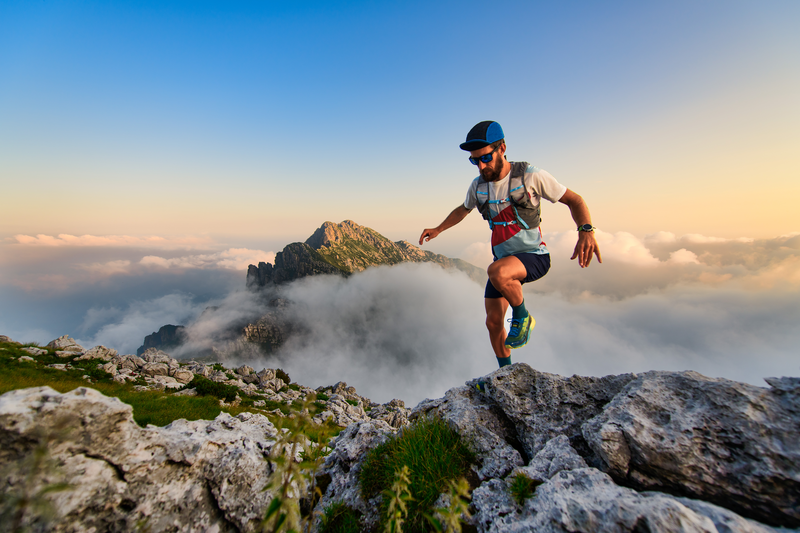
95% of researchers rate our articles as excellent or good
Learn more about the work of our research integrity team to safeguard the quality of each article we publish.
Find out more
METHODS article
Front. Mar. Sci. , 23 May 2022
Sec. Marine Pollution
Volume 9 - 2022 | https://doi.org/10.3389/fmars.2022.874248
The Italian approach to determining background values (BGVs) of metals and trace elements in marine sediments according to the national legislation transposing the water framework directive (WFD) has been illustrated. This study may be helpful for all countries that need to establish local BGVs for the correct assessment of contamination in areas with wide geological and geochemical variability and, particularly, for those that need assessing the good chemical status according to the WFD. The first step was clearly defining the BGV as a concentration value that allows for discriminating between natural and anthropogenic contributions and establishing the concept of the marine geochemical province (MGP). Successively, criteria for delimiting the MGPs were provided, similar to those adopted for the geochemical mapping of the territory. Finally, an integrated method based on the constitution of a dataset from unpolluted sediments obtained from pre-industrial levels was chosen for determining the BGV.
The Italian peninsula has some thousand kilometers of coastline, and since the second half of the 20th century, a large part has been subjected to a strong impact due to the growth of urban, industrial, mining, and agricultural activities, and the presence of many harbors, which have determined increasing contamination of the marine environment. The supply of contaminants may occur for direct discharge to the sea, runoff from contaminated soils, input from river mouths, or via atmospheric deposition, making the sediments the final sink of toxic, persistent, and bioaccumulative contaminants (Kowalewska et al., 2011). In some cases, although the supply of contaminants ceased owing to the closure of the industrial facilities or the implementation of environmental legislation, the contamination persists in deeper sediments and can be recognizable in sediment cores (Croudace et al., 2015). In other cases, the contribution is still active for both the continuation of polluting activities and waste materials that work as a secondary source (Romano et al., 2017). In all these cases, a variable thickness of sediment is contaminated, according to the duration of the contributions and the sedimentation rate. Some of the most common contaminants detectable in sediments are of exclusive anthropogenic origin, while others, such as metals and trace elements (MTEs), are also naturally present in the terrigenous component, deriving from the minerals of rocks acting as sedimentary sources (Li and Schoonmaker, 2003).
As a consequence of widespread contamination in the last decades, the need for specific legislation providing threshold values for natural concentrations to assess sediment contamination arose at a national level (Ausili et al., 2020). At the European level, the Water Framework Directive (2000/60/EC) defined the strategy to hinder the chemical pollution of water bodies through the adoption of the Environmental Quality Standards (EQS). Successively, the Directive 2008/105/EC required member states to define at a national level the EQSs of some substances, including some trace elements, also for sediments. However, the adoption of a single value for each parameter, valid for the whole Italian seas, maybe the origin of unreliable environmental assessment due to the high geochemical heterogeneity of sediments, deriving from the geological complexity of the mainland. Italy is located at the boundary of some tectonic plates and is involved in many past and still active geological processes at a regional and local scale. It is characterized by two orogens which, on the whole, affect nearly the entire territory: the Alps to the North, due to the thrusting toward west and north-west of the Adriatic plate, and the Apennines along the peninsula and in Sicily, due to the subduction of the same plate toward the west (Doglioni and Flores, 1997). Due to this geological setting (Figure 1), several structural units and magmatic provinces, with different chemism, outcrop in the mainland, representing the main sources for sediments, enriching them for one or more elements and determining geochemical anomalies (De Vivo et al., 2008). For example, Hg enrichments affect the sediments of the northern Adriatic and central Tyrrhenian seas, while Cr and Ni are enriched in the northern Tyrrhenian and Ligurian Seas sediments; As enrichments characterize sediments of specific sectors of the central and southern Tyrrhenian Sea, while Cd, Zn, and Pb enrich the sediments of the southern Sardinian Sea (Barghigiani et al., 1996; Bertolotto et al., 2005; Covelli et al., 2006; Piazzolla et al., 2015; Lopes-Rocha et al., 2017; Giglioli et al., 2020; Romano et al., 2020; Sarti et al., 2020 among others).
Figure 1 Italy’s simplified geological map; the chemical symbols of enrichments elements in marine sediments have been added (modified from Bosellini, 2017).
Considering these aspects, the Italian law transposing the European directive introduced the option of identifying the marine geochemical provinces (MGPs) and establishing in each one “local” BGVs that replace the ones identified by the national decrees. Through a scientifically-based approach, their determination is needed only for marine areas with BGV of one or more elements higher than EQSs. Then, the first issue posed by this law consisted in defining the borders of the marine geochemical anomalies related to the geochemical provinces of the land (Spagnoli et al., 2014; Romano et al., 2017; Trifuoggi et al., 2017); however, no examples were available from scientific literature for this purpose. Differently, many methods have historically been applied for the determination of BGVs, that can be categorized in two distinct types, one geochemical (direct) and the other statistical (indirect) (Matschullat et al., 2000; Galuszka, 2007). The direct method may be applied at a global or local scale and includes all the works dealing with fixed (mean or median) concentrations from samples not affected by anthropogenic contribution, both from pre-industrial sediments (e.g., Tanner et al., 2000; Xu et al., 2009; Guo and Yang, 2016), or of pristine areas. The indirect method applies different statistical techniques, such as regression, relative cumulative frequency curves, and the iterative 2σ method for analyzing the distribution of natural concentrations and identifying the contaminated ones as outliers (Matschullat et al., 2000). This paper aims to illustrate the methodological path defined in Italy by the National Network System for Environmental Protection to determine MGPs and their local BGVs for trace elements to be used in the framework of environmental legislation instead of nationally defined values (Romano et al., 2018). In this path, the first aim was to provide the elements for the conceptual, unambiguous definitions of MGP and BGV. Consequently, the methodological approach was defined to delimit the borders of MGP and identify a suitable one, taking into account earlier literature, for building a suitable concentration dataset and processing these data for determining BGVs, according to the given definitions.
Defining the concept of the terrestrial geochemical province is an indispensable element for the subsequent identification of the MGP because it constitutes the principal origin of the material constituting the marine-coastal sediments.
The first definitions of the geochemical province were exclusively based on the homogeneity concept of some characteristics. According to Fersman (1934, 1939), the geochemical province was a geochemically homogeneous area containing a well-defined association of chemical elements. Similarly, Beus and Grigorian (1977) defined the geochemical provinces as units with common geological and geochemical evolution characteristics. The geochemical province was also considered a region characterized by a particular mineral association or one or more specific mineralizations (Parker, 1984).
However, the homogeneity of the geochemical characteristics is not the only requirement to define a geochemical province, distinguishable from the surrounding areas. The introduction of the concept of “anomaly”, as a segment of the earth’s crust with a chemical composition significantly different from the average (Hawkes, 1957; Rose et al., 1979), was fundamental for making the geochemical province easily recognizable. Evidence of the existence of a geochemical province was commonly found by comparison with the composition of igneous rocks; according to Hawkes (1957), variations in the concentration of minor elements could provide reliable criteria to identify it. Anselmi et al. (1978) defined the geochemical provinces as areas of the earth’s crust in which the content of rare elements was persistently anomalous, so introducing the temporal concept in the definition.
Govett (1983) defined the geochemical anomaly as an abnormal content of an element or combination of elements in a particular type of sample due to a specific analytical method. In this case, both uniformity of matrix and analytical method were considered in the definition. These principles were extended to a geochemical province scale, considering the geochemical province as the abnormal distribution of one or some elements in a particular type of sample, as defined by a specific analytical technique (Bölviken et al., 1990). This logical path, which transfers the definition of the geochemical anomaly to the geochemical province, is possible because the geochemical dispersion model has a fractal nature. This means that similar units have a similar shape, albeit at a different scale (Bölviken et al., 1992).
The definition of Bölviken et al. (1990) also highlighted the concept of “abnormal concentration”, making it necessary to define the normal value, corresponding to the global BGV, for quantifying the significant difference characterizing the geochemical province. This value is generally identified by calculating the average crustal composition (Turekian and Wedepohl, 1961; Taylor, 1964; Wedepohl, 1995). The concepts of anomaly, uniformity of matrix, and analytical methods may be transferred to the definition of MGP identified as a sector of sea-bottom characterized by sediments with persistent, anomalously high natural concentrations of specific elements, deriving from the supply from a terrestrial geochemical province, determined through modern, commonly used techniques such as graphite furnace atomic absorption spectrometry (GFAAS) and/or inductively coupled plasma optical emission spectroscopy (ICP-OES).
A precise and unambiguous definition of the concept of BGV is fundamental to identifying the methods for its determination in a marine geochemical province. There are several definitions of BGV in the scientific literature. Some include numerous specific concepts, such as the type of matrix or exotic processes, while others provide a more general definition. Although some definitions such as “ambient background” include both natural and diffuse anthropogenic sources (Crane et al., 2021), only the contribution of natural origin is considered in the concept of (geochemical) BGV (Birch, 2017). For some authors, the BGV implies the absence of any anomalies, and, consequently, neither the natural ones may be considered in the determination of this value (Gough, 1993; Matschullat et al., 2000). More recently, the BGV was defined as a theoretical natural concentration of a substance in marine sediments, considering temporal and spatial variables, determined by direct, indirect, and integrated methods (Galuszka, 2007; Galuszka and Migaszewski, 2011). In this work, the BGV in marine sediments is defined, according to Matschullat et al. (2000), as the value allowing the distinction between the exclusively natural concentration from that influenced by anthropogenic supply, deprived of any anomalies.
The logical path that leads to the definition of the MGP and the determination of the BGVs starts from the hypothesis that there is a geochemical anomaly in the marine sediments of a certain area, based on the existence of a geochemical anomaly on the mainland, documented by pre-existing studies. Once they arrive at the sea, sedimentary contributions from these areas, mainly through waterways, are redistributed starting from the river mouth by sea currents and then deposited in a more or less extensive area which depends on numerous factors. Then, there is the need to collect information through bibliographic and/or field studies on the factors influencing the extent and shape of the MGP to define its potential borders. Finally, based on the bibliographic results, it will be necessary to define an investigation strategy for collecting specific data to define the MGP.
Some aspects of natural processes, both in the terrestrial and marine environment, contribute to the definition of the potential MGP between the limit of the lower action of the waves and the continental shelf border in the absence of anthropogenic modifications. For this reason, they should be considered before any other action.
The geology of the mainland, particularly the chemism of different outcropping lithotypes, and the characteristics of the hydrographic basin, such as its maturity and extension, are important elements for understanding how they may have influenced the chemical and mineralogical composition of marine sediments.
Then, the geochemical characteristics of sediment particles and their entire route, from the rocks of origin to the consequent transport to the sea by the rivers to the sea currents that redistribute them according to the grain-size, up to compositional variations due to geochemical processes such as, for example, dissolution and/or precipitation of solid phases, which occur in the marine environment, are the main aspects to be considered.
The textural and geochemical characteristics of river sediments, associated with the quantification/estimation of suspended solid transport, can be of fundamental importance for a correct evaluation of any enrichment of metals and trace elements in marine sediments. Also, the use of the soil and the vegetation structure existing on the entire hydrographic basin that affects the erodibility of the surface due to meteoric events and runoff are useful elements to consider. Finally, an estimate of the actual solid transport operated by waterways, through multiparametric empirical models, will help assess the reliability of the criteria adopted for this selection and verify if any significant watercourses may not have been considered.
Once the geochemical aspects of the terrestrial environment have been deepened, some processes of the marine environment should be considered. Among the factors that contribute to the formation of sediment in the marine environment, there is certainly the contribution provided by the water column, by possible eutrophication phenomena, as well as by atmospheric depositions responsible not only for the deposition of radionuclides but also the atmospheric contribution of inorganic elements, organic compounds, and nutrients deposited by precipitation. Another very important aspect is related to the hydrodynamic features of the area and the different scales of influence, such as, for example, coastal currents generated by the interaction of wave motion with the seabed.
The knowledge about the topography, bathymetry, and wavy climate of the coast, is fundamental for better understanding the potential influence of the river supply in the marine area. The hydrodynamic currents, generated by the three-dimensional movement over a wide scale of water masses and set in motion along the entire water column by temperature and salinity gradients and on the surface by tides and wind, substantially decide the dispersion of the turbid plumes once offshore. On the whole, the spatial variability of the MGPs is determined by the overlap of two phenomena: the more heterogeneous short-range “coastal” deposition, mainly influenced by local variability of the territory, and the long-range deposition of the turbid plume, whose extension and spatial homogeneity is directly related to the importance of river basins, given that the solid transport of a large basin will affect more physiographic units and will predominate beyond a certain distance from the shore (Ricci Lucchi and Mutti, 1980). Therefore, the comprehension of these two components is of basic importance to identify the extension of the MGP. In addition, specific calculation models are really important, able to integrate all the information into a 3D-hydrodynamic model and simulate the dynamics of mesoscale currents under the action of significant forcing. These are:
− large-scale marine circulation;
− meteorological forcing;
− liquid flow input from watercourses that affect the movement of water masses, both in terms of speed and salinity.
Once the essential information related to elements and processes of both the terrestrial and marine environment that influence the extension of the MGPs has been acquired, it will be necessary to integrate this information with the geochemical data of sediments, with particular attention to those elements which could be responsible for the geochemical anomalies in the area.
In the scientific literature, there are no examples of field studies finalized to the geochemical characterization of marine sediments finalized to the definition of a MGP, while many papers reported the geochemical mapping of the territory. However, it seems reasonable to adapt to the marine environment the general criteria used for the geochemical mapping of the territory (Bölviken et al., 1986; Ottonello and Serva, 2003; Salminen et al., 2005; De Vivo et al., 2009). The investigation strategy subdivides the area into square meshes of equal size where one or more stations are sampled. This approach can be easily transposed to the marine environment, adapting it to the scale of interest and the different morphological and environmental characteristics, also using existing data with the same requirements as those acquired by the field study.
Very variable scales have been adopted for geochemical mapping of the mainland (Garrett et al., 2008). They range from one station every 1-15 km2 for mining exploration, to very low densities, such as one station every 2,500-10,000 km2, for environmental mapping projects on a national and international scale. Reimann et al. (2010) defined, for the sampling grid, the following orders of magnitude:
− Global: < 1 station every 5,000 km2;
− Continental: from 1 station every 5,000 km2 up to 1 every 500 km2;
− Regional: from 1 station every 500 km2 to 1 every 2 km2;
− Local: from 1 to 100 stations every km2;
− Detailed: > 100 stations every km2.
For the definition of sampling density, aimed at defining borders of the MGPs, a regional scale seems appropriate because the processes responsible for the formation and preservation of mineralizations, including the processes that determine the mobilization of their components, transport, accumulation, and preservation in subsequent geological history, occur on a regional to global scale (Wyborn et al., 1994; Reimann et al., 2015). Furthermore, several studies have shown that a low-resolution survey can be adequate in the interpretation of the geological evolution of large regions and the selection of representative areas (Reimann and Melezhik, 2001; Smith and Reimann, 2008; Reimann et al., 2010) due to the fractal nature of geochemical landscapes (Bölviken et al., 1992). This concept, valid for continental areas, can also be applied to the marine environment since the MGP is closely related to continental inputs. In the marine environment, the fractal geometry determined by the topography in the continental areas is not maintained, but the marine processes do not reduce the scale of competence of the geochemical province; on the contrary, it is enlarged and homogenized by the dispersion of contributions to the sea by the coastal dynamics. Therefore, to define the limits of a MGP, a survey at a regional scale can be applied, taking into account the morphology of the seabed, the existing geochemical information, and the potential extension of the area subject to a geochemical anomaly.
Based on the concept that, as described in section 3.1.1, there is a greater variability of the chemism of marine sediments in the areas close to the coastline and a gradual effect of homogenization of the sediments towards the continental margin areas, the sampling scheme needs to be denser in the coastal sector than offshore. Consequently, the portion of the seabed closer to the coast could be sampled along a line, presumably within the bathymetric of 20 m, compatibly with the morphology of the continental shelf, with a variable density from 1 to 10 stations per km. Beyond 20 m of depth, it is recommendable to adopt a regular mesh with different areal densities at increasing depths, between 20 and 50 m depth and between 50 m depth and the limit of the shelf.
A sediment core, sufficiently long to identify pre-industrial levels, must be collected in each mesh, or may be acquired environmental data with similar requirements from previous characterizations. Each core must be sub-sampled by levels not thicker than 2 cm, which must be analyzed for grain size and MTEs to reconstruct vertical concentrations profiles, identifying natural and anthropogenic contributions. For this purpose, the study of the core by an XRF core scanner, which provides the concentration of a wide range of elements, may alternatively be used. This device can provide a large amount of data in a very short time and with a minimum of sample preparation and not destructive analysis (Croudace et al., 2015; Rothwell and Croudace, 2015a; Rothwell and Croudace, 2015b).
Determining a real background of MTEs is of basic importance for recognizing the presence of contamination, defined as the presence of a substance where it should not be or at concentrations above the natural one (Chapman, 2007). The BGVs are used in the calculation of some indices aimed at the quantification of contamination, such as the Geoaccumulation Index (Müller, 1969), Contamination Factor (Hakanson, 1980), Pollution Load Index (Tomlinson et al., 1980), and Enrichment Factor (Middleton and Grant, 1990). Identifying local BGVs is essential in MGPs, because of abnormally high natural concentrations for some elements above the mean global ones.
Geochemists have no general agreement on how geochemical background values should be established; direct, indirect, and integrated methods have been applied (Galuszka and Migaszewski, 2011). The direct one also called geochemical, is based on a single value, determined as a mean or median concentration from exclusively uncontaminated samples obtained from pre-industrial levels of sediment cores (historical aspect) or pristine areas (contemporary aspect). The indirect method uses statistics to identify and remove outliers or spatial analysis. However, both methods have intrinsic limits: the geochemical one does not consider the natural variability of MTEs concentrations, while the statistical one is strongly conditioned by the initial dataset that, in densely populated areas, might not include sediments deprived of MTEs anthropogenic enrichment (Birch, 2017). For this reason, the integrated method, processing with statistical methods concentrations from pristine areas, was considered the best option (Galuszka and Migaszewski, 2011, Figure 2).
Figure 2 The integrated method to determine BGVs (from Galuszka and Migaszewski, 2011).
The integrated method has been applied in many cases, even if the dataset included the variable proportion of contaminated samples. Different statistical techniques have been applied, all substantially aimed at the identification of the threshold between natural and anthropogenically enriched concentrations (Marmolejo-Rodriguez et al., 2007; Surricchio et al., 2019); however, it was recognized that they need a dataset obtained from samples with scarce anthropogenic input to identify reliable BGVs (Sprovieri et al., 2006). Moreover, surface sediment samples assumed to be clean were recognized as metal-enriched compared to pre-industrial core samples making them unreliable for determining BGVs (Aloupi and Angelidis, 2001). Consequently, it was deduced that this approach would not be applicable in a framework of environmental legislation because, due to the high anthropization of the Italian coast, it would not be ensured that most samples are not affected by anthropogenic contribution.
The approach based on sediment cores to determine BGVs in highly anthropized areas appears preferable to the one based on surface sediments because the sediment cores can provide concentration data showing exclusively natural metal contribution because of their pre-industrial age. Several studies based on sediment cores applied the direct (geochemical) methods considering as BGVs the mean or median concentrations of samples from lower core sections deposited in pre-industrial times (Tanner et al., 2000; Cobelo-García and Prego, 2003; Zourarah et al., 2007; Xu et al., 2009; Guo and Yang, 2016). However, this method does not consider the natural variability, and values above the average or the median are considered enrichment. Differently, making a regression line, together with the 95% prediction interval, by plotting the concentration of an element against a normalizer one, allows the identification of BGVs, considering the sediment variability (Covelli and Fontolan, 1997; Liu et al., 2003). In some cases, the regression technique was applied to a dataset, selected using radiochronological dating and statistical methods, to reflect the concentration distributions of natural levels, eliminating the anthropogenic ones (Apitz et al., 2009). More recently, Shahabi-Ghahfarokhi et al. (2021) applied a similar integrated approach, which determined BGVs by considering the pre-industrial samples from sediment cores and applying the median + 2 MAD (Median Absolute Deviation). This approach may be considered as an improvement of the integrated method of Galuszka and Migaszewski (2011) because it considers the natural variability of pre-industrial levels (historical aspect), providing the guarantee to work on a dataset of MTEs concentrations exclusively deriving from natural contributions (Figure 3).
Figure 3 The integrated method used to determine BGVs by the Italian National Network System for Environmental Protection (modified from Galuszka and Migaszewski, 2011).
From the review of scientific literature in the above section, it appears clear that the best choice for determining local BGVs is an integrated approach using unpolluted, pre-industrial samples from sediment cores. This approach guarantees reliable BGVs which can replace the national EQSs. Furthermore, the start of the MTEs supply to marine sediments from anthropogenic activities, such as mining or industries, is recognizable in the sediment cores by typical concentration profiles, which abruptly increase in the upper section where generally show wider variability than in the lower core. This event dates back to variable historical periods along the Italian coasts, ranging from the middle of the 19th to the middle of the 20th century (Romano et al., 2017; Romano et al., 2021).
The approach adopted by the National Network System for Environmental Protection guidelines is based on the geochronological (210Pb, 137Cs), chemical (MTEs), and granulometric data from the same cores used for the definition of MGPs. Because the MGP has been defined as a marine area ranging from the shoreline to the continental shelf limit, it should be considered that variation of sediment texture and mineralogy characterize these samples, from coarser sandy sediments collected close to the coast to clayey ones from offshore bottom. This implies that MTEs concentrations may vary for supply changes both from natural and anthropogenic sources and for changes in sediment texture, mineralogy, and organic matter content (Dung et al., 2013). Normalization procedures, based on the separation of the fine (<63 μm) fraction or the use of conservative elements as geochemical normalizers, are generally used for removing the variability due to different sediment characteristics in studies finalized to contamination assessment (Kersten and Smedes, 2002). Differently, to define local BGVs, the highest natural variability of concentrations in the MGP area should be considered, including one due to grain size and mineralogy changes. This is ensured thanks to the characteristics of the adopted strategy, which is aimed at the systematic sampling of the whole MGP; as a consequence, the collected samples will be representative of the concentration variability as a result of changes of natural contribution, as well as of textural and mineralogical variability of the province itself. The need for a single value at the legislative level for environmental assessment, which considers the natural variability, lets us discard regression techniques and direct us towards simple, easily applicable statistical methods. The study of concentration profiles, associated with the geochronology and integrated with historical information related to the anthropogenic activities in the area, led to identifying the un-polluted, pre-industrial section. The integrated approach applied to determine BGVs in the Orbetello Lagoon (Romano et al., 2015) was recognized as suitable for being adopted by the national guidelines.
The Orbetello Lagoon is an enclosed area of approximately 25 km2 and is constituted by two well-connected basins where several environmental surveys highlighted strong contamination due to past and present anthropogenic activities, such as former industries, historical mining, fish farming, and tourist facilities. The need for anthropogenic contamination assessment was the reason for determining the local BGVs, in particular, of Hg and As, responsible for a geochemical anomaly in the mainland (Lattanzi et al., 2017; Fornasaro et al., 2022), but also in surface marine sediments (Barghigiani et al., 1996).
In the case of Orbetello, which belongs to the well-known geochemical province of Monte Amiata (Tuscany), there was no need to define the borders of the MGP because of the semi-enclosed shape of the lagoon. According to a regional sampling density (one station every 2.5 km2), a total of 10 sediment cores were collected to study grain size variability and MTEs concentration along the depth of the cores determined by means of the ICP-OES technique, except Hg, which was analyzed through DMA-80 (Ausili et al., 2011). Among these, four cores were also studied for geochronological dating using of 210Pb and 137Cs isotopes (Figure 4). These two independent methods provided similar sedimentation rates for all the cores, which ranged, on the whole, 0.36-0.44 and 0.29-0.46 cm y-1, respectively. Then, a constant sedimentation rate of 0.36 cm y-1 was assumed in the Orbetello Lagoon for the whole length of cores, also based on the homogeneous sediment texture (Romano et al., 2015).
Figure 4 Concentration profiles of Pb in the four cores; the upper, anthropogenically enriched sections are highlighted by grey bars. On the left, the position of the sediment cores in the Orbetello lagoon (Romano et al., 2015).
These last ones, selected because they were considered representative of the chemical and textural variability of the area, were used for making a dataset of MTEs concentrations for the determination of BGVs. First, the study of core profiles, supported by descriptive multivariate statistics (Principal Component Analysis - PCA), identified the anthropogenic contribution of MTEs (Figure 5). The PCA, based on standardized chemical and grain size data, displayed two main components, accounting for 65% of the variance on the whole. The PC1 discriminated the sandy sediment fraction, with negative values, from the silty and clayey ones and the MTEs, with positive values. The PC2 differentiated silt, clay, Cr, Ni, and fine sediment fractions at the negative side, pointing to the exclusive geogenic origin of these two elements from the other MTEs, which were at the positive side and supposed to be anthropogenically influenced. These MTEs, also based on the typical shape of concentration profiles, were confirmed as anthropogenically enriched, and among them, the Pb concentration profiles showed the earliest and most marked increase in the upper sections of the four cores. For this character, Pb was considered the best marker of anthropogenic influence and used, also considering the geochronology, to recognize the anthropogenically influenced core levels to be removed from the dataset to determine BGVs. Consequently, the upper core sections, corresponding to a time interval ranging from 1900 for core OR96 to 1950 for cores OR94, OR98, and OR100, were removed from the initial dataset (Figure 5). More in detail, 47 out of 102 samples, which were considered anthropogenically influenced, were excluded from the dataset, while anthropogenic contribution in older times was considered negligible. Moreover, the outliers identified by boxplots were removed because they were attributable to natural causes or ancient anthropogenic activities. In particular, a Hg outlier was recognized in all four cores and interpreted, due to its age dating back to 1850, to the documented historical mining in the Monte Argentario. At this point, the dataset included only samples exclusively affected by a natural geogenic contribution, which can be considered responsible for a normal or log-normal distribution of data (Birch, 2017). Then, from a final dataset including 36 samples the BGVs were calculated using the formula “mean + 2σ” where 97% of cases were below such threshold. Using the described approach, based on cores representative of natural sedimentological and MTEs variability of the area, the local BGVs, exclusively valid for the Orbetello Lagoon, were determined (Table 1). Most MTEs were very close to the global averages, pointing to the validity of the applied method to recover natural concentration values, while higher values were obtained for As and mostly for Hg, which are naturally enriched in the area (Ferrara et al., 1998; Protano et al., 1998; Grassi and Netti, 2000). The Anthropogenically-influenced Background Values (ABV) were also determined to quantify the effect of anthropogenic contribution on the concentration data (Table 1). The ABVs were considerably higher than global averages, except Cr and Ni, respectively, slightly higher and lower than these reference values. Also, a comparison between ABVs and BGVs, highlighted that the first were as far higher than the second ones, excluding Cr and Ni, which were the only elements exclusively of natural origin in the Orbetello Lagoon. The ABV of Pb is more than double than BGV, revealing the highest anthropogenic enrichment for this element that was reliably used as a marker of the anthropogenic influence to define the thickness of the upper, anthropogenically enriched core sections to be not considered in the dataset (Romano et al., 2015).
Figure 5 Scatter plot of Principal Component Analysis on standardized MTEs concentrations and grain size data in the four sediment cores; elements affected by anthropogenic enrichment are recognizable because they are not correlated to the fine sediment fractions (Romano et al., 2015).
Table 1 Orbetello Lagoon: MTEs parameters determined from the dataset including both natural and anthropogenically influenced data (Mean Anthropogenically-influenced Value - MAV; Anthropogenically-influenced Background Value - ABV), are compared with those determined on the exclusively natural concentration data of the same dataset (Mean Natural Value – MNV; local Background Value - BGV) reported by Romano et al. (2015).
The EQSs are tools applicable in national or supranational legislation for the environmental assessment and the definition of the chemical status of the coastal marine areas. Because MTEs may be supplied to the marine environment and finally stored in sediments from natural and anthropogenic sources, their quality standard should be the upper threshold of natural concentration, thus distinguishing between the natural contribution and anthropogenic enrichment. However, because the environmental assessment based on these values supports the decision-makers for adopting environmental policies, an assessment based on rigid quality standards could be unreliable. EQSs for heavy metals lower than local natural concentrations cause overestimation of pollution levels, with possible application of improper remediation actions and waste of public resources.
Peculiar territories, such as the Italian one, extensively affected by geological processes and highly diversified by mineralogical viewpoint, need to apply a flexible environmental strategy to adopt local threshold values replacing the EQSs of MTEs for the definition of the chemical status. The WFD has acknowledged this need for areas affected by natural metal enrichments. The National Network System for Environmental Protection provided a scientifically-based method for determining a single concentration value, corresponding to the BGV, for those MTEs affected by natural enrichment. This value is exclusively applicable locally in areas with evidence-supported geochemical anomalies.
The conceptual model applied to address this requirement was to use scientific literature as the basis for the methodological choices. It started from providing the clear definition of MGP and BGV and was developed through the methods for the spatial definition of the first one and the calculation of the second one from a dataset obtained from sediment cores representative both of sedimentological and geochemical variability within the marine province. An advanced version of the integrated approach considering the historical aspect (pre-industrial levels of geochronologically characterized sediment cores) and statistical frequency analysis of a dataset of natural metal concentrations, cleaned of any outliers, was considered the most suitable method to estimate an accurate BGV. Because of the local use of this value and a sampling strategy that allowed to collect data representative of the local natural variability, no normalization procedures or statistical regression techniques were considered necessary to determine the BGV.
The proposed approach was intentionally conceived as a tool applicable in a standardized way to the whole territory but at the same time flexible and adaptable to the different geological and geochemical conditions present at the local level. However, the reliability of the determined BGVs has been demonstrated by comparison of these values with those determined through the same method, without removing anthropogenically-influenced data, and with global average concentrations.
Pre-existing geochemical data may reduce the considerable effort in terms of sampling and analytical activity required to define MGPs and determine their BGVs; however, they must comply with the criteria described in this work. Moreover, the determination of reliable local BGVs can be considered an investment for the future as, once defined, it supports all upcoming environmental policies.
The need to determine local BGVs for MTEs in marine sediments of naturally enriched areas to substitute the EQSs in the framework of environmental legislation led to establishing the principles and methods to achieve this aim. In this context, the first definition of MGP has been provided, and the BGV has been defined as the upper threshold of natural concentration variability. Based on these definitions, the methods for the spatial delimitation of MGPs and the determination of their BGVs considered the dated sediment core as the only suitable tool for detecting natural concentrations in the coastal zone of industrialized countries, where real pristine areas are virtually absent. Consequently, an integrated geochemical-statistical method for determining the BGVs, considering a concentration dataset from core levels of the pre-industrial age, has been developed.
This approach, adopted in Italy by the environmental authorities, may be considered a scientifically-based and flexible method applicable in other countries with similar geological and geochemical characteristics to meet the increasingly demanding environmental issues, providing reliable environmental assessment for the application of suitable policies.
The original contributions presented in the study are included in the article/supplementary material. Further inquiries can be directed to the corresponding author.
All authors listed have made a substantial, direct and intellectual contribution to the work, and approved it for publication. Each author contributed equally to all sections of the manuscript.
The authors declare that the research was conducted in the absence of any commercial or financial relationships that could be construed as a potential conflict of interest.
All claims expressed in this article are solely those of the authors and do not necessarily represent those of their affiliated organizations, or those of the publisher, the editors and the reviewers. Any product that may be evaluated in this article, or claim that may be made by its manufacturer, is not guaranteed or endorsed by the publisher.
Aloupi M., Angelidis M. O. (2001). Normalization to Lithium for the Assessment of Metal Contamination in Coastal Sediment Cores of the Aegean Sea, Greece. Mar. Environ. Res. 52, 1–12. doi: 10.1016/S0141-1136(00)00255-5
Anselmi B., Benvegnù F., Brondi A., Ferretti O. (1978). “Contributo Allo Studio Dell’influenza Della Geologia E Della Geochimica Dei Bacini Emersi Sulla Composizione Di Sedimenti Marini. Indagine Sistematica Sugli Apporti Fluviali Dell’intero Territorio Nazionale,” in Proceeding of 3rd Conference AIOL, Sorrento, Italy, December 18-20. 279–293.
Apitz S. E., Degetto S., Cantaluppi C. (2009). The Use of Statistical Methods to Separate Natural Background and Anthropogenic Concentrations of Trace Elements in Radio-Chronologically Selected Surface Sediments of the Venice Lagoon. Mar. Pollut. Bull. 58, 402–414. doi: 10.1016/j.marpolbul.2008.10.007
Ausili A., Bergamin L., Romano E. (2020). Environmental Status of Italian Coastal Marine Areas Affected by Long History of Contamination. Front. Environ. Sci. 8, 34. doi: 10.3389/fenvs.2020.00034
Ausili A., Romano E., Bergamin L., Maggi C. (2011). Determinazione Dei Valori Di Fondo Dei Sedimenti Lagunari. Technical Report Rome:Ispra. 31 pp.
Barghigiani C., Ristori T., Lopez Arenas J. (1996). Mercury in Marine Sediment From a Contaminated Area of the Northern Tyrrhenian Sea: < 20 μM Grain-Size Fraction and Total Sample Analysis. Sci. Total Environ. 192, 63–73. doi: 10.1016/0048-9697(96)05292-8
Bertolotto R. M., Tortarolo B., Frignani M., Bellucci L. G., Albanese S., Cuneo C., et al. (2005). Heavy Metals in Surficial Coastal Sediments of the Ligurian Sea. Mar. Pollut. Bull. 50, 344–359. doi: 10.1016/j.marpolbul.2004.12.002
Beus A. A., Grigorian S. V. (1977). Geochemical Exploration Methods for Mineral Deposits. Ed. Wilmette I. L. (Wilmette, Illinois:Applied Publishing Ltd.), 287 pp.
Birch G. F. (2017). Determination of Sediment Metal Background Concentrations and Enrichment in Marine Environments - A Critical Review. Sci. Total Environ. 580, 813–831. doi: 10.1016/j.scitotenv.2016.12.028
Bölviken B., Bergstrom J., Björklund A., Kontio M., Lehmuspelto P., Lindholm T., et al. (1986). Geochemical Atlas of Northern Fennosandia, Scale 1:4 million. Geol. Survs. Finland, Norway and Sweden. 20 pp., 155 maps.
Bölviken B., Kullerud G., Loucks R. R. (1990). Geochemical and Metallogenic Provinces: A Discussion Initiated by Results From Geochemical Mapping Across Northern Fennoscandia. J. Geochem. Explor. 39, 49–90. doi: 10.1016/0375-6742(90)90069-M
Bölviken B., Stokke P. R., Feder J., Jössang T. (1992). The Fractal Nature of Geochemical Landscapes. J. Geochem. Explor. 43, 91–109. doi: 10.1016/0375-6742(92)90001-O
Bosellini A. (2017). “Outline of the Geology of Italy,” in Landscapes and Landforms of Italy. World Geomorphological Landscapes. Eds. Soldati M., Marchetti M. (Cham: Springer), 21–27.
Chapman P. M. (2007). Determining When Contamination is Pollution - Weight of Evidence Determinations for Sediments and Effluents. Environ. Int. 33, 492–501. doi: 10.1016/j.envint.2006.09.001
Cobelo-García A., Prego R. (2003). Heavy Metal Sedimentary Record in a Galician Ria (NW Spain): Background Values and Recent Contamination. Mar Pollut. Bull. 46, 1253–1262. doi: 10.1016/S0025-326X(03)00168-1
Covelli S., Fontolan G. (1997). Application of a Normalization Procedure in Determining Regional Geochemical Baselines. Environ. Geol. 30, 34–45. doi: 10.1007/s002540050130
Covelli S., Fontolan G., Faganeli J., Ogrinc N. (2006). Anthropogenic Markers in the Holocene Stratigraphic Sequence of the Gulf of Trieste (Northern Adriatic Sea). Mar. Geol. 230, 29–51. doi: 10.1016/j.margeo.2006.03.013
Crane J. L., Bijak A. L., Maier M. A., Nord M. A. (2021). Development of Current Ambient Background Threshold Values for Sediment Quality Parameters in U.S. Lakes on a Regional and Statewide Basis. Sci. Total Environ. 793, 148630. doi: 10.1016/j.scitotenv.2021.148630
Croudace I. W., Romano E., Ausili A., Bergamin L., Rothwell R. G. (2015). “X-Ray Core Scanners as an Environmental Forensic Tool: A Case Study of Polluted Harbor Sediment (Augusta Bay, Sicily),” in Micro-XRF Studies of Sediment Core. Eds. Croudace I. W., Rothwell R. G. (Berlin, Germany: Springer), 393–421.
De Vivo B., Bove M. A., Lima A., Albanese S., Cicchella D., Grezzi G., et al. (2009). Atlante Geochimico-Ambientale D’italia (Rome:Aracne Editrice), 512 pp.
De Vivo B., Lima A., Bove M. A., Albanese S., Cicchella D., Sabatini G., et al. (2008). Environmental Geochemical Maps of Italy From the FOREGS Database. Geochem. Explor. Env. A 8, 267–277. doi: 10.1144/1467-7873/08-175
Doglioni C., Flores G. (1997). “Italy,” in Encyclopedia of European and Asian Regional Geology. Eds. Moores E. M., Fairbridge R. W. (New York, USA: Chapman & Hall), 414–435.
Dung T. T. T., Cappuyns V., Swennen R., Pung N. K. (2013). From Geochemical Background Determination to Pollution Assessment of Heavy Metals in Sediments and Soils. Rev. Environ. Sci. Biotechnol. 12, 335–353. doi: 10.1007/s11157-013-9315-1
Ferrara R., Mazzolai B., Edner H., Svanberg S., Wallinder E. (1998). Atmospheric Mercury Sources in the Mt. Amiata Area, Italy. Sci. Total Environ. 213, 13–23. doi: 10.1016/S0048-9697(98)00067-9
Fornasaro S., Morelli G., Rimondi V., Fagotti C., Friani R., Lattanzi P., et al. (2022). Mercury Distribution Around the Siele Hg Mine (Mt. Amiata District, Italy) Twenty Years After Reclamation: Spatial and Temporal Variability in Soil, Stream Sediments, and Air. J. Geochem. Explor. 232, 106886. doi: 10.1016/j.gexplo.2021.106886
Galuszka A. (2007). A Review of Geochemical Background Concepts and an Example Using Data From Poland. Environ. Geol. 52, 861–870. doi: 10.1007/s00254-006-0528-2
Galuszka A., Migaszewski Z. M. (2011). Geochemical Background – An Environmental Perspective. Mineralogia 42 (1), 7–17. doi: 10.2478/v10002-011-0002-y
Garrett R. G., Reimann C., Smith D. B., Xie X. (2008). From Geochemical Prospecting to International Geochemical Mapping: A Historical Overview. Geochem. Explor. Env. A 8, 205–217. doi: 10.1144/1467-7873/08-174
Giglioli S., Colombo L., Contestabile P., Musco L., Armiento G., Somma R., et al. (2020). Source Apportionment Assessment of Marine Sediment Contamination in a Post-Industrial Area (Bagnoli, Naples). Water 12, 2181. doi: 10.3390/w12082181
Gough L. P. (1993). Understanding Our Fragile Environment. Lessons From Geochemical Studies. US Geological Survey Circular 1105, 1–34. doi: 10.3133/cir1105
Govett G. J. S. (1983). Rock Geochemistry in Mineral Exploration (Amsterdam, Netherland: Elsevier), 461 pp.
Grassi S., Netti R. (2000). Sea Water Intrusion and Mercury Pollution of Some Coastal Aquifers in the Province of Grosseto (Southern Tuscany - Italy). J. Hydrol. 237, 198–211. doi: 10.1016/S0022-1694(00)00307-3
Guo Y., Yang S. (2016). Heavy Metal Enrichment in the Changjiang (Yangtze River) Catchment and in the Inner Shelf of the East China Sea Over the Last 150 Years. Sci. Total Environ. 543, 105–115. doi: 10.1016/j.scitotenv.2015.11.012
Hakanson L. (1980). Ecological Risk Index for Aquatic Pollution Control. A Sedimentological Approach. Water Res. 14, 975–1001. doi: 10.1016/0043-1354(80)90143-8
Hawkes H. E. (1957). Principles of Geochemical Prospecting. Geological Survey Bull. 1000-F, 225–355.
Kersten M., Smedes F. (2002). Normalization Procedures for Sediment Contaminants in Spatial and Temporal Trend Monitoring. J. Environ. Monit. 4, 109–115. doi: 10.1039/b108102k
Kowalewska G., Belzunce-Segarra M. J., Schubert B., Heininger P., Heise S. (2011). “The Role of Sediments in Coastal Monitoring,” in Chemical Marine Monitoring: Policy Framework and Analytical Trends. Eds. Quevauviller P., Roose P., Verreet G. (Hoboken, NJ. USA: John Wiley & Sons), 77–395.
Lattanzi P., Rimondi V., Chiarantini L., Colica A., Benvenuti M., Costagliola P., et al. (2017). Mercury Dispersion Through Streams Draining the Mt. Amiata District, Southern Tuscany, Italy. Proc. Earth Planetary Sci. 17, 468–471. doi: 10.1016/j.proeps.2016.12.118
Li Y. H., Schoonmaker J. (2003). “Chemical Composition and Mineralogy of Marine Sediments,” in Treatise on Geochemistry, Sediments, Diagenesis, and Sedimentary Rocks, vol. Volume 3 . Ed. Mackenzie F. T. (Oxford, UK: Elsevier-Pergamon), 1–35.
Liu W. X., Li X. D., Shen Z. G., Wang D. C., Wai O. W. H., Li Y. S. (2003). Multivariate Statistical Study of Heavy Metal Enrichment in Sediments of the Pearl River Estuary. Environ. Pollut. 121, 377–388. doi: 10.1016/S0269-7491(02)00234-8
Lopes-Rocha M., Langone L., Miserocchi S., Giordano P., Guerra R. (2017). Spatial Patterns and Temporal Trends of Trace Metal Mass Budgets in the Western Adriatic Sediments (Mediterranean Sea). Sci. Total Environ. 599-600, 1022–1033. doi: 10.1016/j.scitotenv.2017.04.114
Marmolejo-Rodriguez A. J., Cobelo-Garcia A., Prego R. (2007). Background Values, Distrubution and Contamination of Metals in the Sediments of Pontevedra Ria (NW Spain). Soil Sediment Contamination 16, 557–568. doi: 10.1080/15320380701623370
Matschullat J., Ottestein R., Reiman C. (2000). Geochemical Background - Can We Calculate It? Environ. Geol. 39, 990–1000. doi: 10.1007/s002549900084
Middleton R., Grant A. (1990). Heavy Metals in the Humble Estuary: Scrobicularia Clay as a Pre Industrial Datum. Proc. Yorkshire Geological Soc. 2, 108–118.
Ottonello G., Serva L. (2003). Geochemical Baselines of Italy. Ed. Pacini, + 1 CD–+ 1Rom, 294 (Pisa: Pacini).
Piazzolla D., Scanu S., Frattarelli F. M., Mancini E., Tiralongo F., Brundo M. V., et al. (2015). Trace-Metal Enrichment and Pollution in Coastal Sediments in the Northern Tyrrhenian Sea, Italy. Arch. Environ. Contam. Toxicol. 69 (4), 470–481. doi: 10.1007/s00244-015-0166-3
Protano G., Riccobono F., Sabatini G. (1998). La Cartografia Geochimica Della Toscana Meridionale. Criteri Di Realizzazione E Rilevanza Ambientale Attraverso Gli Esempi Di Hg, As, Sb, Pb E Cd. Memorie Descrittive della Carta Geologica d'Italia 55, 119–140.
Reimann C., Ladenberger A., Birke M., de Caritat P. (2015). Low Density Geochemical Mapping and Mineral Exploration: Application of the Mineral System Concept. Geochem. Explor. Env. A 16 (1), 48–61. doi: 10.1144/geochem2014-327
Reimann C., Matschullat J., Birke M., Salminen R. (2010). Antimony in the Environment - Lessons From Geochemical Mapping. Appl. Geochemistry 25, 175–198. doi: 10.1016/j.apgeochem.2009.11.011
Reimann C., Melezhik V. (2001). Metallogenic Provinces, Geochemical Provinces and Regional Geology – What Causes Large-Scale Patterns in Low-Density Geochemical Maps of the C-Horizon of Podzols in Arctic Europe? Appl. Geochemistry 16, 963–984.
Ricci Lucchi F., Mutti E. (1980). Sedimentologia - Parte III Ambienti Sedimentari E Facies, Clueb. (Bologna: Clueb), 545 pp.
Romano E., Bergamin L., Canepa M., Maggi C., Ausili A., Gruppo di Lavoro (2018). Definizione Delle Province Geochimiche a Mare E Dei Relativi Valori Di Fondo Nei Sedimenti Marini. Linee Guida SNPA 06/18. (Rome: Ispra), 134 pp.
Romano E., Bergamin L., Croudace I. W., Ausili A., Maggi C., Gabellini M. (2015). Establishing Geochemical Background Levels of Selected Trace Elements in Areas Having Geochemical Anomalies: The Case Study of the Orbetello Lagoon (Tuscany, Italy). Environ. Pollut. 202, 96–103. doi: 10.1016/j.envpol.2015.03.017
Romano E., Bergamin L., Croudace I. W., Pierfranceschi G., Sesta G., Ausili A. (2021). Measuring Anthropogenic Impacts on an Industrialised Coastal Marine Area Using Chemical and Textural Signatures in Sediments: A Case Study of Augusta Harbour (Sicily, Italy). Sci. Total Environ. 755, 142683. doi: 10.1016/j.scitotenv.2020.142683
Romano E., Bergamin L., Pierfranceschi G., Maggi C., Berducci M. T., Ausili A. (2020). Conflicting Outcomes of an Integrated Approach to Sediment Quality Assessment in a Sardinian Coastal Area Subjected to Mining Activities. J. Soils Sediments 20, 2630–2640. doi: 10.1007/s11368-019-02489-y
Romano E., De Giudici G., Bergamin L., Andreucci S., Maggi C., Pierfranceschi G., et al. (2017). The Marine Sedimentary Record of Natural and Anthropogenic Contribution From the Sulcis-Iglesiente Mining District (Sardinia, Italy). Mar. Pollut. Bull. 122, 331–343. doi: 10.1016/j.marpolbul.2017.06.070
Rose A. W., Hawkes H. E., Webb J. S. (1979). Geochemistry in Mineral Exploration. 2nd ed (London: Academic Press), 657 pp.
Rothwell G., Croudace I. W. (2015a). “Micro-XRF Studies of Sediment Cores: A Perspective on Capability and Application in Environmental Sciences,” in Micro-XRF Studies of Sediment Cores, Chapter 1. Eds. Croudace I. W., Guy Rothwell R., (Berlin: Springer), 1–21.
Rothwell G., Croudace I. W. (2015b). “Twenty Years of XRF Core Scanning Marine Sediments: What do Geochemical Proxies Tell Us?,” in Micro-XRF Studies of Sediment Cores, Chapter 2. Eds. Ian W., Croudace, Guy Rothwell R., (Berlin: Springer), 25–102.
Salminen R., Batista M. J., Demetriades A., Lis J., Tarvainen T. (2005). Geochemical Atlas of Europe. Part 1 - Background Information, Methodology and Maps (Helsinki: Geological Survey of Finland, Espoo)ISBN 951-690-921-3.
Sarti G., Sammartino I., Amorosi A. (2020). Geochemical Anomalies of Potentially Hazardous Elements Reflect Catchment Geology: An Example From the Tyrrhenian Coast of Italy. Sci. Total Environ. 714, 136870. doi: 10.1016/j.scitotenv.2020.136870
Shahabi-Ghahfarokhi S., Åstrom M., Josefsson S., Apler A., Ketzer M. (2021). Background Concentrations and Extent of Cu, As, Co, and U Contamination in Baltic Sea Sediments. J. Sea Res. 176, 102100. doi: 10.1016/j.seares.2021.102100
Smith D. B., Reimann C. (2008). Low-Density Geochemical Mapping and the Robustness of Geochemical Pattern. Geochem. Explor. Env. A 8, 219–227. doi: 10.1144/1467-7873/08-171
Spagnoli F., Dinelli E., Giordano P., Marcaccio M., Zaffagnini F., Frascari F. (2014). Sedimentological, Biogeochemical and Mineralogical Facies of Northern and CentralWestern Adriatic Sea. J. Mar. Syst. 139, 183–203. doi: 10.1016/j.jmarsys.2014.05.021
Sprovieri M., Sammartino S., Salvagio Manta D., Marsella E., Ferraro L. (2006). Heavy Metals in Top Core Sediments From the Southern Campania Shelf (Italy): Hints to Define Large-Scale Geochemical Backgrounds. Chem. Ecol. 22 (1), 65–91. doi: 10.1080/02757540500456716
Surricchio G., Pompilio L., Arizzi Novelli A., Scamosci E., Marinangeli L., Tonucci L., et al. (2019). Evaluation of Heavy Metals Background in the Adriatic Sea Sediments of Abruzzo Region, Italy. Sci. Total Environ. 684, 445–457. doi: 10.1016/j.scitotenv.2019.05.350
Tanner P. A., Leong L. S., Pan S. M. (2000). Contamination of Heavy Metals in Marine Sediment Cores From Victoria Harbour, Hong Kong. Mar. Pollut. Bull. 40, 769–779. doi: 10.1016/S0025-326X(00)00025-4
Taylor S. R. (1964). Abundance of Chemical Elements in the Continental Crust: A New Table. Geochim. Cosmochim. Acta 28 (8), 1273–1285. doi: 10.1016/0016-7037(64)90129-2
Tomlinson D. C., Wilson J. G., Harris C. R., Jeffery D. W. (1980). Problems in the Assessment of Heavy Metals Levels in Estuaries and the Formation of a Pollution Index. Helgolander Wissenschaftliche Meeresuntersuchungen 33, 566–575. doi: 10.1007/BF02414780
Trifuoggi M., Donadio C., Mangoni O., Ferrara L., Bolinesi F., Nastro R. A., et al. (2017). Distribution and Enrichment of Trace Metals in Surface Marine Sediments in the Gulf of Pozzuoli and Off the Coast of the Brownfield Metallurgical Site of Ilva of Bagnoli (Campania, Italy). Mar. Pollut. Bull. 136, 374–384. doi: 10.1016/j.marpolbul.2018.09.034
Turekian K. K., Wedepohl K. H. (1961). Distribution of the Elements in Some Major Units of the Earth’s Crust. Geol. Soc A. Bull. 72, 175–192. doi: 10.1130/0016-7606(1961)72[175:DOTEIS]2.0.CO;2
Wedepohl K. H. (1995). The Composition of the Continental Crust. Geochim. Cosmochim. Acta 59 (7), 1217–1232. doi: 10.1016/0016-7037(95)00038-2
Wyborn L. A. I., Heinrich C. A., Jaques A. L. (1994). “Australian Proterozoic Mineral Systems: Essential Ingredients and Mappable Criteria,” in The Australian Institute of Mining and Metallurgy (AusIMM) Annual Conference, Darwin, 5-9 August 1994. 109–115.
Xu B., Yang X., Gu Z., Zhang Y., Chen Y., Lv Y. (2009). The Trend and Extent of Heavy Metal Accumulation Over Last One Tundre Years in the Liadong Bay, China. Chemosphere 75, 442–446. doi: 10.1016/j.chemosphere.2008.12.067
Keywords: background values, geochemical provinces, marine sediments, sediment cores, sediment contamination
Citation: Romano E, Ausili A and Bergamin L (2022) Coastal Marine Geochemical Provinces and Background Values in Sediments: A Methodological Approach. Front. Mar. Sci. 9:874248. doi: 10.3389/fmars.2022.874248
Received: 11 February 2022; Accepted: 25 April 2022;
Published: 23 May 2022.
Edited by:
Hans Uwe Dahms, Kaohsiung Medical University, TaiwanReviewed by:
Javier Franco, AZTI, SpainCopyright © 2022 Romano, Ausili and Bergamin. This is an open-access article distributed under the terms of the Creative Commons Attribution License (CC BY). The use, distribution or reproduction in other forums is permitted, provided the original author(s) and the copyright owner(s) are credited and that the original publication in this journal is cited, in accordance with accepted academic practice. No use, distribution or reproduction is permitted which does not comply with these terms.
*Correspondence: Elena Romano, ZWxlbmEucm9tYW5vQGlzcHJhbWJpZW50ZS5pdA==
Disclaimer: All claims expressed in this article are solely those of the authors and do not necessarily represent those of their affiliated organizations, or those of the publisher, the editors and the reviewers. Any product that may be evaluated in this article or claim that may be made by its manufacturer is not guaranteed or endorsed by the publisher.
Research integrity at Frontiers
Learn more about the work of our research integrity team to safeguard the quality of each article we publish.