- 1Department of Biological Sciences, Florida Atlantic University, Boca Raton, FL, United States
- 2Florida Atlantic University - Harbor Branch Oceanographic Institute, Fort Pierce, FL, United States
Recurring brown tide algal blooms of the pelagophyte Aureoumbra lagunensis in the northern Indian River, Mosquito, and Banana River lagoons on the Atlantic coast of central Florida, USA, have resulted in marked ecological impacts. Recent studies have investigated whether suspension feeding invertebrates could contribute to top-down control of these and other bloom-forming phytoplankton. While most studies focused on the effects A. lagunensis has on on bivalve filter feeding in the Indian River Lagoon, this study focused on feeding by the solitary pleated tunicate Styela plicata. Hourly filtration rates (FR) of individual S. plicata were measured after exposure to 5.0 x 105 cells mL–1 of either A. lagunensis or another pelagophyte Aureococcus anophagefferens. The latter species has not been observed in Florida estuaries but can also inhibit feeding. Tisochrysis lutea, an alga commonly used as feed in aquaculture was used as a control. Differences in hourly FR of either brown tide species compared to the control alga were statistically significant (p < 0.0001). Mean hourly FR of tunicates exposed to Aureoumbra lagunensis ranged from -282 mL hr−1 to 542 mL hr−1, whereas tunicates exposed to the control species Tisochrysis lutea had FR from 285 mL hr−1 to 2648 mL hr−1. Mean hourly FR of tunicates exposed to Aureococcus anophagefferens ranged from 343 mL hr−1 to 593 mL hr−1 compared to tunicates exposed to T. lutea which ranged from 600 mL hr−1 to 2482 mL hr−1. Further, to test whether cell density and a longer exposure time affected FR, individual S. plicata were initially exposed to cell densities of 103, 104, or 105 cells mL–1 of either A. lagunensis or T. lutea (control) for 24 hours. Subsequent measurement of hourly FR and showed cell density removal was not significantly different (p = 0.28 and 0.77, respectively). These results add to the body of literature on how organisms in the Indian River Lagoon filter-feeder community respond to brown tide exposure. The pleated tunicate may be an additional species contributing to top-down control of these bloom-forming microalgae.
Introduction
For over a decade, the northern Indian River Lagoon (IRL) and its sub-regions, the north IRL, Banana River Lagoon, and Mosquito Lagoon have suffered marked ecological impacts as a result of recurring harmful algal blooms. Coincident with repeated blooms of the pelagophyte Aureoumbra lagunensis Stockwell, DeYoe, Hargraves et Johnson (DeYoe et al., 1997), first observed in Mosquito Lagoon and the north IRL in 2012 (Gobler et al., 2013), have been widespread loss of seagrasses and drift macroalgae (Phlips et al., 2021), reduced larval recruitment of oysters (Walters et al., 2021), bivalve (Gobler and Sunda, 2012) and fish (Gobler et al., 2013) kills, and unusual mortality events of marine mammals (Florida Fish & Wildlife Conservation Commission, 2021) and birds (Lapointe et al., 2015).
This brown-tide forming pelagophyte is now considered a dominant constituent of the IRL phytoplankton community despite its relatively recent introduction to Florida coastal waters, with significant blooms observed in 2012, 2013, 2015, 2016, and 2018 (Phlips et al., 2019).
In response to eutrophication and loss of filter-feeders world-wide, numerous studies have investigated the ability of oysters, tunicates, and other grazers to attenuate nutrients (e.g., Cerco and Noel, 2007; Buzzelli et al., 2013; Kellogg et al., 2013; Bricker et al., 2018), bacteria (e.g., Draughon et al., 2008) and affect top-down control on bloom-forming phytoplankton (including brown tide, e.g., Galimany et al., 2017; Galimany et al., 2020; Galimany et al., 2021). While considered non-toxic, brown tides formed by Aureoumbra lagunensis, and another pelagophyte Aureococcus anophagefferens Hargraves et Sieburth (DeYoe et al., 1997), affect bivalve and zooplankton feeding due to their cellular structure (e.g., mucilaginous secretions), accumulated biomass, or a combination of these factors (Anderson et al., 2002). The exopolymeric secretions (EPS) of these brown tide species interfered with the ciliary beating required for movement of captured particles to the gut in bivalves (Gainey and Shumway, 1991; Robbins et al., 2010) and adhered to cilia on the surface of protozoa, affecting both motility and feeding (Liu and Buskey, 2000). Even when ingested, the EPS may shield cells from digestion (Bersano et al., 2002). Evidence for feeding and growth inhibition in the hard clam (Mercenaria mercenaria) and the blue mussel (Mytilus edulis) to different strains of Aureococcus anophagefferens was observed by Bricelj et al. (2001).
Several studies suggest top-down control of brown tide may be possible in the IRL. Bricelj et al. (2001) found that juvenile hard clams had normal feeding exposed to Aureococcus anophagefferens at cell densities < 3.5 x 104 cells mL-1, although clearance rates were depressed above this threshold. Eastern oysters (Crassostrea virginica) from the IRL had decreased clearance rates (from 2.19 to ≤ 0.12 L hr−1 g−1) when exposed to A. lagunensis (mean 3.26 × 106 ± 0.20 × 106 (SE) cells ml−1), but absorption and efficiency was comparable to non-bloom conditions suggesting oysters could maintain their feeding requirements on brown tide (Galimany et al., 2017). Feeding experiments conducted on bivalve species widely distributed in the IRL include Eastern oysters, hooked mussels (Ischadium recurvum), charru mussels (Mytella charruana), green mussels (Perna viridis), Atlantic rangia (Rangia cuneata), and hard clams. These species were exposed to isotopically (15N) labeled microalgae, including Aureoumbra lagunensis and other picoplanktonic bloom-forming species isolated from the 2011 IRL “superbloom” (Galimany et al., 2020). While clearance rates differed among bivalves and microalgal treatments, bivalve tissues indicated assimilation of 15N-enriched bloom-forming microalgae, indicating the importance of a biodiverse community of filter-feeders and their potential to force top-down control of phytoplankton in the IRL (Galimany et al., 2020).
Another filter-feeder found ubiquitously in the IRL is the pleated tunicate, Styela plicata (Lesueur). Styela plicata is a solitary ascidian and one of the most introduced tunicate species worldwide (Baker et al., 2004; Pineda et al., 2013). There is no consensus regarding its native range although it is generally thought to be from the northwest Pacific (Carlton and Ruckelshaus, 1997; Lambert and Lambert, 1998; Lambert, 2001; Pineda et al., 2011; Pineda et al., 2013). Bingham (1992) and Carlton and Ruckelshaus (1997) list S. plicata as an introduced species in Florida. It is widespread and abundant in the IRL near inlets and the interior of the lagoon (Mook, 1983).
Tunicate suspension feeding is characterized by pumping water via movement of cilia along the stigmatal openings of a branchial basket lining the pharynx, through the inhalant siphon into the pharynx (Petersen, 2007). An endostyle continuously produces a mucus net, whereby captured particles are rolled into a food string and carried to the oesophagus (Petersen, 2007). There is no evidence of particle selection once taken into the pharynx: any particles entering the pharynx can be captured by the mucus net, and experimental studies indicate no difference between particles in suspension and those ingested, except for very large particles or their own eggs and larvae (Randløv and Riisgård, 1979; Robbins, 1983; Klumpp, 1984; Stuart and Klumpp, 1984; Bingham and Walters, 1989; Ribes et al., 1998). Particle selection can occur at the inhalant siphon aperture where specially arranged tentacles prevent larger particles from entering the pharynx. Regulation of suspension feeding in ascidians is mediated by temperature as well as gut fullness (Petersen et al., 1999; Petersen, 2007).
The present study aimed to determine if the pleated tunicate Styela plicata contributes to top-down control of brown tide in the IRL. Feeding experiments were conducted at varying cell densities of Aureoumbra lagunensis compared to a control microalga. Additionally, a feeding experiment using Aureococcus anophagefferens was conducted. Although not yet observed in Florida, Aureococcus anophagefferens blooms may be a future threat to the IRL, as it has spread to other estuaries along the Atlantic coast of the United States by similar transport mechanisms as Aureoumbra lagunensis (e.g., ship bilge water).
Materials and Methods
The present study examined the filtration rate of the pleated tunicate Styela plicata fed varying cell densities of either Aureoumbra lagunensis or Aureococcus anophagefferens, brown tide-forming pelagophytes. All experiments included a control in which tunicates were fed Tisochrysis lutea Bendif et Probert (previously classified as Isochrysis sp. [clone T-Iso]; Bendif et al., 2013), a microalga regularly used as bivalve feed in aquaculture facilities and feeding experiments, which is of comparable cell size (4–6 μm); and a negative control that included replicate treatment beakers with the microalgae, but no tunicate, which accounted for sedimentation or adherence of cells to the beaker walls. Experiments were conducted in the aquaculture laboratory at Harbor Branch Oceanographic Institute (HBOI), Florida Atlantic University, Fort Pierce, Florida between July 2014 and July 2015.
Tunicate Specimen Collection and Holding
Styela plicata were collected from the HBOI ship channel, removed from subtidal twisted-nylon ropes. The tunicates were transported from the field in 12 L seawater (salinity 29–31) to the HBOI aquaculture laboratory within 10–20 minutes after collection. Specimens were placed in a shallow 344 L tank (305 cm long, 60 cm wide, 45 cm deep) filled to 30 cm with filtered sea water (FSW; 1 µm filtered, UV-irradiated, salinity 29–30.7) and aerated with several air stones. Tunicates were acclimated for two to four hours and then cleaned to remove commensal and macro-fouling organisms and debris using forceps and a soft brush.
Tunicates were held for approximately 48–72 hours prior to experimentation and fed Tisochrysis lutea at a density of 103 cells mL-1 day-1 to minimize gut contents. Individuals with open siphons and consistent coloration over the holding period were selected for the filtration experiments. To prevent movement of individuals during the experiment, selected specimens were secured to individual plastic grids (10 cm x 10 cm with 1.6 cm openings) with monofilament line lassoed around the body away from the incurrent and excurrent siphons. Recovery of the tunicates was rapid as specimens were observed to have open siphons within minutes of attachment and placement back in water.
Microalgae Cultures
Cultures of Tisochrysis lutea were provided by the HBOI aquaculture lab and grown using 0.22 µm filter-sterilized f/2 medium (Florida Aqua Farms, Inc., Dade City, Florida) in the HBOI algae culture facility at ambient temperature (~25°C) under fluorescent light. Cultures of Aureoumbra lagunensis (strain CCMP 1510, isolated from Bird Island Station, Laguna Madre, Texas) and Aureococcus anophagefferens (strain CCMP 1984, isolated from Great South Bay, Long Island, New York) were provided by Dr. Christopher Gobler School of Marine and Atmospheric Sciences, Stony Brook University, Southampton, NY, USA. These two species were cultured separately using 0.22 µm filter-sterilized h/2 medium. The h/2 medium is a derivative of L1 medium that includes 500 mM NH4Cl (National Center for Marine Algae and Microbiota, East Boothbay, Maine). Silicate stock solution was omitted and 1% penicillin/streptomycin (10,000 units mL-1; Hyclone, Logan, Utah) added to starter cultures to minimize bacterial growth. Cultures were scaled to 6L and aerated with CO2-enriched air at ambient temperature under fluorescent light until start of the experiment.
Experiment 1: Filtration Rate When Exposed to Aureoumbra lagunensis
Twenty individual tunicates (16.75–30.83g whole animal wet weight (WAWW)) were randomly placed in individual beakers of 6 L of FSW (1 µm filtered, UV-irradiated, salinity 32.1). The 20 beakers were arranged in unbalanced, randomized blocks in four 305 cm long, shallow (45 cm deep) tanks filled to 30 cm with FSW as a water bath (28.8°C). There were 10 replicates for each algae species treatment and four negative controls for each species.
Cultured Aureoumbra lagunensis and Tisochrysis lutea (control species) were separately passed through sieves with 20 µm openings in order to break up or remove cell clumps. Cell counts of filtered cultures were performed using a hemocytometer and phase contrast microscope. Algae were added to their respective treatment beaker and mixed to homogenize to result in a density of ~5.0 x 105 cells mL-1. Beakers were moderately aerated to provide circulation and minimize sedimentation of algal cells.
A 10 mL water sample was collected from each beaker at the start of the experiment (Hour 0) and then hourly for five hours. Samples were fixed with 1 mL 10% buffered formalin and stored at 4°C until cell counts were performed (the dilution caused by adding formalin was corrected for during density calculations).
In order to determine if there were correlations between weight and FR, after the last water sample, tunicate specimens were removed from their respective beakers, and placed on a paper towel to air-dry. Specimens were weighed for whole animal wet weight (WAWW), then placed in individual aluminum trays, dried in an oven at 95°C for 48 hours, and held at 40°C until whole animal dry weight (WADW) was measured.
Quadruplicate cell counts were performed for each hourly sample and averaged for the calculation of filtration rate. Filtration rate, defined as the volume of water cleared of a particular particle (i.e., algal cells) over time, was calculated as in Draughon et al. (2010). In brief, Coughlan’s formula (Coughlan, 1969), a version of Quayle’s formula modified for the adjustment of particle sedimentation, was used to calculate filtration rate (FR):
where FR = filtration rate in mL hr−1; V0 = volume of water remaining in experimental beaker after withdrawal of sample at T0; Vt =volume of water in experimental beaker before withdrawal of sample at time t; n = number of animals; t = duration of experiment; SE0, SEt = suspension loads in cells or events mL−1 in experimental beaker at T0 and t; SC20, SC2t = suspension loads in cells or events mL−1 in control beaker at T0 and t.
It was determined post hoc in the review of sedimentation controls for this and subsequent experiments that these values would be removed from Coughlan’s formula (Coughlan, 1969) for all calculations of FR (except for T. lutea controls in the first FR experiment) as cell densities in these beakers did not indicate sedimentation occurred, likely due to constant aeration.
The a priori null hypothesis (H0) was that no significant difference exists between individual hourly or individual mean filtration rates of S. plicata between algae treatments. The response variable filtration rate was analyzed using generalized linear model (GLM) repeated measures analysis of variance (RMANOVA; critical alpha = 0.05) in SAS.
Experiment 2: Filtration Rate When Exposed to Aureococcus anophagefferens
The previous experimental design was replicated to determine the filtration rate of S. plicata (10.85–28.66g WAWW) exposed to Aureococcus anophagefferens compared to the filtration rate of tunicates exposed to Tisochrysis lutea (control). The a priori H0 was no significant difference exists between individual hourly or individual mean filtration rates of S. plicata between algae treatments. The response variable filtration rate was analyzed using GLM RMANOVA (critical alpha = 0.05) in SAS.
Experiment 3: Filtration Rate When Exposed to Different Starting Densities of Aureoumbra lagunensis
Individual tunicates were exposed to different starting cell densities (103, 104, and 105 cells mL-1) of Aureoumbra lagunensis or Tisochrysis lutea (control) to determine if cell density had an effect on filtration rate (FR). Each treatment was conducted in triplicate and included negative controls in triplicate to account for sedimentation. Individual tunicates (10.85–28.66g WAWW) were placed in 7.5 L beakers with 6 L FSW (1 µm filtered, UV-irradiated, salinity ~30) and algae added to meet the treatment densities noted above. Beakers were arranged as a balanced, randomized block design in 305 cm long shallow tanks in 30 cm of water which served as a water bath.
Samples were collected at start of the experiment (Hour 0) and again after 24 hours (Hour 24). Cell counts were performed with a hemocytometer and phase-contrast microscope. Quadruplicate cell counts were performed for each sample and averaged for the calculation of FR. The a priori H0 was that no significant difference exists between individual average FR for algal species treatment, or algal density within treatments. The response variable filtration rate was analyzed by GLM two-way ANOVA (critical alpha = 0.05) in SAS for overall mean FR between treatments.
Results
Experiment 1: Filtration Rate When Exposed to Aureoumbra lagunensis
Mean hourly filtration rate (FR) of tunicates exposed to Aureoumbra lagunensis ranged from -282 mL hr−1 to 542 mL hr−1, whereas tunicates exposed to the control species Tisochrysis lutea had FR from 285 mL hr−1 to 2648 mL hr−1 (negative FR indicates an increase in cell density within the beakers) (Figure 1). There was no relationship between WAWW and mean FR for either the A. lagunensis or T. lutea treatments (R2 = 0.02 and 0.05, respectively) nor with WADW and mean FR (R2 = 0.002 and 0.06, respectively); WAWW and WADW were positively correlated (R2 = 0.96).
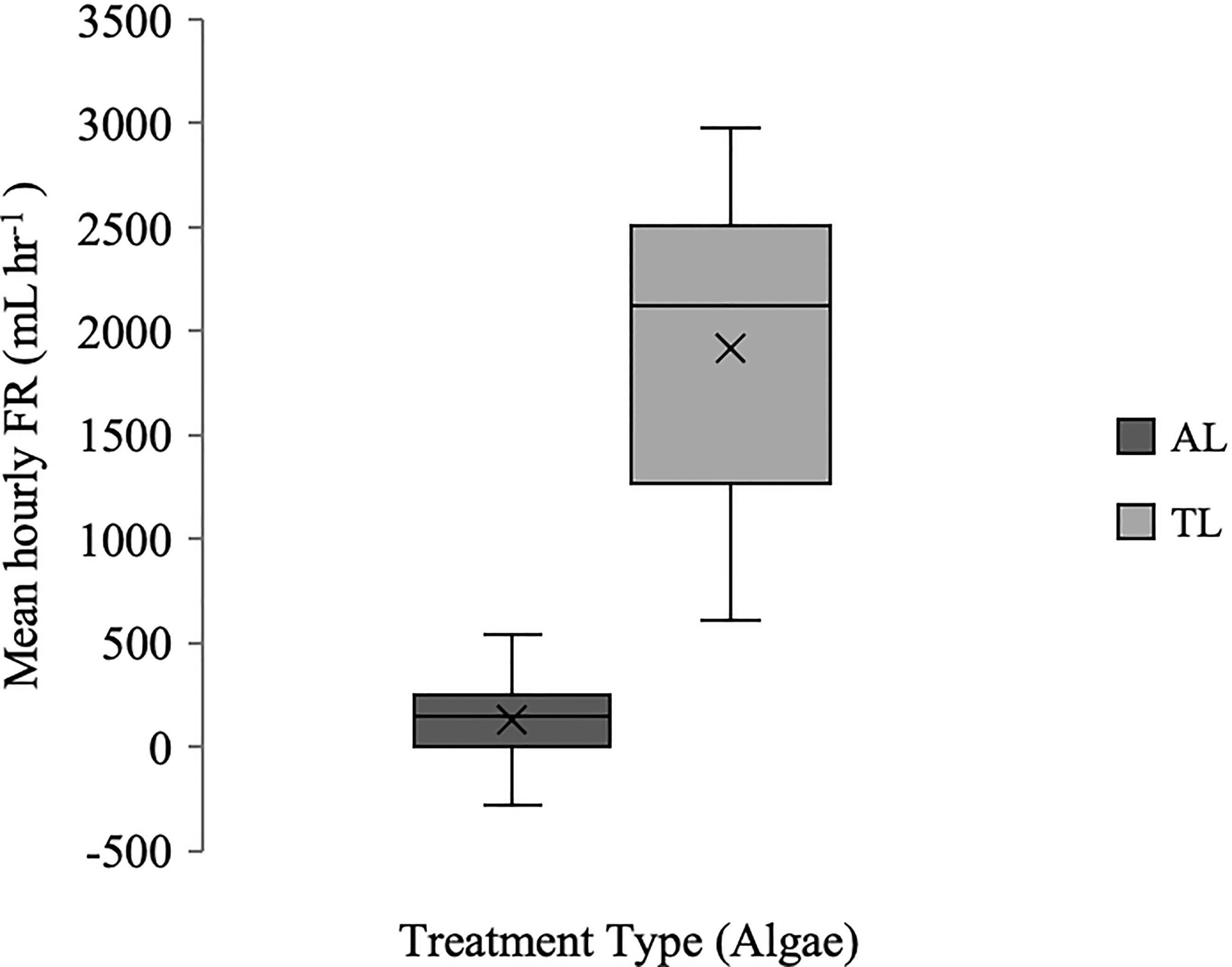
Figure 1 Boxplot (x = mean, n=10) of hourly FR (mL hr-1) for Styela plicata exposed to Aureoumbra lagunensis (AL) or Tisochrysis lutea (TL, control).
Over the five-hour period, cell densities of A. lagunensis in the negative controls (those used to account for sedimentation of cells and excluded a tunicate specimen) fluctuated around a mean of 4.9 x 105 cells mL-1 (± 1.4 x 104 cells mL-1 s.d.). Cell densities of T. lutea in the sedimentation controls gradually decreased from a mean of 4.9 x 105 cells mL-1 (± 3.5 x 104 cells mL-1 s.d.) at Hour 0 to a mean of 4.0 x 105 cells mL-1 (± 3.0 x 104 cells mL-1 s.d.) at Hour 5, indicating some, but minimal sedimentation. Sedimentation, or fluctuation of hourly cell density in the controls, was accounted for in the calculation of filtration rate of tunicates exposed to T. lutea.
Differences in mean FR for S. plicata exposed to either A. lagunensis or T. lutea were significant (GLM: F = 43.85; p < 0.0001; Table 1) with means (± s.d.) of 128 ± 226 mL hr−1 and 1634 ± 797 mL hr−1, respectively, rejecting the null hypothesis. In containers containing a tunicate, there was no marked decrease in A. lagunensis cell density over the five hours and cell density remained steady from Hour 3 to Hour 5 at approximately 5.0 x 105 cells mL−1 (Figure 2). In the T. lutea treatments there was a steady decrease from 5.4 x 105 cells mL−1 at Hour 0 to 1.6 x 105 cells mL−1 at Hour 5 (Figure 2).

Table 1 GLM (RMANOVA) table summarizing analysis of FR of the pleated tunicate Styela plicata by microalgae treatments of the brown tide-forming pelagophyte Aureoumbra lagunensis, and control species Tisochrysis lutea (Experiment 1).
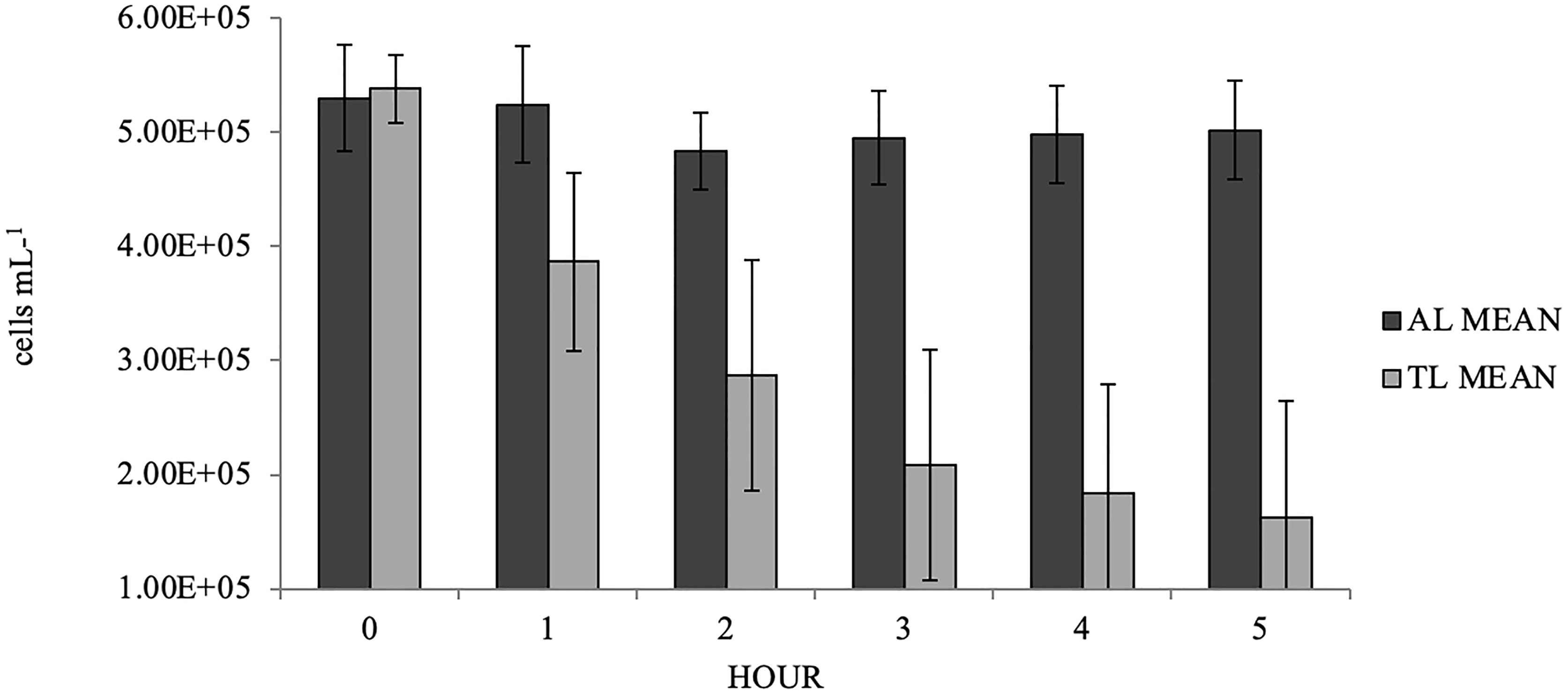
Figure 2 Mean (± s.d., n=10) hourly microalgae cell densities for Styela plicata exposed to Aureoumbra lagunensis (AL) or Tisochrysis lutea (TL, control).
Experiment 2: Filtration Rate When Exposed to Aureococcus anophagefferens
Mean hourly filtration rate (FR) of tunicates exposed to Aureococcus anophagefferens ranged from 343 mL hr−1 to 593 mL hr−1 compared to tunicates exposed to T. lutea which ranged from 600 mL hr−1 to 2482 mL hr−1 (Figure 3). There was no relationship between WAWW and mean FR for either the A. anophagefferens or T. lutea treatments (R2 = 0.006 and 0.08, respectively); nor with WADW and mean FR (R2 = 0.01 and 0.02, respectively); WAWW and WADW were positively correlated (R2 = 0.95).
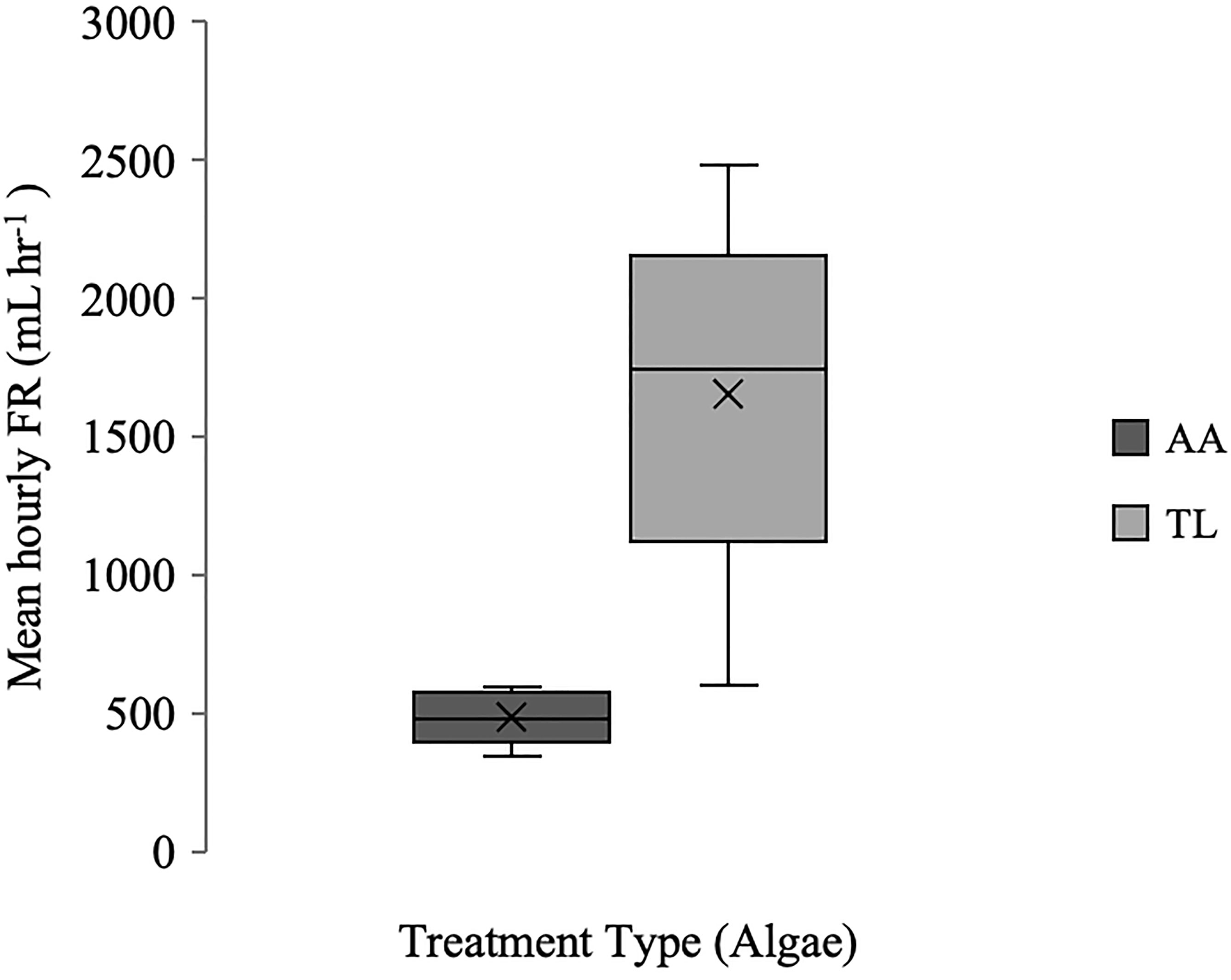
Figure 3 Boxplot (x = mean, n=10) of hourly FR (mL hr-1) for Styela plicata exposed to Aureococcus anophagefferens (AA) or Tisochrysis lutea (TL, control).
Over the five-hour period, the cell densities of A. anophagefferens in the negative controls fluctuated around a mean of 5.0 x 105 cells mL−1 (± 1.4 x 104 cells mL-1 s.d.) with no obvious sedimentation. The cell densities of T. lutea in the negative controls fluctuated around a mean of 4.8 x 105 cells mL−1 (± 6.2 x 104 cells mL-1 s.d.) although hourly fluctuations indicate possible poor mixing, cellular motility, sedimentation, or fluctuation of hourly cell density in the controls, and was accounted for in the calculation of filtration rate. Differences in mean FR of S. plicata exposed to A. anophagefferens and T. lutea were significant (GLM: F = 32.60; p < 0.0001; Table 2), with means ( ± s.d.) of 485 ± 278 mL hr−1 and 1650 ± 733 mL hr−1, respectively, rejecting the null hypothesis. There was a moderate decrease (~23%) in A. anophagefferens cell density over the five hours (5.6 x 105 cells mL−1 at Hour 0 to 4.3 x 105 cells mL−1 at Hour 5, Figure 4). The decrease in T. lutea (control) cell density was greater (~76%; 5.0 x 105 cells mL−1 at Hour 0 to 1.2 x 105 cells mL−1 at Hour 5, Figure 4).

Table 2 GLM (RMANOVA) table summarizing analysis of FR of the pleated tunicate Styela plicata by microalgae treatments of the brown tide-forming pelagophyte Aureococcus anophagefferens, and control species Tisochrysis lutea (Experiment 2).
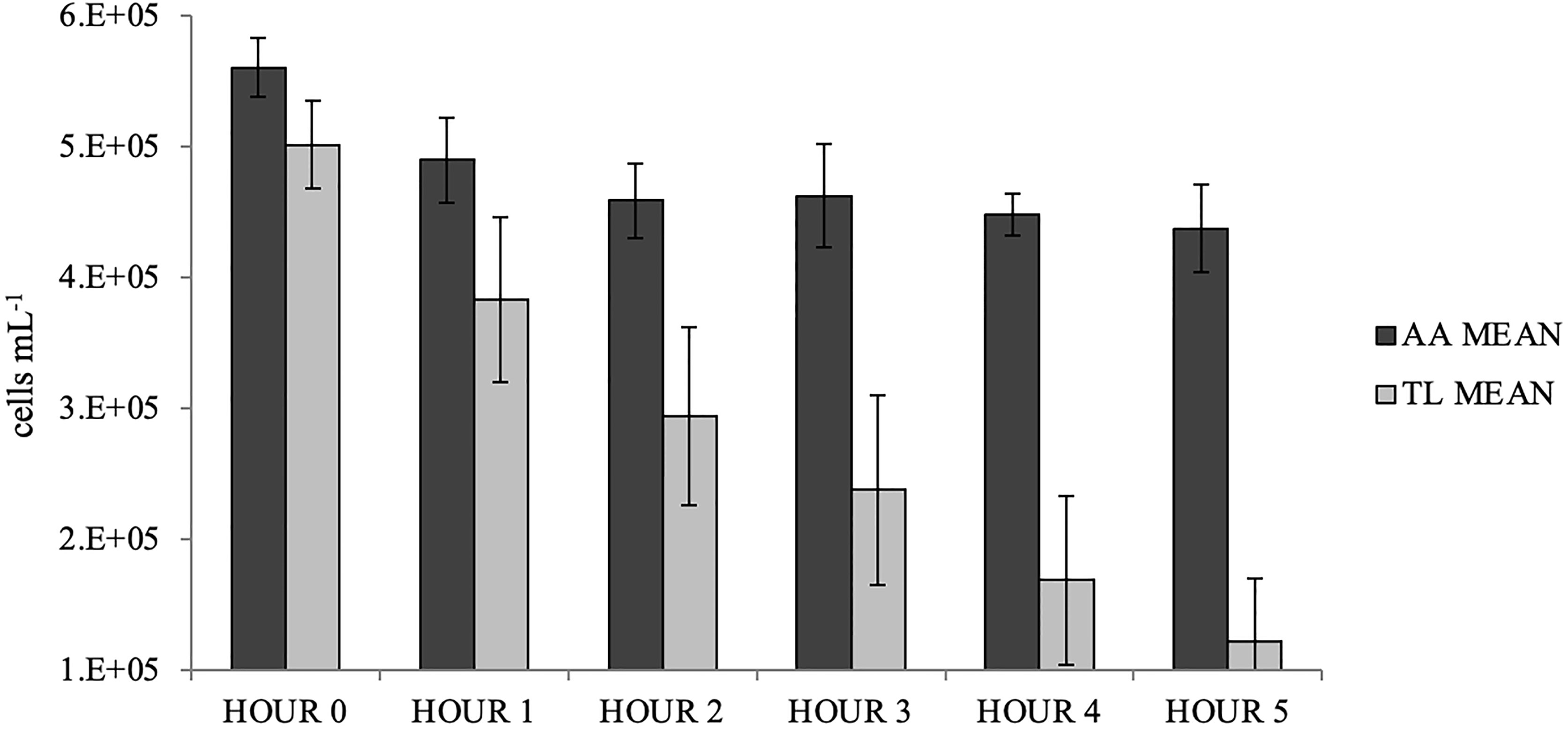
Figure 4 Mean (± s.d., n=10) hourly microalgae cell densities for Styela plicata exposed to Aureococcus anophagefferens (AA) or Tisochrysis lutea (TL, control).
Experiment 3: Filtration Rate When Exposed to Different Starting Densities of Aureoumbra lagunensis
The hourly filtration rate (FR) ranges for individual tunicates exposed for 24 hr to Aureoumbra lagunensis at starting concentrations of 103, 104, and 105 cells mL-1 were 361 mL hr-1 to 2670 mL hr-1, 991 mL hr-1 to 1151 mL hr-1, and 309 mL hr-1 to 718 mL hr-1, respectively (Figure 5). The hourly FR for tunicates exposed to T. lutea at starting concentrations of 103, 104, and 105 cells mL-1 were 153 mL hr-1 to 737 mL hr-1, 451 mL hr-1 to 912 mL hr-1, and 193 mL hr-1 to 1379 mL hr-1, respectively (Figure 5). Over the 24 hours, the sedimentation controls did not decrease in cell density in comparable rates as the experimental beakers, therefore it is assumed the latter was due to filtration.
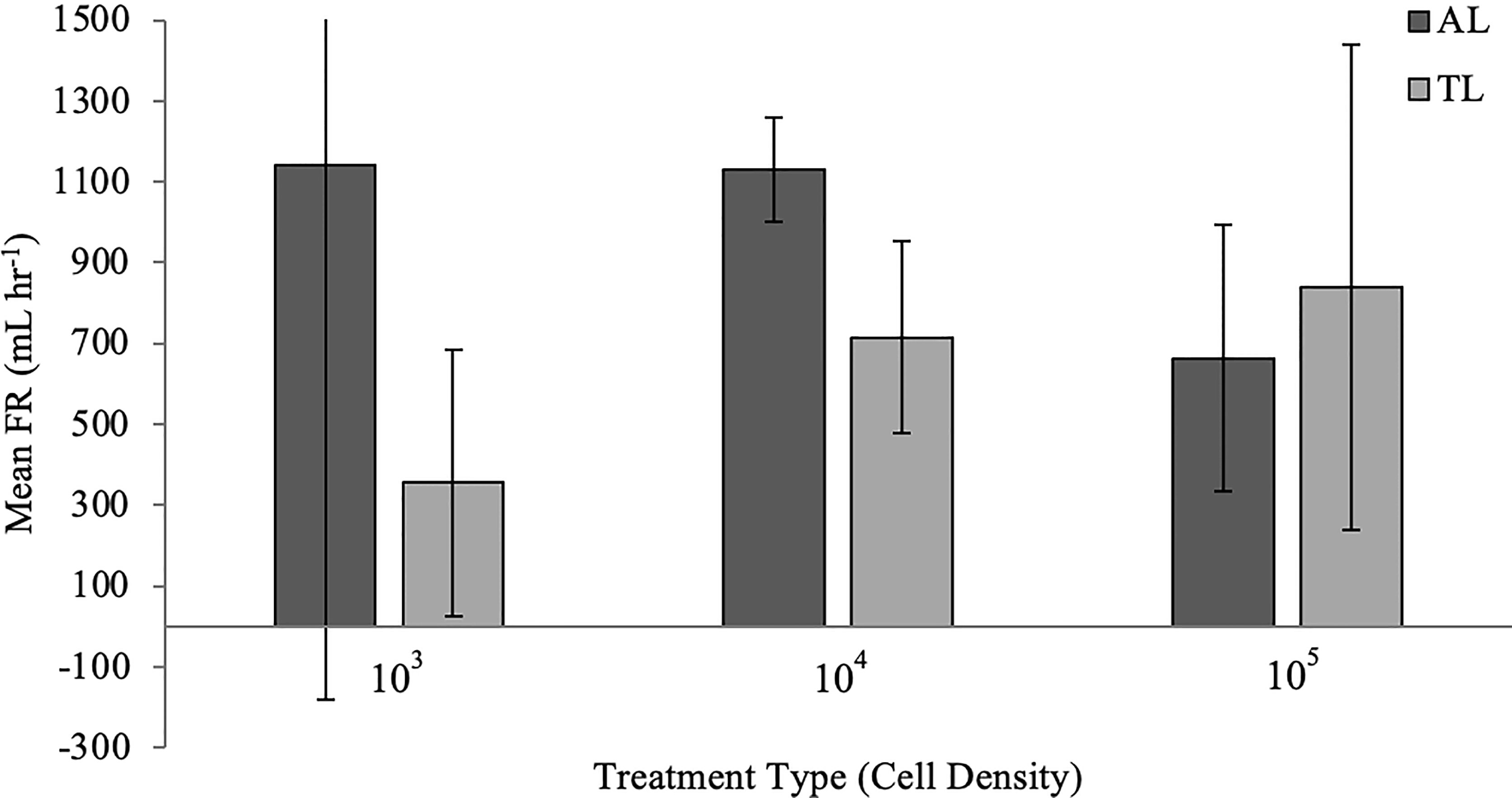
Figure 5 Mean (± s.d., n=3) FR (mL hr-1) for Styela plicata exposed to Aureoumbra lagunesis (AL) or Tisochrysis lutea (TL, control) at varying starting cell densities (x-axis; cells mL-1).
Mean FR for S. plicata exposed to either A. lagunensis or T. lutea (GLM: F = 1.30; p = 0.28; Table 3) and between different starting concentrations (GLM: F = 0.27; p = 0.77; Table 3) were not statistically significant, and therefore the null hypothesis was not rejected.

Table 3 GLM (two-way ANOVA) table summarizing analysis of FR of the pleated tunicate Styela plicata by microalgae treatment and cell density of the brown tide-forming pelagophyte Aureoumbra lagunensis, and control species Tisochrysis lutea (Experiment 3).
Discussion
Although the pleated tunicate Styela plicata had a significant decline in filtration rate (FR) when exposed to Aureoumbra lagunensis for five hours, these filter-feeders had comparable FR to the control when exposed for 24 hrs, effectively filtering brown tide cells across several cell densities. Tunicate size did not affect FR across all experiments. For example, in Experiment 1 the tunicate with the greatest mean FR exposed to A. lagunensis (542 mL hr-1) weighed 33 grams (g) whereas the largest tunicate (45.4 g) had a mean FR of only 52 mL hr-1. The tunicate with the greatest mean FR exposed to Tisochrysis lutea (2975 mL hr-1) weighed 16.8 g whereas the largest tunicate (51.2 g) had a mean FR of 2094 mL hr-1. These results are consistent with Draughon et al. (2010) who found there was no relationship between average hourly filtration rate and whole animal weight (R2 = 0.0001) or dry organ weight (R2 = 0.0067) of S. plicata fed either the bacteria Escherichia coli or the microalgae Nannochloropsis sp. They recommended that FR for the pleated tunicate not be reported based on live or dry weight. In the present study, mean FR of S. plicata fed T. lutea (the control) at 5.0 x 105 cells mL-1 across the three experiments often exceeded 2000 mL hr-1 animal-1. Laboratory measurements by Draughon et al. (2010) of S. plicata FR exposed to Nannochloropsis sp. or E. coli at 105 cells mL−1 filtered 3065 mL hr−1 animal−1 ( ± 1284 mL hr−1 s.d.) and 4654 mL hr−1 animal−1 (± 810 mL hr−1 s.d.), respectively.
The present study did not employ the use of stable isotopes to determine whether tunicates were assimilating nutrients of consumed particles, although feces were produced by tunicates which indicated digestion of material. It is expected that tunicates were not feeding at a consistent rate throughout the 24 hour exposure period, as suggested by the standard deviation of mean FR, especially at low concentrations of A. lagunensis (103 cells mL-1) (Figure 5). Slowing of ciliary beating and lower filtration rate in tunicates could be a result of cellular characteristics of A. lagunensis (i.e., the EPS). Some pico- and nanoplankton develop surface features to increase their mobility through mucus, effectively reducing capture efficiency by mucus-based filters (Jacobi et al., 2020) such as those in tunicates; however, both pelagophytes used in this study are non-motile so this is not assumed to be an evasive mechanism in Experiment 1 or 2. To strengthen Experiment 3, it should be replicated with hourly water samples and calculations of FR in order to determine treatment-specific feeding behavior. The samples taken only at Hour 0 and Hour 24 obscures feeding behavior and averages out the estimated hourly FR. Further, while the experimental treatments exposing tunicates to pelagophytes at 105 cells mL-1 is representative of median cell densities observed during the 2012 brown tide bloom in the IRL (ranging 104 and 106 cells mL-1; Gobler et al., 2013) additional treatments representing peak cell densities of IRL brown tides (> 106 cells mL-1; Gobler et al., 2013; Phlips et al., 2021) would have contributed valuable information to this study.
The sedimentation controls in Experiment 3 indicate that removal of cells was a result of the presence of tunicate filtration rather than sedimentation; the moderate aeration provided in all beakers allowed the unflagellated, non-motile brown tide cells (DeYoe et al., 1997) to remain in suspension rather than settling. Tunicates may also spontaneously squirt as a method of clearing the branchial basket of waste material or in the case of overloading (Petersen 2007); while not observed, periodic squirting may have contributed to water movement throughout the experimental beakers to prevent settling, or may explain hour-to-hour fluctuations in cell densities, highly variable hourly FR, or instances of calculated negative FR.
There are various methods for measuring and calculating the filter feeding capacity of invertebrate suspension feeders in the lab and in the field which can confound direct comparisons between studies. For example, Fiala-Médioni (1978a) calculated the “pumping rate” (speed of water flow through siphons) of S. plicata as 3.30 cm sec-1 and filtration rate as 4.9 L h-1 gm-1 total dry weight at a of 19-22°C (Fiala-Médioni, 1978a) and between 5.3 and 10.7 cm sec-1 and 10.5 and 11.5 L h-1 gm-1 total dry weight at temperatures 15°C (Fiala-Médioni, 1978b). Sumerel and Finelli (2014) measured both “flow speed” (cm s-1) and clearance rate (L hr-1) for S. plicata. Galimany et al. (2017) employed the biodeposition method (Iglesias et al., 1998), a method used in studying bivalve feeding, which uses inorganic matter of water as a tracer of ingestion, egestion, and rejection feeding processes. Use of bivalve clearance of A. lagunensis in their study (in L hr-1), defined by the volume of seawater passing through the gills per unit of time, may be used for rough comparison with the present study, acknowledging the methodological differences: the oyster Crassostrea virginica had a mean FR measured in situ at sites in the Indian River Lagoon between 2 and 3 L hr-1; the hard clam Mercenaria mercenaria was less efficient at filtering high seston loads, with mean FR lower at <1 L hr-1.
The reduction in FR observed in Experiment 1 of the present study was consistent with reductions of FR by bivalves important to the IRL (Bricelj et al., 2001; Gobler et al., 2013). The ecological function of suspension feeding invertebrates is important for maintaining water quality, and thus feeding behavior when exposed to algal blooms is of critical importance. Oysters serve an important role in reef formation and provide heterogeneous habitat for other organisms (Boudreaux et al., 2006). Aureoumbra lagunensis blooms have caused declines in oyster spat recruitment in Mosquito Lagoon, the northern-most segment of the IRL and one of the main subregions most affected by brown tide (Makris, 2016; Walters et al., 2021). Buskey et al. (1997) and Gobler et al. (2013) implicate the role of an extra-cellular polysaccharide sheath surrounding cells of A. lagunensis as cause for the disruption of grazing by filter-feeders. Liu and Buskey (2000) demonstrated that A. lagunensis with thick EPS was not suitable food for the protist Euplotes sp. and other hypotrichous ciliates; the protist Aspidisca sp. had reduced filtration rates and growth rates when exposed to the thick-EPS. Gainey and Shumway (1991) and Bricelj et al. (2001) implicate a dopamine-mimetic bioactive compound associated with the extra-cellular polysaccharide sheath of Aureococcus anophagefferens with the reduction of activity of gill lateral cilia in bivalves necessary to generate movement of water through the gills. In tunicates, cilia are responsible for the movement of trapped particles in a mucus net toward the oesophagus (MacGinitie, 1939; Petersen 1997), so while a similar mechanism may have been operative in the short-term experiments of the present study, identifying the mechanism for feeding inhibition and what explains the comparable (not statistically significant difference of) FR between treatments at 24 hours in Experiment 3 is only hypothesized in the present study and warrants further investigation. While a 24 hour experiment exposing tunicates to different starting cell densities of A. anophagefferens was not conducted, we hypothesize that similar results would be observed.
Despite pelagophyte impacts on suspension feeding behavior, studies indicate that bivalves can feed on A. lagunensis and may be a mechanism for top-down control of blooms in the IRL. Galimany et al. (2020) exposed several IRL bivalves including eastern oysters (C. virginica), hooked mussels (Ischadium recurvum), charru mussels (Mytella charruana), green mussels (Perna viridis), Atlantic rangia (Rangia cuneata), and hard clams (M. mercenaria) to a mixed culture at 3.0 x 104 cells mL-1 that included 15N-enriched A. lagunensis. Clearance rates did vary between species, but isotopic labelling of bivalve tissue indicated that all species assimilated brown tide cells. In a follow-on study, eastern oysters, hooked mussels, and hard clams were exposed to isotopically labeled (13C and 15N) A. lagunensis at cell densities of 104 and 106 cells mL-1. Clearance rates declined with increasing algal density, but bivalves assimilated the greatest number of cells at bloom concentrations (106 cells mL-1) (Galimany et al., 2021). Interspecific variation in filtration rate indicates the importance of a biodiverse suspension feeding community in the IRL.
A conservative estimate of filtration capacity at lagoon-scale using their laboratory-based measures of filtration rate of the Eastern oyster and hard clam (the most common species targeted for restoration efforts) estimate filtration of 0.002% the volume of the IRL at brown tide concentrations of 104 cells mL-1. An additional 1% is estimated based on restored oyster reefs found in Mosquito Lagoon only but doesn’t consider the suite of filter feeding organisms throughout the ecosystem (Galimany et al., 2021). The authors caution that laboratory-based estimates are merely a proxy for conditions in the field, but still provide evidence for these nature-based solutions of improving water quality. Draughon et al. (2008) investigated the use of S. plicata as a bioremediator and estimated a population of 200 S. plicata could filter >600 L hr-1 of algae or bacteria. Styela plicata not only removed E. coli at cell densities of 106 cells mL-1 but killed all cells, rendering them unable to replicate (Draughon et al., 2010). No published estimates of S. plicata densities in the IRL are available from which extrapolations of total volume of the IRL that could be filtered during a brown tide can be made; however, a simple calculation using mean FR from the present study estimates that 200 S. plicata could filter 117.4 L hr-1 (or approximately 2,818 L day-1) of Aureoumbra lagunensis at cell densities exceeding 105 cells mL-1.
Despite various methodological approaches, numerous studies measuring suspension feeding of invertebrate organisms found in the IRL demonstrate the capacity to exert top-down control of phytoplankton. Suspension-feeding bivalves are capable of efficiently capturing particles as small as 1 μm (Møhlenberg and Riisgård, 1978; Galimany et al., 2020), as can ascidians (Jacobi et al., 2020). Further, Jacobi et al. (2020) found that ascidians could retain particles at submicron sizes (using microspheres and planktonic cells measuring as small as 0.3 μm); although the microspheres were captured at greater efficiencies than phytoplankton of comparable size, possibly due to evasive characteristics of phytoplankton. In their study, S. plicata had a capture efficiency rate of >25% of Prochlorococcus sp., which has a cell diameter of ~0.6 μm; and a capture efficiency rate of >50% of Synechococcus sp. and another eukaryotic microalgae “NanoEuk,” which had median cell diameters of ~1.05 μm, and ~6 μm, respectively (Jacobi et al., 2020). Galimany et al. (2020) found that bivalves in their study could also consume and assimilate Synechococcus sp. (although their strain was larger at 5.74 ± 07 μm diameter) but resulted in lower clearance rates. Other physical or chemical surface properties of Synechococcus sp. may explain its low preference by bivalves (Rosa et al., 2017).
The lack of any known particle selection mechanism in ascidians, and their ability to capture and retain sub-micron sized particles, warrants additional investigation and direct comparisons of filtration and assimilation of bloom-forming phytoplankton with that of bivalves present in the IRL. Styela plicata and other tunicates are ubiquitous in the IRL and likely contribute to improvements in water quality. The present study adds additional support that top-down control by extant or restored populations of a diverse suite of suspension feeding organisms could be used as one tool in the “restoration tool box.”
Data Availability Statement
The raw data supporting the conclusions of this article will be made available by the authors, without undue reservation.
Author Contributions
PK conceived of and conducted experiments as part of her Master of Science Thesis at Florida Atlantic University. JS and JH served as committee members and HBOI Summer Internship advisor and major professor, respectively. JS provided key training and guidance on use of lab and aquaculture facilities, as well as procurement of algal specimens and manuscript review. PK took the lead on design and execution of the experiments, data analysis, and writing the manuscript. All authors contributed to the article and approved the submitted version.
Funding
This project was funded by the Link Foundation and Harbor Branch Foundation as part of the Harbor Branch Oceanographic Institute Summer Internship, and Harbor Branch Graduate Research Fellowship, respectively. The authors acknowledge and thank the IRL Council, host of the Indian River Lagoon National Estuary Program (IRLNEP), and the U.S. EPA for providing encouragement and funding to support publication of this manuscript. The funding was provided through a EPA Region 4 supplemental funding grant to the IRLNEP.
Conflict of Interest
The authors declare that the research was conducted in the absence of any commercial or financial relationships that could be construed as a potential conflict of interest.
Publisher’s Note
All claims expressed in this article are solely those of the authors and do not necessarily represent those of their affiliated organizations, or those of the publisher, the editors and the reviewers. Any product that may be evaluated in this article, or claim that may be made by its manufacturer, is not guaranteed or endorsed by the publisher.
Acknowledgments
The authors thank the many staff in the aquaculture laboratory for space and access to equipment and facilities, and Dr. E. Salewski for support of statistical analysis.
Supplementary Material
The Supplementary Material for this article can be found online at: https://www.frontiersin.org/articles/10.3389/fmars.2022.866177/full#supplementary-material
References
Anderson D. M., Glibert P. M., Burkholder J. M. (2002). Harmful Algal Blooms and Eutrophication: Nutrient Sources, Composition, and Consequences. Estuaries 25 (4), 704–726. doi: 10.1007/BF02804901
Baker P., Baker S. M., Fajans J. (2004). Nonindigenous Marine Species in the Greater Tampa Bay Ecosystem (Gainesville, FL, USA: Tampa Bay Estuary Program Technical Publication #02-04), 131.
Bendif E. L., Probert I., Schroeder D. C., de Vargas C. (2013). On the Description of Tisochrysis Lutea Gen. Et. Sp. Nov. And Isochrysis Nuda Sp. Nov. In the Isochrysidales and the Transfer of Dicrateria to the Prymnesiales (Haptophyta). J. Appl. Phycol. 25, 1763–1776. doi: 10.1007/s10811-013-0037-0
Bersano J. G. F., Buskey E. J., Villareal T. A. (2002). Viability of the Texas Brown Tide Alga, Aureoumbra Lagunensis, in Fecal Pellets of the Copepod Acartia Tonsa. Plankton. Biol. Ecol. 49 (2), 88–92.
Bingham B. L. (1992). Life Histories in an Epifaunal Community: Coupling of Adult and Larval Processes. Ecology 73 (6), 2245–2259. doi: 10.2307/1941472
Bingham B. L., Walters L. J. (1989). Solitary Ascidians as Predators of Invertebrate Larvae: Evidence From Gut Analyses and Plankton Samples. J. Exp. Mar. Biol. Ecol. 131, 147–159. doi: 10.1016/0022-0981(89)90004-X
Boudreaux M. L., Stiner J. L., Walters L. J. (2006). Biodiversity of Sessile and Motile Macrofauna on Intertidal Oyster Reefs in Mosquito Lagoon, Florida. J. Shellf. Res. 25 (3), 1079–1089. doi: 10.2983/0730-8000(2006)25[1079:BOSAMM]2.0.CO;2
Bricelj V. M., MacQuarrie S. P., Schaffner R. A. (2001). Differential Effects of Aureococcus Anophagefferens Isolates (“Brown Tide”) in Unialgal and Mixed Suspensions on Bivalve Feeding. Mar. Biol. 139, 605-616. doi: 10.1007/s002270100612
Bricker S. B., Ferreira J. G., Zhu C., Rose J. M., Galimany E., Wikfors G., et al. (2018). Role of Shellfish Aquaculture in the Reduction of Eutrophication in an Urban Estuary. Environ. Sci. Technol. 52 (1), 173–183. doi: 10.1021/acs.est.7b03970
Buskey E. J., Montagna P. A., Amos A. F., Whitledge T. E. (1997). Disruption of Grazer Populations as a Contributing Factor to the Initiation of the Texas Brown Tide Algal Bloom. Limnol. Oceanog. 42, 1215–1222. doi: 10.4319/lo.1997.42.5_part_2.1215
Buzzelli C., Parker M., Geiger S., Wan Y., Doering P., Haunert D. (2013). Predicting System-Scale Impacts of Oyster Clearance on Phytoplankton Productivity in a Small Subtropical Estuary. Environ. Model. Assess. 18, 185–198. doi: 10.1007/s10666-012-9338-y
Carlton J. T., Ruckelshaus M. H. (1997). “Nonindigenous Marine Invertebrates and Algae,” in Strangers in Paradise. Eds. Simberloff D., Schmitz D. C., Brown T. C. (Washington, D.C: Island Press), 187–201. 467p.
Cerco C. F., Noel M. R. (2007). Can Oyster Restoration Reverse Cultural Eutrophication in Chesapeake Bay? Estuar. Coast. 30 (2), 331-343. doi: 10.1007/BF02700175
Coughlan J. (1969). The Estimation of Filtering Rate From the Clearance of Suspensions. Mar. Biol. 2 (4), 356–358. doi: 10.1007/BF00355716
DeYoe H. R., Stockwell D. A., Bidigare R. R., Latasa M., Johnson P. W., Hargraves P. E., et al. (1997). Description and Characterization of the Algal Species Aureoumbra Lagunensis Gen. Et. Sp. Nov. And Referral of Aureoumbra and Aureococcus to the Pelagophyceae. J. Phycol. 33, 1042–1048. doi: 10.1111/j.0022-3646.1997.01042.x
Draughon L. D., Scarpa J., Hartmann J. X. (2010). Are Filtration Rates for the Rough Tunicate Styela Plicata Independent of Weight or Size? J. Environ. Sci. Health - Toxic/Hazard. 45 (2), 168–176. doi: 10.1080/10934520903429816
Draughon L. D., Scarpa J., Keating P., Hartmann J. X. (2008). “Potential Estuarine Water Quality Improvement via Marine Invertebrate Bioremediation,” in Environmental Awareness and Management. Eds. Theophanides M., Theophanides T. (Athens, Greece: Athens Institute for Education and Research), 91–112. 240 pp.
Fiala-Médioni A. (1978a). Filter-Feeding Ethology of Benthic Invertebrates (Ascidians). III. Recording of Water Current in Situ — Rate and Rhythm of Pumping. Mar. Biol. 45, 185–190. doi: 10.1007/BF00390554
Fiala-Médioni A. (1978b). Filter-Feeding Ethology of Benthic Invertebrates (Ascidians). IV. Pumping Rate, Filtration Rate, Filtration Efficiency. Mar. Biol. 48, 243–249. doi: 10.1007/BF00397151
Florida Fish & Wildlife Conservation Commission (2021) Manatee Mortality Event Along the East Coast: 2020–2021. Available at: https://myfwc.com/research/manatee/rescue-mortality-response/ume/ (Accessed December 30, 2021).
Gainey L. F., Shumway S. E. (1991). The Physiological Effect of Aureococcus Anophagefferens (Brown Tide) on the Lateral Cilia of Bivalve Mollusks. Biol. Bull. 181, 298–306. doi: 10.2307/1542101
Galimany E., Lunt J., Freeman C. J., Houk J., Sauvage T., Santos L., et al. (2020). Bivalve Feeding Responses to Microalgal Bloom Species in the Indian River Lagoon: The Potential for Top-Down Control. Estuar. Coast. 43, 1519–1532. doi: 10.1007/s12237-020-00746-9
Galimany E., Lunt J., Freeman C. J., Reed S., Segura-Garcia I., Paul V. J. (2017). Feeding Behavior of Eastern Oysters Crassostrea Virginica and Hard Clams Mercenaria Mercenaria in Shallow Estuaries. Mar. Ecol. Prog. Ser. 567, 125–137. doi: 10.3354/meps12050
Galimany E., Lunt J., Freeman C. J., Segura-Garcia I., Mossop M., Domingos A., et al. (2021). Bivalve Feeding on the Brown Tide Aureoumbra Lagunensis in a Shallow Coastal Environment. Front. Mar. Sci. 8. doi: 10.3389/fmars.2021.714816
Gobler C. J., Koch F., Kang Y., Berry D. L., Tang Y. Z., Lasi M., et al. (2013). Expansion of Harmful Brown Tides Caused by the Pelagophyte, Aureoumbra Lagunensis DeYoe Et Stockwell, to the US East Coast. Harm. Algae. 27, 29–41. doi: 10.1016/j.hal.2013.04.004
Gobler C. J., Sunda W. G. (2012). Ecosystem Disruptive Algal Blooms of the Brown Tide Species, Aureococcus Anophagefferens and Aureoumbra lagunensis. Harmful Algae 14, 36–45. doi: 10.1016/j.hal.2011.10.013
Iglesias J. I. P., Urrutia M. B., Navarro E., Ibarrola I. (1998). Measuring Feeding and Absorption in Suspension-Feeding Bivalves: An Appraisal of the Biodeposition Method. J. Exp. Mar. Biol. Ecol. 219, 71–86. doi: 10.1016/S0022-0981(97)00175-5
Jacobi Y., Shenkar N., Ward J. E., Rosa M., Ramon G. Z., Shavit U., et al. (2020). Evasive Plankton: Size-Independent Particle Capture by Ascidians. Limnol. Oceanogr. 9999, 1–12. doi: 10.1002/lno.11658
Kellogg M. L., Cornwell J. C., Owens M. S., Paynter K. T. (2013). Denitrification and Nutrient Assimilation on a Restored Oyster Reef. Mar. Ecol. Press. Ser. 480, 1-19. doi: 10.3354/meps10331
Klumpp D. W. (1984). Nutritional Ecology of the Ascidian Pyura Stolonifera: Influence of Body Size, Food Quantity and Quality on Filter-Feeding, Respiration, Assimilation Efficiency and Energy Balance. Mar. Ecol. Prog. Ser. 19, 269–284. doi: 10.3354/meps019269
Lambert G. (2001). “A Global Overview of Ascidian Introductions and Their Possible Impact on the Endemic Fauna,” in The Biology of Ascidians. Eds. Sawada H., Tokosawa H., Lambert C. D. (Tokyo, Japan: Springer-Verlag), 249–257. 470p.
Lambert C. C., Lambert G. (1998). Non-Indigenous Ascidians in Southern California Harbors and Marinas. Mar. Biol. 130, 675–688. doi: 10.1007/s002270050289
Lapointe B. E., Herren L. W., Debortoli D. D., Vogel M. A. (2015). Evidence of Sewage-Driven Eutrophication and Harmful Algal Blooms in Florida’s Indian River Lagoon. Harm. Algae. 43, 82–102. doi: 10.1016/j.hal.2015.01.004
Liu H., Buskey E. J. (2000). The Exopolymer Secretions (EPS) Layer Surrounding Aureoumbra Lagunensis Cells Affects Growth, Grazing, and Behavior of Protozoa. Limnol. Oceanog. 45, 1187–1191. doi: 10.4319/lo.2000.45.5.1187
Møhlenberg F., Riisgård H. U. (1978). Efficiency of Particle Retention in 13 Species of Suspension Feeding Bivalves. Ophelia 17 (2), 239–246. doi: 10.1080/00785326.1978.10425487
MacGinitie G. E. (1939). The Method of Feeding of Tunicates. Biol. Bull. 77 (3), 443–447. doi: 10.2307/1537654
Makris P. (2016). Effects of Harmful Algal Blooms Caused by Aureoumbra Lagunensis (Brown Tide) on Larval and Juvenile Life Stages of the Eastern Oyster (Crassostrea Virginica). Department of Biological Sciences, University of Central Florida, Orlanda, FL, United States: Electronic Theses and Dissertations 2004-2019, 5319.
Petersen J. K. (2007). Ascidian Suspension Feeding. J. Exp. Mar. Biol. Ecol. 342, 127–137. doi: 10.1016/j.jembe.2006.10.023
Petersen J. K., Mayer S., Knudsen M.Å. (1999). Beat Frequency of Cilia in the Branchial Basket of the Ascidian Ciona Intestinalis in Relation to Temperature and Algal Cell Concentration. Mar. Biol. 133, 185–192. doi: 10.1007/s002270050457
Phlips E. J., Badylak S., Nelson N. (2019). “Harmful Algal Blooms in the Indian River Lagoon, North,” in Annual Report to the Indian River Lagoon National Estuarine Program, Contract 2018-03 (Sebastian, Florida).
Phlips E. J., Badylak S., Nelson N. G., Hall L. M., Jacoby C. A., Lasi M. A., et al. (2021). Cyclical Patterns and a Regime Shift in the Character of Phytoplankton Blooms in a Restricted Sub-Tropical Lagoon, Indian River Lagoon, Florida, United States. Front. Mar. Sci. 8. doi: 10.3389/fmars.2021.730934
Pineda M. C., Lopez-Legentil S., Turon X. (2011). The Whereabouts of an Ancient Wanderer: Global Phylogeography of the Solitary Ascidian Styela Plicata. PloS One 6 (9), 1–14. doi: 10.1371/journal.pone.0025495
Pineda M. C., Lopez-Legentil S., Turon X. (2013). Year-Round Reproduction in a Seasonal Sea: Biological Cycle of the Introduced Ascidian Styela Plicata in the Western Mediterranean. Mar. Biol. 160, 221–230. doi: 10.1007/s00227-012-2082-7
Randløv A., Riisgård H. U. (1979). Efficiency of Particle Retention and Filtration Rate in Four Species of Ascidians. Mar. Ecol. Prog. Ser. 1, 55–59. doi: 10.3354/meps001055
Ribes M., Coma R., Gili J. M. (1998). Seasonal Variation of in Situ Feeding Rates by the Temperate Ascidian Halocynthia Papillosa. Mar. Ecol. Prog. Ser. 175, 210–213. doi: 10.3354/meps175201
Robbins I. J. (1983). The Effects of Body Size, Temperature, and Suspension Density on the Filtration and Ingestion of Inorganic Particulate Suspensions by Ascidians. J. Exp. Mar. Biol. Ecol. 70, 65–78. doi: 10.1016/0022-0981(83)90149-1
Robbins H. M., Bricelj M. V., Ward J. E. (2010). In Vivo Effects of Brown Tide on the Feeding Function of the Gill of the Northern Quahog Mercenaria Mercenaria. Biol. Bull. 219, 61–71. doi: 10.1086/BBLv219n1p61
Rosa M., Ward J. E., Holohan B. A., Shumway S. E., Wikfors G. H. (2017). Physicochemical Surface Properties of Microalgae and Their Combined Effects on Particle Selection by Suspension-Feeding Bivalve Molluscs. J. Exp. Mar. Biol. Ecol. 486, 59–68. doi: 10.1016/j.jembe.2016.09.007
Stuart V., Klumpp D. W. (1984). Evidence for Food-Resource Partitioning by Kelp-Bed Filter Feeders. Mar. Ecol. Prog. Ser. 16, 27–37. doi: 10.3354/meps016027
Sumerel A. N., Finelli C. M. (2014). Particle Size, Flow Speed, and Body Size Interactions Determine Feeding Rates of a Solitary Ascidian Styela Plicata: A Flume Experiment. Mar. Ecol. Prog. Ser. 495, 193–204. doi: 10.3354/meps10571
Keywords: Indian River Lagoon, estuary, brown tide, harmful algal blooms, top-down control, filtration rate, suspension feeding, ascidian (Styela plicata)
Citation: Klarmann PA, Scarpa J and Hartmann JX (2022) Filtration Rate of the Solitary, Pleated Tunicate Styela plicata on the Brown Tide-Forming Pelagophytes Aureoumbra lagunensis and Aureococcus anophagefferens. Front. Mar. Sci. 9:866177. doi: 10.3389/fmars.2022.866177
Received: 30 January 2022; Accepted: 19 April 2022;
Published: 29 August 2022.
Edited by:
Kevin Brett Johnson, Florida Institute of Technology, United StatesReviewed by:
Xiao Ma, Chinese Academy of Sciences (CAS), ChinaHolly Sweat, Smithsonian Marine Station (SMS), United States
Copyright © 2022 Klarmann, Scarpa and Hartmann. This is an open-access article distributed under the terms of the Creative Commons Attribution License (CC BY). The use, distribution or reproduction in other forums is permitted, provided the original author(s) and the copyright owner(s) are credited and that the original publication in this journal is cited, in accordance with accepted academic practice. No use, distribution or reproduction is permitted which does not comply with these terms.
*Correspondence: Phyllis A. Klarmann, cGtsYXJtYW5Ac2Z3bWQuZ292