- 1Department of Biology University of Turku, Turku, Finland
- 2Natural Resources Institute Finland (Luke), Enonkoski, Finland
Domestication and selective breeding for rapid-growth have impaired the cardiorespiratory system of salmonids, which might compromise their capacity to tolerate environmental stressors, such as heat waves. Exercise training by swimming has been proposed as a potential tool to enhance growth, cardiac function and disease resilience in farmed fish and thereby improves aquaculture production. However, whether exercise training could also improve cardiac robustness against heat waves, which are becoming more common and cause severe challenges to aquaculture, remains unknown. Here, we trained juvenile rainbow trout at three different training velocities: 0.06 m*s-1 (or 0.9 body lengths per second [bl*s-1]; control group), 0.11 m*s-1 (or 1.7 bl*s-1; medium speed group) and 0.17 m*s-1 (or 2.7 bl*s-1; high speed group) for 5 weeks, 6h per day, 5 days per week. Measuring maximal heart rate (fHmax) during acute warming, we demonstrated that training at 1.7 bl*s-1 was optimal in order to increase the temperature at which fHmax reached its peak (Tpeak) as well as the upper thermal tolerance of the cardiovascular function (arrhythmia temperature, TARR), up to 3.6°C as compared to the control fish. However, more intensive training did not provide similar improvement on thermal tolerance. Both training regimes enhanced the ventricular citrate synthase activity which may provide higher aerobic energy production capacity for ventricles. Further mechanistic studies are needed to understand the complex interactions between training intensities and changes in thermal tolerance. Although not conclusive on that point, our findings present a valid training programme for hatchery salmonids to increase their cardiac thermal tolerance and consequently probably also their capacity to tolerate heat waves, which has a direct application for aquaculture.
Introduction
Aquaculture is one the most rapidly growing sectors in the food production industry (FAO, 2020) and it is expected to keep expanding to meet the world’s increasing demand for animal-based protein. Due to this rising demand in fish consumption, the aquaculture industry, especially with regard to salmonids species, is facing several challenges (Ahmed et al., 2019), including emerging diseases (Pulkkinen et al., 2010), lack of genetic diversity, low survival rates (Skaala et al., 2019) and low robustness of fish to respond environmental stressors (Castro et al., 2011; Zhang et al., 2016). Furthermore, the aquaculture sector is expected to be significantly affected by anthropogenic climate change which may lead to suboptimal rearing conditions, that further challenge aquaculture production (Blanchard et al., 2017; Barange et al., 2018; Reid et al., 2019; Collins et al., 2020; Calado et al., 2021; Maulu et al., 2021; Oyinlola et al., 2022). Heat waves are, for instance, causing massive mortality events in aquaculture as the fish cannot escape the rapidly warming waters (Froehlich et al., 2018; Wade et al., 2019; FAO, 2020; Gamperl et al., 2021). Especially in hatcheries, rising water temperature is a concern for juvenile fish since they are often reared in shallow tanks with wide air/water interface exposing them to acute warming. For these reasons, there is an urgent need to optimize and implement better efficiency and productivity in the aquaculture sector, in order to guarantee the sustainability of the industry and animal welfare.
The thermal tolerance of fish, especially salmonids, is thought to be limited by the reduction of the absolute aerobic scope (AAS) - the difference between the minimum (i.e. standard metabolic rate, SMR) and maximum metabolic rate (MMR). When the temperature rises, the AAS reaches its peak at an optimal temperature of aerobic scope (Topt). Thereafter the AAS plateaus and then it starts to decline due to impairments of cardiorespiratory system (McKenzie et al., 2021b). The critical thermal limits are reached when AAS is zero and there is no room for aerobic activities. In salmonids, the decline in AAS is mainly due to impairment of maximum heart rate (fHmax), which is associated to reduction in MMR and, thus, AAS at temperatures beyond Topt (Eliason and Anttila, 2017). At upper critical temperatures, besides a reduction in fHmax, fish start to show cardiac arrhythmias which might lead to death if warming continues (Casselman et al., 2012; Anttila et al., 2017; Gilbert and Farrell, 2021; van der Walt et al., 2021). Farmed fish could be especially challenged by environmental warming since previous studies have shown that selection for fast-growth and sedentary aquaculture conditions are often associated with cardiac deformities and impaired cardiac performance (Gamperl and Farrell, 2004; Brijs et al., 2020; Frisk et al., 2020). The decreased cardiac performance of farmed fish might also hinder their responses to heat waves (Gamperl and Farrell, 2004; Eliason et al., 2013; Eliason and Anttila, 2017).
In this context, aerobic exercise training could be a potential tool to improve aquaculture production by enhancing the welfare of farmed fish and mitigating the detrimental effects of intensive farming (Palstra and Planas, 2013; Palstra et al., 2020; McKenzie et al., 2021a). Several studies have shown that aerobic exercise training, depending on the intensity and duration of the training, can improve different physiological traits of fish including cardiovascular performance (Farrell et al., 1990; Gallaugher et al., 2001; Castro et al., 2011; Palstra et al., 2014; Rovira et al., 2018; Chen et al., 2021) by increasing the cardiac output and heart rate, cardiac muscle growth and hematocrit level (Farrell et al., 1990; Farrell et al., 1991; Palstra et al., 2015; Papadopoulou et al., 2022). However, so far the potential benefits of aerobic exercise training on the capacity of fish to face environmental stressors has been overlooked. To our knowledge, there is only one previous study that has shown the potential benefit of aerobic exercise training, in this case to improve hypoxia tolerance in goldfish (Fu et al., 2011). Despite the promising evidences of the benefits of aerobic exercise training on cardiac performance (Farrell et al., 1991; Gallaugher et al., 2001; Farrell, 2007; Castro et al., 2011; Papadopoulou et al., 2022), any effects on the robustness of the cardiac system against environmental stressors, such as thermal stress, have not been shown yet.
On this basis, our first aim was to design a swimming exercise-training programme for farmed rainbow trout (Oncorhynchus mykiss) that could improve the fHmax responses to high temperature and therefore cardiac thermal tolerance. Our particular interest in fHmax was because increasing the heart rate toward the maximal is one of the immediate physiological responses that helps fish face rapid changes in water temperature (Eliason and Anttila, 2017). The fHmax measurements provide an estimate of upper temperature performance of fishes during acute warming by providing functional transition temperatures, e.g. Arrhenius breakpoint temperature (TABP) - the temperature at which the fHmax first slows down its incremental rate with temperature - and arrhythmia temperature (TARR) - temperature at which fHmax starts to become arrhythmic (Farrell, 2016). The TABP and TARR give estimates within 1-2°C for the optimum temperature of aerobic scope (TOPT) and critical thermal maximum (CTMAX), respectively (Casselman et al., 2012; Ferreira et al., 2014; Anttila et al., 2017; Gilbert and Farrell, 2021; van der Walt et al., 2021).
The second aim of the study was to identify the mechanisms, induced by the training, that could be involved with possible changes in cardiac performance and thermal tolerance. The activity of key aerobic (citrate synthase, CS) and anaerobic (lactate dehydrogenase, LDH) energetic enzymes were used as a comprehensive proxy to assess any energy-metabolic modifications in the ventricles in response to the training programme. Additionally, we measured the protein levels of sarco(endo)plasmic reticulum Ca2+-ATPase (SERCA), hypoxia-inducible factor (HIF) and vascular endothelial growth factor (VEGF) from the whole ventricle as well as the hematocrit (HCT) and haemoglobin (Hb) concentrations from the blood. We focused on these proteins since SERCA is a calcium channel involved in the excitation-contraction coupling (E-C coupling) in myocytes and it has been shown to be related to high cardiovascular performance in sockeye salmon (Oncorhynchus nerka) (Anttila et al., 2019). On the other hand, HIF and VEGF are involved with molecular pathways that will lead to increase in capillary density in muscles (Palstra et al., 2014) and therefore provide more oxygen for exercising muscles. We hypothesized that training could increase the need of oxygen supply to the ventricle and therefore induce angiogenesis of new coronary vessels, that can be evaluated by measuring the levels of HIF and VEGF. Similarly, we measured Hb and HCT since increased hematocrit and hemoglobin levels after training might improve oxygen provision to the muscles. Furthermore, training has been previously shown to enhance swimming muscle vascularization, oxygen diffusion to the mitochondria, E-C coupling and the management of Ca2+ currents (Anttila et al., 2006; Anttila et al., 2008). Therefore, we measured these parameters in ventricles.
Overall, this study will provide new and broader perspectives regarding the potential benefits of aerobic exercise training on the welfare and cardiac robustness of farmed fish in the context of climate change and with direct application to the aquaculture sector. Specifically, we aimed to develop a training program suitable for hatchery facilities using energetically sustainable way for generating the water flow. Therefore, the studies were conducted in regular hatchery facilities using rainbow trout that is one of the most widely cultured salmonid in the world (FAO, 2020).
Material and Methods
Ethics Statement
The current study followed Finnish laws and ethical guidelines regarding animal welfare and was approved by the Regional State Administrative Agency (license number ESAVI/11880/2019).
Animals
The experiments were conducted at the hatchery of the Natural Resources Institute Finland (Luke, Enonkoski, Finland) with juvenile rainbow trout born in June 2019. The fish were reared in a circular tank (1000 L) for 2 months under seasonal fluctuation of temperature, oxygen and light conditions and water flow of 0.06 m*s-1 until the beginning of the experiment, at the end of August 2019. The fish were fed ad libitum with a 0.5-1.5 mm commercial pellet (Raisioaqua, Raisio, Finland) with an automatic feeding machine (Itumic, Itumic Oy, Jyskä, Finland).
Training Program
Before starting the five-week-long training programme, the fish (n=105) were anesthetized with 60 ppm buffered MS-222 (Finquel®, Argent Chemical Laboratories, Redmond, Washington, USA) and their body mass and fork length were measured (4.45 ± 0.14 g; 6.89 ± 0.29 cm). At the beginning of the training experiment, the fish were equally divided into three treatment groups (n=35 per treatment): 1) water flow velocity of 0,06m*s-1 (or 0.9 body length*seconds-1 [b1*s-1] which was normally in use in the hatchery facilities and therefore considered as control group (C); 2) medium intensity (M) of training with 0.11 m*s-1 (or 1.7 b1*s-1) water flow velocity and 3) high intensity (H) of training with 0.17m*s-1 (or 2.9 b1*s-1) water flow velocity (Table S1). These velocities were selected since earlier studies have shown that training with around 1.5 b1*s-1 is optimal for swimming performance of the fish (Anttila et al., 2006; Anttila et al., 2008) and lower velocity will not improve the cardiac thermal tolerance (Papadopoulou et al., 2022). The absolute speed in m*s-1 remained constant over the course of the experiment resulting in decreasing b1*s-1 as the fish grew. The average values of speeds in body length*seconds-1 between week 1 and week 5 were 0.7, 1.3, 2.1 b1*s-1 for control, medium and high speed, respectively.
Each tank was provided by two inputs of water, the control speed inflow during the resting hour, the training inflow was specifically opened during the training hours. The water speed was achieved by regulating the inflow of the water flow already existing in the main water pipes of the hatchery. One third of each tank was partially covered by a black plastic fabric in order to provide a shelter zone where the fish preferentially swim in schools (personal observation) even though the fish were free to choose their swimming location. The velocities of the water flow were measured three times per week in three different spots correspondent to the zone where the fish were swimming in school (Figure S2; Table S1) with a velocitymeter (MiniAir20®+ MiniController MC20, Schiltknecht Messtechnik, Gossau, Switzerland). The training took place for 6 h per day (8:00-14:00), 5 days per week. During the resting period (18 h per day and during weekends) the water flow was 0.06 m*s-1 for all the treatments. The fish were fed ad libitum modulating the daily feeding rate of continuous automatic feeders. All the tanks were identical regular aquaculture circular tanks (80*10 cm, 50 L). The temperature and oxygen level followed the seasonal natural fluctuations during the training programme (Table S1 and Figure S1). One week before the measurement started, the water temperature stabilized around the average of 10.7 ± 0.3°C, and it stayed stable at the average of 10.6 ± 0.4°C during the week of the fHmax measurements. Therefore, all the fish were acclimated for more than 1 week to the same temperature of 10°C.
Measurement of fHmax During Acute Warming
After five weeks of training, the temperature-specific maximum heart rate (fHmax) was measured according to Casselman et al. (2012) (see details in Supplementary Information). To obtain the fHmax that could last for the entire measurement duration, it was induced pharmacologically by sequential injections of atropine and isoproterenol. To stop skeletal muscle movements and therefore to prevent electrical artefacts in the ECG signal, a maintenance dose of anesthetic (50 ppm MS-222) was used in the fHmax measurement setup. To avoid bias related to the day of the measurement, the fHmax measurements were conducted in randomized order and fish belonging to same group were measured in two different days. The pharmacologically induced fHmax was measured during an acute warming challenge with a stepwise temperature increase rate of 1°C/6 min. The stepwise warming rate of 1°C/6 min was chosen since it is the minimum rate that provides proper trade-off between stabilized heart rate at the end of each stepwise increment and fast measurement. The measurements started at 10°C (corresponding to the average water temperature one week before the measurements started see Figure S1) and continued until a cardiac arrhythmia was first detected, i.e. when the QRS complex was missing from the ECG trace indicating arrhythmia in ventricular systole (Figure S3). The temperature was recorded, giving the arrhythmia temperature (TARR) for each fish. Then, the fish was euthanized by blow to the head. The mass and length of the fish was measured (Table 1) and the ventricle was dissected, weighed and flash frozen in liquid nitrogen for western blot analysis. Based on the ECG data traces, we calculated the fHmax by measuring the duration for 15 continuous R-R peaks at the end of each temperature step. The TABP, considered a proxy for the TOPT of an organism (Casselman et al., 2012), was calculated according to Yeager and Ultsch (1989). The fHmax values were also used to calculate additional transition temperatures that characterized the thermal performance curve of the fHmax,: 1) the temperature where the highest fHmax was achieved (Tpeak), 2) upper thermal scope (TS =TARR-TABP) defined as the range of temperatures between the TABP and the TARR, that informs about the upper thermal window for the heart rate and 3) heart resilience (TR =TARR-Tpeak) defined as the range of temperature between the Tpeak and the TARR which provides the information about how long the heart was able to function before collapsing at TARR.
Molecular Analyses
The effect of training programmes on the relative levels of SERCA, HIF and VEGF in the whole ventricle was analyzed with western blots method according to Mottola et al. (2020) (see Supplementary Information). The primary antibodies used were SERCA, ab 91032 (Abcam, Cambridge, UK); HIF, ab2185 (Abcam) and VEGF, ab209835 (Abcam) at concentrations of 1:2000. The gels and membranes were scanned using a ChemicDoc MP Imaging system (Bio-Rad). Relative protein concentrations were calculated with Image Lab 6 (Bio-Rad) by relating the intensity of the bands of SERCA, HIF and VEGF to the intensity of the total amount of protein in the sample in the gel.
The hematocrit and the hemoglobin concentration were measured from fish that did not experience the fHmax measurement (defined so on as naïve fish). The fish were euthanized in 200 ppm buffered MS-222, biometrics were measured and the blood sample was quickly taken from a caudal vein with a heparinized syringe. A portion of the blood was collected in the hematocrit capillaries and the hematocrit was read after centrifugation for 10 min in a capillary-centrifuge (Hettich zentrifugen, Tuttlingen, Germany). Ten μl of the collected blood was also pipetted into tubes containing 1 ml of cyanide solution (0.6 mM K3[Fe(CN)6], 0.77 mM KCN, 1.2 mM KH2PO4) for hemoglobin analyses. The hemoglobin concentration was measured at 540 nm with an EnSpire 2300 Multilabel Reader (Perkin Elmer) and the concentrations were calculated according to Clark et al. (2008).
Ventricles from naïve fish were also dissected and flash frozen with liquid nitrogen. Citrate synthase (CS, EC 2.3.3.1) and lactate dehydrogenase (LDH, EC 1.1.1.27) activities were measured from the samples of the whole ventricle following the protocol established by Dalziel and Schulte (2012) (see Supplementary Information). Enzyme activity was measured as µmol*min-1*mg protein-1. The protein concentration for the calculations was spectrophotometrically measured with a bicinchoninic acid assay kit (ThermoFisher, Waltham, MA, USA). The LDH/CS ratio was calculated by dividing the value of the LDH activity/CS activity.
Statistical Analysis
The values provided in the study are presented as means and s.e. and p < 0.05 was chose to indicate statistical significance. Linear mixed models (LMM) with training group as fixed effect and day of sampling as random factor were used to analyze the effect training on (1) the biometry of all the fish: mass, length, condition factor [(Fish mass (g) * (Fish length (cm))-3)*100], ventricle mass and relative ventricle mass [RVM=(Ventricle Mass (g)*(Fish mass (g)-1)*100]; (2) the cardiac thermal performance (TARR, TABP, Tpeak, TS, TR, highest peak of fHmax during acute warming), and (3) the molecular level variables (proteins levels of SERCA, HIF and VEGF, enzyme activity of CS and LDH and the LDH/CS ratio, HTC and [Hb]). For those variables where the random factor (day) did not had effect or improved AICs, the random factor was removed from LMM. The final model for CS included the training group as a fixed factor and the day of the sampling as a random factor. The final LMM model used to analyse the effect of training on the fHmax during acute warming, included the training groups and temperature steps (10-24°C) as the main factors, the temperature steps as repeated measure and the day of measurement as a random factor. The effects of the atropine and isoproterenol injections on the fH was tested with a LMM including the training groups and injections type as the main factors and also as repeated measure. Sidak post hoc tests were used to the test the differences between the groups. Statistical analyses were conducted using SPSS ver. 26 (IBM Corp., Version 26.0. Armonk, NY, USA).
Results
Cardiac Performance
The medium intensity training improved significantly the upper critical cardiac thermal tolerance by 3.6°C (defined as arrhythmias temperature - TARR) (Figures 1; 2C; Table S2) (F=7.051 p=0.003; C vs M Sidak p=0.003; M vs H Sidak p=0.039) as compared to the control and by 2.6°C as compared to the high intensity training. However, the control and high intensity training did not differ significantly from each other (C vs H Sidak p=0.656). The training did not increase the TABP – the estimate for optimal temperature (F=1.271 p=0.294, Figure 2A; Table S2). The fish trained with the medium intensity achieved the maximal heart rate peak 2.6°C higher temperature (Tpeak)(Figure 2B) (F=5.557 p=0.008; C vs M Sidak p=0.009; M vs H Sidak p=0.064; C vs H p=0.797) than the control fish but not as compared to those from the high intensity training group (Table S2). Fish trained at a medium intensity were also able to significantly extend their thermal scope by 2.8°C (TS=TARR-TABP) (Figure 2E; Table S2) as compared to the control group and by 2.1°C as compared to the high intensity training group (F=6.208 p=0.005; C vs M Sidak p=0.006; M vs H Sidak p=0.043; C vs H Sidak p=0.803). None of the above parameters differed between the control and high intensity training groups (p>0.05). No significant differences were found in the highest fHmax during acute warming (fHpeak) (F=2.228 p=0.124) (Figure 1), or in heart thermal resilience (TR=TARR-Tpeak) (Figure 2D) (F=0.766 p=0.473) (Table S2). The fHmax increased significantly in all the groups during acute warming but there were no significant differences in fHmax values between the groups in the temperature steps of the acute warming (10-24°C) (Figure 1) (Training group F=2.571 p=0.111; Temperature F=340.93 p<0.001; Training group * Temperature F=0.304 p=0.998). At 10°C, the heart rate before the injection was not significantly different between the groups (Training groups F=1.560, p=0.222; Training groups*Injections F= 0.516, p=0.725). Pharmacological stimulation significantly affected the fH (Injections F=11.208, p<0.001). Atropine increased the heart rate from 66.7 ± 3.6 (before injection) to 80.29 ± 1.3 bpm (after atropine injection) (Sidak, p=0.003). On the other hand, isoproterenol injection did not significantly elevate the heart rate after atropine injection (Sidakp=0.176) and heart rate remained at 83.1 ± 0.6 bpm after isoproterenol injection.
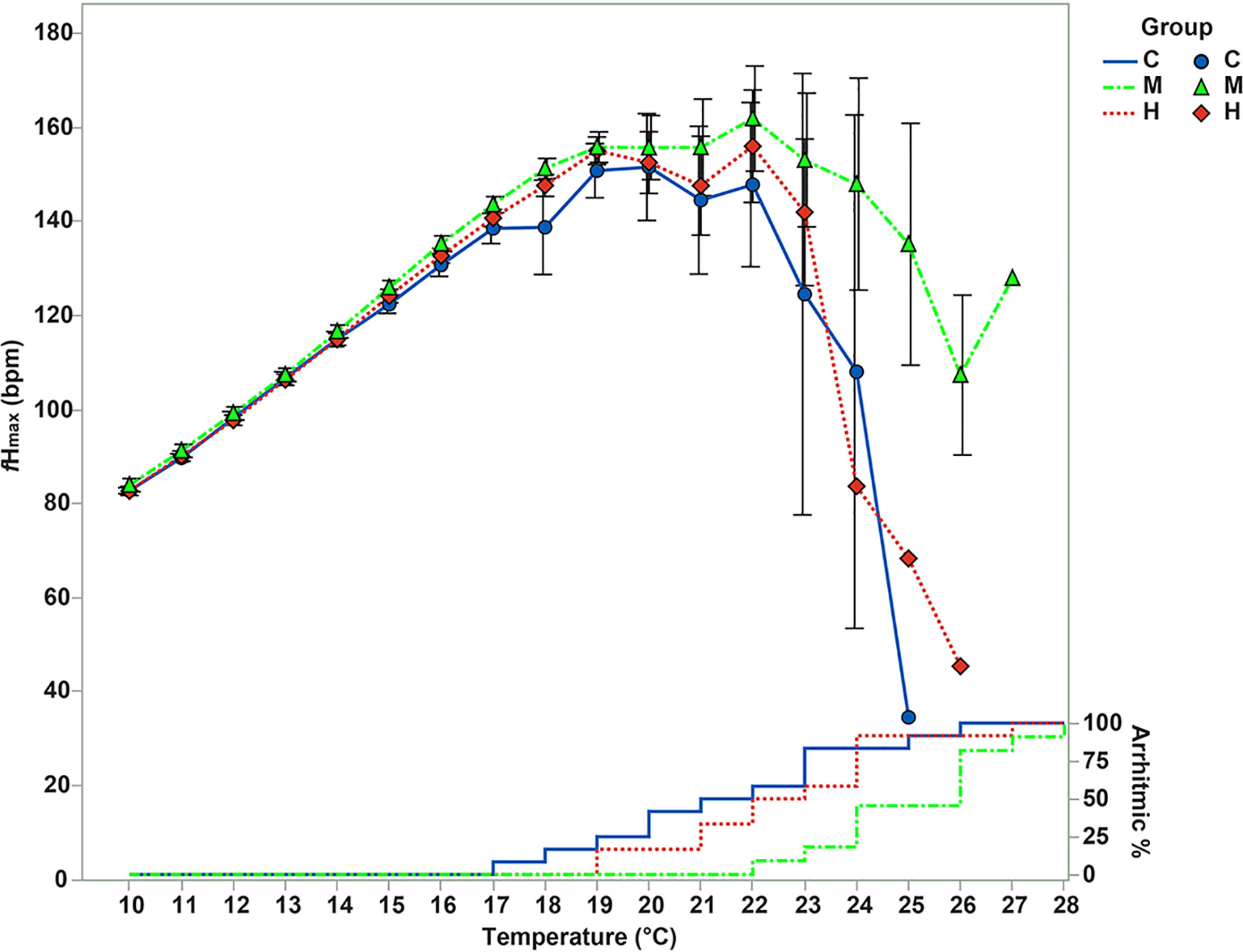
Figure 1 The effects of training programmes on the maximal heart rate (fHmax) during acute warming. The average ( ± s.e.) fHmax of the three training groups (Control=C, blue circles, n=12; Medium intensity=M, green triangles, n=11; High intensity=H, red squares, n=12) during acute warming challenge. The lines on the right side represent the percent of the fish that developed cardiac arrhythmia at the corresponding temperature steps.
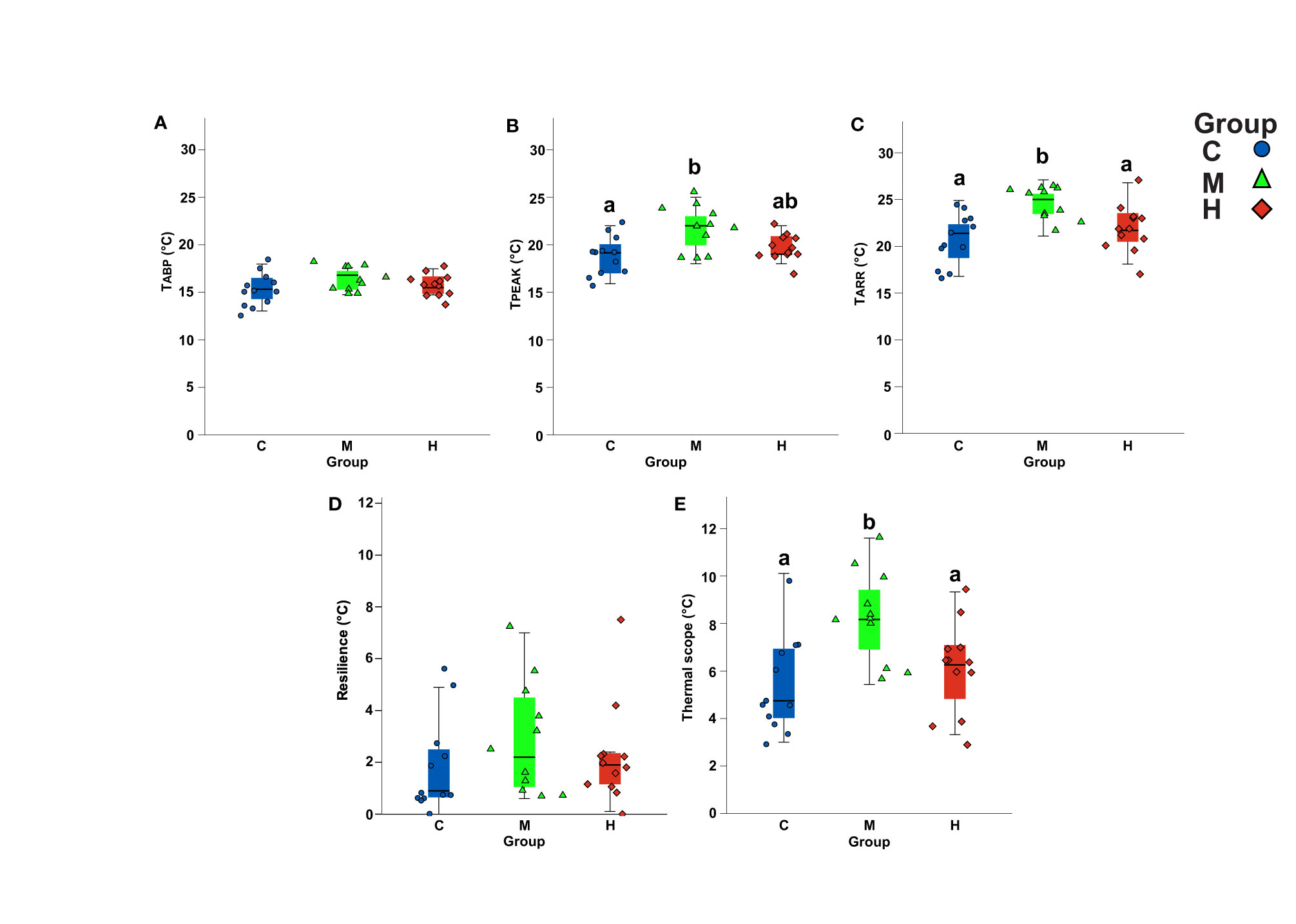
Figure 2 The effects of training programmes on the cardiac transition temperatures. Boxplots of the Arrhenius break point temperature (TABP) the fHmax (A), the temperature where the fish achieved the peak of the fHmax (Tpeak) (B), the temperature where the fish developed the arrhythmia (TARR) (C), the scope of the heart thermal resilience (D), and the thermal scope of the heart function (E). The different letters represent significant difference (p < 0.05) among the groups (Control=C, blue circles, n=12; Medium intensity=M, green triangles, n=11; High intensity=H, red squares, n=12).
Biometry
The training programmes did not significantly affect the mass or the length of the fish (mass: F= 0.429 p=0.653; length: F= 0.103 p=0.902); nor did the training affect the ventricle mass (F=0.810 p=0.449) and the relative ventricle mass (F=1.365 p=0.284) (Table 1). However, the fish from the high intensity training group had a significantly lower condition factor as compared to control groups (F=7.632 p=0.001; C vs H Sidak p=0.001; C vs M Sidak p=0.222; M vs H Sidak p=0.134) (Table 1).
Enzyme Activities
Exercise training significantly affected the CS activity of the ventricles (F=5.386 p=0.014) (Figure 3B; Table S3). Fish trained at medium and high intensity had respectively 22% (Sidak p=0.036) and 21% (Sidak p=0.030) higher CS activity as compared to the control group. Medium and high intensity groups did not differ significantly (Sidak p=0.986). LDH activity also changed significantly in response to training (F=4.791 p=0.019) (Figure 3A; Table S3). High intensity training group had significantly lower LDH activity as compared to the control group (Sidak p=0.018) but not as compared to the medium intensity group (Sidak p=0.235). The medium and control group did not differ significantly from each other (Sidak p=0.534). The LDH/CS activity ratio was significantly lower in high intensity groups as compared to the control group (F=4.945 p=0.017; H vs C group Sidak p=0.016) (Figure 3C; Table S3). However, the medium did not differ from control and high intensity training group (M vs C Sidak p=0.718; M vs C Sidak p=0.132).
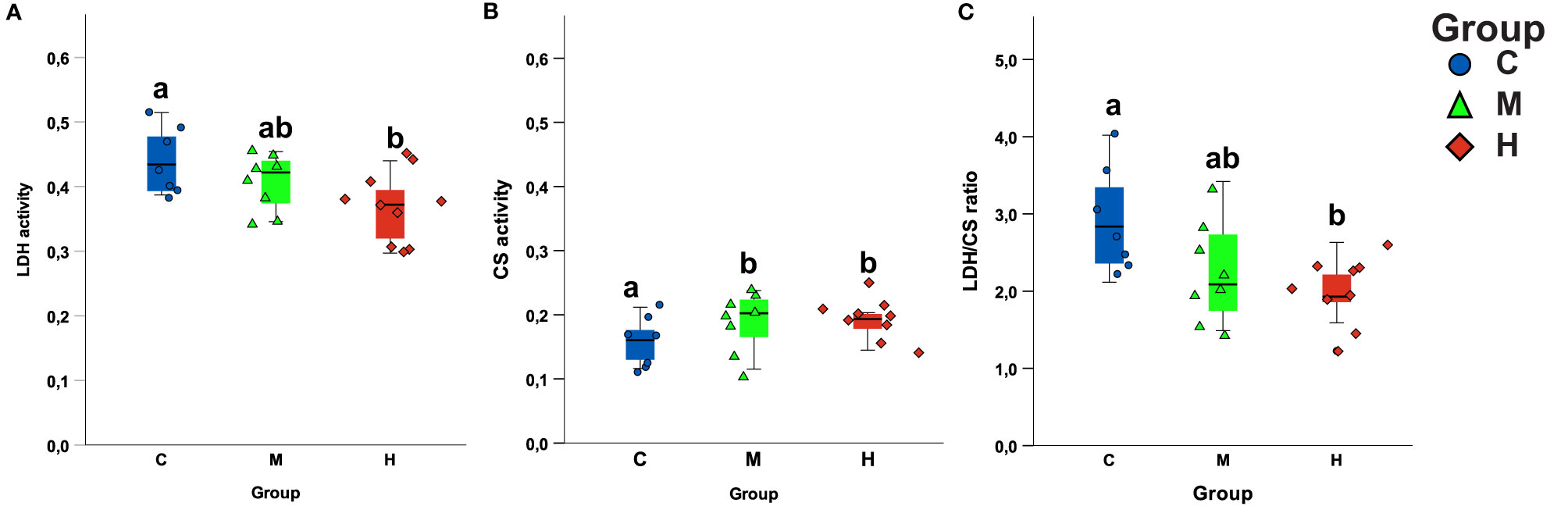
Figure 3 The effects of training programmes on the enzymes activity in the ventricle. Boxplot of the maximal activity of the ventricular (A) lactate dehydrogenase (LDH) and (B) citrates synthase (CS) among training groups. (C) LDH/CS ratio. Different letters represent significant difference among the groups.
Protein and Hematological Measurements
There were no significant differences in the ventricular relative levels of SERCA (F=0.104 p=0.902), HIF (F=0.660 p=0.523) or VEGF (F=0.290 p=0.750) among the groups (Table S3) (Figure S4 gels). The Hematocrit (F=1.563 p=0.233) and Hemoglobin concentrations (F=0.711 p=0.505) did not differ among the experimental groups either (Table S3).
Discussion
This study demonstrates for the first time that aerobic exercise training increases the cardiac upper thermal tolerance (arrhythmia temperature - TARR) and the upper Thermal scope (TS) of the maximal heart rate. However, only the medium intensity training was observed to widen the upper end of the thermal window where the heart can still beat efficiently. In salmonids especially, the cardiac function is one of the most susceptible physiological systems to high environmental temperatures (Eliason and Anttila, 2017). Therefore, as suggested also by Anttila et al. (2014), being able to improve the thermal tolerance of the cardiac function might improve the thermal tolerance of the whole fish and possibly their capacity to respond to future heat waves caused by climate change. Furthermore, the exercise training does not improve only the cardiac function but training has been previously shown to increase the survival rate of fish during infection disease challenges and enhance the immune capacity (Castro et al., 2013; Hou et al., 2022). Our findings, while preliminary, may be extremely relevant for the aquaculture sector, e.g. hatcheries and nursery facilities, in the current global warming scenario. Rainbow trout is widely farmed around the world and one of the most important aquaculture fish species. Therefore, exercise training is not only a useful tool to improve trout heart performance, but it could also be an easy and low-cost solution to the urgent need to enhance the sustainability and reduce the economical loses due to massive death events associated with heat waves (FAO, 2020). Exercise swimming training is particularly applicable in hatcheries, but also in recirculating aquaculture systems (RAS) that are increasingly being used in the global aquaculture sector (Gorle et al., 2018).
The most interesting and important result of this study is that the exercise training programme at a medium intensity was able to increase the TARR by 3.6°C as compared to the control group. Coherent with TARR, the thermal scope (TS) and Tpeakwere all significantly higher in the medium intensity group as compared to the controls. Medium intensity training, therefore, seems to widen the upper thermal window of the cardiac performance without changing the TABP, proxy of the TOPT (Casselman et al., 2012). These results mean that medium training intensity could improve the thermal window without compromising the TOPT and therefore without substantially changing the usual temperatures that the farmers are using to rise their fish. From a physiological point of view, the higher upper thermal tolerance of the cardiovascular system (TARR) might provide a higher tolerance to acute warming in the whole organism (Ferreira et al., 2014; Gilbert et al., 2020). Extensive research has shown that sustained aerobic exercise swimming can enhance the cardiac performance of fishes, i.e. by improving twitch tension, cardiac pumping capacity, contraction rate (Rissoli et al., 2017) and stroke volume (Farrell et al., 1990; Farrell et al., 1991). Therefore, it is possible that as a consequence of these systemic changes, the health status of the cardiovascular system improves. A healthy heart might be beneficial in metabolically demanding situations, such as high water temperature. Furthermore, improvement in the health status would be especially important for farmed fish having high susceptibility for cardiac deformities and arteriosclerotic lesions (Ekström et al., 2019; Brijs et al., 2020). However, the training did not influence the fHmax response to acute warming in any of the temperature steps (10-25°C). Similarly a previous experiment with Chinook salmon (Oncorhynchus tshawytscha), showed that the heart rate during critical swimming speed (Ucrit) did not differ between the trained and untrained fish (Gallaugher et al., 2001). This is in accordance with human exercise training programme results, where aerobic training mainly affects the resting or the routine heart rate and not the maximal rate (D’Souza et al., 2014).
The high intensity training did not induce a significant enhancement of the TARR. Furthermore, the high intensity training significantly decreased the condition factor of the fish as compared to the controls indicating that swimming at this speed was energy demanding. On the other hand, the control fish might have been more obese than fish in high speed group. Nevertheless, these results suggested that excessive high intensity training cannot induce similar beneficial effects on cardiac thermal tolerance as medium intensity training because fish might have altered the energy balance and allocate energy for other functions. High training intensity might cause an additive stress that could diminish the otherwise beneficial effects of training to face acute warming. Indeed, while an optimal training has been shown to reduce the cortisol levels in fish (Woodward and Smith, 1985; Boesgaard et al., 1993; Herbert et al., 2011; Palstra et al., 2020), the exercise training at high speed (2.5 bl*s-1; length 32.4 cm) increased the cortisol level in Atlantic salmon (Salmo salar) (Timmerhaus et al., 2021). A recent study reported that high cortisol levels are associated with lower TARR and lower CTmax in stressed subordinate rainbow trout as compare to dominant ones although fH displayed the same trend during acute warming in both groups (Bard et al., 2021). Although the cortisol levels were not measured in the current study, the lower TARR in the high intensity group as compared to the medium intensity group could possibly be related to the high cortisol level induced by excessive high intensity training. The optimal intensity seem to be, however, species specific since previous studies have reported that training with ≥2 bl*s-1 was already considered an excessively high speed for Atlantic salmon (length 32.4 cm) (Timmerhaus et al., 2021) and European whitefish (Coregonus lavaretus, length 22.2 cm) as it was in our study for rainbow trout (length at the end of training 10.6 cm) - however, it was found to be the best for sea trout (Salmo trutta, length 15.4 cm) (Anttila et al., 2008). When applying the training protocols for fish production, what needs to be also considered is training volume (i.e. intensity* training duration*sets). For example, protocols can be obtained by varying several aspects of the training i.e. water speed, daily duration of the training, daily resting period and overall duration of the training. Different combinations of these parameters might induce different outcomes. Therefore, the training protocol has to be optimal for the species and selected based on the goals, since not all the training programs can be suitable for a defined task/goal, as reported in the literature on human sport physiology (MacInnis and Gibala, 2017). Future training experiments should be planned carefully based on the “desired aim” (i.e. improved growth, delay in maturation, higher tolerance to stressors) and on the physiology of the fish species by setting the training protocols with the optimal combinations of intensity, volume and resting duration. Future studies should also take into consideration the periodization of the training programme, such as what is the best period of the year for training or how long the effects of training last after training period ends.
The second aim of the study was to identify the mechanisms induced by training that could explain the widening of the cardiac thermal window in the medium intensity group (higher TARR and TS). Both medium and high intensity training increased the maximal activity of the citrate synthase that may provide higher aerobic capacity for ATP production. Furthermore, the LDH activity of high intensity training group decreased by 16% as compared to the controls indicating that the ventricles of the high intensity group preferentially used the aerobic pathway to produce ATP. The heart is a high energy demanding organ as it needs to maintain a rhythmic contraction in order to support the living functions. During a single bout of exercise, the heart energy consumption increases (as a result of increased heart rate), in order to keep pace with the increased oxygen consumption of the swimming muscles (Claireaux et al., 2005; Steinhausen et al., 2008). An expected response to long term training is, therefore, optimising the cardiac energy production and minimizing the energy consumption during the resting period (Farrell et al., 1990; He et al., 2013). Indeed, we showed that exercise training stimulates an increase in CS activity. A similar increase in the aerobic metabolic enzyme CS and a decrease in LDH activity has been shown in the ventricles of trained rainbow trout (Farrell et al., 1990) and in the red muscle of trained Atlantic salmon (Anttila et al., 2006; Anttila et al., 2008; Zhang et al., 2016). Additionally, although the hematological variables [Hb] and HCT were not significantly different between the groups, the trained group displayed a slight trend towards a higher Hb and HCT which might improve the oxygen carrying capacity of blood to exercising muscles. In previous studies exercise training has been shown to improve the Hb (Gallaugher et al., 2001). It is necessary to remember that the molecular changes induced by training are often small, but all together they might provide significant improvement in performance at the whole organism level (Davison, 1997; Gamperl and Farrell, 2004). The observed changes with training towards possibly enhanced aerobic energy production capacity could give an advantage in a situation where the energy costs of living increases, such as during exercise, but also during acute warming during summer heat waves (Kühnhold et al., 2019) or migration (Eliason et al., 2011; Mottola et al., 2020). However, since high intensity training group did not have elevated cardiac thermal tolerance, even though their CS activity was enhanced, some other pathways seem to be involved in widening the cardiac thermal window.
Our study also investigated the effect of exercise training on the level of HIF and VEGF, proteins involved in the angiogenesis and oxygen provision to the muscles. This is because the acute increase in energy consumption induced by exercise training might promote an inner hypoxic environment (hypoxemia) (Farrell and Richards, 2009) in the heart that may lead to an over-expression of HIF and VEGF (Palstra et al., 2014) and consequently trigger a higher ventricle vascularization in order to improve the oxygen supply. However, the findings of the current study do not support this hypothesis since training did not induce a change in the protein levels of HIF and VEGF. These results partially reflect those of Castro et al. (2013) who showed that exercise training triggered a higher transcription activity of genes related to VEGF, but immunofluorescent analysis did not detect a difference in VEGF protein expression at the tissue level. There may be several possible explanations for this result. For example, HIF and VEGF are usually markers that respond acutely to hypoxia or hypoxemia and are involved in the regulation mechanisms that provide oxygen homeostasis and induce angiogenesis (Nikinmaa and Rees, 2005). Therefore, their levels have been likely higher at the beginning of training but reduced back to a physiological level once the organism acclimatized to the training. Furthermore, the analyses were conducted from the whole ventricle while the capillaries are located only in the compact layer. This could have prevented us from seeing the changes of angiogenesis in compact layer.
Exercise training could also remodel the E-C coupling mechanism. Therefore, we focused on the ventricular SERCA level. The main energy consumption in cardiomyocytes is due to the E-C coupling and SERCA is one of the most highly ATP-consuming calcium channels that supports the contraction (Schramm et al., 1994). Previous studies in fish have associated high SERCA expression or high activity with athletics and improved skeletal and cardiac muscle contractility (Shiels and Galli, 2014; Anttila et al., 2019). However, our results did not show any significant effect of exercise training on the protein level of SERCA. These results are in line with SERCA results reported by Castro et al. (2013) showing that exercise training did not have a significant influence on the mRNA expression of SERCA. However, it triggered a higher transcription activity of other genes related to E-C coupling, i.e. voltage dependent L-type Ca2+ channel alpha 1D subunit (DHPRa1D dihydropyridine receptor) and calsequestrin (CALSEQ1). Nevertheless, Rissoli et al. (2017) reported that Matrinxã (Brycon amazonicus, length 25.5 cm) trained to 0.4 bl*s-1 for 60 days showed a lower SERCA expression and higher expression in NCX as compared to controls. Overall, a significant amount of future work is required to identify the molecular mechanisms through which moderate exercise training improves the cardiac thermal tolerance in rainbow trout.
In the current study we did not control the temperature of water in the tanks since the aim was to investigate the effects of training in real aquaculture settings. Therefore, we cannot address the possible interaction between the training and the seasonal decrease in temperature. Nevertheless, the temperature, which declined during the middle of the training period and stabilized at the end, influenced all the fish similarly. Therefore, the temperature decline should not be a confounding factor. Especially because cardiac thermal tolerance decreased with cold acclimation (see review in Eliason and Anttila, 2017), therefore the cold acclimation might have even mitigated the improvement in cardiac thermal tolerance that was associated with medium intensity training.
In conclusion, this study demonstrated for the first time that a medium intensity exercise training programme can improve the upper thermal tolerance of a cardiovascular system, one of the most sensitive physiological systems to high temperatures in fishes. These findings lead to a significant advance in the field since it opens the doors to a new way to improve the thermal tolerance of the fishes. Not only did we observe that the medium intensity group achieved a 3.6°C higher TARR as compared to the controls but also that training enhanced the CS activity in the ventricle. However, the molecular mechanisms underpinning the beneficial effect of training to improve cardiac thermal tolerance remain mainly unclear. By conducting the training in regular hatchery tanks we provided direct applicability to the hatchery setting where also temperature fluctuates seasonally. A natural progression of this work is to scale up the training programme evaluating the applicability to adult fish, such as brood-stocks, and to assess the whole organism thermal tolerance and survival rate during real heat waves. Nevertheless, these findings will provide a considerable number of beneficial applications for the salmonid aquaculture sector, especially in the context of the imminent global warming scenario; these applications will also benefit the production of fish stocks for conservation programmes. Considering the market predictions of an increased demand for farmed fish and the possibility of a higher frequency of heat waves in the future, this exercise training can be considered a good and a viable solution to these two aquaculture challenges.
Data Availability Statement
The raw data supporting the conclusions of this article will be available under request to qualified researchers.
Ethics Statement
The animal study was reviewed and approved by Regional State Administrative Agency (license number ESAVI/11880/2019).
Author Contributions
LP: Conceptualization, Data curation, Formal analysis, Investigation, Visualization, Writing – original draft. ES: Funding acquisition, Investigation, Project administration, Resources, Writing – review & editing. AS: Investigation, Resources, Writing – review & editing. KA: Conceptualization, Formal analysis, Funding acquisition, Investigation, Project administration, Supervision, Writing – original draft. All authors contributed to the article and approved the submitted version.
Funding
This work was supported by Kone Foundation.
Conflict of Interest
The authors declare that the research was conducted in the absence of any commercial or financial relationships that could be construed as a potential conflict of interest.
Publisher’s Note
All claims expressed in this article are solely those of the authors and do not necessarily represent those of their affiliated organizations, or those of the publisher, the editors and the reviewers. Any product that may be evaluated in this article, or claim that may be made by its manufacturer, is not guaranteed or endorsed by the publisher.
Acknowledgments
We thank the staff of LUKE in Enonkoski for the fish care and practical help. We thank Tytti Uurasmaa and Henni Kankare for the technical assistance. We thank Pierpaolo Congia for helpful comments. We thank Elizabeth Nyman for the language assistance and proofreading.
Supplementary Material
The Supplementary Material for this article can be found online at: https://www.frontiersin.org/articles/10.3389/fmars.2022.912720/full#supplementary-material
Glossary
References
Ahmed N., Thompson S., Glaser M. (2019). Global Aquaculture Productivity, Environmental Sustainability, and Climate Change Adaptability. Environ. Manage. 63, 159–172. doi: 10.1007/s00267-018-1117-3
Anttila K., Farrell A. P., Patterson D. A., Hinch S. G., Eliason E. J. (2019). Cardiac SERCA Activity in Sockeye Salmon Populations: An Adaptive Response to Migration Conditions. Can. J. Fish. Aquat. Sci. 76, 1–5. doi: 10.1139/cjfas-2018-0334
Anttila K., Jørgensen S. M., Casselman M. T., Timmerhaus G., Farrell A. P., Takle H. (2014). Association Between Swimming Performance, Cardiorespiratory Morphometry, and Thermal Tolerance in Atlantic Salmon (Salmo Salar L.). Front. Mar. Sci. 1. doi: 10.3389/fmars.2014.00076
Anttila K., Järvilehto M., Mänttäri S. (2008). The Swimming Performance of Brown Trout and Whitefish: The Effects of Exercise on Ca2+ Handling and Oxidative Capacity of Swimming Muscles. J. Comp. Physiol. B. Biochem. Syst. Environ. Physiol. 178, 465–475. doi: 10.1007/s00360-007-0239-3
Anttila K., Maünttaüri S., Jaürvilehto M. (2006). Effects of Different Training Protocols on Ca2+ Handling and Oxidative Capacity in Skeletal Muscle of Atlantic Salmon ( Salmo Salar L.). J. Exp. Biol. 209, 2971–2978. doi: 10.1242/jeb.02341
Anttila K., Mauduit F., Le Floch S., Claireaux G., Nikinmaa M. (2017). Influence of Crude Oil Exposure on Cardiac Function and Thermal Tolerance of Juvenile Rainbow Trout and European Sea Bass. Environ. Sci. Pollut. Res. 24, 19624–19634. doi: 10.1007/s11356-017-9609-x
Barange M. B., Beveridge M. C. M., Cochrane K. L., et al. (2018) Impacts of Climate Change on Fisheries and Aquaculture: Synthesis of Currrent Knowledge, Adaptation and Mitigation Options (FAO). Available at: https://agris.fao.org/agris-search/search.do?recordID=XF2018002008 (Accessed September 17, 2021).
Bard B., Dodge A., Joyce W., Lawrence M., Cooke S. J., Gilmour K. M. (2021). Elevated Cortisol Lowers Thermal Tolerance But Results in Limited Cardiac Remodelling in Rainbow Trout (Oncorhynchus Mykiss) Experiencing Chronic Social Stress. J. Exp. Biol. 224, jeb238683. doi: 10.1242/JEB.238683
Blanchard J. L., Watson R. A., Fulton E. A., Cottrell R. S., Nash K. L., Bryndum-Buchholz A., et al. (2017). Linked Sustainability Challenges and Trade-Offs Among Fisheries, Aquaculture and Agriculture. Nat. Ecol. Evol. 1, 1240–1249. doi: 10.1038/s41559-017-0258-8
Boesgaard L., Nielsen M. E., Rosenkilde P. (1993). Moderate Exercise Decreases Plasma Cortisol Levels in Atlantic Salmon (Salmo Salar). Comp. Biochem. Physiol. Part A. Physiol. 106, 641–643. doi: 10.1016/0300-9629(93)90373-C
Brijs J., Hjelmstedt P., Berg C., Johansen I. B., Sundh H., Roques J. A. C., et al. (2020). Prevalence and Severity of Cardiac Abnormalities and Arteriosclerosis in Farmed Rainbow Trout (Oncorhynchus Mykiss). Aquaculture 526, 735417. doi: 10.1016/J.AQUACULTURE.2020.735417
Calado R., Mota V. C., Madeira D., Leal M. C. (2021). Summer Is Coming! Tackling Ocean Warming in Atlantic Salmon Cage Farming. Anim 11, 1800. doi: 10.3390/ANI11061800
Casselman M. T., Anttila K., Farrell A. P. (2012). Using Maximum Heart Rate as a Rapid Screening Tool to Determine Optimum Temperature for Aerobic Scope in Pacific Salmon Oncorhynchus Spp. J. Fish. Biol. 80, 358–377. doi: 10.1111/j.1095-8649.2011.03182.x
Castro V., Grisdale-Helland B., Helland S. J., Kristensen T., Jørgensen S. M., Helgerud J., et al. (2011). Aerobic Training Stimulates Growth and Promotes Disease Resistance in Atlantic Salmon (Salmo Salar). Comp. Biochem. Physiol. Part A. Mol. Integr. Physiol. 160, 278–290. doi: 10.1016/j.cbpa.2011.06.013
Castro V., Grisdale-Helland B., Jørgensen S. M., Helgerud J., Claireaux G., Farrell A. P., et al. (2013). Disease Resistance Is Related to Inherent Swimming Performance in Atlantic Salmon. BMC Physiol. 13, 1. doi: 10.1186/1472-6793-13-1
Chen Z., Zhou Z., Peng X., Sun C., Yang D., Li C., et al. (2021). Cardioprotective Responses to Aerobic Exercise-Induced Physiological Hypertrophy in Zebrafish Heart. J. Physiol. Sci. 71, 33. doi: 10.1186/s12576-021-00818-w
Claireaux G., McKenzie D. J., Genge A. G., Chatelier A., Aubin J., Farrell A. P. (2005). Linking Swimming Performance, Cardiac Pumping Ability and Cardiac Anatomy in Rainbow Trout. J. Exp. Biol. 208, 1775–1784. doi: 10.1242/jeb.01587
Clark T. D., Eliason E. J., Sandblom E., Hinch S. G., Farrell A. P. (2008). Calibration of a Hand-Held Haemoglobin Analyser for Use on Fish Blood. J. Fish. Biol. 73, 2587–2595. doi: 10.1111/j.1095-8649.2008.02109.x
Collins C., Bresnan E., Brown L., Falconer L., Guilder J., Jones L., et al. (2020). Impacts of Climate Change on Aquaculture. MCCIP Sci. Rev. 2020, 482–520. doi: 10.14465/2020.arc21.aqu
Dalziel A. C., Schulte P. M. (2012). Correlates of Prolonged Swimming Performance in F2 Hybrids of Migratory and Non-Migratory Threespine Stickleback. J. Exp. Biol. 215, 3587–3596. doi: 10.1242/jeb.071951
Davison W. (1997). The Effects of Exercise Training on Teleost Fish, a Review of Recent Literature. Comp. Biochem. Physiol. Part A. Physiol. 117, 67–75. doi: 10.1016/S0300-9629(96)00284-8
D’Souza A., Bucchi A., Johnsen A. B., Logantha S. J. R. J., Monfredi O., Yanni J., et al. (2014). Exercise Training Reduces Resting Heart Rate via Downregulation of the Funny Channel HCN4. Nat. Commun. 5, 3775. doi: 10.1038/ncomms4775
Ekström A., Gräns A., Sandblom E. (2019). Can´t Beat the Heat? Importance of Cardiac Control and Coronary Perfusion for Heat Tolerance in Rainbow Trout. J. Comp. Physiol. B. 2019. 1896. 189, 757–769. doi: 10.1007/S00360-019-01243-7
Eliason E. J., Anttila K. (2017). “Temperature and the Cardiovascular System,” in Fish Physiology. Eds. Brauner C. J., Gamperl A. K., Gillis T. E., Farrell A. P. (San Diego: Elsevier), 235–297. doi: 10.1016/bs.fp.2017.09.003
Eliason E. J., Clark T. D., Hague M. J., Hanson L. M., Gallagher Z. S., Jeffries K. M., et al. (2011). Differences in Thermal Tolerance Among Sockeye Salmon Populations. Science 332, 109–112. doi: 10.1126/science.1199158
Eliason E. J., Clark T. D., Hinch S. G., Farrell A. P. (2013). Cardiorespiratory Collapse at High Temperature in Swimming Adult Sockeye Salmon. Conserv. Physiol. 1, cot008. doi: 10.1093/conphys/cot008
FAO (2020). The State of World Fisheries and Aquaculture 2020 Sustainability in action (Rome: FAO). doi: https://doi.org/10.4060/ca9229en
Farrell A. P. (2007). Cardiorespiratory Performance During Prolonged Swimming Tests With Salmonids: A Perspective on Temperature Effects and Potential Analytical Pitfalls. Philos. Trans. R. Soc. B. Biol. Sci. 362, 2017–2030. doi: 10.1098/rstb.2007.2111
Farrell A. P. (2016). Pragmatic Perspective on Aerobic Scope: Peaking, Plummeting, Pejus and Apportioning. J. Fish. Biol. 88, 322–343. doi: 10.1111/jfb.12789
Farrell A. P., Johansen J. A., Steffensen J. F., Moyes C. D., West T. G., Suarez R. K. (1990). Effects of Exercise Training and Coronary Ablation on Swimming Performance, Heart Size, and Cardiac Enzymes in Rainbow Trout, Oncorhynchus Mykiss. Can. J. Zool. 68, 1174–1179. doi: 10.1139/z90-174
Farrell A. P., Johansen J. A., Suarez R. K. (1991). Effects of Exercise-Training on Cardiac Performance and Muscle Enzymes in Rainbow Trout,Oncorhynchus Mykiss. Fish. Physiol. Biochem. 9, 303–312. doi: 10.1007/BF02265151
Farrell A. P., Richards J. G. (2009). “Defining Hypoxia: An Integrative Synthesis of the Responses of Fish to Hypoxia,” in Fish Physiology. Eds. Richards J. G., Farrell A. P., Brauner C. J. (London: Academic Press), 487–503. doi: 10.1016/S1546-5098(08)00011-3
Ferreira E. O., Anttila K., Farrell A. P. (2014). Thermal Optima and Tolerance in the Eurythermic Goldfish (Carassius Auratus): Relationships Between Whole-Animal Aerobic Capacity and Maximum Heart Rate. Physiol. Biochem. Zool. 87, 599–611. doi: 10.1086/677317
Frisk M., Høyland M., Zhang L., Vindas M. A., Øverli Ø., Johansen I. B. (2020). Intensive Smolt Production is Associated With Deviating Cardiac Morphology in Atlantic Salmon (Salmo Salar L.). Aquaculture 529, 735615. doi: 10.1016/j.aquaculture.2020.735615
Froehlich H. E., Gentry R. R., Halpern B. S. (2018). Global Change in Marine Aquaculture Production Potential Under Climate Change. Nat. Ecol. Evol. 2, 1745–1750. doi: 10.1038/s41559-018-0669-1
Fu S. J., Brauner C. J., Cao Z. D., Richards J. G., Peng J. L., Dhillon R., et al. (2011). The Effect of Acclimation to Hypoxia and Sustained Exercise on Subsequent Hypoxia Tolerance and Swimming Performance in Goldfish (Carassius Auratus). J. Exp. Biol. 214, 2080–2088. doi: 10.1242/jeb.053132
Gallaugher P. E., Thorarensen H., Kiessling A., Farrell A. P. (2001). Effects of High Intensity Exercise Training on Cardiovascular Function, Oxygen Uptake, Internal Oxygen Transport and Osmotic Balance in Chinook Salmon (Oncorhynchus Tshawytscha) During Critical Speed Swimming. J. Exp. Biol. 204, 2861–2872. doi: 10.1242/jeb.204.16.2861
Gamperl A. K., Farrell A. P. (2004). Cardiac Plasticity in Fishes: Environmental Influences and Intraspecific Differences. J. Exp. Biol. 207, 2539–2550. doi: 10.1242/jeb.01057
Gamperl A. K., Zrini Z. A., Sandrelli R. M. (2021). Atlantic Salmon (Salmo Salar) Cage-Site Distribution, Behavior, and Physiology During a Newfoundland Heat Wave. Front. Physiol. 12. doi: 10.3389/FPHYS.2021.719594/BIBTEX
Gilbert M. J. H., Farrell A. P. (2021). The Thermal Acclimation Potential of Maximum Heart Rate and Cardiac Heat Tolerance in Arctic Char (Salvelinus Alpinus), a Northern Cold-Water Specialist. J. Therm. Biol. 95, 102816. doi: 10.1016/j.jtherbio.2020.102816
Gilbert M. J. H., Harris L. N., Malley B. K., Schimnowski A., Moore J.-S., Farrell A. P. (2020). The Thermal Limits of Cardiorespiratory Performance in Anadromous Arctic Char (Salvelinus Alpinus): A Field-Based Investigation Using a Remote Mobile Laboratory. Conserv. Physiol. 8, coaa036. doi: 10.1093/conphys/coaa036
Gorle J. M. R., Terjesen B. F., Mota V. C., Summerfelt S. (2018). Water Velocity in Commercial RAS Culture Tanks for Atlantic Salmon Smolt Production. Aquac. Eng. 81, 89–100. doi: 10.1016/j.aquaeng.2018.03.001
Herbert N. A., Kadri S., Huntingford F. A. (2011). A Moving Light Stimulus Elicits a Sustained Swimming Response in Farmed Atlantic Salmon, Salmo Salar L. Fish. Physiol. Biochem. 372, 317–325. doi: 10.1007/S10695-011-9499-7
He W., Xia W., Cao Z.-D., Fu S.-J. (2013). The Effect of Prolonged Exercise Training on Swimming Performance and the Underlying Biochemical Mechanisms in Juvenile Common Carp (Cyprinus Carpio). Comp. Biochem. Physiol. Part A. Mol. Integr. Physiol. 166, 308–315. doi: 10.1016/j.cbpa.2013.07.003
Hou Q., Fu S., Huang T., Li X., Shi X. (2022). Effects of Aerobic Exercise Training on the Growth, Swimming Performance, Antipredation Ability and Immune Parameters of Juvenile Rock Carp (Procypris Rabaudi). Animals 12, 257. doi: 10.3390/ani12030257
Kühnhold H., Novais S. C., Alves L. M. F., Kamyab E., Lemos M. F. L., Slater M. J., et al. (2019). Acclimation Capability Inferred by Metabolic Performance in Two Sea Cucumber Species From Different Latitudes. J. Therm. Biol. 84, 407–413. doi: 10.1016/j.jtherbio.2019.07.019
MacInnis M. J., Gibala M. J. (2017). Physiological Adaptations to Interval Training and the Role of Exercise Intensity. J. Physiol. 595, 2915–2930. doi: 10.1113/JP273196
Maulu S., Hasimuna O. J., Haambiya L. H., Monde C., Musuka C. G., Makorwa T. H., et al. (2021). Climate Change Effects on Aquaculture Production: Sustainability Implications, Mitigation, and Adaptations. Front. Sustain. Food Syst. 0. doi: 10.3389/FSUFS.2021.609097
McKenzie D. J., Palstra A. P., Planas J., MacKenzie S., Bégout M., Thorarensen H., et al. (2021a). Aerobic Swimming in Intensive Finfish Aquaculture: Applications for Production, Mitigation and Selection. Rev. Aquac. 13, 138–155. doi: 10.1111/raq.12467
McKenzie D. J., Zhang Y., Eliason E. J., Schulte P. M., Claireaux G., Blasco F. R., et al. (2021b). Intraspecific Variation in Tolerance of Warming in Fishes. J. Fish. Biol. 98, 1536–1555. doi: 10.1111/jfb.14620
Mottola G., Kristensen T., Anttila K. (2020). Compromised Thermal Tolerance of Cardiovascular Capacity in Upstream Migrating Arctic Char and Brown Trout—Are Hot Summers Threatening Migrating Salmonids? Conserv. Physiol. 8, coaa101. doi: 10.1093/conphys/coaa101
Nikinmaa M., Rees B. B. (2005). Oxygen-Dependent Gene Expression in Fishes. Am. J. Physiol. Integr. Comp. Physiol. 288, R1079–R1090. doi: 10.1152/ajpregu.00626.2004
Oyinlola M. A., Reygondeau G., Wabnitz C. C. C., Frölicher T. L., Lam V. W. Y., Cheung W. W. L. (2022). Projecting Global Mariculture Production and Adaptation Pathways Under Climate Change. Glob. Change Biol. 28, 1315–1331. doi: 10.1111/GCB.15991
Palstra A. P., Mes D., Kusters K., Roques J. A. C., Flik G., Kloet K., et al. (2015). Forced Sustained Swimming Exercise at Optimal Speed Enhances Growth of Juvenile Yellowtail Kingfish (Seriola Lalandi). Front. Physiol. 5. doi: 10.3389/fphys.2014.00506
Palstra A. P., Planas J. V. (2013). Swimming Physiology of Fish: Towards Using Exercise to Farm a Fit Fish in Sustainable Aquaculture (Berlin Heidelberg: Springer). doi: 10.1007/978-3-642-31049-2
Palstra A. P., Roque A., Kruijt L., Jéhannet P., Pérez-Sánchez J., Dirks R. P. (2020). Physiological Effects of Water Flow Induced Swimming Exercise in Seabream Sparus Aurata. Front. Physiol. 11. doi: 10.3389/FPHYS.2020.610049/ABSTRACT
Palstra A. P., Rovira M., Rizo-Roca D., Torrella J. R., Spaink H. P., Planas J. V. (2014). Swimming-Induced Exercise Promotes Hypertrophy and Vascularization of Fast Skeletal Muscle Fibres and Activation of Myogenic and Angiogenic Transcriptional Programs in Adult Zebrafish. BMC Genomics 15, 1136. doi: 10.1186/1471-2164-15-1136
Papadopoulou A., Pettinau L., Seppänen E., Sikanen A., Anttila K. (2022). The Interactive Effects of Exercise Training and Functional Feeds on the Cardiovascular Performance of Rainbow Trout (Oncorhynchus Mykiss) at High Temperatures. Curr. Res. Physiol. 5, 142–150. doi: 10.1016/j.crphys.2022.02.005
Pulkkinen K., Suomalainen L. R., Read A. F., Ebert D., Rintamäki P., Valtonen E. T. (2010). Intensive Fish Farming and the Evolution of Pathogen Virulence: The Case of Columnaris Disease in Finland. Proc. R. Soc B. Biol. Sci. 277, 593–600. doi: 10.1098/rspb.2009.1659
Reid G. K., Gurney-Smith H. J., Flaherty M., Garber A. F., Forster I., Brewer-Dalton K., et al. (2019). Climate Change and Aquaculture: Considering Adaptation Potential. Aquac. Environ. Interact. 11, 603–624. doi: 10.3354/aei00333
Rissoli R. Z., Vasconcelos E., da S., Rantin F. T., Kalinin A. L. (2017). Effects of Exercise Training on Excitation-Contraction Coupling, Calcium Dynamics and Protein Expression in the Heart of the Neotropical Fish Brycon Amazonicus. Comp. Biochem. Physiol. Part A. Mol. Integr. Physiol. 214, 85–93. doi: 10.1016/j.cbpa.2017.09.016
Rovira M., Borràs D. M., Marques I. J., Puig C., Planas J. V. (2018). Physiological Responses to Swimming-Induced Exercise in the Adult Zebrafish Regenerating Heart. Front. Physiol. 9. doi: 10.3389/fphys.2018.01362
Schramm M., Klieber H. G., Daut J. (1994). The Energy Expenditure of Actomyosin-ATPase, Ca(2+)-ATPase and Na+,K(+)-ATPase in Guinea-Pig Cardiac Ventricular Muscle. J. Physiol. 481, 647–662. doi: 10.1113/jphysiol.1994.sp020471
Shiels H. A., Galli G. L. J. (2014). The Sarcoplasmic Reticulum and the Evolution of the Vertebrate Heart. Physiol. (Bethesda). 29, 456–469. doi: 10.1152/physiol.00015.2014
Skaala Ø., Besnier F., Borgstrøm R., Barlaup B., Sørvik A. G., Normann E., et al. (2019). An Extensive Common-Garden Study With Domesticated and Wild Atlantic Salmon in the Wild Reveals Impact on Smolt Production and Shifts in Fitness Traits. Evol. Appl. 12, 1001–1016. doi: 10.1111/EVA.12777
Steinhausen M. F., Sandblom E., Eliason E. J., Verhille C., Farrell A. P. (2008). The Effect of Acute Temperature Increases on the Cardiorespiratory Performance of Resting and Swimming Sockeye Salmon (Oncorhynchus Nerka). J. Exp. Biol. 211, 3915–3926. doi: 10.1242/jeb.019281
Timmerhaus G., Lazado C. C., Cabillon N. A. R., Reiten B. K. M., Johansen L. H. (2021). The Optimum Velocity for Atlantic Salmon Post-Smolts in RAS Is a Compromise Between Muscle Growth and Fish Welfare. Aquaculture 532, 736076. doi: 10.1016/J.AQUACULTURE.2020.736076
van der Walt K.-A., Potts W. M., Porri F., Winkler A. C., Duncan M. I., Skeeles M. R., et al. (2021). Marine Heatwaves Exceed Cardiac Thermal Limits of Adult Sparid Fish (Diplodus Capensis, Smith 1884). Front. Mar. Sci. 8. doi: 10.3389/fmars.2021.702463
Wade N. M., Clark T. D., Maynard B. T., Atherton S., Wilkinson R. J., Smullen R. P., et al. (2019). Effects of an Unprecedented Summer Heatwave on the Growth Performance, Flesh Colour and Plasma Biochemistry of Marine Cage-Farmed Atlantic Salmon (Salmo Salar). J. Therm. Biol. 80, 64–74. doi: 10.1016/j.jtherbio.2018.12.021
Woodward J. J., Smith L. S. (1985). Exercise Training and the Stress Response in Rainbow Trout, Salmo Gairdneri Richardson. J. Fish. Biol. 26, 435–447. doi: 10.1111/J.1095-8649.1985.TB04283.X
Yeager D. P., Ultsch G. R. (1989). Physiological Regulation and Conformation: A BASIC Program for the Determination of Critical Points. Physiol. Zool. 62, 888–907. doi: 10.1086/physzool.62.4.30157935
Keywords: aquaculture, cardiac arrhythmia, citrate synthase, climate change, fish, heart rate, swimming training, thermal tolerance
Citation: Pettinau L, Seppänen E, Sikanen A and Anttila K (2022) Aerobic Exercise Training With Optimal Intensity Increases Cardiac Thermal Tolerance in Juvenile Rainbow Trout. Front. Mar. Sci. 9:912720. doi: 10.3389/fmars.2022.912720
Received: 04 April 2022; Accepted: 17 May 2022;
Published: 22 June 2022.
Edited by:
Leonardo Julián Magnoni, Plant and Food Research, New ZealandReviewed by:
Peter Vilhelm Skov, Technical University of Denmark, DenmarkArjan P. Palstra, Wageningen University and Research, Netherlands
Copyright © 2022 Pettinau, Seppänen, Sikanen and Anttila. This is an open-access article distributed under the terms of the Creative Commons Attribution License (CC BY). The use, distribution or reproduction in other forums is permitted, provided the original author(s) and the copyright owner(s) are credited and that the original publication in this journal is cited, in accordance with accepted academic practice. No use, distribution or reproduction is permitted which does not comply with these terms.
*Correspondence: Luca Pettinau, bHVjYS5wZXR0aW5hdUB1dHUuZmk=