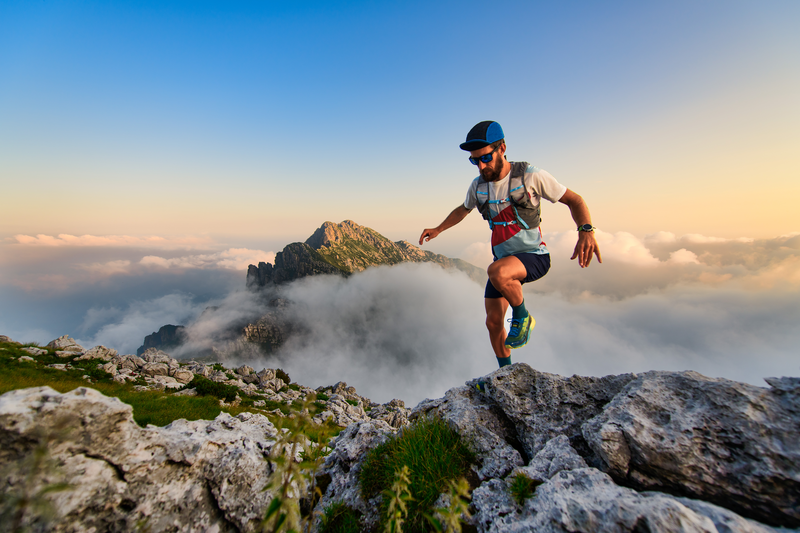
94% of researchers rate our articles as excellent or good
Learn more about the work of our research integrity team to safeguard the quality of each article we publish.
Find out more
REVIEW article
Front. Mar. Sci. , 26 October 2022
Sec. Deep-Sea Environments and Ecology
Volume 9 - 2022 | https://doi.org/10.3389/fmars.2022.898828
This article is part of the Research Topic Understanding Ocean Ridges, a New Frontier for Science and Development View all 18 articles
In February 2018, the Government of Poland and the International Seabed Authority signed a 15-year contract for exploration of polymetallic sulfide deposits on a section of the Mid-Atlantic Ridge extending between the Hayes, Atlantic and Kane transform faults (32°45.378’ N, 39°57.760’ W to 26°14.411’ N, 44°18.008’ W). The contractor is obliged to collect data on the contract area environment and its ecosystem components. In this context, it is important that the contractor establishes a sound starting point which further baseline investigations can be referred to. Such a starting point involves assessment of currently held information and, most importantly, knowledge gaps on the ecosystem components in the area of exploration (and of potential future exploitation). Of major importance here is the knowledge on benthic communities, as it is the benthos that will be most affected by any human intervention in the area of interest. Based on available published evidence, we have reviewed the present state of knowledge on benthic communities in the Polish exploration contract area (PECA). In the process, we have identified important knowledge gaps that will need to be addressed during exploration surveys. These include, but are not limited to, the distribution and structure of benthic communities throughout the contract area, the spatial and temporal variability of those communities, possible differences between communities inhabiting active and inactive vent fields, connectivity issues and the recovery potential. Special consideration should be given to Lost City, a geologically and ecologically unique hydrothermal field which has been a focus of international research and an important conservation target.
The Mid-Atlantic Ridge (MAR) is a part of the global system of mid-oceanic ridges formed at the contact between crustal plates (Karson et al., 2015a). Because of the Earth’s crust characteristics, the ridges are sites of considerable seismic and tectonic instability, manifested as, inter alia, hydrothermal activity which is particularly intense at active hydrothermal fields (Karson et al., 2015a; Karson et al., 2015b). A hydrothermal vent field is a term applied to an area supporting a cluster of vent sites, i.e. sites where heated seawater, modified into an ore-forming fluid, is ejected from the seafloor through conduits and chimneys (Weaver et al., 2021). A vent field may comprise active vent sites, inactive (or extinct) vents and sedimented slopes and plains, i.e., areas covered by soft sediments (Weaver et al., 2021). With regard to the latter, Fabri et al. (2011) distinguished between hydrothermal and pelagic sediments. A good schematic representation of a vent field and its different habitats (precipitation chimneys, basalts, soft sediments) can be found in Weaver and Billett (2019). The geological and geochemical processes associated with the hydrothermal activity induce precipitation, deposition and accumulation of minerals of commercial interest at the venting sites and in their vicinity (Hannington et al., 2011; Boschen et al., 2013; Menini and Van Dover, 2019). In view of their value for meeting the predicted global demand for minerals important in various technological applications (Petersen et al., 2016; Kawano and Furuya, 2022), deposits of polymetallic sulfides (PMS), the major mineral type of commercial interest at mid-ocean ridges, have become a focus of interest for the future deep-sea mining (Cherkashov, 2017; Van Dover et al., 2018). This entails contracts for PMS exploration in international waters with the International Seabed Authority (ISA), an institution “….mandated under the UN Convention on the Law of the Sea to organize, regulate and control all mineral-related activities in the international seabed area for the benefit of mankind as a whole.” (www.isa.org.jm).
In February 2018, the Government of Poland and the ISA signed a 15-year contract for exploration of PMS deposits on a section of the MAR extending between the Hayes, Atlantic and Kane transform faults (32°45.378’ N, 39°57.760’ W to 26°14.411’ N, 44°18.008’ W) (Figure 1).
Figure 1 Location of the Polish contract area (PECA) on the Mid-Atlantic Ridge; LC, Lost City; BS, Broken Spur; dark squares mark exploration blocks; dotted yellow line marks the boundary of Portugal’s Extended Continental Shelf (based on imagery available from NOAA, http:///www.ngdc.noaa.gov/mgg/image/2minrelief.html and ISA, https://isa.org.jm/files/files/documents/Public%20information%20on%20contracts%20Poland.pdf).
The depth range of the Polish exploration contract area (PECA), as determined from querying the GEBCO portal (www.gebco.net; accessed on 18 Aug. 2022) ranges from about 800 to about 4990 m beyond the axial rift; the mid-axial valley depth ranges from about 800 to about 5300 m, decreasing from north to south.
The PECA is known to encompass two confirmed active hydrothermal fields: the Broken Spur and Lost City (Beaulieu and Szafranski, 2019) as well as two inferred active fields (Beaulieu et al., 2013; Weaver et al., 2021). Some more detailed information on the topography, habitats and benthic fauna (primarily the macro- and megabenthos) can be encountered regarding the Broken Spur (Cruz et al., 2022) and the Lost City (see below), but the generally the area remains greatly unexplored. At the moment of writing (August 2022), the first exploration cruise organized by the Polish contractor is in progress to collect geophysical information on the PECA, including detailed bathymetry (M. Tomczak, pers. comm.).
Contract provisions1 oblige the contractor to collect data on the contract area environment and its ecosystem as detailed by the ISA in its “Recommendations for the Guidance of Contractors for the Assessment of the possible environmental impacts arising from exploration for marine minerals in the Area” (ISA, 2019). In addition, a contractor is obliged to implement certain environmental protection measures (Van Dover et al., 2011; Van Dover et al., 2018). Such measures include area-based (AB) spatial planning whereby impact reference zones (IRZs) and preservation reference zones (PRZs) for future mining activities are defined (ISA, 2017; Jones et al., 2020). More generally, there are AB measures such as Marine Protected Areas (MPAs), e.g. an MPA encompassing the Lucky Strike and Menez Gwen hydrothermal fields in the Portuguese national jurisdiction area (Calado et al., 2011; Menini and Van Dover, 2019), and ecosystem-based conservation (e.g. Ortiz et al., 2017). There are also non-AB measures, such as evidence-based conservation (e.g. Mogensen et al., 2022), species- or multispecies-based conservation (Mace et al., 2007; Campagna et al., 2008; Vals et al., 2015) and rules-based approach (e.g. Farley, 2009). In addition, specific sites may be identified as being in need of protection (Dunn et al., 2018). Work is being currently carried out on developing a Regional Environmental Management Plan (REMP) for the MAR, to be implemented by the ISA and its contractors (Weaver et al., 2021).
Given that the resource of interest, i.e. PMS, is an inherent component of the MAR seafloor ecosystem, PMS exploration and potential exploitation will primarily affect the benthic part of the ecosystem (Gollner et al., 2017; Dunn et al., 2018). It is therefore vital for the contractor to collect information on the benthic communities in the contract area. It is also important that contractors recognize gaps in their knowledge of the biological communities they are likely to encounter during exploration, test mining and eventually the exploitation of PMS.
With this necessity in mind, we have reviewed the present state of knowledge on benthic communities in the PECA, based on information contained in publicly available sources, as no dedicated field-oriented research has been as yet undertaken by the Polish contractor. We follow a conventional size-based ecological classification of the benthos (e.g. Gage and Tyler, 1991), whereby organisms associated with the seafloor are considered as representing the meio-, macro-, and megabenthos. It will be noticed, however, that the between-classes boundaries are not always sharp (or are purely pragmatic), hence some taxa are referred to in more than one category.
This paper is based on literature data mining, whereby data on benthic communities in the PECA have been sought in, and extracted from, book chapters and peer-reviewed papers stemming from information collected during research cruises to the MAR (Thaler and Amon, 2019). As can be seen from Table 1, information on the PECA benthos is limited, fragmentary and patchy. Basically, data on the PECA benthos have been collected almost exclusively at the two active hydrothermal vent fields, the Broken Spur and the Lost City. In this context it is worth mentioning that fairly extensive data on benthic communities in the PECA, those in the Broken Spur vent field in particular, have been contributed by Russian authors investigating the MAR ecosystem, based on materials collected during cruises up to 2003 (Gebruk and Mironov, 2006) (Table 1). Boschen-Rose and Colaço (2021) looked for similarities/dissimilarities in the composition of benthic communities across the hydrothermal vent fields in studies reported up to 2020. We performed a similar analysis for the meiobenthos, not covered by Boschen-Rose and Colaço (2021) data, using the non-metric multidimensional scaling (MDS) routine of the PRIMER v. 7 software package (Clarke and Gorley, 2015). To narrow the uncertainty margin of the analysis, which might have been introduced by using taxa of various ranks, the input data matrix included taxa identified to at least the genus level only.
Table 1 A summary of data sources on the PECA benthos (environmental characteristics, sampling methodology when reported, and analyses performed.
Like other ecological groupings, the MAR meiobenthos (benthic meiofauna, i.e. organisms commonly considered – in the deep sea – as falling within the 32-300 µm size range; cf. Thiel, 1975) is greatly understudied (Boschen-Rose and Colaço, 2021), including in the PECA. Most research on the hydrothermal vent meiofauna has been so far carried out in the Pacific ridge areas (e.g. Gollner et al., 2010; Zeppilli et al., 2018, and references therein). There have been few meiobenthos-focused studies in the MAR in general (Zekely et al., 2006; Flint, 2007; Ivanenko et al., 2011; Cuvelier et al., 2014; Sarrazin et al., 2015; Tchesunov, 2015; Zeppilli et al., 2015; Plum et al., 2017; Spedicato et al., 2020). However, information can be extracted by taking a broader view of MAR benthic communities, notably from the work of Galkin (2006) and Gebruk and Mironov (2006). The MAR meiobenthos has been so far reported from a number of known active hydrothermal fields (Menez Gwen, Lucky Strike, Rainbow, Lost City, Broken Spur, TAG, Snake Pit, Logatchev), two of which (Lost City and Broken Spur) are situated within the PECA. In addition to the low number of studies, the sampling effort reported has also been very low; usually researchers have had access to only 2 - 12 samples. Based on such a limited amount of material, it seems premature at the present stage to expect the researchers to provide analyses employing even basic indicators of meiobenthic diversity (rarefaction curves, abundance/diversity relationship estimates). We are at a very early stage of acquiring familiarity with the meiobenthos on the MAR in general, and in the PECA in particular. In this context, it would be highly desirable if future studies addressed habitat-related variability of meiobenthic communities. It seems quite plausible to expect differences in composition and abundances of the meiobenthos sampled from bio-ecological structures such as bivalve aggregations (as in Tchesunov, 2015), from the typical hard substrate, and from soft sediments on the flanks of the fields (Weaver et al., 2021).
Eleven major meiobenthic taxa have been reported from the PECA. The taxonomic richness in the PECA appears to be low in comparison to other areas of the MAR, but this is most likely to be the result of gross undersampling in the area rather than from any underlying biological patterns. Nematodes, ostracods and bivalves were found at the Lost City, while nematodes and siphonostomatoid, harpacticoid and cyclopoid copepods were recorded at the Broken Spur. In addition, few taxa were identified to a lower taxonomic level (family and/or genus), with the Broken Spur and Lost City featuring 13 (nematodes only) and 8 (the nematodes and copepods) such taxa, respectively (Tables 2A, B). Notably, the Lost City nematode assemblage features the genus Oncholaimus, which appears to be very abundant (Tchesunov, 2015; Zeppilli et al., 2018). The genus is regarded as specialising in feeding on free-living chemoautotrophic microorganisms and harbouring an epibiotic microbial community dominated by the Gammaproteobacteria, including vent symbionts (Zeppilli et al., 2018).
Table 2 Meiofaunal taxa reported from the hydrothermal vent fields in the Polish contract area (Lost City, Broken Spur) and elsewhere on MAR; A) non-nematode taxa; B) nematode taxa (data from Gebruk and Mironov, 2006; Flint, 2007; Ivanenko et al., 2011; Tchesunov, 2015; Zeppilli et al., 2015; Sarrazin et al., 2015; Zeppilli et al., 2019; Spedicato et al., 2020); +, taxon recorded; -, taxon not recorded.
In addition, three species of the siphonostomatoid copepod family Dirivultidae were recorded at the Broken Spur. These copepods are regarded as endemic to hydrothermal vents worldwide and are particularly well adapted to life in the vicinity of hot venting hydrothermal fluids (Zeppilli et al., 2018).
As shown by as few as 10 quantitative MAR meiofaunal studies that have been published, meiofaunal assemblages elsewhere on the MAR are dominated by nematodes and harpacticoid copepods, but mainly nematodes. However, at one non-vent site at the TAG hydrothermal field the proportions between these two taxa were reversed (Flint, 2007). Because of the nematode dominance, most of the meiofaunal studies on the MAR focused on nematodes, as shown by the relevant lists of meiofaunal taxa. This is also the case in the PECA (cf. Tables 2A, B).
As shown in Figure 2, the similarity in faunistic composition between meiobenthic assemblages reported so far from different MAR vent fields is quite low. The Broken Spur and Lost City assemblages appear to be more similar to each other (and to the assemblage at the Rainbow) than to those from the remaining fields. The Broken Spur, Lost City and Rainbow fields are all at a similar depth, which might in part explain the apparent similarity. It has to be remembered, however, that the similarity analysis shown in Figure 2 was carried out on a limited amount of data found in a low number of published accounts.
Figure 2 An MDS plot of similarity/dissimilarity (presence-absence transformation; group-average sorting) between the composition of meiobenthic assemblages at different MAR vent fields (presence-absence transformation; Bray-Curtis similarity); Vent field name symbols: R, Rainbow; BS, Broken Spur; Lci, Lost City; MG, Menez Gwen; LS, Lucky Strike; SP, Snake Pit; TAG, Trans-Atlantic Geotraverse; Log, Logatchev.
Macrofaunal benthic communities (i.e. comprising organisms >300 µm; cf. Thiel, 1975) of the MAR have been studied less extensively than the assemblages of megafaunal invertebrates (see below), resulting in a very poor general knowledge. Some detailed studies have been carried out at other localities on the MAR, notably in the Charlie-Gibbs Fracture Zone further north (Alt et al., 2013; Dilman, 2013; Shields et al., 2013; Bell et al., 2016; Alt et al., 2019) and at various hydrothermal vents [Lucky Strike, Menez Gwen, Rainbow, Snake Pit, Logatchev and Trans-Atlantic Geotraverse (TAG)] (e.g. Bellan-Santini and Thurston, 1996; Sigvaldadottir and Desbruyères, 2003; Myers and Cunha, 2004; Cunha and Wilson, 2006; Larsen et al., 2006; Bellan-Santini, 2007; Corbera et al., 2008; Riou et al., 2010; Sarrazin et al., 2015; Bebianno et al., 2018; Klunder et al., 2020).
In the PECA, macrobenthic communities have been reported to date only from the Broken Spur and Lost City hydrothermal vent fields (Tyler et al., 1995; Desbruyères et al., 2000; Kelley et al., 2005; Kelley et al., 2007; Goroslavskaya and Galkin, 2011; Rybakova and Galkin, 2015; Boschen-Rose and Colaço, 2021; Cruz et al., 2022) (see Table 3). A total of 22 species were reported from the Broken Spur (Boschen-Rose and Colaço, 2021). The fauna includes the bivalve Bathymodiolus puteoserpentis (alternatively reported also as the megabenthos; see below), the ampharetid polychaete Amathys lutzi (currently Amphisamytha lutzi) and the ophiuroid Ophioctenella acies (Rybakova and Galkin, 2015) as well as nektobenthic shrimps and polychaetes that can grow large enough to be classified as the megafauna (Desbruyères et al., 2000). A recent review (Boschen-Rose and Colaço, 2021) demonstrated a similarity between the Broken Spur fauna and benthic communities associated with other vent fields including the TAG, Snake Pit, Logatchev and Ashadze-1.
Table 3 Non-meiofaunal taxa reported from the PECA vent fields (Lost City and Broken Spur) (data from Copley et al., 1997; Kelley et al., 2005; Gebruk and Mironov, 2006; Vereshchaka and Vinogradov, 2006; Boschen-Rose and Colaço, 2021); +, taxon recorded; -, taxon not recorded.
Owing to the limitations of physical sampling of the MAR benthos due to the rough terrain, especially in proximity to the mid-axial valley, most data on MAR benthic communities relate to larger organisms (megafauna) seen in photographic and video surveys using Remotely Operated Vehicles (ROVs) (e.g. Alt et al., 2019; Boschen-Rose and Colaço, 2021). Yet, even for the megafauna, data in the PECA are restricted to just two small hydrothermal vent areas. The few records of megafaunal species found at the Broken Spur and Lost City vents, however, allow some observations to be made using information on the same species at other locations on the MAR (e.g. Perez et al., 2012; Priede et al., 2013; Thaler and Amon, 2019; Boschen-Rose and Colaço, 2021 and references therein). Yet, the general knowledge about benthic megafauna of the PECA is still insufficient, as it lacks, inter alia, basic data on some components of benthic communities and is limited to the two vent fields already mentioned, the Broken Spur and Lost City (cf. Table 3).
The megafauna at the Broken Spur shows greater affinity to hydrothermal vent fields in deeper water further south than to the shallower hydrothermal sites of Menez Gwen and Lucky Strike to the north (Fabri et al., 2011). Recently, however, Cruz et al. (2022) referred to a general similarity between the Broken Spur fauna and that encountered elsewhere on the MAR. As reported by Boschen-Rose and Colaço (2021), as few as 9 benthic megafaunal taxa have been recorded so far from Broken Spur, including a cnidarian (Maractis rimicarivora), three molluscs: Phymorhynchus ovatus, Bathymodiolus azoricus and B. puteoserpentis, four decapods: Alvinocaris markensis, Mirorcaris fortunata, Rimicaris exoculata and Segonzacia mesatlantica, and an ophiuroid (Ophioctenella acies). In addition, the Broken Spur was also reported to be significantly impoverished in terms of megabenthic abundance, compared to other vent fields, e.g. the TAG, Snake Pit or Rainbow (Desbruyères et al., 2000). However, the apparent lower number of taxa and lower abundance of the megafauna at the Broken Spur might be due to the lower sampling intensity at this vent field.
The habitat preferences of individual megafaunal species within the Broken Spur field were briefly treated by Desbruyères et al. (2000) who reported R. exoculata as a species dominant on black smoker walls and M. fortunata aggregating at the foot of chimneys, while the two Bathymodiolus species and O. acies were distributed in the surrounding blocs within the active field area. Mirocaris fortunata, one of the dominants at the Lucky Strike field, is an example of “social” (aggregations > 100 ind/m2) and epibiont-dependent shrimps (Zbinden et al., 2018), grouping in the axis of a warm (20-25°C) flow of the hydrothermal current (Shillito et al., 2006; Matabos et al., 2015). The species was observed to occur across a wide range of habitats, often in association with beds of the mussel Bathymodiolus azoricus, and to graze on microorganisms or detrital remains (Gebruk and Galkin, 1997; Matabos et al., 2015). The bythograeid crab Segonzacia mesatlantica was variously described as a scavenger or a carnivore (predator; cf. Boschen-Rose and Colaço, 2021), but its feeding behaviors observed by Matabos et al. (2015) were far from unequivocal.
The Broken Spur vent field is of a great biogeographical importance as a boundary between the northern and the southern vent faunas on the MAR (O’Mullan et al., 2001; Dunn et al., 2018). Regarding the mytilid assemblages, the Broken Spur is also a hybridization zone between the two mussel species (Bathymodiolus azoricus and B. puteoserpentis); in other words, it has the highest proportion of mussel hybrid individuals along the MAR (Dunn et al., 2018).
The shrimp assemblages at the Broken Spur are dominated by Rimicaris exoculata, although shrimp swarms in the area are not as dense as those often observed at, e.g. the TAG (Rybakova and Galkin, 2015). The species’ populations are reported to be stable for a relatively short time (about 15 months) (Copley et al., 1997) as well as in a long-term (decadal) perspective, as found at, e.g. the Lucky Strike (Cuvelier et al., 2011).
Four nektobenthic invertebrate species were sampled from the PECA, all representing the endemic caridean shrimps from the superfamily Bresilioidea, i.e. Alvinocaris markensis, Rimicaris chacei, R. exoculata, and Mirocaris fortunata [(= M. keldyshi (Komai and Segonzac, 2003)] (Mironov et al., 2002; Galkin, 2006; Gebruk and Mironov, 2006). These species are the dominant fauna in images of the seafloor. They have been reported from geologically active sites of the northern MAR, from Deyin (15.02° S) to Moytirra (45.3° N), within a wide depth range (1740–4200 m), their presence revealing clear spatial and demographical patterns (Zbinden and Cambon-Bonavita, 2020). The occurrence of the highly specialized Rimicaris species, whose trophic relationships are dependent on epibiotic microorganisms, is tightly associated with hydrothermal vent orifices, the optimal ambient temperatures ranging within 3.4-22.5°C (Gebruk and Galkin, 1997; Gebruk et al., 2000; Zbinden and Cambon-Bonavita, 2020). The densest swarms of R. exoculata (up to 3000 ind./m2) were recorded at the Logatchev, and also at the Broken Spur (Gebruk et al., 2000). The swarms are maintained by a highly modified, eye-like organ adapted to the black-body radiation emitted by smokers (Williams and Rona, 1986; Pelli and Chamberlain, 1989; Nuckley et al., 1996), the organ enabling the shrimps to stay close to, but at a safe distance from, black-smoker orifices emitting the hot plume.
Functional aspects of benthic communities on the MAR are known only partially. An interesting approach has been recently presented by Alfaro-Lucas et al. (2020) who addressed functional diversity of the Lucky Strike vent field fauna and found it enhanced at sites directly affected by hydrothermal activity and reduced at surrounding sites. An important conclusion of their study was that “Low functional richness and environmental filtering suggest that surrounding areas, with their very heterogeneous species and functional assemblages, may be especially vulnerable to environmental changes related to natural and anthropogenic impacts, including deep-sea mining”. This type of reasoning, however, has not evolved into a generalization encompassing other MAR areas yet. Here we touch upon certain aspects of functioning of vent field benthos which are important as baseline information and for which there exist only limited data from the PECA.
Distribution patterns of individual benthic taxa in the wider MAR, and in the PECA in particular, have not been resolved in detail, although – with regard to the MAR – there exist a number of interesting observations. For example, populations of vent-associated shrimps reveal a highly structured spatial segregation and demographic patterns (Shank et al., 1998b; Gebruk et al., 2000; Methou et al., 2022). While mature females of R. exoculata occur in abundance in areas close to fast flowing hydrothermal fluids, juveniles, in contrast, are found in clusters only some distance from the active vents. Mature males and females of the species form clusters clinging to rocky surfaces in localised areas, becoming prey for sea anemones occupying the space on the edges of black-smokers (Gebruk et al., 2000; Hernández-Ávila et al., 2022; Methou et al., 2022). The reasons for these spatial differences along the thermal gradient have not been fully resolved, but a similar spatial pattern is apparent in several vent invertebrate taxa, such as Kiwa tyleri in the Antarctic and Calyptogena sp. in the Pacific (Marsh et al., 2015). The proposed explanations for this segregation include habitat-thermal requirements, intraspecific and interspecific competition for space and resources or a succession effect (Shank et al., 1998a; Marsh et al., 2015). Juveniles that still hold food reserves from their larval period do not need to compete for access to the microorganism primary producers which use the hydrothermal minerals, essential for adults which are fully dependent on epibionts. It has been proposed that the eye-like organ renders R. exoculata a stronger competitor to R. chacei, a species occurring as solitary individuals or forming small aggregations of some tens to hundreds individuals in more peripheral zones at, e.g. the Snake Pit and TAG (Gebruk et al., 2000; Methou et al., 2022). Alvinocaris markensis, an opportunistic feeder, also stays in peripheral zones and at the foot of hyrothermal vents (Gebruk and Galkin, 1997; Lunina and Vereshchaka, 2014).
Data on the benthic abundance and biomass from the PECA are generally scant, whereas overviews have been presented for other areas (e.g. Galkin, 2006). Those overviews concern primarily the dominant and foundation species at hydrothermal vents, such as the bresilioid shrimps (see above). The abundance and biomass reported for R. exoculata at the Broken Spur were on the order of 2500-3000 ind./m2 and up to 1-1.5 kg/m2, respectively. Another group of foundation and dominant species is formed by mytilid bivalves a representative of which, Bathymodiolus puteoserpentis, forms usually dense and extensive beds, with over 70 kg/m2 biomass at the Logatchev, but is much less abundant at the Broken Spur where it occurs in localized patches only (Galkin, 2006). The lower abundance at the Broken Spur is probably related to this vent acting as a zone of hybridization between B. azoricus and B. puteoserpentis (see above).
Van Dover (2014) described the hydrothermal vent ecosystems as dominated by holobiont taxa, i.e. organisms that host symbiotic chemoautotrophic microorganisms. These taxa often occur as the foundation species forming complex 3-dimensional habitats (Gerdes et al., 2019), e.g. mytilid and vesicomyid bivalves and polychaetes of the genus Chaetopterus which occur in large numbers away from active smokers. Aggregations of these taxa are used as a substratum, refugia and a habitat for epizoic microorganisms, juvenile invertebrates, and other associated organisms, including the meiobenthos (nematodes, harpacticoids, halacarids) which occasionally, e.g. at the Broken Spur, are found in abundance. There are also primary consumers (e.g. the limpets Peltospira smaragdina and Lepetodrilus atlanticus grazing on microbial biofilms) as well as secondary and tertiary consumers such as scavenging and predatory crustaceans and fishes.
The total biomass values of aggregations of R. exoculata or B. puteoserpentis, shown above, are typically very high at vents. Beyond the active hydrothermal vent area, faunal abundances and biomass are usually much lower, the megafauna being represented by solitary large anemones (e.g. Maractis rimicarivora) and gorgonian corals (Van Dover, 2014).
The soft sediment-covered areas away from active venting support fairly abundant meiobenthic communities compared with abundances found in other deep-sea environments. Abundances at the TAG and Snake Pit hydrothermal vent fields are 11-35 and 72-86 ind/10cm2 respectively (Spedicato et al., 2020).
Faunal density responses appear to be a function of stress level. For example, at Middle Valley (2410 m, the Juan de Fuca hydrothermal vent field in the Pacific), very hot sediments (e.g. 94°C at 5 cm into the sediment column) support very few macrofauna, whereas moderately warm sediments inhabited by vesicomyid bivalves may support elevated macrofaunal densities (16,500 ind./m2) relative to those in microbial mats (6,840 ind./m2), hot sediments (1,690 ind./m2), and control (not heated) sediments (2,218 ind./m2) (Bernardino et al., 2012). The only more detailed study demonstrated that, generally, the abundance and biomass of the macrofauna in the soft sediments seem to be at a level similar to that in other deep sea sites at the same depth range (Priede et al., 2013). It is worth noting that the samples analyzed by Priede et al. (2013) were all collected some distance away from the mid-axial valley. No other comparative data are as yet available either from the MAR in general or from the PECA in particular.
Two different trophic pathways have been reported at the Broken Spur and Lost City in the PECA, one present in the in-axis basalt-hosted systems (e.g. Broken Spur) and the other in the off-axis ultramafic-hosted systems (e.g. Lost City). Both systems are based on conversion of the hydrothermal energy into biomass of chemosynthetic bacteria, but differ in specific sources of metabolic energy utilized by primary producers: oxidation of sulfur compounds (basalt-hosted systems; McCollom and Shock, 1997) or reactions involving H2 and CH4 (sulfide-poor ultramafic systems; McCollom, 2007). Thermodynamic modeling indicates that metabolic reactions utilized as energy sources in the ultramafic-hosted systems can supply about twice as much chemical energy and potentially support much higher biomass than analogous systems hosted in basaltic rocks (McCollom, 2007).
There are two main types of primary consumers at the PECA hydrothermal vents studied to date; 1) those hosting endosymbionts (e.g. Bathymodiolus mussels) and 2) those feeding on free-living bacteria (e.g. Rimicaris shrimps). In addition, different types of predators, based on the type of primary consumers present, complete very simple food webs typical of hydrothermal vents (Gebruk et al., 2000; Vereshchaka et al., 2000; Colaço et al., 2002; Colaço et al., 2007). However, as observed by Matabos et al. (2015), some trophic relationships, e.g. those involving the bythograeid crab Segonzacia mesatlantica appear uncertain, although Boschen-Rose and Colaço (2021) – referring to studies using stable isotopes – treat the species as a predator.
Endemism at hydrothermal vents can be considered from two viewpoints: one concerns vent-obligate fauna at hydrothermal vents in general, and the other – endemism at specific individual vent fields. When looked at from the first viewpoint, the vent-obligate fauna on the MAR shows a moderate degree of endemism only. The majority of such taxa are widely distributed along the MAR due to their good swimming ability, planktonic larvae and/or passive migrations (Jollivet, 1996; Teixeira et al., 2013; Hilário et al., 2015). At the present state of knowledge, only five families are considered unique for individual vent fields: the sea anemones Actinostolidae (found at TAG), the gastropods Neolepetopsidae (Lucky Strike), the Neopilinidae (Menez Gwen), and two amphipod families — Amphilochidae (Lucky Strike) and Stegocephalidae (Snake Pit). No information from the PECA in this respect is available.
Of the 61 vent-obligate taxa found at 7 sites on the MAR (Menez Gwen, Lucky Strike, Rainbow, Broken Spur, TAG, Snake Pit and Logatchev) (Mironov et al., 2002), about half (30 species or 49%) are limited in their occurrence to a single field. Most shared vent-obligatory species occurred in the Menez Gwen, Lucky Strike and Rainbow, perhaps because those three vent fields – most accessible due to their vicinity to the Azores and located at relatively shallower depths – received disproportionately large attention from researchers. Generally, a high level of endemism is observed in crustacean amphipods and alvinocarid shrimps, with four species (80%) recorded at only a single site. Incidentally, a new shrimp species, Keldyshicaris vavilovi was discovered in 2001 at the Broken Spur vent field (Lunina and Vereshchaka, 2014). Since it has not been so far reported from any other location, it has to be regarded as field-endemic.
A high degree of endemism (58%) was reported for the Lost City field macrofauna (Kelley et al., 2005) (see below).
Endemic taxa are also known within the macro- and meiofauna. For example, in the latter, the siphonostomatoid copepod family Dirivultidae is known exclusively from vent habitats (e.g. Gollner et al., 2010). Four known vent species of the Halacaridea (Acarina) and nine of the 13 (69.2%) species of the Dirivultidae (Copepoda) are known from only one location on the MAR.
When discussing the available knowledge on the PECA benthos, a special consideration is due to the Lost City. It is a geologically and ecologically distinct hydrothermal field within the MAR (Kelley et al., 2005) which has become a focus of vigorous international research (e.g. Früh-Green et al., 2015). It differs from other hydrothermal fields in its age (spanning about 30,000 years, i.e. older than other MAR structures (Früh-Green et al., 2003), geological setting (serpentinite rather than basalts) and characteristics of the hydrothermal fluid (high alkalinity, lower fluid temperature) (e.g. López-Garcia et al., 2007). The unique characteristics of the Lost City (Denny et al., 2015) and its being one of the few carbonate vent fields discovered so far (Lecoeuvre et al., 2021) resulted in the field becoming an important conservation target, recognised as an Ecologically and Biologically Significant Area (EBSA) under the Convention on Biological Diversity (CBD) (Casson et al., 2019; Johnson, 2019) and as a potential UNESCO World Heritage Site in the high seas (Freestone et al., 2016). As the Lost City does not host any mineral resources of interest, it is not likely to be targeted for mining, but the neighboring areas might be. The Lost City might therefore be affected by consequences of possible mining activities (e.g. sediment plumes, contamination) (Johnson, 2019), hence the need of a more detailed knowledge.
Most of biological research in the Lost City has concerned its microbiology (Boetius, 2005; Dulov et al., 2005), with a particular attention paid to the sub-bottom biome (e.g. Brazelton et al., 2006; Orcutt et al., 2011; Lang and Brazelton, 2020). Studies on the Lost City archaea and bacteria revealed shifts on millennial time scales (Brazelton et al., 2010), associated with preadaptation of various microbial taxa to environmental conditions. On the other hand, only a few studies have targeted benthic communities. The limited data available show the Lost City macro- and megabenthos to be very different in composition from corresponding assemblages in other fields (e.g. Fabri et al., 2011; Boschen-Rose and Colaço, 2021; cf. Table 3), most probably due to the lack of chemoautotrophic symbiont-supporting fauna (see below). The initial surveys indicated few macroorganisms, with a notable absence of large bivalves known from other vent fields. However, beds of dead mytilid bivalve shells were found in a nearby inactive field named Old Village (Lein et al., 2007). With time, as more dives were performed and a larger area was being inspected, diverse but very scattered macro- and megafaunal assemblages, including large eurybathic fish, were revealed. In 2005, Shank and Buckman2 as well as Kelley et al. (2005) reported the presence of more than 70 “potential species” representing the macrobenthos (gastropods, bivalves, amphipods, numerous polychaetes with at least 5 new species) and meiofauna (nematodes, ostracods) as well as fish (e.g. the wreckfish Polyprion americanus, the cut-throat eel Synaphobranchus kaupi).
The biomass (wet weight) at the Lost City was apparently lower compared to a typical hydrothermal vent on the MAR. The large mobile megafauna (the fish mentioned as well as large geryonid crabs, visible around active vents) were the largest biomass components (Kelley et al., 2005).
Kelley et al. (2005) as well as Shank and Buckman2 reported also on a sharp difference between the fauna of vent and non-vent habitats at the Lost City, whereby typical vent areas are sparsely populated, whereas non-venting habitats less than a few meters away (e.g. on the sides of inactive solidified carbonate structures, sedimented areas, and breccia cap rock just to the north of the field) are dominated by hard corals (Desmophyllum pertusum, known formerly as Lophelia pertusa), octocorals (gorgonians), galatheid crabs, turrid gastropods, foraminifera, pteropods, urchins, asteroids, ophiuroids, and “typical” deep-sea barnacles.
It is very likely that the diverse and endemic (endemicity estimated at about 58%; Kelley et al., 2005) local macrofauna feed mainly on microbial mats, both on the surface and in the inner structures of serpentinite chimneys (Kelley et al., 2007). In contrast to other vent fields, the Lost City fauna did not show the presence of chemoautotrophic symbionts because most of the reduced energy at the Lost City comes from hydrogen, and no animals have been reported to support hydrogen oxidizers as symbionts (Boetius, 2005). However, Bathymodiolus azoricus, known to host methane- and sulfur-oxidizing symbionts elsewhere on the MAR was reported from the Lost City (cf. Table 3), but it is not known yet whether its survival there depends on chemosymbiosis. On the other hand, as already indicated and as can be judged from the limited data on the meiobenthic assemblages available, the meiofauna composition shows a somewhat closer affinity to the other active vent field in the PECA (Broken Spur) as well as to the Rainbow field than to the remaining hydrothermal vent fields studied (cf. Figure 2). It remains to be seen, however, to what extent the similarity is a result of the limited database rather than a manifestation of a true distributional pattern.
As can be seen from the “knowns” discussed above, the knowledge gaps with respect to the benthic communities in the PECA, like in the entire MAR (Boschen-Rose and Colaço, 2021), are considerable (see a summary, with respect to the relevant ISA guidelines and recommendations for the contractors, in Table 4).
Table 4 Knowledge gaps in view of the ISA guidelines and recommendations (ISA, 2019).
First of all, there is poor published knowledge on the range of habitats present in the area. Detailed seabed mapping of habitats (cf. Gerdes et al., 2019) is a top priority for the effective planning of both PMS resource exploration and environmental sampling campaigns. High-resolution seabed mapping should include the identification of active and inactive hydrothermal vent fields (Van Dover, 2019; Menini and Van Dover, 2019; Jamieson and Gartman, 2020; Van Dover et al., 2020). Knowledge of extinct hydrothermal fields might be particularly important because they may be the first targets of mining activities (Van Dover et al., 2020).
In line with research needs identified recently by Amon et al. (2022), the broadening of the knowledge base on benthic communities in the PECA requires high-resolution sampling and analysis of all benthic size categories (meio-, macro-, and megabenthos as well as the nektobenthos), using both direct-access gear (e.g. cores) and visual access tools (underwater cameras). To address outstanding issues on within-PECA connectivity of benthic communities, it will be necessary to collect temporally and spatially resolved data on the larval abundance and larval dispersal patterns (Vrijenhoek, 2010; Hilário et al., 2015; Baco et al., 2016).
The research gaps regarding the PECA’s meiobenthos are huge. As the meiobenthos is a good proxy of many natural and anthropogenic processes in the deep sea (Zeppilli et al., 2016; Zeppilli et al., 2018), there is a need to increase knowledge on the composition, abundance, and functional relationships within the meiobenthic communities and on their interactions with other benthic compartments. At hydrothermal vent sites worldwide, the meiobenthos has been found to be associated with various habitats, from inactive bare basalts (Gollner et al., 2010) and sediments (Vanreusel et al., 1997) to diffuse vent flow areas colonised by macrofaunal assemblages (Zekely et al., 2006; Sarrazin et al., 2015). When working on nematodes in the Pacific’s Fiji-Lau area, Vanreusel et al. (1997) found a qualitative dissimilarity between the nematode faunas of hydrothermal vent field sediment and in the nearby abyss, with no vent-field taxa being recorded in the abyssal sediment. As no between-habitats comparisons have been conducted on the MAR and given the prevalence of sedimentary habitats there (cf. Riehl et al., 2020), it is important (also from the standpoint of connectivity) to determine how similar/dissimilar the assemblages are between the ridge itself and the surrounding deep-sea areas. In this context, it is necessary that the contractors use best practices in studying the meiofauna, such as TV-guided coring devices (Varliero et al., 2019; Haeckel and Linke, 2021), especially in and around sediment patches near rocky surfaces in the mid-axial valley, and eDNA assays. All the contractors should be tooling up if they are seriously considering mining for PMS. This includes technologies for environmental baseline studies and subsequent monitoring activities if mining is allowed to proceed.
At present, knowledge of the PECA macrobenthos is extremely limited. Current knowledge relates to data collected in other parts of the MAR, often at a great distance from the PECA, and at different depths. Knowledge on the most important and diverse macrofaunal taxa, such as polychaetes, peracarid crustaceans, bivalves and gastropods is very poor along the whole MAR, and there have been only a few quantitative assessments of the standing stock and diversity. Macrobenthos studies on mid-ocean ridges have been limited owing to the need to use the so-called contact samplers, such as dredges, epibenthic sledges, grabs and corers. However, newer technologies, such as TV-guided corers (see above) and Remotely Operated Vehicles (ROVs) are now allowing greater precision sampling in areas of difficult terrain. A particularly wide knowledge gap concerns the fauna associated with soft sediments (Bergstad et al., 2008; Gebruk et al., 2010; Shields and Blanco-Perez, 2013; Priede et al., 2013). This habitat is a very important component of the MAR region (Niedzielski et al., 2013; Denny et al., 2015; Dutkiewicz et al., 2015), most likely including the PECA. Some studies suggest that the contribution of endemic species in some groups may, even in areas located away from the hydrothermal vent ecosystems, reach several per cent (e.g. Molodtsova et al., 2008; Tabachnick and Collins, 2008; Braga-Henriques et al., 2013). Therefore, studies of the soft-bottom ecosystems located outside the actual vent fields should be amongst the priorities of future research programs in the PECA.
Taxonomic studies based on both morphological and molecular data are vital for biodiversity estimates and ecological analyses (Frutos et al., 2022; Kaiser et al., 2022), such as assessing connectivity between sites within the PECA and more widely on the MAR. Therefore, different complementary sampling techniques (e.g. epibenthic sledges and box corers), including technologies for collecting fauna that are becoming standard systems in areas of rough terrain (e.g. TV-guided box corers and ROVs) should be used to allow biodiversity inventory and taxonomic studies as well as quantitative research that links the information about the benthic biota with data about the environmental factors shaping their communities (Jóźwiak et al., 2020). Earlier studies from the MAR demonstrated that the rate at which new species are described, although increasing with time, is still very low (e.g. Tyler et al., 1995; Sigvaldadottir and Desbruyères, 2003; Myers and Cunha, 2004; Cunha and Wilson, 2006; Larsen et al., 2006; Bellan-Santini, 2007; Corbera et al., 2008; Budaeva, 2012). The identification of a wide range of different taxa in the PECA area, most of them new to science, is a particular challenge for a contractor. Developing the availability of taxonomic expertise to allow the delivery of timely environmental studies is of key importance. Although there are efforts to create relevant taxonomic clearing houses (e.g. the recent ISA’s Sustainable Seabed Knowledge Initiative, www.isa.org/sski), there are difficulties in getting responses on commercial timescales without investing in local expertise by individual contractors. In many cases, contractors have mobilised national universities in supporting deep-sea mining exploration needs and future monitoring tasks.
The scale of the undescribed biodiversity is most probably huge. For example, about 50% of scavenging amphipods collected in the vicinity of the Charlie-Gibbs Fracture Zone proved to be new to science (Horton et al., 2013). The study on the polychaete fauna collected using a megacorer (a type of multiple corer) in the same MAR area (Shields and Blanco-Perez, 2013) resulted in recording 133 species in just 11 samples; almost none could be assigned to already described species. Moreover, the majority of them were singletons. It is worth mentioning that today, almost ten years after that collection was obtained, no taxonomic descriptions were published other than those of representatives of the family Polynoidae (Shields et al., 2013). The perceived high diversity, and probably also a high level of rarity, may create problems with finding appropriate taxonomic expertise and with securing a reasonable sampling effort needed to draw meaningful conclusions. Since large seafloor areas of the PECA have never been sampled, a very high number of species new to science is expected, not only amongst the most speciose and abundant taxonomic groups such as polychaetes or molluscs (as well as other taxa undergoing development via planktonic larvae), but also amongst organisms with direct development, limited dispersal abilities and low abundance, such as peracarids (tanaidaceans, cumaceans and isopods). Despite their low abundance and biomass, they constitute a very important component of the deep-sea communities worldwide (Stępień et al., 2021; Jóźwiak et al., 2022). Along with polychaetes, they form a core of the marine macrobenthic assemblages at bathyal depths and deeper (McCallum et al., 2015; Brandt et al., 2018; Washburn et al., 2021). Their limited mobility (no planktonic larvae, burrowing life style) restricts dispersal, for which reasons these crustaceans are considered a good indicator of environmental conditions and potentially an ideal surrogate for assessing recovery potential of disturbed ecosystems (Jennings et al., 2018; Jakiel et al., 2018; Riehl et al., 2018; Jakiel et al., 2019). Recent molecular studies demonstrated also that the problem of endemism, cryptic diversity and connectivity along the MAR is very complex and requires further studies (Teixeira et al., 2013; Yahagi et al., 2019) which can be very important for further planning of basic taxonomic analyses but also for studies of distribution patterns and community ecology on various temporal and spatial scales. Resolving the connectivity problem is of a particular importance for contractors, as it is through connectivity that areas disturbed by mining might be recolonized and repopulated (Hilário et al., 2015).
Future studies should also include other groups of benthic animals. For example, Jones et al. (2013) showed enteropneusts (Enteropneusta), a taxon belonging to the most underestimated deep-sea organisms worldwide (Tassia et al., 2016), to be important bioturbators at MAR’s bathyal depths. Since the available species lists of various benthic taxa are often scattered among various publications, including short taxonomic notes (e.g. Bellan-Santini and Thurston, 1996; Gebruk et al., 2010; Goroslavskaya and Galkin, 2011; Dilman, 2013; Shields and Blanco-Perez, 2013; Kongsrud et al., 2013), there is also a great need to incorporate the results of the current and future studies into publicly available databases.
Knowledge on environmental controls of the PECA’s benthic assemblages is sorely lacking. It is necessary to find out if environmental controls such as those referred to by Boschen-Rose and Colaço (2021) and Priede et al. (2022), i.e. depth, surface primary production and POC flux, bathymetry and chimney type are likely to influence the distributions of fauna within in the PECA, Knowledge on biology and habitat requirements of individual benthic taxa is incomplete. Fauna at other locations on the MAR have shown a wide variety of associations, interactions and behaviors of individual taxa. Examples are furnished by an association between polychaetes and bivalves (Bebianno et al., 2018) in which the polychaete, Branchipolynoe seepensis, kleptoparasitic in and commensal with Bathymodiolus azoricus, plays a role in metal detoxification of the bivalve, and the association is likely an adaptation to metal concentrations at the vent sites studied (Menez Gwen and Lucky Strike) as well as by an apparently commensal association between polynoid polychaetes (Eunoe bathydomus) and holothurians Deima validum (Shields et al., 2013). These examples demonstrate a unique nature of biological interactions, particularly in the vent systems. Studies of the reproductive behaviour, physiology, and development have also been infrequent, a particular attention being paid to the vent fauna (e.g. Blake and Van Dover, 2005; Riou et al., 2010; Marticorena et al., 2020; Cruz et al., 2022; Hernández-Ávila et al., 2022). The anticipated complexity of mutual interactions as well as a high diversity of trophic and ecological groups shows also a great need for functional analyses that assess the level of niche occupancy in the communities and allow to estimate functional redundancy – a knowledge especially needed in ecosystem considered vulnerable to anthropogenic impacts. Another challenge is presented by studies on biology of individual taxa. Since such approach is often logistically difficult and requires more specialized techniques and planning, it would probably be very difficult to conduct in the near future, although the results might provide important insights into the functioning of the MAR benthic communities.
It has to be borne in mind that the general knowledge on the benthic communities on the MAR, and the PECA in particular, is based on only a relatively low number of published reports. For example, Boschen-Rose and Colaço (2021) based their analyses on 52 peer-reviewed papers (prior to exclusion of some as not meeting the predetermined criteria); Weaver et al. (2021) analysed benthic communities on information from about 110 peer-reviewed papers, whereas we searched for information on the PECA benthos in 90 available publications. Therefore, any generalisations and more comprehensive conclusions regarding the distribution patterns and factors influencing the benthic communities are very tentative and need verification by further studies based on a higher sampling effort. Moreover, methodological constraints have to be taken into consideration. For example, observations of the megafauna, usually conducted from ROVs, produce low-resolution taxonomic assessments leading to underestimation of the actual diversity (e.g. Alt et al., 2019). However, the technical advances in imaging technology (e.g. Dumke et al., 2018) and annotation software (Nuno Gomes-Pereira et al., 2016) have allowed a progress to be made in this respect. Also, methods are at present at hand with which to estimate the megafaunal biomass from underwater imagery (Benoist et al., 2019). Nevertheless, it is still very difficult to identify factors shaping spatial patterns of the megabenthos and to predict possible responses of the communities to anthropogenic impacts (Dunn et al., 2018).
Earlier research in other parts of the MAR suggests also the necessity of exploring microhabitat distribution and analysis of nutrient input that may influence the composition of nektobenthic megafaunal aggregations (Morris et al., 2012; Alt et al., 2019) as well as depth related zonation (Gebruk and Krylova, 2013; Molodtsova et al., 2017). The role of sessile megafaunal communities must also be viewed through their importance as ecosystems engineers that may shape the overall biodiversity by increasing the habitat complexity and providing microhabitats for other invertebrates (Buhl-Mortensen et al., 2008; Alt et al., 2019).
Weaver et al. (2021) have recently published a detailed summary of knowledge on biogeography and habitat-specificity available from published accounts of the MAR fauna. With respect to geographic patterns, they commented on a very unequal coverage of different parts of the MAR, the areas north of the Azores and the vent field off the Azores having received most attention. Regarding the habitats, they distinguished hard (non-hydrothermal, hydrothermally active and hydrothermally inactive) substrata, and areas covered by soft sediment; in some areas, the sediment layer is of a considerable thickness. The non-hydrothermal substrata on the MAR were found to support cold-water corals and sponges, along with their associated fauna (macro- and meiofauna) of which little is known generally; this lack of knowledge concerns the PECA as well. More attention has been given to the fauna of hydrothermally active substrata, with some biogeographic trends detected (see below). Endemicity of the fauna supported by hydrothermally active substrata is not entirely clear. Although a number of taxa have been reported to be endemic to hydrothermal vent substrata in general (e.g. dirivultid copepods; Gollner et al., 2010) or to individual fields (see above), the absence of organisms in scientific observations may be a result of insufficient sampling effort (Weaver et al., 2021). With respect to the benthic invertebrate fauna of soft sediments along the MAR, most information can be found in accounts on the Russian Exploration Area (separated from the PECA by the French Exploration Area; cf. Figure 5.1 of Weaver et al., 2021). The trawling campaigns there revealed the presence of at least 136 megafaunal species (Molodtsova et al., 2017), including hermit crabs, ophiuroids and asteroids, bryozoans, brachiopods, ascidians and pennatulids. As remarked by Weaver et al. (2021), the distribution of macrofaunal and meiofaunal invertebrates, not captured by trawls, is very poorly known from soft sediments along the MAR and virtually no regional patterns can be discerned using the currently available data.
As highlighted by Bell et al. (2016), small scale (a distance lower than 10 km) habitat availability is amongst the most poorly understood aspects of ecological interactions in the MAR region. Recent results from the MAR demonstrated that many important interactions between benthic organisms occur at much smaller scales (Shields et al., 2013; Bebianno et al., 2018). It seems important then to understand processes occurring within a range of meters, at the microhabitat level, because large-scale zoogeographic patterns might result from smaller-scale effects. It seems that our knowledge is very strongly affected by sampling bias, or undersampling, so assessing the strength of any trend observed becomes difficult. For example, Shields and Blanco-Perez (2013) based their analysis of polychaete diversity and distribution patterns on 11 samples from as few as 4 stations widely scattered almost along the entire length of the Charlie-Gibbs Fracture Zone; the species accumulation curves they produced turned out very steep, demonstrating the magnitude of undersampling. The recent and most comprehensive analysis of literature datasets from MAR vent systems (Boschen-Rose and Colaço, 2021) was based on the presence-absence data extracted from studies employing different sampling approaches and very broadly defined environmental factors. One of the most important studies of vent meiofauna was based on no more than 10 qualitative samples per site (Tchesunov, 2015). Our whole knowledge about recovery processes in the MAR vent systems is based on just one small-scale study (macrofauna removal in eight 50 x 50 cm quadrats) at the Lucky Strike (Marticorena et al., 2021). The general paucity of quantitative data precludes modeling or multivariate analyses directly coupled with environmental factors, and allows only for some basic analyses of species distribution patterns, with no in-depth explanation of causes underlying the patterns observed. At the same time, a discussion on relationships between the whole MAR species pool and the local, vent- or habitat-specific, species pools is a challenge resulting from both 1) an almost complete lack of information on genetic connectivity and 2) the patchy distribution of sampling stations. At present it is impossible to assess the level of species rarity and/or patchiness and even calculations of basic diversity indices remain uncertain. Comprehensive studies of community ecology, diversity assessments, and post-disturbance recovery processes in the PECA or on the wider MAR are needed and will necessitate rethinking the policy and future management actions, especially in relation to Environmental Impact Assessments (EIAs) required for test mining and for the eventual exploitation of PMS. Compared to research conducted in shallow waters, the higher costs and logistic difficulties associated with deep-sea studies mean that considerable investment in time and resources is required to conduct meaningful studies that can be used to justify an EIA. Comprehensive EIAs are required to demonstrate that unique biotas are not under-appreciated or overlooked. Lack of statistically significant quantitative data poses a risk to contractors if the international community rejects any EIA and Environmental Management and Monitoring Plan. The paucity of data on the duration of larval stages as well as settlement and post settlement processes strongly affect our understanding of resilience mechanisms and recovery processes as well as the planning of conservation strategies such as those discussed by Dunn et al. (2018). Detection and understanding of patterns, and conclusions on the strength of those patterns, calls for a sound recognition of local-regional species pool relationships (Witman, 2013) and comprehensive environmental baseline data.
In this context, it is necessary to refer again to Boschen-Rose and Colaço (2021) who commented on certain biogeographic changes related to the presence and abundance of different taxa reported from various hydrothermal fields along the northern MAR. They found a switch in the relative abundance (domination) of the macro- and megafauna as one moved along the MAR from the north to the Equator. While Peltospira sp. limpets and Mirocaris sp. shrimps dominated the northernmost sites, the dominance structure changed to the highest relative abundances of Mirocaris fortunata and Bathymodiolus azoricus just south of the Azores, M. fortunata domination giving way to Rimicaris exoculata in deeper water further south (including the Broken Spur). B. azoricus was first co-located with and then replaced by B. puteoserpentis. As already mentioned, the Broken Spur represents a hybridization zone for the two bathymodiolid species. Boschen-Rose and Colaço (2021) extracted the environmental variables which correlate best with faunal similarities they revealed between the vent fields, and found a combination of the maximum reported depth as well as the chimney type (carbonate vs. sulfide) to have the strongest explanatory power. Water depth is a known environmental driver of benthic faunal distributions, but it co-varies with gradients in a number of other environmental variables (e.g. temperature, pressure, food availability). Depth-related changes in faunal community, including zonation observed in the northern part of the MAR (Alt et al., 2013) are a net result of differences in physiological tolerance limits to those gradients. The effects of depth on faunal communities in the PECA are unknown. Conclusions regarding the chimney type may appear, generally, valid for the PECA macro- and megafauna (carbonate chimneys at the Lost City and sulfide chimneys at the Broken Spur). However, due to the paucity of data and biases resulting from it (e.g. a relatively detailed study of nematode assemblages at the Lost City compared to the Broken Spur; cf. Table 2 and Tchesunov, 2015; a higher meiofaunal similarity of the Lost City and Broken Spur relative to other fields; cf. Figure 2), the conclusions drawn require validation through new exploration-oriented baseline surveys.
Regarding environmental variables affecting the distribution and composition of benthic communities on the MAR, Priede et al. (2022) have recently provided a thorough analysis of these with respect to biomass of the vent field megafauna (including fish). They examined effects of net primary production, particulate organic carbon (POC) flux, depth, and substrate heterogeneity. However, their analysis draws upon data acquired primarily in the northern sector of the northern MAR (between 40 and 60° N), and the authors admit that they had limited data on the benthic fauna of the part of the MAR between 12° N and 33° N, covered by the ISA exploration contracts. The authors predict that, owing to a lower POC flux south of the Azores, compared to more northern areas and those at greater depths, the biomass in the contract areas could be at least an order of magnitude lower that at the north. Further, the authors stress a severe lack of data in the Ocean Biodiversity Information System (OBIS) south of 33° N, which restricts generalizing on biogeographic trends.
Still another aspect of mid-oceanic ridge benthos, important for any baseline assessment as well as for distinguishing between natural and anthropogenic effects (Radziejewska et al., 2022) is the temporal variability. The low sampling effort to date on the MAR section covered by the ISA exploration contracts, including the PECA, has resulted also in our poor knowledge of temporal variability in the benthos. There is some information on the temporal variability (or lack of it) in benthic invertebrate assemblages at hydrothermally-active hard substrata. For example, some taxa have been observed to have seasonal reproduction patterns (e.g. bathymodiolids; Dixon et al., 2006), whereas no such patterns have been reported for Rimicaris exoculata (Copley et al., 2007). Sarrazin et al. (2014) observed changes in bathymodiolid abundance between the years, whereas Audenhaege et al. (2022) provide evidence of a long-term (7 years) stability of hydrothermal vent mussel communities. Matabos et al. (2015), too, reported on stable spatial distribution patterns in the shrimp Mirocaris fortunata and the crab Segonzacia mesatlantica. Cuvelier et al. (2017) demonstrated significant multi-day periodicities in polynoid polychaetes, suggesting effects of tidal cycles. As the species mentioned have been reported from the PECA (cf. Table 3), those effects may be taking place there as well. To mention a study in the PECA, Copley et al. (1997) reported vent shrimp populations at the Broken Spur to be stable over a 15-month period. No study addressed temporal variability at the hydrothermally inactive sulfide habitat and in the soft-sediment MAR faunas (Weaver et al., 2021), except for some evidence of temporal variability seen in the large foraminifera Syringamminidae, in polychaetes and sea anemones north of the Azores (Gebruk and Krylova, 2013), related to the POC flux.
Despite the gradual increase in our understanding of the MAR ecosystems (Dunn et al., 2018; Boschen-Rose and Colaço, 2021; Weaver et al., 2021), the inferences that might be drawn from the existing knowledge suffer from problems associated with a low sampling effort or scarcity of temporal analyses. The effects of future mining can only be speculated about, since data on resilience and recovery processes are scarce or non-existent, although some experimental work has been conducted in certain MAR areas suggesting that recovery processes are slow. In particular, Marticorena et al. (2021) studied recovery patterns in 13 Bathymodiolus azoricus assemblages at the Lucky Strike vent field, following artificial clearance of the fauna. Taxonomic richness, but not faunal densities or community structure, was observed to recover within 2 years post-disturbance, and a shift in faunal composition towards gastropod domination was detected. In addition, mobile predators arriving in early-colonisation stages could have slowed down the recovery of abundance, particularly with respect to the major foundation species, B. azoricus whose larvae and juveniles were impacted by the predator pressure. Thus, the recovery processes could have been slowed down by altered biological interactions.
In addition, an appropriate future assessment of impacts produced by mining activities could benefit from molecular studies focusing on the analysis of species composition and diversity (Klunder et al., 2020), such studies being rare in the whole MAR (cf. Table 4).
From our review of the present state of knowledge on benthic communities in the Polish exploration contract area it is evident that this knowledge is far from complete. Many knowledge gaps remain and studies are needed to investigate 1) connectivity between vent fields, 2) affinities between MAR/PECA areas and other, better studied, ridge areas, 3) symbiotic relationships, 4) distinctions between benthic communities in active and inactive PMS fields. The available data suggest that although the PECA benthos is grossly understudied, it is a diverse and abundant component of a very complex system that needs further, much more comprehensive research based on a higher sampling effort, accompanied by the analysis of various environmental factors. At the moment, we have probably managed to get a glimpse on a tip of an iceberg. Therefore, potential future research in the PECA, likely associated with exploration activities, should involve efforts aimed at broadening the benthic ecology knowledge base of PMS exploration. This knowledge will also be important as a source of data for delineation of PRZs and IRZs in the PECA and to form the basis for the Environmental Impact Statement and Environmental Management and Monitoring Plan required for an exploitation contract.
All authors contributed to the article and approved the submitted version.
We are grateful to Dr. Brygida Wawrzyniak-Wydrowska for the assistance with the artwork, to Mr Jakub Miluch for his help with the GEBCO data as well as to three reviewers and Editor David Billett for prompting us to delve deeper in the material and for their valuable input which helped us to improve the original submission.
The authors declare that the research was conducted in the absence of any commercial or financial relationships that could be construed as a potential conflict of interest.
All claims expressed in this article are solely those of the authors and do not necessarily represent those of their affiliated organizations, or those of the publisher, the editors and the reviewers. Any product that may be evaluated in this article, or claim that may be made by its manufacturer, is not guaranteed or endorsed by the publisher.
Alfaro-Lucas J. M., Pradillon F., Zeppilli D., Michel L. N., Martinez-Arbizu P., Tanaka H., et al. (2020). High environmental stress and productivity increase functional diversity along a deep-sea hydrothermal vent gradient. Ecology 101, e03144. doi: 10.1002/ecy.3144
Alt C. H. S., Kremenetskaia (Rogacheva) A., Gebruk A. V., Gooday A. J., Jones D. O. B. (2019). Bathyal benthic megafauna from the Mid-Atlantic Ridge in the region of the Charlie-Gibbs Fracture Zone based on remotely operated vehicle observations. Deep-Sea Res. I 145, 1–12. doi: 10.1016/j.dsr.2018.12.006
Alt C. H. S., Rogacheva A., Boorman B., Hughes J. A., Billett D. S. M., Gooday A. J., et al. (2013). Trawled megafaunal invertebrate assemblages from bathyal depth of the Mid-Atlantic Ridge (48o-54o N). Deep-Sea Res. II 98, 326–340. doi: 10.1016/j.dsr2.2013.02.003
Amon D. J., Gollner S., Morato T., Smith C. R., Chen C., Christiansen S., et al. (2022). Assessment of scientific gaps related to the effective environmental management of deep-seabed mining. Mar. Pol. 138, 105006. doi: 10.1016/j.marpol.2022.105006
Audenhaege L., Van, Matabos M., Brind’Amour A., Drugmand J., Laës-Huon A., Sarradin P.-M., et al. (2022). Long-term monitoring reveals unprecedented stability of a vent mussel assemblage on the Mid-Atlantic Ridge. Prog. Oceanogr. 204, 102791. doi: 10.1016/j.pocean.2022.102791
Baco A. R., Etter R. J., Ribeiro P. A., von der Heyden S., Beerli P., Kinlan B. P. (2016). A synthesis of genetic connectivity in deep-sea fauna and implications for marine reserve design. Molec. Ecol. 25, 3276–3298. doi: 10.1111/mec.13689
Beaulieu S. E., Baker E. T., German C. R., Maffei A. (2013). An authoritative global database for active submarine hydrothermal vent fields. Geochem. Geophys. Geosys. 14, 4892–4905. doi: 10.1002/2013GC004998
Beaulieu S. E., Szafranski K. (2019) InterRidge global database of active submarine hydrothermal vent fields, version 3.4 (2019). Available at: http://vents-data.interridge.org.
Bebianno M. J., Cardoso C., Gomes T., Blasco J., Santos R. S., Colaço A. (2018). Metal interactions between the polychaete Branchipolynoe seepensis and the mussel Bathymodiolus azoricus from Mid-Atlantic-Ridge hydrothermal vent fields. Mar. Env. Res. 135, 70–81. doi: 10.1016/j.marenvres.2018.01.017
Bell J. B., Alt C. H. S., Jones D. O. B. (2016). Benthic megafauna on steep slopes at the northern Mid-Atlantic Ridge. Mar. Ecol. 37, 1290–1302. doi: 10.1111/maec.12319
Bellan-Santini D. (2007). New amphipods of hydrothermal vent environments on the Mid-Atlantic Ridge, Azores Triple Junction zone. J. Nat. Hist. 41, 567–596. doi: 10.1080/00222930701262537
Bellan-Santini D., Thurston M. H. (1996). Amphipoda of the hydrothermal vents along the Mid-Atlantic Ridge. J. Nat. Hist. 30, 685–702. doi: 10.1080/00222939600770381
Benoist N. M. A., Bett B. J., Morris K. J., Ruhl H. A. (2019). A generalised volumetric method to estimate the biomass of photographically surveyed benthic megafauna. Prog. Oceanog. 178, 102188. doi: 10.1016/j.pocean.2019.102188
Bergstad O. A., Falkenhaug T., Astthorsson O. S., Byrkjedal I., Gebruk A. V., Piatkowski U., et al. (2008). Towards improved understanding of the diversity and abundance patterns of the mid-ocean ridge macro- and megafauna. Deep-Sea Res. II 55, 1–5. doi: 10.1016/j.dsr2.2007.10.001
Bernardino A. F., Levin L. A., Thurber A. R., Smith C. R. (2012). Comparative composition, diversity and trophic ecology of sediment macrofauna at vents, seeps and organic falls. PloS One 7, e33515. doi: 10.1371/journal.pone.0033515
Blake E. A., Van Dover C. L. (2005). The reproductive biology of Amathys lutzi, an ampharetid polychaete from hydrothermal vents on the Mid-Atlantic Ridge. Inv. Biol. 124, 254–264. doi: 10.1111/j.1744-7410.2005.00022.x
Boschen-Rose R. E., Colaço A. (2021). Northern Mid-Atlantic Ridge hydrothermal habitats: A systematic review of knowledge status for environmental management. Front. Mar. Sci. 8. doi: 10.3389/fmars.2021.657358
Boschen R. E., Rowden A. A., Clark M. R., Gardner J. P. A. (2013). Mining of deep-sea seafloor massive sulfides: A review of the deposits, their benthic communities, impacts from mining, regulatory frameworks and management strategies. Ocean Coast. Manage. 84, 54e67. doi: 10.1016/j.ocecoaman.2013.07.005
Braga-Henriques A., Porteiro F. M., Ribeiro P. A., de Matos V., Sampaio I., Ocaña O., et al. (2013). Diversity, distribution and spatial structure of the cold-water coral fauna of the Azores (NE Atlantic). Biogeosci. 10, 4009–4036. doi: 10.5194/bg-10-4009-2013
Brandt A., Frutos I., Bober S., Brix S., Brenke N., Guggolz T., et al. (2018). Composition of abyssal macrofauna along the Vema Fracture Zone and the hadal Puerto Rico Trench, northern tropical Atlantic. Deep-Sea Res. Part II Top. Stud. Oceanogr. 148, 35–44. doi: 10.1016/J.DSR2.2017.07.014
Brazelton W. J., Ludwig K. A., Sogin M. L., Andreishcheva E. N., Kelley D. S., Shen C.-C., et al. (2010). Archaea and bacteria with surprising microdiversity show shifts in dominance over 1,000-year time scales in hydrothermal chimneys. PNAS 107 (4), 1612–1617. doi: 10.1073/pnas.0905369107
Brazelton W. J., Schrenk M. O., Kelley D. S., Baros J. A. (2006). Methane- and sulfur-metabolizing microbial communities dominate the Lost City hydrothermal field ecosystem. Appl. Env. Microbiol. 72, 6257–6270. doi: 10.1128/AEM.00574-06
Budaeva N. (2012). Leptoecia midatlantica, a new species of the deep-sea quill-worms (Polychaeta: Onuphidae: Hyalinoeciinae) from the Mid-Atlantic Ridge. Zootaxa 3176, 45–60. doi: 10.11646/zootaxa.3176.1.2
Buhl-Mortensen P., Buhl-Mortensen L., Gebruk A. V., Krylova E. M. (2008). Occurrence of deep-water corals on the Mid-Atlantic Ridge based on MAR-ECO data. Deep Sea Res. II Top. Stud. Oceanogr. 55, 142–152. doi: 10.1016/j.dsr2.2007.09.018
Calado H., Ng K., Lopes C., Paramio L. (2011). Introducing a legal management instrument for offshore marine protected areas in the Azores – the Azores Marine Park. Env. Sci. Pol. 14, 1175–1187. doi: 10.1007/978-3-030-87982-2_11
Campagna C., Sanderson E. W., Coppolillo P. B., Falabella V., Piola A. R., Strindberg S., et al. (2008). A species approach to marine ecosystem conservation. Aquat. Conserv. Mar. Freshw. Ecosyst. 17, S122–S147. doi: 10.1002/aqc.918
Casson L., Losada S., Tsenikli S., Santillo D., Currie D., Sharma G., et al. (2019). In deep water: The emerging threat of deep sea mining (Greenpeace International). Available at: https://issuu.com/greenpeaceinternational/docs/indeep-water-201.
Cherkashov G. (2017). “Seafloor massive sulfide deposits: Distribution and prospecting,” in Deep-Sea mining. resource potential, technical and environmental considerations. Ed. Sharma R. (Cham: Springer), 143–187. doi: 10.1007/978-3-319-52557-0_4
Colaço A., Dehairs F., Desbruyères D. (2002). Nutritional relations of deep-sea hydrothermal fields at the Mid-Atlantic Ridge: A stable isotope approach. Deep Sea Res. I 49, 395–412. doi: 10.1016/S0967-0637(01)00060-7
Colaço A., Desbruyères D., Guezennec J. (2007). Polar lipid fatty acids as indicators of trophic associations in a deep-sea vent system community. Mar. Ecol. 28, 15–24. doi: 10.1111/j.1439-0485.2006.00123.x
Copley J. T. P., Jorgensen P. B. K., Sohn R. A. (2007). Assessment of decadal-scale ecological change at a deep mid-Atlantic hydrothermal vent and reproductive time-series in the shrimp Rimicaris exoculata. J. Mar. Biol. Ass. UK 87, 859–867. doi: 10.1017/S0025315407056512
Copley J. T. P., Tyler P. A., Murton B. J., Van Dover C. L. (1997). Spatial and interannual variation in the faunal distribution at Broken Spur vent field (29°N, Mid-Atlantic Ridge). Mar. Biol. 129, 723–733. doi: 10.1007/s002270050215
Corbera J., Segonzac M., Cunha M. R. (2008). A new deep-sea genus of Nannastacidae (Crustacea, Cumacea) from the Lucky Strike hydrothermal vent field (Azores Triple Junction, Mid-Atlantic Ridge). Mar. Biol. Res. 4, 180–192. doi: 10.1080/17451000801898576
Cruz M., Le Bris N., Colaço A. (2022). Reproductive traits of the vent crab Segonzacia mesatlantica (Guinot, 1989) from the Mid-Atlantic Ridge. Front. Mar. Sci. 9. doi: 10.3389/fmars.2022.900990
Cunha M. R., Wilson G. D. F. (2006). The North Atlantic genus Heteromesus (Crustacea: Isopoda: Asellota: Ischnomesidae). Zootaxa 1192, 3–76. doi: 10.11646/zootaxa.1192.1
Cuvelier D., Beesau J., Ivanenko V. N., Zeppilli D., Sarradin P. M., Sarrazin J. (2014). First insights into macro- and meiofaunal colonisation patterns on paired wood/slate substrata at Atlantic deep-sea hydrothermal vents. Deep Sea Res. I 87, 70–81. doi: 10.1016/j.dsr.2014.02.008
Cuvelier D., Legendre P., Laës-Huon A., Sarradin P. M., Sarrazin J. (2017). Biological and environmental rhythms in (dark) deep-sea hydrothermal ecosystems. Biogeosci. 14, 2955–2977. doi: 10.5194/bg-14-2955-2017
Cuvelier D., Sarrazin J., Colaço A., Copley J., Glover A., Tyler P., et al. (2011). Community dynamics over 14 years at the Eiffel Tower hydrothermal edifice on the Mid-Atlantic Ridge. Limnol. Oceanog. 56, 1624–1640. doi: 10.4319/lo.2011.56.5.1624
Denny A. R., Kelley D. S., Früh-Green G. L. (2015). Geologic evolution of the Lost City hydrothermal field. Geochem. Geophys. Geosyst. 17, 375–394. doi: 10.1002/2015GC005869
Desbruyères D., Almeida A., Biscoito M., Comtet T., Khripounoff A., Le Bris N., et al. (2000). A review of the distribution of hydrothermal vent communities along the northern Mid-Atlantic Ridge: Dispersal vs. environmental controls. Hydrobiologia 440, 201–216. doi: 10.1023/A:1004175211848
Dilman A. B. (2013). Asteroid fauna of the northern Mid-Atlantic Ridge: additional records. Mar. Biol. Res. 9, 563–586. doi: 10.1080/17451000.2012.749993
Dixon D. R., Lowe D. M., Miller P. I., Villemin G. R., Colaço A., Serrao-Santos R., et al. (2006). Evidence of seasonal reproduction in the Atlantic vent mussel Bathymodiolus azoricus, and an apparent link with the timing of photosynthetic primary production. J. Mar. Biol. Ass. UK 86, 1363–1371. doi: 10.1017/S0025315406014391
Dulov L. E., Lein A. Y., Dubinina G. A., Pimenov N. V. (2005). Microbial processes at the Lost City vent field, Mid-Atlantic Ridge. Microbiology 74, 97–103. doi: 10.1007/s11021-005-0035-6
Dumke I., Purser A., Marcon Y., Nornes S. M., Johnsen G., Ludvigsen M., et al. (2018). Underwater hyperspectral imaging as an in situ taxonomic tool for deep-sea megafauna. Sci. Rep. 8, 12860. doi: 10.1038/s41598-018-31261-4
Dunn D. C., Van Dover C. L., Etter R. J., Smith C. R., Levin L. A., Morato T., et al. (2018). A strategy for the conservation of biodiversity on mid-ocean ridges from deep-sea mining. Sci. Adv. 4, eaar4313. doi: 10.1126/sciadv.aar4313
Dutkiewicz A., Müller R. D., O'Callaghan S., Jónasson H. (2015). Census of seafloor sediments in the world's ocean. Geology 43, 795–798. doi: 10.1130/G36883.1
Fabri M. C., Bargain A., Briand P., Gebruk A., Fouquet Y., Morineaux M., et al. (2011). The hydrothermal vent community of a new deep-sea field, Ashadze-1, 12°58’N on the Mid-Atlantic Ridge. J. Mar. Biol. Ass. U. K. 91, 1–13. doi: 10.1017/S0025315410000731
Farley J. (2010). Conservation through the economics lens. Env. Manag 45, 26–38. doi: 10.1007/s00267-008-9232-1
Flint H. C. (2007). Diversity of meiofauna at deep-sea hydrothermal vents and cold seeps with particular reference to nematodes (Southampton: National Oceanography Centre).
Freestone D., Laffoley D., Douvere F., Badman T. (2016). World heritage in the high seas: An idea whose time has come (IUCN). Available at: https://unesdoc.unesco.org/ark:/48223/pf0000245467.
Früh-Green G., Kelley D. S., Bernasconi S., Karson J. A., Ludwig K. A., Butterfield D. A., et al. (2003). 30,000 years of hydrothermal activity at the Lost City vent field. Science 301, 495–498. doi: 10.1126/science.1085582
Früh-Green G. L., Orcutt B. N., Green S. (2015). Expedition 357 scientific prospectus: Atlantis massif serpentinization and life International Ocean Discovery Program. doi: 10.14379/iodp.sp.327.2015
Frutos I., Kaiser S., Pułaski Ł., Studzian M., Błażewicz M. (2022). Challenges and advances in the taxonomy of deep-sea peracarida: From traditional to modern methods. Front. Mar. Sci. 9. doi: 10.3389/fmars.2022.799191
Gage J. D., Tyler P. A. (1991). Deep-Sea biology. A natural history of organisms at the deep-sea floor (Cambridge: Cambridge University Press) 9, 504.
Galkin S. V. (2006). “Prostranstvennaya struktura gidrotermalnykh soobshchestv sredinno-atlanticheskogo khrebta,” in Ekosistemy atlanticheskikh gidroterm [Ecosystems of the Atlantic hydrothermal vents]. Eds. Vinogradov M. E., Vereshchaka A. L. (Moskva: Nauka), 163–202.
Gebruk A. V., Budaeva N. E., King N. J. (2010). Bathyal benthic fauna of the Mid-Atlantic Ridge between the Azores and the Reykjanes Ridge. J. Mar. Biol. Ass. U. K. 90, 1–14. doi: 10.1017/S0025315409991111
Gebruk A. V., Galkin S. V. (1997). Ecology and biogeography of the hydrothermal vent fauna of the Mid-Atlantic Ridge. Adv. Mar. Biol. 32, 93–144. doi: 10.1016/s0065-2881(08)60016-4
Gebruk A. V., Krylova E. M. (2013). Megafauna of the Charlie-Gibbs Fracture Zone (northern Mid-Atlantic Ridge) based on video observations. J. Mar. Biol. Ass. U. K. 93, 1143–1150. doi: 10.1017/S0025315412001890
Gebruk A. V., Mironov A. N. (2006). “Biogeografiya gidroterm sredinno-atlanticheskogo khrebta,” in Ekosistemy atlanticheskikh gidroterm [Ecosystems of the Atlantic hydrothermal vents]. Eds. Vinogradov M. E., Vereshchaka A. L. (Moskva: Nauka), 119–162.
Gebruk A. V., Southward E. C., Kennedy H., Southward A. J. (2000). Food sources, behaviour, and distribution of hydrothermal vent shrimps at the Mid-Atlantic Ridge. J. Mar. Biol. Ass. U. K. 80, 485–499. doi: 10.1017/S0025315400002186
Gerdes K., Martinez Arbizu P., Schwarz-Schampera U., Schwentner M., Kihara T. C. (2019). Detailed mapping of hydrothermal vent fauna: A 3D reconstruction approach based on video imagery. Front. Mar. Sci. 6. doi: 10.3389/fmars.2019.00096
Gollner S., Kaiser S., Menzel L., Jones D. O. B., Brown A., Mestre N. C., et al. (2017). Resilience of benthic deep-sea fauna to mining activities. Mar. Environ. Res. 129, 76–101. doi: 10.1016/j.marenvres.2017.04.010
Gollner S., Riemer B., Martínez Arbizu P., Le Bris N., Bright M. (2010). Diversity of meiofauna from the 9°50′N East Pacific Rise across a gradient of hydrothermal fluid emissions. PloS One 5, e12321. doi: 10.1371/journal.pone.0012321
Goroslavskaya E. I., Galkin S. V. (2011). Benthic fauna associated with mussel beds and shrimp swarms at hydrothermal fields on the Mid-Atlantic Ridge. Oceanology 51, 69–79. doi: 10.1134/S0001437011010048
Haeckel M., Linke P. (2021). RV SONNE fahrtbericht /Cruise report SO268. assessing the impacts of nodule mining on the deep-sea environment. NoduleMonitoring. Manzanillo (Mexico) – Vancouver (Canada). 17.02. – 27.05.2019. GEOMAR Rep N. Ser. 059. doi: 10.3289/GEOMAR_REP_NS_59_2021
Hannington M., Jamieson J., Monecke T., Petersen S., Beaulieu S. (2011). The abundance of seafloor massive sulfide deposits. Geology 39, 1155–1158. doi: 10.1130/G32468.1
Hernández-Ávila I., Cambon-Bonavita M.-A., Sarrazin J., Pradillon F. (2022). Population structure and reproduction of the alvinocaridid shrimp Rimicaris exoculata on the Mid-Atlantic Ridge: Variations between habitats and vent fields. Deep-Sea Res. I 186, 103827. doi: 10.1016/j.dsr.2022.103827
Hilário A., Metaxas A., Gaudron S. M., Howell K. L., Mercier A., Mestre N. C., et al. (2015). Estimating dispersal distance in the deep sea: Challenges and applications to marine reserves. Front. Mar. Sci. 2. doi: 10.3389/fmars.2015.00006
Horton T., Thurston M. H., Duffy G. A. (2013). Community composition of scavenging amphipods at bathyal depths on the Mid-Atlantic Ridge. Deep Sea Res. II Top. Stud. Oceanogr. 98, 352–359. doi: 10.1016/j.dsr2.2013.01.032
ISA (2019). Recommendations for the guidance of contractors for the assessment of the possible environmental impacts arising from exploration for marine minerals in the area.
ISA (2017). ISBA/25/LTC/6. International Seabed Authority, Kingston. “Report of ISA workshop on the design of impact reference zones and preservation reference zones,” in (Report of ISA Workshop on the Design of “Impact Reference Zones” and “Preservation Reference Zones” in Deep- Sea Mining Contract Areas. ISA Technical Study), 41. Available at: https://isa.org.jm/files/files/documents/TechnicalStudy21-ebk_0.pdf.
Ivanenko V. N., Corgosinho P. H. C., Ferrari F., Sarradin P.-M., Sarrazin J. (2011). Microhabitat distribution of Smacigastes micheli (Copepoda: Harpacticoida: Tegastidae) from deep-sea hydrothermal vents at the Mid-Atlantic Ridge, 37° N (Lucky Strike), with a morphological description of its nauplius. Mar. Ecol. 33, 246–256. doi: 10.1111/j.1439-0485.2011.00484.x
Jakiel A., Palero F., Błażewicz M. (2019). Deep ocean seascape and Pseudotanaidae (Crustacea: Tanaidacea) diversity at the Clarion-Clipperton Fracture Zone. Sci. Rep. 9, 17305. doi: 10.1038/s41598-019-51434
Jakiel A., Stępień A., Błażewicz M. (2018). A tip of the iceberg – Pseudotanaidae (Tanaidacea) diversity in the North Atlantic. Mar. Biodiv. 48, 859–895. doi: 10.1007/s12526-018-0881-x
Jamieson J. W., Gartman A. (2020). Defining active, inactive, and extinct seafloor massive sulfide deposits. Mar. Pol. 117, 103926. doi: 10.1016/j.marpol.2020.103926
Jennings R. M., Brix S., Bober S., Svavarsson J., Driskell A. (2018). More diverse than expected: distributional patterns of Oecidiobranchus Hessler, 1970 (Isopoda, Asellota) on the Greenland-Iceland-Faeroe Ridge based on molecular markers. Mar. Biodiv. 48, 845–957. doi: 10.1007/s12526-018-0857-x
Johnson D. E. (2019). Protecting the Lost City hydrothermal vent system: All is not lost, or is it? Mar. Pol. 107, 103593. doi: 10.1016/j.marpol.2019.103593
Jollivet D. (1996). Specific and genetic diversity at deep-sea hydrothermal vents: an overview. Biodivers. Conserv. 5, 1619–1653. doi: 10.1007/BF00052119
Jones D. O. B., Alt C. H. S., Priede I. G., Reid W. D. K., Wigham B. D., Billett D. S. M., et al. (2013). Deep-sea surface-dwelling enteropneusts from the Mid-Atlantic Ridge: Their ecology, distribution and mode of life. Deep Sea Res. II Top. Stud. Oceanogr. 98, 374–387. doi: 10.1016/j.dsr2.2013.05.009
Jones D. O. B., Ardron J. A., Colaço A., Durden J. M. (2020). Environmental considerations for impact and preservation reference zones for deep-sea polymetallic nodule mining. Mar. Pol. 118, 103312. doi: 10.1016/j.marpol.2018.10.025
Jóźwiak P., Pabis K., Brandt A., Blazewicz M. (2020). Epibenthic sled versus giant box corer – comparison of sampling gears for tanaidacean species richness assessment in the abyssal benthic ecosystem. Prog. Oceanog. 181, 102255. doi: 10.1016/j.pocean.2019.102255
Jóźwiak P., Pabis K., Sobczyk R., Serigstad B. (2022). A paradise for rare species: Tanaidacean fauna of the West African continental margin. Front. Mar. Sci. 9. doi: 10.3389/fmars.2022.779134
Kaiser S., Błażewicz M., Kocot K. M., Leduc D., Riehl T., Rouse G. W. (2022). Editorial: Recent and emerging innovations in deep-sea taxonomy to enhance biodiversity assessment and conservation. Front. Mar. Sci. 9. doi: 10.3389/fmars.2022.989245
Karson J. A., Kelley D. S., Fornari D. J., Perfit M. R., Shank T. M. (2015a). “Diversity in seafloor spreading,” in Discovering the deep. a photographic atlas of the seafloor and ocean crust. Eds. Karson J. A., Kelley D. S., Fornari D. J., Perfit M. R., Shank T. M. (Cambridge: Cambridge University Press), 56–86.
Karson J. A., Kelley D. S., Fornari D. J., Perfit M. R., Shank T. M. (2015b). “Hydrothermal vents,” in Discovering the deep. a photographic atlas of the seafloor and ocean crust. Eds. Karson J. A., Kelley D. S., Fornari D. J., Perfit M. R., Shank T. M. (Cambridge: Cambridge University Press), 87–120.
Kawano S., Furuya H. (2022). “Mining and processing of seafloor massive sulfides: Experiences and challenges,” in Perspectives on deep-sea mining. sustainability, technology, environmental policy and management. Ed. Sharma R. (Cham: Springer), 167–198. doi: 10.1007/978-3-030-87982-2_7
Kelley D. S., Früh-Green G. L., Karson J. A., Ludwig K. A. (2007). The Lost City hydrothermal field revisited. Oceanography 20, 90–99. doi: 10.5670/oceanog.2007.09
Kelley D. S., Karson J. A., Früh-Green G. L., Yoerger D. R., Shank T. M., Butterfield D. A., et al. (2005). A serpentinite-hosted ecosystem: The Lost City hydrothermal field. Science 307, 1428–1433. doi: 10.1126/science.1102556
Klunder L., Stigter H., de, Lavaleye M. S. S., van Bleijswijk J. D. L., van der Veer H. W., Reichart G. J., et al. (2020). A molecular approach to explore the background benthic fauna around a hydrothermal vent and their larvae: Implications for future mining of deep-sea SMS deposits. Front. Mar. Sci. 7. doi: 10.3389/fmars.2020.00134
Kongsrud J. A., Budaeva N., Barnich R., Oug E., Bakken T. (2013). Benthic polychaetes from the northern Mid-Atlantic Ridge between the Azores and the Reykjanes Ridge. Mar. Biol. Res. 9, 516–546. doi: 10.1080/17451000.2012.749997
Lang S. Q., Brazelton W. J. (2020). Habitability of the marine serpentinite subsurface: A case study of the Lost City hydrothermal field. Phil. Trans. R. Soc. A378, 20180429. doi: 10.1098/rsta.2018.0429
Larsen K., Błażewicz-Paszkowycz M., Cunha M. R. (2006). Tanaidacean (Crustacea: Peracarida) fauna from chemically reduced habitats – the Lucky Strike hydrothermal vent system, Mid-Atlantic Ridge. Zootaxa 1187, 1–36. doi: 10.11646/zootaxa.1187.1.1
Lecoeuvre A., Ménez B., Cannat M., Chavagnac V., Gérard E. (2021). Microbial ecology of the newly discovered serpentinite-hosted Old City hydrothermal field (Southwest Indian Ridge). ISME J. 15, 818–832. doi: 10.1038/s41396-020-00816-7
Lein A., Galkin S. V., Maslennikov V. V., Bogdanov, Yu A., Bogdanova, et al. (2007). A new type of carbonate rocks on the ocean floor (Mid-Atlantic Ridge, 30°07′ N). Dokl. Earth Sci. 412, 136–140. doi: 10.1134/S1028334X0701031X
López-Garcia P., Vereshchaka A., Moreira D. (2007). Eukaryotic diversity associated with carbonates and fluid-seawater interface in Lost City hydrothermal field. Envir. Microbiol. 9, 546–554. doi: 10.1111/j.1462-2920.2006.01158.x
Lunina A. A., Vereshchaka A. L. (2014). Distribution of hydrothermal alvinocaridid shrimps: Effect of geomorphology and specialization to extreme biotopes. PloS One 9, e92802. doi: 10.1371/journal.pone.0092802
Mace G. M., Possingham H. P., Leader-Williams N. (2007). “Prioritizing choices in conservation,” in Key topics in conservation biology. Eds. Macdonald D. M., Service K. (Oxford: Blackwell Publications), 17–34.
Marsh L., Copley J. T., Tyler P. A., Thatje S. (2015). In hot and cold water: Differential life-history traits are key to success in contrasting thermal deep-sea environments. J. Anim. Ecol. 84, 898–913. doi: 10.1111/1365-2656.12337
Marticorena J., Matabos M., Ramirez-Llodra E., Cathalot C., Laes-Huon A., Leroux R., et al. (2021). Recovery of hydrothermal vent communities in response to an induced disturbance at the Lucky Strike vent field (Mid-Atlantic Ridge). Mar. Environ. Res. 168, 105316. doi: 10.1016/j.marenvres.2021.105316
Marticorena J., Matabos M., Sarrazin. J., Ramirez-Llodra E. (2020). Contrasting reproductive biology of two hydrothermal gastropods from the Mid-Atlantic Ridge: implications for resilience of vent communities. Mar. Biol. 167, 1–19. doi: 10.1007/s00227-020-03721-x
Matabos M., Cuvelier D., Brouard J., Shillito B., Ravaux J., Zbinden M., et al. (2015). Behavioural study of two hydrothermal crustacean decapods: Mirocaris fortunata and Segonzacia mesatlantica, from the Lucky Strike vent field (Mid-Atlantic Ridge). Deep Sea Res. II 121, 146–158. doi: 10.1016/j.dsr2.2015.04.008
McCallum A. W., Woolley S., Błażewicz-Paszkowycz M., Browne J., Gerken S., Kloser R., et al. (2015). Productivity enhances benthic species richness along an oligotrophic Indian Ocean continental margin. Glob. Ecol. Biogeogr 24, 462–471. doi: 10.1111/geb.12255
McCollom T. M. (2007). Geochemical constraints on sources of metabolic energy for chemolithoautotrophy in ultramafic-hosted deep-sea hydrothermal systems. Astrobiology 7, 933–950. doi: 10.1089/ast.2006.0119
McCollom T. M., Shock E. L. (1997). Geochemical constraints on chemolithoautotrophic metabolism by microorganisms in seafloor hydrothermal systems. Geochim Cosmochim. Acta 61, 4375–4391. doi: 10.1016/S0016-7037(97)00241-X
Menini E., Van Dover C. L. (2019). An atlas of protected hydrothermal vents. Mar. Pol. 108, 103654. doi: 10.1016/j.marpol.2019.103654
Methou P., Hernández-Ávila I., Cathalot C., Cambon-Bonavita M., Pradillon F. (2022). Population structure and environmental niches of Rimicaris shrimps from the Mid-Atlantic Ridge. Mar. Ecol. Prog. Ser. 684, 1–20. doi: 10.3354/meps13986
Mironov A. N., Gebruk A. V., Moskalev L. I. (2002). “Geografiya gidrotermalnykh soobshchestv i obligatnykh gidrotermalnykh taksonov [Biogeography of hydrothermal vent communities and obligate hydrothermal taxa],” in Biologiya gidrotermalnykh sistem [Biology of hydrothermal systems]. Ed. Gebruk A. V. (Moscow: KMK Scientific Press), 410–455.
Mogensen L. M. W., Mei Z., Hao Y., Harrison X. A., Wang D., Turvey S. T. (2022). Precautionary principle or evidence-based conservation? Assessing the information content of threat data for the Yangtze finless porpoise. Front. Mar. Sci. 8. doi: 10.3389/fmars.2021.791484
Molodtsova T. N., Galkin S. V., Kobylianksy S. G., Simakova U. V., Vedenin A. A., Dobretsova I. G., et al. (2017). First data on benthic and fish communities from the Mid-Atlantic Ridge, 16°40′-17°14′N. Deep Sea Res. II Top. Stud. Oceanogr. 137, 69–77. doi: 10.1016/j.dsr2.2016.10.006
Molodtsova T., Sanamyan N. P., Keller N. B. (2008). Anthozoa from the northern Mid-Atlantic Ridge and Charlie-Gibbs Fracture Zone. Mar. Biol. Res. 4, 112–130. doi: 10.1080/17451000701821744
Morris K. J., Tyler P. A., Murton B., Rogers A. D. (2012). Lower bathyal and abyssal distribution of coral in the axial volcanic ridge of the Mid-Atlantic Ridge at 45°N. Deep-Sea Res. I 62, 32–39. doi: 10.1016/j.dsr.2011.11.009
Myers A. A., Cunha M. R. (2004). New and little known corophiidean amphipods from the 'Lucky Strike' hydrothermal vent, Mid-Atlantic Ridge. J. Mar. Biol. Ass. U. K. 84, 1019–1025. doi: 10.1017/S0025315404010343h
Niedzielski T., Høines A., Shields M. A., Linley T. D., Priede I. G. (2013). A multi-scale investigation into seafloor topography of the northern Mid-Atlantic Ridge based on geographic information system analysis. Deep Sea Res. II Top. Stud. Oceanogr. 98, 231–243. doi: 10.1016/j.dsr2.2013.10.006
Nuckley D. J., Jinks R. N., Battelle B. A., Herzog E. D., Kass L., Renninger G. H., et al. (1996). Retinal anatomy of a new species of bresiliid shrimp from a hydrothermal vent field on the Mid-Atlantic Ridge. Biol. Bull. 190, 98–110. doi: 10.2307/1542679
Nuno Gomes-Pereira J., Auger V., Beisiegel K., Benjamin R., Bergmann M., Bowden D., et al. (2016). Current and future trends in marine image annotation software. Prog. Oceanog. 149, 106–129. doi: 10.1016/j.pocean.2016.07.005
O’Mullan G. D., Maas P. A. Y., Lutz R. A., Vrijenhoek R. C. (2001). A hybrid zone between hydrothermal vent mussels (Bivalvia: Mytilidae) from the Mid-Atlantic Ridge. Molec. Ecol. 10, 2819–2831. doi: 10.1046/j.0962-1083.2001.01401.x
Orcutt B. N., Sylvan J. B., Knab N. J., Edwards K. J. (2011). Microbial ecology of the dark ocean above, at, and below the seafloor. Microbiol. Molec. Biol. Rev. 75, 361–422. doi: 10.1128/MMBR.00039-10
Ortiz M., Hermosillo Nuñez B., Gonzalez J., Rodgriguez-Zaragoza F., Gómez J., Jordan F. (2017). Quantifying keystone species complexes: Ecosystem-based conservation management in the King George Island (Antarctic Peninsula). Ecol. Ind. 81, 453–460. doi: 10.1016/j.ecolind.2017.06.016
Pelli D. G., Chamberlain S. C. (1989). The visibility of 350°C black-body radiation by the shrimp Rimicaris exoculata and man. Nature 337, 460–461. doi: 10.1038/337460a0
Perez J. A. A., dos Santos Alves E., Clark M. R., Bergstad O. A., Gebruk A., Azevedo Cardoso I., et al. (2012). Patterns of life on the Southern Mid-Atlantic Ridge: Compiling what is known and addressing future research. Oceanography 25, 16–31. doi: 10.5670/oceanog.2012.102
Petersen S., Krätschell A., Augustin N., Jamieson J., Hein J. R., Hannington M. D. (2016). News from the seabed – geological characteristics and resource potential of deep-sea mineral resources. Mar. Pol. 70, 175–187. doi: 10.1016/j.marpol.2016.03.012
Plum C., Pradillon F., Fujiwara Y., Sarrazin J. (2017). Copepod colonization of organic and inorganic substrata at a deep-sea hydrothermal vent site on the Mid-Atlantic Ridge. Deep-Sea Res. II 137, 335–348. doi: 10.1016/j.dsr2.2016.06.008
Priede I. G., Bergstad O. A., Miller P. I., Vecchione M., Gebruk A., Falkenhaug T., et al. (2013). Does presence of a mid-ocean ridge enhance biomass and biodiversity? PloS One 8, e61550. doi: 10.1371/journal.pone.0061550
Priede I. G., Muller-Karger F. E., Niedzielski T., Gebruk A. V., Jones D. O. B., Colaço A. (2022). Drivers of biomass and biodiversity of non-chemosynthetic benthic fauna of the Mid-Atlantic Ridge in the North Atlantic. Front. Mar. Sci. 9. doi: 10.3389/fmars.2022.866654
Radziejewska T., Mianowicz K., Abramowski T. (2022). “Natural variability versus anthropogenic impacts on deep-sea ecosystems of importance for deep-sea mining,” in Perspectives on deep-sea mining. sustainability, technology, environmental policy and management. Ed. Sharma R. (Cham: Springer), 281–311. doi: 10.1007/978-3-030-87982-2_11
Riehl T., Lins L., Brandt A. (2018). The effects of depth, distance, and the Mid-Atlantic Ridge on genetic differentiation of abyssal and hadal isopods (Macrostylidae). Deep Sea Res. II Top. Stud. Oceanogr. 148, 74–90. doi: 10.1016/j.dsr2.2017.10.005
Riehl T., Wölfl A.-C., Augustin N., Devey C. W., Brandt A. (2020). Discovery of widely available abyssal rock patches reveals overlooked habitat type and prompts rethinking deep-sea biodiversity. Proc. Nat. Acad. Sci. U.S.A. 117, 15450–15459. doi: 10.1073/pnas.1920706117
Riou V., Duperron S., Dehairs H. F., Bouillon S., Martin I., Colaço A., et al. (2010). Variation in physiological indicators in Bathymodiolus azoricus (Bivalvia: Mytilidae) at the Menez Gwen Mid-Atlantic Ridge deep-sea hydrothermal vent site within a year. Mar. Environ. Res. 70, 264–271. doi: 10.1016/j.marenvres.2010.05.008
Rybakova E., Galkin S. (2015). Hydrothermal assemblages associated with different foundation species on the East Pacific Rise and Mid-Atlantic Ridge, with a special focus on mytilids. Mar. Ecol. 36, 45–61. doi: 10.1111/maec.12262
Sarrazin J., Cuvelier D., Peton L., Legendre P., Sarradin P. M. (2014). High-resolution dynamics of a deep-sea hydrothermal vent mussel assemblage monitored by the EMSO-Azores MoMAR observatory. Deep-Sea Res. I 90, 62–75. doi: 10.1016/j.dsr.2014.04.004
Sarrazin J., Legendre P., De Busserolles F., Fabri M. C., Guilini K., Ivanenko V. N., et al. (2015). Biodiversity patterns, environmental drivers and indicator species on a high-temperature hydrothermal edifice, Mid-Atlantic Ridge. Deep-Sea Res. II Top. Stud. Oceanogr. 121, 177–192. doi: 10.1016/j.dsr2.2015.04.013
Shank T. M., Fornari D. J., Von Damm K. L., Lilley M. D., Haymon R. M., Lutz R. A. (1998a). Temporal and spatial patterns of biological community development at nascent deep-sea hydrothermal vents (9°50’N, East Pacific Rise). Deep-Sea Res. II Top. Stud. Oceanogr. 45, 465–515. doi: 10.1016/S0967-0645(97)00089-1
Shank T. M., Lutz R. A., Vrijenhoek R. C. (1998b). Molecular systematics of shrimp (Decapoda: Bresiliidae) from deep-sea hydrothermal vents, I: Enigmatic “small orange” shrimp from the Mid-Atlantic Ridge are juvenile Rimicaris exoculata. Molec. Mar. Biol. Biotechnol. 7, 88–96.
Shields M. A., Blanco-Perez R. (2013). Polychaete abundance, biomass and diversity patterns at the Mid-Atlantic Ridge, North Atlantic Ocean. Deep Sea Res. II 98, 315–325. doi: 10.1016/j.dsr2.2013.04.010
Shields M. A., Glover A. G., Wiklund H. (2013). Polynoid polychaetes of the Mid-Atlantic Ridge and a new holothurian association. Mar. Biol. Res. 9, 547–553. doi: 10.1080/17451000.2012.749992
Shillito B., Le Bris N., Hourdez S., Ravaux J., Cottin D., Caprais J. C., et al. (2006). Temperature resistance studies on the deep-sea vent shrimp Mirocaris fortunata. J. Exp. Biol. 209, 945–955. doi: 10.1242/jeb.02102
Sigvaldadottir E., Desbruyères D. (2003). Two new species of Spionidae (Annelida: Polychaeta) from Mid-Atlantic Ridge hydrothermal vents. Cah. Biol. Mar. 44, 219–225.
Spedicato A., Sánchez N., Pastor L., Menot L., Zeppilli D. (2020). Meiofauna community in soft sediments at TAG and Snake Pit hydrothermal vent fields. Front. Mar. Sci. 7. doi: 10.3389/fmars.2020.00200
Stępień A., Pabis K., Sobczyk R., Serigstad B. (2021). High species richness and extremely low abundance of cumacean communities along the shelf and slope of the Gulf of Guinea (West Africa). Front. Mar. Sci. 8. doi: 10.3389/fmars.2021.703547
Tabachnick K., Collins A. G. (2008). Glass sponges (Porifera, Hexactinellida) of the northern Mid-Atlantic Ridge. Mar. Biol. Res. 4, 25–47. doi: 10.1080/17451000701847848
Tassia M. G., Cannon J. T., Konikoff C. E., Shenkar N., Halanych K. M., Swalla B. J. (2016). The global diversity of Hemichordata. PloS One 11 (10), e0162564. doi: 10.1371/journal.pone.0162564
Tchesunov A. V. (2015). Free-living nematode species (Nematoda) dwelling in hydrothermal sites of the North Mid-Atlantic Ridge. Helgol. Mar. Res. 69, 343–384. doi: 10.1007/s10152-015-0443-6
Teixeira S., Olu K., Decker C., Cunha R. L., Fuchs S., Hourdez S., et al. (2013). High connectivity across the fragmented chemosynthetic ecosystems of the deep Atlantic equatorial belt: Efficient dispersal mechanisms or questionable endemism? Molec. Ecol. 22, 4663–4680. doi: 10.1111/mec.12419
Thaler A. D., Amon D. (2019). 262 voyages beneath the sea: A global assessment of macro- and megafaunal biodiversity and research effort at deep-sea hydrothermal vents. PeerJ 7, e7397. doi: 10.7717/peerj.7397
Tyler P. A., Paterson G. J. L., Sibuet M., Guille A. F. (1995). A new genus of ophiuroid (Echinodermata: Ophiuroidea) from hydrothermal mounds along the Mid-Atlantic Ridge. J. Mar. Biol. Ass. U. K. 75, 977–986. doi: 10.1017/S0025315400038303
Vals A., Coll M., Christensen V. (2015). Keystone species: Toward an operational concept for marine biodiversity conservation. Ecol. Monogr. 85, 29–47. doi: 10.1890/14-0306.1
Van Dover C. L. (2014). Impacts of anthropogenic disturbances at deep-sea hydrothermal vent ecosystems: A review. Mar. Environ. Res. 102, 59–72. doi: 10.1016/j.marenvres.2014.03.008
Van Dover C. L. (2019). Inactive sulfide ecosystems in the deep sea: A review. Front. Mar. Sci. 6. doi: 10.3389/fmars.2019.00461
Van Dover C. L., Arnaud-Haond S., Gianni M., Helmreich S., Huber J. A., Jaeckel A. L., et al. (2018). Scientific rationale and international obligations for protection of active hydrothermal vent ecosystems from deep-sea mining. Mar. Pol. 90, 20–28. doi: 10.1016/j.marpol.2018.01.020
Van Dover C. L., Colaço A., Collins P. C., Croot P., Metaxas A., Murton B. J., et al. (2020). Research is needed to inform environmental management of hydrothermally inactive and extinct polymetallic sulfide (PMS) deposits. Mar. Pol. 121, 104183. doi: 10.1016/j.marpol.2020.104183
Van Dover C. L., Smith C. R., Ardron J., Arnaud S., Beaudoin Y., Bezaury J., et al. (2011). Environmental management of deep-sea chemosynthetic ecosystems: Justification of and consideration for a spatially-based approach. ISA Tech. Study 9 Kingston Jamaica: Int. Seabed Authority.
Vanreusel A., Bossche I., van den, Thiermann F. (1997). Free-living marine nematodes from hydrothermal sediments: Similarities with communities from diverse reduced habitats. Mar. Ecol. Prog. Ser. 157, 207–219. doi: 10.3354/meps157207
Varliero G., Blenhold C., Schmid F., Boetius A., Molari M. (2019). Microbial diversity and connectivity in deep-sea sediments of the South Atlantic polar front. Front. Microbiol. 10. doi: 10.3389/fmicb.2019.00665
Vereshchaka A. L., Vinogradov M. E. (2006). “Struktura bentopelagicheskoy komponenty gidrotermalnykh soobschchestv,” in Ekosistemy atlanticheskikh gidroterm [Ecosystems of the Atlantic hydrothermal vents]. Eds. Vinogradov M. E., Vereshchaka A. L. (Moskva: Nauka), 275–290.
Vereshchaka A., Vinogradov G., Lein A. Y., Dalton S., Dehairs F. (2000). Carbon and nitrogen isotopic composition of the fauna from the Broken Spur hydrothermal vent field. Mar. Biol. 136, 11–17. doi: 10.1007/s002270050002
Vrijenhoek R. C. (2010). Genetic diversity and connectivity of deep-sea hydrothermal vent metapopulations. Mol. Ecol. 19, 4391–4411. doi: 10.1111/j.1365-294X.2010.04789.x
Washburn T. W., Menot L., Bonifácio P., Pape E., Błażewicz M., Bribiesca-Contreras G., et al. (2021). Patterns of macrofaunal biodiversity across the Clarion-Clipperton Zone: An area targeted for seabed mining. Front. Mar. Sci. 8. doi: 10.3389/fmars.2021.626571
Weaver P. P. E., Billett D. (2019). “Environmental impacts of nodule, sulphide and crust mining: An overview,” in Environmental issues of deep-sea mining. Ed. Sharma R. (Cham: Springer), 27–62. doi: 10.1007/978-3-030-12696-4_3
Weaver P. P. E., Boschen-Rose R. E., Dale A. C., Jones D., Billett D. S. M., Colaço A., et al. (2021). Regional environmental assessment of the northern Mid-Atlantic Ridge. ISA Tech. Study 28 Int. Seabed Authority Kingston Jamaica: Int. Seabed Authority.
Williams A. B., Rona P. A. (1986). Two new caridean shrimps (Bresiliidae) from a hydrothermal field on the Mid-Atlantic Ridge. J. Crust. Biol. 6, 446–462. doi: 10.2307/1548184
Witman J. D. (2013). Are regional effects on local diversity more important in marine than in terrestrial communities? Oikos 122, 300–305. doi: 10.2307/41937670
Yahagi T., Fukumori H., Warén A., Kano Y. (2019). Population connectivity of hydrothermal-vent limpets along the northern Mid-Atlantic Ridge (Gastropoda: Neritimorpha: Phenacolepadidae). J. Mar. Biol. Ass. U.K. 99, 179–185. doi: 10.1017/S0025315417001898
Zbinden M., Cambon-Bonavita M. A. (2020). Rimicaris exoculata: Biology and ecology of a shrimp from deep-sea hydrothermal vents associated with ectosymbiotic bacteria. Mar. Ecol. Prog. Ser. 652, 187–222. doi: 10.3354/meps13467
Zbinden M., Gallet A., Szafranski K. M., Machon J., Ravaux J., Léger N., et al. (2018). Blow your nose, shrimp! Unexpectedly dense bacterial communities occur on the antennae and antennules of hydrothermal vent shrimp. Front. Mar. Sci. 5. doi: 10.3389/fmars.2018.00357
Zekely J., Van Dover C. L., Nemeschkal H. L., Bright M. (2006). Hydrothermal vent meiobenthos associated with mytilid mussel aggregations from the Mid-Atlantic Ridge and the East Pacific Rise. Deep Sea Res. I 53, 1363–1378. doi: 10.1016/j.dsr.2006.05.010
Zeppilli D., Bellec L., Cambon-Bonavita M.-A., Decraemer W., Fontaneto D., Fuchs S., et al. (2019). Ecology and trophic role of Oncholaimus dyvae sp. nov. (Nematoda: Oncholaimidae) from the Lucky Strike hydrothermal vent field (Mid-Atlantic Ridge). BMC Zool 4, 6. doi: 10.1186/s40850-019-0044-yx
Zeppilli D., Leduc D., Fontanier C., Fontaneto D., Fuchs S., Gooday A. J., et al. (2018). Characteristics of meiofauna in extreme marine ecosystems: A review. Mar. Biodiv. 48, 35–71. doi: 10.1007/s12526-017-0815-z
Zeppilli D., Pusceddu A., Trincardi F., Danovaro R. (2016). Seafloor heterogeneity influences the biodiversity–ecosystem functioning relationships in the deep sea. Sci. Rep. 6, 26352. doi: 10.1038/srep26352
Keywords: benthos, Mid-Atlantic Ridge, hydrothermal vents, polymetallic sulfide exploration, International Seabed Authority
Citation: Radziejewska T, Błażewicz M, Włodarska-Kowalczuk M, Jóźwiak P, Pabis K and Węsławski JM (2022) Benthic biology in the Polish exploration contract area of the Mid-Atlantic Ridge: The knowns and the unknowns. A review. Front. Mar. Sci. 9:898828. doi: 10.3389/fmars.2022.898828
Received: 17 March 2022; Accepted: 28 September 2022;
Published: 26 October 2022.
Edited by:
David Billett, Deep Seas Environmental Solutions Ltd., United KingdomReviewed by:
Jeroen Ingels, Florida State University, United StatesCopyright © 2022 Radziejewska, Błażewicz, Włodarska-Kowalczuk, Jóźwiak, Pabis and Węsławski. This is an open-access article distributed under the terms of the Creative Commons Attribution License (CC BY). The use, distribution or reproduction in other forums is permitted, provided the original author(s) and the copyright owner(s) are credited and that the original publication in this journal is cited, in accordance with accepted academic practice. No use, distribution or reproduction is permitted which does not comply with these terms.
*Correspondence: Teresa Radziejewska, dGVyZXNhLnJhZHppZWpld3NrYUB1c3ouZWR1LnBs
Disclaimer: All claims expressed in this article are solely those of the authors and do not necessarily represent those of their affiliated organizations, or those of the publisher, the editors and the reviewers. Any product that may be evaluated in this article or claim that may be made by its manufacturer is not guaranteed or endorsed by the publisher.
Research integrity at Frontiers
Learn more about the work of our research integrity team to safeguard the quality of each article we publish.