- 1Univ Rennes, Normandie Univ, National Center for Scientifc Research, EthoS (Éthologie animale et humaine) - Joint Research Unit 6552, F-35000, Rennes, France
- 2Département Scientifique et Pédagogique, Planète Sauvage, Port-Saint-Père, France
Study of animal communication and its potential social role implies associating signals to an emitter. This has been a major limitation in the study of cetacean communication as they produce sounds underwater with no distinctive behavioral signs. Different techniques have been used to identify callers, but all proved to have ethical or practical limitations. Bio-logging technology has recently provided new hopes, but tags developed so far are costly and do not allow sufficiently reliable discrimination between calls made by the tagged individual and those made by the surrounding individuals. We propose a new device developed at reasonable cost while providing reliable recordings. We tested caller identification through recordings of vocal production of a group of captive bottlenose dolphins under controlled and spontaneous contexts. Our device proved to identify callers through visual examination of sonograms and quantitative measures of amplitudes, even if tagged emitters are 0.4 m apart (regardless of body orientations). Although this device is not able to identify emitters in an entire group when all individuals are not equipped, it enables efficient exclusion of individuals who were not the caller, suggesting that identification of a caller would be reliable if all the individuals were equipped. This is to our knowledge the first description of a promising low-cost safe recording device allowing individual identification of emitters for captive dolphins. With some improvements, this device could become an interesting tool to increase our knowledge of dolphin acoustic communication.
Introduction
Environmental constraints imposed by turbid aquatic habitats have been a driving force for the evolution of cetaceans’ acoustic communication (Janik, 1999). In this environment with limited visibility, sounds travel quickly and can convey messages over long-distances where other sensory systems can be limited (Schusterman, 1980; Kuznetzov, 1990; Johnson et al., 2009). Hence, to cope with the need of effective communication adapted to their aquatic lifestyle, cetaceans evolved a variety of acoustic signals to convey information and coordinate their activities (Tyack, 2000; Johnson et al., 2009).
Study of animal communication and its potential social role implies associating signals with an emitter’s identity (Quick et al., 2008). Identifying emitters has been one of the major factors limiting the study of cetaceans’ acoustic communication (Nowacek et al., 2016). Specifically, it is difficult to identify callers when animals move fast and spend long period of times underwater, often in relatively dark environments (Tyack, 2000; Andreas et al., 2021). Furthermore, even when the water is clear enough, the absence of distinctive signs of sound production, such as systematic mouth opening or air exhalation, makes it almost impossible to identify callers (Thomas et al., 2002). The anatomy of cetacean vocal production is atypical among mammals, since they do not use vocal folds but vibrate “monkey lips” located in the nasal cavity (Cranford et al., 1996).
Therefore, although the interest for bioacoustics research on cetaceans keeps growing, this field remains under-explored. Both logistical and technological limitations in identifying emitters within a social group make it difficult to investigate all the aspects of cetaceans’ communication (Nowacek et al., 2016). During the past 60 years, different methodologies have been tested to solve the problem of identification and to investigate in depth cetacean bioacoustics. The first attempts involved isolating the focal animal through temporary captures (Lilly and Miller, 1961; Caldwell et al., 1990), but although this method increases the probability to identify callers, it implies stressful conditions and alteration of the animal’s spontaneous behavior (Thomas et al., 2002). A less invasive technique associated vocal emission with the production of bubble streams (McCowan, 1995; Herzing, 1996). Unfortunately, these sounds are not representative of the vocal repertoire (Fripp, 1999; Thomas et al., 2002). Therefore, passive acoustic localization was employed as a non-invasive solution. It compares the timings of arrival of a vocalization at several hydrophones to localize its source and identify the caller (i.e. triangulation) (Janik et al., 2000). This technique requires a very sensitive and expensive system consisting of several finely calibrated hydrophones positioned in the environment to record all of the sounds to be localized (Thomas et al., 2002). Despite the advantage of being noninvasive, only sounds produced when animals are clearly physically separated can be studied in this manner, otherwise it becomes impossible to identify callers. So, it needs conditions that are rarely encountered (Janik et al., 2000). Subsequent improvements in the field of passive acoustic localization have led to associating it with a machine-learning approach to automatically localize the sound source (not in real-life conditions, Woodward et al., 2020) or with video recordings to increase the probability of identification of caller (Thomas et al., 2002). Attempts to identify callers using a combination of audio-video data were also made with a human maneuverable device (Lopez-Marulanda et al., 2017). However, its use depends on the clarity of the water, a relative short distance from the subjects and needs the presence of a human, a potential source of interference for non-habituated populations (Lopez-Marulanda et al., 2017).
As the ideal scenario is to be able to identify the emitter constantly, bio-logging technology appears as the most promising method (Ropert-Coudert and Wilson, 2005). It consists in equipping animals with wearable devices (so-called tags) in order to collect data even when visual assessments are not possible (Shorter et al., 2014). The first tag version used was the vocalight, a device that produces light diodes when the tagged individual emits a vocalization (Tyack, 1985). Identification was facilitated by coupling light signals with sounds recorded by fixed hydrophones, but this association was seldom possible, even if only two individuals were tagged and several observers constantly monitored light emissions (Tyack, 1985). Tyack and Recchia (1991) tagged animals with dataloggers that collected information on the loudness of vocalizations. The purpose was to identify callers after comparison with recordings by fixed hydrophones. However, these dataloggers had several technical limitations and did not allow identification when untagged individuals were in the vicinity (Tyack and Recchia, 1991). Later, acoustic recording systems were integrated directly in a tag, but the need for pressure-resistant housing initially limited their use to non-cetacean species (Fletcher et al., 1996). Subsequent technological improvements enabled integration of both hydrophones and sensors (depth, temperature, speed) in tags to collect data from the animals’ perspective. A striking example is the D-Tag (Johnson and Tyack, 2003), a device conceived to collect audio and orientation data. Although nowadays this version is one of the most employed, its large dimensions and the difficulty to attach them to fast moving animals limit its use for small cetaceans. Also, its high cost and the complexity of the associated analysis tools make it less accessible for researchers and, consequently, it is difficult to use it simultaneously on several individuals and investigate communication aspects (Johnson et al., 2009). D-Tags are however used to investigate ecological aspects (e.g. effects of exposure to noise, Johnson and Tyack, 2003; Johnson et al., 2009). Its limited use for topics such as social behavior and communication is due to the limitation of tags’ acoustic sensors to identify whether a recorded vocalization comes from the tagged animal or from another untagged individual in the vicinity (Johnson et al., 2009). Despite important improvements in tag technology that led to advanced versions of D-Tags usable for different applications including small cetaceans (e.g. echolocation, baseline bioacoustics and diving) (Jones et al., 2013; Zhang et al., 2020), there is definitely a need for more cost effective and easy to use devices.
Therefore, there is still a crucial need for techniques that combine limited research costs and guarantee individual identification of emitters. In the present study, we describe a novel device: a commercial recorder encapsulated and fixed temporarily with a suction cup. This device was developed at a very reasonable cost while providing safe and reliable recordings of equipped bottlenose dolphins. We tested the efficiency and limits of this device in two recording contexts. One was a controlled situation with human-induced acoustic behavior of pairs of dolphins at different distances and with different body orientations (to consider the high degree of directionality of some of the acoustic emissions in these species, Branstetter et al., 2012). The other one sampled spontaneous acoustic behaviors of dolphins free to move in their pools and collected sounds associated with social interactions. Their prevalence in zoos (Wells and Scott, 2018), high sociality and extensive vocal repertoire (Janik, 2009) make bottlenose dolphins interesting for testing new methodologies. The potentiality of this device to identify callers reliably opens new exciting lines for the study of bottlenose dolphins’ acoustic communication and, possibly, that of cetaceans in general. Whereas extensive studies of dolphins’ acoustic communication have mostly provided insights into their vocal repertoire and their use of vocalizations at the group level (Janik et al., 2000; Lima et al., 2017), intra-individual acoustic variability and behavioral contexts in which calls are used require more investigation to be fully understood (Jones et al., 2020).
Materials and methods
Subjects and data collection
The study was conducted on a group of nine captive-born dolphins, housed at Planète Sauvage Animal Park (Port Saint-Père, France), composed of six males and three females. Three dolphins were born on site; the others arrived several years ago (see age and their life history track in Supplementary Figure 1). In this facility, dolphins had free access to a system of four interconnected pools of different sizes (Supplementary Figure 2). Dolphins were fed with a various amount of fish (from 4 to 18 kg) per individual each day delivered during free feedings, training (lasting 5-30min) or enrichment sessions. Data were collected for nine weeks from September to November 2020. Observations and experiments were conducted both during and outside training sessions. For experiments during training sessions dolphins were equipped only for the time necessary to conduct each test (maximum 20 min). During spontaneous observations, they were equipped for as long as possible, according to their willingness to carry the device (maximum 20 min). Since it was not possible to tag the entire group, only the four dolphins already trained to be tagged participated in this study.
Recording systems
A tag consisted in a mini acoustic recorder (KKmoon SK-892, frequency 48 kHz, recording rate 192 kbps) placed in a polylactic acid waterproof housing 3D-printed at Ethos Lab (Figure 1A). Capsules were 11cm long, weighed 20g and had a hydrodynamic shape (similar to their optimal shape in Shorter et al., 2014). They were produced in different colors to facilitate identification from above when cameras (see below) were positioned on dolphins. Tags were fixed dorsally using a non-invasive suction cup (diameter 8.5 cm, Cetacean Research Technology Ltd) widely used for tag attachments and that have proved to be efficient and harmless (Shorter et al., 2014). To standardize recordings, tags were always located in the same position behind the blowhole and with the same orientation towards the dolphin’s head. Specifically, the tag had an internal compartment created to receive the recorder and ensure that it remained fixed in the capsule (Figure 1B) (to avoid possible impact noises). The recorder was always placed so that the microphone was towards the animal (i.e. cephalic orientation, to increase the quality of the audio recordings) (Figure 1C). These positions and orientations were chosen after pilot recordings conducted to identify the most promising tag position for our purpose. From June 2018 to January 2019, the four individuals participating in the study had been trained to wear our device through operant conditioning using positive reinforcement. The method consisted in encouraging subjects through successive learning steps: 1) accepting to be touched with the suction cup, 2) accepting the pressure of the suction cup, 3) wearing the suction cup while remaining immobile, and finally 4) leaving with the attached suction cup for a progressively increasing but standardized period of time before being called back. The entire training procedure was conducted by experienced caregivers, was noninvasive and respectful of the willingness of the dolphin to participate. The following step was undertaken only when the previous one had been achieved without any sign of discomfort or unusual behavior. At the time of our observations, the subjects had reached the stage of free movement for 20-minutes sessions. Once equipped, the dolphins thus behaved as usual.
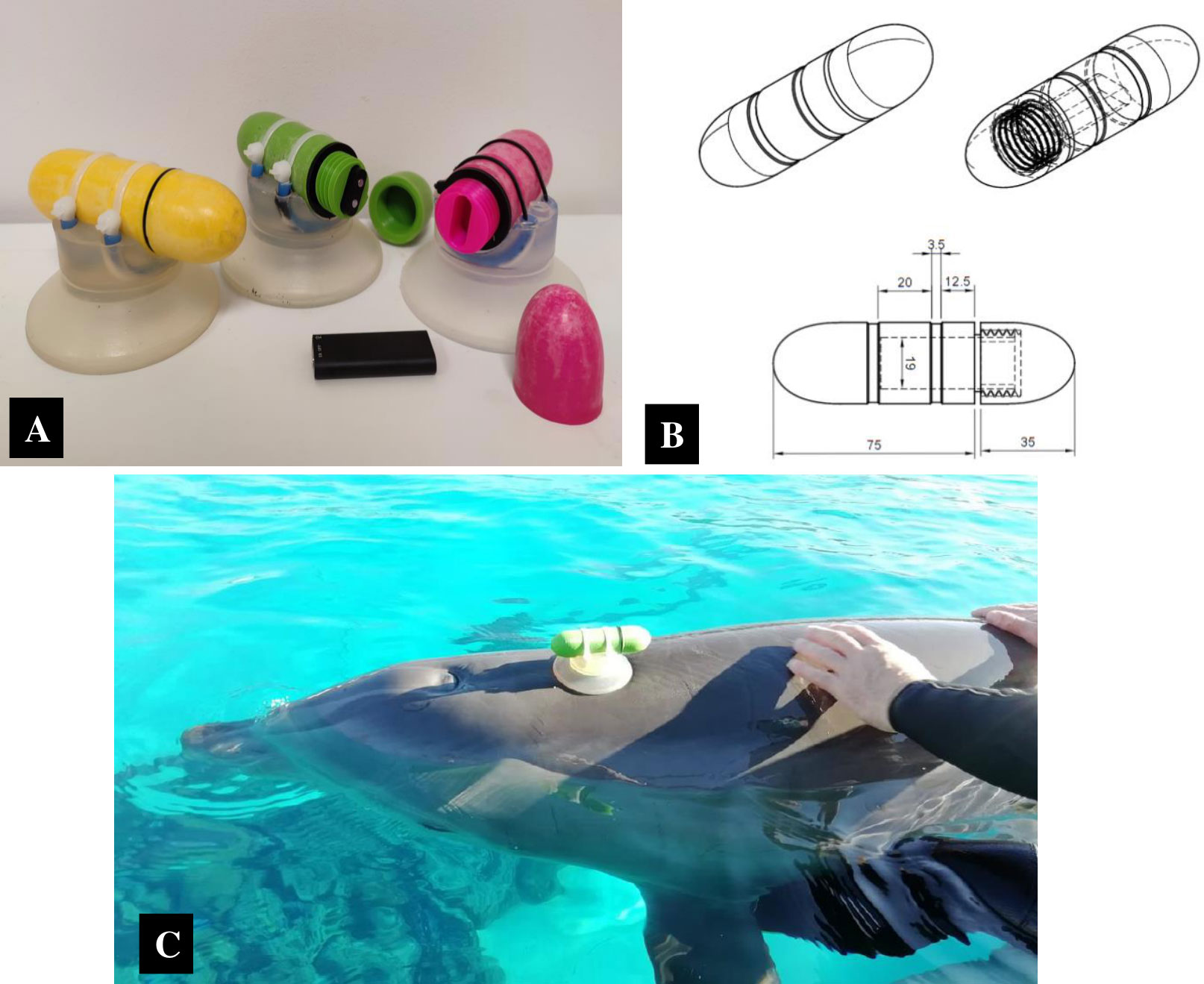
Figure 1 (A) Devices employed for the study. They consist in small recorders housed in 3D-printed capsules placed on suction cups that fixed them on the back of a subject. Different colors facilitated identification of an equipped dolphin. (B) Schematic diagram of the capsules housing recorders. (C) Dolphin equipped with the device. To standardize the data, the device was always placed behind the blowhole with the recording part oriented towards dolphin’s head.
The fixed audio system employed consisted in two Aquarian Scientific AS-1 hydrophones (linear range 1 Hz to 100 kHz ±2dB) connected to PA-4 preamplifiers (gain 26 dB). The hydrophones were connected to a Steinberg UR44 sound card (192 kHz, USB audio interface) and to a laptop during the recording sessions. The video recording system (two cameras Jvc Quad Proof Everio R) was placed so as to have a panoramic view of the entire basin. These data aimed to follow tagged dolphins’ movements and to identify their position in the pools, thus obtaining supporting data useful for callers’ identification (Supplementary Figure 2). In order to collect data from different animals simultaneously, the entire system was synchronized prior to each session. After activating and placing recorders in their waterproof capsule, the tags were synchronized by tapping simultaneously on all the capsules. This sound was thus recorded by each device making it possible to align a posteriori their spectrograms for analyses. Similarly, tags were synchronized with hydrophones by tapping each device on the hydrophones and this sound was used to align their spectrograms. Finally, to synchronize tags with the video system, they were tapped with each other in front of the cameras.
Recordings and tests of reliability
To verify whether our tag represents an advanced tool for caller identification, we tested its capabilities under both controlled and spontaneous conditions.
First, we tagged two dolphins simultaneously to investigate whether the voice of the holder could be distinguished clearly from that of the other individual in the recordings. To control the conditions of this test and check potential limits of our device, we took advantage of dolphins’ abilities to perform behaviors upon request (learned after specific training, Lima et al., 2018). Precisely, they come to the edge of the pool to stay in designated positions and emit a vocalization in response to specific signals. In order to test our tags under as many conditions as possible, different distances and orientations among any possible pair of subjects involved were considered. Recordings were conducted for nine different inter-individual distances between 50m (maximum distance possible in the same pool in this facility) and 0.40m (minimum distance possible, dolphins being then in close contact), precisely at: 50m; 25m; 15m; 10m; 7m; 5m; 2m; 1m; 0.40m. These distances were recognizable by markers on the bottom of the pool. Each distance was tested when dolphins were facing each other or were parallel. Frequency and duration (10-20 min) of the experiments varied from day to day depending on the daily schedules of the training sessions. The vocalization considered for this test is a low-pitched intense sound (called “song”), which differs from any naturally emitted species-specific calls described to date. Trials were conducted with two dolphins at a time while the rest of the group was in other types of training sessions. Thus, interference by other dolphins or presence of vocalizations emitted by other individuals were limited and easily distinguishable acoustically. During a test, two individuals carried simultaneously a device and, after the caregivers had them stationary in every distance and orientation to be tested, they displayed the signal to ask dolphins to vocalize. Precisely, when one dolphin was asked to vocalize, the other was kept silent and vice versa. So, both individuals alternatively vocalized and remained silent during a session. A total of 51 trials were conducted, each distance and each orientation under analysis were tested at least twice. To check conditions close to real ones, we investigated the case when individuals were moving. More precisely, while one dolphin was asked to vocalize, the other approached it on request from between 0.40m and 2m. These additional recordings were conducted twice for each of the two possible orientations.
Recordings of spontaneous acoustic production were then performed to investigate whether the tag allowed us to identify the vocalizations of tagged individuals within the entire group of dolphins free to move and spontaneously emitting species-specific whistles. To increase the reliability of callers’ identification, we combined data obtained from the different devices, including the fixed hydrophones and cameras. For this condition, recordings were performed when dolphins were not involved in public presentations or training sessions (randomly scheduled) and three individuals of the possible four were tagged simultaneously (due to the limited number of devices available at that time). Precisely, hydrophones and especially cameras were used to obtain information on dolphins’ positions (Thomas et al., 2002). Seventeen sessions, averaging 16 minutes yielding 3h50 of recordings and 136 whistles, were analyzed. Dolphins’ positions were constantly monitored during the observations. Individuals were recognized due to particular distinctive marks (Würsig et al., 1990) as well as the tags which had different colors.
Data analysis
Acoustic analyses were carried out via Audacity software (version 2.4.2). Spectrograms were analyzed for qualitative and quantitative comparisons of the sounds recorded by tags and hydrophones. Video data were analyzed on Windows Media Player software and their soundtracks were imported on Audacity for alignment with acoustic data. The combination of audio-video data was useful to identify individual emitters during observations of spontaneous production. Thus, when one sound was detected on the spectrograms, the video recordings of each of both cameras were analyzed in order to have the relative position of each dolphin compared to the group members, which, combined with the measures of the sounds’ relative intensities, allowed hypotheses on the probability that one dolphin was the emitter. Supporting annotations made by the observer were also used for the same purpose. Precisely, after analysis of this array of data, and for each sound production, 5 levels of certainty about the emitter’s identity (and identity of non-emitters) were possible: i) “YES” the target individual was clearly the caller; ii) “VERY LIKELY”: the information gathered indicate the probable identity of the emitter, without excluding totally that another dolphin could be the emitter; iii) “POSSIBLE” there was no element allowing to say that the dolphin was or was not the emitter; iv) “UNLIKELY”: the information gathered suggest that the dolphin is not the emitter but cannot totally exclude it v) “NO” the target individual was clearly not the caller.
The statistical analyses were performed using R (version 3.5.3; https://www.R-project.org): To get to the bottom of possible effects of distance, orientation or interaction distance-orientation, a F-test was performed. We used the AovSum function of R package “FactoMineR” (Le et al., 2008).
Results
Controlled tests
In all the conditions tested, vocalizations were always recorded by both tags carried by the dolphins involved in a test. Both qualitative analyses of spectrograms and quantitative analyses of amplitude spectra showed that we were always able to identify which tagged individual emitted a vocalization (Figure 2A): the maximum amplitude of a signal recorded (in dB) was always higher for the vocalization emitted by the tagged dolphin than for any other vocalization recorded (Figure 2B). Such qualitative and quantitative discriminations were possible for all nine distances and for both orientations tested. However, differences in intensity varied with the distance between the tagged emitter and the other emitter, differences increasing when distance increased, especially when dolphins were oriented face to face (Figure 3). Statistical analyses revealed however (Supplementary Table 1) that only the distance (and not the orientation or the interaction orientation-distance) had a significant effect (F1,1 = 10.73, p = 0.001) on the difference of acoustic intensity registered (measured as the maximum amplitude recorded on the signal).
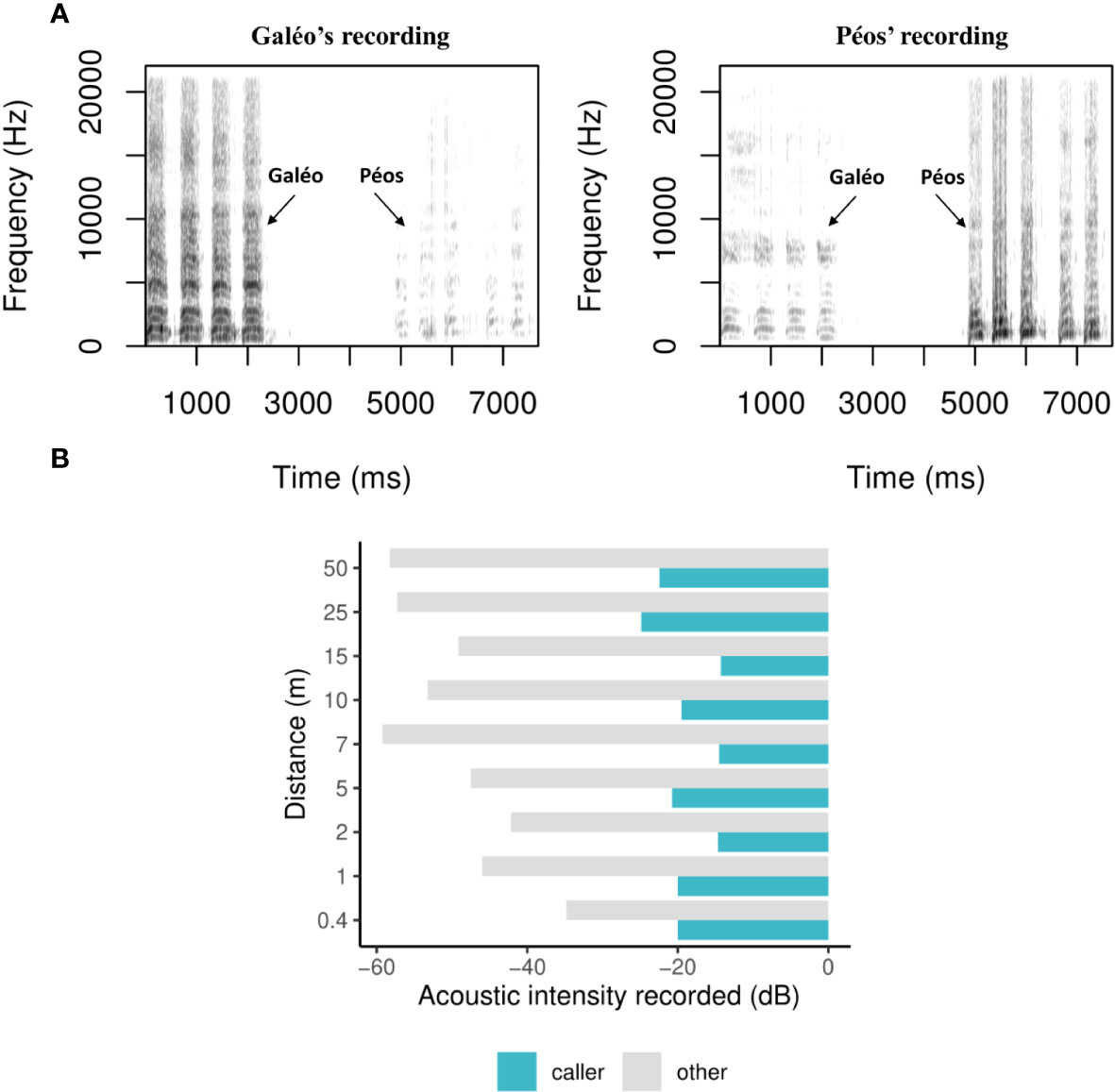
Figure 2 First test: (A) Qualitative analyses of spectrograms showed that vocalizations emitted by the tagged individual were always identifiable and distinguishable from other vocalizations recorded. These two spectrograms were obtained from the tag attached on Galéo (left spectrogram) and Péos (right spectrogram) while they were at a distance of 0.4m and parallel. When Galéo vocalized, his vocalizations were recorded with higher acoustic intensity in his spectrogram (left) than in Péos’ spectrogram (right) and vice versa, when Péos’ vocalizations were analyzed. (B) Bar plots showing that the maximum amplitude (in dB) of vocalizations emitted by tagged dolphins (blue bars) were always recorded with higher acoustic intensity across the nine distances tested.
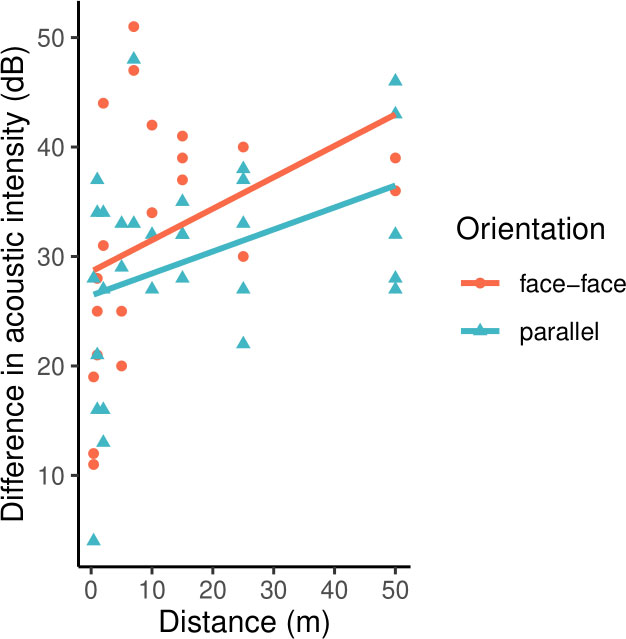
Figure 3 Acoustic intensity recorded by devices at the nine test distances (50m; 25m; 15m; 10m; 7m; 5m; 2m; 1m; 0.40m) and two orientations (face to face, parallel) tested. The scatter plot shows that the difference in acoustic intensity (measured on the maximum amplitude recorded on the signal) increases as the distance separating individuals increases, regardless of the orientation.
Recordings of spontaneous production
Analyses of spectrograms showed that a given spontaneous whistle was recorded with a clear gradient of acoustic intensity by hydrophones and tags. The different acoustic intensities were useful to predict whether the tagged individual emitted a given whistle or not (Figure 4). By combining this comparison with data on dolphins’ positions, it was possible to formulate predictions concerning a callers’ identity. When considering the entire group, a total of 1224 predictions was formulated, with the most represented prediction being POSSIBLE (51.63%) followed, in decreasing order, by: NO (25.98%), UNLIKELY (19.93%), VERY LIKELY (2.37%) and YES (0.08%). However, when considering predictions only for tagged individuals (119 occurrences per individual), they accounted almost exclusively for predictions YES and NO (Supplementary Figure 3). Specifically, 94% of predictions NO and 100% of the predictions YES corresponded to the individuals carrying a device. Therefore, our results showed that the device allowed us to identify if a tagged dolphin had emitted or not a given vocalization thus making possible to exclude from the list of potential callers.
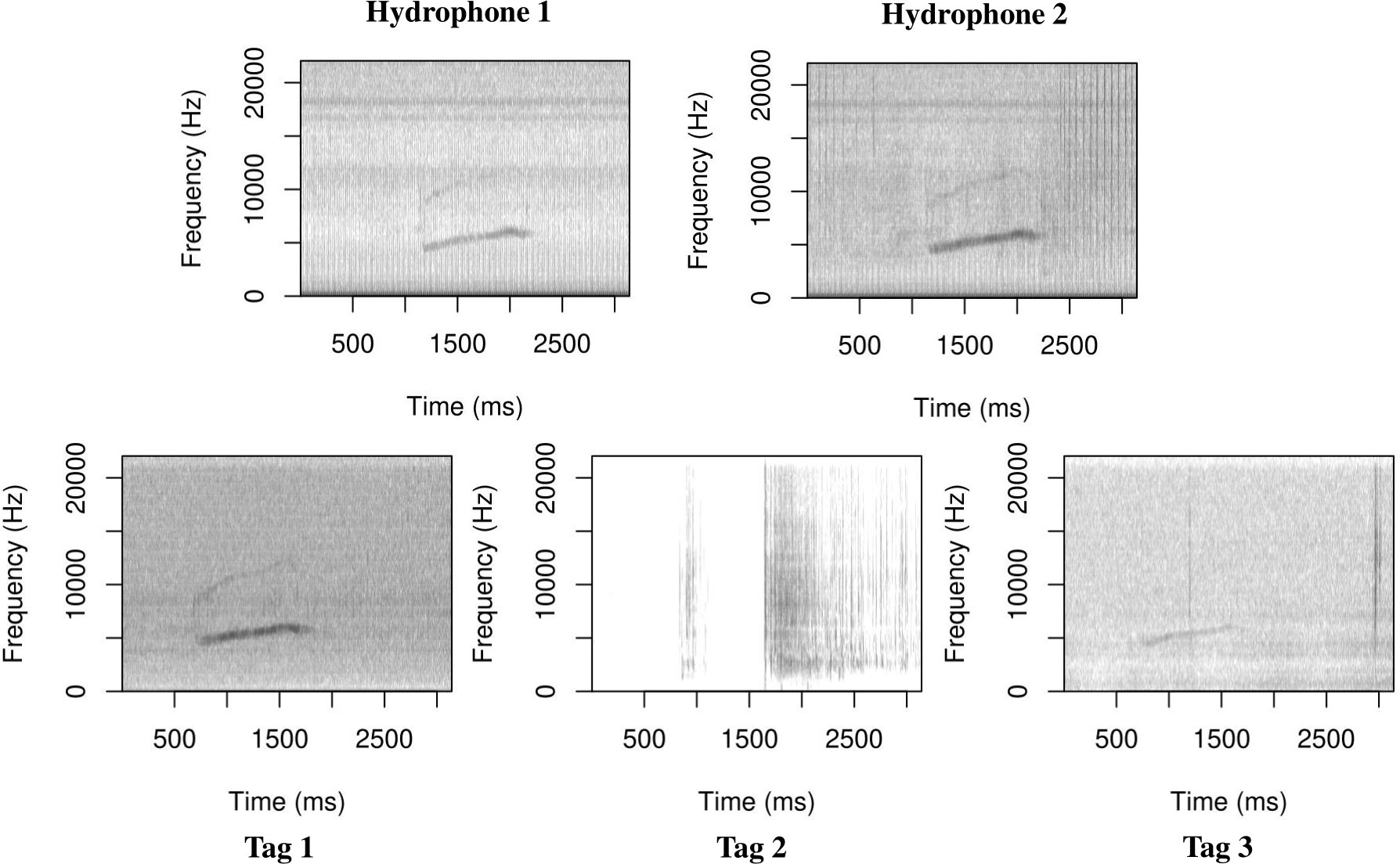
Figure 4 Hydrophone spectrograms (above) and tag spectrograms (below) show that a given whistle was recorded with a clear gradient of acoustic intensity. Qualitative comparisons were useful to formulate predictions concerning a caller’s identity. In the case presented here, the individual equipped with Tag 1 was the best candidate to be the caller.
Discussion
The novel device described here is based on the same concept as the D-Tag (Johnson and Tyack, 2003), but presents the advantage of being easy to manufacture at an extremely low cost. Our findings demonstrated that it is suitable for identifying the emitter and overcome limitations characterizing the study of dolphins’ acoustic communication (Tyack, 2000; Andreas et al., 2021).
Tests of acoustic production under controlled conditions showed that it was always possible to distinguish vocalizations emitted by tagged dolphins (callers) from those of other individuals. Recognition of callers’ vocalizations was possible for both qualitative and quantitative acoustic analyses of spectrograms, even when other emitters were at very short distance (0.40m) and whatever the dolphins’ orientations (face to face/parallel). One of the main problems that limited the use of tag for cetacean acoustic communication up to now was the difficulty to distinguish between vocalizations emitted by tagged animals and those coming from conspecifics at proximity (Johnson et al., 2009). Interestingly, our device shows high potential to overcome this limitation, even for the minimum distance possible (0.40m, dolphins in body contact). Generally, the smaller the distance between sound sources, the more difficult it is to discriminate among various vocalizations recorded. Considering that we analyzed highly intense vocalizations at very short distances, such difficulty was supposedly high. Nevertheless, this discrimination always remained possible, thus demonstrating that the high capabilities endowed by our device can overcome limitations related to identification of callers (Johnson et al., 2009; Kaplan et al., 2014). We acknowledge that sound propagation may differ from one vocal type to another (Branstetter et al., 2012), and that therefore the results of our pilot study cannot be directly generalized to the whole repertoire. Nevertheless, the ability of the dolphins to emit a particular type of call on command allowed us to carry out these highly controlled and standardized experimental sessions.
Furthermore, we tested our tag also under spontaneous conditions to verify whether identification of callers was possible within an entire group of dolphins emitting species-specific vocalizations. Unfortunately, the equipment with tags was not sufficient to identify callers within the entire group free to vocalize spontaneously as it was not possible to identify callers with certainty. However, results showed that our device recorded, with discriminant intensities, whistles emitted by focal and non-focal animals, thus allowing us to conclude whether or not tagged individuals had emitted a given whistle. Concerning identification of callers within a social group, our device makes it possible to exclude with certainty individuals who have not emitted a given vocalization recorded with hydrophones in the pool. Therefore, improvement of our procedure by tagging all group members simultaneously will make their identification easily feasible.
Moreover, our device proves to overcome some difficulties encountered by some techniques developed previously. More precisely, it avoids identification of callers by procedures stressful for the animal, such as isolation (Sayigh et al., 1990), as the animals are free to move and the tag equipment is associated with a less invasive procedure respectful of the individual’s acceptance/willingness to be tagged. This process is not dangerous for the animal and the use of a single suction cup, widely employed on cetaceans, reduces the skin loading and attachment damages (Shorter et al., 2017), even if it is used for long sessions and on free-ranging cetaceans (up to one day, Akamatsu et al., 2005). Then, contrary to telemetry that requires to calibrate finely and spread out in the environment highly sensitive and expensive apparatus (Thomas et al., 2002), our technique involves cheap devices that only need to be synchronized with one another before equipping an animal. Additionally, our device does not depend on multimodal recording systems for identifying a caller. In this way, it is not necessary to use multiple systems, as required by techniques that use a combination of audio and video data, thus avoiding possible biases due to mismatch between audio recordings and video images (Thomas et al., 2002) or unclear images due to limited visibility in turbid water (Lopez-Marulanda et al., 2017). Moreover, contrary to technologies that require the direct presence of observers in the water (Lopez-Marulanda et al., 2017), our tag can leave the animal by itself and free to behave spontaneously far from possible stressors induced by human presence. Although the current version of our device is only suitable for studies on captive groups, we are confident that improvements could be considered to extend its use to certain wild populations by adding deployment and retrieval options.
In conclusion, our device is promising for identifying individual emitters and overcomes a difficulty that characterized studies on dolphins’ acoustic communication so far. By using our tag, it would be possible to explore communication aspects at the individual level thus making it possible to describe the vocal production of an individual and assess both intra- and inter-individual acoustic variability. Furthermore, it will be possible to identify vocalizations shared between group members and the behavioral contexts in which the different types of vocalizations are emitted, and thereby to improve our understanding of their possible functions.
Data availability statement
The raw data supporting the conclusions of this article will be made available by the authors, without undue reservation.
Ethics statement
The animal study was reviewed and approved by Comité Rennais d’Ethique en matière d’Expérimentation Animale (i.e. Rennes Ethical comity for experiments using animals; CREEA decision # 2021122112361534_v8).
Author contributions
AL and MH conceived the study. AT and MB performed the experiment. MB supervised the animal training, KM provided technical support during the experiment and manufactured the capsules. AT, AG, AL, MH performed statistical analysis. AG, AL, MH drafted the manuscript. All the authors revised the final version of the manuscript. All authors contributed to the article and approved the submitted version.
Funding
This study was funded by the Research and Technologies National Association (CIFRE N°2020/1453), Planète Sauvage Animal Park, National Center for Scientific Research (National Center for Scientifc Research) and University of Rennes 1.
Acknowledgments
We would like to thank all the people who contributed to the methodological reflections during the first stages of this study and in particular Jean-Pierre Caudal, Alice De Moura Lima, Dorothee Kremers, Stéphane Louazon, Pablo Pezzino and Jean-Pierre Richard. We are also grateful to all animal caretakers for their enthusiastic contribution to animal training. We deeply thank Ann Cloarec and Michele Iannotti for the English corrections. We wish also to thank the two reviewers for their helpful suggestions.
Conflict of interest
The authors declare that the research was conducted in the absence of any commercial or financial relationships that could be construed as a potential conflict of interest.
Publisher’s note
All claims expressed in this article are solely those of the authors and do not necessarily represent those of their affiliated organizations, or those of the publisher, the editors and the reviewers. Any product that may be evaluated in this article, or claim that may be made by its manufacturer, is not guaranteed or endorsed by the publisher.
Supplementary material
The Supplementary Material for this article can be found online at: https://www.frontiersin.org/articles/10.3389/fmars.2022.915168/full#supplementary-material
References
Akamatsu T., Matsuda A., Suzuki S., Wang D., Wang K., Suzuki M., et al. (2005). New stereo acoustic data logger for free-ranging dolphins and porpoises. Mar. Technol. Soc J. 39 (2), 3–9. doi: 10.4031/002533205787443980
Andreas J., Beguš G., Bronstein M. M., Diamant R., Delaney D., Gero S., et al. (2021) Cetacean translation initiative: a roadmap to deciphering the communication of sperm whales (Accessed April 17, 2021).
Branstetter B. K., Moore P. W., Finneran J. J., Tormey M. N., Aihara H. (2012). Directional properties of bottlenose dolphin (Tursiops truncatus) clicks, burst-pulse, and whistle sounds. J. Acoust. Soc Am. 131 (2), 1613–1621. doi: 10.1121/1.3676694
Caldwell M. C., Caldwell D. K., Tyack P. L. (1990). “A review of the signature whistle hypothesis for the Atlantic bottlenose dolphin, tursiops truncatus,” in The bottlenose dolphin: Recent progress in research. Eds. Leatherwood S., Reeves R. R. (San Diego: Academic Press), 199–234.
Cranford T. W., Amundin M., and Norris K. S. (1996). Functional morphology and homology in the odontocete nasal complex: implications for sound generation. J. Morphol. 228 (3), 223–285. doi: 10.1002/(sici)1097-4687(199606)228:3<223::aid-jmor1>3.0.co;2-3
Fletcher S., Le Boeuf B. J., Costa D. P., Tyack P. L., Blackwell S. B. (1996). Onboard acoustic recording from diving northern elephant seals. J. Acoust. Soc Am. 100 (4), 2531–2539. doi: 10.1121/1.417361
Fripp D. R. (1999). “Techniques for studying vocal learning in bottlenose dolphins, tursiops truncatus,” in Ph.D. Dissertation (Woods Hole and Cambridge: MA: Woods Hole Oceanographic Institution/Massachusetts Institute of Technology).
Herzing D. L. (1996). Sounds and associated underwater behavior of free-ranging Atlantic spotted dolphins, Stenella frontalis, and bottlenose dolphins, Tursiops truncatus. Aquat. Mammals 22, 61–79. doi: 10.12966/abc.02.02.2015
Janik V. M. (1999). Origins and implications of vocal learning in bottlenosedolphins, in mammalian social learning: Comparative and ecological perspectives. Eds. Box H. O., Gibson K. R. (Cambridge: Cambridge University Press), 308–326.
Janik V. M. (2009). Acoustic communication in delphinids. Adv. Study Behav. 40, 123–157. doi: 10.1016/S0065-3454(09)40004-4
Janik V. M., Van Parijs S. M., Thompson P. M. (2000). A two-dimensional acoustic localization system for marine mammals. Mar. Mamm. Sci. 16 (2), 437–447. doi: 10.1111/j.1748-7692.2000.tb00935.x
Johnson M., de Soto N. A., Madsen P. T. (2009). Studying the behaviour and sensory ecology of marine mammals using acoustic recording tags: a review. Mar. Ecol. Prog. Ser. 395, 55–73. doi: 10.3354/meps08255
Johnson M. P., Tyack P. L. (2003). A digital acoustic recording tag for measuring the response of wild marine mammals to sound. IEEE J. Ocean. 28 (1), 3–12. doi: 10.1109/joe.2002.808212
Jones T., Van Houtan K. S., Bostrom B. L., Ostafichuk P., Mikkelsen J., Tezcan E., et al. (2013). Calculating the ecological impacts of animal borne instruments on aquatic organisms. Methods Ecol. Evol. 4, 1178–1186. doi: 10.1111/2041-210X.12109
Jones B., Zapetis M., Samuelson M. M., Ridgway S. (2020). ). sounds produced by bottlenose dolphins (Tursiops): a review of the defining characteristics and acoustic criteria of the dolphin vocal repertoire. Bioacoustics 299 (4), 399–440. doi: 10.1080/09524622.2019.1613265
Kaplan M. B., Aran Mooney T., Sayigh L. S., Baird R. W. (2014). Repeated call types in Hawaiian melon-headed whales (Peponocephala electra). J. Acoust. Soc Am. 136 (3), 1394–1401. doi: 10.1121/1.4892759
Kuznetzov V. B. (1990). “Chemical sense of dolphins: quasi-olfaction,” in Sensory abilities of cetaceans. Eds. Thomas J. A., Kastelein R. A. (New York: Plenum Press), 481–503. doi: 10.1007/978-1-4899-0858-2_34
Le S., Josse J., Husson F. (2008). FactoMineR: An r package for multivariate analysis. J. Stat. Software 25 (1), 1–18. doi: 10.18637/jss.v025.i01
Lilly J. C., Miller A. M. (1961). Sounds emitted by the bottlenose dolphin. Science 133, 1689–1693. doi: 10.1126/science.133.3465.1689
Lima A., Lemasson A., Boye M., Hausberger M. (2017). Vocal activities reflect the temporal distribution of bottlenose dolphin social and non-social activity in a zoological park. Zoo Biol. 36 (6), 351–359. doi: 10.1002/zoo.21387
Lima A., Sébilleau M., Boye M., Durand C., Hausberger M., Lemasson A. (2018). Captive bottlenose dolphins do discriminate human-made sounds both underwater and in the air. Front. Psychol. 9. doi: 10.3389/fpsyg.2018.00055
Lopez-Marulanda J., Adam O., Blanchard T., Vallée M., Cazau D., Delfour F. (2017). First results of an underwater 360° HD audio-video device for etho-acoustical studies on bottlenose dolphins (Tursiops truncatus). Aquat. Mammals 43, 2. doi: 10.1578/AM.43.2.2017.162
McCowan B. (1995). A new quantitative technique for categorizing whistles using simulated signals and whistles from captive bottlenose dolphins (Delphinidae, tursiops truncatus). Ethology 100, 177–193. doi: 10.1111/j.1439-0310.1995.tb00324.x
Nowacek D. P., Christiansen F., Bejder L., Goldbogen J. A., Friedlaender A. S. (2016). Studying cetacean behaviour: new technological approaches and conservation applications. Anim. Behav. 120, 235–244. doi: 10.1016/j.anbehav.2016.07.019
Quick N. J., Rendell L. E., Janik V. M. (2008). A mobile acoustic localization system for the study of free-ranging dolphins during focal follows. Mar. Mamm. Sci. 24 (4), 979–989. doi: 10.1111/j.1748-7692.2008.00231.x
Ropert-Coudert Y., Wilson R. P. (2005). Trends and perspectives in animal-attached remote sensing. Front. Ecol. Environ. 3 (8), 437–444. doi: 10.1890/1540-9295(2005)003[0437:tapiar]2.0.co;2
Sayigh L. S., Tyack P. L., Wells R. S., Scott M. D. (1990). Signature whistles of free-ranging bottlenose dolphins Tursiops truncatus: Stability and mother-offspring comparisons. Behav. Ecol. Sociobiol. 26, 247–260. doi: 10.1007/bf00178318
Schusterman R. J. (1980). “Behavioral methodology in echolocation by marine animals,” in Animal sonar systems. Eds. Busnel R. G., Fish Plenum J. F. (Boston: MA: Springer), 11–41. doi: 10.1007/978-1-4684-7254-7_2
Shorter A. K., Murray M. M., Johnson M., Moore M., Howle L. E. (2014). Drag of suction cup tags on swimming animals: modeling and measurement. Mar. Mamm. Sci. 30 (2), 726–746. doi: 10.1111/mms.12083
Shorter A. K., Shao Y., Ojeda L., Barton K., Rocho-Levine J., van der Hoop J., et al. (2017). A day in the life of a dolphin: Using bio-logging tags for improved animal health and well-being. Mar. Mamm. Sci. 33 (3), 785–802. doi: 10.1111/mms.12408
Thomas R. E., Fristrup K. M., Tyack P. L. (2002). Linking the sounds of dolphins to their locations and behavior using video and multichannel acoustic recordings. J. Acoust. Soc Am. 112 (4), 1692–1701. doi: 10.1121/1.1494805
Tyack P. (1985). An optical telemetry device to identify which dolphin produces a sound. J. Acoust. Soc Am. 78 (5), 1892–1895. doi: 10.1121/1.392777
Tyack P. L. (2000). “Functional aspects of cetacean communication,” in Cetacean societies: field studies of dolphins and whales. Eds. Mann J., Connor R. C., Tyack P. L., Whitehead H. (Chicago, IL: University of Chicago Press), 270–307.
Tyack P. L., Recchia C. A. (1991). A datalogger to identify vocalizing dolphins. J. Acoust. 90 (3), 1668–1671. doi: 10.1121/1.401908
Wells R. S., Scott M. D. (2018). “Bottlenose dolphin: Common bottlenose dolphin (Tursiops truncatus),” in Encyclopedia of marine mammals. Eds. Würsig B., Thewissen J. G. M., Kovacs K. (San Diego, CA: Elsevier, Inc), 118–125.
Woodward S. F., Reiss D., Magnasco M. O. (2020). Learning to localize sounds in a highly reverberant environment: Machine-learning tracking of dolphin whistle-like sounds in a pool. PloS One 15 (6), e0235155. doi: 10.1371/journal.pone.0235155
Würsig B., Kieckhefer T. R., Jefferson T. A. (1990). “Visual displays for communication in cetaceans,” in Sensory abilities of cetaceans. Eds. Thomas J., Kastelein R. (New York: Plenum Press), 545–559.
Keywords: vocal communication, individual identification, biologging devices, acoustic tag, bioacoustics, cetaceans, delphinids, odontocetes
Citation: Gallo A, Thieffry A, Boye M, Monmasson K, Hausberger M and Lemasson A (2022) Identification of individual bottlenose dolphins (Tursiops truncatus) emitters using a cheap wearable acoustic tag. Front. Mar. Sci. 9:915168. doi: 10.3389/fmars.2022.915168
Received: 07 April 2022; Accepted: 03 August 2022;
Published: 18 August 2022.
Edited by:
Tracy A. Romano, Sea Research Foundation, Inc. d/b/a Mystic Aquarium, United StatesReviewed by:
Salvatore Siciliano, Fundação Oswaldo Cruz (Fiocruz), BrazilLaela Sayigh, Woods Hole Oceanographic Institution, United States
Copyright © 2022 Gallo, Thieffry, Boye, Monmasson, Hausberger and Lemasson. This is an open-access article distributed under the terms of the Creative Commons Attribution License (CC BY). The use, distribution or reproduction in other forums is permitted, provided the original author(s) and the copyright owner(s) are credited and that the original publication in this journal is cited, in accordance with accepted academic practice. No use, distribution or reproduction is permitted which does not comply with these terms.
*Correspondence: Alessandro Gallo, alessandro.gallo@univ-rennes1.fr
†These authors share senior authorship