- 1Instituto de Investigação em Ciências do Mar - Okeanos, Universidade dos Açores, Horta, Portugal
- 2Institute of Marine Research (IMAR), Horta , Portugal
- 3Laboratoire d’Ecogéchimie des Environnements Benthiques (LECOB) Sorbonne University, Centre National de la Recherche Scientifique (CNRS), Banyuls, France
Characteristics of the life-history biology of hydrothermal vent species are a prerequisite to understanding the dispersal, population connectivity, and ecology of these insular populations. The vent crab Segonzacia mesatlantica (Guinot, 1989; Brachyuran: Bythograeidae) is one of the most dominant endemic predators at deep-sea hydrothermal vents along the Mid-Atlantic Ridge (MAR). However, the biological life-history characteristics remain poorly understood for this species. The objective of this study was to reveal relevant biological characteristics of the reproductive ecology of S. mesatlantica, contributing to a better understanding of its ecology and the importance of the maintenance of healthy populations. The reproductive ecology of S. mesatlantica from the Broken Spur vent field such as the in situ behavior of brooding female crabs, gametogenesis, fecundity, and the embryonic and larvae development was studied. In contrast to non-brooding crabs, brooding crabs of S. mesatlantica were observed at the vent periphery exhibiting a sequence of specific brooding behaviors, suggesting that they might be providing oxygen to their embryo mass. Results from histological analysis reveal the presence of oocytes in different stages of development within the gonad. Once extruded, the mature oocytes form an egg mass underneath the abdomen. Similar to other bythograeid crabs, the egg mass of S. mesatlantica was composed of a high number of relatively small eggs in different stages of embryonic development. The eggs hatch as a first zoea that undergoes at least four stages of development.
Introduction
Deep-sea hydrothermal vents were first discovered along the Galapagos Rift in 1977 and are located along mid-ocean ridges, back-arc spreading centers, and volcanic seamounts (Lonsdale, 1977; Corliss et al., 1979; Grassle et al., 1979; Segonzac, 1992; Van Dover, 1995). As in most ecosystems, the colonization processes of hydrothermal vents are closely related to reproduction, larval dispersal, recruitment, and migration from surrounding environments (Mullineaux and France 1995; Gaudron et al., 2010; Mullineaux et al., 2018 and references therein). It has been widely recognized that knowledge of the early life traits of vent organisms is essential to understanding the ecological processes that influence the establishment and persistence of vent populations (Tyler and Young, 1999). Furthermore, studying the reproductive biology of vent organisms is a requirement to understand the sensitivity of these organisms to environmental challenges caused by anthropogenic impacts (Van Dover et al., 2011, Van Dover et al., 2014). However, major gaps of knowledge persist on the reproductive biology of these species (Tyler and Young, 1999; Hilário et al., 2009).
Brachyuran crabs of the family Bythograeidae are an important component of the hydrothermal biodiversity worldwide, owing to their predatory aspects in the hydrothermal vent communities (Dittel et al., 2005). Currently, the family consists of 14 species assigned to 6 genera that include Allograea (Guinot et al., 2002), Austinograea (Hessler and Martin 1989), Bythograea (Williams, 1980), Cyanograea (de Saint Laurent 1984; Tsuchida and Hashimoto, 2002), Gandalfus (Takeda et al., 2000) and Segonzacia (Guinot, 1997). So far, knowledge of the life-history traits of the family Byhtograeidae is limited to three species of the genera Bythograea (Van Dover et al., 1985; Epifanio et al., 1999; Jinks et al., 2002; Guinot and Hurtado, 2003; Perovich et al., 2003; Martin and Dittel, 2007; Hilário et al., 2009) and one specie of the genera Gandalfus (Tsuchida et al., 1998; Hamasaki et al., 2010; Nakajima et al., 2010). The authors suggested that bythograeid crabs had similar reproductive patterns as other brachyuran crabs, including the male deposition of sperm packets in the seminal receptacles of the female, providing the potential to fertilize more than one batch of eggs (Van Dover et al., 1985; Jinks et al., 2002; Perovich et al., 2003). Clusters of fertilized eggs are deposited on the abdominal pleopods and brooded externally until hatching (Van Dover et al., 1985; Jinks et al., 2002; Perovich et al., 2003). Furthermore, brooding crabs are rarely seen near the vent sites indicating that they might migrate to the vent periphery to brood and hatch their eggs (Hilário et al., 2009). The eggs hatch as planktotrophic larvae that includes an unknown number of zoeal stages and megalopae stages (Williams, 1980; Van Dover et al., 1985). Indeed, there are several lines of evidence showing that bythograeid crabs disperse via the planktonic larval stage in the water column and have limited swimming range as adults (e.g., Epifanio et al., 1999; Jinks et al., 2002; Dittel et al., 2005). Therefore, the number of studies of the life history traits of the genera Bythograea is increasing. However, the reproductive ecology of the genus Segonzacia remains poorly understood. This genus is monotypic with Segonzacia mesatlantica (Guinot, 1997) with a large distribution throughout the vents along the Mid-Atlantic Ridge (MAR), including at the Menez Gwen, Lucky Strike, Snake Pit, Logatchev, and Broken Spur (Guinot et al., 2002; Martin and Haney, 2005; McLay, 2007, and Mateos et al., 2012) with depths ranging from 850 m to 4080 m. Similar to other vent fields along MAR, crabs of S. mesatlantica at the Broken Spur vent field (depth of 3090 m), is found at hydrothermal structures with a maximum density of 6.66 to 10 individual/m2 (Segonzac, 1992; Murton et al., 1995; Van Dover, 1995; Gebruk et al., 1997; Matabos et al., 2015; Cuvelier et al., 2017);, being the dominant endemic predators (Desbruyeres et al., 2000; Colaço et al., 2002).
The main objective of this study was to provide new knowledge on the reproductive traits of S. mesatlantica from the Broken Spur vent field by including in situ behavior of non-brooding and brooding crabs as well as the reproductive patterns of the brooding crabs, such as the development of oocytes, embryos and larvae, and the fecundity. To the best of our knowledge, there is no information about the life-history biology of this species.
Materials and Methods
Study Site
The Broken Spur vent field (Figure 1) was first discovered in June 1993 and is situated at 29°10’N, 43°10.4’W with a depth range from 3050 to 3875 m at the axial summit graben of the neovolcanic ridge along the Northern Mid-Atlantic Ridge (MAR; South of Azores, Van Dover, 1995; Murton et al., 1995). It consists of more than ten hydrothermal edifices, most of which are active. The edifices are chimneys ranging from a few meters to a few tens of meters in height and up to a few meters in diameter, and they rise above the volcanic basement of the axial ridge (Copley et al., 1997). The active hydrothermal sites are either steep-sided mounds or chimneys, topped with beehive type anhydrite structures and the areas between the sites contain pillow basalts with a dusting of pelagic sediment (Murton et al., 1995). At the time of discovery, the sulphide mineralogy, vent fluid geochemistry, and level of hydrothermal activity was described as similar to other vents at MAR (Murton et al., 1995). The fauna at Broken Spur also displays taxonomic similarities to other MAR that including bresillid shrimp (Rimicaris exoculata, Rimicaris chacei, Mirocaris fortunata, brachyuran crabs (S. mesatlantica), anemones (Actinian sp.), ophiuroids (Ophioctenlla acies), bivalves (Bathymodiolus sp.), gastropods (Phymorhynchus moskalevi), polychaetes (Spionid sp.; Chaetopterus sp.) and fish (Synaphobranchid sp.; Murton and Van Dover, 1993).
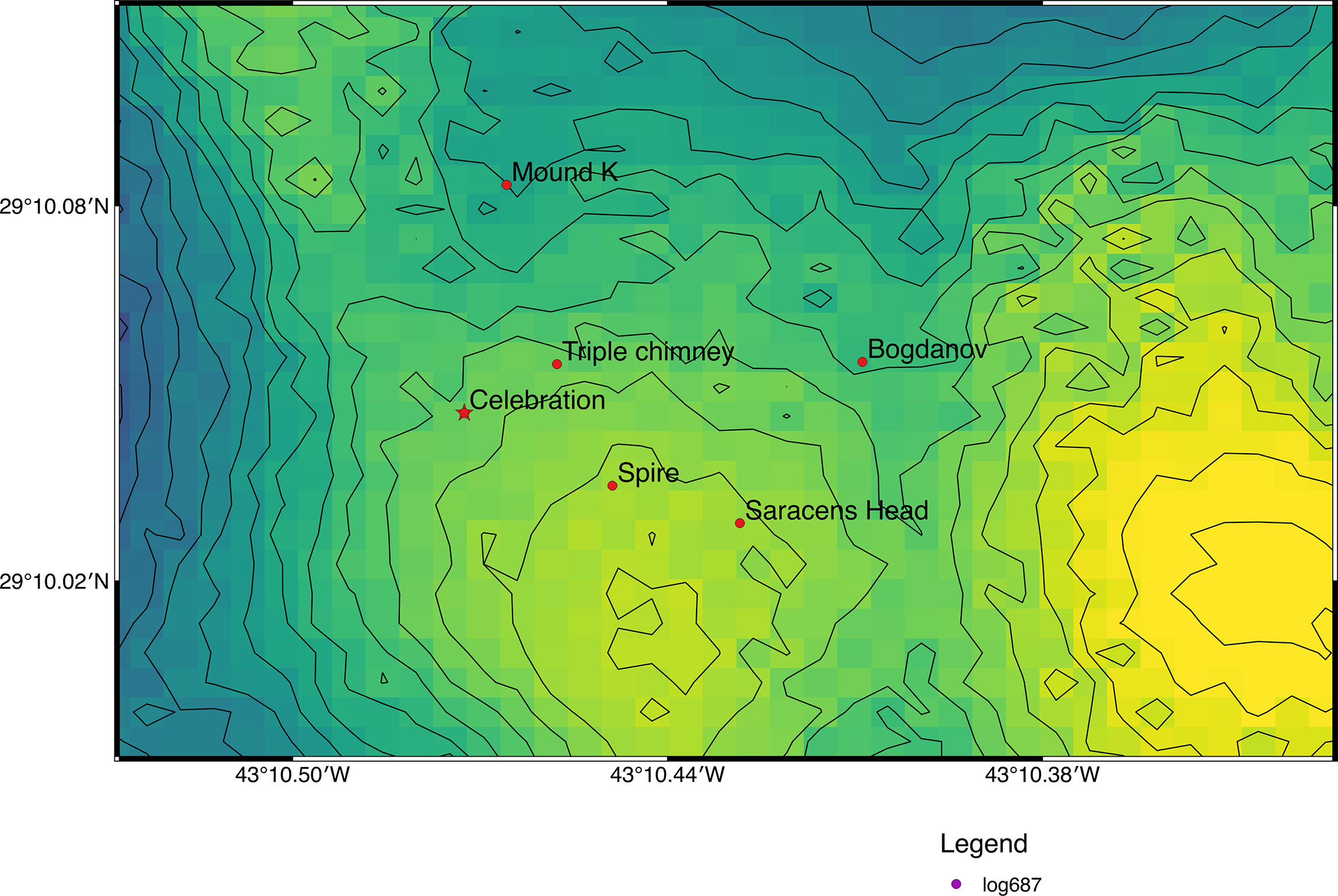
Figure 1 Map of the location of the collection site in the Broken Spur vent field (Map prepared with Q-GIS 2.18.20. Bathymetry and vent field positions from the TRANSECT cruise (Le Bris and the TRANSECT science party, 2018; https://doi.org/10.17600/18000513).
In situ Observations on the Behavior of Brooding and Non-brooding Crabs
In July 2018 the TRANSECT cruise (DOI: 10.17600/18000513) studied the Broken spur vent field (Le Bris et al., 2018). Video transect surveys were performed by the Victor 6000 remotely operated vehicle (ROV) equipped with an HD video camera (Sony FCB-H11). To estimate the abundance and quantify the in situ behavior of crabs, 88 hours of video footage was analyzed. The crab’s total abundance and behavior were calculated and identified based on high-definition photographs and video images. The behavior of crabs was registered according to Matabos et al. (2015), i.e., Predator behavior, inter-specific and intra-specific territorial behavior, intra-specific aggressive behavior, and eating. The behavior of brooding crabs was registered according to Baeza and Fernández (2002), i.e., standing position, abdominal flapping, manipulation of the embryo mass with the chelae (chelae poking), manipulation of the embryo mass using the pereiopods (pereiopod poking) and maxilliped beating.
Field Sampling and Sample Treatment
Only two crabs of Segonzacia mesatlantica (Figure 2) were collected on 27 July 2018 from biological sampling dives (TRANSECT VICTOR dive 692/9) using the remotely operated vehicle (ROV) Victor 6000 ROV equipped with a claw and manipulator arm. After being collected, were fixed in 4% formaldehyde before being transferred to 70% ethanol for reproductive analysis. The standard measure of body size for S. mesaltantica, carapace widths (CW), and carapace length (CL) were determined to the nearest 0.01 mm by vernier calipers.
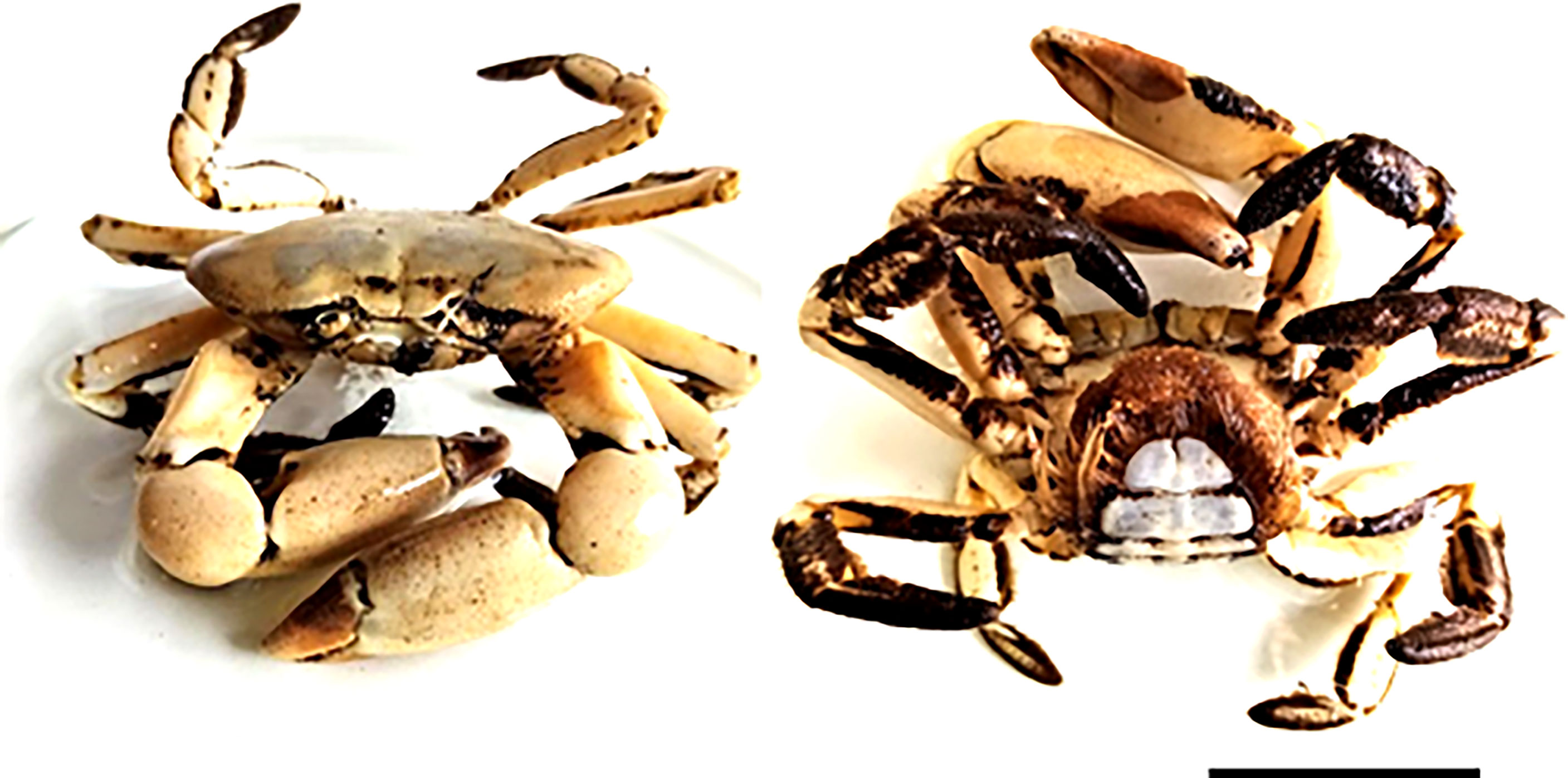
Figure 2 Brooding crabs of Segonzacia mesatlantica in both ventral and dorsal view. Scale bars = 15 mm.
Ovarian Structure and Oocyte Development
To obtain the oocyte size-frequency distributions, the ovaries were removed by dissection from both female crabs and a section of gonad was processed for histology (Colaço et al., 2006). Briefly, the gonad was transferred to a Bouin’s solution for 48h, dehydrated through a series of graded alcohol solutions, and cleared in xylene. The tissues were embedded in paraffin wax and sectioned by a microtome at 5 μm. Sections were mounted on glass slides and stained with hemotoxylin and eosin (Hilário et al., 2009; Marsh et al., 2015). Stained slides were examined under a Leica CTR600 microscope, and for each individual, the diameter of 87 and 146 oocytes that had been sectioned through the nuclei were measured using the program LAS core. Oocytes within a given ovary were grouped into size classes in increments of 10 μm, ranging from 10 to 145 μm (Hilário et al., 2009). The percentage of oocytes in each category was then calculated for each crab and the resulting oocyte size-frequency diagrams were constructed and examined for synchrony at the individual level (Perovich et al., 2003; Hilário et al., 2009).
Fecundity, Embryonic and Larvae Development
To estimate the punctual real individual fecundity, i.e., the number of eggs spawned by an individual during a single spawning event, the egg mass of both brooding crabs was removed from the abdominal pleopods and both eggs and empty eggs (i.e., eggs that were already hatched) were enumerated under a binocular dissecting microscope. The non-ruptured eggs that had retained the embryonic membrane were measured using LAS core software from Leica MZ16FA dissecting microscope and the following shape parameters and indices were calculated: Area (A, mm 2); maximum diameter (D, mm), minimum diameter (d, mm), roundness
[4/A(πD^2)], and aspect ratio (D/d; Tuset et al., 2011). Egg morphology was described and classified in 6 stages of development according to Tuset et al. (2011); Triay-Portella et al. (2014) and Martínez-Rivera et al. (2020) for other deep-sea crabs. Eggs were separated and enumerated according to the development stage. The larvae were classified in four stages of development following the classification of Arshad et al. (2006) for other brachyurans crabs and were enumerated, separated, and measured according to the larvae stage.
Results
Behavior of Crabs
A total of 1613 S. mesatlantica occurrences were counted in 88h of video analysis. While non-brooding crabs were more abundant near the vent sites, brooding crabs were just observed at the vent periphery. Near the vent structures, 235 S. mesatlantica displayed different behaviors (Table 1; Figure 3). Of these, 60 involved repetitive furtive movements followed by rapid threatening motions accompanied by claw activity, and sometimes a shrimp was captured (predator behavior), 5 involved the crab’s movement of several centimeters and/or waving their claws towards a shrimp or crab that had ventured too close (territorial behavior), 35 involved claw attacks (intra-specific aggressive behavior), 49 involve the use of chelae to scratch the areas and/or to break up larger food items, taking directly the food to the oral appendages (eating), and 86 involved crabs at the standing position without making any movement (quietly standing; Table 1; Figure 3). At the vent periphery, 29 S. mesatlantica displayed non-brooding behavior (eight events of predator behavior, two events of territorial behavior, 5 events of aggressive behavior, 7 events of eating, and 7 events of quietly standing). At the vent periphery, 32 brooding crabs exhibited a combination of four different behaviors that were not observed in non-brooding crabs (Table 1; Figure 3). The sequence of four behaviors consisted of standing position, abdominal flapping (Supplementary Video 1), chelae and/or pereiopod probing (Supplementary Video 2), and maxilliped beating (Supplementary Video 3). The standing position was the first behavior of the sequence exhibited by brooding crabs and consisted of females that raised their body pereiopods while maintaining one or both chelae firmly attached to the substrate. When in the standing position, the abdomen was gently lifted from the substrate and females extended their abdomen back and forwards rhythmically beating their embryo mass. Sometimes, after abdominal flapping, females repeatedly introduced a single pereiopod, several or even all of them and/or their chelae into the embryo mass, probing their embryos. Furthermore, maxillipeds were vigorously beaten during abdominal flapping, but most frequently before or after abdominal flapping.
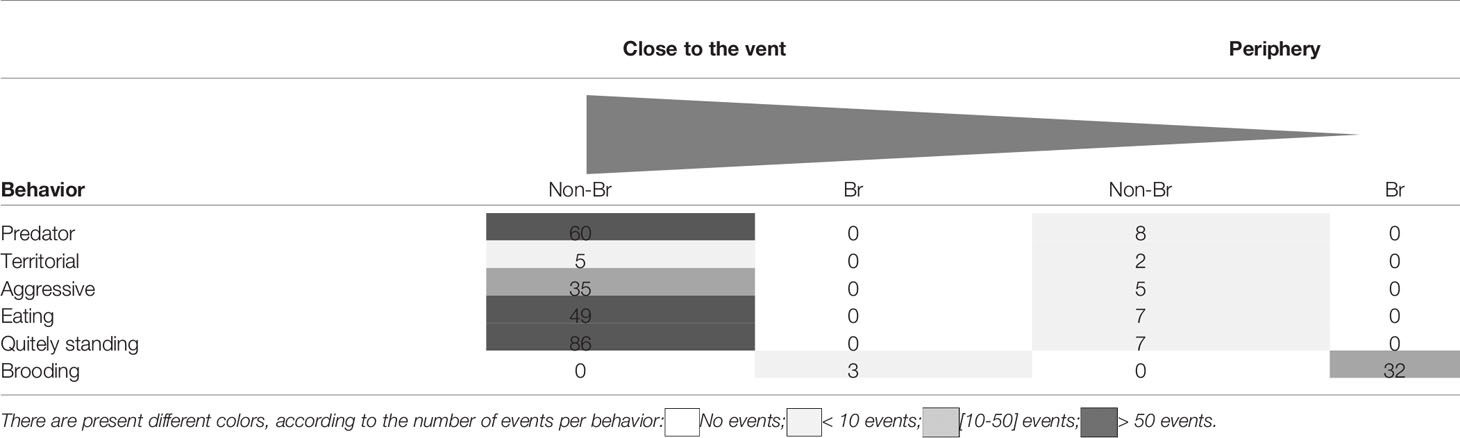
Table 1 Behavior of non-brooding (Non-Br) and brooding (Br) crabs of Segonzacia mesatlantica close to the vent and at the vent periphery, including predator, territorial, aggressive, eating, quietly standing, and brooding behavior.
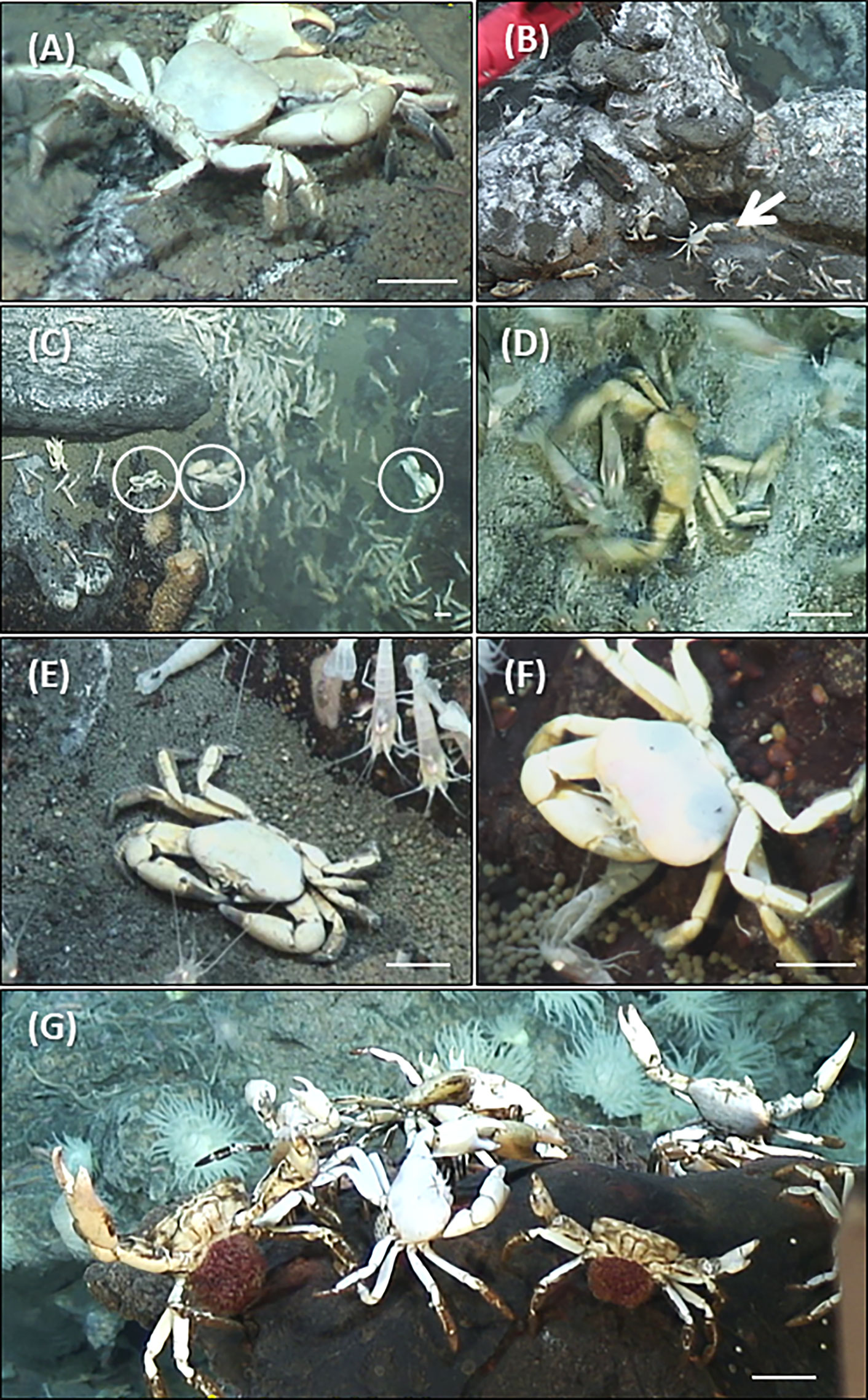
Figure 3 Behavior of non-brooding and brooding crabs of Segonzacia mesatlantica close to the vent (A– F) and at the vent periphery (G). (A) aggressive behavior, (B) territorial behavior – white arrow, (C) quietly standing – white circles, (D) predator behavior, (E, F) eating, (G) brooding behavior. Scale bar = 15 mm.
Ovarian Structure and Ovarian Development
Brooding crabs of S. mesatlantica have m-shape paired ovaries, situated in the cephalothorax, and proliferate within the hepatopancreas. The ovaries were beige and the histological analysis indicated that the ovaries consisted of several layers of developing oocytes enveloped by a thin gonad wall. The youngest oocytes measured about 12.77 ± 1.33 μm in diameter developed into previtellogenic oocytes. Previtellogenic oocytes contained little cytoplasm and nuclei were large concerning cell volume (Figure 4). Vitellogenesis was identified by the presence of yolk granules appearing in the cytoplasm and begins when cells reach about 70 μm in diameter. Early vitellogenic oocytes (70 to 110 μm diameter) changed from round to ovoid shape and contained more cytoplasm (Figure 4). In vitellogenic oocytes (higher than 110 μm) the cytoplasm occupies all the volume of the oocyte and was granular in appearance (Figure 4). The maximum oocyte size of S. mesatlantica was 144 μm in diameter. Atretic oocytes were also present within both gonads, which were characterized by a degenerated ooplasm with vacuoles, a thick surrounding membrane surrounded by follicle cells, and an amorphic shape (Figure 4). According to the oocyte size-frequency distribution, the ovaries of both brooding crabs contained mainly oogonial cells and previtellogenic oocytes (approximately 55%) followed by early-vitellogenic oocytes (approximately 40%) and a few vitellogenic oocytes (approximately 5%; Figure 5).
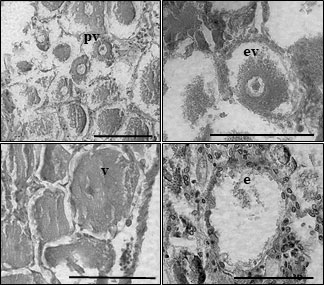
Figure 4 Light micrographs of histological sections through the ovary of Segonzacia mesatlantica using Leica Leica CTR600 microscope, program LAS core. Previtellogenic oocytes (pv); early vitellogenic oocytes (ev); vitellogenic oocyte (v) and empty oocyte (e). Scale bar = 100 μm.
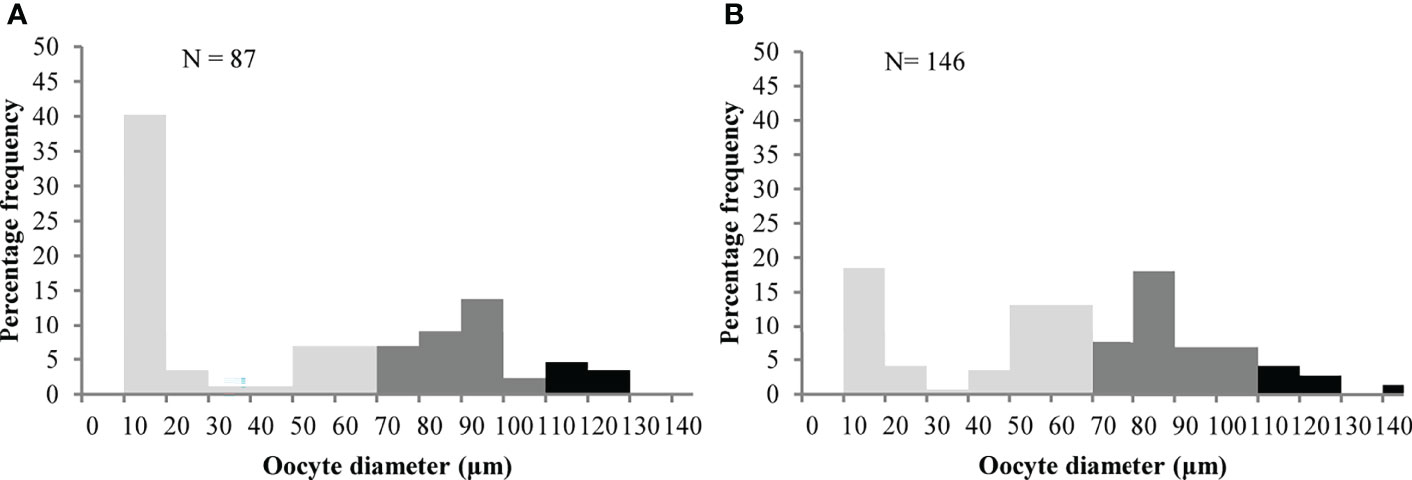
Figure 5 Oocyte size-frequency distribution for each female of Segonzacia mesatlantica with 35.3 mm CW (A) and 48.2 mm CW (B) : oogonia and previtellogenic oocytes;
Early previtellogenic oocytes;
vitellogenic oocytes.
Fecundity, Embryonic Development, and Larvae Development
The 48.2 mm CW female bared 23 696 eggs (23097 eggs that had already hatched and 599 embryos still attached to the pleopods; Table 2), while the 35.3 mm CW female bared 17 671 eggs (7804 eggs that had already hatched and 9867 embryos still attached to pleopods; Table 2). The eggs presented a spherical shape and a mean diameter of 0.566 mm. It described 6 stages of embryonic development within the egg mass in each individual that was based on the morphological characteristics and the dimensions of the eggs (Tables 2, 3; Figure 5; Supplementary Table 1). The eggs increased from newly spawned ones (Stage I: maximum diameter = 0.459 ± 0.034 mm; minimum diameter = 0.416 ± 0.022 mm and area = 0.664 ± 0.097 mm2) to hatching (stage VI: maximum diameter = 0.735 ± 0.040 mm; minimum diameter = 0.656 ± 0.030 mm and area = 1.700 ± 0.183 mm2; Table 2; Supplementary Table 1).

Table 2 Carapace width (CW, mm), carapace length (CL, mm), body weight (BW, g), number of embryos, number of hatched eggs, punctual real individual fecundity, and the number of embryos in each stage of embryonic development (I, II, III, IV, V and VI) for each individual analyzed of the specie Segonzacia mesatlantica.
The recently extruded embryos of S. mesatlantica were filled with yolk with a translucent yellow color that became an opaque dark-pink color as the yolk was used by the embryo (Table 3; Figure 6).
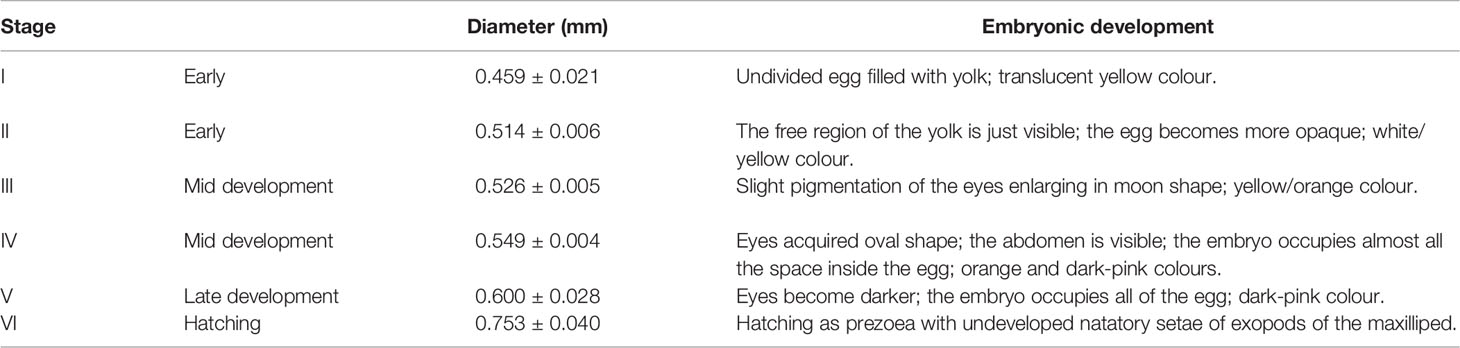
Table 3 Description of the stages of embryonic development, and the respective maximum diameter (mm) of Segonzacia mesatlantica according to Arshad et al. (2006); Tuset et al. (2011) and Martínez-Rivera et al. (2020).
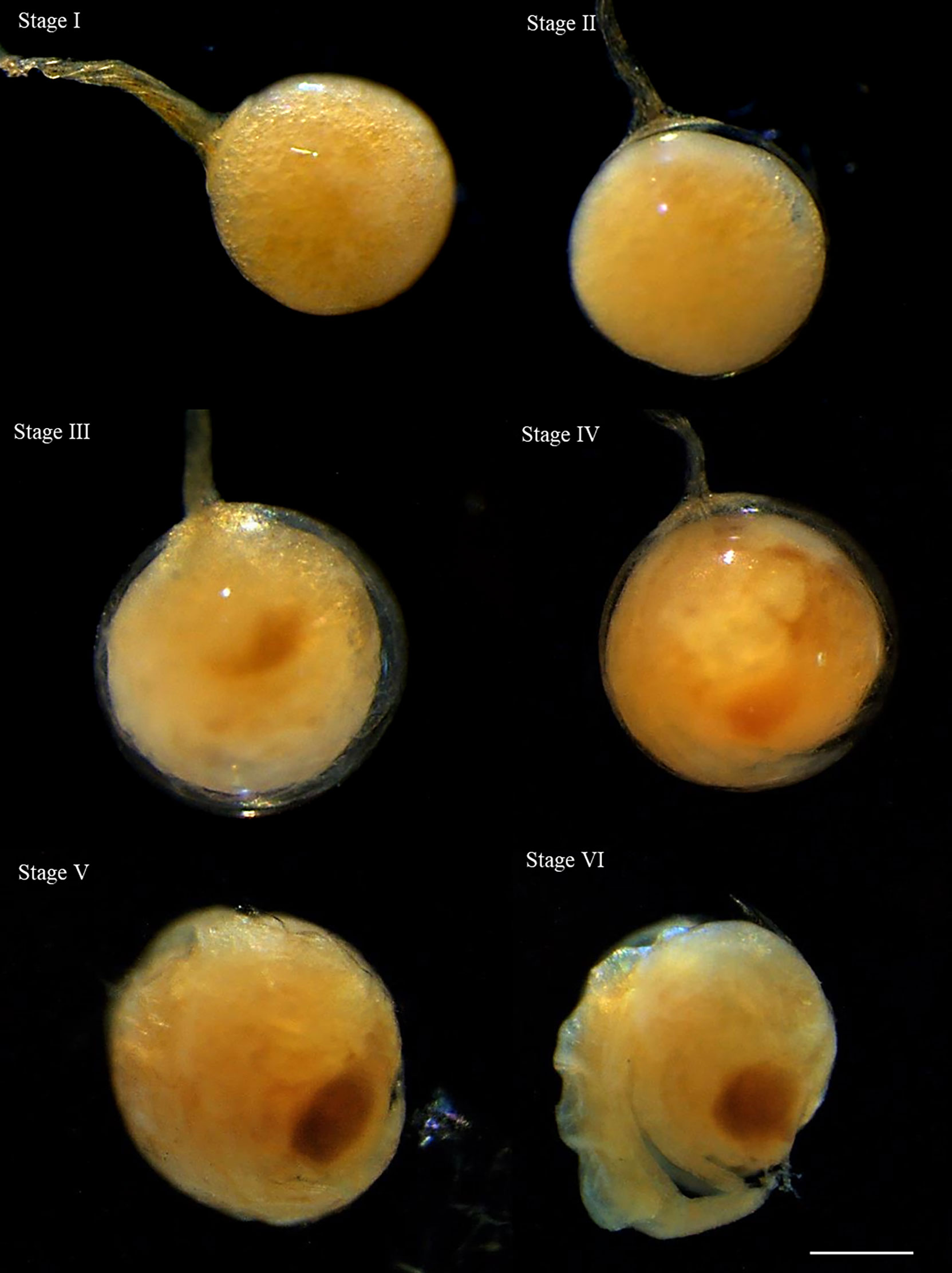
Figure 6 The stages of embryonic development described for Segonzacia mesatlantica taken under Leica MZ16FA. There are present 6 development stages (I, II, III, IV, V, and VI). Scale bars: I - VI = 200 μm.
Within the brood of both individuals analyzed, it was observed larvae in four stages of development (zoea I, II, III, and IV) with the body length increasing and the abdomen becoming more developed from zoea I (1.389 ± 0.081 mm) to zoea IV (1.801 ± 0.049 mm; Table 4; Figure 7). The larvae were tangled within the brood, but free of the embryonic membrane, and all the larvae presented compound pigmented eyes, the first and the second maxillipeds and the telson in a fork position (Figure 7). It was found 11 larvae in the 48.2 mm CW female (8 at the first stage, two at the second stage, and one at the third stage) and 1903 larvae in the 35.8 mm CW (436 at the first stage, 206 at the second stage, 143 at the third stage and 110 at the fourth stage). In addition, some larvae (170 zoea: three in the first stage, 13 in the second stage, 26 in the third stage, and 128 in the fourth stage) and two megalopae (Supplementary Figure 1) were collected at the water column near the vent sites.
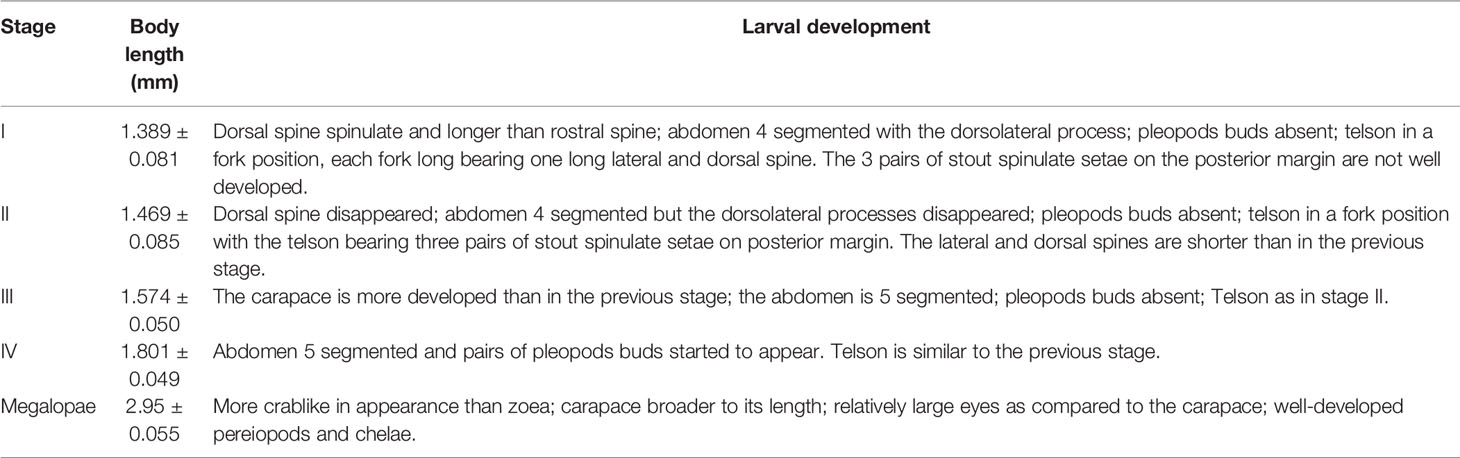
Table 4 Description of the larval development stages of Segonzacia mesatlantica according to Arshad et al. (2006); and Kado et al. (2010).
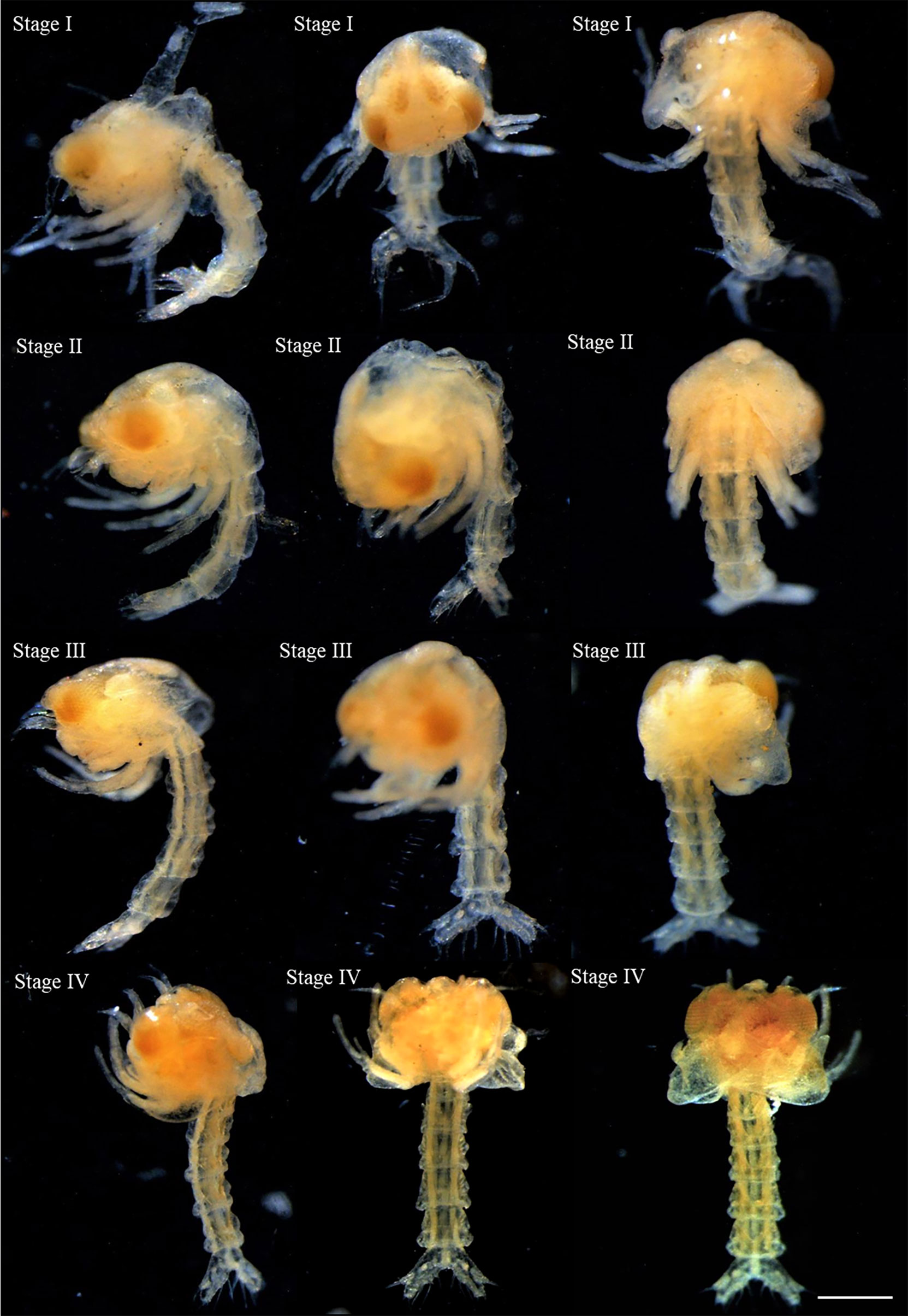
Figure 7 The stages of larval development described for Segonzacia mesatlantica taken under Leica MZ16FA dissecting microscope. There are presently four stages of larval development (I, II, III, and IV) lateral view, ventral view, and dorsal view. Scale bars: I-IV = 200 μm.
Discussion
This study provides for the first time some aspects of the behavior and reproductive ecology of the vent crab Segonzacia mesatlantica.
Behavior of Crabs
The observed non-brooding crabs of S. mesatlantica were found closer to the vents. The presented behaviors changed from a static position with solitary behavior to both territorial and predator behaviors as it was observed in the Lucky Strike vent field (Matabos et al., 2015). In contrast to non-brooding crabs, brooding crabs of S. mesatlantica were only observed outside the vent, positioned at higher structures. The movement of the brooders to the vent periphery was also reported for other bythograeid crabs including Bythograea thermydron (Williams, 1980; Brachyura: Bythograeidae) from the Galapagos Rift, and Bythograea laubieri (Guinot and Segonzac, 1997; Brachyura: Bythograeidae) and Bythograea vrijenhoeki (Guinot and Hurtado, 2003; Brachyura: Bythograeidae) from Southern East Pacific Rise (Wear, 1974; Perovich et al., 2003; Hilário et al., 2009). It is not clear why brooding crabs leave the vent orifice region to brood and hatch their eggs. It is hypothesized that the hydrothermal environment close to fluid exits [i.e., diffuse flow characterized by hypoxic conditions, high temperatures (Le Bris et al., 2019; Supplementary Table 2) combined with vent fluids rich in sulfide and heavy metals (Hourdez and Jollivet, 2021)] might be toxic to the eggs and larvae (Perovich et al., 2003). The vent periphery is characterized by weak flows of vent fluids and the presence of inactive structures, i.e., structures where the hydrothermal activity ceased resulting in structures without the flow of chemically modified fluids (Le Bris and the TRANSECT Science Party, 2018). Therefore, the risk of females being exposed to high temperatures and vent fluids is minimal. Both the mild fluids and the topographic feature might favor the vertical migration of larvae to a higher position in the water column (Le Bris, 2018). The females from the shallow-water hydrothermal vent crab Xenograpsus testudinatus migrate to the vent periphery, to release their larvae and avoid the larvae contacting high toxic plumes (Hung et al, 2019). The hydrothermal vent crab S. mesatlantica is well adapted to these challenging conditions, using ventilation as a compensatory mechanism while the heart rate remains stable down to this critical oxygen tension (Hourdez, 2018). Thus, brooding crabs might migrate to the vent periphery, where the surrounding water is colder and oxygen-rich (Le Bris et al., 2019; Supplementary Table 2), to be able to ventilate the broods.
The behaviors of S. mesatlantica brooding crabs were observed in other brachyuran crabs (Fernández et al. 2000; Baeza and Fernández, 2002; Brante et al., 2003; Fernández et al., 2006; Fernández and Brante et al., 2003), but were never reported for other bythograeid crabs. Despite no specific measure of oxygen demands for brooding crabs being determined in this study, their behavior may be associated with the increasing and assessment of oxygen conditions in the embryo mass (Baeza and Fernández, 2002; Fernández and Brante, 2003; Fernández et al., 2015). The provision of more oxygen was already observed on the brooding crabs of Cancer setosus. They probed their egg mass with chelae and pereiopods and when the dissolved oxygen in the egg mass declined, C. setosus increased the abdominal flapping, especially at the latter development stages when the oxygen demand of the embryos increased, which suggests an active brooding behavior (Fernández et al. 2000; Brante et al., 2003). The abdominal movement of brooding crabs can also have the function to facilitate larvae release as it can cause the rupture of the membranes surrounding the eggs (Rittschof and Cohen 2004). Oxygen availability in egg masses of several marine invertebrate species has been suggested to be an important factor affecting the modes of development of marine invertebrates (Lee and Strathmann, 1998). Oxygen limitation in embryo aggregations has been observed, as well as retarded development of inner embryos, emphasizing the importance of this factor (Cohen and Strathmann, 1996).
Life-History Traits
The general aspects of ovarian morphology and the general intra-ovarian gametogenic process of S. mesatlantica from oogonia that developed into previtellogenic oocytes, followed by early-vitellogenic and vitellogenic oocytes, were similar to other bythograeid crabs such as B. thermydron, B. laubieri and B. vrijenhoeki (Perovich et al., 2003; Hilário et al., 2009). In addition, the oocyte sizes of S. mesatlantica (previtellogenic: lower than 70 μm, early-vittelogenic: 70 to 110 μm, and vitellogenic: higher than 110 μm) were also comparable to B. laubieri and B. vrijenhoeki (previtellogenic: lower than 60 μm, early-vitellogenic: 60 to 100 μm and vitellogenic: higher than 100 μm; Hilário et al., 2009). Although the oocyte size frequency revealed a dominance of previtellogenic and early-vitellogenic oocytes, the presence of oocytes in different stages of development within the gonad of each brooding crab suggested that the oocytes of S. mesatlantica were maturing at different times. Therefore, the studied S. mesatlantica females revealed continuous gametogenesis at the individual level (Perovich et al., 2003), which was similar to B. laubieri and B. vrijenhoeki (Hilário et al., 2009).
Once extruded, mature oocytes form an egg mass attached to the pleopods underneath the abdomen (Marsh et al., 2015). The low sampling does not allow for a correlation between fecundity and size. However, the egg mass of the female with 48.2 mm CW was composed of a higher number of eggs and empty eggs than the female with 35.3 mm CW, The fecundity and the size of the eggs of S. mesatlantica were comparable with other bythograeids crabs such as B. thermydron (48.5 mm CW: 33 505 eggs mean 0.540 mm diameter; Van Dover et al., 1995; Supplementary Table 3), Within the egg mass of both females of S. mesatlantica, the eggs were at different stages of development, which suggests that the embryonic development is continuous at the individual level, similar to other deep-sea crabs such as Chaceon affinis (Milne-Edwards and Bouvier, 1894; Brachyura: Geryonidae; Tuset et al., 2011), and Paromola cuvieri (Risso, 1816; Brachyura: Homolidae; Triay-Portella et al., 2014; Supplementary Table 3). The number of embryonic stages seems to be similar among deep-sea crabs (Tuset et al., 2011; Martínez-Rivera et al., 2020; Supplementary Table 3) however, changes in color patterns during the embryonic development, seem to differ greatly. During the 6 stages of embryonic development of S. mesatlantica, the eggs were initially translucent yellow followed by orange and dark pink, while the eggs of Chaecon fenneri (Manning and Holthuis, 1984; Brachyura: Geryonidae) from Western Atlantic are initially light purple, and become dark purple and purple-brown. In addition, the eggs of Chaecon quinquedens (Smith, 1879; Brachyura: Geryonidae) from Western Atlantic are initially red-orange and become brown, followed by purple and finally black (Wigley et al., 1975; Haefner, 1978; Erdman and Blake, 1988 in Tuset et al., 2011). The color of the eggs might be linked to metal oxide (Guinot 1990) and food sources for the crabs (DeBevoise et al., 1990). The hydrothermal vent bacteria are known to produce carotenoids (orange-red) pigments (DeBevoise et al., 1990; Nègre-Sadargues et al., 1997; Nègre-Sadargues et al., 2000; Marteinsson et al., 2010) Being S. mesatlantica at the top of the hydrothermal food chain (Colaço et al., 2002; Portail et al., 2018), the will have access to those pigments (DeBevoise et al., 1990; Nègre-Sadargues et al., 1997; Nègre-Sadargues et al., 2000).
Within the brood of S. mesatlantica, it was observed the presence of larvae in different stages of development. These larvae were free of the embryonic membrane but entangled in the egg mass. Although only the most prominent features of the larval development stages were described in this study, the larvae development seems to comprise at least four zoeal stages with larvae increasing in length from the first zoea to the fourth zoea and a stage of megalopae. The size of the first zoea and megalopae of S. mesatlantica was similar to the first zoea of both Grandalfus yunohana (Takeda, Hashimoto and Ohta 2000; Brachyura: Bythograeidae) and B. thermydron (approximately 1.5 mm total length; Supplementary Table 3) and the megalopae of B. thermydron (between 2.5 to 7.5 mm CW; Martin and Dittel, 2007), respectively. The number of larval stages of S. mesatlantica was similar to other deep-sea crabs such as C. afinis (four or more zoeal stages and megalopae; Tuset et al., 2011; Supplementary Table 3). However, the only known larval development characteristics, including the number of stages and morphology are only known for G. yunohana which presented five to six planktotrophic zoeal stages (Hamasaki et al., 2010). Only the first zoea and the postlarval megalopae stage of B. thermydron were observed in the water column near the vent sites (Van Dover et al., 1995). The large difference in the respective sizes of the first zoea and the postlarval megalopae stage suggests that the normal larval development of B. thermydron may include several zoeal stages before the megalopae stage (Van Dover et al., 1984; Van Dover et al., 1995). The collection of larvae and megalopae of S. mesatlantica at the water column near the vent sites suggests that larvae might undergo a planktotrophic development, that was similar to the development of other bythograeid crabs such as B. thermydron (e.g., Epifanio et al., 1999; Jinks et al., 2002; Dittel et al., 2008).
It was the first time that brooder crabs of S. mesatlantica were observed. Mature males with mature spermatocytes of this species were observed in June (Tudge et al., 1998). This seems to indicate that the reproductive season might be early summer, despite the continuous development of gonads observed in the studied samples.
In summary, reproductive patterns observed in S. mesatlantica seem not to differ from other species of the family Bythograeidae. Brooding crabs gather outside of the direct influence of the vent field to brood and hatch their eggs into larvae that might go through a planktotrophic development. The behavior of brooding crabs provides evidence that oxygen might be a limiting factor for embryonic development. However, further studies involving an evaluation of the relationship between the female brooding behavior and the oxygen concentration in their egg mass are needed. The ovary microstructure and the general pattern of vitellogenesis of S. mesatlantica are characteristic of brachyuran decapods. As in other deep-sea crabs, both females had oocytes maturing at a different time within the gonads and embryos at different stages of development within the egg mass, suggesting that the gametogenesis and the embryonic development are continuous at the individual level. To infer the reproductive rhythms at a population level, further studies involving a higher number of samples collected at different periods are needed. Furthermore, to understand better the larvae development and the larvae dispersal, future studies with a detailed description of the larvae development and also involving a geochemical model to study the population connectivity are needed.
This study highlights some important aspects of the early life traits of S. mesatlantica that contribute to a better understanding of their reproductive ecology. Knowledge of the reproductive strategies may help establish mitigation strategies to maintain healthy populations. For example, brooding crabs of S. mesatlantica exhibited an active brooding behavior and continuous gametogenesis and embryogenesis, which are energy costly. As a result, there will be limited energy available to the female individual for recovery upon a possible disturbance. In addition, one of the consequences of climate change is a decrease in oxygen concentration in the deep sea (Breitburg et al., 2018; Oschlies et al., 2018). This might be a problem if oxygen will be a limiting factor for embryonic development.
Data Availability Statement
The original contributions presented in the study are included in the article/Supplementary Material. Further inquiries can be directed to the corresponding author.
Author Contributions
AC and MC led the writing process, AC collected the material during the TRANSECT cruise, MC analyzed the samples, NB led the cruise, organized dive plans, and built the Broken Spur shapefile. All authors participated in writing and editing the text and approved the final, submitted manuscript.
Funding
The TRANSECT cruise (DOI: DOI: 10.17600/18000513, chief scientist NLB) was supported by the French Oceanographic Fleet operated by Ifremer for the Sorbonne University and CNRS (scientific program lead and on-board team). MC is financed bythe program Estagiar-L, n° PL2028710, and was supported by the European Union’s Horizon 2020 research and innovation program, under the iAtlantic project (Grant Agreement No 818123). This output reflects only the author’s view and the European Union cannot be held responsible for any use that may be made of the information contained therein. AC received support from the Operational Program AZORES 2020, through the Fund 01-0145-FEDER-000140 “MarAZ Researchers: Consolidate a body of researchers in Marine Sciences in the Azores” of the European Union. AC was also supported by the FCT-IP Program Stimulus of Scientific Employment (CEECIND/00101/2021). AC and MC also acknowledge funds through the FCT – Foundation for Science and Technology, I.P., under the project OKEANOS UIDB/05634/2020 and UIDP/05634/2020.
Conflict of Interest
The authors declare that the research was conducted in the absence of any commercial or financial relationships that could be construed as a potential conflict of interest.
Publisher’s Note
All claims expressed in this article are solely those of the authors and do not necessarily represent those of their affiliated organizations, or those of the publisher, the editors and the reviewers. Any product that may be evaluated in this article, or claim that may be made by its manufacturer, is not guaranteed or endorsed by the publisher.
Acknowledgments
To the TRANSECT 2018 cruise science party and the Atalante R/V and Victor crew (DOI: 10.17600/18000513); to Domitília Rosa for assistance with histological analysis. To Pierre Le Meur for on-board assistance for bathymetry.
Supplementary Material
The Supplementary Material for this article can be found online at: https://www.frontiersin.org/articles/10.3389/fmars.2022.900990/full#supplementary-material
References
Arshad A., Efrizal K. M., Saad C. R. (2006). Study on Fecundity, Embryology and Larval Development of Blue Swimmic Crab Portunus Pelagicus (Linnaeus 1758) Under Laboratory Conditions. Res. J. Fisheries Hydrobiology 1 (1), 35–44.
Baeza J. A., Fernández M. (2002). Active Brood Care in Cancer Setosus (Crustacea: Decapoda): The Relationship Between Female Behaviour, Embryo Oxygen Consumption and the Cost of Brooding. Funct. Ecol. 16 (2), 241–251. doi: 10.1046/j.1365-2435.2002.00616.x
Brante A., Fernández M., Eckerle L., Marck F., Pörtner H. O., Arntz W. (2003). Reproductive Investment in the Crabs Cancer Setosus Along a Latitudinal Cline: Egg Production, Embryo Losses and Embryo Ventilation. Mar. Ecol. Prog. Ser. 251, 221–232. doi: 10.3354/meps251221
Breitburg D., Levin L. A., Oschlies A., Grégoire M., Chavez F. P., Conley D. J., et al. (2018). Declining Oxygen in the Global Ocean and Coastal Waters. Science 359 (6371). doi: 10.1126/science.aam7240
Cohen C. S., Strathmann R. R. (1996). Embryos at the Edge of Tolerance: Effects of Environment and Structure of Egg Masses on Supply of Oxygen to Embryos. Biol. Bull. 190 (1), 8–15. doi: 10.2307/1542671
Colaço A., Dehairs F., Desbruyères D. (2002). Nutritional Relations of Deep-Sea Hydrothermal Fields at the Mid-Atlantic Ridge: A Stable Isotope Approach. Deep Sea Res. Part I: Oceanographic Res. Papers 49 (2), 395–412. doi: 10.1016/S0967-0637(01)00060-7
Colaço A., Martins I., Laranjo M., Pires L., Leal C., Prieto C., et al. (2006). Annual Spawning of the Hydrothermal Vent Mussel, Bathymodiolus Azoricus, Under Controlled Aquarium Conditions at Atmospheric Pressure. J. Exp. Mar. Biol. Ecol., 333, 166–171.
Copley J. T. P., Tyler P. A., Murton B. J., Van Dover C. L. (1997). Spatial and Interannual Variation in the Faunal Distribution at Broken Spur Vent Field (29 N, Mid-Atlantic Ridge). Mar. Biol. 129 (4), 723–733. doi: 10.1007/s002270050215
Corliss J. B., Dymond J., Gordon L. I., Edmond J. M., Von Herzen R. P., Ballard R. D., et al. (1979). Submarine Thermal Springs on the Galapagos Rift. Science 203 (4385), 1073–1083. doi: 10.1126/science.203.4385.1073
Cuvelier D., Legendre P., Laës-Huon A., Sarradin P. M., Sarrazin J. (2017). Biological and Environmental Rhythms in (Dark) Deep-Sea Hydrothermal Ecosystems. Biogeosciences 14, 2955–2977. doi: 10.5194/bg-14-2955-2017
DeBevoise A. E., Childress J. J., Withers N. W. (1990). Carotenoids Indicate Differences in Diet of the Hydrothermal Vent Crab Bythograea Thermydron (Brachyura). Mar. Biol. 105 (1), 109–115. doi: 10.1007/BF01344276
Desbruyeres D., Almeida A., Biscoito M., Comtet T., Khripounoff A., Le Bris N., et al. (2000). “A Review of the Distribution of Hydrothermal Vent Communities Along the Northern Mid-Atlantic Ridge: Dispersal vs. Environmental Controls,” Hydrobiologia 440(1-3), 201–216. doi: 10.1023/A:1004175211848.
Dittel A. I., Epifanio C. E., Perovich G. (2005). Food Sources for the Early Life History Stages of the Hydrothermal Vent Crab Bythograea Thermydron: A Stable Isotope Approach. Hydrobiologia 544 (1), 339–346. doi: 10.1007/s10750-005-1699-7
Dittel A. I., Perovich G., Epifanio C. E. (2008). Biology of the Vent Crab Bythograea Thermydron: A Brief Review. J. Shellfish Res. 27 (1), 63–77. doi: 10.2983/0730-8000(2008)27[63:BOTVCB]2.0.CO;2
Epifanio C. E., Perovich G., Dittel A. I., Cary S. C. (1999). Development and Behavior of Megalopa Larvae and Juveniles of the Hydrothermal Vent Crab Bythograea Thermydron Marine Ecology Progress Series 185, 147–154.
Erdman R.B., Blake N.J. (1988). Reproductive Biology of Female Golden Crabs Geryon Fenneri Manning and Holthuis, From Southeastern Florida. Journal of Crustacean Biology, 8, 392–400. doi: 10.2307/1548278
Fernández M., Bock C., Pörtner H. (2000). The Cost of Being a Caring Mother: The Ignored Factor in the Reproduction of Marine Invertebrates. Ecology Letters 3, 487–494. doi: 10.1111/j.1461-0248.2000.00172.x
Fernández M., Brante A. (2003). Brood Care in Crachyuran Crabs: The Effect of Oxygen Provision on Reproductive Costs. Rev. Chil. Hist. Natural 76, 157–168. doi: 10.4067/S0716-078X2003000200003
Fernández M., Calderón R., Cifuentes M., Pappalardo P. (2006). Brooding Behaviour and Cost of Brooding in Small Body Size Brachyuran Crabs. Mar. Ecol. Prog. Ser. 309, 213–220. doi: 10.3354/meps309213
Gaudron S. M., Pradillon F., Pailleret M., Duperron S., Le Bris N., Gaill F. (2010). Colonization of Organic Substrates Deployed in Deep-Sea Reducing Habitats by Symbiotic Species and Associated Fauna Marine Environmental Research 70, 1–12.
Gebruk A. V., Galkin S. V., Vereshchaka A. L., Moskalev L. I., Southward A. J. (1997). Ecology and Biogeography of the Hydrothermal Vent Fauna of the Mid-Atlantic Ridge. Advances in Marine Biology 32(32), 93–144. doi: 10.1016/S0065-2881(08)60016-4.
Grassle J. F., Berg C. J., Chilmdress J. J., Hessler R. R., Jannasch H. J., Karl D. M., et al. (1979). Galàpagos 79: Initial Findings of a Deep-Sea Biological Quest Vol. 22 (Oceanus), 2–10.
Guinot D. (1997). “Segonzacia Mesatlantica Williams 1980,” in Handbook of Deep-Sea Hydrothermal Vent Fauna, vol. 214 . Eds. Desbruyères D., Segonzac M. (France: Brest: IFREMER).
Guinot D., Segonzac M. (1997). Description D'un Crabe Hydrothermal Nouveau Du Genre Bythograea (Crustacea Decapoda Brachyura) Et Remarques Sur Les Bythograeidae De La Dorsale Du Pacifique Oriental. Zoosystema-Paris, 19, 121–150.
Guinot D., Hurtado L.A., Vrijenhoek R. (2002). New Genus and Species of Brachyuran Crab From the Southern East Pacific Rise (Crustacea Decapoda Brachyura Bythograeidae). Comptes Rendus Biologies, 325(11), 1143–1152. doi: 10.1016/S1631-0691(02)01520-2"10.1016/S1631-0691(02)01520-2
Guinot D., Hurtado L. A. (2003). Two New Species of Hydrothermal Vent Crabs of the Genus Bythograea From the Southern East Pacific Rise and From the Galapagos Rift (Crustacea, Decapoda, Brachyura, Bythograeidae). Comptes Rendus Biologies 326 (4), 423–439. doi: 10.1016/S1631-0691(03)00126-4
Haefner P.A. Jr. (1978) . Seasonal Aspects of the Biology, Distribution and Relative Abundance of the Deep-Sea Red Crab Geryon Quinquedens Smith, in the Vicinity of the Norfolk Canyon, Western North Atlantic. Proceedings of the National Shellfisheries Association. 68, 49–62.
Hamasaki K., Nakajima K., Tsuchida S., Kado R., Kitada S. (2010). Number and Duration of Zoeal Stages of the Hydrothermal Vent Crab Gandalfus Yunohana From Laboratory Reared Specimens. J. Crustacean Biol. 30 (2), 236–240. doi: 10.1651/09-3199.1
Hilário A., Vilar S., Cunha R. M., Tyler P. (2009). Reproductive Aspects of Two Bythograeid Crab Species From Hydrothermal Vents in Pacific-Antarctic Ridge. Mar. Ecol. Prog. Ser. 378, 153–160. doi: 10.3354/meps07858
Hines A. H. (1990). “Commentary on Life History and Ecology of Deep-Sea Crabs of the Family Geryonidae,” in Geryonid Crabs and Associated Continental Slope Fauna: A Research Workshop Report. Florida Sea Grant College Technical Paper, vol. 58. Eds. W. Florida Sea Grant College Program Publications, USA
Hourdez S. (2018). Cardiac Response of the Hydrothermal Vent Crab Segonzacia Mesatlantica to Variable Temperature and Oxygen Levels. Deep Sea Res. Part I: Oceanographic Res. Papers 137, 57–65. doi: 10.1016/j.dsr.2018.03.004
Hourdez S., Jollivet D. (2021). “Metazoan Adaptation to Deep-Sea Hydrothermal Vents,” in Life in Extreme Environments. Insights in Biological Capability, Ecological Reviews (United Kingdom: Cambridge University Press), 42–67. United Kingdom
Hung J. J., Peng S. H., Chen A. C. T., Wei T. P., Hwang J. S. (2019). Reproductive Adaptations of the Hydrothermal Vent Crab Xenograpus Testudinatus: An Isotopic Approach. PloS One 14 (2), e0211516. doi: 10.1371/journal.pone.0211516
Jinks R. N., Markley T. L., Taylor E. E., Perovich G., Dittel A. I., Epifanio C. E., et al. (2002). Adaptive Visual Metamorphosis in a Deep-Sea Hydrothermal Vent Crab. Nature 420 (6911), 68–70. doi: 10.1038/nature01144
Kado R., Hamasaki K., Kitada S., Tshuchida S., Nakajima K. (2010). First Zoeal Stage of the Hydrothermal Vent Crab, Gandalfus Yunohana (Decapoda, Brachyura, Bythograeidae). Crustaceana, 83(5), 525–537. doi: 10.1163/001121610X491022
Le Bris N., The TRANSECT Science Party. (2018). Transfert D’Energie Dans Les Ecosystèmes Chimiosynthétiques En Contexte Ultramafique. TRANSECT Cruise, L’atalante R/V Victor6000. doi: 10.17600/18000513
Le Bris N., Yücel M., Das A., Sievert S. M., LokaBharathi P., Girguis P. R. (2019). Hydrothermal Energy Transfer and Organic Carbon Production at the Deep Seafloor. Front. Mar. Sci. 5, 531. doi: 10.3389/fmars.2018.00531
Lee C., Strathmann R. R. (1998). Scaling of Gelatinous Clutches: Effects of Sibling Competition for Oxygen on Clutch Size and Parental Investment Per Offspring. Am. Nat. 151 (4), 293–310. doi: 10.1086/286120
Lonsdale P. F. (1977). Clustering of Suspension-Feeding Macrobenthos Near Abyssal Hydrothermal Vents at Oceanic Spreading Centers. Deep-Sea Res. 24, 857–863. doi: 10.1016/0146-6291(77)90478-7
Marsh L., Copley J. T., Tyler P. A., Thatje S. (2015). In Hot and Cold Water: Differential Life-History Traits are Key to Success in Contrasting Thermal Deep-Sea Environments. J. Anim. Ecol. 84 (4), 898–913. doi: 10.1111/1365-2656.12337
Marteinsson V. T., Bjornsdottir S. H., Bienvenu N., Kristjansson J. K., Birrien J. L. (2010). Rhodothermus Profundi Sp. Nov., a Thermophilic Bacterium Isolated From a Deep-Sea Hydrothermal Vent in the Pacific Ocean. Int. J. systematic evolutionary Microbiol. 60 (12), 2729–2734. doi: 10.1099/ijs.0.012724-0
Martin J. W., Dittel A. (2007). The Megalopa Stage of the Hydrothermal Vent Crab Genus Bythograea (Crustacea, Decapoda, Bythograeidae). Zoosystema-Paris 29 (2), 365–379.
Martínez-Rivera S., Stevens B. G. (2020). Embryonic Development and Fecundity of the Red Deep-Sea Crab Chaecon Quinquedens (Smith 1879) (Decapoda:Brachyura: Geryonidae) in the Mid-Atlantic Bigh Determined by Image Analysis. J. Crustacean Biol. 40 (3), 230–236. doi: 10.1093/jcbiol/ruaa017
Martin J. W., Haney T. A. (2005). Decapod Crustaceans From Hydrothermal Vents and Cold Seeps: A Review Through 2005. Zoological J. Linn. Soc. 145 (5), 445–522. doi: 10.1111/j.1096-3642.2005.00178.x
Matabos M., Cuvelier D., Brouard J., Shillito B., Ravaux J., Zbinden M., et al. (2015). Behavioural Study of Two Hydrothermal Crustacean Decapods: Mirocaris Fortunata and Segonzacia Mesatlantica, From the Lucky Strike Vent Field (Mid-Atlantic Ridge). Deep-Sea Res. Part II: Topical Stud. Oceanography 121, 146–158. doi: 10.1016/j.dsr2.2015.04.008
Mateos M., Hurtado L. A., Santamaria C. A., Leignel V., Guinot D. (2012). Molecular Systematics of the Deep-Sea Hydrothermal Vent Endemic Brachyuran Family Bythograeidae: A Comparison of Three Bayesian Species Tree Methods. PloS One 7 (3), e32066. doi: 10.1371/journal.pone.0032066
McLay C. (2007). New Crabs From Hydrothermal Vents of the Kermadec Ridge Submarine Volcanoes, New Zealand: Gandalfus Gen. Nov. (Bythograeidae) and Xenograpsus (Varunidae) (Decapoda, Brachyura). Zootaxa 1524 (1), 1–22. doi: 10.5281/zenodo.177502
Milne-Edwards A., Bouvier E. L. (1894). Crustacés Décapodes Provenant Des Campagnes Du Yacht l'Hirondelle (1886, 1887, 1888). I. Brachyures Et Anomoures. Résultats des Campagnes Scientifiques accompliés sur son Yacht par Albert Ier Prince Souverain de Monaco. 7, 3–112; Pls. 1-11.
Mullineaux L.S., France S.C. (1995). Dispersal Mechanisms of Deep-Sea Hydrothermal Vent Fauna. Washington DC American Geophysical Union Geophysical Monograph Series, 91, 408–424. doi: 10.1029/GM091p0408
Mullineaux L.S., Metaxas A., Beaulieu S.E., Bright M., Gollner S., Grupe B.M., et al. (2018). Exploring the Ecology of Deep-Sea Hydrothermal Vents in a Metacommunity Framework. Front. Mar. Sci. 5,49. doi: 10.3389/fmars.2018.00049
Murton B. J., Van Dover C. L. (1993). ALVIN Dives on the Broken Spur Hydrothermal Vent Field at 29 10′ N on the Mid-Atlantic Ridge. Bridge Newsletter, 5, 11–14.
Murton B. J., Van Dover C., Southward E. (1995). Geological Setting and Ecology of the Broken Spur Hydrothermal Vent Field: 29°10’ N. On the Mid-Atlantic Ridge in Hydrothermal Vents and Processes, vol. 87 Eds. MParson C. L. W. L., Dixon D. R. (UK: Geological Society, London, London, Special Publications), 33–41. UK
Nakajima K., Hamasaki K., Tshuchida S., Kado R., Kitada S. (2010). First Zoeal Stage of the Hydrothermal Vent Crab, Gandalfus Yunohana (Decapoda, Brachyura, Bythograeidae). Crustaceana 83 (5), 525– 537. doi: 10.1163/001121610X491022
Nègre-Sadargues G., Castillo R., Segonzac M. (2000). Carotenoid Pigments and Trophic Behaviour of Deep-Sea Shrimps (Crustacea, Decapoda, Alvinocarididae) From a Hydrothermal Area of the Mid-Atlantic Ridge. Comp. Biochem. Physiol. Part A: Mol. Integr. Physiol. 127 (3), 293–300. doi: 10.1016/S1095-6433(00)00258-0
Nègre-Sadargues G., Segonzac M., Castillo R. (1997). Carotenoid Pigments and Trophic Behaviour of Crustacea From the Snake Pit Hydrothermal Area (Mid-Atlantic Ridge, 23 Degree N). Cahiers Biologie Mar. 2, 134–135.
Oschlies A., Brandt P., Stramma L., Schmidtko S. (2018). Drivers and Mechanisms of Ocean Deoxygenation. Nat. Geosci. 11 (7), 467–473. doi: 10.1038/s41561-018-0152-2
Perovich G. M., Epifanio C. E., Dittel A. I., Tyler P. A. (2003). Spatial and Temporal Patterns in Development of Eggs in the Vent Crab Bythograea Thermydron. Mar. Ecol. Prog. Ser. 251, 211–220. doi: 10.3354/meps251211
Portail M., Brandily C., Cathalot C., Colaço A., Gélinas Y., Husson B., et al. (2018). Food-Web Complexity Across Hydrothermal Vents on the Azores Triple Junction. Deep Sea Res. Part I: Oceanographic Res. Papers 131, 101–120. doi: 10.1016/j.dsr.2017.11.010
Rittschof D., Cohen J.H. (2004). Crustacean Peptide and Peptide-Like Pheromones and Kairomones. Peptides, 25(9), 1503–1516. doi: 10.1016/j.peptides.2003.10.024
Segonzac M. (1992). Les Peuplements Associés À L’hydrothermalisme Océanique Du Snake Pit (Dorsale Médio-Atlantique; 23° N 3480 M): Composition Et Microdistribution De La Mégafaune. Comptes rendus l’Académie Des. Sci. Série 3 Sci. la vie 314 (13), 593–600.
Takeda M., Hashimoto J., Ohta S. (2000). A New Species of the Family Bythograeidae (Crustacea, Decapoda, Brachyura) From the Hydrothermal Vents Along Volcanic Front of the Philippine Sea Plate Bulletin of the National Science Museum–A. Zoology, 26, 159–172.
Triay-Portella R., González J. A., Santana J. I., García-Martín V., Romero M., Jiménez-Martín S., et al. (2014). Reproductive Pattern and Egg Development of the Deep-Sea Crab Paromola Cuvieri (Brachyura, Homolidae) Around the Canary Islands (NE Atlantic). Deep-Sea Res. Part I: Oceanographic Res. Papers 85, 1–14. doi: 10.1016/j.dsr.2013.12.001
Tsuchida S., Fujikura K., Hashimoto J., Fujiwara Y., Hunt J. C., Lindsay J. (1998). Molting of Bythograeid Crabs Under Laboratory Conditions. JAMSTEC J. Deep-Sea Res. 14, 515–520.
Tsuchida S., Hashimoto J. (2002). A New Species of Bythograeid Crab, Austinograea Rodriguezensis (Decapoda, Brachyura), Associated With Active Hydrothermal Vents From the Indian Ocean. J. Crustacean Biol. 22 (3), 642–650. doi: 10.1163/20021975-99990276
Tudge C. C., Jamieson B. G. M., Segonzac M., Guinot D. (1998). Spermatozoal Ultrastructure in Three Species of Hydrothermal Vent Crab, in the Genera Bythograea, Austinograea and Segonzacia (Decapoda, Brachyura, Bythograeidae). Invertebrate Reprod. Dev. 34 (1), 13–23. doi: 10.1080/07924259.1998.9652349
Tuset M. V., Espinosa D. I., Garcia-Mederos A., Santanta J. I., Gonález J. A. (2011). Egg Development and Fecundity Estimation in the Deep-Sea Red Crab, Chaceon Affinis (Geryonidae) Off the Canary Islands (NE Atlantic). Fisheries Res. 109 (2-3), 373–378. doi: 10.1016/j.fishres.2011.02.021
Tyler P. A., Young C. M. (1999). Reproduction and Dispersal at Vents and Cold Seeps. J. Mar. Biol. Assoc. United Kingdom 79 (2), 193–208. doi: 10.1017/S0025315499000235
Van Dover C. L. (1995a). “Ecology of Mid-Atlantic Ridge Hydrothermal Vents,” Hydrothermal Vents and Processes, eds. Parson L. M, Walker C. L., Dixon D. R. (UK: Geological Society Special Publication 87), 257–294. doi: 10.1144/GSL.SP.1995.087.01.21
Van Dover C. L., Factor J. R., Williams A. B., Berg C. J. Jr (1995). Reproductive patterns of Decapod Crustaceans From Hydrothermal Vents. Bulletin of the Biological Society of Washington 6, 223–7
Van Dover C. L. (2014). Impacts of Anthropogenic Disturbances at Deep-Sea Hydrothermal Vent Ecosystems: A Review. Mar. Environ. Res. 102, 59–72. doi: 10.1016/j.marenvres.2014.03.008
Van Dover C. L., Factor J. R., Williams A. B., Berg C. J. (1985). Reproductive Patterns of Decapod Crustaceans From Hydrothermal Vents. Bulletin of the Biological Society of Washington, 6, 223–227.
Van Dover C. L., Williams A. B., Factor J. R. (1984). The First Zoeal Stage of a Hydrothermal Vent Crab (Decapoda: Brachyura: Bythograeidae). Proc. Biol. Soc. Washington 97 (2), 413–418.
Ecology of Deep-Sea Hydrothermal Vents in a Metacommunity Framework. Front. Mar. Sci. 5, 49. doi: 10.3389/fmars.2018.00049
Wear R. G. (1974). Incubation in British Decapod Crustacea and the Effects of Temperature on the Rate and Success of Embryonic Development. J. Mar. Biol. Assoc. United Kingdom 54 (3), 745–776. doi: 10.1017/S0025315400022918
Wigley R.L., Theroux R. B., Murray H. E. (1975). Deepsea Red Crab, Geryon Quinquedens, Survey Off the Northeastern United States. Mar. Fish. Rev., 37(8), 1–27.
Keywords: Broken Spur, bythograea, ecology, hydrothermal vent, larvae, life-history biology, deep sea
Citation: Cruz M, Le Bris N and Colaço A (2022) Reproductive Traits of the Vent Crab Segonzacia mesatlantica (Guinot, 1989) From the Mid-Atlantic Ridge. Front. Mar. Sci. 9:900990. doi: 10.3389/fmars.2022.900990
Received: 21 March 2022; Accepted: 30 May 2022;
Published: 13 July 2022.
Edited by:
Jiang-Shiou Hwang, National Taiwan Ocean University, TaiwanReviewed by:
Maite Mascaro, National Autonomous University of Mexico, MexicoHiromi Kayama Watanabe, Japan Agency for Marine-Earth Science and Technology (JAMSTEC), Japan
Copyright © 2022 Cruz, Le Bris and Colaço. This is an open-access article distributed under the terms of the Creative Commons Attribution License (CC BY). The use, distribution or reproduction in other forums is permitted, provided the original author(s) and the copyright owner(s) are credited and that the original publication in this journal is cited, in accordance with accepted academic practice. No use, distribution or reproduction is permitted which does not comply with these terms.
*Correspondence: Ana Colaço, maria.aa.colaco@uac.pt