- 1Divisão de Geologia Marinha, Instituto Hidrográfico, Lisboa, Portugal
- 2CIBIO-Centro de Investigação em Biodiversidade e Recursos Genéticos, InBio Laboratório Associado, Pólo dos Açores, Portugal
- 3BIOPOLIS Program in Genomics, Biodiversity and Land Planning, CIBIO, Vairão, Portugal
- 4MPB - Marine Palaeontology and Biogeography lab, University of the Azores, Açores, Portugal
- 5SMNS – Staatliches Museum für Naturkunde Stuttgart, Rosenstein 1, Stuttgart, Germany
- 6Museo de Ciencias Naturales de Tenerife, Organismo Autónomo de Museos y Centros, Santa Cruz de Tenerife, Spain
- 7Universidade de Lisboa, Faculdade de Ciências, Departamento de Geologia, Lisboa, Portugal
- 8Universidade de Lisboa, Faculdade de Ciências, Instituto Dom Luiz, Lisboa, Portugal
- 9Department of Geosciences, Williams College, Williamstown, MA, United States
- 10Área de Paleontología, Facultad de Biología, Universidad de La Laguna, San Cristóbal de La Laguna, Spain
- 11Instituto Geológico y Minero de España (IGME-CSIC), Unidad Territorial de Canarias, Las Palmas de Gran Canaria, Spain
- 12Departamento de Biologia, Faculdade de Ciências da Universidade do Porto, Portugal, Faculdade de Ciências da Universidade do Porto, Porto, Portugal
Rhodoliths occur extensively around the shores of Fuerteventura Island in the Canary Archipelago, with Lithothamnion cf. corallioides being the most prominent species. A large number of rhodoliths end up washed onshore, the debris from which contributes to the formation of sediments constituting modern beaches. In a previous study by one of the co-authors (MEJ), the northern coast of Fuerteventura was shown to comprise various types of rhodolith deposits such as beach, platform overwash, tidal pools, coastal dunes, and others. An extraordinary example of stranded rhodoliths is located near Caleta del Bajo de Mejillones, approximately 3 km west of Corralejo, on the north coast of the island. The deposit forms a supratidal beach 120 m long and 10 m wide that sits above the landward termination of an extensive wave-cut platform eroded in basalt and exposed at low tide to a width of 130 m perpendicular to shore. Here, rhodoliths are very small (<3 cm) resembling popcorn, and the locality is known as the “Popcorn Beach”. Other examples are berms up to 150 m long and 9 m wide at Caleta del Bajo de Mejillones, or an exposed beach at Playa del Hierro with an area of more than 1500 m2 covered entirely of very coarse rhodolith sand. Extensive living rhodolith beds were found at a water depth of 22 m.
1 Introduction
The North coast of Fuerteventura Island in the Canaries is well known for its beaches of stranded rhodoliths. Here, rhodoliths are very small (<3cm) resembling popcorn and the area is known locally as “Popcorn Beach”. Nevertheless, little is still known about which species contribute to the formation of these rhodoliths and which physical and/or biological factors control their occurrence.
Rhodoliths and rhodolith beds (RBs) correspond to unattached nodules of coralline red algae (Rhodophyta) and are essential ecosystem engineers, yielding structurally complex habitats that harbour distinctive high-diversity faunal and floral assemblages, as a consequence of their branching and interlocking nature (Steller et al., 2003; Foster et al., 2007; Teichert, 2014; Riosmena-Rodríguez, 2017; Fredericq et al., 2019). Organisms living on RBs are better protected against predators, and commercially important species (e.g., fish, crustaceans, molluscs) benefit from the existence of such habitats (Teichert, 2014). In this manner, rhodoliths are crucial for the establishment and maintenance of biodiversity and, thus, contribute to major ecosystem functions (Fredericq et al., 2019; Stelzer et al., 2021).
The growth, geographic distribution and sustainability of rhodoliths are controlled by several factors, of which light, temperature, sedimentation, hydrodynamic regime, existence of marine barriers, and abundance of corals are vital (Wilson et al., 2004; Riosmena-Rodríguez, 2017; Rebelo et al., 2021). Depth and correlated wave-induced turbulence and light decrease are also known to directly influence physical processes, as well as salinity (Otero-Ferrer et al., 2020 and references therein). Although rhodoliths are resilient under a variety of environmental disturbances, they can be severely impacted by storms (with onshore transport; cf. Rebelo et al., 2016; Rebelo et al., 2018), harvesting, ocean acidification and global warming (Martin et al., 2014; Sañé et al., 2016). RBs are commonly found at depths where light is sufficient for growth and water motion and bioturbation are sufficient to move the rhodoliths and prevent burial and anoxia from sedimentation (Foster et al., 2013).
Reefless island shelves, such as characteristic in the Canaries, can be particularly dynamic marine environments due to their exposure to strung surf, storms, volcanism, and mass wasting (Stillman, 1999; Acosta et al., 2004; Rodriguez-Martin et al., 2022). These type of shelves are also narrower and steeper than those typically found around continents, a condition that facilitates sediment transport on the entire shelf and contributes significantly to environmental instability (Ávila et al., 2008; Quartau et al., 2012; Ávila et al., 2015a; Rebelo et al., 2016; Johnson et al., 2017a; Ávila et al., 2019; Zhao et al., 2022). RBs are known to occur between 20 to 100 m depth around most of the Canary Islands (Afonso-Carrillo, 2021 and references therein). Shallower RBs develop from ~2m depth detrital bottoms between Fuerteventura, Lanzarote and Lobos, where their nodules can reach up to 20 cm in diameter (Reyes et al., 2005; Afonso-Carrillo, 2021). Nonetheless, studies regarding insular living rhodoliths are so far very few, especially in the northeast Atlantic archipelagos (e.g., Rosas-Alquicira et al., 2009; Rebelo et al., 2018; Otero-Ferrer et al., 2020). It is still relatively unknown how these rhodoliths cope with environmental pressures, and it is hence the focus of this study.
In this work, we report on the distribution of rhodolith-forming species and consider those factors controlling RBs around the shores of Fuerteventura Island, adding to a better understanding of insular rhodolith formation and deposition. We also stress the importance to preserve RBs as biodiversity hotspots and call for a conscientious effort in the protection and maintenance of these valuable biological resources.
2 Geographic and environmental setting
Lanzarote and Fuerteventura are the easternmost islands in the Canary Archipelago (Figure 1). Both constitute the emerged part of the Canary Ridge, an elongated volcanic edifice trending NNE-SSW (Acosta et al., 2004). Coastal erosion and sea level changes commonly generate wide insular shelves (Quartau et al., 2010; Ramalho et al., 2013; Quartau et al., 2016), where channels sometimes develop between two islands, as in the case between Lanzarote and Fuerteventura Islands. Fuerteventura, the oldest of the Canary Islands, dates to 23 Ma together with integrated Jurassic to Cretaceous sea-floor sediments (Steiner et al., 1998; Stillman, 1999; Acosta et al., 2004; Bogaard, 2013). The shield-building stage on Fuerteventura started about 17–16 Ma and concluded at approximately 9 Ma (Coello et al., 1992). Post-erosional volcanism has occurred sporadically on Fuerteventura over the last 5 Ma. An example is the Bayuyo eruptive fissure that dates 135 ka (Casillas and Torres, 2011; Troll and Carracedo, 2016) with its basaltic lava flows contributing to the Fuerteventura NW coastline. Biogenic sand deposits occur along the coast as the result of currents and wind transport from the shelf to the coast and inland (Roettig et al., 2017; Roettig et al., 2020).
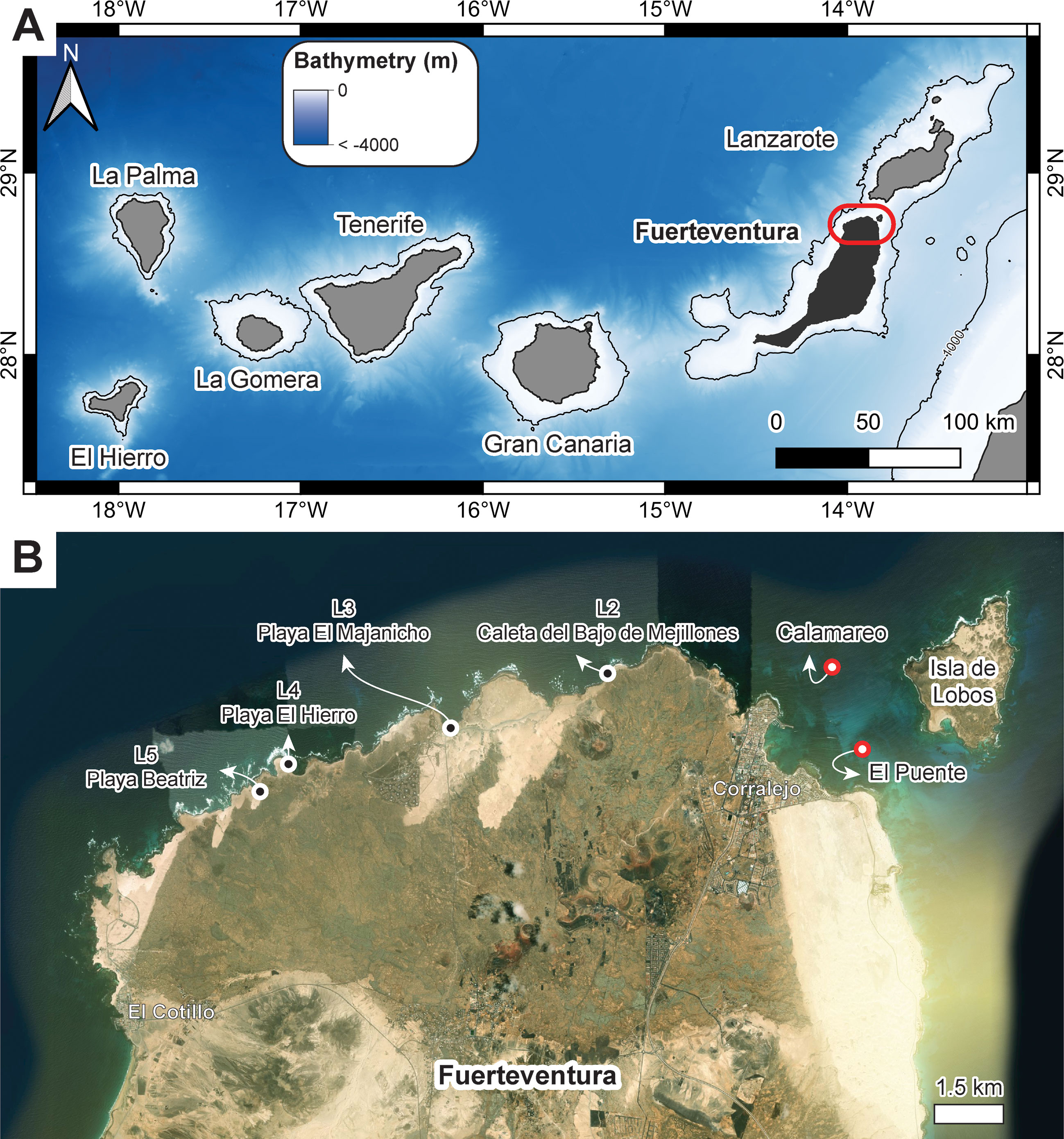
Figure 1 (A) Map of islands in the Canaries highlighting the study area in Fuerteventura, (B) Aerial view of the north coast of Fuerteventura Island showing the location of study sites between the towns of Corralejo and El Cotillo: L2 – Caleta del Bajo de Mejillones; L3 – Majanicho; L4 – Playa El Hierro; L5 – Caleta de Beatriz; Diving spot Calamareo; Diving spot El Puente, Bathymetry was retrieved from Gobierno de Canarias (https://opendata.sitcan.es/dataset/modelo-digital-de-terreno-mdt-de-25x25-metros). Aerial photo from AppleMap (2022).
The area under study lies on the north shore of Fuerteventura Island, and is separated from Lanzarote by a 10-14 km wide channel, La Bocaina strait, with an average depth of 40 m. Nowadays, the majority of the waves hitting the Canary Islands come from NE, N and NW (although the NE is predominant), showing mean values of significant wave height of 2 m that may reach 5-7 m during storms (Rusu and Onea, 2016; Gonçalves et al., 2020). The north-easterly trade-winds dominate on the Canary Islands (Azorin-Molina et al., 2017).
The rhodoliths studied, herein, were collected on an intertidal shore platform that extends for approximately 15 km along the north coast of Fuerteventura, from Corralejo in the NE to El Cotillo in the NW part of the island, including L2 - Caleta del Bajo de Mejillones (Figure 1B). This platform was cut by marine erosion, and at low tide presents a width of 130 m, and is exposed to the strong action of the NW, N and NE waves during sea storms. Rhodoliths are found both forming accumulations to organogenic sands of the supratidal beach and in small tide pools.
3 Methods
Field studies were conducted during the 1st Workshop of Marine Palaeontology and Littoral Geology in Canary Islands: Fuerteventura, between 6 and 14 of November of 2021. The north coast of Fuerteventura was canvassed for sites with stranded rhodoliths. A limited number of rhodoliths was collected for taxonomic studies at Caleta del Bajo de Mejillones, Majanicho, Playa El Hierro and Caleta de Beatriz, locations 2, 3, 4 and 5 respectively, indicated by Johnson et al. (2012) (Figure 1). Locality 1 (from Johnson et al., 2012) corresponds to a sandy beach in the eastern part of the island and was not here considered. Additionally, scuba diving was performed at Calamareo (Lat: 28.7516, Long: -13.8549) and El Puente (Lat: 28.7347, Long: -13.8471), two diving spots also at the NE coast of Fuerteventura (Figures 1, 2) to report on living rhodoliths and environmental conditions. Sampling of rhodoliths was not possible on this site.
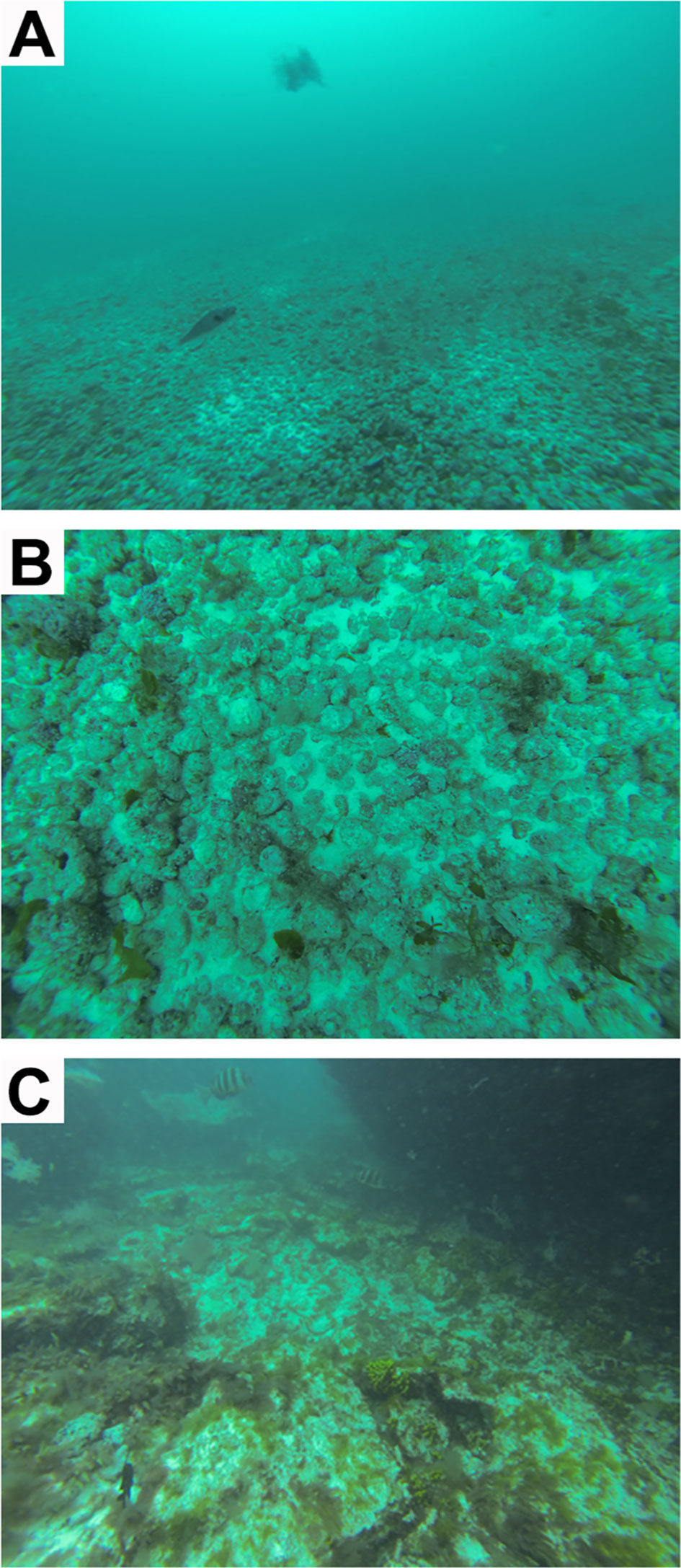
Figure 2 Images acquired by scuba diving. (A) RB over a sandy sea bottom at Calamareo, (B) detail of the RB at Calamareo, (C) rocky sea bottom at El Puente without any visible rhodoliths.
Eleven thin sections (4 of 4.8 x 2.8 cm; 4 of 4.8 x 4.8 cm; 2 of 9.5 x 4.8 cm; and 1 of 9.5 x 7.5 cm) of selected rhodoliths were studied under a compound polarizing microscope (Leica DM750P) equipped with a digital camera (Leica ICC50W). Anatomical and taxonomical terminologies applied to coralline algae follow the works by Braga et al. (1993); Irvine and Chamberlain (1994); Rasser and Piller (1999) and Hrabovský et al. (2016). Growth form terminology conforms to Woelkerling et al. (1993). Morphological classification follows Bosence (1983). Cell and conceptacle dimensions were measured according to Rasser and Piller (1999) using Image J. Mean (M) and standard deviation (SD) were calculated for both cells and conceptacles, whenever the number of measurements allowed (n= 5). All material is stored in the Department of Palaeontology at the State Natural History Museum Stuttgart, Germany.
4 Results
4.1 In situ rhodolith bed
At Calamareo, a diving site located less than 2 km north of Corralejo consists of a rocky platform that is 12 m deep, descending vertically to 20-22 m and featuring caves, vaults and passageways (Figure 1B). Here is a sizeable RB at around 22 m depth on a sandy bottom. Rhodoliths vary greatly in shape and size from 2 to 8 cm (n = 18) (Figures 2A, B). The lateral extension of the RB is unknown, but it may reach several hundred square meters, perhaps even square kilometres. The density of rhodoliths varies from closer packing with rhodoliths stacked over each other, to loose rhodoliths lying on sandy substrate. The RBs comprise smaller patches with soft algae; no invertebrates could be observed in the field.
On El Puente, less than 2 km east of Calamareo, and less than 1 km from the coast, abrupt lava formations occur with caves, chimneys, small canyons, cornices and an impressive bridge. The depth in the studied area is 7 to 10 m, and no rhodoliths were observed on the rocky bottom covered with fleshy algae and sponges. Sediment accumulations are restricted to depressions forming sedimentary traps (Figure 2C).
4.2 Stranded rhodolith accumulations
4.2.1 Taphonomic grades
Over a coastal distance of 17 km between the towns of Corralejo in the east and El Cotillo in the west (Figure 1B), the north shore of Fuerteventura offers an extraordinary insight regarding the development and variation in carbonate sediments derived from modern rhodoliths (Figure 1).
Introduced by Brandt (1989), the concept of taphonomic grades was originally applied to fossil assemblages dominated by marine invertebrates with four classes (A to D) representing deterioration in fabric from a starting point (A) of an original community preserved in situ with little or no evidence of shell disarticulation, breakage, corrasion, or disruption from original growth position. Maximum depreciation (D), amounts to the formation of what otherwise might be called a shell coquina with more than 75 percent attrition in the categories of fossil breakage, corrasion, and orientation and a possible change in the proportion of matrix to shell content of 50 to 75 percent. The two intermediate grades (B and C) are separated, for example, by the degree of breakage estimated at a level below or above 50 percent. Effectively, the range of taphonomic grades so formulated traces a continuum between a biocenosis, or life assemblage, and a thanatocenosis, i.e., a death assemblage, in the latter case, severely damaged. The application of this classification to modern coralline red algae and by extension to fossilized deposits of rhodoliths, was undertaken in a review paper by Johnson et al. (2017b) covering the Macaronesian region with the best examples derived from Fuerteventura Island.
4.2.2 Rhodolith sites
At L2 - Caleta del Bajo de Mejillones, located about 3 km east Corralejo (Figures 1B, 3A), a massive accumulation of rhodoliths occurs as an overwash deposit in a supratidal setting above the adjacent intertidal rock platform. Because the basalt platform at this location is up to 130 m wide and characteristically clean at low tide, the living rhodoliths that arrived onshore were transported a relatively short distance from offshore. Due to the small size of the rhodoliths, between 1 to 3 cm in diameter, and their lumpy morphology, the locality has been called “Popcorn Beach”. Based on dimensions recorded by Johnson et al. (2012), the beach deposit composed of stranded rhodoliths is 10 m wide and extends for a distance of 120 m parallel to the shore. Using a sample quadrate with an area of 0.25 m2, the exposed surface layer corresponds to a density of about 5,000 rhodoliths/m2 (Figure 4). It is estimated that the exposed surface amounts to roughly six million rhodoliths, which is a fraction of the entire assemblage, because an exploratory pit excavated into the beach reveals two layers, each 5 cm thick, separated by a layer of carbonate sand (Johnson et al., 2017b). Overall, only a quarter of the rhodoliths are whole, whereas 65 percent are broken or worn, and at least 10 percent of the surface is strewn by basalt pebbles. According to the scheme proposed by Brandt (1989), a taphonomic grade of C may be applied to the deposit at L2 - Caleta del Bajo de Mejillones.
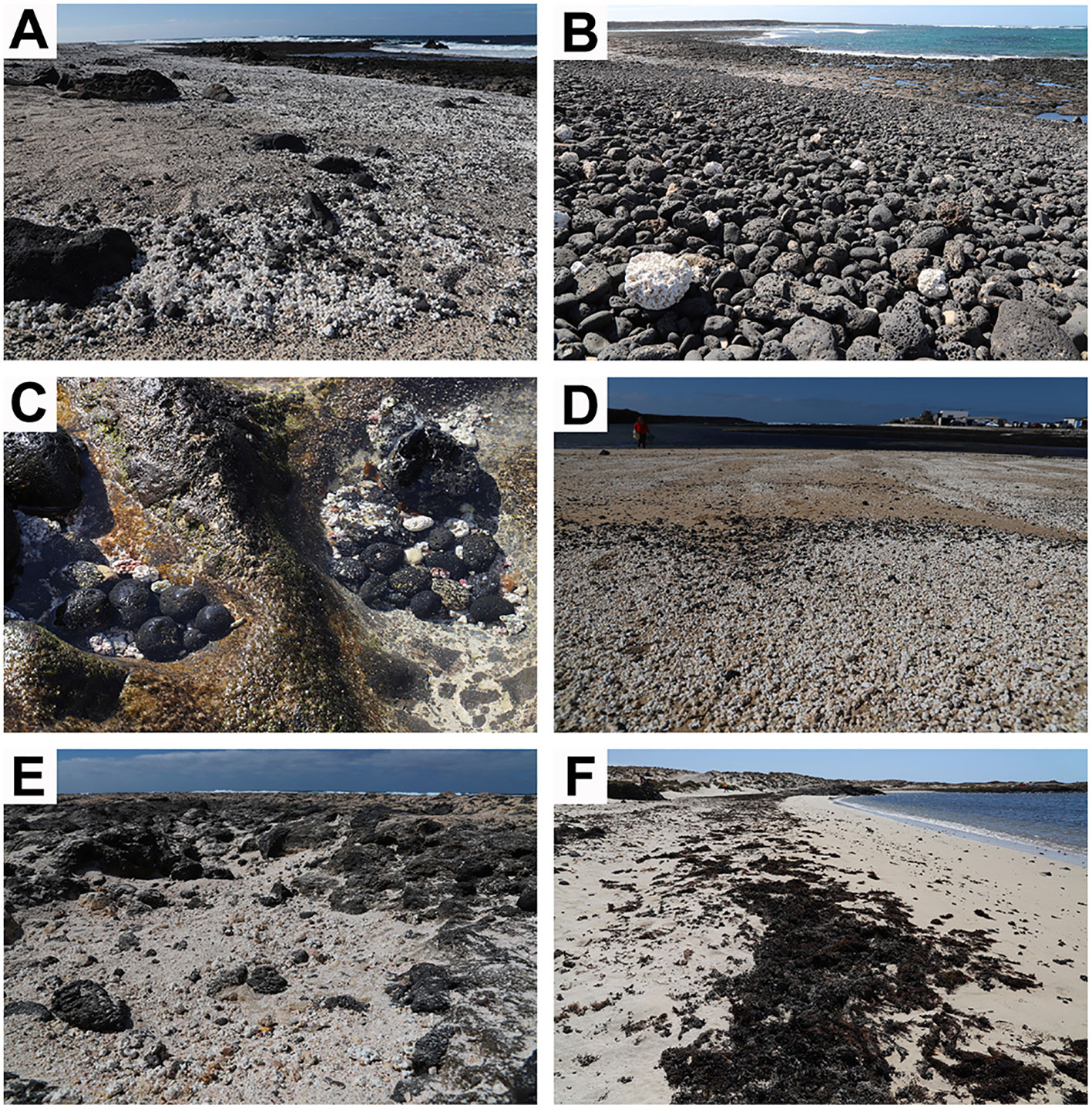
Figure 3 Field photos of stranded rhodoliths. (A) L2 - Caleta del Bajo de Mejillones, (B) L3 - Majanicho berm, (C) L3 - Majanicho intertidal pool, (D) L3 - Playa El Majanicho, (E) L4 - Playa El Hierro, (F) L5 - Caleta de Beatriz.
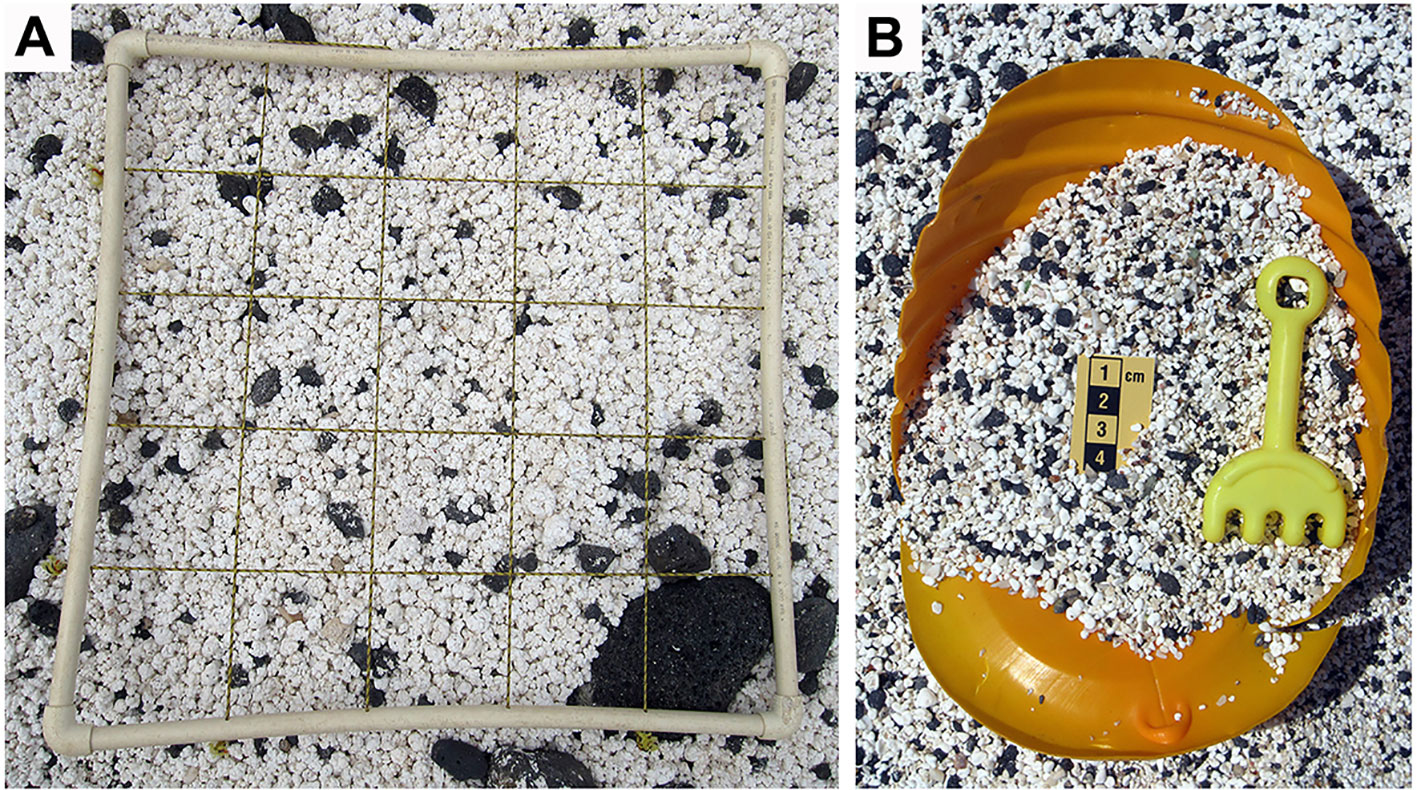
Figure 4 (A) Standard sample quadrat (50 x 50 cm) on “popcorn beach” at L2 - Caleta del Bajo de Mejillones on the north shore of Fuerteventura Island. Each square is 10 cm x 10 cm. Approximately 200 rhodoliths may be counted per unit, (B) close-up view of finely degraded rhodolith material–from L4 - Playa El Hierro (Figures 2C, 3E).
Farther west near L3 - Majanicho (Figures 1B, 3B–D), another overwash deposit of rhodoliths forms a berm elevated 1.4 m above the adjacent rock platform. This deposit is quite similar to the one at L2 - Caleta del Bajo de Mejillones regarding the average size and the degree of breakage of the rhodoliths. It was therefore classified as a taphonomic grade of C. Notably, when visited in July 2011 (Johnson et al., 2012), some traces of brown algae were found still attached to individual rhodoliths, giving some indication of a more complex algal community in situ. This indicates that coralline red algae and foliose brown algae thrived together in shallow, euphotic waters. However, no inclusion of marine invertebrates such as barnacles or small bivalves was observed, either at L2 - Caleta del Bajo de Mejillones or L3 - Majanicho.
At L4 - Playa El Hierro (Figures 1B, 3E), the beach is formed exclusively of very coarse carbonate sand (1 to 2 mm in diameter) derived entirely from the breakdown of rhodoliths. Many of the fragments may be recognized as the somewhat bulbous distal tips of tightly branched rhodoliths (Figure 4B). L5- Caleta de Beatriz (Figures 1B, 3F), shows more evidence of wrack with brown algae mixed with assorted rhodoliths.
In general, the grainsize of carbonate sand decreases progressively over distance to L5 - Caleta de Beatriz where, on a seasonal basis, the wrackline formed by brown algae is even more extensive. Under any consideration, a taphonomic grade of D would be appropriate for these settings. A dune field covering 4 hectares is located inland directly south of the Caleta del Marrajo, near the village of El Cotillo (Figure 1B). Here, the diameter of carbonate sand grains amounts to coarse-to-medium sand (0.25 to 1.0 mm), which reflects blindingly white under the sun. Asymmetrical ripple marks indicate sand migration in a southerly direction, which fits well with an interpretation of fine rhodolith sand lifted from the shore and blown inland. The taphonomic grade for such a dune deposit in the Brandt (1989) scheme merits an extreme D assignment. Carbonate dunes derived exclusively of crushed rhodolith detritus are seldom met in the modern world, but a good example is reported by Sewell et al. (2007) on Isla Coronados in Mexico’s Gulf of California. Pleistocene dunes dominated by rhodolith debris also are known to occur on Maio and São Nicolau in the Cabo Verde Archipelago (Johnson et al., 2013).
4.3 Rhodoliths’ internal composition
In L2 - Caleta del Bajo de Mejillones rhodoliths are mostly monospecific, with rare borings and encrustations. At L3-Majanicho the rhodoliths living in the intertidal pools have the largest dimensions, reaching up to 8 cm. Rhodoliths here are multispecific and have branching density IV, abundant encrustations, and show small borings on the thallus surface. At L4 - Playa El Hierro rhodoliths are monospecific, and heavily bored with some encrustations. At L5 - Caleta de Beatriz rhodoliths are multispecific and have branching density III; borings are abundant and few encrusters may be found (Table 1; Figure 5).

Table 1 Localities and selected features from the rhodoliths of the north shore of Fuerteventura Island.
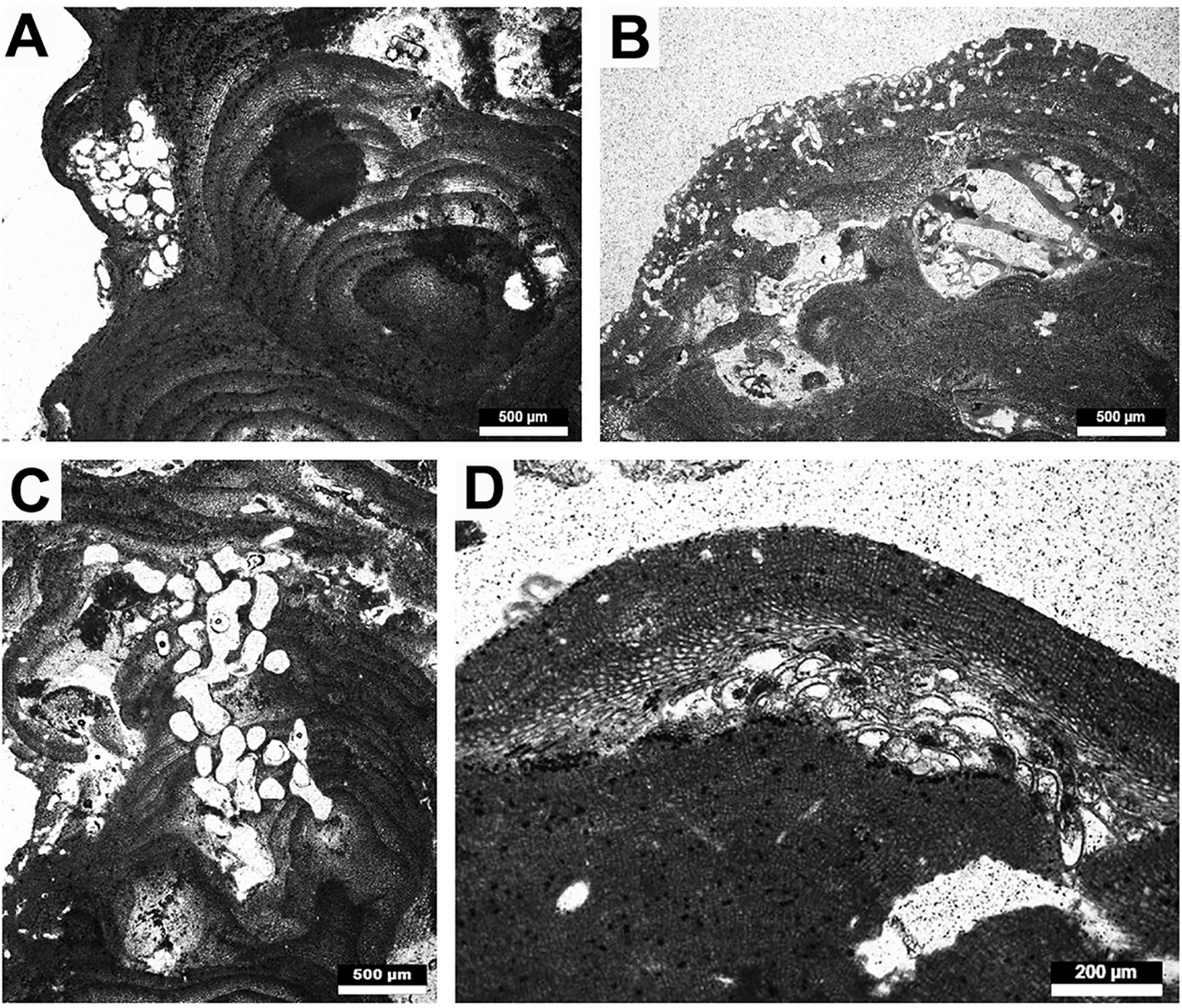
Figure 5 Borings and encrusters present on the Fuerteventura rhodoliths. (A) Encrusting foraminifer? interspersed in the coralline thalli, (B) small-size borings at the coralline thallus extremity and other encrusters, (C) medium-size borings throughout a coralline thallus, (D) bryozoan? in between the coralline thalli.
4.4 Coralline taxonomy
Phylum Rhodophyta Wettstein, 1901
Class Florideophyceae Cronquist, 1960
Subclass Corallinophycidae Le Gall and Saunders, 2007
Order Hapalidiales Nelson et al., 2015
Family Hapalidiaceae Gray, 1864
Genus Lithothamnion Heydrich, 1897, nom. et typ. cons.
Lithothamnion cf. corallioides (P. Crouan and H. Crouan) Crouan HM and Crouan PL, 1867
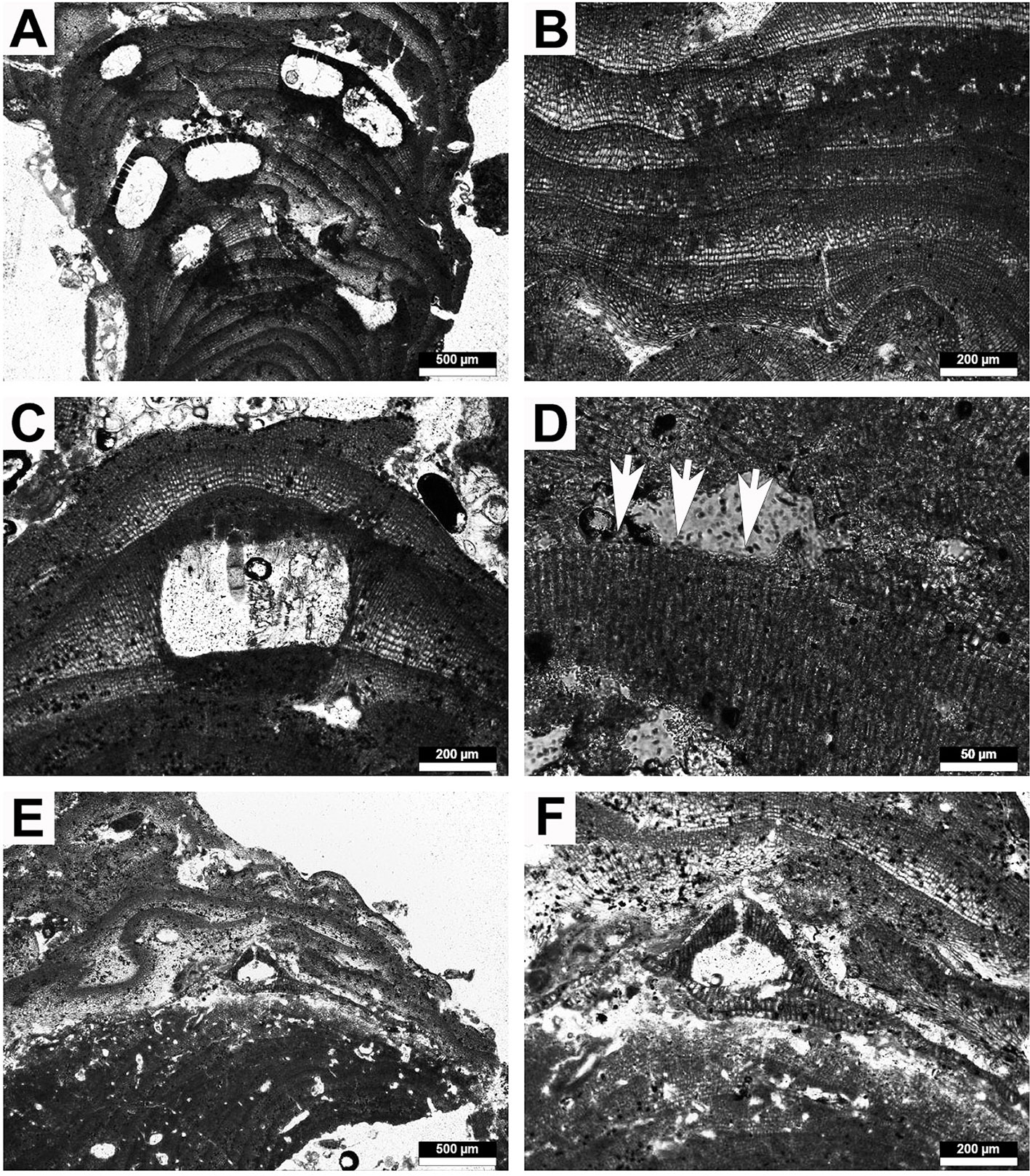
Figure 6 Representative rhodolith forming crustose coralline algae. (A–D) Lithothamnion cf. corallioides. (A) Portion of thallus with multiporate conceptacles, (B) detail of zoned peripheral region, (C) multiporate conceptacle with zoned tetraspore, (D) detail of epithallial cells (arrows). (E, F) Lithoporella sp. (E) Thallus of Lithoporella interspersed between other coralline thalli, (F) detail of uniporate conceptacle.
Studied thin sections: Loc. 2; Loc. 3 Beachway; Loc. 3/1 intertidal; Loc. 3/2 intertidal; Loc. 3/3 intertidal; Loc. 4/1; Loc. 4/2; Loc. 5/2; Loc. 5/3
Description: Growth form encrusting to warty. Thallus monomerous, dorsiventral with non-coaxial core filaments. Protuberances are up to 3 mm in thickness and 2.5 mm in length. Core region is 61-145 µm thick; core cells are 13-19 µm (M = 16; SD = 2) in length and 5-8 µm (M = 7; SD = 1) in diameter. The peripheral region is zoned, formed by 5-11 rows of rectangular cells 5-9 µm (M = 7; SD = 1) in diameter and 9-20 µm (M = 14; SD = 3) in length. Epithallial cells are flat, rounded and flared. Subepithallial cells are as long as or longer than cells subtending them. Cell fusions are present both in the core and peripheral regions. Several multiporate conceptacles throughout the thallus, completely raise above thallus surface. Rectangular to triangular voids above some of the conceptacles’ roof. Conceptacles are 427-908 µm (M = 537; SD = 106) in diameter and 191-337 µm (M = 251; SD = 34) in height. The pore canals are 11-37 µm (M = 17; SD = 4) in diameter and 39-83 µm (M = 64; SD = 10) in height; the roofs are flattened, convex, without rim and composed of 4-7 cell layers. Two conceptacles have remaining spores that are zonately divided.
Remarks: The epithallial cells and the long subepithallial cells indicate the genus Lithothamnion. The conceptacles shape and rims, and the zonation in the peripheral region seems compatible with those described by Adey and McKibbin (1970); Irvine and Chamberlain (1994), and Mendoza and Cabioch (1998). This species is the most common rhodolith former, being present in all localities from Bajo de Mejillones to Caleta de Beatriz. Because the species was identified on a morpho-anatomical base, and our measurements are slightly different from the ones by Afonso-Carrillo and Gil-Rodríguez (1982) we opted to keep it as Lithothamnion cf. corallioides.
Geographic distribution: According to Rebelo et al. (2021), Lithothamnion corallioides (P. Crouan and H. Crouan) P. Crouan and H. Crouan is reported from the following biogeographic provinces, as defined by Spalding et al. (2007) with modifications from Freitas et al. (2019): Northern European Seas, the Mediterranean Sea and the Lusitania Province, i.e., the South European Atlantic Shelf ecoregion, the Azores Archipelago ecoregion, the Webbnesia ecoregion (which integrates the archipelagos of Madeira, Selvagens and Canary Islands) and the Saharan Upwelling ecoregion.
Order Corallinales Silva and Johansen, 1986
Family Mastophoraceae Townsend and Huisman, 2018
Subfamily Mastophoroideae Setchell, 1943
Genus Lithoporella (Foslie) Foslie, 1909
Lithoporella sp.
Studied thin sections: Loc. 3/2 intertidal, Loc. 3/3 intertidal; Loc. 5/4
Description: Growth form encrusting. Thallus dorsiventral and dimerous; core region unistratose, filaments composed of palisade cells. Thallus 1-3 cells thick. Cells of adjacent filaments joined by cell fusions. Cells 6-16 μm (M = 11; SD = 3) in length and 18-36 μm (M = 25; SD = 6) in diameter. Where conceptacles are formed, a small, multi-layered, dorsal region is composed by postigenous filaments, trichocytes not observed. Length of postigenous cells 16-19 μm, diameter 7-12 μm. Tetra/bisporangial conceptacles lacking a columella and completely raised above thallus surface. Cells surrounding the only visible pore canal, perpendicular to the roof surface. Conceptacles 253-297 μm in diameter and 109-121 μm in height. The pore canal is cylindrical in shape and is 38 μm in diameter and 94 μm in height.
Remarks: Lithoporella and Mastophora are the most closely related genera in the Mastophorioideae, and vegetatively no significant differences are known. However in Mastophora a central columella is present (Guiry and Guiry, 2022). Because no columella was found in the studied material, Lithoporella is the designated species.
Geographic distribution: A search on AlgaeBase yielded a total of 8 valid, extant species: Lithoporella atlantica (Foslie) Foslie, reported from the tropical and subtropical western Atlantic (Wynne, 2017); Lithoporella bermudensis (Foslie) W. H. Adey, reported from Bermudas (Taylor, 1960) and from the tropical and subtropical western Atlantic (Wynne, 2017); Lithoporella indica (Foslie) Adey, reported from Réunion Island (Silva et al., 1996) and from the Southeast Asia (Silva et al., 1987); Lithoporella lapidea (Foslie) Foslie, reported from the Caspian Sea (Foslie, 1909); Lithoporella melobesioides (Foslie) Foslie, with a wide geographic range, being reported from the Mediterranean Sea (Bosence, 1983), eastern Atlantic Ocean (Cabo Verde Archipelago; Prud'homme van Reine et al., 2005), Indian Ocean (Silva et al., 1996) and Pacific Ocean (Womersley and Bailey, 1970); Lithoporella quadratica Ishijima, a fossil species described from Japan by Ishijima (1933); Lithoporella ryukyuensis W.Ishijima, another fossil species described from the Yukyu Islands by Ishijima (1938); and finally, Lithoporella sauvageaui (Foslie) Adey, reported from Canary Islands (Haroun et al., 2002) and from Cabo Verde Archipelago (John et al., 2004). Lithoporella sauvageaui (Foslie) Adey is known to occur in Canaries on the islands of Lanzarote, Gran Canaria and Tenerife (Haroun et al., 2002), however no species of Lithoporella has been reported, at least to the authors knowledge, at Fuerteventura Island.
Family Hydrolithaceae Townsend and Huisman, 2018
Subfamily Hydrolithoideae Kato et al., 2011
Genus Hydrolithon (Foslie) Foslie, 1909
Hydrolithon sp.
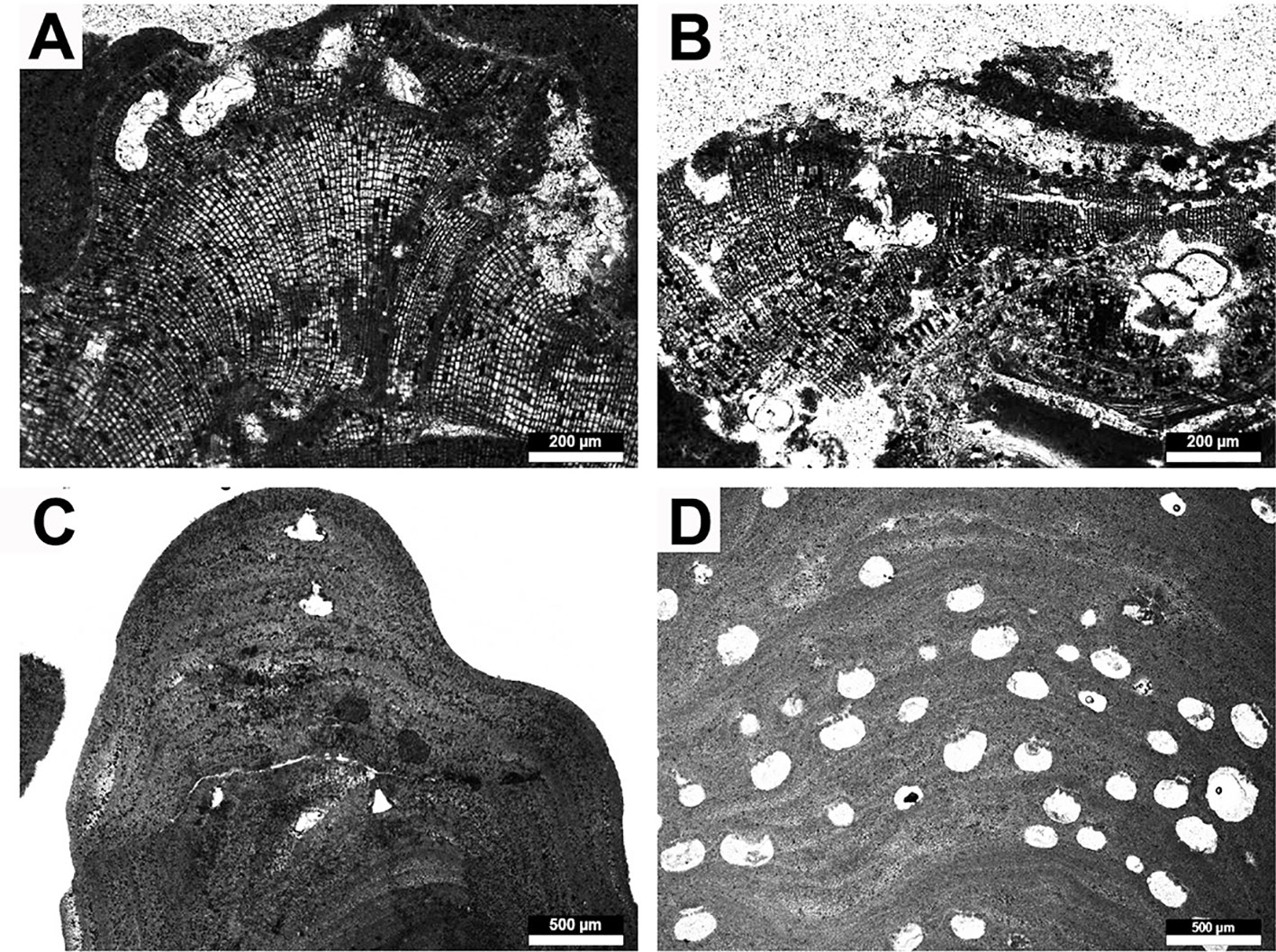
Figure 7 Representative rhodolith forming crustose coralline algae. (A, B) Hydrolithon sp., (A) dimerous thallus with uniporate conceptacles, (B) detail of uniporate conceptacle, (C, D) taxa of uncertain generic placement, (C) thallus with uniporate conceptacles, (D) several multiporate conceptacles dispersed throughout the thallus.
Studied thin section: Loc. 5/4
Description: Growth form encrusting. Thallus dimerous in construction; core region not a palisade. Thallus is 0.4-1 mm thick. Cells of primigenous filaments are 5-9 µm (M = 7; SD = 1) in diameter and 10-14 µm (M = 12; SD = 13) in lenght. Cells of postigenous filaments are rectangular in shape, show a very well defined alignment and are 7-14 µm (M = 11; SD = 2) in diameter and 14-23 µm (M = 18; SD = 3) in length. Rare cell fusions occur in postigenous filaments. Conceptacles are uniporate with a columella, 186-201 µm in diameter and 72-82 µm in height. The pore canal is cylindrical, 24 µm in diameter and 64 µm in height. Filaments surrounding the pore canal are perpendicular to the conceptacle roof.
Remarks: The thallus dimerous organization, the lack of palisade cells in the primigenous filaments, the presence of cells fusions and the filaments surrounding the pore canal oriented perpendicularly to the conceptacle roof indicates the genus Hydrolithon. Because of the absence of further preserved diagnostic characters it was not possible to identify the species.
Geographic distribution: A search on AlgaeBase yielded a total of 22 valid, extant species, of which Rebelo et al. (2021) indicate two as forming rhodoliths: Hydrolithon boergesenii Foslie (Foslie) and Hydrolithon breviclavium (Foslie) Foslie. Both species have a wide geographic distribution, the former being reported from the Western Central Atlantic (Caribbean and Gulf of Mexico), South America (Brazil), Eastern Atlantic (São Tomé and Príncipe Archipelago), Indian Ocean and Pacific Ocean, whereas Hydrolithon breviclavium is reported from the Western Atlantic, Indian Ocean (Seychelles) and Pacific Ocean (Guiry and Guiry, 2022). Hydrolithon boreale (Foslie) Chamberlain, H. cruciatum (Bressam) Chamberlain and H. farinosum (Lamouroux) Penrose and Chamberlain and H. onkodes (Heydrich) Penrose and Woelkerling are reported for Fuerteventura Island (Haroun et al., 2002).
Taxa of uncertain generic placement
Studied thin section: Loc. 2
Unidentified taxon 1: One thallus with two uniporate conceptacles. One conceptacle well rounded, 179 µm in diameter and 92 µm in height. The pore canal is cylindrical, 42 µm in diameter and 73 µm in length. The filaments surrounding the pore canal seem to protrude into the pore. One conceptacle flask shaped, 236 µm in diameter and 72 µm in height. The pore canal is triangular, 100 µm in diameter and 103 µm in length. The poor preservation of the thallus does not allow further identification.
Studied thin section: Loc. 5/1
Unidentified taxon 2: One thallus with several small multiporate conceptacles 230-280 µm (M = 246; SD = 17) in diameter and 112-164 µm (M = 139; SD = 19) in height. The roofs are 32-47 µm thick. The poor preservation of the thallus does not allow further identification.
5 Discussion
5.1 Factors controlling rhodoliths distribution along the northern coast of Fuerteventura
The current knowledge on the extension of the RBs around the Canarian Archipelago is yet incomplete, although efforts are being made in this regard (Otero-Ferrer et al., 2020). Previous studies on rhodoliths from Gran Canaria Island have shown that the local environment has a strong influence on their development, especially as regards water motion and depth (Otero-Ferrer et al., 2020). Dominant wind and wave directions in the Canary Islands are mainly from the NE (Rusu and Onea, 2016; Azorin-Molina et al., 2017). Hence, predominant longshore currents should occur from East to West, although some waves also come from the NW. Rhodolith shape, branching pattern and sphericity are strongly affected by different intensities of water motion (Sciberras et al., 2009 and references therein). All rhodoliths studied show a closely branched morphology suggesting these are formed in a high energy environment. In addition, these were highly eroded rhodoliths showing a degree of breakage of C to D in the Brandt (1989) scheme. This suggests that stormy waves are able to transport and break rhodoliths carried to the nearshore of beaches on the north shores of Fuerteventura. Waves can reach up to 5 m near Fuerteventura (Rusu and Onea, 2016) and are likely able to transport them shoreward towards the beaches as bedload or in suspension aided by the flotation of attached brown algae. This latter effect is not accounted for by the transport mechanisms provided in the review by Masselink et al. (2011). Transport of sediments storm-induced is also a known pattern from the geological past and that appears to be frequent in volcanic oceanic islands [e.g., the Pliocene of Santa Maria Island in the Azores (Meireles et al., 2013; Ávila et al., 2015b; Rebelo et al., 2016; Johnson et al., 2017a)]. Non-algal encrusters, such as foraminifers and bryozoans, are frequently overgrown by the corallines suggesting a temporal disturbance in rhodolith growth that allowed the encrusters to grow only for a short time before the red algae prevailed again. Temporal disturbance also seems to explain why both monospecific and multispecific rhodoliths have borings, many at the extremity of the thallus before their final deposition onshore. We assume that the disturbances were caused by storms that are capable of transporting rhodolith beds into deeper and/or shallower environments, as well as parallel to the shore. It is not known which wave direction is more important for the onshore transport, though waves coming from NW have the longest fetch, hence these are likely to contribute more for this process. This is merely a hypothesis, since the places of rhodolith beds under life conditions has yet to be thoroughly mapped and therefore require future field studies. The Calamareo and El Puente were the only two diving spots we were able to explore during this study and therefore the extension of the rhodolith beds on the north coast of Fuerteventura is still unknown. Calamareo is shown here as a random example of how the rhodolith beds look in situ, and we believe that the rhodoliths come from the submarine part of the beaches where they were found. As it can be seen in Figure 8 the shore platform down to -10 m appears to be a smooth rocky platform, the probable place of development of the rhodoliths. Accordingly, a predictive model is here proposed to show the deposition of the rhodoliths on the north coast of Fuerteventura Island (Figure 9).
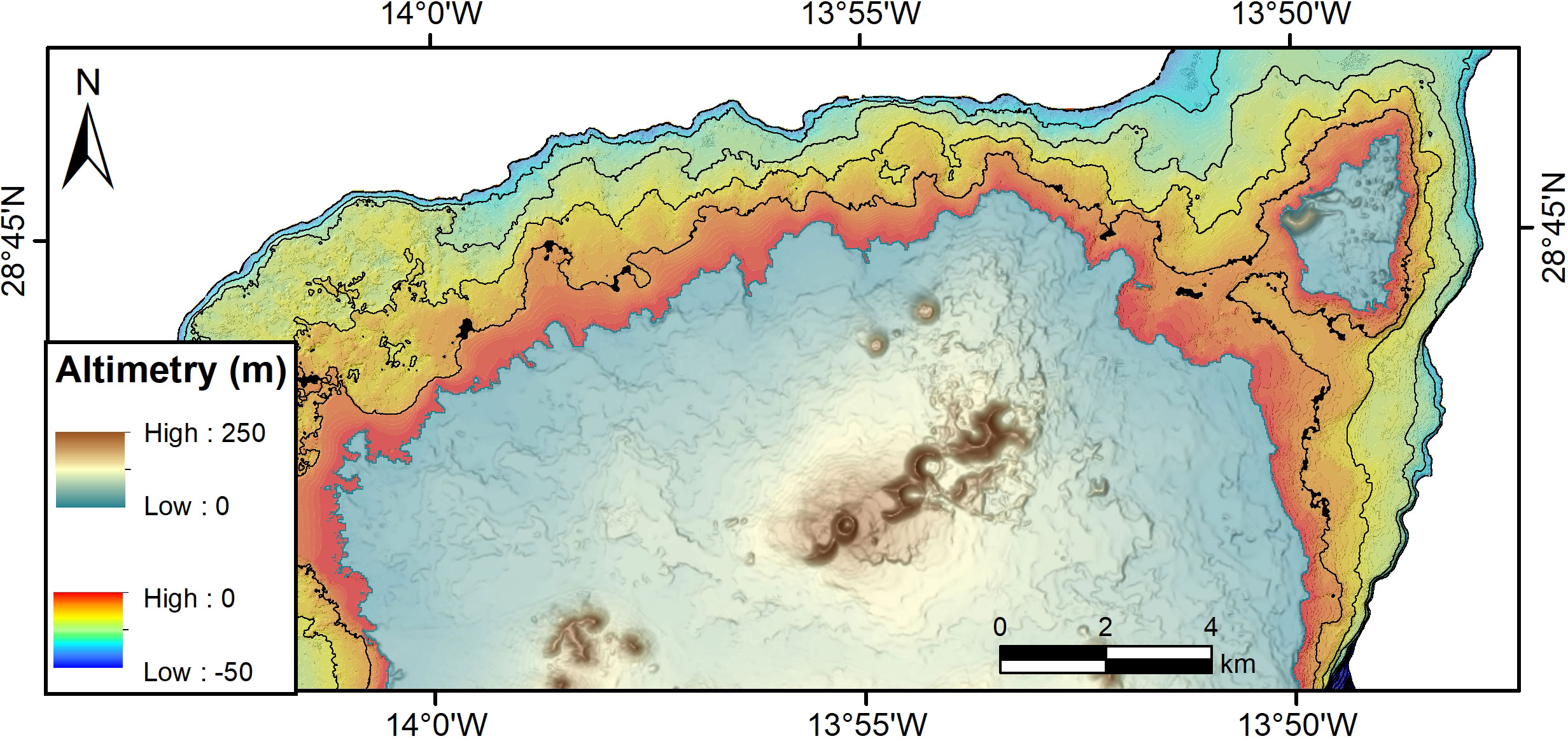
Figure 8 Bathymetry of the north coast of Fuerteventura Island screening the possible place of development of the rhodolith beds. Bathymetry lines are every 10 meters.
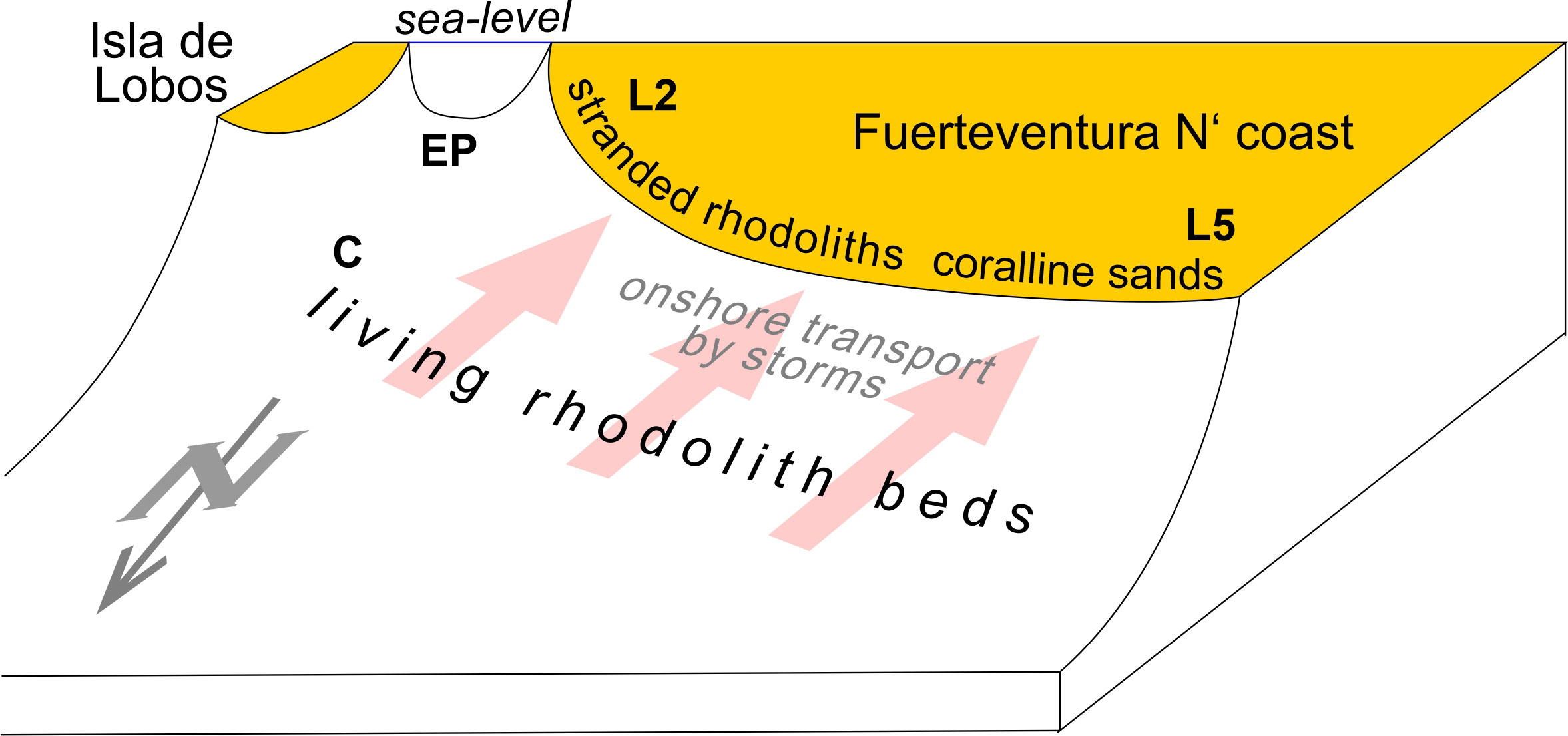
Figure 9 Model for the deposition of the rhodoliths on the north coast of Fuerteventura Island. L2 - Caleta del Bajo de Mejillones, L5 - Caleta de Beatriz, C – Calamareo, EP – El Puente.
5.2. Factors promoting rhodolith transport landward the northern coast of Fuerteventura
At L2 - Caleta del Bajo de Mejillones the deposit of rhodoliths being 120 m long and 10 m wide (as described in Johnson et al., 2012) traces out to the west, where the whole rhodoliths vanish. This demonstrates that the bedload with the rhodoliths has petered out. Figure 10A shows the extension of the beach to the west of L2 – Caleta del Bajo de Mejillones with the exposed rock platform in the right side. The dashed lines mark how the mixed basalt and rhodolith sand in successive events terminate to the west, indicating that the source of the material came from the east. The main explanation for this would be a storm event concurrent with the strong NE trade winds. The way that the rhodolith deposits form excursions onto the rock platform (far right) also indicate that the source of waves is from the east. A small trench made at the beach (Figure 10B) shows that the rhodoliths at the surface represent a single event draped over a pre-existing deposit of beach sand. The overlying rhodolith drift is maximum 5 cm thick while the sand below is no less than 25 cm thick with few rhodoliths mixed. The rhodolith drift on the top represents the most recent event on the beach, since these rhodoliths are positioned well above mean sea level in the supratidal zone.
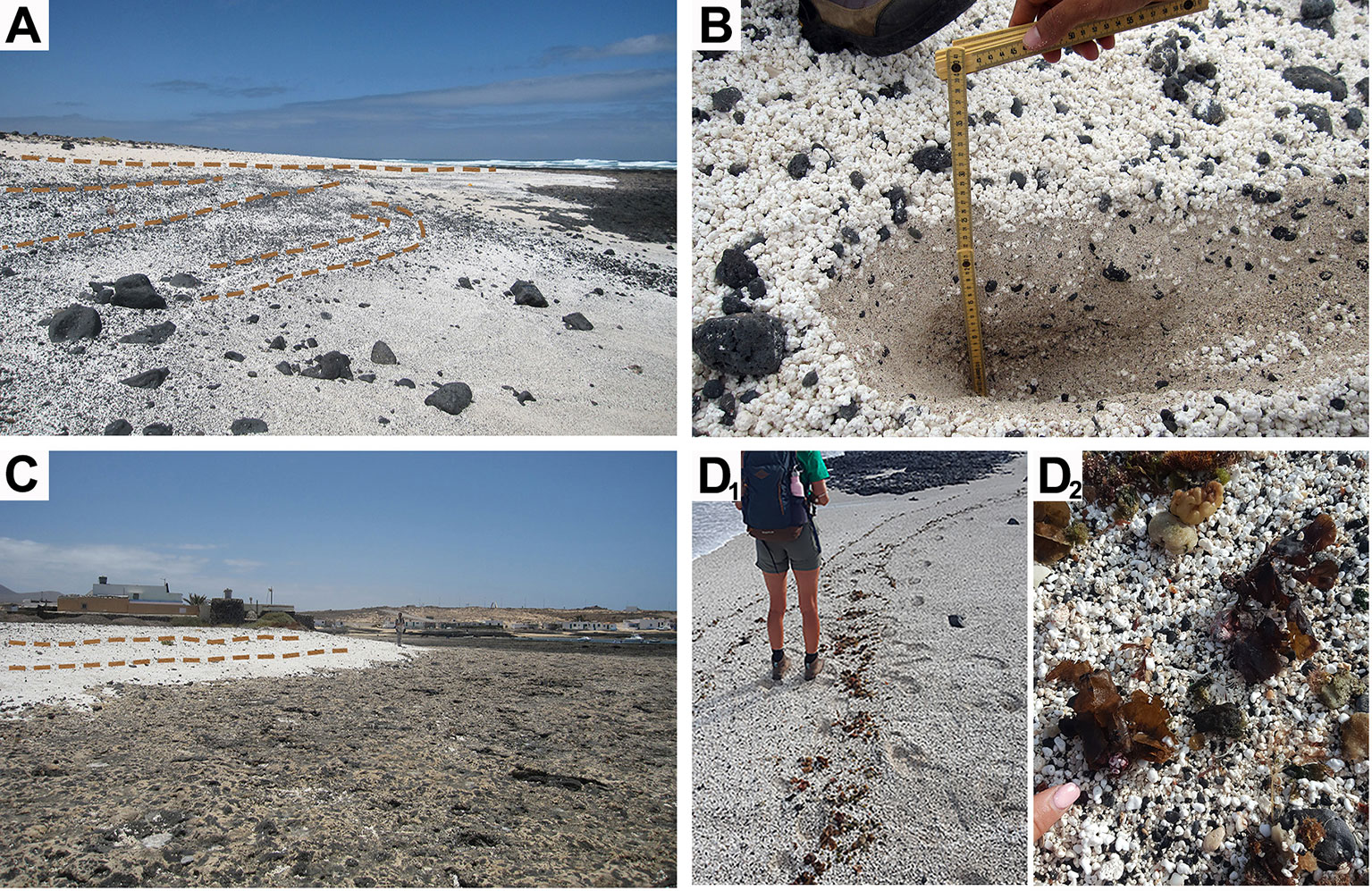
Figure 10 (A) Stranded rhodoliths at L2 - Caleta del Bajo de Mejillones. Image is at the far (distal end) of the 120-m long deposit; dashed lines show how the mixed basalt and rhodolith sand in successive events terminate to the west. (B) detail of small trench trench made at L2 - Caleta del Bajo de Mejillones evidencing the rhodolith drift overlying the sand. (C) Stranded rhodoliths at L3 – Majanicho. Dashed lines show layering indicated by stringers of eroded basalt. (D1) Stranded extant rhodoliths attached to brown algae at Playa El Hierro. (D2) Detail of stranded rhodoliths attached to brown algae.
At L3 - Majanicho stranded rhodoliths also peter out to the west, where they fail to spill into the embayment (Figure 10C). The dashed lines indicate layering represented by stringers of eroded basalt. Figure 10C shows the extent of the distinctive basalt rock platform at extreme low tide. Given the local geography, it is not possible that this deposit came from the west toward the east. Furthermore, individual rhodoliths from L2 - Caleta del Bajo de Mejillones, L3 - Majanicho and L4 - Playa El Hierro still retain an attachment of single strands or blades of brown algae (Figure 10D). This is a well-known phenomenon that in Brazil is called the “arribadas” (Dias and Villaça, 2012), suggesting that the bedload of stranded rhodoliths is quite recent.
Following the definitions of Masselink et al. (2011) regarding the modes of sediment transport, the rhodolith transport on the north coast of Fuerteventura signifies extreme turbulence which the degradation of rhodoliths occurred during saltation. The reason for an interpretation of bedload transport at the highest energy index is that the very wide offshore rock platforms are almost entirely free of sediments except for a few cases where rhodoliths are trapped in small tidal pools. Much stronger currents (eddies) had to have been involved as opposed to the normal traction associated with movement of a bedload. Another reason for this is that mixed among the dominant organic sediments from the rhodoliths, there are larger pebbles and even small cobbles eroded from basalt. It would require stronger wave action to move these heavier clasts in concert with the organic clasts. Thus, the extensive wave cut rock platforms were scoured by strong storm currents, essentially moving along shore from east to west, during which offshore sediments of both rhodoliths and basalt origins were fully swept across the rock platforms to end up in a supratidal setting well above the normal tide line.
5.3 Taphonomic grades
The reduction of rhodolithic shore materials from a grade of C as demonstrated on Popcorn Beach at L2 - Caleta del Bajo de Mejillones to a severely diminished grade of D in the sand dunes near El Cotillo (Figure 1B) reflects the progressive mechanical abrasion of stranded coralline algae under direct influence of onshore wave transport and further beach deflation due to onshore winds. The several pocket beaches between these two areas are dominated by carbonate sand production that shows a clear decrease in average grain size from very coarse grains at L4 - Playa El Hierro (Figure 4B) to finer and finer grains with distance to the west. Overall, the scenario reflects the energy of long-shore currents related to the strong action of wind-driven, moving from east to west across the north coast of Fuerteventura Island and crossing the shore in a diagonal direction. The strongest currents appear at a frequency unrelated to a mere seasonal pattern, although the brisk northeast trade winds are a near constant presence in the region. The exploratory excavation on the beach at L2 - Caleta del Bajo de Mejillones shows that rhodolith deposits are renewed with successive storm events. There exists a general relationship between rhodolith size and the amount of time during which an individual rhodolith continues to grow in diameter by accretion. Mostly less than 3 cm in diameter, the relatively small size of rhodoliths that dominate Popcorn Beach indicates they are harvested during storms that arrive at intervals several years apart. The absence of rhodoliths of an appreciably larger size up to 8 cm in diameter suggests that few if any survive long enough to reach that size in waters directly offshore down to a depth of 25 m along the north coast.
5.4 Coralline algae taxonomic composition
Lithothamnion coralliodes was present at all localities of the present study. L. coralliodes is also very common around the islands of Cabo Verde and Madeira (Foslie, 1908; Cabioch, 1974; Guiry and Guiry, 2022), and is distributed in all the islands of the Canary Archipelago, with the exception of La Palma and La Gomera (Afonso-Carrillo and Gil-Rodríguez, 1982). L. corallioides and Phymatolithon calcareum are coralline algae species that are protected by the Habitats Directive (92/43/EEC) as key habitat providers, crucial for the support of numerous other species (Pardo et al., 2014; Hernándéz-Kantún et al., 2017). The fact that the rhodoliths are composed by species of Lithothamnion, Hydrolithon and Lithoporella which are generally recognized as typical of deep-water (Aguirre et al., 2017 and references therein), could explain the absence of rhodoliths in the shallow diving spot El Puente, not only because of the substrate type but as a result of depth as well. Lithoporella is a new record for Fuerteventura (Haroun et al., 2002), which could be due to the incomplete knowledge of the taxonomic inventory of Canary Islands’ coralline algae.
5.5 Importance of rhodoliths and conservation implications
We take the opportunity of this study to highlight the negative-impact of rhodolith plundering from the beaches of Fuerteventura Island. Although rhodolith beds have been recognized in various European and international frameworks through the adoption of a collection of protection instruments such as Directives, Regulations and Conventions (Basso et al., 2016), more work in this regard is still needed for the Canary Islands. Each year, substantial amount of rhodoliths is removed from the beaches by tourists and locals which is a threat to these ecosystems, as the debris of dead rhodoliths contributes greatly to the sediments that form the contemporary beaches. Local authorities have been working against this trend and trying to return, whenever possible, all materials back to their place of origin. Large boards are displayed throughout the beaches explaining what these “popcorn” are and how their removal is forbidden, nevertheless the plundering is still very evident (Figure 11). We therefore urge the implementation of more protection and management efforts of the local rhodolith beds, and these should be regarded as ecosystem engineers that generate a complex habitat for a high biodiversity of species. For an effective conservation management, more detailed studies of the distribution, biodiversity and community structure of the Fuerteventura RBs beds are needed.
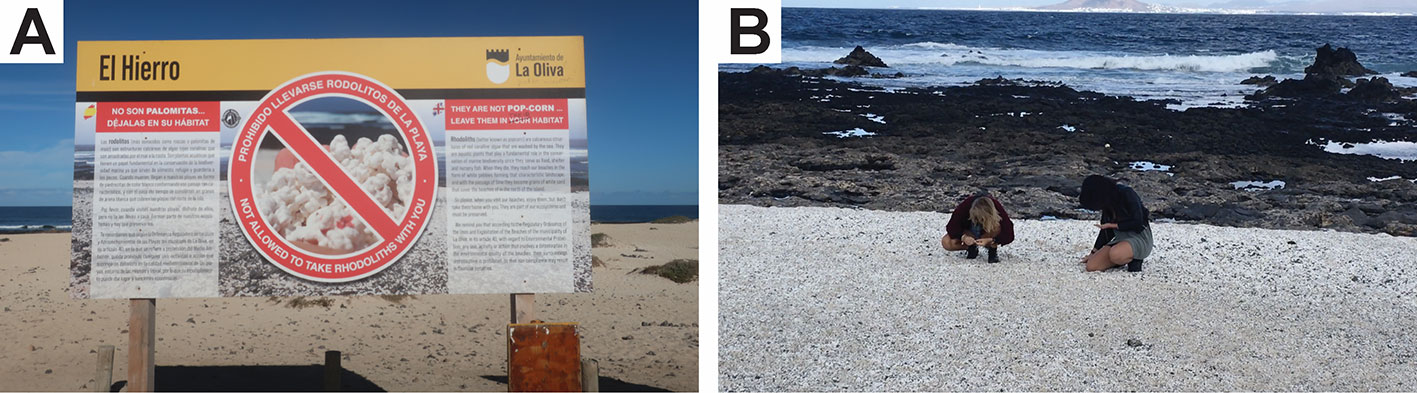
Figure 11 (A) Example of a prohibition sign for rhodolith picking at L4 - Playa El Hierro. (B) Rhodolith plundering at L2 – Caleta del Bajo de Mejillones. Photo courtesy of Félix de la Rosa.
6 Conclusion
Foremost, this study contributes to the first analysis of species composition of the rhodoliths from the north shore of Fuerteventura Island and contributes to a better understanding of the distribution of taxa in different settings. Our results show that a beach deposit composed of stranded rhodoliths can accumulate up to 5000 rhodoliths/m2. Lithothamnion cf. corallioides is the most common species detected in this study. It is also the most representative species from the sea bottom around the Canaries. Although present in the islands of Lanzarote, Gran Canaria and Tenerife the genus Lithoporella is a new record for the island of Fuerteventura. We believe that given the size of the rhodoliths (coarse sand) and their high degree of breakage found on the beaches, these were transported shoreward by stormy waves coming from the NW. This work contributes to a more detailed approach on the present knowledge of rhodoliths from Fuerteventura Island and offers a baseline for further investigation, namely on the role of rhodolith beds as key habitat providers in insular settings. Further field studies are necessary, however, to understand the in-place formation of rhodoliths beds and their associated flora and fauna.
Data availability statement
The raw data supporting the conclusions of this article will be made available by the authors, without undue reservation.
Author contributions
ACR and EM-G conceived the idea and conceptualized it. MEJ, MWR, and ACR contributed to sample collecting and processing. All authors contributed to the article and approved the submitted version.
Acknowledgments
This study was endeavoured by the 1st Workshop of Marine Palaeontology and Littoral Geology in Canary Islands: Fuerteventura (1° MPLG-F) led by E. Martín-González. We thank Alejandro de Vera, Sandra Kaiser and Gregor Rasser for help in field work; Scuba diving center Punta Amanay for assistance during the dives; and Christoph Wimmer-Pfeil (Staatlcihes Museum für Naturkunde Stuttgart, Germany) for thin sections preparation. We thank Ministerio de Agricultura, Pesca y Alimentacion (MAPAMA), Dirección General de Sostenibilidad de la Costa y del Mar y al Ministerio para la Transición Ecológica for bathymetry data from “Ecocartografías mapping Project” (https://www.miteco.gob.es/es/costas/temas/proteccion-costa/ecocartografias/default.aspx). ACR was supported by a Post-doctoral grant SFRH/BPD/117810/2016 from the Portuguese Science Foundation (FCT). CSM was supported by a PhD grant M3.1.a/F/100/2015 from The Regional Government of the Azores (FRCT). LB was supported by a PhD grant SFRH/BD/135918/2018 from the Portuguese Science Foundation (FCT). SPA acknowledges his “Investigador FCT” contract (IF/00465/2015), funded by FCT. This work was co-funded by the project NORTE-01-0246-FEDER-000063, supported by Norte Portugal Regional Operational Programme (NORTE2020), under the PORTUGAL 2020 Partnership Agreement, through the European Regional Development Fund (ERDF). The authors also would like to acknowledge research support from National Funds through FCT - Foundation for Science and Technology under the project UIDB/50027/2020 and through DRCT under the project ACORES-01-0145_FEDER-000078 - VRPROTO: Virtual Reality PROTOtype: the geological history of “Pedra-que-pica, and under DRCTM1.1.a/005/ 519 Funcionamento-C-/2016 (CIBIO-A) project.
Conflict of interest
The authors declare that the research was conducted in the absence of any commercial or financial relationships that could be construed as a potential conflict of interest.
Publisher’s note
All claims expressed in this article are solely those of the authors and do not necessarily represent those of their affiliated organizations, or those of the publisher, the editors and the reviewers. Any product that may be evaluated in this article, or claim that may be made by its manufacturer, is not guaranteed or endorsed by the publisher.
References
Acosta J., Uchupi E., Muñoz A., Herranz P., Palomo C., Ballesteros M., ZEE Working Group (2004). “Geologic evolution of the Canarian Islands of Lanzarote, Fuerteventura, Gran Canaria and La Gomera and comparison of landslides at these Islands with those at tenerife, La Palma and El Hierro,” Marine Geophysical Researches 0, 1–38. doi: 10.1007/1-4020-4352-X_1
Adey W. H., McKibbin D. (1970). Studies on the maerl species Phymatolithon calcareum (Pallas) nov. comb. and Lithothamnion corallioides crouan in the ria de vigo. Botanica Marina 13, 100–106.
Afonso-Carrillo J. (2021). Las algas coralinas (Rhodophyta) ante la acidificación del océano con especial referencia a las islas canarias. Rev. Scientia Insularum 4, 145–204. doi: 10.25145/j.SI.2021.04.08
Afonso-Carrillo J., Gil-Rodríguez M. C. (1982). Sobre la presencia de un fondo de “maerl“ en Islas Canarias. Collectanea Botanica 13, 703–708.
Aguirre J., Braga J. C., Bassi D. (2017). “Rhodoliths and rhodolith beds in the rock record,” in Rhodolith/Maërl beds: a global perspective. coastal research library, vol. vol. 15 . Eds. Riosmena-Rodríguez R., Nelson W., Aguirre J. (Cham: Springer). doi: 10.1007/978-3-319-29315-8_5
Ávila S. P., Madeira P., da Silva C. M., Cachão M., Landau B., Quartau R., et al. (2008). Local disappearance of bivalves in the Azores during the last glaciation. J. Quaternary Sci. 23, 777–785.
Ávila S. P., Melo C., Sá N., Quartau R., Rijsdijk K., Ramalho R. S., et al. (2019). Towards a “Sea-level sensitive marine Island biogeography“ model: the impact of glacio-eustatic oscillations in global marine Island biogeographic patterns. Biol. Rev. 94 (3), 1116–1142.
Ávila S. P., Melo C., Silva L., Ramalho R., Quartau R., Hipólito A., et al. (2015a). A review of the MIS 5e highstand deposits from Santa Maria Island (Azores, NE atlantic): palaeobiodiversity, palaeoecology and palaeobiogeography. Quaternary Sci. Rev. 114, 126–148.
Ávila S. P., Ramalho R., Habermann J., Quartau R., Kroh A., Berning B., et al. (2015b). Palaeoecology, taphonomy, and preservation of a lower pliocene shell bed (coquina) from a volcanic oceanic Island (Santa Maria Island, Azores, NE Atlantic Ocean). Palaeogeography Palaeoclimatology Palaeoecol. 430, 57–73.
Azorin-Molina C., Menendez M., McVicar T. R., Acevedo A., Vicente-Serrano S. M., Cuevas E., et al. (2017). Wind speed variability over the Canary Islands 1948-2014: focusing on trend differences at the land-ocean interface and below-above the trade-wind inversion layer. Climate Dynamics 50, 4061–4081.
Basso D., Babbini L., Kaleb S., Bracchi V. A., Falace A. (2016). Monitoring deep Mediterranean rhodolith beds. Aquat. Conservation: Mar. Freshw. Ecosyst. 26, 549–561.
Bogaard P. (2013). The origin of the Canary Island Seamount Province – new ages of old seamounts. Sci. Rep. 3, 2107.
Braga J. C., Bosence D. W. J., Steneck R. S. (1993). New anatomical characters in fossil coralline algae and their taxonomic implications. Palaeontology 36, 535–547.
Brandt D. S. (1989). Taphonomic grades as a classification for fossiliferous assemblages and implications for paleoecology. Palaios 4, 303–309.
Cabioch J. (1974). Un fond de maerl de l’Archipel de Madère et son peuplement végétal. Bull. la Société Phycologique France 19, 74–82.
Casillas R., Torres J. M. (2011). Inventario de recursos vulcanológicos de fuerteventura (Cabildo de Fuerteventura), 155 p.
Coello J., Cantagrel J.-M., Hernán F., Fúster J.-M., Ibarrola E., Ancochea E., et al. (1992). Evolution of the eastern volcanic ridge of the Canary Islands based on new K-AR data. J. Volcanology Geothermal Res. 53, 251–274.
Crouan H. M., Crouan P. L. (1867). Florule du Finistère. Klincksieck F., (Paris). x+262pp. doi: 10.5962/bhl.title.11601
Dias G. T. M., Villaça R. C. (2012). Coralline algae depositional environments on the Brazilian Central-South-Eastern shelf. J. Coast. Res. 28, 270–279.
Foslie M. (1908). Die Lithothamnien der Deutschen Südpolar-Expedition. Deut. Süd. Exp. Botanik (De Gruyter) 8, 205–220.
Foslie M. (1909). “Algologiske notiser, VI.” Kongelige norske videnskabers selskabs skrifter(De Gruyter), 1909 (2) , 1–63.
Foster M., Amado-Filho G. M., Kamenos N. A., Riosmena-Rodríguez R., Steller D. L. (2013). “Rhodoliths and rhodolith beds,” in Smithsonian Contributions to the marine sciences no. 39. Ed. Lange M. (Smithsonian Institution), pp 143–pp 155. doi: 10.5479/si.1943667X.39
Foster M., McConnico L., Lundsten L., Wadsworth T., Kimball T., Brooks L., et al. (2007). The diversity and natural history of Lithothamnion muelleri - Sargassum horridum community in the Gulf of California. Cienc. Marinas 33, 367–384.
Fredericq S., Krayesky-Self S., Sauvage T., Richards J., Kittle R., Arakaki N., et al. (2019). The critical importance of rhodoliths in the life cycle completion of both macro- and microalgae, and as holobionts for the establishment and maintenance of marine biodiversity. Front. Mar. Sci. 5. doi: 10.3389/fmars.2018.00502
Freitas R., Romeiras M., Silva L., Cordeiro R., Madeira P., González J. A., et al. (2019). Restructuring of the “Macaronesia“ biogeographic unit: a marine multi-taxon biogeographical approach. Sci. Rep. 9, 15792. doi: 10.1038/s41598-019-51786-6
Gonçalves M., Martinho P., Guedes Soares C. (2020). Wave energy assessment based on a 33-year hindcast for the Canary Islands. Renewable Energy 152, 259–269.
Guiry M. D., Guiry G. M. (2022). World-wide electronic publication (Galway: National University of Ireland). Available at: https://www.algaebase.org. 21st of February, 2022.
Haroun R. J., Gil-Rodríguez M. C., Díaz de Castro J., Prud'homme van Reine W. F. (2002). A checklist of the marine plants from the Canary Islands (central eastern Atlantic Ocean). Botanica Marina 45, 139–169.
Hernández-Kantún J. J., Hall-Spencer J. M., Grall J., Adey W., Rindi F., Maggs C. A., et al. (2017). “North Atlantic rhodolith beds,” in Rhodolith/Maërl beds: a global perspective. coastal research library, vol. vol. 15 . Eds. Riosmena-Rodríguez R., Nelson W., Aguirre J. (Cham: Springer). doi: 10.1007/978-3-319-29315-8_10
Hrabovský J., Basso D., Doláková N. (2016). Diagnostic characters in fossil coralline algae (Corallinophycidae: Rhodophyta) from the Miocene of southern Moravia (Carpathian Foredeep, Czech Replubic). J. Systematic Palaeontology 14, 499–525.
Irvine L. M., Chamberlain Y. M. (1994). “Seaweeds of the British Isles,” in Rhodophyta, part 2B corallinales, hildenbrandiales, vol. vol. 1. (London: HMSO), 276 pp.
Ishijima W. (1933). On three species of corallinaceae lately obtained from the Megamiyama Limestone, Sagara District, Prov. Totomi Japan. Japanese J. Geology Geogr. 11, 27–30.
Ishijima W. (1938). New species of calcareous algae from several Tertiary and later limestones from various localities of the Ryukyu Islands. Japanese J. Geology Geogr. 15, 13–16.
John D. M., Prud'homme van Reine W. F., Lawson G. W., Kostermans T. B., Price J. H. (2004). A taxonomic and geographical catalogue of the seaweeds of the western coast of Africa and adjacent Islands. Beihefte zur Nova Hedwigia 127, 1–339.
Johnson M. E., Baarli B. G., Cachão M., da Silva C. M., Ledesma-Vázquez J., Mayoral E. J., et al. (2012). Rhodoliths, uniformitarianism, and Darwin: Pleistocene and Recent carbonate deposits in the Cape Verde and canary Archipelagos. Palaeogeography Palaeoclimatology Palaeoeology 329-330, 83–100.
Johnson M. E., Baarli B. G., da Silva C. M., Cachão M., Ramalho R. S., Ledesma-Vázquez J., et al. (2013). Coastal dunes with high content of rhodolith (coralline red algae) bioclasts: Pleistocene formations on Maio and São Nicolau in the Cape Verde Archipelago. Aeolian Res. 8, 1–9.
Johnson M. E., Uchman A., Costa P. J. M., Ramalho R. S., Ávila S. P. (2017a). Intense hurricane transport sand onshore: example from the Pliocene Malbusca section on Santa Maria Island (Azores, Portugal). Mar. Geology 385, 244–249. doi: 10.1016/j.margeo.2017.02.002
Johnson M. E., Ledesma-Vázquez J., Ramalho R. S., da Silva C. M., Rebelo A. C., Santos A., et al. (2017b). “Taphonomic range and sedimentary dynamics of modern and fossil rhodolith beds: Macaronesian realm (North Atlantic Ocean),” in Rhodolith/Maërl beds: A global perspective. coastal research library, vol. 15 . Eds. Riosmena-Rodríguez R., Nelson W., Aguirre J. (Springer), 368 p. doi: 10.1007/978-3-319-29315-8_9
Kato A, Baba M., Suda S. (2011). Revision of the Mastophoroideae (Corallinales, Rhodophyta) and polyphyly in nongeniculate species widely distributed on Pacific coral reefs. Journal of Phycology 47 (3), 662–672.
Le Gall L., Saunders G. W. (2007). A nuclear phylogeny of the Florideophyceae (Rhodophyta) inferred from combined EF2, small subunit and large subunit ribosomal DNA: establishing the new red algal subclass Corallinophycidae. Molecular Phylogenetics and Evolution 43, 1118–1130.
Martin C. S., Giannoulaki M., De Leo F., Scardi M., Salomidi M., Knittweis L., et al. (2014). Coralligenous and maërl habitats: predictive modelling to identify their spatial distributions across the Mediterranean Sea. Sci. Rep. 4, 5073. doi: 10.1038/srep05073
Masselink G., Hughes M., Knight J., Houser C. (2011). “Sediments, boundary layers and transport,” in Introduction to coastal processes and geomorphology. Eds. Davidson-Arnott R., Bauer B. (Cambridge University Press).
Meireles R. P., Quartau R., Ramalho R., Madeira J., Rebelo A. C., Zanon V., et al. (2013). Depositional processes on oceanic Island shelves – evidence from storm-generated neogene deposits from the mid-north Atlantic. Sedimentology 60, 1769–1785.
Mendoza M. L., Cabioch J. (1998). Étude comparée de la reproduction de Phymatolithon calcareum (Pallas) adey & McKibbin et Lithothamnion coralliodes (P. & H. Crouan) P. & H. Crouan (Corallinales, Rhodophyta), et reconsidérations sur la définition des genres. Can. J. Bot. 76, 1433–1445.
Nelson W. A., Sutherland J. E., Farr T. J., Hart D. R., Neill K. F., Kim H. J., Yoon H. S. (2015). Multi-gene phylogenetic analyses of New Zealand coralline algae: Corallinapetra Novaezelandiae gen. et sp. nov. and recognition of the Hapalidiales ord. nov. Journal of Phycology 51 (3), 454–468.
Otero-Ferrer F., Cosme M., Tuya F., Espino F., Haroun R. (2020). Effect of depth and seasonality on the functioning of rhodolith seabeds. Estuarine Coast. Shelf Sci 235, 106579. doi: 10.1016/j.ecss.2019.106579
Pardo C., López L., Peña V., Hernández-Kantún J. J., Le Gall L., Bárbara I., et al. (2014). A multilocus species delimitation reveals a striking number of maërl species in the OSPAR region. PloS One 9, e104073.
Prud'homme van Reine W. F., Haroun R. J., Kostermans L. B. T. (2005). “Checklists on seaweeds in the Atlantic Ocean and in the Cape Verde Archipelago,” in IV simpósio fauna e flora das ilhas atlânticas, Praia 9-13 setembro 2002 (Praia, Ilha de Santiago, República de Cabo Verde: Ministério do Ambiente, Agricultura e Pescas), 13–26.
Quartau R., Madeira J., Mitchell N. C., Tempera F., Silva P. F., Brandão F. (2016). Reply to comment by Marques et al. on “The insular shelves of the Faial-Pico Ridge (Azores Archipelago): A morphological record of its evolution”. Geochemistry Geophysics Geosystems 17, 633–641.
Quartau R., Tempera F., Mitchell N. C., Pinheiro L. M., Duarte H., Brito P. O., et al. (2012). Morphology of the Faial Island shelf (Azores): The interplay between volcanic, erosional, depositional, tectonic and mass-wasting processes. Geochemistry Geophysics Geosystems 13, Q04012. doi: 10.1029/2011GC003987
Quartau R., Trenhaile A. S., Mitchell N. C., Tempera F. (2010). Development of volcanic insular shelves: Insights from observations and modelling of Faial Island in the Azores Archipelago. Mar. Geology 275, 66–83.
Ramalho R. S., Quartau R., Trenhaile A. S., Mitchell N. C., Woodroffe C. D., Ávila S. P. (2013). Coastal evolution on volcanic oceanic Islands: A complex interplay between volcanism, erosion, sedimentation, sea-level change and biogenic production. Earth-Science Rev. 127, 140–170.
Rasser M. W., Piller W. E. (1999). Application of neontological taxonomic concepts to late Eocene Alpine Foreland Basin in Upper Austria: component analysis, facies, and palecology. Facies 42, 59–92.
Rebelo A. C., Johnson M. E., Quartau R., Rasser M. W., Melo C. S., Neto A. I., et al. (2018). Modern rhodoliths from the insular shelf of Pico in the Azores (Northeast Atlantic Ocean). Estuarine Coast. Shelf Sci. 210, 7–17.
Rebelo A. C., Johnson M. E., Rasser M. W., Silva L., Melo C. S., Ávila S. P. (2021). Global biodiversity and biogeography of rhodolith-forming species. Front. Biogeography 13 (1), e50646. doi: 10.21425/F5FBG50646
Rebelo A. C., Rasser M. W., Kroh A., Johnson M. E., Ramalho R. S., Melo C., et al. (2016). Rocking around a volcanic Island shelf: Pliocene rhodolith beds from Malbusca, Santa Maria Island (Azores, NE Atlantic). Facies 62, 22. doi: 10.1007/s10347-016-0473-9
Reyes J., Sansón M., Afonso-Carrillo J. (2005). Flora y vegetación marina. Algas y sebas. Patrimonio natural de la isla de Fuerteventura, Cabildo de Fuerteventura. Ed. Rodríguez Delgado O. (Tenerife: Gobierno de Canarias y Centro de la Cultura Popular Canaria), 117–140.
Riosmena-Rodríguez R. (2017). “Natural history of rhodolith/maërl beds: their role in near-shore biodiversity and management,” in Rhodolith/maërl beds: a global perspective. Eds. Riosmena-Rodríguez R., Nelson W., Aguirre J. (Cham: Coastal Research Library, Springer), 3–26. doi: 10.1007/978-3-319-29315-8_1
Rodriguez-Martin J., Cruz-Perez N., Santamarta J. C. (2022). Maritime climate in the Canary Islands and its implications for the construction of coastal infrastructures. Civil Eng. Journal-Tehran 8, 24–32.
Roettig C. B., Kolb T., Wolf D., Baumgart P., Richter C., Schleicher A., et al. (2017). Complexity of Quaternary aeolian dynamics (Canary Islands). Palaeogeography Palaeoclimatology Palaeoecol. 472, 146–162.
Roettig C. B., Kolb T., Zöller L., Zech M., Faust D. (2020). A detailed chrono-stratigraphical record of canarian dune archives - interplay of sand supply and volcanism. J. Arid Environments 183, 104240.
Rosas-Alquicira E. F., Riosmena-Rodríguez R., Couto R. P., Neto A. I. (2009). New additions to the Azorean algal flora, with ecological observations on rhodolith formations. Cahiers Biologie Mar. 50, 143–151.
Rusu E., Onea F. (2016). Estimation of the wave energy conversion efficiency in the Atlantic Ocean close to the European Islands. Renewable Energy 85, 687–703.
Sañé E., Chiocci F. L., Basso D., Martorelli E. (2016). Environmental factors controlling the distribution of rhodoliths: An integrated study based on seafloor sampling, ROV and side scan sonar data, offshore the W-Pontine Archipelago. Continental Shelf Res. 129, 10–22.
Sciberras M., Rizzo M., Mifsud J. R., Camilleri K., Borg J. A., Lanfranco E., et al. (2009). Habitat structure and biological characteristics of a maerl bed off the northeastern coast of the Maltese Islands (central Mediterranean). Mar. Biodiversity 39, 251–264.
Setchell W. A. (1943). Mastophora and the Mastophoreae: genus and subfamily of Corallinaceae. Proceedings of the National Academy of Science of the United States of America 29, 127–135.
Sewell A. A., Johnson M. E., Backus D. H., Ledesma-Vázquez J. (2007). Rhodolith detritus impounded by a coastal dune on Isla Coronados, Gulf of California. Cienc. Marinas 33, 483–494.
Silva P. C., Basson P. W., Moe R. L. (1996). Catalogue of the benthic marine algae of the Indian ocean Vol. 79 (University of California Publications in Botany), 1–1259.
Silva P. C., Meñez E. G., Moe R. L. (1987). Catalog of the benthic marine algae of the Philippines. Smithsonian Contributions to Mar. Sci. 27, 1–179. doi: 10.5479/si.1943667X.27.1
Silva P. C., Johansen H. W. (1986). A reappraisal of the order Corallinales (Rhodophyceae). British Phycological Journal 21, 245–254.
Spalding M. D., Fox H. E., Allen G. R., Davidson N., Ferdaña Z. A., Finlayson M., et al. (2007). Marine ecoregions of the world: A bioregionalization of coastal and shelf areas. BioScience 57, 573–583.
Steiner C., Hobson A., Favre P., Stampfli G. M., Hernandez J. (1998). Mesozoic sequence of Fuerteventura (Canary Islands): Witness of Early Jurassic sea-floor spreading in the central Atlantic. Geological Society of America Bulletin 110 (10), 1304–1317.
Steller D. L., Riosmena-Rodríguez R., Foster M., Roberts C. (2003). Rhodolith bed diversity in the Gulf of California: the importance of rhodolith structure and consequences of disturbance. Aquat. Conservation: Mar. Freshw. Ecosyst. 13, S5–S20.
Stelzer P. S., Mazzuco A. C. A., Gomes L. E., Martins J., Netto S., Bernardino A. F. (2021). “Taxonomic and functional diversity of benthic macrofauna associated with rhodolith beds in SE Brazil,” in PeerJ 9, e11903. doi: 10.7717/peerj.11903
Stillman C. J. (1999). Giant Miocene landslides and the evolution of Fuerteventura, Canary Islands. J. Volcanology Geothermal Res. 94, 89–104.
Taylor W. R. (1960). Marine algae of the eastern tropical and subtropical coasts of the americas (Ann Arbor: The University of Michigan Press), 870 pp.
Teichert S. (2014). Hollow rhodoliths increase Svalbard’s shelf biodiversity. Sci. Rep. 4, 6972. doi: 10.1038/srep06972
Townsend R. A., Huisman J. M. (2018) Coralline algae. In: Algae of Australia. Marine benthic algae of north-western Australia. 2. Red Algae. Ed. Huisman J.M. (Canberra: CSIRO Publishing), 86–146.
Troll V. R., Carracedo J. C. (2016). The geology of the Canary Islands (Elsevier), 621 pp. doi: 10.1016/C2015-0-04268-X
Wilson S., Blake C., Berges J. A., Maggs C. (2004). Environmental tolerances of free-living coralline algae (maerl): implications for European marine conservation. Biol. Conserv. 120, 279–289.
Woelkerling W. J., Irvine L. M., Harvey A. S. (1993). Growth-forms in non-geniculate coralline red algae (Corallines, Rhodophyta). Aust. Systematical Bot. 6, 277–293.
Womersley H. B. S., Bailey A. (1970). Marine algae of the Solomon Islands. Philos. Trans. R. Soc. London B. Biol. Sci. 259, 257–352.
Wynne M. J. (2017). A checklist of benthic marine algae of the tropical and subtropical western Atlantic: fourth revision. Nova Hedwigia Beiheft 145, 1–202.
Keywords: coralline algae, modern beaches, taphonomy, marine biodiversity, volcanic islands, Canary Islands
Citation: Rebelo AC, Martín-González E, Melo CS, Johnson ME, González-Rodríguez A, Galindo I, Quartau R, Baptista L, Ávila SP and Rasser MW (2022) Rhodolith beds and their onshore transport in Fuerteventura Island (Canary Archipelago, Spain). Front. Mar. Sci. 9:917883. doi: 10.3389/fmars.2022.917883
Received: 11 April 2022; Accepted: 03 October 2022;
Published: 25 October 2022.
Edited by:
Stefano Aliani, National Research Council (CNR), ItalyReviewed by:
Alan Giraldo, University of Valle, ColombiaSimone Simeone, National Research Council (CNR), Italy
Copyright © 2022 Rebelo, Martín-González, Melo, Johnson, González-Rodríguez, Galindo, Quartau, Baptista, Ávila and Rasser. This is an open-access article distributed under the terms of the Creative Commons Attribution License (CC BY). The use, distribution or reproduction in other forums is permitted, provided the original author(s) and the copyright owner(s) are credited and that the original publication in this journal is cited, in accordance with accepted academic practice. No use, distribution or reproduction is permitted which does not comply with these terms.
*Correspondence: Ana Cristina Rebelo, acfurtadorebelo@gmail.com