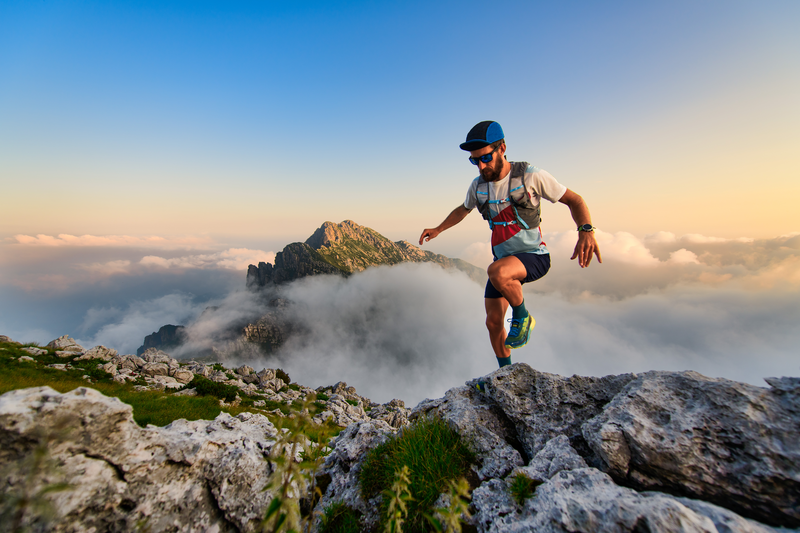
95% of researchers rate our articles as excellent or good
Learn more about the work of our research integrity team to safeguard the quality of each article we publish.
Find out more
ORIGINAL RESEARCH article
Front. Mar. Sci. , 09 June 2022
Sec. Marine Fisheries, Aquaculture and Living Resources
Volume 9 - 2022 | https://doi.org/10.3389/fmars.2022.924569
This article is part of the Research Topic New Progresses and Effects of Functional Feed Additives on Marine Aquatic Animals View all 13 articles
This study evaluated the effects of nano-curcumin on growth performance, mucosal immunity, antioxidant response, glucose metabolism, and endoplasmic reticulum stress in largemouth bass. Three groups (three replicates/group) of 270 fish with the mean body weight of 7.00 ± 0.02 g were fed with diets containing 0% (control), 0.1%, and 0.2% nano-curcumin for 60 days. The results showed that dietary supplementation of nano-curcumin had no significant effects on the growth performance, body composition, lysozyme (LYZ), and alkaline phosphatase (AKP) in skin mucus, superoxide dismutase (SOD), and catalase (CAT) activity in serum of largemouth bass. However, dietary supplementation with 0.2% nano-curcumin significantly increased peroxidase (POD) activity in skin mucus and decreased the serum MDA activity compared with the control group. Moreover, dietary supplementation of 0.2% nano-curcumin significantly decreased the levels of serum glucose and liver glycogen, which may be mainly related to the increased gene expression of glucose transporter 2 (GLUT2), glucokinase (GK), phosphofructokinase (PFK), and pyruvate kinase (PK). At the same time, the hepatic gene expression of glucose-regulated protein78 (GRP78), activating transcription factor6 (ATF6), inositol-requiring enzyme 1 (IRE), and eukaryotic initiation factor 2α (eIF2α) in the 0.2% nano-curcumin group were rapidly suppressed, indicating that adding 0.2% nano-curcumin to the diet can alleviate endoplasmic reticulum stress (ERS) in fish. In conclusion, diets supplemented with 0.2% nano-curcumin effectively improved mucosal immunity, antioxidant properties, and glucose metabolism and alleviated ERS induced by long-term carbohydrate intake in largemouth bass.
Curcumin, known as diferuloylmethane, is a water-insoluble phenolic compound extracted from the rhizome of Curcuma longa. Consistently, curcumin has attracted extensive attention in aquaculture, because of its wide range of pharmacological properties, such as anti-inflammatory, antioxidant, antitumor, antivirus, hypoglycemic, and lipid-lowering effects (Nm et al., 2018; Alagawany et al., 2021; Ji et al., 2021). In recent years, Mahmoud et al. (2017) and Ajani et al. (2020) have revealed that the supplementation of curcumin in feed could enhance the growth performance and disease resistance of Oreochromis niloticus (Mahmoud et al., 2017; Ajani et al., 2020). Yonar et al. (2019) showed that the addition of curcumin in the feed could improve the growth, immunity, and antioxidant capacity of Oncorhynchus mykiss (Yonar et al., 2019). Zhao et al. (2021b) reported that curcumin could reduce chlorpyrifos-induced apoptosis and liver damage in largemouth bass (Zhao et al., 2021b). However, curcumin has limited clinical potential, due to its extremely low oral bioavailability due to its poor aqueous solubility and its chemical instability, having an oral bioavailability of only 1% in rats (Yang et al., 2007; Hewlings and Kalman, 2017). To overcome the solubility issues of curcumin and facilitate its intracellular delivery, several approaches have been developed to increase its bioavailability, including liposomal curcumin (Feng et al., 2017), curcumin nanoparticles (Bisht et al., 2007), and curcumin-phospholipid complexes (Maiti et al., 2007). Recent studies showed that nano-curcumin could potentially improve glucose uptake and insulin sensitivity in diabetic mice (Afifi et al., 2020; Shehata et al., 2020). Marchiori et al. (2019) showed that the addition of 200 mg/kg curcumin/nanocurcumin can effectively improve the growth performance and liver health of broiler chickens reared under cold stress conditions (Marchiori et al., 2019). Yu et al. (2016; 2018) found that Zn(II)-curcumin has a protective effect on the liver on Litopenaeus vannamei (Yu et al., 2016; Yu et al., 2018). However, there is still a paucity of research on nano-curcumin in carnivorous fish, and further studies are needed.
Largemouth bass (Micropterus salmoides) is a carnivorous species, which has become one of the most commercially important species in China, and the total production of largemouth bass in 2020 reached more than 600,000 t (Ministry of Agriculture and Rural Affairs of China, 2021). As a carnivorous fish species, largemouth bass is considered to be a typical model to investigate glucose-induced liver disease due to its poor utilization of carbohydrates; it would exhibit pronounced hyperglycemia after consuming carbohydrates for long periods of time (Guo et al., 2020, Chen P. et al., 2021). Long-term carbohydrate intake would lead to liver damage and glycogen accumulation, as well as increase the occurrence of oxidative stress and endoplasmic reticulum stress (Liu et al., 2017; Guo et al., 2020). Taking into account the beneficial effects of curcumin, the purpose of this study was to evaluate the effects of nano-curcumin on the growth performance, hepatoprotective potential, antioxidant response, glucose metabolism, and endoplasmic reticulum stress of largemouth bass. This will be helpful for providing a theoretical basis for reasonable supplementation of nano-curcumin in largemouth bass feed.
Formulation of the experimental diets (control (without nano-curcumin), 0.1% nano-curcumin, and 0.2% nano-curcumin) is listed in Table 1. The method of diet preparation was carried out with reference to the previous literature report (Xie S. W. et al., 2020). In brief, all the powdered ingredients were precisely weighed and thoroughly mixed, then the lipids and water were added. The twin-screw cooking extruder (HQ, Zhaoqing, China) was used to extrude the wet mixture into 2.5-mm-diameter pellets, and the pellets were air-dried to a moisture content of about 10%, and then stored in a refrigerator at -20°C until use.
Largemouth bass were provided by a local hatchery (Foshan, China). The fish were adapted to an indoor recirculating aquaculture system and fed with commercial diet for 2 weeks. After the acclimation period, 270 fish of similar body weight (7.00 ± 0.02 g) were randomly divided into three groups; each group had three replicates (30 fish/tank). During the 60-day breeding experiment, fish were hand fed twice a day to visual satiation at 8:30 and 16:30, and feed consumption was recorded daily. Throughout the experimental period, the water temperature was 25°C–31°C, dissolved oxygen > 6.0 mg/l, pH 7.0 ± 0.5, and NH4+-N 0.15–0.20 mg/l.
At the end of the feeding trial, all fish were fasted for 24 h and then anesthetized with MS-222 (tricaine methanesulfonate, Sigma, USA). After being counted and weighed, skin mucus of the fish was collected using the method of Hoseinifar et al. (2014) with slight modifications. Briefly, 10 fish from each tank were randomly collected and transferred to a polyethylene bag containing 100 ml of 50 mM NaCl. The bag was gently shaken for 2 min to collect skin mucus. The mucus samples were immediately transferred to 50-ml sterile centrifuge tubes and centrifuged at 1,500 rmp/min under 4°C for 10 min to obtain the sample supernatant, and then the supernatant was stored at -80°C for further analysis (Hoseinifar et al., 2014).
Blood samples from nine fish were collected from the caudal vein with disposable medical syringes. Three fish were dissected for liver samples, which were used for histological analysis and gene expression analysis or biochemical index; all the samples were immediately placed into liquid nitrogen and then stored at −80°C before the analysis. Blood samples were centrifuged (4,000 rmp/min, 15 min) at 4°C, then supernatants from six fish were mixed and used to measure hemolymph biochemical indices and glucose metabolic indices; all samples were immediately frozen and stored at −80°C until analysis. The muscle of six fish was collected for glycogen analysis and nutrient composition determination.
Moisture, crude protein, crude fat, and crude ash of experimental diet, whole body, and muscle were determined by the method of AOAC (AOAC, 2003). The moisture content was determined by the constant temperature drying weight loss method at 105°C. The crude protein content was determined by the Kjeldahl method. The crude fat content was determined by the Soxhlet extraction method. The crude ash content was determined by the muffle furnace ashing method.
The glucose oxidase-peroxidase (GOD-POD) method was used to determine the serum glucose of experimental animals. The content of glucose in the sample can be calculated by measuring the absorbance of the blue substance, which was generated by the hydrogen peroxide and o-toluidine generated by the decomposition of glucose under the action of peroxidase.
Determination of glycogen in the liver and muscle was done using the anthraquinone method (Liu et al., 2017).
Lysozyme (LYZ) hydrolyzed peptidoglycan on the bacterial wall so that it increased the light transmission of the turbid bacterial solution. Therefore, the content of lysozyme could be speculated according to the change in turbidity, which was determined by the absorbance at 520 nm. The determination of peroxidase (POD) was based on the principle that peroxidase catalyzes hydrogen peroxide reaction, and its enzyme activity was obtained by measuring the change of absorbance at 420 nm. Alkaline phosphatase (AKP) was determined by using its decomposable disodium diphenyl phosphate to produce free phenol, which reacted with 4-aminoantipyrine in alkaline solution to produce red quinone derivatives. The enzyme activity could be determined according to the depth of red. All kits were purchased from Nanjing Jiancheng Institute of Biological Engineering.
The activities of superoxide dismutase (SOD), catalase (CAT), and malondialdehyde (MDA) in serum were performed as described by Chen et al. (Chen Y. K. et al., 2021).
Liver histological examination was performed as described by Yu et al. In brief, liver tissue samples were fixed in Bouin solution and embedded in paraffin wax. The embedded liver was cut into 5-µm sections with a microtome and finally stained with hematoxylin and eosin to make the liver tissue sections (Yu et al., 2018).
Total RNA from liver was extracted using TransZol Up Plus RNA Kit, and RNA was reversely transcribed to cDNA using EasyScript® One-Step gDNA Removal and cDNA Synthesis SuperMix (TransGen Biotech, China). Real-time quantitative PCR (RT-qPCR) was performed using the SYBR® Premix Ex Taq™ System in a QuantStudio3 machine (Applied Biosystems, Foster City, CA, USA) with the following program: 94°C for 30 s; 40 cycles of 94°C for 5 s, 60°C for 30 s, 95°C for 15 s, 60°C for 60 s, and 95°C for 1 s. Gene expression was determined using 2-ΔΔCt. All primers used in this study are listed in Table 2.
The parameters in this study were calculated as per the following formula:
where it is the experimental duration in days.
The data are presented as means ± SEM. All experimental data are used with SPSS 22.0 (SPSS, Chicago, IL, USA) for one-way analysis of variance (one-way ANOVA); when the difference was significant, Duncan’s multiple-comparison test was performed, and the significance level was set to P < 0.05.
Addition of nano-curcumin in the feed had no significant effect on the survival rate of juvenile largemouth bass. The survival rate of each group was above 99% (P > 0.05) (Table 3). No significant difference was observed in WGR, SGR, and FCR among the fish in all groups (P > 0.05).
Table 3 Growth performance, feed efficiency, and survival rate of largemouth bass fed different experimental diets for 60 days.
Nano-curcumin had no significant effect on the approximate composition of the whole body and muscles of fish, including crude protein and crude fat (P > 0.05) (Table 4).
Table 4 Whole-body and muscle composition of largemouth bass fed different experimental diets for 60 days.
As set out in Figure 1, the serum glucose levels and liver glycogen in the 0.2% nano-curcumin group were significantly lower than those in the control group and 0.1% nano-curcumin group (P < 0.05). There was no significant difference in muscle glycogen levels among groups (P > 0.05).
Figure 1 Effects of dietary nano-curcumin on serum glucose (A), liver glycogen (B), and muscle glycogen (C) in largemouth bass. Values (mean ± standard error of the mean, SEM) in bars that have the same letter are not significantly different (P > 0.05; Duncan’s test) between treatments (N = 6).
As is shown in Figure 2, the activities of LYZ and AKP in the skin mucus of largemouth bass in the 0.2% nano-curcumin group were higher than those in the control group, but there were no significant differences between the groups (P > 0.05). Meanwhile, the POD content of the nano-curcumin group was significantly higher than that of the control group (P < 0.05).
Figure 2 Effect of dietary nano-curcumin on lysozyme (A), peroxidase (B), and alkaline phosphatase (C) in the liver of largemouth bass. Values (mean ± standard error of the mean, SEM) in bars that have the same letter are not significantly different (P > 0.05; Duncan’s test) between treatments (N = 6).
As is presented in Table 5, treatments of nano-curcumin did not affect the activity of SOD and CAT in serum (P > 0.05), and with the increase in nano-curcumin content, the activity of MDA significantly decreased in the 0.2% nano-curcumin group (P < 0.05).
Table 5 Antioxidant enzymes in serum of largemouth bass fed different experimental diets for 60 days.
The HE-stained paraffin sections of the liver from fish in all groups are shown in Figure 3. The results of the hematoxylin–eosin staining section showed that the fish in the control group had obvious inflammatory cell infiltration compared with the fish in nano-curcumin treatment groups. In addition, the morphology of hepatocytes in the control group and 0.1% nano-curcumin group was similar, with hypertrophy, vacuolization, and loss of nucleus of hepatocytes.
Figure 3 Effect of dietary nano-curcumin on the liver histology (hematoxylin and eosin staining, original magnification ×400) of largemouth bass after 60 days. (A) Liver from fish fed diet 0% nano-curcumin. (B) Liver from fish fed diet 0.1% nano-curcumin. (C) Liver from fish fed diet 0.2% nano-curcumin.
The effect of nano-curcumin on the expression of genes related to glucose metabolism in the liver of largemouth bass is shown in Figure 4. The results showed that with the supplementation of nano-curcumin, the mRNA expression of GK, PFK, and PK in fish fed 0.2% nano-curcumin significantly increased compared with the control group and 0.1% nano-curcumin group (P < 0.05). The mRNA expression of G6Pase and Fbp1 did not show a significant change among the three groups (P > 0.05), while the mRNA expression of PECPK and GLUT2 was significantly increased in the 0.1% nano-curcumin group compared with the control group (P < 0.05).
Figure 4 Effect of dietary nano-curcumin on glucose transporter 2 (glut2) (A), glucokinase (gk) (B), phosphofructokinase liver type (pfk) (C), pyruvate kinase (pk) (D), glucose-6-phosphatase catalytic subunit (G6pase) (E), fructose-bisphosphatase1 (fbp1) (F), and phosphoenolpyruvate carboxykinase (Pepck) (G) related gene mRNA levels in the liver of largemouth bass. Values (mean ± standard error of the mean, SEM) in bars that have the same letter are not significantly different (P > 0.05; Duncan’s test) between treatments (N = 9).
The relative expression of endoplasmic reticulum stress-related genes in the liver of largemouth bass is shown in Figure 5. Compared with the control group, the relative expression of GRP78, ATF6, IRE, and eIF2α in the 0.2% nano-curcumin group was significantly downregulated (P < 0.05).
Figure 5 Effect of dietary nano-curcumin on glucose-regulated protein78 (GRP78) (A), activating transcription Factor6 (ATF6) (B), inositol-requiring enzyme (IRE) (C), and eukaryotic initiation factor 2α (eIF2α) (D) related gene mRNA levels in the liver of largemouth bass. Values (mean ± standard error of the mean, SEM) in bars that have the same letter are not significantly different (P > 0.05; Duncan’s test) between treatments (N = 9).
In the present study, dietary nano-curcumin did not affect the growth performance of largemouth bass, which is consistent with Pirani et al. (2021) who found that dietary curcumin nanomicelles also had no effect on the growth performance of Cyprinus carpio (Pirani et al., 2021). However, the improvement of growth performance was observed in other animals with dietary nano-curcumin supplementation, such as mice, chicken, and white shrimp (Yu et al., 2016; Yu et al., 2018; Marchiori et al., 2019; Afifi et al., 2020; Bhoopathy et al., 2021). There is no consensus on plausible explanations for this discrepancy, which may be related to the size, species, feed composition, culture environment, or difference of nanocarriers.
It is well known that medicinal plants and their extracts can enhance the mucosal immunity of fish (Doan et al., 2020). As the first layer of the innate immune system, fish skin mucus contains different innate immune components, such as lysozyme, protease, alkaline phosphatase, lectin, catalase, and complement (Lazado and Caipang, 2015). In the present study, dietary supplementation of nano-curcumin could increase the activities of LYZ, POD, and AKP in skin mucus of largemouth bass. The same results were found in carp (Giri et al., 2019), Larimichthys crocea (Yu et al., 2015), and Labeo rohita (Sukumaran et al., 2016), indicating that nano-curcumin, as well as curcumin, could improve the mucosal immunity of fish, which might be related to its immune stimulation characteristics, but the exact mechanism of stimulating mucosal immunity of fish still needs to be further explored.
Superoxide dismutase (SOD) and catalase (CAT) are the evaluation indicators of the body’s antioxidant capacity, which are widely present in animals and can protect cells and prevent peroxidation (Lesser, 2006). Malondialdehyde (MDA) is a breakdown product of lipid peroxidation; it can indirectly reflect the degree of cell damage (Koruk et al., 2004). In this study, compared with the control group, the 0.2% nano-curcumin group did not significantly increase the activity of SOD and CAT in serum but significantly decreased the activity of MDA, which echoed the results of liver histopathology, indicating that the appropriate amount of nano-curcumin can improve the antioxidant ability of largemouth bass. The results were in accordance with the previous study, which confirmed that curcumin could improve the antioxidant ability of fish, such as grass carp (Jiang et al., 2016), rainbow trout (Yonar et al., 2019), and Nile tilapia (Mahmoud et al., 2017). This may be due to the molecular structure of curcumin which contains phenolic hydroxyl with strong ability to remove free radicals, and curcumin also significantly increased the activity of antioxidant enzymes, such as SOD and CAT (Ramassamy, 2006; Hewlings and Kalman, 2017; Moniruzzaman and Min, 2020). Moreover, previous studies have shown that nanoencapsulation can enhance the scavenging ability of curcumin on free radicals (Coradini et al., 2014), which may be one of the reasons why nano-curcumin improves the antioxidant capacity of largemouth bass.
As a facilitative glucose transporter, glucose transporter 2 (GLUT2) could promote the bidirectional transfer of glucose across the cell membrane. Together with sodium-glucose cotransporter 2, GLUT2 completes the absorption and transport of glucose, which is the first step in glucose metabolism (Klover and Mooney, 2004). In the present study, dietary addition of 0.1% nano-curcumin significantly upregulated the relative expression of GLUT2 in the liver of fish, indicating that glucose transporters transported glucose to hepatocytes for glycolysis. As the only metabolic pathway leading to glucose catabolism in all organisms, including fish, the important rate-limiting enzymes of glycolysis included GK, PFK, and PK (Seiliez et al., 2013; Ren et al., 2021). Our study found that the relative gene expressions of GK, PFK, and PK were significantly increased in the 0.2% nano-curcumin group compared with the control group, demonstrating that nano-curcumin could reduce serum glucose levels by promoting the expression of key rate-limiting enzyme genes in glycolysis. Similarly, previous studies have also reported that nano-curcumin upregulated the mRNA expression of GK and GLUT2 in diabetic mice (Afifi et al., 2020). Dietary supplementation of curcumin significantly reduced blood glucose levels and improved the liver glycogen content in diabetic db/db mice (Seo et al., 2008). In addition, the change in liver glycogen content is related to gluconeogenesis, which refers to the conversion of non-sugar substances into glucose or glycogen, and G6Pase, Fbp1, and PEPCK are important rate-limiting enzymes in the gluconeogenesis process (Cowey et al., 1977). In the present study, the addition of 0.2% nano-curcumin to the diet did not significantly reduce the relative expression of G6Pase, Fbp1, and PEPCK genes but significantly reduced the content of liver glycogen compared with the control group. These results were partially consistent with previous studies that curcumin could downregulate G6Pase and PEPCK gene expression in diabetic mice and inhibit gluconeogenesis in the liver (Kim et al., 2009; Zhao et al., 2017). Additional studies demonstrated that curcumin could inhibit glucose production in isolated mouse hepatocytes (Fujiwara et al., 2008). Based on the above results, we speculated that nano-curcumin could promote glucose transport and glycolysis, which may be the principal reason for the decrease in serum glucose and liver glycogen content.
Previous studies have shown that excessive carbohydrate intake can induce endoplasmic reticulum stress (ERS) in largemouth bass, which is caused by the accumulation of a large number of unfolded or misfolded proteins (Zhao et al., 2021a). GRP78 is considered to be the marker protein of ERS (Jiang et al., 2017). When the body is in the ERS state, the overexpression of GRP78 can promote the dissociation and activation of ATF6, IRE1, and protein kinase R (PKR)-like endoplasmic reticulum kinase (PERK), while the dissociated and activated PERK can promote the phosphorylation of eIF2α (Bánhegyi et al., 2007). In mice and cell models, curcumin has been proven to markedly alleviate ERS induced by multiple factors, such as lipopolysaccharide (Zhang et al., 2018, Xie X. et al., 2020), diabetes (Fan et al., 2013; Rashid et al., 2017), and alcohol (Mohan et al., 2019; Wang et al., 2019). Similarly, our study showed that dietary supplementation of 0.2% nano-curcumin significantly reduced the relative gene expression of GRP78, IRE, eIF2α, and ATF6 in the liver of largemouth bass, suggesting that nano-curcumin may alleviate endoplasmic reticulum stress, which is induced by long-term intake of carbohydrates. Due to the low number of studies on nano-curcumin, further research is needed to confirm our findings.
In conclusion, the study showed that dietary nano-curcumin had no significant effect on the growth performance and approximate components of largemouth bass, but the non-specific immunity was improved by increasing the activities of mucosal immune indexes. Adding 0.2% nano-curcumin to the diet can alleviate oxidative stress and endoplasmic reticulum stress induced by long-term carbohydrate intake diet, and at the same time, it can reduce serum glucose levels by increasing the expression of liver glucose transport and glycolysis genes, and improve glucose homeostasis.
The original contributions presented in the study are included in the article/supplementary material. Further inquiries can be directed to the corresponding author.
The study was approved by the animal research committees of Foshan University Animal Ethics Committee (approval number: 2020056).
XB and YyY designed the experiments. XB executed the experiments, analyzed the data, and wrote the manuscript. MC, YhY, and HL contributed to the sampling. YyY, HY, YY, and ND revised the manuscript. All authors contributed to the article and approved the submitted version.
This research was supported by the fund of the Guangdong Provincial Key Laboratory of Animal Molecular Design and Precise Breeding (2019B030301010), the Guangdong Basic and Applied Basic Research Foundation (2019A1515110068), and the Key-Area Research and Development Program of Guangdong Province (2019B110209005).
The authors declare that the research was conducted in the absence of any commercial or financial relationships that could be construed as a potential conflict of interest.
All claims expressed in this article are solely those of the authors and do not necessarily represent those of their affiliated organizations, or those of the publisher, the editors and the reviewers. Any product that may be evaluated in this article, or claim that may be made by its manufacturer, is not guaranteed or endorsed by the publisher.
The authors thank the Guangdong Key Laboratory of Animal Molecular Design and Precision Breeding for technical support with the research system.
Afifi M., Alkaladi A., Abomughaid M. M., Abdelazim A. M. (2020). Nanocurcumin Improved Glucose Metabolism in Streptozotocin-Induced Diabetic Rats: A Comparison Study With Gliclazide. Environ. Sci. Pollut. Res. Int. 27, 25271–25277. doi: 10.1007/s11356-020-08941-8
Ajani E. K., Orisasona O., Kazeem O. K., Osho F. E., Adeyemo A. O., Omitoyin B. O., et al. (2020). Growth Performance, Gut Ecology, Immunocompetence and Resistance of Oreochromis Niloticus Juveniles Fed Dietary Curcumin Longa. Ribarstvo. 78, 145–156. doi: 10.2478/cjf-2020-0014
Alagawany M., Farag M. R., Abdelnour S. A., Dawood M. A. O., Elnesr S. S., Dhama K. (2021). Curcumin and its Different Forms: A Review on Fish Nutrition. Aquaculture. 532, 726030. doi: 10.1016/j.aquaculture.2020.736030
AOAC (2003). Official Methods of Analysis of the Association of Official’s Analytical Chemists. 363 17th Edition, Association of Official Analytical Chemists (AOAC), Arlington, Virginia.
Bánhegyi G., Baumeister P., Benedetti A., Dong D., Fu Y., Lee A. S., et al. (2007). Endoplasmic Reticulum Stress. Ann. N. Y. Acad. Sci. 1113, 58–71. doi: 10.1196/annals.1391.007
Bhoopathy S., Inbakandan D., Rajendran T., Chandrasekaran K., Prabha S. B., Reddy B. A., et al. (2021). Dietary Supplementation of Curcumin-Loaded Chitosan Nanoparticles Stimulates Immune Response in the White Leg Shrimp Litopenaeus Vannamei Challenged With Vibrio Harveyi. Fish. Shellf. Immun. 117, 188–191. doi: 10.1016/j.fsi.2021.08.002
Bisht S., Feldmann G., Soni S., Ravi R., Karikar C., Maitra A., et al. (2007). Polymeric Nanoparticle-Encapsulated Curcumin (“Nanocurcumin”): A Novel Strategy for Human Cancer Therapy. J. Nanobiotechnol. 5, 3. doi: 10.1186/1477-3155-5-3
Chen Y. K., Chi S. Y., Zhang S., Dong X. H., Yang Q. H., Liu H. Y., et al. (2021). Replacement of Fish Meal With Methanotroph (Methylococcus Capsulatus, Bath) Bacteria Meal in the Diets of Pacific White Shrimp (Litopenaeus Vannamei). Aquaculture. 541, 736801. doi: 10.1016/j.aquaculture.2021.736801
Chen P., Wu X. F., Gu X., Han J., Xue M., Liang X. F. (2021). FoxO1 in Micropterus Salmoides: Molecular Characterization and its Roles in Glucose Metabolism by Glucose or Insulin-Glucose Loading. Gen. Comp. Endocrinol. 310, 113811. doi: 10.1016/j.ygcen.2021.113811
Coradini K., Lima F. O., Oliveira C. M., Chaves P. S., Athayde M. L., Carvalho L. M., et al. (2014). Co-Encapsulation of Resveratrol and Curcumin in Lipid-Core Nanocapsules Improves Their In Vitro Antioxidant Effects. Eur. J. Pharm. Biopharm. 88, 178–185. doi: 10.1016/j.ejpb.2014.04.009
Cowey C. B., Knox D., Walton M. J., Adron J. W. (1977). The Regulation of Gluconeogenesis by Diet and Insulin in Rainbow Trout (Salmo Gairdneri). Br. J. Nutr. 38, 463–470. doi: 10.1079/bjn19770111
Doan H. V., Hoseinifar S. H., Jaturasitha S., Dawood M. A. O., Harikrishnan R. (2020). The Effects of Berberine Powder Supplementation on Growth Performance, Skin Mucus Immune Response, Serum Immunity, and Disease Resistance of Nile Tilapia (Oreochromis Niloticus) Fingerlings. Aquaculture. 520, 734927. doi: 10.1016/j.aquaculture.2020.734927
Fan Y., Meng X. H., Chen W. L., Zhu L., Shen Y. L., Chen Y. Y. (2013). Protective Effect of Curcumin Against High Glucose-Induced Endoplasmic Reticulum Stress by Downregulation of COX-2 Expression in Huvecs. Cell Biol. Int. 32, S14. doi: 10.1016/j.cellbi.2008.01.069
Feng T., Wei Y. M., Lee R. J., Zhao L. (2017). Liposomal Curcumin and its Application in Cancer. Int. J. Nanomed. 12, 6027–6044. doi: 10.2147/ijn.S132434
Fujiwara H., Hosokawa M., Zhou X., Fujimoto S., Fukuda K., Toyoda K., et al. (2008). Curcumin Inhibits Glucose Production in Isolated Mice Hepatocytes. Diabetes Res. Clin. Pract. 80, 185–191. doi: 10.1016/j.diabres.2007.12.004
Giri S. S., Sukumaran V., Park S. C. (2019). Effects of Bioactive Substance From Turmeric on Growth, Skin Mucosal Immunity and Antioxidant Factors in Common Carp, Cyprinus Carpio. Fish. Shellf. Immun. 92, 612–620. doi: 10.1016/j.fsi.2019.06.053
Guo J. L., Kuang W. M., Zhong Y. F., Zhou Y. L., Chen Y. J., Lin S. M. (2020). Effects of Supplemental Dietary Bile Acids on Growth, Liver Function and Immunity of Juvenile Largemouth Bass (Micropterus Salmoides) Fed High-Starch Diet - ScienceDirect. Fish. Shellf. Immun. 97, 602–607. doi: 10.1016/j.fsi.2019.12.087
Hewlings S. J., Kalman D. S. (2017). Curcumin: A Review of Its Effects on Human Health. Foods. 6, 92. doi: 10.3390/foods6100092
Hoseinifar S. H., Sharifian M., Vesaghi M. J., Khalili M., Esteban M. A. (2014). The Effects of Dietary Xylooligosaccharide on Mucosal Parameters, Intestinal Microbiota and Morphology and Growth Performance of Caspian White Fish (Rutilus Frisii Kutum) Fry - ScienceDirect. Fish. Shellf. Immun. 39, 231–236. doi: 10.1016/j.fsi.2014.05.009
Jiang Q., Chen S., Ren W. K., Liu G., Yao K., Wu G. y., et al. (2017). Escherichia Coli Aggravates Endoplasmic Reticulum Stress and Triggers CHOP-Dependent Apoptosis in Weaned Pigs. Amino Acids 49, 2073–2082. doi: 10.1007/s00726-017-2492-4
Jiang J., Wu X. Y., Zhou X. Q., Feng L., Liu Y., Jiang W. D., et al. (2016). Effects of Dietary Curcumin Supplementation on Growth Performance, Intestinal Digestive Enzyme Activities and Antioxidant Capacity of Crucian Carp Carassius Auratus. Aquaculture. 463, 174–180. doi: 10.1016/j.aquaculture.2016.05.040
Ji R. L., Xiang X. J., Li X. S., Mai K. S., Ai Q. H. (2021). Effects of Dietary Curcumin on Growth, Antioxidant Capacity, Fatty Acid Composition and Expression of Lipid Metabolism-Related Genes of Large Yellow Croaker Fed a High-Fat Diet. Br. J. Nutr. 126, 345–354. doi: 10.1017/s0007114520004171
Kim T., Davis J., Zhang A. J., He X., Mathews S. T. (2009). Curcumin Activates AMPK and Suppresses Gluconeogenic Gene Expression in Hepatoma Cells. Biochem. Biophys. Res. Commun. 388, 377–382. doi: 10.1016/j.bbrc.2009.08.018
Klover P. J., Mooney R. A. (2004). Hepatocytes: Critical for Glucose Homeostasis. Int. J. Biochem. Cell Biol. 36, 753–758. doi: 10.1016/j.biocel.2003.10.002
Koruk M., Taysi S., Savas M. C., Yilmaz O., Akcay F., Karakok M. (2004). Oxidative Stress and Enzymatic Antioxidant Status in Patients With Nonalcoholic Steatohepatitis. Ann. Clin. Lab. Sci. 34, 57–62.
Lazado C. C., Caipang C. M. A. (2015). Nutritional Impacts on Fish Mucosa: Immunostimulants, Pre- and Probiotics. Mucosal Health Aquacult. 211-272. doi: 10.1016/B978-0-12-417186-2.00009-1
Lesser M. P. (2006). Oxidative Stress in Marine Environments: Biochemistry and Physiological Ecology. Annu. Rev. Physiol. 68, 253–278. doi: 10.1146/annurev.physiol.68.040104.110001
Li S. L., Sang C. Y., Turchini G. M., Wang A., Zhang J. C., Chen N. S. (2020). Starch in Aquafeeds: The Benefits of a High Amylose to Amylopectin Ratio and Resistant Starch Content in Diets for the Carnivorous Fish, Largemouth Bass (Micropterus Salmoides). Br. J. Nutr. 124, 1145–1155. doi: 10.1017/S0007114520002214
Liu Z. K., Chen N. S., Wang M. L., Lian X. Y., Yin J. (2017). Suitable Dietary Starch Source and Supplementation Level for Largemouth Bass (Micropterus Salmoides). J. Fish. Sci. China. 24, 317–331. doi: 10.3724/SP.J.1118.2017.16111
Mahmoud H. K., Al-Sagheer A. A., Reda F. M., Mahgoub S. A., Ayyat M. S. (2017). Dietary Curcumin Supplement Influence on Growth, Immunity, Antioxidant Status, and Resistance to Aeromonas Hydrophila in Oreochromis Niloticus. Aquaculture. 475, 16–23. doi: 10.1016/j.aquaculture.2017.03.043
Maiti K., Mukherjee K., Gantait A., Saha B. P., Mukherjee P. K. (2007). Curcumin-Phospholipid Complex: Preparation, Therapeutic Evaluation and Pharmacokinetic Study in Rats. Int. J. Pharm. 330, 155–163. doi: 10.1016/j.ijpharm.2006.09.025
Marchiori M. S., Oliveira R. C., Souza C. F., Baldissera M. D., Ribeiro Q. M., Wagner R., et al. (2019). Curcumin in the Diet of Quail in Cold Stress Improves Performance and Egg Quality. Anim. Feed. Sci. Tech. 254, 114192. doi: 10.1016/j.anifeedsci.2019.05.015
Ministry of Agriculture and Rural Affairs of China (2021). China Fishery Statistics Yearbook (Beijing: China Agriculture Press).
Mohan R., Jose S., Sukumaran S., Asha S., Sheethal S., John G., et al. (2019). Curcumin-Galactomannosides Mitigate Alcohol-Induced Liver Damage by Inhibiting Oxidative Stress, Hepatic Inflammation, and Enhance Bioavailability on TLR4/MMP Events Compared to Curcumin. J. Biochem. Mol. Toxic. 33, e22315. doi: 10.1002/jbt.22315
Moniruzzaman M., Min T. (2020). Curcumin, Curcumin Nanoparticles and Curcumin Nanospheres: A Review on Their Pharmacodynamics Based on Monogastric Farm Animal, Poultry and Fish Nutrition. Pharmaceutics. 12, 447. doi: 10.3390/pharmaceutics12050447
Nm J., Joseph A., Maliakel B., Im K. (2018). Dietary Addition of a Standardized Extract of Turmeric (TurmaFEEDTM) Improves Growth Performance and Carcass Quality of Broilers. J. Anim. Sci. Technol. 601, 8. doi: 10.1186/s40781-018-0167-7
Pirani F., Moradi S., Ashouri S., Johari S. A., Ghaderi E., Kim H. P., et al. (2021). Dietary Supplementation With Curcumin Nanomicelles, Curcumin, and Turmeric Affects Growth Performance and Silver Nanoparticle Toxicity in Cyprinus Carpio. Environ. Sci. Pollut. Res. Int. 28, 64706–64718. doi: 10.1007/s11356-021-15538-2
Ramassamy C. (2006). Emerging Role of Polyphenolic Compounds in the Treatment of Neurodegenerative Diseases: A Review of Their Intracellular Targets. Eur. J. Pharmacol. 545, 51–64. doi: 10.1016/j.ejphar.2006.06.025
Rashid K., Chowdhury S., Ghosh S., Sil P. C. (2017). Curcumin Attenuates Oxidative Stress Induced Nfκb Mediated Inflammation and Endoplasmic Reticulum Dependent Apoptosis of Splenocytes in Diabetes. Biochem. Pharmacol. 143, 140–155. doi: 10.1016/j.bcp.2017.07.009
Ren X., Wang L. X., Chen Z. L., Hou D. Z., Xue Y., Diao X. M., et al. (2021). Foxtail Millet Improves Blood Glucose Metabolism in Diabetic Rats Through PI3K/AKT and NF-κb Signaling Pathways Mediated by Gut Microbiota. Nutrients. 13, 1837. doi: 10.3390/nu13061837
Seiliez I., Médale F., Aguirre P., Larquier M., Lanneretonne L., Alami-Durante H., et al. (2013). Postprandial Regulation of Growth- and Metabolism-Related Factors in Zebrafish. Zebrafish. 10, 237–248. doi: 10.1089/zeb.2012.0835
Seo K. I., Choi M. S., Jung U. J., Kim H. J., Yeo J., Jeon S. M., et al. (2008). Effect of Curcumin Supplementation on Blood Glucose, Plasma Insulin, and Glucose Homeostasis Related Enzyme Activities in Diabetic Db/Db Mice. Mol. Nutr. Food Res. 52, 995–1004. doi: 10.1002/mnfr.200700184
Shehata Y., Mansour M., Shadad S., Metwally M., Arisha A. (2020). Anti-Diabetic Effects of Curcumin-Magnesium Oxide Nanoparticles Conjugate in Type 2 Diabetic Rats. Zagazig. Veter. J. 48, 263–272. doi: 10.21608/zvjz.2020.23007.1098
Sukumaran V., Park S. C., Giri S. S. (2016). Role of Dietary Ginger Zingiber Officinale in Improving Growth Performances and Immune Functions of Labeo Rohita Fingerlings. Fish. Shellf. Immun. 57, 362–370. doi: 10.1016/j.fsi.2016.08.056
Wang B. Y., Gao X. L., Liu B. G., Li Y. C., Bai M., Zhang Z. Q., et al. (2019). Protective Effects of Curcumin Against Chronic Alcohol-Induced Liver Injury in Mice Through Modulating Mitochondrial Dysfunction and Inhibiting Endoplasmic Reticulum Stress. Food Nutr. Res. 63. doi: 10.29219/fnr.v63.3567
Xie X., Peng X. Y., Wang X. H. (2020). Effects of Curcumin on Endoplasmic Reticulum Stress PERK-CHOP Signaling Pathway in Pancreas of Type 2 Diabetic Rats. Int. J. Tradition. Chin. Med. 42, 871–875. doi: 10.3760/cma.j.cn115398-20190916-00088
Xie S. W., Yin P., Tian L. X., Liu Y. J., Niu J. (2020). Lipid Metabolism and Plasma Metabolomics of Juvenile Largemouth Bass Micropterus Salmoides Were Affected by Dietary Oxidized Fish Oil. Aquaculture. 522, 735158. doi: 10.1016/j.aquaculture.2020.735158
Yang K. Y., Lin L. C., Tseng T. Y., Wang S. C., Tsai T. H. (2007). Oral Bioavailabilit of Curcurnin in Rat and the Herbal Analysis From Curcuma Longa by LC-Ms/MS. J. Chromatogr. B. Analyt. Technol. BioMed. Life Sci. 853, 183–189. doi: 10.1016/j.jchromb.2007.03.010
Yonar M. E., Yonar S. M., İspir Ü., Ural M.Ş. (2019). Effects of Curcumin on Haematological Values, Immunity, Antioxidant Status and Resistance of Rainbow Trout (Oncorhynchus Mykiss) Against Aeromonas Salmonicida Subsp. Achromogenes. Fish. Shellf. Immun. 89, 83–90. doi: 10.1016/j.fsi.2019.03.038
Yu Y. Y., Chen S. J., Chen M., Tian L. X., Niu J., Liu Y. J., et al. (2016). Effect of Cadmium-Polluted Diet on Growth, Salinity Stress, Hepatotoxicity of Juvenile Pacific White Shrimp (Litopenaeus Vannamei): Protective Effect of Zn(II)-Curcumin. Ecotoxicol. Environ. Saf. 125, 176–183. doi: 10.1016/j.ecoenv.2015.11.043
Yu J., Chen Q. T., Li S. Y., Hu L. J., Hu K., Zhang J. N., et al. (2015). Effects of Curcumin on Growth and non-Specific Immunity of Pseudosciaena Crocea. J. South. Agriculture. 46, 7. doi: 10.3969/j:issn.2095-1191.2015.7.1315
Yu Y. Y., Niu J., Yin P., Mei X. T., Liu Y. J., Tian L. X., et al. (2018). Detoxification and Immunoprotection of Zn(II)-Curcumin in Juvenile Pacific White Shrimp (Litopenaeus Vannamei) Feed With Aflatoxin B1. Fish. Shellf. Immun. 80, 480–486. doi: 10.1016/j.fsi.2018.05.034
Zhang M., Tang J. J., Xie Y. Y., Zhang J., Zhang Q. H., Yang X., et al. (2018). Effect of Curcumin on Endoplasmic Reticulum Stress of Alveolar Epithelial Cells in a Rat Model of Chronic Obstructive Pulmonary Disease. J. Xi’an. Jiaotong. Univ. (Medical. Sciences). 393, 5. doi: 10.7652/jdyxb201803014
Zhang Y. M., Xie S. W., Wei H. L., Zheng L., Liu Z., Fang H., et al. (2020). High Dietary Starch Impaired Growth Performance, Liver Histology and Hepatic Glucose Metabolism of Juvenile Largemouth Bass, Micropterus Salmoides. Aquacult. Nutr. 26, 1083–1095. doi: 10.1111/anu.13066
Zhao L. L., Liang J., Chen F. K., Tang X. H., Liao L., Liu Q., et al. (2021a). High Carbohydrate Diet Induced Endoplasmic Reticulum Stress and Oxidative Stress, Promoted Inflammation and Apoptosis, Impaired Intestinal Barrier of Juvenile Largemouth Bass (Micropterus Salmoides). Fish. Shellf. Immun. 119, 308–317. doi: 10.1016/j.fsi.2021.10.019
Zhao X. J., Li L. Y., Li L., Tang C. ,. Y., Li H. Z., Liu J. T., et al. (2017). Improving Effect of Curcumin Analogue H8 on Glucose and Lipid Metabolism Disorder in Db/Db Mice. Herald Med. 36, 1354–1358. doi: 10.3870/j.issn.1004-0781.2017.12.005
Keywords: nano-curcumin, immunity, antioxidant, glucose, endoplasmic reticulum stress, largemouth bass
Citation: Bao X, Chen M, Yue Y, Liu H, Yang Y, Yu H, Yu Y and Duan N (2022) Effects of Dietary Nano-Curcumin Supplementation on Growth Performance, Glucose Metabolism, and Endoplasmic Reticulum Stress in Juvenile Largemouth Bass, Micropterus salmoides. Front. Mar. Sci. 9:924569. doi: 10.3389/fmars.2022.924569
Received: 20 April 2022; Accepted: 10 May 2022;
Published: 09 June 2022.
Edited by:
Shiwei Xie, Guangdong Ocean University, ChinaReviewed by:
Xianquan Chen, Guangdong University of Petrochemical Technology, ChinaCopyright © 2022 Bao, Chen, Yue, Liu, Yang, Yu, Yu and Duan. This is an open-access article distributed under the terms of the Creative Commons Attribution License (CC BY). The use, distribution or reproduction in other forums is permitted, provided the original author(s) and the copyright owner(s) are credited and that the original publication in this journal is cited, in accordance with accepted academic practice. No use, distribution or reproduction is permitted which does not comply with these terms.
*Correspondence: Yingying Yu, eXV5eTk5OUB5ZWFoLm5ldA==
Disclaimer: All claims expressed in this article are solely those of the authors and do not necessarily represent those of their affiliated organizations, or those of the publisher, the editors and the reviewers. Any product that may be evaluated in this article or claim that may be made by its manufacturer is not guaranteed or endorsed by the publisher.
Research integrity at Frontiers
Learn more about the work of our research integrity team to safeguard the quality of each article we publish.