured on gastropods (Lau et al., 2012) were applied to ethanol- (Δ13C = 0.48‰, Δ15N = 0.47‰) or formalin-preserved (Δ13C = 1.01‰, Δ15N = 0.53‰) specimens.
Statistical Analyses
Faunal abundances were converted to densities by dividing the total number of individuals by the estimated surface area, and to relative abundance within each sample (Table 2). All analyses were computed using R (R Core Team, 2020). Associated community diversity was assessed using species richness as well as Shannon and Simpson diversity indexes. Species accumulation curves were computed based on the resampling of individuals (rarefaction; Hurlbert, 1971) for each site by combining replicates using Vegan 2.5-6 (Oksanen et al., 2019). A Venn diagram displaying common species among the three vent fields was drawn. A hierarchical clustering of gastropod assemblage samples, based on their species composition, was computed based on Bray-Curtis dissimilarities on square-root transformed data using group average linking. For each environmental variable, mean and standard deviation were calculated when possible (Table 1). Correlations between environmental variables were tested using a Pearson correlation coefficient on log-transformed data, and a principal component analysis (PCA) was conducted on the standardized means and standard deviations of temperature and sulfide concentrations to visualize site ordination in a two-dimensional space. Finally, a distance-based redundancy analysis (RDA) was done on Hellinger-transformed abundance data (Legendre and Gallagher, 2001) using temperature and sulfide concentration data only in order to maintain a significant number of samples representative of all sampling locations on the three vent fields. Note that at MG, gastropod assemblages were only collected at the MG3 site where no sulfide data were available. Consequently, and because temperature data were similar, chemical data from the nearby MG2 site were used to characterize environmental conditions of the assemblage from this vent field in the redundancy analysis.
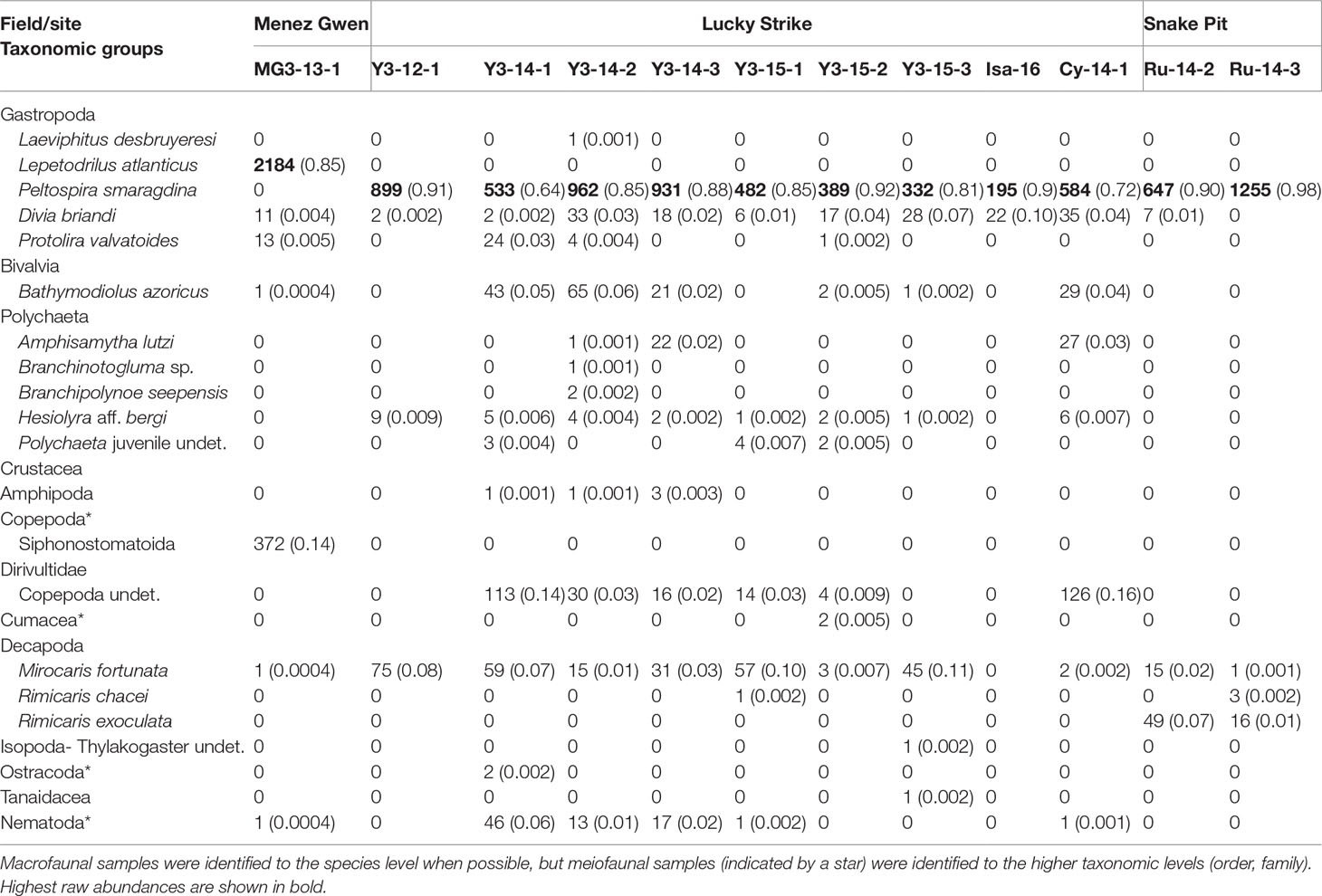
Table 2 Raw and relative (in parentheses) abundances of the different macrofaunal (300 µM) and meiofaunal (20 µM) taxa within the 12 sampled gastropod assemblages.
Results
Community Composition and Diversity
Our results confirm that all samples were dominated by a single species of gastropod that made up 64% to 98% of the total abundance (Tables 2, Tables 3). The species Peltospira smaragdina dominated the LS and SP samples, and Lepetodrilus atlanticus dominated those from MG (Figure 3). The second-most dominant taxa were either alvinocaridid shrimps (in 50% of the samples), copepods (25%) or other vent species (remaining 25%). Nematodes were relatively abundant in only a few samples, particularly on Y3. A few taxa (Laeviphitus desbruyeresi, Branchinotogluma sp., Branchipolynoe seepensis, Cumacea, Isopoda, Ostracoda and Tanaidacea) were only present as singletons or doubletons (Table 2). Among the four most abundant gastropod species, Divia briandi was the only species present in samples from all three vent fields. For the three others, L. atlanticus was only present in the MG samples, P. smaragdina was present at the two other fields, but not at MG, and Protolira valvatoides was absent from SP samples. The total number of individuals collected were the highest on MG (2 583 individuals) and the smallest on Isabel (LS, 217 individuals; Table 3). P. smaragdina densities varied from 19 786 ind./m2 in one sample from Les Ruches to 74 966 ind./m2 in one sample from Y3. Total faunal densities showed high spatial variability within the same site, being up to three times higher at some sampling sites (e.g. Y3; Table 3) and was the highest at MG3, reaching 82 152 ind./m2 (Table 3).
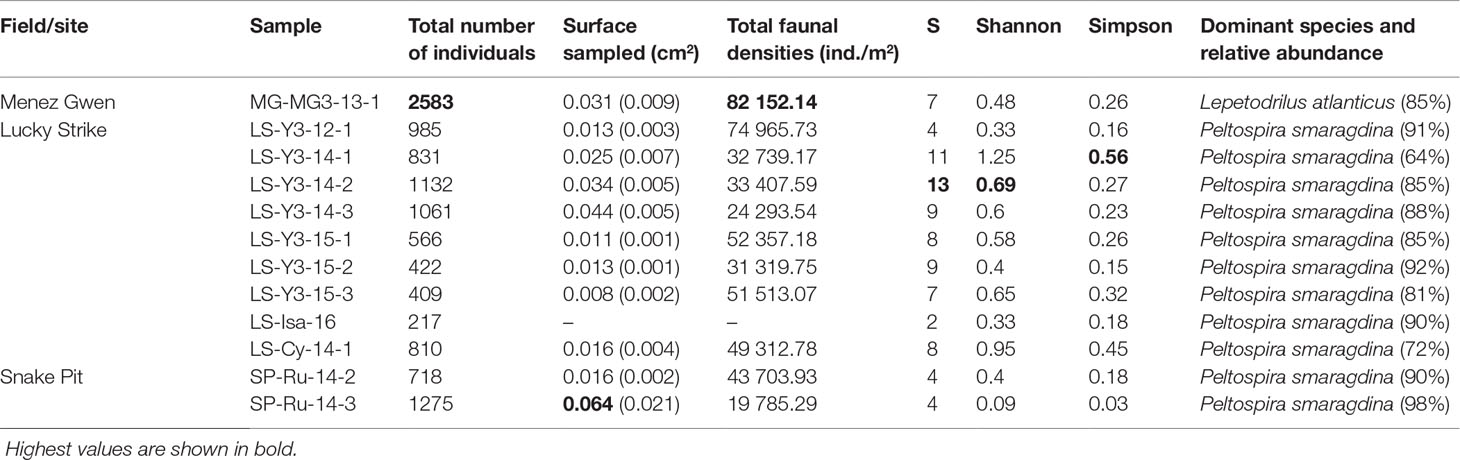
Table 3 Global characteristics of the macro- and meiofaunal communities in the 12 faunal samples considered in the present study with the total number of individuals, the surface area sampled, faunal densities, various diversity variables (S: species richness, Shannon, Simpson) and the relative abundance of the dominant species.
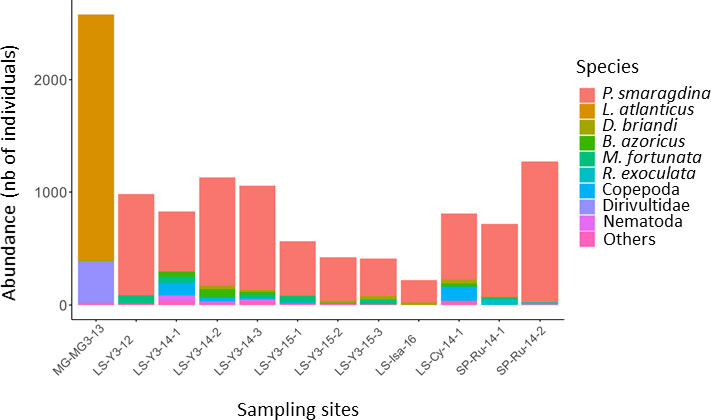
Figure 3 Bar chart of faunal composition (species accounting for >5% of the total relative abundance) in gastropod assemblages collected in three hydrothermal vent fields along the northern Mid-Atlantic Ridge. MG, Menez Gwen; LS, Lucky Strike; SP, Snake Pit. P. smaragdina: Peltospira smaragdina; L. atlanticus: Lepetodrilus atlanticus;D. briandi: Divia briandi; B. azoricus: Bathymodiolus azoricus; M. fortunata: Mirocaris fortunata; R. exoculata: Rimicaris exoculata.
Only two species were shared between the gastropod assemblages of the three vent fields: the shrimp Mirocaris fortunata and gastropod D. briandi (Table 2 and Figure S1). Each of these taxa was present in all samples but one (Table 2). LS and MG gastropod assemblages shared a total of five species, whereas LS and SP shared four species (Figure S1). LS samples harbored the highest number of “exclusive” species (n=12, Figure S1). In this vent field, species richness varied from 2 at Isabel to a maximum of 13 at Y3 (Table 3). Similar to densities, richness was variable within the same site, varying from 4 to 13 species at Y3. The highest Shannon and Simpson indexes were found on Y3, and the lowest on Les Ruches, despite the high number of organisms collected and large surface area sampled on SP-RU-14-2 (Table 3). In fact, the largest sampled surface area at this site corresponds to the lowest densities and diversities (Table 3). In LS, Isabel had a very low species richness and diversity, and gastropod assemblages from Cypress had intermediate diversity values. Overall, Y3 had higher diversity than the two other sites of this field (LS, Table 3), but also the highest number of samples (n = 7) compared with Cypress and Isabel, each sampled only once. The rarefaction curves did not reach an asymptote for any of the sampled sites, with the exception of Les Ruches, indicating that the diversity of most sampled sites was not fully characterized (Figure 4). Except for Isabel, the gastropod assemblages from LS (Y3 & Cypress) appeared to have higher diversity than that from MG and SP. However, these two fields were only represented by a single sampling site. As expected, results of the hierarchical /clustering showed a clear distinction of the MG gastropod assemblages from those of the two other vent fields (Figure 5). Assemblages dominated by P. smaragdina did not cluster according to the vent field, but separated samples collected at LS in 2014 from the others. Because the MG gastropod assemblages strongly differ and were dominated by a different species, the two assemblage types will be further characterized independently.
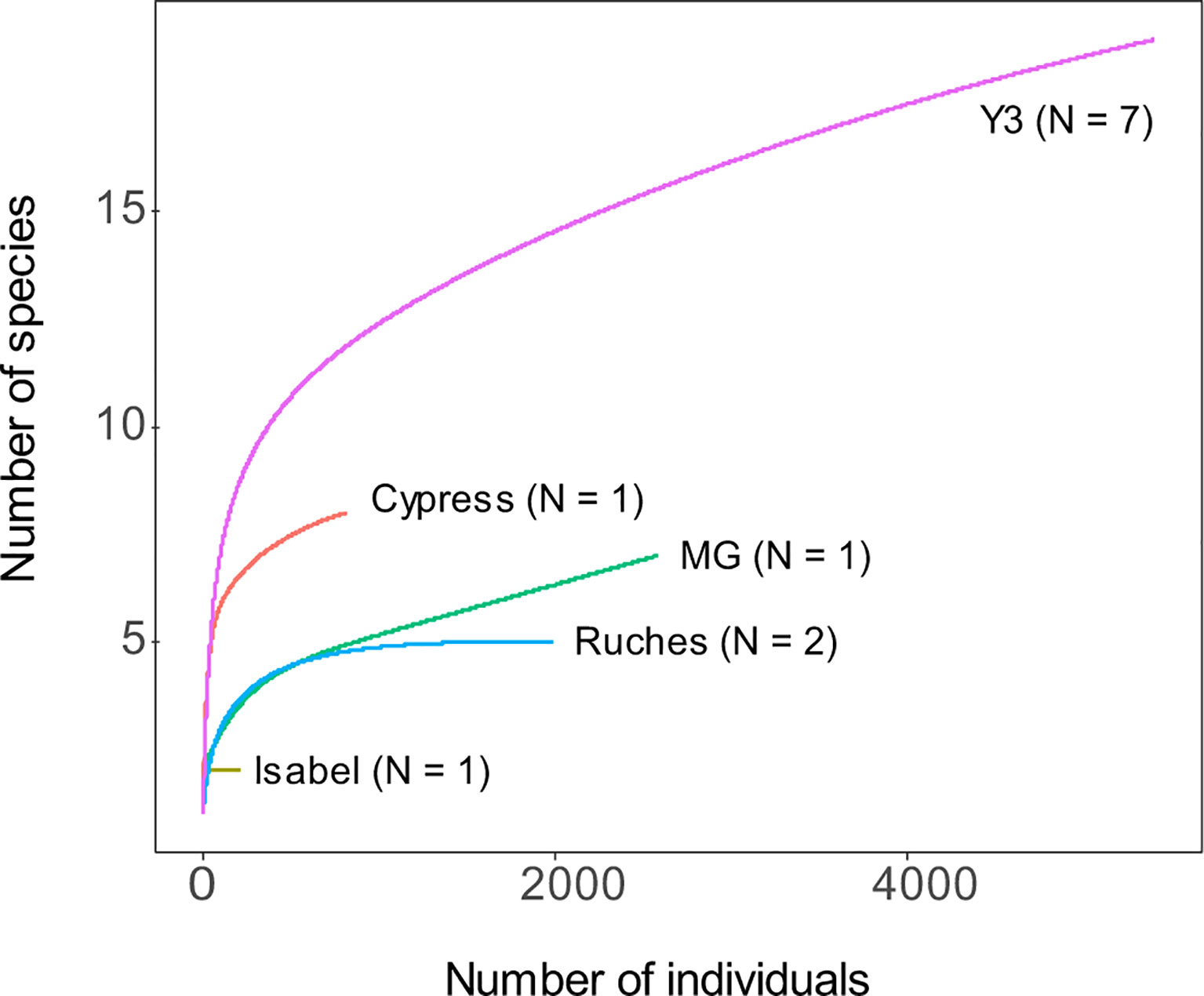
Figure 4 Species accumulation curves based on rarefaction computed for each site among the three hydrothermal vent fields: Menez Gwen (MG3), Lucky Strike (Cypress, Isabel and Y3) and Snake Pit (Les Ruches).
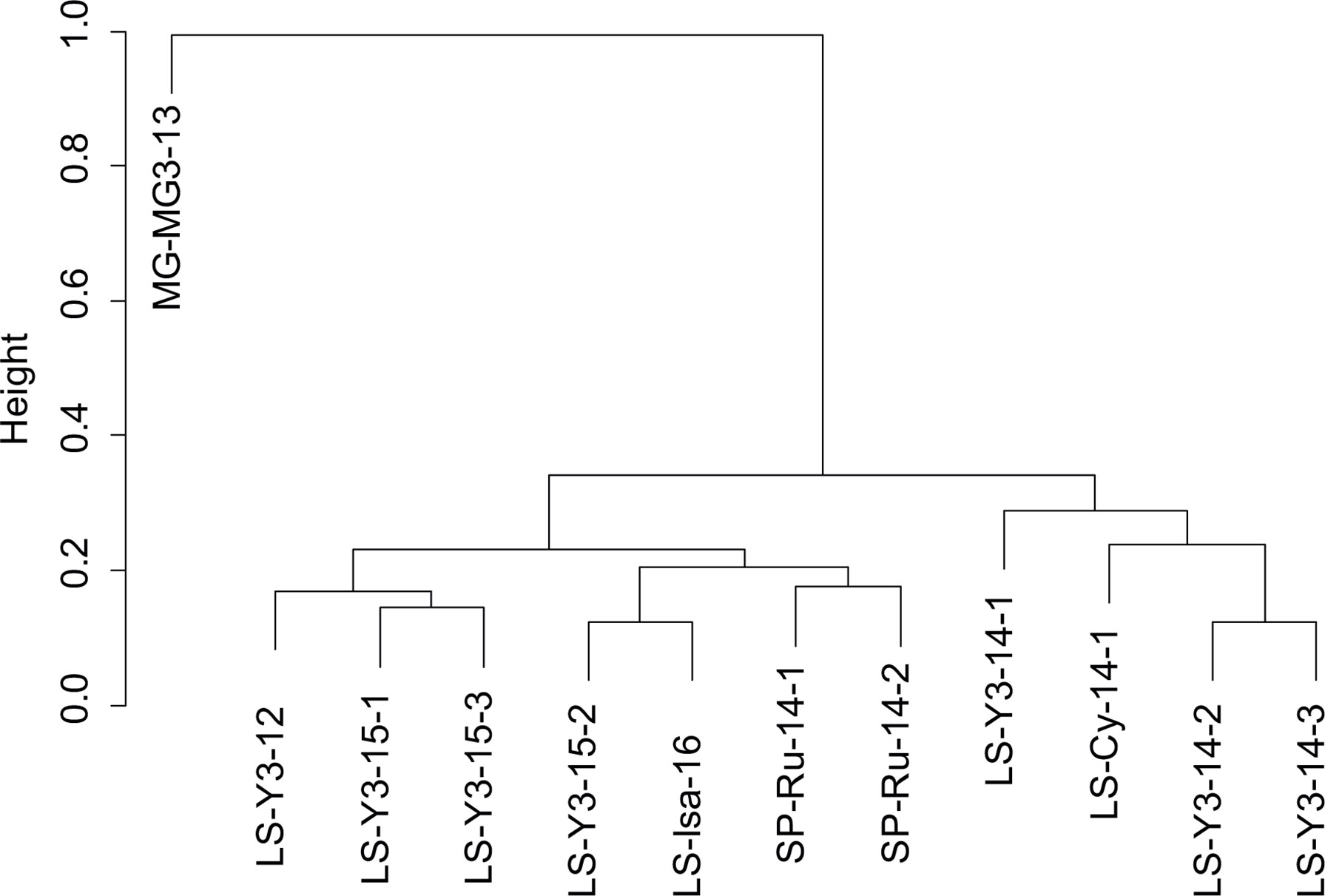
Figure 5 Group average sorting dendrogram based on species composition using the Bray-Curtis similarity among the 12 gastropod samples along the northern Mid-Atlantic Ridge.
Habitat Characterization
Lepetodrilus azoricus assemblages – These gastropod assemblages at MG were found on a very friable granular substratum, covered by whitish material (probably microbial mats) and surrounded by small shimmering chimneys (<50 cm). Clouds of grayish material formed during sampling and a dark black groove was left afterwards (see Supplementary Video S1, https://doi.org/10.24351/88698). These assemblages were surrounded by B. azoricus mussel assemblages, a few meters away. The mean temperatures measured in the two sampled assemblages at MG varied from 8.8 to 8.9°C, which is more or less the temperature of bottom seawater. Corresponding habitat conditions are given in Table 1. Methane and hydrogen sulfide concentrations were particularly high compared with those at the two other vent sites.
Peltospira smaragdina assemblages – Visually, P. smaragdina assemblages from LS were different from one other. At Y3, their habitats resembled those found at MG for B. azoricus: very friable substratum, covered with microbial mats near small active chimneys. At this site, the assemblages were not found directly on the edifice, but rather on a large flange lying on the seafloor and covered by a mix of gastropod/mussel assemblages. Individuals were mostly concentrated at the rim of the flange, where there were intense shimmering fluids (see Supplementary Video S2, https://doi.org/10.24351/88699). At Isabel and Cypress, P. smaragdina assemblages were more similar to those of SP, being found on the edifice walls in areas of intense shimmering. M. fortunata shrimps were abundant around them at Isabel. In SP, P. smaragdina colonized vertical wall surfaces on tall active edifices. At one sampling site, the gastropods were found on a beehive-like structure, in shimmering fluids and surrounded by R. exoculata shrimps (see Supplementary Video S3, https://doi.org/10.24351/88700). At the other site, they were in extremely dense patches and covered large areas of the edifice with abundant shimmering fluid (see Supplementary Video S3, https://doi.org/10.24351/88700). Overall, the gastropod assemblages in SP were much more visible and extended than those from MG or LS.
The mean temperatures, given by the ROV temperature probe (PT200, frequency: 1 Hz), in the P. smaragdina assemblages varied from 5.7°C to 20.4°C in LS and from 2.6°C to 5.2°C in SP (Table 1). Given that the bottom temperatures in the two vent fields were quite different, temperature anomaly appears to be a better proxy than mean temperature to assess fluid inputs. These anomalies varied between 0.7°C at Les Ruches (SP) to 15.8°C at Y3 (LS, Table 1). At Y3, temperature measured by the i-buttons (4 months of acquisition) directly at the surface of the substrata was three times higher (mean 25.42 ± 2.6°C, n = 6) than that measured by the ROV probe 1-2 cm above the surface (mean 7.83 ± 1.9°C, n=17). High spatial variability in mean temperatures (from 17.25 to 37.34°C) was also observed within the same assemblage between closely spaced i-buttons (Figure 6 and Table S2). Temperatures also varied with time, the highest variation between minimum and maximum values being found on HT2.12 (43.04°C, Table S2).
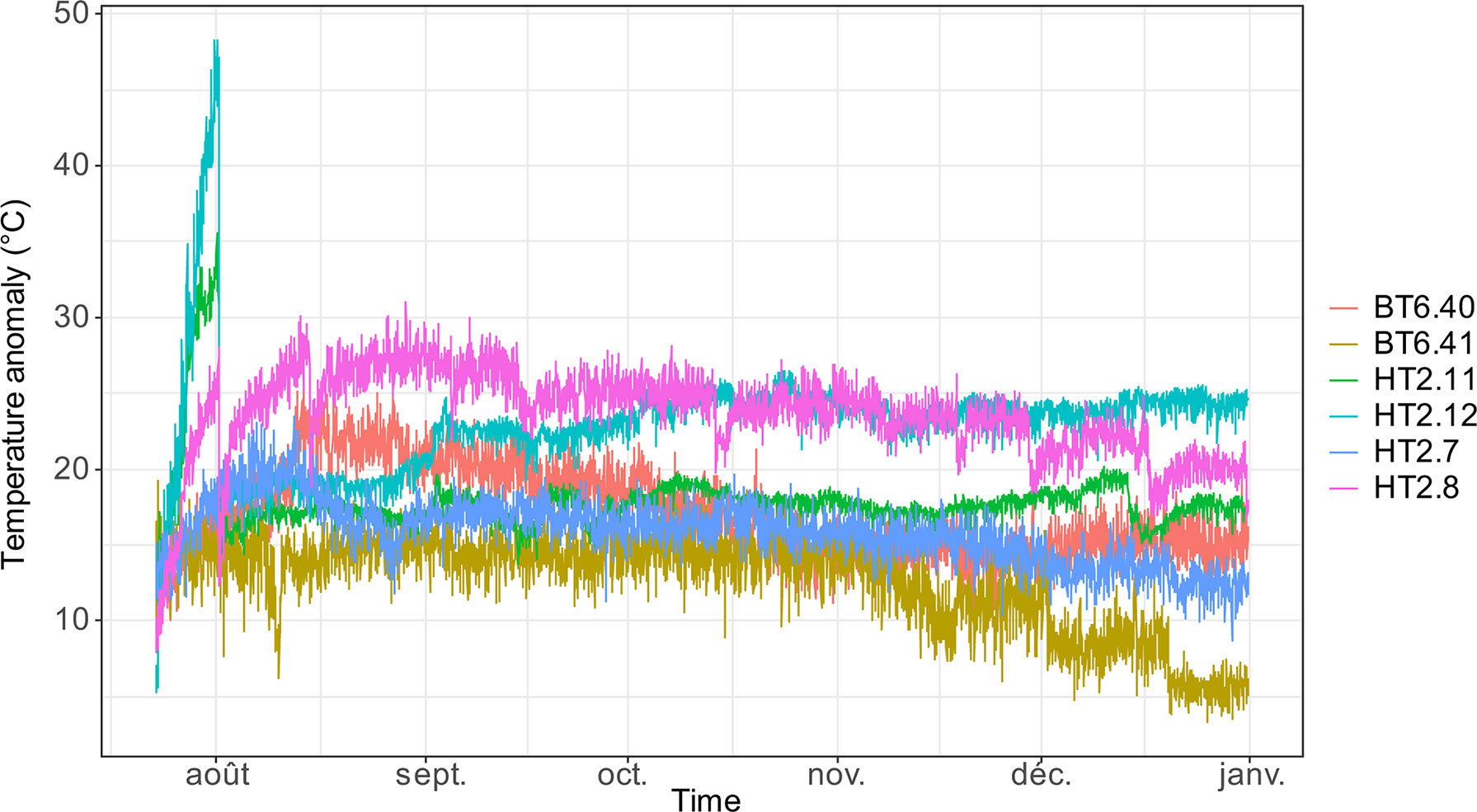
Figure 6 Evolution of temperature anomaly (difference between measured temperature and background bottom seawater temperature at the site) measured with six i-buttons® temperature probes at Y3 (Lucky Strike vent field, Mid-Atlantic Ridge) from 23 July to 31 December 2014. Labels indicate probe name.
The lowest concentrations of hydrogen sulfide, methane and magnesium concentrations were found at Les Ruches (SP), but pH (ca. 7.63) and dissolved iron concentrations were higher at this site than at the LS sites (Table 1). Highest and lowest concentrations of oxygen were measured at Les Ruches (SP). Higher concentrations of hydrogen sulfide, methane and magnesium were found at Cypress (LS, Table 1). Y3 harbored both the highest and lowest concentrations of manganese. For total and particulate iron concentrations, only data from LS are available, varying from 4.1 to 23.7 µM (Fe total) and 0.5 to 2.9 µM (particulate Fe; Table 1).
The PCA explains 77.6% of the variance in environmental conditions (Figure 7). The first axis represents 51.4% of the variance and is associated with average temperature and hydrogen sulfide concentrations as well as with temperature anomaly. The second axis explains 26.2% of the variance and is associated with the standard deviations of temperature and hydrogen sulfide. The PCA does not differentiate the two vent fields, nor the two LS sites or sampling years.
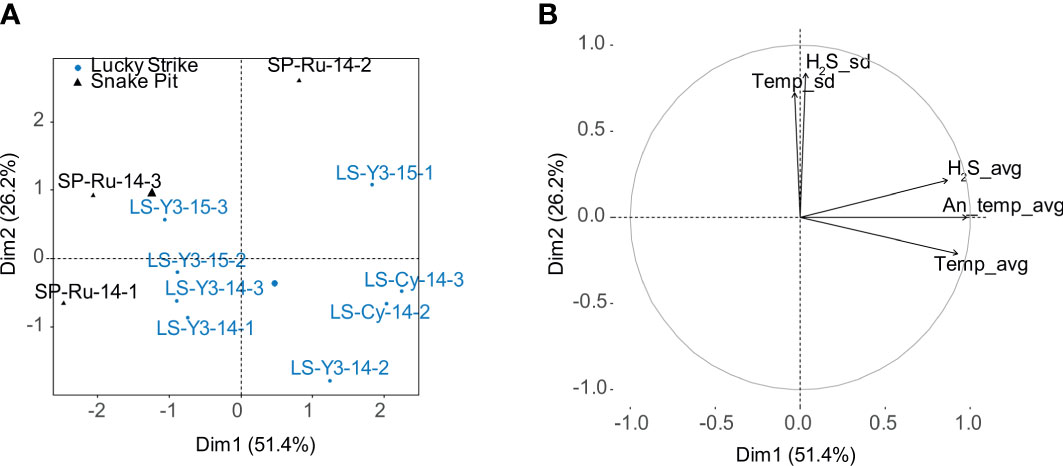
Figure 7 Principal component analysis (PCA) on environmental data measured in gastropod assemblages dominated by Peltospira smaragdina at the Lucky Strike and Snake Pit vent fields along the Mid-Atlantic Ridge. (A) the distribution of sites in the new space formed by the two first principal components; the big circle and triangle represent the centroid for each vent site. (B) the correlation circle shows the contribution of each variable to the explained variance. H2S_avg & H2S_sd: average and standard deviation of in situ hydrogen sulfide concentrations, Temp-avg: average temperature, An_temp_avg: average temperature anomaly calculated against background seawater temperature and Temp_sd: temperature standard deviation.
The RDA on environmental conditions was not significant, supporting a certain homogeneity in environmental conditions within the P. smaragdina assemblages from the LS and SP vent fields. Mean measured environmental conditions within gastropod assemblages of these two vent fields are presented in Figure 8.
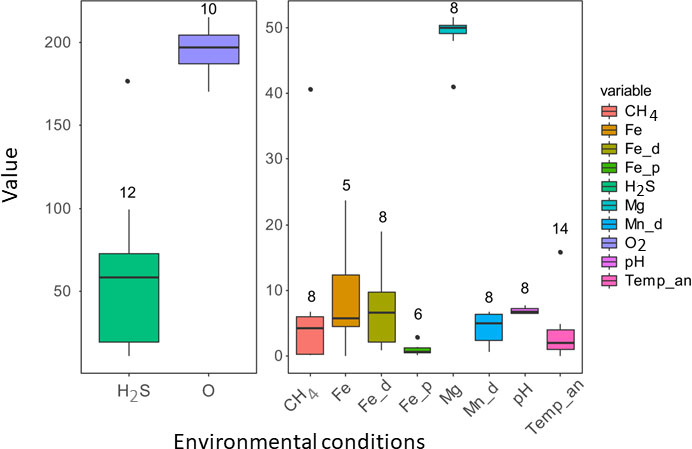
Figure 8 Whisker plots illustrating environmental conditions within assemblages of the gastropod Peltospira smaragdina at the Lucky Strike and Snake Pit vent fields along the Mid-Atlantic Ridge. Given that the values were quite high for hydrogen sulfide (H2S) and oxygen (O2), we provide two figures. The line corresponds to the median, the box (second and third quantiles) include 50% of the measured data and the upper and lower 25% respectively are represented by vertical lines on each box. Individual dots are outliers. H2S: in situ hydrogen sulfide concentrations, O2: oxygen concentrations, CH4: methane concentrations, Fe: in situ total iron concentrations, Fe_d: total dissolved iron concentrations, Fe_p: total particulate iron concentrations, Mg: magnesium concentrations, Mn: manganese concentrations, Temp_an: temperature (°C) anomaly calculated against background seawater temperatures. H2S, O2, CH4, Fe, Fe_d, Fe_p, Mg, and Mn_d are in µM. Numbers on graphs represent the number of measurements taken.
Stable Isotope Compositions of Dominant Gastropod Species
δ13C values of the four species of gastropods were highly variable, ranging from -22.5 to -8.8 ‰ (Figure 9 and Table S3). Regardless of site, δ13C values were, from the most to the least negative, -18.9 ± 2.0 ‰ for P. valvatoides, -14.9 ± 2.4 ‰ for L. atlanticus, -13.6 ± 1.9 ‰ for D. briandi and -10.8 ± 0.8 ‰ for P. smaragdina (mean ± SD in each case). In addition to these general trends, species-specific inter-site variations also seemed to occur (Figure 9). Δ13C of P. valvatoides seemed lower at MG than at Y3. D. briandi showed comparable δ13C at MG and Y3 (LS), but less negative values at SP and, to a lesser extent, at Isabel (LS). Δ13C of P. smaragdina were similar at Y3 and Isabel (LS), but seemed slightly less negative at SP. Finally, δ13C ranges seemed wider at MG than in the two other vent fields. Δ15N values of the four species of gastropods ranged from 2.4 to 7.7 ‰ (Figure 9 and Table S3). Regardless of site distinction, δ15N values were, from the lowest to the highest, 5.1 ± 0.9 ‰ for P. valvatoides, 5.4 ± 1.5 ‰ for L. atlanticus, 6.1 ± 0.6 ‰ for P. smaragdina and 6.8 ± 0.6 ‰ for D. briandi. Overall, δ15N seemed to vary less across species and sites than δ13C. Nevertheless, in MG, SP and Isabel, δ15N seemed to be higher for D. briandi than for other species (Figure 9). This trend was not observed at Y3.
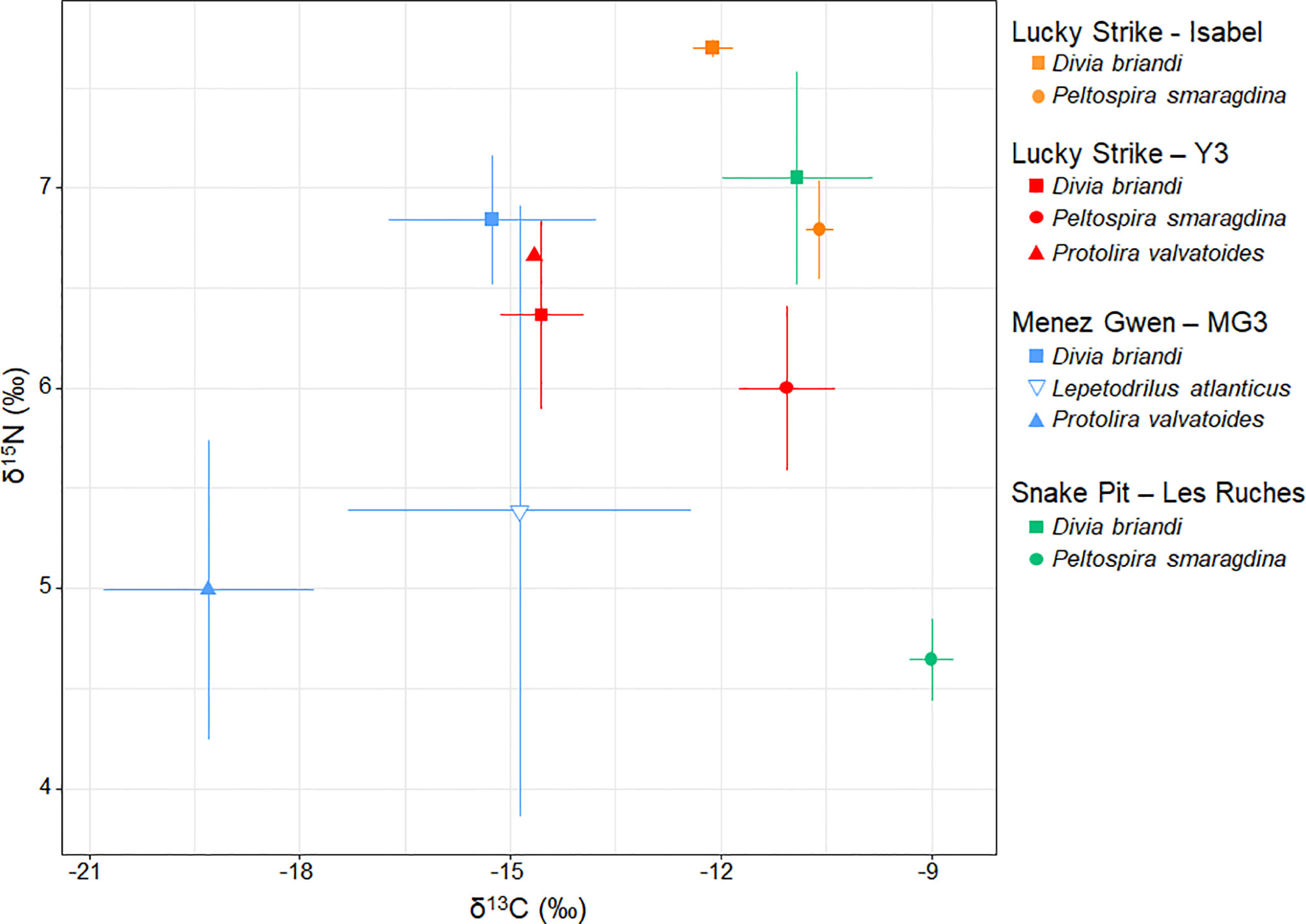
Figure 9 Stable isotope ratios of carbon (δ13C) and nitrogen (δ15N) of the four dominant vent gastropod species from different sites in the three studied vent fields: Menez Gwen (MG3), Lucky Strike (Isabel & Y3) and Snake Pit (Les Ruches) on the northern Mid-Atlantic Ridge. Points are means, error bars are standard deviations.
Environmental Conditions Within the Different Assemblages on the nMAR
In Table S1, based on this study and previously published data, we compiled environmental conditions associated with the three assemblages of the nMAR and for each field. The observed patterns differed from one field to the other. In MG, the mean temperatures were similar between the gastropod (L. atlanticus) and mussel (B. azoricus) assemblages, but the mean hydrogen sulfide concentrations were more than three times higher in the gastropod assemblage. Unfortunately, no data was obtained in the shrimp assemblage in this field. In LS, the P. smaragdina assemblage occupied the highest temperatures and sulfide concentrations compared with the mussel (B. azoricus) and shrimp (Mirocaris fortunata) assemblages. However, with the exception of Cuvelier et al. (2011a), the conditions reported here for the shrimp assemblage corresponds to those of the warmer mussel habitats (in which shrimp dominated), because a proper characterization of shrimp habitats still needs to be done at this field. Habitat characterization of the three faunal assemblages in SP was done the same year, during the Bicose cruise. Results show that environmental conditions were more differentiated than the two other vent fields: the gastropod (P. smaragdina) assemblage occupied intermediate temperature and sulfide concentration habitats (Table S1). The mussel (B. puteoserpentis) assemblages were characterized by lower concentrations of hydrogen sulfide and the shrimp (R. exoculata) colonized the most variable habitats with higher temperatures and sulfide concentrations. In SP, oxygen concentrations were lower in the shrimp (161.1 µM), intermediate in the gastropod (170.6 – 186.4 µM) and higher in the mussel (201.1 – 244.3 µM) assemblages.
Discussion
This study provides the first description of the ecological characteristics of gastropod assemblages that are recurrent in three vent fields – Menez Gwen, Lucky Strike and Snake Pit – along the nMAR, from 830 m to 3500 m depth. Our results confirm that the sampled assemblages were characterized by a strong dominance of a single gastropod species and by distinct environmental conditions compared with the two other dominant assemblages of the MAR, the mussel and shrimp assemblages. Furthermore, a retrospective examination of references and imagery from dives in other vent fields revealed evidence for the presence of distinct gastropod assemblages in at least five other MAR vent fields, including Broken Spur, Rainbow (Jollivet, pers. comm.), TAG (pers. obs.), Moytirra (Wheeler et al., 2013; Collins et al., 2020) and Loki’s Castle.
Community Structure at the Three Vent Fields
Two distinct gastropod assemblages were characterized, one dominated by Lepetodrilus atlanticus (Lepetellida: Lepetodrilidae; (Warén and Bouchet, 2001) at MG and the other dominated by Peltospira smaragdina (Neomphalida: Peltospiridae; Warén and Bouchet, 2001) at the LS and SP vent fields. Both genera are endemic to deep-sea chemosynthetic habitats. The former is the most specious with 16 species; however, Peltospira has only four described species (WoRMS Editorial Board, 2022). To date, these two species had only been described as associated fauna of Bathymodiolus assemblages in the studied vent fields (Sarrazin et al., 2015; Husson et al., 2017; Sarrazin et al., 2020). In the mussel assemblages described in LS, P. smaragdina was reported in very low densities and associated with intermediate temperature habitats, and L. atlanticus can reach high densities (Husson et al., 2017), particularly in cold microhabitats (Sarrazin et al., 2015; Sarrazin et al., 2020). Here, in contrast, we describe new assemblages dominated and structured by these two small macrofaunal gastropods, P. smaragdina and L. atlanticus.
These new gastropod assemblages are characterized by low species richness (<13 taxa) and a large proportion (>60%) of singletons or doubletons. They share structural similarities with faunal assemblages from warmer habitats at other vent sites, which are visually dominated by a few species and exhibit low species diversity [e.g. alvinellid assemblages on the EPR (Gollner et al., 2010) and NEPR (Tsurumi and Tunnicliffe, 2003), alvinocaridid assemblages on the MAR (Hernández-Ávila et al., 2022), high-flow siboglinid assemblages on the NEPR (Sarrazin and Juniper, 1999)]. However, the gastropod species of this study differ from the large symbiotic species living in the vent fields of the western Pacific and Indian oceans, because they are much smaller in size (<1 cm compared with 8.5 – 9.5 cm for Alviniconcha hessleri and Ifremeria nautilei (Desbruyères et al., 2006). Moreover, contrary to the larger vent gastropods, their association with symbionts has not yet been demonstrated, although suspected for P. smaragdina (Collins et al., 2020).
Habitat Characterization
Like faunal composition, environmental conditions were very different at the MG habitats compared with the two other vent fields. The clear separation of MG from other vent fields (i.e. LS & Rainbow) along the MAR was previously reported by Sarrazin et al. (2020) in mussel assemblages, showing both distinct environmental conditions and patterns of abundance and diversity. The mean temperatures (8.8 – 8.9°C) in the MG gastropod assemblages were similar to those reported for bottom temperatures at this field (Sarrazin et al., 2020). At LS, mean temperatures were more variable, probably resulting from the higher number of sampling sites and years compared with MG and SP, but always at least >1°C above ambient temperature (~4.5°C). At SP, the mean temperatures were similar to those reported for bottom water temperatures (~3.6°C), but slightly exceeding them for one sample (+1.6°C). However, these temperatures may not represent those experienced by the gastropods as suggested by the time-series measurements done with the i-button probes at Y3 (LS), which largely exceeded the temperature values measured by the submersible. The videos highlighted the presence of shimmering fluids within the sampled assemblages, suggesting warmer temperatures than those actually measured. Measurements using the submersible probe were performed few centimeters above the gastropod assemblages at three discrete locations and are likely not representative of the temperatures experienced by the organisms over time. Indeed, temperatures can display variations of up to 20°C within a few centimeters in response to turbulence, mixing and biological processes (Le Bris et al., 2005; Lee et al., 2015). Similarly, a recent study showed that temperature values obtained by probes deployed directly on the substratum were significantly higher than those obtained with the submersible sensor few centimeters above the fauna (Marticorena et al., 2021). This difference questions once again the use of single-point temperature measurements to define the niche of vent species. However, submersible measurements can be used to compare different assemblages from different habitats/fields, as it is the case here.
Our results do not show significant differences in environmental conditions between LS and SP habitats, although temperature anomalies were higher in LS and methane concentrations generally lower in SP. Concentrations of hydrogen sulfide were very variable at LS, between edifices and also within the same site, but were within the (large) range found in SP. With these large intra-field variations and without a more substantial (and comparable) number of samples, it remains difficult to further compare gastropod habitats between the two fields. Our comparison of the environmental data from the three key assemblages along the MAR (mussels, gastropods, shrimp) suggests that these small gastropod assemblages occupy an intermediate niche between those of the alvinocaridid shrimp and mussels. This niche separation is visible at SP (dominated by Rimicaris species), but additional environmental data in the warmer areas colonized by shrimp assemblages would be necessary in LS (dominated by Mirocaris fortunata) to confirm the position of the three assemblage types within the temperature gradient.
The absence of P. smaragdina in mussel assemblages in the shallower MG field (Sarrazin et al., 2020) is intriguing and may be linked to bathymetric limits as observed for many deep-sea taxa (McClain and Etter, 2005). Interestingly, P. smaragdina assemblages seem increasingly dominant at the deeper vent fields. In fact, their high abundance at the 2095 m Moytirra vent field (Wheeler et al., 2013) and their recent observation at other deeper fields such as Rainbow (2300 m) and Broken Spur (3b 100 m; Jollivet & Le Bris, comm. pers.) support a possible depth effect on this species’ distribution range. Another possibility is that L. atlanticus has outcompeted P. smaragdina at shallower depths. In fact, the relative dominance of one species can depend on its abilities to outcompete other species over a given range of environmental conditions as previously shown for Lepetodrilus elevatus on the EPR (Matabos et al., 2008). Alternatively, differences in MG abiotic conditions, such as its high sulfide/methane concentrations, may have prevented colonization by P. smaragdina. Differences in the concentrations of the reduced chemicals used for chemosynthesis (such as hydrogen sulfide, methane, iron and hydrogen) may also result in distinct patterns of resource availability. In fact, the availability of different chemical elements may have an impact on the composition of microbial communities (De Busserolles et al., 2009). Our results suggest a different basal carbon source (see below) for the two species supporting the existence of resource partitioning, as previously observed at other vents (Levesque et al., 2003; Lelièvre et al., 2018).
Energy Fluxes and Ecological Interactions
Determining trends in stable isotope compositions of consumers in deep-sea hydrothermal vents can be a challenging task, because trophic effects (changes in consumer isotopic compositions due to actual differences in feeding habits) and baseline effects (changes in consumer isotopic compositions due to changes in isotopic compositions of baseline items) are frequently confounded. Variations in environmental conditions are known to influence baseline compositions at multiple scales (De Busserolles et al., 2009; Beinart et al., 2012). Here, the differences between vent fields and/or sites, with consumers from MG being more 13C-depleted compared to those of SP that were generally the most 13C-enriched, are probably largely due to such effects. Nevertheless, other than these baseline shifts, species-specific patterns could be observed.
P. smaragdina had high δ13C values at all sites, ranging from -9.0 to -11.1‰ on average. These values are consistent with reliance on chemosynthetic bacteria favoring the reductive tricarboxylic acid (rTCA) cycle for their metabolism (Hügler and Sievert, 2011). At Les Ruches (SP) and Isabel (LS), Divia briandi δ13C values were slightly more negative than those of P. smaragdina (-1.5 to -2‰). At Y3 however, the mean difference in carbon isotopic composition between D. briandi and P. smaragdina was greater (-3.5‰). Although all these values remain in the range of rTCA-fueled microorganisms, the differences suggest that the two species feed on different resources (either different microorganisms or similar microorganisms living in different habitats), and that the degree of ecological divergence between these two species may be site-specific. At MG, both D. briandi and L. atlanticus had similar average δ13C, whose values (-15.3 and -14.9‰, respectively) were found at the most negative end of the commonly accepted range for rTCA sulfide oxidizers (roughly -10 to -15‰; Hügler and Sievert, 2011). Conversely, at this site, Protolira valvatoides had the least negative δ13C (-19.3‰), and was found outside this range. This discrepancy suggests that this species does not depend solely on rTCA sulfide oxidizers for its nutrition. Based on its δ13C, at MG, P. valvatoides may combine this resource with sulfide oxidizers that use the Calvin–Benson–Basham (CBB) cycle, methane oxidizers or even photosynthesis-derived organic matter (Portail et al., 2018). Although it has been reported in shrimps, namely Rimicaris exoculata, strong reliance on rTCA sulfide oxidizers is not common in fauna from mussel beds of the same sites, which instead seem to feed on microorganisms fueled by the CBB cycle (Portail et al., 2018; Methou et al., 2020). Δ15N values for all species varied from 4.54 to 7.05‰, with few, if any, consistent observed patterns. While these values may indicate minor trophic position differences, they may also indicate differences in the type of inorganic nitrogen used by the producers on which each species relies (nitrate vs. ammonium; Methou et al., 2020).
Overall, from a functional point of view, our results suggest that all gastropods sampled here are grazers/deposit-feeders; however, the interspecific differences in isotope composition point out subtle variations in their feeding habits and/or feeding habitats. Resource partitioning among species of the same group or feeding guild (i.e. grazers using their radula to consume microbial mats) associated with high biomass is common in vent assemblages and highlight the importance of the diversity of free-living microbial communities in structuring vent communities (Lelièvre et al., 2018). Microorganisms represent most of the living organic carbon in the vent environment (Karl, 1995), and free-living microbes, growing on hard surfaces or proliferating in hydrothermal fluids, offer an abundant food source. Nevertheless, other modes of carbon and nutrient acquisition may also be present in the studied gastropods. Recently, Collins et al. (2020) hypothesized that the observed association between Sulfurimonas biofilm and P. smaragdina at the Moytirra vent field likely represents a holobiontic relationship. These hypotheses still need to be confirmed.
Comparison With Other Vent Gastropod Assemblages
In the present study, in MG, L. atlanticus low-diversity assemblages were found in newly formed habitats, characterized by a friable substratum and vigorous venting. Lepetodrilus limpets are highly successful at vents, reaching densities of several hundred thousands of individuals at certain sites (Warén et al., 2006). They colonize a large variety of substrata in diffuse-flow emissions areas and usually benefit from the 3D structure formed by engineer species including siboglinid tubeworms (Govenar et al., 2005), mussel shells (Van Dover et al., 2002; Matabos et al., 2011), large symbiotic gastropods (Podowski et al., 2009) and stalked barnacles (Marsh et al., 2012). However, in the MAR mussel assemblages, Lepetodrilus densities appear to be lower than those observed in Pacific vents, reaching maximal densities of ~14 000 ind./m2 on the Eiffel Tower edifice (LS, Sarrazin et al., 2020). At MG gastropod assemblages, L. atlanticus reached densities (~69 800 ind./m2) that are similar to those reported by Marsh et al. (2012) for Lepetodrilus concentricus on the East Scotia Ridge (ESR, up to ~57 000 ind./m2) and higher to those reported by Govenar et al. (2005) on the EPR for the denser L. elevatus species (~4 900 ind./m2). On the northern EPR and Juan de Fuca ridge, lepetodrilids are dominant in zones of intermediate fluid intensity (Sarrazin et al., 1997; Shank et al., 1998; Sarrazin et al., 1999; Bates et al., 2005). Mills et al. (2007) report a distinct distribution between various Lepetodrilus species on the EPR. Some such as L. pustulosus, L. elevatus, L. cristatus are associated with warm areas (up to 20°C, Matabos et al., 2008), whereas others (L. ovalis) are associated with the cooler areas (1.8 – 5.9°C), with a niche overlap between the two groups. These examples illustrate the wide range of habitats Lepetodrilus species can occupy (Mills et al., 2007). Moreover, they appear to be able to outcompete other species in optimal environmental conditions (Matabos et al., 2008). The abundance and large distribution of this genus may be related, among other factors, to its feeding plasticity and high mobility. In fact, these limpets exhibit various feeding strategies including grazing with their radula, active suspension feeding and even symbiotic relationships (Bates, 2007; Gaudron et al., 2015) and they are able to adjust their position in response to environmental changes (Bates et al., 2005). Their continuous reproduction and planktonic dispersal strategy also make them effective early colonizers (Kelly and Metaxas, 2007; Matabos et al., 2008; Tyler et al., 2008; Bayer et al., 2011; Nakamura et al., 2014) as observed by their rapid colonization after an eruption at the EPR (Bayer et al., 2011).
Peltospira (McLean, 1989) is a genus of small gastropods only found in active deep-sea hydrothermal vents. P. smaragdina (Warén and Bouchet, 2001) is the type species for the nMAR where it was originally reported to occur in aggregations of 12 to 50 individuals (Warén and Bouchet, 2001). The three other species of the genus (P. operculata, P. lamellifera and P. delicata) have been observed at hydrothermal vent sites on the EPR where they occupy high temperature sulfide-rich habitats (Shank et al., 1998; Matabos et al., 2008). On the MAR, Peltospira gastropods within mussel assemblages seem to colonize an intermediate niche between the hottest habitats and the milder ones (Husson et al., 2017). Their relative proximity to black smokers and diffusing zones supports this assumption. On the EPR, these three Peltospira species are even found in the hottest “alvinellid” zone (Mills et al., 2007). These authors suggest that Peltospira species may exploit microhabitats with higher inputs of hydrothermal fluids, even when found within siboglinid or mussel assemblages. This distribution may potentially reflect their needs for higher concentrations of reduced chemicals such as hydrogen sulfide. At the Moytira vent field, P. smaragdina gastropods are distributed into discrete grazing zones at far higher concentrations (~50 000 ind./m2) than previously observed for the species (Collins et al., 2020). Similar to what was observed in our study, these zones were delineated by cracks of white precipitations, allowing the diffusion of vent fluids. These anhydrite-like mineral deposits are known to form at high temperatures (>150°C) supporting their association with warmer areas.
Role of Gastropods in nMAR Communities
A recent study in LS showed the importance of gastropods early in the recolonization processes following a disturbance (Marticorena et al., 2021). They might take advantage of the microbial films forming on “bare” substratum early in the succession process (Sarrazin et al., 1997; Mullineaux et al., 2010; Mullineaux et al., 2012). As the substratum stabilizes and fluid flow diminishes (e.g. see Juan de Fuca Ridge succession model, Sarrazin et al., 2002), gastropods may increase in abundance until they are replaced by large engineer species typical of low temperature habitats such as mussels. In the LS vent field, mussel assemblages are considered as climax communities (Cuvelier et al., 2014). The decadal stability of environmental conditions (at least at LS) and the maturity of sulfide edifices would explain the relative rarity of these early-successional assemblages in the other two deeper vent fields studied (LS & SP). They appear to be more abundant and to colonize larger areas at greater depths, potentially associated with the presence of newly formed substrata. To our knowledge, and after discussion with several colleagues, we can confirm that these gastropod assemblages are present in at least 50% of the known nMAR vent fields (Marcon et al., 2013; Wheeler et al., 2013; Collins et al., 2020). Their recurrence and distribution suggest that they probably play an important role in the functioning and dynamics of vent communities of the MAR and may even constitute one of the first step of Atlantic vent ecological succession.
Data Availability Statement
The datasets and video images presented in this study can be found in online repositories. Metadata from the various cruises are provided here: CAMBON-BONAVITA Marie-Anne (2014) BICOSE cruise, RV Pourquoi pas?, https://doi.org/10.17600/14000100 CANNAT Mathilde, SARRADIN Pierre-Marie (2012) MOMARSAT2012 cruise, RV Thalassa, https://doi.org/10.17600/12040050 CANNAT Mathilde, SARRADIN Pierre-Marie (2016) MOMARSAT2016 cruise, RV L’Atalante, https://doi.org/10.17600/16001200 LALLIER François (2013) BIOBAZ 2013 cruise, RV Pourquoi pas?, https://doi.org/10.17600/13030030 SARRADIN Pierre-Marie, CANNAT Mathilde (2015) MOMARSAT2015 cruise, RV Pourquoi pas?, https://doi.org/10.17600/15000200 SARRADIN Pierre-Marie, CANNAT Mathilde (2014) MOMARSAT2014 cruise, RV Pourquoi pas?, https://doi.org/10.17600/14000300.
Author Contributions
JS and MM conceived the ideas and designed the methodology. JS, MM, CC and AL collected the samples on board during oceanographic cruises. JS, CC, AL, JM, LM and MM processed and analyzed the data. MM did the statistical analyses. JS, CC, AL, JM, NM and MM discussed and interpreted the results. JS wrote the first draft of the manuscript and all authors commented on previous versions of the manuscript. All authors read and approved the final manuscript.
Funding
This work was supported by the “Laboratoire d’Excellence” LabexMER (ANR-10-LABX-19) and co-funded by a grant from the French government under the “Investissements d’Avenir” program. The project is part of the EMSO-Azores regional node within EMSO-France (http://www.emso-fr.org) and EMSO ERIC Research Infrastructure (http://emso.eu/). The research program was partly funded by an ANR research grant (ANR Lucky Scales ANR-14-CE02-0008-02).
Conflict of Interest
The authors declare that the research was conducted in the absence of any commercial or financial relationships that could be construed as a potential conflict of interest.
Publisher’s Note
All claims expressed in this article are solely those of the authors and do not necessarily represent those of their affiliated organizations, or those of the publisher, the editors and the reviewers. Any product that may be evaluated in this article, or claim that may be made by its manufacturer, is not guaranteed or endorsed by the publisher.
Acknowledgments
We would like to dedicate this paper to Benoît Pernet-Coudrier, our colleague who passed away in 2016. We thank the captain of the R/Vs Thalassa, L’Atalante and Pourquoi pas? and their crews for their steadfast collaboration in the success of the various cruises. We are particularly grateful to Pierre-Marie Sarradin, Mathilde Cannat, François Lallier and Marie-Anne Cambon, chief scientists of the cruises who greatly supported our sampling program. We are also grateful to the ROV Victor6000 pilots for their patience and constant support. We warmly thank the LEP technical team for its valuable help both at sea and in the lab. Two master students contributed to sorting the fauna: Roxane Augen-Langonne (M1, 2014) and Bruno Labelle (M2, 2015). PhD student L. Van Audenhaege helped with the video editing. The manuscript was professionally edited by Carolyn Engel-Gautier.
Supplementary Material
The Supplementary Material for this article can be found online at: https://www.frontiersin.org/articles/10.3389/fmars.2022.925419/full#supplementary-material
References
Bates A. E. (2007). Persistence, Morphology, and Nutritional State of a Gastropod Hosted Bacterial Symbiosis in Different Levels of Hydrothermal Vent Flux. Mar. Biol. 152, 557–568. doi: 10.1007/s00227-007-0709-x
Bates A. E., Tunnicliffe V., Lee R. W. (2005). Role of Thermal Conditions in Habitat Selection by Hydrothermal Vent Gastropods. Mar. Ecol. Prog. Ser. 305, 1–15. doi: 10.3354/meps305001
Bayer S. R., Mullineaux L. S., Waller R. G., Solow A. R. (2011). Reproductive Traits of Pioneer Gastropod Species Colonizing Deep-Sea Hydrothermal Vents After an Eruption. Mar. Biol. 158, 181–192. doi: 10.1007/s00227-010-1550-1
Beaulieu S. E., Szafranski K. M. (2020). InterRidge Global Database of Active Submarine Hydrothermal Vent Fields Version 3.4. doi: 10.1594/PANGAEA.917894
Beinart R. A., Sanders J. G., Faure B., Sylva S. P., Lee R. W., Becker E. L., et al. (2012). Evidence for the Role of Endosymbionts in Regional-Scale Habitat Partitioning by Hydrothermal Vent Symbioses. Proc. Natl. Acad. Sci. 109 (47), E3241–E3250 doi: 10.1073/pnas.1202690109
Cannat M., Sarradin P., Blandin J., Escartın J., Colaço A. (2011). MoMar-Demo at Lucky Strike. A Near-Real Time Multidisciplinary Observatory of Hydrothermal Processes and Ecosystems at the Mid-Atlantic Ridge (San Francisco: AGU Fall meeting). Abstract OS22A-05.
Charlou J., Donval J., Douville E., Jean-Baptiste P., Radford-Knoery J., Fouquet Y., et al. (2000). Geochemical Signatures and the Evolution of Menez Gwen (37 50′ N) and Lucky Strike (37 17 ′ N) Hydrothermal Fluids, South of the Azores Triple Junction on the Mid. Chem. geology 171, 49–75. doi: 10.1016/S0009-2541(00)00244-8
Collins P. C., Hunter W. R., Carlsson J., Carlsson J. (2020). Fortuitous Insights Into the Ecology of a Recently Charted Deep-Sea Hydrothermal Vent, Using Snails’ Feet. Deep-Sea Res. Part I: Oceanogr. Res. Papers 163, 103358. doi: 10.1016/j.dsr.2020.103358
Coplen T. B. (2011). Guidelines and Recommended Terms for Expression of Stable-Isotope-Ratio and Gas-Ratio Measurement Results. Rapid Commun. Mass Spectrom. 25, 2538–2560. doi: 10.1002/rcm.5129
Copley J. T. P., Tyler P. A., Murton B. J., Van Dover C. L. (1997). Spatial and Inter-Annual Variation in the Faunal Distribution at Broken Spur Vent Field (29°N, Mid-Atlantic Ridge). Mar. Biol. 129, 723–733. doi: 10.1007/s002270050215
Cuvelier D., Legendre P., Laes A., Sarradin P. M., Sarrazin J. (2014). Rhythms and Community Dynamics of a Hydrothermal Tubeworm Assemblage at Main Endeavour Field - a Multidisciplinary Deep-Sea Observatory Approach. PLoS One 9 (5), e96924. doi: 10.1371/journal.pone.0096924
Cuvelier D., Sarradin P., Sarrazin J., Colaço A., Copley J. T. P., Desbruyères D., et al. (2011a). Hydrothermal Faunal Assemblages and Habitat Characterisation at the Eiffel Tower Edifice (Lucky Strike, Mid-Atlantic Ridge). Mar. Ecol. 32, 243–255. doi: 10.1111/j.1439-0485.2010.00431.x
Cuvelier D., Sarrazin J., Colaco A., Copley J., Desbruyeres D., Glover A. G.,, Tyler P., Santos R.S.(2009). Distribution and Spatial Variation of Hydrothermal Faunal Assemblages at Lucky Strike (Mid-Atlantic Ridge) Revealed by High-Resolution Video Image Analysis. Deep Sea Res. Part I: Oceanogr. Res. Papers 56 (11), 2026–2040 doi: 10.1016/j.dsr.2009.06.006
Cuvelier D., Sarrazin J., Colaço A., Copley J. T., Glover A. G., Tyler P. A., et al. (2011b). Community Dynamics Over 14 Years at the Eiffel Tower Hydrothermal Edifice on the Mid-Atlantic Ridge. Limnology Oceanogr. 56, 1624–1640. doi: 10.4319/lo.2011.56.5.16241624
De Busserolles F., Sarrazin J., Gauthier O., Gélinas Y., Fabri M. C., Sarradin P. M., et al. (2009). Are Spatial Variations in the Diets of Hydrothermal Fauna Linked to Local Environmental Conditions? Deep-Sea Res. Part II Topical Stud. Oceanogr. 56 (19–20), 1649–1664. doi: 10.1016/j.dsr2.2009.05.011
Desbruyères D., Almeida A. J., Biscoito M., Comtet T., Khripounoff A., le Bris N., et al. (2000). A Review of the Distribution of Hydrothermal Vent Communities Along the Northern Mid-Atlantic Ridge: Dispersal vs. Environmental Controls. Hydrobiologia 440, 201–216. doi: 10.1023/A:1004175211848
Desbruyères D., Biscoito M., Caprais J.-C., Colaço A., Comtet T., Crassous P., et al. (2001). Variations in Deep-Sea Hydrothermal Vent Communities on the Mid-Atlantic Ridge Near the Azores Plateau. Deep-Sea Res. Part I: Oceanogr. Res. Papers 48, 1325–1346. doi: 10.1016/S0967-0637%2800%2900083-2
Desbruyères D., Segonzac M., Bright M. (Eds) (2006) Handbook of Deep-Sea Hydrothermal Vent Fauna.Linz, Austria: Land Oberösterreich, Biologiezentrum der Oberösterreichische Landesmuseen,
Fouquet Y., Wafik A., Cambon P., Mevel C., Meyer G., Gente P. (1993). Tectonic Setting and Mineralogical and Geochemical Zonation in the Snake Pit Sulfide Deposit (Mid-Atlantic Ridge at 23°N). Economic geology 88, 2018–2036. doi: 10.2113/gsecongeo.88.8.2018
Gaudron S. M., Marqué L., Thiébaut E., Riera P., Duperron S., Zbinden M. (2015). How are Microbial and Detrital Sources Partitioned Among and Within Gastropods Species at East Pacific Rise Hydrothermal Vents? Mar. Ecol. 36, 18–34. doi: 10.1111/maec.12260
Gebruk A. V., Galkin S., Vereshchaka A., Moskalev L., Southward A. J. (1997). Ecology and Biogeography of the Hydrothermal Vent Fauna of the Mid-Atlantic Ridge. Adv. Mar. Biol. 32, 93–144. doi: 10.1016/S0065-2881(08)60016-4
Gollner S., Colaço A., Gebruk A., Halpin P. N., Higgs N., Menini E., et al. (2021). Application of Scientific Criteria for Identifying Hydrothermal Ecosystems in Need of Protection. Mar. Policy 132, 104641. doi: 10.1016/j.marpol.2021.104641
Gollner S., Riemer B., Martínez Arbizu P., Le Bris N., Bright M. (2010). Diversity of Meiofauna From the 9°50’n East Pacific Rise Across a Gradient of Hydrothermal Fluid Emissions. PLoS One 5, e12321. doi: 10.1371/journal.pone.0012321
Govenar B. (2012). Energy Transfer Through Food Webs at Hydrothermal Vents: Linking the Lithosphere to the Biosphere. Oceanography 25, 246–255. doi: 10.5670/oceanog.2012.23
Govenar B., Fisher C. R. (2007). Experimental Evidence of Habitat Provision by Aggregations of Riftia Pachyptila at Hydrothermal Vents on the East Pacific Rise. Mar. Ecol. 28, 3–14. doi: 10.1111/j.1439-0485.2007.00148.x
Govenar B., le Bris N., Gollner S., Glanville J., Aperghis A., Hourdez S., et al. (2005). Epifaunal Community Structure Associated With Riftia Pachyptila Aggregations in Chemically Different Hydrothermal Vent Habitats. Mar. Ecol. Prog. Ser. 305, 67–77. doi: 10.3354/meps305067
Hernández-Ávila I., Cambon-Bonavita M.-A., Sarrazin J., Pradillon F. (2022). Population Structure and Reproduction of the Alvinocaridid Shrimp Rimicaris Exoculata on the Mid-Atlantic Ridge: Variations between Habitats and Vent Fields. Deep-Sea Res. I: Oceanogr. Res. Pap. 186 (103827), 14. doi: 10.1016/j.dsr.2022.103827
Hügler M., Sievert S. M. (2011). Beyond the Calvin Cycle: Autotrophic Carbon Fixation in the Ocean. Annu. Rev. Mar. Sci. 3, 261–289. doi: 10.1146/annurev-marine-120709-142712
Hurlbert S. H. (1971). The Nonconcept of Species Diversity: A Critique and Alternative Parameters. Ecology 52, 577–586. doi: 10.2307/1934145
Husson B., Sarradin P., Zeppilli D., Sarrazin J. (2017). Picturing Thermal Niches and Biomass of Hydrothermal Vent Species. Deep-Sea Res. Part II: Topical Stud. Oceanogr. 137, 6–25. doi: 10.1016/j.dsr2.2016.05.028
Jannasch H. W. (1985). The Chemosynthetic Support of Life and the Microbial Diversity at Deep-Sea Hydrothermal Vents. Proc. R. Soc. B: Biol. Sci. 225, 277–297. doi: 10.1098/rspb.1985.0062
Karl D. M. (1995). “Ecology of Free-Living, Hydrothermal Vent Microbial Communities,” in The Microbiology of Deep-Sea Hydrothermal Vents, vol. 1995) . Ed. Karl D. M. (Boca Raton, FL: CRC Press Inc.), 35–124.
Karson J. A., Thompson G., Humphris S. E., Edmond J. M., Bryan W. B., Brown J. R., et al. (1987). Along-Axis Variations in Seafloor Spreading in the MARK Area. Nature 328, 681–685. doi: 10.1038/328681a0
Kelly N. E., Metaxas A. (2007). Influence of Habitat on the Reproductive Biology of the Deep-Sea Hydrothermal Vent Limpet Lepetodrilus Fucensis (Vetigastropoda: Mollusca) From the Northeast Pacific. Mar. Biol. 151, 649–662. doi: 10.1007/s00227-006-0505-z
Langmuir C. H., Humphris S. E., Fornari D. J., Van Dover C. L., Von Damm K., Tivey M. K., et al. (1997). Hydrothermal Vents Near a Mantle Hot Spot: The Lucky Strike Vent Field at 37°N on the Mid-Atlantic Ridge. Earth Planetary Sci. Lett. 148, 69–91. doi: 10.1016/S0012-821X(97)00027-7
Lau D. C. P., Leung K. M.Y., Dudgeon D. (2012). Preservation Effects on C/N Ratios and Stable Isotope Signatures of Freshwater Fishes and Benthic Macroinvertebrates. Limnology Oceanogr.: Methods 10, 75–89. doi: 10.4319/lom.2012.10.75
Le Bris N., Zbinden M., Gaill F. (2005). Processes Controlling the Physico-Chemical Micro-Environments Associated With Pompeii Worms. Deep-Sea Res. Part I: Oceanogr. Res. Papers 52, 1071–1083. doi: 10.1016/j.dsr.2005.01.003
Lee R. W., Robert K., Matabos M., Bates A. E., Juniper S. K. (2015). Temporal and Spatial Variation in Temperature Experienced by Macrofauna at Main Endeavour Hydrothermal Vent Field. Deep-Sea Res. Part I: Oceanogr. Res. Papers 106, 154–166. doi: 10.1016/j.dsr.2015.10.004
Legendre P., Gallagher E. (2001). Ecologically Meaningful Transformations for Ordination of Species Data. Oecologia 129, 271–280. doi: 10.1007/s004420100716
Lelievre Y., Legendre P., Matabos M., Mihaly S., Lee R. W., Sarradin P.-M., et al. (2017). Astronomical and Atmospheric Impacts on Deep-Sea Hydrothermal Vent Invertebrates. Proc. Of R. Soc. B-biological Sci. 284 (1852), 20162123. doi: 10.1098/rspb.2016.2123
Lelièvre Y., Sarrazin J., Marticorena J., Schaal G., Day T., Legendre P., Hourdez S., Matabos M., (2018). Biodiversity and Trophic Ecology of Hydrothermal Vent Fauna Associated With Tubeworm Assemblages on the Juan De Fuca Ridge. Biogeosciences 15. 2629–2647doi: 10.5194/bg-15-2629-2018
Levesque C., Juniper S. K., Marcus J. (2003). Food Resource Partitioning and Competition Among Alvinellid Polychaetes of Juan De Fuca Ridge Hydrothermal Vents. Mar. Ecol. Prog. Ser. 246, 173–182. doi: 10.3354/meps246173
Luther G. W., Gartman A., Yücel M., Madison A., Moore T., Nees H., et al. (2012). Chemistry, Temperature, and Faunal Distributions at Diffuse-Flow Hydrothermal Vents: Comparison of Two Geologically Distinct Ridge Systems. Oceanography 25, 234–245. doi: 10.5670/oceanog.2012.22
Luther G. W., Rozan T. F., Taillefert M., Nuzzio D. B., Di Meo C., Shank T. M., et al. (2001). Chemical Speciation Drives Hydrothermal Vent Ecology. Nature. 410, 813–816. doi: 10.1038/35071069
Maas P. A. Y., O’Mullan G. D., Lutz R. A., Vrijenhoek R. C. (1999). Genetic and Morphometric Characterization of Mussels (Bivalvia: Mytilidae) From Mid-Atlantic Hydrothermal Vents. Bioogical Bull. 196, 265–272. doi: 10.2307/1542951
McLean J.H. (1989). New Archaeogastropod Limpets from Hydrothermal Eents: New Family Peltospiridae, New Superfamily Peltospiracea. Zool. Scr. 18(1), 49–66.
Marcon Y., Sahling H., Borowski C., dos Santos Ferreira C., Thal J., Bohrmann G. (2013). Megafaunal Distribution and Assessment of Total Methane and Sulfide Consumption by Mussel Beds at Menez Gwen Hydrothermal Vent, Based on Geo-Referenced Photomosaics. Deep-Sea Res. Part I: Oceanogr. Res. Papers 75, 93–109. doi: 10.1016/j.dsr.2013.01.008
Marsh L., Copley J. T., Huvenne V. A. I., Linse K., Reid W. D. K., Rogers A. D., et al. (2012). Microdistribution of Faunal Assemblages at Deep-Sea Hydrothermal Vents in the Southern Ocean. PLoS One 7, e48348. doi: 10.1371/journal.pone.0048348
Marticorena J., Matabos M., Ramirez-Llodra E., Cathalot C., Laes-Huon A., Leroux R., et al. (2021). Recovery of Hydrothermal Vent Communities in Response to an Induced Disturbance at the Lucky Strike Vent Field (Mid-Atlantic Ridge). Mar. Environ. Res. 168 (105316), 14 doi: 10.1016/j.marenvres.2021.105316
Martins I., Cosson R. P., Riou V., Sarradin P., Sarrazin J., Santos R. S., et al. (2011). Relationship Between Metal Levels in the Vent Mussel Bathymodiolus Azoricus and Local Microhabitat Chemical Characteristics of Eiffel Tower (Lucky Strike). Deep-Sea Res. Part I: Oceanogr. Res. Papers 58, 306–315. doi: 10.1016/j.dsr.2011.01.002
Matabos M., Le Bris N., Pendlebury S., Thiébaut E. (2008). Role of Physico-Chemical Environment on Gastropod Assemblages at Hydrothermal Vents on the East Pacific Rise (13 N/EPR). J. Mar. Biol. Assoc. UK 88, 995–1008. doi: 10.1017/S002531540800163X
Matabos M., Plouviez S., Hourdez S., Desbruyères D., Legendre P., Warén A., et al. (2011). Faunal Changes and Geographic Crypticism Indicate the Occurrence of a Biogeographic Transition Zone Along the Southern East Pacific Rise. J. Biogeography 38, 575–594. doi: 10.1111/j.1365-2699.2010.02418.x
McClain R., Etter R. J. (2005). Mid-Domain Models as Predictors of Species Diversity Patterns: Bathymetric Diversity Gradients in the Deep Sea. Oikos 109 (3), 555–566. doi: 10.1111/j.0030-1299.2005.13529.x
Methou P., Hernández-Ávila I., Aube J., Cueff-Gauchard V., Gayet N., Amand L., et al. (2019). Is it First the Egg or the Shrimp? – Diversity and Variation in Microbial Communities Colonizing Broods of the Vent Shrimp Rimicaris Exoculata During Embryonic Development. Front. Microbiol. 10. doi: 10.3389/fmicb.2019.00808
Methou P., Michel L. N., Segonzac M., Cambon-Bonavita M.-A., Pradillon F. (2020). Integrative Taxonomy Revisits the Ontogeny and Trophic Niches of Rimicaris Vent Shrimps. R. Soc. Open Sci., 7(7), 200837 (13p.). doi: 10.1098/rsos.200837
Mills S. W., Mullineaux L. S., Tyler P. A. (2007). Habitat Associations in Gastropod Species at East Pacific Rise Hydrothermal Vents (9 Degrees 50’n). Biol. Bull. 212, 185–194. doi: 10.2307/25066601
Mullineaux L. S., Adams D. K., Mills S. W., Beaulieu S. E. (2010). Larvae From Afar Colonize Deep-Sea Hydrothermal Vents After a Catastrophic Eruption. Proc. Natl. Acad. Sci. 107, 7829–7834. doi: 10.1073/pnas.0913187107
Mullineaux L. S., Le Bris N., Mills S. W., Henri P., Bayer S. R., Secrist R. G., et al. (2012). Detecting the Influence of Initial Pioneers on Succession at Deep-Sea Vents. PLoS One 7, e50015. doi: 10.1371/journal.pone.0050015
Mullineaux L. S., Peterson C. H., Micheli F., Mills S. W. (2003). Successional Mechanisms Varies Along a Gradient in Hydrothermal Fluid Flux at Deep-Sea Vents. Ecol. Monogr. 73, 523–542. doi: 10.1890/02-0674
Nakamura M., Watanabe H., Sasaki T., Ishibashi J.-I., Fujikura K., Mitarai S. (2014). Life History Traits of Lepetodrilus Nux in the Okinawa Trough, Based Upon Gametogenesis, Shell Size, and Genetic Variability. Mar. Ecol. Prog. Ser. 505, 119–130. doi: 10.3354/meps10779
Nye V., Copley J. T. P., Tyler P. A. (2013). Spatial Variation in the Population Structure and Reproductive Biology of Rimicaris Hybisae (Caridea: Alvinocarididae) at Hydrothermal Vents on the Mid-Cayman Spreading Centre. PLoS One 8, e60319. doi: 10.1371/journal.pone.0060319
Oksanen J., Blanchet F.G., Kindt R., Legendre P., Minchin P., O’Hara R.B., et al(2013). Vegan: Community Ecology Package. in R Package Version. 2.0-10. http://CRAN.R-project.org/package=vegan. CRAN.
Ondréas H., Cannat M., Fouquet Y., Normand A., Sarradin P.M., Sarrazin J. (2009). Recent Volcanic Events and the Distribution of Hydrothermal Venting at the Lucky Strike Hydrothermal Field, Mid-Atlantic Ridge. Geochemistry Geophysics Geosystems 10 (2),1–18. doi: 10.1029/2008GC002171
Podowski E. L., Moore T. S., Zelnio K. A., Luther G. W., Fisher C. R. (2009) Distribution of Diffuse Flow Megafauna in Two Sites on the Eastern Lau Spreading Center, Tonga. Deep-Sea Res. I, 56, 2041–2056. doi: 10.1016/j.dsr.2009.07.002
Podowski E. L., Ma S., Luther G. W., Wardrop D., Fisher C. R. (2010). Biotic and Abiotic Factors Affecting Distributions of Megafauna in Diffuse Flow on Andesite and Basalt Along the Eastern Lau Spreading Center, Tonga. Mar. Ecol. Prog. Ser. 418, 25–45. doi: 10.3354/meps08797
Portail M., Brandily C., Cathalot C., Colaço A., Gélinas Y., Husson B., et al. (2018). Food-Web Complexity Across Hydrothermal Vents on the Azores Triple Junction. Deep Sea Res. Part I: Oceanogr. Res. Papers 131, 101–120. doi: 10.1016/j.dsr.2017.11.010
R Core Team (2020). R: A Language and Environment for Statistical Computing (Vienna, Austria). Available at: http://www.R-project.org/.
Sancho G., Fisher C. R., Mills S. W., Micheli F., Johnson G. A., Lenihan H. S., et al. (2005). Selective Predation by the Zoarcid Fish Thermarces Cerberus at Hydrothermal Vents. Deep Sea Res. Part I: Oceanogr. Res. Papers 52, 837–844. doi: 10.1016/J.DSR.2004.12.002
Sarradin P., Caprais J.-C., Riso R., Kerouel R., Aminot A. (1999). Chemical Environment of the Hydrothermal Mussel Communities in the Lucky Strike and Menez Gwen Vent Fields, Mid Atlantic Ridge. Cahiers Biologie Mar. 40, 93–104.
Sarradin P., Waeles M., Bernagout S., Le Gall C., Sarrazin J., Riso R. (2009). Speciation of Dissolved Copper Within an Active Hydrothermal Edifice on the Lucky Strike Vent Field (MAR, 37°N). Sci. Total Environ. 407, 869–878. doi: 10.1016/j.scitotenv.2008.09.056
Sarrazin J., Juniper S. K. (1999). Biological Characteristics of a Hydrothermal Edifice Mosaic Community. Mar. Ecol. Prog. Ser. 185 (1999), 1–19. doi: 10.3354/meps185001
Sarrazin J., Juniper S. K., Massoth G., Legendre P. (1999). Physical and Chemical Factors Influencing Species Distributions on Hydrothermal Sulfide Edifices of the Juan De Fuca Ridge, Northeast Pacific. Mar. Ecol. Prog. Ser. 190, 89–112. doi: 10.3354/meps185001
Sarrazin J., Legendre P., de Busserolles F., Fabri M.-C., Guilini K., Ivanenko V. N., et al. (2015). Biodiversity Patterns, Environmental Drivers and Indicator Species on a High-Temperature Hydrothermal Edifice, Mid-Atlantic Ridge. Deep Sea Res. Part II: Topical Stud. Oceanogr. 121, 177–192. doi: 10.1016/j.dsr2.2015.04.013
Sarrazin J., Levesque C., Juniper S., Tivey M. (2002). Mosaic Community Dynamics on Juan De Fuca Ridge Sulphide Edifices: Substratum, Temperature and Implications for Trophic Structure. Cahiers Biologie Mar. 43 (3-4), 275–279. https://archimer.ifremer.fr/doc/00000/893/
Sarrazin J., Portail M., Legrand E., Cathalot C., Laes A., Lahaye N., et al. (2020). Endogenous Versus Exogenous Factors: What Matters for Vent Mussel Communities? Deep-Sea Res. Part I: Oceanogr. Res. Papers 160, 103260. doi: 10.1016/j.dsr.2020.103260
Sarrazin J., Robigou V., Juniper S. K., Delaney J. R. (1997). Biological and Geological Dynamics Over Four Years on a High-Temperature Sulfide Structure at the Juan De Fuca Ridge Hydrothermal Observatory. Mar. Ecol. Prog. Ser. 153, 5–24. doi: 10.3354/meps153005
Schmidt C., le Bris N., Gaill F. (2008). Interactions of Deep-Sea Vent Invertebrates With Their Environment: The Case of Rimicaris Exoculata. J. Shellfish Res. 27, 79–90. doi: 10.2983/0730-8000(2008)27[79:IODVIW]2.0.CO;2
Schneider C. A., Rasband W. S., Eliceiri K. W. (2012). NIH Image to ImageJ: 25 Years of Image Analysis. Nat. Methods. 9, 671–675. doi: 10.1038/nmeth.2089
Segonzac M., De Saint-Laurent M., Casanova B.1993Enigma of the Trophic Adaptation of the Shrimp Alvinocarididae in Hydrothermal Areas Along the Mid-Atlantic Ridge. Cah. Biol. Mar.344535–571
Sen A., Becker E. L., Podowski E. L., Wickes L. N., Ma S., Mullaugh K. M., et al. (2013). Distribution of Mega Fauna on Sulfide Edifices on the Eastern Lau Spreading Center and Valu Fa Ridge. Deep Sea Res. Part I: Oceanogr. Res. Papers 72, 48–60. doi: 10.1016/j.dsr.2012.11.003
Shank T. M., Fornari D. J., Von Damm K. L., Lilley M. D., Haymon R. M., Lutz R. A. (1998). Temporal and Spatial Patterns of Biological Community Development at Nascent Deep-Sea Hydrothermal Vents (9°50’n, East Pacific Rise). Deep-Sea Res. Part II: Topical Stud. Oceanogr. 45, 465–515. doi: 10.1016/S0967-0645(97)00089-1
Singh S. C., Crawford W. C., Carton H., Seher T., Combier V., Cannat M., et al. (2006). Discovery of a Magma Chamber and Faults Beneath a Mid-Atlantic Ridge Hydrothermal Field. Nature 442, 1029–1032. doi: 10.1038/nature05105
Tsurumi M., Tunnicliffe V. (2003). Tubeworm-Associated Communities at Hydrothermal Vents on the Juan De Fuca Ridge, Northeast Pacific. Deep Sea Res. I 50 (2003), 611–629. doi: 10.1016/S0967-0637(03)00039-6
Tunnicliffe V. (1991). The Biology of Hydrothermal Vents - Ecology and Evolution. Oceanogr. Mar. Biol. 29, 319–407.
Tyler P. A., Pendlebury S., Mills S. W., Mullineaux L. S., Eckelbarger K. J., Baker M. C., et al. (2008). Reproduction of Gastropods From Vents on the East Pacific Rise and the Mid- Atlantic Ridge. J. Shellfish Res. 27, 107–118. doi: 10.2983/0730-8000(2008)27[107:ROGFVO]2.0.CO;2
Van Dover C. L. (2002). Community Structure of Mussel Beds at Deep-Sea Hydrothermal Vents. Mar. Ecol. Prog. Ser. 230, 137–158. doi: 10.3354/meps230137
Van Dover C. L. (2003). Variation in Community Structure Within Hydrothermal Vent Mussel Beds of the East Pacific Rise. Mar. Ecol. Prog. Ser. 253, 55–66. doi: 10.3354/meps253055
Van Dover C. L., Doerries M. B. (2005). Community Structure in Mussel Beds at Logatchev Hydrothermal Vents and a Comparison of Macrofaunal Species Richness on Slow- and Fast-Spreading Mid-Ocean Ridges. Mar. Ecol. 26 (2), 110–120. doi: 10.1111/j.1439-0485.2005.00047.x
Van Dover C. L., German C. R., Speer K. G., Parson L. M., Vrijenhoek R. C. (2002). Evolution and Biogeography of Deep-Sea Vent and Seep Invertebrates. Science (New York N.Y.) 295, 1253–1257. doi: 10.1126/science.1067361
Vuillemin R., Le Roux D., Dorval P., Bucas K., Laes-Huon A., Hamon M., et al. (2009). CHEMINI: A New in Situ CHEmical MINIaturized Analyzer. Deep Sea Res. Part I: Oceanogr. Res. Paper 56 (8), 1391–1399. doi: 10.1016/j.dsr.2009.02.002
Warén A., Bouchet P. (2001). Gastropoda and Monoplacophora From Hydrothermal Vents and Seeps: New Taxa and Records. Veliger 44, 116–231.
Warén A., Bouchet P., von Cosel R. (2006). “Lepetodrilus McLean 1988, “Dimorphic Limpets,” in Handbook of Deep-Sea Hydrothermal Vent Fauna. Eds. Desbruyères D., Segonzac M., Bright M. (Linz: Biologiezentrum der Oberösterreichischen Landesmuseen).
Wheeler A. J., Murton B., Copley J., Lim A., Carlsson J., Collins P. C., et al. (2013). Moytirra: Discovery of the First Known Deep-Sea Hydrothermal Vent Field on the Slow-Spreading Mid-Atlantic Ridge North of the Azores. Geochemistry Geophysics Geosystems 14, 4170–4184. doi: 10.1002/ggge.20243
WoRMS Editorial Board (2022). World Register of Marine Species. Available at: https://www.marinespecies.org at: (Accessed July 04, 2022).
Keywords: gastropod, new assemblage, habitat, environmental conditions, diversity, hydrothermal vent, Mid-Atlantic Ridge
Citation: Sarrazin J, Cathalot C, Laes A, Marticorena J, Michel LN and Matabos M (2022) Integrated Study of New Faunal Assemblages Dominated by Gastropods at Three Vent Fields Along the Mid-Atlantic Ridge: Diversity, Structure, Composition and Trophic Interactions. Front. Mar. Sci. 9:925419. doi: 10.3389/fmars.2022.925419
Received: 21 April 2022; Accepted: 10 June 2022;
Published: 26 July 2022.
Edited by:
Elva G. Escobar-Briones, National Autonomous University of Mexico, MexicoReviewed by:
Daniel Pech, El Colegio de la Frontera Sur, MexicoTakuya Yahagi, The University of Tokyo, Japan
Copyright © 2022 Sarrazin, Cathalot, Laes, Marticorena, Michel and Matabos. This is an open-access article distributed under the terms of the Creative Commons Attribution License (CC BY). The use, distribution or reproduction in other forums is permitted, provided the original author(s) and the copyright owner(s) are credited and that the original publication in this journal is cited, in accordance with accepted academic practice. No use, distribution or reproduction is permitted which does not comply with these terms.
*Correspondence: Jozée Sarrazin, am96ZWUuc2FycmF6aW5AaWZyZW1lci5mcg==