- 1División de Investigación Pesquera, Instituto de Fomento Pesquero, Valparaíso, Chile
- 2Escuela de Ciencias del Mar, Pontificia Universidad Católica de Valparaíso, Valparaíso, Chile
- 3Faculty of Life and Environmental Sciences, University of Iceland, Reykjavík, Iceland
Ages of Chilean jack mackerel (Trachurus murphyi) in the southern Pacific Ocean were validated using three methods: 1) daily microincrement readings in sagittal otoliths of young-of-the year (YOY) fish to validate the first annulus; 2) modal progression of strong year-classes (PSYC) to validate the first, second and third annuli, and 3) bomb radiocarbon analysis of otolith cores to validate the absolute age in older fish >38 cm fork length (FL). A Laird-Gompertz (LG) model was fitted to fork length (cm) at age (days) relationship in fish ranging from 3.4 to 25.0 cm FL and from 56 to 550 days in age. The LG model estimated a mean FL of 22 cm at the end of the first year of life, which was large compared with the mean FL estimated through conventional ageing in whole otoliths. The comparison between daily age with annual growth bands from whole otoliths, showed a false increment formed at 185 ± 34 days and a second translucent increment formed at 352 ± 79 days, corresponding to the first annulus for fish with the mean FL of 21.4 ± 1.8 cm. The PSYC coinciding with the recruits of 2008 (age 0), was tracked through three subsequent years with high accuracy, attaining 23, 27 and 30 cm FL in 2009, 2010 and 2011, respectively. These modes identified as age 1, age 2 and age 3 in the PSYC, matched to the mean length of fish with 2, 4 and 5 translucent increments in sagittal otoliths. Results from bomb radiocarbon analysis confirmed that most of the ages were correct, because the Δ14C content of adult otolith core matched the reference chronology. The absolute mean ages for the assayed otoliths, based on the comparison with the reference chronology, ranged from 7 to 13 years for sizes between 39 and 60 cm FL. The age validation for T. murphy as addressed in the current study provided crucial information for enhancing stock assessment output of this important transboundary commercial species.
Introduction
Age determination is a key stage in the process of stock assessment of aquatic living resources, especially in fish populations undergoing fishery exploitation (Fowler and Ling, 2010; Botsford et al., 2011; Siskey et al., 2016; Quist and Isermann, 2017). Most fish age determination is carried out by counting periodic growth bands, assumed to be formed annually, recorded in calcified structures such as otoliths, scales, spines and other structures (e.g., Panfili et al., 2002; Carbonara et al., 2020; Kindong et al., 2020). Millions of hard structures are aged each management cycle in fishery laboratories worldwide (e.g., Campana and Thorrold, 2001; Panfili et al., 2002; Winkler et al., 2019), with age determination methods often varying among species. However, different methods often result in different age interpretations, thus requiring validation of the ageing method to confirm its accuracy (Campana, 2001; Ong et al., 2020; Passerotti et al., 2020). Regular comparisons within and among age readers are subsequently needed to confirm that the annual growth bands are being assigned consistently (e.g., Morales-Nin et al., 2002; Stransky et al., 2005; Hussy, 2010; Wakefield et al., 2017). Furthermore, in transboundary resources shared by several countries, calibration exercises between laboratories are necessary to ensure consistency in the age data provided for multinational stock assessment (Power et al., 2006; Wakefield et al., 2017).
The Chilean jack mackerel (CJM) Trachurus murphyi, is a highly migratory and commercially important carangid, which is widely distributed across the Pacific Ocean from south-central Chile to New Zealand and Tasmania (Evseenko, 1987; Serra, 1991; Elizarov et al., 1993; Arcos et al., 2001; Taylor, 2002). In the southeastern Pacific, this species occurs from the Galapagos Islands and southern Ecuador to southern Chile. Catches in Chile alone peaked at 4.6 million tons in 1995, which ranked it among the world’s largest fisheries, although catches have declined in subsequent years to 277 000 t in 2017 (Aranís et al., 2018). Since mid-1980s, the CJM has been one of the most important commercial fishes managed since 2013 by the South Pacific Regional Fisheries Management Organization (SPRFMO), an inter-governmental group committed to the long-term conservation and sustainable use of fishery resources in the South Pacific Ocean (Parada et al., 2017). However, despite the commercial importance of this highly migratory pelagic carangid, key issues concerning its population biology and ecology remain unaddressed.
Knowledge about the age composition of the CJM catches is critical to an understanding of its population dynamics and to the accuracy of the stock assessment, but uncertainties in the age determination method persist (Cerna et al., 2016; Horn and Maolagáin, 2020). All age monitoring is currently based on external examination of whole otoliths, a method which can be rapid but of low accuracy in many moderate and long-lived fishes because it tends to underestimate age and longevity (Campana, 2001). The accuracy of the whole otolith ageing method was inferred many years ago for the CJM, based on studies which reported annual translucent zone formation in young fish each winter based on marginal increment analysis, although the marginal increments were unresolvable for larger fish (Castillo and Arrizaga, 1987; Serra and Gili, 1995). Subsequent studies reported acceptable precision (reproducibility) of age readers in Chile to at least Age 10 (Cerna et al., 2016), and a recent study of age determination in T. murphy in New Zealand waters, reported a precision level of 4.6% (average percentage error), using transverse sections of sagittal otoliths (Horn and Maolagáin, 2020). However, no study to date has validated the accuracy of either the ageing method or the absolute age of CJM in the south Pacific. Moreover, no study to date has validated first annulus formation in this species, despite the importance of this validation step for providing a correctly defined starting point, without which age determinations could be consistently biased (Campana, 2001). Validation of the first annulus in young fish poses special problems, and counts of daily growth increments (micro-increments) in otoliths has proven its reliability in some species of fishes (Waldron, 1994; Campana, 2001).
Tracking strong year classes, termed “progression of strong year-classes” (PSYC), is an age corroboration method where the modal age of a recruitment pulse (determined through annulus counts) is followed across subsequent years (Campana, 2001). If the recruitment pulses are well defined and there is no appreciable age-structured migration or natural mortality, this method can provide a strong confirmation of annuli periodicity (Donald et al., 1992; Campana, 2001). Although PSYC has been widely applied on an informal basis by stock assessment biologists, only a few studies have used it more rigorously to support the validity of the otolith-based ageing method (e.g., Donald et al., 1992; Morison et al., 1998). More recently, Uriarte et al. (2016) successfully validated the age of European anchovy otoliths in the Bay of Biscay by monitoring very strong year-classes in successive spring catches and surveys, demonstrating the applicability of this approach even in a small pelagic fish, where recruitment variability can be very high.
Bomb derived radiocarbon from nuclear testing provides one of the best age validation approaches available for long-lived fishes, and has been used to successfully validate absolute age in many fish species over the last three decades (e.g., Campana, 1997; Campana, 2001; Kastelle et al., 2016; King et al., 2017; Ong et al., 2020; Passerotti et al., 2020). This method is based on the abrupt increase in atmospheric Δ14C in the late 1950s due to the onset of atmospheric nuclear testing (Kalish, 1993). Thus, the period is analogous to a large-scale chemical tagging experiment, wherein all otolith cores of fish hatched before 1958 contain relatively little Δ14C, all those hatched after 1968 contain elevated levels, and fish born in the transition period contain intermediate levels (Campana, 2001). Hence, if the age of capture is determined through counts of otolith growth increments, an assumed year of formation of the otolith core can be calculated. If the growth increments were aged correctly, there should be a close match between the assumed years of increase in the otolith core Δ14C and the known period of increase from a reference Δ14C chronology. Since there has been no appreciable decay of radiocarbon in recent decades, the bomb radiocarbon approach can be applied to any species where the otoliths of fish hatched before about 1970 have been archived, such as is the case for T. murphy.
The objective of the present study was to apply several modern and rigorous age validation and corroboration methods to CJM in order to determine the validity of current ageing methods and thus enhance the accuracy of the current stock assessment. The age validation methods were applied sequentially so as to confirm the correct and accurate age assignment to the key life history stages in order of appearance: first, otolith microstructure analysis was used to confirm the interpretation of the first- deposited annulus; secondly, length frequency progression analysis was used to confirm the interpretation of the first three age classes; and, finally, bomb radiocarbon assays were used to confirm the interpretation and absolute age of the adult fishes.
Materials and Methods
Validation of the First Annulus
The identification of the first otolith annulus was confirmed using sagittal otolith microstructure in 45 juvenile CJM of lengths between 3 and 25 cm FL. Thirty-five fish smaller than 20 cm FL were collected aboard a scientific vessel off the northwestern coast of Chile between December 2011 and March 2015, while an additional 10 fish between 21 and 25 cm FL were caught by a commercial seine fisheries vessel off the coast of central Chile (Figure 1).
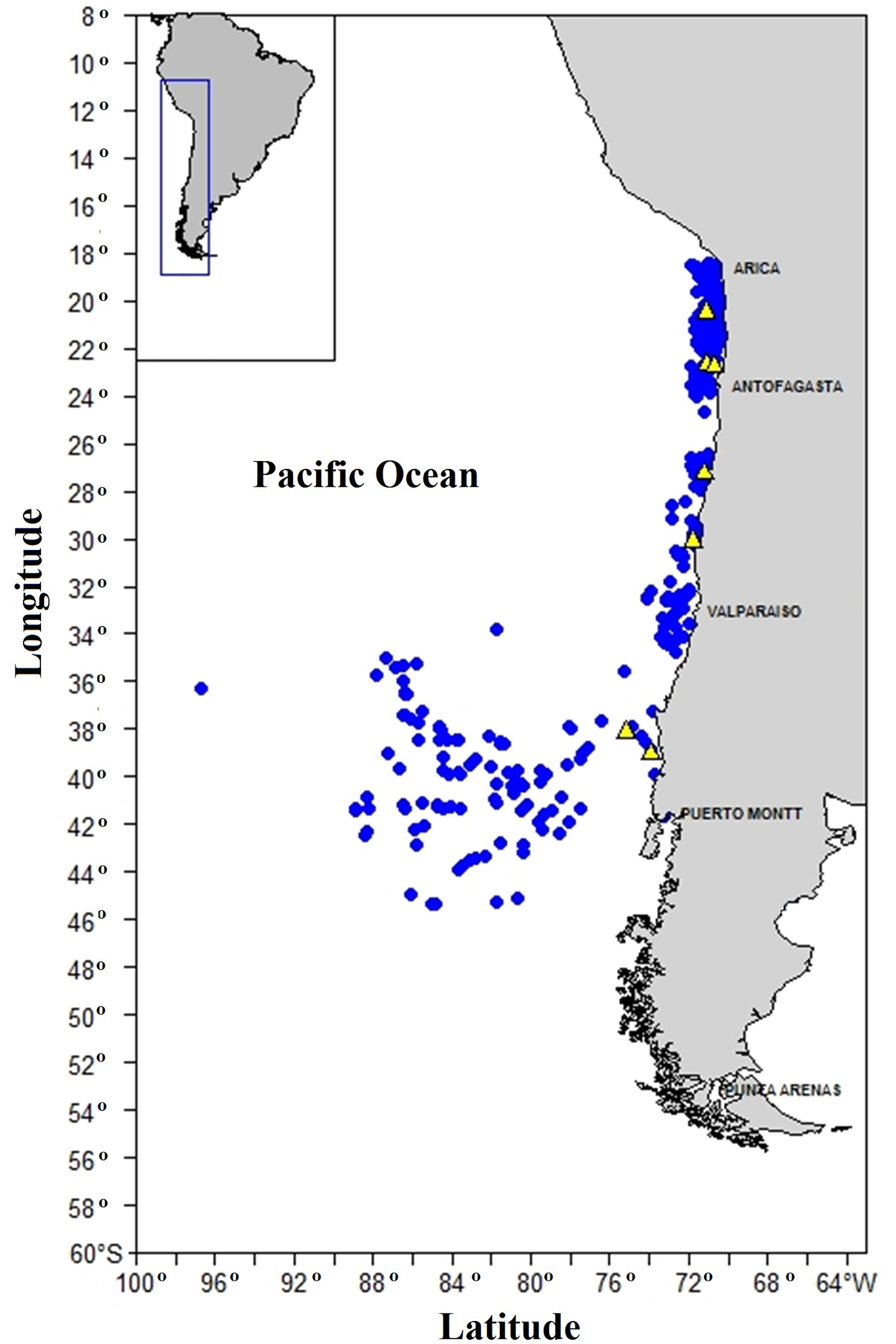
Figure 1 Illustration of the spatial distribution of sampling points for juvenile Trachurus murphyi, collected off Chilean coast, for validation of the first annuli (yellow dots) and for juvenile and adult fish used for modal-progression analysis (blue dots).
Otolith microstructure was examined in otoliths which were mounted in epoxy resin on a glass slide and polished in the sagittal plane using 30- µm and 1-µm grit lapping film. Otoliths that showed a clear and uninterrupted sequence of daily growth increments from the primordium to the postrostral edge were photographed at various locations along the growth axis with a QImagen Evolution 5.0 camera at 400X magnification to obtain images with 5 mega pixels of resolution. Growth increments around the primordium were photographed and examined at 1000X magnification. The resulting images were combined along the longitudinal plane of each otolith using the tiling tool of ImagePro Plus 5.1 software (Mediacybernetics Inc., Bethesda, MD, USA). Growth increments in fish up to 27 cm FL have previously been validated to form daily in laboratory rearing experiments (Araya et al., 2003; Araya et al., 2019). A distinctive microstructural feature in the CJM otolith was the presence of accessory primordia, which divided the sagittal plane of the otolith into primary and secondary growth zones. In some areas of the otoliths, sub-daily and double increments were visible and interpreted, following the criteria of Campana and Jones (1992).
The growth increment sequence was counted in combined images by two independent age readers at different times, and the mean of the two records was used as the total daily age. Daily ageing precision was calculated with the coefficient of variation (CV) (Campana, 2001). Hatch date was calculated as the daily age subtracted from the catch date, with the resulting hatch date frequency distribution interpreted as an approximation of spawning date distribution.
The Laird–Gompertz (LG) model was used to estimate the length (Lt)-at-age (X) relationship for juveniles, commonly used to characterize early growth patterns in many teleost fish species. The function has three parameters: L∞ is the asymptotic length; X0 is the inflection point of the curve and the age at which absolute growth rate begins to decline; and α is the instantaneous growth rate at age X0 (Campana and Jones, 1992):
The LG model was fitted using the maximum likelihood method, through the function “nls”, available in the stats package of R software (Ihaka and Gentleman, 1996).
Progression of Strong Year- Classes (PSYC)
Modal PSYC was used as an alternative method to corroborate the first and second annuli. Length–frequency (Fork Length; FL) data at 1-cm intervals were obtained from commercial fishery samples collected monthly January 2008 to December 2011(Figure 1;Table 1). The recruitment failures between 2009 and 2011 permitted the mode to be tracked across time. Length frequency modes of the catch were identified using mixture analysis, as implemented though the mixdist package for R (MacDonald and Du, 2004). The algorithm iteratively estimated the proportion, mean and standard deviation of each mode through a maximum likelihood-based decomposition of length–frequency data into age classes (Macdonald and Pitcher, 1979).
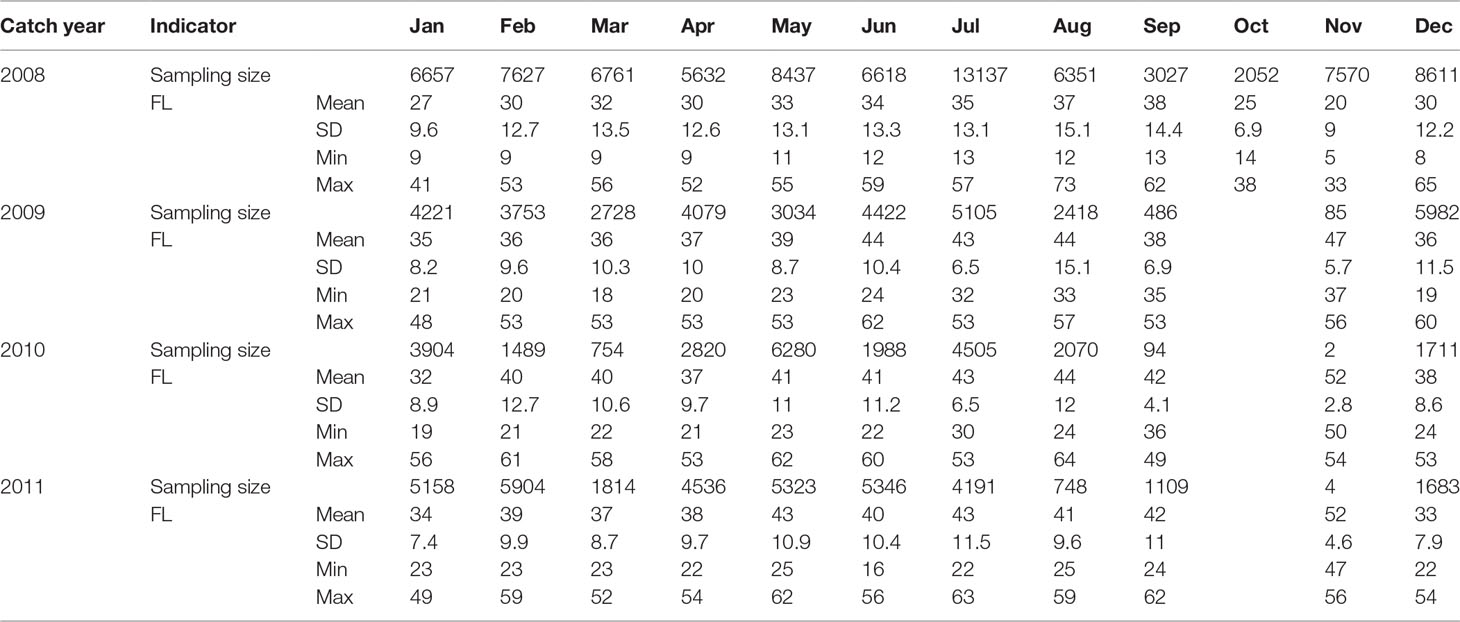
Table 1 Descriptive statistics of database of fork length (FL) by year and month of Trachurus murphy, from commercial fishery samples off Chilean coast, (SD, 1 standard deviation).
Validation of Absolute Age Using Bomb Radiocarbon
Bomb radiocarbon assays were used to test the accuracy of age determination of 21 CJM adults (mean ± SE; 47 ± 1.4 cm fork length) collected off the coast of Chile between 1971 and 1982. Age estimates for the 21 fish were available both from examination of whole left otoliths and transverse sections of the right otolith. Left otoliths were immersed whole in glycerin in a petri dish on a black background, and were then examined under a stereoscopic microscope at a magnification of 10X using reflected light. Right otoliths were firstly embedded in a slow-drying hard epoxy (Araldite epoxy GY502 and hardener HY956 in 5:1 weight ratio) and a section was obtained cutting through the core (~450 μm thickness) using twin blades separated by a spacer on an Isomet low-speed diamond-bladed saw. The section was lightly polished to improve visibility, and photographed at resolution of 2048 x 2048 under a microscope at 16-40X magnification using reflected light, then digitally enhanced. The age interpretations were based on counts of presumed annual growth increments on the digital photos. Translucent zones were regarded as annuli following the recommendations described by Morales-Nin and Panfili, 2002.
Otolith cores for bomb radiocarbon age validation were isolated from three adjacent 1-mm thick transverse sections of the right otolith (also used for section age determination), polished lightly in order to view the growth sequence. Otolith cores were isolated from the central section as a solid piece with a Merchantek computer-controlled micromilling machine using 300-μm diameter steel cutting bits and burrs. Additional core material from the same otolith was isolated from the two adjacent sections but restricted to the innermost two growth increments so as to allow for the offset of these lateral sections from the primordium. This procedure of obtaining material from multiple sections per otolith was necessary to maximize the amount of sample material available for assay from each otolith. Occasionally, the mean weight of isolated core material was lower than 3 mg. In such cases, otolith cores from 1-2 additional fish of the same age and collection year were extracted and pooled so as to bring the sample weight up to the minimum of 3 mg necessary for radiocarbon assay. The date of sample formation was calculated as the year of fish collection minus the age of the fish, plus one half the number of growth increments extracted in the core. After sonification in Super Q water and drying, the sample was weighed to the nearest 0.1 mg in preparation for 14C assay with accelerator mass spectrometry (AMS). AMS assays also provided δ13C (0/00) values, which were used to correct for isotopic fractionation effects and provide information on the source of the carbon. Radiocarbon values were subsequently reported as Δ14C, which is the per mil (0/00) deviation of the sample from the radiocarbon concentration of 19th-century wood, corrected for sample decay prior to 1950 according to methods outlined by Stuiver and Polach (1977). The mean standard deviation of the individual radiocarbon assays was about 5 0/00.
Radiocarbon Reference Chronology
Radiocarbon chronologies based on known-age, young-of-the-year otoliths are typically used as accurate reference time series of 14C uptake into carbonates from the target environment. Since no juvenile otoliths were available to describe the Chilean reference chronology prior to about 1964, the reference chronology based on juvenile Pacific halibut (Hippoglossus hippoglossus) (Pinner and Wischniowski, 2004) from the northwest Pacific Ocean was used as a basis for comparison. This chronology was scaled to match the pre-bomb and peak values apparent in the CJM chronologies. The transformations applied to scale the Pacific halibut chronology to CJM were:
Results
Validation of First Annulus
In fish from 15 to 25 cm FL, a complete sequence of daily microincrements were observed from the primordium to the post-rostrum edge of sagittal otoliths (Figure 2). The back-calculated hatch dates (HD) from otolith microstructure analysis extended from August to February with a maximum in October-November (Figure 3). Daily age for juveniles and sub-adults used for validation of the first annulus ranged from 51 to 550 days, for fish ranging from 3.4 to 25.0 cm in FL. The average coefficient variation (CV) of ageing was 6.0%.
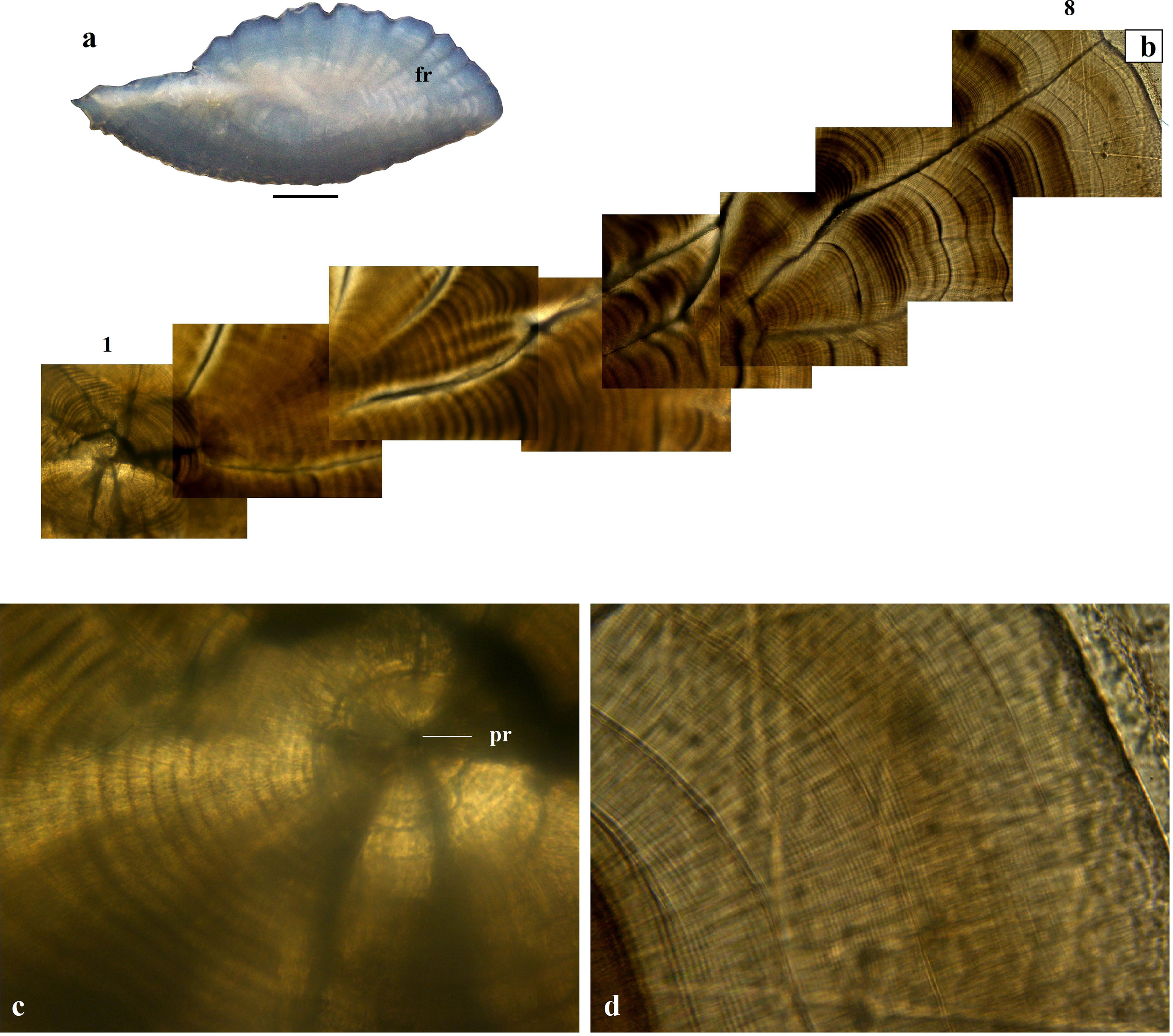
Figure 2 Macro (A) and microstructure (B) of a sagittal otolith from a 18 cm FL juvenile Trachurus murphyi, illustrating the sequence of micro-increments from the primordium (pr) to the post-rostrum edge. The images (C) and (D) correspond to a zoom for sequences 1 and 8, respectively, associated to the zones of fastest and lowest growth. ap, accessory primordia; fr, first false increment. Scale bar= 1 mm for the whole otolith.
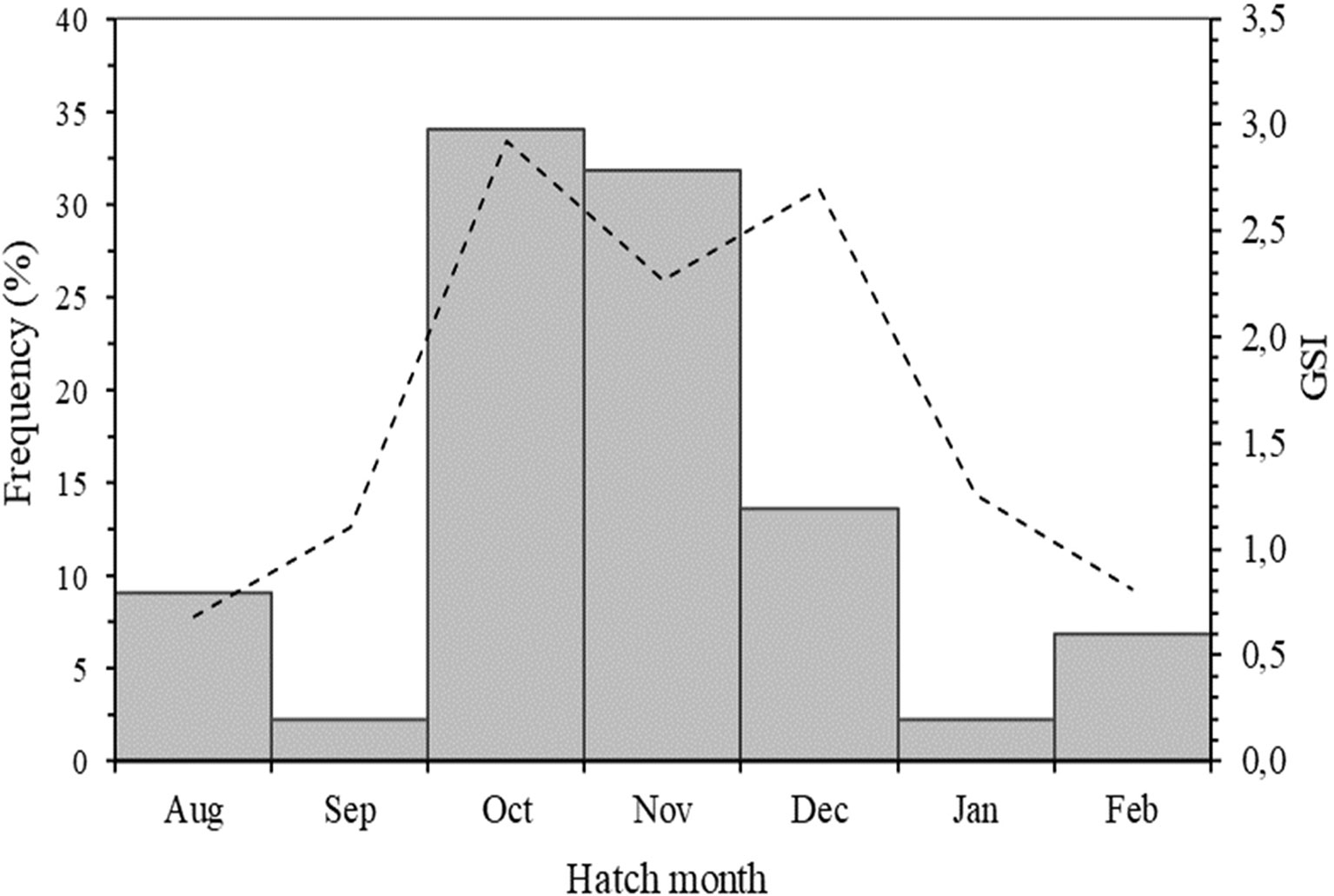
Figure 3 Frequency distribution of hatch months based on daily increment counts of juvenile Trachurus murphyi otoliths collected between Arica and Coquimbo in Chile. The dashed line represents the female gonadosomatic index between 2010-2015 in the same region (Aranís et al., 2018).
The Laird-Gompertz growth model provided a good fit to the age-length relationship of juvenile and young adult CJM (3.4-25.0 cm FL; ages of 56-550 days) between Arica and San Antonio (Figures 3,4). The coefficient of determination (r2 = 0.80) was high, the parameters were significant (Table 2), and the residuals were normally distributed (Shapiro-Wilk normality test, SW=0.98; P=0.79). The model predicted a fork length of 22 cm for a fish aged 365 days. Table 2 summarizes the comparison between the number of microincrements counted from the primordium to the distal edge of each translucent zone. The second translucent zone was fully formed at the end of the first year of life. Conversely, the first and third translucent increment were formed far before the end of the first and second year of life, respectively (Table 3). The age at formation of the fourth translucent increment could not be validated in the present study because daily microincrement analysis was not carried out in fish > 25 cm FL.
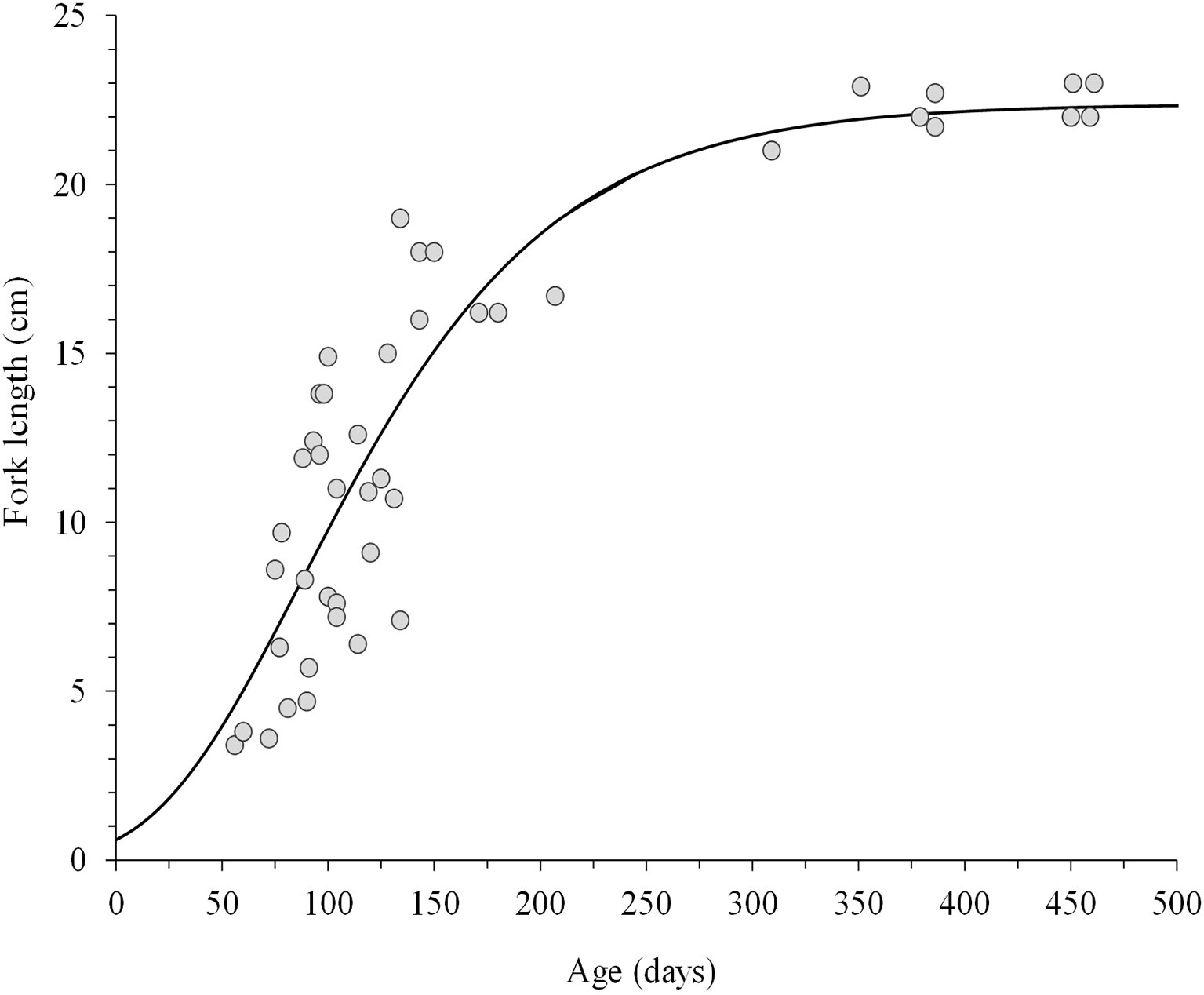
Figure 4 Laird–Gompertz model fitted to the age (daily) – fork length relationship of juvenile Trachurus murphyi, caught between Arica and Coquimbo.
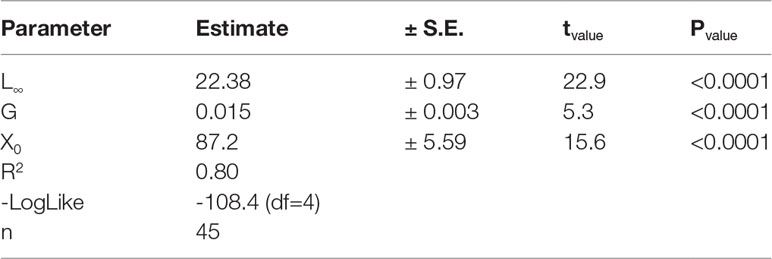
Table 2 Estimated parameters from a Laird–Gompertz model fitted to the age–length relationship of Trachurus murphyi< 25 cm fork length.

Table 3 Basic statistics of mean daily age and length at the end of translucent zone formation in sagittal otoliths of Trachurus murphyi ≤ 25 cm in fork length.
Progression of Strong Year-Classes
The annual progression of the first length frequency mode, corresponding to the 2008 year-class, was easily followed through subsequent years because recruitment of individuals<20 cm failed between 2009 and 2011 (Figure 5). Significant differences were found in the mean lengths of the first modal group through the years analyzed (ANOVA, P<0.05), with modal values increasing from 14.6 to 30.0 cm FL across the years 2008 to 2011 (Table 4; Figure 5).
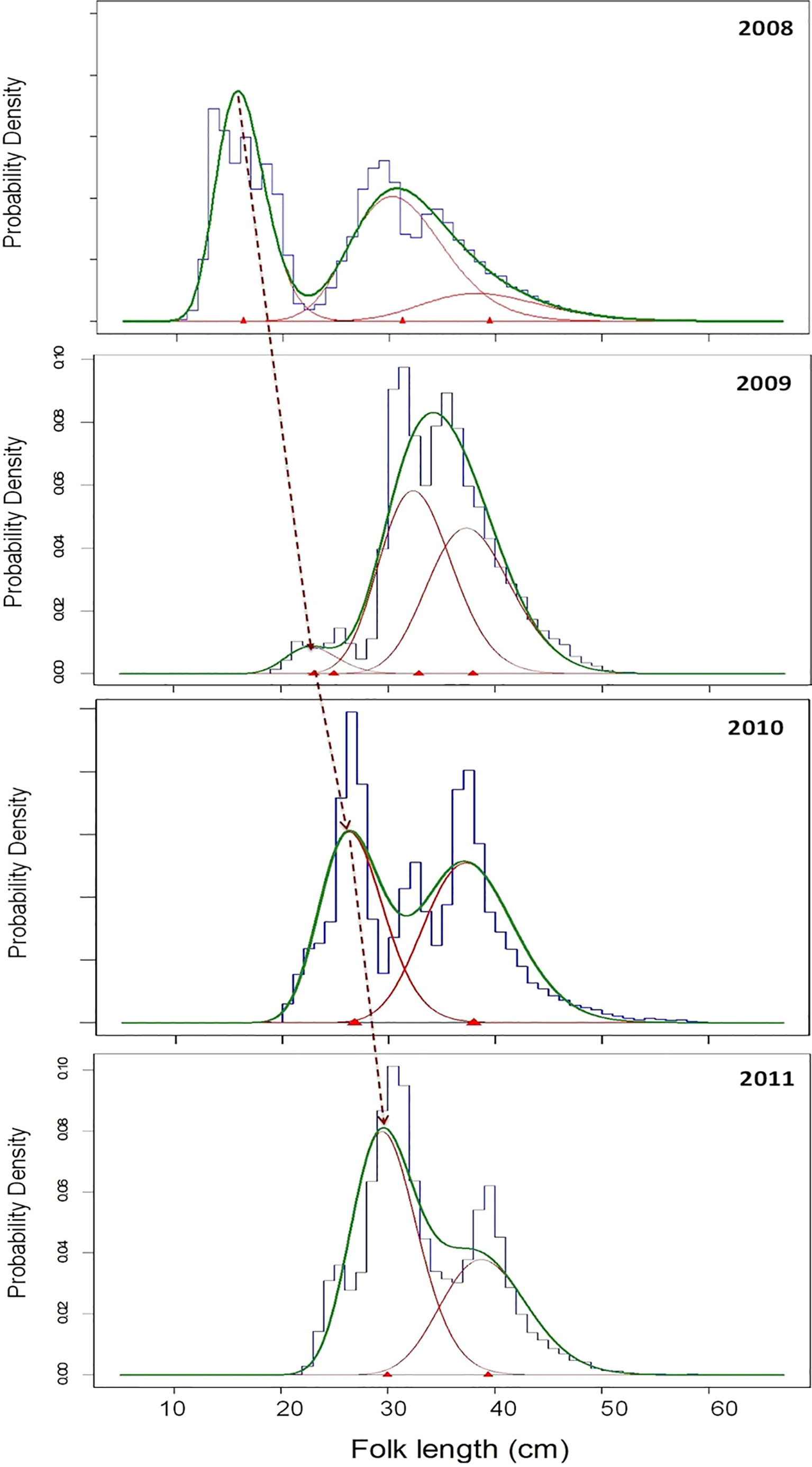
Figure 5 Length–frequency distributions (bars), principal mode estimates (red curve) and total predicted fit (green curve) obtained from a modal-progression analysis of Trachurus murphyi, collected during 2008 to 2011 from Chilean waters. Modal progression of one year class from year to year is shown by the dashed line.
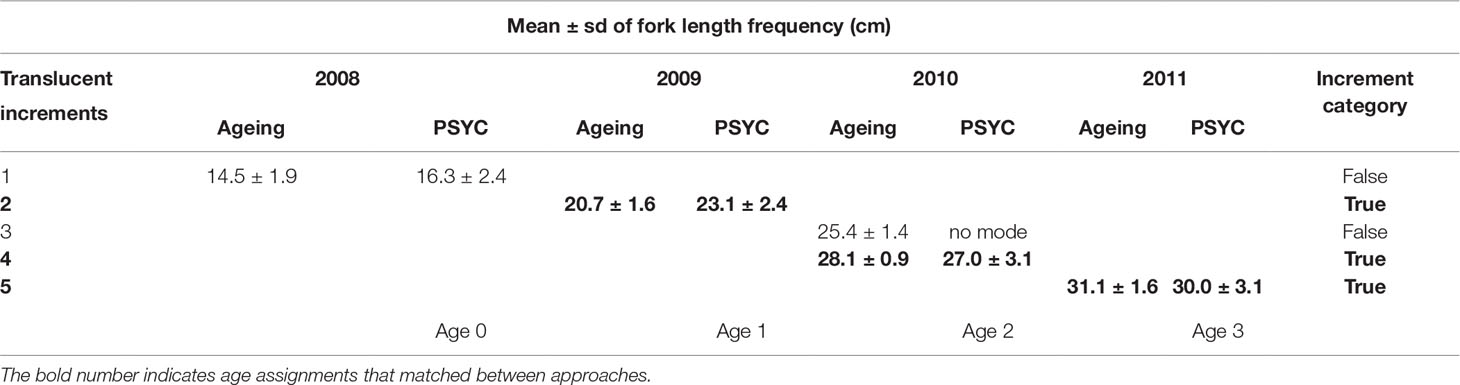
Table 4 Estimated fork length [mean and standard deviation (sd)] from an annual modal progression of a strong year class (PSYC), compared with mean length of aged fish for each sagittal otolith translucent zone in jack mackerel for the period 2008 to 2011 from Chilean fisheries.
The first mode at 14.6 cm FL, corresponding to recruitment of fish of age 0, matched with the mean FL of fish aged with microincrement analysis, which had a mean age of 185 ± 34 days. The mean length of subsequent modes identified as age 1, age 2 and age 3 in the PSYC for 2009, 2010 and 2011, respectively, closely matched the mean length of fish with 2, 4 and 5 translucent increments (Table 3; Figure 5). There was no mode corresponding to a length of fish with 3 translucent zones.
Radiocarbon Age Validation
If the annulus-based ageing is correct, the radiocarbon chronology described by the adult otolith cores should be similar to that of the reference chronology. A comparison of the reference and adult core chronologies from the CJM indicated that the ranges were consistent with each other (Table 5).
Radiocarbon chronology time series plots were prepared to compare the bomb radiocarbon content of the CJM reference chronology with that of otolith cores of adult fish (Figures 6A, B). The period of increase for the majority of otoliths appears to be similar between the sectioned age chronology and the Δ14C smoothed scaled Pacific halibut reference chronology (Figure 6B). Thus, the CJM section ages lying close to the scaled smoothing curve must have been correctly aged, at least on average. More importantly, none of the sectioned otoliths seem to have been seriously over-aged, which would have been evident as radiocarbon values which were much higher than that of the surrounding environment (Figures 6B, 7). In contrast, some of the whole-otolith ages appear to be too old (Figures 6A): three fish with anomalously low radiocarbon values for a presumed year of formation after 1968 were evident in both the sectioned and whole otolith plots (Figures 6A, B), indicative of substantial under-ageing.
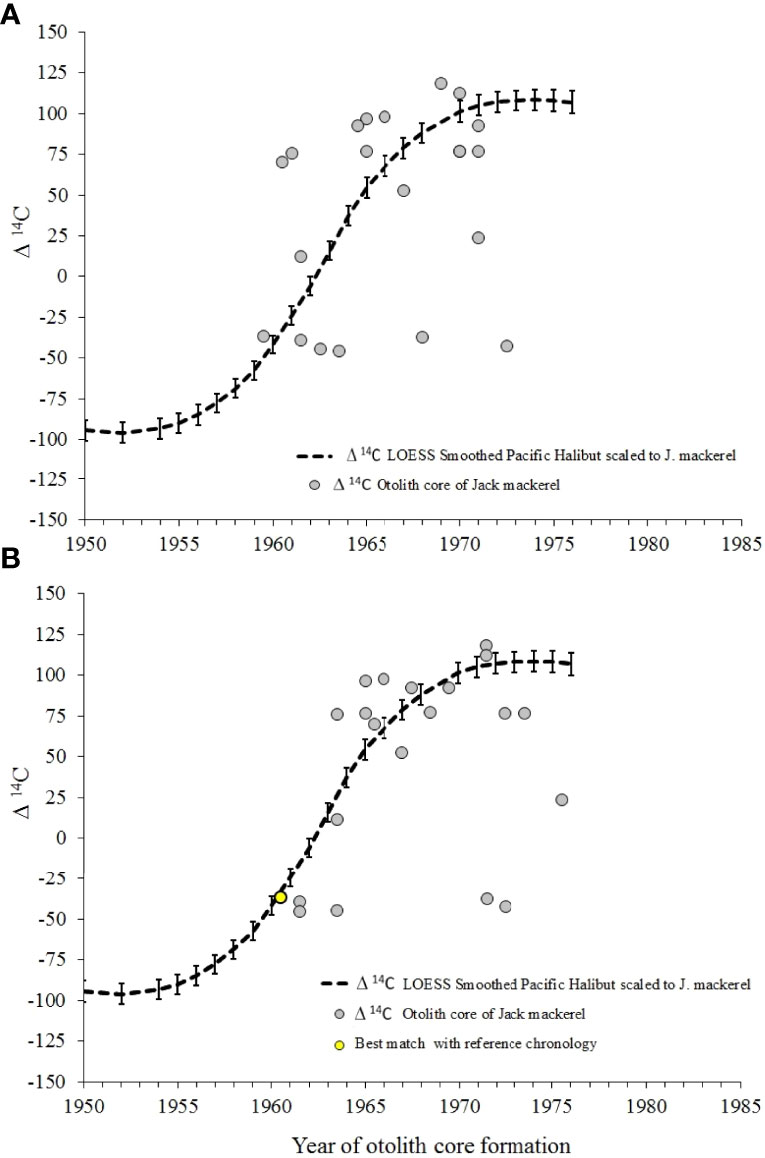
Figure 6 Radiocarbon reference chronology for Pacific halibut and Δ14C in otolith’s cores of adult Trachurus murphyi versus year of formation inferred from counts of the growth increment from: (A) whole otolith reading and (B) Otolith transverse section reading. The yellow point shows the best match between year core formation with the reference chronology, for the sectioned otolith of Figure 7.
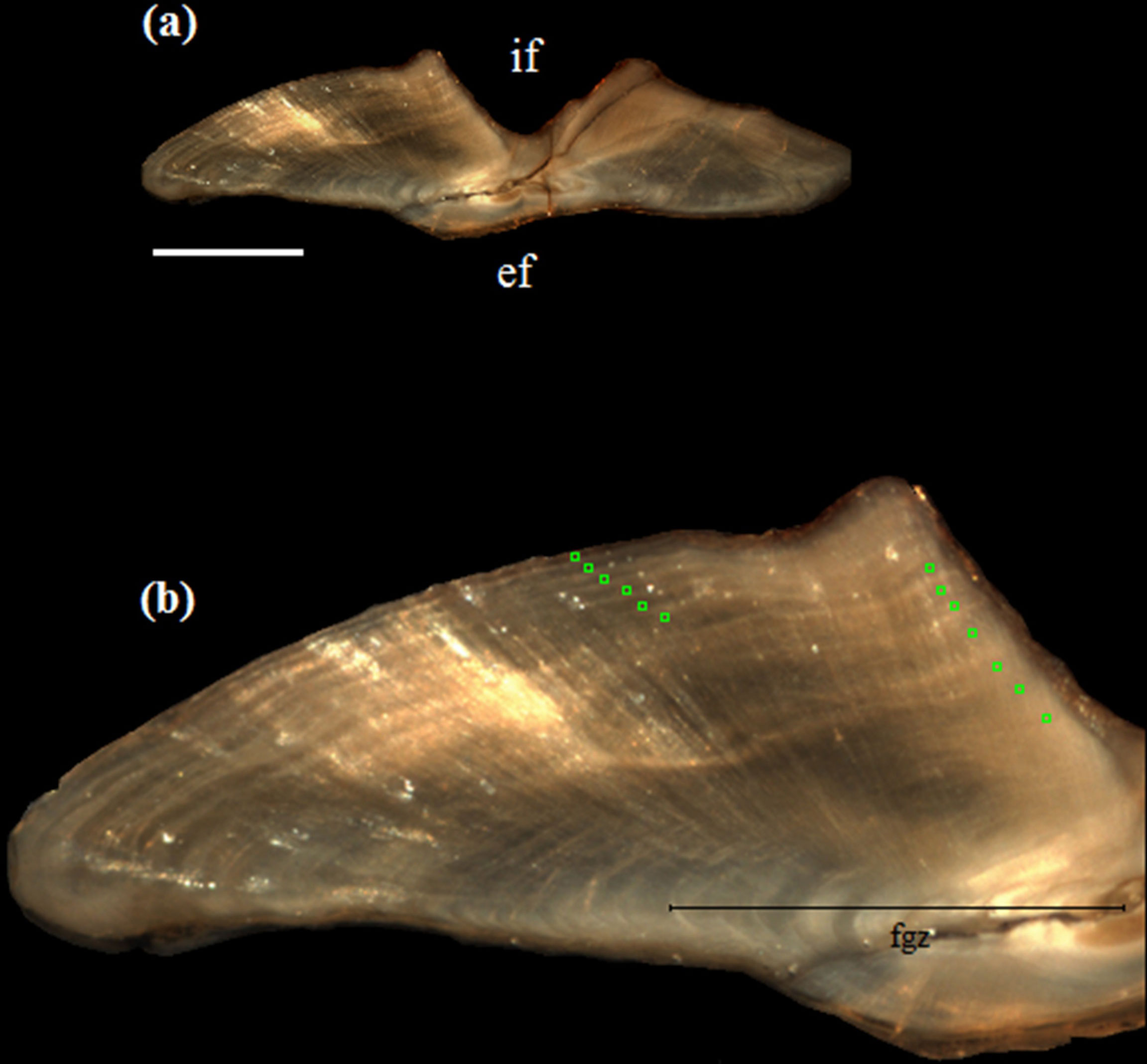
Figure 7 Complete (A) and zoomed (B) images of a cross-section of a sagittal otolith of Trachurus murphy, bomb dated as a 13-year-old. specimen (green squares), showing the best match to the scaled radiocarbon chronology (i.e., yellow dot in Figure 6B). fgz, first growth zone of sub-annual increments; Scale bar= 1mm.
Discussion
The Laird-Gompertz growth curve for the length interval between 3 and 16 cm was similar to the reported by Goicochea et al. (2013) for CJM caught off the Peruvian coast; however, the predicted mean length at 365 days was 17 cm FL for Peru, and 22 cm FL for Chile (this study), which is indicative of growth differences between the stocks. Nevertheless, both our study and that of Goicochea et al. (2013) document a higher growth rate in juvenile and young adult CJM in the southeast Pacific Ocean than has been previously suggested for this species. Previously, a length of about 13 cm TL had been suggested for YOY at the end of their first year of life, but based on unvalidated methods (Castillo and Arrizaga, 1987; Kochkin, 1994; Dioses, 2013). In the current study the mean length reached at the first year of life was close to the length-at-maturity (L50) of 22.7 cm FL (Leal et al., 2013), suggesting that CJM reaches maturity at the end of its first year of life. Clearly, the reliability of these estimations depends upon the validation of the periodicity of formation of primary micro-increments, all of which has been confirmed in T. murphyi both juvenile (<13 cm TL; Araya et al., 2019) and adult (>27 cm FL; Araya et al., 2003) Furthermore, the hatch date distributions based on otolith microstructure coincided with the spawning season reported for this species off the Chilean coast (Leal et al., 2013; Aranís et al., 2018), which is further evidence of the accuracy of YOY CJM age determinations in this study.
There are few descriptions of the otolith microstructure in carangid species, other than the study of Waldron and Kerstan (2001) for the horse mackerel Trachurus trachurus, collected off the south west coast of South Africa. There, the authors reported the absence of a translucent zone in otolith of juveniles younger than 274 days old, with a FL between 5.1–14.3 cm. The same study used scanning electron microscopy of the otolith microstructure to validate the ages of horse mackerel up to an age of four years. Hence, our study corroborates the results reported by Waldron and Kerstan (2001), confirming the presence of resolvable microincrements to at least the first translucent zone, despite the allometry that seem to occur at the end of the first year of life. Such a pattern is opposite to that of slow-growing species where micro-increments in the translucent zone become unresolvable after approximately the sixth month of YOY life (Waldron, 1994, Radtke et al., 1985, Beckman and Calfee, 2014).
A noticeable finding was the early formation of a first translucent zone between days 100 and 180, after a phase of accelerated growth. Clearly, this first translucent zone cannot be regarded as the first annulus for this species, and must be ignored in the annual age determination. Conversely, the second prominent translucent zone ended its formation on average at 369 days. This second translucent zone appears to represent the first true annulus for CJM, and is consistent with the length modes evident from following the distinct 2008 year-class for three years, through several years of almost no new recruitment. The mean length of the 2008 year-class in 2009 was very close to the mean length obtained by counting daily increments (22 cm FL). Hence, the remaining length modes in the following years would correspond to the second and third age classes, with mean lengths of 27 and 30 cm FL, respectively. Such a conclusion seems to be reliable because this approach met the requirement of following a well-defined recruitment pulse, where no apparent age-structured migration occurs (e.g., Donald et al., 1992; Beamish and McFarlane, 1995; Morison et al., 1998; Campana, 2001; Uriarte et al., 2016). Although adults (> 25 cm) CJM are known to migrate at the end of the austral winter to spawn in offshore waters (80-90 °W), young juveniles encompassing the first recruitment pulse, remain either in nursery or feeding areas off the Chilean coast, until migrating into offshore areas for first spawning (Arcos et al., 2001; Parada et al., 2017).
It is important to note that many species form the translucent zone (annulus) during periods of slow growth, which in some species may be linked to winter (i.e., the cold season), and in other species is the result of energy cost associated with spawning (e.g., Pearson, 1996; Suyama et al., 2006). Therefore, it is reasonable to infer that when the spawning season coincides totally or partially with the cold season, a well-marked and consistent annulus would be formed. However, T. murphy in Chilean waters spawns continuously for about four months each spring, with maximum reproductive activity concentrated every year between October and December (e.g., Grechina, 1998; Leal et al., 2013). This lag may explain the existence of the first (non-annual) translucent band, which would correspond to a period of slow growth associated with the first winter season; whereas the first annulus would be associated with the first spawning event. This same phenomenon apparently occurred in the following year, because the fourth translucent band corresponded to the second true annulus, as demonstrated by the modal-progression of the strong year-class. In addition, the consistency between the size of the 2008 class fish and the average length of fish with five translucent otolith bands is indicative that this fifth band would correspond to the third true annulus. Presumably, as growth rate declines, the occurrence of two translucent zones per year would become undetectable in the sequence of translucent bands, triggering higher reliability in age determination at later ages. This finding also confirms the value of the PSYC method used in some other fast-growing species (e.g., Donald et al., 1992; Beamish and McFarlane, 1995; Morison et al., 1998; Campana, 2001; Uriarte et al., 2016), where the presence of additional “false increments” would make it difficult to apply traditional techniques.
The bomb radiocarbon method has successfully been applied not only in long-lived fishes (Kalish, 1995a; Kalish, 1995b; Kalish et al., 1996; Campana, 1997; Kalish et al., 1997; Campana, 1997; Campana and Jones, 1998; Campana, 1999; Ong et al., 2020; Passerotti et al., 2020), but also in the Atlantic herring, a short-lived (<10 yr) pelagic fish (Melvin and Campana, 2010) and for species with a moderate longevity (<23 yr) as in Trachurus declives of New Zealand (Lyle et al., 2000) and T. murphy in the present study. In CJM a good approximation of absolute age was obtained with the bomb radiocarbon method for fish over 40 cm FL, which validated on average the absolute age for fish between 7 and 13 years-old. Although this method did not validate the age of fish< 7 years-old, there was good consistency between the otolith microstructure analysis and PSYC, which verified the age determination of the younger specimens. Only one previous study by Beentjes et al. (2013) addressed age determination issues in the Trachurus genus using bomb radiocarbon. Those authors reported high levels of Δ14C (110.57 ± 0.4‰) for fish< 20-year-old born after 1966, which was close to the estimate obtained in the present study.
The year of formation of the otolith core was estimated from readings of both whole and sectioned otoliths, but ages estimated from sections matched better with the radiocarbon reference chronology. Since radiocarbon values above zero did not exist in surface marine waters prior to about 1958, elevated otolith core radiocarbon values in fish whose apparent age of birth (based on counts of growth increments) was prior to 1958 must have been incorrectly aged with increments (Campana, 2001). Three such fish were identified using whole otoliths, but none with sectioned otoliths. In contrast, anomalously low otolith radiocarbon values in the post-bomb era are explicable either by under-ageing or residence in very deep or upwelled waters. Three fish were apparently under-aged using both whole and sectioned otoliths, but deepwater residence is another possible explanation (Campana et al., 2016). Sagittal otoliths of CJM become concave as the fish grows, which make the surface identification of annuli more difficult along the otolith edge, particularly in older fish. This problem did not occur in transverse sections, which is probably responsible for their more accurate age determinations, but does not explain why there were more over-aged whole otoliths. To date, use of transverse sections to identify annuli in the internal sagittal face have become the preferred method for otolith-based age determination in both closely-related (e.g., Jack mackerels (Horn, 1993; Lyle et al., 2000; Beentjes et al., 2013); Cardinalfish Epigonus crassicaudus (Ojeda et al., 2010); Atlantic striped bass (Liao et al., 2013); bluefish (Robillard et al., 2009) and un-related species with similar otolith morphologies (e.g., Polyprion americanus (Brick-Peres and Haimovici, 2004); Sebastes ruberrimus, (Allen et al., 2002)). Hence, based on the results of the present study, and in light of the other studies, transverse sections of sagittal otoliths should be recommended instead of surface reading for determining the age of CJM.
The results of the present study are consistent with previous works addressing age validation in Trachurus species. For example, Horn (1993) verified annulus formation in Trachurus novaezelandiae and T. declivis from New Zealand using PSYC. Age determination of Horse mackerel (T. trachurus) was verified using marginal increment analysis and the first four annuli with unvalidated primary microincrement counts (Waldron and Kerstan, 2001). More recently, Jurado-Ruzafa and Santamaría (2018) and Katayama et al. (2019) validated the periodicity of annulus formation in T. picturatus and T. japonicus respectively, using marginal increment analysis and frequency distribution of edge type.
T. murphy growth, estimated from the validated age data, revealed fast growth during the first two years. This rapid growth has not been previously reported, due to the lack of age validation, and was confounded by the presence of false increments whose identity could not be confirmed using traditional age verification approaches. These new evidences match well with the results recently reported by Horn and Maolagáin (2020), where a high occurrence of sub-annual zones were observed within the first two annuli, using transverse sections of sagittal otoliths of T. murphy from New Zealand waters. The occurrence of false increments is also common in other Trachurus species, where the high difficulty in identifying annuli is known. This situation has been recently described in the ICES WKARHOM workshops (Bellodi et al., 2020), where the reproducibility exercises Trachurus trachurus, T. mediterraneus) and. T. picturatus showed very low precision (CV of 19, 40 and 36%, respectively). Some of the main difficulties noted were: (i) the identification of the first winter increment versus several false increments, (ii) an indistinct winter increment, (iii) a false (reproductive) increment detected between the first and second winter increments, (iv) overlapping of the transparent zones after the 3th or between the winter increment in T. mediterraneus, and (v) overlapping of the transparent zones after the second winter increment in T. picturatus and again between the 4th and 5th winter increment in horse mackerel (Bellodi et al., 2020). The T. murphyi otoliths from the southeastern Pacific Ocean present similar difficulties of age interpretation, which has resulted in a CV of 20% in intercalibration exercises being the main disagreements among readers the assignment of the first and second annuli (Cerna et al., 2016). Therefore, for this kind of species it is considered mandatory to create catalogs and collections of reference otoliths based on validated age. The application of three simultaneous age validation methods in the current study is a critical step in achieving this goal and thus contributes to the sustainable management of this important transboundary migratory carangid. In conclusion, age has been validated for CJM for the first time in the present study, which will increase the levels of precision and accuracy in routine aging monitoring for this important commercial species.
Data Availability Statement
The raw data supporting the conclusions of this article will be made available by the authors, without undue reservation.
Ethics Statement
Ethical review and approval was not required for the animal study because this study only used fish extracted through commercial capture, which is duly formalized and authorized by the corresponding authorities in Chile.
Author Contributions
FC led this research and performed the analytical procedures for modal progression analysis. FC also wrote a first draft of the MS, supported by SC and GP, who made contributions in all sections of the MS. SC was also responsible for radiocarbon analysis and GM worked in laboratory procedures associated to otolith microstructure of juveniles. CV and LM, worked in processing otolith and ageing adult fish. All authors contributed to the article and approved the submitted version.
Funding
The present study was funded by the projects FIPA 2014-32 and INNOVA-CORFO 05CN11IP.
Conflict of Interest
The authors declare that the research was conducted in the absence of any commercial or financial relationships that could be construed as a potential conflict of interest.
Publisher’s Note
All claims expressed in this article are solely those of the authors and do not necessarily represent those of their affiliated organizations, or those of the publisher, the editors and the reviewers. Any product that may be evaluated in this article, or claim that may be made by its manufacturer, is not guaranteed or endorsed by the publisher.
Acknowledgments
The authors thank to the heads of ASIPA Projects Gabriela Bohm and Antonio Aranís. We also thank to Vilma Ojeda, Head of the INNOVA project and to the IFOP scientific observers.
References
Allen H. A., Gregor M., Cailliet A., Kenneth H., Coale A., Kristen M., et al. (2002). Radiometric Age Validation of the Yelloweye Rockfish (Sebastes Ruberrimus) From Southeastern Alaska. Mar. Freshw. Res. 53, 139–146. doi: 10.1071/MF01126
Aranís A., Gómez A., Walker K., Muñoz L., Caballero L., Eisele G., et al. (2018). Convenio De Desempeño 2017. Programa De Seguimiento De Las Principales Pesquerías Pelágicas De La Zona Centro-Sur De Chile, V-XI Regiones, Año 2017. Subsecretaría Economía y EMT Inst. Fom. Pesq. Valparaíso Chile. Informe Final. 330
Araya M., Marianela M., Segovia E., Peñailillo J., Avilés M., Chisen K., et al. (2019). Validación De La Formación De Los Anillos De Crecimiento Diario De Jurel. Proyecto FIPA N° 2017-61. Informe Final., 170.
Araya M., Medina M., Arancibia H. (2003). Preliminary Result of Empirical Validation of Daily Increments in Otoliths of Jack Mackerel Trachurus Symetricus (Ayres 1855) Marked With Oxytetracycline. Scientia Marina 67, 47–475. doi: 10.3989/scimar.2003.67n4471
Arcos D. F., Cubillos L., Nuñez S. (2001). The Jack Mackerel Fishery and El Niño 1997–98 Effects Off Chile. Prog. Oceanogr. 49, 597–617. doi: 10.1016/S0079-6611(01)00043-X
Beamish R. J., McFarlane G. A. (1995). “A Discussion of the Importance of Aging Errors, and an Application to Walleye Pollock: The World’s Largest Fishery,” in In Recent Developments in Fish Otolith Research. Eds. Secor D. H., Dean J. M., Campana S. E. (Columbia: University of South Carolina Press), 545–565.
Beckman D. W., Calfee J. W. (2014). Timing of First Annulus Formation in White Sucker Otoliths. N. Am. J. Fish 34, 1187–1189. doi: 10.1080/02755947.2014.951809
Beentjes M. P., Neil H. L., Taylor P. R., Marriot P. (2013). “Further Studies on Age Validation of Murphy’s Mackerel (Trachurus Symmetricus Murphyi),” in New Zealand Fisheries Assessment Report 2013/14. ISSN 1179-5352, 38.
Bellodi A., Defruit G. B., Carbonara P., Cox L., Chantre C., Davies J., et al. (2020). ICES Workshop on Age Validation Studies of Small Pelagic Species (WKVALPEL). ICES Sci. Rep. 2, 15. doi: 10.17895/ices.pub.5966
Botsford L. W., Holland M. D., Samhouri J. F., White J. W., Hasting S. A. (2011). Importance of Age Structure in Models of the Response of Upper Trophic Levels to Fishing and Climate Change. ICES J. Mar. Sci. 68, 1270–1283. doi: 10.1093/icesjms/fsr042
Brick-Peres M., Haimovici M. (2004). Age and Growth of Southwestern Atlantic Wreckfish Polyprion Americanus. Fisher. Res. 66, 1577–1169. doi: 10.1016/S0165-7836(03)00207-8
Campana S. E. (1997). Use of Radiocarbon From Nuclear Fallout as a Dated Marker in the Otoliths of Haddock, Melanogrammus Aeglefinus. Mar. Ecol. Prog. Ser. 15, 49–56. doi: 10.3354/meps150049
Campana S. E. (1999). Chemistry and Composition of Fish Otoliths: Pathways, Mechanisms and Applications. Mar. Ecol. Prog. Ser. 188, 263–297. doi: 10.3354/meps188263
Campana S. E. (2001). Accuracy, Precision and Quality Control in Age Determination, Including a Review of the Use and Abuse of Age Validation Methods. J. Fish Biol. 59, 197–242. doi: 10.1111/j.1095-8649.2001.tb00127.x
Campana S. E., Jones C. M. (1992). Analysis of Otolith Microstructure Data, P 73-100. In D.K. Stevenson and SE Campana (Ed) Otolith Microstructure Examination and Analysis. Can. Spec. Publ. Fich. Aquat. Sci. 117, 126. doi: 10.13140/RG.2.2.22258.61127
Campana S. E., Jones C. M. (1998). Radiocarbon From Nuclear Testing Applied to Age Validation of Black Drum, Pogonias Cromis. Fish. Bull. 96, 185–192.
Campana S. E., Thorrold S. R. (2001). Otoliths, Increments, and Elements: Keys to a Comprehensive Understanding of Fish Populations? Can. J. Fish. Aquat. Sci. 58, 30–38. doi: 10.1139/f00-177
Campana S. E., Valentin A. E., MacLellan S. E., Groot J. B. (2016). Image-Enhanced Burnt Otoliths, Bomb Radiocarbon and the Growth Dynamics of Redfish (Sebastes Mentella and S. Fasciatus) Off the Eastern Coast of Canada. Mar. Freshw. Res. 67, 925–936. doi: 10.1071/MF15002
Carbonara P., Bellodi A., Palmisano M., Mulas A., Porcu C., Zup W., et al. (2020). Growth and Age Validation of the Thornback Ray (Raja Clavata Linnaeus 1758) in the South Adriatic Sea (Central Mediterranean). Front. Mar. Sci. 7. doi: 10.3389/fmars.2020.586094
Castillo G., Arrizaga A. (1987). Edad Y Crecimiento Del Chilean Jack Mackerel Trachurus Symmetricus Murphyi (Nichols 1920) En Aguas De Chile Central. Biol. Pesq. 16, 19–33.
Cerna F., Valero C., Moyano G., Muñoz L. (2016). Protocolo De Lectura De Otolitos De Jurel. I. nforme Final FIP N° 2014-32., 147.
Dioses T. (2013). “Edad Y Crecimiento Del Jurel Trachurus Murphyi, (Nichols 1920) En El Perú,” in Ecología, Pesquería Y Conservación Del Jurel (Trachurus Murphyi) En El Perú. Rev. Peru. Biol. Número Especial, vol. 20 . Eds. Csirke J., Guevara-Carrasco R., Espino M.(Perú: Universidad Nacional Mayor de San Marcos), 045–052.
Donald D. B., Babaluk J. A., Craig J. F., Musker W. A. (1992). Evaluation of the Scale and Operculum Methods to Determine Age of Adult Goldeyes With Special Reference to a Dominant Year-Class. Tran. Amer. Fisher. Soc 121, 792–796. doi: 10.1577/1548-8659(1992)121<0792:EOTSAO>2.3.CO;2
Elizarov A. A., Grechina A. S., Kotenev B. N., Kuzetsov A. N. (1993). Peruvian Jack Mackerel, Trachurus Symmetricus Murphyi, in the Open Waters of the Southern Pacific. J. Ichth. 33, 86–104.
Evseenko S. A. (1987). Reproducción De Jurel Del Perú Trachurus Symmetricus Murphyi (Nichols) En El Pacífico Sur. Voprosy Ichthyologii 27, 264–273.
Fowler A. J., Ling J. K. (2010). Ageing Studies Done 50 Years Apart for an Inshore Fish Species From Southern Australia-Contribution Towards Determining Current Stock Status. Environ. Biol. Fishes 89, 253–265. doi: 10.1007/s10641-010-9659-0
Goicochea C., Mostacero J., Moquillaza P., Dioses T., Topiño Y., Guevara-Carrasco R. (2013). Validación Del Ritmo De Formación De Los Anillos De Crecimiento En Otolitos Del Jurel Trachurus Murphyi Nichols 1920. Rev. Peru. Biol. 20, 53–60. doi: 10.15381/rpb.v20i1.2619
Grechina A. (1998). “Historia De Investigaciones Y Aspectos Básicos De La Ecología Del Jurel (Trachurus Symmetricus Murphyi (Nichols)) En Alta Mar Del Pacífico Sur,” in Biología Y Ecología Del Jurel En Aguas Chilenas. Ed. Arcos D. (Concepción, Chile: Editorial Aníbal Pinto), 11–34.
Horn P. L. (1993). Growth, Age Structure, and Productivity of Jack Mackerels (Trachurus Spp.) in New Zealand Waters. N. Z. J. Mar. Freshw. Res. 27, 145–155. doi: 10.1080/00288330.1993.9516553
Horn P. L., Maolagáin M. O. (2020). The Growth and Age Structure of Chilean Jack Mackerel (Trachurus Murphyi) Following Its Influx to New Zealand Waters. J. Fish Biol. 98, 1144–1154. doi: 10.1111/jfb.14647
Hussy K. (2010). Why is Age Determination of Baltic Cod (Gadus Morhua) So Difficult? ICES J. Mar. Sci. 67, 1198–1205.
Ihaka R., Gentleman R. (1996). R: A Language for Data Analysis and Graphics. J. Comput. Graph. Stat. 5, 299–314.
Jurado-Ruzafa A., Santamaría M. T. G. (2018). Age, Growth and Natural Mortality of Blue Jack Mackerel Trachurus Picturatus (Carangidae) From the Canary Islands, Spain (NW Africa). Afr. J. Mar. Sci. 40, 451–460. doi: 10.2989/1814232X.2018.1550019
Kalish J. M. (1993). Pre- and Post-Bomb Radiocarbon in Fish Otoliths. Earth Planet. Sci. Lett. Letters. 114, 549–554. doi: 10.1016/0012-821X(93)90082-K
Kalish J. M. (1995a). Application of the Bomb Radiocarbon Chronometer to the Validation of Redfish Centroberyx Affinis Age. Can. J. Fish. Aquat. Sci. 52, 1399–1405. doi: 10.1139/f95-135
Kalish J. M. (1995b). “Radiocarbon and Fish Biology,” in In Recent Developments in Fish Otolith Research. Eds. Secor D. H., Dean J. M., Campana S. E. (Columbia: University of South Carolina Press), 637–653.
Kalish J. M., Johnston J. M., Gunn J. S., Clear N. P. (1996). Use of the Bomb Radiocarbon Chronometer to Determine Age of Southern Bluefin Tuna Thunnus Maccoyii. Mar. Ecol. Prog. Ser. 143, 1–8. doi: 10.3354/meps143001
Kalish J. M., Johnston J. M., Smith D. C., Morison A. K., Robertson S. G. (1997). Use of the Bomb Radiocarbon Chronometer for Age Validation in the Blue Grenadier Macruronus Novazelandiae. Mar. Biol. 128, 557–563. doi: 10.1007/s002270050121
Kastelle C. R., Helser T. E., Wischniowski S. G., Loher T., Goetz B. J., Kautzi L. A. (2016). Incorporation of Bomb-Produced 14C Into Fish Otoliths: A Novel Approach for Evaluating Age Validation and Bias With an Application to Yellowfin Sole and Northern Rockfish. Ecol. Model. 320, 79–91. doi: 10.1016/j.ecolmodel.2015.09.013
Katayama S., Yamada H., Onodera K., Masuda Y. (2019). Age and Growth From Oita and Miyagi Prefectures of Japanese Jack Mackerel Trachurus Japonicus. Fish. Sci. 85, 475–481. doi: 10.1007/s12562-019-01302-6
Kindong R., Wang H., Wu F., Dai X., Tian S. (2020). Age, Growth, and Sexual Maturity of the Crocodile Shark, Pseudocarcharias Kamoharai, From the Eastern Atlantic Ocean. Front. Mar. Sci. 7, 586024. doi: 10.3389/fmars.2020.586024
King J. R., Helser T., Gburski C., Ebert D. A., Cailliet G., Kastelle C. R. (2017). Bomb Radiocarbon Analyses Validate and Inform Age Determination of Longnose Skate (Raja Rhina) and Big Skate (Beringraja Binoculata) in the North Pacific Ocean. Fish. Res. 193, 195–206. doi: 10.1016/j.fishres.2017.04.004
Kochkin P. N. (1994). Age Determination and Estimate Growth Rate for the Peruvian Jack Mackerel, Trachurus Symmetricus Murhyi. J. Ichthyol. 34, 39–50.
Leal E., Díaz E., Saavedra-Nievas J. C., Claramunt G. (2013). Ciclo Reproductivo, Longitud Y Edad De Madurez De Jurel Trachurus Murphyi, En La Costa De Chile. Rev. Biol. Mar. Oceanogr. 48, 601–611. doi: 10.4067/S0718-19572013000300016
Liao H., Sharov A. F., Jones C. M., Nelson G. A. (2013). Quantifying the Effects of Aging Bias in Atlantic Striped Bass Stock Assessment. Trans. Am. Fish. Soc 142, 193–207. doi: 10.1080/00028487.2012.705255
Lyle J. M., Krusic-Golub K., Morison A. K. (2000). “Age and Growth of Jack Mackerel and the Age Structure of the Jack Mackerel Purse Seine Catch,” in FRDC Project N°1995/034, Final Report. Published by the Tasmanian Aquaculkture and Fisheries Institute ( Australia: University of Tasmania), ISBN: 0-7246-4760-0, 49.
MacDonald P., Du J. (2004) Mixdist: Finite Mixture Distribution Models (Version 0.5-2). Available at: http://www.math.mcmaster.ca/peter/mix/mix.html.
Macdonald P., Pitcher T. J. (1979). Age-Groups From Size-Frequency Data: A Versatile and Efficient Method of Analyzing Distribution Mixtures. J. Fish. Res. Board. Can. 36, 987–1001. doi: 10.1139/f79-137
Melvin G. D., Campana S. E. (2010). High-Resolution Bomb Dating for Testing the Accuracy of Age Interpretations for a Short-Lived Pelagic Fish, the Atlantic Herring. Environ. Biol. Fishes 89, 297–311. doi: 10.1007/s10641-010-9663-4
Morales-Nin B., Canha A., Casas M., Figuereido I., Gordo L. S., Gordon J. D. M., et al. (2002). Intercalibration of Age Readings of Deepwater Black Scabbardfish, Aphanopus Carbo (Lowe 1839). ICES J. Mar. Sci. 59, 352–364. doi: 10.1006/jmsc.2001.1154
Morales-Nin B., Panfili J., (2002). "Age Estimation" in Manual of Fish Sclerochronology, eds. Panfili J., De Pontual H., Troadec H., Wright P. J. ( Brest, France: lfremer-IRD), 91–98.
Morison A. K., Coutin P. C., Robertson S. G. (1998). Age Determination of Black Bream, Acanthopagrus Butcheri (Sparidae), From the Gippsland Lakes of Southeastern Australia Indicates Slow Growth and Episodic Recruitment. Mar. Freshw. Res. 49, 491–498. doi: 10.1071/MF97237
Ojeda V., Wiff R., Labrín C., Contreras F. (2010). ). Longevity of Cardinalfish Epigonus Crassicaudus in Chile: Is it Similar to That of Its Relatives? Rev. Biol. Mar. Oceanogr. 45, 507–511. doi: 10.4067/S0718-19572010000300015
Ong J. J. L., Meekan M. G., Hsu H. H., Fanning L. P., Campana S. E. (2020). Annual Bands in Vertebrae Validated by Bomb Radiocarbon Assays Provide Estimates of Age and Growth of Whale Sharks. Front. Mar. Sci. 7, 188. doi: 10.3389/fmars.2020.00188
Panfili J., De Pontual H., Troadec H., Wrigh P. J. (2002) Manual of Fish Sclerochronology. Ifremer. Available at: https://archimer.ifremer.fr/doc/00017/12801/.
Parada C., Gretchina A., Sebastián V., Belmadani A., Combes C., Ernst B., et al. (2017). Expanding the Conceptual Framework of the Spatial Population Structure and Life History of Jack Mackerel in the Eastern South Pacific: An Oceanic Seamount Region as Potential Spawning/Nursery Habitat. ICES J. Mar. Sci. 74, 2398–2414. doi: 10.1093/icesjms/fsx065
Passerotti M. S., Andrews A. H., Natanson L. J. (2020). Inferring Life History Characteristics of the Oceanic Whitetip Shark Carcharhinus Longimanus From Vertebral Bomb Radiocarbon. Front. Mar. Sci. 7, 581775. doi: 10.3389/fmars.2020.581775
Pearson D. E. (1996). Timing of Hyaline-Zone Formation as Related to Sex, Location, and Year of Capture in Otoliths of the Widow Rockfish, Sebastes Entomelas. Fish. Bull. 94, 190–197.
Pinner K. R., Wischniowski S. G. (2004). Pacific Halibut Chronology of Bomb Radiocarbon in Otoliths From 1944 to 1981 and a Validation of Ageing Methods. J. Fish Biol. 64, 1060–1071. doi: 10.1111/j.1095-8649.2004.0371.x
Power G. R., King P. A., Kelly C. J., McGrath D., Mullins E., Gullaksen O. (2006). Precision and Bias in the Age Determination of Blue Whiting, Micromesistius Poutassou (Risso 1810), Within and Between Age-Readers. Fish. Res. 80, 312–321. doi: 10.1016/j.fishres.2006.03.031
Quist M. C., Isermann D. A. (2017). Age and Growth of Fishes: Principles and Techniques(USA: American Fisheries Society).
Radtke R.L., Fine M.L., Bell J. (1985) Somatic and otolith growth in the oyster toadfish (Opsanus tau L.). J. Exp. Ma.r Biol. Ecol. 90, 259–75.
Robillard E., Reiss C. S., Jones C. M. (2009). Age-Validation and Growth of Bluefish (Pomatomus Saltatrix) Along the East Coast of the United States. Fish. Res. 95, 65–75. doi: 10.1016/j.fishres.2008.07.012
Serra R. (1991). Important Life History Aspects of the Chilean Jack Mackerel. Trachurus symmetricus murphyi. Investigaciones Pesqueras 36, 67–83.
Serra R., Gili R. (1995). “Identificación De Anillos Anuales En Otolitos De Chilean Jack Mackerel,” in Informe De Taller, IFOP. Junio 1995.(Chile: Correspond to a technical report of IFOP)
Siskey M. R., Wilberg M. J., Allman R. J., Barnett B. K., Secor D. H. (2016). Forty Years of Fishing: Changes in Age Structure and Stock Mixing in Northwestern Atlantic Bluefin Tuna (Thunnus Thynnus) Associated With Size-Selective and Long-Term Exploitation. ICES J. Mar. Sci. 73, 2518v2528. doi: 10.1093/icesjms/fsw115
Stransky C., Gudmundsdottir S., Sigurdsson T., Lemvig S., Nedreaas K., Saborido-Rey F. (2005). Age Determination and Growth of Atlantic Redfish (Sebastes Marinus and S. Mentella): Bias and Precision of Age Readers and Otolith Preparation Methods. ICES J. Mar. Sci. 62, 655–670. doi: 10.1016/j.icesjms.2005.01.018
Stuiver M., Polach H. A. (1977). Reporting of C-14 Data. Radiocarbon 19, 355–363. doi: 10.1017/S0033822200003672
Suyama S., Oshima K., Nakagami M., Ueno Y. (2006). Seasonal Change in the Relationship Between Otolith Radius and Body Length in Age-Zero Pacific Saury Cololabis Saira. Fis. Sci. 75, 325v333. doi: 10.1007/s12562-008-0039-z
Taylor P. R. (2002). Stock Structure and Population Biology of the Peruvian Jack Mackerel, Trachurus Symmetricus Murphyi. New Zealand Fisheries Assessment Report 2002/21 (Wellington, New Zealand: Ministry of Fisheries).
Uriarte A., Rico I., Villamor B., Duhamel E., Duenas C., Aldanondo N., et al. (2016). Validation of Age Determination Using Otoliths of the European Anchovy (Engraulis Encrasicolus L.) in the Bay of Biscay. Mar. Freshw. Res. 67, 951–966. doi: 10.1071/MF15092
Wakefield C. B., O’Malley J. M., Williams A. J., Taylor B. M., Nichols R. S., Halafihi T., et al. (2017). Ageing Bias and Precision for Deep-Water Snappers: Evaluating Nascent Otolith Preparation Methods Using Novel Multivariate Comparisons Among Readers and Growth Parameter Estimates. ICES J. Mar. Sci. 74, 193–203. doi: 10.1093/icesjms/fsw162
Waldron M. (1994). Validation of Annuli of the South African Anchovy, Engraulis Capensis, Using Daily Otolith Growth Increments. ICES J. Mar. Sci. 51, 233–234. doi: 10.1006/jmsc.1994.1022
Waldron M., Kerstan M. (2001). Age Validation in Horse Mackerel (Trachurus Trachurus) Otoliths. ICES J. Mar. Sci. 58, 806–813. doi: 10.1006/jmsc.2001.1071
Keywords: Chilean jack mackerel, age validation, daily increment, modal progression analysis, radiocarbon analysis, growth model
Citation: Cerna F, Moyano G, Valero C, Muñoz L, Plaza G and Campana SE (2022) Bomb Radiocarbon, Otolith Daily Increments and Length Modes Validate Age Interpretations of Chilean Jack Mackerel (Trachurus murphyi). Front. Mar. Sci. 9:906583. doi: 10.3389/fmars.2022.906583
Received: 28 March 2022; Accepted: 15 June 2022;
Published: 20 July 2022.
Edited by:
Oscar Sosa-Nishizaki, Center for Scientific Research and Higher Education in Ensenada (CICESE), MexicoReviewed by:
Nathan Smith, Texas Parks and Wildlife Department, United StatesYasuhiro Kamimura, Japan Fisheries Research and Education Agency (FRA), Japan
Alba Jurado-Ruzafa, Centro Oceanográfico de Canarias (CN IEO, CSIC), Spain
Copyright © 2022 Cerna, Moyano, Valero, Muñoz, Plaza and Campana. This is an open-access article distributed under the terms of the Creative Commons Attribution License (CC BY). The use, distribution or reproduction in other forums is permitted, provided the original author(s) and the copyright owner(s) are credited and that the original publication in this journal is cited, in accordance with accepted academic practice. No use, distribution or reproduction is permitted which does not comply with these terms.
*Correspondence: Guido Plaza, Z3VpZG8ucGxhemFAcHVjdi5jbA==