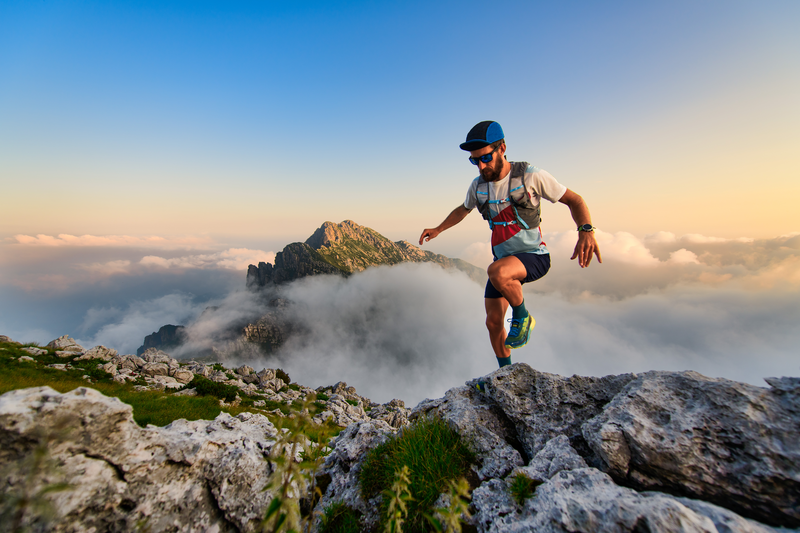
94% of researchers rate our articles as excellent or good
Learn more about the work of our research integrity team to safeguard the quality of each article we publish.
Find out more
ORIGINAL RESEARCH article
Front. Mar. Sci. , 10 August 2022
Sec. Marine Ecosystem Ecology
Volume 9 - 2022 | https://doi.org/10.3389/fmars.2022.922389
This article is part of the Research Topic Coralline Algae: Past, Present, and Future Perspectives View all 10 articles
Phymatolithon Foslie is one of the most studied and ecologically important genera of crustose coralline algae (CCA) due to their dominant abundance in various marine ecosystems worldwide. The taxonomy of the genus is complex and has been revised and updated many times based on morphological and molecular analyses. We report on a crustose coralline algal species collected in June 2011 via snorkeling in the subtidal zone along the beach Abu Qir on the Mediterranean coast of Egypt, as part of a larger macroalgal diversity survey in the region. The species shows significant sequence divergences (3.5%–14.8% in rbcL; 2.9%–11% in psbA) from other closely related Phymatolithon taxa. Morpho-anatomically, this species possesses the characters considered collectively diagnostic of the genus Phymatolithon, namely, thalli non-geniculate epithelial cells and non-photosynthetic and domed-shaped meristematic cells, usually as short with progressive elongation of their perithallial derivatives. Based on molecular and morphological analyses, we determined that these specimens encompass a new, distinct species that we herein name Phymatolithon abuqirensis. Including this new species, the total number of described Phymatolithon species found in the Mediterranean Sea is now six.
Species of Phymatolithon Foslie (Hapalidiales) are some of the most studied, ecologically important taxa due to their dominant abundance in maërl ecosystems worldwide (Pardo et al., 2014; Peña et al., 2014; Peña et al., 2015; Pardo et al., 2019). The worldwide distribution of Phymatolithon is vast, with the Arctic and Subarctic, the Atlantic Boreal, Lusitanian, and Mediterranean members primarily suggesting to be of Tethyan origin (pre-Mediterranean) (Adey and Steneck, 2001; Adey and Hayek, 2005; Adey and Hayek, 2011; Adey et al., 2013; Adey et al., 2018). Interestingly, no members of Phymatolithon have been collected in the northern Pacific, which have been confirmed through DNA sequencing (Adey et al., 2018).
Currently, there are 35 species of Phymatolithon and 20 infraspecific names, of which 22 are currently accepted taxonomically (Guiry and Guiry, 2021). The taxonomy and understanding of the genus Phymatolithon has been revised throughout the past two centuries. Phymatolithon was established by Foslie (1898) using a single species, Phymatolithon polymorphum (L.) Fosl. mscr., without referencing any specimen or description, and transferring Linnaeus’s designation of Millepora polymorpha (Linnaeus, 1767) to P. polymorpha (Woelkerling and Irvine, 1986). Historic samples of Phymatolithon have been named and substituted among many genera such as Millepora, Nullipora, Apora, Corallium, Agardhia, Juergensia, and Eleutherospora (as summarized in Woelkerling and Irvine, 1986). Phymatolithon was finally lectotypified by Woelkerling and Irvine (1986), almost a century after Foslie’s original description of the genus. Prior to this, one of the hardest challenges in the designation of Phymatolithon was that over 40 diagnostic character combinations had been used to describe the genus (Foslie, 1898; Kylin, 1956; Adey, 1964; Adey, 1970; Adey and Adey, 1973; Adey and Macintyre, 1973). For example, a newly proposed Phymatolithon species, P. atlanticum, is morphologically unique in the genus so far by exhibiting the presence of pitted pore plates of tetrasporangial/bisporangial conceptacle roofs (Jeong et al., 2021). Thus, caution should be noted when using this character as the authors point out that it shows strong evidence of convergent evolution among a phylogenetically distant genus, Lithothamnion (Hapalidiales). Moreover, specimens of Lithothamnion calcareum have been transferred to the genus Phymatolithon, thus adding to the taxonomic confusion (Guiry & Guiry, 2021). Other examples include Lithothamnion repandum, which was proposed as a new name for L. lenormandii f. australe (Foslie, 1904). Wilks and Woelkerling (1994) transferred the species to Phymatolithon repandum and treated L. asperulum as a heterotypic synonym. Adey (1970) separates the genera Phymatolithon and Lithothamnion based on three morphological characters, i.e., i) shape of distal walls of terminal epithelial cells, ii) relative length of subepithelial meristem cells, and iii) cell elongation type. It must be noted that no gametangial plants of the type species were examined in this typification (Adey, 1970). Adey et al. (2001) suggested the reinstatement of the genus Leptophytum, separating this genus from Phymatolithon based on differences in reproductive features (origin of gonimoblasts, position of conceptacle primordia, spermatangial systems, and the presence/absence of asexual conceptacle pore cells). Species delimitation using both morphological characters and molecular data still presents challenges. When looking at sequence divergence among other members of Phymatolithon, the interspecific variations range between 6.4% and18.8% for rbcL, 3.3% and 13% for psbA, and 6.5% and 16.8% for COI-5P (Gabrielson et al., 2011; Torrano-Silva et al., 2018; Jeong et al., 2019; Jeong et al., 2021).
With the advances of DNA sequencing technologies and our modern understanding of cryptic diversity, it is no longer possible to conduct morphological analyses alone to accurately identify species or even genera for both geniculate and non-geniculate CCA (Gabrielson et al., 2011; Martone et al., 2012; Hind et al., 2014; Hind et al., 2015; Van der Merwe et al., 2015; Richards et al., 2017; Richards et al., 2020; Richards et al., 2022; Puckree-Padua et al., 2020). Gabrielson et al. (2011) established an integrated taxonomic approach in which DNA sequencing and morphological analyses of type specimens are used from the same specimens. For example, previously, specimens of Lithothamnion ferox were transferred to the genus Mesophyllum (Adey, 1970) and have since been transferred to Phymatolithon as P. ferox thanks to DNA sequencing (Van der Merwe and Maneveldt, 2014; Maneveldt et al., 2020). According to Adey et al. (2015), morphological characters unique to Clathromorphum (previously confused as Phymatolithon) consist of a multilayered, photosynthetic epithallium and a double mode of calcification enabling the formation of massive carbonate structures with multiyear longevity. Molecular work also showed a complex relationship with northern species of Hapalidiaceae using a three-gene analysis (SSU, psbA, rbcL) (Adey et al., 2015). Most recently, a new genus, Phymatolithopsis, has been described by Jeong et al. (2022) for some species previously assigned to Phymatolithon. This new genus is sister to Mesophyllum and located in a clade distinct from Phymatolithon. Phymatolithopsis is differentiated from Phymatolithon by morphological features such as the origin of the conceptacle primordia and the distribution of gonimoblast filaments (Jeong et al., 2022). The description of this new genus included the taxonomic transfer of Phymatolithon prolixum and P. repandum to Phymatolithopsis prolixa and P. repanda, respectively (Jeong et al., 2022).
Specimens were collected in June 2011 via snorkeling in the Mediterranean Sea, Alexandria, Abu Qir, Egypt (31˚ 19.3345’ N, 30˚ 3.6198’ E) (Figure 1), from the subtidal habitat as part of a larger macroalgal diversity survey around the Mediterranean and Red Seas. Specimens were preserved by desiccating in silica gel and deposited in the University of Louisiana at Lafayette Herbarium (LAF). Approximately, 100 mg of silica desiccated tissue was ground into a fine powder immediately prior to DNA extraction.
Figure 1 Specimen collection map of Phymatolithon abuqirensis vouchers TS 757 and TS 759 collected in the Mediterranean Sea, Alexandria, Abu Qir, Egypt.
DNA was extracted from the newly collected specimens using the Quick-DNA Plant/Seed Miniprep Kit (Zymo Research, Irvine, CA, USA). Markers chosen for PCR and sequencing included the plastid-encoded genes psbA (encodes photosystem II reaction center protein D1 gene) and rbcL (encodes the large subunit of the enzyme ribulose-1,5-bisphosphate carboxylase/oxygenase), and the nuclear-encoded LSU (partial 28S rDNA) as well as SSU (partial 18S rDNA). The PCR performed followed the protocols and primers described in Richards et al. (2014). PCR products were cleaned by the addition of 2 µl of ExoSAP-IT™ (USB, Cleveland, Ohio) per 5 µl of amplified DNA product. Reactions were incubated at 37°C for 15 min, followed by inactivation of ExoSAP-IT™ at 80°C for 15 min. Purified PCR products were subsequently cycle-sequenced using the BrightDye® Terminator Cycle Sequencing Kit (Molecular Cloning Laboratories [MCLAB], South San Francisco, CA, USA). Resulting cycle sequence reactions were purified with ETOH/EDTA precipitation and were sequenced in-house at the UL Lafayette campus on an ABI Model 3130xl Genetic Analyzer. The resulting chromatograms were assembled and edited using Sequencher 5.1 (Gene Codes Corp., Ann Arbor, MI, USA) and exported as individual “.FASTA” files. Species identification, specimen collection information, and GenBank accession numbers are listed in Supplementary Table 1.
Sequences for psbA and rbcL were aligned separately using MUSCLE and then analyzed downstream as single-gene analyses or concatenated using Sequence Matrix v. 1.8 (Vaidya et al., 2011). The DNA matrices were exported as Philip “.PHY” format and for analyses with phylogenetic tools available on the CIPRES server, namely PartitionFinder 2 (Lanfear et al., 2017), to determine the best fitting model of evolution and data partition and tree reconstruction with RAxML (Stamatakis, 2014). The single gene alignments of psbA and rbcL both resulted in the selection of GTR+G model with three partitions based on the Akaike Information Criterion corrected (AICc) and Akaike information criterion (AIC) scores. The concatenated alignments including psbA and rbcL resulted in the selection of GTR+G models with three partitions each with the first, second, and third codon per gene, based on the AICc and AIC scores. For both datasets, RAxML searches consisted of 1000 independent restarts with the above models and partition schemes with 1000 independent searches to find the tree with the lowest likelihood score and 1000 Bootstrap (BS) replications. The Newick file was imported into FigTree 1.4.2 (Rambaut and Drummond, 2012) as a starting point for further editing in Microsoft Publisher (i.e., tree structure export for label editing, respectively).
The single gene alignments were 959 bp for psbA and 1,494 bp for rbcL, while the concatenated alignment was 2,453 bp. The psbA alignments included 19 sequences of Phymatolithon with 11 additional sequences in the Hapalidiales ingroup and two sequences in the Sporolithales outgroup; rbcL included 21 sequences of Phymatolithon and 11 additional sequences in the Hapalidiales ingroup and two sequences in the Sporolithales outgroup; and the concatenated tree of psbA and rbcL consisted of 32 sequences of Phymatolithon and 19 additional sequences in the Hapalidiales ingroup, and two sequences in the Sporolithales outgroup.
Estimation of evolutionary distances from nucleotide sequences was done in MEGA X using the Jukes-Cantor pairwise distance model. This analysis involved 34 nucleotide sequences. All ambiguous positions were removed for each sequence pair (pairwise deletion option). There were a total of 507 positions in the final dataset (Supplementary Table 2). Species delimitation analysis for each gene was performed separately using Assemble Species by Automatic Partitioning (ASAP) (Puillandre et al., 2021) using the Jukes-Cantor substitution model for the following parameters: split groups below 0.01 probability, highlighting genetic distances between 0.005 and 0.05.
Sample preparation and scanning electron microscope (SEM) were adapted and modified according to the protocol of Richards et al. (2016) as follows: portions of the thallus from silica gel-dried specimens were removed using a razor blade and forceps. Crustose specimens were sectioned by performing vertical fractures (cutting from the thallus surface to the substratum), whereas protuberances were sectioned longitudinally (through the middle of the protuberance from the tip to the base) and transversely (through the lateral sides of protuberance). Specimens were sectioned manually using a new single-edge razor blade for each fracture and were mounted using liquid graphite and coated with 15 nm of gold. To ensure even distribution of the gold over the three-dimensional features in the sections, coating was performed in two applications. First, 8 nm of gold was applied with the stub lying flat on the stage of the coating chamber. After the first application, the specimen was tilted using a coin placed underneath the stub and a second application of 7 nm of gold was performed. Specimens were viewed using a Hitachi S-3000N SEM at a voltage of 15 kV, housed in the Microscopy Center at UL Lafayette, following the manufacturer’s instructions. Cell dimensions were measured from SEM micrographs following the protocols of Irvine and Chamberlain (1994) and Adey et al. (2005). Terminology follows Woelkerling (1988) and Adey et al. (2015).
The sequences obtained for TS 757 and TS 759 were identical for each of psbA; rbcL could only be generated for TS 757. Both single and concatenated gene analyses indicated Phymatolithon abuqirensis (TS 757 and TS 759) as sister to Phymatolithon nantuckensis (Figures 2–4). The pairwise distance for rbcL between P. abuqirensis and P. nantuckensis was 3.5%; with P. ferox it ranged from 5.5% to 5.7%; between it and P. koreanum it was 7.0%; between it and P. rugulosum, P. lenormandii, and P. purpureum it was 9.2%; and between it and P. dosungii and P. squamulosum it was 11.3%. When looking at the sequences of Phymatolithon sequences, P. atlanticum is 12.4% different to P. abuqirensis, P. lustitanicum 14.5%, and P. lamii 14.8%. Other taxa in the Hapalidiales range from 12.9% difference (Phymatolithopsis prolixa = ‘Lithothamnion’/Phymatolithon proxilum) to 17.5% (Neopolyporolithon reclinatum), and the Sporolithales outgroups are 18.5% different from P. abuqirensis (Supplementary Table 2).
Figure 2 ML analyses of psbA sequences (959 bp). Numbers at branches indicate bootstrap values out of 1,000 replicates. * denotes full support.
Figure 3 ML analyses of rbcL sequences (1494 bp). Numbers at branches indicate bootstrap values out of 1,000 replicates. * denotes full support.
Figure 4 ML analyses of concatenated psbA and rbcL sequences (2,453 bp). Numbers at branches indicate bootstrap values out of 1,000 replicates.* denotes full support.
When comparing pairwise distances for the psbA gene, TS 757 and TS 759 were 100% identical; there is a 2.9% difference between the sister species, P. nantuckensis, and 3.1% between P. ferox and P. koreanum. In the closest clade, differences range from 5.1% (P. calcareum) to 6.3% (P. margoundulatus and P. purpureum). P. lamii, P. lustanicum, and P. rugulosum all differ from the TS 757 and TS 759 specimens by 9.8%, 10.1%, and 11%, respectively. Other taxa in the Hapalidiales range from 9.1% (“Synarthrophyton” patena) to 13.7% (Clathromorphum compactum), and the outgroup Sporolithales differs by 14.0% for both taxa (Supplementary Table 3).
Phymatolithon abuqirensisR.P.Kittle and T.Sauvage sp. nov. (Figures 5, 6)
Figure 5 Scanning electron microscopy images of Phymatolithon abuqirensis voucher TS 757 (LAF 7722). (A) Habit view of thallus, scale bar = 1 cm. (B) Section view of thallus, scale bar = 200 µm. (C) Section view showing hypothallium (bracket) with monomerous thallus construction and hypothallial cells’ direction of growth (white arrow), scale bar = 100 µm. (D) Magnified view of perithallium with adjacent perithallial cells linked by cell fusions in the x-axis (f) and z-axis (*), scale bar = 20 µm. (E) Section showing domed epithelial cells (e), meristematic cells (m), and tear-dropped shaped perithallial cells (p), scale bar = 30 µm. (F) Magnified view of surface cells, emphasizing the domed epithelial cells (e), scale bar = 20 µm.
Figure 6 Scanning electron microscopy images of Phymatolithon abuqirensis voucher TS 759 (LAF 7723). (A) Habit view of thallus, scale bar = 1 cm. (B) Section view showing monomerous thallus construction with hypothallial cells grown parallel to substratum (bottom bracket) and perithallial filaments arching upward (top bracket), scale bar = 200 µm. (C) Magnified view of hypothallium over substratum, scale bar = 100 µm. (D) Magnified view of perithallium with adjacent perithallial cells linked by cell fusions in the x-axis (f) and z- axis (*), scale bar = 20 µm. (E) Section showing domed epithelial cells (e), meristematic cells (m), and teardrop-shaped perithallial cells (p), scale bar = 30 µm. (F) Magnified view showing surface cells, emphasizing the domed epithelial cells (e), meristematic cells (m), and teardrop-shaped perithallial cells (p), scale bar = 20 µm.
Holotype: TS 757 (LAF 7722): Abu Qir, Egypt (31˚ 19.3345’ N, 30˚ 3.6198’ E), Mediterranean Sea, 8.vii.2011, depth <2m, leg. TS.
Isotype: TS 759 (LAF 7723): Abu Qir, Egypt (31˚ 19.3345’ N, 30˚ 3.6198’ E), Mediterranean Sea, 8.vii.2011, depth <2m, leg. TS.
Etymology: The specific epithet refers to the area the sample was collected from, Abu Qir, Egypt.
Description:
DNA sequence data: rbcL and psbA sequences diagnostic for this species. rbcL: TS 757 = ON376984; psbA: TS 757 = ON376951, TS 759 = ON376952; LSU and SSU sequences are also provided. LSU: TS 759 = ON362134; SSU: TS 757 = ON362158.
Habit and vegetative anatomy:
Thallus habit non-geniculate, forming epilithic crust on rock (Figures 5, 6); thallus construction monomerous with hypothallial cells rectangular to elongate with rounded corners, approximately 10 to 19 µm long and 5 to 10 µm wide; perithallium with cell fusions abundant in both x- and z-axes (Figures 5, 6); secondary pit connections absent between cells (Figures 5, 6); perithallial cells obovate (tear-drop shaped), 4 to 6 µm wide by 4 to 7 µm tall; intercalary meristematic cells short, 4 to 6 µm wide by 3.0 to 5 µm tall (Figures 5, 6); epithallium comprised of a single layer of epithelial cells dome-shaped 3.0 to 5.5 µm wide by 1.5 to 2.5 µm tall (Figures 5, 6).
Reproductive Morphology: No reproductive structures were observed in samples of P. abuqirensis vouchers TS 757 or TS 759.
Our phylogenetic analyses show that Phymatolithon vouchers TS 757 and TS 759 encompass a single, distinct species within Phymatolithon that we here name P. abuqirensis. The species shows significant sequence divergences (3.5%–14.8% in rbcL; 2.9 to 11% in psbA) from other closely related Phymatolithon species. These sequence divergence values correlated well with other coralline algal studies (Gabrielson et al., 2011; Torrano-Silva et al., 2018; Jeong et al., 2019; Jeong et al., 2021) that had reported interspecific variations for rbcL 6.4%–18.8% and for psbA 3.3%–13%. Further delimitation methods should be used with more genes to determine species and generic boundaries. Fragments of 18S and LSU were generated but were not phylogenetically informative enough given the lack of type specimens sequenced for these genes, and limited sequences to compare hindered the interpretation of results.
Morpho-anatomically, P. abuqirensis possesses the characters considered collectively to be diagnostic of the genus Phymatolithon, namely, thalli non-geniculate epithelial cells and non-photosynthetic and domed-shaped meristematic cells that are usually as short with progressive elongation of the perithallial derivatives. However, many of these morphological characters are shared with other genera of distantly related CCA, so interpretation using only morphology should be used with caution (as summarized in Supplemental Table 4). There are five reported species of Phymatolithon other than P. abuqirensis that have been collected in the Mediterranean Sea, namely, P. calcareum, P. lamii, P. lenormandii, P. lusitanicum, and P. purpureum (Irvine and Chamberlain, 1994; Kaleb et al., 2012; Peña et al., 2015; Wolf et al., 2016; Cormaci et al., 2017), four of which have been found in a wide biogeographical distribution outside of the Mediterranean Sea, such as the Atlantic Sea, northern Spain and France, Arctic Norway, eastern North America (Newfoundland, Canada to Massachusetts), and Asia (Adey and Adey, 1973; Chamberlain, 1991; Irvine and Chamberlain, 1994; Hernández-Kantún et al., 2014; Hernández-Kantún et al., 2015; Peña et al., 2015; Adey et al., 2018). Despite initially hypothesizing P. abuqirensis having a Tethyan distribution like other taxa found in the Red and Mediterranean Seas, the species closest to P. abuqirensis is P. nantuckensis, a taxon that has only been reported in Nantucket Island in the western North Atlantic (Adey et al., 2018; Guiry and Guiry, 2021). Adey et al. (2018) hypothesized that the existence of P. nantuckensis could be a relict species or possibly introduced by the heavy use of Nantucket Harbor as a whaling port during the 18th and 19th centuries, but no samples have been reported in the Northern Atlantic. Alternatively, this species might have just been overlooked and previously misidentified by initially being lumped with other species. As sequencing technologies advance in conjunction with a better understanding of phylogenetically informative morphological characteristics, a better understanding of Phymatolithon as a whole will become more evident. The knowledge of coralline algal diversity has greatly improved and led to both the reclassification of taxa and the discovery of new species. More in-depth biogeographical analyses should be conducted in the future.
CCA have been estimated to exist in the Mediterranean for ~140 My (Chatalov et al., 2015). More frequent environmental disturbances and anthropogenic stressors are impacting coralline algal ecosystems and adjacent habitats worldwide (Coll et al., 2010; Blanfuné, 2016; Basso et al., 2018; Quéré et al., 2019). CCA such as P. abuqirensis are at risk of being displaced due to the dynamic nature of calcium carbonate in the cell walls being intrinsically linked to global climate change and ocean acidification. The Mediterranean Basin has been coined a “climate change hotspot” where climate models are consistently projecting regional warming at rates 20% above the global means (Hilmi et al. 2022). CCA in the Mediterranean have already shown to be vulnerable to elevated temperatures and pCO2 experimental conditions (Martin and Gattuso, 2009; McCoy and Kamenos, 2015) leading to 2- to 3-fold increase in the percentage of death and dissolution of thalli. When examining the effects of climate change on early life stages of coralline, it was found that they led to lower reproductive success and recruitment (Cumani et al., 2010; Porzio et al., 2011; Kroeker et al., 2012).
Rindi et al. (2019) stated that populations of the same species in the eastern versus western Mediterranean may respond differently to future climatic changes. One reason might be because of the variability in oceanographic conditions within the basins, such as the influence of the Atlantic Ocean in the western Mediterranean to the overall spatial sea surface temperatures and sea surface salinities that are controlled by the distribution of the colder Black Sea Waters, and the advection of the warmer Levantine Waters of the Aegean Sea (Estournel et al., 2021).
This study helps the global diversity research of CCA by adding to the number of described species of Phymatolithon for the Mediterranean region and by contributing to understanding cryptic coralline algal species on a global scale. Multiple markers (rbcL, psbA, SSU, and LSU) are provided for Phymatolithon abuqirensis to aid future studies to better understand the phylogenetic relationships and species delimitations of the genus. Phymatolithon abuqirensis could also have been introduced in the Mediterranean Sea due to the close proximity to the Red Sea. Since the distribution of P. abuqirensis is unknown outside of Abu Qir, Egypt, additional sampling is needed to assess the full biogeographical range of this species.
The datasets presented in this study can be found in online repositories. The names of the repository/repositories and accession number(s) can be found in the article/Supplementary Material.
RK, JR, WS, TS, DG, and SF conceived the study. TS collected the samples; RK and JR conducted the laboratory work; RK performed the data analyses. RK, JR, and SF wrote the manuscript with contributions from WS, TS, and DG. All authors contributed to the article and approved the submitted version.
This work was funded by NSF grant DEB-1754504.
We greatly acknowledge the support from NSF research grant DEB1754504. RK would like to thank the Phycological Society of America Grant-In-Aid Research and the University of Louisiana at Lafayette Graduate Student Organization for partially funding this research. We thank Tom Pesacreta Mike Purpera, and Lily Ann Hume at the UL Lafayette Microscopy Center for their help with SEM imaging, and Ally Nyugen for help with PCR and initial sequencing.
The authors declare that the research was conducted in the absence of any commercial or financial relationships that could be construed as a potential conflict of interest.
All claims expressed in this article are solely those of the authors and do not necessarily represent those of their affiliated organizations, or those of the publisher, the editors and the reviewers. Any product that may be evaluated in this article, or claim that may be made by its manufacturer, is not guaranteed or endorsed by the publisher.
The Supplementary Material for this article can be found online at: https://www.frontiersin.org/articles/10.3389/fmars.2022.922389/full#supplementary-material
Supplementary Table 1 | List of specimens and their information used in the molecular analyses. Type/topotype material are in bold; Taxa are listed in alphabetical order; ‒ indicates no sequence available; Genera in single quotes represent genus names of uncertain status.
Supplementary Table 2 | Sequence divergence values of psbA sequences.
Supplementary Table 3 | Sequence divergence values of rbcL sequences.
Supplementary Table 4 | Comparison table of vegetative morphological characters of P. abuqirensis and closely related taxa.
Adey W. H. (1964). The genus Phymatolithon in the gulf of Maine. Hydrobiologia 24, 377–420. doi: 10.1007/BF00170412
Adey W. H. (1970). The effects of light and temperature on growth rate in boreal-subarctic crustose corallines. J. Phycol. 6, 269–276. doi: 10.1111/j.1529-8817.1970.tb02392.x
Adey W. H., Adey P. J. (1973). Studies on the biosystematics and ecology of the epilithic crustose corallinaceae of the British isles. Br. Phycol. J. 8, 343–407. doi: 10.1080/00071617300650381
Adey W. H., Athanasiadis A., Lebednik P. (2001). Re-instatement of Leptophytum and its type Leptophytum laeve: Taxonomy and biogeography of the genera Leptophytum and Phymatolithon (Corallinales, Rhodophyta). Eur. J. Phycol. 36, 191–203. doi: 10.1080/09670260110001735338
Adey W. H., Chamberlain Y. M., Irvine L. M. (2005). An SEM-based analysis of the morphology, anatomy, and reproduction of Lithothamnion tophiforme (Esper) unger (Corallinales, rhodophyta) with a comparative study of associated north Atlantic Arctic/Subarctic melobesioideae. J. Phycol. 41, 1010–1024. doi: 10.1111/j.1529-8817.2005.00123.x
Adey W. H., Halfar J., Williams B. (2013). The coralline genus Clathromorphum foslie emend. adey: biological, physiological, and ecological factors controlling carbonate production in an arctic-subarctic climate archive. Smithson. Contr. Mar. Sci. 40, 1–41. doi: 10.5479/si.1943667X.40.1
Adey W. H., Hayek L. C. (2005). The biogeographic structure of the western north Atlantic rocky intertidal. Crypt. Algol. 26, 35–66.
Adey W. H., Hayek L. A. C. (2011). Elucidating marine biogeography with macrophytes: quantitative analysis of the north Atlantic supports the thermogeographic model and demonstrates a distinct subarctic region in the northwestern Atlantic. Northeast. Natur. 18, 1–128. doi: 10.1656/045.018.m801
Adey W. H., Hernández-Kantún J. J., Gabrielson P. W., Nash M. C., Hayek L. C. (2018). Phymatolithon (Melobesioideae, hapalidiales) in the boreal-subarctic transition zone of the north Atlantic. Smithson. Inst. Sch. Press 40, 1–90. doi: 10.5479/si.1943-667X.41
Adey W. H., Hernández-Kantún J. J., Johnson G., Gabrielson P. W. (2015). DNA Sequencing, anatomy, and calcification patterns support a monophyletic, subarctic, carbonate reef-forming Clathromorphum (Hapalidiaceae, corallinales, rhodophyta). J. Phycol. 51, 189–203. doi: 10.1111/jpy.12266
Adey W. H., Macintyre I. G. (1973). Crustose coralline algae: a re-evaluation in the geological sciences. Geol. Soc Am. Bull. 84, 883–904. doi: 10.1130/0016-7606(1973)84%3C883:CCAARI%3E2.0.CO;2
Adey W. H., Steneck R. S. (2001). Thermogeography over time creates biogeographic regions: a temperature/space/time-integrated model and an abundance-weighted test for benthic marine algae. J. Phycol. 37, 677–698. doi: 10.1046/j.1529-8817.2001.00176.x
Basso D., Caronni S., Caragnano A., Hereu B., Angeletti L., Bracchi V. (2018). “Evidence of coralline white patch disease in a rhodolith bed of the egadi islands,” in Abstract book, VI international rhodolith workshop, vol. 60. (Roscoff, France: CNRS Sorbonne Université Station Biologique de Roscoff).
Blanfuné A. (2016). Global change in the NW Mediterranean Sea: the fate of forests of cystoseira and sargassum, lithophyllum rims and blooms of ostreopsis (Ph.D. thesis) (Marseilles, France: Université d'Aix-Marseille).
Chamberlain Y. M. (1991). Historical and taxonomic studies in the genus Titanoderma (Rhodophyta, corallinales) in the British isles. Bull. Brit. Museum (Nat. Hist.) Bot. Ser. 21, 1–80.
Chatalov A., Bonev N., Ivanova D. (2015). Depositional characteristics and constraints on the mid-valanginian demise of a carbonate platform in the intra-tethyan domain, circum-rhodope belt, northern Greece. Cret. Res. 55, 84–115. doi: 10.1016/j.cretres.2015.02.001
Coll M., Piroddi C., Steenbek J., Kaschner K., Lasram F. B. R., Aguzzi J., et al. (2010). The biodiversity of the mediterraneran Sea: estimates, patterns and threats. PloS One 5, e11842. doi: 10.1371/journal.pone.0011842
Cormaci M., Furnari G., Alongi G. (2017). Flora marina bentonica del mediterraneo rhodophyta (Rhodymeniophycidae escluse). Bollet. Accad. Gioenia Sci. Natur. Catania 50, 1–391. Available at: http://proceedings.ct.infn.it/gioenia/index.php/gioenia/article/view/10/10
Cumani F., Bradassi F., Di Pascoli A., Bressan G. (2010). Marine acidification effects on reproduction and growth rates of corallinaceae spores (Rhodophyta). Rapp. Commun. Int. Mer Medit. 39, 735.
Estournel C., Marsaleix P., Ulses C. (2021). A new assessment of the circulation of Atlantic and intermediate waters in the Eastern Mediterranean. Prog. Oceanogr. 198, 102673. doi: 10.1016/j.pocean.2021.102673
Foslie M. H. (1898). Systematical survey of the lithothamnia. det kongel. norske videnskab. selskabs skr. 20 pp. (Rondiem, Norway: Aktietrykkeriet).
Foslie M. H. (1904). “Lithothamnioneae, melobesieae, mastophoreae,” in The corallinaceae of the siboga expedition, siboga-expeditie LXI, 10–77. (Leiden, Netherlands: E.J. Brill)
Gabrielson P. W., Miller K. A., Martone P. T. (2011). Morphometric and molecular analyses confirm two distinct species of Calliarthron (Corallinales, rhodophyta), a genus endemic to the northeast pacific. Phycologia 50, 298–316. doi: 10.2216/10-42.1
Guiry M. D., Guiry G. M. (2021) AlgaeBase (Galway: World-wide electronic publication, National University of Ireland). Available at: https://www.algaebase.org (Accessed December 20, 2021).
Hernández-Kantún J. J., Riosmena-Rodriguez R., Adey W. H., Rindi F. (2014). Analysis of the cox 2-3 spacer region for population diversity and taxonomic implications in rhodolith-forming species (Rhodophyta: Corallinales). Phytotaxa 190, 331–354. doi: 10.11646/phytotaxa.190.1.20
Hernández-Kantún J. J., Riosmena-Rodriguez R., Hall-Spencer J. M., Peña V., Maggs C. A., Rindi F. (2015). Phylogenetic analysis of rhodolith formation in the corallinales (Rhodophyta). Eur. J. Phycol. 50, 46–61. doi: 10.1080/09670262.2014.984347
Hilmi N., Ali E., Carnicer Cols J., Cramer W., Georgopoulou E., Le Cozannet G. (2022). Vienna, Austria:IPCC AR6 WGII Cross-Chapter Paper 4: Mediterranean Region, EGU General Assembly 2022. doi: doi.org/10.5194/egusphere-egu22-10590
Hind K. R., Gabrielson P. W., Lindstrom S. C., Martone P. T. (2014). Misleading morphologies and the importance of sequencing type specimens for resolving coralline taxonomy (Corallinales, rhodophyta): Pachyarthron cretaceum is Corallina officinalis. J. Phycol. 50, 760–764. doi: 10.1111/jpy.12205
Hind K. R., Miller K. A., Young M., Jensen C., Gabrielson P. W., Martone P. T. (2015). Resolving cryptic species of Bossiella (Corallinales, rhodophyta) using contemporary and historical DNA. Am. J. Bot. 102, 1912–1930. doi: 10.3732/ajb.1500308
Irvine L. M., Chamberlain Y. M. (1994). Seaweeds of the British isles, vol. 1. Rhodophyta,Part 2B corallinales, hildenbrandiales (London: Her Majesty's Stationery Office), 276 pp.
Jeong S. Y., Diaz-Pulido G., Maneveldt G. W., Gabrielson P. W., Nelson W. A., Won B. Y., et al. (2022). Phymatolithopsis gen. nov. (hapalidiales, corallinophycidae, rhodophyta) based on molecular and morpho-anatomical evidence. J. Phycol. 58, 161–178. doi: 10.1111/jpy.13227
Jeong S. Y., Diaz-Pulido G., Won B. Y., Cho T. O. (2021). Phymatolithon atlanticum sp. nov. (Hapalidiales, rhodophyta) from the northeast Atlantic ocean. Phycologia 60, 200–209. doi: 10.1080/00318884.2021.1885197
Jeong S., Won B., Cho T. O. (2019). Two new encrusting species from the genus Phymatolithon (Hapalidiales, corallinophycidae, rhodophyta) from Korea. Phycologia 58, 592–604. doi: 10.1080/00318884.2019.1625608
Kaleb S., Falace A., Woelkerling W. (2012). Phymatolithon lamii (Hapalidiaceae, corallinales, rhodophyta): a first report for the Mediterranean Sea. Botanica Marina 55, 377–385. doi: 10.1515/bot-2012-0141
Kroeker K. J., Micheli F., Gambi M. C. (2012). Ocean acidification causes ecosystem shifts via altered competitive interactions. Nat. Clim. Change 3, 156–159. doi: 10.1038/nclimate1680
Lanfear R., Frandsen P. B., Wright A. M., Senfeld T., Calcott B. (2017). PartitionFinder 2: new methods for selecting partitioned models of evolution for molecular and morphological phylogenetic analyses. Mol. Biol. Evol. 34, 772–773. doi: 10.1093/molbev/msw260
Linnaeus C. (1767). Systema naturae per regna tria naturae: secundum classes, ordines, genera, species, cum characteribus, differentiis, synonymis, locis Vol. Vol. 1, No. part 1 (Stockholm: Laurentii Salvii), 532.
Maneveldt G. W., Jeong S. Y., Cho T. O., Hughey J. R., Gabrielson P. W. (2020). Reassessment of misapplied names, Phymatolithon ferox and P. repandum (Hapalidiales, corallinophycidae, rhodophyta) in south Africa, based on DNA sequencing of type and recently collected material. Phycologia 59, 449–455. doi: 10.1080/00318884.2020.1800298
Martin S., Gattuso J. P. (2009). Response of Mediterranean coralline algae to ocean acidification and elevated temperature. Glob. Change Biol. 15, 2089–2100. doi: 10.1111/j.1365-2486.2009.01874.x
Martone P. T., Lindstrom S. C., Miller K. A., Gabrielson P. W. (2012). Chiharaea and Yamadaia (Corallinales, rhodophyta) represent reduced and recently derived articulated coralline morphologies. J. Phycol. 48, 859–868. doi: 10.1111/j.1529-8817.2012.01190.x
McCoy S. J., Kamenos N. A. (2015). Coralline algae (Rhodophyta) in a changing world: integrating ecological, physiological, and geochemical responses to global change. J. Phycol. 51, 6–24. doi: 10.1111/jpy.12262
Pardo C., Guillemin M. L., Peña V., Bárbara I., Valero M., Barreiro R. (2019). Local coastal configuration rather than latitudinal gradient shape clonal diversity and genetic structure of Phymatolithon calcareum maerl beds in north European Atlantic. Front. Mar. Sci. 6. doi: 10.3389/fmars.2019.00149
Pardo C., Lopez L., Pena V., Hernández-Kantún J., Le Gall L., Barbara I., et al. (2014). A multilocus species delimitation reveals a striking number of species of coralline algae forming maerl in the OSPAR maritime area. PloS One 9 (8), e104073. doi: 10.1371/journal.pone.0104073
Peña V., Hernández-Kantún J. J., Grall J., Pardo C., López L., Bárbara I., et al. (2014). Detection of gametophytes in the maerl-forming species Phymatolithon calcareum (Melobesioideae, corallinales) assessed by DNA barcoding. Cryptog. Algol. 35, 15–25. doi: 10.7872/crya.v35.iss1.2014.15
Peña V., Pardo C., López L., Carro B., Hernandez-Kantun J., Adey W. H., et al. (2015). Phymatolithon lusitanicum sp. nov. (Hapalidiales, rhodophyta): the third most abundant maerl-forming species in the Atlantic Iberian peninsula. Cryptog. Algol. 36, 429–459. doi: 10.7872/crya/v36.iss4.2015.429
Porzio L., Buia M. C., Hall-Spencer J. M. (2011). Effects of ocean acidification on macroalgal communities. J. Exp. Mar. Biol. Ecol. 400, 278–287. doi: 10.1016/j.jembe.2011.02.011
Puckree-Padua C. A., Gabrielson P. W., Hughey J. R., Maneveldt G. W. (2020). DNA Sequencing of type material reveals Pneophyllum marlothii comb. nov. from south Africa and P. discoideum comb. nov. (Chamberlainoideae, corallinales, rhodophyta) from Argentina. J. Phycol. 56, 1625–1641. doi: 10.1111/jpy.13047
Puillandre N., Brouillet S., Achaz G. (2021). ASAP: assemble species by automatic partitioning. Mol. Ecol. Res. 21, 609–620. doi: 10.1111/1755-0998.13281
Quéré G., Intertaglia L., Payri C., Garland P. E. (2019). Disease specific bacterial communities in a coralline alga of the northwestern Mediterranean Sea: a combined culture dependent and -independent approach. Front. Microbiol. 10. doi: 10.3389/fmicb.2019.01850
Rambaut A., Drummond A. (2012). “FigTree: Tree figure drawing tool, v1. 4.2,” in Inst. of evol. biol (Univ. Edinburgh).
Richards J. L., Gabrielson P. W., Fredericq S. (2014). New insights into the genus Lithophyllum (Lithophylloideae, corallinaceae, corallinales) from deepwater rhodolith beds offshore the NW gulf of mexico. Phytotaxa 190, 162–175. doi: 10.11646/phytotaxa.190.1.11
Richards J. L., Kittle III R. P., Abshire J. R., Fuselier D., Schmidt W. E., Gurgel C. F. D., et al. (2020). Range extension of Mesophyllum erubescens (Foslie) me. lemoine (Hapalidiales, rhodophyta); first report from mesophotic rhodolith beds in the northwestern gulf of Mexico offshore Louisiana and Texas, including the flower garden banks national marine sanctuary. Check List 16, 513–519. doi: 10.15560/16.3.513
Richards J. L., Kittle III R. P., Schmidt W. E., Sauvage T., Gurgel C. F. D., Gabriel D., et al. (2022). Assessment of rhodolith diversity in the northwestern Gulf of Mexico including the description of Sporolithon gracile sp. nov. (Sporolithales, Rhodophyta), and three new species of Roseolithon (Hapalidiales, Rhodophyta). Front. Mar. Sci. 9, 906679. doi: 10.3389/fmars.2022.906679
Richards J. L., Sauvage T., Schmidt W. E., Fredericq S., Hughey J. R., Gabrielson P. W. (2017). The coralline genera Sporolithon and Heydrichia (Sporolithales, rhodophyta) clarified by sequencing type material of their generitypes and other species. J. Phycol. 53, 1044–1059. doi: 10.1111/jpy.12562
Richards J. L., Viei ra-Pinto T., Schmidt W. E., Sauvage T., Gabrielson P. W., Oliveira M. C., et al. (2016). Molecular and morphological diversity of Lithothamnion spp. (Hapalidiales, rhodophyta) from deepwater rhodolith beds in the northwestern gulf of Mexico. Phytotaxa 278, 81–114. doi: 10.11646/phytotaxa.278.2.1
Rindi F., Braga J. C., Martin S., Peña V., Le Gall L., Caragnano A., et al. (2019). Coralline algae in a changing Mediterranean Sea: How can we predict their future, if we do not know their present? Front. Mar. Sci. 6. doi: 10.3389/fmars.2019.00723
Stamatakis A. (2014). RAxML version 8: a tool for phylogenetic analysis and post-analysis of large phylogenies. Bioinformatics 30, 1312–1313. doi: 10.1093/bioinformatics/btu033
Torrano-Silva B. N., Vieira B. R., Riosmena-Rodriguez R., Oliveira M. C. (2018). Guidelines for DNA barcoding of coralline algae, focusing on lithophylloideae (Corallinales) from Brazil. Bot. Mar. 61, 127–140. doi: 10.1515/bot-2017-0040
Vaidya G., Lohman D. J., Meier R. (2011). SequenceMatrix: concatenation software for the fast assembly of multi-gene datasets with character set and codon information. Cladistics 27, 171–180. doi: 10.1111/j.1096-0031.2010.00329.x
Van der Merwe E., Maneveldt G. W. (2014). The genus Phymatolithon (Hapalidiaceae, corallinales, rhodophyta) in south Africa, including species previously ascribed to Leptophytum. S. Afr. J Bot. 90, 170–192. doi: 10.1016/j.sajb.2013.11.004
Van der Merwe E., Miklasz K., Channing A., Maneveldt G. W., Gabrielson P. W. (2015). DNA Sequencing resolves species of Spongites (Corallinales, rhodophyta) in the northeast pacific and south Africa, including s. agulhensis sp. nov. Phycologia 54, 471–490. doi: 10.2216/15-38.1
Wilks K. M., Woelkerling W. J. (1994). An account of southern Australian species of Phymatolithon (Corallinaceae, rhodophyta) with comments on Leptophytum. Aust. Syst. Bot. 7, 183–223. doi: 10.1071/SB9940183
Woelkerling W. J. (1988). The coralline red algae: An analysis of the genera and subfamilies of nongeniculate corallinaceae (London and Oxford: University Press. British Museum (Natural History) Oxford).
Woelkerling W. J., Irvine L. M. (1986). The typification and status of Phymatolithon (Corallinaceae, rhodophyta). Brit. Phycol. J. 21, 55–80. doi: 10.1080/00071618600650071
Keywords: anatomy, coralline algae, CCA, marine biodiversity, Egypt, Mediterranean Sea
Citation: Kittle RP III, Richards JL, Sauvage T, Gabriel D, Schmidt WE and Fredericq S (2022) A new species of Phymatolithon Foslie, P. abuqirensis (Hapalidiaceae, Hapalidiales), from Mediterranean Egypt. Front. Mar. Sci. 9:922389. doi: 10.3389/fmars.2022.922389
Received: 17 April 2022; Accepted: 08 July 2022;
Published: 10 August 2022.
Edited by:
Kathryn Schoenrock, National University of Ireland Galway, IrelandReviewed by:
Wendy Nelson, The University of Auckland, New ZealandCopyright © 2022 Kittle, Richards, Sauvage, Gabriel, Schmidt and Fredericq. This is an open-access article distributed under the terms of the Creative Commons Attribution License (CC BY). The use, distribution or reproduction in other forums is permitted, provided the original author(s) and the copyright owner(s) are credited and that the original publication in this journal is cited, in accordance with accepted academic practice. No use, distribution or reproduction is permitted which does not comply with these terms.
*Correspondence: Ronald P. Kittle III, Um9uYWxkLktpdHRsZTFAbG91aXNpYW5hLmVkdQ==
Disclaimer: All claims expressed in this article are solely those of the authors and do not necessarily represent those of their affiliated organizations, or those of the publisher, the editors and the reviewers. Any product that may be evaluated in this article or claim that may be made by its manufacturer is not guaranteed or endorsed by the publisher.
Research integrity at Frontiers
Learn more about the work of our research integrity team to safeguard the quality of each article we publish.