- 1Conservation Programs and Partnerships Department, Seattle Aquarium, Seattle, WA, United States
- 2Life Sciences Department, Seattle Aquarium, Seattle, WA, United States
- 3Hawaii Division of Aquatic Resources, Honokohau Marina, Kailua-Kona, HI, United States
- 4Marine Laboratory, California State Polytechnic University Humboldt, Arcata, CA, United States
Hawai′i coral reefs are essential ecosystems providing resources in the form of food and recreation as well as stabilizing nearshore biodiversity. The Seattle Aquarium has exhibited Hawai′i reef fishes and corals since the mid-1980s to educate guests about these critical ecosystems. In 2009, and in collaboration with Hawai′i’s Division of Aquatic Resources (DAR) and Washington State University, the aquarium expanded its conservation work in Hawai′i through annual surveying of eight reefs along the west coast of Hawai′i via SCUBA-based diver operated video (DOV). Five of the sites are in areas partially closed to most fishing while three sites are in areas partially open to most fishing. 100-meter DOV surveys took place a meter above a horizontal or vertical reef, and survey locations were marked with GPS and fixed underwater markings to enable annual surveys to occur in the same locations. Counts of fish species were subsequently made from the archived video. Over the 11 year dataset we documented increased total abundance at all sites and periods of increasing and decreasing species richness. Multivariate analyses comparing fish community structure before (2009-2012) and after an anomalous warm-water event (2013-2019) documented a persistent shift in community structure. This coincides with a documented marine heat wave in Hawai′i and associated coral bleaching events between 2013-2016. These results suggest that our long-term monitoring program captured a phase shift in community structure associated with changing environmental conditions. These persistent shifts may thus indicate hysteresis at relatively short temporal scales, and ongoing monitoring is required to observe whether the systems shift back to the pre-2013 community structure. As coral reef ecosystems face a multitude of stressors from warming waters to marine pollution, long-term monitoring programs are essential to illuminate trends that may inform conservation and management strategies to preserve these imperiled ecosystems.
Introduction
Coral reefs ecosystems are areas of high productivity and biodiversity in tropical and sub-tropical oceans. They are an important resource, providing critical habitat for many biologically and commercially important fish and invertebrate species with coral health thought to serve as indicators of ecosystem health (Marshall et al., 2019). The coral reefs off the west coast of the Big Island of Hawai′i (hereafter referred to as West Hawai′i) are some of the most diverse and productive in the main Hawaiian Islands and are also popular for many types of fishing and snorkel/dive tourism (Walsh et al., 2018). In addition, the global trade in fish for hobbyists has increased significantly since the early 1970s with west Hawaiian reefs being a primary source for the tropical marine ornamental fish trade (Young, 1997: Walsh, 2014).
In 1985, the state of Hawai′i recognized the potential negative impact of the removal of large numbers of fish on coral reef ecosystems and, in 1991, established a network of marine protected areas (MPAs) termed Fish Replenishment Areas (FRAs) prohibiting take for the ornamental fish trade in West Hawai′i (Tissot et al., 2004). These areas combined with previously established Fishery Management Areas (FMAs) and Marine Life Conservation Districts (MLCDs) closed 35.2% of West Hawai′i to aquarium collecting. Since 1999, the West Hawai′i Aquarium Project (WHAP) has been monitoring West Hawai′i reefs to document the effectiveness of the FRAs (Tissot and Hallacher, 2003; Tissot et al., 2004) and to measure the effect of the ornamental fish trade on reef ecosystems (Walsh, 2014). Results from previous monitoring of South Kohala’s Puakō FRA over approximately three decades documented many fish species declining over time (Walsh et al., 2018). The study compared data from reference underwater visual count (UVC) collected from 1979-1981 and data collected from 2007-2008 using similar methods (Walsh et al., 2018). Ninety percent of the 35 most abundant fish families such as Yellow Tang, Zebrasoma flavescens, and Achilles Tang, Acanthurus achilles, were found to have decreased during the two time periods (Walsh et al., 2018). The observed declines were thought to have been driven by anthropogenic stressors such as habitat decline associated with poor water quality from adjacent residential housing and climate change such as warm water events which lead to coral bleaching and resulting loss of quality reef habitat (Walsh et al., 2018). Additional monitoring of FRAs between 1999-2018 using similar methods documented that the FRAs in West Hawai′i were successful in increasing some fish species such as Yellow Tang, Zebrasoma flavescens, and Goldring Tang, Ctenochaetus Truncatus, since their creation by the Division of Aquatic Resources (DAR) in 1998 (Walsh et al., 2019). Since 2017, the courts have closed all take for the aquarium trade while subsistence and recreation fishing continue in West Hawai′i (Umberger, 2017).
Monitoring of reef fish has been the primary method to determine success of FRAs and MPAs in West Hawai′i and elsewhere, however the methods used are variable. The applicability and limitations of various techniques for estimating fish abundance, diversity and changes over time have been previously studied (Russell et al., 1978; Sale and Douglas, 1981; Brock, 1982; DeMartini and Roberts, 1982; Sale and Sharp, 1983; Kimmel, 1985; Sanderson and Solonsky, 1986; Bortone and Kimmel, 1991; Wilson et al., 2018). These techniques range from the use of traditional fishing gear (nets, traps, and hook-and-line) to non-invasive visual observations. Diver based underwater visual survey (UVS) techniques have been used to assess the abundance of reef fish since the 1950s (Brock, 1954; Odum and Odum, 1955; Estes and Gilbert, 1978; Marsh and Sinclair, 1989). The effectiveness of Hawai′i’s MPAs and FRAs have been measured primarily using UVS techniques. Accuracy of the visual techniques is affected by many variables such as visibility and currents as well as each diver’s familiarity with fish identification (Williams et al., 2006). Remote observation via underwater video cameras deployed from vessels or carried by divers has over time become an increasingly popular method for estimating fish abundance and distribution (Smith and Tyler, 1973; Alevizon and Brooks, 1975; Powles and Barans, 1980; Wilson et al., 2018).
The two most employed methods to monitor fish non-invasively have been UVS and diver operated video (DOV). The two methods have been found to be comparable when used to estimate fish abundance (Langlois et al., 2010; Wilson et al., 2018). The major disadvantage of UVS is the skill required of the diver on the survey to quickly identify fish to species and estimate numbers of fish accurately on a slate while conducting the dive (Wilson et al., 2018). Errors and inter-observer variability in estimates of fish diversity and abundance has been found to be high using this method and has implications for comparisons of datasets between different observers and over time and space (Harvey et al., 2004). Harman et al., 2003 and Westera et al., 2003 suggested the best method to eliminate variability between divers on a UVS was to have a diver swim an underwater video camera wearing a full-face mask with a microphone to record audio observations of fish onto the videotapes, thus combining some of the UVS and DOV aspects. In addition, other variables that remain constant over time such as surveys that are completed during the same time of day (e.g., between pre-defined hours of the day) at the same time of year, as well as consistency of divers and data collectors have been found to eliminate much of the variability (Edgar et al., 2004; Emslie et al., 2018). The major advantage of DOV is that it is easily repeatable, non-invasive, and provides a permanent record of the survey, which can be repeatedly viewed to verify data and may provide new information for future research and/or may be used for educational purposes. The major disadvantage of DOV transects is that water conditions may limit visibility, rugosity of structures that may limit fish detections and the considerable time spent extracting fish data from archived video imagery (Wilson et al., 2018). However even with these limitations, DOV along strip transects have proven to be, and are regarded as, a precise method of fish diversity and abundance estimation (Cappo and Brown, 1996; Cappo et al., 2003; Wilson et al., 2018).The Seattle Aquarium has exhibited Hawai′i reef fishes and corals since the mid-1980s to educate guests about these critical ecosystems. In 2009 the aquarium began 100 m DOV surveys in West Hawai′i in 2009 to expand the aquarium’s conservation and education work in the region. Here, we describe our unique method of replicated 100 meters (m) transects DOV coupled with an audio slate, as well as the initial eleven years of data collected on fish diversity and abundance on eight reef systems in West Hawai′i. Our questions were: 1) Were the 100 m DOV transects sufficient to document fish abundance, diversity and community structure over time? 2) Were replicate surveys necessary to capture community structure?
Methods
In 2009, the Seattle Aquarium, in cooperation with Hawai′i’s DAR and Washington State University, began conducting annual surveys of West Hawai′i coral reefs using DOV in four study regions inside and outside MPAs. The two MPAs were Puakō (a FMA that prohibited collection of aquatic life for aquarium purposes; sites 1, 2 and 5) and Old Kona Airport, OLD KONA, (a Marine Life Conservation District [MLCD] that prohibited all take; sites 3 and 4), two areas open to all extractive activities to the north of Puako, Māhukona (sites 6 and 7) and site 8 south of OLD KONA added later in 2014 which was removed from further analysis because of limited data (five years compared to eleven years) (Figure 1). Study sites were located within these areas to determine the relative effectiveness of the FRAs and MLCDs as well as to document changes in fish diversity and abundance over time (see Table 1 for site descriptions). Each site has unique features easily identifiable by the trained diver that make repeatable surveys possible such as a vertical coral reef or rock/lava shelf that is at least 100 m long (sites 1-5) or bottom features such as sand channels next to reefs (Sites 6) or old mooring chains (site 7) that are relatively unbroken for exact and repeatable routes to follow. All sites were surveyed in late January or early February between 2009-2019 with the following exceptions : Sites 6-7 have fewer years of data, nine compared to eleven, because we added them in 2010 and could not survey in 2015 because of prohibitive ocean conditions.
Diver Based Underwater Video Documentation
The method used was diver based underwater video documentation, DOV, along strip transects comparable to but also unique to those established in the late 1990s and early 2000’s in similar locations (Tissot and Hallacher, 2003; Tissot et al., 2004). Our surveys are unique in that the transects described here are longer (100 m vs 30 m) and use audio slates and cameras compared to diver handheld slates. Our surveys fall into the strip transect category, where two divers swim for a defined distance (100 m) counting all individuals within a strip of pre-determined width (fish are counted within 4-5 m of a forward-facing camera lens horizontally held in relation to the bottom contour representing a maximum volume of water ca. 30-58 cubic m in all dimensions of camera) using non-instantaneous fish counts from archived videos conducted after surveys. The goal was to use non-invasive DOV with full face masks and microphones to record audio fish identification, numerical fish counts and distance from the zero point onto the video acting as an archived audio slate (Harman et al., 2003; Westera et al., 2003; Harvey et al., 2004). The audio slate was used to assist in counting the fish but was not the sole method to document fish in that all fish counted were visually located off the archived video down to fish as small as 2.5 cm. This method allowed for counts of fish abundance and diversity annually and provided a permanent record of the reef for repeated analysis, future research, and educational purposes.
Sites were located each year using underwater still pictures documenting recognizable reef features (e.g., a mooring block, large rocks, unique coral assemblages, sand channel and/or mooring chains) and a GPS location collected at the water surface directly above the survey start location. When transects were conducted, a 4 lb. SCUBA weight attached to a buoyant marker approximately 1 m off the sea floor temporarily marks the center point of the survey. Dive teams begin the survey by attaching one end of the transect tape to a structure near the weighted line. The transects were conducted in 4 steps: Step 1: The divers swam 50 m in one direction away from the starting point along the reef face 1 m off the bottom and approximately 1 m from the reef deploying the transect tape and recording digital video. The videographer was positioned closest to the reef with the transect tape diver swimming alongside an additional meter away from the reef. The transect tape diver stayed behind the camera field of view of the camera lens and usually swam a shoulder length behind the video diver. All divers wore full face masks with communication systems allowing safety checks and discussion between divers as well as for the recording of audio observations of fish species on the digital video, similar to a slate transect except the documentation is orally on the tape. The transect-tape diver recorded fish noted during the dive and also stated an audible distance every 10 m along the tape (10m, 20m, 30m, 40m and 50m). Step 2: At 50 m from the start point, divers turned around and returned to the marker, continuing to count off every 10 m along the transect line collecting data. Step 3: Started once back at the marker, the divers continued the survey for another 50 m in the opposite direction along the reef. Step 4: At 50 m in this other direction the divers returned back to the marker. The complete transect was 100 m (two 50 m forward and two 50 m reverse) and took approximately 20 minutes to complete (Figure S1 for visual interpretation of method). This procedure allowed for two views of the reef face (forward and reverse) and allowed the data to be measured for changes in fish behavior such as attraction or avoidance due to the presence of divers or gear. Ideally a transect was duplicated during a single dive for two to three complete 100-meter transects, conditions and air supply dependent. The goal was to complete four 100-meter replicate transect samples per site per year (T1-T4) using up to two dive teams to capture schooling and cryptical fish species. Transects were separated by at least 20 minutes up to two to three days. Video transects were conducted at approximately the same time of year (late January into early February) and were conducted between 1000 and 1500 hours for optimal video quality and consistency of conditions (light and presence of diurnal fish).Lens combinations for all cameras and housings were selected to maximize the available field of view while still being able to identify fish. The underwater video camera system used between 2009-2011 was a Sony™ VX2100 mini DV camera housed in a Light and Motion™ Bluefin VX2100 Elite Travel Package. Accessories included a Light and Motion™ Bluefin Compact Monitor (VX2100) and a Light and Motion™ UWA 100° Wide Angle Lens. Underwater video camera systems used between 2012-2019 were a Sony αNEX 5N, α5000 or α6500 Digital camera using a Sony SEL16F28 16mm f2.8 (83° angle of view) in a Nauticam NEX 5N, NA-5000 or NA-A6500 underwater housing with a 4.33” fisheye port. The 100° accessory wide lens on the Light and Motion housing was used as the initial benchmark for field of view for this research. Furthermore, the aspect ratio of the recording format changed from 4:3 to 16:9 when the switch was made to the Sony α series cameras. Reducing the field of view from 100° to 83° most closely matched the performance of the original lens system used from 2009 to 2011. From 2012 to 2019 the camera lens and dome port specification has remained unchanged between the Sony α series cameras giving a consistent field of view on this video platform with no detectable effect on fish ids. Lasers or other reference tools were not used to attempt to size fish.
Underwater communications consisted of diver worn full facemasks equipped with a wireless transceiver and either a direct hardwire link to the camera housing (Bluefin) or a wireless receiver Ocean Technology Systems Inc. (OTS) RX-100 mounted external to the camera housing (Nauticam) for recording of diver audio. Masks were either Interspiro Divator MkII or OTS Guardian Full Face Masks (GFFM) equipped with OTS earphones and microphone. Divers wore OTS wireless transceivers (SSB-2010) to provide audio communication between divers and the camera system. The wireless transceivers were used in a voice-activated mode allowing hands-free communication between the divers and the camera recording system. This allowed each diver to manage either the video camera or transect tape without interrupting the survey process. Continuous recording of diver speech onto the video file enables diver observations to be made at the rate the diver can clearly speak. Underwater communications allowed for a more consistent rate of travel than conventional transect surveys as there was no need to stop swimming or look away from the survey area to record written data to a slate.A 100M fiberglass surveyor’s tape reel graded in metric units was used to measure distance. Direct drive reels without mechanical gearing were chosen to improve reliability and ease of operation underwater. Tapes were marked at 10 m increments with contrasting color labels to highlight the distance callout locations for the dive teams. One hundred meter tapes were chosen even though individual transect legs are only 50 m in length. This minimized the number of mechanical rotations needed when deploying and recovering the tapes by increasing the internal diameter of the spool. It also provided spare tape that could be used if the initial 50 m of the tape was damaged on a survey or if further distance was needed.
Post-Processing
Fish data was collected off archived video on a large screen monitor using commercially available video players (Windows Media Player, Power DVD, Quicktime Player and VLC Media player). Three person counting teams consisted of two staff with professional expertise on the identification of Hawai′i reef fish and one additional member to tally the counts. Counting teams were primarily comprised of the pool of divers that collected the data in the field and thus had some prior knowledge of the surveys. Only fish that were clearly identifiable to the species level on the video were included in the counts regardless of if it was called verbally on the archived footage. The audio counts and identification from divers in the field were used only to assist fish ID but were not used as the sole means to identify a fish. Accurate fish identification to the species level was a function of the size of fish and proximity to the camera with smaller species needing to be closer to the videographer for valid identification (such as damsel fish close to 2.5 cm) and larger fish easily identifiable much farther from the videographer (such as triggerfish). We pooled Longnose Butterflyfish as Forcipiger spp., to include both F. longirostris (Longnose Butterflyfish) and F. flavissimus (Forceps Fish) because of uncertainty in
To document the marine heat wave these reefs experienced during the study period, post 2013, we collected average sea surface temperature (SST) per site per year from Environmental data downloaded from publicly available datasets from NOAA. Hourly sea surface temperature (°C) data were downloaded from historical NOAA Tides and Currents, Kawaihae Station (No. 1617433), and averaged per year.
Species Abundance, Richness, and Diversity
Data from forward and reverse passes of transects were both tallied and used for initial statistical comparisons, which were found to be significantly different (One way ANOVA, DF=1, F=50.19, p<0.0001) with the forward view documenting more fish than reverse, thus data from the forward passes were retained for abundance, richness and multivariate visualizations and analyses. To visualize mean abundance and species richness, the four transects were averaged with a loess-smoother (via geom_smooth in the ggplot2 package (Wickham, 2016) with span=1 in R producing a site-average of the four transects across the 11-year time series. 95% confidence intervals were calculated to evaluate within-site variation among the four transects as well as among-site and -location variation in mean abundance and richness. The four replicate transects were retained in these analyses given the potential for encountering cryptical or schooling fish that any single transect might miss. Species-richness curves—the number of species encountered per additional individual sampled)—and species-accumulation curves—the number of species encountered per additional transect sampled—were calculated using the rarecurves and specaccum functions, respectively, in the vegan package (Dixon, 2003) for R.
Non-Metric Multidimensional Scaling (nMDS)
Multivariate analyses were performed to extract and visualize the predominate patterns of community structure using the vegan package (Dixon, 2004) in R. The sample unit was kept at the scale of the individual transect. To evaluate how one versus all four transects contrast in terms of the patterns of community structure captured, we conducted our nMDS analysis on two data frames: one containing observations of all four transects (T1-T4), and another containing only transect 1 (T1), the first pass of the forward data for each 100m transect. This one- versus four-transect contrast enabled us to evaluate whether four transects were necessary to capture the cryptical and schooling species that the video and derived data that anyone transect may miss. Species were retained for multivariate analyses if their frequency of occurrence (regardless of abundance) exceeded 2% along the 261 transects of the T1-T4 data frame this reduced the community matrix from 115 to 81 species (Figure S2). All three 2017 site 5 transects were dropped due to inclement sea conditions and the resulting poor video/data quality. Abundances in the 81-dimensional community matrix were retained at the natural scale; a log base-10 transformation was explored, but nMDS stress increased beyond acceptable levels (see Results). Given the tradeoff between (1) un-transformed data where highly abundant species may drive dissimilarity matrix/ordination patterns versus (2) transformed data with a high stress ordination (indicating a mismatch between the original 81-dimensions and subsequent 2-dimensional ordination), we accepted the potential for the former to retain a more interpretable (lower stress) ordination. We used the Bray-Curtis distance metric to calculate a dissimilarity matrix for our n = 261 rows (T1-T4) and n = 72 rows (T1 only), and the metaNDS function in the vegan package to perform nMDS analysis.
PERMANOVA and Homogeneity of Dispersion
To test for significant differences in the position of the centroids comprising groups of sample units (transects grouped by, e.g., site, location), we performed PERMANOVA (Permutational Multivariate Analysis of Variance) via the adonis function in the vegan package. PERMANOVA’s pseudo-F statistic can be significant due to “true” differences in centroid position among groups, or due to differences in the variance of point-spread (dispersion) among groups. To test homogeneity of dispersion among groups, we used the betadisper function in vegan, followed by anova. PERMANOVA was used to evaluate centroid differences with the T1-T4 data only, as the T1 only data contained comparatively fewer points.
To test for group differences i.e., distinct arrangements of community structure among the three overarching locations—Puakō, Old Kona, and Māhukona—we used PERMANOVA with a model where location was a factor. Within each of the three locations, we separately tested an additive model with site, transects nested within site (and thereby transect permutations generating a nonparametric p-value occurred only within-site), and a grouping variable—PrePost (also nested within site)—that designated transects as pre- or post-2013, i.e., before and after the anomalous warming and coral bleaching event. This model allowed us to sequentially evaluate the extent to which (1) sites differed within a location, (2) transects differed within a site, and (3) grouping sample units within a site based on year in relation to the warming event all contributed (or not) to distinct point-clusters (distinct centroid positions).
Results
Abundance, Richness, and Diversity
A total of 261 100 m transect surveys were conducted along the reefs of west Hawai′i, with 104,415 individuals observed across 115 different fish species (See Table 1 for surveys descriptions, surveys per site and depth range and Figure S2 for the 81 species documented from archived video that were included in the multivariate analyses). Fish were documented only if they could be visually located on the video. The audio slate made by divers taking the video was used to assist in the documentation of fish but was not used as the sole method to identify species.
Fish species showed shifts in abundance across years, and many were generally trending upwards over the study time period (Figure 2A). Broadly, abundances were highest at Old Kona and Puakō, and lowest at Māhukona (Figure 2A). Starting in 2013 there was a sustained increase in abundance across all sites with a spike in abundance in 2016 (Figures 2A, 5). During 2009-12, community structure was dominated by a number of highly abundant species at most sites, with Blackfin Chromis, Chromis vanderbilti, Yellow Tang, Zebrasoma flavescens, Lavender Tang, Acanthurus nigrofuscus, Goldring Tang, Ctenochaetus strigosus, Niger trigger, Odonus niger, and Agile Chromis, Chromis agilis, the most common species, making up 73% of overall abundances across years (Figure 5). During subsequent shifts in composition starting in 2013, except for the Goldring Tang, Ctenochaetus strigosus, and Blackfin Chromis, Chromis vanderbilti, these same species increased in abundance in the community and were joined by Yellowstripe Goatfish, Mulloidichthys flavolineatus, Hawaiian Sargent Major, Abudefduf abdominalis, and Agile Chromis, Chromis agilis, (Figure 5). For species comprising < 1% of total abundance, abundances after 2013 were nearly twice as large compared to the 2009-12 baseline. Species exhibiting this pattern were Sailfin Tang, Zebrasoma veliferum, Ringtail Surgeonfish, Acanthurus blochii, Bullethead Parrotfish, Chlorurus sordidus, Arc-eye Hawkfish, Paracirrhites arcatus, Blackside Hawkfish, Paracirrhites forsteri, Blue Goatfish, Parupeneus cyclostomus, Ornate Wrasse, Halichoeres ornatissimus, and Cornetfish, Fistularia commersonii, (Figure S2).
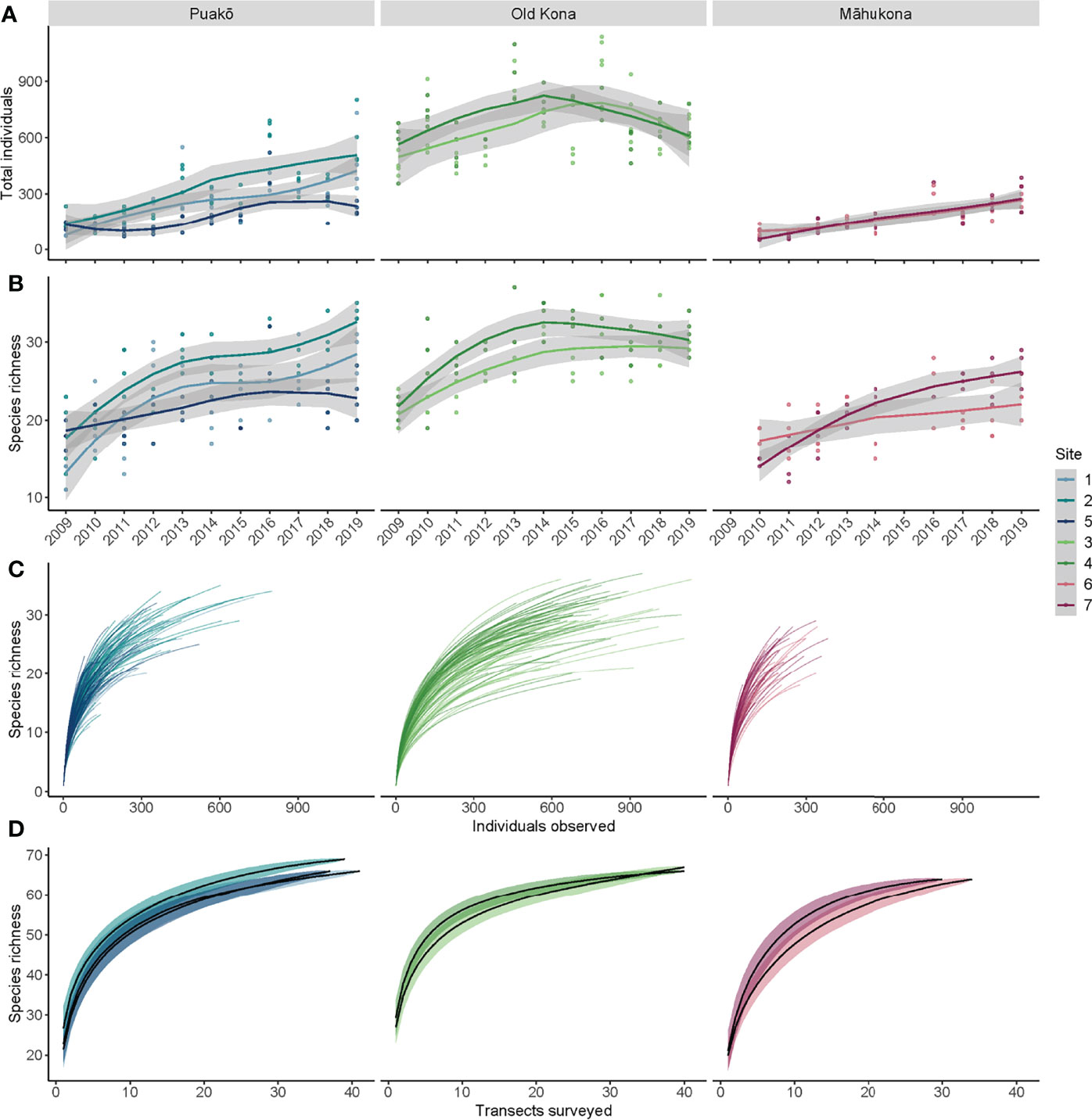
Figure 2 (A) Total individuals sampled per transect (dots) across the time series. The solid lines in (A, B) are loess-smoothed (span=1) via geom_smooth in ggplot2 per site, with gray shaded 95% confidence intervals. (B) Species richness per transect (dots) once more loess-smoothed per site. (C) Species richness curves—the relationship between the numbers of species versus individuals sampled—via rarecurves in the vegan package for R. Each line is a transect (colored by site and grouped by location) that has been rarefied (within a row) to calculate the expected species richness as additional individuals are sampled. The different line lengths reflect the different abundances sampled per transect. (D) Species accumulation curves (also known as species-area or species-effort curves)—the relationship between the numbers of species (the accumulation of species) versus the number of transects sampled—via specaccum in the vegan package for R following Coleman et al. (1982). All transects within a site were grouped (i.e., all within-site transects across time were grouped) for these calculations. Transects were added in random order within specaccum with n=1000 permutations. The shaded confidence intervals are +/- 1 standard deviation.
Site-averaged species richness likewise trended upwards throughout the time series. Puakō and Old Kona exhibited the highest richness and also exhibited differences between-sites within location (non-overlapping 95% confidence intervals and 1 standard deviation confidence intervals in Figures 2B, D, respectively). The species richness curves (Figure 2C) indicate the 100m transects adequately captured the asymptotic relationship between individuals counted versus new species recorded. This asymptotic relationship is most visible at Old Kona and also Puakō, and least so at Māhukona, where there were too few individuals present to produce a comparable asymptotic relationship between individuals counted and increases in richness (Figure 2C). The slope of the species richness curves provides an indication of the appropriateness of the 100 m scale of sampling and an additional metric by which to contrast fish communities among-sites and -locations. The steep slope of the species accumulation curves (or species-area curves) indicate there is a substantial gain in species richness when conducting one versus four—or more—replicate transects, with the asymptotic rise occurring after approximately 10 transects (Figure 2D).
Average sea surface temperature was similar at all sites thus they sites were combined in Figure S3. Annual average temperatures started to increase in 2010 and 2011 but then rose dramatically after 2013 with a peak in 2015 and remained sustained high above the 2009-2012 baseline (Figure S3).
Multivariate Analyses
Both analyses with 261 (T1-T4) and 72 (T1 only) transect sample units converged upon a 2-dimensional ordination of sample units in species-space, with stress = 0.15 for both the T1-T4 (Figure 3A) and T1-only analysis (Figure 3B). When testing a log base-10 transformation (on the T1-T4 data), stress increased to 0.21, and caution is warranted when interpreting ordinations with stress > 0.20 (Kruskal, 1964). Overall, for the T1-T4 data, the three locations—Puakō, Māhukona, and Old Kona—exhibit distinct clusters within the nMDS ordination: PERMANOVA (pseudo F2,258 = 75.3, R2 = 0.368, p-value < 0.001) (Figure 3A). Within each location, the PrePost warm water event starting in 2013 was significant (Figure 4), indicating separate point clusters, i.e., distinct patterns of community structure before and after the warming event (the three locations are individually elaborated upon below).
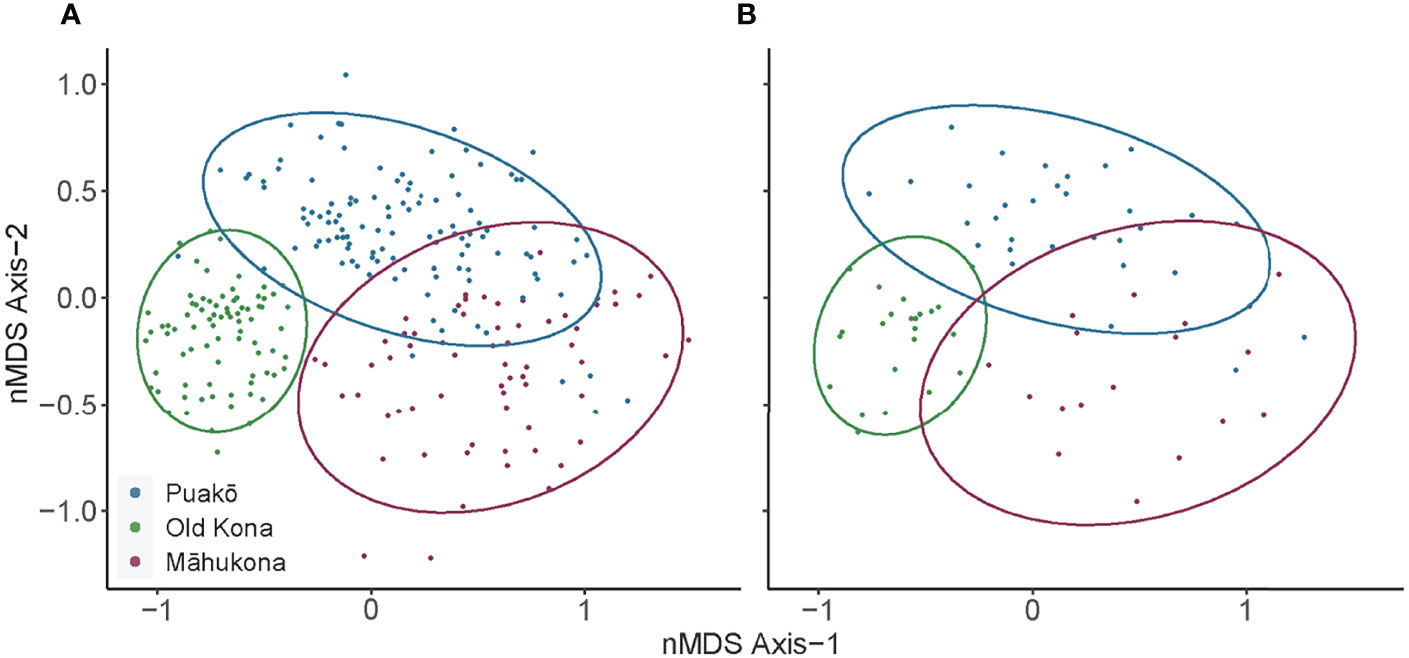
Figure 3 (A) The n-261 sample unit (T1-T4) 2-dimensional nMDS ordination grouped by the three locations: Puako, Old Kona, and Mahukona. Dots (the sample unit) represent individual transects (stress=0.15, Bray-Curtis dissimilarity metric). Ellipses produced in ggplot2 (Wickham, 2016) using methods from Fox and Weisberg (2011). Old Kona forms a cluster apart from both Puako and Mahukona, who share some overlap. (B) The n-72 sample unit (T1 only) 2-dimensional nMDS ordination, also grouped by the three locations. The strong concordance between (A, B) indicate that the T1 only data captured the same broad patterns of community structure relative to the T1-T4 data.
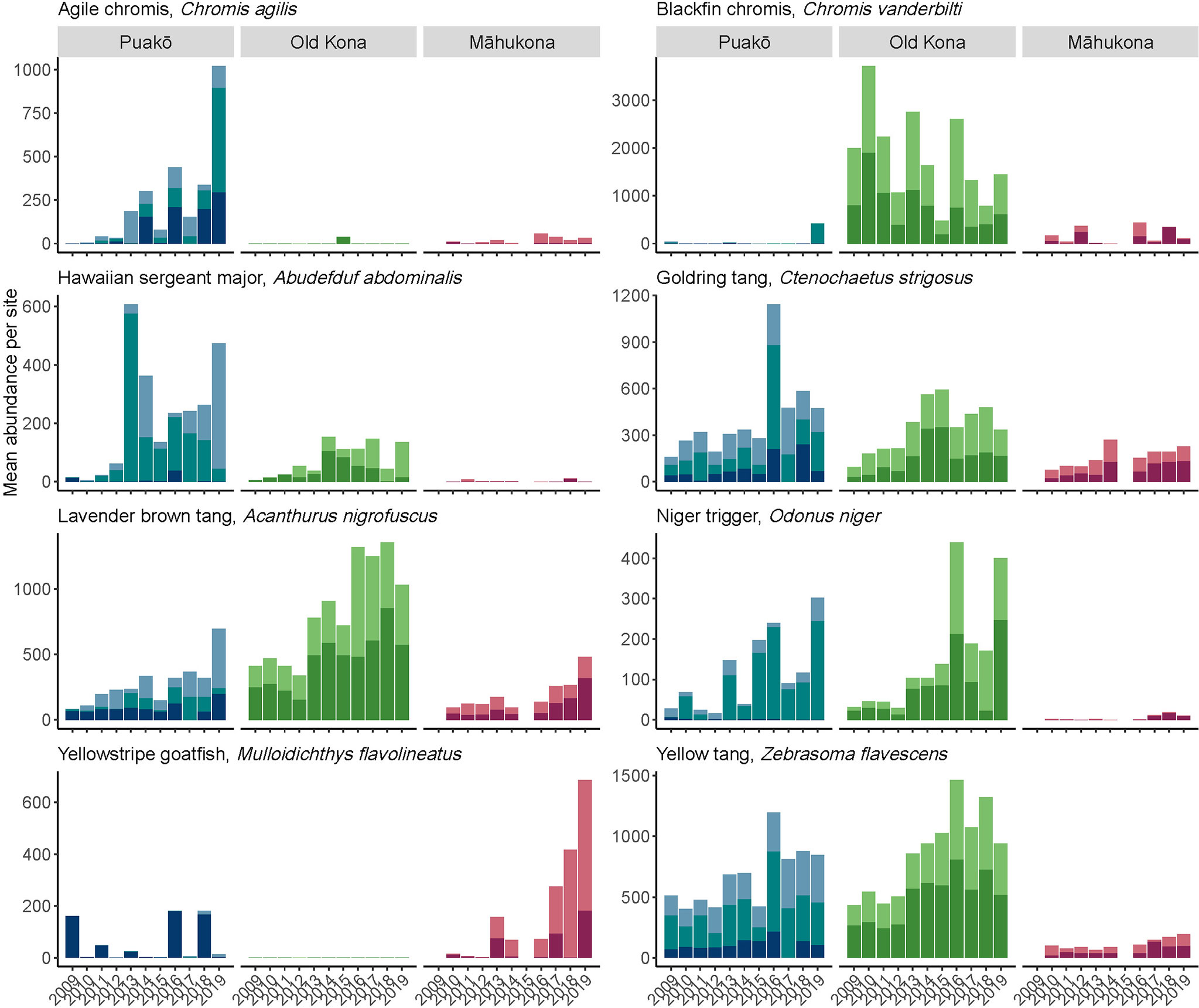
Figure 4 Column 1: the nMDS ordination of each site using all four transects. Column 2: the nMDS ordination using just T1. In both rows the PrePost factor was visualized with color, designating sample as prior-to (blue) or after (red) the anomalous heat event that started in 2013. All Puakõ sites shifted towards the left in species-space (A–C, H–J), Old Kona exhibited the least amount of change in community structure, i.e., it exhibited tight point clusters across a relatively small area of species-space (D, E, K, L). While Mãhukona exhibited several differences relative to Puakõ and Old Kona. At Site 6, no change in community structure was visibly associated with the warming event (F, M). At Site 7 however there were large fluctuations in community structure pre-2013 (in a part of species-space shared by Puakõ’s Site 5), before shifting to the left in species-space whereupon the large fluctuations in community structure largely ceased (G, N).
The four transects within a site per year did not significantly differ (Figure S4). The T1 only data exhibited very similar patterns of community structure compared to the T1-T4 data (Figure 4 column 2, H-N and A-G respectively). While the T1 only data lacked an appropriate sample size for PERMANOVA, coloring the sample units by pre- and post-2013 increase in temperature reveals nearly identical changes in ordination space relative to the T1-T4 data (Figures 4H–N). We thus conclude that a single transect captures the broad patterns of community structure distilled by nMDS analysis.Puakō, Old Kona, and Māhukona formed distinct clusters visualized with ellipses for both the T1—T4 and T1 only data (Figures 3A, B respectively). Puakō and Old Kona exhibit very little overlap, whereas Puakō and Māhukona exhibit substantial overlap in community structure. The PERMANOVA analysis comparing T1-T4 data for Puakō, Old Kona, and Māhukona was significant (pseudo F2,258 = 75.3, R2 = 0.37, p-value < 0.001), though so was the analysis of dispersion (F2,258 = 72.8, p-value < 0.001). This indicates that while the centroids comprising the three locations significantly differ, so too does point dispersion, and thus the latter may have contributed to the significance of the former. This result however is unsurprising given that Old Kona exhibits much less dispersion, i.e., smaller changes in community structure that produced a tighter point cluster, relative to the broader shifts (larger dispersion) exhibited by Puakō and Māhukona.
Sites 1 and 2 at Puakō exhibited similar patterns of community structure, whereas Site 5 often occupied a distinct region positively associated with axis-1 (the x-axis) and negatively associated with axis-2 (the y-axis) (Figures 4A–C, H–J). This latter region was predominantly observed prior to the warming event, after which (i.e., post-2013) all Puakō sites shifted towards the left in species-space. In the PERMANOVA model for Puakō, site was significant (pseudo-F2,102 = 17.6, R2 = 0.20, p-value < 0.001), transect was not (pseudo-F9,102 = 0.55, R2 = 0.03, p-value = 0.99), and PrePost was significant (pseudo-F3,102 = 11.2, R2 = 0.19, p-value < 0.001). Dispersion was marginally significant within Puakō (F1,115 = 4.2, p-value = 0.04).Old Kona exhibited the least amount of change in community structure, i.e., it exhibited tight point clusters across a relatively small area of species-space (Figures 4D, E, K, L). Given the observed changes at the other two locations—and changing environmental conditions overlapping with the monitoring—Old Kona appears to be the most resilient of the three locations to changes in fish community structure pre and post warming event. That being said, both sites exhibited a small but persistent shift upwards i.e., positively associated with axis-2, post-2013 (Figures 4D, E, K, L). These shifts are reflected in the PERMANOVA analysis, with site significant (pseudo-F1,70 = 13.6, R2 = 0.10, p-value < 0.001), transect not significant (pseudo-F6,70 = 0.82, R2 = 0.038, p-value = 0.68), and PrePost significant (pseudo-F2,70 = 21.1, R2 = 0.32, p-value < 0.001). Dispersion did not significantly differ between the two Old Kona sites when partitioned by PrePost (F1,78 = 0.23, p-value = 0.63), thus we confidently conclude that the differences in point-spread colored by PrePost are indeed due to differences in centroid position. That is to say, fish community structure exhibited a statistically significance change post-2013. We can further derive from the ordination that this community shift has not reversed.
The portion of the ordination containing Māhukona exhibited several differences relative to Puakō and Old Kona. At Site 6, no change in community structure was visibly associated with the warming event (Figures 4F, M). Site 7 however exhibits large fluctuations in community structure pre-2013 (in a part of species-space shared by Puakō’s Site 5), before shifting to the left in species-space whereupon the large fluctuations in community structure largely ceased (Figures 4G, N). In the PERMANOVA model, site was significant (pseudo-F1,54 = 5.59, R2 = 0.07, p-value < 0.001), transect was not (pseudo-F6,54 = 0.81, R2 = 0.06, p-value = 0.78), and PrePost was significant (pseudo-F2,54 = 9.8, R2 = 0.23, p-value < 0.001). Dispersion did not significantly differ when comparing the groups of PrePost (F1,62 = 0.34, p-value = 0.56).
Discussion
This project employed a unique DOV method that was successful in quantitative and qualitative documentation of fish abundance, diversity and community structure over time. The method employed here resulted in data collection that was independent of the observers’ perceptions or limitations during the dive which is common in UVS but was also limited by the cameras ability to capture fish species based on underwater visibility and cryptical fish. Post processing of the archived video to count fish assisted by the audio slate resulted in all identifiable fish counted off the video down to approximately 2.5 cm, not only the index species, those that are unusual in occurrence or number or that are easily observable during the dive, which tends to be typical with UVS. The 100 m transects were robust enough to capture species richness. However, this gain in richness from one to four transects per site per year contrasts with our finding (discussed below) that a single transect adequately captures the broad patterns of community structure. This implies a tradeoff between survey effort and obtaining general patterns of community structure versus a more comprehensive snapshot of species richness.
Transect replicates were repeatable and resulted in non-significant differences within sites per year (Figures 3B, 4, S4). The technique employed here used multiple (up to 4) replicates of the survey sites each year to capture schooling, cryptical and rare fish species moving throughout the transect (Figure S4 nMDS ordination showing each replicate transect). Although this replication of transects within sites did result in greater resolution in the nMDS ordination it was not necessary to capture community structure or community shifts which were clearly identified in the first transect (T1) alone (Figure 3B, 4H–N, S4).There were significant differences between areas, most notably between the northern areas, Māhukona and Puakō, and the southern area, Old Kona (Figure 3). Both of the northern areas were under different management with Māhukona open to aquarium collections until 2017, lay net use and spearfishing and Puakō open to spear fishing only. The most protected site over time was Old Kona which had the most fish species documented, the highest species diversity and richness and was the most stable or resilient over time (Figures 3, 4D, E, K, L).Shifts in species abundance started most notably in 2013 with increases in Blackfin Chromis, Agile Chromis, Chromis agilis, Yellow tang, Zebrasoma flavescens, Goldring Tangs, Ctenochaetus strigosus, Lavender Tangs, Acanthurus nirofuscus, Niger Trigger, Melichthys niger, and Hawaiian Sargent Majors, Abudefduf abdominalis, as well as a number of rarer species (Figure 5, S2). These trends are similar to WHAP data from 1999 to 2018 showing increasing populations of Yellow tang, Zebrasoma flavescens, and Goldring Tangs, Ctenochaetus strigosus, in both FRAs and MPA, and could be the result of the fish recruitment in 2014 which is when we start to see community changes (Walsh et al., 2019). The fish trends documented here are opposite of what was found in the earlier survey dataset collected in 1979-1981 and 2007-2008 using similar methods documenting 90% declines in the 35 most abundant fish families including Yellow Tang, Zebrasoma flavescens, in Puakō (Walsh et al., 2018). However, this earlier dataset and the dataset presented here are not directly comparable as the earlier dataset used 50 m transects rather than 100 m transects, used UVS techniques rather than the DOV, surveyed six different habitat zones and were conducted over different time periods with this study starting just after the 2007-2008 surveys. The six different zones in the earlier study were from inshore to offshore: Surge Zone, Shallow, Coral Rich Zone, Shallow, Basalt reef platform, Cliff Base Zone, Deep, Coral Rich Zone and Sand-Coral Interface Zone with some zones (e.g., nearshore) likely more affected by some types of anthropogenic impacts, e.g. water quality (Tissot et al., 2004). The dataset presented here surveyed in the area most closely aligned to the Cliff Base Zone and the trends follow the later WHAP dataset results clearly showing increases in many species over time that may be due to a combination of factors the likely include active management, environmental factors and recruitment events (Walsh et al., 2018).
One of the most interesting results in this dataset was the movement of sites through two-dimensional ordination space indicating a shift in community structure starting in 2013 (Figure 4). This coincides with a documented marine heat wave in Hawai′i and associated coral bleaching events between 2013-2016 (Kramer et al., 2016; Coral Bleaching Recovery Plan, 2017). These results suggest that our novel 100 m DOV method was suitable for documenting community structure that was likely driven by changing environmental conditions (Scheffer et al., 2001; Dudgeon et al., 2010; Bestelmeyer et al., 2011). Notably, once water conditions slightly cooled post-2018, community structure did not reverse completely to 2012 ordination space. This indicates hysteresis at relatively short temporal scales (Van de Leemput et al., 2016). All the reef systems monitored responded similarly to the marine heatwave event starting in 2013 and peaking in 2016 (Figure S3) regardless of management regulations (open, semi-open or closed). However even in this alternate state many fish species increased after 2012, primarily herbivores and planktivores, and have remained high. This increase is likely the result of increased algae on these reef systems post warming waters and coral bleaching (Morais et al., 2020; Olsen et al., 2022 in review). Continued long term monitoring is required to document full recovery or continued shifts in state in these reef systems.
Conclusion
The novel DOV survey presented here was able to document changes in fish abundance, diversity, richness and community structure over time. Multiple replicates of transects were done, up to 4 separated by at least 20 minutes, to capture the cryptical fish through the transect. Multivariate Non-metric multidimensional scaling (nMDS) suggests that although the four replicate transects resulted in greater resolution in the nMDS ordination it was not necessary to capture community structure or community shifts which were clearly identified in the first transect (T1) alone, thus one transect per site is sufficient to capture community changes. The dataset reported here suggests that the coral reef fish communities were heavily perturbed by the marine heat wave, and that this altered state persists at present. Whether this phase shift persists or eventually reverses to a pre-2013 community state requires ongoing monitoring. The results presented here also highlights the power of long-term datasets. We did not see shifts in systems until 2013, four years into the study, and we have yet to document a shift back after 11 years of the study. In the face of climate change, we do not know how often these stressors such as marine heat waves will affect these coral reef ecosystems. Nor do we know how these systems will react to repeated pressures, or whether the “disturbed” state will become the new normal. Only consistent monitoring over time will show us what the future holds for these reef ecosystems, but the data here suggest that there is a consistent response in these systems regardless of their location or management in West Hawai′i.
Data Availability Statement
The datasets analyzed for this study can be found in Github. https://github.com/zhrandell/Seattle_Aquarium_Hawaii_coral_reef_fish_monitoring.
Ethics Statement
Written informed consent was obtained from the individual(s) for the publication of any potentially identifiable images or data included in this article.
Author Contributions
SL: conceptualization, data curation and acquisition, formal analysis, funding acquisition, investigation, methodology, project administration, resources, supervision, validation, visualization, writing-original draft, writing-review, and editing. JC: conceptualization, data acquisition, formal analysis, investigation, methodology, resources, validation, visualization, writing-original draft, writing-review, and editing. AO: data acquisition, formal analysis, resources, writing-original draft, writing-review, and editing. WW: conceptualization, resources, writing-original draft, writing-review, and editing. CT: resources, writing-original draft, writing-review, and editing. BT: conceptualization, resources, methodology, formal analysis, writing-original draft, writing-review, and editing. ZR: resources, methodology, formal analysis, writing-original draft, writing-review, and editing. All authors contributed to the article and approved the submitted version.
Funding
This research was funded by the Seattle Aquarium, the Foley Frischkorn Conservation Fund, and the FY16 Coral Reef Conservation Program, Domestic Coral Reef Conservation Grants, Award # NA16NOS4820053.
Conflict of Interest
The authors declare that the research was conducted in the absence of any commercial or financial relationships that could be construed as a potential conflict of interest.
Publisher’s Note
All claims expressed in this article are solely those of the authors and do not necessarily represent those of their affiliated organizations, or those of the publisher, the editors and the reviewers. Any product that may be evaluated in this article, or claim that may be made by its manufacturer, is not guaranteed or endorsed by the publisher.
Acknowledgments
Special thanks go to the Seattle Aquarium’s Conservation Programs and Partnerships and Life Sciences Teams (Tim Carpenter, Andrew Sim, Alan Tomita, Bryan McNeil, Joel Hollander, Kaela Wuesthoff, Chris VanDamme) for collecting the data and analyzing many video hours. Thanks to Pete McCormick and Mark and April Johnston for donated boat use and captain services. Thanks to Dom and Marie Addario, Mike Budd and Karen Anderson for providing housing.
Supplementary Material
The Supplementary Material for this article can be found online at: https://www.frontiersin.org/articles/10.3389/fmars.2022.892261/full#supplementary-material
Supplementary Figure 1 | A visual interpretation of the how we conducted the surveys. Divers (camera operator and reel diver) start at a unique spot on the benthos and swim along reef face or channel for a repeatable strip transect route. Step 1: Divers swim forward away from the start 50 m in one direction filming. Step 2: divers turn around to return to start point filming the reverse direction. Step 3: Divers then swim 50 m forward in the opposite direction filming. Step 4: Diver turn around and then swim back to start point filming the reverse. The total transect is 100 m in the forward direction and 100 m in the reverse direction. This is then repeated within either 20 minutes or a few days up to four times to capture rarely seen and cryptic fish species.
Supplementary Figure 2 | All 81 fish species comprising our multivariate analyses. Transects were averaged to produce a mean estimate at the site level.
Supplementary Figure 3 | Sea Surface Temperature (SST) downloaded from publicly available datasets from NOAA. Hourly sea surface temperature (°C) data were downloaded from historical NOAA Tides and Currents, Kawaihae Station (No. 1617433), and averaged per site per year. All sites had similar temperature data thus all sites were combined.
Supplementary Figure 4 | nMDS ordination with all four transects. None were significantly different thus we conclude that T1 is sufficient to capture community structure.
References
Alevizon W. S., Brooks M. G. (1975). The Comparative Structure of Two Western Atlantic Reef Fish Assemblages. Bull. Mar. Sci. 25, 482–490.
Bestelmeyer B. T., Ellison A. M., Fraser W. R., Gorman K. B., Holbrook S. J., Laney C. M., et al. (2011). Analysis of Abrupt Transitions in Ecological Systems. Ecosphere 2 (12), 129. doi: 10.1890/ES11-00216.1
Bortone S. A., Kimmel J. J. (1991). “Environmental Assessment and Monitoring of Artificial Habitats”, in Artificial Habitat for Marine and Freshwater Fisheries. Eds. Seaman W. Jr., Sprague L. M. (New York, NY: Academic Press), 177–236.
Brock V. E. (1954). A Preliminary Report on a Method of Estimating Reef Fish Populations. J. Wild. Manage. 18, 297–308. doi: 10.2307/3797016
Brock R. E. (1982). A Critique of the Visual Census Method for Assessing Coral Reef Fish Populations. Bull. Mar. Sci. 32, 269–276.
Cappo M., Brown I. W. (1996). Evaluating of Sampling Methods for Reef Fish Populations of Commercial and Recreational Interest (Townsville: CRC Reef Research Centre Ltd.). Technical Report No. 6.
Cappo M. A., Harvey E., Malcolm H., Speare P. (2003). “Potential of Video Techniques to Monitor Diversity, Abundance and Size of Fish in Studies of Marine Protected Areas,” in Aquatic Protected Areas-What Works Best and How do We Know, Queensland, University of Queensland vol. 1. , pp.455–pp.464.
Coleman B. D., Mares M. A., Willis M. R., Hsieh Y. (1982). Randomness, Area and Species Richness. Ecol. 63, 1121–1133. doi: 10.2307/1937249
Coral Bleaching Recovery Plan (2017). Available at: https://dlnr.hawaii.gov/dar/files/2017/04/Coral_Bleaching_Recovery_Plan_final.pdf.
DeMartini E. E., Roberts D. (1982). An Empirical Test of Biases in the Rapid Visual Technique for Species-Time Censuses of Reef Fish Assemblages. Mar. Biol. 70, 129–134. doi: 10.1007/BF00397676
Dixon P. (2003). VEGAN, a Package of R Functions for Community Ecology. J. Vegetation. Sci. 14, 927–930. doi: 10.1111/j.1654-1103.2003.tb02228.x
Dudgeon S. R., Aronson R. B., Bruno J. F., Precht W. F. (2010). Phase Shifts and Stable States on Coral Reefs. Mar. Ecol. Prog. Ser. 413, 201–216. doi: 10.3354/meps08751
Edgar G. J., Barrett N. S., Morton A. J. (2004). Biases Associated With the Use of Underwater Visual Census Techniques to Quantify the Density and Size-Structure of Fish Populations. J. Exper. Mar. Bio. Ecol. 308 (2), 269–290. doi: 10.1016/j.jembe.2004.03.004
Emslie M. J., Cheal A. J., MacNeil M. A., Miller I. R., Sweatman H. P. (2018). Reef Fish Communities are Spooked by Scuba Surveys and may Take Hours to Recover. Peer. J., 6, e4886. doi: 10.7717/peerj.4886
Estes J. A., Gilbert J. R. (1978). Evaluation of Aerial Survey of Pacific Walruses (Odobenus Rosmarus Divergens). J. Fish. Res. B Can. 35, 1130–1140. doi: 10.1139/f78-178
Harman N., Harvey E., Kendrick G. A. (2003). Differences in Fish Assemblages From Different Reef Habitats in Hamelin B, South-Western Australia. Mar. Fresh. Res. 54, 177–184. doi: 10.1071/MF02040
Harvey E., Fletcher D., Shortis M. R., Kendrick G. A. (2004). A Comparison of Underwater Visual Distance Estimates Made by Scuba Divers and a Stereo-Video System: Implications for Underwater Visual Census of Reef Fish Abundance. Mar. Fresh. Res. 55, 573–580. doi: 10.1071/MF03130
Kimmel J. (1985). A New Species-Time Method for Visual Assessment of Fishes and its Comparison With Established Methods. Environ. Biol. Fishes. 12, 23–32. doi: 10.1007/BF00007707
Kramer K., Cotton S., Lamson M., Walsh W. (2016). “Bleaching and Catastrophic Mortality of Reef-Building Corals Along West Hawai′i Island: Findings and Future Directions,” in Proceedings of the 13th International Coral Reef Symposium, Honolulu, 229–241.
Kruskal J. B. (1964). Multidimensional Scaling by Optimizing Goodness of Fit to a Nonmetric Hypothesis. Psychometrika. 29, 1–27. doi: 10.1007/BF02289565
Langlois T. J., Harvey E. S., Fitzpatrick B., Meeuwig J. J., Shedrawi G., Watson D. L. (2010). Cost-Efficient Sampling of Fish Assemblages: Comparison of Baited Video Stations and Diver Video Transects. Aqu. Biol. 9 (2), 155–168. doi: 10.3354/ab00235
Marshall N., Marshall P., Curnock M., Pert P., Smith A., Visperas B. (2019). Identifying Indicators of Aesthetics in the Great Barrier Reef for the Purposes of Management. PLoS One 14 (2), e0210196. doi: 10.1371/journal.pone.0210196
Marsh H., Sinclair D. F. (1989). Correcting for Visibility Bias in Strip Transect Aerial Surveys of Aquatic Fauna. J. Wild. Manage. 53, 1017–1024. doi: 10.2307/3809604
Morais R. A., Depczynski M., Fulton C., Marnane M., Narvaez P., Huertas V., et al. (2020). Severe Coral Loss Shifts Energetic Dynamics on a Coral Reef. Funct. Ecol. 34, 1507–1518. doi: 10.1111/1365-2435.13568
Odum H. T., Odum E. P. (1955). Trophic Structure and Productivity of a Windward Coral Reef Community on Eniwetok Atoll, Marshall Islands. Ecol. Mono. 25, 291–320. doi: 10.2307/1943285
Olsen A. Y., Larson S., Padilla-Gamiño J. L., Klinger T. (2022). Changes in Fish Assemblage After a Marine Heatwave in West Hawai′i Island. Mar. Ecol. Prog. Ser.
Powles H., Barans C. (1980). Bottomfish Monitoring in Sponge-Coral Areas Off the Southeastern United States. Mar. Fish. Rev. 42 (5), 21–35.
R Core Team (2020). R: A Language and Environment for Statistical Computing (Vienna, Austria: R Foundation for Statistical Computing). Available at: https://www.R-project.org/.
Russell B. C., Talbot F. H., Anderson G. R. V., Goldman B. (1978). ““Collection and Sampling of Reef Fishes,”,” in Coral Reefs: Research Methods. Eds. Stoddart D. R., Johannes R. E. (Paris: UNESCO), 329–345.
Sale P. F., Douglas W. A. (1981). Precision and Accuracy of Visual Census Technique for Fishes Assemblages on Coral Patch Reefs. Environ. Biol. Fish. 6, 333–339. doi: 10.1007/BF00005761
Sale P. F., Sharp B. J. (1983). Correction for Bias in Visual Transect Censuses of Coral Reef Fishes. Coral Reefs. 2, 37–42. doi: 10.1007/BF00304730
Sanderson S. L., Solonsky A. C. (1986). Comparison of a Rapid Visual and a Strip Transect Technique for Censusing Reef Fish Assemblages. Bull. Mar. Sci. 39, 119–129.
Scheffer M., Carpenter S., Foley J. A., Folke C., Walker B. (2001). Catastrophic Shifts in Ecosystems. Nature 413, 53–59. doi: 10.1038/35098000
Smith C. L., Tyler J. C. (1973). Population Ecology of a Bahamian Suprabenthic Shore Fish Assemblage. Am. Mus. Novit, 2528.
Tissot B. N., Hallacher L. E. (2003). Effects of Aquarium Collectors on Coral Reef Fishes in Kona, Hawai′I. Cons. Bio. 17 (6), 1759–1768.
Tissot B. N., Walsh W. J., Hallacher L. E. (2004). Evaluating Effectiveness of a Marine Protected Area Network in West Hawai′i to Increase Productivity of an Aquarium Fishery. Pac. Sci. 58 (2), 175–188. doi: 10.1353/psc.2004.0024
Umberger V. (2017). Department of Land & Natural Resources ( Hawai′i Supreme Court). Available at: https://law.justia.com/cases/hawaii/supreme-court/2017/scwc-13-0002125.html.
Van de Leemput I. A., Hughes T. P., Van Nes E. H., Scheffer M. (2016). Multiple Feedbacks and the Prevalence of Alternate Stable States on Coral Reefs. Coral Reefs 35 (3), 857–865. doi: 10.1007/s00338-016-1439-7
Walsh W. (2014). Report to the Thirtieth Legislature 2015, Regular Session Report on the Findings and Recommendations of Effectiveness of the West Hawai′i Regional Fishery Management Area (State of Hawai′i: Department of Land and Natural Resources), 51. Available at: https://dlnr.hawaii.gov/dar/files/2015/01/ar_hrs188_2015.pdf.
Walsh W. J., Cotton S., Jackson L., Kramer L., Lamson M., Marcoux S., et al. (2019). Findings and Recommendations of Effectiveness of Thewest Hawai′i Regional Fishery Management Area (WHRFMA) (State of Hawai′i: Department of Land and Natural Resources). Available at: https://dlnr.hawaii.gov/dar/files/2020/05/ar_hrs188_2020.pdf.
Walsh W. J., Zamzow J. P., Kramer L. (2018). Continued Long-Term Decline of the Coral Reef Biota at Puakō and Pauoa, West Hawai′i, (1979–2008) ( Hawai′i Division of Aquatic Resources Report) Department of Land and Natural Resources (DLNR), Division of Aquatic Resources (DAR), Kona, Hawaii.
Westera M., Lavery P., Hyndes G. (2003). Differnces in Recreationally Targeted Fishes Between Protected Ad Fished Areas of a Coral Reef Marine Park. J. exper. Mar. bio. Ecol. 294, 145–168. doi: 10.1016/S0022-0981(3)00268-5
Wickham H. (2016). Ggplot2: Elegant Graphics for Data Analysis (Verlag New York: Springer). Available at: https://ggplot2.tidyverse.org.
Williams I. D., Walsh W. J., Tissot B. N., Hallacher L. E. (2006). Impact of Observers’ Experience Level on Counts of Fishes in Underwater Visual Surveys. Mar. Ecol. Prog. Ser. 310, 185–191. doi: 10.3354/meps310185
Wilson S. K., Graham N. A. J., Holmes T. H., MacNeil M. A., Ryan N. M. (2018). Visual Versus Video Methods for Estimating Reef Fish Biomass. Ecol. Ind. 85, 146–152. doi: 10.1016/j.ecolind.2017.10.038
Young L. G. L. (1997). ““Sustainability Issues in the Trade for Wild and Cultured Aquarium Species,”,” in Marketing and Shipping Live Aquatic Products, ‘96, Seattle, Washington, 13-15 October 1996 (Ithaca, New York, NY: Northeastern Regional Agricultural Engineering Service Cooperative Extension), 145–151.
Keywords: West Hawai′i, SCUBA, diver operated survey, DOV, climate change, monitoring, coral reefs
Citation: Larson S, Christiansen J, Olsen AY, Walsh WJ, Teague CH, Tissot B and Randell Z (2022) A Unique 100 Meter Underwater Survey Method Documents Changes in Abundance, Richness, and Community Structure of Hawaiʹi Reef Fishes. Front. Mar. Sci. 9:892261. doi: 10.3389/fmars.2022.892261
Received: 08 March 2022; Accepted: 13 June 2022;
Published: 18 July 2022.
Edited by:
Aldo Cróquer, The Nature Conservancy, Dominican RepublicReviewed by:
Jorge Garcia-Sais, Reef Research, Inc., Puerto RicoCesar Augusto Marcelino Mendes Cordeiro, Universidade Estadual do Norte Fluminense Darcy Ribeiro, Brazil
Copyright © 2022 Larson, Christiansen, Olsen, Walsh, Teague, Tissot and Randell. This is an open-access article distributed under the terms of the Creative Commons Attribution License (CC BY). The use, distribution or reproduction in other forums is permitted, provided the original author(s) and the copyright owner(s) are credited and that the original publication in this journal is cited, in accordance with accepted academic practice. No use, distribution or reproduction is permitted which does not comply with these terms.
*Correspondence: Shawn Larson, Uy5sYXJzb25Ac2VhdHRsZWFxdWFyaXVtLm9yZw==
†ORCID: Shawn Larson, orcid.org/0000-0003-0376-1051
Amy Y. Olsen, orcid.org/0000-0002-2752-8337
Zachary Randell, orcid.org/0000-0003-2342-1652