- 1College of Sciences and Engineering, Flinders University, Adelaide, SA, Australia
- 2School of Electrical Engineering, Computing and Mathematical Sciences, Curtin University, Bentley, WA, Australia
- 3School of Agriculture, Food and Wine, University of Adelaide, Adelaide, SA, Australia
- 4Conservation Department, Phillip Island Nature Parks, Cowes, VIC, Australia
Droughts in many regions of the world are increasing in frequency and severity which, coupled with effects from anthropogenic water extraction and diversion, are reducing river discharges. Yet to date, few studies have investigated the impacts of hydrological droughts (i.e., reduced river outflows to the ocean) on seabirds. Here, we examined the consequences of the “Millennium Drought” on the local decline of an iconic Australian seabird, the little penguin (Eudyptula minor). We analysed monthly and annual penguin numbers in relation to river outflow, rainfall, the characteristics of the coastal waters (sea surface temperatures and chlorophyll-a concentrations), and local abundance of key predators and prey species. We found a negative association between monthly penguin numbers and both sea surface temperatures and river outflow. Annual penguin numbers were positively associated with southern garfish numbers (our local indicator of food availability) but negatively associated with annual chlorophyll-a concentrations. Our findings emphasizing the need for further research into the effect of hydrological droughts on seabird populations and for improved river management that account for potential downstream impacts on the coastal environment receiving freshwater from rivers.
Introduction
Seabirds are important bio-indicators within the marine environment (e.g., Croxall et al., 2002; Lascelles et al., 2012) and are currently one of the most threatened group of birds, with 110 of 359 seabird species threatened globally (reviewed in Dias et al., 2019). Seabirds are highly sensitive to changes in their food resources (Reid and Croxall, 2001; Crawford, 2007; Frederiksen et al., 2007; Chiaradia et al., 2010), and disappearance of their primary prey often result in decreased breeding success and population size (Croxall et al., 1999; Dann et al., 2000; Lynnes et al., 2004; Österblom et al., 2006). Climatic or oceanographic conditions can have contrasting consequences for seabird species (e.g., Newton et al., 2009; Chambers et al., 2011; Krüger and Petry, 2011; Tavares et al., 2016). For example, upwelling processes, and resulting cold surface waters, may act differently on various prey species and thus may be beneficial to some seabirds (by increasing their prey availability) but increase mortality in other seabird species due to reduced food productivity (see Newton et al., 2009) or thermoregulatory stress. Understanding the impacts that climatic and oceanographic factors can have on seabird population decline is thus crucial but challenging.
Rainfall patterns are expected to change across the world as a result of climate change (Milly et al., 2005; Power et al., 2013). Droughts, in particular, are becoming more frequent and extreme in many regions of the world (Dai, 2013), resulting in reduced water outflow to the ocean and decreased oceanic productivity across multiple trophic levels (Lamberth et al., 2009; Lee et al., 2012; Arnell and Gosling, 2013; Auricht et al., 2018). Human interventions, such as water extraction and diversion, can greatly exacerbate climatic effects of drought, and even lead to hydrological droughts that are independent of climatic effects (Wada et al., 2013). Increased nutrient loading from freshwater outflows into the ocean stimulates phytoplankton and zooplankton production (Morgan et al., 2005), often leading to large aggregations of larval fishes and their predators near river estuaries (Cyrus and Blaber, 1987; Cyrus and Blaber, 1992; Grimes and Kingsford, 1996; Gillanders and Kingsford, 2002). Therefore, knowledge of the link between freshwater outflow and coastal trophic communities is critical but this relationship is often poorly understood (Gillanders and Kingsford, 2002; Gillson, 2011).
The Murray River is the largest river in Australia and a major source of freshwater entering coastal waters in South Australia. The Murray River discharges into two shallow and large freshwater lakes (Lakes Alexandrina and Albert also known as the “Lower Lakes”, regulated by a series of barrages), mixing in a shallow estuarine-lagoon (the Coorong) before reaching the mouth of the river (thereafter referred to as the “Murray Mouth”) (Figure 1). Freshwater outflow into the coastal environment from the Murray River has declined significantly over the past century due to intensive drought periods and increased extraction for human use (Maheshwari et al., 1995; Thom et al., 2020). Human demands have reduced the average annual outflow at the Murray Mouth by 61%, meaning that water now ceases to flow to the mouth 40% of the time compared to 5% before increased extraction for human use occurred (Csiro, 2008). In addition, between 2001 and 2010, Australia experienced one of the worst droughts recorded since European settlement, known as the “Millennium Drought”. This combined pressure resulted in reduction of both seawater exchange and freshwater outflows (Mosley et al., 2012; Geddes et al., 2016), as well as decreasing coastal productivity in the area (Auricht et al., 2018).
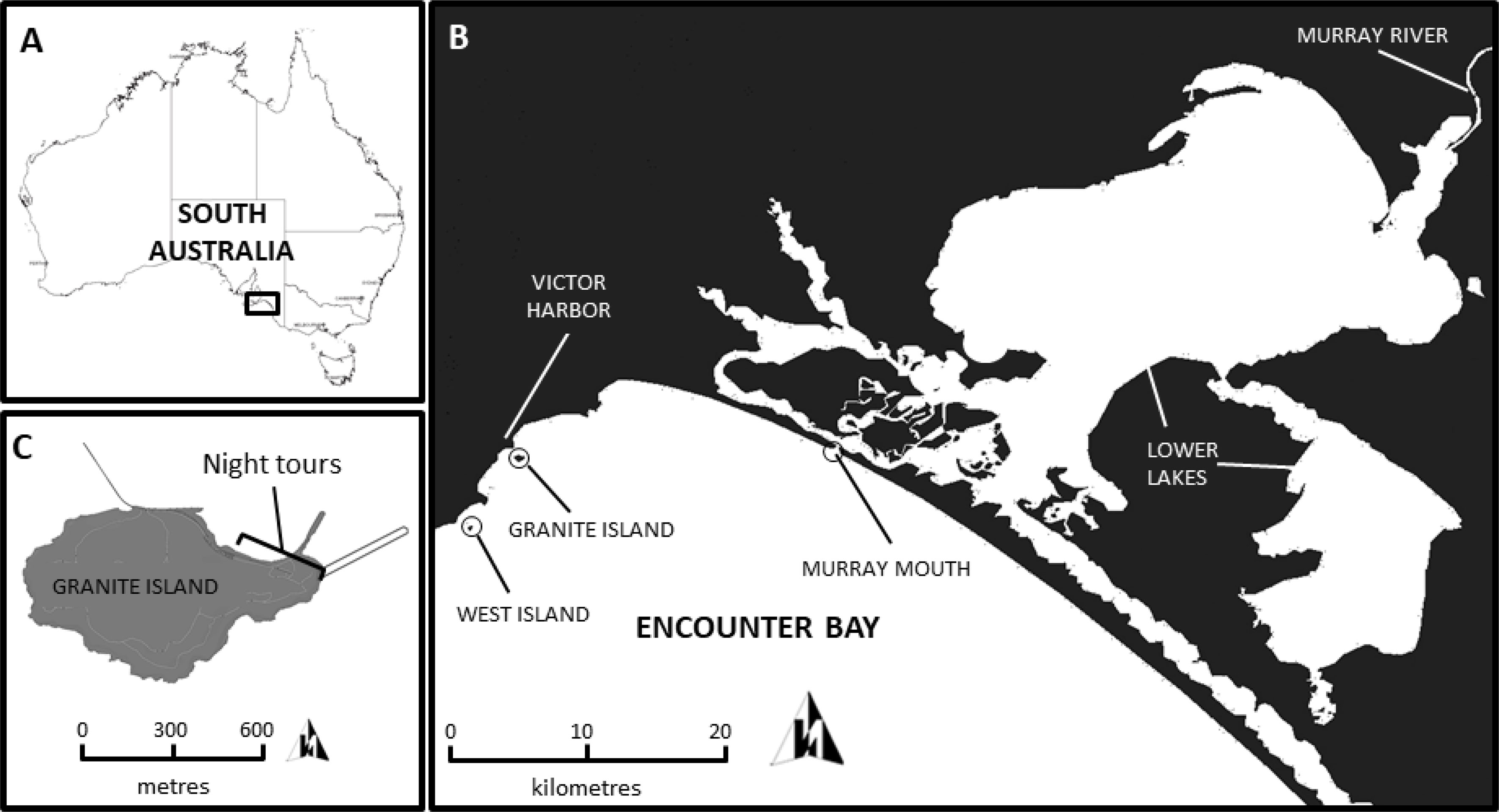
Figure 1 Maps of the location of (A) the study area in Australia, (B) Granite Island in relation to the Murray River and West Island and (C) the night tours on Granite Island.
Little penguins (Eudyptula minor) are colonial seabirds that become central-place foragers during breeding, with most of their prey being captured within less than 60 km of their colony when provisioning chicks (Collins et al., 1999; Bool et al., 2007; Hoskins et al., 2008). As a consequence, their breeding success is strongly correlated to food availability (Chiaradia and Nisbet, 2006) and large colonies are expected to be located near areas of high productivity, such as freshwater inputs or upwelling (see also Fortescue, 1999; Poupart et al., 2017). Tracking studies at several colonies in Victoria have shown that many little penguins have a tendency to forage near estuarine environments or freshwater outflows (Collins et al., 1999; Hoskins et al., 2008; Preston et al., 2008; Kowalczyk et al., 2015). Kowalczyk et al. (2015) in particular found that little penguins within Port Phillip Bay (Victoria) foraged closer to the Yarra River mouth during drought years, but increased their foraging range in years of high rainfall – when outflows from the Yarra River increased – likely to follow the dispersed nutrients (as nutrients play a crucial role in regulating primary production and hence the food web; Mortimer et al., 1999; Cruzado et al., 2002; Sommer et al., 2002) and thus maximize resource acquisition.
Little penguins are generalist feeders that rely mainly on clupeidae fish species, such as anchovy (Engraulis spp.) and pilchards (Sardinops sagax) (Klomp and Wooller, 1988; Cullen et al., 1992; Chiaradia et al., 2003; Chiaradia et al., 2012). In South Australia, anchovies are the most frequently consumed prey species and account for 31-67% of the little penguin estimated prey biomass (Bool et al., 2007; Wiebkin, 2012). Both anchovies (Wedderburn and Suitor, 2012) and little penguins (Colombelli-Négrel, unpublished data) have been recorded in the Coorong and Lower Lakes system of the Murray River, and the Murray Mouth is well within the foraging range of the little penguins breeding in Encounter Bay (20-60 km; average 41 ± 4 km; Figure 1; Bool et al., 2007). Therefore, it is likely that some dependence between the Encounter Bay penguins and productivity of the Murray River estuary exists. This hypothesis is further supported by the fact that penguin numbers in Encounter Bay have experienced major declines in the early 2000s (Colombelli-Négrel and Kleindorfer, 2014; Colombelli-Négrel, 2015a), which coincides with the Millennium Drought (2001-2010). While many factors have been suggested to explain these declines in Encounter Bay – such as predation on land or at sea, low reproductive success or juvenile survival, as well as parasite abundance (Bool et al., 2007; Wiebkin, 2011; Colombelli-Négrel, 2015b; Colombelli-Négrel, 2015a; Colombelli-Négrel, 2016) – only one study to date investigated the role of these suggested factors. Specifically, Colombelli-Négrel (2015a) reported that juvenile survival, but not reproductive success, was the most critical variable affecting little penguin population trends on Granite Island. Yet the relationship between penguin numbers in Encounter Bay and Murray River outflows has never been explored, which is the aim of this study. Based on the findings from Auricht et al. (2018), we hypothesised a significant and positive relationship between little penguin numbers and river outflow, via primary productivity and thus food availability (see conceptual model in Figure 2).
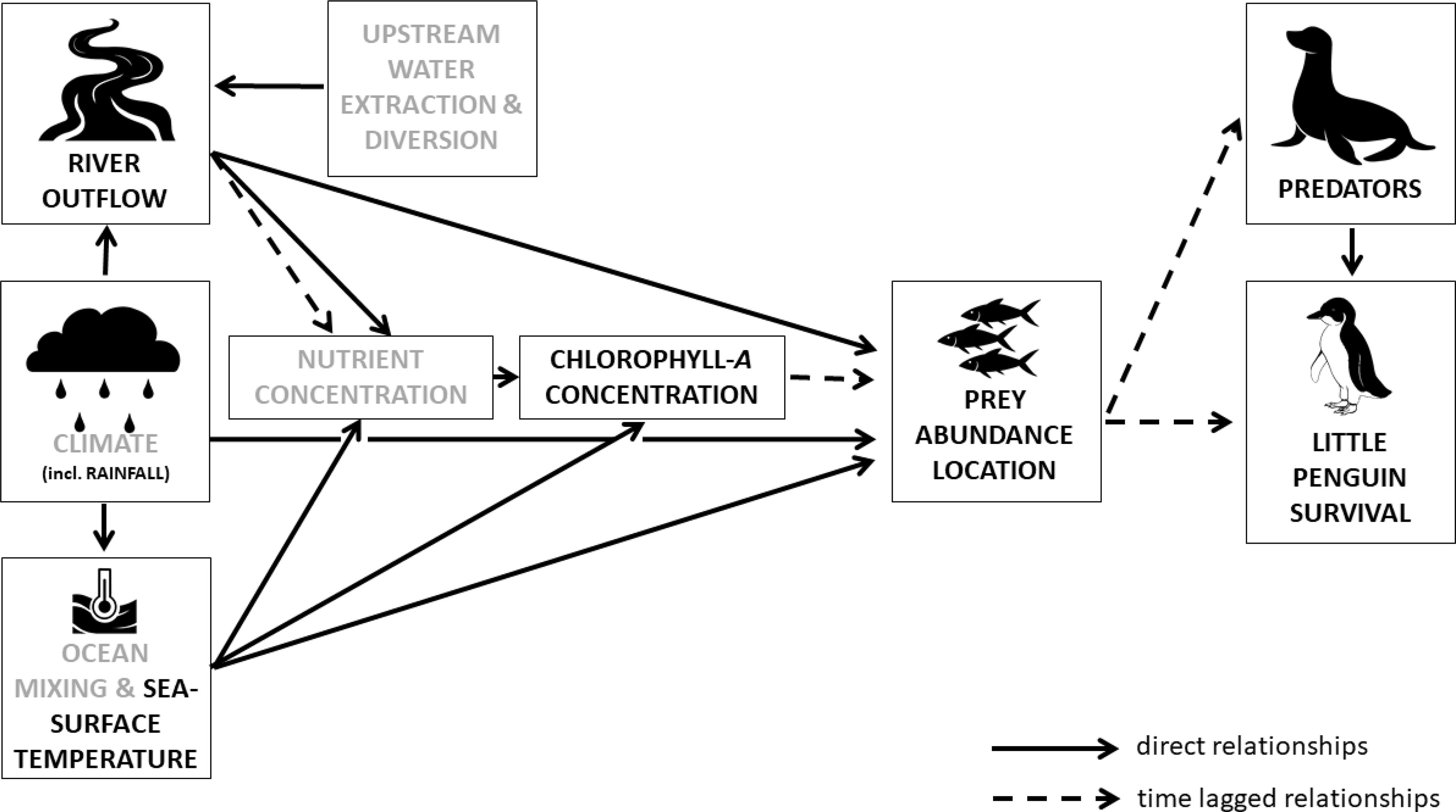
Figure 2 Conceptual model detailing the relationships between the environmental variables included our study (rainfall, sea surface temperatures, and chlorophyll-a concentrations), food availability (prey abundance and location), little penguin survival, and their predators (long-nosed fur seals). Straight arrows represent direct relationships, while dashed arrows represent time lagged relationships. We also present in grey other variables (upstream water extraction and diversion, other climate variables, ocean mixing processes and nutrient concentration) that were not included in our models but affect food availability and environmental variables.
Material and Methods
Study Area
Penguin numbers (yearly and daily numbers; see below: penguin numbers) were collected on Granite Island (Encounter Bay) and environmental data (rainfall, sea surface temperatures, chlorophyll-a concentrations, food availability, and predator abundance; see below: predictor variables) were collected around the coastal ocean zone between Granite Island and the Murray River (Figure 1; see also Figure 2). Granite Island (35°37’S, 138°36’E) is a small island (approximately 500m wide and 900m long) off Victor Harbor, connected to the mainland by a bridge causeway. Little penguin numbers on Granite Island, the largest population in Encounter Bay, have fallen from 1548 individuals in 2001 to 146 in 2010 (Wiebkin, 2011; Colombelli-Négrel, 2017). Since 2012, after the end of the Millennium Drought when a period of higher river flows occurred, the population stabilised to approximately 20-30 individuals (Colombelli-Négrel, 2020). All the other colonies within Encounter Bay (Wright Island, West Island, Seal Island and Pullen Island) are now considered extinct (Colombelli-Négrel and Kleindorfer, 2014). As its name suggests, Granite Island has a granite rock coastline. Little penguins come ashore at dusk to breed and moult in naturally excavated burrows and artificial nests (i.e., concrete tunnels and arrangement of rocks) located all around the island. The Murray Mouth is located approx. 20 km by sea from Granite Island (Figure 1).
Penguin Numbers
We used two datasets comprising daily and annual penguin numbers: the daily data span was January 2003 to December 2018; the annual data span was January 1981 to December 2018 (specific periods for which data were obtained are specified in brackets throughout the methods). Penguin numbers were obtained via (1) nightly counts (daily data 2003-2018) and (2) annual population census (annual data 1981-2018). The nightly penguin counts report on the total number of adult little penguins counted by the Penguin Tour Guides on the North Shore of Granite Island during the Little Penguin Tours (see Figure 1). Tours were conducted for visitors to spot little penguins as they return from the ocean at sunset and ran all year. Additional nightly counts were conducted by the Flinders University research team in weeks when no penguin tours were scheduled. These additional counts by Flinders University were conducted under the guidance of one of the Penguin Tour Guides and followed the same guidelines as the Penguin Tours; hence the data collected by the two teams were comparable. Counts were conducted within two hours after dark and lasted approximately 1 to 1.5 hours.
Population census data prior to 2001 were collected by R. Brandle over five sections of the island and interpolated for the entire colony by D. Colombelli-Négrel. Maps of these surveys confirmed that the area monitored before 2001 corresponded to half of the area surveyed since 2001. Surveys before 2001 established that the density of little penguin across the island did not vary until 2006. Therefore, the numbers obtained by R. Brandle were doubled for the interpolation. From 2001 onwards, annual population censuses were carried out each year (except in 2004) by a large team of local volunteers and a penguin ecologist. Each year, the island was divided into separate smaller sections and each section was thoroughly searched for presence or absence of penguin nests. Once a nest was found, its status was noted as active or inactive. A nest was recorded as active if it contained eggs, chicks, or adults, or had clear evidence of penguin presence such as fresh droppings, a strong penguin smell or recent nest excavation. A nest was recorded as inactive if none of the above criteria were found or if it had cobwebs at the entrance indicating that no adult penguin was regularly entering or exiting the nest. Nests were also marked with talcum powder to avoid double counting by different teams of volunteers. Censuses were conducted during the peak of the breeding season to ensure comparability of the data between years, and total population numbers were estimated as two adult penguins per active nest found. Little penguins are asynchronous breeders that can breed any time between April/May and January/February of the following year in South Australia (Wiebkin, 2012; Colombelli-Négrel, 2015a; Johnson and Colombelli-Négrel, 2020). As a result, the beginning and duration of each breeding season (and hence the peak of the breeding season) is highly variable among years (Allen et al., 2011; Wiebkin, 2012; Colombelli-Négrel, 2018; Johnson and Colombelli-Négrel, 2020). During our study, the peak of breeding on Granite Island shifted from winter/spring (~July-September) to spring/summer (~October-December) following 2012 (Colombelli-Négrel, 2018).
The reported decreases in penguin numbers were not due to migration to other colonies based on mark-recapture data collected on Granite and West Islands between 1998 and 2003, which showed that only 0.9% of the 1255 marked individuals moved between the two islands, with no individual breeding on another island than their island of origin (see (Colombelli-Négrel, 2015a). Additional mark-recapture data in the area showed that little penguin adult survival rate in South Australia was less than 10% during this period (see also (Colombelli-Négrel, 2015a; Colombelli-Négrel et al., 2020). In addition, all adjacent colonies became extinct during the monitoring period (Wiebkin, 2011; Colombelli-Négrel and Kleindorfer, 2014).
Predictor Variables
The dynamics of marine productivity are intricate as many variables can interact (Behrenfeld et al., 2006). Therefore, to understand the relationship between penguin numbers and the Murray River outflow, we included other environmental variables in addition to river flow in our analyses to determine which variable(s) had the most influence on penguin numbers (see conceptual model in Figure 2). We specifically focused on rainfall, sea surface temperatures, and chlorophyll-a concentrations as these have been shown to influence the dispersion and abundance of fish species (Congdon et al., 2007; Nicolas et al., 2014; Syed and Ahmed, 2015; Auricht et al., 2018), and as a result, seabird breeding success and survival (e.g., Pinaud and Weimerskirch, 2002; Monticelli et al., 2007; Le Bohec et al., 2008; Cullen et al., 2009; Sidhu et al., 2012; Mills et al., 2020). In addition, chlorophyll-a concentrations are recognised indices of phytoplankton abundance and biomass (primary productivity) and often serve as indicators for prey availability (Monticelli et al., 2007; Lo-Yat et al., 2011). Similarly, sea surface temperatures are good indices of climatic variability as seabirds are known to be sensitive to extreme temperatures because of thermal stress (e.g., Hochscheid et al., 2002; Oswald and Arnold, 2012; Meyer, 2014) and often correlate with chlorophyll-a concentrations (Radiarta and Saitoh, 2008; Kavak and Karadogan, 2012). Rainfall, sea surface temperatures, and chlorophyll-a concentrations have all been found to correlate with survival in little penguins in other studies (Sidhu et al., 2012; Agnew et al., 2015; Ganendran et al., 2016). We present our conceptual model of how all the variables interact in Figure 2. While chlorophyll-a concentrations have a near immediate response to nutrients from river outflow (Black et al., 2016), there may be a time lag of up to 12 months between primary productivity and actual prey availability for marine top predators, such as seabirds (Price et al., 2020). Therefore, we include in Figure 2, and in our analyses, both the direct and time lagged relationships.
Daily outflow estimations (in mega-litres, ML; 1981-2018) from the Murray Mouth (based on the sum of estimated flows from all the barrages) were obtained from the Department of Environment, Water and Natural Resources (South Australia) (see also Auricht et al., 2018). Daily rainfall data (mm; 1981-2018) were obtained from the Australian Bureau of Meteorology database on the following website http://www.bom.gov.au/climate/data/using Victor Harbor meteorological station (distance: 2.8 km from Granite Island). Sea surface temperatures (°C; 1995-2018) and chlorophyll-a concentrations (mg/m³; 2002-2018) were only available monthly. Data on sea surface temperatures within a 20 km radius of ocean surrounding Granite Island were sourced from the Integrated Marine Observing System (IMOS; see http://imos.org.au/home.html). Chlorophyll-a concentration estimations used in this study were derived as monthly composite satellite imagery from NASA’s Moderate Resolution Imaging Spectroradiometer (MODIS), a sensor on board the polar-orbiting satellite ‘Aqua’. MODIS–Aqua Level-3 chlorophyll-a products (at approximately 4 km spatial resolution) are free to access on the NASA Ocean Colour website (https://oceancolor.gsfc.nasa.gov). Although daily chlorophyll-a products are available from this source, cloud cover can result in large numbers of missing pixels. Instead, monthly composite imagery was used, where each image is an average of all clear observations over each calendar month. The average chlorophyll-a concertation was calculated in a region stretching 20 km south-west from the Murray Mouth (see Auricht et al., 2018 for additional details on the methodology), which captures the ocean region surrounding Granite Island.
For the annual data analysis, the environmental data were averaged for the three months prior to the moulting period as this is a critical period for little penguins’ survival and includes a significant part of the breeding period (Ganendran et al., 2016). The beginning and duration of each moulting period on Granite Island varied among years but generally started in December-January and finished in March-April (Colombelli-Négrel, 2018). For our analyses, we adjusted this three months period based on the respective timing of the moult for each of our study years. We also included (1) the annual number of long-nosed fur seals (Arctocephalus forsteri) as an indicator of predation risk (Wiebkin, 2011) and (2) the annual amount of southern garfish (Hyporhamphus melanochir) commercially caught in the southern Gulf St Vincent as an indicator of food availability. Garfishes have been recorded in the diet of little penguins in other studies (Klomp and Wooller, 1988; Montague and Cullen, 1988; Cullen et al., 1992) and are the second most frequent prey item found in the diet of little penguins in South Australia (20%; Bool et al., 2007). Local and relevant data on their most frequent prey item (sardines) were not available. Long-nosed fur seal and southern garfish data were only available as annual datasets. Data on the annual southern garfish commercial catch (tonnes; 2007-2017) were obtained from the Fisheries Research and Development Corporation (https://fish.gov.au/report/191-Southern-Garfish-2018). Annual long-nosed fur seal population influence was also considered (recorded on non-consecutive years between 1991 and 2018), using data representing the highest number of seals observed during the penguin breeding season on West Island, located 5 km from Granite Island (see Figure 1). These observations were recorded by a local community member during repeated boat surveys, and by the local rangers as part of regular surveys conducted for the Department for Environment and Water.
Statistical Analyses
All analyses were conducted in R 4.1.1 (R Core Team, 2019) using the packages Astsa, dplyr, forecast, fpp2, fpp3, ggplot2, GGally, imputeTS, MASS, timeDate and trend. We used standard time series regression (Shumway and Stoffer, 2017) and multiple hypothesis testing (i.e., hypotheses being tested simultaneously) to understand the associations between the time series of the predictors (rainfall, sea surface temperatures, chlorophyll-a concentrations, food availability, and predator abundance) and the decline of the little penguins (our outcome of interest), using daily (converted to monthly; see below) and annual penguin numbers. The main aim of a regression analysis is to investigate whether a change in an outcome variable can be explained by a set of selected predictors, while controlling for multiple potential confounding factors (Bhaskaran et al., 2013). Time series regression is being applied to estimate the effects of the predictors over time based on their effects on the previous period of time. Poisson regression could not be used as the variables were correlated, especially the predictor variables. Standard assumptions for multiple linear regression apply for time series regression (Hyndman and Athanasopoulos, 2018): the relationships between the variables should be linear, the error terms should be independent and identically normally distributed with a zero mean and a constant variance. To find the best model, we followed three steps: model specification (also referred to as model identification; during this step, we established the trends of the data, any correlation and seasonality, and selected a set of candidate models through variable or model selection process), model fitting (also referred to as model estimation; during this step, we estimated the parameters of the models and tested for the significance of the parameter estimates), and model diagnostics (during this step, we checked the assumptions of normality (QQ plot), constant variance (residual plots) and independence (ACF of residuals; the Auto-Correlation Function, which measures whether earlier data in the series have some relation to later data). Furthermore, we estimated the goodness-of-fit by comparing adjusted R2 and the Akaike Information Criterion (AIC). The best model is the one with the lowest AIC and the highest R2).
Missing data in time series regressions introduce bias. As the data collection was irregular in our study (see Methods and Figure 3), instead of removing incomplete records, we created a modified dataset for both the annual and daily penguin data using interpolation method following Moritz et al. (2015) as the time series plots of the variables showed either trend or seasonality. For the daily data, missing datapoints were estimated using an interpolation method, except for 2004 and 2011, for which too many penguin datapoints were missing to apply this method. Missing datapoints for 2004 and 2011 were therefore estimated using data from 2005 and 2012 respectively, as patterns observed in 2004 and 2011 were likely to be similar to those observed in 2005 and 2012. For the annual data, missing datapoints for the penguin (1991-1999, 2004) or predictor data (long-nosed fur seals: prior to 1991, 1995-1998, 2001-2003, 2005, 2007; garfishes: prior to 2007, sea surface temperatures: prior to 1995; and chlorophyll-a concentrations: prior to 2002) were estimated using either interpolation or the moving average method. To test for the robustness of our interpolations, we used the forecast and imputeTS R packages (Hyndman and Khandakar, 2008; Moritz et al., 2015; Moritz and Bartz-Beielstein, 2017). The ImputeTS package is designed specifically to address imputation for univariate time series. We use the interpolation method following Moritz et al. (2015) as the time series plots of the variables showed either trend or seasonality. We first analysed the distribution of the missing vales using the plots for each variable (penguin data, sea surface temperatures, rainfall, chlorophyll-a concentrations, and river outflow) using the function plotNA.distribution within the ImputeTS package. We then did the imputation for univariate time series, plotted the results, and compared the functions na.interpolation and na.kalman within ImputeTS R package. We decided to use the results from na.interpolation as they were similar to na.kalman but simpler and faster computationally. We then aggregated the daily data (penguin count, river outflow and rainfall) to monthly data using the average function. Determining trends prior to building time series models is a critical first step for time series analyses. Therefore, we confirmed declining trends in penguin numbers in the time series with non-parametric Mann-Kendall trend tests (Mann, 1945; Kendall, 1975), which are used to check whether data collected over time have constant increasing or decreasing trends (i.e., Mann-Kendall trend tests check for difference in trends between earlier and later data). Statistics obtained from the Mann-Kendall trend tests are determined by the sequences and the ranks of the time series rather than the original values. Both datasets (monthly and annual) were then explored using exploratory data analysis and correlation analysis; variables, such as penguin counts and river outflow, exhibiting nonconstant variance were log-transformed (i.e., to stabilize the variance). After model fitting, we performed model diagnostics on the residuals by checking the ACF of the residuals to ensure independence of the data, along with normality and constant variance (Table 1). Seasonality existed for the monthly rainfall and chlorophyll-a concentrations data; and such seasonality has been considered in the modelling. Variable selection method, the forward selection based on Akaike Information Criterion, was implemented to choose the subset of the predictors that was “best” in a given sense (Sheather, 2009). While we acknowledge that variable selection may affect the properties of the estimators, as well as the standard inferential procedures such as tests and confidence intervals (i.e., the regression coefficients obtained after variable selection are often biased and the P-values obtained from F- and t -statistics are generally much smaller than their true values; Sheather, 2009), no model selection is immune to these difficulties (Sheather, 2009). In this study, we used three criteria to evaluate the subsets of predictor variables: the Akaike’s Information Criterion, the Deviance and the Residual Deviance (Burnham and Anderson, 2002). The corrected AIC, AICc (a bias corrected version of the AIC) is used for the annual data as the sample size was small. As stated above, we conducted two analyses for the annual penguin numbers: one including the direct relationships between the variables and one including the time lagged relationships described in Figure 2.
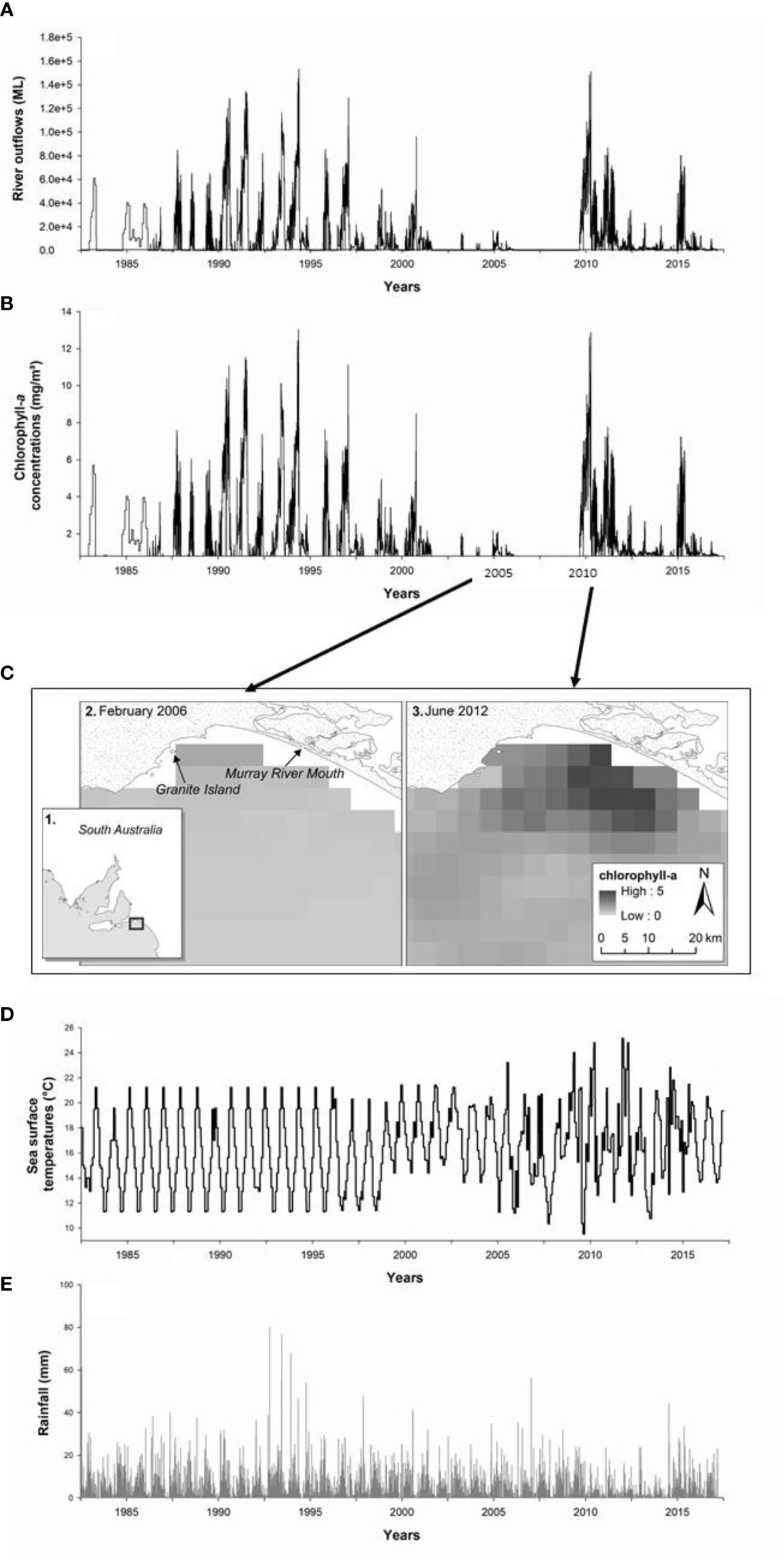
Figure 3 (A) Daily river outflows (ML) from the Murray Mouth to the coastal ocean. (B) Monthly chlorophyll-a concentrations (mg/m³) within a 20 km radius of the Murray Mouth (C) Frame (1) shows the location extent of a comparison of MODIS-Aqua (monthly composite) satellite imagery products showing chlorophyll-a concentrations in during (2) a low flow period of February 2006 and (3) a high flow, post drought period in June 2012. (D) Monthly sea surface temperatures (°C) of the ocean surrounding Granite Island within a 20 km radius. (E) Daily rainfall (mm) for Granite Island. Data for (A, B, D, E) are presented from January 1981 to December 2018.
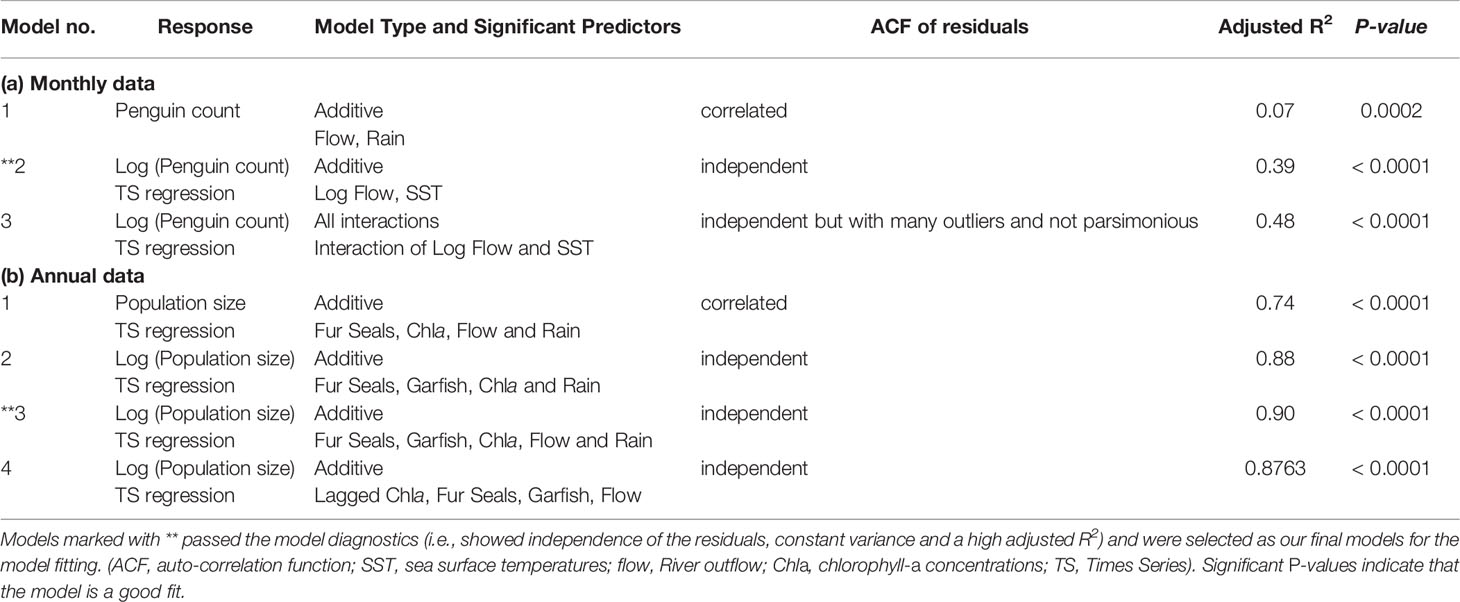
Table 1 Best fitted models examining the variation in (a) monthly and (b) annual little penguin numbers in relation to river outflow, sea surface temperatures, rainfall, chlorophyll-a concentrations (monthly and annual numbers), as well as long-nosed fur seal numbers and southern garfish numbers (annual numbers only).
Results
There was a clear reduction in daily river outflows from the Murray Mouth to the coastal ocean during the Millennium Drought, with minor discharges occurring between 2003 and 2006 and zero discharge between 2007 and 2010 (Figure 3A). High river outflows started to occur again following 2011-2012 (Figure 3A), which coincided with a stabilisation in the decline of little penguin numbers on Granite Island (Figure 4). Chlorophyll-a concentrations in the ocean around the Murray Mouth and Granite Island were elevated in years with high river outflows and low in years when outflows were reduced (Figures 3B, C). A comparison of the chlorophyll-a concentrations in a year of extreme-drought period with no river outflow (low chlorophyll-a concentrations; 2006) vs a period of high river outflow (high chlorophyll-a concentrations; 2012) is presented in Figure 3C. Monthly sea surface temperature and daily rainfall temporal patterns are presented in Figures 3D, E respectively. There was a strong and distinct declining trend for both the annual and monthly little penguin numbers over time (monthly data z = -10.56, N = 192, P < 0.0001; annual data: z = -5.70, N = 38, P < 0.0001; Figures 4A, B). For the monthly penguin numbers, while the data showed a small and significant increasing trend after 2010 (z = 7.65, N = 95, P < 0.0001; Figure 4A), the overall declining trend (comparing before and after 2010) was stronger than the small increasing trend after 2010 (z = -8.56, N = 97, P < 0.0001; Figure 4A).

Figure 4 (A) Monthly data: Average number per year of little penguins observed during the North Shore night tours between January 2003 and December 2018. (B) Annual data: Estimated population size of little penguins on Granite Island between 1981 and 2018. Missing data represent years when no census or count occurred.
The best model explaining the monthly penguin numbers included river outflow and sea surface temperatures (Table 2A). The model explained 39% of the variation in the data. Monthly penguin numbers were predicted to decrease by a multiplied factor of 0.94 for every 1 unit (°C) increase of sea surface temperatures (β = 0.94, 95% CI: 0.90; 0.97; Figure 5A) and by a multiplied factor of 0.86 for every 1 unit (ML) increase of river outflow (β = 0.86, 95% CI: 0.84, 0.89; Figure 5B). Analysing the direct relationships between the variables (see Figure 2), the best model explaining the decline of the annual penguin numbers included all predictor variables (river outflow, chlorophyll-a concentrations, rainfall, numbers of long-nosed fur seals and southern garfish commercial catch; Table 2B). The model explained 74% of the variation in the data and the strongest predictors were the numbers of southern garfish and chlorophyll-a concentrations. Annual penguin numbers were predicted to increase in the model by a factor of 1.4 [= exp (0.33)] with increasing of 1 unit (tones) of southern garfish numbers (β = 0.33, 95% CI: 0.17, 0.50; Figure 6A) but predicted to decrease by a factor of 0.37 [= exp (-1.01)] with increasing of 1 unit (mg/m³) of chlorophyll-a concentrations (β = -1.01, 95% CI: -1.65, -3.68; Figure 6B). Annual penguin numbers were also predicted to increase with increasing river outflow (β < 0.001, 95% CI: 7.44e-07, 1.68e-05) and increasing rainfall (β = 0.01, 95% CI: 0.002, 0.03), but to decrease with increasing long-nosed fur seal numbers (β = -0.02, 95% CI: -0.003, -0.01). Adding the time lagged relationships described in Figure 2 did not change our results as the best model explaining the data remained model 3 (Table 1). While the assumption of independence was satisfied in model 4 (Table 1; with the time lagged relationships), the assumptions of constant variance and normality were not satisfied. In addition, the adjusted R2 for model 4 was lower than for model 3 and the AIC and residual deviance of model 4 were higher than model 3 (Table 2).
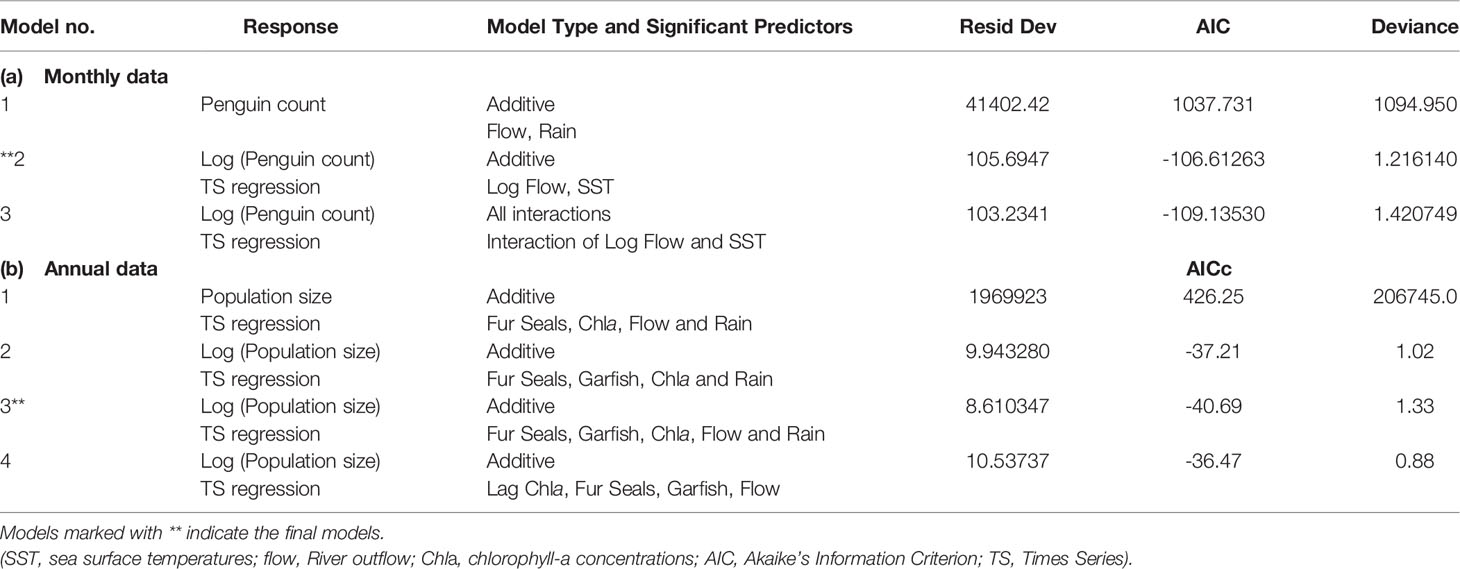
Table 2 Best models explaining little penguin numbers on Granite Island. Data are presented for (a) the monthly dataset and (b) the annual dataset.
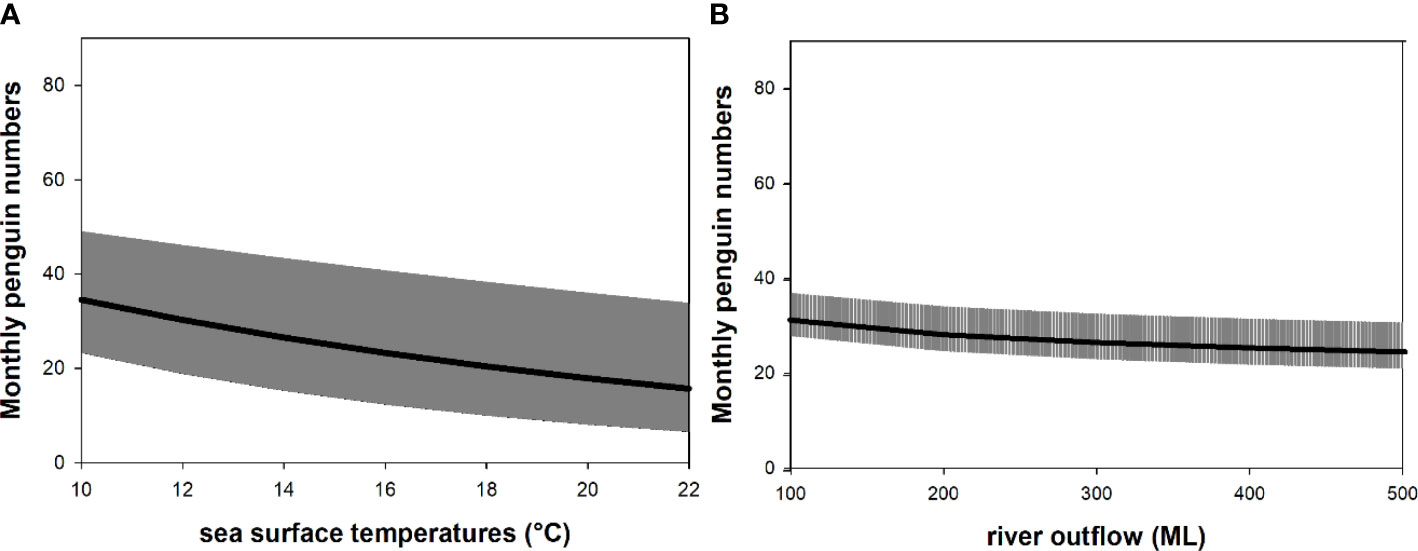
Figure 5 Relationship between the monthly average little penguin numbers on Granite Island (South Australia) and (A) sea surface temperatures (°C) and (B) river outflow (ML). Each plot represents the effect of a single predictor on the monthly number of little penguins assuming all other predictors remain constant. The slope of the relationship is represented by the black line; shaded areas indicate 95% confidence intervals.
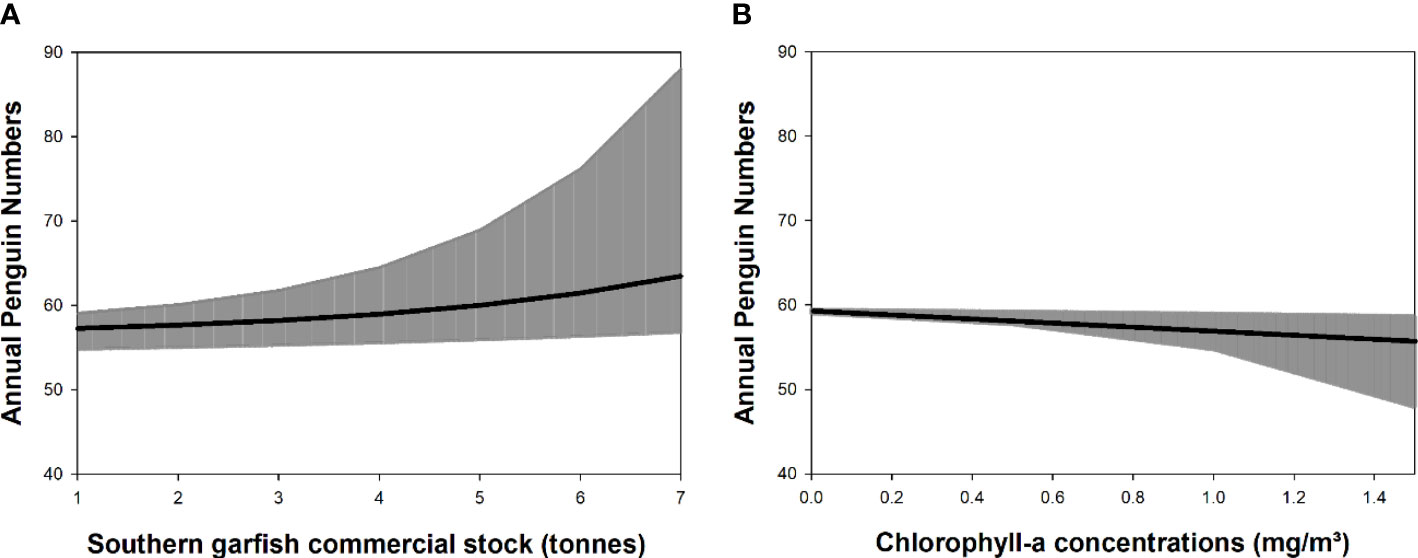
Figure 6 Relationship between the annual little penguin numbers on Granite Island (South Australia) and (A) southern garfish commercial catch numbers (tonnes) or (B) chlorophyll-a concentrations (mg/m³). Each plot represents the effect of a single predictor on the annual number of little penguins assuming all other predictors remain constant. The slope of the relationship is represented by the black line; shaded areas indicate 95% confidence intervals.
Discussion
Seabirds around the world can serve as sentinels of environmental changes and help us understand how they are affecting wildlife (e.g., Wanless et al., 2007; Divoky et al., 2015; Thomsen and Green, 2019). In this study, we showed that (1) monthly little penguin numbers, an iconic and locally breeding Australian seabird, were negatively associated with sea surface temperatures and river outflow, and that (2) annual penguin numbers were positively associated with southern garfish numbers (our local indicator of food availability) but negatively associated with chlorophyll-a concentrations. The results of this study suggest that decisions regarding river water management should consider, not only human and terrestrial environmental requirements, but also the long-term impacts that this may have for the coastal environment outside the river system (see also Auricht et al., 2018; Hallett et al., 2018; Thom et al., 2020).
Chlorophyll-a concentrations are considered a reliable measure of primary productivity in an area (and hence food availability) because they are a proxy for phytoplankton production, which forms the base of the food chain as prey for zooplankton, fish larvae and other heterotrophic organisms (Monticelli et al., 2007; Lo-Yat et al., 2011; Lander et al., 2013). A previous study showed that decreasing Murray River outflow during the Millennium Drought had reduced primary productivity in adjacent coastal waters, and postulated there was likely consequences for higher trophic levels (Auricht et al., 2018). Specifically, the authors showed that primary productivity collapsed in the near-shore region during the Millennium Drought, and that the primary productivity plume was up to 60 km from the coast in years of high river outflows (Auricht et al., 2018), which would encompass Granite Island (Figure 1 and 3B). In our study, we found a negative relationship between chlorophyll-a concentrations and annual penguin numbers, which contrasts with a previous study on little penguins showing that annual survival increased with increasing chlorophyll-a concentrations (Agnew et al., 2015). As previously stated, chlorophyll-a concentrations have a near immediate response to nutrients from river outflow (Black et al., 2016), while there may be a time lag of up to 12 months between primary productivity and actual prey availability for marine top predators, such as seabirds (Price et al., 2020). Yet additional analyses including time lagged relationships (see Results as well as Tables 1, 2) did not better explain the variation in penguin numbers. Interestingly, there was also a negative relationship between annual southern garfish numbers and chlorophyll-a concentrations. It is possible that other environmental variables, not accounted for in our study, may have impacted food availability (and hence this negative relationship) as small pelagic fishes (such as those preyed upon by little penguins) are often highly dependent on plankton-based food webs, which are very sensitive to short-term environmental changes in salinity (see Kelly et al., 2016). Furthermore, increased chlorophyll-a concentrations would increase the production of fish larvae, thereby also increasing the presence of predators and fishing intensity (see below) in the area, which in turn could have long-term negative impacts on little penguins. Finally, this negative relationship may be due to the low capacity of recovery of the Granite Island little penguin population as annual penguin numbers remained low and continued to decline after 2010 when chlorophyll-a concentrations started to increase. Indeed, previous study showed that the decline of the little penguins on Granite Island was linked to low juvenile survival (less than 2%; Colombelli-Négrel, 2015a). Delayed sexual maturity (in little penguins, individuals do not breed until they are two or three years old; Priddel et al., 2008) and a decrease in juvenile survival can add significant lagged effects on population size and recovery, especially for small population where birth and death rates can be extremely random (e.g., Legrendre et al., 1999). This suggests that the observed low juvenile survival may have had a stronger negative effect on little penguin numbers than any of the potential benefits gained from the increased river outflow (and consequent chlorophyll-a concentrations) once the population reached critical levels. Further investigation into the food web and association between South Australian little penguin numbers and chlorophyll-a concentrations are needed to fully understand their causal and temporal relationships.
Monthly little penguin numbers were negatively associated with sea surface temperatures and river outflow. This aligns with studies in South Australia (Johnson and Colombelli-Négrel, 2020) and Western Australia (Cannell et al., 2012) showing that little penguins had a lower breeding success when sea surface temperatures were higher in the months before breeding. However, studies in Victoria showed that foraging effort and first year survival in little penguins were positively associated with sea surface temperatures (Sidhu et al., 2012; Berlincourt and Arnould, 2015) and little penguins had a greater breeding success when sea surface temperatures were higher in the autumn preceding spring breeding (Cullen et al., 2009). The negative association with river outflow also contrasts with our annual results, as annual little penguin numbers were positively associated with river outflow. Monthly penguin numbers would have been affected by the overall annual decline of the population but also the probability that individuals be present on land at certain time of the year. Hence the contrasting results may be explained if greater numbers of penguins were fishing at sea for longer periods during times of high river outflow. Results similar to this were found by Kowalczyk et al. (2015) in Victoria where penguins increased their foraging range when the outflow from the Yarra River increased.
Seals are recognised predators of penguins worldwide (e.g., Hofmeyr and Bester, 1971; Du Toit et al., 2004; Johnson et al., 2006; Lee et al., 2019; Bester et al., 2020). In this study, we found that annual little penguin numbers decreased when long-nosed fur seal numbers increased, supporting the idea that seal predation may have influenced the decline of little penguins in South Australia (Bool et al., 2007; Wiebkin, 2011). Yet the relationship between penguin and long nosed fur seal numbers was close to 1:1, indicating that a decrease in penguin numbers due to seal predation would be very slow. While the available data may have underestimated the number of seals in the study area, previous studies have shown that the relative importance of little penguins in long-nosed fur seal diets varies significantly across regions (Bool et al., 2007; Baylis and Nichols, 2009; Reinhold, 2014), suggesting that little penguin availability is not the main factor driving predation. It is therefore possible that, in years of low river outflow when primary production was low and food resources may have been scarce, long-nosed fur seals switched to preying on little penguins (as seen in Antarctic fur seals (Arctocephalus gazella) and chinstrap penguins (Pygoscelis antarctica) when krill abundance was low; Daneri et al., 2008). An increase in long-nosed fur seal numbers may also have resulted in reduced food availability for little penguins as seals can compete with penguins for food resources (Croll and Tershy, 1998; Lowther et al., 2020) and long-nosed fur seals also consume southern garfishes in South Australia (Reinhold, 2014). These hypotheses should be further tested with additional tracking and diet studies.
Our model predicted an increase in annual penguin numbers with increasing southern garfish numbers, indicating that a decrease in food availability may have been responsible for the annual decline of the little penguin populations in the area (on Granite Island but also on Wright, West, Seal and Pullen islands; see Colombelli-Négrel and Kleindorfer, 2014). A previous study showed that juvenile survival was the most important driver of little penguin population trends on Granite Island (Colombelli-Négrel, 2015a). While no information is available for South Australian little penguins, studies in Victoria have suggested that mortality of juveniles in their first years occurred mostly at sea (Reilly and Cullen, 1982; Dann, 1991), likely due to a combined effect of parasites and starvation (Harrigan, 1992). These studies and our results suggest that low juvenile survival on Granite Island (less than 2%; Colombelli-Négrel, 2015a) may be driven by low food availability (see also Reilly and Cullen, 1982; Dann, 1988), which in turn would drive the observed populations declines. A study conducted in 2006 on Granite and West islands showed that garfishes were the second most frequent prey item consumed by little penguins (Bool et al., 2007). Yet, the foraging behaviour and diet of South Australian little penguins is little studied with only two studies to date (Bool et al., 2007; Wiebkin, 2012), and additional dietary studies would be beneficial, especially considering that southern garfishes are a commercially fished species in South Australia. Further research is therefore warranted to determine if commercial catch of southern garfishes may have negatively impacted little penguins.
In conclusion, we found a strong association between little penguin numbers and southern garfish numbers (our local indicator of food availability), which may explain the local decline observed in penguin numbers in Encounter Bay during the Millennium Drought. The fact that the Granite Island little penguin population still has not recovered in 2020 (Colombelli-Négrel, 2020), despite larger outflows in 2012-2013 and at the end of 2016, suggests that the population may have reached some critical reduction in the number of breeding birds during the drought period. While this study focused on a single colony, further decrease in little penguin numbers (as recorded in South Australia; Colombelli-Négrel and Kleindorfer, 2014; Johnson and Colombelli-Négrel, 2020, this study) may be observed in the future because of climate change and/or reduced rainfall/river outflow, considering their high dependence with estuarine environments and freshwater outflows (Collins et al., 1999; Hoskins et al., 2008; Preston et al., 2008; Kowalczyk et al., 2015). Much of the research to date on seabirds and climate change have focused on the impacts of increased ocean temperatures (e.g., Sandvik et al., 2005; Chambers et al., 2011; Descamps et al., 2017; Johnson and Colombelli-Négrel, 2020). Similar to other studies on Arctic systems showing the importance of glacial outflows on marine productivity as well as seabird foraging and populations (Grémillet et al., 2015; Urbanski et al., 2017; Bertrand et al., 2021; see also Michel et al., 2015), our findings highlight that other factors need to be taken into consideration, such as the potential importance of river outflow for the health and resilience of the coastal ecosystem, and our results should be considered in future river management strategies. Given the wide distribution of seabirds, their key role in ecosystems, and the fact that droughts are becoming more and more frequent (Dai, 2013), future studies, both within Australia and elsewhere, are needed to identify which species may be affected by hydrological droughts to further enhance both seabird conservation and river management.
Data Availability Statement
The raw data supporting the conclusions of this article will be made available by the authors, without undue reservation.
Ethics Statement
The animal study was reviewed and approved by Flinders University Ethics Committee (E388-E449).
Author Contributions
All authors listed have made a substantial, direct, and intellectual contribution to the work and approved it for publication.
Funding
We would like to thank the Adelaide and Mt Lofty Natural Resources Management Board, the Nature Conservation Society of South Australia, Birds SA, the Field Naturalists Society of South Australia, and the Lirabenda Endowment Research Fund for their financial support. The idea for this paper came from some work funded by the South Australian Department of Environment and Water (DEW) to Peter Dann.
Conflict of Interest
The authors declare that the research was conducted in the absence of any commercial or financial relationships that could be construed as a potential conflict of interest.
Publisher’s Note
All claims expressed in this article are solely those of the authors and do not necessarily represent those of their affiliated organizations, or those of the publisher, the editors and the reviewers. Any product that may be evaluated in this article, or claim that may be made by its manufacturer, is not guaranteed or endorsed by the publisher.
Acknowledgments
Specific thanks to (1) Robert Randle for collecting the data between 1981 and 1990, (2) Natalie Gilbert for collecting the data between 2001 and 2012, (3) Nathalie Bool and Vanessa Owens for collecting the data in 2012 and (4) Ian Milnes and the local rangers for collecting the long-nosed fur seal numbers on West Island. Many thanks to all the volunteers who helped collect the data over the years and to all the people who have contributed to the project. Special thanks to Stephen Hedges and all the night tours guides. Many thanks to Lauren Common for her illustrations for Figure 2. Finally, we thank Tony Flaherty for his continued support.
References
Agnew P., Lalas C., Wright J., Dawson S. (2015). Variation in Breeding Success and Survival of Little Penguins Eudyptula minor in Response to Environmental Variation. Mar. Ecol. Prog. Ser. 541, 219–229. doi: 10.3354/meps11538
Allen W. J., Helps F. W., Molles L. E. (2011). Factors Affecting Breeding Success of the Flea Bay White-Flippered Penguin (Eudyptula minor Albosignata) Colony. N. Z. J. Ecol. 35, 199–208.
Arnell N. W., Gosling S. N. (2013). The Impacts of Climate Change on River Flow Regimes at the Global Scale. J. Hydrobiol. 486, 351–364. doi: 10.1016/j.jhydrol.2013.02.010
Auricht H. C., Clarke K. D., Lewis M. M., Mosley L. M. (2018). Have Droughts and Increased Water Extraction From the Murray River (Australia) Reduced Coastal Ocean Productivity? Mar. Freshw. Res. 69, 343–356. doi: 10.1071/MF17226
Baylis A. M., Nichols P. D. (2009). Milk Fatty Acids Predict the Foraging Locations of the New Zealand Fur Seal: Continental Shelf Versus Oceanic Waters. Mar. Ecol. Prog. Ser. 380, 271–286. doi: 10.3354/meps07919
Behrenfeld M. J., O’malley R. T., Siegel D. A., Mcclain C. R., Sarmiento J. L., Feldman G. C., et al. (2006). Climate-Driven Trends in Contemporary Ocean Productivity. Nature 444, 752–755. doi: 10.1038/nature05317
Berlincourt M., Arnould J. P. (2015). Influence of Environmental Conditions on Foraging Behaviour and its Consequences on Reproductive Performance in Little Penguins. Mar. Biol. 162, 1485–1501. doi: 10.1007/s00227-015-2685-x
Bertrand P., Strøm H., Bêty J., Steen H., Kohler J, Vihtakari M, et al. (2021). Feeding at the Front Line: Interannual Variation in the Use of Glacier Fronts by Foraging Black-Legged Kittiwakes. Mar. Ecol. Prog. Ser. 677, 197–208. doi: 10.3354/meps13869
Bester M., Dilley B., Davies D., Glass T. (2020). Sub-Antarctic Fur Seals Depredate Northern Rockhopper Penguins at Nightingale Island, Tristan Da Cunha. Polar. Biol. 43, 1–3. doi: 10.1007/s00300-020-02688-1
Bhaskaran K., Gasparrini A., Hajat S., Smeeth L., Armstrong B. (2013). Time Series Regression Studies in Environmental Epidemiology. Int. J. Epidemiol. 42, 1187–1195. doi: 10.1093/ije/dyt092
Black K. P., Longmore A. R., Hamer P. A., Lee R., Swearer S. E., Jenkins G. P. (2016). Linking Nutrient Inputs, Phytoplankton Composition, Zooplankton Dynamics and the Recruitment of Pink Snapper, Chrysophrys auratus, in a Temperate Bay. Estuar. Coast. Shelf. Sci. 183, 150–162. doi: 10.1016/j.ecss.2016.10.032
Bool N. M., Page B., Goldsworthy S. D. (2007). “What is Causing the Decline of Little Penguins (Eudyptula minor) on Granite Island, South Australia?,” in Report to PIRSA Fisheries and Aquaculture (Adelaide: South Australian Research and Development Institute (Aquatic Sciences).
Burnham K. P., Anderson D. R. (2002). “A Practical Information-Theoretic Approach,” in Model Selection and Multimodel Inference, 2nd ed, vol. 2. (New York: Springer).
Cannell B. L., Chambers L. E., Wooller R. D., Bradley J. S. (2012). Poorer Breeding by Little Penguins Near Perth, Western Australia is Correlated With Above Average Sea Surface Temperatures and a Stronger Leeuwin Current. Mar. Freshw. Res. 63, 914–925. doi: 10.1071/MF12139
Chambers L. E., Devney C. A., Congdon B. C., Dunlop N., Woehler E. J., Dann P. (2011). Observed and Predicted Effects of Climate on Australian Seabirds. Emu 111, 235–251. doi: 10.1071/MU10033
Chiaradia A., Costalunga A., Kerry K. (2003). The Diet of Little Penguins (Eudyptula minor) at Phillip Island, Victoria, in the Absence of a Major Prey - Pilchard (Sardinops sagax). Emu 103, 43–48. doi: 10.1071/MU02020
Chiaradia A., Forero M. G., Hobson K. A., Cullen J. M. (2010). Changes in Diet and Trophic Position of a Top Predator 10 Years After a Mass Mortality of a Key Prey. ICES. J. Mar. Sci. 67, 1710–1720. doi: 10.1093/icesjms/fsq067
Chiaradia A., Forero M. G., Hobson K. A., Swearer S. E., Hume F., Renwick L., et al. (2012). Diet Segregation Between Two Colonies of Little Penguins Eudyptula minor in Southeast Australia. Austral. Ecol. 37, 610–619. doi: 10.1111/j.1442-9993.2011.02323.x
Chiaradia A., Nisbet I. C. T. (2006). Plasticity in Parental Provisioning and Chick Growth in Little Penguins Eudyptula minor in Years of High and Low Breeding Success. Ardea 94, 257–270.
Collins M., Cullen J. M., Dann P. (1999). Seasonal and Annual Foraging Movements of Little Penguins From Phillip Island, Victoria. Wildl. Res. 26, 705–721. doi: 10.1071/WR98003
Colombelli-Négrel D. (2015a). Low Survival Rather Than Breeding Success Explains Little Penguin Population Decline on Granite Island. Mar. Freshw. Res. 66, 1057–1065. doi: 10.1071/MF14098
Colombelli-Négrel D. (2015b). “Penguin Monitoring and Conservation Activities in the Gulf St Vincent (July 2014 - June 2015),” in Report to the Adelaide and Mt Lofty Natural Resources Management Board (Adelaide: Flinders University).
Colombelli-Négrel D. (2016). “Penguin Monitoring and Conservation Activities in Gulf St Vincent (July 2015 - June 2016),” in Report to the Adelaide and Mt Lofty Natural Resources Management Board (Adelaide: Flinders University).
Colombelli-Négrel D. (2017). “Penguin Monitoring and Conservation Activities in the Gulf St Vincent (July 2016 - June 2017),” in Report to the Adelaide and Mt Lofty Natural Resources Management Board (Adelaide: Flinders University).
Colombelli-Négrel D. (2018). “Penguin Monitoring and Conservation Activities in the Gulf St Vincent (July 2017 - June 2018),” in Report to the Adelaide and Mt Lofty Natural Resources Management Board (Adelaide: Flinders University).
Colombelli-Négrel D. (2020). “Penguin Monitoring and Conservation Activities in the Gulf St Vincent (June 2019 - May 2020),” in Report to the Adelaide and Mt Lofty Natural Resources Management Board (Adelaide: Flinders University).
Colombelli-Négrel D., Kleindorfer S. (2014). “Penguin Monitoring and Conservation Activities in the Gulf St Vincent (July 2013 - June 2014),” in Report to the Adelaide and Mt Lofty Natural Resources Management Board (Adelaide: Flinders University).
Colombelli-Négrel D., Slender A., Bertozzi T., Bradford T., Gardner M. G. (2020). Caution Should Be Used When Interpreting Estimations of Population Structure: A Reply to. J. Hered. 111, 510–511. doi: 10.1093/jhered/esaa033
Congdon B., Erwin C., Peck D., Baker G., Double M., O’neill P. (2007). “Vulnerability of Seabirds on the Great Barrier Reef to Climate Change,” in Climate Change and the Great Barrier Reef, vol. 427. Eds. Johnson J., Marshall P. (Townsville, Australia: Great Barrier Reef Marine Park Authority).
Crawford R. J. (2007). Food, Fishing and Seabirds in the Benguela Upwelling System. J. Ornithol. 148, 253–260. doi: 10.1007/s10336-007-0228-z
Croll D., Tershy B. (1998). Penguins, Fur Seals, and Fishing: Prey Requirements and Potential Competition in the South Shetland Islands, Antarctica. Polar. Biol. 19, 365–374. doi: 10.1007/s003000050261
Croxall J., Reid K., Prince P. (1999). Diet, Provisioning and Productivity Responses of Marine Predators to Differences in Availability of Antarctic Krill. Mar. Ecol. Prog. Ser. 177, 115–131. doi: 10.3354/meps177115
Croxall J. P., Trathan P., Murphy E. (2002). Environmental Change and Antarctic Seabird Populations. Science 297, 1510–1514. doi: 10.1126/science.1071987
Cruzado A., Velásquez Z., Del Carmen Pérez M. A., Bahamón N., Grimaldo N. S., Ridolfi F. (2002). Nutrient Fluxes From the Ebro River and Subsequent Across-Shelf Dispersion. Cont. Shelf. Res. 22, 349–360. doi: 10.1016/S0278-4343(01)00060-7
Csiro (2008). “Water Availability in the Murray–Darling Basin,” in Summary of a Report From CSIRO to the Australian Government (Australia: CSIRO, Canberra, ACT).
Cullen J. M., Chambers L. E., Coutin P. C., Dann P. (2009). Predicting Onset and Success of Breeding in Little Penguins Eudyptula minor From Ocean Temperatures. Mar. Ecol. Prog. Ser. 378, 269–278. doi: 10.3354/meps07881
Cullen J., Montague T., Hull C. (1992). Food of Little Penguins Eudyptula minor in Victoria: Comparison of Three Localities Between 1985 and 1988. Emu 91, 318–341. doi: 10.1071/MU9910318
Cyrus D., Blaber S. (1987). The Influence of Turbidity on Juvenile Marine Fishes in Estuaries. Part 1. Field Studies at Lake St. Lucia on the Southeastern Coast of Africa. J. Exp. Mar. Biol. Ecol. 109, 53–70. doi: 10.1016/0022-0981(87)90185-7
Cyrus D. P., Blaber S. J. (1992). Turbidity and Salinity in a Tropical Northern Australian Estuary and Their Influence on Fish Distribution. Estuarine. Coastal. Shelf. Sci. 35, 545–563. doi: 10.1016/S0272-7714(05)80038-1
Dai A. (2013). Increasing Drought Under Global Warming in Observations and Models. Nat. Clim. Change. 3, 52–58. doi: 10.1038/nclimate1633
Daneri G., Carlini A., Harrington A., Balboni L., Hernandez C. (2008). Interannual Variation in the Diet of non-Breeding Male Antarctic Fur Seals, Arctocephalus gazella, at Isla 25 De Mayo/King George Island. Polar. Biol. 31, 1365. doi: 10.1007/s00300-008-0475-3
Dann P. (1988). An Experimental Manipulation of Clutch Size in the Little Penguin Eudyptula minor. Emu 88, 101–103. doi: 10.1071/MU9880101
Dann P. (1991). Distribution, Population Trends and Factors Influencing the Population Size of Little Penguins Eudyptula minor on Phillip Island, Victoria. Emu 91, 263–272. doi: 10.1071/MU9910263
Dann P., Norman F. I., Cullen J. M., Neira F. J., Chiaradia A. (2000). Mortality and Breeding Failure of Little Penguins, Eudyptula minor, in Victoria 1995-96, Following a Widespread Mortality of Pilchard, Sardinops sagax. Mar. Freshw. Res. 51, 355–362. doi: 10.1071/MF99114
Descamps S., Anker-Nilssen T., Barrett R. T., Irons D. B., Merkel F., Robertson G. J., et al. (2017). Circumpolar Dynamics of a Marine Top-Predator Track Ocean Warming Rates. Glob. Change Biol. 23, 3770–3780. doi: 10.1111/gcb.13715
Dias M. P., Martin R., Pearmain E. J., Burfield I. J., Small C., Phillips R. A., et al. (2019). Threats to Seabirds: A Global Assessment. Biol. Conserv. 237, 525–537. doi: 10.1016/j.biocon.2019.06.033
Divoky G. J., Lukacs P. M., Druckenmiller M. L. (2015). Effects of Recent Decreases in Arctic Sea Ice on an Ice-Associated Marine Bird. Prog. Oceanogr. 136, 151–161. doi: 10.1016/j.pocean.2015.05.010
Du Toit M., Bartlett P., Bester M., Roux J. (2004). Seabird Predation by Individual Seals at Ichaboe Island, Namibia. S. Afr. J. Wildl. Res. 34, 45–54.
Fortescue M. (1999). Temporal and Spatial Variation in Breeding Success of the Little Penguin Eudyptula minor on the East Coast of Australia. Mar. Ornithol. 27, 21–28.
Frederiksen M., Mavor R. A., Wanless S. (2007). Seabirds as Environmental Indicators: The Advantages of Combining Data Sets. Mar. Ecol. Prog. Ser. 352, 205–212. doi: 10.3354/meps07071
Ganendran L. B., Sidhu L., Catchpole E., Chambers L., Dann P. (2016). Effects of Ambient Air Temperature, Humidity and Rainfall on Annual Survival of Adult Little Penguins Eudyptula minor in Southeastern Australia. Int. J. Biometeorol. 60, 1237–1245. doi: 10.1007/s00484-015-1119-2
Geddes M., Shiel R., Francis J. (2016). Zooplankton in the Murray Estuary and Coorong During Flow and No-Flow Periods. Trans. R. Soc. S. Aust. 140, 74–89. doi: 10.1080/03721426.2016.1151497
Gillanders B., Kingsford M. (2002). “Impact of Changes in Flow of Freshwater on Estuarine and Open Coastal Habitats and the Associated Organisms,” in Oceanography and Marine Biology: An Annual Review, vol. Volume 40 . Eds. Gibson R., Barnes M., Atkinson R. (New York: Taylor & Francis), 233–309.
Gillson J. (2011). Freshwater Flow and Fisheries Production in Estuarine and Coastal Systems: Where a Drop of Rain Is Not Lost. Rev. Fish. Sci. 19, 168–186. doi: 10.1080/10641262.2011.560690
Grémillet D., Fort J., Amélineau F., Zakharova E., Le Bot T., Sala E., et al. (2015). Arctic Warming: Nonlinear Impacts of Sea-Ice and Glacier Melt on Seabird Foraging. Glob. Change Biol. 21, 1116–1123. doi: 10.1111/gcb.12811
Grimes C. B., Kingsford M. J. (1996). How do Riverine Plumes of Different Sizes Influence Fish Larvae: Do They Enhance Recruitment? Mar. Freshw. Res. 47, 191–208. doi: 10.1071/MF9960191
Hallett C. S., Hobday A. J., Tweedley J. R., Thompson P. A., Mcmahon K., Valesini F. J. (2018). Observed and Predicted Impacts of Climate Change on the Estuaries of South-Western Australia, a Mediterranean Climate Region. Reg. Environ. Change 18, 1357–1373. doi: 10.1007/s10113-017-1264-8
Harrigan K. E. (1992). Causes of Mortality of Little Penguins Eudyptula minor in Victoria. Emu 91, 273–277. doi: 10.1071/MU9910273
Hochscheid S., Grémillet D., Wanless S., Du Plessis M. A. (2002). Black and White Under the South African Sun: Are Juvenile Cape Gannets Heat Stressed? J. Therm. Biol. 27, 325–332. doi: 10.1016/S0306-4565(01)00097-3
Hofmeyr G., Bester M. (1971). Predation on King Penguins by Antarctic Fur Seals. South Afr. J. Antarctic. Res. 23, 71–74. http://hdl.handle.net/123456789/7623
Hoskins A. J., Dann P., Ropert-Coudert Y., Kato A., Chiaradia A., Costa D. P., et al. (2008). Foraging Behaviour and Habitat Selection of the Little Penguin Eudyptula minor During Early Chick Rearing in Bass Strait, Australia. Mar. Ecol. Prog. Ser. 366, 293–303. doi: 10.3354/meps07507
Hyndman R. J., Athanasopoulos G. (2018). “Forecasting,” in Principles and Practice (Melbourne, Australia: OTexts).
Hyndman R. J., Khandakar Y. (2008). Automatic Time Series Forecasting: The Forecast Package for R. J. Stat. Soft. 26, 1–22. doi: 10.18637/jss.v027.i03
Johnson B., Colombelli-Négrel D. (2020). Breeding Success in Southern Australian Little Penguins is Negatively Correlated With High Wind Speeds and Sea Surface Temperatures. Condor 123, 1–15. doi: 10.1093/ornithapp/duaa062
Johnson R., Venter A., Bester M., Oosthuizen W. (2006). Seabird Predation by White Shark, Carcharodon carcharias, and Cape Fur Seal, Arctocephalus pusillus pusillus, at Dyer Island. S. Afr. J. Wildl. Res. 36, 23–32. https://hdl.handle.net/10520/EJC117231
Kavak M. T., Karadogan S. (2012). The Relationship Between Sea Surface Temperature and Chlorophyll Concentration of Phytoplanktons in the Black Sea Using Remote Sensing Techniques. J. Environ. Biol. 33, 493.
Kelly P., Clementson L., Davies C., Corney S., Swadling K. (2016). Zooplankton Responses to Increasing Sea Surface Temperatures in the Southeastern Australia Global Marine Hotspot. Estuar. Coast. Shelf. Sci. 180, 242–257. doi: 10.1016/j.ecss.2016.07.019
Klomp N., Wooller R. (1988). Diet of Little Penguins, Eudyptula minor, From Penguin Island, Western Australia. Mar. Freshw. Res. 39, 633–639. doi: 10.1071/MF9880633
Kowalczyk N. D., Reina R. D., Preston T. J., Chiaradia A. (2015). Environmental Variability Drives Shifts in the Foraging Behaviour and Reproductive Success of an Inshore Seabird. Oecologia 178, 967–979. doi: 10.1007/s00442-015-3294-6
Krüger L., Petry M. V. (2011). On the Relation of Antarctic and Subantarctic Seabirds With Abiotic Variables of South and Southeast Brazil. Oecol. Australis. 15, 51–58. doi: 10.4257/oeco.2011.1501.05
Lamberth S. J., Drapeau L., Branch G. M. (2009). The Effects of Altered Freshwater Inflows on Catch Rates of Non-Estuarine-Dependent Fish in a Multispecies Nearshore Linefishery. Estuar. Coast. Shelf. Sci. 84, 527–538. doi: 10.1016/j.ecss.2009.07.021
Lander M. E., Fritz L. W., Johnson D. S., Logsdon M. G. (2013). Population Trends of Steller Sea Lions (Eumetopias jubatus) With Respect to Remote Sensing Measures of Chlorophyll-a in Critical Habitat. Mar. Biol. 160, 195–209. doi: 10.1007/s00227-012-2077-4
Lascelles B. G., Langham G. M., Ronconi R. A., Reid J. B. (2012). From Hotspots to Site Protection: Identifying Marine Protected Areas for Seabirds Around the Globe. Biol. Conserv. 156, 5–14. doi: 10.1016/j.biocon.2011.12.008
Le Bohec C., Durant J. M., Gauthier-Clerc M., Stenseth N. C., Park Y.-H., Pradel R., et al. (2008). King Penguin Population Threatened by Southern Ocean Warming. PNAS 105, 2493–2497. doi: 10.1073/pnas.0712031105
Lee R. S., Black K. P., Bosserel C., Greer D. (2012). Present and Future Prolonged Drought Impacts on a Large Temperate Embayment: Port Phillip Bay, Australia. Ocean. Dyn. 62, 907–922. doi: 10.1007/s10236-012-0538-4
Lee W. Y., Jung J.-W., Chung H., Kim J.-H. (2019). Weddell Seal Feeds on Adelie Penguins in the Ross Sea, Antarctica. Polar. Biol. 42, 1621–1624. doi: 10.1007/s00300-019-02539-8
Legendre S., Clobert J., Møller A. P., Sorci G. (1999). Demographic Stochasticity and Social Mating System in the Process of Extinction of Small Populations: The Case of Passerines Introduced to New Zealand. Am. Nat. 153, 449–463. doi: 10.1086/303195
Lowther A., Staniland I., Lydersen C., Kovacs K. (2020). Male Antarctic Fur Seals: Neglected Food Competitors of Bioindicator Species in the Context of an Increasing Antarctic Krill Fishery. Sci. Rep. 10, 1–12. doi: 10.1038/s41598-020-75148-9
Lo-Yat A., Simpson S. D., Meekan M., Lecchini D., Martinez E., Galzin R. (2011). Extreme Climatic Events Reduce Ocean Productivity and Larval Supply in a Tropical Reef Ecosystem. Glob. Change Biol. 17, 1695–1702. doi: 10.1111/j.1365-2486.2010.02355.x
Lynnes A., Reid K., Croxall J. (2004). Diet and Reproductive Success of Adélie and Chinstrap Penguins: Linking Response of Predators to Prey Population Dynamics. Polar. Biol. 27, 544–554. doi: 10.1007/s00300-004-0617-1
Maheshwari B., Walker K., Mcmahon T. (1995). Effects of Regulation on the Flow Regime of the River Murray, Australia. Regulated. Rivers.: Res. Manage. 10, 15–38. doi: 10.1002/rrr.3450100103
Mann H. B. (1945). Nonparametric Tests Against Trend. Econometrica 13, 245–259. doi: 10.2307/1907187
Meyer C. (2014). The Endangered Bank Cormorant Phalacrocorax neglectus: The Heat Is on. Master Thesis. South Africa: University of Cape Town.
Michel C., Hamilton M. C., Hansen E., Barber D., Reigstad M., Iacozza J., et al. (2015). Arctic Ocean Outflow Shelves in the Changing Arctic: A Review and Perspectives. Prog. Oceanogr. 139, 66–88. doi: 10.1016/j.pocean.2015.08.007
Mills W. F., Xavier J. C., Bearhop S., Cherel Y., Votier S. C., Waluda C. M., et al. (2020). Long-Term Trends in Albatross Diets in Relation to Prey Availability and Breeding Success. Mar. Biol. 167, 29. doi: 10.1007/s00227-019-3630-1
Milly P. C., Dunne K. A., Vecchia A. V. (2005). Global Pattern of Trends in Streamflow and Water Availability in a Changing Climate. Nature 438, 347–350. doi: 10.1038/nature04312
Montague T., Cullen J. (1988). The Diet of the Little Penguin Eudyptula minor at Phillip Island, Victoria. Emu 88, 138–149. doi: 10.1071/MU9880138
Monticelli D., Ramos J. A., Quartly G. D. (2007). Effects of Annual Changes in Primary Productivity and Ocean Indices on Breeding Performance of Tropical Roseate Terns in the Western Indian Ocean. Mar. Ecol. Prog. Ser. 351, 273–286. doi: 10.3354/meps07119
Morgan C. A., De Robertis A., Zabel R. W. (2005). Columbia River Plume Fronts. I. Hydrography, Zooplankton Distribution, and Community Composition. Mar. Ecol. Prog. Ser. 299, 19–31. doi: 10.3354/meps299019
Moritz S., Bartz-Beielstein T. (2017). ImputeTS: Time Series Missing Value Imputation in R. R. J. 9, 1. doi: 10.32614/RJ-2017-009
Moritz S., Sardá A., Bartz-Beielstein T., Zaefferer M., Stork J. (2015). Comparison of Different Methods for Univariate Time Series Imputation in R. arXiv. 1510.03924. doi: 10.48550/arXiv.1510.03924
Mortimer R., Krom M., Watson P., Frickers P., Davey J., Clifton R. (1999). Sediment–Water Exchange of Nutrients in the Intertidal Zone of the Humber Estuary, UK. Mar. Pollut. Bull. 37, 261–279. doi: 10.1016/S0025-326X(99)00053-3
Mosley L. M., Zammit B., Leyden E., Heneker T. M., Hipsey M. R., Skinner D., et al. (2012). The Impact of Extreme Low Flows on the Water Quality of the Lower Murray River and Lakes (South Australia). Water Resour. Manage. 26, 3923–3946. doi: 10.1007/s11269-012-0113-2
Newton K. M., Croll D. A., Nevins H. M., Benson S. R., Harvey J. T., Tershy B. R. (2009). At-Sea Mortality of Seabirds Based on Beachcast and Offshore Surveys. Mar. Ecol. Prog. Ser. 392, 295–305. doi: 10.3354/meps08152
Nicolas D., Rochette S., Llope M., Licandro P. (2014). Spatio-Temporal Variability of the North Sea Cod Recruitment in Relation to Temperature and Zooplankton. PloS One 9, e88447. doi: 10.1371/journal.pone.0088447
Österblom H., Casini M., Olsson O., Bignert A. (2006). Fish, Seabirds and Trophic Cascades in the Baltic Sea. Mar. Ecol. Prog. Ser. 323, 233–238. doi: 10.3354/meps323233
Oswald S. A., Arnold J. M. (2012). Direct Impacts of Climatic Warming on Heat Stress in Endothermic Species: Seabirds as Bioindicators of Changing Thermoregulatory Constraints. Integr. Zool. 7, 121–136. doi: 10.1111/j.1749-4877.2012.00287.x
Pinaud D., Weimerskirch H. (2002). Ultimate and Proximate Factors Affecting the Breeding Performance of a Marine Top-Predator. Oikos 99, 141–150. doi: 10.1034/j.1600-0706.2002.990114.x
Poupart T. A., Waugh S. M., Bost C., Bost C.-A., Dennis T., Lane R., et al. (2017). Variability in the Foraging Range of Eudyptula minor Across Breeding Sites in Central New Zealand. N. Z. J. Ecol. 44, 225–244. doi: 10.1080/03014223.2017.1302970
Power S., Delage F., Chung C., Kociuba G., Keay K. (2013). Robust Twenty-First-Century Projections of El Niño and Related Precipitation Variability. Nature 502, 541–545. doi: 10.1038/nature12580
Preston T. J., Ropert-Coudert Y., Kato A., Chiaradia A., Kirkwood R., Dann P., et al. (2008). Foraging Behaviour of Little Penguins Eudyptula minor in an Artificially Modified Environment. Endanger. Spec. Res. 4, 95–103. doi: 10.3354/esr00069
Price C. A., Hartmann K., Emery T. J., Woehler E. J., Mcmahon C. R., Hindell M. A. (2020). Climate Variability and Breeding Parameters of a Transhemispheric Migratory Seabird Over Seven Decades. Mar. Ecol. Prog. Ser. 642, 191–205. doi: 10.3354/meps13328
Priddel D., Carlile N., Wheeler R. (2008). Population Size, Breeding Success and Provenance of a Mainland Colony of Little Penguins (Eudyptula minor). Emu 108, 35–41. doi: 10.1071/MU07038
R Core Team. (2019). R: A Language and Environment for Statistical Computing (Vienna, Austria: R Foundation for Statistical Computing).
Radiarta I. N., Saitoh S. I. (2008). Satellite-Derived Measurements of Spatial and Temporal Chlorophyll-a Variability in Funka Bay, Southwestern Hokkaido, Japan. Estuar. Coast. Shelf. Sci. 79, 400–408. doi: 10.1016/j.ecss.2008.04.017
Reid K., Croxall J. P. (2001). Environmental Response of Upper Trophic-Level Predators Reveals a System Change in an Antarctic Marine Ecosystem. Proc. R. Soc. Lond. B. 268, 377–384. doi: 10.1098/rspb.2000.1371
Reilly P., Cullen J. (1982). The Little Penguin Eudyptula minor in Victoria. III. Dispersal of Chicks and Survival After Banding. Emu 82, 137–142. doi: 10.1071/MU9820137
Reinhold S.-L. (2014). “An Investigation of Long-Nosed Fur Seal Diet,” in Identifying the Importance of Commercially Fished Species and Predation on Little Penguins (Flinders University: Honours Thesis).
Sandvik H., Erikstad K. E., Barrett R. T., Yoccoz N. G. (2005). The Effect of Climate on Adult Survival in Five Species of North Atlantic Seabirds. J. Anim. Ecol. 74, 817–831. doi: 10.1111/j.1365-2656.2005.00981.x
Sheather S. (2009). “A Modern Approach to Regression With R,” in Springer Ebooks (New York: Springer).
Shumway R. H., Stoffer D. S. (2017). “ARIMA Models,” in Time Series Analysis and Its Applications. Eds. Shumway R. H., Stoffer D. S. (Switzerland: Springer), 75–163.
Sidhu L. A., Dann P., Chambers L., Catchpole E. A. (2012). Seasonal Ocean Temperature and the Survival of First-Year Little Penguins Eudyptula minor in South-Eastern Australia. Mar. Ecol. Prog. Ser. 454, 263–272. doi: 10.3354/meps09709
Sommer U., Stibor H., Katechakis A., Sommer F., Hansen T. (2002). “Pelagic Food Web Configurations at Different Levels of Nutrient Richness and Their Implications for the Ratio Fish Production: Primary Production,” in Sustainable Increase of Marine Harvesting: Fundamental Mechanisms and New Concepts. Eds. Olav V., Yngvar O. (Dordrecht: Springer), 11–20.
Syed N. A., Ahmed S. G. (2015). Study of Impact of Climatic Variability on the Sea Surface Temperature and Chlorophyll-A Concentration Using Statistical Analysis on Satellite Derived Data for The Arabian Sea. Int. J. Marine. Sci. 5, 1–11. doi: 10.5376/ijms.2015.05.0002
Tavares D. C., Fulgencio De Moura J., Siciliano S. (2016). Environmental Predictors of Seabird Wrecks in a Tropical Coastal Area. PloS One 11, e0168717. doi: 10.1371/journal.pone.0168717
Thom B., Rocheta E., Steinfeld C., Harvey N., Pittock J., Cowell P. (2020). The Role of Coastal Processes in the Management of the Mouth of the River Murray, Australia: Present and Future Challenges. River. Res. Appl. 36, 656–667. doi: 10.1002/rra.3551
Thomsen S. K., Green D. J. (2019). Predator-Mediated Effects of Severe Drought Associated With Poor Reproductive Success of a Seabird in a Cross-Ecosystem Cascade. Glob. Change Biol. 25, 1642–1652. doi: 10.1111/gcb.14595
Urbanski J., Stempniewicz L., Węsławski J., Dragańska-Deja K, Wochna A, Goc M, et al. (2017). Subglacial Discharges Create Fluctuating Foraging Hotspots for Sea Birds in Tidewater Glacier Bays. Sci. Rep. 7, 43999. doi: 10.1038/srep43999
Wada Y., Van Beek L. P., Wanders N., Bierkens M. F. (2013). Human Water Consumption Intensifies Hydrological Drought Worldwide. Environ. Res. Lett. 8, 034036. doi: 10.1088/1748-9326/8/3/034036
Wanless S., Frederiksen M., Daunt F., Scott B., Harris M. (2007). Black-Legged Kittiwakes as Indicators of Environmental Change in the North Sea: Evidence From Long-Term Studies. Prog. Oceanogr. 72, 30–38. doi: 10.1016/j.pocean.2006.07.007
Wedderburn S., Suitor L. (2012). “South Australian River Murray Regional Wetlands Fish Assessment 2003–2012,” in Report to the South Australian Murray–Darling Basin Natural Resources Management Board (Adelaide: University of Adelaide).
Wiebkin A. S. (2011). “Conservation Management Priorities for Little Penguin Populations in Gulf St Vincent,” in Report to Adelaide and Mount Lofty Ranges Natural Resources Management Board (Adelaide: SARDI Publication No. F2011/000188-1).
Keywords: climate change, predation, population decline, seabirds, drought
Citation: Colombelli-Négrel D, Nur D, Auricht HCC, Clarke KD, Mosley LM and Dann P (2022) Combined Effects of Hydrological Drought and Reduced Food Availability on the Decline of the Little Penguins in South Australia. Front. Mar. Sci. 9:875259. doi: 10.3389/fmars.2022.875259
Received: 14 February 2022; Accepted: 28 April 2022;
Published: 31 May 2022.
Edited by:
Tracey T. Sutton, Nova Southeastern University, United StatesReviewed by:
Alejandro Simeone, Andres Bello University, ChileSebastien Descamps, Norwegian Polar Institute, Norway
Copyright © 2022 Colombelli-Négrel, Nur, Auricht, Clarke, Mosley and Dann. This is an open-access article distributed under the terms of the Creative Commons Attribution License (CC BY). The use, distribution or reproduction in other forums is permitted, provided the original author(s) and the copyright owner(s) are credited and that the original publication in this journal is cited, in accordance with accepted academic practice. No use, distribution or reproduction is permitted which does not comply with these terms.
*Correspondence: Diane Colombelli-Négrel, diane.colombelli-negrel@flinders.edu.au
†ORCID: Diane Colombelli-Négrel, orcid.org/0000-0002-9572-1120
Darfiana Nur, orcid.org/0000-0002-7690-1097
Peter Dann, orcid.org/0000-0002-3044-701X