- 1CAS Key Laboratory of Marine Ecology and Environmental Sciences, Institute of Oceanology, Chinese Academy of Sciences, Qingdao, China
- 2College of Marine Science, University of Chinese Academy of Sciences, Qingdao, China
- 3Laboratory of Marine Ecology and Environmental Sciences, Qingdao National Laboratory for Marine Science and Technology, Qingdao, China
- 4Jiaozhou Bay National Marine Ecosystem Research Station, Institute of Oceanology, Chinese Academy of Sciences, Qingdao, China
Aurelia coerulea, a type of scyphozoan jellyfish, has massively appeared in the coastal waters of China in recent years and caused great damage, but the asexual reproduction of A. coerulea polyp predicted by local temperature could not correspond well to the field abundance of jellyfish in summer. To understand the influence of winter and spring conditions on the reproductive process and to provide the basis for jellyfish population prediction, A. coerulea polyps, originally reared from planula larvae of medusae collected from Jiaozhou Bay (120.2°E, 36.1°N), were investigated at orthometric 3 overwintering temperatures (2, 5, and 8°C), 3 spring warming speeds (1°C increment every 5 days, 7.5 days, and 10 days), and 3 feeding frequencies (once per 9 days, 6 days, and 3 days), representing the range of environment conditions in winter and spring where they exist. Polyps had earlier strobilation, higher strobilation frequency, and more ephyra and bud production during warmer winter, but had intensive strobilation after colder winter when the temperature began to rise in spring. When warming speed was fast in spring, the percentage of the first strobilation was higher and ephyrae were released more intensively. However, when warming speed was slow in spring, the polyp could conduct secondary strobilation, resulting in higher strobilation frequency and more ephyrae with a longer period. High feeding frequency helped polyps accumulate more energy and promote asexual reproduction, especially beneficial to the second strobilation. This experiment shows that winter and spring temperature in addition to food availability has a significant effect on the asexual reproduction of polyp. However, these may not be only factors necessarily lead to jellyfish outbreaks in the natural environment. In the context of global warming, whether reproduction of polyps will eventually lead to the outbreak of jellyfish needs to be multifacetedly analyzed based on the complex situation in the field, such as the concentration and timing of jellyfish appearance, and their interactions with other species.
1 Introduction
In the past half-century, jellyfish blooms have been reported in many regions of the world (Mills, 2001; Purcell, 2005; Schrope, 2012), which causes serious ecological and economic problems in tourism, aquaculture, coastal facilities, marine fisheries, ecosystem health, and human life safety (Purcell et al., 2007; Qiu, 2014). As a consequence, the causes of jellyfish outbreaks have become the focus of research (Condon et al., 2012; Fernandez-Alias et al., 2021), which was widely attributed to a number of factors such as eutrophication, fishing activities, aquaculture, marine construction, ocean shipping, and ecosystem change, especially the rise in temperature with global warming (Mills, 2001; Purcell, 2012).
Analysis through field investigations and long-term historical datasets found that there are strong corresponding relationships between jellyfish population dynamics and climate factors (Mills, 2001; Purcell, 2005; Sun et al., 2012a). In the Irish Sea, climatic index could explain 68% of the variability in jellyfish abundance (Lynam et al., 2011). In the North Sea, the increase in jellyfish since 1958 saw a positive correlation in the North Atlantic Oscillation (NAO) index (Attrill et al., 2007). At the Mondego Estuary, there has been an increase in jellyfish since 2005, with temperature being the main controlling factor (Primo et al., 2012). Research on the Strait of Messina showed that rising water temperatures can promote jellyfish outbreaks by affecting their metabolism, growth, and reproduction rates (Rosa et al., 2013). Given that numerous studies found that global warming is accelerating, and the global climate system is undergoing rapid and widespread changes for the foreseeable future (IPCC, 2021), jellyfish populations would be expected to be affected.
In the wild water off the coast of China, seasonal warming and cooling can trigger the scyphozoan polyp populations to strobilate and release ephyrae, and then develop into a population of jellyfish. The main period of strobilation occurs following a temperature increase in spring after a wintering period (Feng et al., 2018); thus, the influence of bottom temperature in winter and spring before the emergence of planktonic jellyfish is particularly important for the prediction of the number of jellyfish in summer. However, until now, it is difficult to make reliable predictions of interannual variations in scyphozoan jellyfish populations off the coast of China based on local hydrological data in winter and spring. Although the majority of opinions support the idea that global warming can promote jellyfish bloom through more budding and strobilation, there is still no definitive agreement or corresponding relations on whether colder or warmer winters could lead to jellyfish blooms because of the complexity of field ecological environments with numerous fouling organisms, different species with particular characteristics, and specific geographical regions (Lynam et al., 2004; Feng et al., 2015a; Loveridge et al., 2021).
The outbreak of jellyfish requires preconditions of high reproduction rate, high growth rate, and low mortality, of which the high reproductive rate is mainly related to the life cycle strategy of jellyfish (Sun et al., 2012b). Most scyphomedusae and many hydromedusae have a metagenic life cycle, which can be divided into two stages. One is a planktonic medusa stage where medusae float in the water and produce planula by sexual reproduction. The other one is a benthic perennial polyp stage where polyps asexually produce more polyps through budding, longitudinal fission, or podocyst formation (Kakinuma, 1975), or produce new jellyfish through strobilation and ephyra release (Lucas, 2001). The asexual reproduction of polyps controlled by benthic environment is crucial to jellyfish bloom, because it determines the number of polyps existing on the sea floor and the number of ephyrae released by polyps through strobilation (Sun, 2012; Qiu, 2014). Previous laboratory studies have shown that in the suitable temperature range for polyps to produce bud, higher temperatures, especially 14–16°C, could encourage budding of polyps, which creates more polyps and expands the polyp population (Willcox et al., 2007; Han and Uye, 2010; Hubot et al., 2017). The relatively suitable temperature range for the strobilation of Aurelia medusa polyp in China is 8–18°C, and the optimum temperature is 13–15°C (Wang et al., 2015; Feng et al., 2018; Wang et al., 2018). In the suitable temperature range for polyps to strobilate and release ephyrae, higher water temperature and longer duration in the suitable temperature are beneficial to earlier strobilation, longer strobilation period, and more ephyrae released (Purcell, 2005; Purcell et al., 2007; Wang et al., 2018). However, more asexual reproduction does not mean jellyfish outbreaks are inevitable, because the timing, number, and concentration of young jellyfish populations are also critical in terms of how they interact with other creatures in the early spring and whether they can take advantage.
Along the north coast of China, scyphozoan Aurelia coerulea is one of the most frequent blooming scyphomedusae species (Wang et al., 2012a; Wang et al., 2020b). It is also the most widespread of all Aurelia species studied to date and has the least genetic variation sampled from multiple sites (Dawson, 2003). A. coerulea usually occurs near artificial marine facilities like bridge piers and ports, or around nuclear power plants, or appear in the coastal waters and estuaries during May to August in temperate regions including the Yellow Sea and Bohai Sea (Wan and Zhang, 2012; Wang et al., 2012b; Wang and Sun, 2015; Wang et al., 2020b), whose aggregations can block fishing nets and block the cooling water intake screen at nuclear power plants (Dong et al., 2010).
Most previous laboratory experiments simulated the life histories of polyps at different fixed temperatures, while few studies have simulated the effects of a dynamic temperature regime in certain seasons. At the same time, the relationship between the amount of asexual reproduction of polyps and jellyfish outbreaks is not well explained or does not correspond. This study explores the response of the asexual reproduction of A. coerulea polyp to global warming in the absence of other biological disturbances in the laboratory, especially the mimicking of temperature variation during winter and subsequent spring warming experienced by polyps prior to and during ephyra release, with different food levels, in order to contribute to a better understanding of jellyfish outbreaks from a basic biological perspective and provide a theoretical basis for the early warning and prediction of jellyfish outbreak in the natural environment.
2 Materials and Methods
2.1 Local Environment
A. coerulea were originally obtained from Jiaozhou Bay (120.2°E, 36.1°N), and the experiment was carried out in the jellyfish laboratory of the Institute of Oceanology, Chinese Academy of Sciences in Qingdao, China. Jiaozhou Bay is a semi-enclosed inner bay in the south of Shandong Peninsula, belonging to the north temperate zone. The average monthly bottom water temperature of Jiaozhou Bay is between 5 and 25°C. In winter, water temperature remains below 10°C from December to March, averaging around 5°C, and then water temperature rises slowly in spring and reaches a peak of 25–27°C in August.
2.2 Polyp Preparation
The polyps of A. coerulea used in this experiment were mixed and random in generation, obtained from a permanent culture in the Institute of Oceanology, Chinese Academy of Sciences, at a constant temperature of 20°C with a salinity of 30–31, and fed with newly hatched Artemia nauplii, and the water was replaced with sand-filtered sea water every day. According to the unpublished data of our research group, the difference in asexual reproduction among different generations of polyps was small. They were originally hatched from mature A. coerulea medusae collected from the Jiaozhou Bay population using artificial asexual reproduction methods (Feng et al., 2017a).
The polyps with a similar size, with 16 tentacles attached on the plastic corrugated plate, were randomly detached carefully with forceps, and every 2 polyps were placed in each well of the 6-well polycarbonate culture plates (growth area: 8.87 cm2, volume: 5 ml). In order to let the polyp attach to the culture plates, there were 10 days without any water exchange or feeding, and the culture temperature was maintained at 20°C ± 1°C by a thermostatic biological incubator (Jiangnan SPX) during that period. Polyps were cultured under dark conditions during the attaching period and the entire experiment duration to prevent light from influencing asexual reproduction.
After 10 days of attaching, all the 6-well plates with successfully attached polyps were randomly divided into 3 groups and placed into 3 thermostatic biological incubators (Jiangnan SPX-288) to cool down to their respective experimental temperatures with a speed of 1°C per day from 20°C until the culture temperature reached 2, 5, and 8°C. During the cooling period, the polyps in the 6-well plate were provided with newly hatched Artemia nauplii and then the water was replaced with freshly prepared sand-filtered sea water at the same temperature after 3 h. Feeding and water exchange were conducted every 3 days. Any directly and stolonally budded polyps produced before the start of the winter period were removed using forceps after naturally separating from the parent polyp.
2.3 Experimental Design
After the polyps cooled down to their respective experimental temperatures, the beginning of the wintering period marked the beginning of the experiment. The experiment was divided into 2 parts: a wintering period for 8 weeks and a spring warming period for around 16 weeks until all the ephyrae have been released by strobila. The experiment consisted of 3 orthogonal factors, and the levels were based on the analysis of historical hydrological data of Jiaozhou Bay: winter temperature (3 levels: 2, 5, and 8°C), warming speed [3 levels: 1°C increment every 5 days (I), 1°C increment every 7.5 days (II), and 1°C increment every 10 days (III)], and feeding frequency [3 levels: once per 3 days (3d), once per 6 days (6d), and once per 9 days (9d)] (Figure 1). There were altogether 27 treatments (3*3*3), and every treatment consisted of 3 replicates, which means three 6-well plates with 30–36 successfully attaching polyps each treatment. Altogether, there were 869 [approximately (30–36)*3*3*3] individuals initially in the experiments.
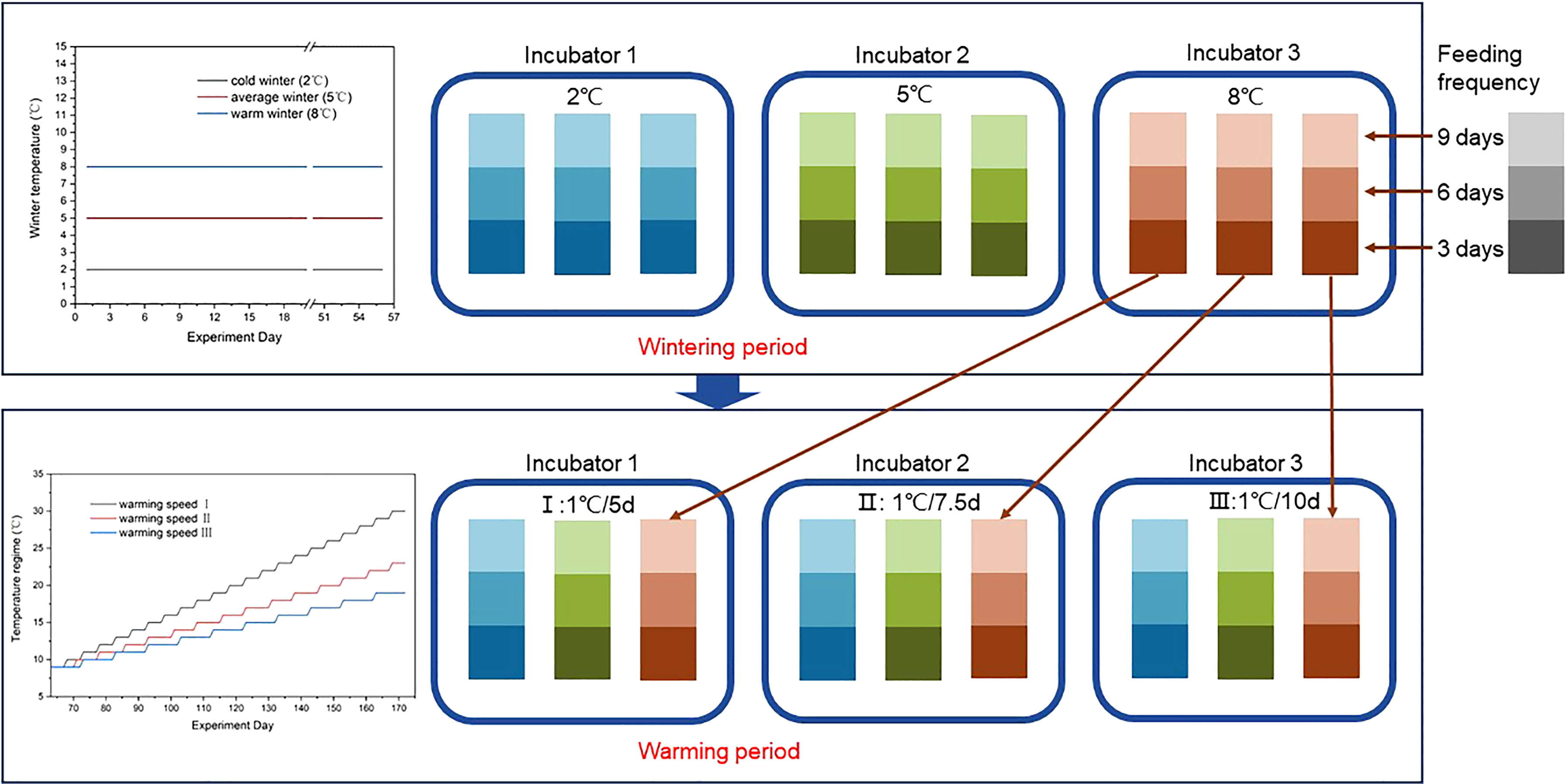
Figure 1 Experimental design. These polyps were first stored at 3 winter temperatures (2°C, 5°C, and 8°C) for 8 weeks, and then experienced the culture of 3 warming speeds (1°C increment every 5 days, 7.5 days, and 10 days). During the whole experiment, the polyps were fed with adequate Artemia nauplii under 3 feeding frequencies (once per 9 days, once per 6 days, and once per 3 days).
Experiment day was used to record time in this experiment, which set the first day of the experiment (the first day of the wintering period) as day 1. The experiment started on February 2, 2021 and ended on July 23, 2021, lasting 172 days.
2.3.1 Wintering Period
The factor “winter temperature” represented different winter conditions in the history of Jiaozhou Bay. According to the historical averages, 5°C was chosen to mimic the average winter temperature. According to the historical extremes in winter, we adjusted 3°C up or down to 8°C or 2°C to simulate a relatively warmer and colder winter, in order to explore the effects of different overwintering temperatures on the strobilation and reproduction of A. coerulea polyps. According to previous reports, strobilation can be induced after the storage at low temperature for more than 1 month (Kawahara et al., 2006) and a 6- to 8-week overwintering period was appropriate for the Aurelia aurita polyp to activate proteins regulating strobilation (Loveridge et al., 2021). To approximately simulate low-temperature duration in winter in offshore areas of Jiaozhou Bay, the winter duration of this experiment was set as 8 weeks (56 days). After culturing at low temperatures, the 2°C and 5°C treatments were warmed up to 8°C at a speed of 1°C per day to allow for spring warming from the same initial temperature.
2.3.2 Warming Period
The factor “warming speed” represented how fast the temperature rises in spring. The polyps in each of the 3 overwintering group were redivided into 3 new groups and placed into 3 incubators to start the spring warming period on day 62. Each new warming group equally contains the polyps from 3 overwintering temperatures. In the case of uniformly rising water temperature from 8°C in spring, the factor “warming speed” represented the duration of the optimal temperature for strobilation of polyps in spring to some extent. When the warming speed is high, the water temperature remains at the optimal temperature range for a very short time, and soon warms up to a higher summer temperature, which is no longer suitable for polyps to strobilate, and vice versa. According to the analysis of historical data of Jiaozhou Bay and the coastal area of Qingdao, the average duration of suitable temperature (8–18°C) for the polyp to strobilate and release the ephyrae was 70.24 days in spring, with a maximum of 85 days and a minimum of 56 days in 25 years from 1996 to 2020. Therefore, in this experiment we define a rise of 1°C increment every 5 days as a sharp warming (I), a rise of 1°C increment every 7.5 days as an ordinary spring warming (II), and a rise of 1°C increment every 10 days as a slow warming (III). The warming period lasts for 110 days until no more strobilation happens and all the ephyrae were released when the slowest warming group reached 19°C. At that time, the other two groups were warmed up to 30 and 23°C, although no valuable data were collected when the upper temperature of strobilation was exceeded.
2.3.3 Feeding Frequency and Polyp Maintenance
Three levels [once per 3 days (3d), once per 6 days (6d), and once per 9 days (9d)] of feeding frequency were designed to investigate the food effect combined with a different temperature regime.
During the wintering and warming periods, the polyps in the 6-well plate were supplied with non-limiting quantities of newly hatched Artemia nauplii for 3 h each time according to the feeding frequency and then water was replaced with freshly prepared sand-filtered sea water at the same temperature. After feeding, the presence of residual Artemia nauplii in the well of the 6-well plates and redness in the gut of the polyps indicated that the feeding was sufficient and ensured non-limiting feeding. Water exchanges were also made every 3 days if the group was not fed. The 6-well plates were quickly returned into the incubator after feeding and changing the water.
2.4 Data Collection and Statistical Calculation
Records were taken every week during the wintering period and every 3 days during the warming period. During both wintering and warming periods, the number of newly strobilated polyps was recorded with the date, and ephyrae released by each strobila were collected with a pipette and counted. New buds were removed carefully with forceps under a dissecting microscope (Olympus SZ61), and the number of buds was recorded until the strobilation started. Microscopic observations and record taking took less than 10 min for each treatment, and the 6-well plates were immediately returned to the incubator after the operation.
For each treatment in the orthogonal experiment, the following parameters were calculated or recorded: (a) strobilation frequency, which represented the mean number of times that the polyp strobilated in that group during the whole experiment; (b) first strobilation percentage and second strobilation percentage, which represent the percentage of first strobilated polyps or second strobilated polyps in all the polyps in that group; (c) strobilation percentage, which was calculated from the number of strobilae divided by polyp numbers; (d) days to first ephyra release, which represents the experiment date that the first ephyra was found to be released; (e) cumulative numbers of ephyrae (ind·polyp−1), which was calculated from the number of cumulative ephyrae divided by initial polyp numbers in the group; (f) ephyrae released (ind·polyp−1), which was calculated from the number of ephyrae released divided by polyp numbers; and (g) bud produced by each polyp before strobilation, which means the average number of buds produced by each polyp in one group before strobilation because the polyp did not produce buds during strobilation and the recovery period. Mean trait values were obtained per experimental unit (a 6-well plate) and used in subsequent statistical analysis. The average value of 3 units in the group was taken to calculate the parameters. Figures were made by Origin 2019b, and the statistical analysis was performed using SPSS 25. Data were checked for independence, normality (Shapiro–Wilk test), outliers, homogeneity, and collinearity before applying statistical models. If the date meets the precondition, three-way ANOVA was used to test the effect of 3 factors (winter temperature, warming speed, and feeding frequency). If the overall ANOVA results were significant, post-hoc test was conducted using the LSD pairwise comparison method to test the differences between experimental combinations. If they did not meet the precondition, the variance was firstly mathematically transformed, but if the transformation did not work, non-parametric analysis (Kruskal–Wallis and Kendall’s W method) was used and Tukey contrasts were used to compare the pairwise comparisons. Generalized linear models (GLMs) were used to analyze the relationship between budding and ephyrae release in order to explore the potential developed tendency of polyp population. Experimental data were expressed as mean ± standard error (SE).
3 Results
3.1 Strobilation Percentage and Strobilation Frequency
Strobilation occurred in all 27 treatments; however, polyps strobilated no more than twice during the whole experiment. The average frequency of strobilation per polyp difiered significantly among winter temperature, feeding frequency, and warming speed (Table 1). The total strobilation frequency was higher at warmer winter temperatures (Figure 2A), slow warming process (Figure 2B), and high feeding frequency (Figure 2C). The maximal average frequency was 1.29 ± 0.07 at the warmest winter temperature (8°C), the slowest warming speed (1°C rise per 10 days), and the highest feeding frequency (once per 3 days) (Figure 2D).
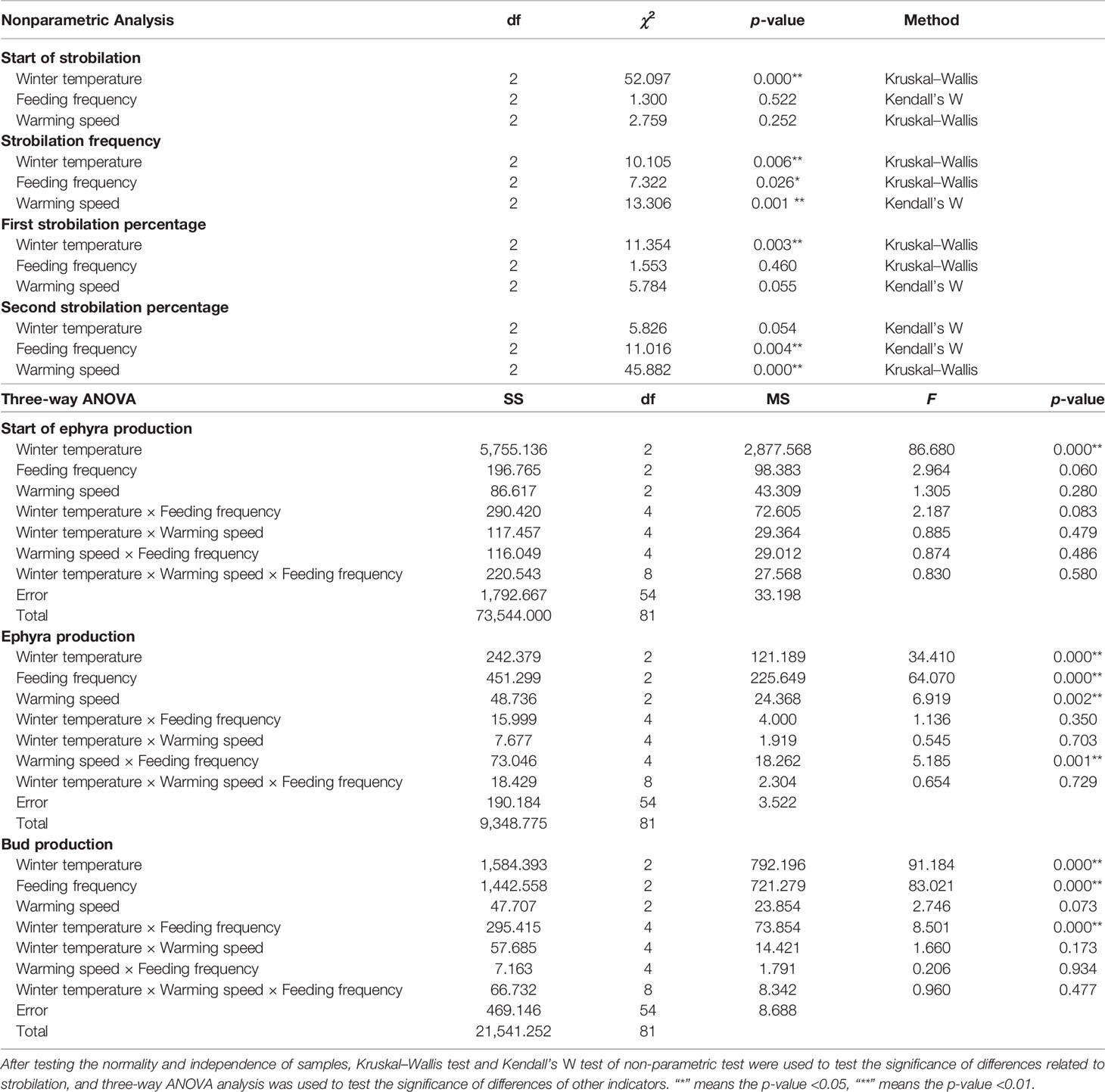
Table 1 Summary of nonparametric analysis and three-way ANOVA results among winter temperature, warming speed, and feeding frequency on the start of strobilation, strobilation frequency, first and second strobilation percentage, start of ephyra production, ephyra production, and bud production.
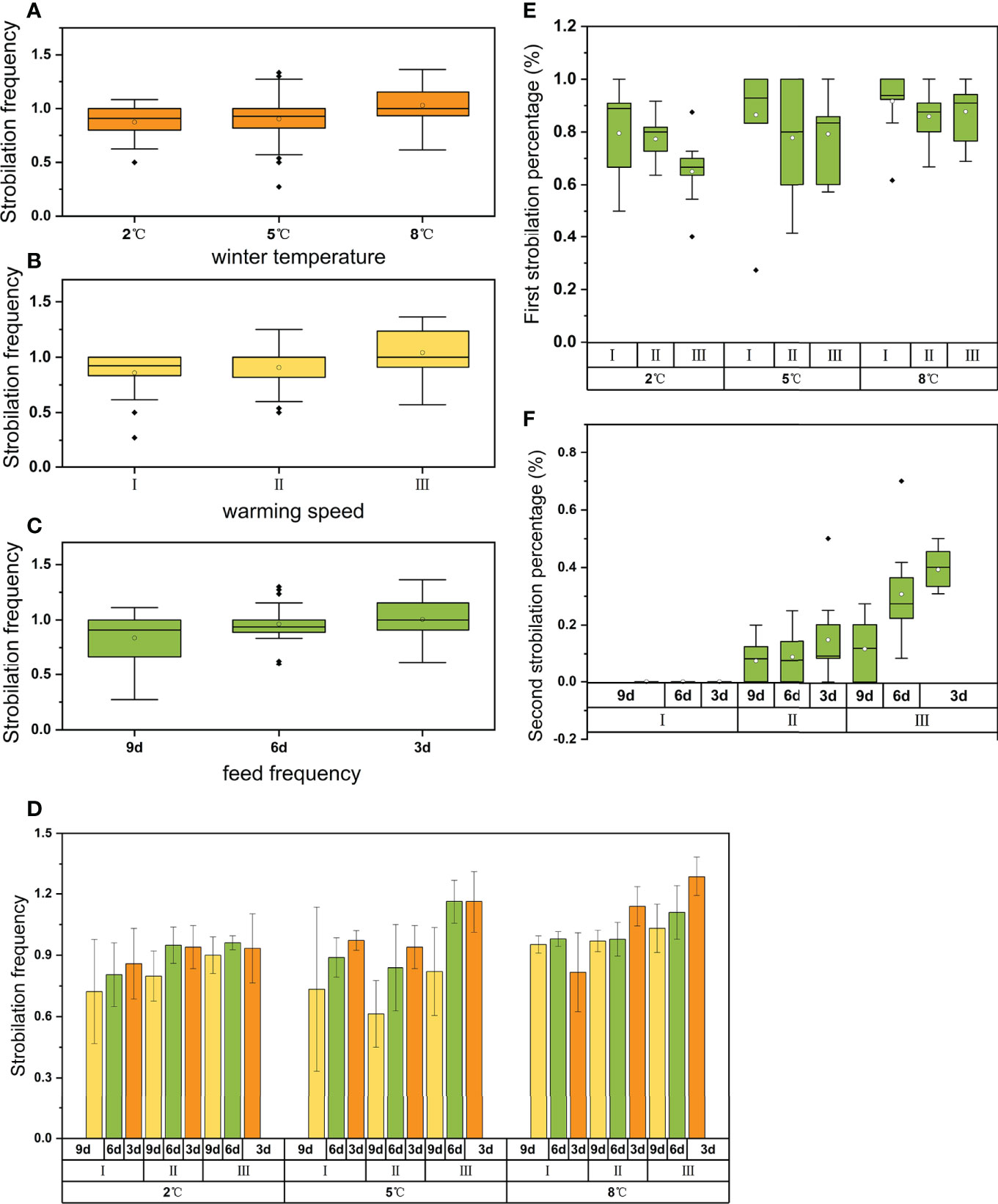
Figure 2 Mean strobilation frequency at 3 winter temperatures (A), 3 warming speeds (B), and 3 feeding frequencies (C). Strobilation frequency in all 27 treatments (D). First strobilation percentage at 3 winter temperatures and 3 warming speeds (E). Second strobilation percentage at 3 warming speeds and 3 feeding frequencies (F). The solid line in each box represents the median and the hollow circle represents the mean. The extremities of the box denote the first and the third quartile and the ends of vertical lines denote the range. Any points beyond the ends of vertical line are outliers.
All the replicates performed first strobilation while only replicates in warm speed II and III performed second strobilation. During the first strobilation, the percentage of strobilated polyps differed significantly among winter temperatures and marginally significantly among warming speeds (Table 1). First strobilation percentages increased with the increase of winter temperature and increase of warming speed (Figure 2E). The lowest value was 65.0 ± 12.9% at the coldest winter temperature (2°C) combined with the slowest warming speed (1°C rise per 10 days), while the maximal mean first strobilation percentage was 91.6 ± 12.6% at the warmest winter temperature (8°C) combined with the fastest warming speed (1°C rise per 5 days) (Figure 2E). The second strobilation percentages differed significantly among feeding frequencies and warming speeds rather than among winter temperatures (Table 1). Second strobilation did not happen in the fastest warming speed, warming speed I. In other warming speed treatments, second strobilation percentage increased with the decrease of warming speed and the increase of feeding frequency (Figure 2F). The maximal mean second strobilation percentage was 39.2 ± 6.8% at the warmest winter temperature (8°C) and the slowest warming speed (1°C rise per 10 days) (Figure 2F). Secondary strobilation reached 35.09% of the total strobilation frequency in the group with the slowest warming speed, and it was 12.96% in the group with the medium warming speed.
3.2 Strobilation Process Under Different Overwintering Conditions
The beginning of strobilation was significantly influenced by winter temperature in that the warmer the winter, the earlier the polyps strobilated (Table 1). The peak of strobilation came also earlier under warmer winter conditions (Figures 3A–C).
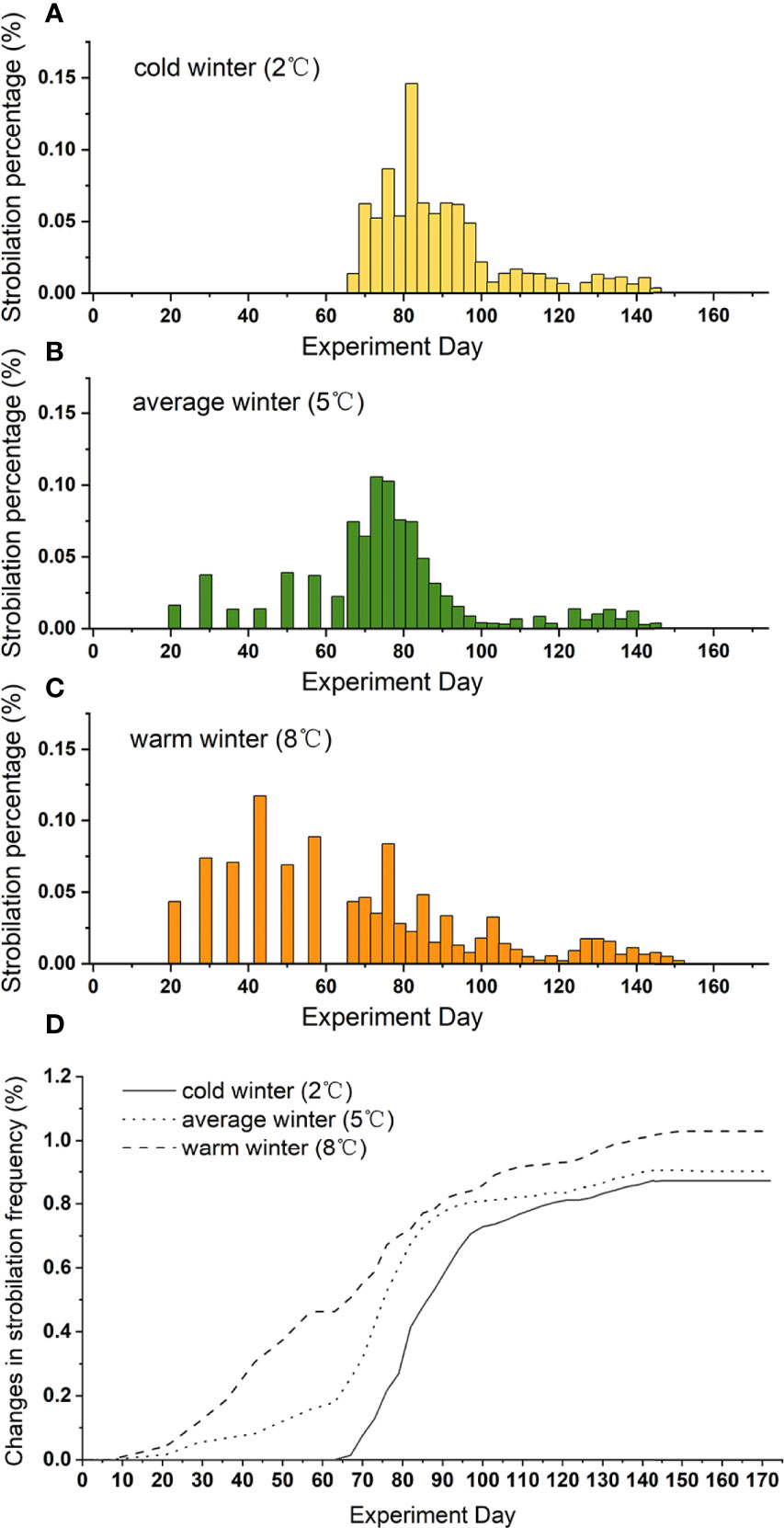
Figure 3 Strobilation percentage with time at different winter temperatures [2°C (A), 5°C (B), and 8°C (C)] and change in strobilation frequency at 3 winter temperatures (D). During the winter period, records were made every 7 days, and during the warming period, records were made every 3 days.
Strobilation occurred faster in cold winter, but most occurred in warm winter. Polyps in the cold winter treatments strobilated more intensively in a short time when temperature started to rise, while a long period was needed to allow most of the polyps to strobilate subsequently at 5°C and 8°C (Figure 3D).
3.3 Ephyra Production
The number of newly liberated ephyrae was significantly influenced by winter temperature, warming speed, and feeding frequency (Table 1). The group that released the most ephyrae was that treated with the combination of the warmest winter temperature (8°C), the slowest warming speed, and the highest feeding frequency, with a maximum of 18.14 ± 0.55 ephyrae per polyp (Figure 4). When the warming speed was high (I), polyps at the low feeding frequency (9d) significantly produced less ephyrae than that at medium and high feeding frequencies (ANOVA, p < 0.01). When the warming speed was slow (III), polyps at the high feeding frequency (3d) significantly produced more ephyrae than that at medium and low feeding frequencies (ANOVA, p < 0.01).
3.4 Effects of Overwintering Temperature and Feed Frequency on the Ephyra Release Process
The higher the wintering temperature, the earlier the polyps released the ephyrae (Table 1) (Figure 5A). There was no significant difference in the speed when they started to release ephyrae (ANOVA, p > 0.05), but the total number of ephyrae released was significantly different (Table 1) among different wintering temperatures (8.07 ± 0.50 ephyrae polyp−1 at 2°C, 9.99 ± 0.73 ephyrae polyp−1 at 5°C, and 12.31 ± 0.64 ephyrae polyp−1 at 8°C) (Figure 5B). The number of ephyrae released in 8°C and 5°C overwintering polyps was 152.99% and 123.73% of that in 2°C overwintering polyps, respectively.
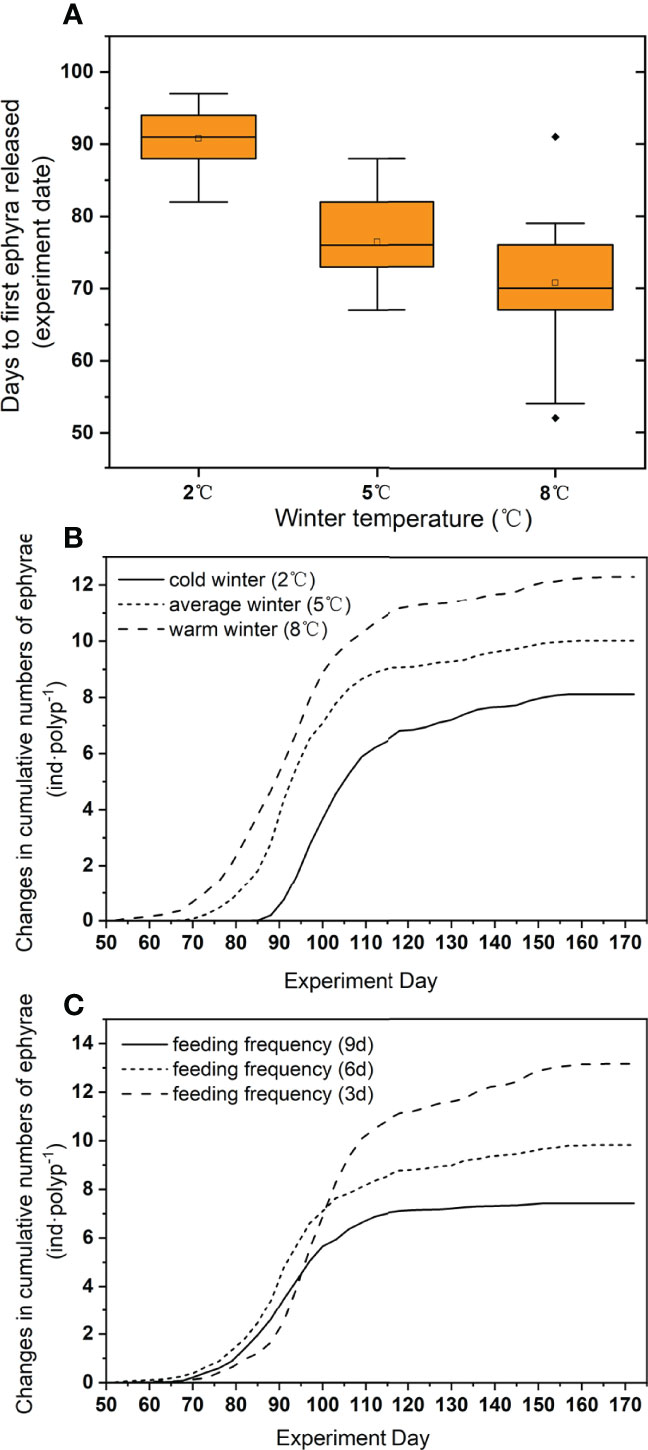
Figure 5 The days to first ephyra released (A) and change in cumulative number of ephyrae at 3 winter temperatures (B) and 3 feeding frequencies (C). The solid line in each box represents the median and the hollow circle represents the mean. The extremities of the box denote the first and the third quartiles and the ends of vertical lines denote the range. Any points beyond the ends of vertical line are outliers.
The total number of ephyrae increased significantly as the feeding frequency increased (Table 1). There was no significant difference in the first 52 days of releasing the ephyrae under different feeding frequencies (ANOVA, p > 0.05), but from day 55, the polyps in the higher feeding frequency treatment continued to release more ephyrae, while those in the lower feeding frequency treatment gradually stopped releasing ephyrae (Figure 5C). The number of ephyrae released by polyps fed every 3 days and every 6 days was 178.87% and 132.28% of those fed every 9 days, respectively.
3.5 Ephyra Releasing Process Under Different Warming Speed Conditions
Fast warming in spring prompted polyps to release ephyrae more quickly in the early stages, but slow warming could offer plenty of time for the polyps to release more ephyrae, and even to develop a second strobilation that eventually released a significantly larger amount of ephyrae (Figures 6A, B) (Table 1). The intensive release of the ephyrae was earlier at faster warming speed with a higher temperature (day 91 at warming speed I with 14–15°C, Figure 6C), while the peak was later in the treatment at slower warming speed with a lower temperature (day 93 at warming speed II with 13°C, Figure 6D, and day 97 at warming speed III with 12°C, Figure 6E), followed by the second peak of release.
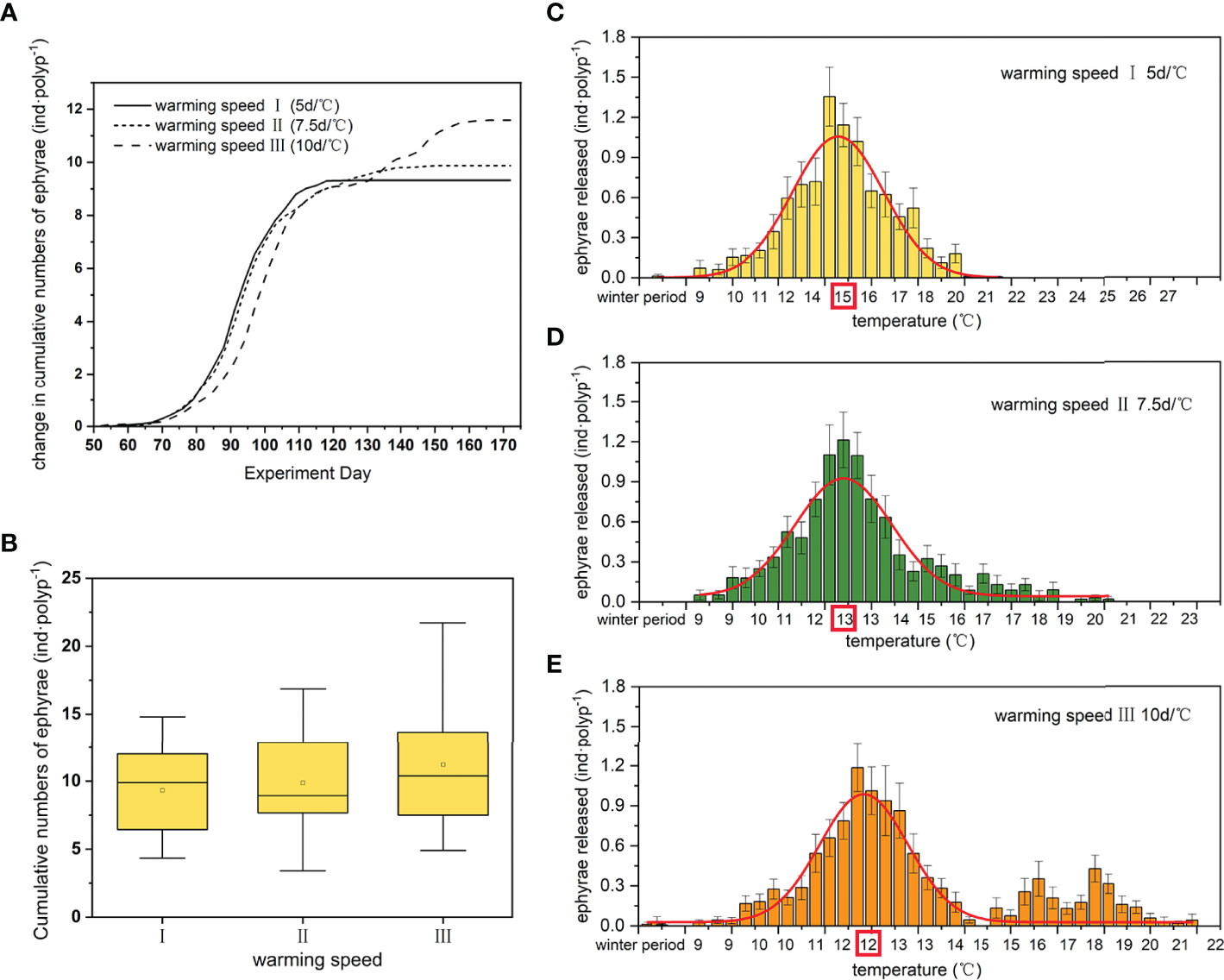
Figure 6 Changes in cumulative numbers of ephyrae at 3 warming speeds (A), the total numbers of ephyrae released per polyp at 3 warming speeds (B), and the numbers of ephyrae released as temperature changes at different warming speeds [warming speed I (C), warming speed II (D), and warming speed III (E)]. The solid line in each box in Figure 6B represents the median and the hollow circle represents the mean. The extremities of the box denote the first and the third quartile and the ends of vertical lines denote the range. Any points beyond the ends of vertical line are outliers. Gauss function was used to make the curves in the bar chart (C–E) (parameters: t-test, p < 0.01. model: ANOVA, p < 0.01), and the red box on the horizontal axis of the bar chart indicates the peak temperature of the concentrated release ephyrae.
3.6 Total Bud Production
Polyp asexual reproduction includes direct budding, stolonic budding, longitudinal fission, planuloid budding, and podocyst formation (Kakinuma, 1975). In our experiment, products of direct budding and stolonic budding were observed and recorded together as buds. Only very few podocysts were found in the group with the fastest warming speed, but the number of podocysts was too small to be statistically analyzed so it was not included in the results for discussion. The number of new produced buds were significantly influenced by winter temperature and feeding frequency, while warming speed had a limited influence on budding (Table 1). The group that produced most buds were those treated with the combination of the warmest winter temperature, medium warming speed, and the highest feeding frequency, with a maximum of 30.77 buds per polyp in 121 days (Figure 7A). Using simple linear regression, we found that the bud variation in the winter warming effects was likely infiuenced by feeding frequency (Figure 7B). The more sufficient the feed, the greater the promoting effect of winter warming on the production of buds.
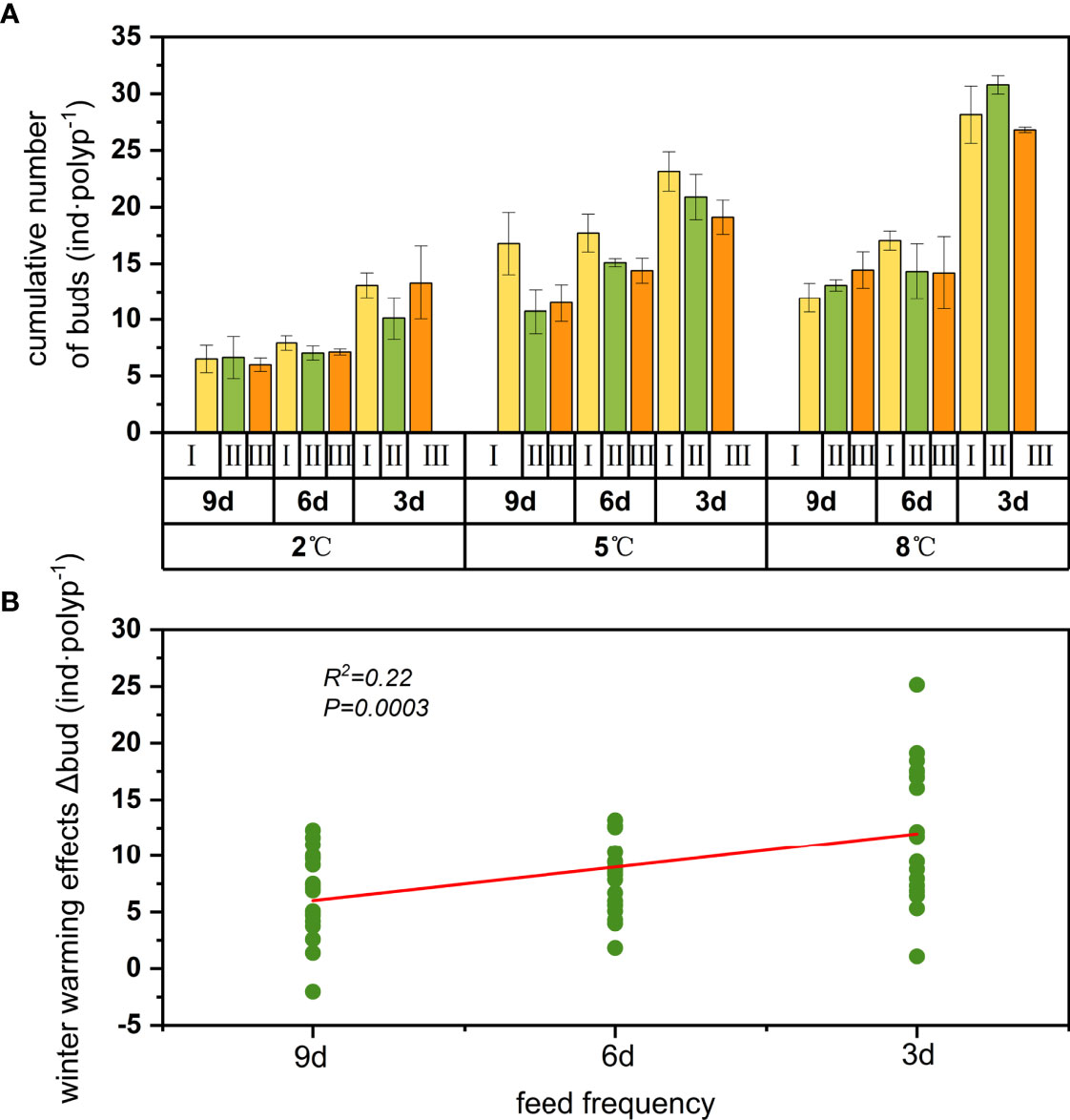
Figure 7 Cumulative numbers of buds released per polyp in 27 treatments (A) and relationship of winter warming effects on cumulative number of buds produced per polyp at different feeding frequencies (B).
3.7 Bud Releasing Process Under Different Overwintering Conditions and Feeding Frequencies
During the wintering period, polyps at 2°C wintering produced almost no buds while polyps at 8°C produced more buds than those at 5°C. When temperature started to elevate, there was no significant difference in the speed of bud production (ANOVA, p > 0.05) (Figure 8A).
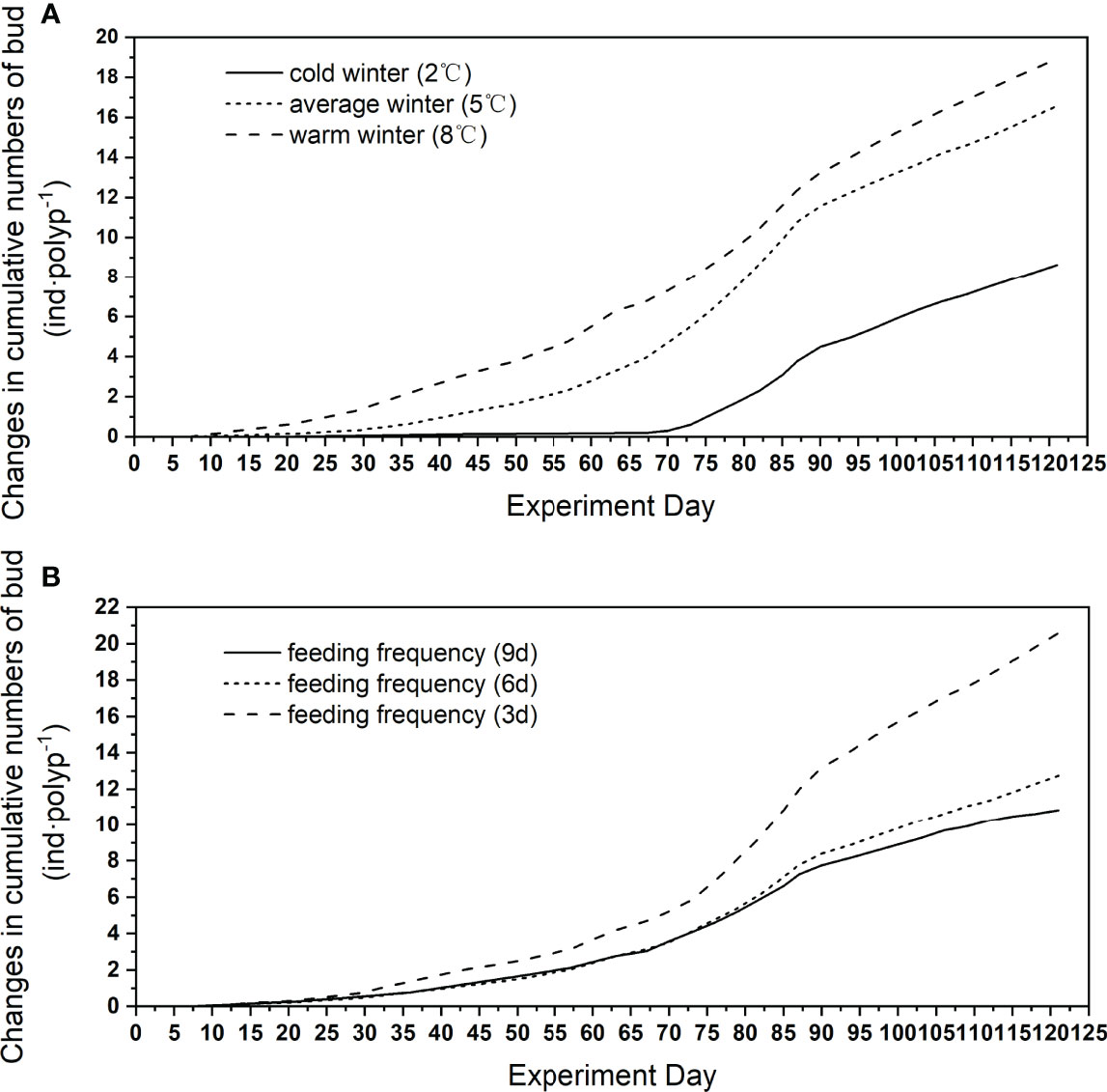
Figure 8 Change in cumulative number of buds at 3 winter temperatures (A) and 3 feeding frequencies (B).
The polyps in the highest feeding frequency treatment produced buds faster than the other treatment and finally released more buds until strobilation (Figure 8B). The number of buds released by polyps fed every 3 days and every 6 days was 190.0% and 179.6% of those fed every 9 days, respectively.
4 Discussion
4.1 The Effect of Wintering Temperature on Asexual Reproduction
In our experiment, the warm winter temperature significantly promoted strobilation, budding, and ephyrae release. Most studies focusing on temperature effects on scyphozoan jellyfish have found that the increase in temperature generally correlates positively with the abundance of jellyfish (Purcell, 2005) and improves the asexual reproduction rates (Purcell et al., 2012). Holst (2012) reported that a warmer winter temperature had several positive effects on strobilation, including longer strobilation period, higher strobilation percentage, and higher ephyra production per polyp in A. aurita. With global warming, rising water temperatures may cause jellyfish populations to shift more from polyps on the seafloor to plankton jellyfish in the water column, causing jellyfish outbreaks. One reason for this positive relationship may be that the wintering period is the preparation period when polyps obtain energy for strobilation and ephyrae release (Wang et al., 2015), and a relatively high winter temperature may be beneficial for polyps to gain more energy with high physiological activity. A previous study found that the efficiency of predation was reduced due to the weaker mobility of polyp’s tentacles at the colder temperature, which would lead to relatively less energy intake (Wang et al., 2018). At very low winter temperatures, polyps’ physiological activities are limited (Höhn et al., 2017). Rising water temperatures can boost jellyfish growth, metabolism, and reproduction (Rosa et al., 2013). At higher temperatures, polyps had shorter digest time (Wang et al., 2021), which indicated that when there was plenty of prey in the surrounding waters, polyps could digest more prey per unit time when water temperature is high.
Our experiment showed that polyps in warmer winter conditions started strobilation earlier. In a previous study, Purcell et al. (2009) found that there was a close relationship between high temperature in winter and strobilation time. In Southampton Water, UK, ephyrae appeared 2 months earlier when minimum winter temperature was warm (6.5°C) than when temperature was normal (5°C) (Lucas, 2001). In Washington, USA, the preparation period of strobilation of Aurelia labiata polyps was halved between 7 and 15°C (Purcell et al., 2007). In Tasmania, Australia, when winter water temperatures were 1°C cooler than the previous year, strobilation started later and ended sooner, and fewer polyps strobilated (Willcox et al., 2008). In the northwestern Mediterranean, Rhizostoma pulmo started to produce ephyra more quickly at 28°C than at 21°C (Purcell et al., 2012).
However, polyps treated at 2°C wintering developed an intensive strobilation when temperature started to rise while polyps at 8°C carried out a milder and slower strobilation process with longer time. It may be because polyps at 2°C overwintering are stimulated enough by low overwintering temperatures, and fully reached the threshold of transcriptional expression of genes controlling strobilation. A long enough wintering period or a low enough wintering temperature can help the polyp shift to the strobilation state (Loveridge et al., 2021), and help the polyp reach the critical low threshold needed to induce upregulation of the CL390 transcript, which are secreted proteins that are strongly upregulated prior to metamorphosis in response to the seasonal temperature changes (Fuchs et al., 2014). For our experiment, 8 weeks of overwintering at 8°C was sufficient to reach the threshold for triggering strobilation of polyps. Meanwhile, the analysis of the historical data of Jiaozhou Bay showed that the water temperature of Jiaozhou Bay can be kept below 10°C for 3–4 months, which has already reached the overwintering condition of local population polyp strobilation; thus, there are no insufficient overwintering problems to limit the strobilation of polyps in the local wild environment or in a laboratory experiment. Combined with the experimental results, it is speculated that after a relatively lower temperature overwintering stimulation, the concentrated and explosive strobilation of polyps in the wild environment may lead to the release of a large number of ephyrae in a short time, quickly gaining a competitive advantage in the plankton ecosystem when developing into a jellyfish population, which is perhaps one of the causes of jellyfish outbreak.
The budding of a polyp can supplement the population size of the polyp itself and, under appropriate conditions, release a large number of ephyrae. Before strobilation, polyps at warmer wintering temperatures produced more buds than those at colder temperatures. Polyps produced nearly no buds at 2°C wintering while polyps at 8°C produced more buds than those at 5°C. However, when the temperature started to rise, the speed of bud production had no significant difference among 3 winter temperatures. Water temperature and energy acquisition in winter only controlled the amount of budding in that winter and had limited effect on budding quantity in the following seasons, which may indicate that budding is an asexual reproduction process that is sensitive to marine environment and responds rapidly to changes in temperature and feed conditions.
4.2 The Effect of Spring Warming Speed on Asexual Reproduction
In addition to increased temperature, annual prolongation of favorable duration in warm years may be a crucial driver of recent jellyfish blooms (Feng et al., 2015b). Our experiments showed that plenty of polyps in slower warming treatments had secondary strobilation, resulting in significant high strobilation frequency and more ephyrae. In the wild waters of Jiaozhou Bay, polyps of A. coerulea were found to strobilate on February 25 when sea water temperature was 3.9°C, and persisted for 105 days until June 9 when sea water temperature was 18.1°C (Feng et al., 2018). In our experiments, the upper temperature limit of the polyp’s strobilation still did not exceed 18°C. For a uniform temperature rise under simulation starting from 8°C, the more slowly the water warms in spring, the longer it stays in the range of 8–18°C. A mild spring warming gives the polyps more time to finish strobilation and release ephyrae rather than quickly during summer, which is no longer suitable for strobilation and ephyrae release. A previous study also found that strobilation can occur continuously with the culture temperature held constant within temperature ranges that favor strobilation (Wang et al., 2018), and an intermediate temperature allows polyps to perform at their optimum, with high survival and propagation rates (Chi et al., 2019). Similar to A. coerulea, Nemopilema nomurai, with frequent outbreaks in the coastal waters of China, also has a benthic life history stage. For the N. nomurai, medusae were more likely to bloom if the duration at relatively cool temperatures (10–16°C) was prolonged in spring (Feng et al., 2020). The ecological model simulations also showed that along the coasts of the Yellow Sea, including the Changjiang Estuary, Jiangsu coast, and Haizhou Bay, the duration in which bottom seawater temperature ranges between 10 and 18°C in spring was shown to strongly correspond to the blooms of N. nomurai from 2006 to 2013; in contrast, the duration was much shorter in non-bloomed years (Zhao et al., 2016).
When water temperatures rose more rapidly in spring, the average percentage of first strobilation was higher, possibly due to the fact that polyps were more stimulated by the quick rising temperature. Studies have also shown that changing temperature is a critical factor in initiating strobilation or determining the reproductive seasons of most scyphomedusa species (Lucas and Lawes, 1998; Purcell et al., 1999).
4.3 The Effect of Food Supply on Asexual Reproduction
In our experiment, the amount of food the polyps consumed was controlled by different feeding frequencies. Feeding frequency had a significant influence on strobilation frequency, second strobilation percentage, ephyra production, and bud production. On the premise that the polyp is fully fed in each feeding, the higher the feeding frequency, the more active the polyp buds and releases ephyrae. This phenomenon was also reported for scyphozoan and hydrozoan jellyfish (Lucas and Lawes, 1998; Purcell et al., 1999; Ma and Purcell, 2005). Wangnan et al. (2015) found that at a high nutrition level, more ephyrae and buds were produced per polyp. Han and Uye (2010) found that production of new polyps by directly budding from the parent stalk and polyps budded from the parent pedal stolon was significantly increased with the increase in food. Purcell stated that with the increase of zooplanktons and protozoans resulting from eutrophication in the field, polyps could obtain more food and grow better, and in turn enhance the strobilation percentage, and ephyra and podocyst production (Purcell et al., 2007; Purcell et al., 2012).
Moreover, our study found that sufficient food supply could significantly enhance the second strobilation, resulting in more ephyrae. Polyps fed once every 9 days produced a lower percentage of secondary strobilation than those fed once every 6 or 3 days. A previous study also found that with enough food, polyps can store energy and strobilate twice, release more ephyrae, and produce more buds, instead of strobilate once and produce less buds under poor food conditions (Wang et al., 2015; Wang et al., 2018).
Food can alter the polyp’s tolerance to uncomfortable temperatures and its reproduction at different temperatures. In our experiment, food can enhance the warming effect on polyp reproduction through budding and ephyrae release. When the warming speed was high (I), polyps at the low feeding frequency (9d) significantly produced less ephyrae than that at medium and high feeding frequencies. This may be due to the fact that when the warming speed was high, polyps needed a lot of energy to reproduce asexually and release ephyrae in a short time, and the lack of food became a limiting factor. On the other hand, when the warming speed was slow (III), polyps at the high feeding frequency (3d) significantly produced more ephyrae than that at medium and low feeding frequencies, which may be explained by the greater frequency of feeding acting as a contributing factor that could help polyps carry out a second strobilation and produce more ephyrae. Sun (2012) reported that under high-temperature conditions, the polyp’s metabolism is active with a large amount of energy required, and the food level has an obvious influence on the growth and reproduction of polyps. Chi et al. (2019) also found that food conditions can significantly alter the temperature tolerance curve of polyps. The ability to adjust ephyra release based on seawater temperature may help ensure that Aurelia offspring are most prevalent with sufficient food.
4.4 The Potential Combined Effect of Winter Temperature, Warming Speed, and Feeding Frequency on the Jellyfish Bloom in the Natural Field of Jiaozhou Bay
The results of our experiment showed that the overwintering temperature and the spring warming speed significantly affected the amount, concentration, and timing of asexual reproduction of polyps, which may have a great significance to the early development of jellyfish population in that year. Firstly, we determined that a warm winter had a positive effect on the total amount of asexual reproduction of polyps, with more ephyrae release and budding. However, a colder winter gave polyps a stronger low-temperature stimulation, which may stimulate the expression of relevant molecules in polyps, leading to more intensive strobilations. Secondly, a spring with slower warming and longer duration of lower temperatures gave polyps enough time to strobilate and release ephyra at the suitable temperature range, so that more ephyrae were released. A rapidly warming spring, however, would result in a concentration of strobilations in polyps. We found that the most ephyrae release and the highest strobilation frequency happened in the treatment with the highest winter temperature and the slowest warming speed, and the highest first strobilation percentage happened in the treatment with the highest winter temperature and the fastest warming speed. Third, preys also played an important role in the whole process. When polyps rapidly and intensively strobilated under temperature control, a large amount of prey was required in a short period of time, and the lack of food became a limiting factor. At the same time, when polyps strobilated multiple times over a longer period of time, more prey acted as a promoter, providing continuous energy for the asexual reproduction of polyps.
Whether jellyfish can reach a level of outbreak depends on a number of factors: (1) how large is the population size of the polyp itself; (2) whether there are enough polyps to strobilate; (3) whether enough ephyrae are released; (4) whether there is sufficient food support for the growth and development of the polyps, ephyrae, and jellyfish; (5) whether there are enough unpreyed ephyrae and juvenile jellyfish to survive; and (6) whether the adult jellyfish population can be harmed on a large scale in a short period of time (Sun, 2012; Sun et al., 2012b), which means that whether the jellyfish will reach a level of outbreak is not only determined by the physical environment, but also affected by the biological environment. Because other organisms living in the habitat of a polyp in situ directly determine whether and to what extent the polyp and the ephyrae are threatened, it is necessary to continue to investigate whether environmental conditions are favorable for the polyp, as well as for its predators and competitors, and how stress changes for the polyp in more complex ecosystems in the wild. Only when jellyfish rapidly gain a competitive advantage in the marine ecosystem during the early stage of population development in spring and successfully develop into large numbers of adult jellyfish can they reach the scale of outbreak in summer.
A rapid and large number of first strobilation helps the ephyra appear in large numbers in early spring and take advantage of the first position, which may lead to the outbreak of jellyfish in that year if the prey and environmental conditions are suitable. The competitive advantage of a rapid increase in jellyfish populations in early spring can come from many sources. For example, fish and jellyfish compete for resources and may prey on each other, especially in their juvenile and planktonic life stages (Angel et al., 2016). There is a strong negative correlation between jellyfish population and fish amount according to studies from all over the world (Purcell and Arai, 2001; Purcell and Sturdevant, 2001; Lynam et al., 2011). The blooming season of jellyfish coincides with the growth, reproduction, and replenishment period of fish population, which will produce huge feeding pressure on fish eggs and larvae (Purcell, 1997). In addition, jellyfish outbreaks need large amounts of prey to support them. The massive consumption of other plankton in the ocean by jellyfish results in a significant decline in zooplankton populations, which inhibits the development of other organisms at the same trophic level, thus gaining a competitive advantage (Schneider and Behrends, 1994; Uye, 2011; Wang et al., 2020a).
In Jiaozhou Bay, the temperature fluctuation increased in the past century, and the temperature increased significantly in winter. In the past 40 years, the seasonal water temperature has also increased, especially in winter (Sun et al., 2011). In spring, the date that the daily mean temperature reached 15°C tended to advance in the past 20 years (W. Zang, unpublished data). According to the limited field survey data, it was difficult to find a significant correlation between the winter and spring hydrological records and the outbreak of jellyfish population during summer in Jiaozhou Bay. However, in recent decades, the number of jellyfish in Jiaozhou Bay has been increasing, especially small jellyfish (Sun et al., 2012a). The relationship between jellyfish and copepods and other zooplankton is complex, and at the polyp stage, jellyfish are also affected by the benthic ecosystem. In Jiaozhou Bay, the main species of biofouling living in the same habitat with polyps are Bryozoa, Pyrosomella verticilliata, Mytilidae, Ostrea gigas Thunberg, Reniclona sp., Balanus, Hydra, sea anemone, Ulva lactuca, and lime bug (MA et al., 2015). A study showed that these biofouling communities could control the number of polyps and the amount of ephyrae by occupying, covering, predation, and food competition (Feng et al., 2017b).
This study focused on the specific season in which polyps reproduce asexually, and studied the effects of temperature changes throughout winter and spring on polyps, rather than the simple response of polyps to short-term laboratory temperature changes. Although mass asexual reproduction of polyps does not necessarily mean jellyfish outbreaks are inevitable, this study still provides a basis for further field studies of communities and ecosystems.
Data Availability Statement
The original contributions presented in the study are included in the article/supplementary material. Further inquiries can be directed to the corresponding authors.
Ethics Statement
This study was carried out in accordance with the current laws in China. There are no legal or ethical restrictions involving jellyfish and zooplankton populations.
Author Contributions
WZ and SS contributed to conception and design of the study, and led the writing of this study. WZ and XC performed the experiment. WZ organized the database, performed the statistical analysis, and finished writing the draft. ZF proofread the draft carefully and gave detailed advice on analysis and writing. All authors contributed critically to the drafts and gave final approval for publication.
Funding
This work was financially supported by the National Natural Science Foundation of China (Nos. 42130411 and 42076166), Key Deployment Projects of the Center for Ocean Mega-Science, Chinese Academy of Sciences (COMS2019Z01), and Mount Tai Scholar Climbing Plan to SS.
Conflict of Interest
The authors declare that the research was conducted in the absence of any commercial or financial relationships that could be construed as a potential conflict of interest.
Publisher’s Note
All claims expressed in this article are solely those of the authors and do not necessarily represent those of their affiliated organizations, or those of the publisher, the editors and the reviewers. Any product that may be evaluated in this article, or claim that may be made by its manufacturer, is not guaranteed or endorsed by the publisher.
Acknowledgments
We thank Miss Zhenghua Zhang for caring for the polyps. We thank Feng Song and Wang Nan for giving advice on the design of the experiment. We are grateful to the reviewers and the editor for their valuable comments.
References
Angel D. L., Edelist D., Freeman S. (2016). Local Perspectives on Regional Challenges: Jellyfish Proliferation and Fish Stock Management Along the Israeli Mediterranean Coast. Regional Environ. Change 16 (2), 315–323. doi: 10.1007/s10113-014-0613-0
Attrill M., Wright J., Edwards M. (2007). Climate-Related Increases in Jellyfish Frequency Suggest a More Gelatinous Future for the North Sea. Limnol Oceanography 52 (1), 480–485.
Chi X., Mueller-Navarra D. C., Hylander S., Sommer U., Javidpour J. (2019). Food Quality Matters: Interplay Among Food Quality, Food Quantity and Temperature Affecting Life History Traits of Aurelia Aurita (Cnidaria: Scyphozoa) Polyps. Sci. Total Environ. 656, 1280–1288.
Condon R. H., Graham W. M., Duarte C. M., Pitt K. A., Lucas C. H., Haddock S. H. D., et al. (2012). Questioning the Rise of Gelatinous Zooplankton in the World's Oceans. BioScience 62 (2), 160–169. doi: 10.1525/bio.2012.62.2.9
Dawson M. N. (2003). Macro-Morphological Variation Among Cryptic Species of the Moon Jellyfish, Aurelia (Cnidaria: Scyphozoa). Marine Biol. 143 (2), 369–379. doi: 10.1007/s00227-003-1070-3
Dong Z., Liu D., Keesing J. (2010). Jellyfish Blooms in China: Dominant Species, Causes and Consequences. Marine Pollution Bull. 60 (7), 954–963.
Feng S., Lin J., Sun S., Zhang F. (2017a). Artificial Substrates Preference for Proliferation and Immigration in Aurelia Aurita(s. L.) Polyps. Chin. J. Oceanol. Limnol. 35 (1), 153–162.
Feng S., Lin J., Sun S., Zhang F., Li C., Xian W. (2020). Combined Effects of Seasonal Warming and Hyposalinity on Strobilation of Nemopilema Nomurai Polyps. J. Exp. Marine Biol. Ecol. 524, 151316.
Feng S., Wang S., Sun S., Zhang F., Zhang G., Liu M., et al. (2018). Strobilation of Three Scyphozoans (Aurelia Coelurea, Nemopilema Nomurai, and Rhopilema Esculentum) in the Field at Jiaozhou Bay, China. Marine Ecol. Prog. Ser. 591, 141–153.
Feng S., Wang S.-W., Zhang G.-T., Sun S., Zhang F. (2017b). Selective Suppression of in Situ Proliferation of Scyphozoan Polyps by Biofouling. Marine Pollution Bull. 114 (2), 1046–1056. doi: 10.1016/j.marpolbul.2016.10.062
Feng S., Zhang F., Sun S., Wang S., Li C. (2015a). Effects of Duration at Low Temperature on Asexual Reproduction in Polyps of the Scyphozoan Nemopilema Nomurai (Scyphozoa: Rhizostomeae). Hydrobiologia 754 (1), 97–111.
Feng S., Zhang G., Sun S., Zhang F., Wang S., Liu M. (2015b). Effects of Temperature Regime and Food Supply on Asexual Reproduction in Cyanea Nozakii and Nemopilema Nomurai. Hydrobiologia 754 (1), 201–214. doi: 10.1007/s10750-015-2279-0
Fernandez-Alias A., Marcos C., Perez-Ruzafa A. (2021). Larger Scyphozoan Species Dwelling in Temperate, Shallow Waters Show Higher Blooming Potential. Marine Pollution Bull. 173. doi: 10.1016/j.marpolbul.2021.113100
Fuchs B., Wang W., Graspeuntner S., Li Y., Insua S., Herbst E.-M., et al. (2014). Regulation of Polyp-To-Jellyfish Transition in Aurelia Aurita. Curr. Biol. 24 (3), 263–273.
Han C.-H., Uye S.-I. (2010). Combined Effects of Food Supply and Temperature on Asexual Reproduction and Somatic Growth of Polyps of the Common Jellyfish Aurelia Aurita s.L. Plankton Benthos Res. 5 (3), 98–105.
Höhn D. P., Lucas C. H., Thatje S. (2017). Respiratory Response to Temperature of Three Populations of Aurelia Aurita Polyps in Northern Europe. PloS One 12 (5), e0177913.
Holst S. (2012). Effects of Climate Warming on Strobilation and Ephyra Production of North Sea Scyphozoan Jellyfish. Hydrobiologia 690, 127–140.
Hubot N., Lucas C. H., Piraino S. (2017). Environmental Control of Asexual Reproduction and Somatic Growth of Aurelia Spp. (Cnidaria, Scyphozoa) Polyps From the Adriatic Sea. PloS One 12 (6), e0178482.
IPCC (2021). “Climate Change 2021: The Physical Science Basis. Contribution of Working Group I to the Sixth Assessment Report of the Intergovernmental Panel on Climate Change,” in Cambridge University Press. Eds. Masson-Delmotte V., Zhai P., Pirani A., Connors S. L., Péan C., Berger S., Caud N., Chen Y., Goldfarb L., Gomis M. I., Huang M., Leitzell K., Lonnoy E., Matthews J. B. R., Maycock T. K., Waterfield T., Yelekçi O., Yu R., Zhou B.. Cambridge University Press, Cambridge, United Kingdom and New York, NY, USA, In press, doi: 10.1017/978100915789
Kakinuma Y. (1975). An Experimental Study of the Life Cycle and Organ Differentiation of Aurelia Aurita Lamarck. Bull. Marine Biol. Station Asamushi Tokyo Univ. 15 (3), 101–113.
Kawahara M., Uye S.-i., Ohtsu K., Iizumi H. (2006). Unusual Population Explosion of the Giant Jellyfish Nemopilema Nomurai (Scyphozoa: Rhizostomeae) in East Asian Waters. Marine Ecol. Prog. Ser. 307, 161–173.
Loveridge A., Lucas C. H., Pitt K. A. (2021). Shorter, Warmer Winters may Inhibit Production of Ephyrae in a Population of the Moon Jellyfish Aurelia Aurita. Hydrobiologia 848 (3), 739–749.
Lucas C. H. (2001). Reproduction and Life History Strategies of the Common Jellyfish, Aurelia Aurita, in Relation to its Ambient Environment. Hydrobiologia 451 (1-3), 229–246.
Lucas C. H., Lawes S. (1998). Sexual Reproduction of the ScyphomedusaAurelia Aurita in Relation to Temperature and Variable Food Supply. Marine Biol. 131 (4), 629–638. doi: 10.1007/s002270050355
Lynam C. P., Hay S. J., Brierley A. S. (2004). Interannual Variability in Abundance of North Sea Jellyfish and Links to the North Atlantic Oscillation. Limnol Oceanography 49 (3), 637–643.
Lynam C. P., Lilley M. K. S., Bastian T., Doyle T. K., Beggs S. E., Hays G. C. (2011). Have Jellyfish in the Irish Sea Benefited From Climate Change and Overfishing? Global Change Biol. 17 (2), 767–782.
Ma X., Purcell J. E. (2005). Temperature, Salinity, and Prey Effects on Polyp Versus Medusa Bud Production by the Invasive Hydrozoan Moerisia Lyonsi. Marine Biol. 147 (1), 225–234. doi: 10.1007/s00227-004-1539-8
MA S., Wang Z., Liu H., Zhao J., Duan J., JianPing X., et al. (2015). Investigation and Analysis of Fouling Organisms in Qingdao Seagulls Floating Dock. J. Guangxi Acad. Sci. 31 (3), 214–218.
Mills C. E. (2001). Jellyfish Blooms: Are Populations Increasing Globally in Response to Changing Ocean Conditions? Hydrobiologia 451 (1-3), 55–68.
Primo A. L., Marques S. C., Falcao J., Crespo D., Pardal M. A., Azeiteiro U. M. (2012). Environmental Forcing on Jellyfish Communities in a Small Temperate Estuary. Marine Environ. Res. 79, 152–159.
Purcell J. E. (1997). Pelagic Cnidarians and Ctenophores as Predators: Selective Predation, Feeding Rates, and Effects on Prey Populations. Annales l'Institute Oceanographique 73 (2), 125–137.
Purcell J. E. (2005). Climate Effects on Formation of Jellyfish and Ctenophore Blooms: A Review. J. Marine Biol. Assoc. United Kingdom 85 (3), 461–476.
Purcell J. E. (2012). Jellyfish and Ctenophore Blooms Coincide With Human Proliferations and Environmental Perturbations. Annu. Rev. Marine Sci. 4 (1), 209–235.
Purcell J. E., Arai M. N. (2001). Interactions of Pelagic Cnidarians and Ctenophores With Fish: A Review. Hydrobiologia 451 (1-3), 27–44.
Purcell J. E., Atienza D., Fuentes V., Olariaga A., Tilves U., Colahan C., et al. (2012). Temperature Effects on Asexual Reproduction Rates of Scyphozoan Species From the Northwest Mediterranean Sea. Hydrobiologia 690 (1), 169–180.
Purcell J. E., Hoover R. A., Schwarck N. T. (2009). Interannual Variation of Strobilation by the Scyphozoan Aurelia Labiata in Relation to Polyp Density, Temperature, Salinity, and Light Conditions in Situ. Mar. Ecol. Prog. Ser. 375, 139–149.
Purcell J. E., Sturdevant M. V. (2001). Prey Selection and Dietary Overlap Among Zooplanktivorous Jellyfish and Juvenile Fishes in Prince William Sound, Alaska. Marine Ecol. Prog. Ser. 210, 67–83. doi: 10.3354/meps210067
Purcell J. E., Uye S.-i., Lo W.-T. (2007). Anthropogenic Causes of Jellyfish Blooms and Their Direct Consequences for Humans: A Review. Mar. Ecol. Prog. Ser. 350 (Nov), 153–174.
Purcell J. E., White J. R., Nemazie D. A., Wright D. A. (1999). Temperature, Salinity and Food Effects on Asexual Reproduction and Abundance of the Scyphozoan Chrysaora Quinquecirrha. Marine Ecol. Prog. Ser. 180 (3), 187–196.
Rosa S., Pansera M., Granata A., Guglielmo L. (2013). Interannual Variability, Growth, Reproduction and Feeding of Pelagia Noctiluca (Cnidaria: Scyphozoa) in the Straits of Messina (Central Mediterranean Sea): Linkages With Temperature and Diet. J. Marine Syst. 111, 97–107.
Schneider G., Behrends G. (1994). Population-Dynamics and the Trophic Role of Aurelia-Aurita Medusae in the Kiel Bight and Western Baltic. Ices J. Marine Sci. 51 (4), 359–367. doi: 10.1006/jmsc.1994.1038
Sun S. (2012). New Perception of Jellyfish Bloom in the East China Sea and YEello Sea. Oceanologia Limnologia Sin. 43 (3), 406–410.
Sun S., Li Y., Sun X. (2012a). Changes in the Small-Jellyfish Community in Recent Decades in Jiaozhou Bay, China. Chin. J. Oceanol. Limnol. 30 (4), 507–518.
Sun S., Sun X., Zhang G., Tang H., Liu Q., Li G. (2011). Long-Term Changes in Major Meteorological and Hydrological Factors in the Jiaozhou Bay. Oceanologia Limnologia Sin. 42 (5), 632–638.
Sun S., Yu Z., Li C., Huang B., Zhuang Z., Wei H., et al. (2012b). Progress in the Jellyfish Bloom Research in the Yellow Sea and East China Sea. Oceanologia Limnologia Sin. 43 (3), 401–405.
Uye S.-i. (2011). Human Forcing of the Copepod-Fish-Jellyfish Triangular Trophic Relationship. Hydrobiologia 666 (1), 71–83. doi: 10.1007/s10750-010-0208-9
Wang B., Dong J., Wang W.-B., Li Y.-L., Li Y.-P., Liu X.-Z., et al. (2012a). The Quantity Distribution of Giant Jellyfish and its Relationship to Seawater Temperature and Salinity in Inshore Waters of the Northern Liaodong Bay Region. Oceanologia Limnologia Sin. 43 (3), 568–578.
Wang N., Li C., Liang Y., Shi Y., Lu J. (2015). Prey Concentration and Temperature Effect on Budding and Strobilation of Aurelia Sp 1 Polyps. Hydrobiologia 754 (1), 125–134.
Wang N., Li C., Wang Y., Feng S. (2018). Carbon Distribution Strategy of Aurelia Coerulea Polyps in the Strobilation Process in Relation to Temperature and Food Supply. J. Oceanol. Limnol. 36 (6), 2216–2230.
Wang Y., Sun S. (2015). Population Dynamics of Aurelia Sp.1 Ephyrae and Medusae in Jiaozhou Bay, China. Hydrobiologia 754 (1), 147–155.
Wang P., Zhang F., Guo D., Sun S. (2021). Diets and Seasonal Ingestion Rates of Aurelia Coerulea (Cnidaria: Scyphozoa) Polyps by in Situ Feeding Experiments in Jiaozhou Bay, China. Front. Marine Sci. 8 (1336).
Wang P., Zhang F., Liu M., Sun S., Xian H. (2020a). Isotopic Evidence for Size-Based Dietary Shifts in the Jellyfish Cyanea Nozakii in the Northern East China Sea. J. Plankton Res. 42 (6), 689–701. doi: 10.1093/plankt/fbaa042
Wang S., Zhang G., Song S., Wang Y., Zhao Z. (2012b). Population Dynamics of Three Scyphozoan Jellyfish Species During Summer of 2011 in Jiaozhou Bay. Oceanologia Limnologia Sin. 43 (3), 471–479.
Wang P., Zhang F., Sun S., Yang T. (2020b). Distribution of Giant Jellyfish in the Bohai Sea in June 2018. Oceanologia Limnologia Sin. 51, 85–94.
Wan A., Zhang G. (2012). Annual Occurrence of Moon Jellyfish Aurelia Sp.1 in the Jiaozhou Bay and its Impacts on Zooplankton Community. Oceanologia Limnologia Sin. 43 (3), 494–501.
Willcox S., Moltschamwskyj N. A., Crawford C. (2007). Asexual Reproduction in Scyphistomae of Aurelia Sp.: Effects of Temperature and Salinity in an Experimental Study. J. Exp. Marine Biol. Ecol. 353 (1), 107–114.
Willcox S., Moltschaniwskyj N. A., Crawford C. M. (2008). Population Dynamics of Natural Colonies of Aurelia Sp. Scyphistomae in Tasmania, Australia. Marine Biol. 154 (4), 661–670.
Keywords: jellyfish bloom, Aurelia coerulea, scyphopolyp, asexual reproduction, temperature, global warming
Citation: Zang W, Zhang F, Chi X and Sun S (2022) Relationship Between Asexual Reproduction of Aurelia coerulea Polyps and Jellyfish Blooms Under the Influence of Temperature Dynamics in Winter and Spring. Front. Mar. Sci. 9:888656. doi: 10.3389/fmars.2022.888656
Received: 03 March 2022; Accepted: 16 May 2022;
Published: 13 June 2022.
Edited by:
Stelios Katsanevakis, University of the Aegean, GreeceReviewed by:
Jinho Chae, Marine Environmental Research and Information Laboratory, South KoreaZhigang Yu, Ocean University of China, China
Copyright © 2022 Zang, Zhang, Chi and Sun. This is an open-access article distributed under the terms of the Creative Commons Attribution License (CC BY). The use, distribution or reproduction in other forums is permitted, provided the original author(s) and the copyright owner(s) are credited and that the original publication in this journal is cited, in accordance with accepted academic practice. No use, distribution or reproduction is permitted which does not comply with these terms.
*Correspondence: Song Sun, c3Vuc29uZ0BxZGlvLmFjLmNu