- 1Tvärminne Zoological Station, University of Helsinki, Hanko, Finland
- 2Baltic Sea Centre, Stockholm University, Stockholm, Sweden
- 3Department of Biology, Miami University, Oxford, OH, United States
Benthic consumers profoundly impact nutrient regeneration in coastal marine ecosystems. The concurrent nutrient imbalance and warming of our coastal seas will change the nutritional requirements and metabolic demands of these consumers, which may affect their ability to recycle nitrogen and phosphorous. Here we explore whether nutrient excretion rates of two benthic consumers, the Baltic clam (Macoma balthica) and the invasive spionid polychaete (Marenzelleria spp.) can be quantified with basic biological traits across seasons using allometric and stoichiometric relationships. We found species-specific N and P excretion rates that positively link to allometric traits, i.e., per individual rates increased with body mass and temperature; thus, high mass-specific excretion rates characterized small relative to large macrofaunal individuals. Interestingly, our body size scaling coefficients diverge from predictions by the metabolic theory of ecology (MTE) and the universal model of excretion. Furthermore, stoichiometric traits and stable isotope signatures (δ 13C and δ 15N) explained a minor additional proportion of variability in excretion rates among species. The excretion rates also varied strongly seasonally, with the highest nutrient recycling rates during summer months, when community NH4-N and PO4-P excretion clearly exceeded net sediment efflux. The seasonal pattern emphasized that changes in temperature and food availability drove metabolic processes and thus excretion rates of the benthic consumers, and indicated that these effects could outweigh the importance of animal biomass. Our results highlight the benefits of using allometric and stoichiometric traits when quantifying species-specific contributions to nutrient recycling in coastal marine environments, and in predicting alteration of function in response to environmental change.
Introduction
The functional trait characteristics of organisms in combination with the environment define ecosystem process rates (Villnäs et al., 2013; Atkinson et al., 2017). By merging ecological stoichiometry with the metabolic theory of ecology (MTE), recent research has provided mechanisms to quantify the influence of consumers on elemental recycling rates on an ecosystem level (Meunier et al., 2017). Still, few studies have addressed the applicability of these theories in resolving species contribution to ecosystem functions within and across taxa under differing environmental conditions (Allgeier et al., 2015; Atkinson et al., 2017, but see Sharitt et al., 2021).
Diverse assemblages of benthic consumers underpin nutrient recycling, a fundamental process of marine ecosystems. The acceleration of human impacts that alter the elemental balance (Carnicer et al., 2015, Peñuelas et al., 2020), temperature, and biodiversity of our coastal seas (Lotze et al., 2006; Cloern et al., 2016), may substantially alter the role of benthic consumers in biogeochemical cycling. Benthic fauna can affect ecosystem nutrient cycling indirectly through bioturbation, and directly through feeding, by retaining nutrients in their tissues, and by supplying nutrients via excretion and egestion (Vanni, 2002). Although many studies, including laboratory experiments (Norling et al., 2007), ex situ (Kauppi et al., 2017; Gammal et al., 2019) and in situ incubations (Villnäs et al., 2013; Norkko et al., 2013) confirm the contribution of benthic biodiversity and traits to sediment nutrient fluxes, they yield frequently complex and context-dependent results, both within and among ecosystems (Gammal et al., 2019). This inconsistency emphasizes the need to quantify the direct contribution of benthic consumers to nutrient recycling in coastal marine habitats, to predict how environmental change alters their impact across temporal and spatial scales (Snelgrove et al., 2014; Lohrer et al., 2015; Vanni & McIntyre, 2016; Allgeier et al., 2017). In this study we show that excretion rates of benthic communities, which represent important fluxes that affect ecosystem-level nutrient dynamics (Atkinson et al., 2017), can be quantified with basic biological traits when using allometric and stoichiometric relationships.
Theoretical ecological frameworks can help to predict the physiological process of excretion of inorganic nutrients, such as ammonium (NH4+) and phosphate (PO43-) (Vanni et al., 2002). The metabolic theory of ecology (MTE; Gillooly et al., 2001; Brown et al., 2004), predicts that consumer metabolic rates, including excretion rates, scale predictably with body size and temperature, thus expressing an allometric relationship (Vanni et al., 2002; Allen & Gillooly, 2009). The allometric scaling of excretion (E) to body mass (M) is expressed as E=aMb, where a and b are constants (Hall et al., 2007). The constant b, often called the allometric scaling coefficient, is < 1 for most aquatic animals as predicted by theory (Gillooly et al., 2001), but varies greatly among taxa (Vanni and McIntyre, 2016). This value implies that excretion rates do not increase proportionally to body size, instead smaller animals should have higher excretion rate per body mass unit (i.e., mass-specific excretion rates) than larger conspecifics (Vanni, 2002; Hall et al., 2007; Alves et al., 2010). Hence, when the biomass of two different communities are the same, a community dominated by many small individuals should exhibit higher excretion rates compared to one where large individuals dominate (Hall et al., 2007). Moreover, for poikilotherms, metabolism and hence excretion rates are temperature dependent and increase as temperatures rise (Allen & Gillooly, 2009).
In addition to MTE, ecological stoichiometry, which describes the balance of elements in an organism and related ecological processes (Sterner and Elser, 2002), likely affects species’ excretion rates. The theory of ecological stoichiometry suggests that animals should be homeostatic in body nutrient composition, at least over short time scales (Persson et al., 2010; but see e.g. Liess & Hillebrand, 2005, Mäkelin and Villnäs 2022). Therefore, the rates and ratios at which animals excrete and egest nutrients should reflect any differences in nutrient composition between animals and their food sources. Whereas the C:N and C:P ratios of consumer tissues can reflect the balance of energy versus nutrient limitation, the N:P ratio of consumers should be directly relevant for predicting excretion rates of these two elements and the ratio at which they are excreted (Frost et al., 2006; Alves et al., 2010; Vanni & McIntyre, 2016). For example, the N:P excretion ratio could potentially be higher in small consumers, because of the anticipated lower N:P ratio in their tissues; small animals often grow faster, increasing their demand for P (allocated to ribosomal RNA during rapid growth; growth rate hypothesis; Elser et al., 2003).
Recent comprehensive data syntheses regarding excretion rates by aquatic animals show that metabolic parameters, such as body mass and temperature, perform better than stoichiometric parameters in predicting animal excretion rates (Allgeier et al., 2015; Vanni & McIntyre, 2016). Notably, these studies also found strong species identity effects on nutrient recycling (Vanni et al., 2002; Allgeier et al., 2015; Vanni & McIntyre, 2016), presumably reflecting species-specific nutrient requirements as well as on ontogenetic shifts in dietary nutrients (Isaac and Carbone, 2010; Allgeier et al., 2015). Stable isotope signals (δ13C and δ15N) of consumer tissues may mirror changes in diets, where δ13C responds to the carbon (energy) source for the organism while δ15N reflects the trophic position of the consumer, which is typically enriched 3.4 ‰ relative to its diet (Post, 2002; Fry, 2006). Following “you are what you eat less excrete” (Fry, 2006), variation in tissue stable isotope signatures can potentially influence both inter- and intraspecific excretion rates, although isotopic composition typically integrates variation in diet over a longer period of time than the time scale of most excretion rate estimates.
The observed intra- and interspecific variation in excretion rates points to the importance of identifying biological traits responsible for changes in animal-driven nutrient dynamics (cf. Enquist et al., 2016; Meunier et al., 2017). In this study, we explore the excretion rates of two dominant species within the Baltic Sea, the bivalve Macoma balthica (Linnaeus 1758) and the invasive polychaete Marenzelleria spp. (Mesnil 1896). We hypothesized that the excretion rates of these consumers would be species-specific and increase with body size, but with an allometric scaling coefficient <1, resulting in higher mass-specific excretion rates in smaller animals. We compared the N and P excretion rates of M. balthica and Marenzelleria spp. to the universal model of excretion rates developed by Vanni & McIntyre (2016). We further explored whether excretion rates and ratios change between seasons, and if variation in allometric or stoichiometric traits or differences in stable isotope signatures of animal tissue could explain such changes. Finally, we explored the magnitude of excreted N and P at the community level, and compared the results with overall sediment nutrient fluxes measured with ex-situ incubations in July.
Methods
Study Site
Our two study sites were situated in the south-western Finnish archipelago, in the brackish-water, non-tidal Baltic Sea. The sites were sampled in July, August, October and January, when bottom water temperatures were 8°C, 18°C, 12°C and 4°C, respectively (Mäkelin & Villnäs 2022, Table S1). Station XXVI (20 m depth) is situated in a relatively open, dynamic archipelago with a connection to the open sea. Upwelling events that bring in nutrient-rich water from deeper areas in the Gulf of Finland occasionally affect this station (Kauppi et al., 2018a). The other site, IH4 (18 m depth) is situated between islands and borders a deeper area that can experience strong seasonal stratification and periodic oxygen deficiency (see Gammal et al., 2017 for geographic positions; XXVI is O3). However, during all sampling occasions at the stations, we observed similar bottom water salinity (5.5-6.5), and oxygenated conditions (>7.8 mg/l; Table S1). Seasonal differences were most apparent in primary production, with reduced Chl a values in surface waters in January (0.3 µg l-1) compared to the other sampling occasions (3.2-7.6 µg l-1; Table S1, Mäkelin & Villnäs 2022). Silty sediments with a surface organic matter content ranging between 6-12.5% characterize both sites (averaging 7.6 ± 1.5% at XXVI and 11.7 ± 0.6% at station IH4, respectively, Table S1). Generally higher carbon and nitrogen content (C:N:P 106:12:1) characterized the uppermost sediment layer (0-1 cm) at the latter site compared to XXVI (C:N:P 73:8:1). In contrast, sediment P content was similar at both sites (0.15 ± 0.02% at IH4 versus 0.14 ± 0.4% at XXVI, percent of dry mass; Table S1).
The tellenid bivalve Macoma balthica dominates the benthic community at both stations (representing, in general, >50% of total macrofaunal abundance and >90% of total dry biomass, that is >60% if excluding its shell). The invasive spionid polychaete complex, Marenzelleria spp. accounts for 6-23% of community abundance, while its contribution to total community biomass varies between 0-3%, or 2-8% when excluding bivalve shells (Figure S1 and Figure 4). M. balthica has high biomass and abundance within the Baltic Sea (Bonsdorff et al., 1995). This facultative deposit- and suspension-feeder (Rossi et al., 2004) plays a key role in ecosystem processes such as energy transfer in the benthic food web and in sediment biogeochemical cycling (Norkko et al., 2013). The invasive spionid polychaete, Marenzelleria spp. was introduced to the southern Baltic Sea in 1985 and has rapidly spread to become a dominant species throughout the entire Baltic Sea (Kauppi et al., 2015). Three species, M. viridis (Verrill 1873), M. neglecta (Sikorski and Bick 2004), and M. arctica (Chamberlin 1920) have been reported in our study area, where these burrowing, sub-surface deposit feeding polychaetes can occur in high densities (Kauppi et al., 2018a).
Nutrient Flux Measurements
We measured nutrient excretion (NH4-N, PO4-P), body (dry) mass and tissue carbon (C), nitrogen (N) and phosphorous (P) concentrations as well as stable isotope signatures (δ13C and δ15N) primarily of M. balthica and Marenzelleria spp. during four different sampling occasions at the two stations. We also measured excretion rates of less dominant species (the priapulid Halicryptus spinulosus, the isopod Saduria entomon, the amphipod Monoporeia affinis and Chironomidae) in the community if they occurred during a sampling event. Benthic sampling utilized a box corer, and we collected three replicate samples to account for variation in benthic abundance and biomass at the sites. The sediment was sieved (0.5 mm) and animals picked for excretion, elemental content, and isotope analyses were gently rinsed before incubating them in 110 ml of filtered seawater.
The animals were kept in dark conditions at in situ temperatures during the excretion incubations (using climate controlled rooms). It is possible that the incubations stressed the animals, noting they had no sediment available or time to acclimate. Therefore our measured rates may represent their maximum excretion potential. For M. balthica, we performed a minimum of nine incubations per sampling time and station, and at least five replicate measurements for Marenzelleria spp. When incubating several animals together, we selected individuals of similar size. We separated M. balthica into the following size classes; <0.5, 0.5-0.9, 1.0-1.4, 1.5-1.9, ≥2 cm shell width, and determined the size class of the polychaete Marenzelleria spp. by measuring the width of the 5th segment; small (<0.9 mm), medium (ca 1 mm), large (>1.1 mm). Although we incubated large individuals of M. balthica (> 2 cm) separately, we grouped up to 35 juveniles (<0.5 cm) (Mäkelin & Villnäs 2022). As a control treatment, we incubated M. balthica shells separately to evaluate the effect of any biofilm on water nutrient concentrations. Before and after 1 to 1.5 h of incubation, the water was sampled with a syringe and filtered (GF/F) to remove feces and pseudofeces prior to freezing (-20°C), pending analyses of excreted dissolved nitrogen (N as NH4-N) and phosphorous (P as PO4-P). Ammonium was determined manually by a spectrometric procedure, following the ISO 7150/1-1984 method. Phosphate determination used the antimony-molybdate method (Koroleff, 1983) on a Thermo Scientific Aquakem 250 analyzer.
After collecting water samples for excretion rate measurements, the animals remained in filtered seawater for a total of 24 h, to allow them to empty their guts. The animals were frozen (-70°C) before dissection to remove bivalve shells. After freeze drying (-60°C during 24h), we measured the dry weights and prepared the specimens for elemental and stable isotope (δ13C and δ15N) analyses as described in Mäkelin and Villnäs (2022).
Additionally, in July, we incubated four intact sediment cores at each sampling site to measure sediment oxygen consumption and solute fluxes (NH4-N, (NO3-+NO2-)-N, PO4-P) across the sediment-water interface. Following collection of split cores (ø 90 mm, obtained with a Gemax twincorer), we sealed the upper section of the cores for use as a flux chamber, incubating the cores for 4 h in darkness at in situ temperature conditions under constant stirring. Water samples obtained at the start and end of the incubation were analyzed for oxygen concentration (Winkler procedure) and for dissolved nutrients (as described above). Differences in concentration between start and end were used to calculate the sediment oxygen and nutrient fluxes (mol m-2 h-1). For further description of the method, see Gammal et al. (2016). After incubation, we sieved the sediment (0.5 mm) and determined the abundance and biomass of the benthic animals in the cores.
Statistical Analyses
Variation in benthic tissue C:N:P content and stable isotope signatures (δ13C and δ15N) between the two species, the two sites and between the four sampling times was analyzed with a three-way permutational analysis of variance (PERMANOVA, PRIMER v7, Anderson et al., 2008) and illustrated with box-plots diagrams. For further information on variation in stoichiometric traits at the study sites see Mäkelin and Villnäs (2022). We calculated excretion rates as the difference between final and initial nutrient concentrations per hour, expressed either per individual (µmol N or P ind-1 h-1) or per dry mass (µmol N or P g-1 h-1). The excretion calculations did not consider the effect of the control incubations (M. balthica shells) because we most often detected only minor changes in nutrient concentrations. Thus, effects of shell biofilm on water nutrient concentrations are included in our results. A three-way permutational analysis of variances (PERMANOVA, PRIMER v7; Anderson et al., 2008) with individual dry mass (g) as co-variate explored differences in excretion rates between species, sites and among sample dates, allowing for interactions. We did not assume homogeneity of slopes because the model included the interaction term, explicitly allowing for different slopes for different levels of the factor (Anderson et al., 2008). Pairwise post-hoc tests pinpointed differences between sampling occasions. A three-way PERMANOVA ensured detection of similar patterns for biomass-corrected excretion rates. Prior to the analyses, PERMDISP tests checked homogeneity of dispersions.
We compared our measured excretion rates for M. balthica and Marenzelleria spp. to estimates obtained using the universal excretion model by Vanni & McIntyre (2016). By applying an extensive global data set to explore excretion rates of aquatic consumers (marine and freshwater), Vanni and McIntyre (2016) found that body mass, water temperature and trophic guild likely yield sufficient general excretion estimates for invertebrates. Specifically, we used the equation for invertebrates feeding on algae or detritus; log10 (N excretion µg ind-1 h-1) = 1.4610 + 0.6840 * log10 (dry mass) + 0.0246 * Temperature (°C) - 0.0389, whereas log10 (P excretion µg ind-1 h-1) = 0.6757 + 0.5656 * log10 (dry mass) + 0.0194 * Temperature (°C) + 0.0173 (for further details see Vanni & McIntyre, 2016). The first factor describes the intercept and the last accounts for the trophic guild of the animal. Note that dry mass for bivalves should exclude the shell (Vanni & McIntyre, 2016).
Distance-based linear models (DistLM in PERMANOVA+ for PRIMER v7, Anderson et al., 2008) determined the importance of variation in allometric (biomass, temperature) and stoichiometric (tissue C, N, P % and ratios) traits as well as stable isotope signatures (δ13C, δ15N) on excretion rates of N and P (µmol ind-1 h-1). An average value per size class of each species at a sampling site and time represented the stoichiometric parameters and stable isotope signatures. Log10 transformations of the predictors reduced skewness. To explore whether predictors varied in importance between the two species we analyzed them together as well as separately.
Finally, we calculated a maximum community excretion potential (µmol NH4-N or PO4-P m-2 h-1) using the biomass-corrected excretion rates for the six dominant species at the two sites (M. balthica, Marenzelleria spp., H. spinulosus, S. entomon, M. affinis and Chironomidae), which comprised over 90% of the total biomass at each sampling occasion. The average excretion rate per size class (µmol g-1 h-1) of a species at a sampling occasion was multiplied by the corresponding biomass per replicate sample. Differences between sites and time-points were analysed with PERMANOVA as described above. In addition, in July we calculated excretion rates for the fauna within the incubated cores and compared their excretion to total sediment nutrient fluxes.
Results
Stoichiometric Traits and Stable Isotope Signatures of the Benthic Animals
Overall, the bivalve M. balthica had a slightly higher tissue carbon content and lower nitrogen and phosphorous content (on average C: 43.3 ± 3.0, N: 8.5 ± 1.3, P: 0.7 ± 0.3% of dry mass) compared to Marenzelleria spp. (C: 42.2 ± 2.8, N: 9.9 ± 0.7, P: 0.8 ± 0.2%, Table S2; p<0.05, for further information, see Mäkelin & Villnäs 2022). Hence, we observed higher C:N and C:P ratios on average for the bivalve, but detected no difference in body N:P ratio among the species (Figure S2 and Table S2; p>0.05). The C:N ratios correlate significantly and positively with body mass, and show significant seasonal variation, with lower C:N and C:P ratios during winter months (Figure S2 and Table S2, Mäkelin & Villnäs 2022). Values of δ15N yielded the most pronounced difference in stable isotope signatures between the two species, with significantly lower values in M. balthica (on average δ15N: 8.0 ± 0.5) compared to the polychaete (δ15N: 9.8 ± 0.6, Figure S2 and Table S2). Seasonal differences in δ13C were also noted, with slightly lower δ13C values measured in July (Figure S2; Mäkelin & Villnäs 2022).
Inter- and Intraspecific Differences in Excretion Rates
The individual excretion rates of N and P co-varied (Table 1) and increased with body mass for both species (Figures 1A, B). Not surprisingly, given its larger size range, greater variation in excreted nutrients per individual characterized M. balthica compared to Marenzelleria spp. (PERMDISP p<0.01 for N excretion ind.-1 h-1). Nonetheless, we detected significant differences in N and P excretion rates between the two species, both when considering individual (Table 1 and Figures 1A, B) and mass-specific nutrient excretion rates (PERMDISP; p>0.05, PERMANOVA; p<0.001, Table S3). Marenzelleria spp. mass-specific excretion rates exceeded those in the bivalve (cf. Figure 1, Table S3), and although the average N:P excretion ratio was highly variable, it was slightly lower for the polychaete compared to the bivalve (Figure 1C and Table 1). We found no significant relationships between the excreted N:P ratio vs. body mass (Figure 1C) or tissue N:P ratio considering both species (Pearson correlation p>0.5). However, we noted an overall difference in excreted N:P between sites, with slightly higher ratios at site XXVI compared to IH4 (Table 1).
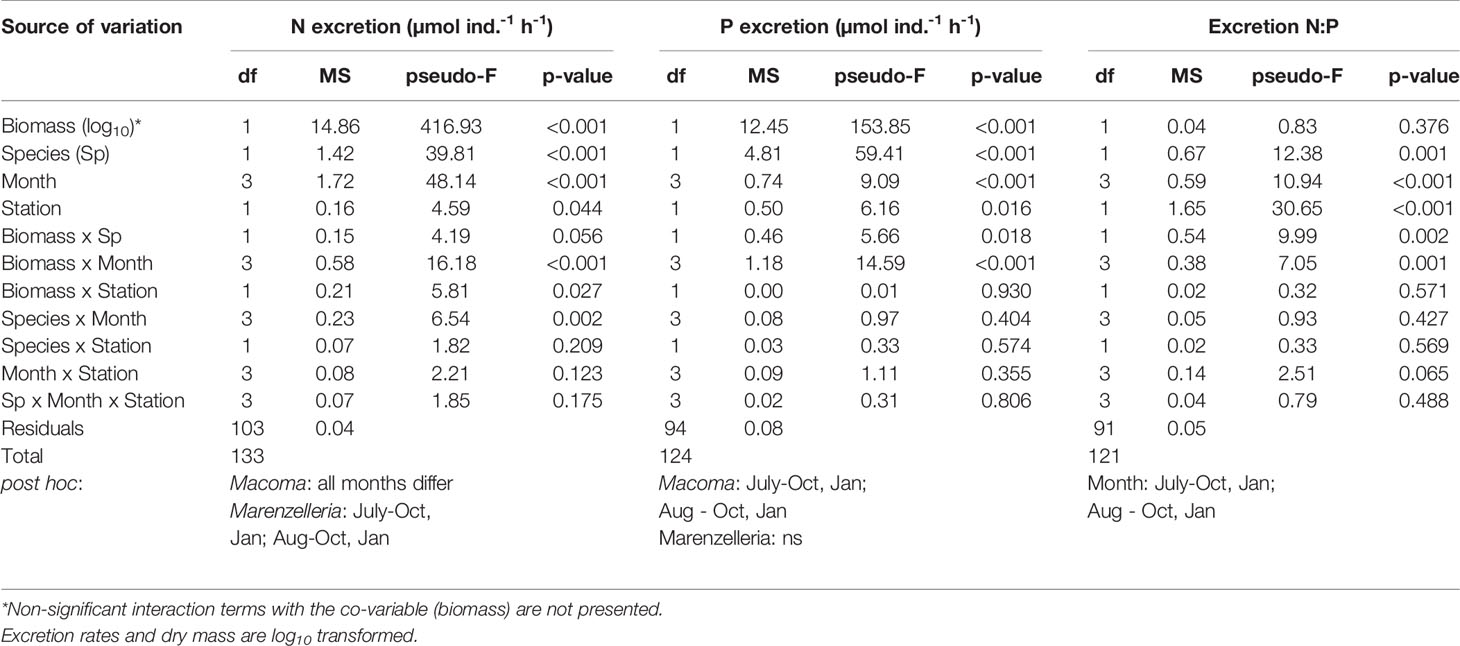
Table 1 Three-way PERMANOVA with individual biomass (dw, g) as co-variate exploring differences in N and P excretion rates (µmol ind.-1 h-1) between species, seasons (month) and sampling stations.
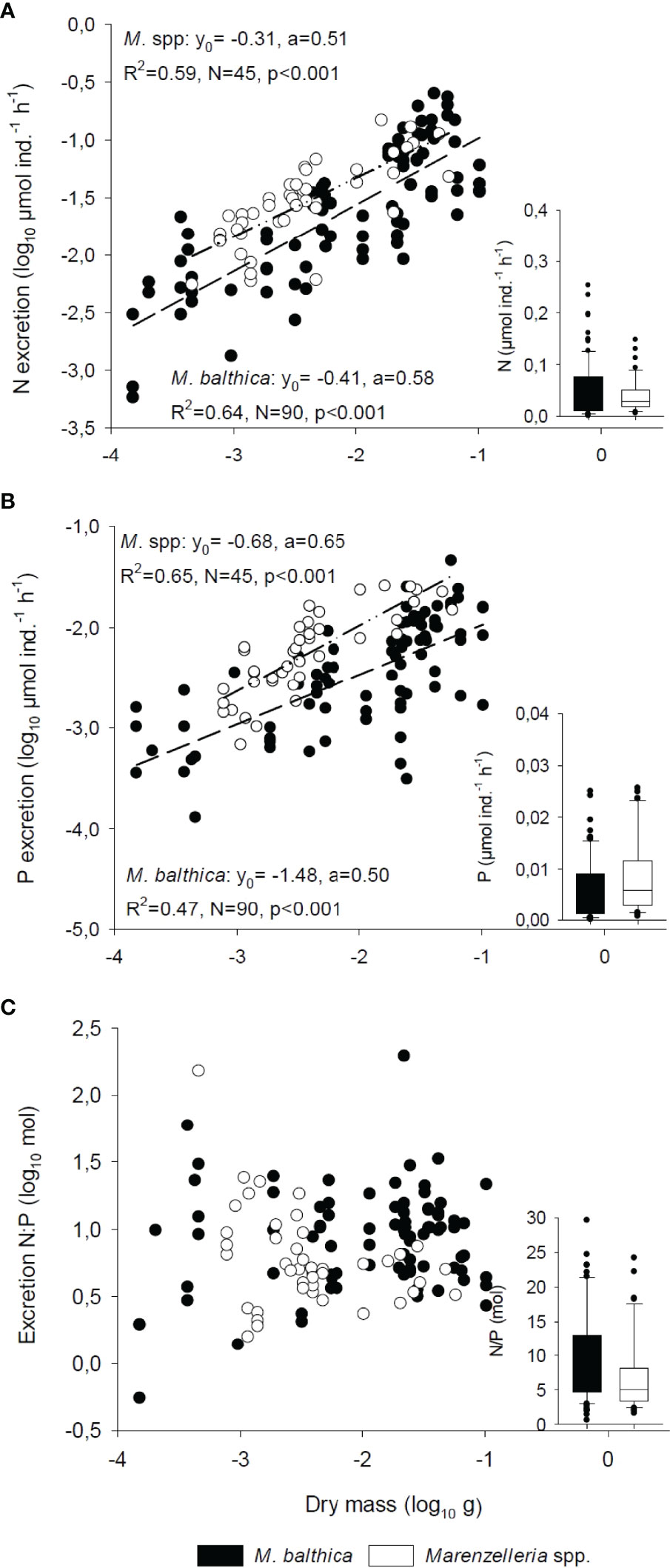
Figure 1 Linear regression models (f = y0+a*x) between excretion rates of (A) nitrogen and (B) phosphorous (log10 µmol ind.-1 h-1) versus individual dry mass (log10 g) for M. balthica and Marenzelleria spp. In (C), the excretion N:P ratio (log10 mol) is plotted against dry mass. The box plot insert in each graphs show the median, the 25th and 75th percentile and error of excretion rates (µmol ind.-1 h-1) and ratio (N:P) for each species.
We found species-specific seasonal differences in excretion rates of both N and P (Table 1, Table S3 and Figures 2A, B). For N excretion, all time-points differed significantly for M. balthica (post hoc p<0.05), whereas the greatest differences for Marenzelleria spp. occurred between the summer (July-August) vs. winter (October-January) months (Table 1 and Table S3). Monthly regressions between N excretion rates (µmol ind.-1 h-1) and dry mass showed the highest slopes in July, whereas the intercept decreased from summer to winter (Table S4). We also detected positive slopes between individual N excretion rates and tissue N content (July: R2 = 0.41, p<0.05, August: R2 = 0.22, p<0.01, October: R2 = 0.58, p<0.001, January R2 = 0.34 p<0.001), whereas the intercept showed a notable decrease from summer to winter months (Figure 2C). Overall, patterns in P excretion rates resembled those in N over time (Figure 2B and Table 1). The highest P excretion rates of M. balthica occurred in summer, with significantly lower rates in January compared to both August and July (Table 1 and Figure 2B). Interestingly, post hoc comparisons detected no seasonal differences in P excretion of Marenzelleria spp. (Table 1). The slopes and intercepts of monthly regressions between individual P excretion rates and body mass decreased from summer to winter (Table S4). We did not observe strong relationships between P excretion rate and P tissue content (Figure 2D), detecting only a weak positive trend in July (R2 = 0.2, p<0.05) and a negative relationship in January (R2 = 0.39, p<0.001) and non-significant relationships for August and October (p>0.05). The excreted N:P ratio was, overall, slightly higher in July and August compared to October and January for the two species (Table 1).
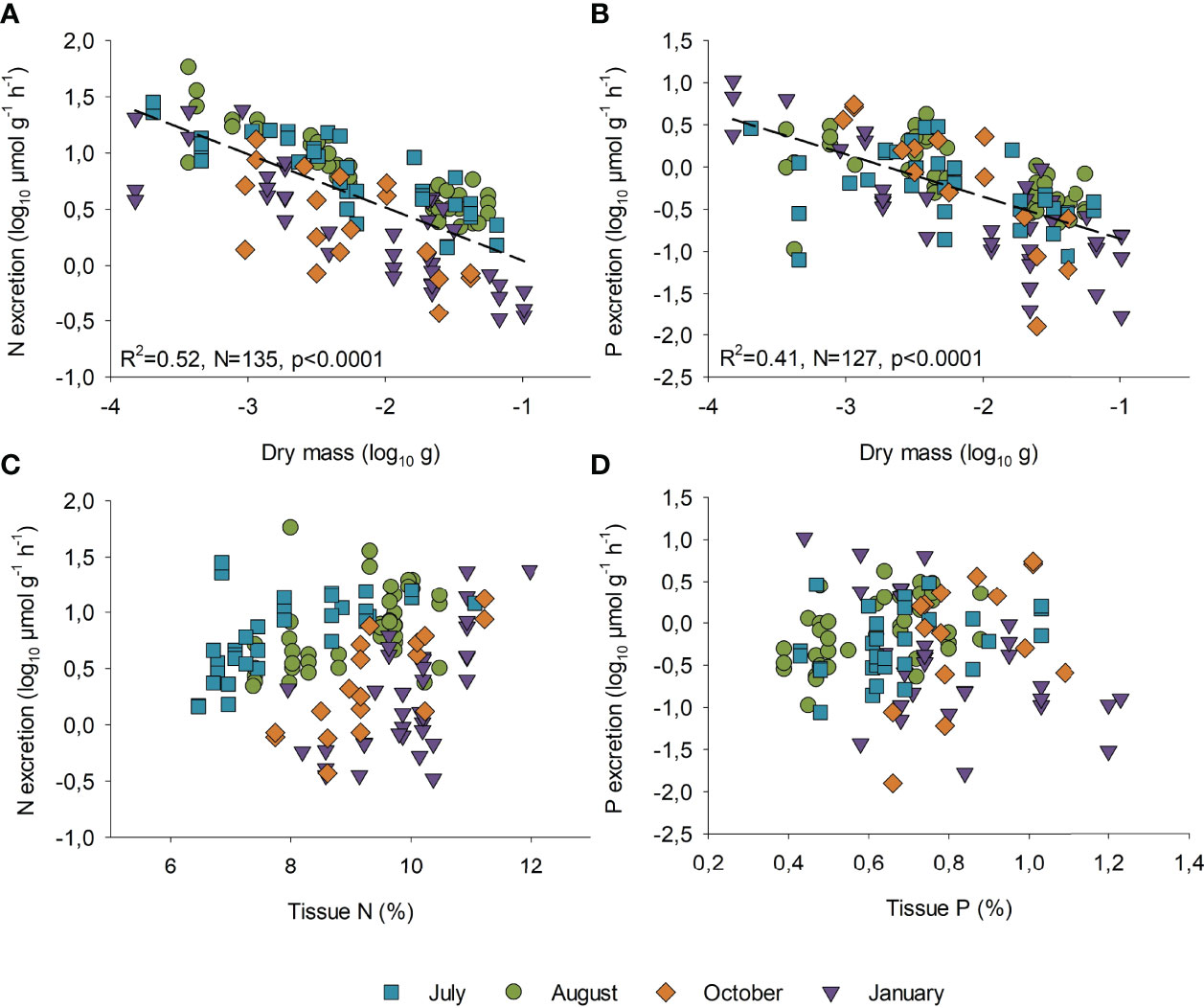
Figure 2 Scatter plots presenting the seasonal differences in biomass-specific (A) nitrogen and (B) phosphorus excretion rates (log10 µmol g-1 h-1) versus dry mass (log10 g) for the two species. The linear regression (f = y0+a*x) is fitted to all data. The relation between nutrient excretion rates to tissue N (%) and P (%) content are presented in (C, D), respectively.
We compared our measured excretion values to the universal excretion model by Vanni and McIntyre (2016). When testing their model on our two dominant species (Figure 3), we found similar slopes for M. balthica measured versus modelled excretion rates (µg N or P ind-1 h-1), but markedly lower intercepts for the measured than for the modelled rates (Figure 3). In contrast, the slope of N and P excretion of Marenzelleria spp. differed from the modelled value (lower for N, higher for P) and the measured N intercept did not converge with the modelled values (Figure 3).
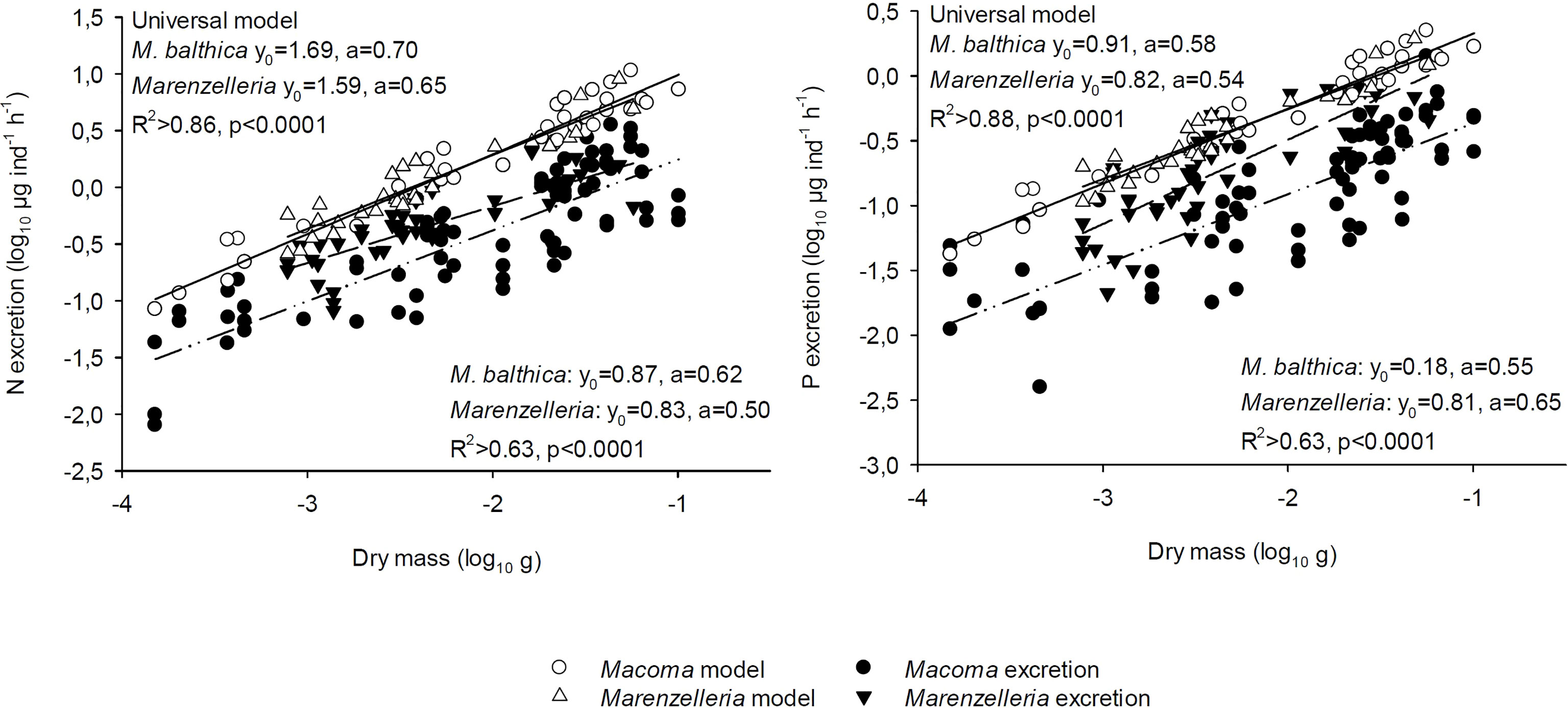
Figure 3 Measured versus modelled excretion rates for M. balthica and Marenzelleria spp. Note that excretion is given as µg ind.-1 h-1 (log10). The universal excretion model by Vanni & McIntyre (2016) was used for calculating excretion rates of both N and P, accounting for variation in individual dry mass as well as temperature. Linear regression models (f= y0+a*x) were used to relate the modelled excretion rates of the species to dry mass (continuous line). Regression for measured excretion values versus dry mass are marked with line-dot for M. balthica, while short dash were used for Marenzelleria spp.
Predictors of Individual Excretion Rates
Allometric variables, particularly size (individual tissue biomass) and temperature were the most important factors explaining variation in species N and P excretion rates. Whereas individual mass alone often accounted for over half of the explained variation, temperature differences had less impact, explaining only 4-14% of the variation (Table 2). In addition, temperature was not a significant predictor of P excretion by Marenzelleria spp., nor of the N:P excretion ratio of the two species. Interestingly, when considering both species, the model included δ15N as an explanatory variable for both N and P excretion (accounting for 4.6 and 12.4% of the total explained variation, respectively). Different stoichiometric variables increased the explained variation foremost in N excretion; specifically, the tissue C:N ratio explained 4.5% of the overall variation in M. balthica N excretion, whereas the model explaining variability in N excretion by Marenzelleria spp. included the tissue N:P ratio and the tissue N content (%) (Table 2, Figure 2C). Regarding P excretion by both species, the overall model included δ13C signature and tissue C content (%), and C content was also a significant predictor variable when considering P excretion of M. balthica (Table 2). We could not find a satisfactory explanatory model for the excreted N:P ratio, but species identity and biomass together explained 12.4% of the variation (Table 2).
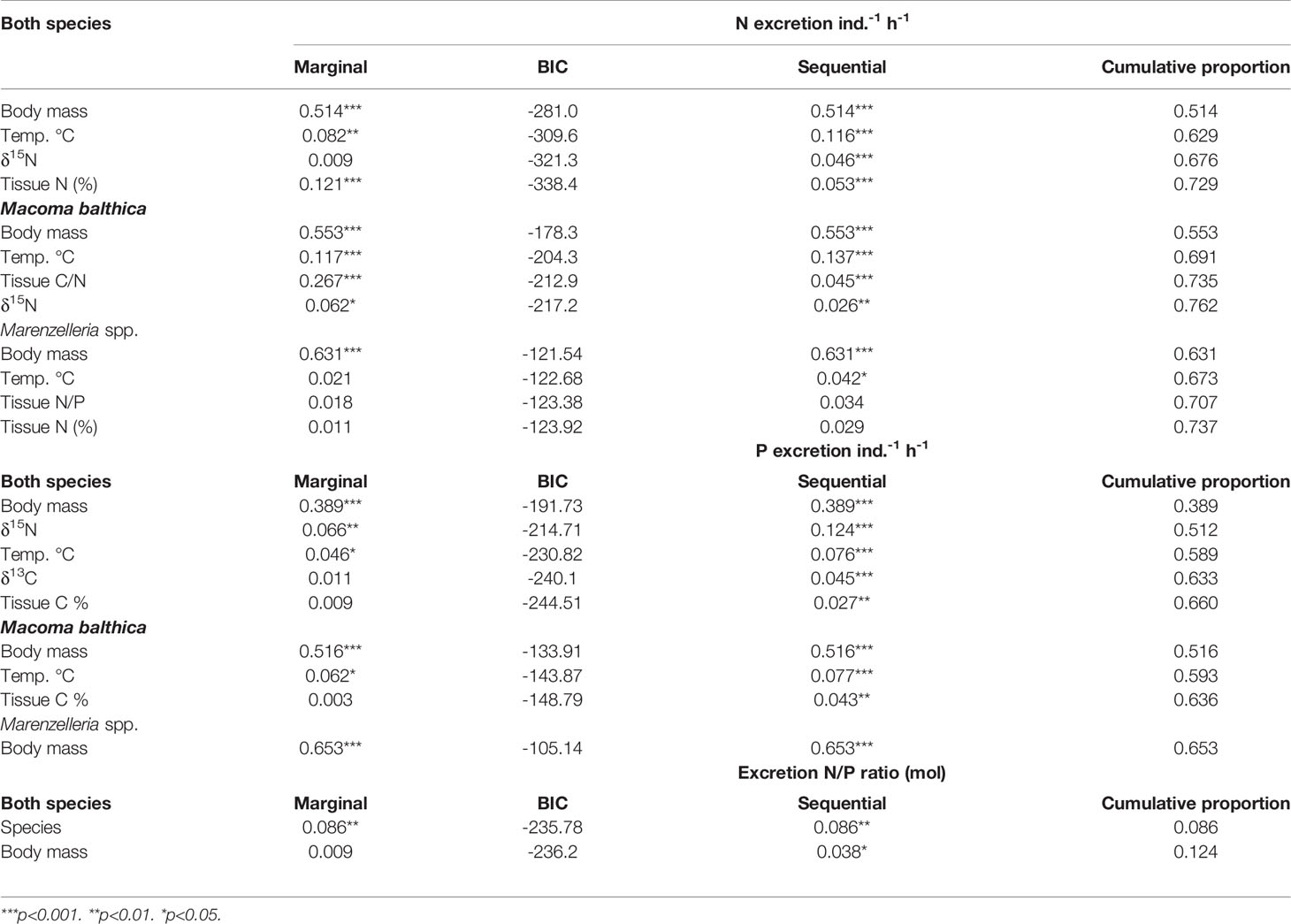
Table 2 Result of distance based linear models (DISTLM) shows the most important predictors for explaining variation in excretion rates (N and P µmol ind.-1 h-1) of Macoma balthica and Marenzelleria spp. together (“both species”, N=135) and separately (M. balthica: N=91, Marenzelleria spp.: N=44).
Seasonal Variation in Community Excretion Rates
Overall, benthic community abundance and biomass were high at both stations in this productive coastal area (Figure S1, Figure 4), but community excretion rates showed clear seasonal differences (PERMANOVA; p<0.001, Table S5). Substantially higher rates occurred in summer (July, August) compared to October and January (Figure 4). Despite high variability in excretion, especially during the summer months, we nonetheless observed a difference in community P excretion rate between stations (Table S5), with a generally higher excretion N:P ratio at station XXVI (on average 12.8 ± 3.3) compared to IH4 (6.0 ± 1.9; October values excluded from both stations). Although M. balthica and Marenzelleria spp. accounted for at least 70% of the total invertebrate community excretion rate at all times, the crustaceans M. affinis and S. entomon as well as Chironomidae also contributed significantly when present (Figure 4). However, we noted a deteriorated community with lower excretion rates at IH4 in October (Figure 4).
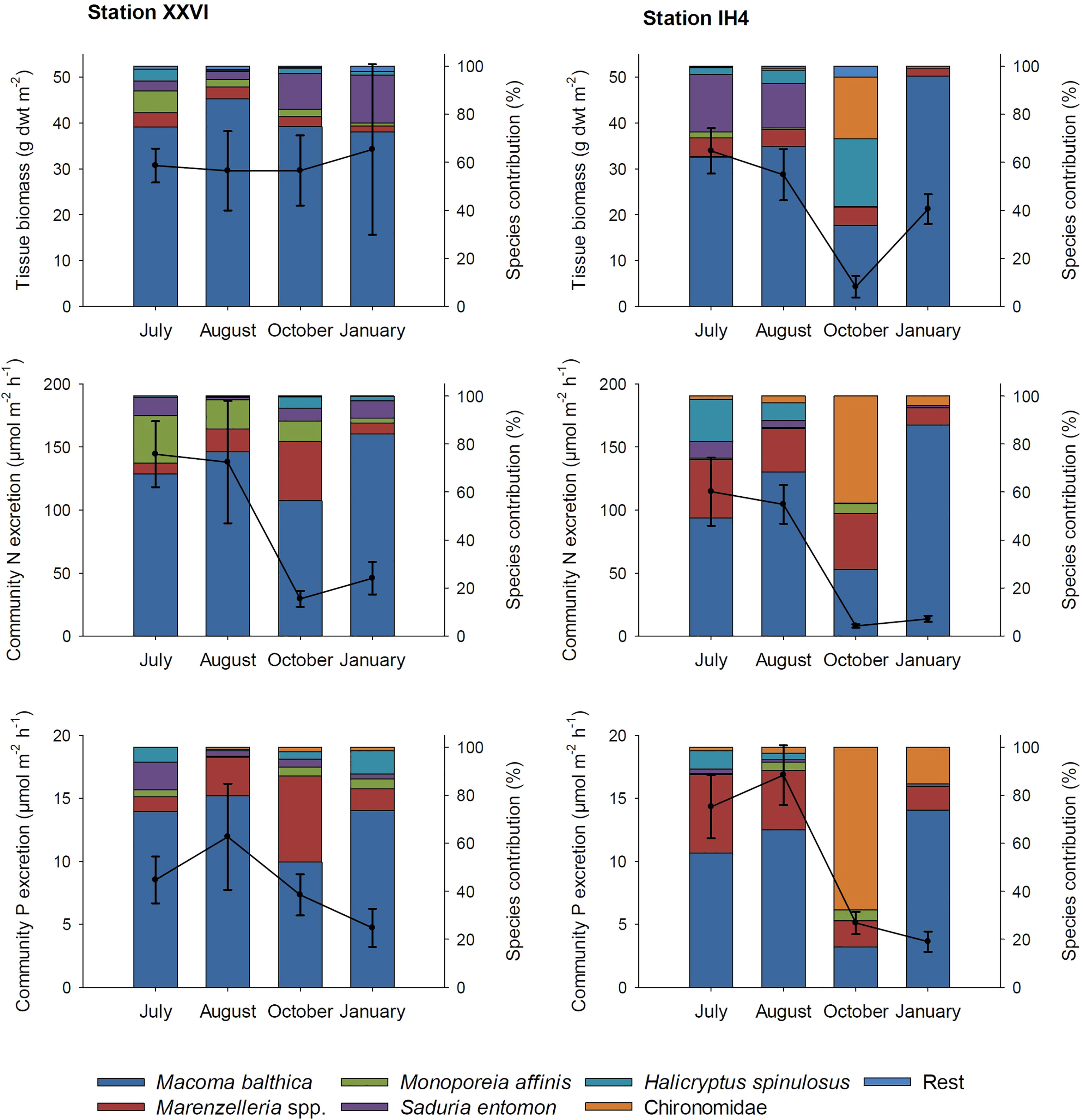
Figure 4 The average tissue biomass of the benthic community (g dwt m-2 ± std) and the maximum excretion potential of N and P (µmol h-1 m-2 ± std) per station and sampling occasion is depicted on the primary y-axis. The contribution of each species to the total biomass and excretion rate (%) is illustrated with columns at the secondary y-axis. Note that biomass does not include bivalve shells.
In July, the benthic community N excretion rates calculated for animals within the flux core incubations were substantially higher (station XXVI: 78.1 ± 44.2, IH4: 44.9 ± 19.2 µmol h-1 m-2) than the measured sediment efflux of NH4-N at both stations (efflux at station XXVI: 18.5 ± 5.4, IH4: 11.4 ± 22.2 µmol h-1 m-2, Figure S3). We measured (NO3-+NO2-)-N effluxes of similar magnitude at the two sites (XXVI: 11.52 ± 2.24, IH4: 7.58 ± 2.15 µmol h-1 m-2, Figure S3). Regarding phosphate, the benthic community P excretion rates in the cores (XXVI: 5.1 ± 2.8, IH4: 10.7 ± 4.7 µmol h-1 m-2) generally resembled the measured P efflux from sediments (XXVI: 8.8 ± 5.3, IH4: 4.6 ± 1.7 µmol h-1 m-2, Figure S3).
Discussion
Our study confirms that benthic consumers profoundly impact nutrient regeneration in productive coastal marine ecosystems. We show high and variable maximum potential excretion rates from aphotic benthic communities, depending on community composition and season. As expected, excretion rates decreased from summer to winter months, but the dominant species, M. balthica and Marenzelleria spp., primarily drove community nutrient recycling rates at all times. In line with current literature (e.g. Vanni et al., 2002; Alves et al., 2010; Allgeier et al., 2015; Vanni & McIntyre, 2016), individual excretion rates of both N and P were species-specific and positively related to increases in traits relevant to metabolic ecology, i.e. to individual body mass and temperature, whereas small individuals had higher mass-specific excretion rates than larger ones. Interestingly, stoichiometric traits and stable isotope signatures explained a minor, but significant, amount of the variability in excretion, particularly between species. Our results emphasize the benefits of using allometric and stoichiometric traits of benthic consumers when quantifying species-specific contributions to nutrient recycling in coastal marine environments, and when predicting how function may respond to environmental change.
The metabolic theory of ecology suggests a scaling coefficient of 0.75 with body mass for predicting physiological rates of organisms (Brown et al., 2004), including excretion rates of N and P (Allen and Gillooly, 2009). On average, scaling coefficients <0.75 characterized our study species, and these coefficients differed between the bivalve (slope: 0.62 for N and 0.55 for P) and the polychaete (0.50 for N, 0.65 for P; Figure 3). Importantly, our data support strong temperature dependence of metabolism and thus excretion rates (Gillooly et al., 2001). The low bottom water temperatures, especially in January (4-5°C) slow down metabolic reactions (Gillooly et al., 2001) compared to summer and autumn months (July: 8°C, October: 12°C, August 18°C), and it also affected the scaling coefficients. This interesting finding implies that temperature effects might depend on animal size, i.e. showing an interactive effect that MTE does not explicitly predict. However, based on an extensive, global data set of ectothermic aquatic invertebrates and vertebrates, Vanni & McIntyre (2016) also reported lower species-specific metabolic scaling coefficients (0.68 for N, 0.57 for P) than predicted by MTE, using data pooled from all aquatic animal species including marine and freshwater invertebrates and vertebrates. Importantly, the slopes calculated by Vanni & McIntyre accounted for variables such as temperature, biomass, trophic guild as well as invertebrate and vertebrate classification. When adapting the universal model to our data, we found that M. balthica yielded a similar slope for measured versus modeled excretion rates, but with markedly lower intercepts for regressions fitted to measured data (Figure 3). Although the universal model of excretion accounts for temperature, the lower energy content (C %) in bivalve tissue during winter in response to lower food availability (Mäkelin & Villnäs 2022) likely leads to decreased metabolism in the bivalve, thereby reducing its excretion rates below the predicted. In contrast, the slopes of N and P excretion by Marenzelleria spp. differed from the modelled slopes.
Previous studies report substantial variation in species-specific scaling coefficients (slopes) when estimating excretion rates across taxa of benthic consumers (Vanni et al., 2002; Allgeier et al., 2015). Therefore, the application of a universal model should be used only as a general approximation (Vanni & McIntyre, 2016). However, the reasons for such differences between species remain unclear. Our results suggests that differences in tissue nitrogen content (N %) as well as δ15N could explain part of the observed variation in N excretion rates between M. balthica and Marenzelleria spp. The polychaete likely has higher nitrogen requirements because its tissue N content averages about 10%, in contrast to a lower percentage in the bivalve (8.5%; Karlson et al., 2015, Mäkelin & Villnäs 2022). Tissue δ15N values, which showed a corresponding enrichment for the polychaete compared to the bivalve support this pattern. Whereas M. balthica suspension or surface feed on newly settled organic matter (Kahma et al., 2020), Marenzelleria spp. utilizes older sedimentary carbon sources (Karlson et al., 2011; Kahma et al., 2020) that contain a higher proportion of microbially processed organic matter that potentially leads to δ15N enrichment (Karlson et al., 2015). Interestingly, Karlson et al. (2015) suggested that δ15N enrichment in M. arctica could also reflect higher isotopic fractionation in this polychaete than in species such as M. balthica, especially if a mismatch occurs between the N content of the food source of this polychaete and its high nitrogen requirements. Indeed, at our sites, we observed a lower sediment N:P than the N:P ratio of polychaete tissue (cf. Figure S2 and Table S1). In line with our results, Allgeier et al. (2015) reported that δ15N could explain a large part of the variance in N and P excretion of aquatic consumers, and emphasized the importance of trophic position. However, collinearity between δ15N and biomass blurred the results and their study included multiple consumer feeding guilds, from algivores to carnivores (Allgeier et al., 2015).
Theoretically, if the biomass-scaling coefficients are similar for both N and P excretion, the slope of body mass vs excretion N:P ratio should be zero (Allgeier et al., 2015; Vanni & McIntyre, 2016). Despite variation in the scaling coefficients both for the bivalve and the polychaete in our study, we found no clear relationship between the excreted N:P and consumer dry mass. In theory the excretion N:P could decrease with body size, because large animals within a species likely have higher tissue N:P (and demand) than small individuals (Elser et al., 2003; Hall et al., 2007). By utilizing a larger dataset, Mäkelin & Villnäs (2022) demonstrated a positive relationship between tissue N:P and dry mass for both M. balthica (r2 = 0.11, p<0.01) and Marenzelleria spp. (r2 = 0.51, p<0.01). However, the more limited data set of this study did not yield such a pattern, presumably as a result of the smaller size range of the animals. We could not detect any difference in tissue N:P between the bivalve and the polychaete (bivalve shell excluded; Table S2). In contrast, the excretion N:P differed significantly between the species and over time, emphasizing that in addition to allometric variables, other species-specific traits as well as environmental variability likely play an important role when predicting animal nutrient recycling rates.
Although our study did not test for the effects of nutrient imbalances between consumer tissue and diet, the pronounced seasonal differences in N and P excretion rates indicate that food quantity and quality affect consumer excretion. Indeed, our model suggested that stoichiometric traits describing the energy content of the consumers (C %) and available food (δ13C) might slightly improve on the main predictor variables (body mass and temperature) of P excretion. Mäkelin & Villnäs (2022) showed a decrease in soft tissue C (%) in both of our study species and a simultaneous increase in N content (%) from May to March. Part of these changes related to temporal changes in food availability, i.e. less fresh food during winter time (Table S1, Rodil et al., 2020) and lower metabolic rates associated with colder temperatures (Mäkelin & Villnäs 2022). A more pronounced decrease in the C:N ratio occurred in the bivalve, whose glycogen, protein, and lipid content declines after spawning in early summer towards winter months (Beukemaa and De Bruin, 1977).
Overall, the biomass of our benthic communities remained high throughout the year, and at levels comparable to the high biomass levels reported for M. balthica during the 2000s in this productive coastal area (Ehrnsten et al., 2019). Still, the importance of physiological changes in response to colder temperatures and food availability becomes clear when considering the community excretion patterns of N and P, which decreased markedly from summer to winter months. Although the community biomass remained stable, excretion rates at station XXVI decreased towards winter (Figure 4 and Figure S1). Variation in community composition can explain some of this pattern; for example, biomass increased at station XXVI in January because of S. entomon, but this isopod has low excretion rates relative to its dry weight (Figure 4). Importantly, the pattern prevailed when we excluded this species from the data set.
The estimated community excretion rate of N, which as mentioned may represent a maximal rate, was about four times higher than fluxes of NH4-N across the sediment-water interface as measured by core incubations in July. The stress experienced by incubated animals associated with handling prior to the incubation likely elevated our measured excretion rates (Whiles et al., 2009), whereas animals in the intact cores might have experienced less stress from handling. Whiles et al. (2009) evaluated the effects of stress versus starvation on the excretion rates of fish and tadpoles and demonstrated that handling stress can increase ammonium excretion rates during short-term incubations. However, several studies report a positive relationship between macrofaunal biomass and sediment NH4+ effluxes (e.g. Gibbs et al., 2005; Norkko et al., 2013), and benthic excretion and activity have been shown to stimulate macrobenthic (Norkko et al., 2001), meiobenthic, and microbial activity and enhance sediment biogeochemical cycling (Henriksen et al., 1983; Stief, 2013; Zilius et al., 2022). In our case, the high excretion rates of NH4-N in comparison to sediment efflux emphasize that the benthic fauna likely enhances nitrification, as indicated by the effluxes of nitrate and nitrite from the sediments (Figure S3). Fecal pellets of Macoma balthica are hotspots for nitrification (Henriksen et al., 1983), and the excreted ammonium also likely promotes the process of nitrification-coupled denitrification (Henriksen et al., 1983; Stief, 2013; Benelli et al., 2017; Hoellein et al., 2017). This process is likely more efficient at organic-rich sites (cf. IH4 to XXVI), given the strong association of nitrifiers and denitrifiers with the organic rich fraction of the sediment (Jäntti et al., 2011). In contrast to N, the benthic community P excretion rates were generally similar to the measured sediment P effluxes, and slightly lower than reported elsewhere in the Gulf of Finland (Berezina et al., 2019). Clearly, our sediment incubations only provide a snap-shot in time, and sediment fluxes result from both environmental and biotic variables and their interactions (cf. Belley and Snelgrove, 2016). Nevertheless, our findings are supported by Kauppi et al. (2017), who showed that the abundance of benthic consumers, such as Marenzelleria spp., can be a particularly important predictor of sediment nutrient fluxes across seasons.
In our coastal study area, the benthic macrofauna processes a major part of organic carbon sedimentation (Ehrnsten et al., 2019). Our study emphasizes the importance of nutrient excretion by benthic communities for nutrient recycling rates in shallow coastal ecosystems, which likely supports primary production. Multiple studies have demonstrated that benthic consumers can change nutrient concentrations and limiting nutrients in aquatic ecosystems (Atkinson et al., 2013). Our study cannot explicitly link excretion rates and ratios to primary production, which we did not measure, but the N:P ratio of benthic excretion was lower than the Redfield ratio (16:1), especially for Marenzelleria spp. Indeed, Berezina et al. (2019) found that Marenzelleria arctia contributed to 79-98% of the total benthic community P excretion rates in the eastern part of the Gulf of Finland. Berezina et al. (2019) suggest that the excretion by this invasive, but now dominant polychaete (Kauppi et al., 2015) may drive primary producers toward N limitation and away from P limitation. In theory, N limitation, together with increasing ocean temperatures can create conditions that favour harmful cyanobacterial blooms. Still, other ecological processes performed by the benthic consumers could counteract such a development. Indeed, the polychaete Marenzelleria spp. is a well-known bioturbator, which can oxidize the sediment and promote overall P binding (Norkko et al., 2012, Berezina et al., 2019). Still, previous work demonstrates the highly context dependent impact of this polychaete on sediment solute fluxes (Kauppi et al., 2018b). Marenzelleria spp. likely increases sediment nutrient cycling, but the broader ramifications of this polychaete genus for nutrient concentration and limitation on an ecosystem level remain unresolved across larger scales in the Baltic Sea.
In conclusion, we showed significant relationships between consumer nutrient recycling rates and allometric traits at a species level, whereas stoichiometric variables and tissue stable isotope signatures (δ15N) helped to describe differences in excretion rates and ratios between our two species. As expected, excretion rates of benthic consumers showed strong seasonality pattern, with highest rates during summer and lowest during winter, emphasizing that changes in temperature and food availability affect metabolic processes of benthic consumers. Thus, pinpointing which physiological traits and environmental variables can enhance our understanding of benthic nutrient recycling at the ecosystem level will require further research. Nevertheless, the biological trait concept is a promising tool for exploring the contribution of benthic consumers to nutrient processes over time and space, and for identifying hotspots of change (Miatta et al., 2021). This potential is especially important in a world where climate warming and environmental change can increase the metabolism of benthic consumers, homogenize the biodiversity of species and traits, and thus disrupt the elemental balance of natural ecosystems.
Data Availability Statement
The raw data supporting the conclusions of this article will be made available by the authors, without undue reservation.
Author Contributions
AV and SM designed the study and conducted the field- and laboratory work. AV, SM and MV structured the ms. AV performed statistical analyses, wrote the first draft of the ms. All authors provided significant intellectual input and contributed to revisions. Funding was obtained by AV. All authors contributed to the article and approved the submitted version.
Conflict of Interest
The authors declare that the research was conducted in the absence of any commercial or financial relationships that could be construed as a potential conflict of interest.
Publisher’s Note
All claims expressed in this article are solely those of the authors and do not necessarily represent those of their affiliated organizations, or those of the publisher, the editors and the reviewers. Any product that may be evaluated in this article, or claim that may be made by its manufacturer, is not guaranteed or endorsed by the publisher.
Funding
This study was funded by the Academy of Finland (Decision 323212), the University of Helsinki three-year research grant to AV, the Sophie von Julin Foundation and the Walter and Andree de Nottbeck Foundation.
Acknowledgments
We thank Dr. Mikko Kiljunen and Nina Honkanen at the University of Jyväskylä for conducting the elemental and SI analyses. We are grateful to the crew of R/V Augusta, laboratory technicians, and all the staff members and interns at Tvärminne Zoological Station for help during this study. Special thanks to Dr. Parima Hajializadeh, Norman Goebeler and Lauri Laitila for assisting with the field sampling and Dr. Laura Kauppi and Dr. Eva Ehrnsten for scientific discussions. We are grateful for constructive comments by the editor Prof. Paul Snelgrove and the reviewers which helped to improve this ms.
Supplementary Material
The Supplementary Material for this article can be found online at: https://www.frontiersin.org/articles/10.3389/fmars.2022.870308/full#supplementary-material
References
Allen A. P., Gillooly J. F. (2009). Towards an Integration of Ecological Stoichiometry and the Metabolic Theory of Ecology to Better Understand Nutrient Cycling. Ecol. Lett. 12, 369–384. doi: 10.1111/j.1461-0248.2009.01302.x
Allgeier J. E., Burkepile D. E., Layman C. A. (2017). Animal Pee in the Sea: Consumer-Mediated Nutrient Dynamics in the World’s Changing Oceans. Global Change Biol. doi: 10.1111/gcb.13625
Allgeier J. E., Wengerb S. J., Rosemond A. D., Schindler D. E., Layman C. A. (2015). Metabolic Theory and Taxonomic Identity Predict Nutrient Recycling in a Diverse Food Web. PNAS, E2640–E2647. doi: 10.1073/pnas.1420819112
Alves J. M., Caliman A., Guariento R. D., Figueiredo-Barros M. P., Carneiro L. S., Farjalla V. F., et al. (2010). Stoichoimetry of Benthic Invertebrate Nutrient Recycling: Interspecific Variation and the Role of Body Mass. Aqua. Ecol. 44, 421–430. doi: 10.1007/s10452-009-9302-3
Anderson M. J., Gorley R. N., Clarke K. R. (2008). PERMANOVA+ for PRIMER: Guide to Software and 7 Statistical Methods (Plymouth, UK: PRIMER-E).
Atkinson C. L., Capps K. A., Rugenski A. T., Vanni M. J. (2017). Consumer-Driven Nutrient Dynamics in Freshwater Ecosystems: From Individuals to Ecosystems. Biol. Rev. 92, 2003–2023. doi: 10.1111/brv.12318
Belley R., Snelgrove P. V. R. (2016). Relative Contributions of Biodiversity and Environment to Benthic Ecosystem Functioning. Front. Mar. Sci. 3. doi: 10.3389/fmars.2016.00242
Benelli S., Bartoli M., Racchetti E., Moraes P. C., Zilius M., Lubiene I., et al. (2017). Rare But Large Bivalves Alter Benthic Respiration and Nutrient Recycling in Riverine Sediments. Aquat. Ecol. 51, 1–16. doi: 10.1007/s10452-016-9590-3
Berezina N. A., Maximov A. A., Vladimirova O. M. (2019). nfluence of Benthic Invertebrates on Phosphorus Flux at the Sediment-Water Interface in the Easternmost Baltic Sea. Mar. Ecol. Prog. 608, 33–43. doi: 10.3354/meps12824
Beukema J. J., De Bruin W. (1977). Seasonal Changes in Dry Weight and Chemical Composition of the Soft Parts of the Tellenid Bivalve Macoma Balthica in the Dutch Wadden Sea. Neth. J. Sea Res. 11, 42–55. doi: 10.1016/0077-7579(77)90020-5
Bonsdorff E., Norkko A., Boström C. (1995). “Recruitment and Population Maintenance of the Bivalve Macoma Balthica (L.) - Factors Affecting Settling Success and Early Survival on Shallow Sandy Bottoms,” in Biology and Ecology of Shallow Coastal Waters (Fredensborg: Olsen & Olsen), 253–260.
Brown J. H., Gillooly J. F., Allen A. P., Savage V. M., West G. (2004). Toward a Metabolic Theory of Ecology. Ecology 85, 1771–1789. doi: 10.1890/03-9000
Carnicer J., Sardans J., Stefanescu C., Ubach A., Bartrons M., Asensio D., et al. (2015). Global Biodiversity, Stoichiometry and Ecosystem Function Responses to Human-Induced C–N–P Imbalances. J. Plant Physiol. 172, 82–91. doi: 10.1016/j.jplph.2014.07.022
Cloern J. E., Abreu P. C., Carstensen J., Chauvaud L., Elmgren R., Grall J., et al. (2016). Human Activities and Climate Variability Drive Fast-Paced Change Across the World's Estuarine–Coastal Ecosystems. Glob. Change Biol. 22, 513–529. doi: 10.1111/gcb.13059
Ehrnsten E., Norkko A., Timmermann K., Gustafsson B. G. (2019). Benthic-Pelagic Coupling in Coastal Seas – Modelling Macrofaunal Biomass and Carbon Processing in Response to Organic Matter Supply. J. Mar. Syst. 196, 36–47. doi: 10.1016/j.jmarsys.2019.04.003
Elser J. J., Acharya K., Kyle M., Cotner J., Makino W., Markow T., et al. (2003). Growth Rate–Stoichiometry Couplings in Diverse Biota. Ecol. Lett. 6, 936–943. doi: 10.1046/j.1461-0248.2003.00518.x
Enquist B. J., Michaletz S., Kerkhoff A. J. (2016). “Toward a General Scaling Theory for Linking Traits, Stoichiometry, and Body Size to Ecosystem Function,” in A Biogeoscience Approach to Ecosystems. Eds. Johnson E. A., Martin Y. E. (UK: Cambridge University Press), 9–46.
Frost P. C., Benstead J. P., Cross W. F., Hillebrand H., Larson J. H., Xenopoulos M. A., et al. (2006). Threshold Elemental Ratios of Carbon and Phosphorus in Aquatic Consumers. Ecol. Lett. 9, 774–779. doi: 10.1111/j.1461-0248.2006.00919.x
Gammal J., Järnström M., Bernard G., Norkko J., Norkko A. (2019). Environmental Context Mediates Biodiversity–Ecosystem Functioning Relationships in Coastal Soft-Sediment Habitats. Ecosystems 22, 137–151. doi: 10.1007/s10021-018-0258-9
Gammal J., Norkko J., Pilditch C. A., Norkko A. (2017). Coastal Hypoxia and the Importance of Benthic Macrofauna Communities for Ecosystem Functioning. Estuar. Coasts. doi: 10.1007/s12237-016-0152-7
Gibbs M., Funnell G., Pickmere S., Norkko A., Hewitt J. (2005). Benthic Nutrient Fluxes Along an Estuarine Gradient: Influence of the Pinnid Bivalve Atrina Zelandica in Summer. Mar. Ecol. Prog. Ser. 288, 151–164. doi: 10.3354/meps288151
Gillooly J. F., Brown J. H., West G. B., Savage V. M., Charnov E. L. (2001). Effects of Size and Temperature on Metabolic Rate. Science 293, 2248–2251. doi: 10.1126/science.1061967
Hall R. O. J., Koch B. J., Marshall M. C., Taylor B. W., Tronstad L. M. (2007). “How Body Size Mediates the Role of Animals in Nutrient Cycling in Aquatic Ecosystems,” in Body Size: The Structure and Function of Aquatic Ecosystems. Eds. Hildrew A. G., Edmonds-Brown R., Raffaelli D. (New York: Cambridge University Press), 286–205.
Hoellein T. J., Zarnoch C. B., Bruesewitz D. A., DeMartini J. (2017). Contributions of Freshwater Mussels (Unionidae) to Nutrient Cycling in an Urban River: Filtration, Recycling, Storage, and Removal. Biogeochem. 135, 307–324. doi: 10.1007/s10533-017-0376-z
Isaac N. J. B., Carbone C. (2010). Why are Metabolic Scaling Exponents So Controversial? Quantifying Variance and Testing Hypotheses. Ecol. Lett. 13, 728–735. doi: 10.1111/j.1461-0248.2010.01461.x
Jäntti H., Stange F., Leskinen E., Hietanen S. (2011). Seasonal Variation in Nitrification and Nitrate-Reduction Pathways in Coastal Sediments in the Gulf of Finland, Baltic Sea. Aquat. Microb. Ecol. 63, 171–181. doi: 10.3354/ame01492
Kahma T. I., Karlson A. M. L., Sun X., Mörth C.-M., Humborg C., Norkko A., et al. (2020). Macroalgae Fuels Coastal Soft-Sediment Macrofauna: A Triple-Isotope Approach Across Spatial Scales. Mar. Environ. Res. 162, 105–163. doi: 10.1016/j.marenvres.2020.105163
Karlson A. M. L., Gorokhova E., Elmgren R. (2015). Do Deposit-Feeders Compete? Isotopic Niche Analysis of an Invasion in a Species-Poor System. Sci. Rep. 5, 9715. doi: 10.1038/srep09715
Karlson A. M. L., Näslund J., Ryden S. B., Elmgren R. (2011). Polychaete Invader Enhances Resource Utilization in a Species Poor System. Oecologia 166, 1055–1065. doi: 10.1007/s00442-011-1936-x
Kauppi L., Bernard G., Bastrop R., Norkko A., Norkko J. (2018b). Increasing Densities of an Invasive Polychaete Enhance Bioturbation With Variable Effects on Solute Fluxes. Sci. Rep. 8, 7619. doi: 10.1038/s41598-018-25989-2
Kauppi L., Norkko J., Ikonen J., Norkko A. (2017). Seasonal Variability in Ecosystem Functions: Quantifying the Contribution of Invasive Species to Nutrient Cycling in Coastal Ecosystems. Mar. Ecol. Prog. Ser. 572, 193–207. doi: 10.3354/meps12171
Kauppi L., Norkko A., Norkko J. (2015). Large-Scale Species Invasion Into a Low-Diversity System: Spatial and Temporal Distribution of the Invasive Polychaetes Marenzelleria Spp. In the Baltic Sea. Biol. Inv. 17, 2055–2074. doi: 10.1007/s10530-015-0860-0
Kauppi L., Norkko A., Norkko J. (2018a). Seasonal Population Dynamics of the Invasive Polychaete Genus Marenzelleria Spp. In Contrasting Soft-Sediment Habitats. J. Sea Res. 131, 46–60. doi: 10.1016/j.seares.2017.10.005
Koroleff F. (1983). Determination of Phosphorus, in Methods of Seawater Analysis, 2nd edition. Eds. Grasshoff K., Ehrhardt M., Kremling K.. Weinheim: Verlag Chemie. pp. 125–139.
Liess A., Hillebrand H. (2005). Stoichiometric Variation in C:N, C:P, and N:P Ratios of Littoral Benthic Invertebrates. J. N. Amer. Benthol. Soc 24, 256–269. doi: 10.1899/04-015.1
Lohrer A., Thrush S., Hewitt J., Kraan K. (2015). The Up-Scaling of Ecosystem Functions in a Heterogeneous World. Sci. Rep. 5, 10349. doi: 10.1038/srep10349
Lotze H. K., Lenihan H. S., Bourque B. J., Bradbury R. H., Cooke R. G., et al. (2006). Depletion, Degradation, and Recovery Potential of Estuaries and Coastal Seas. Science 5781, 1806–1809. doi: 10.1126/science.1128035
Mäkelin S., Villnäs A. (2002). Food Sources Drive Temporal Variation in Elemental Stoichiometry of Benthic Consumers. Limnol. Oceanogr. 67, 784–799. doi: 10.1002/lno.12034
Meunier C. L., Boersma M., El-Sabaawi R., Halvorson H. M., Herstoff E. M., Van de Waal D. B., et al. (2017). From Elements to Function: Toward Unifying Ecological Stoichiometry and Trait-Based Ecology. Front. Environ. Sci. 5, 18. doi: 10.3389/fenvs.2017.00018
Miatta M., Bates A. E., Snelgrove P. V. R. (2021). Incorporating Biological Traits Into Conservation Strategies. Annu. Rev. Mar. Sci. 13, 421–443. doi: 10.1146/annurev-marine-032320-094121
Norkko A., Hewitt J. E., Thrush S. F., Funnell G. A. (2001). Benthic–pelagic Coupling and Suspension-Feeding Bivalves: Linking Site-Specific Sediment Flux and Biodeposition to Benthic Community Structure. Limnol. Oceanogr. 46, 2067–2072. doi: 10.4319/lo.2001.46.8.2067
Norkko J., Reed D. C., Timmermann K., Norkko A., Gustafsson B. G., Bonsdorff E., et al. (2012). A Welcome can of Worms? Hypoxia Mitigation by an Invasive Species. Glob. Change Biol. 18, 422–434. doi: 10.1111/j.1365-2486.2011.02513.x
Norkko A., Villnäs A., Norkko J., Valanko S., Pilditch C. (2013). Size Matters: Implications of the Loss of Large Individuals for Ecosystem Function. Sci. Rep. 3, 2646. doi: 10.1038/srep02646
Norling K., Rosenberg R., Hulth S., Grémare A., Bonsdorff E. (2007). Importance of Functional Biodiversity and Species-Specific Traits of Benthic Fauna for Ecosystem Functions in Marine Sediments. Mar. Ecol. Prog. Ser. 332, 11–23. doi: 10.3354/meps332011
Peñuelas J., Janssens I. A., Ciais P., Obersteiner M., Sardans J. (2020). Anthropogenic Global Shifts in Biospheric N and P Concentrations and Ratios and Their Impacts on Biodiversity, Ecosystem Productivity, Food Security, and Human Health. Glob. Change Biol. 26, 1962–1985. doi: 10.1111/gcb.14981
Persson J., Fink P., Goto A., Hood J. M., Jonas J., Kato S. (2010). To be or Not to be What You Eat: Regulation of Stoichiometric Homeostasis Among Autotrophs and Heterotrophs. Oikos 119, 741–751. doi: 10.1111/j.1600-0706.2009.18545.x
Pilati A., Vanni M. J. (2007). Ontogeny, Diet Shifts, and Nutrient Stoichiometry in Fish. Oikos 116, 1663–1674. doi: 10.1111/j.0030-1299.2007.15970.x
Post D. M. (2002). Using Stable Isotopes to Estimate Trohpic Position: Models, Methods, and Assumptions. Ecology 83, 703–718. doi: 10.1890/0012-9658(2002)083[0703:USITET]2.0.CO;2
Rodil I.F., Lucena-Moya P., Tamelander T., Norkko J., Norkko A. (2020). Seasonal Variability in Benthic–Pelagic Coupling: Quantifying Organic Matter Inputs to the Seafloor and Benthic Macrofauna Using a Multi-Marker Approach. Front. Mar. Sci. 7, 1–18. doi: 10.3389/fmars.2020.00404
Rossi F., Herman P. M. J., Middelburg J. J. (2004). Interspecific and Intraspecific Variation of δ13c and δ15n in Deposit- and Suspension-Feeding Bivalves (Macoma Balthica and Cerastoderma Edule): Evidence of Ontogenetic Changes in Feeding Mode of Macoma Balthica. Limnol. Oceanogr. 49, 408–414. doi: 10.4319/lo.2004.49.2.0408
Sharitt C. A., Gonzalez M. J., Williamson T. J., Vanni M. J. (2021). Nutrient Excretion by Fish Supports a Variable But Significant Proportion of Lake Primary Productivity Over 15 Years. Ecology, e03364. doi: 10.1002/ecy.3364
Snelgrove P. V. R., Thrush S. F., Wall D. H., Norkko A. (2014). Real World Biodiversity-Ecosystem Functioning: A Seafloor Perspective. TREE 29, 398–405. doi: 10.1016/j.tree.2014.05.002
Sterner R. W., Elser J. J. (2002). “Ecological Stoichiometry,” in The Biology of Elements From Molecules to the Biosphere (New Jersey, USA: Princeton University Press).
Stief P. (2013). Stimulation of Microbial Nitrogen Cycling in Aquatic Ecosystems by Benthic Macrofauna: Mechanisms and Environmental Implications. Biogeosciences 10, 7829–7846. doi: 10.5194/bg-10-7829-2013
Vanni M. J. (2002). Nutrient Cycling by Animals in Freshwater Ecosystems. Annu. Rev. Ecol. Syst. 33, 341–370. doi: 10.1146/annurev.ecolsys.33.010802.150519
Vanni M. J., Flecker A. S., Hood J. M., Headworth J. L. (2002). Stoichiometry of Nutrient Recycling by Vertebrates in a Tropical Stream: Linking Species Identity and Ecosystem Processes. Ecol. Lett. 5, 285–293. doi: 10.1046/j.1461-0248.2002.00314.x
Vanni M. J., McIntyre P. B. (2016). Predicting Nutrient Excretion of Aquatic Animals With Metabolic Ecology and Ecological Stoichiometry: A Global Synthesis. Ecology 97, 3460–3471. doi: 10.1002/ecy.1582
Villnäs A., Norkko J., Hietanen S., Josefson A. B., Lukkari K., Norkko A. (2013). The Role of Recurrent Disturbances for Ecosystem Multifunctionality. Ecology 94, 2275–2287. doi: 10.1890/12-1716.1
Whiles M. R., Huryn A. D., Taylor B. W., Reeve J. D. (2009). Influence of Handling Stress and Fasting on Estimates of Ammonium Excretion by Tadpoles and Fish: Recommendations for Designing Excretion Experiments. Limnol. Oceanogr.: Methods 7, 1–7. doi: 10.4319/lom.2009.7.1
Keywords: nutrient recycling, coastal ecosystems, Baltic Sea, biological traits, seasonal change, stoichiometry, macrofaunal communities
Citation: Villnäs A, Mäkelin S and Vanni MJ (2022) Allometric and Stoichiometric Traits Predict Nutrient Excretion Rates by Benthic Consumers. Front. Mar. Sci. 9:870308. doi: 10.3389/fmars.2022.870308
Received: 06 February 2022; Accepted: 27 May 2022;
Published: 01 July 2022.
Edited by:
Paul Snelgrove, Memorial University of Newfoundland, CanadaReviewed by:
Neus Campanyà-Llovet, University of the Azores, PortugalAndrew Martin Lohrer, National Institute of Water and Atmospheric Research (NIWA), New Zealand
Copyright © 2022 Villnäs, Mäkelin and Vanni. This is an open-access article distributed under the terms of the Creative Commons Attribution License (CC BY). The use, distribution or reproduction in other forums is permitted, provided the original author(s) and the copyright owner(s) are credited and that the original publication in this journal is cited, in accordance with accepted academic practice. No use, distribution or reproduction is permitted which does not comply with these terms.
*Correspondence: Anna Villnäs, anna.villnas@helsinki.fi