- 1State Key Laboratory of Biocontrol, Southern Marine Science and Engineering Guangdong Laboratory (Zhuhai), School of Life Sciences, Sun Yat-Sen University, Guangzhou, China
- 2Institute for the Oceans and Fisheries, The University of British Columbia, Vancouver, BC, Canada
Fishery resources are threatened by environmental changes and anthropogenic pressures, particularly in coastal ecosystems. It is crucial to understand the changes of fish communities and their responses to environmental changes and human disturbances to formulate rational fisheries and ecosystem-based management. The Pearl River Estuary (PRE) is a typical sub-tropic coastal ecosystem located in the center of the Guangdong-Hong Kong-Macao Greater Bay Area in the northern South China Sea. The demersal fish in the PRE is traditionally targeted as commercial fishing and severely impacted by overexploitation and hypoxia in the last few decades. In this study, we analyze the fish survey data during the period of 2020~2021 using multivariate statistics to investigate the impacts of human disturbances on the species and functional dynamics of the demersal fish community in the PRE. The results reveal that dissolved oxygen and temperature have significant correlations with the functional traits of the demersal fish community. The impacts of hypoxia on the demersal fish vary with species and locations. We found that the mean functional redundancy of the demersal fish community in the PRE was high across three surveys, but the functional diversity was low in this region. The abundance and richness of the demersal fish community increased during the summer fishing moratorium in the South China Sea in 2021, but the functional diversity did not increase significantly. We conclude that the high functional redundancy in the PRE might not be sufficient to buffer against environmental disturbances because of its low functional diversity. Our study highlights the complicated interactions between the demersal fish community and disturbances in the PRE. Understanding the traits structure and functional diversity of the fish community can help elucidate the factors determining the dynamic responses of the fish community to disturbances.
Introduction
Over-exploitation, climate changes, pollution and other anthropogenic pressures are threatening the sustainability of global marine ecosystems and fishery resources (Rouyer et al., 2008; Cheung et al., 2013; Frelat et al., 2018). These human pressures play important roles in shaping the spatial distribution of marine species (Perry et al., 2005; Poloczanska et al., 2016; Frelat et al., 2018), impacting the temporal dynamics of communities and ecosystem functions (Rouyer et al., 2008; Mollmann et al., 2009; Brind’Amour et al., 2011; Asefa et al., 2017).
The resilience of communities to environmental changes and disturbances depends on the biological traits, species and functional diversity of communities (Cadotte, 2017; Craven et al., 2018; McLean et al., 2019). Species’ persistence is dependent on environmental conditions and the adaptability of their biological traits. Functional diversity of the community, which is dependent on the functional traits of species, decreases in more extreme environmental conditions where a subset of traits can adapt (Mouillot et al., 2014; McLean et al., 2019). When several species perform similar functions, functional redundancy of communities occurs. It can prevent the loss of communities’ functions caused by the declines in the species and functional diversity (Yachi and Loreau, 1999; Fonseca and Ganade, 2001). Thus, community studies should focus on not only the change of species but also the change in community function and even the interrelation between functional diversity and distributions.
In the marine realm, estuaries are important transitional regions for the material exchanges between land and ocean ecosystems, and generally host remarkable diversity of fishes. Fish communities sustain key ecosystem processes and provide fishery resources in estuary ecosystems. However, the fish communities in estuaries face high pressure due to overfishing and changes in environmental conditions (Duan et al., 2009; Cornwall and Eddy, 2015; Gobler and Baumann, 2016). Acidification and hypoxia in bottom water in temperate and tropical estuaries were frequently reported (Gobler and Baumann, 2016; Marshall et al., 2017).The Pearl River Estuary (PRE) is a typical subtropical estuary ecosystem, a traditional fishing ground and an important nursery ground for many species (Jia et al., 2005). In the last decades, human pressures have deteriorated the water conditions and fish community in the PRE (Jia et al., 2005; Ke et al., 2007). For instance, the hypoxia in the bottom water expanded the intensity and influence range since being discovered in 1985, which directly impacts the demersal fish community in the PRE (Yin et al., 2004; Wang et al., 2018; Cui et al., 2019). Demersal fishes are the traditional commercial fishes in the PRE, and have experienced over-exploitation since 1978 because of the high increase in the number of fishing boats and the advance in the fishing technology (Jia et al., 2005). The PRE can be a typical overexploited estuary ecosystem to analyze the dynamics of the demersal fish community and the response to distributions.In the last two decades, multivariate statistical analysis have been developed and provide ecologists with tools to investigate the relationships between species, functional traits and environmental conditions (Dray et al., 2003; Villeger et al., 2008; Laliberte and Legendre, 2010; Dray et al., 2014; Frelat et al., 2018). In this study, we used multivariate statistical analysis to analyze the temporal and spatial variations of species, the dynamics of trait structure, the functional diversity and the functional redundancy of the demersal fish community, and further explore the impacts of environmental variation on the demersal fish community in the PRE.
Materials and Methods
Fish Abundance Data
We focus on the demersal fish community in the PRE. Since 2020, we conducted three surveys, two in summer (August 2020 and July 2021) and another in winter (December 2020). The summer survey in 2021 was carried out during the fishing moratorium in the South China Sea, including the PRE. The bottom trawl boat used in our surveys is about 120 tons with an engine power of 138 kW. The surveys were conducted at 4 knots for about 30 minutes on average. The surveys covered most of the shallow areas of the PRE (Figure 1). Three surveys include 66 hauls, around 20-24 hauls per survey in the same spatial coverage of the PRE. Fish catches were stored frozen by sites and taken to the laboratory for species identification. We removed the rare and pelagic fish species, which are less than 0.01% of the total abundance of the recorded species in our surveys. Finally, we got 80 fish species from 66 hauls in the PRE.
Fish Traits Dataset and Environmental Variables in the PRE
Ecological traits, which are associated to the energy transfer, the environmental filtering and biological interactions, are usually taken into consideration in community studies because they can be used to describe the ecosystem processes and species’ responses to environmental changes (McLean et al., 2018; McLean et al., 2019). we integrated six ecological traits to examine the dynamic processes of communities, including body size, trophic level, the resilience ability of species, salinity preference and habitat preference in the water column position (Table S6). The functional traits we used in this study represent the trophic ecology, morphologic characteristics, life history and habitat preference of fish species, referring to Mouillota et al. (2014). The salinity preference and water column position of the species are adopted to describe the ecological traits of fish species in estuary ecosystems. The detailed information on species traits was obtained from the Fishbase (https://www.fishbase.se/search.php). Environmental variables can shape the structure of fish communities since species tend to live in their preferred environment (Frelat et al., 2018). Temperature, dissolved oxygen (DO) and pH of the bottom water and the average depth of each site are taken into account in this study.
RLQ Analysis and Fourth-Corner Method
The multivariate statistic methodologies allow ecologists to investigate the impacts of environmental variables on the temporal, spatial and functional dynamics of communities simultaneously (Frelat et al., 2018). We applied the RLQ analysis and fourth-corner method to clarify the relationships among the fish distribution, the community composition, the species traits and environmental variables in the PRE. The RLQ analysis and the fourth-corner approach are the most integrated methods for analyzing trait–environment relationships (Kleyer et al., 2012). The RLQ analysis is an extension method of co-inertia analysis to simultaneously perform the ordinations of three matrices, the abundance data (called A or L), the matrix of species by biological or behavioral traits (called B or Q) and the matrix of sites by habitat characteristics (called C or R) (Figure 2). The fourth-corner method uses the formula, D=Q'L'R or D=R' LQ, to calculate the matrix D and test the significance of the species traits and environmental characteristics (Legendre and Legendre, 2012; Borcard et al., 2018).
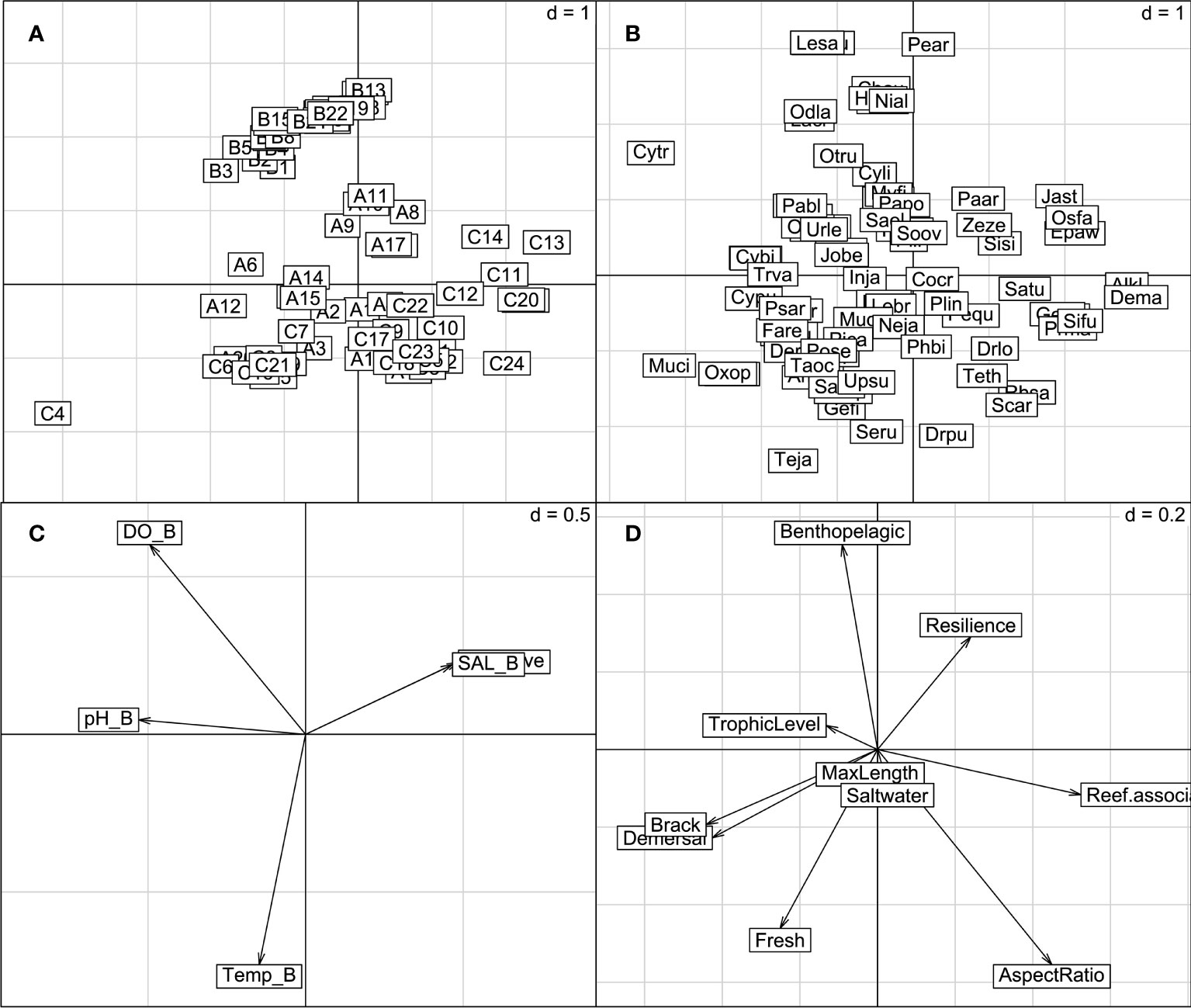
Figure 2 Results of the RLQ analysis on the demersal fish community in the PRE: (A) the site (L) scores, (B) the species (Q) scores, (C) the environmental variables and (D) the species traits. Ai, Bi and Ci in (A) are the sampling sites in August 2020, December 2020 and July 2021, respectively (Table S1, S2 and S3). The acronyms with four letters in (B) are the species in the PRE (Table S4). The variates in (C) are the environmental variables, where DO_B is the DO in the bottom water, pH_D is the pH in the bottom water, Temp_D is the temperature in the bottom water and Depth_Ave is the average depth of the sampling site. The acronyms in (D) are the functional traits of species (Table S6).
Diversity Indices
Beta Diversity
Beta diversity can reflect the spatial variance of the community composition in a region by measuring variations in species composition and abundance among all sites (Anderson et al., 2006). We use a method independent from alpha and gamma diversity to estimate the beta diversity, which is the total variance of the site-by-species table Y, Var(Y), called BDTotal (Borcard et al., 2018). The BDTotal is written as
where the SSTotal is the total sum-of-squares of Y (Legendre and De Caceres, 2013; Borcard et al., 2018).
Beta diversity results from the processes of species replacement and richness difference (species gain and loss) (Williams, 1996; Lennon et al., 2001; Borcard et al., 2018). In this study, Podani’s Jaccard-based indices were adopted to describe the richness difference and replacement values of the sampling sites. We used the triangle plots to show the richness difference, replacement and the corresponding similarity of all pairs of the sites. The points in triangle plots represent the triplet of values of a pair of sites, which correspond to the similarity (1-D), replacement (Repl) and richness difference (RichDiff) (Legendre, 2014).
Functional Diversity and Functional Redundancy Indices
Four distance-based multi-trait diversity indices, including functional richness (FRic), functional evenness (Feve), functional divergence (Fdiv) and functional dispersion (Fdis), were proposed by Villeger et al. (2008) and Laliberte and Legendre. (2010). They were independent components in the functional diversity of the demersal fish community in the PRE and are related to or can be used to measure the functional space of a given community, the regularity of the abundance distribution in the multidimensional function space and species abundances distributing within the functional trait space (Villeger et al., 2008; Laliberte and Legendre, 2010).
The inverse Simpson diversity (TD) can represent the potential maximum value of the functional diversity in a community (de Bello et al., 2010; Borcard et al., 2018). The difference between the inverse Simpson diversity and the functional diversity (FD) can be used to measure functional redundancy (FR) (de Bello et al., 2007). Functional diversity is described by using the Rao coefficient, which is calculated as the sum of trait dissimilarities between pairs of species, multiplied by species abundance (Leps et al., 2007). The difference between species i and species j (dij) is conducted by the dissimilarities in functional traits.
Because the number of traits may influence the results of the functional indices (Guillemot et al., 2011), we also performed sensitivity analyses to test the robustness of the functional indices by rerunning all analyses with all combinations of five traits out of six. We did not reduce the number of traits to less than five to process the sensitivity analyses to keep the important dimensions of the functional space of fish assemblage (McLean et al., 2019).
Results
Traits and Distributions of the Demersal Fish Community Linked With Environmental Variables in the PRE
A total of 6930 individuals representing 80 species were sampled in our study (Table S1–S3). There are 36 common species found in the three surveys (Figure S1), but the dominant species are different in each survey. The most abundant species were Ambassis gymnocephalus, Johnius belangerii and Decapterus maruadsi for the three surveys, respectively (Table S5). The sampling sites were divided into summer and winter sub-assemblages, based on the Ward hierarchical clustering. The environmental variables in the summer are characterized by higher water temperature and lower oxygen concentrations, compared to those in winter (Figure 2). DO of bottom water reduced sharply during the summer investigation in 2021. Most of the sites underwent hypoxia processes (Table S3), and the DO of sites C9, C11, C12, C18, C19, C20, C23 and C24 were even less than 2.0 mg/l (Table S3).
The main relationships between fish traits and environmental variables can be explained by the first two RLQ axes. The right (positive) part of the first RLQ axis identifies species of reef-associated habitat preference, such as Decapterus maruadsi, Priacanthus macracanthus, Siganus fuscescens and Saurida tumbil (Figures 2A, C), which are mainly located at the hypoxia areas in the summer of 2021 (Figure 2B). The left part of the first RLQ axis distinguished the species of high trophic levels and habitat preference of the brackish and demersal. The second RLQ axis profiles the assemblages of the winter and summer sampling sites. The positive part associated the winter sampling sites with high salinity and low temperature. The related species, Cynoglossus lineolatus, Lepturacanthus savala and Odontamblyopus lacepedii, were mainly caught in these sites (Figures 2A, C). The negative part of the axis was associated with the summer sampling sites, and the species Drepane punctate, Photopectoralis bindus, Secutor ruconius and Upeneus sulphureus distributed throughout these sites.The D matrix shows detailed interpretations of the traits of the demersal fish and environment variables in the PRE. According to the results, the temperature and DO of the bottom water were strongly linked with the traits of benthopelagic, the preference for saltwater habitat and the aspect ratio of the caudal fin of the demersal fish in the PRE (Figure 3). For instance, the trait of preference for saltwater habitat is positively associated with the temperature in the bottom water but negatively related with the DO in the bottom water. The aspect ratio of the caudal fin of fishes, as the only morphological trait taken into consideration, is negatively associated with the DO of the bottom water. It indicates that the species with a small aspect ratio tend to distribute in non-hypoxic areas. Based on the analysis results of RLQ, the species with a small aspect ratio, such as the Cynoglossus lineolatus and Cynoglossus puncticeps, were mainly caught in the winter of 2020, and these species were also found in the sites with high DO in summer (Figure 2).
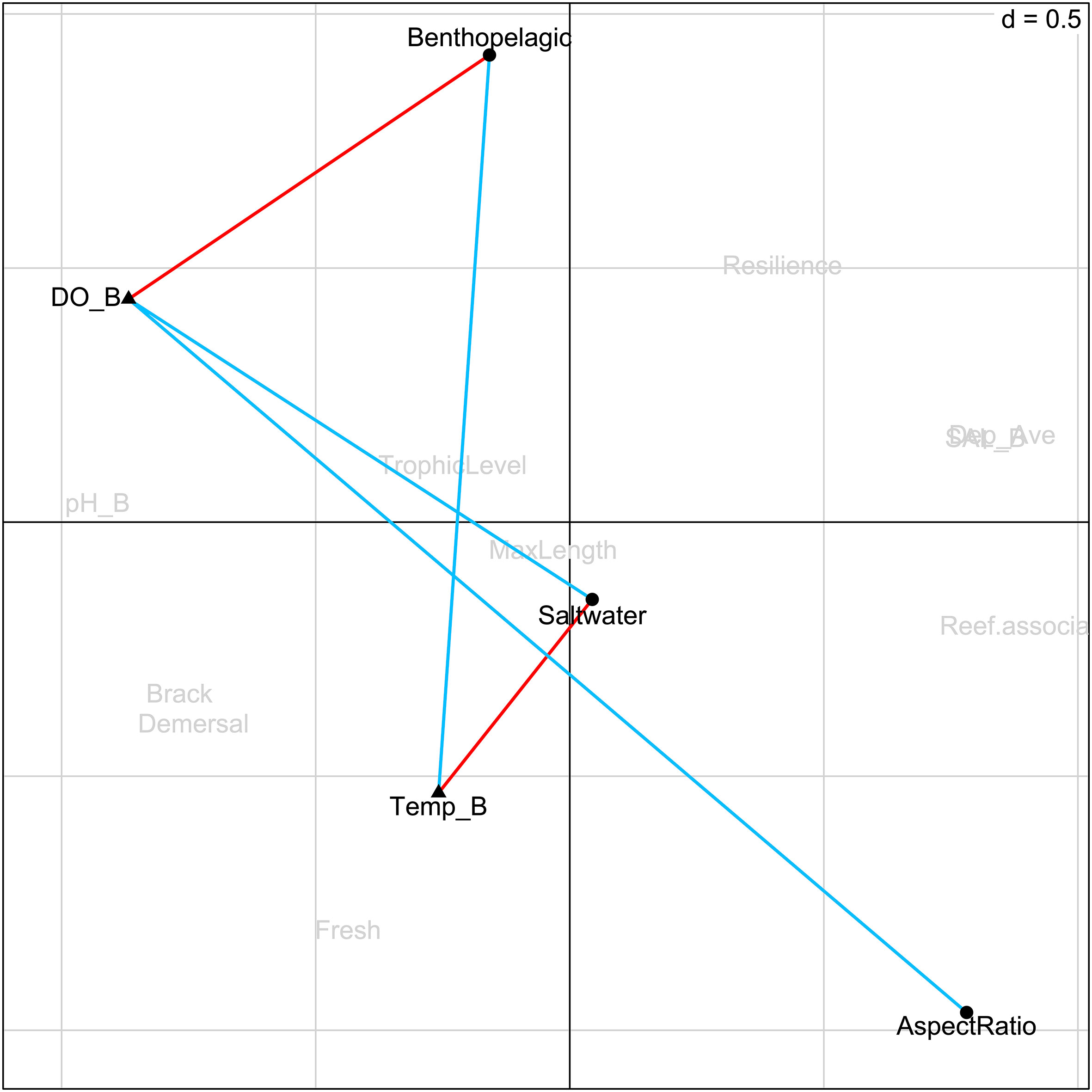
Figure 3 Biplot of the fish traits and environmental variables from the RLQ analysis. The significant positive correlations between fish traits and environmental variables are represented by red cells and negative ones by blue cells, which are obtained from the multiple testing using the FDR (false discovery rate) procedure with the α = 0.05 level. DO_B is the DO in the bottom water; Temp_D is the temperature in the bottom water; Benthopelagic is the trait of species for water column position; Saltwater is the trait of species of salinity preference; AspectRatio is the trait of species of the aspect ratio of the caudal fin. See Table S6 for acronyms of traits.
Community Structure and Functional Diversity of the Demersal Fish in the PRE
The beta diversity indices, representing the spatial variations of the demersal fish structure, are 0.71, 0.63, and 0.79 for the summer and winter in 2020 and the summer in 2021, respectively (Table 1). According to the indices of pairwise sites in the similarity, replacement and richness difference, the among-site variations of the demersal fish community were dominated by replacement with the means of 0.5 in Figure 4A and 0.479 in Figure 4B for the winter and summer in 2020, respectively. The mean value of richness differences in the summer of 2021 was 0.471, higher than the mean value of replacement 0.396 (Figure 4C). Even though there was a relatively higher abundance of fish in the summer, we did not catch any fish in site C19 where the DO reduced to 0.08 mg/L. The environmental factor of the most significant variation is the reduction of DO in the summer of 2021. The results indicate that the hypoxia and anoxia do impact the richness of the fish assemblage in the sampling sites. The impacts of hypoxia depend on the species’ tolerance to hypoxia (Wu, 2002).
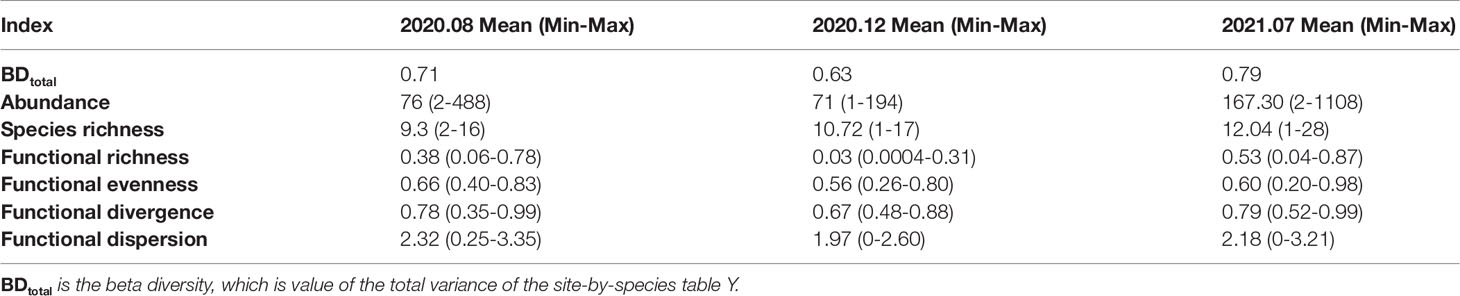
Table 1 The species diversity and the functional diversity indices of the demersal fish community in the PRE.
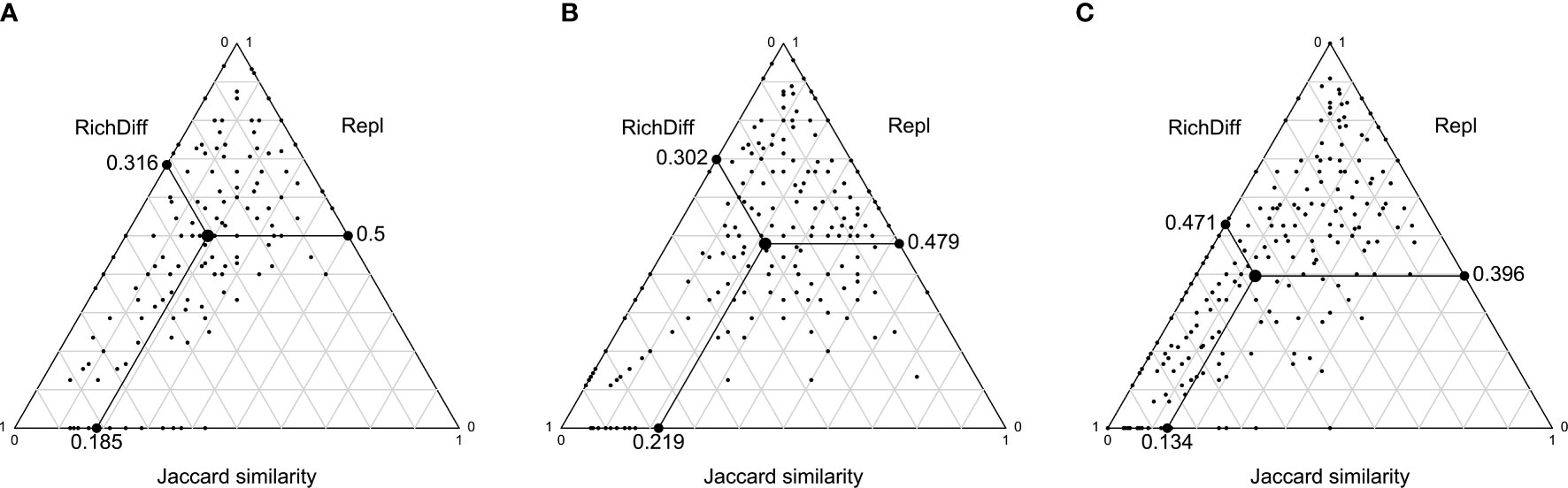
Figure 4 Triangle plots of all pairs of sites in the PRE demersal fish data set for the three surveys, based on the Podani indices of richness, abundance difference, replacement and corresponding similarities. The larger black dots in each plot is the mean values of the indices, as well as the position of a “mean pair of sites”. RichDiff is the richness difference. Repl is the replacement.
The alpha functional diversity indices (functional richness, functional evenness, functional divergence and functional dispersion) fluctuated with wide ranges in the PRE (Table 1). The mean values of the alpha functional diversity indices of the demersal fish community in summer were generally higher than those in winter (Table 1). Functional richness indices were significantly low in winter, which indicated that the functional trait space of the demersal fish community was small in winter. The functional richness increased in summer with the increase of abundance and richness of species. According to the sensitivity analyses (Figure S2), our findings on the trends of the functional diversity are robust in the PRE.The mean functional diversity of the demersal fish community was low across all the surveys in the PRE (Figure 5). The mean functional redundancy of the demersal fish community was high in the PRE (Figure 5), indicating that many species in this region showed similar functional traits. The functional diversity and the functional redundancyof the demersal fish community in the PRE were characterized by a typical seasonal pattern. The abundance and richness of species increased during the summer fishing moratorium in the South China Sea in 2021. However, there was no significant difference in the functional diversity of the demersal fish community in the PRE during the summer fishing moratorium of 2021 and out of the fishing moratorium in the summer of 2020. On the contrary, the mean functional redundancy decreased in the summer of 2021 during the summer fishing moratorium, showing a mismatch with the increase of the abundance and richness of species. The trends of abundance and richness of species in and out of the fishing moratorium indicate that the summer fishing moratorium in the South China Sea does benefit some demersal fish species. Nevertheless, for the functional diversity of the demersal fish community, there is no significant benefits from the fishing moratorium.
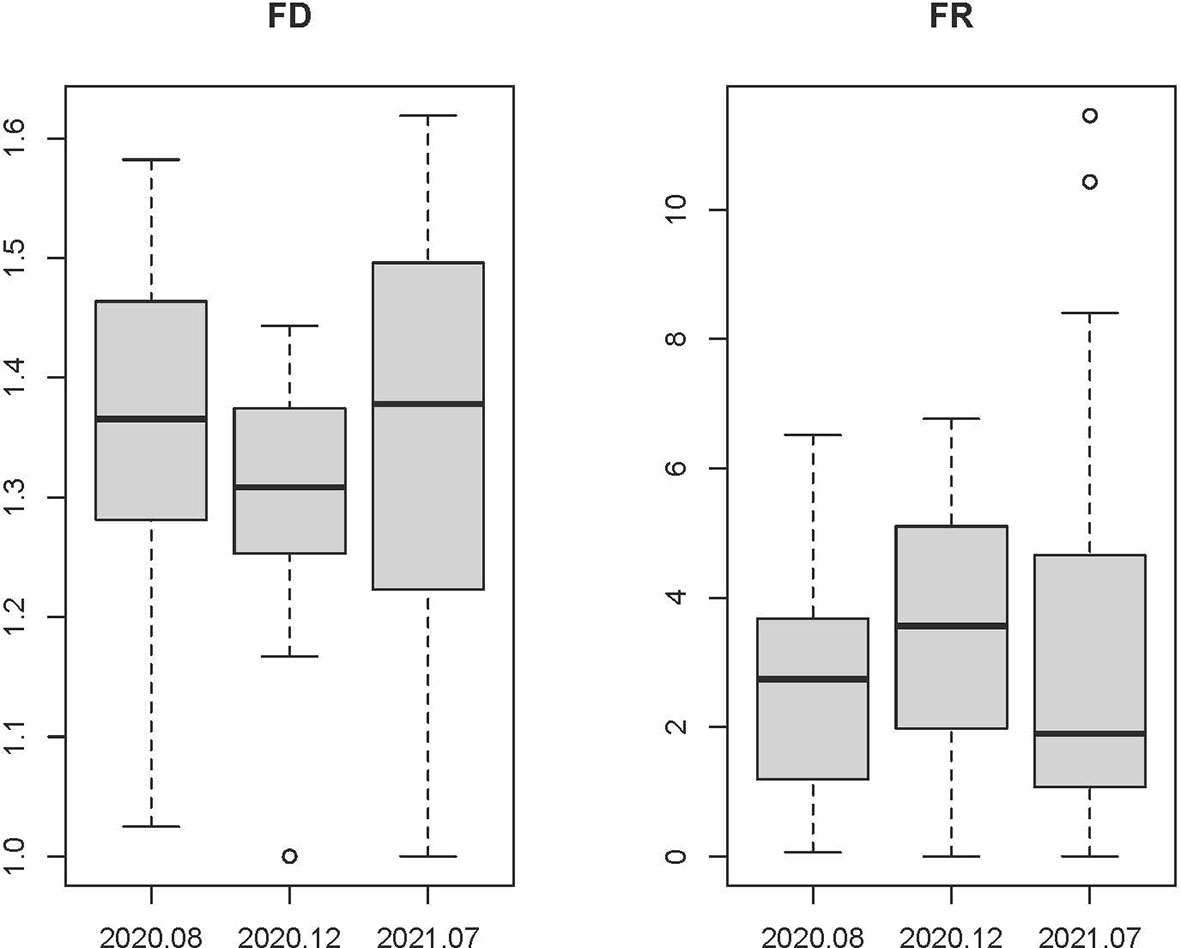
Figure 5 Variations in the functional diversity (FD) and functional redundancy (FR) indices of the demersal fish communities in the PRE.
Discussion
Based on the multivariate analyses and the statistical tests, the sampling sites were separated into the summer cluster and the winter cluster in the PRE. The abundance, richness of fish species and the composition of the demersal fish community in the PRE change dynamically in summer and winter. The temperature of the bottom water is significantly associated with the functional traits of the demersal fish community. Since our surveys are limited to 2020-2021, the change in water temperature mainly represents the seasonal changes in these two years. However, our findings suggest that temperature is an important factor shaping demersal fish communities in the PRE. Thus, the past and future ocean warming in the South China Sea region are likely to impact the PRE fish community (Cheung et al., 2013; Cheung et al., 2016). The PRE is an important fish breeding ground in the Chinese coastal areas. The seasonal fluctuation of the water temperature could affect the species’ reproduction processes that impact fish communities beyond the PRE (Jia et al., 2005). Previous studies also demonstrated that ocean warming would be the main threat to the fish communities and fishery resources in the PRE in the next decades (Zeng et al., 2019). Continuous surveys in the future can provide time-series data that help understand the influences of inter-annual changes of water temperature on the fish communities in the PRE.
Hypoxia in coastal waters impacts the fish community compositions by changing their distributions and abundances all around the world (Breitburg et al., 2018). During our survey period in the summer of 2021, there were serious hypoxia processes in the PRE. In some hypoxia sites, the abundance and richness of the demersal fish did not reduce, while the abundance and richness of the demersal fish reduced sharply in other hypoxia sites such as in the C19, no fish being found. Hypoxia affects the physiological and ecological processes of species, then reduces species distribution in ecosystems (Wu, 2002; Vaquer-Sunyer and Duarte, 2008; Seibel, 2011; Hughes et al., 2015). Fish species of high tolerance to hypoxia can expand their distributions in the hypoxic areas. Furthermore, hypoxia can alter the predator-prey relationship and foraging dynamics of fish communities, so some species can benefit from decreasing the predation risks of hypoxia progress (Chan et al., 2008; A. A. Keller et al., 2010; A. A. Keller et al., 2015). Zeng et al. (2019) pointed out that the DO decrease was an important potential factor to the ecosystem and fishery resources in the PRE. The fish species and functional groups in the PRE have different sensitivity to the DO change. The impacts of the hypoxia on the demersal fish in the PRE are complicated and vary among species and locations.According to the insurance hypothesis, the functional redundancy of communities provides communities with a buffer against the impacts of environmental variables, for many species perform similar functions in communities (Sanders et al., 2018). There would be potential declines in the functional diversity, following the loss of species in ecosystems with little functional redundancy among species (Guillemot et al., 2011; Mouillot et al., 2014). In the PRE, the average functional redundancy of the sampling sites was high, while the average functional diversity was low across the three surveys. The abundance and richness of the demersal fish increased during the summer fishing moratorium in 2021, but the functional diversity of the demersal fish community did not significantly change in this period. In the PRE, the high functional redundancy of the demersal fish community may not be able to endure the impacts of environmental changes and anthropogenic pressure with a low functional diversity.Functional diversity and functional redundancy represent the difference and similarity of interspecific functional traits, and affect the ecosystem function through the ecological complementarity and insurance effect, respectively (Yao et al., 2016). However, there is no necessary connection between the higher trait diversity and higher functional redundancy (Mouillot et al., 2013; McLean et al., 2019). Given the low functional diversity and high functional redundancy in the PRE, there would be alternative explanations for the functional redundancy of such highly exploited coastal ecosystems. In the PRE, the high functional redundancy of the demersal fish community might be attributed to the heavy fishing intensity. Anthropogenic stresses on communities may lead to the homogenization of the trait structure of communities to adapt to environmental changes and perturbations (Diaz and Cabido, 2001; Sasaki et al., 2009; Yao et al., 2016). There has been overexploitation of the fish resources for decades in the PRE (Jia et al., 2005; Duan et al., 2009). With the impact of high fishing intensity, the small fish species with short generation and high resilience, which share similar traits, would become the dominant species (Pauly et al., 1998; Ling et al., 2009; Fisher et al., 2010). The compositions of the organismal traits in ecosystems also impact the resistivity of communities to the disturbances (McLean et al., 2019). McLean et al. (2019) found that the increase of the sensitivity of the climatically vulnerable traits (e.g., small, fast-growing species) caused fish communities in the English Channel and Seychelles Islands ecosystem sensitive to climate change (McLean et al., 2019). In the PRE, this kind of apparent insurance provided by the high redundancy with smaller fish species may hide a high vulnerability to the disturbances of environmental variables and fish pressure.Our study is limited by the data collected over two years. It is hard to extend the survey data to long-time series data, since such fish community data in the PRE is scarce and not publicly available. Our study focuses on the seasonal and spatial changes of the taxa, traits and functional diversity of the demersal fish community in the PRE. Although the survey data does not allow us to examine the long-term trends in functional diversity and vulnerability, the observed patterns are valuable to understand the response of the demersal fish community to environmental stresses such as hypoxia in the PRE. Our findings can also contribute to the development of ecosystem models to understand the trophodynamics of the PRE ecosystems and their responses to environmental change (Duan et al., 2009; Zeng et al., 2019). Furthermore, because fishing is the main factor shaping the structure of demersal fish in the PRE, further analysis is needed to find out the effects of the functional redundancy on the stability of the demersal fish community and address the relationship between the functional redundancy and the stability of communities.
Supplementary Data
We provide the details of the species richness, abundance and dissolved oxygen of the bottom water of sampling sites, the ecological traits used in this study, and the sensitivity analyses of the average functional diversity of sampling sites in the supplementary material.
Data Availability Statement
The original contributions presented in the study are included in the article/Supplementary Material. Further inquiries can be directed to the corresponding author.
Author Contributions
ZYZ: building the research framework of this study and administering the sampling survey; analyzing the survey data; concluding the results and findings of this study; writing and revising the manuscript; WC: Highlighting and sublimating the findings of this study; HL: Participating in making the survey plan and data analysis; HY: assisting in collecting field fishery data; SB: assisting in collecting field fishery data; HYL: assisting in collecting field fishery data; XLC: assisting in collecting field fishery data Su: assisting in collecting field fishery data; XL: assisting in collecting field fishery data; QXC: assisting in collecting environmental parameters; ZLZ: assisting in collecting environmental parameters; XW: assisting in collecting environmental parameters; JHC: Collecting environmental parameters; GFL: in charge of the sampling survey and supervising this study, is the corresponding author of this work. All authors contributed to the article and approved the submitted version.
Funding
This study is supported by the Fundamental Research Funds for the Central Universities, Sun Yat-sen University (No. 22qntd2618), the Innovation Group Project of Southern Marine Science and Engineering Guangdong Laboratory (Zhuhai) (No.311021004), the Guangdong Basic and Applied Basic Research Foundation (No.2019B1515120065), the National Key R&D Program of China (2019YFD0901205) and the NSFC-Guangdong Joint Fund (No. U1901209).
Conflict of Interest
The authors declare that the research was conducted in the absence of any commercial or financial relationships that could be construed as a potential conflict of interest.
Publisher’s Note
All claims expressed in this article are solely those of the authors and do not necessarily represent those of their affiliated organizations, or those of the publisher, the editors and the reviewers. Any product that may be evaluated in this article, or claim that may be made by its manufacturer, is not guaranteed or endorsed by the publisher.
Acknowledgments
We are grateful to Shiyu Li, Shaotian Li and Jiatang Hu, who provided very useful suggestion.
Supplementary Material
The Supplementary Material for this article can be found online at: https://www.frontiersin.org/articles/10.3389/fmars.2022.921595/full#supplementary-material
References
Anderson M. J., Ellingsen K. E.,, McArdle B. H. (2006). Multivariate Dispersion as a Measure of Beta Diversity. Ecol. Lett. 9 (6), 683–693. doi: 10.1111/j.1461-0248.2006.00926.x
Asefa M., Cao M., Zhang G. C., Ci X. Q., Li J.,, Yang J. (2017). Environmental Filtering Structures Tree Functional Traits Combination and Lineages Across Space in Tropical Tree Assemblages. Sci. Rep. 7, 132. doi: 10.1038/s41598-017-00166-z
Breitburg D., Levin Lisa A., Oschlies A., Grégoire M., Chavez Francisco P., Conley Daniel J.,, Zhang J. (2018). Declining Oxygen in the Global Ocean and Coastal Waters. Science, 359(6371), eaam7240. doi: 10.1126/science.aam7240
Brind’Amour A., Boisclair D., Dray S.,, Legendre P. (2011). Relationships Between Species Feeding Traits and Environmental Conditions in Fish Communities: A Three-Matrix Approach. Ecol. Appl. 21 (2), 363–377. doi: 10.1890/09-2178.1
Cadotte M. W. (2017). Functional Traits Explain Ecosystem Function Through Opposing Mechanisms. Ecol. Lett. 20 (8), 989–996. doi: 10.1111/ele.12796
Chan F., Barth J. A., Lubchenco J., Kirincich A., Weeks H., Peterson W. T., et al. (2008). Emergence of Anoxia in the California Current Large Marine Ecosystem. Science 319 (5865), 920–920. doi: 10.1126/science.1149016
Chen C., Zhu Z., Li Y., Yao T., Pan S., Wei X., et al. (2016). Effects of Interspecific Trait Dissimilarity and Species Evenness on the Relationship Between Species Diversity and Functional Diversity in an Alpine Meadow. Acta Ecol. Sinca. 36 (3), 661−674. doi: 10.5846/stxb201405070903
Cheung W. W. L., Reygondeau G.,, Frölicher T. L. (2016). Large Benefits to Marine Fisheries of Meeting the 1.5 C Global Warming Target. Science 354 (6319), 1591–1594. doi: 10.1126/science.aag2331
Cheung W. W., Watson R.,, Pauly D. (2013). Signature of Ocean Warming in Global Fisheries Catch. Nature 497 (7449), 365–368. doi: 10.1038/nature12156
Cornwall C. E., Eddy T. D. (2015). Effects of Near-Future Ocean Acidification, Fishing, and Marine Protection on a Temperate Coastal Ecosystem. Conserv. Biol. 29 (1), 207–215. doi: 10.1111/cobi.12394
Craven D., Eisenhauer N., Pearse W. D., Hautier Y., Isbell F., Roscher C., et al. (2018). Multiple Facets of Biodiversity Drive the Diversity-Stability Relationship. Nat. Ecol. Evol. 2 (10), 1579–1587. doi: 10.1038/s41559-018-0647-7
Cui Y. S., Wu J. X., Ren J.,, Xu J. (2019). Physical Dynamics Structures and Oxygen Budget of Summer Hypoxia in the Pearl River Estuary. Limnol. Oceanog. 64 (1), 131–148. doi: 10.1002/lno.11025
de Bello F., Lavergne S., Meynard C. N., Leps J.,, Thuiller W. (2010). The Partitioning of Diversity: Showing Theseus a Way Out of the Labyrinth. J. Vegetat. Sci. 21 (5), 992–1000. doi: 10.1111/j.1654-1103.2010.01195.x
de Bello F., Leps J., Lavorel S.,, Moretti M. (2007). Importance of Species Abundance for Assessment of Trait Composition: An Example Based on Pollinator Communities. Community, Ecol. 8 (2), 163–170.
Diaz S., Cabido M. (2001). Vive La Difference: Plant Functional Diversity Matters to Ecosystem Processes. Trends Ecol. Evol. 16 (11), 646–655. doi: 10.1016/s0169-5347(01)02283-2
Dray S., Chessel D.,, Thioulouse J. (2003). Co-Inertia Analysis and the Linking of Ecological Data Tables. Ecology 84 (11), 3078–3089. doi: 10.1890/03-0178
Dray S., Choler P., Doledec S., Peres-Neto P. R., Thuiller W., Pavoine S., et al. (2014). Combining the Fourth-Corner and the RLQ Methods for Assessing Trait Responses to Environmental Variation. Ecology 95 (1), 14–21. doi: 10.1890/13-0196.1
Duan L. J., Li S. Y., Liu Y., Moreau J.,, Christensen V. (2009). Modeling Changes in the Coastal Ecosystem of the Pearl River Estuary From 1981 to 1998. Ecol. Model. 220 (20), 2802–2818.
Fisher J. A. D., Frank K. T.,, Leggett W. C. (2010). Global Variation in Marine Fish Body Size and its Role in Biodiversity-Ecosystem Functioning. Mar. Ecol. Prog. Ser. 405, 1–13. doi: 10.3354/meps08601
Fonseca C. R.,, Ganade G. (2001). Species Functional Redundancy, Random Extinctions and the Stability of Ecosystems., Eco. 89 (1), 118–125. doi: 10.1046/j.1365-2745.2001.00528.x
Frelat R., Orio A., Casini M., Lehmann A., Merigot B., Otto S. A., et al. (2018). A Three-Dimensional View on Biodiversity Changes: Spatial, Temporal, and Functional Perspectives on Fish Communities in the Baltic Sea. ICES. J. Mar. Sci. 75 (7), 2463–2475. doi: 10.1093/icesjms/fsy027
Gobler C. J., Baumann H. (2016). Hypoxia and Acidification in Ocean Ecosystems: Coupled Dynamics and Effects on Marine Life. Biology Lett. 12 (5), 20150976. doi: 10.1098/rsbl.2015.0976
Guillemot N., Kulbicki M., Chabanet P., Vigliola L. (2011). Functional Redundancy Patterns Reveal Non-Random Assembly Rules in a Species-Rich Marine Assemblage. PLoS ONE 6 (10), e26735. doi: 10.1371/journal.pone.0026735
Hughes B. B., Levey M. D., Fountain M. C., Carlisle A. B., Chavez F. P., Gleason M. G. (2015). Climate Mediates Hypoxic Stress on Fish Diversity and Nursery Function at the Land-Sea Interface. Proc. Natl. Acad. Sci. U.S.A. 112 (26), 8025–8030. doi: 10.1073/pnas.1505815112
Jia X. P., Li C. H., Qiu Y. S. (2005). Survey and Evaluation of Guangdong Marine Fishery Resources and the Measures for Sustainable Utilization. Chinese marine publication.
Ke D. S., Guan B., Yu H. S., Z. (2007). Environmental Pollution and Study Trend in Pearl River Estuary. Mar. Environ Res. (05), 488–491. ( In Chinese)
Keller A. A., Ciannelli L., Wakefield W. W., Simon V., Barth J. A., Pierce S. D. (2015). Occurrence of Demersal Fishes in Relation to Near-Bottom Oxygen Levels Within the California Current Large Marine Ecosystem. Fish. Oceanog. 24 (2), 162–176. doi: 10.1111/fog.12100
Keller A. A., Simon V., Chan F., Wakefield W. W., Clarke M. E., Barth J. A., et al. (2010). Demersal Fish and Invertebrate Biomass in Relation to an Offshore Hypoxic Zone Along the US West Coast. Fish. Oceanog. 19 (1), 76–87. doi: 10.1111/j.1365-2419.2009.00529.x
Kleyer M., Dray S., Bello F., Lepš J., Pakeman R. J., Strauss B., Thuiller W., Lavorel S. (2012). Assessing Species and Community Functional Responses to Environmental Gradients: Which Multivariate Methods? J. Veg. Sci. 23, 805−821. doi: 10.1111/j.1654-1103.2012.01402.x
Laliberte E., Legendre P. (2010). A Distance-Based Framework for Measuring Functional Diversity From Multiple Traits. Ecology 91 (1), 299–305. doi: 10.1890/08-2244.1
Legendre P. (2014). Interpreting the Replacement and Richness Difference Components of Beta Diversity. Global Ecol. Biogeog. 23 (11), 1324–1334. doi: 10.1111/geb.12207
Legendre P., De Caceres M. (2013). Beta Diversity as the Variance of Community Data: Dissimilarity Coefficients and Partitioning. Ecol. Lett. 16 (8), 951–963. doi: 10.1111/ele.12141
Lennon J. J., Koleff P., Greenwood J. J. D., Gaston K. J. (2001). The Geographical Structure of British Bird Distributions: Diversity, Spatial Turnover and Scale. J. Anim. Ecol. 70 (6), 966–979. doi: 10.1046/j.0021-8790.2001.00563.x
Leps J., de Bello F., Lavorel S., Berman S. (2007). Quantifying and Interpreting Functional Diversity of Natural Communities: Practical Considerations Matter. Preslia 79 (1), 100–100.
Ling S. D., Johnson C. R., Frusher S. D., Ridgway K. R. (2009). Overfishing Reduces Resilience of Kelp Beds to Climate-Driven Catastrophic Phase Shift. Proc. Natl. Acad. Sci. U.S.A. 106 (52), 22341–22345. doi: 10.1073/pnas.0907529106
Marshall K. N., Kaplan I. C., Hodgson E. E., Hermann A., Busch D. S., McElhany P., et al. (2017). Risks of Ocean Acidification in the California Current Food Web and Fisheries: Ecosystem Model Projections. Glob. Chang. Biol. 23 (4), 1525–1539. doi: 10.1111/gcb.13594
McLean M., Auber A., Graham N. A. J., Houk P., Villeger S., Violle C., et al. (2019). Trait Structure and Redundancy Determine Sensitivity to Disturbance in Marine Fish Communities. Glob. Chang. Biol. 25 (10), 3424–3437. doi: 10.1111/gcb.14662
McLean M., Mouillot D., Lindegren M., Engelhard G., Villeger S., Marchal P., et al. (2018). A Climate-Driven Functional Inversion of Connected Marine Ecosystems. Curr. Biol. 28 (22), 3654–3660. doi: 10.1016/j.cub.2018.09.050
Mollmann C., Diekmann R., Muller-Karulis B., Kornilovs G., Plikshs M., Axe P. (2009). Reorganization of a Large Marine Ecosystem Due to Atmospheric and Anthropogenic Pressure: A Discontinuous Regime Shift in the Central Baltic Sea. Glob. Chang. Biol. 15 (6), 1377–1393. doi: 10.1111/j.1365-2486.2008.01814.x
Mouillot D., Graham N. A. J., Villeger S., Mason N. W. H., Bellwood D. R. (2013). A Functional Approach Reveals Community Responses to Disturbances. Trends Ecol. Evol. 28 (3), 167–177. doi: 10.1016/j.tree.2012.10.004
Mouillot D., Villeger S., Parravicini V., Kulbicki M., Arias-Gonzalez J. E., Bender M., et al. (2014). Functional Over-Redundancy and High Functional Vulnerability in Global Fish Faunas on Tropical Reefs. Proc. Natl. Acad. Sci. U.S.A. 111 (38), 13757–13762. doi: 10.1073/pnas.1317625111
Pauly D., Christensen V. V., Dalsgaard J., Froese R., Torres F. Jr. (1998). Fishing Down Marine Food Webs. Science 279 (5352), 860–863. doi: 10.1126/science.279.5352.860
Perry A. L., Low P. J., Ellis J. R., Reynolds J. D. (2005). Climate Change and Distribution Shifts in Marine Fishes. Science 308 (5730), 1912–1915. doi: 10.1126/science.1111322
Poloczanska E. S., Burrows M. T., Brown C. J., Molinos J. G., Halpern B. S., Hoegh-Guldberg O., et al. (2016). Responses of Marine Organisms to Climate Change Across Oceans. Front. Mar. Sci. 362., doi:10.3389/fmars.2016.00062
Rouyer T., Fromentin J. M., Menard F., Calzelles B., Briand K., Pianet R., et al. (2008). Complex Interplays Among Population Dynamics, Environmental Forcing, and Exploitation in Fisheries. Proc. Natl. Acad. Sci. U.S.A. 105 (14), 5420–5425. doi: 10.1073/pnas.0709034105
Sanders D., Thebault E., Kehoe R., van Veen F. J. F. (2018). Trophic Redundancy Reduces Vulnerability to Extinction Cascades. Proc. Natl. Acad. Sci. U.S.A. 115 (10), 2419–2424. doi: 10.1073/pnas.1716825115
Sasaki T., Okubo S., Okayasu T., Jamsran U., Ohkuro T., Takeuchi K. (2009). Two-Phase Functional Redundancy in Plant Communities Along a Grazing Gradient in Mongolian Rangelands. Ecology 90 (9), 2598–2608. doi: 10.1890/08-1850.1
Seibel B. A. (2011). Critical Oxygen Levels and Metabolic Suppression in Oceanic Oxygen Minimum Zones. J. Exp. Biol. 214 (2), 326–336. doi: 10.1242/jeb.049171
Vaquer-Sunyer R., Duarte C. M. (2008). Thresholds of Hypoxia for Marine Biodiversity. Proc. Natl. Acad. Sci. U.S.A. 105 (40), 15452–15457. doi: 10.1073/pnas.0803833105
Villeger S., Mason N. W. H., Mouillot D. (2008). New Multidimensional Functional Diversity Indices for a Multifaceted Framework in Functional Ecology. Ecology 89 (8), 2290–2301. doi: 10.1890/07-1206.1
Wang B., Hu J., Li S., Yu L., Huang J. (2018). Impacts of Anthropogenic Inputs on Hypoxia and Oxygen Dynamics in the Pearl River Estuary. Biogeosciences 15 (20), 6105–6125. doi: 10.5194/bg-15-6105-2018
Williams P. H. (1996). Mapping Variations in the Strength and Breadth of Biogeographic Transition Zones Using Species Turnover. Proc. R. Soc. London. Ser. B. Biol. Sci. 263 (1370), 579–588. doi:10.1098/rspb.1996.0087
Wu R. S. S. (2002). Hypoxia: From Molecular Responses to Ecosystem Responses. Mar. pollut. Bull. 45 (1), 35–45. doi: 10.1016/s0025-326x(02)00061-9
Yachi S., Loreau M. (1999). Biodiversity and Ecosystem Productivity in a Fluctuating Environment: The Insurance Hypothesis. Proc. Natl. Acad. Sci. U.S.A. 96 (4), 1463–1468. doi: 10.1073/pnas.96.4.1463
Yao T., Zhu Z., Li Y., Pan S., Kong B., Wei X., et al. (2016). Effects Offunctional Diversity and Functional Redundancy on the Community Stability of an Alpine Meadow. Acta Ecol. Sinca. 36 (6), 1547–1558.
Yin K., Lin Z., Ke Z. (2004). Temporal and Spatial Distribution of Dissolved Oxygen in the Pearl River Estuary and Adjacent Coastal Waters. Continent. Shelf. Res. 24 (16), 1935–1948. doi: 10.1016/j.csr.2004.06.017
Keywords: demersal fish community, functional diversity, functional redundancy, hypoxia, Pearl River Estuary (PRE)
Citation: Zeng Z, Cheung WWL, Lai H, Yi H, Bi S, Li H, Chen X, Su Y, Liu X, Chen Q, Zhang Z, Wei X, Chen J and Li G (2022) Species and Functional Dynamics of the Demersal Fish Community and Responses to Disturbances in the Pearl River Estuary. Front. Mar. Sci. 9:921595. doi: 10.3389/fmars.2022.921595
Received: 16 April 2022; Accepted: 31 May 2022;
Published: 13 July 2022.
Edited by:
Jun Xu, Institute of Hydrobiology (CAS), ChinaReviewed by:
Meilin Wu, South China Sea Institute of Oceanology (CAS), ChinaLi Liu, South China Agricultural University, China
Copyright © 2022 Zeng, Cheung, Lai, Yi, Bi, Li, Chen, Su, Liu, Chen, Zhang, Wei, Chen and Li. This is an open-access article distributed under the terms of the Creative Commons Attribution License (CC BY). The use, distribution or reproduction in other forums is permitted, provided the original author(s) and the copyright owner(s) are credited and that the original publication in this journal is cited, in accordance with accepted academic practice. No use, distribution or reproduction is permitted which does not comply with these terms.
*Correspondence: Guifeng Li, bGlndWlmQG1haWwuc3lzdS5lZHUuY24=