Corrigendum: Methodologies for patellid limpets’ aquaculture: From broodstock management to juveniles
- 1Mariculture Center of Calheta (CMC), Regional Directorate for the Sea, Calheta, Portugal
- 2Centro Interdisciplinar de Investigação Marinha e Ambiental (CIIMAR), Terminal de Cruzeiros do Porto de Leixões, Universidade do Porto, Matosinhos, Portugal
- 3OKEANOS - Instituto de Investigação em Ciências do Mar da Universidade dos Açores, Horta, Portugal
- 4Fundação Gaspar Frutuoso (FGF) - Universidade dos Açores, Ponta, Delgada, Portugal
- 5AquaLab - Laboratório Experimental de Aquicultura, IMAR - Instituto do Mar, Horta, Portugal
- 6Grupo de Investigación en Acuicultura (GIA), Instituto Universitario de Acuicultura Sostenible y Ecosistemas Marinos (IU-ECOAQUA), Universidad de Las Palmas de Gran Canaria (ULPGC), Carretera de Taliarte s/n, Las Palmas de Gran Canaria, Spain
The production of cultured limpets is a recent research field contributing to aquaculture diversification, focusing on low trophic species while reducing the carbon footprint. Limpets are gastropods that colonize rocky substrates and are mostly present on tidal and subtidal shores. This animal group is in high commercial demand and is endangered in several regions. The aquaculture production of limpets has been traditionally challenging. The most successful reproduction method has been gonadal dissection, as artificial spawning induction has shown limited success to date. Moreover, methods for larval culture, settlement, and juvenile growth have been poorly developed and remain largely unknown. In recent years, advances in this field have led to the optimization of methods to enhance larval production, larval culture, settlement induction of competent larvae, and management of post-larvae and juveniles. The present manuscript reviews these advances, obtained within the framework of AQUAINVERT project, focusing on broodstock management, gametes release, larval production, larviculture, settlement, and grow-out of post-larvae, and providing an update on the actual state of the art in limpets’ aquaculture.
Introduction
True limpets (hereafter referred to as limpets) are a monophyletic group of gastropods belonging to the subclass Patellogastropoda (Harasewych and McArthur, 2000; Nakano and Ozawa, 2007). Limpets play a key top-down influence on the structure of rocky shore communities (Branch, 1981; Branch, 1985; Menge, 2000; Burgos-Rubio et al., 2015) as powerful grazers that prevent the recruitment of macroalgae by consuming their sporelings and propagules (Branch, 1971; Branch, 1981; Branch, 1985; Jernakoff, 1985; Benedetti-Cecchi, 2000; Bulleri et al., 2000; Coleman et al., 2006; Lorenzen, 2007). Limpets also contribute to the stability of the red encrusting coralline algae assemblages, a worldwide group of red algae that dominate rocky shorelines (Steneck, 1986; Dethier, 1994) and play an important role in carbon sequestration, reef building, invertebrate recruitment, and fish nursery grounds (McCoy and Kamenos, 2015). These encrusting algae evolved to tolerate intense animal herbivory pressure (limpets, chitons, and sea urchins) that removes algal competitors (Underwood, 1980; Branch, 1981; Steneck, 1982; Branch, 1985; Steneck, 1990; Piazzi et al., 2016).
The exploitation of limpets as fishery resources has been reported to exist since 10,000 years into the paleontological records of distant parts of the world, such as South Africa (Klein, 1979; Klein and Steele, 2013), California (Erlandson et al., 2011), North Spain (Muñoz-Colmenero et al., 2012), and several Mediterranean sites (Stiner et al., 1999; Colonese et al., 2011). Currently, the exploitation of limpets continues for either ornamental, food, or fishing bait collection purposes (Espinosa and Rivera-Ingraham, 2017; Firth, 2021). The exploited taxa include the genera Cellana (Lasiak, 1993; Harada et al., 1997; McCoy, 2008), Cymbula (Branch and Odendaal, 2003), Lottia (Pombo and Escofet, 1996; Sagarin et al., 2007), Patella (Guerra-García et al., 2004; Guallart et al., 2013c; Henriques et al., 2017; Sousa et al., 2019a; Sousa et al., 2020a), and Scutellastra (Carballo et al., 2020; Valdez-Cibrián et al., 2021). Overfishing has led to a detrimental impact on several populations of limpets worldwide, resulting in smaller specimens and population sizes, a reduced female:male ratio, and an overall plummet of biomass and reproductive/recruitment output (Branch, 1975; Branch and Odendaal, 2003; Guerra-García et al., 2004; Espinosa et al., 2006; Espinosa et al., 2009a; Sousa et al., 2019b; Sousa et al., 2020b). Consequently, several limpet populations are currently declared vulnerable, endangered, or extinct (Espinosa et al., 2014; Espinosa and Rivera-Ingraham, 2017; Henriques et al., 2017; Luque et al., 2018; Carballo et al., 2020). Different governmental authorities have implemented mitigation measures to reduce the overfishing impact, mostly based on the application of Marine Protected Areas (MPAs) and capture restrictions (Branch and Odendaal, 2003; Espinosa and Rivera-Ingraham, 2017; Sousa et al., 2019a; Sousa et al., 2020a).
Aquaculture could offer an opportunity to reduce the impact of overfishing on limpet populations. Moreover, limpets represent a low trophic group with a reduced carbon footprint, which is especially interesting for the diversification of aquaculture. The limpets could also be incorporated as one of the trophic levels to integrated multi-trophic aquaculture (IMTA) systems, similar to the abalone that has revealed potential for IMTA production in land-based (Nobre et al., 2010) and offshore mariculture systems (Viera et al., 2016). The ability to culture limpets might also provide an opportunity for restoration projects of endangered species (Guallart et al., 2020a; Ferranti et al., 2022). Today, limpets aquaculture is still in its infancy and at the experimental level, as “there are many issues in limpet aquaculture because of their sensitive nature and complex environmental and biological requirements, most of which is still unknown in laboratory environments” (Mau and Jha, 2018). Moreover, scientific literature is especially scarce regarding larval culture methods, settlement requirements, and post-larval and juvenile grow-out experiences (Nhan, 2014; Ferranti et al., 2018; Mau and Jha, 2018; Guallart et al., 2020b; Ferranti et al., 2022).
The AQUAINVERT project (INTERREG MAC 2014-2020) aims to enhance and promote the aquaculture of marine invertebrates in the Macaronesia region. One major goal of the project is the development of aquaculture protocols for the congeneric patellid limpet species Patella aspera Röding, 1798 (Figures 1A–C), and Patella candei d’Orbigny, 1840 (Figures 1D–F). These native species from the European Macaronesia (including the Azores, Madeira, and Canary archipelagos) (Weber and Hawkins, 2002; Henriques et al., 2012; Sousa et al., 2017) represent important cultural, gastronomic, and economic resources. Nevertheless, both species endure relevant overfishing pressure, so legislative, restrictive, and protective measures have been promoted to reduce the anthropogenic impact on the native populations in Azores (Ferraz et al., 2001; Martins et al., 2011; Diogo et al., 2016; Martins et al., 2017), Madeira (Fernandes et al., 2019; Sousa et al., 2019a; Sousa et al., 2019b; Sousa et al., 2020a), and the Canary Islands (Navarro et al., 2005; López et al., 2012; González-Lorenzo et al., 2015; Parker et al., 2020).
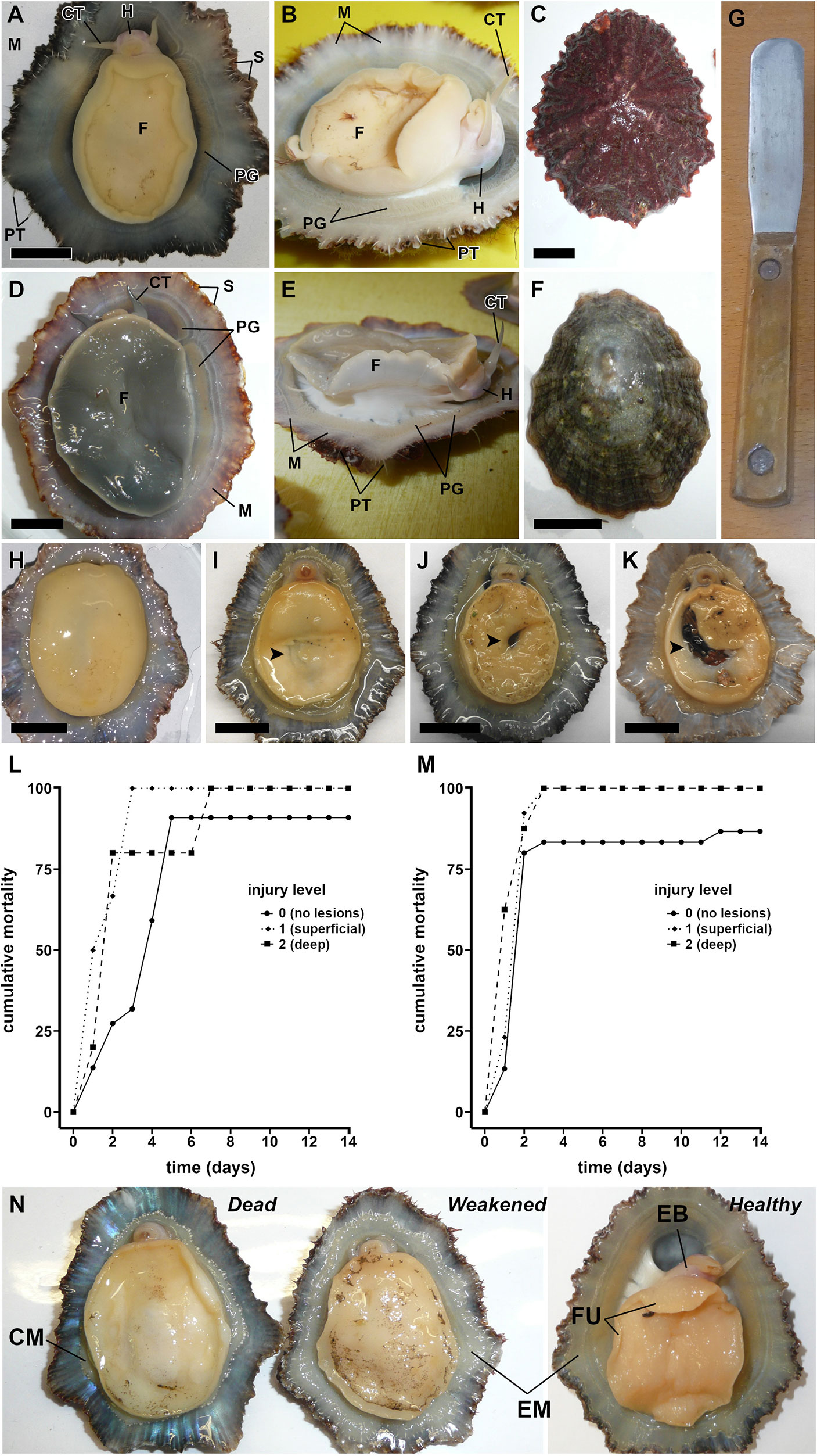
Figure 1 Limpet species studied in the present study (A–F). Patella aspera Röding, 1798 from Madeira (A–C): ventral view (A), lateral view (B), and dorsal view of a shell covered by encrusting coralline algae (C). Patella candei d’Orbigny, 1840 from Madeira (D–F): ventral view (D), lateral view (E), and dorsal view of a shell with scarce epibionts (F). Blunt knife known as “lapeira” used for the traditional fishing of limpets (G). Impact of physical damage on broodstock survival (H–M). Category level of the physical injuries using P. aspera as example (H–K): level 0, no visible injuries (H); level 1, superficial lesions (I); level 2, deep lesions (J); level 3, evisceration and/or decapitation (K). Cumulative mortality during the first 2 weeks after the capture (L, M): P. aspera (L) and P. candei (M). Health condition of the specimens using P. aspera as example (N). Scale bar = 10 mm. CM, contracted mantle; CT, cephalic tentacles; EB, extended body; EM, extended mantle; F, foot; FU, foot undulations; H, head; PG, pallial gills; PT, circumpallial tentacles; S, shell.
The present manuscript thoroughly describes suitable methodologies developed for the culture of both limpet species, regarding broodstock management, larval production, and animal culture up to the grow-out phase. It reviews the methodologies already developed for other limpet species with an update and optimization in the framework of the AQUAINVERT project and introduces methodologies for settlement induction and post-larval management. Experimental data to validate the protocols and provide insight into the early life biology of the patellid limpets are included.
Materials and Methods
Limpets’ Collection and Research Facilities
The research work took place at two distinct facilities: the Mariculture Center of Calheta (CMC; Madeira) and the Experimental Aquaculture Laboratory (AquaLab; Azores). In both facilities, adult specimens of P. aspera and P. candei were captured during the reproductive period (October to April) (Góis et al., 2010; Henriques et al., 2012; Sousa et al., 2017). The specimens collected at CMC facilities were used for assays 1–3, 5–16, and 18, and those collected at AquaLab facilities were used for assays 4 and 17.
General Procedures
A total of 18 assays were realized with the following purposes: assays 1 to 2 deal with “Recollection and management of the broodstock”; assays 3 to 12 deal with “Gametes and larval production methods”; assays 13 to 15 deal with “Larval development and larviculture methods”; assays 16 to 17 deal with “Settlement and metamorphosis in limpets”; and assay 18 deals with “Management of the post-larvae and grow-out”.
The adults dissected for larval production (assays 7–12), larviculture (assays 13–15), settlement (assay 16), and post-larval management (assay 18) were characterized using the average shell length, total mass, and gonadosomatic index (Supplementary Table 1 for details). The gonadosomatic index was calculated following Sousa et al. (2017): GSI = GM × (TM − SM)-1 × 100, where GM is the gonadal mass, TM is the total mass, and SM is the shell mass.
For alkaline bath, incubation, and larval culture, we used glass beakers (600 ml) (Figures 2A, B) and commercial plastic cups (80–100 ml) (Figures 2D, E). For settlement, we used culture cell plates (Figure 2G), Petri dishes (Figure 2H), and tanks with transparent fiberglass plates (Figure 2F). The water employed was filtered (5–20 µm) seawater sterilized with ultraviolet (acronym: FSS).
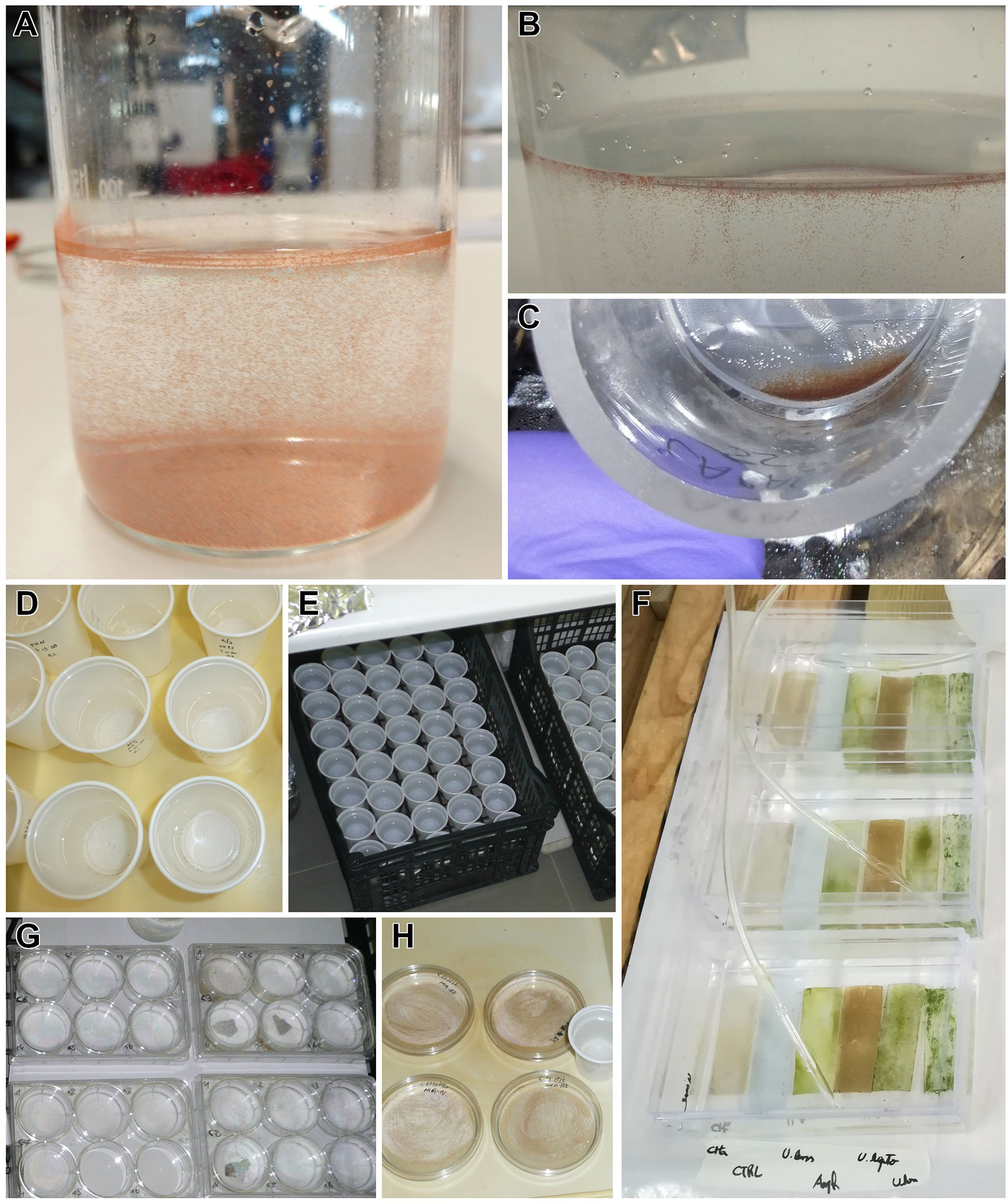
Figure 2 Different containers used for the culture of limpets. Glass beaker (600 ml), used for alkaline treatment, fertilization, incubation, and larval culture (A, B): glass beaker showing a pool of trochophores (A), and trochophores swarming near the water surface (B). Mesh (55 µm) used to gather the trochophores collected by siphoning (C). Plastic cups (80–100 ml) used for alkaline treatment, fertilization, incubation, and larval culture (D, E). Tanks (1 L) with fiberglass plates covered by different biofilms used for settlement assays and grow-out of post-larvae (F). Culture cell plates with different substrates used for settlement assays (G). Petri dishes with N. incerta biofilms used for settlement assays and grow-out of post-larvae (H).
Larval morphology was used as a criterion to distinguish between viable and deformed larvae, e.g., trochophores (assays 6–15; Figures 9A–C, J–K) and pediveligers (assays 14–15; Figures 9G–I, L–M). Larval descriptions are detailed in “Section 3. Larval development and larviculture”.
Assays 1–2: Recollection and Management of the Broodstock
Assay 1. Impact of physical damage on broodstock survival. The influence of physical injuries on the survival of P. aspera (n = 45) and P. candei (n = 45) in captivity was evaluated. This assay was performed between November 2019 and February 2020. The specimens were tagged (PVC sheets glued with cyanoacrylate; Figures 3D, G, J). Injuries were categorized into four levels: 0 = no visible injuries, 1 = superficial lesions, 2 = deep lesions, and 3 = evisceration and/or decapitation (Figures 1H–K). The specimens were placed in the culture tanks, and mortality was monitored daily to calculate the relative cumulative mortality for each injury level. A bias in this study was that a majority of healthy specimens (level 0; Figure 1N) were removed prematurely for experimental purposes.
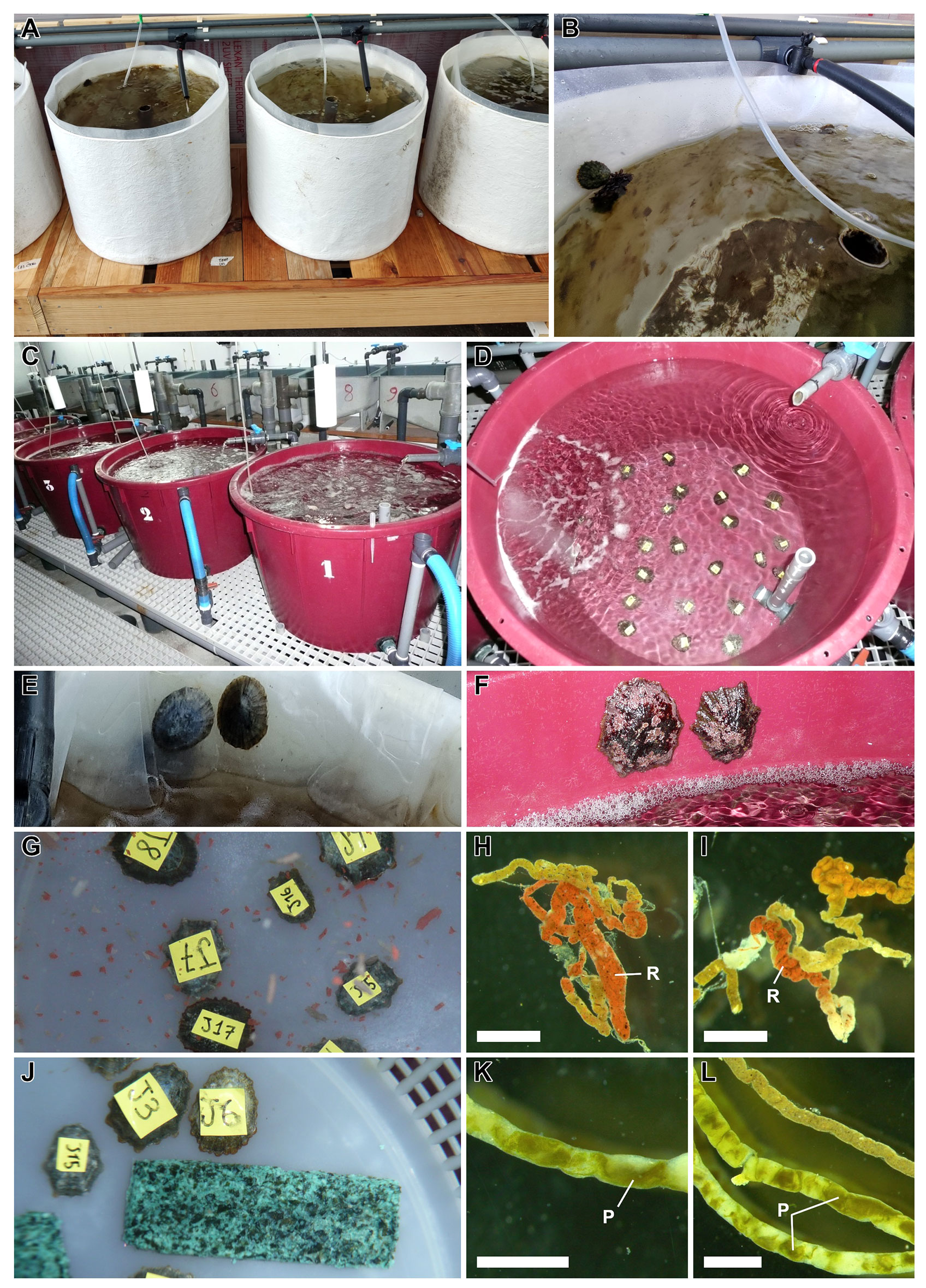
Figure 3 Culture tanks used for the maintenance of adult limpets and feeding trials. Culture tanks from the AquaLab facilities, Azores (A, B). Culture tanks from the CMC facilities, Madeira (C, D). Limpets attached to the culture tank walls above the water level (E, F). Feeding trials for adult specimens (G–L). Commercial fish flakes: flakes available for the adult limpets (G), and resulting feces (H, I). Artificial flour-based meal: prepared meal available for the adult limpets (J), and resulting feces (K, L). Scale bar = 1 mm. R, red coloration derived from a fish flake; P, Porphyra piece from the artificial meal.
Assay 2. Feeding trials on adult limpets. Acclimated adults of P. aspera and P. candei were placed in plastic baskets located inside the culture tanks. The food was kept for 2 days (Figures 3G, J) and tested: commercial fish flakes (ActivPet, Pingo Doce, Portugal) (Figure 3G) and artificial meals based on a flour mixture inspired by those developed for Cellana sandwicensis by Nhan (2014) (Supplementary Table 2; Figure 3J). The feces were examined using a dissecting microscope (Figures 3H, I, K, L).
Assays 3–12: Gametes and Larval Production Methods
Assay 3. Female fecundity. Female fecundity was calculated as total oocyte production per female in P. aspera (n = 73) and P. candei (n = 37) sampled from October 2020 to April 2021. The shell length was measured, and the gonadosomatic index was calculated. Each female gonad was placed inside a measuring cylinder, leveled up to 100 ml with FSS, and dropped in a beaker. The oocytes from the gonad were extracted (Figures 5I–J), and three samples (1 ml volume) were collected and fixed with 300 µl of formaldehyde 37%. The oocytes from the samples were counted to calculate oocyte production.
Assay 4. Spawning induction assays. This assay evaluates different treatments for spawning induction in P. aspera and P. candei. Specimens were collected from intertidal areas in Faial Island (Azores) and acclimatized for 2 weeks in 35-L tanks. Half an hour before the trial, specimens were placed on a tray, left upward, and covered with a wet towel. Trials were performed in an acclimatized room using 500 ml of transparent boxes as experimental containers. Water flow was kept running using filtered (1 µm) seawater (acronym: FSW) at ambient temperature (17.3 ± 0.4°C). All treatments had 8 independent replicates (individual limpets), except for one trial, which was conducted with 5 individuals for each treatment (Table 1). The treatments lasted between 02:00 and 04:30 h or until the first individuals started to spawn. At the end of each trial, the sex ratio was estimated by dissection of 25% of the treated specimens and calculated as: Nmale × (Nmale + Nfemale)−1 (Table 1).
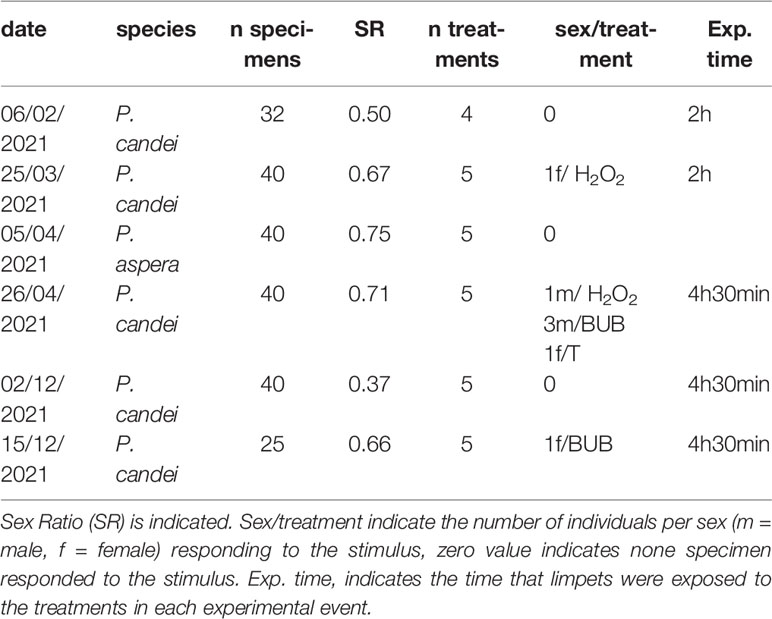
Table 1 Results of induction tests performed with different treatments: bubbling (BUB), thermic shock (T), hydrogen peroxide (H2O2), ultraviolet radiation (UV) and control (CNT). .
The protocol was mainly based on Ferranti et al. (2018) and included five treatments: intense bubbling, UV-light irradiation, hydrogen peroxide (H2O2), thermal shock, and a negative control group. The bubbling treatment used specimens placed in a cylindrical colander inside the boxes with FSW and was generated by aquarium air stones positioned under the colander. The UV-light irradiation treatment was tested as it induces spawning in different abalone species (Ebert and Houk, 1984; Moss et al., 1995). The specimens were placed in a flow-through system with UV-treated FSW with a calculated irradiation of 600 mW h L−1. In the thermal shock treatment, the specimens were placed in boxes with FSW whose temperature was increased by 4–5°C. The temperature was maintained at a constant level using a thermostat that was switched off at the end of the treatment. Hydrogen peroxide treatment employed an experimental box filled with FSW in which the pH was increased to 9.1 using 2 M Tris. The specimens were placed in those boxes for 15 min before the addition of 3.2 ml of freshly prepared 10% H2O2 solution. The specimens of the control treatment were placed in experimental boxes filled with FSW. At the end of all treatments, seawater was changed in all boxes with FSW and maintained at room temperature until the end of the trial, which lasted 7 ± 1 h.
Assay 5. Measurement of the diameter of oocytes. The oocytes from ten specimens per limpet species (P. aspera and P. candei) were photographed immediately after their extraction using a dissecting microscope (Leica M165, Leica Microsystems, Wetzlar, Germany) connected to a camera and image analysis software (LAS V4.12; Leica Microsystems, Wetzlar, Germany). The oocytes from the same specimens were also measured after receiving an alkaline treatment (NaOH, pH 8.9, 3 h). The minimum and maximum Feret’s diameters were measured for 40 oocytes per specimen using the image analysis software ImageJ 1.53k to calculate the average Feret’s diameter, following Dopchiz et al. (2018).
Assay 6. Variability in female fertility. Larval production (as a ratio of viable trochophores) was analyzed individually in P. aspera (n = 40) and P. candei (n = 16) females sampled from November 2020 to April 2021. Two treatments were applied to the oocytes: control (FSS) and alkaline treatment (NaOH, pH 8.9, 3 h). The specimens were processed in batches of eight females and four males. The males were used to prepare four different sperm pools: ABC, ABD, ACD, and BCD; each letter represents a different male. Each sperm pool was used to fertilize two females. Fertilization was performed at a density of 105 sperm cells ml−1, and incubation lasted 24 h (16 ± 2°C). Three replicates per treatment and per female were realized. Samples were fixed in formaldehyde 6%–8% and identified as viable trochophores (VT; Figures 9A–C), deformed specimens (Figures 9J, K), and unfertilized oocytes (Figure 5O–S). The ratio of viable trochophores was calculated as VT × total specimens-1. Pairwise comparisons of the ratio of viable trochophores between control and alkaline treatments were performed in each female to calculate the percentage of specimens showing significant differences. The influence of the sperm pool was analyzed by pairwise comparisons between females fertilized with the same sperm pool.
Assays 7–8. Alkaline agent (NaOH vs. NH4OH). This experiment was repeated twice to study the influence of different alkaline treatments on the larval production of P. aspera. The following factors were combined: two alkaline bases (NaOH and NH4OH), two pH values (8.4 and 8.9), and six times (10, 20, 30, 60, 120 and 180 min), resulting in 24 combined treatments. In addition, a control treatment (FSS, pH 8.0) was applied at the same times specified before (six control treatments). Three replicates per combination were used. The oocytes were rinsed at the end of each corresponding time and kept in FSS. Fertilization was simultaneous for all oocytes (105 sperm cells ml−1). Incubation lasted 24 h (17 ± 1°C). The specimens were fixed and examined to calculate the ratio of viable trochophores, ratio of normal development, and ratio of fertilization (Cañizares et al., 2021).
Assays 9–12. Sperm density. For consistency of the results, evaluation of the optimal sperm density in P. aspera was done in four separate assays, applying six treatments using logarithmic increments of sperm concentration: 10N sperm cells ml−1; being n = 2, 3, 4, 5, 6, and 7. Four replicates per treatment were used. The oocytes were alkaline treated (NaOH, pH 8.4, 3 h). Incubation lasted 24 h (16 ± 1°C). The specimens were fixed and examined to calculate the ratio of viable trochophores, ratio of normal development, and ratio of fertilization (Cañizares et al., 2021).
Assays 13–15: Larval Development and Larviculture Methods
Assays 13–15. Validation of the larviculture protocol. Assay 13 compared the viability of the trochophores (24 h post-fertilization) obtained from non-alkalinized oocytes (control using FSS) and from alkalinized oocytes (NaOH, pH 8.9, 3 h). The trochophores were distributed in eight plastic cups per treatment; four cups per treatment were sampled immediately and fixed to calculate (1) the initial density of trochophores and (2) the ratio of viable trochophores. The remaining cups were cultured for 48 h and fixed to calculate (1) the final density of pediveligers and (2) the ratio of viable pediveligers as viable pediveligers × total specimens−1. Student’s t-test (p < 0.05) was used to analyze pairwise comparisons of the ratios of viable larvae and the densities of larvae.
Assays 14 and 15 were performed with oocytes from two different treatments: control (FSS) and alkaline bath (NH4OH, pH 9, 10 min) and were divided into two sequential parts. First, for each treatment, the oocytes were distributed in four plastic cups (40 ml FSS) and three glass beakers (500 ml FSS) using 100 oocytes ml−1. The beakers were sampled to calculate the initial quantity of oocytes in each beaker. Fertilization used 105 sperm cells ml−1, and incubation lasted 24 h (16 ± 1°C). The plastic cups were sampled and fixed to calculate the ratio of viable trochophores. In each glass beaker, the upper 400 ml was siphoned, and specimens were gathered using a 55-µm mesh, resuspended in 100 ml of FSS and sampled to calculate the ratio of collected trochophores relative to the initial quantity of oocytes.
At continuation, the collected trochophores from each treatment were pooled and distributed in eight plastic cups (40 ml FSS) and four glass beakers (500 ml FSS) using 4 ± 1 trochophores ml−1. Four cups per treatment were sampled and fixed as described in assay 13. The beakers were sampled to calculate the initial quantity of trochophores per beaker. The remaining cups and the beakers were cultured for 48 h. Then, the plastic cups were sampled and fixed following assay 13. In each glass beaker, the upper 400 ml was siphoned, and specimens were gathered using a 55-µm mesh, resuspended in 50 ml FSS, and sampled to calculate the ratio of collected pediveligers relative to the initial quantity of trochophores.
Assays 16–17: Settlement and Metamorphosis in Limpets
Assay 16 studied the settlement in P. candei following the methodology described by Castejón et al. (2022). The pediveligers were obtained from alkalinized oocytes (NaOH, pH 8.9, 3 h) and trochophores cultured in glass beakers (12 ± 4 larvae ml−1). The assay was performed by placing pediveligers (72 h post-fertilization) on cell culture plates (Figure 2G) using 8 ml of FSS and 4 ± 2 larvae ml−1. The assay lasted 13 days. Six treatments with six replicates each were tested: (1) negative control; (2) shell pieces covered by light pink coralline crusts (143 ± 27 mm2); (3) diatom Halamphora coffeaeformis biofilm; (4) diatom Navicula incerta biofilm; (5) free swimming haptophycean Pavlova sp.; and (6) combined H. coffeaeformis and Pavlova sp. The assay was monitored daily to calculate the ratio of swimming larvae, ratio of crawling larvae, ratio of settled specimens, and ratio of dead specimens. Settlement success was analyzed at Day 13 as the ratio of juveniles (post-larvae with teleoconch).
Assay 17 used gametes of P. candei obtained from a spawning induction in April 2020 (one female and three males; Table 1). The oocytes were suitable for fertilization, so alkaline treatment was not required (Nunes et al., 2021). Fertilization was performed using 29 ± 6 oocytes ml−1 and 4.7 × 105 sperm cells ml−1 and lasted 3 h. Pediveligers 72 h post-fertilization were placed in three rectangular tanks (1 L) with FSS at room temperature (17 ± 1°C). The treatments were performed on transparent fiberglass plates (2 × 7 cm; Figure 2F) in which different biofilms were grown: Amphora sp. (BEA 1588B), Chaetoceros sp. (BEA 0419B), Ulvella lens (CS-801-19), Ulvella leptochaete (BEA 0702B), and Ulva lactuca, in addition to a plate without biofilm as a negative control. The tanks were filled with 400 ml of FSS, placing ca. 200 pediveligers per tank. For 1 week, 50 ml of FSW was added daily to maintain healthy experimental conditions. From Day 8 to Day 64 post-fertilization, the tanks were topped up to 1 L and switched to a flow-through system at constant temperature (17 ± 1°C).
Assay 18: Petri Dishes as Culture Containers for Limpet Post-Larvae
Assay 18. Petri dishes with grown N. incerta biofilms were tested as culture containers for limpet post-larvae. The same algal culture was used as a settlement inducer in both P. aspera and P. candei. The pediveligers (72 h post-fertilization) were obtained from alkalinized oocytes (NH4OH, pH 9, 10 min). Larvae were cultured in glass beakers (4 ± 1 larvae ml−1). The culture media used for N. incerta was removed before the start of the assay and replaced with 25 ml of FSS. The initial density was 3 ± 1 pediveligers ml−1. The assay was monitored weekly.
Statistical Analyses
All statistical analyses were performed using the statistical software R version 4.1.0. Yuen’s test for trimmed means (package “WRS2 1.1–3”) was used in assays 5–6 as a nonparametric approach for pairwise comparisons (null hypothesis rejected when p < 0.01). The homogeneity of the variances was analyzed using Levene’s test (package “car 3.0-12”), and the normality of the residuals was analyzed using the Shapiro–Wilk normality test (null hypothesis rejected when p < 0.05). The Tukey HSD was used in assays 7–8 to establish the significant differences relative to the treatment with the highest value following Pérez et al. (2016); in assay 6, two combined treatments (NaOH × pH 8.9 × 3 h; NH4OH × pH 8.4 × 3 h) were removed from the statistical analysis because two replicates from each treatment were accidentally lost. The Tukey HSD was used in assays 9–12 as a parametric method for pairwise comparisons among treatments (null hypothesis rejected when p < 0.05). The Games–Howell test (package “rstatix 0.7.0”) was used in assays 9–10 as an alternative for the Tukey HSD test when the data were normal but not the homogeneity of variances (null hypothesis rejected when p < 0.01). Student’s t-test was used in assays 13–15 as a parametric method to analyze pairwise comparisons (null hypothesis rejected when p < 0.05). Assay 16 analyzed the ratio of juveniles performing the post-hoc Tukey HSD test (null hypothesis rejected when p < 0.05) on arcsine square root transformed data to fit the normality and homoscedasticity assumptions. The HAL+PAV treatment was removed since zero values were obtained in all replicates.
Results and Discussion
Recollection and Management of the Broodstock
Recollection and Transport
To date, adult limpets can only be obtained from wild populations because technologies to produce captive bred animals have yet to be developed (Mau and Jha, 2018). Wild limpets form an isolation barrier consisting of the limpets’ shell, the substrate, and the mucus attachment (Davies, 1969; Branch, 1971; Wolcott, 1973), with the purpose of surviving different levels of environmental stressors such as insolation, temperature, and dehydration in the littoral zone (Orton, 1929; Davies, 1969; Branch, 1971; Wolcott, 1973; Harley et al., 2009). Breeders, either for production or for experimental purposes, might consider that simple detachment exposes the limpets to external stressors, increasing the risk of mortality (Mau and Jha, 2018). Wild specimens arrived at the culture facilities within a few hours after capture (usually less than 2 h) and were directly delivered by the fishermen in bags or nets. In contrast, for long transport, it has been suggested to cover the specimens with pieces of cloth soaked with seawater and place them inside portable refrigerators with coolers (Guallart et al., 2020b; Ferranti et al., 2022).
The physical damage that occurred during the capture influenced the survival in culture conditions (assay 1): specimens eviscerated or decapitated did not survive longer than 24 h, while injured specimens showed 100% mortality in approximately 1 week (Figures 1L, M). Non-injured specimens were the only ones that survived longer than 2 weeks, showing the necessity to avoid physical injuries to minimize mortality (Guallart et al., 2013a; Guallart et al., 2013b; Mau and Jha, 2018). Distinct levels of health status were identified (Figure 1N): dead specimens show a contracted mantle, absence of mantle reflexes to physical contact, and no activity; weakened specimens have a partially extended mantle and mantle reflexes but little or no activity; healthy specimens show an extended mantle, mantle reflexes, and notorious activity (Video 1).
Tank Acclimation
Broodstocks of P. aspera and P. candei were maintained using the same conditions for both species. At the AquaLab facilities (Azores), the adults were kept at a density ranging from 15 to 30 specimens in 55-L cylindrical tanks covered by plastic liners naturally colonized by algae (Figures 3A, B). At CMC facilities (Madeira), the adults were kept at a density of 30 to 35 specimens in 200-L cylindrical tanks; in this case, neither plastic liners nor algal growths were employed (Figures 3C, D). The environmental conditions were the same at both installations and for both limpet species: flow-through system (1–4 L min−1), at ambient temperature (19 ± 2°C) and salinity (37 ± 1 g L-1), and under a photoperiod of 12 h light:12 h dark. Aeration was provided to maintain oxygen saturation above 90%. Shallow tanks with flowing water at ambient temperature have also been used to keep different limpets’ species (Hodgson et al., 2007; Ribeiro, 2008; Mau et al., 2018). Other authors preferred recirculation systems (Ferranti et al., 2018; Nakano et al., 2020; Ferranti et al., 2022).
Cleaning and Maintenance
Tank cleaning was performed routinely (every 1–2 days during the first week and every 2–3 days during the second week) to remove feces, mucus, and dead specimens.
The limpets are prone to injuries; consequently, it is suggested to minimize the manipulation as much as possible. If animal manipulations are needed, we suggest to use a blunt knife (Figure 1G) to exert a smooth pressure below the shell to detach the specimens, supporting previous studies (Guallart et al., 2013a). The use of plastic liners is another measure that facilitates the detachment of the broodstock (Figures 3A, B, E), as suggested by previous studies (Nhan, 2014; Mau et al., 2018; Mau and Jha, 2018). P. aspera and P. candei can move above the upper water level (Figures 3E, F). This behavior did not cause associated problems, yet other authors preferred to place artificial turfs at the water level to limit animal movement (Nhan, 2014; Mau et al., 2018; Mau and Jha, 2018) or to generate artificial tides to reduce air exposure (Guallart et al., 2020b; Ferranti et al., 2022).
Broodstock of P. aspera and P. candei were not fed due to the unavailability of diets for these species. However, short feeding trials showed that limpets can accept certain food items (assay 2; Figures 3G, J). The examination of the feces revealed content related to the commercial fish flakes (Figures 3H, I) and the flour-based meals (Figures 3K, L). These observations support the limpets as generally opportunistic feeders with an unrestricted diet (Branch, 1971; Della Santina et al., 1993; Burgos-Rubio et al., 2015), which would facilitate the development of diets for aquaculture purposes.
Gametes and Larval Production Methods
The first limiting factor to start the production of limpets is the availability of mature specimens. Limpets are generally protandrous hermaphrodites in which the smaller and younger specimens tend to mature as males, while the larger and older specimens tend to mature as females (Orton et al., 1956; Wright and Lindberg, 1982; Creese et al., 1990; Espinosa et al., 2006; Lindberg, 2007; Espinosa et al., 2009b; Sousa et al., 2019b). Overfishing affects the sex ratio toward a higher proportion of males and smaller, less productive females (Martins et al., 2017; Sousa et al., 2019b), a factor to be considered when working with specimens from exploited areas. However, the most important factor is the reproductive season. In Azores, the populations of P. candei reproduce during the whole year, peaking in summer (Curdia et al., 2005). In Madeira, the populations P. aspera and P. candei are winter breeders (Góis et al., 2010; Henriques et al., 2012; Sousa et al., 2017), seriously limiting the acquisition of reproductive stocks due to the frequent adverse sea conditions (Torres and Andrade, 2010).
Sex Determination
Limpets have been traditionally considered animals with no secondary sex characteristics, so sex determination has relied on invasive techniques such as biopsies or dissection (Dodd, 1956; Blackmore, 1969; Rao, 1973; Wright and Lindberg, 1979; Wright and Lindberg, 1982; Guallart et al., 2013b; Ferranti et al., 2022). Nevertheless, we found some visual cues that enable sex identification. In P. aspera, the foot should be highly pressed toward the animal body to identify the hue of the ventral mid-left side of the foot (Figures 4A, D): dark hue in females (Figures 4A, B) and pale hue in males (Figures 4C, D). From the selected batch (n = 15), both males (n = 5) and females (n = 9) were successfully identified, with only a single unidentified specimen due to unclear features. In P. candei, the foot should be highly extended upward while clearly showing the abductor musculature, with the purpose of identifying the hue of the lateral left side of the foot (Figures 4E–H): females were harder to identify, showing no clear coloration changes (Figures 4E, F), while males showed a cream-color hue (Figures 4G, H). From the randomly selected batch (n = 21), 100% of males (n = 5) were successfully identified, while 87.5% of females (n = 8) were identified. The remaining specimens (n = 8) did not show clear visual cues. This method is based on the observation of the gonad through the animal body and could be useful for the management of specimens from endangered populations.
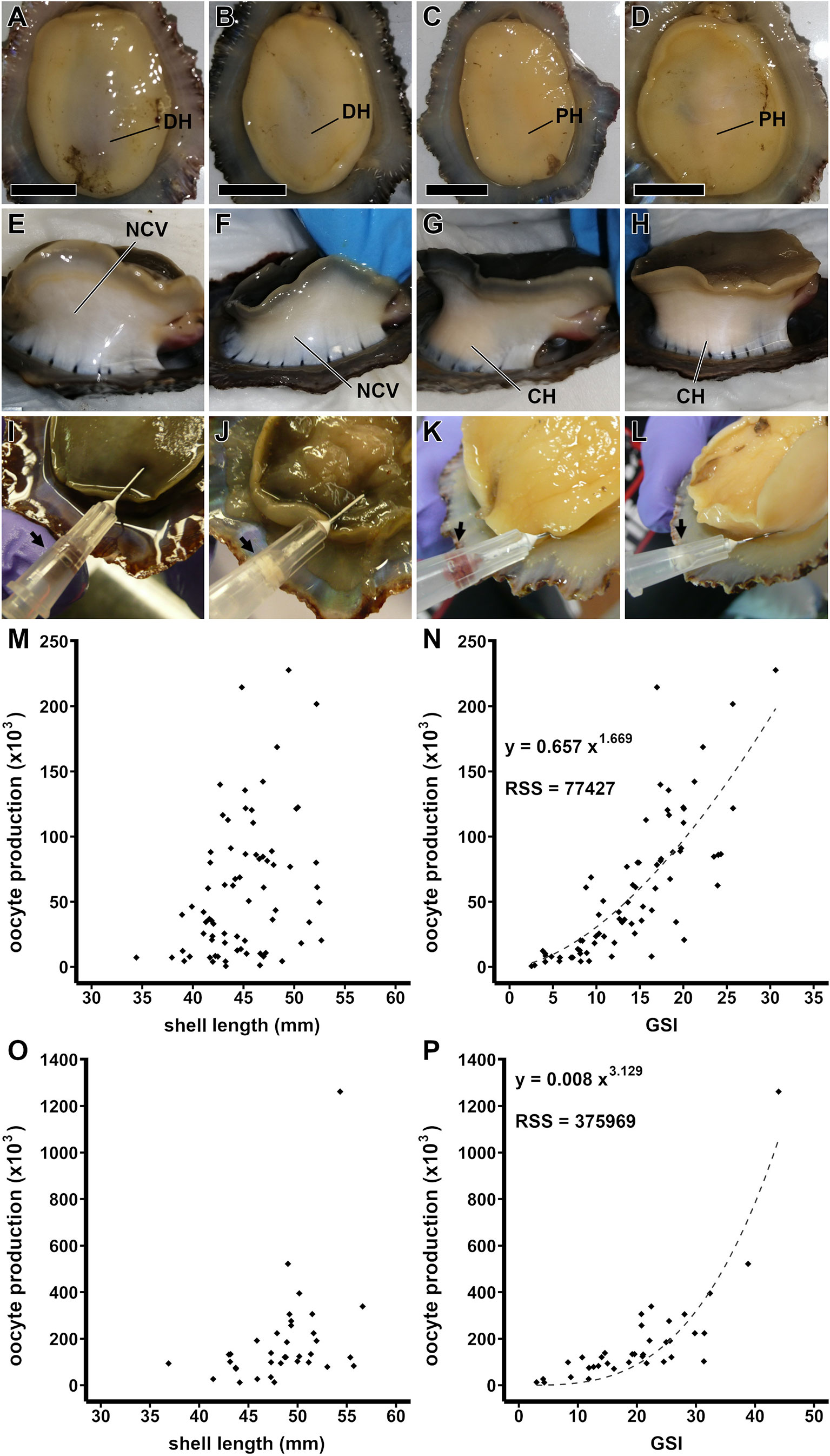
Figure 4 Characters identified for the sex determination of limpets by visual examination. P. aspera, coloration of the ventral side of the foot when highly contracted (A–D): females, dark hue (A, B), and males, pale hue (C, D). P. candei, coloration of the lateral-left side of the foot when highly extended (E–H): females, no color variation (E, F), and males, cream-color hue (G, H). Biopsy as method to determine the sex in limpets. Biopsy realized through the ventral-left side of the foot in P. candei (I, J): female (I) and male (J). Biopsy realized through the left side of the foot basis in P. aspera (K, L): female (K) and male (L). Female fecundity as oocyte production (× 103) per specimen (M–P). P. aspera (M, N): oocyte production per female ordered by shell length (M) and by gonadosomatic index (N). P. candei (O, P): oocyte production per female ordered by shell length (O) and by gonadosomatic index (P). Scale bar = 10 mm. CH, cream-color hue; DH, dark hue; NCV, no color variation; PH, pale hue.
The visual determination was shown to be neither infallible nor applicable for all the specimens. The gonadal biopsy designed by Wright and Lindberg (1979) is a non-lethal and minimally invasive alternative. It is performed using a syringe with a hypodermic needle (0.5 × 16 mm) and pinching through the foot or the mantle (Figures 4I–L). Guallart et al. (2013b) reported that a quick puncture through the mantle allows a survival rate higher than 90%.
Female Fecundity
The fecundity (assay 3) in females of P. aspera was on average 59,000 oocytes, ranging from less than 1,000 to ca. 280,000 oocytes for specimens ranging from 34 to 53 mm shell length (Figures 4M, N), while in females of P. candei, it was on average 186,000 oocytes, ranging from ca. 12,000 to ca. 1.2 × 106 oocytes for specimens ranging from 37- to 57-mm shell length (Figures 4O, P). The greater fecundity values increased with the animal size, yet the correlation was not clear (Figures 4M, O), probably masked by differential gonadal development (Figures 4N, P). In this sense, fecundity increased with animal size in the limpet Patella ferruginea (Espinosa et al., 2006; Guallart et al., 2020b).
Regarding the gamete release for the limpets, two methodologies are available: (1) spawning induction and (2) dissection of the adult specimens to extract the gonads.
Spawning Induction Methods
Spawning induction techniques are based on the exposure of mature specimens to physical or chemical factors able to induce gamete release and are used successfully in marine gastropods with aquaculture interest, such as abalone (Morse et al., 1977; Uki and Kikuchi, 1984; Moss et al., 1995; Courtois de Vicose et al., 2007). The factors tested in limpets include air desiccation, chemical exposition (H2O2, KCl), osmolarity shock, thermal shock, vigorous aeration, and different combinations of these; however, only a small fraction of stimulated adults actually released gametes (Kay and Emlet, 2002; Ferranti et al., 2018; Ferranti et al., 2022). Spawning induction has been tested in P. aspera and P. candei (assay 4), with the higher number of gametes released reported in the bubbling treatments (Table 1), a physical factor that could simulate the environmental conditions favorable for limpets’ reproduction (Kay and Emlet, 2002; Ferranti et al., 2018). Recently, Ferranti et al. (2022) developed a potentially successful protocol for P. ferruginea consisting of maintaining specimens upturned in cold (5°C) and dry conditions, performing a gonad biopsy followed by cold bubbling for at least 1 h (temperature 5°C lower than ambient seawater temperature), air drying upturned specimens at room temperature, and performing a final immersion in seawater at environmental temperature and without aeration, waiting for spawning. Spawning success is probably influenced by gonadal maturation stages, as mature specimens are the most prone to release gametes (Uki and Kikuchi, 1984). Magnetic resonance is a promising non-lethal technique to determine gonadal maturation in endangered limpet populations (Guallart et al., 2020a).
As an alternative approach, Mau et al. (2018) tested the viability of intramuscular injections of salmon-gonadotropin-releasing hormone analog (250 ng g−1 for priming and 500 ng g−1 for resolving) in the limpet C. sandwicensis, reporting a spawning success between 10% and 13%. Ferranti et al. (2022) used injections of human chorionic gonadotropin (5 µl g−1) and luteinizing hormone-releasing hormone (1 µg g−1) in P. ferruginea, but spawning was unsuccessful except for a single male individual. The use of analogous hormones is an interesting approach that requires more studies in different limpet species using different hormones and concentrations.
Dissection and Fertilization In Vitro
The most traditional method to produce limpets’ larvae is based on the dissection of mature specimens to extract the gonad and obtain gametes (Smith, 1935; Dodd, 1957). The dissection proceeded with specimens upturned over a flat surface. A scalpel was used to cut the perimeter of the foot musculature from the left to the right side of the animal. The foot was removed, exposing the gonads (Figures 5A–D). Sex is determined by gonad coloration. The females of P. aspera and P. candei showed a red–orange and brown–purple colored gonad, respectively (Figures 5A, C), with the oocytes visible to the naked eye. The males of both species showed a cream-white gonad and seminal fluid (Figures 5B, D).
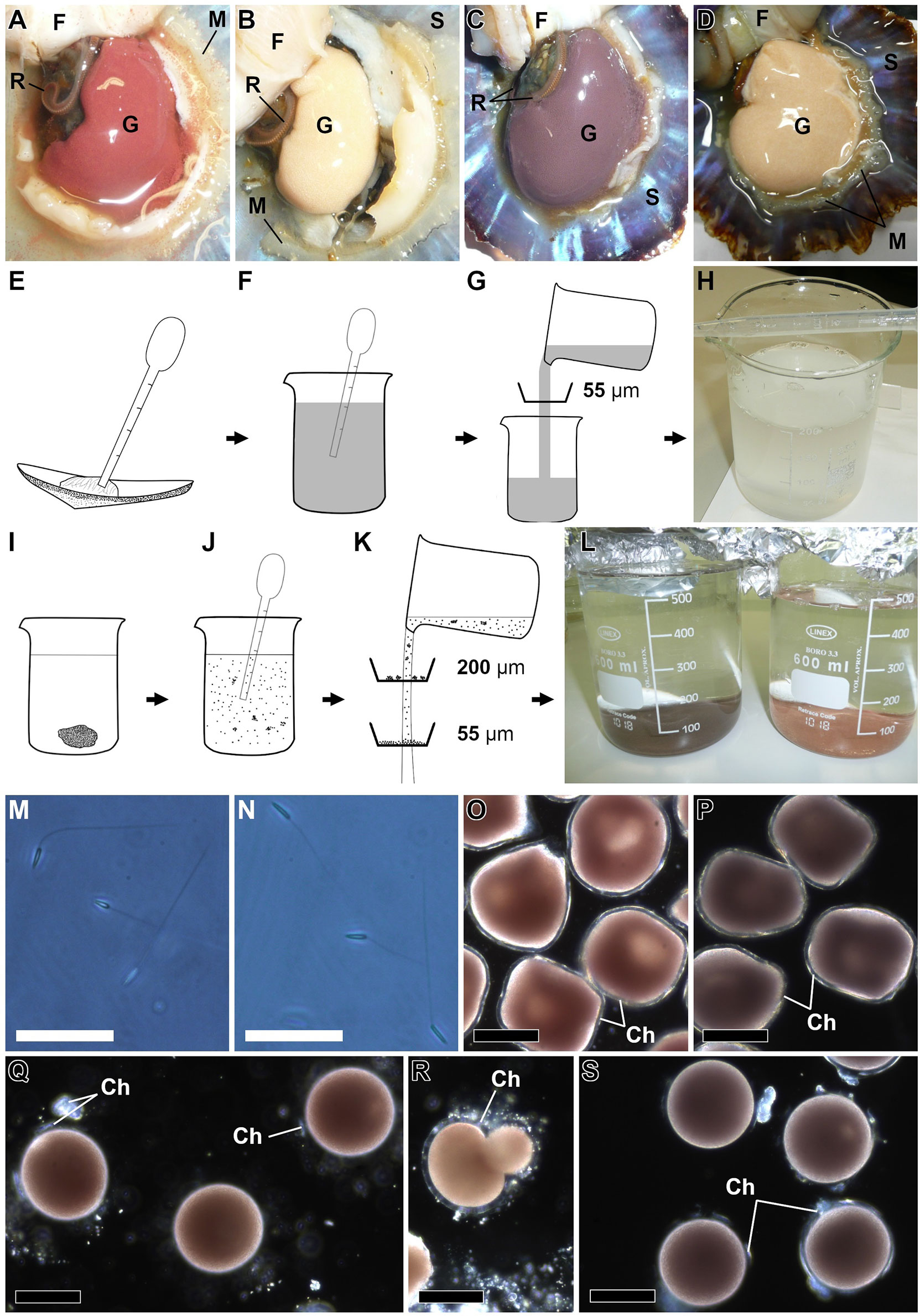
Figure 5 Method for obtaining the gametes of the limpet species P. aspera and P. candei. Gonad of P. aspera exposed by dissection (A, B): female (A) and male (B). Gonad of P. candei exposed by dissection (C, D): female (C) and male (D). Method for obtaining the sperm (E–H): sperm collected using a Pasteur’s pipette (E), dilution of the sperm in seawater (F), filtration (55 µm) of the sperm solution (G), and example for sperm solution (H). Method for obtaining the oocytes (I–L): female gonad inside a glass beaker (I), oocytes released using a Pasteur’s pipette (J), filtration using a 200- and a 55-µm mesh (K), and example of pool of oocytes of P. aspera (right) and P. candei (left) (L). Sperm cells (M, N): P. aspera (M) and P. candei (N). Oocytes just after the extraction showing polyhedral shape and the chorion membrane (O, P): P. aspera (O) and P. candei (P). Oocytes just after the alkaline treatment showing spherical shape and the degradation of the chorion membrane (Q, S): P. aspera (Q) and P. candei (S). Oocyte of P. aspera deformed due to the alkaline treatment (R). Scale bar = 25 µm (M, N), 100 µm (O–S). Ch, chorion; F, foot; G, gonad; M, mantle; R, radula; S, shell.
The protocol to obtain the gametes described hereby is an improved version of the one used in previous studies (Cañizares et al., 2021; Castejón et al., 2021). The sperm were collected directly from the male gonad with a plastic Pasteur pipette (Figure 5E) and immediately diluted in FSS (Figure 5F). This step should be carefully performed to avoid damaging the underlying organs and collecting debris. It is recommended to pool the sperm of several males to enhance the chances for successful fertilization and genetic variability. The sperm solution can be filtered through a 55- to 100-µm mesh to remove large debris (Figures 5G). The sperm started to activate immediately after dilution in seawater. This method enabled sperm solutions of 50–200 ml with a density ranging from 107 to more than 108 sperm cells ml−1 (Figures 5H, M, N). Following Cañizares et al. (2021), the sperm can be placed in the fridge (5 ± 2°C) for more than 6 h without adverse consequences for fertilization. Active sperm cells have been reported up to 24 h after being diluted in seawater at 16 ± 1°C.
The oocytes were extracted by applying repeated suction-release pressure efforts using a plastic Pasteur pipette to break the female gonad inside a glass beaker with FSS (Figures 5I, J). The resulting solution was a mixture of oocytes, hemolymph, and tissue debris (Figure 5J). The diameter of the oocytes was 156 ± 11 µm in P. aspera and 161 ± 10 µm in P. candei (Figures 5O, P; assay 5). Then, the oocytes were washed using a 200-µm mesh to retain large debris and a 55-µm mesh to retain the oocytes (Figure 5K). It is recommended to pool the oocytes of several females (Figure 5L), as fertility is highly variable (Figure 6). Limpets’ oocytes are denser than seawater and immediately sink, so the supernatant was removed by siphoning and replaced with FSS, which increases the water quality for the incubation (Ferranti et al., 2022).
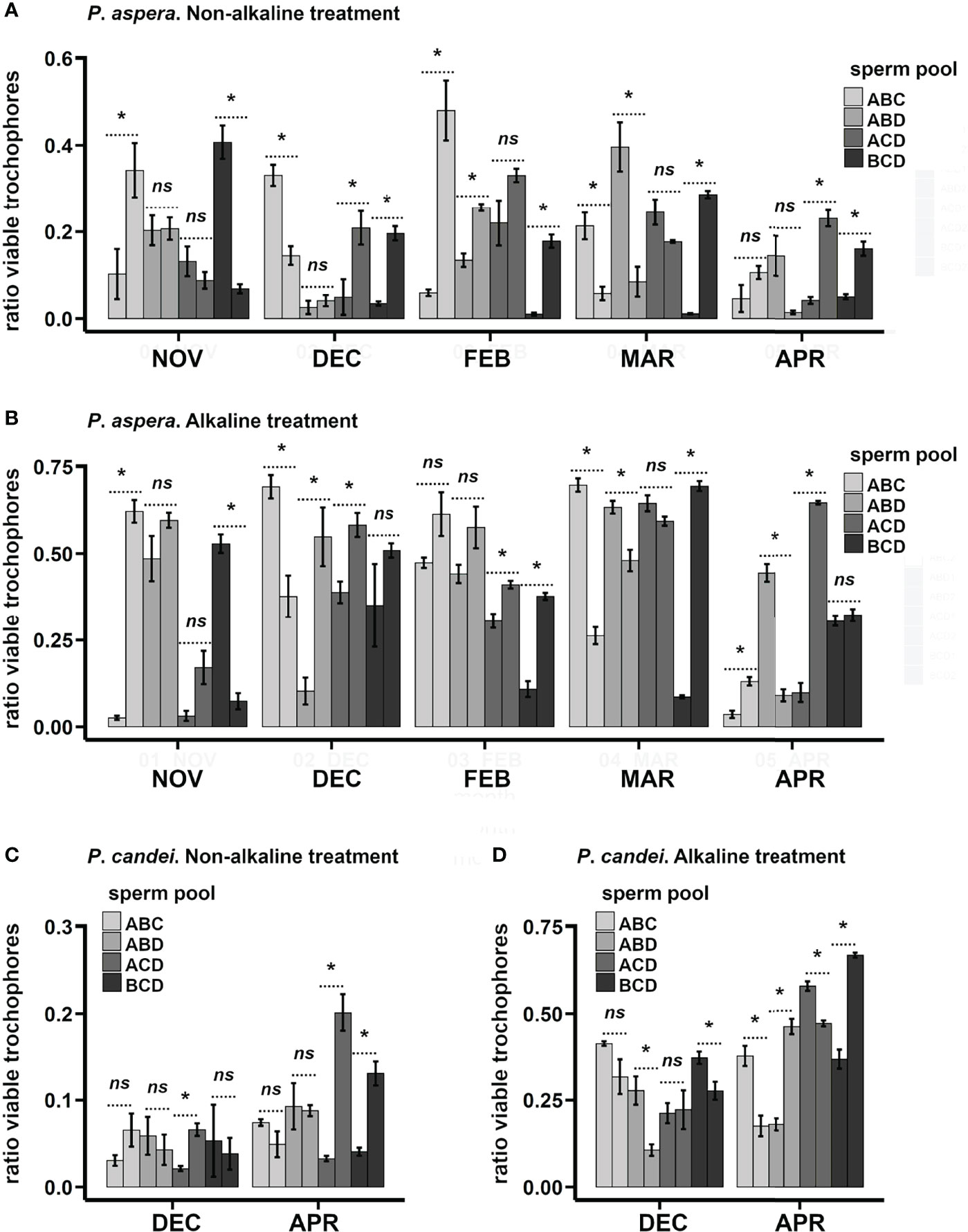
Figure 6 Individual variation of the fertility among female limpets, studied as a ratio of viable trochophores, and comparison between non-alkaline (control) and alkaline treatments. Eight females were analyzed each month. Each bar represents the average ± SD ratio of viable trochophores obtained in each female. The females of each species follow the same order in non-alkaline and alkaline treatments. The differences in ratio of viable trochophores within pairs of females fertilized using the same sperm pool were analyzed statistically (n.s., not significant differences; *p < 0.01). Ratio of viable trochophores in different females of P. aspera (A, B): non-alkaline treatment (A) and alkaline treatment (B). Ratio of viable trochophores in different females of P. candei (C, D): non-alkaline treatment (C) and alkaline treatment (D).
The recently extracted limpet oocytes show a polyhedral shape and a membrane covering called the chorion (Figures 5O, P), exhibiting low fertility. Since the study by Corpuz (1981), it has been known that fertilization success can be enhanced using an artificial maturation treatment consisting of an alkaline seawater bath, generally consisting of NaOH solutions at pH 9.0–9.5 for several hours (Aquino De Souza et al., 2009; Pérez et al., 2016; Guallart et al., 2020b; Cañizares et al., 2021; Castejón et al., 2021), or based on NH4OH solutions at pH 8.5–9.0 lasting no more than 10 min (Wanninger et al., 1999; Gould et al., 2001; Hodgson et al., 2007; Ribeiro, 2008; Pérez et al., 2016; Seabra et al., 2019; Nakano et al., 2020). The alkaline treatment promoted chorion removal and the acquisition of a spherical shape and significantly reduced the oocyte diameter to 141 ± 6 µm in P. aspera (Yuen’s test, p-value = 0) and 146 ± 6 µm in P. candei (Yuen’s test, p-value = 0) (Figures 5Q, S; assay 5) when using NaOH at pH 8.4–9.0 for 3 h on these species (Cañizares et al., 2021; Castejón et al., 2021; Castejón et al., 2022). The duration of the alkaline bath should be controlled to avoid the degradation of the oocytes (Figure 5R) and to limit the ratio of abnormal development (Figures 9J, K) (Cañizares et al., 2021; Castejón et al., 2021). For this reason, the oocytes should be washed to remove the alkaline agent after the finalization of the bath; we recommend using a 55-µm mesh and abundant FSS. The density of oocytes used during the alkaline bath ranged from 100 to 500 oocytes ml−1, representing from 4 to 50 oocytes mm2 at the bottom of the containers.
The fertilization of the oocytes of P. aspera and P. candei was realized by adding the sperm directly to the oocytes at a density of 105–106 sperm cells ml−1 (Cañizares et al., 2021; Castejón et al., 2021; Castejón et al., 2022), which was within the range used for other patellid limpet species (Hodgson et al., 2007; Ferranti et al., 2018; Guallart et al., 2020b; Ferranti et al., 2022). The fertilization period is highly variable in the literature, from 30 min for Patella ulyssiponensis (Hodgson et al., 2007) to 3 h for Patella vulgata (Pérez et al., 2016), and intermediate values for other patellids (González-Novoa, 2014; Seabra et al., 2019; Guallart et al., 2020b). However, the sperm can be left with the oocytes during the entire incubation period (24 ± 2 h) without adverse effects on the culture, such as increased polyspermy (Cañizares et al., 2021; Castejón et al., 2021; Castejón et al., 2022), saving additional rinses and losses of oocytes. The incubation was realized in static conditions, i.e., neither aeration nor stirring was provided. The density of oocytes used during the incubation ranged from 30 to 150 oocytes ml−1, representing from 1 to 15 oocytes mm2 at the bottom of the containers.
Variability in Female Fertility
Individual fertility of the females was studied in P. aspera (n = 40) and P. candei (n = 16) (assay 6). Current results are preliminary as a study is still ongoing when publishing this manuscript, yet this information could be valuable in the current context. Both species showed a high variability in the ratio of viable trochophores obtained in the control and alkaline treatments (Figure 6). The alkaline treatment significantly increased the ratio of viable trochophores in 55% of the specimens in P. aspera (Figures 6A, B) and 81% of the specimens in P. candei (Figures 6C, D). The sperm pool cannot explain such variability because significant differences occurred in the majority of the pairwise comparisons between females fertilized with the same sperm pool: 65% in P. aspera (Figures 6A, B) and 75% in P. candei when using alkaline treatment (Figure 6D). Altogether, these results support that female quality is a key factor to be considered for obtaining good larval production yields.
Alkaline Agent (NaOH vs. NH4OH)
Ammonium hydroxide (NH4OH) has been used as an alkaline agent in several limpet species, showing greater larval production yields while requiring shorter application times than sodium hydroxide (NaOH) (Wanninger et al., 1999; Gould et al., 2001; Hodgson et al., 2007; Pérez et al., 2016; Seabra et al., 2019; Nakano et al., 2020). The effectiveness of NH4OH to produce viable trochophores in P. aspera was studied (assays 7–8). The optimal treatment was NH4OH at pH 8.9 for 10 min, which was not significantly different from the highest value (Figure 7; Supplementary Figure 1). NH4OH at pH 8.9 should not be used longer than 30 min because it showed adverse effects on larval production (Figures 7A, B; Supplementary Figures 1A, B).
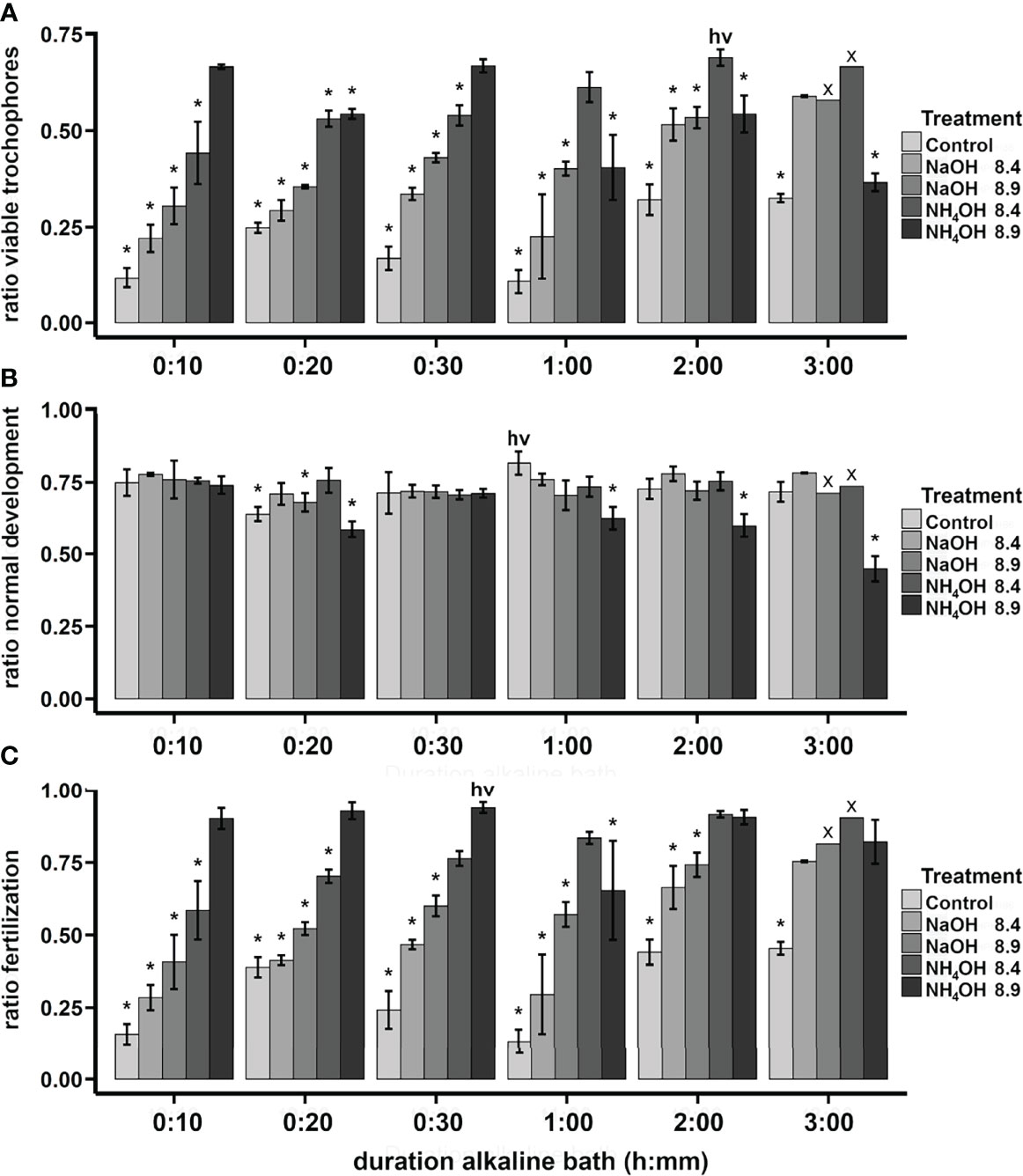
Figure 7 P. aspera. First test to study the influence of different alkaline baths on the larval production. Treatments combined different alkaline agents (NaOH and NH4OH), pH (8.4 and 8.9), and bath durations (10, 20, 30, 60, 120, and 180 min), plus negative control treatments. Bars indicate average ± SD. Asterisks indicate significant differences (p < 0.05) with the treatment with highest value (hv). Marked treatments (x) were excluded from the analyses due to the lack of replicates. Ratio of viable trochophores (A), ratio of normal development (B), and ratio of fertilization (C).
Sperm Concentration
The optimal sperm concentration for larval production (as a ratio of viable trochophores) was tested on P. aspera (assays 9–12). The optimal sperm concentration was 105 sperm cells ml−1 (Figure 8A). The ratio of normal development usually decreased with higher sperm concentrations (Figure 8B), while the ratio of fertilization decreased with lower sperm concentrations (Figure 8C). Similar results were described in P. ferruginea (Guallart et al., 2020b).
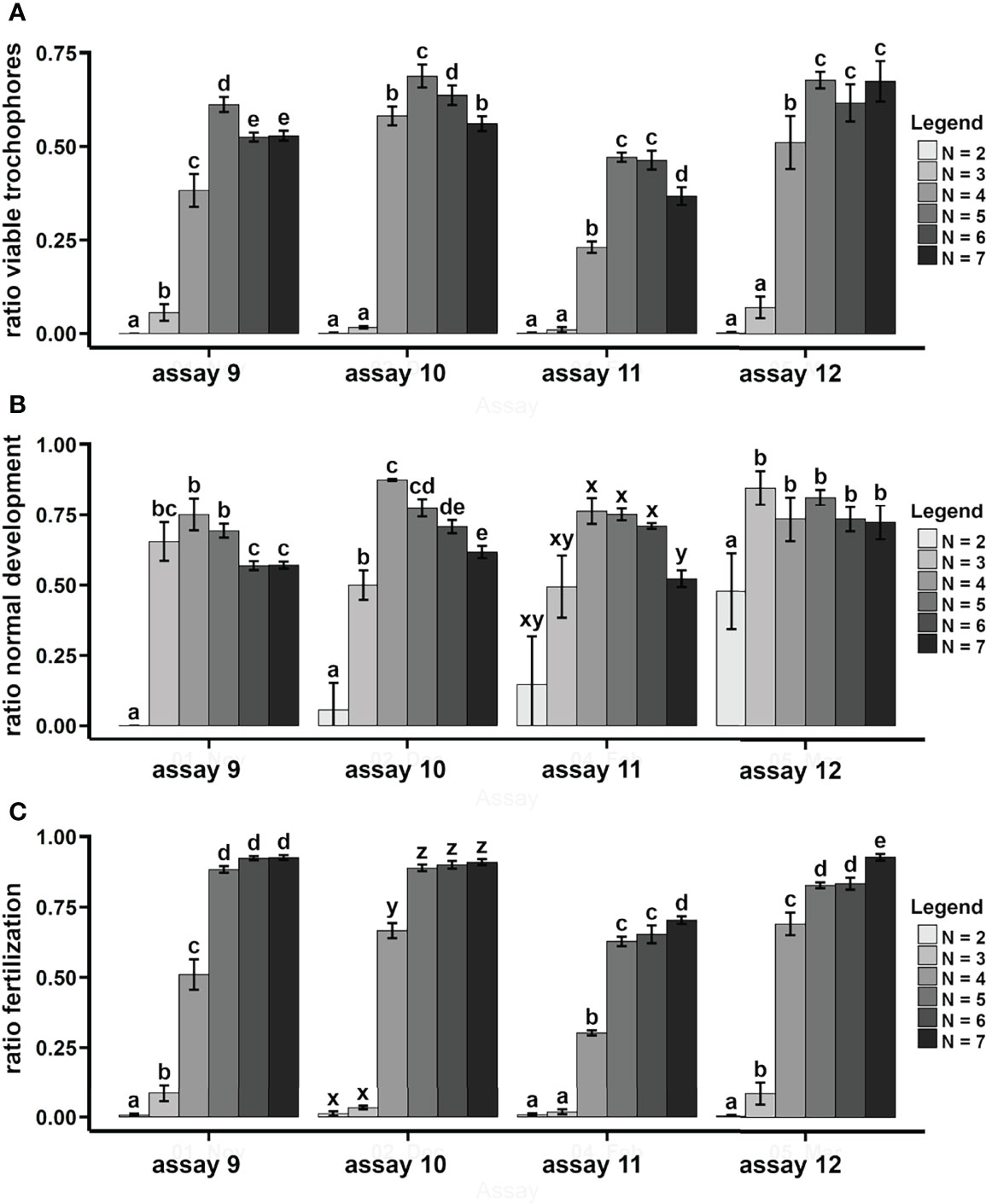
Figure 8 P. aspera. Influence of the sperm concentration on the larval production. Treatments were based on logarithmic increments on the sperm concentration: 10N sperm cells ml−1; N = 2, 3, 4, 5, 6 and 7. Bars indicate average ± SD. Different letters indicate significant differences among treatments (p < 0.05). Ratio of viable trochophores (A), ratio of normal development (B), and ratio of fertilization (C).
Larval Development and Larviculture
The larval development of P. aspera and P. candei is similar, in terms of both larval morphology and developmental time (Figure 9), except for the body coloration, which resembles that of the oocytes (Figures 5O–S). Incubation and larval culture were performed at 17 ± 1°C, and trochophores were observed 17 h post-fertilization. Trochophores showed an oval shape, an apical tuft of cilia, and prototroch cilia (Figures 9A–C). The veliger stage was observed 48 h post-fertilization, showing an oval velum and globular protoconch (Figures 9D–F). The pediveliger (last larval stage) was observed 72 h post-fertilization, showing eyespots, cephalic tentacles, foot, and operculum (Figures 9G–I). The larval morphology of P. aspera and P. candei and the timing of development resemble those described in other limpet species, e.g., Lottia asmi (Kay and Emlet, 2002), L. digitalis (Kay and Emlet, 2002), L. persona (Kolbin and Kulikova, 2011), L. tenuisculpta (Nakano et al., 2020), Patella depressa (Ribeiro, 2008), P. ferruginea (Guallart et al., 2020b; Ferranti et al., 2022), P. ulyssiponensis (Ribeiro, 2008), and P. vulgata (Aquino De Souza et al., 2009).
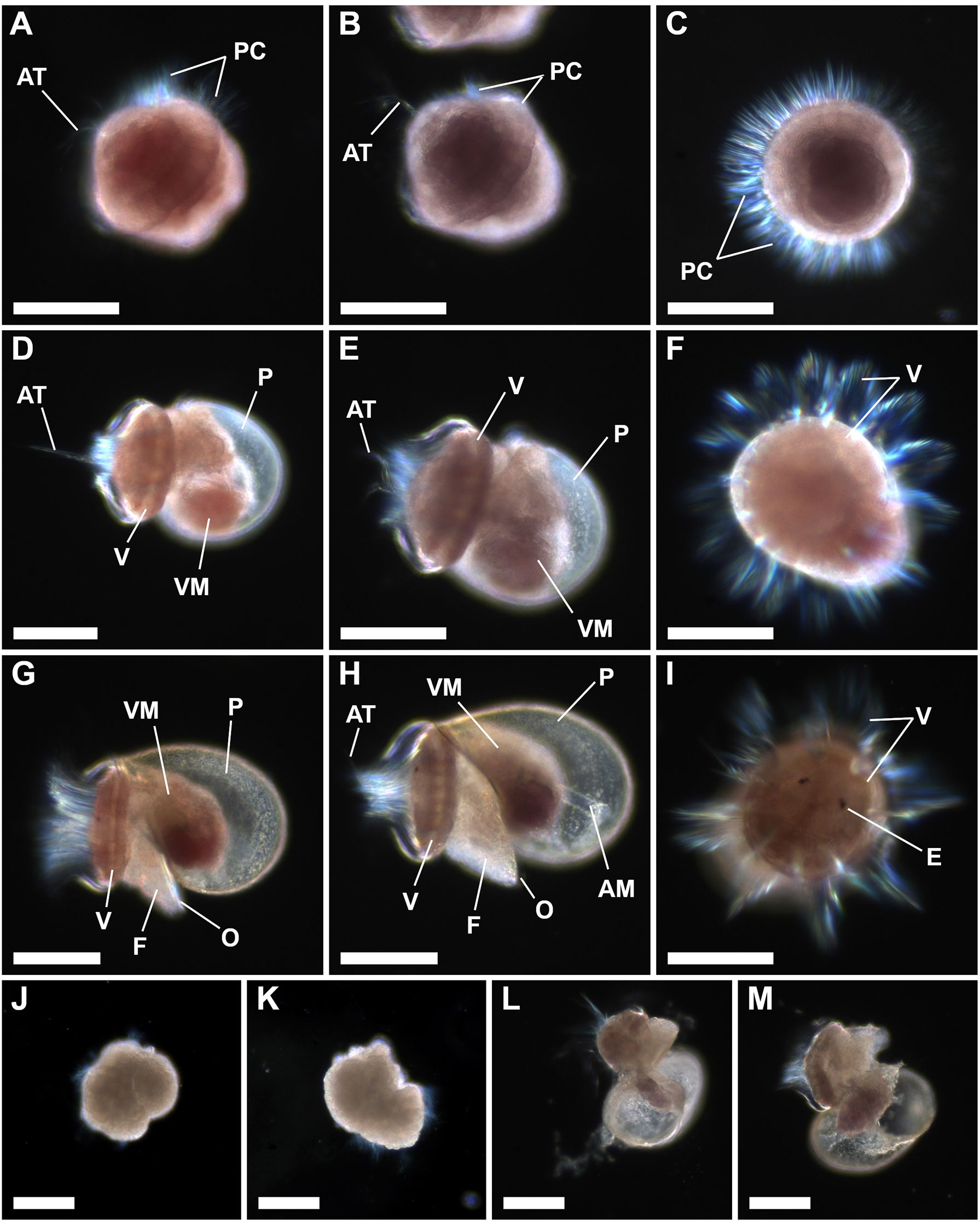
Figure 9 Larval development of the limpet species P. aspera and P. candei. Trochophore stage ca. 17–24 h post-fertilization (A–C): P. aspera in lateral view (A), P. candei in lateral view (B), and P. candei in frontal view (C). Veliger stage ca. 40–48 h post-fertilization (D–F): P. aspera in lateral view (D), P. candei in lateral view (E), and P. aspera in frontal view (F). Pediveliger stage ca. 72 h post-fertilization (G–I): P. aspera in lateral view (G), P. candei in lateral view (H), and P. aspera in frontal view (I). Deformed trochophores (J, K). Deformed pediveligers (L, M). Scale bar = 100 µm. AM, abductor muscles; AT, apical tuft; E, eyespot; P, protoconch; PC, prototroch cilia; V, velum; VM, visceral mass.
Larviculture Methodology
Larval culture of both P. aspera and P. candei started with trochophores within 24 ± 2 h post-fertilization. The trochophores were found to generally swarm near the water surface (Figures 2A, B; Video 2), allowing for siphoning using a plastic tube (4 mm diameter). The siphon output was released over a 55-µm mesh to capture the trochophores, and it is important to keep the mesh partially immersed to minimize the air exposure of the larvae (Figure 2C; Video 3). Siphoning the bottom of the culture containers should be avoided, as it is composed of debris, abnormal larvae, and unfertilized oocytes. In this sense, good results were obtained by siphoning the first 400 ml of seawater from beakers filled with 500 ml of seawater.
The same type of containers used for the alkaline treatment and the incubation were used successfully for larviculture during several trials. Previous studies used larval densities ranging from 1 larvae ml−1 (Ribeiro, 2008), 3–7 larvae ml−1 (Seabra et al., 2019), to 10–15 larvae ml−1 (Mau et al., 2018; Castejón et al., 2022; Ferranti et al., 2022). Nevertheless, particularly good results were obtained when using an initial density of approximately 5 larvae ml−1. The conditions for the larval culture were as follows: filtered seawater sterilized by ultraviolet or autoclaved (acronym: FSS), no aeration or water circulation (static), temperature ranging from 15 to 18°C, and natural salinity (36 ± 1 g L−1). Some authors have tried feeding limpet larvae to increase their survival and to test whether it was essential to feed them (Dodd, 1957; Ribeiro, 2008; Ferranti et al., 2018; Nhan and Ako, 2019). However, P. aspera and P. candei larvae were not fed, as they are probably lecitotrophic animals (Castejón et al., 2022), as in other limpet species (Seabra et al., 2019; Guallart et al., 2020b). The trochophores placed in the larviculture conditions previously described reached the pediveliger stage at 48 h (equivalent to 72 h post-fertilization). The pediveligers swim in the water column, so the same procedure used to collect the trochophores (siphoning and gathering using a 55-µm mesh) can be employed. It is recommended to collect the pediveligers at Day 3 post-fertilization because the crawling behavior of older larvae would interfere with the efficiency of the present methodology.
The present larviculture protocol shares certain similarities with previous protocols. In P. ferruginea, the trochophores were transferred (by siphoning or decantation) to different vessels, providing clean seawater for culture (Kay and Emlet, 2002; Ferranti et al., 2018; Guallart et al., 2020b; Ferranti et al., 2022). Some authors used a mesh to transfer the larvae to the culture containers (Ribeiro, 2008; Ferranti et al., 2018), while other authors preferred to remove the debris of the bottom instead of the larvae (Nakano et al., 2020). The water for larviculture can be exchanged routinely for maintenance (Ribeiro, 2008; Ferranti et al., 2018; Seabra et al., 2019); this step was not required for our research. The use of antibiotics such as streptomycin and penicillin to prevent fungal and microbial infections has been used and proposed (Wanninger et al., 1999), but larval development was completed successfully without using them.
Validation of the Larviculture Protocol
The present larviculture protocol has been used successfully for obtaining limpets larvae ready to settle and metamorphose (Castejón et al., 2022). Regardless of this good result, P. aspera was used as a model to test its reliability in terms of production yields.
Assay 13 showed that the final ratio of viable pediveligers was similar to the initial ratio of viable trochophores, with little or no variation between the initial and final larval densities (Table 2). Moreover, the final ratio of viable pediveligers did not vary with the original treatment of the oocytes (control: 0.71 ± 0.03; alkaline: 0.76 ± 0.03; t-test p = 0.06).
Assays 14–15 showed a significantly increased ratio of viable trochophores when alkaline treatment was applied (assay 14: control = 0.28 ± 0.05, alkaline = 0.72 ± 0.02, t-test p < 0.001; assay 15: control = 0.11 ± 0.01, alkaline = 0.35 ± 0.08, U-test p = 0.03), as reported in previous studies (Cañizares et al., 2021; Castejón et al., 2021). Regarding the efficiency of the siphoning method, the ratio of collected trochophores relative to the initial quantity of oocytes was greater when alkaline treatment was applied (assay 14: control = 0.31 ± 0.03, alkaline = 0.50 ± 0.08, p = 0.04; assay 15: control = 0.11 ± 0.03, alkaline = 0.36 ± 0.05, t-test p < 0.001).
In assays 14–15, the larviculture in plastic cups obtained similar results to that described in assay 13. The ratio of viable larvae was similar between the starting trochophores and the final pediveligers, without negative influence by the treatment of the oocytes (assay 14: t-test p = 0.07; assay 15: t-test p = 0.77; Table 2). Regarding the reliability of the glass beakers for the larval culture, the ratio of collected pediveligers relative to the initial quantity of trochophores was similar between treatments (assay 14: control = 0.64 ± 0.14, alkaline = 0.67 ± 0.15, t-test p = 0.82; assay 15: control = 0.41 ± 0.11, alkaline = 0.32 ± 0.08, t-test p = 0.22). Moreover, in assay 15, the quality of the pediveligers collected from the water column was greater than those from the bottom (control: column = 1.00 ± 0.01, bottom = 0.84 ± 0.03, t-test p < 0.001; alkaline: column = 0.99 ± 0.01, bottom = 0.77 ± 0.12, t-test p = 0.03).
Several conclusions can be extrapolated from these assays. The morphology is a valid criterion to recognize the viability of the trochophores. The reduced variation in larval density during culture suggests minimal mortality, as reported in Cellana exarata (Corpuz, 1981). The alkaline bath has no “carry over” effects on the resulting trochophores, supporting the safety of this technique to increase larval production. The limpets’ larvae might also be highly resilient, being able to tolerate several management techniques, including siphoning, filtering, pipetting, stirring, and resuspension. The first factor affecting larval production was female fertility, as it influences the production of viable trochophores. The second factor was the pediveliger behavior, as the quantity of swimming pediveligers influenced the efficiency of the siphoning. For laboratory studies, the stimulation of swimming in pediveligers would be useful for better production yields, i.e., the pediveligers from the water column showed a better quality.
Settlement and Metamorphosis in Limpets
Settlement and recruitment are key processes in which the planktonic larvae of benthic species must find a suitable site on the benthos to settle and metamorphose to the first post-larval stage (Rodríguez et al., 1993; Jenkins et al., 2009). The loss of the velum, the pediveliger swimming organ, has been used to define the onset of metamorphosis in several marine gastropods (McGee and Targett, 1989; Inestrosa et al., 1992; Searcy-Bernal et al., 1992; Davis, 1994; Roberts and Nicholson, 1997; Gallardo and Sánchez, 2001; Zhao and Qian, 2002; Salas‐Garza et al., 2009; Courtois de Vicose et al., 2010), including different limpet species, e.g., P. aspera (Castejón et al., 2022), P. caerulea (Dodd, 1957; Wanninger et al., 1999), P. ferruginea (Guallart et al., 2020b; Ferranti et al., 2022), and P. vulgata (Dodd, 1957; Wanninger et al., 1999). In limpets, teleoconches are another key characteristic whose presence is restricted to post-larvae (Kay and Emlet, 2002; Ferranti et al., 2018; Nakano et al., 2020; Castejón et al., 2022; Ferranti et al., 2022).
P. aspera and P. candei showed morphological and behavioral similarities during the settlement and metamorphic processes (Figure 10). The pediveligers showed several behaviors: swimming propelled by the velum cilia (Figures 10 A, E; Video 4), crawling using the foot (Figures 10B, F; Video 5), withdrawal inside the shell and enclosed by the operculum (Figures 10C, G), and resting inside the shell while supported by the foot (Figures 10D, H). The early post-larvae is the first post-larval and post-metamorphic stage (Castejón et al., 2022), identified by the loss of the velum and the operculum, and commonly found resting over different surfaces (Figures 10I, J, L, M; Video 6). The sooner settlement-competency might occur approximately 6 days post-fertilization, since early post-larvae were rarely observed sooner (Castejón et al., 2022). The morphology and behavior of the early post-larvae suggest a distinctive stage involving transformative changes for benthic life, including the development of the digestive structures required to ingest and digest particulate food (Castejón et al., 2022). The next post-larval stage is the juvenile stage (post-larvae with teleoconches), which is reported to be ca. 72 h post-settlement. The juveniles have a wider head, teleoconch, and marked digestive system (Figures 10K, N) (Castejón et al., 2022). The juveniles, as active grazers (Videos 7–8), represent the starting point for the grow-out phase.
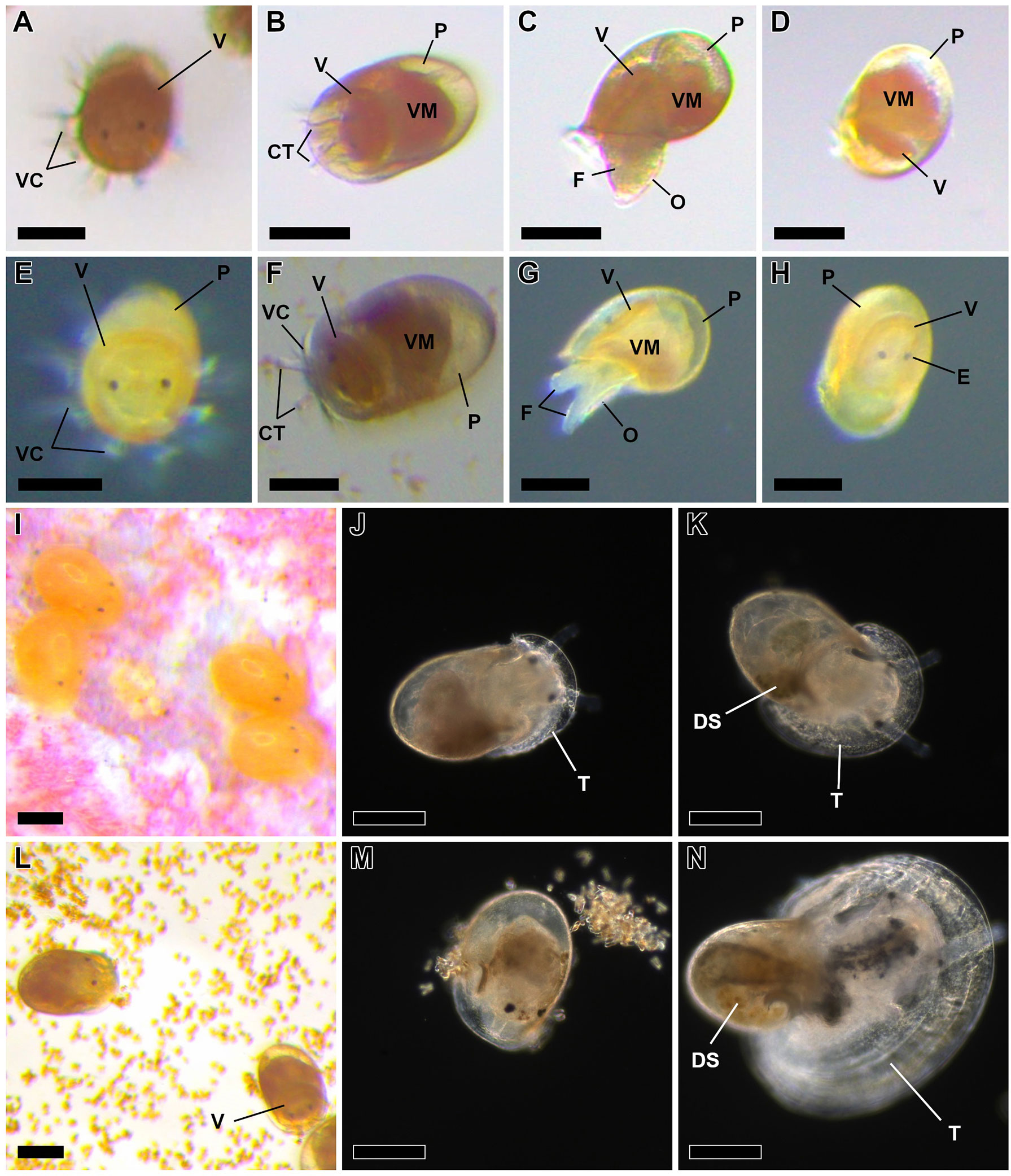
Figure 10 Settlement and metamorphosis in the limpet species P. aspera and P. candei. Key characters for the identification of the pediveligers and typical behaviors of this stage (A–H). P. aspera 4 days post-fertilization (A–D): swimming (A), crawling (B), withdraw (C), and resting (D). P. candei 6 days post-fertilization (E–H): swimming (E), crawling (F), withdraw (G), and resting (H). Comparison between the pediveliger and the post-larval stages (I–N). P. aspera (I–K): group of early post-larvae settled on encrusting coralline algae, 7 days post-fertilization (I); early post-larvae, 8 days post-fertilization (J); juvenile, 15 days post-fertilization (K). P. candei (L–N): early post-larvae (left) and crawling pediveliger (right) on Navicula incerta biofilm, 7 days post-fertilization (L); early post-larvae, 39 days post-fertilization (M); juvenile, 39 days post-fertilization (N). Scale bar = 100 µm. CT, cephalic tentacle; DS, digestive system; E, eyespot; F, foot; O, operculum; P, protoconch; T, teleoconch; V, velum; VC, velum cilia; VM, visceral mass.
Traditionally, the settlement process in limpets has been considered a bottleneck associated with high mortality (Corpuz, 1981), with little or no knowledge regarding their requirements and associated settlement cues (Mau and Jha, 2018). Considering the fundamental role of settlement and metamorphosis in the successful development of limpets aquaculture, the search for adequate settlement inducers was a major objective of the present research.
Settlement and Metamorphosis in P. aspera
Castejón et al. (2022) studied the suitability of several substrates as settlement inducers for P. aspera larvae. The results showed that encrusting coralline algae (CCA) of the order Corallinales shortened the timing for the earliest settlers, increased the daily number of settled specimens, and increased the final ratio of juveniles. In contrast, the same algal strains used for assay 16 did not show any influence on the settlement.
Settlement and Metamorphosis in P. candei
The results obtained in CMC (Madeira; assay 16) showed that N. incerta biofilms significantly promoted a greater ratio of juveniles (Figure 11A), reduced swimming activity (Figure 11B), peaked crawling activity (Figure 11C), and increased the ratio of settlers over time (Figure 11D). The CCA showed the second highest value for juveniles, but it was not significantly different from the control (Figure 11A). The CCA also reduced swimming activity (Figure 11B) and increased the ratio of settlers over time (Figure 11D). Moreover, the treatments of N. incerta biofilms and CCA showed a low mortality (Figure 11E). Overall, these results showed that biofilms of N. incerta can be useful as settlement substrates for the limpet P. candei, while the encrusting coralline algae showed promising potential to be elucidated in future studies.
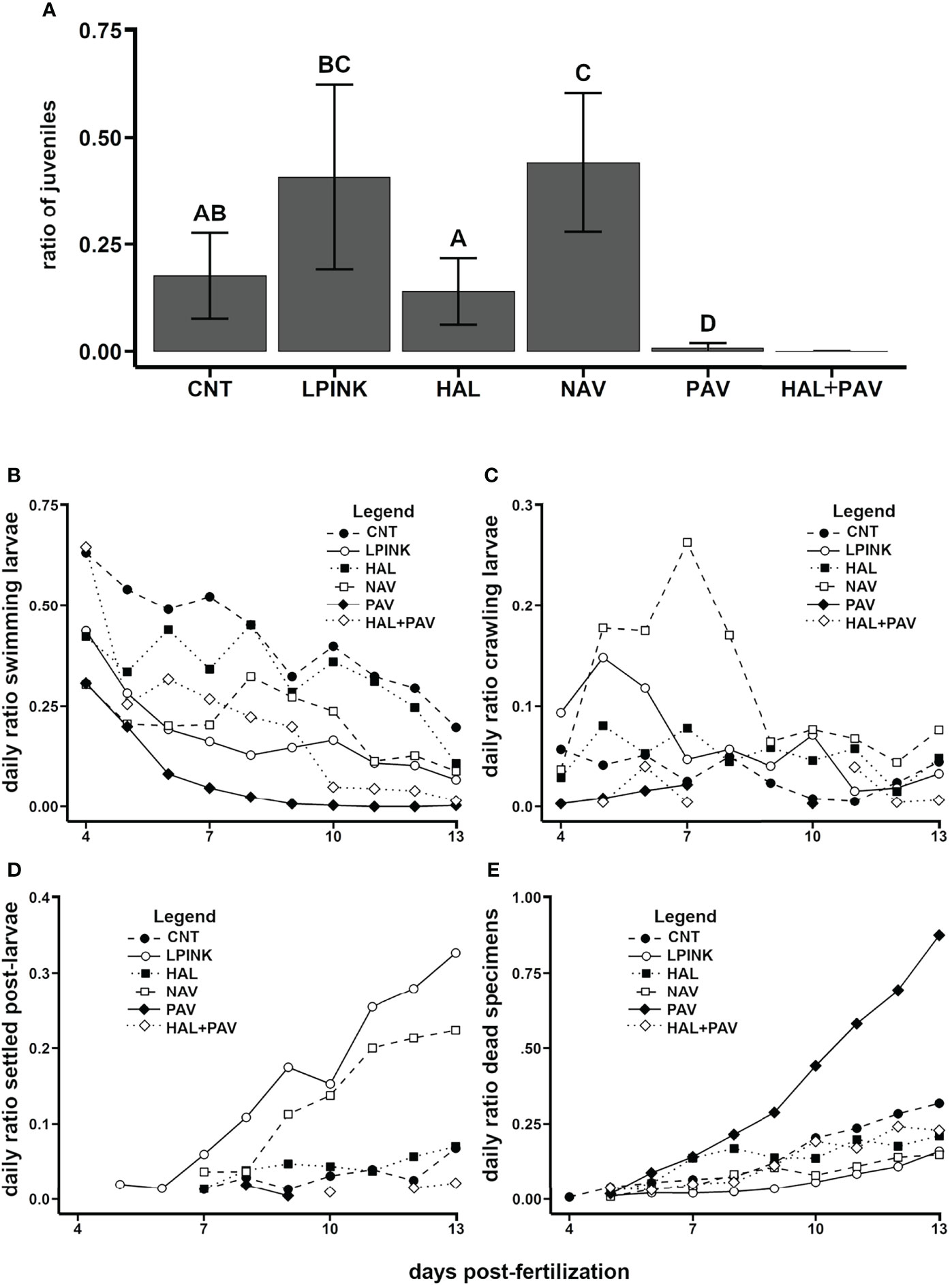
Figure 11 P. candei. Influence of different cultured algal strains and encrusting coralline algae on the settlement (study realized in Madeira). Ratio of juveniles obtained at the end of the assay, bars indicate average ± SD and different letters indicate significant differences (p < 0.01) (A). Daily average ratio: swimming (B) and crawling pediveligers (C), settled specimens (early post-larvae + juveniles) (D) and dead specimens (empty shells) (E). CNT, negative control treatment; HAL, diatom Halamphora coffeaeformis biofilm; HAL+PAV, combination of the treatments HAL and PAV; LPINK, coralline algae formed by light pink crusts; NAV, diatom Navicula incerta biofilm; PAV, free swimming algae Pavlova sp.
The results obtained in AquaLab (Azores; assay 17) reported a crawling phase extended up to Day 17 post-fertilization (Figure 12A), in which the pediveligers were recorded on all biofilms showing an exploratory behavior with no evident preference. Settlement and metamorphosis occurred between 17 and 30 days post-fertilization (Figure 12B), similar to the results reported in P. ferruginea when using cultured algae as a settlement substrate (Ferranti et al., 2022). The juveniles were recorded only on plates covered with Amphora sp. (1.7 ± 1.5 specimens), Ulvella lens (4.0 ± 3.5 specimens), and Ulvella leptochaete (0.3 ± 0.6 specimens) (Figure 12B; Video 8). After 2 months (64 days post-fertilization), the juveniles (n = 14) increased greatly in size (shell size length = 1.76 ± 0.46 mm), being observed exclusively on plates with U. lens (Figures 12B, 13C).
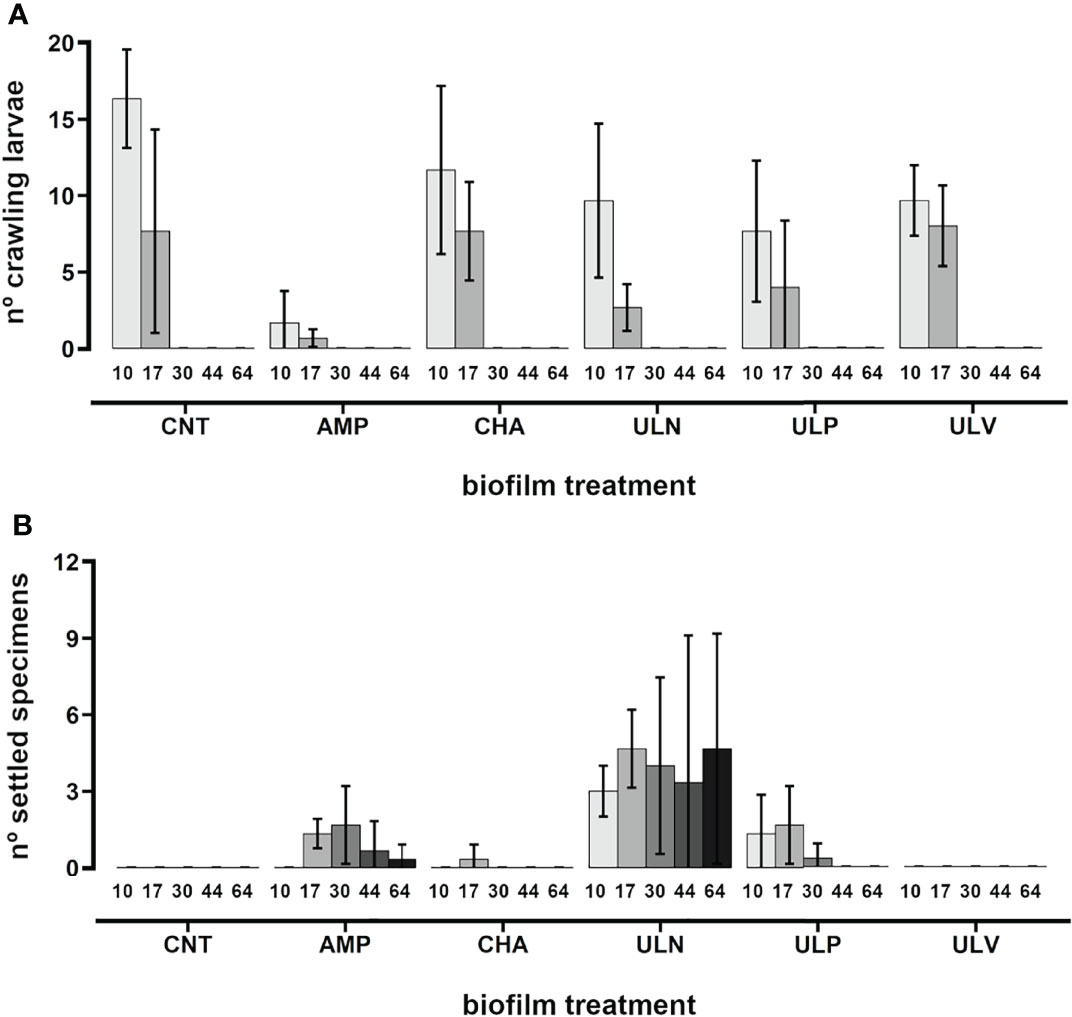
Figure 12 P. candei. Influence of different cultured algal strains on the settlement (study realized in Azores). Pediveliger larvae (no. of crawling pediveligers (A) and metamorphosed post-larvae and juveniles (no. of settled specimens (B) recorded during different days post-fertilization (10, 17, 30, 44, and 64 days) on different cultured algal biofilms. Bars indicate average ± SD. AMP, diatom Amphora sp.; CHA, diatom Chaetoceros sp.; CNT, negative control treatment; ULN, chlorophyte Ulvella lens; ULP, chlorophyte Ulvella leptochaete; ULV, chlorophyte Ulva sp.
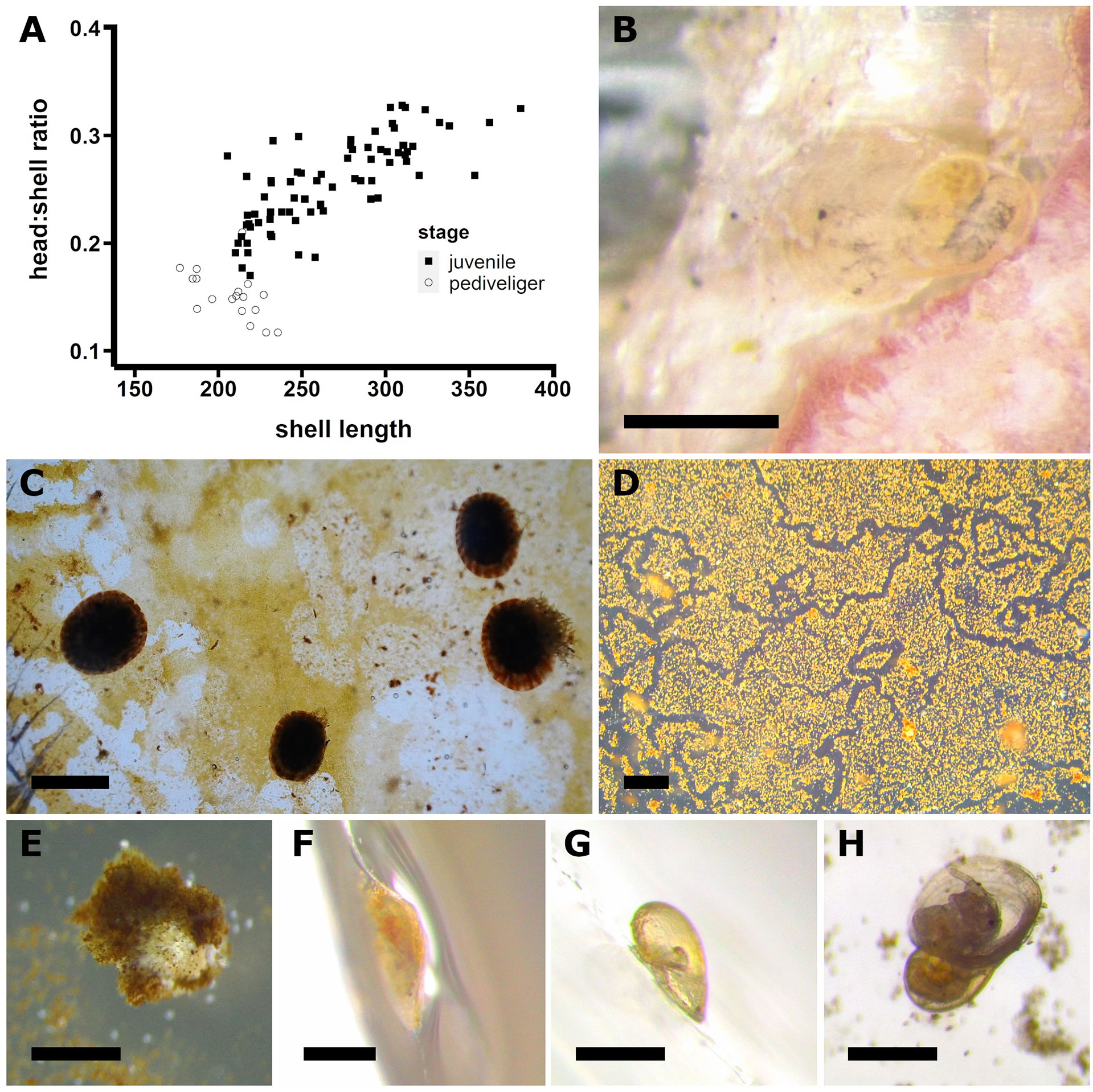
Figure 13 Post-metamorphic morphologic and behavioral changes observed in limpets. Variation of the head:shell ratio in relationship with the animal growth in P. aspera; head size was measured as eye distance relative to the shell length and shell length was measured as: protoconch + teleoconch (A). P. aspera juvenile 10 days post-settlement showing body pigmentation (B). P. candei juveniles around 2 months post-settlement showing shell pigmentation and grazing behavior on Ulvella lens biofilm (C). Grazing marks left by the juveniles of P. candei on Navicula incerta biofilm, 7 days post-settlement (D). P. aspera juvenile with the shell totally covered by surrounding environmental elements, ca. 7 weeks post-settlement (E). P. candei juvenile placed vertically and reaching the water level (F). P. candei juvenile desiccated due to stay above the water level (G). P. aspera juvenile accidentally upturned, 1 month post-settlement (H). Scale bar = 200 µm (B, F–H), 500 µm (E), and 1 mm (C, D).
Altogether, these results reveal important information for future studies focused on the settlement of different limpet species. Limpets’ larvae originally obtained from spawning induction (Nunes et al., 2021) or alkalinized oocytes (Castejón et al., 2022) develop successfully, reaching post-larval stages. The settlement response was triggered in P. aspera exclusively by encrusting coralline algae, whose effectiveness is influenced by the following factors: species, area coverage, and health status (Castejón et al., 2022). The lack of one or more of these factors could explain the unclear effectiveness of the CCA as a settlement inducer in P. candei. The importance of the Corallinales cannot be neglected, since this algal group can trigger mass settlement events (Video 6) and are recognized settlement inducers for several limpet species (Ribeiro, 2008) and other marine gastropods (Roberts and Nicholson, 1997; Daume et al., 1999; Moss, 1999; Roberts et al., 2004). In P. candei, the settlement response was triggered by biofilms formed by the diatom N. incerta and the chlorophyte U. lens. Such results are promising, as they are fast-growing algae that are easy to culture. Moreover, the post-larvae cultured with biofilms of U. lens biofilms reported fast growth, as they are tenfold the shell length in 2 months, implying the possibility of using this alga as an effective feeding source for the development of grow-out culture systems for limpets.
Management of the Post-Larvae and Grow-Out
The scientific literature provides scarce information about the management of the early post-larvae and juveniles of limpets, partly due to the limited success reaching these stages (Ferranti et al., 2018; Mau and Jha, 2018; Guallart et al., 2020b; Ferranti et al., 2022). This section resumes the major findings obtained during the present research in relation to the management of the post-larvae using P. aspera and P. candei as model species, which could be helpful for the development of improved protocols.
Post-metamorphic development includes several morphological changes. The head widening is proportionally greater than the animal growth in length (Figures 10I–N and 13A). The teleoconch starts as a thin line (Figure 10J), which gradually encloses posteriorly as a plane shield surrounding the animal body (Figures 10K, N). Body pigmentation was reported approximately 10 days post-settlement (Figure 13B), and shell pigmentation was reported 2 months post-settlement (Figure 13C). The timing for those developmental changes is more affected by the time after the settlement rather than animal age, i.e., specimens with the same post-fertilization age can show extreme differences if metamorphosis is delayed over time (Figures 10M, N).
The juveniles of P. aspera and P. candei showed features and behaviors to be considered for either production or experimental purposes. The widening of the head mentioned before (Figure 13A) probably implies the development of the radula and other mouth structures required to actively graze the surroundings, which include shedding of encrusting coralline algae (Castejón et al., 2022) and cultured biofilms (Figures 13C, D; Videos 7–8), suggesting non-selective grazing, as observed in adults (Branch, 1971; Della Santina et al., 1993; Burgos-Rubio et al., 2015). Several specimens were found to be partially or totally covered by different environmental elements resembling a cryptic strategy to avoid predation (Figure 13E; Video 7). The juvenile activity was restricted to a 2D environment where the specimens attach tightly and firmly to the surface below. The juveniles showed special affinity for the vertical surfaces, which increases the risk of mortality by desiccation (Figures 13F, G).
Direct manipulation of juveniles is not recommended since no reliable methods for animal detachment are available. Moreover, juveniles lying on the back of their shell are unable to recover their normal position (Figure 13H; Video 9). If individual management is needed, selective pipetting (using a plastic pipette with the tip cut) showed limited success on already detached specimens (Video 10). The juveniles attach firmly to the surface when threatened, so the optimal solution would be to move the surfaces instead of the specimens themselves.
Petri Dishes as Culture Containers for Limpet Post-Larvae (Assay 18)
In P. candei, approximately 1 month after the start of the assay (Day 30 post-fertilization), the ratio of juveniles was 0.37 ± 0.04, corresponding to 111 juveniles distributed on four Petri dishes. Swimming pediveligers and early post-larvae were reported as well. This observation suggests that limpets can maintain the pediveliger stage for a long time without disabling metamorphosis, as reported by Ferranti et al. (2022), which would allow long dispersal in wild conditions. More than 80% of the juveniles were observed over the vertical wall of the Petri dishes, limiting the possibilities to measure the animal growth (Figure 13F) and increasing the mortality by desiccation (Figure 13G). The assay was terminated because the biofilm destabilized without recovery. In conclusion, we do not recommend the Petri dishes as culture containers for limpet post-larvae. Alternatively, the Petri dishes have been used as additional surfaces placed inside larger culture containers (Guallart et al., 2017; Guallart et al., 2020b; Ferranti et al., 2022). The use of vertical plates in culture tanks is an alternative to be tested.
Although the same algal culture and management conditions were used for P. aspera and P. candei, P. aspera did not show any settlement response in N. incerta biofilms, coinciding with a previous study (Castejón et al., 2022).
Challenges and Future Goals
Traditionally, several bottlenecks were present in limpets when considering adult management, gamete release, larviculture and settlement, and metamorphosis induction. The present manuscript represents a significant advance in all these fields, marking a starting point for the aquaculture of different limpet species. Several methodologies were tested, adapted, and optimized for the native species of the Macaronesia region, P. aspera and P. candei, i.e., a methodology for experimental larval production is presented, establishes a larviculture protocol, presents successful settlement inducers, and shows the management and feeding requirements of the post-metamorphic stages. In a few years, it might be possible to close the life cycle of different limpet species and establish large-scale production.
However, researchers have a profuse field to be explored. We acknowledge those authors who previously worked hard on the subject and highlighted many of the specializations and limpets’ requirements. The spawning methods should be polished and optimized; in this sense, the studies realized in P. ferruginea show potential, and the use of hormonal injections should be deeply studied. The determination of female fertility would be important to determine oocyte quality and expected larval productivity. Larviculture methods need to be expanded to allow reliable large-scale production of larvae. The settlement and the grow-out of juveniles are fully experimental, requiring more research before reaching large-scale production levels. In this sense, some of the techniques used for abalone could be adapted for limpets.
Data Availability Statement
The raw data supporting the conclusions of this article will be made available by the authors upon reasonable request.
Ethics Statement
The authors confirm that the ethical policies of the journal, as noted on the journal’s author guidelines page, have been adhered to. All applicable international, national, and/or institutional guidelines for the care and use of animals were followed.
Author Contributions
DC: animal management, experimental design, experiment realization, sampling, analysis of samples, statistical analyses, pictures design, and drafted paper. MG: animal management, experiment realization, sampling, analysis of samples, and drafted paper review. JC: animal management, experimental design, sampling, analysis of samples, and drafted paper review. CN: animal management, experiment realization, sampling, analysis of samples, and drafted paper review. MG: animal management, experimental design, experiment realization, sampling, analysis of samples, statistical analyses, pictures design, drafted paper review, and project elaboration. EI: drafted paper review, project elaboration, coordination, and direction. GV: drafted paper review, project elaboration, coordination, and direction. NN: drafted paper review and project elaboration. CA: drafted paper review, project elaboration, coordination, and direction. All authors contributed to the article and approved the submitted version.
Funding
Financial support was provided by the projects: 1) “AQUAINVERT - Development of sustainable, integrated and innovative aquaculture in Macaronesia. Research and Development to promote the production of marine invertebrates of commercial interest (MAC/1. 1a/282)” founded by FEDER under the INTERREG MAC 2014-2020 program; and 2) “ISLANDAP ADVANCED - R+D+I towards aquaponic development in the up islands and the circular economy. Interregional Forward Challenges (MAC2/1.1a/299)”. DC was supported by a grant under the project AQUAINVERT.
Conflict of Interest
The handling editor SL declared a past co-authorship with the author CA.
The remaining authors declare that the research was conducted in the absence of any commercial or financial relationships that could be construed as a potential conflict of interest.
Publisher’s Note
All claims expressed in this article are solely those of the authors and do not necessarily represent those of their affiliated organizations, or those of the publisher, the editors and the reviewers. Any product that may be evaluated in this article, or claim that may be made by its manufacturer, is not guaranteed or endorsed by the publisher.
Acknowledgments
The sampling of limpets for scientific purposes during closed season was authorized by the Fisheries Directorate, Secretary for the Sea and Fisheries, Regional Government of Madeira. The authors would like to thank the technicians at the Mariculture Center of Calheta (CMC) and at the Experimental Laboratory of Aquaculture (AquaLab) for their assistance. The authors would like to thank the local fishermen of Calheta for helping provide the specimens. The authors would also like to thank Alfonso A. Ramos, Francisca Giménez, Carmen Barberá, and Andrés Izquierdo-Muñoz, for the opportunity to use the installations of the Research Marine Centre in Santa Pola (Spain).
Supplementary Material
The Supplementary Material for this article can be found online at: https://www.frontiersin.org/articles/10.3389/fmars.2022.884262/full#supplementary-material
Supplementary Figure 1 | P. aspera. Second test to study the influence of different alkaline baths on the larval production. Treatments combined different alkaline agents (NaOH and NH4OH), pH (8.4 and 8.9), and bath durations (10, 20, 30, 60, 120, and 180 min), plus negative control treatments. Bars indicate average ± SD. Asterisks indicate significant differences (p < 0.05) with the treatment with highest value (hv). Ratio of viable trochophores (A), ratio of normal development (B), and ratio of fertilization (C).
References
Aquino De Souza R., Tyler P., Hawkins S. J. (2009). Artificial Oocyte Maturation in Patella Depressa and Patella Vulgata Using NaOH-Alkalinized Seawater. Mar. Biol. Res. 5 (5), 503–510. doi: 10.1080/17451000802603645
Benedetti-Cecchi L. (2000). Predicting Direct and Indirect Interactions During Succession in a Mid-Littoral Rocky Shore Assemblage. Ecol. Monogr. 70 (1), 45–72. doi: 10.1890/0012-9615(2000)070[0045:PDAIID]2.0.CO;2
Blackmore D. (1969). Studies of Patella Vulgata L. I. Growth, Reproduction and Zonal Distribution. J. Exp. Mar. Biol. 3 (2), 200–213. doi: 10.1016/0022-0981(69)90018-5
Branch G. M. (1971). The Ecology of Patella Linnaeus From the Cape Peninsula, South Africa I. Zonation, Movements and Feeding. Afr. Zoology 6 (1), 1–38. doi: 10.1080/00445096.1971.11447402
Branch G. M. (1975). Notes on the Ecology of Patella Concolor and Cellana Capensis, and the Effects of Human Consumption on Limpet Populations. Afr. Zoology 10 (1), 75–85. doi: 10.1080/00445096.1975.11447494
Branch G. M. (1981). The Biology of Limpets: Physical Factors, Energy Flow, and Ecological Interactions. Oceanog. Mar. Biol.: Annu. Rev. 192, 235–280.
Branch G. M. (1985). “Limpets: Evolution and Adaptation,” in The Mollusca. Eds. Trueman E., Clarke M. (New York: Academic Press, Inc), 187–220.
Branch G., Odendaal F. (2003). The Effects of Marine Protected Areas on the Population Dynamics of a South African Limpet, Cymbula Oculus, Relative to the Influence of Wave Action. Biol. Conserv. 114 (2), 255–269. doi: 10.1016/S0006-3207(03)00045-4
Bulleri F., Menconi M., Cinelli F., Benedetti-Cecchi L. (2000). Grazing by Two Species of Limpets on Artificial Reefs in the Northwest Mediterranean. J. Exp. Mar. Biol. Ecol. 255 (1), 1–19. doi: 10.1016/S0022-0981(00)00275-6
Burgos-Rubio V., de la Rosa J., Altamirano M., Espinosa F. (2015). The Role of Patellid Limpets as Omnivorous Grazers: A New Insight Into Intertidal Ecology. Mar. Biol. 162 (10), 2093–2106. doi: 10.1007/s00227-015-2739-0
Cañizares J. M., Castejón D., Haroun R., Nogueira N., Andrade C. A. P. (2021). Enhancing Oocyte Maturation and Fertilisation in the Black-Foot Limpet Patella Candei D′Orbigny 1840 (Patellidae, Mollusca). Aquacul. Rep. 21, 100856. doi: 10.1016/j.aqrep.2021.100856
Carballo J. L., Yáñez B., Bautista-Guerrero E., García-Gómez J. C., Espinosa F., Tortolero-Langarica J. J., et al. (2020). Decimation of a Population of the Endangered Species Scutellastra Mexicana (Broderip and Sowerby 1829) (Mollusca, Gastropoda) in the Marías Island (Eastern Ocean Pacific) Biosphere Reserve. Aquat. Conservation: Mar. Freshw. Ecosyst. 30 (1), 20–30. doi: 10.1002/aqc.3239
Castejón D., Cañizares J. M., Nogueira N., Andrade C. A. P. (2021). Artificial Maturation and Larval Production of the Limpet Patella Aspera Röding 1798(Patellogastropoda, Mollusca): Enhancing Fertilization Success of Oocytes Using NaOH-Alkalinized Seawater. Aquacul. Res. 52 (5), 1904–1914. doi: 10.1111/are.15039
Castejón D., Nogueira N., Andrade C. A. P. (2022). Limpet Larvae (Patella Aspera Röding 1798), Obtained by Gonad Dissection and Fecundation In Vitro, Settled and Metamorphosed on Crustose Coralline Algae. J. Mar. Biol. Assoc. UK 101(7), 991–1002. doi: 10.1017/S0025315421000916
Coleman R. A., Underwood A. J., Benedetti-Cecchi L., Åberg P., Arenas F., Arrontes J., et al. (2006). A Continental Scale Evaluation of the Role of Limpet Grazing on Rocky Shores. Oecologia 147 (3), 556–564. doi: 10.1007/s00442-005-0296-9
Colonese A. C., Mannino M. A., Mayer D. B.-Y., Fa D., Finlayson J., Lubell D., et al. (2011). Marine Mollusc Exploitation in Mediterranean Prehistory: An Overview. Quat. Int. 239 (1-2), 86–103. doi: 10.1016/j.quaint.2010.09.001
Corpuz G. C. (1981). Laboratory Culture of Cellana Exarata Reeve (Gastropoda: Prosobranchia, Patellidae). Aquaculture 24, 219–231. doi: 10.1016/0044-8486(81)90058-2
Courtois de Vicose G., Viera M., Bilbao A., Izquierdo M. (2007). Embryonic and Larval Development of Haliotis Tuberculata Coccinea Reeve: An Indexed Micro-Photographic Sequence. J. Shellfish Res. 26 (3), 847–854. doi: 10.2983/0730-8000(2007)26[847:EALDOH]2.0.CO;2
Courtois de Vicose G., Viera M., Bilbao A., Izquierdo M. (2010). Larval Settlement of Haliotis Tuberculata Coccinea in Response to Different Inductive Cues and the Effect of Larval Density on Settlement, Early Growth, and Survival. J. Shellfish Res. 29 (3), 587–591. doi: 10.2983/035.029.0306
Creese R., Schiel D., Kingsford M. (1990). Sex Change in a Giant Endemic Limpet, Patella Kermadecensis, From the Kermadec Islands. Mar. Biol. 104 (3), 419–426. doi: 10.1007/BF01314345
Curdia J., Rodrigues A. S., Martins A., Costa M. (2005). The Reproductive Cycle of Patella Candei Gomesii Drouët 1858 (Mollusca: Patellogastropoda), an Azorean Endemic Subspecies. Invertebr. Reprod. Dev. 48 (1-3), 137–145. doi: 10.1080/07924259.2005.9652180
Daume S., Brand-Gardner S., Woelkerling W. J. (1999). Preferential Settlement of Abalone Larvae: Diatom Films vs. non-Geniculate Coralline Red Algae. Aquaculture 174 (3), 243–254. doi: 10.1016/S0044-8486(99)00003-4
Davies P. S. (1969). Physiological Ecology of Patella. III. Desiccation Effects. J. Mar. Biol. Assoc. United Kingdom 49 (2), 291–304. doi: 10.1017/S0025315400035918
Davis M. (1994). Short-Term Competence in Larvae of Queen Conch Strombus Gigas: Shifts in Behavior, Morphology and Metamorphic Response. Mar. Ecol. Prog. Ser. 104, 101–101. doi: 10.3354/meps104101
Della Santina P., Sonni C., Sartoni G., Chelazzi G. (1993). Food Availability and Diet Composition of Three Coexisting Mediterranean Limpets (Patella Spp.). Mar. Biol. 116 (1), 87–95. doi: 10.1007/BF00350735
Dethier M. N. (1994). The Ecology of Intertidal Algal Crusts: Variation Within a Functional Group. J. Exp. Mar. Biol. Ecol. 177 (1), 37–71. doi: 10.1016/0022-0981(94)90143-0
Diogo H., Pereira J. G., Schmiing M. (2016). Catch Me If You can: Non-Compliance of Limpet Protection in the Azores. Mar. Policy 63, 92–99. doi: 10.1016/j.marpol.2015.10.007
Dodd J. M. (1956). Studies on the Biology of Limpets: III. Hermaphroditism in the Three British Species of Patella. J. Mar. Biol. Assoc. United Kingdom 35 (2), 327–340. doi: 10.1017/S002531540001016X
Dodd J. M. (1957). Artificial Fertilisation, Larval Development and Metamorphosis in Patella Vulgata L. And Patella Caerulea L. Pubblicazioni della Stazione Zoologica di Napoli 29, 172–185.
Dopchiz L. P., Ansaldo M., Genovese G. (2018). Gonadal Histology and Gametogenesis of the Antarctic Limpet Nacella Concinna (Patellogastropoda, Nacellidae) Collected at Potter Cove, 25 De Mayo (King George) Island, During Austral Summer. Polar Biol. 41 (9), 1751–1762. doi: 10.1007/s00300-018-2314-5
Ebert E. E., Houk J. L. (1984). Elements and Innovations in the Cultivation of Red Abalone Haliotis Rufescens. Aquaculture 39 (1), 375–392. doi: 10.1016/0044-8486(84)90279-5
Erlandson J. M., Braje T. J., Rick T. C., Jew N. P., Kennett D. J., Dwyer N., et al. (2011). 10,000 Years of Human Predation and Size Changes in the Owl Limpet (Lottia Gigantea) on San Miguel Island, California. J. Archaeol. Sci. 38 (5), 1127–1134. doi: 10.1016/j.jas.2010.12.009
Espinosa F., Guerra-Garcia J. M., Fa D., Garcia-Gomez J. C. (2006). Aspects of Reproduction and Their Implications for the Conservation of the Endangered Limpet, Patella Ferruginea. Invertebr. Reprod. Dev. 49 (1-2), 85–92. doi: 10.1080/07924259.2006.9652197
Espinosa F., Rivera-Ingraham G. A. (2017). “Chapter Three - Biological Conservation of Giant Limpets: The Implications of Large Size,” in Advances in Marine Biology. Ed. Curry B. E. ( United Kingdom & United States: Academic Press), 105–155.
Espinosa F., Rivera-Ingraham G., Fa D., García-Gómez J. (2009a). Effect of Human Pressure on Population Size Structures of the Endangered Ferruginean Limpet: Toward Future Management Measures. J. Coastal Res. 25(4 (4 (254), 857–863. doi: 10.2112/08-1005.1
Espinosa F., Rivera-Ingraham G., García-Gómez J. C. (2009b). Gonochorism or Protandrous Hermaphroditism? Evidence of Sex Change in the Endangered Limpet Patella Ferruginea. Mar. Biodivers. Rec. 2, e153. doi: 10.1017/S1755267209990790
Espinosa F., Rivera-Ingraham G. A., Maestre M., González A. R., Bazairi H., García-Gómez J. C. (2014). Updated Global Distribution of the Threatened Marine Limpet Patella Ferruginea (Gastropoda: Patellidae): An Example of Biodiversity Loss in the Mediterranean. Oryx 48 (2), 266–275. doi: 10.1017/S0030605312000580
Fernandes I., Fernandes T., Cordeiro N. (2019). Nutritional Value and Fatty Acid Profile of Two Wild Edible Limpets From the Madeira Archipelago. Eur. Food Res. Technol. 245 (4), 895–905. doi: 10.1007/s00217-019-03234-y
Ferranti M. P., Guallart J., Fanciulli G., Panzalis P. A., Chiantore M. (2022). Advancements Towards Restoration of the Endangered Limpet Patella Ferruginea Gmelin 1791 Through Controlled Reproduction. Aquacul. Res. 53 (3), 782–798. doi: 10.1111/are.15614
Ferranti M. P., Monteggia D., Asnaghi V., Chiantore M. (2018). Artificial Reproduction Protocol, From Spawning to Metamorphosis, Through Noninvasive Methods in Patella Caerulea Linnaeus 1758. Aquacul. Res. 49 (10), 3386–3391. doi: 10.1111/are.13802
Ferraz R. R., Menezes G. M., Santos R. S. (2001). Limpet (Patella Spp.) (Mollusca: Gastropoda) Exploitation in the Azores, During the Period 1993-1998. Arquipélago. Life Mar. Sci. Supplement 2 (Part B), 59–65.
Firth L. B. (2021). What Have Limpets Ever Done for Us? On the Past and Present Provisioning and Cultural Services of Limpets. Int. Rev. Environ. Hist. 7 (2), 5–45. doi: 10.22459/IREH.07.02.2021.01
Gallardo C. S., Sánchez K. A. (2001). Induction of Metamorphosis and its Effect on the Growth and Survival of Postmetamorphic Juveniles of Chorus Giganteus (Gastropoda: Muricidae). Aquaculture 201 (3-4), 241–250. doi: 10.1016/S0044-8486(01)00611-1
González-Lorenzo G., Hernández E. M., Pérez-Dionis G., Hernández A. B., Santos B. G., Diez J. B. (2015). Ineffective Conservation Threatens Patella Candei, an Endangered Limpet Endemic to the Macaronesian Islands. Biol. Conserv. 192, 428–435. doi: 10.1016/j.biocon.2015.11.005
González-Novoa P. R. (2014). Fecundación in vitro de gametos de lapa. Final Undergraduate Project, Universidade da Coruña.
Gould M. C., Stephano J. L., Ortíz-Barrón B., Pérez-Quezada I. (2001). Maturation and Fertilization in Lottia Gigantea Oocytes: Intracellular Ph, Ca2+, and Electrophysiology. J. Exp. Zoology 290 (4), 411–420. doi: 10.1002/jez.1082
Guallart J., Acevedo I., Calvo Revuelta M., Machordom A. (2013a). Protocolo No Letal Para La Obtención De Muestras De Tejido (Para Estudios Genéticos) En La Lapa Amenazada Patella Ferruginea (Mollusca, Patellidae). Iberus 31 (2), 171–174.
Guallart J., Calvo Revuelta M., Acevedo I., Templado J. (2013b). Two-Way Sex Change in the Endangered Limpet Patella Ferruginea (Mollusca, Gastropoda). Invertebr. Reprod. Dev. 57, 247–253. doi: 10.1080/07924259.2012.754794
Guallart J., Ferranti M. P., Bacigalupo L., Chiantore M. (2020a). In Vivo Magnetic Resonance Imaging to Assess the Sexual Maturity of the Endangered Limpet Patella Ferruginea. J. Molluscan Stud. 86 (4), 422–426. doi: 10.1093/mollus/eyaa021
Guallart J., Luque Á.A., Acevedo I., Calvo M. (2013c). Distribución Y Censo Actualizado De La Lapa Ferrugínea (Patella Ferruginea Gmelin 1791) En El Litoral De Melilla (Mediterráneo Suroccidental). Iberus 31 (1), 21–51.
Guallart J., Pena J. B., Luque A. A., Templado J. (2017). Where Have All the Youngest Gone? The Postlarval and Young Stages of the Mediterranean Endangered Limpet Patella Ferruginea Gmelin 1791. Mediterr. Mar. Sci. 18 (3), 385–392. doi: 10.12681/mms.2076
Guallart J., Peña J., Pérez-Larruscaín J., Luque Á., Templado J. (2020b). Filling Gaps: Closing the Life Cycle of the Endangered Mediterranean Limpet Patella Ferruginea Gmelin 1791 (Gastropoda, Patellidae). Mediterr. Mar. Sci. 21 (2), 400–419. doi: 10.12681/mms.22508
Guerra-García J. M., Corzo J., Espinosa F., García-Gómez J. C. (2004). Assessing Habitat Use of the Endangered Marine Mollusc Patella Ferruginea (Gastropoda, Patellidae) in Northern Africa: Preliminary Results and Implications for Conservation. Biol. Conserv. 116 (3), 319–326. doi: 10.1016/S0006-3207(03)00201-5
Harada K., Miyasaki T., Maeda H., Satoh K. (1997). Statistical Estimation of Probable Feeding Attractive Components in Fishing Baits for Abalone. Aquacul. Sci. 45 (4), 533–537. doi: 10.11233/aquaculturesci1953.47.455
Harasewych M. G., McArthur A. (2000). A Molecular Phylogeny of the Patellogastropoda (Mollusca: Gastropoda). Mar. Biol. 137 (2), 183–194. doi: 10.1007/s002270000332
Harley C. D. G., Denny M. W., Mach K. J., Miller L. P. (2009). Thermal Stress and Morphological Adaptations in Limpets. Funct. Ecol. 23 (2), 292–301. doi: 10.1111/j.1365-2435.2008.01496.x
Henriques P., Delgado J., Sousa R., Ray S. (2017). “Patellid Limpets: An Overview of the Biology and Conservation of Keystone Species of the Rocky Shores,” in Organismal and Molecular Malacology ( London: Intech Croatia), 71–95.
Henriques P., Sousa R., Pinto A., Delgado J., Faria G., Alves A., et al. (2012). Life History Traits of the Exploited Limpet Patella Candei (Mollusca: Patellogastropoda) of the North-Eastern Atlantic. J. Mar. Biol. Assoc. United Kingdom 92 (6), 1379–1387. doi: 10.1017/S0025315411001068
Hodgson A. N., Le Quesne W. J., Hawkins S. J., Bishop J. D. (2007). Factors Affecting Fertilization Success in Two Species of Patellid Limpet (Mollusca: Gastropoda) and Development of Fertilization Kinetics Models. Mar. Biol. 150 (3), 415–426. doi: 10.1007/s00227-006-0354-9
Inestrosa N. C., Gonzalez M., Campos E. O. (1992). Metamorfosis De Larvas Planctonicas De Concholepas Concholepas ("Loco") (Mollusca; Gastropoda; Muricidae). Rev. Biología Marina y Oceanografía 27 (2), 249–263.
Jenkins S. R., Marshall D., Fraschetti S. (2009). “Settlement and Recruitment,” in Marine Hard Bottom Communities: Patterns, Dynamics, Diversity, and Change. Ed. Wahl M. (Berlin, Heidelberg: Springer Berlin Heidelberg), 177–190.
Jernakoff P. (1985). Interactions Between the Limpet Patelloida Latistrigata and Algae on an Intertidal Rock Platform. Mar. Ecol. Prog. series. Oldendorf 23 (1), 71–78.
Kay M. C., Emlet R. B. (2002). Laboratory Spawning, Larval Development, and Metamorphosis of the Limpets Lottia Digitalis and Lottia Asmi (Patellogastropoda, Lottiidae). Invertebr. Biol. 121 (1), 11–24. doi: 10.1111/j.1744-7410.2002.tb00125.x
Klein R. G. (1979). Stone Age Exploitation of Animals in Southern Africa: Middle Stone Age People Living in Southern Africa More Than 30,000 Years Ago Exploited Local Animals Less Effectively Than the Later Stone Age People Who Succeeded Them. Am. Sci. 67 (2), 151–160.
Klein R. G., Steele T. E. (2013). Archaeological Shellfish Size and Later Human Evolution in Africa. Proc. Natl. Acad. Sci. 110 (27), 10910–10915. doi: 10.1073/pnas.1304750110%
Kolbin K. G., Kulikova V. A. (2011). Reproduction and Larval Development of the Limpet Lottia Persona (Rathke 1833) (Gastropoda: Lottiidae). Russian J. Mar. Biol. 37 (3), 239–242. doi: 10.1134/s1063074011030072
Lasiak T. (1993). The Shellfish-Gathering Practices of Indigenous Coastal People in Transkei: Patterns, Preferences and Perceptions. South Afr. J. Ethnol. 16 (4), 115–120. doi: 10520/AJA02580144_669
Lindberg D. R. (2007). Reproduction, Ecology, and Evolution of the Indo-Pacific Limpet Scutellastra Flexuosa. Bull. Mar. Sci. 81 (2), 219–234.
López C., Poladura A., Hernández J. C., Martín L., Concepción L., Sangil C., et al. (2012). Contrasting Effects of Protection From Harvesting in Populations of Two Limpet Species in a Recently Established Marine Protected Area. Scientia. Mar. 76 (4), 799–807. doi: 10.3989/scimar.03601.15C
Lorenzen S. (2007). The Limpet Patella Vulgata L. At Night in Air: Effective Feeding on Ascophyllum Nodosum Monocultures and Stranded Seaweeds. J. Molluscan Stud. 73 (3), 267–274. doi: 10.1093/mollus/eym022
Luque Á.A., Guallart J., González J. T., Pola M. (2018). Recopilación Y Análisis De La Información Científica Disponible Sobre Patella Ferruginea (Spain: Sociedad Española de Malacología).
Martins G. M., Borges C. D., Vale M., Ribeiro P. A., Ferraz R. R., Martins H. R., et al. (2017). Exploitation Promotes Earlier Sex Change in a Protandrous Patellid Limpet, Patella Aspera Röding 1798. Ecol. Evol. 7 (10), 3616–3622. doi: 10.1002/ece3.2925
Martins G. M., Jenkins S. R., Hawkins S. J., Neto A. I., Medeiros A. R., Thompson R. C. (2011). Illegal Harvesting Affects the Success of Fishing Closure Areas. J. Mar. Biol. Assoc. United Kingdom 91 (4), 929–937. doi: 10.1017/S0025315410001189
Mau A., Bingham J.-P., Soller F., Jha R. (2018). Maturation, Spawning, and Larval Development in Captive Yellowfoot Limpets (Cellana Sandwicensis). Invertebrate Reprod. Dev. 62 (4), 239–247. doi: 10.1080/07924259.2018.1505670
Mau A., Jha R. (2018). Aquaculture of Two Commercially Important Molluscs (Abalone and Limpet): Existing Knowledge and Future Prospects. Rev. Aquacul. 10 (3), 611–625. doi: 10.1111/raq.12190
McCoy M. D. (2008). Hawaiian Limpet Harvesting in Historical Perspective: A Review of Modern and Archaeological Data on Genus Cellana Spp. From the Kalaupapa Peninsula, Moloka’i Island. Pac. Sci. 62 (1), 21–38. doi: 10.2984/1534-6188(2008)62[21:HLHIHP]2.0.CO;2
McCoy S. J., Kamenos N. A. (2015). Coralline Algae (Rhodophyta) in a Changing World: Integrating Ecological, Physiological, and Geochemical Responses to Global Change. J. phycol. 51 (1), 6–24. doi: 10.1111/jpy.12262
McGee B. L., Targett N. M. (1989). Larval Habitat Selection in Crepidula (L.) and its Effect on Adult Distribution Patterns. J. Exp. Mar. Biol. Ecol. 131 (3), 195–214. doi: 10.1016/0022-0981(89)90112-3
Menge B. A. (2000). Top-Down and Bottom-Up Community Regulation in Marine Rocky Intertidal Habitats. J. Exp. Mar. Biol. Ecol. 250 (1), 257–289. doi: 10.1016/S0022-0981(00)00200-8
Morse D. E., Duncan H., Hooker N., Morse A. (1977). Hydrogen Peroxide Induces Spawning in Mollusks, With Activation of Prostaglandin Endoperoxide Synthetase. Science 196 (4287), 298–300. doi: 10.1126/science.403609
Moss G. A. (1999). Factors Affecting Settlement and Early Post-Settlement Survival of the New Zealand Abalone Haliotis Australis. New Z. J. Mar. Freshw. Res. 33 (2), 271–278. doi: 10.1080/00288330.1999.9516876
Moss G. A., Illingworth J., Tong L. J. (1995). Comparing Two Simple Methods to Induce Spawning in the New Zealand Abalone (Paua), Haliotis Iris. New Z. J. Mar. Freshw. Res. 29 (3), 329–333. doi: 10.1080/00288330.1995.9516667
Muñoz-Colmenero M., Turrero P., Horreo J. L., Garcia-Vazquez E. (2012). Evolution of Limpet Assemblages Driven by Environmental Changes and Harvesting in North Iberia. Mar. Ecol. Prog. Ser. 466, 121–131. doi: 10.3354/meps09906
Nakano T., Nakayama R., Takahashi Y. (2020). Artificial Fertilisation and Early Development of a Limpet Lottia Tenuisculpta (Patellogastropoda: Lottiidae). Molluscan Res. 40 (1), 52–59. doi: 10.1080/13235818.2019.1688752
Nakano T., Ozawa T. (2007). Worldwide Phylogeography of Limpets of the Order Patellogastropoda: Molecular, Morphological and Palaeontological Evidence. J. Molluscan Stud. 73 (1), 79–99. doi: 10.1093/mollus/eym001
Navarro P. G., Ramírez R., Tuya F., Fernandez-gil C., Sanchez-jerez P., Haroun R. J. (2005). Hierarchical Analysis of Spatial Distribution Patterns of Patellid Limpets in the Canary Islands. J. Molluscan Stud. 71 (1), 67–73. doi: 10.1093/mollus/eyi009%J
Nhan H. T. (2014). Development of Aquaculture Technology for the Hawaiian Opihi Cellana Spp (Honolulu: University of Hawaii).
Nhan H. T., Ako H. (2019). Reproductive Biology, Seed Production, and Culture of the Hawaiian Limpet Cellana Sandwicensis (Pease 1861). Invertebr. Ecophysiol. Manage. 1–19. doi: 10.5772/intechopen.87128
Nobre A., Robertson-Andersson D., Neori A., Sankar K. (2010). Ecological–economic Assessment of Aquaculture Options: Comparison Between Abalone Monoculture and Integrated Multi-Trophic Aquaculture of Abalone and Seaweeds. Aquaculture 306 (1-4), 116–126. doi: 10.1016/j.aquaculture.2010.06.002
Nunes C., Ramirez A., Rodeia J., De Girolamo M., Isidro E. (2021). “Invasive vs. non-Invasive Methods of Reproduction in Patella Candei (D’orbigny 1840): From Fertilization to Settlement,” in Aquaculture Europe 2021 – Oceans of Opportunity (Funchal, Madeira, Portugal: European Aquaculture Society).
Orton J. H. (1929). Observations on Patella Vulgata. Part III. Habitat and Habits. J. Mar. Biol. Assoc. United Kingdom 16 (1), 277–288. doi: 10.1017/S0025315400029805
Orton J. H., Southward A. J., Dodd J. M. (1956). Studies on the Biology of Limpets: II. The Breeding of Patella Vulgata L. @ in Britain. J. Mar. Biol. Assoc. United Kingdom 35 (1), 149–176. doi: 10.1017/S0025315400009036
Parker W., Yanes Y., Mesa Hernández E., Hernández Marrero J. C., Pais J., Soto Contreras N., et al. (2020). Shellfish Exploitation in the Western Canary Islands Over the Last Two Millennia. Environ. Archaeol. 25 (1), 14–36. doi: 10.1080/14614103.2018.1497821
Pérez S., Fernández N., Ribeiro P. A. (2016). Standardization of a Patella Spp.(Mollusca, Gastropoda) Embryo–Larval Bioassay and Advantages of its Use in Marine Ecotoxicology. Ecotoxicol. Environ. Saf. 127, 175–186. doi: 10.1016/j.ecoenv.2016.01.025
Piazzi L., Bulleri F., Ceccherelli G. (2016). Limpets Compensate Sea Urchin Decline and Enhance the Stability of Rocky Subtidal Barrens. Mar. Environ. Res. 115, 49–55. doi: 10.1016/j.marenvres.2016.01.009
Pinto A. R., Alves A., Faria G., Reis R., Delgado J. (2010). “Reproductive Biology of Patella Candei D’orbigny 1840 in Madeira Archipelago,” in XVI Simposio Iberico De Estudios De Biologia Marina(Alicante: Departamento de Ciencias del Mar y Biología Aplicada de la Universidad de Alicante).
Pombo O. A., Escofet A. (1996). Effect of Exploitation on the Limpet Lottia Gigantea: A Field Study in Baja California (Mexico) and California U.S.A. Pac. Sci. 50 (4), 393–403. doi: 10125/2914
Rao M. B. (1973). Sex Phenomenon and Reproductive Cycle in the Limpet Cellana Radiata (Born) (Gastropoda: Prosobranchia). J. Exp. Mar. Biol. Ecol. 12 (3), 263–278. doi: 10.1016/0022-0981(73)90057-9
Ribeiro P. (2008). Dispersal and Connectivity of Northeastern Atlantic Patellid Limpets: A Multidisciplinary Approach. Thesis (Southampton: University of Southampton).
Roberts R. D., Kaspar H. F., Barker R. J. (2004). Settlement of Abalone (Haliotis Iris) Larvae in Response to Five Species of Coralline Algae. J. Shellfish Res. 23 (4), 975–988.
Roberts R. D., Nicholson C. M. (1997). Variable Response From Abalone Larvae (Haliotis Iris, H. Virginea) to a Range of Settlement Cues. Molluscan Res. 18 (2), 131–141. doi: 10.1080/13235818.1997.10673687
Rodríguez S., Ojeda F. P., Inestrosa N. C. (1993). Settlement of Benthic Marine Invertebrates. Mar. Ecol. Prog. Ser. 97, 197–207. doi: 10.3354/meps097193
Sagarin R. D., Ambrose R. F., Becker B. J., Engle J. M., Kido J., Lee S. F., et al. (2007). Ecological Impacts on the Limpet Lottia Gigantea Populations: Human Pressure Over a Broad Scale on Island and Mainland Intertidal Zones. Mar. Biol. 150 (3), 399–413. doi: 10.1007/s00227-006-0341-1
Salas‐Garza A., Parés-Sierra G., Gómez-Rigalt R., Carpizo-Ituarte E. (2009). The Larval Development, Metamorphosis and Juvenile Growth of the Turban Snail Lithopoma (Astraea) Undosa (Wood 1828) (Gastropoda: Turbinidae). J. World Aquacul. Soc. 40 (4), 460–471. doi: 10.1111/j.1749-7345.2009.00268.x
Seabra M. I., Cruz T., Fernandes J. N., Silva T., Hawkins S. (2019). Recruitment of the Limpet Patella Ulyssiponensis and its Relationship With Crustose Coralline Algae: Patterns of Juvenile Distribution and Larval Settlement. J. Mar. Biol. Assoc. United Kingdom 99 (8), 1787–1796. doi: 10.1017/S0025315419000869
Searcy-Bernal R., Salas-Garza A. E., Flores-Aguilar R. A., Hinojosa-Rivera P. R. (1992). Simultaneous Comparison of Methods for Settlement and Metamorphosis Induction in the Red Abalone (Haliotis Rufescens). Aquaculture 105 (3-4), 241–250. doi: 10.1016/0044-8486(92)90090-8
Smith F. G. W. (1935). III-The Development of Patella Vulgata. Philosophical Transactions of the Royal Society of London. Ser. B Biol. Sci. 225 (520), 95–125. doi: 10.1098/rstb.1935.0008
Sousa R., Delgado J., Pinto A. R., Henriques P. (2017). Growth and Reproduction of the North-Eastern Atlantic Keystone Species Patella Aspera (Mollusca: Patellogastropoda). Helgoland Mar. Res. 71 (1), 8. doi: 10.1186/s10152-017-0488-9
Sousa R., Henriques P., Vasconcelos J., Pinto A. R., Delgado J., Riera R. (2020a). The Protection Effects of Marine Protected Areas on Exploited Molluscs From an Oceanic Archipelago. Aquat. Conservation: Mar. Freshw. Ecosystems 30 (4), 717–729. doi: 10.1002/aqc.3285
Sousa R., Pinto A., Vasconcelos J., Riera R. (2020b). Does Harvesting Affect the Relative Growth in Patella Aspera Röding 1798? Eur. Zoological J. 87 (1), 395–401. doi: 10.1080/24750263.2020.1791266
Sousa R., Vasconcelos J., Henriques P., Pinto A. R., Delgado J., Riera R. (2019a). Long-Term Population Status of Two Harvested Intertidal Grazers (Patella Aspera and Patella Candei), Before, (1996–2006) and After, (2007–2017) the Implementation of Management Measures. J. sea Res. 144, 33–38. doi: 10.1016/j.seares.2018.11.002
Sousa R., Vasconcelos J., Riera R., Pinto A. R., Delgado J., Henriques P. (2019b). Potential Impact of Harvesting Management Measures on the Reproductive Parameters of the Limpets Patella Aspera and Patella Candei From Madeira Island. Estuarine Coastal Shelf Sci. 226, 106264. doi: 10.1016/j.ecss.2019.106264
Steneck R. S. (1982). A Limpet-Coralline Alga Association: Adaptations and Defenses Between a Selective Herbivore and its Prey. Ecology 63 (2), 507–522. doi: 10.2307/1938967
Steneck R. S. (1986). The Ecology of Coralline Algal Crusts: Convergent Patterns and Adaptative Strategies. Annu. Rev. Ecol. systematics 17 (1), 273–303. doi: 10.1146/annurev.es.17.110186.001421
Steneck R. S. (1990). “Herbivory and the Evolution of Nongeniculate Coralline Algae (Rhodophyta, Corallinales) in the North Atlantic and North Pacific,” in Evolutionary Biogeography of the Marine Algae of the North Atlantic. Eds. Garbary D. J., South G. R. (Berlin Heidelberg: Springer), 107–129.
Stiner M. C., Munro N. D., Surovell T. A., Tchernov E., Bar-Yosef O. (1999). Paleolithic Population Growth Pulses Evidenced by Small Animal Exploitation. Science 283 (5399), 190–194. doi: 10.1126/science.283.5399.190
Torres C., Andrade C. (2010). Processo De Decisão De Análise Espacial Na Selecção De Áreas Óptimas Para a Aquacultura Marinha: O Exemplo Da Ilha Da Madeira. Rev. Gestão Costeira Integrada-Journal Integ. Coastal Zone Manage. 10 (3), 321–330. doi: 10.5894/rgci180
Uki N., Kikuchi S. (1984). Regulation of Maturation and Spawning of an Abalone, Haliotis (Gastropoda) by External Environmental Factors. Aquaculture 39 (1), 247–261. doi: 10.1016/0044-8486(84)90270-9
Underwood A. J. (1980). The Effects of Grazing by Gastropods and Physical Factors on the Upper Limits of Distribution of Intertidal Macroalgae. Oecologia 46 (2), 201–213. doi: 10.1007/BF00540127
Valdez-Cibrián A., Michel-Morfín J. E., Gómez-Torres M., Landa-Jaime V., Gómez-Gastelum L., Carballo J. L. (2021). Population Status of the Endangered Giant Mexican Limpet Scutellastra Mexicana in the Central Mexican Pacific. Aquat. Conservation: Mar. Freshw. Ecosyst. 31 (3), 531–542. doi: 10.1002/aqc.3480
Viera M., Courtois de Viçose G., Fernández-Palacios H., Izquierdo M. (2016). Grow-Out Culture of Abalone Haliotis Tuberculata Coccinea Reeve, Fed Land-Based IMTA Produced Macroalgae, in a Combined Fish/Abalone Offshore Mariculture System: Effect of Stocking Density. Aquacul. Res. 47 (1), 71–81. doi: 10.1111/are.12467
Wanninger A., Ruthensteiner B., Lobenwein S., Salvenmoser W., Dictus W. J., Haszprunar G. (1999). Development of the Musculature in the Limpet Patella (Mollusca, Patellogastropoda). Dev. Genes Evol. 209 (4), 226–238. doi: 10.1007/s004270050247
Weber L. I., Hawkins S. J. (2002). Evolution of the Limpet Patella Candei d'Orbigny (Mollusca, Patellidae) in Atlantic Archipelagos: Human Intervention and Natural Processes. Biol. J. Linn. Soc. 77 (3), 341–353. doi: 10.1046/j.1095-8312.2002.00102.x
Wolcott T. G. (1973). Physiological Ecology and Intertidal Zonation in Limpets (Acmaea): A Critical Look at "Limiting Factors". Biol. Bull. 145 (2), 389–422. doi: 10.2307/1540048
Wright W. G., Lindberg D. R. (1979). A non-Fatal Method of Sex Determination for Patellacean Gastropods. J. Mar. Biol. Assoc. United Kingdom 59 (3), 803–803. doi: 10.1017/S0025315400045793
Wright W. G., Lindberg D. R. (1982). Direct Observation of Sex Change in the Patellacean Limpet Lottia Gigantea. J. Mar. Biol. Assoc. United Kingdom 62 (3), 737–738. doi: 10.1017/S0025315400019895
Keywords: patellids, larval production, larviculture, settlement induction, juvenile production
Citation: Castejón D, García L, Cañizares JM, De Girolamo M, Nunes C, Isidro E, Courtois de Viçose G, Nogueira N and Andrade CAP (2022) Methodologies for Patellid Limpets’ Aquaculture: From Broodstock Management to Juveniles. Front. Mar. Sci. 9:884262. doi: 10.3389/fmars.2022.884262
Received: 26 February 2022; Accepted: 05 May 2022;
Published: 15 June 2022.
Edited by:
Sílvia Lourenço, Instituto Politécnico de Leiria, PortugalReviewed by:
Maria Paola Ferranti, University of Genoa, ItalyMaria Flavia Gravina, University of Rome “Tor Vergata”, Italy
Copyright © 2022 Castejón, García, Cañizares, De Girolamo, Nunes, Isidro, Courtois de Viçose, Nogueira and Andrade. This is an open-access article distributed under the terms of the Creative Commons Attribution License (CC BY). The use, distribution or reproduction in other forums is permitted, provided the original author(s) and the copyright owner(s) are credited and that the original publication in this journal is cited, in accordance with accepted academic practice. No use, distribution or reproduction is permitted which does not comply with these terms.
*Correspondence: Diego Castejón, diego.castejon.dcb@gmail.com