- 1Nordcee, Department of Biology, University of Southern Denmark, Odense, Denmark
- 2Chemical Oceanography department, GEOMAR Helmholtz Centre for Ocean Research Kiel, Kiel, Germany
- 3Institute for Geosciences, Kiel University, Kiel, Germany
- 4Department of Geosciences and Natural Resource Management (IGN), Geology, University of Copenhagen, Copenhagen, Denmark
- 5Institute for Carbon Cycles, Helmholtz Center Hereon, Geesthacht, Germany
- 6Danish Institute for Advanced Science (DIAS), University of Southern Denmark, Odense, Denmark
Oxygen minimum zones (OMZs) have been suggested as a suitable niche for the oxygen-sensitive process of biological fixation of dinitrogen (N2) gas. However, most N2 fixation rates reported from such waters are low. This low N2 fixation activity has been proposed to result from the unusual community of N2 fixers, in which cyanobacteria were typically underrepresented. The Northern Benguela Upwelling System (North BUS) is part of one of the most productive marine ecosystems and hosts a well-developed OMZ. Although previous observations indicated low to absent N2 fixation rates, the community composition of diazotrophs needed to understand the North BUS has not been described. Here, we present a first detailed analysis of the diazotrophic diversity in the North BUS OMZ and the Angola tropical zone (ATZ), based on genetic data and isotope speciation. Consistent with a previous study, we detected a slight N deficit in the OMZ, but isotope data did not indicate any active or past N2 fixation. The diazotroph community in the North BUS was dominated by non-cyanobacterial microbes clustering with members of gamma-proteobacteria, as is typical for other OMZ regions. However, we found a strikingly high diversity of Cluster III diazotrophs not yet described in other OMZs. In contrast to previous observations, we could also identify cyanobacteria of the clades Trichodesmium sp., UCYN-A and Cyanothece sp., in surface waters connected to or above the OMZ, which were potentially active as shown by the presence of genes and transcripts of the key functional marker gene for N2 fixation, nifH. While the detection of diazotrophs and the absence of active N2 fixation (based on isotopic speciation) are consistent with other OMZ observations, the detected regional variation in the diversity and presence of cyanobacteria indicate that we still are far from understanding the role of diazotrophs in OMZs, which, however, is relevant for understanding the N cycle in OMZ waters, as well for predicting the future development of OMZ biogeochemistry in a changing ocean.
1 Introduction
Nitrogen (N) is an essential nutrient, and limiting for primary production and carbon (C) uptake from the atmosphere in large parts of the ocean (Redfield, 1958; Capone and Carpenter, 1982; Gruber, 2005). Specialized microbes, so-called diazotrophs, can circumvent this shortage due to their ability to fix dinitrogen gas (N2). Classically, cyanobacteria were considered the most important diazotrophs in the ocean, i.e., the filamentous genus Trichodesmium and unicellular genera, including Crocosphaera sp. and Candidatus Atelocyanobacteriym thalassa (UCYN-A) (Moisander et al., 2010). However, due to their need for light, their habitat is largely restricted to sunlit surface waters. In recent years, a large diversity of non-cyanobacterial diazotrophs has been discovered, many of them from oxygen minimum zone (OMZ) waters (Fernandez et al., 2011; Hamersley et al., 2011; Jayakumar et al., 2012; Löscher et al., 2014; Turk-Kubo et al., 2014; Cheung et al., 2016; Jayakumar et al., 2017; Jayakumar and Ward, 2020; Löscher et al., 2020). These waters are characterized by massive N loss, creating a theoretical need for N2 fixation by providing excess phosphorous (Ingall and Jahnke, 1997; Deutsch et al., 2007; Kalvelage et al., 2013). Yet studies have confirmed that N2 fixation is typically minor in such regions (Wang et al., 2019). In addition, anoxic conditions have been shown to reduce the energy constraints of N2 fixation as compared to nitrate uptake, which suggests that N2 fixation might be an attractive way of N acquisition in oxygen (O2)-depleted waters (Großkopf and LaRoche, 2012). Indeed, based on the analysis of the key functional marker gene for N2 fixation, nifH, a broad diversity of mostly non-cyanobacterial diazotrophs was found in OMZ waters of the Eastern Tropical North Pacific (ETNP), the Eastern Tropical South Pacific (ETSP), the Arabian Sea (AS), and the Bay of Bengal (BoB) (Fernandez et al., 2011; Halm et al., 2011; Hamersley et al., 2011; Jayakumar et al., 2012; Bonnet et al., 2013; Farnelid et al., 2013; Löscher et al., 2014; Cheung et al., 2016; Löscher et al., 2016a; Jayakumar et al., 2017; Moisander et al., 2017; Christiansen and Löscher, 2019; Löscher et al., 2020; Li et al., 2021).
A recent report of diazotrophs in OMZ waters revealed a biogeographically distinct and diverse community of N2 fixers throughout the OMZs of the ETNP, ETSP, and AS (Jayakumar and Ward, 2020), but dominated by only a few operational taxonomic units (OTUs) (Jayakumar and Ward, 2020). Interestingly, despite a certain degree of geographical variation in the overall community structure of N2 fixers, the identified heterotrophic diazotrophs represented similar metabolic types in all OMZ waters (Jayakumar and Ward, 2020). For instance, proteobacteria-like sequences related to nifH Cluster I (Zehr et al., 2003) include alpha-proteobacterial methanotrophs and gamma-proteobacterial N2 fixers such as Vibrio diazotrophicus and Pseudomonas stutzeri, which have been reported in the OMZ waters of the ETNP, ETSP, AS and BoB (Fernandez et al., 2011; Löscher et al., 2014; Turk-Kubo et al., 2014; Cheung et al., 2016; Jayakumar et al., 2017). Epsilon-proteobacteria related to Arcobacter nitrofigilis (Löscher et al., 2014) and sequences related to Azotobacter vinelandii and Teridinibacter tunerae have been reported (Turk-Kubo et al., 2014) from the ETSP and BoB (Löscher et al., 2020). Besides Cluster I sequences, delta-proteobacterial nifH and other more rare nifH types of Clusters II, III, and IV (Zehr et al., 2003) have been identified in OMZs, including anaerobic sulphate-reducers in the ETNP (Jayakumar et al., 2017), the ETSP (Löscher et al., 2014; Turk-Kubo et al., 2014; Christiansen and Löscher, 2019), the AS (Jayakumar et al., 2012) and the BoB (Löscher et al., 2020). Cluster II is rarely found and has only been reported in ETSP (Fernandez et al., 2011). Cluster IV, commonly associated with non-functional nif-types or paralogue genes, has been reported from the ETNP (Cheung et al., 2016; Jayakumar et al., 2017), the ETSP (Löscher et al., 2014), the AS (Jayakumar et al., 2012) and BoB OMZ waters (Löscher et al., 2020).
Interestingly, while diazotrophs are usually present and diverse in OMZ waters, they only seem to fix N2 at minimal rates (Bonnet et al., 2013; Dekaezemacker et al., 2013; Löscher et al., 2016b; Jayakumar et al., 2017; Chang et al., 2019; Löscher et al., 2020). One explanation might be the absence of cyanobacterial N2 fixers from those waters. Only a few sequences related to cyanobacteria have been reported. This includes Trichodesmium (<5 sequences) in the ETNP, ETSP, AS and BoB (Jayakumar et al., 2012; Turk-Kubo et al., 2014; Jayakumar et al., 2017; Löscher et al., 2020), UCYN-B, related to Crocosphaera in the ETSP (Löscher et al., 2014) and UCYN-A in the ETSP (Turk-Kubo et al., 2014; Li et al., 2021). In addition, UCYN-B related sequences were detected in the AS based on 16S rRNA analysis (Mazard et al., 2004).
To date, the diazotrophic composition of the OMZ waters in the Northern Benguela upwelling system (North BUS) has not been extensively studied. The Benguela current system spans from 14°S to 35°S is one of four major eastern boundary upwelling regions, and is recognized as the most productive upwelling zone in the global ocean (Bakun, 1996; Carr, 2001). The Benguela current system consists of northern (14–26°S) and southern (26–35°S) sectors with active upwelling which are separated by the Lüderitz upwelling cell (25–27°S). North of 14°S, the coastal areas are part of the Angola tropical zone (ATZ). Upwelling zones in this area are not affected by winds as coastal upwelling zones typically are. North BUS is characterized by equatorward winds, low water temperatures, high phytoplankton biomass, and seasonally O2 depleted waters with locally intense hydrogen sulfide (H2S) events and massive N loss (Tyrrell and Lucas, 2002; Kuypers et al., 2005; Monteiro and van der Plas, 2006; Bartholomae and van der Plas, 2007; Hutchings et al., 2009; Nagel et al., 2013; Vizy et al., 2018). At 17°S, the warm tropical waters coming from the north are mixed with cold waters from the south. This is also known as the Angola-Benguela Frontal Zone (Hutchings et al., 2009). This study focused on the ATZ and North BUS. Waters off North BUS are characterized by N loss, together with increased fluxes of phosphate from sediments underlying an OMZ to the water column (Ingall and Jahnke, 1997). Creating a low N:P ratio similar to other OMZ regions, this would classically be considered favorable for N2 fixation (Wasmund et al., 2005; Flohr et al., 2014). Previous studies have identified N2 fixation from below the detection limit to up to 8 nmol N L-1 d-1 in surface waters (>70 m) (Staal et al., 2007; Sohm et al., 2011; Wasmund et al., 2015). While previous studies (Staal et al., 2007; Sohm et al., 2011) might have even overestimated N2 fixation rates dues to the possible use of contaminated 15N2 gas (Dabundo et al., 2014), all rates presented in those studies were comparable to other reports of N2 fixation in OMZ waters with rates of 0.4 nmol L-1 d-1 to 3.5 nmol L-1 d-1 found in the ETNP and ETSP (Fernandez et al., 2011; Bonnet et al., 2013; Löscher et al., 2014; Jayakumar et al., 2017). N2 fixation was also low or undetectable in the OMZ waters of BoB, (Löscher et al., 2020; Saxena et al., 2020) and has not been measured in the OMZ of the AS, but diazotrophs have been found active (Jayakumar et al., 2012). A similar environment has measured 0.07 nmol L-1 d-1 in the Southern California Bight (Hamersley et al., 2011) and 0.44 nmol L-1 d-1 in the Baltic Sea (Farnelid et al., 2013).
The last decade has revealed a distinct diversity of heterotrophic diazotrophs in OMZ waters (Bonnet et al., 2013; Dekaezemacker et al., 2013; Löscher et al., 2016b; Jayakumar et al., 2017; Chang et al., 2019; Löscher et al., 2020). Their role and importance, however, in these OMZ waters are not clear. Understanding the community composition of diazotrophs in these waters is the first step to shed light on their potential contribution to N2 fixation. Here, we present a first report of the diazotrophic community composition and distribution present in the OMZ of the Northern BUS and the ATZ using a combination of isotope analysis, and sequencing of the biomarker gene, nifH. Results were compared and compiled into a statistical analysis with similar sequencing techniques from OMZs in the ETNP, ETSP and AS (Jayakumar and Ward, 2020).
2 Materials and Methods
2.1 Seawater Sampling
Samples were collected during the cruise M148/1 on the German research vessel RV Meteor from 24th of May to 29th of June 2018. The survey crossed a large area with contrasting environmental properties (e.g., temperature) from the ATZ (South ATZ (10-16°S) and North ATZ (north from 5°S) to North BUS (17-23°S). Details regarding the individual stations can be seen in Table 1 and the Supplementary Material, Table S1. Sampling was carried out by means of a CTD-Rosette system equipped with double sensors for salinity and temperature (Sea-Bird SBE 43), as well as sensors for turbidity and fluorescence (ECO FLNTURTD, Wetlabs) and O2 (Sea-Bird Electronics, Bellevue, WA). The O2 sensor was calibrated by manual Winkler titration. Seawater samples for nutrient analyses were obtained from 10 Niskin bottles attached to the CTD-Rosette. Nitrate , nitrite , and concentrations (detection limits: 0.01 µM were determined on board with a QuAAtro auto-analyzer (SEAL Analytical GmbH, Germany) immediately after sampling following Grasshoff (1999). Ammonium concentrations were measured photometrically following Grasshoff (1999) (detection limit: 0.05 µM .
2.2 Nucleic Acid Extraction
Seawater samples for nucleic acid extraction were collected from Niskin bottles in a volume of 0.5 -2 L (exact volumes were recorded) and filtered on Millipore Durapore membrane filters (Millipore, Billaterica, USA) with a pore diameter of 0.2 µm using a gentle vacuum. After a maximum of 20 min of filtration time, filters were stored at -80°C until further analysis. Filters were flash-frozen in liquid nitrogen and nucleic acids (DNA and RNA) were extracted using the Qiagen DNA/RNA AllPrep Kit (Qiagen, Hildesheim, DE) as described in Löscher et al. (2012). The nucleic acid concentration and quality were checked spectrophotometrically on a MySpec spectrofluorometer (VWR, Darmstadt, Germany). Genomic DNA was eliminated from RNA by treating it with DNase I (Invitrogen, Thermo Fisher Scientific United Kingdom). RNA was checked for purity by a nifH nested PCR. As no DNA contamination could be seen, RNA was transcribed to cDNA using Qiagen QuantiTect Reverse Transcription Kit (Qiagen, Hildesheim, DE).
2.3 Molecular Methods
Environmental DNA and cDNA samples (48 samples in total) were subjected to nifH PCR-amplification using a nested PCR with primers and conditions described by Zani et al. (2000). Both nested PCR runs were carried out with Taq DNA polymerase (Thermo Scientific, Waltham USA). For the first PCR round, Bovine Serum Albumin (BSA, 1.6 µg in 25 µl) (Thermo Scientific, Waltham, USA) were added to the PCR mix. nifH amplicons were purified from an agarose gel using the E.Z.N.A. Gel Extraction Kit (Omega Bio-Tek, Norcross, USA). In total, 21 nifH products were recovered covering waters from ATZ (northern part off Congo (5°S), southern part off Angola (10-16°S)), North BUS (off Namibia (17-23°S)) (Figure 1) and different O2 ranges (oxic waters >100 µM O2, oxycline 20-100 µM O2, suboxic 5-20 µM O2, and OMZ <5 µM O2. nifH amplicons were Topo TA cloned (Thermo Fisher Scientific, Waltham, US). Sequencing was carried out as a service of the Institute of Clinical Molecular Biology in Kiel, Germany. In total, 958 nifH sequences (191 transcripts and 767 gene sequences) were obtained. Sequences were quality-checked and trimmed to a length of 321 bp in MEGAX (Kumar et al., 2018). The sequences were submitted to NCBI with accession ID OK082431-OK083221, OL412005-OL412134 and OM453718 - OM453821.
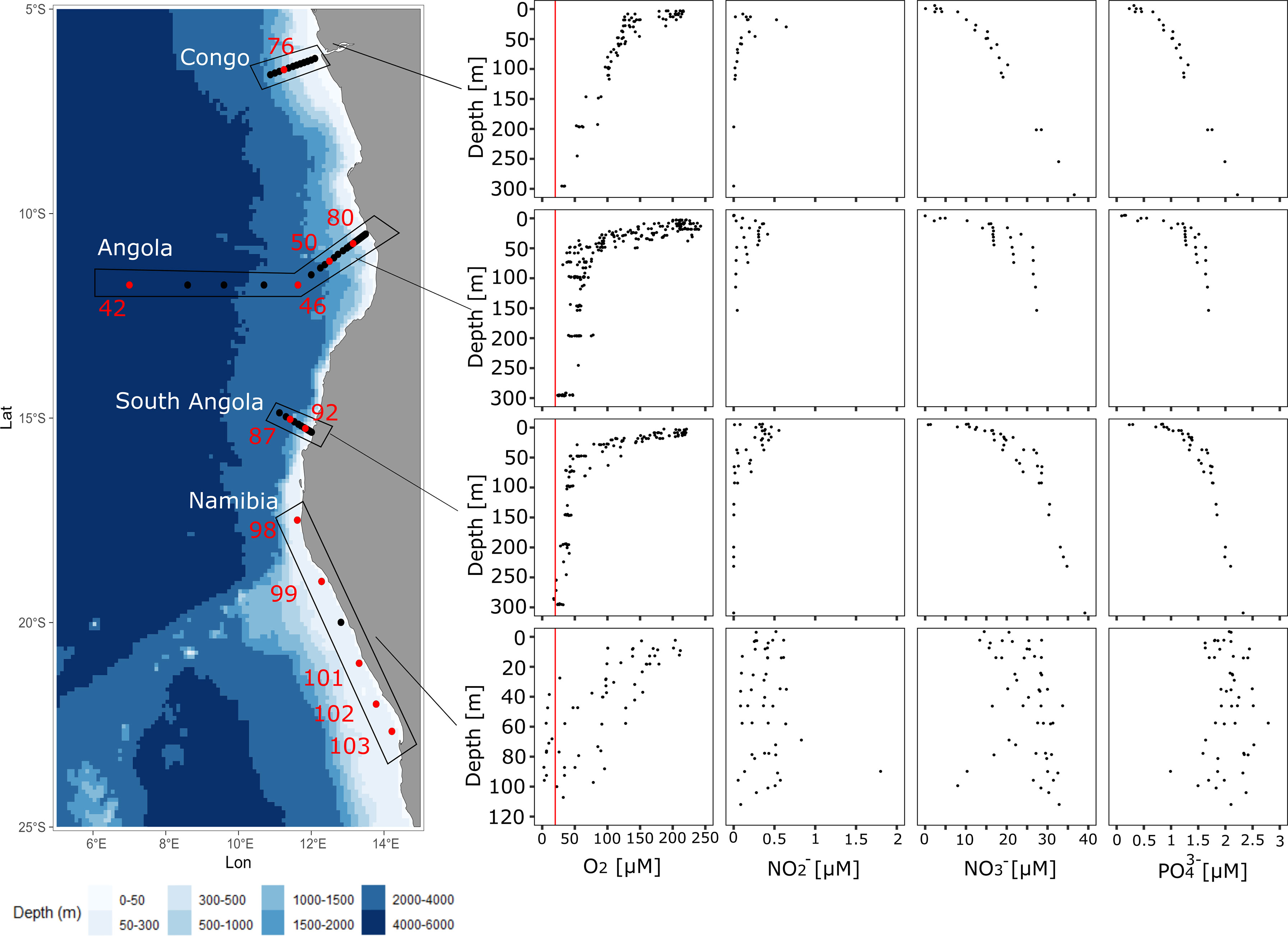
Figure 1 Left panel: Topographical map of the North ATZ (north of 10°S) and South ATZ (between 10 and 15°S) and the North BUS (15-25°S). The four coastal sections of the cruise track are indicated with black dots. Red dots indicate stations from which nifH amplicons were generated. Right panels: Oxygen and nutrient depth profiles from each transect as indicated with black boxes on the map. The red line indicates an O2 concentration of 20 µM.
2.4 Phylogenetic Analysis and OTU Generation
nifH amplicons were translated into amino acids and aligned in MEGAX (Kumar et al., 2018). Based on the nucleotide alignment OTUs were defined using Mothur (Schloss et al., 2009). For comparability, OTUs were generated following Jayakumar and Ward (2020). Briefly, a distance matrix was created using pairwise.seqs followed by assigning sequences to OTUs using cluster.classic at the default setting (furthest neighbor clustering algorithm). nifH richness was calculated using the command rarefaction.single, from each location and O2 feature. OTUs were defined at a threshold of 0.03% which is similar to the level for species definition used for 16S rDNA (Gaby et al., 2018). Different alignment constraints (delay divergent cut-off (%) at 30, 60, 80, 95) and gap penalties (10 and 20) were tested. There were no observed changes in OTU outcome. In total, 82 OTUs were generated, which were aligned with published nifH sequences using Methanosarcina lacustris (AAL02156) as the outer group. A maximum-likelihood phylogenetic tree was constructed using the Poisson model with 1000 bootstraps replicate. Finally, a phylogenetic tree was visualized in iTOL (Letunic and Bork, 2019)
2.5 Natural Abundance of δ15N in the Particulate Organic Material (POM) and
For each sample, 2 L of seawater was filtered onto pre-combusted (450°C, 4-6 hours) 45 mm 0.7 GF/F filters (GE Healthcare Life Sciences, Whatman, USA) at 200 mbar vacuum. Prior to analysis, filters were stored in a -20°C freezer. Natural abundances of δ15N in POM were determined as follows. Filters were acidified over fuming HCl overnight, oven-dried, and duplicates were analyzed on an Elemental Analyzer Flash EA 1112 series (Thermo Fisher, USA) coupled to an isotope ratio mass spectrometer (Finnigan Delta Plus XP, Thermo Fisher, USA) against a caffeine standard.
Isotope ratios of (plus ) were determined by the denitrifier method (Casciotti et al., 2002; Sigman et al., 2001). This method is based on a mass spectrometric measurement of isotopic ratios of N2O produced by the bacterium Pseudomonas aureofaciens. Briefly, 20 nmol of were injected in a 20 mL vial containing P. aureofaciens. Two international standards were used (IAEA δ15N= +4.7 ‰, USGS34 δ15N = −1.8 ‰) for a regression-based correction of isotope values. For further quality assurance, an internal standard was measured with each batch of samples. The standard deviation for δ15N was better than 0.2 ‰. Samples were measured on a Delta Plus XP mass spectrometer coupled to a Gasbench II (Thermo Fisher, USA).
2.6 Statistical Analysis
The most abundant OTUs from North BUS along with environmental factors , isotope , depth, temperature, turbulence, salinity and fluorescence) were used for a redundancy analysis (RDA) using the vegan package in R (Oksanen et al., 2020). Briefly, environmental factors from OTUs mixed from different stations were included by taking the average between the stations contributing above 5% to the OTU. Environmental factors were Hellinger-transformed using decostand. Then, the most parsimonious RDA model was determined using vegan’s function ordistep. The RDA model for OTUs derived from this study included depth, , salinity, O2, and as explanatory variables. Turbulence, fluorescence, and was removed as those parameters turned out to be repetitive. For cross-comparison between OMZ regions, OTUs from the ETNP, ETSP and AS taken from Jayakumar and Ward, 2020 were included in an additional RDA. Compiling OTUs from all OMZ waters, temperature, depth, O2, and were identified as explanatory variables. Raw data can be accessed in Table S5.
3 Results and Discussion
3.1 Hydrochemistry in the ATZ and North BUS
We investigated the community diversity and distribution of N2 fixers in the ATZ and the OMZ off the North BUS and compared our results to previous studies on OMZ N2 fixers. During the time of the cruise, we observed a meridional gradient in O2 with decreasing concentrations from 5˚S to 16˚S. O2 concentrations in the ATZ reached a minimum of around 20 µM O2 at 300 m water depth with an oxycline (20-100 µM O2) starting at around 50-100 m (Figure 1). Only in the Northern BUS, a strong OMZ with O2 concentrations below 5 µM O2 could be detected (Figure 1).
Along with decreased O2 concentrations in South ATZ (10-16 °S), a small secondary maximum was detected at a water depth of 75 m (Figure 1), possibly indicative for N loss processes, such as denitrification or anammox (Kalvelage et al., 2013). Comparable to the ATZ (5-16 °S), concentrations in the Northern BUS were generally elevated with concentrations of up to 0.8 µM and a single datapoint of 1.8 µM at a depth of 87 m (Figure 1; Table 1). and showed a vertical distribution with concentrations increasing below the oxycline reaching concentrations of up to 39 µM and 2.5 µM, respectively, in our deepest samples from 300 m water depth in the ATZ. In the Northern Benguela upwelling system OMZ waters, where O2 concentrations were below 20 µM, some samples showed a decrease of over depth along with increased concentrations, again suggesting beginning denitrification within the water column or the sediment below (Table 1; Figure 1). Overall, the northern BUS seemed to be N limited compared to the ATZ, as indicated by the negative intercept of the trendline for the N:P ratio (Figure 2A). The presence of N loss processes is corroborated by the detected surplus of compared to fixed N assuming Redfield stoichiometry (Redfield et al., 1963) (Figure 2A). Alternatively, the surplus might result from enhanced P flux at anoxia (Ingall and Jahnke, 1997).
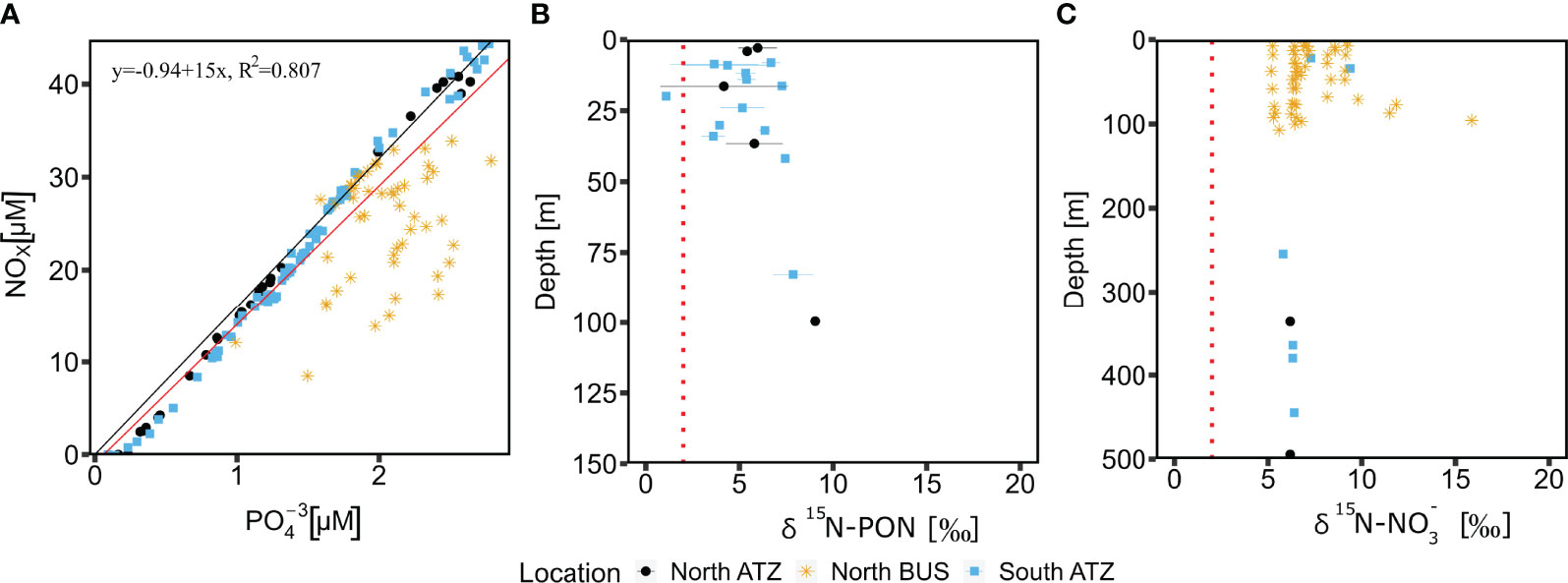
Figure 2 NOx over concentrations from all sampled stations (A). The N:P ratio is around the Redfield ratio of N:P = 16:1 (black line). The linear regression equation (red line) has a negative intercept suggesting a slight N limitation. Samples from the North BUS OMZ are an exception, as those show an undersaturation of N suggesting active N loss processes. δ15N-PON (B) and (C) shows no indication of active N2 fixation but rater N-loss (denitrification, anammox).
Classically, a surplus of over fixed nitrogen in combination with low O2 concentrations would be considered favorable for O2-sensitive diazotrophs (Wasmund et al., 2005; Deutsch et al., 2007; Flohr et al., 2014). However, our δ15N-PON (3‰ to 7‰) and (5‰ to 17‰) signatures indicates an absence of N2 fixation (Figures 2B, C).Our data rather points towards active denitrification in the north BUS OMZ water, as active N2 fixation would be expected to result in a lighter δ15N signature of -2‰ – 2‰ (Delwiche and Steyn, 1970; Montoyal et al., 2002; Dähnke and Thamdrup, 2013). Cautiously, one must note that δ15N signatures are a mixed signal of many processes and might be impacted by other factors and processes, including denitrification, annamox or N import via dust and rivers. Our measurements are, however, in line with a previous study from the North BUS reporting an isotope enrichment from 1 - 10‰ in the OMZ waters of this region (Nagel et al., 2013) and from other OMZ regions with δ15N-PON signatures from 2‰ - 8‰ (Kumar et al., 2004) or 5-8‰ (Löscher et al., 2020) in the BoB and 4‰ -11.6‰ in the ETNP (Voss et al., 2001).
3.2 Diazotrophic Diversity in the Northern Benguela Upwelling System
While we did not find any clear evidence for active N2 fixation in either the North/South ATZ or the Northern BUS during our cruise, we found the genetic potential and presence of transcripts for N2 fixation using the key functional marker gene nifH. Altogether, we obtained 958 nifH amplicon sequences, which include 191 transcripts from an area covering the North ATZ (waters off Congo 6°S) (133 sequences), the South ATZ (waters off Angola [11°S-15°S)] (320 sequences), the North BUS (waters off Namibia [17-22°S)] (505 sequences) and oxic features, oxic (>100 µM O2) (435 sequences), oxycline (20-100 µM O2), suboxic (5-20 µM O2) and OMZ (<5 µM O2). See Tables S1, S2 for details.
Compared to a previous study, which used a similar approach and a higher number of input sequences for nifH sequence analysis from OMZs of the ETNP, ETSP, and AS (505 from the North BUS OMZ compared to 787 sequences combined from the ETSP, ETSP and AS, (Jayakumar and Ward, 2020)), we found a higher diversity based on the number of OTUs (in total 82 OTUs combining both ATZ and North BUS compared to 59 OTUs in the previous study) (Figures S1, S2). This diversity might be somewhat biased as our samples were derived from a wider range of biogeochemical conditions, including the ATZ and the higher obtained total number of sequences. If taking only sequences from the North BUS suboxic (5-20 µM O2) and OMZ (<5 µM O2) conditions into account, a similar diversity (41 OTUs in this study and 38 OTUs in Jayakumar and Ward, 2020) can be observed in both studies (Table S2; Figure S1). Interestingly, we found the highest N2 fixer diversity in waters of the Northern BUS, which also showed strong OMZ conditions with O2 concentration below 5 µM O2 (Figure 1; Table S1). However, we also recovered more sequences from those waters as compared to our other study sites and compared to the individual OMZs explored by Jayakumar and Ward (2020). The 15 most abundant OTUs and their phylogeny are shown in Table 2. Nine of the OTUs were associated with nifH Cluster I, which consists of cyanobacteria and proteobacteria mainly (Table 2; Figure 4). The remaining six OTUs were associated with Cluster III (Table 2; Figure 5).
3.2.1 Cyanobacterial Diazotrophs in the Northern Benguela Upwelling System
With above 99% coverage and identity, the most abundant OTU (OTU-1), consisting of 284 sequences, was associated with the filamentous cyanobacterium Trichodesmium IMS101 on DNA and protein level (Table 2; Figure 4). OTU-1 was mainly found in samples from the South and North ATZ (65% and 22%, respectively) and to a lesser extent in samples from the OMZ in the North BUS (13%, Figure 3; Table S4). Of those 284 sequences. 23.5% were derived from cDNA and were found in surface waters of the North ATZ (13% cDNA) and the North BUS (10% cDNA) (Tables S3, S4). Only below 0.1% were derived from cDNA extracted in the South ATZ (Tables S3, S4). Earlier studies found no evidence of Trichodesmium sp. and blooms were not detectable by satellite imaging (Shannon and Pillar, 1986; Pitcher et al., 1991; Tyrrell et al., 2003; Westberry and Siegel, 2006; Sohm et al., 2011). More recent studies, however, detected Trichodesmium only in low biomass or in a concentration of 1−10 colonies per m3 (Nagel et al., 2013; Wasmund et al., 2015). Low numbers did not appear to be a result of iron (Fe), vitamin or other trace metal limitations in those earlier studies, but rather of low water temperatures (20-25°C), which limited the growth and activity of Trichodesmium (Staal et al., 2007; Wasmund et al., 2015; Tang and Cassar, 2019) (Figure S3).
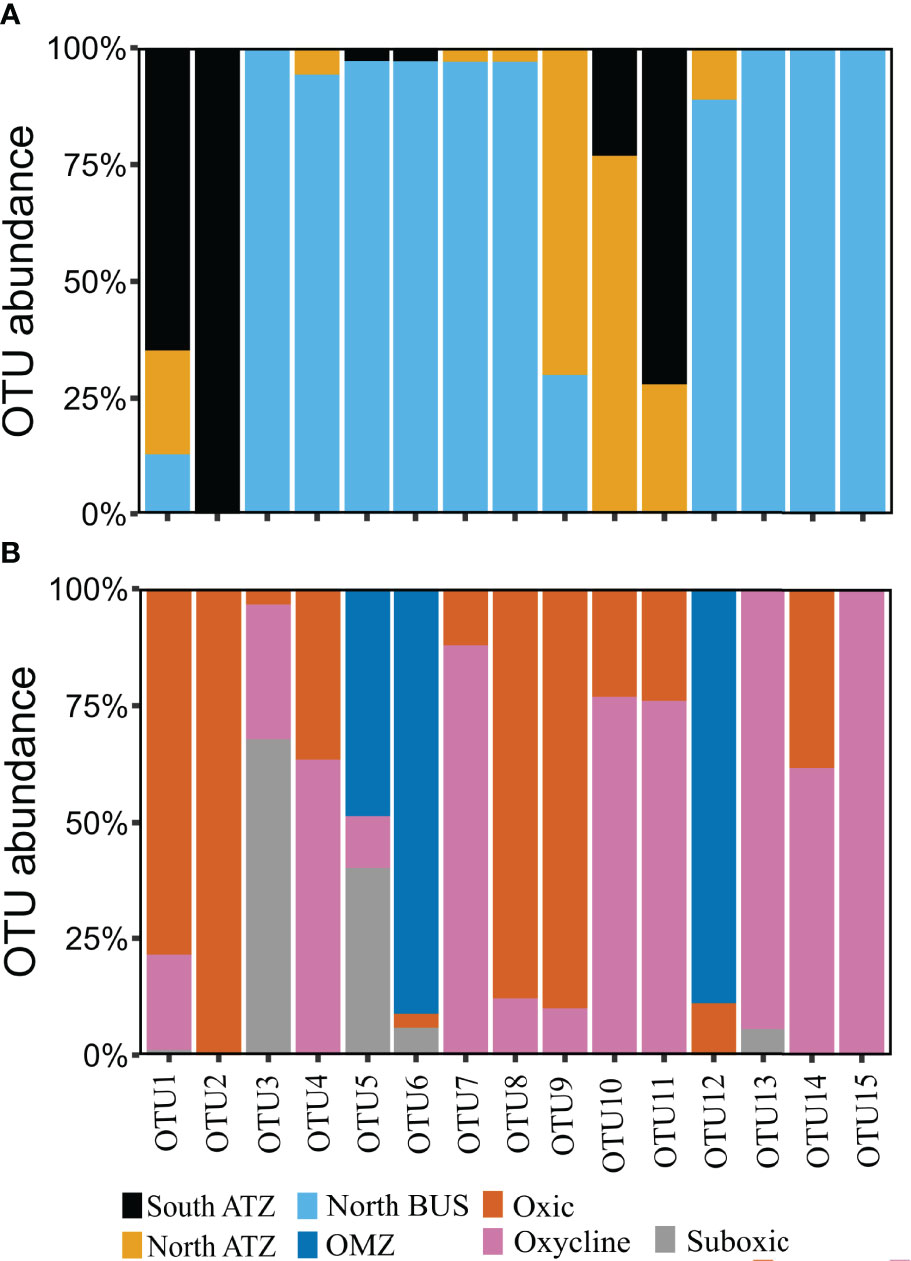
Figure 3 Histogram of the 15 most abundant OTUs (above 10 sequences) divided by location (A) and O2 feature (B). OTUs were defined at a threshold of 3% nucleotide differences and are presented in descending abundance with OTU1 being the most abundant. ATZ stands for Angola Tropical zone and BUS for Benguela Upwelling System.
The small unicellular, cosmopolitan cyanobacterium UCYN-A (OTU-9) was detected in the oxic waters of the North ATZ (70%) and North BUS (30%) (Table 2; Figure 3) with >99% coverage and identity. UCYN-A were not detected in the South ATZ. OTU-9 consisted of 90% transcripts and was found in surface waters down to 30 m water depths (58% cDNA from the North ATZ and 30% from the North BUS), suggesting UCYN-A contributing to N2 fixation (Tables S3, S4). Thus, highlighting the importance of this clade for N2 fixation. UCYN-A has been found all over the globe, from polar to tropical regions, often playing a major role in N2 fixation (Short and Zehr, 2007; Martínez-Pérez et al., 2016; Harding et al., 2018; Tang and Cassar, 2019; Gradoville et al., 2020; Mills et al., 2020). Previously, there was one report of UCYN-A from the Benguela upwelling system where it has been found at water depths of 40-110 m off Angola (14.75°S) (Sohm et al., 2011). Otherwise, UCYN-A has not been found in OMZs except for the ETSP and the BoB (Turk-Kubo et al., 2014; Li et al., 2021).
Cyanothece sp. WH8904 (OTU-14) was detected only in the North BUS and 15% of the sequences were derived from cDNA, suggesting that Cyanothece sp. may be active in the North BUS. Additionally, we detected four cyanobacterial OTUs, one clustering closely with Dolichospermum spp. (previously known as Anabaena sp. [Wacklin et al., 2009)] (OTU-18) and three singletons (OTU-61, OTU-66, OTU-76) related to Trichodesmium thiebautii (Figure 4). In this study, we found a regional difference in cyanobacterial diazotroph presence and nifH gene expression within the Benguela current system (ATZ and North BUS). Moreover, we report for the first time not only the abundance of nifH genes but also the detection of nifH transcripts related to Trichodesmium, Cyanothece and UCYN-A in surface waters adjacent to an OMZ.
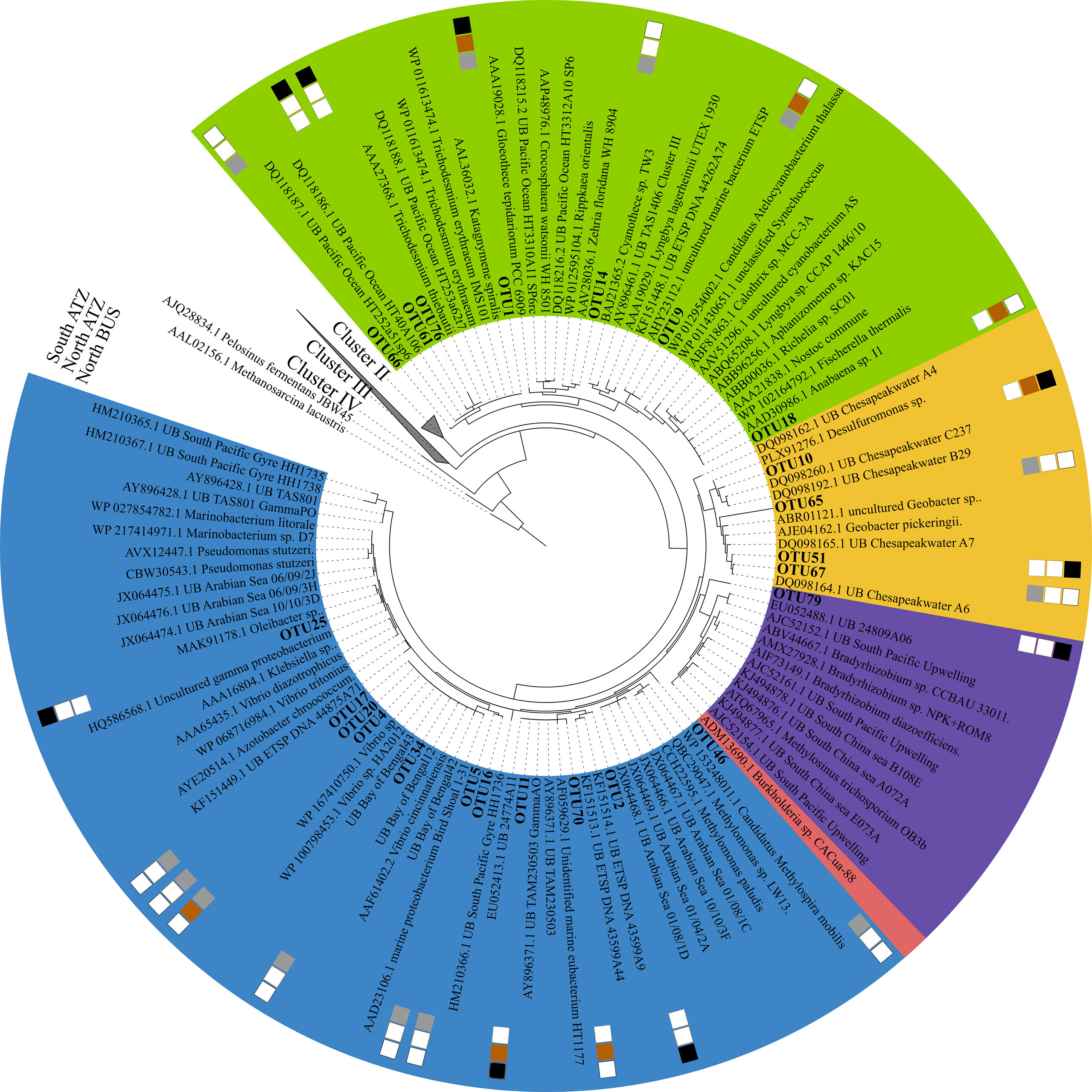
Figure 4 Maximum likelihood tree of Cluster I nifH amino acid sequences. The phylogenetic tree is based on a Poisson model with 1000 bootstraps. OTUs are shown in bold nearest to their closest relative. Locations from where sequences were derived from are shown in the outer heatmap, fark brown represents the Northern Angola Tropical Zone, black indicates the Southern Angola Tropical Zone and grey indicates the Northern Benguela Upwelling Zone. Cyanobacteria are shown in green, gamma-proteobacteria are shown in blue, epsilon-proteobacteria are shown in yellow, alpha-proteobacteria are shown in red and beta-proteobacteria are shown in purple.
3.2.2 Heterotrophic Diazotrophs in the Northern Benguela Upwelling System
Besides cyanobacterial N2 fixers, we found several OTUs related to common OMZ heterotrophic diazotrophs (Table 2; Figure 4). Based on protein level, several gamma-proteobacteria were detected (OTU-2, 4, 5 and 11) and were related to Azotobacter sp., Vibrio sp. and Pseudomonas stutzeri (P. stutzeri) (above 90% identity and coverage) and nifH sequences from the BoB OMZ and gamma-proteobacterial sequences from the South Pacific (Moisander et al., 2014). (Table 2; Figure 4). Related sequences have been reported within both ETNP, ETSP, AS and BOB (e.g., Jayakumar and Ward, 2020). Based on DNA comparison (identity score of 80%), OTU-2 and 7 were related to alpha-proteobacteria, Magnetococcus marinus and Bradyrhizobium respectively. Based on protein comparison OTU-2 were related to Azotobacter sp. with 100% identity. Phylogenetic analysis further establishes OTU-2 being related to gamma-proteobacteria. OTU-7 did not cluster to alpha-proteobacteria or nifH cluster I, but rather with nifH cluster III sequences related to Pontiellaceae.
Like in other OMZ waters, we detected sequences related to Vibrio sp. (OTU-4) and P. stutzeri (OTU-5) in the North BUS (94% and 97%, respectively). Alpha-proteobacterial sequences have been reported the OMZs of the ETNP, ETSP, AS and BoB (e.g., M. palustris, S. melioti and S. azotifegens), but were not confidently detected in this study, nor were beta-proteobacterial sequences (Figure 4) (Halm et al., 2011; Hamersley et al., 2011; Jayakumar et al., 2012; Farnelid et al., 2013; Turk-Kubo et al., 2014; Cheung et al., 2016; Jayakumar et al., 2017). 23 out of 82 OTUs belonged to nifH Cluster I. 13 of those OTUs were found in the North BUS (Figure 4). These proteobacteria include diazotrophs commonly reported in OMZs (Jayakumar et al., 2012; Löscher et al., 2014; Cheung et al., 2016; Löscher et al., 2020), low O2 waters of the Southern Californian Bight (Hamersley et al., 2011) and anoxic waters in the Baltic Sea (Farnelid et al., 2013). Gamma-proteobacteria of the Atlantic clade (OTU-11 and -70) (Langlois et al., 2008) (Figure 4) were only detected and expressed their nifH gene in the ATZ. As previously suggested, they are not commonly active in OMZ or low-O2 waters (Langlois et al., 2015; Moisander et al., 2017). While gamma-proteobacteria M. paludis, a methanotroph (Danilova et al., 2013) have been reported in both ETNP, ETSP and AS (Fernandez et al., 2011; Cheung et al., 2016; Jayakumar and Ward, 2020). We did not find any evidence of methanotrophs in the North BUS. Unlike in the AS and ETNP, we found four OTUs clustering among epsilon-proteobacteria which has also been reported before in ETSP and BoB. However, only two singletons were identified in the North BUS (OTU-65 and 67) (Table S3). The more abundant OTU-10 clustered with Chesapeakwater and shares high similarity to Desulfuromonas sp. (above 95% identity) and was only found in the ATZ (north 77%, south 23%).
Overall, compared to other OMZ regions (Jayakumar and Ward, 2020), we found a similarly small diversity of heterotrophic diazotrophs in the North BUS OMZ waters. In contrast to most other OMZ waters, we found potentially active UCYN-A and Trichodesmium in OMZ-adjacent surface waters. While the role of heterotrophic microbes is unclear, it does not seem that heterotrophic Cluster I diazotrophs play a major role in N2 fixation in the Northern Benguela upwelling system. Rather, cyanobacterial diazotrophs might play a bigger role due to their presence and activity in the OMZ surface waters.
3.2.3 Delta-Proteobacterial Diazotrophs in the Northern Benguela Upwelling System
In addition to Cluster I diazotrophs, we found a vast diversity of Cluster III sequences (59 OTUs out of 82 OTUs) related to Delta-proteobacteria, Bacteroides, Chlorobi, Spirochaetes, Verrucomicrobiae/Kiritimatiellales and Thiospirochaetes (Figure 5). Notably, we did not detect any Cluster II and Cluster IV sequences, which represent e.g., archaeal sequences or non-functional N2 fixers. Only four out of 59 OTUs consisted of sequences from stations in the ATZ (Figure 5). Among the top 15 OTUs associated with Cluster III, only OTU-10 was found in the ATZ (OTU-10: south 23%, north 77%).
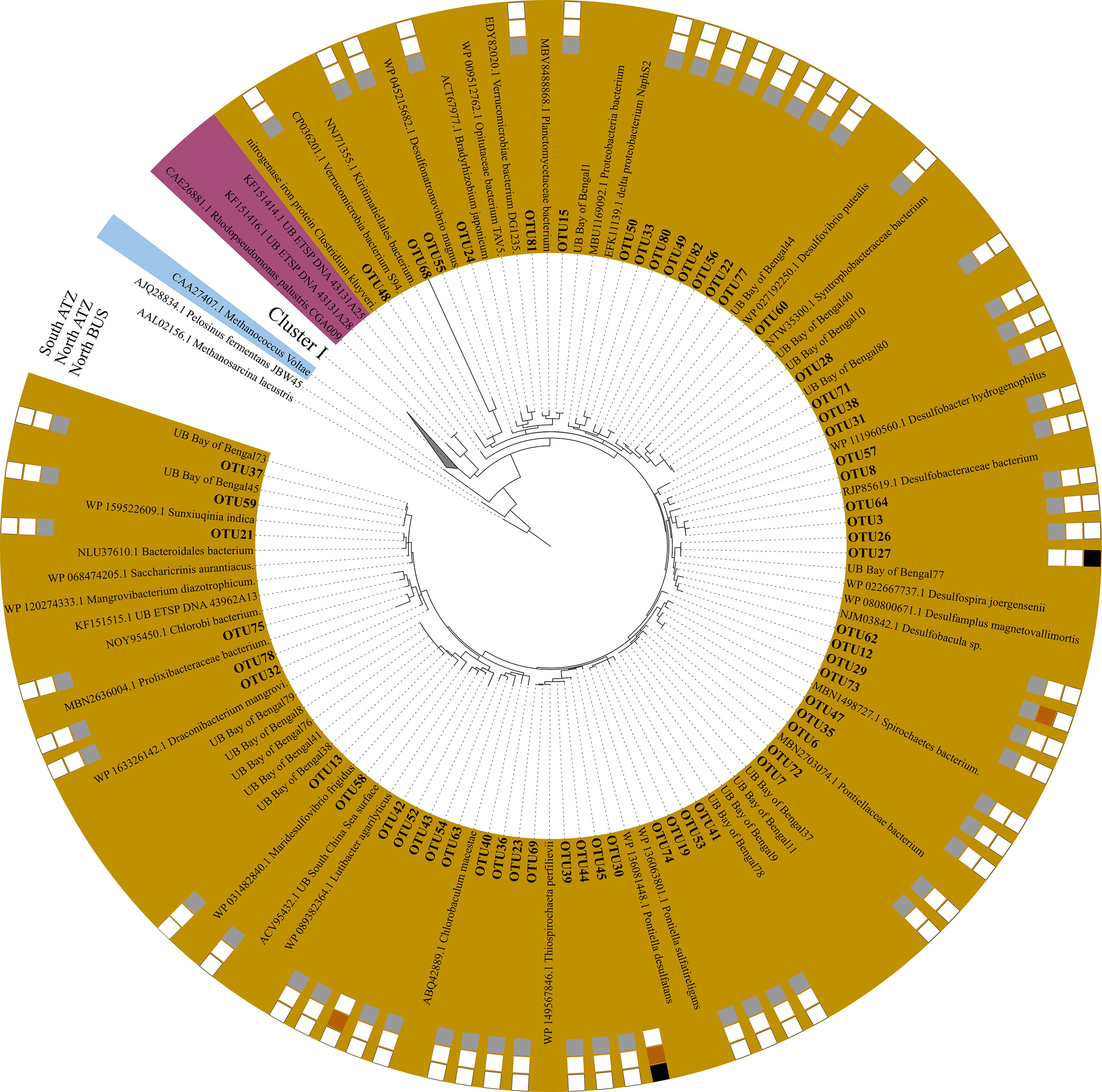
Figure 5 Maximum likelihood tree of Cluster II, III, and IV nifH-amino acid sequences. The phylogenetic tree is based on a Poisson model with 1000 bootstraps. OTUs are shown in bold nearest to their next cultivated relatives. Cluster II is shown in purple, Cluster III in brown and Cluster IV in blue.
OTU-3, 6, 7, 8, 10, 12 and 13 scored above 90% of identity on protein level and closely related to Desulfobacteraceae sp., Solidesulfovibrio sp., Pontiellaceae sp., Lutibacter sp., Desulfuromonas sp., Maridesulfovibrio sp. and Desulfobacula sp., respectively (Table 2). OTU-3 was mainly found in suboxic waters (68%), while OTU-10 and 13 were found in the oxycline (77% and 94%, respectively), and OTU-6 and 12 were found in the OMZ (91% and 88%) (Table 2, S4 and Figure 3, 5). Despite a wide range of Cluster III sequences, only OTU-13, in the top 15 OTUs, contained transcript sequences (5.5%). OUT-13 were only found in North BUS in oxycline and suboxic conditions (94.5% and 5.5%, respectively). The low number of nifH transcripts is somewhat expected in OMZs as N2 fixation rates are typically low, too. Although there were few high identity scores (based on DNA) among the top 15 OTUs to known species, four out of six were either on DNA or protein level affiliated with sulphate-reducing microbes (Table 2). This speaks for the importance of sulphate reduction associated with N2 fixation in North BUS. Such co-occurrence resulting in a coupling between sulphate-reduction and N2 fixation has been previously described in OMZ waters and underlying sediments (Bertics et al., 2013; Gier et al., 2016), mostly represented by sequences clustering closely with Desulfovibrio [Figure 5, (Fernandez et al., 2011; Löscher et al., 2014; Turk-Kubo et al., 2014)]. Indeed, this coupling seems to be consistence between all OMZ waters stressing the importance of this co-occurrence (Jayakumar and Ward, 2020).
Besides sulphate-reducing clades, we also found sulfide-oxidizing clades, such as Thiospirochaeta and Spirochaetes (Figure 5). OTU-6 consisted mainly of OMZ derived sequences (91%) is related to Sulfurospirillum sp., or Solidesulfovibrio sp. (Table 2), while the phylogenetic analysis determined it as being closely related to the sulfide-oxidizing group Spirochaetes (Figure 5). OTU-23, the most abundant OTU related to Thiospirochaeta, was only found in the North BUS in the OMZ region. Chlorobi and Spirochaetes identified in our study, have previously been described in the ETNP, ETSP and anoxic waters of the Baltic Sea (Farnelid et al., 2013; Christiansen and Löscher, 2019; Jayakumar and Ward, 2020) (Figure 5). Our identified Bacteroidetes sequences were related to sequences from the BoB (Figure 5) and have previously been reported in e.g., the sulfidic OMZ off the coast off Peru (Schunck et al., 2013). Also, a recent 16S rRNA-based study from the Southern Benguela upwelling system revealed a similar community with Bacteroides and sulfate-reducing clades present in low-O2 waters (Rocke et al., 2020). Overall, the diversity of Cluster III microbes could result from locally occurring H2S events favoring anaerobic sulphate-reducing and sulfide-oxidizing microbes. Like for Cluster I diazotrophs, there is a distinct difference between the North BUS OMZ (waters off Namibia) and the ATZ (waters off Congo and Angola).
3.3 Biogeochemical Controls on Diazotroph Distribution and Diversity
The waters off North BUS were the only ones during this cruise with an OMZ with O2 concentrations below 5 µM in its core waters. As one main goal of this study was to explore OMZ diazotrophs in North BUS, an RDA based on OTUs (from the top 15), and environmental variables was carried out from those waters specifically. A model containing depth, salinity, the isotope signature of could explain up to 99% of the total variability (Figures 6A, B). While a clear difference in OTUs between the ATZ and the North BUS (Figure 3) could be observed, the geographical factors within North BUS seems also evident in the redundancy analysis.
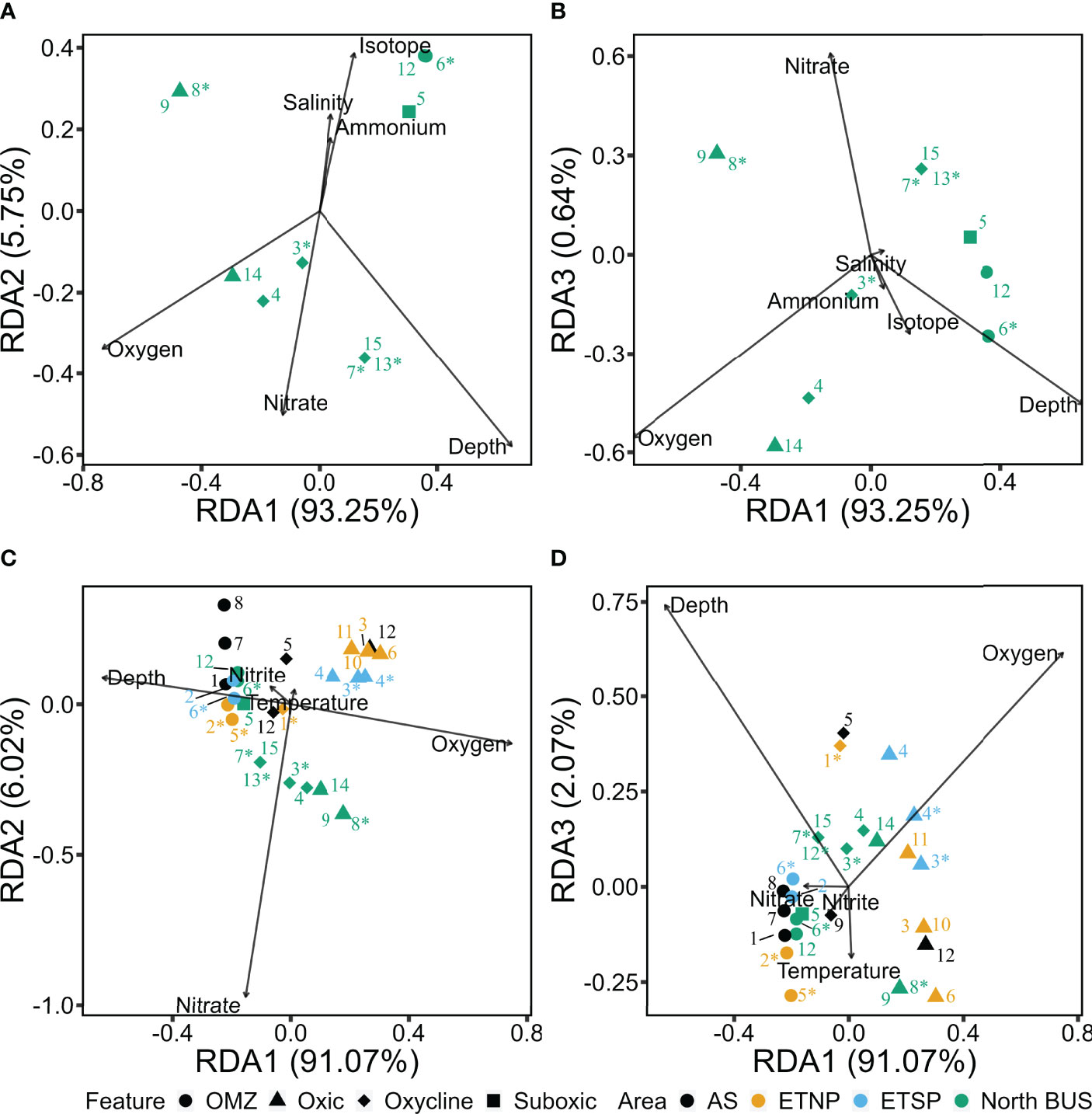
Figure 6 Redundancy analysis (RDA) plot illustrating the relationship between biogeochemical variables and OTUs within the coastal waters the Northern Benguela Upwelling System (North BUS, waters off Namibia) (A, B) and between different OMZ areas (C, D). Numbers indicate OTU identifiers, different shapes denote the O2 feature. Numbers with * are OTUs belonging to Cluster III, other OTUs belong to Cluster I. The Arabian Sea is denoted in black, the ETNP in yellow, the ETSP in blue and waters off Namibia in green. (A, B) show only the top 15 abundant OTUs derived majorly from Namibia (>5%). (C, D) show OTUs generated from the ETNP, ETSP and AS (Jayakumar and Ward, 2020) along with OTUs from the Northern Benguela Upwelling system.
The diazotroph community was divided into three clusters which positively correlated with either O2 (OTU-4 and 14), (OTU-7, -13 and -15) and along with (OTU-5, -6 and -12). OTU-4 (Vibrio sp.) and 14 (Cyanothece sp.) were derived mainly from waters with >20 µM O2 and positively correlated with O2. OTU-7 (Pontiellaceae sp.), -13 (Maridesulfovibrio sp.) and -15 (Plantomyceataceae sp.), derived from oxycline features, were positively correlated to depth and and negatively to and . While including the third component (RDA3), OTU-7, -13 and -15 showed the same tendency and were positively correlated to but negatively correlated to O2, (Figures 6A, B). OTU-5 (P. stutzeri), -6 (Solidesulfovibrio sp.) and -12 (Desulfobacula sp.) were identified as suboxic and OMZ- derived OTUs. They correlated positively with along with and negatively to and O2. The remaining OTUs (8, 9 and 3) could not be sufficiently explained by our statistical approach. Thus, the diverse explanatory factors for OTU-8 (Lutibacter sp.), -9 (UCYN-A), and -3 (Desulfobacteraceae sp.) suggest a lack of factors (e.g., organic matter or Fe) that could help to further explain the dataset. Interestingly, our statistical approach suggests that plays a role in separating OTUs derived from oxycline waters (~20-100 µM O2) from OTUs derived from suboxic/OMZ waters (<5 and 5-20 µM O2) (Figures 6A, B). OTU-5, -6 and -12 correlating to and indicate that they are present in denitrifying waters enriched and in potentially sulfidic waters typically enriched with . While this correlation does not indicate coupling of denitrification and N2 fixation in OMZs as previously proposed (Deutsch et al., 2007), it suggests a spatial coupling between the habitat of diazotrophs within OMZs and denitrification, and a possible link to sulfate-reduction (Jayakumar and Ward, 2020). Lastly, when comparing across OMZ waters, OTUs derived from OMZ core correlate positively to and negatively to O2 and (Figures 6C, D). OTUs derived from the North BUS correlated positively to (Figure 6C). We see between OMZ regions (ETNP, ETSP, AS) a similar pattern where OMZ OTUs correlated positively to and oxic derived OTUs with O2 or positively with temperature, as it showed collinearity with depth (Figures 6C, D).
Compared to other OMZ areas, the most striking difference is the positive correlation between high- and low-O2 derived OTUs from the North BUS (Figures 6A, B). Assimilation of has been suggested to be energetically not of advantage as compared to N2 fixation in low O2 environments (Großkopf and LaRoche, 2012). However, it is unclear whether this explains the observed difference between suboxic and OMZ OTUs. The correlation of diazotroph presence to might also be indicative of a correlation with any other compound in the same water mass. As the signal could be sedimentary, it could be similar to a signal of dissolved Fe concentrations from coastal upwelling, or of organic matter possibly stimulating diazotrophic growth (Löscher et al., 2014; Benavides et al., 2015; Benavides et al., 2020). As typical for OMZs, the diazotroph community collected during this cruise consisted mainly of heterotrophic microbes. While we see a segregation of the community in response to macronutrients, it is important to consider that this might rather be an indicator for a general niche within those waters than for directly. It has previously been suggested that heterotrophic N2 fixation is independent of the Redfield-based N deficiency as long as and iron (Fe) is available (Löscher et al., 2014; Bombar et al., 2016).
Fe can always be considered an important factor for N2 fixation because the Fe requirement of diazotrophs is 60 times that of other microbes (Berman-Frank et al., 2001). In the Northern BUS, the sedimentary release of Fe is rather low (less than 1 wt.% Fe compared to other OMZ regions between 2-3 wt.% Fe) (Scholz et al., 2016; Böning et al., 2020). This potential Fe limitation might explain why Trichodesmium was mainly detected in the ATZ and to a lesser extent the North BUS. It might also explain low N2 fixation rates (Staal et al., 2007; Sohm et al., 2011; Wasmund et al., 2015) and δ15N-PON, along with , being outside of the range indicative for N2 fixation (Figure 2) (Nagel et al., 2013). Sohm et al., 2011 measured up to 2.4 nM Fe in the North BUS and 0.08-0.34 nM in surface waters of the ATZ (north of 14°S). Compared to ETSP OMZ waters with Fe concentrations of 10-60 nmol kg-1 and upwelled surface waters with Fe concentrations of up to 267 nmol kg-1, the concentrations measured in the Benguela region indeed appear to be very low (Löscher et al., 2014). On the other hand, nutrient addition experiments did not increase N2 fixation in Benguela waters (Staal et al., 2007; Wasmund et al., 2015). Thus, other factors that could limit N2 fixation should be taken into account, such as micronutrients (e.g. molybdenum, zinc, vitamins), macronutrients (e.g., organic matter) or physical factors such as light, temperature, or turbulence (Arrigo, 2005; Saito et al., 2008). Though based on our RDA model, turbulence did not explain the dataset significantly, while temperature only explained a minor part.
4 Conclusion
In this study, we explored the diazotrophic community and distribution along the ATZ and the North BUS spanning from the waters off Congo (5°S) to Namibia (23°S). Typically, OMZ regions across the world contain a considerable nifH diversity in both surface and OMZ waters, thus it was of little surprise that we could also recover a broad diversity of Cluster I and III diazotrophs. However, in contrast to other OMZs, we did not find any representatives of other nifH clusters. Interestingly, Trichodesmium, Cyanothece and UCYN-A were both present and potentially active in the ATZ (Coast off Congo and Angola). Moreover, we report a distinct pattern of cyanobacterial in the OMZ waters of the North BUS. While Trichodesmium was mainly found in the ATZ; it was, although to a lesser extent, also found potentially active in OMZ surface waters of the North BUS. UCYN-A was detected in the North BUS and the ATZ in both the gene and the transcript pool, even in surface waters of the OMZ waters. Lastly, Cyanothece were only found in the North BUS surface waters, potentially active. Cluster I heterotrophic diazotrophs were related to gamma-proteobacteria such as P. stutzeri, Vibrio sp., and Azotobacter sp. and were mainly found in the North BUS OMZ waters. Unlike in other OMZs, we did not detect any alpha – or beta-proteobacterial diazotrophs. The most striking difference to other OMZs was the vast diversity of Cluster III diazotrophs, consisting of delta-proteobacteria, Bacteroides, Chlorobium, Spirochaetes and Thiospirochaeta. All of them were found in the OMZ environment.
Combining our data with reports from other OMZ regions, we observe a similar pattern with OMZ derived OTUs responding positively to the secondary peak. However, looking at the North BUS OMZ waters, did not play a significant role. Contrary, we did observe a positive correlation with to OTUs derived from low-O2 waters (~20 µM O2). While assimilation of is energetically unfavorable in low-O2 waters, the correlation might indicate a response to either upwelled Fe or organic matter, stimulating diazotrophic growth. OMZ derived OTUs positively correlated with and which might support the idea of spatially coupled denitrification to N2 fixation and a link between sulfate-reduction and N2 fixation.
While we did not measure N2 fixation rates, earlier reports confirm low rates in waters above 70 m in line with our δ15N isotope speciation data. Like in other OMZs, low or absent rates are related to a specific OMZ diazotroph community with cyanobacteria being underrepresented and heterotrophic diazotrophs fixing N2 only at low rates. Therefore, the diversity of diazotrophs especially of Cluster III in the North BUS suggests that they find a niche in OMZ waters not because of their ability to fix N2, but because of other metabolic capabilities, e.g., sulphate reduction or oxidation. Thus, it is still unclear why those microbes keep their genetic ability to fix N2, as they rarely seem to use it.
Data Availability Statement
The sequences were submitted to NCBI with accession ID OK082431-OK083221, OL412005-OL412134 and OM453718 - OM453821.
Author Contributions
CL, CR, and DA-M designed the study. JC-C, TS, CR, and DA-M carried out field and laboratory experiments. CR performed statistical and molecular analysis. JC-C and TS carried out isotopic measurement. CR wrote the manuscript with contributions from all co-authors. All authors contributed to the article and approved the submitted version.
Funding
Financial support was provided by the German Federal Ministry of Education and Research within the collaborative project ‘Southwest African Coastal Upwelling System and Benguela Niños II (SACUS)’, the Villum Foundation (grants #16518 to Don Canfield and #29411 to CL), grants #15397 to NP.
Conflict of Interest
The authors declare that the research was conducted in the absence of any commercial or financial relationships that could be construed as a potential conflict of interest.
Publisher’s Note
All claims expressed in this article are solely those of the authors and do not necessarily represent those of their affiliated organizations, or those of the publisher, the editors and the reviewers. Any product that may be evaluated in this article, or claim that may be made by its manufacturer, is not guaranteed or endorsed by the publisher.
Acknowledgments
We are grateful to Captain Rainer Hammacher and his crew and the Chief Scientist of M148, Marcus Dengler, for excellent collaboration and centralized services. We thank Hanna Campen (GEOMAR) and Danial Bastian (Hereon) for nutrient and oxygen analyses. Likewise, we thank Erik Laursen (SDU) for assistance with molecular analyses and Zarah Kofoed (SDU) for assistance with isotopic measurements. The ship time on board R/V METEOR was provided by the German Research Council (DFG) within the core program METEOR/MERIAN. We also thank Amal Jayakumar and Bess Ward for sharing metadata and for helpful and straightforward communication. We further acknowledge the Nordcee labs, GEOMAR, and DIAS for core support.
Supplementary Material
The Supplementary Material for this article can be found online at: https://www.frontiersin.org/articles/10.3389/fmars.2022.868261/full#supplementary-material
References
Arrigo K. R. (2005). Marine Microorganisms and Global Nutrient Cycles. Nature 438, 122. doi: 10.1038/nature04265
Bakun A. (1996). “Patterns in the Ocean: Ocean Processes and Marine Population Dynamics,” in Calif. Sea Grant, Coop. with Cent. Investig. Biol (La Paz, Mexico: California Sea Grant, in cooperation with Centro de Investigaciones Biologicas del Noroeste), 332 pp.
Bartholomae C., van der Plas A. (2007). Towards the Development of Environmental Indices for the Namibian Shelf, With Particular Reference to Fisheries Management. Afr. J. Mar. Sci. 29, 25–35. doi: 10.2989/AJMS.2007.29.1.2.67
Benavides M., Duhamel S., Van Wambeke F., Shoemaker K. M., Moisander P. H., Salamon E., et al. (2020). Dissolved Organic Matter Stimulates N2 Fixation and nifH Gene Expression in Trichodesmium. FEMS Microbiol. Lett. 367, 1–8. doi: 10.1093/femsle/fnaa034
Benavides M., Moisander P. H., Berthelot H., Dittmar T., Grosso O., Bonnet S. (2015). Mesopelagic N2 Fixation Related to Organic Matter Composition in the Solomon and Bismarck Seas (Southwest Pacific). PLoS One 10, 1–20. doi: 10.1371/journal.pone.0143775
Berman-Frank I., Cullen J. T., Shaked Y., Sherrell R. M., Falkowski P. G. (2001). Iron Availability, Cellular Iron Quotas, and Nitrogen Fixation in Trichodesmium. Limnol. Oceanogr. 46, 1249–1260. doi: 10.4319/lo.2001.46.6.1249
Bertics V. J., Löscher C. R., Salonen I., Dale A. W., Gier J., Schmitz R. A., et al. (2013). Occurrence of Benthic Microbial Nitrogen Fixation Coupled to Sulfate Reduction in the Seasonally Hypoxic Eckernförde Bay, Baltic Sea. Biogeosciences 10, 1243–1258. doi: 10.5194/bg-10-1243-2013
Bombar D., Paerl R. W., Riemann L. (2016). Marine Non-Cyanobacterial Diazotrophs: Moving Beyond Molecular Detection. Trends Microbiol. 24, 916–927. doi: 10.1016/j.tim.2016.07.002
Böning P., Schnetger B., Belz L., Ferdelman T., Brumsack H. J., Pahnke K. (2020). Sedimentary Iron Cycling in the Benguela Upwelling System Off Namibia. Earth Planet. Sci. Lett. 538, 116212. doi: 10.1016/j.epsl.2020.116212
Bonnet S., Dekaezemacker J., Turk-Kubo K. A., Moutin T., Hamersley R. M., Grosso O., et al. (2013). Aphotic N2 Fixation in the Eastern Tropical South Pacific Ocean. PLoS One 8, 1–14. doi: 10.1371/journal.pone.0081265
Capone D., Carpenter E. J. (1982). Nitrogen Fixation in the Marine Environment Science (New York, N.Y.) 4565, 1140–1142. doi: 10.1126/science.217.4565.1140
Carr M. E. (2001). Estimation of Potential Productivity in Eastern Boundary Currents Using Remote Sensing. Deep. Res. Part II. Top. Stud. Oceanogr. 49, 59–80. doi: 10.1016/S0967-0645(01)00094-7
Casciotti K. L., Sigman D. M., Galanter Hastings M., Böhlke J. K., Hilkert A. (2002) Measurement of the Oxygen Isotopic Composition of Nitrate in Seawater and Freshwater Using the Denitrifier Method. Anal. Chem. 74 (19), 4905–4912, doi: 10.1021/ac020113w.
Chang B. X., Jayakumar A., Widner B., Bernhardt P., Mordy C. W., Mulholland M. R., et al. (2019). Low Rates of Dinitrogen Fixation in the Eastern Tropical South Pacific. Limnol. Oceanogr. 64, 1913–1923. doi: 10.1002/lno.11159
Cheung S., Xia X., Guo C., Liu H. (2016). Diazotroph Community Structure in the Deep Oxygen Minimum Zone of the Costa Rica Dome. J. Plankt. Res. 38, 380–391. doi: 10.1093/plankt/fbw003
Christiansen C. F., Löscher C. R. (2019). Facets of Diazotrophy in the OMZ Off Peru Revisited- What We Couldn’t See From a Single Marker Gene Approach. BioarXiv. doi: 10.1101/558072
Dabundo R., Lehmann M. F., Treibergs L., Tobias C. R., Altabet M. A., Moisander P. H., et al. (2014). The Contamination of Commercial15n2 Gas Stocks With15n-Labeled Nitrate and Ammonium and Consequences for Nitrogen Fixation Measurements. PLoS One 9, e110335. doi: 10.1371/journal.pone.0110335
Dähnke K., Thamdrup B. (2013). Nitrogen Isotope Dynamics and Fractionation During Sedimentary Denitrification in Boknis Eck, Baltic Sea. Biogeosci. Discuss. 10, 681–709. doi: 10.5194/bgd-10-681-2013
Danilova O. V., Kulichevskaya I. S., Rozova O. N., Detkova E. N., Bodelier P. L. E., Trotsenko Y. A., et al. (2013). Methylomonas Paludis Sp. Nov., the First Acidtolerant Member of the Genus Methylomonas, From an Acidic Wetland. Int. J. Syst. Evol. Microbiol. 63, 2282–2289. doi: 10.1099/ijs.0.045658-0
Dekaezemacker J., Bonnet S., Grosso O., Moutin T., Bressac M., Capone D. G. (2013). Evidence of Active Dinitrogen Fixation in Surface Waters of the Eastern Tropical South Pacific During El Niño and La Niña Events and Evaluation of its Potential Nutrient Controls. Global Biogeochem. Cycle. 27, 768–779. doi: 10.1002/gbc.20063
Delwiche C. C., Steyn P. L. (1970). Nitrogen Isotope Fractionation in Soils and Microbial Reactions. Environ. Sci. Technol. 4, 929–935. doi: 10.1021/es60046a004
Deutsch C., Sarmiento J. L., Sigman D. M., Gruber N., Dunne J. P. (2007). Spatial Coupling of Nitrogen Inputs and Losses in the Ocean. Nature 445, 163–167. doi: 10.1038/nature05392
Farnelid H., Bentzon-Tilia M., Andersson A. F., Bertilsson S., Jost G., Labrenz M., et al. (2013). Active Nitrogen-Fixing Heterotrophic Bacteria at and Below the Chemocline of the Central Baltic Sea. ISME. J. 7, 1413–1423. doi: 10.1038/ismej.2013.26
Fernandez C., Farías L., Ulloa O. (2011). Nitrogen Fixation in Denitrified Marine Waters. PLoS One 6, e20539. doi: 10.1371/journal.pone.0020539
Flohr A., van der Plas A. K., Emeis K. C., Mohrholz V., Rixen T. (2014). Spatio-Temporal Patterns of C : N : P Ratios in the Northern Benguela Upwelling System. Biogeosciences 11, 885–897. doi: 10.5194/bg-11-885-2014
Gaby J. C., Rishishwar L., Valderrama-Aguirre L. C., Green S. J., Valderrama-Aguirre A., Jordan I. K., et al. (2018). Diazotroph Community Characterization via a Highthroughput nifH Amplicon Sequencing and Analysis Pipeline. Appl. Environ. Microbiol. 84, e01512–e01517. doi: 10.1128/AEM.01512-17
Gier J., Sommer S., Löscher C. R., Dale A. W., Schmitz R. A., Treude T. (2016). Nitrogen Fixation in Sediments Along a Depth Transect Through the Peruvian Oxygen Minimum Zone. Biogeosciences 13, 4065–4080. doi: 10.5194/bg-13-4065-2016
Gradoville M. R., Farnelid H., White A. E., Turk-Kubo K. A., Stewart B., Ribalet F., et al. (2020). Latitudinal Constraints on the Abundance and Activity of the Cyanobacterium UCYN-A and Other Marine Diazotrophs Methods of Seawater Analysis, in the North Paci Fi C. Limnol. Oceanogr. 9999, 1–18. doi: 10.1002/lno.11423
Grasshoff G., Kremling K., Erhardt M. (1999). (Weinheim: Wiley VCH, Weinheim), 3rd Edn., ISBN 3527295895.
Großkopf T., LaRoche J. (2012). Direct and Indirect Costs of Dinitrogen Fixation in Crocosphaera Watsonii WH8501 and Possible Implications for the Nitrogen Cycle. Front. Microbiol. 3. doi: 10.3389/fmicb.2012.00236
Halm H., Lam P., Ferdelman T. G., Lavik G., Dittmar T., Laroche J., et al. (2011). Heterotrophic Organisms Dominate Nitrogen Fixation in the South Pacific Gyre. ISME. J. 6, 1238–1249. doi: 10.1038/ismej.2011.182
Hamersley M. R., Turk K. A., Leinweber A., Gruber N., Zehr J. P., Gunderson T., et al. (2011). Nitrogen Fixation Within the Water Column Associated With Two Hypoxic Basins in the Southern California Bight. Aquat. Microb. Ecol. 63, 193–205. doi: 10.3354/ame01494
Harding K., Turk-Kubo K. A., Sipler R. E., Mills M. M., Bronk D. A., Zehr J. P. (2018). Symbiotic Unicellular Cyanobacteria Fix Nitrogen in the Arctic Ocean. Proc. Natl. Acad. Sci. U. S. A. 115, 13371–13375. doi: 10.1073/pnas.1813658115
Hutchings L., van der Lingen C. D., Shannon L. J., Crawford R. J. M., Verheye H. M. S., Bartholomae C. H., et al. (2009). The Benguela Current: An Ecosystem of Four Components. Prog. Oceanogr. 83, 15–32. doi: 10.1016/j.pocean.2009.07.046
Ingall E., Jahnke R. (1997). Influence of Water-Column Anoxia on the Elemental Fractionation of Carbon and Phosphorus During Sediment Diagenesis. Mar. Geol. 139, 219–229. doi: 10.1016/S0025-3227(96)00112-0
Jayakumar A., Al-Rshaidat M. M. D., Ward B. B., Mulholland M. R. (2012). Diversity, Distribution, and Expression of Diazotroph nifH Genes in Oxygen-Deficient Waters of the Arabian Sea. FEMS Microbiol. Ecol. 82, 597–606. doi: 10.1111/j.1574-6941.2012.01430.x
Jayakumar A., Chang B. X., Widner B., Bernhardt P., Mulholland M. R., Ward B. B. (2017). Biological Nitrogen Fixation in the Oxygen-Minimum Region of the Eastern Tropical North Pacific Ocean. ISME. J. 11, 2356–2367. doi: 10.1038/ismej.2017.97
Jayakumar A., Ward B. B. (2020). Diversity and Distribution of Nitrogen Fixation Genes in the Oxygen Minimum Zones of the World Oceans. Biogeosciences 17, 5953–5966. doi: 10.5194/bg-17-5953-2020
Kalvelage T., Lavik G., Lam P., Contreras S., Arteaga L., Löscher C. R., et al. (2013). Nitrogen Cycling Driven by Organic Matter Export in the South Pacific Oxygen Minimum Zone. Nat. Geosci. 6, 228–234. doi: 10.1038/ngeo1739
Kumar S., Ramesh R., Bhosle N. B., Sardesai S., Sheshshayee M. S. (2004). Natural Isotopic Composition of Nitrogen in Suspended Particulate Matter in the Bay of Bengal. Biogeosci. Discuss 1, 63–70. doi: 10.5194/bgd-1-87-2004
Kumar S., Stecher G., Li M., Knyaz C., Tamura K. (2018). MEGA X: Molecular Evolutionary Genetics Analysis Across Computing Platforms. Mol. Biol. Evol. 35, 1547–1549. doi: 10.1093/molbev/msy096
Kuypers M. M. M., Lavik G., Woebken D., Schmid M., Fuchs B. M., Amann R., et al. (2005). Massive Nitrogen Loss From the Benguela Upwelling System Through Anaerobic Ammonium Oxidation. Proc. Natl. Acad. Sci. U. S. A. 102, 6478–6483. doi: 10.1073/pnas.0502088102
Langlois R., Großkopf T., Mills M., Takeda S., LaRoche J. (2015). Widespread Distribution and Expression of Gamma A (UMB), an Uncultured, Diazotrophic, γ-Proteobacterial nifH Phylotype. PLoS One 10, 1–18. doi: 10.1371/journal.pone.0128912
Langlois R. J., Hümmer D., LaRoche J. (2008). Abundances and Distributions of the Dominant nifH Phylotypes in the Northern Atlantic Ocean. Appl. Environ. Microbiol. 74, 1922–1931. doi: 10.1128/AEM.01720-07
Letunic I., Bork P. (2019). Interactive Tree of Life (iTOL) V4: Recent Updates and New Developments. Nucleic Acids Res. 47, 256–259. doi: 10.1093/nar/gkz239
Li L., Wu C., Huang D., Ding C., Wei Y., Sun J. (2021). Integrating Stochastic and Deterministic Process in the Biogeography of N2-Fixing Cyanobacterium Candidatus Atelocyanobacterium Thalassa. Front. Microbiol. 12. doi: 10.3389/fmicb.2021.654646
Löscher C. R., Bange H. W., Schmitz R. A., Callbeck C. M., Engel A., Hauss H., et al. (2016a). Water Column Biogeochemistry of Oxygen Minimum Zones in the Eastern Tropical North Atlantic and Eastern Tropical South Pacific Oceans. Biogeosciences 13, 3585–3606. doi: 10.5194/bg-13-3585-2016
Löscher C. R., Bourbonnais A., Dekaezemacker J., Charoenpong C. N., Altabet M. A., Bange H. W., et al. (2016b). N2 Fixation in Eddies of the Eastern Tropical South Pacific Ocean. Biogeosciences 13, 2889–2899. doi: 10.5194/bg-13-2889-2016
Löscher C. R., Großkopf T., Desai F. D., Gill D., Schunck H., Croot P. L., et al. (2014). Facets of Diazotrophy in the Oxygen Minimum Zone Waters Off Peru. ISME. J. 8, 1–13. doi: 10.1038/ismej.2014.71
Löscher C. R., Kock A., Könneke M., LaRoche J., Bange H. W., Schmitz R. A. (2012). Production of Oceanic Nitrous Oxide by Ammonia-Oxidizing Archaea. Biogeosciences 9, 2419–2429. doi: 10.5194/bg-9-2419-2012
Löscher C. R., Mohr W., Bange H. W., Canfield D. E. (2020). No Nitrogen Fixation in the Bay of Bengal? Biogeosciences 17, 851–864. doi: 10.5194/bg-17-851-2020
Martínez-Pérez C., Mohr W., Löscher C., Dekaezemacker J., Littmann S., Yilmaz P., et al. (2016). The Small Unicellular Diazotrophic Symbiont, UCYN-A, is a Key Player in the Marine Nitrogen Cycle. Nat. Microbiol. 1, 16163. doi: 10.1038/nmicrobiol.2016.163
Mazard S. L., Fuller N. J., Orcutt K. M., Bridle O., Scanlan D. J. (2004). PCR Analysis of the Distribution of Unicellular Cyanobacterial Diazotrophs in the Arabian Sea. Appl. Environ. Microbiol. 70, 7355–7364. doi: 10.1128/AEM.70.12.7355-7364.2004
Mills M. M., Turk-Kubo K. A., van Dijken G. L., Henke B. A., Harding K., Wilson S. T., et al. (2020). Unusual Marine Cyanobacteria/Haptophyte Symbiosis Relies on N2 Fixation Even in N-Rich Environments. ISME. J. 14, 2395–2406. doi: 10.1038/s41396-020-0691-6
Moisander P. H., Beinart R. A., Hewson I., White A. E., Johnson K. S., Carlson C. A., et al. (2010). Unicellular Cyanobacterial Distributions Broaden the Oceanic N2 Fixation Domain Am. Assoc. Adv. Sci. 327, 1512–1514. doi: 10.1126/science.1185468
Moisander P. H., Benavides M., Bonnet S., Berman-Frank I., White A. E., Riemann L. (2017). Chasing After non-Cyanobacterial Nitrogen Fixation in Marine Pelagic Environments. Front. Microbiol. 8. doi: 10.3389/fmicb.2017.01736
Moisander P. H., Serros T., Paerl R. W., Beinart R. A., Zehr J. P. (2014). Gammaproteobacterial Diazotrophs and nifH Gene Expression in Surface Waters of the South Pacific Ocean. ISME. J. 8, 1962–1973. doi: 10.1038/ismej.2014.49
Monteiro P. M. S., van der Plas A. K. (2006). 5 Low Oxygen Water (LOW) Variability in the Benguela System: Key Processes and Forcing Scales Relevant to Forecasting. Larg. Mar. Ecosyst. 14, 71–90. doi: 10.1016/S1570-0461(06)80010-8
Montoyal J. P., Carpenter E. J., Capone D. G. (2002). Nitrogen Fixation and Nitrogen Isotope Abundances in Zooplankton of the Oligotrophic North Atlantic. Limnol. Oceanogr. 47, 1617–1628. doi: 10.4319/lo.2002.47.6.1617
Nagel B., Emeis K. C., Flohr A., Rixen T., Schlarbaum T., Mohrholz V., et al. (2013). N-Cycling and Balancing of the N-Deficit Generated in the Oxygen Minimum Zone Over the Namibian Shelf - An Isotope-Based Approach. J. Geophys. Res. Biogeosci. 118, 361–371. doi: 10.1002/jgrg.20040
Oksanen F. J., Blanchet G., Friendly M., Roeland Kindt P., Legendre D., McGlinn P. R., et al. (2020). Vegan: Community Ecology Package. version 2.5.7, Available at: http://cran.r-project.org/, http://vegan.r-forge.r-project.org/
Pitcher G. C., Walker D. R., Mitchell-Innes B. A., Moloney C. L. (1991). Short-Term Variability During an Anchor Station Study in the Southern Benguela Upwelling System: A Simulation Model. Prog. Oceanogr. 28, 39–64. doi: 10.1016/0079-6611(91)90023-F
Redfield A. (1958). The Biological Control of Chemical Factors in the Environment Sigma Xi, The Scientific Research Honor Society 46, 205–221. doi: 10.1515/9783112312308-007
Redfield A. C., Ketchum B. H., Richards F. A. (1963). The Influence of Organisms on the Composition of Sea Water. sea 2, 26–77.
Rocke E., Cheung S., Gebe Z., Dames N. R., Liu H., Moloney C. L. (2020). Marine Microbial Community Composition During the Upwelling Season in the Southern Benguela. Front. Mar. Sci. 7. doi: 10.3389/fmars.2020.00255
Saito M. A., Tyler G. J., Ritt J. T. (2008). Some Thoughts on the Concept of Colimitation : Three Definitions and the Importance of Bioavailability. Limnol. Oceanogr. 53, 276–290. doi: 10.4319/lo.2008.53.1.0276
Saxena H., Sahoo D., Khan M. A., Kumar S., Sudheer A. K., Singh A. (2020). Dinitrogen Fixation Rates in the Bay of Bengal During Summer Monsoon. Environ. Res. Commun. 2, 051007. doi: 10.1088/2515-7620/ab89fa
Schloss P. D., Westcott S. L., Ryabin T, Hall J. R., Hartmann M, Hollister E. B., et al. (2009). Introducing Mothur. Appl. Env. Microbiol. 75, 7537–7541. doi: 10.1128/AEM.01541-09.
Scholz F., Löscher C. R., Fiskal A., Sommer S., Hensen C., Lomnitz U., et al. (2016). Nitrate-Dependent Iron Oxidation Limits Iron Transport in Anoxic Ocean Regions. Earth Planet. Sci. Lett. 454, 272–281. doi: 10.1016/j.epsl.2016.09.025
Schunck H., Lavik G., Desai D. K., Großkopf T., Kalvelage T., Löscher C. R., et al. (2013). Giant Hydrogen Sulfide Plume in the Oxygen Minimum Zone Off Peru Supports Chemolithoautotrophy. PLoS One 8, e68661. doi: 10.1371/journal.pone.0068661
Shannon L. V., Pillar S. C. (1986). “The Benguela Ecosystem. Part III. Plankton,” in Oceanogr. Mar. Biol London: CRC Press, 65–170 .
Short S. M., Zehr J. P. (2007). Nitrogenase Gene Expression in the Chesapeake Bay Estuary. Environ. Microbiol. 9, 1591–1596. doi: 10.1111/j.1462-2920.2007.01258.x
Sigman D. M., Casciotti K. L., Andreani M., Barford C., Galanter M., Böhlke J. K. (2001). A Bacterial Method for the Nitrogen Isotopic Analysis of Nitrate in Seawater and Freshwater. Anal. Chem. 73 (17), 4145–4153. doi: 10.1021/ac010088e.
Sohm J. A., Hilton J. A., Noble A. E., Zehr J. P., Saito M. A., Webb E. A. (2011). Nitrogen Fixation in the South Atlantic Gyre and the Benguela Upwelling System. Geophys. Res. Lett. 38, 1–7. doi: 10.1029/2011GL048315
Staal M., Hekkert S. T. L., Brummer G. J., Veldhuis M., Sikkens C., Persijn S., et al. (2007). Nitrogen Fixation Along a North-South Transect in the Eastern Atlantic Ocean. Limnol. Oceanogr. 52, 1305–1316. doi: 10.4319/lo.2007.52.4.1305
Tang W., Cassar N. (2019). Data-Driven Modeling of the Distribution of Diazotrophs in the Global Ocean. Geophys. Res. Lett. 46, 12258–12269. doi: 10.1029/2019GL084376
Turk-Kubo K. A., Karamchandani M., Capone D. G., Zehr J. P. (2014). The Paradox of Marine Heterotrophic Nitrogen Fixation: Abundances of Heterotrophic Diazotrophs do Not Account for Nitrogen Fixation Rates in the Eastern Tropical South Pacific. Environ. Microbiol. 16, 3095–3114. doi: 10.1111/1462-2920.12346
Tyrrell T., Lucas M. I. (2002). Geochemical Evidence of Denitrification in the Benguela Upwelling System. Cont. Shelf. Res. 22, 2497–2511. doi: 10.1016/S0278-4343(02)00077-8
Tyrrell T., Marañón E., Poulton A. J., Bowie A. R., Harbour D. S., Woodward E. M. S. (2003). Large-Scale Latitudinal Distribution of Trichodesmium Spp. In the Atlantic Ocean. J. Plankt. Res. 25, 405–416. doi: 10.1093/plankt/25.4.405
Vizy E. K., Cook K. H., Sun X. (2018). Decadal Change of the South Atlantic Ocean Angola–Benguela Frontal Zone Since 1980. Clim. Dyn. 51, 3251–3273. doi: 10.1007/s00382-018-4077-7
Voss M., Dippner J. W., Montoya J. P. (2001). Nitrogen Isotope Patterns in the Oxygen-Deficient Waters of the Eastern Tropical North Pacific Ocean. Deep. Res. Part I. Oceanogr. Res. Pap. 48, 1905–1921. doi: 10.1016/S0967-0637(00)00110-2
Wacklin P., Hoffmann L., Komárek J. (2009). Nomenclatural Validation of the Genetically Revised Cyanobacterial Genus Dolichospermum (Ralfs Ex Bornet Et Flahault) Comb, Nova. Fottea 9, 59–64. doi: 10.5507/fot.2009.005
Wang W. L., Moore J. K., Martiny A. C., Primeau F. W. (2019). Convergent Estimates of Marine Nitrogen Fixation. Nature 566, 205–211. doi: 10.1038/s41586-019-0911-2
Wasmund N., Lass H. U., Nausch G. (2005). Distribution of Nutrients, Chlorophyll and Phytoplankton Primary Production in Relation to Hydrographic Structures Bordering the Benguela-Angolan Frontal Region. Afr. J. Mar. Sci. 27, 177–190. doi: 10.2989/18142320509504077
Wasmund N., Struck U., Hansen A., Flohr A., Nausch G., Grüttmüller A., et al. (2015). Missing Nitrogen Fixation in the Benguela Region. Deep. Res. Part I. Oceanogr. Res. Pap. 106, 30–41. doi: 10.1016/j.dsr.2015.10.007
Westberry T. K., Siegel D. A. (2006). Spatial and Temporal Distribution of Trichodesmium Blooms in the World’s Oceans. Glob. Biogeochem. Cycle. 20, GB4016. doi: 10.1029/2005GB002673
Zani S., Mellon M. T., Collier J. L., Zehr J. P. (2000). Expression of nifH Genes in Natural Microbial Assemblages in Lake George, New York, Detected by Reverse Transcriptase PCR. Appl. Environ. Microbiol. 66, 3119. doi: 10.1128/AEM.66.7.3119-3124.2000
Keywords: nitrogen fixation, diazotrophs diversity, oxygen minimum zone (OMZ), Benguela coastal upwelling system, Angola Basin
Citation: Reeder CF, Arévalo-Martínez DL, Carreres-Calabuig JA, Sanders T, Posth NR and Löscher CR (2022) High Diazotrophic Diversity but Low N2 Fixation Activity in the Northern Benguela Upwelling System Confirming the Enigma of Nitrogen Fixation in Oxygen Minimum Zone Waters. Front. Mar. Sci. 9:868261. doi: 10.3389/fmars.2022.868261
Received: 02 February 2022; Accepted: 22 April 2022;
Published: 26 May 2022.
Edited by:
Amal Jayakumar, Princeton University, United StatesReviewed by:
Camila Fernandez, UMR7621 Laboratoire d’océanographie microbienne (LOMIC), FranceMaren Voss, Leibniz Institute for Baltic Sea Research (LG), Germany
Copyright © 2022 Reeder, Arévalo-Martínez, Carreres-Calabuig, Sanders, Posth and Löscher. This is an open-access article distributed under the terms of the Creative Commons Attribution License (CC BY). The use, distribution or reproduction in other forums is permitted, provided the original author(s) and the copyright owner(s) are credited and that the original publication in this journal is cited, in accordance with accepted academic practice. No use, distribution or reproduction is permitted which does not comply with these terms.
*Correspondence: Christian Furbo Reeder, crfurbo@biology.sdu.dk