Corrigendum: #OceanOptimism: Balancing the narrative about the future of the ocean
- 1AZTI, Marine Research, Basque Research and Technology Alliance (BRTA), Pasaia, Spain
- 2Faculty of Marine Sciences, King Abdulaziz University, Jeddah, Saudi Arabia
- 3Department of Biological & Marine Sciences, University of Hull, Hull, United Kingdom
- 4International Estuarine & Coastal Specialists (IECS) Ltd., Leven, United Kingdom
- 5Department of Journalism, University of the Basque Country (UPV/EHU), Leioa, Spain
- 6Hopkins Marine Station and Stanford Center for Ocean Solutions, Stanford University, Pacific Grove, CA, United States
- 7Institute of Marine Research, Tromsø, Norway
- 8National Museum of Natural History, Smithsonian Institution, Washington, DC, United States
The ocean is facing multiple pressures from human activities, including the effects of climate change. Science has a prominent role in identifying problems and communicating these to society. However, scientists are also increasingly taking an active role in developing solutions, including strategies for adapting to and mitigating climate change, increasing food security, and reducing pollution. Transmitting these solutions to society changes our narrative about the ocean and motivates actions. The United Nations triple initiatives for this decade—the Sustainable Development Goals, the Decade on Ocean Science for Sustainable Development, and the Decade of Ecosystem Restoration—provide the momentum for this change in narrative and focus. Here, we reflect on the search for solutions and the need for better ways of communicating science in a positive way. We synthesize insights from a summer school held during the COVID-19 pandemic and present some examples of successes and failures and the lessons learned from these.
1 Introduction
A healthy ocean is essential for human and societal health and wellbeing (Costanza et al., 2017; Borja et al., 2020a). It provides energy, livelihood, and food for half the global population and climate regulation for all. It is home to an extraordinary diversity of life and ecosystems, much of it still unexplored and unstudied (Costello, 2015). Accounting for 70% of the Earth’s surface and over 90% of its habitable space, the ocean offers unrecognized and untapped potential for solving some of our greatest challenges.
Recently, the United Nations (UN) Decade of Ocean Science for Sustainable Development (UNDOS, 2021–2030) was launched (Ryabinin, 2021), with a vision of “The science we need for the ocean we want” and a mission of “Transformative ocean science solutions for sustainable development, connecting people and our ocean”. The keywords here are “science solutions” to reverse the decline in ocean health while maintaining the services and benefits from the ocean, particularly under a changing climate. This decline, in terms of habitat disturbance, biodiversity loss, resource overexploitation, climate change, contamination, acidification, and invasive species, has been documented by the UN’s Second World Ocean Assessment (WOAII) (United Nations, 2021a; United Nations, 2021b), which identifies key factors threatening the ocean (Table 1).
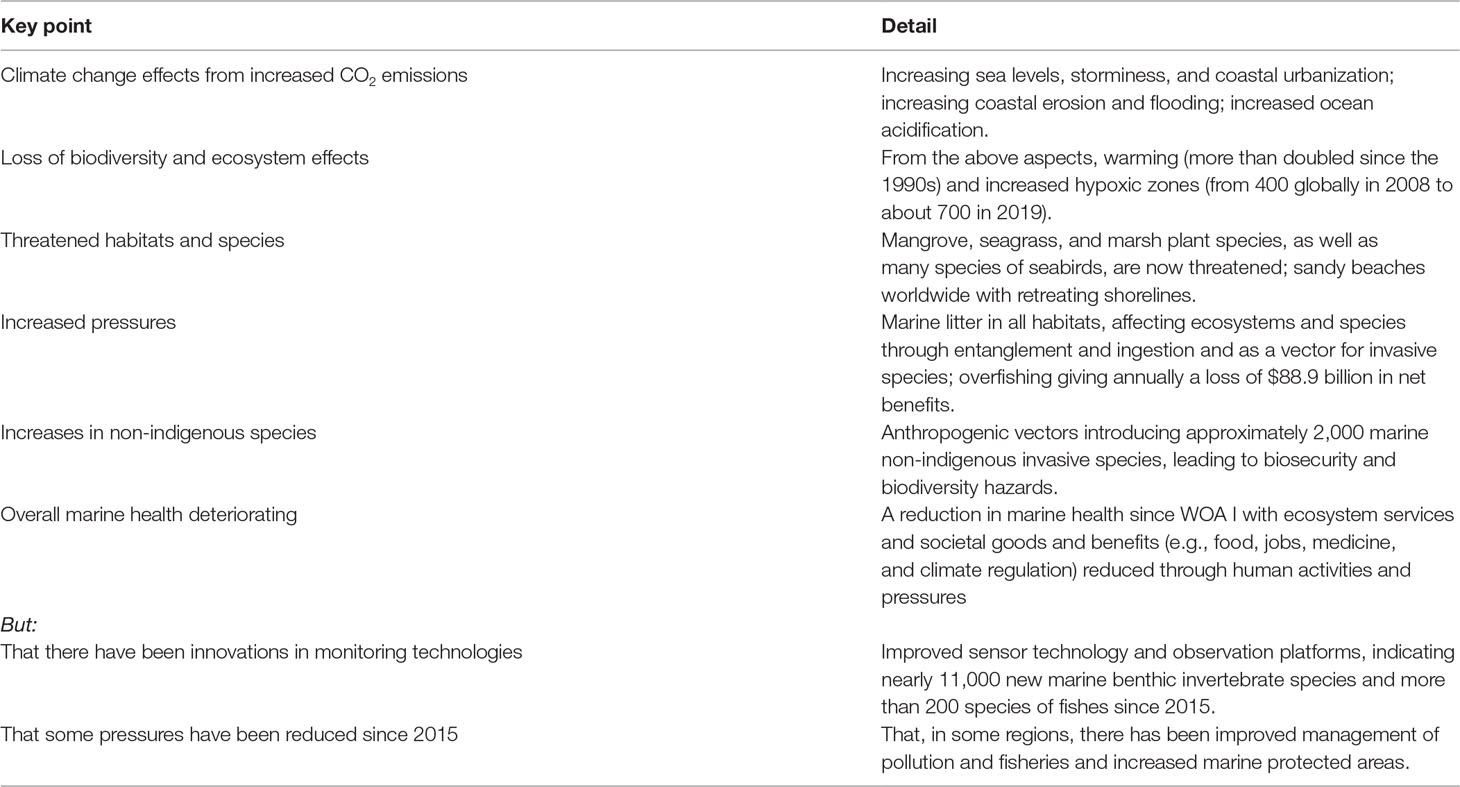
Table 1 Key points threatening the ocean, after United Nations(2021a; 2021b). WOA I, First World Ocean Assessment.
The WOAII lays the foundation for the implementation of the 2030 UN Agenda for Sustainable Development Goals (SDG; United Nations, 2016). It provides a comprehensive and integrated review of scientific information on the state of the marine environment, emphasizing SDG14 (Life Below Water). The aim is to support better stewardship of our ocean, and as such, it coincides with the commencement of the UNDOS (Ryabinin, 2021). Both of these also coincide with the start of the UN Ocean Decade of Ecosystem Restoration 2021–2030 (UNDER), which also outlines high ideals for remedying the damage to global ecosystems, including the marine system (Waltham et al., 2020). These initiatives, together with others such as the G7 Future of the Seas Initiative1 and the ocean emphasis in COP26 in Glasgow, led to 2021 being termed “a super year for the ocean”.
The abovementioned threats to the ocean (or “ocean calamities”) have been echoed widely by the media, leading to a growing concern that the state of the ocean is fatally compromised and driving society into pessimism (Duarte et al., 2015b; Duarte et al., 2020). This shows that the ocean has suffered from many impacts and losses, and enormous challenges remain (Jouffray et al., 2020); some authors (e.g., Knowlton, 2021) have highlighted the increasing evidence of the progress made in developing and implementing solutions, and advocate for an “ocean optimism”. The goal of this emphasis on “ocean optimism” is to put a focus on solutions and successes, which will help them to become the norm rather than the exception (Knowlton, 2021), and to use message-framing strategies to enhance marine conservation (Kolandai-Matchett and Armoudian, 2020).
In connection with UNDOS, a solution-oriented model is emerging, based on a theory of change that stipulates “knowing the ocean” will contribute to sustainable and equitable development (Singh et al., 2021). This approach includes the endorsement, in June 2021, of the UNDOS Program “Global Ecosystem for Ocean Solutions (GEOS)” that aims at developing, testing, and deploying equitable, durable, and scalable ocean-based solutions for addressing complex ocean health and climate challenges. Achieving the SDGs will require rebuilding marine life-support systems that deliver the ecosystem services, from which the benefits that society receives from a healthy ocean arise (Duarte et al., 2020). Most of the solutions that are being proposed are nature-based (Oen, 2019; Davies et al., 2021) and consider that the ocean is key to achieving climate and societal goals (Hoegh-Guldberg et al., 2019b).
However, to achieve these goals, we need to change the narrative about the ocean to encourage us (governments, business, society, media, and scientists) to change our minds and behaviors. We need to move from only raising problems by documenting or predicting calamities to outlining pathways for scalable solutions (Kolandai-Matchett et al., 2021a). To that end, Lubchenco and Gaines (2019) propose a new ocean narrative: “The ocean is not too big to fail, nor is it too big to fix, but it is too big and important to ignore”.
Thus, although “doom and gloom” scenarios have been prominent in the environmental literature since the 1960s (Borja and Elliott, 2021), there is an increasing focus on what can be done about the challenges we face. For ocean conservation, an early manifestation of this trend was the first International Marine Conservation Congress, whose formal program in 2009 was preceded by a day-long symposium hosted by the Smithsonian Institution entitled “Beyond the Obituaries: Success Stories in Ocean Conservation”. Five years later, the twitter campaign #OceanOptimism was launched, and the 2020s began with the publication of two reviews focused on what was working and what more could be done to restore ocean health (Duarte et al., 2020; Knowlton, 2021).
Much of the early inspiration for these efforts came from the realization that ocean professionals, especially younger ones, wanted to do more than simply document the decline of marine ecosystems and species (Knowlton, 2017), which is also central to the UNDOS (Ryabinin, 2021). In this context, coinciding with World Ocean Day, from June, 8 to 10, 2021, the authors of this article organized in San Sebastian (Spain) a virtual summer school to discuss, with selected speakers and 50 attendees, how “ocean optimism”, and this new narrative could become operational. The idea underpinning this focus on ocean solutions is not that we have addressed all or even most of the threats responsible for the dramatic degradation of ocean life and ecosystems that has occurred over the past decades and centuries. Rather, despite the alarming trends, some progress has been made and that a better future depends not only on new strategies not yet attempted but also on replicating and scaling up the achievements to date. For this to happen, recognition and understanding of what has worked is essential. Globally, there are many examples of small-scale restoration, but the challenge is now to ensure these can be up-scaled for the benefit of the planet (Waltham et al., 2020). This overview presents the main facts, evidence, and outcomes debated around the concept of “ocean optimism” during the summer school. Hence, we focus on the topics debated, which provide examples of positive actions that can generate a change in the narrative: sustainable food from the ocean, ecosystem conservation and restoration, reduction of pollution from marine litter, and climate mitigation and adaptation.
2 Four Priority Topics for Positive Action
2.1 Sustainable Food From the Ocean
2.1.1 Role of Healthy Ocean and Blue Foods in the Global Food System
Progress in conserving biodiversity and mitigating climate change impacts needs to be accompanied with ensuring food and nutrition security, healthy diets, and sustainable food systems for a growing global population (Leape et al., 2021). Most notably, 71% of tropical and sub-tropical deforestation comes from agriculture, with these together creating a quarter of anthropogenic greenhouse gas (GHG) emissions (Pendrill et al., 2019). Population growth and global environmental change are challenging the global food supply, which will need to support as many as 10 billion people by 2050. Currently, half of the global population is malnourished, 795 million people lack sufficient food for a healthy life, 2 billion people suffer from some form of micronutrient deficiency, and 161 million children under the age of 5 are undernourished, while, at the same time, paradoxically, an estimated 1.9 billion adults are overweight or obese2. More daunting challenges lie ahead: in addition to a growing global population, rising incomes and an increasing middle class in developing countries are predicted to increase the demand for animal protein by over 80% by 2050. These challenges are increasing inequality and disparities in access to food and income worldwide, aggravating rapidly increasing risks from climate change.
Aquatic or “blue” foods—all species of fish, shellfish, and algae harvested and grown from marine and freshwater ecosystems—are already an important part of the global food system. They feed 3 billion people; sustain livelihoods for 800 million people; are rich in protein, vitamins, and omega-3 fatty acids and other important nutrients; and are produced with relatively low environmental footprint compared with land agriculture (Golden et al., 2021).
Blue foods are highly diverse, their supply from wild capture fisheries leveled off in the 1990s, and sustained production is threatened by climate change (FAO, 2020). Aquaculture production has increased exponentially, but continued expansion also poses challenges from environmental impacts, as well as access and distribution, as most production is increasingly concentrated in a few countries [principally, China and other Asian countries, primarily from inland waters (FAO, 2020; Naylor et al., 2021)].
The Blue Food Assessment—led by Stanford’s Center for Ocean Solutions and Center on Food Security and the Environment, in collaboration with the Stockholm Resilience Centre and Springer Nature—brought together more than 100 leading researchers around the world to address the future of blue foods and their role in building a healthy, sustainable, and equitable global food system. This initiative conducted the first concerted analysis of the role of blue foods in the global food system, assessed opportunities and trade-offs, and produced roadmaps and partnerships to realize their potential. This included the contribution of blue foods to human nutrition, the environmental footprint and limits of blue food production, expected trends in blue food demand, climate threats and risk reduction strategies, the role of small-scale fishers and farmers, and paths to achieving more equitable food systems. These insights were brought to the UN Food System Summit, held in September 2021,3 that, for the first time, included blue food in its action tracks.
Analyses and modeling projections indicate the blue food demand could double by 2050, with China, currently the largest consumer, accounting for over half of the growth in total fish consumption (Naylor et al., 2021). Given the limited potential for further growth in wild-fisheries production, most of current and future demand will need to be met by a more sustainable aquaculture (e.g., through innovation, feed improvements, and diversification).
An extensive compilation and analysis of the nutritional content of more than 3,500 aquatic species indicates that many blue foods are richer in nutrients than terrestrial meats, particularly for omega-3 fatty acids, calcium, and vitamin B12. A single serving of many species of small pelagic fish provides more than the daily recommendations for those nutrients (Golden et al., 2021). Compared with chicken, trout has approximately 19 times more omega-3 fatty acids, oysters and mussels have 76 times more vitamin B12 and five times more iron, and carp has nine times more calcium.
There is an increasing potential for harnessing the diversity of blue foods to address malnutrition while also giving health and economic benefits. In countries with high micronutrient deficiencies, supply chains and availability can be strengthened by improving aquaculture and fisheries management, enhancing sustainable aquaculture, and building more equitable trade networks. The national dietary guidelines and nutrition policies should prioritize blue foods where culturally and socially appropriate, and governments could include blue foods in social welfare programs, such as food assistance and school meal programs, e.g., Peru, Vietnam, and Cambodia (Leape et al., 2021).
Fisheries and aquaculture can pose major environmental stressors. However, many blue foods already perform well compared with other foods in terms of their contribution to global warming, eutrophication, land use, and freshwater consumption (Gephart et al., 2021). Farmed bivalves and wild-caught small pelagic fish produce the least GHG emissions. Farmed salmon, trout, carp, catfish, and tilapia perform similarly or better than chicken—often considered the most efficient meat.
There is also the potential for future gains by improving existing production systems (e.g., by restoring healthy stocks, reducing capture fisheries emissions by optimizing gear, reducing the amount of feed in aquaculture, and changing the farmed and capture species) (Peck et al., 2020). This includes benefits in shifting species and production systems to those with a lower environmental footprint (e.g., small pelagics and unfed aquaculture species) (Gephart et al., 2021).
Realizing the potential of blue foods in building healthy, nature-positive, and resilient food systems will require that industries, private business, and governments embed blue foods in food system governance, harness the nutritional diversity of blue foods in food security and policy, and take measures to reduce food waste. Governments need to expand investment in small-scale actors, support sustainable development and diversification of their sector, and ensure that trade and economic policy takes account of their roles in providing equitable economic opportunity and nutrition (Leape et al., 2021).
2.1.2 Examples for Optimism on Fisheries Recovery
Despite the recent opportunities to develop more sustainable and equitable blue food systems, the public perception of global fisheries has been shaped by high-impact studies (Pauly et al., 1998; Myers and Worm, 2003; Worm et al., 2006), revealing declines in the state of seas and the sustainability of fisheries, leading, in turn, to calls to avoid fish consumption entirely (The Guardian4, Seaspiracy5). Although the challenges faced by fisheries and their impacts on ecosystems are widespread, the tendency toward oversimplification and a “doom and gloom” narrative has eclipsed other assessments (Worm et al., 2009; Branch et al., 2011) that showed large geographic and taxonomic variation in the state of fish stocks and highlighted some positive trends in at least some areas. Recent research (Zimmermann and Werner, 2019; Hilborn et al., 2020; Hilborn et al., 2021) has found encouraging trends toward improved sustainability of fisheries over the past two decades in some stocks and regions, notably the Northeast Atlantic Ocean. These positive trends do not imply that all issues are solved, but indicate that improvement is possible.
The Northeast Atlantic Ocean has been exploited for centuries, with very high fishing pressure since the 1950s. Since then, overcapitalized fishing fleets and a lack of regulation resulted in the loss of large fish species (Sguotti et al., 2016), followed by a decline in many commercial species (Rijnsdorp et al., 1996; Zimmermann and Werner, 2019). However, in the early 2000s, persistent overfishing prompted policy changes (EU, 2002) to improve the management of fish stocks and facilitate their rebuilding. After initial stagnation (Froese and Proelß, 2010), signs of a trend reversal started to emerge (Cardinale et al., 2013; Fernandes and Cook, 2013), with clear improvements in their sustainability in recent years (Zimmermann and Werner, 2019; Hilborn et al., 2021).
Despite stock-specific variation, a clear link between implementing science-based management, reductions in fishing pressure, and increases in stock sizes has been shown (Zimmermann and Werner, 2019). European fisheries provide examples of how biodiversity loss and overexploitation can be reversed when science-based management is implemented and enforced. However, they also highlight that rebuilding fish stocks requires a persistent management effort, success can depend on changes in the environment and ecosystems, and there is a need for transnational coordination and collaboration, such as the International Council for the Exploration of the Sea (ICES). In the following, we outline two examples and discuss the broader impacts of climate change.
-Atlantic Herring
The Norwegian spring-spawning (NSS) stock of Atlantic herring (Clupea harengus) has been fished for centuries and has been scientifically investigated for more than 100 years, providing some of the earliest case studies in marine research (Hjort and Lea, 1911). The increasing fishing pressure after World War II and a period of poor recruitment resulted in a large-scale decline of the stock in the 1960s, decreasing from a biomass estimated above 10 million tons to around 16,000 tons (Dragesund et al., 1997; Toresen and Østvedt, 2000). Although the collapse of NSS herring highlighted the risks and repercussions of overfishing, it also revealed the challenges of natural fluctuations for fisheries management. As with other Atlantic herring stocks (Trochta et al., 2020), NSS herring is known for cyclic dynamics in stock biomass, driven by environmental factors that control the stock productivity and distribution (Dragesund et al., 1980). Population dynamics are driven by a few strong year classes produced by a range of factors (Zimmermann et al., 2019; Tiedemann et al., 2020; Garcia et al., 2021), whereas periods of intermediate to poor recruitment cause declines in stock biomass.
Natural fluctuations in productivity require adaptive fisheries management that adjusts the fishing pressure to the stock trajectory and are a particular challenge when attempting to rebuild a stock. Although a fishing moratorium was enforced after the collapse of NSS herring, it took around 15 years for it to recover. The spawning stock biomass increase to levels above which could be harvested sustainably, up to 7 million tons in 2009, followed a slow decline due to a decade of largely weak year classes. However, compared with the 1960s, substantially improved management that includes large-scale monitoring efforts and a management plan aimed at maintaining sustainable harvest levels (ICES, 2018) provides safeguards against overexploitation.
-Atlantic Bluefin Tuna
Atlantic bluefin tuna (Thunnus thynnus) is an iconic and highly valued species that can reach body sizes of more than 3 m and 900 kg and is managed as two separate stocks: eastern bluefin tuna (EBFT) in the eastern Atlantic has its main spawning grounds in the Mediterranean Sea, whereas western bluefin tuna (WBFT) spawns in the Gulf of Mexico and migrates along the eastern coast of the Americas (Fromentin and Powers, 2005), although recent studies found that cross-Atlantic migration and mixing occur (Rooker et al., 2019). The combined factors of high value, extremely wide distribution, a multinational fishery with fishing fleets from almost 40 countries involved in the EBFT fishery, and substantial amounts of illegal and unreported fishing resulted in a perfect storm for the management of this species. This led to overfishing and declines in both stocks from the 1960s onward (Fromentin and Powers, 2005; Failletaz et al., 2019), with the threat to extinction. However, although the public narrative of a highly endangered species persists, there is evidence that Atlantic bluefin tuna has recovered over the past 10 years, with EBFT considered as sustainably managed after returning to high stock levels6.
Its recovery was the product of a science-based management plan, facilitated by increasing public pressure and calls to ban the trade of Atlantic bluefin tuna under the CITES agreement (Nayar, 2010), improved compliance and enforcement (Gonçalves, 2021), as well as favorable environmental conditions (Failletaz et al., 2019). Improvement in stock condition was unexpectedly rapid, as indicated by the increase in spawning stock size and return of migration patterns to the level of pre-overfishing periods. Large schools began to return to Norwegian waters from 2012 onward, after EBFT had largely disappeared after the mid-1960s (Nøttestad et al., 2020). Although mainly found in temperate to warm water, Atlantic bluefin tuna has been observed in recent years as far north as Svalbard in Arctic waters, probably following Atlantic mackerel (Scomber scombrus) as an important prey species that has expanded its own range during the past decade. The increase in stock biomass has also resulted in increasing total allowable catches (TACs) after the initial reductions around 2008 to allow for a recovery, returning to similar levels as in the past while largely preventing illegal or unreported catches that exceed the TAC.
-Climate Winners? Benefits and Challenges of Range Expansion
Although climate change is mostly associated with negative impacts on marine ecosystems (Hoegh-Guldberg and Bruno, 2010), fish stocks (Plagányi, 2019), and fisheries (Mendenhall et al., 2020), for many fish stocks, impacts are mixed or positive (Peck et al., 2020; Kjesbu et al., 2021). Environmental shifts can be beneficial when food availability increases through higher ecosystem productivity or habitat expansion, or a combination thereof. Combined with effective management, such changes can result in large increases of stock biomasses and fisheries yields. Notable examples for such a development are Northeast Atlantic mackerel, Northeast Arctic (NEA) cod (Gadus morhua), and Northern hake (Merluccius merluccius). All three stocks were below limit reference points in the early 2000s but increased notably over the subsequent years, with mackerel, hake, and NEA cod increasing more than 2.5-, 6-, and 10-fold, respectively, from their prior low point to the following biomass peak.
Despite their different habitats and distribution, the three stocks were all adversely affected by unsustainable fishing pressure during the 1990s, representing the general state of most European fisheries at the time. Policy changes at the beginning of the 2000s and subsequent improvements in management gave all three stocks the opportunity to use favorable environmental conditions and expand their feeding range, enabling the observed increases in stock sizes. Northeast Atlantic mackerel has traditionally depended on extensive migrations between the spawning areas along the shelf break from Portuguese to Scottish waters as well as in the North Sea (Trenkel et al., 2014) to the feeding grounds in the Norwegian Sea between Norway and Iceland (Brunel et al., 2018). As its range is largely limited by temperature, higher temperatures allowed an increasing mackerel stock to expand its feeding migrations substantially to include areas further north and west, up to the Greenland Sea (Olafsdottir et al., 2019; Nikolioudakis et al., 2019). Similar positive feedbacks have also driven the large increases of Northern hake, expanding its northern range into the North Sea and Norwegian Sea (Westgaard et al., 2017), whereas NEA cod decreased in the southern North Sea but benefited from higher temperatures in higher latitudes and extended feeding ranges in the Barents Sea (Fall et al., 2018).
However, the three examples show how positive developments can create new management challenges through species interactions (Zimmermann and Yamazaki, 2017; van Gemert and Andersen, 2018), and quota conflicts following distribution shifts (Gullestad et al., 2020) while causing challenges and opportunities for fishers that need to modify their target species (Peck et al., 2020).
-Lessons Learned
These cases have provided some important lessons: (i) it is possible to rebuild a stock even after a major collapse, and relatively fast; (ii) a recovery often also requires beneficial conditions such as a strong year class; (iii) even large, straddling, and fluctuating stocks fished by fleets from multiple nations can be managed sustainably if science-based management measures are strictly implemented and enforced; (iv) science-based policies not only can provide higher resistance and resilience against adverse climate effects (Brander, 2010) and offset their impacts (Gaines et al., 2018) but also can create the necessary conditions to reap the potential benefits of positive climate effects; and (v) fish stocks shared by several countries highlight that political decision-making and quota negotiations remain major challenges for sustainable fisheries.
2.2 Ecosystem Restoration
Given the scale of environmental damage that has occurred, there is increasing interest in the role for restoration in marine conservation, despite concerns about scalability and cost, as shown by the establishment of the UNDER 2021-2030. It aims to greatly increase the restoration of degraded ecosystems to combat the climate crisis and enhance food security, water supply, and biodiversity (Waltham et al., 2020). Ecosystem restoration is fundamental to achieving the SDGs, mainly those on climate change, poverty eradication, food security, water and biodiversity conservation, as well as post-2020 biodiversity targets (Arneth et al., 2020; CBD, 2020). UNDER shows the need to accelerate existing global restoration goals (to restore 3.50 M km2 of degraded ecosystems by 2030). Currently, 57 countries, subnational governments, and private organizations have committed to bring >2.90 M km2 of degraded land under restoration by 2030, and notably, it focuses on targeted ecosystems, including ocean and coasts.
There is a growing number of examples of successful marine restoration, and their analysis holds promise for increasing restoration effectiveness (Saunders et al., 2020). Successful restoration can have multiple benefits, such as for food security and climate change. One recent notable example concerns the seagrass habitats off the coast of Virginia in the US, which involved a 20-year effort and the planting of over 70 million seeds of the eelgrass Zostera marina (Orth et al., 2020). Similar recovery trends have been detected in Europe (de los Santos et al., 2019) and elsewhere (Dunic et al., 2021). The result has been not only the recovery of the eelgrass beds but also the ecosystem services and societal benefits that they provide (Orth et al., 2020), including an increase in water clarity, improved capture of carbon and nitrogen in the sediments, and an increase in biomass of both invertebrates (including the commercially important sea scallop) and finfish. This is like worldwide examples of oyster restoration, leading to both ecosystem rehabilitation: direct economic benefits and spillover effects on fin-fisheries (Gilby et al., 2018). It is notable that such attempts are now being scaled-up to meaningful and effective levels (Pogoda et al., 2020).
One particularly well-studied example of restoration concerns the Basque region in the north of Spain, with its 12 small estuaries ranging in lengths from 2 to 22 km and extending over 150 km of coastline (Borja et al., 2006a). Given that most ocean problems, and therefore solutions, relate to the estuarine and coastal activities, it is important to consider such areas when discussing ocean improvements (Defeo and Elliott, 2021). Industrialization, urbanization, and population growth, beginning in the mid-19th century, removed 15% to 88% of the intertidal estuarine habitat, depending on the estuary, as well as creating many other human pressures (Borja et al., 2006a) such as nutrient discharges, water and sediment pollution by metals and organic compounds, water abstraction, sediment dredging, shoreline reinforcement, moorings (ports), and the introduction of alien species (Borja et al., 2006a). The region reached its maximum degradation around the 1970s to 1980s, with anoxic and azoic areas in some estuaries (Borja et al., 2016).
However, since then, diverse management measures were implemented (i.e., wastewater treatment and habitat restoration), and systematic monitoring was organized (numerous physico-chemical variables in water, sediment and biomonitors, as well as different ecosystem components, such as phytoplankton, macroalgae, seagrasses, macroinvertebrates, and fishes), allowing marine ecosystem recovery to be documented (Borja et al., 2006b; Borja et al., 2016, Borja et al., 2016b).
Most of the indicators showing improvement (e.g., nutrients and oxygen) are directly related to the effectiveness of the wastewater treatment plants, as well as the changes in industrial production systems, including the economic downturn that saw industries close (Borja et al., 2006b; Borja et al., 2016. Phytoplankton, chlorophyll a, and cell counts show more significant worsening trends than improving ones probably due to the initially turbid waters, which prevented phytoplankton growth, with a subsequent increase in chlorophyll concentration when the water turbidity had been reduced (Borja et al., 2016). For macroinvertebrates, five study parameters showed a much higher percentage of significant improving trends than worsening ones. In the case of fish, none of the five parameters worsened, with diversity and demersal community richness and fish richness showing higher degrees of improvement.
A more detailed analysis of one system, the Nervión estuary, documented not only the physical and biological recovery but also the recovery of ecosystem services and societal benefits, namely, recreational fishing and the use of beaches for sunbathing and sports. These services have been studied under three perspectives: environmental (Pouso et al., 2019), social (Pouso et al., 2018a; Pouso et al., 2018b), and economic (Pouso et al., 2018c; Pouso et al., 2021). The increase in recreational fishing has followed the physico-chemical recovery of the system, especially in terms of dissolved oxygen and also in the recolonization of the inner estuary by fish (Pouso et al., 2019), with increasing individual catches. It is notable that the perception of fishers does not match with the reality, because 69% of the fishers think that there is a decrease in fish abundance and 36% consider that there is no change in fish richness (Pouso et al., 2018b). In contrast, 75% of beach users consider that the quality of the water has improved with time, and 62% of visitors practice aquatic activities in the beaches because of this (Pouso et al., 2018a).
It is necessary to translate the environmental improvement into measures understandable by policymakers, often as a financial benefit. Using the travel cost method, Pouso et al. (2020) studied the amount that visitors are willing to pay to reach the recreation site and determined that beachgoers would spend on average 7.05 € trip−1, or an annual total of 3.5 M€ year−1. In the case of recreational fishing, these values are 449 € year−1 fisher-1 and 1.1 M€ year−1, respectively. Given that the beach maintenance costs 0.67 M€ year−1, and the wastewater treatment costs 23.7 M€ year−1, just these two benefits cover 100% of the beach maintenance and 20% of the water treatment costs (Pouso et al., 2020). As there are many other ecosystem services and societal benefits not included in the analysis (e.g., food provision, carbon sequestration, nursery functions, pollutants cycling, and other cultural benefits), it is likely that the economic benefits of the estuary restoration could be much higher than the investments expended in recent decades.
In essence, these actions in the Basque Country provide some valuable lessons: (i) long-term monitoring networks, covering multiple ecosystem components, provide appropriate knowledge to take informed management decisions to restore marine systems; (ii) the recovery of degraded marine systems can take decades, although the water column variables can recover faster than sediments and organisms used for biomonitoring, and the ecosystem components depend on each other for recovery, both structure and function; and (iii) the recovery of the societal benefits from restored ecosystem services shows that only a few of them are required to cover most of the investments undertaken in restoration.
Although there is a long history of ecological restoration in coastal and marine areas (e.g., Borja et al., 2010; Duarte et al., 2015a; Duarte et al., 2020), there are also examples of what has been unsuccessful because there was a poor understanding of the physical processes (e.g., geomorphology and hydrology) that create the conditions for biological systems to develop (Elliott et al., 2016). The latter studies led to the recognition that the essence of successful and sustainable ecological restoration is in acknowledging the role of ecohydrology, as the links between the ecology and the physical system (Wolanski and Elliott, 2015).
The future of successful ocean restoration may mean translating the lessons from estuaries and coasts to ocean areas. However, in many cases, there may be a lesser ability to manipulate the system, and so it may mean removing stressors and allowing areas to recover without direct assistance. Increasingly, restoration and the alleviation of problems due to urban and industrial developments may involve compensating for the loss of natural habitats (Elliott et al., 2020). This will include six compensatory options: (i) restoring the habitat to ensure the maintenance of its conservation value and compliance with the conservation objectives of the site; (ii) improving the remaining habitats proportional to that is lost due to the development projects; (iii) preservation of habitat stock, specifically measures to prevent further erosion of the coherence of the network; (iv) creating a reserve (including strong restrictions in land use); (v) providing incentives for certain economic activities that sustain key ecological functions; and/or (vi) reduction of (other) pressures, usually upon species, either through action on a single source or through co-ordinated action on all pressures.
2.3 Solutions to Marine Litter
Marine litter has been identified as a challenge to ocean health, is present in all marine environments (Morales-Caselles et al., 2021), and has become a priority issue for policymakers and governments (Maes et al., 2019). It is listed within the UN SDGs (United Nations, 2016), being the subject of a number of national and regional initiatives such as the EU Marine Strategy Framework Directive. Although not necessarily the largest threat to ocean health (Borja and Elliott, 2019; Tiller et al., 2019), marine litter has also become a pressing issue for the general public, and high public awareness and media attention have led to the creation of numerous entrepreneurial start-up initiatives to address the problem of marine litter, although little academic research has documented their effectiveness (Dijkstra et al., 2021).
Marine litter may be regarded as a land-based problem (an estimated 78% has terrestrial origin), and the largest share of plastic (95%) is found in surface waters and on shorelines (83%) (Morales-Caselles et al., 2021). The composition of litter at the ocean surface changes from take-out consumer items nearshore to a dominance of fishing-related items offshore, with single-use items even more predominant in low-income countries. Most marine litter emanates from 1,000 rivers worldwide (Meijer et al., 2021) and so the efficient implementation of solutions and policymaking requires a good understanding of the distribution, abundance, and sources of marine litter.
Various solutions have been designed to prevent or mitigate the presence and effects of macrolitter (items >2.5 cm) in the marine environment (Table 2), and more technological, governance, and management solutions can be found elsewhere (Löhr et al., 2017; SCP/RAC, 2017; Dijkstra et al., 2021). Upstream preventive solutions are preferable to downstream mitigation (Fabres et al., 2016; UNEP, 2016), but downstream policy-oriented solutions are more commonly implemented. Although there is a plethora of monitoring initiatives, these are not management actions and only indicate the need for (and efficacy of) actions (Bellou et al., 2021). Transboundary governance and collaboration also play a key role in addressing marine litter, and some projects7 have demonstrated the value of such collaboration (LIFE LEMA, 2020).
Most solutions have focused on coastal macrolitter (Morales-Caselles et al., 2021), but few solutions have reached mature technical readiness and market availability (Bellou et al., 2021). In terms of microplastics (< 0.5 cm), efforts are being made both at industrial and governance levels (e.g., bans on microbeads) (Stoll et al., 2020; Gago et al., 2020), and there is promising work on identifying and genetically modifying plastic-degrading organisms and enzymes8. However, collection at sea is complex, so that further development of promising solutions that capture plastics before they enter the ocean, such as a gelatinous solution to capture microplastics from wastewater treatment plants using a filter made of jellyfish mucus (Freeman et al., 2020), remains a high priority.
2.3.1 Preventive Solutions
There are numerous examples of applying the circular economy approach, which targets design, production, consumption, and end-of-life management. Taking fishing gear as an example, the solutions have focused on: (i) recovering and recycling, to create high-value products such as clothing, accessories, or components for aquaculture gear (Basurko et al., 2020); (ii) using alternative materials, such as bio-based polymers for gillnets (Grimaldo et al., 2020) or organic materials to build fish aggregating devices used by the tropical tuna fisheries (Zudaire et al., 2020); (iii) developing designs that need less or alternative materials or that can be easily dismantled to facilitate recycling; or (iv) creating circular design standards for fishing and aquaculture gear (MRAG, 2020).
There are also efforts to create behavioral change. Beach cleaning events are popular initiatives to raise awareness and may possibly result in behavior change (Willis et al., 2018). More effective approaches, including problem-structuring frameworks to assess the causes, consequences, and responses to an environmental problem in a holistic and meaningful way, can be used to determine the most efficient measures and means to communicate, educate, and raise awareness (Elliott et al., 2017). The ResponSEAble project is a good example applied to solving the problem of microplastics and cosmetics (ResponSEAble, 2021).
2.3.2 Mitigation Solutions
Effective mitigation solutions require stakeholder engagement, legislation, the use of market-based instruments, best environmental practices, and best available techniques (UNEP, 2016). They can be divided in two groups: (i) monitoring of the presence of marine litter at rivers, coastal areas, and open seas (a priori solutions), and (ii) collection of marine litter from the environment (a posteriori solutions) (some examples are shown in Table 2). As with prevention measures designed to act on sources, such as the measures applied to waste-water treatment plants (Schuhen and Sturm, 2020), home laundry machines (SCP/RAC, 2017), or the end-of-life fishing net recovery schemes (Basurko et al., 2020), mitigation solutions are not source-specific. However, the only sustainable strategy requires human behavioral changes to prevent the discharge in the first place.
Spatial and temporal monitoring are needed to assess the level of marine litter pollution and provide objective information for the design of mitigation solutions and the promotion of adaptative management, as well as for assessing their effectiveness. They come in a variety of forms: (i) modeling the fate of marine litter in the ocean to determine the pathways, sink, and accumulation areas of marine litter (e.g., patches, ocean gyres, and windrows) using, for example, Lagrangian particle tracking combined with trackers and validated with surface drifters or observations (van Sebille et al., 2012; Lebreton et al., 2018; Declerck et al., 2019); (ii) remote sensing to detect litter accumulations at sea and undertake beach mappings for monitoring purposes (Biermann et al., 2020; Salgado-Hernanz et al., 2021), for example, combining technology from satellite imagery or drones with image processing using intelligent algorithms; and (iii) videometry systems for riverine litter monitoring (González et al., 2016), these are used, for example, in Asian, French, and Spanish rivers to inform the management action of local authorities (van Lieshout et al., 2020; Ruiz et al., 2020a).
There have been various collection efforts to remove litter from marine environments (LIFE LEMA, 2020; Dijkstra et al., 2021), including vessel or land-based structures, and should be environmentally friendly. Borja and Elliott (2019) emphasize the need for a holistic and multidisciplinary approach to solving the challenge of ocean plastics, which considers the ecological, economic, technological, societal, legal, administrative, political, cultural, ethical, and communication aspects.
“Fishing for litter” schemes engage the fishing sector in the protection of the marine environment and raising awareness; currently, 10 European countries are participating in the Fishing for Litter initiative (KIMO, 2021). Efforts involve either “active Fishing for Litter” [UNEP(DEPI)/MED, 2016] with specialized marine litter cleaning boats usually operating in coastal surface waters (LIFE LEMA, 2020) or “passive Fishing for Litter” by volunteer fishers (KIMO, 2021) usually targeting seafloor litter. Riverine litter can be trapped by booms (LIFE LEMA, 2020; CLAIMS, 2021) or bubble curtains (The Great Bubble Barrier, 2021) and later removed mechanically or by hand. A combined autonomous technology also exists (e.g., the Interceptor of The Ocean Cleanup), and related efforts occur in harbors (e.g., Mr Trash Wheel®), where smaller scale mechanisms have also been deployed (e.g., Seabin, 2021). Solutions for gyres in the open ocean are led by The Ocean Cleanup, which has developed a U-shaped barrier that guides the plastic into a retention zone, which, once full, is hauled on board a vessel (The Ocean Cleanup, 2021). The effectiveness of some of these collection efforts is enhanced by drawing on monitoring information (LIFE LEMA, 2020; Delpey, 2021).
2.3.3 Lessons Learned From Marine Litter
Marine litter is a clear example of pollution that is not exclusively derived from Blue Economy–related sectors (UNDP, 2018; Morales-Caselles et al., 2021). Despite the exponential growth in the development of marine litter solutions, embedded within the regulatory framework and funding schemes, the implementation of solutions has become the weakest part of ocean management due to its complexity. This results from the fact that governance is fragmented across jurisdictions, sectors, and products (Dauvergne, 2018); the currently existing framework does not deal adequately with all the key sources and entry points of marine litter (UNEP, 2016); and there are no one-size-fits-all solutions (Williams and Rangel-Buitrago, 2019). In particular, it is a problem requiring land-based solutions even if the effects and receptors are in the marine environment. Furthermore, the selection of solutions and who pays for them is not straightforward, and thus, understanding of the costs and benefits of these actions needs improving to boost their implementation by governments (Andrés et al., 2021). Similarly, although scientific advances and industrial and policy developments have been significant during the last decade (UNEP, 2016), the effectiveness of solutions remains to be assessed. More than half a century of tackling pollution problems (Borja and Elliott, 2021) has shown that “prevention is better than the cure” and that all sectors of society must be involved in finding solutions. Unlike many other contaminants, plastics will continue to be a much-needed and valuable commodity so that their wise-use and wide-disposal is imperative—hence, the mantra of “reduce, reuse, repair, rot, and recycle” must be applied to litter, which eventually, at present, ends in the oceans.
2.4 Ocean-Based Solutions for Climate Mitigation and Adaptation
Current emission reduction pledges under the 2015 Paris Agreement are insufficient to limit warming to the 2°C target in 2100 relative to pre-industrial levels (IPCC, 2019). Increased ambition, with additional actions, is required to avoid the unmanageable as well as to manage the unavoidable impacts from climate change and extremes already being experienced (Gattuso et al., 2018; Gattuso et al., 2019; Gattuso et al., 2021), including their effects on ocean biodiversity (Poloczanska et al., 2016; Pörtner et al., 2021).
The oceans are not only showing the adverse consequences of climate change but are also now being regarded as a solution space. Gattuso et al. (2018; 2019) assessed ocean-based actions, through literature review and expert surveys, for their potential contributions to climate change mitigation and adaptation. These solutions were grouped into four main categories: addressing the causes of climate change [i.e., reducing anthropogenic GHG emissions or increasing the long-term removal of GHG, primarily CO2, commonly termed CDR (carbon dioxide removal)], protection of biota and ecosystems (habitats, species, resources, etc.), manipulation of biological and ecological adaptation, and solar radiation management. Gattuso et al. (2019) and other analyses concluded that the greatest benefit is derived by combining global and local solutions. Some “decisive” measures have been already implemented, are highly effective in reducing emissions, and have known but accepted costs (Perrow, 2019). These include renewable energy, especially offshore fixed and floating wind turbines, which has grown threefold in less than 5 years (although they can have other environmental impacts; Galparsoro et al., 2021; Lloret et al., 2021). Other “low regret” measures are also already implemented and feasible and provide many co-benefits, although they have limited effectiveness in addressing climate change drivers at global scales. For example, the belowground or long-lived parts of coastal vegetation such as mangroves, salt marsh, and seagrass are important for carbon storage, but their global climate mitigation potential is constrained by their limited global extent although they provide coastal protection and nursery habitat (Duarte, 2016; Duarte et al., 2020).
Similarly, GESAMP (2019) assessed ocean-based geoengineering approaches that focused on technologies for storing CO2 (at depth or in sub-sea strata), absorbing more carbon (through increases in plant or algal biomass and productivity with carbon then sinking to deep water), or deflecting heat from the surface. Many of these techniques are still at a very early or experimental stage or have not been demonstrated to be scalable to a level needed to make a globally relevant difference to atmospheric CO2 levels. Nevertheless, increasing plant and algal biomass remains a potentially important form of ecoengineering (rather than geoengineering), part of a range of nature-based solutions giving habitat benefits with ancillary benefits in short- to medium-term carbon storage.
Some of the ocean and coastal techniques for climate adaptation and mitigation have been implemented and refined over many decades, although not always specifically designed to address climate change impacts. For example, marine protected areas (MPAs) may have substantial climate benefits (Roberts et al., 2017) and are approaching an overall 7.4% of the global ocean, although still far from the UN objective of 30% by 2030 (Grorud-Colvert et al., 2021).
Blue carbon is an increasingly used term in policy and climate change discussions and is taken to mean all carbon associated with aquatic systems, especially coasts and oceans (Hoegh-Guldberg et al., 2019a). Ecoengineering solutions such as maintaining or increasing certain types of habitats offer high potential as a means of increasing “blue carbon,” and enhancing and maintaining coastal vegetation can give what may be termed triple wins—for biodiversity, coastal protection, and carbon storage (Elliott et al., 2019). However, it is cautioned that, at present, the term “blue carbon” includes short-term and labile storage (such as by surface short-lived vegetation, e.g., saltmarshes) as well as long-term sediment sequestration and storage in long-lived vegetation such as mangroves—only the long-term refractory storage would have a marked contribution to climate change amelioration.
Hoegh-Guldberg et al. (2019a) propose five opportunities for action to promote the ocean as a solution to climate change. It is possible that a combination of these ocean-based climate actions that have the greatest effectiveness and co-benefits and the lowest potential negative impacts could reduce global GHG emissions by up to 4 billion tons of CO2 equivalents in 2030 and by up to 11 billion tons in 2050 (Hoegh-Guldberg et al., 2019b), thereby contributing as much as 21% of the emission reduction required by 2050 to limit warming to 1.5°C and 25% for a 2°C target.
3 Discussion
3.1 Changing the Narrative
Science and scientists must not only identify the problems in the ocean but also move to documenting existing solutions and proposing new ones (Figure 1). Changing the narrative in scientific publications and media from negative views and despair to a positive view based on scientific, technical, knowledge-based (including indigenous and local knowledge), societal, and nature-based solutions is required (Knowlton, 2017; Lubchenco and Gaines, 2019; Knowlton, 2021) (Figure 1). This provides hope that it might be possible to approach climate change mitigation targets and address other societal challenges, including ensuring food security; resilient coastal economies and communities; and human health, equity, and justice.
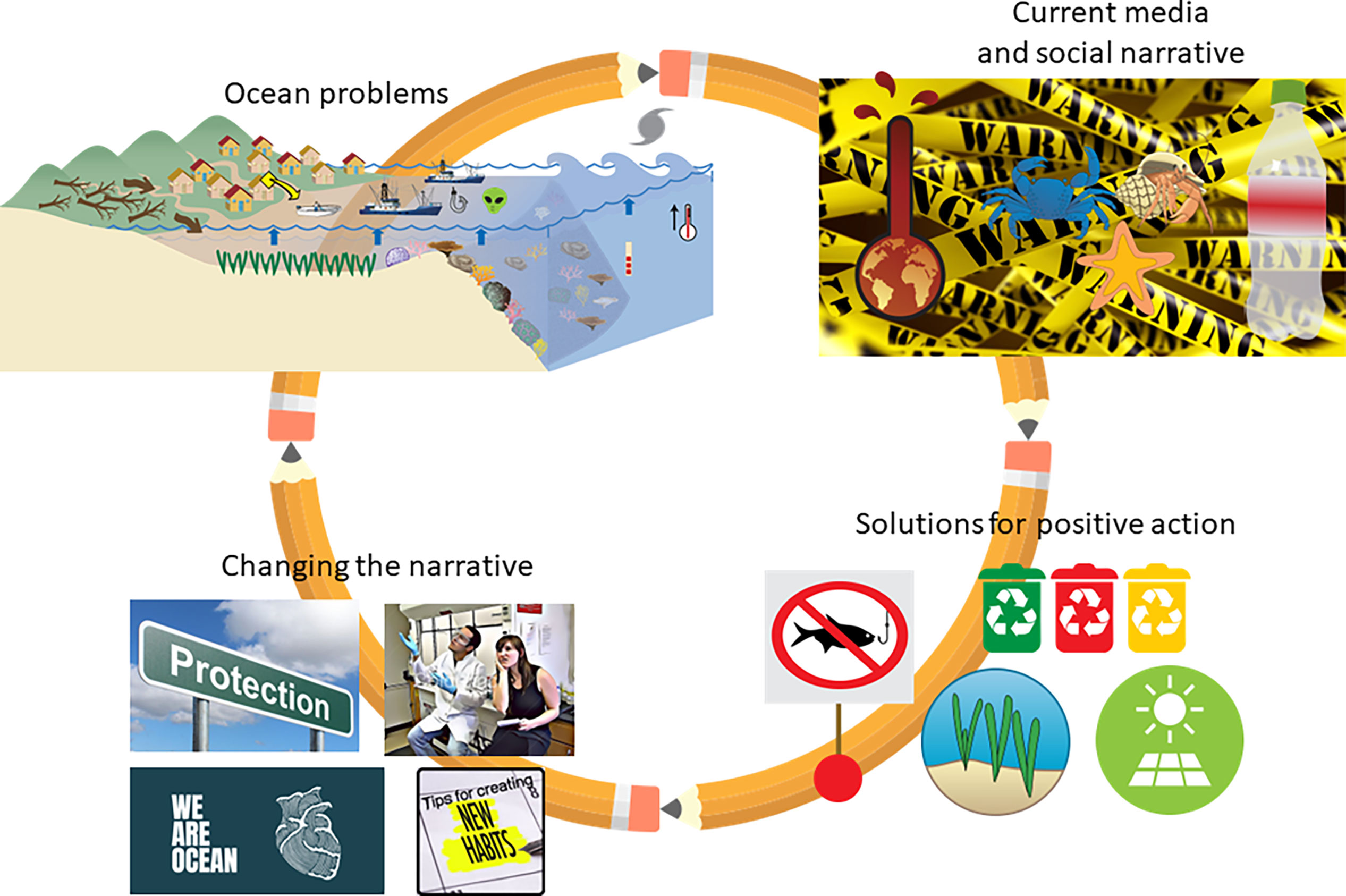
Figure 1 Actions to tackle problems and solutions for the current ocean problems, changing the narrative. These problems include, among others, coastal development, land use in the catchment, sea level rise, pollution, overfishing, acidification, warming, increasing storms, and invasive species (this is a modified version of a figure by Tracey Saxby, Integration and Application Network; https://ian.umces.edu/media-library). The most common problems shown currently by media are illustrated by icons on climate change, biodiversity loss (both by Tracey Saxby), and plastics (by Kim Kraeer and Lucy Van Essen-Fishman, Integration and Application Network; https://ian.umces.edu/media-library), over a Power Point resource and CC BY license. The four topics studied are fish management, plastics solutions, restoration, and climate change mitigation (renewables icon), all icons by Jane Hawkey, Integration and Application Network (https://ian.umces.edu/media-library). Finally, to change the narrative, some issues discussed include protection/conservation, need to dialogue between journalists and scientists, ocean literacy (initiative We Are Ocean), and change the behavior (all from Power Point resources under license CC BY-NC-ND).
The change in narrative should also focus on the connection between ocean health and human health (Borja et al., 2020a). Human wellbeing must be a central concern in all ocean conservation efforts, a fact made explicit by the focus of the UNDOS, the UNDER, and the SDGs, especially SDG14. This focus on human wellbeing is increasingly also being interpreted in the context of not only who benefits but also how conservation is achieved, encompassing intergenerational equity and full and effective participation of indigenous peoples and local communities (CBD, 2020).
In this context, journalists and scientists must work together in disseminating these messages (Kolandai-Matchett et al., 2021b) (Figure 1). This can be challenging as scientists and journalists use different languages, formats, and levels of required detail and have different time constraints (Elliott et al., 2017; Kolandai-Matchett et al., 2021a). Scientists have more time to prepare and publish their articles and are relatively specialized in their expertise, and the novelty of their findings is usually assessed by their colleagues (although some high-profile journals focus on especially newsworthy topics). In contrast, journalists normally work with short deadlines, have a more general and often more limited knowledge of scientific issues, communicate with a wide variety of audiences, have greater restrictions on the size and level of detail allowed, and are looking for stories that might be considered novel and newsworthy by society at large. In addition, the general public, and indeed politicians, may only require or be able to accommodate brief stories, sound bites, headlines, or short news bulletins (Elliott et al., 2017).
Scientists also have to be aware that all news media have their own agendas and that different outlets have declared or undeclared biases, with some being more environmentally aware than others. Hence, a journalist may have a pre-prepared “story” and will mainly look for those scientists who support this narrative. Bad news is often more newsworthy than good news, and the absence of an impact is rarely considered newsworthy. However, the latter also occurs in scientific journals—for example, it is difficult to publish when there was no result from an experiment.
Despite this, scientists and journalists have much in common, including a shared interest in combatting fake news (Elías, 2019) and in communicating not only problems but also solutions. In describing successful conservation outcomes, positive messages must be rooted in facts. Society must be aware of the broader impacts on the ocean (which journalists consider as news), and both scientists and journalists must analyze the causes, identifying and providing solutions to them. However, facts alone are not sufficient. To transmit these stories, scientists and journalists need to find a new focus, following the recommendations from Taylor & Francis9 (i) a major breakthrough in the field (e.g., the Mekong Delta mangrove forest is the largest habitat restoration undertaken to date); (ii) impact on society and relevance to the everyday lives of people (e.g., a study found that contact with blue spaces during the COVID-19 pandemic lockdown was beneficial for mental health); (iii) recommendation for change to improve practice or policy (e.g., changes in policies have increased the populations of some cetaceans); and (iv) connection to current events or popular ideas (e.g., there is an urgent need to reduce meat and dairy consumption to meet climate targets). The use of personal stories can be particularly effective (Leslie et al., 2013) although not normally a part of scientific papers (e.g., what led to the interest in this topic, what obstacles were encountered). Eliciting an emotional reaction can be helpful, especially when trying to encourage people to act (e.g., the problem of marine plastic pollution, a negative issue, can be used to get volunteers to pick up garbage and to engage society in general). To achieve this, it is most effective to communicate with a national or local perspective, because news items that are close to people are more interesting.
3.2 Prospects for the Future
As shown here, the ocean is facing important challenges in need of solutions within this decade (Borja et al., 2020b). Because of management actions taken in the past three decades, some habitats and species are recovering, and some new solutions are emerging, as shown in the previous sections, not only for those problems but also for wider marine pollution issues, using integrated approaches and providing integrated solutions (Figure 1). Despite this, most conservation successes remain small compared with the scale of the challenges at hand. For example, only approximately 2.7% of the ocean is highly protected (Sala et al., 2021). Initiatives, such as that of 30-by-30 (to protect at least 30% of the environment by 2030)10, try to cover this gap, although some studies determine that conserving threatened marine species and biodiversity requires 40% of ocean protection (Jefferson et al., 2021). Thus, extensive benefits could be achieved by replicating and/or scaling-up the solutions whose effectiveness has already been demonstrated. As with all science, however, ocean conservation does not stand still, and no review of “ocean optimism” can be complete without a consideration of how new approaches could contribute to ocean health in the future.
Just as we speak of the industrial revolution and the information revolution, we must consider ocean conservation in the context of other ongoing technical revolutions, specifically the carbon revolution, the food revolution, the data revolution, the genetic revolution, and the financial revolution. These include the rapid expansion of ocean-based tidal, wave, and offshore wind energy; decarbonizing the marine shipping and transport sector; our growing ability to track fishing activity across the globe; the use of genetic methods to monitor and enhance ocean health (Gattuso et al., 2018; Duarte et al., 2020; Novak et al., 2020; Gattuso et al., 2021; Gold et al., 2021); and the establishment of “Blue Bonds” to finance marine protection [e.g., in the Seychelles, (McFarland, 2021)]. One area still needing as yet undeveloped approaches, despite active research, is the challenge posed by the ever-escalating amounts of plastic pollution, for which no clear solution is yet in sight (see Section 2.3).
The revolutions we have spoken of up to now are technical revolutions. In the end, however, what is truly needed for transformative change in ocean conservation, and conservation broadly, is a kind of social revolution, e.g., a huge commitment to shouldering the costs of the energy transition, protection, and restoration on a global scale, and major changes in diet. In this, we emphasize that scientists are an integral part of society and that marine scientists have a major role to play, by engaging with society at large, not only in identifying problems but also in providing and communicating solutions (Kolandai-Matchett et al., 2021a; Kolandai-Matchett et al., 2021b). To do this, ocean conservation professionals need to focus on three steps: (i) take their science and transform it into a narrative that can inspire others to join in the effort; (ii) acknowledge that setbacks and trade-offs will occur, but not to let them become paralyzing; and (iii) retain a focus on the end goal, which is not the problem but the solution. These steps probably can be attained through the UNDOS and UNDER, as well as the SDGs, using ocean literacy to connect people to their oceans (Claudet et al., 2019; Borja et al., 2020c). Making society aware of the problems of the ocean, as well as the solutions that science can bring, will facilitate a change of narrative to positive views (Figure 1).
Despite the positive narrative embraced here, we are concerned that the COVID-19 pandemic and the aggression to Ukraine are changing societal priorities. It is questioned whether society can accommodate several crises—for example, in the midst of a health crisis and, as we speak, a major European war affecting global food security, can the public also give attention to climate change and especially problems in the oceans? The latter may be a case of “out of sight, out of mind” in that long-term concerns about ocean acidification, deoxygenation, warming, and loss of habitats are displaced when there are immediate threats to health. There is the danger that, although environmental catastrophes have been predicted since the 1960s (Borja and Elliott, 2021), the public still sees “sun, sea, and sand” when they go on holiday and so they are not concerned (and could still fulfil those holiday wishes even with an abiotic environment)!. This illustrates the importance that scientists provide solutions rather than focusing only on yet more identification of problems (Figure 1). We cannot keep calling for more monitoring if there are no actions proposed if the monitoring shows adverse trends.
A further concern is whether ocean science will be funded sufficiently to achieve the greater knowledge necessary to define the problems and solutions. If not, then the cause for optimism and solutions to satisfy it will not be reached. For example, despite the importance of the oceans, the mismatch in most countries in funding for space and ocean research has been highlighted previously (Borja and Elliott, 2018), and it is likely that financial resources will now have to be diverted from environmental research and monitoring to paying for the effects of the pandemic. It is of note and concern that UNDOS and UNDER will not be accompanied by funding to countries to achieve the desired aims. Similarly, we note that different countries have both different priorities and different financial resources to tackle the environmental challenges. For example, wealthy countries will be able to keep raising their dykes to cope with sea-level rise and flooding (Manhattan and London will not be allowed to flood), whereas poorer countries will suffer displaced climate migrants (e.g., the Sundarbans in Bangladesh will get inundated and its people will have to move) (Elliott et al., 2019).
During the summer school in San Sebastian, these ideas were discussed, and a suite of actions and solutions were identified by the participants (Table 3). These actions try to bring together scientists from different backgrounds, journalists, and the society at large, to change our narrative about the ocean problems and achieve a healthy and sustainable ocean in coming few decades (Lubchenco and Gaines, 2019; Duarte et al., 2020; Knowlton, 2021). Some actions focus on successes and aim to replicate and scale what works. Building on successes in, for example, fisheries management, pollution reduction, and ecosystem protection is crucial to achieving positive impacts at regional and global scales. There is also a suite of additional solutions, which will require new approaches, capacity, investments, and governance. For example, there is a vast potential in new technologies, improved international cooperation, and in directly linking ocean and human health, but upscaling these from local schemes to globally effective levels is perhaps the greatest challenge. Underlying all solutions, there is the critical imperative of developing and implementing solutions in equitable, inclusive, and ethical ways to conserve ocean and biodiversity (CBD, 2020). A global ocean optimism movement that is solution-focused and sustained and motivated by these principles is urgently needed to address the grand challenges of our time, in the ocean and beyond.
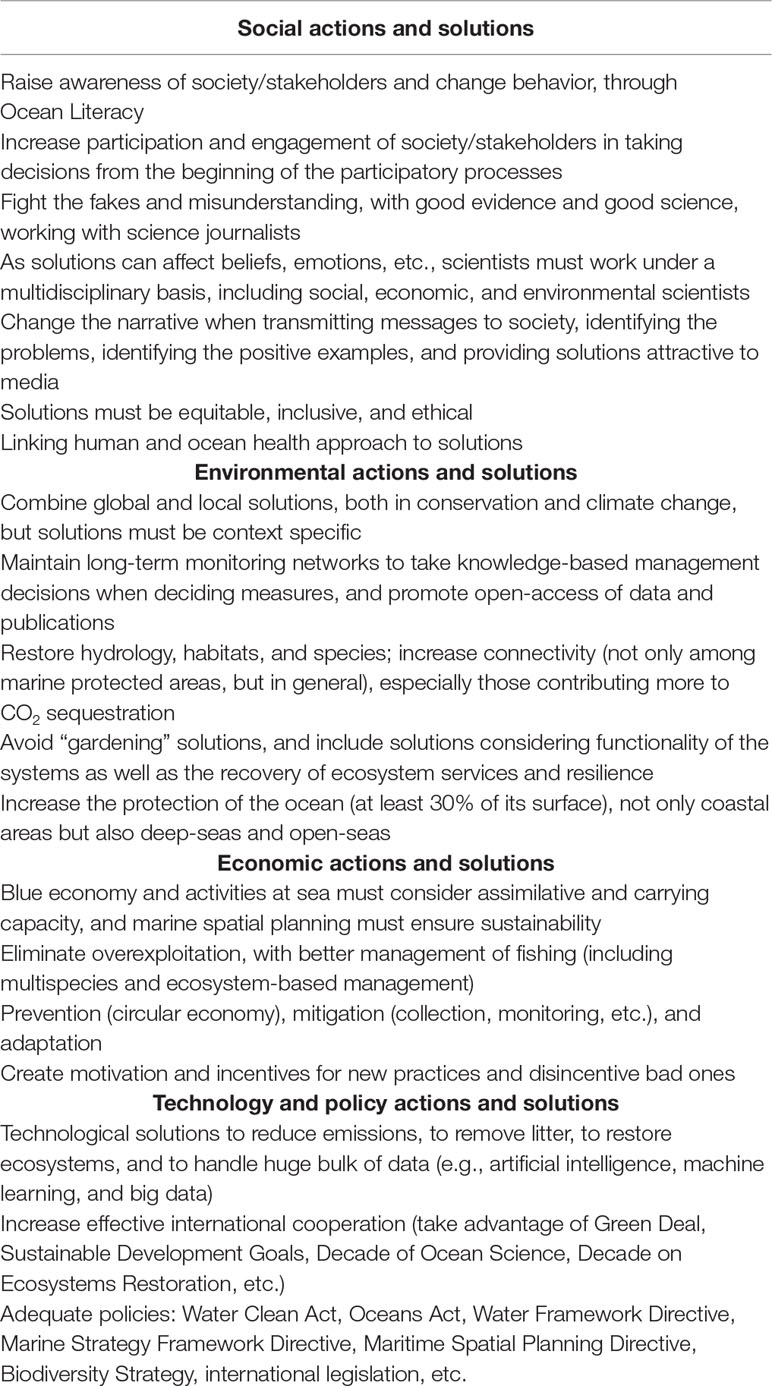
Table 3 Some actions and solutions for problems in the ocean, which can be implemented by scientists, raised by the participants in the summer school in San Sebastian (Spain), in June 2021.
Data Availability Statement
The original contributions presented in the study are included in the article/supplementary material. Further inquiries can be directed to the corresponding author.
Author Contributions
AB developed the idea of the summer school and the paper. Each author wrote one of the sections under his/her expertise. AB wrote the first complete draft of the article and all authors contributed equally to the discussion and conclusions and in writing the final manuscript. All authors read and approved the final manuscript.
Funding
This manuscript is the result of an AZTI’s Summer School, celebrated in San Sebastian (Spain), from June 8 to 10, 2021, funded by AZTI (www.azti.es), the Aquarium of San Sebastian, the European Environment Agency (EEA), and Frontiers in Marine Science. AB was supported through a convention between AZTI and the Basque Water Agency, for monitoring and assessing marine waters, and Consorcio de Aguas Bilbao-Bizkaia has provided also some data. FM acknowledges The Ocean Solutions Initiative and the Blue Food Assessment. FZ acknowledges funding from the Research Council of Norway (project no. 324159).
Conflict of Interest
Author ME is the unpaid Director of International Estuarine & Coastal Specialists Ltd. He and the remaining authors declare that the research was conducted in the absence of any commercial or financial relationships that could be construed as a potential conflict of interest.
Publisher’s Note
All claims expressed in this article are solely those of the authors and do not necessarily represent those of their affiliated organizations, or those of the publisher, the editors and the reviewers. Any product that may be evaluated in this article, or claim that may be made by its manufacturer, is not guaranteed or endorsed by the publisher.
Acknowledgments
This is contribution number 1105 from AZTI’s Marine Research Division.
Footnotes
- ^ https://www.g7fsoi.org/activities/
- ^ https://bluefood.earth
- ^ https://www.un.org/en/food-systems-summit
- ^ https://www.theguardian.com/commentisfree/2019/may/09/seas-stop-eating-fish-fishing-industry-government
- ^ https://www.seaspiracy.org/
- ^ https://www.iccat.int/Documents/SCRS/ExecSum/BFT_ENG.pdf
- ^ www.lifelema.eu
- ^ https://www.theguardian.com/environment/2022/feb/05/how-super-enzymes-that-eat-plastics-could-curb-our-waste-problem
- ^ https://editorresources.taylorandfrancis.com/the-editors-role/increase-journal-visibility-impact/media-relations/newsworthy-research/
- ^ https://www.nrdc.org/30x30-nrdcs-commitment-protect-nature-and-life-earth
References
Andrés M., Delpey M., Ruiz I., Declerck, (2021). Measuring and Comparing Solutions for Floating Marine Litter Removal: Lessons Learned in the South-East Coast of the Bay of Biscay From an Economic Perspective. Mar. Pol. 127, 104450. doi: 10.1016/j.marpol.2021.104450
Andriolo U., Gonçalves G., Rangel-Buitrago N., et al. (2021). Drones for Litter Mapping: An Inter-Operator Concordance Test in Marking Beached Items on Aerial Images. Mar. pollut. Bull. 169, 112542. doi: 10.1016/j.marpolbul.2021.112542
Arneth A., Shin Y.-J., Leadley P., et al. (2020). Post-2020 Biodiversity Targets Need to Embrace Climate Change. Proc. Natl. Acad. Sci. 117, 30882–30891. doi: 10.1073/pnas.2009584117
Basurko O. C., Zudaire I., Larreta J., Suarez M. J., et al. (2020). Second Chances for Fishing and Aquaculture Gears. Proc. BAMAR Workshop Mar. Litter (BAMAR 2020), 52. https://isms.cat/wp-content/uploads/2020/09/libro-BAMAR-ISMS-2020.pdf
Bellou N., Gambardella C., Karantzalos K., et al. (2021). Global Assessment of Innovative Solutions to Tackle Marine Litter. Nat. Sustainability 4, 516–524. doi: 10.1038/s41893-021-00726-2
Biermann L., Clewley D., Martinez-Vicente V., Topouzelis K. (2020). Finding Plastic Patches in Coastal Waters Using Optical Satellite Data. Sci. Rep. 10, 5364. doi: 10.1038/s41598-020-62298-z
Borja A., Andersen J. H., Arvanitidis C. D., et al. (2020b). Past and Future Grand Challenges in Marine Ecosystem Ecology. Front. Mar. Sci. 7. doi: 10.3389/fmars.2020.00362
Borja Á., Chust G., Rodríguez J. G., et al. (2016). ‘The Past is the Future of the Present’: Learning From Long-Time Series of Marine Monitoring. Sci. Total Environ. 566–567, 698–711. doi: 10.1016/j.scitotenv.2016.05.111
Borja Á., Dauer D., Elliott M., Simenstad C. (2010). Medium- and Long-Term Recovery of Estuarine and Coastal Ecosystems: Patterns, Rates and Restoration Effectiveness. Estuaries Coasts 33, 1249–1260. doi: 10.1007/s12237-010-9347-5
Borja A., Elliott M. (2018). There is No Planet B: A Healthy Earth Requires Greater Parity Between Space and Marine Research. Mar. pollut. Bull. 13, 28–30. doi: 10.1016/j.marpolbul.2018.03.015
Borja A., Elliott M. (2019). So When Will We Have Enough Papers on Microplastics and Ocean Litter? Mar. pollut. Bull. 146, 312–316. doi: 10.1016/j.marpolbul.2019.05.069
Borja A., Elliott M. (2021). From an Economic Crisis to a Pandemic Crisis: The Need for Accurate Marine Monitoring Data to Take Informed Management Decisions. Adv. Mar. Biol. 89, 79–114. doi: 10.1016/bs.amb.2021.08.002
Borja A., Galparsoro I., Solaun O., et al. (2006a). The European Water Framework Directive and the DPSIR, a Methodological Approach to Assess the Risk of Failing to Achieve Good Ecological Status. Estuar. Coast. Shelf Sci. 66, 84–96. doi: 10.1016/j.ecss.2005.07.021
Borja A., Muxika I., Franco J. (2006b). Long-Term Recovery of Soft-Bottom Benthos Following Urban and Industrial Sewage Treatment in the Nervión Estuary (Southern Bay of Biscay). Mar. Ecol. Prog. Ser. 313, 43–55. doi: 10.3354/meps313043
Borja A., Santoro F., Scowcroft G., Fletcher S., Strosser P. (2020c). Editorial: Connecting People to Their Oceans: Issues and Options for Effective Ocean Literacy. Front. Mar. Sci. 6. doi: 10.3389/fmars.2019.00837
Borja A., White M. P., Berdalet E., et al. (2020a). Moving Toward an Agenda on Ocean Health and Human Health in Europe. Front. Mar. Sci. 7. doi: 10.3389/fmars.2020.00037
Branch T. A., Jensen O. P., Ricard D., Ye Y., Hilborn R. (2011). Contrasting Global Trends in Marine Fishery Status Obtained From Catches and From Stock Assessments. Conserv. Biol. 25, 777–786. doi: 10.1111/j.1523-1739.2011.01687.x
Brander K. (2010). Impacts of Climate Change on Fisheries. J. Mar. Syst. 79, 389–402. doi: 10.1016/j.jmarsys.2008.12.015
Brunel T., Van Damme C. J., Samson M., Dickey-Collas M. (2018). Quantifying the Influence of Geography and Environment on the Northeast Atlantic Mackerel Spawning Distribution. Fisheries Oceanography 27, 159–173. doi: 10.1111/fog.12242
Cardinale M., Dörner H., Abella A., et al. (2013). Rebuilding EU Fish Stocks and Fisheries, a Process Under Way? Mar. Policy 39, 43–52. doi: 10.1016/j.marpol.2012.10.002
CBD (2020). “Update of the Zero Draft of the Post-2020 Global Biodiversity Framework,” in Convention on Biological Diversity, 9 pp., United Nations, New York, CBD/POST2020/PREP/2/1.
CLAIMS (2021) Successful Installation and Trial of CLAIM’s Marine Litter Containment Floating Boom. Available at: https://www.claim-h2020project.eu/successful-installation-and-trial-of-claims-marine-litter-containment-floating-boom/ (Accessed 29 July 2021).
Claudet J., Bopp L., Cheung W. W. L., Devillers R., Escobar-Briones E., Haugan P., et al. (2019). A Roadmap for Using the UN Decade of Ocean Science for Sustainable Development in Support of Science, Policy, and Action. One Earth. doi: 10.1016/j.oneear.2019.1010.1012
Costanza R., de Groot R., Braat L., et al. (2017). Twenty Years of Ecosystem Services: How Far Have We Come and How Far do We Still Need to Go? Ecosystem Serv. 28, 1–16. doi: 10.1016/j.ecoser.2017.09.008
Costello M. J. (2015). Biodiversity: The Known, Unknown, and Rates of Extinction. Curr. Biol. 25, R368–R371. doi: 10.1016/j.cub.2015.03.051
Cózar A., Aliani S., Basurko O. C., et al. (2021). Marine Litter Windrows: A Strategic Target to Understand and Manage the Ocean Plastic Pollution. Front. in Mar. Sci. 8. doi: 10.3389/fmars.2021.571796
Dauvergne P. (2018). Why is the Global Governance of Plastic Failing the Oceans? Global Environ. Change 51, 22–31. doi: 10.1016/j.gloenvcha.2018.05.002
Davies C., Chen W. Y., Sanesi G., Lafortezza R. (2021). The European Union Roadmap for Implementing Nature-Based Solutions: A Review. Environ. Sci. Policy 121, 49–67. doi: 10.1016/j.envsci.2021.03.018
Declerck A., Delpey M., Rubio A., et al. (2019). Transport of Floating Marine Litter in the Coastal Area of the South-Eastern Bay of Biscay: A Lagrangian Approach Using Modelling and Observations. J. Operational Oceanography 12, S111–S125. doi: 10.1080/1755876X.2019.1611708
Defeo O., Elliott M., (2021). The ’Triple Whammy’ of coasts under threat – why we should be worried! Marine Pollution Bulletin, 163: 111832. doi: 10.1016/j.marpolbul.2020.111832
de los Santos C. B., Krause-Jensen D., Alcoverro T., et al. (2019). Recent Trend Reversal for Declining European Seagrass Meadows. Nat. Commun. 10, 3356. doi: 10.1038/s41467-019-11340-4
Delpey M. (2021). “FML-Track. An Operational Service to Support the Reduction of Floating Marine Litter in the Coastal Area,” in Copernicus Marine Service General Assembly, , January 26-28, 2021.
de Vries R., Egger M., Mani T., Lebreton L. (2021). “Vessel-Based Optical Data and Artificial Intelligence for Sampling Mega-Plastic Concentrations on the High Seas,” in EGU General Assembly 2021, , 19–30 Apr 2021. EGU21–15204. doi: 10.5194/egusphere-egu21-15204
Dijkstra H., van Beukering P., Brouwer R. (2021). In the Business of Dirty Oceans: Overview of Startups and Entrepreneurs Managing Marine Plastic. Mar. pollut. Bull. 162, 111880. doi: 10.1016/j.marpolbul.2020.111880
Dragesund O., Hamre J., Ulltang Ø. (1980). Biology and Population Dynamics of the Norwegian Spring-Spawning Herring. Rapports procès-verbaux Des. réunions 177, 43–71. http://hdl.handle.net/11250/108448
Dragesund O., Johannessen A., Ulltang Ø. (1997). Variation in Migration and Abundance of Norwegian Spring Spawning Herring (Clupea Harengus L.). Sarsia 82, 97–105. doi: 10.1080/00364827.1997.10413643
Duarte C. M. (2016). Reviews and Syntheses: Hidden Forests, the Role of Vegetated Coastal Habitats on the Ocean Carbon Budget. Biogeosciences Discusssions 2016, 1–17. doi: 10.5194/bg-2016-339
Duarte C. M., Agusti S., Barbier E., et al. (2020). Rebuilding Marine Life. Nature 580, 39–51. doi: 10.1038/s41586-020-2146-7
Duarte C., Borja A., Carstensen J., Elliott M., Krause-Jensen D., Marbà N. (2015a). Paradigms in the Recovery of Estuarine and Coastal Ecosystems. Estuaries Coasts 38, 1202–1212. doi: 10.1007/s12237-013-9750-9
Duarte C. M., Fulweiler R. W., Lovelock C. E., et al. (2015b). Reconsidering Ocean Calamities. BioScience 65, 130–139. doi: 10.1093/biosci/biu198
Dunic J. C., Brown C. J., Connolly R. M., Turschwell M. P., Côté I. M. (2021). Long-Term Declines and Recovery of Meadow Area Across the World’s Seagrass Bioregions. Global Change Biol. 27, 4096–4109. doi: 10.1111/gcb.15684
Ecoalf (2021) Upcycling the Oceans. Available at: https://ecoalf.com/es/p/upcycling-the-oceans-15 (Accessed 29 July 2021).
Elías C. (2019). Science on the Ropes. Decline of Scientific Culture in the Era of Fake News ( Springer Nature Switzerland), Cham, 330 pp.
Elliott M., Barnard S., Boyes S. J., et al. (2020). “The Framework and Options for Compensation for Marine Annex 1 Habitats for Offshore Wind Farms,” in Unpublished Report YBB875-F-2020, Report for Ørsted Wind Power NS, Strategic Environment Team (Contract No. 0456) (University of Hull and International Estuarine & Coastal Specialists (IECS), Hull, pp 1–p115.
Elliott M., Day J. W., Ramachandran R., Wolanski E. (2019). “Chapter 1-A Synthesis: What Future for Coasts, Estuaries, Deltas, and Other Transitional Habitats in 2050 and Beyond?,” in Coasts and Estuaries: The Future. Eds. Wolanski E., Day J. W., Elliott M., Ramachandran R., Eds R. (Amsterdam: Elsevier), p1–28, ISBN: ISBN 978-0-12-814003-1.
Elliott M., Mander L., Mazik K., et al. (2016). Ecoengineering With Ecohydrology: Successes and Failures in Estuarine Restoration. Estuar. Coast. Shelf Sci. 176, 12–35. doi: 10.1016/j.ecss.2016.04.003
Elliott M., Snoeijs-Leijonmalm P., Barnard S. (2017). ‘The Dissemination Diamond’ and Paradoxes of Science-to-Science and Science-to-Policy Communication: Lessons From Large Marine Research Programmes. Mar. pollut. Bull. 125, 1–3. doi: 10.1016/j.marpolbul.2017.08.022
EU (2002). Regulation (EC) No 2371/2002 of 20 December 2002 on the Conservation and Sustainable Exploitation of Fisheries Resources Under the Common Fisheries Policy. Off. J. Eur. Union, 59.
Fabres J., Savelli H., Schoolmeester T., Rucevska I., Baker E. (2016). Marine Litter Vital Graphics (UN-Environment, GRID-Arendal).
Failletaz R., Beaugrand G., Goberville E., Kirby R. R. (2019). Atlantic Multidecadal Oscillations Drive the Basin-Scale Distribution of Atlantic Bluefin Tuna. Sci. Adv. 5, eaar6993. doi: 10.1126/sciadv.aar6993
Fall J., Ciannelli L., Skaret G., Johannesen E. (2018). Seasonal Dynamics of Spatial Distributions and Overlap Between Northeast Arctic Cod (Gadus Morhua) and Capelin (Mallotus Villosus) in the Barents Sea. PLoS One 13, e0205921. doi: 10.1371/journal.pone.0205921
FAO (2020) The State of World Fisheries and Aquaculture 2020. Sustainability in Action. Available at: http://www.fao.org/documents/card/en/c/ca9229en.
Fernandes P. G., Cook R. M. (2013). Reversal of Fish Stock Decline in the Northeast Atlantic. Curr. Biol. 23, 1432–1437. doi: 10.1016/j.cub.2013.06.016
Freeman S., Booth A. M., Sabbah I., et al. (2020). Between Source and Sea: The Role of Wastewater Treatment in Reducing Marine Microplastics. J. Environ. Manage. 266, 110642. doi: 10.1016/j.jenvman.2020.110642
Froese R., Proelß A. (2010). Rebuilding Fish Stocks No Later Than 2015: Will Europe Meet the Deadline? Fish Fisheries 11, 194–202. doi: 10.1111/j.1467-2979.2009.00349.x
Fromentin J.-M., Powers J. E. (2005). Atlantic Bluefin Tuna: Population Dynamics, Ecology, Fisheries and Management. Fish Fisheries 6, 281–306. doi: 10.1111/j.1467-2979.2005.00197.x
Gago J., Booth A. M., Tiller R., Maes T., Larreta J. (2020). “Microplastics Pollution and Regulation,” in Handbook of Microplastics in the Environment. Eds. Rocha-Santos T., Costa M., Mouneyrac C. (Cham: Springer International Publishing), 1–27.
Gaines S. D., Costello C., Owashi, (2018). Improved Fisheries Management Could Offset Many Negative Effects of Climate Change. Sci. Adv. 4, eaao1378. doi: 10.1126/sciadv.aao1378
Galparsoro I., Korta M., Subirana I., Borja Á., Menchaca I., Solaun O., et al. (2021). A New Framework and Tool for Ecological Risk Assessment of Wave Energy Converters Projects. Renewable Sustain. Energy Rev. 151, 111539. doi: 10.1016/j.rser.2021.111539
Garcia T., Planque B., Arneberg P., Bogstad B., Skagseth Ø., Tiedemann M. (2021). An Appraisal of the Drivers of Norwegian Spring-Spawning Herring (Clupea Harengus) Recruitment. Fish. Oceanogr. 30, 159–173. doi: 10.1111/fog.12510
Gattuso J.-P., Magnan A. K., Bopp L., et al. (2018). Ocean Solutions to Address Climate Change and its Effects on Marine Ecosystems. Front. Mar. Sci. 5. doi: 10.3389/fmars.2018.00337
Gattuso J.-P., Magnan A. K., Gallo N., et al. (2019). “Opportunities for Increasing Ocean Action in Climate Strategies,” in Iddri Policy Brief, 1–4. Available at: https://www.iddri.org/en/publications-and-events/policy-brief/opportunities-increasing-ocean-action-climate-strategies. 02/19.
Gattuso J.-P., Williamson P., Duarte C. M., Magnan A. K. (2021). The Potential for Ocean-Based Climate Action: Negative Emissions Technologies and Beyond. Front. Climate 2. doi: 10.3389/fclim.2020.575716
Gephart J. A., Henriksson P. J. G., Parker R. W. R., et al. (2021). Environmental Performance of Blue Foods. Nature 597, 360–365. doi: 10.1038/s41586-021-03889-2
GESAMP (2019). “High Level Review of a Wide Range of Proposed Marine Geoengineering Techniques,” in IMO/FAO/UNESCO-IOC/UNIDO/WMO/IAEA/UN/UN Environment/UNDP/ISA Joint Group of Experts on the Scientific Aspects of Marine Environmental Protection. International Maritime Organization, London, Eds. Boyd P. W., Vivian C. M. G., 144 p. Rep. Stud. GESAMP No. 98.
Gilby B. L., Olds A. D., Peterson C. H., et al. (2018). Maximising the Benefits of Oyster Reef Restoration for Finfish and Their Fisheries. Fish Fisheries 19 (5), 931–947. doi: 10.1111/faf.12301
Golden C. D., Koehn J. Z., Shepon A., et al. (2021). Aquatic Foods to Nourish Nations. Nature 598, 315–320. doi: 10.1038/s41586-021-03917-1
Gold Z., Sprague J., Kushner D. J., Marin E. Z., Barber P. H. (2021). eDNA Metabarcoding as a Biomonitoring Tool for Marine Protected Areas. PLoS One 16, e0238557. doi: 10.1371/journal.pone.0238557
Gonçalves L. R. (2021). “ICCAT and the Cooperation for Eastern Bluefin Tuna Management,” in Regional Fisheries Management Organizations: The Interplay Between Governance and Science (Cham: Springer International Publishing), 39–60. doi: 10.1007/978-3-030-70362-2_3
González D., Hanke G., Tweehuysen G., et al. (2016). “Riverine Litter Monitoring - Options and Recommendations,” in MSFD GES TG Marine Litter Thematic Report. EUR 28307. Publications Office of the European Union, Luxembourgdoi: 10.2788/461233
Grimaldo E., Herrmann B., Jacques N., Vollstad J., Su B. (2020). Effect of Mechanical Properties of Monofilament Twines on the Catch Efficiency of Biodegradable Gillnets. PLoS One 15, e0234224. doi: 10.1371/journal.pone.0234224
Grorud-Colvert K., Sullivan-Stack J., Roberts C., et al. (2021). The MPA Guide: A Framework to Achieve Global Goals for the Ocean. Science 373, eabf0861. doi: 10.1126/science.abf0861
Gullestad P., Sundby S., Kjesbu O. S. (2020). Management of Transboundary and Straddling Fish Stocks in the Northeast Atlantic in View of Climate-Induced Shifts in Spatial Distribution. Fish Fisheries 21, 1008–1026. doi: 10.1111/faf.12485
Hennen M., Arias M., Aliani S., Cózar A., Jacobs C., et al. (2019). “EO Tracking of Marine Debris in the Mediterranean Sea From Public Satellites,” in Final Report, vol. 31. (Paris: European Space Agency). ESA Contract No. 4000124861/18/I-NB.
Hilborn R., Amoroso R. O., Anderson C. M., et al. (2020). Effective Fisheries Management Instrumental in Improving Fish Stock Status. Proc. Natl. Acad. Sci. 117, 2218–2224. doi: 10.1073/pnas.1909726116
Hilborn R., Hively D. J., Loke N. B., et al. (2021). Global Status of Groundfish Stocks. Fish Fisheries. 22, 911–928, doi: 10.1111/faf.12560
Hjort J., Lea E. (1911). II. Some Results of the International Herring-Investigations 1907–1911. J. du Conseil 1, 8–34. doi: 10.1093/icesjms/s1.61.8
Hoegh-Guldberg O., Bruno J. F. (2010). The Impact of Climate Change on the World’s Marine Ecosystems. Science 328, 1523–1528.
Hoegh-Guldberg O., Caldeira K., Chopin T., et al. (2019a). “The Ocean as a Solution to Climate Change: Five Opportunities for Action,” in Report (Washington, DC: World Resources Institute), 116 pp. Available at: http://www.oceanpanel.org/climate.
Hoegh-Guldberg O., Northrop E., Lubchenco J. (2019b). The Ocean is Key to Achieving Climate and Societal Goals. Science 365, 1372–1374. doi: 10.1126/science.1189930
ICES (2018). “Report of the Workshop on the Determination of Reference Points for Norwegian Spring Spawning Herring (WKNSSHREF),” in ICES CM 2018/ACOM. International Council for the Exploration of the Sea, Copenhagen. Available at:https://www.ices.dk/sites/pub/Publication%20Reports/Expert%20Group%20Report/acom/2018/WKNSSHREF/WKNSSHREF%20Report%202018.pdf. 45.83.
IPCC (2019) Summary for Policymakers. In: IPCC Special Report on the Ocean and Cryosphere in a Changing Climate. Available at: https://www.ipcc.ch/srocc/chapter/summary-for-policymakers/.
Jefferson T., Costello M. J., Zhao Q., Lundquist C. J. (2021). Conserving Threatened Marine Species and Biodiversity Requires 40% Ocean Protection. Biol. Conserv. 264, 109368. doi: 10.1016/j.biocon.2021.109368
Jouffray J.-B., Blasiak R., Norström A. V., Österblom H., Nyström M. (2020). The Blue Acceleration: The Trajectory of Human Expansion Into the Ocean. One Earth 2, 43–54. doi: 10.1016/j.oneear.2019.12.016
Kikaki A., Karantzalos K., Power C. A., Raitsos D. E. (2020). Remotely Sensing the Source and Transport of Marine Plastic Debris in Bay Islands of Honduras (Caribbean Sea). Remote Sens. 12, 1727. doi: 10.3390/rs12111727
KIMO (2021) Fishing for Litter. Available at: https://fishingforlitter.org/ (Accessed 29 July 2021).
Kjesbu O. S., Sundby S., Sandø A. B., et al. (2021). Highly Mixed Impacts of Near-Future Climate Change on Stock Productivity Proxies in the North-East Atlantic. Fish Fisheries. 23, 601–615. doi: 10.1111/faf.12635
Knowlton N. (2021). Ocean Optimism: Moving Beyond the Obituaries in Marine Conservation. Annu. Rev. Mar. Sci. 13. 479−499 doi: 10.1146/annurev-marine-040220-101608
Kolandai-Matchett K., Armoudian M. (2020). Message Framing Strategies for Effective Marine Conservation Communication. Aquat. Conservation: Mar. Freshw. Ecosyst. 30, 2441–2463. doi: 10.1002/aqc.3349
Kolandai-Matchett K., Armoudian M., Li E. (2021a). Communicating Complex Ocean Issues: How Strategically Framed Messages Affect Awareness and Motivation When Conveyed Using Narrative vs. Expository Language. Aquat. Conservation: Mar. Freshw. Ecosyst. 31, 870–887. doi: 10.1002/aqc.3484
Kolandai-Matchett K., Armoudian M., Thrush S., et al. (2021b). Marine Ecosystem Science and the Media: Exploring Ways to Improve News Coverage Through Journalist–Scientist Working Relations. Aquat. Conservation: Mar. Freshw. Ecosyst. 31, 3034–3055. doi: 10.1002/aqc.3708
Leape J., Micheli F., Tigchelaar M., et al. (2021). “The Vital Roles of Blue Foods in the Global Food System,” in Policy Brief to the UNFSS Scientific Group. United Nations, New York, Available at: https://sc-fss2021.org/wp-content/uploads/2021/04/FSS_Brief_Blue_Economy_MT.pdf.
Lebreton L., Slat B., Ferrari F., Sainte-Rose B., et al. (2018). Evidence That the Great Pacific Garbage Patch is Rapidly Accumulating Plastic. Sci. Rep. 8, 4666. doi: 10.1038/s41598-018-22939-w
Leslie H. M., Goldman E., McLeod K. L., et al. (2013). How Good Science and Stories can Go Hand-in-Hand. Conserv. Biol. 27, 1126–1129. doi: 10.1111/cobi.12080
LIFE LEMA (2020) LIFE LEMA’s Synthesis Report. Available at: https://www.lifelema.eu/wp-content/uploads/2020/03/LIFE_LEMA_Final_Technical_report_v10-1_compressed.pdf.
Lloret J., Turiel A., Solé J., Berdalet E., Sabatés A., Olivares A., et al. (2021). Unraveling the Ecological Impacts of Large-Scale Offshore Wind Farms in the Mediterranean Sea. Sci. Total Environ. 824, 153803. doi: 10.1016/j.scitotenv.2022.153803
Löhr A., Savelli H., Beunen R., et al. (2017). Solutions for Global Marine Litter Pollution. Curr. Opin. Environ. Sustainability 28, 90–99. doi: 10.1016/j.cosust.2017.08.009
Lubchenco J., Gaines S. D. (2019). A New Narrative for the Ocean. Science 364, 911–911. doi: 10.1126/science.aay2241
Maes T., Perry J., Alliji K., Clarke C., Birchenough S. N. R. (2019). Shades of Grey: Marine Litter Research Developments in Europe. Mar. pollut. Bull. 146, 274–281. doi: 10.1016/j.marpolbul.2019.06.019
Martín-Rodríguez F., Rodríguez-Barreiro L., Fernández-Bastos M., Martínez Iglesias G. (2017). “LitterDrone: Monitorización De Basura Marina Empleando Drones Y Análisis De Imagen,” in Proceedings of URSI-2018, Granada, 5-7 September 2018.
McFarland B. J. (2021). “Blue Bonds and Seascape Bonds,” in Conservation of Tropical Coral Reefs (Cham: Palgrave Macmillan). doi: 10.1007/978-3-030-57012-5_15
Meijer L. J. J., van Emmerik T., van der Ent R., Schmidt C., Lebreton L. (2021). More Than 1000 Rivers Account for 80% of Global Riverine Plastic Emissions Into the Ocean. Sci. Adv. 7, eaaz5803. doi: 10.1126/sciadv.aaz5803
Mendenhall E., Hendrix C., Nyman E., et al. (2020). Climate Change Increases the Risk of Fisheries Conflict. Mar. Policy 117, 103954. doi: 10.1016/j.marpol.2020.103954
Miladinova S., Macias D., Stips A., Garcia-Gorriz E. (2020). Identifying Distribution and Accumulation Patterns of Floating Marine Debris in the Black Sea. Mar. pollut. Bull. 153, 110964. doi: 10.1016/j.marpolbul.2020.110964
Morales-Caselles C., Viejo J., Martí E., et al. (2021). An Inshore–Offshore Sorting System Revealed From Global Classification of Ocean Litter. Nat. Sustainab. 4, 484–493. doi: 10.1038/s41893-021-00720-8
MRAG (2020). “Study on Circular Design of the Fishing Gear for Reduction of Environmental Impacts,” in Final Report. EASME/EMFF/2018/011 Specific Contract No.1, 74. European Commission, Brussels. Available at: https://cinea.ec.europa.eu/system/files/2021-03/StudyCircularDesing-EMFF2018.pdf.
Myers R. A., Worm B. (2003). Rapid Worldwide Depletion of Predatory Fish Communities. Nature 423, 280–283. doi: 10.1038/nature01610
Nøttestad L., Boge E., Ferter K. (2020). The Comeback of Atlantic Bluefin Tuna (Thunnus Thynnus) to Norwegian Waters. Fisheries Res. 231, 105689. doi: 10.1016/j.fishres.2020.105689
Naylor R. L., Hardy R. W., Buschmann A. H., et al. (2021). A 20-Year Retrospective Review of Global Aquaculture. Nature 591, 551–563. doi: 10.1038/s41586-021-03308-6
Nikolioudakis N., Skaug H. J., Olafsdottir A. H., et al. (2019). Drivers of the Summer-Distribution of Northeast Atlantic Mackerel (Scomber Scombrus) in the Nordic Seas From 2011 to 2017; a Bayesian Hierarchical Modelling Approach. ICES J. Mar. Sci. 76, 530–548. doi: 10.1093/icesjms/fsy085
Novak B. J., Fraser D., Maloney T. H. (2020). Transforming Ocean Conservation: Applying the Genetic Rescue Toolkit. Genes 11, 209. doi: 10.3390/genes11020209
Oen A. M. (2019). Nature-Based Solutions are Gaining Momentum From International Initiatives Promoting Environmental, Social, and Economic Benefits. Integrated Environ. Assess. Manage. 15, 830–831. doi: 10.1002/ieam.4203
Olafsdottir A. H., Utne K. R., Jacobsen J. A., et al. (2019). Geographical Expansion of Northeast Atlantic Mackerel (Scomber Scombrus) in the Nordic Seas From 2007 to 2016 was Primarily Driven by Stock Size and Constrained by Low Temperatures. Deep Sea Res. Part II: Topical Stud. Oceanography 159, 152–168. doi: 10.1016/j.dsr2.2018.05.023
Orth R. J., Lefcheck J. S., McGlathery K. S., et al. (2020). Restoration of Seagrass Habitat Leads to Rapid Recovery of Coastal Ecosystem Services. Sci. Adv. 6, eabc6434. doi: 10.1126/sciadv.abc6434
Pauly D., Christensen V., Dalsgaard J., Froese R., Torres F. Jr. (1998). Fishing Down Marine Food Webs. Science 279, 860–863. doi: 10.1126/science.279.5352.860
Peck M. A., Catalán I. A., Damalas D., et al. (2020). Climate Change and European Fisheries and Aquaculture: ‘CERES’ Project Synthesis Report. doi: 10.25592/uhhfdm.804
Pendrill F., Persson U. M., Godar J., et al. (2019). Agricultural and Forestry Trade Drives Large Share of Tropical Deforestation Emissions. Global Environ. Change 56, 1–10. doi: 10.1016/j.gloenvcha.2019.03.002
Perrow M. (Ed.) (2019). Wildlife and Windfarms - Conflicts and Solutions, Volume 3: Offshore: Potential Effects (Exeter, UK: Pelagic Publishing), ISBN: ISBN 978-1-78427-127-5.
Plagányi É. (2019). Climate Change Impacts on Fisheries. Science 363, 930–931. doi: 10.1126/science.aaw5824
Pogoda B., Boudry P., Bromley C., et al. (2020). NORA Moving Forward: Developing an Oyster Restoration Network in Europe to Support the Berlin Oyster Recommendation. Aquat. Conservation: Mar. Freshw. Ecosyst. 30, 2031–2037. doi: 10.1002/aqc.3447
Poloczanska E. S., Burrows M. T., Brown C. J., et al. (2016). Responses of Marine Organisms to Climate Change Across Oceans. Front. Mar. Sci. 3. doi: 10.3389/fmars.2016.00062
Pörtner H. O., Scholes R. J., Agard J., et al. (2021). Scientific Outcome of the IPBES-IPCC Co-Sponsored Workshop on Biodiversity and Climate Change (Report). Intergovernmental Science-Policy Platform on Biodiversity and Ecosystem Services (IPBES), Bonn. 256 pp doi: 10.5281/zenodo.4659158
Pouso S., Borja Á., Martín J., Uyarra M. C. (2019). The Capacity of Estuary Restoration to Enhance Ecosystem Services: System Dynamics Modelling to Simulate Recreational Fishing Benefits. Estuarine Coast. Shelf Sci. 217, 226–236. doi: 10.1016/j.ecss.2018.11.026
Pouso S., Borja Á., Uyarra M. C. (2020). An Interdisciplinary Approach for Valuing Changes After Ecological Restoration in Marine Cultural Ecosystem Services. Front. Mar. Sci. 7. doi: 10.3389/fmars.2020.00715
Pouso S., Ferrini S., Turner R. K., Borja Á., Uyarra M. C. (2021). Monetary Valuation of Recreational Fishing in a Restored Estuary and Implications for Future Management Measures. ICES J. Mar. Sci. 77, 2295–2303. doi: 10.1093/icesjms/fsz091
Pouso S., Ferrini S., Turner R. K., Uyarra M. C., Borja Á. (2018c). Financial Inputs for Ecosystem Service Outputs: Beach Recreation Recovery After Investments in Ecological Restoration. Front. Mar. Sci. 5. doi: 10.3389/fmars.2018.00375
Pouso S., Uyarra M. C., Borja Á. (2018a). The Recovery of Estuarine Quality and the Perceived Increase of Cultural Ecosystem Services by Beach Users: A Case Study From Northern Spain. J. Environ. Manage. 212, 450–461. doi: 10.1016/j.jenvman.2018.02.033
Pouso S., Uyarra M. C., Borja Á. (2018b). Recreational Fishers’ Perceptions and Behaviour Towards Cultural Ecosystem Services in Response to the Nerbioi Estuary Ecosystem Restoration. Estuarine Coast. Shelf Sci. 208, 96–106. doi: 10.1016/j.ecss.2018.04.033
ResponSEAble (2021) Microplastics and Cosmetics. Available at: https://www.responseable.eu/key-stories/microplastics-cosmetics (Accessed 16 July 2021).
Rijnsdorp A. D., van Leeuwen P. I., Daan N., Heessen H. J. L. (1996). Changes in Abundance of Demersal Fish Species in the North Sea Between 1906–1909 and 1990–1995. ICES J. Mar. Sci. 53, 1054–1062. doi: 10.1006/jmsc.1996.0132
Roberts C. M., O’Leary B. C., McCauley D. J., et al. (2017). Marine Reserves can Mitigate and Promote Adaptation to Climate Change. Proc. Natl. Acad. Sci. 114, 6167–6175. doi: 10.1073/pnas.1701262114
Rooker J. R., Fraile I., Liu H., et al. (2019). Wide-Ranging Temporal Variation in Transoceanic Movement and Population Mixing of Bluefin Tuna in the North Atlantic Ocean. Front. Mar. Sci. 6. doi: 10.3389/fmars.2019.00398
Ruiz I., Basurko O. C., Epelde I., et al. (2020a). “Monitoring Floating Riverine Pollution by Advance Technology,” in EGU General Assembly, Vienna, Austria. doi: 10.5194/egusphere-egu2020-22613
Ruiz I., Basurko O. C., Rubio A., et al. (2020b). Litter Windrows in the South-East Coast of the Bay of Biscay: An Ocean Process Enabling Effective Active Fishing for Litter. Front. Mar. Sci. 7. doi: 10.3389/fmars.2020.00308
Ryabinin V. (2021). United Nations Decade of Ocean Science for Sustainable Development 2021-2030, Implementation Plan (United Nations) New York, 56 pp. Available at: https://www.oceandecade.org/wp-content/uploads//2021/09/337567-Ocean%20Decade%20Implementation%20Plan%20-%20Full%20Document.
Sala E., Mayorga J., Bradley D., et al. (2021). Protecting the Global Ocean for Biodiversity, Food and Climate. Nature. doi: 10.1038/s41586-021-03371-z
Salgado-Hernanz P. M., Bauzà J., Alomar C., Compa M., Romero L., Deudero S. (2021). Assessment of Marine Litter Through Remote Sensing: Recent Approaches and Future Goals. Mar. pollut. Bull. 168, 112347. doi: 10.1016/j.marpolbul.2021.112347
Saunders M. I., Doropoulos C., Bayraktarov E., et al. (2020). Bright Spots in Coastal Marine Ecosystem Restoration. Curr. Biol. 24, R1500–R1510. doi: 10.1016/j.cub.2020.10.056
Schuhen K., Sturm M. T. (2020). “Microplastic Pollution and Reduction Strategies,” in Handbook of Microplastics in the Environment. Eds. Rocha-Santos T., Costa M., Mouneyrac C. (Cham: Springer International Publishing), 1–33.
SCP/RAC (2017). 25 Innovative and Inspiring Solutions to Combat Plastic Marine Litter in the Mediterranean Region (Barcelona: Regional Activity Centre for Sustainable Consumption and Production (SCP/RAC).
Seabin (2021) Seabin Project. Available at: https://seabinproject.com/the-seabin-v5/ (Accessed 28 July 2021).
Sguotti C., Lynam C. P., García-Carreras B., Ellis J. R., Engelhard G. H. (2016). Distribution of Skates and Sharks in the North Sea: 112 Years of Change. Global Change Biol. 22, 2729–2743. doi: 10.1111/gcb.13316
Singh G. G., Harden-Davies H., Allison E. H., et al. (2021). Opinion: Will Understanding the Ocean Lead to “The Ocean We Want”? Proc. Natl. Acad. Sci. 118, e2100205118. doi: 10.1073/pnas.2100205118
Soto-Navarro J., Jordá G., Deudero S., et al. (2020). 3D Hotspots of Marine Litter in the Mediterranean: A Modeling Study. Mar. pollut. Bull. 155, 111159. doi: 10.1016/j.marpolbul.2020.111159
Stoll T., Stoett P., Vince J., Hardesty B. D. (2020). “Governance and Measures for the Prevention of Marine Debris,” in Handbook of Microplastics in the Environment. Eds. Rocha-Santos T., Costa M., Mouneyrac C. (Cham: Springer International Publishing), 1–23.
TERNUA (2021b) Sustainable Innovation Redcycle. Available at: https://www.ternua.com/com/sustainability-seacycle (Accessed 29 July 2021).
The Great Bubble Barrier (2021) Our Technology: The Great Bubble Barrier. Available at: https://thegreatbubblebarrier.com/technology/ (Accessed 29 July 2021).
The Ocean Cleanup (2021) How it Works the Interceptor. Available at: https://theoceancleanup.com/oceans/ (Accessed 29 July 2021).
Tiedemann M., Nash R. D. M., Stenevik E. K., et al. (2020). Environmental Influences on Norwegian Spring-Spawning Herring (Clupea Harengus L.) Larvae Reveal Recent Constraints in Recruitment Success. ICES J. Mar. Sci. 78, 640–652. doi: 10.1093/icesjms/fsaa072
Tiller R., Arenas F., Galdies C., et al. (2019). Who Cares About Ocean Acidification in the Plasticene? Ocean Coast. Manage. 174, 170–180. doi: 10.1016/j.ocecoaman.2019.03.020
Topouzelis K., Papageorgiou D., Karagaitanakis A., Papakonstantinou A., Arias Ballesteros M. (2020). Remote Sensing of Sea Surface Artificial Floating Plastic Targets With Sentinel-2 and Unmanned Aerial Systems (Plastic Litter Project 2019). Remote Sens. 12, 2013. doi: 10.3390/rs12122013
Toresen R., Østvedt O. J. (2000). Variation in Abundance of Norwegian Spring-Spawning Herring (Clupea Harengus, Clupeidae) Throughout the 20th Century and the Influence of Climatic Fluctuations. Fish Fisheries 1, 231–256. doi: 10.1046/j.1467-2979.2000.00022.x
Trenkel V. M., Huse G., MacKenzie B. R., et al. (2014). Comparative Ecology of Widely Distributed Pelagic Fish Species in the North Atlantic: Implications for Modelling Climate and Fisheries Impacts. Prog. Oceanography 129, 219–243. doi: 10.1016/j.pocean.2014.04.030
Trochta J. T., Branch T. A., Shelton A. O., Hay D. E. (2020). The Highs and Lows of Herring: A Meta-Analysis of Patterns and Factors in Herring Collapse and Recovery. Fish Fisheries 21, 639–662. doi: 10.1111/faf.12452
UNDP (2018). “Leveraging the Blue Economy for Inclusive and Sustainable Growth,” in Sustainable Blue Economy Conference. United Nations. 7.
UNEP(DEPI)/MED (2016). Implementing the Marine Litter Regional Plan in the Mediterranean (Fishing for Litter Guidelines, Assessment Report, Baselines Values, and Reduction Targets) (Nairobi: UNEP). Available at: https://wedocs.unep.org/bitstream/handle/20.500.11822/6072/16ig22_28_22_10_eng.pdf
UNEP (2016). Marine Plastic Debris and Microplastics — Global Lessons and Research to Inspire Action and Guide Policy Change (Nairobi: United Nations Environment Programme). Available at: https://wedocs.unep.org/handle/20.500.11822/7720.
United Nations (2016). Report of the Inter-Agency and Expert Group on Sustainable Development Goal Indicators. (E/CN.3/2016/2/Rev.1) (New York: United Nations Economic and Social Council), 49 pp. Available at: https://digitallibrary.un.org/record/821651.
United Nations (2021a). The Second World Ocean Assessment. Volume I (United Nations publication), New York. 570 pp. Available at: https://www.un.org/regularprocess/sites/www.un.org.regularprocess/files/2011859-e-woa-ii-vol-i.pdf, ISBN: ISBN: 978-92-1-1-130422-0.
United Nations (2021b). The Second World Ocean Assessment. Volume Ii (United Nations publication), New York, 520 pp. Available at: https://www.un.org/regularprocess/sites/www.un.org.regularprocess/files/2011859-e-woa-ii-vol-ii.pdf, ISBN: ISBN: 978-92-1-1-130422-0.
van Gemert R., Andersen K. H. (2018). Challenges to Fisheries Advice and Management Due to Stock Recovery. ICES J. Mar. Sci. 75 (6), 1864–1870. doi: 10.1093/icesjms/fsy084
van Lieshout C., van Oeveren K., van Emmerik T., Postma E. (2020). Automated River Plastic Monitoring Using Deep Learning and Cameras. Earth Space Sci. 7, e2019EA000960. doi: 10.1029/2019EA000960
van Sebille E., England M. H., Froyland G. (2012). Origin, Dynamics and Evolution of Ocean Garbage Patches From Observed Surface Drifters. Environ. Res. Lett. 7, 044040. doi: 10.1088/1748-9326/7/4/044040
Waltham N. J., Elliott M., Lee S. Y., et al. (2020). UN Decade on Ecosystem Restoration 2021–2030—What Chance for Success in Restoring Coastal Ecosystems? Front. Mar. Sci. 7. doi: 10.3389/fmars.2020.00071
Waterfront Partnership (2021) Mr Trash Wheel. Available at: https://www.mrtrashwheel.com/technology/ (Accessed 29 July 2021).
Westgaard J.-I., Staby A., Aanestad Godiksen J., et al. (2017). Large and Fine Scale Population Structure in European Hake (Merluccius Merluccius) in the Northeast Atlantic. ICES J. Mar. Sci. 74, 1300–1310. doi: 10.1093/icesjms/fsw249
Williams A. T., Rangel-Buitrago N. (2019). Marine Litter: Solutions for a Major Environmental Problem. J. Coast. Res. 35 (3), 648–663. doi: 10.2112/JCOASTRES-D-18-00096.1
Willis K., Maureaud C., Wilcox C., Hardesty B. D. (2018). How Successful are Waste Abatement Campaigns and Government Policies at Reducing Plastic Waste Into the Marine Environment? Mar. Policy 96, 243–249. doi: 10.1016/j.marpol.2017.11.037
Wolanski E., Elliott M. (2015). Estuarine Ecohydrology: An Introduction (Amsterdam: Elsevier), p322, ISBN: ISBN 978-0-444-63398-9.
Worm B., Barbier E. B., Beaumont N., et al. (2006). Impacts of Biodiversity Loss on Ocean Ecosystem Services. Science 314, 787. doi: 10.1126/science.1132294
Worm B., Hilborn R., Baum J. K., et al. (2009). Rebuilding Global Fisheries. Science 325, 578. doi: 10.1126/science.1173146
Zimmermann F., Claireaux M., Enberg K. (2019). Common Trends in Recruitment Dynamics of North-East Atlantic Fish Stocks and Their Links to Environment, Ecology and Management. Fish Fisheries 20, 518–536. doi: 10.1111/faf.12360
Zimmermann F., Werner K.-M. (2019). Improved Management is the Main Driver Behind Recovery of Northeast Atlantic Fish Stocks. Front. Ecol. Environ. 17, 93–99. doi: 10.1002/fee.2002
Zimmermann F., Yamazaki S. (2017). Exploring Conflicting Management Objectives in Rebuilding of Multi-Stock Fisheries. Ocean Coast. Manage. 138, 124–137. doi: 10.1016/j.ocecoaman.2017.01.014
Zudaire I., Andrés M M., Larreta J., Basurko O. C. (2021). “Innovación En El Sector Atunero Congelador a Través De La Economía Circular: Reciclado Y Transformación De Artes De Pesca (Project SAREBIO),” in Report for the Basque Government,Pasaia, 305 pp.
Zudaire I., Tolotti M. T., Murua J., et al. (2020). “Testing Designs and Identify Options to Mitigate Impacts of Drifting Fads on the Ecosystem. Second Interim Report,” in European Commission. Specific Contract No. 7 EASME/EMFF/2017/1.3.2.6 Under Framework Contract No. EASME/EMFF/2016/008, Publications Office of the European Union, Luxembourg193 pp. doi: 10.2826/79656
Keywords: ocean health, status assessment, restoration, recovery, ocean solutions, decade of ocean science, decade on ecosystem restoration, sustainable development goals
Citation: Borja A, Elliott M, Basurko OC, Fernández Muerza A, Micheli F, Zimmermann F and Knowlton N (2022) #OceanOptimism: Balancing the Narrative About the Future of the Ocean. Front. Mar. Sci. 9:886027. doi: 10.3389/fmars.2022.886027
Received: 28 February 2022; Accepted: 13 June 2022;
Published: 22 July 2022.
Edited by:
Martin Edwards, Plymouth Marine Laboratory, United KingdomReviewed by:
Michelle Jillian Devlin, Fisheries and Aquaculture Science (CEFAS), United KingdomRafael Sarda, Spanish National Research Council (CSIC), Spain
Copyright © 2022 Borja, Elliott, Basurko, Fernández Muerza, Micheli, Zimmermann and Knowlton. This is an open-access article distributed under the terms of the Creative Commons Attribution License (CC BY). The use, distribution or reproduction in other forums is permitted, provided the original author(s) and the copyright owner(s) are credited and that the original publication in this journal is cited, in accordance with accepted academic practice. No use, distribution or reproduction is permitted which does not comply with these terms.
*Correspondence: Angel Borja, aborja@azti.es