- 1Key Laboratory of South China Sea Fishery Resources Exploitation and Utilization, Ministry of Agriculture and Rural Affairs; South China Sea Fisheries Research Institute, Chinese Academy of Fishery Sciences, Guangzhou, China
- 2Guangdong Provincial Engineer Technology Research Center of Marine Biological Seed Industry, Guangzhou, China
- 3Tropical Aquaculture Research and Development Center, South China Sea Fisheries Research Institute, Chinese Academy of Fishery Sciences, Sanya, China
- 4Sanya Tropical Fisheries Research Institute, Sanya, China
Tumour necrosis factor-α (TNFα) is a multifarious mediator of lymphoid tissue growth and antimicrobial defence mechanisms, and it acts as a pro-inflammatory regulator. The function of TNFα in parasite infection and the underlying mechanism through which nuclear factor-κB (NF-κB) regulates TNFα remain largely unclear in teleosts. In the present study, TNFα (ToTNFα) from golden pompano (Trachinotus ovatus) was identified, and its sequence features and expression levels were determined. The genomic DNA sequence is composed of 1,130 bp, consists of four exons and three introns, and encodes 341 amino acid polypeptides. The putative protein sequence shares 34.7%–61.9% identity with fish TNFα and possesses a TNF family signature and two conserved cysteine residues. Moreover, the expressions of ToNF-κB and ToTNFα are constitutively expressed in all examined tissues, with higher levels observed in the immune relevant tissues. Both ToNF-κB and ToTNFα transcription was increased in the local infection sites (skin and gill) and system immune tissues (liver, spleen and head kidney) after Cryptocaryon irritans stimulation. In addition, to investigate whether ToNF-κB is a regulator of ToTNFα, promoter analysis was performed. The region from -970 to +79 bp is known as the core promoter by different truncated mutants of ToTNFα. Subsequently, the activity of the ToTNFα-p2 promoter was dramatically reduced after targeted mutation of the M6-binding site. Additionally, an electrophoretic mobile shift assay (EMSA) verified that ToNF-κB interacted with the M6-binding site in the ToTNFα promoter region to control the expression of ToTNFα. In conclusion, the present study provides the positive regulation of TNFα transcription by NF-κB and contributes to a better understanding of the transcriptional mechanism of TNFα in fish.
Introduction
TNFα is a pleiotropic cytokine that controls pathological processes and multiple physiological processes, including the progression of chronic inflammatory diseases and cell necrosis, apoptosis, and survival (Takada et al., 2007; Chu, 2013). Both in vivo and in vitro, TNFα can regulate inflammatory cytokines, such as four interleukin (IL)-1/6/8/17C, indicating its core role in the mediation of the inflammatory response (Wollenberg et al., 1993; Amiot et al., 1997; Hong et al., 2013). Moreover, TNFα is a type-II transmembrane protein produced by multifarious cells, including natural killer, neutrophils, monocytes, macrophages, and T/B-lymphocyte cells (Tracey and Cerami, 1993; Ma, 2001; Goetz et al., 2004). In a cell-to-cell contact fashion, the biological functions of the transmembrane TNFα precursor are different from the characteristics of soluble TNFα (Horiuchi et al., 2010).
In fish, TNFα has been found in more than 20 species, such as in important seawater species, Japanese flounder (Paralichthys olivaceus) (Hirono et al., 2000), gilthead seabream (Sparus aurata) (García-Castillo et al., 2002), rainbow trout (Oncorhynchus mykiss) (Zou et al., 2002; Hong et al., 2013), catfish (Ictalurus punctatus) (Zou et al., 2003), turbot (Psetta maxima) (Ordás et al., 2007), sea bass (Dicentrarchus labrax) (Nascimento et al., 2007), bluefin tuna (Thunnus orientalis) (Kadowaki et al., 2009), striped trumpeter (Latris lineata) (Covello et al., 2009), orange-spotted grouper (Epinephelus coioides) (Lam et al., 2011), rock bream (Oplegnathus fasciatus) (Kim et al., 2011; Ko et al., 2022), fugu (Fugu rubripes) (Biswas et al., 2015), large yellow croaker (Larimichthys crocea) (Huang et al., 2020), and pufferfish (Takifugu obscurus) (Kong et al., 2021), and in important freshwater species, common carp (Cyprinus carpio) (Forlenza et al., 2009; Zhao et al., 2012), zebrafish (Danio rerio) (Savan et al., 2005), ayu (Plecoglossus altivelis) (Uenobe et al., 2007), goldfish (Carassius auratus) (Grayfer et al., 2008), grass carp (Ctenopharyngodon idella) (Zhang et al., 2012), and snakehead (Channa argus) (Cui et al., 2020). Furthermore, two TNFα isoforms have been characterized in Atlantic bluefin tuna Thunnus thynnus (Lepen Pleić et al., 2014), T. orientalis (Kadowaki et al., 2009), E. coioides (Lam et al., 2011), and C. auratus (Grayfer et al., 2008), three TNFα isoforms in O. mykiss (Hong et al., 2013), and even four in C. carpio (Savan and Sakai, 2004; Zhao et al., 2012). Moreover, all fish TNFα molecules possess typical structures of the TNF family, containing a TACE restriction site at position S71/L72, a transmembrane domain, a TNF family signature (I118-F131) (Cui et al., 2020; Huang et al., 2020), and two conserved cysteine residues (C39 and C179) that are essential for disulphide bridges in the tertiary structure of TNFα (Savan et al., 2005). Nevertheless, another gene in the TNF-β family, which also exists in mammals, shares 50% homology at the protein level (Bodmer et al., 2002).
The transcription factor nuclear factor-κB (NF-κB) includes several subunits, such as RelA, RelB, c-Rel, NF-κB1 (p50 and its precursor p105), and NF-κB2 (p52 and its precursor p100), which are evolutionarily conserved and play an important role in the regulation of numerous basic cellular processes (Cohen et al., 2006; Wang et al., 2013). It can be induced by various stimuli, such as cytokines, growth factors, mitogens, and viral/bacterial pathogens (Kravtsova-Ivantsiv et al., 2009; Lapid et al., 2017). Furthermore, some research has shown that apoptosis is inhibited, and TNFα is activated in HEK293T cells after overexpression of the sea cucumber Holothuria leucospilota NF-κB (He et al., 2021). Consequently, to confirm whether a similar regulatory relationship exists in golden pompano Trachinotus ovatus, the sequence characterization, expression pattern, and transcriptional regulation of ToTNFα by ToNF-κB (p105) were determined. The present study examining ToNF-κB (p105) will be useful for tracing the TNFα system in marine fishes and promoting the development of the anti-parasite industry in the future.
Materials and Methods
Cryptocaryon irritans Challenge and Sampling
Healthy golden pompano (98 ± 15 g), purchased from the Linshui Marine Fish Farm of Hainan Province, China, were maintained in a fresh seawater system at 28 ± 2°C, accompanied by 25‰ salinity. The fish were allowed to feed on commercial feed (crude protein and crude fat >37% and >7%, respectively, Hengxin, Zhanjiang, China) for 2 weeks before the experiment. In Lingshui Marine Fish Farm, no disease outbreaks was recorded during the course of breeding. The gills and mucus from 10 fish were observed using a light microscope to ensure there were no C. irritans theronts, and their serum was tested for the presence or absence of parasite infection.
One hundred and twenty healthy pompano were challenged with C. irritans theronts at a dose of 600 theronts/fish according to previous research (Dan et al., 2006; Zhu et al., 2020). Fifty fish were known as the control group. The skin, gill, liver, spleen, and head kidney were collected from six fish after 0 h, 6 h, 12 h, 1 day, 2 days, and 3 days of infection. The same tissues from uninfected pompano were defined as the negative control at each time point. Three replicates were collected at each time point. We used MS222 (0.1 g/l; Sigma, Alcobendas, Spain) to narcotize the pompano before dissection. All samples were immediately frozen in liquid nitrogen and then stored at -80°C until use.
RNA Extraction and Gene Cloning
A mortar and pestle were used to homogenize the tissues, accompanied by addition into liquid nitrogen. Then, total RNA (1 µg) was isolated from each sample with the HiPure Fibrous RNA Plus Kit (Magen, Guangzhou, China) according to the manufacturer’s protocol and stored at -80°C. cDNA was synthesized by Cloned AMV First-Strand cDNA Synthesis Kit (Invitrogen, Carlsbad, CA, USA) following the manufacturer’s protocol.
Plasmid Construction, Cell Culture and Dual-Luciferase Reporter Assays
Total genomic DNA was isolated from T. ovatus muscle and is known as a template for augmenting alternative promoter sequences (Sun et al., 2013). To confirm the interaction effect of ToTNFα with ToNF-κB, five truncated mutants from the ToTNFα promoter were amplified by specific primers with XhoI and KpnI restriction sites (Table 1). Subsequently, the five truncated fragments [denoted pGL3-basic-TNFα-p1 (-1,969 to +79), pGL3-basic-TNFα-p2 (-970 to +79), pGL3-basic-TNFα-p3 (-736 to +79), pGL3-basic-TNFα-p4 (-446 to +79) and pGL3-basic-TNFα-p5 (-150 to +79)] were subcloned into the pGL3-basic luciferase reporter plasmid (Promega, Madison, WI, USA) with the corresponding restriction sites.
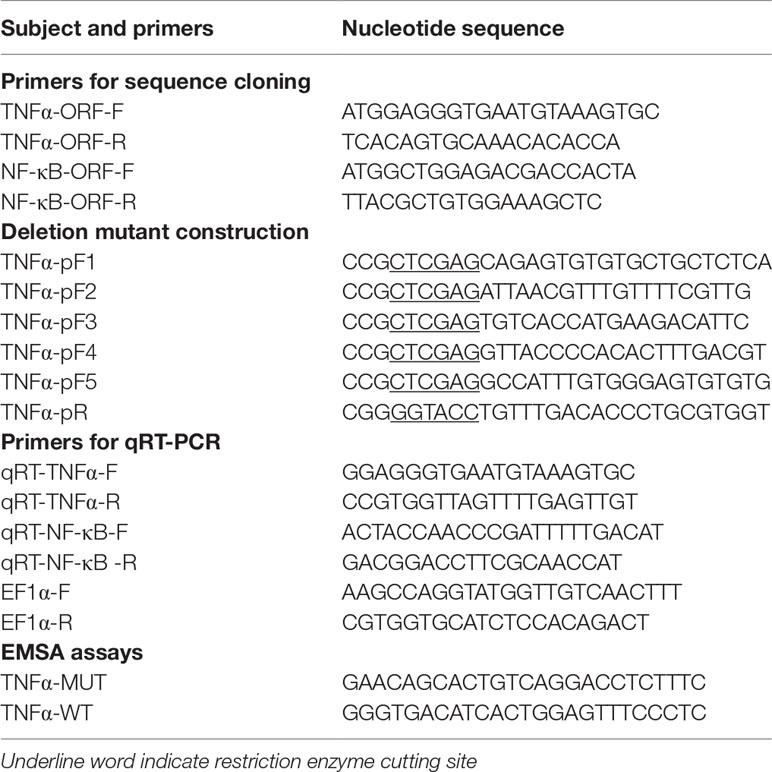
Table 1 Primers used for sequence cloning, deletion mutant construction, mRNA construction and qRT-PCR.
To validate the potential role of the ToNF-κB binding sites on the core ToTNFα promoter, six assumed recombinant plasmids of mutations were constructed. To predict the underlying binding sites of ToNF-κB on the ToTNFα promoter, we used the transcription factor-binding site prediction (TFBS)-JASPAR database (http://jaspar.genereg.net/), TRANSFAC®, and MatInspector® software. Moreover, according to the manufacturer’s protocol, truncated mutants of the pGL3-basic-TNFα-P2 promoter were generated by a Muta-Direct™ site-directed mutagenesis kit (SBS Genetech, Shanghai, China). The pGL3-basic-TNFα-P2 promoter was considered wild-type. To acquire the six mutants, the procedure of PCR augmentation was applied as in a previous study (Dong et al., 2016). The six point mutants directly deleted the prediction of six binding sites, which included M1 (-804 to -783 bp), M2 (-682 to -661 bp), M3 (-530 to -509 bp), M4 (-433 to -412 bp), M5 (-197 to -176 bp) and M6 (-116 to -92 bp) from the wild-type promoter. The homologous TF-binding site sequences are shown in Figure 1A.
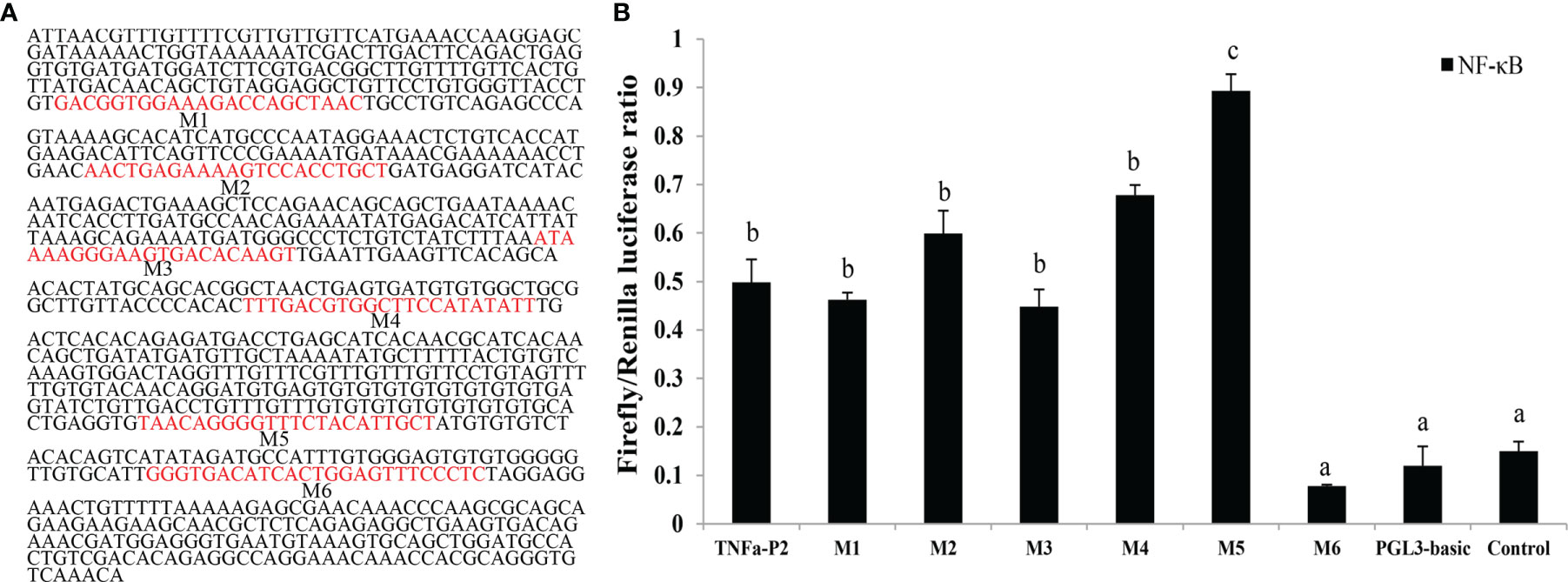
Figure 1 Construction of truncated mutants for the identification of predicted transcription factor (TF) binding sites in the ToTNFα promoter. (A) The nucleotide sequence and predicted binding sites in the core region of the ToTNFα promoter. (B) Effects of three mutants on To TNFα-P2 promoter activity. Binding sites are shown with boxes. Mutations of promoter sequences are listed in Table 2. Data are presented as the means of three replicates ± SE. Different letters indicate significant differences (p < 0.05).
The Renilla luciferase plasmid pRL-TK (Promega, USA) was used as an internal control. The TransGen Plasmid Mini Kit (Beijing, China) was used to isolate recombinant plasmids. Human embryonic kidney (HEK293T) (GeneCreate, Wuhan, China) cell cultures and transfection experiments were performed according to the methods described by Li et al. (2017). To verify whether ToNF-κB could upregulate ToTNFα in vitro, HEK293T cells overexpressing pcDNA3.1-NF-κB and pcDNA3.1-Flag were used.
Electrophoretic Mobility Shift Assay
The EMSA experimental method was performed according to a previously described procedure (Yu et al., 2010). Briefly, for DNA/protein conjugation reactions, the lysates of HEK293T cells transfected with pcDNA3.1-Flag-NF-κB were provided. To mark the wild-type and mutated oligonucleotides (Table S2), the EMSA Probe Biotin Labelling Kit (Beyotime, Shanghai, China) was used according to the manufacturer’s instructions. DNA/protein-binding reactions were accomplished using an EMSA/Gel-Shift Kit (Beyotime, China) at 25°C. To exclusively understand the DNA/protein-binding reactions, competition assays were implemented with 100× excessive non-marked mutated or wild-type probes. Thus, the completed reactions were divided on non-denaturing 4% PAGE gels for 20 min. A LightShift® Chemiluminescent EMSA Kit (Pierce, Waltham, MA, USA) was used to develop the proteins by the autoradiography method.
Quantitative Real-Time PCR and Statistical Analysis
Quantitative real-time polymerase chain reaction (qRT-PCR) was used to analyse the expression patterns of ToNF-κB and ToTNFα in five infected tissues (skin, gill, liver, spleen, head kidney) from T. ovatus. The specific primers for ToNF-κB and ToTNFα and the housekeeping gene EF-1α (elongation factor 1, alpha) are shown in Table 1. The qRT-PCR procedure was executed as previously described (Zhang et al., 2018). To evaluate the relative expression of those genes, the 2–ΔΔCT method was used (Livak and Schmittgen, 2001). The data from different tissues and groups were analysed by the Duncan test using one-way ANOVA. Data are presented as the means of three replicates ± SD, and the level of statistical significance was set at p < 0.05.
Results
Sequence Characterization of ToTNFα
The open reading frame (ORF) of ToTNFα (GenBank accession number: OM777150, Figure 2) was 726 bp with a predicted theoretical isoelectric point (PI) of 6.13 and a molecular weight (Mw) of 27.18 kDa. Similar to most fish TNFα molecules, ToTNFα has a TACE restriction site at position S71/L72 and a TNF family signature (I118-F131; Figure 2 and Figure S1). Two conserved cysteine residues, C138 and C183, are found in ToTNFα sequences; C138 is only conserved in teleost fish TNFα, but C183 exists in all known species TNFα (Figure 2 and Figure S1). The assumed total aa sequence of ToTNFα shares higher identity with Oreochromis niloticus TNFα (61.9%), Oryzias latipes TNFα (59.3%), Gasterosteus aculeatus TNFα (58.8%), Poecilia formosa TNFα (56.2%), while it has only 25.6% identity to Homo sapiens TNFα1 (Table S1). Moreover, the ORF of ToNF-κB (GenBank accession number: OM777151, Figure S2) was 2,826 bp with a predicted theoretical isoelectric point (PI) of 4.84 and a molecular weight (Mw) of 100.98 kDa.
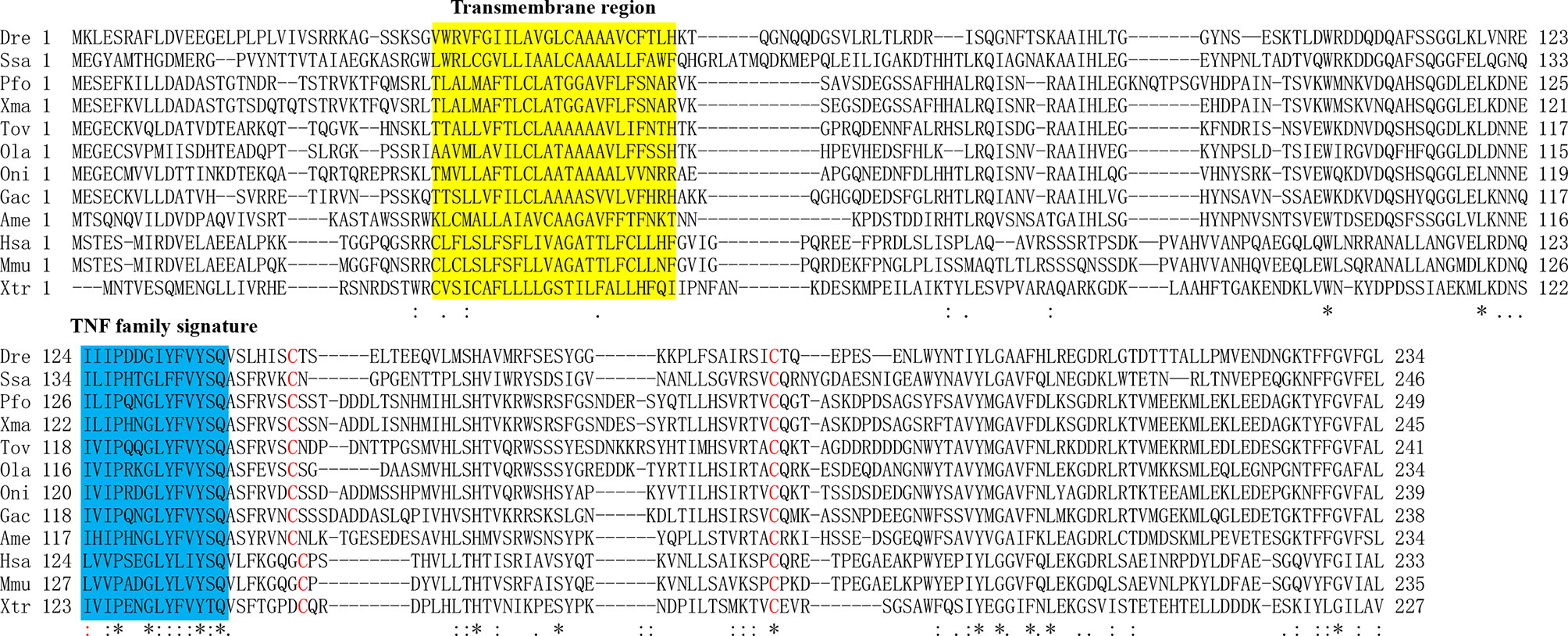
Figure 2 Alignments of TNFα amino acid sequences from different species. The TNF signature motif is shown in the blue box. The transmembrane region is shown in the yellow box. Cysteines conserved in TNFα are marked by red. Identical (asterisks) and similar (: or.) residues identified by the CLUSTAL W program are indicated. The Latin abbreviation and accession numbers are listed in Table S1.
ToTNFα Structural and Phylogenetic Analysis
The genomic sequence of ToTNFα is 1,130 bp, containing four exons and three introns (Figure 3 and Figure S3). All the 5′/3′ ends of the introns displayed typical sequence characteristics (GT/introns/AG). The distributions and lengths of the introns and exons of metazoan TNFα genes are shown in Table S2.
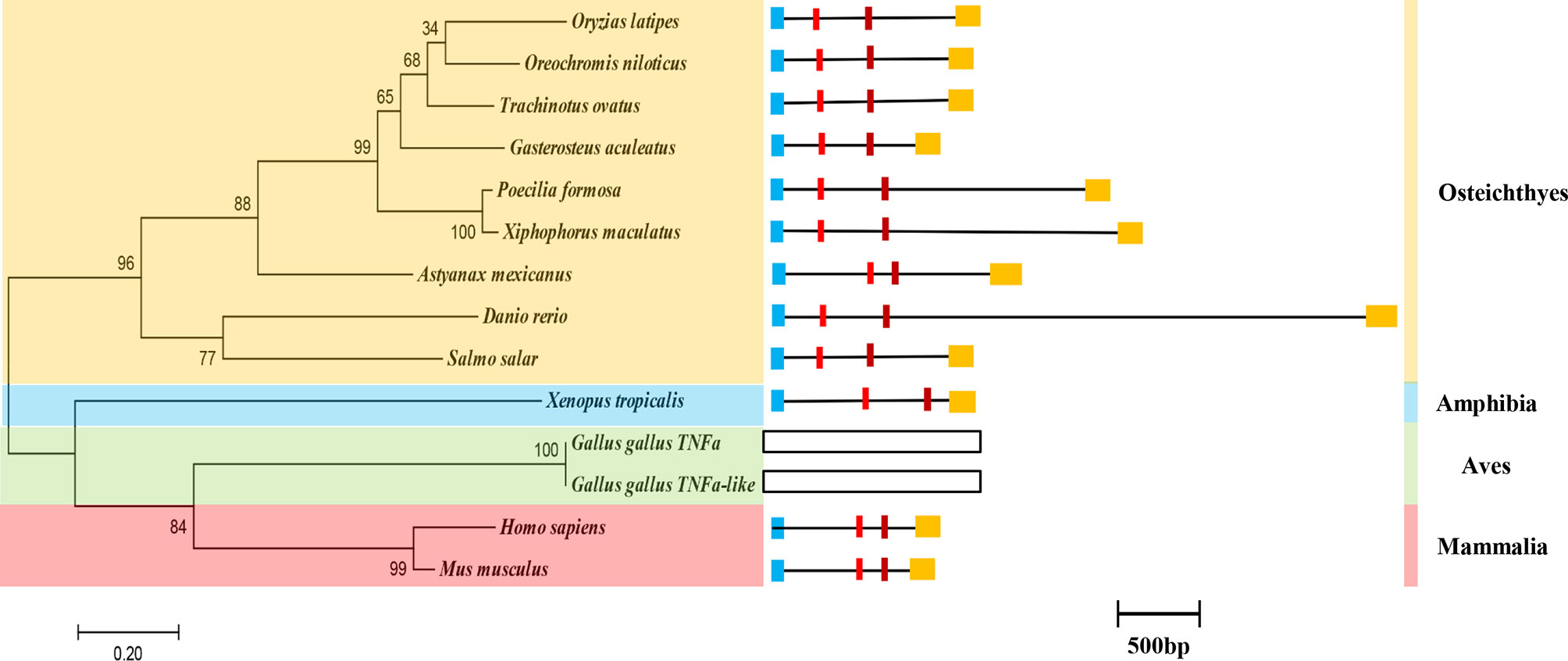
Figure 3 The phylogenetic relationship and genome structure of the ToTNFα gene. Genome structure analysis of ToTNFα genes according to their phylogenetic relationship. Lengths of exons and introns of each TNFα gene are displayed proportionally. Different colour boxes and lines represent exons and introns, respectively. The identical colour boxes represent homologous sequences.
Additionally, the phylogenetic relationship of TNFα has been determined in vertebrates (Figure 3). The computer program Clustal W alignment and MEGA 6.0 software were used to construct a phylogenetic tree of TNFα aa sequences with a maximum likelihood (ML) method. In the phylogenetic tree, ToTNFα is grouped together with O. niloticus and G. aculeatus, and the homology with ToTNFα, from close to distant, is other Osteichthyes, Amphibia, Aves and Mammalia (Figure 3). This result was consistent with the traditional taxonomic relationship of the above species.
Tissue Expression of ToNF-κB and ToTNFα
To confirm the role of ToNF-κB and ToTNFα in various tissues, the constitutive expression of two genes in gill, head-kidney, brain, small intestine, spleen, fin, liver, white muscle, stomach, blood and male and female gonads was detected by qRT-PCR (Figure 4). The highest expression level of ToNF-κB was in the gill, head-kidney, skin and small intestine, while a low expression was observed in the liver and white muscle (p < 0.05) (Figure 4A). Moreover, the highest expression level of ToTNFα was also in the head-kidney, gill, skin and small intestine, while a low expression was observed in the stomach, liver and blood (p < 0.05) (Figure 4B).
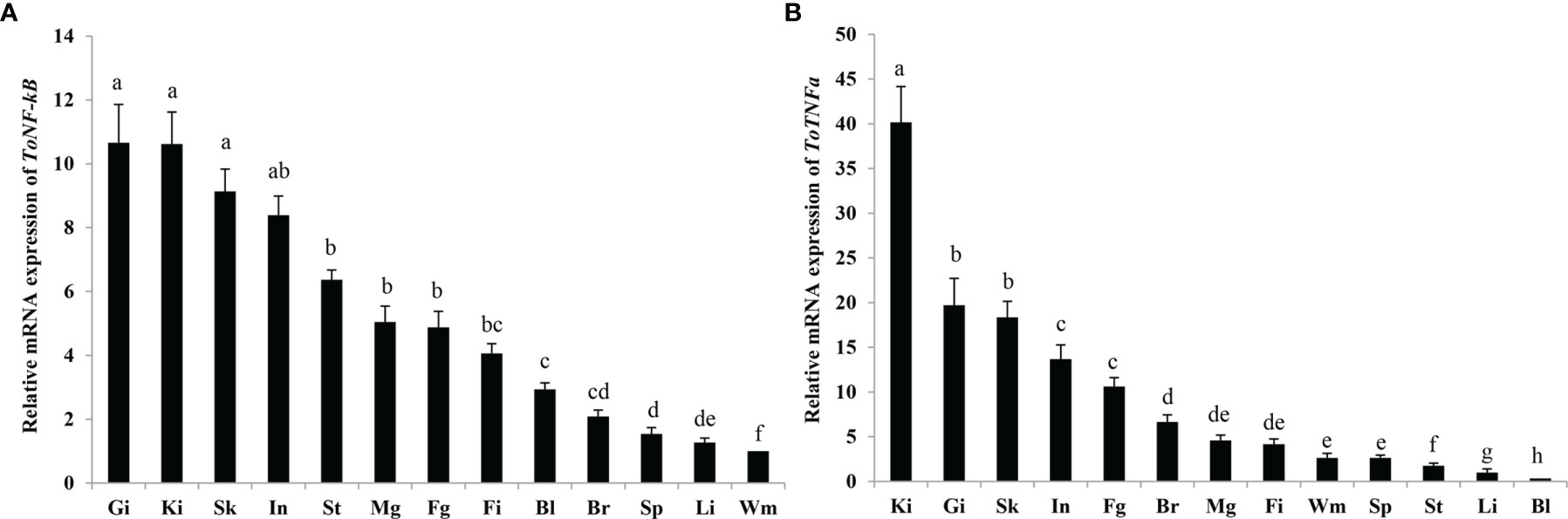
Figure 4 Expression patterns of ToNF-κB (A) and ToTNFα (B) gene transcripts in various tissues. The 12 tissues are skin (Sk), brain (Br), blood (Bl), gill (Gi), fin (Fi), head-kidney (Ki), small intestine (In), stomach (St), spleen (Sp), liver (Li), white muscle (Wm), female gonad (Fg), and male gonad (Mg). Different letters indicate significant differences (p < 0.05).
To analyse the possible role of ToNF-κB and ToTNFα in defence against parasite infection, the mRNA levels of ToNF-κB (Figure 5A) and ToTNFα (Figure 5B) were determined in local infection sites (skin and gills) and system immune tissue (liver, spleen and head kidney) after C. irritans challenge.
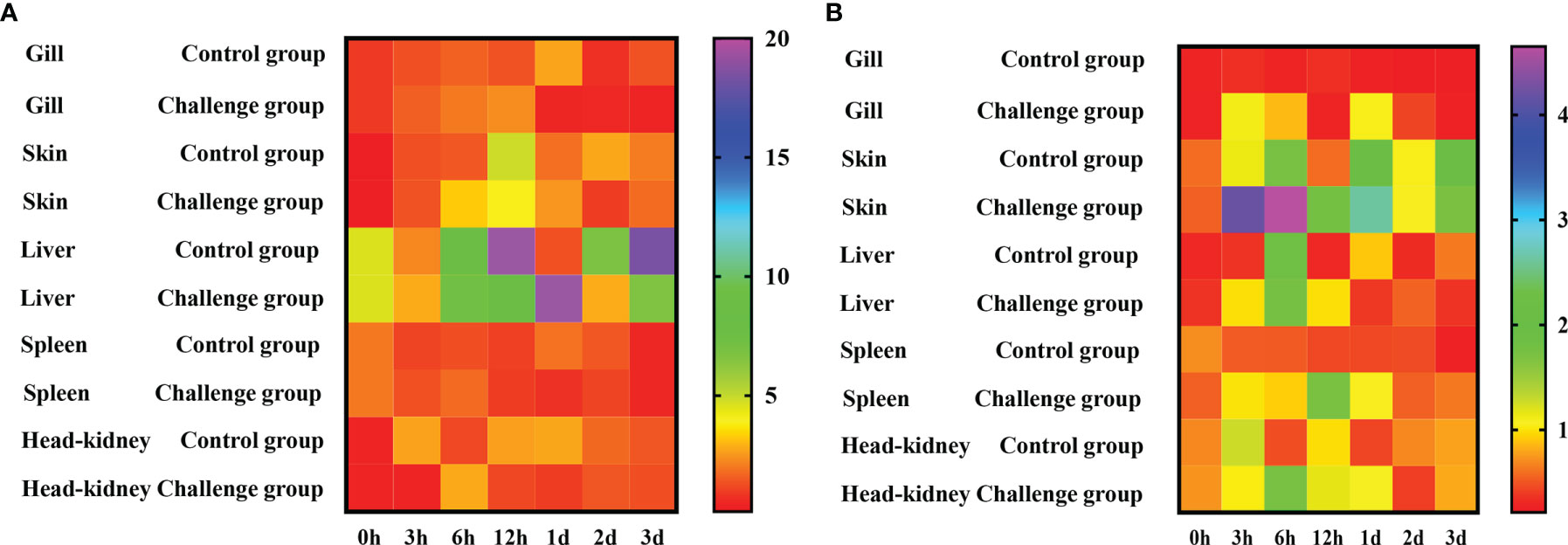
Figure 5 Temporal expression of ToNF-κB (A) and ToTNFα (B) mRNA is detected in gill, skin, liver, spleen, and kidney by qRT-PCR after infection with C. irritans (0, 3, 6, 12 hpi, 1 day, 2 days, 3 days). EF-1a is used as the internal control to calibrate the cDNA templates for all the samples. The heatmap is constructed by GraphPad Prism software.
The expression of ToNF-κB was upregulated in those five tissues after C. irritans stimulation. In the gill, ToNF-κB was upregulated at 3–12 h, and the peak of expression (2.49-fold relative to the uninfected control) was observed at 12 h. In the skin, ToNF-κB was increased from 3 to 12 h, with the peak of expression at 12 h, which was 31.9-fold relative to the uninfected control. In the liver, ToNF-κB was upregulated at 3 h, 6 h, 12 h and 1 day and then returned to normal levels. In the spleen, ToNF-κB was upregulated from 3 to 6 h and then returned to normal levels. In the head kidney, the peak of ToNF-κB expression was 2.49-fold relative to the uninfected control at 12 h (Figure 5A). Moreover, after C. irritans induction, the expression of ToTNFα was also increased in those five tissues. In the gill, ToTNFα was upregulated at 3 and 6 h and then returned to normal levels. There was also a second peak of ToTNFα expression at day 1, which then returned to normal levels. In the skin, ToTNFα was upregulated from 3 h to 1 day, and the peak of expression (7.74-fold relative to the uninfected control) was observed at 6 h. In the spleen, ToTNFα was upregulated from 3 h to 1 day and then dramatically decreased at 2 and 3 days. In the head kidney, ToTNFα expression was significantly increased at 6 h and 1 day, with the peak expression (2.29-fold relative to the uninfected control) at 6 h (Figure 5B). ToTNFα was upregulated at early time points in both systemic immune tissues and local infection sites, implying that ToTNFα might be involved in host defence against C. irritans by a combination of systemic and mucosal immunity.
ToNF-κB Promotes ToTNFα Expression
A total of 2,048 bp of the 5′-flanking sequence of the ToTNFα gene was obtained and identified as the candidate promoter. To investigate the promoter activity of ToTNFα in response to ToNF-κB in HEK293T cells, a series of truncated mutants, which included pGL3-basic-TNFα-p1, pGL3-basic-TNFα-p2, pGL3-basic-TNFα-p3, pGL3-basic-TNFα-p4 and pGL3-basic-TNFα-p5, were constructed (Figure 6A). The expression level of TNFα-P2 was higher than the activity of other mutants with the ToNF-κB response (Figure 6B), suggesting that the centre promoter region was present between -970 and +79 bp, which contained the NF-κB-binding sites.
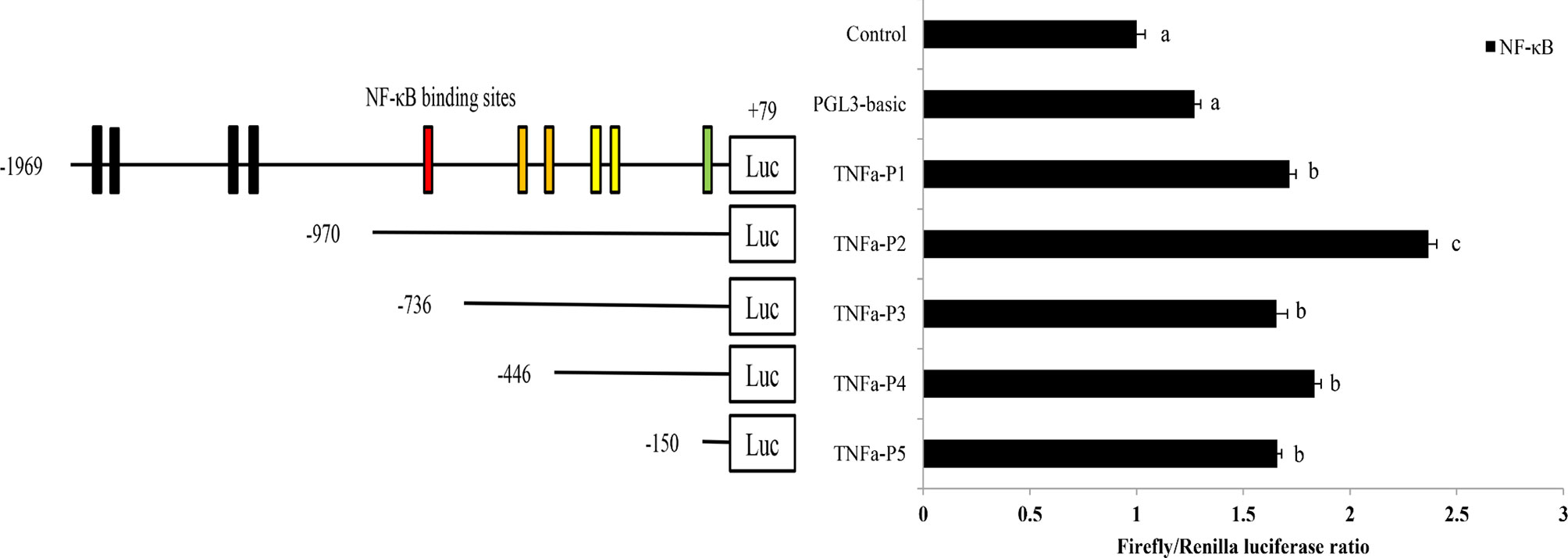
Figure 6 Promoter activity analysis of the ToTNFα gene. The structure and transcriptional activity of the ToTNFα promoter. Five recombinant plasmids, denoted TNFα-P1 (-1,969 to +79), TNFα-P2 (-970 to +79), TNFα-P3 (-736 to +79), TNFα-P4 (-446 to +79) and TNFα-P5 (-150 to +79), were constructed and transfected, along with the transcription factor ToNF-κB, into HEK 293T cells. Dual-luciferase activity was driven by the ToTNFα promoter upon the transfection of pcDNA3.1-NF-κB or pcDNA3.1 into HEK 293T cells. Data are presented as the means of three replicates ± SE. Different letters indicate significant differences (p < 0.05).
To further pinpoint the ToNF-κB-binding sites in the ToTNFα promoter, the binding sites were predicted and mutated (Figure 1A; Table 2). Exogenous cells were co-transfected with ToNF-κB and mutant vectors (M1, M2, M3, M4, M5 or M6) or the empty vector (pGL3-basic). Notably, the results indicated that only mutations of the M5 (-197 to -176 bp) and M6 (-116 to -92 bp) binding sites caused dramatic increases and reductions in promoter activity (Figure 1B), respectively. Furthermore, no significant difference was found between the wild type (TNFα-p2) and M1, M2, M3 or M4. It was suggested that the M5 mutation site in the ToTNFα-P2 promoter was an important site for triggering ToTNFα-upregulated expression by ToNF-κB.
To further confirm the ToNF-κB-binding motif in the ToTNFα promoter, an EMSA was performed. Based on the predicted ToNF-κB-binding site, oligonucleotide probes were synthesized (Table S2) and incubated with HEK293T cell lysates, including recombinant NF-κB, in vitro. Recombinant NF-κB bound to the oligonucleotide probes of the predicted NF-κB-binding site in the ToTNFα promoter. Nevertheless, mutations in the NF-κB-binding site resulted in the dissociation of the DNA-rNF-κB complex (Figure 7), suggesting that NF-κB specifically interacted with the M5 motif in the ToTNFα promoter. The formation of the DNA-rNF-κB complex was specific, as it could only be blocked by excessive unlabelled control probes (100×).
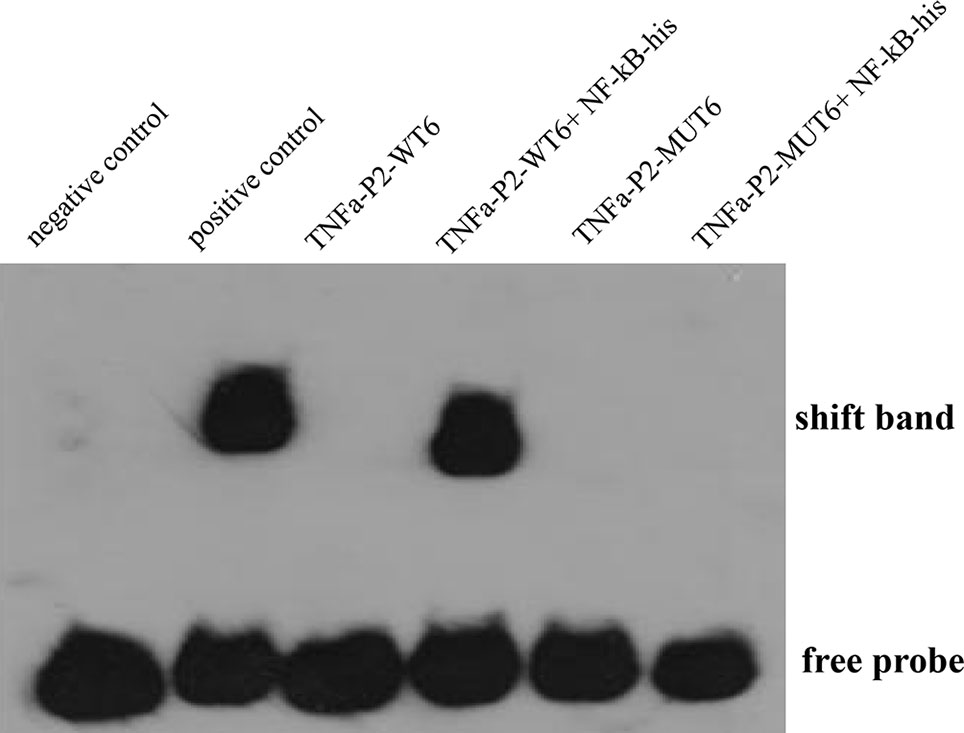
Figure 7 Binding reactions of ToNF-κB and the ToTNFα promoter. Biotin-labelled EMSA probes are incubated with lysates of HEK293T cells containing NF-κB protein. WT, wild-type probe; MUT, mutated probe. Positive control indicates ToTNFα+Protein; negative control indicates ToTNFα-WT.
Discussion
A member of this family, TNFa, is a major mediator of proinflammatory and antimicrobial defence mechanisms and is able to eliminate various pathogens by inducing a variety of cellular responses, such as phagocytosis and chemotaxis, and is considered an excellent biomarker and health indicator for both mammals and fish (Frederick et al., 2004; Kohchi et al., 2006). Indeed, NF-κB plays an important role in the regulation of TNFα pathway genes. In the present study, to further comprehend the molecular level of TNFα in teleosts, we first characterized TNFα from T. ovatus, with particular attention to the genomic structure, expression levels and regulation by NF-κB.
Similar to other TNFAs in vertebrates, the deduced aa sequence of ToTNFα contained a TACE restriction site at position S71/L72 and a TNF family signature (I118-F131; Figure 2 and Figure S1) (Cui et al., 2020; Huang et al., 2020). Two conserved cysteine residues, one of which is only conserved in teleost fish TNFα and the other of which exists in all known species TNFα. Therefore, conserved structural domains in fish TNFα molecules may suggest their functional similarity. Moreover, the assumed total aa sequence of ToTNFα shares higher identity with O. niloticus TNFα (61.9%), O. latipes TNFα (59.3%) and G. aculeatus TNFα (58.8%), which is analogous to the result of phylogenetic analysis. The evolutionary relationship was in accordance with the findings of conventional taxonomy, showing that ToTNFα displayed a close genetic relationship with Perciformes. Furthermore, similar to the TNFα gene in all detected vertebrates, the genomic sequence of ToTNFα is also composed of four exons and three introns. These results indicated that the genomic structure of vertebrate TNFαs was evolutionarily conserved.
In the present study, ToTNFα transcripts were constitutively expressed in all tested tissues of healthy fish, which was similar to the expression of TNFα in other teleosts (Hirono et al., 2000; García-Castillo et al., 2002; Zou et al., 2003; Savan and Sakai, 2004; Hong et al., 2013; Biswas et al., 2015; Cui et al., 2020; Huang et al., 2020; Kong et al., 2021; Ko et al., 2022). The mRNA level of ToTNFα was rich in the head-kidney, gill, skin and small intestine and poor in the stomach, liver and blood. Similarly, the highest expression levels of trout TNFα1/2/3 (Hong et al., 2013) and Thunnus orientalists TNFα2 (Kadowaki et al., 2009) were also determined in the gill. Therefore, a high expression of TNFα exists in immune tissues. Moreover, both TNFα1 and TNFα2 transcripts also had the lowest expression in the stomach (Huang et al., 2020). The reason for the diversity in TNFα expression patterns in different teleosts might be due to the multiple immune systems of fishes (Lepen Pleić et al., 2014). Additionally, the ToNF-κB transcript was found to be widely expressed in all tested tissues of healthy fish. It is likely that ToNF-κB is an important transcription factor (He et al., 2021). ToNF-κB expression was highest in the intestine, suggesting that the intestine is an important tissue associated with the immune defence response to various stresses in pompanos (Zhang et al., 2018; He et al., 2021).
Previous studies have declared that viruses [grass carp reovirus (GCRV)], poly I: C, and bacteria (LPS, Vibrio alginolyticus, Photobacterium damselae, Aeromonas schubertii, A. hydrophila, Nocardia seriolae) and peptidoglycan can generate antiviral and antibacterial responses in teleosts, where NF-κB and TNFα play a role (Nascimento et al., 2007; Wang et al., 2013; Huang et al., 2020; He et al., 2021; Kong et al., 2021). Nevertheless, in fish, few studies have been performed to explain the role of TNFα in response to parasite stimulation. Moreover, C. irritans is one of the major threats to T. ovatus (Dan et al., 2006). Consequently, to analyse the possible role of ToTNFα in the defence against parasite infection, the mRNA level of ToTNFα was determined in local infection sites (skin and gills) and system immune tissues (liver, spleen and head-kidney) after C. irritans challenge. In our previous study, IRF2 was upregulated at early time points in both systemic immune tissues and local infection sites in T. ovatus (Zhu et al., 2020). Overexpressing ToIRF2 in vitro notably increased the transcription of several type II IFN/IRF-based signalling pathway genes (Zhu et al., 2020). In the present study, both ToNF-κB and ToTNFα expression was upregulated in those five tissues after C. irritans stimulation, implying that ToNF-κB and ToTNFα might be involved in host defence against C. irritans by a combination of systemic and mucosal immunity. To some extent, this finding was consistent with findings in E. coioides and T. ovatus (Lam et al., 2013; Zhu et al., 2020).
Overexpression of H. leucospilota NF-κB could increase TNFα expression (He et al., 2021). In T. ovatus, it remains unclear whether ToNF-κB regulates the transcription of ToTNFα. To determine the promoter activity of ToTNFα in response to the transcription factor ToNF-κB in HEK293T cells, a series of progressive deletion constructs provided by our laboratory were used in the present study. The results of the dual-luciferase activity assay showed that the promoter activity of TNFα-P2 (-970 to +79 bp) was highest among the five plasmids in response to ToNF-κB, suggesting that the region from -970 to +79 bp contained the core promoter. Moreover, the expression levels of TNFα-P2 were 2.37-fold higher than those of the empty vector (pGL3-basic) in response to ToNF-κB. These results indicated that the core promoter region, which included the NF-κB binding sites, requires further analysis.
To further elucidate the binding of ToNF-κB to the ToTNFα promoter sequence, point mutations and EMSAs were analysed. In the present study, deletion of the TNFα-p2 M6 (-116 to -92 bp) binding site prominently reduced the promoter activity of ToTNFα. The EMSA also showed that ToNF-κB specifically bound to the ToTNFα promoter at the binding M6 site. ToNF-κB could bind a motif (GGGTGACATCACTGGAGTTTCCCTC) but not a mutant motif (GAACAGCACTGTCAGGACCTCTTTC) in vitro. Briefly, ToNF-κB could control ToTNFα expression by binding the M6-binding sites in fish. This study could provide basic information for the study of TNFα promoters in marine fish.
Conclusion
The expression characteristics and regulatory function of ToNF-κB and ToTNFα were reported. We verified that ToNF-κB and ToTNFα transcripts were increased in both local infection sites (skin and gill) and system immune tissues (liver, spleen and head-kidney) after challenge with C. irritans. Moreover, the positive regulatory function of ToNF-κB on ToTNFα expression was also determined. Thus, a positive feedback mechanism mediated by TNFα-induced ToNF-κB activation was proposed in T. ovatus. Our findings might help further understand the regulatory functions of TNFα in fish.
Data Availability Statement
The original contributions presented in the study are included in the article/Supplementary Material. Further inquiries can be directed to the corresponding author.
Ethics Statement
The animal study was reviewed and approved by the Animal Care and Use Committee of South China Sea Fisheries Research Institute, Chinese Academy of Fishery Sciences.
Author Contributions
S-GJ and D-CZ contributed to the conception and design of the study. K-CZ and B-SL organized the database. H-YG and LG performed the experiments. B-SL and NZ performed the bioinformatics and statistical analysis. K-CZ wrote the manuscript. All authors contributed to the manuscript revision and read and approved the submitted version.
Funding
This study was supported by the National Natural Science Foundation of China (U20A2064), Hainan Yazhou Bay Seed Laboratory (B21HJ0702), Guangdong Rural Revitalization Strategy Special Funds (Fishery Industry Development) (YueCaiNong [2020]4), Central Public-interest Scientific Institution Basal Research Fund CAFS (NO.2020TD29), China Agriculture Research System of MOF and MARA (CARS-47), and Guangdong Provincial Special Fund for Modern Agriculture Industry Technology Innovation Teams (2019KJ143).
Conflict of Interest
The authors declare that the research was conducted in the absence of any commercial or financial relationships that could be construed as a potential conflict of interest.
Publisher’s Note
All claims expressed in this article are solely those of the authors and do not necessarily represent those of their affiliated organizations, or those of the publisher, the editors and the reviewers. Any product that may be evaluated in this article, or claim that may be made by its manufacturer, is not guaranteed or endorsed by the publisher.
Supplementary Material
The Supplementary Material for this article can be found online at: https://www.frontiersin.org/articles/10.3389/fmars.2022.884892/full#supplementary-material
References
Amiot F., Fitting C., Tracey K. J., Cavaillon J.-M., Dautry F. (1997). Lipopolysaccharide-Induced Cytokine Cascade and Lethality in LTa/TNFa- Deficient Mice Mol. Med. (Cambridge, Mass.) 183, 864–875. doi: 10.1007/BF03401722
Biswas G., Kinoshita S., Kono T., Hikima J., Sakai M. (2015). Evolutionary Evidence of Tumor Necrosis Factor Super Family Members in the Japanese Pufferfish (Takifugu Rubripes): Comprehensive Genomic Identification and Expression Analysis. Mar. Genomics 22, 25–36. doi: 10.1016/j.margen.2015.03.003
Bodmer J. L., Schneider P., Tschopp J. (2002). The Molecular Architecture of the TNF Superfamily. Trends Biochem. Sci. 27, 19–26. doi: 10.1016/S0968-0004(01)01995-8
Cohen S., Lahav-Baratz S., Ciechanover A. (2006). Two Distinct Ubiquitin-Dependent Mechanisms are Involved in NF-κb P105 Proteolysis. Biochem. Biophys. Res. Commun. 345, 7–13. doi: 10.1016/j.bbrc.2006.04.036
Covello J. M., Bird S., Morrison R. N., Battaglene S. C., Secombes C. J., Nowak B. F. (2009). Cloning and Expression Analysis of Three Striped Trumpeter (Latris Lineata) Proinflammatory Cytokines, TNF-α, IL-1β and IL-8, in Response to Infection by the Ectoparasitic, Chondracanthus Goldsmidi. Fish. Shellfish. Immunol. 26, 773–786. doi: 10.1016/j.fsi.2009.03.012
Cui Z. W., Kong L. L., Zhao F., Tan A. P., Deng Y. T., Jiang L. (2020). Two Types of TNF-Alpha and Their Receptors in Snakehead (Channa Argus): Functions in Antibacterial Innate Immunity. Fish. Shellfish. Immunol. 104, 470–477. doi: 10.1016/j.fsi.2020.05.059
Dan X. M., Li A. X., Lin X. T., Teng N., Zhu X. Q. (2006). A Standardized Method to Propagate Cryptocaryon Irritans, on a Susceptible Host Pompano Trachinotus Ovatus. Aquaculture 258, 127–133. doi: 10.1016/j.aquaculture.2006.04.026
Dong Y., Wang S. Q., Chen J. L., Zhang Q. H., Liu Y., You C. H., et al. (2016). Hepatocyte Nuclear Factor 4α (Hnf4α) is a Transcription Factor of Vertebrate Fatty Acyl Desaturase Gene as Identified in Marine Teleost Siganus Canaliculatus. PLoS One 11, e0160361. doi: org/10.1371/journal.pone.0160361
Forlenza M., Magez S., Scharsack J. P., Westphal A., Savelkoul H. F. J., Wiegertjes G. F. (2009). Receptor-Mediated and Lectin-Like Activities of Carp (Cyprinus Carpio) TNF-α. J. Immunol. 183, 5319–5332. doi: 10.4049/jimmunol.0901780
Frederick W. G., Planas J. V., MacKenzie S. (2004). Tumor Necrosis Factor. Dev. Comp. Immunol. 28, 487–497. doi: 10.1016/j.dci.2003.09.008
García-Castillo J., Pelegrín P., Mulero V., Meseguer J. (2002). Molecular Cloning and Expression Analysis of Tumor Necrosis Factor α From a Marine Fish Reveal its Constitutive Expression and Ubiquitous Nature. Immunogenetics 54, 200–207. doi: 10.1007/s00251-002-0451-y
Goetz F. W., Planas J. V., MacKenzie S. (2004). Tumor Necrosis Factors. Dev. Comp. Immunol. 28, 487–497. doi: 10.1016/j.dci.2003.09.008
Grayfer L., Walsh J. G., Belosevic M. (2008). Characterization and Functional Analysis of Goldfish (Carassius Auratus L.) Tumor Necrosis Factor-Alpha. Dev. Comp. Immunol. 32, 532–543. doi: 10.1016/j.dci.2007.09.009
He J. Y., Li P. H., Huang X., Sun Y. H., He X. P., Huang W., et al. (2021). Molecular Cloning, Expression and Functional Analysis of NF-Kb1 P105 From Sea Cucumber Holothuria Leucospilota. Dev. Comp. Immunol. 114, 103801. doi: 10.1016/j.dci.2020.103801
Hirono I., Nam B., Kurobe T., Aok T. (2000). Molecular Cloning, Characterization, and Expression of TNF cDNA and Gene From Japanese Flounder Paralychthys. J. Immunol. 165, 4423–4427. doi: 10.4049/jimmunol.165.8.4423
Hong S., Li R., Xu Q., Secombes C. J., Wang T. (2013). Two Types of TNF-A Exist in Teleost Fish: Phylogeny, Expression, and Bioactivity Analysis of Type-II TNF-A3 in Rainbow Trout Oncorhynchus Mykiss. J. Immunol. 191, 5959–5972. doi: 10.4049/jimmunol.1301584
Horiuchi T., Mitoma H., Harashima S., Tsukamoto H., Shimoda T. (2010). Transmembrane TNF-α: Structure, Function and Interaction With Anti-TNF Agents. Rheumatology 49, 1215–1228. doi: 10.1093/rheumatology/keq031
Huang M. Y., Mu P. F., Li X. F., Ren Q. L., Zhang X. Y., Mu Y. N., et al. (2020). Functions of TNF-Alpha 1 and TNF-Alpha 2 in Large Yellow Croaker (Larimichthys Crocea) in Monocyte/Macrophage Activation. Dev. Comp. Immunol. 105, 103576. doi: 10.1016/j.dci.2019.103576
Kadowaki T., Harada H., Sawada Y., Kohchi C., Soma G., Takahashi Y., et al. (2009). Two Types of Tumor Necrosis Factor-α in Bluefin Tuna (Thunnus Orientalis) Genes: Molecular Cloning and Expression Profile in Response to Several Immunological Stimulants. Fish. Shellfish. Immunol. 27, 585–594. doi: 10.1016/j.fsi.2008.12.006
Kim W. L., Kim M. S., Kim K. H. (2011). Molecular Cloning of Rock Bream’s (Oplegnathus Fasciatus) Tumor Necrosis Factor Receptor-Associated Factor 2 and its Role in NF-κb Activation. Fish. Shellfish. Immunol. 30, 1178–1183. doi: 10.1016/j.fsi.2011.02.007
Kohchi C., Inagawa H., Nishizawa T., Yamaguchi T., Nagai S., Soma G. (2006). Applications of Lipopolysaccharide Derived From Pantoea Agglomerans (IP-PA1) for Health Care Based on Macrophage Network Theory. J. Biosci. Bioeng. 10, 485–496. doi: 10.1263/jbb.102.485
Ko S., Lim J., Hong S. (2022). Functional Characterization of a Novel Tumor Necrosis Factor Gene (TNF-New) in Rock Bream (Oplegnathus Fasciatus). Dev. Comp. Immunol. 127, 104269. doi: 10.1016/j.dci.2021.104269
Kong L. H., Qian K., Wu S. W., Li B. X., Guo Z., Yin X. X., et al. (2021). Functional Characterization of TNF-Alpha in Pufferfish (Takifugu Obscurus) in Immune Response and Apoptosis Against Aeromonas Hydrophila. J. Fish. Dis. 44 (9), 1343–1353. doi: 10.1111/jfd.13393
Kravtsova-Ivantsiv Y., Cohen S., Ciechanover A. (2009). Modifcation by Single Ubiquitin Moieties Rather Than Polyubiquitination is Suffcient for Proteasomal Processing of the P105 NF-κb Precursor. Mol. Cell. 33, 496–504. doi: 10.1016/j.molcel.2009.01.023
Lam F. W., Wu S., Lin S., Lin C., Chen Y., Wang H., et al. (2011). The Expression of Two Novel Orange-Spotted Grouper (Epinephelus Coioides) TNF Genes in Peripheral Blood Leukocytes, Various Organs, and Fish Larvae. Fish. Shellfish. Immunol. 30, 618–629. doi: 10.1016/j.fsi.2010.12.011
Lapid D., Lahav-Baratz S., Cohen S. (2017). A20 Inhibits Both the Degradation and Limited Processing of the NF-κb P105 Precursor: A Novel Additional Layer to its Regulator Role. Biochem. Bioph. Res. Co. 493, 52–57. doi: 10.1016/j.bbrc.2017.09.075
Lepen Pleić I., Secombes C. J., Bird S., Mladineo I. (2014). Characterization of Three Proinflammatory Cytokines, Tnfα1, Tnfα2 and IL-1β, in Cage-Reared Atlantic Bluefin Tuna Thunnus Thynnus. Fish. Shellfish. Immunol. 36, 98–112. doi: 10.1016/j.fsi.2013.10.011
Li S. L., Monroig O., Wang T. J. (2017). Functional Characterization and Differential Nutritional Regulation of Putative Elovl5 and Elovl4 Elongases in Large Yellow Croaker (Larimichthys Crocea). Sci. Rep. 7, 2303. doi: 10.1038/s41598-017-02646-8
Livak K. J., Schmittgen T. D. (2001). Analysis of Relative Gene Expression Data Using Real-Time Quantitative PCR and the 2-ΔΔCT Method. Methods 25, 402–408. doi: 10.1006/meth.2001.1262
Ma X. J. (2001). TNF-α and IL-12: A Balancing Act in Macrophage Functioning. Microb. Infect. 3, 121–129. doi: 10.1016/S1286-4579(00)01359-9
Nascimento D. S., Pereira P. J. B., Reis M. I. R., Do Vale A., Zou J., Silva M. T., et al. (2007). Molecular Cloning and Expression Analysis of Sea Bass (Dicentrarchus Labrax L.) Tumor Necrosis Factor-α (TNF-α). Fish. Shellfish. Immunol. 23, 701–710. doi: 10.1016/j.fsi.2007.02.003
Ordás M. C., Costa M. M., Roca F. J., López-Castejón G., Mulero V., Meseguer J., et al. (2007). Turbot TNFα Gene: Molecular Characterization and Biological Activity of the Recombinant Protein. Mol. Immunol. 44, 389–400. doi: 10.1016/j.molimm.2006.02.028
Savan R., Kono T., Igawa D., Sakai M. (2005). A Novel Tumor Necrosis Factor (TNF) Gene Present in Tandem With the TNF-Alpha Gene on the Same Chromosome in Teleosts. Immunogenetics 57, 140–150. doi: 10.1007/s00251-005-0768-4
Savan R., Sakai M. (2004). Presence of Multiple Isoforms of TNF Alpha in Carp (Cyprinus Carpio L.): Genomic and Expression Analysis. Fish. Shellfish. Immunol. 17, 87–94. doi: 10.1016/j.fsi.2003.11.001
Sun L. Y., Zhang D. C., Jiang S. G., Guo H. Y., Zhu C. Y. (2013). Isolation and Characterization of 21 Polymorphic Microstatellites in Golden Pompano Trachinotus Ovatus. Conserv. Genet. Resour. 5, 1107–1109. doi: 10.1007/s12686-013-9942-4
Takada Y., Sung B., Sethi G., Chaturvedi M. M., Aggarwal B. B. (2007). Evidence That Genetic Deletion of the TNF Receptor P60 or P80 Inhibits Fas Mediated Apoptosis in Macrophages. Biochem. Pharmacol. 74, 1057–1064. doi: 10.1016/j.bcp.2007.07.005
Tracey K. J., Cerami A. (1993). Tumor Necrosis Factor, Other Cytokines and Disease. Annu. Rev. Cell Biol. 9, 317–343. doi: 10.1146/annurev.cb.09.110193.001533
Uenobe M., Kohchi C., Yoshioka N., Yuasa A., Inagawa H., Morii K., et al. (2007). Cloning and Characterization of a TNF-Like Protein of Plecoglossus Altivelis (Ayu Fish). Mol. Immunol. 44, 1115–1122. doi: 10.1016/j.molimm.2006.07.281
Wang T., Sun Y., Jin L., Thacker P., Li S., Xu Y. (2013). Aj-Rel and Aj-P105, Two Evolutionary Conserved NF-κb Homologues in Sea Cucumber (Apostichopus Japonicus) and Their Involvement in LPS Induced Immunity. Fish. Shellfsh. Immunol. 34, 17–22. doi: 10.1016/j.fsi.2012.09.006
Wollenberg G. K., DeForge L. E., Bolgos G., Remick D. G. (1993). Differential Expression of Tumor Necrosis Factor and Interleukin-6 by Peritoneal Macrophages In Vivo and in Culture. Am. J. Pathol. 143, 1121–1130.
Yu S, Mu Y., Ao J., Chen X., (2010). Peroxiredoxin IV Regulates Pro-Inflammatory Responses in Large Yellow Croaker (Pseudosciaena crocea) and Protects Against Bacterial Challenge. J Proteome Res. 9, 1424–36.
Zhang A., Chen D., Wei H., Du L., Zhao T., Wang X., et al. (2012). Functional Characterization of TNF-α in Grass Carp Head Kidney Leukocytes: Induction and Involvement in the Regulation of NF-κb Signaling. Fish. Shellfish. Immunol. 33, 1123–1132. doi: 10.1016/j.fsi.2012.08.029
Zhang L. L., Xu D. L., Cui M. (2018). The Guanine Nucleotide-Binding Protein α Subunit Protein ChGnaq Positively Regulates Hsc70 Transcription in Crassostrea Hongkongensis. Biochem. Bioph. Res. Co. 499, 215–220. doi: 10.1016/j.bbrc.2018.03.130
Zhao X., Duan D., Feng X., Chen Y., Sun Z., Jia S., et al. (2012). Molecular Cloning and Expression Analysis of Common Carp Cyprinus Carpio Tumor Necrosis Factor-α. Fish. Sci. 78, 1229–1236. doi: 10.1007/s12562-012-0563-8
Zhu K. C., Liu B. S., Zhang N., Guo H. Y., Guo L., Jiang S. G., et al. (2020). Interferon Regulatory Factor 2 Plays a Positive Role in Interferon Gamma Expression in Golden Pompano, Trachinotus Ovatus (Linnaeus 1758). Fish. Shellfish. Immunol. 96, 107–113. doi: 10.1016/j.fsi.2019.12.006
Zou J., Secombes C. J., Long S., Miller N., Clem L. W., Chinchar V. G. (2003). Molecular Identification and Expression Analysis of Tumor Necrosis Factor in Channel Catfish Ictalurus Punctatus. Dev. Comp. Immunol. 27, 845–858. doi: 10.1016/S0145-305X(03)00085-5
Keywords: Trachinotus ovatus, promoter activity, transcription factor, TNFα, NF-κB
Citation: Zhu K-C, Guo H-Y, Guo L, Liu B-S, Zhang N, Jiang S-G and Zhang D-C (2022) Nuclear Factor-κB Plays a Positive Role in TNFα Expression in Golden Pompano, Trachinotus ovatus (Linnaeus 1758). Front. Mar. Sci. 9:884892. doi: 10.3389/fmars.2022.884892
Received: 27 February 2022; Accepted: 31 May 2022;
Published: 07 July 2022.
Edited by:
Youji Wang, Shanghai Ocean University, ChinaReviewed by:
Xinhua Chen, Fujian Agriculture and Forestry University, ChinaChao Li, Qingdao Agricultural University, China
Xiaoyan Xu, Shanghai Ocean University, China
Zhitao Qi, Yancheng Institute of Technology, China
Copyright © 2022 Zhu, Guo, Guo, Liu, Zhang, Jiang and Zhang. This is an open-access article distributed under the terms of the Creative Commons Attribution License (CC BY). The use, distribution or reproduction in other forums is permitted, provided the original author(s) and the copyright owner(s) are credited and that the original publication in this journal is cited, in accordance with accepted academic practice. No use, distribution or reproduction is permitted which does not comply with these terms.
*Correspondence: Dian-Chang Zhang, emhhbmdkY2hAc2NzZnJpLmFjLmNu