- 1Institute for Advanced Sustainability Studies (IASS), Potsdam, Germany
- 2Ocean Exploration Trust, Honolulu, HI, United States
- 3Middlebury Institute of International Studies at Monterey, Monterey, CA, United States
- 4Center for Ecology and Sustainable Management of Oceanic Islands (ESMOI), Departamento de Biología Marina, Facultad de Ciencias del Mar, Universidad Católica del Norte, Coquimbo, Chile
- 5National Geographic Pristine Seas, Washington, DC, United States
- 6Hawaii Institute of Marine Biology, University of Hawaii, Kaneohe, HI, United States
- 7Centre for Biodiversity and Conservation Science, School of Earth and Environmental Sciences, University of Queensland, St Lucia, QLD, Australia
- 8World Commission on Protected Areas, International Union for Conservation of Nature, (WCPA-IUCN) Santiago, Chile
- 9Department of Protected Areas, Ministry of the Environment, Santiago, Chile
- 10Departamento de Ciencias Ecológicas, Facultad de Ciencias, Universidad de Chile, Santiago, Chile
- 11The Nature Conservancy, London, United Kingdom
Connectivity is essential to consider in area-based marine conservation measures because it greatly impacts vital ecological processes (e.g., recycling nutrients, regulating temperature, sequestering carbon, and supporting the life cycle of species). However, it is generally not well studied or integrated into conservation measures, particularly in the Southeast Pacific. This region is home to the Salas y Gómez and Nazca ridges, two adjacent seamount chains that stretch between the Peruvian coast and Rapa Nui (i.e., Easter Island). The area has been recognized for its exceptional natural and cultural significance as well as one of the most important global areas to protect. However, over 73% of the ridges are in areas beyond national jurisdiction, where they are unprotected and under threat. This study provides a synthesis of what we know about connectivity in the Salas y Gómez and Nazca ridges and provides practical recommendations for integrating this knowledge into conservation and management measures for the region. While data on connectivity is still limited, it should not constrain the development of conservation measures, which should be co-designed and co-developed, leveraging the expertise of international working groups throughout the region. Due to their high rates of endemism, as well as importance as migratory corridors, enacting large-scale conservation measures would address important conservation considerations for both connectivity and endemism. Enacting such large-scale conservation measures would not only elevate the Salas y Gómez and Nazca ridges as an exemplary case study, providing lessons for other marine regions seeking to implement similar measures, but could also help raise awareness on the severity of externally originating threats like climate change and plastic pollution to global audiences.
1 Introduction
Connectivity refers to the linkages of organisms, propagules, nutrients, or pollutants between one area to another, and has fundamental implications for ocean health (Balbar and Metaxas, 2019; Metaxas et al., 2020). Connectivity includes structural connections which are based on distances and conditions between similar habitats or species (Dunn et al., 2019) as well as functional connections which are the movement of individuals, species, energy, or materials across ocean spaces, connecting ecosystems (Popova et al., 2019; Metaxas et al., 2020). Beyond ecological connections, ocean spaces are connected through pressures stemming from human activities (e.g., fishing, pollution, climate change) as well as human and cultural connections.
Connectivity involves the exchange across all ocean layers (Popova et al., 2019; Metaxas et al., 2020). Thus, management needs to consider the ocean as a three-dimensional space that changes over time, and the linkages between ocean, land, and atmosphere (Ortuño Crespo et al., 2020). Some marine species migrate across entire ocean basins or connect basins (Dunn et al., 2019). Other species connect the ocean through vertical migrations which ties together the seabed and water column and is also crucial in Marine Protected Area (MPA) design (Ban et al., 2014; O’Leary and Roberts, 2018). Similarly, understanding how climate change impacts the ocean differently across depths is critical to developing conservation measures that are coherent and resilient (Brito-Morales et al., 2022).
Connectivity is essential to consider in area-based conservation measures because it greatly impacts vital ecological processes (e.g., recycling nutrients, regulating temperature, sequestering carbon, and supporting the life cycle of species) (Carr et al., 2017; Balbar and Metaxas, 2019; Dunn et al., 2019; Metaxas et al., 2020). For instance, if a species protected through a targeted area-based conservation measure receives food from a source which is under threat outside the protected area, the effectiveness of the conservation measure will be undermined (Metaxas et al., 2020).
The detrimental effects of habitat degradation and fragmentation can be mitigated if connectivity is properly integrated into conservation and management measures (Carr et al., 2017). Furthermore, measures designed to protect and maintain connectivity not only benefit the species that live there, but can also increase ecosystem resilience and benefits to humans (O’Leary and Roberts, 2018; Dunn et al., 2019; Metaxas et al., 2020). MPAs are commonly viewed as an effective area-based conservation measure to protect and restore marine biodiversity (Dunn et al., 2019; Ortuño Crespo et al., 2020; Lausche et al., 2021). Connectivity, which supports species to thrive and ecosystems to be maintained, is essential to designing effective and resilient networks of MPAs (Dunn et al., 2019; Lausche et al., 2021).
Connectivity is not well studied in the Southeast Pacific or elsewhere, particularly in relation to MPAs (Guarderas et al., 2008). Marine conservation efforts in this region are generally focused on either coastal areas in the continental margins, or waters surrounding offshore islands under the jurisdiction of South American countries (Figure 1). However, there is little information about connectivity between these coastal areas and offshore islands, thus hindering conservation efforts, particularly in areas beyond national jurisdiction (ABNJ).
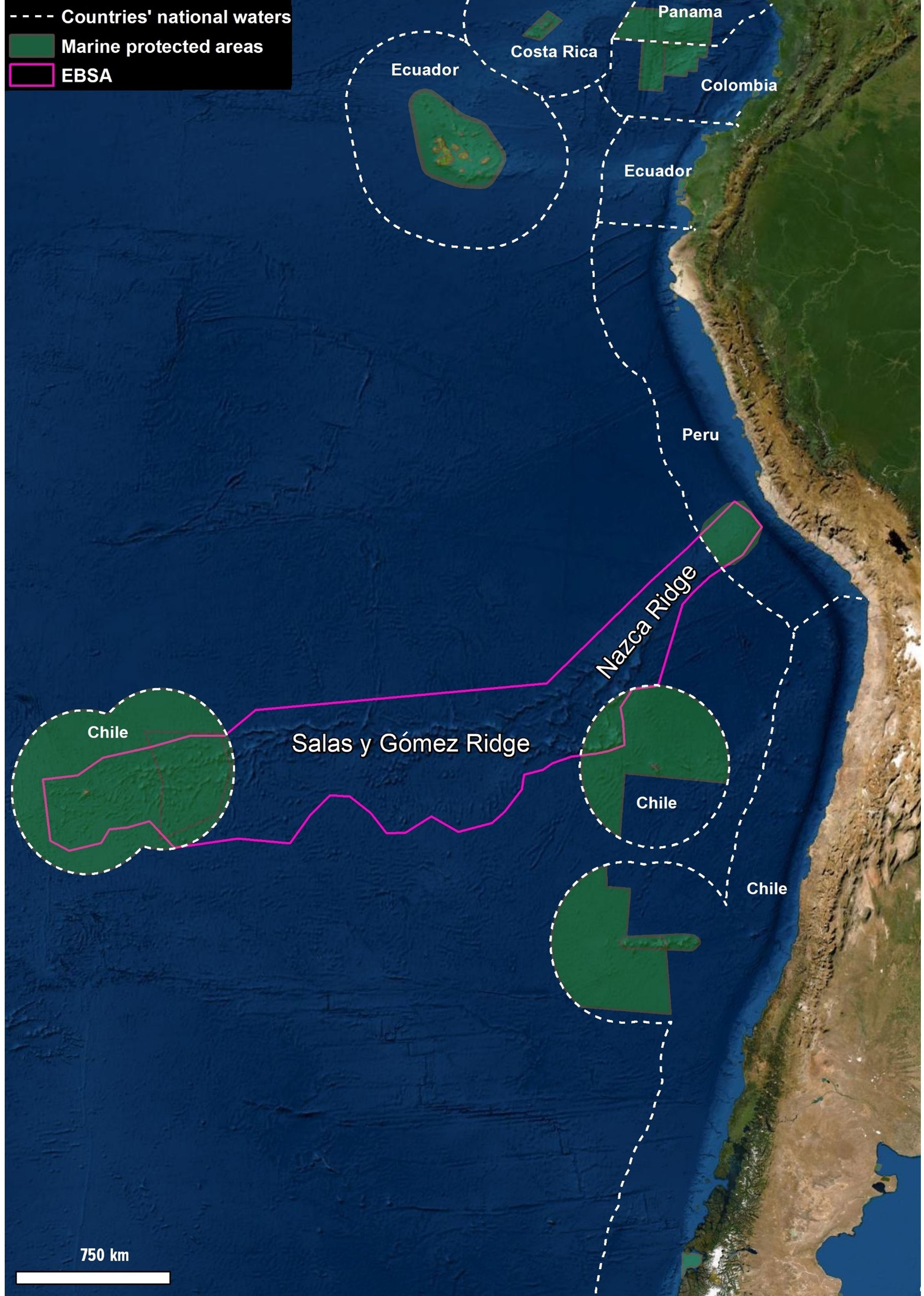
Figure 1 Map showing the location of MPAs around the Salas y Gómez and Nazca ridges. Over 73% of Salas y Gómez and Nazca ridges lie in ABNJ where they are unprotected.
ABNJ have recently gained attention as States are negotiating a legally binding agreement to conserve and sustainably use marine biodiversity beyond national jurisdiction (often referred to as the BBNJ Agreement). The negotiations are still ongoing, with the fifth negotiation in August 2022 failing to reach an agreement. Several recent studies identified priority ocean areas for conservation (Lucifora et al., 2011; Selig et al., 2014; Jones et al., 2020; Maxwell et al., 2020; Visalli et al., 2020; Wagner et al., 2020; Beal et al., 2021; Davies et al., 2021; Sala et al., 2021) based on varying and diverse criteria, including in ABNJ. Some of these priority areas in ABNJ have gained attention from governments seeking to advance conservation outside their national jurisdictions, resulting in the designation of new MPAs (Davies et al., 2021).
Located mostly in ABNJ off South America, the Salas y Gómez and Nazca ridges have been recognized as one of the most important areas to protect globally (reviewed in Wagner et al., 2021a; Wagner et al., 2021b). There has been progress in protecting portions of the ridges that lie within national jurisdictions of Chile (Friedlander and Gaymer, 2021; Wagner et al., 2021b) and, to a lesser degree, Peru (MINAM, 2021). However, over 73% of the ridges are located in ABNJ, where they are under threat from overfishing, plastic pollution, and climate change, with potential seabed mining looming in the future (Toro et al., 2020; García et al., 2020; Wagner et al., 2021b). Both cobalt-rich crusts and polymetallic nodules are found in this region see Miller et al. (2018) and references therein. Despite the recognition that these ridges have a high natural and cultural significance, they currently do not have any protections in ABNJ, which represents the largest and most threatened portion of the ridges (Figure 1).
One obstacle hindering conservation of the Salas y Gómez and Nazca ridges is a lack of understanding of how its ecosystems are connected to those of adjacent States. While many scientific assessments have noted the importance of this region for the connectivity of highly mobile species (Shillinger et al., 2008; Gálvez-Larach, 2009; Block et al., 2011; Hucke-Gaete et al., 2014; CBD, 2017; Pazmiño et al., 2018; Álvarez-Varas et al., 2021a; Álvarez-Varas et al., 2021b), there have been no reviews on connectivity across this region. The purpose of this study is to review relevant information about the connectivity of the Salas y Gómez and Nazca ridges and provide recommendations for integrating this knowledge into conservation and management measures in the region.
2 The Salas y Gómez and Nazca ridges
The Salas y Gómez and Nazca ridges are two adjacent seamount chains that stretch across over 2,900 km between the subduction zone off the Peruvian coast and Rapa Nui (also known as Easter Island). The ridges consist of over 110 seamounts, many of which peak close to the surface and host photosynthetic communities (Easton et al., 2017; Wagner et al., 2021a). The ridges host ecosystems with some of the highest rates of marine endemism in the world and are a haven to over 80 threatened or endangered species (reviewed in Wagner et al., 2021b). In addition to providing critical habitat for many benthic organisms, the ridges serve as essential migration corridors for many highly mobile species (Shillinger et al., 2008; Gálvez-Larach, 2009; Block et al., 2011; Hucke-Gaete et al., 2014; CBD, 2017; Serratosa et al., 2020; Álvarez-Varas et al., 2021a). The main human activity taking place in this region is fishing (Wagner et al., 2021b), which is managed by the Inter-American Tropical Tuna Commission (IATTC) for highly-mobile species and by the South Pacific Regional Fisheries Management Organisation (SPRFMO) for non-highly migratory fish species (see Section 3.2.1 below).
The natural and cultural significance of this region, as well as its vulnerability to overexploitation, has inspired many to raise awareness about its importance, uniqueness, and fragility (reviewed in Wagner et al., 2021b; Delgado et al., 2022). In 2011, both the Global Ocean Biodiversity Initiative and the Global Census of Marine Life on Seamounts recognized the global importance of this region (Clark et al., 2011). In 2014, the Convention on Biological Diversity (CBD) identified the Salas y Gómez and Nazca ridges as an Ecologically or Biologically Significant Area (EBSA), a status given to areas deemed worthy of protection or increased management. The Salas y Gómez and Nazca ridges were also recognized as a Hope Spot by Mission Blue, and all places where the ridges rise above sea level (Rapa Nui, Salas y Gómez Island and Desventuradas Islands) are considered Important Bird Areas (IBAs) by Birdlife International, as well as Key Biodiversity Areas (KBAs) by the 13 member organizations of the KBA partnership. Salas y Gómez Island and the entire area surrounding the ridges is known for its rich history of human seafaring and cultural significance (Delgado et al., 2022).
These distinctions, along with the many scientific assessments that underpinned them, have laid the foundation for the call to protect the Salas y Gómez and Nazca ridges. Within Chilean waters of the ridges, there are three established MPAs (the Nazca-Desventuradas Marine Park, the Motu Motiro Hiva Marine Park, and the Rapa Nui Multiple-Use Coastal Marine Protected Area), whereas Peruvian waters include one MPA that protects the seafloor portion of the ridges (Nazca Ridge National Reserve; Figure 1). While these recent efforts by Chile and Peru provide important advances to safeguarding the unique biodiversity and cultural resources of the region, the majority of the ridges are located in ABNJ. In October 2020, a group of 24 experts from various academic and non-governmental organizations presented a proposal for a fishing closure in the area of SPRFMO (Wagner et al., 2020). In April 2021, the Chilean Government announced that it would start efforts to create a fully protected MPA in this region at the Virtual Leaders Climate Summit (MINREL, 2021) , which was followed by multiple presentations to SPRFMO by Chile highlighting the significance of the ridges (Ministry of Foreign Affairs of Chile 2021; Gaymar et al. 2022).
3 Connectivity in the Salas y Gómez and Nazca ridges region
3.1 Natural connectivity
3.1.1 Biodiversity and oceanography
The biodiversity of the Salas y Gómez and Nazca ridges contains the highest level of marine endemism found anywhere on Earth (reviewed in Wagner et al., 2021b). This unique biodiversity is a result of circulation patterns, such as the South Pacific Gyre, which isolate the region (Von Dassow and Collado-Fabbri, 2014). The Atacama Trench, which plunges over 8,000 m deep off the Chilean and Peruvian Coast, acts as a barrier to the movement of many species between South America and the ridges. Similarly, the strong Humboldt Current, which travels north along the South American coast, acts as an additional barrier for species recruitment from the South American coast (Friedlander et al., 2016), and are further affected by the El Niño Southern Oscillation and the Pacific Decadal Oscillation (Yañez et al., 2017).
The eastern portion of the ridges intersects a region that has some of the most oxygen-poor waters in the world (Fuenzalida et al., 2009; Ulloa and Pantoja, 2009), which has been speculated to serve as yet another dispersal barrier for deep-sea fauna (Wagner et al., 2021a). Collectively, these features have reduced connectivity between the South American Continent and the Salas y Gómez and Nazca ridges. In fact, the biodiversity of the ridges is more like that found in distant places in the Western Indo-Pacific than to that of the South American Continent or nearby islands (reviewed in Wagner et al., 2021b). The marine flora of Rapa Nui is more similar to that found over 15,000 km away in Vietnam than to that found on the Juan Fernandez Archipelago, located just under 3,000 km away (Santelices and Meneses, 2000).
3.1.2 Genetic and larval connectivity
Oceanic circulation has profound effects on larval dispersal and biological connectivity for many species inhabiting the Salas y Gómez and Nazca ridges. Although there is a predominant eastern flow in the currents in the region, occasionally westward currents prevail. Notwithstanding the prevailing currents in the area, the species composition of the Salas y Gómez and Nazca ridges are mainly composed of species related to those from the Western Indo-Pacific, but also include some from the Eastern Tropical Pacific (Andrade et al., 2014). Reef-building corals in shallow water are a good example of this mixed influence, as the ridges contain some coral species that are related to those found in Colombia, Ecuador, and Panama (Glynn et al., 2007; Easton et al., 2019). It is important to highlight that the Salas y Gómez and Nazca ridges are the only place in the Southeast Pacific, and one of the few places in ABNJ globally, where seamounts peak in waters shallow enough (<200m) to host photosynthetic reef-building corals (CBD, 2017; Easton et al., 2017; Wagner et al., 2020; Wagner et al., 2021a; Wagner et al., 2021b; Zapata-Hernández et al., 2021). These reef-building corals in turn are important ecosystem engineers that create habitat for a wide variety of associated species (Wagner et al., 2020; Georgian et al., 2021).
Recent studies have examined the connectivity of two reef-associated species, the Easter Island spiny lobster Panulirus pascuensis Reed, 1954 and the Easter island rudderfish Kyphosus sandwicensis (Sauvage, 1880), on the western end of the Salas y Gómez Ridge using variability of microsatellite loci (Meerhoff et al., 2018; Valencia et al., 2021). These studies found weak connectivity amongst marine fauna populations located on Rapa Nui and Salas y Gómez Island (415 km away), findings that are consistent with other studies that indicate weak connectivity within the ridges for many reef taxa (Comité Oceanográfico Nacional de Chile, 2017; Friedlander et al., 2021). In contrast, high genetic connectivity between the Desventuradas Islands and the Juan Fernandez Archipelago was described for the Juan Fernandez rock lobster Jasus frontalis (Milne Edwards, 1837) using mtDNA COI variability (Porobić et al., 2013) and the diadematid sea urchin Centrostephanus sylviae Fell 1975 using Single Nucleotide Polymorphism (SNPs) (Veliz et al., 2021). Like several other endemic species from this region, both the lobster and the sea urchin species are currently only known from the Desventuradas and Juan Fernandez Islands, indicating that eastern portion of the ridges has stronger connectivity to the Juan Fernandez Archipelago than to the western side of the ridges. A recent study projected increases in connectivity between Juan Fernandez and the Desventuradas Islands, and along the Sala y Gomez Ridge as a result of a warmer climate in the near future (Dewitte et al., 2021).
3.1.3 Animal connectivity
While there are important dispersal barriers preventing certain species reaching the ridges, these are overcome by some highly mobile species. In fact, the ridges themselves have been described as movement corridors for some migratory species, including the endangered blue whale (Balaenoptera musculus), the critically endangered leatherback turtle (Dermochelys coriacea), the critically endangered waved albatross (Phoebastria irrorata), as well as many other seabirds (Shillinger et al., 2008; Block et al., 2011; CBD, 2017; Easton et al., 2018; Boteler et al., 2019; Serratosa et al., 2020).
Blue whales are known for their large migrations from cold-water feeding grounds to warm water calving grounds. One subpopulation, which some contend is a discreet subspecies, regularly pass through the Salas y Gómez and Nazca ridges during their migration from the southern tip of Chile to the Galapagos Islands (Hucke-Gaete et al., 2014; Torres-Florez et al., 2014; Buchan et al., 2017). Blue whales have been shown to feed and rest on seamounts in the North Atlantic (Lesage et al., 2018), and it is likely that this is also the case for the Salas y Gómez and Nazca ridges (CBD, 2017). Humpback whales have a similar migration pattern, traveling from Antarctic feeding grounds to warmer waters near the Galapagos to breed, transiting through the Salas y Gómez and Nazca ridges annually (Acevedo et al., 2013). Several other species of marine mammals have been observed migrating through the Salas y Gómez and Nazca ridges, including the Cuvier’s beaked whale Ziphius cavirostris, sperm whale Physeter macrocephalus, Blainville’s beaked whale Mesoplodon densirostris, minke whale Balaenoptera bonaerensis or B. acutorostrata, false killer whale Pseudorca crassidens, pilot whale Globicephala sp., bottlenose dolphin Tursiops truncatus, common dolphin Delphinus sp., leopard seal Hydrurga leptonyx, and southern elephant seal Mirounga leonina (Hucke-Gaete et al., 2014), many of which are top-predators. Leatherback turtle and and the green turtle Chelonia mydas migrate through this region in order to lay eggs on neighbouring beaches and may also forage on seamounts (Shillinger et al., 2008; Boyle et al., 2009; Block et al., 2011; Álvarez-Varas et al., 2021b). Several species of Terns, Petrels and Boobies nest on the islands of Rapa Nui, Salas y Gómez, and the Desventuradas, and are known to feed in waters of the Salas y Gómez and Nazca ridges (Portflitt-Toro et al., 2020). This includes the emblematic Sooty Tern (Onychoprion fuscatus), a sacred bird in Rapa Nui culture.
Twelve species of seabirds are known to breed on Rapa Nui while eleven species have been observed breeding on Salas y Gómez Island. The Kermadec petrel, Christmas shearwater (Puffinus nativitatis), red-tailed Tropicbird (Phaethon rubricauda), great frigatebird (Fregata minor), masked booby (Sula dactylatra), brown noddy (Anous stolidus), grey noddy (Anous albivitta), common white tern (Gygis alba), and sooty tern all breed on both Rapa Nui and Salas y Gómez Island (Flores et al., 2014). Sala y Gómez Island is considered an Important Bird and Biodiversity Area (IBA) due to the presence of breeding populations of the masked booby and grey noddy. The ridges also intersect five proposed IBAs, which are being considered because they include nodes of migratory routes including incubation areas for Waved Albatross, as well as non-breeding areas for Chatham Albatross (Thalassarche eremita) and Salvin’s Albatross (Thalassarche salvini), and Cook’s petrel (Pterodroma cookii), Chatham petrel (Pterodroma axillaris) and white-chinned petrel (Procellaria aequinoctialis).
The Chatham Albatross travels thousands of kilometers each year to get from their breeding grounds on the Pyramid, a small outcropping in New Zealand’s Chatham Islands, to South America traveling above the ridges (Nicholls and Robertson, 2007). The Chatham Petrel is also known to migrate from New Zealand across the Salas y Gómez and Nazca ridges each year to feed in its non-breeding season (Rayner et al., 2012). Several top predators occur on both sides of the southern Pacific, including the Salas y Gómez and Nazca ridges. This includes the Galapagos shark (Carcharhinus galapagensis) (Morales et al., 2019; Pazmiño et al., 2018), the yellowtail amberjack (Seriola lalandi) (Premachandra et al., 2017; Morales et al., 2019) and the yellowfin tuna (Thunnus albacares) (Pecoraro et al., 2018), all of which likely use the ridges as migration corridors. Swordfish (Xiphias gladius) move from over the ridges north across the Galapagos Rise, representing another connection between the ridges and the area around the Galapagos Islands (Abascal et al., 2010; Evans et al., 2014).
3.2 Human connectivity
3.2.1 Human and cultural connectivity
Despite being uninhabited, the waters of the Salas y Gómez and Nazca ridges have an exceptionally rich history of human seafaring and cultural significance (reviewed in Delgado et al., 2022). This ranges from indigenous Polynesian cultures who first ventured to this region close to a thousand years ago, to the period of European colonial exploration, as well as the rise of the modern global economy. Voyaging, the transportation of commodities, and fishing have occurred throughout the human history of this region. As a result, there are many yet-to-be discovered shipwrecks, as well as numerous accounts in the written and oral history that highlight the profound connections of many cultures to this region (Delgado et al., 2022). In relation to the human colonization of Rapa Nui Island, recent evidence suggests prehistoric contact of Polynesian individuals with Native American individuals (approx. AD 1200) contemporaneous with the settlement of remote Oceania. This suggests that a single contact event occurred in eastern Polynesia, before the settlement of Rapa Nui, between Polynesian individuals and a Native American group related to the indigenous inhabitants of present-day Colombia (Ioannidis et al., 2020). In modern times, vessels could be sources of biological connectivity along the Salas y Gómez and Nazca ridges, by transporting invasive species in ballast water or as hull fouling (Carlton, 1985; Cariton and Geller, 1993; Bax et al., 2003; Bailey, 2015). Indeed, many cultures, particularly Rapa Nui culture, have profound connections to these remote waters. It is important that this knowledge is perpetuated via research, education, and outreach activities, so that people continue to appreciate both the natural and cultural significance of the region. Figure 2.
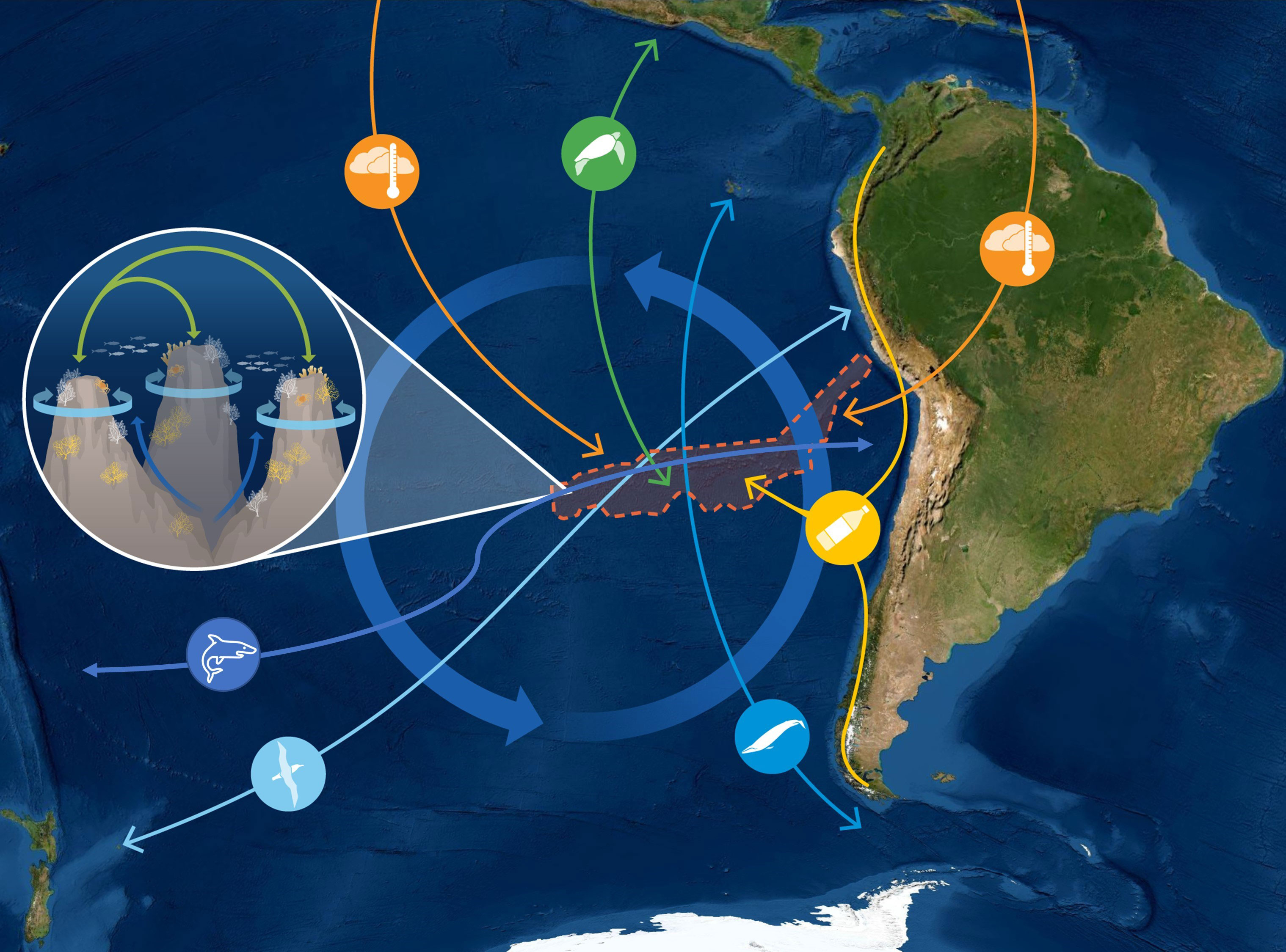
Figure 2 Diagram showing how the natural and cultural significance of the Salas y Gómez and Nazca ridges are influenced by connectivity via movement of organisms, nutrients, and pollutants.
3.2.2 Connectivity in governance arrangements
The concept of connectivity is found in many multilateral agreements (Dunn et al., 2019), and particularly revolves around (1) international cooperation for highly migratory species (e.g., CMS, 1979; UNCLOS, 1982), (2) management of straddling and highly migratory fish stocks (UNFSA, art. 5 and in the conventions of regional fisheries management organizations (RFMOs)), (3) conservation of albatrosses and petrels (ACAP, 2001), (4) conservation of cetaceans through whale sanctuaries; (5) application of precautionary and ecosystem approaches (e.g., CITES, 1973; CMS, 1979; CBD, 1992; UNFSA, 1995; and in RFMO and regional seas organization (RSO) Conventions); (6) minimisation of pollution and preventing transboundary environmental damage (e.g., London Convention, 1972; London Convention Protocols, 1978; MARPOL Convention, 1978; UNCLOS, 1982; CBD, 1992; UNFSA, 1995, and in RFMO and RSO Conventions); and (7) establishment of protected areas and ecological networks (e.g., CBD, 1992; and under the International Seabed Authority (ISA), and in RSO Conventions). In 2021, the importance of connectivity was formally recognised by the United Nations General Assembly, stressing the need to maintain ecosystem connectivity through the development of transboundary cooperation, the application of EIAs, the application of ecosystem-based approaches, and the establishment of transboundary protected areas and ecological corridors (UNGA, 2021). Within the Salas y Gómez and Nazca ridges, both SPRFMO and IATTC have legal provisions that include the concept of connectivity, particularly with regards to the adoption of measures for target fish stocks and associated species, pollution minimisation, and the application of the precautionary approach (e.g., IATTC art. VII; SPRFMO art. 3) (IATTC, 1949; SPRFMO, 2009). SPRFMO also has a specific provision on the application of the ecosystem approach (SPRFMO, art. 3.2) (SPRFMO, 2015). The South Pacific Permanent Commission (CPPS), through the 1981 Lima Convention, also has legal provisions for pollution minimisation in ABNJ (Lima Convention, 1981).
3.3 External threats to connectivity
3.3.1 Plastic pollutants and marine debris
The Salas y Gómez and Nazca ridges are located near the South Pacific Gyre, a circulation feature known for its high concentrations of plastic pollution and other marine debris (Eriksen et al., 2014; Boteler et al., 2019; Luna-Jorquera et al., 2019; van Gennip et al., 2019). These pollutants originate mostly from the South American coast (Thiel et al., 2018; van Gennip et al., 2019) and are then concentrated in the circulating waters of the gyre. High levels of plastic pollution impact many species of marine mammals, sharks, turtles, seabirds, and fishes, through entanglement or ingestion (Thiel et al., 2018). Moreover, floating anthropogenic debris is known to be an agent for transporting non-indigenous species (Rech et al., 2016; Rech et al., 2018; Rech et al., 2021), thus increasing the risk of biological invasions throughout the region.
3.3.2 Fishing
Currently, fishing is the dominant human activity that occurs in the waters of the Salas y Gómez and Nazca ridges (Wagner et al., 2021b). The main species caught in the Southeast Pacific region remain: Anchoveta (Engraulis ringens), Jumbo flying squid (Dosidicus gigas), Araucanian herring (Clupea bentincki), Chilean jack mackerel (Trachurus murphyi), Chilean kelp (Durvillaea antarctica, Scomber japonicus), Skipjack tuna (Katsuwonus pelamis), and Chub mackerel (Scomber japonicus) (FAO, 2020). Fishing effort is still considered moderate, and is attributable mostly to vessels flagged by China, Spain, Japan, Taiwan, and the Republic of Korea, which collectively account for over 96% of the fishing effort (reviewed in Wagner et al., 2021b). It is important to highlight that fishing by bordering countries to the Salas y Goméz and Nazca ridges fish mainly takes place within their national jurisdiction. Fishing effort in ABNJ is mainly undertaken by distant water fishing nations. However, nearby countries are most likely to be negatively impacted by the depletion of ABNJ fisheries species that could otherwise be available in adjacent national waters. The SPRFMO Convention includes specific provisions to protect Vulnerable Marine Ecosystems (VMEs) and has defined ten benthic invertebrate taxa that are regarded as indicators of VMEs for the South Pacific (SPRFMO, 2020).
3.3.3 Deep-sea mining
Though there is currently no deep seabed mining taking place in ABNJ, several countries and international consortia hold contracts with the International Seabed Authority (ISA) to explore areas within the Pacific, Indian, and Atlantic oceans. It is expected that deep seabed mining will have severe and long-lasting impacts on the marine environment, which has prompted several countries and organisations to call for a moratorium on deep seabed mining (Christiansen et al., 2022) While there have been no ISA contracts issued to explore for deep-sea minerals in the Salas y Gomez and Nazca ridges, the occurrence of seabed minerals in this region may attract interest in the future.
3.3.4 Climate change
Climate change is another external-originating threat and is one of the most important ocean threats globally (IPCC, 2019; Hoegh-Guldberg, 2019). While climate change is impacting ocean ecosystems everywhere, some areas are considered particularly susceptible, including the Salas y Gómez and Nazca ridges. This is because the region contains some of the most nutrient-poor waters in the world, with communities that are adapted to these conditions (Von Dassow and Collado-Fabbri, 2014). As climate changes, with temperature, salinity, and oxygen shifts, some of these communities may no longer be able to tolerate local conditions, resulting in potential range shifts, habitat loss, decreased biodiversity, and decreased resilience (Cheung and Levin, 2018; Dewitte et al., 2021; Wagner et al., 2021b). These consequences will be compounded by the El Niño Southern Oscillation and the Pacific Decadal Oscillation, which already have widespread impacts throughout the region (Yañez et al., 2017). The Southeast Pacific Subtropical Anticyclone, which is the dominant forcing mechanism of currents in the region, has already experienced a poleward shift due to climate change, a shift that is projected to continue (Yañez et al., 2017). It is important to highlight that future warming climates will increase south-easterly trade winds in the Southeastern Pacific (Dewitte et al., 2021). As a result, connectivity between the Juan Fernandez and Desventuradas Islands, and along the Sala y Gomez Ridge, is projected to increase (Dewitte et al., 2021).
4 Integrating connectivity into management and conservation
Although the importance of maintaining connectivity is well recognised globally, quantitative methods for identifying areas of high connectivity are rarely utilized in the design of area-based conservation efforts (Magris et al., 2014). There are a handful of MPAs which included connectivity as a criteria in their design, most of which are located in Australia or the United States (Balbar and Metaxas, 2019). The complexity of connectivity (i.e., ocean dynamics and processes), however, means that there is no one-size-fits-all solution, but rather a suite of scientific tools, management approaches, and governance arrangements that will need to be applied. Identifying specific conservation and management objectives upfront, will be critical to determining how to best integrate connectivity. In this regard, IUCN has produced guidelines on marine connectivity (Lausche et al., 2021), which support the design of MPAs and MPAs networks using the best available science in the face of climate change.
4.1 Tools for understanding connectivity
4.1.1 Baseline studies
Baseline biodiversity and ocean circulation studies will remain fundamental to understanding connectivity and will need to be complemented with new tools to generate information needed by decision makers (Borja et al., 2016; Gjerde et al., 2021). There are only a few studies that have surveyed biodiversity across the large range of the Salas y Gómez and Nazca ridges (Comité Oceanográfico Nacional de Chile, 2017; Friedlander et al., 2021). These studies found unique species compositions with few species shared between opposite ends of the ridges, highlighting the need to enact large-scale conservation measures to conserve representative biodiversity. This is particularly important due to the exceptionally high proportion of endemic species and fragility of this region. Loss of habitat in the Salas y Gómez and Nazca ridges could therefore lead to species extinctions.
4.1.2 DNA-based analyses
DNA-based analyses are a promising approach for connectivity research. Genomic analyses are useful to detect species or population geographical delimitation (e.g., Veliz et al., 2021), effective population size (Pazmiño et al., 2017) and also symmetry/asymmetry in connectivity among populations in a metapopulation context (Liggins et al., 2020). Another approach is the analysis of environmental DNA (eDNA), which organisms release into the environment and thereby allow their detection without sampling the organisms themselves (Levin et al., 2019). This approach still requires baseline knowledge of species identity and longevity of the DNA signal in the environment (Levin et al., 2019), but can cost-effectively complement in situ sampling (Gjerde et al., 2021).
4.1.3 Acoustic monitoring
Acoustic monitoring is another approach that is useful to survey large spatial scales, and can provide information about seafloor topography, currents, temperature, abundance, and type of marine life (Howe et al., 2019). Combining acoustic monitoring with DNA techniques offers significant promise for enhancing ocean observations and addressing questions about long-range connectivity (Howe et al., 2019). These approaches can also be complemented by satellite data to monitor phytoplankton and harmful algal blooms, including their spatial and temporal dynamics (Borja et al., 2016).
4.1.4 Underwater vehicles
Advanced technologies like human occupied vehicles (HOVs), remotely operated vehicles (ROVs), autonomous underwater vehicles (AUVs), and towed camera systems have been used to survey deep-water areas (Dominguez-Carrió et al., 2021). Unfortunately, these approaches typically require expensive instruments, specialized technicians, and costly ship time, thereby being restrictive (Dominguez-Carrió et al., 2021). Cost-effective drop cameras developed by the National Geographic Society (Turchik et al., 2015) were used to conduct the first surveys on both ends of the Salas y Gómez and Nazca ridges (Figure 3; Friedlander et al., 2021). Future surveys using such cost-effective methods have the potential to improve baseline knowledge of the deep-water fauna and connectivity of the region. However, these tools still face challenges of identifying species from visual imagery of alone. Additionally, biodiversity estimates based on imagery often under-represent biodiversity, requiring baseline data (e.g., through species collection).
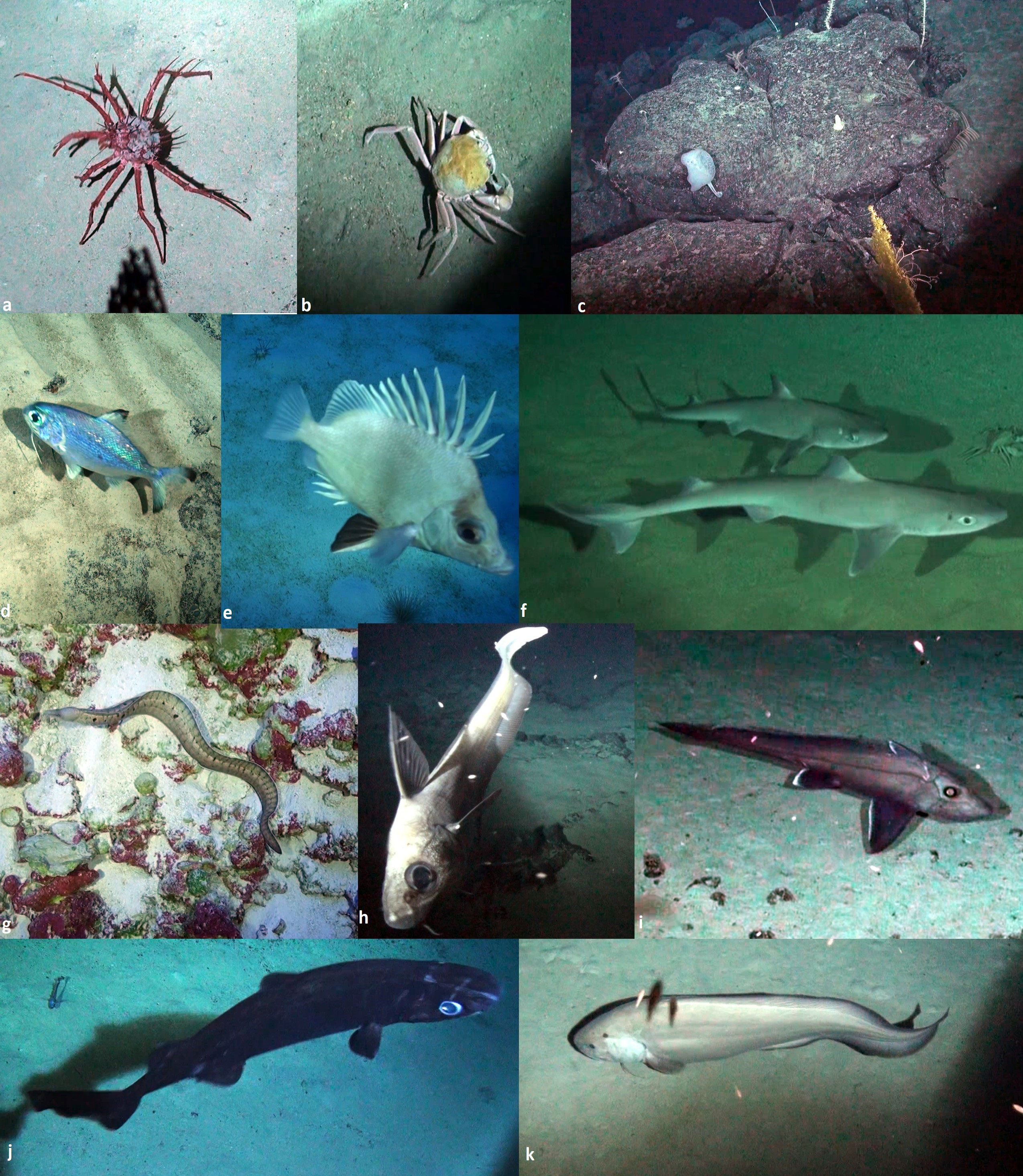
Figure 3 Examples of deep-water fauna documented using cost-effective drop cameras during the first ever study that surveyed both ends of the Salas y Gómez and Nazca ridges. From top left to bottom right: (A) Lithodidae, (B) Chaceon chilensis, (C) various deep-sea corals and sponges, (D) Polymixia salagomeziensis, (E) Pentaceros decacanthus, (F) Squalus sp., (G) Gymnothorax bathyphilus, (H) Coryphaenoides sp., (I) Hydrolagus sp., (J) Etmopterus sp., and (K) Spectrunculus sp. (Friedlander et al., 2021; images courtesy of National Geographic Pristine Seas and National Geographic Exploration Technology Lab).
4.1.5 Machine learning methods
Progress has also been made with machine learning methods for analysing large datasets. This has implications for the Salas y Gómez and Nazca ridges, since many historical datasets (see Wagner et al., 2021b; Delgado et al., 2022) have not yet been examined in exhaustive detail and could therefore be mined to glean new insights. One important caveat is that many available datasets do not have the fine-scale resolution, or do not capture the complexity of habitats needed to apply such methods (Saleh et al., 2020). Another challenge is that much of the analysis with machine learning methods still rely on costly human labour (e.g., to prepare the photos for analysis) which does support the models to learn how to detect species in natural settings (Saleh et al., 2020).
4.1.6 Animal borne devises
Other technologies, such as animal-borne devices, can be used to study species movement and behaviour. These are rapidly advancing and providing increasingly accurate information (Davies et al., 2021). Tracking data of birds, mammals, fishes, and turtles is increasingly becoming available, and is highly relevant to understanding how to integrate connectivity into conservation and management measures (Dunn et al., 2019; Hays et al., 2019; Davies et al., 2021). Connectivity data is currently available for a few species that migrate to the Salas y Gómez and Nazca ridges (Shillinger et al., 2008; Block et al., 2011). Future studies, including those that tag animals on the ridges, will be essential to improve our understanding of their movement and connectivity within the region. Such approaches have successfully been used to study movement patterns of Galapagos shark (Carcharhinus galapagensis) and yellowtail amberjack (Seriola lalandi) in the waters around Salas y Gómez Island (Morales et al., 2021). New knowledge platforms such as the Migratory Connectivity in the Ocean (MiCO) system will help make connectivity information available to researchers, decision makers, and the public (Dunn et al., 2019).
4.1.7 Modelling
Collected data can be combined with modelling to predict spatial distribution patterns across broader scales, including in areas that have not yet been surveyed (Borja et al., 2016; Oppel et al., 2018; Georgian et al., 2021; Gjerde et al., 2021). Modelling is increasingly being used to predict the distribution, composition, and structure of marine ecosystems, including climate-driven changes (Gjerde et al., 2021). A recent study modelled the distribution of corals and sponges across the Salas y Gómez and Nazca ridges and found that these taxa are widespread across the ridges and found that these taxa are widespread across the ridges (Figure 4; Georgian et al., 2021). Corals and sponges provide important habitats for many associated species and are also highly susceptible to impacts; protecting these vulnerable marine ecosystems (VMEs) is therefore an important component of the framework for managing high seas bottom fisheries under the United Nations General Assembly Sustainable Fisheries Resolution (Jones and Lockhart, 2011; Georgian et al., 2021). Promising approaches include combining long-term monitoring studies with population connectivity models. Such approaches can assess potential area-based management tools (ABMTs), including MPAs, in terms of their relative contributions and their dependence on regional ecosystem characteristics (Carr et al., 2019).
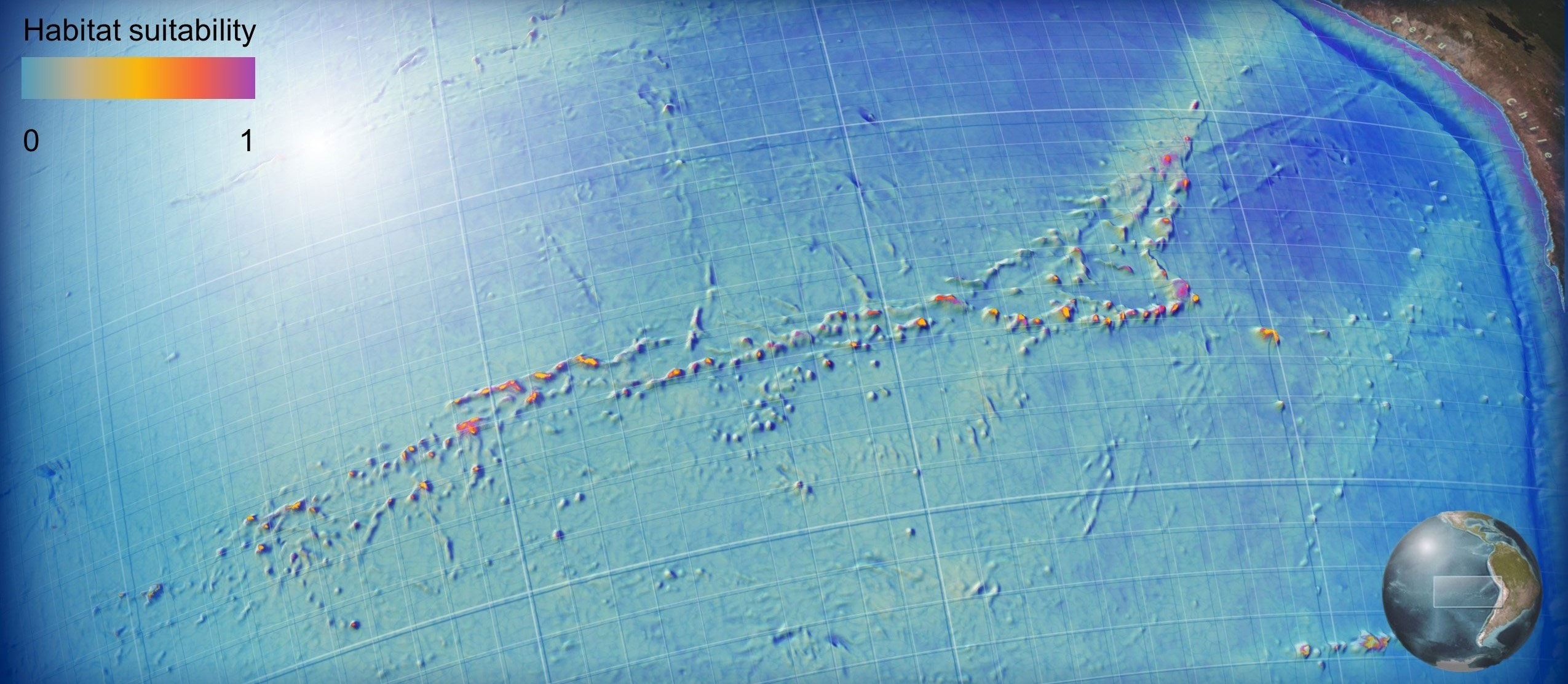
Figure 4 Map showing results of a recent modelling study (Georgian et al., 2021) that noted that every seamount of the Salas y Gómez and Nazca ridges contains highly suitable habitat for habitat-forming corals and sponges. Warmer colours indicate more suitable habitat. (Credit: John Nelson).
4.1.8 Metrics for connectivity criteria
Research results can be used to understand connectivity patterns and create criteria for the region to support management decisions and in particular the design of MPAs. There is no agreed upon approach amongst scientists by which to establish connectivity patterns for the design of MPAs, while some classifications have been suggested: modelling, tagging, genetics, and simple observation (Balbar and Metaxas, 2019 citing Bryan-Brown et al., 2017). Balbar and Metaxas, 2019 provide a comprehensive review of the different methods and approaches available to decision makers to integrate connectivity criteria into the design of MPAs and provide a number of recommendations: “determine whether to prioritise connectivity as an ecological criterion; identify the role of an MPA in supporting connectivity; identify appropriate spatial and temporal scales; and improve regional knowledge of patterns of connectivity.” The synthesis provided in this paper could be a first step in creating a connectivity index for the region, as no such index exists, focusing on aspects of ecological and cultural connectivity and considering pressures such as fishing and climate change.
4.1.9 Interdisciplinary and international collaboration
Biodiversity and oceanographic data alone are not enough to understand ecosystem vulnerability and needs to be combined with spatial data on threats and human activities to inform decision making (Hays et al., 2019). Due to the complexity of connectivity and the variety of scientific methods to study it, interdisciplinary and international collaborations will be critical to improve our understanding of connectivity. As tools for collecting and analysing scientific information continue to advance, it will be essential that scientists find effective ways to translate research findings into conservation benefits. This requires a successful science-policy interface to communicate research findings to policy makers, as well as the public at large.
4.2 Management approaches to include connectivity in decision making
Conservation efforts need to include surface to seabed protection (O’Leary and Roberts, 2018), and explicitly integrate climate adaptation objectives (Tittensor et al., 2019). Particular challenges to incorporating connectivity in ABNJ conservation include the scarcity of spatial and temporal data (Dunn et al., 2019; Visalli et al., 2020), as well as challenges related to monitoring, control, and enforcement in remote regions (Wilhelm et al., 2014; Ortuño Crespo et al., 2020).
Data obtained using multiple scientific methods and from various sources can create a more complete understanding, thereby supporting the better integration of connectivity into management decisions. For instance, genetic, acoustic and telemetry data have been used to describe connectivity for blue whales (Torres-Florez et al., 2014; Buchan et al., 2015; Torres-Florez et al. 2015), but additional work is needed to aggregate this information into integrated models (e.g., through MiCO). Holistic studies through strategic environmental assessments (SEA) or regional environmental assessments (REA) can create a comprehensive basis for understanding marine ecosystems (Gjerde et al., 2021), as well as assess conditions important for conservation, such as species distributions, temporal variability, trophic relationships, ecosystem function, oceanographic connectivity, and resilience. First steps towards conducting such assessments focused on the status of marine biodiversity and human activities in the region have been completed (Boteler et al., 2019) and could be used to expand upon.
While environmental assessments typically focus on a defined area, connectivity is not an area-based characteristic, making it more difficult to integrate it into management and policy decisions (Balbar and Metaxas, 2019). It is therefore necessary to include data that ranges beyond the geographical and temporal scope of an environmental assessment to guide decision making. Such decisions will need to be made on a case-by-case basis depending on the known and potential dispersal of focal species and impacts. This is further complicated because connectivity patterns may vary over time, requiring regular environmental assessments to assess temporal changes (Balbar and Metaxas, 2019). Environmental assessments can also identify knowledge gaps and guide future research initiatives. As noted previously, connectivity data for some species of whales, turtles, and seabirds indicate that these highly mobile species regularly use the Salas y Gómez and Nazca ridges for foraging and resting. Consequently, areas that are likely to include these focal species should be prioritized for increased protection.
Holistic environmental assessments can help underpin management decisions about the role and function of connectivity. It is important to include migratory connectivity with MPA site selection criteria and network design criteria (Dunn et al., 2019). Identifying connectivity corridors can build coherence between fragmented ecosystems, or buffer zones from potentially damaging external influences (Bennett and Mulongoy, 2006). Connectivity metrics (to quantify connectivity of biodiversity of an area such as habitats or species) can help specify desired conservation objectives and actions, as well as prioritize areas for inclusion in MPA networks (Keeley et al., 2021). Spatial tools, such as marine spatial planning, can also help to evaluate the role of connectivity (Balbar and Metaxas, 2019). The use of marine spatial planning in ABNJ remains limited, while exploratory studies for the application of area-based planning tools such as marine spatial planning have been explored for the Southeast Pacific (UNEP-WCMC, 2019). Such spatial tools must be combined with information on human activities and stressors, including impacts of climate change, to identify threats to connectivity and allow a multi-sector approach. Screening of potential activities through environmental impact assessments (EIAs) can quantify impacts where activities are taking place, but also more widely to where impacts might occur in the future (Tessnow-von Wysocki, 2021). Existing EIAs within the broader South Pacific stem from sectoral organizations such as SPRFMO and IATTC. Other organizations which could require EIAs in the region include the International Seabed Authority (mining) and the International Maritime Organization (shipping); however, activities regulated by these organizations are currently very limited in the region (Wagner et al., 2021b). EIAs can also provide an understanding about whether activities taking place within national jurisdiction are leading to impacts in ABNJ, and vice versa (Livingstone and Jose, 2021). This is particularly relevant to cross-jurisdictional impacts of potential human activities. In regard to human impacts, it is important to highlight that fishing and other extractive activities are still at relatively low levels in the Salas y Gómez and Nazca ridges, so there is a time-sensitive opportunity to proactively conserve the unique natural and cultural resources of the region, while having minimal impacts on industries.
Holistic assessments that include connectivity will be critical to inform management decisions. Priorities for managing connectivity, including ecological, economic, and social considerations, will need to be documented in management plans, including respective roles and time plans for achieving conservation objectives (Balbar and Metaxas, 2019). Only when a management plan lays out the scientific assessment and associated management activities in a transparent manner, can stakeholders evaluate management effectiveness over time. Management planning is a key challenge for remote MPAs (Gaymer et al., 2014; Wilhelm et al., 2014), but recent years have seen a major advance in the generation of management plans. All the MPAs created in Chilean waters of the Salas y Gomez and Nazca ridges (see Figure 1) now have completed management plans, although these still have not been officialised by the Government (Gaymer et al., 2018; Gaymer et al., 2021a; Gaymer et al., 2021b; Gaymer et al., 2021c). These management plans highlight the importance of connectivity in maintaining the life cycles in key habitats and ecosystem services.
Transboundary and cross-sectoral cooperation are key to ensuring that connectivity is fully considered within ocean governance. Spatial planning tools and holistic environmental assessments are key to ensure that connectivity is optimized for biodiversity conservation. The inclusion of ‘ecological connectivity [and/or coherence]’ as one of the criteria for the identification of future ABMTs under the proposed BBNJ Agreement will help ensure that connectivity is considered by relevant stakeholders when establishing ABMTs, including species corridors, MPA networks, linkage zones, permeability areas, and climate corridors.
5 Discussion
Integrating connectivity into conservation for the Salas y Gómez and Nazca ridges will be critically important to conserve the natural and cultural resources of this unique region. ABNJ have gained attention in recent years through the negotiations for the BBNJ Agreement and are increasingly understood as critical to achieve global sustainability goals. Closely connected to coastal States in South America, the Salas y Gómez and Nazca ridges are important for global marine biodiversity and provide numerous benefits to humanity, including climate regulation, food security, cultural values, and other ecosystem services.
This review is a first step in conducting a baseline study on connectivity of the region. While this study summarizes information from various publicly available sources, a more in-depth holistic regional environmental assessment should be developed to coalesce additional information that may be available and is not yet in the public domain. Conducting such a study will be logistically complex, as regional characteristics will need to be considered across numerous jurisdictions, disciplines (e.g., ecological, physical, chemical, economic, and social), as well as take into account changes over time and beyond the immediate geographic focal area. Such a regional environmental assessment will require a collaborative effort, bringing together individuals from different countries, expertise, and experience. This exercise should build upon relevant working groups in the region (e.g., within CPPS, SPRFMO, IATTC, International Union for Conservation of Nature, World Commission on Protected Areas, Deep Ocean Stewardship Initiative, UN Decade for Ocean Science, ESMOI, and others), and coalesce information from existing data platforms.
A regional environmental assessment will be critical to identifying information gaps in relation to connectivity and help identify the tools and approaches needed to fill these gaps. Such assessments will represent important first steps at integrating connectivity in the design and implementation of conservation approaches for the region. Available data should provide a starting point to include connectivity in conservation measures. Data collection should be a continuous objective, which requires ongoing contributions to work towards a fuller understanding of connectivity. Through such an iterative process, key characteristics of connectivity will begin to emerge, and ultimately, with communication across stakeholder groups, connectivity priorities will be established and integrated into management measures. It will therefore be necessary to develop a suite of indicators by which to understand and assess connectivity within the region and beyond, thereby underpinning decision making and monitoring of management results. It is important to note that connectivity is an important criterion for conservation efforts, such as a network of MPAs, across large spatial scales but should also be complemented with other considerations like endemism. Due to the high rate of endemism (Comité Oceanográfico Nacional de Chile, 2017; Friedlander et al., 2021; Tapia-Guerra et al., 2021) as well as, the importance of the ridges as migratory corridors for highly mobile species, creating a large-scale MPA in this region would address several conservation considerations. In addition to having advantages in terms of connectivity and ecological integrity, large-scale MPAs also have lower establishment costs per unit area (Toonen et al., 2013; Wilhelm et al., 2014).
Regional conservation objectives for the Salas y Gómez and Nazca ridges should be established based on identified priorities, and these should explicitly deal with connectivity. Objectives should focus on conserving areas that are key to maintaining connectivity and restoring degraded areas that may be impeding connectivity. Management approaches such as dynamic closures, migratory corridors, and buffer zones could be considered along with existing or new ABMTs to achieve such objectives. Regional action plans for connectivity should be considered to establish a management process such as roles of key stakeholders, a timeline for implementation, as well as monitoring and enforcement mechanisms. These efforts will also need to be reviewed over time and updated based on new information.
Some limited legal provisions exist for connectivity, but more could be done to bring together stakeholders within the region to jointly address the management of connectivity. Another opportunity is to establish regional or global targets for connectivity, potentially through the Convention on Biological Diversity’s post-2020 Global Biodiversity Framework. However, as connectivity is not strictly an area-based target as other ecological criteria such as representation, it is difficult to develop quantitative, spatial objectives.
Connectivity is widely recognised as a critical ecological feature but remains under-addressed in management strategies. Nevertheless, there are a range of opportunities from new scientific approaches, to developing management efforts, or addressing governance issues, which could build upon existing efforts to advance marine management within the region. Such efforts will require political will and support from many different actors and will therefore take time to develop. However, continued efforts to integrate connectivity into conservation efforts for the Salas y Gómez and Nazca ridges will not only improve the health of its ecosystems, but also elevate the region as an exemplary model for cross-sectoral collaboration and cooperation.
6 Recommendations and conclusion
Integrating connectivity into conservation efforts in the Salas y Gómez and Nazca ridges will provide benefits for biodiversity, climate regulation, food security, cultural services, as well as advance global sustainability goals. Based on available information from previous studies, conservation approaches, and governance arrangements, the following recommendations emerge:
● The Salas y Gómez and Nazca ridges are of exceptional natural and cultural significance and should therefore be protected using the best-available conservation measures. Human activities that cause harm to the health of the Salas y Gómez and Nazca ridges should be restricted. A zone expanding beyond the immediate area of the ridges should be established to create a buffer between the Salas y Gómez and Nazca ridges and human activities within the region.
● Despite being remote, uninhabited, and not yet targeted by extractive activities in a major way, the Salas y Gómez and Nazca ridges are being impacted by externally originating threats like plastic pollution and climate change. These threats need to be reduced in collaboration with many stakeholders, including through regional and global policy fora. In particular, States within the region could create a coalition of countries to call upon global action to address this global threat.
● The otherwise pristine ecosystems of the Salas y Gómez and Nazca ridges can provide important examples to showcase the severity of these externally originating threats to global audiences.
● Due to their high rates of endemism, as well as their importance as migratory corridors, enacting large-scale conservation measures in the Salas y Gómez and Nazca ridges, such as the establishment of a large-scale marine protected area and the identification of this region as a vulnerable marine ecosystem (VME), would address important conservation considerations for both connectivity and representation.
● Data on connectivity is still limited but should not constrain the development of conservation measures. A precautionary approach should be implemented wherever understanding is considered insufficient to ensure that human activities do not cause significant or irreversible harm to biodiversity. This is particularly relevant to industrial activities like fishing and mining that have not been fully developed around the ridges, and could therefore be restricted proactively, without impacting industries.
● Data for some species of whales, turtles, and seabirds indicate that these highly mobile species regularly use the Salas y Gómez and Nazca ridges for foraging and resting. Consequently, areas that are likely to include these focal species should be prioritized for increased protection. It is therefore necessary to include data that ranges beyond the geographical and temporal scope of the region into environmental assessments to guide decision making.
● While remote, the Salas y Gómez and Nazca ridges are connected to adjacent coastal States in many ways. Actors from these States should be brought together to co-design and co-deliver conservation measures that integrate connectivity.
● A holistic assessment of the region should be conducted and build upon relevant working groups in the region (e.g., within CPPS, SPRFMO, IATTC, International Union for Conservation of Nature, World Commission on Protected Areas, Deep Ocean Stewardship Initiative, UN Decade for Ocean Science, ESMOI, and others), and coalesce information from existing data platforms.
● International and interdisciplinary collaboration will be needed to not only conserve the Salas y Gómez and Nazca ridges, but also help fill knowledge gaps relating to connectivity. Observational data should be combined with modelling to predict spatial distribution patterns across broader scales, including in areas that have not yet been surveyed. Emerging technologies like genomic sequencing, eDNA, acoustic methods, and deep-sea camera systems will need to be integrated with baseline biodiversity and ocean circulation studies to improve our understanding of connectivity. Research should be strategically paired with education and outreach activities, so that people continue to appreciate both the natural and cultural significance of the region.
● Research should contribute to development of comprehensive indicators for understanding and assessing natural and cultural connectivity within the region and beyond. Indicators can be paired with information and knowledge about pressures such as climate change and human activities. A suite of indicators will underpin decision making such as for the identification and design of management measures i.e., MPAs and MPA networks and support the assessment of management decisions.
● States, organizations, civil society, and scientists should coordinate efforts to share knowledge on the region including questions of connectivity. A holistic assessment co-developed by various stakeholders will help create the basis for establishing regional management and conservation targets, including connectivity considerations.
● The Salas y Gómez and Nazca ridges could act as an exemplary case study for integrating connectivity into conservation. Lessons learned could be documented and shared broadly to support initiatives in other locations around the globe.
Author contributions
BB: Conceptualization, Formal analysis, Writing original draft, Writing - review and editing. DW: Conceptualization, Data curation, Formal analysis, Visualization, Writing - original draft, Writing - review and editing. CD: Conceptualization, Formal analysis, Writing original draft, Writing -review and editing. ES, Writing original draft. CG: Writing - review and editing. AF: Writing - review and editing. DD: Writing - review and editing. FV: Writing - review and editing. DV: Writing - review and editing. CH: Writing - review and editing. All authors contributed to the article and approved the submitted version.
Funding
This work was funded in part by the Paul M. Angell Family Foundation, Alan Eustace, and Tom and Currie Barron. Support was also provided by the German Federal Ministry of Education and Research (BMBF) through its Research for Sustainable Development program (FONA), and the Federal State of Brandenburg.
Acknowledgments
We further thank the Coral Reefs of the High Seas Coalition for providing invaluable support to this effort, and in particular A. McGowern, A. Wilhelm, L. van der Meer, R. Pyle, L. Morgan, N. Clark, E. Karan, and A. Smith for all their thoughtful contributions to this work.
Conflict of interest
The authors declare that the research was conducted in the absence of any commercial or financial relationships that could be construed as a potential conflict of interest.
Publisher’s note
All claims expressed in this article are solely those of the authors and do not necessarily represent those of their affiliated organizations, or those of the publisher, the editors and the reviewers. Any product that may be evaluated in this article, or claim that may be made by its manufacturer, is not guaranteed or endorsed by the publisher.
References
Abascal F. J., Mejuto J., Quintans M., Ramos-Cartelle A. (2010). Horizontal and vertical movements of swordfish in the southeast pacific. ICES J. Mar. Sci. 67, 466–474. doi: 10.1093/icesjms/fsp252
ACAP (2001). Agreement on the Conservation of Albatrosses and Petrels, opened for signature 19 June 2001, ATS 5 (entered into force 1 February 2004).
Acevedo J., Haro D., Dalla Rosa L., Aguayo-Lobo A., Hucke-Gaete R., Secchi E., et al. (2013). Evidence of spatial structuring of eastern south pacific humpback whale feeding grounds. Endang Species Res. 22, 33–38. doi: 10.3354/esr00536
Álvarez-Varas R., Heidemeyer ,. M., Riginos C., Benítez H. A., Reséndiz E., Lara-Uc M., et al. (2021a). Integrating morphological and genetic data at different spatial scales in a cosmopolitan marine turtle species: Challenges for management and conservation. Zool. J. Linn. Soc 191, 434–453. doi: 10.1093/zoolinnean/zlaa066
Álvarez-Varas R., Rojas-Hernández N., Heidemeyer M., Riginos C., Benítez H. A., Araya-Donoso R., et al. (2021b). Green, yellow or black? genetic differentiation and adaptation signatures in a highly migratory marine turtle. Proc. R. Soc B Biol. Sci. 288. doi: 10.1098/rspb.2021.0754
Andrade I., Hormazábal S., Correa-Ramírez M. (2014). Variabilidad espacio-temporal de la clorofila-a satelital en la provincia de isla de pascua, océano pacífico suroriental. Lat. Am. J. Aquat. Res. 42, 871–887. doi: 10.3856/vol42-issue4-fulltext-13
Bailey S. A. (2015). An overview of thirty years of research on ballast water as a vector for aquatic invasive species to freshwater and marine environments. Aquat. Ecosyst. Heal. Manage. 18, 261–268. doi: 10.1080/14634988.2015.1027129
Balbar A. C., Metaxas A. (2019). The current application of ecological connectivity in the design of marine protected areas. Glob. Ecol. Conserv. 17. doi: 10.1016/j.gecco.2019.e00569
Ban N. C., Maxwell S. M., Dunn D. C., Hobday A. J., Bax N. J., Ardron J., et al. (2014). Better integration of sectoral planning and management approaches for the interlinked ecology of the open oceans. Mar. Policy 49, 127–136. doi: 10.1016/j.marpol.2013.11.024
Bax N., Williamson A., Aguero M., Gonzalez E., Geeves W. (2003). Marine invasive alien species: A threat to global biodiversity. Mar. Policy 27, 313–323. doi: 10.1016/S0308-597X(03)00041-1
Beal M., Dias M. P., Phillips R. A., Oppel S., Hazin C., Permian E. J., et al. (2021). Global political responsibility for the conservation of albatrosses and large petrels. Sci. Adv. 7(10), 753–774. doi: 10.1126/sciadv.abd7225
Bennett G., Mulongoy K. J. (2006). Review of experience with ecological networks, corridors and buffer zones. Secretariat Convention Biol. Divers. 100
Block B. A., Jonsen I. D., Jorgensen S. J., Winship A. J., Shaffer S. A., Bograd S. J., et al. (2011). Tracking apex marine predator movements in a dynamic ocean. Nature 475, 86–90. doi: 10.1038/nature10082
Borja A., Elliott M., Andersen J. H., Berg T., Carstensen J., Halpern B. S., et al. (2016). Overview of integrative assessment of marine systems: The ecosystem approach in practice. Front. Mar. Sci. 3. doi: 10.3389/fmars.2016.00020
Boteler B., Wanless R., Dias M., Packeiser T., Awad A., Yannicelli B., et al. (2019). “Ecological baselines of the southeast Atlantic and southeast pacific: Status of marine biodiversity and anthropogenic pressures in areas beyond national jurisdiction,” in STRONG high seas proj Potsdam, Germany. doi: 10.2312/iass.2019.061
Boyle M. C., FitzSimmons N. N., Limpus C. J., Kelez S., Vélez-Zuazo X., Waycott M. (2009). Evidence for transoceanic migrations by loggerhead sea turtles in the southern pacific ocean. Proc. R Soc. B Biol. Sci. 276, 1993–1999. doi: 10.1098/rspb.2008.1931
Brito-Morales I., Schoeman D., Klein C., Dunn D. C., Everett J., García Molinos J., et al. (2021). Climate-smart, 3-d protected areas in the high seas. 1–18.
Brito-Morales I., Schoeman D. S., Everett J. D., et al. (2022). Towards climate-smart, three-dimensional protected areas for biodiversity conservation in the high seas. Nat. Clim. Chang 12, 402–407.
Bryan-Brown D. N., Brown C. J., Hughes J. M., Connolly. R. M. (2017). Patterns and trends in marine population connectivity research. Mar. Ecol. Prog. Ser. 585, 243–256.
Buchan S., Hucke-Gaete R., Stafford K. M., Clark C. W. (2017). Occasional acoustic presence of Antarctic blue whales on a feeding ground in southern Chile. Mar. Mammal Sci. 34, 440–458. doi: 10.1111/mms.12441
Buchan S., Stafford K. M., Hucke-Gaete R. (2015). Seasonal occurrence of southeast pacific blue whale songs in southern Chile and the eastern tropical pacific. Mar. Mammal Sci. 31. doi: 10.1111/mms.12173
Cariton J. T., Geller J. B. (1993). Ecological roulette: The global transport of nonindigenous marine organisms. Science 261, 78–82. doi: 10.1126/science.261.5117.78
Carlton J. T. (1985). Transoceanic and interoceanic dispersal of coastal marine organisms: The biology of ballast water. Oceanog. Mar. Biol. - Annu. Rev., 313–371.
Carr M. H., Robinson S. P., Wahle C., Davis G., Kroll S., Murray S., et al. (2017). The central importance of ecological spatial connectivity to effective coastal marine protected areas and to meeting the challenges of climate change in the marine environment. Aquat. Conserv. Mar. Freshw. Ecosyst. 27, 6–29. doi: 10.1002/aqc.2800
Carr M. H., Wilson White J., Saarman E., Lubchenco J., Milligan K., Caselle J. E. (2019). Marine protected areas exemplify the evolution of science and policy. Oceanography 32, 94–103. doi: 10.5670/oceanog.2019.315
CBD (1992). Convention on Biological Diversity, opened for signature 5 June 1992, ATS 32 (entered into force 29 December 1993).
CBD (2017)Ecologically or biologically significant areas (EBSAs). Available at: https://chm.cbd.int/database/record?documentID=204100.
Cheung W., Levin L. (2018). “Model-averaging time of emergence of climate change in each RFMO area,” in Deep-ocean climate change impacts on habitat, fish and fisheries. Eds. Levin L., Baker M., Thompson A. (Rome: FAO Fisheries and Aquaculture Technical Paper, No. 638), 186.
Christiansen S., Durussel C., Guilhon M., Singh P., Unger S. (2022). Towards an ecosystem approach to management in areas beyond national jurisdiction: REMPs for deep seabed mining and the proposed BBNJ instrument. Front. Mar. Sci. 9. doi: 10.3389/fmars.2022.720146
Clark M. R., Dunstan P. K., Guinotte J., Niklitschek E., O'Hara T., Rowden A. A., et al. (2011). Identifying ecologically and biologically significant areas on seamounts. IUCN, 2011–2056.
Comité Oceanográfico Nacional de Chile (2017). Crucero CIMAR 22 islas oceánicas (13 de octubre al 14 de noviembre de 2016) resultados preliminares Directemar, Armada de Chile, Valparaíso, Chile. 130.
Davies T. E., Carneiro A. P. B., Campos B., Hazin C., Dunn D. C., Gjerde K. M., et al. (2021). Tracking data and the conservation of the high seas: Opportunities and challenges. J. Appl. Ecol. 58, 2703–2710. doi: 10.1111/1365-2664.14032
Delgado J. P., Brennan M. L., Rapu Haoa S. A., Rapu Leong J. H., Gaymer C. F., Carabias D., et al. (2022). The hidden landscape: Maritime cultural heritage of the Salas y Gómez and Nazca ridges with implications for conservation on the high seas. Mar. Policy 136, 104877. doi: 10.1016/j.marpol.2021.104877
Dewitte B., Conejero C., Ramos M., Bravo L., Garçon V., Parada C., et al. (2021). Understanding the impact of climate change on the oceanic circulation in the Chilean island ecoregions. Aquat. Conserv. Mar. Freshw. Ecosyst. 31, 245–259. doi: 10.1002/aqc.3506
Dominguez-Carrió C., Fontes J., Morato A. (2021). A cost-effective video system for a rapid appraisal of deep-sea benthic habitats: The azor drift-cam. Methods Ecol. Evol. 12, 1379–1388. doi: 10.1111/2041-210X.13617
Dunn D. C., Harrison A. L., Curtice C., DeLand S., Donnelly B., Fujioka E. I., et al. (2019). The importance of migratory connectivity for global ocean policy. Proc. R. Soc B Biol. Sci. 286. doi: 10.1098/rspb.2019.1472
Easton E. E., Sellanes J., Gaymer C. F., Morales N., Gorny M., Berken E. (2017). Diversity of deep-sea fishes of the Easter island ecoregion. Deep Sea Res. Part II Top. Stud. Oceanogr. 137, 78–88. doi: 10.1016/j.dsr2.2016.12.006
Easton E. E., Sellanes J., Gorny M. (2018). First record of the yellowfin soldierfish, myripristis chryseres Jordan and everman In the Easter island ecoregion. Pacific Sci. 72, 143–148. doi: 10.2984/72.1.9
Easton E. E., Gorny M., Mecho A., Sellanes J., Gaymer C. F., Spalding H. L., et al. (2019). Chile and the Salas y Gomez ́ Ridge. Mesophotic Coral Ecosystems. Coral Reefs World (Cham) 12, 477–490.
Eriksen M., Lebreton L. C., Carson H. S., Thiel M., Moore C. J., Borerro J. C., et al. (2014). Plastic pollution in the world’s oceans: More than 5 trillion plastic pieces weighing over 250,000 tons afloat at Sea. PloS One 9, 1–15. doi: 10.1371/journal.pone.0111913
Evans K., Abascal D., Kolody T., Sippel J., Holdsworth J., Maru P. (2014). The horizontal and vertical dynamics of swordfish in the south pacific. Ocean. J. Exp. Mar. Bio. Ecol. 450, 55–67. doi: 10.1016/j.jembe.2013.10.025
FAO (2020). FAO fisheries and aquaculture department, statistics and information service FishStatJ: Universal software for fishery statistical time series.
Flores M. A., Schlatter R. P., Hucke-Gaete R. (2014). Las aves marinas de isla de pascua, isla Salas y Gómez e islas desventuradas, océano pacífico suroriental. Lat. Am. J. Aquat. Res. 42, 752–759. doi: 10.3856/vol42-issue4-fulltext-6
Friedlander A. M., Ballesteros E., Caselle J. E., Gaymer C. F., Palma A. T., Petit I., et al. (2016). Marine biodiversity in Juan fernández and desventuradas islands, Chile: Global endemism hotspots. PloS One, 11, e0145059. doi: 10.1371/journal.pone.0145059
Friedlander A. M., Gaymer C. F. (2021). Progress, opportunities and challenges for marine conservation in the pacific islands. Aquat. Conserv. Mar. Freshw. Ecosyst. 31, 221–231. doi: 10.1002/aqc.3464
Friedlander A. M., Goodell W., Giddens J., Easton E. E., Wagner D. (2021). Deep-sea biodiversity at the extremes of the Salas y Gómez and Nazca ridges with implications for conservation. PloS One 16, 1–27. doi: 10.1371/journal.pone.0253213
Fuenzalida R., Schneider W., Garcés-Vargas J., Bravo L., Lange C. (2009). Vertical and horizontal extension of the oxygen minimum zone in the eastern south pacific ocean. Deep Sea Res. Part II Top. Stud. Oceanogr. 56, 992–1003. doi: 10.1016/j.dsr2.2008.11.001
Gálvez-Larach M. (2009). Seamounts of Nazca and Salas y Gómez: a review for management and conservation purposes. Lat. Am. J. Aquat. Res. 373, 479–500.
García M., Correa Drubi J., Maksaev V., Townley Callejas B. (2020). Potential mineral resources of the Chilean offshore: An overview. Andean Geol. 47, 1–13. doi: 10.5027/andgeov47n1-3260
Gaymer C. F., Garay-Flühmann R., Aburto J., Varas-Belemmi K., Petit-Vega R., Olivares M., et al. (2021a). Diseño de un Plan de Manejo para el Área Marina Costera Protegida de Múltiples Usos Mar de Juan Fernández (AMCP-MU MJF) y Planes Generales de Administración para Parques Marinos Contiguos. Licitación ID: 608897-76-le19. Informe Final. ESMOI - Facultad de Ciencias del Mar- Universidad Católica del Norte. 1-208.
Gaymer C. F., Garay-Flühmann R., Sfeir R., Aburto J., Petit-Vega R., Wilhelm A., et al. (2021b). “Propuesta valorizada de plan general de administración áreas marinas protegidas de Rapa Nui: Parque Marino Motu Motiro Hiva.“ Informe Final proyecto “Estudio para el diseño y elaboración del Plan General de Administración del Parque Marino Motu Motiro Hiva”, ID 4728-6-LP18 CUI 2018-12-FAP-5. ESMOI -Universidad Católica del Norte. 1-230.
Gaymer C. F., Garay-Flühmann R., Sfeir R., Aburto J., Petit-Vega R., Wilhelm A., et al. (2021c). “Propuesta valorizada de plan general de administración áreas marinas protegidas de Rapa Nui: Área Marina Costera Protegida de Múltiples Usos Rapa Nui”. Informe Final proyecto “Elaboración de propuesta de plan de administración del Área Marina Costera Protegida de Múltiples Usos Rapa Nui”, Ministerio del Medio Ambiente, Chile. ESMOI - Universidad Católica del Norte. 207 pp.
Gaymer C., Garay-Fluhmann R., Sfeir R., Vega-Schweizer C., Luna-Jorquera G., Petit-Vega I. (2018). Bases técnicas para la gestión del parque Marino Nazca - desventuradas y propuesta de plan general de administración. Subsecretaría de Pesca, Gobierno de Chile, Valparaíso, Chile 1–204.
Gaymer C. F., Stadel A. V., Ban N. C., Cárcamo P., Ierna J., Lieberknecht L. M. (2014). Merging top-down and bottom-up approaches in marine protected areas planning: Experiences from around the globe. Aquat. Conserv. Mar. Freshw. Ecosyst. 24, 128–144. doi: 10.1002/aqc.2508
Gaymer C. F., Wagner D., Álvarez R., Bravo L., Dewitte B., Easton E., et al. (2022). Available at: https://www.sprfmo.int/assets/02-SC10/Meeting-Papers/SC10-Doc30-Importance-of-the-Salas-y-Gomez-and-Nazca-ridges-CL.pdf.
Georgian S., Morgan L., Wagner D. (2021). The modeled distribution of corals and sponges surrounding the Salas y Gómez and Nazca ridges with implications for high seas conservation. PeerJ 9, 1–35. doi: 10.7717/peerj.11972
Gjerde K. M., Wright G., Durussel C. (2021). Strengthening high seas governance through enhanced environmental assessment processes: A case study of mesopelagic fisheries and options for a future BBNJ treaty. STRONG High Seas Proj. 56. doi: 10.48440/iass.2021.001
Glynn P. W., Wellington G. M., Riegl B., Olson D., Borneman E., Wieters E. A. (2007). Diversity and biogeography of the scleractinian coral fauna of Easter island (Rapa nui). Pacific Sci. 61, 67–90. doi: 10.1353/psc.2007.0005
Guarderas A. P., Hacker S. D., Lubchenco J. (2008). Current status of marine protected areas in Latin America and the Caribbean. Conserv. Biol. 22, 1630–1640. doi: 10.1111/j.1523-1739.2008.01023.x
Hays G. C., Worboys G. L., Keeley A., Woodley S., Lausche B., Locke H., et al. (2019). Translating marine animal tracking data into conservation policy and management. Trends Ecol. Evol. 34, 459–473. doi: 10.1016/j.tree.2019.01.009
Hoegh-Guldberg O. (2019). The ocean as a solution to climate change: Five opportunities for action. World Resour. Inst., 1–116. Available at: https://oceanpanel.org/publication/the-ocean-as-a-solution-to-climate-change-five-opportunities-for-action/.
Howe B. M., Miksis-Olds J., Rehm E., Sagen H., Worcester P. F., Haralabus G. (2019). Observing the oceans acoustically. Front. Mar. Sci. 6. doi: 10.3389/fmars.2019.00426
Hucke-Gaete R., Lobo A., Yancovic-Pakarati S., Flores M. (2014). Mamíferos marinos de la isla de pascua (Rapa nui) e isla Salas y Gómez (Motu motiro hiva), Chile: Una revisión y nuevos registros. Lat. Am. J. Aquat. Res. 42, 743–751. doi: 10.3856/vol42-issue4-fulltext-5
IATTC (1949). Convention for the Strengthening of the Inter-American Tropical Tuna Commission established by the 1949 Convention between the United States of America and the Republic of Costa Rica, opened for signature 27 June 2003 (entered into force 27 August 2010).
Ioannidis A. G., Blanco-Portillo J., Sandoval K., Hagelberg E., Miquel-Poblete J. F., Moreno-Mayar J. V., et al. (2020). Native American gene flow into Polynesia predating Easter island settlement. Nature 583, 572–577. doi: 10.1038/s41586-020-2487-2
IPCC (2019). Summary for Policymakers. In: Pörtner H.-O., Roberts D.C., Masson-Delmotte V., Zhai P., Tignor M., Poloczanska E., et al eds. IPCC Special Report on the Ocean and Cryosphere in a Changing Climate Cambridge, UK and New York, NY, USA: Cambridge University Press, 3–35. doi: 10.1017/9781009157964.001
Jones K. R., Klein C. J., Grantham H. S., Possingham H. P., Halpern B. S., Burgess N. D., et al. (2020). Area requirements to safeguard earth’s marine species. One Earth 22, 188–196. doi: 10.1016/j.oneear.2020.01.010
Jones C. D., Lockhart S. J. (2011). Detecting vulnerable marine ecosystems in the southern ocean using research trawls and underwater imagery. Mar. Policy 35, 732–736. doi: 10.1016/j.marpol.2011.02.004
Keeley A. T. H., Beier P., Jenness J. S. (2021). Connectivity metrics for conservation planning and monitoring. Biol. Conserv. 255, 109008. doi: 10.1016/j.biocon.2021.109008
Lausche B., Laur A., Collins M. (2021). Marine connectivity conservation 'Rules of thumb' for MPA and MPA network design. version 1.0. IUCN WCPA connectivity conservation specialist group's marine connectivity working group.
Lesage V., Gosselin J.-F., Lawson J. W., McQuinn I., Moors-Murphy H., Plourde S., et al. (2018). Habitats important to blue whales (Balaenoptera musculus) in the western north Atlantic. DFO Can. Sci. Advis. Sec. Res. Doc 50.
Levin L., Betts B. J., Gates A. R., Heimbach P., Howe B. M., Janssen F., et al. (2019). Global observing needs in the deep ocean. Front. Mar. Sci. 6241. doi: 10.3389/fmars.2019.00241
Liggins L., Treml E. A., Riginos C. (2020). Seascape genomics: Contextualizing adaptive and neutral genomic variation in the ocean environment BT - population genomics: Marine organisms. Eds. Oleksiak M. F., Rajora O. P. (Cham, Switzerland: Cham: Springer International Publishing), 171–218. doi: 10.1007/13836_2019_68
Lima Convention (1981). Convenio para la Protección del Medio Marino y la Zona Costera del Pacífico Sudeste [Convention for the Protection of the Marine Environment and Coastal Area of the Southeast Pacific], opened for signature 12 November 1981 (entered into force 19 May 1986).
Livingstone O., Jose J. P. (2021) Connectivity of the high seas to coastal waters. Available at: http://www.highseasalliance.org/2021/05/21/connectivity-of-the-high-seas-to-coastal-waters/.
London Convention (1972). Convention on the Prevention of Marine Pollution by Dumping of Wastes and Other Matter, opened for signature 13 November 1972, ATS 16 (entered into force 30 August 1975).
London Convention Protocols (1978). Protocol to the Convention on the Prevention of Marine Pollution by Dumping of Wastes and Other Matter, 1972, opened for signature 7 November 1996, 36 ILM 1 (entered into force 24 March 2006) amended in 2006.
Lucifora L. O., García V. B., Worm B. (2011). Global diversity hotspots and conservation priorities for sharks. PloS One 6. doi: 10.1371/journal.pone.0019356
Luna-Jorquera G., Thiel M., Portflitt-Toro M., Dewitte B. (2019). Marine protected areas invaded by floating anthropogenic litter: An example from the south pacific. Aquat. Conserv. Mar. Freshw. Ecosyst. 29. doi: 10.1002/aqc.3095
Magris R. A., Pressey R. L., Weeks R., Ban N. C. (2014). Integrating connectivity and climate change into marine conservation planning. Biol. Conserv. 170, 7–221. doi: 10.1016/j.biocon.2013.12.032
MARPOL Convention (1978). International Convention for the Prevention of Pollution from Ships as modified by the Protocol of 1978, opened for signature 17 February 1978, ATS 9 (entered into force 2 October 1983).
Maxwell S. L., Cazalis V., Dudley N., Hoffmann M., Rodrigues A. S. L., Stolton S., et al. (2020). Area-based conservation in the twenty-first century. Nature 586, 217–227. doi: 10.1038/s41586-020-2773-z
Meerhoff E., Yannicelli B., Dewitte B., Díaz-Cabrera E., Vega Retter C., Ramos M., et al. (2018). Asymmetric connectivity of the lobster panulirus pascuensis in remote islands of the southern pacific: Importance for its management and conservation. Bull. Mar. Sci. 94 (22), 753–774. doi: 10.5343/bms.2017.1114
Metaxas A., Snelgrove P., Dunn D. C., Baker M., Sharman H., Harden-Davies H., et al. (2020). “Ecological connectivity: Implications for ocean governance,” in Deep ocean stewardship. Available at: https://www.dosi-project.org/wp-content/uploads/DOSI-Connectivity_brief_Feb2020.pdf.
Miller K. A., Thompson K. F., Johnston P., Santillo D. (2018). An overview of seabed mining including the current state of development, environmental impacts, and knowledge gaps. Front. Mar. Sci. 10. doi: 10.3389/fmars.2017.00418
MINREL (2021). “President Piñera announces Chile will advance a proposal to fully protect an area of the high seas in the southeastern pacific, the first of its kind,” in Chile Foregin affairs press release. Ministerio de Relaciones exteriores de Chile, Santiago, Chile. Available at: https://minrel.gob.cl/minrel/site/docs/20210422/20210422114339/press_release_chile_leaders_summit_on_climate.pdf.
Ministry of Foreign Affairs of Chile (2021). Available at: https://www.sprfmo.int/assets/2021-SC9/SC9-Doc31-rev1-Paper-on-the-High-Seas-of-Nazca-and-Salas-y-Gomez-Submarine-Ridges.pdf.
Morales N. A., Easton E. E., Friedlander A. M., Harvey E. S., Garcia R., Gaymer C. F. (2019). Spatial and seasonal differences in the top predators of Easter island: Essential data for implementing the new rapa nui multiple-uses marine protected area. Aquat. Conserv. Mar. Freshw. Ecosyst. 29, 118–129. doi: 10.1002/aqc.3068
Morales N. A., Heidemeyer M., Bauer R., Hernández S., Acuña E., Friedlander A. M., et al. (2021). Residential movements of top predators in chile’s most isolated marine protected area: Implications for the conservation of the Galapagos shark, carcharhinus galapagensis, and the yellowtail amberjack, seriola lalandi. Aquat. Conserv. Mar. Freshw. Ecosyst. 31, 340–355. doi: 10.1002/aqc.3472
Nicholls D., Robertson C. J. R. (2007). Assessing flight characteristics for the chatham albatross (Thalassarche eremita) from satellite tracking. Notornis 54, 168–179.
O’Leary B. C., Roberts C. M. (2018). Ecological connectivity across ocean depths: Implications for protected area design. Glob. Ecol. Conserv. 15. doi: 10.1016/j.gecco.2018.e00431
Oppel S., Bolton M., Carneiro A. P. B., Dias M. P., Green J. A., Masello J. F., et al. (2018). Spatial scales of marine conservation management for breeding seabirds. Mar. Policy 98, 37–46. doi: 10.1016/j.marpol.2018.08.024
Ortuño Crespo G., Mossop J., Dunn D., Gjerde K., Hazen E., Reygondeau G., et al. (2020). Beyond static spatial management: Scientific and legal considerations for dynamic management in the high seas. Mar. Policy 122. doi: 10.1016/j.marpol.2020.104102
Pazmiño D., Maes G. E., Green M. E., Simpfendorfer C. A., Hoyos-Padilla E. M., Duffy C. J. A., et al. (2018). Strong trans-pacific break and local conservation units in the Galapagos shark (Carcharhinus galapagensis) revealed by genome-wide cytonuclear markers. Heredity 120, 407–421. doi: 10.1038/s41437-017-0025-2
Pazmiño D. A., Maes G. E., Simpfendorfer C. A., Salinas de León P., van Herwerden L. (2017). Genome-wide SNPs reveal low effective population size within confined management units of the highly vagile Galapagos shark (Carcharhinus galapagensis). Cons. Gen. 18, 1151–1163. doi: 10.1007/s10592-017-0967-1
Pecoraro C., Babbucci M., Franch R., Rico C., Ch P., Chassot E., et al. (2018). The population genomics of yellowfin tuna (Thunnus albacares) at global geographic scale challenges current stock delineation. Sci. Rep. 8, 13890. doi: 10.1038/s41598-018-32331-3
Popova E., Vousden D., Sauer W. H. H., Mohammed E. Y., Allain V., Downey-Breedt N., et al. (2019). Ecological connectivity between the areas beyond national jurisdiction and coastal waters: Safeguarding interests of coastal communities in developing countries. Mar. Policy 104, 90–102. doi: 10.1016/j.marpol.2019.02.050
Porobić J., Canales-Aguirre C. B., Ernst B., Galleguillos R., Hernández C. E. (2013). Biogeography and historical demography of the juan fernández rock lobster, Jasus frontalis (Milne edwards 1837). J. Hered. 104, 223–233. doi: 10.1093/jhered/ess141
Portflitt-Toro M., Luna Bravo N., Plaza R. P., Varela A. I., Serratosa J., Luna-Jorquera G. (2020). Aves Marinas en las islas oceánicas chilenas: Un patrimonio de biodiversidad por conservar. La Chiricoca 13–27.
Premachandra H. K. A., la Cruz F., Takeuchi L. D., Miller Y., Fielder S., O’Connor W., et al. (2017). Genomic DNA variation confirmed Seriola lalandi comprises three different populations in the pacific, but with recent divergence. Sci. Rep. 7, 9386. doi: 10.1038/s41598-017-07419-x
Rayner M. J., Taylor G. A., Gummer H. D., Phillips R. A., Sagar P. M., Shaffer S. A., et al. (2012). The breeding cycle, year-round distribution and activity patterns of the endangered chatham petrel (Pterodroma axillaris). Emu - Austral Ornithol. 1122, 107–116. doi: 10.1071/MU11066
Rech S., Borrell Y., García-Vazquez E. (2016). Marine litter as a vector for non-native species: What we need to know. Mar. pollut. Bull. 113, 40–43. doi: 10.1016/j.marpolbul.2016.08.032
Rech S., Gusmao J. B., Kiessling T., Hidalgo-Ruz V., Meerhoff E., Gatta-Rosemary M., et al. (2021). A desert in the ocean – depauperate fouling communities on marine litter in the hyper-oligotrophic south pacific subtropical gyre. Sci. Total Environ. 759. doi: 10.1016/j.scitotenv.2020.143545
Rech S., Thiel M., Borrell Pichs Y. J., García-Vazquez E. (2018). Travelling light: Fouling biota on macroplastics arriving on beaches of remote rapa nui (Easter island) in the south pacific subtropical gyre. Mar. pollut. Bull. 137, 119–128. doi: 10.1016/j.marpolbul.2018.10.015
Sala E., Mayorga J., Bradley D., Cabral R. B., Atwood T. B., Auber A., et al. (2021). Protecting the global ocean for biodiversity, food and climate. Nature 592, 397–402. doi: 10.1038/s41586-021-03371-z
Saleh A., Laradji I. H., Konovalov D. A., Bradley M., Vazquez D., Sheaves M. (2020). A realistic fish-habitat dataset to evaluate algorithms for underwater visual analysis. Sci. Rep. 10, 1–10. doi: 10.1038/s41598-020-71639-x
Santelices B., Meneses I. (2000). A reassessment of the phytogeographic characterization of temperate pacific south America. Rev. Chil. Hist. Nat. 73, 605–614. doi: 10.4067/s0716-078x2000000400005
Selig E. R., Turner W. R., Troëng S., Wallace B. P., Halpern B. S., Kaschner K., et al. (2014). Global priorities for marine biodiversity conservation. PloS One 9 (1), e82898. doi: 10.1371/journal.pone.0082898
Serratosa J., Hyrenbach K. D., Miranda-Urbina D., Portflitt-Toro M., Luna N., Luna-Jorquera G. (2020). Environmental drivers of seabird At-Sea distribution in the Eastern south pacific ocean: Assemblage composition across a longitudinal productivity gradient. Front. Mar. Sci. 6. doi: 10.3389/fmars.2019.00838
Shillinger G. L., Palacios D. M., Bailey H., Bograd S. J., Swithenbank A. M., Gaspar P., et al. (2008). Persistent leatherback turtle migrations present opportunities for conservation. PloS Biol. 6 (7), e171. doi: 10.1371/journal.pbio.0060171
SPRFMO (2009). Convention on the conservation and management of high seas fishery resources in the south pacific ocean. Hoboken New Jersey, USA: New Zealand Yánez John Wiley & Sons Ltd,
SPRFMO (2015). Available at: http://www.sprfmo.int/assets/Basic-Documents/Convention-web-12-Feb-2018.pdf.
SPRFMO (2020) CMM 03-2020 conservation and management measure for the management of bottom fishing in the SPRFMO convention area. Available at: https://www.sprfmo.int/assets/Fisheries/Conservation-and-Management-Measures/2020-CMMs/CMM-03-2020-Bottom-Fishing-31Mar20.pdf.
Tapia-Guerra J. M., Mecho A., Easton E. E., Gallardo M.D.L.Á., Gorny M., Sellanes J. (2021). First description of deep benthic habitats and communities of oceanic islands and seamounts of the Nazca desventuradas marine park, Chile. Sci. Rep. 11 (1), 1–16.
Tessnow-von Wysocki T. (2021) Assessing the human’s footprint on ocean biodiversity. maripoldata. Available at: https://www.maripoldata.eu/assessing-the-humans-footprint-on-ocean-biodiversity/.
Thiel M., Luna-Jorquera G., álvarez-Varas R., Gallardo C., Hinojosa I. A., Luna N., et al. (2018). Impacts of marine plastic pollution from continental coasts to subtropical gyres-fish, seabirds, and other vertebrates in the SE pacific. Front. Mar. Sci. 5. doi: 10.3389/fmars.2018.00238
Tittensor D. P., Beger M., Boerder K., Boyce D. G., Cavanagh R. D., Cosandey-Godin A., et al. (2019). Integrating climate adaptation and biodiversity conservation in the global ocean. Sci. Adv. 5, 1–16. doi: 10.1126/sciadv.aay9969
Toonen R. J., Wilhelm T. A., Maxwell S. M., Wagner D., Bowen B. W., Sheppard C. R. C., et al. (2013). One size does not fit all: The emerging frontier in large-scale marine conservation. Mar. pollut. Bull. 77, 7–10. doi: 10.1016/j.marpolbul.2013.10.039
Toro N., Jeldres R. I., Órdenes J. A., Robles P., Navarra A. (2020). Manganese nodules in Chile, an alternative for the production of Co and Mn in the future–a review. Minerals 10, 1–19. doi: 10.3390/min10080674
Torres-Florez J. P., Hucke-Gaete R., LeDuc R., Lang A., Taylor B., Pimper L. E., et al. (2014). Blue whale population structure along the eastern south pacific ocean: evidence of more than one population. Mol. Ecol. 23, 5998–6010. doi: 10.1111/mec.12990
Torres-Florez J. P., Olson P. A., Bedriñana-Romano L., Rosenbaum H., Ruiz J., LeDuc R., et al. (2015). First documented migratory destination for eastern South Pacific blue whales. Marine Mammal Science. 31 (4), 1580–1586.
Turchik A. J., Berkenpas E. J., Henning B. S., Shepard C. M. (2015). The deep ocean dropcam: A highly deployable benthic survey tool. in OCEANS 2015 - (Washington: MTS/IEEE), 1–8. doi: 10.23919/OCEANS.2015.7401978
Ulloa O., Pantoja S. (2009). The oxygen minimum zone of the eastern south pacific. Deep Sea Res. Part II Top. Stud. Oceanogr. 56, 987–991. doi: 10.1016/j.dsr2.2008.12.004
UNCLOS (1982). United Nations Convention on the Law of the Sea, opened for signature 10 December 1982, ATS 31 (entered into force 16 November 1994).
UNEP-WCMC (2019). A marine spatial planning framework for areas beyond national jurisdiction. technical document produced as part of the GEF ABNJ deep seas project (Cambridge (UK: UN Environment Programme World Conservation Monitoring Centre), 45.
UNFSA (1995). United Nations Agreement for the Implementation of the Provisions of the United Nations Convention on the Law of the Sea of 10 December 1982 Relating to the Conservation and Management of Straddling Fish Stocks and Highly Migratory Fish Stocks, opened for signature 8 September 1995, ATS 8 (entered into force 11 December 2001).
UNGA (2021). United Nations General Assembly, Resolution Adopted by the General Assembly, GA Res 75/271, 75th sess, Agenda Item 19, A/RES/75/271 (20 April 2021).
Valencia E., Meerhoff E., Díaz-Cabrera E., Guerrero F., Roja-Hernández N., Vega-Retter C., et al. (2021). Weak connectivity and population cohesiveness in rudderfish kyphosus sandwicensis (Teleostei: Kyphosidae) inhabiting remote oceanic islands. Aquat. Conserv. Mar. Freshw. Ecosyst. 31, 356–366. doi: 10.1002/aqc.3438
van Gennip S. J., Dewitte B., Garçon V., Thiel M., Popova E., Drillet Y., et al. (2019). In search for the sources of plastic marine litter that contaminates the Easter island ecoregion. Sci. Rep. 9, 1–13. doi: 10.1038/s41598-019-56012-x
Veliz D., Rojas-Hernández N., Fibla P., Dewitte B., Cornejo-Guzmán S., Parada C. (2021). High levels of connectivity over large distances in the diadematid sea urchin centrostephanus sylviae. PloS One 16 (11), e0259595.
Visalli M. E., Best B. D., Cabral R. B., Cheung W. W. L., Clark N. A., Garilao C., et al. (2020). Data-driven approach for highlighting priority areas for protection in marine areas beyond national jurisdiction. Mar. Policy 122. doi: 10.1016/j.marpol.2020.103927
Von Dassow P., Collado-Fabbri S. (2014). Oceanografía biológica, ciclos biogeoquímicos y funcionamiento del ecosistema pelágico de la región centro-este del giro del pacífico sur: Centrado en isla de pascua e isla Salas y Gómez. Lat. Am. J. Aquat. Res. 42, 703–742. doi: 10.3856/vol42-issue4-fulltext-4
Wagner D., Friedlander A. M., Pyle R. L., Brooks C. M., Gjerde K. M., Wilhelm T. (2020). Coral reefs of the high seas: Hidden biodiversity hotspots in need of protection. Front. Mar. Sci. 7. doi: 10.3389/fmars.2020.567428
Wagner D., Friedlander A. M., Pyle R. L., Gjerde K. M., Wilhelm T. A., Easton E. E., et al. (2021a). “Conserving the last ocean frontiers,” in GIS for science volume 3 – maps for saving the planet. Eds. Wright, Harder (Redlands, California USA : ESRI Press), 94–105.
Wagner D., van der Meer L., Gorny M., Sellanes J., Gaymer C. F., Soto E. H., et al. (2021b). The Salas y Gómez and Nazca ridges: A review of the importance, opportunities and challenges for protecting a global diversity hotspot on the high seas. Mar. Policy 126. doi: 10.1016/j.marpol.2020.104377
Wilhelm T. A., Sheppard C. R. C., Sheppard A. L. S., Gaymer C. F., Parks J., Wagner D., et al. (2014). Large Marine protected areas - advantages and challenges of going big. Aquat. Conserv. Mar. Freshw. Ecosyst. 24, 24–30. doi: 10.1002/aqc.2499
Yáñez E., Lagos N. A., Norambuena R., Silva C., Letelier J., Muck K.-P., et al. (2017). “Impacts of climate change on marine fisheries and aquaculture in Chile,” in Climate change impacts on fisheries and aquaculture: A global analysis. John Wiley & Sons Ltd, Hoboken New Jersey, USA. doi: 10.1002/9781119154051.ch10
Keywords: connectivity, Salas y Gómez and Nazca ridges, marine conservation, marine policy, areas beyond national jurisdiction (ABNJ)
Citation: Boteler B, Wagner D, Durussel C, Stokes E, Gaymer CF, Friedlander AM, Dunn DC, Vargas FP, Veliz D and Hazin C (2022) Borderless conservation: Integrating connectivity into high seas conservation efforts for the Salas y Gómez and Nazca ridges. Front. Mar. Sci. 9:915983. doi: 10.3389/fmars.2022.915983
Received: 08 April 2022; Accepted: 14 September 2022;
Published: 11 October 2022.
Edited by:
Alexandra Anh-Thu Weber, Swiss Federal Institute of Aquatic Science and Technology, SwitzerlandReviewed by:
Sergi Taboada, Autonomous University of Madrid, SpainCandice Bobby Untiedt, Commonwealth Scientific and Industrial Research Organisation (CSIRO), Australia
Copyright © 2022 Boteler, Wagner, Durussel, Stokes, Gaymer, Friedlander, Dunn, Vargas, Veliz and Hazin. This is an open-access article distributed under the terms of the Creative Commons Attribution License (CC BY). The use, distribution or reproduction in other forums is permitted, provided the original author(s) and the copyright owner(s) are credited and that the original publication in this journal is cited, in accordance with accepted academic practice. No use, distribution or reproduction is permitted which does not comply with these terms.
*Correspondence: Ben Boteler, ben.boteler@iass-potsdam.de; Daniel Wagner, daniel@oceanexplorationtrust.org