- 1State Key Laboratory of Marine Resource Utilization in South China Sea, Hainan University, Haikou, China
- 2College of Marine Science, Hainan University, Haikou, China
- 3Guangxi Key Laboratory of Aquatic Genetic Breeding and Healthy Aquaculture, Guangxi Academy of Fisheries Sciences, Nanning, China
Pinctada fucata martensii and P. maxima are two main traditional pearl oyster species that can produce seawater pearls. Our previous study showed a higher clearance rate (CR) and growth performance in P. f. martensii than in P. maxima fed with Isochrysis galbana. In this study, the P. f. martensii and P. maxima juveniles of two sizes (large and small) were fed with six different microalgae diets [I. galbana (I), Platymonas subcordiformis (P), Chaetoceros muelleri I, I+P, I+C, and P+C] to evaluate the differences in growth, feeding, and metabolism between two pearl oyster species. After 60 d of the rearing period, P. f. martensii and P. maxima fed with mixed microalgae showed a significantly higher relative growth rate (RGR) than those fed with single microalgae (P< 0.05). The RGRs were significantly higher in P. f. martensii than those in P. maxima fed with the same diets (P< 0.05). The RGRs showed a decreasing tendency with the growth in both pearl oyster species. The CRs of pearl oysters fed with mixed microalgae were significantly higher than those fed with single microalgae (P< 0.05), and the CRs of P. f. martensii were significantly higher than those of P. maxima fed with the same diets (P< 0.05). Significantly lower respiration rates (RRs) were observed in small-size P. f. martensii groups fed with I, P, and I+P diets and all large P. f. martensii groups compared to P. maxima fed with the same diets (P< 0.05). Higher activities of amylase, cellulase, lipase, and pepsin in P. f. martensiiwere observed compared to P. maxima fed with the same diets at two sizes. The pepsin activities in P. maxima decreased with the growth, while there were no consistent pepsin activities of P. f. martensii with the growth. The carbonic anhydrase activities in P. maxima were significantly higher than those in P. f. martensii fed with the same diets (P< 0.05). The carbonic anhydrase activities were highest in the I+C diet group, followed by C+P and I+P, I, C, and P groups. Significant differences were observed among different diet groups in the same pearl oyster species (P< 0.05). Our results suggest that the lower CR and activities of digestive enzymes and higher RRs and activities of carbonic anhydrase may cause a lower growth rate of P. maxima compared to P. f. martensii.
Introduction
Pearl oysters are farmed throughout the Indo-Pacific region (Southgate and Lucas, 2008; McDougall et al., 2016; Gu et al., 2020; He et al., 2021). Of these, Pinctada fucata martensii and P. maxima are the two main farmed species used for producing nucleated round pearls (Du et al., 2017; Hao et al., 2018; Massault et al., 2021; Zhang et al., 2021). The maximum shell height of P. f. martensii is 60–70 mm, while that of P. maxima ranges from 200 to 250 mm (Yukihira et al., 1998; Yang et al., 2018). P. maxima is primarily cultured for the high-value production of large-scale pearls, and P. f. martensii is cultured for small-scale pearls (Hao et al., 2018; Zhang et al., 2021). Both species are commercially important oyster species in pearl industries (Southgate and Lucas, 2008).
In China, P. f. martensii and P. maxima are the traditional pear oyster species for seawater pearl fishing and are cultured in the same offshore areas, mainly in Guangdong, Guangxi, and Hainan provinces (Hao et al., 2018; Zhang et al., 2021). However, mortality was observed highly in P. maxima farming, especially at the growth stage of 3–6 cm in shell height than P. f. martensii (Hamzah et al., 2011; Liang et al., 2016). Until now, the causes of the high mortality rate of P. maxima are ascribed to diseases, environmental degradation, micro-algal composition, and so on (Pass et al., 1987; Taylor et al., 2004; Kvingedal et al., 2010; Crockford and Jones, 2012; Deng et al., 2013a and Deng et al., 2013b). In our previous study, a higher clearance rate (CR) and growth rate in P. f. martensii were observed compared to P. maxima fed with Isochrysis galbana (Yang et al., 2021; Zhang et al., 2021). We inferred that the lower CR and feed conversion ratio (FCR) to microalgae caused a low growth rate in P. maxima.
An understanding of the changes in food intake and energy budget within an organism can help study the different growth performances in P. f. martensii and P. maxima. Several studies have shown that the higher growth rates of oysters are attributed to higher feeding rates and lower metabolic costs (Tamayo et al., 2014; Hao et al., 2018; Hall et al., 2020). Zhang et al. (2021) reported that the fast-growing selective strain of P. f. martensii showed a significantly higher CR and lower oxygen consumption and ammonia excretion rates (ARs) than unselected groups. In Pacific oyster (Crassostrea gigas), higher energy gain rates coupled with lower metabolic costs of growth were reported in fast growers (Tamayo et al., 2014). Similarly, a relatively higher food-processing capacity in faster-growing mussels and a generally higher metabolic cost of feeding and growth in slower-growing individuals were observed in green-lipped mussel (Perna canaliculus) (Ibarrola et al., 2017). In addition, changes in the activities of digestive enzymes have been considered reliable indicators of food digestive efficiency and nutritional status in bivalves (Palais et al., 2012; Ibarrola et al., 2017; Zhang et al., 2021). The amylase, lipase, and pepsin activities of the fasting-growing strain of P. f. martensii were significantly higher than those of a normal cultured population (Zhang et al., 2021). In bivalves, carbonic anhydrase is an important indicator of biological mineralization, which shows a positive relationship with food intake (Medakovi´c, 2000; Cardoso et al., 2019; Zhao et al., 2020). In black-lip pearl oyster P. margaritifera, oysters fed with the highest food level had thicker aragonite tables and a higher mineralization rate (Linard et al., 2011).
In this study, the growth performance, physiological energetics, and activities of the digestive enzymes and carbonic anhydrase of P. f. martensii and P. maxima fed with different microalgae diets were detected to test whether the food intake and digestion influences the growth rate of the oyster species.
Materials and Methods
Microalgal Diet Culture
The microalgae used in this study were I. galbana (I, 5.51 ± 0.22 μm in cell diameter), Platymonas subcordiformis (P, 9.93 ± 0.94 μm in cell diameter), and Chaetoceros muelleri (C, 4.5 ± 0.25 μm in cell diameter). All microalgae were obtained from the Algal Species Laboratory of Hainan University, Haikou, Hainan province, China. Microalgae were cultured in 50-L white plastic buckets using a semi-continuous batch culturing system and Ningbo University #3 improvement solution (Chen et al., 2021). Air with 2% CO2 was bubbled into the buckets to support growth. The algae were grown using a continuous culture regime under a light/dark cycle of 12/12 h at 24 ± 1°C for 7–10 d; the cell densities of I, P, and C were ~6 × 106, ~2 × 105, and ~3 × 106 cells/ml, respectively.
Proximate Composition of Microalgae
For biochemical analysis, each microalgal species was grown in triplicate 10-L flasks. Microalgae were centrifuged (5,000 rpm, 30 min) when cultures reached the late logarithmic growth phase. The sediments were washed with 0.5 M of ammonium formate and recentrifuged at 5,000 rpm for 30 min. Then, the microalgae concentrates were lyophilized at −60°C in a vacuum freeze dryer (Scientz-10 N, Ningbo, China). Dried microalgae were ground into powder using a tissue lyser (Tissuelyser-48, Shanghai Jingxin Industrial Development Co., Ltd., Shanghai, China). The crude protein content was determined by the Kjeldahl method using a fully automated Kjeldahl nitrogen/protein analyzer (FOSS-Soxtec 2050, Hoganäs, Sweden) after acid digestion in a Tecator digestor 2020. Crude lipid was extracted with petroleum ether (Sinopharm Chemical Reagent Co. LTD, Shanghai, China) as described by Li et al. (2020), and crude ash was determined gravimetrically in a muffle furnace by incineration at 550°C for 24 h.
Pearl Oyster Collection
On May 1, 2020, two pearl oyster species, P. f. martensii and P. maxima, were selected to conduct mass breeding experiments at a shellfish hatchery in Beihai, Guangxi province, China. Larvae were reared according to the procedures described by Zhang et al. (2021). After 30 d, juveniles from each species were transferred to coastal areas with a raft culture in Beihai (21°26′~21°55′ N, 108°50′~109°47′ E), Guangxi province, China.
In October 2020 and June 2021, 5-month [15.87 ± 0.84 mm (0.06 ± 0.02-g dry soft tissue weight) and 14.57 ± 1.04 mm (0.04 ± 0.01-g dry soft tissue weight) in shell height for P. f. martensii and P. maxima, respectively] and 13-month [45.46 ± 2.34 mm (0.23 ± 0.03-g dry soft tissue weight) and 44.57 ± 2.64 mm (0.12 ± 0.02-g dry soft tissue weight) in shell height for P. f. martensii and P. maxima, respectively] pearl oysters were collected, transported to the laboratory, acclimatized for 1 week before the feeding experiment, and then maintained at 26 ± 2°C in 80 cm × 40 cm × 50 cm tanks containing filtered running seawater (with a salinity of 30 ± 2 ppt) with recirculating water systems. During the acclimatization period, these pearl oysters were fed with a moderate amount of a superfluous mixture of microalgae (I+P+C) twice a day (7:00 and 17:00), and a half volume of seawater was daily replaced.
Experimental Design
Five-month and 13-month P. maxima and P. f. martensii were fed with six different microalgae diets (I, P, C, I+P, I+C, and P+C). The culture experiment was maintained indoors for 60 d. For 5-month P. maxima and P. f. martensii, 540 pearl oysters of each species were randomly divided into six groups and fed with six different diets (I, P, C, I+P, I+C, and P+C). Oysters fed with the same diets were divided into three parallel groups and cultured in three 160-L tanks. The densities of six microalgae diets were 30×104 cells·ml-1 for I, 10×104 cells/ml for P, 30×104 cells·ml-1 for C, (15×104 + 5×104) cells·ml-1 for I+P, (15×104 + 15×104) cells·ml-1 for I+C, and (5×104 + 15 ×104) cells·ml-1 for P+C, respectively. Before feeding, the concentration of each cultured microalgae was measured with a hemocytometer (Marienfeld, Devan Instrument Co., LTD, Wuxi, China) under a microscope. For 13-month P. f. martensii and P. maxima, 270 pearl oysters of each species were randomly divided into six groups and cultured as 5-month oysters. The culture conditions were as follows: pearl oysters were fed twice a day (7:00 and 17:00) with different microalgae. Half-volume seawater was changed daily, and all the water was changed once a week. The water temperature, salinity, and dissolved oxygen were 26 ± 2°C, 30 ± 2 ppt, and ≥ 4 mg·ml-1, respectively.
After 60-day rearing, pearl oysters were starved for 24 h before sampling. For each tank, the remaining pearl oysters were counted, and the shell height of each pearl oyster was measured. Four pearl oysters of each tank were randomly sampled for physiological energetic analysis. The remained individuals of each tank were sacrificed, and the mantle and visceral mass of each individual were sampled and stored at -80°C.
Growth Performance
The shell height of all pearl oysters was measured at the beginning and end of the experiment. The shell height was measured as the vertical distance from the umbo to the ventral edge.
The survival rate (SR) and relative growth rate (RGR) were evaluated as follows:
Physiological Energetic Analysis
After 60-d rearing, four pearl oysters per tank were sampled for CR, respiration rate (RR), and AR analyses. The species and concentrations of microalgae used in each group were the same as in the rearing experiment. After physiological energetic analysis, the soft tissues of pearl oysters were sampled and dried at 65°C for 24 h to obtain their dry tissue weight.
Clearance Rate
For detecting the CR in each group, three pearl oysters were randomly collected and placed in 1-L beakers. Each treatment was replicated thrice. The microalgae species and concentration in each group were the same as in the rearing experiment. One blank beaker with no pearl oyster was set as the control. After 3 h, the concentration of algae was calculated under a microscope using a hemocytometer. The CR (L·h-1·g-1) was calculated according to the method by Wang et al. (2015), and the equation is given as follows:
where V is the volume beaker, and CT − C0 is the microalgae concentrations at the initial and T time. Sd is the variable coefficient of microalgae concentrations in the control group. N is the number of pearl oysters in the beaker, and T is the elapsed time (h). DW is the dry weight of soft tissues of pearl oysters.
Respiration Rate (VO2)
The RR detection was performed after 3-h feeding. Each treatment was replicated thrice. Three pearl oysters were incubated in sealed 800-ml cylindrical chambers containing seawater with oxygen probes (YSI®5730). The oxygen concentration was recorded at regular intervals (∼1 h) until it dropped below 30% of the initial value. One parallel chamber without a pearl oyster was used as a control. The RR (mg·h-1·g-1) was calculated following the equation
where V is the volume of the cylindrical chamber, CO0 and COT are the oxygen concentrations of seawater at initial and T time, and DW is the dry weight of soft tissues of pearl oysters.
Ammonia Excretion Rate (VNH4-N)
The AR detection was performed after 3-h feeding. Each treatment was replicated thrice. AR was determined by placing individual pearl oysters in closed chambers containing 250 ml of air-saturated seawater filtered using 0.22-μm Millipore membranes. One chamber without pearl oysters was used as a control. After 60 min, the water samples were taken from each chamber and stored at −20°C until analysis according to the phenol-hypochlorite method described by Solorzano (1969). The AR (mg·h-1g-1) was calculated following the equation
where V is the volume of the chamber, VNH4-NT and VNH4-N0 are the ammonia concentrations of seawater at initial and T time, and DW is the dry weight of soft tissues of pearl oysters.
Detection of Digestive Enzyme Activities
The frozen visceral mass of pearl oysters was weighed, thawed at 4°C, and homogenized (5,000 rpm, 10 min) with 0.9% precooled and sterilized normal saline at a ratio of 1:9 (tissue: saline). These samples were centrifuged at 2,500 rpm for 10 min, and the supernatant was collected in a 1.5-ml Eppendorf tube for digestive enzyme activity analysis. The activities of amylase (Amylase Assay Kit, iodine-starch colorimetry), cellulase [Cellulase (CL) Test Kit, colorimetry], lipase (Lipase Assay Kit, colorimetry), and pepsin (Pepsin Assay Kit, colorimetry) were determined using the respective kits following the manufacturer’s instructions (Jiancheng Biological Engineering Institute, Nanjing, China).
Detection of Carbonic Anhydrase Activities
The frozen mantle of each sampled pearl oyster was weighed and treated as the visceral mass mentioned above. Carbonic anhydrase activities were measured using the classical phenol red method (Roy et al., 2012). The samples were read spectrophotometrically at 450 nm, and results were expressed in units per milligram of protein (U g−1 protein).
Statistical Analysis
Data were presented as mean ± standard deviation (SD). All statistical analyses were conducted using Data Processing System (DPS) statistical software. The comparison among the proximate composition of three microalgae was made using one-way analysis of variance (ANOVA), followed by the least significant difference (LSD) test. The data of growth performance, physiological energetics, digestive enzyme, and carbonic anhydrase activities were analyzed by two-way analysis of variance (ANOVA), and means were subsequently separated by Tukey’s test. A value of P< 0.05 was considered statistically significant.
Results
Proximate Compositions in Three Microalgal Diets
As shown in Table 1, there were significant variations in the proximate compositions among three microalgae (P< 0.05). The contents of crude protein in the I and C groups were 51.06% and 50.01% of dry weight, respectively, which showed significantly higher than those in the P group (P< 0.05). Similarly, the highest lipid content was observed in the I (12.77%) group, followed by C (10.96%) and P (8.42%) groups, and significant differences were found in the I vs. P group (P< 0.05).
Survival Rate and Growth Performance of P. f. martensii and P. maxima Fed With Different Microalgae
As shown in Table 2, the SR and RGR of pearl oysters of two sizes were significantly affected by diet and species (P< 0.05). For small-size pearl oysters, the growth of shell heights (GHs) of P. f. martensii and P. maxima fed with different microalgae ranged from 2.32 mm to 10.04 mm and from 5.69 mm to 11.04 mm, respectively, and the highest GHs in both P. f. martensii and P. maxima were fed with the I+C group (Figure 1). The RGRs of P. f. martensii and P. maxima fed with mixed microalgae were significantly higher than those of the same pearl oyster species fed with single microalgae (P< 0.05). The RGRs of P. f. martensii were significantly higher than those of P. maxima fed with the same diets (P< 0.05) (Table 2). The SRs of P. f. martensii and P. maxima fed with P were 66.67% and 50.00%, respectively, which were significantly lower than other groups (P< 0.05) (Table 2).
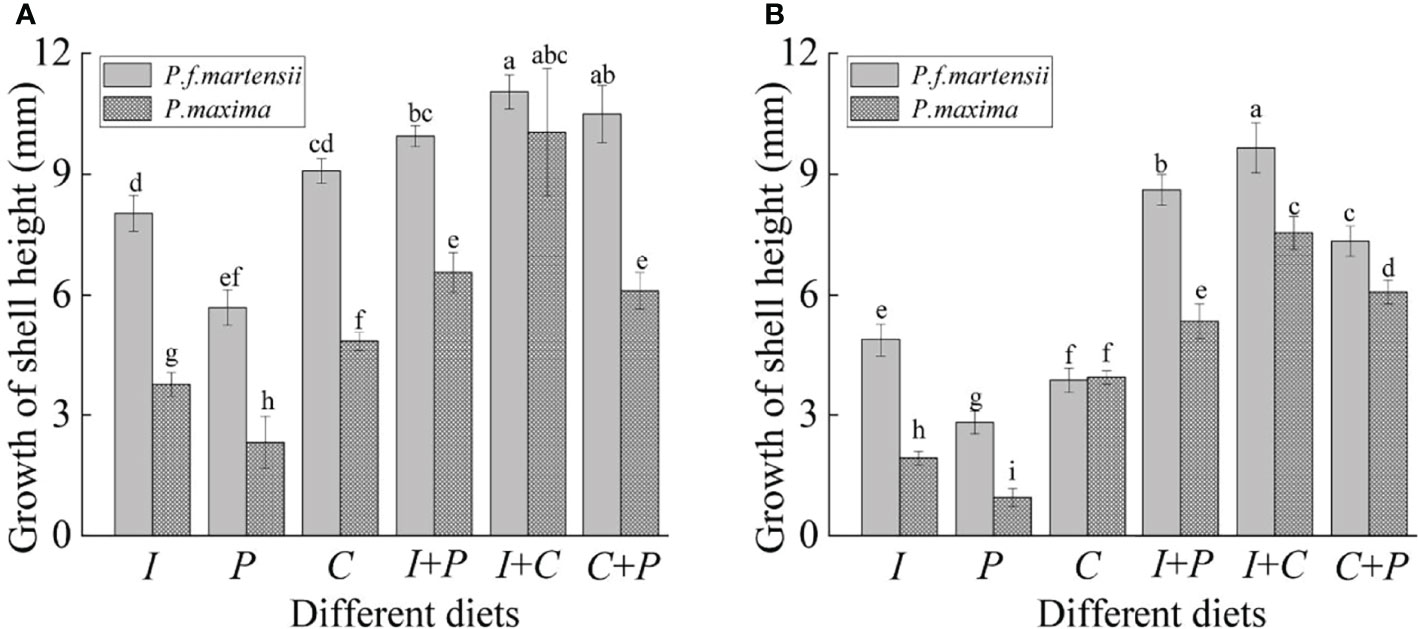
Figure 1 Growth of shell height of P. f. martensii and P. maxima of different sizes [small (A) and large (B)] fed with different microalgae. Different letters represent significant differences (P< 0.05).
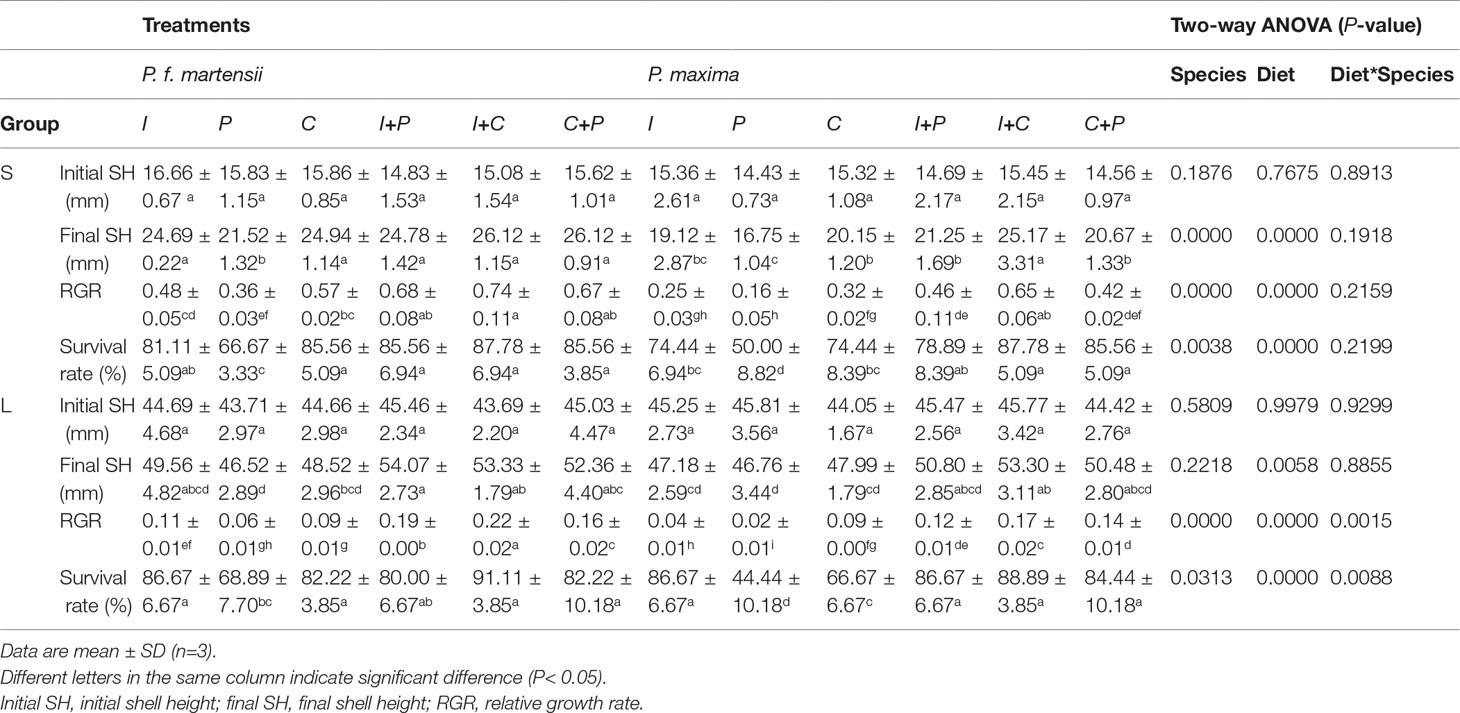
Table 2 Growth performance of P. f. martensii and P. maxima of two sizes fed with different microalgae for 60 d.
For large-size pearl oysters, although the GH, RGR, and SR among different microalgae showed a similar tendency to the small-size pearl oysters’ experiment, obvious decreases in GH and RGR were observed (Table 2, Figure 1). The SRs of P. maxima fed with P and C were 44.44% and 66.67%, which showed an obvious decrease compared to small-size P. maxima fed with the same diets (Table 2).
Clearance Rate, Respiration Rate, and Ammonia Excretion Rate
The CRs of P. f. martensii and P. maxima fed with different diets are shown in Table 3. For small-size pearl oysters, the CRs of P. f. martensii and P. maxima were 0.58–1.82 L·h-1·g-1 and 0.25–1.62 L·h-1·g-1, respectively. Compared to the six different microalgae diets for P. f. martensii and P. maxima, the CRs were the highest in I+C treatments (1.82 and 1.62 L·h-1·g-1, respectively). The CRs were significantly affected by pearl oyster species and diets (P< 0.05). The CRs of pearl oysters fed with mixed microalgae were significantly higher than those of pearl oysters fed with single microalgae (P< 0.05), and the CRs of P. f. martensii were significantly higher than those of P. maxima fed with the same diet (P< 0.05). The same tendency was also observed in large-size P. f. martensii and P. maxima groups.
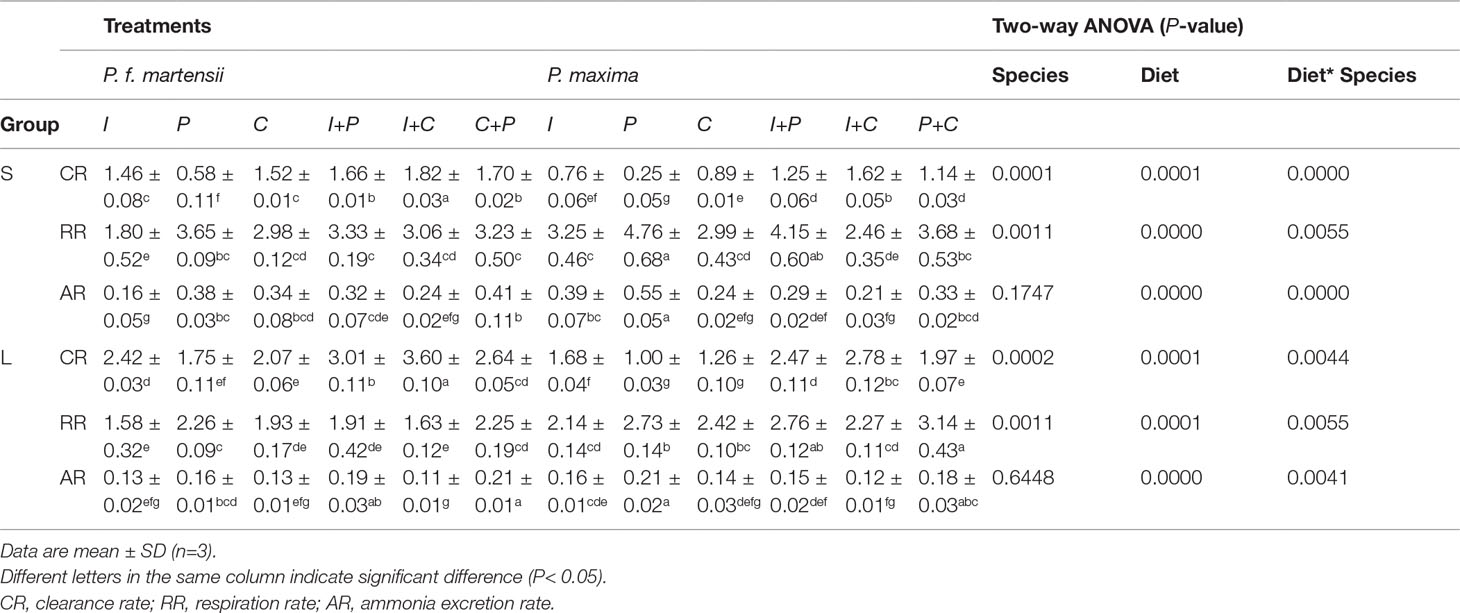
Table 3 Physiological determinations of P. f. martensii and P. maxima of two sizes fed with different microalgae.
The RRs of small-size P. f. martensii and P. maxima were 1.80–3.33 mg·h-1g-1 and 2.46–4.76 mg·h-1·g-1, respectively, and were significantly affected by both pearl oyster species and diets (P< 0.05) (Table 3). The highest RRs were observed in the P-treated groups of P. f. martensii (3.65 mg·h-1·g-1) and P. maxima (4.76 mg·h-1·g-1), respectively, followed by the I+P-treated groups of these two pearl oyster species. In some microalgae-feeding groups (e.g., I, P, and I+P), significant differences in RRs were observed between P. f. martensii and P. maxima fed with the same diets (P< 0.05). For large-size pearl oysters, the RRs of P. f. martensii were significantly lower than those of P. maxima fed with the same diets (1.58–2.46 mg·h-1·g-1 and 2.14-3.14 mg·h-1·g-1 for P. f. martensii and P. maxima, respectively). Obvious decreases in RR were observed in both P. f. martensii and P. maxima than in small-size pearl oyster groups.
The ARs were not significantly affected by pearl oyster species in both small and large sizes (P = 0.1741 and 0.6448, respectively), while significant differences were observed among different diet groups (P< 0.05) (Table 2). In P. f. martensii, the ARs of small- and large-size oysters were 0.16–0.41 mg·h-1·g-1and 0.11–0.21 mg·h-1·g-1, respectively. The ARs of P. f. martensii fed with C+P were the highest in both size groups. In P. maxima, the ARs of small- and large-size groups were 0.21–0.55 mg·h-1·g-1 and 0.12–0.21 mg·h-1·g-1, respectively. The top three highest ARs of P. maxima of both sizes were fed with P, I, and P+C. Obvious decreases of ARs in both P. f. martensii and P. maxima were observed with the growth.
Digestive Enzyme Activities
The digestive enzyme activities of pearl oysters are shown in Table 4. The four digestive enzyme activities of pearl oysters were significantly affected by species and diets (P< 0.05). The four digestive enzyme activities in P. f. martensii and P. maxima fed with mixed microalgae almost showed an obvious increase compared to the same pearl oyster species fed with single microalgae. The amylase, cellulase, and pepsin activities in P. f. martensii and P. maxima fed with P were the lowest, and significant differences were observed when comparing P-treated pearl oysters with other diet groups (P< 0.05), except for the amylase activity of the I vs. P group in small-size P. f. martensii.
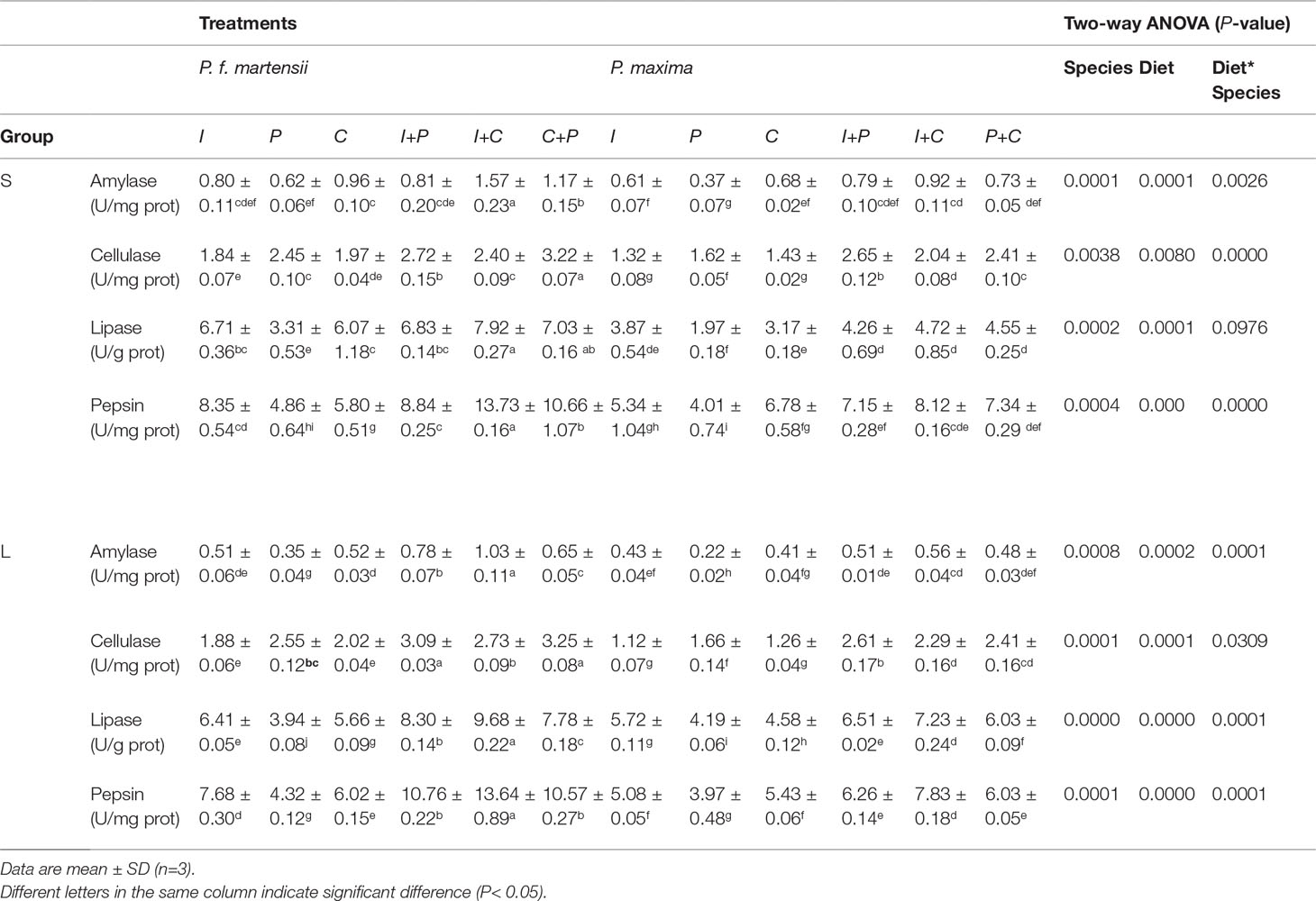
Table 4 Digestive enzyme activities in intestinal tissues of P. f. martensii and P. maxima of two sizes fed with different microalgae.
Obvious decreases in amylase activities in P. f. martensii and P. maxima were observed with their growth. In P. maxima, the lipase activities increased with the growth, while the opposite tendency of pepsin activities was observed. In P. f. martensii, no consistent lipase and pepsin activities were observed with their growth in six diet groups. Furthermore, higher activities of amylase, cellulase, lipase, and pepsin in P. f. martensii were observed compared to P. maxima fed with the same diets.
Carbonic Anhydrase Activities
As shown in Figure 2, the carbonic anhydrase activities in P. maxima were significantly higher than those in P. f. martensii fed with the same diets (P< 0.05). For small-size P. f. martensii and P. maxima, the carbonic anhydrase activities were highest in the I+C diet groups (0.31 and 0.36 U·g-1 prot for P. f. martensii and P. maxima, respectively), followed by C+P and I+P, I, C, and P, and significant differences were observed among different diet groups in the same pearl oyster species (P< 0.05). The same tendency of carbonic anhydrase activities was also observed in large-size P. f. martensii and P. maxima groups.
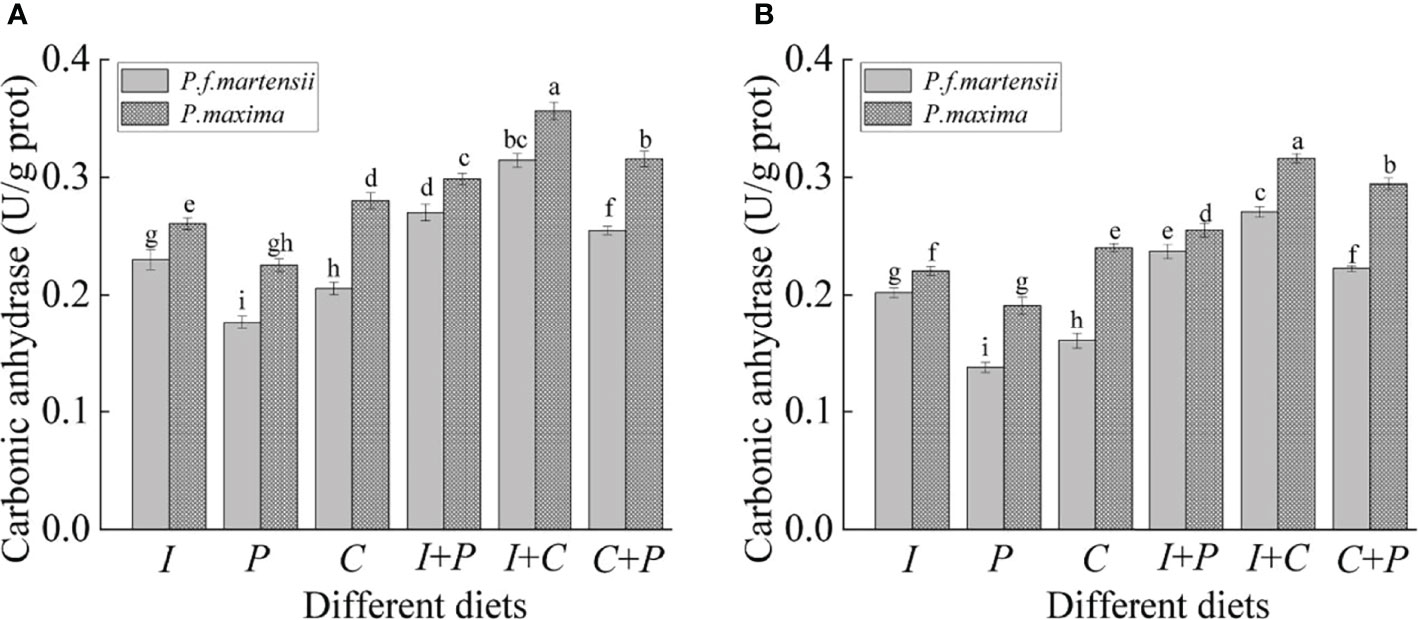
Figure 2 Carbonic anhydrase activities of P. f. martensii and P. maxima of different sizes [small (A) and large (B)] fed with different microalgae. Means ± SD are presented (n = 6). Different letters represent significant differences (P< 0.05).
Discussion
Microalgae are the main diet for bivalve mollusks in both wild and artificial culture conditions (Guedes and Malcata, 2012). Although two oyster species fed with six different diets showed significant increases in shell height, an obvious feeding preference for these two oyster species was observed in this study, which showed that mixed microalgae were better than the single ones, and the I or C group was better than the P group. Although many studies have reported suitable algae species in bivalve diets, a combination of flagellates and diatoms is better in an indoor culture (Galley et al., 2010; Hassan et al., 2021). Pacific oyster had better growth when fed with equal proportions of flagellates and diatoms compared to a high proportion of flagellates or diatoms (Rico-Villa et al., 2006). A mixed diet (Isochrysis sp. and C. muelleri) outperformed a monospecies diet in the blue mussel (Mytilus edulis) culture (Galley et al., 2010).
Bivalves have the ability to select their food in both natural and artificial conditions (Ward and Shumway, 2004). Many factors, such as cell size, cell surface, digestibility, toxic metabolite production, and gross biochemical composition, determine the suitability of microalgae as food for bivalves (Gouda et al., 2006; Rosa et al., 2017). Suitable particle sizes for P. f. martensii and P. maxima are 2.5–10 μm and >4 μm, respectively (Yukihira and Klumpp, 1999; Tomaru et al., 2002). Although the microalgae of all sizes used in this study could be easily ingested, small-size microalgae [I. galbana (I, ~5.51 μm) and C. muelleri (C, ~4.5 μm)] were more suitable for these two pearl oyster species compared to the large one [P. subcordiformis (P, ~9.93 μm)]. The nutritive quality of microalgae for bivalves is also determined by their biochemical composition (Martínez-Fernández et al., 2006). The biochemical composition of microalgae varies among species and according to culture conditions (Valenzuela-Espinoza et al., 2002; Martínez-Fernández et al., 2006). Protein is the largest organic component of microalgae. Lipid, especially polyunsaturated fatty acids, is also an essential nutrient. Previous studies showed that high dietary protein and lipid in microalgae were associated with a good growth of juvenile bivalves, such as blue mussel M. edulis, Pacific oyster C. gigas, Caribbean pearl oyster P. aimbricata (Leonardos and Lucas, 2000; Knuckey et al., 2002; Lodeiros et al., 2017). In our study, the crude protein and lipid in the I and C groups were higher than those in the P group, which indicated that more nutrients are obtained by pearl oysters from I and C treatments.
When comparing the differences in the growth rate and survival between P. f. martensii and P. maxima, significantly higher growth rates were observed in P. f. martensii at both two sizes compared to P. maxima fed with the same diets. Bivalve species have different strategies of feeding selection and energy budgets to adapt to different environments (Yukihira et al., 1998; Wang et al., 2000; Strohmeier et al., 2012). For example, Ibarrola et al. (2012) reported that the CR of mytilid mussel M. chilensis was the highest, followed by giant mussel Choromytilus chorus and ribbed mussel Aulacomya ater; the absorbed energy in P. margaritifera was significantly higher than that in P. maxima at 19°C, while the opposite results were observed at 28°C and 32°C (Yukihira et al., 2000). In our study, significantly higher CRs and lower RRs were observed in P. f. martensii than those in P. maxima fed with the same diets, although the ARs varied between these two oyster species. These results indicated more food intake and less energy consumption in P. f. martensii than in P. maxima. Furthermore, the activities of amylase, cellulase, lipase, and pepsin in P. f. martensii were also higher than those in P. maxima, which meant that P. f. martensii had a stronger digestive ability than P. maxima. Therefore, higher growth performances were detected in P. f. martensii than those in P. maxima.
The higher growth rates in P. f. martensii might be ascribed to the relatively higher adaptability to complex and volatile environments than those in P. maxima. P. maxima prefers to live in the oligotrophic tropical waters of coral reefs and atolls, while P. f. martensii prefers to live in coastal areas of higher latitudes and is more tolerant to extreme temperature and nutrient inputs (Yang et al., 2021; Zhang et al., 2021). The natural diets for pearl oysters were more complex than artificial diets. The higher SR in P. f. martensii was observed in coastal areas suspended on a raft compared to P. maxima, which showed the same tendency as our results (Hwang et al., 2007; Liang et al., 2016; Zhang et al., 2021). Therefore, the sensitivities to environmental changes at the juvenile stage are the main cause of the mortality of P. maxima.
The growth rate of bivalve species shows a decreasing tendency with their increased size, which is attributed to their energy budget changes at different life stages (Ibarrola et al., 2017; Hao et al., 2018; Liu et al., 2020). In our study, both the GH and the RGR of large-size P. f. martensii and P. maxima fed with the same microalgae significantly decreased than small-size groups. When comparing the energy budget parameters, the CRs of two pearl oyster species significantly increased, from 0.58–1.82 L·h-1·g-1 to 1.75–3.60 L·h-1·g-1 for P. f. martensii and from 0.25–1.62 L·h-1·g-1 to 1.00–2.78 L·h-1·g-1 for P. maxima, respectively. The CRs in P. f. martensii and P. maxima were almost the same as in the previous studies by Yukihira et al. (1998); Zhang et al. (2021), and Yang et al. (2021). Furthermore, the RRs and ARs of each pearl oyster species decreased with the increase in size, which was detected in other bivalve species, such as green-lipped mussel and pacific oyster (Tamayo et al., 2014; Ibarrola et al., 2017). The lower mass exponents for CR compared to RR and AR are the causes of the decrease in growth rate with the increase in body size (Rueda and Smaal, 2004; Ibarrola et al., 2017).
The feeding habits vary at different life stages in many marine organisms, and their digestive enzyme activities change because of their food preference (Kolkovski, 2001; Prudence et al., 2006; Ibarrola et al., 2012). In our study, the cellulase activities of P. f. martensii and P. maxima had no changes between the two sizes, while there was an obvious decrease in amylase activities in both two pearl oyster species, which indicated that carbohydrate requirements decreased with the increase in size in both P. f. martensii and P. maxima. Similar results have also been observed in M. chilensis of different sizes (Ibarrola et al., 2012). As for the lipase and pepsin activities, there were almost no changes in P. f. martensii with the increase in its size, while obvious increases for lipase and decreases for pepsin were observed in large groups of P. maxima compared to small-size groups. These obvious changes in lipase and pepsin activities indicated that the protein and lipid requirements of P. maxima changed dramatically with the size growth (Labarta et al., 2002; Zhang et al., 2021). However, the diets used in this study were unchanged; therefore, some microalgae groups, such as P. subcordiformis and C. muelleri, could not meet the nutritional requirements of large-size P. maxima, which led to a lower growth rate, even death.
The carbonic anhydrase activity changes markedly with different growth performances in the oyster mantle and is considered one of the important indicators of biological mineralization (Kawai, 1955; Ivanina et al., 2017). The carbonic anhydrase activity showed a similar tendency to the growth rate within the same pearl oyster species fed with different diets or at different life stages in this study. It is known that P. maxima has thicker shells than P. f. martensii (Gervis and Sims, 1992), which means that a higher proportion of total energy is used for the calcification of P. maxima. Significantly higher carbonic anhydrase activities were highly observed in P. maxima than those in P. f. martensii.
Conclusion
In conclusion, P. f. martensii and P. maxima fed with mixed microalgae showed higher growth and SR than those fed with single microalgae. Furthermore, the growth rate of these two pearl oyster species significantly decreased with the increased size. When comparing the growth performance between P. f. martensii and P. maxima, the RGR values in P. f. martensii were significantly higher than those in P. maxima fed with the same microalgae, which might be caused by the higher CR, lower RR values, and higher digestive enzyme activities in P. f. martensii.
Data Availability Statement
The original contributions presented in the study are included in the article/supplementary material. Further inquiries can be directed to the corresponding author.
Ethics Statement
The animal study was reviewed and approved by The Committee and Laboratory Animal Department of Hainan University.
Author Contributions
BY performed the experiment and wrote the original draft. ZG worked with the software and supervision. XZ performed the experiment. YY wrote the paper. AW revised the paper. CL designed the experiment and wrote the paper. All authors contributed to the article and approved the submitted version.
Funding
This research was supported financially by the Key Research and Development Project of Hainan Province (ZDYF2021XDNY277, ZDYF2021SHFZ269) and the National Key Research and Development Program of China (2019YFD0901301, 2018YFD0900704).
Conflict of Interest
The authors declare that the research was conducted in the absence of any commercial or financial relationships that could be construed as a potential conflict of interest.
Publisher’s Note
All claims expressed in this article are solely those of the authors and do not necessarily represent those of their affiliated organizations, or those of the publisher, the editors and the reviewers. Any product that may be evaluated in this article, or claim that may be made by its manufacturer, is not guaranteed or endorsed by the publisher.
References
Cardoso J. C., Ferreira V., Zhang X., Anjos L., Félix R. C., Batista F. M. (2019). Evolution and Diversity of Alpha-Carbonic Anhydrases in the Mantle of the Mediterranean Mussel (Mytilus Galloprovincialis). Sci. Rep. 9, 1–14. doi: 10.1038/s41598-019-46913-2
Chen Q., Jiang X. M., Han Q. X., Sheng P., Chai Y. H., Peng R. B., et al. (2021). Growth, Calcium Content, Proximate Composition, and Fatty Acid Composition of Triangle Sail Mussel (Hyriopsis Cumingii) Fed Five Different Microalgal Diets. Aquaculture 530, 735719. doi: 10.1016/j.aquaculture.2020.735719
Crockford M., Jones B. (2012). Aquatic Animal Health Subprogram: Investigation of Chlamydiales-Like Organisms in Pearl Oysters, Pinctada Maxima ( Government of Western Australian, Department of Fisheries). Perth.
Deng Y., Fu S., Liang F., Du X., Xie S. (2013a). Growth and Survival of Pearl Oyster Pinctada Maxima, Spat Reared Under Different Environmental Conditions. J. Shellfish. Res. 32 (3), 675–679. doi: 10.2983/035.032.0308
Deng Y., Shao F., Liang F., Xie S. (2013b). Effects of Stocking Density, Diet, and Water Exchange on Growth and Survival of Pearl Oyster Pinctada Maxima Larvae. Aquacult. Int. 21, 1185–1194. doi: 10.1007/s10499-013-9622-0
Du X. D., Fan G. Y., Jiao Y., Zhang H., Guo X. M., Huang R. L., et al. (2017). The Pearl Oyster Pinctada Fucata Martensii Genome and Multi-Omic Analyses Provide Insights Into Biomineralization. GigaScience. 8, 1–12. doi: 10.1093/gigascience/gix059
Galley T. H., Batista F. M., Braithwaite R., King J., Beaumont A. R. (2010). Optimisation of Larval Culture of the Mussel Mytilus Edulis (L.). Aquac. Int. 18, 315–325. doi: 10.1007/s10499-009-9245-7
Gervis M. H., Sims N. A. (1992). The Biology and Culture of Pearl Oysters (Bivalvia: Pteriidae). ICLARM Stud. Rev. Manila, Philippines. 49.
Gouda R., Kenchington E., Hatcher B., Vercaemer B. (2006). Effects of Locally-Isolated Micro-Phytoplankton Diets on Growth and Survival of Sea Scallop (Placopecten Magellanicus) Larvae. Aquaculture 259 (1-4), 169–180. doi: 10.1016/j.aquaculture.2006.03.050
Guedes A. C., Malcata F. X. (2012). Nutritional Value and Uses of Microalgae in Aquaculture. Aquaculture 10 (1516), 59–78. doi: 10.5772/30576
Gu Z. F., Wei H. J., Cheng F., Wang A. M., Liu C. S. (2020). Effects of Air Exposure Time and Temperature on Physiological Energetics and Oxidative Stress of Winged Pearl Oyster (Pteria Penguin). Aquacult. Rep. 17, 100384. doi: 10.1016/j.aqrep.2020.100384
Hall S. A., Méthé D., Stewart-Clark S. E., Clark K. F., Tremblay Fraser T. (2020). Comparison of absorption efficiency and metabolic rate between wild and aquaculture oysters (Crassostrea virginica). Aquacult. Rep. 16, 100263. doi: 10.1016/j.aqrep.2019.100263
Hamzah M. S., Nababan B., Nababan B. (2011). The Effect of Seasons and Depths on Growth and Survival Rate of Pearl Oyster (Pinctada Maxima) in Kodek Bay, North Lombok. J. Ilmu. Teknol. Kelaut. 3 (2), 48–61. doi: 10.29244/jitkt.v3i2.7821
Hao R. J., Wang Z. M., Yang C. Y., Deng Y. W., Zheng Z., Wang Q. H., et al. (2018). Metabolomic Responses of Juvenile Pearl Oyster Pinctada Maxima to Different Growth Performances. Aquaculture 491, 258–265. doi: 10.1016/j.aquaculture.2018.03.050
Hassan M. M., Parks V., Laramore S. (2021). Optimizing Microalgae Diets for Hard Clam, Mercenaria Mercenaria, Larvae Culture. Aquacult. Rep. 20, 100716. doi: 10.1016/j.aqrep.2021.100716
He G., Liu X., Xu Y., Liang J., Deng Y., Zhang Y., et al. (2021). Repeated Exposure to Simulated Marine Heatwaves Enhances the Thermal Tolerance in Pearl Oysters. Aquat. Toxicol. 239, 105959. doi: 10.1016/j.aquatox.2021.105959
Hwang J. J., Yamakawa T., Aoki I. (2007). Growth of Wild Pearl Oysters Pinctada Fucata, Pinctada Margaritifera and Pinctada Sugillata (Bivalvia: Pteriidae) in Taiwan. Fish. Sci. 73 (1), 132–141. doi: 10.1111/j.1444-2906.2007.01311.x
Ibarrola I., Arambalza U., Navarro J. M., Urrutia M. B., Navarro E. (2012). Allometric Relationships in Feeding and Digestion in the Chilean ytilids Mytilus chilensis (Hupé), Choromytilus chorus (Molina) and Aulacomya ater (Molina): A Comparative Study.J. Exp. Mar. Biol. Ecol.426–427, 18–27. doi: 10.1016/j.jembe.2012.05.012
Ibarrola I., Hilton Z., Ragg N. L. (2017). Physiological Basis of Inter-Population, Inter-Familiar and Intra-Familiar Differences in Growth Rate in the Green-Lipped Mussel Perna Canaliculus. Aquaculture 479, 544–555. doi: 10.1016/j.aquaculture.2017.06.031
Ivanina A. V., Falfushynska H. I., Beniash E., Piontkivska H., Sokolova I. M. (2017). Biomineralization-Related Specialization of Hemocytes and Mantle Tissues of the Pacific Oyster Crassostrea Gigas. J. Exp. Biol. 220 (18), 3209–3221. doi: 10.1242/jeb.160861
Kawai D. K. (1955). Carbonic Anhydrase in Pearl Oyster-Ii. Changes of the Enzyme Activity in Relation to Growth and Seasons, Publ. Seto Mar. Biol. Lab.5, 89–94, Publications of the Seto Marine Biological Laboratory.
Knuckey R. M., Brown M. R., Barrett S. M., Hallegr G. M. (2002). Effisolation of New Nanoplanktonic Diatom Strains and Their Evaluation as Diets for Juvenile Pacific Oysters (Crassostrea Gigas). Aquaculture 211 (1–4), 253–274. doi: 10.1016/S0044-8486(02)00010-8
Kolkovski S. (2001). Digestive Enzymes in Fish Larvae and Juveniles—Implications and Applications to Formulated Diets. Aquaculture 200 (1-2), 181–201. doi: 10.1016/S0044-8486(01)00700-1
Kvingedal R., Evans B. S., Lind C. E., Taylor J. J., Dupont-Nivet M., Jerry D. R. (2010). Population and Family Growth Response to Different Rearing Location, Heritability Estimates and Genotype×Environment Interaction in the Silver-Lip Pearl Oyster (Pinctada Maxima). Aquaculture 304 (1-4), 1–6. doi: 10.1016/j.aquaculture.2010.02.035
Labarta U., Fernández-Reiriz M. J., Navarro J. M., Velasco A. (2002). Enzymatic Digestive Activity in Epifaunal (Mytilus Chilensis) and Infaunal (Mulinia Edulis) Bivalves in Response to Changes in Food Regimes in a Natural Environment.Marine Biol. 140, 669–676.doi: 10.1007/s00227-001-0742-0
Leonardos N., Lucas I. A. N. (2000). The Nutritional Value of Algae Grown Under Different Culture Conditions for Mytilus Edulis L. Larvae. Aquaculture 182, 301–315. doi: 10.1016/S0044-8486(99)00269-0
Liang F., Xie S., Fu S., Li J., Deng Y. (2016). Growth Pattern and Biometric Relationship of Pearl Oyster Pinctada Maxima Cultured in Beibu Bay, China. J. Appl. Aquacult. 28 (2), 110–118. doi: 10.1080/10454438.2016.1172535
Li C. L., Liu E., Li T. Y., Wang A. M., Liu C. S., Gu Z. F. (2020). Suitability of Three Seaweeds as Feed in Culturing Strawberry Conch Strombus Luhuanus. Aquaculture 519, 734–761. doi: 10.1016/j.aquaculture.2019.734761
Linard C., Gueguen Y., Moriceau J., Soyez C., Hui B., Raoux A., et al. (2011). Calcein Staining of Calcified Structures in Pearl Oyster Pinctada Margaritifera and the Effect of Food Resource Level on Shell Growth. Aquaculture 313, 149–155. doi: 10.1016/j.aquaculture.2011.01.008
Liu C. S., Li X. B., Wu C. L., Wang A. M., Gu Z. F. (2020). Effects of Three Light Intensities on the Survival, Growth Performance and Biochemical Composition of Two Size Giant Clams Tridacna Crocea in the Southern China Sea. Aquaculture 528, 735548. doi: 10.1016/j.aquaculture.2020.735548
Lodeiros C. J., Freites L., Márquez A., Glem M. E., Guevara M., Saucedo P. E. (2017). Comparative Growth and Survival of Spat of the Caribbean Pearl Oyster, Pinctada Imbricata Cultivated Indoor With Microalgae Diets and Outdoor With Natural Diet. Aquacult. Nutr. 23 (3), 511–522. doi: 10.1111/anu.12419
Martínez-Fernández E., Acosta-Salmón H., Southgate P. C. (2006). The Nutritional Value of Seven Species of Tropical Microalgae for Black-Lip Pearl Oyster (Pinctada Margaritifera, L.) Larvae. Aquaculture 257, 491–503. doi: 10.1016/j.aquaculture.2006.03.022
Massault C., Jones D. B., Zenger K. R., Strugnell J. M., Barnard J. M., Jerry D. R. (2021). A SNP Parentage Assignment Panel for the Silver Lipped Pearl Oyster (Pinctada Maxima). Aquacult. Rep. 20, 100687. doi: 10.1016/j.aqrep.2021.100687
McDougall C., Moase P., Degnan B. M. (2016). Host and Donor Influence on Pearls Produced by the Silver-Lip Pearl Oyster, Pinctada Maxima. Aquaculture 450, 313–320. doi: 10.1016/j.aquaculture.2015.08.008
Medakovi´c D. (2000). Carbonic Anhydrase Activity and Biomineralization Process in Embryos, Larvae and Adult Blue Mussels Mytilus Edulis L. Helgol. Mar. Res. 54, 1–6. doi: 10.1007/s101520050030
Palais F., Dedourge G. O., Beaudon A., Pain D. S., Trapp J., Geffard O., et al. (2012). One-Year Monitoring of Core Biomarker and Digestive Enzyme Responses in Transplanted Zebra Mussels (Dreissena Polymorpha). Ecotoxicology 21 (3), 888–905. doi: 10.1007/s10646-012-0851-1
Pass D. A., Dybdahl R., Mannion M. M. (1987). Investigations Into the Causes of Mortality of the Pearl Oyster, Pinctada Maxima (Jamson), in Western Australia.Aquaculture 65, 2, 149–169. doi: 10.1016/0044-8486(87)90259-6
Prudence M., Moal J., Boudry P., Daniel J. Y., Quere C., Jeffroy F., et al. (2006). An Amylase Gene Polymorphism Is Associated With Growth Differences in the Pacific Cupped Oyster Crassostrea Gigas. Anim. Genet. 37 (4), 348–351. doi: 10.1111/j.1365-2052.2006.01481.x
Rico-Villa B., Le Coz J. R., Mingant C., Robert R. (2006). Influence of Phytoplankton Diet Mixtures on Microalgae Consumption, Larval Development and Settlement of the Pacific Oyster Crassostrea Gigas (Thunberg). Aquaculture 256, 377–388. doi: 10.1016/j.aquaculture.2006.02.015
Rosa M., Ward J. E., Holohan B. A., Shumway S. E., Wikfors G. H. (2017). Physicochemical Surface Properties of Microalgae and Their Combined Effects on Particle Selection by Suspension-Feeding Bivalve Molluscs. J. Exp. Mar. Biol. Ecol. 486, 59–68. doi: 10.1016/j.jembe.2016.09.007
Rueda J. L., Smaal A. C. (2004). Variation of the Physiological Energetics of the Bivalve Spisula Subtruncata (Da Costa 1778) Within an Annual Cycle. J. Exp. Mar. Biol. Ecol. 301, 141–157. doi: 10.1016/j.jembe.2003.09.018
Solorzano L. (1969). Determination of Ammonia in Natural Water by the Phenolhypochlorite Method. Limnol. Oceanogr. 14, 799–801. doi: 10.4319/lo.1969.14.5.0799
Strohmeier T., Strand Ø., Alunno-Bruscia M., Duinker A., Cranford P. J. (2012). Variability in Particle Retention Efficiency by the Mussel Mytilus Edulis. J. Exp. Mar. Biol. Ecol. 412, 96–102. doi: 10.1016/j.jembe.2011.11.006
Tamayo D., Ibarrola I., Urrutxurtu I., Navarro E. (2014). Physiological Basis of Extreme Growth Rate Differences in the Spat of Oyster (Crassostrea Gigas). Mar. Biol. 161, 1627–1637. doi: 10.1007/s00227-014-2447-1
Taylor J. J., Southgate P. C., Rose R. A. (2004). Effects of Salinity on Growth and Survival of Silver-Lip Pearl Oyster, Pinctada Maxima, Spat. J. Shellfish. Res. 23 (2), 375–378. doi: 61USC/12126121780002621
Tomaru Y., Udaka N., Kawabata Z. I., Nakano S. I. (2002). Seasonal Change of Seston Size Distribution and Phytoplankton Composition in Bivalve Pearl Oyster Pinctada Fucata Martensii Culture Farm. Hydrobiologia 481, 181–185. doi: 10.1023/A:1021232832390
Valenzuela-Espinoza E., Millan-Nunez R., Nunez-Cebrero F. (2002). Protein Carbohydrate, Lipid and Chlorophyll a Content in Isochrysis Aff. Galbana (Clone T-Iso) Cultured With a Low Cost Alternative to the F/2 Medium. Aquac. Eng. 25, 207–216. doi: 10.1016/S0144-8609(01)00084-X
Wang A., Deng F., Zhang X., Yan B., Zhang M. (2000). RAPD Analysis on genetic diversity of Pinctada martensii Dunker. Wuhan University J. 46, 467–470.
Wang Y. J., Li L. S., Hu M. H., Lu W. Q. (2015). Physiological Energetics of the Thick Shell Mussel Mytilus Coruscus Exposed to Seawater Acidification and Thermal Stress. Sci. Total. Environ. 514, 261–272. doi: 10.1016/j.scitotenv.2015.01.092
Ward J. E., Shumway S. E. (2004). Separating the Grain From the Chaff: Particle Selection in Suspension- and Deposit-Feeding Bivalves. J. Exp. Mar. Biol. Ecol. 300, 83–130. doi: 10.1016/j.jembe.2004.03.002
Yang S., Li X., Vasquez H. E., Wang A., Shi Y., Li J., et al. (2021). Comparative Profiling of Survival, Growth and Intestinal Microbial Community of Pearl Oyster Pinctada Maxima Juvenile in the Industrial Farming: The Feasibility of Live Microalga Instead by Pray-Dried Microalgae Powder. Front. Mar. Sci. 8. doi: 10.3389/fmars.2021.800627
Yang J. M., Luo S. J., Li J. H., Zheng Z., Du X. D., Deng Y. W. (2018). Transcriptome Analysis of Growth Heterosis in Pearl Oyster Pinctada Fucata Martensii. FEBS. Open Bio. 8, 1794–1803. doi: 10.1002/2211-5463.12502
Yukihira H., Klumpp D. W. (1999). Feeding Adaptations of the Pearl Oysters, Pinctada Margaritifera and P. Maxima, to Variations in Natural Particulates. Mar. Ecol. Prog. Series. 182, 161–173. doi: 10.3354/meps182161
Yukihira H., Klumpp D. W., Lucas J. S. (1998). Comparative Effects of Microalgal Species and Food Concentration on Suspension Feeding and Energy Budgets of the Pearl Oysters Pinctada Margaritifera and P. Maxima (Bivalvia: Pteriidae). Mar. Ecol. Prog. Ser. 171, 71–84. doi: 10.3354/meps171071
Yukihira H., Lucas J. S., Klumpp D. W. (2000). Comparative Effects of Temperature on Suspension Feeding and Energy Budgets of the Pearl Oysters Pinctada Margaritifera and P. Maxima. Mar. Ecol. Prog. Ser. 195, 179–188. doi: 10.3354/meps195179
Zhang X. Z., Ye B. C., Gu Z. F., Li M., Yang S. G., Wang A. M., et al. (2021). Comparison in Growth, Feeding, and Metabolism Between a Fast-Growing Selective Strain and a Cultured Population of Pearl Oyster (Pinctada Fucata Martensii). Front. Mar. Sci. 8. doi: 10.3389/fmars.2021.770702
Keywords: pearl oyster, microalgae, growth performance, physiological energetics, digestive enzymes, carbonic anhydrase
Citation: Ye B, Gu Z, Zhang X, Yang Y, Wang A and Liu C (2022) Comparative Effects of Microalgal Species on Growth, Feeding, and Metabolism of Pearl Oysters, Pinctada fucata martensii and Pinctada maxima. Front. Mar. Sci. 9:895386. doi: 10.3389/fmars.2022.895386
Received: 13 March 2022; Accepted: 20 June 2022;
Published: 19 July 2022.
Edited by:
Mohamed Salah Azaza, National Institute of Marine S cience and Technology, TunisiaReviewed by:
Walid Medhioub, Institut National des Sciences et Technologies de la Mer, TunisiaSabrine Boucetta, University of Annaba, Algeria
Copyright © 2022 Ye, Gu, Zhang, Yang, Wang and Liu. This is an open-access article distributed under the terms of the Creative Commons Attribution License (CC BY). The use, distribution or reproduction in other forums is permitted, provided the original author(s) and the copyright owner(s) are credited and that the original publication in this journal is cited, in accordance with accepted academic practice. No use, distribution or reproduction is permitted which does not comply with these terms.
*Correspondence: Chunsheng Liu, lcs5113@163.com
†These authors have contributed equally to this work