- 1Stazione Zoologica Anton Dohrn, Department of Marine Animal Conservation and Public Engagement, Naples, Italy
- 2Stazione Zoologica Anton Dohrn, Department of Marine Biotechnologies, Naples, Italy
- 3Stazione Zoologica Anton Dohrn, Department of Research Infrastructures for Marine Biological Resources, Naples, Italy
- 4Stazione Zoologica Anton Dohrn, Department of Integrative Marine Ecology, Sicily Marine Center, Messina, Italy
The Ocean Decade (OD) is the name of a United Nations (UN) initiative devoted to ocean science for sustainable development. It started in 2021 and will provide an opportunity to create a new foundation, across the science-policy interface, to strengthen the sustainable management of oceans and coasts and, at same time, bring benefits for future generations. The OD will establish a common strategy to achieve the objectives of the 2030 Agenda for Sustainable Development and the other European Union (EU) and international agreements, such as the EU Marine Strategy Framework Directive (MSFD), the Marine Biodiversity Strategy and the UN World Assessment I and II aimed at preserving ocean health. Furthermore, the OD has several expected outcomes that concern different aspects of marine environment, including its enormous values for humans. Several entities will combine efforts to achieve these goals and science is at the forefront of the sustainable blue economy. Marine research is solving complex challenges through interdisciplinary approaches, revolutionizing our life and our interaction with the ocean. This review discusses recent advancements in science related to the OD outcomes. The role of new technology for ocean exploration and monitoring, the importance of omics science and biotechnology to deal with ocean pollution, and other innovative solutions are discussed. All of these are inspired by the idea of using marine resources in a sustainable way and without impacting in a negative way on marine ecosystems. The role of science communication is therefore considered a crucial issue to spread the OD messages and to reach the general public and stakeholders. Only by the integration of science, governance, industry and public, the OD will have any chance to succeed.
1 Introduction
The ocean is a vast body of saltwater on the Earth’s surface interconnected with human activity and well-being, and marine ecosystems are both a source of food and energy, and a fundamental actor in regulating climate and in the carbon sink process. However, the ocean is largely impacted by anthropic activities, such as pollution, overfishing, global warming, anoxia and acidification, that seriously threaten the ocean’s current state and conservation. To respond to these threats, it is urgent to develop and adopt new solutions that involve stakeholders in different territorial contexts (local, regional, national and global). Many preparatory actions have been started in recent years, leading to the 2021 launch of the UN Decade of Ocean Science. What follows is a brief history, summarizing the novel concepts introduced. In 2014, the EU Commission launched the Maritime Spatial Planning (MSP)1 which is ‘a process by which the relevant Member State’s authorities analyze and organize human activities in marine areas to achieve ecological, economic and social objectives. Generally, MSP foresees a consolidative process to face the growing demand for maritime space from traditional and emerging sectors while conserving the correct performance of the marine ecosystems. In 2016, the Intergovernmental Oceanographic Commission of UNESCO started to develop this concept involving all the interested parties. In 2020, the new EU Biodiversity Strategy for 20302 has been launched in order to reinforce the defense of marine ecosystems and to reinstate them to accomplish “good environmental status”, including through the increase of secure areas and the institution of severely protected areas for habitats and fish stocks salvage. The Strategy highlights the necessity for an ecosystem-based methodology to manage human activities at sea. It also envisages the reduction or at least the elimination of by-catch for increasing the protection of sea mammals and to avoid the tackling practices for reducing the seabed damage. Finally, during the 72nd Session of the United Nations General Assembly the UN Decade of Ocean Science for Sustainable Development (2021–2030) or ‘Ocean Decade (OD)’ was proclaimed by the motto: “The science we need for the ocean we want”.
The UN raised the role of marine science from lab scale to social and political sphere, involving the society into the ideas of sustainable development and marine conservation, connecting people and oceans. The OD is strictly linked to the 2030 Agenda adopted by the UN in 2015 with its 17 Sustainable Development Goals3 and to many others EU and international agreements aimed at preserving the ocean environment. In 2021 the United Nation published the World Assessment II, which describes the five drivers that apply pressure on the ocean; drivers are characterized as social, demographic and economic developments in societies, including changes in lifestyles and associated consumption and production patterns that apply pressures to the ocean. Relationships between drivers and pressures (and their impacts) are complex and dynamic, with interconnections leading to cumulative interactions. The OD aims at increasing the understanding of marine processes and reducing human pressures, restoring ecosystems for the benefit of future generations, and promoting scientific research to support countries’ actions towards sustainability. In order to achieve these ambitions, a true revolution of science is needed, focusing on solutions for the society, developing an integrated approach, using multi- and inter-disciplinary techniques and with audacious and farsighted challenges. Science should be inclusive and planned as a “multi-stakeholder environment to be relevant and responsive across the entire value-chain; shared and communicated in forms that are widely understood across society and that trigger excitement about the ocean and behavior change”4. The OD has identified seven outcomes (see Figure 1) that describe the state of the ocean at the end of the decade (“the ocean we want”).
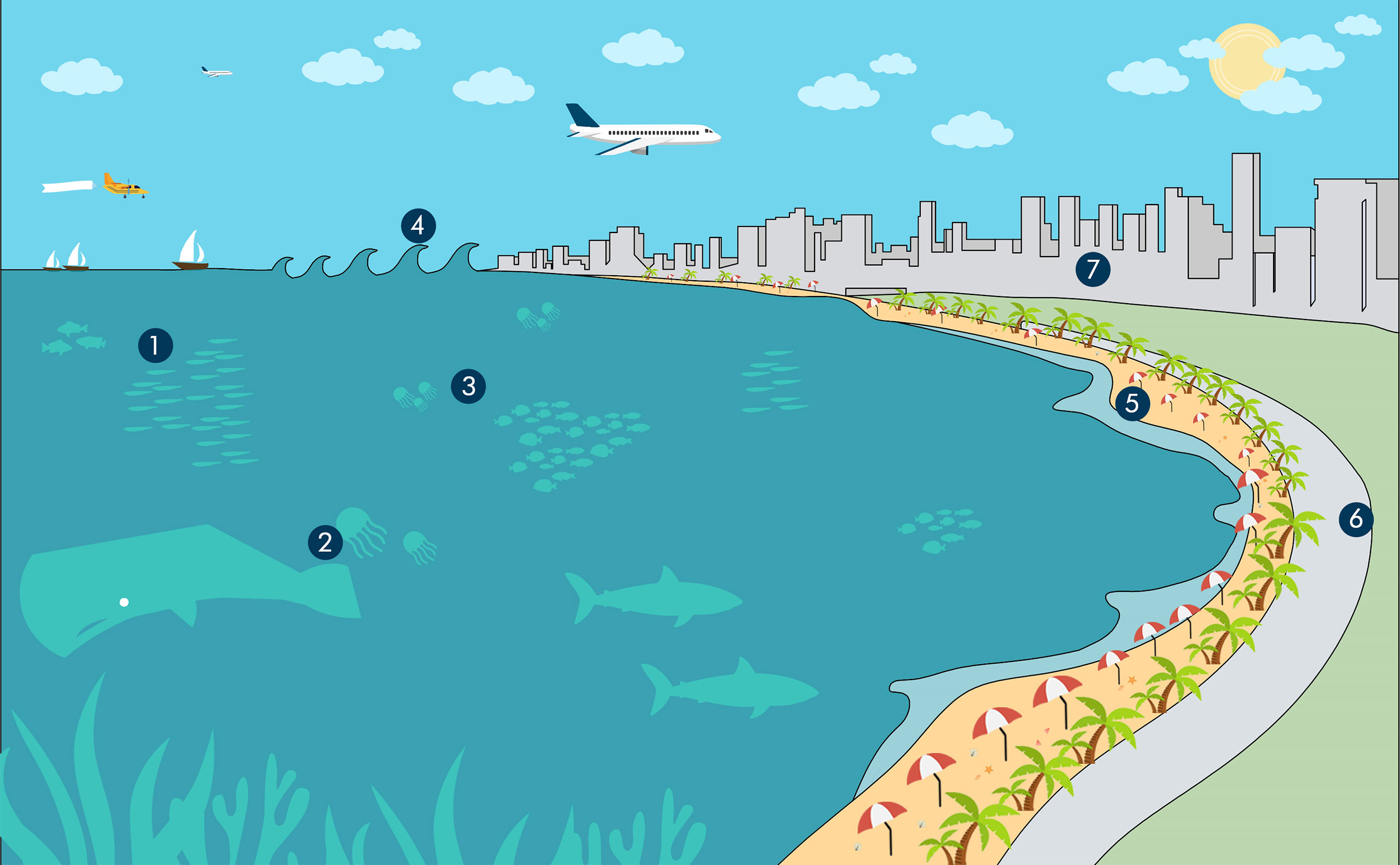
Figure 1 The areas where the OD outcomes are expected to be addressed. The actions of the first three outcomes take place in the Ocean as they relate to its conservation. Outcomes 4 and 5 take place on the coast as they relate to the interaction between the Ocean and human society. Outcomes 6 and 7 are achieved in the city, understood as community, as they concern information sharing and communication on the Ocean.
The first three outcomes are: (1) “A clean ocean”, (2) “A healthy and resilient ocean”, (3) “A productive ocean”. These are dedicated to the well-being of the marine environment, the removal of pollution, the protection and understanding of fragile habitats and the defense of ocean resources for the benefit of nature but also for the good of humans and animals that rely on them. The outcomes (4) “A predicted ocean” and (5) “A safe ocean” are focused instead on a better understanding of the ocean, promoting its exploration, and predicting ocean’s hazards, and developing solutions in order to minimize their impact. The last two outcomes are mostly related to data management and communication: outcome (6) “An accessible ocean” aims at promoting access to information, technology, and innovation regarding the ocean among all the stakeholders (community, states, industries, scientists); while the outcome (7) “An inspiring and engaging ocean” promotes the better understanding of ocean value and ocean literature, and their importance to the whole society.
These outcomes are specifically linked to the Sustainable Development goals that will shape and support the scientific efforts worldwide and of course, the OD is linked to many targets of the SDG 14: Life Below Water.
Specifically:
SDG 14.1: By 2025, prevent and significantly reduce marine pollution of all kinds, in particular from land- based activities, including marine debris and nutrient pollution.
SDG 14.5: By 2020, conserve at least 10 percent of coastal and marine areas, consistent with national and international law and based on the best available scientific information.
SDG 14.7: By 2030, increase the economic benefits to small island developing States and least developed countries from the sustainable use of marine resources.
SDG 14.a: Increase scientific knowledge, develop research capacity, and transfer marine technology, in order to improve ocean health and to enhance the contribution of marine biodiversity in developing countries.
SDG 14.3: Minimize and address the impacts of ocean acidification, including through enhanced scientific cooperation at all levels.
The OD will involve the main actors of our society (general public, governance, industry, science) and its success will in fact guarantee long-term sustainability and benefits both for the oceans and the social community (Figure 2). In this review, we present the status quo of marine science focusing on its perspectives in relation to the OD outcomes. Recently accomplished progresses are discussed, particularly in the field of: (i) marine exploration (focusing deep-sea), (ii) non-invasive methods to monitor marine organisms and habitats, (iii) marine biotechnology to develop sustainable solutions, (iv) technology to remotely observe extreme ecosystems, (v) alert systems to predict ocean hazards, (vi) conservation actions to protect all livelihood that depends on marine environment, (vii) communication tools to share scientific results to society by increasing public knowledge and awareness for all of us as stakeholders of the ocean.
2 Scientific Effort to Address OD Outcomes
2.1 Outcome 1: A Clean Ocean
(Where sources of pollution are identified, and reduced or removed)
Marine pollution has reached alarming levels in the last decades. Marine sediments of coastal areas act as sink and storage for large amounts of toxic chemical contaminants, typically persistent organic pollutants (POPs) and heavy metals. These molecules tend to bioaccumulate and biomagnify through the marine food web and have been associated with several devastating effects to the ecosystems (Landrigan et al., 2020; Sanganyado et al., 2021). In open waters, major risks are represented by oil spills, caused by oil extraction and/or transportation. Plastic pollution is also considered to be one of the most dangerous hazards the ocean is facing due to the uncontrolled use and mismanagement of waste (Cózar et al., 2014; Law et al., 2014; Sharifinia et al., 2020). It is expected that 20 million tons of plastic will be released in the ocean by 2030, and this represents a major threat for the marine environment (Gewert et al., 2015). In the past, the strategies employed relied on sediment removal and treatment, but these approaches had several disadvantages, such as limited space capacity and high cost, and their application can sometimes be impractical. Thanks to the latest advances in marine biotechnology, in situ bioremediation strategies have proven their efficacy for the removal of pollutants, particularly of hydrocarbons (Skinder et al., 2020).
Bioremediation technologies include biosurfactant amendments, biostimulation and bioaugmentation. Biosurfactants are amphipathic molecules produced by microorganisms that are extensively used for bioremediation of hydrocarbons as they enhance oil solubilization, increasing hydrocarbons availability to microorganisms able to degrade these pollutants (Kubicki et al., 2019). Biosurfactants are gradually replacing synthetic surfactants in many fields because of their low toxicity, high biodegradability and tolerance to extreme conditions (Olasanmi and Thring, 2018; Naughton et al., 2019). Identification of new biosurfactants with better properties, as well as identification of their biosynthetic pathways, that can lead to enhanced production in heterologous hosts, will be crucial for further exploiting these molecules in bioremediation. Through biostimulation, metabolic activity of endogenous microorganisms is increased. This is achieved by providing nutrients (carbon, nitrogen or phosphorus sources) that support the growth of the bacteria already present in the contaminated site (Andreolli et al., 2015; Paniagua-Michel, 2015). Biostimulation is regarded as an effective remediation strategy with the advantage of not-altering the taxonomic structure of the bacterial community; a limitation to this strategy is related to nutrients delivery, as they could be easily dispersed in water (Gertler et al., 2015). The poor presence of microorganisms with adequate degradative/assimilative capabilities in the polluted area may be another limiting factor. Bioaugmentation can in fact overcome these limitations: the addition to the pollution site of specialized microbes selected in laboratory conditions has proven to be a very effective approach for the removal of pollutants (Da Silva and Alvarez, 2010; Fernandez-Luqueno et al., 2011). Microorganisms can be supplied as a single strain or as a consortium of different strains with specialized ability (hydrocarbons degradation, biosurfactants production, biofilm formation). However, the success of the bioaugmentation process depends mostly on the adaptation of the microbial consortia to the contaminated environment. Very often the bacteria tend to disperse in the environment or cannot survive in it due to both abiotic and biotic factors. Encapsulation of microorganisms is a good strategy that consists of entrapping living microorganisms within a semi-permeable gel or carrier materials. In this state, biological and physical stabilities are ensured by reducing challenges such as sudden variations of temperature or pH. Overall, encapsulation is associated with high biomass concentration and enhanced cell survival, and it has been successfully investigated and employed (Shi et al., 2014; Surkatti and El-Naas, 2014; Dellagnezze et al., 2016).
Another potential advantage of bioaugmentation is the possibility to introduce genetically modified strains. The advances in our knowledge of pollutants degradation metabolism and in genetic engineering allow us to design and construct better strains for this task. So far, many engineered bacteria with augmented abilities of pollutant degradation have been constructed, significantly improving the efficiency in degradation of pollutants (Liu et al., 2019). Engineered microorganisms could offer a viable way of tackling the problem of plastic and microplastic pollution in the oceans. Many recent reports have clearly indicated that some microorganisms possess enzymes that can help to degrade plastic polymers (Amobonye et al., 2021); hence, integration of these genes or pathways in suitable optimized microbial hosts could be pivotal for plastic bioremediation. The major limitation for plastic degradation is the still poor knowledge of plastic-degrading enzymes (PDEs), so the identification of new microorganisms acting on the plastic polymers is expected to drive the discovery of biocatalysts with functional features never described so far. Cutinases, esterases and lipases are able to hydrolyze ester bonds in polystyrene (PS), polyurethane (PUR) and polyethylene terephthalate (PET) (Schmidt et al., 2016), whereas lignin-degrading enzymes (e.g., laccases, manganese and lignin peroxidases) are involved in the degradation of the C-C backbone of polyethylene (PE) (Krueger et al., 2015). Recently, the screening of microbial communities from environmental samples contaminated by PET led to the isolation of the bacterium Ideonella sakaiensis 201-F6 that a) produces a PET hydrolase, which hydrolyzes PET into mono (2-hydroxyethyl) terephthalic acid (MHET), and a MHET hydrolase that degrades MHET to terephthalic acid and ethylene glycol, and b) can grow on PET (Yoshida et al., 2016). Biotechnology can also offer solutions to the plastic problem acting at its source, i.e., waste generation. Biodegradable polymers that have a very low or null impact on the environment include among others polylactic acid (PLA) and polyhydroxyalkanoate (PHA). PHAs are entirely a product of microbial metabolism, while PLA is produced through a combination of fermentation (to produce lactic acid) and chemistry (Cheng et al., 2009). Their use is still limited due to uncompetitive pricing compared to fossil-based plastics, but the continuing advances in metabolic engineering are expected to close the gap, allowing to design novel pathways to both known and novel biodegradable polymers.
For bioremediation processes, microorganisms are the principal biological entities used. Specifically, bacteria and microalgae are widely employed for bioremediation of PAHs and heavy metals due to their fast growth, their versatile metabolism and their natural occurrence in all the areas of the ocean. However, systems employing macroorganisms already exist and have found many applications. Macroalgae can accumulate heavy metals, through different mechanisms and to degrade emerging contaminants, primarily synthetic organic chemicals (Kumar et al., 2016; Zeraatkar et al., 2016; Ayele et al., 2021). Therefore, they represent a promising solution for the treatment of municipal or industrial wastewater. This water is characterized by poor quality and high levels of anthropic contamination with a broad range of chemicals (Muñoz et al., 2009). These effluents end into rivers, lakes, aquifers and coastal waters, supplying the marine environment with hazardous chemicals that can have direct or indirect effects on the entire ecosystem. Macroalgae-based technologies for wastewater treatment have already been implemented in many countries such as the USA, UK and Australia, using different approaches that have the great advantage to yield high volumes of algal biomass that can be used as source of compounds, or fertilizers or even energy (Piccini et al., 2019; Wollmann et al., 2019). More recent implementations regard the synergistic utilization of micro- and macroalgae for heavy metals bioremediation and the on-field application of engineered organisms (Lee et al., 2022). Genetic modification of macroalgae focuses on their pollutant absorption capabilities, as well as on modifying the metabolic pathways that degrade hazardous pollutants. As for the utilization of engineering microorganisms, their employment is seriously threatened by rigid legislation in some countries (particularly in the EU), which is based more on political views rather than scientific ones. Seeing the immense potential of these technologies, the authors hope for a radical change in the public perception of OMGs, unlocking their utilization for OD outcomes. Other macroorganisms that are being investigated for bioremediation uses are sponges. These metazoans are natural active filters that can sift hundreds of liters of water daily, feeding on the ultraplankton fraction and dissolved organic matter in the surrounding seawater (De Goeij et al., 2008; Morganti et al., 2019). Moreover, microorganims associated with sponges, defined endosymbionts, have shown the capacity to accumulate or degrade many organic pollutants and toxic metals (Bauvais et al., 2015). Thanks to these abilities, sponges could be employed as bioremediation agents for removal of toxic bacteria present in coastal areas via municipal wastewater, improving aquaculture or mariculture systems (Milanese et al., 2003; Zhang et al., 2010).
2.2 Outcome 2: A Healthy and Resilient Ocean
(Where marine ecosystems are understood, protected, restored and managed)
Cleaning up the ocean, however, is only a first step in ocean conservation. In the last few years, many scientific studies have shown the dramatic depletion of ocean resources and its biodiversity (Danovaro et al., 2020). The preservation of marine ecosystems must rely on comprehensive and powerful systems to monitor its state. However, monitoring and safeguarding this vast environment, particularly off-shore and deep-pelagic areas, still denote a significant challenge (Howell et al., 2020). The scientific community has yet to understand basic biological information on thousands of rare and elusive marine organisms (Danovaro et al., 2014). Research in marine science and environmental protection requires expeditions that are costly to carry out and very often influenced by extreme climatic and environmental conditions (Howell et al., 2021). Therefore, the acquisition of data from poorly known areas is still a difficult endeavor, and in the near future will represent the limit to overcome for marine conservation. Consequently, ocean monitoring is rapidly innovating, in accordance with the global trend toward more independent systems able to collect huge amounts of data in near real-time, with higher resolution and during long-term and large-scale monitoring. International programs now aim to fill this gap by mapping the ocean habitats (Mayer et al., 2018), and multiple types of observatories are deployed underwater and available to the community of international experts (Whitt et al., 2020). Underwater vehicles, such as UAV (Unmanned Autonomous Vehicle), ROV (Remotely Operated Vehicle), and MUV (Manned Underwater Vehicle), have been used for the past twenty years mainly for physical oceanography and biogeochemical studies, targeting the pressing issue of global warming.
The improvement of Autonomous Observation Systems (AOS) is a key element to increase the ability to explore and learn more about ocean environments (Figure 3D), allowing them to work safely and without directly exposing personnel (Whitt et al., 2020). New sensors capable of acquiring information on the diversity and relative abundance of ecological communities need to be integrated within these platforms, so that they can simultaneously produce effective results using state-of-the-art environmental monitoring devices (Hawkins et al., 2020). Our current ability to run comprehensive observations of the marine ecosystems is limited on ecological applications; current in situ autonomous platforms need to be able to acquire data on important facets such as marine biodiversity (Scholin et al., 2019). Nevertheless, computational advances have enabled the development of automated data analysis techniques (i.e. machine learning approaches) to monitor ecosystems and marine organisms, enabling large amounts of data to be analyzed quickly and reliably and to produce standardized measurements (Caruso et al., 2020a). Furthermore, expensive robots and vehicles are being developed with the ability to overcome the challenge of the deep sea, to observe and manipulate living organisms in dangerous environments and to allow communication with each other while remotely operated (Figure 3A). The possibility to use both new autonomous systems and non-invasive techniques, reducing effort of marine ship-based surveys and increasing efficiency of sampling processes, will be necessary to monitor different marine ecosystems and drastically mitigate human impacts on a healthy ocean.
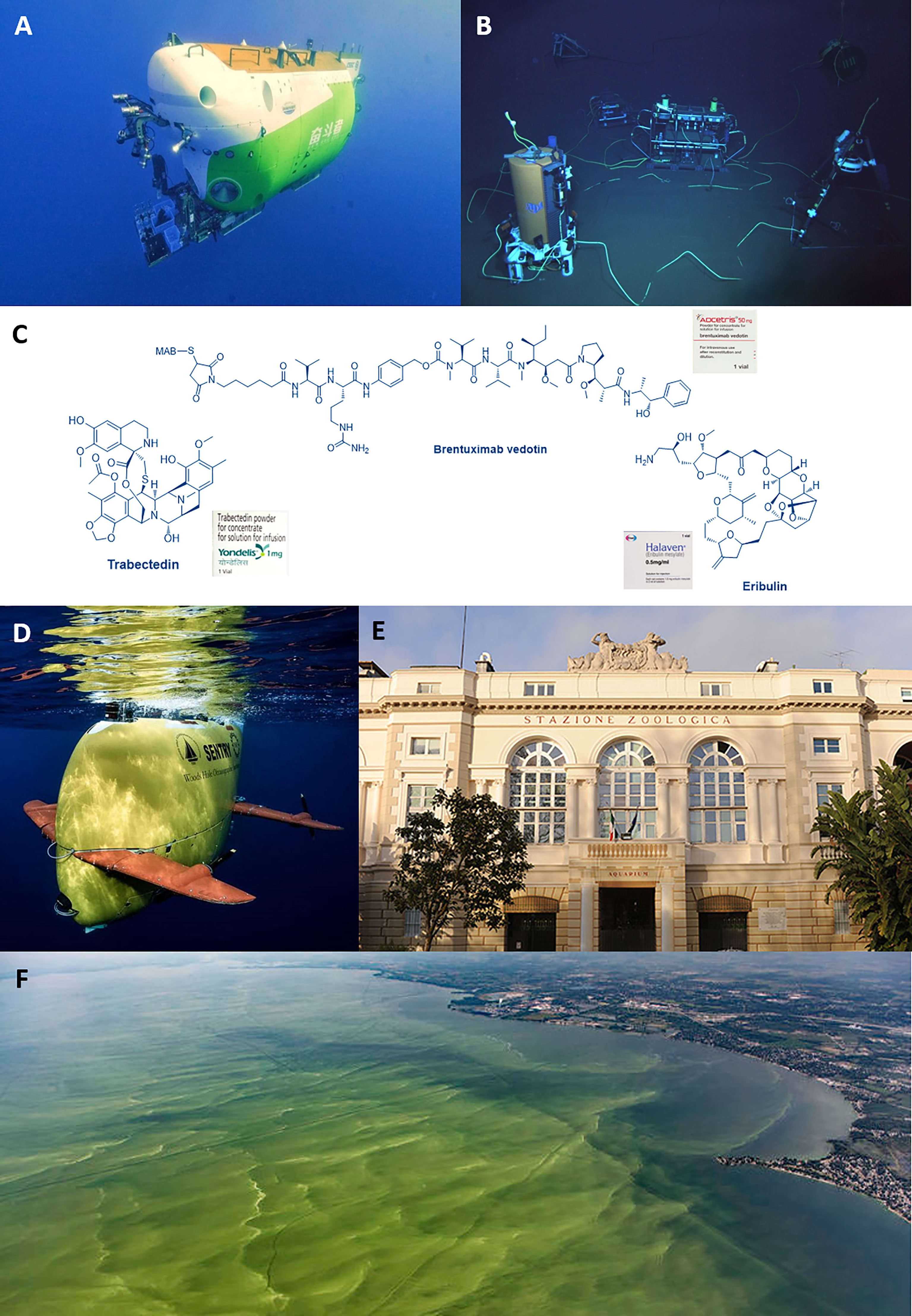
Figure 3 Ocean challenges and opportunities (A) The deep-sea manned submersible called “Fendouzhe” (//english.cas.cn/) successfully landed on the Mariana Trench, the deepest site of the Earth (B) The ALOHA Cabled Observatory (//www.hawaii.edu/), the world’s deepest cabled observatory; (C) Marine-natural-product-inspired pharmaceuticals. Trabectedin, bretuximab and eribulin are the active principles of Yondelis, Halaven and Adcetris, respectively, and are used for cancer treatment; (D) Picture of the Sentry AUV (//www.whoi.edu/), a fully autonomous underwater vehicle capable of exploring the Ocean down to 6,000 meters; (E) The front gate of Stazione Zoologica Anton Dohrn, a world-wide famous marine research center and the oldest public Aquarium in Europe; (F) Aerial view of an algal bloom (//www.nrdc.org/harmful-algal-blooms).
For example, Passive Acoustic Monitoring (PAM) and Environmental DNA Monitoring (eDNA) are two different emerging non-invasive methods to assess biodiversity, distribution and abundance of marine organisms without direct observations. PAM applications are opening up new scientific prospects in ocean monitoring and exploration, even in deep-pelagic waters (Caruso et al., 2015). This is a recent investigation technique spreading around the globe as a key sampling approach for acquiring ecological information, including the effect of human activities and habitat degradation (Howe et al., 2019). Array of hydrophones are used to listen and survey underwater acoustic sources providing data on spatio-temporal distributions of natural phenomena (e.g., wind, rain, ice), sound producing organisms (e.g., crustaceans, fishes, marine mammals) and sources of anthropogenic noise (e.g., ships, sonar, seismic air guns, pile-driving) (Caruso et al., 2020b; Xu et al., 2020). eDNA is another key sampling method on the rise for the future of marine biology and ecology (Stefanni et al., 2018): the possibility of analyzing a mixture of genomic DNA from different organisms – found in an environmental sample such as seawater – reduce the costs and impacts of traditional survey, thus becoming an extremely powerful tool for genetically monitoring diversity, distribution and abundance of both microbes and higher complex organisms, and it is considered a good proxy for off-shore biodiversity since the direct identification of marine species (Yamahara et al., 2019),
Marine biodiversity is proving even more susceptible than previously believed, with increasing biodiversity losses observed in relation to rising temperatures due to global warming (Smale et al., 2019; Antão et al., 2020). Throughout the EU, the current political agenda is increasingly concerned about the reduction of environmental damage by protecting and restoring natural ecosystems, with promising and ambitious plans to reduce the impact of human activities (European Green Deal) and with a commitment to restore degraded ecosystems and conserving biodiversity (European Biodiversity Strategy for 2030). Transition to a sustainable society urgently requires to develop and organize observation systems of marine biodiversity integrated with physico-chemical sensor networks (Heymans et al., 2020). Regional coverage of established long-term biodiversity datasets, combining traditional methods and emerging techniques, is needed (Claudet et al., 2020).Therefore, marine biodiversity observation networks (BONs), through international coordinated approaches (e.g. EMBRC, EMO-BON, GEO-BON), has an important role to converge the research effort and produce meaningful data expanding the local and national activities to a broader scale and global level. The BONs seek to establish a process for standard biodiversity measurements around the world. Shared datasets to understand how biodiversity is changing locally and how these changes are related to global dynamics, and to provide information to help define options for government policies relevant to the conservation of marine resources. Finally, data acquisition must take a rigorous approach to protocols and quality control to ensure the replicability of experiments and validation of ocean models using in-situ observations. For example, the European Marine Omics Biodiversity Observation Network (EMO BON) is an EU contribution to the global observation of omics which, following appropriate data and metadata standards, offers insights into the genetic composition of marine biodiversity.
In addition, marine animal telemetry through tagging is also an important emerging field benefiting from new technologies and automatisation of data processing, with crucial implications for animal conservation and management (Allen and Singh, 2016; Hays et al., 2016). Bio-logging technology has improved over the past several years and tags are increasingly able to measure a range of movements, behaviors and physiological parameters of different marine organisms (Flaspohler et al., 2019; Savoca et al., 2021), with also environmental data for days or weeks at a time (Martín López et al., 2022). For example, AniBOS (Animal Borne Ocean Sensors) is an emerging network of the Global Ocean Observing System (GOOS), as a project of the OD program “Ocean Observing Co-Design – Evolving ocean observing for a sustainable future” 5. AniBOS is providing freely available oceanographic measurements across the oceans collected through bio-logging tags. These non-invasive and key tools are enabling the direct observation of the animals in the wild and providing both physical and ecological data in ocean regions directly at the scale and resolution at which animals move (Harcourt et al., 2019). Therefore, animal movements (i.e., migration routes, home range, habitat limits), their fine-scale behaviors (i.e., dynamic body acceleration, energy expenditure, foraging rates, dive patterns) and co-located environmental data (i.e., temperature, conductivity, dissolved oxygen, wind speed) can be examined improving our ability to observe wildlife and predict global climate processes for societal benefit.
There are also marine ecosystems that can be safely explored by diving operators (e.g. mesophotic grounds, marine caves), where autonomous tools are unnecessary and not cost-effective in relation to research objectives and available fundings. The advancement of scientific diving through new technologies and approaches (e.g. technical diving, Closed Circuit Rebreathers) is expanding its range and enhancing the monitoring of understudied coastal or restricted/remote environments. The activity of divers is essential nowadays both for the supply of in-situ data and samples, and for the deployment, implementation, maintenance and recovery of marine instruments. Scientific diving is a highly productive support in the progress of European marine research. The European Scientific Diving Panel (ESDP) was affiliated to the Marine Board of the European Science Foundation in 2008 (Benjamin and Mackintosh, 2016) and is currently encouraging underwater scientific excellence being part of the MARS Network (The European Network of Marine Stations). The ESDP has defined two levels of professional qualifications: the European Scientific Diver (ESD) and Advanced European Scientific Diver (AESD).
Finally, active habitat restoration is an important concept that is expected to play a vital role for the health and resilience of marine ecosystems in the near future. While in constant expansion, this field of research is still in development, hence new discoveries and models are proposed and tested every so often. Today, one of the emerging concepts underlying habitat restoration is the definition of which factor is more important for a successful restoration. Basically, it seems that the context (i.e., where the action is performed) is more important than the way the restoration is carried out. Furthermore, for a successful restoration, the re-establishment of previous habitats and the removal (if possible) of any stressor is more important than the creation of new areas (full details in (Fraschetti et al., 2021)).
2.3 Outcome 3: A Productive Ocean
(Supporting sustainable food supply and a sustainable Ocean economy)
From the point of view of human society, ocean conservation (see 2.1 and 2.2) is also fundamental from a nutritional and economic aspect. Today, the ocean makes a significant contribution to food security and nutrition, and it is expected to play an even bigger role in the global food system. However, current food arrangement is responsible of the 60% of loss of biodiversity in terms of global terrestrial resources, with a 30% related to the overexploitation of commercial fishing populations (Costello et al., 2020). (FAO 20186; UNEP 20167). The status of conservation of some species ranges from unknown for many of the smaller tuna species, such as bullet tuna, bonito, and little tunny (ICCAT, 2016, 20188), to fully exploit or recover from depletion for other species (i.e., mackerel, bluefin tuna, swordfish) (ICCAT, 2018). Fisheries subsidies are mainly focused to large-scale industrial fishing fleets (Schuhbauer et al., 2017), with a scanty attention towards small-scale fisheries which can make this sector less economically viable (Schuhbauer and Sumaila, 2016). Criminal activities in the fishing sector, such as market frauds and illegal, unreported and unregulated (IUU) fishing, also affected the marine environment and the associated ecosystems. A specific strategy for ocean governance is required to achieve a sustainable ocean economy with the aim of assessing local food resources, including seafood, for each country, as well as to promote all fishery resources, including fisheries discards and by-catches, and to reduce imports/export systems. Today, fish, crustaceans and mollusks provide only 17% of edible meat; sustainable fisheries and mariculture together should be able to deliver 36–74% higher yields by 2050 (increasing from 21 million to 44 million metric tons), meeting 12–25% of the extra meat needed to cope with the dramatic increase of human world population (expected to reach 10 billion by 2050), that will call for more and more proteins and essential nutrients (Costello et al., 2020).
To increase aquatic food production for a growing world population, and make it in a sustainable way, it is necessary to improve fisheries assessment and management systems in all regions by ensuring that the best available scientific advice is duly considered in decision-making processes. Science can also support the continued growth of aquaculture, particularly through innovative practices which ensure social, environmental, and economic sustainability. It is important that these processes take place in synergy with MSP activities that have been developing in recent years with the support and promotion of UNESCO and the MSP global initiative. MSPs are procedures that bring together multiple users of the ocean – including energy, industry, government, conservation, and recreation – to make informed and coordinated decisions about how to sustainably use marine resources. MSP generally uses maps to create a more comprehensive picture of a marine area, identifying where and how it is being used, and which natural resources and habitat exist there. Through the planning and mapping process of a marine ecosystem, planners can consider the cumulative effect of maritime industries on our seas, seek to make industries more sustainable, and proactively minimize conflicts between industries seeking to use the same sea area. The intended result of MSP is a more coordinated and sustainable approach to how our oceans are used – ensuring that marine resources and services are used, but within clear environmental limits to ensure marine ecosystems remain healthy and biodiversity is conserved.
The identification of new edible species could also be seen as a possible solution for the needed food increase in the near future. Deep-sea fishes and crustaceans, and edible sea plants, are underestimated in the human diet even if their properties in terms of protein and micronutrients are well recognized. Likewise, smaller tuna species in several European countries could help to diversify the socio-economic portfolio for local and artisanal fisheries and culinary options for local consumers. However, the population, biomass and exploitation levels of these species, relatively to sustainable fisheries management objectives (e. g., FMSY- Fishing Maximum Sustainable Yield, and BMSY- Biomass Maximum Sustainable Yield), are generally unknown because of limited data (ICCAT, 2016, 2018). Consequently, it is unclear whether populations of these species are already at risk of collapse due to past overexploitation or could support increased exploitation and marketing effort. A change in food demand, managed by several actors along the value chain, will help the seafood industry to reduce its overall CO2 emissions by identifying new opportunities for economic growth based on locally caught and processed species, whose value chains have lower CO2 emissions than those sourced and processed somewhere else.
The ocean also provides services for a wide range of human needs other than food, in particular health. Ocean biodiversity represents an untapped source of useful substances to cope with some of today’s global health threats, such as cancer, antibiotic-resistant infections, and epidemic diseases. The unrivaled degree of marine biodiversity is paralleled with biochemical diversity at a molecular level, thereby making marine organisms and microorganisms a reservoir with virtually unlimited biosynthetic potential. In recent years, the number of promising marine natural products (MNPs) is increasing rapidly, with approximately 28,500 novel chemical entities identified by the end of 2016 (Jiménez 2018). These molecules unveiled precious biological activities, such as anticancer, antibacterial, antifungal, antiviral, anti-inflammatory and immunomodulatory properties (Della Sala et al., 2018) (Figure 3C). In this scenario, the renaissance of MNPs has passed through the omics technologies, which have accelerated discovery of metabolites and their biosynthetic gene clusters (BGCs), by integration of (meta)genomic and metabolomic data. Harnessing the natural biosynthetic routes for complex natural products provides the tools to create sustainable sources of bioactive compounds which are rare in nature. Therefore, mining genomes and metabolomes from marine organisms in an integrated manner leads to identification of BGCs, which in turn can be exploited for production of bioactive compounds in native or heterologous hosts, either through microbial fermentation and/or genetic engineering.
Moreover, as cultured bacteria represent a very small fraction of total estimated microbial biodiversity in the ocean, the metagenomic age has made the uncultivated majority accessible for exploration and identification of BGCs, thus bypassing current limitations experienced by cultivation-based methods (Wilson et al., 2014). With the advent of next-generation sequencing methods and the drop of costs, high-throughput sequencing of metagenomic DNA samples has become more and more common, thus enabling fast detection of BGCs for bioactive MNPs (Sudek et al., 2007; Donia et al., 2011). In-silico platforms, such as antiSMASH (Blin et al., 2019) and PRISM (Skinnider et al., 2020), provide a consolidated strategy to screen huge amounts of metagenomic contigs and have accelerated the genome mining process, as being specifically addressed to genome-wide detection of BGCs and structural prediction of the relevant secondary metabolites. Through prediction of BGCs, genome mining may: provide a rationale for targeted isolation of natural products and unlock “hidden” natural products encoded by silent gene clusters (Ziemert et al., 2016). Identification of BGCs should pave the way for heterologous expression of MNPs, which is essential to functionally characterize natural biosynthetic routes, unlock products of cryptic genes, and enable sustainable production of MNPs of pharmaceutical and nutraceutical interest. However, heterologous expression at the moment still remains a significant bottleneck in natural product development. The development of tools and strains of so-called “microbial cell factories”, finely designed for MNPs production, will be the challenge of the next decade to finally exploit at full the potential of marine metabolites.
2.4 Outcome 4: A Predicted Ocean
(Where society understands and can respond to changing ocean conditions)
To find out where and how to take appropriate conservation actions to protect our oceans and seas and to use marine resources in a sustainable way, we should focus our efforts on valid scientific data and research. Data collection and analysis are the basis for the development of models and strategies to address ocean health issues. In general, Marine Information Technology (MarineIT) addresses observation, communication and/or control tools for the maritime domain. This involves gathering, transmission, processing, and merging of signals from multiple sensors installed in devices, platforms, observatories and vessels. Traditionally, this topic has been referred to in the context of acoustic, optical, and electromagnetic sensing of the ocean environment, most notably sonar/radar processing and satellite remote sensing. Nowadays, MarineIT is accompanied by the advancements in cabled or wireless ocean observation networks, and it is now a specialized branch of the information technologies. MarineIT plays an important role in many underwater applications, such as marine science research, environmental exploration, resource exploitation, security and defense (Xu et al., 2019). An IT-based marine environment is needed to provide efficient monitoring and protection protocols and to address some critical issues including autonomy, adaptability, scalability, simplicity and self-healing of data sampling processes. The following specific requirements for a hardly accessible environment should be considered during design, development and deployment of new sensors and instrumentations (Xu et al., 2019):
• high resistance: sensors need to have very high levels of water and pressure resistance. Equipment also needs against physical phenomena of the ocean, such as. currents, waves, tides, storms
• low energy consumption and energy harvesting: energy conservation and harvesting measures need to be considered due to long communication distances and a constantly moving environment;
• data streaming: acquisition of data from multi-parametric stations, supporting a variety of sensors and array options, and transmitting in near-real time full bandwidth data to on-shore laboratories, or performing in situ processing and transmitting metadata through satellite link.
Marine bioinformatics refers to the use of information and network technologies to collect, store, integrate, analyze, interpret and disseminate data about marine organisms such as their distribution, description, systematic classification, phylogeny, biomolecular structure and genomics. The bioinformatics field offers specialized analytical technology tools for both laboratory and networking technology fields to achieve the goal of finding natural bioactive compounds from marine organisms as potential drugs, antifouling compounds, biomaterials etc. It has the highest implication in marine based ecology, biology, biotechnology and molecular biology, which are integrated for the gene-based discovery and development of marine drugs (Liguori et al., 2020; Wilms, 2021). The marine bioinformatics includes marine genomics and marine proteomics. The main challenge for the future is to fill the gap between the ability to accumulate data, catalog and reconstruct the structure of a genome, and the need to understand its expression and the regulatory networks.
In addition, the effects of climate change and biodiversity loss are strongly affecting many coastal areas around the world. Many coastal cities suffer high tide flooding thresholds, rising sea level inundating the near-shore regions and contributing to coastal flooding and eroded shorelines. Therefore, coastal infrastructures start to be more vulnerable, also in relation to possible damages generated by extreme weather events. These events have evident socio-economic impacts, as they can affect human health with the risks deriving from exposure to poor water quality, pathogens and harmful chemicals. For example, Harmful Algal Blooms (HABs) occur when colonies of cyanobacteria or algae overgrow and may have dramatic impacts on public health, tourism, fisheries, and ecosystems (Figure 3F). HABs may also produce toxic secondary metabolites, such as cyanotoxins (e.g., microcystins, saxitoxins, anatoxins), causing fish mortality and risks for human health, including skin irritation, nephro-, lung- and hepatotoxicity (Teta et al., 2021). Furthermore, HABs reduce oxygen suply and light availability in surface waters, thereby resulting in dead zones and mass mortality of sea animals. The monitoring of these alarming phenomena is increasing in the last decades, and multidisciplinary approaches have been set up to allow their early detection and analysis (early warning systems). In particular, remote and proximal sensing provide useful data to monitor HABs dynamics by measuring light reflected from water surfaces at specific wavelengths. Indeed, exploiting the unique spectral reflectance signatures of photosynthetic pigments, chlorophyll and carotenoids can be used to track algal blooms while phycobiliproteins (phycoerythrin and phycocyanin) to evaluate cyanobacteria spreading (Teta et al., 2017). Although HABs occur naturally under specific conditions of light, nutrients and temperature, anthropogenic factors contribute to the onset of such phenomena, through climate change, pollution and excessive nutrient input to water bodies (cultural eutrophication) due to urban and agricultural activities (Teta et al., 2016). Satellite sensors (i.e., remote sensing) ensure to cover large temporal and spatial scales while aircrafts and drones equipped with multispectral camera (proximal sensing), have more powerful resolution on a circumscribed area, and, therefore, greater sensitivity. Through spectral anomalies detection, remote/proximal sensing imagery may restrict sampling areas to perform in situ environmental studies, including chemical and metagenomic analyses to assess bloom composition and toxicity. These are the future technological challenges to properly respond to these hazardous events.
Another striking example of the changing global ocean conditions is noise pollution. The increase in human activities at sea has resulted in doubling the noise levels every decade during the last sixty years (Xu et al., 2020), and many studies have described the anthropogenic noise as an important stressor for marine animals, causing behavioral changes, hearing loss and masking of communication (Erbe et al., 2019; Popper and Hawkins, 2019). Marine soundscapes are rapidly changing not only in relation to the increase in anthropogenic sources, but also for the decreasing in abundance of sound-producing animals, and the altered contributions of geophysical sources due to the effects of global warming (Duarte et al., 2021). What is urgently needed to reduce the noise pressure on the marine environment is to bridge the gap between science and industry, improving innovative solutions, regulating the global market and promoting behavioral changes for local communities living near the coasts. Moreover, the collection of new information will be essential, since the sound components of many marine habitats are still unknown and critical information on the ecology of marine organisms can be acquired by passive listening of the ocean. For this reason, underwater sound has been recently added by the Biology and Ecosystem Panel of the Global Ocean Observing System (GOOS) as “Essential Ocean Variable” (EOV) (T/yack et al., 2021). GOOS is led by the Intergovernmental Oceanographic Commission (IOC) of UNESCO and supports a community of international, regional and national ocean observing initiatives9. Its main goal is to provide the essential information necessary for sustainable development and human well-being. An example of GOOS’ international collaboration is the ARGO program, a series of thousands of inter-calibrated vertical profilers that measure ocean parameters providing key data through satellite links when on the surface10.
2.5 Outcome 5: A Safe Ocean
(Where life and livelihoods are protected from Ocean-related hazards)
A well-monitored and guarded ocean is also a safer one. We have already seen how Maritime Spatial Planning (MSP) is evolving in defining mechanisms for the management of sea and ocean in a changing environment. It is necessary to rely on long-term data series, over a period of time that is frequently beyond a human life in order to facilitate the acquisition of information by scientists that are developing predictive models for the policy makers (Oliveri et al., 2022). Moreover, unpredictable events may affect ecosystems, such as the arrival of a new species, a tornado, a new ocean current, a volcanic eruption; such events can drastically change the balance and function of an ecosystem and are part of the evolutionary history of the Earth. Different life forms shape marine habitats, and our abilities to observe the effects of these changes are still very limited: precise and systematic observation systems are necessary. The data generated in the outcomes 2.2 and 2.4 will be pivotal to provide services to society such as the forecasting of hazards (i.e., harmful algal blooms and bioprospecting) for the Blue Bioeconomy. Technological tools for capturing different kinds of information have been greatly enhanced in the last decade, and together with these technological developments now we can access a data storage capacity that was impossible just a few years ago. Nevertheless, the skills and algorithms needed to correctly interpret these huge datasets, and to analyze metadata databases, are still a weak point. It is necessary to move from data collection to the understanding of biological phenomena that underlie them, to finally develop predictive models that can be useful for the protection of biodiversity, the environment and human health.
Implementing ocean risk reduction for increased safety can only be based on scientific achievements (Cappelletto et al., 2021). A multidisciplinary approach to observe, monitor and model, will promote more effective and sustainable management and development policies. The implementation of best-practice policies for industries and fisheries towards sustainable use of marine resources is necessary to reduce human impact; this can be achieved through the implementation of MSP principles (see 2.3), as a public process of analyzing and allocating the spatial and temporal distribution of human activities in marine areas to achieve ecological, economic, and social objectives that are usually specified through a political process11. In this direction many initiatives have already started. In the Mediterranean area, the SciNMeet Programme (“Science We Need for the Mediterranean Sea We Want”) (Cappelletto et al., 2021) is aimed to address major challenges and gaps in scientific knowledge to manage regional impacts in consideration of emerging environmental issues. The Biomolecular Ocean Observing Network is developing a global system that will allow science and society to understand and protect ocean life and will also help communities to detect biological hazards. This is an endorsed programme of the OD and will be a key component of next-generation ocean observing systems12.
Concerning HABs, several countermeasures have already been adopted to mitigate them. These strategies aim to reduce the number of toxic species or withdraw secreted, toxic secondary metabolites from the water column. Physical methods for aquaculture facilities have been based on: a) increasing water mixture to hamper stratification, b) bloom disruption by sonication, or c) creating a separation between the bloom and aquaculture animals (Gallardo-Rodríguez et al., 2019). Moreover, algicidal agents, including copper sulphate, hydrogen peroxide, hypochlorite, anthraquinones and low molecular weight water-soluble chitosans, have been described in recent years, showing good efficacy and specificity in removing toxic algal species (Park et al., 2016; Pal et al., 2020). Promising microorganisms, mainly algicidal bacteria, have emerged as useful biotic tools to control HABs in water ecosystems (Matthijs et al., 2016). As an alternative, physical–chemical methods, consisting in inducing bloom flocculation and sedimentation by flocculating agents, have been shown to be effective in removing algal species, nutrients and toxins. In this perspective, the OD could provide the chance for the implementation of new controlling methods, through an in-depth analysis of the economic and environmental impacts.
2.6 Outcome 6: An Accessible Ocean
(With open and equitable access to data, information and technology and innovation)
As stated in the previous outcomes, the success of the OD will rely on the capability to collect new data using new technologies and methods. The acquisition of reliable scientific data will lend credence to any claim for pollution prevention, conservation and rehabilitation of our oceans, creating tools to communicate strong messages to the public. Moreover, more evidence is needed to face environmental problems, to identify and establish appropriate mitigation and restoration actions and to provide information for decision makers, allowing effective sustainable management of marine resources. The target is represented by institutional authorities and all the stakeholders that are involved in the utilization of marine ecosystem services (e.g., fishermen associations, tourism categories), including the civil society. Complex phenomena, such as those that regulate life on our planet, by their nature, cannot be understood by analyzing a single parameter. A network of regional facilities and multidisciplinary observatories are necessary to explore the oceans, to better understand the phenomena happening within the marine ecosystems, and to monitor their role in relation to the evolution of the Earth system (Figure 3B). Platforms equipped with multiple sensors need to be placed along the water column and on the seafloor, measuring different physical, biogeochemical and ecological parameters and addressing alarming issues such as climate change and geo-hazards extreme events (Lo Bue et al., 2021). The synthesis among different disciplines will enable far more holistic insights into evaluation of marine ecosystems, critical for the future of marine policy.
The creation of a central data portal and a series of information hubs that exchange evidence about marine life is essential to organize and assimilate multidisciplinary datasets, and to produce knowledge in an open and concise form for scientists, conservationists, general public and policy makers. It is necessary to enforce the data network, such as the European Marine Observation and Data Network (EMODnet), in order to provide access to indications of spatiotemporal distribution of marine species in a multi-use manner13. Nowadays, not all world’s regions and types of data are equally represented and biological data are much less prevalent compared to physico-chemical datasets, with the lack of - for example - a new generation of taxonomists (Pinheiro et al., 2019). In this direction, EMODnet Biology is built upon the World Register of Marine Species (WoRMS) and the Ocean Biodiversity Information System (OBIS). The WoRMS is providing an authoritative classification and database of marine organisms14, and OBIS is establishing a comprehensive and online gateway to the world’s ocean biodiversity and biogeographic data 15. As an example, these data are important to support the scientific evidence necessary for the creation of marine protected areas (MPAs) and to monitor whether what’s established is effectively protected (Claudet et al., 2020). Therefore, well-organized scientific data are needed if we want to protect our oceans and levels of protection should be increased in relation to active monitoring activities to ensure we deliver tangible benefits for biodiversity conservation.
Data sharing is also essential to develop a policy of Open Science for a large and diverse group of users, from scientists and industries to institutions and policy makers. However, many technical aspects remain to be solved regarding the management of large-scale infrastructures and big data, and the improvement of autonomous observatories. For instance, machine learning is used in Ocean science to identify objects from images (e.g., automatically identifying certain types of coral) and to learn from numeric patterns of long-term data series (OECD 2020). These will be indispensable to support decision making for practical use in marine spatial planning processes. Innovation, interoperability, data standards and best practices are necessary, but sustainable management is the key for a healthy and resilient ocean (Buck et al., 2019; Liu et al., 2019). Once interoperable infrastructures are in place, technologies would certainly offer tools for the effective analysis of large marine dataset able to examine long-term changes in environmental conditions, as well as fine-scale geographical and temporal variations. Improving these research infrastructures is a theme of primary importance for the EU. The Program Horizon Europe 16, planning to fund research in the period 2021-2027, has the goal to improve European research infrastructures with a dedicated work program, organized into five destinations. In particular, the destination INFRANET - Network Connectivity in Research and Education, focuses on improving networks and relative services to better connect researchers, to help the sharing of data and information, regardless of the location of the users, in order to achieve excellent research.
The establishment of specific data management plans are also another fundamental aspect in the future of marine research. After the publication of scientific data, subsequent possibility of “data reuse” by the international community is essential to improve knowledge discovery and future innovation. Four principles – Findability, Accessibility, Interoperability, and Reusability (Wilkinson et al., 2016) are guiding academia at European level. Moreover, the Information and Communication Technologies (ICT) are fundamental to manage, transmit and communicate data using new performing technologies able to exchange information from remote and different sensors, to increase computing and storage capacities. The goal will be the integration of data from different fields of science to fully understand the natural phenomena that regulate life in the Oceans.
2.7 Outcome 7: An Inspiring and Engaging Ocean
(Where society understands and values the Ocean in relation to human well-being and sustainable development)
Scientific research cannot be considered complete without the active dissemination of its results. Indeed, while the scientific press will cover such a need for peer-to-peer communication, the necessity to convey new discoveries and their consequences to the general public is undeniable. This is even truer in this moment in history, in which we witness a growing dissent and mistrust towards science and scientists. The failure of the limited model in the so-called PUS (Public Understanding of Science), in which the public audience was basically seen as a container to be filled by oversimplified basic scientific concepts, has brought that part of the scientific community involved in communication to reconsider how to actually communicate science. The PUS model was rapidly converted in PEST (Public Engagement with Science and Technology) and PawS (Public Awareness of Science), in which researchers finally accepted that communication is a two-ways process, that should actually engage and involve the audience in order to increase public awareness and consensus on the great themes of scientific research. Scientific dissemination is a dialogue, not a (often boring) monologue, and scientists from all disciplines should be aware of this very important, and still too often ignored, issue.
In this perspective, public structures of scientific interest and communication, such as museums, zoos, aquaria and public scientific libraries, as well as recent social media-based communication, become fundamental for the two-way communication of science, and the increase of environmental and scientific awareness of people. In the light of the dawning OD, science communication and dissemination become an undeniable part of research, and at the same time an irrefutable need for scientists and the general public alike. Scientific libraries are centers of information and active transfer of knowledge and represent an important tool to increase awareness of research progress among scientists and citizens. Their public access is an important contribution to the OD passing through awareness and behavioral changes towards a more sustainable attitude in order to preserve marine environment and biodiversity. Museums can constitute the repository of marine biological collections. The evolution of biodiversity in time is addressed by exploiting platforms of long-term monitoring (decadal scale), crating museum collections and archives (centennial scale) and by cooperating with archeologists and paleobiologists (millennial and geological time scales). Moreover, given the current rate of extinction for certain species, it is urgent to collect demographic data essential to develop risk assessment for their disappearance. The data collection by aquaria, zoos and museums represents an opportunity for comparative and conservation biology and can be used to fill some of these gaps (Conde et al., 2019).
Natural History Museums are changing, are evolving in order to survive – in an interesting parallel with all living beings. The original scope of showing wonders of the world, often bordering myths and legends (e.g., the “Chambers of Wonders”, that survived well into the modern age) or rows upon rows of systematically ordered specimens, is no longer compatible with the contemporary view of scientific communication. New strategies need to be developed in order to transform such places into interfaces between science and the public (Dick, 2017). Aside from their changing role, museums and aquaria have to embrace the idea of using narrative and storytelling to interact with the public and to convey ideas and concepts, always creating a strong interaction between the visitor and the display (Figure 3E). Interaction is not necessarily immediate and automatic, although the use of new technologies (especially techs such as Augmented Reality and Virtual Reality) in museums and aquaria is becoming more and more important today (Vermeeren et al., 2018). Interaction can be created with narrative, or with peculiar display of items, or by letting the public itself form bonds with what is before the eyes (Davidsson and Jakobsson, 2012). Museums, zoos and aquarium communities fulfill several objectives of environmental conservation, education and entertainment, the so-called edutainment, by attracting their visitors through their enchanting exhibits. Large aquaria are energy-intensive facilities and need to guarantee a low environmental impact to generate economic savings. So, the shift of their focus towards smaller-bodied native local species within scientific research centers is a coherent and trustworthy contribution to OD dissemination and public engagement.
In order to increase public awareness towards natural history and biodiversity conservation within a global change that is so hardly impacting upon our lives, museums and aquaria must step into their new roles of leaders of scientific dissemination and focal points for public awareness. Modern aquaria actively contribute to the recovery of threatened species through ex situ breeding within institutions and through substantial contributions to in situ conservation projects in natural habitats (Conde et al., 2011; Mooney et al., 2020). Steps have already been taken towards this goal; for instance, the formal partnership between ICOM Nathist (the International Committee for Museums and Collections of Natural History of the International Council of Museums) and WAZA (World Association of Zoos and Aquariums) represents a cornerstone of this new “interface building” that will see museums and zoo/aquaria take the lead in promoting good practice and behavioral changes through science popularization (Barongi et al., 2015). The success of the Biodiversity is Us campaign, launched by WAZA and deeply involving the ICOM Nathist, shows how much the close relationship between two only apparently different types of institutions can actually achieve in terms of biodiversity knowledge and preservation. Communication campaigns raise awareness on programs to protect autochthonous species engaging local communities and stakeholders with overlapping interests. Recovery and rescue of stranded animal programs promote synergy with local authorities and launch targeted management policies, even supporting the establishment of protected areas. Scientific libraries develop newly digitalized peer reviewed publications that can be distributed through on-line platforms, thus amplifying the access opportunities by users in real-time. Meanwhile, in the last decade the proliferation of social media-based communication has proven successful in terms of dissemination of scientific information as well as on many other themes17. The recognized limitation of this extremely fast method of information transmission and broadcasting is related to the lack of peer review processes. The general posting system can sometimes even generate ill effects such as miscommunication, fear and panic or disseminate wrong interpretations of the research results creating aversive or disruptive concepts that can further amplify the negative behaviors even more (Barr-Walker et al., 2021).
“The Ocean … is our life support system, and it begins to falter due to human activities on land “ (Stel, 2021). These words perfectly frame the current situation of our world, and better than any other manage to convey the urge for ocean literacy. Although this concept is only relatively new (for a full summary on ocean literacy see (Payne and Marrero, 2021) and references within), between the OD and the dramatic conditions of our oceans the need for a worldwide understanding of at least the basic principles of marine science and their utmost importance in the light of critical ecosystem failure we are facing are now clearer than ever. Unfortunately, education systems do not seem to always fully support the needs evidenced by ocean literacy, and each country still has its own pace and setting; however, as Marrero and coworkers (Marrero et al., 2019) pointed out, the urge to cooperate between all the ocean literacy networks. Therefore, museums, zoos and aquaria are in the position to answer the call for this cooperation, and this will be one of the challenges we will all be facing in the upcoming years. However, there is a downside with such places: learning there is “informal”, therefore it is hardly measurable (Seraphin et al., 2019). Again, we urge to fully cooperate with scientific and educational institutions to give the best experience available in terms of ocean literacy and promote not only better understanding but a greater awareness of the ocean and relative issues, and from there develop a true behavioral change. Similarly, citizen science is constantly evolving and is essential in actively engaging the public, both in terms of biodiversity monitoring (Arvanitidis et al., 2011) or environmental research (Garcia-Soto et al., 2021).
Finally, in order to promote behavioral change in their citizens, policy makers should concentrate on the importance of internal motivation that positively affects conscientious behaviors towards biodiversity protection. This influence motivates people to change their perceptions and attitudes and reveals its importance versus pro-environmental behaviors. Moreover, the trust in the government and institutions can promote respect for the policies dictated by policy makers, thus ensuring also positive behavioral changes (Nguyen-Van et al., 2021). These findings not only imply that policymakers should include social influence programs in their communication but also that entrusted scientific institutions can act as information carriers and foster environmental awareness toward eco-friendly behaviors.
3 Conclusion
The Ocean is a living system that has accompanied humans since the origin of our species. Some people approach it as an endless strip-mine of all types of resources such as fisheries products, alternative fuel, medicine, nutraceutical products, or a platform for intercontinental transports, tourism and leisure. Others had the opportunity to only see its surface without the knowledge of the processes taking place below the seawater interface. Although the OD may somehow seem a utilitarian view limiting the preservation of the Ocean to a resource to sustain and guarantee a future of well-being for our species, it is in reality a challenging opportunity for technological development and knowledge implementation to better sustain all forms of life on our planet. OD outcomes to be strategically addressed are represented by the need to understand the processes that occur within marine ecosystems in order to allow biodiversity protection, use the resources in a sustainable way, counteract climate change and improve quality of life. Habitat preservation and sustainability are both “institutional and individual generalized responsibility” to be implemented by a consistent and effective respect for the environment in everyday life.
However, we can only provide the innovative solutions needed to achieve the OD outcomes by focusing our efforts on scientific research (Table 1). In particular, biotechnology is in a leading position. Multidisciplinary by definition, it is proving its value providing important answers in many outcomes. The use of macro and micro-organism is already crucial for the bioremediation of the polluted ocean. The discovery and the subsequent implementation of new or engineered biocatalysts with better bioremediation properties is the principal challenge for the next few years, together with the genetic modification of organisms. Biotechnology has an important role in valorizing the ocean potential for new molecules for human and animal health. The improvement of technologies such as metagenomics, metatranscriptomic and metabolomics has unlocked the hidden treasure of the ocean, and at the same time has given new instruments for environmental monitoring. This will be crucial for many OD outcomes, since a constant and efficient control of as many as possible ocean parameters will allow us to properly protect the ocean environment. In general, technological progress will be essential to this end through the development of new non-invasive, autonomous and eco-compatible technologies for the exploration and monitoring of the oceans. Innovative platforms and sensors capable of providing information in near real-time, and global data portals to allow easy access to organized databases for all categories of users. Finally, the role of scientific research is to highlight and convert these innovations into opportunities, increasing the understanding of biological mechanisms, evolution of sea life and spreading public awareness about ocean issues. Currently, research projects need specific dissemination activities and communication plans, with the involvement of social community and stakeholders.
Actually, despite the scientific evidence on the different threats generated by humans on marine ecosystems, many countries face enormous challenges to take effective environmental actions due to their socio-economic vulnerability, political uncertainty and technical-scientific capabilities (Muelbert et al., 2021). The information required by policy makers should be available in an accessible and inclusive way, taking into account data on specific areas and overcoming regional imbalances in terms of economic development, technological level, building capability, societal involvement and governmental control (Muelbert et al., 2021). EU governance must certainly strengthen measures for the protection of the marine ecosystems (e.g. environmental laws, protected areas), but also support the creation of new infrastructures (including digital ones) in which the public can easily acquire scientific knowledge and evolve an ecological consciousness. Research institutes and related knowledge must become the entrusted reference points that will guide the challenges of the human being, in the decade we are facing. Even with the SARS-CoV2 pandemic still raging on our planet, these institutions are doing their best to bring reliable scientific knowledge and up-to-date information into the homes of many people (Gili et al., 2021), perhaps reaching an even wider virtual audience than ever before.
Author Contributions
DP and CG conceived the manuscript. PT and FC organized the manuscript structure. All authors contributed by writing and revising the text.
Conflict of Interest
The authors declare that the research was conducted in the absence of any commercial or financial relationships that could be construed as a potential conflict of interest.
Publisher’s Note
All claims expressed in this article are solely those of the authors and do not necessarily represent those of their affiliated organizations, or those of the publisher, the editors and the reviewers. Any product that may be evaluated in this article, or claim that may be made by its manufacturer, is not guaranteed or endorsed by the publisher.
Acknowledgments
The authors are grateful to Sara Tedesco for providing the graphics for Figure 1.
Footnotes
- ^ https://ec.europa.eu/oceans-and-fisheries/ocean/blue-economy/maritime-spatial-planning_it.
- ^ https://ec.europa.eu/environment/strategy/biodiversity-strategy-2030_en.
- ^ https://sdgs.un.org/goals.
- ^ https://www.oceandecade.org/.
- ^ https://www.oceandecade.org/actions/ocean-observing-co-design-evolvingocean-observing-for-a-sustainable-future/.
- ^ www.fao.org/3/i9540en/i9540en.pdf.
- ^ www.resourcepanel.org/reports/food-systemsand-natural-resources.
- ^ https://iccat.int/Data/REP_EN_10-11_I_1_Annex_6_Confidentiality.pdf.
- ^ www.goosocean.org.
- ^ https://argo.ucsd.edu/.
- ^ https://unesdoc.unesco.org/ark:/48223/pf0000186559.
- ^ https://www.obon-ocean.org/.
- ^ https://emodnet.ec.europa.eu/en.
- ^ https://www.marinespecies.org/index.php.
- ^ https://obis.org/.
- ^ https://ec.europa.eu/info/research-and-innovation/funding/fundingopportunities/funding-programmes-and-open-calls/horizon-europe_en.
- ^ http://www.ict-21.ch/com-ict/IMG/pdf/learning-2.0-EU-17pages-JRC56958.pdf.
References
Allen A. M., Singh N. J. (2016). Linking Movement Ecology With Wildlife Management and Conservation. Front. Ecol. Evol. 3, 155. doi: 10.3389/fevo.2015.00155
Amobonye A., Bhagwat P., Singh S., Pillai S. (2021). Plastic Biodegradation: Frontline Microbes and Their Enzymes. Sci. Total Environ. 759, 143536. doi: 10.1016/j.scitotenv.2020.143536
Andreolli M., Lampis S., Brignoli P., Vallini G. (2015). Bioaugmentation and Biostimulation as Strategies for the Bioremediation of a Burned Woodland Soil Contaminated by Toxic Hydrocarbons: A Comparative Study. J. Environ. Manage 153, 121–131. doi: 10.1016/j.jenvman.2015.02.007
Antão L. H., Bates A. E., Blowes S. A., Waldock C., Supp S. R., Magurran A. E., et al. (2020). Temperature-Related Biodiversity Change Across Temperate Marine and Terrestrial Systems. Nat. Ecol. Evol. 4, 927–933. doi: 10.1038/s41559-020-1185-7
Arvanitidis C., Faulwetter S., Chatzigeorgiou G., Penev L., Bánki O., Dailianis T., et al. (2011). Engaging the Broader Community in Biodiversity Research: The Concept of the COMBER Pilot Project for Divers in ViBRANT. ZooKeys 150, 211–229. doi: 10.3897/zookeys.150.2149
Ayele A., Getachew D., Kamaraj M., Suresh A. (2021). Phycoremediation of Synthetic Dyes: An Effective and Eco-Friendly Algal Technology for the Dye Abatement. J. Chem. 2021, 9923643. doi: 10.1155/2021/9923643
Barongi R., Fisken F. A., Gusset M., Parker M. (2015). Committing to Conservation : The World Zoo and Aquarium Conservation Strategy (Gland, Switzerland: World Association of Zoos and Aquariums (WAZA).
Barr-Walker J., Depiñeres T., Ossom-Williamson P., Mengesha B., Berglas N. F. (2021). Countering Misinformation About Abortion: The Role of Health Sciences Librarians. Am. J. Public Health 111, 1753–1756. doi: 10.2105/AJPH.2021.306471
Bauvais C., Zirah S., Piette L., Chaspoul F., Domart-Coulon I., Chapon V., et al. (2015). Sponging Up Metals: Bacteria Associated With the Marine Sponge Spongia Officinalis. Mar. Environ. Res. 104, 20–30. doi: 10.1016/j.marenvres.2014.12.005
Benjamin J., Mackintosh R. (2016). Regulating Scientific Diving and Underwater Archaeology: Legal and Historical Considerations. Int. J. Nautical Archaeology 45, 153–169. doi: 10.1111/1095-9270.12141
Blin K., Shaw S., Steinke K., Villebro R., Ziemert N., Lee S. Y., et al. (2019). antiSMASH 5.0: Updates to the Secondary Metabolite Genome Mining Pipeline. Nucleic Acids Res. 47, W81–W87. doi: 10.1093/nar/gkz310
Buck J. J. H., Bainbridge S. J., Burger E. F., Kraberg A. C., Casari M., Casey K. S., et al. (2019). Ocean Data Product Integration Through Innovation-The Next Level of Data Interoperability. Front. Mar. Sci. 6, 32. doi: 10.3389/fmars.2019.00032
Cappelletto M, Santoleri R, Lorenza Evangelista L, Galgani F, Garcés E, Giorgetti A.. (2021). The Mediterranean Sea We Want. Ocean Coast. Res. 69 (suppl), e21031. doi: 10.1590/2675-2824069.21019mc
Caruso F., Dong L., Lin M., Liu M., Gong Z., Xu W., et al. (2020a). Monitoring of a Nearshore Small Dolphin Species Using Passive Acoustic Platforms and Supervised Machine Learning Techniques. Front. Mar. Sci. 7, 267. doi: 10.3389/fmars.2020.00267
Caruso F., Dong L., Lin M., Liu M., Xu W., Li S. (2020b). Influence of Acoustic Habitat Variation on Indo-Pacific Humpback Dolphin (Sousa Chinensis) in Shallow Waters of Hainan Island, China. J. Acoustical Soc. America 147, 3871–3882. doi: 10.1121/10.0001384
Caruso F., Sciacca V., Bellia G., De Domenico E., Larosa G., Papale E., et al. (2015). Size Distribution of Sperm Whales Acoustically Identified During Long Term Deep-Sea Monitoring in the Ionian Sea. PLos One 10, e0144503. doi: 10.1371/journal.pone.0144503
Cheng Y., Deng S., Chen P., Ruan R. (2009). Polylactic Acid (PLA) Synthesis and Modifications: A Review. Front. Chem. China 4, 259–264. doi: 10.1007/s11458-009-0092-x
Claudet J., Loiseau C., Sostres M., Zupan M. (2020). Underprotected Marine Protected Areas in a Global Biodiversity Hotspot. One Earth 2, 380–384. doi: 10.1016/j.oneear.2020.03.008
Conde D. A., Flesness N., Colchero F., Jones O. R., Scheuerlein A. (2011). An Emerging Role of Zoos to Conserve Biodiversity. Science 331, 1390–1391. doi: 10.1126/science.1200674
Conde D. A., Staerk J., Colchero F., Da Silva R., Schöley J., Baden H. M., et al. (2019). Data Gaps and Opportunities for Comparative and Conservation Biology. PNAS 116, 9658–9664. doi: 10.1073/pnas.1816367116
Costello C., Cao L., Gelcich S., Cisneros-Mata M.Á., Free C. M., Froehlich H. E., et al. (2020). The Future of Food From the Sea. Nature 588, 95–100. doi: 10.1038/s41586-020-2616-y
Cózar A., Echevarría F., González-Gordillo J. I., Irigoien X., Ubeda B., Hernández-León S., et al. (2014). Plastic Debris in the Open Ocean. Proc. Natl. Acad. Sci. U.S.A. 111, 10239–10244. doi: 10.1073/pnas.1314705111
Danovaro R., Fanelli E., Aguzzi J., Billett D., Carugati L., Corinaldesi C., et al. (2020). Ecological Variables for Developing a Global Deep-Ocean Monitoring and Conservation Strategy. Nat. Ecol. Evol. 4, 181–192. doi: 10.1038/s41559-019-1091-z
Danovaro R., Snelgrove P. V., Tyler P. (2014). Challenging the Paradigms of Deep-Sea Ecology. Trends Ecol. Evol. 29, 465–475. doi: 10.1016/j.tree.2014.06.002
Da Silva M. L. B., Alvarez P. J. J. (2010). “"Bioaugmentation,",” in Handbook of Hydrocarbon and Lipid Microbiology, 4531–4544. Springer: Dordrecht
Davidsson E., Jakobsson A. (2012). Understanding Interactions at Science Centers and Museums (Rotterdam Netherlands: Sense Publishers).
De Goeij J. M., Moodley L., Houtekamer M., Carballeira N. M., Van Duyl F. C. (2008). Tracing 13C-Enriched Dissolved and Particulate Organic Carbon in the Bacteria-Containing Coral Reef Sponge Halisarca Caerulea: Evidence for DOM-Feeding. Limnol. Oceanogr. 53, 1376–1386. doi: 10.4319/lo.2008.53.4.1376
Dellagnezze B. M., Vasconcellos S. P., Angelim A. L., Melo V. M. M., Santisi S., Cappello S., et al. (2016). Bioaugmentation Strategy Employing a Microbial Consortium Immobilized in Chitosan Beads for Oil Degradation in Mesocosm Scale. Mar. pollut. Bull. 107, 107–117. doi: 10.1016/j.marpolbul.2016.04.011
Della Sala G., Agriesti F., Mazzoccoli C., Tataranni T., Costantino V., Piccoli C. (2018). Clogging the Ubiquitin-Proteasome Machinery With Marine Natural Products: Last Decade Update. Mar. Drugs 16 (12), 467. doi: 10.3390/md16120467
Dick G. (2017). “"Natural History Museums, Zoos and Acquariums,",” in The Future of Natural History Museums. Ed. Dorfman E. (London: Routledge), 157–167.
Donia M. S., Fricke W. F., Partensky F., Cox J., Elshahawi S. I., White J. R., et al. (2011). Complex Microbiome Underlying Secondary and Primary Metabolism in the Tunicate-Prochloron Symbiosis. Proc. Natl. Acad. Sci. 108, E1423–E1432. doi: 10.1073/pnas.1111712108
Duarte C. M., Chapuis L., Collin S. P., Costa D. P., Devassy R. P., Eguiluz V. M., et al. (2021). The Soundscape of the Anthropocene Ocean. Science 371 (6529), eaba4658. doi: 10.1126/science.aba4658
Erbe C., Dähne M., Gordon J., Herata H., Houser D. S., Koschinski S., et al. (2019). Managing the Effects of Noise From Ship Traffic, Seismic Surveying and Construction on Marine Mammals in Antarctica. Front. Mar. Sci. 6, 647. doi: 10.3389/fmars.2019.00647
Fernandez-Luqueno F., Valenzuela-Encinas C., Marsch R., Martinez-Suarez C., Vazquez-Nunez E., Dendooven L. (2011). Microbial Communities to Mitigate Contamination of PAHs in Soil–Possibilities and Challenges: A Review. Environ. Sci. pollut. Res. Int. 18, 12–30. doi: 10.1007/s11356-010-0371-6
Flaspohler G. E., Caruso F., Mooney T. A., Katija K., Fontes J., Afonso P., et al. (2019). Quantifying the Swimming Gaits of Veined Squid (Loligo Forbesi I) Using Bio-Logging Tags. J. Exp. Biol. 222 (24), jeb198226. doi: 10.1242/jeb.198226
Fraschetti S., Mcowen C., Papa L., Papadopoulou N., Bilan M., Boström C., et al. (2021). Where Is More Important Than How in Coastal and Marine Ecosystems Restoration. Front. Mar. Sci. 8, 626843. doi: 10.3389/fmars.2021.626843
Gallardo-Rodríguez J. J., Astuya-Villalón A., Llanos-Rivera A., Avello-Fontalba V., Ulloa-Jofré V. (2019). A Critical Review on Control Methods for Harmful Algal Blooms. Rev. Aquacult 11, 661–684. doi: 10.1111/raq.12251
Garcia-Soto C., Seys J. J. C., Zielinski O., Busch J. A., Luna S. I., Baez J. C., et al. (2021). Marine Citizen Science: Current State in Europe and New Technological Developments. Front. Mar. Sci. 8, 621472. doi: 10.3389/fmars.2021.621472
Gertler C., Bargiela R., Mapelli F., Han X., Chen J., Hai T., et al. (2015). Conversion of Uric Acid Into Ammonium in Oil-Degrading Marine Microbial Communities: A Possible Role of Halomonads. Microb. Ecol. 70, 724–740. doi: 10.1007/s00248-015-0606-7
Gewert B., Plassmann M. M., Macleod M. (2015). Pathways for Degradation of Plastic Polymers Floating in the Marine Environment. Environ. Science: Processes Impacts 17, 1513–1521. doi: 10.1039/C5EM00207A
Gili C., Vasconi M., Gagliardi F. (2021). Impact of SARS-CoV-2 on Aquaria: An Italian Perspective. J. Appl. Anim. Ethics Res. 3 (1), 74–90. doi: 10.1163/25889567-BJA10015
Harcourt R., Sequeira A. M. M., Zhang X., Roquet F., Komatsu K., Heupel M., et al. (2019). Animal-Borne Telemetry: An Integral Component of the Ocean Observing Toolkit. Front. Mar. Sci. 6, 326. doi: 10.3389/fmars.2019.00326
Hawkins S. J., Allcock A. L., Bates A. E., Evans A. J., Firth L. B., Mcquaid C. D., et al. (2020). Oceanography and Marine Biology: An Annual Review. 1st ed (London, UK: CRC Press).
Hays G. C., Ferreira L. C., Sequeira A. M. M., Meekan M. G., Duarte C. M., Bailey H., et al. (2016). Key Questions in Marine Megafauna Movement Ecology. Trends Ecol. Evol. 31, 463–475. doi: 10.1016/j.tree.2016.02.015
Heymans J. J., Bundy A., Christensen V., Coll M., De Mutsert K., Fulton E. A., et al. (2020). The Ocean Decade: A True Ecosystem Modeling Challenge. Front. Mar. Sci. 7, 554573. doi: 10.3389/fmars.2020.554573
Howell K. L., Hilário A., Allcock A. L., Bailey D. M., Baker M., Clark M. R., et al. (2020). A Blueprint for an Inclusive, Global Deep-Sea Ocean Decade Field Program. Front. Mar. Sci. 7, 584861. doi: 10.3389/fmars.2020.584861
Howell K. L., Hilário A., Allcock A. L., Bailey D., Baker M., Clark M. R., et al. (2021). A Decade to Study Deep-Sea Life. Nat. Ecol. Evol. 5, 265–267. doi: 10.1038/s41559-020-01352-5
Howe B. M., Miksis-Olds J., Rehm E., Sagen H., Worcester P. F., Haralabus G. (2019). Observing the Oceans Acoustically. Front. Mar. Sci. 6, 426. doi: 10.3389/fmars.2019.00426
Jiménez C. (2018). Marine Natural Products in Medicinal Chemistry. ACS Medicinal Chemistry Letters 2018. 9(10), 959–961. doi: 10.1021/acsmedchemlett.8b00368
Krueger M. C., Harms H., Schlosser D. (2015). Prospects for Microbiological Solutions to Environmental Pollution With Plastics. Appl. Microbiol. Biotechnol. 99, 8857–8874. doi: 10.1007/s00253-015-6879-4
Kubicki S., Bollinger A., Katzke N., Jaeger K. E., Loeschcke A., Thies S. (2019). Marine Biosurfactants: Biosynthesis, Structural Diversity and Biotechnological Applications. Mar. Drugs 17 (7), 408. doi: 10.3390/md17070408
Kumar S., Stecher G., Tamura K. (2016). MEGA7: Molecular Evolutionary Genetics Analysis Version 7.0 for Bigger Datasets. Mol. Biol. Evol. 33, 1870–1874. doi: 10.1093/molbev/msw054
Landrigan P. J., Stegeman J. J., Fleming L. E., Allemand D., Anderson D. M., Backer L. C., et al. (2020). Human Health and Ocean Pollution. Ann. Glob Health 86, 151. doi: 10.5334/aogh.2831
Law K. L., Morét-Ferguson S. E., Goodwin D. S., Zettler E. R., Deforce E., Kukulka T., et al. (2014). Distribution of Surface Plastic Debris in the Eastern Pacific Ocean From an 11-Year Data Set. Environ. Sci. Technol. 48, 4732–4738. doi: 10.1021/es4053076
Lee X. J., Ong H. C., Ooi J., Yu K. L., Tham T. C., Chen W.-H., et al. (2022). Engineered Macroalgal and Microalgal Adsorbents: Synthesis Routes and Adsorptive Performance on Hazardous Water Contaminants. J. Hazardous Materials 423, 126921. doi: 10.1016/j.jhazmat.2021.126921
Liguori L., Monticelli M., Allocca M., Cubellis M. V., Hay Mele B. (2020). Bioinformatics Tools for Marine Biotechnology: A Practical Tutorial With a Metagenomic Approach. BMC Bioinf. 21, 348. doi: 10.1186/s12859-020-03560-z
Liu L., Bilal M., Duan X., Iqbal H. M. N. (2019). Mitigation of Environmental Pollution by Genetically Engineered Bacteria - Current Challenges and Future Perspectives. Sci. Total Environ. 667, 444–454. doi: 10.1016/j.scitotenv.2019.02.390
Lo Bue N., Best M. M.R., Embriaco D., Abeysirigunawardena D., Beranzoli L., Dewey R. K., et al (2021). The Importance of Marine Research Infrastructures in Capturing Processes and Impacts of Extreme Events. Front. Mar. Sci. 8:626668. doi: 10.3389/fmars.2021.626668
Marrero M. E., Payne D. L., Breidahl H. (2019). The Case for Collaboration to Foster Global Ocean Literacy. Front. Mar. Sci. 6, 325. doi: 10.3389/fmars.2019.00325
Martín López L. M., Aguilar De Soto N., Madsen P. T., Johnson M. (2022). Overall Dynamic Body Acceleration Measures Activity Differently on Large Versus Small Aquatic Animals. Methods Ecol. Evol. 13, 447–458. doi: 10.1111/2041-210X.13751
Matthijs H. C. P., Jančula D., Visser P. M., Maršálek B. (2016). Existing and Emerging Cyanocidal Compounds: New Perspectives for Cyanobacterial Bloom Mitigation. Aquat. Ecol. 50, 443–460. doi: 10.1007/s10452-016-9577-0
Mayer L., Jakobsson M., Allen G., Dorschel B., Falconer R., Ferrini V., et al. (2018). The Nippon Foundation—GEBCO Seabed 2030 Project: The Quest to See the World’s Oceans Completely Mapped by 2030. Geosciences 8 (2), 63. doi: 10.3390/geosciences8020063
Milanese M., Chelossi E., Manconi R., Sarà A., Sidri M., Pronzato R. (2003). The Marine Sponge Chondrilla Nucula Schmidt 1862 as an Elective Candidate for Bioremediation in Integrated Aquaculture. Biomolecular Eng. 20, 363–368. doi: 10.1016/S1389-0344(03)00052-2
Mooney T. A., Di Iorio L., Lammers M., Lin T.-H., Nedelec S. L., Parsons M., et al. (2020). Listening Forward: Approaching Marine Biodiversity Assessments Using Acoustic Methods. R. Soc Open Sci. 7, 201287. doi: 10.1098/rsos.201287
Morganti T. M., Ribes M., Yahel G., Coma R. (2019). Size Is the Major Determinant of Pumping Rates in Marine Sponges. Front. Physiol. 10, 1474. doi: 10.3389/fphys.2019.01474
Muelbert M. M. C., Copertino M., Cotrim da Cunha L., Lewis M. N., Polejack A., A.d.C Peña-Puch, et al. (2021). The Ocean and Cryosphere in a Changing Climate in Latin America: Knowledge Gaps and the Urgency to Translate Science Into Action. Front. Climate 3, 748344. doi: 10.3389/fclim.2021.748344
Muñoz I., Gómez-Ramos M. J., Agüera A., Fernández-Alba A. R., García-Reyes J. F., Molina-Díaz A. (2009). Chemical Evaluation of Contaminants in Wastewater Effluents and the Environmental Risk of Reusing Effluents in Agriculture. TrAC Trends Analytical Chem. 28, 676–694. doi: 10.1016/j.trac.2009.03.007
Naughton P. J., Marchant R., Naughton V., Banat I. M. (2019). Microbial Biosurfactants: Current Trends and Applications in Agricultural and Biomedical Industries. J. Appl. Microbiol. 127, 12–28. doi: 10.1111/jam.14243
Nguyen-Van P., Stenger A., Tiet T. (2021). Social Incentive Factors in Interventions Promoting Sustainable Behaviors: A Meta-Analysis. PLos One 16, e0260932. doi: 10.1371/journal.pone.0260932
Olasanmi I. O., Thring R. W. (2018). The Role of Biosurfactants in the Continued Drive for Environmental Sustainability. Sustainability 10 (12), 4817. doi: 10.3390/su10124817
Oliveri P., Simoncelli S., Di Pietro P., Fratianni C., Mattia G., Delrosso D., et al. (2022). SOURCE: Sea Observations Utility for Reprocessing, Calibration and Evaluation. Front. Mar. Sci. 8, 750387. doi: 10.3389/fmars.2021.750387
Pal M., Yesankar P. J., Dwivedi A., Qureshi A. (2020). Biotic Control of Harmful Algal Blooms (HABs): A Brief Review. J. Environ. Manage 268, 110687. doi: 10.1016/j.jenvman.2020.110687
Paniagua-Michel J. (2015). Marine Bioremediation - A Sustainable Biotechnology of Petroleum Hydrocarbons Biodegradation in Coastal and Marine Environments. J. Bioremediation Biodegradation 6, 273. doi: 10.4172/2155-6199.1000273
Park S.-C., Choi C., Jeong G.-W., Lee H.-S., Choi S.-J., Kim W.-S., et al. (2016). Algicidal Effects of Free-Amine Water-Soluble Chitosan to Marine Harmful Algal Species. J. Ind. Eng. Chem. 34, 139–145. doi: 10.1016/j.jiec.2015.11.002
Payne D. L., Marrero M. E. (2021). “"Ocean Literacy: From a Ripple to a Wave,",” in Ocean Literacy: Understanding the Ocean. Eds. Koutsopoulos K. C., Stel J. H. (Cham: Springer International Publishing), 21–39.
Piccini M., Raikova S., Allen M. J., Chuck C. J. (2019). A Synergistic Use of Microalgae and Macroalgae for Heavy Metal Bioremediation and Bioenergy Production Through Hydrothermal Liquefaction. Sustain. Energy Fuels 3, 292–301. doi: 10.1039/C8SE00408K
Pinheiro H. T., Moreau C. S., Daly M., Rocha L. A. (2019). Will DNA Barcoding Meet Taxonomic Needs? Science 365, 873–874. doi: 10.1126/science.aay7174
Popper A. N., Hawkins A. D. (2019). An Overview of Fish Bioacoustics and the Impacts of Anthropogenic Sounds on Fishes. J. Fish Biol. 94, 692–713. doi: 10.1111/jfb.13948
Sanganyado E., Chingono K. E., Gwenzi W., Chaukura N., Liu W. (2021). Organic Pollutants in Deep Sea: Occurrence, Fate, and Ecological Implications. Water Res. 205, 117658. doi: 10.1016/j.watres.2021.117658
Savoca M. S., Czapanskiy M. F., Kahane-Rapport S. R., Gough W. T., Fahlbusch J. A., Bierlich K. C., et al. (2021). Baleen Whale Prey Consumption Based on High-Resolution Foraging Measurements. Nature 599, 85–90. doi: 10.1038/s41586-021-03991-5
Schmidt J., Wei R., Oeser T., Belisário-Ferrari M. R., Barth M., Then J., et al. (2016). Effect of Tris, MOPS, and Phosphate Buffers on the Hydrolysis of Polyethylene Terephthalate Films by Polyester Hydrolases. FEBS Open Bio 6 (9), 919–927. doi: 10.1002/2211-5463.12097
Scholin C. A., Preston C. M., Yamahara K., Ussler W., Birch J. M., Goodwin K. D., et al. (2019). The Quest to Develop Ecogenomic Sensors: A 25-Year History of the Environmental Sample Processor (ESP) as a Case Study. Oceanography 30 4, 100–13. doi: 10.5670/oceanog.2017.427
Schuhbauer A., Chuenpagdee R., Cheung W. W. L., Greer K., Sumaila U. R. (2017). How Subsidies Affect the Economic Viability of Small-Scale Fisheries. Mar. Policy 82, 114–121. doi: 10.1016/j.marpol.2017.05.013
Schuhbauer A., Sumaila U. (2016). Economic Viability and Small-Scale Fisheries — A Review. Ecol. Economics 124, 69–75. doi: 10.1016/j.ecolecon.2016.01.018
Seraphin K. D., East J. L., Whitley L. N. (2019). Book Review: Exemplary Practices in Marine Science Education. Front. Mar. Sci. 6, 6. doi: 10.3389/fmars.2019.00006
Sharifinia M., Bahmanbeigloo Z. A., Keshavarzifard M., Khanjani M. H., Lyons B. P. (2020). Microplastic Pollution as a Grand Challenge in Marine Research: A Closer Look at Their Adverse Impacts on the Immune and Reproductive Systems. Ecotoxicol Environ. Saf. 204, 111109. doi: 10.1016/j.ecoenv.2020.111109
Shi S., Qu Y., Ma F., Zhou J. (2014). Bioremediation of Coking Wastewater Containing Carbazole, Dibenzofuran and Dibenzothiophene by Immobilized Naphthalene-Cultivated Arthrobacter Sp. W1 in Magnetic Gellan Gum. Bioresour Technol. 166, 79–86. doi: 10.1016/j.biortech.2014.05.036
Skinder B. M., Uqab B., Ganai B. A. (2020). “"Bioremediation: A Sustainable and Emerging Tool for Restoration of Polluted Aquatic Ecosystem,",” in Fresh Water Pollution Dynamics and Remediation. Eds. Qadri H., Bhat R. A., Mehmood M. A., Dar G. H. (Singapore: Springer Singapore), 143–165.
Skinnider M. A., Johnston C. W., Gunabalasingam M., Merwin N. J., Kieliszek A. M., Maclellan R. J., et al. (2020). Comprehensive Prediction of Secondary Metabolite Structure and Biological Activity From Microbial Genome Sequences. Nat. Commun. 11, 6058. doi: 10.1038/s41467-020-19986-1
Smale D. A., Wernberg T., Oliver E. C. J., Thomsen M., Harvey B. P., Straub S. C., et al. (2019). Marine Heatwaves Threaten Global Biodiversity and the Provision of Ecosystem Services. Nat. Climate Change 9, 306–312. doi: 10.1038/s41558-019-0412-1
Stefanni S., Stanković D., Borme D., De Olazabal A., Juretić T., Pallavicini A., et al. (2018). Multi-Marker Metabarcoding Approach to Study Mesozooplankton at Basin Scale. Sci. Rep. 8, 12085–12085. doi: 10.1038/s41598-018-30157-7
Stel J. H. (2021). “"Ocean Literacy: Background, Future Drivers, and Opportunities,",” in Ocean Literacy: Understanding the Ocean. Eds. Koutsopoulos K. C., Stel J. H. (Cham: Springer International Publishing), 3–20.
Sudek S., Lopanik N. B., Waggoner L. E., Hildebrand M., Anderson C., Liu H., et al. (2007). Identification of the Putative Bryostatin Polyketide Synthase Gene Cluster From "Candidatus Endobugula Sertula", the Uncultivated Microbial Symbiont of the Marine Bryozoan Bugula Neritina. J. Nat. Prod 70, 67–74. doi: 10.1021/np060361d
Surkatti R., El-Naas M. H. (2014). Biological Treatment of Wastewater Contaminated With P-Cresol Using Pseudomonas Putida Immobilized in Polyvinyl Alcohol (PVA) Gel. J. Water Process Eng. 1, 84–90. doi: 10.1016/j.jwpe.2014.03.008
Teta R., Della Sala G., Mangoni A., Lega M., Costantino V. (2016). Tracing Cyanobacterial Blooms to Assess the Impact of Wastewaters Discharges on Coastal Areas and Lakes. Int. J. Sustain. Dev. Plann. 11, 804–811. doi: 10.2495/SDP-V11-N5-804-811
Teta R., Romano V., Sala G. D., Picchio S., Sterlich C. D., Mangoni A., et al. (2017). Cyanobacteria as Indicators of Water Quality in Campania Coasts, Italy: A Monitoring Strategy Combining Remote/Proximal Sensing and in Situ Data. Environ. Res. Lett. 12, 024001. doi: 10.1088/1748-9326/aa5649
Teta R., Sala G. D., Esposito G., Stornaiuolo M., Scarpato S., Casazza M., et al. (2021). Monitoring Cyanobacterial Blooms During the COVID-19 Pandemic in Campania, Italy: The Case of Lake Avernus. Toxins 13 (7), 471. doi: 10.3390/toxins13070471
Tyack P. L., Miksis-Olds-Olds J., Urban J. E. R., Ausubel J. (2021). Measuring Ambient Ocean Sound During the COVID-19 Pandemic. Eos Transactions Am. Geophysical Union 102. doi: 10.1029/2021EO155447
Vermeeren A. P. O. S., Calvi L., Sabiescu A., Trocchianesi R., Stuedahl D., Giaccardi E., et al. (2018). “"Future Museum Experience Design: Crowds, Ecosystems and Novel Technologies,",” in Museum Experience Design, 1–16. Springer
Whitt C., Pearlman J., Polagye B., Caimi F., Muller-Karger F., Copping A., et al. (2020). Future Vision for Autonomous Ocean Observations. Front. Mar. Sci. 7, 697. doi: 10.3389/fmars.2020.00697
Wilkinson M. D., Dumontier M., Aalbersberg I. J., Appleton G., Axton M., Baak A., et al. (2016). The FAIR Guiding Principles for Scientific Data Management and Stewardship. Sci. Data 3, 160018. doi: 10.1038/sdata.2016.18
Wilms S. N. (2021). A Beginner’s Guide on Integrating *Omics Approaches to Study Marine Microbial Communities: Details and Discussions From Sample Collection to Bioinformatics Analysis. Front. Mar. Sci. 8, 692538. doi: 10.3389/fmars.2021.692538
Wilson M. C., Mori T., Rückert C., Uria A. R., Helf M. J., Takada K., et al. (2014). An Environmental Bacterial Taxon With a Large and Distinct Metabolic Repertoire. Nature 506, 58–62. doi: 10.1038/nature12959
Wollmann F., Dietze S., Ackermann J.-U., Bley T., Walther T., Steingroewer J., et al. (2019). Microalgae Wastewater Treatment: Biological and Technological Approaches. Eng. Life Sci. 19860–871. doi: 10.1002/elsc.201900071
Xu W., Dong L., Caruso F., Gong Z., Li S. (2020). Long-Term and Large-Scale Spatiotemporal Patterns of Soundscape in a Tropical Habitat of the Indo-Pacific Humpback Dolphin (Sousa Chinensis). PLos One 15, e0236938. doi: 10.1371/journal.pone.0236938
Xu G., Shi Y., Sun X., Shen W. (2019). Internet Things Mar. Environ. Monitoring: A Review Sensors 19 (7), 1711. doi: 10.3390/s19071711
Yamahara K. M., Preston C. M., Birch J., Walz K., Marin R., Jensen S., et al. (2019). In Situ Autonomous Acquisition and Preservation of Marine Environmental DNA Using an Autonomous Underwater Vehicle. Front. Mar. Sci. 6, 373. doi: 10.3389/fmars.2019.00373
Yoshida S., Hiraga K., Takehana T., Taniguchi I., Yamaji H., Maeda Y., et al. (2016). A Bacterium That Degrades and Assimilates Poly(Ethylene Terephthalate). Science 351, 1196–1199. doi: 10.1126/science.aad6359
Zeraatkar A. K., Ahmadzadeh H., Talebi A. F., Moheimani N. R., Mchenry M. P. (2016). Potential Use of Algae for Heavy Metal Bioremediation, a Critical Review. J. Environ. Manage 181, 817–831. doi: 10.1016/j.jenvman.2016.06.059
Zhang X., Zhang W., Xue L., Zhang B., Jin M., Fu W. (2010). Bioremediation of Bacteria Pollution Using the Marine Sponge Hymeniacidon Perlevis in the Intensive Mariculture Water System of Turbot Scophthalmus Maximus. Biotechnol. Bioeng 105, 59–68. doi: 10.1002/bit.22522
Keywords: Ocean Decade, marine biodiversity, environmental sustainability, marine technology, marine biotechnology, marine omics
Citation: Caruso F, Tedesco P, Della Sala G, Palma Esposito F, Signore M, Canese S, Romeo T, Borra M, Gili C and de Pascale D (2022) Science and Dissemination for the UN Ocean Decade Outcomes: Current Trends and Future Perspectives. Front. Mar. Sci. 9:863647. doi: 10.3389/fmars.2022.863647
Received: 27 January 2022; Accepted: 27 May 2022;
Published: 29 June 2022.
Edited by:
Jose Henrique Muelbert, Universidade Federal do Rio Grande, BrazilReviewed by:
Thanos Dailianis, Hellenic Centre for Marine Research, GreeceTymon Zielinski, Institute of Oceanology, Polish Academy of Sciences, Poland
Copyright © 2022 Caruso, Tedesco, Della Sala, Palma Esposito, Signore, Canese, Romeo, Borra, Gili and de Pascale. This is an open-access article distributed under the terms of the Creative Commons Attribution License (CC BY). The use, distribution or reproduction in other forums is permitted, provided the original author(s) and the copyright owner(s) are credited and that the original publication in this journal is cited, in accordance with accepted academic practice. No use, distribution or reproduction is permitted which does not comply with these terms.
*Correspondence: Claudia Gili, Y2xhdWRpYS5naWxpQHN6bi5pdA==; Donatella de Pascale, ZG9uYXRlbGxhLmRlcGFzY2FsZUBzem4uaXQ=
†These authors have contributed equally to this work and share first authorship
‡These authors have contributed equally to this work and share last authorship