- Third Institute of Oceanography, Ministry of Natural Resources, Xiamen, China
The Oncaeidae is an important family of small copepods in marine ecosystems and is characterized by high diversity and abundance. The family was established by Giesbrecht in the late 19th century. Since then, 115 oncaeid species have been discovered and described. However, Oncaeidae species are small-sized and show considerable morphological similarity. The family includes a great number of sibling species and intraspecific form variants. Consequently, there are large knowledge gaps regarding their taxonomy and diversity, and their importance in marine ecosystems may have been underestimated. In this study, we have charted the progress of oncaeid taxonomy and diversity studies worldwide. We have identified the major challenges faced by the researchers and have described the marine science techniques. We also provide an overview of the distribution and ecology of the family Oncaeidae. Progress has been made toward understanding the distribution, habitat preference, and feeding behavior of oncaeids. The knowledge of oncaeids taxonomy has also been considerably increased by improvements in the research methodology, such as the use of molecular biology techniques and interdisciplinary approaches in tandem with the traditional taxonomic methods.
The significance of Oncaeidae research
Copepods play an important role in marine ecosystems as they are the most diverse and abundant marine zooplankton. They typically account for 70% of the abundance and 30% of the zooplankton biomass in open tropical oceans (Mckinnon and Duggan, 2014). Small copepods (body length < 1 mm) have very short life cycles, high reproduction rates, and high annual productivities. In marine ecosystems, their utilization for primary productivity (17.63%–69.92%) is far greater than that of large copepods (3.18%–18.43%) (Wang et al., 2002). Hence, small copepods play a much more important role in energy transfer than larger organisms.
Oncaeidae is an important family of small marine copepods, which are most being smaller than 600 μm (Böttger-Schnack and Schnack, 2009; Böttger-Schnack and Schnack, 2015; Heron, 1976; Malt, 1983). They are widely distributed, inhabiting seas from the equator to the pole, and also can be found in all vertical layers from the surface to the deep sea (Böttger-Schnack and Schnack, 2015). However, as a result of the small size and focus of studies on the photic zone, many oncaeids that occur in the mesopelagic and bathypelagic zones are yet to be discovered (Malt, 1982). This has severely hindered the research on species diversity of the family Oncaeidae. Consequently, the importance of this family in marine environments may be underestimated.
Advances in Oncaeidae taxonomy
The family Oncaeidae belongs to the suborder Poecilostomatoida of the subclass Copepoda. At present, a total of 115 oncaeids have been described worldwide (Razouls et al., 2020). In the late 19th century, Giesbrecht established the family Oncaeidae and described many of its species during a study of planktonic copepods in the Gulf of Naples (Di Capua et al., 2017). Since then, a great variety of oncaeids have been discovered and described (Figure 1). Heron described 26 Oncaeidae species from the Southwest Pacific-Antarctica area, established the genus Epicalymma, and described 22 new species in 1977 (Heron, 1976). Thereafter, Malt (Malt, 1982) described the morphology of one new species and two new combined species of the genus Oncaea and studied the phylogenetic relationships of species within the genus Oncaea based on their character states. In the next year, 17 species belonging to the genus Oncaea from the North Atlantic were described by him. This research also reported that Oncaea venusta is ubiquitous between 65°N and 50°S (Malt, 1983). Six years later, another 17 species of Oncaea were recorded in the Mediterranean Sea by Malt (Malt et al., 1989), three of which were newly added from the Mediterranean faunal list. They also observed that Oncaea species were most abundant from December to January of the following year, and from March to May. Go et al. (Go, 1994) found that Oncaea species occur near Jeju Island in the East China Sea all year round and have the highest abundances in spring (May to June) and autumn (October to November). Böttger-Schnack (Böttger-Schnack, 1999; Böttger-Schnack, 2001; Böttger-Schnack, 2003; Böttger-Böttger-Schnack, 2002; Böttger-Schnack and Schnack, 2015) described several new Oncaea species in the Red Sea and studied the taxonomic features of 112 species of Oncaea. As studies in this area continued to progress, disputes began to arise about oncaeid genera. For instance, Böttger-Schnack (Böttger-Schnack, 1999) established the genus Triconia based on a study of Oncaeidae in the Red Sea and suggested that some Oncaea species should be categorized under this genus. However, Heron and Frost (Heron and Frost, 2000) rejected the genus Triconia. Nonetheless, Boxshall and Halsey (2004) still used the findings of Böttger-Schnack to classify the family Oncaeidae into the genera Archioncaea, Conaea, Epicalymma, Monothula, Oncaea, Spinoncaea, and Triconia, according to their morphological characteristics. Di Capua et al. (Di Capua et al., 2017) used a phylogenetic tree based on mitochondrial cytochrome c oxidase subunit I (COI) sequences to study the evolution of Oncaeidae species, which raised doubts about the validity of the genus Triconia. They then suggested that Triconia should be demoted to a subgenus.
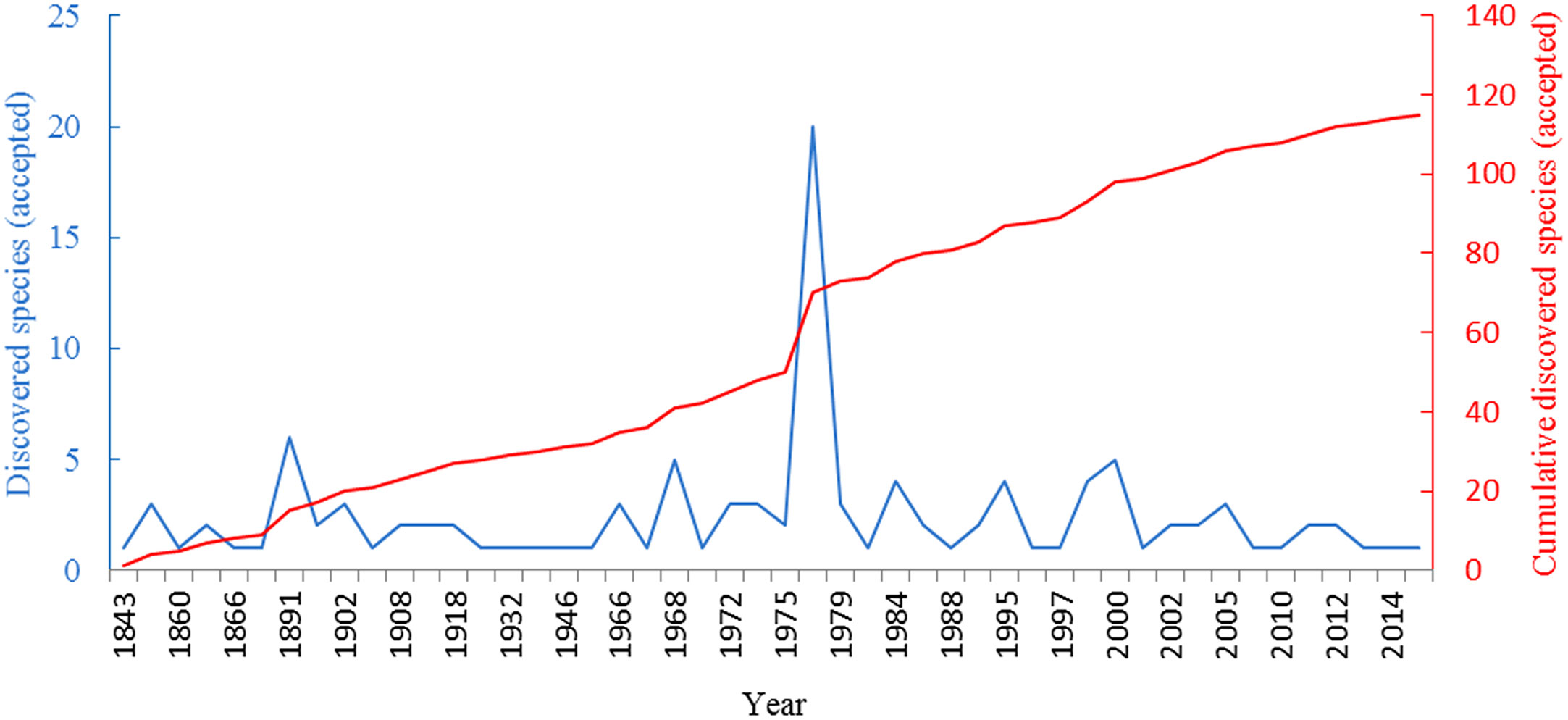
Figure 1 Trend chart of the number of discovered species and cumulative discovered species in Oncaeidae over the years.
At present, Boxshall and Halsey’s classification system is still used internationally, which divides the family Oncaeidae into 7 genera (Table 2), among which Oncaea has the largest number of species. The genus Oncaea contains 72 species (including one species affinis), mainly distributed in the Mediterranean Sea, Black Sea, Indian Ocean and Red Sea. Triconia followed by 27 species, which are widely distributed in the Mediterranean Sea, Black Sea, Indian Ocean, Red Sea, and the Pacific Ocean. Epicalymma recorded 7 species worldwide, including 5 species in the Arctic Ocean and 4 species in the Pacific Ocean (Table 1). The genera Conaea, Spinoncaea, Archioncaea and Monothula have 4, 3, 1, and 1 recorded species, respectively. According to the geographical distribution data of Oncaeidae species provided by the Global Biodiversity Information Facility (GBIF, https://www.gbif.org/species/5048), the cumulative number of Oncaeidae species was calculated in 1-degree grid (Figure 2). From the perspective of global distribution, Oncaeidae can be found in various sea areas, and the largest cumulative number of species found in the Mediterranean Sea and the Black Sea. The reason may be that most research on Oncaeidae was concentrated in the Mediterranean and Black Seas.
Challenges faced by studies on the diversity of Oncaeidae species and their technical trends
Oncaeidae is highly diverse, with many specimens distinguishable only by small structural differences in their appendages. This has led to the misidentification and underestimation of the actual species diversity of Oncaeidae (Böttger-Schnack et al., 2004). Oncaeidae also includes a large number of sibling species (Böttger-Schnack and Machida, 2011), which further complicates taxonomic studies of the family.
For example, O. venusta Philippi, 1843, is ubiquitous in the Atlantic, Pacific, and Indian Oceans (Malt, 1983) and also occurs in the Yellow Sea, East China Sea, and the South China Sea. The earliest known record of this species dates back to 1843 (Philippi, 1843). Eventually, different forms of O. venusta were discovered by conducting marine surveys in the Indian Ocean and the Red Sea (Böttger-Schnack et al., 1989; Böttger-Schnack and Huys, 2001), the Atlantic Ocean (Ferrari, 1975; Boxshall, 1977), and the Northwest Pacific Ocean (Heron and Bradford-Grieve, 1995; Heron, 2002, Heron, 1976). Farran (1929); Sewell (1947), and Tanaka (1960) established two different form variants of O. venusta —a larger forma typica and smaller forma venella, based on their body lengths and the ratios between the lengths of their posterior segments. In 1929, Farran described the morphology of these two forms of O. venusta from the Atlantic Ocean. He described the f. venella differs from the f. typica form by its smaller size and more uniformly oval and narrower cephalothorax. And the forebody of f. venella is also slightly longer in proportion to the hind body than in the f. typica. Thereafter, Sewell described the large and small forms of O. venusta in detail. The f. typica variant has a ratio of 59:41 between the lengths of their anterior and posterior regions, a ratio of 59:29 between the length and breadth of the anterior region, and a ratio of 13:46:7:7:9:18 between the lengths of the six segments of the posterior region (including the caudal rami), whereas f. venella has a ratio of 60:40 between the length of the anterior and posterior regions of the body, a ratio of 60:24 between the length and breadth of the anterior region, and a ratio of 13:43:7:8:9:20 between the lengths of the segments of the posterior region. Sewell also noted that these forms have different spawning seasons. Subsequently, Tanaka reported that the appendages of f. typica and f. venella are indistinguishable, but the appendages the of latter are slenderer than the former. For a long time, both form variants were recorded as O. venusta. In 2002, Heron performed detailed observations and analyses on O. venusta samples collected near the coast of Liberia and the Gulf of Mexico and found that the appendage structure of O. venusta var. venella is slightly different from that of the larger variant. The venella variant has a mid-dorsal dilation in the lateral view of the second pediger and setae on the fifth leg of the males. Based on these characteristics, Heron concluded that these variants are not the same species and, for the first time, promoted O. f. venella to the species level.
Many other oncaeids also have intra-species variants similar to O. venusta. This undoubtedly increases the difficulty of studying and describing oncaeid taxonomy. For instance, Sewell (1947) and Tanaka (1960) divided Oncaea media into large (forma major) and small (forma minor) variants based on the body lengths of males and females. However, these form variants do not have any significant differences in their appendages, and they only exhibit intraspecific differences in their body sizes. Based on the lengths of males and females and the form of their bodies, Chihara and Murano (1997) divided O. mediterranea samples collected from the seas of Japan into small, typical, and large forms. Chihara and Murano (1997) and Farran (1936) identified three forms of Triconia conifera. based on the differences in the cephalothorax of the females, the dorsal projection of the second thoracic segment, and their body length: (1) the ‘stocky’ form, which has a significant dorsal projection of the second thoracic segment; (2) the ‘minus’ form, which has a slight dorsal projection of the second thoracic segment; and (3) the ‘bumped’ form, which has dorsal projections in the second thoracic segment and cephalothorax.
It can be difficult to differentiate between closely related taxa, such as congeneric and cryptic species, based on morphology (Matarese et al., 2011; Kwun, 2018). Furthermore, copepod taxonomists have different levels of expertise and capabilities, making their experience a dependent variable in the morphological identification of oncaeids. As a result of these limitations, the information on the community structure and vertical distribution of the family Oncaeidae is scarce and limited (Hopcroft et al., 1998; Böttger-Schnack et al., 2004). In addition, the available biological data are often based on higher taxonomic categories, such as genera or even families (Sutton et al., 2001). The traditional taxonomic approach is time-consuming, requires effort, and depends on the experience and subjective opinions of the researcher. Furthermore, its application is also constrained by the quantity and quality of the collected samples. These inherent limitations of the morphology-based identification systems and the decline in the number of taxonomists necessitate the adoption of a molecular approach to identify species (Di Capua et al., 2017). Hence, there is a consensus among researchers that the future of taxonomy and species diversity research lies in the establishment of molecular markers that are related to morphological characteristics (Böttger-Schnack and Machida, 2011). This would result in rapid, accurate, and objective identification of species.
DNA barcoding is a kind of molecular biology technique, which widely used in animal evolutionary genetics, molecular ecology, genetic diversity studies, and conservation studies (Goetze, 2003; Hebert et al., 2003a; Hebert et al., 2003b; Kress et al., 2015) and has also been used to study the family Oncaeidae. For example, to facilitate the identification of Oncaeidae in the Mediterranean Sea, Böttger-Schnack and Machida (Böttger-Schnack and Machida, 2011) used a combination of morphological taxonomy and molecular biology techniques. A total of 67 preidentified specimens were analyzed using approximately 650 and 500 bp region of the mitochondrial COI and 12S small ribosomal RNA (12S rRNA) gene sequence, and 24 oncaeid species were successfully identified. This study also compared the genetic data of three species of the similis-subgroup, Triconia minuta, and Triconia. umerus and Triconia sp. 8, which have been interpreted as sibling species based on their morphological characters. Triconia sp.8 was genetically confirmed as a distinct species as the three species showed the same genetic distance to other known subgroups. Using the COI gene method, this study analyzed the taxonomic status of two form variants of Oncaea mediterranea. It is difficult to assess whether two forms can represent genuine species when they cannot be distinguished based on morphological methods as the two forms differed only concerning the appearance of both sexes and the length to width ratio of the caudal ramus in females (Böttger-Schnack and Huys, 1997; Böttger-Schnack and Machida, 2011). The genetic analyses confirmed that the two forms represent genuine species and proved the usefulness of COI sequencing for species discrimination.
Elvers et al. (Elvers et al., 2006) clarified the relationship between O. venusta and O. f. venella by sequencing their mitochondrial cytochrome b (Cyt b) gene and internal transcribed spacer 1 (ITS1) and concluded that they are distinct species. The cyt b sequences of the genus Oncaea showed a much higher level of variation than ITS1. Hence, the results obtained by using two different genes were concordant. Four genetic clades could be differentiated. A small-size and a large-size group of Oncaea were unambiguously assigned to two distinct clades or lineages, indicating that the two morphs of O. venusta have to be defined as different species. Additional morphological studies and more sensitive nuclear markers are required for the medium-size group to clarify their taxonomic status. Iole Di Capua et al. (Di Capua et al., 2017) identified six oncaeid species using ITS rDNA and COI, which are complexes of closely related species (Heron and Frost, 2000; Böttger-Schnack and Huys, 2001; Böttger-Schnack and Machida, 2011). The phylogenetic analyses based on these two genomic regions corroborated the species identification based on morphological characters and validated the sisterhood of the genera Triconia and the Oncaea sensu stricto. The result also suggests the possibility to demote Triconia to sub-genus or allocating Oncaea sp. 7, Oncaea parabathyalis, Oncaea shmelevi, and Oncaea prendeli into another genus.
The resolution of these controversies has demonstrated the promise of molecular biology techniques for the study of cryptic species in the family Oncaeidae. Further improvements in molecular biology techniques will enhance the speed and accuracy of taxonomic studies on the family of Oncaeidae. It may then become possible to infer the evolutionary history, geological isolation, and genetic differentiation of oncaeid species. This shall provide basic data and technical support for the study of oncaeid taxonomy and diversity, thus driving advances in these areas. Although the number of available COI sequences for marine planktonic copepods is relatively high, obtaining COI fragments from oncaeids using the universal primers commonly employed for copepods proved to be difficult (Machida et al., 2004; Böttger-Schnack and Machida, 2011). As the DNA barcode data on the family Oncaeidae contains large gaps, the classification of oncaeids based on molecular biology techniques is still in an exploratory stage. For instance, the GenBank database only contains 29 DNA barcodes of the family Oncaeidae, which represent 16 species. The Barcode of Life Data System (BOLD) database only contains 46 DNA barcodes of 16 species from the family Oncaeidae (Figure 3). Most of these data were collected from the Mediterranean Sea (Figure 4), whereas data from other marine regions are extremely scarce. Therefore, there is a great discrepancy between the DNA barcode data and the species diversity of the family Oncaeidae.
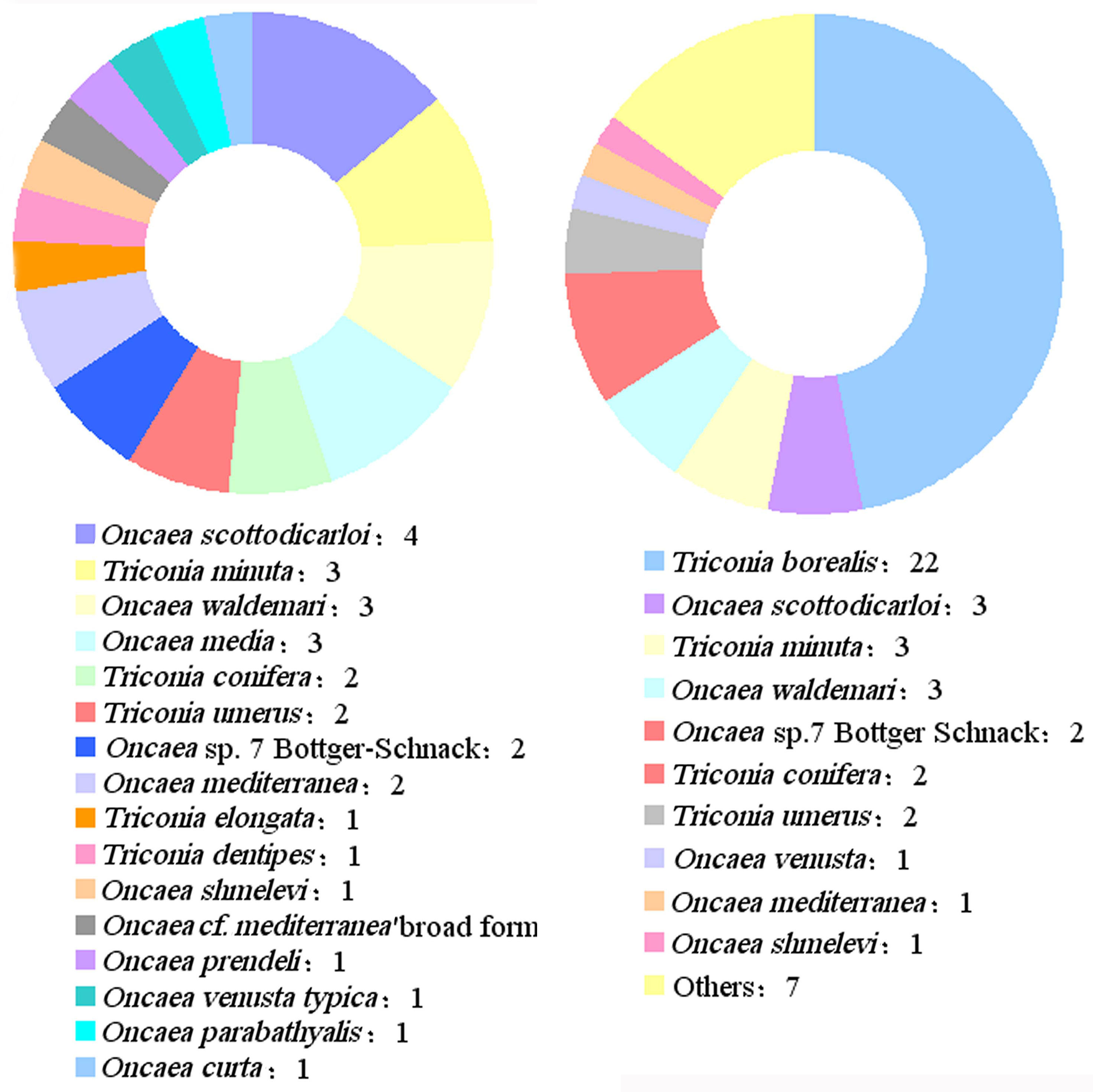
Figure 3 The number of DNA barcodes of Oncaeidae in the Database of Genbank (right) and BOLD (lift).
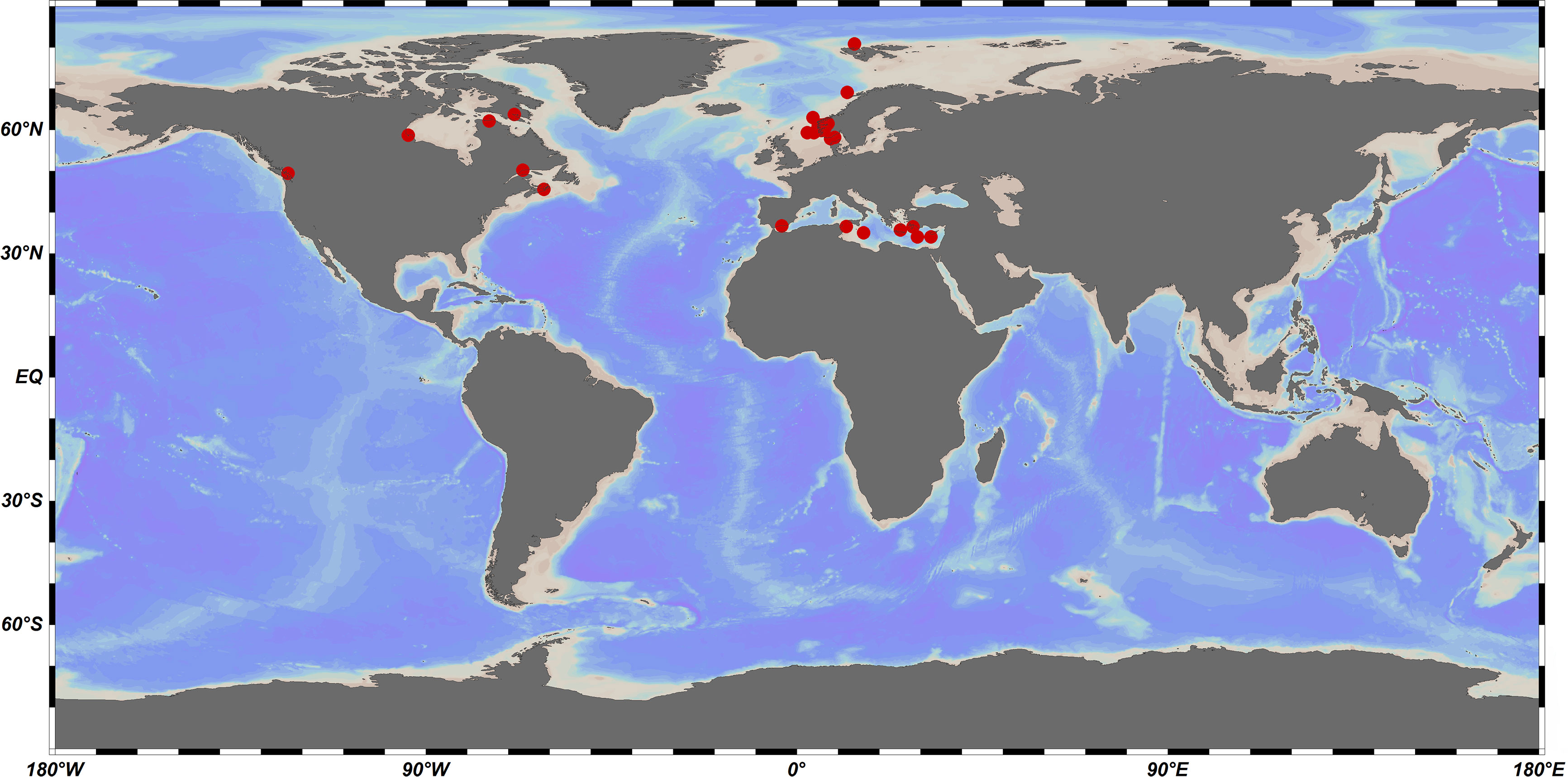
Figure 4 The distribution map of Oncaeidae species with DNA barcoding in different sea areas of the world.
Finally, we propose that DNA barcodes should be sequenced from different copepods to improve the database, which will broaden our knowledge regarding the main inhabitants of the pelagic realm, and subsequently, enable future studies based on secondary structure-derived stem phylogeny (Schultz and Wolf, 2009; Di Capua et al., 2017).
Studies on the distribution and habitat of the family Oncaeidae
The Oncaeidae family is widely distributed in the Atlantic Ocean (Ferrari, 1975; Boxshall, 1977), the Indian Ocean (Böttger-Schnack et al., 1989; Böttger-Schnack and Huys, 2001), the Pacific Ocean (Heron and Bradford-Grieve, 1995; Heron, 2002), the Mediterranean Sea (Malt, 1983; Malt et al., 1989) and polar waters (Heron, 1976; Kattner et al., 2003).
Many studies describe the vertical distribution of Oncaeidae species in water. Malt (1983) recorded the distribution areas and water layers inhabited by 17 species of Oncaeidae. Paffenhöfer (Paffenhöfer, 1983) observed that the abundance of Oncaea species increased with an increase in depth in the Northeast Florida shelf. Böttger-Schnack (Böttger-Schnack, 1996) collected micro metazoans in the Arabian Sea using a fine mesh of 0.05 mm size and studied their abundance and vertical distribution. He found that the species diversity of Oncaeidae increased remarkably in the area below 1,050 m in the deep-sea layers. He collected a total of 69 species of Oncaeidae, out of which two-thirds were confined to this layer. When Paffenhöfer and Mazzocchi (Paffenhöfer and Mazzocchi, 2003) studied the vertical distribution of plankton copepods in Bermuda waters, they found that the genus Oncaea was almost absent in the upper mixed layer and upper thermocline. The vertical distribution showed an increase in abundance with depth. The species abundance showed diurnal changes, and the abundance at night was 1.5–15 times higher than that during the day. Böttger-Schnack (Böttger-Schnack, 1992) studied the female and male individuals of Oncaea tregoubovi in the middle of the Red Sea in autumn. It was found that the females were evenly distributed at a depth of 250–450 m and no diurnal difference in the vertical distribution was observed. The males were also distributed at similar depths as females during the day, but they tended to move downward at night, with high distribution in the water layer at 400–450 m depth. Lo et al. (Lo et al., 2004) observed the diurnal vertical movement of plankton copepods in the upwelling area of Cotton Valley in northern Taiwan and observed that O. venusta, O. mediterranea, and Oncaea conifera showed a diurnal vertical movement pattern. The individuals of these species descended during the day and ascended at night, and they reached different depths at different rates based on the species. Itho et al. (Itoh et al., 2014) found that most species of Oncaeidae in the Northwest Pacific Ocean migrated downwards from the surface layer between August and November when the thermocline decreased significantly.
Many studies have focused on the ecological habits of Oncaeidae, particularly on feeding habits. For example, Ohtsuka and Kubo (Ohtsuka and Kubo, 1991) found that O. mediterranea can ingest particulate organic matter found in marine snow. Further, Oncaea species can also use gelatinous zooplankters such as Thaliacea as a potential substrate for survival (Böttger-Schnack et al., 1989). Ohtsuka et al. (Ohtsuka et al., 1993) studied the vertical distribution characteristics of most Oncaea species and found that their abundance was significantly positively correlated with the abundance of Appendicularia because some Oncaea species can use the “appendicularian houses” as their living environment and food source. Steinberg et al. (Steinberg et al., 1994) found that O. conifera and Oncaea similus can also inhabit the houses of Bathochordaeus spp. with an average number of occurrences per house as high as 64.6. In a study of intestinal contents of Oncaea by Ohtsuka et al. (Ohtsuka et al., 1996), gender differences were detected in the proportion of housing in Ascidian—on average, the detection rate of females 61.0% was higher than that of males (42.4%). The most significant gender difference was observed for O. venusta and Oncaea clevei. Diatoms, dinoflagellates, copepodids, copepods nauplii, and radiolarian were also detected in the intestinal contents of Oncaea species. Among them, copepodids and copepods nauplii were only detected in the intestinal tract of larger species (> 0.7 mm), whereas radiolarian was only detected in a few species. Further, the detection rate in female O. conifera was up to 60%. Metz (Metz, 1998), when studying Oncaea curvata in the Southern Ocean, found that 18% of the carbon in O. curvata, which was cultured in the natural environment, was obtained by ingesting Phaeocystis. When Phaeocystis explosive growth, the daily intake of Phaeocystis could reach 35% of the carbon content in the body. Go et al. (Go et al., 1998) studied each ontogenetic stage of Oncaea and found that in the later stage (larvae and mature individuals of copepods of the fifth stage), they often appeared at the same time as Sagitta and Oikopleura, and could prey on Sagitta. Kattner et al. (Kattner et al., 2003) found that the food source for Oncaea species in the polar regions is mainly organic debris particles or polymers and not fresh phytoplankton and inferred that these species can be omnivorous or carnivorous. Wu et al. (Wu et al., 2004) also observed non-selective feeding in the intestinal contents of O. venusta, O. mediterranea, and O. conifera in the southern Taiwan Strait, and found the major components of the diets of the 3 copepods were the diatoms Chaetoceros sp. and Thalassiothrix sp., although radiolarian, microzooplanktonic, and copepod debris was also found in the gut contents.
In addition, relevant studies have been undertaken to reveal the population characteristics and behavioral characteristics of Oncaeidae. Boxshall (Boxshall, 1977) collected specimens of O. mediterranea and O. conifera from the Waters of the Cape Verde Islands using a 0.3 mm net and found that males were considerably less prevalent in these two populations—males constituted less than 5% of the adult stock. Moore and Sander} (Moore and Sander, 1983) collected Oncaea species throughout the year from the west coast of Barbados using a 0.25 mm mesh. The samples contained approximately the same number of males and females. Similarly, Böttger-Schnack et al. (Böttger-Schnack et al., 1989) also obtained a sex ratio of approximately 1:1 for Oncaeidae species, which were collected from the Red Sea using a 0.1 mm mesh size. They speculated that the difference in the mesh size resulted in the discrepancy in the sex ratio of the collected species, and they hypothesized that the sex ratio is related to the body size of the family. The gender differences among Oncaea species become less apparent with a decrease in the average size of the species. Small-sized Oncaea ivlevi has approximately similar sized males and females. In contrast, the males of the larger O. mediterranea and O. conifera are 30% to 40% smaller than the females. Consequently, the males of these species may not be collected if larger nets are used, which may account for the low percentage of males of O. mediterranea and O. conifera obtained by Boxshall. Paffenhofer (Paffenhöfer, 1993) found that at a high level of food consumption, the food intake of O. mediterranea is approximately 100% of body weight at an index increase of 0.26 in the early stage, and the average reproductive rate of O. mediterranea in the field is 5.3–13.3 nauplii per day, and the average life span is 29–41 days. At the same time, the author suggests that increasing the number of eggs, the O. mediterranea can maintain a low metabolic cost and may account for the prevalence of O. mediterranea in the sea. Böttger-Schnack et al. (Böttger-Schnack et al., 1989) found that the egg diameter of the Oncaea species was not proportional to the female body length and that small species of Oncaea carried far fewer eggs than the large ones. For example, each oocyst in Oncaea vodjanitskii and O. ivlevi carries only one to three eggs. However, the diameter of these eggs is almost identical to that of larger species. Females smaller than 0.5 mm produce eggs of fairly constant size regardless of the body length, and the minimum diameter of eggs may be about 0.04 mm. Hwang and Turner (Hwang and Turner, 1995) observed the movement patterns of several plankton copepods off the coast of Taiwan and found that O. venusta has long periods of immobility, but once they began to swim, they progress in a series of small jumps and often swim in circles while frequently changing their movement and rest modes. Paffenhöfer et al. (Paffenhöfer et al., 1996) compared the activity cycle and speed of eight species of plankton copepods on the southeastern continental shelf of the United States. O. mediterranea (an order of magnitude higher than Temora stylifera, which with the slowest movement speed) has the highest rate of movement. Seuront et al. (Seuront et al., 2004) studied the swimming path, swimming speed and other behavioral characteristics of O. venusta and reported the interaction between its behavior and environment.
Outlook on future research
Oncaeidae is an important family of small copepods found in marine ecosystems. Oncaeidae species are small in size and often have similar morphologies. This family contains many sibling species as well as intraspecific variants, and only 115 species of Oncaeidae have been described to date. At present, the studies of Oncaeidae are deepening, but the limitations of taxonomy and sampling methods hindered the biodiversity research, and the role in the marine environment was severely underestimated. Future research needs to constantly extend in depth and breadth, this paper puts forward suggestions from three aspects.
The species of Oncaeidae show high morphological similarity, and many of them can only be distinguished by structural differences in the appendages. The morphological taxonomy of Oncaeidae is time-consuming and labor-intensive, and relies heavily on researchers’ personal experience and subjective understanding. Moreover, the morphological taxonomy of Oncaeidae is also hindered by the quantity and quality of specimens. Therefore, it will be the future trend to establish a rapid, accurate, and relatively objective molecular identification basis based on traditional taxonomic and molecular biological methods. It is believed that the speed and accuracy of the classification of Oncaeidae can be improved through optimization technology, solving the species evolution history, geographical isolation, and genetic differentiation. This will provide basic data and technical support for species classification and biodiversity analysis of Oncaeidae, and promote the classification research process.
Oncaeidae is widely distributed from the equatorial to the polar oceans on the horizontal scale and from the ocean surface to the deep sea on the vertical scale. However, previous studies on the planktonic flora and fauna usually focused on the epipelagic zone. The studies that are conducted in the deep sea, particularly in the vast oligotrophic regions of the ocean and the mesopelagic and bathypelagic zones, are extremely rare. Therefore, expanding taxonomic researches of Oncaeidae on geographic areas, particularly in the coastal zone, edge and deep-sea, will accelerate the development of taxonomy and species diversity of Oncaeidae, and the discovery of new species. The specimens of marine organisms are the precondition of taxonomic research, which is related to the limitation of sampling technology, equipment and funds. We need to pay attention to the renewal of sampling technology and the improvement of sampling equipment, and promote the use of diverse sampling nets, such as the WP2 plankton net suitable for the collection of small and medium-sized zooplankton, and the Multinet is used to collect more comprehensive samples. At the same time, improving the analysis equipment in the laboratory. Therefore, marine ecologists will pay more attention to the middle and small copepods, and the taxonomic study of the family Oncaeidae must be deepened, and its species diversity can be continuously explored and discovered.
Author contributions
Conceptualization: BX and CW. Funding acquisition: CW, BX, and RS. Project administration: CW, XC, and YW. Writing -original draft: RS. Writing review & editing: BX, CW, and PX. All authors contributed to the article and approved the submitted version.
Funding
This study was supported by Global climate change and ocean atmosphere interaction research II [grant number GASI-01-SIND-STwin], Scientific Research Foundation of Third Institute of Oceanography, Ministry of Natural Resources [grant number 2017009], The Marine Biological Sample Museum[grant number GASI-02-YPK-SW].
Acknowledgments
The authors would like to thank Prof. Guangshan Lian for the support and valuable comments on the manuscript.
Conflict of interest
The authors declare that the research was conducted in the absence of any commercial or financial relationships that could be construed as a potential conflict of interest.
Publisher’s note
All claims expressed in this article are solely those of the authors and do not necessarily represent those of their affiliated organizations, or those of the publisher, the editors and the reviewers. Any product that may be evaluated in this article, or claim that may be made by its manufacturer, is not guaranteed or endorsed by the publisher.
References
Böttger-Schnack R. (1992). Community structure and vertical distribution of cyclopoid and poecilostomatoid copepods in the red sea. III. re-evaluation for separating a new species of oncaea. Mar. Ecol. Prog. Ser. 80, 301–304. doi: 10.3354/meps080301
Böttger-Schnack R. (1996). Vertical structure of small metazoan plankton, especially noncalanoid copepods. i. deep Arabian Sea. J. Plankton Res. 18 (7), 1073–1101. doi: 10.1093/plankt/18.7.1073
Böttger-Schnack R. (1999). Taxonomy of oncaeidae (Copepoda, poecilostomatoida) from the red Sea: I. 11 species of triconia gen. nov. and a redescription of t. similis (SARS) from Norwegian waters Vol. 96) (Hamburg: Mitteilungen aus dem Hamburgischen Zoologischen Museum und Institut), 37–128.
Böttger-Schnack R. (2001). Taxonomy of oncaeidae (Copepoda, poecilostomatoida) from the red sea. II. seven species of oncaea s. str. Bull. Nat. Hist. Mus. Lond. (Zool.) 67, 25–84.
Böttger-Schnack R. (2002). Taxonomy of oncaeidae (Copepoda, poecilostomatoida) from the red sea. VI. morphology and zoogeography of oncaea bispinosa sp. nov., a sistertaxon of o. zernovi shmeleva. J. Plankton Res. 24 (11), 1107–1129. doi: 10.1093/plankt/24.11.1107
Böttger-Schnack R. (2003). Taxonomy of oncaeidae (Copepoda, poecilostomatoida) from the red sea. v. three species of spinoncaea gen. nov. (ivlevi-group), with notes on zoogeographical distribution. Zoological J. Linn. Soc. 137 (2), 187–226. doi: 10.1046/j.1096-3642.2003.00056.x
Böttger-Schnack R., Huys R. (1997). Morphological observations on oncaea mediterranea (Claus 1863)(Copepoda, poecilostomatoida) with a comparison of red Sea and eastern Mediterranean populations. Bull. Natural Hist Mus., Zool Ser. 63 (2), 137–147.
Böttger-Schnack R., Huys R. (2001). Taxonomy of oncaeidae (Copepoda, poecilostomatoida) from the red sea. III. morphology and phylogenetic position of oncaea subtilis giesbrecht 1892. Hydrobiologia 453 (1), 467–481. doi: 10.1023/A:1013114706718
Böttger-Schnack R., Lenz J., Weikert H. (2004). Are taxonomic details of relevance to ecologists? an example from oncaeid microcopepods of the red Sea. Mar. Biol. 144 (6), 1127–1140. doi: 10.1007/s00227-003-1272-8
Böttger-Schnack R., Machida R. J. (2011). Comparison of morphological and molecular traits for species identification and taxonomic grouping of oncaeid copepods. Hydrobiologia 666 (1), 111–125. doi: 10.1007/s10750-010-0094-1
Böttger-Schnack R., Schnack D. (2009). Taxonomic diversity and identification problems of oncaeid microcopepods in the Mediterranean Sea. Mar. Biodiversity 39 (2), 131–145. doi: 10.1007/s12526-009-0013-8
Böttger-Schnack R., Schnack D. (2015). Development of an interactive identification key for oncaeidae (Copepoda: Cyclopoida). J. Natural History 49, 1–15. doi: 10.1080/00222933.2015.1022614
Böttger-Schnack R., Schnack D., Weikert H. (1989). Biological observations on small cyclopoid copepods in the red Sea. J. Plankton Res. 11 (5), 1089–1101. doi: 10.1093/plankt/11.5.1089
Boxshall G. (1977). The planktonic copepods of the notheastern Atlantic ocean: Some taxonomic observations on the oncaeidae (Cyclopoida). Bull. Br. Museum (Natural History) Zoology 31 (3), 53.
Boxshall A., Halsey S. H. (2004). An introduction to copepod diversity (New York: Cold Spring Harbor Laboratory Press).
Chihara M., Murano M. (1997). An illustrated guide to marine plankton in Japan (Tokyo: Tokai University Press).
Di Capua I., Maffucci F., Pannone R., Mazzocchi M. G., Biffali E., Amato A. (2017). Molecular phylogeny of oncaeidae (Copepoda) using nuclear ribosomal internal transcribed spacer (ITS rDNA). PloS One 12 (4), e0175662. doi: 10.1371/journal.pone.0175662
Elvers D., Bottger-Schnack R., Blohm D., Hagen W. (2006). Sympatric size variants of the microcopepod oncaea venusta exhibit distinct lineages in DNA sequences. Mar. Biol. 149 (3), 503–513. doi: 10.1007/s00227-005-0234-8
Farran G. P. (1929). Crustacea. part x. copepoda. natural history report. British Antarctic (Terra Nova) expedition. 1910. Zoology 8, 203–306.
Ferrari F. D. (1975). Taxonomic notes of the genus oncaea (Copepoda: Cyclopoida) from the gulf of Mexico and northern Caribbean Sea. Proc. Biol. Soc Wash. 88, 217–232.
Go Y. (1994). Seasonal fluctuation of pelagic copepods on the coast of cheju island, Korea. Bull. Mar. Res. Inst. Cheju Nat'l Univ. 18, 15–26.
Goetze E. (2003). Cryptic speciation on the high seas; global phylogenetics of the copepod family eucalanidae. Proc. Biol. Sci. 270 (1531), 2321–2331. doi: 10.1098/rspb.2003.2505
Go Y. B., Oh B. C., Terazaki M. (1998). Feeding behavior of the poecilostomatoid copepods oncaea spp. on chaetognaths. J. Mar. Systems. 15 (1-4), 475–482. doi: 10.1016/S0924-7963(97)00038-9
Hebert P., Cywinska A., Ball S. L., Dewaard J. (2003a). Biological identification through DNA barcodes. Proc. R. Soc. London. Ser. B: Biol. Sci. 270 (1512), 313–321. doi: 10.1098/rspb.2002.2218
Hebert P. D., Ratnasingham S., De Waard J. R. (2003b). Barcoding animal life: cytochrome c oxidase subunit 1 divergences among closely related species. Proc. R. Soc. London. Ser. B: Biol. Sci. 270 (suppl 1), S96–S99. doi: 10.1098/rsbl.2003.0025
Heron G. A. (1976). Twenty‐Six Species of Oncaeidae (Copepoda: Cyclopoida) from the Southwest Pacific‐Antarctica Area[J]. Biology of the Antarctic Seas VI 26, 37–96.
Heron G. (2002). Oncaea frosti, a new species (Copepoda: Poecilostomatoida) from the Liberian coast and the gulf of Mexico. Hydrobiologia 480 (1), 145–154. doi: 10.1023/A:1021297304421
Heron G. A., Bradford-Grieve J. M. (1995). The marine fauna of new Zealand: pelagic copepoda: Poecilostomatoida: Oncaeidae. Oceanographic Literature Rev. 3 (43), 278.
Heron G. A., Frost B. W. (2000). Copepods of the family oncaeidae (Crustacea : Poecilostomatoida) in the northeast pacific ocean and inland coastal waters of Washington state. Proc. Biol. Soc. Washington 113 (4), 1015–1063.
Hopcroft R., Roff J., Lombard D. (1998). Production of tropical copepods in Kingston harbour, Jamaica: The importance of small species. Mar. Biol. 130 (4), 593–604. doi: 10.1007/s002270050281
Hwang J. S., Turner J. T. (1995). Behaviour of cyclopoid, harpacticoid, and calanoid copepods from coastal waters of Taiwan. Mar. Ecol. 16 (3), 207–216. doi: 10.1111/j.1439-0485.1995.tb00406.x
Itoh H., Nakata K., Sasaki K., Ichikawa T., Hidaka K. (2014). Seasonal and diel changes in the vertical distribution of oncaeid copepods in the epipelagic zone of the kuroshio extension region. Plankton Benthos Res. 9 (1), 1–14. doi: 10.3800/pbr.9.1
Kattner G., Albers C., Graeve M., Schnack-Schiel S. (2003). Fatty acid and alcohol composition of the small polar copepods, oithona and oncaea: Indication on feeding modes. Polar Biol. 26 (10), 666–671. doi: 10.1007/s00300-003-0540-x
Kress W. J., García-Robledo C., Uriarte M., Erickson D. L. (2015). DNA Barcodes for ecology, evolution, and conservation. Trends Ecol. Evol. 30 (1), 25–35. doi: 10.1016/j.tree.2014.10.008
Kwun H. J. (2018). Species identification of juvenile fishes of the genus pseudoblennius using mitochondrial DNA barcoding. Mitochondrial DNA Part B 3 (1), 405–408. doi: 10.1080/23802359.2018.1456982
Lo W. T., Shih C. T., Hwang J. S. (2004). Diel vertical migration of the planktonic copepods at an upwelling station north of Taiwan, western north pacific. J. Plankton Res. 26 (1), 89–97. doi: 10.1093/plankt/fbh004
Machida R. J., Miya M. U., Nishida M., Nishida S. (2004). Large-Scale gene rearrangements in the mitochondrial genomes of two calanoid copepods eucalanus bungii and neocalanus cristatus (Crustacea), with notes on new versatile primers for the srRNA and COI genes. Gene 332, 71–78. doi: 10.1016/j.gene.2004.01.019
Malt S. J. (1982). New and little known species of oncaeidae (Cyclopoida) from the northeastern Atlantic. ZOOL 42 (3), 185–205.
Malt S. J. (1983). Copepoda, oncaea. fiches didentification Du zooplancton, 169/170/171. Andr Fred Host & Fils, 1–11.
Malt S. J., Lakkis S., Ziedane R. (1989). The copepod genus oncaea (Poecilostomatoidea) from the Lebanon: taxonomic and ecological observations. J. Plankton Res. 11 (5), 949–969. doi: 10.1093/plankt/11.5.949
Matarese A. C., Spies I. B., Busby M. S., Orr J. W. (2011). Early larvae of zesticelus profundorum (family cottidae) identified using DNA barcoding. Ichthyological Res. 58 (2), 170–174. doi: 10.1007/s10228-010-0198-7
Mckinnon A. D., Duggan S. (2014). “Community ecology of pelagic copepods in tropical coastal waters,” in Copepods: Diversity, habitat and behavior. Ed. Seuront L. (Escocia: Nova Scotia Publishers, Inc.), 25–49.
Metz C. (1998). Feeding of oncaea curvata (Poecilostomatoida, copepoda). Mar. Ecol. Prog. Ser. 169, 229–235. doi: 10.3354/meps169229
Moore E. A., Sander F. (1983). Physioecology of tropical marine copepods. II. sex ratios. crustaceana. 113–122. (Netherlands: Brill Academic Publishers)
Ohtsuka S., Boettger Schnack R., Okada M., Onbé T. (1996). In situ feeding habits of oncaea (Copepoda: Poecilostomatoida) from the upper 250 m of the central red Sea, with special reference to consumption of appendicularian houses (Hikone Japan: Bulletin of Plankton Society of Japan).
Ohtsuka S., Kubo N. (1991). Larvaceans and their houses as important food for some pelagic copepods (Hikone Japan: Bull. Plankton Soc. Japan), 535–551.
Ohtsuka S., Kubo N., Okada M., Gushima K. (1993). Attachment and feeding of pelagic copepods on larvacean houses. J. Oceanography 49 (1), 115–120. doi: 10.1007/BF02234012
Paffenhöfer G. A. (1983). Vertical zooplankton distribution on the northeastern Florida shelf and its relation to temperature and food abundance. J. Plankton Res. 5 (1), 15–33. doi: 10.1093/plankt/5.1.15
Paffenhöfer G. A. (1993). On the ecology of marine cyclopoid copepods (Crustacea, copepoda). J. Plankton Res. 15 (1), 37–55. doi: 10.1093/plankt/15.1.37
Paffenhöfer G. A., Mazzocchi M. (2003). Vertical distribution of subtropical epiplanktonic copepods. J. Plankton Res. 25 (9), 1139–1156. doi: 10.1093/plankt/25.9.1139
Paffenhöfer G. A., Strickler J., Lewis K., Richman S. (1996). Motion behavior of nauplii and early copepodid stages of marine planktonic copepods. J. Plankton Res. 18 (9), 1699–1715. doi: 10.1093/plankt/18.9.1699
Philippi A. (1843). Fernere beobachtungen über die copepoden des mittelmeeres. Archiv für Naturgeschichte 9 (1), 54–71.
Razouls C., de Bovée F., Kouwenberg J., Desreumaux N. (2020) Diversity and geographic distribution of marine planktonic copepods 2005–2017. Available at: http://copepodes.obs-banyuls.fr/en/ (Accessed 2020-08-15).
Schultz J., Wolf M. (2009). ITS2 sequence–structure analysis in phylogenetics: a how-to manual for molecular systematics. Mol. Phylogenet. Evol. 52 (2), 520–523. doi: 10.1016/j.ympev.2009.01.008
Seuront L., Hwang J. S., Tseng L. C., Schmitt F., Souissi S., Wong C. K. (2004). Individual variability in the swimming behavior of the sub-tropical copepod oncaea venusta (Copepoda: Poecilostomatoida). Mar. Ecology-progress Ser. 283, 199–217. doi: 10.3354/meps283199
Sewell R. B. S. (1947). The free-swimming planktonic copepod. systematic account. the John Murray expedition 1933–34 scientific reports Vol. Volume VIII (London: British Museum (Natural History).
Steinberg D. K., Silver M. W., Pilskaln C. H., Coale S. L., Paduan J. B. (1994). Midwater zooplankton communities on pelagic detritus (giant larvacean houses) in Monterey bay, California. Limnology Oceanography 39 (7), 1606–1620. doi: 10.4319/lo.1994.39.7.1606
Sutton T., Hopkins T., Remsen A., Burghart S. (2001). Multisensor sampling of pelagic ecosystem variables in a coastal environment to estimate zooplankton grazing impact. Continental Shelf Res. 21 (1), 69–87. doi: 10.1016/S0278-4343(00)00074-1
Tanaka O. (1960). Pelagic copepods. biological results of the Japanese Antarctic research expedition. special publications from the seto marine biological laboratory, Vol. 10. 1–177. Polar Section of the National Science Museum (Tokyo, Japan)
Wang R., Zhang H., Wang K., Zuo T. (2002). Function performed by small copepods in marine ecosystem. Oceanol. Limnol. Sin. 33, 453–460. doi: 10.3321/j.issn:0029-814X.2002.05.001
Keywords: taxonomy, species diversity, species distribution, oncaeidae, small copepods
Citation: Sun RX, Wang YG, Wang CG, Xiang P, Chen XY and Xing BP (2022) Research advance in the taxonomy and ecology of Oncaeidae Giesbrecht, 1893. Front. Mar. Sci. 9:919877. doi: 10.3389/fmars.2022.919877
Received: 14 April 2022; Accepted: 12 July 2022;
Published: 03 August 2022.
Edited by:
Li-Zhe Cai, Xiamen University, ChinaReviewed by:
Daniel Pech, El Colegio de la Frontera Sur, MexicoJun Sun, China University of Geosciences Wuhan, China
Copyright © 2022 Sun, Wang, Wang, Xiang, Chen and Xing. This is an open-access article distributed under the terms of the Creative Commons Attribution License (CC BY). The use, distribution or reproduction in other forums is permitted, provided the original author(s) and the copyright owner(s) are credited and that the original publication in this journal is cited, in accordance with accepted academic practice. No use, distribution or reproduction is permitted which does not comply with these terms.
*Correspondence: Bingpeng Xing, bluprin@tio.org.cn
†ORCID: Rouxin Sun, orcid.org/0000-0003-0774-5868
Yanguo Wang, orcid.org/0000-0002-8995-6468
Peng Xiang, orcid.org/0000-0002-3193-0775
Xiaoyin Chen, orcid.org/0000-0002-6665-7588
Bingpeng Xing, orcid.org/0000-0002-4963-6574