- 1Institute of Oceanology “Fridtjof Nansen” – Bulgarian Academy of Sciences, Varna, Bulgaria
- 2Water Research Institute, Unit Talassografico “A. Cerruti”, National Research Council CNR-IRSA, Taranto, Italy
- 3National Research Institute of Fisheries Science, Yokohama, Japan
Plankton cyst abundance and distribution is controlled by multiple factors. The stress linked to the fluctuations and variations of the environmental conditions in the water column is a major vector of encystment and intraspecific variability is an important adaptive strategy. The present study aims to disclose a link between the spatial distribution and abundance of different cyst morphotypes of Scrippsiella acuminata complex in surface sediments collected in the Black Sea at 34 sites and selected environmental variables. With this purpose, a basin scale data set was analyzed for patterns of intraspecific spatial heterogeneity. Redundancy analysis (RDA) was implemented to identify explanatory environmental variables associated with the cyst morphotypes abundance. Environmental multiyear data were used to ensure better approximation of a model that links environmental gradients with cyst abundance. Our results show that all S. acuminata cysts morphotypes are significantly correlated to one or a combination of the environmental variables, i.e., salinity, temperature and nutrients (nitrates and phosphates). The geographical distribution of Scrippsiella blooms in the Black Sea indicates that the interplay between the planktonic and benthic habitat of the dinoflagellate gives to S. acuminata the advantage to dominate in the plankton communities.
Introduction
Many dinoflagellates produce benthic cysts as a part of their life cycle (reviewed in Belmonte and Rubino, 2019). The resting cysts accumulate in the sediments and serve as potential seed banks that secure the survival of the species over time (Lundholm et al., 2011; Ribeiro et al., 2013). They also play a crucial role in bloom initiation, especially important in the case of harmful algal bloom species (HABs) (Anderson et al., 2003; Garcés et al., 2004). Encystment is considered as an adaptive strategy the species play out to respond to environmental stresses driven by variations of environmental conditions, such as temperature, salinity, nutrient concentration or daylength. These variations can also influence the patterns of spatial distribution of dinocysts (Anderson et al., 1984; Devillers and de Vernal, 2000; Sgrosso et al., 2001; Grigorszky et al., 2006; Richter et al., 2007; Zonneveld and Susek, 2007; Kremp et al., 2009; Lundgren and Granéli, 2011; Shin et al., 2018). Furthermore, intraspecific morphological variability among cysts produced by some species, including dinoflagellates like Scrippsiella spp., has been associated to variations in environmental conditions, both in cultures (Kokinos and Anderson, 1995) and in the field (Olli and Anderson, 2002; Zonneveld and Susek, 2007; Mertens et al., 2009) and this intraspecific variability can be read as a quantitative measure of environmental variations (Mertens et al., 2009; Mertens et al., 2012a; Persson et al., 2013; Shin et al., 2013; Hoyle et al., 2019).
The cosmopolitan dinoflagellate Scrippsiella acuminata (Ehrenberg, 1836) Kretschmann, Elbrächter, Zinssmeister, S. Soehner, Kirsch, Kusber & Gottschling, 2015 (the currently accepted name of Scrippsiella trochoidea (Stein, 1883) Loeblich III, 1976), is one of the dominant species in the phytoplankton community in the Black Sea, responsible for bloom events in the coastal areas of this basin (Nesterova et al., 2008; Terenko and Terenko, 2009; Moncheva et al., 2019 and the references therein). Even the cysts of this species are widespread in the basin, being dominant in the western region off the Bulgarian coast (Rubino et al., 2010). Their presence is reported also from many sites in the South (Mudie et al., 2017) and in the Northwest off the Ukrainian coast where the abundance increases with the depth in the shelf zone (Nikonova, 2010; Nikonova, 2015; Mudie et al., 2021).
Many studies, aimed to clarify the taxonomic characters both of thecae and cysts of S. acuminata and the cyst-theca relationships, showed that actually this species should be considered as a member of a complex (Montresor et al., 2003; Gottschling et al., 2005) with cryptic species genetically differentiated but morphologically indistinguishable so far (Zinssmeister et al., 2011; Kretschmann et al., 2014).
Based on the high morphological variability of the S. acuminata cysts already observed in the Black Sea (Dzhembekova et al., 2020), and in the Mediterranean (Ferraro et al., 2017; Rubino et al., 2017) and reported also from the East China Sea (Gu et al., 2008), the aim of the present study was to clarify whether the geographic distribution of the different cyst morphotypes of S. acuminata in the surface sediments of many coastal and shelf sites of the Black Sea, could be related to other environmental variables, besides hypoxia and pH, as reported by Shin et al. (2013) and Ishikawa et al. (2019) from the Pacific Ocean. Accordingly, sea surface temperature (SST), sea surface salinity (SSS), currents speed (CS), station depth, nitrates (NO3) and phosphates (PO4) were analyzed as plausible drivers of the spatial distribution and abundance of S. acuminata cyst morphotypes.
Materials and Methods
Sampling
The study area covers Ukrainian, Romanian, Bulgarian, Turkish and Georgian waters. Surface sediment samples (top 2 cm; N = 48) were collected during different campaigns carried out in spring and/or summer seasons, from April 2008 to June 2016, at 34 sites both at coastal (C), shelf (S) and open sea (O) areas (depth 13.1 – 2100 m), using a multicorer or a Van Veen Grab sampler. In addition, material collected by a sediment trap deployed at 1,000 m in the deep sea (bottom: 2,000 m depth) was analyzed (Figure 1; Table 1) (details about the sediment trap are given in Gogou et al., 2014). All the sediment samples were stored in the dark without preservatives at 4°C until processing.
Sediment Treatment, Qualitative and Quantitative Analysis of Cysts
From each sample, an aliquot of homogenized sediment (from 2.0 to 2.2 cm3) was used for the analyses of cysts while a parallel aliquot (≈ 10 cm 3) was collected to calculate the water content. The wet aliquots were weighed and screened through a 10 µm mesh (Endecotts Limited steel sieves, ISO3310-1, London, England) using natural filtered (0.45 µm) seawater (Montresor et al., 2010). The material retained onto the sieve was ultrasonicated for 1 min at low frequency and screened again through a sieve battery (200, 75 and 20 µm mesh sizes). A fine-grained fraction (20–75 µm) mainly containing protistan cysts, was obtained. The material retained onto the 75 and 200 µm mesh was not considered in this study. No chemicals were used to dissolve sediment particles in order to preserve calcareous cyst walls.
Qualitative and quantitative analyses were carried out under an inverted microscope (Zeiss Axiovert 200M) equipped with a Leica MC170 HD digital camera at x320-400 magnification. Both full cysts with cytoplasmic content (i.e., presumably viable) and empty, already germinated cysts were enumerated; 200 viable cysts were counted at least for each sample to obtain abundance values as homogeneous as possible and evaluate rare species too. To estimate the water content of sediment an aliquot from each sample (≈ 10 cm3) was weighed and dried out overnight at 70°C. Quantitative data are reported as cysts g-1 of dry sediment.
All resting stage morphotypes were identified based on published descriptions. For organic dinocysts, the images and the key provided in Appendix B by Mudie et al. (2017) were used. The different resting stage morphotypes of S. acuminata were identified based on published descriptions (Gu et al., 2008; Shin et al., 2013; Shin et al., 2014) and germination experiments (as described below). They are distinguished here based on their size and wall features (i.e., calcified or uncalcified walls, presence and type of processes) and named with acronyms considering these features as:
SaR= S. acuminata rough
SaU= S. acuminata uncalcified
SaL= S. acuminata large
SaM= S. acuminata medium
SaS= S. acuminata small.
To confirm the taxonomical identification, germination experiments were performed on single cysts isolated into Nunclon MicroWell plates (Nalge Nunc International, Roskilde, Denmark) containing ≈ 1 ml of natural sterilized seawater. Particular attention was given to the naked forms (i.e., without calcareous structures) with a smooth wall and those with a size smaller than that typical of S. acuminata. A total of more than 100 cysts of these types were isolated, considering the samples from all the campaigns, to obtain germination. All the isolated cysts were incubated at 20°C, equinoctial photoperiod and 80 μE m-2 s-1 irradiance and examined daily until germination. The choice of the incubation conditions was based on previous studies of S. acuminata excystment (Ferraro et al., 2017; Rubino et al., 2017).
Environmental Data
A multiyear (1998-2016) data set of six environmental variables near surface temperature (SST), salinity (SSS), currents’ speed (CS), station depth, nitrates (NO3) and phosphates (PO4) concentrations) was constructed based on monthly Black Sea reanalysis products available from the Copernicus Marine Environment Monitoring Service (CMEMS https://resources.marine.copernicus.eu/?option=com_csw&task=results). The time interval of the data set was calibrated considering the time span represented by the sediment surface layer collected for the cyst analysis. The physical parameters (CMEMS product id: BLKSEA_REANALYSIS_PHYS_007_004) were derived from a hydrodynamic model (NEMO, v3.6) implemented over the whole Black Sea basin (Madec, 2012). The horizontal grid resolution of the model is 1/36° in zonal resolution, 1/27° in meridional resolution. The latter provides data for temperature, salinity, horizontal velocity (zonal and meridional components) for the Black Sea domain since 1992. Nutrients (NO3 and PO4) were obtained from CMEMS product id: BLKSEA_REANALYSIS_BIO_007_005- BAMHBI, with the same grid resolution as NEMO model (Grégoire et al., 2008; Grégoire & Soetaert, 2010; Capet et al., 2016). A multiyear (1998–2016) environmental (explanatory) dataset covering the period from April to September, corresponding with the seasons of sediment sampling campaigns for cyst collection, was extracted.
Statistical Analyses
The data set for the analysis was based on the abundance of the different Scrippsiella acuminata cyst morphotypes as registered at each coastal and shelf sampling sites during the entire survey period (N = 34 samples excluding open sea stations and the trap data). For the stations with repetitive sampling (narrow Bulgarian coastal and shelf area – stations from 12 to 20) the data were averaged for the purpose of the analyses. Detrended canonical analysis (DCA) was employed for the estimation of gradient lengths and feasibility of linear or unimodal methods, respectively. Redundancy analysis (RDA) was implemented to identify explanatory environmental variables, associated with cyst morphotypes abundance (log-transformed data). Initially, all the six environmental variables were explored by RDA to check their influence on the cyst abundance. The variables “depth” and “current” resulted not statistically significant from this preliminary analysis, so they were excluded to improve the overall model statistical significance. The variance inflection factors of the variables were calculated as a diagnostic tool for multicollinearity of data. Analysis of variance (ANOVA) was used as permutation test to assess the overall RDA model significance, RDA axes and the explanatory variables significance.
Bi-dimensional representation of the statistical comparison among the samples and the seasonal maxima of monthly mean values of environmental variables (SST, SSS, NO3 and PO4) was performed by means of non-parametric multidimensional scaling (NMDS). The analysis of similarities (ANOSIM) was carried out to statistically verify the segregation of the sampling sites in relation to the environmental variables.
All analyses and graphic representations were performed using the statistics and programming software R 3.6.2 (R Core Team, 2019), packages ‘vegan’ (Oksanen, 2015), available through the CRAN repository (www.r-project.org). All maps were produced using ArcGIS software version 10.2.2 (ESRI 2011).
Results
Cyst Distribution and Concentrations
Total dinocyst abundance varied largely during the study period, ranging from 5 cysts g-1 (st. 13, June 2008) to 5,981 cysts g-1 (st. 1, May 2016) for viable forms and from 6 cysts g-1 (st. 21, June 2008) to 3,963 cysts g-1 (st. 31, May 2016) for empty forms. Cysts of Scrippsiella spp. (i.e., also including species different from S. acuminata) were recorded at all stations representing between 29 and 100% of the total abundance of viable cysts and between 30 and 94% of empty cysts total number, with viable cysts prevailing in the majority of the samples. Altogether, 13 different taxa were distinguished within the genus Scrippsiella with six identified to species level (S. acuminata, S. kirschiae, S. lachrymosa, S. ramonii, S. spinifera, S. trifida). S. acuminata cysts were widespread throughout the entire study area (Figure 2) and dominated in the sediments, with 46 to 100% of Scrippsiella viable forms.
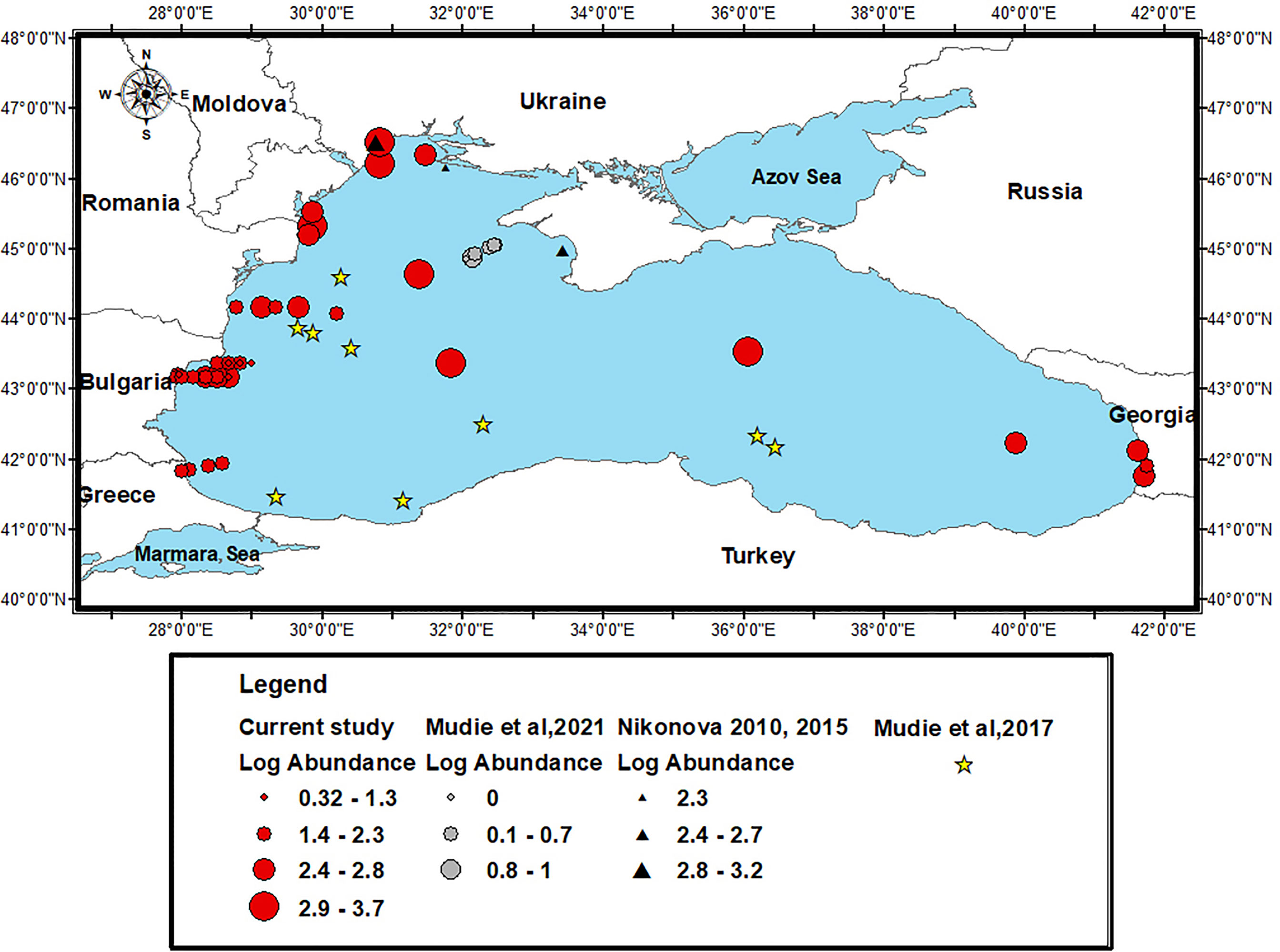
Figure 2 Distribution of S. acuminata complex cysts in the Black Sea sediments (data according to Mudie et al., 2017 are not quantitative and are shown only as presence locations).
Similar to total dinocysts, the cyst densities of S. acuminata complex were highly variable. The lowest values were registered in June 2008 at the Bulgarian shelf station 13 and at the coastal site 21, along the Varna transect, with 3 and 5 cysts g-1 respectively, while the highest densities were observed in May 2016 at the open sea station 31, with 4,275 cysts g-1 for full forms and 2,500 cysts g-1 for empty ones. Viable cyst proportions exceeded 50% (up to 100% – st. 14 in June 2008) of the total cyst abundance in about 68% of the samples, and in 58% of the samples for the empty cysts. Spatially, S. acuminata complex cysts were more abundant at open sea (347–4,275 cysts g-1). In the shelf area, the abundance varied between 3 and 1,985 full cysts g-1, and for coastal stations from 5 to 2,556 full cysts g-1.
Finally, cysts of S. acuminata complex were abundant in the sediment trap deployed at a depth of 1,000 m as well, accounting up to 83% of the Scrippsiella cysts and 48% of the total dinocyst abundance.
Scrippsiella acuminata Cysts Morphotypes – Identification and Distribution
Five cyst morphotypes of Scrippsiella acuminata were observed in the sediment samples (Figure 3, 1a-7; Table 2).
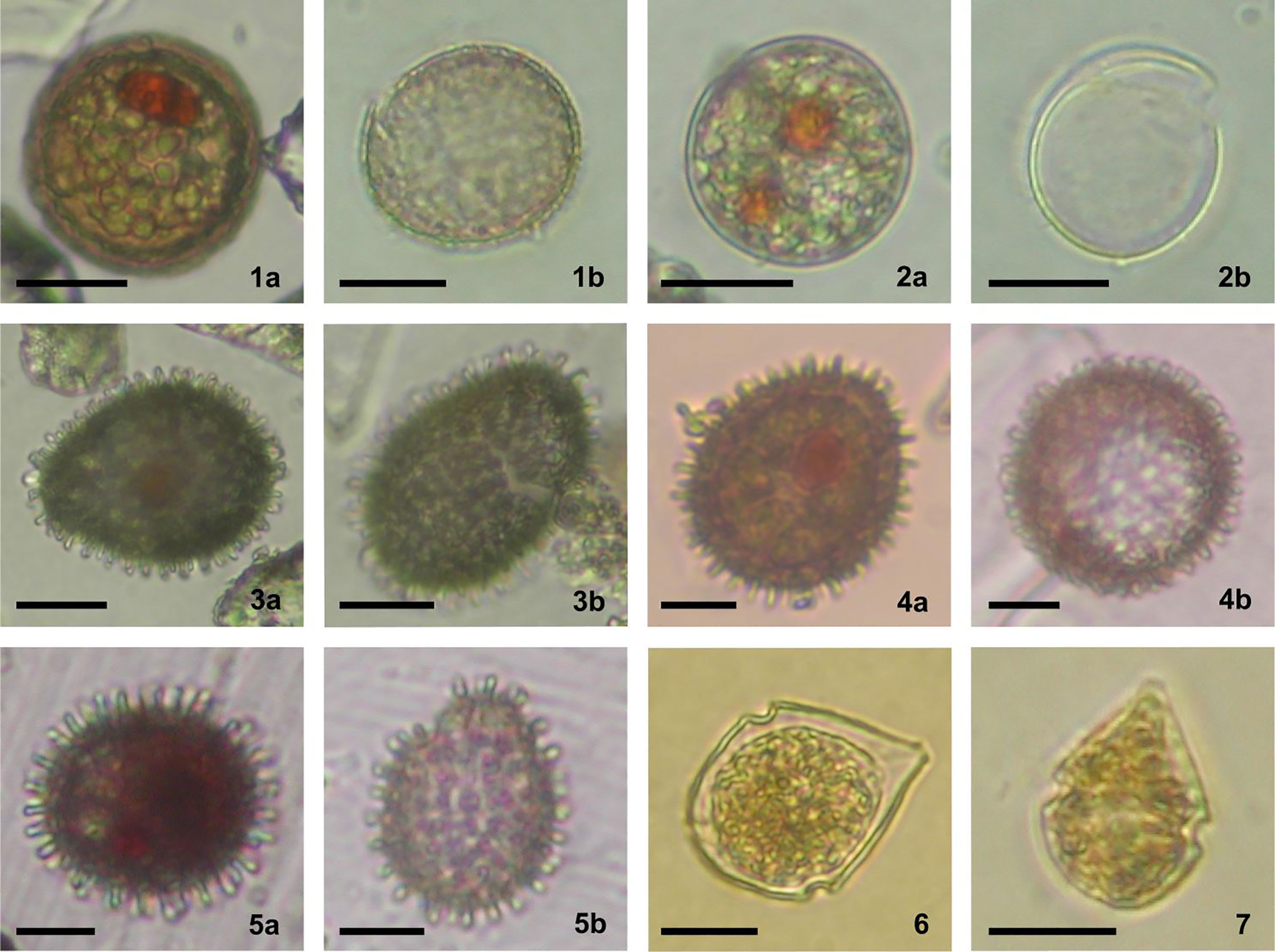
Figure 3 Different cyst morphotypes produced by Scrippsiella acuminata complex, identified in the surface sediments of the Black Sea; a and b refer to viable and germinated cysts respectively; scale bars = 20 µm, except 4a, b and 5a, b scale bars = 10 µm. 1. SaR (1a, b) – S. acuminata rough type; 2. SaU (2a, b) – S. acuminata uncalcified type; 3. SaL (3a, b) – S. acuminata large type; 4. SaM (4a, b) S. acuminata medium type; 5. SaS (5a, b) – S. acuminata small type; 6. Vegetative stage produced by germination of SaR; 7. Vegetative stage produced by germination of SaM.
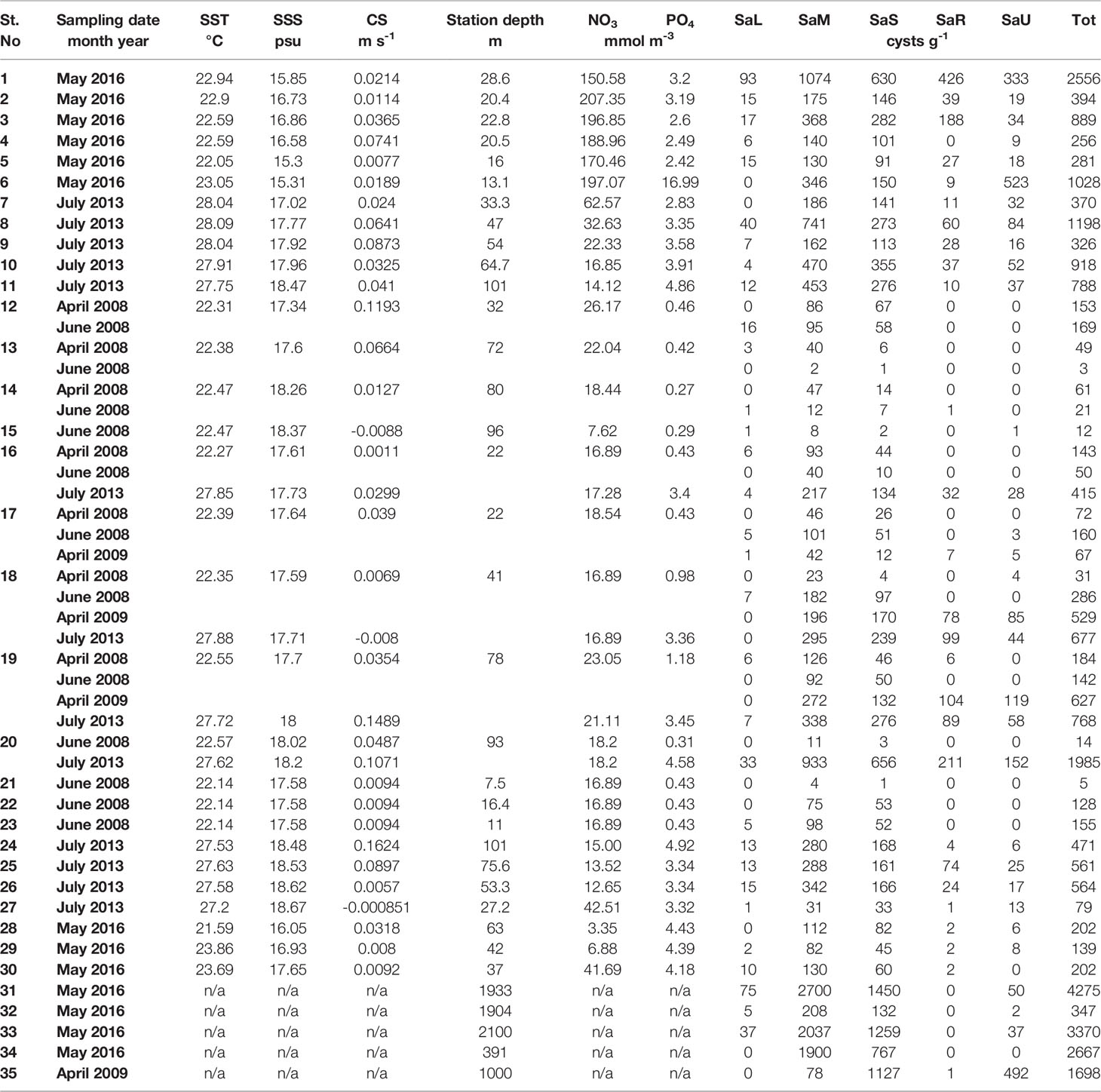
Table 2 Environmental variables (sea surface temperature, SST; sea surface salinity, SSS; currents’ speed, CS; station depth; nitrates, NO3;and phosphates, PO4) and abundance of viable S. acuminata cyst morphotypes (SaL, S. acuminata large; SaM, S. acuminata medium; SaS, S. acuminata small; SaR, S. acuminata rough; SaU, S. acuminata uncalcified; Tot , total).
SaR (Scrippsiella acuminata rough type) (Figure 3, 1a, b)
Spherical, from light to dark brown in color, 30-40 µm in diameter. The calcareous layer has a rough surface with many irregular cobblestone-like crystals. A red body is always visible inside, with many other greenish granules. The archeopyle is chasmic and epicystal.
More than 70% of the incubated cysts (n = 11) germinated producing active stages identified as Scrippsiella acuminata by light microscopy.
SaU (Scrippsiella acuminata uncalcified type) (Figure 3, 2a, b)
Spherical, light brown in color, 32-38 µm in diameter. The wall is uncalcified, made by a thick organic layer, resistant to dissolution in HCl 10%. One or two large red or sometimes orange bodies are always well visible. The archeopyle is chasmic epicystal.
More than 75% of the incubated cysts (n = 23) germinated producing active stages identified as Scrippsiella acuminata by light microscopy.
SaL (Scrippsiella acuminata large type) (Figure 3a, b)
Spherical to mostly ovoid, dark brown, with a prominent red body clearly visible inside. The wall is covered by typical rod-like capitate or spiny calcareous processes. The body size ranges from 45 µm to 55 µm for the major axis and 30 µm to 40 µm for the minor axis (excluding processes). The archeopyle is chasmic epicystal, in form of a zig-zag split along some plate boundaries. The epicyst usually remains attached. More than 80% of the incubated cysts (n = 15) germinated producing active stages identified as Scrippsiella acuminata by light microscopy. This morphotype is similar to that produced by Scrippsiella erinaceus, recently signaled in the Black Sea, along the Romanian coast (Žerdoner Čalasan et al., 2019).
SaM (Scrippsiella acuminata medium type) (Figure 3, 4a, b)
Spherical to ovoid, dark brown, with a prominent red body clearly visible below the wall. This is covered by typical rod-like capitate or spiny calcareous processes. The body size ranges from 26 µm to 31 µm for the minor axis and 30 µm to 40 µm for the major axis (excluding processes). The archeopyle is meso-epicystal, in form of a zig-zag split. There is no detachable operculum.
More than 70% of the incubated cysts (n = 18) germinated producing active stages identified as Scrippsiella acuminata by light microscopy.
SaS (Scrippsiella acuminata small type) (Figure 3, 5a, b)
Spherical to ovoid, dark brown, with a prominent red body clearly visible below the wall. This is made by calcareous capitate, sometimes spiny, processes. The body size ranges from 20 µm to 26 µm in diameter (excluding processes). The archeopyle is epicystal, in form of a zig-zag split and without an operculum that can detach. Nearly 50% of the incubated cysts (n = 10) germinated producing active stages identified as Scrippsiella acuminata by light microscopy.
Generally, more than two different S. acuminata morphotypes co-occurred in the studied samples. The morphotypes SaM and SaS resulted the most abundant, accounting for 59% and 30% of the total density of S. acuminata cysts. The abundance of SaL was much lower (only 2%) while SaR and SaU densities resulted variable, but they were observed in a high number of sites, i.e., 57% and 67%, respectively.
Concerning the spatial distribution, the highest density of SaR viable cysts (426 cysts g-1) was observed at the Ukrainian station 1, while it resulted less abundant in Georgian and Turkish areas. The range of abundance of this morphotype in coastal area was between 0 and 426 cysts g-1, whereas at the deeper stations the maximum abundance was lower (between 0 and 211 cysts g-1 for shelf and between 0 and 1 cysts g-1 for open sea). Worth to note, SaR and SaU viable cysts were observed mainly during the summer surveys. Although its variable density at the sampling sites, SaU viable cysts were particularly abundant at the Ukrainian station 6 (523 cysts g-1) (Figure 4). In the coastal area the SaU abundance was between 0 and 523 cysts g-1, similarly in the open sea it varied from 0 to 496, while in the shelf the maximum value was lower (0 – 152 cysts g-1).
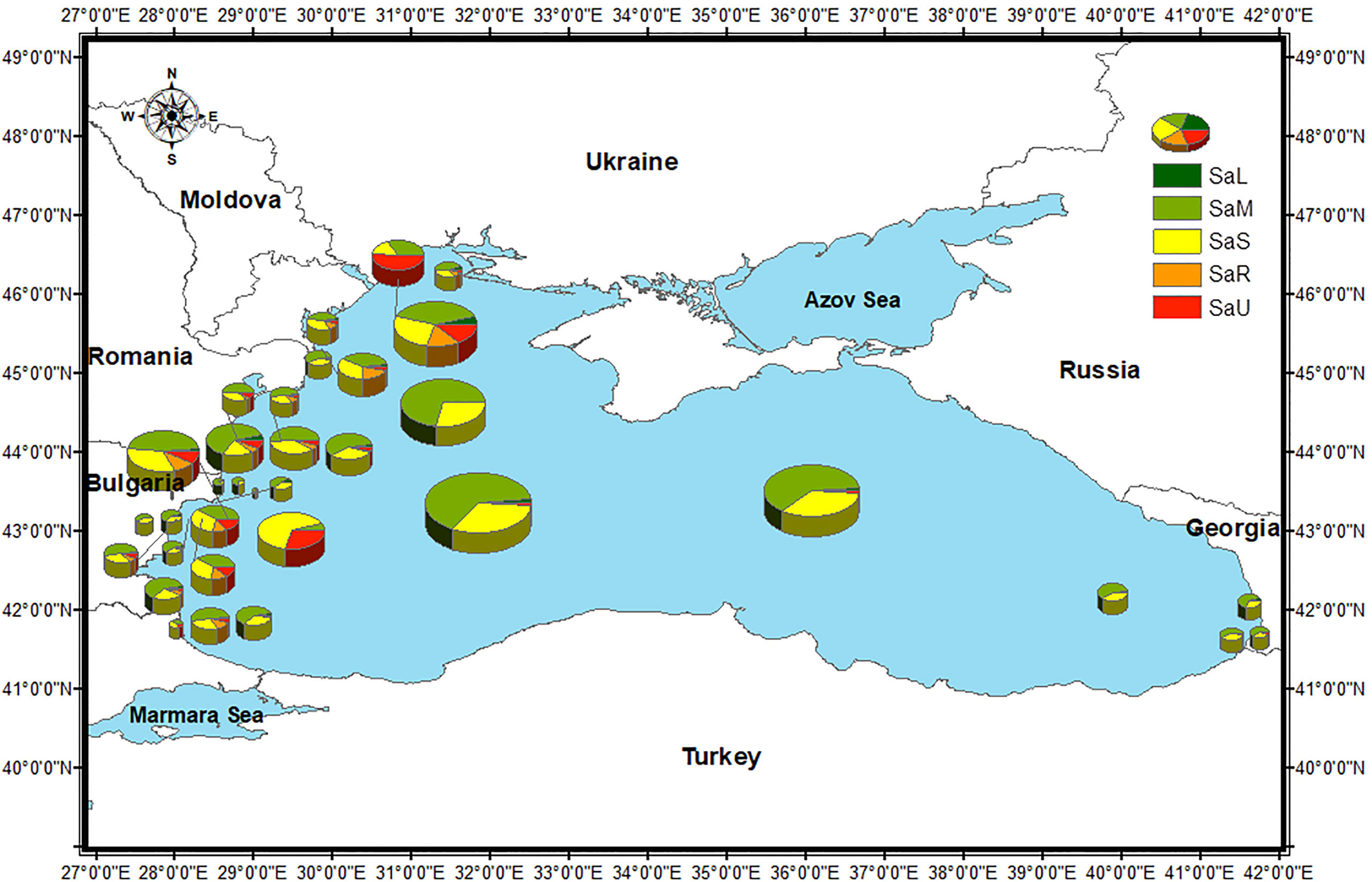
Figure 4 S. acuminata morphotypes distribution and % of the total abundance of S. acuminata viable cysts in the Black Sea, by sampling stations (for the stations with repetitive sampling, the maximum abundance is shown). Size of the pies corresponds to total S. acuminata cyst abundance (Table 2).
For SaL type the maximum recorded abundance was lowest for shelf area (0 – 33 cysts g-1) whereas the values for coastal and open sea stations were close (0 – 93 cysts g-1 and 0 – 75 cysts g-1, respectively). SaM and SaS tended to increase offshore (78 – 2700 cysts g-1 and 132 – 1450 cysts g-1, respectively). At coastal stations, they ranged between 4 and 1074 cysts g-1 for SaM and from 1 to 630 cysts g-1 for SaS. In shelf area, SaM abundance varied between 2 and 933 cysts g-1 and SaS between 1 and 656 cysts g-1.
Among the four different morphotypes collected in the trap, i.e., SaR, SaU, SaM and SaS, the “small type” was the dominant one (66% of all S. acuminata cysts), followed by the “uncalcified type” (29%) while the presence of the “medium” and “rough” types was much lower (5%).
Environmental Factors and Statistical Analyses
The summary of the environmental variables’ statistics is presented in Table 3, showing rather limited ranges of variation of salinity and temperature.
According to the NMDS ordination (ANOSIM R = 0.69 and p = 0.0001), the sampling sites, together with the seasonal maxima of the monthly values of the considered environmental variables are segregated into three clearly differentiated clusters (C1, C2 and C3) (Figure 5).
Cluster C1 comprised Ukrainian stations only (1–5) where NO3 concentrations were higher than all other sampling sites (150.6–207.3 mmol NO3 m-3). Station 6 (also located in the Ukrainian area) segregated alone due to high NO3 concentration together with the highest PO4 concentration (16.99 mmol PO4 m-3). Cluster C2 comprised all the Bulgarian sites sampled in spring and was defined by small ranges of variation of SST (22.14–22.94°C) and SSS (17.34–18.37 PSU) and relatively low NO3 and PO4 concentrations (7.62–22.04 mmol NO3 m-3 and 0.27–1.18 mmol PO4 m-3). The third cluster (C3) comprised the Romanian, Turkish, Georgian and Open Sea sites, plus the three Bulgarian sites sampled in the summer of 2013 and was correlated to higher variability of SST (21.59–28.09°C), SSS (16.05-18.67 PSU), NO3 (3.35–62.57 mmol NO3 m-3) and PO4 (2.83–4.92 mmol PO4 m-3). The segragation of sites into clusters was more strongly related to the nutrient concentrations rather than SST and SSS, which fluctuated within relatively short ranges, suggesting that eutrophication level is most likely acting as a strong selective environmental driver.
Detrended correspondence analysis (DCA) results proved that species abundance data are homogeneous and thus more suitable for linear ordination methods (1st ordination axis length is 1.21 in DC units or gradient length is < 3, respectively the most appropriate ordination method is RDA).
Variance Inflation Factors were estimated for environmental variables to test data for multicollinearity and avoid model misinterpretation. RDA provided a statistically significant model (p = 0.001, R2 = 0.43, = 0.35) with the first two axes explaining roughly 35% of the total variance in morphotype concentrations (RDA1 – 26.21%, p = 0.001, and RDA2 – 7.14%. p = 0.03). Salinity (p = 0.001), temperature (p = 0.010), phosphates (p = 0.022) and nitrates (p = 0.034) were found to significantly contribute to the variance of cyst concentrations (SSS – 10.83%, SST – 4.18%, PO4 and NO3 with roughly 4% each to the variance explained by RDA1). The RDA triplot (Figure 6) shows that SaM and SaL abundance is strongly associated with SSS, SaS and SaR with SST and NO3, while SaU is associated with NO3 and PO4 concentrations and SST.
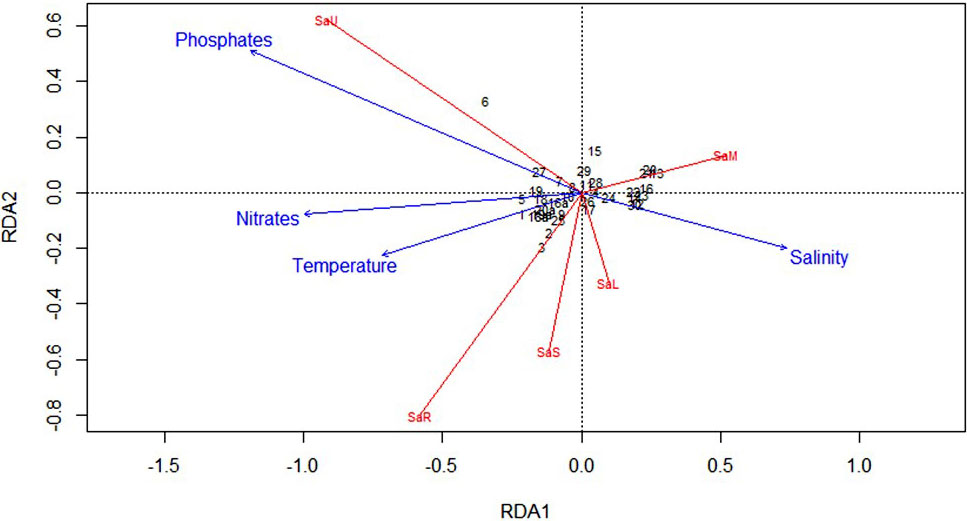
Figure 6 RDA Correlation Triplot (scaling type 2 – lc) between environmental multiyear explanatory dataset and the corresponding response cysts matrix with fitted site scores.
Discussion
The present study represents one of the largest basin-scale surveys (depth 13.1–2100 m) of Scrippsiella acuminata (the currently accepted name of S. trochoidea) cyst distribution in the Black Sea conducted to this date. As a consequence, a great variability in cysts abundance throughout the study area was observed, both for the whole dinoflagellate encysted community and the S. acuminata complex. Even considering the natural variability linked to the different seasons of sampling, the huge amplitude of densities observed (ranging from 5 to 5,981 cysts g-1 dry weight of sediment for viable forms), most likely could be associated with the different abiotic and biotic conditions occurring within the Black Sea study region, as demonstrated in the recent literature. In a comprehensive study of cysts distribution from 43 samples at water depths of 71–905 m on the outer Ukrainian Shelf and upper continental slope, Mudie et al. (2021) reported the presence of Scrippsiella spp. cysts at almost all the investigated sites with densities ranging between 2-89 cysts g-1 dry weight of sediment. The cysts of S. acuminata (as S. trochoidea) were recorded at 6 sites only with very low abundance (1–10 cysts g-1 dry weight of sediment) (Figure 2). Nikonova (2010; 2015) found much higher densities (190–1,690 cysts g-1 dry weight of sediment), at three stations in the same area close to those observed in our study (Stations 1, 5 and 6) (Table 2; Figure 2). Over the investigated 34 sites, our samples recorded a presence of the S. acuminata cysts widespread throughout the entire area and particularly higher relative abundance (46 to 100% of Scrippsiella viable forms) as compared to the data (less than 5%) documented by Mudie et al. (2017). The study of Mudie et al. (2017) was based on information from different datasets considering data from top 2 cm of sediment, similar to the current study. However, the use of hydrochloric acid impose difficulties in the differentiation of the cyst morphotypes of species with organic walls such as S. trochoidea (= S. acuminata), as highlighted in the paper (Mudie et al., 2017). Even if the highest densities were detected at the deep open sea stations (up to 4,275 cysts g-1), high values were also observed at the coastal stations (up to 2,556 cysts g-1) and at some shelf stations, suggesting no distinct pattern of spatial distribution related to depth and/or distance from the coast. Similarly, Mudie et al. (2021) exploring the relationship to sediment grain size, abiotic and biotic oceanographic parameters, including methanogenesis, found that Scrippsiella cysts (mostly cysts of S. acuminata) tend to be more abundant at higher depths on the continental slope, but this species could be also dominant on the continental shelf-break around 200 m depth.
Cysts identified in surface sediment samples are usually assumed as recently produced in relation to the sedimentary regime of the area. Nevertheless, the natural encysted community represents an integration in time and space of the species encystment/excystment dynamics and consists of different “dormancy aged” assemblages (Kim and Han, 2000) expression of different time intervals with a succession of highly variable environmental conditions. They reflect the dominant ecological processes for plankton over several years rather than a seasonal signature (Dale, 1976), that imposes a further complication to explain dinocyst distribution patterns with environmental specific conditions at the time of sampling (Devillers and de Vernal, 2000). Taking into account various factors such as sedimentation, sampling method, and amount of bioturbation, Mudie et al. (2017) estimated that the time span represented by the Black Sea surface sediment samples is between less than five years to a few decades. Therefore, in the present study, environmental multiyear data were used in the statistical analysis, to account for the disparity between the time scale of the cyst record and the time scale of the environmental factors (Ribeiro and Amorim, 2008; García-Moreiras et al., 2021) and to ensure better approximation of the model linking environmental gradients with cysts abundance data.
In this study, Redundant discriminant analysis (RDA) helped to explore the relationships between the environmental variables and the S. acuminata cyst assemblages. All the cysts morphotypes were found to be associated to a single or a combination of environmental variables, confirming that cysts beds are repositories of ecophysiological diversity for the planktonic communities (Ribeiro et al., 2013). The links revealed by the applied multivariate analyses between temperature, salinity, phosphates, and nitrates and the spatial distribution of cyst morphotypes conform to findings in other studies (e.g., Sala-Pérez et al., 2020); salinity was generally reported to induce primarily variation in cyst process morphology of some Gonyaulacacean dinoflagellate species (e.g., Ellegaard, 2000; Mertens et al., 2009). In particular, process length variation has been observed to mostly change with water density (as a function of salinity and temperature) (Mertens et al., 2011; Mertens et al., 2012a; Mertens et al., 2012b). However, other variables have been mentioned as drivers of intraspecific variation, such as water column deoxygenation (Pross, 2001; Mudie et al., 2021), temperature and nutrients, associated with intraspecific cyst body size variation (Mertens, 2013; Mousing et al., 2017). In our study, the diagnostic of morphological variability of the cyst produced by S. acuminata was particularly related to the cyst wall surface structure (calcification and length of the processes), cyst body shape and size. The literature provides contradictory links between nutrients levels and Scrippsiella cyst morphotypes. Accordingly, the naked-type (i.e. non-calcified) cysts of S. acuminata were perceived as a morphological response to pH in the sediments and it has been suggested that they could be an indicator for eutrophication or acidification (Shin et al., 2013). On the contrary, Wang et al. (2007) reported high rates of formation of the naked cyst-type of S. acuminata during the bloom and shortly after the bloom, perceived to be related to the cellular nutrient limitation, while Gu et al. (2008) did not find any effect of nutrients on calcification in laboratory conditions.
Our results suggest that the formation of different morphotypes of cysts of S. acuminata complex within the water depths studied is regulated by specific environmental conditions (salinity, temperature, nitrates and phosphates) and even if some morphotypes appeared to have more confined geographical distribution, our data did not explain the existence of explicit ecological niches. The ecological niche theory (Pocheville, 2015) assumes that differences in species composition among communities are caused by heterogeneity in the environment or by limiting resources and environmental filtering of species according to their environmental requirements, such as climate, oceanographic conditions, both in pelagic and coastal areas, and competition for resources such as nutrients for marine algae, as a result of biotic interactions (Cadotte and Tucker, 2017).
In order to add new information about the relation between environmental drivers and S. acuminata morphotypes a very cautious approach should be used to interpret the data, considering the high cryptic diversity within the Scrippsiella species complex (Montresor et al., 2003). Essentially, the taxonomy of species within S. acuminata complex is still quite challenging and problematic. DNA-based results showed the presence of two S. acuminata ribotypes in the Romanian Black Sea assigned ambiguously as S. cf. erinaceus and S. aff. acuminata (Žerdoner Čalasan et al., 2019). Thus, additional molecular analyses would be vital (Žerdoner Čalasan et al., 2019) to clarify if the morphotypes are ecotypes, produced under distinctive environmental conditions, indicating different survival strategies of a single species (Sgrosso et al., 2001), or they represent a variety of cryptic species (Zinssmeister et al., 2011).
We found out that the Black Sea sediments harbor phenotypically diverse seed banks of S. acuminata complex as described also in other geographic areas (Montresor et al., 2003; Gu et al., 2008; Rubino et al., 2016; Rubino et al., 2017). Noteworthy, S. acuminata morphotypes dominated in the sediment trap sample, collected at 1,000 depth, confirming the high efficiency of the species in cyst production (Montresor et al., 1994; Wang et al., 2004; Morozova et al., 2016). The environmental and hydrodynamic conditions in the water column can influence the resting stage assemblages as species composition and abundance, and the resting stage encystment/excystment dynamics, by coupling the bentho-pelagic compartments (Casabianca et al., 2020). The conditions leading to bloom seeding and initiation are still not fully understood, although seeding from planktonic populations is considered the most plausible hypothesis (García-Moreiras et al., 2021 and the references therein). The high S. acuminata complex cyst densities documented in our study suggest the build-up and growth in time of significant cyst beds. They play a crucial role in the dinoflagellate bloom dynamics, seeding the planktonic blooms and refueling the communities in the water column (Kremp, 2001; Rubino and Belmonte, 2021). Moreover, they ensure the persistence of the species in the environment, serving as a benthic reservoir of biodiversity (Belmonte and Rubino, 2019). The geographical spread of Scrippsiella blooms in the Black Sea indicates that most likely the interplay between the planktonic and benthic habitat is advantageous and common for S. acuminata, allowing it to dominate in the plankton communities and proliferating to bloom densities (Nesterova et al., 2008; Moncheva et al., 2019). Outbursts of S. acuminata have been observed basin-wide since the ‘70s in abundance ranging between 7.8 x 105–125.4 x 106 cells/L – in Odessa Bay (Ukraine) in 2005 (8.0 x 106 cells/L, Terenko and Terenko, 2009) and Crimea in 2011 (4.4 x 106 cells/L, Bryantseva and Gorbunov, 2012), in Romanian waters (25.8–125.4 x 106 cells/L in the period 1973–2005, Bodeanu, 2002; Nesterova et al., 2008), in Varna Bay (Bulgaria) (1.9 x 106 cells/L, Moncheva et al., 1995) in Turkish waters – Bay of Sinop (7.8 x 105 cells/L during 1995–1996, Turkoglu and Koray, 2002) and Trabson (1.34 x 106 cells/L in 2000, Feyzioğlu and Oğut, 2006), and in Russian waters (Novorosiysk) (8.6 x 106 cells/L in 2005–2006, Yasakova, 2010).
Although the new finding of different cyst morphotypes and ecological affinity to the environmental conditions and regional distribution advances our understanding of S. acuminata complex, many uncertainties remain about their ecological role in the benthic seed banks and plankton dynamics above all. Moreover, there are unanswered questions about the persistence and expansion of homogenous/heterogeneous vegetative populations in the water column in relation to the dynamics and distribution of cyst beds in the sediment. Further research focusing on the pelagic/benthic coupling however seems promising for large-scale monitoring and tracking phytoplankton community alterations and climate change effects.
Data Availability Statement
The original contributions presented in the study are included in the article/supplementary material. Further inquiries can be directed to the corresponding author.
Author Contributions
SM, ND, and IZ conceived the study. ND, SM, FR, and IZ wrote the manuscript. FR and MB analysed the cysts. SM and NS carried out the samplings and organized the research cruises. VS extracted and prepared the environmental data and maps. IZ carried out statistical analyses. All authors commented on the manuscript. All authors contributed to the article and approved the submitted version.
Funding
This study was supported by the National Science Fund, Ministry of Education and Science (MES), Bulgaria under project “Phytoplankton cysts – an intricacy between a “memory” or a “potential” for Black sea biodiversity and algal blooms” (Grant number DN01/8, 16.12.2016) and the National Science Program “Environmental Protection and Reduction of Risks of Adverse Events and Natural Disasters”, approved by the Resolution of the Council of Ministers № 577/17.08.2018 and supported by the Ministry of Education and Science (MES) of Bulgaria (Agreement № D01-230/06.12.2018).
Conflict of Interest
The authors declare that the research was conducted in the absence of any commercial or financial relationships that could be construed as a potential conflict of interest.
Publisher’s Note
All claims expressed in this article are solely those of the authors and do not necessarily represent those of their affiliated organizations, or those of the publisher, the editors and the reviewers. Any product that may be evaluated in this article, or claim that may be made by its manufacturer, is not guaranteed or endorsed by the publisher.
Acknowledgments
We thank Peta Mudie (NRCan Geological Survey Canada Atlantic) for critically reading an earlier version of the ms, providing valuable comments and many useful suggestions that greatly improved this manuscript.
References
Anderson D. M., Fukuyo Y., Matsuoka K. (2003). “Cyst Methodologies” in Manual on Harmful Marine Microalgae. Eds. Hallegraeff G. M., Anderson D. M., Cembella A. D. (Paris: UNESCO Publishing), 165–209.
Anderson D. M., Kulis D., Binder B. (1984). Sexuality and Cyst Formation in the Dinoflagellate Gonyaulax Tamarensis: Cyst Yield in Batch Cultures. J. Phycol. 20, 418–425. doi: 10.1111/j.0022-3646.1984.00418.x
Belmonte G., Rubino F. (2019). “Resting Cysts From Coastal Marine Plankton” in Oceanography and Marine Biology: An Annual Review. Eds. Hawkins S. J., Allcock A. L., Bates A. E., Firth L. B., Smith I. P., Swearer S. E., Todd P. A. (Boca Raton: CRC Press), 1–88.
Bodeanu N. (2002). Algal Blooms in Romanian Black Sea Waters in the Last Two Decades of the XXth Century. Cercet. Mar. 34, 7–22.
Bryantseva Y., Gorbunov V. (2012). Spatial Distribution Phytoplankton Basic Parameters at the Northern Black Sea. Optim. Prot. Ecosyst. 7, 126–137.
Cadotte M., Tucker C. (2017). Should Environmental Filtering be Abandoned? Trends Ecol. Evol. 32, 429–437. doi: 10.1016/j.tree.2017.03.004
Capet A., Meysman F. J. R., Akoumianaki I., Soetaert K. (2016). Integrating Sediment Biogeochemistry Into 3D Oceanic Models: A Study of Benthic-Pelagic Coupling in the Black Sea. Ocean. Modell. 101, 83–100. doi: 10.1016/j.ocemod.2016.03.006
Casabianca S., Capellacci S., Ricci F., Andreoni F., Russo T., Scardi M., et al. (2020). Structure and Environmental Drivers of Phytoplanktonic Resting Stage Assemblages in the Central Mediterranean Sea. Mar. Ecol. Prog. Ser. 639, 73–89. doi: 10.3354/meps13244
Dale B. (1976). Cyst Formation, Sedimentation, and Preservation, Factors Affecting Dinoflagellate Assemblages in Recent Sediments From Trondheimsfjord, Norway. Rev. Palaeobot. Palynot. 22, 39–60. doi: 10.1016/0034-6667(76)90010-5
Devillers R., de Vernal A. (2000). Distribution of Dinoflagellate Cysts in Surface Sediments of the Northern North Atlantic in Relation to Nutrient Content and Productivity in Surface Waters. Mar. Geol. 166, 103–124. doi: 10.1016/S0025-3227(00)00007-4
Dzhembekova N., Rubino F., Nagai S., Zlateva I., Slabakova N., Ivanova P., et al. (2020). Comparative Analysis of Morphological and Molecular Approaches Integrated Into the Study of the Dinoflagellate Biodiversity Within the Recently Deposited Black Sea Sediments – Benefits and Drawbacks. Biodivers. Data J. 8, e55172. doi: 10.3897/BDJ.8.e55172
Ellegaard M. (2000). Variations in Dinoflagellate Cyst Morphology Under Conditions of Changing Salinity During the Last 2000 Years in the Limfjord, Denmark. Rev. Palaeobot. Palynol. 109, 65–81. doi: 10.1016/S0034-6667(99)00045-7
Ferraro L., Rubino F., Belmonte M., Da Prato S., Greco M., Frontalini F. (2017). A Multidisciplinary Approach to Study Confined Marine Basins: The Holobenthic and Merobenthic Assemblages in the Mar Piccolo of Taranto (Ionian Sea, Mediterranean). Mar. Biodivers. 47, 887–911. doi: 10.1007/s12526-016-0523-0
Feyzioğlu A. M., Oğut H. (2006). Red Tide Observations Along the Eastern Black Sea Coast of Turkey. Turk. J. Bot. 30, 375–379.
Garcés E., Bravo I., Vila M., Figueroa R. I., Masó M., Sampedro N. (2004). Relationship Between Vegetative Cells and Cyst Production During Alexandrium Minutum Bloom in Arenys De Mar Harbour (NW Mediterranean). J. Plankton. Res. 26, 637–645. doi: 10.1093/plankt/fbh065
García-Moreiras I., Oliveira A., Santos A. I., Oliveira P. B., Amorim A. (2021). Environmental Factors Affecting Spatial Dinoflagellate Cyst Distribution in Surface Sediments Off Aveiro-Figueira Da Foz (Atlantic Iberian Margin). Front. Mar. Sci. 8. doi: 10.3389/fmars.2021.699483
Gogou A., Sanchez-Vidal A., Durrieu de Madron X., Stavrakakis S., Calafat A. M., Stabholz M., et al. (2014). Carbon Flux to the Deep in Three Open Sites of the Southern European Seas (SES). J. Mar. Syst. 129, 224–233. doi: 10.1016/j.jmarsys.2013.05.013
Gottschling M., Knop R., Plötner J., Kirsch M., Willems H., Keupp H. (2005). A Molecular Phylogeny of Scrippsiella Sensu Lato (Calciodinellaceae, Dinophyta) With Interpretations on Morphology and Distribution. Eur. J. Phycol. 40, 207–220. doi: 10.1080/09670260500109046
Grégoire M., Raick C., Soetaert K. (2008). Numerical Modeling of the Deep Black Sea Ecosystem Functioning During the Late 80’s (Eutrophication Phase). Prog. Oceanogr. 76, 286–333. doi: 10.1016/j.pocean.2008.01.002
Grégoire M., Soetaert K. (2010). Carbon, Nitrogen, Oxygen and Sulfide Budgets in the Black Sea: A Biogeochemical Model of the Whole Water Column Coupling the Oxic and Anoxic Parts. Ecol. Model. 221, 2287–2301. doi: 10.1016/j.ecolmodel.2010.06.007
Grigorszky I., Kiss K. T., Beres V., Bacsi I., Márta M., Máthé C., et al. (2006). The Effects of Temperature, Nitrogen, and Phosphorus on the Encystment of Peridinium Cinctum, Stein (Dinophyta). Hydrobiologia 563, 527–535. doi: 10.1007/s10750-006-0037-z
Gu H., Sun J., Kooistra W. H., Zeng R. (2008). Phylogenetic Position and Morphology of Thecae and Cysts of Scrippsiella (Dinophyceae) Species in the East China Sea 1. J. Phycol. 44, 478–494. doi: 10.1111/j.1529-8817.2008.00478.x
Hoyle T. M., Sala-Pérez M., Sangiorgi F. (2019). Where Should We Draw the Lines Between Dinocyst “Species”? Morphological Continua in Black Sea Dinocysts. J. Micropalaeontol. 38, 55–65. doi: 10.5194/jm-38-55-2019
Ishikawa A., Wakabayashi H., Kim Y. O. (2019). A Biological Tool for Indicating Hypoxia in Coastal Waters: Calcareous Walled-Type to Naked-Type Cysts of Scrippsiella Trochoidea (Dinophyceae). Plankton. Benthos. Res. 14, 161–169. doi: 10.3800/pbr.14.161
Kim Y. O., Han M. S. (2000). Seasonal Relationships Between Cyst Germination and Vegetative Population of Scrippsiella Trochoidea (Dinophyceae). Mar. Ecol. Prog. Ser. 204, 111–118. doi: 10.3354/MEPS204111
Kokinos J. P., Anderson D. M. (1995). Morphological Development of Resting Cysts in Cultures of the Marine Dinoflagellate Lingulodinium Polyedrum (=L. Machaerophorum). Palynology 19, 143–166. doi: 10.1080/01916122.1995.9989457
Kremp A. (2001). Effects of Cyst Resuspension on Germination and Seeding of Two Bloom-Forming Dinoflagellates in the Baltic Sea. Mar. Ecol. Prog. Ser. 216, 57–66. doi: 10.3354/meps216057
Kremp A., Rengefors K., Montresor M. (2009). Species Specific Encystment Patterns in Three Baltic Cold-Water Dinoflagellates: The Role of Multiple Cues in Resting Cyst Formation. Limnol. Oceanogr. 54, 1125–1138. doi: 10.4319/lo.2009.54.4.1125
Kretschmann J., Elbrächter M., Zinssmeister C., Soehner S., Kirsch M., Kusber W. H., et al. (2015). Taxonomic Clarification of the Dinophyte Peridinium Acuminatum Ehrenb., ≡ Scrippsiella Acuminata, Comb. Nov. (Thoracosphaeraceae, Peridiniales). Phytotaxa 220, 239–256. doi: 10.11646/phytotaxa.220.3.3
Kretschmann J., Zinssmeister C., Gottschling M. (2014). Taxonomic Clarification of the Dinophyte Rhabdosphaera Erinaceus Kamptner, ≡ Scrippsiella Erinaceus Comb. Nov. (Thoracosphaeraceae, Peridiniales). System. Biodivers. 12, 393–404. doi: 10.1080/14772000.2014.934406
Lundgren V., Granéli E. (2011). Influence of Altered Light Conditions and Grazers on Scrippsiella Trochoidea (Dinophyceae) Cyst Formation. Aquat. Microb. Ecol. 63, 231–243. doi: 10.3354/ame01497
Lundholm N., Ribeiro S., Andersen T. J., Koch T., Godhe A., Ekelund F., et al. (2011). Buried Alive – Germination of Up to a Century-Old Protist Resting Stages. Phycologia 50, 629–640. doi: 10.2216/11-16.1
Mertens K. N. (2013). ““Morphological Variation in Dinoflagellate Cysts: Current Status and Future Challenges.”,” in AASP-CAP-NAMS-CIMPDINO10 Joint Meeting (San Francisco, USA: Canadian Association of Palynologists), 137–137.
Mertens K. N., Bradley L. R., Takano Y., Mudie P. J., Marret F., Aksu A. E., et al. (2012a). Quantitative Estimation of Holocene Surface Salinity Variation in the Black Sea Using Dinoflagellate Cyst Process Length. Quater. Sci. Rev. 39, 45–59. doi: 10.1016/j.quascirev.2012.01.026
Mertens K. N., Bringué M., Van Nieuwenhove N., Takano Y., Pospelova V., Rochon A., et al. (2012b). Process Length Variation of the Cyst of the Dinoflagellate Protoceratium Reticulatum in the North Pacific and Baltic-Skagerrak Region: Calibration as an Annual Density Proxy and First Evidence of Pseudo-Cryptic Speciation. J. Quater. Sci. 27, 734–744. doi: 10.1002/jqs.2564
Mertens K. N., Dale B., Ellegaard M., Jansson I., Godhe A., Kremp A., et al. (2011). Process Length Variation in Cysts of the Dinoflagellate Protoceratium Reticulatum, From Surface Sediments of the Baltic–Kattegat–Skagerrak Estuarine System: A Regional Salinity Proxy. Boreas 40, 242–255. doi: 10.1111/j.1502-3885.2010.00193.x
Mertens K. N., Ribeiro S., Bouimetarhan I., Caner H., Nebout N. C., Dale B., et al. (2009). Process Length Variation in Cysts of a Dinoflagellate, Lingulodinium Machaerophorum, in Surface Sediments: Investigating its Potential as Salinity Proxy. Mar. Micropaleontol. 70, 54–69. doi: 10.1016/j.marmicro.2008.10.004
Moncheva S., Boicenco L., Mikaelyan A. S., Zotov A., Dereziuk N., Gvarishvili C., et al. (2019). “Chapter 1.3.2. Phytoplankton,” in State of the Environment of the Black Se-2014/5). Ed. Krutov A. (Istanbul, Turkey: Publications of the Commission on the Protection of the Black Sea against Pollution (BSC) 2019), 225–284. Available at: http://www.blacksea-commission.org/Inf.%20and%20Resources/Publications/SOE2014/, ISBN: 978-605-84837-0-5, 225-283.
Moncheva S., Petrova-Karadjova V., Palazov A. (1995). “Harmful Algal Blooms Along the Bulgarian Black Sea Coast and Possible Patterns of Fish and Zoobenthic Mortalities” in Harmful Marine Algal Blooms . Ed. Lassus P. (Lavoisier, Paris: Harmful marine algal blooms), 193–298.
Montresor M., Bastianini M., Cucchiari E., Giacobbe M., Penna A., Rubino R., et al. (2010). “Capitolo 26. Stadi Di Resistenza Del Plankton” in Metodologie Di Studio Del Plancton Marino. Eds. Socal G., Buttino I., Cabrini M., Mangoni O., Penna A., Totti C. (Rome: ISPRA - Istituto Superiore per la protezione e la ricerca ambientale), 258–273.
Montresor M., Montesarchio E., Marino D., Zingone A. (1994). Calcareous Dinoflagellate Cysts in Marine Sediments of the Gulf of Naples (Mediterranean Sea). Rev. Palaeobot. Palynol. 84, 45–56. doi: 10.1016/0034-6667(94)90040-X
Montresor M., Sgrosso S., Procaccini G., Kooistra W. H. (2003). Intraspecific Diversity in Scrippsiella Trochoidea (Dinophyceae): Evidence for Cryptic Species. Phycologia 42, 56–70. doi: 10.2216/i0031-8884-42-1-56.1
Morozova T. V., Orlova T. Y., Efimova K. V., Lazaryuk A. Y., Burov B. A. (2016). Scrippsiella Trochoidea Cysts in Recent Sediments From Amur Bay, Sea of Japan: Distribution and Phylogeny. Bot. Mar. 59, 159–172. doi: 10.1515/bot-2015-0057
Mousing E. A., Ribeiro S., Chisholm C., Kuijpers A., Moros M., Ellegaard M. (2017). Size Differences of Arctic Marine Protists Between Two Climate Periods – Using the Paleoecological Record to Assess the Importance of Within-Species Trait Variation. Ecol. Evol. 7, 3–13. doi: 10.1002/ece3.2592.
Mudie P. J., Marret F., Mertens K. N., Shumilovskikh L., Leroy S. A. (2017). Atlas of Modern Dinoflagellate Cyst Distributions in the Black Sea Corridor: From Aegean to Aral Seas, Including Marmara, Black, Azov and Caspian Seas. Mar. Micropaleontol. 134, 1–152. doi: 10.1016/j.marmicro.2017.05.004
Mudie P. J., Yanko-Hombach V. V., Mudryk I. (2021). Palynomorphs in Surface Sediments of the North-Western Black Sea as Indicators of Environmental Conditions. Quat. Int. 590, 122–145. doi: 10.1016/j.quaint.2020.05.014
Nesterova D., Moncheva S., Mikaelyan A., Vershinin A., Akatov V., Boicenco L., et al. (2008). “Chapter 5.The State of Phytoplankton” in State of the Environment of the Black Se-2006/7). Ed. Oguz T. (Istanbul, Turkey: Black Sea Commission Publications 2008-3), 133–167. Available at: http://www.blacksea-commission.org/_publ-SOE2009.asp.
Nikonova S. (2010). The Dinoflagellate Cysts of Odessa and Tendra Regions of the Northwestern Part of the Black Sea. Sci. Iss. Ternopil. Natl. Pedagogic. Univ. Series.: Biol. 3, 190–192.
Nikonova S. (2015). Spatial Distribution of Dinoflagellates Cysts in the Northern Part of the Black Sea. Sci. Iss. Ternopil. Volody. Hnatiuk. Natl. Pedagogic. Univ. Series.: Biol. 64, 3-4, 503–506.
Oksanen J. (2015) Vegan: An Introduction to Ordination. Available at: http://cran.r-project.org/web/packages/vegan/vignettes/intro-vegan.pdf.
Olli K., Anderson D. M. (2002). High Encystment Success of the Dinoflagellate Scrippsiella Cf. Lachrymose in Culture Experiment. J. Phycol. 38, 145–156. doi: 10.1046/j.1529-8817.2002.01113.x
Persson A., Smith B. C., Morton S., Shuler A., Wikfors G. H. (2013). Sexual Life Stages and Temperature Dependent Morphological Changes Allow Cryptic Occurrence of the Florida Red Tide Dinoflagellate Karenia Brevis. Harm. Algae. 30, 1–9. doi: 10.1016/j.hal.2013.08.004
Pocheville A. (2015). “The Ecological Niche: History and Recent Controversies” in Handbook of Evolutionary Thinking in the Sciences. Eds. Heams T., Huneman P., Lecointre G., Silberstein M. (Dordrecht: Springer Science+Business Media Dordrecht), 543–586. doi: 10.1007/978-94-017-9014-7_26
Pross J. (2001). Paleo-Oxygenation in Tertiary Epeiric Seas: Evidence From Dinoflagellate Cysts. Palaeogeogr. Palaeoclimatol. Palaeoecol. 166, 369–381. doi: 10.1016/S0031-0182(00)00219-4
R Core Team (2019). R: A Language and Environment for Statistical Computing (Vienna, Austria: R Foundation for Statistical Computing). Available at: https://www.R-project.org/.
Ribeiro S., Amorim A. (2008). Environmental Drivers of Temporal Succession in Recent Dinoflagellate Cyst Assemblages From a Coastal Site in the NorthEast Atlantic (Lisbon Bay, Portugal). Mar. Micropaleontol. 68, 156–178. doi: 10.1016/j.marmicro.2008.01.013
Ribeiro S., Berge T., Lundholm N., Ellegaard M. (2013). Hundred Years of Environmental Change and Phytoplankton Ecophysiological Variability Archived in Coastal Sediments. PloS One 8, e61184. doi: 10.1371/journal.pone.0061184
Richter D., Vink A., Zonneveld K. A., Kuhlmann H., Willems H. (2007). Calcareous Dinoflagellate Cyst Distributions in Surface Sediments From Upwelling Areas Off NW Africa, and Their Relationships With Environmental Parameters of the Upper Water Column. Mar. Micropaleontol. 63, 201–228. doi: 10.1016/j.marmicro.2006.12.002
Rubino F., Belmonte G. (2021). Habitat Shift for Plankton: The Living Side of Benthic-Pelagic Coupling in the Mar Piccolo of Taranto (Southern Italy, Ionian Sea). Water 13, 3619. doi: 10.3390/w13243619
Rubino F., Cibic T., Belmonte M., Rogelja M. (2016). Microbenthic Community Structure and Trophic Status of Sediments in the Mar Piccolo of Taranto (Mediterranean, Ionian Sea). Environ. Sci. Pollut. Res. 23, 12624–12644. doi: 10.1007/s11356-015-5526-z
Rubino F., Moncheva S., Belmonte M., Slabakova N., Kamburska L. (2010). Resting Stages Produced by Plankton in the Black Sea — Biodiversity and Ecological Perspective. Rap. Comm. Int. Pour. l’Exploration. Scientif. La. Mer. Mediter. 39, 399.
Sala-Pérez M., Lattuada M., Flecker R., Anesio A., Leroy S. A. G. (2020). Dinoflagellate Cyst Assemblages as Indicators of Environmental Conditions and Shipping Activities in Coastal Areas of the Black and Caspian Seas. Reg. Stud. Mar. Sci. 39, 101472. doi: 10.1016/j.rsma.2020.101472
Sgrosso S., Esposito F., Montresor M. (2001). Temperature and Daylength Regulate Encystment in Calcareous Cyst-Forming Dinoflagellates. Mar. Ecol. Prog. Ser. 211, 77–87. doi: 10.3354/meps211077
Shin H. H., Jung S. W., Jang M. C., Kim Y. O. (2013). Effect of pH on the Morphology and Viability of Scrippsiella Trochoidea Cysts in the Hypoxic Zone of a Eutrophied Area. Harm. Algae. 28, 37–45. doi: 10.1016/j.hal.2013.05.011
Shin H. H., Li Z., Kim Y. O., Jung S. W., Han M. S. (2014). Morphological Features and Viability of Scrippsiella Trochoidea Cysts Isolated From Fecal Pellets of the Polychaete. Capitella Harm. Algae. sp37, 47–52. doi: 10.1016/j.hal.2014.05.005
Shin H. H., Li Z., Lim D., Lee K. W., Seo M. H., Lim W. A. (2018). Seasonal Production of Dinoflagellate Cysts in Relation to Environmental Characteristics in Jinhae-Masan Bay, Korea: One-Year Sediment Trap Observation. Estuar. Coast. Shelf. Sci. 215, 83–93. doi: 10.1016/j.ecss.2018.09.031
Terenko L., Terenko G. (2009). Dynamics of Scrippsiella Trochoidea (Stein) Balech 1988 (Dinophyceae) Blooms in Odessa Bay of the Black Sea (Ukraine). Oceanol. Hydrobiol. Stud. 38, 107–112.
Turkoglu M., Koray T. (2002). Phytoplankton Species’ Succession and Nutrients in the Southern Black Sea (Bay of Sinop). Turk. J. Bot. 26, 235–252.
Wang Z., Matsuoka K., Qi Y., Chen J., Lu S. (2004). Dinoflagellate Cyst Records in Recent Sediments From Daya Bay, South China Sea. Psychol. Res. 52, 396–407. doi: 10.1111/j.1440-183.2004.00357.x
Wang Z., Qi Y., Yang Y. (2007). Cyst Formation: An Important Mechanism for the Termination of Scrippsiella Trochoidea (Dinophyceae) Bloom. J. Plankton. Res. 29, 209–218. doi: 10.1093/plankt/fbm008
Yasakova O. N. (2010). “Annual Dynamics of Toxic Phytoplankton in Novorossiysk Bay” in ICHA14 Conference Proceedings Crete. Crete, International Society for the Study of Harmful Algae and Intergovernmental Oceanographic Commission of UNESCO, 132.
Žerdoner Čalasan A., Kretschmann J., Filipowicz N. H., Irimia R. E., Kirsch M., Gottschling M. (2019). Towards Global Distribution Maps of Unicellular Organisms Such as Calcareous Dinophytes Based on DNA Sequence Information. Mar. Biodivers. 49 (2), 749–758. doi: 10.1007/s12526-018-0848-y
Zinssmeister C., Soehner S., Facher E., Kirsch M., Meier K. S., Gottschling M. (2011). Catch Me If You can: The Taxonomic Identity of Scrippsiella Trochoidea (F. Stein) AR Loebl. (Thoracosphaeraceae, Dinophyceae). System. Biodivers. 9, 145–157. doi: 10.1080/14772000.2011.586071
Keywords: Black Sea, Scrippsiella acuminata, cyst abundance, cyst distribution, environmental variables, redundancy analysis
Citation: Dzhembekova N, Rubino F, Belmonte M, Zlateva I, Slabakova N, Ivanova P, Slabakova V, Nagai S and Moncheva S (2022) Distribution of Different Scrippsiella acuminata (Dinophyta) Cyst Morphotypes in Surface Sediments of the Black Sea: A Basin Scale Approach. Front. Mar. Sci. 9:864214. doi: 10.3389/fmars.2022.864214
Received: 28 January 2022; Accepted: 04 April 2022;
Published: 28 April 2022.
Edited by:
Alberto Basset, University of Salento, ItalyReviewed by:
Haifeng Gu, State Oceanic Administration, ChinaJelena Godrijan, Rudjer Boskovic Institute, Croatia
Copyright © 2022 Dzhembekova, Rubino, Belmonte, Zlateva, Slabakova, Ivanova, Slabakova, Nagai and Moncheva. This is an open-access article distributed under the terms of the Creative Commons Attribution License (CC BY). The use, distribution or reproduction in other forums is permitted, provided the original author(s) and the copyright owner(s) are credited and that the original publication in this journal is cited, in accordance with accepted academic practice. No use, distribution or reproduction is permitted which does not comply with these terms.
*Correspondence: Snejana Moncheva, snejanam@abv.bg