- 1Ocean College, Zhejiang University, Zhoushan, China
- 2National Marine Environment Monitoring Center, Ministry of Ecology and Environment, Dalian, China
- 3Ocean School, Yantai University, Yantai, China
From late November 2021, a large-scale Phaeocystis globosa bloom occurred for the first time in the southern Yellow Sea, covering an area of over 630 km2 and lasting for nearly 40 days. Two investigations, combined with satellite remote sensing, were successively conducted on December 11 and 23, 2021 to study the bloom dynamics and impacts. During the bloom, the average temperature decreased from 11.8°C to 10.0°C and salinity increased slightly from 30.9 to 31.3. The pH remained consistently at 8.22, while dissolved oxygen and chlorophyll-a content decreased dramatically from 7.23 mg L-1 to 5.82 mg L-1, 8.72 μg L-1 to 2.35 μg L-1, respectively. The concentration of nitrate and silicate decreased moderately, whereas nitrite, ammonium and phosphate increased obviously, especially the ammonium content, elevated from 0.90 μmol L-1 to 1.39 μmol L-1 during two investigations. Meanwhile, the P. globosa colony densities varied between 10 colonies L-1 and 185 colonies L-1, with a mean of 70.0 colonies L-1 on Dec. 11, and decreased dramatically to an average of 1.46 colonies L-1 on Dec. 23. Similarly, single cells decreased from 3.6×106 cells L-1 to 1.05×106 cells L-1 accordingly. Moreover, diameter of the colonies varied from 0.5 cm to 2.0 cm, with an average diameter of 1.0 cm, and no colonies smaller than 0.5 cm was observed in the bloom. Correlation analysis indicated temperature and salinity related with the colony abundance positively (P<0.001) and negatively (P<0.05), respectively, and silicate and nitrite concentration showed significant positive effects on solitary cell density (P<0.05). No mortality of marine organisms was observed and the haemolytic activity of Phaeocystis samples was low, varying between 13.07 Hu L-1 and 19.22 Hu L-1. Based on phylogenetic analysis, this P. globosa strain may represent a possible different ecotype which prefers low temperature and is less or nontoxicity.
Introduction
Haptophyte Phaeocystis globosa Scherffel is a cosmopolitan bloom-forming species, which can form nearly monospecific dense blooms in the world’s oceans, including the north temperate, tropical and subtropical waters (Schoemann et al., 2005; Shen and Qi, 2021). Massive blooms of P. globosa have been reported in many systems, particularly those nutrient-rich areas (e.g., North Sea, English Channel, Arabian Sea, and South China Sea) (Lancelot et al., 1998; Shen et al., 2018). Its blooms are considered harmful algal blooms (HABs) which are associated with dramatic ecosystem changes and negative impacts on fisheries and fish farming (Lancelot et al., 1987; Chen et al., 1999; Verity et al., 2007). They have also been responsible for causing large-scale fish kill, net-clogging and subsequent consequence (Rousseau et al., 2013; Yu et al., 2017). All these impacts are primarily due to a heteromorphic life cycle event that transforms between solitary cell and gelatinous colonial stages where hundreds of or thousands of cells are embedded in a polysaccharide matrix (Rousseau et al., 2007; Verity et al., 2007). Although numerous studies have focused on the ecology and physiology of Phaeocystis blooms worldwide during the past several decades, it is still difficult to generalize the physical-biological interactions in the coastal system (Smith et al., 2021).
Since the first large-scale bloom outbreak in the southeast coast of China in late 1997, P. globosa has expanded rapidly in the coastal areas of China and often cause substantial economic losses in aquaculture fisheries (Shen et al., 2018; Yu et al., 2020). By 2020, totally 97 Phaeocystis bloom events have been recorded, covering an area of nearly 20,000 km2, which is mostly distributed in the northern South China Sea (SCS), and the Bohai Sea (BS) is the next (Wang et al., 2021). However, despite the high frequency of blooms along the eutrophic coasts of China, no P. globosa bloom was recorded in the southern Yellow Sea and the northern East China Sea near the Yangtze River Estuary in the past 30 years. It is a rather unique phenomenon since these coastal areas are located just between the SCS and BS, where Phaeocystis blooms occurred frequently (Shen et al., 2018).
In late November 2021, a large-scale Phaeocystis bloom dominated by giant colonies broke out in the southern Yellow Sea for the first time, covering an area up to 1543 km2 (estimated by North Sea Forecast Center of State Oceanic Administration, China). According to morphological and molecular characteristics, the causative species was identified as Phaeocystis globosa. This bloom event has a distinguishing feature as it occurred in winter in Yellow Sea and the temperature was much lower than that of tropical and subtropical areas (Shen et al., 2004; Xu et al., 2017; Wang et al., 2021). To better understand the bloom dynamics and its influencing factors, in situ investigations on phytoplankton succession, colony numbers and size, nutrient concentrations as well as other environmental factors were conducted during the bloom. Given its large coverage area, satellite remote sensing technology was combined to track and analyse the development of the bloom, hoping to reveal the dynamic characteristics and influencing factors of the first occurrence of P. globosa bloom in the southern Yellow Sea.
Materials and Methods
Study Area, Field Sampling and Processing
The bloom area is within the southern Yellow Sea (SYS) and closes to Qingdao’s west coasts. The water depth is less than 20 m in most part of the bloom area and there are no major rivers discharged and it is profoundly influenced by two water regimes: the Yellow Sea Warm Current (YSWC) and the southward coastal current (Xiong et al., 2019). The YSWC is distinctly warmer and saltier than the ambient water, which affects the hydrography and biogeochemistry of the shallow water dramatically (Su, 2005). Our sampling sites are near Dongjiakou Harbor, one of the busiest harbors in the world, with an average water depth of about 15 m. During the past 30 years, this region is usually characterized by largely eutrophic conditions, i.e. high concentration of inorganic nutrients. Consequently, the dynamical variation and biogeochemical processes of nutrients and physical features in the region are very complicated (Xing et al., 2015).
Due to the large scale of the bloom, field samples were successively collected from 9 selected stations to cover different areas during the bloom on December 11 and 23, 2021, respectively. Stations A1-A2 were close to Dongjiakou Harbor, A3-A5 were near the mariculture area and A6-A9 were offshore stations, respectively (Figure 1).
At each station, water samples were collected from the surface and the 10 m depth using a 5-L Niskin bottle and in situ environmental factors (temperature, salinity, Dissolved Oxygen (DO) and pH) were measured by a YSI 6600 multi-parameter water quality monitor (Yellow Springs Instrument Co., USA). After collection, water samples for dissolved nutrients and chlorophyll a (Chl a) determination were filtered through 0.45 μm cellulose filters and both the water and filters were preserved at -20°C in darkness before further laboratory processing. The nutrient (nitrate, nitrite, ammonia, phosphate and silicate) concentrations were analyzed by a nutrient-analyzer (SEAL AA500, Germany) according to the standard method. Chlorophyll a concentration was measured according to Parsons et al. (1984) on a Turner Design 10-AU fluorometer.
Phytoplankton Analysis
Water samples for phytoplankton analysis (500 ml) were fixed with formaldehyde in 2% final concentration, and then concentrated to 20 ml using the Utermöhl method. Cells were counted at 100×~1000× magnification on an Olympus microscope (BX53). For Phaeocystis colony, three subsamples (volume was determined after colony measurements) was taken gently using a petri dish (120mm in diameter) before fixation to measure the colony number and diameters. The diameters of individual colonies were measured by a ruler which was placed under the Petri dish (resolution 0.2 mm).
Molecular Identification
Colonies were collected during the first investigation and washed by sterilized seawater several times. The cells were then centrifuged at 10,000 rpm and used for DNA extraction. HP Plant DNA Kit (OMEGA Bio-Tek, Inc., US) was used to extract the total DNA according to the manufacture’s instructions and the SSU rDNA gene was amplified using the primers 18S comF1: (5′-GCTTGT CTCAAAGATTAAGCCATGC-3′) and 18S comR1: (5′-CACCTACGGAAACCTTGTTACGAC-3′) as described in Qin et al., 2016). The PCR products were then purified and sequenced by Shanghai Sangon Biological Engineering Co., Ltd. The 18S rDNA sequence was compared to Phaeocystis spp. sequences in GenBank (Table 1). Maximum-likelihood (ML) method was used for phylogenetic analyses and Prymnesium Parvum K081 (Prymnesiophyceae) was used as the outgroup. Sequences were aligned using CLUSTALW and MEGAX was used to construct the phylogenetic tree with a bootstrap value of 1000.
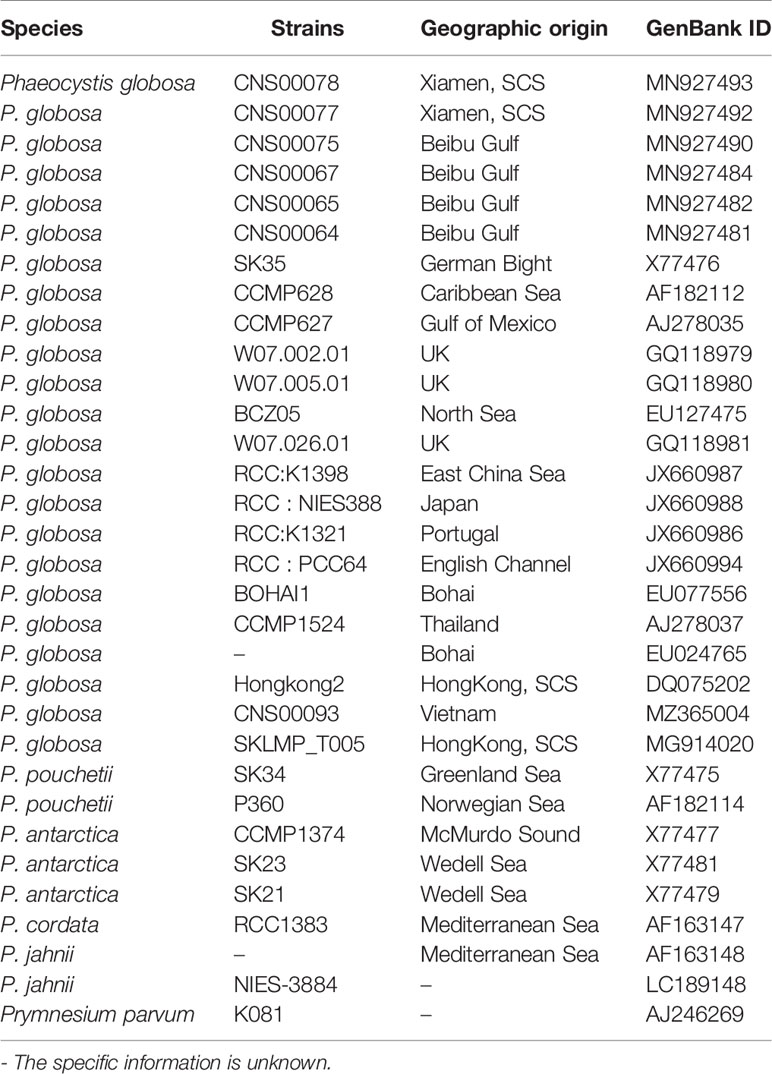
Table 1 Geographic origins and SSU rDNA sequence GenBank Acc. No. of algal strains used in the phylogenetic analysis.
Remote Sensing
The GF-1 and Sentinel-2 data was downloaded from the China Center for Resources Satellite Date and Application, and the European space agency, respectively. The watercolor information discriminant method is used to interpret the bloom and the true color image is synthesized by the red, green, and blue wavebands. The macroscopic observation is carried out first to master the overall characteristics of the image. Based on established interpretation symbols, classification samples database and related dataset, the algal bloom and its distribution was identified and calculated according to the bloom color characteristics (appearing in brown or black) and its surrounding water body using the human-computer interaction method in the ENVI software.
Data Analysis
The distribution of the environmental factors and Phaeocystis globosa abundance were determined using Surfer 13.0 (Golden Software, Inc.). Correlation analysis between these parameters was performed with Pearson coefficient analysis (Statistics 26, SPSS), and results were presented as significant with the symbol P* (<0.05) and P** (<0.01), respectively. To understand which environmental parameter has the greatest influence on Phaeocystis assemblage, principal components analysis (PCA) was employed to analyze a matrix of the environmental variables including temperature, salinity, pH, DO, and nutrients (SiO3, PO4, NH4, NO3 and NO2). All concentration data were transformed and normalized, and multivariate analyses was carried out using Origin software.
Haemolytic Activity Analysis
Haemolytic activity was analyzed on filtered P. globosa colony samples which were collected through a plankton net (77 μm meshed net) during the first investigaion according to the erythrocyte lysis assay method (van Rijssel et al., 2007).
Results
Bloom Description (Location, Timing, and Coverage)
The onset of the Phaeocystis globosa bloom in the SYS was around November 20, 2021, when high-density colonies were suddenly observed by naked eyes near the sampling area (fisherman, per. comm.). The remote sensing imagery showed the development of the bloom in coverage and spatial information from November 27 to December 27, 2021 (Figure 2). The bloom broke out near the southern coastal line with a coverage area of 6 km2 on November 23, 2021 (Table 2), then it developed dramatically to a maximum coverage of 630 km2 on December 2, 2021 (Figure 2). After that, the bloom lasted for nearly 40 days and disappeared due to a severe cold wave on December 27, 2021 (Figure 2).
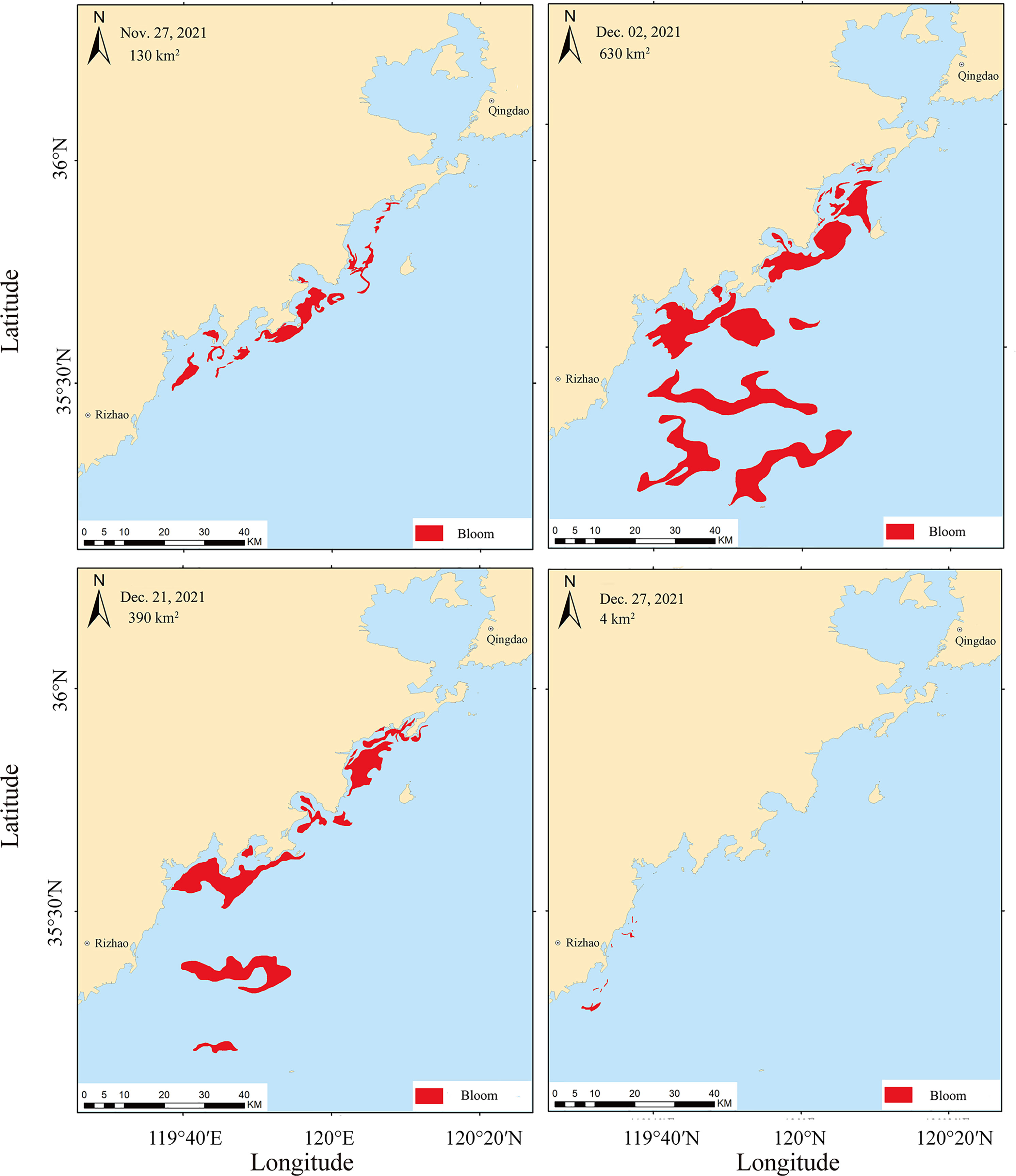
Figure 2 Remote sensing imagery of the Phaeocystis globosa bloom showing the coverage area (red patch) development from November 27 to December 27, 2021.
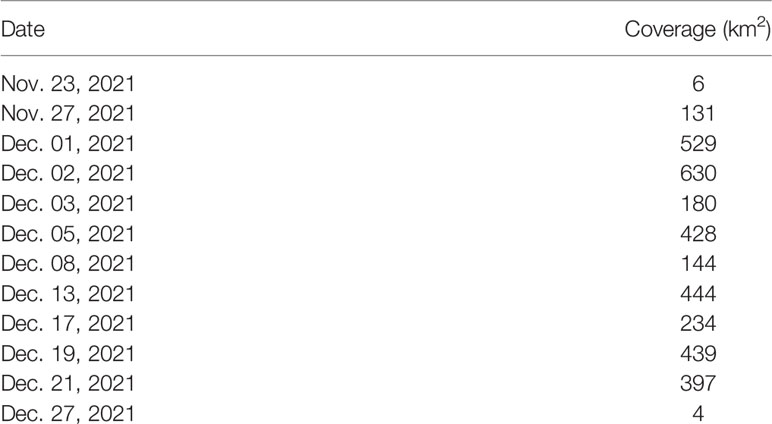
Table 2 Estimated coverage of the Phaeocystis globosa bloom based on remote sensing imagery along the southern Yellow Sea from November 23 to December 27, 2021.
In general, the water temperature of the SYS in the winter of 2021 fluctuated greatly (Figure 3). Before the bloom, the water temperature in 2021 was lower than those of 2019 and 2020 due to a severe cold wave in middle November (Figure 3). Then a large-scale Phaeocystis bloom occurred around Nov. 20, 2021 and lasted for over 30 days. During the bloom, the temperature kept relatively consistent and higher than those of previous years until December 26, when the water temperature decreased sharply from 12.0°C to 8.0°C due to a severe cold wave (Figure 3). Consequently, the algal bloom decayed ultimately.
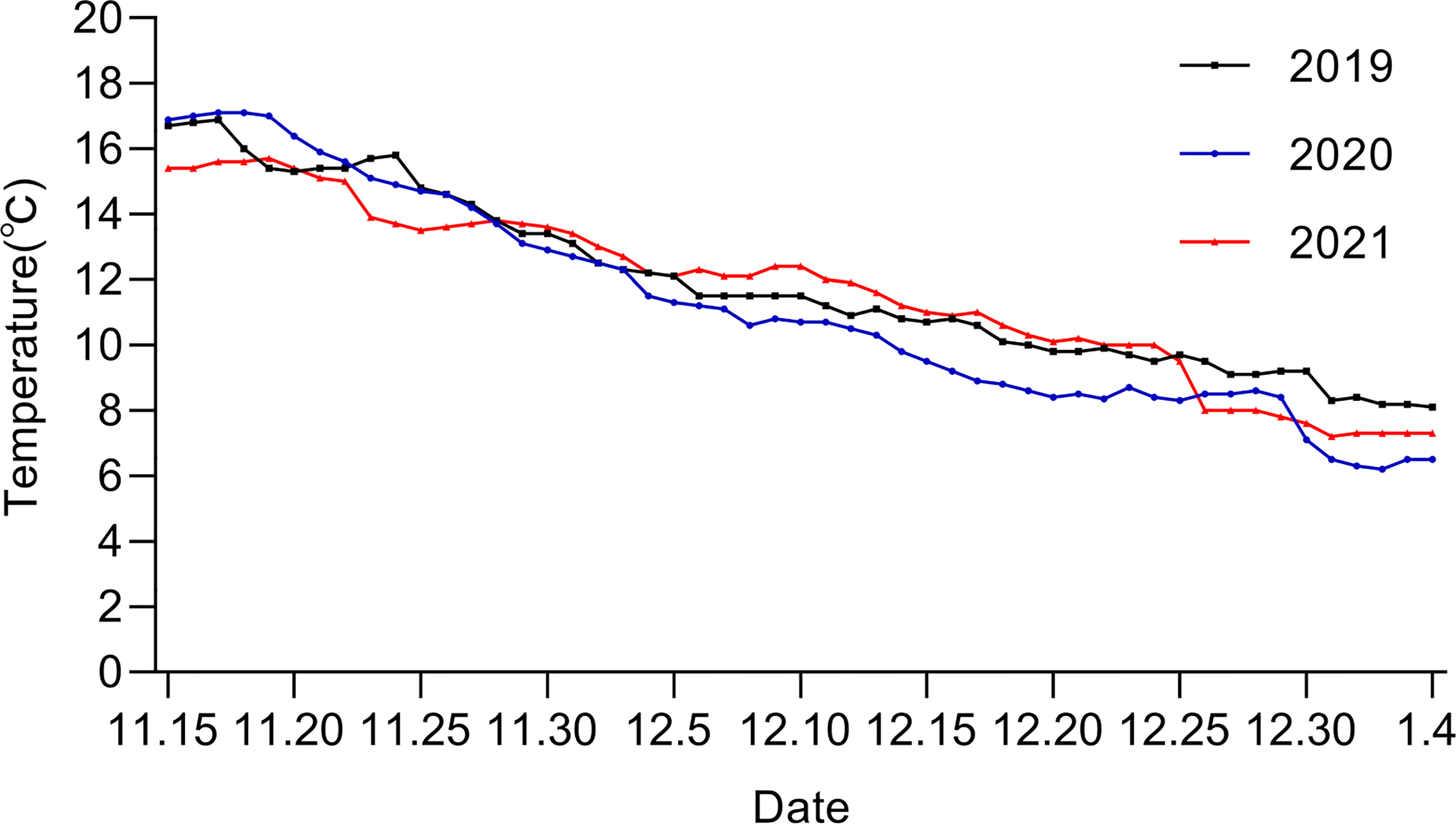
Figure 3 Sea surface temperature of the southern Yellow Sea in winter (from November to January) of 2019-2021 (data from Qingdao Marine forecast and marine early warning information release platform http://www.qdmf.org.cn/).
In Situ Measurement of the Environmental Factors
Two investigations were successively conducted during the middle and late phases of the Phaeocystis bloom on December 11 and 23, 2021, respectively. During the development of the bloom, the average temperature of the water column in sampling stations was 11.8°C on Dec. 11, and decreased to 10.0 °C on Dec. 23, 2021 (Table 3). The salinity increased slightly from 30.9 to 31.3 and the pH remained consistently at 8.22, while the DO decreased dramatically from 7.23 mg L-1 to 5.82 mg L-1. Meanwhile, the Chla content dropped sharply from 8.72 μg L-1 to 2.35 μg L-1 during two investigations (Table 3).
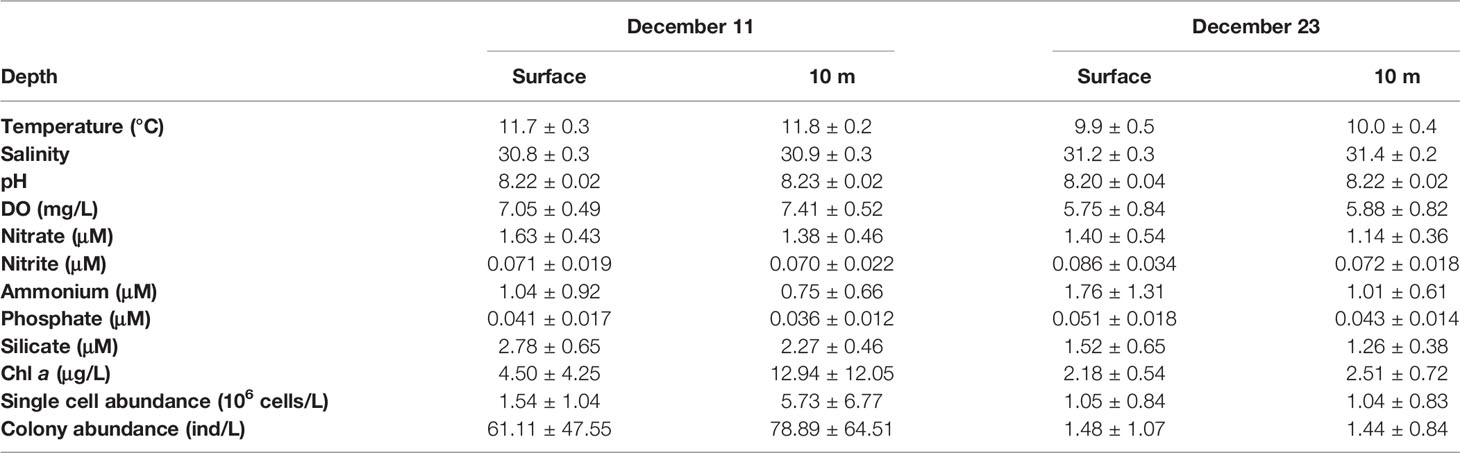
Table 3 Environmental parameters (Average ± SD) of in situ investigations during the Phaeocystis globosa bloom in the coastal water of southern Yellow Sea in December 2021.
As the bloom progressed, the average concentrations of nitrate and silicate in the water column decreased moderately throughout the survey area (Table 2), whereas nitrite, ammonium, and phosphate contents increased obviously after the peak of the bloom, in particular the concentration of ammonium which elevated from 0.90 μmol L-1 to 1.39 μmol L-1 in the decline period (Table 3). In vertical distribution, the nutrient concentrations were generally higher in the surface water than in the 10 m layer at most stations except stations A1 and A8 (Figure 4). Horizontal distributions of the nutrients showed spatial variation with the highest concentrations mostly occurring in the north-western part of the survey area, especially during the second investigation (Figure 4).
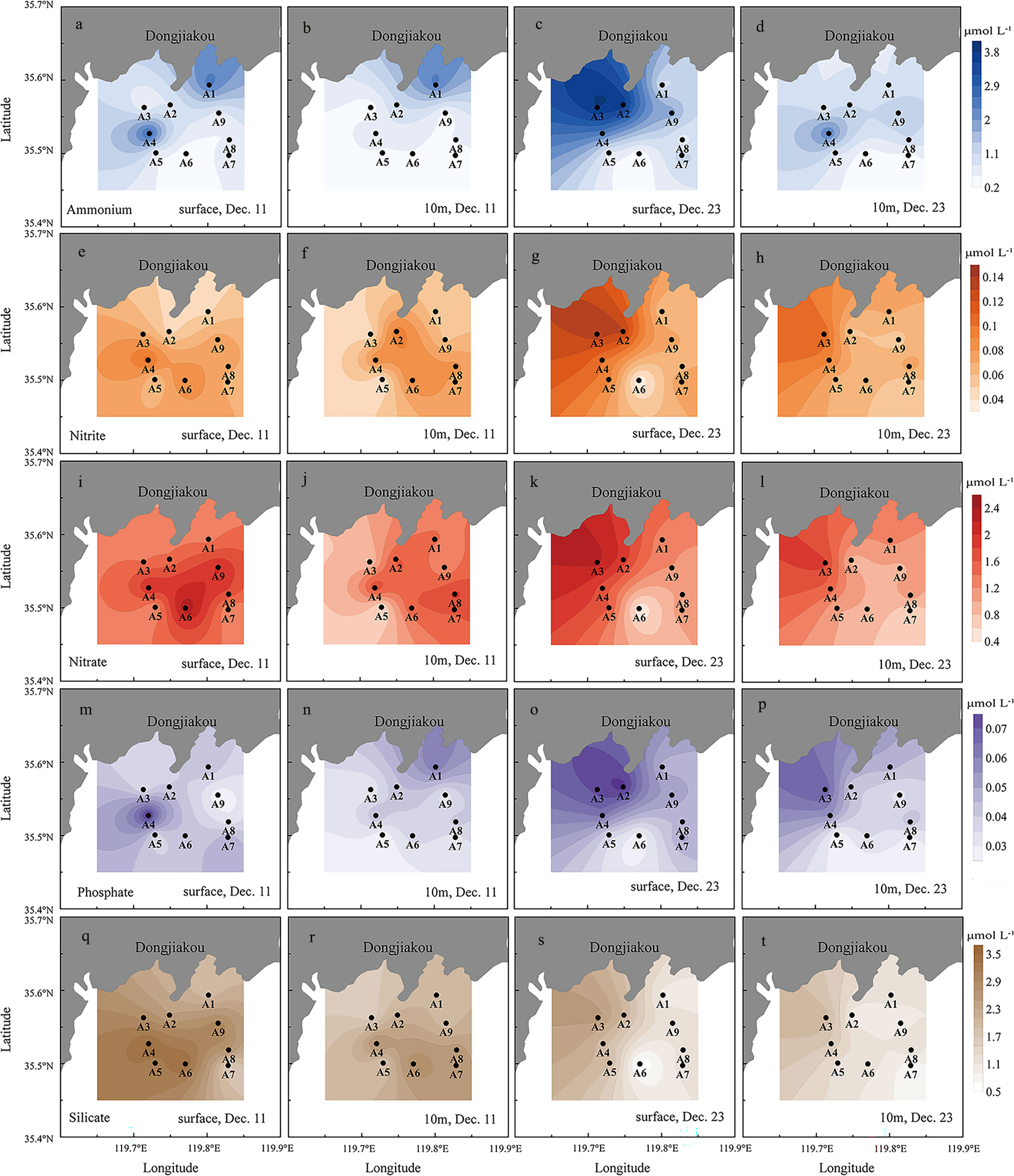
Figure 4 Horizontal and vertical distribution of nutrient concentrations in the surface and the 10m layer in the sampling stations (Ammonium: a-d; Nitrite: c-h; Nitrate: i-l; Phosphate: m-p; Silicate; q-t).
Dynamics of the Phaeocystis globosa Bloom
Phaeocystis globosa completely dominated the community as large colonies both in abundance and biomass during the bloom. The size of the colonies varied from 0.5 cm to 2.0 cm, with an average diameter of 1.0 cm, and no colonies smaller than 0.5 cm were observed in the bloom. During the first investigation, the colony numbers varied between 10 colonies L-1 to 185 colonies L-1, with a mean of 70.0 ± 55.7 colonies L-1 through the water column (Table 2 and Figure 5). In surface water, the colony density ranged from 20 colonies L-1 to 121 colonies L-1, with an average of 61.1 ± 47.6 colonies L-1, while at the 10 m water depth, the colony numbers shifted between 10 colonies L-1 and 185 colonies L-1 (mean 78.9 ± 65.5 colonies L-1), which was much higher than those of the surface (Table 3 and Figure 5). Besides the colony, single-cell density was recorded between 0.24×106 cells L-1 and 17.1×106 cells L-1, with a mean of 3.6×106 cells L-1. Similarly, the single-cell density in surface water (1.54×106 cells L-1) was also lower than that of the 10 m layer (5.73×106 cells L-1) (Table 3).
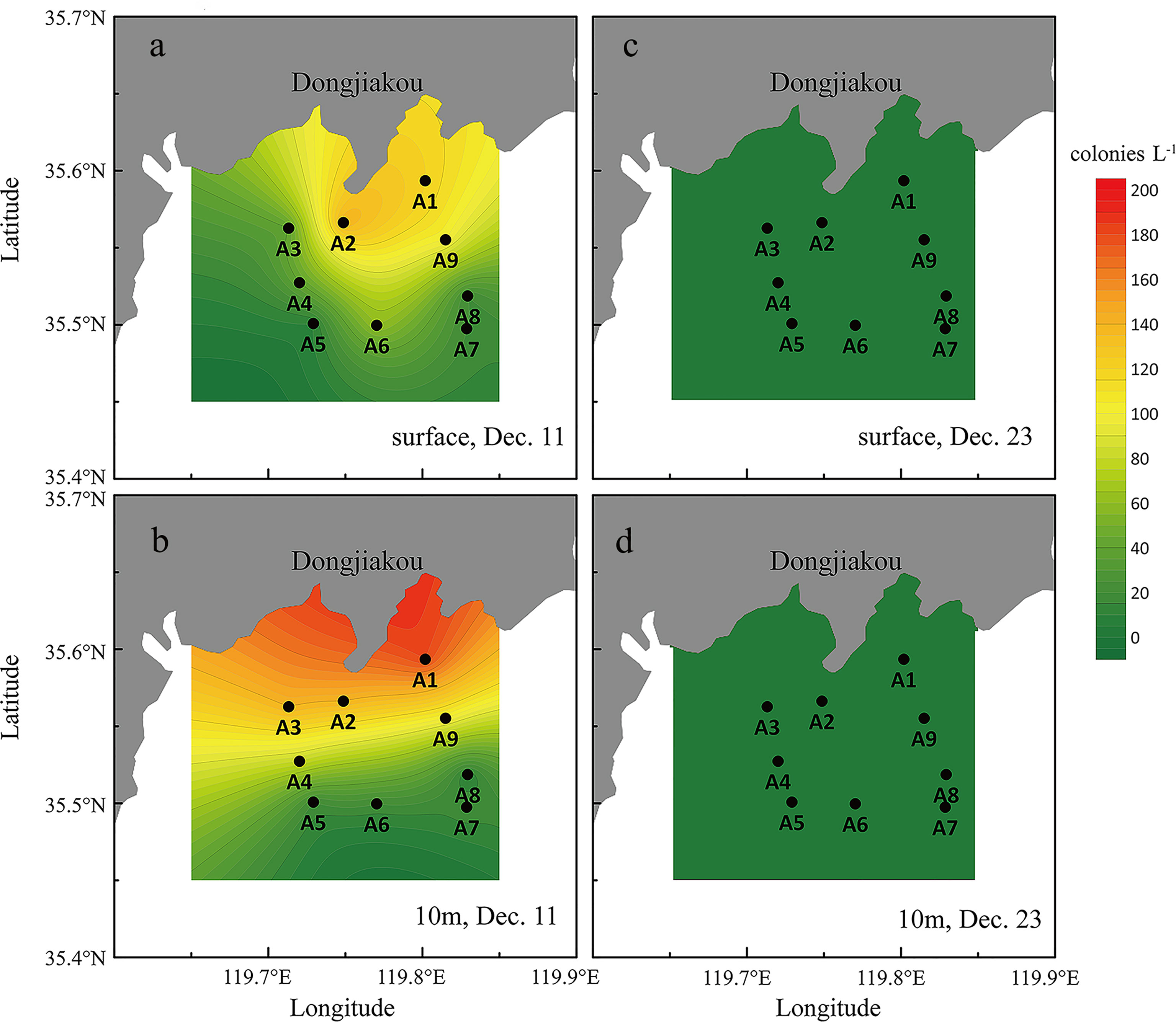
Figure 5 Phaeocystis globosa colony distribution in the surface and the 10m layer (A): surface, Dec. 11; (B): 10m layer, Dec. 11; (C): surface, Dec. 23; (D): 10m layer, Dec. 23.
The second investigation was conducted during the decline of the bloom, when the colony density decreased dramatically to an average of 1.48 colonies L-1 and 1.44 colonies L-1 in the surface nd the 10 m water depth, respectively. Meanwhile, the density of single cell in the water column was around 1.05×106 cells L-1 (Table 3).
Species Identification
Base on phylogenetic analysis, our strain (SYS-01) is confirmed as Phaeocystis globosa and shows the highest similarity (99.7%) with P. globosa strains isolated from the Chinese coasts, including the South China Sea and the Bohai Sea, belong to the same sub-group within the P. globosa clade but with a minor sequence divergence (0.3%) (Figure 6).
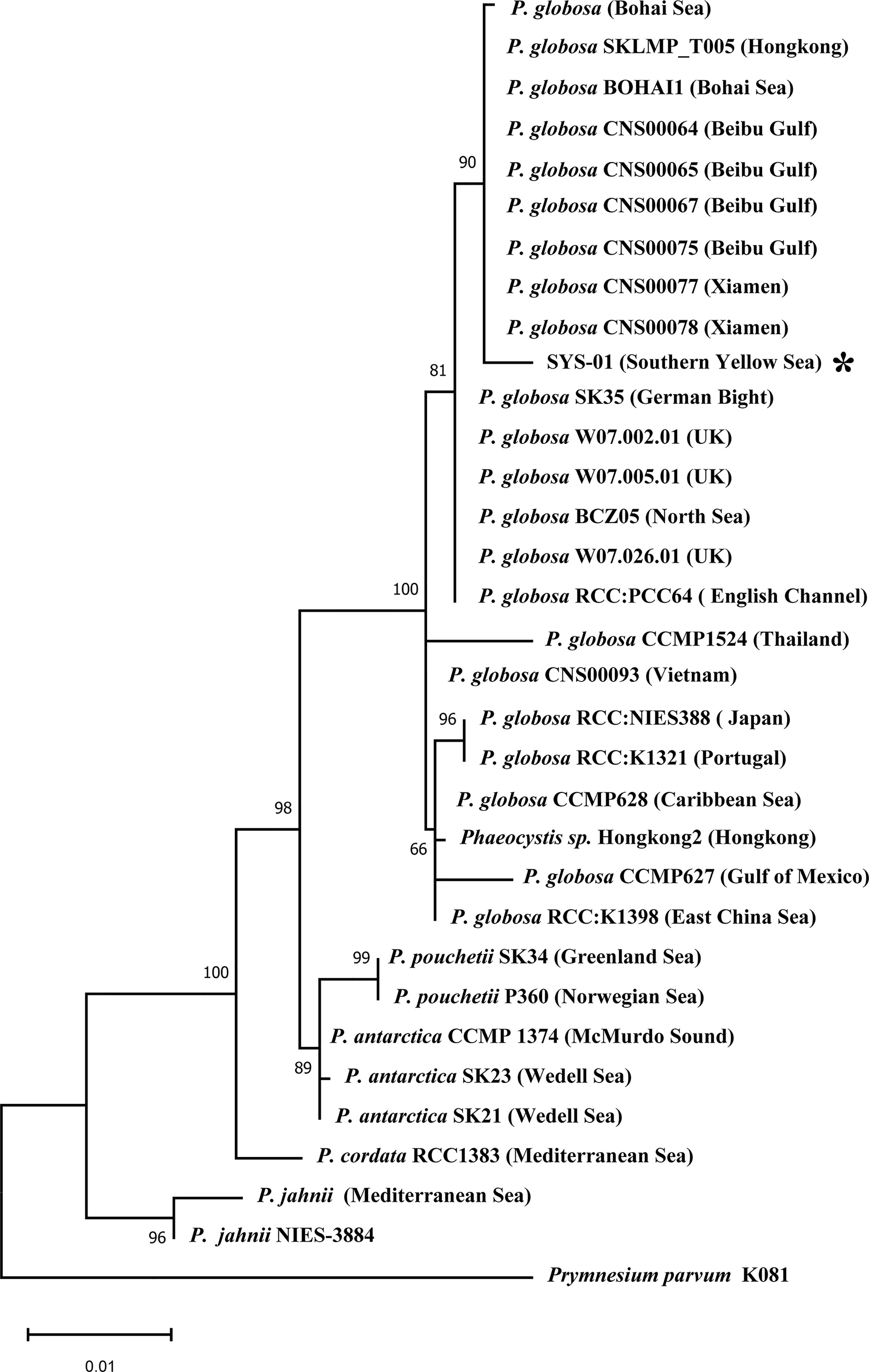
Figure 6 Phylogeny of Phaeocystis based on 18S rDNA partial sequence sequences (1478 characters after data processing). The phylogenetic tree was inferred by the maximum likelihood (ML) method, using a Tamura-Nei model of DNA substitution. Bootstrap values are indicated above internodes and were estimated from 1000 replicates. The scale bar indicates 0.01% sequence divergence. The symbol * indicates the SYS strain isolated from the southern Yellow Sea.
Statistical Analysis
Temperature and salinity were found to be positively (P<0.001, n=36) and negatively (P<0.05, n=36) related with the P. globosa colony abundance, respectively, whereas silicate (P<0.001, n=36) and nitrite concentration (P<0.05, n=36) showed significant positive effects on solitary cell density during the investigations.
Multivariate analysis (PCA) showed that the first two components combined accounted for approximately 72% of the total variance. The first principle component (PC1, explaining 39.4% of the total variance) was positive for phosphate, inorganic nitrogen (ammonia, nitrite, and nitrate), but negative for temperature and DO content. The second principle component (PC2, explaining 32.4% of the total variance) was mainly due to variations in silicate level, salinity, and temperature (Figure 7). Samples from the middle bloom were featured with high temperature, DO and high silicate concentration, while in the late bloom, samples were characterized by high salinity, high concentrations of ammonium and phosphate, but low temperature and low silicate content on the contrary (Figure 7).
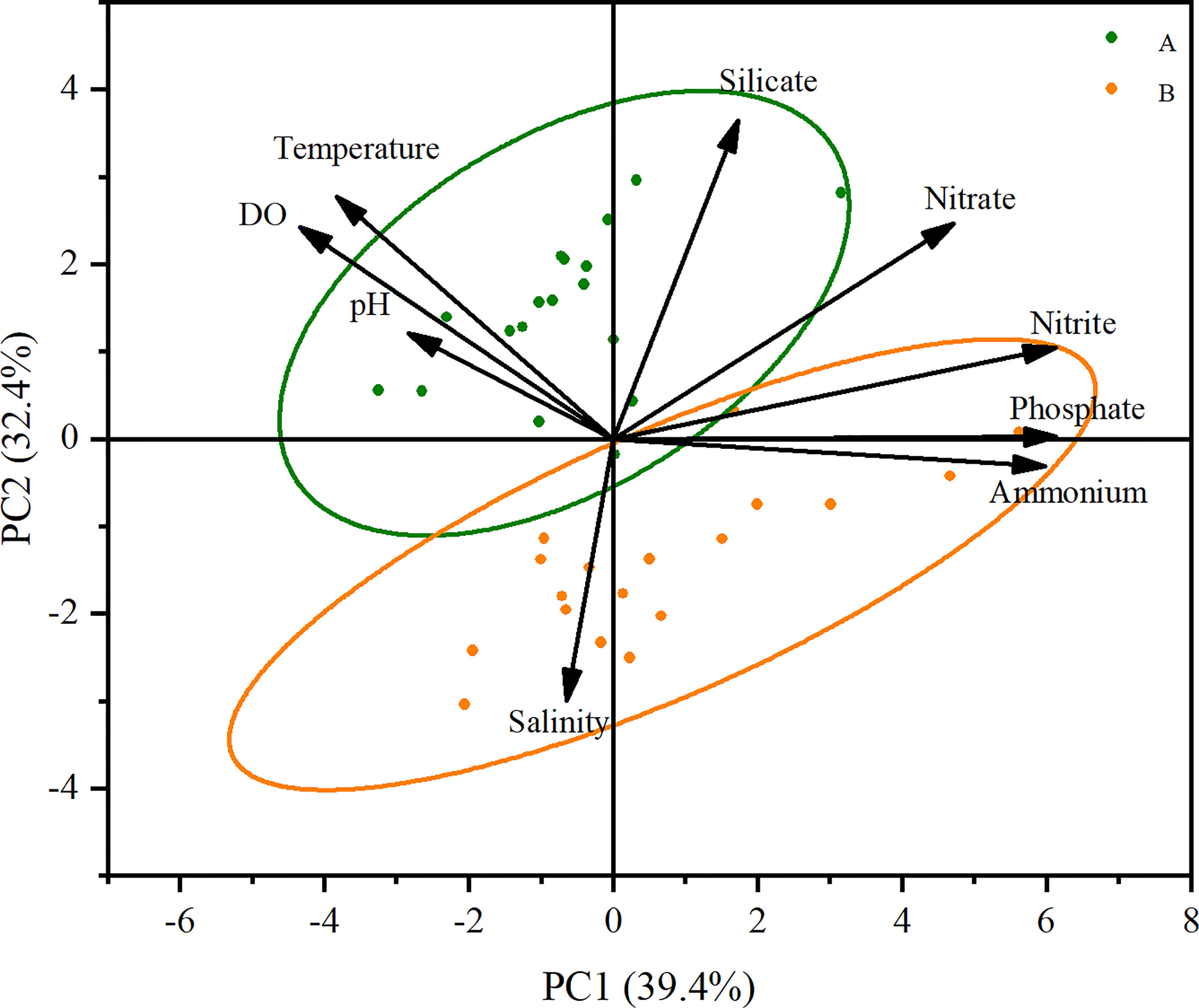
Figure 7 Principal component analysis of the environmental factors (A: green circles indicate samples from the middle bloom on December 11, 2021; B: orange circles indicate samples from the late bloom on December 23, 2021).
Bloom Impacts and Haemolytic Activity of Phaeocystis globosa
No mortality of marine organisms during the bloom was observed both in free-living fishes and caged bivalves and molluscs, but the structure and function of the ecosystem have changed substantially. The haemolytic activity measured on the filtered colony samples collected during the first investigation was very low, which varied between 13.07 HuL-1 and 19.22 Hu L-1.
Discussion
Environmental Regulation
This is the first record of Phaeocystis globosa bloom in the SYS and also the first occurrence of algal bloom in winter (November to December) along the Shandong coast since 1952 (Zhou et al., 2020). This bloom broke out in late November 2021 suddenly and an estimated coverage area 1543 km2 along Qingdao west coast was ever reported on December 4-5, 2021 (estimated by North Sea Forecast Center of State Oceanic Administration, China). Several distinguishing features, such as large coverage, long duration, extremely high biomass, huge colony, and in particular the low temperature have differentiated this bloom from other Phaeocystis bloom events. During the investigations, the water temperature ranged from 9.1°C to 12.2°C, which was much lower than those of P. globosa blooms in the South China Sea (15°C to 27°C) (Wang et al., 2021), and also lower than the recorded temperature for the Bohai Sea P. globosa blooms (~15°C to 20.8°C; Tu et al., 2011). For high-latitude North Sea, P. globosa blooms usually occurred between early April to early June and the optimal temperature for the blooms was 15°C to 20°C (Schoemann et al., 2005), sometimes lower than 15°C (Seuront et al., 2006)., P. globosa is a warm-water species with the growth temperature range in culture was 2-30°C and its bloom is usually concentrated in an area with high water temperature and high nutrients in temperate and tropical seas (Schoemann et al., 2005; Medlin and Zingone, 2007; Shen and Qi, 2021). However, the first occurrence of P. globosa bloom in the SYS from late November to December 2021, expanded the temperature ranges of P. globosa blooms significantly.
A suitable temperature is an important factor affecting the occurrence and extinction of Phaeocystis globosa bloom. Studies have shown that appropriate temperature can accelerate the cell division of P. globosa, leading to the rapid formation of colonies from solitary cells, and then transform into new colonies (Rousseau et al., 1994; Tian, 2010; Rousseau et al., 2013), while temperature increase is not conducive to the formation of colonies (Wang et al., 2010), indicating that stable temperature was more important for the occurrence and maintenance of P. globosa bloom. In this study, compared with previous years, the temperature remained relatively higher and consistent throughout the bloom period, providing a suitable environmental condition for the bloom. The ability of P. globosa to grow at a wide range of temperature allows its bloom development in the winter season and this should be one main reason for the occurrence of P. globosa bloom in the winter Yellow Sea, and our correlation results also confirmed the significant effect of temperature.
Besides stable temperature, the development of Phaeocystis bloom can be generally controlled by nutrient levels (He et al., 2019; Xu et al., 2019). For example, early spring massive blooms in Antarctic waters are terminated once nutrients become depleted (Schoemann et al., 2005). In the present study, the only temperature was found to be positively related to the colony abundance, whereas silicate and nitrite showed significant positive effects on solitary-cell density during the investigations. As the bloom progressed, the concentrations of nitrate and silicate decreased moderately, whereas nitrite, ammonium and phosphate contents increased obviously, in particular the ammonium concentration which elevated from 0.90 μmol L-1 to 1.39 μmol L-1, suggesting sufficient nutrient supply in these coastal areas. In fact, the Yellow Sea is usually characterized by high eutrophication in the past few decades, especially during the summer when massive green tides occurs (Xing et al., 2015; Tian et al., 2020), indicating that nutrients are not limiting factors in the SYS. Similarly, the abundance and length of Phaeocystis bloom in the North Sea were not correlated with the increasing eutrophication (Schoemann et al., 2005). Therefore, other mechanisms rather than nutrient driving should be taken into account in the future studies.
Biological Factors
In addition to environmental factors, biological factors are essential in the occurrence of algal bloom, including the bloom-forming organism and its specific features (Lin et al., 2019; Glibert, 2020; Yu et al., 2020). For Phaeocystis globosa, of particular importance is polymorphic life cycle alternating between solitary cells of 3-9 μm in diameter and gelatinous colonies usually of several mm up to 3cm (Peperzak et al., 2000; Chen et al., 2003). The occurrence of P. globosa bloom is primarily a life cycle event that transforms from single cells to colonies under eutrophication conditions in a short time and forms a nearly monospecific bloom in the marine ecosystem (Verity et al., 2007). Therefore, the transition mechanisms and influencing factors are important to understand the bloom development of this species (Reigstad and Wassmann, 2007).
P. globosa occurred exclusively in the community as large colonies both in abundance and biomass during the bloom. The maximum colony concentration of 185 colonies L-1 and 30.3 μg L-1 Chl a concentration were observed during the first investigation, which was similar to a maximum record in the North Sea Dutch coastal area (20×107 cells L-1, 35 μg L-1 Chl a, respectively, Schoemann et al., 2005). A maximum colony density was recorded as 496 colonies L-1 near the Fenghe estuary during the first investigation and the Chl a content was 24.3 μg L-1 (data not included in the above statistical analysis). Although the solitary cell abundance of P. globosa was up to 1.7×107 cells L-1 in the present study, the in situ seawater was almost colorless and transparent, indicating the single-cell density was very low in natural condition and the relatively high cell density was most likely due to the disruption of the colony during sample manipulation. In addition, considering the fact that no colonies smaller than 0.5 cm existed during the bloom, it was speculated that the proliferation of Phaeocystis mainly depended on the division of colony directly, rather than the formation from solitary cells (Rousseau et al., 2007).
Besides life-history strategy, it has been demonstrated that P. globosa is a species complex composed of at least three subspecies (Medlin and Zingone, 2007; Smith et al., 2014). Although this SYS strain shows the highest similarity with the P. globosa strains isolated from the South China Sea and the Bohai Sea, a minor sequence divergence (0.3%) was detected based on 18S rDNA sequence. Moreover, high genetic diversity of the strains along the China coasts were revealed based on high-resolution molecular markers (See Song et al., 2021 and references therein). Therefore, the P. globosa SYS strain may represent a possible different ecotype that prefers low growth temperature or has other physiological features. Although no P. globosa record has ever been reported in previous surveys in the SYS (Wang, 2003; Qu et al., 2009), it can’t be ruled out the suspicion of the local origin of this organism at present. Therefore, further regional research needed to be done to elucidate its characteristics, bloom dynamics and mechanism if as a new ecotype despite its origin.
Bloom Impacts
Phaeocystis blooms are considered harmful algal blooms as high concentration colonies have been associated with a variety of ecosystem changes and negative effects on fisheries and fish farming (Lancelot et al., 1987; Schoemann et al., 2005; Veldhuis and Wassmann, 2005; Nejstgaard et al., 2007). The first occurrence of P. globosa bloom in the South China Sea has ever resulted in massive fish kill in 1997 (Chen et al., 1999) and that strain has been reported to produce certain toxic substances, including hemolytic glycolipids and other ichthyotoxins (He et al., 1999; Peng et al., 2005; van Rijssel et al., 2007; Yang et al., 2009). Fortunately, no mortality of marine organisms throughout the bloom was observed both in free-living fishes and caged bivalves and molluscs in the SYS area. Most importantly, the hemolytic activity measured on the P. globosa colony collected during the bloom was very low, indicating less toxicity or nontoxicity of the SYS strain. Similarly, the P. globosa blooms that occurred in the temperate Bohai Sea also caused no damage to marine life before (Qu et al., 2008; Tu et al., 2011). It has been demonstrated that temperature was the most prominent factor in toxin production and the P. globosa toxic strain isolated from the South China Sea accumulated less toxin during rapid growth under low temperature (Guo et al., 2007). Moreover, synthesis of the toxins mainly occurred in the stationary and senescent phase, and rarely in the logarithmic phase of the bloom (Peng et al., 2005).
Since Phaeocystis-dominated ecosystems are generally associated with commercially important stocks of crustaceans, molluscs, fishes and mammals, dense blooms have been responsible for causing net-clogging and alteration of fish taste (Levasseur et al., 1994). Due to its large-scale and high density, the structure and function of the ecosystem have changed substantially, and no fish was caught during this period and some coastal trawl instruments and nets were blocked seriously and damaged in the SYS (fishermen, per. comm.). Therefore, future work should be focused on its ecological impacts in particular the extremely high biomass and related biogeochemistry contributions to the local ecosystem.
Conclusion
This is the first report of large-scale Phaeocystis globosa bloom in the SYS which occurred from late November to December 2021, covering an area of over 630 km2 and lasting for nearly 40 days. Several distinguishing features, including huge colony, extremely high biomass, and especially the low temperature have differentiated it from other bloom events. This bloom occurred in winter and the temperature (9.1-12.2°C) was much lower than that of tropical and subtropical areas (15-27°C). It was dominated completely by giant colonies, with the sizes varying from 0.5 cm to 2.0 cm and the maximum Chl a concentration was up to 30 µg L−1 during the bloom. Temperature showed a significant positive effects on the colony abundance, while no significant correlations were detected between levels of nutrients and colonies. Therefore, other mechanisms rather than nutrient driving should be taken into account in the future studies. Moreover, based on phylogenetic analysis, the P. globosa SYS strain may represent a possible different ecotype that prefers low growth temperature or has other physiological features (i.e. less toxicity or nontoxicity under low temperature). Therefore, further long-term investigation needed to be done to better understand the bloom dynamics and mechanism of this possibly new ecotype P. globosa in the southern Yellow Sea.
Data Availability Statement
The raw data supporting the conclusions of this article will be made available by the authors, without undue reservation.
Author Contributions
DL and PS conceptualized the study. YX, QS, FC, YM, DL, MT, and PS acquired the data. YX, QS, and PS performed the statistical analysis. YX and QS generated the figures. DL and PS drafted the manuscript. All authors contributed to the article and approved the submitted version.
Funding
This study was supported by the National Natural Science Foundation of China (41976114) and Open Fund of Laboratory for Marine Ecology and Environmental Science, Qingdao National Laboratory for Marine Science and Technology (LMEES201803).
Conflict of Interest
The authors declare that the research was conducted in the absence of any commercial or financial relationships that could be construed as a potential conflict of interest.
Publisher’s Note
All claims expressed in this article are solely those of the authors and do not necessarily represent those of their affiliated organizations, or those of the publisher, the editors and the reviewers. Any product that may be evaluated in this article, or claim that may be made by its manufacturer, is not guaranteed or endorsed by the publisher.
Acknowledgments
Thanks to Dr. Tao Jiang who provided useful information of this bloom events. Our team members in Yantai University helped to process the samples in the laboratory.
References
Chen Y., Shao P., Wang N., Zhou H., Qu L., Medlin L. K. (2003). Molecular Identification of Bloom-Forming Species Phaeocystis Globosa (Prymnesiophyta)and its Dispersal Based on rDNA ITS Sequence Analysis. Acta Oceanol. Sin. 22, 243–253. doi: 10.1029/2002JC001507
Chen J., Xu N., Jiang T., Wang Y., Wang Z., Qi Y. (1999). A Report of Phaeocystis Globosa Bloom in Coastal Water of Southeast China. J. Jinan. Univ. (Natural. Science). 20, 124–129. doi: 10.1088/0256-307X/16/12/025
Glibert P. M. (2020). Harmful Algae at the Complex Nexus of Eutrophication and Climate Change. Harmful. Algae. 91, 101583. doi: 10.1016/j.hal.2019.03.001
Guo J., Yang W., Liu J. (2007). Effects of Salinity, Temperature and Light Intensity on the Growth and Toxin Production of Phaeocystis Globosa. Acta Sci. Circumstantiae. 27, 1341–1346. doi: 10.1139/y71-060
He J., Shi Z., Zhang Y., Liu Y., Jiang T., Qi Y. (1999). Morphological Characteristics and Toxins of Phaeocystis Cf. Pouchetii (Prymenesiophyceae). Oceanol. Limnol. Sin. 30, 172–179.
He C., Song S. Q., Li C. W. (2019). The Spatial-Temperal Distribution of Phaeocystis Globosa Colonies and Related Affecting Factors in Guangxi Beibu Gulf. Oceanol. Limnol. Sin. 50 (3), 630–643. doi: 10.11693/hyhz20180800192
Lancelot C., Billen G., Sournia A., Weisse T., Colijn F., Veldhuis M. J., et al. (1987). Phaeocystis Blooms and Nutrient Enrichment in the Continental Coastal Zones of the North Sea. Ambio 16, 38–46. doi: 10.1016/0198-0254(87)90379-7
Lancelot C., Keller M. D., Rousseau V., Smith W. O., Mathot S. (1998). “Autecology of the Marine Haptophyte Phaeocystis Sp,” in Physiological Ecology of Harmful Algal Blooms, vol. vol. G 41 . Eds. Anderson D. M., Cembella A. D., Hallagraeff G. M. (Berlin: Springer Verlag), 209–224.
Levasseur M., Keller M., Bonneau E., D’amours D., Bellows W. (1994). Oceanographic Basis of a DMS-Related Atlantic Cod (Gadus Morhua) Fishery Problem: Blackberry Feed. Can. J. Fish. Aquat. Sci. 51, 881–889. doi: 10.1139/f94-087
Lin S., Ji N., Luo H. (2019). Recent Progress in Marine Harmful Algal Bloom Research. Oceanol. Limnol. Sin. 50, 495–510. doi: 10.11693/hyhz20180800191
Medlin L., Zingone A. (2007). A Taxonomic Review of the Genus Phaeocystis. Biogeochemistry 83, 3–18. doi: 10.1016/0198-0254(83)96596-2
Nejstgaard J. C., Tang K. W., Steinke M., Dutz J., Koski M., Antajan E., et al. (2007). Zooplankton Grazing on Phaeocystis: A Quantitative Review and Future Challenges. Biogeochemistry 83, 147–172. doi: 10.1007/s10533-007-9098-y
Parsons T., Maita Y., Lalli C. (1984). A Manual of Chemical & Biological Methods for Seawater Analysis (New York: Pergamon Press).
Peng X. C., Yang W. D., Liu J. S., Peng Z. Y., Lu S. H., Ding W. Z. (2005). Characterization of the Hemolytic Properties of an Extract From Phaeocystis Globosa Scherffel. J. Integr. Plant Biol. 47, 165–171. doi: 10.1111/j.1744-7909.2005.00039.x
Peperzak L., Colijn F., Vrieling E., Gieskes W., Peeters J. (2000). Observations of Flagellates in Colonies of Phaeocystis Globosa (Prymnesiophyceae); a Hypothesis for Their Position in the Life Cycle. J. Plankton. Res. 22, 2181–2203. doi: 10.1093/plankt/22.12.2181
Qin X. L., Lai J. X., Chen B., Jiang F. J., Xu M. B. (2016). Molecular Identification of Phaeocystis From Beibu Gulf Based on 18S rDNA Sequences. J. Trop. Subtrop. Bot. 24, 2, 176–181. doi: 10.11926/j.issn.1005-3395.2016.02.008
Qu J., Gong X. Z., Zhuang H., Zhao Y., Dong S. (2009). Phytoplankton Community Structure in Coastal Water of Jiaonan and Adjacent Water in Summer and Winter. Trans. Oceanol. Limnol. 3, 143–154. doi: 10.13984/j.cnki.cn37-1141.2009.03.002
Qu L., Lu S., Gao C., Sun P., Sun X. (2008). Structure and Sequence Analysis of 18s rDNA and ITS Gene of Phaeocystis Isolate From the Bohai Sea. Adv. Mar. Sci. 26 (2), 200–206. doi: 10.1007/s11442-008-0073-x
Reigstad M., Wassmann P.. (2007). Does Phaeocystis spp. Contribute Significantly to Vertical Export of Biogenic Matter? Biogeochemistry 83, 217–233. doi: 10.1007/s10533-007-9093-3
Rousseau V., Chrétiennot-Dinet M. J., Jacobsen A., Verity P., Whipple S. (2007). The Life Cycle of Phaeocystis: State of Knowledge and Presumptive Role in Ecology. Biogeochemistry 83, 29–47. doi: 10.1007/s10533-007-9085-3
Rousseau V., Lantoine F., Rodriguez F., LeGall F., Chrétiennot-Dinet M. J., Lancelot C. (2013). Characterization of Phaeocystis Globosa (Prymnesiophyceae), the Blooming Species in the Southern North Sea. J. Sea. Res. 76, 105–113. doi: 10.1016/j.seares.2012.07.011
Rousseau V., Vaulot D., Casotti R., Cariou V., Lenz J., Gunkel J., et al. (1994). The Life Cycle of Phaeocystis (Prymnesiophycaea): Evidence and Hypotheses. J. Mar. Syst. 5, 23–39. doi: 10.1016/0924-7963(94)90014-0
Schoemann V., Becquevort S., Stefels J., Rousseau V., Lancelot C. (2005). Phaeocystis Blooms in the Global Ocean and Their Controlling Mechanisms: A Review. J. Sea. Res. 53, 43–66. doi: 10.1016/j.seares.2004.01.008
Seuront L., Vincent D., Mitchell J. G.. (2006). Biologically Induced Modification of Seawater Viscosity in the Eastern English Channel During a Phaeocystis Dlobosa Spring Bloom. J. Mar. Syst. 61, 118–133. doi: 10.1016/j.jmarsys.2005.04.010
Shen P., Qi Y. (2021). Research Progress on Species Diversity and Distribution of the Genes Phaeocystis. Oceanol. Limnol. Sin. 52, 1–15. doi: 10.11693/hyhz20200300085
Shen P., Qi Y., Ou L. (2018). Phaeocystis Globosa in Coastal China: Taxonomy, Distribution, and its Blooms. Mar. Sci. 42, 146–162. doi: 10.11759/hykx20171225004
Shen P., van Rijssel M., Wang Y., Lu S., Qi Y. (2004). “Toxic Phaeocystis Globosa Strains From China Grow at Remarkably High Temperatures,” in Harmful Algae 2002. Eds. Steidinger K. A., Landsberg J. H., Tomas C. R., Vargo G. A. (St. Petersburg: Florida Fish and Wildlife Conservation Commission, Florida Institute of Oceanography and Intergovernmental Oceanographic Commission of UNESCO), 396–398.
Smith D. R., Arrigo K. R., Alderkamp A. C., Allen A. E. (2014). Massive Difference in Synonymous Substitution Rates Among Mitochondrial, Plastid, and Nuclear Genes of Phaeocystis Algae. Mol. Phylogen. Evol. 71, 36–40. doi: 10.1016/j.ympev.2013.10.018
Smith W. O. Jr., Zhang W. G., Hirzel A., Stanley R. M., Meyer M. G., Sosik H., et al. (2021). A Regional, Early Spring Bloom of Phaeocystis Pouchetii on the New England Continental Shelf. J. Geophys. Res.: Oceans. 126, e2020JC016856. doi: 10.1029/2020JC016856
Song H., Chen Y., Gibson K., Liu S., Yu Z., Chen N. (2021). High Genetic Diversity of the Harmful Algal Bloom Species Phaeocystis Globosa Revealed Using the Molecular Marker COX1. Harmful. Algae. 107, 102065. doi: 10.1016/j.hal.2021.102065
Tian J. (2010). Effects of Environmental Factors on the Colony Formation of Phaeocystis Globosa. Guangzhou: (Jinan University).
Tian H., Liu Q., Goes J., Gomes H., Yang M. (2020). Temporal and Spatial Changes in Chlorophyll-a Concentrations in the Yellow Sea From 2002 to 2018 Based on MODIS Data. Mar. Sci. Bull. 39, 101–110. doi: 10.11840/j.issn.1001-6392.2020.01.011
Tu J., Zhang Q., Xu Y., Hu Y., Shi H. (2011). Preliminary Analysis of Phaeocystis Globosa Scherffel Red Tide in Tianjin Coastal Sea Areas of Bohai Bay. Mar. Sci. Bull. 30, 334–337. doi: 10.3969/j.issn.1001-6392.2011.03.016
van Rijssel M. V., Alderkamp A. C., Nejstgaard J. C., Sazhin A. F., Verity P. G. (2007). Haemolytic Activity of Live Phaeocystis Pouchetii During Mesocosm Blooms. Biogeochemistry 83, 189–200. doi: 10.1007/978-1-4020-6214-8_14
Veldhuis M. J., Wassmann P. (2005). Bloom Dynamics and Biological Control of a High Biomass HAB Species in European Coastal Waters: A Phaeocystis Case Study. Harmful. Algae. 4, 805–809. doi: 10.1016/j.hal.2004.12.004
Verity P. G., Brussaard C. P., Nejstgaard J. C., Leeuwe M. A., Lancelot C., Medlin L. K. (2007). Current Understanding of Phaeocystis Ecology and Biogeochemistry, and Perspectives for Future Research. Biogeochemistry 83, 311–330. doi: 10.1007/978-1-4020-6214-8_21
Wang J. (2003). Study on Phytoplankton in the Yellow Sea in Autumn and Winter. Mar. Freshwater. Res. 24, 15–23. doi: 10.3969/j.issn.1000-7075.2003.01.004
Wang K., Chen B., Gao Y., Lin H. (2021). Harmful Algal Blooms Caused by Phaeocystis Globosa From 1997 to 2018 in Chinese Coastal Waters. Mar. Pollut. Bull. 173, 112949. doi: 10.1016/j.marpolbul.2021.112949
Wang X. D., Tang K. W., Wang Y., Smith W. O. (2010). Temperature Effects on Growth, Colony Development and Carbon Partitioning in Three Phaeocystis Species. Aquat. Biol. 9, 239–249. doi: 10.3354/ab00256
Xing Q., Tosi L., Braga F., Gao X., Gao M. (2015). Interpreting the Progressive Eutrophication Behind the World’s Largest Macroalgal Blooms With Water Quality and Ocean Color Data. Nat. Hazards. 78, 7–21. doi: 10.1007/s11069-015-1694-x
Xiong X., Hu X., Guo Y., Yu L., Chen L., Xue Y. (2019). Existence, Morphology and Structure of the Yellow Sea Warm Current Branch Approaching Waters Offshore Qingdao, China. Sci. China.: Earth Sci. 62, 1167–1180. doi: 10.1007/s11430-018-9331-1
Xu N., Huang B., Hu Z., Tang Y., Duan S., Zhang C. (2017). Effects of Temperature, Salinity, and Irradiance on the Growth of Harmful Algal Bloom Species Phaeocystis Globosa Scherffel(Prymnesiophyceae) Isolated From the South China Sea. Chin. J. Oceanol. Limnol. 35, 557–565. doi: 10.1007/s00343-017-5352-x
Xu Y. X., Zhang T., Zhou J. (2019). Historical Occurrence of Algal Blooms in the Northern Beibu Gulf of China and Implications for Future Trends. Front. Microbiol. 10. doi: 10.3389/fmicb.2019.00451
Yang W., Shang W., Liu J. (2009). Acute Toxicities of Phaeocystis Globosa on Five Aquatic Ani Mals. J. Trop. Subtrop. Bot. 17, 68–73. doi: 10.3969/j.issn.1005-3395.2009.1.2107
Yu R., Lü S., Qi Y., Zhou M. (2020). Progress and Perspectives of Harmful Algal Bloom Studies in China. Oceanol. Limnol. Sin. 51, 768–788. doi: 10.11693/hyhz20200400127
Yu Z., Song X., Cao X., Liu Y. (2017). Mitigation of Harmful Algal Blooms Using Modified Clays: Theory, Mechanisms, and Applications. Harmful. Algae. 69, 48–64. doi: 10.1016/j.hal.2017.09.004
Keywords: Phaeocystis globosa, bloom, first record, southern Yellow Sea, remote sensing
Citation: Li D, Xue Y, Song Q, Cui F, Ma Y, Tong M and Shen P (2022) First Report on Large-Scale Phaeocystis globosa Bloom in the Southern Yellow Sea, China. Front. Mar. Sci. 9:880984. doi: 10.3389/fmars.2022.880984
Received: 22 February 2022; Accepted: 14 March 2022;
Published: 07 April 2022.
Edited by:
Peng Jin, University of Guangzhou, ChinaReviewed by:
Haifeng Gu, State Oceanic Administration, ChinaDayong Liang, Guangzhou University, China
Copyright © 2022 Li, Xue, Song, Cui, Ma, Tong and Shen. This is an open-access article distributed under the terms of the Creative Commons Attribution License (CC BY). The use, distribution or reproduction in other forums is permitted, provided the original author(s) and the copyright owner(s) are credited and that the original publication in this journal is cited, in accordance with accepted academic practice. No use, distribution or reproduction is permitted which does not comply with these terms.
*Correspondence: Pingping Shen, cHNoZW5AeXR1LmVkdS5jbg==