- Department of Biology, Laboratório para a Inovação e Sustentabilidade dos Recursos Biológicos Marinhos da Universidade de Aveiro (ECOMARE), Centro de Estudos do Ambiente e do Mar (CESAM) - Centre for Environmental and Marine Studies, University of Aveiro, Aveiro, Portugal
Biofouling communities are broadly distributed and there is a growing need to understand, monitor, and prevent their dispersal and colonization. Ascidians are a major group of fouling organisms but have remained poorly studied in this context. Furthermore, the search for improved sustainable practices regarding shipping networks, biofouling management, treatments, and monitorization has made headway rapidly. The present study surveyed and established a baseline for the ascidian biofouling community in a coastal lagoon, by operationalizing the concept of artificial substrate units (ASU) through a customized 3-D unit with the shape of a triangular-based pyramid, a nature-based structure that simulates natural habitats, made with oyster shells sourced from local aquaculture farms. The ASU were grouped into a five-replicate star-shaped, to be collected at each sampling moment. Throughout the 295 days (from May to December of 2020) of the present study covering five different locations of Ria de Aveiro (Portugal) coastal lagoon, a total of 12 species of ascidians were collected. While Ascidiella aspersa, Microcosmus sp., and Molgula sp. 1 were registered in all the locations surveyed, the remaining nine ascidian species were dominant only in specific locations of the coastal lagoon. Values of total abundance presented an overall increasing trend in all locations surveyed, with maximum values corresponding to summer periods. Two locations (Oyster Farm and Integrated Multi-Trophic Aquaculture Farm) recorded the highest abundance values. The present findings demonstrated that the ASU employed using oyster shells, a widely available co-product of oyster farming, can be considered an efficient support structure for short- or long-term monitoring of the ascidian community, as well as fouling communities in general. Hydrodynamics, seasonality, and nutrient-enriched waters were the main contributors to the establishment of ascidians. For the first time, Clavelina lepadiformis and A. aspersa were collected and reported in coastal waters of mainland Portugal. While preventing the settlement of fouling communities can be extremely difficult, an improved understanding of existing communities of these organisms can undoubtedly contribute to the development of improved management practices to control them. An updated list of all ascidian species recorded to date from coastal waters of mainland Portugal is also presented.
Introduction
Ascidians are amongst the most significant groups of fouling organisms worldwide, a feature that has increased the interest of the research community in these organisms (Fitridge et al., 2012; Taylor et al., 2014). They are sessile filter-feeders that display fast growth rates (Millar, 1952) and inhabit a variety of substrates (Millar, 1971; Lambert, 2007). They can also be recognized as a potential co-cultured/extractive species for Integrated Multi-Trophic Aquaculture (IMTA) with potential added value as bioresources (Marques et al., 2022). Ascidians present a short lifespan and their lecithotrophic larval development makes them an important bioindicator of anthropogenic transport (Marins et al., 2010). Their larvae can tolerate long-distance dispersal as a result of human intervention (Marshall et al., 2003) through ballast waters or translocations of cultivated shellfish for aquaculture purposes (Lambert, 2001; Locke et al., 2007). Ascidians can quickly spread and colonize extensive areas and new ecosystems, occupying artificial and natural substrates (Millar, 1971; Lambert, 2007). Fouling ascidians settle on aquaculture gear, piers, floating docks, marinas, and wharf piles from commercial ports causing deleterious impacts on those locations (Dumont et al., 2011; Koplovitz et al., 2016). Furthermore, ascidian fouling on mussel farms can negatively impact the growth of these bivalves (Guenther et al., 2006; Bullard et al., 2013), increase their mortality (Forrest et al., 2007; Bannister et al., 2019), induce shell deformities (Taylor et al., 1997), and by increasing the weight on aquaculture infrastructures (Ramsay et al., 2008a; Rodriguez and Ibarra-Obando, 2008) can cause negative ecological and economic impacts (Carver et al., 2003; Lutz-Collins et al., 2009; Lacoste et al., 2016).
Controlling and mitigating biofouling is a large financial burden to the aquaculture industry. Estimates indicate that 20-30% of additional costs are spent annually on biofouling control, with these figures varying with cultured species and location (Lacoste and Gaertner-Mazouni, 2015). Thus, aquaculture activities demand the development of innovative technological solutions that target fouling organisms, to reduce their impacts and achieve more sustainable farming approaches. However, biofouling control in aquaculture must be assessed with caution, as the removal process can also be stressful and damaging for species being cultured (Lacoste and Gaertner-Mazouni, 2015). Expectations are that these methods will evolve as the aquaculture industry moves forward (Bannister et al., 2019).
Therefore, one of the measures to assist this matter includes the establishment of monitorization programs and carrying out local surveys, as these will allow an early detection and enable a rapid response. Implementation of monitoring programs is normally managed by international organizations, including the Convention for the Protection of the Marine Environment of the North-East Atlantic (OSPAR) (Lehtiniemi et al., 2015). Throughout the years, different monitoring programs for fouling communities have been addressed, such as metabarcoding analysis (Azevedo et al., 2020), the maintenance and surveillance of marine (Gewing and Shenkar, 2017), proteomic profiling (Kuplik et al., 2019), and the use of oyster shells as a habitat collector (Outinen et al., 2019), all of which can significantly serve as an effective support structure for the early-detection of biofouling species. As such, it is essential to understand the biofouling communities, with emphasis on the ascidians community's composition, abundance, and distribution, and identify the major environmental drivers that shape the settlement dynamics of these communities for further implementing enhanced mitigation actions.
The present study aims to investigate three research questions: i) can customized 3-D ASU structures made of oyster shells support the monitoring of ecosystem shifts on ascidians diversity? ii) using Ria de Aveiro, coastal lagoon (Portugal) as a case study, can these 3-D ASU be employed to survey and establish a baseline for the ascidian biofouling community? iii) can these 3-D ASU also be used in an aquaculture facility?
The main objective was to monitor and establish a benchmark for the ascidian biofouling community by using an innovative customized 3-D star-shaped structure composed of five replicated ASU using oyster shells as a nature-based substrate.
Material and Methods
Study Area
The study area is located in the shallow coastal lagoon Ria de Aveiro, Portugal (Figure 1), which forms four major channels (Ovar Channel, 29 km to the North; Espinheiro Channel, a highly modified water body, which corresponds to the Vouga estuary; the Ílhavo Channel, 15 km to the southeast; and the Mira Channel, 20 km to the south characterized by a typical estuarine gradient); and connects to the Atlantic Ocean through a narrow artificial navigation channel (Lopes et al., 2017). Our experimental design consists of five distinct research locations distributed within Mira Channel and the premises of a facility that receives water from the Ílhavo Channel. The first sampling location is located at the Ocean boundary of the Ria de Aveiro, which receives the greatest influence from marine waters and features 1.3 km long, 350 m wide, and 20 m deep. The tidal currents velocities can reach 1 m s−1 and progressively gets weaker in the many innermost canals (Martins et al., 2010). Three sampling locations are allocated in the Mira Channel, characterized by the existence of intertidal zones, namely mudflats and salt marshes, where recreational and aquaculture activities occur, such as extensive natural banks of shellfish; "Fishing Harbour" (FH) (40° 37' 57.3" N. 8° 43' 57.8" W), where commercial vessels resort for shelter, landing catches of coastal fisheries and has a maximum capacity of 136 medium size fishery boats; "Marina" (M) (40° 37' 13.4" N. 8° 44' 54.2" W), where small recreational boats can dock on floating structures, with the capacity of 130 berths, and "Oyster Farm" (OF) (40° 37' 06.7" N. 8° 44' 28.2" W), is established within a rack-and-bag culture oyster farm of Crassostrea gigas. This method is highly dependent on the tidal range. Ílhavo Channel, with a 15 km length, is characterized by partially mixed waters depending on the volume of freshwater inflow from the Boco river. The fifth sampling location is in this channel "Integrated Multi-Trophic Aquaculture Farm" (IMTA), (40°36'44"N 8°40'3"W). A land-based semi-intensive fish farm that operates under an IMTA framework. At this facility, seabass and seabream are produced in earth ponds, with their nutrient-rich effluents being supplied to farm macroalgae in concrete tanks.
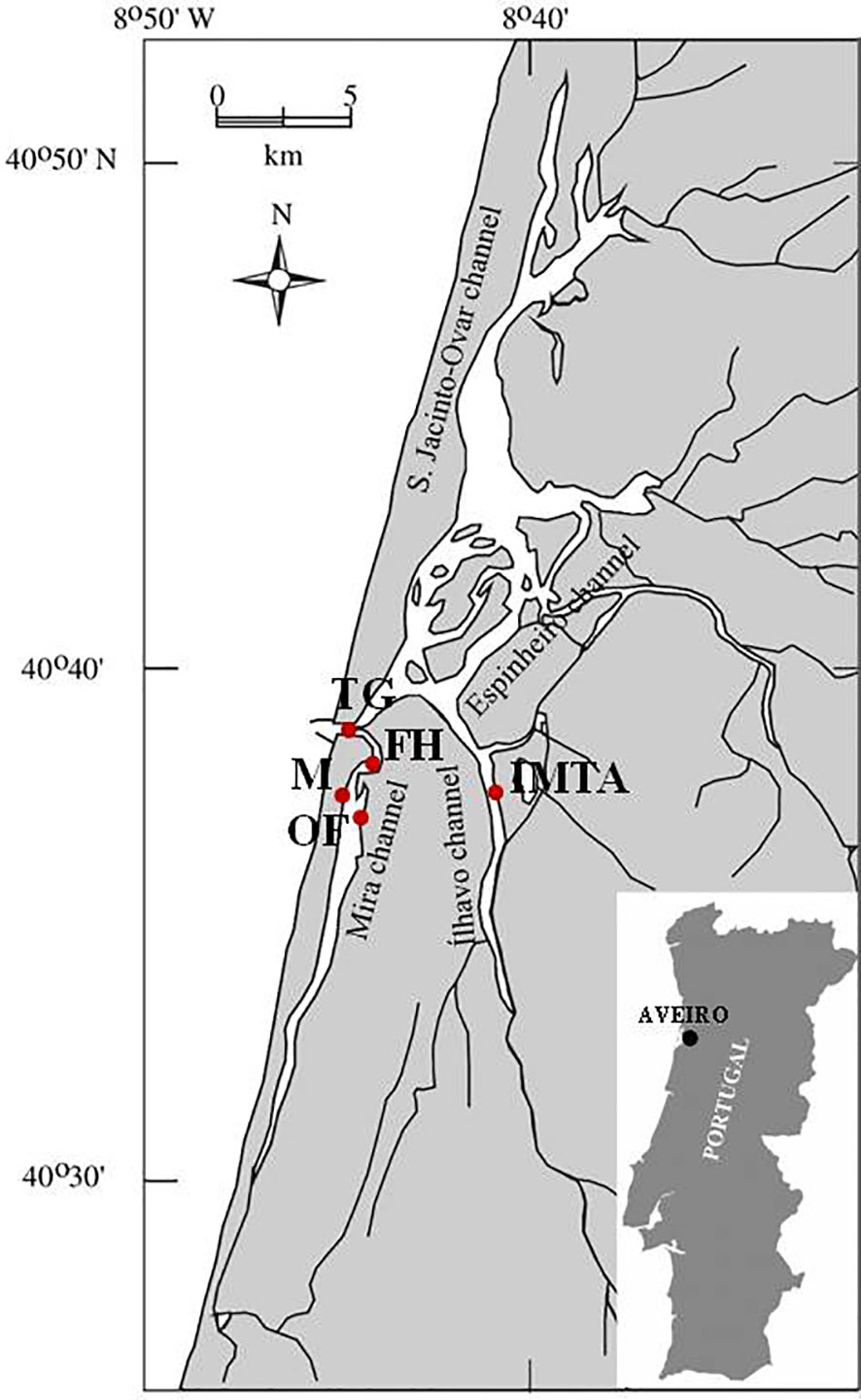
Figure 1 Ria de Aveiro coastal lagoon and the four sampling sites located in the Mira channel and the fifth sampling site located in Ílhavo channel, surveyed in the present study (red dots). TG, Tide Gauge; FH, Fishing Harbour; M, Marina; OF, Oyster Farm; IMTA, Integrated Multi-Trophic Aquaculture.
Customized 3-D Artificial Substrate Units
Oyster shell-based units were developed to serve as a nature-based structure for ascidian larvae to settle (Figure 2). Initially, from a net roll with a 0.01 m mesh (Figure 2A), a rectangle with 0.2 m in length and 0.4 m in height was cropped (Figure 2B). Afterward, the rectangle was folded in half and the lateral parts were sewn together with a nylon fishing line (Figure 2C). Next, the superior edge was opened, 30 oyster shells (10 large + 20 small) were placed within each triangular-based pyramid (Figures 2D, E), and the opposite sides were joined and sewn together forming the final triangular-based pyramid (Figure 2F). Oyster shells (from Crassostrea gigas) were sourced from an oyster farm at Ria de Aveiro, where this is an abundant co-product of oyster farming due to mortality during grow-out. To achieve the final star-shaped composed of five replicated ASU, five triangular-based pyramids were connected using a polypropylene nautical cord and a zip tie fastened through the center edges (Figure 2H). Each pyramid was weighed to ensure similar weights amongst replicates. The final weight varied between 270 – 310 g with an average weight of 285 g. Each pyramid measured 0.2 m in length and 0.2 m in height and holds 1 L volume-wise. A total of 175 triangular-based pyramids were created, which were used to assemble 35 star-shaped composed of five replicated ASU.
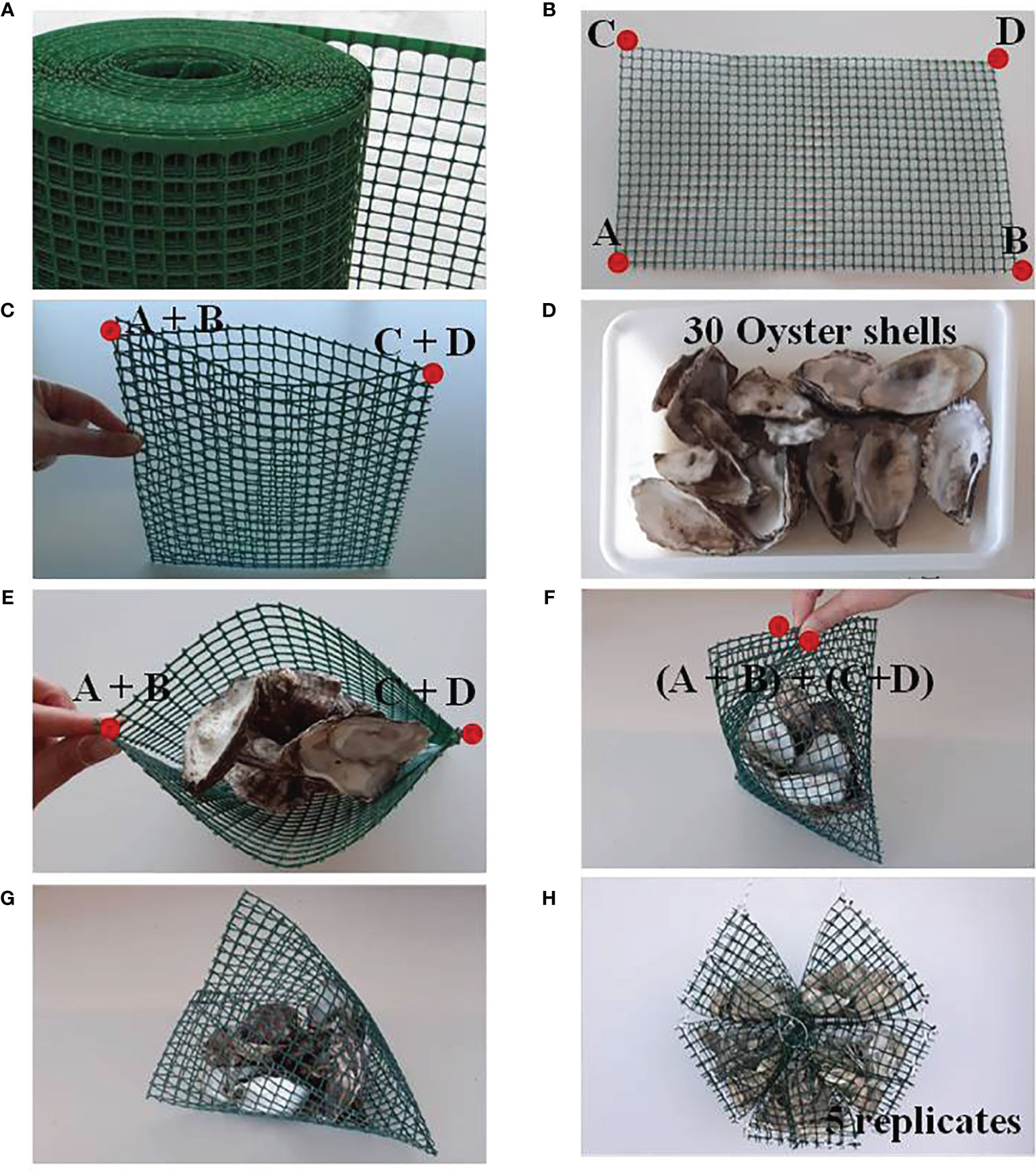
Figure 2 Representation of the steps to set up the triangular-based pyramid (A–G) and the final star-shaped artificial substrate unit (ASU) consisting of five replicates (H).
Sampling and Laboratory Procedures
During February 2020 (late winter), seven star-shaped units, each composed of five replicated ASU, were deployed at each of the five research sites. These were randomly widespread and attached to existing structures, remaining fully or partially submerged during tides. During the experimental period, no intervention whatsoever (including cleaning) was performed on the ASU. The first sampling procedure occurred in May 2020, after 92 days, and it was repeated every five weeks until December 2020 (late autumn), with the final unit being sampled 295 days after being deployed. At each sampling moment, five ASU (corresponding to one star-shaped unit) was retrieved from each location, transported in a cooler with local water, and immediately processed upon arrival at the laboratory. From each star-shaped unit collected, every one of the five triangular-based pyramids was processed individually, thus allowing to retrieve five independent replicates from each location.
Nutrient Analysis
Salinity, water temperature (◦C), pH, and dissolved oxygen (mg L-1) were measured in situ (Table SI1) during each sampling event. Water samples, collected in triplicates, were transported under refrigerated conditions and immediately filtered with pre-weighed filters (Ahlstrom Munksjö GF/C, Ø47 mm dehydrated at 105°C). Afterward, filters were dried at 60°C for 48 h (suspended particulate matter: SPM) and combusted at 450°C for 5h (ash-free dry weight: AFDW), particulate organic matter (POM) was calculated by subtracting SPM and AFDW, following the EPA Method 160.2. Water aliquots were stored frozen at -20°C until analysis. A Skalar San++ Continuous Flow Analyzer (Skalar Analytical, Breda, The Netherlands) was used to determine dissolved ammonium (NH4-N, mg L-1), nitrogen oxides (N-NOx, mg L-1) and orthophosphate (P-PO4, mg L-1) concentrations, using Skalar's standard automated methods for NH4-N (Modified Berthelot reaction for ammonia determination), N-NOx (Total UV digestible nitrogen/nitrate + nitrite/nitrite) and P-PO4 (Total UV digestible phosphate/orthophosphate).
Ascidian Sampling
Biological samples were transported in a cooler with local water and immediately processed upon arrival at the laboratory. Ascidians from each replicate were retrieved from the net and the oyster shells, being subsequently counted. Ascidians were carefully removed manually and placed in a Petri dish with seawater. Most ascidians exhibit a tactile response during measuring and may contract; therefore, for a more accurate measurement, ascidians were spread out on a tray and left for 15 minutes before measuring was performed using graph paper. Individual mass (wet weight) was registered for each replicate. Colonial ascidians for which removal from the net and oyster shells was not possible were photographed and coverage area was measured using the software ImageJ. Subsequently, ascidians were stored at -80°C and subsequently freeze-dried to determine their total biomass. Ascidians were identified to the lowest possible taxa, using identification guides and dichotomous keys (Alder and Hancock, 1905; Hayward et al., 1990).
Diversity Indicators
A set of five indices was determined (Table SI2): 1) to evaluate the number of species present in each location (species richness); 2) and 3) species richness and evenness were determined using Pielou's Evenness and Margalef's Richness Index (Margalef, 1968; Pielou, 1969), as these allow to evaluate the level of homogeneity within the ascidian community at each location; 4) and 5) to characterize species diversity we employed Shannon's and Simpson's index, as these account for both abundance and evenness of the species present at each sampling location (Simpson, 1949; Shannon and Weaver, 1963).
Statistical Analyses
Data from the total abundance were log(x+1) transformed to reduce the skewness of our original data and a Bray-Curtis similarity matrix (Bray and Curtis, 1957) was assembled. A two-way PERMANOVA design was created with "location" and "sampling period" being used as fixed factors. Whenever significant differences were observed, two pairwise test analyses were performed: the first to infer which sampling locations presented significant differences between the total abundance of ascidians collected; and the second to determine which sampling periods within each location presented significant differences. The statistical significance of variance components was tested using 999 permutations of unrestricted permutations of data, with an a priori chosen significance level of α=0.05. The Monte Carlo P value (P(MC)) was used whenever permutations were less than 100.
A multidimensional scaling (MDS) was performed to visualize the overall patterns and relationships between the biological matrices surveyed. Before the statistical analysis of environmental parameters (PO43-, NH4+, NOx, and POM), a resemblance matrix based on Euclidean distances was calculated and data were log(x+1) transformed, again, to reduce the skewness of our original data. Afterward, all parameters followed normalization. The relationship between all environmental variables (PO43-, NH4+, NOx, and POM) and the distribution of the ascidian community was explored by carrying out a Distance-Based Linear Model analysis (DistLM) with "Best" as the selection procedure and "BIC" (Bayesian information criterion) as the selection criterion. All multivariate analyses were performed using PRIMER 6 + PERMANOVA© software (software package from Plymouth Marine Laboratory, UK) (Anderson et al., 2008).
Results
A total of eight genera and 12 species of ascidians were collected from the ASU during the present study from all five locations (Figure 3). Three colonial ascidians Botryllus schlosseri (Pallas, 1766), Botrylloides violaceus (Oka, 1927), Clavelina lepadiformis (Müller, 1776) and nine solitary ascidian species, Ascidiella aspersa (Müller, 1776), Ciona intestinalis (Linnaeus, 1767), Styela plicata (Lesueur, 1823), Styela sp. (Fleming, 1822), Microcosmus sp. (Heller, 1877), Molgula sp. 1 (Forbes, 1848), Molgula sp. 2 (Forbes, 1848) were identified (with two solitary ascidians having remained unidentified, due to their small size or slightly damaged body). The total number of ascidians species retrieved in each sampled location ranged from five to eight species at the end of the trial (Table 1). The ascidian species A. aspersa, Microcosmus sp., and Molgula sp. 1 were recorded in all sampling locations (Table 1). Nonetheless, these species displayed some variations in their abundance and biomass values (Figure 4). Microcosmus sp. and Molgula sp. 1 recorded the highest values of both abundance and biomass throughout the study period, in all sampling locations, with a particular highlight in Mira Channel locations (FH, M, and OF) (Table 2). Despite Ciona intestinalis being collected from four out of the five studied locations, this species registered abundance and biomass values at the inner Ílhavo Channel IMTA farm (Figure 4E), of 50.4% and 84.5% total respectively (Table 2). At the ocean boundary TG location, Clavelina lepadiformis was dominant with 43% of the total abundance while Microscosmus sp. and Molgula sp. 1 dominated the biomass values with a combining percentage of 85% (Table 2). Styela plicata was solely retrieved from both farm locations at Mira and Ílhavo Channels (OF and IMTA), while Styela sp. was retrieved from the port locations at Mira Channel (FH and M) (Table 1).
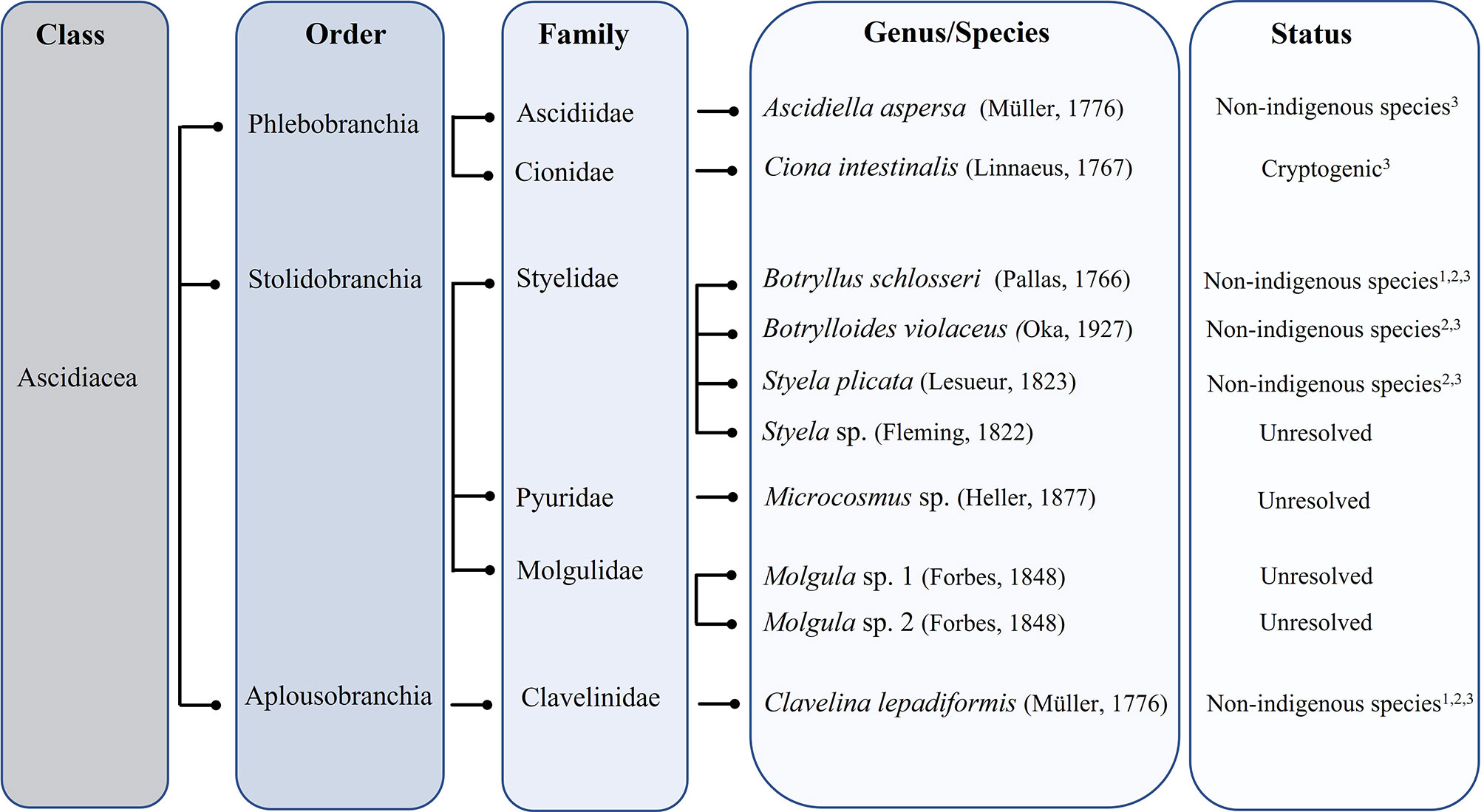
Figure 3 Taxonomic tree of the ten identified species retrieved from the Ria de Aveiro. Superscript numbers indicate reference: 1- Canning-Clode et al. (2013); 2 – Chainho et al. (2015); 3 – Ramalhosa et al. (2021).
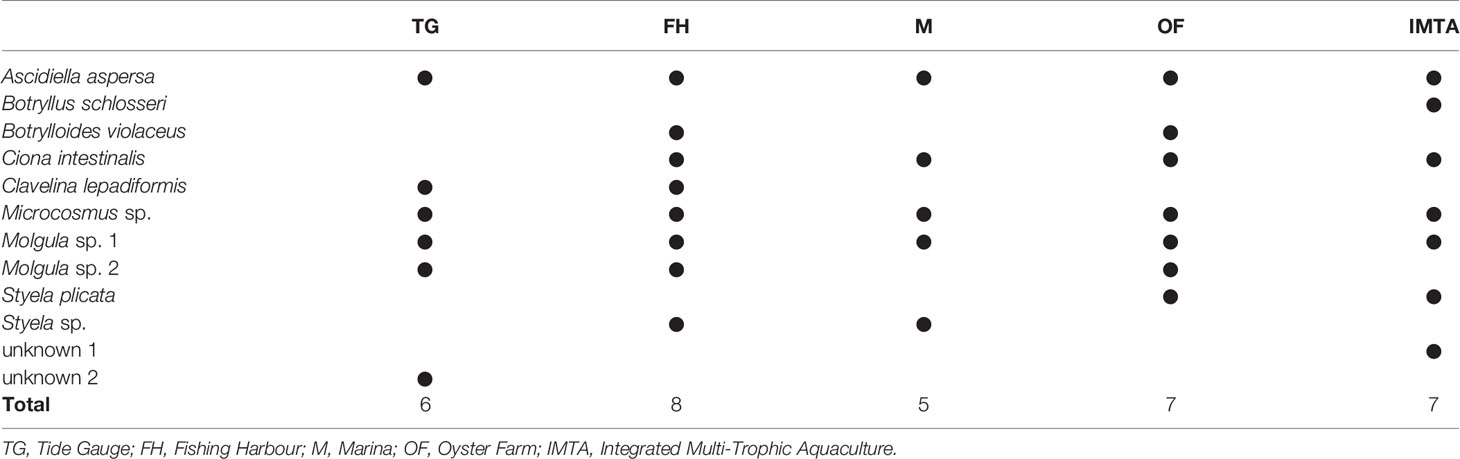
Table 1 Presence/absence table of the collected ascidians throughout the duration of the experiment and their respective location within Ria de Aveiro.
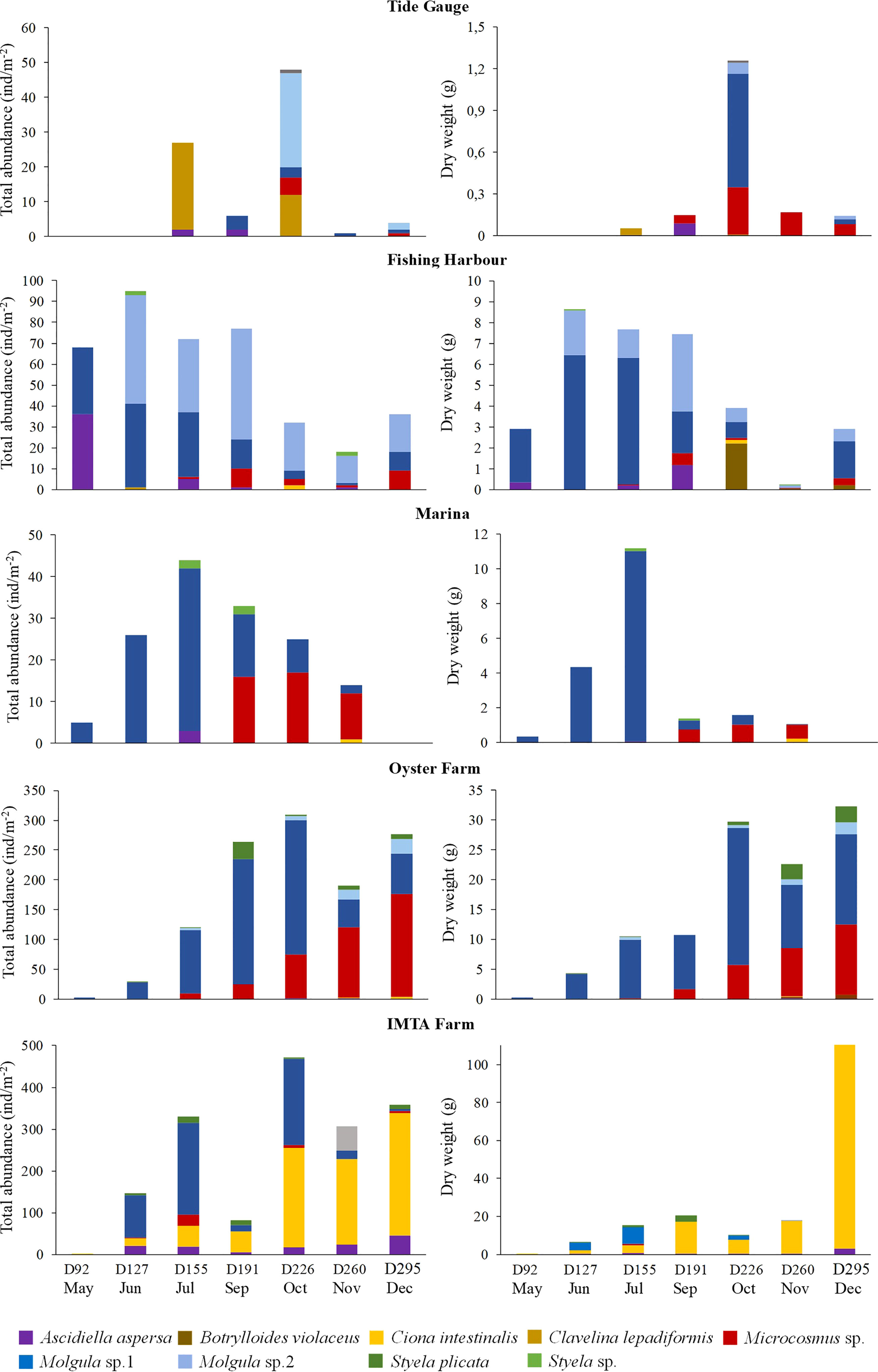
Figure 4 Total abundance (ind.m-2) and dry weight (g) registered for each collected ascidian throughout the duration of the experiment, from each sampling location. D, day.
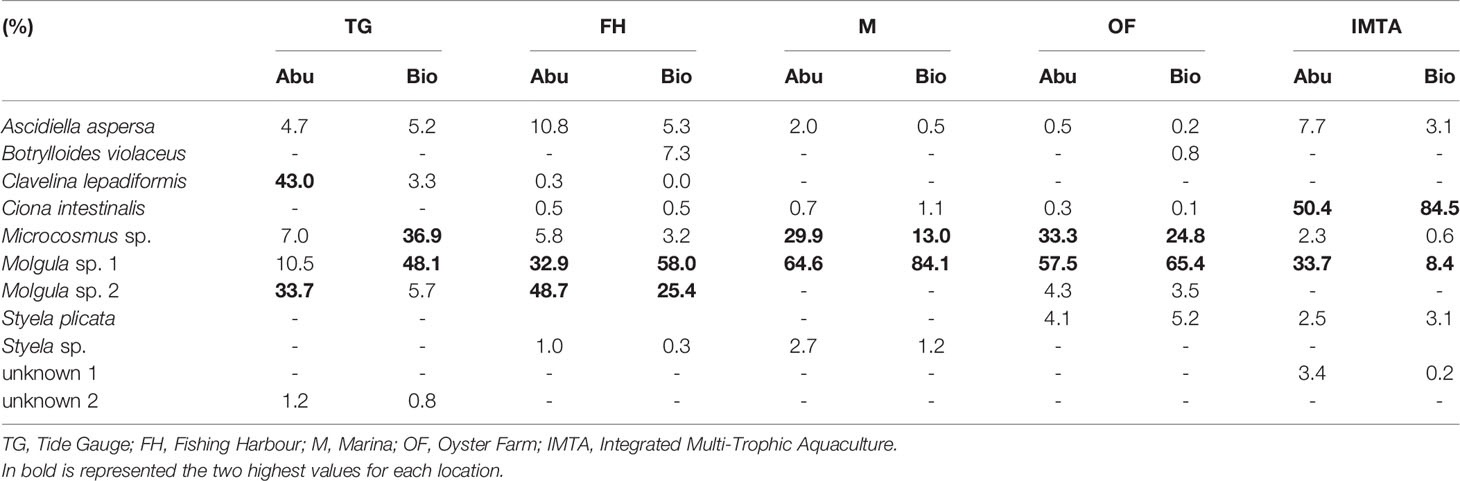
Table 2 Percentage of the total abundance and total biomass registered for each collected ascidian from each location throughout the duration of the experiment.
A particular trend towards an increase in total abundance over time, followed by a subsequent decrease was observed, with maximum values being recorded during sampling days 155 to 226 (from July to October) (Figure 4). However, this trend is not so evident concerning biomass values. In Mira Channel locations (FH, M, and OF), the species that presented the highest abundance also presented higher biomass values (Table 2). Interestingly, in all studied locations, the same combination of two species consistently dominated total abundance and total biomass, except for TG location: C. lepadiformis and Molgula sp. 2 represented 76.7% of the total abundance while Microcosmus sp. and Molgula sp. 1 represented 85% of the total biomass. In the remaining locations: at FH Molgula sp. 1 and Molgula sp. 2 represented 81.7% of the total abundance and 83.4% of the total biomass; at M and OF locations, Microcosmus sp. and Molgula sp. 1 dominated 94.6% and 90.8%, respectively of the total abundance and 97.2% and 90.2%, respectively of the total biomass; at IMTA site, C. intestinalis and Molgula sp. 1 represented 84% of the total abundance and 92.9% of the total biomass. Ascidian Botryllus schlosseri was only collected at IMTA, registering a maximum area of 0.014 m2 at sampling day 127. Similar values of the coverage area of Botrylloides violaceus were recorded at locations FH and OF, however, these were recorded at different sampling periods (0.0032 m2 at sampling day 295 (December) and 0.0033 m2 at sampling day 226 (October), respectively). Overall, significant differences were detected inthe total abundance of the ascidian community between each sampling site and sampling period (Table 3). Furthermore, pairwise test analysis (Table SI3) revealed that abundance values of C. intestinalis were significantly different between IMTA and the remaining locations, (IMTA/TG p=0.001; IMTA/FH p=0.001; IMTA/M p=0.001; IMTA/OF p=0.001), as was C. lepadiformis between the ocean boundary TG and other locations (TG/FH p=0.04; TG/M p=0.012; TG/OF p=0.015; TG/IMTA p=0.013). Likewise, the two species within the genus Molgula presented abundance values with significant differences amongst all locations.

Table 3 Results of the two-factor permutational multivariate analysis of variance (PERMANOVA) of the Log(x+1) transformed data for the ascidian species collected throughout the present study in the Ria de Aveiro.
The MDS ordination analysis showed a clear separation of the total abundance of the ascidian community between all five locations (Figure 5). Furthermore, a separation between the farm sites (OF and IMTA) with the remaining sampling locations is quite evident. The size and direction of species vectors indicate that C. intestinalis and A. aspersa are the main contributors to the IMTA site, whereas Clavelina lepadiformis is responsible for the separation of the ocean boundary TG, but with lesser influence. Molgula sp. 2 is the main contributor to the FH location, Microcosmus sp. and Molgula sp. 1 are equally dominant at M and OF and are represented in the MDS ordination plot as such.
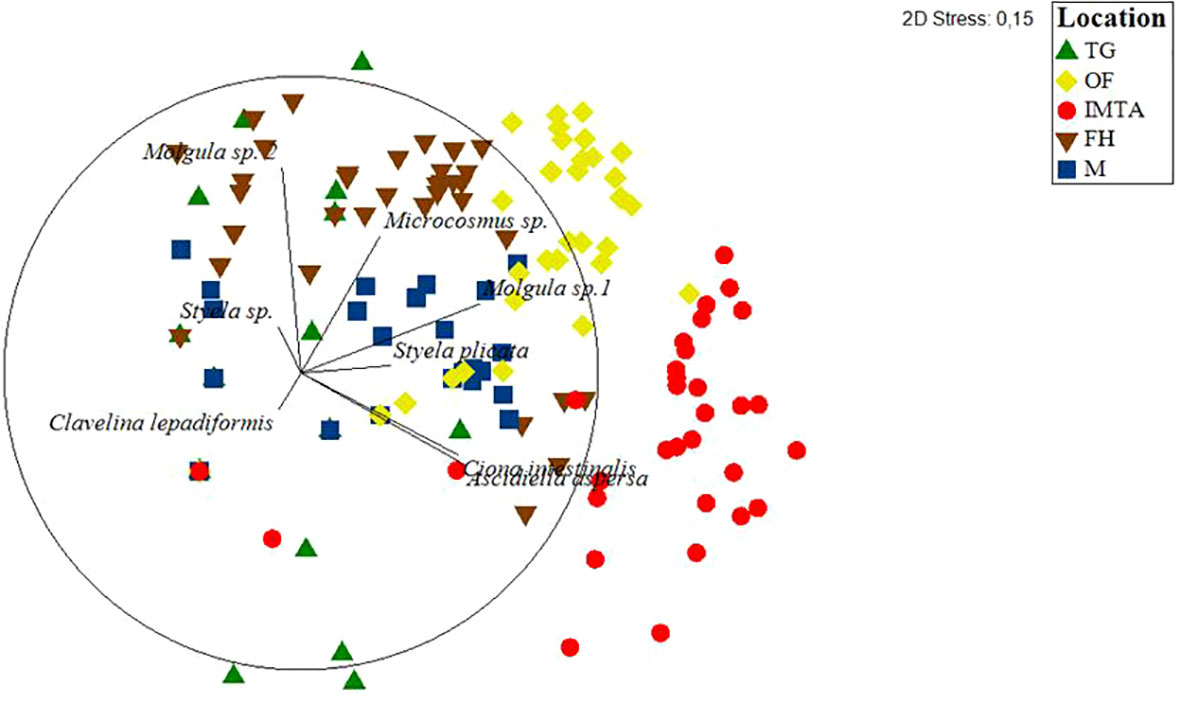
Figure 5 Multidimensional scaling plot (MDS) with each dot representing the abundance values for each ascidian retrieved from each of the sampled locations and their spatial distribution. TG, Tide Gauge; FH, Fishing Harbour; M, Marina; OF, Oyster Farm; IMTA, Integrated Multi-Trophic Aquaculture.
The analysis of nutrient concentrations provided from the water samples retrieved from each sampling period demonstrated that PO43- and NH4+ displayed a similar pattern. The OF and IMTA sites presented the highest mean values, both with statistically significant differences (1.68µmol/L and 1.69µmol/L for PO43; 17.35 µmol/L and 21.86 µmol/L for NH4+ respectively) (Figure 6). Furthermore, DistLM analysis unveiled that NH4+ best explained the variations in ascidians' total abundance amongst the farm locations (OF and IMTA) while NOx best explained for the remaining locations.
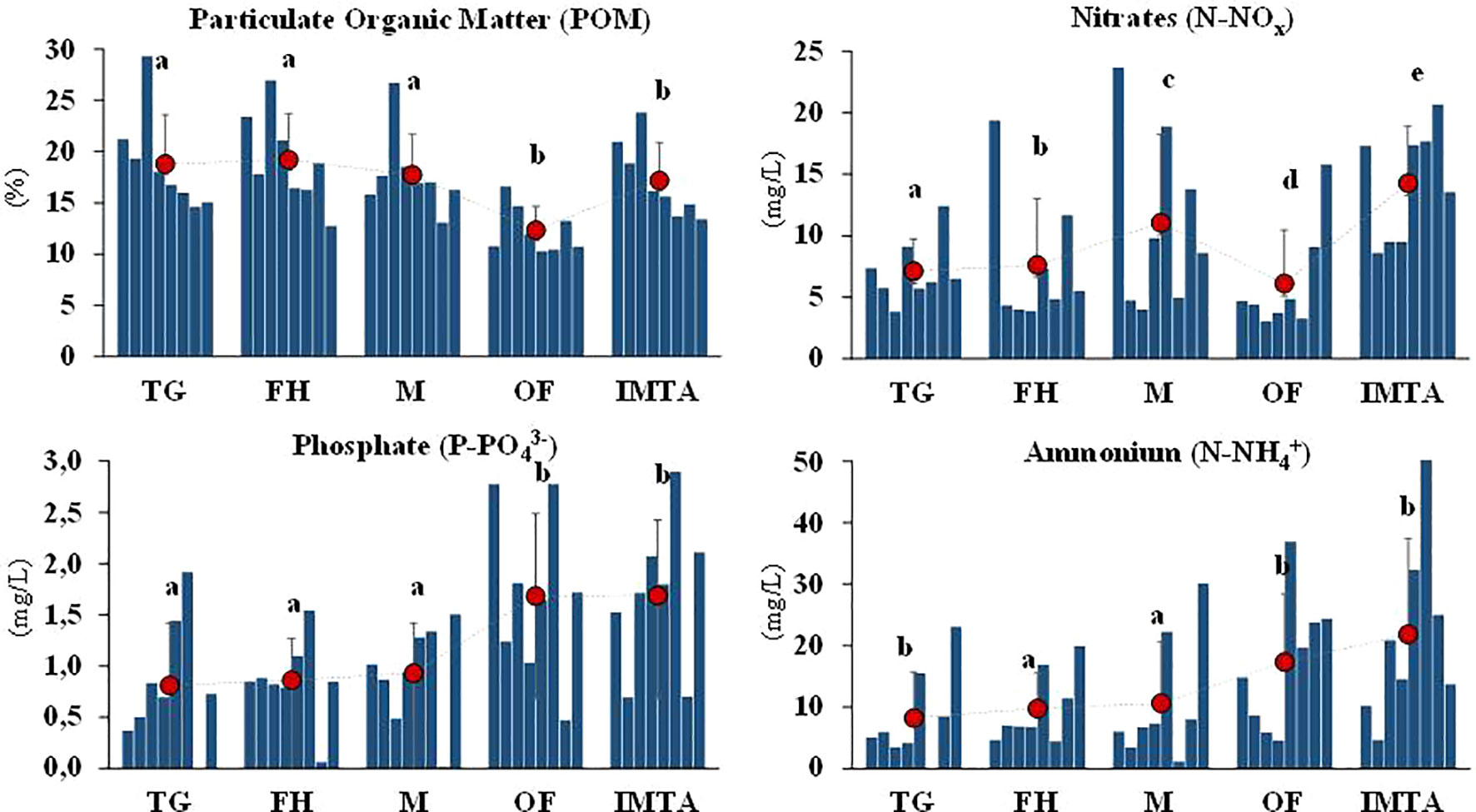
Figure 6 Environmental parameters [POM (%), NOx (µmol/L), PO43- (µmol/L), NH4+ (µmol/L)] registered at each sampled location throughout the duration of the experiment, in the Ria de Aveiro. Red dots represent the mean average with the respective standard deviation. Letters represent statistically significant differences. TG, Tide Gauge; FH, Fishing Harbour; M, Marina; OF, Oyster Farm; IMTA, Integrated Multi-Trophic Aquaculture.
Ecological diversity indices Shannon and Simpson revealed an equal pattern throughout the five locations, in which TG presented the highest values of biodiversity, followed by FH, IMTA, OF, and finally M with the lowest index values. Margalef's index indicated that the FH is the location with the highest species richness. Pielou's index demonstrated that TG and IMTA represent the two locations in which the ascidian communities are the most homogeneous (Table SI4). Lastly, species richness indicated that the highest number of ascidian species recorded was eight within the FH location, while the lowest was five, within the M location.
A detailed list of ascidians reported from coastal waters from mainland Portugal with an indication of their specific location and where were they collected is provided (Table 4). A more detailed list of all ascidian species reported to date in Portuguese waters (mainland Portugal along with Madeira and Azores archipelagos) is also presented (Table SI5). A total of 75 ascidian species were recorded in mainland Portuguese waters to date, being distributed over 40 different genera, 55% of which belong to order Stolidobranchia, 22.5% to order Aplousobranchia, and the remaining 22.5% to order Phlebobranchia. Moreover, 68% of all recorded species are solitary ascidians, with the other 32% being colonial organisms.
Discussion
The presence of A. aspersa, Microcosmus sp., and Molgula sp. 1 in all five locations demonstrates that, despite some variations in the abundance and biomass values throughout samplings, these ascidians are highly tolerant to the environmental fluctuations present in coastal ecosystems, such as Ria de Aveiro, a mesotidal coastal lagoon. Furthermore, A. aspersa and species belonging to the genus Microcosmus (M. squamiger, M. plana) are considered to be non-indigenous (NIS) species in Portugal (Figure 3), with invasive behavior (Chainho et al., 2015; Ramalhosa et al., 2021) and exhibit a wide distribution (Lambert et al., 2010). The sampling locations at Mira Channel (FH, M, and OF), registered the highest abundance of these three ascidians species, indicating the presence of favorable conditions for their settlement and growth.
However, species-specific traits must be taken into consideration. Abdul Jaffar et al. (2016) described that ascidians' distribution may not be influenced by hydrodynamic factors but rather by the type and availability of substrates. However, considering the distribution of the species recorded in our study, the hydrodynamic factors present in Ria de Aveiro (Lopes et al., 2017) may well be an explanation for such distribution. The highest total number of ascidians were collected at the OF and IMTA location (intertidal areas with poor or low water flow, (Dias et al., 2000), while the lowest number of ascidians were recorded at the TG, FH, and M locations (subtidal areas that exhibit a higher water flow). Therefore, these findings point out a strong relationship between water circulation and larval establishment in the existing infrastructures.
In coastal ecosystems, namely hosting boating and shipping activities, boats and ship's hulls and ship ballast water are commonly recognized for their potential as vectors of the introduction of biofouling organisms consequently making places such as marinas, ports, and wharves extremely susceptible to the fouling activities (Davidson et al., 2010; Hoxha et al., 2018). Furthermore, inshore aquaculture infrastructures can be targeted as well due to the loading of nutrients and the availability of artificial subtracts favorable for biofoulers organisms (Lambert, 2007; Atalah et al., 2014; Atalah et al., 2020; Loureiro et al., 2021). In the Ria de Aveiro, colonial ascidian B. violaceus was registered only at the FH and OF sites, in line with what previous authors have reported regarding the pathways of introduction (Carver et al., 2006; Bock et al., 2011; Palanisamy et al., 2018). Interestingly, B. schlosseri was only registered at the IMTA site. These two species have been recognized as NIS in Portugal (Figure 3) (Canning-Clode et al., 2013; Chainho et al., 2015; Ramalhosa et al., 2021).
Ciona intestinalis total abundance dominated at the IMTA location, representing 50% of the total ascidian community collected throughout the present study, whereas at the remaining locations (FH, M, and OF) it presented extremely low abundance values (0.5%, 0.7%, 0.3%, respectively). Additionally, this location recorded the highest concentrations of dissolved inorganic nutrients. This evidence suggests that the nutrient-enriched waters produced by the fish cultivation system are favoring the growth and biomass accumulation of this species (Chatzoglou et al., 2020). On the other hand, the OF location overall registered high abundances, but on what concerns POM values, this location recorded the lowest values. A possible explanation for such finding is the existing oyster production at this location that may contribute to a reduction of the POM from the water and consequently increase the ammonia signal (Dame et al., 1984).
The general trend of the increase in abundance values was observed in all the studied locations during sampling days 155 to 226 (July to October), which in turn corresponds to the period of ascidians settlement (Coma et al., 2000; Lambert, 2007). Several studies have verified that seasonality is a key element in the development of marine fouling communities (Lindeyer and Gittenberger, 2011; Sievers et al., 2013; Lezzi and Giangrande, 2018; Fortič et al., 2021) and that larval availability also varies with the season (Shenkar et al., 2008).
Our results are compatible with those found by other authors, who have demonstrated that ascidians can present different interspecific recruitment periods year-round. More specifically, Valentine et al. (2016) verified that recruits of colonial B. violaceus can be observed from September to October. Solitary C. intestinalis reaches a recruitment peak in August and can continue until late November (Ramsay et al., 2008b; Ramsay et al., 2009; Valentine et al., 2016). Lindeyer and Gittenberger (2011) documented the succession of native versus non-native fouling communities and verified that Molgula socialis, Styela clava, and B. violaceus settled mostly from June to December, while C. intestinalis, A. aspersa, and B. schlosseri settled from March to December. Therefore, in locations where predictable seasonal fouling patterns are present, the moment of retrieval of the substrate used to collect marine fouling communities is paramount.
Some biofouling populations can proliferate very rapidly and then gradually retreat. This is especially true for C. intestinalis and S. clava. (Watts et al., 2015). However, environmental factors such as temperature and salinity (Vercaemer et al., 2011; Valentine et al., 2016), light (Gulliksen, 2011; Al-Sofyani and Satheesh, 2019), rainfall (Gama et al., 2006), substrate availability (Osman and Whitlatch, 1995) among others, may also play a significant role in the recruitment process. For example, according to Valentine et al. (2016), ascidians C. intestinalis and A. aspersa have a negative response to temperatures above 21 °C, but other ascidians such as S. clava are less sensitive to temperature fluctuations. Ascidian colonization has been positively correlated with warmer water temperatures (Rodriguez and Ibarra-Obando, 2008), and at the five locations selected for this experiment, temperatures varied on average from 15.4°C-18.1°C, indicating that ascidian colonization is benefiting from these temperatures.
Ecological indicators are mainly used as supporting information regarding a targeted ecosystem and to evaluate possible impacts on those ecosystems. These indexes provide data about an ecosystem, namely the biodiversity status (Karydis and Tsirtsis, 1996). The Simpson index considered a dominance indicator, revealed that three locations (TG, FH, and IMTA) presented dominant species, C. lepadiformis, Molgula sp., and C. intestinalis, respectively. Despite the Shannon-Weiner index accounting for both species richness and its evenness, analogous results to the Simpson index were obtained. Moreover, the Pielou index displayed the locations TG and IMTA with the most uniformed ascidian community, possibly because of the abundancies registered at these locations of C. lepadiformis and C. intestinalis, respectively. Although the differences in species richness between each location were minor and Gamito (2010) revealed that this index is strongly affected by sampling effort and caution must be taken into consideration, the FH location was indicated as the location with the highest number of species. This evidence shows that it cannot be ruled out that this port, located in a loading dock and most probably other ports belonging to the Ria de Aveiro, are more susceptible to biofouling activities and constitute pathways for fouling introductions.
Notwithstanding, ascidian biofouling in marinas and ports should be greater when compared to natural locations as described by (Marins et al., 2010; McNaught and Norden, 2011). In our study, such observations were not entirely met. A possible explanation for such findings is that, in some way, the presence of our star-shaped ASU mimics the artificial infrastructures that are normally present in marinas and ports, and therefore artificializing natural locations. Furthermore, at OF and IMTA locations the presence of aquaculture activities may have also contributed to higher abundance values. Also, possibly a longer experimental trial would be needed to detect a higher biofouling presence under these artificial conditions.
Furthermore, the availability of non-colonized substrates and/or new artificial substrates can influence the recruitment and settlement of ascidian larvae (Osman and Whitlatch, 1995). In the present study, the substrate used (non-colonized oyster shells within a star-shaped unit) was the same in all locations. Therefore the element of preference for one type of substrate over another was eliminated, as other authors have previously described (Stoner, 1994; Chase et al., 2016). Future research involving the investigation of ecological ascidian succession from each location and the effectiveness of this ASU would imply a different experimental approach, such as longer experimental sampling periods, identification of the surrounding fauna and respective specie status, more frequent records of environmental variations, and ultimately standardization and method validation.
Previous review studies on antifouling techniques (Fitridge et al., 2012; Sievers et al., 2017; Bannister et al., 2019) revealed that the great majority of the methods that are employed are based on reactive treatments rather than proactive prevention of the fouling organisms. The most popular methods are water pressure, air exposure, coating technology, physical removal, biological control using grazers, heat, and acetic acid. Consequently, many farmers are having reservations concerning these methods due to the negative outcomes, such as efficiency, stock fitness, costs and profits, and environmental impact. However, few antifouling preventive methods are being discussed. Strategies such as the use of metals (copper, nickel, and tin) may promote negative impacts (Fitridge et al., 2012); despite investigations indicating that little environmental impact is caused by the use of natural compounds that inhibit larval metamorphosis, no commercial-scale trials to test the effectiveness have been achieved (Bannister et al., 2019); encapsulation technique is mostly applied to boat hulls, pontoons, and piles, and as the selective breeding of fouling resistant stock may be a prosperous option (Sievers et al., 2017), nevertheless, these methods can be time-consuming.
Our research supports the repurposing of oyster shells that otherwise would end up in a land-fill or inadequate disposal (Ramakrishna et al., 2018; Chilakala et al., 2019). it is an environmentally friendly practice and reduces the costs for farmers. For instance, in the UK, the disposal of oyster shell waste can cost £80 per ton (Morris et al., 2019). Repurposing oyster shell waste has been an increasingly studied topic with innumerous applications, such as biological filtration in marine aquariums (Cohen et al., 2021), desulfurization/denitrification sorbents (Jung et al., 2007), a substrate to collect non-indigenous ascidians species (Outinen et al., 2019), used as an artificial stone (Silva et al., 2019), restoration of oyster reefs (Burrows et al., 2005), eutrophication control (Kwon et al., 2017), reduction of environmental toxicity and as natural resources (e.g. limestone) (Chilakala et al., 2019).
The star-shaped ASU is easy-to-use, it can be employed not only by farmers but also by local authorities and can be deployed and retrieved at any time. Moreover, the ASU can be reused, just by letting it dry in the sun where all fouling organisms will shed off. This feature is a valuable asset if there is no intention of using ascidian biomass for other purposes. However, there is the possibility of extra benefits for farmers through the added value that ascidians' biomass may provide as they are rich in omega-3 fatty acids (Dagorn et al., 2010; Marques et al., 2021) and can be used as an aquafeed supplement (White et al., 2019).
sp.To date, knowledge of the ascidian diversity, distribution, and status in mainland Portugal is scarce however, a recent study on fouling ascidians was carried out in Madeira Islands (Ramalhosa et al., 2021). Although 75 different species have already been reported for mainland Portuguese coastal waters, 68% of them have been recorded by Ramos Esplá (1988) more than 30 years ago. It is therefore likely that due to ongoing climate change and anthropogenic activity new species may have established in the meantime and others may no longer be present. It is unquestionable that, at least for Portuguese waters, an updated survey of ascidian species occurring in this region is needed.
Conclusions
The present study revealed that the customized 3-D star-shaped ASU applied is effective for the monitorization of ecosystem shifts the ascidian diversity and it can be used to survey and establish a baseline for the ascidian biofouling community. In addition, this structure can be applied in aquaculture facilities as well, allowing for the monitorization of ascidians fouling behavior. This study represents the first attempt to survey the coastal lagoon of Ria de Aveiro addressing the ascidian biofouling community, its distribution, and composition, resorting to oyster shells, an abundant co-product of oyster farming.
Evidence suggests that the geographic distribution of the ascidian community is conditioned by hydrodynamic variations, seasonality, and by nutrient availability. Furthermore, ascidians settled most in aquaculture environment locations, such as oyster production and at the IMTA farm facility.
Therefore, two species (C. lepadiformis and A. aspersa) were, for the first time, collected and reported for mainland Portugal.
Using oyster shells as a substrate makes this unit low-cost, non-toxic, easy-to-handle, reusable, non-species-specific and a more sustainable product.The ASU can be considered an efficient support structure for the short- or long-term monitoring of the ascidian community, as well as fouling communities in general. While preventing biofouling is quite a complicated and complex endeavor, knowledge of existing communities in a given location can undoubtedly contribute towards improved management of this issue.
Data Availability Statement
The original contributions presented in the study are included in the article/Supplementary Material. Further inquiries can be directed to the corresponding author.
Author Contributions
LM contributed to the development of the original design, participated in all field campaigns, sample collection, and processing, contributed to writing the original draft, reviewing, and editing the final version. GT participated in field campaigns, sample collection, and processing, data analysis, contributed on editing the final version. RC provided supervision of the writing, reviewing, editing and validation. AL contributed to the development of the original design, supervision of the writing, reviewing, and editing process, validation and funding acquisition. All authors contributed to the article and approved the submitted version.
Funding
We are thankful to the Integrated Program of SR&TD 'Smart Valorization of Endogenous Marine Biological Resources Under a Changing Climate' (reference Centro-01-0145-FEDER-000018) and to the PORBIOTA project (POCI-01-0145-FEDER-022127) financed by the ‘‘Programa Operacional de Competitividade e Internacionalização’’ and ‘‘Programa Operacional Regional de Lisboa, FEDER’’, and by the ‘‘Fundação para a Ciência e a Tecnologia, I.P. (FCT)’’ through national funds (PIDAC). LM was also funded by national funds by the FCT under the PhD grant (PD/BD/127918/2016). We acknowledge financial support to CESAM by FCT/MCTES (UIDP/50017/2020+UIDB/50017/2020+LA/P/0094/2020), through national funds.
Conflict of Interest
The authors declare that the research was conducted in the absence of any commercial or financial relationships that could be construed as a potential conflict of interest.
Publisher’s Note
All claims expressed in this article are solely those of the authors and do not necessarily represent those of their affiliated organizations, or those of the publisher, the editors and the reviewers. Any product that may be evaluated in this article, or claim that may be made by its manufacturer, is not guaranteed or endorsed by the publisher.
Supplementary Material
The Supplementary Material for this article can be found online at: https://www.frontiersin.org/articles/10.3389/fmars.2022.921094/full#supplementary-material
References
Abdul Jaffar H., Soban Akram A., Kaleem Arshan M. L., Sivakumar V., Tamilselvi M. (2016). Distribution and Invasiveness of a Colonial Ascidian, Didemnum Psammathodes, Along the Southern Indian Coastal Water. Oceanologia 58, 212–220. doi: 10.1016/j.oceano.2016.04.002
Affinito O., Andreakis N., Caputi L., Marino R., Pannone R., Sordino P., et al. (2015). High Connectivity and Directional Gene Flow in European Atlantic and Mediterranean Populations of Ciona Intestinalis Sp. A. Mar. Ecol. 36, 1230–1243. doi: 10.1111/maec.12226
Alder J., Hancock A. (1905). The British Tunicata; an Unfinished Monograph. Ed. Hopkinson J. (London: Printed for the Ray society). doi: 10.5962/bhl.title.1824
Al-Sofyani A. M. A., Satheesh S. (2019). Recruitment Patterns of the Solitary Ascidian Phallusia Nigra Savigny 1816 on Artificial Substrates Submerged in the Central Red Sea, Saudi Arabia. Oceanol. Hydrobiol. Stud. 48, 262–269. doi: 10.2478/ohs-2019-0023
Anderson M. J., Gorley R. N., Clarke K. R. (2008). PERMANOVA+for PRIMER: Guide to Software and Statistical Methods. 218.
Atalah J., Fletcher L. M., Davidson I. C., South P. M. (2020). Artificial Habitat and Biofouling Species Distributions in an Aquaculture Seascape. Aquac. Environ. Interact. 12, 495–509. doi: 10.3354/aei00380
Atalah J., Newcombe E. M., Hopkins G. A., Forrest B. M. (2014). Potential Biocontrol Agents for Biofouling on Artificial Structures. Biofouling 30, 999–1010. doi: 10.1080/08927014.2014.956734
Azevedo J., Antunes J. T., Machado A. M., Vasconcelos V., Leão P. N., Froufe E. (2020). Monitoring of Biofouling Communities in a Portuguese Port Using a Combined Morphological and Metabarcoding Approach. Sci. Rep. 10, 1–16. doi: 10.1038/s41598-020-70307-4
Bannister J., Sievers M., Bush F., Bloecher N. (2019). Biofouling in Marine Aquaculture: A Review of Recent Research and Developments. Biofouling 35, 631–648. doi: 10.1080/08927014.2019.1640214
Ben-Shlomo R., Paz G., Rinkevich B. (2006). Postglacial-Period and Recent Invasions Shape the Population Genetics of Botryllid Ascidians Along European Atlantic Coasts. Ecosystems 9, 1118–1127. doi: 10.1007/s10021-006-0141-y
Bock D., Zhan A., Lejeusne C., MacIsaac H., Cristescu M. (2011). Looking at Both Sides of the Invasion: Patterns of Colonization in the Violet Tunicate Botrylloides Violaceus. Mol. Ecol. 20, 503–516. doi: 10.1111/j.1365-294X.2010.04971.x
Bray J. R., Curtis J. T. (1957). An Ordination of the Upland Forest Communities of Southern Wisconsin. Ecol. Monogr. 27, 325–349. doi: 10.2307/1942268
Bullard S. G., Davis C. V., Shumway S. E. (2013). Seasonal Patterns of Ascidian Settlement at an Aquaculture Facility in the Damariscotte River, Maine. J. Shellfish Res. 32, 255–264. doi: 10.2983/035.032.0202
Burrows F., Harding J. M., Mann R., Dame R., Coen L. (2005). Restoration Monitoring of Oyster Reefs. Sci. Restor. Monit. Coast. Habitats 2, 4.1.
Canning-Clode J., Fofonoff P., McCann L., Carlton J. T., Ruiz G. (2013). Marine Invasions on a Subtropical Island: Fouling Studies and New Records in a Recent Marina on Madeira Island (Eastern Atlantic Ocean). Aquat. Invasions 8, 261–270. doi: 10.3391/ai.2013.8.3.02
Carver C. E., Chisholm A., Mallet A. L. (2003). Strategies to Mitigate the Impact of Ciona Intestinalis (L.) Biofouling on Shellfish Production. J. Shellfish Res. 22, 621–631.
Carver C., Mallet A. L., Vercaemer B. (2006). Biological Synopsis of the Colonial Tunicates, Botryllus Schlosseri and Botrylloides Violaceus. Can. Manuscr. Rep. Fish. Aquat. Sci. 42, v+42.
Chainho P., Fernandes A., Amorim A., Ávila S. P., Canning-Clode J., Castro J. J., et al. (2015). Non-Indigenous Species in Portuguese Coastal Areas, Coastal Lagoons, Estuaries and Islands. Estuar. Coast. Shelf Sci. 167, 199–211. doi: 10.1016/j.ecss.2015.06.019
Chase A. L., Dijkstra J. A., Harris L. G. (2016). The Influence of Substrate Material on Ascidian Larval Settlement. Mar. Pollut. Bull. 106, 35–42. doi: 10.1016/j.marpolbul.2016.03.049
Chatzoglou E., Kechagia P., Tsopelakos A., Miliou H., Slembrouck J. (2020). Co-Culture of Ulva Sp. And Dicentrarchus Labrax in Recirculating Aquaculture System: Effects on Growth, Retention of Nutrients and Fatty Acid Profile. Aquat. Living Resour. 33, 1–13. doi: 10.1051/alr/2020023
Chilakala R., Thannaree C., Shin E. J., Thenepalli T., Ahn J. W. (2019). Sustainable Solutions for Oyster Shell Waste Recycling in Thailand and the Philippines. Recycling 4, 1–10. doi: 10.3390/recycling4030035
Cohen F. P. A., Cabral A. E., Lillebø A. I., Calado R. (2021). Relieving Pressure From Coral Reefs: Artificial Oyster Rocks can Replace Reef Rocks Used for Biological Filtration in Marine Aquariums. J. Clean. Prod. 325, 129326. doi: 10.1016/J.JCLEPRO.2021.129326
Coma R., Ribes M., Gili J., Zabala M. (2000). Seasonality in Coastal Benthic Ecosystems. Trends Ecol. Evol. 15, 448–453. doi: 10.1016/S0169-5347(00)01970-4
Dagorn F., Dumay J., Monniot C., Rabesaotra V., Barnathan G. (2010). Phospholipid Distribution and Phospholipid Fatty Acids of the Tropical Tunicates Eudistoma Sp. And Leptoclinides Uniorbis. Lipids 45, 253–261. doi: 10.1007/s11745-010-3389-0
Dame R. F., Zingmark R. G., Haskin E. (1984). Oyster Reefs as Processors of Estuarine Materials. J. Exp. Mar. Bio. Ecol. 83, 239–247. doi: 10.1016/S0022-0981(84)80003-9
Davidson I. C., Zabin C. J., Chang A. L., Brown C. W., Sytsma M. D., Ruiz G. M. (2010). Recreational Boats as Potential Vectors of Marine Organisms at an Invasion Hotspot. Aquat. Biol. 11, 179–191. doi: 10.3354/ab00302
Davis M. H., Davis M. E. (2005). Styela Clava (Tunicata: Ascidiacea) - A New Addition to the Fauna of the Portuguese Coast. J. Mar. Biol. Assoc. United Kingdom 85, 403–404. doi: 10.1017/S002531540501132Xh
Dias J. M., Lopes J. F., Dekeyser I. (2000). Tidal Propagation in Ria De Aveiro Lagoon, Portugal. Phys. Chem. Earth Part B Hydrol. Ocean. Atmos. 25, 369–374. doi: 10.1016/S1464-1909(00)00028-9
Dumont C., Gaymer C. F., Thiel M. (2011). Predation Contributes to Invasion Resistance of Benthic Communities Against the non-Indigenous Tunicate Ciona Intestinalis. Biol. Invasions 13, 2023–2034. doi: 10.1007/s10530-011-0018-7
Fitridge I., Dempster T., Guenther J., Nys R. (2012). The Impact and Control of Biofouling in Marine Aquaculture: A Review. Biofouling 28, 649–669. doi: 10.1080/08927014.2012.700478
Forrest B. M., Hopkins G. A., Dodgshun T. J., Gardner J. P. A. (2007). Efficacy of Acetic Acid Treatments in the Management of Marine Biofouling. Aquaculture 262, 319–332. doi: 10.1016/j.aquaculture.2006.11.006
Fortič A., Mavrič B., Pitacco V., Lipej L. (2021). Temporal Changes of a Fouling Community: Colonization Patterns of the Benthic Epifauna in the Shallow Northern Adriatic Sea. Reg. Stud. Mar. Sci. 45, 101818. doi: 10.1016/j.rsma.2021.101818
Gama P. B., Leonel R. M. V., Hernández M. I. M., Mothes B. (2006). Recruitment and Colonization of Colonial Ascidians (Tunicata: Ascidiacea) on Intertidal Rocks in Northeastern Brazil. Iheringia - Ser. Zool. 96, 165–172. doi: 10.1590/S0073-47212006000200005
Gamito S. (2010). Caution is Needed When Applying Margalef Diversity Index. Ecol. Indic. 10, 550–551. doi: 10.1016/j.ecolind.2009.07.006
Gewing M. T., Shenkar N. (2017). Monitoring the Magnitude of Marine Vessel Infestation by non-Indigenous Ascidians in the Mediterranean. Mar. Pollut. Bull. 121, 52–59. doi: 10.1016/j.marpolbul.2017.05.041
Guenther J., Southgate P. C., Nys R. (2006). The Effect of Age and Shell Size on Accumulation of Fouling Organisms on the Akoya Pearl Oyster Pinctada Fucata (Gould). Aquaculture 253, 366–373. doi: 10.1016/j.aquaculture.2005.08.003
Gulliksen B. (2011). Spawning, Larval Settlement, Growth, Biomass, and Distribution of Ciona Intestinalis L. (Tunicata) in Borgenfjorden, North-Tröndelag, Norway. Sarsia 51, 83–96. doi: 10.1080/00364827.1972.10411225
Hayward P. J., Ryland J. S., John S. (1990). The Marine Fauna of the British Isles and North-West Europe. Eds. Hayward P. J., Ryland J. S. (Urbana: University College of Swansea).
Hoxha T., Crookes S., Lejeusne C., Dick J. T. A., Chang X., Bouchemousse S., et al. (2018). Comparative Feeding Rates of Native and Invasive Ascidians. Mar. Pollut. Bull. 135, 1067–1071. doi: 10.1016/j.marpolbul.2018.08.039
Jung J.-H., Yoo K.-S., Kim H.-G., Lee H.-K., Shon B.-H. (2007). Reuse of Waste Oyster Shells as a SO2/NOx Removal Absorbent. J. Ind. Eng. Chem. 13, 512–517. doi: 10.5772/33887
Karydis M., Tsirtsis G. (1996). Ecological Indices: A Biometric Approach for Assessing Eutrophication Levels in the Marine Environment. Sci. Total Environ. 186, 209–219. doi: 10.1016/0048-9697(96)05114-5
Koplovitz G., Shmuel Y., Shenkar N. (2016). Floating Docks in Tropical Environments - A Reservoir for the Opportunistic Ascidian Herdmania Momus. Manage. Biol. Invasions 7, 43–50. doi: 10.3391/mbi.2016.7.1.06
Kuplik Z., Novak L., Shenkar N. (2019). Proteomic Profiling of Ascidians as a Tool for Biomonitoring Marine Environments. PLoS One 14, e0215005. doi: 10.1371/JOURNAL.PONE.0215005
Kwon H., Lee C., Jun B., Yun J., Weon S.-Y., Koopman B. (2017). Recycling Waste Oyster Shells for Eutrophication Control. Resour. Conserv. Recycl. 41, 75–82. doi: 10.1016/j.resconrec.2003.08.005
Lacoste E., Gaertner-Mazouni N. (2015). Biofouling Impact on Production and Ecosystem Functioning: A Review for Bivalve Aquaculture. Rev. Aquac. 7, 187–196. doi: 10.1111/raq.12063
Lacoste É., Raimbault P., Harmelin-vivien M., Gaertner-mazouni N. (2016). Trophic Relationships Between the Farmed Pearl Oyster Pinctada Margaritifera and its Epibionts Revealed by Stable Isotopes and Feeding Experiments. Aquac. Environ. Interact. 8, 55–66. doi: 10.3354/aei00157
Lambert G. (2001). A Global Overview of Ascidian Introductions and Their Possible Impact on the Endemic Fauna. Biol. Ascidians, 249–257. doi: 10.1007/978-4-431-66982-1_40
Lambert G. (2007). Invasive Sea Squirts: A Growing Global Problem. J. Exp. Mar. Bio. Ecol. 342, 3–4. doi: 10.1016/j.jembe.2006.10.009
Lambert G., Shenkar N., Swalla B. J. (2010). First Pacific Record of the North Atlantic Ascidian Molgula Citrina – Bioinvasion or Circumpolar Distribution? Aquat. Invasions 5, 369–378. doi: 10.3391/ai.2010.5.4.06
Lehtiniemi M., Ojaveer H., David M., Galil B., Gollasch S., McKenzie C., et al. (2015). Dose of Truth - Monitoring Marine non-Indigenous Species to Serve Legislative Requirements. Mar. Policy 54, 26–35. doi: 10.1016/J.MARPOL.2014.12.015
Lezzi M., Giangrande A. (2018). Seasonal and Bathymetric Effects on Macrofouling Invertebrates’ Primary Succession in a Mediterraenan non-Indigenous Species Hotspot Area. Mediterr. Mar. Sci. 19, 572–588. doi: 10.12681/MMS.14786
Lindeyer F., Gittenberger A. (2011). Ascidians in the Succession of Marine Fouling Communities. Aquat. Invasions 6, 421–434. doi: 10.3391/ai.2011.6.4.07
Locke A., Hanson J. M., Ellis K. M., Thompson J., Rochette R. (2007). Invasion of the Southern Gulf of St. Lawrence by the Clubbed Tunicate (Styela Clava, Herdman): Potential Mechanisms for Invasions of Prince Edward Island Estuaries. J. Exp. Mar. Bio. Ecol. 342, 69–77. doi: 10.1016/j.jembe.2006.10.016
Lopes M. L., Marques B., Dias J. M., Soares A. M. V. M., Lillebø A. I. (2017). Challenges for the WFD Second Management Cycle After the Implementation of a Regional Multi-Municipality Sanitation System in a Coastal Lagoon (Ria De Aveiro, Portugal). Sci. Total Environ. 586, 215–225. doi: 10.1016/J.SCITOTENV.2017.01.205
Loureiro T. G., Peters K., Robinson T. B. (2021). Dropping Plates to Pick Up Aliens: Towards a Standardised Approach for Monitoring Alien Fouling Species. Afr. J. Mar. Sci. 43, 483–497. doi: 10.2989/1814232X.2021.1989488
Lutz-Collins V., Ramsay A., Quijón P. A., Davidson J. (2009). Invasive Tunicates Fouling Mussel Lines: Evidence of Their Impact on Native Tunicates and Other Epifaunal Invertebrates. Aquat. Invasions 4, 213–220. doi: 10.3391/ai.2009.4.1.22
Margalef R. (1968). “Perspectives in Ecological Theory,” (Chicago: The University of Chicago Press), 111.
Marins F. O., Novaes R. L. M., Rocha R. M., Junqueira A. O. R. (2010). Non Indigenous Ascidians in Port and Natural Environments in a Tropical Brazilian Bay. Zoologia 27, 213–221. doi: 10.1590/S1984-46702010000200009
Marques L., Calado R., Lillebø A. I. (2022). Potential of Ascidians as Extractive Species and Their Added Value in Marine Integrated Multitrophic Aquaculture Systems–from Pests to Valuable Blue Bioresources. Front. Mar. Sci. 9. doi: 10.3389/fmars.2022.849870
Marques L., Domingues M. R., Costa E., Abreu M. H., Lillebø A. I., Calado R. (2021). Screening for Health-Promoting Fatty Acids in Ascidians and Seaweeds Grown Under the Influence of Fish Farming Activities. Mar. Drugs 19, 1–13. doi: 10.3390/md19080469
Marshall D. J., Pechenik J. A., Keough M. J. (2003). Larval Activity Levels and Delayed Metamorphosis Affect Post-Larval Performance in the Colonial Ascidian Diplosoma Listerianum. Mar. Ecol. Prog. Ser. 246, 153–162. doi: 10.3354/meps246153
Martins V., da Silva E. F., Sequeira C., Rocha F., Duarte A. C. (2010). Evaluation of the Ecological Effects of Heavy Metals on the Assemblages of Benthic Foraminifera of the Canals of Aveiro (Portugal). Estuar. Coast. Shelf Sci. 87, 293–304. doi: 10.1016/j.ecss.2010.01.011
McNaught D. C., Norden W. S. (2011). Generalized Regional Spatial Patterns of Larval Recruitment of Invasive Ascidians, Mussels, and Other Organisms Along the Coast of Maine. Aquat. Invasions 6, 519–523. doi: 10.3391/ai.2011.6.4.18
Millar R. H. (1952). The Annual Growth and Reproductive Cycle in Four Ascidians. J. Mar. Biol. Assoc. United Kingdom 31, 41–61. doi: 10.1017/S0025315400003672
Millar R. H. (1971). The Biology of Ascidians (Dunstaffnage Marine Research Laboratory, Oban, Argyll, Scotland: Elsevier). doi: 10.1016/S0065-2881(08)60341-7
Morris J. P., Backeljau T., Chapelle G. (2019). Shells From Aquaculture: A Valuable Biomaterial, Not a Nuisance Waste Product. Rev. Aquac. 11, 42–57. doi: 10.1111/raq.12225
Nagar A., Huys R., Bishop J. D. D. (2010). Widespread Occurrence of the Southern Hemisphere Ascidian Corella Eumyota Traustedt 1882 on the Atlantic Coast of Iberia. Aquat. Invasions 5, 169–173. doi: 10.3391/ai.2010.5.2.06
Oliveira F., Almeida C. (2009). Ascidonia Flavomaculata (Heller 1864), a New Record for the Portuguese Marine Invertebrate Fauna (Decapoda, Pontoniidae). Crustaceana 82, 63–67. doi: 10.1163/156854008X363740
Osman R. W., Whitlatch R. B. (1995). The Influence of Resident Adults on Larval Settlement: Experiments With Four Species of Ascidians. J. Exp. Mar. Bio. Ecol. 190, 199–220. doi: 10.1016/0022-0981(95)00036-Q
Outinen O., Forsström T., Yli-Rosti J., Vesakoski O., Lehtiniemi M. (2019). Monitoring of Sessile and Mobile Epifauna – Considerations for non-Indigenous Species. Mar. Pollut. Bull. 141, 332–342. doi: 10.1016/j.marpolbul.2019.02.055
Palanisamy S. K., Thomas O. P., Mccormack G. (2018). Bio-Invasive Ascidians in Ireland: A Threat for the Shellfish Industry But Also a Source of High Added Value Products. Bioengineered 9, 55–60. doi: 10.1080/21655979.2017.1392421
Peck L. S., Clark M. S., Power D., Reis J., Batista F. M., Harper E. M. (2015). Acidification Effects on Biofouling Communities: Winners and Losers. Glob. Change Biol. 21, 1907–1913. doi: 10.1111/gcb.12841
Pielou E. C. (1969). An Introduction to Mathematical Ecology. Wiley Intersci. 286. doi: 10.1002/BIMJ.19710130308
Ramakrishna C., Thenepalli T., Young Nam S., Kim C., Whan Ahn J. (2018). Oyster Shell Waste is Alternative Sources for Calcium Carbonate (CaCO3) Instead of Natural Limestone. J. Energy Eng. 27, 59–64. doi: 10.5855/ENERGY.2018.27.1.059
Ramalhosa P., Gestoso I., Rocha R. M., Lambert G., Canning-Clode J. (2021). Ascidian Biodiversity in the Shallow Waters of the Madeira Archipelago: Fouling Studies on Artificial Substrates and New Records. Reg. Stud. Mar. Sci. 43, 101672. doi: 10.1016/j.rsma.2021.101672
Ramos Esplá A. A. (1988). Ascidias Litorales Del Mediterraneo, Faunistica, Ecologia Y Biogeografia. España: Universitat de Barcelona
Ramos-Esplá A. A., Turon X., Vazquez E. (1993). Aplidium Sagresensis N. Sp. (Ascidiacea, Polyclinidae) From the Atlantic Coast of the Iberian Peninsula. Ophelia 38, 97–105. doi: 10.1080/00785326.1993.10429891
Ramsay A., Davidson J., Bourque D., Stryhn H. (2009). Recruitment Patterns and Population Development of the Invasive Ascidian Ciona Intestinalis in Prince Edward Island, Canada. Aquat. Invasions 4, 169–176. doi: 10.3391/ai.2009.4.1.17
Ramsay A., Davidson J., Landry T., Arsenault G. (2008a). Process of Invasiveness Among Exotic Tunicates in Prince Edward Island, Canada. Biol. Invasions 10, 1311–1316. doi: 10.1007/s10530-007-9205-y
Ramsay A., Davidson J., Landry T., Stryhn H. (2008b). The Effect of Mussel Seed Density on Tunicate Settlement and Growth for the Cultured Mussel, Mytilus Edulis. Aquaculture 275, 194–200. doi: 10.1016/j.aquaculture.2008.01.024
Reming. (1822). The Philosophy of Zoology: or a General View of the Structure, Functions and Classification of Animals. Edinburgh 2, 1–618.
Rodriguez L. F., Ibarra-Obando S. E. (2008). Cover and Colonization of Commercial Oyster (Crassostrea Gigas) Shells by Fouling Organisms in San Quintin Bay, Mexico. J. Shellfish Res. 27, 337–343. doi: 10.2983/0730-8000(2008)27[337:CACOCO]2.0.CO;2
Ruiz S. (2015). The Status of the Invasive Sea Squirts and Barnacles Found in the Marinas and Ports of Algarve, Southern Portugal. Universidade do Algarve, Faculdade de Ciências do Mar e do Ambiented
Saldanha L. V. C. (1974) Estudo do Povoamento Dos Horizontes Superiores Da Rocha Litoral Costa Da Arrábida (Portugal). Available at: https://books.google.pt/books/about/Estudo_do_povoamento_dos_horizontes_supe.html?id=y5MRNAAACAAJ&redir_esc=y (Accessed April 6, 2022).
Shannon C. E., Weaver W. W. (1963). “The Mathematical Theory of Communications.,” in Scientific Research Publishing (Urbana: University of Illinois Press), 117.
Shenkar N., Zeldman Y., Loya Y. (2008). Ascidian Recruitment Patterns on an Artificial Reef in Eilat (Red Sea). Biofouling 24, 119–128. doi: 10.1080/08927010801902083
Sievers M., Fitridge I., Bui S., Dempster T. (2017). To Treat or Not to Treat: A Quantitative Review of the Effect of Biofouling and Control Methods in Shellfish Aquaculture to Evaluate the Necessity of Removal. Biofouling 7014, 1–13. doi: 10.1080/08927014.2017.1361937
Sievers M., Fitridge I., Dempster T., Keough M. J. (2013). Biofouling Leads to Reduced Shell Growth and Flesh Weight in the Cultured Mussel Mytilus Galloprovincialis. Biofouling, 37–41. doi: 10.1080/08927014.2012.749869
Silva T., Mesquita-Guimarães J., Henriques B., Silva F.S., Fredel M.C., et al (2019). The Potential Use of Oyster Shell Waste in New Value-Added By-Product. Biofouling 8, 13. doi: 10.3390/resources8010013
Stoner D. S. (1994). Larvae of a Colonial Ascidian Use a non-Contact Mode of Substratum Selection on a Coral Reef. Mar. Biol. 121, 319–326. doi: 10.1007/BF00346740
Taylor J. J., Southgate P. C., Rose R. A. (1997). Fouling Animals and Their Effect on the Growth of Silver-Lip Pearl Oysters, Pinctada Maxima (Jameson) in Suspended Culture. Aquaculture 153, 31–40. doi: 10.1016/S0044-8486(97)00014-8
Turon X., Nishikawa T., Rius M. (2007). Spread of Microcosmus Squamiger (Ascidiacea: Pyuridae) in the Mediterranean Sea and Adjacent Waters. J. Exp. Mar. Bio. Ecol. 342, 185–188. doi: 10.1016/J.JEMBE.2006.10.040
Valentine P., Carman M. R., Blackwood D., Bullard S. (2016). Observations of Recruitment and Colonization by Tunicates and Associated Invertebrates Using Giant One-Meter2 Recruitment Plates at Woods Hole, Massachusetts. Manage. Biol. Invasions 7, 115–130. doi: 10.3391/mbi.2016.7.1.14
Vercaemer B., Sephton D., Nicolas J. M., Howes S., Keays J. (2011). Ciona Intestinalis Environmental Control Points: Field and Laboratory Investigations. Aquat. Invas 6, 477–490. doi: 10.3391/ai.2011.6.4.13
Watts A. M., Goldstien S. J., Hopkins G. A. (2015). Characterising Biofouling Communities on Mussel Farms Along an Environmental Gradient: A Step Towards Improved Risk Management. Aquac. Environ. Interact. 8, 15–30. doi: 10.3354/aei00159
Keywords: settlement, coastal lagoon, artificial substrate, monitorization, fouling community, tunicates
Citation: Marques L, Teixeira G, Calado R and Lillebø AI (2022) Using Oyster Shells for Customized 3-D Structures for Monitoring Ecosystem Shifts on Ascidians Diversity. Front. Mar. Sci. 9:921094. doi: 10.3389/fmars.2022.921094
Received: 15 April 2022; Accepted: 18 May 2022;
Published: 20 June 2022.
Edited by:
Michael Phillips, WorldFish (Malaysia), MalaysiaReviewed by:
Matthew Lee, Universidad de Los Lagos, ChileJasmine Ferrario, University of Pavia, Italy
Copyright © 2022 Marques, Teixeira, Calado and Lillebø. This is an open-access article distributed under the terms of the Creative Commons Attribution License (CC BY). The use, distribution or reproduction in other forums is permitted, provided the original author(s) and the copyright owner(s) are credited and that the original publication in this journal is cited, in accordance with accepted academic practice. No use, distribution or reproduction is permitted which does not comply with these terms.
*Correspondence: Luisa Marques, luisa.marques@ua.pt; Ana Isabel Lillebø, lillebo@ua.pt