- 1Nordcee, Department of Biology, University of Southern Denmark, Odense, Denmark
- 2Danish Institute for Advanced Study, University of Southern Denmark, Odense, Denmark
Biological dinitrogen (N2) fixation is the pathway making the large pool of atmospheric N2 available to marine life. Besides direct rate measurements, a common approach to explore the potential for N2 fixation in the ocean is a screening-based targeting the key functional marker gene nifH, coding for a subunit of the nitrogenase reductase. As novel sequencing techniques improved, our understanding of the diversity of marine N2 fixers grew exponentially. However, one aspect of N2 fixation in the ocean is often underexplored, which are the two alternative types of the key enzyme of N2 fixation, the nitrogenase. Altogether there are three isoenzymes, the most common Mo-Fe nitrogenase Nif, the Fe-Fe nitrogenase Anf, and the V-Fe nitrogenase Vnf, which differ regarding their genetic organization, as well as their metal co-enzymes. While Mo is only available in the presence of at least traces of oxygen (O2), V and Fe are available if O2 is absent. Therefore, low O2 and anoxic ocean environments could be an ideal place to explore the diversity of the different isotypes of the nitrogenases. Most phylogenetic studies, however, were only based on the functional marker gene nifH, encoding for a subunit of the Nif nitrogenase, and thus limited in representing the diversity of alternative nitrogenases. Here, we screened metagenomes and -transcriptomes from O2 minimum zones off Peru, from the Bay of Bengal, and the anoxic Saanich Inlet to explore the diversity of genes involved in N2 fixation. We identified genes related to all three nitrogenases, and a generally increased diversity as compared to our previous nifH based on studies from OMZ waters. While we could not confirm gene expression of alternative nitrogenases from our transcriptomic, we detected diazotrophs harboring the genetic potential for alternative nitrogenases. We suggest that alternative nitrogenases may not be used under conditions present in those waters, however, depending on trace metal availability they may become active under future ocean deoxygenation.
Introduction
Biological fixation of dinitrogen gas (N2) is quantitatively the most important external supply of nitrogen (N) to the Ocean. Only certain N2 fixing microbes, called diazotrophs, can perform this highly energy costly enzymatic reaction. First pioneering studies involving large scale sequencing surveys, based on the then-available Sanger sequencing technique, identified the paraphyletic nature of diazotrophs throughout the archaeal and bacterial kingdoms (Zehr et al., 1998; Zani et al., 2000; Zehr and Turner, 2001). More recent studies using high throughput sequencing approaches [e.g., (Farnelid et al., 2011; Cheung et al., 2016; Gaby et al., 2018)] broadened the tree of diazotrophs and more clades could be added to the diversity of N2 fixers in the Ocean, however, it appears that the initial trees did not fundamentally change concerning their main cluster structure.
One major reason may be in the nature of the available genetic screening methods, which are mostly based on selectively targeting the nifH gene, defined as a key functional marker for the operon encoding for the enzyme dinitrogenase reductase (Yun and Szalay, 1984). The operon contains, however, two additional structural genes, nifD and nifK, altogether the nif regulon comprises seven operons (Brill, 1980). nifH genes are often represented in small numbers in the marine realm, and PCR-based detection requires subsequent amplification steps thus introducing certain biases. Thus, to approach the diversity of diazotrophs in the environment, it may be helpful to consider other parts of the Nif operon to obtain a more complete picture. An additional problem regarding the molecular screening for nitrogenases is that the common nitrogenase (encoded by nif) is only one out of three nitrogenases (Bishop et al., 1980; Joerger et al., 1988; Kennedy et al., 1991).
Two alternative nitrogenases were described, one of which is the Anf nitrogenase, which is characterized by an iron-iron (Fe) co-enzyme, the other nitrogenase, Vnf, is a vanadium (V)-Fe nitrogenase. The classic Nif nitrogenase has a Fe-Molybdenum (Mo) cofactor. The difference regarding those metal cofactors is of particular interest in anoxic or generally O2 depleted environments. Mo –in contrast to Fe and V- is only available when at least traces of O2 are present (Bertine and Turekian, 1973; Collier, 1985; Morford and Emerson, 1999; Anbar and Knoll, 2002). This is important with regard to the predicted loss of O2 in today’s Oceans in a warming world (Stramma et al., 2008; Keeling et al., 2010; Schmidtko et al., 2017) which might possibly lead to Mo not always being available, disabling Nif nitrogenases and facilitate the use of alternative nitrogenases. The reason for the evolutionary development of three different nitrogenases is still debated. The general conclusion, however, is that the two alternative nitrogenases originated from nif (Raymond et al., 2004; Boyd et al., 2011; Boyd and Peters, 2013; Boyd et al., 2015), which is supported, e.g., by a similar genetic structure and alternative nitrogenases being dependent on nif-machinery for biosynthesis (Kennedy and Dean, 1992).
Alternative nitrogenases are frequently detected and active in terrestrial environments (Bellenger et al., 2020). However, information on the ecological role of alternative nitrogenases and their activity and presence in marine settings is scarce. A previous study based on whole-genome mining and PacBio from coastal environments identified a 20 fold increase in diversity of diazotrophs when including genes encoding for alternative nitrogenases anf and vnf (McRose et al., 2017) suggesting the importance of those nitrogenases for obtaining a conclusive picture of the diazotroph community in an environment. The contribution of alternative nitrogenases to N2 fixation in environments including cyanolichens, microbial mats, anaerobic sediments has been further corroborated by studies based on isotope fractionation using an isotopic acetylene reduction assay able to distinguish canonical Mo and alternative nitrogenase activities based on carbon isotope fractionation during acetylene reduction to ethylene (Zhang et al., 2016). Based on this assay, Zhang et al., 2016 determined alternative nitrogenases to contribute 20-55% to bulk N2 fixation rates in salt marshes.
Due to the above-mentioned potential advantage of diazotrophs with alternative nitrogenases, marine OMZs might turn into suitable niches for those microbes. In the light of Ocean deoxygenation (Stramma et al., 2008; Keeling et al., 2010; Schmidtko et al., 2017) and increasing frequency of regional severe anoxic and sulfidic events (Lennartz et al., 2014) diazotrophs possessing those alternative nitrogenases may therefore increasingly obtain advantage, because Mo may become limiting under anoxia, thus disabling the functionality of the nif-nitrogenase (Helz et al., 1996; Bellenger et al., 2020; Bennett and Canfield, 2020). Still, information on the presence and distribution of alternative nitrogenases in OMZ waters is to date not available.
In this study, we explored the presence of the three different nitrogenases in OMZs with different intensities. We compared nitrogenases in the OMZ off Peru, which is one of the most prominent examples for expanding and progressing deoxygenation (Stramma et al., 2010) and displays coastal sulfidic anoxia (Schunck et al., 2013; Löscher et al., 2015; Callbeck et al., 2018), Saanich Inlet (SI), a seasonally anoxic fjord (Carter, 1932; Carter, 1934; Anderson and Devol, 1973; Torres-Beltrán et al., 2017), and the Bay of Bengal (BoB) OMZ as part of the Northern Indian Ocean, which has been described to maintain traces of oxygen in its OMZ core waters (Bristow et al., 2017).
Materials and Methods
We re-analyzed metagenomic and -transcriptomic datasets collected on a cruise to the eastern tropical South Pacific in 2009/2010. The cruise was carried out in the framework of the collaborative research center SFB 754 ‘Climate-biogeochemistry interactions in tropical Oceans’ on the German research vessel RV Meteor. The samples were collected as previously described (Schunck et al., 2013; Löscher et al., 2014) on the shelf on station #19, 12°21.88´S, 77°10.00´W, where the water column was anoxic from 20 m down to the sediment (124 m) and hydrogen sulfide (H2S) was present in the anoxic zone reaching concentrations up to 5 µmol L-1. Metagenomic dataset for from BoB were collected from Löscher et al., 2020 with location 17°N, 88.2°E. Metagenomic dataset from SI (48.5°N, 123.5°W) are accessible through JGI IMG/G portal as indicated in Hawley et al., 2017.
Seawater Sampling
Samples for salinity, O2 and nutrient analysis, including nitrate, nitrite, ammonia, and phosphate (NO3-, NO2-, NH4+ and PO43-), respectively were collected from a pump-CTD system. On M77/3 this allowed to combine the classical conductivity-temperature-density sensor measurements with O2, fluorescence, turbidity, and acoustic doppler counter profiler measurements, and importantly with continuous water sampling over a water column of maximum 350 m depth in high resolution. Seabird sensor O2 measurements were calibrated with Winkler method measurements; salinity and nutrients were measured directly after sampling according to Grasshoff et al., 1999 using an autoanalyzer. Samples for nucleic acid extraction were prefiltered through 10 µm pore size filters (Whatman Nuclepore Track-Etch) and cells were collected on 0.22 µm pore size filters (Durapore Membrane filters, Millipore) using a vacuum pump, with filtration times not exceeding 20 min. Filters were frozen and stored at -80°C.
Molecular Methods
Molecular analysis for this study is based on a publicly available metagenomic and –transcriptomic dataset, originally presented in Schunck et al., 2013 with a focus on chemolithoautotrophic lifestyles in sulfidic OMZ waters. Sequencing resulted in 1,888,768 (DNA) and 1,560,959 (RNA) sequences with an average length of 392 base pairs, accounting for 757,439,211 and 599,103,110 base pairs of sequence information, respectively. Sequence datasets are publicly available from the metagenomics analysis server (MG-RAST) under accession numbers 4460677.3, 4450892.3, 4450891.3, 4460736.3, 4461588.3, 4460676.3, 4452038.3, 4460734.3, 4452039.3, 4452042.3, 4460735.3, 4460734.3 and 4452043.3.
Bioinformatic Methods
Metagenomic and transcriptomic raw-reads from the Peruvian shelf, BoB and SI were uploaded and processed on MG-RAST, an open-submission portal for analyzing metagenomic/transcriptomic dataset, such as annotation and functional reconstruction (Keegan et al., 2016). We screened for nitrogenase genes using COG (Cluster of Orthologous genes) database and were subsequently exported. A total of 8677 nif-related and 1112 alternative-related genes were exported from SI, 529 nif-related and 124 alternative-related genes from the Peruvian Shelf, and 116 nif-related and 27 alternative-related genes from the BoB. Exported nitrogenase genes were subject to a BLAST search on the NCBI Genbank database to create a reference library. Mega 7 was used for phylogenetic analysis (Neighbor-joining method) using the reference library and exported nitrogenase genes (Kumar et al., 2016). Clades were defined based on phylogenetic analysis. In order to constrain differences to our previous study of the same samples (Löscher et al., 2014), which were based on nifH Sanger sequencing, we constructed Neighbor-joining trees. Gene/transcript relative abundances were estimated by normalizing counts of nitrogenase genes to the housekeeping gene, rpoB.
In order to identify parameters determining the distribution of N2 fixers in these sulfidic waters, we applied simple correlation analysis and a principal component analysis (PCA, Table S1 and Figure S2). This dataset was compared to a metagenomic dataset available from the BoB from a situation with an OMZ with only traces of O2 left in its core waters, low productivity and a typical OMZ diazotroph community as identified by Sanger sequencing of nifH (Löscher et al., 2020), and from the SI from Oct 2011 where O2 concentrations were below 5 µmol L-1 (Hawley et al., 2017).
Results and Discussion
Hydrochemistry Features
In this study, we used full metagenomes and –transcriptomes from an environment sharing certain characteristics with an ancient Ocean, in order to explore the functional diversity of N2 fixers, and the expression of genes involved in it. We compared the results of this presumably unbiased dataset to previous PCR-based studies targeting the classical functional marker for N2 fixation, nifH. Sampling took place on the shelf off Peru, in a patch of water that was sulfidic at the time. Sulfidic conditions were reported in other studies as well (Galán et al., 2014; Callbeck et al., 2018), thus this condition could be a permanent feature. The distribution of nutrients and chemical properties can be seen in Schunck et al., 2013. Briefly, the path which was sulfidic during our cruise is visibly O2 depleted even in this integrated plot. The water column was anoxic from 18 m downwards and hydrogen sulfide (H2S) was detected along the vertical profile from 27 m downwards (Figures 3C, D). At the same depth (27 m) NO3- and NO2- were depleted and NH4+was accumulated throughout the anoxic water column (Figure 1). Iron (Fe) concentrations were reportedly high reaching concentrations up to about 267 nmol kg-1 in the water column, mostly in the bioavailable form of Fe(II) (Schlosser et al., 2018) in line with earlier reports from that same area (Hong and Kester, 1986).
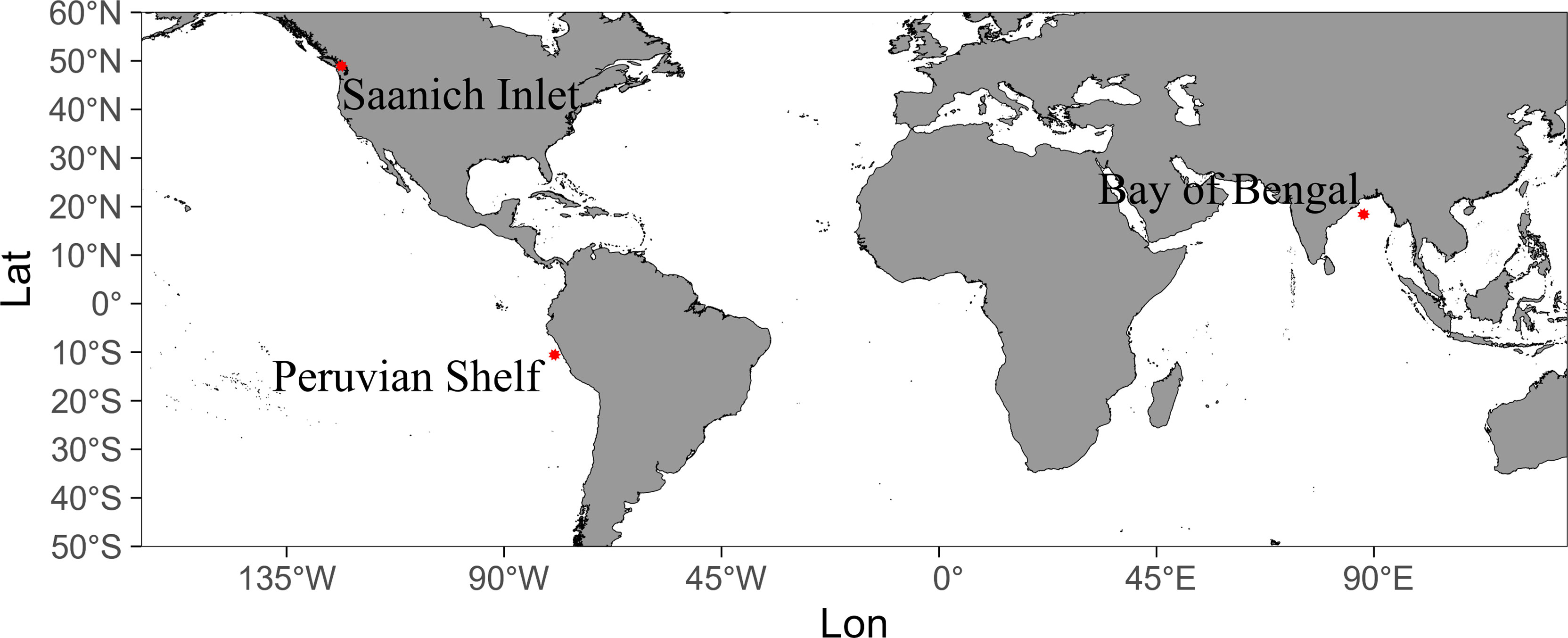
Figure 1 Location of stations from where samples were analyzed from. Stations are indicated by a red star. Peruvian Shelf at 12.2°S, 77°W. Saanich inlet at 48.5°N, 123.5°W. Bay of Bengal at 17°N, 88.2°E.
Composition of Diazotrophs off the Peruvian Shelf
In our previous study from 2014 (Löscher et al., 2014) based on Sanger sequencing of the nifH gene, we identified a community of seven previously unknown and two described clades of N2 fixers in the OMZ waters off Peru. Organisms matching those clades could be recovered from our metagenomes, thus supporting our previous study regarding the validity of sequence presence (Figure 2). In addition to those previously described N2 fixers, we identified several diazotroph clades on the genus level from the combined metagenomes and transcriptomes, thus suggesting that the previously used Sanger sequencing did not entirely cover the present diversity. Taking all nif, anf, and vnf genes into consideration, we identified additional N2 fixers amongst ß-, ɣ-, and ɗ- Proteobacteria, green and purple sulfur bacteria, Firmicutes, Verrumicrobia, Crenarchaeota and Euryarchaeota.
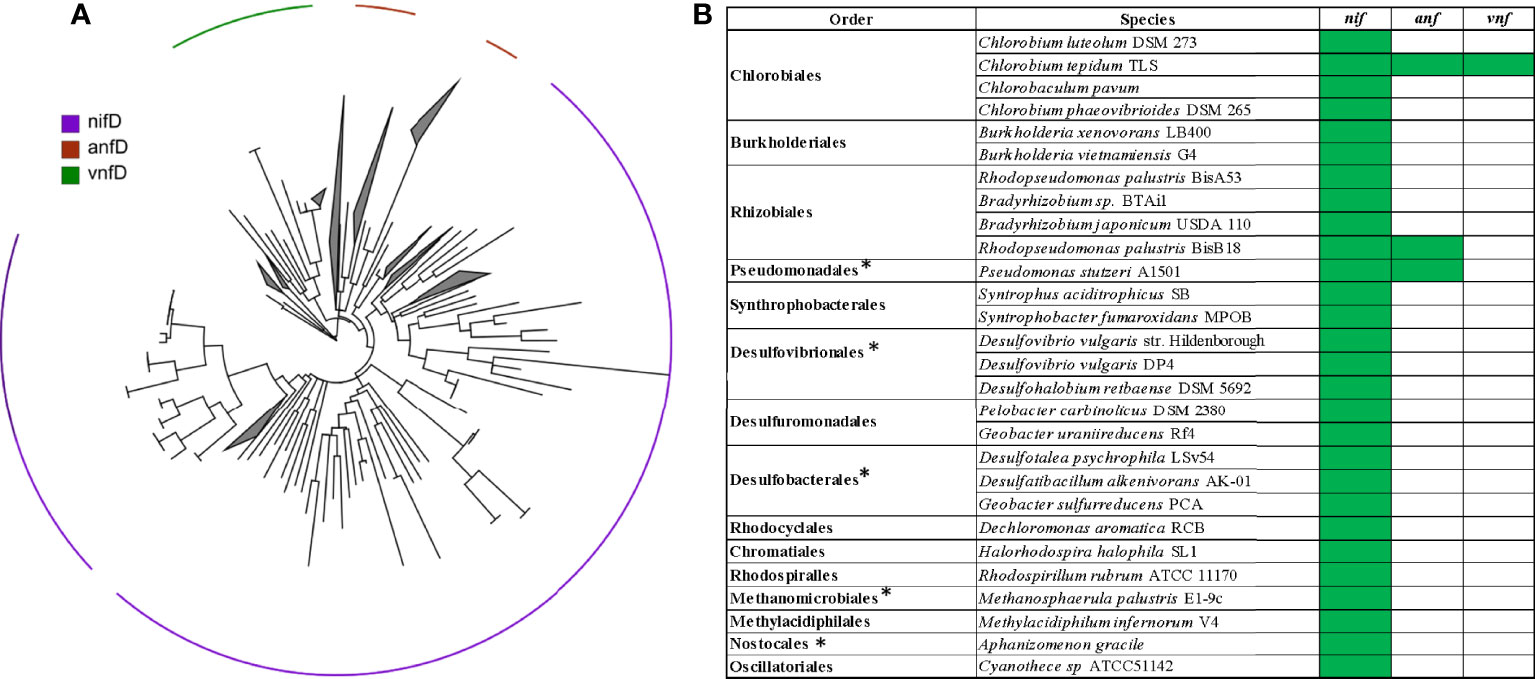
Figure 2 Presence of nitrogenase genes in the metagenomes and -transcriptomes. (A) The maximum likelihood tree shows the detected diversity based on nifD, anfD, and vnfD. (B) Species closest related to the detected diazotrophs, the presence of nif, anf, and vnf is indicated in green. Note that the majority of diazotrophs possesses nif-nitrogenase genes only. * mark genera, of which we found representatives in our previous nifH-based study.
Importantly, we could not identify the traditionally genetic marker gene for nitrogenases (nifH), but rather, rather nifA, were the most abundant nitrogenase gene identified (Tables S2A, B). This, indeed, leaves us wondering how far any of the genes identified in this or other studies are translated into functional nitrogenases. N2 fixation has been shown to occur in those OMZ waters, however, rates deviated strongly and ranged from below the detection limit (Bonnet et al., 2013; Dekaezemacker et al., 2013; Turk-Kubo et al., 2014) to comparably high rates of up to 24.8 ± 8.4 nmol N L-1 d-1 (Löscher et al., 2014; Löscher et al., 2016) (Figure 3D). The latter, however possibly having been corrupted by the use of a potentially contaminated gas stock (Dabundo et al., 2014). In our previous studies, we could not clearly correlate a specific clade of N2 fixers to the high rates in N2 fixation in the euphotic zone in the sulfidic patch. Our data, however, shows the presence of N2 fixers within the genera of both Burkholderiales, Rhizobiales, and Myxococcales in the euphotic zone (Figure 3A and Figure S1), possibly contributing to N2 fixation in surface waters.
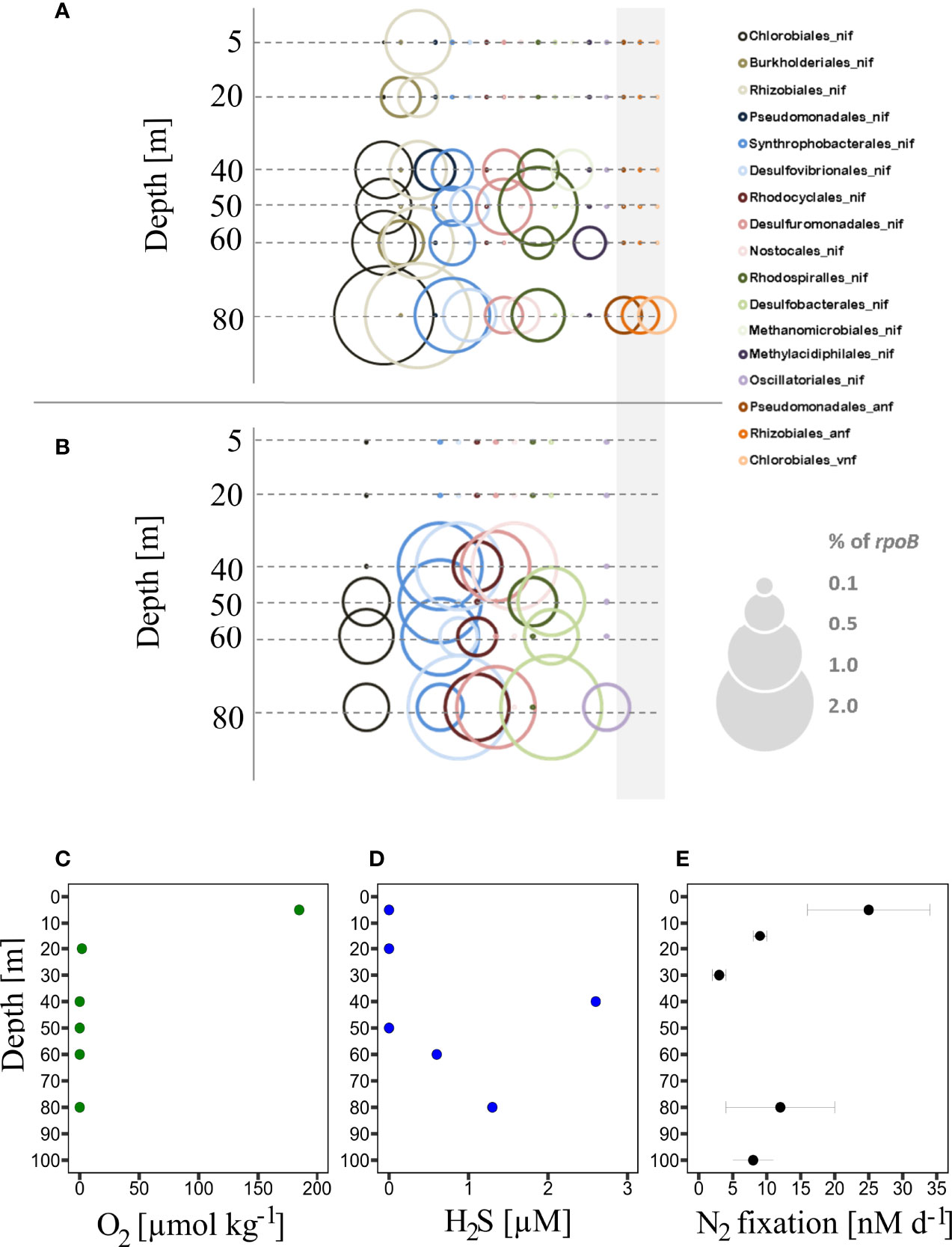
Figure 3 Phylogenetic representation of organisms possessing nitrogenase genes of the nif, anf, or vnf type (all genes of the operons pooled) in metagenomes (A) and -transcriptomes (B) from the sulfidic station. Genera identified from annotations of protein-coding genes (in the NCBI database) in both our metagenomes and –transcriptomes. Nitrogenase gene abundances and expression are shown relative to the putative single copy per organism of RNA polymerase subunit B (rpoB). The grey rectangle indicates the alternative nitrogenases anf and vnf, note that no expression of either of them was found. Vertical profiles from the Peruvian Shelf of O2 (C), H2S (D) and N2 fixation rate (E) are modified from Löscher et al., 2014.
There were two descriptions of this area developing sulfidic anoxia (Schunck et al., 2013; Callbeck et al., 2018). Historical reports of Peruvian fishermen on the characteristic smell and black fishing gear (Schunck et al., 2013), and at least two earlier descriptions of sulfidic anoxia in those waters point towards re-occurring sulfidic anoxia in this region (Burtt, 1852; Dugdale et al., 1977). We identified clades typically observed in anoxic environments, such as clades related to Desulfovibrio and Chlorobiales (Farnelid et al., 2013; Löscher et al., 2014; Jayakumar and Ward, 2020). A large fraction, of N2 fixers in those sulfidic waters, as previously described (Fernandez et al., 2011; Löscher et al., 2014), are microbes involved in sulfur cycling, and similar to those found in organic carbon-rich sediments [e.g., (Bertics et al., 2013; Gier et al., 2016)]. While those N2 fixers do not seem to be quantitatively important for N2 fixation rates, they still find a niche and might become more important in the future with intensifying, expanding OMZs and more frequent events of sulfidic anoxia (Stramma et al., 2008; Lennartz et al., 2014).
Activity of Diazotrophs off the Peruvian Shelf
The metatranscriptomic analysis showed no expression of genes coding for either of the two alternative nitrogenases. nif transcripts could be detected at water depths below 40 m downwards (Figure 3B) affiliated with the genera of Chlorobiales, Syntrophobacterales, Desulfovibrionales, Rhodocyclales, Desulfuromonadales, and Desulfobacterales. The presence and transcriptional activity of the sulfate-reducing Desulfovibrionales and Desulfobacterales, as well as the sulfur-respiring Desulfuromonadales, is in line with our previous study, as well as with a sediment-focused study on N2 fixation from the same area, where those clades have been identified important amongst N2 fixers (Gier et al., 2016).
Green sulfur bacteria (Chlorobiales), sulfate-reducing Syntrophobacterales, and Rhodocyclales (mostly classified closest to Dechloromonas clades, which can denitrify) have, however, previously never been described to play any role in N2 fixation in the Peruvian OMZ. Except for the identified Rhodocyclales, all those newly identified N2 fixing clades are capable of metabolizing sulfur compounds, thus hinting towards a link between N2 fixation and sulfur cycling. However, a statistical correlation to the concentration of H2S could only be observed for transcripts of Desulfovibrionales and Desulfuromonadales, thus speaking for a potential link of N2 fixation and H2S turnover only in those clades (Figure 3D, Table S1).
Previous studies reported both, massive denitrification rates, as well as extreme nitrous oxide production from the sulfidic shelf area off Peru (Kalvelage et al., 2013; Arévalo-Martínez et al., 2015). We identified Rhodocyclales, in our dataset related to Dechloromonas, which are described as denitrifiers, producing the greenhouse gas nitrous oxide as an end-product in their denitrification chain (Horn et al., 2005). Principle component analysis revealed transcript abundances of Rhodocyclales to be linearly correlated to nitrous oxide concentrations (Table S1B) which supports the idea of spatially coupled denitrification and N2 fixation, as previously suggested (Deutsch et al., 2007). However, this correlation is limited by sampling size and further, nitrous oxide concentrations are a result of combined multiple processes. Thus, a connection between denitrification and N2 fixation remains speculative and will still require a deeper focused analysis in those waters in the future. nif transcripts of Desulfovibrionales and Desulfobacterales were dominant at 80 m depth, which is where N2 fixation was at its maximum at anoxic conditions. Those clades were identified as well via nifH amplicon-based screening and peaked in abundance at the same depth in our previous study, however, only in the gene, but not in the transcript pool.
Based on the dominant abundance of transcripts related to Desulfovibrionales and Desulfobacterales, those clades seem to play an important role in N2 fixation in those sulfidic waters, possibly contributing to the described N2 fixation rates. In this sense, using full metagenomes/-transcriptomes did, compared to the nifH-only based approach, only provide novel insights into identifying active diazotrophs to a certain extent. The power of a metagenome/-transcriptome based approach seems to rather be important in exploring the diversity of possibly underrepresented clades, as well as clades discriminated against by the common nifH primers (Zehr et al., 1998).
Alternative Nitrogenases and Diazotrophs Across OMZ Waters
In addition to nif, we identified alternative nitrogenases in OMZ waters off Peru, SI and the BoB. We detected anf genes associated with Pseudomonadales and Rhizobiales, and Chlorobiales, and vnf genes associated with Rhizobiales, Chlorobiales, and low abundances of Rhodospririllales, Acidothiobacillales, Methylacidiphilales and Desulfovibrionales (Figures 2, 3A and Figures S1).If compared to other O2 depleted ocean water bodies with available full metagenomic datasets (BoB, SI), the diversity of alternative nitrogenases seems similar, with Pseudomonadales, Rhizobiales and Chlorobiales consistently present in the anf pool, and Rhizobiales, Chlorobiales, and Rhodospirillales dominating the vnf pool of sequences (Figure S1). Because alternative N2 fixers always also have genes coding for the nif nitrogenase, it cannot be expected that the diversity of alternative nitrogenase genes exceeds the diversity of nif genes.
One obvious effect of the limitations of nifH-based mining is the previous lack of a description of alternative nitrogenases. In our samples, we could identify at least some clades possessing genes for anf and vnf consistently through OMZs of different intensity thus raising the question of whether those genes are maintained in the genome for at least occasional use when Mo is limiting for the expression of nif. This, however, cannot be answered from our dataset and is generally a question we can only speculate about.
The turnover of trace metals, in general, is largely redox sensitive (Morford and Emerson, 1999), and has been reported to be impacted by ENSO-dependent changes in redox conditions at the sediment-water interface in this area. With a cyclic alternation between oxic conditions favoring V and Mo fluxes to the water column and anoxic conditions re-precipitating V and Mo to the sediment, both V and Mo accumulate in shelf sediments in the Peruvian OMZ (Scholz et al., 2011). Further, a reduction of V to V(III) by H2S has been demonstrated experimentally, thus explaining the redox-dependent accumulation in sulfate-reducing sediments (Wanty and Goldhaber, 1992). Under sulfidic conditions, Mo(VI) is reduced to Mo(IV) and precipitated to the sediments (Crusius et al., 1996). While this precipitation at anoxic and sulfidic conditions in principle removes Mo and V from the water column, it promotes the formation of a reservoir of those trace metals in the sediments (Bennett and Canfield, 2020), enabling their availability in the water column via upwelling at least occasionally when redox conditions allow for it. Given the decrease in Mo and V availability at anoxic-sulfidic conditions, possessing the genes for an additional Fe-Fe nitrogenase may thus be advantageous, both regarding ocean anoxic events in Earth history and future ocean deoxygenation likewise. Thus, one could suggest that while anf and vnf may presently not play a major role for N2 fixation in OMZs they may become important under a possible future euxinia scenario.
It has only recently been shown that even under Mo limitation alternative N2 fixers will try to acquire enough of this trace metal to still sustain their nif nitrogenase instead of expressing the alternative nitrogenases (Philippi et al., 2021). Thus, the presence of alternative nitrogenases, while being a given, is not explainable for us right now. Thou, they may play a substantial role in N2 fixation in costal sediment environments, particular in sediments (Zhang et al., 2016; McRose et al., 2017).
Conclusion
This study addresses the diazotrophic community, based on a whole-metagenome and – transcriptome screening. It reports an increased diversity of N2 fixing microbes in the sulfidic shelf water off Peru, as compared to the previous target-gene based studies from the same waters. In addition to a generally higher diversity, genes encoding for alternative nitrogenases, which were previously not subject of any study on N2 fixation in those OMZs, were detected. The ecological meaning and evolutionary history of those alternative nitrogenases are debated, however, their presence in OMZ waters would possibly become relevant under scenarios of extreme and persistent anoxia, which may become important in a future ocean challenged by progressive deoxygenation.
Data Availability Statement
The datasets presented in this study can be found in online repositories. The names of the repository/repositories and accession number(s) can be found in the article/Supplementary Material
Author Contributions
CL and CR designed the study. CR carried out bioinformatic analysis. CL and CR wrote the manuscript. All authors contributed to the article and approved the submitted version.
Funding
The cruise was funded by the German Research Foundation within the Collaborative Research Center SFB754. Funding was further received from the European Union in the framework of the H2020 program (Marie Curie IF, # 704272 to CL), and from the Villum Foundation (Grant No. 16518 to D. Canfield and Grant No. 29411 to CL).
Conflict of Interest
The authors declare that the research was conducted in the absence of any commercial or financial relationships that could be construed as a potential conflict of interest.
Publisher’s Note
All claims expressed in this article are solely those of the authors and do not necessarily represent those of their affiliated organizations, or those of the publisher, the editors and the reviewers. Any product that may be evaluated in this article, or claim that may be made by its manufacturer, is not guaranteed or endorsed by the publisher.
Acknowledgments
We acknowledge the captain, crew and the chief scientist, Martin Frank, of the RV Meteor cruise M77/3. We thank the Peruvian government for giving access to their territorial waters. G. Klockgether, A. Ellrott, V. León Fernández, and P. Fritsche, we thank you for technical support during the cruise. We further thank Will Bennett for helpful discussion of trace metal availability.
Supplementary Material
The Supplementary Material for this article can be found online at: https://www.frontiersin.org/articles/10.3389/fmars.2022.875582/full#supplementary-material
References
Anbar A. D., Knoll A. H. (2002). Proterozoic Ocean Chemistry and Evolution: A Bioinorganic Bridge? Science 297, 1137–1142. doi: 10.1126/science.1069651
Anderson J. J., Devol A. H. (1973). Deep Water Renewal in Saanich Inlet, an Intermittently Anoxic Basin. Estuar. Coast. Mar. Sci. 1, 1–10. doi: 10.1016/0302-3524(73)90052-2
Arévalo-Martínez D. L., Kock A., Löscher C. R., Schmitz R. A., Bange H. W. (2015). Evidence of Massive Nitrous Oxide Emissions From the Tropical South Pacific Ocean. Nat. Geosci. 8, 530–533. doi: 10.1038/ngeo2469
Bellenger J. P., Darnajoux R., Zhang X., Kraepiel A. M. L. (2020). Biological Nitrogen Fixation by Alternative Nitrogenases in Terrestrial Ecosystems: A Review. Biogeochemistry 149, 53–73. doi: 10.1007/s10533-020-00666-7
Bennett W. W., Canfield D. E. (2020). Redox-Sensitive Trace Metals as Paleoredox Proxies: A Review and Analysis of Data From Modern Sediments. Earth-Sci. Rev. 204, 103175. doi: 10.1016/j.earscirev.2020.103175
Bertics V. J., Löscher C. R., Salonen I., Dale A. W., Gier J., Schmitz R. A., et al. (2013). Occurrence of Benthic Microbial Nitrogen Fixation Coupled to Sulfate Reduction in the Seasonally Hypoxic Eckernförde Bay, Baltic Sea. Biogeosciences 10, 1243–1258. doi: 10.5194/bg-10-1243-2013
Bertine K. K., Turekian K. K. (1973). Molybdenum in Marine Deposits. Geochim. Cosmochim. Acta 37, 1415–1434. doi: 10.1016/0016-7037(73)90080-X
Bishop P. E., Jarlenski D. M., Hetherington D. R. (1980). Evidence for an Alternative Nitrogen Fixation System in Azotobacter Vinelandii. Proc. Natl. Acad. Sci. U. S. A. 77, 7342–7346. doi: 10.1073/pnas.77.12.7342
Bonnet S., Dekaezemacker J., Turk-Kubo K. A., Moutin T., Hamersley R. M., Grosso O., et al. (2013). Aphotic N2 Fixation in the Eastern Tropical South Pacific Ocean. PloS One 8, 1–14. doi: 10.1371/journal.pone.0081265
Boyd E. S., Anbar A. D., Miller S., Hamilton T. L., Lavin M., Peters J. W. (2011). A Late Methanogen Origin for Molybdenum-Dependent Nitrogenase. Geobiology 9, 221–232. doi: 10.1111/j.1472-4669.2011.00278.x
Boyd E. S., Garcia Costas A. M., Hamilton T. L., Mus F., Peters J. W. (2015). Evolution of Molybdenum Nitrogenase During the Transition From Anaerobic to Aerobic Metabolism. J. Bacteriol. 197 (9), 1690–1699. doi: 10.1128/JB.02611-14
Boyd E. S., Peters J. W. (2013). New Insights Into the Evolutionary History of Biological Nitrogen Fixation. Front. Microbiol. 4. doi: 10.3389/fmicb.2013.00201
Brill W. J. (1980). Biochemical Genetics of Nitrogen Fixation. Microbiol. Rev. 44, 449–467. doi: 10.1128/mr.44.3.449-467.1980
Bristow L. A., Callbeck C. M., Larsen M., Altabet M. A., Dekaezemacker J., Forth M., et al. (2017). N2 Production Rates Limited by Nitrite Availability in the Bay of Bengal Oxygen Minimum Zone. Nat. Geosci. 10, 24–29. doi: 10.1038/ngeo2847
Burtt J. L. (1852). On Fish Destroyed by Sulphuretted Hydrogen in the Bay of Callao. Am. J. Sci. 2, 433–434.
Callbeck C. M., Lavik G., Ferdelman T. G., Fuchs B., Gruber-Vodicka H. R., Hach P. F., et al. (2018). Oxygen Minimum Zone Cryptic Sulfur Cycling Sustained by Offshore Transport of Key Sulfur Oxidizing Bacteria. Nat. Commun. 9, 1729. doi: 10.1038/s41467-018-04041-x
Carter N. M. (1932). The Oceanography of the Fjords of Southern British Columbia. J. Fish. Res. Bd. Canada Prog. Rept. Pac. Coast. Sta 12, 7–11.
Carter N. M. (1934). Physiography and Oceanography of Some British Columbia Fjords. J. Proc. Fifth. Pac. Sci. Cong. 1, 721.
Cheung S., Xia X., Guo C., Liu H. (2016). Diazotroph Community Structure in the Deep Oxygen Minimum Zone of the Costa Rica Dome. J. Plankton. Res. 38, 380–391. doi: 10.1093/plankt/fbw003
Collier R. W. (1985). Molybdenum in the Northeast Pacific Ocean. Limnol Oceanogr. 30, 1351–1354. doi: 10.4319/lo.1985.30.6.1351
Crusius J., Calvert S., Pedersen T., Sage D. (1996). Rhenium and Molybdenum Enrichments in Sediments as Indicators of Oxic, Suboxic and Sulfidic Conditions of Deposition. Earth Planet. Sci. Lett. 145, 65–78. doi: 10.1016/s0012-821x(96)00204-x
Dabundo R., Lehmann M. F., Treibergs L., Tobias C. R., Altabet M. A., Moisander P. H., et al. (2014). The Contamination of Commercial 15n2 Gas Stocks With 15N–Labeled Nitrate and Ammonium and Consequences for Nitrogen Fixation Measurements. PloS One 9, e110335. doi: 10.1371/journal.pone.0110335
Dekaezemacker J., Bonnet S., Grosso O., Moutin T., Bressac M., Capone D. G. (2013). Evidence of Active Dinitrogen Fixation in Surface Waters of the Eastern Tropical South Pacific During El Niño and La Niña Events and Evaluation of its Potential Nutrient Controls. Global Biogeochem. Cycles 27, 768–779. doi: 10.1002/gbc.20063
Deutsch C., Sarmiento J. L., Sigman D. M., Gruber N., Dunne J. P. (2007). Spatial Coupling of Nitrogen Inputs and Losses in the Ocean. Nature 445, 163–167. doi: 10.1038/nature05392
Dugdale R. C., Goering J. J., Barber R. T., Smith R. L., Packard T. T. (1977). Denitrification and Hydrogen Sulfide in the Peru Upwelling Region During 1976. Deep. Res. 24, 601–608. doi: 10.1016/0146-6291(77)90530-6
Farnelid H., Andersson A. F., Bertilsson S., Al-Soud W. A., Hansen L. H., Sørensen S., et al. (2011). Nitrogenase Gene Amplicons From Global Marine Surface Waters Are Dominated by Genes of Non-Cyanobacteria. PloS One 6, e19223. doi: 10.1371/journal.pone.0019223
Farnelid H., Bentzon-Tilia M., Andersson A. F., Bertilsson S., Jost G., Labrenz M., et al. (2013). Active Nitrogen-Fixing Heterotrophic Bacteria at and Below the Chemocline of the Central Baltic Sea. ISME J. 7, 1413–1423. doi: 10.1038/ismej.2013.26
Fernandez C., Farías L., Ulloa O. (2011). Nitrogen Fixation in Denitrified Marine Waters. PloS One 6, e20539. doi: 10.1371/journal.pone.0020539
Gaby J. C., Rishishwar L., Valderrama-Aguirre L. C., Green S. J., Valderrama-Aguirre A., Jordan I. K., et al. (2018). Diazotroph Community Characterization via a Highthroughput nifH Amplicon Sequencing and Analysis Pipeline. Appl. Environ. Microbiol. 84, e01512–17. doi: 10.1128/AEM.01512-17
Galán A., Faúndez J., Thamdrup B., Santibáñez J. F., Farías L. (2014). Temporal Dynamics of Nitrogen Loss in the Coastal Upwelling Ecosystem Off Central Chile: Evidence of Autotrophic Denitrification Through Sulfide Oxidation. Lmnol. Ocean. 59, 1865–1878. doi: 10.4319/lo.2014.59.6.1865
Gier J., Sommer S., Löscher C. R., Dale A. W., Schmitz R. A., Treude T. (2016). Nitrogen Fixation in Sediments Along a Depth Transect Through the Peruvian Oxygen Minimum Zone. Biogeosciences 13, 4065–4080. doi: 10.5194/bg-13-4065-2016
Grasshoff G., Kremling K., Erhardt M. (1999). Methods of Seawater Analysis. 3ed (Weinheim: Wiley VCH).
Hawley A. K., Torres-Beltrán M., Zaikova E., Walsh D. A., Mueller A., Scofield M., et al. (2017). A Compendium of Multi-Omic Sequence Information From the Saanich Inlet Water Column. Sci. Data 4, 170160. doi: 10.1038/sdata.2017.160
Helz G. R., Miller C. V., Charnock J. M., Mosselmans J. F. W., Pattrick R. A. D., Garner C. D., et al. (1996). Mechanism of Molybdenum Removal From the Sea and its Concentration in Black Shales: EXAFS Evidence. Geochim. Cosmochim. Acta 60, 3631–3642. doi: 10.1016/0016-7037(96)00195-0
Hong H., Kester D. R. (1986). Redox State of Iron in the Offshore Waters of Peru1. Limnol. Oceanogr. 31, 512–524. doi: 10.4319/lo.1986.31.3.0512
Horn M. A., Ihssen J., Matthies C., Schramm A., Acker G., Drake H. L. (2005). Dechloromonas Denitrificans Sp. Nov., Flavobacterium Denitrificans Sp. Nov., Paenibacillus Anaericanus Sp. Nov. And Paenibacillus Terrae Strain MH72, N2O-Producing Bacteria Isolated From the Gut of the Earthworm Aporrectodea Caliginosa. Int. J. Syst. Evol. Microbiol. 55, 1255–1265. doi: 10.1099/ijs.0.63484-0
Jayakumar A., Ward B. B. (2020). Diversity and Distribution of Nitrogen Fixation Genes in the Oxygen Minimum Zones of the World Oceans. Biogeosciences 17, 5953–5966. doi: 10.5194/bg-17-5953-2020
Joerger R. D., Bishop P. E., Evans H. J. (1988). Bacterial Alternative Nitrogen Fixation Systems. CRC Crit. Rev. Microbiol. 16, 1–14. doi: 10.3109/10408418809104465
Kalvelage T., Lavik G., Lam P., Contreras S., Arteaga L., Löscher C. R., et al. (2013). Nitrogen Cycling Driven by Organic Matter Export in the South Pacific Oxygen Minimum Zone. Nat. Geosci. 6, 228–234. doi: 10.1038/ngeo1739
Keegan K. P., Glass E. M., Meyer F. (2016). MG-RAST, a Metagenomics Service for Analysis of Microbial Community Structure and Function. Methods Mol. Biol. 1399, 207–233. doi: 10.1007/978-1-4939-3369-3_13
Keeling R. F., Körtzinger A., Gruber N. (2010). Ocean Deoxygenation in a Warming World. Ann. Rev. Mar. Sci. 2, 199–229. doi: 10.1146/annurev.marine.010908.163855
Kennedy C., Bali A., Blanco G., Contreras A., Drummond M., Merrick M., et al. (1991). “Regulation of Expression of Genes for Three Nitrogenases in Azotobacter Vinelandii,” in Nitrogen Fixation (Dordrecht: Springer Netherlands), 13–23. doi: 10.1007/978-94-011-3486-6_2
Kennedy C. I., Dean D. (1992). The Nifu, niPS and nifV Gene Products are Required for Activity of All Three Nitrogenases of Azotobacter Vinelandii. Molec. Gen. Genet. 231, 494–498. doi: 10.1007/BF00292722
Kumar S., Stecher G., Tamura K. (2016). MEGA7: Molecular Evolutionary Genetics Analysis Version 7.0 for Bigger Datasets. Mol. Biol. Evol. 33, 1870–1874. doi: 10.1093/molbev/msw054
Lennartz S. T., Lehmann A., Herrford J., Malien F., Hansen H. P., Biester H., et al. (2014). Long-Term Trends at the Boknis Eck Time Series Station (Baltic Sea), 1957-2013: Does Climate Change Counteract the Decline in Eutrophication? Biogeosciences 11, 6323–6339. doi: 10.5194/bg-11-6323-2014
Löscher C. R., Bange H. W., Schmitz R. A., Callbeck C. M., Engel A., Hauss H., et al. (2015). Water Column Biogeochemistry of Oxygen Minimum Zones in the Eastern Tropical North Atlantic and Eastern Tropical South Pacific Oceans. Biogeosci. Discuss. 12, 4495–4556. doi: 10.5194/bgd-12-4495-2015
Löscher C., Bourbonnais A., Dekaezemacker J., Charoenpong C., Altabet M., Bange H., et al. (2016). N2 Fixation in Eddies of the Eastern Tropical South Pacific Ocean. Biogeosciences 13, 2889–2899. doi: 10.5194/bg-13-2889-2016
Löscher C. R., Großkopf T., Desai F., Gill D., Schunck H., Croot P., et al. (2014). Facets of Diazotrophy in the Oxygen Minimum Zone Off Peru. ISME J. 8, 2180–2192. doi: 10.1038/ismej.2014.71
Löscher C. R., Mohr W., Bange H. W., Canfield D. E. (2020). No Nitrogen Fixation in the Bay of Bengal? Biogeosciences 17, 851–864. doi: 10.5194/bg-17-851-2020
McRose D. L., Zhang X., Kraepiel A. M. L., Morel F. M. M. (2017). Diversity and Activity of Alternative Nitrogenases in Sequenced Genomes and Coastal Environments. Front. Microbiol. 8. doi: 10.3389/fmicb.2017.00267
Morford J. L., Emerson S. (1999). The Geochemistry of Redox Sensitive Trace Metals in Sediments. Geochim. Cosmochim. Acta 63, 1735–1750. doi: 10.1016/S0016-7037(99)00126-X
Philippi M., Kitzinger K., Berg J. S., Tschitschko B., Kidane A. T., Littmann S., et al. (2021). Purple Sulfur Bacteria Fix N2 via Molybdenum-Nitrogenase in a Low Molybdenum Proterozoic Ocean Analogue. Nat. Commun. 12, 1–12. doi: 10.1038/s41467-021-25000-z
Raymond J., Siefert J. L., Staples C. R., Blankenship R. E. (2004). The Natural History of Nitrogen Fixation. Mol. Biol. Evol. 21, 541–554. doi: 10.1093/molbev/msh047
Schlosser C., Streu P., Frank M., Lavik G., Croot P. L., Dengler M., et al. (2018). H2S Events in the Peruvian Oxygen Minimum Zone Facilitate Enhanced Dissolved Fe Concentrations. Sci. Rep. 8, 12642. doi: 10.1038/s41598-018-30580-w
Schmidtko S., Stramma L., Visbeck M. (2017). Decline in Global Oceanic Oxygen Content During the Past Five Decades. Nature 542, 335–339. doi: 10.1038/nature21399
Scholz F., Hensen C., Noffke A., Rohde A., Liebetrau V., Wallmann K. (2011). Early Diagenesis of Redox-Sensitive Trace Metals in the Peru Upwelling Area – Response to ENSO-Related Oxygen Fluctuations in the Water Column. Geochim. Cosmochim. Acta 75, 7257–7276. doi: 10.1016/j.gca.2011.08.007
Schunck H., Lavik G., Desai D. K., Großkopf T., Kalvelage T., Löscher C. R., et al. (2013). Giant Hydrogen Sulfide Plume in the Oxygen Minimum Zone Off Peru Supports Chemolithoautotrophy. PloS One 8, e68661. doi: 10.1371/journal.pone.0068661
Stramma L., Johnson G. C., Sprintall J., Mohrholz V. (2008). Expanding Oxygen-Minimum Zones in the Tropical Oceans. Science 320, 655–658. doi: 10.1126/science.1153847
Stramma L., Schmidtko S., Levin L. A., Johnson G. C. (2010). Ocean Oxygen Minima Expansions and Their Biological Impacts. Deep. Res. Part I-Oceanogr. Res. Pap. 57, 587–595. doi: 10.1016/j.dsr.2010.01.005
Torres-Beltrán M., Hawley A. K., Capelle D., Zaikova E., Walsh D. A., Mueller A., et al. (2017). A Compendium of Geochemical Information From the Saanich Inlet Water Column. Sci. Data 4, 1–11. doi: 10.1038/sdata.2017.159
Turk-Kubo K. A., Karamchandani M., Capone D. G., Zehr J. P. (2014). The Paradox of Marine Heterotrophic Nitrogen Fixation: Abundances of Heterotrophic Diazotrophs do Not Account for Nitrogen Fixation Rates in the Eastern Tropical South Pacific. Environ. Microbiol. 16, 3095–3114. doi: 10.1111/1462-2920.12346
Wanty R. B., Goldhaber M. B. (1992). Thermodynamics and Kinetics of Reactions Involving Vanadium in Natural Systems: Accumulation of Vanadium in Sedimentary Rocks. Geochim. Cosmochim. Acta 56, 1471–1483. doi: 10.1016/0016-7037(92)90217-7
Yun A. C., Szalay A. A. (1984). Structural Genes of Dinitrogenase and Dinitrogenase Reductase are Transcribed From Two Separate Promoters in the Broad Host Range Cowpea Rhizobium Strain Irc78. Proc. Natl. Acad. Sci. U. S. A. 81, 7358–7362. doi: 10.1073/pnas.81.23.7358
Zani S., Mellon M. T., Collier J. L., Zehr J. P. (2000). Expression of nifH Genes in Natural Microbial Assemblages in Lake George, New York, Detected by Reverse Transcriptase PCR. Appl. Environ. Microbiol. 66, 3119–3124. doi: 10.1128/AEM.66.7.3119-3124.2000
Zehr J. P., Mellon M. T., Zani S. (1998). New Nitrogen-Fixing Microorganisms Detected in Oligotrophic Oceans by Amplification of Nitrogenase (Nifh) Genes. Appl. Environ. Microbiol. 64, 3444–3450. doi: 10.1128/AEM.64.9.3444-3450.1998
Zehr J. P., Turner P. J. (2001). “Nitrogen Fixation: Nitrogenase Genes and Gene Expression,” in Methods in Microbiology, vol. 30. (San Diego: Academic Press Inc), 271–286.
Keywords: alternative nitrogenases, nitrogen fixation, oxygen minimum zone (OMZ), diazotrophs diversity, bioinfomatic analysis
Citation: Reeder CF and Löscher CR (2022) Nitrogenases in Oxygen Minimum Zone Waters. Front. Mar. Sci. 9:875582. doi: 10.3389/fmars.2022.875582
Received: 14 February 2022; Accepted: 25 May 2022;
Published: 04 July 2022.
Edited by:
Annie Bourbonnais, University of South Carolina, United StatesReviewed by:
Takuhei Shiozaki, The University of Tokyo, JapanAnthony Bertagnolli, Montana State University, United States
Shunyan Cheung, University of California, Santa Cruz, United States
Copyright © 2022 Reeder and Löscher. This is an open-access article distributed under the terms of the Creative Commons Attribution License (CC BY). The use, distribution or reproduction in other forums is permitted, provided the original author(s) and the copyright owner(s) are credited and that the original publication in this journal is cited, in accordance with accepted academic practice. No use, distribution or reproduction is permitted which does not comply with these terms.
*Correspondence: Carolin Regina Löscher, cloescher@biology.sdu.dk