- 1Key Laboratory of Experimental Marine Biology, Institute of Oceanology, Chinese Academy of Sciences, Qingdao, China
- 2Laboratory for Marine Fisheries Science and Food Production Processes, Pilot National Laboratory for Marine Science and Technology, Qingdao, China
- 3National and Local Joint Engineering Laboratory of Ecological Mariculture, Qingdao, China
Worldwide, the bivalve aquaculture industry has realized or recognized the potential gains from selective breeding programs using phenotypic and pedigree data. Yesso scallop Patinopecten yessoensis are among the most important commercial shellfish in China. A family-based breeding program to investigate the genetic variations for growth and survival-related traits at suspended and bottom environments of sea-based culture systems was reported in this study. We proposed and conducted a novel phenotyping technique to longitudinally evaluate the shell heights in the yesso scallop. At harvest after rearing for 20 months, the individual shell heights at 6, 10, 16, and 20 months were simultaneously obtained by the growth rings in the outer shells of the animals. Meanwhile, the body weight (BW) of the survived individuals at harvest was also recorded. Variance components and genetic parameters for growth and survival-related traits were estimated using an animal and threshold model, respectively. In the suspended environment, the heritability estimates for BW and shell heights at specific ages ranged from moderate to high (0.328–0.853). The estimated correlations between shell heights at contiguous ages were consistently high, ranging from 0.890 to 0.958 but decreased with increasing intervals between ages (0.496–0.828). The estimated correlations between shell heights at contiguous ages and BW at harvest were similar, ranging from 0.535 to 0.983. The heritability estimates for individual survival at harvest were at a low level of 0.128 by the probit-threshold model. While at the bottom environment, estimates of heritability for growth and survival-related traits were similar but slightly lower than those at the suspended environment. Furthermore, the genetic correlation for BW between the two environments was very small, which probably indicates genotype-by-environment interaction effects for growth in the yesso scallop. The study can provide prior information, which might develop a new idea for selection in this species. The results are discussed concerning selection work with yesso scallop, and solutions for accurate estimation of genetic parameters and increasing genetic gain are also outlined.
Introduction
Selective breeding, the benefits of which can be cumulative and permanent, is an attractive approach in genetic improvement in aquaculture species (Gjedrem, 2010). Estimates of genetic gain per generation for economically important traits such as growth rate are reported high of average 13%, as demonstrated for major farmed species like Atlantic salmon and Nile tilapia (Gjedrem, 2012). Although the molecular breeding method was reported in animals in the genomics era, the best linear unbiased prediction method traditionally based on pedigree records and phenotypic data still comprises one of the main approaches in selective breeding programs so far, especially in aquaculture species (Boudry et al., 2022). The prerequisite of the traditional family-based selection is the accurate estimates of the genetic parameters including heritability and genetic correlations for the target traits. Factually, the genetic parameter estimation is vital to breeders to make an effective breeding plan, predict the genetic gains, and conduct a cost-benefit analysis in the program. In molluscan species especially in bivalves, the genetic parameter estimations were relatively widely reported in oysters, scallops, clams, and mussels (Alcapán et al., 2007; de Melo et al., 2016; Barros et al., 2018; Tan et al., 2020).
Scallops are one of the most ecologically and economically important bivalve groups in the world (Shumway and Parsons, 2006). They are a high-value aquaculture group, with an annual farmed yield of about 2 million metric tons accounting for more than 10% of aquaculture production for the major molluscan species groups in 2018 worldwide (Food and Agriculture Organisation [FAO], 2020). Yesso or Japanese scallop, Patinopecten yessoensis, was introduced from Japan to Dalian areas, China in the 1980s (Liu, 1983). The aquaculture of yesso scallops has been growing rapidly since its introduction because of the larger sizes and higher market prices compared with the native scallop species in China. It has become a major aquaculture industry in northern China, mostly in Liaoning and northern Shandong Province. Two main culture forms in sea-based culture were suitable for yesso scallop farming in China. Lantern nets on suspended longlines, the dominant form of grow-out for native scallops, are also implemented for yesso scallop culture. The scallops can also be released for on-bottom culture without any protective gear. During the past decade, the on-bottom culture of yesso scallops has gained popularity and greatly expanded in China. Although several studies were conducted to compare the merits and demerits between the two culture modes in yesso scallop, there remains controversy in which mode is more productive in China.
However, in recent years, outbreaks of mass mortality and slow growth rate are major threats to sustainable production and future expansion of yesso scallop aquaculture since 2007 in China (Ren et al., 2013). Mortalities of farmed stocks in this species have continued to 2014, however, no specific pathogens have been found associated with the mortalities. Although several selective breeding programs were initiated by varying organizations or companies in China (Wang et al., 2014), it seems that no sufficient data of genetic evaluations were reported on target traits in this species.
Here, we present estimates of heritability for shell heights, BW, and survival after growing full-sib families under two grow-out conditions (environments). We also try to evaluate the genotype by environment interaction effects for BW between different environments. This study would provide a theoretical and practical context for developing selective breeding programs for yesso scallop farming in China.
Materials and Methods
Population, Mating, and Production of Families
The materials used in the study were from a scallop hatchery, Zoneco Group Co., Ltd., China. The base population of the selection experiments was established by two yesso scallop stocks, A and B. According to the hatchery records from Zoneco Company, stock A was introduced from Aomori, Japan in 1982. After breeding for 14 generations every 2 years since introduction, individuals from stock A were randomly chosen as broodstock. While, stock B was produced for 2 generations after being introduced in 2009, recently. Scallop stocks A and B are both commercially cultured using suspended longline in Changhai County, Dalian, China before being selected as broodstock in the study. To our knowledge, no experimental or field trials were conducted to evaluate the production performance or features between the two stocks previously. We used the two stocks as the base population for broadening the genetic base and detecting possible heterosis for the target traits in the study. Full- and half-sib families were produced by a 2 × 2 factorial mating design, in which two dams were mated to the same sire, and two sires were mated to the same dam. After fertilization and hatching, successfully established full-sibs were carefully separately in each 100 L tank.
Fertilized eggs, embryos, and larvae were reared following the procedures described by Wang and Wang (1993). The D-shaped and umbo larvae in each full-sib were cultured in appropriate 15°C seawater with aeration in succession. The density of larvae and the concentrations of feeds were maintained and adjusted to a suitable level to keep the larvae growing comfortably. In brief, the density of larvae in the tanks was maintained at 8 larvae/ml and reduced to 1–2 larvae/ml with the development of the larvae to metamorphosis by adjusting the water volume. About 30 days after fertilization, the collectors adhered to juvenile scallops were transferred to the field in the sea from nursery to grow-out stage. As the animals developed in the nursery stage, stocking densities in each full-sibs were adjusted for consistent rearing environmental conditions. Density adjustment was made in all families three times in the nursery stage. This practice is essential to adjust for possible bias resulting from environmental differences in the subsequent genetic evaluation. Finally, only 43 full-sibs with sufficient sample numbers were established when juvenile scallops with shell heights of ∼3 cm got to the grow-out stage. The numbers of mating pairs, sires, and dams used to generate the full families are presented in Table 1.
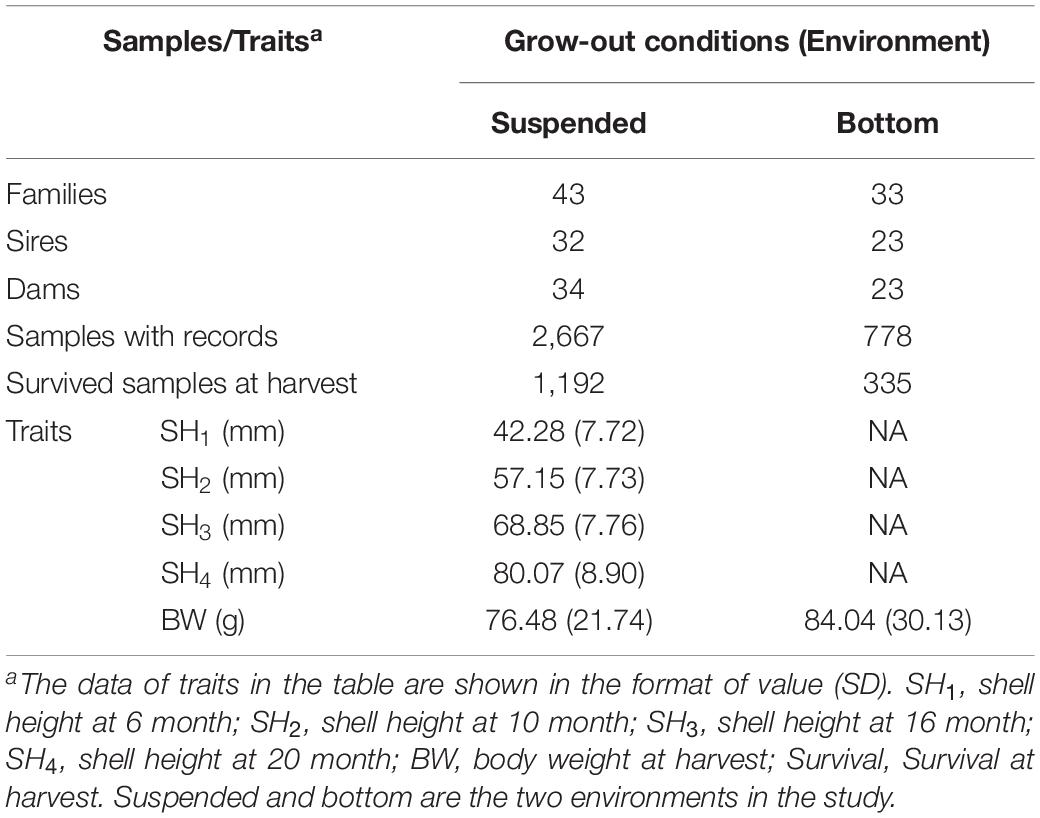
Table 1. Summary statistics of families, sample number, and phenotypic values of traits in yesso scallops in the study.
Growth and Survival Tests at Suspended and Bottom Environments
Scallops with a shell height of ∼2.5 cm were tagged with digital numbered tags tied by a hole drilled in the ear part of the animal (Katherine et al., 2001). After tagging and acclimating for no mortality occurred, the scallops in each of the full-sib families were transferred to two sites closely located in Zhangzi Island of the North Yellow Sea, where yesso scallop is widely cultured in China, for adult growth periods (Guo and Luo, 2016). The lantern net suspension in longlines culture mode in one site and an on-bottom culture mode in the other site were utilized as two environments to measure the growth and survival performance of the full-sibs at the grow-out stage were separately conducted.
At the suspended environment, a total of 2,667 randomly selected individuals from the 43 full-sib families were placed in 13 lantern nets with the same density (20 scallops were placed in each layer of the lantern nets) and placed in the same natural water to minimize the effects of culture conditions. While, at the on-bottom environment, we placed netting cages in the bottom of the sea on the other site in this study. Compared with the routine way in yesso scallop on-bottom culture in open areas, this is to guarantee the recapture of the experimental animals at harvest. The netting cage consisted of a 2 m × 1 m × 0.45 m (L × W × D) rectangular frame made of rebar (ϕ = 1 cm), we placed two nets around each cage with a mesh size of 2 cm for holding scallops. In this study, seven netting cages as scallop bottom culture equipment were anchored into the seabed at a water depth of 20 m. However, during the culture at the bottom environment, one netting cage was crashed by rocks and destroyed, while two netting cages occurred predators of starfish (Asterias amurensis), nearly all the scallops totally occurred mortality. Therefore, a total of only 780 scallop samples from the 33 full-sib families in four cages were included in data collection at the bottom culture environment.
At harvest after grow-out in the suspended environment, the numbers of live and dead scallops at each site for each full-sib family were counted to obtain the survival data (0/1). Final sizes (the shell heights SH4) and weights of the scallops (BW) were determined by measuring all the survived individuals at the site. Besides, the three number of growth rings present in our survived samples allowed recognition of shell height growth chronologies (Figure 1). With the help of the growth rings which we inferred occurrence at the age of 6, 10, and 16 months, we finally obtained the data of SH1, SH2, and SH3 together with SH4, simultaneously (Figure 1). Scallop shell heights were defined as the maximum distance from the hinge line to the growth rings or shell edges and measured using electronic vernier calipers to an accuracy of 0.01 mm. BW was recorded using an electronic balance to an accuracy of 0.01 g. To guarantee the accuracy of shell height data obtained by the new method, preliminary measurements were conducted during the culture at 6 months. Measurements were carried out to obtain the shell heights of 100 randomly chosen scallops at 6 months, which are seen as “true values.” While at harvest, shell height data indicated by the growth ring at 6 months were recorded again to the survived animals from the 100 randomly chosen scallops. The means of shell heights obtained at harvest and “true values” were compared using Tukey’s test (Zar, 1984). Additionally, Tukey’s test revealed no significant difference between data recorded by the growth ring method and “true values” (P > 0.05). It indicated that the accuracy of shell height data obtained by the growth ring method was guaranteed.
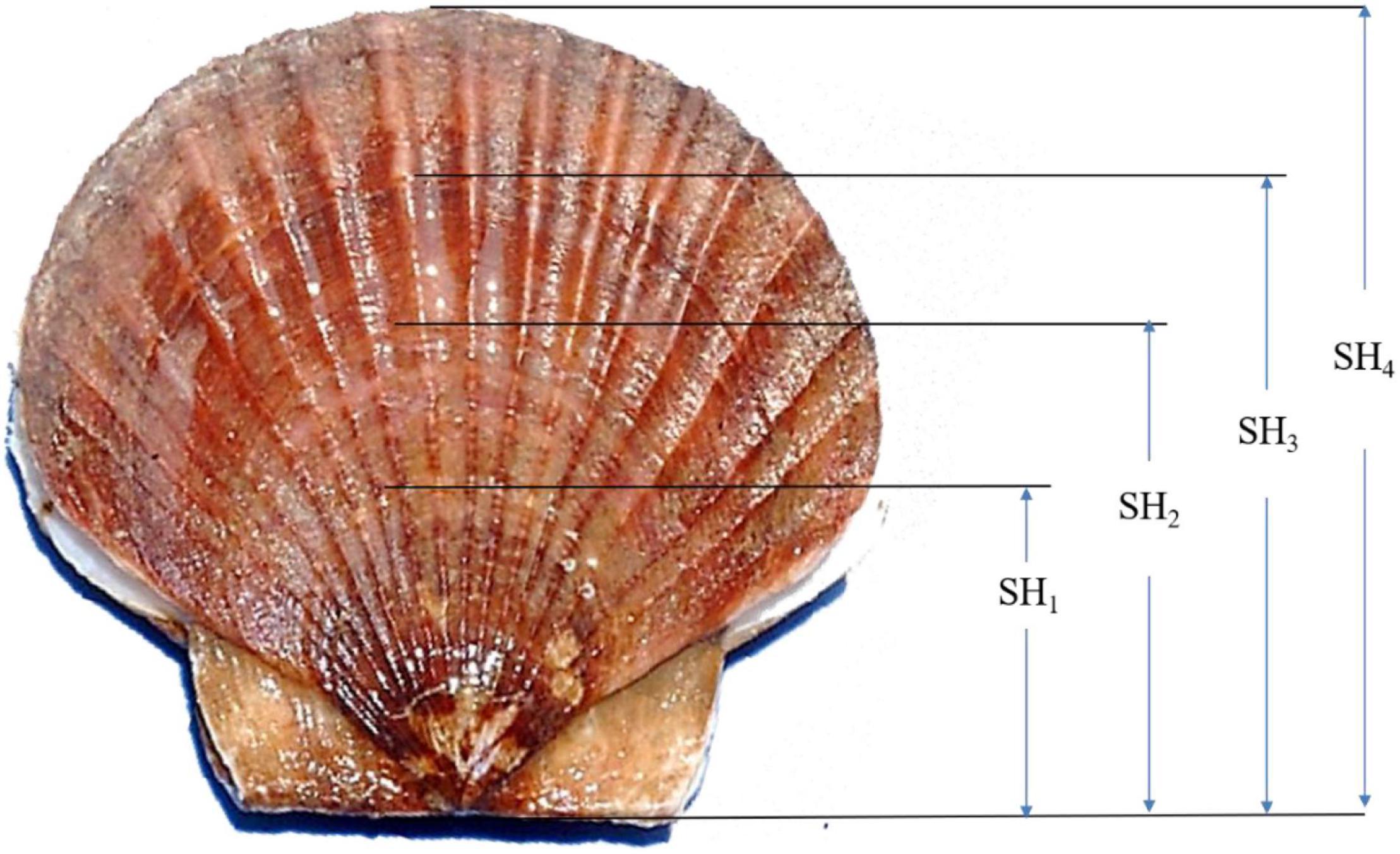
Figure 1. Shell height growth was indicated by the rings in the outer shells of the yesso scallop in this study. SH1, shell height at 6 months; SH2, shell height at 10 months; SH3, shell height at 16 months; SH4, shell height at 20 months.
While, at harvest after grow-out at the bottom environment, all the number of live scallops in only four cages were collected and counted to obtain the survival data. The final individual BW of the scallops (BW) was determined by measuring all the survived individuals at the site. Gender information including male, female, or undistinguished of all survived samples at the two culture conditions was also recorded at harvest.
Data Analysis
Genetic analyses for growth-related traits including SH1, SH2, SH3, SH4, and BW in the suspended culture condition were performed using a multivariate animal model as follows:
where y is the vector of phenotypes of each trait; β is the vector of fixed effects, including overall mean, crosses (AA, AB, BA, or BB), and sex (male, female, or undistinguished); a is the vector of random additive genetic effects, following a normal distribution of N(0, A), where σ2a is the additive genetic variance and A is the numerator relationship matrix based on pedigree information; c is the vector of common effects to full-sibs with the distribution of N(0, I), where is the variance of common effects to full-sibs and I is the identity matrix; e is the vector of random residuals with the distribution of N(0, I), where is the residual variance; X, W, and Z1 are incidence matrices linking β, c, and a to y, respectively. Ward-F-tests were conducted to detect the significance of the fixed effects, and the fixed effects should be removed if no significance was detected (P < 0.05). If the multivariate genetic analyses including all the traits cannot achieve convergence by the restricted maximum likelihood (REML) method, bivariate genetic analyses between any of two traits using an animal model were carried out.
Genetic analyses for growth-related traits including BW at the suspended and bottom environments were using a bivariate animal model to detect the genotype by environment interaction indicated by genetic correlation levels as Formula (1). Traits of BW at the two culture environments were seen as different variates as shown in G by E analysis proposed by Falconer and Mackay (1996).
Survival traits at each of the two environments were treated as a binary trait since survived and dead samples at harvest were assigned values of 1 and 0, respectively. Genetic analyses for survival traits in each of the two environments were using a threshold logit model to estimate the genetic components and narrow-sense heritability, respectively.
The assumed single-trait model for the underlying distribution of the liability (If) for analysis of each binary trait was
where β is a vector of fixed effects as displayed in Formula (1); s and d is the vector of sire and dam genetic effects; e is a vector of residual effects, and X, Z2 , and Z3 are incidence matrices that link fixed and genetic effects to liabilities, respectively. The response in Formula (2) was modeled with a probit link function approach, thus, the = .
Heritabilities (h2) in the animal model for growth-related traits including scallop SH and BW were calculated as , while heritabilities (h2) in the sire-dam model for scallop survival-related traits using probit-link function were calculated .
The multivariate or bivariate animal models were used to estimate the genetic and phenotypic correlation between growth-related traits in suspended culture conditions, and between BW traits in the two culture conditions. The genetic correlation (rg) formula is:
where σa12 is the genetic covariance between trait 1 and trait 2, and are the additive genetic variance of traits 1 and 2, respectively. The phenotypic correlation (rp) formula is:
where σe12 is the residual covariance between trait 1 and trait 2, and are the residual variance of traits 1 and 2, respectively.
Results
Phenotype Statistics for Scallop Growth and Survival at Harvest
After 20-month rearing at the two environments in the sea-based culture system, the total number of survived scallops was 1,192 at the suspended environment, accounting for the survival rate of 44.69%. While, the total number of surviving scallops in the remaining four netting cages at the bottom environment was 335, accounting for the survival rate of 43.06%. The average BW of the yesso scallop was 76.48 g at the suspended environment and 84.04 g at the bottom environment, respectively. At harvest, according to the growth rings in the outer shells of yesso scallops, the shell heights at varying ages were also shown in Table 1. The mean and SD for shell heights increased while the coefficient of variation (proportion of SD to mean) decreased from 18 to 11% with advancing age.
At the family level, there were large variations in the average BW of yesso scallop cultured at both environments, respectively. Additionally, it seemed that large difference in average BW between the same families in the two environments (Figure 2). The phenotypic variations were similar in terms of survival rates of the animals at the suspended and bottom environments at the family level (Figure 2).
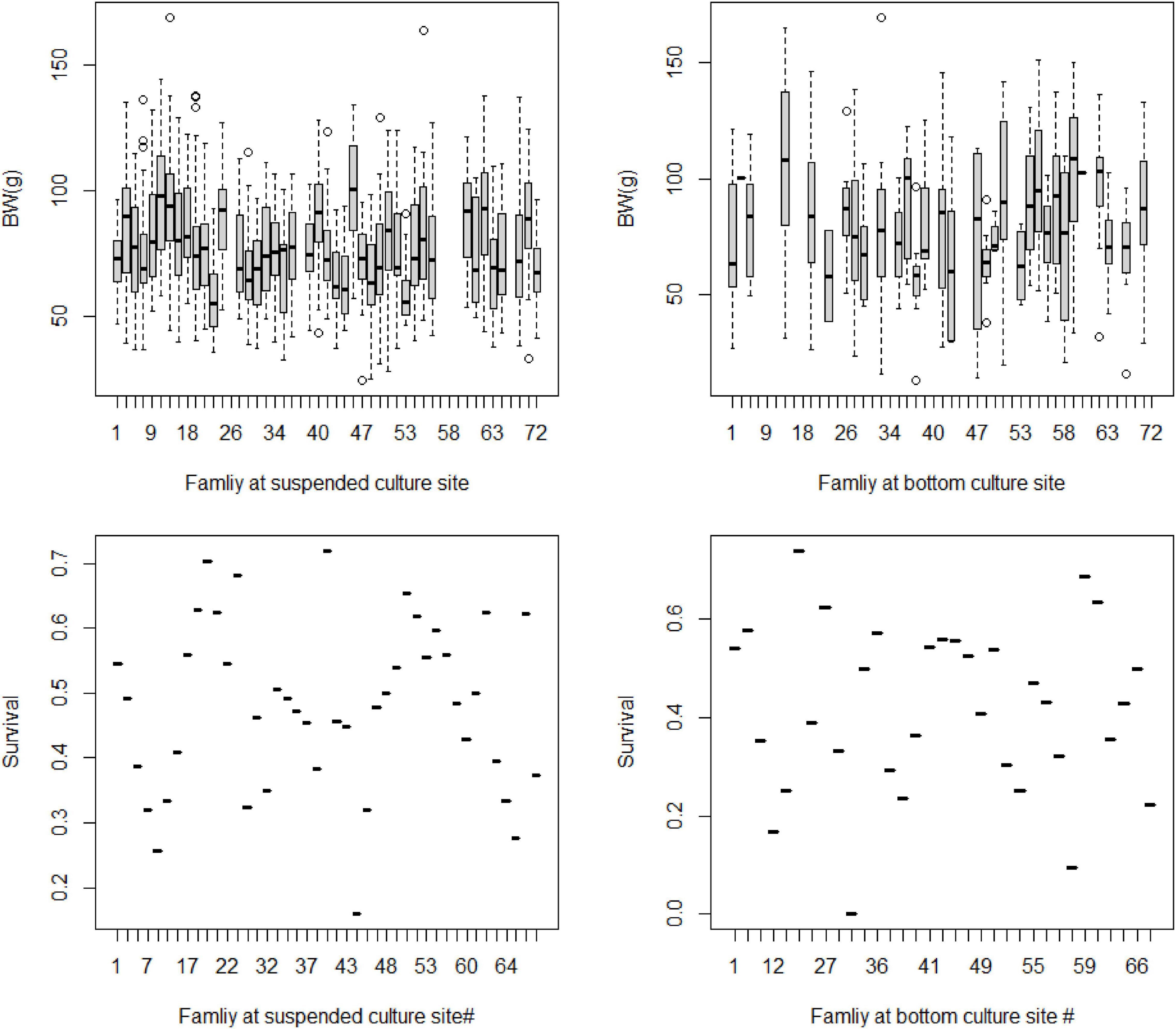
Figure 2. Body weight (BW, g) and survival rate (Survival, × 100%) of yesso scallop full-sib families at suspended and bottom culture environments.
Genetic Parameter Estimates for Scallop Growth and Survival at Suspended and Bottom Environments
In the study, a cross between two populations, A and B, and scallop sex at harvest were treated as fixed effects in REML analysis. Wald-F statistical analysis was conducted and showed that these two factors had no significant effect on scallop growth and survival at harvest (P > 0.05). Therefore, the two factors were removed in the subsequent analysis using REML in the mixed linear model.
Estimated variance components from multivariate models including SH1, SH2, SH3, SH4, and BW traits could not obtain a converged result by the REML method. Therefore, we re-analyzed the longitudinal data of SH1, SH2, SH3, and SH4 from a multivariate model to get a reliable estimation of narrow-sense heritability and genetic correlation between any two of the traits. For the genetic correlation analysis between each one of the shell heights at varying ages and BW at harvest, a bivariate model using REML was conducted. Since the common effects to full-sib family indicated by the Z-ratio in components were very small, we removed it in the model, and therefore, only additive and residue effects were treated as random effects in the mixed linear model.
At the suspended environment, the estimated heritability of scallop shell heights at varying ages was of the medium to the high level, ranging from 0.410 at 20 months to 0.853 at 6 months as shown in Table 2. Genetic correlations between SH from 6 to 20 months were significantly different from zero (Table 2, above the diagonal). Genetic correlations between contiguous ages were high ranging from 0.496 between 6 and 20 months to 0.958 between 6 and 10 months. As the length of the interval increased, genetic correlations tended to decrease. At harvest, the estimated heritability of scallop BW was of medium level (0.328, Table 2), while the estimated heritability of scallop survival was of low level (0.128, Table 2). Genetic correlations between BW at harvest to shell height at varying ages were also significantly different from zero (Table 2, above the diagonal). The genetic correlations between BW and shell height at varying ages were high, ranging from 0.535 between shell height at 6 months and BW at harvest to 0.983 between shell height at 20 months and BW at harvest. Similarly, as the length of the interval increased, genetic correlations between BW at harvest to shell height tended to decrease. The genetic correlation between BW and survival at harvest was of a low level (0.398) and was not significantly different from zero.
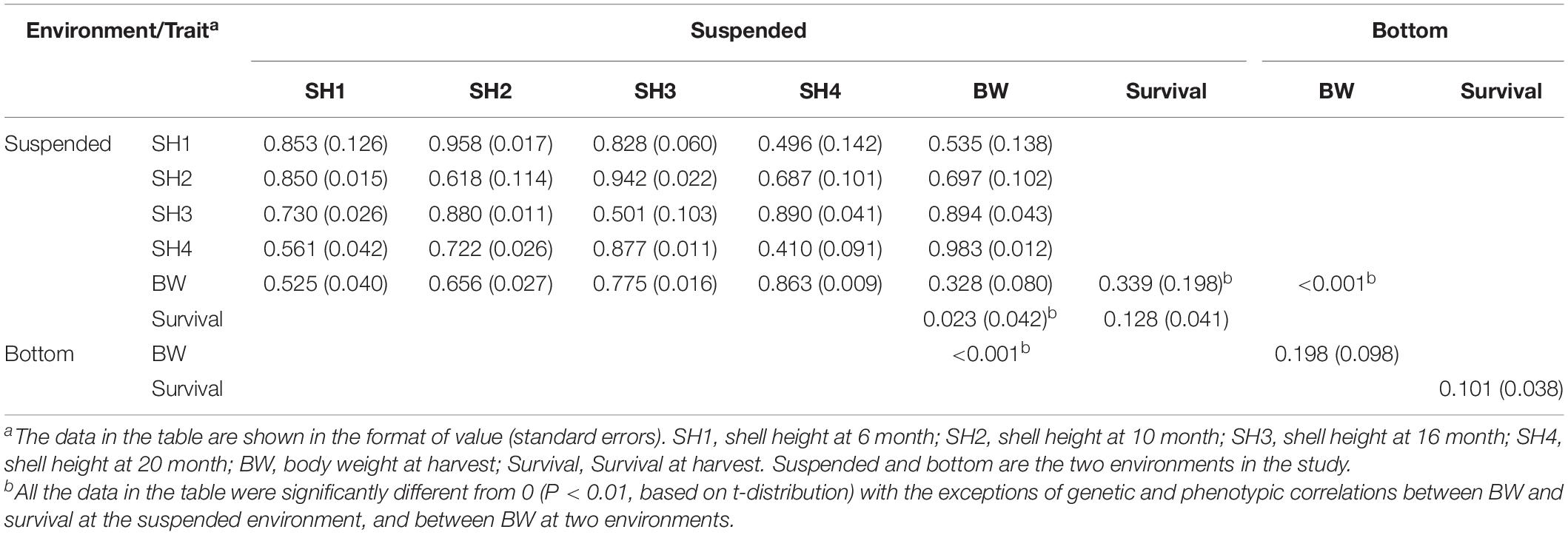
Table 2. Estimates of heritabilities (the diagonal), genetic correlation (above the diagonal), and phenotypic correlation (below the diagonal) between varying traits in yesso scallop at two environments.
At the bottom culture environment, the estimated heritability of scallop BW was 0.198 (Table 2), while the estimated heritability of scallop survival was of low level (0.101, Table 2). Both estimates were significantly different from zero, however, lower than those at the suspended culture environment.
G × E interaction in terms of scallop BW at two culture environments was indicated by the estimate of genetic correlation for BW between the environments. The genetic correlation for BW between the environments was very small (<0.001, Table 2), which indicates a considerable genotype by environment interaction for growth in yesso scallop.
Discussion
Because of their high economic importance, genetic improvement in scallop species is continuously attracting attention in recent years (Zheng et al., 2004; Wang et al., 2017, 2018; Tan et al., 2020). Heritability reflects the genetic ability of traits, which is an important parameter for selective breeding (Song et al., 2022). In this study, animal or individual models by the REML method were used to estimate narrow-sense heritability for the growth-related traits of shell heights and BW, and the results showed that the heritability was at a moderate to a high level in yesso scallop in sea-based aquaculture system. Similar results were obtained by Barros et al. (2018), in the Caribbean scallop, Argopecten nucleus, with estimated heritability larger than 0.26 from growth traits. Wang et al. (2018) also reported that the single-nucleotide polymorphism-based heritability for shell length, shell height, shell width, and whole weight in Zhikong scallop, Chlamys farreri, was of high level, from 0.28 to 0.54, respectively. The moderate to high heritability for shell height, BW in this study indicates that the potential for genetic improvement of these traits is large, and it is necessary to perform selective breeding in yesso scallop in the environment of a sea-based aquaculture system. While the estimated heritabilities for survival trait in yesso scallop after rearing for 20 months were relatively low at both environments (suspended and bottom conditions). Generally, the additive genetic variation for survival or stress tolerance-related trait is reported low in aquaculture species, such as shrimp (Li et al., 2015) and oyster (de Melo et al., 2016). It might indicate the large confounded non-additive effects including environmental effects for survival-related traits and the difficulty to obtain considerable gains through selective breeding for survival traits in yesso scallop. Furthermore, at the bottom environment, estimates of heritability for growth and survival-related traits were similar but slightly lower than those at the suspended environment. Comparatively, the bottom environment seems to be harsher to scallops indicated by the destroyed cages and predating during the culture period than the suspended environment. The higher environmental variations at the bottom environment for the target traits probably might be the reason for the lower level of estimated heritability than that at the suspended environment.
A narrow genetic base or limited additive genetic variance of the foundation population will lead to a fast accumulation of inbreeding at a high level and decrease the genetic gain during selection. In this study, we used two stocks A and B as broodstock for the sake of broadening the genetic base. Intraspecies and interspecies crossbreeding were reported to be an effective means to improve the growth and survival traits in aquaculture species, such as salmon (Bryden et al., 2004), prawn (Thanh et al., 2010), oyster (Hedgecock and Davis, 2007), and scallop (Xing et al., 2022). However, the Wald-F-test from the mixed linear model showed that the crossing effects by the two stocks (A and B) were not significant for all the traits. This is maybe due, at least in part, to low genetic variation in the introduced yesso scallop stocks of A and B. Although there existed a difference in domesticated generations after being introduced into China, the lack of genetic variation due to small effective population size and poor genetic management after introduction might be responsible for the results.
Common environmental effects to full-sibs were reported important in a family-based selective breeding program (Gjedrem et al., 2012). Estimation of variance components including the additive variance and common environmental variance was vital to the selection accuracy for target traits. However, in this study, common environmental variances in both environments (suspended and bottom conditions) were estimated very small, which is different from published studies (Gjerde et al., 2004; Luan et al., 2012). This is maybe due to the absence of genetic ties between half-sib families from different sires (Table 1). In this study, there were 73 full-sib scallop families before metamorphosis, however, about 30 families were culled for parallel environmental control. Since no sufficient samples were partitioned from the same full-sib, this is the reason that the inconsistent number of families were used in the two environments. The lack or loss of genetic ties between families may have contributed to the fact that the common environmental effects for BW and survival could not be partitioned effectively in this study.
In the genetic breeding program in animals, growth and developmental traits at a specific age (in the day, month, or year), such as the time to market are the main targets of selection improvement. As such, genetic evaluation of the growth and developmental process can increase the efficiency of selection compared to that of traits measured at specific ages (Schaeffer, 2004; He et al., 2017). However, the repeated records of growth or body size on an individual in aquaculture animals are apparently a labor and time-cost task in genetic evaluation (Hollenbeck and Johnston, 2018). Besides, repeated measurements at specific ages can often cause stress to farmed animals, especially aquaculture animals (Boudry et al., 2022). In this study, we put forward and conducted a longitudinal evaluation of shell heights at harvest, obtaining four shell height data by the growth rings in the outer shells of the yesso scallop. The characteristics of the growth cycle of the yesso scallop were reported per the water temperature (Wang et al., 1986; Wang and Wang, 1993). It is reported that the suitable temperature range for yesso scallops is 5–20°C, and about 15°C is the most suitable growth temperature in China. The upper and lower limits of the water temperature are 23 and 0°C, respectively (Wang et al., 1986). Thereafter, two rapid growth periods for yesso scallop in northern China usually occur in late spring and early winter. Additionally, shell rings are usually shaped in a suspended culture environment when the animals stop growth during the high water temperature period (>23°C) in late August and low-temperature period (<0°C) in February (Wang et al., 1986). In this study, we conducted spawning of the broodstock at the hatchery in February and reared the animals for 20 months. Therefore, a total of three growth rings corresponds to the time when the scallop stopped growing. It is clear that the points of the growth rings are determined to be 6, 10, and 16 months, respectively, in the study. In fact, the fine periodic growth patterns on shell surfaces have been widely used for studies in the ecology and evolution of scallops (Xing et al., 2017). Due to seasonal changes in the physical and chemical properties of seawater in the environment, the rates of secretion and deposition of shell materials are varied, resulting in a periodic appearance of growth patterns on shells with different densities in the internal structure and chemical elements (Michio and Hiromichi, 2013). Apparently, the growth rings in the outer shells of the yesso scallop cannot change with time, which guarantees the accuracy of shell height data recorded by the growth ring method at harvest in this study.
There are merits and demerits of the shell growth ring method for longitudinally scallop shell height recording in this study. First, one merit of the method was easy to conduct and labor and time cost-effective. Furthermore, compared with the traditional measurements at each of the specific ages on scallops, many of which might occur mortality subsequently, the data collected at harvest were from the survived animals and more valuable. Finally, with the help of shell rings or other shell markers, more repeated records of shell growth data can be obtained by elaborately recording such as the computed tomography (CT) approach (Xing et al., 2017). In this situation, recent developments in mixed model theory and advances in computer software now allow for the implementation of random effects in the statistical model and the modeling of the covariance structure of the data (König et al., 2006), e.g., random regression model reported in aquaculture animals (He et al., 2017). However, the shortcoming of the method might be that heritability for shell height at an earlier age was overestimated (e.g., h2 ∼ 0.853 for SH1 at 6 months). This estimate is expected to be biased upward due to possible effects that have not partitioned from additive genetic effect (Eknath et al., 2007). The possible effects might be the maternal or common environmental effect which is more important in the early days after fertilization.
Good environmental control is the key to the design of all selective breeding experiments. Selection is effective in non-stress environments because they are reproducible; selection is also successful in reproducible stress environments but not irreproducible ones (Hufstetler et al., 2007). According to the theory of quantitative genetics, heritability is relative and varies with the environment. In addition, when the environmental variance is large, it will increase the difficulty of estimating the genetic variance. Estimates of heritability depend on the environment, which is variable over time and space. The goal of environmental control is to minimize environmental variation and maximize the ratio of genetic variation in phenotypic variation, thereby increasing the accuracy of breeding selection and improving genetic gain. For marine mollusks including the yesso scallop, it is very hard to conduct good environmental control, especially in a sea-based culture system. The survival results in this study (∼ 40% at harvest) also indicated the difficult task to conduct good environmental control in a scallop selection program. Environmental control will likely be an important area of research in scallop selective breeding programs in the future.
In this study, a very low level of genetic correlation between scallop BW at the two environments (suspended vs. bottom) indicated the occurrence of G × E interaction for BW trait. Low genetic correlation between environments indicates significant G × E interaction according to the study of Falconer and Mackay (1996). It means that the potential different genes are responsible for the phenotypic variation of a target trait in different environments. According to Robertson (1959) and Sae-Lim et al. (2016), the genetic correlation between environments less than 0.8 indicates the occurrence of G × E interaction in aquaculture animals. Our results indicate that selecting yesso scallop individuals of greater genetic merit evaluated at suspended sea-based culture environment may reduce the accuracy of selection when transferred to the animals reared at the bottom-based environment. However, Sae-Lim et al. (2016) suggested at least 100 families per environment are required for target traits to G × E interaction study in aquaculture. In this study, only a limited number of 33–43 full-sib families were assessed in the two environments (suspended vs. bottom) for G × E interaction evaluation. It seems that no sufficient genetic ties existed between the environments. This is might be one of the reasons for the low level (<0.01) of genetic correlation for the scallop growth between the two environments analyzed by the multitrait mixed model. Compared with the reported high levels of genetic correlation (>0.8) for growth at varying environments in aquaculture (refer to the review by Sae-Lim et al., 2016), the estimate in this study apparently biased downward. More data especially more full-sib families should be provided to get a more reliable G × E interaction evaluation in the future. Furthermore, the sample size in a specific full-sib was also reported as important to heritability and genetic correlation estimates, especially for survival traits in marine mollusks with vary large phenotypic variation within full-sib families (Kube et al., 2007). The differences in the survival rate among different families were shown in Figure 2. Large variation in terms of survival rate among different families within and between the environments was detected. However, we have not displayed the genetic correlation estimates for the scallop survival traits between the environments. Owing to the insufficient samples to each full-sib per environment with the only average number of 23–62 individuals per full-sibs, and the low level of heritability estimates for survival traits at both environments, it is reasonable that the estimate of G × E interaction effects analyzed by the multitrait mixed model will be of very low confidence.
Genomic selection has been found to outperform traditional selection in bivalve species, however, one of the major limitations of genomic selection is the cost, especially genotyping a large number of animals (Hollenbeck and Johnston, 2018). A family-based selection program is still an important approach in yesso scallop. Estimates of genetic parameters including heritability and genetic correlation for growth and survival traits are still important in the genomic selection era such as they can provide prior information to scallop selection program.
Conclusion
We found a considerable level of additive genetic variation for shell height and BW measured in yesso scallop at suspended and bottom sea-based culture environments, which indicated that the traits will show a favorable response to selection in this species. A longitudinal evaluation of shell heights was carried out and proved to be feasible in yesso scallop genetic evaluation. The G × E interaction effects between BW measured in suspended vs. bottom environments will decrease the accuracy of selection for growth in bottom culture, the major culture mode in China. In addition, good environmental control can be used to improve heritability and estimated breeding value accuracy and accelerate the rate of genetic gain in the yesso scallop.
Data Availability Statement
The original contributions presented in the study are included in the article/supplementary material, further inquiries can be directed to the corresponding author/s.
Author Contributions
FW conceived the study, conducted the data analysis, and drafted the manuscript. FW, CL, and JZ carried out the field and laboratory work. FW and GZ discussed the draft manuscript. All authors approved the manuscript for publication.
Funding
This study was supported by the National Key R&D Program of China (No. 2019YFD0900801), the National Science Foundation of China (No. 31972790), and the China Agriculture Research System of MOF and MARA (CARS-49).
Conflict of Interest
The authors declare that the research was conducted in the absence of any commercial or financial relationships that could be construed as a potential conflict of interest.
Publisher’s Note
All claims expressed in this article are solely those of the authors and do not necessarily represent those of their affiliated organizations, or those of the publisher, the editors and the reviewers. Any product that may be evaluated in this article, or claim that may be made by its manufacturer, is not guaranteed or endorsed by the publisher.
Acknowledgments
We thank all members of the Lab of Marine Mollusc Aquaculture and Biotechnology, IOCAS for valuable discussions. We are grateful to Zoneco Group Co., Ltd., for support during our study.
References
Alcapán, A. C., Nespolo, R. F., and Toro, J. E. (2007). Heritability of body size in the Chilean blue mussel (Mytilus chilensis Hupé 1854): effects of environment and ageing. Aquac. Res. 38, 313–320. doi: 10.1111/j.1365-2109.2007.01678.x
Barros, J., Velasco, L. A., and Winkler, F. M. (2018). Heritability, genetic correlations and genotype by environment interactions in productive traits of the Caribbean scallop, Argopecten nucleus (Mollusca: Bivalvia). Aquaculture 488, 39–48. doi: 10.1016/j.aquaculture.2018.01.011
Boudry, P., Allal, F., Aslam, M. L., Bargelloni, L., Bean, T. P., Brard-Fudulea, S., et al. (2022). Current status and potential of genomic selection to improve selective breeding in the main aquaculture species of International Council for the exploration of the Sea (ICES) member countries. Aquac. Rep. 20:100700. doi: 10.1016/j.aqrep.2021.100700
Bryden, C. A., Heath, J. W., and Heath, D. D. (2004). Performance and heterosis in farmed and wild Chinook salmon (Oncorhynchus tshawytscha) hybrid and purebred crosses. Aquaculture 235, 249–261. doi: 10.1016/j.aquaculture.2004.01.027
de Melo, C. M. R., Durland, E., and Langdon, C. (2016). Improvements in desirable traits of the Pacific oyster, Crassostrea gigas, as a result of five generations of selection on the West Coast, USA. Aquaculture 460, 105–115. doi: 10.1016/j.aquaculture.2016.04.017
Eknath, A. E., Bentsen, H. B., Ponzoni, R. W., Rye, M., Nguyen, N. H., Thodesen, J., et al. (2007). Genetic improvement of farmed tilapias: composition and genetic parameters of a synthetic base population of Oreochromis niloticus for selective breeding. Aquaculture 273, 1–14. doi: 10.1016/j.aquaculture.2007.09.015
Falconer, D. S., and Mackay, T. F. C. (1996). Introduction to Quantitative Genetics, fourth Edn. Harlow: Prentice Hall, Longman Group Limited.
Food and Agriculture Organisation [FAO] (2020). Fishery and Aquaculture Statistics. Global Production by Production Source 1950-2018 (FishstatJ). Rome: FAO Fisheries and Aquaculture Department.
Gjedrem, T. (2010). The first family-based breeding program in aquaculture. Rev. Aquac. 2, 2–15. doi: 10.1111/j.1753-5131.2010.01011.x
Gjedrem, T. (2012). Genetic improvement for the development of efficient global aquaculture: a personal opinion review. Aquaculture 344-349, 12–22. doi: 10.1016/j.aquaculture.2012.03.003
Gjedrem, T., Robinson, N., and Rye, M. (2012). The importance of selective breeding in aquaculture to meet future demand for animal protein: a review. Aquaculture 350-353, 117–129. doi: 10.1016/j.aquaculture.2012.04.008
Gjerde, B., Terjesen, B. F., Barr, Y., Lein, I., and Thorland, I. (2004). Genetic variation for juvenile growth and survival in Atlantic cod (Gadus morhua). Aquaculture 236, 167–177. doi: 10.1016/j.aquaculture.2004.03.004
Guo, X., and Luo, Y. (2016). Scallops and scallop aquaculture in China. Dev. Aquac. Fish. Sci. 40, 937–952.
He, J., Zhao, Y., Zhao, J., Gao, J., Han, D., Xu, P., et al. (2017). Multivariate random regression analysis for body weight and main morphological traits in genetically improved farmed tilapia (Oreochromis niloticus). Genet. Sel. Evol. 49:80.
Hedgecock, D., and Davis, J. P. (2007). Heterosis for yield and crossbreeding of the Pacific oyster Crassostrea gigas. Aquaculture 272(Suppl. 1) S17–S29. doi: 10.1016/j.aquaculture.2007.07.226
Hollenbeck, C. M., and Johnston, I. A. (2018). Genomic tools and selective breeding in molluscs. Front. Genet. 9:253. doi: 10.3389/fgene.2018.00253
Hufstetler, E. V., Boerma, H. R., Carter, T. E., and Earl, H. J. (2007). Genotypic variation for three physiological traits affecting drought tolerance in soybean. Crop Sci. 47, 25–35. doi: 10.2135/cropsci2006.04.0243
Katherine, A., Ross, K. A., Thorpe, J. P., Norton, T. A., and Brand, A. R. (2001). An assessment of some methods for tagging the great scallop, Pecten maximus. J. Mar. Biol. Assoc. U. K. 81, 975–977. doi: 10.1017/S0025315401004921
König, S., Köhn, F., Kuwan, K., Simianer, H., and Gauly, M. (2006). Use of repeated measures analysis for evaluation of genetic background of dairy cattle behavior in automatic milking systems. J. Dairy Sci. 89, 3636–3644. doi: 10.3168/jds.S0022-0302(06)72403-1
Kube, P. D., Appleyard, S. A., and Elliott, N. G. (2007). Selective breeding greenlip abalone (Haliotis laevigata): preliminary results and issues. J. Shellfish Res. 26, 821–824. doi: 10.2983/07308000
Li, W., Luan, S., Luo, K., Sui, J., Xu, X., Tan, J., et al. (2015). Genetic parameters and genotype by environment interaction for cold tolerance, body weight and survival of the Pacific white shrimp Penaeus vannamei at different temperatures. Aquaculture 441, 8–15. doi: 10.1016/j.aquaculture.2015.02.013
Luan, S., Yang, G., Wang, J., Luo, K., Zhang, Y., Gao, Q., et al. (2012). Genetic parameters and response to selection for harvest body weight of the giant freshwater prawn Macrobrachium rosenbergii. Aquaculture 362–363, 88–96. doi: 10.1016/j.aquaculture.2012.05.011
Michio, S., and Hiromichi, N. (2013). Mollusk shell structures and their formation mechanism. Can. J. Zool. 91, 529–531. doi: 10.1139/cjz-2012-0333
Ren, W., Chen, H., Renault, T., Cai, Y., Bai, C., Wang, C., et al. (2013). Complete genome sequence of acute viral necrosis virus associated with massive mortality outbreaks in the Chinese scallop, Chlamys farreri. Virol. J. 10, 110–116. doi: 10.1186/1743-422X-10-110
Robertson, A. (1959). The sampling variance of the genetic correlation coefficient. Biometrics 15, 469–485. doi: 10.1007/BF00285414
Sae-Lim, P., Gjerde, B., Nielsen, H. M., Mulder, H., and Kause, A. (2016). A review of genotype-by-environment interaction and micro-environmental sensitivity in aquaculture species. Rev. Aquac. 8, 369–393. doi: 10.1111/raq.12098
Schaeffer, L. R. (2004). Application of random regression models in animal breeding. Livest. Prod. Sci. 86, 35–45. doi: 10.1016/S0301-6226(03)00151-9
Shumway, S. E., and Parsons, G. J. (2006). Scallops: biology, ecology and aquaculture. Dev. Aquac. Fish. Sci. 164, 280–281.
Song, H., Xu, S., Luo, K., Hu, M., Luan, S., Shao, H., et al. (2022). Estimation of genetic parameters for growth and egg related traits in Russian sturgeon (Acipenser gueldenstaedtii). Aquaculture 546:737299. doi: 10.1016/j.aquaculture.2021.737299
Tan, K., Zhang, H., Lim, L., and Zheng, H. (2020). Selection breeding program of Nan’ao Golden Scallop Chlamys nobilis with higher nutritional values and less susceptible to stress. Aquaculture 517:734769. doi: 10.1016/j.aquaculture.2019.734769
Thanh, N. M., Nguyen, N. H., Ponzoni, R. W., Vu, N. H., Barnes, A. C., and Mather, P. B. (2010). Estimates of strain additive and non-additive genetic effects for growth traits in a diallel cross of three strains of giant freshwater prawn (Macrobrachium rosenbergii) in Vietnam. Aquaculture 299, 30–36. doi: 10.1016/j.aquaculture.2009.12.011
Wang, C., Liu, B., Liu, X., Ma, B., Zhao, Y., Zhao, X., et al. (2017). Selection of a new scallop strain, the Bohai Red, from the hybrid between the bay scallop and the Peruvian scallop. Aquaculture 479, 250–255. doi: 10.1016/j.aquaculture.2017.05.045
Wang, Q. Z., Li, S., Fu, C., Zhang, M., Teng, W., Liu, Z., et al. (2014). Establishment of high temperature resistance families and use of laboratory assays to predict subsequent survival in juvenile stage of the Japanese scallop (Mizuhopecten yessoensis). J. Fish. China 38, 371–377. (in Chinese), doi: 10.3724/SP.J.1231.2014.48971
Wang, Q., Kou, B., Liu, Y., and Li, W. (1986). A primary approach to the subject of rearing spats of Japanese scallop (Pecten yessoensis) at optimum temperature. Mar. Sci. China 10, 49–50. (in Chinese),
Wang, R., and Wang, Z. (1993). Science of Marine Shellfish Culture. Qingdao: China Ocean University Press.
Wang, Y., Sun, G., Zeng, Q., Chen, Z., Hu, X., Li, H., et al. (2018). Predicting growth traits with genomic selection methods in Zhikong Scallop (Chlamys farreri). Mar. Biotechnol. 20, 769–779. doi: 10.1007/s10126-018-9847-z
Xing, Q., Wei, T., Chen, Z., Wang, Y., Lu, Y., Wang, S., et al. (2017). Using a multiscale image processing method to characterize the periodic growth patterns on scallop shells. Ecol. Evol. 7, 1616–1626. doi: 10.1002/ece3.2789
Xing, Q., Yang, Z., Zhu, X., Liu, J., Huang, X., Hua, J., et al. (2022). Interspecific hybridization between Patinopecten yessoensis (♀) and P. caurinus (♂) with heterosis in growth and temperature tolerance. Aquaculture 547:737489. doi: 10.1016/j.aquaculture.2021.737489
Keywords: yesso scallop Patinopecten yessoensis, heritability, genetic correlation, shell growth, sea-based culture
Citation: Wu F, Liu C, Zhang J and Zhang G (2022) Genetic Evaluation of Growth and Survival-Related Traits in Yesso Scallop Patinopecten yessoensis in Sea-Based Culture System. Front. Mar. Sci. 9:865736. doi: 10.3389/fmars.2022.865736
Received: 30 January 2022; Accepted: 17 February 2022;
Published: 23 March 2022.
Edited by:
Liqiang Zhao, Guangdong Ocean University, ChinaReviewed by:
Jitao Li, Chinese Academy of Fishery Sciences (CAFS), ChinaXingzhi Zhang, Guangxi Academy of Fishery Sciences, China
Liang Jian, Tianjin Agricultural University, China
Copyright © 2022 Wu, Liu, Zhang and Zhang. This is an open-access article distributed under the terms of the Creative Commons Attribution License (CC BY). The use, distribution or reproduction in other forums is permitted, provided the original author(s) and the copyright owner(s) are credited and that the original publication in this journal is cited, in accordance with accepted academic practice. No use, distribution or reproduction is permitted which does not comply with these terms.
*Correspondence: Fucun Wu, d3VmdWN1bkBxZGlvLmFjLmNu