- 1Zhejiang Marine Fisheries Research Institute, Zhoushan, China
- 2Marine and Fisheries Institute of Zhejiang Ocean University, Zhoushan, China
- 3Scientific Observation and Experimental Station of Fishery Resources of Key Fishing Grounds, Ministry of Agriculture and Rural Affairs of the People’s Republic of China, Zhoushan, China
- 4Key Laboratory of Sustainable Utilization of Technology Research for Fishery Resources of Zhejiang Province, Zhoushan, China
Sciaenid fishes are usually associated with large freshwater inputs and are the most important economic fish on the coastal shelf off mainland China. To compare the differences in ecological niche and resource sharing among different populations of Sciaenidae species, we collected samples of Larimichthys polyactis, Collichthys lucidus, Johnius belangerii, Nibea albiflora, and Miichthys miiuy from Zhoushan Archipelago waters from 2019 to 2021 and investigated the carbon and nitrogen isotopic values in muscle tissues, the contribution of each food resource, and trophic niche widths and overlaps. Significant differences were observed in both isotopes in the muscles of the five Sciaenid species. Zooplankton was a key food resource for all Sciaenid species. In addition to zooplankton, J. belangerii, N. albiflora, and M. miiuy also fed on benthos organisms. C. lucidus presented a wide trophic niche width and had extensive habitat use. The trophic niche occupied by N. albiflora and M. miiuy was narrow; they presented a high trophic level, with a high degree of trophic niche overlap. This study showed that sciaenid fishes have overlapping trophic niches due to their common feeding on zooplankton, and differences in body size, migration, habitat, and feeding choices led to the reasonable sharing of resources among the five sciaenid fishes, allowing the coexistence of these species.
Introduction
Marine sciaenid fishes are cosmopolitan, and they are important for fisheries worldwide. These fishes are usually associated with large freshwater inputs, and are mainly distributed in the Atlantic, Indian, and Pacific oceans (Longhurst and Pauly, 1987; McConnell and Lowemcconnell, 1987). In Asia, sciaenid fishes are mainly distributed in the northeast, including the west coast of South Korea, the Bohai Sea, and the East China Sea. Larimichthys polyactis (Sciaenidae: Perciformes), is one of the most commercially important fish in South Korea and China, where it has long been used as a source of food and medicine (Choi and Kim, 2020). In China, L. polyactis and other sciaenid fishes are prized because of their delicious meat and high trophic value. Thus, this is an economically important fish species on the coastal shelf of mainland China (Chen et al., 1997).
As one of the four major marine products in China, 400,000 tonnes of L. polyactis were harvested in 2010 (Ministry of Agriculture and Rural Affairs of the People’s Republic of China, 2009-2018). In recent years, due to overexploitation and overfishing of marine resources, the L. polyactis population has severely declined (Lin, 2004). Since the 1990s, China has implemented fishery revitalisation policies, such as fishing bans in summer as well as the proliferation and release of commercial fishes; despite these efforts, the yield of L. polyactis continues to decline every year, and individual size miniaturisation is prevalent among the populations in Zhoushan fishing farm (Wang et al., 2021). Furthermore, populations of a comparable species Larimichthys crocea, have not rebounded after overfishing, and wild L.crocea has almost disappeared (Zhao et al., 2002).
Fishing pressure can lead to different indirect impacts, depending on the species. One of these processes is the competition release process (Walker and Hislop, 1998; Dulvy et al., 2000). For example, large species become locally extinct due to overfishing, whereas the populations of small species increase abundantly (Jin, 2000). According to the China Fishery Statistics Yearbook, during a 10-year survey from 2008 to 2017, the number of Nibea albiflora caught in China’s offshore fisheries decreased by 22.81% while that of C. lucidus increased by 21.9% (Ministry of Agriculture and Rural Affairs of the People’s Republic of China, 2009-2018). Moreover, overfishing causes changes in the trophic structure of food webs and trophic niches of species; for example, the food source of the hairtail can change, diversity of nutrient sources can decrease, and trophic niches can become smaller (He et al., 2021).
Niche overlap among species defines species exclusion or coexistence when competition takes place (De Roos et al., 2008). Thus, niche overlap can cause adjustments in habitat use and diet (Oelze et al., 2014; Lush et al., 2017; Merkle et al., 2017) as well as species marginalisation and disappearance (Simon and Townsend, 2003; Beaudrot et al., 2013). In contrast, niche diversification, in which competing species focus on different resources or exploit the same resource with spatial or temporal variation (i.e., spatial or temporal niche partitioning), favours species coexistence (Tilman, 1982; Chesson, 2000).
L. polyactis, Collichthys lucidus, Johnius belangerii, N. albiflora, and Miichthys miiuy are sciaenid fishes with similar body sizes and ecological habits. Most are medium-sized, and can prey on small fishes (Deng and Yang, 1997). However, these fishes can also serve as potential prey for bigger predators. Among these five species of Sciaenidae, C. lucidus is a euryhaline fish that is distributed in estuaries and near islands (Zhuang et al., 2006); the other four species winter in the open sea, and approach the Zhoushan offshore in spring and summer to feed and spawn (Lin et al., 2013; Wang et al., 2012a; Xu and Chen, 2009; Zhong et al., 2010). The morphological similarity of these fishes and interactions resulting from the presence of such similar species in the same community can lead to competition for limited resources (Pianka, 1981). In general, the more ecologically similar two species are, the more likely intense competition is. Intense interspecific competition may lead to exclusion of one species (Wisheu, 1998). However, it is common to find ecologically similar species living together in the same community, indicating that different strategies allow coexistence. Resource partitioning, such as foraging on different food items or at diverse locations or times, minimises interspecific competition and facilitates sympatric coexistence (Schoener, 1974).
Stable isotope analysis, which was first used in diet studies over 40 years ago (DeNiro and Epstein, 1987; Matthews and Bier, 1983), has become a frequently used tool in ecology. Through stable isotopes of carbon (δ13C) and nitrogen (δ15N), we can determine food resources and trophic positions (Crawford et al., 2008). This approach is powerful in its application to communities; for example, for the analysis of resource partitioning and trophic niche dimensions (Polis, 1984; Sheppard et al., 2018; Balčiauskas et al., 2019; Costa et al., 2020; Manlick and Pauli, 2020; De Camargo et al., 2021). Stable isotope ratios facilitate the identification of dietary changes (Koike et al., 2016) and the influence of habitat conditions (Hopkins III and Kurle, 2016). Though isotopic niche is not completely analogous to trophic niche (Hette‐Tronquart, 2019), stable isotopes can provide a quantitative indicator of trophic niches as long as all the contributing factors are fully considered in the interpretation of isotope values (Marshall et al., 2019).
In this study we used stable isotope analysis to determine the status of five sciaenid fishes, L. polyactis, C. lucidus, J. belangerii, N. albiflora, and M. miiuy in the Zhoushan Archipelago. The archipelago is located southeast of the mouth of the Yangtze River and east of Hangzhou Bay. The waters which connect the Yangtze River and the ocean are scattered with thousands of islands, with strong habitat heterogeneity. The river’s diluting water brings rich nutrients, and the island can block the ocean current, forming turbulence and whirlpools. In addition, the coastal cold current from the north and the Taiwan warm current from the south are in confluence near the islands (Hou et al., 2013). This accelerates the circulation of nutrients, thus, the waters of the Zhoushan Archipelago have high primary productivity, abundant bait organisms, and are feeding and spawning grounds for many offshore fish (Yu et al., 2010). In this study, based on stable isotopes analysis, we investigated the trophic niche width of five types of sciaenid fishes in the Zhoushan Archipelago waters, the differences in resource sharing, and interspecific competition to determine the status of those species in the area. Our results provide some basic parameters for the study of the structure, function, and stability of the Zhoushan fishing ground fish community.
Materials and Methods
Research Area
The study was conducted in the east of Hangzhou Bay, southeast of the Yangtze River Estuary, and northeast of Zhejiang Province (29° 00’ – 31° 00’ N and 121° 30’ – 124° 00’ E; Figure 1). Due to the convergence of Taiwan warm and coastal cold currents, Zhoushan Archipelago waters is rich in prey, and it provides a suitable environment for local aquatic animals.
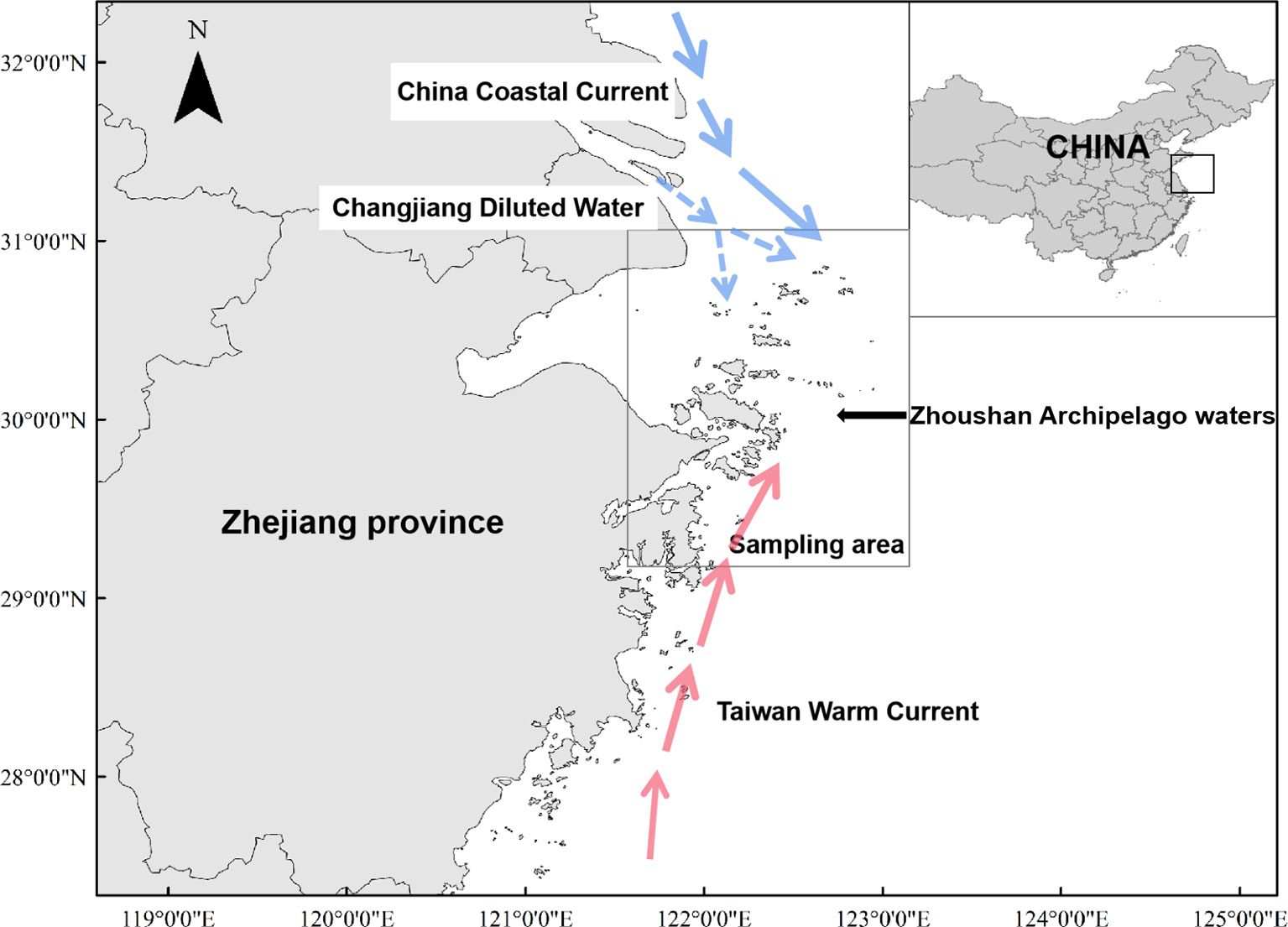
Figure 1 Area of Zhoushan Archipelago where sciaenid fish species were collected between 2019 and 2021 for assessment of δ13C and δ15N values.
Sampling
Samples of sciaenid species and their potential prey species were collected from a trawl survey of fishery resources in Zhoushan Archipelago during spring and autumn of 2019–2021. A total of 199 sciaenid fish, including 59 L. polyactis, 51 C. lucidus, 40 J. belangerii, 31 N. albiflora, and 18 M. miiuy, were collected. A total of 89 prey samples of 13 species were collected. Referring to the results of the stomach content analysis (Wang et al., 2012b; Lin et al., 2013; Wang, 2015; Wang et al., 2020; Zhang et al., 2020), we classified the potential prey of five sciaenid fishes into three main ecological groups: nekton, zoobenthos and zooplankton. Nekton include small fishes such as Amblychaeturichthys hexanema and Benthosema pterotum; zoobenthos mainly include small shrimps and crabs such as Palaemon gravieri and Charybdis bimaculata; zooplankton include copepods and krill. All of these prey organisms are widely distributed in Zhoushan Archipelago waters and are easily ingested by other predators. Nekton and zoobenthos were collected by trawl surveys, and the zooplankton such as copepods and krill were collected using a shallow water I-type plankton net (505 μm). The collected samples were refrigerated on a fishing boat and transported to the laboratory via cold chain logistics after the fishing boat landed. The samples were then thawed in the laboratory, and biological parameters such as body length (mm), total length (mm), body weight (g), pure weight (g), and stomach fullness were measured (General Administration of Quality Supervision, Inspection and Quarantine of the People’s Republic of China, Standardization Administration, 2008).
We collected muscles of fishes, abdominal muscles of shrimps, and the whole body of small planktonic crustaceans, such as copepods. To prevent the C isotopes in crustacean shells from affecting experimental results, small planktonic crustaceans were acidified with 1 mol·L−1 HCl until no bubbles were generated. The muscle samples were wrapped in tin foil, dried in an Alpha 1-2LDPLUS freeze dryer (Beijing BMH Instruments Co. Ltd., Shanghai, China) for 24 h, and fully ground into powder. The powder was placed in a 2 mL centrifuge tube and stored under dry conditions until further analysis.
Stable Isotope Analysis
All samples were analysed for stable carbon and nitrogen isotope ratios using an EA-HT Elemental Analyser (Thermo Fisher Scientific, Inc., Bremen, Germany) and DELTA V Advantage Isotope Ratio Mass Spectrometer (Thermo Fisher Scientific). The samples were combusted in an elemental analyser to generate CO2 and N2. The mass spectrometer detected the ratio of 13C to 12C of CO2 and compared it with an international standard (Pee Dee Belemnite or PDB) to calculate the δ13C value of each sample. The ratio of 15N to 14N was compared with the international standard (atmospheric N2) to calculate the δ15N value of a sample.
Calculation method of isotope abundance:
where X is 13C or 15N and Rsa and Rst are 13C/12C or 15N/14N of the unknown and standard samples, respectively. To ensure the precision and accuracy of the test results, 3 international standard samples were put in after every ten samples to calibrate the carbon and nitrogen stable isotopes. 10 replicate tests were performed on the same sample, the accuracy of δ13C and δ15N values was less than ±0.1‰ and less than ±0.2‰, respectively.
Data Processing
One-way analysis of variance (ANOVA) was used to test significant differences in the stable carbon and nitrogen isotope values among the five sciaenid fishes (α = 0.05). First, the data were tested for normality and homogeneity of variance. If any of the above requirements were not met, a non-parametric test (Kruskal-Wallis H test) was performed. Pearson correlation analysis was used to test the correlation between δ13C and δ15N values of individuals of the five sciaenid fishes and their body length. Statistical analysis was performed using SPSS version 10.0 (SPSS Inc., Chicago, IL, USA).
Trophic level was calculated according to the following formula (Jake Vander Zanden and Fetzer, 2007):
where TL represents the trophic level estimated using stable isotope; δ15Nc is the δ15N ratio of consumers, and δ15Nb is the δ15N ratio of the baseline organism. The baseline organism selected in this study was Calanus sinicus, which is present year-round in the Zhoushan Archipelago waters and has a monotonous diet (δ15N value is 5.65‰) (Yang, 1997; Xu et al., 2005); TEF is the nitrogen enrichment at a trophic level; we used TEF = 3.4‰ based on Minagawa and Wada (Minagawa and Wada, 1984); and λ represents the trophic level of the selected baseline organism, which is 2.
To assess the contribution of different food sources to the isotopic signature of each target fish, separate Bayesian stable isotope mixing models (Moore and Semmens, 2008) with a specified number of putative sources were run for the sections of sea area using the R software package SIAR (R CoreTeam, 2015; Stock and Semmens, 2016). SIAR model fitting was via Markov chain Monte Carlo (MCMC) to estimate parameters from observed data and user-specified prior distributions (Parnell et al., 2010). We ran the model for 200,000 iterations, and checked whether the estimated 95% credibility intervals for each proportion contained the original generated proportions (Parnell et al., 2010).
To quantify differences in isotopic niche use among the five species, the probability of a group appearing within the niche region (space) of another group was estimated by using the R package nicheROVER with 95% credible intervals based on 10,000 iterations (Swanson et al., 2015).
To describe the trophic niche ellipses and estimate the trophic niche widths and overlaps of different species, we calculated the standard ellipse area (Yeakel et al.) and corrected for small sample size (SEAc) with the Stable Isotope Bayesian Ellipses (SIBER) package in R (Jackson et al., 2011). The SEAc was set to contain 40% of isotopic observations of each group. The overlap between SEAc was used to quantify the overlapping area of stable isotope niches between fish species (Jackson et al., 2011). An overlap ratio greater than 1 indicates high overlap, and below 0.30 indicates low overlap (Yeakel et al., 2015).
Results
Stable Isotopes in Sciaenidae
The highest average value of both δ13C and δ15N were found in M. miiuy, and the lowest average value of both δ13C and δ15N were found in C. lucidus. N. albiflora had a broader range of δ13C and δ15N than other fishes, while M. miiuy had the narrowest range of δ13C and δ15N compared with other fishes (Table 1). Stable isotopes of the five sciaenid species communities were significantly different (Kruskal-Wallis H, P = 0.000 < 0.050), which may be due to differences in low trophic-level species (L. polyactis and C. lucidus) and high trophic-level species (J. belangerii, N. albiflora, and M. miiuy) (Table 2).

Table 1 δ13C and δ15N values observed for five sciaenid fish species in Zhoushan Archipelago waters collected between 2019 and 2021.
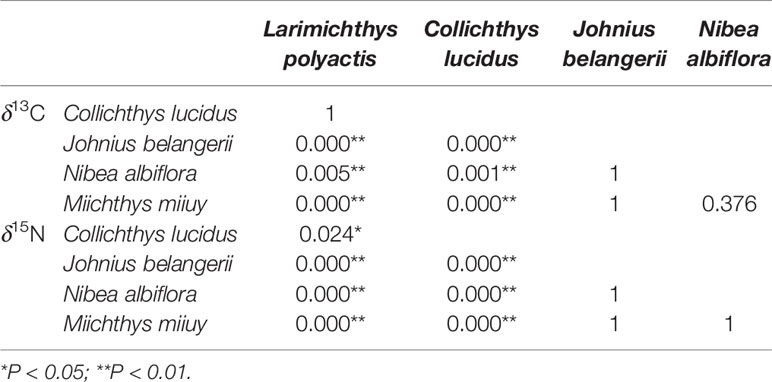
Table 2 Comparison of δ13C and δ15N values of five sciaenid fish species collected from the Zhoushan Archipelago between 2019 and 2021 based on the Kruskal-Wallis H test.

Table 3 δ13C and δ15N values of the major prey groups of five sciaenid fish species collected from the Zhoushan Archipelago between 2019 and 2021.
Stable Isotopes in Other Groups
One-way ANOVA showed that there were significant differences in the stable isotopes of the three prey groups (P < 0.01). Among them, zoobenthos had the highest δ13C value, nekton had the highest δ15N value, and zooplankton had the lowest δ13C and δ15N values (Table 3).
Stable Isotopes vs. Length
Pearson correlation analysis showed a significant positive correlation between the body length and δ13C values of N. albiflora (R2 = 0.500, P < 0.01), and a positive correlation between the body length and δ15N values of J. belangerii (R2 = 0.436, P < 0.01). No significant correlations were observed between the body length and δ13C and δ15N values of the other three fishes (Figure 2).
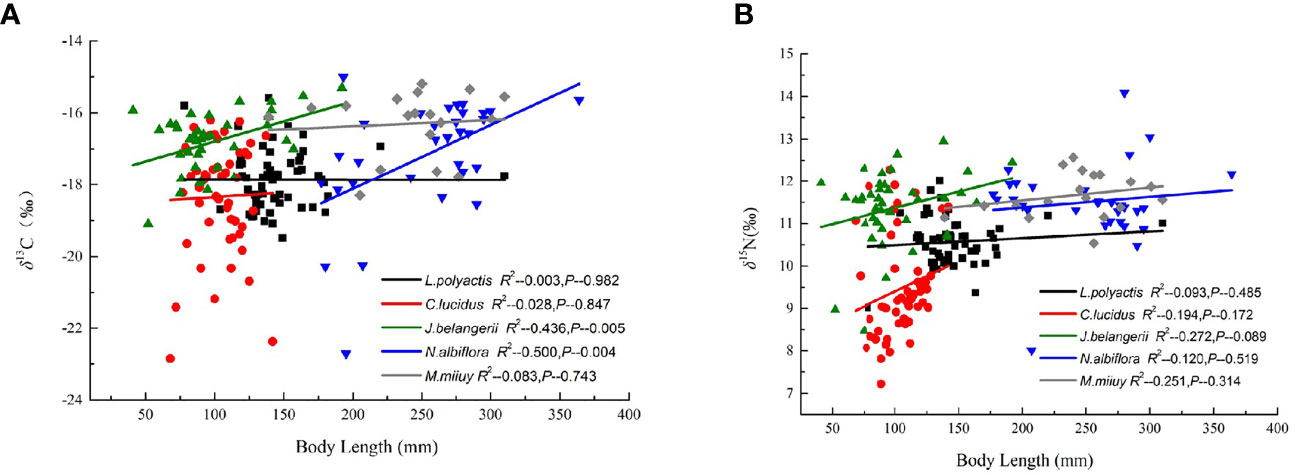
Figure 2 Correlation of body length with δ13C and δ15N values of five sciaenid fish species collected form the Zhoushan Archipelago between 2019 and 2021.
SIAR Model
The SIAR model showed that the five sciaenid fishes relied on zooplankton and zoobenthos as their food source, with fishes as the least preferred option. L. polyactis and C. lucidus were at a lower trophic level, zooplankton contributed more than 80% as their food source, and C. lucidus relied more on zooplankton than L. polyactis. In addition to zooplankton, J. belangerii, N. albiflora and M. miiuy also fed on zoobenthos. Among these three species, M. miiuy fed the most on zoobenthos, followed by J. belangerii, and N. albiflora the least (Figure 3).
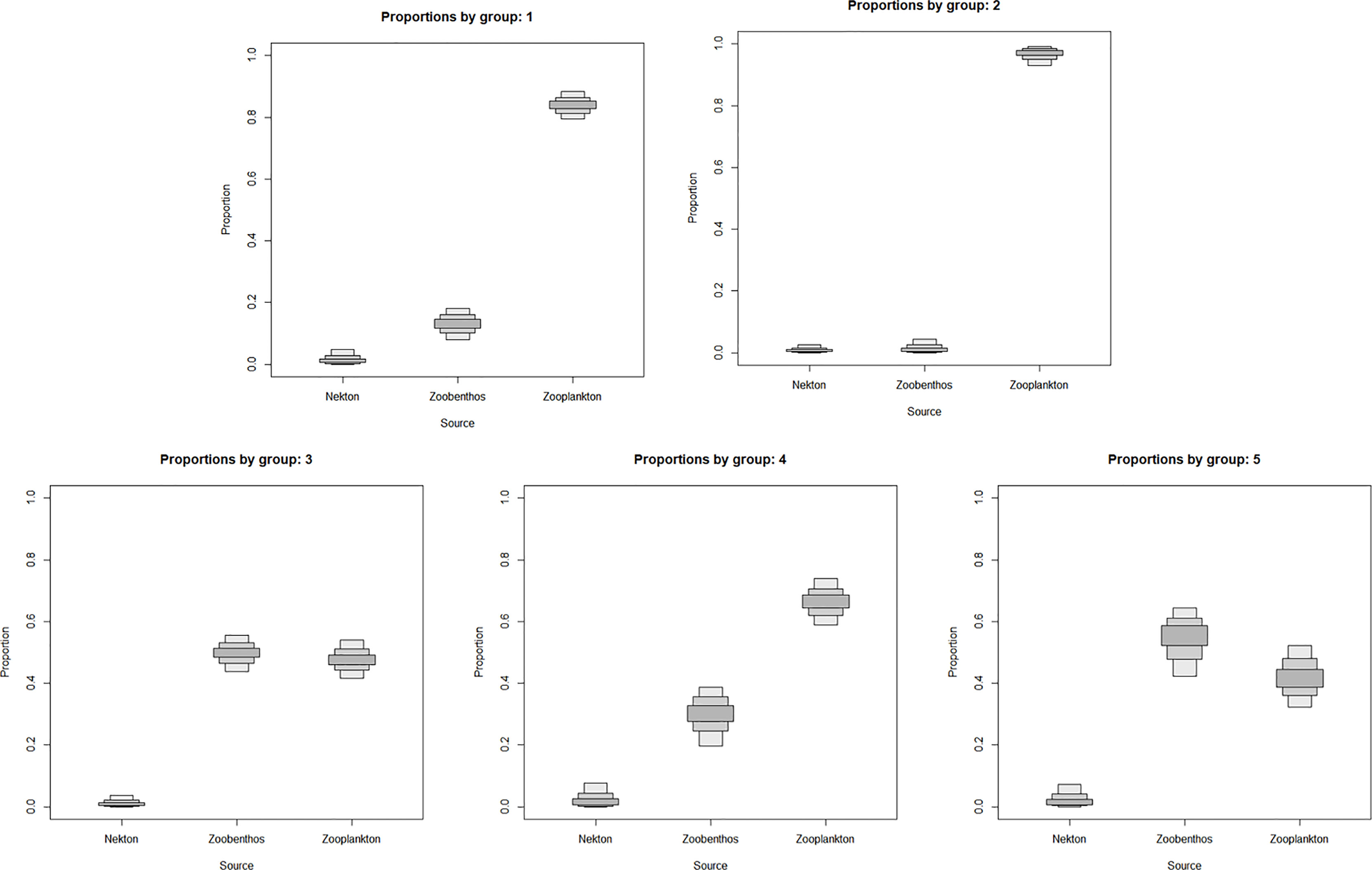
Figure 3 Contributions of different food sources to five sciaenid fishes collected from the Zhoushan Archipelago between 2019 and 2021. Group 1 is Larimichthys polyactis; Group 2 is Collichthys lucidus; Group 3 is Johnius belangerii; Group 4 is Nibea albiflora; Group 5 is Miichthys miiuy.
Trophic Niches
For each species of fish and every pair of isotopes, 10 random elliptical projections of the trophic niche regions were created (Figure 4). Smoothed histograms (density plots) and scatterplots indicate no violation of the assumption of normality. Ratios of δ13C and δ15N varied more widely in C. lucidus and N. albiflora than in the other three species, which would obviously contribute to a larger overall (Figure 4).
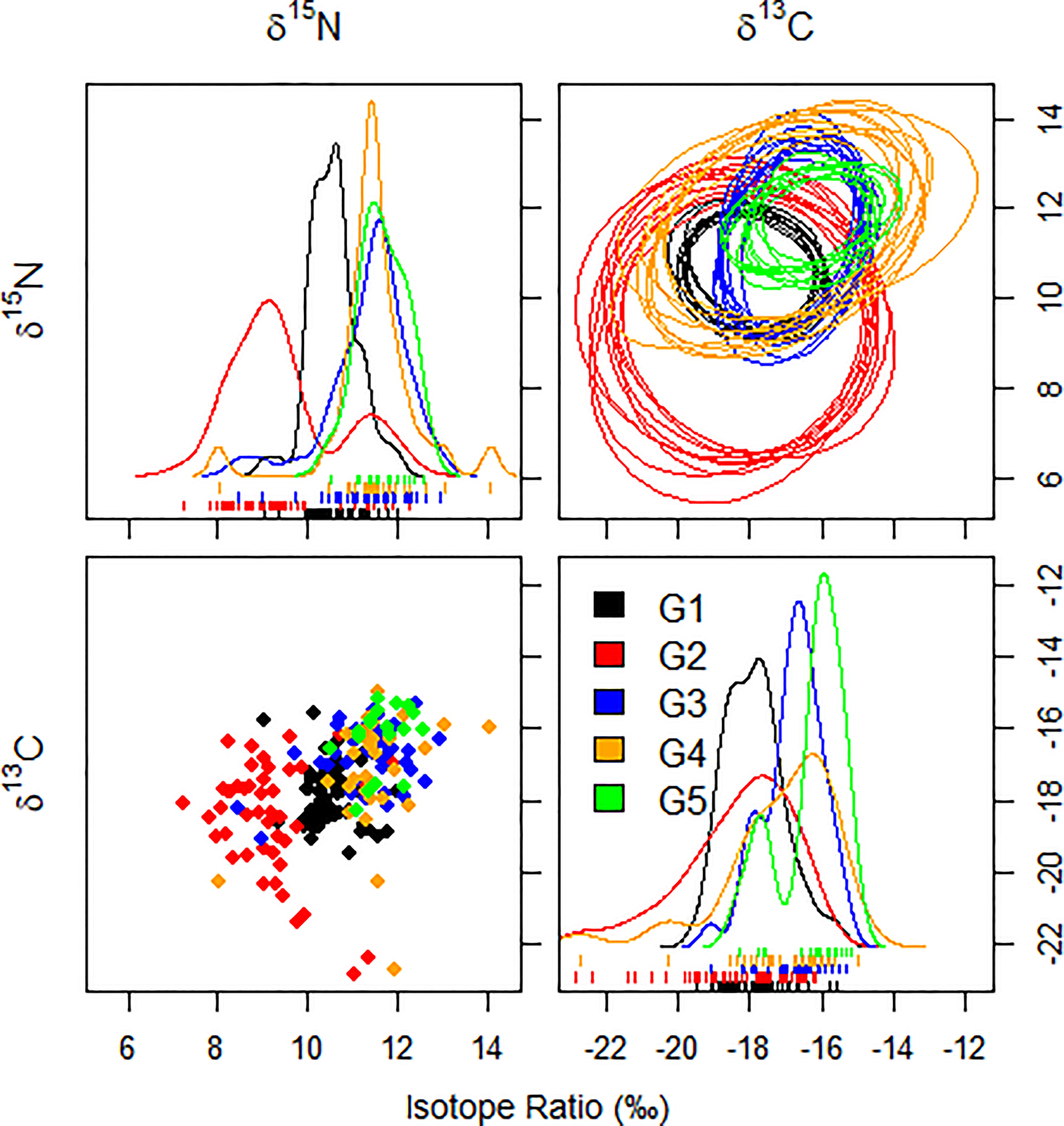
Figure 4 Ten random elliptical projections of the trophic niche region (NR) for each species of sciaenid fish collected from the Zhoushan Archipelago between 2019 and 2020, as well as a pair of isotopes (elliptical plots). Also displayed are one-dimensional density plots (lines) and two-dimensional scatterplots.
The posterior distributions of the overlap metric were also assessed (Figure 5). The nicheROVER analysis revealed the probability of sciaenid individuals to be found in the same niche region of different sciaenid species. The isotope niche of L. polyactis showed a high probability to be found in the niche regions of C. lucidus, J. belangerii and N. albiflora. The isotope niche of C. lucidus was low in the niche regions of the other four species. The isotope niche of J. belangerii was high in the niche regions of the other four species and showed an extremely high probability to be found in the niche regions of N. albiflora. the isotope niche of M. miiuy was low in the niche regions of L. polyactis and C. lucidus, but high in J. belangerii and N. albiflora.
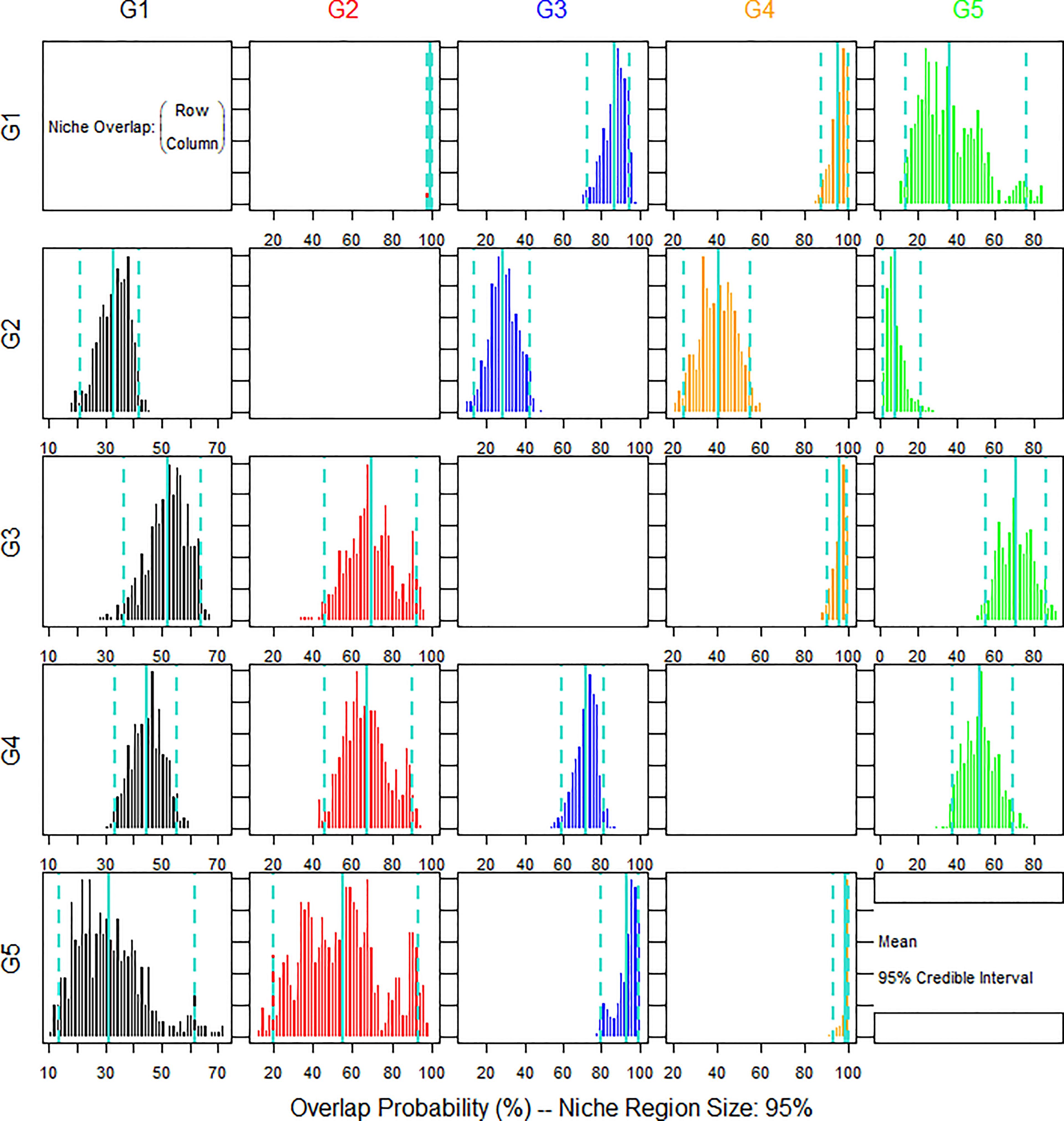
Figure 5 Bayesian plot of the posterior probability distribution of niche region metric (%) for five species of sciaenid fish collected from the Zhoushan Archipelago between 2019 and 2021 estimated by nicheROVER analysis. The posterior means and 95% credible intervals are displayed in turquoise colour.
The isotopic niche width of five sciaenid indicated that C. lucidus had the largest isotopic niche area, followed by N. albiflora, J. belangerii, M. miiuy, and L. polyactis had the smallest isotopic niche area (Figure 6). Though J. belangerii showed some isotope niche overlap with N. albiflora and M. miiuy, overall, we found little isotope niche overlap between the five sciaenid fishes.
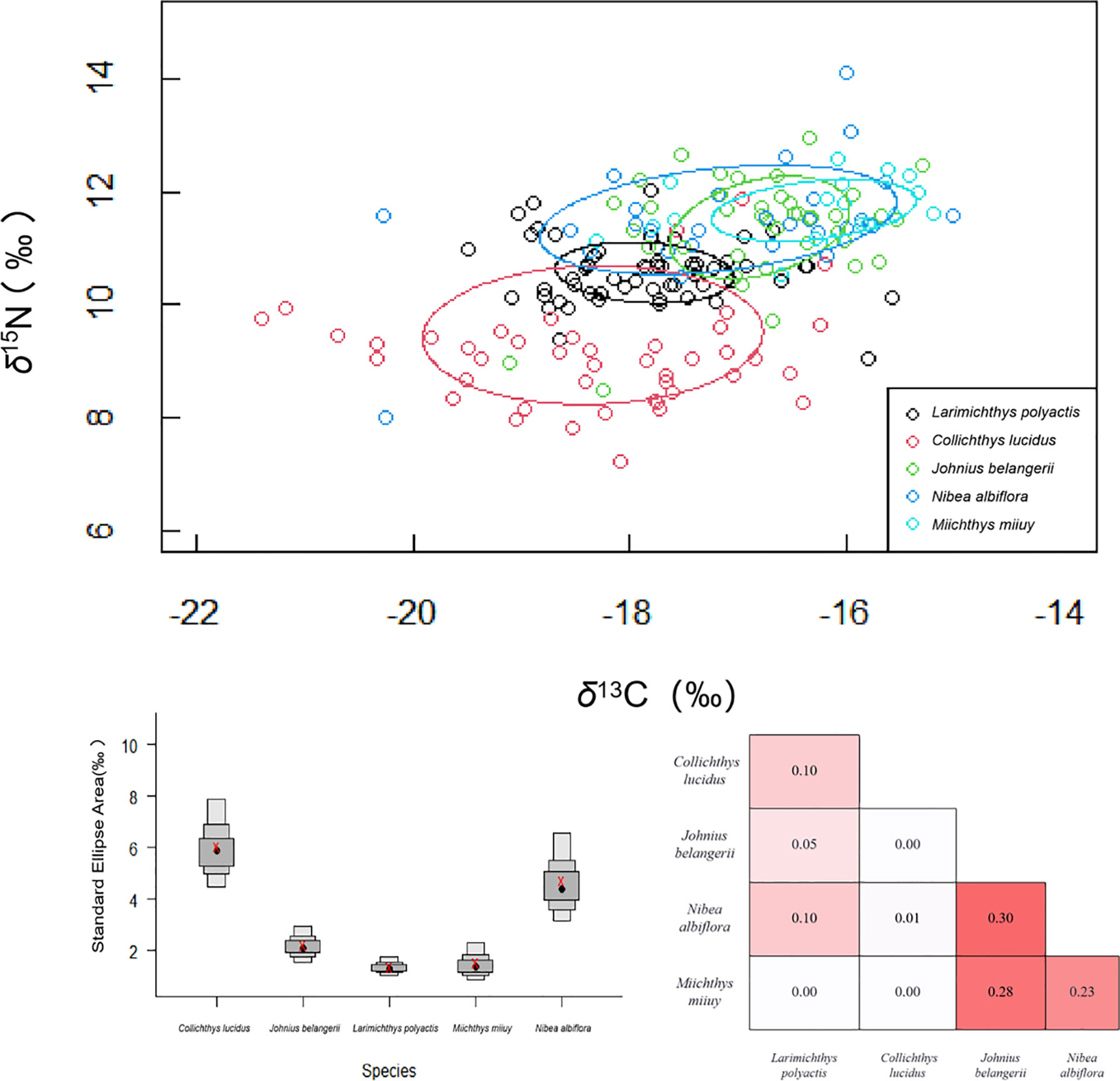
Figure 6 Corrected standard ellipse area (SEAc) representing the trophic niche width of five sciaenid fish species collected from the Zhoushan Archipelago between 2019 and 2021. SEAc represents the trophic niche width of five sciaenid fishes. Overlap (numbers inside boxes) of corrected standard ellipse areas (SEAcs) of sciaenid fishes estimated using Stable Isotope Bayesian Ellipses (SIBER) in R. Colour intensity increases with SEAc overlap.
Discussion
In this study, the analysis of stable C and N isotopes provided insights into the trophic ecology of five co-occurring and highly abundant sciaenid species in the Zhoushan Archipelago waters. Our results suggest that the coexistence of five sciaenid species in the Zhoushan Archipelago waters may be enabled by spatial and food diversification, as indicated by the diversification of isotopic niches between the sciaenid species.
Generally, during the growth of organisms, carbon and nitrogen stable isotopes are continuously enriched in the body. According to the “optimal feeding theory”, as individuals grow, the food organisms ingested by consumers gradually become larger (Xue et al., 2004). A large number of studies have shown that there is a correlation between the stable isotope composition of marine organisms and their own growth stages (Wilson et al., 2009). In our study, we found significant positive relationships between δ13C and δ15N with body length in two of five sciaenid species. The δ13C value of N. albiflora varies with the developmental stage. The larger the body length, the larger the δ13C value of the N. albiflora. The δ13C value in the organism came from the food it ingested, indicating that the food source of the N. albiflora changed greatly during the growth process. The δ15N value of J. belangerii increases with the increase of body length. The δ15N value in the organism indicates the trophic level, indicating that the trophic position of J. belangerii changed substantially during the growth. Ontogenetic shifts in diet are frequently observed in sciaenid species, with the consumption of prey that is larger and at a higher trophic-level attributable to metabolic requirements of larger individuals and changes in foraging ability due to an increase in gape and swimming speed (He et al., 2012; Wei et al., 2018). Wangkai et al. found that J. belangerii juveniles mainly feed on amphipods, while adults mainly feed on fish and shrimp (Wang et al., 2012b). Lin et al. (2013) found that the food intake of N. albiflora also changed to a certain extent during the growth process. The N. albiflora with a body length of 80–220 mm mainly fed on small shrimps, while those with a body length of more than 220 mm mainly fed on fish and mantis shrimp (Lin et al., 2013). However, in this study, the isotope ratios in three of the five sciaenid fishes, L. polyactis, C. lucidus, and M. miiuy, did not change significantly with the increase of body length, which agrees with the findings of Wilson et al. (2009). Changes in stable isotope composition are related to the organisms ingested, and for species with complex feeding habits, stable isotope ratios may not be identified.
Our results suggest that different species feed at different trophic levels. L. polyactis and C. lucidus are small, and they mainly feed on zooplankton. N. albiflora and M. miiuy are larger in size, and they feed more on zoobenthos. This conclusion can also be verified via existing reports on stomach content analysis (He et al., 2012; Wang et al., 2012c; Wei et al., 2018; Wang et al., 2020; Zhang et al., 2020). J. belangerii is a unique species; although it is small, it has a high trophic level, which may be due to its upper jaw being longer than the lower jaw and the low mouth position, it has a specific selectivity towards prey organisms, preying more on benthic organisms (Zhang et al., 2020).
Furthermore, our results suggest that the widest trophic niche was characteristic of the dominant species C. lucidus and N. albiflora. Moreover, their ranges of δ13C and δ15N values exceeded that of other species by approximately two times, and the total area of isotopic niche exceeded that of other fishes by 2–6 times. C. lucidus showed a broad range of δ13C values as compared to other organisms with similar size ranges, such as L. polyactis. This finding suggests that C. lucidus has a wider habitat use or greater range of movement and more feeding resources than L. polyactis. This may be due to the fact that C. lucidus is distributed in estuaries and oceans, with strong habitat heterogeneity, and the stable isotope ratio of its living environment varies greatly. (Tilley et al., 2013; Yeakel et al., 2015). Within a species, differences in diet, trophic position, and habitat use can be related to age (size), sex-specific energy requirements, vulnerability to predators, and reproduction. Such differences affect the structure and dynamics of populations, communities, and ecosystems (Hammerschlag-Peyer et al., 2011; Hussey et al., 2011; Kiszka et al., 2014).
We observed a degree of isotopic niche overlap between J. belangerii, N. albiflora, and M. miiuy, which suggests that these species co-occur in the same space and share feeding resources. These three sciaenid species showed a decreased overlap with L. polyactis and C. lucidus, suggesting some degree of resource segregation. J. belangerii and N. albiflora spend their lives near the reef, according to stomach content studies (Wang et al., 2012b; Zhang et al., 2020), and share their main choice of prey (Amphipoda and Decapoda) although in different proportions, while other prey appear less frequently in their diet. In contrast, L. polyactis and C. lucidus feed on relatively lower trophic-level prey (He et al., 2012; Wei et al., 2018), such as zooplankton and other low trophic level prey. This explains their lower δ15N as compared to the other three species. These factors could clarify the reasons for the relatively low isotopic niche overlap of L. polyactis and C. lucidus with the other three species. The main prey for each species was different, suggesting some degree of resource partitioning between these species as a possible strategy to reduce interspecific competition.
Our study found that an abundance of prey groups supported the large fishery resources in Zhoushan Archipelago waters, and the coexistence of five species of Sciaenidae in Zhoushan fisheries resulted from the separation of feeding selection and the partitioning of trophic ecological niches. The limitation of this study is that the analysis of differences in feeding choice should be combined with stomach content analysis to improve the reliability of the results.
Data Availability Statement
The raw data supporting the conclusions of this article will be made available by the authors, without undue reservation.
Ethics Statement
The samples used in this study are from ocean trawl surveys approved by the Marine Fisheries Service, so ethical review and approval was not required for this study.
Author Contributions
Conceptualization, JW; methodology, JW; software, JW; validation, YX; formal analysis, JW; investigation, FC; resources, RY; data curation, R-JJ; writing—original draft preparation, JW; writing—review and editing, JW; visualization, JW; supervision, Y-dZ; project administration, R-JJ. and HX X.; funding acquisition, R-JJ, Y-dZ, and H-XX; All authors have read and agreed to the published version of the manuscript.
Funding
This research was funded by the National Key R&D Program of China (grant numbers 2018YFD0900904 and 2019YFD0901204); and the Public Welfare Technology Application Research Project of Zhejiang Province (grant number: LGN20C190012).
Conflict of Interest
The authors declare that the research was conducted in the absence of any commercial or financial relationships that could be construed as a potential conflict of interest.
Publisher’s Note
All claims expressed in this article are solely those of the authors and do not necessarily represent those of their affiliated organizations, or those of the publisher, the editors and the reviewers. Any product that may be evaluated in this article, or claim that may be made by its manufacturer, is not guaranteed or endorsed by the publisher.
Acknowledgments
We would like to express our gratitude to the staff of the Resource Research Office of Zhejiang Marine Fisheries Research Institute for their great help during the data collection process. We also thank the reviewers for their valuable comments and suggestions.
References
Balčiauskas L., Skipitytė R., Balčiauskienė L., Jasiulionis M. (2019). Resource Partitioning Confirmed by Isotopic Signatures Allows Small Mammals to Share Seasonally Flooded Meadows. Ecol. Evol. 9, 5479–5489. doi: 10.1002/ece3.5144
Beaudrot L., Struebig M. J., Meijaard E., van Balen S., Husson S., Marshall A. J. (2013). Co-Occurrence Patterns of Bornean Vertebrates Suggest Competitive Exclusion Is Strongest Among Distantly Related Species. Oecologia 173, 1053–1062. doi: 10.1007/s00442-013-2679-7
Chen W. Z., Li C. S., Hu F. (1997). A Review of the Fisheries Resource Status in East China Sea. J. Fish. Sci. China. 4, 39–43. doi: 10.3321/j.issn:1005-8737.1997.03.008
Chesson P. (2000). Mechanisms of Maintenance of Species Diversity. Annu. Rev. Ecol. Syst. 31, 343–366. doi: 10.1146/annurev.ecolsys.31.1.343
Choi M. J., Kim D. H. (2020). Assessment and Management of Small Yellow Croaker (Larimichthys Polyactis) Stocks in South Korea. Sustainability 12, 8257. doi: 10.3390/su12198257
Costa A. F., Botta S., Siciliano S., Giarrizzo T. (2020). Resource Partitioning Among Stranded Aquatic Mammals From Amazon and Northeastern Coast of Brazil Revealed Through Carbon and Nitrogen Stable Isotopes. Sci. Rep. 10, 1–13. doi: 10.1038/s41598-020-69516-8
Crawford K., Mcdonald R. A., Bearhop S. (2008). Applications of Stable Isotope Techniques to the Ecology of Mammals. Mamm. Rev. 38, 87–107. doi: 10.1111/j.1365-2907.2008.00120.x
De Camargo N. F., Reis G. G., Camargo A. C. L., Nardoto G. B., Kneitel J. M., Vieira E. M. (2021). Seasonal Isotopic Niche of a Rodent: High Between-Individual Variation But No Changes in Individual Niche Width During the Rich-Resource Period. Biotropica 53, 966–975. doi: 10.1111/btp.12921
Deng J. Y., Yang J. M. (1997). Species Interaction and Food Web of Major Predatory Species in the Bohai Sea. J. Fish. Sci. China 4, 2–8. doi: 10.3321/j.issn:1005-8737.1997.04.001
DeNiro M. J., Epstein S. (1978). Influence of Diet on the Distribution of Carbon Isotopes in Animals. Geochimi. Cosmochim. Acta 42, 495–506. doi: 10.1016/0016-7037(78)90199-0
De Roos A. M., Schellekens T., Kooten T. V., Persson L. (2008). Stage-Specific Predator Species Help Each Other to Persist While Competing for a Single Prey. Proc. Natl. Acad. Sci. U. S. A. 105, 13930–13935. doi: 10.1073/pnas.0803834105
Dulvy N. K., Metcalfe J. D., Glanville J., Pawson M. G., Reynolds J. D. (2000). Fishery Stability, Local Extinctions, and Shifts in Community Structure in Skates. Conserv. Biol. 14, 283–293. doi: 10.1046/j.1523-1739.2000.98540.x
General Administration of Quality Supervision, Inspection and Quarantine of the People’s Republic of China, Standardization Administration (2008). “Gb/T 12763.6-2007 Specifications for Oceanographic-Part 6,” in Marine Biological Survey (Beijing: Standards Press of China).
Hammerschlag-Peyer C. M., Yeager L. A., Araújo M. S., Layman C. A. (2011). A Hypothesis-Testing Framework for Studies Investigating Ontogenetic Niche Shifts Using Stable Isotope Ratios. PLoS One 6, e27104. doi: 10.1371/journal.pone.0027104
He X. B., Li B., Wang J. X., Yi M. R., Kang B., Yan Y. R. (2021). Changes in the Trophic Niche of Trichiurus Japonicus in the Beibu Gulf in Different Periods. Chin. J. Appl. Ecol. 32, 683–690. doi: 10.13287/j.1001-9332.202102.036
He Z. T., Zhang Y. Z., Xue L. J., Jin H. W., Zhou Y. D. (2012). Seasonal and Ontogenetic Diet Composition Variation of Collichthys Lucidus in Inshore Waters in the North of East China Sea. Mar. Fish. 34, 270–276. doi: 10.13233/j.cnki.mar.fish.2012.03.010
Hette-Tronquart N. (2019). Isotopic Niche Is Not Equal to Trophic Niche. Ecol. Let. 22 (11), 1987–1989. doi: 10.1111/ele.13218
Hopkins J. B. III, Kurle C. M. (2016). Measuring the Realized Niches of Animals Using Stable Isotopes: From Rats to Bears. Methods Ecol. Evol. 7, 210–221. doi: 10.1111/2041-210X.12446
Hou W. F., Yu C. G., Chen X. Q. (2013). Temperature Distribution in Zhoushan Fishing Ground. J. Ningbo. Univ. (NSEE). 26, 31–34. doi: CNKI:SUN:NBDZ.0.2013-03-008
Hussey N. E., Dudley S. F. J., Mccarthy I. D., Cliff G., Fisk A. (2011). Stable Isotope Profiles of Large Marine Predators: Viable Indicators of Trophic Position, Diet, and Movement in Sharks? Can. J. Fish. Aquat. Sci. 68, 2029–2045. doi: 10.1139/f2011-115
Jackson A. L., Inger R., Parnell A. C., Bearhop S. (2011). Comparing Isotopic Niche Widths Among and Within Communities: SIBER — Stable Isotope Bayesian Ellipses in R. J. Anim. Ecol. 80, 595–602. doi: 10.1111/j.1365-2656.2011.01806.x
Jake Vander Zanden M., Fetzer W. W. (2007). Global Patterns of Aquatic Food Chain Length. Oikos 116, 1378–1388. doi: 10.1111/j.0030-1299.2007.16036.x
Jin X. S. (2000). The Dynamics of Major Fishery Resources in the Bohai Sea. J. Fish. Sci. China. 7, 22–26. doi: 10.3321/j.issn:1005-8737.2000.04.006
Kiszka J. J., Charlot K., Hussey N. E., Heithaus M. R., Simon-Bouhet B., Humber F., et al. (2014). Trophic Ecology of Common Elasmobranchs Exploited by Artisanal Shark Fisheries Off South-Western Madagascar. Aquat. Biol. 23, 29–38. doi: 10.3354/ab00602
Koike S., Nakashita R., Kozakai C., Nakajima A., Nemoto Y., Yamazaki K. (2016). Baseline Characterization of the Diet and Stable Isotope Signatures of Bears That Consume Natural Foods in Central Japan. Eur. J. Wildl. Res. 62, 23–31. doi: 10.1007/s10344-015-0969-6
Lin L. S. (2004). Analysis on Extant Abundance of Small Yellow Croaker Pseudosciaena Polyactis in the East China Sea. Mar. Fish. 26, 18–23. doi: 10.3969/j.issn.1004-2490.2004.01.004
Lin N., Jiang Y. Z., Yuan X. W., Ling J. Z., Yang L. L., Li S. F. (2013). Reproductive Biology of Nibea Albiflora in Xiangshan Bay. J. Fish. Sci. China 35 (04), 389–395. doi: 10.13233/j.cnki.mar.fish.2013.04.003
Lush L., Ward A. I., Wheeler P. (2017). Dietary Niche Partitioning Between Sympatric Brown Hares and Rabbits. J. Zool. 303, 36–45. doi: 10.1111/jzo.12461
Manlick P. J., Pauli J. N. (2020). Human Disturbance Increases Trophic Niche Overlap in Terrestrial Carnivore Communities. Proc. Natl. Acad. Sci. U. S. A. 117, 26842–26848. doi: 10.1073/pnas.2012774117
Marshall H. H., Inger R., Jackson A. L., McDonald R. A., Thompson F. J., Cant M. A. (2019). Stable Isotopes Are Quantitative Indicators of Trophic Niche. Ecol. Lett. 22, 1990–1992. doi: 10.1111/ele.13374
Matthews D. E., Bier D. M. (1983). Stable Isotope Methods for Nutritional Investigation. Annu. Rev. Nutr. 3, 309–339. doi: 10.1146/annurev.nu.03.070183.001521
McConnell R., Lowemcconnell R. H. (1987). Ecological Studies in Tropical Fish Communities Vol. 1987 (England: Cambridge University Press).
Merkle J. A., Polfus J. L., Derbridge J. J., Heinemeyer K. S. (2017). Dietary Niche Partitioning Among Black Bears, Grizzly Bears, and Wolves in a Multiprey Ecosystem. Can. J. Zool. 95, 663–671. doi: 10.1139/cjz-2016-0258
Minagawa M., Wada E. (1984). Stepwise Enrichment of 15N Along Food Chains: Further Evidence and the Relation Between δ15n and Animal Age. Geochimi. Cosmochim. Acta 48, 1135–1140. doi: 10.1016/0016-7037(84)90204-7
Ministry of Agriculture and Rural Affairs of the People’s Republic of China. (2009-2018). China Fishery Statistical Yearbook 2009–2018 (Beijing, China: China Agriculture Press).
Moore J. W., Semmens B. X. (2008). Incorporating Uncertainty and Prior Information Into Stable Isotope Mixing Models. Ecol. Lett. 11, 470–480. doi: 10.1111/j.1461-0248.2008.01163.x
Oelze V. M., Head J. S., Robbins M. M., Richards M., Boesch C. (2014). Niche Differentiation and Dietary Seasonality Among Sympatric Gorillas and Chimpanzees in Loango National Park (Gabon) Revealed by Stable Isotope Analysis. J. Hum. Evol. 66, 95–106. doi: 10.1016/j.jhevol.2013.10.003
Parnell A. C., Inger R., Bearhop S., Jackson A. L. (2010). Source Partitioning Using Stable Isotopes: Coping With Too Much Variation. PLoS One 5 (3), e9672. doi: 10.1371/journal.pone.0009672
Pianka E. R. (1981). “Competition and Niche Theory,” in Theoretical Ecology, 2nd ed. Ed. May R. M. (Oxford OX2 0EL, UK: Blackwell Science), 167–196.
Polis G. A. (1984). Age Structure Component of Niche Width and Intraspecific Resource Partitioning: Can Age Groups Function as Ecological Species? Am. Nat. 123, 541–564. doi: 10.1086/284221
R Core Team. (2015). R: A Language and Environment for Statistical Computing. (Vienna, Austria: University of Auckland).
Schoener T. W. (1974). Resource Partitioning in Ecological Communities. Science 185, 27–39. doi: 10.1126/science.185.4145.27
Sheppard C. E., Inger R., McDonald R. A., Barker S., Jackson A. L., Thompson F. J., et al. (2018). Intragroup Competition Predicts Individual Foraging Specialisation in a Group-Living Mammal. Ecol. Lett. 21, 665–637. doi: 10.1111/ele.12933
Simon K. S., Townsend C. R. (2003). Impacts of Freshwater Invaders at Different Levels of Ecological Organisation, With Emphasis on Salmonids and Ecosystem Consequences. Freshw. Biol. 48, 982–994. doi: 10.1046/j.1365-2427.2003.01069.x
Stock B. C., Semmens B. X. (2016). Unifying Error Structures in Commonly Used Biotracer Mixing Models. Ecology 97, 2562–2569. doi: 10.1002/ecy.1517
Swanson H. K., Lysy M., Power M., Stasko A. D., Johnson J. D., Reist J. D. (2015). A New Probabilistic Method for Quantifying N-Dimensional Ecological Niches and Niche Overlap. Ecology 96, 318–324. doi: 10.1890/14-0235.1
Tilley A., López-Angarita J., Turner J. R. (2013). Diet Reconstruction and Resource Partitioning of a Caribbean Marine Mesopredator Using Stable Isotope Bayesian Modelling. PloS One 8, e79560. doi: 10.1371/journal.pone.0079560
Tilman D. (1982). “Resource Competition and Community Structure,” in Monographs in Population Biology Series (Princeton: Princeton University Press).
Walker P. A., Hislop R. G. (1998). Sensitive Skates or Resilient Rays? Spatial and Temporal Shifts in Ray Species Composition in the Central and North-Western North Sea Between 1930 and the Present Day. I.C.E.S. J. Mar. Sci. 55, 392–402. doi: 10.1006/jmsc.1997.0325
Wang J. F. (2015). The Preliminary Study of Food Composition and Feeding Habits of Collichthy lucidus in the Yangtze Estuary (Nanjing, China:Nanjing Agricultural University).
Wang Y. L., Hu C. L., Li Z. H., Jiang R. J., Zhou Y. D., Zhang L. L., et al. (2021). Population Structure and Resource Change of Larimichthys Polyactis in Spring in Zhoushan Fishery Spawning Ground Protection Area, China. Yingyong. Shengtai. Xuebao. 09, 3349–3356. doi: 10.13287/j.1001-9332.202109.036
Wang C. Q., Tang J. H., Xiong Y., Wang Y. P., Shi J. J., Yan X., et al. (2020). Feeding Habits of Miichthys Miiuy in Jiangsu Coastal Waters. J. Zhejiang. Ocean. Univ. (Nat. Sci. A) 39, 372–378. doi: 10.3969/j.issn.1008-830X.2020.04.014
Wang K., Zhang S. Y., Wang Z. H., Xu M., Zhao J. (2012b). Feeding Habits of Small Yellow Croaker Off Ma’an Archipelago. Acta Hydrobiol. Sin. 36, 1188–1192. doi: 10.3724/SP.J.1035.2012.01188
Wang K., Zhang S. Y., Wang Z. H., Zhao J., Xu M. (2012a). A Preliminary Study on Fishery Biology of Johnius Belangerii Off Ma’an Archipelago. J. Fish. China 36, 228–237. doi: 10.3724/SP.J.1231.2012.27691
Wang K., Zhang S. Y., Wang Z. H., Zhao J., Xu M., Lin J. (2012c). Dietary Composition and Food Competition of Six Main Fish Species in Rocky Reef Habitat Off Gouqi Island. Yingyong. Shengtai. Xuebao. 23, 536–544.
Wei X. J., Zhang B., Shan X. J., Jin X. S., Ren Y. P. (2018). Feeding Habits of Small Yellow Croaker Larimichthys Polyactis in the Bohai Sea. J. Fish. Sci. China 25, 142–151. doi: 10.3724/SP.J.1118.2018.18163
Wilson R. M., Chanton J., Lewis G., Nowacek D. (2009). Isotopic Variation (δ15n, δ13c, and δ34s) With Body Size in Post-Larval Estuarine Consumers. Estuar. Coast. Shelf. Sci. 83, 307–312. doi: 10.1016/j.ecss.2009.04.006
Wisheu I. C. (1998). How Organisms Partition Habitats: Different Types of Community Organization can Produce Identical Patterns. Oikos 83, 246–258. doi: 10.2307/3546836
Xu Z. L., Chen J. J. (2009). Analysis on Migratory Routine of Larimichthy Polyactis. J. Fish. Sci. China. 16, 931–940. doi: 10.3321/j.issn:1005-8737.2009.06.014
Xue Y., Jin X. S., Zhang B., Zhang C. L. (2004). Diet Composition and Seasonal Variation in Feeding Habits of Small Yellow Croaker Pseudosciaena Polyactis Bleeker in the Central Yellow Sea. J. Fish. Sci. China. 11, 237–243. doi: 10.3321/j.issn:1005-8737.2004.03.011
Xu Z. L., Shen X. Q., Ma S. W. (2005). Ecological Characteristics of Dominant Zooplankton Species in Spring and Summer Near Yangtze River Estuary. Mar. Sci. 29, 13–19. doi: CNKI:SUN:HYKX.0.2005-12-003
Yang J. M. (1997). Preliminary Study on the Feeding of Calanus Sinicus of the Bohai Sea. Oceanol. Limnol. Sin. 4, 376–382. doi: 10.3321/j.issn:0029-814X.1997.04.007
Yeakel J. D., Bhat U., Elliott Smith E. A., Newsome S. D. (2015). Exploring the Isotopic Niche: Isotopic Variance, Physiological Incorporation, and the Temporal Dynamics of Foraging. Front. Ecol. Evol. 4, 1. doi: 10.3389/fevo.2016.00001
Yu C. G., Chen Q. Z., Chen X. N., Ning P., Zheng J. (2010). Species Composition and Quantity Distribution of Fishes in the Zhoushan Fishing Ground and Its Adjacent Waters. Oceanol. Limnol. Sin. 41, 410–417. doi: 10.11693/hyhz201003018018
Zhang Y. L., Xu B. D., Zhang C. L., Ji Y. P., Ren Y. P., Cheng Y., et al. (2020). Spatial Heterogeneity in the Feeding Habits and Feeding Ground Distribution of Johnius Belangerii in Haizhou Bay During Spring. J. Fish. Sci. China 27, 315–326. doi: CNKI:SUN:ZSCK.0.2020-03-006
Zhao S. L., Wang R. X., Liu X. S. (2002). Reasons of Exhaustion of Resources of Pseudosciaena Crocea in Zhoushan Fishing Ground and the Measures of Protection and Proliferation. J. Zhejiang. Ocean. Univ. (Nat. Sci. A) 21, 160–165. doi: 10.3969/j.issn.1008-830X.2002.02.018
Zhong X. M., Tang J. H., Zhang H., Zhong F., Zhong J. S., Wu L., et al. (2010). Spatial and Temporal Distribution Characteristics of Miichthys Miiuy in Jiangsu Coastal Area. Haiyang. Xuebao. 32 (03), 95–106. doi: CNKI:SUN:SEAC.0.2010-03-012
Keywords: sciaenid fish, isotope analysis, trophic niche, niche overlap, food source
Citation: Wang J, Jiang R-J, Xiao Y, Yin R, Chen F, Zhou Y-d and Xu H-X (2022) Trophic Niche Partitioning of Five Sciaenidae Species Sampled in Zhoushan Archipelago Waters via Stable Isotope Analysis. Front. Mar. Sci. 9:880123. doi: 10.3389/fmars.2022.880123
Received: 21 February 2022; Accepted: 16 May 2022;
Published: 17 June 2022.
Edited by:
Jun Xu, Institute of Hydrobiology (CAS), ChinaReviewed by:
Alberto Sánchez-González, Instituto Politécnico Nacional (IPN), MexicoXuefeng Wang, Guangdong Ocean University, China
Copyright © 2022 Wang, Jiang, Xiao, Yin, Chen, Zhou and Xu. This is an open-access article distributed under the terms of the Creative Commons Attribution License (CC BY). The use, distribution or reproduction in other forums is permitted, provided the original author(s) and the copyright owner(s) are credited and that the original publication in this journal is cited, in accordance with accepted academic practice. No use, distribution or reproduction is permitted which does not comply with these terms.
*Correspondence: Ri-Jin Jiang, jiangridge@163.com