- 1Jiangsu Key Laboratory of Marine Bioresources and Environment, Jiangsu Ocean University, Lianyungang, China
- 2Co-Innovation Center of Jiangsu Marine Bio-industry Technology, Jiangsu Ocean University, Lianyungang, China
- 3Jiangsu Key Laboratory of Marine Biotechnology, Jiangsu Ocean University, Lianyungang, China
- 4Key Laboratory of Marine Ecosystem and Biogeochemistry, Second Institute of Oceanography, Ministry of Natural Resources, Hangzhou, China
Clams are commercially important species and are a vital component of the offshore ecosystem. Investigations on microplastics (MPs) in clams have attracted public attention recently, for its ecological and food safety risks. However, knowledge gaps still exist regarding MPs in commercial clams from the intertidal zone of the South Yellow Sea (SYS), China, an important clam habitat. In this study, to investigate the distribution of MPs in clams of the SYS, four species of clams were collected from nine sites in different tide zones (high, middle, and low tides) in three coastal areas (Nantong, Yancheng and Lianyungang). The abundance and characteristics of MPs, including size, shape, color and composition, were determined using microscope and micro-Fourier transform infrared (FTIR) spectroscopy. Furthermore, to evaluate the MPs purification ability of clams, the abundance of MPs in various tissues was examined by microscopic observation after being purified in filtered seawater for 72 h. The results showed that there were significant differences in the abundance of MPs in clams from different regions, with the most serious pollution in the high-tide zone. Moreover, the lowest MPs abundance was found in Yancheng (5.07 ± 2.73 items/individual, 1.16 ± 0.78 items/g), while Lianyungang was found with the most abundant MPs (7.52 ± 2.68 items/individual, 3.94 ± 3.00 items/g). The size of MPs in Ruditapes philippinarum (1,329.99 μm) was significantly higher than in other species. The MPs in clams were characterized by fiber in shape, black-grey, blue-green and white-transparent in color and rayon in composition. Moreover, it was found that the MPs originally present in various tissues tended to migrate and accumulate into the mantle of the clam during depuration, and after 72 h of depuration, removing the mantle could reduce nearly half amount of microplastics in clams. Overall, our study revealed the microplastic distribution in commercial clams from the intertidal zone of the SYS and provided suggestions for the safety of aquatic production.
Introduction
Since the 1950s, plastic products have been mass-produced and widely used in human society due to their light weight, low cost and durability (Barnes et al., 2009). Annual global plastic production increased exponentially and reached 367 million tons in 2020 (Plastics Europe, 2021). It has been estimated that 60% of all plastics that have been produced were discarded in landfills or in the natural environment, and 12,000 million tons of plastic waste will accumulate in the environment by 2050 if current trends continue (Geyer et al., 2017). Microplastics (MPs) were defined as plastics with a particle size of less than 5 mm in the environment (Thompson et al., 2004). When classified according to the origin of microplastics, they can be divided into primary and secondary microplastics (Koehler et al., 2015). Primary microplastics are originally manufactured as microscopic particles, for example, those used in polyethylene microbeads for facial-cleansers or cosmetics, drug delivery media, industrial abrasives for sandblasting, or in plastic pre-production pellets (Cole et al., 2011; Horton et al., 2017). Secondary microplastics result from the gradual fragmentation of large plastic debris under the effect of physical, chemical and biological processes (Weinstein et al., 2016). Both primary and secondary microplastics are ubiquitous in marine environments and available for organisms due to the diverse densities of polymers (Lusher et al., 2013). In addition, MPs are not only pollutants by themselves, but also have a high adsorption capacity for chemical contaminants (Lee et al., 2014) such as heavy metals (Ta and Babel, 2020; Li et al., 2020b) and polycyclic aromatic hydrocarbons (PAHs) (Tang et al., 2018), and bring them into the food web, causing potential hazards to human health (Hou et al., 2020).
The intertidal zone is the transition zone between land and ocean, which has more human activity than other marine environments and can receive MPs from both terrestrial and marine sources (Cole et al., 2011; Zhang et al., 2020; Zhang et al., 2022). Therefore, microplastic pollution in the intertidal zone has been a matter of grave concern. Wang et al. (2020) found that the abundance of MPs in intertidal sediments of the coast in China was 309 ± 81 items/kg dry weight, and rayon (RY), polypropylene (PP), polyethylene terephthalate (PET) and polyethylene (PE) were the major types of MPs. Wu et al. (2020) found that the MPs abundance in the surface sediments of the intertidal zone during the neap tide cycles was higher than that in the spring tide cycles and that the abundance of microplastics in all sediment samples was negatively correlated with the strength of hydrological processes i.e., submergence time and flow velocity. Clams are the most common organisms in the intertidal zone, and their habits (burrowing and filtering water) determine their close contact with sediments and water bodies (Guo and Xu, 2016). In the intertidal zone, the abundance of MPs in sediments showed differences at different locations, and clams are widely distributed in various regions (Heo et al., 2013; Díaz-Jaramillo et al., 2021). Accordingly, by quantifying the amount of MPs in clams, we can understand the contaminant level in the intertidal zone and provide a reliable warning signal for environmental monitoring of MPs.
The South Yellow Sea (SYS) has a coastline of more than 888.945 km and is rich in marine biological resources. In 2019, the annual catch of marine shellfish in the SYS exceeded 37,905 tons (Bureau of Fisheries, Ministry of Agriculture and Rural Affairs. P. R. China, 2020), among which the most common shellfish were clams. Furthermore, as low-trophic level species, clams have the risk of MPs accumulating in the body and entering the human body through consumption, especially the coastal population (Leung et al., 2021). Currently, there is no relevant report on the per capita intake of clams from the coastal areas of the SYS. Since clams are molluscs, China’s per capita intake of molluscs in 2018 was 9.93 kg/person/year according to the relevant data from FAOSTAT (FAOSTAT, 2018). This study revealed the occurrence, quantity and types of MPs in four common commercial clams at different tide zones in the intertidal zone of the SYS. The distribution and migration of MPs in organs during the self-purification process were studied to assess the risk of microplastic pollution caused by consuming clams. The results of this study provide new recommendations for reducing food health risks associated with consuming wild clams.
Materials and methods
Study area
A total of nine intertidal sites (S1-S9) in three coastal areas of the South Yellow Sea, adjacent to the three developed urban cities, i.e., Nantong, Yancheng, Lianyungang, are selected to collect the clam samples in September 2019 (Figure 1). These areas are important clam habitats with frequent human activities and thus are more likely to receive microplastic pollution. Each area contains three sites, with each site sampled in three situations as high, middle, and low tide zones. Additionally, sampling was conducted in each area for three days, while for the low tide sampling, only one station was collected each day.
Sample collection and preparation
The sample collection was carried out at the lowest tide, and the sediments isolated from the sea surface were collected in the order from the low tide zone to the high tide zone. Three sediment quadrats of 25 cm × 25 cm × 30 cm were randomly selected in the high tide, middle tide and low tide areas at each site, and then the organisms were screened out using a sieve with a 1 mm aperture. A total of 276 clams were collected, which could be divided into four species, including Mactra veneriformis, Ruditapes philippinarum, Meretrix pethechialis, and Cyclina sinensis. After sieving, the clams were quickly sealed into aluminum foil bags and transferred in a cooler (-5°C) to the laboratory for storage at -20°C pending processing and analysis. Furthermore, an additional 30 clams of each species were collected in Lianyungang for a depuration experiment. Half of them were dissected and digested immediately after being transferred to the laboratory, and the other half was kept in filtered seawater (20 L seawater, 15 individual per tank, aerated throughout the process) for 72 h at 20°C before dissection and digestion. The seawater used to purify the clams was collected from the intertidal zone of Lianyungang, with a salinity of 29‰, and then filtered with glass microfiber membrane (pore size 2.7 μm, Whatman Grade GF/D) and aerated for 24 hours, to avoid microplastic pollution and prevent the death of clams due to lack of oxygen in the seawater.
Quality assurance and control
All chemical reagents and deionized water were filtered with glass microfiber membrane (pore size 2.7 μm, Whatman Grade GF/D) prior to use. All instruments and equipment used in the experiment were rinsed with deionized water and then cleaned three times with alcohol (75%). Since MPs are widely distributed in the indoor environment (Gasperi et al., 2018), the number of laboratory operators was minimized, and outdoor air circulation was reduced to avoid airborne MPs contamination in the laboratory. Operators scrubbed their hands and forearms with alcohol (75%) three times before the experiment, and were equipped with white cotton lab coats, disposable latex gloves and masks throughout the experiment. Five procedural blanks without clam samples were set up during the processing of each batch (8-12 clams/batch) to correct for MPs contamination under laboratory conditions.
Dissection and digestion
After thawing, clam shells were rinsed using distilled water to remove the influence of foreign matter such as sediment, and individual shell length, height and width were measured using vernier calipers. The total weight and soft tissue weight (wet weight) of the clams were measured with a precision electronic balance (BS124S, Sartorius electronic balance, Beijing). The clams were placed on a metal tray and the soft tissues were separated from the shell using a scalpel, dissecting scissors and forceps, where the samples in the depuration experiment were separated in order of foot, mantle, gills and viscera. The separated tissues were immediately transferred to a beaker and 100 mL of 10% KOH was added and covered with aluminum foil. They were then placed in an oscillating incubator (DKZ-3, Shanghai Yiheng, China) for 24h at 60°C, 90 rpm for digestion (Ding et al., 2021). The digestion solution was filtered onto glass microfiber membrane (pore size 2.7 μm, Whatman Grade GF/D) using a filter device with a Büchner funnel (AP-01P, Autoscience, China). The glass microfiber membrane was then placed in a clean petri dish with a lid and dried completely at 40°C for further observation.
Observation and identification of MPs
A Nikon SMZ 1500 N stereo microscope (Nikon, Japan) with a charge coupled device (CCD) camera was used to observe, photograph and measure (taking the largest cross section of MP particles as the particle size) suspected MP particles and classify them according to their shape, size and color. Due to the large number of suspected MPs observed (1,700 pieces), approximately 20% of the filters were randomly selected from each region for analysis using the transmittance mode of a micro-Fourier Transformed Infrared Spectroscope (Nicolet iN10, Thermo Fisher Scientific, USA). The specific parameters were spectral range of 650-4000 cm-1, resolution of 8 cm-1, pore size range from 50×50 to 150×150 mm, scanning times of 64. The polymers were subsequently identified using OMNIC software with comparison to standard spectral libraries, and polymers with spectral matches higher than 70% were accepted as MPs (Thompson et al., 2004; Su et al., 2020). All data used in this study to calculate abundance and to determine color, shape and size were based on visual identification.
Statistical analysis
SPSS v.23 software was used for data analysis, and data from each treatment conformed to a normal distribution (Shapiro-Wilk, P > 0.05) and variance could be considered equal (Levene’s test, P > 0.05). Differences in the abundance of MPs before and after self-depuration in each species of the clam were assessed using t-test (paired-samples t-test). One-way analysis of variance (ANOVA) was used to assess differences in MPs abundance in clams from different areas, tide zones and species. A two-way ANOVA was used to evaluate the effects of species and areas on the size of MPs in clams. Least significant difference was conducted for ANOVA post hoc investigation. A 95% confidence interval was set for all tests.
Results
Characteristics of clams
A total of 276 clam samples were collected from nine stations in three areas (Table 1). M. veneriformis was 113 items (25 items for high-tide zone, 34 items for middle-tide zone, 54 items for low-tide zone), R. philippinarum was 61 items (11 items for high-tide zone, 22 items for middle-tide zone, 28 items for low-tide zone), M. pethechialis was 42 (9 items for high-tide zone, 20 items for middle-tide zone, 13 items for low-tide zone) and C. sinensis was 60 (20 items for high-tide zone, 26 items for middle-tide zone, 14 items for low-tide zone). The weight, soft tissue weight, shell length, shell height and shell width of clams was 11.58 ± 5.67 g, 4.23 ± 2.52 cm, 3.83 ± 0.55 cm, 2.60 ± 1.15 cm and 2.28 ± 0.93 cm, respectively.
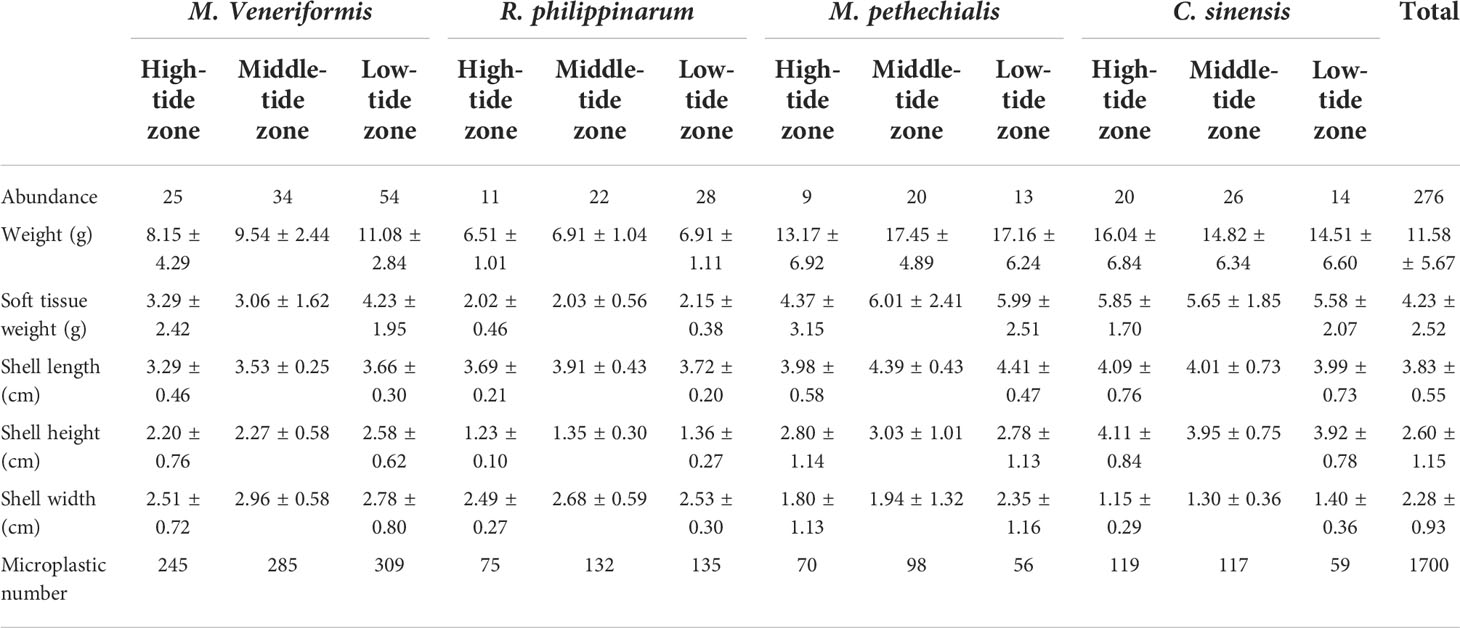
Table 1 A summary of the characteristics of the clam samples. Biological parameters are expressed as mean ± SD.
Spatial and interspecific distribution of microplastics in clams
The potential contamination from air and reagents was ignored because our procedural blanks (0.15 ± 0.38 items/batch) were inappreciable compared to the abundance level in the clams (6.16 ± 2.87 items/batch). A total of 1,700 MPs particles were isolated from 276 clams, and the distribution of MPs in clams from different intertidal zone of SYS was first shown and presented as mean ± SD (Figure 2). When normalized to items/individual, the highest abundance of MPs was recorded in M. veneriformis at high-tide zone in Nantong (11 ± 3 items/individual) and the lowest abundance was found in M. pethechialis at low-tide zone in Yancheng (3.4 ± 0.89 items/individual). In terms of items/g, M. veneriformis at high-tide zone in Lianyungang (9.54 ± 4.42 items/g) and M. pethechialis at low-tide zone in Yancheng (0.46 ± 0.18 items/g) had the highest and lowest MPs abundance, respectively. Whether it’s items/individual or items/g, one-way ANOVA showed that MPs abundance in different regions was significantly different for each species of clam, and clams in Lianyungang had the highest MPs abundance (Figure S1). Additionally, the MPs abundance in each species of clams in Lianyungang is significantly higher than that in Nantong and Yancheng except M. veneriformis when considering items/individual. M. veneriformis from Nantong had significantly higher MPs abundance than that from Yancheng, while the other three hadn’t.
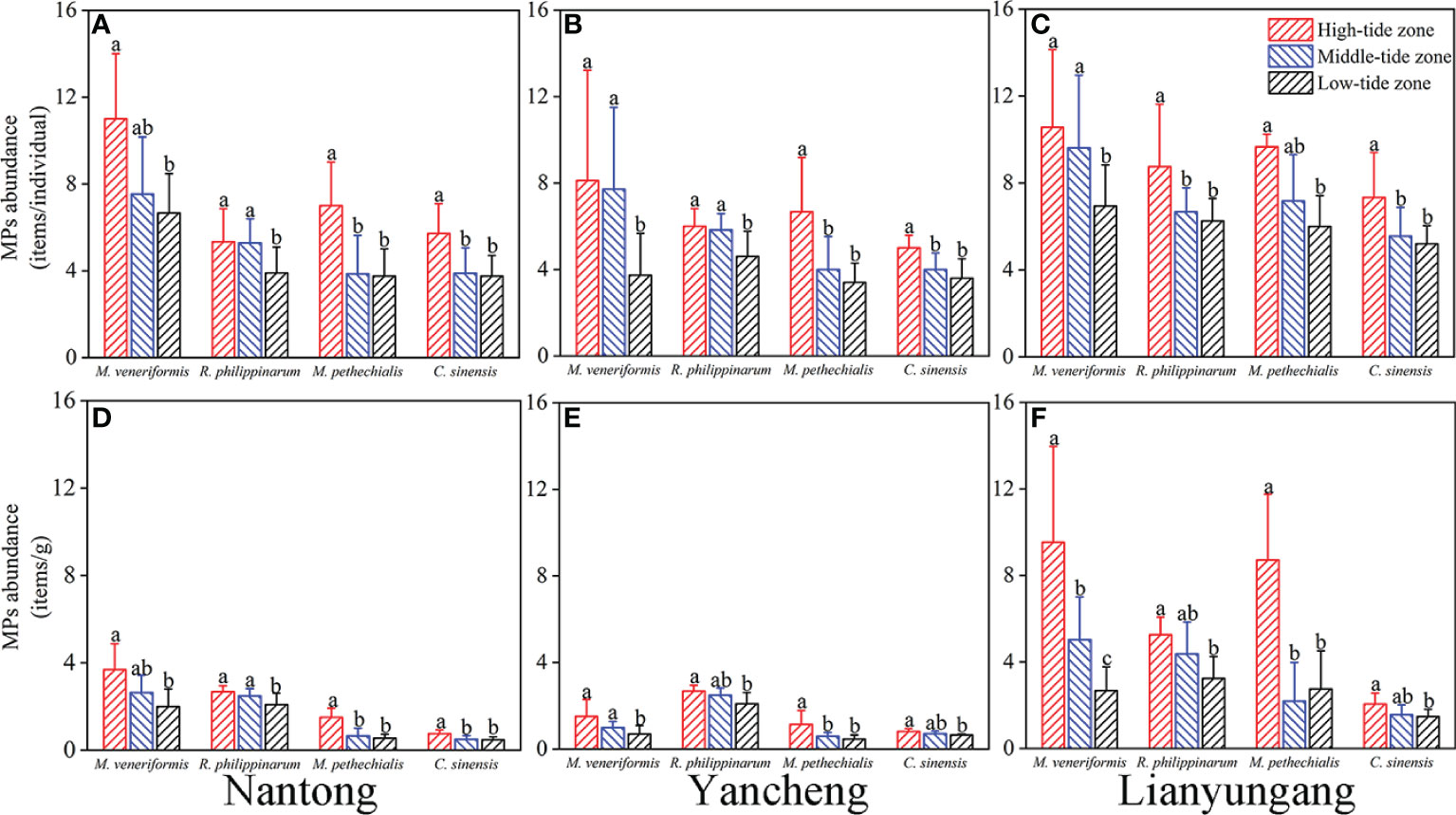
Figure 2 Abundance of MPs (mean ± SD) in different tide zones (high-tide zone, middle-tide zone and low-tide zone) of four species of clams from different sites in three intertidal zone of South Yellow Sea normalized to items per individual (A–C) and items per gram wet weight (D–F). Different letters above the bars indicate significant differences between tide zones of the same species in the same area (One-way ANOVA, P < 0.05).
Distribution and concentration of microplastics in different tide zones were also investigated. Figure 2 showed the microplastic abundance of clams collected at different tide zones within the same species in the same area. The MPs abundance of clams of high-tide zone was significantly higher than that of low-tide zone regardless of the areas and species, items/individual or items/g. Moreover, the MPs abundance in clams from the middle-tide zone is generally between the high-tide zone and low-tide zone. The MPs abundance of M. veneriformis at three tide zones in Lianyungang (9.54 ± 4.42 items/g, 5.03 ± 1.98 items/g and 2.67 ± 1.10 items/g) were significantly different from each other.
The abundance of MPs in different species of clams in each region was shown in Figure S2. When normalized to items/individual, the abundance in M. veneriformis in Nantong (7.76 ± 2.79 items/individual) was significantly higher than that in the other three clams. There was no significant difference between the four clams in Yancheng. The abundance of MPs in M. veneriformis in Lianyungang (8.67 ± 3.20 items/individual) was significantly higher than that in C. sinensis (6.00 ± 1.69 items/individual), while R. philippinarum (6.90 ± 1.73 items/individual) and M. pethechialis (7.38 ± 2.10 items/individual) were between M. veneriformis and C. sinensis. In terms of items/g, MPs abundance in M. veneriformis (2.53 ± 1.06 items/g) and R. philippinarum (2.15 ± 0.66 items/g) were significantly higher than that in M. pethechialis (0.81 ± 0.49 items/g) and C. sinensis (0.59 ± 0.20 items/g) in Nantong. In contrast, the abundance of MPs in M. veneriformis in Yancheng (0.97 ± 0.59 items/g) decreased, and MPs abundance in R. philippinarum (2.32 ± 0.49 items/g) was significantly higher than the other three species. In Lianyungang, the abundance of MPs in M. veneriformis (5.04 ± 3.63 items/g) and R. philippinarum (4.11 ± 1.39 items/g) was significantly higher than that in C. sinensis (1.68 ± 0.50 items/g), and MPs abundance in M. pethechialis (3.87 ± 3.37 items/g) was in the middle level.
Distribution of MPs in different tissues during depuration
In order to explore the migration of microplastics in clams, the variation tendency of MPs abundance in different organs after depuration was investigated (Figure 3). We found that the abundance of MPs in the viscera of four species of clams was significantly higher than that in the other three organs before depuration, and the MPs abundance in the viscera was significantly reduced after depuration. Through depuration, the MPs abundance in the gills and foot decreased slightly, and the abundance of MPs in the mantle showed an upward trend, while the MPs abundance in the mantle of C. sinensis increased significantly. Moreover, the distribution of MPs in each organ of M. veneriformis and R. philippinarum tended to be even after depuration, with significant differences only between viscera and foot. After depuration, the abundance of MPs in the mantle and viscera of M. pethechialis and C. sinensis was significantly higher than that in the gills and foot.
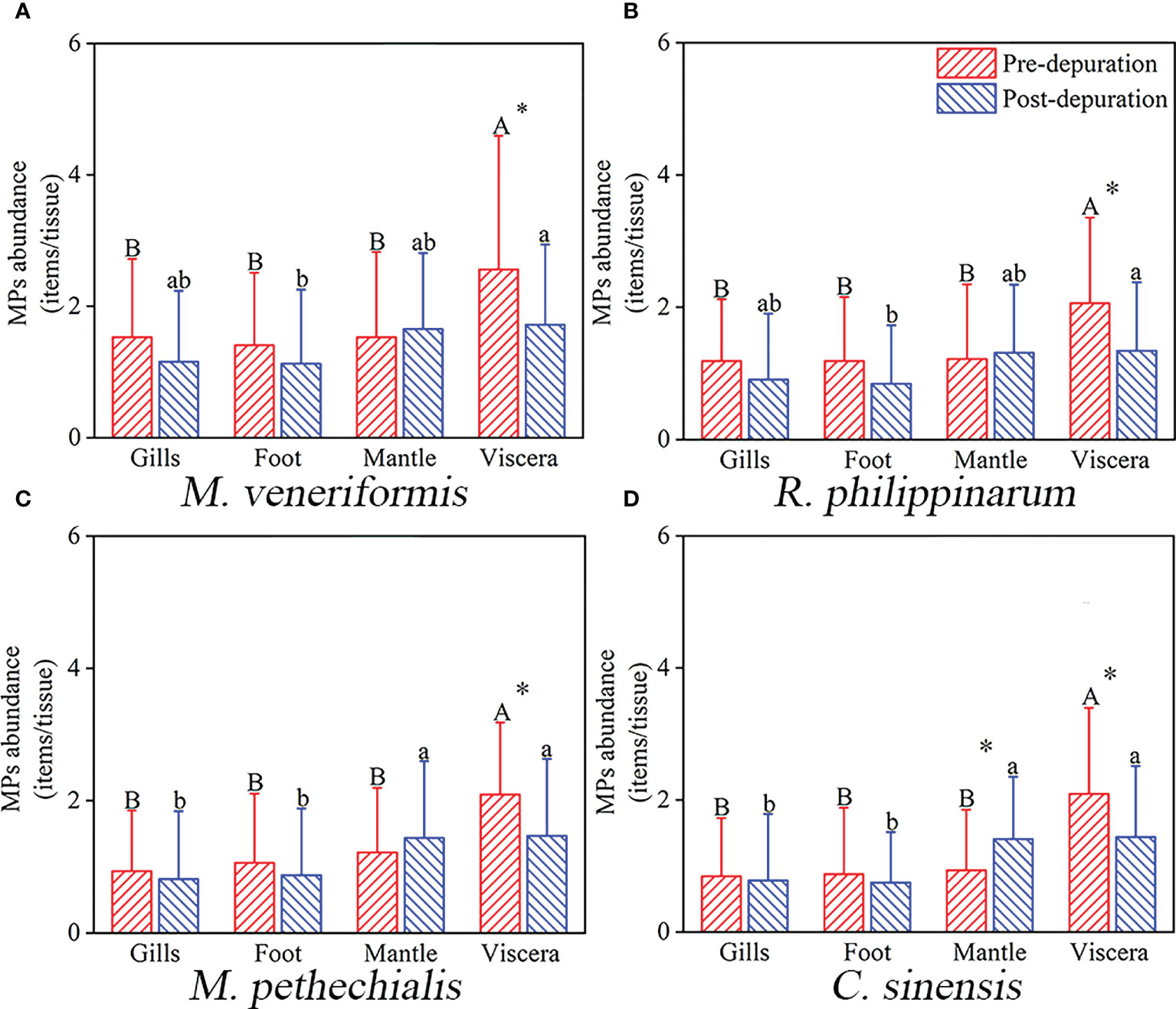
Figure 3 Abundance of MPs (mean ± SD) in different tissues (gills, foot, mantle and viscera) of M. Veneriformis (A), R. philippinarum (B), M. pethechialis (C) and C. sinensis (D) from the intertidal zone of South Yellow Sea. The different capital letters above the bars indicate the significant differences between the tissues before depuration, while lowercase letters indicate post-depuration (One-way ANOVA, P < 0.05). * indicates that the tissues have changed significantly after depuration (paired-samples t-test, P < 0.05).
Shape, color, size and polymer composition of MPs in clams
Four morphotypes of MPs were detected in 276 clam samples, including fiber, film, fragment and microbead (Figure 4). In Nantong, the most abundant morphotype was fiber (96.67%), followed by fragment (2.96%) and film (0.37%), but no microbead was detected. Moreover, all four morphotypes were detected in Yancheng and Lianyungang. The morphology distribution of microplastics in clam in Yancheng was fiber (91.54%), fragment (6.07%), microbead (1.74%) and film (0.65%), while fiber (96.57%), fragment (2.86%), film (0.43%) and microbead (0.14%) were found in clam in Lianyungang.
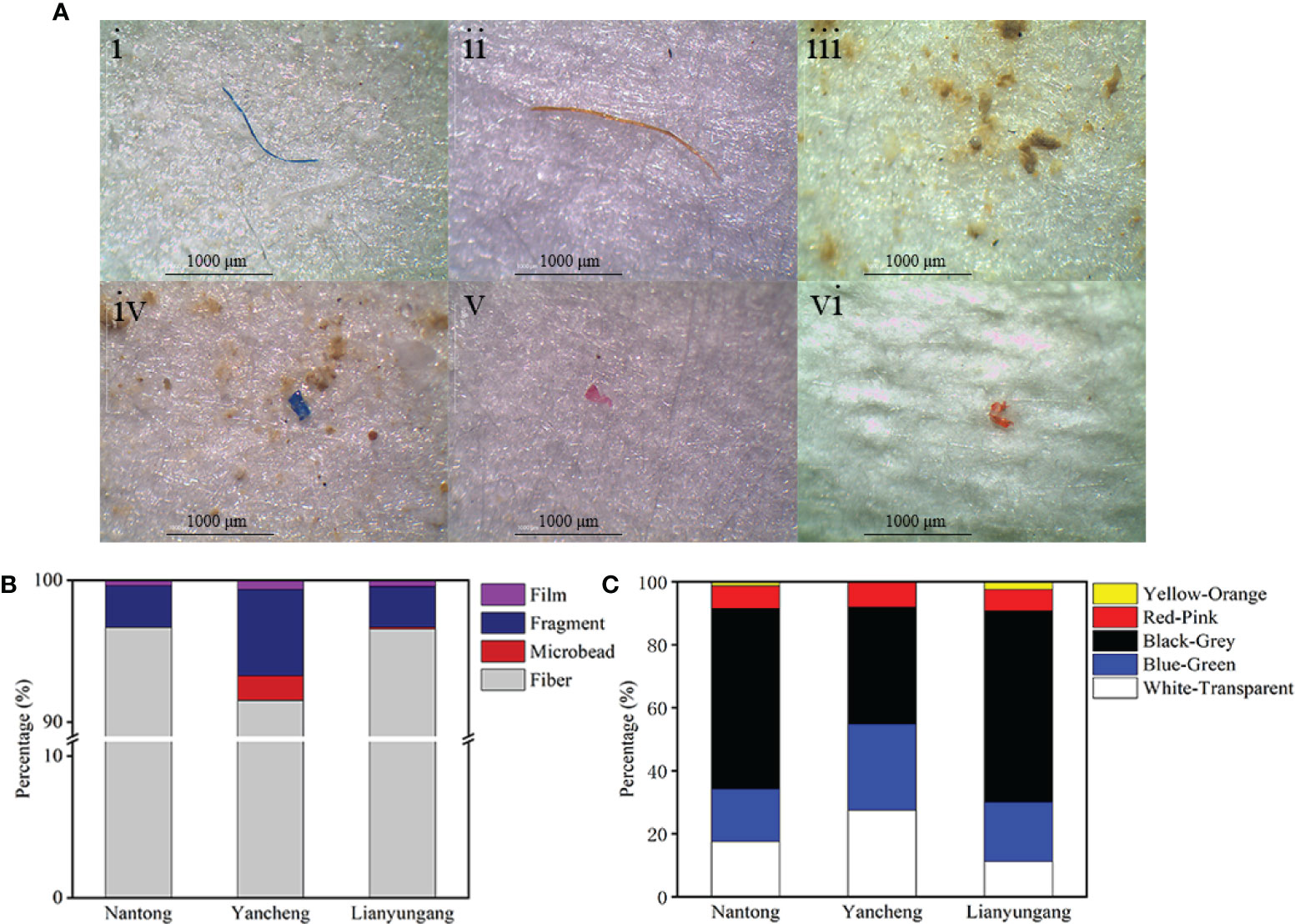
Figure 4 Micrographs of several typical MPs (A) and the shape (B) and color (C) distribution of MPs observed in clam samples. (i) blue fiber, (ii) orange fiber, (iii) white microbead, (iv) blue fragment, (v) red fragment, (vi) red film.
Color proportions of MPs in clams from different regions were also calculated (Figure 4). Four colors were observed in clams in all three regions, including black, white, blue and red, with additional yellow detected in Nantong and Lianyungang. In Yancheng, the color with the highest proportion was black (37.09%), followed by white (27.55%) and blue (27.33%), while red (8.03%) had the lowest proportion. Black was the dominant form in clam in Nantong (57.22%) and Lianyungang (60.66%), and the proportion of other colors in Nantong from high to low was white (17.59%), blue (16.67%), red (7.22%) and yellow (1.30%), while in Lianyungang it was blue (18.74%), white (11.30%), red (6.87%) and yellow (2.43%).
The particle size distribution of MPs in different species and regions of clams was shown in Figure 5. When measured the MPs in all clam samples, the particle size of MPs ranged from 28.86 to 4,993.24 μm, with the smallest being found in M. veneriformis in Yancheng and the largest being found in M. pethechialis in Lianyungang. Two-way ANOVA indicated that species (F=6.818, df=2, P <0.001) had a significant impact on the particle size of MPs, while region (1.062, df=2, p=0.346) did not. Post-hoc LSD test showed that the average MPs particle size in R. philippinarum (1,329.99 μm) was significantly higher than the other three species. The abundance of MPs decreased exponentially with the increase of particle size (y=e6.578-0.001x, R2 = 0.9657). The proportion of MPs with a size of 0-1,000 μm was 58.41%, and those with a size of 2,500-5,000 μm accounted for 9.06%.
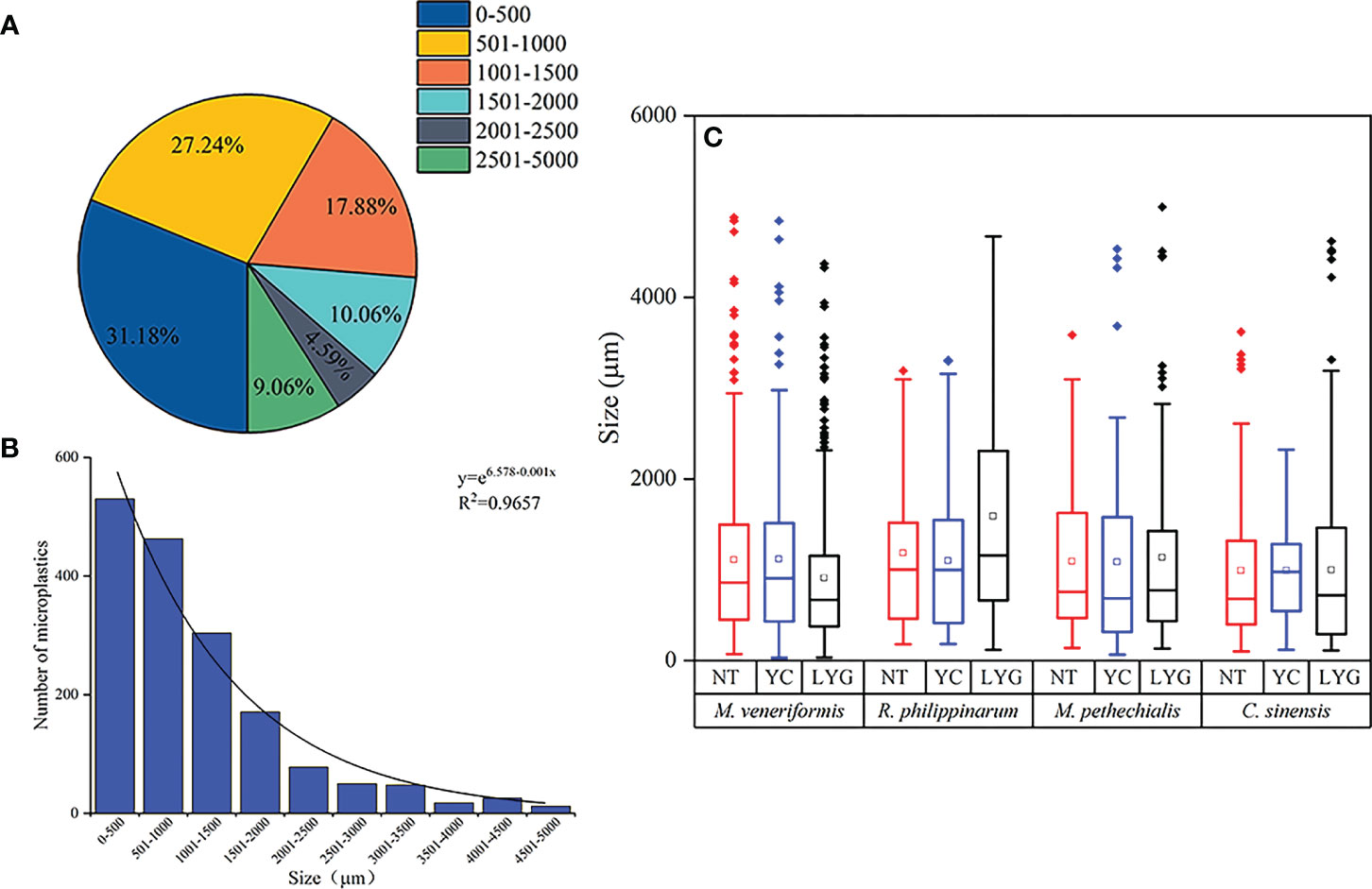
Figure 5 Particle size distribution of MPs identified in all clam samples (A, B) and four species from three intertidal zone (C) of South Yellow Sea.
Three hundred potential microplastics were identified and 229 items were identified as polymers. A total of six polymers were detected (Figure 6), and their proportions in descending order were rayon (RY, 41.48%), polyethylene terephthalate/polyester (PET/Polyester, 22.27%), cellophane (CP, 12.66%), polypropylene (PP, 9.17%), polyethylene (PE, 8.73%), nylon (3.06%) and others (2.62%).
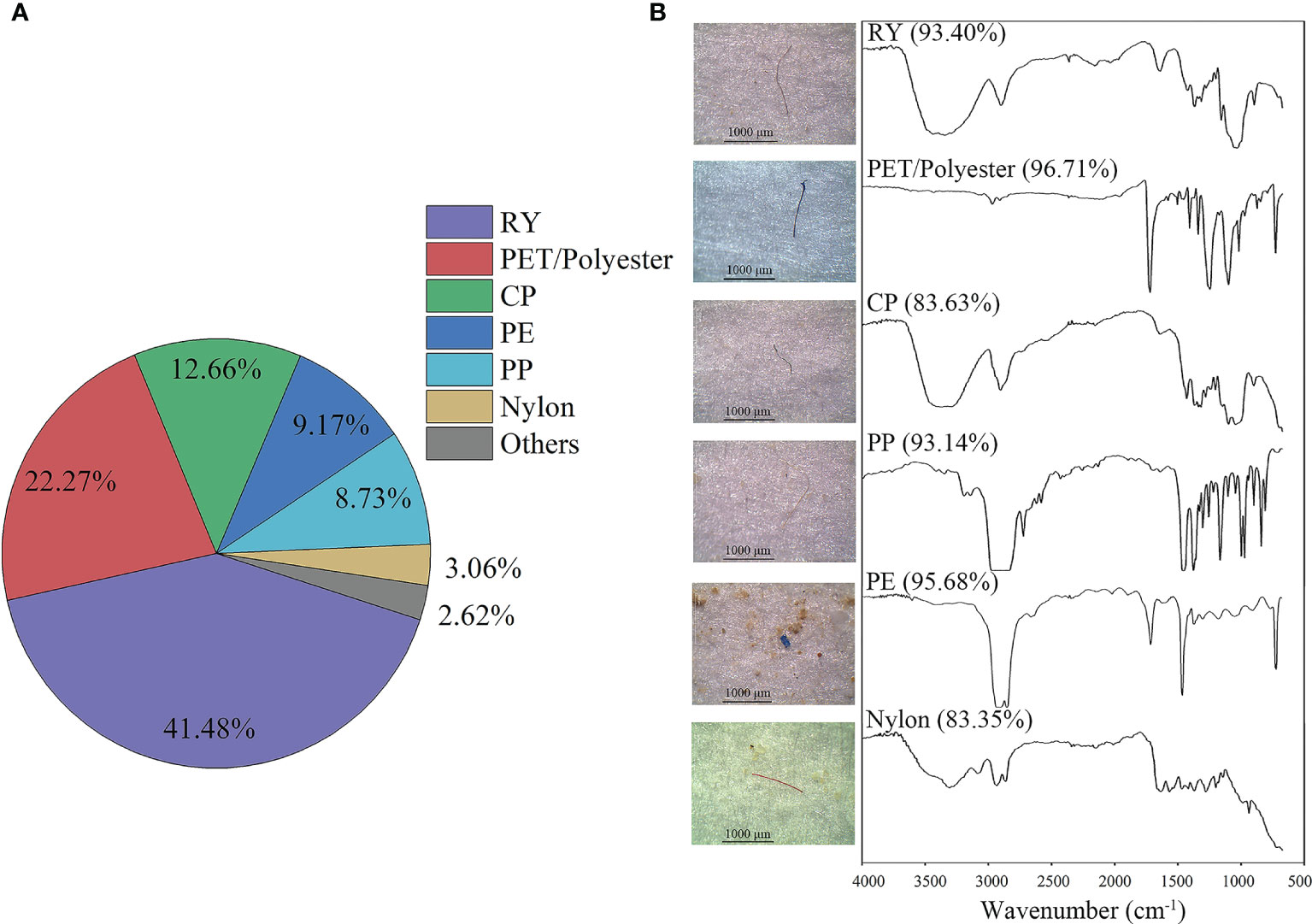
Figure 6 Type distribution (A) and material spectra (B) identified by μ-FTIR microscope of the MPs observed in clam samples.
Discussion
MPs pollution level of commercial clams
In this study, the MPs pollution of four major commercial clams from the intertidal zone of SYS was studied. The results showed that all species of clams were found to ingest MPs regardless of geographic location and tide zone. The average abundance of MPs in clams was 6.16 ± 2.87 items/individual (2.30 ± 2.56 items/g), and ranged from 4.92 ± 1.58 items/individual (1.00 ± 0.58 items/g) in C. sinensis to 7.42 ± 3.52 items/individual (2.90 ± 2.79 items/g) in M. veneriformis. Previous studies have confirmed the presence of microplastics in crabs and fish in the SYS, with abundances ranging from 2.00 ± 2.00 - 9.81 ± 8.08 items/individual (0.80 ± 1.09 - 22.71 ± 24.56 items/g) and 13.54 ± 2.09 items/individual- 22.21 ± 1.70 items/individual (1.61 ± 0.56 items/g- 11.19 ± 1.28 items/g), respectively (Feng et al., 2019; Zhang et al., 2021). They had higher levels of microplastic pollution than clams, possibly due to the fact that clams are a low-trophic level species and consume less food.
Our investigation showed that the level of microplastic pollution in clams in Lianyungang (7.52 ± 2.68 items/individual and 3.94 ± 3.00 items/g) area was higher than those in Yancheng (5.07 ± 2.73 items/individual and 1.16 ± 0.78 items/g) and Nantong (5.87 ± 2.68 items/individual and 1.76 ± 1.15 items/g). Current studies have confirmed that river input, shipping, and mariculture are important sources of microplastics (Cau et al., 2019; Feng et al., 2020b; Li et al., 2020a; Liu et al., 2021), while Lianyungang has a port with very intensive human activities, many rivers entering the sea, and mariculture areas, which may be the reason for the high pollution level in Lianyungang area. In addition, the abundance of MPs in the clams collected in the high tide area was significantly higher than that in the low tide area. This may be caused by more frequent human activities in high tide zones, such as fishing port operations, storage of breeding equipment, and disposal of domestic garbage, which will cause more serious microplastic pollution.
The abundance of MPs in molluscs from this and previous studies is summarized in Table 2. When compared with molluscs in other coastal areas in China, we found that the four species of clams from the intertidal zone of SYS had an intermediate level of MPs contamination. The abundance of MPs in the clams from the intertidal zone of SYS was higher than that in the four molluscs from the Liaohe Estuary (1.42 ± 1.41 items/g), the Crassostrea hongkongensis from the Maowei Sea (4.7 ± 0.3 items/individual, 0.8 ± 0.2 items/g) and the four bivalves from the Qingdao coast (0.5-3.3 items/individual) (Zhu et al., 2019; Ding et al., 2021; Wang et al., 2021a). Among them, when calculated as items/g, the MPs abundance in R. philippinarum from the coast of Qingdao (4.5-20.1 items/g) was higher than this study (Ding et al., 2021). In addition, the level of MPs pollution in clams from the SYS was comparable to those of mussels along the coast of China (1.5-7.6 items/individual, 0.9-4.6 items/g), and lower than that of the nine species of bivalves in the Chinese market (4.3-57.2 items/individual, 2.1-10.5 items/g) (Li et al., 2015; Li et al., 2016). Compared to molluscs in other regions of the world, clams from the intertidal zone of SYS had higher contamination levels than Donax cuneatus from India (0.29-2.7 items/individual, 0.64-1.3 items/g), Mytilus edulis from Belgium (0.26-0.51 items/g) and six species of molluscs from Bizerta (1.03 ± 0.36 items/g); lower than five species of molluscs from the Persian Gulf of Iran (3.7-17.7 items/individual, 0.2-21 items/g) and wild M. edulis from Canada (34 items/individual) (De Witte et al., 2014; Mathalon and Hill, 2014; Naji et al., 2018; Abidli et al., 2019; Narmatha Sathish et al., 2020). Overall, the MPs pollution of clams from the intertidal zone of SYS was at a relatively high level in the world.
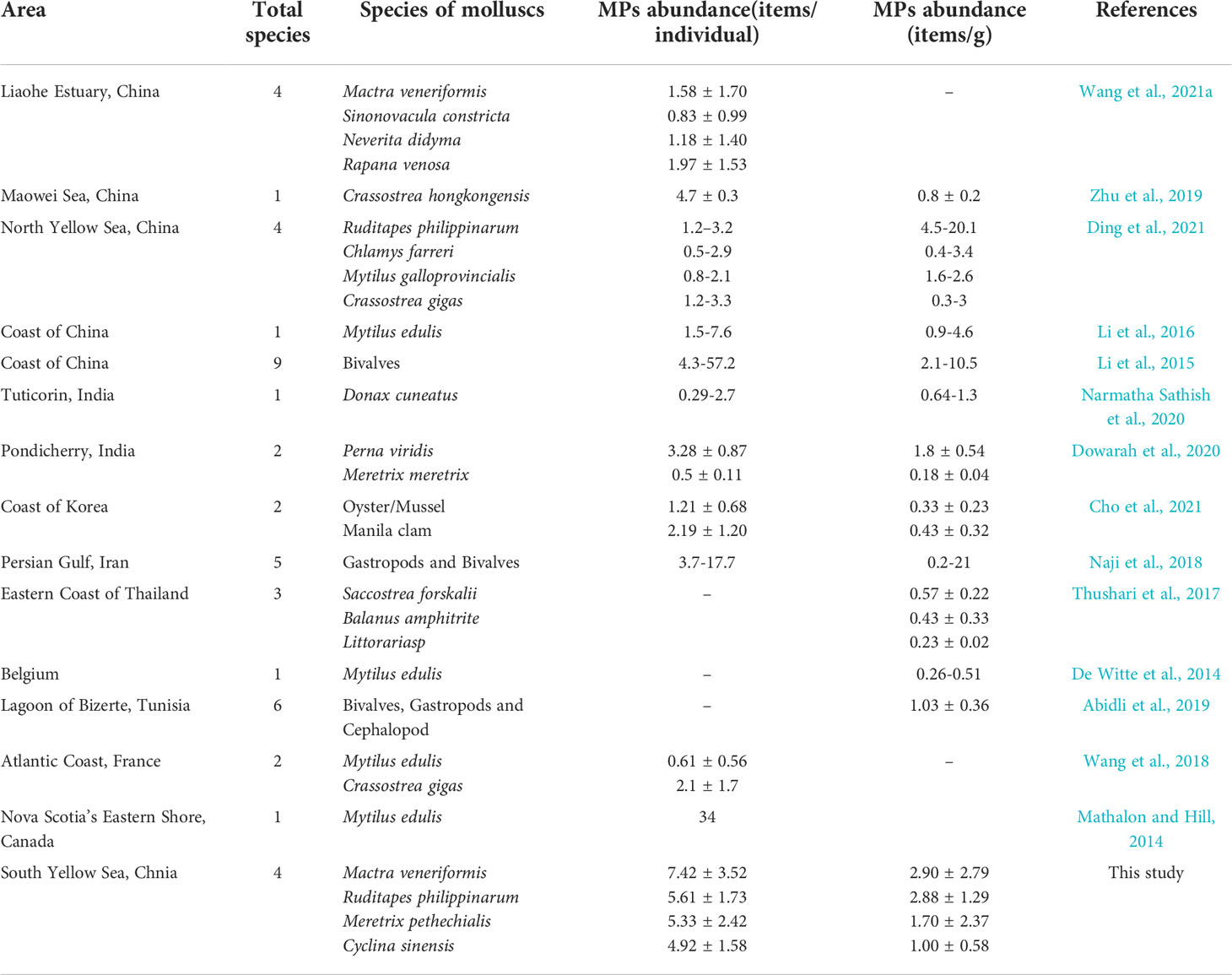
Table 2 A summary of MPs abundances (mean values) found in main molluscs in this and previous studies.
Characterization of MPs in clams
Previous studies have shown that fibers are the most abundant microplastic shape in the Yellow Sea, and similar results have been demonstrated in many studies related to MPs contamination in organisms (Wang et al., 2018; Zhu et al., 2018; Feng et al., 2019; Feng et al., 2020a; Zhang et al., 2020). Fibers dominated all microplastics detected in clams from the intertidal zone of SYS, which is similar to other studies on marine bivalves, fish and crabs (Feng et al., 2019; Ding et al., 2021; Zhang et al., 2021). In this study, the proportion of microplastic particles with a particle size of less than 1000 μm accounted for more than half, which may be related to the distribution of MPs in the marine environment (Wang et al., 2018; Sun et al., 2018). In addition, previous studies have shown that small particles of MPs are more readily ingested by organisms (Browne et al., 2008). The particle size of MPs in R. philippinarum was significantly higher than that of the other three species, which may be due to its smaller volume. Previous studies have shown that the filtration rate of clams increases with decreasing volume (Narmatha Sathish et al., 2020), thereby acquiring more plastic particles with larger particle sizes and making them difficult to expel. In terms of color and material, the predominant colors in this study were black-grey, blue-green and white-transparent, and the predominant material was RY followed by PET/Polyester and CP, which are similar to the characteristics of the microplastics found in the sediments of Hazhou Bay, Lianyungang (Li et al., 2020c). The color and material of MPs in bivalves varied in different areas, which may be due to the fact that bivalves are filter-feeding organisms with no tendency to capture MPs, and their microplastic characteristics may be related to environment media (Phuong et al., 2018; Abidli et al., 2019; Cho et al., 2021). The stress response of bivalves when they encounter danger and their digging habit lead to close contact with the sediment, and in addition, when the sediment was disturbed, MPs in the sediment were re-suspended into the overlying water column and filtered by them (Mathalon and Hill, 2014; Oberbeckmann et al., 2015; Christoph et al., 2017). RY and CP are semi-synthetic cellulose, which, although not traditional petroleum-based plastics, have recently been recognized as plastics (Li et al., 2016; Li et al., 2018a). In recent years, RY has been used in a wide range of applications such as garment manufacturing, upholstery and industry, while CP is often used instead of petroleum-based plastics in the packaging of goods as well as in coatings (Brasier, 1986; Benyathiar et al., 2015; Yang et al., 2015). Polyester is an important fabric type and PET is an imitation of polyester, so they may be derived from domestic sewage (Wang et al., 2019). The presence of PP, PE and Nylon was also detected in this study, and since the SYS has four large fishing grounds (Lüsi Fishing Ground, Dasha fishing ground, Fishing grounds of Changjiang estuary and Haizhou Bay fishing ground) with a developed fishing industry, they may originate from aquaculture appliances (Park et al., 2004; Andrady, 2011).
The risk of microplastics in commercial clams
According to the calculation of the abundance of MPs in clams in this study, the per capita amount of MPs entering the human body through clam consumption in China was 22,839 items/person/year (FAOSTAT, 2018). Due to the differences in dietary habits between regions and species of molluscs, the above data has certain deviations. Therefore, when studying the MPs entering the human body through clam consumption in the coastal areas of the SYS, we investigated the consumption of the four clam species in this study by a total of 314 people in three areas and obtained a result of 15,801.69 g (total weight)/person/year. Converting from the proportions in this study, the soft tissue intake of clams obtained was 5,777.99 g/person/year, resulting in a calculated amount of 13,289.38 items/person/year of MPs entering the human body through clam consumption per capita in the coastal areas of the SYS. Compared to the exposure levels of MPs brought by consumption of molluscs in other regions, this study has similar levels with 11,970 items/person/year in Italy (Mytilus galloprovincialis) (Cho et al., 2019), 11,000 items/person/year in France (M. edulis and Crassostrea gigas) (Wang et al., 2018) and 176-10380 items/person/year in Hong Kong (Perna viridis) (Leung et al., 2021), which is higher than India’s 397.79 items/person/year (P.viridis and Meretrix meretrix) (Dowarah et al., 2020) and Iran’s 4,800 items/person/year (5 species of molluscs) (Naji et al., 2018). It can be seen that the annual per capita intake of MPs through the consumption of clams by the coastal population from the coastal areas of the SYS is at a relatively high level worldwide.
MPs ingestion by clam can have a range of negative effects. Exposure to MPs affects the clam’s filtration and alters energy allocation (Sussarellu et al., 2016; Green et al., 2017; Woods et al., 2018). Exposure of clams to MPs can lead to significant reductions in total oocyte number, oocyte diameter and sperm velocity, and embryogenesis can be affected (Sussarellu et al., 2016; Luan et al., 2019);. As an important primary consumer in offshore ecosystem, clams are a food source for crabs, fish and seabirds, and the negative effects of MPs exposure are detrimental to ecosystem stability and energy transfer (Li et al., 2019; Li et al., 2022; Zhou et al., 2022).
Removal of MPs in clams
Seafood is an important source of protein intake for coastal populations, and clams, as commercially important organisms, are at high risk of MPs contamination (Leung et al., 2021). In order to reduce the risk of human exposure to MPs through the consumption of clams, we investigated the self-purification of MPs by clams and preliminarily explored the pattern of MPs changes between different organs in clams. The residence time of MPs in bivalves is related to various factors, e.g., density, size, material and shape (Brillant and MacDonald, 2000; Li et al., 2018b);. It is verified that MPs in bivalves have a high excretion rate within 72 h in many studies (Woods et al., 2018; Sendra et al., 2020; Wang et al., 2021b). Based on this phenomenon, 72 h was selected as the depuration time in this study. The results of the purification experiment in this study showed that the abundance of MPs in the gills, foot and viscera decreased, while the abundance in the mantle increased after clams were cultured with filtered seawater for 72 h. As the first tissues that exposed to MPs, the removal rates of MPs in gills and foot were lower than that in viscera, which may most likely due to the recapture of expelled MPs. Bivalves expel microplastics by producing pseudofeces through rejection mechanisms for inedible particles (Baudrimont et al., 2019). The mantle is the only route for excretion (Sendra et al., 2020), so the accumulation of MPs in the mantle has a certain hysteresis, which leads to a decrease in the abundance of MPs in other tissues after 72 h, but an increase in the abundance of MPs in the mantle. The results are similar to the study on the ingestion of MPs by Mytilus coruscus, which were exposed to MPs particles of different particle sizes for 87 h and purified with artificial seawater free of MPs particles for 87 h, and found that the abundance of MPs in the digestive tract decreased significantly after purification while the MPs abundance in mantle increased significantly (Wang et al., 2021b). The above results show that clams can self-purify MPs in their bodies, and we found that the abundance of MPs per gram of soft tissue in the purified clams decreased by 0.35 items (the removal rate was 16.35%), and this value reached 0.89 items (the removal rate was 41.90%) after the removal of the mantle. The biological characteristics of the clams collected in this study indicate that the average mass of the mantle was only 0.76 ± 0.44 g, which was of low economic value. In summary, we recommend using clean artificial seawater to temporarily raise clams for 72 h before consumption, and remove the mantle when eating. This method may reduce the risk of human exposure to MPs in the coastal areas of the SYS caused by clam consumption by 5,142.41 items/person/year.
Conclusion
This study identified the presence of MPs in clams in the SYS, with the most contamination at the high-tide zone. The abundance of MPs was the highest in the clams from Lianyungang, and the MPs size was largest in R. philippinarum (1,329.99 μm). These MPs were dominated by fiber in shape, black-grey, blue-green and white-transparent in color and rayon in composition. Additionally, purifying clams in filtered seawater for 72 h and removing the mantle before consumption can effectively reduce the intake of MPs in the human body. Taken together, the effect of MPs on the human body and the mechanism of migration in different tissues of clams need further research.
Data availability statement
The raw data supporting the conclusions of this article will be made available by the authors, without undue reservation.
Author contributions
RT: conceptualization, methodology, writing – original draft, writing - review and editing, supervision, and funding acquisition. TZ: investigation, methodology, formal analysis, and visualization. KS: conceptualization, methodology, and writing - review and editing. YC: investigation. YS: investigation. WH: methodology. ZF: conceptualization, formal analysis, writing – original draft, writing - review and editing, supervision, and funding acquisition. All authors contributed to the article and approved the submitted version.
Funding
This work was supported by Jiangsu Industry University Research Cooperation Project (BY2020428), Open Project of Key Laboratory of Coastal Salt Marsh Ecology and Resources, Ministry of Natural Resources (KLCSMERMNR2021105), Provincial Policy Guidance Program (North Jiangsu Science and Technology Special Project) (SZ-LYG202131), Universities Natural Science Research Project of Jiangsu Province (20KJB170029), Jiangsu Province Postdoctoral Research Foundation (2021K212B), Postdoctoral Research Foundation of Lianyungang (LYG2021002), Postgraduate Research & Practice Innovation Program of Jiangsu Province, China (Grant No. KYCX20_2882) and the Priority Academic Program Development of Jiangsu Higher Education Institutions.
Acknowledgments
The authors thank all of the members of S105 for their support for the study.
Conflict of interest
The authors declare that the research was conducted in the absence of any commercial or financial relationships that could be construed as a potential conflict of interest.
Publisher’s note
All claims expressed in this article are solely those of the authors and do not necessarily represent those of their affiliated organizations, or those of the publisher, the editors and the reviewers. Any product that may be evaluated in this article, or claim that may be made by its manufacturer, is not guaranteed or endorsed by the publisher.
Supplementary material
The Supplementary Material for this article can be found online at: https://www.frontiersin.org/articles/10.3389/fmars.2022.905923/full#supplementary-material
References
Abidli S., Lahbib Y., Trigui El Menif N. (2019). Microplastics in commercial molluscs from the lagoon of bizerte (Northern Tunisia). Mar. pollut. Bull. 142, 243–252. doi: 10.1016/j.marpolbul.2019.03.048
Andrady A. L. (2011). Microplastics in the marine environment. Mar. pollut. Bull. 62, 1596–1605. doi: 10.1016/j.marpolbul.2011.05.030
Barnes D. K., Galgani F., Thompson R. C., Barlaz M. (2009). Accumulation and fragmentation of plastic debris in global environments. Philos. Trans. R Soc. Lond B Biol. Sci. 364 (1526), 1985–1998. doi: 10.1098/rstb.2008.0205
Baudrimont M., Arini A., Guégan C., Venel Z., Gigault J., Pedrono B., et al. (2019). Ecotoxicity of polyethylene nanoplastics from the north Atlantic oceanic gyre on freshwater and marine organisms (microalgae and filter-feeding bivalves). Environ. Sci. pollut. Res. 27, 3746–3755. doi: 10.1007/s11356-019-04668-3
Benyathiar P., Selke S., Auras R. (2015). Effect of irradiation on the biodegradation of cellophane films. J. Polym Environ. 23 (4), 449–458. doi: 10.1007/s10924-015-0740-9
Brasier J. (1986). Cellophane–the deceptively versatile non-plastic. Mater Design. 7 (2), 65–67. doi: 10.1016/S0261-3069(86)80003-6
Brillant M. G. S., MacDonald B. A. (2000). Postingestive selection in the sea scallop, placopecten magellanicus (Gmelin): the role of particle size and density. J. Exp. Mar. Biol. Ecol. 253, 211–227. doi: 10.1016/s0022-0981(00)00258-6
Browne M. A., Dissanayake A., Galloway T. S., Lowe D. M., Thompson R. C. (2008). Ingested microscopic plastic translocates to the circulatory system of the mussel, mytilus edulis (L). Environ. Sci. Technol. 42 (13), 5026–5031. doi: 10.1021/es800249a
Bureau of Fisheries, Ministry of Agriculture and Rural Affairs. P. R. China. (2020). China Fishery statistical yearbook (Beijing: China Agriculture Press).
Cau A., Avio C. G., Dessì C., Follesa M. C., Moccia D., Regoli F., et al. (2019). Microplastics in the crustaceans nephrops norvegicus and aristeus antennatus: Flagship species for deep-sea environments? Environ. pollut. 255 (Pt 1), 113107. doi: 10.1016/j.envpol.2019.113107
Cho Y., Shim W. J., Jang M., Han G. M., Hong S. H. (2019). Abundance and characteristics of microplastics in market bivalves from south Korea. Environ. pollut. 245, 1107–1116. doi: 10.1016/j.envpol.2018.11.091
Cho Y., Shim W. J., Jang M., Han G. M., Hong S. H. (2021). Nationwide monitoring of microplastics in bivalves from the coastal environment of Korea. Environ. pollut. 270, 116175. doi: 10.1016/j.envpol.2020.116175
Christoph D. R., Jahnke A., Gorokhova E., Kühnel D., Mechthild S. J. (2017). Impacts of biofilm formation on the fate and potential effects of microplastic in the aquatic environment. Environ. Sci. Technol. Lett. 4 (7), 258–267. doi: 10.1021/acs.estlett.7b00164
Cole M., Lindeque P., Halsband C., Galloway T. S. (2011). Microplastics as contaminants in the marine environment: a review. Mar. pollut. Bull. 62, 2588–2597. doi: 10.1016/j.marpolbul.2011.09.025
De Witte B., Devriese L., Bekaert K., Hoffman S., Vandermeersch G., Cooreman K., et al. (2014). Quality assessment of the blue mussel (Mytilus edulis): comparison between commercial and wild types. Mar. pollut. Bull. 85 (1), 146–155. doi: 10.1016/j.marpolbul.2014.06.006
Díaz-Jaramillo M., Islas M. S., Gonzalez M. (2021). Spatial distribution patterns and identification of microplastics on intertidal sediments from urban and semi-natural SW Atlantic estuaries. Environ. pollut. 273, 116398. doi: 10.1016/j.envpol.2020.116398
Ding J., Sun C., He C., Li J., Ju P., Li F. (2021). Microplastics in four bivalve species and basis for using bivalves as bioindicators of microplastic pollution. Sci. Total Environ. 782, 146830. doi: 10.1016/j.scitotenv.2021.146830
Dowarah K., Patchaiyappan A., Thirunavukkarasu C., Jayakumar S., Devipriya S. P. (2020). Quantification of microplastics using Nile red in two bivalve species perna viridis and meretrix meretrix from three estuaries in pondicherry, India and microplastic uptake by local communities through bivalve diet. Mar. pollut. Bull. 153, 110982. doi: 10.1016/j.marpolbul.2020.110982
FAOSTAT (2018). . Data from: Food and agriculture organization food balance sheet. https://www.fao.org/faostat/en/#data/FBS
Feng Z., Wang R., Zhang T., Wang J., Huang W., Li J., et al. (2020a). Microplastics in specific tissues of wild sea urchins along the coastal areas of northern China. Sci. Total Environ. 728, 138660. doi: 10.1016/j.scitotenv.2020.138660
Feng Z., Zhang T., Li Y., He X., Wang R., Xu J., et al. (2019). The accumulation of microplastics in fish from an important fish farm and mariculture area, haizhou bay, China. Sci. Total Environ. 696, 133948. doi: 10.1016/j.scitotenv.2019.133948
Feng Z., Zhang T., Wang J., Huang W., Wang R., Xu J., et al. (2020b). Spatio-temporal features of microplastics pollution in macroalgae growing in an important mariculture area, China. Sci. Total Environ. 719, 137490. doi: 10.1016/j.scitotenv.2020.137490
Gasperi J., Wright S. L., Dris R., Collard F., Mandin C., Guerrouache M., et al. (2018). Microplastics in air: are we breathing it in? Curr. Opin. Environ. Sci. Health 1, 1–5. doi: 10.1016/j.coesh.2017.10.002
Geyer R., Jambeck J. R., Law K. L. (2017). Production, use, and fate of all plastics ever made. Sci. Adv. 3 (7), 1700782. doi: 10.1126/sciadv.1700782
Green D. S., Boots B., O'Connor N. E., Thompson R. (2017). Microplastics affect the ecological functioning of an important biogenic habitat. Environ. Sci. Technol. 51 (1), 68–77. doi: 10.1021/acs.est.6b04496
Guo C., Xu S. (2016). Research development of the mactridae. J. Biol. 33 (01), 86–91+103. doi: 10.3969/j.issn.2095-1736.2016.01.086
Heo N. W., Hong S. H., Han G. M., Hong S., Lee J., Song Y. K., et al. (2013). Distribution of small plastic debris in cross-section and high strandline on heungnam beach, south Korea. Ocean Sci. J. 48 (2), 225–233. doi: 10.1007/s12601-013-0019-9
Horton A. A., Walton A., Spurgeon D. J., Lahive E., Svendsen C. (2017). Microplastics in freshwater and terrestrial environments: Evaluating the current understanding to identify the knowledge gaps and future research priorities. Sci. Total Environ. 586, 127–141. doi: 10.1016/j.scitotenv.2017.01.190
Hou R., Wu Y., Xu J., Gao K. (2020). Solar UV radiation exacerbates photoinhibition of a diatom by antifouling agents irgarol 1051 and diuron. J. Appl. Phycol. 32, 1243–1251. doi: 10.1007/s10811-020-02048-w
Koehler A., Anderson A., Andrady A., Arthur C., Baker J., Bouwman H., et al. (2015). Sources, fate and effects of microplastics in the marine environment: A global assessment. London: International Maritime Organization. doi: 10.13140/RG.2.1.3803.7925
Lee H., Shim W. J., Kwon J. H. (2014). Sorption capacity of plastic debris for hydrophobic organic chemicals. Sci. Total Environ. 470-471, 1545–1552. doi: 10.1016/j.scitotenv.2013.08.023
Leung M. M., Ho Y. W., Maboloc E. A., Lee C. H., Wang Y., Hu M., et al. (2021). Determination of microplastics in the edible green-lipped mussel perna viridis using an automated mapping technique of raman microspectroscopy. J. Hazard Mater. 420, 126541. doi: 10.1016/j.jhazmat.2021.126541
Li F., Fan J., Hu L., Beardall J., Xu J. (2019). Physiological and biochemical responses of thalassiosira weissflogii (diatom) to seawater acidification and alkalization. ICES J. Mar. Sci. 76 (6), 1850–1859.
Li Q., Feng Z., Zhang T., Ma C., Shi H. (2020a). Microplastics in the commercial seaweed nori. J. Hazard. Mater. 388, 122060. doi: 10.1016/j.jhazmat.2020.122060
Li Z., Gao C., Yang J., Wu L., Zhang S., Liu Y., et al. (2020c). Distribution characteristics of microplastics in surface water and sediments of haizhou bay, lianyungang. Environ. Sci. 41 (07), 3212–3221. doi: 10.13227/j.hjkx.201910005
Li F., Li H. X., Xu T., Li S., Xu J. (2022). Seawater acidification exacerbates the negative effects of UVR on the growth of the bloom-forming diatom skeletonema costatum. Front. Mar. Sci. 9. doi: 10.3389/fmars.2022.905255
Li W., Lo H. S., Wong H. M., Zhou M., Wong C. Y., Tam N. F., et al. (2020b). Heavy metals contamination of sedimentary microplastics in Hong Kong. Mar. pollut. Bull. 153, 110977. doi: 10.1016/j.marpolbul.2020.110977
Li J., Lusher A., Rotchell J. M., Company S. D., Turra A., Bråte I. L. N., et al. (2018b). Using mussel as a global bioindicator of coastal microplastic pollution. Environ. pollut. 244, 522–533. doi: 10.1016/j.envpol.2018.10.032
Li H. X., Ma L. S., Lin L., Ni Z. X., Xu X. R., Shi H. H., et al. (2018a). Microplastics in oysters saccostrea cucullata along the pearl river estuary, China. Environ. pollut. 236, 619–625. doi: 10.1016/j.envpol.2018.01.083
Li J., Qu X., Su L., Zhang W., Yang D., Kolandhasamy P., et al. (2016). Microplastics in mussels along the coastal waters of China. Environ. pollut. 214, 177–184. doi: 10.1016/j.envpol.2016.04.012
Liu K., Zhang Z., Wu H., Wang J., Wang R., Zhang T., et al. (2021). Accumulation of microplastics in a downstream area of a semi-enclosed bay: Implications of input from coastal currents. Sci. Total Environ. 791, 14828. doi: 10.1016/j.scitotenv.2021.148280
Li J., Yang D., Li L., Jabeen K., Shi H. (2015). Microplastics in commercial bivalves from China. Environ. pollut. 207, 190–195. doi: 10.1016/j.envpol.2015.09.018
Luan L., Wang X., Zheng H., Liu L., Luo X., Li F. (2019). Differential toxicity of functionalized polystyrene microplastics to clams (Meretrix meretrix) at three key development stages of life history. Mar. pollut. Bull. 139, 346–354. doi: 10.1016/j.marpolbul.2019.01.003
Lusher A. L., McHugh M., Thompson R. C. (2013). Occurrence of microplastics in the gastrointestinal tract of pelagic and demersal fish from the English channel. Mar. pollut. Bull. 67 (1-2), 94–99. doi: 10.1016/j.marpolbul.2012.11.028
Mathalon A., Hill P. (2014). Microplastic fibers in the intertidal ecosystem surrounding Halifax harbor, Nova Scotia. Mar. pollut. Bull. 81 (1), 69–79. doi: 10.1016/j.marpolbul.2014.02.018
Naji A., Nuri M., Vethaak A. D. (2018). Microplastics contamination in molluscs from the northern part of the Persian gulf. Environ. pollut. 235, 113–120. doi: 10.1016/j.envpol.2017.12.046
Narmatha Sathish M., Immaculate Jeyasanta K., Patterson J. (2020). Monitoring of microplastics in the clam donax cuneatus and its habitat in tuticorin coast of gulf of mannar (GoM), India. Environ. pollut. 266 (Pt 1), 115219. doi: 10.1016/j.envpol.2020.115219
Oberbeckmann S., Loder M. G. J., Labrenz M. (2015). Marine microplastic-associated biofilms - a review. Environ. Chem. 12 (5), 551. doi: 10.1071/en15069
Park C. H., Kang Y. K., Im S. S. (2004). Biodegradability of cellulose fabrics. J. Appl. Polym. Sci. 94 (1), 248–253. doi: 10.1002/app.20879
Phuong N. N., Poirier L., Pham Q. T., Lagarde F., Zalouk-Vergnoux A. (2018). Factors influencing the microplastic contamination of bivalves from the French Atlantic coast: Location, season and/or mode of life? Mar. pollut. Bull. 129 (2), 664–674. doi: 10.1016/j.marpolbul.2017.10.054
Plastics Europe (2021). Plastics-the facts 2020-an analysis of European plastics production, demand and waste data. Frankfurt: Plastics Europe.
Sendra M., Saco A., Yeste M. P., Romero A., Novoa B., Figueras A. (2020). Nanoplastics: From tissue accumulation to cell translocation into mytilus galloprovincialis hemocytes. resilience of immune cells exposed to nanoplastics and nanoplastics plus vibrio splendidus combination. J. Hazard Mater. 388, 121788. doi: 10.1016/j.jhazmat.2019.121788
Sun X., Liang J., Zhu M., Zhao Y., Zhang B. (2018). Microplastics in seawater and zooplankton from the yellow Sea. Environ. pollut. 242 (Pt A), 585–595. doi: 10.1016/j.envpol.2018.07.014
Su L., Sharp S. M., Pettigrove V. J., Craig N. J., Nan B., Du F., et al. (2020). Superimposed microplastic pollution in a coastal metropolis. Water Res. 168, 115140. doi: 10.1016/j.watres.2019.115140
Sussarellu R., Suquet M., Thomas Y., Lambert C., Fabioux C., Pernet M. E., et al. (2016). Oyster reproduction is affected by exposure to polystyrene microplastics. Proc. Natl. Acad. Sci. U S A. 113 (9), 2430–2435. doi: 10.1073/pnas.1519019113
Ta A. T., Babel S. (2020). Microplastics pollution with heavy metals in the aquaculture zone of the chao phraya river estuary, Thailand. Mar. pollut. Bull. 161 (Pt A), 111747. doi: 10.1016/j.marpolbul.2020.111747
Tang G., Liu M., Zhou Q., He H., Chen K., Zhang H., et al. (2018). Microplastics and polycyclic aromatic hydrocarbons (PAHs) in xiamen coastal areas: Implications for anthropogenic impacts. Sci. Total Environ. 634, 811–820. doi: 10.1016/j.scitotenv.2018.03.336
Thompson R. C., Olsen Y., Mitchell R. P., Davis A., Rowland S. J., John A. W., et al. (2004). Lost at sea: where is all the plastic? Sci. 304 (5672), 838. doi: 10.1126/science.1094559
Thushari G. G. N., Senevirathna J. D. M., Yakupitiyage A., Chavanich S. (2017). Effects of microplastics on sessile invertebrates in the eastern coast of Thailand: An approach to coastal zone conservation. Mar. pollut. Bull. 124 (1), 349–355. doi: 10.1016/j.marpolbul.2017.06.010
Wang S., Hu M., Zheng J., Huang W., Shang Y., Kar-Hei Fang J., et al. (2021b). Ingestion of nano/micro plastic particles by the mussel mytilus coruscus is size dependent. Chemosphere. 263, 127957. doi: 10.1016/j.chemosphere.2020.127957
Wang Q., Shan E., Zhang B., Teng J., Wu D., Yang X., et al. (2020). Microplastic pollution in intertidal sediments along the coastline of China. Environ. pollut. 263 (Pt A), 114428. doi: 10.1016/j.envpol.2020.114428
Wang F., Wu H., Wu W., Wang L., Liu J., An L., et al. (2021a). Microplastic characteristics in organisms of different trophic levels from liaohe estuary, China. Sci. Total Environ. 789, 148027. doi: 10.1016/j.scitotenv.2021.148027
Wang T., Zou X., Li B., Yao Y., Li J., Hui H., et al. (2018). Microplastics in a wind farm area: A case study at the rudong offshore wind farm, yellow Sea, China. Mar. pollut. Bull. 128, 466–474. doi: 10.1016/j.marpolbul.2018.01.050
Wang T., Zou X., Li B., Yao Y., Zang Z., Li Y., et al. (2019). Preliminary study of the source apportionment and diversity of microplastics: Taking floating microplastics in the south China Sea as an example. Environ. pollut. 245, 965–974. doi: 10.1016/j.envpol.2018.10.110
Weinstein J. E., Crocker B. K., Gray A. D. (2016). From macroplastic to microplastic: Degradation of high-density polyethylene, polypropylene, and polystyrene in a salt marsh habitat. Environ. Toxicol. Chem. 35 (7), 1632–1640. doi: 10.1002/etc.3432
Woods M. N., Stack M. E., Fields D. M., Shaw S. D., Matrai P. A. (2018). Microplastic fiber uptake, ingestion, and egestion rates in the blue mussel (Mytilus edulis). Mar. pollut. Bull. 137, 638–645. doi: 10.1016/j.marpolbul.2018.10.061
Wu F., Pennings S. C., Tong C., Xu Y. (2020). Variation in microplastics composition at small spatial and temporal scales in a tidal flat of the Yangtze estuary, China. Sci. Total Environ. 699, 134252. doi: 10.1016/j.scitotenv.2019.134252
Yang D., Shi H., Li L., Li J., Jabeen K., Kolandhasamy P. (2015). Microplastic pollution in table salts from China. Environ. Sci. Technol. 49 (22), 13622–13627. doi: 10.1021/acs.est.5b03163
Zhang T., Feng Z., Luo C., Sun Y., Li J., Xu J., et al. (2020). Fluorescence characterization and microbial degradation of dissolved organic matter (DOM) leached from salt marsh plants in the yellow river delta. J. Plant Ecol. 13 (5), 525–537. doi: 10.1093/jpe/rtaa040
Zhang T., Song K., Meng L., Tang R., Song T., Huang W., et al. (2022). Distribution and characteristics of microplastics in barnacles and wild bivalves on the coast of the yellow Sea, China. Front. Mar. Sci. 8. doi: 10.3389/fmars.2021.789615
Zhang T., Sun Y., Song K., Du W., Huang W., Gu Z., et al. (2021). Microplastics in different tissues of wild crabs at three important fishing grounds in China. Chemosphere. 271, 129479. doi: 10.1016/j.chemosphere.2020.129479
Zhou W., Wu H., Huang J., Wang J., Zhen W., Wang J., et al. (2022). Elevated-CO2 and nutrient limitation synergistically reduce the growth and photosynthetic performances of a commercial macroalga gracilariopsis lemaneiformis. Aquaculture 550, 737878. doi: 10.1016/j.aquaculture.2021.737878
Zhu L., Bai H., Chen B., Sun X., Qu K., Xia B. (2018). Microplastic pollution in north yellow Sea, China: Observations on occurrence, distribution and identification. Sci. Total Environ. 636, 20–29. doi: 10.1016/j.scitotenv.2018.04.182
Keywords: clam, Yellow Sea, microplastics, pollution, health risk
Citation: Tang R, Zhang T, Song K, Sun Y, Chen Y, Huang W and Feng Z (2022) Microplastics in commercial clams from the intertidal zone of the South Yellow Sea, China. Front. Mar. Sci. 9:905923. doi: 10.3389/fmars.2022.905923
Received: 28 March 2022; Accepted: 04 July 2022;
Published: 25 July 2022.
Edited by:
Kyung-Hoon Shin, Hanyang University, South KoreaReviewed by:
Xuemei Sun, Yellow Sea Fisheries Research Institute (CAFS), ChinaMohammad Zakeri, Khorramshahr Marine Science and Technology University, Iran
Mitsuharu Yagi, Nagasaki University, Japan
Copyright © 2022 Tang, Zhang, Song, Sun, Chen, Huang and Feng. This is an open-access article distributed under the terms of the Creative Commons Attribution License (CC BY). The use, distribution or reproduction in other forums is permitted, provided the original author(s) and the copyright owner(s) are credited and that the original publication in this journal is cited, in accordance with accepted academic practice. No use, distribution or reproduction is permitted which does not comply with these terms.
*Correspondence: Zhihua Feng, fengzhihua@jou.edu.cn