Corrigendum: Optimizing cryopreservation of sea cucumber (Apostichopus japonicus) sperm using a programmable freezer and computer-assisted sperm analysis
- 1Chinese Academy of Sciences (CAS) Key Laboratory of Marine Ecology and Environmental Sciences, Institute of Oceanology, Chinese Academy of Sciences, Qingdao, China
- 2Laboratory for Marine Ecology and Environmental Science, Qingdao National Laboratory for Marine Science and Technology, Qingdao, China
- 3Center for Ocean Mega-Science, Chinese Academy of Sciences, Qingdao, China
- 4Chinese Academy of Sciences (CAS) Engineering Laboratory for Marine Ranching, Institute of Oceanology, Chinese Academy of Sciences, Qingdao, China
- 5University of Chinese Academy of Sciences, Beijing, China
- 6Shandong Province Key Laboratory of Experimental Marine Biology, Qingdao, China
- 7The Innovation of Seed Design, Chinese Academy of Sciences, Wuhan, China
The sea cucumber Apostichopus japonicus has high nutritional, medicinal, and economic value. However, factors such as overexploitation, climate change, and environmental pollution have resulted in serious germplasm degradation in both farmed and wild A. japonicus, and it has been listed as endangered on the IUCN (International Union for Conservation of Nature) Red List of Threatened Species. Cryopreservation is an important method to protect germplasm resources and solve the problem of germplasm degradation. Using a programmable freezer and computer-aided sperm analysis, we comprehensively studied and screened the factors that affect the post-thaw motility of A. japonicus sperm during cryopreservation. Based on our results, we propose the following optimal cryopreservation procedure for A. japonicus sperm: cryo-diluent composition of 12.5% dimethyl sulfoxide and 0.1 mol/L glucose, with filter-sterilized (the filter mesh size: 0.45μm) natural seawater (NSW) as the extender; 1:5 mixing ratio of sperm and cryo-diluent; cooling rate and thawing temperatures of 10°C/min and 20°C, respectively. The post-thaw motility of sperm treated using the optimal procedure was > 65%, the fertilization rate (in the blastocyst stage) was nearly 80%, and the hatching rate (in the early auricularia larva stage) was > 65%. Additionally, frozen sperm that had been cryopreserved for 1 year retained a considerable post-thaw motility and fertilization rate compared to recently cryopreserved sperm. We detected obvious differences in sperm freezability among individual A. japonicus, and cryopreservation caused some damage to the sperm structure. In conclusion, our optimized procedure make large-scale cryopreservation of A. japonicus sperm possible, and our results provide valuable information that could be applied to research and conservation of A. japonicus.
1 Introduction
Sea cucumbers are important marine species with an annual net global production of nearly 220,000 tons, yielding a gross value of over $7 billion US dollars (Liu et al., 2020). The sea cucumber Apostichopus japonicus is considered to be the most nutritious edible sea cucumber with a high nutrional and medicinal value (Bordbar et al., 2011; Ru et al., 2019; Xing et al., 2021). A. japonicus is widely cultivated in China and has gradually become one of the most important fishery resources in Asia (Yang et al., 2015). However, factors such as overexploitation, climate change, and environmental pollution have resulted in serious germplasm degradation in both farmed and wild A. japonicus, and it has been listed as endangered on the IUCN (International Union for Conservation of Nature) Red List of Threatened Species. Germplasm degradation in farmed A. japonicus mainly causes problems such as slow growth, frequent disease outbreaks, and low survival rates, which result in billions in economic losses in China every year (Xia et al., 2012; Wang et al., 2014b). Therefore, preservation of A. japonicus germplasm resources has become an urgent task.
The development of cryopreservation protocols is important for protecting germplasm resources and solving the problem of germplasm degradation. Cryopreservation has many advantages, such as establishment of biobanks; providing year-round access to high quality material; breaking through geographic isolation; realizing remote hybridization; and protecting endangered species (Paredes, 2015; Paredes, 2016; Paredes et al., 2019). Compared with germplasm resources such as eggs and embryos, spermatozoa are easier to use in cryopreservation research due to their simpler structure (Tsai and Lin, 2012; Paredes et al., 2019; Campos et al., 2021). Generally, the sperm cryopreservation process includes sperm collection, quality evaluation, cryo-diluent (extender and cryoprotectant agent (CPA)) preparation, mixing of cryo-diluent with semen, cooling, storage, thawing, and sperm quality evaluation after thawing. Among these steps, the type and concentration of CPAs (cryoprotectant agents), the type of extenders, the mixing ratio of sperm and cryo-diluent, the cooling procedure, and the thawing procedure greatly impact the quality of the sperm after thawing, and the most suitable conditions for these factors vary by species (Dunn and Mclachlan, 1973; Akarasanon et al., 2004; Paredes, 2015).
In contrast to the abundance of cryopreservation studies focused on mammals, fish, and plants, few studies of marine invertebrates have been reported (Paredes, 2015). Of the exiting studies, mollusks (mainly oysters) and echinoids (mainly sea urchins) together account for 72% of the research subjects (Liu et al., 2015; Paredes, 2015; Paredes, 2016; Paredes et al., 2019). At present, only two studies of cryopreservation of sea cucumber sperm have been published (Shao et al., 2006; Mizuno et al., 2019). Shao et al. (2006) reported that the optimal freezing rate, sperm motility, and fertilization rate after thawing were 35°C/min, 76.7 ± 2.9%, and 60.2 ± 5.4% respectively, whereas Mizuno et al. (2019) reported 10.4°C/min, 19.3 ± 1.1%, and 89.8 ± 1.7%, respectively.
These significantly different results may be related to their experimental facilities and experimental methods, which are subject to more subjective factors. To date, most studies of cryopreservation of echinoderm sperm have controlled the freezing rate by adjusting the distance of the sample from the liquid nitrogen surface, and assessment of sperm motility has been conducted visually using an optical microscope. These methods have too many subjective factors and potential procedural errors to accurately assess the quality of frozen sperm (Dunn and Mclachlan, 1973; Asahina and Takahashi, 1978; Adams et al., 2004; Shao et al., 2006; Jalali and Crawford, 2012; Fabbrocini et al., 2014; Paredes, 2015; Paredes, 2016; Mizuno et al., 2019; Paredes et al., 2019).
To eliminate the influence of subjective factors and errors as much as possible, we used a programmable control rate freezer to control the freezing rate of sperm and computer-assisted sperm analysis (CASA) to quantify sperm motility (Paredes, 2015; Wu et al., 2019). We evaluated key parameters in the cryopreservation of sea cucumber sperm to identify the optimal cryopreservation method for A. japonicus sperm. Fertilization rate and hatching rate of frozen sperm with different post-thawing motility were examined, and the application value of frozen sperm that had been cryopreserved for one year was tested. Our results can be used to establish a standardized protocol for A. japonicus sperm cryopreservation technology and a dedicated cryobank for A. japonicus sperm.
2 Materials and methods
2.1 Materials
Sexually mature A. japonicus with a weight of 300–450 g were collected from the sea area of Yantai, China during the spawning season (May–July). About 40 sea cucumber (about 10 ind/m3) were maintained in two 2,000 L tanks (2×1×1 m3) filled with circulating seawater at 10-15°C in our laboratory. Continuous aeration was provided to ensure adequate oxygen and 40% of the seawater in each container was changed daily to guarantee good water quality. The culture water quality parameters were: temperature
10-15°C, salinity 30 ± 2 ppt, pH 7.8-8.2, and dissolved oxygen > 8mg·L−1. Sea cucumber was fed at 10 a.m. and 22 p.m. every day. The sea cucumbers feces were collected by siphon before changing the water. The uneaten food was also removed by siphoning.
2.2 Sperm collection and motility test
We removed sea cucumbers from the maintenance tanks for gamete collection and placed them individually in 20 L buckets connected to an air pump. For each spawning session, we injected 500 µL of 5 mg/L of a peptide (NGIWYamide, Wuhan Dangang Biotechnology Co., Ltd, Hubei, China) into the abdomen of three to five sea cucumbers. About 40 minutes after the injection, the sea cucumbers expelled gametes from the genital pore; females ejected granular eggs, and males ejected white, smoke-like semen (Kato et al., 2009). As soon as white semen was visible, which identified the specimen as male, the animal was immediately selected and dissected to obtain the testis. We used a sterile scalpel to dissect the sea cucumber, removed the complete testis, and dried its surface. We then cut the testis into pieces with scissors, placed the pieces into a sterile 50 mL centrifuge tube and filtered them through a 0.048 mm mesh in the tube, and placed the obtained semen in a 4°C constant temperature incubator until use. The whole process was conducted on crushed ice (Shao et al., 2006).
Sperm were activated by a 1:1000 dilution with activator. The composition of the activator was filter-sterilized (the filter mesh size: 0.45μm) NSW containing 5 wt% albumin (EVERY GREEN, Zhejiang, China) (Paredes et al., 2013; Mizuno et al., 2019). After the sperm were activated for about 30 s, we added 10 μL of the mixed solution to the sperm counting plate and placed it in the CASA system (ML-500JZ, Nanning Songjintianlun Biotechnology Co., Ltd, Nanning, China) to quantify sperm motility. Each sample was measured three times, and data from at least two fields of view were recorded each time. The CASA system was equipped with MAILANG sperm automatic analytical software (Nanning Songjintianlun Biotechnology Co., Ltd, Nanning, China), a high contrast sperm optical imaging lighting device, and a MAILANG computer sperm scanning counting board (Wu et al., 2019). The acquisition parameters were set as follows: frame rate = 60/s; total captured images = 60; microscope 100x magnification. We analyzed the following semen characteristics using the CASA system: a) sperm density (SD): the number of spermatozoid(spz) per unit volume in semen (spz/mL); b) sperm motility rate (SMR): motile sperm count as a percentage of total sperm count; c) sperm curvilinear velocity (VCL-tm, µm/sec): the ratio of the distance traveled by the sperm along the two-dimensional movement curve seen in the microscope to time; d) straight line velocity (VSL-tm, µm/sec): the average of the straight-line distance traveled by the sperm in the tested time range versus the time value; and e) beat cross frequency (BCF): whips per second) (Fabbrocini et al., 2016; Van Der Horst et al., 2018).
2.3 Protocol for sperm cryopreservation experiment
According to previous relevant experimental results (Adams et al., 2004; Shao et al., 2006; Jalali and Crawford, 2012; Fabbrocini et al., 2014; Liu et al., 2015; Paredes, 2015; Paredes, 2016; Mizuno et al., 2019; Paredes et al., 2019), we first established the general procedure for the sea cucumber sperm cryopreservation experiment using a programmable control rate freezer (CBS Micro-Digitcool, IMV Technologies (Shanghai) Co., Ltd) (http://www.cryobiosystem.cn). The steps were as follows:
(1) We prepared the cryo-diluent in advance and stored it in a 4°C constant temperature incubator until use. We made the cryo-diluent by mixing the extender and cryoprotectant in a certain volume ratio. For example, mix 45ml NSW and 5ml DMSO to make 50ml of 15% DMSO as cryo-diluent.
(2) Using fresh semen with sperm motility > 85%, we mixed fresh semen and cryo-diluent according to a certain dilution ratio into 2 mL cryotubes. The process was conducted on crushed ice. For example, mix 80ml fresh semen and 400ml cryo-diluent into 2 mL cryotubes at a dilution ratio of 1:5.
(3) We mixed the sample in the cryotube, immediately placed it in the programmable cooling instrument, and ran the cooling program. The cooling procedure was as follows: sample equilibration at 0°C for 5 min, cooling to -80° at a specific cooling rate, equilibration at -80°C for 5 min, cooling to -150°C at a cooling rate of 20°C/min, equilibration at -150°C for 5 min, and storage in liquid nitrogen (-196°C) for medium and long-term storage.
(4) The cryotube was removed from the liquid nitrogen after at least 2 h, and the sample was thawed in a water bath at 20°C, shaken gently, removed immediately when only a small amount of solids remained, and shaken in air until completely thawed. Sperm vitality then was checked immediately. A certain volume of thawed frozen semen was evenly mixed with the activator so that the total dilution reached 600 times. For example, mix 10ul thawed frozen semen and 990ul activator at a dilution ratio of 1:5. After the sperm were diluted and activated for about 30 s, 10 μL of the activated frozen semen was added to the sperm counting plate, and the sperm motility analysis was performed with CASA.
2.4 Screening of the optimal parameters for sperm cryopreservation
Based on the basic protocol of sea cucumber sperm mother liquor cryopreservation described above, we screened each key part to select the optimal parameters for sperm cryopreservation. Each screening experiment was carried out in sequence, and the next experiment was performed after analyzing the results of the previous experiment. The semen used in each screening experiment came from only one sea cucumber.
2.4.1 Screening for the optimal extender
We tested seven different extenders [D15, Cortland, MPRS, artificial seawater (ASW), filter-sterilized (the filter mesh size: 0.45μm) NSW, Hank’s Balanced Salt Solution (HBSS), and calcium-free Hank’s (D-Hank’s)], all of which are commonly used extenders in the cryopreservation of fish and invertebrate sperm (Adams et al., 2004; Shao et al., 2006; Fan et al., 2014; Figueroa et al., 2018; Vuthiphandchai et al., 2021) (Table 1). In this experiment, we used dimethyl sulfoxide (DMSO) as the cryoprotectant; cryo-diluent was prepared with a concentration of 10%; the mixing ratio of semen and cryo-diluent was 1:5; the cooling rate was 15°C/min; and the thawing temperature was 20°C. There were six replicates in each experimental group. Table 1 shows the formulas of the six extenders.
2.4.2 Screening for optimal cooling rate
In this experiment, we tested five cooling rates: 10°C/min, 15°C/min, 20°C/min, 25°C/min, and 30°C/min (Paredes, 2015; Paredes, 2016; Paredes et al., 2019). We used NSW and DMSO as extender and cryoprotectant to prepare cryo-diluent with a concentration of 10%, and the mixing ratio of semen and cryo-diluent was 1:5. The thawing temperature was 20°C. There were four replicates in each experimental group.
2.4.3 Screening for optimal dilution ratio
The dilution ratio is the mixing ratio of sperm and cryo-diluent. In this experiment, we tested six dilution ratios: 1:1, 1:3, 1:5, 1:7, 1:9, and 1:19. We used NSW and DMSO as extender and cryoprotectant to prepare cryo-diluent with a concentration of 10%, the cooling rate was 10°C/min, and the thawing temperature was 20°C. There were four replicates in each experimental group.
2.4.4 Screening for optimal thawing temperature
In this experiment, we tested three thawing temperatures: 20°C, 37°C, and 50°C. We used NSW and DMSO as extender and cryoprotectant to prepare cryo-diluent with a concentration of 10%, the cooling rate was 10°C/min, and the mixing ratio of semen and cryo-diluent was 1:5. There were six replicates in each experimental group.
2.4.5 Screening for optimal cryoprotectant type and concentration
We tested six commonly used CPAs: DMSO, propylene glycol (PG), ethylene glycol (EG), glycerol (GLY), methanol (MeOH), and dimethylacetamide (DMA) (Paredes, 2015; Paredes, 2016; Paredes et al., 2019). We used NSW as the extender to prepare cryo-diluent with concentrations of 5%, 10%, 15%, and 20% for each CPA. The mixing ratio of semen and cryo-diluent was 1:5, the cooling rate was 10°C/min, and the thawing temperature was 20°C. There were four replicates in each experimental group, and further screening will be carried out if necessary.
2.4.6 Screening for optimal co-CPAs
We tested three co-CPAs (non-permeable CPAs: sucrose, glucose (Glu), trehalose) at 0.025 mol/L, 0.05 mol/L, 0.1 mol/L, and 0.2 mol/L, and egg yolk powder at 10%, 20% (W/V). We used NSW and DMSO as the extender and cryoprotectant to prepare cryo-diluent with a concentration of 12.5%, the cooling rate was 10°C/min, the dilution ratio was 1:5, and the thawing temperature was 20°C. There were four replicates in each experimental group. The control group did not add co-CPAs and other conditions remained unchanged.
2.4.7 Screening for optimal frozen storage volume and type of cryotube
We tested 0.5 mL of cryo-diluent in a 0.5 mL cryotube and 0.5 mL, 1 mL, and 2 mL of cryo-diluent in a 2 mL cryotube. We used NSW and DMSO as the extender and cryoprotectant and prepared cryo-diluent with a concentration of 12.5%. The cooling rate was 10°C/min, the dilution ratio was 1:5, and the thawing temperature was 20°C. There were four replicates in each experimental group.
2.5 Fertilization experiment using frozen sperm with different cryopreservation formulas
Based on the results of the screening experiments described above, we conducted fertilization rate and hatching rate experiments using sperm from eight different groups of sperm: groups of sperm from five frozen formulas with different frozen sperm motility (A: 12.5% DMSO+ 0.1 mol/L Glu; B: 12.5% DMSO; C: 20% PG; D: 10% EG; and E: 20% DMA); sperm from formulas A and B that were cryopreserved for 1 year (A-1yr, B-1yr); and fresh unfrozen sperm (control group). There were three replicates in each experimental group.
After female sea cucumbers described in section 2.2 released eggs, we collected and rinsed fresh eggs to prepare the mother solution and calculate the egg concentration. Fertilization experiments were carried out within 2 h after eggs were released. We prepared fresh unfrozen sperm in advance and we thawed the frozen sperm, and then we calculated the concentration of sperm using CASA. Finally, fresh semen and frozen semen were separately mixed with eggs in 2 L beakers at a sperm-to-egg ratio of 10,000:1 (Shao et al., 2006). The beakers were filled with 2 L of filtered NSW and continuously aerated, and a heating rod was used to keep the water temperature at a constant temperature of 20°C. The fertilization rate and the hatching rate were calculated at 10 h and 52 h after fertilization, and random samples were taken from each beaker for measurement at each time point. The fertilization rate was calculated as the percentage of embryos in the blastocyst stage to the total number of cells in the field of view. The hatching rate was calculated as the percentage of the number of early auricularia larva to the total number of cells in the field of view.
2.6 Ultrastructure of fresh and cryopreserved sperm
Samples from fresh and thawed cryopreserved sperm treated with frozen formula A described above were sent to Wuhan Service Biotechnology Co., Ltd. for examination of ultrastructure using scanning electron microscopy (SEM) and transmission electron microscopy (TEM). Please refer to the website for the related operation steps (https://www.servicebio.cn/data-detail?id=4301&code=DJSYBG).
2.7 Statistical analysis
All data are presented as the mean ± standard error (SE). Statistical analysis was performed using IBM SPSS software 23.0 (Armonk, NY, USA). The percentage data for various treatment and control groups, such as SMR, fertilization rate, and hatching rate, were arcsine-square root transformed, and the normality of the distribution of the data was evaluated using the Shapiro-Wilk test. The equality of the groups was assessed using the F test or Bartlett’s test. The Tukey multiple comparison test and Student’s t-test were conducted to examine differences among the groups. For cases in which the data did not pass the normality test or the equality test, the statistical significance of the differences among the groups was calculated using the non-parametric Kruskal-Wallis H test. All significance levels were set at P < 0.05.
3 Results
3.1 Information about different sea cucumber individuals
Table 2 lists relevant information about seven sea cucumber individuals and their sperm before and after cryopreservation. Gross weight (GW) showed a correlation with body wall weight (BWW) and testis weight (TW) (R = 0.63; R = 0.70, respectively), and TW had the strongest correlation with semen volume (SV). Therefore, we initially assessed the relationship between SV and GW of sea cucumbers (y = 0.19x – 51.82, R =0.71, y = SV, x = GW) so that we could use the gross weight of sea cucumbers at the mature stage to estimate the amount of semen excreted. Fresh sperm motility (FSM) and fresh sperm density (FSD) were not strongly correlated with GW (R=0.39, R=0.32). Additionally, there was no correlation between FSM and FSD or between FSM and post-thawing sperm motility (TSM). We found that the cryopreservation process significantly reduced sperm density (P < 0.05): the density in fresh samples was 2.0–5.0×1010 Pcs/mL compared to 1.0–2.0×1010 Pcs/mL in thawed samples. The fresh sperm velocity was significantly higher than that of post-thawing sperm. The curvilinear velocity and straight-line velocity of A. japonicus fresh sperm were generally in the range of 80-100 µm/s and 50-70 µm/s respectively. The curvilinear velocity and straight-line velocity of A. japonicus frozen sperm were generally in the range of 40-90 µm/s and 30-60 µm/s respectively.
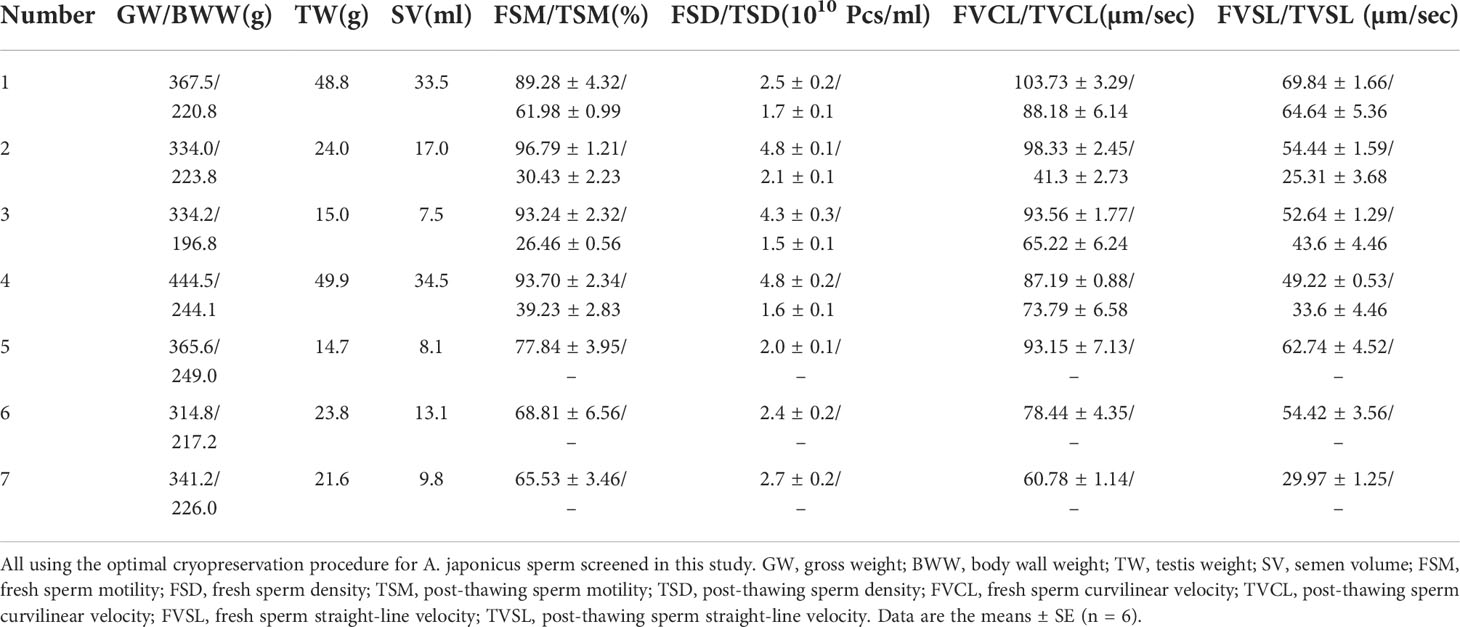
Table 2 Information about different sea cucumber individuals and their sperm before and after cryopreservation.
3.2 Optimal extender
Table 2 and Figure 1 show the composition of the six extenders tested and the results of the screening experiment, respectively. The post-thaw motility was highest when the extender was NSW (45.97% ± 2.30%), followed by ASW (40.97% ± 1.32%) and D15 (32.05% ± 2.23%). Cryopreserved spermatozoa showed lower motilities (< 15%) when the other three extenders were used.
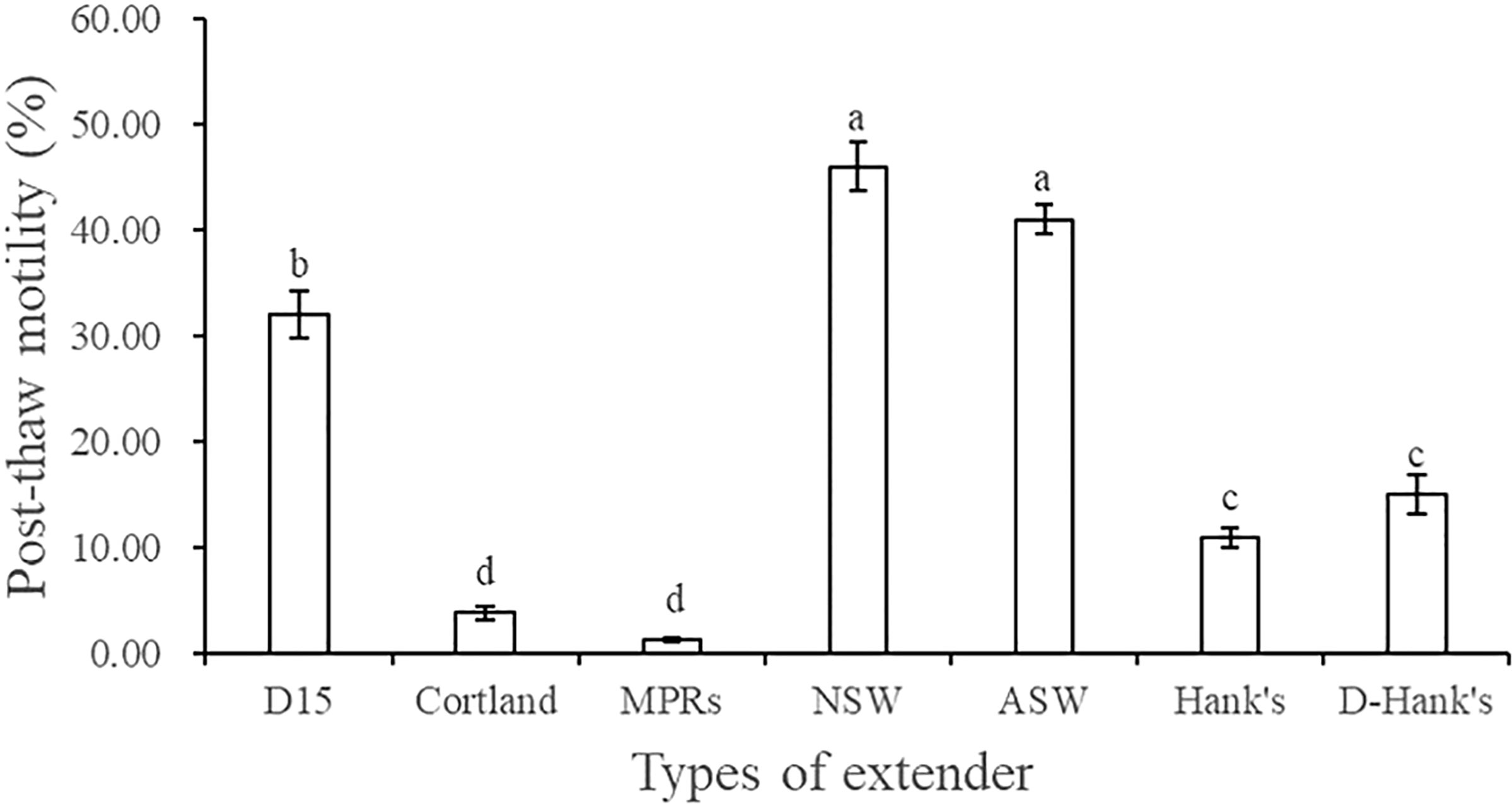
Figure 1 Effect of extender type on the post-thaw motility of A. japonicus spermatozoa. Data are presented as means ± SE (n = 6). Different letters indicate significant differences (P < 0.05). Data were not transformed when performing one-way ANOVA.
3.3 Optimal cooling rate
Figure 2 shows the post-thaw motility of sperm that were cryopreserved using five different cooling rates. As the cooling rate increases, the sperm motility significantly decreased gradually (P < 0.05). The post-thaw motility was highest at the cooling rate of 10°C/min (49.43% ± 3.63%), followed by 15°C/min (45.66% ± 3.98%), and these motility values did not differ significantly (P > 0.05).
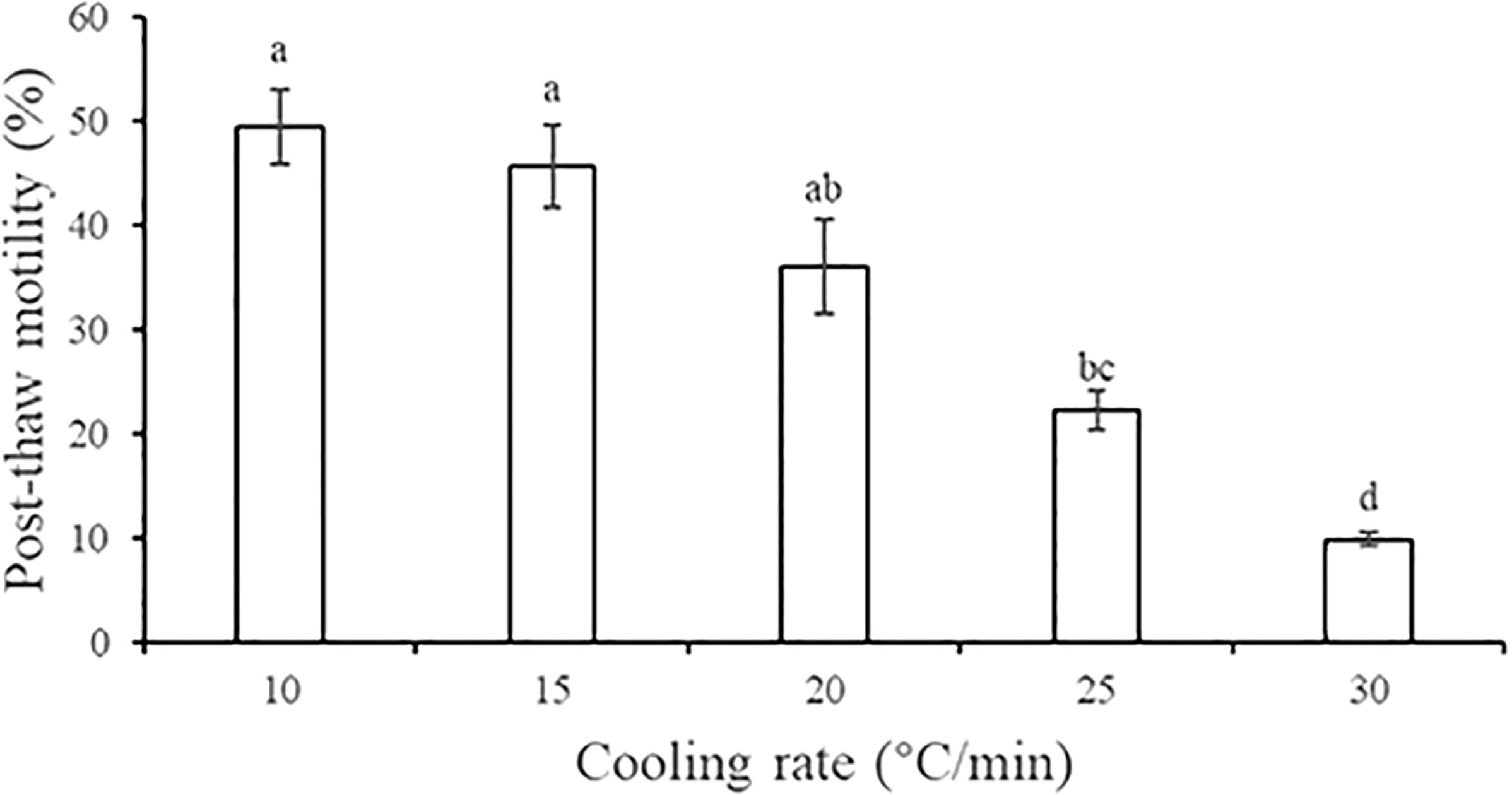
Figure 2 Effect of five cooling rates on the post-thaw motility of A. japonicus spermatozoa. Data are presented as the means ± SE (n = 4). Different letters indicate significant differences (P < 0.05). Data were not transformed when performing one-way ANOVA.
3.4 Optimal dilution ratio
When we tested six different dilution ratios, we found that post-thaw motility was highest when the mixing ratio of sperm and cryo-diluent was 1:5 (29.79% ± 1.70%), followed by 1:7 (27.84% ± 1.48%), 1:9 (26.23% ± 1.21%), and 1:3 (24.54% ± 1.60%), and there was no significant difference in motility among these groups (P > 0.05) (Figure 3). When the dilution ratio was too small (1:19) or too large (1:1), lower sperm motilities were recorded (13.51% ± 2.04% and 15.60% ± 0.58%, respectively). Therefore, we used 1:5 as the dilution ratio in subsequent screening experiments.
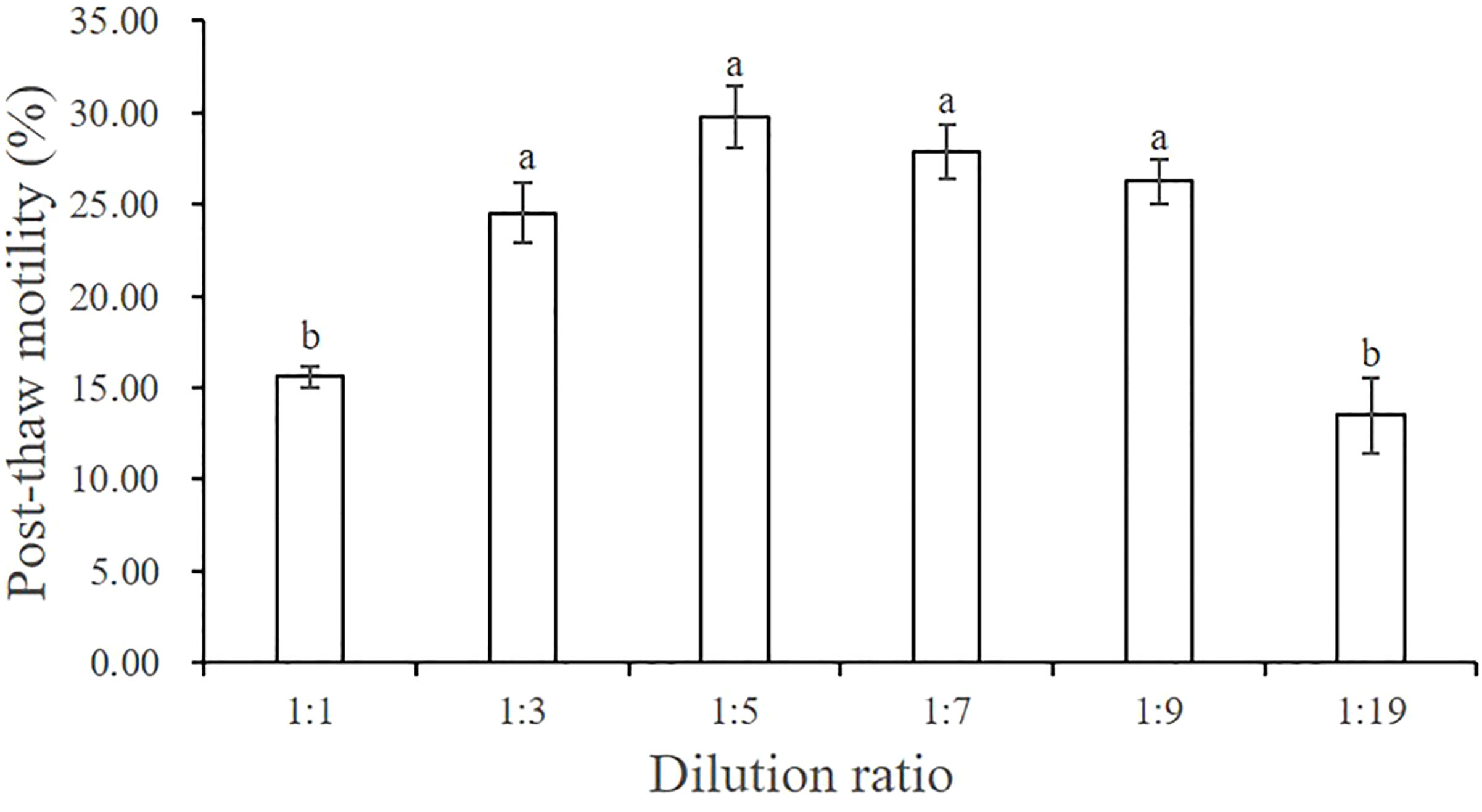
Figure 3 Effect of six dilution ratios on the post-thaw motility of A. japonicus spermatozoa. Data are presented as the means ± SE (n = 4). Different letters indicate significant differences (P < 0.05). Data were not transformed when performing one-way ANOVA.
3.5 Optimal thawing temperature
We found that higher thawing temperature resulted in lower post-thaw sperm motility (Figure 4). The post-thaw motility was highest when the thawing temperature was 20°C (27.23% ± 1.83%), which was significantly higher than that at 37°C (18.84% ± 1.79%) and 50°C (14.59% ± 1.19%) (P < 0.05).
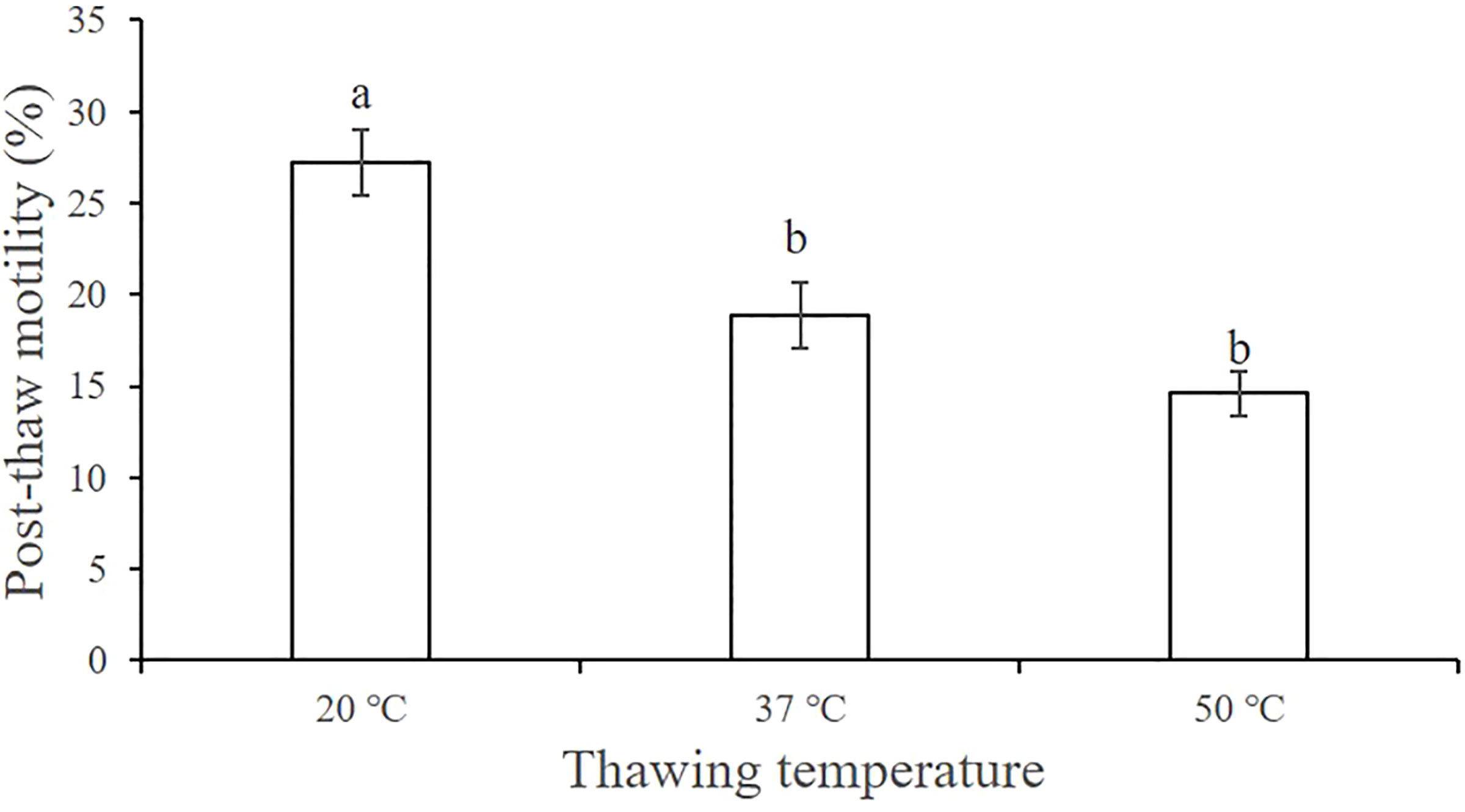
Figure 4 Effect of the thawing temperature on the post-thaw motility of A. japonicus spermatozoa. Data are presented as the means ± SE (n = 6). Different letters indicate significant differences (P < 0.05). Data were not transformed when performing one-way ANOVA.
3.6 Optimal cryoprotectant type and concentration
Figure 5A shows the post-thaw motility of spermatozoa that were cryopreserved with diluents containing one of six kinds of CPAs at one of four concentrations. The motility was highest when using 10% DMSO as the CPA (16.17% ± 0.50%), followed by 15% DMSO (14.91% ± 0.60%), and these motility values did not differ significantly (P > 0.05). Among the other CPAs, the 20% PG (7.84% ± 0.61%), 10% EG (4.98% ± 0.98%), and 20% DMA (6.52% ± 0.84%) treatment groups had relatively high post-thaw sperm motility. Although the sea cucumber sperm used in this experiment had poor freezing resistance such that post-thaw sperm motility was not > 20%, we could still infer from the trend of the results that DMSO was the most suitable of the six antifreeze agents. Therefore, we conducted another screening experiment to identify the optimal concentration of DMSO and found that 12.5% DMSO yielded higher sperm motility than 10% DMSO and 15% DMSO (Figure 5B).
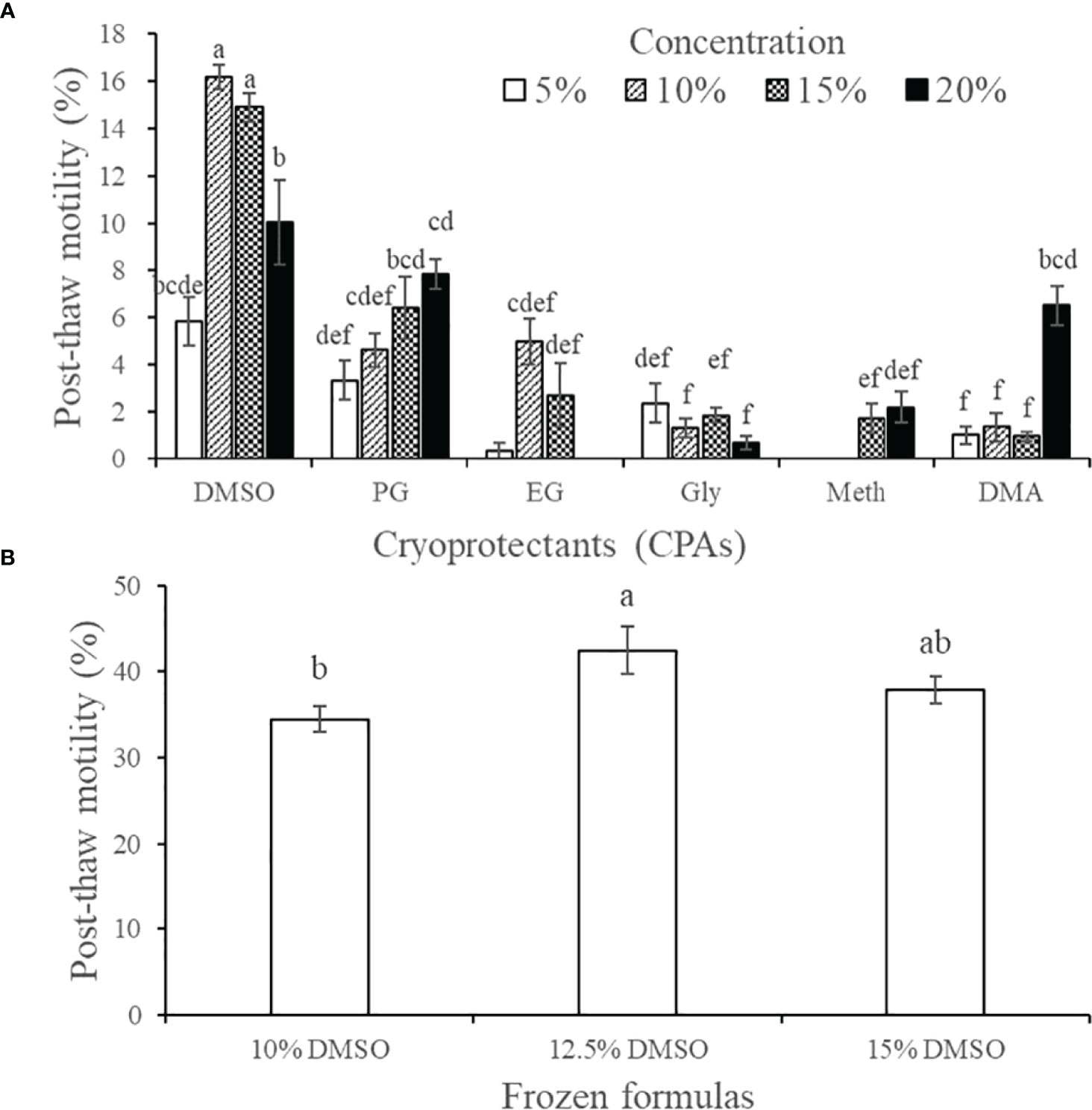
Figure 5 Effect of cryoprotective agents on the post-thaw motility of A. japonicus spermatozoa. (A) Preliminary screening results of six CPAs at four concentrations; (B) Further screening results of the optimal concentration of DMSO. Data are presented as the means ± SE (n = 4). Different letters indicate significant differences (P < 0.05). Data were not transformed when performing one-way ANOVA. Note: One-way ANOVA was performed on all treatment groups in A with a normal distribution, and discarding obviously invalid treatment group data.
3.7 Optimal co-CPAs
Compared with the post-thaw sperm motility of the control group (38.34% ± 2.69%), the non-permeable co-CPA and concentration that achieved the highest post-thaw sperm motility was 0.1 mol/L Glu (65.07% ± 3.67%, P < 0.001), followed by 0.05 mol/L Glu (50.78% ± 1.98%, P < 0.05) (Figure 6). The post-thaw sperm motility of the other treatment groups was not significantly different from that of the control group (P > 0.05). The two concentrations of yolk (10% and 20%, W/V) did not achieve the expected effect as co-CPAs, and the post-thaw sperm was basically non-viable (< 2%).
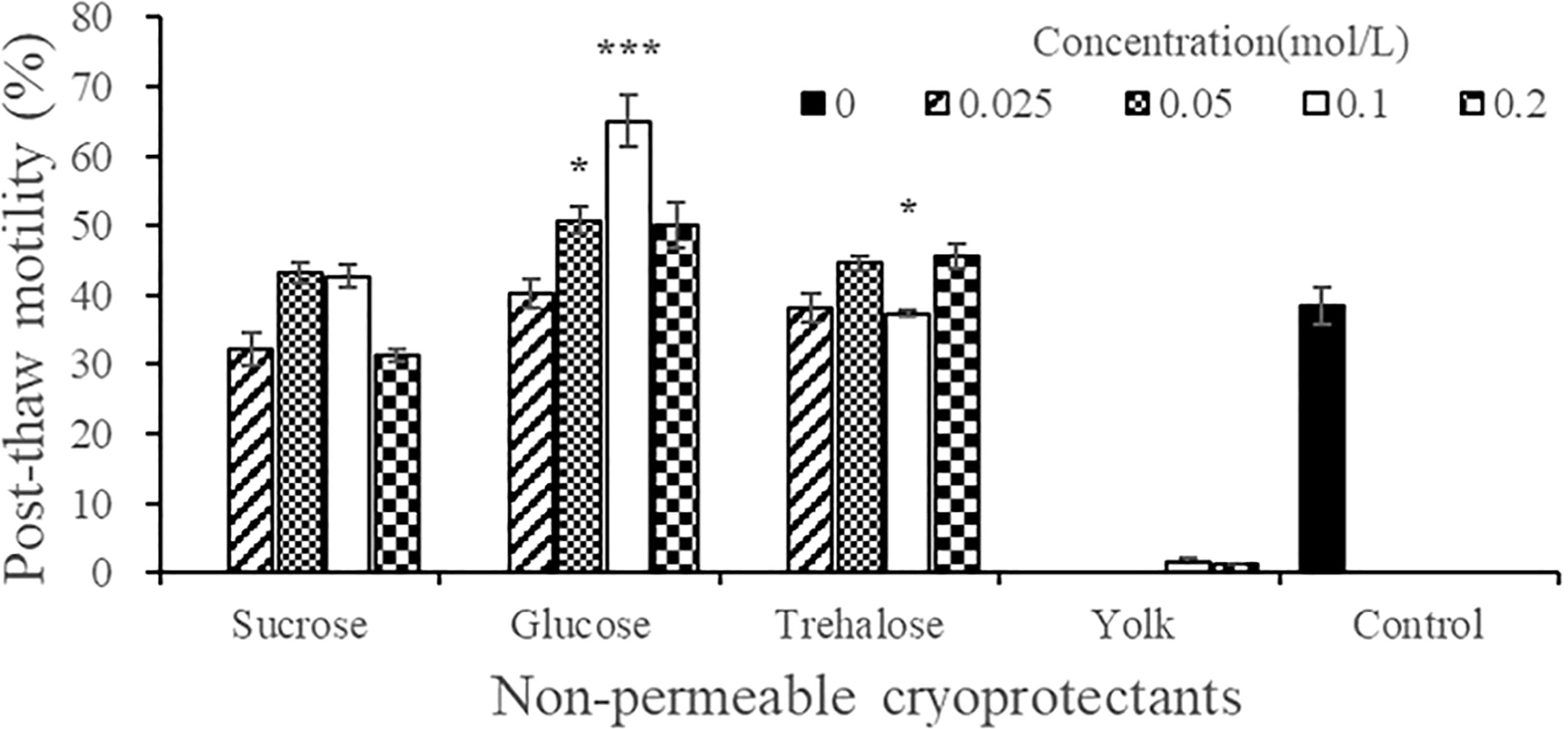
Figure 6 Effect of the types and concentrations of four non-permeable cryoprotectant agents on the post-thaw motility of A. japonicus spermatozoa. Note: The egg yolk powder uses the mass concentration (W/V), 0.1 represents 10% (0.1 kg/L), and 0.2 represents 20% (0.2 kg/L). Data are presented as the means ± SE (n = 4). Asterisks indicate significant differences between each experimental group and the control group (*P < 0.05, ***P < 0.001). One-way ANOVA was performed between the four concentration treatment groups of the three carbohydrates and the control group respectively, and data were not transformed.
3.8 Optimal frozen storage volume and type of cryotube
Figure 7 shows the post-thaw motility of sperm that were cryopreserved with different frozen storage volumes and types of cryotube. No significant difference in the cryopreservation effect was detected between the 0.5 mL and the 2 mL cryotubes (P > 0.05), for which the post-thaw motility values of sperm were 57.44% ± 2.52% and 56.27% ± 6.41%, respectively, when filled with 0.5 mL of cryo-diluent. Additionally, post-thaw sperm motility did not differ significantly when the 2 mL cryotube was filled with different volumes of cryo-diluent.
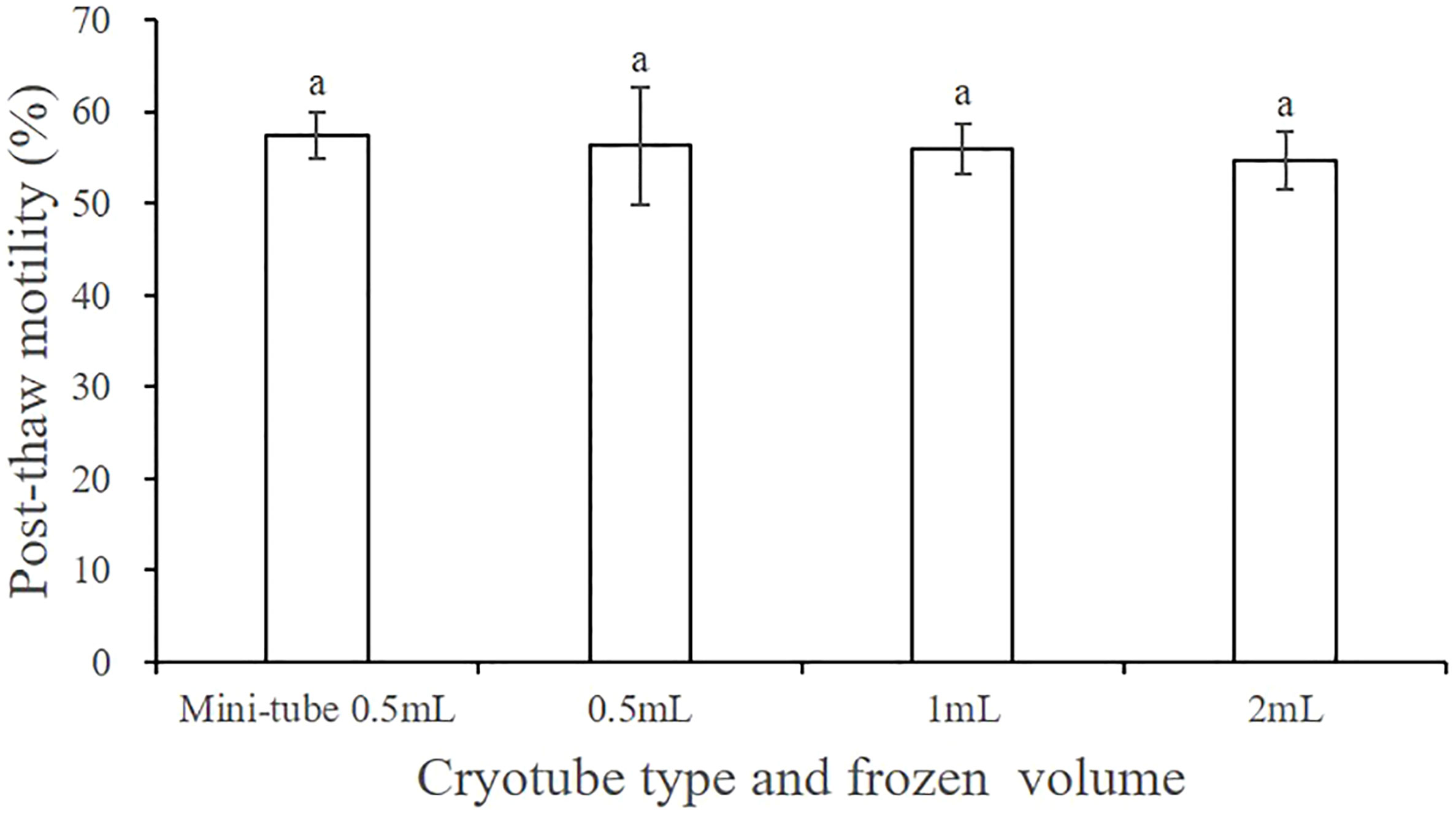
Figure 7 Effect of the cryotube types and frozen storage volume on the post-thaw motility of A. japonicus spermatozoa. Data are presented as the means ± SE (n = 4). Different letters indicate significant differences (P < 0.05). Data were not transformed when performing one-way ANOVA.
3.9 Fertilization experiment using sperm frozen with different cryopreservation formulas
Based on the experiments described in sections 3.2–3.8, the optimal procedures for cryopreservation of A. japonicus sperm is as follows: cryo-diluent composition of 12.5% DMSO and 0.1 mol/L Glu using filter-sterilized (the filter mesh size: 0.45μm) NSW as the extender; 1:5 mixing ratio of sperm and cryo-diluent; cooling rate and thawing temperatures of 10°C/min and 20°C, respectively.
Sperm treated with the five different cryopreservation formulas had different post-thaw motility (Figure 8A). The motility was highest when using formula A (68.92% ± 0.97%), followed by formula B (63.04% ± 1.17%), and there was no significant difference between them (P > 0.05). Sperm treated with formulas C and E also achieved relatively high post-thaw motility (40.43% ± 7.33% and 34.45% ± 1.48%, respectively), but the values were significantly lower than those of formulas A and B (P < 0.05). The post-thaw motility of the sperm treated with formula D was extremely low (5.24% ± 1.43%), and the value was significantly different from those of the other formulas (P < 0.05). We also found that frozen sperm maintained a certain degree of vitality after one year of storage, and the corresponding post-thaw sperm motility values for formula A-1yr and formula B-1yr were 40.65% ± 1.08% and 36.08% ± 1.79%, respectively. The post-thaw motility of sperm cryopreserved with formula B after 1 year was not significantly different from its motility value a year earlier (38.34% ± 2.67%) (Figure 6).
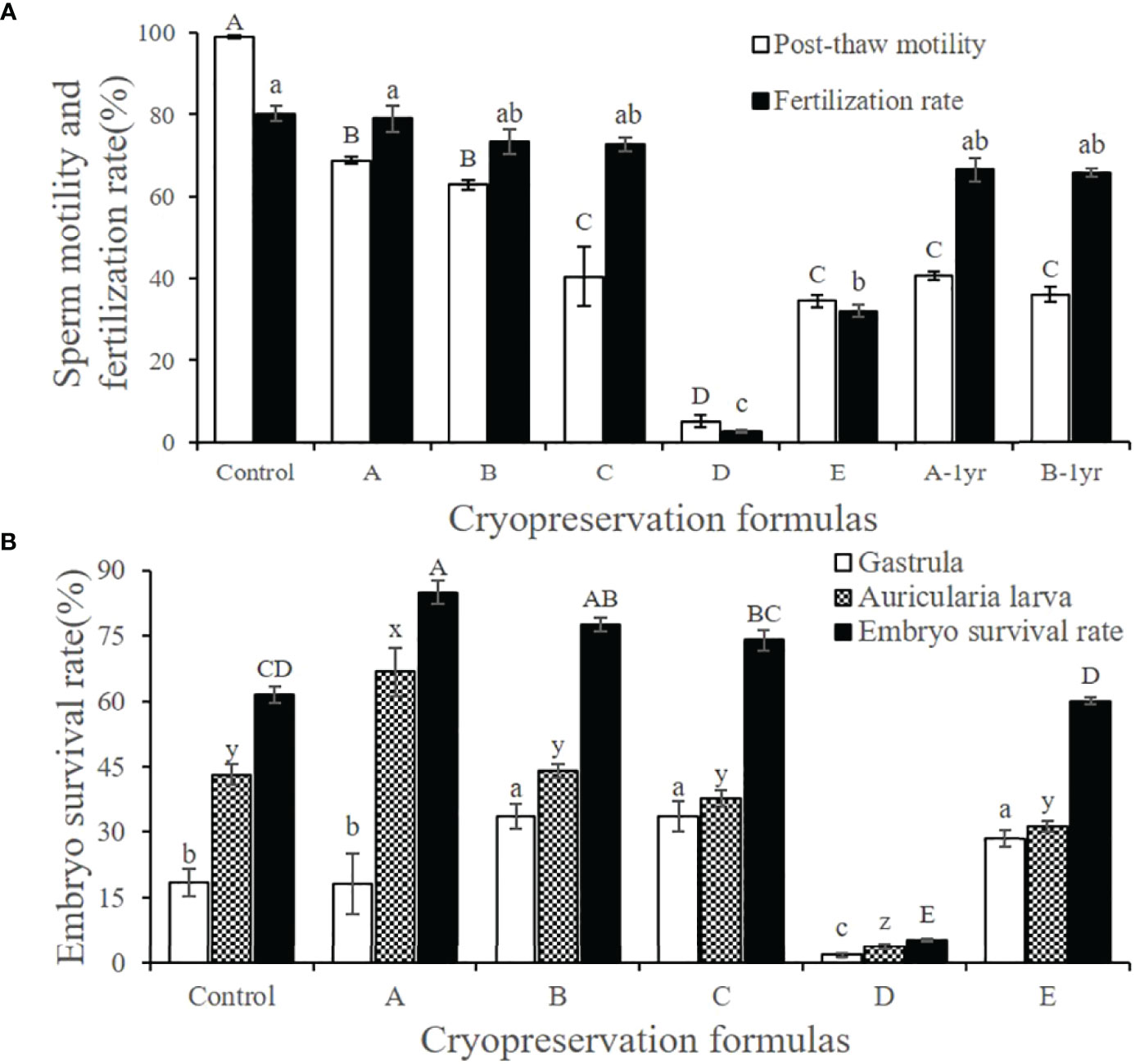
Figure 8 Effect of cryopreservation formulas with different post-thaw sperm motility on fertilization rate and hatching rate. (A) Post-thaw sperm motility and fertilization rate under different cryopreservation formulas. (B) Hatching rate of frozen spermatozoa under different cryopreservation formulas. Different cryopreservation formulas were (Control) Fresh unfrozen sperm; (A) 12.5% DMSO + 0.1 mol/L Glu; (B) 12.5% DMSO; (C) 20% DMA; (D) 10% EG; (E) 20% PG; (A-1yr) One-year cryopreservation of sea cucumber sperm with formula A; (B-1yr) One-year cryopreservation of sea cucumber sperm with formula (B) The rest of the freezing procedure was the same and was the optimum procedure. The fertilization rate and the hatching rate were calculated at 10 h (blastocyst stage) and 52 h (early auricularia larva stage) after fertilization. Data are presented as the means ± SE (n = 6). Different letters indicate significant differences (P < 0.05). Data were not transformed when performing one-way ANOVA.
After thawing, the fertilization rate of the cryopreserved sperm treated with formula A (79.03% ± 3.22%) was almost the same as that of fresh sperm (80.22% ± 1.90%), even though the motility of the fresh sperm was 99.00% ± 1.90% (Figure 8A). The fertilization rates of frozen sperm treated with formula B (73.34% ± 3.01%), formula C (72.86% ± 1.69%), formula A-1yr (66.67% ± 2.85%), and formula B-1yr (65.69% ± 1.04%) did not differ significantly from one another (P > 0.05). Although the post-thaw motility of the sperm treated with formula E was not significantly different from that of formula C, its fertilization rate was significantly lower than that of the other three formulas (P > 0.05), and a large number of deformed embryos appeared in this group. Formula D had the lowest fertilization rate due to the lowest post-thaw motility, and a large number of eggs were observed to be unfertilized and necrotic.
We measured the hatching rate 52 h after fertilization, so we observed both early auricularia larvae as well as some gastrula embryos. We counted both and used the sum of the two as the embryo survival rate (Figure 8B). The low post-thaw sperm motility and low fertilization rate of the formula D treatment group resulted in a low hatching rate, so we did not include the data in further analyses. Cryopreserved sperm treated with formula A had the highest hatching rate (66.74% ± 5.61%) and embryo survival rate (84.85% ± 2.70%), and the values were significantly higher than those of the other treatment groups (P < 0.05). Unexpectedly, the fresh sperm control group had a significantly lower hatching rate (43.03% ± 2.33%) than the formula A group (P < 0.05) and a significantly lower gastrula ratio (18.46% ± 3.19%) than the other treatment groups (P < 0.05). The water in the control group was turbid, with a lot of foam, and we speculate that this may be associated with the addition of an excessive number of sperm. Although the hatching rates of the formula B (44.06% ± 1.58%), formula C (37.51% ± 1.90%), and formula E (31.43% ± 1.30%) groups were significantly lower than those of formula A (P < 0.05), they had a relatively high proportion of gastrulae, resulting in a higher embryo survival rate, which means that the larvae had the potential to continue to develop.
3.10 Ultrastructure of fresh and cryopreserved sperm
Figure 9 shows the ultrastructure of fresh and cryopreserved A. japonicus sperm. SEM results showed that the head, middle, and tail of fresh sperm were intact and continuous (Figure 9A), whereas the head and middle of frozen sperm were ruptured and the tail was broken (Figure 9B). The proportions of morphologically normal sperm and morphologically deformed sperm in fresh semen were 89.0% and 11.0%, respectively. While those in cryopreserved sperm were 58.0% and 42.0%, respectively. TEM micrographs showed that the sperm membrane of fresh sperm was complete, the head and neck were continuous, the nucleus chromatin had high electron density, the proximal centriole structure was normal, the number of double microtubules was normal, all of them were symmetrically distributed in nine pairs, and there was no obvious separation between the central microtubules and the double microtubules (Figures 9C, E). In cryopreserved sperm, the sperm membrane was largely damaged, the organelles were free, the head was irregular, the nucleus was irregular in shape, the chromatin was condensed, the sub-acrosomal space was visible between nuclei, the sperm tail structure was swollen and the membrane was discontinuous, mitochondria were obviously swollen, the mitochondrial membrane was dissolved, cristae disappeared, most of the doublet microtubules were separated, and the sub-microtubule structure was absent (Figures 9D, F). These results showed that the cryopreservation process caused structural damage to A. japonicus sperm.
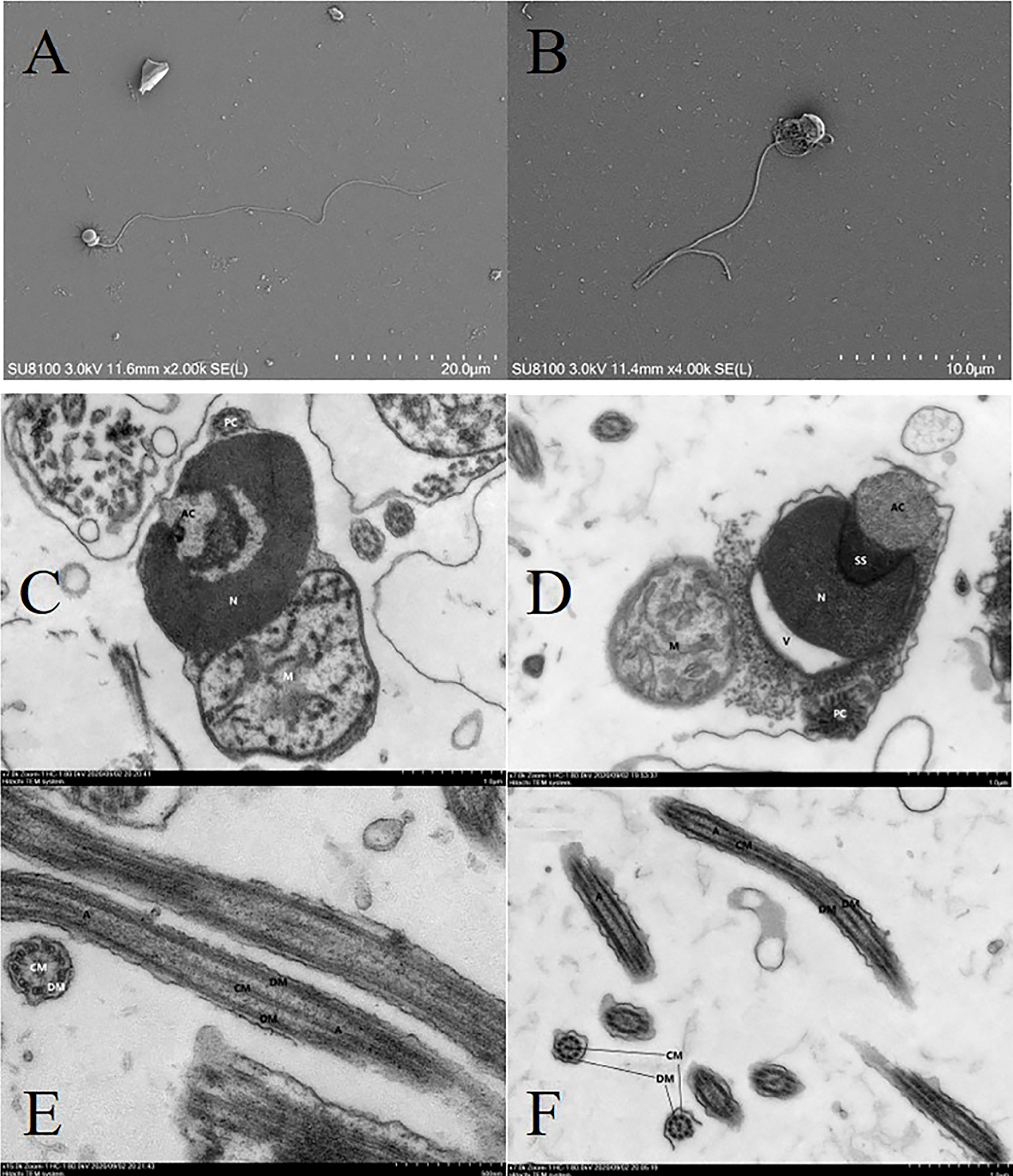
Figure 9 Electron micrographs showing structures of fresh and cryopreserved sperm of A. japonicus. SEM results: (A) fresh sperm; (B) cryopreserved sperm. TEM results: (C) head of fresh sperm; (D) head of cryopreserved sperm; (E) tail of fresh sperm; (F) tail of cryopreserved sperm. AC, acrosome; N, nucleus; M, mitochondrion; PC, proximal centriole; SS, subacrosome space; V, vesicle; A, axoneme; CM, central microtubules; DM, double microtubules; SEM, scanning electron microscopy.
4 Discussion
4.1 The cryopreservation procedure for A. japonicus sperm
4.1.1 Extender
It is generally believed that an extender used for semen cryopreservation must be able to inhibit sperm motility and maintain the balance of cell electrolytes and osmotic pressure (Dong et al., 2005a; Liu et al., 2015). In cryopreservation studies of marine fish and mollusk sperm, Hank’s balanced salt solution without Ca2+ is often used as the extender (Dong et al., 2005a). However, ASW or filtered NSW has been widely used as extender in echinoderm sperm cryopreservation research, but little is known about the effects of other potential extenders (Dunn and Mclachlan, 1973; Adams et al., 2004; Shao et al., 2006; Jalali and Crawford, 2012; Fabbrocini et al., 2014). It is worth noting that echinoderm sperm can be activated by ASW or filtered NSW, which is obviously contrary to the need for the extender to inhibit sperm motility (Shao et al., 2006).
In this study, we tested the effects of seven kinds of extenders on the motility of cryopreserved A. japonicus sperm and still found that using NSW as the extender resulted in the highest post-thaw motility (%), followed by ASW. NSW or ASW was suitable as the extender for echinoderm sperm cryopreservation may because the low amount of seawater used in the experiment that it did not activate all of the sperm and the longer survival time of the sperm after activation (Shao et al., 2006; Gallego et al., 2014; Fabbrocini et al., 2016). Shao et al. (2006) found that ASW concentration < 30% cannot activate A. japonicus sperm. Gallego et al. (2014) found that the sperm motilities and velocities of the sessile species (sea urchin and ascidian) were able to maintain at its initial value for a long time (about 45 min) after activation. Fabbrocini et al. (2016) found that sea urchin sperm could maintain high motility for 1 h after activation and maintain relative motility for 24 h. Although NSW is currently the most commonly used extender for cryopreservation of echinoderm sperm, we believe that we should continue to study the activation mechanism of sperm and develop new diluents to reduce the energy consumption of sperm (Liu et al., 2015).
4.1.2 Freezing techniques and cooling rate
Techniques to freeze sperm can be categorized as non-programmable (liquid nitrogen vapor) and programmable. Non-programmable freezing technique usually control the cooling rate by adjusting the distance of the sample from the liquid nitrogen (LN). Programmable freezing requires special equipment to control the cooling rate(s) precisely (Liu et al., 2015; Paredes, 2015). Programmable freezing offers more precise temperature control and more accurate results than non-programmable freezing. To date, programmable freezing has been used frequently in studies of fish and mollusks, but it has rarely been used in cryopreservation research of echinoderm sperm.
In this study, we used a programmable control rate freezer to control the entire process of equilibrium and cooling, and found that frozen sperm had better post-thaw motility when the cooling rate was 10°C/min and 15°C/min and that post-thaw motility decreased as the cooling rate increased (Figure 2). Mizuno et al. (2019) reported that the post-thaw motility of frozen sperm was highest when the height of the sample from the liquid nitrogen level was 15 cm and the cooling rate was 10.4°C/min, which is basically consistent with our findings. In many previous echinoderm sperm cryopreservation studies, the optimal cooling rates ranged from 5°C/min to 50°C/min (Dunn and Mclachlan, 1973; Adams et al., 2004; Shao et al., 2006; Jalali and Crawford, 2012). However, these values were all based on non-programmable freezing techniques and different freezing devices, so the results are not comparable. Excessively rapid cooling may increase osmotic shock, cause more ice crystal damage, have irreversible effects on cells, and result in a sharp decline in sperm motility. In contrast, a cooling rate that is too slow will increase the toxicity of CPAs (Mazur, 1990; Gao and Critser, 2000; Pegg, 2002). Therefore, we will conduct further experiments to fully consider CPA toxicity, control the equilibration time, and combine different cooling rates to further optimize the cryopreservation technology for sea cucumber sperm. Our results can also be used to guide non-programmable freezing techniques of A. japonicus sperm.
4.1.3 Dilution and Thawing
Low levels of sperm dilution may lead to detrimental agglutination of sperm after thawing, whereas high levels of sperm dilution may result in rapid depletion of sperm energy, changes in physiology, and reduced protective components in semen (Paniagua-Chavez et al., 1998; Dong et al., 2005a). Studies have shown that the optimal dilution ratio for cryopreservation of echinoderm sperm ranges from 1:3 to 1:20 (Dunn and Mclachlan, 1973; Asahina and Takahashi, 1978; Adams et al., 2004; Shao et al., 2006; Fabbrocini et al., 2014). In this study, we found that dilution ratio in the range of 1:3 to 1:9 resulted in better post-thaw motility of frozen sperm compared to other ratios (Figure 3). Because sperm densities in fresh semen taken from individual gonads vary, it is reasonable to assume that the optimal cryopreservation dilution ratio for a species is actually a range (Asahina and Takahashi, 1978; Adams et al., 2004). To more effectively compare and optimize experimental protocols for sperm cryopreservation in future studies, we plan to dilute the sperm to a specific concentration and then carry out related optimization experiments.
Liu et al. (2015) divided the thawing temperature for cryopreservation of marine mollusk sperm into three ranges: low temperature (< 29°C), medium temperature (30°C–49°C), and high temperature (> 50°C). According to this standard, the thawing temperature of previous studies of cryopreservation of echinoderm sperm were low and medium temperatures, ranging from room temperature and 15°C to 45°C in a water bath (Dunn and Mclachlan, 1973; Adams et al., 2004; Shao et al., 2006; Jalali and Crawford, 2012; Fabbrocini et al., 2014; Mizuno et al., 2019). In this study, we found that the post-thaw motility of cryopreserved sperm from A. japonicus was highest at thawing temperature of 20°C (Figure 4). This result was similar to that previous studies (Anchordoguy et al., 1988; Mizuno et al., 2019). In contrast, Shao et al. (2006) reported that 37°C was the appropriate thawing temperature for cryopreservation of A. japonicus sperm. We believe that it may be more appropriate to use 20°C as the thawing temperature for cryopreservation of A. japonicus sperm because this temperature is the optimum temperature for sperm-egg union, and sperm thawed at this temperature are less stimulated (Ru et al., 2019). Thawing is the inverse process of freezing, and it may result in cryo-damage such as devitrification and recrystallization (He et al., 2004; Liu et al., 2015; Paredes, 2016; Guo and Weng, 2020). To date, little is known about proper thawing temperature selection for sperm cryopreservation in echinoderms, so the thawing process should be the focus of future research (Paredes, 2015).
4.1.4 CPAs and co-CPAs
In general, DMSO is the most used CPA for sperm cryopreservation. The addition methodology is highly variable, but the concentration range is usually 1–2 M (or 5%– 20%) (Liu et al., 2015; Paredes, 2015; Paredes, 2016). However, different sperm have specific optimal cryoprotectant and concentration ranges (Guo and Weng, 2020). For example, GLY has been used more successfully in the cryopreservation of shrimp and crab sperm, and it provides a better cryoprotective effect than DMSO on the semen of the horseshoe crab Limulus polyphermus (Behlmer and Brown, 1984) and the prawn Macrobrachium rosenbergii (Akarasanon et al., 2004). In invertebrates such as sea urchins and oysters, DMSO is the main permeating cryoprotectant used to preserve sperm, although EG and PG are also commonly used (Liu et al., 2015; Paredes, 2015; Paredes et al., 2019). However, DMSO can also be toxic, and its toxicity increases with increasing concentration (Nascimento et al., 2005; Paredes and Bellas, 2009; Tsai and Lin, 2012). The combination of permeating and non-permeating CPAs can improve sperm motility (Hassan et al., 2015).
In this study, we found that 12.5% DMSO + 0.1 mol/L Glu was the most suitable cryoprotectant formula for cryopreservation of sea cucumber sperm, which was in accordance with previously published reports (Paredes, 2015; Paredes, 2016; Tsai et al., 2018). Shao et al. (2006) reported that the optimal CPA for cryopreservation of A. japonicus sperm was 15% DMSO, but they only compared the concentrations of 15% and 20%. Mizuno et al. (2019) reported that the optimal CPA for cryopreservation of A. japonicus sperm was 20% DMSO. However, the composition of their extender was 20% fetal bovine serum and 80% ASW, which may have affected the cryoprotectant osmolality, thus requiring a higher concentration of DMSO for freezing-dehydration. Sugar molecules typically interact with the lipid bilayer during the freezing phase to maintain plasma membrane integrity when cells undergo dehydration. When combined with other permeable cryoprotectants, sugar prolonged and enhanced cellular post-thaw viability, but the type and concentration of sugar should be optimized according to the species (Thurston et al., 2002; Dong et al., 2005b; Liu et al., 2014; Tsai et al., 2018). Future studies should assess the toxic effects and toxicity thresholds of different types and concentrations of CPAs on A. japonicus sperm in order to further improve the cryopreservation procedure.
4.1.5 Cryopreservation container
Straws (0.25–0.5 µL) and tubes (0.5–2 mL) are commonly used containers for sperm cryopreservation, and both have advantages (Liu et al., 2015; Paredes, 2015; Guo and Weng, 2020). Straws occupy less space and allow higher cooling and thawing rates, but they can only store small amounts of samples and are a bit cumbersome to handle.
Cryotubes have greater storage volume and can hold cryopreserved tissue or tissue fragments, which can be useful in aquaculture production. However, they have lower cooling and thawing rates (He et al., 2004; Paredes, 2016; Paredes et al., 2019). In this study, we found that the type of cryotube (0.5 mL or 2 mL) and cryopreservation volume (0.5-2 mL) had no significant effect on post-thaw motility, which makes it possible to obtain large quantities of frozen sea cucumber sperm and to improve production practice. In future research, we will study cryopreservation of sea cucumber embryos and the effect of freezing containers (cryotubes or straws) on the survival rate of embryos after thawing (Paredes, 2016; Paredes et al., 2019).
4.2 Quality of fresh and frozen A. japonicus sperm
4.2.1 Individual differences in sperm freezability
In this study, we found significant individual differences in sperm quality and sperm freezability of A. japonicus. Mature sea cucumbers with a heavy weight did not necessarily have higher sperm motility and sperm density than those with a lighter weight (Table 2). Similarly, different A. japonicus individuals with high fresh sperm motility would not necessarily have high post-thaw sperm motility (Table 2). It is normal for different individuals of the same species to have differences (Adams et al., 2004; Dere et al., 2016; Hussain et al., 2016). In this study, the semen used in each screening experiment came from only one sea cucumber to study the individual differences in sea cucumber sperm freezability. However, the current findings of this study are not sufficient to explain what factors determine the sperm freezability. In future studies, we will fully evaluate various factors such as nutrients in the seminal plasma, enzyme activity, transcriptome and proteomics of different sea cucumber individual sperm, and combine them with the quality of the thawed sperm to find out what are the factors that determine the freezability of A. japonicus sperm (Wang et al., 2014a).
4.2.2 Fertilization rate and hatching rate
Fertilization rate and hatching rate experiments are key steps to test the quality of frozen sperm (Liu et al., 2015; Paredes, 2015). Most previous studies of cryopreservation of echinoderm sperm have provided data on the fertilization rate (50%–95%) of frozen sperm, but few data on hatching rate are available (Liu et al., 2015; Paredes, 2015; Paredes, 2016; Paredes et al., 2019). Dunn and Mclachlan (1973) reported that the sperm motility of Strongylocentrotus drobachiensis was obviously compromised after cryopreservation, but could achieve 95% fertilization by increasing the sperm-oocyte ratio. Asahina and Takahashi (1978) also observed a significant decrease in post-thawing sperm motility and a 75% fertilization rate in Strongylocentrotus intermedius, Hemicentrotus pulcherrimus, and Strongylocentrotus nudus. Mizuno et al. (2019) reported that the maximum post-thaw motility of A. japonicus sperm after cryopreservation was 19.3%, and the fertilization rate was 89.8% (compared to 92.7% for fresh sperm). In our study, the maximum post-thaw motility of A. japonicus sperm treated with the optimal cryopreservation procedures was 68.92% ± 0.97%, the fertilization rate was 79.03% ± 3.22%, and the hatching rate was 66.74% ± 5.61% (Figure 8). Although our fertilization rate did not reach 90%, we think that related to the time point we assessed it. Dunn and Mclachlan (1973) calculated the fertilization rate based on whether or not the fertilization membrane was formed, and Shao et al. (2006) calculated it 1 h post-fertilization based on whether or not the second polar body was extruded. Mizuno et al. (2019) calculated the fertilization rate 3 h post-fertilization based on whether or not the fertilized egg was cleaved. In our study, we calculated the fertilization rate and hatching rate at 10 h (in the blastocyst stage) and 52 h (in the early auricularia larva stage) after fertilization, which is significantly later than other studies. As the duration of the artificial incubation time increased, the risk to embryo survival increased, and if the incubation conditions were unfavorable, the embryo may experience growth arrest (Asahina and Takahashi, 1978). In addition, when we counted the hatching rate, we observed both early auricularia larvae and some embryos in the gastrula stage. These factors may explain why our values are small and conservative.
It is worth noting that the fertilization rate and hatching rate of fresh sperm (control group) in our study were relatively low, as they should be > 90%. We believe that these results were due to the addition of too many sperm and too sub-optimal egg quality. Because the motility of sperm is affected by cryopreservation, a large volume of sperm generally is added to ensure fertilization. However, too much semen can negatively impact water quality, so the proper sperm-egg ratio is very important (Dunn and Mclachlan, 1973; Adams et al., 2004; Fabbrocini et al., 2014; Paredes et al., 2019). Generally speaking, the concentration of cryopreserved sperm required to fertilize eggs has been estimated to be 30 times higher than that of unfrozen sperm (Paredes, 2015). Shao et al. (2006) reported that the fertilization rate and hatching rate of the 10,000:1 sperm-to-egg ratio achieved better results than the 1000:1 ratio for cryopreserved sperm of A. japonicus. In our study, we used the same 10,000:1 sperm-to-egg ratio for fresh semen and frozen semen in the fertilization rate experiment. Because the sperm concentration of fresh semen was nearly double that of frozen semen (Table 2), the fresh sperm control group may have contained too much sperm, resulting in poor water quality and affecting embryo growth. This may explain the suboptimal fertilization and hatchability results in the fresh sperm treatment group (Adams et al., 2004; Fabbrocini and D'adamo, 2017). In future studies, we will optimize the sperm-egg ratio for fresh sperm and frozen sperm and determine how to prolong and maintain the quality of sea cucumber eggs. We believe that if the egg quality is good and the sperm-to-egg ratio is suitable, the fertilization rate and hatching rate of the frozen sperm cryopreserved using our optimized procedure will improve.
In this study, we also examined the fertilization and hatchability of frozen sperm with different post-thaw motility values that were cryopreserved using non-optimal formulas (20% DMA or 20% PG). Based on the results, we propose that frozen sperm with post-thaw motility > 40% can be used in production practice, which lays the foundation for further studies of the protection mechanism of different types of CPAs. We also considered preservation time and found that cryopreservation for 1 year had no significant effect on the post-thaw motility of frozen sperm. This part of our research is ongoing, and future steps will including studying the effects of cryopreservation process and time on the transcriptome and proteome of A. japonicus sperm and exploring the effect of cryopreservation technology on A. japonicus sperm from a molecular perspective (Figure 8).
4.2.3 Ultrastructure
We attribute the observed decline in sperm motility and fertilization rate to the structural damage to sperm caused by the cryopreservation process (Diaz et al., 2019; Cerdeira et al., 2020). Our comparison of the ultrastructure of fresh sperm and cryopreserved sperm showed that the main damage occurred in the head, mitochondria, and membranes of A. japonicus sperm (Figure 9). In future studies, we will assess the damage ratio, enzyme activity changes, and DNA integrity of A. japonicus sperm after cryopreservation to comprehensively describe the effect of cryopreservation on A. japonicus sperm.
5 Conclusion
In this study, we used a programmable control rate freezer and CASA to comprehensively screen and evaluate for optimal values of key parameters involved in the cryopreservation of A. japonicus sperm. When we applied our established optimal method for cryopreservation of A. japonicus sperm, the post-thaw motility of sperm was > 65%, the fertilization rate (in the blastocyst stage) was nearly 80%, and the hatching rate (in the early auricularia larva stage) was > 65%. These results showed that our method had practical value for production. We also found that A. japonicus sperm that had been cryopreserved for 1 year retained a considerable post-thaw motility and fertilization rate compared to recently cryopreserved sperm. However, the cryopreservation process caused damage to the ultrastructure of A. japonicus sperm. Herein we have fully discussed individual differences in freezability of A. japonicus sperm, the direction of future development of A. japonicus sperm cryopreservation technology, and the problems that still need to be solved. Our results provide valuable information for future research and conservation of A. japonicus.
Data availability statement
The raw data supporting the conclusions of this article will be made available by the authors, without undue reservation.
Author contributions
SX, SLL, LBZ, CGL, LNS, JCS, LLX, and HSY conceived and designed the experiments. SX, SLL, JCS, and CXJ performed the experiments. SX, CXJ, and HSY analyzed the data and drafted the manuscript. SX, SLL, JCS, and HSY contributed to writing, review, and editing. SLL and HSY contributed to securing the funding. All authors contributed to the article and approved the submitted version.
Funding
This work was supported by National Key Research and Development Project (2018YFD0901602), the Agricultural Seed Project of Shandong Province (2020LZGC015), Marine S&T Fund of Shandong Province for Pilot National Laboratory for Marine Science and Technology (Qingdao) (2018SDKJ0502), Chinese Academy of Sciences “Modern Marine Ranching Construction Principles and Engineering Technology Innovation Cross Team” Project (Y82327101L), the Strategic Priority Research Program of the Chinese Academy of Sciences (XDA24030304) and International Partners Program of Chinese Academy of Sciences (133137KYSB20180069). The funders had no role in experiment design, data processing and analysis, and decision to publish or preparation of the manuscript.
Acknowledgments
We thank Qinghua Liu, Xueying Wang, Xiaoshang Ru, and Da Huo for their help and advice on experimental design.
Conflict of interest
The authors declare that the research was conducted in the absence of any commercial or financial relationships that could be construed as a potential conflict of interest.
Publisher’s note
All claims expressed in this article are solely those of the authors and do not necessarily represent those of their affiliated organizations, or those of the publisher, the editors and the reviewers. Any product that may be evaluated in this article, or claim that may be made by its manufacturer, is not guaranteed or endorsed by the publisher.
References
Adams S. L., Hessian P. A., Mladenov P. V. (2004). Cryopreservation of sea urchin (Evechinus chloroticus) sperm. Cryoletters 25, 287–299. doi: 10.1201/9780849380549.ch41
Akarasanon K., Damrongphol P., Poolsanguan W. (2004). Long-term cryopreservation of spermatophore of the giant freshwater prawn, Macrobrachium rosenbergii (de man). Aquacult. Res. 35, 1415–1420. doi: 10.1111/j.1365-2109.2004.01163.x
Anchordoguy T., Crowe J. H., Griffin F. J., Clark W. H. (1988). Cryopreservation of sperm from the marine shrimp sicyonia-ingentis. Cryobiology 25, 238–243. doi: 10.1016/0011-2240(88)90031-4
Asahina E., Takahashi T. (1978). Freezing tolerance in embryos and spermatozoa of sea-urchin. Cryobiology 15, 122–127. doi: 10.1016/0011-2240(78)90016-0
Behlmer S. D., Brown G. (1984). Viability of cryopreserved spermatozoa of the horseshoe-crab, limulus-polyphemus-L. Int. J. Invertebr. Reprod. Dev. 7, 193–199. doi: 10.1080/01688170.1984.10510089
Bordbar S., Anwar F., Saari N. (2011). High-value components and bioactives from Sea cucumbers for functional foods-a review. Mar. Drugs 9, 1761–1805. doi: 10.3390/md9101761
Campos S., Troncoso J., Paredes E. (2021). Major challenges in cryopreservation of sea urchin eggs. Cryobiology 98, 1–4. doi: 10.1016/j.cryobiol.2020.11.008
Cerdeira J., Sanchez-Calabuig M. J., Perez-Gutierrez J. F., Hijon M., Castano C., Santiago-Moreno J. (2020). Cryopreservation effects on canine sperm morphometric variables and ultrastructure: Comparison between vitrification and conventional freezing. Cryobiology 95, 164–170. doi: 10.1016/j.cryobiol.2020.03.007
Dere E., Huse S., Hwang K., Sigman M., Boekelheide K. (2016). Intra- and inter-individual differences in human sperm DNA methylation. Andrology 4, 832–842. doi: 10.1111/andr.12170
Diaz R., Lee-Estevez M., Quinones J., Dumorne K., Short S., Ulloa-Rodriguez P., et al. (2019). Changes in Atlantic salmon (Salmo salar) sperm morphology and membrane lipid composition related to cold storage and cryopreservation. Anim. Reprod. Sci. 204, 50–59. doi: 10.1016/j.anireprosci.2019.03.004
Dong Q. X., Eudeline B., Huang C. J., Allen S. K., Tiersch T. R. (2005b). Commercial-scale sperm cryopreservation of diploid and tetraploid pacific oysters, Crassostrea gigas. Cryobiology 50, 1–16. doi: 10.1016/j.cryobiol.2004.09.003
Dong Q. X., Huang C. J., Eudeline B., Tiersch T. R. (2005a). Systematic factor optimization for cryopreservation of shipped sperm samples of diploid pacific oysters, Crassostrea gigas. Cryobiology 51, 176–197. doi: 10.1016/j.cryobiol.2005.06.007
Dunn R. S., Mclachlan J. (1973). Cryopreservation of echinoderm sperm. Can. J. Zool. 51, 666–669. doi: 10.1139/z73-100
Fabbrocini A., D'adamo R. (2017). Motility of sea urchin Paracentrotus lividus spermatozoa in the post-activation phase. Aquacult. Res. 48, 5526–5532. doi: 10.1111/are.13373
Fabbrocini A., D'adamo R., Pelosi S., Oliveira L. F. J., Silvestri F., Sansone G. (2014). Gamete cryobanks for laboratory research: Developing a rapid and easy-to-perform protocol for the cryopreservation of the sea urchin Paracentrotus lividus (Lmk 1816) spermatozoa. Cryobiology 69, 149–156. doi: 10.1016/j.cryobiol.2014.06.009
Fabbrocini A., Maurizio D., D'adamo R. (2016). Sperm motility patterns as a tool for evaluating differences in sperm quality across gonad development stages in the sea urchin Paracentrotus lividus (Lmk 1816). Aquaculture 452, 115–119. doi: 10.1016/j.aquaculture.2015.10.033
Fan B., Liu X. C., Meng Z. N., Tan B. H., Wang L., Zhang H. F., et al. (2014). Cryopreservation of giant grouper Epinephelus lanceolatus (Bloch 1790) sperm. J. Appl. Ichthyol. 30, 334–339. doi: 10.1111/jai.12321
Figueroa E., Farias J. G., Lee-Estevez M., Valdebenito I., Risopatron J., Magnotti C., et al. (2018). Sperm cryopreservation with supplementation of alpha-tocopherol and ascorbic acid in freezing media increase sperm function and fertility rate in Atlantic salmon (Salmo salar). Aquaculture 493, 1–8. doi: 10.1016/j.aquaculture.2018.04.046
Gallego V., Perez L., Asturiano J. F., Yoshida M. (2014). Sperm motility parameters and spermatozoa morphometric characterization in marine species: A study of swimmer and sessile species. Theriogenology 82, 668–676. doi: 10.1016/j.theriogenology.2014.05.026
Gao D., Critser J. K. (2000). Mechanisms of cryoinjury in living cells. ILAR J. 41, 187–196. doi: 10.1093/ilar.41.4.187
Guo J. H., Weng C. F. (2020). Current status and prospects of cryopreservation in aquatic crustaceans and other invertebrates. J. Crust. Biol. 40, 343–350. doi: 10.1093/jcbiol/ruaa034
Hassan M. M., Qin J. G., Li X. (2015). Sperm cryopreservation in oysters: A review of its current status and potentials for future application in aquaculture. Aquaculture 438, 24–32. doi: 10.1016/j.aquaculture.2014.12.037
He Y. M., Dong Q. X., Tiersch T. R., Devireddy R. V. (2004). Variation in the membrane transport properties and predicted optimal rates of freezing for spermatozoa of diploid and tetraploid pacific oyster, Crassostrea gigas. Biol. Reprod. 70, 1428–1437. doi: 10.1095/biolreprod.103.025296
Hussain Y. H., Guasto J. S., Zimmer R. K., Stocker R., Riffell J. A. (2016). Sperm chemotaxis promotes individual fertilization success in sea urchins. J. Exp. Biol. 219, 1458–1466. doi: 10.1242/jeb.134924
Jalali A., Crawford B. (2012). A freezing technique that maintains viability of sperm from the starfish Pisaster ochraceus. Invertebr. Reprod. Dev. 56, 242–248. doi: 10.1080/07924259.2011.601125
Kato S., Tsurumaru S., Taga M., Yamane T., Shibata Y., Ohno K., et al. (2009). Neuronal peptides induce oocyte maturation and gamete spawning of sea cucumber, Apostichopus japonicus. Dev. Biol. 326, 169–176. doi: 10.1016/j.ydbio.2008.11.003
Liu Y., Li X., Robinson N., Qin J. (2015). Sperm cryopreservation in marine mollusk: a review. Aquacult. Int. 23, 1505–1524. doi: 10.1007/s10499-015-9900-0
Liu Z., Li D., Song L., Liu Y., Yu M., Zhang M., et al. (2020). Effects of proteolysis and oxidation on mechanical properties of sea cucumber (Stichopus japonicus) during thermal processing and storage and their control. Food Chem. 330, 127248. doi: 10.1016/j.foodchem.2020.127248
Liu Y. B., Xu T., Robinson N., Qin J. G., Li X. X. (2014). Cryopreservation of sperm in farmed Australian greenlip abalone Haliotis laevigata. Cryobiology 68, 185–193. doi: 10.1016/j.cryobiol.2014.01.002
Mazur P. (1990). Equilibrium, quasi-equilibrium, and nonequilibrium freezing of mammalian embryos. Cell Biophysics 17, 53–92. doi: 10.1007/bf02989804
Mizuno Y., Fujiwara A., Yamano K., Ohta H. (2019). Motility and fertility of cryopreserved spermatozoa of the Japanese sea cucumber Apostichopus japonicus. Aquacult. Res. 50, 106–115. doi: 10.1111/are.13872
Nascimento I. A., Leite M., De Araujo M. M. S., Sansone G., Pereira S. A., Santo M. D. (2005). Selection of cryoprotectants based on their toxic effects on oyster gametes and embryos. Cryobiology 51, 113–117. doi: 10.1016/j.cryobiol.2005.04.006
Paniagua-Chavez C. G., Buchanan J. T., Tiersch T. R. (1998). Effect of extender solutions and dilution on motility and fertilizing ability of Eastern oyster sperm. J. Shellfish Res. 17, 231–237.
Paredes E. (2015). Exploring the evolution of marine invertebrate cryopreservation - landmarks, state of the art and future lines of research. Cryobiology 71, 198–209. doi: 10.1016/j.cryobiol.2015.08.011
Paredes E. (2016). Biobanking of a marine invertebrate model organism: The Sea urchin. J. Mar. Sci. Eng. 4(1), 7. doi: 10.3390/jmse4010007
Paredes E., Adams S. L., Vignier J. (2019). “Cryopreservation of sea urchin sperm and early life stages,” in Echinoderms, pt a. Methods in Cell Biology Eds. Foltz K. R., Hamdoun A., 47–69. doi: 10.1016/bs.mcb.2018.11.008
Paredes E., Bellas J. (2009). Cryopreservation of sea urchin embryos (Paracentrotus lividus) applied to marine ecotoxicological studies. Cryobiology 59, 344–350. doi: 10.1016/j.cryobiol.2009.09.010
Paredes E., Bellas J., Adams S. L. (2013). Comparative cryopreservation study of trochophore larvae from two species of bivalves: Pacific oyster (Crassostrea gigas) and blue mussel (Mytilus galloproviricialis). Cryobiology 67, 274–279. doi: 10.1016/j.cryobiol.2013.08.007
Pegg D. E. (2002). The history and principles of cryopreservation. Semin. Reprod. Med. 20, 5–13. doi: 10.1055/s-2002-23515
Ru X., Zhang L., Li X., Liu S., Yang H. (2019). Development strategies for the sea cucumber industry in China. J. Oceanol. Limnol. 37, 300–312. doi: 10.1007/s00343-019-7344-5
Shao M. Y., Zhang Z. F., Yu L., Hu J. J., Kang K. H. (2006). Cryopreservation of sea cucumber Apostichopus japonicus (Selenka) sperm. Aquacult. Res. 37, 1450–1457. doi: 10.1111/j.1365-2109.2006.01581.x
Thurston L. M., Watson P. F., Holt W. V. (2002). Semen cryopreservation: A genetic explanation for species and individual variation? Cryoletters 23, 255–262.
Tsai S., Chong G., Meng P. J., Lin C. (2018). Sugars as supplemental cryoprotectants for marine organisms. Rev. Aquac. 10, 703–715. doi: 10.1111/raq.12195
Tsai S., Lin C. (2012). Advantages and applications of cryopreservation in fisheries science. Braz. Arch. Biol. Technol. 55, 425–433. doi: 10.1590/s1516-89132012000300014
Van Der Horst G., Bennett M., Bishop J. D. D. (2018). CASA in invertebrates. Reprod. Fertil. Dev. 30, 907–918. doi: 10.1071/rd17470
Vuthiphandchai V., Boonthai T., Nimrat S. (2021). Chilled storage of Asian seabass, lates calcarifer Bloch semen: Effects of ions, extenders and storage periods on sperm quality and fertilization ability. J. Appl. Ichthyol. 37, 593–600. doi: 10.1111/jai.14218
Wang Y., Rong X., Liao M. (2014b). Technical monograph for health culture and disease control of sea cucumber (Beijing: China Agriculture Press).
Wang P., Wang Y. F., Wang H., Wang C. W., Zan L. S., Hu J. H., et al. (2014a). HSP90 expression correlation with the freezing resistance of bull sperm. Zygote 22, 239–245. doi: 10.1017/s096719941300004x
Wu Y., Guo L., Liu Z., Wei H., Zhou Y., Tan J., et al. (2019). Microelements in seminal and serum plasma are associated with fresh semen quality in Yorkshire boars. Theriogenology 132, 88–94. doi: 10.1016/j.theriogenology.2019.04.002
Xia S., Yang H., Li Y., Liu S., Zhou Y., Zhang L. (2012). Effects of different seaweed diets on growth, digestibility, and ammonia-nitrogen production of the sea cucumber Apostichopus japonicus (Selenka). Aquaculture 338-341, 304–308. doi: 10.1016/j.aquaculture.2012.01.010
Xing L., Sun L., Liu S., Zhang L., Yang H. (2021). Comparative metabolomic analysis of the body wall from four varieties of the sea cucumber Apostichopus japonicus. Food Chem. 352, 129339. doi: 10.1016/j.foodchem.2021.129339
Keywords: Apostichopus japonicus, sperm, cryopreservation, motility, CASA, programmable freezer
Citation: Xu S, Liu S, Sun J, Zhang L, Lin C, Sun L, Xing L, Jiang C and Yang H (2022) Optimizing cryopreservation of sea cucumber (Apostichopus japonicus) sperm using a programmable freezer and computer-assisted sperm analysis. Front. Mar. Sci. 9:917045. doi: 10.3389/fmars.2022.917045
Received: 10 April 2022; Accepted: 01 July 2022;
Published: 01 August 2022.
Edited by:
Mercedes González-Wangüemert, University of Algarve, PortugalReviewed by:
Nathalie Rose Le François, Espace pour la vie, CanadaSusana Galante-Oliveira, University of Aveiro, Portugal
Copyright © 2022 Xu, Liu, Sun, Zhang, Lin, Sun, Xing, Jiang and Yang. This is an open-access article distributed under the terms of the Creative Commons Attribution License (CC BY). The use, distribution or reproduction in other forums is permitted, provided the original author(s) and the copyright owner(s) are credited and that the original publication in this journal is cited, in accordance with accepted academic practice. No use, distribution or reproduction is permitted which does not comply with these terms.
*Correspondence: Hongsheng Yang, hshyang@ms.qdio.ac.cn