- Animal Ecology, Zoological Institute and Museum, University of Greifswald, Greifswald, Germany
Shallow aquatic environments are characterized by strong environmental variability. For ectotherms, temperature is the main driver of metabolic activity, thus also shaping performance. Ingestion rates in mysids are fast responses, influenced by metabolic and behavioral activity. We examined ingestion rates of the mysid Neomysis integer, collected in the Baltic Sea, after one-week exposure to different constant and fluctuating temperature regimes (5, 10, 15, 20°C and 9 ± 5, 14 ± 5°C, respectively). To investigate possible differences between sexes, thermal performance curves (TPCs) were established for female and male mysids based on ingestion rates measured at constant temperatures. TPCs of ingestion rates at constant temperatures differed between sexes, with female mysids showing a higher total ingestion rate as well as a higher thermal optimum compared to male mysids. Females showed reduced ingestion rates when exposed to fluctuating temperatures around their thermal optimum, whereas ingestion of male mysids was not reduced when exposed to fluctuating temperatures. The observed sex-specific differences might be related to potentially higher lipid and energy demands of the females. We suggest future studies should investigate males and females to improve our understanding about impacts of environmental variability on natural populations.
Introduction
Natural environments are characterized by variability at various temporal and spatial scales. Variability of abiotic factors in shallow brackish habitats can occur as fluctuations in temperature, salinity, nutrients, light availability, oxygen, and pH (Boyd & Hutchins, 2012; Franz et al., 2019). Variability is induced by various processes, such as seasons, day-night cycles, radiation, tides, currents, wind, and up- and downwelling, or even by the biological activity of organisms (Feely et al., 2008; Hurd et al., 2011; Cornwall et al., 2013; Saderne et al., 2013). The temporal scales at which variability affects environmental conditions range from seconds/minutes over days up to months (e.g. Gunderson et al., 2016), and are especially important in relation to the timescale organisms experience them (Jackson et al., 2021).
Temperature is the primary driver of metabolic rates in ectotherms, thus affecting both physiological and fitness traits of marine ectotherms. An increase in temperature accelerates most physiological processes within tolerance limits (Newell and Branch, 1980). Traditionally, the performance of ectotherms at different temperatures is determined by exposing organisms to constant temperature treatments along a thermal gradient (Huey and Stevenson, 1979; Schulte et al., 2011). From this, thermal performance curves (TPCs) can be deduced that may vary in shape depending on the measured trait, but performance usually peaks around a thermal optimum, followed by a rapid decline (Dowd et al., 2015). Different nonlinear mathematical models have been used to fit the thermal performance data, ranging from negative quadratic to more complex functions (Angilletta, 2006). Such TPCs and the mathematical principle of nonlinear averaging, often also known as Jensen’s Inequality (Supplementary Box 1; Jensen, 1906; Ruel and Ayres, 1999), can be used to predict performance in variable environments if time-dependent effects do not play a large role. For instance, population growth rates of a green alga under fluctuating temperatures could be sufficiently predicted by non-linear averaging using a TPC measured at constant temperatures (Bernhardt et al., 2018). Another study on the bivalve Mytilus, combining two experiments at different time scales, could predict long-term performance at fluctuating temperatures on the basis of short-term functioning (feeding) measured at constant temperatures using nonlinear averaging (Vajedsamiei et al., 2021). These studies also confirm the theory based on Jensen’s Inequality that performance is smaller in fluctuating compared to constant temperatures (of the same mean) when fluctuations range in the concave part of the generally non-linear, unimodal TPC (Supplementary Box 1; Jensen, 1906).
Despite these examples of successfully using Jensen’s Inequality to predict performance in fluctuating environments, limitations of this method have been described. Koussoroplis et al. (2019) showed that non-linear averaging is suitable only at particular fluctuation frequencies to predict effects of resource variability. Additionally, duration and order of exposure to temperature events can affect ectotherm performance (Kingsolver et al., 2015), as well as other time-dependent effects, such as stress, acclimation, compensation, use of reserves, or the interplay among several (co-)varying factors (Koussoroplis et al., 2017). Stress responses can be beneficial short-term but may reduce organism performance at longer time scales, whilst acclimation may amplify or buffer fluctuation effects, depending on the frequency of change in relation to acclimation speed of the organism (Kingsolver et al., 2015; Koussoroplis et al., 2017). Predicting the direction of time-dependent effects and differentiating between time-dependent effects and Jensen’s Inequality is still a challenge (Kingsolver et al., 2015).
For the next decades, extreme events like storms, heavy precipitation or marine heatwaves are predicted to occur with increasing magnitude and frequency (IPCC, 2021). This will change established variability patterns of temperature, but the effects on organisms are largely unexplored. Therefore, there is a need to incorporate effects of fluctuations when studying species’ functioning and performance in a changing world with variability (Vasseur et al., 2014). Fluctuating temperature showed substantial effects on the performance of marine organisms (Bernhardt et al., 2018; Vajedsamiei et al., 2021), which can differ between species depending on the shape of the TPC and whether the thermal mean is on the convex, concave or linear part of the TPC. For instance, Morón Lugo et al. (2020) observed decreased feeding of the sea star Asterias rubens under warming and an additional increased stress response under thermal fluctuation. In contrast, feeding of the crab Hemigrapsus takanoi was unaffected by thermal fluctuations. This suggests that thermal fluctuations have been situated in the linear part of H. taganoi’s TPC, leading to no effect, whereas they have been situated in the concave part of A. rubens’. Whether responses to fluctuating temperatures also differ within aquatic species, e.g. amongst sexes, genotypes or ontogenetic stages, remains to be explored. In particular, we are not aware of any study investigating sex-specific differences in fluctuation effects on performance of marine invertebrates so far. However, if sex-specific differences in response to changing temperature regimes exist, changes in the sex ratio and thus to consequences for population dynamics and ultimately food web functioning are expected. To fill this knowledge gap, we investigate the effect of thermal fluctuations on the performance of male and female Neomysis integer as important littoral mysid species of Baltic Sea food webs.
We collected male and female mysids from a brackish estuary of the Baltic Sea and measured their ingestion rates after exposing them to different constant and fluctuating temperatures in the laboratory. Here, ingestion rate serves as an estimate of the organism’s performance, combining short-term responses of its metabolic capacity and behavior that ultimately affect longer-term responses such as growth and reproduction. Based on the mysids’ responses measured at constant temperatures, a TPC was established (TPCConstant) and used to calculate a TPC that predicts performance under fluctuating temperature conditions (TPCFluctuation) using non-linear averaging. We also measured ingestion rates of mysids that experienced daily temperature fluctuations and compared these responses to the two different TPC types. We hypothesize that ingestion rates show a unimodal response across the temperature gradient as typically observed for many other performance traits. We further hypothesize that ingestion rates of mature females should be higher than those of males as mature females require more energy for egg production. Since metabolic rates, and thus the energy demand of N. integer, are higher in warmer temperatures (Weisse and Rudstam 1989, Fockedey et al., 2006), we expect more pronounced differences in females’ and males’ energy demands, and consequently ingestion rates, at warmer temperatures. We hypothesize lower ingestion rates of mysids that experienced temperature fluctuations compared to constant temperatures based on Jensen’s Inequality principle, which predicts lower mean performance in fluctuating environments within the concave range of the TPC. Any deviation from the prediction based on Jensen’s Inequality (i.e., our TPCFluctuation), whether positive or negative, will point to effects of biological processes, such as stress responses or short-term acclimation.
Methods
The Mysid Neomysis integer
Mysids are an important component in the food web of coastal zones, linking benthic and pelagic systems by nutrient exchange and biomass transfer (Roast et al., 1998). They can switch between two feeding modes (suspension and raptorial feeding), allowing them to feed on detritus and phytoplankton, but also to actively select zooplankton (Viitasalo and Rautio, 1998). In turn, they serve as a protein-rich (>70% of dry weight, Raymont et al., 1968) food source for higher trophic levels. We used Neomysis integer Leach, 1814 (Mysidacea: mysidae) in our experiments. N. integer occurs in the hyperbenthic zones of estuaries and is also abundant in shallow coastal waters of the Baltic Sea (Margoñski and Maciejewska, 1999). In estuaries and brackish water systems, such as the Greifswalder Bodden, thermal variability occurs on both daily and seasonal scales (Seifert, 1938; Schiewer, 2008).
Collection and Laboratory Conditions
N. integer were collected at water depths <0.5 m in a shallow bay (coordinates: 54.097495, 13.455123) of the Greifswalder Bodden, in the southwest of the Baltic Sea on 04th May 2021. At collection, the water temperature was 11.3°C and the salinity was 7.5 psu. The bay is very shallow (<5 m), making it likely that mysids can experience strong thermal variability at short time scales. To avoid acute stress responses, especially in the warmer temperature treatments of the experiment, 150 mysids were kept in a climate chamber at constant 15°C for the next 16 days. Mysids stayed in aerated 40 L-aquaria and were fed ad libitum with freshly-hatched Artemia salina nauplii and fish pellets (MultiFit). The culture medium consisted of a 50:50% mixture of artificial seawater (Tropic Marin Pro-Reef Salt) and filtered (0.2 µm) water from the sample site. The salinity was set to 10 psu, somewhat higher than the ambient value, to establish a reference for future experiments and for comparative purposes with other experimental studies (e.g. Roast et al., 1999; Roast et al., 2000). At least 50% of this culture medium was exchanged every 3-4 days. The light regime in the climate chamber and during experiments was set to a 12:12 h dark:light photoperiod.
Temperature Treatments
Before the ingestion measurements, mysids were exposed simultaneously for 7 days to 4 constant temperature treatments and to 2 fluctuating temperature treatments (Figure 1). They were kept in 6 L-aquaria placed in different climate cabinets under the respective treatment temperature (1 climate cabinet per treatment) and fed ad libitum with freshly-hatched A. salina nauplii. Mysids in constant treatments were kept on 5 (♂ n = 13; ♀ n = 4), 10 (♂ n = 15; ♀ n = 2), 15 (♂ n = 5; ♀ n = 1), or 20°C (♂ n = 3; ♀ n = 10), whereas mysids in the two fluctuating treatments experienced 9 ± 5°C (♂ n = 12; ♀ n = 5) and 14 ± 5°C (♂ n = 11; ♀ n = 6) with a frequency of once per day (see Figure 2 for shape of the temperature fluctuation profile). The amplitude of ± 5°C was chosen to elicit a response and is likely larger than what the animals would experience on a diurnal basis in the environment. Highly controllable and precise climate cabinets were used (± 0.5 Kelvin) to avoid potential differences caused by cabinet identity.
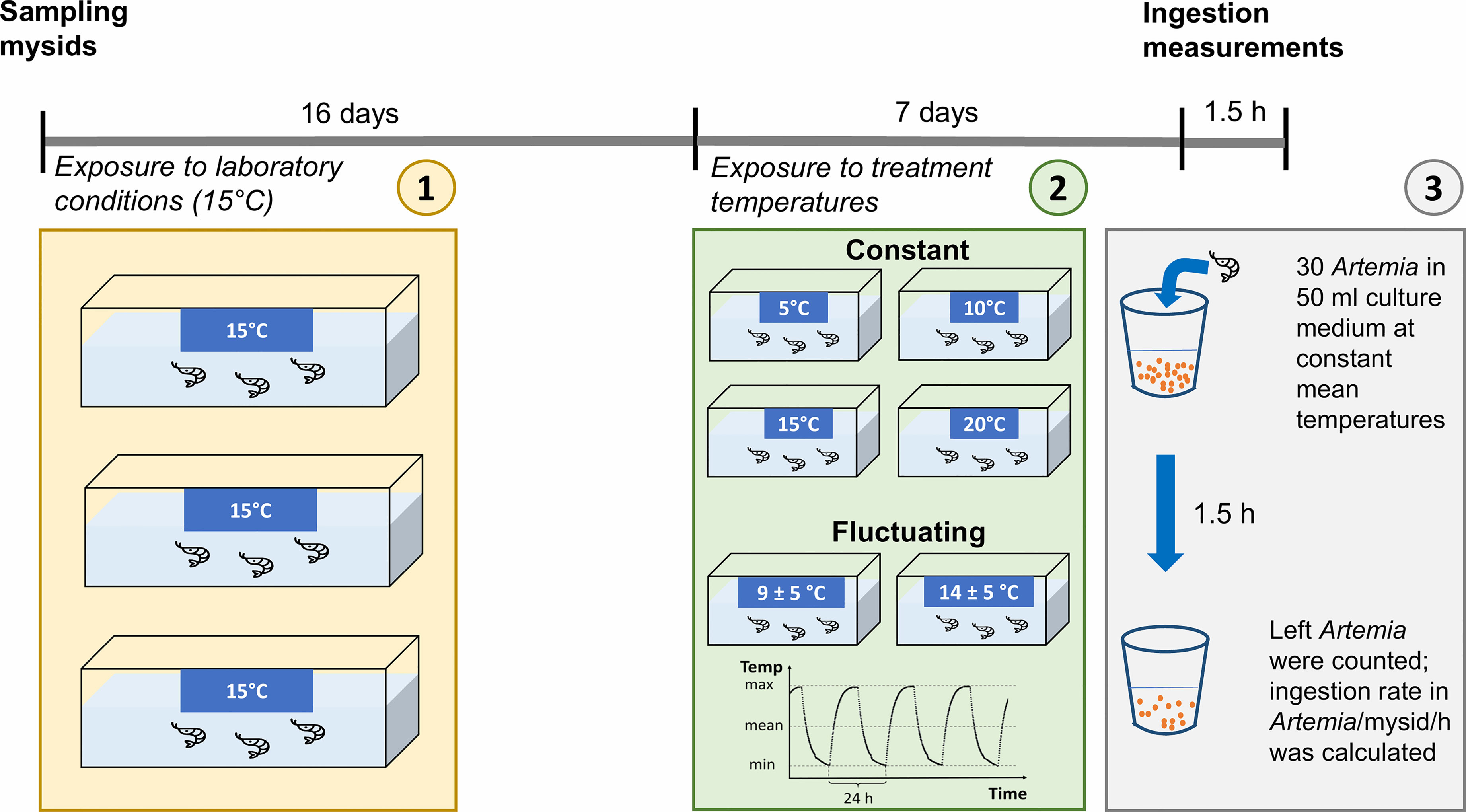
Figure 1 Scheme of the experimental set-up and time schedule. Mysids were exposed to laboratory conditions for 16 days at constant 15°C before they were randomly distributed and exposed to the treatment temperature regimes. For the ingestion experiment, mysids were placed individually in beakers containing 30 Artemia nauplii. After 1.5 h, mysids were removed and remaining Artemia were counted for the calculation of the ingestion rates (Artemia/mysid/hour).
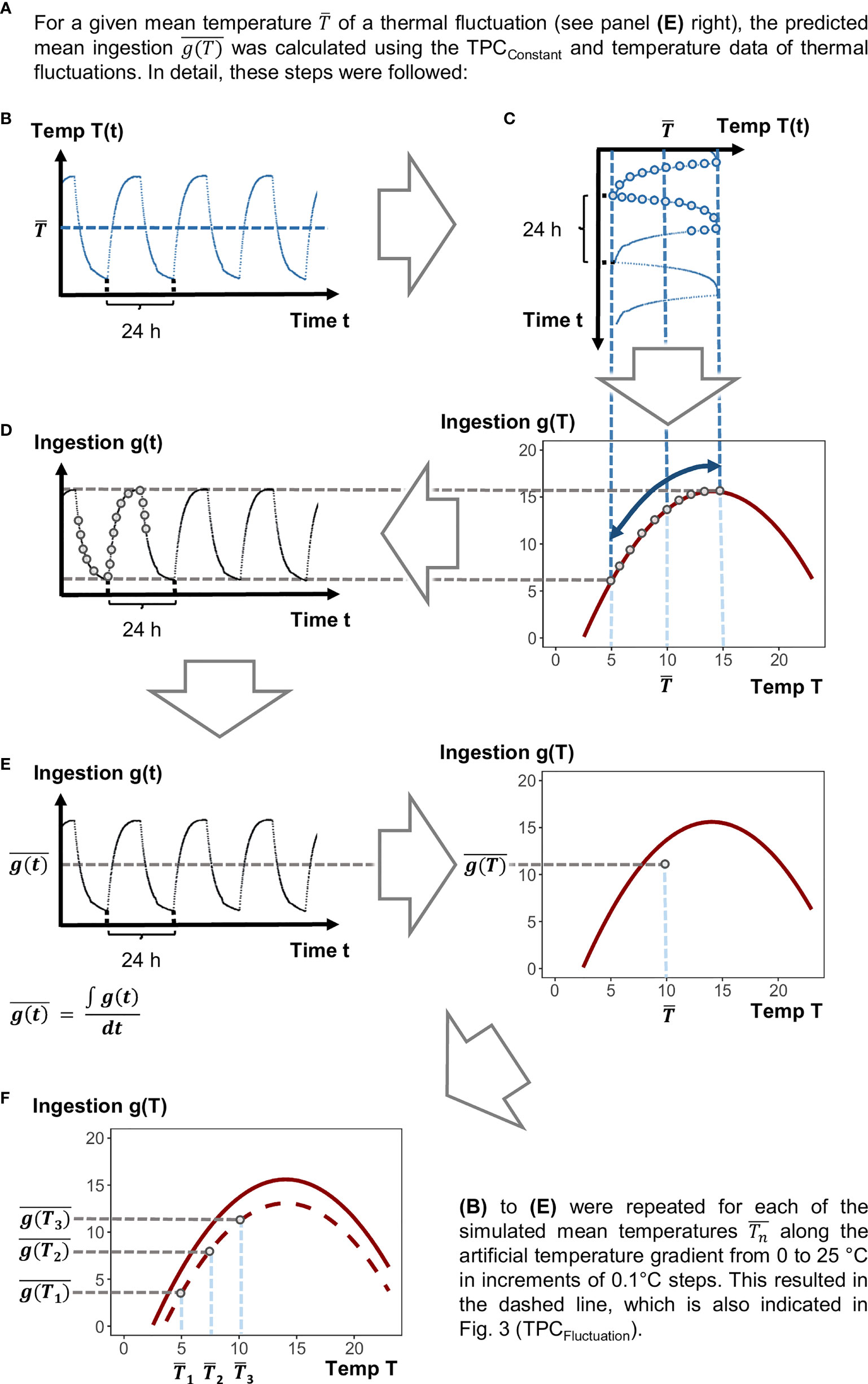
Figure 2 Schematic figure showing how to predict ingestion rates at fluctuating conditions on the basis of ingestion rates measured at constant temperatures. References to and explanations for labels (A–F) are given in the main text.
Ingestion Rate Measurements
Vivid N. integer were starved for one day prior to the measurements of ingestion rates (Lindén and Kuosa, 2004). Ingestion rates of N. integer individuals from both the constant and fluctuating treatments were measured in the respective constant mean temperatures of 5, 10, 15, and 20°C, and in case of the fluctuating treatments when temperature was close to the respective mean value. One climate cabinet was used for each constant mean temperature. Ingestion rates of mysids from the fluctuating treatments were measured in the same climate cabinets as ingestion rates of mysids from the respective constant temperature treatment to ensure comparability. For the measurements, N. integer were placed individually in small beakers containing 50 ml of the culture medium described above at an initial food density of 30 A. salina nauplii. After 1.5 h, N. integer individuals were removed and stored in ethanol for later sex and length determination. The remaining A. salina nauplii were counted, and ingestion rates per mysid per hour were calculated (Figure 1). The sex of the mysids was identified based on sexual characteristics using a stereo-microscope. All females had a developed marsupium. Body length (from the base of eyestalks to the end of the last abdominal segment, excluding uropods and telson; Fockedey et al., 2005) was determined from photographs using the software ImageJ (mean body length ± SD, females: 11.11 ± 1.65 mm, males: 9.46 ± 0.87 mm). Since all mysids used in the experiment were adults and were sampled in May, i.e. at the beginning of the growing season providing ample of food, we expected all individuals being in a reproductive state.
Data Analyses
Data analyses were performed in R version 4.0.2 (R Core Team, 2020). Individual ingestion rates at constant temperatures were analyzed using Analysis of Covariance (ANCOVA) with a negative quadratic function of temperature as continuous independent variable and sex of animals as factor. Preliminary inspection of the data revealed that our data were distributed rather in the upper part of the TPC above a potential inflection point where the second derivative of the TPC is always negative, i.e. the concave downward part of the TPC. For this region, a negative quadratic function is a reasonable simplification and fits the typical unimodal responses in ectotherm TPCs (Angilletta, 2006). The full model was simplified based on AIC using the R function step(). A potential decrease of the ingestion rates measured under fluctuating temperatures was tested against the performance prediction of the ANCOVA for the respective constant temperature with a one-sided, one sample t-test. We also tested for any deviation from the performance predicted under fluctuating temperature by performing a two-sided, one sample t-test.
To calculate ingestion rates predicted at fluctuating temperature regimes, we used the deviations of the actually realized temperatures (measured in the climate cabinet) from the mean temperature of the 9 ± 5°C fluctuation treatment. This treatment was chosen exemplary since the realized temperature deviations from the mean in the 14 ± 5°C treatment were very similar and resulted in similar calculated ingestion rates at the end. In detail, we conducted the following steps (Figure 2):
Preparation of Simulated Temperature Data
1.) The temperature fluctuation regime realized in the 9 ± 5°C treatment was logged every 5 min during the experiment (Onset, HOBO Pendant logger). From this temperature profile, the realized mean temperature was calculated. was subtracted from every single value of the realized temperature profile to obtain deviations from the mean temperature across time in the fluctuation regime.
2.) A thermal gradient ranging from 0 to 25°C in increments of 0.1°C steps was simulated. The single values of this gradient were used as simulated mean temperatures for the predictions of ingestions rates under fluctuating temperature (Figure 2F).
3.) To each of these , we added the deviations across time which were calculated in step 1. This procedure provided simulated fluctuating temperature profiles with the same deviations from the mean temperature as in our experimental treatments, but for all along the simulated thermal gradient (0 to 25°C).
Calculation of Ingestion Rates Predicted at Fluctuating Temperature
4.) For every temperature of each of these temperature fluctuation profiles (cf. Figure 2B), we calculated the predicted instantaneous performance g(T) (Figure 2C, lower plot), based on the TPCConstant, over time in steps of every 5 mins, g(t) (Figure 2D).
5.) From these predicted values across the temperature fluctuation regime, we calculated the mean ingestion rate over time (, Figure 2E). The , was calculated for each along the simulated thermal gradient described in step 2 (Figure 2F). The resulting mean ingestion rates under fluctuating temperature , were added as TPCFluctuation to the figure showing also the TPCConstant.
Results
Sex-Specific Ingestion Rates at Constant Temperatures
Under constant temperature conditions, ingestion rates of N. integer showed a unimodal relationship to temperature for both sexes (Figure 3A), characterizing a TPCConstant that can be well described by a negative quadratic function (ANCOVA; F1,48 = 5.02, p = 0.030). The female TPCConstant reached a higher maximum than the male TPCConstant (Figure 3A, sex: F1,48 = 5.44, p = 0.024), and the thermal optimum (14.1°C and 10.7°C for females and males, respectively) was shifted towards higher temperatures (interaction Temp × Sex, F1,48 = 9.03, p = 0.004).
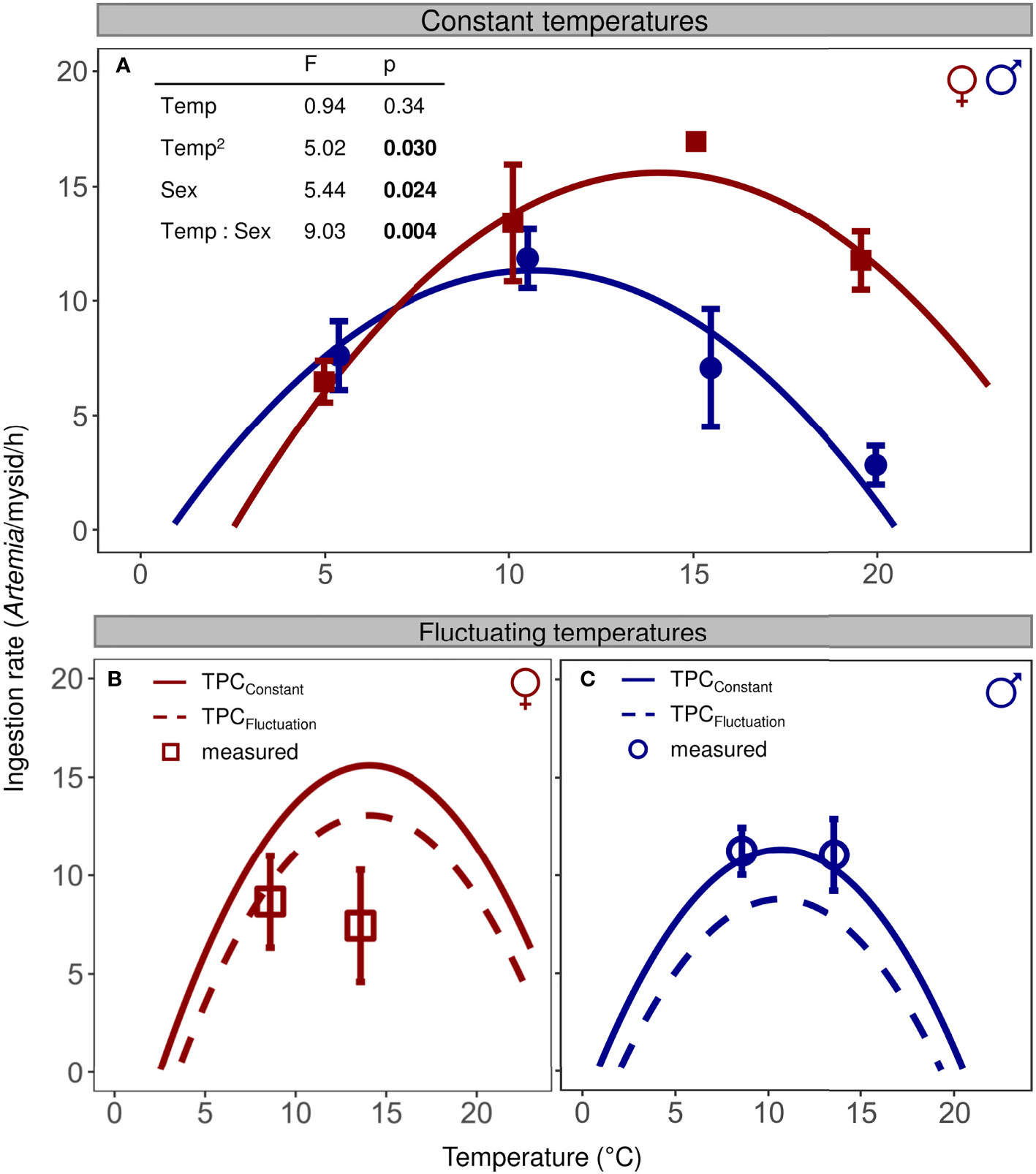
Figure 3 Sex-specific ingestion rates of N. integer exposed to (A) constant temperatures (filled symbols, mean ± SE) and (B, C) fluctuating temperature conditions (open symbols). (A) TPCConstant for females (red squares) and males (blue circles) were calculated based on estimates of an ANCOVA model with a negative quadratic function (see table inlet) fitted through ingestion rates measured at constant temperature. (B, C) Ingestion rates predicted for fluctuating temperature conditions (TPCFluctuation) were calculated using non-linear averaging based on the TPCsConstant.
Thermal Fluctuation Effect on Sex-Specific Ingestion Rates
The measured ingestion rates of female mysids exposed to the 14 ± 5°C regime (Figure 3B, open symbol), close to their thermal optimum, were lower than the ingestion value predicted by the TPCConstant (one-sided t-test, p = 0.018), but did not deviate positively or negatively from the value of the TPCFluctuation (two-sided t-test, p = 0.11). For female mysids exposed to the 9 ± 5°C regime, ingestion rates neither decreased compared to the value of the TPCConstant (one-sided t-test, p = 0.11) nor did they deviate from the corresponding TPCFluctuation value (two-sided t-test, p = 0.72). In contrast to females, the ingestion rates of male mysids exposed to fluctuating temperatures (Figure 3C) were very similar to the values predicted by the TPCConstant for both corresponding temperatures (9 and 14°C, one-sided t-tests, p > 0.63) and did also not deviate from the two values of the TPCFluctuation (two-sided t-tests, p > 0.10).
Discussion
Summary of Results
Our study showed first results that temperature fluctuations can affect ingestion rates, a process influenced by metabolism and behavior of marine crustaceans, and that differences in responses even occur between females and males of the same population. In our case, we found evidence for differences in sex-specific ingestion rates of N. integer. At constant temperatures, the thermal optimum of ingestion rates based on fitted TPCs was higher for females than for males, and at warmer temperatures, females showed higher ingestion rates than males. Temperature fluctuations of ± 5°C around the observed thermal optimum led to reduced ingestion rates for females, but not for males, when compared to ingestion rates observed at constant temperature.
Sex-Specific Ingestion Rates Under Constant Temperatures
In our study, ingestion rates of female and male mysids show a unimodal pattern across the tested temperature range, reflecting the expected non-linear nature of TPCs (e.g. Angilletta, 2006; Dowd et al., 2015). Whilst the TPCConstant for females and males showed similar responses to lower temperatures, sex-specific differences were apparent at higher temperatures (Figure 3A). Female mysids showed both a higher thermal optimum and higher total ingestion at the optimum temperature compared to males. Simmons & Knight (1975) described sex-specific differences in performance for the mysid Neomysis intermedia under various temperature and salinity that were weight-dependent. The respiratory response of N. intermedia was also dependent on the status of gravidity (Simmons and Knight, 1975). Similarly, Simmons and Knight (1975) indicated linkages between the respiration of mysids and the level of reproduction. The higher ingestion rates of females observed at higher temperatures in our study could be therefore related to the reproductive status of the used individuals. For females, such a relation between a higher ingestion and a higher energy demand for reproduction, in form of glycogen, has also been discussed for other marine invertebrates, such as the oyster Pinctada (Chávez-Villalba et al., 2013). Further, Winkler and Greve (2002) found that mysids’ reproduction rates and growth factors for smaller females seem to be higher at 15°C than at 10°C, resulting in shorter intermoult periods, which could lead to higher energy demands and thus to higher ingestion rates at 15°C compared to 10°C, as observed in our study. In the context of reproduction, a higher lipid demand, necessary for gamete production, may have led to the observed higher thermal optimum of females and their higher total ingestion of Artemia. Support for this assumption arises from a previous study showing that ovigerous females of N. integer contained almost double the amount of lipids compared to males due to the lipid-rich eggs (Linford, 1965). The lipid content and the energy budget were dependent on the season and therefore also influenced by variations in prey and temperature (Linford, 1965; Verslycke and Janssen, 2002). The lipid amount also correlates with the wet weight of an animal, with sex-specific relative lipid concentrations (Linford, 1965). This may also have led to the observed higher ingestion rates of females compared to males in our study, as female N. integer were slightly larger than males. However, the relationship between ingestion rates and length in the different temperature treatments was neither significant for females nor for males (p > 0.4). Alternatively, differences in ingestion rates between males and females may be influenced by differences in thermal tolerance. For instance, lower thermal tolerance in males compared to females may explain lower ingestion rates of males at higher temperatures. So far, however, nothing is known about sex-specific differences in thermal tolerance of mysids based on fitness-related traits such as growth rate or survival, making it difficult to draw conclusions about this option.
Similar to our observed ingestion rates for females, Roast et al. (2000) observed a significant increase of egestion rates for N. integer with increasing temperature up to 15°C. In another study, Roast et al. (1999) found an interactive effect of temperature and sex on respiration, with males showing a higher oxygen consumption than females. Even though we also found a significant interaction between temperature and sex, our results showed the opposite pattern, with higher ingestion rates of females observed at higher temperature. However, Roast et al. (1999) did not measure feeding in their study, and therefore the direct link between respiration and feeding rates in N. integer remains unclear. In studies using the blue mussel Mytilus, it was shown that respiration and feeding are two different functional traits, which at times are decoupled from each other, suggesting that they should be compared with caution (Vajedsamiei et al., 2021).
Females Are Negatively Affected by Thermal Fluctuations Around Their Optimum, Whilst Males Are Not
Ingestion rates of female N. integer exposed to temperature fluctuations around their optimum were lower than ingestion rates at constant temperature, but not lower than ingestion rates predicted under thermal fluctuations, suggesting females behaved as predicted by Jensen’s Inequality. In contrast, ingestion rates of male mysids exposed to fluctuating temperatures were similar to the values of the TPCConstant (Figure 3C). This suggests that males are not affected by time-dependent effects of thermal fluctuations, whilst females were affected by such effects at their thermal optimum. Based on our current knowledge, we can only speculate that males instantaneously respond to the changing conditions and therefore show a TPCconstant-predicted ingestion rate, while females deviate as they either are capable of cognitively integrating their feeding behavior across the temperature fluctuations or suffer from some time-dependent effect, such as a stress-related response.
The longer-term thermal histories of females and males used in our study should be similar as N. integer lives in swarms (Lindén, 2007), and our animals were collected from the same swarm. Swarms might experience similar temperatures in the field, but could also show sex-dependent habitat preferences (Vesakoski et al., 2008). The extent of thermal variability the mysids actually experience in the study region, in particular in high spatial and temporal resolution, is a matter of further investigations.
Perspectives and Conclusions
There are many different types of thermal variability in natural environments. Thermal fluctuations are not as regular or periodic as in laboratory studies, but rather stochastic (e.g. Pansch et al., 2018) and vertical or horizontal movements of mobile ectotherms can change the amplitudes and frequencies of fluctuations they experience (Koussoroplis et al., 2019). Further complexity is also added by the interplay of temperature with other factors, such as acidification, hypoxia, food availability, that may all vary in time and space (Cornwall et al., 2013; Comeau et al., 2014; Koussoroplis and Wacker, 2016). Thus, there is a lot to learn about predicting ectotherm performance in naturally fluctuating multifactorial systems, especially in the context of changing variability patterns and frequencies of extreme events due to climate change. To our knowledge, this study is the first investigating a TPC for mysids’ ingestion rates under constant temperatures, and combining it with ingestion rates under fluctuating conditions. We observed differences in ingestion rates between females and males in warmer temperatures at constant conditions and that females responded to temperature fluctuations whilst males did not. Investigations on the underlying mechanisms, that may be related to the reproductive status or other physiological differences in females and males, are of considerable interest for future research. We further advise caution when using TPCs based on measurements in constant conditions to predict performance in fluctuating environments, since time-dependent effects such as stress-induced damage or compensation can influence the ectotherms’ responses at variable conditions. Since we observed differences in sex-specific ingestion rates within one species, we propose that future studies should put more emphasis on studying potential differences between female and male individuals of studied populations. This would allow a better understanding of variation observed in thermal performance curves and possible effects of fluctuating conditions, and is crucial for improved predictions of species’ performance under climate change.
Data Availability Statement
The original contributions presented in the study are included in the article/Supplementary Material. Further inquiries can be directed to the corresponding authors.
Author Contributions
All authors contributed to conception and design of the study. LMH and KB acquired the data. All authors performed the statistical analysis. LMH and KB wrote the first draft of the manuscript. All authors contributed to manuscript revision, read, and approved the submitted version.
Conflict of Interest
The authors declare that the research was conducted in the absence of any commercial or financial relationships that could be construed as a potential conflict of interest.
Publisher’s Note
All claims expressed in this article are solely those of the authors and do not necessarily represent those of their affiliated organizations, or those of the publisher, the editors and the reviewers. Any product that may be evaluated in this article, or claim that may be made by its manufacturer, is not guaranteed or endorsed by the publisher.
Acknowledgments
We thank Hamam Aflok for help in the laboratory and Christin Park who ensured that all laboratory work could be conducted in time and as planned. KB and LMH are associated with the DFG graduate college and Research Training Group (RTG 2010) ‘Biological RESPONSEs to Novel and Changing Environments’. We acknowledge support for the Article Processing Charge by the German Research Foundation and the Open Access Publication Fund of the University of Greifswald. We are grateful to the reviewers for their constructive comments which improved previous versions of the manuscript.
Supplementary Material
The Supplementary Material for this article can be found online at: https://www.frontiersin.org/articles/10.3389/fmars.2022.883265/full#supplementary-material
References
Angilletta M. J. (2006). Estimating and Comparing Thermal Performance Curves. J. Therm. Biol. 31, 541–545. doi: 10.1016/j.jtherbio.2006.06.002
Bernhardt J. R., Sunday J. M., Thompson P. L., O’Connor M. I. (2018). Nonlinear Averaging of Thermal Experience Predicts Population Growth Rates in a Thermally Variable Environment. Proc. R. Soc. B.: Biol. Sci. 285, 20181076. doi: 10.1098/rspb.2018.1076
Boyd P. W., Hutchins D. A. (2012). Understanding the Responses of Ocean Biota to a Complex Matrix of Cumulative Anthropogenic Change. Mar. Ecol. Prog. Ser. 470, 125–135. doi: 10.3354/meps10121
Chávez-Villalba J., Soyez C., Aurentz H., Le Moullac G. (2013). Physiological Responses of Female and Male Black-Lip Pearl Oysters (Pinctada Margaritifera) to Different Temperatures and Concentrations of Food. Aquat. Liv. Resour. 26, 263–271. doi: 10.1051/alr/2013059
Comeau S., Edmunds P. J., Spindel N. B., Carpenter R. C. (2014). Diel Pco2 Oscillations Modulate the Response of the Coral Acropora Hyacinthus to Ocean Acidification. Mar. Ecol. Prog. Ser. 501, 99–111. doi: 10.3354/meps10690
Cornwall C. E., Hepburn C. D., McGraw C. M., Currie K. I., Pilditch C. A., Hunter K. A., et al. (2013). Diurnal Fluctuations in Seawater pH Influence the Response of a Calcifying Macroalga to Ocean Acidification. Proc. R. Soc. B.: Biol. Sci. 280, 20132201. doi: 10.1098/rspb.2013.2201
Dowd W. W., King F. A., Denny M. W. (2015). Thermal Variation, Thermal Extremes and the Physiological Performance of Individuals. J. Exp. Biol. 218, 1956–1967. doi: 10.1242/jeb.114926
Feely R. A., Sabine C. L., Hernandez-Ayon J. M., Ianson D., Hales B. (2008). Evidence for Upwelling of Corrosive “Acidified” Water Onto the Continental Shelf. Science 320, 1490–1492. doi: 10.1126/science.1155676
Fockedey N., Ghekiere A., Bruwiere S., Janssen C., Vincx M. (2006). Effect of Salinity and Temperature on the Intra-Marsupial Development of the Brackish Water Mysid Neomysis integer (Crustacea: Mysidacea). Mar. Biol. 148, 1339–1356. doi: 10.1007/s00227-005-0160-9
Fockedey N., Mees J., Vangheluwe M., Verslycke T., Janssen C. R., Vincx M. (2005). Temperature and Salinity Effects on Post-Marsupial Growth of Neomysis Integer (Crustacea: Mysidacea). J. Exp. Mar. Biol. Ecol. 326, 27–47. doi: 10.1016/j.jembe.2005.05.005
Franz M., Lieberum C., Bock G., Karez R. (2019). Environmental Parameters of Shallow Water Habitats in the SW Baltic Sea. Earth Syst. Sci. Data 11, 947–957. doi: 10.5194/essd-11-947-2019
Gunderson A. R., Armstrong E. J., Stillman J. H. (2016). Multiple Stressors in a Changing World: The Need for an Improved Perspective on Physiological Responses to the Dynamic Marine Environment. Ann. Rev. Mar. Sci. 8, 357–378. doi: 10.1146/annurev-marine-122414-033953
Huey R. B., Stevenson R. D. (1979). Integrating Thermal Physiology and Ecology of Ectotherms: A Discussion of Approaches. Am. Zool. 19, 357–366. doi: 10.1093/icb/19.1.357
Hurd C. L., Cornwall C. E., Currie K., Hepburn C. D., McGraw C. M., Hunter K. A., et al. (2011). Metabolically Induced pH Fluctuations by Some Coastal Calcifiers Exceed Projected 22nd Century Ocean Acidification: A Mechanism for Differential Susceptibility? Global Change Biol. 17, 3254–3262. doi: 10.1111/j.1365-2486.2011.02473.x
IPCC (2021). “Summary for Policymakers”, in Climate Change 2021: The Physical Science Basis. Contribution of Working Group I to the Sixth Assessment Report of the Intergovernmental Panel on Climate Change. Eds. Masson-Delmotte V., Zhai P., Pirani A., Connors S. L., Péan C., Berger S., Caud N., Chen Y., Goldfarb L., Gomis M. I., Huang M., Leitzell K., Lonnoy E., Matthews J. B. R., Maycock T. K., Waterfield T., Yelekçi O., Yu R., Zhou B. (Cambridge, United Kingdom and New York, NY, USA: Cambridge University Press) pp. 3–32. doi: 10.1017/9781009157896.001
Jackson M. C., Pawar S., Woodward G. (2021). The Temporal Dynamics of Multiple Stressor Effects: From Individuals to Ecosystems. Trends Ecol. Evol. 36, 402–410. doi: 10.1016/j.tree.2021.01.005
Jensen J. L. W. V. (1906). Sur Les Fonctions Convexes Et Les Inégalités Entre Les Valeurs Moyennes. Acta Math. 30, 175–193. doi: 10.1007/BF02418571
Kingsolver J. G., Higgins J. K., Augustine K. E. (2015). Fluctuating Temperatures and Ectotherm Growth: Distinguishing Non-Linear and Time-Dependent Effects. J. Exp. Biol. 218, 2218–2225. doi: 10.1242/jeb.120733
Koussoroplis A.-M., Pincebourde S., Wacker A. (2017). Understanding and Predicting Physiological Performance of Organisms in Fluctuating and Multifactorial Environments. Ecol. Monogr. 87, 178–197. doi: 10.1002/ecm.1247
Koussoroplis A.-M., Schälicke S., Raatz M., Bach M., Wacker A. (2019). Feeding in the Frequency Domain: Coarser-Grained Environments Increase Consumer Sensitivity to Resource Variability, Covariance and Phase. Ecol. Lett. 22, 1104–1114. doi: 10.1111/ele.13267
Koussoroplis A.-M., Wacker A. (2016). Covariance Modulates the Effect of Joint Temperature and Food Variance on Ectotherm Life-History Traits. Ecol. Lett. 19, 143–152. doi: 10.1111/ele.12546
Lindén E. (2007). The More the Merrier: Swarming as an Antipredator Strategy in the Mysid Neomysis Integer. Aquat. Ecol. 41, 299–307. doi: 10.1007/s10452-006-9055-1
Lindén E., Kuosa H. (2004). Effects of Grazing and Excretion by Pelagic Mysids (Mysis Spp.) on the Size Structure and Biomass of the Phytoplankton Community. Hydrobiologia 514, 73–78. doi: 10.1023/B:hydr.0000018207.42449.fb
Linford E. (1965). Biochemical Studies on Marine Zooplankton: II. Variations in the Lipid Content of Some Mysidacea. ICES J. Mar. Sci. 30, 16–27. doi: 10.1093/icesjms/30.1.16
Margoñski P., Maciejewska K. (1999). The Distribution, Abundance and Biomass of Mysis Mixta and Neomysis Integer (Crustacea: Mysidacea) in the Open Waters of the Southern Baltic Sea. Bull. Sea. Fish. Inst. Gdynia. 147, 23–35.
Morón Lugo S. C., Baumeister M., Nour O. M., Wolf F., Stumpp M., Pansch C. (2020). Warming and Temperature Variability Determine the Performance of Two Invertebrate Predators. Sci. Rep. 10, 6780. doi: 10.1038/s41598-020-63679-0
Newell R. C., Branch G. (1980). The Influence of Temperature on the Maintenance of Metabolic Energy Balance in Marine Invertebrates. Adv. Mar. Biol. 17, 329–396. doi: 10.1016/S0065-2881(08)60304-1
Pansch C., Scotti M., Barboza F. R., Al-Janabi B., Brakel J., Briski E., et al. (2018). Heat Waves and Their Significance for a Temperate Benthic Community: A Near-Natural Experimental Approach. Global Change Biol. 24, 4357–4367. doi: 10.1111/gcb.14282
Raymont J. E. G., Austin J., Linford E. (1968). Biochemical Studies on Marine Zooplankton V. The Composition of the Major Biochemical Fractions in Neomysis Integer. J. Mar. Biol. Assoc. Unit. Kingd. 48, 735–760. doi: 10.1017/S002531540001924X
R Core Team (2020). “R: A language and environment for statistical computing, R Foundation for Statistical Computing. (Vienna, Austria). Available at: https://www.R-project.org/.
Roast S. D., Widdows J., Jones M. B. (1998). The Position Maintenance Behaviour of Neomysis Integer (Peracarida: Mysidacea) in Response to Current Velocity, Substratum and Salinity. J. Exp. Mar. Biol. Ecol. 220, 25–45. doi: 10.1016/S0022-0981(97)00082-8
Roast S. D., Widdows J., Jones M. B. (1999). Respiratory Responses of the Estuarine Mysid Neomysis Integer (Peracarida: Mysidacea) in Relation to a Variable Environment. Mar. Biol. 133, 643–649. doi: 10.1007/s002270050504
Roast S. D., Widdows J., Jones M. B. (2000). Egestion Rates of the Estuarine Mysid Neomysis Integer (Peracarida: Mysidacea) in Relation to a Variable Environment. J. Exp. Mar. Biol. Ecol. 245, 69–81. doi: 10.1016/S0022-0981(99)00152-5
Ruel J. J., Ayres M. P. (1999). Jensen’s Inequality Predicts Effects of Environmental Variation. Trends Ecol. Evol. 14, 361–366. doi: 10.1016/S0169-5347(99)01664-X
Saderne V., Fietzek P., Herman P. M. J. (2013). Extreme Variations of Pco2 and pH in a Macrophyte Meadow of the Baltic Sea in Summer: Evidence of the Effect of Photosynthesis and Local Upwelling. PloS One 8, e62689. doi: 10.1371/journal.pone.0062689
Schulte P. M., Healy T. M., Fangue N. A. (2011). Thermal Performance Curves, Phenotypic Plasticity, and the Time Scales of Temperature Exposure. Integr. Comp. Biol. 51, 691–702. doi: 10.1093/icb/icr097
Seifert R. (1938). Die Bodenfauna Des Greifswalder Boddens. Ein Beitrag Zur Ökologie Der Brackwasserfauna. Z. Für. Morpholog. Und. Ökolog. Der. Tiere. 34, 221–271. doi: 10.1007/BF00408759
Simmons M. A., Knight A. W. (1975). Respiratory Response of Neomysis Intermedia (Crustacea: Mysidacea) to Changes in Salinity, Temperature and Season. Comp. Biochem. Physiol. – Part A.: Physiol. 50, 181–193. doi: 10.1016/S0010-406X(75)80223-4
Vajedsamiei J., Melzner F., Raatz M., Morón S., Pansch C. (2021). Cyclic Thermal Fluctuations can be Burden or Relief for an Ectotherm Depending on Fluctuations’ Average and Amplitude. Funct. Ecol. 35, 2483– 2496. doi: 10.1111/1365-2435.13889
Vasseur D. A., DeLong J. P., Gilbert B., Greig H. S., Harley C. D. G., McCann K. S., et al. (2014). Increased Temperature Variation Poses a Greater Risk to Species Than Climate Warming. Proc. R. Soc. B.: Biol. Sci. 281, 20132612. doi: 10.1098/rspb.2013.2612
Verslycke T., Janssen C. R. (2002). Effects of a Changing Abiotic Environment on the Energy Metabolism in the Estuarine Mysid Shrimp Neomysis Integer (Crustacea: Mysidacea). J. Exp. Mar. Biol. Ecol. 279, 61–72. doi: 10.1016/S0022-0981(02)00339-8
Vesakoski O., Boström C., Ramsay T., Jormalainen V. (2008). Sexual and Local Divergence in Host Exploitation in the Marine Herbivore Idotea Baltica (Isopoda). J. Exp. Mar. Biol. Ecol. 367, 118–126. doi: 10.1016/j.jembe.2008.09.006
Viitasalo M., Rautio M. (1998). Zooplanktivory by Praunus Flexuosus (Crustacea: Mysidacea): Functional Responses and Prey Selection in Relation to Prey Escape Responses. Mar. Ecol. Prog. Ser. 174, 77–87. doi: 10.3354/meps174077
Weisse T., Rudstam L. G. (1989). Excretion and Respiration Rates of Neomysis integer (Mysidaceae): Effects of Temperature, Sex and Starvation. Hydrobiologia 178, 253–258. doi: 10.1007/BF00006032
Keywords: nonlinear averaging, fluctuations, variable environments, thermal performance curves, sex-specific effects, time-dependent effects, brackish Mysida
Citation: Hennigs LM, Bergunder K, Sperfeld E and Wacker A (2022) Thermal Fluctuations Yield Sex-Specific Differences of Ingestion Rates of the Littoral Mysid Neomysis integer. Front. Mar. Sci. 9:883265. doi: 10.3389/fmars.2022.883265
Received: 24 February 2022; Accepted: 28 April 2022;
Published: 21 June 2022.
Edited by:
Christopher Edward Cornwall, Victoria University of Wellington, New ZealandReviewed by:
Jonathan Y.S. Leung, University of Adelaide, AustraliaKaitlyn Lowder, The Ocean Foundation, United States
Copyright © 2022 Hennigs, Bergunder, Sperfeld and Wacker. This is an open-access article distributed under the terms of the Creative Commons Attribution License (CC BY). The use, distribution or reproduction in other forums is permitted, provided the original author(s) and the copyright owner(s) are credited and that the original publication in this journal is cited, in accordance with accepted academic practice. No use, distribution or reproduction is permitted which does not comply with these terms.
*Correspondence: Laura M. Hennigs, laura.hennigs@uni-greifswald.de; Konstanze Bergunder, konstanze.bergunder@uni-greifswald.de
†These authors have contributed equally to this work and share first authorship