- 1Centre for Research into Ecological and Environmental Modelling, University of St Andrews, St Andrews, United Kingdom
- 2Scottish Oceans Institute, University of St Andrews, St Andrews, United Kingdom
- 3Scottish Association for Marine Science, Oban, United Kingdom
- 4NatureScot, Oban, United Kingdom
- 5School of Mathematics and Statistics, University of Glasgow, Glasgow, United Kingdom
- 6Marine Laboratory, Marine Scotland Science, Aberdeen, United Kingdom
Catch-and-release angling is widespread, but the impacts of this practice for captured individuals are understudied, especially among elasmobranchs. Studies on sub-lethal behavioural impacts are particularly sparse, despite their importance for the interpretation of biologging data and for assessments of species’ tolerance to capture. In this study, the behavioural responses of flapper skate (Dipturus intermedius) to catch-and-release angling were described for the first time, using archival observations (depth and temperature) for 21 tag deployment/retrieval events and five recreational angling events that occurred during tagged individuals’ time at liberty from charter vessels off the west coast of Scotland in 2016–17. During capture (8–50 minutes), the changes in depth and temperature experienced by individuals typically exceeded natural variability. Post-release, behavioural change was apparent from visual inspection, regression and functional data analysis of the time series. Immediately following release, movements into deeper water and short periods of low vertical activity (usually 1–2 hours in duration) were common. However, overall average vertical activity was typically around 38% higher in the 12 hours following release than in undisturbed activity. A small number of individuals (n = 3, 14%) exhibited irregular post-release behaviour in the form of rapid, transient re-ascents towards the surface following release. Collectively, the evidence for limited, short-term behavioural changes suggests that flapper skate behaviour is relatively resilient to catch-and-release angling from charter vessels, but irregular post-release behaviour in 14% of individuals is sufficiently notable to indicate that further research is required on the impacts of this practice. This study clearly demonstrates the value of biologging data and behavioural analyses for examining the impacts of disturbance and separating ‘disturbed’ and ‘undisturbed’ behaviours in studies of animal movement.
Introduction
Catch-and-release angling is widespread (Cooke and Schramm, 2007). This activity can have substantial socioeconomic, conservation and scientific benefits, including the contribution of information on population ecology (Arlinghaus and Cooke, 2009). However, these benefits can come with conservation concerns (Cooke et al., 2014). While these have been studied extensively for teleosts, the impacts of catch-and-release angling on elasmobranchs have received less attention (Arlinghaus et al., 2007; Gallagher et al., 2017; Musyl and Gilman, 2019).
The impacts of capture on individual elasmobranchs (namely, sharks) have been examined principally from physical, physiological and behavioural perspectives. These impacts may be sub-lethal, cumulative and/or lethal over short or long timeframes. Physical damage caused by hooks is often the most obvious (Brownscombe et al., 2017). Physiological impacts, such as metabolic acidosis, have also been documented (Brill et al., 2008; Mohan et al., 2020; Weber et al., 2020). However, less attention has been paid to behavioural responses (Gallagher et al., 2017), despite their potential influence on the interpretation of biologging data (Hoolihan et al., 2011) and their utility as indicators of species’ tolerance to capture (Guida et al., 2017; Mohan et al., 2020; Whitney et al., 2021).
To date, most research on the behavioural responses of elasmobranchs to capture has been conducted in commercial fisheries settings and focused on specific behavioural metrics, such as reflex impairment indices or behavioural release scores, that indicate release condition and survival prospects (Hyatt et al., 2016; Ellis et al., 2017). Studies in recreational catch-and-release angling settings that have examined behaviour have also tended to focus on specific indicators of survival (Heberer et al., 2010; Danylchuk et al., 2014; Sepulveda et al., 2015; Mohan et al., 2020; Weber et al., 2020). Meanwhile, few studies have examined behaviour per se during angling or post-release (Hoolihan et al., 2011; Whitney et al., 2016; Whitney et al., 2017). Furthermore, existing research has focused almost exclusively on sharks, while the behavioural responses of batoids such as skate (Rajidae) to catch-and-release angling remain unstudied.
One technology that can be used to examine behaviour in relation to capture via hook and line is depth sensors such as archival tags. These principally collect high-resolution data on vertical movement (i.e. depth use and vertical activity), from which metrics describing behavioural responses during and following capture have been developed in several settings (Hoolihan et al., 2011; Guida et al., 2016; Whitney et al., 2016; Guida et al., 2017). During capture, fight time is a key variable that can be defined from depth observations. Fight time is underpinned by the static force required to pull an animal to the surface (which depends on body size and shape), but it is also affected by resistance from hooked individuals, alongside other variables (Gallagher et al., 2016). Consequently, statistical modelling of fight time can indicate the conditions that affect individuals’ propensity to resist capture (alongside the importance of other variables). For example, fight time may be longer at particular times of day or year in association with the effects of environmental variables, such as temperature and dissolved oxygen levels, on physiological performance (Lear et al., 2019). Prior experience may also influence the response to capture, as demonstrated for other forms of disturbance (Jordan et al., 2013). These possibilities are important because empirical studies show that fight time is a consistent correlate of physiological indicators of stress (Danylchuk et al., 2014; Gallagher et al., 2014; French et al., 2015; Whitney et al., 2017). Consequently, models of fight time can indicate the circumstances under which individuals may be more or less susceptible to capture-related impacts, even in the absence of physiological parameters. During capture, the rate and magnitude of the changes that individuals experience in depth or environmental conditions, such as temperature, relative to the experiences of undisturbed individuals, are also indicative of the potential impacts of catch-and-release, since particularly rapid ascents and temperature change may exacerbate anaerobic exertion, cause thermal shock or induce barotrauma (Garcia et al., 2015; Cook et al., 2019). For example, evidence for systemic gas embolism following capture from deep sea trawls has been documented in sleeper sharks (Somniosus rostratus), suggesting that in some circumstances elasmobranchs may be vulnerable to barotrauma, despite the lack of internal air spaces (Garcia et al., 2015).
Post-release, depth observations can reveal changes in movement, such as reduced or elevated vertical activity, that reflect behavioural changes, including switches between resting and more active behaviours (Hoolihan et al., 2011; Danylchuk et al., 2014; Rogers et al., 2017). Similarly, unusual movements, such as prolonged use of a constant depth or erratic changes in depth, may indicate irregular post-release behaviours (IPRBs), such as depth-holding, hyperactive or escape behaviour (Hoolihan et al., 2011). For benthic or demersal species in bathymetrically heterogeneous environments, these responses may be closely related to overall activity levels and patterns of space use, and collectively they can provide an indicator of the extent to which individuals are disturbed by capture (Whitney et al., 2016). However, current research suggests that behavioural responses to capture are often individual-, species- and environment-specific, which challenges a priori predictions for the impacts of catch-and-release on unstudied species.
The flapper skate (Dipturus intermedius) is a large, benthic, Critically Endangered elasmobranch that is prized by recreational anglers (Neat et al., 2015; Ellis et al., 2021). Once widespread, this species was extirpated from parts of its former range (Brander, 1981), but it remains locally abundant in an area off the west coast of Scotland where recreational angling and tagging prompted the designation of the Loch Sunart to the Sound of Jura Marine Protected Area (LStSJ MPA) for its conservation (Neat et al., 2015). However, the responses of skate to catch-and-release angling in this area remain poorly studied.
The possible responses of flapper skate to catch-and-release angling can be exhibited in four primary stages. In the contact phase (1), skate bite the bait and then typically dig into the sediment, forming a seal which only breaks after a period (typically less than 10 minutes in duration) of tension from a rod and line. In the ascent phase (2), skate are pulled to the surface. Some fish make strong downwards movements during this phase. In the surface phase (3), individuals are brought onto angling vessels (or the shore) before release. Post-release (4), information is restricted to capture-recapture and photo-identification studies (Neat et al., 2015; Benjamins et al., 2018; Skatespotter, 2021). In the most recent report of recapture records for the west coast of Scotland, 40% of individuals (n = 631) are identified as having been recaptured at least once (Skatespotter, 2021). Evidence for numerous, repeated captures is also recorded for specific individuals, with one individual having been recaptured 22 times. However, while this research shows that individuals can survive catch-and-release angling (at least in some circumstances), the short-term, sub-lethal impacts of capture, including on behaviour, remain unknown.
The aim of this study is to investigate the behavioural responses of flapper skate to catch-and-release angling, and their potential implications for our understanding of the wider impacts of this practice, using biologging data from archival tags. There are two main objectives:
(1) To quantify fight time, its drivers and the change in depth and temperature that individuals experience during angling as metrics of the potential stress induced by this practice.
(2) To examine individual movement patterns, systematic changes in depth use and vertical activity, and IPRB as indicators of post-release behavioural change.
Materials and Methods
Study Area
The LStSJ MPA is situated on the west coast of Scotland (Figure 1). The bathymetric environment is complex, including shallow (< 50 m) areas alongside glacially over-deepened channels up to 290 m in depth (Howe et al., 2014). Most recorded angling (post 2016) occurs from a small number (2–3) of charter vessels in three areas over relatively deep (> 100 m) water (Lavender et al., 2021a) (Figure S1). Water temperature varies seasonally from a minimum of approximately 7.5°C in March to a maximum of 16.0°C in September, with the surface waters warming by 1–2°C more than the layers below in summer. Seasonal variation in air temperature is greater, typically extending from -2°C in winter to 22°C in summer.
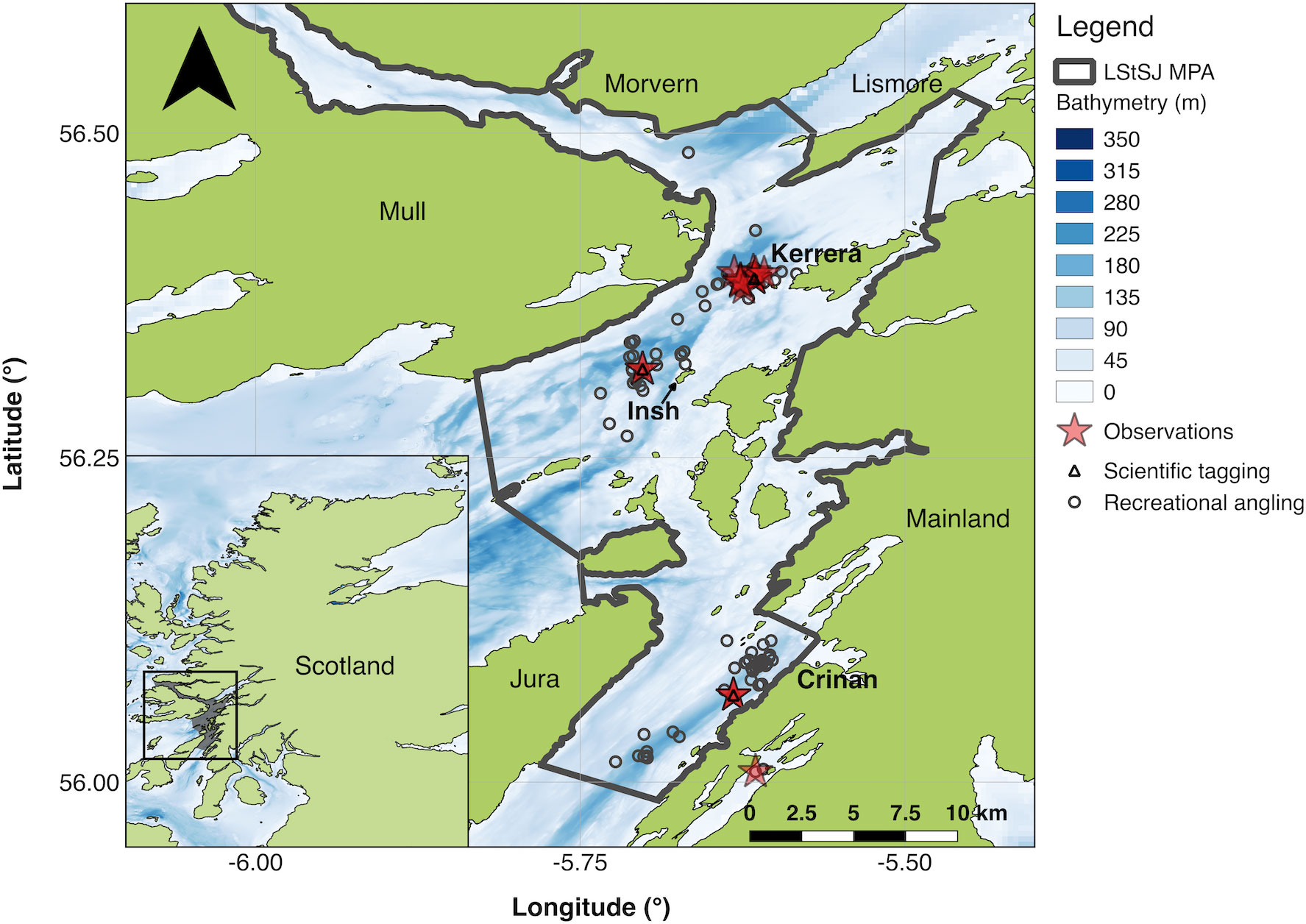
Figure 1 The study site and catch-and-release records. In the inset, the location of the study site in Scotland is shown in grey and enclosed. In the main figure, the Loch Sunart to the Sound of Jura Marine Protected Area is shown. The three main angling sites (Kerrera, Insh and Crinan) are noted in bold. Points mark recreational angling and scientific tagging events recorded in the capture-recapture (n = 1,166) and Movement Ecology of Flapper Skate archival (Star Oddi milli-TD) tagging (n = 45) databases, as described in Lavender et al. (2021a). Stars mark capture events associated with archival data used in this study (n = 26). The background shows the bathymetry (m) around Scotland at 15-arc resolution and the MPA at mixed 5 x 5 m and 15 arc second resolution, with high-resolution data from Howe et al. (2014) shown where available and lower resolution data from the General Bathymetric Chart of the Oceans (GEBCO Compilation Group, 2020) shown otherwise. Coastline data were sourced from the Database of Global Administrative Areas (Global Administrative Areas, 2022).
Tagging
In the MPA, 45 individuals were caught and tagged with Star Oddi milli-TD archival tags in March 2016–April 2017 (Table S1) as part of research conducted by the Movement Ecology of Flapper Skate project on depth use (Thorburn et al., 2021), vertical movement (Lavender et al., 2021b) and residency (Lavender et al., 2021a). Skate were caught from two anchored charter vessels using 50–80 Ib class rods with lever drag multiplier reels. A braided main line was used that terminated in a 3 m 250 Ib rubbing length. A standard lead weight (12 oz) was attached via a sliding boom so that it could drop off in the case of a broken line. The tackle terminated in a 10/0 O’Shaughnessy hook with the barb removed. Whole mackerel (Scomber scombrus) were used as bait. Once captured, on the vessel, individual sex, total length (snout tip to tail tip) and disc width (wing tip to wing tip), measured with a tape measure over the dorsal surface, were recorded. Maturation status (immature, mature) was later inferred using a model for maturation status with total length (see Supporting Information §1.1). After measurement, individuals were tagged with archival tags on the leading edge of the left wing. Each tag was attached to the dorsal surface on top of a silicon pad via two stainless steel pins pushed through the wing and secured on the ventral surface with another silicon pad and a base plate. Tags were programmed to record depth (m), to a resolution of 0.24 m and an accuracy of 4.77 m, and temperature (°C), to a resolution of 0.032°C and an accuracy of 0.1°C, every two minutes. A total of 45 tags were deployed on the basis that this number should provide sufficient data to investigate depth use, vertical movement and residency, given a 40% recapture rate (Lavender et al., 2021a; Lavender et al., 2021b; Skatespotter, 2021; Thorburn et al., 2021). During their time at liberty, individuals could be caught and released by recreational anglers. Data were recovered from individuals that were recaptured and had their tags removed, either by recreational anglers or as part of ongoing monitoring. In this study, these data were made available from previous studies and used opportunistically to investigate the capture process and behavioural responses to catch-and-release.
Catch-and-Release Events
Three sources of information on the capture process and the behavioural responses of flapper skate to catch-and-release angling were identified within recovered time series: tag deployment events; recreational catch-and-release angling events that occurred during individuals’ time at liberty; and tag retrieval events (Figure S2). For recreational catch-and-release events that occurred during individuals’ time at liberty, only confirmed events, recorded in a capture-recapture database maintained by NatureScot, Marine Scotland Science and the Scottish Association for Marine Science, were incorporated in analyses. However, all near-surface (≤ 5 m) movements in individuals’ depth time series were inspected visually for evidence of unrecorded capture events.
The Capture Experience
Recovered data were analysed in R, version 4.0.2 (R Core Team, 2020). The capture process was investigated using data around recreational angling events that occurred during individuals’ time at liberty and immediately preceding tag retrieval (Figure S2). For each event, the start time was defined as the time of last depth observation preceding the capture ascent and the surface time as the time of the first observation at the surface. Fight time was estimated from the difference between the start and surface times as an indicator of the potential stress induced by capture. To investigate putative drivers of capture fights, fight time was considered in relation to sex (‘M’, ‘F’), body ‘size’ (specifically, dorsal surface area, m2), the number of previous captures (‘n’), the time (days) since the last capture (‘gap’) and the angle of the sun above the horizon (°), temperature (°C) and depth (m) at the onset of each capture event, while accounting for the effects of the tide with a metric of the rate of change in tidal elevation, using a generalised linear model of the form:
where i indexes capture events (see Supporting Information §1.2). It is recognised that other variables, such as angler experience and environmental conditions, may affect fight time, but in this study these additional possibilities could not be directly investigated (see Supporting Information §1.2). For recreational events, following capture fights, handling time was estimated as the difference between the surface and end time, with the latter defined as the time of the last depth observation preceding the rapid descent phase that followed individual release (Figure S2).
During capture, the total changes in depth and temperature that each individual experienced were compared to the distribution of changes in the rest of each individual’s time series as additional indicators of the potential impacts of capture. The ‘rest of each individual’s time series’ was defined to exclude all observations from two hours before capture events until 12 hours post-release: this ensured that analyses were comparable across all individuals and that any time spent on the hook pre-ascent or within the ‘post-release’ period considered in subsequent analyses (see below) was excluded. Outside of capture events, both (a) the change in depth and temperature between sequential observations and (b) the total, sustained change in depth and temperature over successive time bins equal in duration to the capture duration were examined, since it was not clear a priori whether sequential or sustained changes would reflect the true extent of the changes experienced under normal conditions.
Post-Release Movements
Observations immediately following tag deployment and recreational angling events that occurred during individuals’ time at liberty (Figure S2) were used to investigate short-term post-release vertical movement patterns as indicators of behaviour. ‘Short-term’ was defined as 12-hours post-release. This time of window was chosen because it was expected that, if capture affects post-release behaviour, at least some signatures of behavioural change would be exhibited shortly following release while, over longer periods, any changes may be masked by natural variability. This approach also permitted investigation of post-release behaviour across all sampled individuals. For each capture event, observed time series before and/or immediately post-release were visualised to identify patterns in depth use or vertical activity (the difference between sequential depth observations) potentially attributable to capture—especially descent into deeper water, low vertical activity and erratic movement—reflective of behavioural change.
Average Changes in Depth and Vertical Activity
To quantify systematic changes in depth use and vertical activity, post-release time series were compared against ‘undisturbed’ time series samples drawn from the rest of each individual’s time series. Each ‘undisturbed’ sample was drawn such that it began at the same time of day as the capture event and lasted for the same duration as the post-release period under consideration (12 hours). Time series were sampled at random according to a uniform distribution. In this way, 50% of the total number of available, non-overlapping 12-hour samples (up to a maximum of 100 samples) was drawn for each individual. (The actual number depended on the length of available data, which differed among individuals due to variation in their time at liberty.) For each sample, the first four observations were excluded to focus on the movements of skate once they had returned to the seabed.
Given substantial variability in vertical movements, the mean depth, the mean absolute vertical activity and the duration of ‘minimal’ (≤ 0.5 m) absolute vertical activity from each 12-hour sample were identified as response variables indicative of average movement patterns. To determine whether or not capture affected these variables, and how responses differed among life-history categories, each response was modelled in a generalised linear modelling framework in relation to a three-way interaction between a factor (‘sample’) distinguishing post-release (‘PR’) and ‘undisturbed’ time series, sex and body size (specifically total length). Following the model for fight time, temperature was included as a metric of seasonality. The model took the form:
where f represents the Gaussian distribution for the mean depth and absolute vertical activity and the Gamma distribution for the duration of minimal absolute vertical activity (see Supporting Information §1.3).
Irregular Post-Release Behaviour
Post-release and ‘undisturbed’ samples were compared qualitatively and using functional principal component analysis (FPCA) to identify IPRB. FPCA is a type of functional data analysis that represents each time series as a smooth function and then applies FPCA to the set of smooths (Ullah and Finch, 2013; Wang et al., 2016). The outcome is a series of harmonics (one for each principal component) that describes the variation among the smooths and a corresponding set of scores for each smooth that maps it onto the harmonics. Distinct time series have mappings that differ substantially from other time series and appear as isolated points on score plots.
FPCA was implemented separately for each individual, since the number of post-release and ‘undisturbed’ time series varied among individuals and behaviour unusual for one individual might not be unusual for another, using the fda package (Ramsay et al., 2020). For each individual, FPCA was implemented for (a) the depth time series and (b) the absolute vertical activity time series. For these analyses, post-release and ‘undisturbed’ depth and absolute vertical activity time series were represented as smooths using a B-spline basis with 25 or 100 basis functions respectively. FPCA was applied to these smooths and the resultant harmonics and scores for the post-release time series relative to those for the ‘undisturbed’ time series were visually inspected for evidence of distinctness (see Supporting Information §1.4).
Results
Capture Events
Archival tags were successfully recovered from 21 individuals (Table S1) following deployment periods of 3–772 (median = 164) days. Five recreational catch-and-release events were recorded during individuals’ time at liberty (Table S2). All events occurred from charter angling vessels. Visual inspection of near-surface movements suggested that two unrecorded catch-and-release events may also have occurred (Figure S3) (see Supporting Information §2.1). Across all confirmed capture events (n = 26), fight time ranged from 8–50 (median = 18) minutes while recreational handling time (n = 5) ranged from 4–6 (median = 4) minutes (Table S2). In the model of fight time, there was indicative evidence that capture fights were longer for larger individuals caught in cooler, deeper water (Figure 2 and Tables S2, S3). However, none of the terms in the model were statistically significant at the 0.05 level (Table S3). Nonetheless, the model explained 60% of the deviance and residual diagnostics were reasonable.
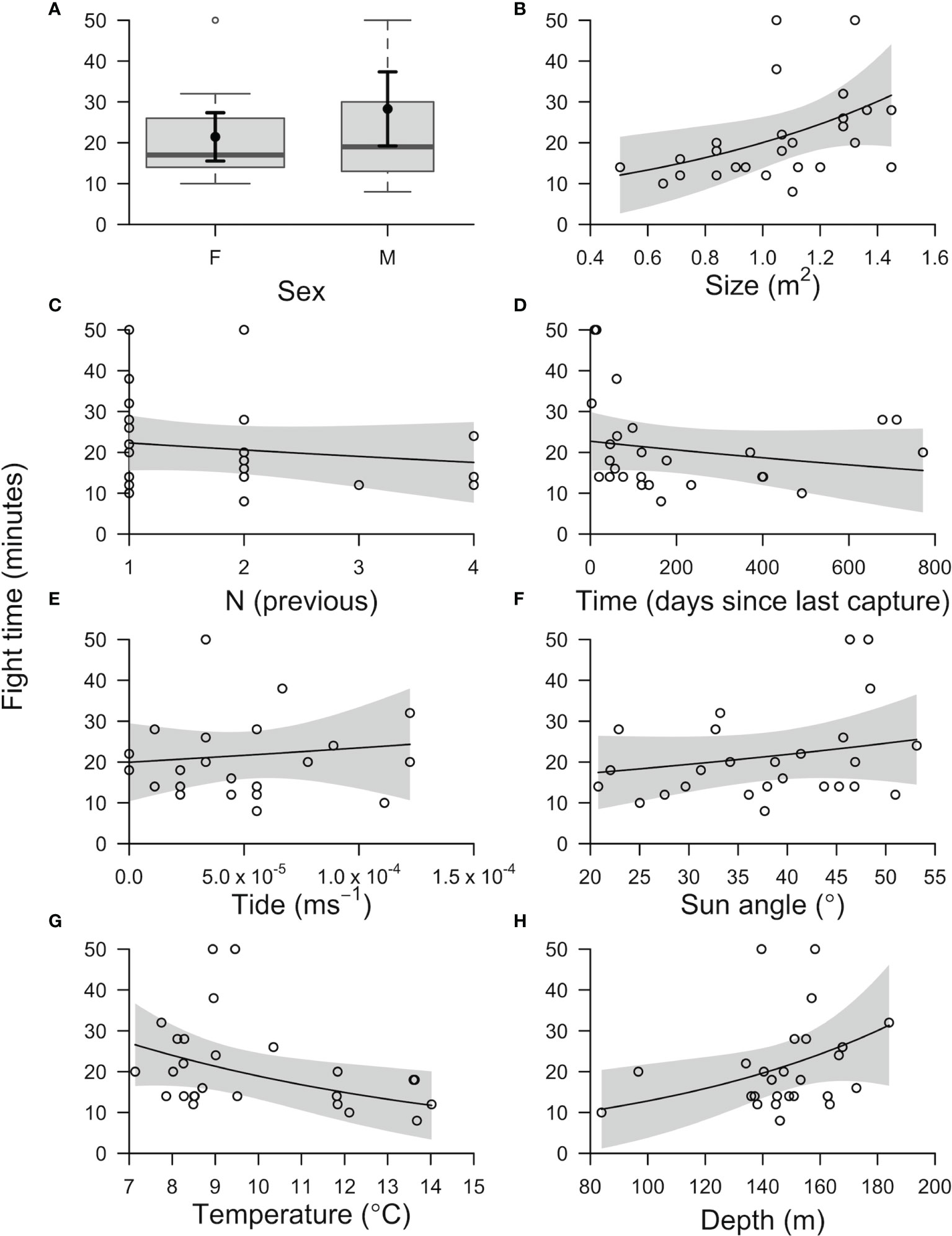
Figure 2 The drivers of fight time during catch-and-release angling. Each plot shows the relationship between fight time and one of the following variables: (A) sex; (B) body size (dorsal surface area); (C) the number of previous capture events; (D) the number of days since the last capture event; (E) the strength of the tide; (F) the sun angle above the horizon (this is lowest early/late in the day/year and highest in the middle of the day/year); (G) the water temperature; and (H) the depth. In (A) boxplot width is proportional to the number of capture events of females (n = 18) and males (n = 8). The lower, middle and upper whiskers in grey represent the 25th, 50th and 75th percentiles respectively. The black points and surrounding lines mark the mean ± 95% confidence intervals. In (B–H) the black lines and grey envelopes mark predictions and 95% confidence intervals from a generalised linear model of fight time, with other variables held at their median values. Note that relationships are shown for all variables in the model but none of the terms in the model were statistically significant at the 0.05 level (Table S3).
During capture fights, changes in depth and temperature generally exceeded naturally observed variation (Table S2 and Figure S4). While sequential changes in depth were generally small outside of capture events, and only exceeded 100 m on 14 occasions, capture ascent distances varied between 80–178 (median = 145) m. Likewise, the distribution of sequential changes in temperature (-3.61–2.46, median = 0.00°C) was generally smaller than the change during capture fights (-0.24–14.77, median = 0.14°C). For the recreational angling events that occurred during individuals’ time at liberty, the additional temperature change during handling varied from -0.57–5.26 (median = 1.05) °C. However, overall, individuals only experienced temperatures that exceeded the maximum bottom temperature (16.00°C) expected in the study area on 2/26 occasions (Table S2).
Post-Release Movement Patterns
A total of 26 post-release time series were identified from 21 tag deployment events and five recreational catch-and-release events that occurred during individuals’ time at liberty (Table S2). Following release, in all cases individuals rapidly descended into deeper water (Figures 3, 4). In 13 cases, individuals exhibited a short hiatus in their descent (e.g., Figure 4H), usually lasting less than half an hour and in some cases resembling a small ‘bounce’ in the depth time series (e.g., Figure 4N), before continuing to descend more gradually into deeper (150–200 m) water over a period of one or more hours [labelled (1) in Figure 4]. Following descent, in 15 cases individuals exhibited very low vertical activity at depths around 150 or 200 m for periods of time lasting from less than hour (e.g., Figure 4B), to around two hours (e.g., Figure 4C), to more than 10 hours (e.g., Figure 4R) [labelled (2)]. The onset of this low vertical activity phase typically occurred within three hours post-release, but it did not become apparent until eight hours post-release for individual 1552 (Figure 4S). In seven time series with relatively short-lived initial resting phases, the phase repeated after a few hours (e.g., Figure 4C) [labelled (3)]. In contrast, more erratic movements (rather than a clear period of low vertical activity) were exhibited in 11 cases (e.g., Figure 4E) [labelled (4)].
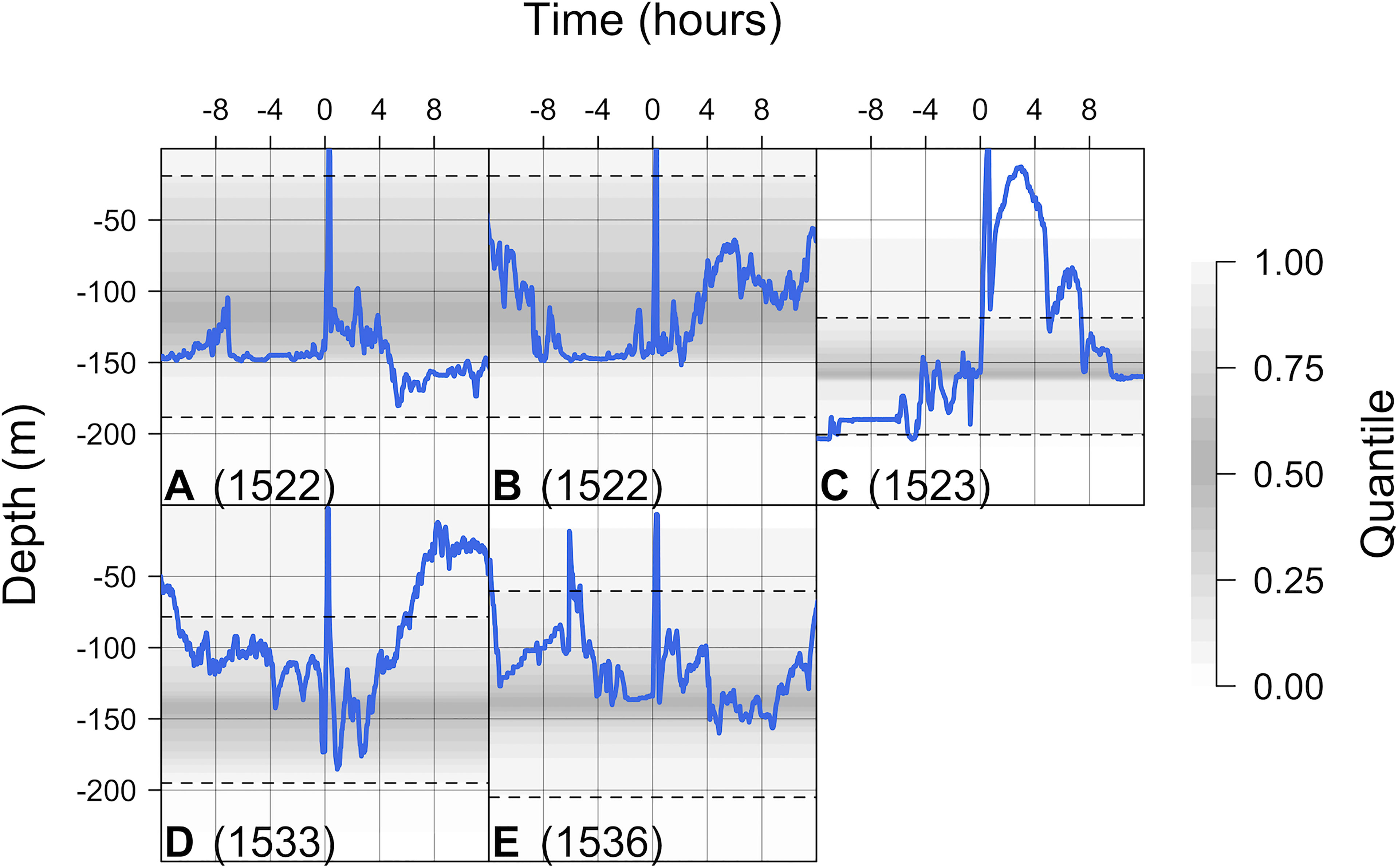
Figure 3 Depth time series around recreational catch-and-release events that occurred during individuals’ time at liberty. Each plot (A–E) plot shows the observed time series (in blue) around a capture event for a specific individual. The background shading marks the quantiles of observed variation in depth based on the rest of each individual’s time series, with quantiles near the middle of the distribution shaded more darkly than quantiles towards the edge of the distribution. The dashed lines mark the 25th and 75th quantiles respectively. Depth observations beyond these lines are considerably shallower or deeper than average, while depth observations beyond the shading are more extreme than observed outside of capture event(s).
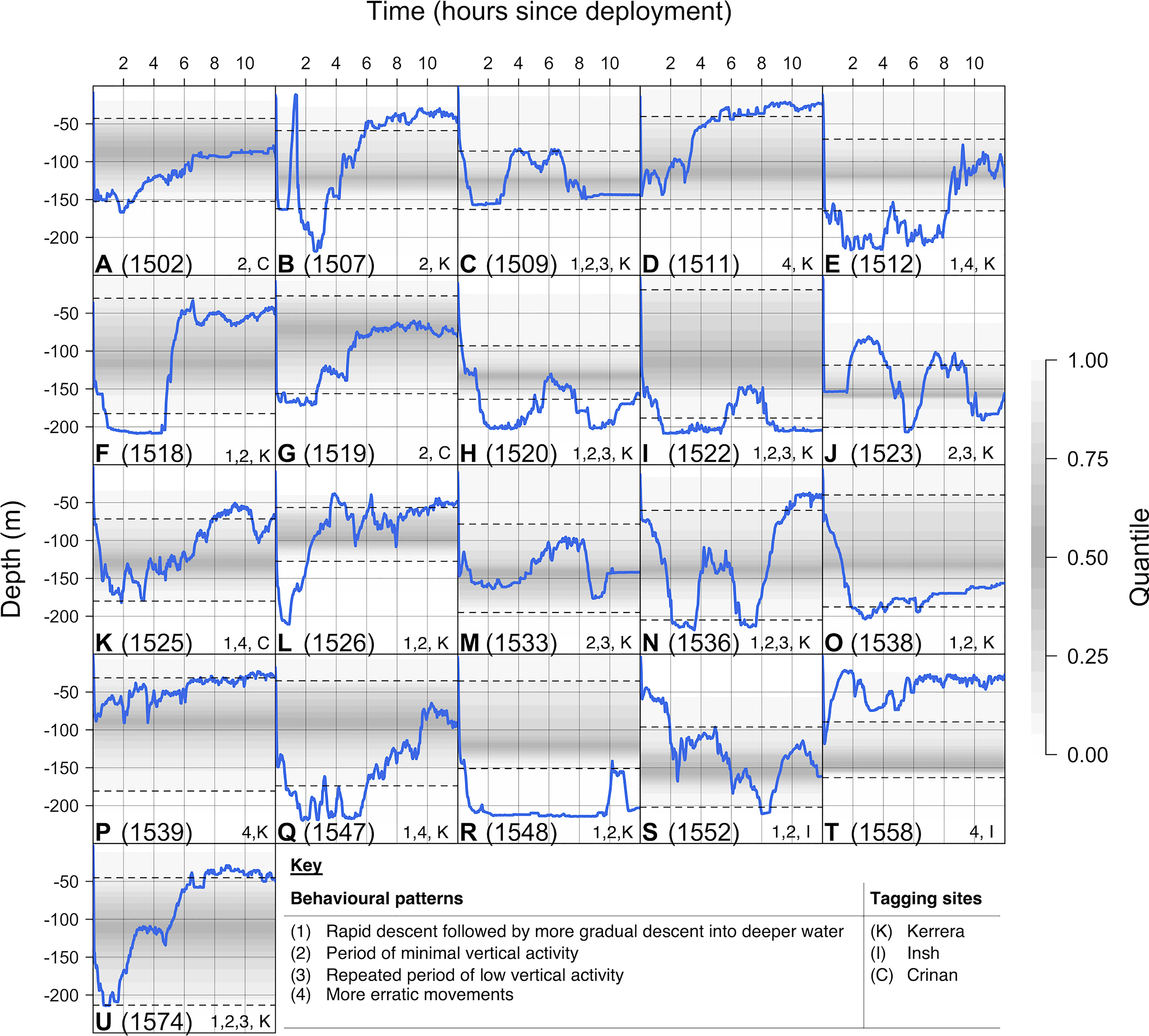
Figure 4 Depth time series following tag deployment. Each plot (A–U) shows the observed time series (in blue) for a specific individual. The background shading marks the quantiles of observed variation in depth based on the rest of each individual’s time series, with quantiles near the middle of the distribution shaded more darkly than quantiles towards the edge of the distribution. The dashed lines mark the 25th and 75th quantiles respectively. Depth observations beyond these lines are considerably shallower or deeper than average, while depth observations beyond the shading are more extreme than observed outside of capture event(s). Numbers and letters mark distinct patterns and tagging locations, respectively.
Shortly following descent, three individuals also undertook distinct, rapid, short-lived re-ascents into very shallow (< 25 m) water. These movements were exhibited by a mature male (1523: Figure 3C) following recreational capture in May 2016 and a mature female (1507: Figure 4B) and an immature female (1558: Figure 4T) following tag deployment in March 2016 (see Supporting Information §2.2).
Over subsequent hours, individual movements were highly variable, including periods of low and high vertical activity in both deep (> 150 m) and shallow (< 50 m) water (Figures 3, 4). However, 11 recently tagged individuals and one individual released by recreational anglers showed a clear pattern of moving shallower with the onset of night (e.g., Figures 3B, 4A).
Average Changes in Depth and Vertical Activity
Average depth use was associated with sex and total length in ‘undisturbed’ samples but there was no evidence that post-release samples differed (Figures 5A, B and Table S4). Similarly, mean absolute vertical activity was associated with sex, length and temperature in ‘undisturbed’ samples, with mean absolute vertical activity noticeably higher for larger individuals in particular. However, these relationships differed post-release (Figures 5C, D and Table S4). The smaller males (1536 and 1520) were more active post-release (Figure 5C). For instance, for individual 1536 the expected mean absolute vertical activity in ‘undisturbed’ samples (1.28 [1.18–1.40 (95% confidence intervals)]) significantly increased post-release (2.57 [1.54–4.31] m). However, this difference was less apparent for the three larger males. In females, mean absolute vertical activity was higher post-release across all sampled lengths (135–218 cm). Taking a female (e.g., 1525) with the median length (201 cm) as an example, the expected mean absolute vertical activity in ‘undisturbed’ samples (1.69 [1.63–1.91]) increased by approximately 38% post-release (2.34 [1.74–2.87] m). Accordingly, compared to ‘undisturbed’ time series, the duration of minimum absolute vertical activity was shorter post-release for the smaller males (43.60 [24.75–76.83] versus 114.59 [107.87–121.70] minutes for individual 1536) and across all sampled sizes of females (50.66 [40.44–63.66] versus 85.91 [83.46–88.44] minutes for a median-sized female) (Figures 5E, F and Table S4). Taken together, across all individuals, the expected mean absolute vertical activity was -12.77–100.92 (median = 37.91) % higher and the duration of minimal absolute vertical activity -8.13–61.94 (median = 42.16) % lower than in undisturbed activity. These models only explained 9.52–14.46% of the variability, but residual diagnostics were acceptable.
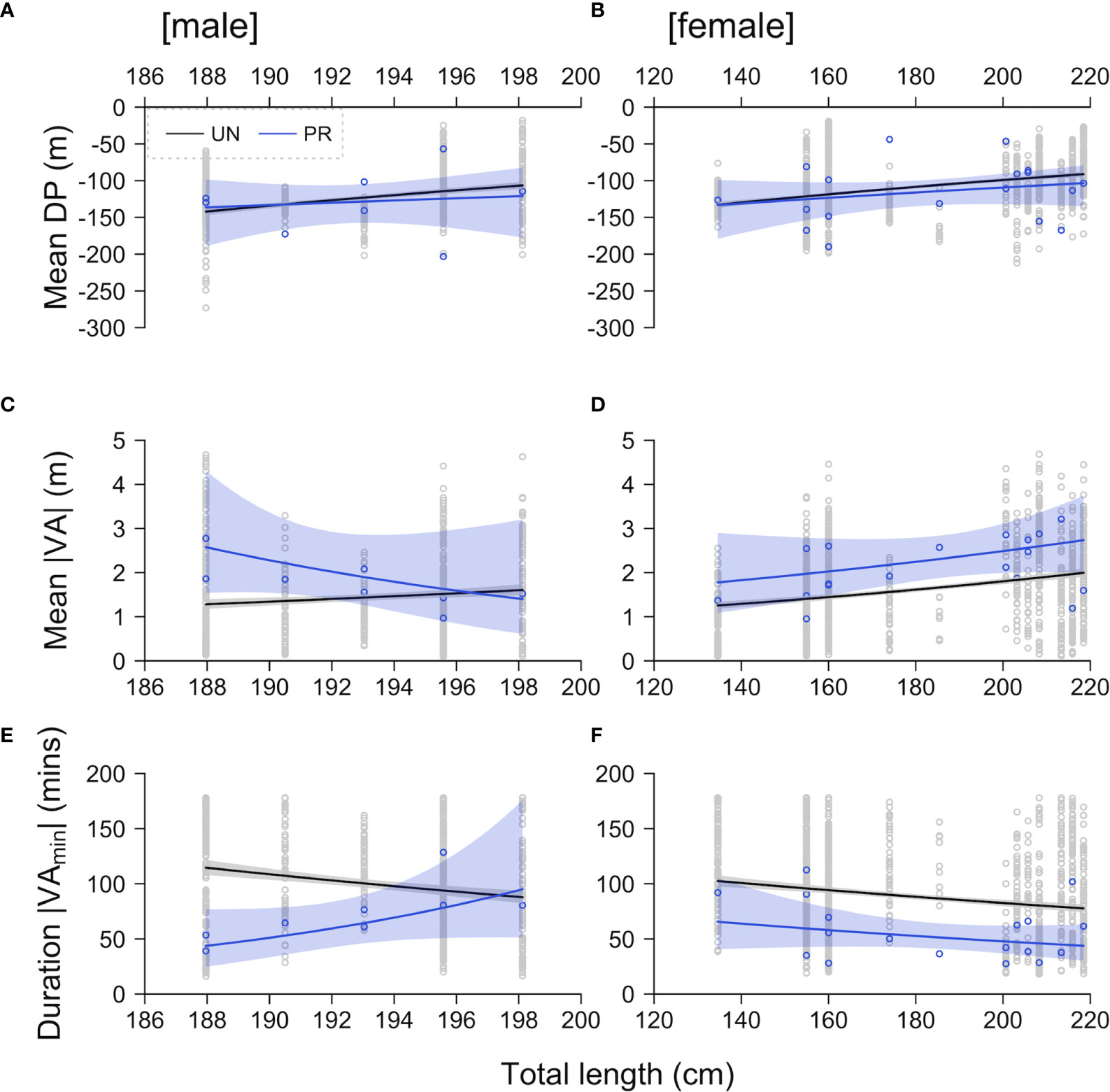
Figure 5 Predictions of the mean depth (A, B), mean absolute vertical activity (C, D) and the duration of ‘minimal’ (≤ 0.5 m) absolute vertical activity (E, F) for males (left-hand side: A, C, E) and females (right-hand side: B, D, F) in ‘undisturbed’ (UN, grey) and ‘post-release’ (PR, blue) 12-hour samples with total length. Points mark observations and the lines and surrounding envelopes mark model predictions and 95% confidence intervals. In all plots, temperature is held at the mean (scaled) value.
Irregular Post-Release Behaviour
Qualitative examination of movement time series did not indicate IPRB, with similar patterns apparent in both ‘undisturbed’ and ‘post-release’ time series (Figure S5). For instance, in ‘undisturbed’ samples there was evidence for periods of minimal vertical activity lasting for hours or days as well as low vertical activity over more prolonged periods (Figure S5A). ‘Undisturbed’ time series also exhibited occasional periods of very high vertical activity (Figures S5B, C). A tendency for movement into shallower water at night was also common among sampled individuals, demonstrating that these movements were not unique to post-release periods (Figure S5D).
In line with the results from qualitative examination, FPCA suggested that most ‘undisturbed’ and ‘post-release’ time series were similar in structure (Figure 6). For most individuals, ‘undisturbed’ and ‘post-release’ samples clustered together, despite substantial variability (e.g., Figure 6A). Even for individuals with moderately extreme principal component (PC) scores (e.g., Figure 6H), there was limited separation of post-release and ‘undisturbed’ time series along the PCs.
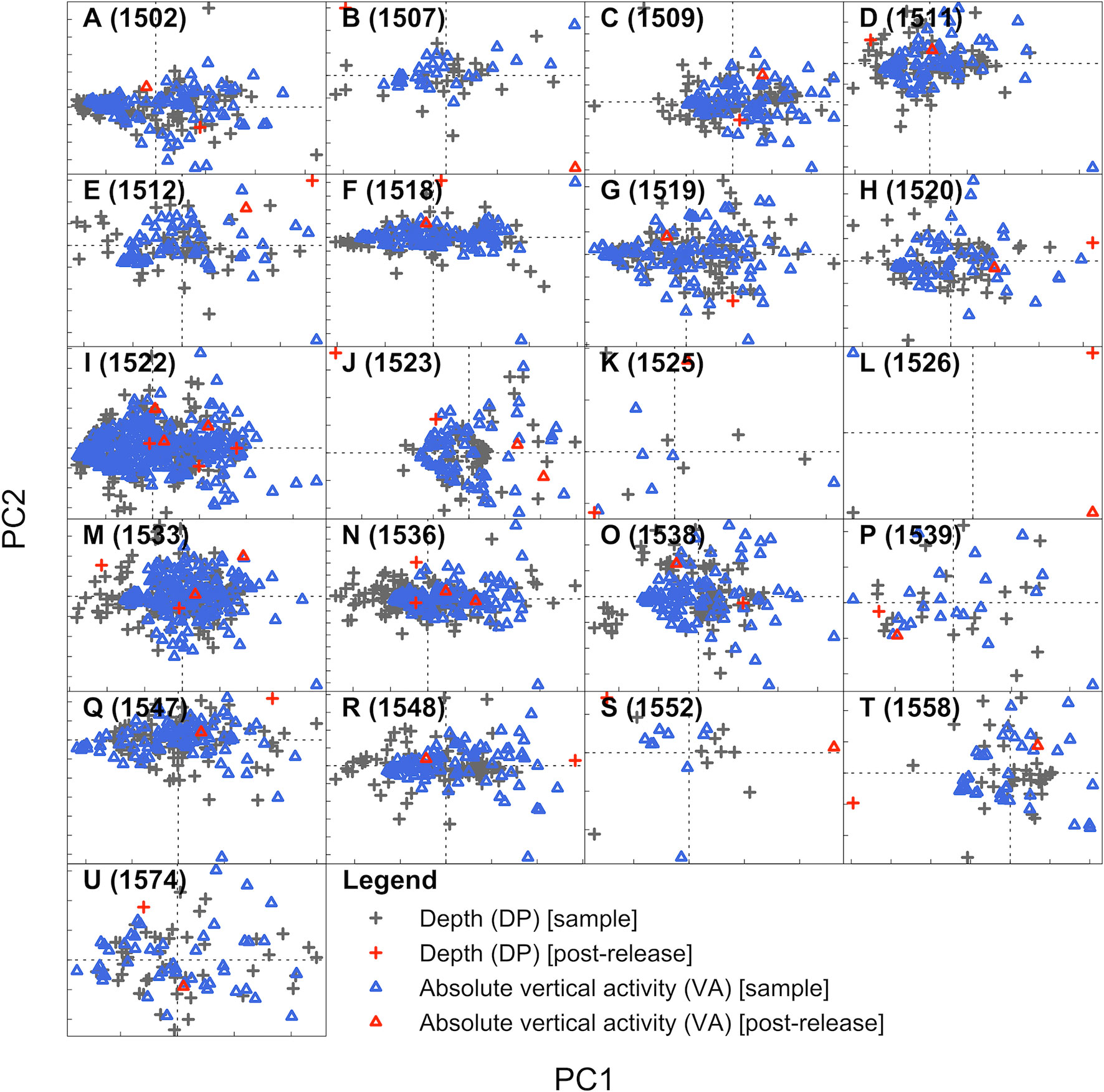
Figure 6 Functional principal component analysis (FPCA) scores for post-release and ‘undisturbed’ depth and absolute vertical activity time series samples. Following the order of Figure 4, each plot shows the scores for a specific individual from FPCA of the (i) depth and (ii) absolute vertical activity time series, with ‘undisturbed’ samples shown in grey and blue, respectively, and post-release time series (following tag deployment and recreational angling, if applicable) shown in red. Note that the number of ‘undisturbed’ samples varies among individuals due to variation in individuals’ time at liberty. All scores are centred and scaled to facilitate comparisons. The dashed horizonal and vertical lines mark adjusted scores on principal component 1 (PC1) and principal component 2 (PC2) of zero. Tick marks (bottom and left for each plot) are spaced one unit apart. The relative placement of scores for different samples indicates distinctness. For PC1, the proportion of the variability explained by FPCA ranged between 0.470–1.000 (median = 0.662) and 0.142–1.000 (median = 0.262) for the depth and absolute vertical activity time series respectively; for PC2, the proportions ranged between 0.000–0.298 (median = 0.124) and 0.000–0.246 (median = 0.094).
However, there was evidence for IPRB from distinct post-release PC scores for three individuals (1523, 1507 and 1558). For these individuals, distinct PC scores were driven by rapid movement into shallow (< 25 m) water, a pattern which appeared to be irregular (Figure S6). There was weaker evidence for IPRB in five other cases (1518, 1525, 1526, 1552 and 1512) but the differences between ‘undisturbed’ and post-release scores were not as strong. For example, individual 1518 also rapidly re-ascended into shallow water post-release (Figure 4F) and this movement was associated with slightly higher PC scores from the depth time series (Figures 6F, S6D). Distinct PC scores for individuals 1525, 1526 and 1552 were suggestive of IPRB, but these were derived from comparison against a small number (< 10) of ‘undisturbed’ samples.
Discussion
To the best of our knowledge, this is the first study on the impacts of catch-and-release angling on flapper skate and one of only a handful on elasmobranchs (and the only study on a skate species) to examine behaviour (Hoolihan et al., 2011; Whitney et al., 2016; Whitney et al., 2017). Following the contact phase, there is indicative evidence that fight time was associated with size, temperature and depth. During capture, the changes that individuals experienced in depth and temperature were extreme in the context of natural variability. For most individuals (n = 15, 71%), tag deployment was followed by a short period (typically 1–2 hours in duration) of minimal vertical activity that resembles recovery behaviour, as observed following capture and handling in other elasmobranchs (Hoolihan et al., 2011; Danylchuk et al., 2014; Raoult et al., 2019), but overall vertical activity was typically higher in the 12-hours post-release. There was limited evidence for IPRB, which suggests that in general flapper skate is behaviourally resilient to catch-and-release angling from charter vessels. However, short-lived IPRB was documented in three individuals, which is consistent with observations of more erratic or active post-release behaviour in other species following capture by recreational and commercial gear (Hoolihan et al., 2011; Whitney et al., 2021). These results demonstrate the multifaceted value of biologging data and how they can be used opportunistically to address knowledge gaps in data-poor systems.
Among elasmobranchs, fight time is a major driver of the impacts of catch-and-release, with documented impacts on physiology (Danylchuk et al., 2014; French et al., 2015; Whitney et al., 2017) and survival (Heberer et al., 2010; Sepulveda et al., 2015) principally attributable to exhaustive exercise (Brill et al., 2008). While studies on rajids are lacking, the estimates for fight time for flapper skate (8–50 minutes) are broadly comparable with those for other large elasmobranchs targeted by anglers, such as common thresher sharks (Alopias vulpinus) (Heberer et al., 2010; Sepulveda et al., 2015), notwithstanding substantial differences in biology and capture context. In the model of fight time for flapper skate, there was indicative evidence that fight times were higher for larger individuals, as observed by anglers and in other settings, which suggests that larger individuals may be more susceptible to capture-related impacts (Danylchuk et al., 2014). Similarly, graphical analysis supported the hypothesis that fight times are longer for individuals caught in cooler, deeper water. However, in line with the limited availability of data, unsurprisingly these terms were not statistically significant and further data are required to clarify their effects. In contrast, there was no evidence that fight times were substantially longer for males compared to females, in relation to recent capture history or at particular times of day.
Collectively, the results from the model of fight time suggest that a large portion of variation in fight time is attributable to unmeasured variables, potentially including environmental conditions (such as dissolved oxygen levels), angler variables (such as gear) and individual characteristics (such as energy levels). For instance, dissolved oxygen levels underpin aerobic activity and are likely to influence individual responses during capture (Musyl and Gilman, 2019). In this study, the effects of dissolved oxygen levels are likely to correlate with temperature, but the shape and strength of this relationship in the study area, and its effects on capture responses, warrant further investigation, as noted elsewhere (Musyl and Gilman, 2019). Other environmental conditions, such as seabed sediment type, suspended sediment loads and localised variation in current speeds not captured by the rate of change in tidal elevation metric used in this study, may also be important. In other settings, angler variables such as gear have been shown to influence fight time (Mullen et al., 2020) and post-release outcomes such as survival (Mohan et al., 2020). In this study, gear was standardised for tag deployment, but the gear used by recreational anglers to capture individuals during their time at liberty and in association with tag retrieval events may have differed and these differences may explain some residual variability. However, it is worth noting that there are clear gear guidelines in the study area and most recorded recreational angling occurs from a small number (2–3) charter vessels, where standard gear is available. Thus, while angler effects on capture responses warrant further investigation, it may be that other variables, such as skate energy levels, are important too. In many taxa, there is also accumulating evidence that personality affects responses to novel situations (Roche et al., 2016). In a study on Port Jackson sharks (Heterodontus portusjacksoni), bolder individuals were more responsive to a stress-inducing stimulus than shyer individuals (Byrnes and Brown, 2016). This raises the intriguing possibility that flapper skate may have distinct personalities that affect capture responses. Taken together, the results demonstrate that a targeted scientific data collection programme is required to understand fully the drivers of fight time in flapper skate. In particular, future sampling should prioritise the collection of data using standardised gear across a more representative range of body sizes, temperatures and depths. Direct measurements of fight time, in situ environmental conditions (such as current speeds) and physiological parameters of the muscular activity induced by capture would greatly support this research.
During capture, the changes that individuals experienced in depth and temperature were relatively extreme given the distribution of natural variation. While these changes could not be related to post-release outcomes for most individuals in this study, evidence from other species suggests that extreme changes in depth and temperature have the potential to impact skate. For example, in sleeper sharks, decompression-like gas bubbles were documented following capture by commercial trawlers in waters 600–800 m deep (Garcia et al., 2015). While catch-and-release angling for flapper skate differs from commercial trawling for sleeper sharks, this suggests that rapid ascents can affect elasmobranchs and that the impacts of rapid capture-induced ascents in flapper skate warrant further investigation. Elevated water temperatures can also influence the impacts of catch-and-release angling (Danylchuk et al., 2014). It is notable that the maximum temperature experienced by a flapper skate (1519) during capture (22.89°C) was 44.0% higher than the typical maximum water temperature in the area (16.00°C), but the consequences of exposure to elevated temperatures remain uncertain.
Following catch-and-release angling, there was evidence for altered post-release movement patterns and behaviour. Progressive descents into deeper water are probably partly attributable to the bathymetric landscape, particularly off Kerrera where the deep trench over which most angling occurred deepens towards the west of the study area. However, individuals may have also sought refuge in deeper water, with similar movements documented in other species following capture by recreational and commercial gear (Hoolihan et al., 2011; Rogers et al., 2017). Particularly in summer, deeper water may provide a suitable resting location in which cooler temperatures and/or shelter from currents facilitate recovery from exhaustive exercise. Indeed, following tagging and descent, 67% of individuals exhibited a period of minimal vertical activity that is suggestive of ‘reorientation’, resting or recovery behaviour, like that observed in other species (Hoolihan et al., 2011; Danylchuk et al., 2014). However, low vertical activity around angling marks could also result from rapid horizontal movement along the bottom of the long, deep trenches found in these locations, so additional (accelerometry) data would be beneficial for clarifying the links between these movements and behaviour (Gleiss et al., 2017).
Despite short-lived periods of low vertical activity post-release, overall average vertical activity in the 12-hours post-release increased. Post-release elevations in vertical activity or ‘hyperactivity’ have been documented in other elasmobranchs (Hoolihan et al., 2011) and teleosts (Cooke and Philipp, 2004). The causes for this remain unclear, but physiological changes, such as elevated adrenaline, and post-recovery ‘escape’ behaviour are possibilities.
However, there was limited evidence for IPRB. This result suggests that flapper skate are generally behaviourally resilient to catch-and-release angling conducted from charter vessels in the LStSJ MPA. This suggestion is consistent with repeated captures of individual skate by anglers (Benjamins et al., 2018; Skatespotter, 2021) and the condition of rajids caught in commercial fisheries, although the capture experience between hooks and nets differs (Little, 1995; Benoît et al., 2010). This resilience may reflect skate biology, angling practices and the study design. Biologically, elasmobranchs with buccal-pump ventilation are generally thought to be relatively resilient to capture (Cook et al., 2019). The adoption of best-practice angling guidelines, such as the use of barbless hooks, may also have minimised capture-related impacts (Skomal, 2007). Aspects of this study’s design may have further contributed towards the apparent resilience of skate. Importantly, only catch-and-release angling from charter vessels was considered, which may be less impactful than shore-based angling in situations where skate are pulled over the substrate for substantial distances. Additionally, archival time series only permit examination of a small number of movement metrics based on depth use and vertical activity which, in turn, only directly reflect behavioural responses to capture (Whitney et al., 2016). A related limitation is the small sample of capture events. This translates into a limited sample of the conditions which might worsen capture-induced stress, including ontogeny, environmental variables, such as temperature, and angling mishaps, such as hooking damage or retention (Cook et al., 2019). For example, despite best practices, individuals are occasionally deep hooked (in the throat or gut) and, in these situations, hooks can be difficult to recover and may be left in skate. An additional consideration is that archival data may positively bias inferred resilience because they can only be recovered from individuals that have survived multiple capture events. However, the recapture rate in this study (46%), in line with the rate documented across all individuals identified in the area (Skatespotter, 2021), suggests that survivability for many skate is high following angling.
Nevertheless, there was evidence for IPRB in at least three skate. This took the form of rapid re-ascents towards the surface. Few studies are available against which to compare this behaviour and its causes are unclear. Some teleosts show qualitatively similar patterns following catch-and-release. For example, Pacific cod (Gadus macrocephalus) often undertake a rapid ‘escape’ dive before re-ascending and then descending once more through a series of ‘bounce’ dives which progressively weaken over a period of days (Nichol and Chilton, 2006). In cod, this behaviour is attributable to the rupture, subsequent repair and refilling of swim bladder. While elasmobranchs lack a swim bladder, their buoyancy is sensitive to pressure changes and similar movements following capture of a leafscale gulper shark (Centrophorus squamosus) by a commercial longline (Rodríguez-Cabello and Sánchez, 2017) suggest that rapid ascents might contribute to a loss of equilibrium. In other elasmobranchs, reports of aberrant behaviour are generally associated with extreme external trauma (Kabasakal, 2010; Rodríguez-Cabello and Sánchez, 2017), but this is unlikely to apply for angled flapper skate. However, this suggests that skate may be more sensitive to capture than previously assumed in some circumstances.
Given limited pre-capture data, there are few obvious factors that might have increased the vulnerability of the individuals that exhibited IPRB to capture-related impacts. Among the five individuals with pre-capture data, it is notable that the individual that exhibited IPRB (1523) had the longest fight time (38 minutes), given the influence of fight time on capture-related impacts in other species (Danylchuk et al., 2014; Sepulveda et al., 2015). Additionally, all individuals that exhibited IPRB had relatively recent capture records (within 46–76 days), though the number of previous captures varied from one (1523) to four (1507). While data are limited, the lack of a clear relationship between previous captures and IPRB supports the view that the behavioural changes of skate following catch-and-release angling are relatively short-lived. In terms of individual characteristics, IPRB was documented in both sex and maturity categories. Further data are required to investigate whether the tendency of each sex or maturity categories to exhibit IPRB varies. Furthermore, IPRB was documented in spring in relatively cool water (8.72–10.13°C) and the temperature change that the individual with data (1523) experienced during capture was small (0.27°C), contrary to the hypothesis that capture resilience might be lower in late summer. There are insufficient data to determine whether the prevalence of IPRB differed between recreational catch-and-release angling events that occurred during individuals’ time at liberty (0.20) and post-tagging (0.14), but preliminary estimates suggest this is not the case. Other aspects of the capture process, such as hooking location, may have affected IPRB but the data required to evaluate these were unavailable.
While IPRB was uncommon, from a conservation perspective it is potentially concerning. Rapid ascents are likely to be energetically costly for skate. On top of the exertion of capture, such ascents may have implications for activities such as foraging (Skomal, 2007). Rapid ascents may also induce barotrauma or worsen symptoms caused by the initial capture process. If a loss of equilibrium underlies rapid ascents, they may reflect increased predation risks for smaller skate, as shown for other taxa (Cooke and Philipp, 2004). Disruption to mating, which is thought to occur in spring when catch-and-release angling is most frequent (Lavender et al., 2021a), may occur too. Taken together, the main message of these results is that flapper skate is typically behaviourally resilient to catch-and-release angling from charter vessels in the LStSJ MPA, but observed post-release behavioural changes inferred from archival data, especially IPRB, are sufficiently notable to indicate that further research is required into the cumulative individual and population-level impacts of this practice. This is essential for accurate population estimates and successful conservation (Neat et al., 2015).
Data Availability Statement
The datasets presented in this article are not readily available because of third party restrictions. Requests to access the datasets should be directed to Marine Scotland Science and NatureScot via James Thorburn, amF0MjFAc3QtYW5kcmV3cy5hYy51aw==.
Ethics Statement
The animal study was reviewed and approved by Ethics Committee of the University of St Andrews (number SEC21024). All regulated procedures involving animals were carried out in compliance with The Animals (Scientific Procedures) Act 1986 under the Home Office Project License number 60/4411 by competent Personal License holders.
Author Contributions
EL led the study conception and design. JT and JD led data collection. EL analysed the data and wrote the first draft of the manuscript. All authors contributed to revisions and approved the final manuscript.
Funding
This work was supported by a PhD Studentship at the University of St Andrews, jointly funded by NatureScot via the Marine Alliance for Science and Technology for Scotland (MASTS), and the Centre for Research into Ecological and Environmental Modelling. Data were collected as part of research funded by NatureScot (project 015960) and Marine Scotland (projects SP004 and SP02B0) via the Movement Ecology of Flapper Skate project. Additional funding was provided from MASTS and Shark Guardian.
Conflict of Interest
The authors declare that the research was conducted in the absence of any commercial or financial relationships that could be construed as a potential conflict of interest.
Publisher’s Note
All claims expressed in this article are solely those of the authors and do not necessarily represent those of their affiliated organizations, or those of the publisher, the editors and the reviewers. Any product that may be evaluated in this article, or claim that may be made by its manufacturer, is not guaranteed or endorsed by the publisher.
Acknowledgments
The authors thank anglers for contributing capture-recapture data; Ronnie Campbell, Roger Eaton and Francis Neat for supporting tagging; and Samuel Iglésias for sharing data and analyses of length–maturity relationships.
Supplementary Material
The Supplementary Material for this article can be found online at: https://www.frontiersin.org/articles/10.3389/fmars.2022.864344/full#supplementary-material
References
Arlinghaus R., Cooke S. J. (2009). “Recreational Fisheries: Socioeconomic Importance, Conservation Issues and Management Challenges,” in Recreational Hunting, Conservation and Rural Livelihoods. Eds. Dickson B., Hutton J., Adams W. M. (Oxford: Blackwell Publishing Ltd). p. 39–58. doi: 10.1002/9781444303179.ch3
Arlinghaus R., Cooke S. J., Lyman J., Policansky D., Schwab A., Suski C., et al. (2007). Understanding the Complexity of Catch-and-Release in Recreational Fishing: An Integrative Synthesis of Global Knowledge From Historical, Ethical, Social, and Biological Perspectives. Rev. Fish. Sci. 15, 75–167. doi: 10.1080/10641260601149432
Benjamins S., Dodd J., Thorburn J., Milway V. A., Campbell R., Bailey D. M. (2018). Evaluating the Potential of Photo-Identification as a Monitoring Tool for Flapper Skate (Dipturus intermedius). Aquat. Conserv. Mar. Freshw. Ecosyst. 28, 1360–1373. doi: 10.1002/aqc.2937
Benoît H. P., Hurlbut T., Chassé J. (2010). Assessing the Factors Influencing Discard Mortality of Demersal Fishes Using a Semi-Quantitative Indicator of Survival Potential. Fish. Res. 106, 436–447. doi: 10.1016/j.fishres.2010.09.018
Brander K. (1981). Disappearance of Common Skate Raia batis From Irish Sea. Nature 290, 48–49. doi: 10.1038/290048a0
Brill R., Bushnell P., Schroff S., Seifert R., Galvin M. (2008). Effects of Anaerobic Exercise Accompanying Catch-and-Release Fishing on Blood-Oxygen Affinity of the Sandbar Shark (Carcharhinus plumbeus, Nardo). J. Exp. Mar. Bio. Ecol. 354, 132–143. doi: 10.1016/j.jembe.2007.10.011
Brownscombe J. W., Danylchuk A. J., Chapman J. M., Gutowsky L. F. G., Cooke S. J. (2017). Best Practices for Catch-and-Release Recreational Fisheries – Angling Tools and Tactics. Fish. Res. 186, 693–705. doi: 10.1016/j.fishres.2016.04.018
Byrnes E. E., Brown C. (2016). Individual Personality Differences in Port Jackson Sharks Heterodontus portusjacksoni. J. Fish Biol. 89, 1142–1157. doi: 10.1111/jfb.12993
Cooke S. J., Hogan Z. S., Butcher P. A., Stokesbury M. J. W., Raghavan R., Gallagher A. J., et al. (2014). Angling for Endangered Fish: Conservation Problem or Conservation Action? Fish Fish. 17, 249–265. doi: 10.1111/faf.12076
Cooke S. J., Philipp D. P. (2004). Behavior and Mortality of Caught-and-Released Bonefish (Albula spp.) in Bahamian Waters With Implications for a Sustainable Recreational Fishery. Biol. Conserv. 118, 599–607. doi: 10.1016/j.biocon.2003.10.009
Cooke S. J., Schramm H. L. (2007). Catch-and-Release Science and its Application to Conservation and Management of Recreational Fisheries. Fish. Manage. Ecol. 14, 73–79. doi: 10.1111/j.1365-2400.2007.00527.x
Cook K. V., Reid A. J., Patterson D. A., Robinson K. A., Chapman J. M., Hinch S. G., et al. (2019). A Synthesis to Understand Responses to Capture Stressors Among Fish Discarded From Commercial Fisheries and Options for Mitigating Their Severity. Fish Fish. 20, 25–43. doi: 10.1111/faf.12322
Danylchuk A. J., Suski C. D., Mandelman J. W., Murchie K. J., Haak C. R., Brooks A. M. L., et al. (2014). Hooking Injury, Physiological Status and Short-Term Mortality of Juvenile Lemon Sharks (Negaprion bevirostris) Following Catch-and-Release Recreational Angling. Conserv. Physiol. 2, cot036. doi: 10.1093/conphys/cot036
Ellis J. R., McCully-Philipps S. R., Sims D., Walls R. H. L., Cheok J., Derrick D., et al. (2021). Dipturus intermedius. IUCN Red List Threat. Species 2021, e .T18903491A68783461. doi: 10.2305/IUCN.UK.2021-2.RLTS.T18903491A68783461.en
Ellis J. R., McCully Phillips S. R., Poisson F. (2017). A Review of Capture and Post-Release Mortality of Elasmobranchs. J. Fish Biol. 90, 653–722. doi: 10.1111/jfb.13197
French R. P., Lyle J., Tracey S., Currie S., Semmens J. M. (2015). High Survivorship After Catch-and-Release Fishing Suggests Physiological Resilience in the Endothermic Shortfin Mako Shark (Isurus oxyrinchus). Conserv. Physiol. 3, cov044. doi: 10.1093/conphys/cov044
Gallagher A. J., Hammerschlag N., Danylchuk A. J., Cooke S. J. (2017). Shark Recreational Fisheries: Status, Challenges, and Research Needs. Ambio 46, 385–398. doi: 10.1007/s13280-016-0856-8
Gallagher A. J., Serafy J. E., Cooke S. J., Hammerschlag N. (2014). Physiological Stress Response, Reflex Impairment, and Survival of Five Sympatric Shark Species Following Experimental Capture and Release. Mar. Ecol. Prog. Ser. 496, 207–218. doi: 10.3354/meps10490
Gallagher A. J., Staaterman E. R., Cooke S. J., Hammerschlag N. (2016). Behavioural Responses to Fisheries Capture Among Sharks Caught Using Experimental Fishery Gear. Can. J. Fish. Aquat. Sci. 74, 1–7. doi: 10.1139/cjfas-2016-0165
Garcia D., Crespo J. L., Guallart J., García-Salinas P., Jiménez M., Brodsky M. (2015). “Systemic Gas Embolism in Sharks. Evidence of Decompression Like Lesions in by-Caught Little Sleeper Sharks Somniosus Rostratus From Deep Sea Trawls,” in Poster 19th Annual Scientific European Elasmobranch Association. doi: 10.13140/RG.2.1.4407.3685
Gleiss A. C., Morgan D. L., Whitty J. M., Keleher J. J., Fossette S., Hays G. C. (2017). Are Vertical Migrations Driven by Circadian Behaviour? Decoupling of Activity and Depth Use in a Large Riverine Elasmobranch, the Freshwater Sawfish (Pristis pristis). Hydrobiologia 787, 181–191. doi: 10.1007/s10750-016-2957-6
Global Administrative Areas (2022). GADM Database of Global Administrative Areas, Version 4.0. Available at: www.gadm.org.
Guida L., Dapp D. R., Huveneers C. P. M., Walker T. I., Reina R. D. (2017). Evaluating Time-Depth Recorders as a Tool to Measure the Behaviour of Sharks Captured on Longlines. J. Exp. Mar. Bio. Ecol. 497, 120–126. doi: 10.1016/j.jembe.2017.09.011
Guida L., Walker T. I., Reina R. D. (2016). Temperature Insensitivity and Behavioural Reduction of the Physiological Stress Response to Longline Capture by the Gummy Shark, Mustelus antarcticus. PloS One 11, e0148829. doi: 10.1371/journal.pone.0148829
Heberer C., Aalbers S. A., Bernal D., Kohin S., DiFiore B., Sepulveda C. A. (2010). Insights Into Catch-and-Release Survivorship and Stress-Induced Blood Biochemistry of Common Thresher Sharks (Alopias vulpinus) Captured in the Southern California Recreational Fishery. Fish. Res. 106, 495–500. doi: 10.1016/j.fishres.2010.09.024
Hoolihan J. P., Luo J., Abascal F. J., Campana S. E., De Metrio G., Dewar H., et al. (2011). Evaluating Post-Release Behaviour Modification in Large Pelagic Fish Deployed With Pop-Up Satellite Archival Tags. ICES J. Mar. Sci. 68, 880–889. doi: 10.1093/icesjms/fsr024
Howe J. A., Anderton R., Arosio R., Dove D., Bradwell T., Crump P., et al. (2014). The Seabed Geomorphology and Geological Structure of the Firth of Lorn, Western Scotland, UK, as Revealed by Multibeam Echo-Sounder Survey. Earth Environ. Sci. Trans. R. Soc Edinburgh 105, 273–284. doi: 10.1017/S1755691015000146
Hyatt M. W., Anderson P. A., O’Donnell P. M. (2016). Behavioral Release Condition Score of Bull and Bonnethead Sharks as a Coarse Indicator of Stress. J. Coast. Res. 32, 1464–1472. doi: 10.2112/JCOASTRES-D-15-00108.1
Jordan L. K., Mandelman J. W., McComb D. M., Fordham S. V., Carlson J. K., Werner T. B. (2013). Linking Sensory Biology and Fisheries Bycatch Reduction in Elasmobranch Fishes: A Review With New Directions for Research. Conserv. Physiol. 1, 1–20. doi: 10.1093/conphys/cot002
Kabasakal H. (2010). Post-Release Behavior and Anthropogenic Injuries of the Bluntnose Sixgill Shark, Hexanchus griseus (Bonnaterr) (Chondrichthyes: Hexanchidae) in Turkish Waters. Ann. Ser. Hist. Nat. 20, 39–46.
Lavender E., Aleynik D., Dodd J., Illian J., James M., Wright P. J., et al. (2021a). Movement Patterns of a Critically Endangered Elasmobranch (Dipturus intermedius) in a Marine Protected Area. Aquat. Conserv. Mar. Freshw. Ecosyst. 32, 348–365. doi: 10.1002/aqc.3753
Lavender E., Aleynik D., Dodd J., Illian J., James M., Wright P. J., et al. (2021b). Environmental Cycles and Individual Variation in the Vertical Movements of a Benthic Elasmobranch. Mar. Biol. 168, 164. doi: 10.1007/s00227-021-03973-1
Lear K. O., Whitney N. M., Morgan D. L., Brewster L. R., Whitty J. M., Poulakis G. R., et al. (2019). Thermal Performance Responses in Free-Ranging Elasmobranchs Depend on Habitat Use and Body Size. Oecologia 191, 829–842. doi: 10.1007/s00442-019-04547-1
Little W. (1995). Common Skate and Tope: First Results of Glasgow Museum’s Tagging Study. Glas. Nat. 22, 455–466.
Mohan J. A., Jones E. R., Hendon J. M., Falterman B., Boswell K. M., Hoffmayer E. R., et al. (2020). Capture Stress and Post-Release Mortality of Blacktip Sharks in Recreational Charter Fisheries of the Gulf of Mexico. Conserv. Physiol. 8, coaa041. doi: 10.1093/conphys/coaa041
Mullen E. J., Schoen A. N., Hauger M. D., Murray L., Anderson W. G. (2020). Angler Experience and Seasonal Effects on the Response of the Lake Sturgeon to Catch-and-Release Angling. Trans. Am. Fish. Soc 149, 709–720. doi: 10.1002/tafs.10266
Musyl M. K., Gilman E. L. (2019). Meta-Analysis of Post-Release Fishing Mortality in Apex Predatory Pelagic Sharks and White Marlin. Fish Fish. 20, 466–500. doi: 10.1111/faf.12358
Neat F., Pinto C., Burrett I., Cowie L., Travis J., Thorburn J., et al. (2015). Site Fidelity, Survival and Conservation Options for the Threatened Flapper Skate (Dipturus cf. intermedia). Aquat. Conserv. Mar. Freshw. Ecosyst. 25, 6–20. doi: 10.1002/aqc.2472
Nichol D. G., Chilton E. A. (2006). Recuperation and Behaviour of Pacific Cod After Barotrauma. ICES J. Mar. Sci. 63, 83–94. doi: 10.1016/j.icesjms.2005.05.021
Ramsay J. O., Graves S., Hooker G. (2020). fda: Functional Data Analysis. R Package Version 5.1.5.1. Available at: https://cran.r-project.org/package=fda.
Raoult V., Williamson J., Smith T., Gaston T. (2019). Effects of on-Deck Holding Conditions and Air Exposure on Post-Release Behaviours of Sharks Revealed by a Remote Operated Vehicle. J. Exp. Mar. Bio. Ecol. 511, 10–18. doi: 10.1016/j.jembe.2018.11.003
R Core Team. (2020). R: A Language and Environment for Statistical Computing. Available at: https://r-project.org.
Roche D. G., Careau V., Binning S. A. (2016). Demystifying Animal Personality (or Not): Why Individual Variation Matters to Experimental Biologists. J. Exp. Biol. 219, 3832–3843. doi: 10.1242/jeb.146712
Rodríguez-Cabello C., Sánchez F. (2017). Catch and Post-Release Mortalities of Deep-Water Sharks Caught by Bottom Longlines in the Cantabrian Sea (NE Atlantic). J. Sea Res. 130, 248–255. doi: 10.1016/j.seares.2017.04.004
Rogers P. J., Knuckey I., Hudson R. J., Lowther A. D., Guida L. (2017). Post-Release Survival, Movement, and Habitat Use of School Shark Galeorhinus galeus in the Great Australian Bight, Southern Australia. Fish. Res. 187, 188–198. doi: 10.1016/j.fishres.2016.11.011
Sepulveda C. A., Heberer C., Aalbers S. A., Spear N., Kinney M., Bernal D., et al. (2015). Post-Release Survivorship Studies on Common Thresher Sharks (Alopias vulpinus) Captured in the Southern California Recreational Fishery. Fish. Res. 161, 102–108. doi: 10.1016/j.fishres.2014.06.014
Skatespotter (2021) Data Summary Report - September 2021. Available at: https://skatespotter.sams.ac.uk/news/2019/SkatespottersummarySep2021.pdf.
Skomal G. B. (2007). Evaluating the Physiological and Physical Consequences of Capture on Post-Release Survivorship in Large Pelagic Fishes. Fish. Manage. Ecol. 14, 81–89. doi: 10.1111/j.1365-2400.2007.00528.x
Thorburn J., Wright P. J., Lavender E., Dodd J., Neat F., Martin J. C. A., et al. (2021). Seasonal and Ontogenetic Variation in Depth Use by a Critically Endangered Benthic Elasmobranch and its Implications for Spatial Management. Front. Mar. Sci. 8. doi: 10.3389/fmars.2021.656368
Ullah S., Finch C. F. (2013). Applications of Functional Data Analysis: A Systematic Review. BMC Med. Res. Methodol. 13, 43. doi: 10.1186/1471-2288-13-43
Wang J.-L., Chiou J.-M., Müller H.-G. (2016). Functional Data Analysis. Annu. Rev. Stat. Its Appl. 3, 257–295. doi: 10.1146/annurev-statistics-041715-033624
Weber D. N., Frazier B. S., Whitney N. M., Gelsleichter J., Sancho G. (2020). Stress Response and Postrelease Mortality of Blacktip Sharks (Carcharhinus limbatus) Captured in Shore-Based and Charter-Boat-Based Recreational Fisheries. Fish. Bull. 118, 297–314. doi: 10.7755/FB.118.3.8
Whitney N. M., Lear K. O., Morris J. J., Hueter R. E., Carlson J. K., Marshall H. M. (2021). Connecting Post-Release Mortality to the Physiological Stress Response of Large Coastal Sharks in a Commercial Longline Fishery. PloS One 16, e0255673. doi: 10.1371/journal.pone.0255673
Whitney N., White C., Anderson P., Hueter R., Skomal G. (2017). The Physiological Stress Response, Postrelease Behavior, and Mortality of Blacktip Sharks (Carcharhinus limbatus) Caught on Circle and J-Hooks in the Florida Recreational Fishery. Fish. Bull. 115, 532–543. doi: 10.7755/FB.115.4.9
Keywords: archival, biologging, disturbance, management, Rajidae, recreational
Citation: Lavender E, Aleynik D, Dodd J, Illian J, James M, Wright PJ, Smout S and Thorburn J (2022) Behavioural Responses of a Large, Benthic Elasmobranch to Catch-and-Release Angling. Front. Mar. Sci. 9:864344. doi: 10.3389/fmars.2022.864344
Received: 28 January 2022; Accepted: 08 March 2022;
Published: 14 April 2022.
Edited by:
Jeffrey C. Mangel, Prodelphinus, PeruReviewed by:
Brett Falterman, Fisheries Research Support LLC, United StatesIan Bouyoucos, University of Manitoba, Canada
Copyright © 2022 Lavender, Aleynik, Dodd, Illian, James, Wright, Smout and Thorburn. This is an open-access article distributed under the terms of the Creative Commons Attribution License (CC BY). The use, distribution or reproduction in other forums is permitted, provided the original author(s) and the copyright owner(s) are credited and that the original publication in this journal is cited, in accordance with accepted academic practice. No use, distribution or reproduction is permitted which does not comply with these terms.
*Correspondence: Edward Lavender, ZWw3MkBzdC1hbmRyZXdzLmFjLnVr
†These authors share last authorship