- 1Key Laboratory of Fisheries Remote Sensing, Ministry of Agriculture and Rural Affairs, East China Sea Fisheries Research Institute, Chinese Academy of Fishery Sciences, Shanghai, China
- 2Fishery and Life Science College, Shanghai Oceanography University, Shanghai, China
Oysters and barnacles are dominant inhabitants of natural and restored oyster reefs around the world, and high areal coverage of barnacles at natural or restored reefs commonly decreases substrate accessibility for oyster settlement. The overall understanding of oyster and barnacle recruitment dynamics provides invaluable information on site selection and strategies employed for oyster reef restoration. This study documented the temporal and spatial patterns of oyster and barnacle recruitment on and near the largest natural intertidal oyster reef (Liyashan) in China during 2019–2020. The oyster Crassostrea sikamea recruitment appeared as a continuous process from June through late November or early December, with the peak in August. Greater recruits of oyster spat occurred on the sheltered inshore at the upstream of the natural oyster reef than on the reef and the nearby open coast (p < 0.05). The barnacle recruitment extended from spring to early winter, with mid-spring and mid-summer peaks. Conversely, higher barnacle recruitment appeared on the natural oyster reef and the nearby open coast than on the sheltered inshore. Across all the monitoring sites, the cumulative recruits of oysters in each of 2019 and 2020 was negatively correlated with those of barnacles (p < 0.05). The inshore sites (SH1 and SH2) with high oyster recruitment and low barnacle recruitment should be recognized as the natural spatfall sites for the natural oyster reef restoration. The separation in the recruitment peak between the oyster and the barnacle indicated that August was the most favorable window for capturing oyster spat through substratum addition to the water around the natural reef.
Introduction
Oyster reef is an important marine habitat that provides a variety of ecosystem services and functions, including water filtration (Grizzle et al., 2006; Grizzle et al., 2008), nitrogen removal (Kellogg et al., 2013; Humphries et al., 2016), habitat provision (Coen et al., 1999; Quan et al., 2009), and shoreline stabilization (Wiberg et al., 2019). Over 85% of oyster reefs around the world have been lost as a result of over-harvesting, disease, pollution, and habitat destruction in the past century (Beck et al., 2011). In response to the loss of natural oyster reefs, ecological restoration efforts are increasingly implemented in North America (Schulte and Burke, 2014), Europe (Preston et al., 2020), Australia (McAfee et al., 2020), and China (Quan et al., 2009; Quan et al., 2012a; Quan et al., 2012b; Quan et al., 2017).
Restoring oyster reefs must address the provision of substrates, broodstock enhancement, and perennial recruitment sufficient for population persistence (Schulte and Burke, 2014). In most cases, oyster habitat restoration often relies on natural recruitment. “Supply-side ecology” emphasizes the importance of recruitment for population replenishment and community process (Wasson et al., 2016). Therefore, understanding recruitment dynamics is essential for the conservation and restoration of oysters and their building reefs. In the USA, long-term monitoring programs have been established to track recruitment dynamics of eastern oyster (Crassostrea virginica) in the Chesapeake Bay (Southworth and Mann, 2004) and in Delaware Bay (Fegley et al., 2003; Powell et al., 2008), and Pacific oyster (Crassostrea gigas) and Olympia oyster (Ostrea lurida) on the west coast (Wasson et al., 2016). The lack of synchrony in large regional scales highlights the need for quantifying local recruitment dynamics of oysters within a particular system (Wasson et al., 2016; Grossman et al., 2020). The overall understanding of local oyster recruitment dynamics can help identify when or where the larval density is greatest, and therefore provides invaluable information on site selection and timing for oyster reef restoration.
Barnacles are dominant inhabitants of natural and restored oyster reefs around the world (Osman et al., 1989; Boudreaux et al., 2009; Johnson and Soltis, 2017). As sessile suspension feeders, barnacles can compete food and space with oysters (Osman et al., 1989; Boudreaux et al., 2009). High areal coverage of barnacles at natural or restored reefs largely decreases substrate accessibility for oyster settlement, and the concomitant lower oyster recruitment terminally results in failure in restoration efforts (Boudreaux et al., 2009). Understanding the duration and intensity of barnacle recruitment is of critical importance to the implementation strategy of oyster-target restoration projects.
The natural reefs formed by Crassostrea spp. historically occupied many estuaries in China (Geng et al., 1991; Quan et al., 2022), but most oyster reefs have been seriously degraded or lost due to coastal development, overfishing, and pollution (Geng et al., 1991; Lau et al., 2020; Quan et al., 2020; Quan et al., 2022). Oyster restoration and enhancement have become increasingly popular in China in recent years (Quan et al., 2009; Quan et al., 2017; Lau et al., 2020), but limited understanding about uncertainty in environmental and biological responses of degraded reef resulted in unsatisfactory efforts. The Liyashan oyster reef, located on the inshore of the southern Yellow sea, is the largest natural intertidal oyster reef in China (Quan et al., 2012c; Quan et al., 2016). Primarily driven by heavy coastal development, approximately 39% of the reef has been lost during 2003–2013 (Quan et al., 2016), and the evident three-dimensional high-relief reefs (approximately 2 m) in the mid-1980s were replaced with the present low-relief bars (less than 0.6 m). From 2013 to 2018, the reef-building oyster Crassostrea sikamea densities have heavily decreased by approximately 90% (Quan et al., 2020).
This study documented the temporal and spatial dynamics in oyster and barnacle recruitment on and near the largest natural intertidal oyster reef in China. Specific objectives were to address two questions about spat recruitment in the Liyashan waters, namely: (1) what were the temporal patterns in oyster and barnacle recruitment within the spawning season? (2) what were the small-scale spatial patterns in oyster and barnacle recruitment in the Liyashan waters? From oyster reef conservation and restoration perspectives, this study was designed to recognize the optimum time and site for supplementing substrates to capture natural oyster spat in the Liyashan waters.
Materials and Methods
Study Area
The Liyashan oyster reef (32° 08′ 22.6″–32° 09′ 22.5″ N, 121° 32′ 33.2″–121° 33′ 22.8″ E, Figure 1) covered 3.3 km2, including the 0.2-km2 natural reef area (footprint), the 0.7-km2 degraded oyster habitat, and the 2.4-km2 intertidal mudflat (Quan et al., 2020). The reef area was composed of 750 reef patches with a vertical relief of 0.2–0.6 m relative to the surrounding degraded habitat or mudflat (Quan et al., 2016; Quan et al., 2020). The tides were semidiurnal, with a range from 2.63 to 3.63 m (National Height Datum 1985) (data from the LYS hydrology monitoring station).
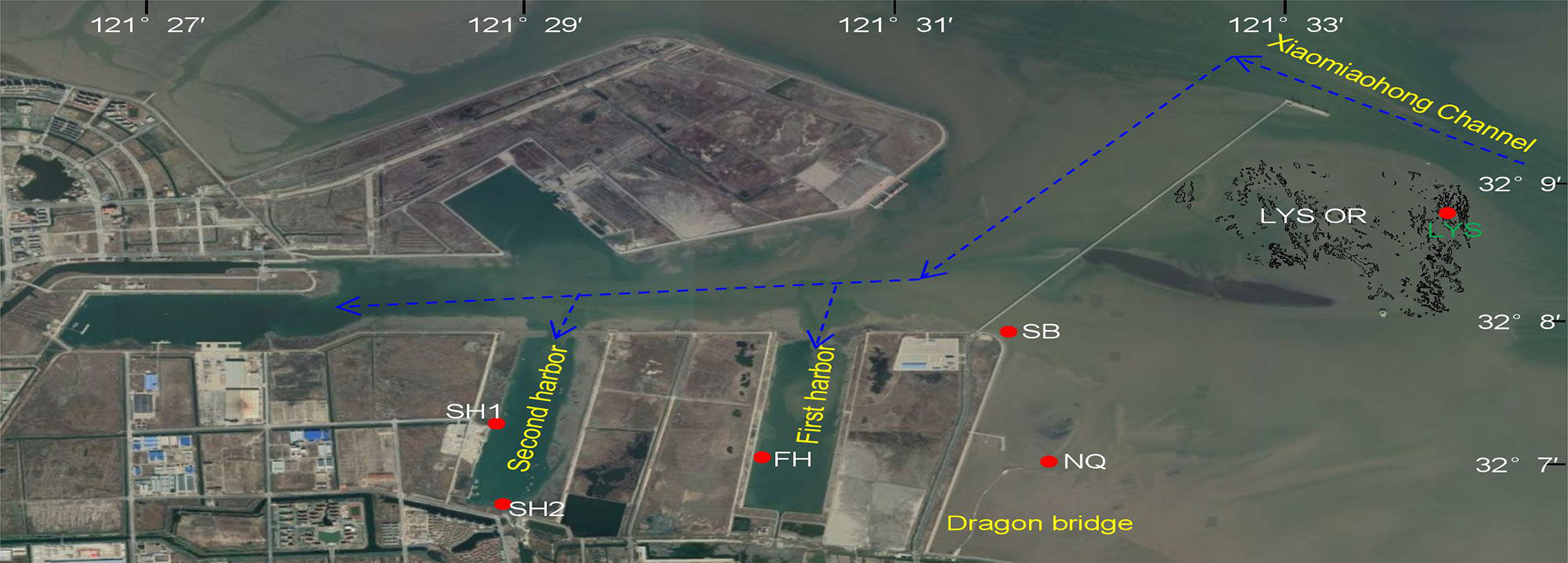
Figure 1 The Liyashan oyster reef (LYS OR) and five no-reef monitoring stations (red circle) for oyster and barnacle recruitment. The arrows indicate the direction of the flooding tide current.
To explore small-scale spatial patterns in the recruitment of oyster and barnacle, one reef station (LYS) on and the five no-reef stations (SB, NQ, FH, SH1, and SH2) near the LYS oyster reef were chosen to monitor oyster and barnacle recruitment. The LYS station is located in the northeastern reef area of the LYS oyster reef. The SB and NQ stations are at the lower intertidal mudflat on open coasts near the natural reef. The FH station is situated in the first harbor that has no river discharge, while the SH1 and SH2 stations in the second harbor are the most inland sites where salinity largely fluctuates due to river discharge. In general, the FH, SH1, and SH2 stations are more sheltered from hydrodynamic and wave exposure relative to the SB, NQ, and LYS stations on open coasts.
Oyster and barnacle recruitment rates were monitored on the LYS and FH stations in 2019 (Figure 1). To find the potential spatfall sites for the natural reef restoration, this study monitored the oyster and barnacle recruitment on the five no-reef stations (NQ, SB, FH, SH1, and SH2) in 2020 (Figure 1).
Sampling Regime
Disarticulated oyster C. sikamea shells with a similar size [shell height: 47.7 ± 1.0 (SE) mm, total surface area: 25.41 ± 0.92 (SE) cm2] from the local shellfish processing plant were used as the settlement substrate. Each experimental unit (shell strings) was created by drilling a hole near the center of 5 weathered left shell valves and stringing them using 1 m of polyethylene wire to provide shells with a 0.1-m vertical interval.
At each monitoring station, the shell strings with 5 replicates were deployed approximately 0.3 m off the bottom and alternated at 5-m intervals along a line parallel to the shore (1.0 m above mean lower low water) on May 15 of 2019 or 2020. Each end of a shell string was attached to 1 steel bar inserted into reef or mud vertically. During monthly or biweekly visits, shell strings were retrieved and replaced using clean experimental units throughout the spawning seasons until December of each year (2019: 7 monitoring periods, a sum of 210 days; 2020: 9 monitoring periods, a sum of 229 days) (Figure 2). After cleaning sediments on the retrieved shell strings using tap water, oyster and barnacle recruits settled on five left valves at each experimental unit were identified and counted with a dissecting microscope, respectively. Surface water temperature and salinity at each monitoring station were simultaneously measured by a salinity meter (YSI).

Figure 2 Schematic maps illustrating the monitoring periods of oyster and barnacle recruitment in 2019 and 2020.
Statistical Analyses
Recruitment rates (spat shell−1 day−1) of oysters or barnacles were calculated by dividing the total number of spats at each experimental unit by the number of shells examined (5 shells) and the number of days within a monitoring period. Before all analyses, the data were log (x + 1) transformed and tested for the normality (Kolmogorov–Smirnov test) and homogeneity of the variances (Cochrane test). If normality and homogeneity were met, a 2-factor (temporal factor: monitoring period, spatial factor: monitoring station) analysis of variance (ANOVA), with Tukey HSD pairwise comparisons (α = 0.05), was performed to examine temporal and spatial dynamics in recruitment rates of oyster or barnacle. If normality and homogeneity were not met, a Kruskal–Wallis one-way ANOVA on ranks with Tukey test (p < 0.05) was used to test temporal or spatial variations in the recruitment.
The cumulative recruitment (spat shell−1) at each station was calculated by the sum of the mean number of spats on each shell throughout the whole monitoring period. As the normality test failed, a Kruskal–Wallis one-way ANOVA on ranks with Tukey test (p < 0.05) was used to test spatial variations in the cumulative recruitment of oyster or barnacle. Correlations in cumulative recruits between the barnacle and the oysters across all the monitoring stations were explored further using Pearson’s product moment correlation coefficients.
Results
Environmental Conditions
During our sampling intervals, water temperatures across all the monitoring stations fluctuated between 8°C and 31°C each year, and were similar among all the monitoring stations (Figure 3). The observed temperatures were highest from mid-July to mid-September (Figure 3). Salinity was consistently lower at the second harbor (SH1 and SH2) than at the FH and three open coast sites (LYS, SB, and NQ) (Figure 3).
Oyster Recruitment
In 2019, the oyster recruitment commenced in mid-June, and terminated earlier at the LYS (mid-November) than at the FH (mid-December) (Figure 4). The Kruskal–Wallis one-way ANOVA on ranks found that there were evident temporal changes in recruitment rates at each of the 2 monitoring stations. During the high recruitment periods (D2–D5 monitoring periods, mid–June–mid-October), greater recruitment rates occurred at the FH than at the LYS (Figure 4, one-way ANOVA on ranks, p < 0.05). The peak recruitment rates (4.5 ± 0.5 spat shell−1 day−1) at the FH were 22 times higher than those (0.2 ± 0.0 spat shell−1 day−1) at the LYS (Figure 4), but the inter-site differences were absent during the low recruitment period (D1, D6, and D7) (Figure 4, one-way ANOVA on ranks, p < 0.05).
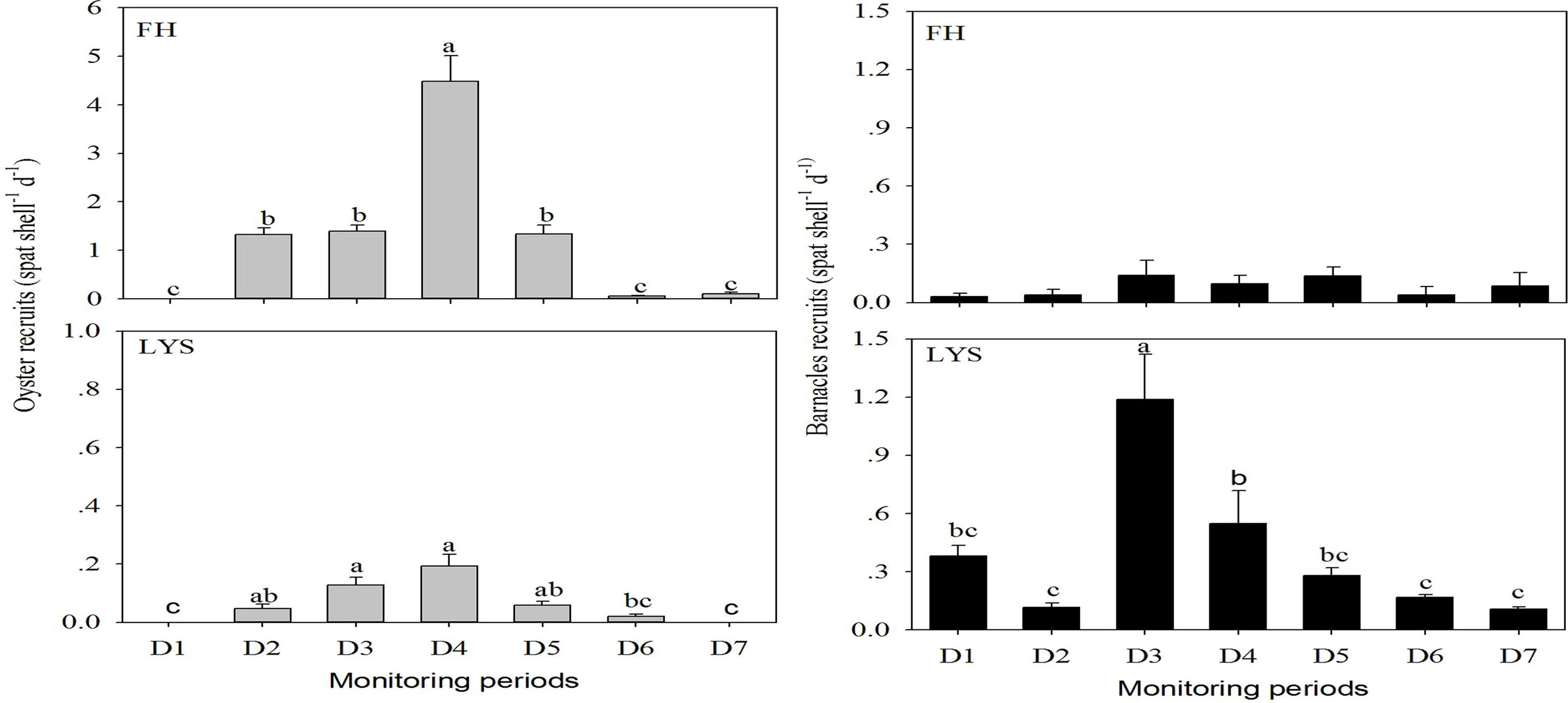
Figure 4 Spatial and temporal variations in the oyster (left panel) and the barnacle (right panel) recruitment (mean ± SE) in the spawning season of 2019. Significant differences were indicated by different lowercase letters.
In 2020, the oyster recruitment at the five stations consistently began in mid-June and peaked in August (Figure 5), but terminated 1 month earlier at the 2 open coast stations (NQ and SB) than at the three sheltered inshore stations (FH, SH1, and SH2) (Figure 5). The recruitment rates showed significant temporal (Figure 5, one-way ANOVA on ranks, p < 0.05) and spatial (one-way ANOVA on ranks, p < 0.05) variations. At each of the five monitoring stations, the peak oyster recruits consistently appeared in August (T4–T5, Figure 5). The maximum recruitment rates (37.0 ± 3.9 spat shell−1 day−1) were documented at the SH2 in the early and mid-August (Figure 5). During high recruitment (from early July to mid-September), the ranks of the oyster recruits among the five stations were as follows: FH = SH1 = SH2 > NQ = SB in the T4 monitoring period (Figure 5, one-way ANOVA on ranks, p < 0.05), SH2 > SH1 > FH = SB > NQ in the T4 monitoring period (Figure 5, one-way ANOVA on ranks, p < 0.05), SH2 = SH1 > SB = FH = NQ in the T5 monitoring period (Figure 5, one-way ANOVA on ranks, p < 0.05), and SH2 > SH1 = FH =SB > NQ in the T6 monitoring period (Figure 5, one-way ANOVA on ranks, p < 0.05). In contrast, the inter-site differences disappeared during the low recruitment (T1–T2 and T7–T9) (Figure 5, one-way ANOVA on ranks, p > 0.05).
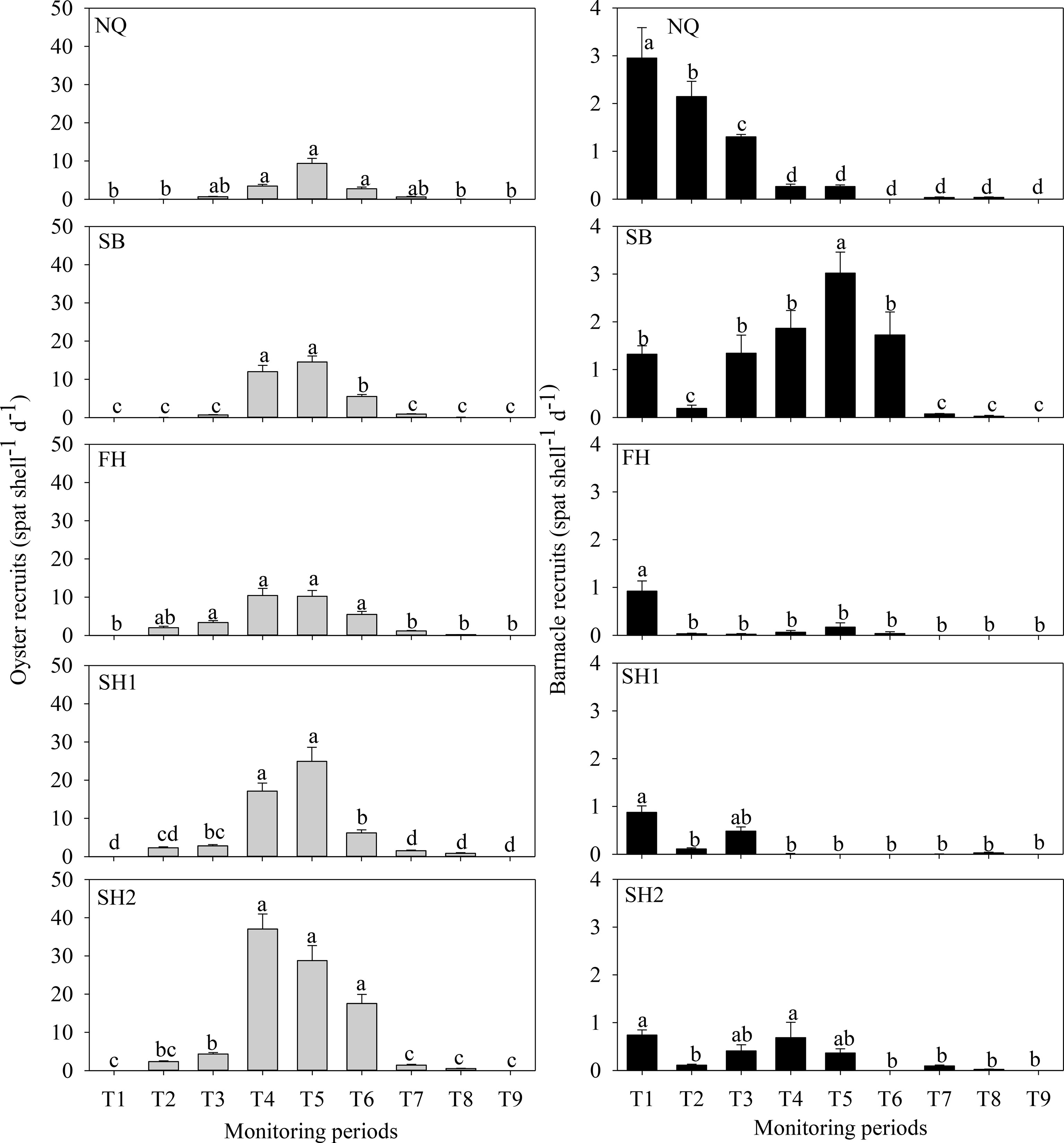
Figure 5 Spatial and temporal variations in the oyster (left panel) and the barnacle (right panel) recruitment (mean ± SE) in the spawning season of 2020. Significant differences were indicated by different lowercase letters (p < 0.05).
Barnacle Recruitment
Within each of 2019 and 2020, the barnacle had earlier and longer recruitment periods than the oyster (Figures 4, 5). Significant temporal and spatial variations were observed in the magnitude of barnacle recruitment, with distinct peaks appearing at each station (Figures 4, 5).
In 2019, the barnacle recruitment at the LYS had significant temporal changes (Figure 4, one-way ANOVA on ranks, p < 0.05), and reached the peak values (1.2 ± 0.2 spat shell−1 day−1) in the D3 period (mid-July to mid-August). In contrast, the recruitment rates at the FH station were consistently low and had no evident peak (Figure 4, one-way ANOVA on ranks, p > 0.05). The LYS had significantly greater barnacle recruitment rates than the FH in the early six monitoring periods (Figure 4, one-way ANOVA on ranks, p < 0.05), while the inter-site differences were absent in the last monitoring period (Figure 4, one-way ANOVA on ranks, p > 0.05).
A two-way ANOVA documented that the barnacle recruitment rates in 2020 showed significant temporal (F8,405 = 30.077, p < 0.001) and spatial (F4,405 = 47.168, p < 0.001) variations, and the interactions (F32,405 = 12.454, p < 0.001) were evident between time and site. Greater barnacle recruits occurred at the 2 open coast stations (NQ and SB) than at the 3 sheltered inshore stations (FH, SH1, and SH2) during the high recruitment periods (T1–T5) (Figure 5, Tukey test, p < 0.05), while the inter-site differences disappeared in the last 4 monitoring periods (T6–T9) (Figure 5, Tukey test, p > 0.05).
Cumulative Oyster and Barnacle Recruitment
In 2019, the FH (average 259 ± 20 spat shell−1) had significantly greater cumulative oyster recruits than the LYS (average 13 ± 2 spat shell−1) (Figure 6A, one-way ANOVA on ranks, H = 14.296, p < 0.001). The cumulative oyster recruits in 2020 also showed evident spatial variations (Figure 6A, one-way ANOVA on ranks, H = 44.732, p < 0.001), with the highest recruits (1,551 ± 79 spat shell−1) at the SH2 and the lowest values (280 ± 17 spat shell−1) at the NQ (Figure 6A, one-way ANOVA on ranks, p < 0.05).
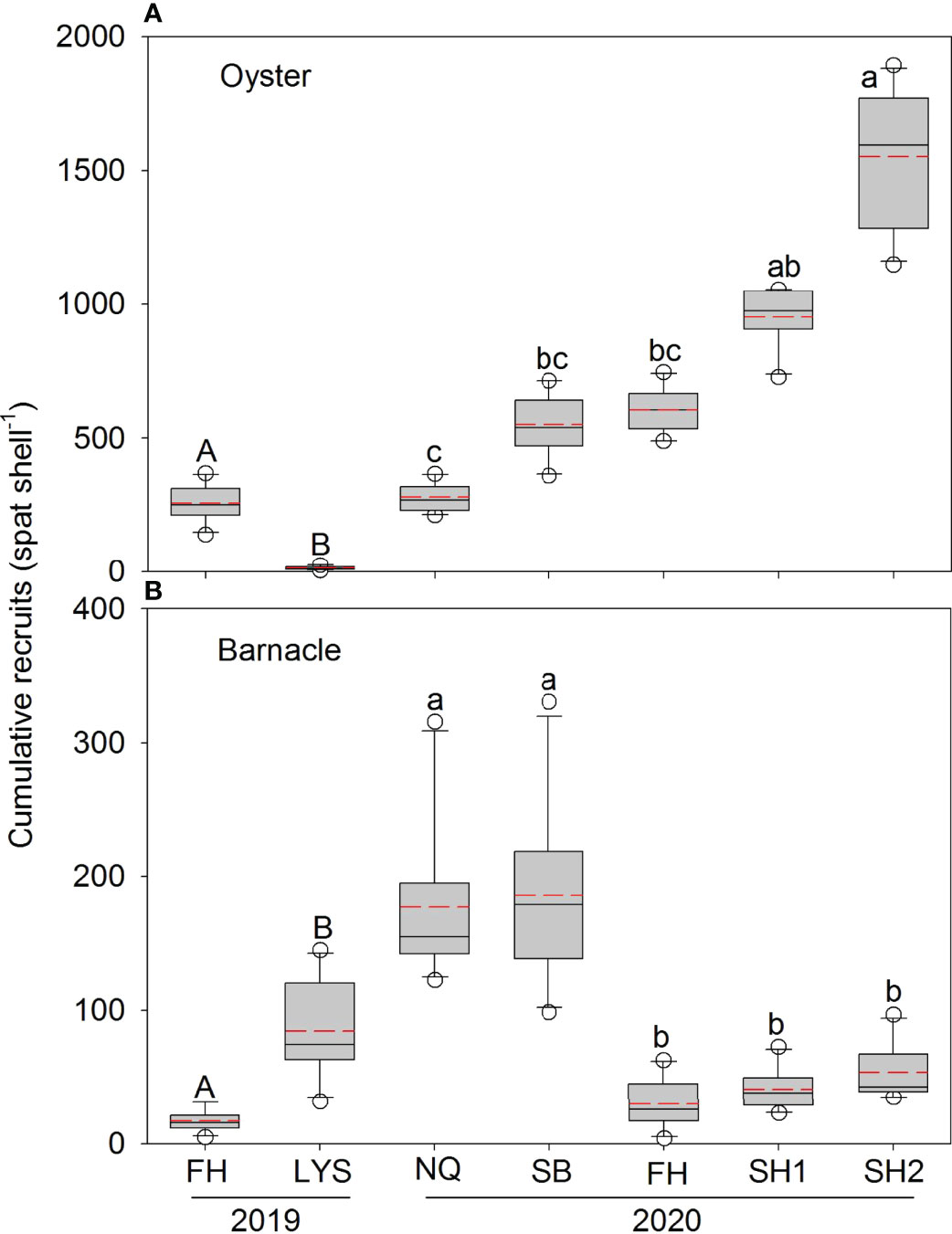
Figure 6 Box plots of the cumulative oyster (A) and barnacle (B) recruitment in 2019 and 2020. The solid line and short dashed lines represent the median and mean recruits, respectively. Significant differences within each year are indicated by different letters on the outlier (p < 0.05).
In contrast with the oyster, the cumulative barnacle recruitment showed converse patterns (Figure 6B). In 2019, the cumulative barnacle recruits were significantly greater at the LYS than at the FH (Figure 6B, one-way ANOVA on ranks, H =14.318, p < 0.001). The ranks of the cumulative barnacle recruits in 2020 were as follows: SB = NQ > FH = SH1 = SH2 (Figure 6B, one-way ANOVA on ranks, H = 38.020, p < 0.001). There were significantly negative correlations in the cumulative recruitment between the oyster and the barnacle in each of the 2 years (Figure 7, 2019: r = −0.851, p < 0.001, n = 20; 2020: r = −0.513, p < 0.001, n = 50).
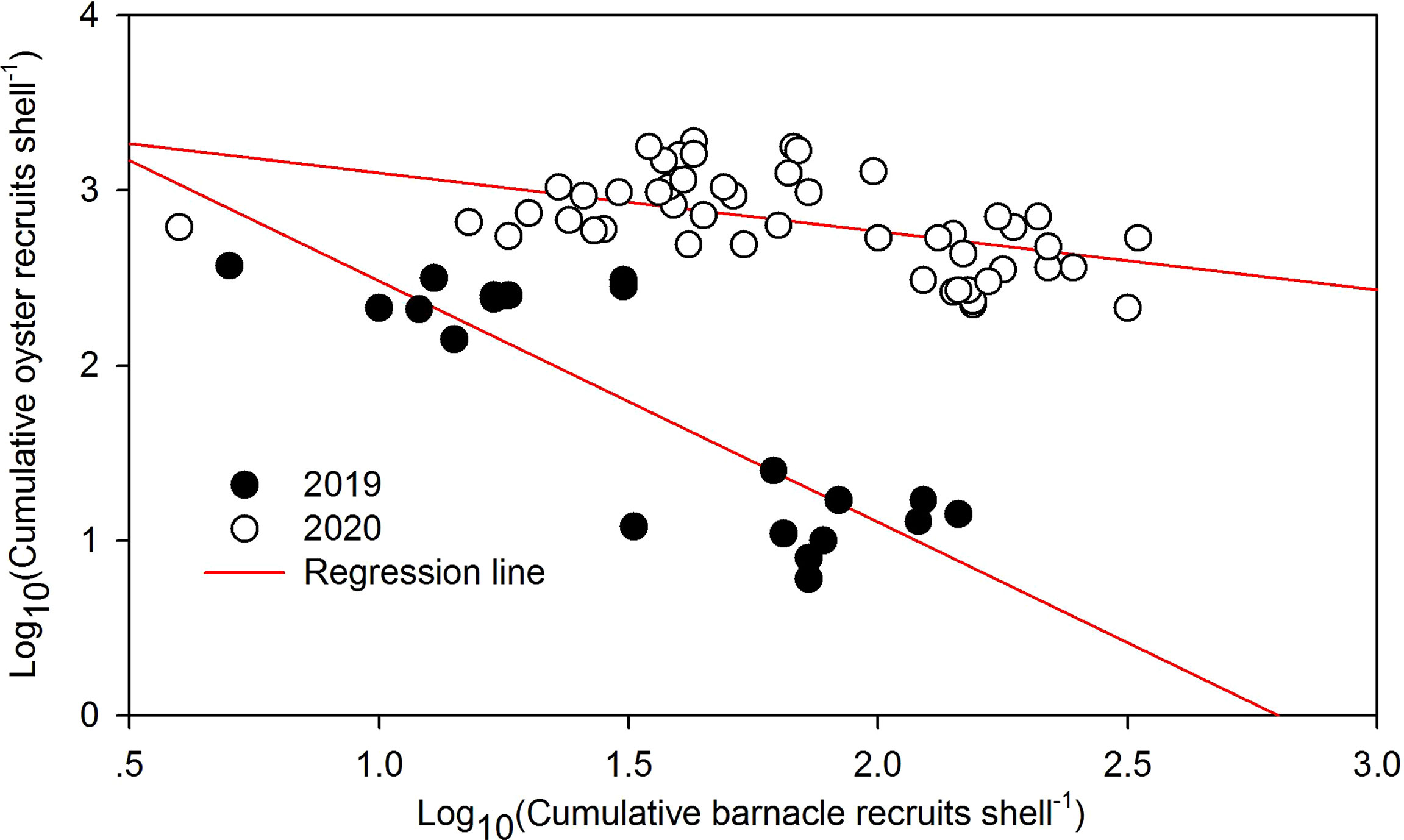
Figure 7 Correlations in cumulative recruits between the barnacle and the oysters across all the monitoring stations. Evident negative associations in the cumulative recruits were present between the oyster and the barnacle (p < 0.001).
Discussion
Experimental ecology can deal with the uncertainty and provide confidence for restoration decision-making. This study documented temporal and spatial dynamics in oyster and barnacle recruitment on and near the largest natural intertidal oyster reef in China, and identified their distinct seasonal recruitment peaks and the potential spatfall site for the natural reef restoration. Our recruitment patterns described here provided a repeatable and low-cost solution for effectively managing and exploiting oyster larval supply for the conservation and restoration of the LYS oyster reef.
Temporal Patterns of Oyster and Barnacle Recruitment
It is of critical importance for the development and implementation of oyster conservation and restoration strategy to understand the spawning and recruitment phenology of oysters. Precisely recognizing the timing and magnitude of oyster spawning and recruitment events is of ecological significance (Valdez and Ruesink, 2017). The oyster C. sikamea recruitment at our study area appeared as a continuous process from June through late November or early December. The overall broader window of recruitment was in contrast with the much narrower patterns observed for C. virginica (May–September) in the Atlantic coast of the USA (Ortega and Sutherland, 1992; O’Beirn et al., 1995; O’Beirn et al., 1996) and O. lurida (June–September) in the Hood Canal of Washington (Valdez and Ruesink, 2017). Typically, the oyster C. sikamea has a larval development of approximately 14 days in a hatchery environment at 23°C (Sun et al., 2020). The initial spawning of oysters at the natural reef should initiate in late May when the minimal water temperature (20°C) for the event arrived at the water. The warmer weather condition at our study site could lengthen oyster recruitment season. The peak recruitment in each of the 2 monitoring years consistently occurred in August and was consistent with those recorded for C. virginica (mid-July through August) on coastal Georgia (O’Beirn et al., 1995; O’Beirn et al., 1996), flat oyster Ostrea angasi (late summer) in South Australia’s Gulf St. Vincent, and C. gigas (mid-August through September) in the Tau lagoon of France (Lagarde et al., 2017). The synchrony in seasonal timing of recruitment peaks has been previously recorded in marine sessile benthic organisms (oyster, barnacle, and mussel) among different bio-geographic regions along the west coast of America (Broitman et al., 2008; Navarrete et al., 2008) and coastal European waters (Philippart et al., 2012). The explanations were that regional oceanographic drivers including sea surface temperature and oceanographic process determined more strongly temporal patterns in oyster recruitment than local dynamics (Wasson et al., 2016).
Temperature is a major factor in the timing of the reproduction of barnacles and remains an important species-specific factor for spawning (Anil et al., 1995; Dattesh et al., 2006; Ziadi et al., 2015). The hatching peak of oysters often coincides with warmer water temperature, but barnacles often breed during relatively colder months and their larval peak is correlated to low temperature (Anil et al., 1995; Dattesh et al., 2006; Ziadi et al., 2015). The present study indicated that the barnacle recruited earlier and longer (early spring to early winter) than the oyster (mid spring to late fall), with two distinct recruitment peaks (mid-spring and mid-summer) that were separate from those (August) of the oyster. In early spring and early summer when oyster settlement was absent or low, the barnacle larvae could rapidly occupy accessible substrate surface and become the dominant species. Previous studies demonstrated that increased barnacle abundances had a negative impact on settlement, growth, and survival of oysters (Osman et al., 1989; Boudreaux et al., 2009). Therefore, this study concluded that August was the most favorable window for adding substrate to capture oyster spat in the water around the LYS oyster reef.
Spatial Patterns of Oyster and Barnacle Recruitment
Considerable spatial variability in oyster recruitment had been noted at both regional (among estuaries) and local (within estuaries) scales (Dye et al., 1990; O’Beirn et al., 1995; O’Beirn et al., 1996; Wasson et al., 2016; Lau et al., 2020). Wasson et al. (2016) investigated spatial scale in Olympia oyster recruitment across 37 sites in eight estuaries along the west coast of North America, and suggested that oyster recruitment was influenced more strongly by local dynamics within estuaries than by regional oceanographic environments. Here, we documented extreme spatial heterogeneity in oyster recruitment on a just several-kilometer scale around the natural reef. The higher oyster recruitment at the sheltered inshore sites may be caused by the poorer wave, lower water velocity, greater phytoplankton abundance and higher larval supply relative to the natural reef and nearby open coast. Bushek (1988) documented a similar result wherein C. virginica dominated pier pilings within 10 m of the shore in Galveston Bay of Texas, while the ivory barnacle Balanus eburneus predominated on pilings beyond 10 m. This pattern of horizontal zonation reflected differential settlement: barnacles and oysters settle in areas of high and low water motion, respectively. High water motion promoted barnacle growth, but had no effect on oyster growth. Adams et al. (1991) noted a similar spatial pattern wherein C. virginica settlement and recruitment were significantly higher in smaller, sheltered tidal creeks throughout the marshes than the larger open bodies of water. The cumulative oyster recruits at the favorable sites (SH2 and SH1) were comparable with those in French traditional breeding basins (Lagarde et al., 2017), the coastal water of Hong Kong (Lau et al., 2020), and the Atlantic coast of North America (Ortega and Sutherland, 1992; O’Beirn et al., 1996).
In contrast with oysters, barnacle larvae settle and recruit preferably on open coasts with stronger wave exposure and higher water motion (Bushek, 1988; Nishizaki and Carriington, 2015). The underlying mechanisms were that a strong hydrographic process increased the chances of filtering food and accessing hard substrate for barnacles. The rates of barnacle recruitment and growth were positively correlated with flow under laboratory experiments and field observation, and increased flow commonly yielded greater settlement densities and higher growth rate of barnacles (Judge and Craig, 1997; Johnson and Soltis, 2017). This study also concluded a consistent result that higher barnacle recruitment appeared on the LYS oyster reef and nearby open coast than on the sheltered inshore. The pattern was highlighted by significant negative relationships in the cumulative recruits between the barnacle and the oyster across all the sampling sites. The separation in horizontal zonation between these two sessile species within the small-scale water indicated their different favorable environmental windows. The higher water velocity, greater concentrations of suspended sediment, and lower phytoplankton abundance at the natural reef decreased the larval settlement and survival of oysters largely, but the high water motion promoted the settlement, growth, and recruitment of the barnacle by increasing the chances of filtering food and accessing hard substrate.
Implications for the LYS Oyster Reef Restoration
This study showed that the sheltered inshore had high oyster and low barnacle recruitment. Conversely, low oyster and high barnacle recruits took place at the natural reef and nearby open coast. Therefore, the inshore (SH1 and SH2) with high oyster recruitment should be recognized as the natural spatfall sites for oyster population restoration at the natural reef. Additionally, the separation in the recruitment peak between the oyster and the barnacle at the study area indicated that August was the most favorable window for capturing oyster spat through substratum addition to the water around the natural reef. Consequently, the LYS oyster reef restoration in the future can benefit from knowledge of the temporal and spatial dynamics in the oyster and barnacle recruitment.
Data Availability Statement
The original contributions presented in the study are included in the article/supplementary material. Further inquiries can be directed to the corresponding author.
Author Contributions
WJ and W-MQ conceived and designed the research. WJ, W-JS, N-NL, R-LF, and W-KZ performed the experiments. WJ and W-MQ analyzed the data. WJ, W-JS, N-NL, R-LF, and W-KZ contributed reagents/materials/analysis tools. WJ and W-MQ wrote and edited the manuscript. All authors contributed to the article and approved the submitted version.
Funding
The research was funded by the National Key R&D Program of China (2020YFD0900805), Special Funds for Natural Resources Development (Marine Science and Technology Innovation) of Jiangsu Province (JSZRHYKJ202109), and the Natural Science Foundation of China (61936014).
Conflict of Interest
The authors declare that the research was conducted in the absence of any commercial or financial relationships that could be construed as a potential conflict of interest.
Publisher’s Note
All claims expressed in this article are solely those of the authors and do not necessarily represent those of their affiliated organizations, or those of the publisher, the editors and the reviewers. Any product that may be evaluated in this article, or claim that may be made by its manufacturer, is not guaranteed or endorsed by the publisher.
References
Adams M. P., Walker R. L., Heffrnan P. B., Reinart R. E. (1991). Eliminating Spat Settlement on Oysters Cultured in Coastal Georgia: A Feasibility Study. J. Shellfish Res. 10, 207–213.
Anil A. C., Chiba K., Okamoto K., Kurokura H. (1995). Influence of Temperature and Salinity on Larval Development of Balanus Amphitrite: Implications in Fouling Ecology. Mar. Ecol. Prog. Ser. 118, 59–166. doi: 10.3354/meps118159
Beck M. B., Brumbaugh R. D., Airoldi L., Carranza A., Coen L. D., Crawford C., et al. (2011). Oyster Reefs at Risk and Recommendations for Conservation, Restoration, and Management. Bioscience 61, 107–116. doi: 10.1525/bio.2011.61.2.5
Boudreaux M. L., Walters L. J., Rittschof D. (2009). Interactions Between Native Barnacles, non-Native Barnacles, and the Eastern Oyster Crassostrea Virginica. Bull. Mar. Sci. 84, 43–57. doi: 10.1146/annurev.marine.010908.163757
Broitman B., Blanchette C., Menge B., Lubchenco J., Krenz C., Foley M., et al. (2008). Spatial and Temporal Patterns of Invertebrate Recruitment Along the West Coast of the United States. Ecol. Monogr. 78, 403–421. doi: 10.1890/06-1805.1
Bushek D. (1988). Settlement as a Major Determinant of Intertidal Oyster and Barnacle Distributions Along a Horizontal Gradient. J. Exp. Mar. Biol. Ecol. 122, 1–18. doi: 10.1016/0022-0981(88)90208-0
Coen L. D., Knott D. M., Wenner E. L., Hadley N. H., Ringwood A. H., Luckenbach M. W., Mann R., Wesson ,. J. A. (1999). “Intertidal Oyster Reef Studies in South Carolina: Design, Sampling and Experimental Focus for Evaluating Habitat Value and Function,” in Oyster Reef Habitat Restoration: A Synopsis and Synthesis of Approaches, vol. pp . Eds. Luckenbach M. W., Mann R., Wesson ,. J. A. (Gloucester Point: VA: Virginia Institute of Marine Science Press), 131–156.
Dattesh V., Desai A. C., Venkat A. K. (2006). Reproduction in Balanus Amphitrite Darwin (Cirripedia: Thoracica): Influence of Temperature and Food Concentration. Mar. Biol. 149, 1431–1441. doi: 10.1007/s00227-006-0315-3
Dye A. H. (1990). Episodic Recruitment of the Rock Oyster Saccostrea Cucullata (Born 1778) on the Transkei Coast. S. Afr. J. Zool. 25 (3), 185–187. doi: 10.1080/02541858.1990.11448209
Fegley S. R., Ford S. E., Kraeuter J. N., Haskin H. H. (2003). The Persistence of New Jersey’s Oyster Seedbeds in the Presence of Disease and Harvest: The Role of Management. J. Shellfish Res. 22, 451–464.
Geng X. S., Fu M. Z., Xu X. S., Li P. Y. (1991). Development, Ecological Characteristics and Paleoenvironmental Significance of Modern Oyster Reefs. Sci. China Ser. B 8), 867–876. doi: CNKI:SUN:JBXK.0.1991-08-013
Grizzle R. E., Greene J. K., Coen L. D. (2008). Seston Removal by Natural and Constructed Intertidal Eastern Oyster (Crassostrea Virginica) Reefs: A Comparison With Previous Laboratory Studies, and the Value of in Situ Methods. Estuar. Coast. 31, 1208–1220. doi: 10.1007/s12237-008-9098-8
Grizzle R. E., Greene J. K., Luckenbach M. W., Coen L. D. (2006). A New in Situ Method for Measuring Seston Uptake by Suspension Feeding Bivalve Molluscs. J. Shellfish Res. 25, 643–649. doi: 10.2983/0730-8000(2006)25[643:ANISMF]2.0.CO;2
Grossman S. K., Grossman E. E., Barber J. S., Gamblewood S. K., Crosby S. C. (2020). Distribution and Transport of Olympia Oyster Ostrea Lurida Larvae in Northern Puget Sound, Washington. J. Shellfish Res. 39, 215–233. doi: 10.2983/035.039.0204
Humphries A. T., Ayvazian S. G., Carey J. C., Hancock B. T., Grabbert S., Cobb D., et al. (2016). Directly Measured Denitrification Reveals Oyster Aquaculture and Restored Oyster Reefs Remove Nitrogen at Comparable High Rates. Front. Mar. Sci. 3, 74. doi: 10.3389/fmars.2016.00074
Johnson K. B., Soltis K. A. (2017). Larval Supply and Recruitment of the Ivory Barnacle Balanus Eburneus (Gould 1841) on Oyster Cultch Used in Oyster-Reef Restoration. J. Crustacean Biol. 37 (3), 1–6. doi: 10.1093/jcbiol/rux015
Judge M. L., Craig S. F. (1997). Positive Flow Dependence in the Initial Colonization of a Fouling Community: Results From in Situ Water Current Manipulations. J. Exp. Mar. Biol. Ecol. 210, 209–222. doi: 10.1016/S0022-0981(96)02691-3
Kellogg M. L., Cornwell J. C., Owens M. S., Paynter K. T. (2013). Feature Article: Denitrification and Nutrient Assimilation on a Restored Oyster Reef. Mar. Ecol. Prog. Ser. 480, 1–19. doi: 10.3354/meps10331
Lagarde F., d’orbcastel E. R., Ubertini M., Mortreux S., Bernard I., Fiandrino A., et al. (2017). Recruitment of the Pacific Oyster Crassostrea Gigas in a Shellfish-Exploited Mediterranean Lagoon: Discovery, Driving Factors and a Favorable Environmental Window. Mar. Ecol. Prog. Ser. 578, 1–17. doi: 10.3354/meps12265
Lau S. C. Y., Thomas M., Hancock B., Russel B. D. (2020). Restoration Potential of Asian Oysters on Heavily Developed Coastlines. Restor. Ecol. 28, 1643–1653. doi: 10.1111/rec.13267
McAfee D., Connell S. D. (2020). Cuing Oyster Recruitment With Shell and Rock: Implications for Timing Reef Restoration. Restor. Ecol. 28, 506–511. doi: 10.1111/rec.13134
Navarrete S. A., Broitman B. R., Menge B. A. (2008). Interhemispheric Comparison of Recruitment to Intertidal Communities: Pattern Persistence and Scales of Variation. Ecology 89, 1308–1322. doi: 10.1890/07-0728.1
Nishizaki M. T., Carriington E. (2015). The Effect of Water Temperature and Velocity on Barnacle Growth: Quantifying the Impact of Multiple Environmental Stressors. J. Therm. Biol. 54, 37–46. doi: 10.1016/j.jtherbio.2015.02.002
O’Beirn F. X., Heffernan P. B., Walker R. L. (1995). Preliminary Recruitment Studies of the Eastern Oyster, Crassostrea Virginica, and Their Potential Applications, in Coastal Georgia. Aquaculture 136, 231–242. doi: 10.1016/0044-8486(95)01059-9
O’Beirn F. X., Heffernan P. B., Walker R. L. (1996). Recruitment of the Eastern Oyster in Coastal Georgia: Patterns and Recommendations. N. Am. J. Fish. Manage. 16, 413–426. doi: 10.1577/1548-8675(1996)016<0413:ROTEOI>2.3.CO;2
Ortega S., Sutherland J. P. (1992). Recruitment and Growth of the Eastern Oyster, Crassostrea Virginica, in North Carolina. Estuaries 15, 158–170. doi: 10.2307/1352689
Osman R. W., Whitlach R. B., Zajac R. N. (1989). Effects of Resident on Recruitment Into a Community: Larva Settlement Versus Post Settlement Mortality in the Oyster Crassostrea Virginica. Mar. Ecol. Prog. Ser. 54, 61–73. doi: 10.3354/meps054061
Philippart C. J., Amaral A., Asmus R., van Bleijswijk J., Bremner J., Buchholz F., et al. (2012). Spatial Synchronies in the Seasonal Occurrence of Larvae of Oysters (Crassostrea Gigas) and Mussels (Mytilus Edulis/Galloprovincialis) in European Coastal Waters. Estua. Coast. Shelf S. 108, 52–63. doi: 10.1016/j.ecss.2012.05.014
Powell E. N., Ashton-Alcox K. A., Kraeuter J. N., Ford S. E., Bushek D. (2008). Long-Term Trends in Oyster Population Dynamics in Delaware Bay: Regime Shifts and Response to Disease. J. Shellfish Res. 27, 729–755. doi: 10.2983/0730-8000(2008)27[729:LTIOPD]2.0.CO;2
Preston J., Fabra M., Helmer L., Johnson E., Harris-Scott E., Hendy I. W. (2020). Interactions of Larval Dynamics and Substrate Preference Have Ecological Significance for Benthic Biodiversity and Ostrea Edulis Linnaeu in the Presence of Crepidula Fornicata. Aquat. Conserv.: Mar. Freshw. Ecosyst. 30, 2133–2149. doi: 10.1002/aqc.3446
Quan W. M, Fan R. L., Li N. N., Sun Z. Y., Jiang W., Wang T. N., et al. (2020). Seasonal and Temporal Changes in the Kumamoto Oyster Crassostrea sikamea Population and Associated Benthic Macrofaunal Communities at an Intertidal Oyster Reef in China. J. Shellfish Res. 39, 207–214. doi:10.2983/035.039.0203
Quan W. M., Feng M., Zhou Z. X., Wu Z. L., Tang F. H., Wang Y. L., et al. (2017). Ecological Assessment of the Oyster Crassostrea Sikamea Population and Associated Benthic Communities on Restored Oyster Reefs Along Jiangsu Province Coast, China. Acta Ecol. Sin. 37 (5), 1709–1718. doi: 10.5846/stxb201510102049
Quan W. M., Humphries A. T., Shen X. Q., Chen Y. Q. (2012a). Oyster and Associated Benthic Macrofaunal Development on a Created Intertidal Oyster (Crassostrea Ariakensis) Reef in the Yangtze River Estuary, China. J. Shellfish Res. 31, 599–610. doi: 10.2983/035.031.0302
Quan W. M., Humphries A. T., Shi L. Y., Chen Y. Q. (2012b). Determination of Trophic Transfer at a Created Intertidal Oyster (Crassostrea Ariakensis) Reef in the Yangtze River Estuary Using Stable Isotope Analyses. Estuar. Coast. 35, 109–120. doi: 10.1007/s12237-011-9414-6
Quan W. M., Humphries A. T., Shi L. Y., Chen Y. Q. (2012c). Determination of Trophic Transfer at a Created Intertidal Oyster (Crassostrea ariakensis) Reef in the Yangtze River Estuary Using Stable Isotope Analyses. Estuar. Coast. 35, 109–120. doi: 10.1007/s12237-011-9414-6
Quan W. M., Zhang Y. L., Qi Z. L., Xu M., Fan R. L., Wang T. N., et al. (2022). Distribution and Ecological Status of Natural Oyster Reefs on the Coast of Caofeidian-Leting, Tangshan, Hebei Province. Acta Ecol. Sin. 42 (3), 1142–1152. (in Chinese with English abstract). doi: 10.5846/stxb202005101172
Quan W. M, Zhou W. F., Ma C. Y., Feng M., Zhou Z. X., Tang F. H., et al. (2016). Ecological status of a natural intertidal oyster reef in Haimen County, Jiangsu Province. Acta Ecologica Sinica. 36:7749–7757 (in Chinese with English abstract). doi: 10.5846/stxb201510102048
Quan W. M., Zhu J. X., Ni Y., Shi L. Y., Chen Y. Q. (2009). Faunal Utilization of Constructed Intertidal Oyster (Crassostrea Rivularis) Reef in the Yangtze River Estuary, China. Ecol. Engin. 35, 1466–1475. doi: 10.1016/j.ecoleng.2009.06.001
Schulte D. M., Burke R. P. (2014). Recruitment Enhancement as an Indicator of Oyster Restoration Success in Chesapeake Bay. Ecol. Restor. 32, 434–440. doi: 10.3368/er.32.4.434
Southworth M., Mann R. (2004). Decadal Scale Changes in Seasonal Patterns of Oyster Recruitment in the Virginia Sub Estuaries of the Chesapeake Bay. J. Shellfish Res. 23, 391–402.
Sun Z. Y. (2020). Preliminary Studies on the Hatchery, Settlement and Predation on Two Reef-Forming Oysters. Master Thesis.Shanghai: Shanghai Oceanography University.
Valdez S. R., Ruesink J. L. (2017). Scales of Recruitment Variability in Warming Waters: Comparing Native and Introduced Oysters in Hood Canal, Washington, USA. Mar. Ecol. 38, e12435. doi: 10.1111/maec.12435
Wasson K., Hughes B. B., Berriman J. S., Chang A. L., Deck A. K., Dinnel P. A., et al. (2016). Coast-Wide Recruitment Dynamics of Olympia Oysters Reveal Limited Synchrony and Multiple Predictors of Failure. Ecology 97, 3503–3516. doi: 10.1002/ecy.1602
Wiberg P. L., Taube S. R., Ferguson A. E., Kremer M. R., Reidenbach M. A. (2019). Wave Attenuation by Oyster Reefs in Shallow Coastal Bays. Estuar. Coast. 42, 331–347. doi: 10.1007/s12237-018-0463-y
Keywords: Crassostrea sikamea, settlement, survival, substrate, Yellow Sea
Citation: Jiang W, Shi W-J, Li N-N, Fan R-L, Zhang W-K and Quan W-M (2022) Oyster and Barnacle Recruitment Dynamics on and Near a Natural Reef in China: Implications for Oyster Reef Restoration. Front. Mar. Sci. 9:905373. doi: 10.3389/fmars.2022.905373
Received: 27 March 2022; Accepted: 30 May 2022;
Published: 06 July 2022.
Edited by:
Susana Carvalho, King Abdullah University of Science and Technology, Saudi ArabiaReviewed by:
Su Yin Chee, Universiti Sains Malaysia, MalaysiaRussell Paul Burke, Christopher Newport University, United States
Copyright © 2022 Jiang, Shi, Li, Fan, Zhang and Quan. This is an open-access article distributed under the terms of the Creative Commons Attribution License (CC BY). The use, distribution or reproduction in other forums is permitted, provided the original author(s) and the copyright owner(s) are credited and that the original publication in this journal is cited, in accordance with accepted academic practice. No use, distribution or reproduction is permitted which does not comply with these terms.
*Correspondence: Wei-Min Quan, cXVhbndlaW1AMTYzLmNvbQ==