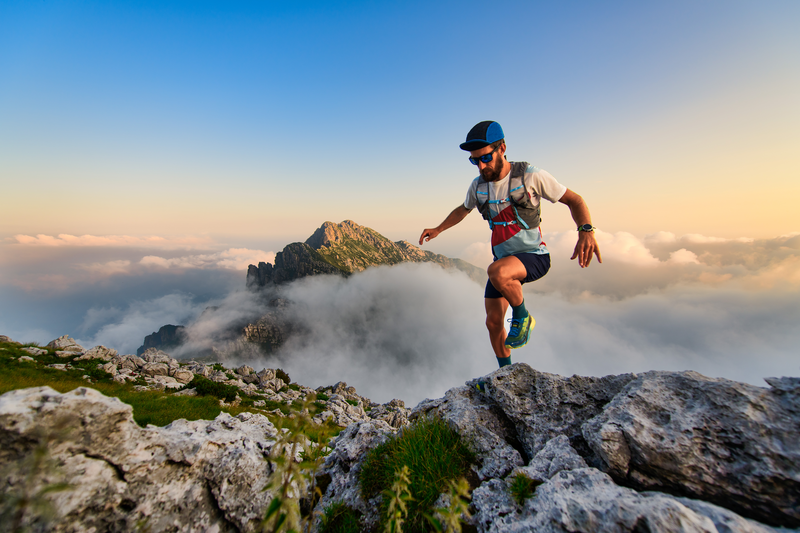
95% of researchers rate our articles as excellent or good
Learn more about the work of our research integrity team to safeguard the quality of each article we publish.
Find out more
ORIGINAL RESEARCH article
Front. Mar. Sci. , 15 June 2022
Sec. Marine Pollution
Volume 9 - 2022 | https://doi.org/10.3389/fmars.2022.923494
This article is part of the Research Topic Coastal Environmental and Ecological Data Analysis View all 30 articles
Heavy metal pollution in the mariculture areas is of considerable attention due to its potential ecological effects and public concern for seafood safety. A better understanding of the current contamination status and historical trend of heavy metals in the ecosystems of mariculture areas has an important implication for the sustainable development of marine ecosystems and for public health concerns. To assess the impact of human activities on heavy metal pollution in the mariculture area, seven metals (Cu, Pb, Zn, Cd, Hg, As, and Cr) and the environmental parameters were seasonally investigated in the surface seawater and sediments in Qinzhou Bay, a typical mariculture bay in South China. Seasonal variations in the concentration of heavy metals were found in both seawater and sediment, which are mainly influenced by seasonal hydrological change, biological activity, and human influence. The concentration of heavy metals in the seawater was at a relatively higher level than that of other mariculture areas in China, while a lower level was found in the sediment. The concentration of Cu increased in both seawater and sediment for the past decades (by nearly 2 times), which is mainly influenced by the mariculture and shipping activities. The concentration of Hg decreased significantly in the seawater for the past 40 years (decreased by 13 times) due to the decrease in production and usage of Hg. However, the concentrations of Pb, Zn, and Cd in seawater showed an increasing trend in the mariculture bay, while the concentrations of Pb, Zn, As, and Cr decreased in the sediment over the past decades. The decrease in pH value (decreasing by 4.7% for the past 20 years) was responsible for the different trend of those metals between seawater and sediment because the decrease in pH could re-release metals from sediments into the water column. Hg and As are the main ecological risk factors in the mariculture bay. This study suggests that environmental changes, such as ocean acidification, affect the distribution of metals in seawater and sediments, which we should be more vigilant and concerned about under the global climate change.
Heavy metal pollution has become a global concern because of their high toxicity, persistence, and bioaccumulation (Duodu et al., 2016; Gong et al., 2018; Alengebawy et al., 2021). The coastal ecological environment is now facing a significant risk due to heavy metal pollution caused by intensive human activities (Wang et al., 2013; Lao et al., 2019; Adyasari et al., 2021; Li et al., 2022). Although many countries have made great efforts to reduce the anthropogenic discharges of heavy metals for the past decades, high levels of heavy metals can still be found in various environmental mediums around the world due to their persistence, particularly in the coastal areas (Chen et al., 2018; Wu et al., 2018; Cai et al., 2019; Kumar et al., 2019; Yan et al., 2022). Excessive amounts of heavy metals in the coastal environment would adversely affect the health of marine organisms and eventually threaten human life (Castro-González and Méndez-Armenta, 2008; Achary et al., 2017; Mishra et al., 2019; Liu et al., 2021).
Seafood from mariculture is an important animal protein source for humans and is a developing industry that greatly contributes to the national economy (Wang et al., 2016). With the global climate change and the continuous expansion of the mariculture scale, various environmental problems have also appeared in the mariculture areas, such as eutrophication (Baquiran and Conaco, 2018; Lao et al., 2021a), hypoxia, ocean acidification (Clements and Chopin, 2017; Ng and Chiu, 2020), and heavy metal pollution (Wang et al., 2016; Liu et al., 2020). Excessive heavy metals are discharged into the mariculture area, which strongly cause accumulation and biomagnification by aquaculture organisms along the water, sediment, and aquatic food (Islam and Tanaka, 2004; Jiang et al., 2018). Moreover, sediments are not only the sink for heavy metals in marine environments but also the source of heavy metals by the disturbance of bottom water and the changes in environmental conditions, such as pH (Ma et al., 2016) because the absorbed heavy metals can be released again with the decrease in pH value in the environment (Kashem and Singh, 2001). Under the stress of anthropogenic input and environmental change, the issue of heavy metal pollution in mariculture areas has become more and more serious. Thus, a better understanding of the current degree of contamination and historical trend of heavy metals in the ecosystems of mariculture areas has an important implication for the seafood industry, the sustainable development of marine ecosystems, and public health concerns.
Qinzhou Bay, located in the northern Beibu Gulf, South China Sea, is one of the most important mariculture grounds in the coast of the South China Sea. The bay is influenced by the discharge of several rivers and tidal influx from outer Beibu Gulf and constitutes a good estuary–bay multi-ecosystem with rich biological diversity (Xu et al., 2020 and Xu et al., 2021). However, with the rapid development of urbanization and industrialization around the coast regions in the Beibu Gulf, a large amount of terrestrial contaminant input resulted in the bay facing many environmental problems now (Gu et al., 2015; Lao et al., 2021a; Lao et al., 2021b and Li et al., 2018; Liu et al., 2020; Lao et al., 2021c). Rivers around the Qinzhou Bay discharged a large amount of terrestrial contaminants into the bay every year, and the contaminant input by the river has shown an increasing trend in the recent two decades (Lao et al., 2020). This resulted in the eutrophication increase over the past 40 years (increased by 18 times) (Lao et al., 2021a), and the pollution of heavy metals in Qinzhou Bay is more serious when compared with that of other bays in Beibu Gulf (Lao et al., 2019; Liu et al., 2020). In addition, a higher level of heavy metal pollution in seawater was found in the Maowei Sea (Upper Qinzhou Bay, Figure 1) among the four main mariculture areas in the Guangxi Beibu Gulf, and the level of some metals, such as Cu, was much higher than that found in the past 20 years (Liu et al., 2020). In sediments, high concentrations of Pb, Cr, and Cu were observed in Qinzhou Bay, which is not suitable for mariculture, endangered species reserves, and nature reserves (Gu et al., 2015). However, systematic studies on heavy metal pollution in seawater and sediments, including the estuary and the mariculture areas, have not been reported. The dynamic processes and the internal factors causing the changes of heavy metals between seawater and sediments in the bay are still unclear, which is significantly important for the sustainable development of the mariculture and seafood industry.
Figure 1 Study area and the sampling stations in the estuarine and the adjacent sea in Qinzhou Bay. The red box in the left is the sampling area, the red dots in the right are the seawater sampling stations, and the blue crosses in the right are the sediment sampling stations. SM, summer monsoon; WM, winter monsoon.
In this study, the seasonal concentrations of metals and other physicochemical parameters in seawater and sediments of the Jingu River Estuary and the adjacent mariculture area in Qinzhou Bay were investigated and combined with the historical data to identify the occurrence, potential sources, historical trend and its influencing factors, and ecological risk of heavy metals in the mariculture bay.
Qinzhou Bay is s semi-enclosed bay in the northern Beibu Gulf, located at the northwest of South China Sea (Figure 1). The area of Qinzhou Bay is 908 km 2, and the water depth is mostly <10 m. The climate in the bay is controlled by the East Asian Monsoon, with the monthly air temperature ranging from 13.9 to 28.6°C (average of 22.5°C) (http://data.cma.cn/site/index.html). The annual rainfall is 2,174 mm, of which over 83% falls during the rainy season (from April to September), and the rainfall increased significantly from April [average of 113.8 mm in April, which is significantly higher than that from October to March (average of 54.8 mm)] and decreased significantly after October (http://data.cma.cn/site/index.html). Due to the unique ecological environment and mixture of freshwater and saltwater, Qinzhou Bay and the Maowei Sea in the north of the bay have been used for mariculture over 50 years, and these regions are the largest natural oyster seeding (~2,340 hm2) and mariculture bay in China (Yang et al., 2019; Xu et al., 2020). The bay represents a significant economic developing area, which has been greatly influenced by anthropogenic activities (Xu et al., 2021).
In this study, two cruises were conducted in the Jingu River Estuary and the adjacent mariculture area in Qinzhou Bay in winter (December 2019, dry season) and spring (the end of April 2020, early rainy season), respectively. A total of 20 seawater stations (20 seawater samples) and 11 sediment stations (11 seawater samples) were investigated during the dry season and early rainy season, respectively (Figure 1). In addition, the historical data of the surface water and sediment in 2010 and 2013 were collected in a similar station in Qinzhou Bay (data obtained from the Marine Environmental Monitoring Centre of Beihai, State Oceanic Administration). Surface seawaters (depth of 0.5 m) were collected using a rosette sampler fitted with a 5-L Niskin bottle and then transferred into acid-cleaned polypropylene bottles. The temperature, salinity, dissolved oxygen (DO), and pH were measured on-site. For the chlorophyll a (Chl a) sample, about 1,000 ml seawater was filtered using glass fiber filters (GF/F, 0.7μm, Whatman) and stored at -20°C until further analysis. The total suspended particulate matter (TSM) samples were filtered through pre-weighed GF/F. For the heavy metal analysis, the seawater samples were filtered through acid-cleaned cellulose acetate filters (0.45-μm pore size and 47-mm diameter, Whatman), and then the filtrate was placed into 250-ml acid-cleaned polyethylene bottles, and HNO3 was added to the filtrate to achieve a pH of approximately 2. Finally, the filtrate was stored at -20°C until further analysis. Surface sediment (0–5 cm) was collected using a grab sampler. The upper sediment samples (1 to 2 cm) were removed using an acid-washed plastic spoon to avoid any pollution from the metallic sampler, and the sediment sample was placed into a polyethylene bag and stored at -20°C until further analysis. Six metals (Cu, Pb, Zn, Cd, Hg, and As) and seven metals (Cu, Pb, Zn, Cd, Hg, As, and Cr) were measured in the seawater and sediment samples, respectively. The pretreatment and laboratory analyses for the heavy metal samples were conducted according to the method by Lao et al. (2019). The measurement of Cu, Pb, Zn, Cd, As, and Cr in the sediment samples were oven-dried at 105°C for ~72 h, and the sediment samples for Hg were dried naturally at room temperature (24°C). After drying, the sediment samples were ground with a pestle and agate mortar and then sieved through an 80-mesh sieve for Hg and a 160-mesh sieve for Cu, Pb, Zn, Cd, As, and Cr.
Salinity was measured using a salinometer (SYA2-2, Beijing, China), and pH in the field was measured using a pH meter (PHS-3C, Shanghai, China). The DO samples were analyzed by the Winkler titration method with a precision of 0.07 mg L-1. The chemical oxygen demand samples were measured by the potassium permanganate oxidation method with a precision of 0.15 mg L-1. The Chl a samples were extracted using 90% acetone solution (v/v) and measured by a spectrophotometer (Turner Designs Fluorometer, Model 10AU, Shanghai, China). The total organic matter (TOC) in the sediment samples were freeze-dried for the analysis, and the level was determined using the potassium dichromate oxidation–ferrous titration method (GAQS-IQ, 2008). The detection limit of TOC was 0.01%. The sediment samples for the grain size were pretreated according to the Chinese National Standard (GB/T12763.8-2007), and the granulometry was determined by a laser diffractometer (Malvern Mastersizer 3000, UK).
The analysis of heavy metal in the seawater and sediment samples in this study was conducted according to Lao et al. (2019). The measurements of Cu, Pb, Zn, and Cd in seawater were used in anodic stripping voltammetry (797 VA Computrace, Wantong, Switzerland). The measurements of Hg and As were used in atomic fluorescence spectrometry (ASF-9530, Beijing Haiguang Instrument, China). The detection limits of Cu, Pb, Zn, Cd, Hg, and As in the seawater samples were 0.6, 0.1, 1.0, 0.01, 0.007, and 0.5 µg L-1, respectively. During the analysis of heavy metals in the sediment, 0.5 g of processed sediment samples was digested with a mixture containing HCl and HNO3 at 95°C for 1 h and then diluted to 50 ml with distilled water before the analysis. Cr, Pb, Zn, Cu, and Cd were measured by atomic absorption spectrometry (ZEENH700P, Jena, German). As and Hg were measured by atomic fluorescence spectrometry (ASF-9530, Beijing Haiguang Instrument, China). The detection limits of Cu, Pb, Zn, Cd, Cr, Hg, and As were 2.0, 1.0, 6.0, 0.04, 2.0, 0.002, and 0.06 µg g-1, respectively.
All glassware during the experimental procedure were soaked in nitric acid solution (HNO3, 1:3) for 7 days and then cleaned with ultra-pure water before use. To ensure high accuracy and precision, the number of duplicate samples, blanks, and certified reference material [GBW-07309 from the State Oceanographic Administration of China and GBW(E)-080040 from the Second Institute of Oceanography, Ministry of Natural Resources of China] accounted for 20% of samples in the batch digestion experiments. The results were all blank-corrected in this study. Duplicate samples were analyzed to check the reproducibility, and the relative difference was <5%. Spike recovery test was conducted to test the analytical procedures, and the recoveries in the standard were 99 ± 4% for Cu, 95 ± 5% for Pb, 102 ± 3% for Zn, 98 ± 3% for Cd, 95 ± 6% for Cr, 103 ± 6% for Hg, and 97 ± 3% for As.
The contaminant degree of heavy metals in seawater was assessed by the ecological risk index (ERI) (Hakanson, 1980). The equation is as follows:
where , , Ci, and Co represent each heavy metal potential ERI, toxic response factor (TRF), heavy metal value in the seawater, and standard value. The Co in this study adopted the National Standard of China for Seawater Quality GB 3097-1997, grades I and II (Table 1). According to this method, <40 and ERI <150 represent a low ecological risk, 40 < <80 and 150 < ERI <300 represent a moderate ecological risk, 80 < <160 and 300 < ERI <600 represent a high ecological risk, 160 < <320 represent a very high ecological risk, and ≥320 and ERI ≥600 represent an extremely high ecological risk. The TRF of Cu, Pb, Zn, Cd, Hg, and As are 5, 5, 1, 30, 40, and 10, respectively (Liu et al., 2020).
Table 1 Summary of the average values of physico-chemical parameters in the seawater of different areas during spring and winter.
The contaminant degree of heavy metals in the sediment was assessed by the pollution load index (PLI), geoaccumulation index (Igeo), and enrichment factor (EF). The background values of Cu, Pb, Zn, Cd, Cr, Hg, and As in the sediment were from the Beibu Gulf, and the values were 15.8, 28.9, 75.8, 0.09, 35.0, 0.029, and 7.8 µg g-1, respectively (Lao et al., 2019).
The PLI, Igeo, and EF values were calculated according to Islam et al. (2015) and Lao et al. (2019). The PLI value was calculated as follows:
where CF denotes the ratio of heavy metal concentration and background value (CF = Cmeasured/Cbackground). A PLI value of 0 represents no contamination, a value of 1 represents the presence of only a baseline level of contamination, and >1 represents progressive deterioration. In addition, a CF value <1 represents a low degree, 1 ≤ CF ≤3 represents a moderate degree, 3 ≤ CF ≤6 represents a considerable degree, and CF ≥6 represents a very high degree (Lao et al., 2019).
The Igeo value was calculated as follows:
where Cn represents the measured value of each metal in the field, and Bn represents the background value of each metal. Igeo ≤0 represents being practically uncontaminated, 0 ≤ Igeo ≤1 represents low contamination, 1 ≤ Igeo ≤2 represents moderate contamination, 2 ≤ Igeo ≤3 represents moderate to heavy contamination, 3 ≤ Igeo ≤4 represents heavy contamination, 4 ≤ Igeo ≤5 represents heavy to extreme contamination, and Igeo ≥5 represents extreme contamination (Islam et al., 2015; Lao et al., 2019).
The variations of the physico-chemical parameters in Qinzhou Bay are presented in Figure 2 and Table 1. The temperature in the mariculture area was higher than that in the estuary area during winter, but the opposite feature was found in spring (Figure 2A). Salinity exhibited an upward trend from the upper estuary to the mariculture area during both seasons, with higher salinity in the dry season (ranging from 27.59 to 30.75) and lower salinity in the early rainy season (ranging from 23.11 to 27.99) (Figure 2B). This suggested that the seawater intruded into the estuary with the decrease in runoff due to the low rainfall in the dry season. The pH and DO values were higher than those in the estuary area during both seasons (Figures 2C, D). The TSM concentration in spring was higher than that in winter, while there was a little difference between the values of the estuary and mariculture area during both seasons (Figure 2E). The Chl a levels were all higher in spring and lower in winter (Figure 2F). During spring, phytoplankton blooms occurred in the mariculture area (Figure 2F). There was a little difference in the pH values in the sediment between winter (average of 7.67) and spring (average of 7.67), but the value in the mariculture area was higher than that in the estuary area during both seasons. Notably, the pH values in Qinzhou Bay decreased during the rainy seasons for the past 20 years, decreasing by 4.7% (Figure 3). The TOC concentrations in the sediment during spring (average of 1.53 µg g-1) were higher than those in winter (average of 1.04 µg g-1). Figure 4 displays the composition of the grain size of the sediments. The composition of the grain size of the sediments is similar during both seasons, and silt- and sand-sized grains mainly exist in the two regions.
Figure 2 Variations of the physico-chemical parameters in the seawater of the estuary and mariculture area in Qinzhou Bay during winter and spring.
Figure 3 Variations of pH values during the rainy seasons for the past 20 years. Data obtained from the Marine Environmental Monitoring Centre of Beihai, State Oceanic Administration.
Figure 4 Seasonal and spatial variations of grain size composition in the surface sediment of the estuary and mariculture area in Qinzhou Bay.
The concentrations of six metals in the surface seawater are presented in Figure 5 and Table 2. The average concentration of heavy metals followed a decreasing order of Zn > Cu > Pb > As > Cd > Hg during both seasons. Generally, the concentrations of Cu, Pb, Zn, Cd, and As in winter were higher than those in spring, while the concentration of Hg in spring was higher than that in winter (Figure 5 and Table 2). The concentration of Cu in the mariculture area was higher than that in the estuary, while Zn exhibited an opposite trend during both seasons (Table 2). The other metals showed a little difference between mariculture and estuary area. The concentrations of Cu, Zn, Cd, Hg, and As were within the range of grade I in seawater, and the concentration of Pb was within the range of grade II in seawater (Table 2). Compared with the other areas, the concentrations of heavy metals were generally lower than those in developed coastal areas, such as the Yellow River Estuary (Wang et al., 2018) and Guangdong coastal area (Zhang et al., 2015), while the level was higher than the other mariculture areas in China (Table 3)—for example, all metals except As are higher than those in Laoshan Bay (Wang et al., 2019); the concentrations of Pb, Zn, and Cd are higher than those in Zhanjiang Bay (Zhang et al., 2018) and Lianzhou Bay in northeastern Beibu Gulf (Liu et al., 2020) and Maowei Sea in the upper Qinzhou Bay (Liu et al., 2020); and all metals except Hg are higher than those in Pearl Bay in northwestern Beibu Gulf (Liu et al., 2020). Notably, despite the fluctuation, the concentrations of Cu, Pb, Zn, and Cd showed an increasing trend in the seawater, while the concentration of Hg decreased over the past 40 years (Table 3).
Figure 5 Variations of heavy metals in the seawater of the estuary and mariculture area in Qinzhou Bay during winter and spring.
Table 2 Summary of the average value of heavy metals in the seawater (μg L-1) and sediment (µg g-1) of different areas in Qinzhou Bay during spring and winter.
The concentrations of seven metals in the surface sediments are presented in Figure 6 and Table 2. The average concentrations of the seven metals revealed a decreasing order of Zn > Cr > Cu > Pb > As > Cd > Hg during the spring, while the concentrations showed a decreasing order of Zn > Pb > Cr > As > Cu > Cd > Hg during the winter (Table 2). The different orders suggested that different metal sources may occur in different seasons. The concentrations of Cu, Zn, As, and Cr in spring were higher than those in winter, while Pb and Hg exhibited higher concentrations in winter but lower concentrations in spring. The concentration of Cd in winter was similar to that in spring. Differently from those in the seawater, the concentrations of heavy metals in the sediment were more complicated between mariculture and the estuary area. The concentration of Cu in the estuary area was higher than that in the mariculture area, while the concentration of Zn showed an opposite trend during both seasons (Table 2). The concentration of Pb in spring were higher in the estuary area, while higher concentrations in winter were found in the mariculture area. Other metals showed a little difference between mariculture and the estuary areas during both seasons. Differently from those in the seawater, the concentrations of heavy metals in the sediment were generally lower than those in other similar coastal mariculture bays and other coastal regions in China (Table 4). The concentrations of all metals in the sediment were within the range of grade I (Table 4). In contrast to the tendency of heavy metals in seawater, except for the concentration of Cu which increased, the concentrations of Pb, Zn, As, and Cr generally decreased in the sediment for the past 20 years, and the concentration of Hg in the sediment has a little change for the past years (Table 4).
Figure 6 Variations of heavy metals in the sediments of the estuary and mariculture area in Qinzhou Bay during winter and spring.
Table 4 Comparison of heavy metal concentrations (μg g-1) in surface sediments with those in other regions.
In the marine environment, the distribution and transportation of heavy metals are greatly influenced by the physicochemical processes (Lao et al., 2019) and the external inputs of heavy metals, including anthropogenic and terrestrial sources, sediment release, biogenic processes, and hydrography (Lao et al., 2019). To better understand the control factors for the distribution of heavy metals in the estuary and adjacent mariculture area in Qinzhou Bay, the relationships between heavy metals and environmental parameters in both seawater and sediment were analyzed, and the results are presented in Tables 5, 6. Zn is not related to the physicochemical factors in the seawater. Hg is negatively correlated with salinity, suggesting that the metal is greatly influenced by the terrestrial input. In contrast, there is a significantly positive relationship between Cu, Pb, Cd, and As, and they showed positive correlations with salinity while showing negative correlations with Hg, suggesting that the level of these metals may be influenced by the intrusion of seawater. Previous studies have found that, under the influence of the circulation of Beibu Gulf, a high concentration of heavy metals in the other areas can be introduced into the coastal seawaters of Guangxi Beibu Gulf (Lao et al., 2019; Liu et al., 2020). Correspondingly, Cu, Pb, Cd, and As showed a significantly positive correlation with temperature, indicating that the intrusion of these metals into the coastal mariculture area mainly occurred in winter (dry season). During the dry season, the runoff around the coastal area decreased sharply (Lao et al., 2020; Lao et al., 2021b), and the seawater could intrude into the bay; thus, the salinity increased. In contrast, Hg showed a positive correlation with temperature, indicating that the increase of runoff in the rainy season (higher temperature) could introduce more land-based Hg into the bay. Hg showed a significantly negative correlation with pH. This suggested that, except for the terrestrial input, the Hg level in the estuary and the bay may also be influenced by the water environment. Pb and As showed a significantly negative correlation with TSM level, indicating that Pb and As may be absorbed into the particulate matter under the higher TSM level in the water. According to the partition coefficient (Kd) in the similar semi-enclosed bay in South China, a high ratio of particulate Pb to dissolved Pb was found (higher than other metals), indicating a stronger affinity between Pb and suspended particles (Zhang et al., 2018). A high partition coefficient of Pb was also found in Yangtze Estuary in East China (Feng et al., 2017). In addition, As would be more easily adsorbed onto TSM under the higher TSM level and salinity in the estuary areas (Feng et al., 2017). Although the salinity slightly decreased in spring, the salinity in the study area was still >23 during that season, which was favored by the metal adsorbed onto TSM (Wang and Liu, 2003). Thus, the lower level of Pb and As that was observed in spring may be influenced by the higher TSM level due to the increase of runoff in that season. In addition, Cu, Pb, Cd, and As showed positive correlations with DO, while Hg showed a negative correlation with DO. Pb showed a negative correlation with Chl a. These results suggest that these metals may present a close relationship with biological activities. The complexation prosperities of heavy metals with organic matter in the marine environment could result in the metal remaining in the water (Baeyens et al., 1998; Lao et al., 2019). In the sediment, Cu, Zn, and Cr showed significantly positive correlations with TOC, indicating that the TOC level greatly influenced their distribution and enrichment. There are significantly positive correlations between Cu, Pb, Cd, and Cr, indicating that there may be similar sources in the sediment. However, Hg showed a negative correlation with Cu, Cd, and TOC, suggesting that other factors influenced the distribution of Hg in the sediment.
Table 5 Correlation analysis between heavy metal concentrations and the physicochemical parameters in surface water.
Table 6 Correlation analysis between heavy metal concentrations and the environmental parameters in sediment.
To further evaluate the correlations and the possible origins of heavy metals in Qinzhou Bay, the principal component analysis (PCA) for heavy metals and the related environmental parameters in the seawater and sediment were analyzed in this study, and the results are presented in Figure 7. In the seawater, three principal components that accounted for 73.458% of the total data variance were identified. PC1 accounted for 47.696% of the variance, with high negative loadings for Hg and salinity and high positive loadings for Cu, Pb, and As. This further confirmed that those metals are influenced by their hydrological characteristics. Hg in the seawater may be influenced by the terrestrial inputs, and Cu, Pb, and As may be influenced by the intrusion of water with higher salinity from the outer bay. Pb is widely used as an antiknock agent in diesel fuel and anti-corrosive compound for shipping, Cu and As are constituents of some algaecides and fungicides, and the high concentrations of these two metals are usually found in mariculture areas (Wang et al., 2018). Thus, mariculture and shipping activities mainly contributed to PC1. PC2 accounted for 16.003%, with high negative loadings for Pb, pH, and Chl a. PC3 accounted for 9.759%, with high positive loadings for Zn, Cd, and Chl a. PC2 and PC3 indicated the influence of biological activities. The growth of phytoplankton would not only absorb some metals but also could induce the decomposition of organic matter that releases the metal into the water. The increase of phytoplankton (fresh and labile organic matter) tended to modify and, more generally, increase the decomposition rate of the more recalcitrant organic matter (Fontaine et al., 2003; Guenet et al., 2010). The increase in decomposition rate would result in more specific metals released into the water (Cotrufo et al., 1995; Luo et al., 2022). Thus, except from the influence of hydrological processes, biological activity is another important factor that influences the distribution of heavy metals in the seawater. In sediments, three principal components that account for 75.513% of the total variance were identified. PC1 accounted for 43.804% of the variance, with high positive loadings for Cu, Zn, Cr, and TOC and high negative loadings for Hg and TOC, further confirming that TOC was the main controlling factor for the distribution of these metals. PC2 accounted for 19.215% of the total variance, with high negative loadings for Cd and As, suggesting the different fate of these two metals in the sediment. Cd is widely used in chemical plants, and chemical material manufacturing discharges wastewater containing high Cd concentrations (Wang et al., 2018). Thus, Cd reflects the input of terrestrial and industrial sources, while As reflects mariculture activities. Generally, mariculture activity keeps away from urban and industrial wastewater outlets. PC3 accounted for 12.494%, with high negative loadings for Pb and pH, indicating that the sediment environment (acidity and alkalinity) was the main factor influencing the enrichment of Pb in the sediment.
Figure 7 Results of principal component analysis of heavy metal concentrations and environmental parameters in the estuary and the adjacent mariculture in Qinzhou Bay.
The concentration of Cu increased in both seawater and sediment of the mariculture area in Qinzhou Bay over the past years (Tables 3, 4). This is similar to the observation in Beibu Gulf (Lao et al., 2019; Liu et al., 2020). Cu is used as fertilizers and as additive in algaecides and agriculture fungicides (Burgos-Núñez et al., 2017; Wang et al., 2018; Naser, 2013). Previous studies suggested that the concentration of Cu showed a significantly increasing trend in both seawater and sediments of the whole Guangxi Beibu Gulf due to the influence of rapid industrial development and the enhancement and expansion of mariculture activities over the past years (Lao et al., 2019; Liu et al., 2020). In addition, due to the great toxicity and harm of Hg, the production of mercury began to decline as early as 1884, and the usage of Hg has also decreased significantly in the past years (Schuster et al., 2002; Pacyna et al., 2006). Thus, the decrease in production and usage of Hg may be responsible for the decrease in Hg concentration in the seawater over the past 40 years. Notably, the concentration of Hg showed a significantly negative correlation to pH, indicating that pH would directly affect the biosorption of metal ions (Atkinson et al., 2007; Ma et al., 2016; Lao et al., 2019).
However, different variation tendencies of some metals were observed in seawater and sediment according to the historical data. The concentrations of Pb, Zn, and Cd showed an increasing trend in the seawater of Qinzhou Bay for the past 40 years (Table 3), while the concentrations of Pb, Zn, As, and Cr in the sediment generally decreased for the past 20 years (Table 4). If the increase of terrestrial input and the enhancement of human activity intensity are the dominant factors that result in the increase of those metals in the mariculture area, the level of the metal in both seawater and sediment should be increased. In contrast, the level of some metals decreased in the sediment, indicating that other factors may control such an opposite trend between the seawater and the sediment. The change in the water environment in Qinzhou Bay may be an important factor causing the different change trends of heavy metals in seawater and sediments. For the past 20 years, the pH value of seawater in Qinzhou Bay has decreased significantly (Figure 3). In addition, the results of correlation and principal component analyses show that the pH value is the important parameter affecting the distribution of heavy metals in both seawater and sediment (Tables 5, 6 and Figure 7)—for example, high negative loadings for Pb and pH were found in both sediment and seawater (Figure 7), indicating that the increased Pb concentration in seawater and the decrease in the sediment may be greatly influenced by the decrease in pH over the past decades. The pH in the aquatic environment and sediment is very important for the mobility of heavy metals because metal availability is relatively low when the pH value is at a low level (Ma et al., 2016). The absorbed metals can be released into the water again with the change of pH (Kashem and Singh, 2001). The release of heavy metals is associated with a lower pH since the low pH can weaken the strength of heavy metal association and impede the retention of heavy metals by particulate matter and sediments, while the higher pH value promotes precipitation and adsorption (Ma et al., 2016; Zhang et al., 2014). Thus, the decrease in pH value would lead to the re-release of heavy metals in particles and sediments into the water column, resulting in the decrease of heavy metals in the sediment and an increase in the seawater.
In the seawater, the in the estuary and the adjacent mariculture area decreased in the order Hg > Cd > Pb > Cu > Zn > As during both seasons. Although the ERI value (60) in spring was higher than that in winter (44), those values were all lower than 150, indicating that the potential risks of the six metals to the aquaculture ecosystem were relatively low. Notably, the value for Hg (44) in spring was higher than 40 (at the level of moderate ecological risk), and the contribution for ERI was 73%, showing a potential ecological risk.
In the sediment, the mean values for the seven metals were in the decreasing order of Hg (2.91) > As (1.53) > Cd (0.98) > Cu (0.59) > Zn (0.53) > Pb (0.49) > Cr (0.37) during winter and As (1.66) > Cu (1.22) > Cd (0.96) > Hg (0.88) > Zn (0.80) > Cr (0.62) > Pb (0.48) during spring. Hg and As in winter and As and Cu in spring exhibited a moderate degree of contamination. The PLI values of those seven metals ranged from 0.70 to 0.96 (an average of 0.79) and from 0.64 to 1.14 (an average of 0.86) during winter and spring, respectively. The PLI level is an important reference index to decision-makers about pollution status and can provide inhabitants with an understanding of the environmental quality of the mariculture area (Islam et al., 2015; Lao et al., 2019). The lower PLI values (<1) in both seasons indicated the presence of only the baseline levels of pollutants.
The Igeo values for Hg are in the range of 0.79 to 1.06 (an average of 0.95), while the average Igeo values for other metals are all lower than 0 in winter. This indicates that Hg is moderately contaminated, while the other metals are uncontaminated in the sediment. In the spring, the Igeo values for As are in the range of -0.05 to 0.33 (an average of 0.15), indicating that As is a potential ecological risk factor during that season. However, the average Igeo values for other metals are all lower than 0, suggesting that those metals are uncontaminated in the sediment during spring. Overall, Hg and As presented a higher risk in both the seawater and sediment of the mariculture area, and the heavy metal contamination in the mariculture area must not be ignored.
Environmental change, such as the decrease in pH and seasonal hypoxia, likely changes the heavy metal status in aquatic systems (Atkinson et al., 2007; Chakraborty et al., 2016; Ma et al., 2016; Lao et al., 2019). Previous studies have found that the DO level is a key factor controlling the stability and lability of metals in the sediment (Chakraborty et al., 2016)—for example, some metals, such as Pb, associated with Fe/Mn-oxyhydroxide phases in the sediment would reduce with the decreasing DO level of the overlying water because of the dissolution of the Fe/Mn-oxyhydroxide phase (Chakraborty et al., 2016). In Qinzhou Bay, the eutrophication degree has increased in the past few decades due to the rapid development of industrialization and urbanization and the continuous expansion of aquaculture activities (Lao et al., 2021a), which may induce seasonal hypoxia in the bay (Howarth et al., 2011). Moreover, the pH value has decreased significantly over the past 20 years in Qinzhou Bay, which may cause more metals to be released into the water environment. In addition, Qinzhou Bay is a sea area with strong hydrodynamic conditions in Beibu Gulf (Chen et al., 2022). Particularly under the influence of the northeast monsoon in winter, the bottom sediment is resuspended into the water column, which may also lead to the increase of heavy metal release in a more acidic environment. The increase of heavy metal concentrations in seawater would lead to the increase of heavy metal absorption by aquaculture organisms, which poses a great threat to the health of organisms and ultimately endangers human health. Especially under global climate change, the acidification of seawater in most aquaculture areas in the world is aggravated (Clements and Chopin, 2017; Ng and Chiu, 2020; Jin et al., 2020; Swezey et al., 2020), which may also lead to the increase of heavy metal released into the seawater. This problem should be more vigilant and concerned in the future.
Seasonal changes in the concentrations of heavy metals were found in Qinzhou Bay. The concentrations of heavy metals in the seawater were higher, while the levels in the sediment were lower than that in other mariculture areas in China. The concentration of Cu in the seawater and sediment increased over the past decades, mainly influenced by the enhancement and expansion of mariculture and shipping activities. The concentration of Hg has decreased significantly in the seawater over the past 40 years due to the decrease in production and usage of Hg. However, the concentrations of Pb, Zn, and Cd in the seawater showed an increasing trend over the past 40 years, while the concentrations of Pb, Zn, As, and Cr in the sediment generally decreased over the past 20 years. The decrease in pH value (by 4.7%) over the past 20 years was responsible for the different trends of those metals between seawater and sediment. Hg and As presented a higher risk in both the seawater and sediment of the mariculture area. Overall, this study suggests that environmental changes, such as decrease in pH, affect the distribution of heavy metals in water and sediments, which we should be more vigilant and concerned about under the global climate change.
The original contributions presented in the study are included in the article/supplementary material. Further inquiries can be directed to the corresponding author.
FC was responsible for the conceptualization. QL prepared and wrote the original draft. QL and FC wrote, reviewed, and edited the manuscript. SC and PH were responsible for the data curation. QS, XLe, CC, QZ, XLu, and XZ were responsible for the experimental operation. QS, XLe, and GL were responsible for field sampling. FC and QL funded the acquisition. All authors contributed to the article and approved the submitted version.
This study was supported by the National Natural Science Foundation of China (U1901213 and 92158201), Guangdong Natural Science Foundation of China (2019B1515120066), Innovation and Entrepreneurship Project of Shantou (2021112176541391), and Guangxi Natural Science Foundation of China (2020GXNSFBA297065).
The authors declare that the research was conducted in the absence of any commercial or financial relationships that could be construed as a potential conflict of interest.
All claims expressed in this article are solely those of the authors and do not necessarily represent those of their affiliated organizations, or those of the publisher, the editors and the reviewers. Any product that may be evaluated in this article, or claim that may be made by its manufacturer, is not guaranteed or endorsed by the publisher.
We would like to thank the Marine Environmental Monitoring Centre of Beihai, State Oceanic Administration, for their support in collecting seawater and sediment samples and their supply in historical data.
Achary M. S., Satpathy K. K., Panigrahi S., Mohanty A. K., Padhi R. K., Biswas S., et al. (2017). Concentration of Heavy Metals in the Food Chain Components of the Nearshore Coastal Waters of Kalpakkam, Southeast Coast of India. Food Contr. 72, 232–243. doi: 10.1016/j.foodcont.2016.04.028
Adyasari D., Pratama M. A., Teguh N. A., Sabdaningsih A., Kusumaningtyas M. A., Dimova N. (2021). Anthropogenic Impact on Indonesian Coastal Water and Ecosystems: Current Status and Future Opportunities. Mar. Poll. Bull. 171, 112689. doi: 10.1016/j.marpolbul.2021.112689
Alengebawy A., Abdelkhalek S. T., Qureshi S. R., Wang M. Q. (2021). Heavy Metals and Pesticides Toxicity in Agricultural Soil and Plants: Ecological Risks and Human Health Implications. Toxics 9 (3), 42. doi: 10.3390/toxics9030042
Atkinson C. A., Jolley D. F., Simpson S. L. (2007). Effect of Overlying Water Ph, Dissolved Oxygen, Salinity and Sediment Disturbances on Metal Release and Sequestration From Metal Contaminated Marine Sediments. Chemosphere 69 (9), 1428–1437. doi: 10.1016/j.chemosphere.2007.04.068
Baeyens W., Elskens M., Gillain G., Goeyens L. (1998). “Biogeochemical Behaviour of Cd, Cu, Pb and Zn in the Scheldt Estuary During the Period 1981–1983,” in In Trace Metals in the Westerschelde Estuary: A Case-Study of a Polluted, Partially Anoxic Estuary (Dordrecht: Springer), 15–44.
Baquiran J. I. P., Conaco C. (2018). Sponge-Microbe Partnerships are Stable Under Eutrophication Pressure From Mariculture. Mar. Poll. Bull. 136, 125–134. doi: 10.1016/j.marpolbul.2018.09.011
Burgos-Núñez S., Navarro-Frómeta A., Marrugo-Negrete J., Enamorado-Montes G., Urango-Cárdenas I. (2017). Polycyclic Aromatic Hydrocarbons and Heavy Metals in the Cispata Bay, Colombia: A Marine Tropical Ecosystem. Mar. Poll. Bull. 120 (1-2), 379–386. doi: 10.1016/j.marpolbul.2017.05.016
Cai L. M., Wang Q. S., Luo J., Chen L. G., Zhu R. L., Wang S., et al. (2019). Heavy Metal Contamination and Health Risk Assessment for Children Near a Large Cu-Smelter in Central China. Sci. Total. Environ. 650, 725–733. doi: 10.1016/j.scitotenv.2018.09.081
Castro-González M. I., Méndez-Armenta M. (2008). Heavy Metals: Implications Associated to Fish Consumption. Environ. Toxicol. Pharmacol. 26 (3), 263–271. doi: 10.1016/j.etap.2008.06.001
Chakraborty P., Chakraborty S., Jayachandran S., Madan R., Sarkar A., Linsy P., et al. (2016). Effects of Bottom Water Dissolved Oxygen Variability on Copper and Lead Fractionation in the Sediments Across the Oxygen Minimum Zone, Western Continental Margin of India. Sci. Total. Environ. 566, 1052–1061. doi: 10.1016/j.scitotenv.2016.05.125
Chen C., Lao Q., Shen Y., Jin G., Chen F., Su Q., et al. (2022). Comparative Study of Nitrogen Cycling Between a Bay With Riverine Input and a Bay Without Riverine Input, Inferred From Stable Isotopes. Front. Mar. Sci 9, 885037. doi: 10.3389/fmars.2022.885037
Chen F., Lin J., Qian B., Wu Z., Huang P., Chen K., et al. (2018). Geochemical Assessment and Spatial Analysis of Heavy Metals in the Surface Sediments in the Eastern Beibu Gulf: A Reflection on the Industrial Development of the South China Coast. Int. J. Environ. Res. Public Health 15 (3), 496. doi: 10.3390/ijerph15030496
Clements J. C., Chopin T. (2017). Ocean Acidification and Marine Aquaculture in North America: Potential Impacts and Mitigation Strategies. Rev. Aquacult. 9 (4), 326–341. doi: 10.1111/raq.12140
Cotrufo M. F., De Santo A. V., Alfani A., Bartoli G., De Cristofaro A. (1995). Effects of Urban Heavy Metal Pollution on Organic Matter Decomposition in Quercus Ilex L. Woods. Environ. Poll. 89 (1), 81–87. doi: 10.1016/0269-7491(94)00041-B
Duodu G. O., Goonetilleke A., Ayoko G. A. (2016). Comparison of Pollution Indices for the Assessment of Heavy Metal in Brisbane River Sediment. Environ. Poll. 219, 1077–1091. doi: 10.1016/j.envpol.2016.09.008
Feng C., Guo X., Yin S., Tian C., Li Y., Shen Z. (2017). Heavy Metal Partitioning of Suspended Particulate Matter–Water and Sediment–Water in the Yangtze Estuary. Chemosphere 185, 717–725. doi: 10.1016/j.chemosphere.2017.07.075
Fontaine S., Mariotti A., Abbadie L. (2003). The Priming Effect of Organic Matter: A Question of Microbial Competition? Soil Biol. Biochem. 35 (6), 837–843. doi: 10.1016/S0038-0717(03)00123-8
Gong Y., Zhao D., Wang Q. (2018). An Overview of Field-Scale Studies on Remediation of Soil Contaminated With Heavy Metals and Metalloids: Technical Progress Over the Last Decade. Water Res. 147, 440–460. doi: 10.1016/j.watres.2018.10.024
Guenet B., Danger M., Abbadie L., Lacroix G. (2010). Priming Effect: Bridging the Gap Between Terrestrial and Aquatic Ecology. Ecology 91 (10), 2850–2861. doi: 10.1890/09-1968.1
Gu Y. G., Lin Q., Yu Z. L., Wang X. N., Ke C. L., Ning J. J. (2015). Speciation and Risk of Heavy Metals in Sediments and Human Health Implications of Heavy Metals in Edible Nekton in Beibu Gulf, China: A Case Study of Qinzhou Bay. Mar. Poll. Bull. 101 (2), 852–859. doi: 10.1016/j.marpolbul.2015.11.019
Hakanson L. (1980). An Ecological Risk Index for Aquatic Pollution Control. A Sedimentological Approach. Water Res. 14 (8), 975–1001.
Howarth R., Chan F., Conley D. J., Garnier J., Doney S. C., Marino R., et al. (2011). Coupled Biogeochemical Cycles: Eutrophication and Hypoxia in Temperate Estuaries and Coastal Marine Ecosystems. Front. Ecol. Environ. 9 (1), 18–26. doi: 10.1890/100008
Islam M. S., Ahmed M. K., Raknuzzaman M., Habibullah-Al-Mamun M., Islam M. K. (2015). Heavy Metal Pollution in Surface Water and Sediment: A Preliminary Assessment of an Urban River in a Developing Country. Ecol. Indic. 48, 282–291. doi: 10.1016/j.ecolind.2014.08.016
Islam M. S., Tanaka M. (2004). Impacts of Pollution on Coastal and Marine Ecosystems Including Coastal and Marine Fisheries and Approach for Management: A Review and Synthesis. Mar. Poll. Bull. 48 (7-8), 624–649. doi: 10.1016/j.marpolbul.2003.12.004
Jiang Z., Xu N., Liu B., Zhou L., Wang J., Wang C., et al. (2018). Metal Concentrations and Risk Assessment in Water, Sediment and Economic Fish Species With Various Habitat Preferences and Trophic Guilds From Lake Caizi, Southeast China. Ecotoxicol. Environ. Saf. 157, 1–8. doi: 10.1016/j.ecoenv.2018.03.078
Jin P., Hutchins D. A., Gao K. (2020). The Impacts of Ocean Acidification on Marine Food Quality and its Potential Food Chain Consequences. Front. Mar. Sci. 7, 543979. doi: 10.3389/fmars.2020.543979
Kashem M. A., Singh B. R. (2001). Metal Availability in Contaminated Soils: I. Effects of Floodingand Organic Matter on Changes in Eh, pH and Solubility of Cd, Ni andZn. Nutri. Cycl. Agroecosyst. 61 (3), 247–255.
Kumar V., Parihar R. D., Sharma A., Bakshi P., Sidhu G. P. S., Bali A. S., et al. (2019). Global Evaluation of Heavy Metal Content in Surface Water Bodies: A Meta-Analysis Using Heavy Metal Pollution Indices and Multivariate Statistical Analyses. Chemosphere 236, 124364. doi: 10.1016/j.chemosphere.2019.124364
Lao Q., Liu G., Gao J., Shen Y., Guo Z., Qing S., et al. (2021a). Study on the Characteristics and Eutrophication of Nutrients in the Mariculture Farms of Qinzhou Bay, South China. Mar. Environ. Sci. 40 (3), 407–416. doi: 10.13634/j.cnki.mes.2021.03.011
Lao Q., Liu G., Shen Y., Su Q., Gao J., Chen F. (2020). Distribution Characteristics and Fluxes of Nutrients in the Rivers of the Beibu Gulf. Haiyang Xuebao 42 (12), 93–100. doi: 10.3969/j.issn.0253−4193.2020.12.010
Lao Q., Liu G., Shen Y., Su Q., Lei X. (2021b). Biogeochemical Processes and Eutrophication Status of Nutrients in the Northern Beibu Gulf, South China. J. Earth Syst. Sci. 130 (4), 1–14. doi: 10.1007/s12040-021-01706-y
Lao Q., Liu G., Zhou X., Chen F., Zhang S. (2021c). Sources of Polychlorinated Biphenyls (PCBs) and Dichlorodiphenyltrichloroethanes (DDTs) Found in Surface Sediment From Coastal Areas of Beibu Gulf: A Reflection on Shipping Activities and Coastal Industries. Mar. Poll. Bull. 167, 112318. doi: 10.1016/j.marpolbul.2021.112318
Lao Q., Su Q., Liu G., Shen Y., Chen F., Lei X., et al. (2019). Spatial Distribution of and Historical Changes in Heavy Metals in the Surface Seawater and Sediments of the Beibu Gulf, China. Mar. Poll. Bull. 146, 427–434. doi: 10.1016/j.marpolbul.2019.06.080
Liu G., Lao Q., Su Q., Shen Y., Chen F., Qing S., et al. (2020). Spatial and Seasonal Characteristics of Dissolved Heavy Metals in the Aquaculture Areas of Beibu Gulf, South China. Hum. Ecol. Risk Assess.: Int. J. 26 (7), 1957–1969. doi: 10.1080/10807039.2019.1629273
Liu S., Shi J., Wang J., Dai Y., Li H., Li J., et al. (2021). Interactions Between Microplastics and Heavy Metals in Aquatic Environments: A Review. Front. Microbiol. 12, 730. doi: 10.3389/fmicb.2021.652520
Li C., Wang H., Liao X., Xiao R., Liu K., Bai J., et al. (2022). Heavy Metal Pollution in Coastal Wetlands: A Systematic Review of Studies Globally Over the Past Three Decades. J. Hazard. Material. 424, 127312. doi: 10.1016/j.jhazmat.2021.127312
Li J., Zhang H., Zhang K., Yang R., Li R., Li Y. (2018). Characterization, Source, and Retention of Microplastic in Sandy Beaches and Mangrove Wetlands of the Qinzhou Bay, China. Mar. Poll. Bull. 136, 401–406. doi: 10.1016/j.marpolbul.2018.09.025
Luo H., Wang Q., Liu Z., Wang S., Long A., Yang Y. (2020). Potential Bioremediation Effects of Seaweed Gracilaria Lemaneiformis on Heavy Metals in Coastal Sediment From a Typical Mariculture Zone. Chemosphere 245, 125636. doi: 10.1016/j.chemosphere.2019.125636
Luo H., Xie S., Dai X., Wang Q., Yang Y. (2022). Biomass Decomposition and Heavy Metal Release From Seaweed Litter, Gracilaria Lemaneiformis, and Secondary Pollution Evaluation. J. Environ. Manage. 310, 114729. doi: 10.1016/j.jenvman.2022.114729
Ma X., Zuo H., Tian M., Zhang L., Meng J., Zhou X., et al. (2016). Assessment of Heavy Metals Contamination in Sediments From Three Adjacent Regions of the Yellow River Using Metal Chemical Fractions and Multivariate Analysis Techniques. Chemosphere 144, 264–272. doi: 10.1016/j.chemosphere.2015.08.026
Mishra S., Bharagava R. N., More N., Yadav A., Zainith S., Mani S., et al. (2019). “). Heavy Metal Contamination: An Alarming Threat to Environment and Human Health,” in In Environmental Biotechnology: For Sustainable Future (Singapore: Springer), 103–125.
Naser H. A. (2013). Assessment and Management of Heavy Metal Pollution in the Marine Environment of the Arabian Gulf: A Review. Mar. Poll. Bull. 72 (1), 6–13. doi: 10.1016/j.marpolbul.2013.04.030
Ng J. C., Chiu J. M. (2020). Changes in Biofilm Bacterial Communities in Response to Combined Effects of Hypoxia, Ocean Acidification and Nutrients From Aquaculture Activity in Three Fathoms Cove. Mar. Poll. Bull. 156, 111256. doi: 10.1016/j.marpolbul.2020.111256
Niu H., Deng W., Wu Q., Chen X. (2009). Potential Toxic Risk of Heavy Metals From Sediment of the Pearl River in South China. J. Environ. Sci. 21, 1053–1058. doi: 10.1016/S1001-0742(08)62381-5
Pacyna E. G., Pacyna J. M., Steenhuisen F., Wilson S. (2006). Global Anthropogenic Mercury Emission Inventory for 2000. Atmos. Environ. 40 (22), 4048–4063. doi: 10.1016/j.atmosenv.2006.03.041
Reimann C., De Caritat P. (2012). Chemical Elements in the Environment: Factsheets for the Geochemist and Environmental Scientist (Springer, Berlin: Springer Science & Business Media), 398 p
Schuster P. F., Krabbenhoft D. P., Naftz D. L., Cecil L. D., Olson M. L., Dewild J. F., et al. (2002). Atmospheric Mercury Deposition During the Last 270 Years: A Glacial Ice Core Record of Natural and Anthropogenic Sources. Environ. Sci. Technol. 36 (11), 2303–2310. doi: 10.1021/es0157503
Swezey D. S., Boles S. E., Aquilino K. M., Stott H. K., Bush D., Whitehead A., et al. (2020). Evolved Differences in Energy Metabolism and Growth Dictate the Impacts of Ocean Acidification on Abalone Aquaculture. Proc. Natl. Acad. Sci. 117 (42), 26513–26519. doi: 10.1073/pnas.2006910117
Wang Z. L., Liu C. Q. (2003). Distribution and Partition Behavior of Heavy Metals Between Dissolved and Acid-Soluble Fractions Along a Salinity Gradient in the Changjiang Estuary, Eastern China. Chem. Geol. 202 (3-4), 383–396. doi: 10.1016/j.chemgeo.2002.05.001
Wang X., Liu L., Zhao L., Xu H., Zhang X. (2019). Assessment of Dissolved Heavy Metals in the Laoshan Bay, China. Mar. Poll. Bull. 149, 110608. doi: 10.1016/j.marpolbul.2019.110608
Wang Y., Wei Y., Guo P., Pan J., Wu Q., Liu N. (2016). Distribution Variation of Heavy Metals in Maricultural Sediments and Their Enrichment, Ecological Risk and Possible Source—A Case Study From Zhelin Bay in Southern China. Mar. Poll. Bull. 113 (1-2), 240–246. doi: 10.1016/j.marpolbul.2016.09.028
Wang S. L., Xu X. R., Sun Y. X., Liu J. L., Li H. B. (2013). Heavy Metal Pollution in Coastal Areas of South China: A Review. Mar. Poll. Bull. 76 (1-2), 7–15. doi: 10.1016/j.marpolbul.2013.08.025
Wang X., Zhao L., Xu H., Zhang X. (2018). Spatial and Seasonal Characteristics of Dissolved Heavy Metals in the Surface Seawater of the Yellow River Estuary, China. Mar. Poll. Bull. 137, 465–473. doi: 10.1016/j.marpolbul.2018.10.052
Wei M., He B. (2004). Change Trend of Water Environment Index in Recent 20a in Qinzhou Bay-III Content Distribution of Trace Metal and its Source Analysis. Mar. Environ. Sci. 23 (1), 29–32. doi: 10.3969/j.issn.1007-6336.2004.01.009
Wu J., Lu J., Li L., Min X., Luo Y. (2018). Pollution, Ecological-Health Risks, and Sources of Heavy Metals in Soil of the Northeastern Qinghai-Tibet Plateau. Chemosphere 201, 234–242. doi: 10.1016/j.chemosphere.2018.02.122
Xu C., Dan S. F., Yang B., Lu D., Kang Z., Huang H., et al. (2021). Biogeochemistry of Dissolved and Particulate Phosphorus Speciation in the Maowei Sea, Northern Beibu Gulf. J. Hydrol. 593, 125822. doi: 10.1016/j.jhydrol.2020.125822
Xu F., Tian X., Yin X., Yan H., Yin F., Liu Z. (2015). Trace Metals in the Surface Sediments of the Eastern Continental Shelf of Hainan Island: Sources and Contamination. Mar. Poll. Bull. 99, 276–283. doi: 10.1016/j.marpolbul.2015.07.055
Xu C., Yang B., Dan S. F., Zhang D., Liao R., Lu D., et al. (2020). Spatiotemporal Variations of Biogenic Elements and Sources of Sedimentary Organic Matter in the Largest Oyster Mariculture Bay (Maowei Sea), Southwest China. Sci. Total. Environ. 730, 139056. doi: 10.1016/j.scitotenv.2020.139056
Yan X., An J., Yin Y., Gao C., Wang B., Wei S. (2022). Heavy Metals Uptake and Translocation of Typical Wetland Plants and Their Ecological Effects on the Coastal Soil of a Contaminated Bay in Northeast China. Sci. Total. Environ. 803, 149871. doi: 10.1016/j.scitotenv.2021.149871
Yang B., Lan R. Z., Lu D. L., Dan S. F., Kang Z. J., Jiang Q. C., et al. (2019). Phosphorus Biogeochemical Cycling in Intertidal Surface Sediments From the Maowei Sea in the Northern Beibu Gulf. Reg. Stud. Mar. Sci. 28, 100624. doi: 10.1016/j.rsma.2019.100624
Zhang C., Yu Z. G., Zeng G. M., Jiang M., Yang Z. Z., Cui F, et al(2014). Effects of Sediment Geochemical Properties on Heavy Metal Bioavailability. Environment international. 73, 270-281. doi: 10.1016/j.envint.2014.08.010.x
Zhang L., Shi Z., Zhang J. P., Jiang Z., Wang F., Huang X. (2015). Spatial and Seasonal Characteristics of Dissolved Heavy Metals in the East and West Guangdong Coastal Waters, South China. Mar. Poll. Bull. 95, 419–426. doi: 10.1016/j.marpolbul.2015.03.035
Zhang L., Ye X., Feng H., Jing Y., Ouyang T., Yu X., et al. (2007). Heavy Metal Contamination in Western Xiamen Bay Sediments and its Vicinity, China. Mar. Poll. Bull. 54, 974–982. doi: 10.1016/j.marpolbul.2007.02.010
Zhang J., Zhou F., Chen C., Sun X., Shi Y., Hui Z., et al. (2018). Spatial Distribution and Correlation Characteristics of Heavy Metals in the Seawater, Suspended Particulate Matter and Sediments in Zhanjiang Bay, China. PloS One 13, e0201414. doi: 10.1371/journal.pone.0201414
Zhao G., Lu Q., Ye S., Yuan H., Ding X., Wang J. (2016). Assessment of Heavy Metal Contamination in Surface Sediments of the West Guangdong Coastal Region, China. Mar. Poll. Bull. 108, 268–274. doi: 10.1016/j.marpolbul.2016.04.057
Keywords: heavy metals, pH, historical trend, mariculture area, ecological risk, Qinzhou Bay
Citation: Lao Q, Cai S, Huang P, Chen F, Su Q, Lei X, Zhou X, Chen C, Zhu Q, Lu X and Liu G (2022) Contaminant Characteristics and Influencing Factors of Heavy Metals in Seawater and Sediments in a Typical Mariculture Bay in South China. Front. Mar. Sci. 9:923494. doi: 10.3389/fmars.2022.923494
Received: 19 April 2022; Accepted: 11 May 2022;
Published: 15 June 2022.
Edited by:
Meilin Wu, Chinese Academy of Sciences, ChinaReviewed by:
Zuhao Zhu, Ministry of Natural Resources, ChinaCopyright © 2022 Lao, Cai, Huang, Chen, Su, Lei, Zhou, Chen, Zhu, Lu and Liu. This is an open-access article distributed under the terms of the Creative Commons Attribution License (CC BY). The use, distribution or reproduction in other forums is permitted, provided the original author(s) and the copyright owner(s) are credited and that the original publication in this journal is cited, in accordance with accepted academic practice. No use, distribution or reproduction is permitted which does not comply with these terms.
*Correspondence: Fajin Chen, ZmpjaGVuQGdkb3UuZWR1LmNu
Disclaimer: All claims expressed in this article are solely those of the authors and do not necessarily represent those of their affiliated organizations, or those of the publisher, the editors and the reviewers. Any product that may be evaluated in this article or claim that may be made by its manufacturer is not guaranteed or endorsed by the publisher.
Research integrity at Frontiers
Learn more about the work of our research integrity team to safeguard the quality of each article we publish.