- 1College of Ecology and Environment, Hainan University, Haikou, China
- 2State Key Laboratory of Marine Resource Utilization in South China Sea, Hainan University, Haikou, China
- 3Hainan Provincial Ecological and Environmental Monitoring Centre, Haikou, China
- 4College of Marine Science, Hainan University, Haikou, China
Mass coral bleaching is a severe threat to coral reefs in the era of global warming, and upwelling areas may serve as thermal refugia for coral reefs by mitigating the effects of heating anomalies. This study seeks to examine the impact of the Qiongdong upwelling (QDU) on the coastal coral reefs of the Hainan Island. The environmental variables (i.e., physiochemical parameters, coastal land use) differed significantly in upwelling and non-upwelling areas. In particular, the presence of colder and more saline water was the important indicator of coastal upwelling. In the QDU, turf algae and macroalgae dominated the algae communities, but habitat-specific environment and coastal development contributed to lower calcified algae, coral larvae replenishment and live corals cover. Lower abundance of coral reefs fishes could be attributed to the disappearance of live corals, increase of algae coverage, low habitat complexity and strong wave exposure at upwelling stations. Upwelling-related environmental parameters, wave exposure, land use and potential local stressors were revealed as major driving factors related to significant spatial changes in stony coral and fish communities. Overall, the present findings suggest that the Qiongdong upwelling has the potential to provide thermal refuge for coral reefs, but is severely affected by local anthropogenic activities.
Introduction
Coral reefs are one of the most productive and biologically diverse ecosystems on Earth (Barnes et al., 2019). They provide a wide range of goods and services (e.g., provision, regulation, cultural and support services) that directly or indirectly influence millions of people around the world (Woodhead et al., 2019). However, coral reefs are increasingly threatened by human disturbance as well as global climate change (Ban et al., 2014). Among the anthropogenic stressors, overfishing, land development, and pollution have been identified as the main reasons that render extensive changes in coral reef ecosystems (Hughes et al., 2003; Ellis et al., 2019). Additionally, global warming is causing coral bleaching to most coral reefs around the world (Hughes et al., 2018). In recent years, many coral reef ecosystems experienced reduction of coverage and loss of structural complexity (Hughes et al., 2018; Ellis et al., 2019). When multiple stressors affect the dynamics and function of the coral reef community, stony corals tend to transform to turf and macroalgae species, and the associated ecosystem services may be seriously damaged (Harborne et al., 2017; Evans et al., 2020).
The rising ocean temperature is a major threat to the future of coral reefs (Chollett and Mumby, 2013). The global average ocean temperature may increase by 3°C by 2100, which create an environment that that may not be tolerated by most coral species (Frieler et al., 2013; Cacciapaglia and van Woesik, 2016). Some researchers suggested that coral reefs may survive better at locations of low thermal stress (Chollett and Mumby, 2013), such as deep areas, strong ocean current areas, upwelling areas, and high latitude areas. These locations are potential targets of priority conservation activities and can serve as coral refugia in the context of ocean warming (Chollett and Mumby, 2013). In particular, upwelling can offset the thermal stress caused by the anomaly of sea surface temperature (SST) when it is strong enough, as the inflow of cold water effectively prevents the SST from becoming lethally high (Tkachenko and Soong, 2017; Lee et al., 2020).
Local upwelling events in summer has the potential to keep temperature below bleaching level and mitigate the effects of heating anomalies by providing cooler, nutrient-enriched waters to coral reefs (Riegl, 2003; Riegl et al., 2019). For example, during a very strong El Niño Southern Oscillation in 2015–2016, topographically driven upwelling, which is a source of cold water and dissolved inorganic nutrients, helped corals endure the heating episode and avoid mortality from coral bleaching in northern Galapagos (Riegl et al., 2019). Similarly, upwelling in the Gulf of Panamá moderated the high temperatures and allowed the corals to escape thermal stress caused by El Niño, and upwelling zones may thus provide temporary and local refugia for the thermal effects of climate change as global warming continues (Randall et al., 2020). Overall, cold water induced by internal waves or upwelling has the capacity to act as a buffer against the major threat posed by thermal stress in a warming ocean (Lee et al., 2020; Wyatt et al., 2020).
The Qiongdong upwelling (QDU) is one of the primary coastal upwelling systems in the South China Sea. The upwelling zone is mainly off the east coast of the Hainan Island and is generally centered in the nearby coastal waters at 18.5°–20.5°N and 110.0°–111.5°E from June to September (Lu et al., 2021). Large-scale coral bleaching in summer of 2020 is the most severe bleaching event reported in China within recent years, western Hainan Island incurred more severe bleaching than QDU (Zhu et al., 2021; Lyu et al., 2022). The QDU zone has the potential to mitigate the effects of harmful heat stress and may serve as a potential thermal refugia for climate change. However, due to the limited observation data, the ecological impact of QDU on the biodiversity of coral reef ecosystem remains inadequately understood. In this work, we explored the spatial distribution of the coastal environment, and the coral communities and their relationships in the QDU zone and the nearby non-upwelling zone. The findings are key to understand the ecological status of the local environment and coral reefs under the influence of upwelling and anthropogenic pressure as they can provide early warning of the identification of priority protected areas.
Materials and methods
Study area
The Hainan Island (18°09’–20°10’N and 108°37’–111°03’E), which is located in southern China and has a coastline of 1944 km (Fu et al., 2021), has a tropical monsoon climate and is the main densely populated area of the Hainan Province of China (Figure 1A). The eastern coast of the Hainan Island is strongly affected by the QDU during summer (Jing et al., 2009). In recent years, the coral reefs experienced rapid urbanization as well as intensive agricultural development [Figure 1B; (Han, 2018)]. The Hainan Island is near the Coral Triangle and is a major coral reef region in China (Dong et al., 2017), and coral reefs used to be abundant in the shallow water (<10 m) along the coast. Unfortunately, human activities including overfishing, land reclamation, coral mining, and aquaculture expansion contributed to the long-term coral degradation in Hainan Island [Figure 1C; (Huang et al., 2021)]. In the year of 2020, there was few coral bleaching events (1.26%) in the QDU zone, whereas average bleaching percentage was 58.4% for the coastal waters of the northwestern Hainan Island [Figure 1D; (Zhu et al., 2021; Lyu et al., 2022)]. In this work, we surveyed the coral reefs in 2021 from July to September at 20 representative sampling stations (Supplementary Table 1). The locations of the sampling sites were chosen based on literature review and field investigation to adequately reflect the distribution of coral reef resource in the coastal area of the Hainan Island. The east coast was under the influence of the QDU (Li et al., 2015), and had 9 stations distributed in Wenchang, Qionghai, and Wanning (Zhu et al., 2022). The northwest coast was not affected by the QDU, and had 11 stations distributed in Changjiang, Danzhou, and Lingao (Supplementary Figure 1).
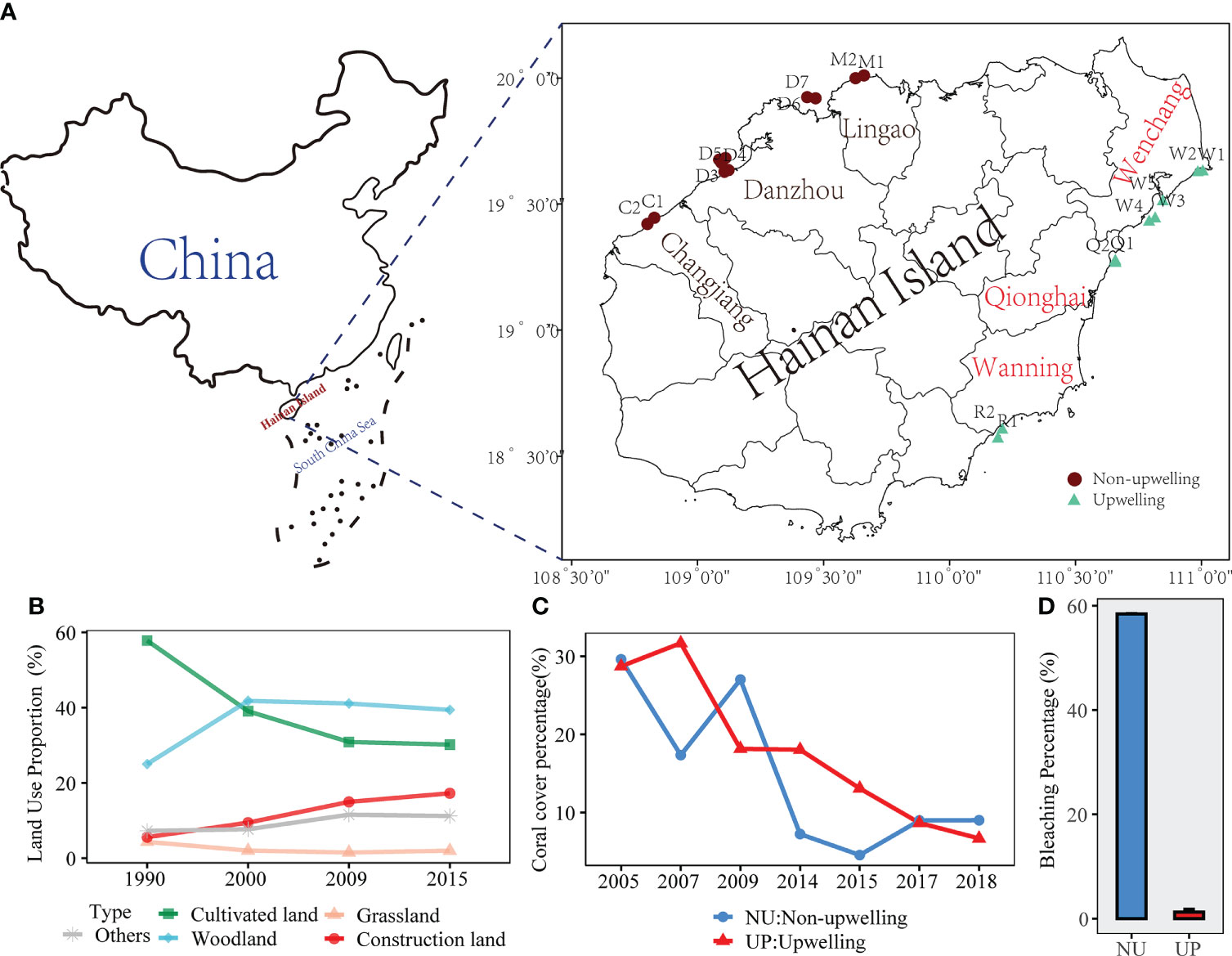
Figure 1 Location of survey sites (A). Change in land use proportion of Hainan Island coastal zone from 1990 to 2015 (B), and the data was from results by Han (2018). Live reef coral coverage at fixed stations in the west (non-upwelling stations) and east (upwelling stations) of Hainan Academy of Ocean and Fisheries Sciences (C), and data was described in a previous study by Huang et al. (2021). The bleaching percentage of northwest coastal areas compared with that of eastern Hainan Island in 2020 (D), and the results were from Zhu et al. (2021) and Lyu et al. (2022).
Survey of benthic and fish community
Considering the abundance and spatial distribution, transects were located in coral reef habitats, at depths ranging from 2–8 m. The fish communities were filmed with an underwater camera along a 60 m nylon line on both sides of the transect (1m) fixed parallel to the coastline. Classification was made at species level for each fish video, and the density of each fish species (ind/100m2) was counted for a 120 m2 area in the sample zone. To determine the reef benthic composition, a 60 m plastic tape was fixed along the cross-section, and a diver swimming in a straight line at 50 cm above the seabed filmed with a camera. The benthic components (i.e., stony coral, dead coral, sand, rock, rubble, soft coral, turf algae, macroalgae, calcified algae, etc.) were counted for every 10 cm of the cross-section (600 data points in total). Relative coverage was calculated as the ratio of the total length of each substrate type or reef building coral to the length of the transect (Facon et al., 2016). Reef-building corals were identified to the species level whenever possible. At least 40 quadrats (0.4 m × 0.7 m) were randomly selected at each photographed cross-section (Edmunds et al., 1998). Juvenile corals (i.e., those<5 cm in height and diameter) were obtained for each transect through analysis of the above photoquadrats (Edmunds et al., 1998).
In situ environments and land use
The geographical coordinate of each sampling site was obtained from GPS. A YSI multi-parameter probe was used to measure salinity, temperature, dissolved oxygen, and pH in situ. Turbidity was measured using an Aqualogger 310TY turbidity recorder. Water samples were collected by scuba divers in 1 L Niskin bottles close to the reef substrate at each site and immediately passed through a glass fiber filter (Whatman GF/F, 0.45 μm) using a suction device. The filtrate was collected for dissolved nutrient analysis, and the filter membrane was folded in half and stored for chlorophyll-a (Chl-a) analysis with a laboratory fluorometer (Turner Designs, United States). All samples were frozen at −20°C before laboratory analysis. Phosphate (DIP), nitrate ( ), nitrite ( ) and ammonium ( ) were measured using an automatic nutrient analyzer (Seal AA3, Germany) (Zhu et al., 2022).
The monthly average sea surface temperature (SST) was obtained from MODIS Aqua level-3 products (https://oceancolor.gsfc.nasa.gov/) for June to September 2021 with a spatial resolution of 4 km × 4 km. We further analyzed the changes of temporal SST from 2002 to 2022 in eastern and northwest coast of Hainan Island with a temporal resolution of 8 days (https://giovanni.gsfc.nasa.gov). ArcGIS 10.3 was used to remove invalid values, and Kriging interpolation was used to map the spatial distribution. Land use was examined for the 6 km × 6 km area upstream of each sampling site (Zhou et al., 2020), and determined from six Landsat 8 remote sensing images (United States Geological Survey, USGS, http://earthexplorer.usgs.gov/) of the Hainan Island in 2021 with a spatial resolution of 30 m. For each image, cloudy areas were removed by ENVI 5.3 to give the cloud-free image after mosaic and clipping (Zhou et al., 2020). The cloud-free image was then subjected to geometric accuracy correction, atmospheric correction, and registration. The inspected areas were divided into 2 km × 2 km square grid cells, and the land use was assigned through knowledge-based supervision and maximum likelihood classification using ArcGIS to one of the five types: grassland, woodland, cultivated land, construction land, and unused land.
Statistical analysis
All statistical analyses were carried out using the R software. The Shannon diversity index was calculated using the “vegan” package for coral and fish. The spatial differences between the non-upwelling zone and the QDU zone were compared through Student’s t test for coral and fish coverage, diversity, and environmental factors. To determine the spatial differences in the composition of reef building coral and the fish communities of different regions, coverage percentage and density data were further populated in a species/sample abundance matrix for NMDS analysis based on the Bray–Curtis distance using the “vegan” and “ggplot2” packages. Permutation multivariate analysis of variance (PERMANOVA) was used to determine if the coral and fish combinations differed significantly across sea areas (Anderson, 2001). Upon detection of significant differences in coral and fish communities, SIMPER analysis was performed based on the Bray–Curtis dissimilarity index to determine if the species has a significant impact on the differences in coral composition. Further analysis generated the multicollinearity of each environmental factor, and highly correlated variables were excluded based on the variance inflation factor (VIF) test using the “car” package. Stepwise VIF selection was repeated to calculate the VIF values for all factors until all VIF values were below the threshold (Wang et al., 2021). Redundancy analysis (RDA) was used to determine the impact of environmental factors on the diversity of coral and fish assemblages. To detect any significant correlation between community structure and environmental factor, the Mantel test and the Procrustes analysis were run using the Bray–Curtis distance of the species composition data and the Euclidean distance of the environmental factor.
Results
Satellite remote sensing of SST
The strongest influence of QDU on the SST of the study areas was in June and July. In June 2021, the east coast of the Hainan Island showed significantly lower temperature with the SST ranging in 26.23–27.34°C for most areas (Supplementary Figure 2), whereas the SST was mostly 30.63–31.74°C in the northwestern coastal waters of the Hainan Island. In the warmer season of July, the SST was still lower in the east (28.94–29.95°C) than in the northwest (30.96–31.97°C). The influence of QDU on the coastal waters weakens in August and September, but the SST was still far lower for the waters of the east coast. In August, the SST was 28.25–29.34°C at Wenchang and 27.16–28.25 at Qionghai and Wanning, but 30.43–31.52°C at Changjiang and 31.52–32.61°C at Danzhou and Lingao. The SST in September was 30.43–31.42°C for Danzhou and Lingao, but 29.54–30.43°C for Changjiang and most of the coastal waters. SST in QDU was higher during the 2010, and 2015/2016 global thermal bleaching events, but they were significantly lower than 30°C (coral bleaching threshold SST) off the east coast (Supplementary Figure 3A). Results of long-term time series from 2002 to 2022 showed that SST of the upwelling season fluctuated in different years, but SST in QDU during most of the upwelling season were significantly lower than 30°C and SST in the northwest coast (Figure 2; Supplementary Figure 3B). Considering coral reefs in Hainan Island suffered unprecedented large-scale coral bleaching that in the summer of 2020, we assessed the changes of temporal SST in eastern and northwest coast during this mark year (Supplementary Figure 3B). Specifically, from June 8 to July 19 in 2020, the SST in the east coast was always lower than 29°C, while for most affected regions, the SST in the northwest Hainan Island had exceeded 31°C. Especially in August and September, the SST in the northwest area exceeded 32°C, but warming condition in QDU was less severe. Overall, the bleaching event from June to September in 2020 prevailed in northwest coast of Hainan Island, whose corresponding average SST was significantly higher than the eastern shore of Hainan Island that experienced less severe bleaching.
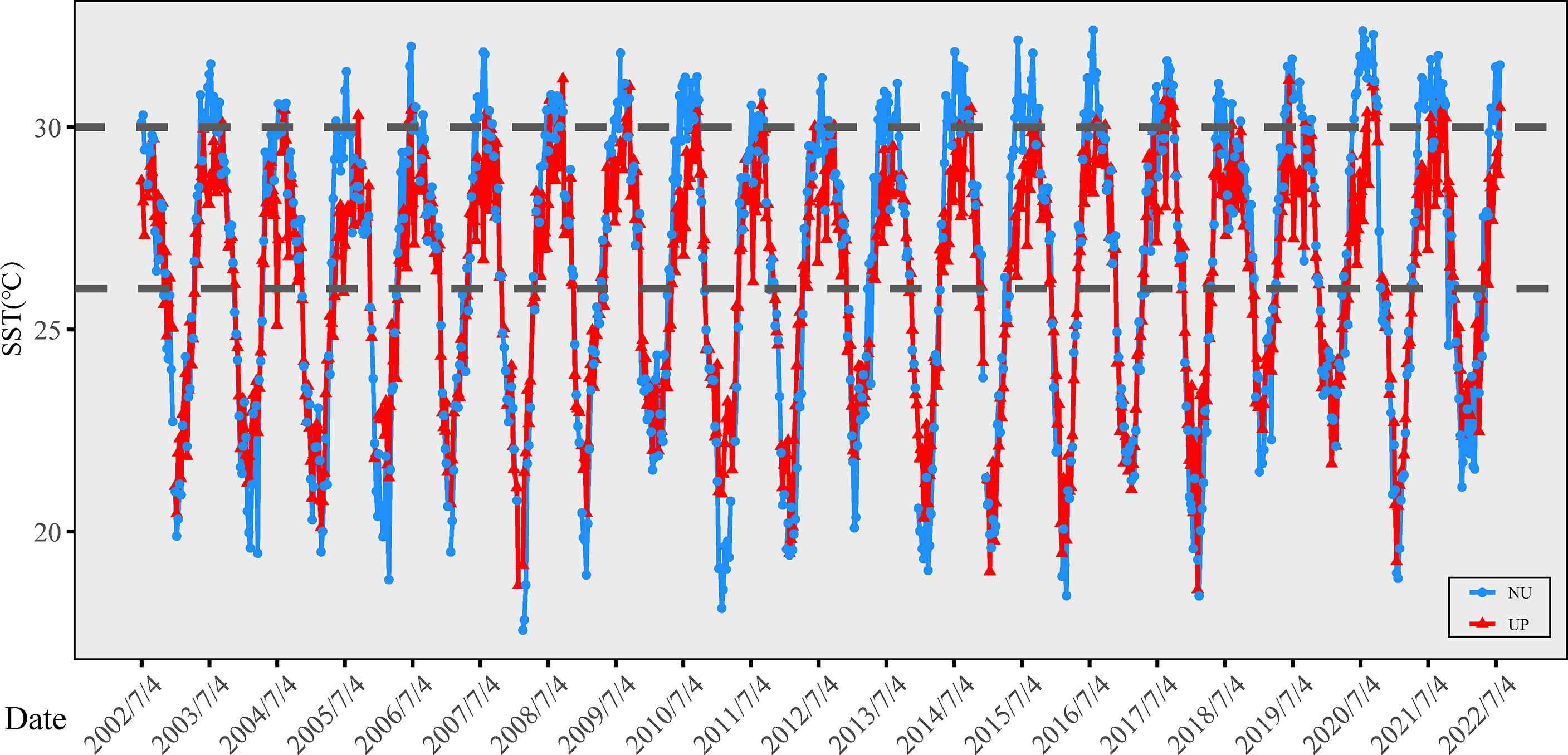
Figure 2 Temporal SST with a resolution of 8 days at the eastern and northwest coast of Hainan Island from 2002 to 2022.
In situ environments and coastal land use
Spatial variations in physicochemical factors were analyzed by PCA to identify differences among different sampling areas (Figure 3A). The temperature gradient measured on August 11, 2021 at the three stations of Wenchang (open sea area near W3-W5) showed that the surface waters were 3.11°C warmer than the seawater at 10 m depth. The low temperature layer (27.70 ± 0.69°C) started when the depth was greater than 6 m, and the water temperature dropped to 25.97 ± 0.28°C at 10 m depth (Figure 3B). The coastal land use was mainly woodland and construction land for the QDU zone, and cultivated land and woodland for the non-upwelling zone (Figure 3C; Supplementary Table 2). The coastal land of the QDU sites had significantly more woodland and construction land but significantly less cultivated land (Figure 3D). The sea water temperature in situ also had the same trend as SST, i.e., much lower in the QDU zone (28.54 ± 0.44°C; mean ± standard error of mean) that in the non-upwelling zone (31.58 ± 0.17°C; Supplementary Table 3). The salinity and pH were much higher in the QDU zone (34.76 ± 0.07‰; 8.48 ± 0.00) than in the non-upwelling zone (32.66 ± 0.06 ‰; 8.27 ± 0.05). The non-upwelling zone had much higher turbidity (1.63 ± 0.14 FTU) than the QDU zone (1.00 ± 0.14), but there was no significant difference in dissolved oxygen and Chl-a between the two areas. Compared with the non-upwelling zone, the QDU zone had slightly higher (0.06 ± 0.01 μmol/L) and DIP (0.06 ± 0.00 μmol/L) as well as slightly lower (4.91 ± 0.23 μmol/L) and (0.16 ± 0.04 μmol/L), but the difference was not statistically significant (Supplementary Figure 4). Finally, we verified whether sampling in different months would have an impact on the regional changes by further analysis of the seawater characteristics from different sampling stages (Supplementary Figure 5). Temperature and salinity fluctuated seasonally at upwelling sites and there were significant differences between upwelling and non-upwelling stations in August. These results suggested that it was feasible to compare the environmental variables of different voyages according to different regions.
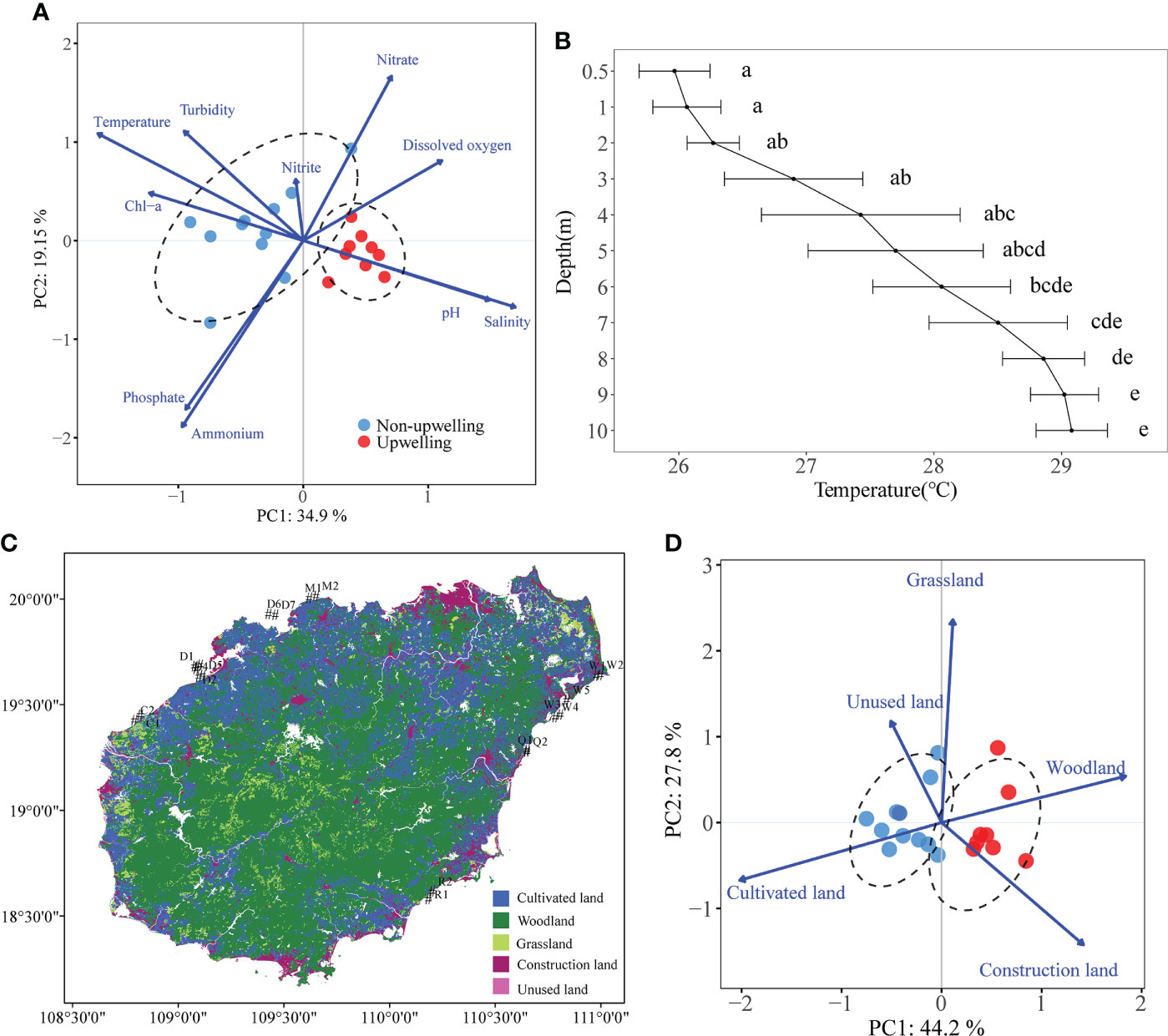
Figure 3 Principal Component Analysis (PCA) of the seawater environmental parameters (A); The temperature gradient of seawater (0.5–10 m) at Wenchang (B); Spatial distribution of coastal land use (C); PCA of the coastal land use of the survey sites (D).
Reef benthic composition and coral communities
Compared with the QDU zone, the non-upwelling zone had a higher coverage of rock and rubble, and a lower coverage of sand and soft coral, none with any significant difference (Figure 4A; Supplementary Table 4). Instead, the upwelling zone had significantly higher coverage of turf algae (21% vs 10%), calcified algae (7% vs 3%), and macroalgae. A total of 105 species of reef building corals were found in the survey. The stations in the QDU zone have 5–42 species, and those in the non-upwelling zone have 6–29 species. The five genera of reef building corals with the highest abundance were Favia, Porites, Turbinaria, Montipora, and Galaxea in the QDU zone, and Porites, Galaxea, Favia, Goniopora, and Acropora in the non-upwelling zone (Figure 4B). The two sea areas had 38 common species, but there were more endemic coral species in the QDU zone. The QDU zone had a much lower coral coverage but a much higher Shannon diversity index (6% and 3.54 ± 0.23) than the non-upwelling zone (12% and 2.99 ± 0.28; Figure 4C, D). The common species with the highest frequency of coral occurrence were Porites lutea, Porites lobata, Favites complanata, Galaxea fascicularis, and Favites flexuosa, all of which were tolerant massive coral. Significant difference exists in the reef building coral communities between the QDU and the non-upwelling zones (ANOSIM, R = 0.4348, P = 0.001; Figure 4E). According to the SIMPER analysis, the species that contributed significantly to the difference of coral communities between the QDU and non-upwelling zones mainly included Porites lutea (13.06%), Galaxea fascicularis (10.90%), Goniopora sp. (6.54%), and Favites complanata (5.26%; Supplementary Table 5). The recruitment of juvenile corals was higher in the non-upwelling zone (3.09 ± 1.28 ind/m2) than in the QDU zone (1.73 ± 0.49 ind/m2).
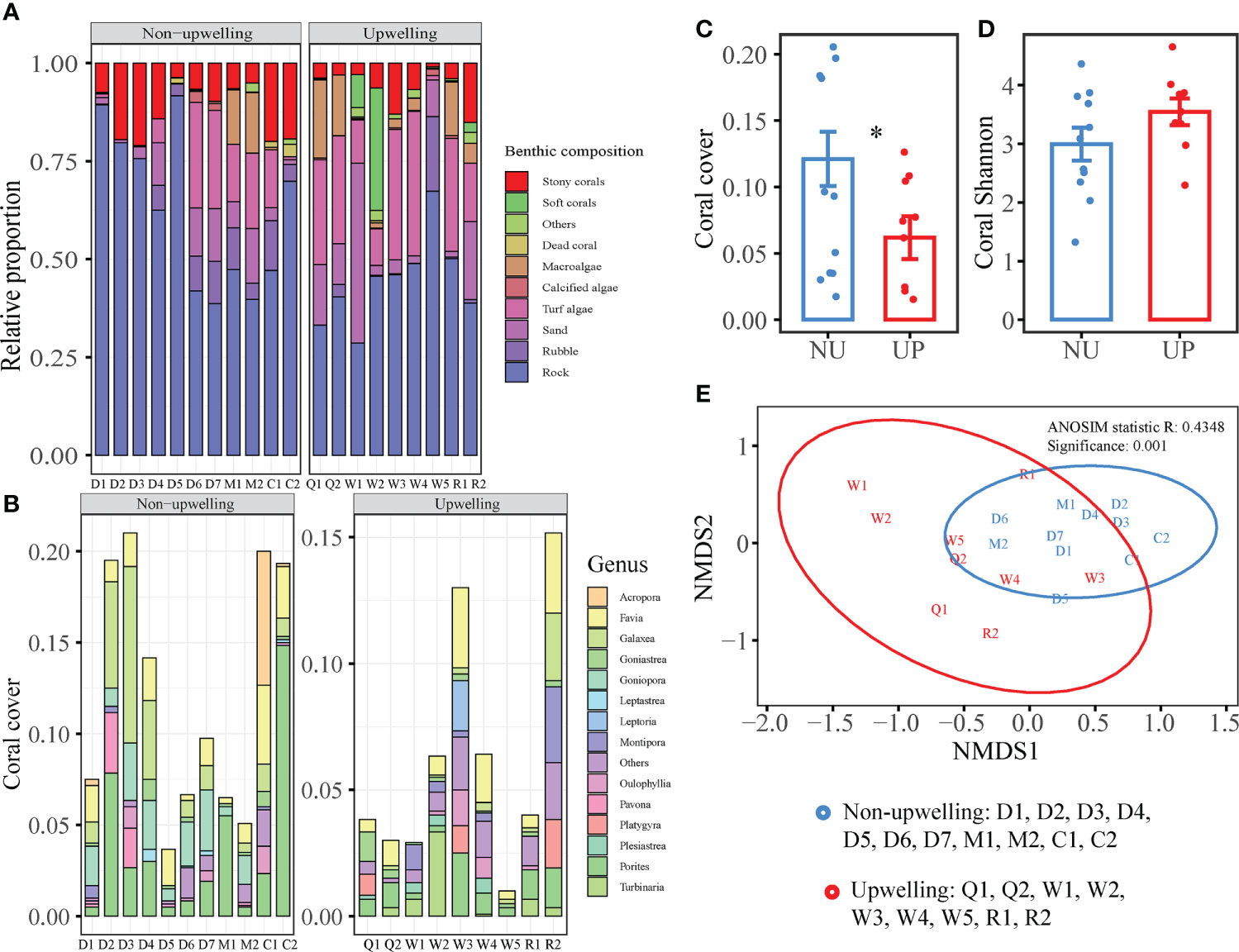
Figure 4 Comparison of benthic composition, coral composition, coral coverage, coral diversity, and coral community of the QDU and non-upwelling zone: (A) Percentage cover of coral reef composition; (B) Percentage composition of hard coral at genus level; (C) Total coral coverage; (D) Coral diversity; (E) Non-metric multidimensional scaling (NMDS) of the Bray–Curtis distances of coral community. The symbol "*"denote p value less than 0.05.
A total of 43 fish species were found from the two coral reef areas. There were 5–10 and 3–11 coral reef fish species at the stations of the QDU zone and the non-upwelling zone, respectively. The five fish genera with the highest abundance were Pomacentrus, Stegastes, Pempheris, Halichoeres, and Pomachromis in the QDU zone, and Neopomacentrus, Pomachromis, Stegastes, Anchoviella, and Pempheris in the non-upwelling zone (Figure 5A). Among them, Pomachromis and Stegastes were significantly more abundant and Pomacentrus was significantly less abundant in the non-upwelling zone. The Shannon diversity index was much higher for the QDU zone (2.34 ± 0.25) than the non-upwelling zone (1.45 ± 0.19; Figure 5B). However, the fish density was much higher in the non-upwelling zone (139.05 ± 39.46 ind/100m2) than in the QDU zone (18.23 ± 4.68 ind/100m2; Figure 5C; Supplementary Table 6). The coral fish community differed significantly between the QDU and non-upwelling zones (ANOSIM, R = 0.4617, P = 0.003; Figure 5D). According to SIMPER analysis, Neopomacentrus Azysron, Stegastes Fasciolatus, Pomachromis Richardsoni, and Pomacentrus Chrysurus accounted for 27.68%, 16.17%, 8.94%, and 4.31% of the difference in the fish community between the QDU and the non-upwelling zones, respectively.
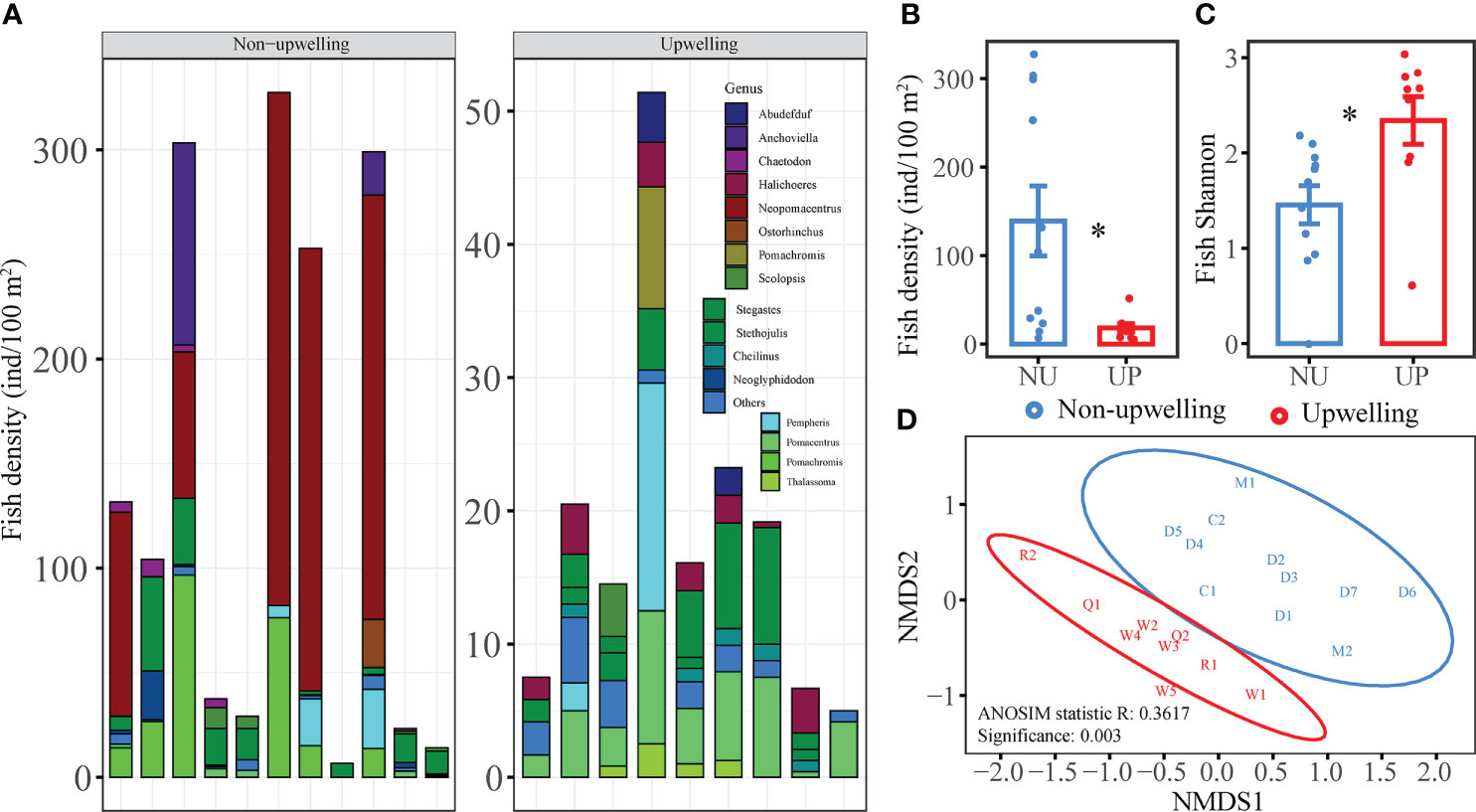
Figure 5 Comparison of fish composition, fish density, fish diversity, and fish community of the QDU and non-upwelling zones: (A) Density of coral reef fish at genus level; (B) Total fish density; (C) Fish diversity; (D) NMDS of Bray–Curtis distances of the fish community. The symbol "*"denote p value less than 0.05.
Driving factors of coral biome composition
The impact of environmental factors on the composition of the coral biome was evaluated through RDA by measuring the correlations between the communities of fish and reef-building corals (species abundance) and environmental factors. The seven environmental variables selected for RDA were turbidity, temperature, salinity, pH, cultivated land, woodland, and construction land (Figure 6A). Environmental variables including dissolved oxygen, temperature, salinity, pH, cultivated land, woodland and construction land were used in the analysis of the association between fish communities and environmental factors (Figure 6B). The overall results of RDA were significant for the coral (F = 1.5251, P = 0.002) and the fish communities (F = 1.9829, P = 0.002). The seven selected environmental variables could explain 47.08% and 53.63% of the overall variation of coral and fish composition, respectively. For the coral community, the first axis and the second axis accounted for 31.23% and 18.90% of the total variance, respectively. For the fish community, the first axis and the second axis accounted for 45.29% and 22.27% of the total variance, respectively. Therefore, the first two axes could well reflect the impact of environmental factors on the differences between the composition of coral and fish communities. The first part of the RDA (rda1) separated coral and fish communities in the QDU zone from the non-upwelling zone.
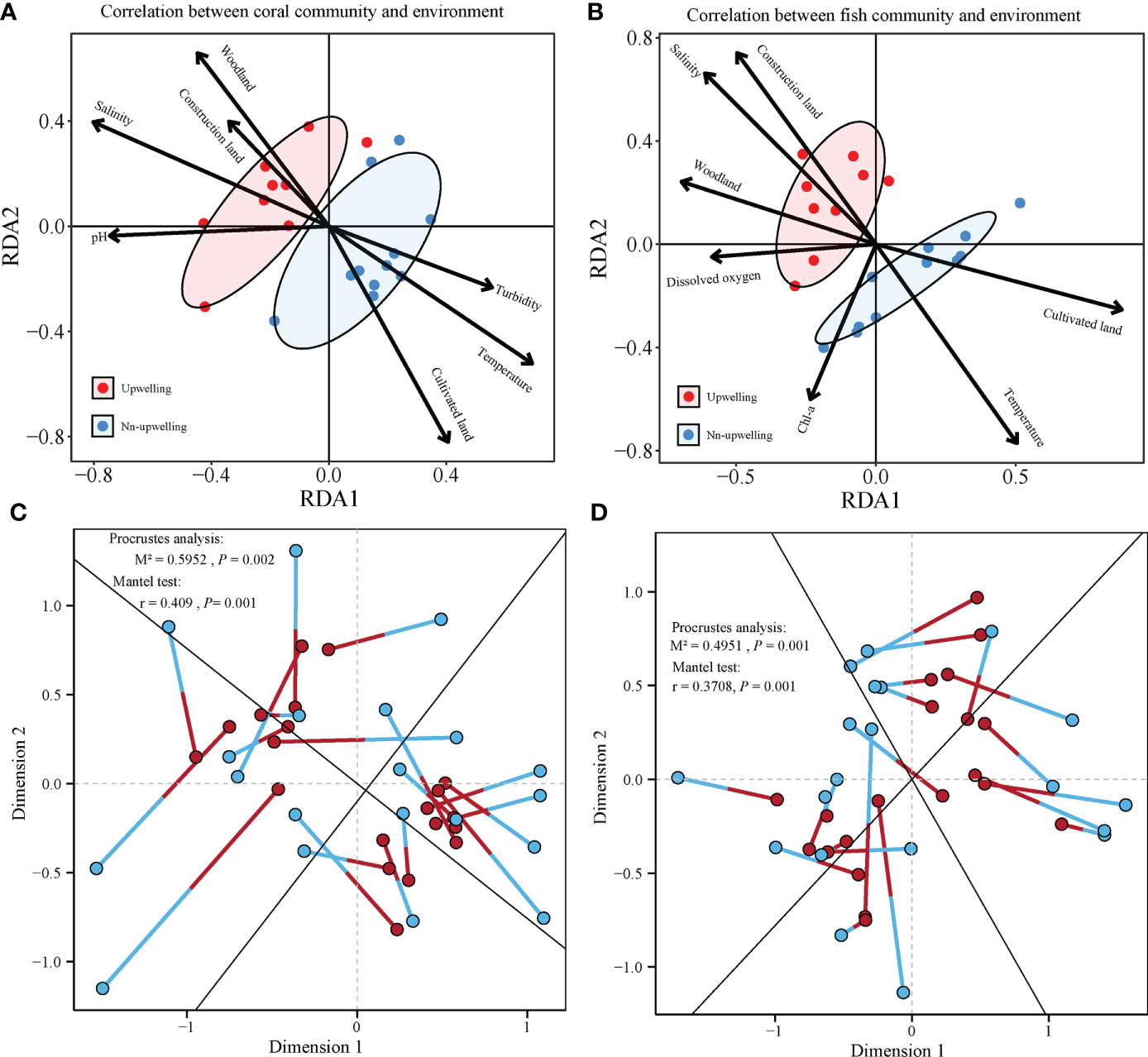
Figure 6 Correlation between environmental factors and coral community. Redundancy analysis (RDA) of (A) hard coral and (B) fish abundance at species level and environmental characteristics. The Procrustes analysis of (C) coral compositions and (D) fish community depicts significant correlations with environmental characteristics.
Furthermore, through forward selection, 999 Monte Carlo tests were carried out to analyze the significant influence of the environmental factors on coral communities. The results showed that temperature (F = 1.8737, p = 0.009), cultivated land (F = 1.7881, p = 0.013), turbidity (F = 1.7562, P = 0.007), salinity (F = 1.5988, P =0.032), and pH (F = 1.5841, P =0.025) can explain most of the differences in coral communities. In addition, temperature (F = 3.5659, p = 0.003), cultivated land (F = 2.1674, P = 0.052), Chl-a (F = 2.2769, P =0.0340), dissolved oxygen (F = 1.7970, P =0.072), and pH (F = 1.5841, P = 0.025) could explain most of the differences in fish communities. The results of the Mantel test and the Procrustes analysis both showed that coral communities (Figure 6C) and fish communities (Figure 6D) were significantly correlated with environmental factors. Specifically, according to the Mantel test, salinity (r = 0.4312, P = 0.001), temperature (r = 0.3247, P = 0.002), pH (r = 0.2250, P = 0.007), cultivated land (r = 0.1982, P = 0.021), and construction land (r = 0.3205, P = 0.007) determined the change in the community structure of reef-building corals, whereas temperature (r =0.1564, P = 0.041), salinity (r = 0.3135, P = 0.002), woodland (r = 0.2444, P = 0.007), construction land (r = 0.2742, P = 0.007), and cultivated land (r = 0.4203, P = 0.001) were significantly correlated with the fish community structure. It could be concluded that environmental factors played an important role in causing the observed spatial variation in coral and fish communities.
Discussion
Spatial characteristics in environmental factors
Existing research on the environmental effects and ecology of QDU has mostly focused on offshore areas, and few studies examined the environmental effects on the coral reefs in coastal shallow water (Li et al., 2015). The remote sensing data and in situ temperature showed that the QDU zone had much lower seawater temperature. The combined analysis of available SST and in situ temperature agreed well with the previous field observations of the coral reefs affected by upwelling off the northeastern Hainan Island (Li et al., 2015). Although the wind-driven QDU is usually seasonal, it is synchronized with the period of thermal anomalies in Hainan Island. In particular, coral reefs in the South China Sea suffered unprecedented large-scale coral bleaching in summer of 2020 owing to anomalous thermal stress associated with summer marine heatwaves (Yao and Wang, 2021). Based on high-resolution SST, we uncovered a pronounced temperature disparity between the eastern and western coast of Hainan Island. For comparison, a large-scale coral bleaching incident occurred in the northwestern zone of the Hainan Island but little in a coastal upwelling zone at the eastern coast where cool water may alleviate heating in 2020 (Zhu et al., 2021; Lyu et al., 2022). Frequency and intensity of coral bleaching events that was associated with anthropogenic global warming have significantly increased (Hughes et al., 2017), but fringing reefs at the east coast whereas they are located in within the reach of summer upwelling benefited from colder waters than northwest reefs in the non-upwelling zone. Therefore, the recent result indicates that QDU zone is a potential thermal refuge for reef-building corals due to the heat alleviation effects of upwelling.
Previous studies have shown that the upwelling can bring rich inorganic nutrients to the coastal waters (Su and Pohlmann, 2009; Hernández and Tapia, 2021). However, in the present study, there was no significant difference between the QDU zone and the non-upwelling zone in nutrients content. Coastal coral reef stations are more vulnerable to the serious impacts of terrestrial material input that arise from high population density and rapid economic development (Li et al., 2015). For example, the western part of the Hainan Island was transformed heavily into cultivated land from 1990 to 2015, and most of the cropland in the western and northeastern parts was transformed into water reservoirs and shrimp aquaculture (Sun et al., 2020). The current study revealed that in 2021, cultivated land was more abundant on the northwestern coast, whereas woodland and construction land were more abundant on the eastern coast. The land use is related to various human activities including urban expansion, land reclamation, and tourism development, etc. Agricultural catchments in the northwestern sea area may alter the flow regimes, raise the water temperature and accentuate its fluctuation, elevate nutrient concentration, and increase primary productivity in the coral reef waters (Lee et al., 2017). Terrestrial runoff such as river discharge and aquaculture effluent can alter the environmental conditions and cause nutrient enrichment (Herbeck et al., 2013), consequently masking the nutrient enrichment effects of the QDU. The present work revealed that the shallow coral reefs at the eastern Hanan Island were affected by the QDU, coastal land use, and human activities.
Benthic community and potential factors
Coral reefs in the Anthropocene will be occupied by thick mats of turf algae and sediments (Bellwood et al., 2019). In the present study, the he relatively high abundance of macroalgae in the coral reefs of the QDU zone can be mainly attributed to the effects of low seawater temperature and elevated inorganic nutrients (Li et al., 2015). Further, wave exposure can drive shallow benthic algal productivity, and even shift the benthic community to a dominance by turf algae (Williams et al., 2013; Roff et al., 2019). Wave action from annual monsoon winds have been shown to drastically alter benthic algal communities by enhancing algal growth and mechanical effects (Becerro et al., 2006), and sheltered reefs were less predisposed to macroalgal overgrowth compared to wave-exposed reefs are more susceptible (Alemu I and Mallela, 2021). On the other hand, coral reefs along the coast are seriously threatened by eutrophication, fisheries, and other anthropogenic impacts (Alcívar-Mendoza et al., 2021), all of which reduced the diversity of macroalgae and increase the coverage of turf algae. The overgrowth of turf algae and the increased in nutrients may disrupt the natural biophysical relationship between macroalgae and environmental factors (Liao et al., 2021). Consequently, although the QDU creates a suitable condition for the growth of macroalgae, they still cannot dominate inshore coral reefs on the east coast of the Hainan Island (Liao et al., 2021). In addition, the QDU and the non-upwelling waters both had very low coverage of calcified algae and coral larvae, far lower the survey in 2010. Low abundance calcified algae may impair the recruitment of juvenile corals and reduce the abundance of adult coral communities (Nelson, 2009). The current findings demonstrated that the algae communities in the QDU zone were dominated by turf algae and macroalgae and had extremely low coverage of calcified algae, a situation that may decrease coral coverage and damage the health of the coral reef ecosystem.
Some studies believe that although upwelling may prevent coral bleaching, it may also cause the outbreak of benthic algae because of the low temperature and increased nutrient concentration, and the benthic algae will expand and compete with corals for space (Riegl et al., 2019). Especially, coral coverage was relatively low for both the QDU and the non-upwelling zones. Notably, record-level flooding in 2010 reduced the coverage of live coral from 15.2% to 9.8% and calcified algae from 28.1% to 1.7% in eastern Hainan Island, and the surviving coral community is likely subject to intensified competition with macroalgae (Huang et al., 2014). Seasonal upwelling leading to significant changes in environmental parameters (such as temperature, salinity, and nutrient availability) and wave exposure can affect coral community composition (Eidens et al., 2015). Our findings were in accordance with former studies, the low temperature caused by the annual upwelling events limits the development of coral reefs on the east coast of Venezuela near these upwelling areas, and marginal coral communities are developed on shallow hard substrates (Rodríguez et al., 2009). Development of coral reefs in the Indo-Pacific is restricted to sites that are sheltered from high wave or upwelling due to temperature-induced decreases and turbulent environments in coral growth during upwelling (Eidens et al., 2015). Therefore, the varying influence of waves was considered to be one of the major controls of the spatial cover and community composition of hermatypic corals along the coast off eastern Hainan (Wang et al., 2013).
Moreover, large and sub-large stress-tolerant species were dominant in the coral communities of the QDU and non-upwelling zones. Nevertheless, the continued decline of offshore corals along the coast of China does not mainly result from climate change (Hughes et al., 2012). More land construction activity in the coastal areas is also an important contribution to cause lower coral coverage along the QDU zone. For instance, urban development is believed to play a dominant role in altering water quality and reduce coral coverage between 1936 and 2004 in Puerto Rico, whereas the changes in the coverage of cropland and forest seem to only have a secondary role (Ramos-Scharrón et al., 2015). The massive or encrusting species such as Porites lutea, Porites lobata, Favites complanata, Galaxea fascicularis were more abundance in the non-upwelling zone and contributed significantly to the difference of the coral communities, likely because they are more resistant to high temperature and elevated nutrient concentration (Duprey et al., 2016). Instead, continuous human activities have a stronger influence on the coastal coral reefs (Hughes et al., 2012), and the impact of these activities can be seen from indicators such as high nutrients, land development in coastal areas, and high algae community coverage during the upwelling period. This study revealed the significant spatial differences in the structure of reef building coral communities in the QDU zone and non-upwelling waters of Hainan Island, which may be attributed to the coastal upwelling events, complex historical background, and strong human activities in the study areas.
Fish community and driving patterns
Reef-building corals are essential for maintaining the diversity of fish communities, and the benthic composition affects the fish community of coral reefs (Pombo-Ayora et al., 2020). The diversity, species abundance, and function of coral reef fish communities generally decrease when there is a significant decline in live coral coverage (Pratchett et al., 2011; Rogers et al., 2017; Richardson et al., 2018). In the current survey, fish density was higher in the in non-upwelling zone where coral coverage was high than in the QDU zone where coral coverage was low. In particular, the abundance of Pomachromis and Stegastes was significantly higher in the non-upwelling zone. Pomacentridae is very vulnerable to the decline of coral coverage and one of the most abundant and ecologically diverse families of coral reef fish (Bonin, 2011). Some Pomacentridae species rely heavily on healthy coral groups for recruitment (Bonin et al., 2011). Consumption of algae by herbivorous fish is one of the most important structural forces in the coral reef benthic communities. When the algae consumption is reduced, coral reefs undergo a phase shift from coral- to macroalgae-dominated systems (Smith, 2008). The phase shift from coral-dominated reefs to barren grounds affects the fish that rely on living corals for food and shelter (Lin et al., 2021). Although the QDU zone had a high algae level, it had very low fish abundance, possibly because these fish species were directly affected by living corals.
In addition, the heterogeneity of the underlying habitat also has a strong impact on the community structure of the coral reef fish (Halford et al., 2004). Fragmentation of habitat may offset the negative effects of habitat loss for coral fish and promote species richness by weakening the competition within and among fish species (Bonin et al., 2011). The coral fish had a higher level of diversity possibly because the fragmentation of coral habitats was greater in the QDU zone than in the non-upwelling zone, even though the latter had higher coral coverage due to habitat complexity. Finally, wave exposure is also considered to be one of the major factors in shaping coral reef fish assemblages (Nunes et al., 2013). Physical structure of coral reefs can be influenced by disturbance from waves and ocean currents, and coral reefs with lower exposure tend to favor complex structural framework for higher fish abundance (Alemu et al., 2021). The reefs in ODU which may be subject to unpredictable variation in wave exposure seem to diminish the chance of establishment of reef fishes without stable habitat conditions (Krajewski and Floeter, 2011). Our findings are supported by the study in the Hawaiian archipelago, suggesting that fish biomass is lowest when water flow increases in locations exposed to swells (Friedlander et al., 2003).
Although the above evidence supports the dependence of fish on coral cover (Sartori et al., 2021), habitat complexity and wave exposure, the fish community is also affected by other environmental factors (e.g., Chl-a concentration, temperature) as well as coastal land use (Ruppert et al., 2018). Chl-a concentration can determine the presence of predatory fish in coral reefs (Williams et al., 2015), and areas with stronger primary production could promote fish growth and survival (Goldstein and Sponaugle, 2020). Rising water temperature generally decreases dissolved oxygen and damages the swimming ability, metabolism, and reproductive activities of fish, and larger individuals that rely on oxygen are more seriously affected (Pörtner and Knust, 2007; Johansen et al., 2014). Surprisingly, the non-upwelling zone had higher seawater temperature and higher fish density. It was possible that some coral reef fish might develop metabolic traits to adapt to higher temperature (Munday et al., 2017), and the low temperature water from upwelling might reduce the activity and feeding performance of tropical fish (Kingsbury et al., 2020). In the case of Bootless Bay, where population is dense in the urbanized area and the species diversity of coral reefs is low, the species richness of coral reef fish may be less than half that of coastal coral reefs in rural areas (Drew et al., 2015). Similarly, the total fish density was much lower in the QDU zone, whose coastal area was mostly construction land, than in the non-upwelling zone, whose coastal area was dominated by agricultural land. In addition, some weak relationships exist between land use and nutrient factors at the local scale; that is, these stressors act relatively independently (Erős et al., 2020).
Conclusion
In this study, comprising analyses of environmental parameters and community composition from coastal coral reefs with (eastern coast of Hainan Island) and without (northwest coast) signatures of upwelling were investigated. Our results suggest that coral reefs of eastern Hainan Island are under the influence of Qiongdong upwelling which coincides with phase of summer heat, and have strong potential as local thermal refugia for corals in a future with raised ocean temperatures. Upwelling-related environmental parameters, wave exposure and potential anthropogenic actions were the major factor structuring benthic communities and fish communities. However, turf algae and macroalgae dominated the algae communities, but calcified algae coverage, recruitment of juvenile corals, reefs fish density and live corals cover were low in QDU. This suggests that potential refuge function is currently compromised, mainly due to the coastal development and human activities as the greatest threat to local corals in QDU. Although our associated data can’t fully explain the effects of upwelling and human activity on coral reef, the results of ecological monitoring can provide early warning of threats to coral reefs in the Anthropocene by evaluating the refugia potential of inshore reefs. Finally, ecosystem-based management and restoration may be the key way to restore the function of thermal refugia for corals from QDU.
Data availability statement
The original contributions presented in the study are included in the article/Supplementary Material. Further inquiries can be directed to the corresponding author.
Author contributions
WZ: Conceptualization, Investigation, Methodology, Writing – original draft. YR, XL: Investigation, Data curation. DH, JX, MZ HY, RC: Investigation. XL: conceptualization, Writing – review & editing, Supervision. All authors contributed to the article and approved the submitted version.
Acknowledgments
This work was financially supported by the National Natural Science Foundation of China (42076108, 42161144006 or 3511/21), the Natural Science Foundation of Hainan Province (421RC488), the Hainan Provincial Key Research and Development Program (ZDYF2020177), and the Foundation of Hainan University [KYQD(ZR)1805]. We are grateful for the assistance from Hainan Provincial Ecological and Environmental Monitoring Centre in the investigation.
Conflict of interest
The authors declare that the research was conducted in the absence of any commercial or financial relationships that could be construed as a potential conflict of interest.
Publisher’s note
All claims expressed in this article are solely those of the authors and do not necessarily represent those of their affiliated organizations, or those of the publisher, the editors and the reviewers. Any product that may be evaluated in this article, or claim that may be made by its manufacturer, is not guaranteed or endorsed by the publisher.
Supplementary material
The Supplementary Material for this article can be found online at: https://www.frontiersin.org/articles/10.3389/fmars.2022.888888/full#supplementary-material
References
Alcívar-Mendoza L. J., Arellano-Verdejo J., Valle M., Cabanillas-Terán N. (2021). Algae on coral rocky reefs as indicators of disturbances along the ecuadorian coast. Reg. Stud. Mar. Sci. 46, 101899. doi: 10.1016/j.rsma.2021.101899
Alemu I J. B., Mallela J. (2021). Recent dynamics on turbid-water corals reefs following the 2010 mass bleaching event in tobago. Mar. Environ. Res. 170, 105411. doi: 10.1016/j.marenvres.2021.105411
Alemu J. B., Ishmael-Lalla M., Mannette R. P., Williams G. J., Agard J. (2021). Hydro-morphological characteristics provide insights into coral reef ecosystem services and disservices. Ecosyst. Serv. 49, 101281. doi: 10.1016/j.ecoser.2021.101281
Anderson M. (2001). A new method for non-parametric multivariate analysis of variance. Austral Ecol. 26, 32–46. doi: 10.1111/j.1442-9993.2001.01070.pp.x
Ban S. S., Graham N. A. J., Connolly S. R. (2014). Evidence for multiple stressor interactions and effects on coral reefs. Global Change Biol. 20, 681–697. doi: 10.1111/gcb.12453
Barnes M. L., Mbaru E., Muthiga N. (2019). Information access and knowledge exchange in co-managed coral reef fisheries. Biol. Conserv. 238, 108198. doi: 10.1016/j.biocon.2019.108198
Becerro M. A., Bonito V., Paul V. J. (2006). Effects of monsoon-driven wave action on coral reefs of Guam and implications for coral recruitment. Coral Reefs 25, 193–9. doi: 10.1007/s00338-005-0080-7
Bellwood D. R., Streit R. P., Brandl S. J., Tebbett S. B. (2019). The meaning of the term ‘function’ in ecology: A coral reef perspective. Funct. Ecol. 33, 948–961. doi: 10.1111/1365-2435.13265
Bonin M. (2012). Specializing on vulnerable habitat: Acropora selectivity among damselfish recruits and the risk of bleaching-induced habitat loss. Coral Reefs 31, 287–297. doi: 10.1007/s00338-011-0843-2
Bonin M. C., Almany G. R., Jones G. P. (2011). Contrasting effects of habitat loss and fragmentation on coral-associated reef fishes. Ecology 92, 1503–1512. doi: 10.1890/10-0627.1
Cacciapaglia C., van Woesik R. (2016). Climate-change refugia: Shading reef corals by turbidity. Global Change Biol. 22, 1145–1154. doi: 10.1111/gcb.13166
Chollett I., Mumby P. J. (2013). Reefs of last resort: Locating and assessing thermal refugia in the wider caribbean. Biol. Conserv. 167, 179–186. doi: 10.1016/j.biocon.2013.08.010
Dong X., Huang H., Zheng N., Pan A., Wang S., Huo C., et al. (2017). Acidification mediated by a river plume and coastal upwelling on a fringing reef at the east coast of Hainan Island, northern South China sea. J. Geophys. Res.: Oceans 122, 7521–7536. doi: 10.1002/2017JC013228
Drew J., Amatangelo K., Hufbauer R. (2015). Quantifying the human impacts on papua new guinea reef fish communities across space and time. PloS One 10, e140682. doi: 10.1371/journal.pone.0140682
Duprey N. N., Yasuhara M., Baker D. M. (2016). Reefs of tomorrow: Eutrophication reduces coral biodiversity in an urbanized seascape. Global Change Biol. 22, 3550–3565. doi: 10.1111/gcb.13432
Edmunds P., Aronson R., Swanson D., Levitan D., Precht W. (1998). Photographic versus visual census techniques for the quantification of juvenile corals. Bull. Mar. Sci. 62, 937–946.
Eidens C., Hauffe T., Bayraktarov E., Wild C., Wilke T. (2015). Multi-scale processes drive benthic community structure in upwelling-affected coral reefs. Front. Mar. Sci. 2. doi: 10.3389/fmars.2015.00002
Ellis J. I., Jamil T., Anlauf H., Coker D. J., Curdia J., Hewitt J., et al. (2019). Multiple stressor effects on coral reef ecosystems. Global Change Biol. 25, 4131–4146. doi: 10.1111/gcb.14819
Erős T., Czeglédi I., Tóth R., Schmera D. (2020). Multiple stressor effects on alpha, beta and zeta diversity of riverine fish. Sci. Total Environ. 748, 141407. doi: 10.1016/j.scitotenv.2020.141407
Evans R. D., Wilson S. K., Fisher R., Ryan N. M., Babcock R., Blakeway D., et al. (2020). Early recovery dynamics of turbid coral reefs after recurring bleaching events. J. Environ. Manage. 268, 110666. doi: 10.1016/j.jenvman.2020.110666
Facon M., Pinault M., Obura D., Pioch S., Pothin K., Bigot L., et al. (2016). A comparative study of the accuracy and effectiveness of line and point intercept transect methods for coral reef monitoring in the southwestern indian ocean islands. Ecol. Indic. 60, 1045–1055. doi: 10.1016/j.ecolind.2015.09.005
Friedlander A. M., Brown E. K., Jokiel P. L., Smith W. R., Rodgers K. S. (2003). Effects of habitat, wave exposure, and marine protected area status on coral reef fish assemblages in the hawaiian archipelago. Coral Reefs 22, 291–305. doi: 10.1007/s00338-003-0317-2
Frieler K., Meinshausen M., Golly A., Mengel M., Lebek K., Donner S. D., et al. (2013). Limiting global warming to 2°C is unlikely to save most coral reefs. Nat. Climate Change 3, 165–170. doi: 10.1038/nclimate1674
Fu T., Zhang L., Yuan X., Chen B., Yan M. (2021). Spatio-temporal patterns and sustainable development of coastal aquaculture in Hainan Island, China: 30 years of evidence from remote sensing. Ocean Coast. Manage. 214, 105897. doi: 10.1016/j.ocecoaman.2021.105897
Goldstein E., Sponaugle S. (2020). Juvenile reef fish growth and survival related to subregional patterns of primary production. Mar. Biol. 167, 16. doi: 10.1007/s00227-019-3627-9
Halford A., Cheal A. J., Ryan D., Williams D. M. (2004). Resilience to large-scale disturbance in coral and fish assemblages on the great barrier reef. Ecology 85, 1892–1905. doi: 10.1890/03-4017
Han X. (2018). Analysis of land use change and driving force in the coastal zone of Hainan Island (Shandong Agricultural University).
Harborne A. R., Rogers A., Bozec Y., Mumby P. J. (2017). Multiple stressors and the functioning of coral reefs. Annu. Rev. Mar. Sci. 9, 445–468. doi: 10.1146/annurev-marine-010816-060551
Herbeck L. S., Unger D., Wu Y., Jennerjahn T. C. (2013). Effluent, nutrient and organic matter export from shrimp and fish ponds causing eutrophication in coastal and back-reef waters of ne Hainan, tropical China. Cont. Shelf Res. 57, 92–104. doi: 10.1016/j.csr.2012.05.006
Hernández A., Tapia F. J. (2021). Connecting spatial structure in subtidal benthic communities with temporal variability in bottom temperature and dissolved oxygen along an upwelling coast. Estuar. Coast. Shelf Sci. 250, 107166. doi: 10.1016/j.ecss.2021.107166
Huang H., Chen Z., Huang L. (2021). Status report of coral reefs of China, (2010-2019) (Beijing: Ocean Press).
Huang H., Yang Y., Li X., Yang J., Lian J., Lei X., et al. (2014). Benthic community changes following the 2010 hainan flood: Implications for reef resilience. Mar. Biol. Res. 10, 601–611. doi: 10.1080/17451000.2013.841942
Hughes T. P., Baird A. H., Bellwood D. R., Card M., Connolly S. R., Folke C., et al. (2003). Climate change, human impacts, and the resilience of coral reefs. Sci. (New York N.Y.) 301, 929–933. doi: 10.1126/science.1085046
Hughes T. P., Barnes M. L., Bellwood D. R., Cinner J. E., Cumming G. S., Jackson J., et al. (2017). Coral reefs in the anthropocene. Nature 546, 82–90. doi: 10.1038/nature22901
Hughes T., Huang H., Young M. (2012). The wicked problem of China’s disappearing coral reefs. Conserv. Biol. 27, 261–269. doi: 10.1111/j.1523-1739.2012.01957.x
Hughes T. P., Kerry J. T., Baird A. H., Connolly S. R., Dietzel A., Eakin C. M., et al. (2018). Global warming transforms coral reef assemblages. Nature 556, 492–496. doi: 10.1038/s41586-018-0041-2
Jing Z., Qi Y., Hua Z., Zhang H. (2009). Numerical study on the summer upwelling system in the northern continental shelf of the South China sea. Cont. Shelf Res. 29, 467–478. doi: 10.1016/j.csr.2008.11.008
Johansen J. L., Messmer V., Coker D. J., Hoey A. S., Pratchett M. S. (2014). Increasing ocean temperatures reduce activity patterns of a large commercially important coral reef fish. Global Change Biol. 20, 1067–1074. doi: 10.1111/gcb.12452
Kingsbury K. M., Gillanders B. M., Booth D. J., Coni E. O. C., Nagelkerken I. (2020). Range-extending coral reef fishes trade-off growth for maintenance of body condition in cooler waters. Sci. Total Environ. 703, 134598. doi: 10.1016/j.scitotenv.2019.134598
Krajewski J. P., Floeter S. R. (2011). Reef fish community structure of the fernando de noronha archipelago (equatorial western atlantic): The influence of exposure and benthic composition. Environ. Biol. Fishes 92, 25. doi: 10.1007/s10641-011-9813-3
Lee I., Fan T., Fu K., Ko D. S. (2020). Temporal variation in daily temperature minima in coral reefs of Nanwan Bay, Southern Taiwan. Sci. Rep. 10, 8656. doi: 10.1038/s41598-020-65194-8
Lee F., Simon K. S., Perry G. L. W. (2017). Increasing agricultural land use is associated with the spread of an invasive fish (gambusia affinis). Sci. Total Environ. 586, 1113–1123. doi: 10.1016/j.scitotenv.2017.02.101
Liao Z., Yu K., Chen B., Huang X., Qin Z., Yu X. (2021). Spatial distribution of benthic algae in the South China sea: Responses to gradually changing environmental factors and ecological impacts on coral communities. Diversity Distrib. 27, 929–943. doi: 10.1111/ddi.13243
Lin Y., Rabaoui L., Basali A. U., Lopez M., Lindo R., Krishnakumar P. K., et al. (2021). Long-term ecological changes in fishes and macro-invertebrates in the world’s warmest coral reefs. Sci. Total Environ. 750, 142254. doi: 10.1016/j.scitotenv.2020.142254
Li X., Wang D., Huang H., Zhang J., Lian J., Yuan X., et al. (2015). Linking benthic community structure to terrestrial runoff and upwelling in the coral reefs of northeastern Hainan Island. Estuar. Coast. Shelf Sci. 156, 92–102. doi: 10.1016/j.ecss.2014.09.021
Lu X., Huang C., Chen F., Zhang S., Lao Q., Chen C., et al. (2021). Carbon and nitrogen isotopic compositions of particulate organic matter in the upwelling zone off the east coast of Hainan Island, China. Mar. pollut. Bull. 167, 112349. doi: 10.1016/j.marpolbul.2021.112349
Lyu Y., Zhou Z., Zhang Y., Chen Z., Deng W., Shi R. (2022). The mass coral bleaching event of inshore corals form South China sea witnessed in 2020: Insight into the causes, process and consequence. Coral Reefs. doi: 10.1007/s00338-022-02284-1
Munday P. L., Donelson J. M., Domingos J. A. (2017). Potential for adaptation to climate change in a coral reef fish. Global Change Biol. 23, 307–317. doi: 10.1111/gcb.13419
Nelson W. (2009). Calcified macroalgae critical to coastal ecosystems and vulnerable to change: A review. Mar. Freshw. Res. 60, 787–801. doi: 10.1071/MF08335
Nunes J. D. A. C., Sampaio C. L. S., Barros F. (2013). How wave exposure, group size and habitat complexity influence foraging and population densities in fishes of the genus halichoeres (perciformes: Labridae) on tropical rocky shores. Mar. Biol. 160, 2383–2394. doi: 10.1007/s00227-013-2233-5
Pombo-Ayora L., Coker D. J., Carvalho S., Short G., Berumen M. L. (2020). Morphological and ecological trait diversity reveal sensitivity of herbivorous fish assemblages to coral reef benthic conditions. Mar. Environ. Res. 162, 105102. doi: 10.1016/j.marenvres.2020.105102
Pörtner H. O., Knust R. (2007). Climate change affects marine fishes through the oxygen limitation of thermal tolerance. Science 315, 95–97. doi: 10.1126/science.1135471
Pratchett M., Hoey A., Wilson S., Messmer V., Graham N., Au N. (2011). Changes in biodiversity and functioning of reef fish assemblages following coral bleaching and coral loss. Diversity 3, 424–452. doi: 10.3390/d3030424
Ramos-Scharrón C. E., Torres-Pulliza D., Hernández-Delgado E. A. (2015). Watershed- and island wide-scale land cover changes in puerto rico, (1930s–2004) and their potential effects on coral reef ecosystems. Sci. Total Environ. 506-507, 241–251. doi: 10.1016/j.scitotenv.2014.11.016
Randall C. J., Toth L. T., Leichter J. J., Maté J. L., Aronson R. B. (2020). Upwelling buffers climate change impacts on coral reefs of the eastern tropical pacific. Ecology 101, e2918. doi: 10.1002/ecy.2918
Richardson L. E., Graham N. A. J., Pratchett M. S., Eurich J. G., Hoey A. S. (2018). Mass coral bleaching causes biotic homogenization of reef fish assemblages. Global Change Biol. 24, 3117–3129. doi: 10.1111/gcb.14119
Riegl B. (2003). Climate change and coral reefs: different effects in two high-latitude areas (arabian gulf, South Africa). Coral Reefs 22, 433–446. doi: 10.1007/s00338-003-0335-0
Riegl B., Glynn P. W., Banks S., Keith I., Rivera F., Vera-Zambrano M., et al. (2019). Heat attenuation and nutrient delivery by localized upwelling avoided coral bleaching mortality in northern galapagos during 2015/2016 enso. Coral Reefs 38, 773–785. doi: 10.1007/s00338-019-01787-8
Rodríguez S., Alvizu A., Tagliafico A., Bastidas C. (2009). Low natural repopulation of marginal coral communities under the influence of upwelling. Hydrobiologia 624, 1–11. doi: 10.1007/s10750-008-9662-z
Roff G., Bejarano S., Priest M., Marshell A., Chollett I., Steneck R. S., et al. (2019). Seascapes as drivers of herbivore assemblages in coral reef ecosystems. Ecol. Monogr. 89, e1336. doi: 10.1002/ecm.1336
Rogers A., Blanchard J., Mumby P. (2018). Fisheries productivity under progressive coral reef degradation. J. Appl. Ecol. 55, 1041–1049. doi: 10.1111/1365-2664.13051
Ruppert J. L. W., Vigliola L., Kulbicki M., Labrosse P., Fortin M., Meekan M. G. (2018). Human activities as a driver of spatial variation in the trophic structure of fish communities on pacific coral reefs. Global Change Biol. 24, e67–e79. doi: 10.1111/gcb.13882
Sartori G., Taylor M. L., Sebastian P., Prasetyo R. (2021). Coral reef carnivorous fish biomass relates to oceanographic features depending on habitat and prey preference. Mar. Environ. Res. 172, 105504. doi: 10.1016/j.marenvres.2021.105504
Smith T. (2008). Temperature effects on herbivory for an indo-pacific parrotfish in panam?: Implications for coral-algal competition. Coral Reefs 27, 397–405. doi: 10.1007/s00338-007-0343-6
Sun R., Wu Z., Chen B., Yang C., Qi D., Lan G., et al. (2020). Effects of land-use change on eco-environmental quality in Hainan Island, China. Ecol. Indic. 109, 105777. doi: 10.1016/j.ecolind.2019.105777
Su J., Pohlmann T. (2009). Wind and topography influence on an upwelling system at the Eastern Hainan Coast. J. Geophys. Res. 114, C06017. doi: 10.1029/2008JC005018
Tkachenko K. S., Soong K. (2017). Dongsha atoll: A potential thermal refuge for reef-building corals in the South China sea. Mar. Environ. Res. 127, 112–125. doi: 10.1016/j.marenvres.2017.04.003
Wang D., Li Y. C., Lan J. X. (2013). Spatial differentiation of coral species related to wave energy along the Changqi Coast, Hainan Island, southern China. Cont. Shelf Res. 57, 117–122. doi: 10.1016/j.csr.2012.12.004
Wang S., Luo B., Qin Y., Zhao J., Wang T., Stewart S. D., et al. (2021). Fish isotopic niches associated with environmental indicators and human disturbance along a disturbed large subtropical river in China. Sci. Total Environ. 750, 141667. doi: 10.1016/j.scitotenv.2020.141667
Williams I., Baum J., Heenan A., Hanson K., Nadon M., Brainard R. (2015). Human, oceanographic and habitat drivers of central and western pacific coral reef fish assemblages. PloS One 10, e120516. doi: 10.1371/journal.pone.0120516
Williams G., Smith J., Conklin E., Gove J., Sala E., Sandin S. (2013). Benthic communities at two remote pacific coral reefs: Effects of reef habitat, depth, and wave energy gradients on spatial patterns. Peerj 1, e81. doi: 10.7717/peerj.81
Woodhead A. J., Hicks C. C., Norström A. V., Williams G. J., Graham N. A. J. (2019). Coral reef ecosystem services in the anthropocene. Funct. Ecol. 33, 1023–1034. doi: 10.1111/1365-2435.13331
Wyatt A. S. J., Leichter J. J., Toth L. T., Miyajima T., Aronson R. B., Nagata T. (2020). Heat accumulation on coral reefs mitigated by internal waves. Nat. Geosci. 13, 28–34. doi: 10.1038/s41561-019-0486-4
Yao Y., Wang C. (2021). Variations in summer marine heatwaves in the South China sea. J. Geophys. Res.: Oceans 126, e2021J–e17792J. doi: 10.1029/2021JC017792
Zhou L., Chen W., Sun J., Liu L., Huang X. (2020). Spatial variation in bacterioplankton communities in the pearl river, South China: Impacts of land use and physicochemical factors. Microorganisms 8, 814. doi: 10.3390/microorganisms8060814
Zhu W., Xia J., Ren Y., Xie M., Yin H., Liu X., et al. (2021). Coastal corals during heat stress and eutrophication: A case study in northwest hainan coastal areas. Mar. pollut. Bull. 173, 113048. doi: 10.1016/j.marpolbul.2021.113048
Keywords: coral reef, qiongdong upwelling, stony coral, coral reef fish, coastal land use, anthropogenic influence
Citation: Zhu W, Ren Y, Liu X, Huang D, Xia J, Zhu M, Yin H, Chen R and Li X (2022) The impact of coastal upwelling on coral reef ecosystem under anthropogenic influence: Coral reef community and its response to environmental factors. Front. Mar. Sci. 9:888888. doi: 10.3389/fmars.2022.888888
Received: 03 March 2022; Accepted: 12 September 2022;
Published: 28 September 2022.
Edited by:
Hajime Kayanne, The University of Tokyo, JapanReviewed by:
Guillermo Horta-Puga, Universidad Nacional Autónoma de México, MexicoGuanghua Wang, Guangxi University, China
Tianran Chen, Key Laboratory of Marginal Sea Geology, South China Sea Institute of Oceanology, Chinese Academy of Sciences (CAS), China
Thamasak – Yeemin, Ramkhamhaeng University, Thailand
Copyright © 2022 Zhu, Ren, Liu, Huang, Xia, Zhu, Yin, Chen and Li. This is an open-access article distributed under the terms of the Creative Commons Attribution License (CC BY). The use, distribution or reproduction in other forums is permitted, provided the original author(s) and the copyright owner(s) are credited and that the original publication in this journal is cited, in accordance with accepted academic practice. No use, distribution or reproduction is permitted which does not comply with these terms.
*Correspondence: Xiubao Li, xiubaoli@hainanu.edu.cn