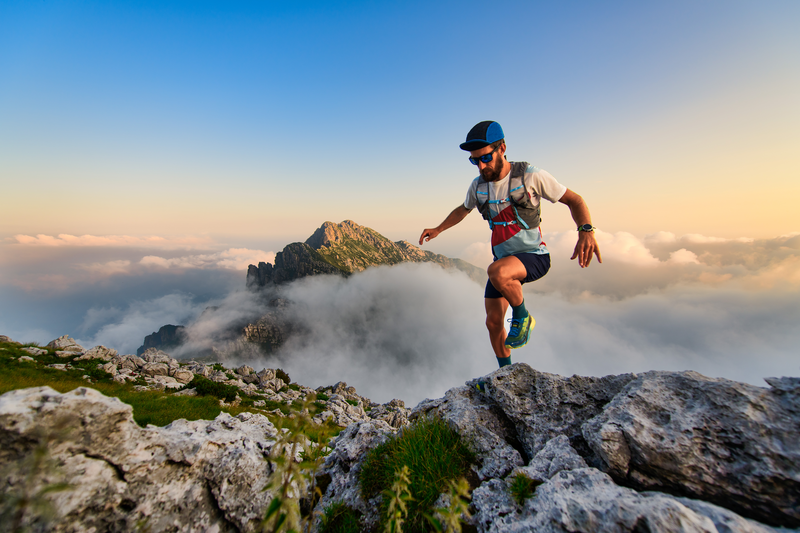
94% of researchers rate our articles as excellent or good
Learn more about the work of our research integrity team to safeguard the quality of each article we publish.
Find out more
ORIGINAL RESEARCH article
Front. Mar. Sci. , 17 May 2022
Sec. Marine Fisheries, Aquaculture and Living Resources
Volume 9 - 2022 | https://doi.org/10.3389/fmars.2022.899768
This article is part of the Research Topic New Progresses and Effects of Functional Feed Additives on Marine Aquatic Animals View all 13 articles
A 10-week feeding trial was conducted to investigate the beneficial effects of bile acids (BAs) on hepatic health status in tongue sole (Cynoglossus semilaevis). Three experimental diets were prepared with different levels of BA inclusion in the commercial basal diet: control group (CT: 0 mg/kg basal diet), low-level group (BA1: 300 mg/kg basal diet), and high-level group (900 mg/kg basal diet). At the end of the feeding trial, growth performance and survival rate were measured, as well as numerous physiological and biochemical parameters of the liver, including four hepatic function indices, five antioxidant indices, five lipid metabolism parameters, and three digestive enzyme activities. Moreover, the mRNA expression levels of three growth-related genes and three immune-related genes in the liver were assayed. Results showed that growth performance and survival were substantially improved in both low- and high-level BA inclusive groups. Further, the tested liver physiological and biochemical parameters combined with the quantitative real-time PCR results revealed enhanced antioxidant capacity, energy metabolism, digestive ability, and immune response. Finally, these findings provide a wider spectrum of the beneficial effects of dietary BAs on liver health in tongue sole.
Bile acids (BAs), synthesized from cholesterol in the liver, can act as emulsifiers to promote lipid digestion and absorption in animals (Hofmann et al., 2010). Other roles of BAs include enhancing glucose absorption and transport, regulating cholesterol levels, and modulating the immune response in the liver and intestine (Houten et al., 2006; Nguyen and Bouscarel, 2008; Fiorucci et al., 2010; Fiorucci and Distrutti, 2015; Xie et al., 2021; Fu et al., 2022; Xu et al., 2022). International research on the BAs as feed additives in livestock and poultry started in the 1970s, and BAs were soon used in aquaculture species in the 1980s. In China, BA-related products have been proved as animal feed additives since 2014 by the Ministry of Agriculture.
Indeed, BAs as feed additives have shown much effectiveness in solving a series of adverse effects caused by unbalanced diets or antinutritional factors in feeds (e.g., high lipid diets, high starch diets, and high plant protein-based diets) (Romano et al., 2020). Recently, the beneficial effects of dietary BAs on liver health have been investigated in many aquaculture species. New studies have shown remarkable promise to enhance liver health and then further improve productivity by dietary BA supplementation in fishes, including large yellow croaker (Larimichthys crocea) (Ding et al., 2020), tiger pufferfish (Takifugu rubripes) (Liao et al., 2020), largemouth bass (Micropterus salmoides) (Yu et al., 2019; Guo et al., 2020; Yin et al., 2021), black seabream (Acanthopagrus schlegelii) (Jin et al., 2019), tilapia (Oreochromis niloticus) (Jiang et al., 2018), and grass carp (Ctenopharyngodon idella) (Zhou et al., 2018).
The liver has long been acknowledged as one of the essential metabolic organs and is extensively involved in maintaining normal energy metabolism in the body through sophisticated processes, such as lipid digestion, glucose homeostasis, and antioxidant defense (McBride and Kelly, 1990; Li et al., 2012; Wu et al., 2013; Xie et al., 2016). Further, the liver is the major lymphoid organ containing many innate immune effectors that undertake immune defense when exposed to infectious diseases in teleost (Zapata et al., 2006). Thus, a good physiological status of the liver contributes to building the hepatic antioxidant and immune defense system, which is significantly important for health maintenance.
In view of these perspectives, it is a salient interest point to reveal the beneficial effects of BAs on the fish hepatic status by dietary supplementation. However, studies focused on this field are still scarce in aquaculture species, especially in flatfishes. Tongue sole (Cynoglossus semilaevis) is an important flatfish that is of high economic values and widely cultured in coastal areas in China (Li et al., 2020; Li et al., 2021b; Li et al., 2021c; Li et al., 2021d). The objectives of this study were mainly focused on the effect of BA administration on liver health. Numerous parameters, including growth performance, physiological and biochemical indices of the liver, and immune gene expression levels, were investigated by dietary BA inclusion through a 10-week feeding trial using tongue sole juveniles. This research sheds light on a potential strategy for improving hepatic health status in tongue sole.
In this study, a diet formulated containing ~53% crude protein, ~8% crude fat, ~3% crude fiber, and ~16% crude ash was used as the basal diet in the control group (CT), while the other two experimental diets were formulated by adding 300 (BA1) and 900 mg/kg (BA2) of BAs (Longchang Group, China; purity 99%; containing 69.9% hyodeoxycholic acid, 18.9% chenodeoxycholic acid, and 7.8% hyocholic acid) to the basal diet. The additive contents of BAs were determined according to previous studies (Li et al., 2021a). The compound of BAs was extracted from viscera of animals like livestock and poultry by our group. The experimental diets were commercially manufactured by our partner (Sangtong Bio-engineering (Weifang) Co. Ltd, Weifang, China), and the main ingredients and proximate compositions of the basal diet are as described in previous studies (Li et al., 2021e).
Juvenile tongue sole fish were obtained from a flatfish aquaculture company in Tangshan, China. After a 15-day acclimation (fed with a control diet), fish were randomly distributed to nine rearing tanks (1 m3), each tank has 180 (N0) individuals, and each group has three triplicate tanks. Then fish were hand-fed to apparent satiation with an amount of 1%–2% of wet body weight twice daily at 06:00 and 18:00 for 10 weeks. For each meal, in most cases, there were little feed residuals within 0.5 h after feeding, but residuals would be eaten up in the extended 0.5 h. The daily amount was fine-tuned weekly according to the total wet weight of the fish in each tank. Throughout the whole experimental period, the physicochemical parameters of water were as follows: temperature 22.5°C–23.5°C, salinity 26‰–30‰, pH 7.6–8.3, ammonia nitrogen <0.06 mg/L, dissolved oxygen 6–8 mg/L, and daily water exchange rate 500%.
At the beginning and end of the feeding trial, 30 individuals from each tank were randomly selected and measured after 24-h starvation to obtain the initial body weight (BWi) and final body weight (BWf). The survival fish (N1) of each tank were counted at the same time. The weight gain rate (WGR), specific growth rate (SGR), hepatosomatic index (HSI), and survival rate (SR) are calculated as follows: ; ; ; . Herein, the BWi is the average of the initially measured body weight of 30 individuals.
At the end of the feeding trial, fish were fasted for 24 h, and then 10 fish from each tank were randomly selected and anesthetized with MS-222 (30 mg/L) for sampling. Briefly, the body weight of 10 fish from each tank was individually measured, and then the whole liver of each fish was dissected and measured for weight promptly. After that, three of these liver samples from each tank were divided in half, separately pooled in 2 ml of sterilized RNase-free tubes, immediately frozen in liquid nitrogen, and then stored at −80°C for later use. All the scientific activities were performed in accordance with the protocols and guidelines approved by the Care and Use of Laboratory Animals of Shandong Longchang Animal Health Product Co., Ltd.
Four hepatic function indices, five lipid metabolism parameters in the liver, five antioxidant parameters in the liver, and three digestive enzyme activity indices in the liver were assayed by commercial kits (Nanjing Jiancheng Bioengineering Institute, Nanjing, China) according to the manufacturer’s instructions. The detailed parameters are listed in Table 1.
For detecting the immune responses in the liver, the expression levels of three immune-related genes (i.e., IL-8, TGF-β1, and TNF-α) and three growth-related genes (insulin-like growth factor (IGF-I), FAS, and G6PD) were analyzed by qRT-PCR. Total RNA of liver samples was extracted using TRIzol Reagent (Takara, Maebashi, Japan) according to the manufacturer’s instructions. The RNA quality and quantity were determined by agarose gel electrophoresis (1.5%) and spectrophotometric analysis (Nanodrop 2000, Thermo Fisher Scientific, Waltham, MA, USA). Then RNA samples were reversely transcribed into complementary DNA for qRT-PCR analysis using Servicebio® RT reagent kit with gDNA Eraser (Servicebio, Wuhan, China). Primers were designed by Premier 5.0 and listed in Table 2. β-Actin gene has been validated as a stable internal reference gene and is widely used in various tissue samples of tongue sole (Li et al., 2010). The qRT-PCR amplification was performed based on QuantStudio™ 5 system (Thermo Scientific, USA). The detailed reaction system and programs were described by Wei et al. (2019). The gene expression levels were normalized by the 2−ΔΔCT method (Livak and Schmittgen, 2001), and the CT was used as the reference group.
Data were shown as mean ± SE. Data expressed in percentage were arcsine square-root transformed and followed by one-way ANOVA to determine significant differences in various parameter indices in R software (v4.1.0). Tukey’s test was used for significance comparison between different dietary groups. The significance level was set as p < 0.05.
The growth performance parameters, HSI, and survival rate are presented in Table 3. The inclusion of BAs in diets substantially enhanced the growth performance of tongue sole; parameters including BWf, WGR, and SGR were significantly improved in both BA supplementary groups, especially in the high addition group (p < 0.05). In the BA1 group, the HSI increased slightly (p > 0.05), while in the BA2 group, it was significantly improved as compared to both the CT and BA1 groups. For SR, a significant improvement was observed only in the BA2 group (p < 0.05).
Table 3 Growth performance, hepatosomatic index, and survival of tongue sole fed diets supplemented with bile acids for 10 weeks.
Effects of dietary BAs on hepatic function indices are shown in Table 4. The urea nitrogen (UN) content in the liver was only significantly increased in the BA2 group (p < 0.05). Compared with the CT group, the albumin (ALB) content in the liver was significantly improved in both dietary BA supplementation groups (p < 0.05). However, when BAs were added to the basal diet, the hepatic alanine transaminase (ALT) activity decreased dramatically (p < 0.05), where the value was the lowest in the BA1 group, while for aspartate aminotransferase (AST) activity, it significantly declined (p < 0.05) when 300 mg/kg of BAs was added to the basal diet (BA1) and further slightly declined (p > 0.05) when 900 mg/kg of BAs was added (BA2).
Five lipid metabolism-related parameters in the liver are summarized in Table 5. The content of total bile acid (TBA) declined significantly (p < 0.05) when a low amount of BA (300 mg/kg, BA1) was added to the basal diet, while the addition level of BAs increased to 900 mg/kg basal diet (BA2), and it went up the same level of the CT group. The content of total cholesterol (TC) was significantly reduced (p < 0.05) in both BA supplementary groups, where it was significantly lower in the BA1 group than in the BA2 group (p < 0.05). The contents of TG and HDL-C in the liver were significantly gradually improved with the increase of BAs in the basal diet (p < 0.05). With the increase of the BA addition, the content of LDL-C in the liver showed first a significant decrease (BA1), followed by a significant rise (BA2) close to the level in the CT group.
The activities of protease, lipase, and amylase in the liver were all gradually significantly boosted with the increase of BA inclusion levels (p < 0.05) (Table 6).
Table 6 Effects of dietary bile acids on the activity levels of protease, lipase, and amylase in liver in tongue sole.
The antioxidative parameters in the liver are listed in Table 7. The significantly improved superoxide dismutase (SOD) activity (p < 0.05) was only observed in high BA addition groups (BA2), and the improvement in the BA1 group was negligible. Catalase (CAT) activity and total antioxidant capacity (TAOC) content in the liver significantly declined when 300 mg/kg of BAs was added to the basal diet (BA1), and the values were further significantly reduced in the 600 mg/kg supplementary group (BA2) (p < 0.05). The activity of GSH-PX showed a significant decline in the low BA supplementation group (BA1) compared to the control group (p < 0.05) but underwent a marked improvement in the BA2 group compared to the other two groups (p < 0.05). The content of malondialdehyde (MDA) in the liver was significantly cut down with the increase of dietary BA supplementation (p < 0.05).
Growth and immune gene expression levels in the liver are illustrated in Figure 1. As shown in Figure 1, the mRNA expression level of G6PD was significantly downregulated (p < 0.05) regardless of low or high BA administration levels; the expression level of FAS was dramatically downregulated in the BA2 group (p < 0.05), while in the BA1 group, it showed an intermediate value (p > 0.05); however, dietary BAs (both the BA1 and BA2 groups) significantly increased the transcription of IGF-I (p < 0.05) when BAs were supplemented in diets. With the increase of supplementation of BAs in the diet, the hepatic mRNA expression levels of IL-8 and TNF-α were significantly downregulated (p < 0.05), while the expression level of TGF-β1 showed the opposite trend, which is significantly upregulated in both BA inclusion groups compared to the CT group (p < 0.05) (Figure 1).
Figure 1 Growth and immune-related gene expression in the liver of tongue sole fed a diet of BAs for 10 weeks. a,b,cValues with different superscript letters in the same row show significant differences (p < 0.05). CT, basal diet; BA1 and BA2, bile acids were supplemented at the level of 300 and 900 mg/kg of basal diet.
As previously mentioned, tongue sole is an important aquaculture sector of flatfish production in China. Due to its high nutritional value, tongue sole is one of the most expensive aquaculture seafood in China. However, to a large extent, the industrialization production of tongue sole was limited by its susceptibility to bacterial diseases usually resulting in a low survival rate under commercial rearing environments. Therefore, improving fish welfare to ensure high productivity by health management is of the highest priority. In this regard, genetic improvement toward disease resistance has been extensively studied by selective breeding geneticists with effort (Li et al., 2019; Hu et al., 2020; Li et al., 2020; Song et al., 2020; Lu et al., 2021). Furthermore, in the long term, improving tongue sole healthy status by functional feed additives (e.g., BAs) inclusion is a practical and effective strategy to produce fine crops.
In China, tongue sole feed has been well formulated and is commercially produced by several renowned manufacturers. In this study, to improve the formulation of tongue sole feeds and provide first-hand data to feed manufacturers, we conducted this fundamental research. Basically, these results will further boost the tongue sole aquaculture industry in China. Overall, according to our results, firstly, the growth performance of tongue sole was substantially improved, as well as the survival rate, although the experimental period is relatively short (only 10 weeks). These results were consistent with those of previous studies on other aquaculture species (Jiang et al., 2018; Zhou et al., 2018; Ding et al., 2020; Guo et al., 2020). Therefore, an extensive investigation of the beneficial effects of BAs on the hepatic health status of tongue sole is of significance for future health management.
The studied hepatic function indices were significantly enhanced or declined with favorable trends. For example, ALT and AST as the main indicators for evaluating hepatic function showed a notable decrease, which implies an improvement status of the liver because the reasonable decline of both parameters indicates a mitigated liver degeneration and injury (Akrami et al., 2015). Further, the abnormal fluctuations of lipid metabolism indices (e.g., triacylglycerol (TG), TC, low-density lipoprotein cholesterol (LDL-C), and high-density lipoprotein cholesterol (HDL-C)) are considered underlying biomarkers of liver damage (Chrostek et al., 2013; Helkin et al., 2016). Thus, the results of assayed lipid metabolism parameters in this study may also imply the ability of BAs to improve lipid metabolism status in tongue sole. However, the increased or decreased contents of lipid metabolism parameters in this study are not exactly the same as in previous studies, which may be discussed case by case because the species and experimental designs are quite different.
Moreover, the antioxidant enzymes are of significance to protect cells and tissues from peroxidation, and they are important indicators that can reflect growth performance and the health status of the body (Mate, 2000; Jin et al., 2017). In this study, significantly enhanced activities of SOD and GSH-PX and lower contents of MDA in the liver were observed when BAs were included in the basal diet, which agrees with previous reports (Jin et al., 2019; Ding et al., 2020). On the contrary, the CAT activity and TAOC content were significantly declined when BAs were added, which are incompatible with the other three studied antioxidant parameters, whereas relevant research about the effect of dietary BAs on liver antioxidant capability is very limited in other aquaculture species. Intensive studies focused on the mechanism of liver antioxidants regulated by dietary BA inclusion are needed.
As we previously mentioned in the Introduction section, the liver is an important organ that plays key roles in food digestion and immune response. In the present study, the activities of protease, lipase, and amylase in the liver were significantly improved in both low and high BA supplementation levels. To a large extent, these findings are in accordance with previous studies (Adhami et al., 2017; Zhou et al., 2018; Ding et al., 2020). Thus, the increased digestive enzyme activity may be a rational explanation for the healthy growth performance of tongue sole. This was corroborated by the upregulation of IGF-I with BA administration. Moreover, interestingly, the other two hepatic fatty acid regulatory enzymes (G6PD and FAS) were downregulated. Previous studies reported that the expression level of FAS was positively correlated with TC content in the body (Semenkovich, 1997; Leng et al., 2012). As indicated in this study, the hepatic TC content and FAS expression shared a consistent downtrend. The substantially decreased expression levels of FAS and G6PD may result in the decelerated accumulation of lipid in the liver and may also imply a reduced risk of fatty liver disease.
TGF-β1, TNF-α, and IL-8 are major cytokines extensively involved in pro-inflammatory reactions, innate immune responses, and wound repair processes (Secombes et al., 2001; Willuweit et al., 2001; Moldoveanu et al., 2009; Atiba et al., 2011; Cho et al., 2011), where the higher expression level of TGF-β1 means an accelerated wound healing and reduced tissue damage, and the lower expression levels of TNF-α and IL-8 indicate the decreased inflammatory responses. In this study, the expression level of TGF-β1 in the liver was significantly upregulated, and the expression levels of TNF-α and IL-8 were significantly downregulated with dietary BA addition. These findings were consistent with those in tilapia when guanidinoacetic acid was included in the diets (Aziza et al., 2020).
This study concludes that dietary BA supplementation in tongue sole markedly improved the health status of the liver. The physiological and biochemical indices closely related to growth, lipid metabolism, immune, and antioxidants were markedly improved. The analytical results revealed well energy metabolisms and immune responses in the liver and finally explained the prominently improved growth performance and survival rate. This study provides a wider spectrum of the beneficial effects of dietary BAs on liver health in tongue sole.
The original contributions presented in the study are included in the article/supplementary material, further inquiries can be directed to the corresponding author/s.
The animal study was reviewed and approved by The Care and Use of Laboratory Animals of Shandong Longchang Animal Health Product Co., Ltd.
SW: methodology, conceptualization, and writing—original draft preparation. WZ: methodology, data curation, visualization, validation, and writing—original draft preparation. AC: visualization and investigation. ZP: supervision, resources, investigation, and writing—reviewing and editing. TL: supervision and writing—reviewing and editing. All authors listed have made a substantial, direct, and intellectual contribution to the work and approved it for publication.
The authors declare that this study received funding from Shandong Longchang Animal Health Product Co., Ltd. The funder was not involved in the study design, collection, analysis, interpretation of data, the writing of this article or the decision to submit it for publication.
Authors SW, WZ, AC, ZP, and TL were employed by Shandong Longchang Animal Health Product Co., Ltd.
The authors declare that this study received funding from Shandong Longchang Animal Health Product Co., Ltd. The funder was not involved in the study design, collection, analysis, interpretation of data, the writing of this article or the decision to submit it for publication.
All claims expressed in this article are solely those of the authors and do not necessarily represent those of their affiliated organizations, or those of the publisher, the editors and the reviewers. Any product that may be evaluated in this article, or claim that may be made by its manufacturer, is not guaranteed or endorsed by the publisher.
We are grateful to Dr. Yangzhen Li and Dr. Yuanri Hu for the feeding trial and other technical support.
Adhami B., Amirkolaie A. K., Oraji H., Kenari R. E. (2017). Growth Performance, Nutrient Digestibility and Lipase Activity in Juvenile Rainbow Trout (Oncorhynchus Mykiss) Fed Fat Powder in Diet Containing Emulsifiers (Cholic Acid and Tween-80). Aquacult. Nutr. 23, 1153–1159. doi: 10.1111/anu.12484
Akrami R., Gharaei A., Mansour M. R., Galeshi A. (2015). Effects of Dietary Onion (Allium Cepa) Powder on Growth, Innate Immune Response and Hemato-Biochemical Parameters of Beluga (Huso Huso Linnaeus 1754) Juvenile. Fish. Shellf. Immunol. 45, 828–834. doi: 10.1016/j.fsi.2015.06.005
Atiba A., Nishimura M., Kakinuma S., Hiraoka T., Goryo M., Shimada Y., et al. (2011). Aloe Vera Oral Administration Accelerates Acute Radiation-Delayed Wound Healing by Stimulating Transforming Growth Factor-β and Fibroblast Growth Factor Production. Am. J. Surg. 201, 809–818. doi: 10.1016/j.amjsurg.2010.06.017
Aziza A., Mahmoud R., Zahran E., Gadalla H. (2020). Dietary Supplementation of Guanidinoacetic Acid Improves Growth, Biochemical Parameters, Antioxidant Capacity and Cytokine Responses in Nile Tilapia (Oreochromis Niloticus). Fish. Shellf. Immunol. 97, 367–374. doi: 10.1016/j.fsi.2019.12.052
Cho K., Adamson L. K., Greenhalgh D. G. (2011). Parallel Self-Induction of TNF-α and Apoptosis in the Thymus of Mice After Burn Injury. J. Surg. Res. 98, 9–15. doi: 10.1006/jsre.2001.6157
Chrostek L., Supronowicz L., Panasiuk A., Cylwik B., Gruszewska E., Flisiak R. (2013). The Effect of the Severity of Liver Cirrhosis on the Level of Lipids and Lipoproteins. Clin. Exp. Med. 14, 417–421. doi: 10.1007/s10238-013-0262-5
Ding T., Xu N., Liu Y., Du J., Xiang X., Xu D., et al. (2020). Effect of Dietary Bile Acid (BA) on the Growth Performance, Body Composition, Antioxidant Responses and Expression of Lipid Metabolism-Related Genes of Juvenile Large Yellow Croaker (Larimichthys Crocea) Fed High-Lipid Diets. Aquaculture 518, 734768. doi: 10.1016/j.aquaculture.2019.734768
Fiorucci S., Cipriani S., Mencarelli A., Renga B., Distrutti E., Baldelli F. (2010). Counterregulatory Role of Bile Acid Activated Receptors in Immunity and Inflammation. Curr. Mol. Med. 106, 579–595. doi: 10.2174/1566210206424385240
Fiorucci S., Distrutti E. (2015). Bile Acid-Activated Receptors, Intestinal Microbiota, and the Treatment of Metabolic Disorders. Trends Mol. Med. 21, 702–714. doi: 10.1016/j.molmed.2015.09.001
Fu J., Yu M., Xu W., Yu S. (2022). Research Progress of Bile Acids in Cancer. Front. Oncol. 11, 778258. doi: 10.3389/fonc.2021.778258
Guo J. L., Kuang W. M., Zhong Y. F., Zhou Y. L., Chen Y. J., Lin S. M. (2020). Effects of Supplemental Dietary Bile Acids on Growth, Liver Function and Immunity of Juvenile Largemouth Bass (Micropterus Salmoides) Fed High-Starch Diet. Fish. Shellf. Immunol. 97, 602–607. doi: 10.1016/j.fsi.2019.12.087
Helkin A., Stein J. J., Lin S., Siddiqui S., Maier K. G., Gahtan V. (2016). Dyslipidemia Part 1—Review of Lipid Metabolism and Vascular Cell Physiology. Vasc. Endovasc. Surg. 50, 107–118. doi: 10.1177/1538574416628654
Hofmann A. F., Hagey L. R., Krasowski M. D. (2010). Bile Salts of Vertebrates: Structural Variation and Possible Evolutionary Significance. J. Lipid Res. 512, 226–246. doi: 10.1194/jlr.R000042
Houten S. M., Watanabe M., Auwerx J. (2006). Endocrine Functions of Bile Acids. EMBO J. 25, 1419–1425. doi: 10.1038/sj.emboj.7601049
Hu Y., Li Y., Li Z., Chen C., Zang J., Li Y., et al. (2020). Novel Insights Into the Selective Breeding for Disease Resistance to Vibriosis by Using Natural Outbreak Survival Data in Chinese Tongue Sole (Cynoglossus Semilaevis). Aquaculture 529, 735670. doi: 10.1016/j.aquaculture.2020.735670
Jiang M., Wen H., Gou G. W., Liu T. L., Lu X., Deng D. F. (2018). Preliminary Study to Evaluate the Effects of Dietary Bile Acids on Growth Performance and Lipid Metabolism of Juvenile Genetically Improved Farmed Tilapia (Oreochromis Niloticus) Fed Plant Ingredient-Based Diets. Aquacult. Nutr. 24, 1175–1183. doi: 10.1111/anu.12656
Jin M., Monroig O., Lu Y., Yuan Y., Li Y., Ding L., et al. (2017). Dietary DHA/EPA Ratio Affected Tissue Fatty Acid Profiles, Antioxidant Capacity, Hematological Characteristics and Expression of Lipid-Related Genes But Not Growth in Juvenile Black Seabream (Acanthopagrus Schlegelii). PloS One 12, e0176216. doi: 10.1371/journal.pone.0176216
Jin M., Pan T., Cheng X., Zhu T. T., Sun P., Zhou F., et al. (2019). Effects of Supplemental Dietary L-carnitine and Bile Acids on Growth Performance, Antioxidant and Immune Ability, Histopathological Changes and Inflammatory Response in Juvenile Black Seabream (Acanthopagrus Schlegelii) Fed High-Fat Diet. Aquaculture 504, 199–209. doi: 10.1016/j.aquaculture.2019.01.063
Leng X. J., Wu X. F., Tian J., Li X. Q., Guan L., Weng D. C. (2012). Molecular Cloning of Fatty Acid Synthase From Grass Carp (Ctenopharyngodon Idella) and the Regulation of its Expression by Dietary Fat Level. Aquac. Nutr. 18, 551–558. doi: 10.1111/j.1365-2095.2011.00917.x
Liao Z., Sun B., Zhang Q., Jia L., Wei Y., Liang M., et al. (2020). Dietary Bile Acids Regulate the Hepatic Lipid Homeostasis in Tiger Puffer Fed Normal or High-Lipid Diets. Aquaculture 519, 734935. doi: 10.1016/j.aquaculture.2020.734935
Li Y., Cheng P., Li M., Hu Y., Cui Z., Zhang C., et al (2021b). Transcriptome Analysis and Candidate Gene Identification Reveals Insights Into the Molecular Mechanisms of Hypermelanosis in Chinese Tongue Sole (Cynoglossus Semilaevis). Aquacult. Fish. doi: 10.1016/j.aaf.2021.02.003
Li Y., Hu Y., Yang Y., Cheng J., Cheng X., Chen S. (2021c). Genetic Parameter Estimates for Female Proportion in Tongue Sole (Cynoglossus Semilaevis). Aquaculture 548, 737652. doi: 10.1016/j.aquaculture.2021.737652
Li Y., Hu Y., Yang Y., Zheng W., Chen C., Li Z. (2020). Selective Breeding for Juvenile Survival in Chinese Tongue Sole (Cynoglossus Semilaevis): Heritability and Selection Response. Aquaculture 531, 735901. doi: 10.1016/j.aaf.2019.12.002
Li Y., Hu Y., Zheng W., Cui Z., Cheng J., Cheng P., et al. (2021d). Insights Into the Heritable Variation of Hypermelanosis in Chinese Tongue Sole (Cynoglossus Semilaevis): Potential for Future Selective Breeding. Aquaculture 539, 736617. doi: 10.1016/j.aquaculture.2021.736617
Li Q., Liu Y., Che Z., Zhu H., Meng G., Hou Y., et al. (2012). Dietary L-arginine Supplementation Alleviates Liver Injury Caused by Escherichia Coli LPS in Weaned Pigs. Innate. Immun. 18, 804–814. doi: 10.1177/1753425912441955
Livak K. J., Schmittgen T. D. (2001). Analysis of Relative Gene Expression Data Using Real-Time Quantitative PCR and the 2–ΔΔct Method. Methods 25, 402–408. doi: 10.1006/meth.2001.1262
Li Y., Wang S., Hu Y., Cheng J., Cheng X., Cheng P., et al. (2021a). Dietary Bile Acid Supplementation Reveals Beneficial Effects on Intestinal Healthy Status of Tongue Sole (Cynoglossus Semilaevis). Fish. Shellf. Immunol. 116, 52–256. doi: 10.1016/j.fsi.2021.06.020
Li Y., Wang L., Yang Y., Li X., Dai H., Chen S. (2019). Genetic Analysis of Disease Resistance to Vibrio Harveyi by Challenge Test in Chinese Tongue Sole (Cynoglossus Semilaevis). Aquaculture 503, 430–435. doi: 10.1016/j.aquaculture.2019.01.011
Li Y., Yang Y., Song L., Wang J., Hu Y., Yang Q., et al. (2021e). Effects of Dietary Supplementation of Lactobacillus Plantarum and Bacillus Subtilis on Growth Performance, Survival, Immune Response, Antioxidant Capacity and Digestive Enzyme Activity in Olive Flounder (Paralichthys Olivaceus). Aquacult. Fish. 6, 283–288. doi: 10.1016/j.aaf.2020.10.006
Li Z., Yang L., Wang J., Shi W., Pawar R. A., Liu Y., et al. (2010). β-Actin Is a Useful Internal Control for Tissue-Specific Gene Expression Studies Using Quantitative Real-Time PCR in the Half-Smooth Tongue Sole Cynoglossus Semilaevis Challenged With LPS or Vibrio Anguillarum. Fish. Shellf. Immunol. 29, 89–93. doi: 10.1016/j.fsi.2010.02.021
Li M., Yang Y., Zheng W., Li Z., Cheng J., Li Y. (2020). Estimation of Heritabilities of Disease Resistance to Edwardsiella Tarda and Genetic Correlations Between Resistance and Growth Traits in Chinese Tongue Sole (Cynoglossus Semilaevis). Aquacult. Fish. 5, 289–293. doi: 10.1016/j.aaf.2019.12.002
Lu S., Zhou Q., Chen Y., Liu Y., Li Y., Wang L., et al. (2021). Development of a 38 K Single Nucleotide Polymorphism Array and Application in Genomic Selection for Resistance Against Vibrio Harveyi in Chinese Tongue Sole, Cynoglossus Semilaevis. Genomics 113, 1838–1844. doi: 10.1016/j.ygeno.2021.03.034
Mate J. M. (2000). Effects of Antioxidant Enzymes in the Molecular Control of Reactive Oxygen Species Toxicology. Toxicology 153, 83–104. doi: 10.1016/S0300-483X(00)00306-1
McBride B. W., Kelly J. M. (1990). Energy Cost of Absorption and Metabolism in the Ruminant Gastrointestinal Tract and Liver: A Review. J. Anim. Sci. 68, 2997–3010. doi: 10.2527/1990.6892997x
Moldoveanu B., Otmishi P., Jani P., Walker J., Sarmiento X., Guardiola J., et al. (2009). Inflammatory Mechanisms in the Lung. Inflamm. Res. 2, 1–11. doi: 10.2147/JIR.S4385
Nguyen A., Bouscarel B. (2008). Bile Acids and Signal Transduction: Role in Glucose Homeostasis. Cell Signal. 20, 2180–2197. doi: 10.1016/j.cellsig.2008.06.014
Romano N., Kumar V., Yang G., Kajbaf K., Rubio M. B., Overturf K., et al. (2020). Bile Acid Metabolism in Fish: Disturbances Caused by Fishmeal Alternatives and Some Mitigating Effects From Dietary Bile Inclusions. Rev. Aquacult. 12, 1792–1817. doi: 10.1111/raq.12410
Secombes C. J., Wang T., Hing S., Peddie S., Crampe M., Laing K. J., et al. (2001). Cytokines and Innate Immunity of Fish. Dev. Comp. Immunol. 25, 713–723. doi: 10.1016/S0145-305X(01)00032-5
Semenkovich C. F. (1997). Regulation of Fatty Acid Synthase (FAS). Prog. Lipid Res. 36, 43–53. doi: 10.1016/S0163-7827(97)00003-9
Song Y., Zheng W., Zhang M., Cheng X., Cheng J., Wang W., et al. (2020). Out-of-Season Artificial Reproduction Techniques of Cultured Female Tongue Sole (Cynoglossus Semilaevis): Broodstock Management, Administration Methods of Hormone Therapy and Artificial Fertilization. Aquaculture 518, 734866. doi: 10.1016/j.aquaculture.2019.734866
Wei M., Xu W., Gan T., Wang L., Zhang H., Zhao F., et al. (2019). Cloning, Expression Prolife, and Immune Characterization of a Novel Stat Family Member (stat5bl) in Chinese Tongue Sole (Cynoglossus Semilaevis). Fish. Shellf. Immunol. 84, 962–969. doi: 10.1016/j.fsi.2018.10.030
Willuweit A., Sass G., Schöneberg A., Eisel U., Tiegs G., Clauss M. (2001). Chronic Inflammation and Protection From Acute Hepatitis in Transgenic Mice Expressing TNF in Endothelial Cells. J. Immunol. 167, 3944–3952. doi: 10.4049/jimmunol.167.7.3944
Wu G., Wu Z., Dai Z., Yang Y., Wang W., Liu C., et al. (2013). Dietary Requirements of “Nutritionally Non-Essential Amino Acids” by Animals and Humans. Amino Acids 44, 1107–1113. doi: 10.1007/s00726-012-1444-2
Xie C., Wang Q., Wang J., Tan B., Fan Z., Deng Z. Y., et al. (2016). Developmental Changes in Hepatic Glucose Metabolism in a Newborn Piglet Model: A Comparative Analysis for Suckling Period and Early Weaning Period. Biochem. Biophys. Res. Commun. 470, 824–830. doi: 10.1016/j.bbrc.2016.01.114
Xie S., Wei D., Tian L., Liu Y. (2021). Dietary Supplementation of Chenodeoxycholic Acid Improved the Growth Performance, Immune Response and Intestinal Health of Juvenile Penaeus Monodon Fed a Low Fish-Meal Diet. Aquacult. Rep. 20, 100773. doi: 10.1016/j.aqrep.2021.100773
Xu J., Xie S., Chi S., Zhang S., Cao J., Tan B. (2022). Protective Effects of Taurocholic Acid on Excessive Hepatic Lipid Accumulation Via Regulation of Bile Acid Metabolism in Grouper. Food Funct. 13, 3050–3062. doi: 10.1039/D1FO04085E
Yin P., Xie S., Zhuang Z., He X., Tang X., Tian L., et al. (2021). Dietary Supplementation of Bile Acid Attenuate Adverse Effects of High-Fat Diet on Growth Performance, Antioxidant Ability, Lipid Accumulation and Intestinal Health in Juvenile Largemouth Bass (Micropterus Salmoides). Aquaculture 531, 735864. doi: 10.1016/j.aquaculture.2020.735864
Yu H., Zhang L., Chen P., Liang X., Cao A., Han J., et al. (2019). Dietary Bile Acids Enhance Growth, and Alleviate Hepatic Fibrosis Induced by a High Starch Diet Via AKT/FOXO1 and cAMP/AMPK/SREBP1 Pathway in Micropterus Salmoides. Front. Physiol. 10, 1430. doi: 10.3389/fphys.2019.01430
Zapata A., Diez B., Cejalvo T., Gutiérrezde F. C., Cortés A. (2006). Ontogeny of the Immune System of Fish. Fish. Shellf. Immunol. 20, 126–136. doi: 10.1016/j.fsi.2004.09.005
Keywords: hepatic health, antioxidant capacity, immune response, digestive enzyme activity, hepatic function
Citation: Wang S, Zhang W, Cao A, Pan Z and Liu T (2022) Dietary Supplementation of Bile Acids in Tongue Sole (Cynoglossus semilaevis): A Promising Strategy to Improve Hepatic Health Status. Front. Mar. Sci. 9:899768. doi: 10.3389/fmars.2022.899768
Received: 19 March 2022; Accepted: 12 April 2022;
Published: 17 May 2022.
Edited by:
Shiwei Xie, Guangdong Ocean University, ChinaReviewed by:
Yingying Yu, Foshan University, ChinaCopyright © 2022 Wang, Zhang, Cao, Pan and Liu. This is an open-access article distributed under the terms of the Creative Commons Attribution License (CC BY). The use, distribution or reproduction in other forums is permitted, provided the original author(s) and the copyright owner(s) are credited and that the original publication in this journal is cited, in accordance with accepted academic practice. No use, distribution or reproduction is permitted which does not comply with these terms.
*Correspondence: Zhongchao Pan, NDA2ODUzMzU3QHFxLmNvbQ==; Tailiang Liu, bHRsNjhAMTYzLmNvbQ==
†These authors have contributed equally to this work
Disclaimer: All claims expressed in this article are solely those of the authors and do not necessarily represent those of their affiliated organizations, or those of the publisher, the editors and the reviewers. Any product that may be evaluated in this article or claim that may be made by its manufacturer is not guaranteed or endorsed by the publisher.
Research integrity at Frontiers
Learn more about the work of our research integrity team to safeguard the quality of each article we publish.