- 1Key Laboratory of Tropical Marine Bio-resources and Ecology, South China Sea Institute of Oceanology, Chinese Academy of Sciences, Guangzhou, China
- 2Southern Marine Science and Engineering Guangdong Laboratory (Guangzhou), Guangzhou, China
- 3University of Chinese Academy of Sciences, Beijing, China
The spatial and seasonal distributions of stable carbon and nitrogen isotopes (δ13C and δ15N) in dominant zooplankton groups were investigated in Jiaozhou Bay. Zooplankton δ13C values ranged from −22.89‰ to −15.86‰, and δ15N values ranged from 3.18‰ to 13.57‰, respectively. The δ13C and δ15N values generally followed the order of small zooplankton < large calanoids < small Sagitta < large Sagitta. Spatial distribution patterns of zooplankton δ13C and δ15N values varied in different seasons. Our results suggested that the spatial variation of δ13C was mainly controlled by terrigenous organic matter (OM) input and phytoplankton biomass, but water temperature may have played a key role in the seasonal variation of δ13C. In spring, the high phytoplankton biomass might increase the δ13C value of small zooplankton in the inner bay. During other seasons, the δ13C values of zooplankton generally increased from the inner bay to the outer bay, which might be associated with the influence of 13C-depleted terrigenous OM carried by the river discharge. Small zooplankton stable isotope values were significantly correlated with that of particulate organic matter (POM). The influence of anthropogenic nutrient input on isotopic baseline can be cascaded to the zooplankton, and the effect might be weak at higher trophic levels. The Bayesian standard ellipse areas of dominant zooplankton groups were generally smallest in the winter, suggesting a narrow niche width during that time. The niche partition between small zooplankton, large calanoids, and Sagitta was most distinct in winter, and followed by summer. The relative trophic level of Sagitta ranged from 2.23 to 4.01, which generally declined from the inner bay to the outer bay during the spring, autumn, and winter seasons. High anthropogenic nutrient loading might reduce the difference in trophic niches among zooplankton groups. This study provided detailed information on the distribution of zooplankton δ13C and δ15N in a coastal bay, which will be useful for understanding the anthropogenic influence on the ecosystem structure and function.
Introduction
Zooplankton play a key role in aquatic ecosystems: they transfer energy and materials from primary producers to higher trophic levels (Hannides et al., 2009). The species composition and abundance of zooplankton can be quickly changed by the variation of environmental factors, and potentially affect ecosystem function and biogeochemical cycling (Dickman et al., 2008). Knowledge of zooplankton trophic structure is necessary to understand food-web function and response to climate change or human activities (Kozak et al., 2020; Nagelkerken et al., 2020).
Carbon and nitrogen stable isotopes (δ13C and δ15N) are widely used to trace the material source and trophic structure in aquatic ecosystems (Post, 2002; Fry, 2006). Generally, the δ13C value increases by approximately 1.5‰, and the δ15N value increases by 2–4‰ per trophic level (Fry, 2006). Due to the fast growth rate and short turnover time, the stable isotope values of zooplankton can quickly change with environmental variation (Rolff, 2000; O’Reilly et al., 2002). Describing the distribution patterns of stable isotopes in pelagic ecosystems is important when assessing the anthropogenic influence on coastal ecosystems. Stable isotopes have the potential to integrate variability in environmental conditions, and help us understand the relationship between eutrophication and the trophic structure of pelagic food web (Fry, 2006; El-Sabaawi et al., 2013). In general, the δ15N values are more sensitive to environmental change. Nitrogen concentration needs to be considered when using δ15N values in biota as indicators of anthropogenic nitrogen inputs (Pruell et al., 2020). The δ15N of zooplankton can also vary over inter-annual scales of climate variability. Décima et al. (2013) reported a significant 15N enrichment of approximately 2‰ for zooplankton during the 1998 El Niño in the southern California Current Ecosystem. The zooplankton isotopic niche suggested that the planktonic food web spans >3 trophic levels, ranging from herbivores to higher predators (El-Sabaawi et al., 2009; Chi et al., 2021). Trophic niche and niche overlap among zooplankton species can well reflect the influence of environmental change on ecosystem function and biogeochemical cycling. Generally, short food webs are expected in eutrophic ecosystems compared to oligotrophic ecosystems (Armengol et al., 2019). However, Kozak et al. (2020) reported that increased primary and secondary productivity results in an increase of food web complexity, and produces longer food chains. The response of zooplankton trophic structure to eutrophication might be different in various marine ecosystems. To date, high-resolution studies of trophic dynamics within zooplankton community are still scarce.
Jiaozhou Bay is a semi-enclosed bay in northern China, bordering on the Yellow Sea. Many studies have suggested that the ecosystem of this bay has been strongly disturbed by intensive anthropogenic activities, including aquaculture, wastewater discharge, land reclamation, and harbor construction (Liu et al., 2005; Yuan et al., 2016; Ke et al., 2020). Jiaozhou Bay has been regarded as an ideal region for studying the influence of human activities on coastal ecosystems and there are abundant reports on the structure and function of ecosystem corresponding to anthropogenic influences (Sun et al., 2012; Yuan et al., 2016; Wang et al., 2021). The size structure of zooplankton was significantly associated with environmental factors in this bay, and the total zooplankton abundance and small size ranks presented a decreasing gradient from the inner bay to the outer part (Wang et al., 2020a). Recently, Wang et al. (2020b) indicated that the population explosion of the jellyfish Aurelia coerulea in Jiaozhou Bay increased the predation pressure on zooplankton and changed the trophic structure of pelagic ecosystem. Most studies only focused on the species composition and abundance of zooplankton. Few studies have estimated the source of particulate organic matter (POM) or sediment organic matter using carbon and nitrogen stable isotopes. Both the δ13C value and C/N ratio suggested a higher contribution of marine organic carbon than terrestrial organic carbon in this bay (Yang et al., 2011). Using carbon stable isotopes, Kang et al. (2017) estimated that marine-sourced OM contributed to 45%–79% of total organic carbon in the sediment of Jiaozhou Bay. Ke et al. (2020) indicated that the 15N of POM was significantly depleted in the northeastern region due to the strong influence of urban sewage from Qingdao City. In comparison, there are still relatively limited data available on δ13C and δ15N of zooplankton in Jiaozhou Bay. The zooplankton trophic position and response to environmental change remain poorly understood.
In this study, we investigated the spatial and seasonal distribution of zooplankton stable isotope values in Jiaozhou Bay. Seasonal variations of stable isotopic signatures in zooplankton remain mostly undescribed in coastal bay, which is important as a baseline reference for trophic structure analyses in marine ecosystem (Kozak et al., 2020). We compared the relative trophic positions of the dominant zooplankton groups in different seasons. The objective of this study was to examine the variations in stable isotope signatures and trophic niches of zooplankton in a coastal bay under anthropogenic influences. The relationships between the zooplankton stable isotopic signatures and environmental variables were also analyzed. The results of this study may assist with assessing the anthropogenic influence on the trophic structure of coastal ecosystems.
Materials and Methods
Study Area
The Jiaozhou Bay is located in northern China. Its area is about 390 km2, and the average water depth is about 7 m. The bay mouth is narrow with about 2.5 km. The tide is a typical semi-diurnal tide with a mean range of 7 m. This bay is under a temperate oceanic monsoon climate. The average annual temperature is 12°C, and the average annual precipitation is 900 mm in this region. The Jiaozhou Bay is surrounded by Qingdao City, and its ecosystem has been strongly impacted by human activities (Liu et al., 2005). Since the 1980s, the species composition of zooplankton in this bay has changed significantly. Both the abundance and species diversity of small jellyfish have increased in Jiaozhou Bay during the past two decades (Sun et al., 2012; Wang et al., 2020b). The long-term investigation suggested that the ecological environment of Jiaozhou Bay has changed under the influence of anthropogenic activities, including algal blooms, biodiversity decline, and ecosystem degradation (Yuan et al., 2016; Zhang et al., 2021).
Sample Collection and Analysis
Seasonal surveys were carried out at 12 stations (S1–S12) in Jiaozhou Bay in spring (April 6–8, 2016), summer (August 17–19, 2017), autumn (November 18–20, 2016), and winter (January 5–8, 2016) (Figure 1). Water samples were collected using a 10-L Niskin bottle at a depth of 0.5 m below the surface. Water temperature, salinity, nutrients, and chlorophyll a (Chl a) concentrations were measured. The sampling and analysis methods of environment variables were introduced in detail in Ke et al. (2020). POM samples were obtained by filtering 0.5–1 L of surface water through pre-combusted Whatman GF/F filters. Zooplankton samples were collected by vertical tows from the bottom to the surface using a conical plankton net (0.5 m diameter, length 2 m, and mesh size 169 μm). The net was hauled 2–4 times at each station with the speed of about 0.5 m s−1. Collected zooplankton specimens were placed in pre-filtrated sea water for about 0.5 h to exclude the gut content. Then, these samples were aggregated using a piece of 180-μm nylon sieve and frozen in liquid nitrogen. When returned to the lab, these zooplankton samples were stored at −20°C until further species identification and separation.
Each zooplankton sample was first sieved using a 500-μm mesh sieve to separate the <500-μm size class that was processed as small zooplankton. Three dominant groups were picked up from the individuals larger than 500 μm, including large calanoids (large CA), small Sagitta and large Sagitta. These groups contributed to most of the zooplankton biomass in the coastal bays of China (Ma et al., 2014; Wang et al., 2020a). The body length of small Sagitta was generally less than 1.2 cm and large Sagitta was larger than 1.5 cm. These dominant groups were collected at most stations during the investigation. Because the collected zooplankton contained less carbonate and acid treatment can significantly affect the δ15N value, these samples were not treated with HCl (Troina et al., 2020). To obtain sufficient samples for stable isotope analysis, several individuals (2-8) of similar size belonging to the same taxonomic group were pooled together. Zooplankton samples were rinsed with distilled water, placed in pre-weighed tin capsules with a 5-mm diameter, and dried at 60°C for 24 h.
Stable Isotopes Analysis and Statistical Analysis
A total of 0.5–2 mg of zooplankton samples was wrapped into tin capsules and sent for the analysis of stable isotope composition. Stable isotope values of POM and zooplankton were determined with a continuous-flow isotope-ratio mass spectrometer (Delta V Advantage, Thermo Fisher Scientific, Waltham, MA, USA) coupled to an elemental analyzer (Flash EA 1112, Thermo Fisher Scientific, Milan, Italy). Isotopic ratios were expressed in δ notation as the deviation in parts per mil (‰) from a standard reference material: δ13C or δ15N (‰) = (Rsample/Rstandard − 1) × 1,000, where R is either 13C/12C or 15N/14N. The reference standards of carbon and nitrogen are the PeeDee Belemnite and atmospheric N2, respectively. The analytical precision for both isotopes is ± 0.2‰. Casein protein was used as a working standard, whose certified values for carbon and nitrogen were determined by EA-IRMS calibrated to IAEA-CH-6 and IAEA-N-1 (IAEA, Vienna).
Prior to the data analysis, a lipid correction of δ13C values was carried out for specimens with high C:N ratios (>3.5) according to Post et al. (2007). The correction formula was as follows: δ13Cnormalized = δ13Cuntreated - 3.32+0.99× C:N, where δ13Cnormalized was used for further analysis in this study, δ13Cuntreated denotes the raw data, and C:N ratio is the bulk carbon-to-nitrogen ratio. The δ15N values of POM showed a significant spatial variation in Jiaozhou Bay (Ke et al., 2020). In this study, the trophic level of small zooplankton (<500 μm) was assigned a value of 2 to eliminate the influence of fluctuations in the δ15N baseline. Relative trophic level calculation: , where RTL is the relative trophic level, and δ15NZoo and δ15NSmall ZP are the values of δ15N in dominant zooplankton groups and small zooplankton, respectively. The trophic enrichment factor of δ15N is assigned as 3.4‰ for each trophic level (Post, 2002).
The isotopic niches of zooplankton community were estimated by bivariate trophic space, in which the δ13C and δ15N values represented the carbon source and trophic level of zooplankton, respectively. The Stable Isotope Bayesian Ellipses in R (SIBER) package in the R-routine was used to estimate the potential isotope niche width and overlap for dominant zooplankton groups during different seasons (Jackson et al., 2011; R Core Team, 2020). The areas of Bayesian standard ellipse were calculated using a bivariate approach of the niche width, as defined by the isotopic space of δ13C vs. δ15N plots. Because small and large Sagitta were usually not collected at one station simultaneously, the average δ13C and δ15N values of small and large Sagitta at each station were used to make the comparison between different zooplankton groups. One-way analysis of variance (ANOVA) was used to check the seasonal and spatial differences in stable isotope values or nutrient concentrations. All statistical analyses were performed with SPSS software package, version 19.0 for Windows (SPSS Inc., Chicago, IL, USA). The map and the contour figures were made by the software Ocean Data View 5.2 (Schlitzer, R., http://odv.awi.de).
Results
Environmental Conditions
The average values of main environmental variables in four seasons are shown in Figure 2. Annual average values of water temperature and salinity were 14.8°C and 30.8, respectively. The variation in water temperature was large in a year. The average sea surface sea temperature (SST) was 6.3°C in winter and 27.4°C in summer. In contrast, sea surface salinity (SSS) was relatively stable in different seasons, but was slightly lower in summer (average 30.1). The seasonal variation of nutrient concentration was great, which was highest in summer and lowest in winter (Figure 2). Annual average DIN, , and concentrations were 13.6 μM, 0.3 μM, and 7.2 μM, respectively. Detailed spatial distributions of environmental variables were previously described in Ke et al. (2020). In brief, the concentrations of nutrients and Chl a generally decreased from the inner to the outer bay, and were very high at station S1 in the northeastern bay (Ke et al., 2020).
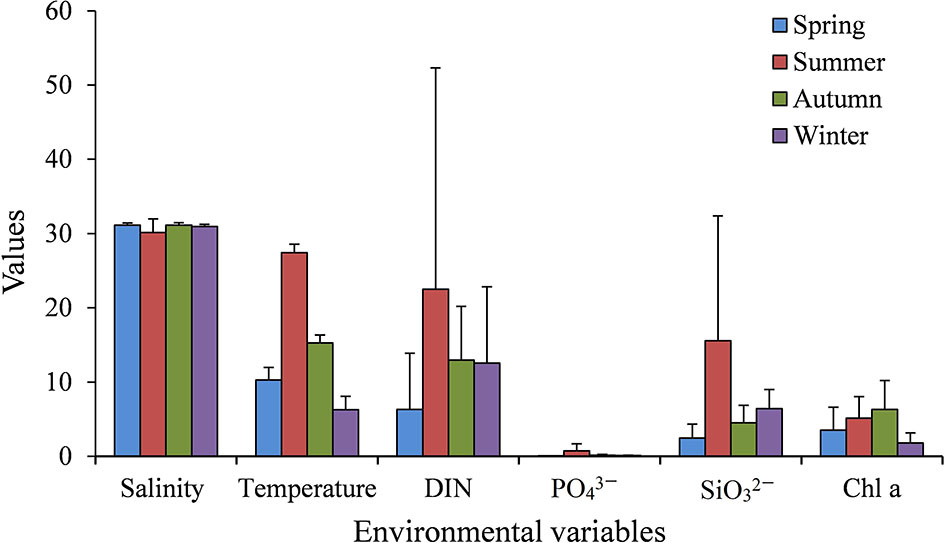
Figure 2 Seasonal average values of key environmental variables, including salinity, temperature, DIN (μM), (μM), (μM), and Chl a (μg L−1) in Jiaozhou Bay.
Spatial and Seasonal Variations of Stable Isotopes in Dominant Zooplankton Groups
In this study, the large calanoids were mainly represented by adult Calanus sinicus, the large Sagitta were mainly represented by adult Sagitta crassa, and the small Sagitta were mainly represented by junior Sagitta crassa and Sagitta bedoti. The spatial distributions of δ13C and δ15N values were similar in these dominant zooplankton groups (Figures 3, 4). The seasonal distribution patterns of zooplankton δ13C values were complicated in Jiaozhou Bay. Lower δ13C values generally occurred in the inner bay during summer, autumn, and winter. However, in spring, the δ13C values of zooplankton were higher in the inner bay especially for the small zooplankton (Figure 3). In autumn, obviously lower 13C values of zooplankton were found at station S6 in the western inner bay. The seasonal average values of δ13C and δ15N of these dominant zooplankton groups are shown in Table 1. The δ13C values of zooplankton ranged from −22.89‰ to −15.86‰. Annual average δ13C value was −19.09‰ for small zooplankton, −18.94‰ for large calanoids, −18.68‰ for small Sagitta, and –17.18‰ for large Sagitta. In winter, small zooplankton displayed the lowest δ13C values, which gradually increased from the inner bay to the outer bay.
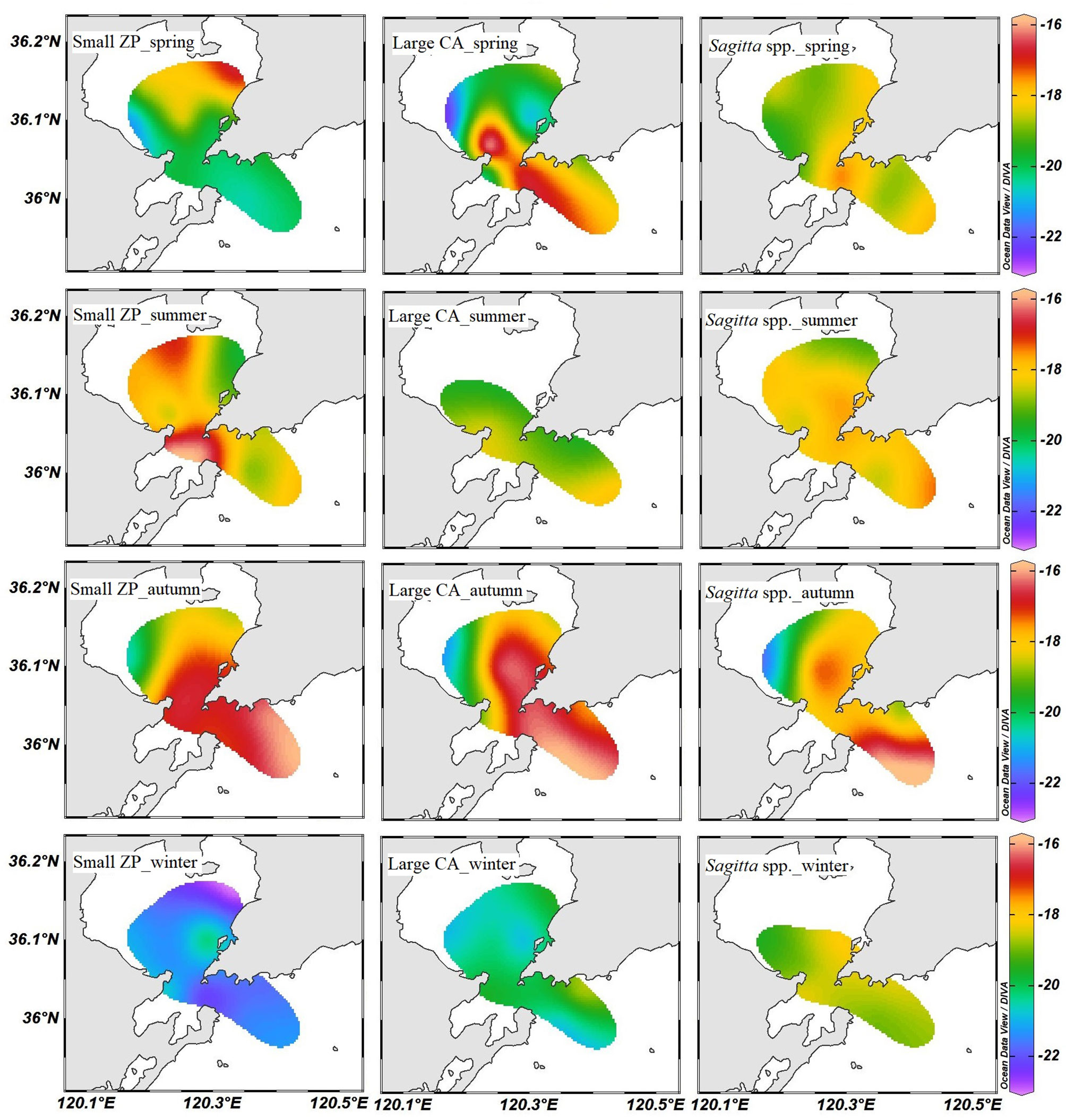
Figure 3 Horizontal distribution of δ13C in small zooplankton (small ZP), large calanoids (large CA), and Sagitta spp. in Jiaozhou Bay.
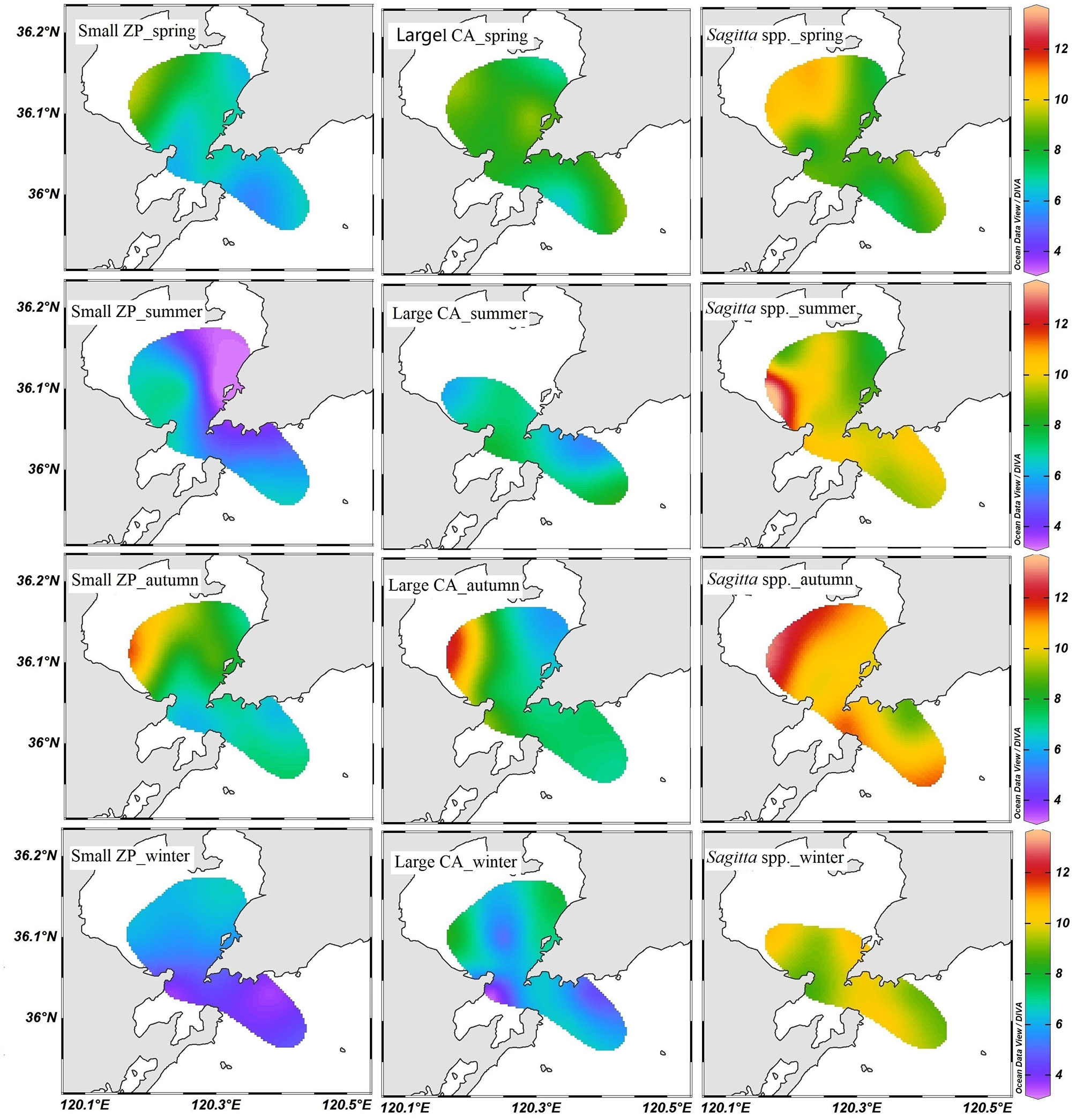
Figure 4 Horizontal distribution of δ15N in small zooplankton (small ZP), large calanoids (large CA), and Sagitta spp. in Jiaozhou Bay.
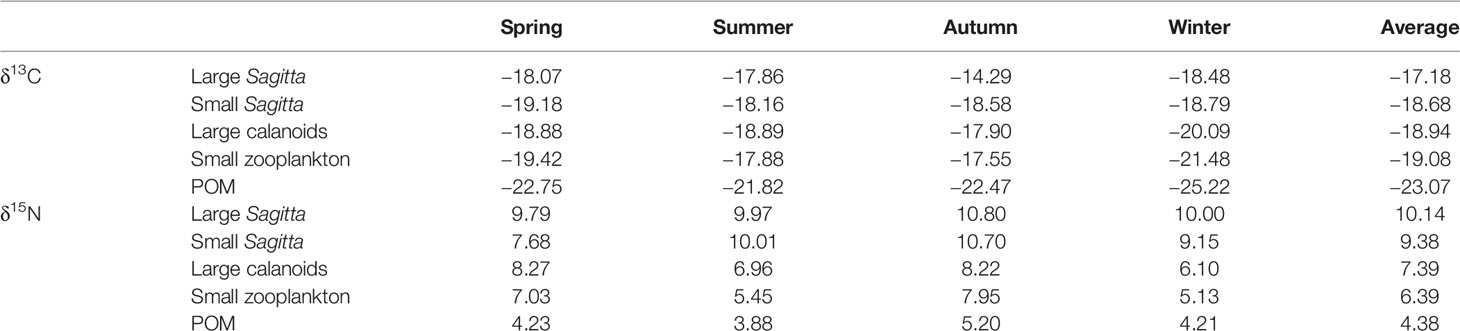
Table 1 Seasonal average values (‰) of δ13C and δ15N in dominant zooplankton groups and suspended particulate organic matter (POM) in Jiaozhou Bay.
Generally, a weak increasing trend in the δ15N values of zooplankton and POM was observed from the inner bay to the outer bay (Figure 4). In most cases, zooplankton showed higher δ15N values in the western inner bay. Only the small zooplankton showed obviously lower δ15N values in the northeastern bay (Figure 4) during summer. The δ15N values of zooplankton ranged from 3.18‰ to 13.57‰. The annual average δ15N values were 6.39‰ for small zooplankton, 7.39‰ for large calanoids, 9.38‰ for small Sagitta, and 10.14‰ for large Sagitta, respectively. In spring, the body size of Sagitta showed large variation ranging from 0.5 to 2.1 cm. The large Sagitta showed significantly higher δ15N values (average 9.79‰) than the small Sagitta (average 7.68‰) in spring (ANOVA, F = 22.51, p < 0.001). However, in other seasons, the difference in δ15N value was very small between them (Table 1).
Seasonal Variations in the Trophic Structure of Zooplankton Community
The bivariate approach of the ecological niche defined by δ13C and δ15N showed the seasonal variation in the trophic niche of zooplankton community (Figure 5). In spring, an intensive overlap occurred among these three zooplankton groups. The niche partition between small zooplankton, large calanoids, and Sagitta was most distinct in winter, and followed by summer. The largest standard ellipse areas were observed during autumn and the smallest ones were observed in winter. The range of zooplankton δ13C values was narrowest in winter, suggesting a simple food source during that period.
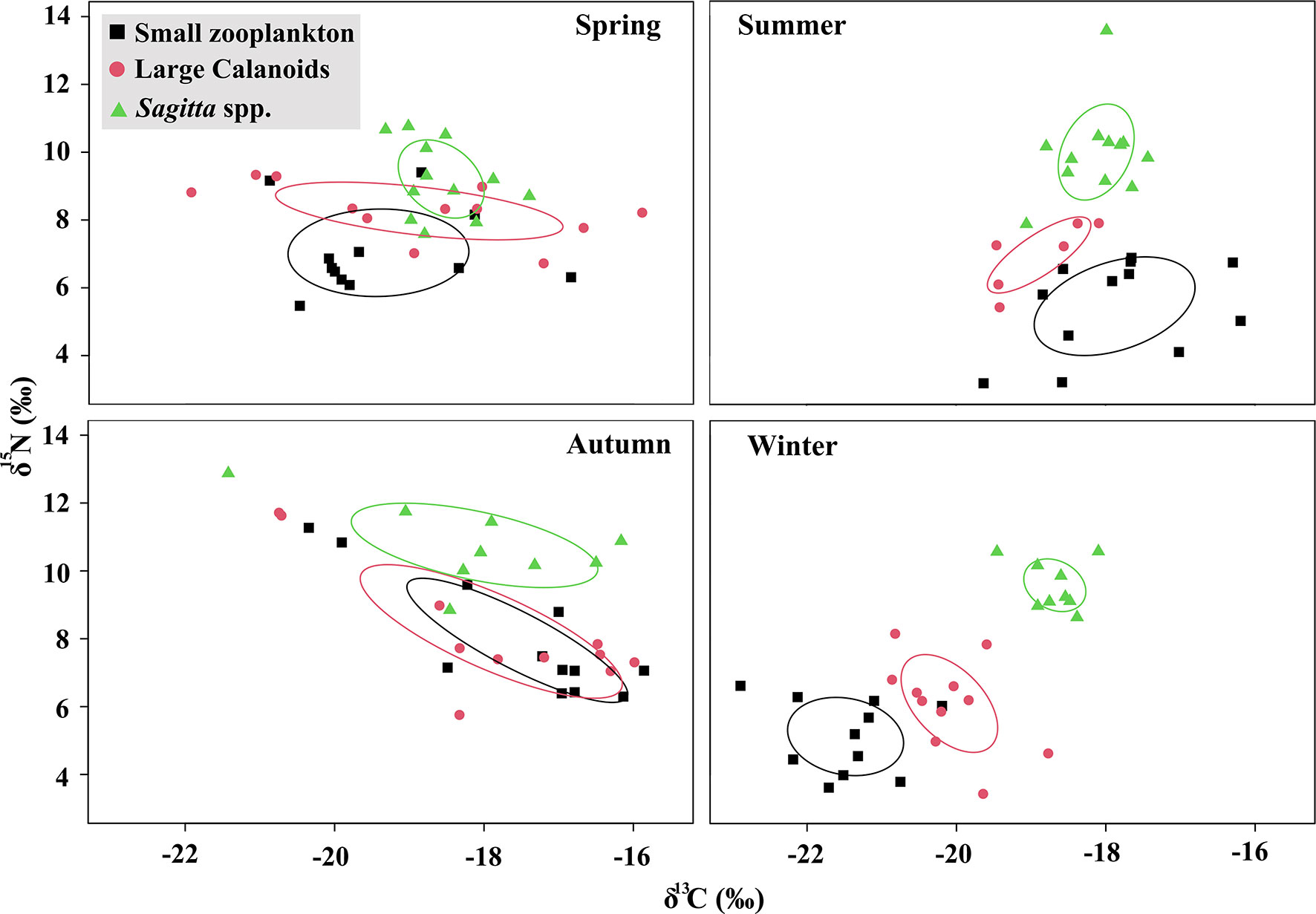
Figure 5 Seasonal variations in the bivariate trophic niche of dominant zooplankton groups represented by Bayesian ellipses in Jiaozhou Bay.
The average RTL of large calanoids was 2.24, which was slightly higher than that of small zooplankton. Sagitta occupied the highest trophic level in the pelagic food web with an annual average RTL of 3.1. The RTL of Sagitta ranged from 2.23 to 4.01, which was closely correlated with body size. Especially in spring, the body size of Sagitta varied greatly, and large Sagitta have significantly higher RTL than small Sagitta (ANOVA, F = 10.16, p < 0.01). The annual average RTLs of small and large Sagitta were 2.97 and 3.14, respectively. According to the spatial distribution patterns, Sagitta generally showed a lower RTL in the inner bay during spring, autumn, and winter seasons (Figure 6). No regular distribution pattern of RTL was found in the summer.
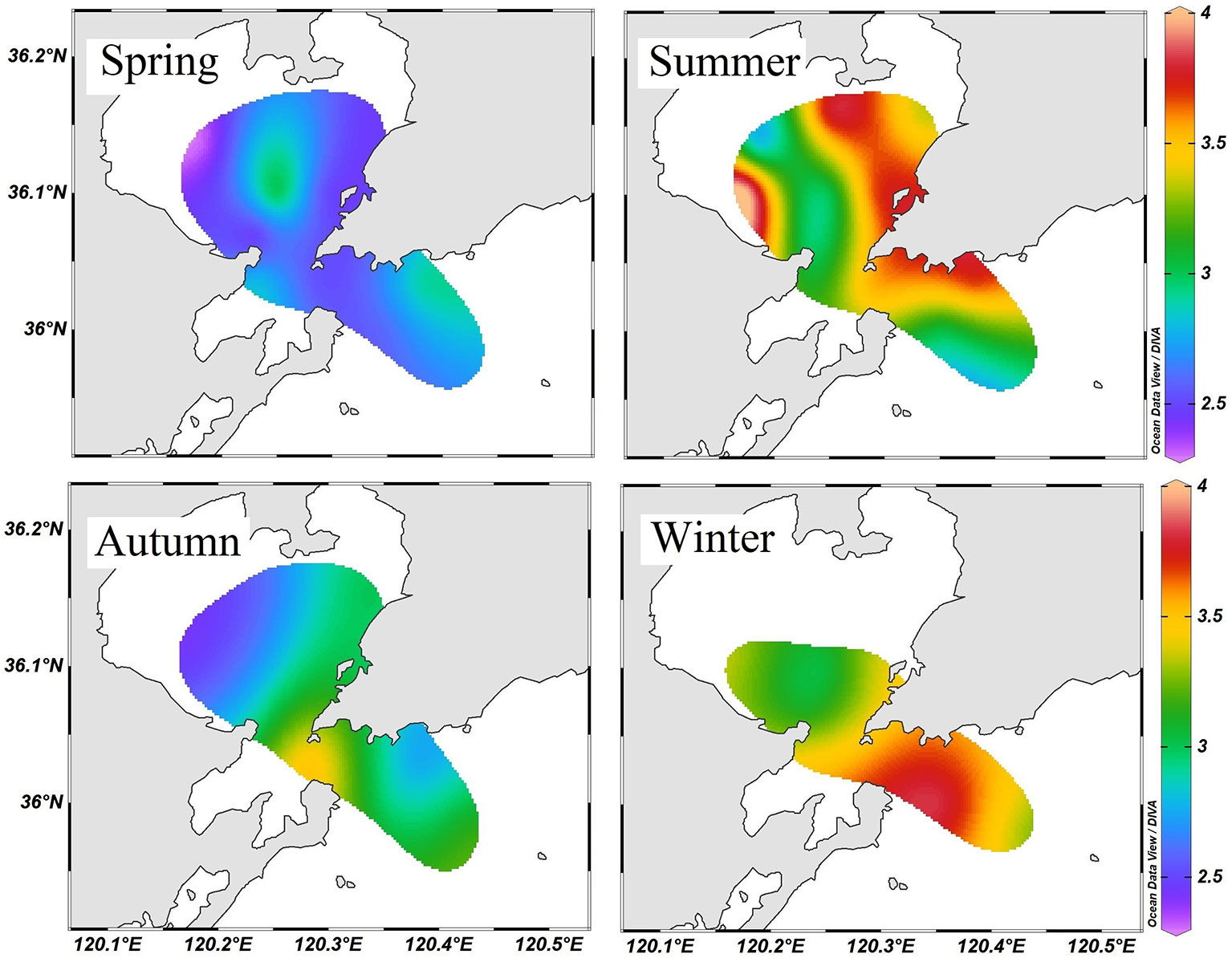
Figure 6 Relative trophic level of Sagitta spp. calculated using small zooplankton (<500 μm) as the baseline (trophic level = 2).
Correlation Between Stable Isotopes and Environmental Variables
The correlation coefficients between the stable isotope values of dominant zooplankton groups and key environmental variables are shown in Figure 7. The δ13C values of POM, small zooplankton, and large calanoids were significantly positively correlated with SST. The δ15N value of small zooplankton was positively correlated with SSS. The δ13C values of large calanoids were significantly positively correlated with concentrations. The δ15N values were generally negatively correlated with nutrients. However, the δ15N of Sagitta showed a weak correlation with environmental variables. In addition, the δ13C and δ15N values of POM and zooplankton generally showed a positive correlation with Chl a.
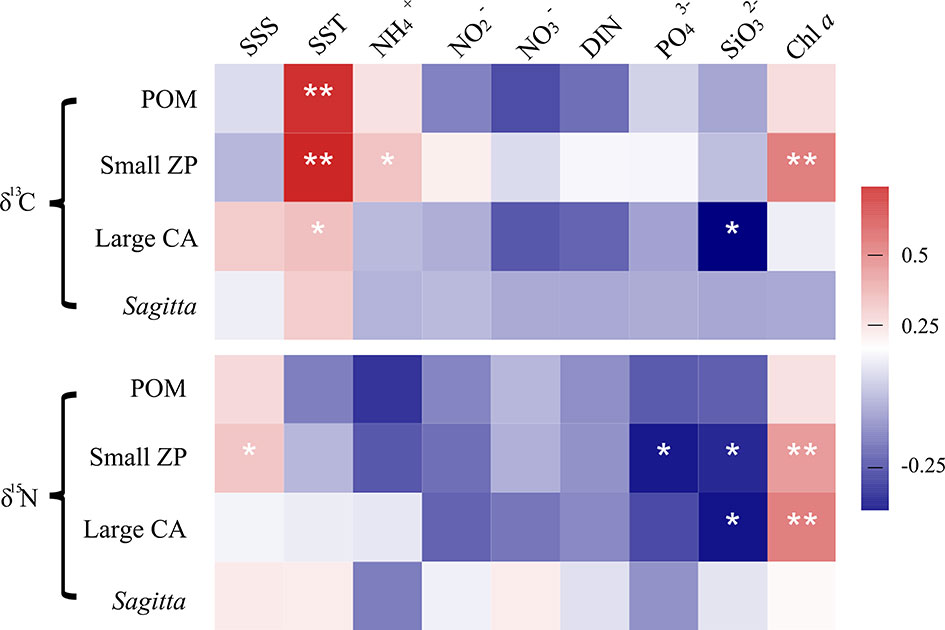
Figure 7 Correlation coefficients between stable isotopes (δ13C and δ15N) and key environmental parameters in Jiaozhou Bay. *p < 0.05, **p < 0.01.
Compared with the δ13C, the δ15N values showed a stronger correlation between POM and each dominant zooplankton group (Figure 8). The δ13C values of POM were significantly correlated with that of small zooplankton (p < 0.01). The δ15N values of POM were significantly correlated with that of small zooplankton, large calanoids, and Sagitta (p < 0.01).
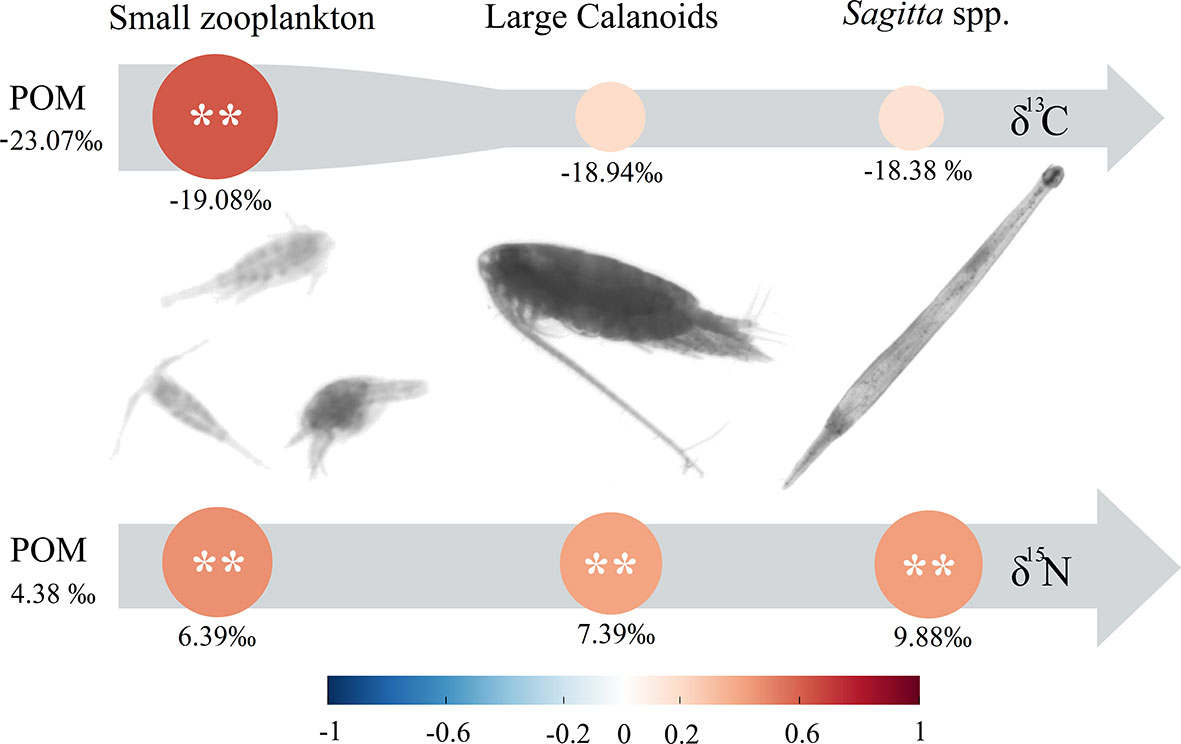
Figure 8 Annual average δ13C and δ15N values and correlation coefficients between POM and dominant zooplankton groups. **p < 0.01.
Discussion
Spatial and Seasonal Variability in δ13C and δ15N of Zooplankton
The stable isotope signatures of zooplankton can well reflect environmental change due to their short life span and relatively fast turnover time (Rolff, 2000; O’Reilly et al., 2002). Variation in the stable isotopes of food items (such as phytoplankton or POM) has been recognized as a mechanism inducing the variability in bulk tissue δ13C and δ15N values of zooplankton in different environments (Montoya et al., 1990; Edje and Chigbu, 2021). Strong correlations of stable isotope values between POM and zooplankton have been well known (Bănaru et al., 2014). In this study, zooplankton generally showed similar spatial distribution patterns of stable isotopes with POM in Jiaozhou Bay. In the inner bay, more terrigenous OM contributed to the POM. As we know, the terrigenous OM is more depleted in 13C (generally < −28‰) than marine organic matter (about -22‰) (Cifuentes and Eldridge, 1998). The general pattern is that the δ13C values of plankton are more negative in estuarine or inshore regions under the influence of riverine/terrestrial waters (Troina et al., 2020). This mechanism can be used to interpret the spatial distribution pattern of δ13C during summer, autumn, and winter in Jiaozhou Bay. However, our results suggested that the δ13C value of small zooplankton was significantly higher in the inner bay in spring. In coastal bays, higher δ13C values of POM are usually associated with higher Chl-a concentrations (Ke et al., 2017). Many studies have indicated that the fast growth rate and high biomass of phytoplankton can increase the δ13C values of POM (Rau et al., 1996; Perry et al., 1999; Bardhan et al., 2015). In Jiaozhou Bay, Ke et al. (2020) suggested that the higher δ13C value of POM in the inner bay during spring was mainly due to the significantly higher phytoplankton biomass under the influence of intensive anthropogenic nutrient input. This result suggested that the δ13C value of zooplankton can be influenced by the food source in Jiaozhou Bay. However, our results showed that only the δ13C values of small zooplankton were significantly correlated with that of POM, suggesting that the cascade effect of δ13C might be weak along the food chain.
Previous studies have indicated that the regional variation of zooplankton stable isotopes was stronger in δ15N than in δ13C (El-Sabaawi et al., 2013). In this study, all the δ15N values of the three dominant zooplankton groups were significantly correlated with that of POM (Figure 8), which suggested that the baseline influence was more significant on the δ15N of consumers. Spatial heterogeneity or seasonal variation in nitrogen nutrients can drive significant and rapid fluctuations in the δ15N values of zooplankton (Syväranta et al., 2006). Many studies suggested that the δ15N value of POM was largely depleted under higher nutrient concentration (Ke et al., 2019; Pruell et al., 2020). Incubation experiments have demonstrated that the δ15N values of primary producers can be changed significantly with the variation of DIN concentration, and these changes can be quickly transferred into aquatic food webs (Pruell et al., 2020). It has been reported that the δ15N value of POM showed significantly lower values in the northeaster Jiaozhou Bay where it is intensively influenced by sewage discharge from Qingdao City (Ke et al., 2020). In this study, the distribution pattern of zooplankton δ15N was generally in accordance with that of POM. This result suggested that the influence of anthropogenic nutrient input on the δ15N value can be effectively transferred to the higher trophic level in the pelagic ecosystem.
Seasonal variation in zooplankton δ13C and δ15N may be due to variations in the composition of diet items and baseline of stable isotopes (Edje and Chigbu, 2021). In general, more 13C-depleted terrigenous OM was transported into the bay during the wet season. Therefore, it was expected that zooplankton should show lower δ13C values in Jiaozhou Bay during summer. However, in this study, the dominant zooplankton groups generally showed the highest δ13C values in the wet summer and the lowest δ13C values in the dry winter. It seemed that the input of terrigenous OM was not the determining factor for the seasonal variation of δ13C in the pelagic ecosystem. El-Sabaawi et al. (2013) indicated that it was possible that any influence of 13C-depleted terrestrial OM on zooplankton δ13C can be obscured by local or regional variability in primary production. The fast growth of phytoplankton might elevate the background δ13C value in the summer. On the other hand, temperature is a significant factor causing the change of δ13C value in phytoplankton, and higher temperature results in higher δ13C value (Goericke and Fry, 1994). In this study, our results showed that the δ13C values of POM, small zooplankton, and large calanoids were significantly positively correlated with water temperature. It suggested that temperature might be the most important driving factor for the seasonal variation of δ13C value in POM and zooplankton.
Seasonal and Regional Variability in Trophic Niche of Zooplankton
The baseline stable isotope values are very important in trophic ecological studies (Magozzi et al., 2017). The fluctuation in zooplankton δ15N values might be attributed to either a variation of δ15N values at the base of the food web or a changed trophic level in different environments (Romero-Romero et al., 2019). The δ15N values of net zooplankton are usually used as the baseline in the study of aquatic food webs. Our results suggested that the δ15N values varied greatly among the different zooplankton groups. Niche partitioning was evident among small zooplankton, large calanoids, and Sagitta. The Sagitta generally occupies the highest trophic level in zooplankton community, feeding preferentially on copepods, and on other small invertebrates and fish larvae (Kimmerer, 1984). In this study, the RTL of Sagitta seemed to increase from the inner bay to the outer bay during the spring, autumn, and winter seasons. Due to the strong anthropogenic influence, the nutrient concentration and phytoplankton biomass were generally higher in the inner Jiaozhou Bay (Liu et al., 2005; Ke et al., 2020). High primary productivity and eutrophication can shorten the food chain length (Armengol et al., 2019). Nevertheless, in summer, the RTL of Sagitta did not show a clear difference between the inner and outer bay. The significant spatial variation of baseline δ15N values in summer might disturb the accurate estimation of zooplankton trophic position.
Seasonal changes in the low levels of pelagic food web can be due to changes in food quality and the active choice of prey type (Kozak et al., 2020). Most copepods are omnivores feeding on a wide range of food items, including phytoplankton, debris, and ciliates (López-Ibarra et al., 2018; Schoo et al., 2018). The composition of food items can change according to the quality and availability of food source. For example, the feeding habit of Calanus pacificus can switch between predatory and suspension feeding modes when the relative abundances of phytoplankton and naupliar prey are varied (Landry, 1981). In this study, the Bayesian standard ellipse areas of large calanoids showed great variation in different seasons. It suggested that large calanoids have sufficient dietary flexibility to alter their trophic position in response to environmental change. The trophic flexibility of calanoid copepods acts as a stabilizing force on the basic levels of marine food webs (Sprules and Bowerman, 1988). This ability of trophic flexibility contributes to the resilience in planktonic systems under spatial and temporal environmental variations.
Scatters of δ13C and δ15N values suggested that the seasonal variation of zooplankton trophic structure was significant in Jiaozhou Bay. In a pelagic ecosystem, the main perturbation effect on trophic structure may be the absolute decline in food biomass, rather than altered proportions of prey (Décima et al., 2013). There is generally a shorter food web in productive regions and a more complex food web in oligotrophic regions (Armengol et al., 2019). Greater resource opportunity can promote the coexistence of species with similar resource use and enhance the functional redundancy of the community (Ying et al., 2020). Our results suggested that the niche overlap of different zooplankton groups was greater in spring. In Jiaozhou Bay, zooplankton generally reached their peak abundance during spring, and both the small and large Sagitta abundances were high in the water. The isotopic niche suggested that the RTL of Sagitta was low in spring, which was close to that of large calanoids (Figure 5). A large number of small Sagitta narrowed the niche partition between Sagitta and large calanoids in spring. Many studies have suggested that non-consistent patterns are assumed in the base of pelagic food for the response to the changes of primary productivity and planktonic biomass (Giering et al., 2019; Kozak et al., 2020). Our results showed that the niche partition among different zooplankton groups was most distinct in the winter, and followed by the summer. This might be caused by the distribution pattern of different groups and food availability in different seasons. In summer, the large calanoids were generally collected in the outer bay (Figure 3). Large calanoids were mainly composed of C. sinicus in Jiaozhou Bay, whose abundance was lower in summer due to the high temperature. The significantly different trophic positions between small zooplankton and large calanoids in summer should be attributed to the difference in distribution region. In winter, the Sagitta spp. cannot be collected at several stations in the inner bay (Figure 3). It suggested that the Sagitta spp. might tend to live in the outer bay during winter and occasionally enter the inner bay with water exchange. The migration of zooplankton between the outer bay and inner bay might greatly affect the trophic structure of zooplankton during summer and winter. On the other hand, the abundance of phytoplankton was low during winter. The shortage of food resource promoted the trophic separation of different zooplankton groups during winter. In summary, seasonal variation of zooplankton trophic structure was controlled by food competition and zooplankton immigration in Jiaozhou Bay. Further study is needed to understand the mechanism of seasonal variation in trophic partitioning.
Conclusion
Our results suggested that the δ13C and δ15N values generally followed the order of small zooplankton < large calanoids < small Sagitta < large Sagitta in Jiaozhou Bay. Spatial variation of zooplankton δ13C was mainly controlled by terrigenous OM input and phytoplankton biomass. Water temperature might play a key role in seasonal variation of δ13C value. Our results showed that only the δ13C values of small zooplankton were significantly correlated with that of POM, suggesting that the cascade effect of δ13C might be weak along the food chain. However, all the δ15N values of dominant zooplankton groups showed a significant correlation with POM. This result suggested that the influence of anthropogenic nutrient input on the δ15N value can be effectively transferred to the higher trophic level in planktonic ecosystem. The Bayesian standard ellipse suggested that the niche partition between small zooplankton, large calanoids, and Sagitta was most distinct in the winter, and followed by the summer. The seasonal variation of zooplankton trophic structure might be controlled by food competition and zooplankton immigration in Jiaozhou Bay. The RTL of Sagitta generally declined from the inner bay to the outer bay except during summer. High phytoplankton biomass and eutrophication might shorten the food chain length in the inner bay. This study provided detailed information on the seasonal patterns in zooplankton δ13C and δ15N that will help to track the change of pelagic trophic structure under anthropogenic influences.
Data Availability Statement
The raw data supporting the conclusions of this article will be made available by the authors, without undue reservation.
Author Contributions
ZK: methodology, investigation, and original draft writing. ZK and RL: data analysis and writing—review and editing. DC and CZ: investigation. YT: supervision and writing—review and editing. All authors contributed to the article and approved the submitted version.
Funding
This work was supported by the National Natural Science Foundation of China (32171548), the Key Special Project for Introduced Talents Team of Southern Marine Science and Engineering Guangdong Laboratory (Guangzhou) (GML2019ZD0401, GML2019ZD0405), the National Basic Research Program of China (973 Program, 2015CB452904), and the Science and Technology planning Project of Guangdong Province of China (2021B1212050023).
Conflict of Interest
The authors declare that the research was conducted in the absence of any commercial or financial relationships that could be construed as a potential conflict of interest.
Publisher’s Note
All claims expressed in this article are solely those of the authors and do not necessarily represent those of their affiliated organizations, or those of the publisher, the editors and the reviewers. Any product that may be evaluated in this article, or claim that may be made by its manufacturer, is not guaranteed or endorsed by the publisher.
Acknowledgments
The authors would like to thank Dr. Shouhui Dai for his assistance in the stable isotope analysis.
References
Armengol L., Calbet A., Franchy G., Rodríguez-Santos A., Hernández-León S. (2019). Planktonic Food Web Structure and Trophic Transfer Efficiency Along a Productivity Gradient in the Tropical and Subtropical Atlantic Ocean. Sci. Rep. 9, 2044. doi: 10.1038/s41598-019-38507-9
Bănaru D., Carlotti F., Barani A., Grégori G., Neffati N., Harmelin-Vivien M. (2014). Seasonal Variation of Stable Isotope Ratios of Size-Fractionated Zooplankton in the Bay of Marseille (NW Mediterranean Sea). J. Plankt. Res. 36 (1), 145–156. doi: 10.1093/plankt/fbt083
Bardhan P., Karapurkar S. G., Shenoy D. M., Kurian S., Sarkar A., Maya M. V., et al. (2015). Carbon and Nitrogen Isotopic Composition of Suspended Particulate Organic Matter in Zuari Estuary, West Coast of India. J. Mar. Syst. 141, 90–97. doi: 10.1016/j.jmarsys.2014.7.009
Chi X. P., Dierking J., Hoving H. J., Lüskow F., Denda A., Christiansen B., et al. (2021). Tackling the Jelly Web: Trophic Ecology of Gelatinous Zooplankton in Oceanic Food Webs of the Eastern Tropical Atlantic Assessed by Stable Isotope Analysis. Limnol. Oceanogr. 66, 289–305. doi: 10.1002/lno.11605
Cifuentes L. A., Eldridge P. M. (1998). A Mass- and Isotope- Balance Model of DOC Mixing in Estuaries. Limnol. Oceanogr. 43, 1872–1882. doi: 10.2307/3037942
Décima M., Landry M. R., Popp B. N. (2013). Environmental Perturbation Effects on Baseline Values and Zooplankton Trophic Flexibility in the Southern California Current Ecosystem. Limnol. Oceanogr. 58 (2), 624–634. doi: 10.4319/lo.2013.58.2.0624
Dickman E. M., Newell J. M., Gonzalez M. N., Vanni M. J. (2008). Light, Nutrients and Food-Chain Length Constrain Planktonic Energy Transfer Efficiency Across Multiple Trophic Levels. Proc. Natl. Acad. Sci. U S A 105, 18408–18412. doi: 10.1073/pnas.0805566105
Edje B. O., Chigbu P. (2021). Carbon and Nitrogen Stable Isotopes of Copepods in a Tidal Estuarine System in Maryland, USA. Reg. Stud. Mar. Sci. 42, 101620. doi: 10.1016/j.rsma.2021.101620
El-Sabaawi R., Dower J. F., Kainz M., Mazumder A. (2009). Characterizing Dietary Variability and Trophic Positions of Coastal Calanoid Copepods: Insight From Stable Isotopes and Fatty Acids. Mar. Biol. 156, 225–237. doi: 10.1007/s00227-008-1073-1
El-Sabaawi R., Trudel M., Mazumder A. (2013). Zooplankton Stable Isotopes as Integrators of Bottom-Up Variability in Coastal Margins: A Case Study From the Strait of Georgia and Adjacent Coastal Regions. Prog. Oceanogr. 115, 76–89. doi: 10.1016/j.pocean.2013.05.010
Giering S. L. C., Wells S. R., Mayers K. M. J., Schuster H., Cornwell L., Fileman E. S., et al. (2019). Seasonal Variation of Zooplankton Community Structure and Trophic Position in the Celtic Sea: A Stable Isotope and Biovolume Spectrum Approach. J. Prog. Oceanogr. 177, 101943. doi: 10.1016/j.pocean.2018.03.012
Goericke R., Fry B. (1994). Variations of Marine Plankton δ13c With Latitude, Temperature, and Dissolved CO2 in the World Ocean. Global Biogeochem. Cy. 8 (1), 85–90. doi: 10.1029/93GB03272
Hannides C. C. S., Popp B. N., Landry M. R., Graham B. S. (2009). Quantification of Zooplankton Trophic Position in the North Pacific Subtropical Gyre Using Stable Nitrogen Isotopes. Limnol. Oceanogr. 54, 50–61. doi: 10.4319/lo.2009.54.1.0050
Jackson A. L., Inger R., Parnell A. C., Bearhop S. (2011). Comparing Isotopic Niche Widths Among and Within Communities: SIBER-Stable Isotope Bayesian Ellipses in R. J. Anim. Ecol. 80, 595–602. doi: 10.1111/j.1365-2656.2011.01806.x
Kang X. M., Song J. M., Yuan H. M., Li X. G., Li N., Duan L. Q. (2017). The Sources and Composition of Organic Matter in Sediments of the Jiaozhou Bay: Implications for Environmental Changes on a Centennial Time Scale. Acta Oceanol. Sin. 36 (11), 68–78. doi: 10.1007/s13131-017-1076-1
Ke Z. X., Chen D. T., Liu J. X., Tan Y. H. (2020). The Effects of Anthropogenic Nutrient Inputs on Stable Carbon and Nitrogen Isotopes in Suspended Particulate Organic Matter in Jiaozhou Bay, China. Cont. Shelf Res. 208, 104244. doi: 10.1016/j.csr.2020.104244
Ke Z. X., Tan Y. H., Huang L. M., Liu J.X., Xiang C.H., Zhao C.Y., et al. (2019). Significantly Depleted 15N in Suspended Particulate Organic Matter Indicating a Strong Influence of Sewage Loading in Daya Bay, China. Sci. Total Environ. 650, 759–768. doi: 10.1016/j.scitotenv.2018.09.076
Ke Z. X., Tan Y. H., Huang L. M., Zhao C. Y., Jiang X. (2017). Spatial Distributions of δ13c, δ15n and C/N Ratios in Suspended Particulate Organic Matter of a Bay Under Serious Anthropogenic Influences: Daya Bay, China. Mar. pollut. Bull. 114, 183–191. doi: 10.1016/j.marpolbul.2016.08.078
Kimmerer W. J. (1984). Selective Predation and its Impact on Prey of Sagitta Enflata (Chaetognatha). Mar. Ecol. Prog. Ser. 15, 55–62. doi: 10.3354/meps015055
Kozak E. R., Franco-Gordo C., Godínez-Domínguez E., Suárez-Morales E., Ambriz-Arreola I. (2020). Seasonal Variability of Stable Isotope Values and Niche Size in Tropical Calanoid Copepods and Zooplankton Size Fractions. Mar. Biol. 167, 37. doi: 10.1007/s00227-020-3653-7
Landry M. R. (1981). Switching Between Herbivory and Carnivory by the Planktonic Marine Copepod Calanus Pacificus. Mar. Biol. 65, 77–82. doi: 10.1007/BF00397070
Liu S. M., Zhang J., Chen H. T., Zhang G. S. (2005). Factors Influencing Nutrient Dynamics in the Eutrophic Jiaozhou Bay, North China. Pro. Oceanogr. 66, 66–85. doi: 10.1016/j.pocean.2005.03.009
López-Ibarra G. A., Bode A., Hernández-Trujillo S., Zetina-Rejón M. J., Arreguín-Sánchez F. (2018). Trophic Position of Twelve Dominant Pelagic Copepods in the Eastern Tropical Pacific Ocean. J. Mar. Syst. 187, 13–22. doi: 10.1016/j.jmarsys.2018.06.009
Magozzi S., Yool A., Vander Zanden H. B., Wunder M. B., Trueman C. (2017). Using Ocean Models to Predict Spatial and Temporal Variation in Marine Carbon Isotopes. N. Ecosphere 8 (5), e01763. doi: 10.1002/ecs2.1763
Ma Y. E., Ke Z. X., Huang L. M., Tan Y. H. (2014). Identification of Human-Induced Perturbations in Daya Bay, China: Evidence From Plankton Size Structure. Cont. Shelf Res. 72, 10–20. doi: 10.1016/j.csr.2013.10.012
Montoya J. P., Horrigan S. G., McCarthy J. J. (1990). Natural Abundance of 15N in Particulate Nitrogen and Zooplankton in the Chesapeake Bay. Mar. Ecol. Prog. Ser. 65, 35–61. doi: 10.3354/meps065035
Nagelkerken I., Goldenberg S. U., Ferreira C. M., Ullah H., Connell S. D. (2020). Trophic Pyramids Reorganize When Food Web Architecture Fails to Adjust to Ocean Change. Science 369, 829–832. doi: 10.1126/science.aax0621
O’Reilly C. M., Hecky R. E., Cohen A. S., Plisnier P. D. (2002). Interpreting Stable Isotopes in Food Webs: Recognizing the Role of Time Averaging at Different Trophic Levels. Limnol. Oceanogr. 47 (1), 306–309. doi: 10.4319/lo.2002.47.1.0306
Perry R. I., Thompson P. A., Mackas D. L., Harrison P. J., Yelland D. R. (1999). Stable Carbon Isotopes as Pelagic Food Web Tracers in Adjacent Shelf and Slope Regions Off British Columbia, Canada. Can. J. Fish. Aquat. Sci. 56, 2477–2486. doi: 10.1139/cjfas-56-12-2477
Post D. M. (2002). Using Stable Isotopes to Estimate Trophic Position: Model, Methods, and Assumptions. Ecology 83, 703–718. doi: 10.1890/0012-9658(2002)083[0703:USITET]2.0.CO;2
Post D. M., Layman C. A., Arrington D. A., Takimoto G., Quattrochi J., Montana C. G. (2007). Getting to the Fat of the Matter: Models, Methods and Assumptions for Dealing With Lipids in Stable Isotope Analyses. Oecologia 152, 179–189. doi: 10.1007/s00442-006-0630-x
Pruell R. J., Taplin B. K., Oczkowski A. J., Grear J. S., Mendoza W. G., Pimenta A. R., et al. (2020). Nitrogen Isotope Fractionation in a Continuous Culture System Containing Phytoplankton and Blue Mussels. Mar. pollut. Bull. 150, 110745. doi: 10.1016/j.marpolbul.2019.110745
Rau G. H., Riebesell U., Wolf-Gladrow D. (1996). A Model of Photosynthetic 13C Fractionation by Marine Phytoplankton Based on Diffusive Molecular CO2 Uptake. Mar. Ecol. Prog. Ser. 133, 275–285. doi: 10.3354/meps133275
R Core Team (2020). “R: A Language and Environment for Statistical Computing,” in R Foundation for Statistical Computing(Vienna, Austria)https://www.R-project.org/.
Rolff C. (2000). Seasonal Variation in δ13c and δ15n of Size Fractionated Plankton at a Coastal Station in the Northern Baltic Proper. Mar. Ecol. Prog. Ser. 203, 47–65. doi: 10.3354/meps203047
Romero-Romero S., Choy C. A., Hannides C. C. S., Popp B. N., Drazen J. C. (2019). Differences in the Trophic Ecology of Micronekton Driven by Diel Vertical Migration. Limnol. Oceanogr. 64, 1473–1483. doi: 10.1002/lno.11128
Schoo K. L., Boersma M., Malzahn A. M., Löder M. G. J., Wiltshire K. H., Aberle N. (2018). Dietary and Seasonal Variability in Trophic Relations at the Base of the North Sea Pelagic Food Web Revealed by Stable Isotope and Fatty Acid Analysis. J. Sea. Res. 141, 61–70. doi: 10.1016/j.seares.2018.08.004
Sprules W. G., Bowerman J. E. (1988). Omnivory and Food Chain Length in Zooplankton Food Webs. Ecology 69 (2), 418–426. doi: 10.2307/1940440
Sun S., Li Y. H., Sun X. X. (2012). Changes in the Small-Jellyfish Community in Recent Decades in Jiaozhou Bay, China. Chin. J. Oceanol. Limn. 30 (4), 507–518. doi: 10.1007/s00343-012-1179-7
Syväranta J., Hämäläinen H., Jones R. I. (2006). Within-Lake Variability in Carbon and Nitrogen Stable Isotope Signatures. Freshw. Biol. 51, 1090–1102. doi: 10.1111/j.1365-2427.2006.01557.x
Troina G. C., Dehairs F., Botta S., Di Tullio J. C. (2020). Zooplankton-Based δ13c and δ15n Isocapes From the Outer Continental Shelf and Slope in the Subtropical Western South Atlantic. Deep-Sea Res. Part I. 159, 103235. doi: 10.1016/j.dsr.2020.103235
Wang W. C., Sun S., Sun X. X., Zhang G. T., Zhang F. (2020a). Spatial Patterns of Zooplankton Size Structure in Relation to Environmental Factors in Jiaozhou Bay, South Yellow Sea. Mar. pollut. Bull. 150, 110698. doi: 10.1016/j.marpolbul.2019.110698
Wang Z. X., Wang H. P., Fan S. L., Xin M., Sun X. (2021). Community Structure and Diversity of Macrobenthos in Jiaozhou Bay. Mar. pollut. Bull. 171, 112781. doi: 10.1016/j.marpolbul.2021.112781
Wang P. P., Zhang F., Sun S., Wang W. C., Wan A. Y., Li C. L. (2020b). Experimental Clearance Rates of Aurelia Coerulea Ephyrae and Medusa, and the Predation Impact on Zooplankton in Jiaozhou Bay. J. Oceanol. Limnol. 38 (4), 1256–1269. doi: 10.1007/s00343-020-0024-7
Yang L. Y., Wu Y., Zhang J., Liu S. M., Deng B. (2011). Burial of Terrestrial and Marine Organic Carbon in Jiaozhou Bay: Different Response to Urbanization. Reg. Environ. Change 11, 707–714. doi: 10.1007/s10113-010-0202-9
Ying R., Cao Y. T., Yin F. M., Guo J. L., Huang J. R., Wang Y. Y., et al. (2020). Trophic Structure and Functional Diversity Reveal Pelagic-Benthic Coupling Dynamic in the Coastal Ecosystem of Daya Bay, China. Ecol. Indic. 113, 106241. doi: 10.1016/j.ecolind.2020.106241
Yuan Y., Song D., Wu W., Liang S., Wang Y., Ren Z. (2016). The Impact of Anthropogenic Activities on Marine Environment in Jiaozhou Bay, Qingdao, China: A Review and a Case Study. Reg. Stud. Mar. Sci. 8, 287–296. doi: 10.1016/j.rsma.2016.01.004
Keywords: zooplankton, trophic structure, food web, stable isotope, anthropogenic influence
Citation: Ke Z, Li R, Chen D, Zhao C and Tan Y (2022) Spatial and Seasonal Variations in the Stable Isotope Values and Trophic Positions of Dominant Zooplankton Groups in Jiaozhou Bay, China. Front. Mar. Sci. 9:900372. doi: 10.3389/fmars.2022.900372
Received: 20 March 2022; Accepted: 17 May 2022;
Published: 22 June 2022.
Edited by:
Jun Xu, Institute of Hydrobiology (CAS), ChinaReviewed by:
Bin Yang, Beibu Gulf University, ChinaAnupam Priyadarshi, Banaras Hindu University, India
Copyright © 2022 Ke, Li, Chen, Zhao and Tan. This is an open-access article distributed under the terms of the Creative Commons Attribution License (CC BY). The use, distribution or reproduction in other forums is permitted, provided the original author(s) and the copyright owner(s) are credited and that the original publication in this journal is cited, in accordance with accepted academic practice. No use, distribution or reproduction is permitted which does not comply with these terms.
*Correspondence: Yehui Tan, tanyh@scsio.ac.cn