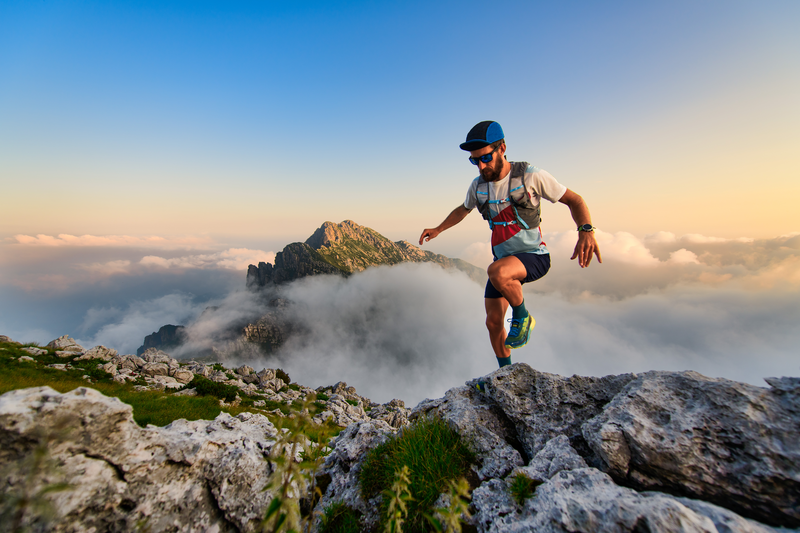
94% of researchers rate our articles as excellent or good
Learn more about the work of our research integrity team to safeguard the quality of each article we publish.
Find out more
ORIGINAL RESEARCH article
Front. Mar. Sci. , 19 April 2022
Sec. Marine Biology
Volume 9 - 2022 | https://doi.org/10.3389/fmars.2022.866906
This article is part of the Research Topic Biological Invasions in the Mediterranean Sea View all 13 articles
Ascidians are the largest and most diverse class of the subphylum Tunicata, and are important components of marine benthic communities. They are also renowned worldwide invaders, of growing concern due to ease of spread and impacts on native biota. We hereby combined bathymetric and habitat mapping, extensive transect and photo-quadrat sampling, and a morphological and molecular study on selected specimens to evaluate taxonomic composition, cover, and distribution pattern of ascidians in a marine reserve of the central-western Mediterranean Sea, the Miseno Lake (Bacoli, Naples, Italy). Twenty-four taxa were found, of which 9 were non-indigenous species (NIS), 5 cryptogenic, and 10 native, delineating the Miseno Lake as a major hotspot of NIS and cryptogenic ascidians. DNA barcoding yielded 114 sequences, confirming identification for 22 taxa but also revealing single to few mismatches per species. Taxonomic insights were offered in the text and the Supplementary Material for several taxa, including the understudied Ascidia colleta and Ascidia malaca. The presence of a new clade was discovered in the Distaplia bermudensis species complex. Didemnum pseudovexillum and Polyclinum constellatum were respectively first recorded in Italy and western Mediterranean, and the same holds true for other taxa whose accurate identification was only possible through molecular evidence. The analysis of photographic archives allowed backdating and georeferencing to the Miseno Lake of the first records of Aplidium accarense and Botrylloides niger in Italy and of P. constellatum in the Mediterranean. Generalized Additive Models revealed that the abundance of NIS increased with availability of hard substrates (rocks or litter), decreased with depth, and was the highest in the channels connecting to other water bodies, followed by the peripheral areas of the lake. No certain evidence of competitive restriction of native ascidians was found. Finally, introduction pathways, potential impacts, and additional insights were discussed for NIS. The present study provides a baseline to evaluate shifts in the ascidian communities in the future, suggests that constant monitoring programs constitute crucial steps to achieve solid NIS management, and confirms marine reserves as widely vulnerable to biological invasions, especially when they coincide with potential hotspots of arrival or spreading such as semi-enclosed basins.
The introduction of marine non-indigenous species (NIS) is a threat to native biodiversity with often severe impacts on biodiversity, economy, and human health (Vilà and Hulme, 2017; Bédry et al., 2021). The European seas are particularly affected by biological invasions, with more than 600 NIS already established in the area (Katsanevakis et al., 2014; Zenetos et al., 2017). Concerned by the issue, the European Union developed a specific Descriptor (D2) of the Marine Strategy Framework Directive (MSFD) to assess NIS pressure on ecosystems and coordinate response strategies (Directive 2008/56/CE). Nevertheless, the descriptor D2 was one of the least investigated so far, with limited dedicated monitoring programs especially in the Mediterranean Sea (European Commission, 2020a; European Commission, 2020b).
The Gulf of Naples, and generally the Campania region (Italy, central-western Mediterranean Sea), is already known as a major hub for NIS spreading in the western parts of the Mediterranean basin (Servello et al., 2019). The city of Naples and its surroundings are one of the most densely populated areas of Italy, which is reflected in a territory with a constantly growing commercial and touristic demand (De Luca and Rosciano, 2020). The area is subjected to inter-continental shipping routes and a wide local traffic, with numerous ferries that interlink Naples harbor with other Mediterranean harbors but also with the popular touristic islands of the region (Capri, Ischia, and Procida) (Appolloni et al., 2018). In addition, a number of small marinas and several aquaculture enterprises (mostly mussel farms) are distributed along its coastline (Esposito et al., 2013; Appolloni et al., 2018), and four marine coastal lagoons are present in the Phlegraean area (Miseno, Fusaro, Lucrino, and Torrefumo) (Camilletti and Lanzi, 2018). Notwithstanding these premises, the study of local NIS still lacks targeted research efforts, and previous records were most often the result of casual or opportunistic findings (Gambi, 2021 and references therein).
Among all potential hotspots for monitoring and/or early detection listed above, marine lagoons are renowned as NIS-favored environments, as filter-feeding species flourish there thanks to the combined effect of anthropogenic disturbance and general eutrophic conditions and high nutrient levels (e.g. Occhipinti-Ambrogi, 2000; Marchini et al., 2015). In the last decade, about 15 NIS have already been discovered in Italy in enclosed and semi-enclosed marine basins (e.g. Occhipinti-Ambrogi et al., 2011; Langeneck et al., 2020), and the same also holds true for the Phlegraean lagoons, which already accounted for important sightings in the recent past (e.g. Crocetta et al., 2013; Hanson et al., 2013; López-Legentil et al., 2015; Montesanto et al., 2021; Montesanto and Mastrototaro in Orfanidis et al., 2021). Within the biota living in lagoons, ascidians (Tunicata: Ascidiacea) are species that play a pivotal role among NIS due to their ease of spread and include taxa that are renowned worldwide invaders (Shenkar and Swalla, 2011; Zhan et al., 2015). They are somehow facilitated in the colonization of new areas by environmental plasticity, being well adapted to live in polluted environments (Lambert and Lambert, 1998; López-Legentil et al., 2015; Streit et al., 2021), and also easily fouling on anthropogenic substrates (Shenkar et al., 2008; Aldred and Clare, 2014; Gewing et al., 2017). Finally, in favorable conditions, ascidians also display an invasive character, resulting in sudden community shifts and dominance of the available substrates (Lambert and Lambert, 1998; Bullard et al., 2007).
Within projects aiming to investigate various aspects of the biodiversity of the Campania region, we hereby first explored the status of the Miseno Lake (Bacoli, Naples) protected area as a potential hotspot for NIS and cryptogenic species. To do so, we focused on the target phylogenetic group mentioned above (ascidians) and evaluated its local taxonomic composition, cover, and distribution pattern through a multidisciplinary approach that includes bathymetric and habitat mapping, a benthic survey carried out with transects and photoquadrats, an integrative taxonomic approach on selected specimens, and the analysis of the data obtained through Generalized Additive Models. Finally, the arrival of NIS in the area was backdated as much as possible through the analysis of photographic archives.
The Miseno Lake (~40.793111N - 14.072287E, central-western Mediterranean Sea) is a brackish-water body of around 40.25 hectares and a total perimeter of 2800 meters, located in the town of Bacoli (Naples, Italy) (Leccese and Speziale, 1967). It is included in the list of Sites of Community Importance (SCI no. IT8030017; European Commission Habitats Directive 92/43/EEC) as a Special Area of Conservation (SAC), and it also lays within the Campi Flegrei Regional Park (https://www.parcodeicampiflegrei.it/). As an area subject to protection, access and recreational activities are only allowed to authorized personnel. The lake has a regular outline, somehow resembling a right-angled triangle with the right angle cut away by a rectangular portion, and in its southern part is separated from the Tyrrhenian Sea by a 200 m-wide stretch of coast (Leccese and Speziale, 1967; ENEA, 2002; Figure 1). The entire lake is bordered by an artificial dock wall (~50–60 cm in height) composed of tuff, a friable volcanic rock. Water exchange with the sea is allowed by two channels, the Miliscola channel (MC), located on the western side, and the Casevecchie channel (CC), located on the eastern side. The MC is 250 m long, has a sandy bottom with scattered rocks and an average depth of ~1.5 m, and faces a stretch of open sea, the Procida Strait. The outer part is characterized by rock boulders laying on a sandy bottom, covered by littoral assemblages dominated by photophilic algae. The CC is 100 m long, has a rocky bottom and an average depth of ~1 m, and opens directly into a sheltered bay that hosts two marinas, namely the Bacoli marina and Marina Piccola, and a maritime police facility operative until 2020. Outside the marinas, two mussel farms are still active nowadays. Little is known on the lake’s bathymetry and main substrates, except for preliminary characterizations of the area made about 50 and 20 years ago (Leccese and Speziale, 1967; Rigillo Troncone, 1975; ENEA, 2002; La Magna et al., 2002).
Figure 1 The Miseno Lake and its position (red square) in the Mediterranean Sea (top right) and in the Gulf of Naples (bottom right). Left image highlighting sampling grid (red squares), longitudinal transects (black lines), dock wall perimeter, channels (CC, Casevecchie channel; MC, Miliscola channel), and simplified bathymetry (for a detailed bathymetric map see Supplementary Figure 1).
Bathymetry of the Miseno Lake was evaluated through transects held parallel to the southern shoreline (~15–20 m from each other) carried out with a 3 m-long inflatable boat equipped with a 10 cv Suzuki engine and a single beam echo sounder (SBES) (HYDROTRAC®, Odom), set at 200 KHz (accuracy 0.01 meter ± 0.1% depth). For safety reasons (boat limitations), depth measurements started from 0.6 m. Each point of measurement was real-time geo-referenced through a DGPS (Differential Global Positioning System) Vector VS330 GNSS (Hemisphere) connected to LandStar (Landstar System, Inc., USA), a satellite transmission service for GPS differential corrections, recording geographic coordinates and depths at 15 points/s. Acquired data were quality checked, filtered (set at 30 cm of distance from each other), and tidally corrected. Bathymetry was finally processed in ArcGis 9.2 (ESRI, Redlands, USA) and georeferenced according to the UTM WGS84 coordinate system (central Meridian: 15°00’00”; false East: 500,000; scale factor: 0.9996).
Fieldwork was carried out in September–November 2021 by three trained operators, two snorkeling and/or scuba diving (R.V. and V.T.) and the third (F.C.) mostly giving support on the inflatable boat. Given the particular outline, the lake was divided into a grid made by squares of ~100×100 m (Figure 1). Then, fieldwork used a combination of different strategies according not only to the topography of the lake but also to the investigated sections, namely the inner lake bottom (subsequently divided into inner and peripheral), the dock wall perimeter, and the two channels.
To investigate the habitat characterization of the inner bottom and the ascidian community living there, seven main latitudinal transects (A–G), which were 100 m apart, with the first (A) and the last (G) being ~50 m away from the dock walls, were investigated through snorkeling/scuba diving using a 100-m line. Each latitudinal transect was divided into 100 m-long sub-transects following the grid, for a total of 49 grid sectors. However, wherever the sector could not host a straight 100-m latitudinal transect (A1, A2, A4, B1, B2, B6, C2, C8, D1, D8, E1, E9, F7, G1, and G7), it was amended so that the total was 100 m (Figure 1). The two observers recorded the following information (within 1.5 m on the left/right of the line): i) main substrate encountered, namely Caulerpa prolifera (Forsskål) J.V. Lamouroux, gravel/dead shells, litter, mud, rocks, and sand; ii) number of specimens and/or colonies found per species; iii) type of substrate where ascidians were found, divided into four categories: rocks, litter, C. prolifera, and other biogenic substrates — mostly natural wood, tubes of the polychaete Chaetopterus variopedatus (Renier, 1804) species complex, and the bryozoan Schizoporella errata (Waters, 1878). For colonial species which form zooid aggregations, such as Polyandrocarpa zorritensis (Van Name, 1931), each cluster was counted separately.
To investigate the community living on the dock wall perimeter, each sector was surveyed through photoquadrats. In particular, three replicates were taken per sector (for a total of 72 photoquadrats, placed approximately at a fixed distance from each other according to the length of the dock wall in the sector), using a PVC frame of 49.5×49.5 cm (total area 2450.25 cm2) fixed to a Sony RX100 mkII camera in a polycarbonate housing equipped with a INON D2000 strobe. Images were taken placing the quadrat below the surface level at the lowest tide. For each quadrat, notes on the main species found in the frame were also taken on a diving whiteboard to facilitate the subsequent laboratory work.
To investigate the communities of the two channels, a combination of both approaches was used. In particular, a single additional 100-m bottom transect was carried out in each channel, whereas the communities of the dock walls were investigated through 12 additional photoquadrats (6 photoquadrats per channel, with 3 photoquadrats at each side of each channel wall).
Finally, to investigate the proper taxonomy of the species encountered not only with visual census, but also with an integrative approach, samples were collected during transects/photoquadrats, but sometimes also opportunistically, for subsequent laboratory work (internal and external morphology and molecular analyses).
Ascidian samples collected were brought to the Laboratory of Benthos of Stazione Zoologica Anton Dohrn (SZN, Naples, Italy) for proper taxonomic identification. When necessary, specimens were analyzed and dissected under a Zeiss Axio Zoom.V16 (Carl Zeiss, Oberkochen, Germany) stereomicroscope. Then, single specimens (in case of solitary ascidians) or a sub-sample of each colony (in case of colonial ascidians) were isolated for molecular analyses. As a general rule (whenever possible), conspecificity between samples was tested by sequencing 5–6 samples per putative species, collected from different places of the lake.
To do so, total genomic DNA was extracted from muscular tissue of single zooids using the DNeasyBlood & Tissue® kit (Qiagen, Hilden, Germany), following the producer’s protocol. Amplifications of a partial region of the cytochrome c oxidase subunit I (COI) gene were obtained through polymerase chain reactions (PCRs) with the following primer sets by Brunetti et al. (2017): dinF (5’-CGTTGRTTTATRTCTACWAATCATAARGA-3’) and Nux1r (5’-GCAGTAAAATAWGCTCGRGARTC-3’). Polymerase chain reactions (PCRs) were carried as follows: first denaturation at 95°C for 5 min, followed by 35 cycles of denaturation at 95°C for 1 min, annealing at 48–50°C for 1 min, extension at 72°C for 1 min, with a final elongation at 72°C for 5 min. The PCR products were purified and sequenced at the SZN Molecular Biology and Sequencing Service through an Automated Capillary Electrophoresis Sequencer 3730 DNA Analyzer (Applied Biosystems, CA, USA), using the BigDye® Terminator v3.1 Cycle Sequencing Kit (Life Technologies, Renfrew, UK).
Sequences obtained were quality checked, assembled, and edited using Unipro UGene v.39 (Okonechnikov et al., 2012). Basic Local Alignment Search Tool (BLAST; https://blast.ncbi.nlm.nih.gov/Blast.cgi) (Morgulis et al., 2008) was then used to assess the identity of each sequence against the GenBank database (last research: 30.XII.2021).
Sequenced and other collected specimens were finally fixed in 99.9% ethanol and deposited in the collection of the Laboratory of Benthos (SZN), under different accession codes (Supplementary Table 1).
Since the sequences obtained for Distaplia bermudensis Van Name, 1902 gave unclear BLAST results (see below), we also carried out phylogenetic analyses in order to assess the phylogenetic position of our specimens within other D. bermudensis collected worldwide.
A total of thirty-two COI partial sequences were used in the final alignment, including the five sequences of D. bermudensis amplified in this study and twenty-one sequences of D. bermudensis retrieved from GenBank (accession numbers reported in Supplementary Tables 1, 2). Six additional representatives of the order Aplousobranchia Lahille, 1886, collected in this study, were used as outgroup: Clavelina lepadiformis (Müller, 1776) (SZN_B_1879ASC15G), Clavelina sabbadini Brunetti, 1987 (SZN_B_2707ASC36A), Didemnum pseudovexillum Turon & Viard, 2020 (SZN_B_1902ASC22A), Lissoclinum weigelei Lafargue, 1968 (SZN_B_3172ASC49A), Aplidium accarense (Millar, 1953) (SZN_B_1822ASC18B), and Polyclinum constellatum Savigny, 1816 (SZN_B_2884ASC43A). The selected sequences were aligned and trimmed with Unipro UGene v.39 (Okonechnikov et al., 2012), and the best-fit evolutionary model (GTR + I + G) was found with JModelTest 2 v.0.1.10 (Darriba et al., 2012), selected with the Akaike information criterion (AIC) method. The maximum likelihood (ML) analyses were run with RAxML v.8.2 (Stamatakis, 2014) using 1000 rapid bootstrap pseudo-replicates. The Bayesian Inference (BI) was performed with MrBayes v.3.2.5 (Ronquist et al., 2012) run for 10 million generations, sampled every 1000 generations, with a standard 25% burn-in. Convergence of the MCMC runs was checked with Tracer v1.7.1 (Rambaut et al., 2018).
Three species delimitation methods were then used on the same alignment to infer Operational Taxonomic Units (OTUs) boundaries within the Distaplia clade: Automated Barcode Gap Discovering (ABGD, Puillandre et al., 2012), Poisson Tree Processes (PTP, Zhang et al., 2013), and Generalized Mixed Yule Coalescent (GMYC, Fujisawa and Barraclough, 2013). The ABGD analysis was performed on the ABGD web platform (https://bioinfo.mnhn.fr/abi/public/abgd/abgdweb.html) with Jukes-Cantor (JC69) model and standard parameters. The PTP and GMYC analyses were run on the Exelixis Lab web server (The Exelixis Lab, https://species.h-its.org). The PTP was run for 500000 generations, 25% burn-in, and 100 thinning based on the rooted ML tree obtained in the aforementioned analysis. The GMYC was performed in single threshold (s-GMYC) method. As input, an ultrametric tree was obtained with BEAST v.1.10.4 (Suchard et al., 2018) based on the same alignment as above.
Once a solid identification of the specimens was achieved, species were classified into the following categories based on the most up to date literature (see Essl et al., 2018): i) native for species with a putative Atlantic-Mediterranean origin; ii) cryptogenic for species with uncertain native range; iii) non-indigenous for species native in other biogeographic sectors and locally introduced through human activities (European Commission, 2014).
Finally, the digital photographic archives (2014–2021) of one of the authors (G.V.), who regularly surveyed and mostly photographed the lagoon biota in the past decade, was screened in order to backdate as much as possible the most probable first introduction event of selected NIS in the Miseno Lake.
Images obtained (photoquadrats) were subsequently analyzed in Photoquad v1.4 (Trygonis and Sini, 2012). Each picture was calibrated according to the known frame measures. After picture calibration, abundance and cover of each species were calculated counting single specimens (for solitary ascidians) and colonies (single separated clusters) for each quadrat. Data obtained from transects and photoquadrats were then collated in a matrix in Excel (Supplementary Data Sheet 1). Data are reported as total number of individuals counted on the seabed and on the dock wall, as well as mean count per transect on the seabed and mean percentage cover on the dock wall ± standard error (SE).
The abundance (number of individuals per transect) of the most commonly encountered NIS ascidians, namely Botrylloides niger Herdman, 1886, D. bermudensis, P. constellatum, and A. accarense, as well as of all NIS and cryptogenic species combined together, was modeled with Generalized Additive Models (GAMs; Hastie and Tibshirani, 1990). Due to severe overdispersion (residual deviance of the fitted models much higher than the residual degrees of freedom), and thus violation of the assumption of equal mean and variance in the response variables, the negative binomial distribution was used instead of the Poisson distribution (Lindén and Mäntyniemi, 2011). The expected values of the number of individuals counted in each transect were related to spatial and environmental covariates, according to the general formulation f(E[counts]) = LPi = c + Σmsm (zmi) + ΣrFri, where f is the link function (in this case link=log), LP is the linear predictor, sm(·) is the one-dimensional smooth function for covariate m, Fr are categorical predictors, and zmi is the value of spatial covariate m for the ith transect. The smooth function sm(·) was represented using penalized regression splines (cubic splines with basis dimension q=10), estimated by penalized iterative least squares (Wood, 2006). The optimum degree of smoothing was defined by generalized cross validation (GCV), where the amount that the effective degree of freedom of each model counts in the GCV score was increased by a factor c=1.4 according to Kim and Gu (2004) to avoid occasional overfitting. The mgcv package implemented in R v4.1.0 was used for model fitting (Wood, 2006; R Core Team, 2021).
Four predictor variables were used for modelling the abundance of NIS on the seafloor, namely: i) depth; ii) hard, the amount of hard substrate items counted in each transect — i.e. the sum of rocks and litter items; iii) section, a categorical variable with three levels representing the distinct seafloor areas investigated in the lake (channel, inner, and peripheral); iv) Caulerpa, a categorical variable with two values, 0 for absence and 1 for presence, indicating the presence/absence of C. prolifera within each transect, as the seagrass can act as substrate for epiphytic ascidians. The degree of collinearity among these variables was measured by calculating the variance inflation factor (VIF) for each covariate based on the negative binomial models. Since all VIF values were <3, there was no substantial multicolinearity among the independent variables and thus were all kept in the analyses. Overall, eight candidate models gi were used in the analyses linked to the following hypotheses: h1 - abundance is affected by depth; h2 - abundance is restricted by the availability of proper substrate (hard+Caulerpa); h3 - the three different sections have varying conditions (other than depth and substrate) — e.g. hydrodynamism, that may affect the abundance of the species (Supplementary Table 3). Furthermore, the abundance of native species on the seafloor was modelled. For this, in addition to the same three hypotheses, a fourth one was investigated: h4 - abundance of native species is restricted by the abundance of NIS and cryptogenic species. An additional predictor variable was included — i.e. the abundance of NIS and cryptogenic species (AlCr), and the set of candidate models for native species included overall sixteen models (Supplementary Table 4). This hypothesis was also tested using the estimated cover of native vs NIS/cryptogenic species along the dock wall perimeter (photoquadrat sampling), based on only four models, the null model, one including the cover of NIS and cryptogenic species as a predictor variable, one including section (peripheral vs channels) as a predictor variable, and one including both predictors.
Akaike’s information criterion was used for model selection (Akaike, 1973; Burnham and Anderson, 2002), and the AIC differences Δi=AICi - AICmin were computed over all candidate models gi, where AICmin is the minimum AIC corresponding to the best model. To quantify the plausibility of each model, given the data and set of candidate models, the ‘Akaike weight’ wi of each model was calculated, where wi = exp(-0.5∆i)/Σj exp(-0.5∆j) (Burnham and Anderson, 2002). The relative importance of each of the hypotheses made was estimated by summing the Akaike weights across all the models in the set that were linked with each hypothesis (Burnham and Anderson, 2002).
The bathymetric survey recorded a total of 228000 reliable depth measurements, interpolated to produce a detailed bathymetric map of the lake (Supplementary Figure 1). The average depth was ~2 m, whereas the maximum depth was ~3.5 m. The habitat characterization revealed the presence of muddy bottoms almost dominated by a dense meadow of the native alga C. prolifera in several grid sectors but mostly in the inner parts of the lake (A1, B2–B5, C3–C7, D2–D7, E2–E6, F2–F6, G3–G7). The meadow only becomes sparse in the majority of the peripheral parts of the lake and underneath the dock wall, nearby freshwater inputs, and in the proximity of the two channels (A2–A4, B1, B6, C1, C2, C8, D1, D8, E1, E7–E9, F1, F7, G1, G2), where the substrate is again unconsolidated but with a wider granulometric range, from mud to gravel. Worth a mention, the sector C1 was mostly characterized by a sand bank with a very low depth, and thus it was excluded from the analysis of the transects-based data, although retained for the dock wall analysis through photoquadrats. The area was also characterized by the presence of sparse rocks (n=455) and widely impacted by litter items (n=1801), mostly originating from commercial or recreational activities in the nearby town of Bacoli. Their presence and distribution was generally influenced by geographic position and conformation of the nearby substrate. Rocks were rare to absent in the inner parts of the lake and mostly found in grid sectors close to the dock wall — C2 (43), D1 (59), D8 (68), E1 (47), F1 (53), G1 (54), as they constitute waste material from the previous levee. Litter items were found in all transects, with the sectors close to the CC being the ones most impacted — D8 (296), E8 (91), and E9 (80).
A total of 24 taxa were observed during our survey, belonging to the three orders of the class Ascidiacea Blainville, 1824 (nine Aplousobranchia, six Phlebobranchia Lahille, 1886, and nine Stolidobranchia Lahille, 1886) (Table 1, Figures 2, 3 and Supplementary Table 1). Of these, ten are native, nine are NIS, and five are cryptogenic species. Distribution in taxonomic orders varied based on the species’ status in the Mediterranean Sea. Among native species, Aplousobranchia and Phlebobranchia accounted for four taxa each, whereas Stolidobranchia only accounted for two. On the contrary, NIS accounted for five Stolidobranchia, followed by three Aplousobranchia, and one Phlebobranchia. Finally, cryptogenic species accounted for two species of Aplousobranchia and Stolidobranchia each, and only one Phlebobranchia (Table 1, Figures 2, 3 and Supplementary Table 1).
Table 1 Surveyed ascidian species in the Miseno Lake, the Casevecchie channel, and the Miliscola channel, with known status (S: C, cryptogenic; N, native; NIS, non-indigenous).
Figure 2 Ascidian species from the Miseno Lake (part 1) (see Table 1). Abbreviations used: C, cryptogenic; N, native; NIS, non-indigenous. Order Aplousobranchia. Family Clavelinidae: (A) Clavelina lepadiformis - C (picture not taken during surveying activities). (B) Clavelina sabbadini - N. Family Didemnidae: (C) Didemnum pseudovexillum - C (alcohol preserved). (D) Lissoclinum perforatum - N. (E) Lissoclinum weigelei - N. (F) Trididemnum cereum - N (picture not taken during surveying activities). Family Holozoidae: (G) Distaplia bermudensis - NIS (overgrowing a tube of the polychaete C. variopedatus). Family Polyclinidae: (H) Aplidium accarense - NIS. (I). Polyclinum constellatum - NIS. Order Phlebobranchia. Family Ascidiidae: (J) Ascidia colleta - N. (K) Ascidia malaca - N. (L) Phallusia fumigata - N.
Figure 3 Ascidian species from the Miseno Lake (part 2) (see Table 1). C, cryptogenic; N, native; NIS, non-indigenous. Order Phlebobranchia. Family Ascidiidae: (A) Phallusia mammillata - N. Family Cionidae: (B) Two specimens of Ciona robusta - NIS, flanked on the left by A. malaca (on litter). Family Perophoridae: (C) Perophora viridis - C, nested within B. niger. Order Stolidobranchia. Family Pyuridae: (D) Microcosmus polymorphus - N. (E) Microcosmus squamiger - NIS, detail of the oral siphon. (F) Pyura dura - N (on litter). Family Styelidae: (G) Botrylloides niger - NIS. (H) Botryllus schlosseri - C. (I) Polyandrocarpa zorritensis - NIS. (J) Styela canopus - C. (K) Styela plicata - NIS (on wood) (picture not taken during surveying activities). (L) Symplegma brakenhielmi - NIS.
The amplification of the COI partial region gave a total of 114 sequences, ranging from 504 to 828 base pairs (bp), with a number of specimens sequenced per species varying from one to six. The morphological identification was supported by BLASTn hits (~95–100% similarity) for 22 species (Supplementary Table 1). However, several ambiguities in the GenBank database were found, as follows: i) single to a few mismatches in four species — Lissoclinum perforatum (Giard, 1872), Phallusia mammillata (Cuvier, 1815), Pyura dura (Heller, 1877), and B. niger — were presumably due to misidentifications or incorrect uploads; ii) mismatches in three species — L. weigelei, A. accarense, and Ciona robusta Hoshino & Tokioka, 1967 — were due to identification not provided at a species level by some authors when uploading sequences in GenBank or lack of GenBank updates after the species complexes were resolved (e.g. Caputi et al., 2007); iii) mismatches in four additional taxa — C. lepadiformis, D. bermudensis, Botryllus schlosseri (Pallas, 1766), and Styela canopus (Savigny, 1816) — were due to the fact that they belong to species complexes (Turon et al., 2003; Nydam et al., 2017; Barros and Rocha, 2021; Salonna et al., 2021; Nuzzo et al., 2022), and thus the sequencing of local specimens allowed clarification of their exact taxonomic allocation and also identification of a new clade in D. bermudensis (see below); for the sake of text clarity, these species are still listed in the subsequent chapters as monotypic taxa; iv) mismatches in two species — P. constellatum and Symplegma brakenhielmi (Michaelsen, 1904) — have already been reported and presumably require further taxonomic work to solve the related taxonomic issues, including sequencing of specimens from the type localities of the taxa involved (Mastrototaro et al., 2019; Montesanto et al., 2022); these species are listed in the subsequent chapters following the most recently updated literature on the Mediterranean biota.
Finally, with regards the two remaining species, mismatches in Ascidia malaca (Traustedt, 1883) may be due to misidentifications or even uncertainties resulting from the use of the COI marker within the genus Ascidia Linnaeus, 1767 (see Streit et al., 2021), whereas Ascidia colleta Monniot & Monniot, 1970 accounted for a new GenBank entry, as it only showed 80.4–81.2% similarity with Ascidia conchilega Müller, 1776 (MN064596–7: Couton et al., 2019). Given taxonomic issues regarding worldwide species of this genus, these taxa are also further illustrated and discussed in Supplementary Data Sheet 2.
BLAST results of D. bermudensis sequences from the Miseno Lake (SZN_B_1743ASC10H–1747ASC10N) gave high similarity (99.71%) to another sequence of a specimen identified as D. bermudensis (MT637947) from Puerto Rico marinas by Streit et al. (2021), but also significant differences (80.33–86.5%) from other D. bermudensis worldwide (Supplementary Table 1).
After sequences-editing steps, we obtained a trimmed alignment (575 bp) of COI partial sequences, with 250 parsimony-informative sites. Both the ML and BI analyses produced congruent tree topologies, hence we showed the best ML tree with bootstrap (bs) and posterior probability (pp) values reported at nodes (Figure 4). Both tree topologies clustered the newly collected D. bermudensis from Miseno with the specimen from Puerto Rico marinas by Streit et al. (2021), forming a different clade with respect to the other samples. This clade, that we first name here as clade C, was well supported (bs=99; pp=1) and the sister (bs=82; pp=0.99) of clade B sensu Evans et al. (2021). Results of the species delimitation analyses also well supported the three-clades grouping well (Figure 4 and Supplementary Data Sheet 3). The ABGD analysis inferred three OTUs within the D. bermudensis sequences in the primary partition. The PTP and GMYC analyses were also consistent, reporting the three-clade grouping in all the analyses carried out, with PTP Bayesian supporting values ranging from 0.18 to 0.88 (Supplementary Data Sheet 3).
Figure 4 Best ML phylogenetic tree obtained from selected COI sequences. Numbers above/below branches represent bootstrap (bs) and bayesian posterior probability (pp) values. Species delimitation outputs are graphically represented through bars: ABGD (Automatic Barcode Gap Discovery), PTP (Poisson Tree Process), and GMYC (Generalized Mixed Yule Coalescent). Colors of clades A and B as in Evans et al. (2021).
The digital photographic archives of the Miseno Lake biota were composed of ~400 pictures, ranging from May 2014 to October 2021. By subsequently excluding widespread NIS ascidians introduced in the entire Mediterranean Sea in historical times (C. robusta and S. plicata), and notwithstanding limitations in identifying ascidians on the basis of underwater photographs, unequivocal evidence was found regarding the presence of at least five NIS before our survey, namely D. bermudensis, A. accarense, P. constellatum, B. niger, and P. zorritensis. In particular, four of them were recorded since 2014, and one (D. bermudensis) since 2019, with three sightings that predate first-record dates and localities in Italy, one of which also accounts for the first sighting in the Mediterranean Sea (Table 2 and Figure 5). It is noteworthy that four of these species were the most common NIS encountered during our survey (see below). Finally, photographic evidence of the impact of D. bermudensis on dock wall communities were also found, and illustrated in Figures 5F, G.
Table 2 First finding date of NIS ascidians in the Miseno Lake (ML) (see Figure 5), with date and locality of first published records from the Mediterranean Sea and Italy (when different).
Figure 5 Photographic evidence backdating NIS arrival in the Miseno Lake and impact of Distaplia bermudensis on dock wall communities (see Table 2). (A) Distaplia bermudensis, October 2019. (B) Aplidium accarense, September 2014. (C) Polyclinum constellatum (with P. zorritensis), June 2014. (D) Botrylloides niger, June 2014. (E) Polyandrocarpa zorritensis, May 2014. (F, G) The Casevecchie channel before and after the arrival of D. bermudensis, with abundant colonies of P. zorritensis and B. niger (left, August 2015) and colonies of D. bermudensis (of different colours) colonizing the same dock wall area (right, March 2021).
Overall, a total of 20197 ascidian individuals/colonies were counted in the Miseno Lake. Of these, 4537 were censused during transects on the seabed of the lake, 11570 were found in the dock wall, and the remaining 4090 in transects and dock walls in the Casevecchie and Miliscola channels (CC=3482; MC=608) (Table 1 and Supplementary Data Sheet 1).
Fifteen species were found in the seabed transects, among which B. niger (n=2696, nm=29 ± 62.3) and D. bermudensis (n=796, nm=8.3 ± 32.8) were the most common (Table 1 and Supplementary Data Sheet 1). The distribution of the four most abundant NIS is depicted in Figure 6. However, the peripheral parts of the lake hosted all species mentioned above, whereas the ascidian diversity was highly reduced in the inner parts of the lake, with only seven species found, among which only B. schlosseri and B. niger ranked high in numbers, which was also reflected in their presence in the transects (33/48 and 28/48, respectively) (Supplementary Data Sheet 1). With regard to substrates: 13 species were found on/below rocks, with B. niger and A. accarense being among the most commonly found, 12 species were found associated with litter, with B. niger and D. bermudensis almost completely dominating the group, five species were found associated with other biogenic substrates, among which D. bermudensis and B. niger were again the most commonly encountered, and two species only were found as epiphytes on C. prolifera leaves, namely B. niger and B. schlosseri (Supplementary Data Sheet 1).
Figure 6 Heatmaps showing abundance (counts per 100-m transect) of the four most common NIS (Distaplia bermudensis, Aplidium accarense, Polyclinum constellatum, and Botrylloides niger) found on the seabed of the Miseno Lake. For a detailed account of the sectors see Figure 1.
Thirteen species were found in the photoquadrats along the dock wall perimeter, among which the NIS B. niger (n=5770, cm= 1.4% ± 0.2%), P. constellatum (n=2115, cm=1.1% ± 0.2%), A. accarense (n=1327, cm=0.3%), and D. bermudensis (n=975, cm=2% ± 0.6%) were the most widespread (Table 1 and Supplementary Data Sheet 1). The distribution of these four species is depicted in Figure 7. Such abundances were also somewhat reflected in the overall presence of the species in the photoquadrats, with B. niger appearing in all photoquadrats (72/72), followed by P. constellatum (68/72), and A. accarense (64/72). On the other hand, D. bermudensis was locally abundant in only few sectors (25/72) (Table 1, Figure 7 and Supplementary Data Sheet 1). The dock wall sectors with the highest mean total cover were E8 (cm=17.0% ± 7.2%), mostly dominated by D. bermudensis (n=214, cm=10.4% ± 3.8%), B. niger (n=855; cm=1.1% ± 0.1%), and P. constellatum (n=132, cm=0.7% ± 0.2%), followed by C8 (cm=14.7% ± 9.2%) and A3 (cm=8.7% ± 4.4%) (Supplementary Data Sheet 1).
Figure 7 Heatmaps showing percent cover per sector of the four most common NIS (Distaplia bermudensis, Aplidium accarense, Polyclinum constellatum, and Botrylloides niger) found on the dock walls of the Miseno Lake. For a detailed account of the sectors see Figure 1.
Fourteen and thirteen species were respectively found in CC and MC, with assemblages differing between them and both channels hosting exclusive taxa. With regards CC, eight species were found in the transect, with D. bermudensis (n=1542) and B. niger (n=794) being the most common, whereas 12 species were found in the photoquadrats, with D. bermudensis (n=680, cm=13.1% ± 2.3%) dominating the assemblages (Table 1 and Supplementary Data Sheet 1). The CC also accounted for the highest cover in a quadrat (c=44.3%) and for the highest mean cover per sector (cm=22.3% ± 4.6%) (Supplementary Data Sheet 1). With regards MC, seven species were found in the transect, whereas 13 species were found in the photoquadrats; B. niger was the most common species in both of them (n=272; n=113, cm=0.6% ± 0.3%) (Table 1 and Supplementary Data Sheet 1). Microcosmus polymorphus Heller, 1877 ranked low in number of specimens found (n=5), but high in cover (cm=0.2% ± 0.2%) due to the big size of the specimens found. The overall mean cover (cm=2.6% ± 0.8%) was much lower than in CC (Supplementary Data Sheet 1).
Through the GAM analyses it was found that, when NIS and cryptogenic species were combined, the best model for their overall abundance on the seafloor was the full model (g7), including all predictor variables (Figure 8 and Supplementary Table 3). Their abundance declined with depth, increased with the availability of hard substrates, and was the highest in the channels, particularly in the CC, and the lowest in the inner zone (Figure 8). The best model for the abundance on the seafloor of all native species combined together was g1 (Supplementary Table 4), with only section as a predictor variable, having substantially higher support than the null model. For native species only h3 had high support, with no strong apparent effect of NIS or cryptogenic species (low support for h4, although it cannot be totally discarded) (Table 3 and Supplementary Figure 2). With regards the dock wall perimeter, the model using the abundance of NIS and cryptogenic species as predictor variable was the best, although its Akaike difference with the null model was only 0.6, and thus h4 does not have substantially higher support than the null hypothesis; hypothesis h3 had even less support (Supplementary Table 4). Hence, although the cover of the native ascidians was negatively correlated to the cover of NIS and cryptogenic species (Supplementary Figure 3), no solid conclusion can be made at this stage.
Figure 8 Estimated terms in the best model for all alien and cryptogenic species abundance on the seafloor. The smooth terms or estimated levels of categorical predictors (unbroken black lines) are given in the linear predictor scale. The corresponding 95% confidence intervals are given as gray shadows/dotted lines. Rug plot on the horizontal axis represents density of data points.
Table 3 Support by the data of the various hypothesis on the factors that affect ascidian abundance, based on Akaike weights (%).
With regards the four dominant NIS encountered in the Miseno Lake, namely D. bermudensis, A. accarense, P. constellatum, and B. niger, when analyzing the seabed, the best models had significantly higher support by the data in comparison to the null model (with Akaike differences >20), indicating that the assumption of completely random distribution of the species in Miseno Lake has no support (Supplementary Table 3). In all four cases, section was included in the best model, which indicates that the conditions among the two channels and the peripheral and the inner zones of the lake were sufficiently different to affect the abundance of NIS ascidians; hypothesis h3 had high support (Table 3). Three of the four species (D. bermudensis, P. constellatum, and B. niger) had the lowest abundance in the inner part of the lake (zero in the case of D. bermudensis), and the highest in the channels and in particular in the CC (Supplementary Figures 4, 6, 7), whereas A. accarense was absent from the channels but still had higher abundance in the peripheral than in the inner zone (Supplementary Figures 5). Depth (hypothesis h1) was negatively correlated with abundance in the cases of B. niger and P. constellatum (Supplementary Figures 6, 7 and Supplementary Table 3); in the case of B. niger, h1 had high support (Table 3). In particular, B. niger and A. accarense seemed to be favored by the existence of appropriate substrates, with higher local abundances with increased availability of rocks and litter items; however, the hypothesis h2 had only medium support for these species (Table 3).
Marine reserves are protected areas aiming to achieve conservation of nature with its associated ecosystem services and cultural values. Notwithstanding legal limitations that are in place in these areas, a growing amount of literature concerns their vulnerability to biological invasions, in particular when they host, coincide with, or are close to potential hotspots of NIS arrival or spreading (Otero et al., 2013; Giakoumi et al., 2019; Bilecenoğlu and Çınar, 2021). Both qualitative and quantitative results of the present study match this statement. The benthic survey carried out here revealed that the Miseno Lake hosts a previously undetected thriving community of ascidians, with native taxa that still rank high in species richness (10 taxa: ~41.5%), but that are fewer than NIS (9 taxa: 37.5%) plus cryptogenic species (5 taxa: ~21%) combined.
Moreover, among NIS, only two (C. robusta and S. plicata) are relatively old introductions and thus almost ubiquitous in the Mediterranean Sea (Caputi et al., 2007; Pineda et al., 2016a), whereas the majority of them are only known from a few records at a regional level, with some taxa that were never found in the study area (P. constellatum, P. zorritensis, and S. brakenhielmi), others that were only known from single opportunistic sightings (D. bermudensis, A. accarense, and B. niger: Montesanto and Mastrototaro in Orfanidis et al., 2021; Montesanto et al., 2021; Della Sala et al., 2022), and Microcosmus squamiger Michaelsen, 1927 which was the only species widely recorded in the Gulf of Naples, but has not been reported again in grey or published literature for more than 20 years (since 1996: Turon et al., 2007). However, the absence of records also holds true at Mediterranean level and is particularly surprising for the four NIS dominating the assemblage. Indeed, the most abundant NIS in the Miseno Lake was B. niger, only known from a few specimens sequenced from Israel (Rubinstein et al., 2013; Griggio et al., 2014) and Italy (Taranto: Salonna et al., 2021; Fusaro Lake, Naples: Della Sala et al., 2022), followed closely by P. constellatum, only known by few localities in the eastern Mediterranean (Halim and Abdel Messeih, 2016; Aydin-Onen, 2018; Montesanto et al., 2022), and A. accarense, the only species apparently more widespread, but only in some areas of the western Mediterranean (López-Legentil et al., 2015; Montesanto et al., 2021). Finally, D. bermudensis is again only known from a few records in Spain (Balearic Islands: Pérès, 1957) and Italy (Taranto: Mastrototaro and Brunetti, 2006; Miseno Lake: Montesanto and Mastrototaro in Orfanidis et al., 2021). The present paper highlights a high local invasiveness for all these species, report P. constellatum for the first time in the western Mediterranean, document the presence of another species (hereby named clade C) in the complex of D. bermudensis, and finally backdate and georeference to the Miseno Lake the first finding of A. accarense and B. niger in Italy and of P. constellatum in the Mediterranean Sea.
Cryptogenic species also accounted for a significant part of the ascidian biota of the lake. In particular, S. canopus and B. schlosseri were widespread, while C. lepadiformis, D. pseudovexillum, and Perophora viridis Verrill, 1871 were rare. The first two taxa are common in shallow sheltered waters and account for at least five different clades each in the Mediterranean (Barros and Rocha, 2021; Salonna et al., 2021). Specimens of S. canopus from the Miseno Lake well match the lineage G10 sensu Barros and Rocha (2021), already known from India, Angola, and Mediterranean Spain, and first recorded here from Italy, whereas specimens of B. schlosseri belong to the subclade A1 sensu Salonna et al. (2021), widely recorded worldwide including in Italy. Clavelina lepadiformis is represented by two clades in the Mediterranean (Turon et al., 2003; Nuzzo et al., 2022), and specimens from the Miseno Lake belong to C. lepadiformis sp. B sensu Nuzzo et al. (2022), commonly found in semi-enclosed basins and only known with certainty in Italy from the nearby Fusaro Lake (Nuzzo et al., 2022). Finally, with regards the two remaining species, P. viridis was described from the northwestern Atlantic (Buzzard’s Bay, Massachusetts, USA) (Verrill, 1871) but is very similar to several congeneric species, thus suggesting that at least part of the Mediterranean historical records may be based on misidentifications and making it difficult to trace a putative colonization history of the basin (Zenetos et al., 2017). This species was already recorded from the area by Neppi (1921), Salfi (1931), and Chimenz et al. (1985), and the Gulf of Naples is also the locality of description of its junior synonym Perophora dellavallei Neppi, 1921. Sequences of specimens from the Miseno Lake are the first based on Mediterranean specimens and proved conspecific with those from the USA deposited in GenBank, thus strengthening the synonymy between P. viridis and P. dellavallei and suggesting that historical records from the Gulf of Naples may be true. Finally, D. pseudovexillum was only known so far from Roscoff (Atlantic France) and Catalonia (Mediterranean Spain) and the present record widens its distribution to Italy. Prior to its formal description, this species was often mixed up with other didemnids, such as Didemnum vexillum Kott, 2002 (see discussions in Turon et al., 2020), and even the present finding was incidental, as the only colony found in the Miseno Lake was initially misidentified in the field as the native didemnid Trididemnum cereum (Giard, 1872).
Finally, some native species found here deserve attention. Among them, four — Phallusia fumigata (Grube, 1864), P. mammillata, M. polymorphus, and P. dura — are almost ubiquitous in Mediterranean coastal areas (e.g. Brunetti and Mastrototaro, 2017), whereas those of the family Didemnidae Giard, 1872 (L. perforatum, L. weigelei, and T. cereum) usually live in fouling communities of harbors and semi-enclosed basins (e.g. Chimenz et al., 1985; Casso et al., 2018), and even their presence in the Miseno Lake may be due to intra-Mediterranean transfers, as already speculated for the occurrence of both species of the genus Lissoclinum Verrill, 1871 on ship hulls in the Gulf of Taranto (Lafargue and Tursi, 1975). Integrative approaches, which include literature reviews and population genetic studies, may shed further light on that. It is noteworthy that the three remaining species (C. sabbadini, A. colleta, and A. malaca) are apparently quite rare in the Mediterranean Sea. The two former species were never recorded in Italy outside their area of description, namely the Adriatic Sea (Brunetti and Mastrototaro, 2017; Mastrototaro and Montesanto, 2021): for A. colleta this may be due to misidentifications with the very similar Ascidia muricata Heller, 1874, while C. sabbadini is almost unmistakable even in the field, suggesting that it may have ecological requirements that limit its distribution. On the other hand, A. malaca was originally described by Traustedt (1883) based on specimens collected in the Gulf of Naples, and considered conspecific with Ascidia depressa sensu Heller (1874) from the Lesina Lagoon (Adriatic Sea), an environment similar to the one investigated here. We figure topotypical specimens for the first time in more than a century. Although taxonomic uncertainties in the genus Ascidia prevented us from reaching any sort of taxonomic conclusion, the implementation of the genetic information on this genus, with two species newly sequenced here, may represent an advance toward resolving its taxonomy.
The present revealing results from an investigated area that is less than 1 km2 in extent are somewhat unexpected, but are likely due to two main previous limitations, namely taxonomic impediments (or general absence of zoologists and taxonomic specialists in the area) and lack of specific monitoring programs. Although the ascidian fauna of the central-western Mediterranean Sea is considered as the most studied in the entire basin (Koukouras et al., 1995), the rich literature published on the Gulf of Naples and nearby areas mostly dates back to a century ago, when early pioneers focusing on Mediterranean biodiversity investigated the local biota (e.g. Della Valle, 1877; Della Valle, 1881; Traustedt, 1883; Salfi, 1929; Salfi, 1931; Parenzan, 1959). Then, in the subsequent historical periods, faunistic and taxonomic work has been often considered old-fashioned, resulting in a lack of proper studies but for a single one assessing seasonal diversity and shifts of ascidian species in Ischia harbor (Chimenz et al., 1985) and few NIS records generated opportunistically by specialists (López-Legentil et al., 2015; Montesanto and Mastrototaro in Orfanidis et al., 2021; Montesanto et al., 2021). All this somehow makes it difficult to discuss the ascidian assemblage found in the Miseno Lake in a local perspective. However, Chimenz et al. (1985) reported 12 species in the investigated area, seven of which — C. lepadiformis, T. cereum, C. intestinalis (now C. robusta), P. viridis, B. schlosseri, S. partita (synonym of S. canopus), and S. plicata — were shared with the Miseno Lake. The absence of the NIS now found dominating the Miseno Lake suggests that they may have arrived only recently in the Gulf of Naples, although no genetic support was available for the 1985 study, and the knowledge of the Mediterranean ascidian biota was more limited, and thus we cannot exclude that putative discrepancies in taxonomically difficult families (e.g. Didemnidae, Ascidiidae Müller, 1776, Styelidae Herdman, 1881, and Pyuridae Hartmeyer, 1908) may be the result of misidentifications. On the other hand, studies at the Mediterranean level are indeed more widespread. Knowledge of ascidian biota in selected areas was gained either through targeted studies (e.g. Gewing et al., 2017; Casso et al., 2018; Arroyo et al., 2021), data gathering for the creation of checklists (e.g. Lafargue et al., 1986; Koukouras et al., 1995; Moreno et al., 2014), or scattered records in surveys of fouling communities (e.g. Leclerc and Viard, 2018; Lezzi et al., 2018; Giangrande et al., 2021). Studies investigating port environments also often focused on NIS, including ascidians (e.g. López-Legentil et al., 2015; Marchini et al., 2015; Tempesti et al., 2020), whereas specific surveys in enclosed and semi-enclosed basins were carried out in several Mediterranean localities (e.g. Pérez-Ruzafa, 1989; Mastrototaro et al., 2008; Chebbi et al., 2010; Davis and Davis, 2010). In general, the native ascidian assemblage found in the Miseno Lake is substantially coherent with the ones reported in the aforementioned studies, whereas no studies reported such a high and contemporary presence of NIS and cryptogenic species in a single and somewhat restricted study site. All this identifies the Miseno Lake as a major hotspot of NIS and cryptogenic ascidian species in the Mediterranean basin, highlights that the spreading of at least some of these taxa went partially overlooked for about a decade, and suggests that the Miseno Lake protected area deserves the establishment of appropriate monitoring programs for new NIS detections at early stages of arrival.
The spatial analyses of the ascidian biota of the Miseno Lake revealed a general homogeneity of assemblages in terms of species composition, with dominant species occurring in almost all investigated transects and areas of the lake, although their cover and abundance often varied on the basis of the topography of the lake as well as of the main benthic substrate and presence/absence of additional substrates available for settlement (whether natural or anthropogenic). Finally, both channels also hosted exclusive taxa, notably different between them.
The dock wall represented the main pool of ascidians in the basin, although showing species-specific distributional patterns and abundances. As an example, A. accarense and B. niger were somewhat widespread in terms of mean cover and number of colonies in the entire lake, whereas P. constellatum was mostly found in the western side of the lake, where colonies also seemed to be bigger in average size, and D. bermudensis displayed a marked segregation, being mainly restricted to sectors close to the CC except for a cluster of colonies found in the southern side of the lake. Other species did not show a clear distribution pattern but were widely scattered, namely P. dura, P. zorritensis, and S. canopus, whereas the natives C. sabbadini and M. polymorphus and the NIS M. squamiger were rarely encountered.
The inner sectors hosting the dense C. prolifera meadow were not a preferred habitat for almost any of the ascidian species found in the Miseno Lake. In particular, although 15 species were censused in the transects and seven in the Caulerpa meadows, only B. niger and B. schlosseri were found as epiphytes on the seaweed, with B. schlosseri being widespread in small scattered colonies evenly distributed within the meadow, while B. niger was limited to the outermost parts of the meadow, thus close to the dock wall where it is dominant. However, where present, B. niger was apparently more aggressive in its colonization, fouling a large part of the leaves. On the other hand, in the peripheral parts of the lake, where the seaweed meadow becomes sparse or ends abruptly, the substrate was mainly sandy/muddy and ascidians were mostly found on/under scattered rocks, litter, or other biogenic substrates, with a community similar to the one living in the dock wall, but with the addition of A. colleta and P. fumigata. As revealed by GAMs, the presence of hard substrates such as rocks and litter also influenced ascidians distribution in the lake. Indeed, although litter was found in all the lake, the sectors near the dock wall were mostly characterized by heavy-weight sunken objects (e.g. tyres, cans, tarpaulins, and hard plastic), whereas the inner part was mostly littered by lower-density drifting objects (e.g. tissues and soft plastic), still often detached from the substrate. Therefore, litter colonization by ascidians mostly occurred in the peripheral parts of the lake, where litter objects soon turn into additional anthropogenic substrates available for the resident fauna. Finally, when present, other kinds of natural substrates were also often overgrown by tunicates, such as sunken woods or polychaete tubes.
Although we did not investigate here chemico-physical parameters such as water salinity or turbidity, the observed differences in ascidians’ distribution are presumably related to the species-specific traits of the species found. Unfortunately, little is known of species’ autoecology for the majority of the taxa found in the Miseno Lake, although several of them, namely A. accarense, P. constellatum, B. niger, and P. zorritensis, are well known to adapt to different environmental conditions (Van Name, 1945; Mastrototaro and Brunetti, 2006; Mastrototaro et al., 2008) and that is presumably why they thrived in almost all the lake from the calm inner side, under rocks or inside crevices, to the unsheltered areas of the channels. Other species, such as P. mammillata, C. robusta, and S. plicata, are known to tolerate calm waters and poor environmental conditions (Chimenz et al., 1985; Mastrototaro et al., 2008; Caputi et al., 2013), and thus their distribution in the lake is likely to be influenced by additional factors. However, the renowned invasiveness of several NIS indeed implies wide environmental tolerances, with species able to adapt to various environments and being able to resist temperature shifts (Shenkar and Loya, 2008; Grey, 2011) and sunlight exposure (Forward et al., 2000), or even to cope with the local environmental metabolome (Palanisamy et al., 2018). With regards to this, antifouling properties due to secondary metabolites are widely reported from the alga Caulerpa prolifera and congeneric species (Smyrniotopoulos et al., 2003; Dobretsov et al., 2006), and thus they may also act locally as inhibitors for larval settlement of ascidians, being thus at the basis of the absence of the majority of the species in the inner parts of the lake. Interestingly, the two only species fouling the leaves (B. niger and B. schlosseri) are phylogenetically closely related, which may led to the speculation that these species possess antagonist strategies against the antifouling molecules produced by the alga. In addition, litter material has already been shown to be a collector for sessile species on unconsolidated bottoms, enhancing the exploitable substrate (Katsanevakis et al., 2007; Crocetta et al., 2020) and influencing the overall biomass of benthic assemblages (Ramirez-Llodra et al., 2013). In our case, litter was mostly fouled by NIS (e.g. D. bermudensis, A. accarense, P. constellatum, and B. niger), thus possibly enhancing their invasiveness in the lake, as also supported by the GAM analyses.
Finally, the two lake channels differed markedly in terms of species diversity. The CC showed an ascidian diversity which is comparable to the rest of the lake, although with a significantly higher NIS cover and dominance. It also hosted four exclusive taxa, namely C. lepadiformis, D. pseudovexillum, T. cereum, and P. viridis. In contrast, the MC showed an overall lower ascidian cover, as well as a significantly different community, with L. perforatum, L. weigelei, and S. brakenhielmi only found there. The importance of the channels as species and biomass enhancers in lagoon habitats was already acknowledged by several studies (e.g. Sordino et al., 1989; Procaccini and Scipione, 1992; Macali et al., 2013), and this is mostly the result of the peculiar hydro-dynamic conditions occurring in these areas and of the dynamic balance between the massive arrival of larvae and the interference that currents may cause to larval settlement (Bingham and Young, 1991; Valentine et al., 2009). The higher ascidian cover, and particularly of NIS, found in the CC dock wall is presumably influenced by the general similarity of conditions between the eastern side of the Miseno Lake, the CC, and the nearby Bacoli Bay, an additional enclosed bay connected to the Miseno Lake through the CC itself. On the other hand, the MC is characterized in its outer part by an open marine environment dominated by photophilic algae, thus suggesting that only this channel has a true role as an ecotone, with a somewhat impoverished presence of brackish-water ascidians colonizing the dock wall. The marked difference observed in transects is, instead, likely due to the different substrate composition (rocky in the CC and sandy with scattered rocks in the MC).
The Mediterranean Sea is widely acknowledged as hotspot of native biodiversity, but at the same time it also hosts a high number of NIS (Coll et al., 2010; Zenetos et al., 2017), arriving in the basin through different pathways and with varying patterns by ecoregion (Galil, 2009; Zenetos et al., 2012; Katsanevakis et al., 2013; Katsanevakis et al., 2014; Convention on Biological Diversity, 2014). However, once NIS arrive in a biogeographic sector, the high functional connectivity in the Mediterranean Sea favors their secondary spread, often aided by small-scale (e.g. fishing and recreational boats) to medium- and large-scale (e.g. shipping) human transport (Katsanevakis et al., 2014; Ferrario et al., 2017), thus obscuring their routes and complicating the tracing of putative introduction pathways unless at early stages of arrival.
The conspicuous community of NIS ascidians found here in syntopy in the Miseno Lake immediately raises the question about their origin. The assemblage was composed of species native to different localities worldwide (from Western Atlantic to Indo-Pacific), thus suggesting that such co-occurrences are the result of different introduction events. However, the majority of them are renowned worldwide invaders that also already colonized other Mediterranean areas, and also sometimes co-occur there (e.g. Mastrototaro et al., 2008; López-Legentil et al., 2015; Casso et al., 2018). In addition, they are widely known to colonize new areas through shipping, whether in ballast water or as fouling communities of ship hulls (Gewing and Shenkar, 2017; Lambert, 2019), or through aquaculture (Mastrototaro et al., 2019; Ramos-Esplá et al., 2020), with the sole exception of P. constellatum, commonly considered to be a Lessepsian migrant (Halim and Abdel Messeih, 2016; Aydin-Onen, 2018; Montesanto et al., 2022). However, even for the latter species, the present record from the Miseno Lake, as well its presence in the area since 2014, would dismiss such hypothesis and rather suggest that transport-stowaway may have played a primary role also here, or that at least a combination of both pathways may have occurred in invading the Mediterranean Sea. Unfortunately, with few exceptions (e.g. Pineda et al., 2011; Pineda et al., 2016a; Pineda et al., 2016b for S. plicata and Rius et al., 2008 for M. squamiger), no detailed genetic information is known at a population level for all these species in the Mediterranean Sea and even worldwide, which makes it difficult to trace putative sources and pathways. This is also particularly relevant in the case of D. bermudensis, whose clade C is also present at least in Puerto Rico (see Streit et al., 2021), but for which no additional information is available from other Mediterranean localities, thus making it unknown whether other lineages of the complex are invading the basin yet. Finally, the general absence of genetic data in support of faunal studies worldwide is also why at least two of the species found here are considered as cryptogenic in the Mediterranean Sea, namely P. viridis and D. pseudovexillum. The former species was already discussed above, whereas, in the case of the latter species, further integrative taxonomic approaches focused on “whitish/creamish didemnids” could easily reveal that its distribution is not only overlooked in the Mediterranean, but even worldwide. Notwithstanding these premises, the known auto-ecology of these species and the presence of mussel farms and two active marinas in the vicinity of the Miseno Lake point towards the speculation that these areas may be the first sites of impact and thus the true reservoir of these species. Further field studies to be carried out in these localities will confirm or dismiss such a hypothesis.
Moreover, although this benthic survey did not focus on species-specific impacts of NIS, and no certain evidence of competitive restriction of native ascidians was found, several additional considerations can be made. As already mentioned above, lack of knowledge and mostly of field studies presumably hindered so far the recognition of true invasiveness of these species in the Mediterranean Sea. However, among these, B. niger is widely known to dominate fouling communities in native and invaded areas (Sheets et al., 2016; Nydam et al., 2021; Ramalhosa et al., 2021), and this also holds true for A. accarense (López-Legentil et al., 2015; Montesanto et al., 2021), M. squamiger (Turon et al., 2007; Rius et al., 2008), P. zorritensis (López-Legentil et al., 2015; Casso et al., 2018; Ramalhosa et al., 2021), and S. brakenhielmi (Mastrototaro et al., 2019; Ramos-Esplá et al., 2020), which are worryingly expanding in the Mediterranean and becoming stable presences in littoral environments. In addition, the eminent invader P. constellatum is also considered a serious potential threat to native communities (Tovar-Hernández et al., 2010; Govindharaj et al., 2022). All these statements well match the high abundances of NIS observed here. Two of these species, namely D. bermudensis and B. niger, were also found to aggressively overgrowing tubes of the polychaete Chaetopterus variopedatus in the sectors close to CC (C8, D8, E7, E8, E9), sometimes totally covering the aperture of the tubes and thus providing evident stress to the native polychaete (see Figure 2G). Such interaction was never reported before in the Mediterranean Sea, but aggressive overgrow on benthic organisms by other members of the genus Distaplia Della Valle, 1881 was already reported for Distaplia viridis Kott, 1957 and Distaplia cf. stylifera (Kowalevsky, 1874), and may possibly lead to mass mortality events of the local biota (Russ, 1982; Moreno-Dávila et al., 2021). Although true impacts of these NIS on C. variopedatus have still to be properly evaluated, the observed occurrence was worrying since most of the tubes observed were affected, and D. bermudensis already showed aggressive behaviour, as also highlighted by comparison of CC dock wall communities before and after its arrival. Finally, the finding of S. brakenhielmi in a typically marine environment in the outermost part of the MC, and its absence in other areas of the lake widely colonized by NIS, somewhat disagrees with previous Mediterranean records of this species, generally confined to mussel farms and port environments (Mastrototaro et al., 2019; Ramos-Esplá et al., 2020), and suggests that this species may easily thrive even outside the areas usually targeted for NIS detection.
The presence of NIS is a constantly growing phenomenon in the Mediterranean Sea, which can only be tackled through coordinated and pan-Mediterranean efforts to control their main pathways and identify priority areas for constant-surveillance for a wide range of taxa. Constant monitoring programs should be linked to an early warning-rapid eradication mechanisms, as eradication in the marine environment can only be feasible at the very early stage of introduction.
The present study, carried out through a multidisciplinary approach, provides the first snapshot of the ascidian biota of the Miseno Lake and in general of the wider region about 40 years after the last investigation, revealing a massive presence of NIS, several of which are only known from a few records throughout the entire Mediterranean basin. Thus, these species are presumably more widespread than suspected, suggesting that it is already too late for a successful eradication and that local introduction pathways of NIS have been acting undisturbed for at least a decade.
Finally, this also constitutes the first study on a previously understudied environment, and provides a strong baseline to evaluate eventual shifts in the ascidian communities in future and to document the arrival of new NIS in the area. It also confirms semi-enclosed basins as hotspots of introduced species and shows that even marine reserves may be widely vulnerable to biological invasions. Further studies aiming to explore this and other phyla are therefore awaited from the Miseno Lake, the Phlegraean area, and more generally the Gulf of Naples, to evaluate the true magnitude of local NIS invasions.
The datasets presented in this study can be found in online repositories. The names of the repository/repositories and accession number(s) can be found in the article/Supplementary Material.
AF and FC proceeded to funding acquisition. FC designed and directed the research. RV, VT, GV, and FC performed fieldwork. RV and VT performed molecular analyses. FT performed bathymetric mapping. SK performed the statistical analyses. RV and FC wrote the main manuscript. All authors reviewed and approved the final manuscript.
Work supported by the following projects: i) POR Campania FESR 2014–2020. Risanamento Ambientale e valorizzazione dei Campi Flegrei. Intervento di risanamento idraulico del bacino del Miseno e riapertura, sistemazione e protezione delle foci del lago Fusaro. Monitoraggio ambientale e caratterizzazione sedimenti del lago Fusaro e Miseno; ii) PO FEAMP Campania 2014–2020. DRD n. 35 of 15th March 2018. Innovazione, sviluppo e sostenibilità nel settore della pesca e dell’acquacoltura per la regione Campania. Misura 2.51. WP5. Task 5.5. Presenza e distribuzione di specie non indigene del macrozoobenthos e del necton in Campania; iii) POR Campania FESR 2014–2020. Technology Platform for Therapeutic Strategies against Cancer. Antitumor Drugs and Vaccines from the Sea (ADViSE). CUP B43D18000240007–SURF 17061BP000000011. WP1. Task 1.1. Tassonomia, distribuzione, abbondanza e stagionalità dei principali organismi macrobentonici con bioattività presenti nel Golfo di Napoli.
The authors declare that the research was conducted in the absence of any commercial or financial relationships that could be construed as a potential conflict of interest.
All claims expressed in this article are solely those of the authors and do not necessarily represent those of their affiliated organizations, or those of the publisher, the editors and the reviewers. Any product that may be evaluated in this article, or claim that may be made by its manufacturer, is not guaranteed or endorsed by the publisher.
Centro Ittico Campano S.p.A. (Bacoli, Napoli) allowed sampling and offered support. Clemente Costigliola and Lega Navale Italiana Bacoli (Napoli) provided the inflatable boat. Mariella Saggiomo (SZN, Napoli) helped during bathymetric mapping. Alessandro Manfredonia (SZN, Napoli) helped during field sampling. Nikoletta Koukourouvli (University of the Aegean, Greece) offered GIS support. Pasqualina Fiorentino (SZN, Napoli) provided literature upon request. Xavier Turon (Blanes, Spain) and two additional referees kindly improved this contribution. We are grateful to all of them.
The Supplementary Material for this article can be found online at: https://www.frontiersin.org/articles/10.3389/fmars.2022.866906/full#supplementary-material
Akaike H. (1973). Maximum Likelihood Identification of Gaussian Autoregressive Moving Average Models. Biometrika 60, 255–265. doi: 10.2307/2334537
Aldred N., Clare A. S. (2014). Mini-Review: Impact and Dynamics of Surface Fouling by Solitary and Compound Ascidians. Biofouling 30, 259–270. doi: 10.1080/08927014.2013.866653
Appolloni L., Sandulli R., Vetrano G., Russo G. F. (2018). A New Approach to Assess Marine Opportunity Costs and Monetary Values-In-Use for Spatial Planning and Conservation; the Case Study of Gulf of Naples, Mediterranean Sea, Italy. Ocean Coast. Manage. 152, 135–144. doi: 10.1016/j.ocecoaman.2017.11.023
Arroyo E., Moya-Urbano E., García-Ruíz C., Esteban A., Ramos-Esplá A. A. (2021). Ascidians (Chordata: Tunicata) From Circalittoral and Upper-Bathyal Soft Bottoms Sampled by Experimental Trawling in the Iberian Mediterranean Sea. Reg. Stud. Mar. Sci. 43, 101669. doi: 10.1016/j.rsma.2021.101669
Aydin-Onen S. (2018). Distribution of Ascidians With a New Record of the Non-Indigenous Species Polyclinum constellatum Savigny 1816 From the Aegean Coast of Turkey. Turkish J. Fish. Aquat. Sci. 18, 1077–1089. doi: 10.4194/1303-2712-v18_9_07
Barros R. C., Rocha R. M. (2021). Genetic Analyses Reveal Cryptic Diversity in the Widely Distributed Styela Canopus (Ascidiacea : Styelidae). Invertebr. Syst. 35, 298–311. doi: 10.1071/is20058
Bédry R., de Haro L., Bentur Y., Senechal N., Galil B. S. (2021). Toxicological Risks on the Human Health of Populations Living Around the Mediterranean Sea Linked to the Invasion of non-Indigenous Marine Species From the Red Sea: A Review. Toxicon 191, 69–82. doi: 10.1016/j.toxicon.2020.12.012
Bilecenoğlu M., Çınar M. E. (2021). Alien Species Threat Across Marine Protected Areas of Turkey — An Updated Inventory. J. Mar. Sci. Eng. 9, 1077. doi: 10.3390/jmse9101077
Bingham B. L., Young C. M. (1991). Larval Behavior of the Ascidian Ecteinascidia Turbinata Herdman; an in Situ Experimental Study of the Effects of Swimming on Dispersal. J. Exp. Mar. Biol. Ecol. 145, 189–204. doi: 10.1016/0022-0981(91)90175-V
Brunetti R., (1978). Polyandrocarpa zorritensis (Van Name) A colonial ascidian new to the Mediterranean record. Vie Milieu. 29, 647–652
Brunetti R., Manni L., Mastrototaro F., Gissi C., Gasparini F. (2017). Fixation, Description and DNA Barcode of a Neotype for Botryllus Schlosseri (Pallas 1766) (Tunicata, Ascidiacea). Zootaxa 4353, 29–50. doi: 10.11646/zootaxa.4353.1.2
Brunetti R., Mastrototaro F. (2017). “Fauna D'italia. Ediz. Illustrata,” in Ascidiacea of the European Waters, vol. LI. (Milano, Italy: Edagricole-New Business Media).
Bullard S. G., Lambert G., Carman M. R., Byrnes J., Whitlatch R. B., Ruiz G., et al. (2007). The Colonial Ascidian Didemnum Sp. A: Current Distribution, Basic Biology and Potential Threat to Marine Communities of the Northeast and West Coasts of North America. J. Exp. Mar. Biol. Ecol. 342, 99–108. doi: 10.1016/j.jembe.2006.10.020
Burnham K. P., Anderson D. R. (2002). Model Selection and Multimodel Inference. A Practical Information-Theoretic Approach. 2nd ed. (Fort Collins: Springer).
Camilletti P., Lanzi G. (2018). Natural and Man-Made Landscape in the Phlegraean Fields: Linking Identity and Potentials for Sustainable Development. Territ. Res. Settlements Environ. 20, 119–140. doi: 10.6092/2281-4574/5822
Caputi L., Andreakis N., Mastrototaro F., Cirino P., Vassillo M., Sordino P. (2007). Cryptic Speciation in a Model Invertebrate Chordate. Proc. Natl. Acad. Sci. U. S. A. 104, 9364–9369. doi: 10.1073/pnas.0610158104
Caputi L., Crocetta F., Toscano F., Sordino P., Cirino P. (2013). Long-Term Demographic and Reproductive Trends in Ciona Intestinalis Sp. A. Mar. Ecol. 36, 118–128. doi: 10.1111/maec.12125
Casso M., Navarro M., Ordóñez V., Fernández-Tejedor M., Pascual M., Turon X. (2018). Seasonal Patterns of Settlement and Growth of Introduced and Native Ascidians in Bivalve Cultures in the Ebro Delta (NE Iberian Peninsula). Reg. Stud. Mar. Sci. 23, 12–22. doi: 10.1016/j.rsma.2017.11.002
Chebbi N., Mastrototaro F., Missaoui H. (2010). Spatial Distribution of Ascidians in Two Tunisian Lagoons of the Mediterranean Sea. Cah. Biol. Mar. 51, 117–127. doi: 10.21411/CBM.A.E590FC4D
Chimenz C., Fresi E., Brunetti E. (1985). Ricerche Sui Popolamenti Bentonici Di Substrato Duro Del Porto D’ischia: Ascidiacei. Cah. Biol. Mar. 26, 15–33. doi: 10.21411/CBM.A.56C31EBC
Coll M., Piroddi C., Steenbeek J., Kaschner K., Lasram F. B. R., Aguzzi J., et al. (2010). The Biodiversity of the Mediterranean Sea: Estimates, Patterns, and Threats. PloS One 5, e11842. doi: 10.1371/journal.pone.0011842
Convention on Biological Diversity (2014). Pathways of Introduction of Invasive Species, Their Prioritization and Management (Montreal, Canada: UNEP/CBD/SBSTT).
Couton M., Comtet T., Le Cam S., Corre E., Viard F. (2019). Metabarcoding on Planktonic Larval Stages: An Efficient Approach for Detecting and Investigating Life Cycle Dynamics of Benthic Aliens. Manage. Biol. Invasions 10, 657–689. doi: 10.3391/mbi.2019.10.4.06
Crocetta F., Macali A., Furfaro G., Cooke S., Villani G., Valdés Á. (2013). Alien Molluscan Species Established Along the Italian Shores: An Update, With Discussions on Some Mediterranean “Alien Species” Categories. Zookeys 277, 91–108. doi: 10.3897/zookeys.277.4362
Crocetta F., Riginella E., Lezzi M., Tanduo V., Balestrieri L., Rizzo L. (2020). Bottom-Trawl Catch Composition in a Highly Polluted Coastal Area Reveals Multifaceted Native Biodiversity and Complex Communities of Fouling Organisms on Litter Discharge. Mar. Environ. Res. 155, 104875. doi: 10.1016/j.marenvres.2020.104875
Darriba D., Taboada G. L., Doallo R., Posada D. (2012). Jmodeltest 2: More Models, New Heuristics and Parallel Computing. Nat. Methods 9, 772. doi: 10.1038/nmeth.2109
Davis M. H., Davis M. E. (2010). The Impact of the Ascidian Styela Clava Herdman on Shellfish Farming in the Bassin De Thau, France. J. Appl. Ichthyol. 26, 12–18. doi: 10.1111/j.1439-0426.2010.01496.x
Della Sala G., Coppola D., Virgili R., Vitale G. A., Tanduo V., Teta R., et al. (2022). Untargeted Metabolomics Yields Insights Into the Lipidome of Botrylloides Niger Herdman 1886, an Invasive Ascidian in the Mediterranean Sea. Front. Mar. Sci 9, 865751. doi: 10.3389/fmars.2022.865751
Della Valle A. (1877). Contribuzioni Alla Storia Naturale Delle Ascidie Composte Del Golfo Di Napoli (Napoli, Italy: Tipografia dei comuni).
Della Valle A. (1881). Nuove Contribuzioni Alla Storia Naturale Delle Ascidie Composte Del Golfo Di Napoli. Atti Accad. Naz. Lincei - Mem. 10, 1–70.
De Luca G., Rosciano M. (2020). Quantile Dependence in Tourism Demand Time Series: Evidence in the Southern Italy Market. Sustainability 12, 3243. doi: 10.3390/SU12083243
Dobretsov S., Dahms H.-U., Harder T. (2006). Allelochemical Defense Against Epibiosis in the Macroalga Caulerpa Racemosa Var. Turbinata. Mar. Ecol. Prog. Ser. 318, 165–175. doi: 10.3354/meps318165
ENEA (2002) Progetto Regi Lagni. Schede Laghi Flegrei – Note Di Primo Inquadramento. Available at: http://www.bologna.enea.it/ambtd/regi-lagni/volume-1/05-4-vol1-miseno.htm.
Esposito M. G., Trinchella F., Scudiero R. (2013). “Mussel Farms in the Gulf of Naples (Campania, Southern Italy): Real Opportunity or a Risk to Consumers?,” in Mussels: Ecology, Life Habits and Control. Eds. Nowak J., Kozlowski M. (Hauppauge, NY: Nova Science Publishers), 149–156.
Essl F., Bacher S., Genovesi P., Hulme P. E., Jeschke J. M., Katsanevakis S., et al. (2018). Which Taxa Are Alien? Criteria, Applications, and Uncertainties. BioScience 68, 496–509. doi: 10.1093/biosci/biy057
European Commission (2014). Regulation (EU) No 1143/2014 of the European Parliament and of the Council of 22 October 2014 on the Prevention and Management of the Introduction and Spread of Invasive Alien Species. Off. J. Eur. Union. L 317/35, 1–21.
European Commission (2020a). Report From the Commission to the European Parliament and the Council on the Implementation of the Marine Strategy Framework Directive (Directive 2008/56/Ec) (Brussels, Belgium: European Commission).
European Commission (2020b). Key Stages and Progress Up to 2019. Accompanying the Report From the Commission to the European Parliament and the Council on the Implementation of the Marine Strategy Framework Directive (Directive 2008/56/Ec) (Brussels, Belgium: European Commission).
Evans J. S., Erwin P. M., Sihaloho H. F., López-Legentil S. (2021). Cryptic Genetic Lineages of a Colonial Ascidian Host Distinct Microbiomes. Zool. Scr. 50, 423–438. doi: 10.1111/zsc.12482
Ferrario J., Caronni S., Occhipinti-Ambrogi A., Marchini A. (2017). Role of Commercial Harbours and Recreational Marinas in the Spread of Non-Indigenous Fouling Species. Biofouling 33, 651–660. doi: 10.1080/08927014.2017.1351958
Forward R. B., Welch J. M., Young C. M. (2000). Light Induced Larval Release of a Colonial Ascidian. J. Exp. Mar. Biol. Ecol. 248, 225–238. doi: 10.1016/S0022-0981(00)00168-4
Fujisawa T., Barraclough T. G. (2013). Delimiting Species Using Single-Locus Data and the Generalized Mixed Yule Coalescent Approach: A Revised Method and Evaluation on Simulated Data Sets. Syst. Biol. 62, 707–724. doi: 10.1093/sysbio/syt033
Galil B. S. (2009). Taking Stock: Inventory of Alien Species in the Mediterranean Sea. Biol. Invasions 11, 359–372. doi: 10.1007/s10530-008-9253-y
Gambi M. C. (2021). “Ubi Bene, Ibi Patria: Specie Marine Aliene Lungo Le Coste Della Campania E Ruolo Del Cambiamento Climatico E Della Citizen Science” in Ambient. Rischio Comun, vol. 17., 45–56. (Naples, Italy: Doppiavoce)
Gewing M. T., López-Legentil S., Shenkar N. (2017). Anthropogenic Factors Influencing Invasive Ascidian Establishment in Natural Environments. Mar. Environ. Res. 131, 236–242. doi: 10.1016/j.marenvres.2017.10.001
Gewing M. T., Shenkar N. (2017). Monitoring the Magnitude of Marine Vessel Infestation by Non-Indigenous Ascidians in the Mediterranean. Mar. Pollut. Bull. 121, 52–59. doi: 10.1016/j.marpolbul.2017.05.041
Giakoumi S., Katsanevakis S., Albano P. G., Azzurro E., Cardoso A. C., Cebrian E., et al. (2019). Management Priorities for Marine Invasive Species. Sci. Total Environ. 688, 976–982. doi: 10.1016/j.scitotenv.2019.06.282
Giangrande A., Arduini D., Borghese J., Del Pasqua M., Lezzi M., Petrocelli A., et al. (2021). Macrobenthic Success of Non-Indigenous Species Related to Substrate Features in the Mar Grande of Taranto, Italy (Mediterranean Sea). BioInvasions Rec. 10, 238–256. doi: 10.3391/bir.2021.10.2.02
Govindharaj M., Sayed N., Hashemi A., Saghar S., Vijayavenkataraman S. (2022). Bioprinting of Bioactive Tissue Scaffolds From Ecologically-Destructive Fouling Tunicates. J. Clean. Prod. 330, 129923. doi: 10.1016/j.jclepro.2021.129923
Grey E. K. (2011). Relative Effects of Environment and Direct Species Interactions on the Population Growth Rate of an Exotic Ascidian. Oecologia 166, 935–947. doi: 10.1007/s00442-011-1931-2
Griggio F., Voskoboynik A., Iannelli F., Justy F., Tilak M. K., Turon X., et al. (2014). Ascidian Mitogenomics: Comparison of Evolutionary Rates in Closely Related Taxa Provides Evidence of Ongoing Speciation Events. Genome Biol. Evol. 6, 591–605. doi: 10.1093/gbe/evu041
Halim Y., Abdel Messeih M. (2016). Aliens in Egyptian Waters. A Checklist of Ascidians of the Suez Canal and the Adjacent Mediterranean Waters. Egypt. J. Aquat. Res. 42, 449–457. doi: 10.1016/j.ejar.2016.08.004
Hanson D., Cooke S., Hirano Y., Malaquias M. A. E., Crocetta F., Valdés Á. (2013). Slipping Through the Cracks: The Taxonomic Impediment Conceals the Origin and Dispersal of Haminoea Japonica, an Invasive Species With Impacts to Human Health. PloS One 8, e77457. doi: 10.1371/journal.pone.0077457
Hastie T. J., Tibshirani R. J. (1990). Generalized Additive Models (London, England: Chapman and Hall).
Heller C. (1874). Untertsuchungen uber die Tunicaten des Adriatischen Meeres. Denkschr. Akad. Wien. 34, 1–20.
Katsanevakis S., Coll M., Piroddi C., Steenbeek J., Lasram F. B. R., Zenetos A., et al. (2014). Invading the Mediterranean Sea: Biodiversity Patterns Shaped by Human Activities. Front. Mar. Sci. 1, 31. doi: 10.3389/fmars.2014.00032
Katsanevakis S., Verriopoulos G., Nikolaidou A., Thessalou-Legaki M. (2007). Effect of Marine Pollution With Litter on the Benthic Megafauna of Coastal Soft Bottoms. Mar. Pollut. Bull. 54, 771–778. doi: 10.1016/j.marpolbul.2006.12.016
Katsanevakis S., Zenetos A., Belchior C., Cardoso A. C. (2013). Invading European Seas: Assessing Pathways of Introduction of Marine Aliens. Ocean Coast. Manage. 76, 64–74. doi: 10.1016/j.ocecoaman.2013.02.024
Kim Y.-J., Gu C. (2004). Smoothing Spline Gaussian Regression: More Scalable Computation via Efficient Approximation. J. R. Stat. Soc B. 66, 337–356. doi: 10.1046/j.1369-7412.2003.05316.x
Koukouras A., Voultsiadou-Koukoura E., Kevrekidis T., Vafidis D. (1995). Ascidian Fauna of the Aegean Sea With a Check List of the Eastern Mediterranean and Black Sea Species. Ann. Inst. Oceanogr. 71, 19–34.
Lafargue F., Tursi A. (1975). Contribution a La Connaissance Des Didemnidae (Ascidies Composées) Des CôTes Des Pouilles (Mer Ionienne Et Mer Adriatique). Vie Milieu 25, 33–48.
Lafargue F., Ramos A. A., Turon X., Banaigs B., Wahl M. (1986). The Littoral Ascidians of the Spanish Mediterranean. 1. From Port Bou to the Islas Medas. Vie Milieu 36, 133–139.
La Magna G., Guarino G., Riccardi N., Giovinazzi R. (2002). Studio Degli Impatti Antropici Sulla Qualità Ambientale Dei Laghi Di Transizione in Campania (Napoli, Italy: Dipartimento Provinciale di Napoli A.R.P.A.C).
Lambert G. (2019). Fouling Ascidians (Chordata: Ascidiacea) of the Galápagos: Santa Cruz and Baltra Islands. Aquat. Invasions 14, 132–149. doi: 10.3391/ai.2019.14.1.05
Lambert C. C., Lambert G. (1998). Non-Indigenous Ascidians in Southern California Harbors and Marinas. Mar. Biol. 130, 675–688. doi: 10.1007/s002270050289
Langeneck J., Lezzi M., Del Pasqua M., Musco L., Gambi M. C., Castelli A., et al. (2020). Non-Indigenous Polychaetes Along the Coasts of Italy: A Critical Review. Mediterr. Mar. Sci. 21, 238–275. doi: 10.12681/mms.21860
Leccese A., Speziale V. (1967). “I Laghi Fusaro E Miseno E L'inquinamento Delle Loro Acque,” in Centro Ittico Tarantino-Campano (Taranto, Italy: Jonica Editrice).
Leclerc J. C., Viard F. (2018). Habitat Formation Prevails Over Predation in Influencing Fouling Communities. Ecol. Evol. 8, 477–492. doi: 10.1002/ece3.3654
Lezzi M., Del Pasqua M., Pierri C., Giangrande A. (2018). Seasonal Non-Indigenous Species Succession in a Marine Macrofouling Invertebrate Community. Biol. Invasions 20, 937–961. doi: 10.1007/s10530-017-1601-3
Lindén A., Mäntyniemi S. (2011). Using the Negative Binomial Distribution to Model Overdispersion in Ecological Count Data. Ecology 92, 1414–1421. doi: 10.1890/10-1831.1
López-Legentil S., Legentil M. L., Erwin P. M., Turon X. (2015). Harbor Networks as Introduction Gateways: Contrasting Distribution Patterns of Native and Introduced Ascidians. Biol. Invasions 17, 1623–1638. doi: 10.1007/s10530-014-0821-z
Macali A., Conde A., Smriglio C., Mariottini P., Crocetta F. (2013). The Evolution of the Molluscan Biota of Sabaudia Lake: A Matter of Human History. Sci. Mar. 77 (4), 649–662. doi: 10.3989/scimar
Marchini A., Ferrario J., Sfriso A., Occhipinti-Ambrogi A. (2015). Current Status and Trends of Biological Invasions in the Lagoon of Venice, a Hotspot of Marine NIS Introductions in the Mediterranean Sea. Biol. Invasions 17, 2943–2962. doi: 10.1007/s10530-015-0922-3
Mastrototaro F., Brunetti R. (2006). The Non-Indigenous Ascidian Distaplia Bermudensis in the Mediterranean: Comparison With the Native Species Distaplia Magnilarva and Distaplia Lucillae Sp. Nov. J. Mar. Biol. Assoc. U. K. 86, 181–185. doi: 10.1017/S0025315406013014
Mastrototaro F., D’Onghia G., Tursi A. (2008). Spatial and Seasonal Distribution of Ascidians in a Semi-Enclosed Basin of the Mediterranean Sea. J. Mar. Biol. Assoc. U. K. 88, 1053–1061. doi: 10.1017/S0025315408001392
Mastrototaro F., Montesanto F. (2021). “Ascidiacea,” in Checklist of the Italian Fauna. Version 1.0. Eds. Bologna M. A., Zapparoli M., Oliverio M., Minelli A., Bonato L., Cianferoni F., Stoch F..Available at https://www.lifewatchitaly.eu/en/initiatives/checklist-fauna-italia-en/checklist/. Last Access 30.XII.2021
Mastrototaro F., Montesanto F., Salonna M., Grieco F., Trainito E., Chimienti G., et al. (2019). Hitch-Hikers of the Sea: Concurrent Morphological and Molecular Identification of Symplegma Brakenhielmi (Tunicata: Ascidiacea) in the Western Mediterranean Sea. Mediterr. Mar. Sci. 20, 197–207. doi: 10.12681/mms.19390
Montesanto F., Chimienti G., Gissi C., Mastrototaro F. (2022). Polyclinum Constellatum (Tunicata, Ascidiacea), An Emerging Non-Indigenous Species of the Mediterranean Sea: Integrated Taxonomy and the Importance of Reliable DNA Barcode Data. Mediterr. Mar. Sci. 23, 69–83. doi: 10.12681/mms.28311
Montesanto F., Chimienti G., Mastrototaro F. (2021). Spread of the Non-Indigenous Ascidian Aplidium Accarense (Millar 1953) in the Eastern Mediterranean Sea : Morphological and Molecular Tools for an Accurate Identification. Mediterr. Mar. Sci. 22, 246–254. doi: 10.12681/mms.24887
Moreno-Dávila B., Gómez-Gutiérrez J., Alcoverro T., Ramírez-Luna S., Sánchez C., Balart E. F., et al. (2021). Mass Mortality of Pen Shell Atrina Maura (Bivalvia: Pinnidae) Due to Abrupt Population Increase of Tunicate (Distaplia Sp.) in a Subtropical Bay, Mexico. Estuar. Coast. Shelf Sci. 260, 107493. doi: 10.1016/j.ecss.2021.107493
Moreno T. R., de Faria S. B., Rocha R. M. (2014). Biogeography of Atlantic and Mediterranean Ascidians. Mar. Biol. 161, 2023–2033. doi: 10.1007/s00227-014-2483-x
Morgulis A., Coulouris G., Raytselis Y., Madden T., Agarwala R., Schäffer A. A. (2008). Database Indexing for Production MegaBLAST Searches. Bioinformatics 24, 1757–1764. doi: 10.1093/bioinformatics/btn322
Neppi V. (1921). Descrizione Di Una Nuova Specie Di Perophora Del Golfo Di Napoli. Pubbl. Staz. Zool. Napoli 3, 33–38.
Nuzzo G., Gallo C., Crocetta F., Romano L., Barra G., Senese G., et al. (2022). Identification of the Marine Alkaloid Lepadin A as Potential Inducer of Immunogenic Cell Death. Biomolecules 12, 246. doi: 10.3390/biom12020246
Nydam M. L., Lemmon A. R., Cherry J. R., Kortyna M. L., Clancy D. L., Hernandez C., et al. (2021). Phylogenomic and Morphological Relationships Among the Botryllid Ascidians (Subphylum Tunicata, Class Ascidiacea, Family Styelidae). Sci. Rep. 11, 8351. doi: 10.1038/s41598-021-87255-2
Nydam M. L., Yanckello L. M., Bialik S. B., Giesbrecht K. B., Nation G. K., Peak J. L. (2017). Introgression in Two Species of Broadcast Spawning Marine Invertebrate. Biol. J. Linn. Soc 120, 879–890. doi: 10.1093/biolinnean/blw012
Occhipinti-Ambrogi A. (2000). Biotic Invasions in a Mediterranean Lagoon. Biol. Invasions 2, 165–176. doi: 10.1023/A:1010004926405
Occhipinti-Ambrogi A., Marchini A., Cantone G., Castelli A., Chimenz C., Cormaci M., et al. (2011). Alien Species Along the Italian Coasts: An Overview. Biol. Invasions 13, 215–237. doi: 10.1007/s10530-010-9803-y
Okonechnikov K., Golosova O., Fursov M. (2012). Unipro UGENE: A Unified Bioinformatics Toolkit. Bioinformatics 28, 1166–1167. doi: 10.1093/bioinformatics/bts091
Orfanidis S., Alvito A., Azzurro E., Badreddine A., Ben Souissi J., Chamorro M., et al. (2021). New Alien Mediterranean Biodiversity Records (March 2021). Mediterr. Mar. Sci. 22, 180–198. doi: 10.12681/mms.25294
Otero M., Cebrian E., Francour P., Galil B., Savini D. (2013). Monitoring Marine Invasive Species in Mediterranean Marine Protected Areas (MPAs): A Strategy and Practical Guide for Managers (Malaga, Spain: Medpan North project, IUCN).
Palanisamy S. K., Thomas O. P., McCormack G. P. (2018). Bio-Invasive Ascidians in Ireland: A Threat for the Shellfish Industry But Also a Source of High Added Value Products. Bioengineered 9, 55–60. doi: 10.1080/21655979.2017.1392421
Pérès J. M. (1957). Ascidies Récoltées Dans Les Parages Des Baléares Par Le Professeur Lacaze-Duthiers (2e Partie) Ibiza Et San Antonio. Vie Milieu 6, 223–234.
Pérez-Ruzafa A. (1989). Estudio Ecológico Y Bionómico De Los Poblamientos Bentónicos Del Mar Menor (Murcia, S. E. De España). [PhD Thesis] (Murcia (Spain: Universidad de Murcia).
Pineda M. C., López-Legentil S., Turon X. (2011). The Whereabouts of an Ancient Wanderer: Global Phylogeography of the Solitary Ascidian Styela Plicata. PloS One 6, e25495. doi: 10.1371/journal.pone.0025495
Pineda M. C., Lorente B., López-Legentil S., Palacín C., Turon X. (2016b). Stochasticity in Space, Persistence in Time: Genetic Heterogeneity in Harbour Populations of the Introduced Ascidian Styela Plicata. PeerJ 4, e2158. doi: 10.7717/peerj.2158
Pineda M. C., Turon X., Pérez-Portela R., López-Legentil S. (2016a). Stable Populations in Unstable Habitats: Temporal Genetic Structure of the Introduced Ascidian Styela Plicata in North Carolina. Mar. Biol. 163, 59. doi: 10.1007/s00227-016-2829-7
Procaccini G., Scipione M. B. (1992). Observations on the Spatio-Temporal Distribution of Crustacean Amphipods in the Fusaro Coastal Lagoon (Central Tyrrhenian Sea, Italy) and Some Notes on Their Presence in Mediterranean Lagoons. Mar. Ecol. 13, 203–224. doi: 10.1111/j.1439-0485.1992.tb00351.x
Puillandre N., Lambert A., Brouillet S., Achaz G. (2012). ABGD, Automatic Barcode Gap Discovery for Primary Species Delimitation. Mol. Ecol. 21, 1864–1877. doi: 10.1111/j.1365-294X.2011.05239.x
Ramalhosa P., Gestoso I., Rocha R. M., Lambert G., Canning-Clode J. (2021). Ascidian Biodiversity in the Shallow Waters of the Madeira Archipelago: Fouling Studies on Artificial Substrates and New Records. Reg. Stud. Mar. Sci. 43, 101672. doi: 10.1016/j.rsma.2021.101672
Rambaut A., Drummond A. J., Xie D., Baele G., Suchard M. A. (2018). Posterior Summarization in Bayesian Phylogenetics Using Tracer 1.7. Syst. Biol. 67, 901–904. doi: 10.1093/sysbio/syy032
Ramirez-Llodra E., De Mol B., Company J. B., Coll M., Sardà F. (2013). Effects of Natural and Anthropogenic Processes in the Distribution of Marine Litter in the Deep Mediterranean Sea. Prog. Oceanogr. 118, 273–287. doi: 10.1016/j.pocean.2013.07.027
Ramos-Esplá A. A., Bitar G., Sghaier Y. R., Çinar M. E., Deidun A., Ferrario J., et al. (2020). Symplegma (Ascidiacea: Styelidae), A Non-Indigenous Genus Spreading Within the Mediterranean Sea: Taxonomy, Routes and Vectors. Aquat. Invasions 15, 44–62. doi: 10.3391/AI.2020.15.1.04
R Core Team (2021). R: A Language and Environment for Statistical Computing (Vienna, Austria: R Foundation for Statistical Computing). Available at: https://www.R-project.org/.
Rigillo Troncone M. (1975). Salvaguardia Di Un Ambiente Idrico, Il Lago Miseno, Nel Contesto Delle Azioni Per L’assetto Territoriale Di Bacoli. Fondaz. Polit. Mezz. Italia, Vol. 100. 1–20. (Naples, Italy: Giannini).
Rius M., Pascual M., Turon X. (2008). Phylogeography of the Widespread Marine Invader Microcosmus Squamiger (Ascidiacea) Reveals High Genetic Diversity of Introduced Populations and Non-Independent Colonizations. Divers. Distrib. 14, 818–828. doi: 10.1111/j.1472-4642.2008.00485.x
Ronquist F., Teslenko M., van der Mark P., Ayres D. L., Darling A., Höhna S., et al. (2012). MrBayes 3.2: Efficient Bayesian Phylogenetic Inference and Model Choice Across a Large Model Space. Syst. Biol. 61, 539–542. doi: 10.1093/sysbio/sys029
Rubinstein N. D., Feldstein T., Shenkar N., Botero-Castro F., Griggio F., Mastrototaro F., et al. (2013). Deep Sequencing of Mixed Total DNA Without Barcodes Allows Efficient Assembly of Highly Plastic Ascidian Mitochondrial Genomes. Genome Biol. Evol. 5, 1185–1199. doi: 10.1093/gbe/evt081
Russ G. R. (1982). Overgrowth in a Marine Epifaumal Community: Competitive Hierarchies and Competitive Networks. Oecologia 53, 12–19. doi: 10.1007/BF00377130
Salonna M., Gasparini F., Huchon D., Montesanto F., Haddas-Sasson M., Ekins M., et al. (2021). An Elongated COI Fragment to Discriminate Botryllid Species and as an Improved Ascidian DNA Barcode. Sci. Rep. 11, 4078. doi: 10.1038/s41598-021-83127-x
Servello G., Andaloro F., Azzurro E., Castriota L., Marcello C., Froglia C., et al. (2019). Marine Alien Species in Italy: A Contribution to the Implementation of Descriptor D2 of the Marine Strategy Framework Directive. Mediterr. Mar. Sci. 20, 1–48. doi: 10.12681/mms.18711
Sheets E. A., Cohen C. S., Ruiz G. M., da Rocha R. M. (2016). Investigating the Widespread Introduction of a Tropical Marine Fouling Species. Ecol. Evol. 6, 2453–2471. doi: 10.1002/ece3.2065
Shenkar N., Loya Y. (2008). The Solitary Ascidian Herdmania Momus: Native (Red Sea) Versus Non-Indigenous (Mediterranean) Populations. Biol. Invasions 10, 1431–1439. doi: 10.1007/s10530-008-9217-2
Shenkar N., Swalla B. J. (2011). Global Diversity of Ascidiacea. PloS One 6, e20657. doi: 10.1371/journal.pone.0020657
Shenkar N., Zeldman Y., Loya Y. (2008). Ascidian Recruitment Patterns on an Artificial Reef in Eilat (Red Sea). Biofouling 24, 119–128. doi: 10.1080/08927010801902083
Smyrniotopoulos V., Abatis D., Tziveleka L.-A., Tsitsimpikou C., Roussis V., Loukis A., et al. (2003). Acetylene Sesquiterpenoid Esters From the Green Alga Caulerpa Prolifera. J. Nat. Prod. 66, 21–24. doi: 10.1021/np0202529
Sordino P., Gambi M. C., Carrada G. C. (1989). Spatio-Temporal Distribution of Polychaetes in an Italian Coastal Lagoon (Lago Fusaro, Naples). Cah. Biol. Mar. 30, 375–391. doi: 10.21411/CBM.A.41441801
Stamatakis A. (2014). RAxML Version 8: A Tool for Phylogenetic Analysis and Post-Analysis of Large Phylogenies. Bioinformatics 30, 1312–1313. doi: 10.1093/bioinformatics/btu033
Streit O. T., Lambert G., Erwin P. M., López-Legentil S. (2021). Diversity and Abundance of Native and Non-Native Ascidians in Puerto Rican Harbors and Marinas. Mar. Poll. Bull. 167, 112262. doi: 10.1016/j.marpolbul.2021.112262
Suchard M. A., Lemey P., Baele G., Ayres D. L., Drummond A. J., Rambaut A. (2018). Bayesian Phylogenetic and Phylodynamic Data Integration Using BEAST 1.10. Virus Evol. 4, vey016. doi: 10.1093/ve/vey016
Tempesti J., Mangano M. C., Langeneck J., Lardicci C., Maltagliati F., Castelli A. (2020). Non-Indigenous Species in Mediterranean Ports: A Knowledge Baseline. Mar. Environ. Res. 161, 105056. doi: 10.1016/j.marenvres.2020.105056
Tovar-Hernández M. A., Suárez-Morales E., Yáñez-Rivera B. (2010). The Parasitic Copepod Haplostomides Hawaiiensis (Cyclopoida) From the Invasive Ascidian Polyclinum Constellatum in the Southern Gulf of California. Bull. Mar. Sci. 86, 637–648.
Traustedt M. P. A. (1883). Die Einfachen Ascidien Des Golfes Von Neapel. Mitth. Zool. Stat. Neapel 4, 448–488.
Trygonis V., Sini M. (2012). PhotoQuad: A Dedicated Seabed Image Processing Software, and a Comparative Error Analysis of Four Photoquadrat Methods. J. Exp. Mar. Biol. Ecol. 424–425, 99–108. doi: 10.1016/j.jembe.2012.04.018
Turon X., Casso M., Pascual M., Viard F. (2020). Looks can be Deceiving: Didemnum Pseudovexillum Sp. Nov. (Ascidiacea) in European Harbours. Mar. Biodivers 50, 48. doi: 10.1007/s12526-020-01083-7
Turon X., Nishikawa T., Rius M. (2007). Spread of Microcosmus Squamiger (Ascidiacea: Pyuridae) in the Mediterranean Sea and Adjacent Waters. J. Exp. Mar. Biol. Ecol. 342, 185–188. doi: 10.1016/j.jembe.2006.10.040
Turon X., Tarjuelo I., Duran S., Pascual M. (2003). Characterising Invasion Processes With Genetic Data: An Atlantic Clade of Clavelina Lepadiformis (Ascidiacea) Introduced Into Mediterranean Harbours. Hydrobiologia 503, 29–35. doi: 10.1023/B:HYDR.0000008481.10705.c2
Valentine P. C., Carman M. R., Dijkstra J., Blackwood D. S. (2009). Larval Recruitment of the Invasive Colonial Ascidian Didemnum Vexillum, Seasonal Water Temperatures in New England Coastal and Offshore Waters, and Implications for Spread of the Species. Aquat. Invasions 4, 153–168. doi: 10.3391/ai.2009.4.1.16
Van Name W. G. (1945). The North and South American Ascidians. Bull. Am. Mus. Nat. Hist. 84, 283–494.
Verrill A. E. (1871). On the Distribution of Marine Animals on the Southern Coast of New England. Am. J. Sci. Arts 3, 357–362. doi: 10.2475/ajs.s3-2.11.357
Vilà M., Hulme P. E. (2017). Impact of Biological Invasions on Ecosystem Services (New York: Springer).
Wood S. N. (2006). Generalized Additive Models: An Introduction With R (Boca Raton, Florida: Chapman and Hall / CRC).
Zenetos A., Çinar M. E., Crocetta F., Golani D., Rosso A., Servello G., et al. (2017). Uncertainties and Validation of Alien Species Catalogues: The Mediterranean as an Example. Estuar. Coast. Shelf Sci. 191, 171–187. doi: 10.1016/j.ecss.2017.03.031
Zenetos A., Gofas S., Morri C., Rosso A., Violanti D., García Raso J. E., et al. (2012). Alien Species in the Mediterranean Sea by 2012. A Contribution to the Application of European Union’s Marine Strategy Framework Directive (MSFD). Part 2. Introduction Trends and Pathways. Mediterr. Mar. Sci. 13, 328–352. doi: 10.12681/mms.327
Zhan A., Briski E., Bock D. G., Ghabooli S., MacIsaac H. J. (2015). Ascidians as Models for Studying Invasion Success. Mar. Biol. 162, 2449–2470. doi: 10.1007/s00227-015-2734-5
Keywords: biological invasions, Tunicata, bathymetric and habitat mapping, DNA barcoding, Phlegraean lagoons, benthic survey, cytochrome c oxidase subunit I (COI), generalized additive models (GAMs)
Citation: Virgili R, Tanduo V, Katsanevakis S, Terlizzi F, Villani G, Fontana A and Crocetta F (2022) The Miseno Lake (Central-Western Mediterranean Sea): An Overlooked Reservoir of Non-Indigenous and Cryptogenic Ascidians in a Marine Reserve. Front. Mar. Sci. 9:866906. doi: 10.3389/fmars.2022.866906
Received: 31 January 2022; Accepted: 17 March 2022;
Published: 19 April 2022.
Edited by:
Jason Michael Hall-Spencer, University of Plymouth, United KingdomReviewed by:
Alfonso Angel Ramos-Esplá, University of Alicante, SpainCopyright © 2022 Virgili, Tanduo, Katsanevakis, Terlizzi, Villani, Fontana and Crocetta. This is an open-access article distributed under the terms of the Creative Commons Attribution License (CC BY). The use, distribution or reproduction in other forums is permitted, provided the original author(s) and the copyright owner(s) are credited and that the original publication in this journal is cited, in accordance with accepted academic practice. No use, distribution or reproduction is permitted which does not comply with these terms.
*Correspondence: Riccardo Virgili, cmljY2FyZG8udmlyZ2lsaUBzem4uaXQ=; Fabio Crocetta, ZmFiaW8uY3JvY2V0dGFAc3puLml0
Disclaimer: All claims expressed in this article are solely those of the authors and do not necessarily represent those of their affiliated organizations, or those of the publisher, the editors and the reviewers. Any product that may be evaluated in this article or claim that may be made by its manufacturer is not guaranteed or endorsed by the publisher.
Research integrity at Frontiers
Learn more about the work of our research integrity team to safeguard the quality of each article we publish.