- 1Department of Geoscience, Faculty of Science, Shizuoka University, Shizuoka, Japan
- 2Department of Aquaculture, National Taiwan Ocean University, Keelung, Taiwan
- 3Center of Excellence for the Oceans, National Taiwan Ocean University, Keelung, Taiwan
- 4Université de Lille, CNRS, Univ. Littoral Côte d’Opale, IRD, UMR 8187, LOG, Laboratoire d’Océanologie et de Géosciences, Station Marine de Wimereux, Lille, France
- 5Creative Science Unit (Geoscience), Faculty of Science, Shizuoka University, Shizuoka, Japan
In this study, an individual-based model (IBM) was established and applied to simulate the effects of salinity variations on the productivity of a promising live feed cyclopoid copepod Apocyclops royi for aquaculture applications. The model integrates the effect of salinity on the different reproductive traits and temperature on female longevity. To calibrate the model developed on the Mobidyc platform, we collected data from previous literature and conducted complementary experiments. The model outputs on total nauplii production match the experimental results. Both showed a progressive increase in nauplii production from 0 up to 21 PSU, beyond which the production decreases. There were no significant differences between the estimated nauplii production and the observed ones for most salinity conditions. We then used the model to estimate the egg and nauplii production of a population initiated with 1,000 females along a salinity gradient from 0 to 39 PSU during 20-d cultivation. Around the optimal salinity of 21 PSU, the egg and nauplii production peaked at 1.8x105 eggs and 1.39x105 nauplii, respectively. A deviation of 7 PSU from the optimal salinity range would lead to a loss of 22 to 25% in egg and nauplii production. The results indicate that implementing the IBM into a life-cycle model provides useful tool for managing the risks of salinity variation on the copepod productivity in aquaculture conditions.
Introduction
Fishing activities have increased concomitantly with the rapidly increasing human population. However, the natural productivity of marine products is limited, and overfishing has kept on increasing over the years, hovering around 30% of fish stocks overexploited this last decade (FAO, 2020). Aquaculture can solve part of those issues and is an effective way to increase seafood supply (Costello et al., 2020). In aquaculture, fish are fed a variety of food to enable them to live and grow. Those foods depend on the developmental stage of the fish. Employing suitable feed in each developmental stage enables aquaculture to produce high-quality fish (Das et al., 2012). In early larval stages, it is common to use Rotifer and Artemia as live feed because they are easy to culture, but copepod nauplii are known to be superior live-feed (Støttrup, 2000; Støttrup, 2003; Conceição et al., 2010; Drillet et al., 2011). Copepods are the natural preys of larvae of many marine species and their nutritional profile is superior to artemia and rotifers (Shields et al., 1999; Payne et al., 2001; Rajkumar and Kumaraguru Vasagam, 2006; van der Meeren et al., 2008; Piccinetti et al., 2014). Several species of copepods could biosynthesize and accumulate polyunsaturated fatty acids (Bell et al., 2003; McKinnon et al., 2003; Dayras et al., 2021; Monroig et al., 2022; see Nielsen et al., 2020 for an exhaustive list of species and references therein), which are important nutrients for the development of fish larvae (Mejri et al., 2021). Additionally, the small size exhibited by the copepod larvae stage makes them the recommended live feed for several marine fishes (Lee et al., 2010; Mitsuzawa et al., 2017; Anzeer et al., 2019; Burgess et al., 2020). The swimming behavior of some copepod species could trigger the urge to forage in small-mouthed larval fish (Buskey, 2005; Mahjoub et al., 2011). All these characteristics have led to increasing interest and effort in the mass cultivation of copepod to serve as a live feed.
Copepods are used extensively in the aquaculture industries of some countries, such as Taiwan (Blanda et al., 2017), China (Zeng et al., 2018), Vietnam (Grønning et al., 2019; Nguyen et al., 2020), Australia (Payne et al., 2001; Camus et al., 2021), and others (van der Meeren et al., 2014; Magouz et al., 2021). Outdoor copepod production is a practical industry in Taiwan (Blanda et al., 2015; Blanda et al., 2017). However, one of the bottlenecks in outdoor copepod culture is the difficulty to maintain stable production, as copepod productivities are under the influences of many drivers, such as temperature (Devreker et al., 2009; Devreker et al., 2012; Liu et al., 2015), food quantity and quality (Pan et al., 2014; Rasdi and Qin, 2018; Tordesillas et al., 2018; Dayras et al., 2020; Aganesova, 2021), stocking density (Alajmi and Zeng, 2014), photoperiod (Camus and Zeng, 2008). In addition, the majority of the outdoor copepod cultures are in subtropical and tropical regions, where sudden rainfall and storm often occur and induce salinity variation. Although brackish copepod could survive at a wide salinity range, salinity can significantly affect the reproductive traits of these tiny crustaceans (Devreker et al., 2009; Devreker et al., 2012; Pan et al., 2016; Nguyen et al., 2020). The response to salinity is linked to the osmotic stress that limits the energy available for other basic physiological functions of copepods (Chen et al., 2006), and it could reduce dramatically their production. Environmental monitoring and on-site management (e.g., introduction of seawater or freshwater for salinity adjustment of the pond water) are both crucial for sustaining a stable copepod production. Although the effects of salinity on copepods have been extensively studied, these experimental results are limited to specific reproductive traits among salinity intervals. For aquaculture management, it would be best to have at one disposal a tool that integrates the effects on the different reproductive traits for estimating the global copepod productivity under a salinity gradient.
Focusing on the individual active components of a system, Individual-Based Models (IBMs) have been more and more proving their efficiency in defining optimal conditions for diverse marine organisms including, shellfish (Nunes et al., 2003), Euphrasia (Dorman et al., 2015), zebrafish (Beaudouin et al., 2015). Individual-based models can provide managerial solutions for the production of well-described species and reduce the cost induced by the classical trial and error method. To our knowledge, only one model has been developed for improving copepod cultivation (Drillet and Lombard, 2015). This model is nevertheless not an IBM and focuses on a free-spawner. To our knowledge, there is no IBM that aims to improve egg-bearing copepod culture.
Among the copepods explored for mass culture, calanoid copepods represent the majority (Rasdi and Qin, 2016). However, Cyclopoid copepods have great potential as live feed due to their small size, high tolerance to salinity and temperature variations, and ability to withstand high densities (Lee et al., 2012; Pan et al., 2016; Lee et al., 2017; Jepsen et al., 2021). Recent research efforts have been made to identify the best external conditions leading to optimize cyclopoid mass production (Lee et al., 2013; Nielsen et al., 2021; Dayras et al., 2021). The key bottleneck for this egg-bearing copepod is the fecundity and nauplii production as we cannot harvest their eggs as it is done for free-spawners like Acartia tonsa or A. bilobata (Drillet et al., 2014; Pan et al., 2021).
Apocyclops royi is a euryhaline cyclopoid copepod cultured commercially as live feed in the Taiwanese aquaculture industry (Su et al., 2005; Ajiboye et al., 2011). This species has a wide range of tolerance of salinity and temperature, facilitating the establishment of mass culture (Lee et al., 2005). Apocyclops royi can also survive on a low polyunsaturated fatty acid diet (Blanda et al., 2015; Nielsen et al., 2020), hence reducing the cost of the copepod diet. Like many cyclopoid copepods, A. royi reproduce sexually. The male uses its antennules to hold on to the female and transfers spermatophores to the female genital opening. The female could store the sperm and use it to fertilize many clutches from one insemination. The eggs are spawned simultaneously in the egg sac and the same-clutch nauplii hatch almost synchronously (Liu et al., 2007; Marten and Reid, 2007). The durations of interclutch time and embryogenic development are important reproductive traits to estimate copepod overall productivity in aquaculture, and they vary with environmental conditions (Liu et al., 2007; Devreker et al., 2009). Several experiments have investigated the effect of salinity on the reproductive traits of A. royi (Lee et al., 2005; Pan et al., 2016; Van Someren Gréve et al., 2020), providing useful information for building an IBM for this species.
In this study, we integrated the different effects of salinity on the reproductive traits of A. royi using an IBM developed on the MOBIDYC platform (Ginot et al., 2002) that simulates the reproductive cycle of egg-bearing copepods (Dur et al., 2009). The model description follows the Overview, Design Concepts, and Details protocol proposed by Grimm et al. (2006; Grimm et al., 2010; Grimm et al., 2020). The experimental results from studies on the effect of salinity on A. royi (Pan et al., 2016; Van Someren Gréve et al., 2020) and from complementary experiments on the reproductive cycle conducted for this study were used for model calibration. Following the calibration, we used the individual-based model to quantify the effect of salinity on the production of a cohort of 1000 A. royi females and to determine the optimal salinity for reproduction.
Materials and Methods
Purpose and Patterns
The model’s higher-level purpose was to determine the optimal salinity for the recruitment of the egg-carrying copepod. More specifically, the model was designed to quantify the effect of salinity on reproductive traits and success in a copepod cohort of 1000 individual females. The model was calibrated for the copepod A. royi. Based on laboratory observations, we evaluated our model by its ability to reproduce two patterns:
At the individual level: A decrease in interclutch duration with increasing salinity up to a maximum value beyond which the interclutch duration increases, a decrease in mortality with increasing salinity up to a limit; an increase in clutch size with increasing salinity until reaching a threshold beyond which the clutch size decline slowly.
At the cohort level: An overall increase in egg and offspring production until an optimal salinity beyond which the recruitment slows down and even stops.
Entities, State Variables, and Scales
Spatial Scale
The model was not spatially explicit. The agents (i.e. A. royi female) were not located in geometrical space.
Temporal Scale
The simulation ran with a time step fixed at three hours and lasted 20 days, which is the maximum life span of an A. royi female at 28°C (unpublished data)
Entities
The model included two kinds of entity predefined in Mobidyc: located agents and non-located agents (Ginot et al., 2002).
The two located agents represent 1) an A. royi individual of the adult female stage, and 2) an individual of the first naupliar stage. As A. royi is an egg carrier, reproduction is relatively complex and involves a multi-phase cycle (Dur et al., 2009). Adult females of A. royi have been credited with several state variables, called attributes in Mobidyc (Table 1). The nauplii are represented in the model only to confirm the hatching success being taken into consideration. The nauplii of a clutch hatch synchronously and die at the second time step following the hatching after being counted. The state variable of nauplii is, therefore “age” only. While the assumption of synchronous hatching does not reflect the biology of copepod, it does not affect the outcome of the present model. Asynchronous hatching does not affect A. royi adult females reproductive process but the nauplii development, which is not considered in the present model. Moreover, according to our observations, A. royi nauplii of a given clutch hatch within a couple of hours. The time step of the model is three hours. Hence, the hatching can be considered synchronous.
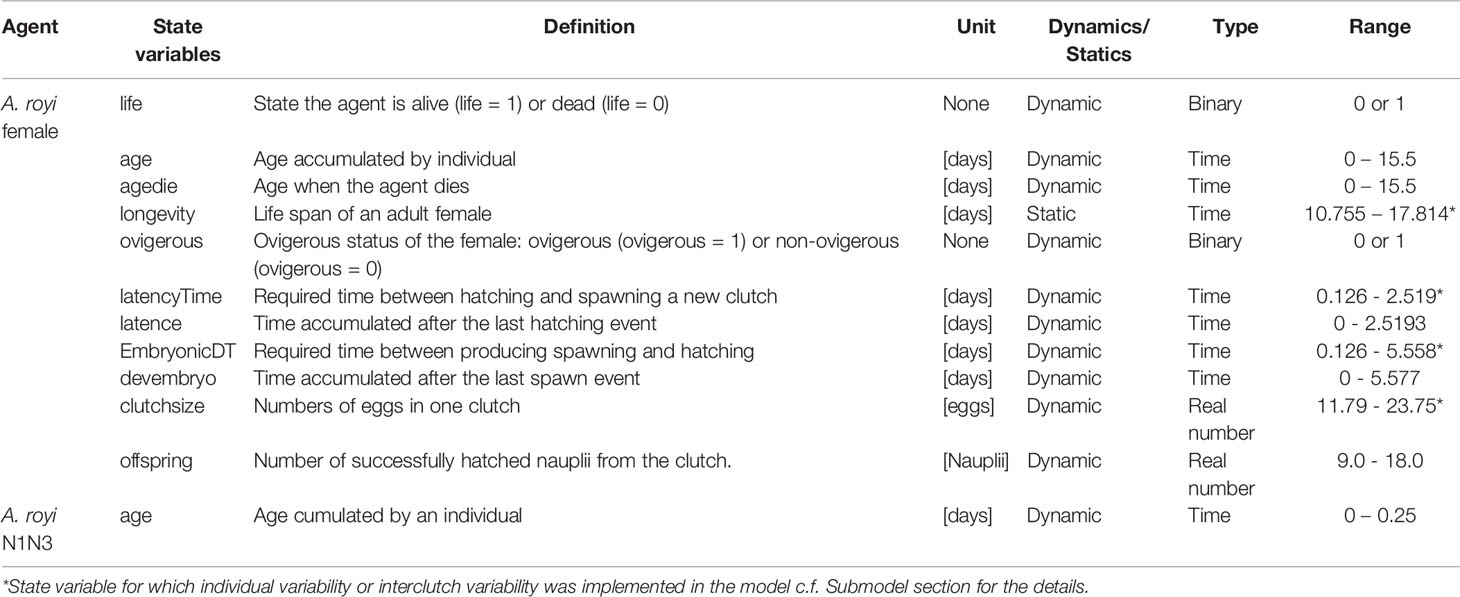
Table 1 State variables used to represent the located agent of Apocyclops royi adult female and A. royi first naupliar stages (N1N3).
The non-located agents of the platform Mobidyc were used to design the model and collect required data from the simulation (Ginot et al., 2002). The first three non-located agents were used to store global variables, reducing the number of attributes carried by the located agents (Table 2). ParamDev contained the parameter values of the normal distribution used to introduce individual variability on a female’s life span. ParamSurv reflected the survival probability of the females and how it varied based on the salinity. ParamRepro stored all parameters of the normal distribution used to introduce individual variability on the clutch size (CS), embryonic development time (EDT), and latency time (LT). ParamRepro also stored the value of the hatching success (HS), which was set constant at 75% according to our lab observations (reanalyzed data of the results presented in Pan et al., 2016). Two additional non-located agents were used to represent the environment and the experimenter. The environment agent (Envi) refers to the overall environment, including temperature (fixed at 28°C) and salinity. The experimenter agent (Scientist) recorded the age of the female at the time of death and the number of eggs and nauplii produced throughout the simulation.
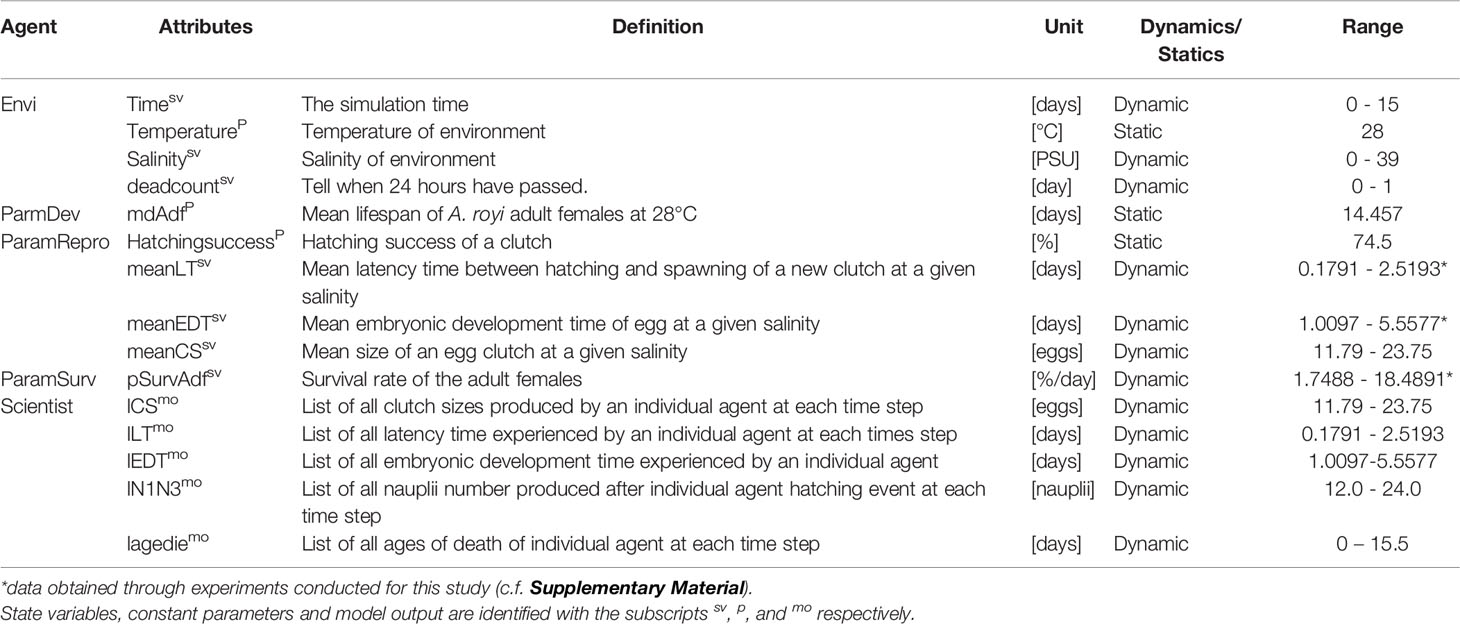
Table 2 Attributes of non-located agents facilitating the computation of the reproductive cycle of the Adult female copepod Apocyclop royi (ParamX), the environment (Envi), and the one used to extract and save the output data (Scientist).
Process Overview and Scheduling
Summary
1. The non-located agent Envi executes its “GrowOlder” submodel, with increments for its state variable “time” of three hours.
2. The non-located agent ParamDev executes its “mdAdf” which defines the mean lifespan of A.royi female under 28°C.3. The non-located agent ParamRepro executes its “SalEffect” submodel, which computes the value of mean CS, mean EDT, and mean LT in relation to the salinity.4. The non-located agent ParamSurv executes its “DefProbSurv” submodel, which calculates the survival probability of the adult females, pSurvAdf, in relation to salinity.5. The adult female agents each execute different tasks simulating the “reproductive cycle”. A schematic representation of the reproductive cycle and the flowchart of the submodel scheduling are provided in Figures 1, 2. The located female agents execute their submodels in the following order:
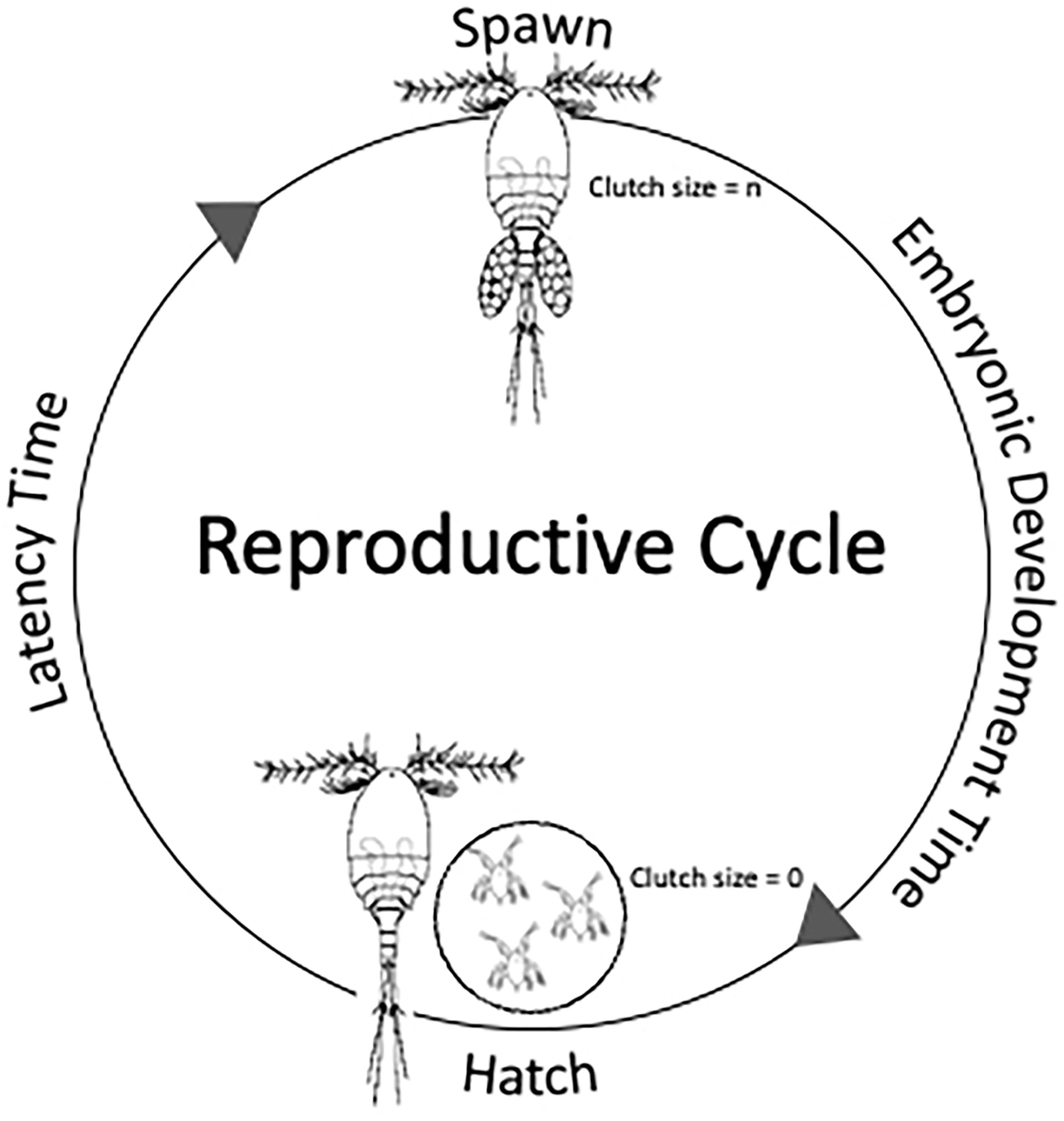
Figure 1 Schematic representation of the reproductive cycle of the cyclopoida copepod Apocyclops royi. Females enter this cycle after molting from the last juvenile stage C5 and perform this cycle until their death defined by their survival probability and longevity. The cycle integrates two main processes: the production (spawning) of a clutch of size n (Clutch size = n) and the hatching of the eggs. The time between hatching and spawning is defined as Latency Time, and between hatching and spawning as Embryonic Development Time.
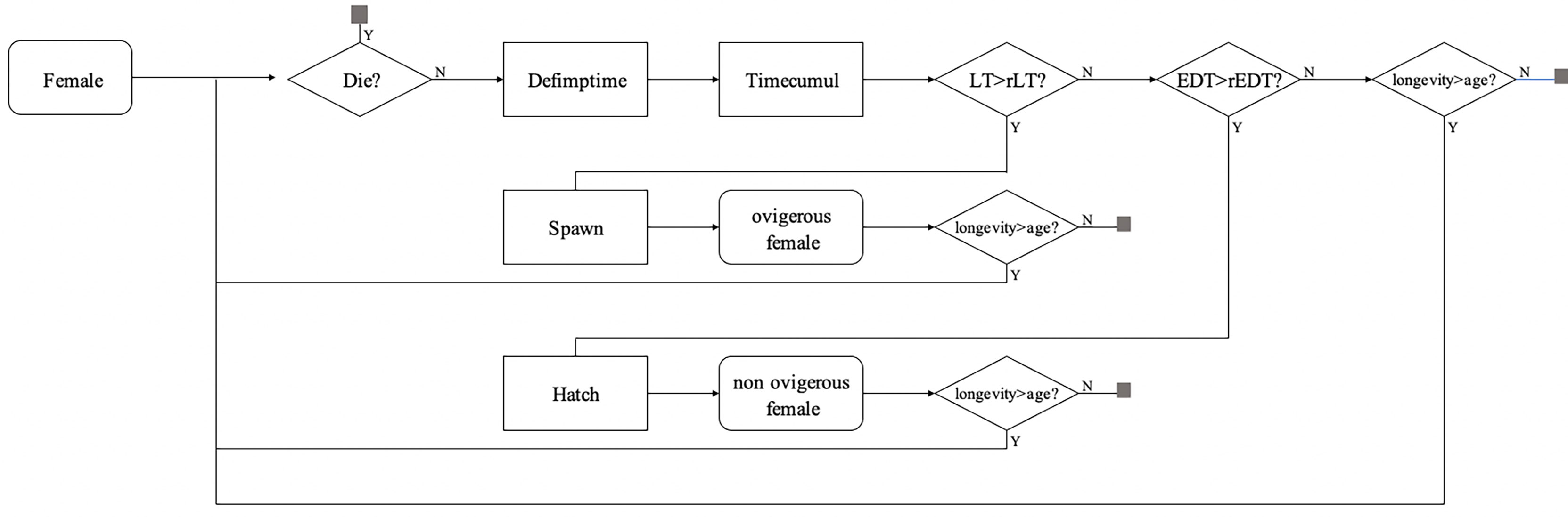
Figure 2 Flow chart representing the scheduling of the model processes used to define the reproductive cycle of the copepod Apocyclops royi. The conditional tasks “Spawn” and “Hatch” respectively require the latency time (LT) and Embryonic Development Time (EDT) to reach a threshold value (rLT and rEDT) for their execution. The details on each task are presented in Table 3.
a. “Survive”, which defines whether the agent will live or die every 24 hours.
b. “DefImpTime”, which defines the longevity of the female when the female age is 0 and the latencyTime to produce the subsequent clutch every time the female is non-ovigerous and has a latence of 0.
c. “TimeCumul”, which increments the female age, latence, and devembryo by one-time step (i.e. three hours).
d. “Spawn”, which sets the ovigerous status to 1, calculates a new value of clutch size and embryoDT and resets the value of latence and devembryo when the proper conditions for spawning are met (cf submodel section).
e. “Hatch”, which creates the nauplius 1 agents and resets to zero the attributes ovigerous, devembryo, latence, and clutchsize.
f. “Die”, which kills the individual when age exceed the longevity
6. The Naupliar 1 agents only survive until there are counted.7. The non-located Scientist (a) save the value of the clutch size and age of death of the female, and (b) counts the number of nauplii produced and kills them.
Rationale
The Envi is scheduled first because the subsequent ParamSurv action depends on salinity. In the female agent, the submodel DefImpTime occurs before TimeCumul to allow the computation of the required latencyTime just after the hatching process (i.e. Reproduction) and the latencyTime of the first clutch. EggProduct has to occur before Reproduce to allow the proper condition and timing for hatching. Finally, Scientist actions take place at the end of the time step.
Design Concepts
Basic principles – In this model, the salinity affected the survival rate of the female, the latency time, the embryonic development time, and the number of eggs per clutch (clutch size, CS). To determine the effects, we used empirical relationships that are described hereafter (see Submodel, below).
Emergence – The key outcomes of the model are the total production and cumulated production of eggs and offspring by a female cohort. All results emerge from the effect of salinity on the survival rate and reproductive traits.
Adaptation – Adaptation is not implemented in the model
Objectives – Objectives measures are not implemented in the model
Learning – Learning is not implemented in the model
Prediction – The model includes no prediction process
Fitness – Individual fitness is determined by a female’s survival rate, longevity, clutch size (CS), latency time (LT), embryonic development time (EDT), and hatching success (HS). Although the consequences of variation in the fitness at a higher level can be quantified, the present study did not address this question.
Sensing – The state variable “ovigerous” defines the proper time to perform appropriate reproductive tasks, e.g. spawning and hatching.
Interactions – Interactions between individuals were not introduced in the present model. We assumed that the time required for a male (not represented in the model) to anchor its spermatophore to a female is included in the latency time. Neither the limitation due to mating nor the failure of egg fertilization was considered here.
Stochasticity – Survival was interpreted as a random variable; a survival rate of 95% means an individual will die only if the generated random number belonging to a uniform probability density between [0,1] is larger than 0.95. Stochasticity is used to assign each individual female a life span (see the Submodel section). The LT, EDT, and CS are also stochastic as their values were drawn randomly from predefined distributions (see the Submodel section) after the hatching process (for LT) and the spawning process (for EDT and CS) of each clutch produced.
Collectives – The model includes no collectives.
Observation – The non-located Scientist (a) save the value of the clutch size and age of death of the female, and (b) counts the number of nauplii produced and kills them. This procedure allowed total egg and offspring production to be described, but also investigate the distribution of different reproductive traits (cf Emergence).
Initialization
We first confirm the proper implementation of the model by running a set of simulations with conditions similar to those of the experiment conducted by Pan et al. (2016), i.e., 8 females, 8 salinities (0 to 35), four replicates, 14 days. We then tested 40 salinity conditions, with salinity ranging from 0 to 39. For this second set of simulations, at initialization, the female cohort consisted of 1000 newly molted adult females (i.e. age = 0), and the simulations last 20 days. This number of individuals was selected after visualization of the stabilization of the variability of the output variables (Supplementary Figure 1). For each condition, the simulated experiments were repeated 15 times (15 replicates) to consider the output variability produced by the same set of parameters and initial conditions (Supplementary Figure 2).
Input
The temperature was fixed at 28°C, and salinity values were constant for each simulation experiment. The model did not use input data to represent time-varying processes.
Submodels
Reproductive Cycle of a Female
To simulate the reproductive cycle of the females, we slightly adapt the model of Dur et al. (2009) to the case of A. royi. Compared to the model of Dur et al. (2009), the individual variability was not represented by a gamma distribution but by a Gaussian distribution. We, therefore, modified the tasks DefImpTime and Spawn. Table 3 presents the different tasks used to simulate the reproductive cycle of A. royi and their respective pseudocode. For the rationales behind these tasks, we report the reader to the paper of Dur et al. (2009).
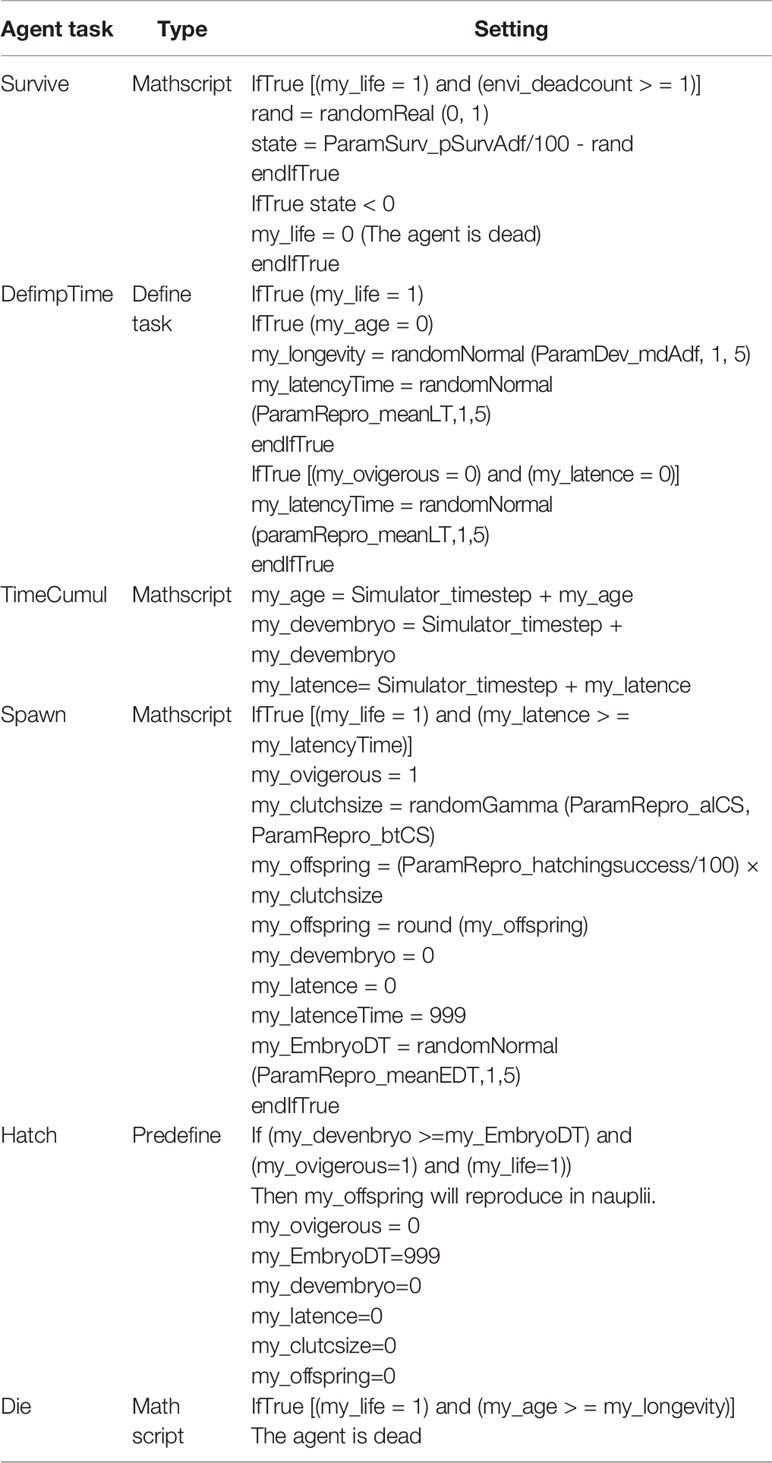
Table 3 Settings of the tasks used to develop the reproductive cycle of an adult female of the copepod Apocyclops royi.
Effect of Salinity on A. royi Reproductive Traits
To implement the effect of salinity on A. royi reproductive traits into the model, we collected information from published data (Pan et al., 2016; Van Someren Gréve et al., 2020) and conducted some experiments for the traits with no available data (i.e., EDT). Details on the experimental procedures and the results used for calibration are presented in supplementary materials (Supplementary Text 1). We subsequently proceeded to the calibration by testing different functions types to express the effect of salinity on those reproductive traits.
Latency Time and Embryonic Development Time.
Preliminary analysis of the experimental results revealed a pattern similar to a temperature-dependent development, i.e. LT and EDT decreased with increasing salinity. Consequently, we tested different commonly used equations to define the effect of temperature on the development of arthropods (Quinn, 2017 – Supplementary Table 1). Among the five function types tested, that of Yamakawa and Matsuda (1997) exhibited the best fit for both LT and EDT (Figure 3).
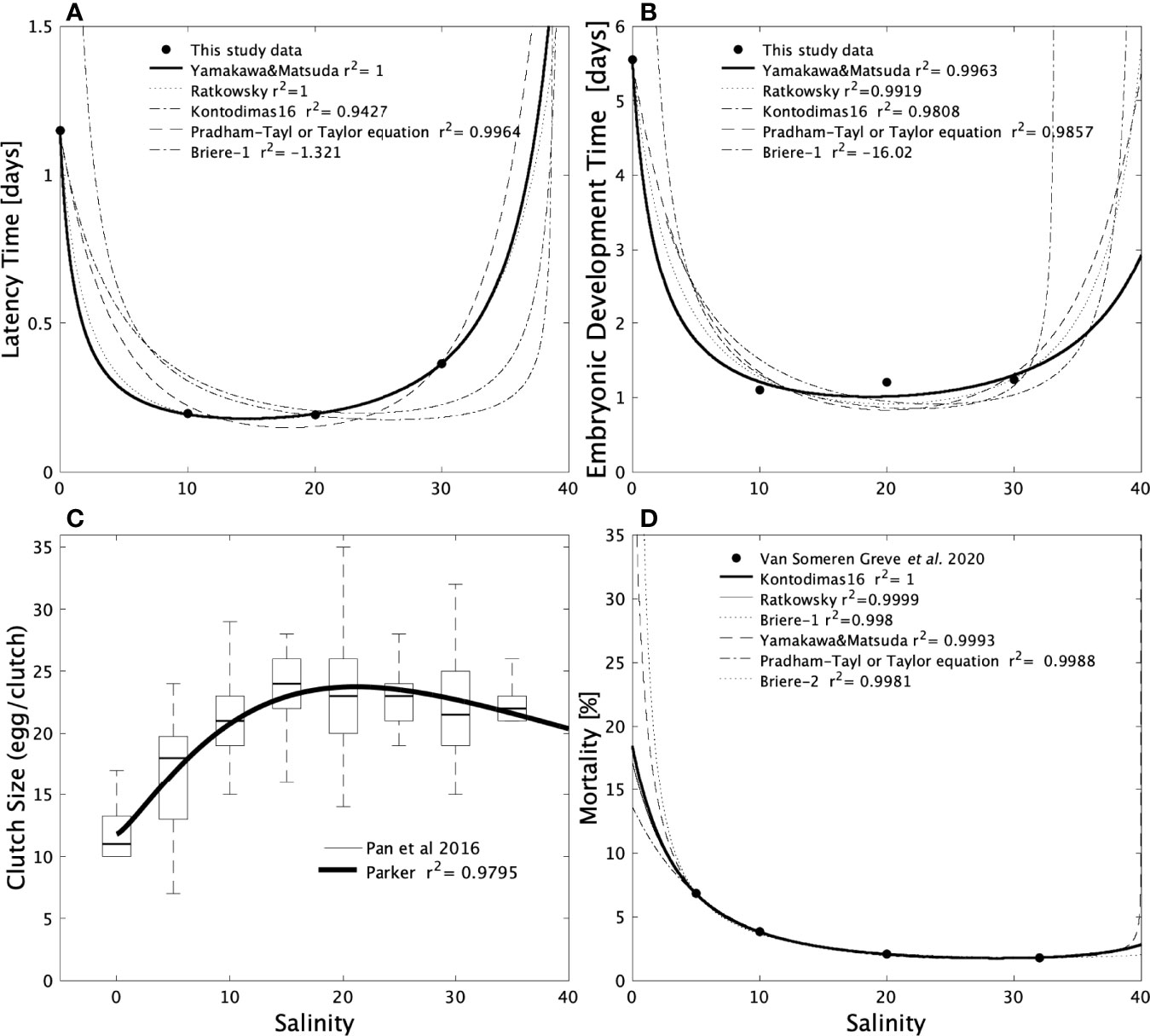
Figure 3 Relationship between salinity and the Latency Time (A) Embryonic Development Time (B), the Clutch Size (C), and the mortality (D). The black continuous lines represent the function implemented in the model to represent the effect of salinity. Details on the functions plotted here are provided in Supplementary Table 1.
The effect of salinity (S) on the mean latency time (meanLT, in days) was expressed as:
and the effect of salinity (S) on the mean embryonic development time (meanEDT, in days) as:
Both mean values were computed by the non-located agent ParamRepro and stored into its attribute meanLT and meanEDT (Table 2).
Clutch Size
For the effect of salinity on the clutch size (CS), we slightly modified the mathematical expression of Parker (1974) to fit the experimental data of Pan et al. (2016). The experimental CS data of Pan et al., 2016 revealed that within the viability range of A. royi there is an optimal salinity for the clutch size. And the equation of Parker is one of the many equations proposed to reproduce the effect of an optimal temperature on different process. We replaced the temperature variables in Parker equation by salinity variables, and added a constant value (c):
where Sm: is the upper limit of salinity beyond which the female does not produce egg (CS(Sm) = 0), So is the optimal salinity for spawning (CS (So) = CSmax, and θ is a parameter of the function shape.
With such an equation, we obtained a good fit on the experimental data of Pan et al., 2016 (Figure 3C).
Survival
Among the six tested equations, that of Kontodimas-16 provided the “best” fit to represent the effect of salinity (S) on mortality data obtained in the lab (c.f. Supplementary Material). This function is characterized by a min and a max value that can be associated with the range of tolerance of A. royi (Table S1; Figure 3D).
In the model, we used survival rate instead of mortality. Hence the probability for a female to survive one day of simulation at salinity S was 100 – Mortality.
The survival (or death) of the female was tested every day (i.e. when Envi_deadcount ≥1). For a high value of survival (e.g. ParamSurv_Adf = 0.95), the probability of drawing a random number larger than ParamSurv_Adf is low; consequently, the individual has a larger probability of survival than dying every day.
Adult Female Longevity
In the present model, we considered the mean longevity of females to be independent of salinity as shown by Lee et al. (2005). The simulations were conducted for a constant temperature of 28°C in accordance with the temperature used for the experiment (Text S1). To define the mean longevity of A. royi female at 28°C, we first fit five common equations used to describe the effect of temperature on development on the data obtained by Lee et al. (2005). The best fit was obtained with the Bĕlehraídek equation (Supplementary Figure 3) which gave mean longevity (ParamDev_mdAdf) of 14.457 days at 28°C. Individuals with 100% daily survival rate reach their maximum age (longevity) defined at the first timestep of the simulation by DefImptime (Table 3).
Individual and Interclutch Variabilities
Individual variability was considered for the female longevity and was modeled with a normal density function of variance equal to one and the mean value of ParamDev_mdAdf. The task DefImpTime was affected by this task.
DefImpTime was also used to implement interclutch variability on the latency time. Similar to the longevity, the latency time for each clutch was randomly sorted from a gaussian distribution with a variance equal to one and a mean value of ParamRepro_meanLT.
The task Spawn was used to take into consideration the interclutch variability in EDT and CS (Table 3). The number of eggs produced by the female at each spawning event is randomly selected in a gamma distribution defined by a shape parameter (α) and the scale parameter (β). The values of were determined using the method described in Souissi (2006) to separate the contribution of salinity from the intrinsic variability (α kept constant and β multiplied by ParamRepro_meanCS which depends on salinity). The number of offspring that will hatch from each clutch represents a part of the randomly sorted CS (i.e. HS x CS). The EDT required for every single clutch was also defined by the task Spawn, and sorted from a gaussian distribution of variance equal to one and mean value equal to ParamRepro_meanEDT.
Statistical Analysis
To confirm the proper implementation of the model, we compared the results obtained by Pan et al. (2016) with the model outputs for the same conditions. The Kolmogorov-Smirnov test confirmed the heteroscedasticity of the data. We, therefore, used the Wilcoxon rank-sum test (aka Wilcoxon T-test, WT) to compare the model results to the experimental results for each tested condition. All statistical tests were conducted at the 95% confidence level. These analyses were performed using the Matlab software (Mathworks Inc., Version, 7.5)
Results
Model Results Compared With Experimental Data
The total nauplii production estimated with the model and experimentally exhibit a similar response to increasing salinity (Figure 4), with an increase in production up to salinity 20 and a decrease beyond. The model results were not significantly different from the experimental results for salinities 10 to 25 (WT, p>0.05). However, the model outputs were significantly higher for salinities 5, 30, and 35 (WT, p<0.05). At salinity 0, the simulated total nauplii production was significantly lower than the one observed experimentally (WT, p<0.05).
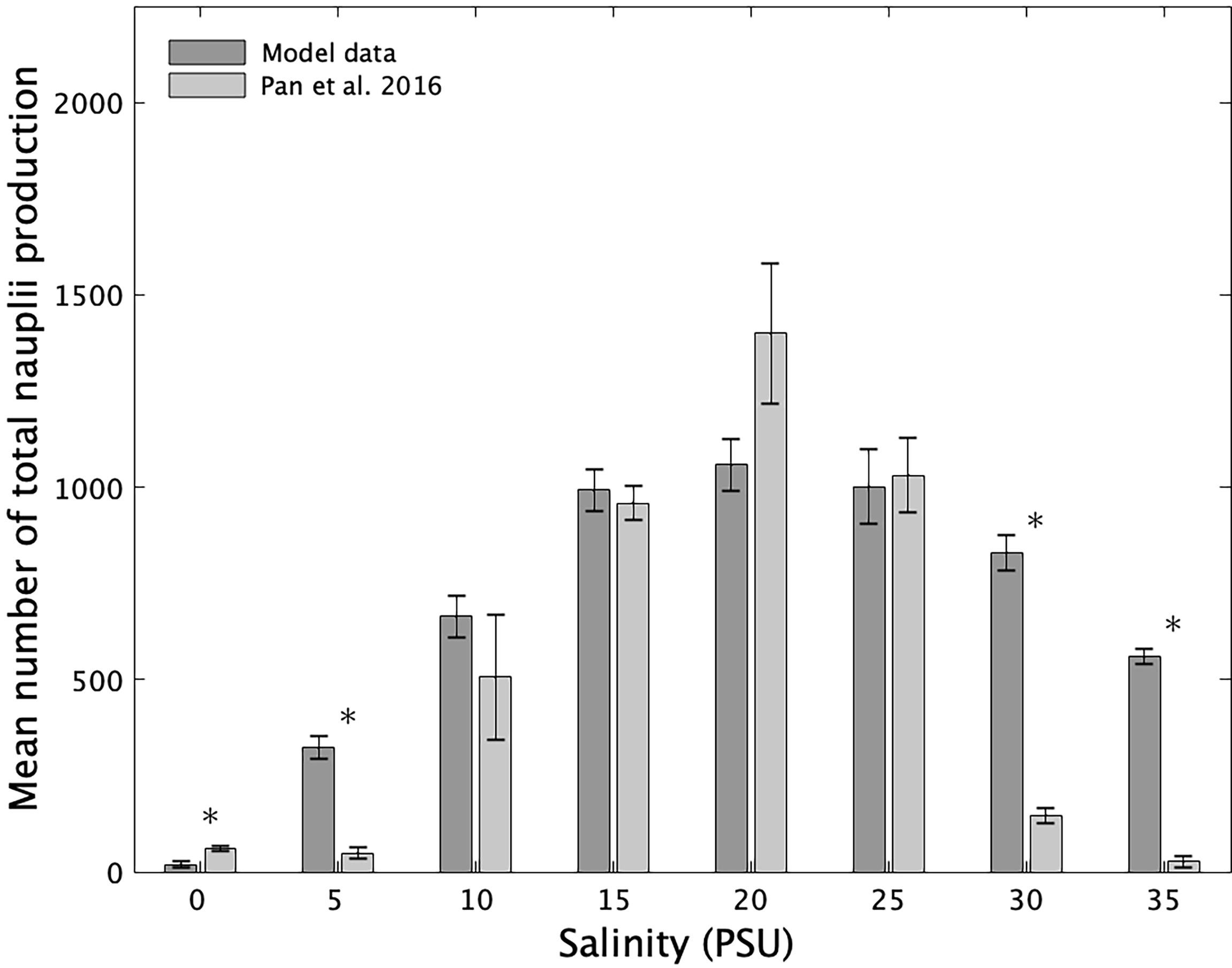
Figure 4 Comparison of experimental (in grey) and simulation (dark grey) results of the total number of nauplii produced by 8 females of A. royi at eight salinities. Vertical bars represent the mean values over four replicates and the error bars represent the standard error. Asterisks indicate a significant difference between experimental and simulation results.
Effect of Salinity on Individual Endpoints
The numerical outputs provided a dynamical explanation for the effect of salinity on the total production of A. royi (Figure 5). Due to the implemented effect of salinity on survival, female longevity was strongly affected by low salinities, decreasing below 10 days for salinities lower than 7. Consequently, the number of clutches a female can produce during its lifetime was reduced at low salinities. The number of clutches increased with increasing salinities, reaching a maximum of about 8 clutches between salinities 15 and 25, and steadily decreasing for higher salinities. This decrease observed at higher salinity was not related to female longevity which stayed relatively constant. Instead, the decrease in clutch number at higher salinity is related to the effect of salinity on the LT and EDT, which became longer when the salinity exceeds 10 for LT and 20 for EDT (Figure 3). The daily egg production, fecundity, and total offspring followed a trend similar to that of clutch number.
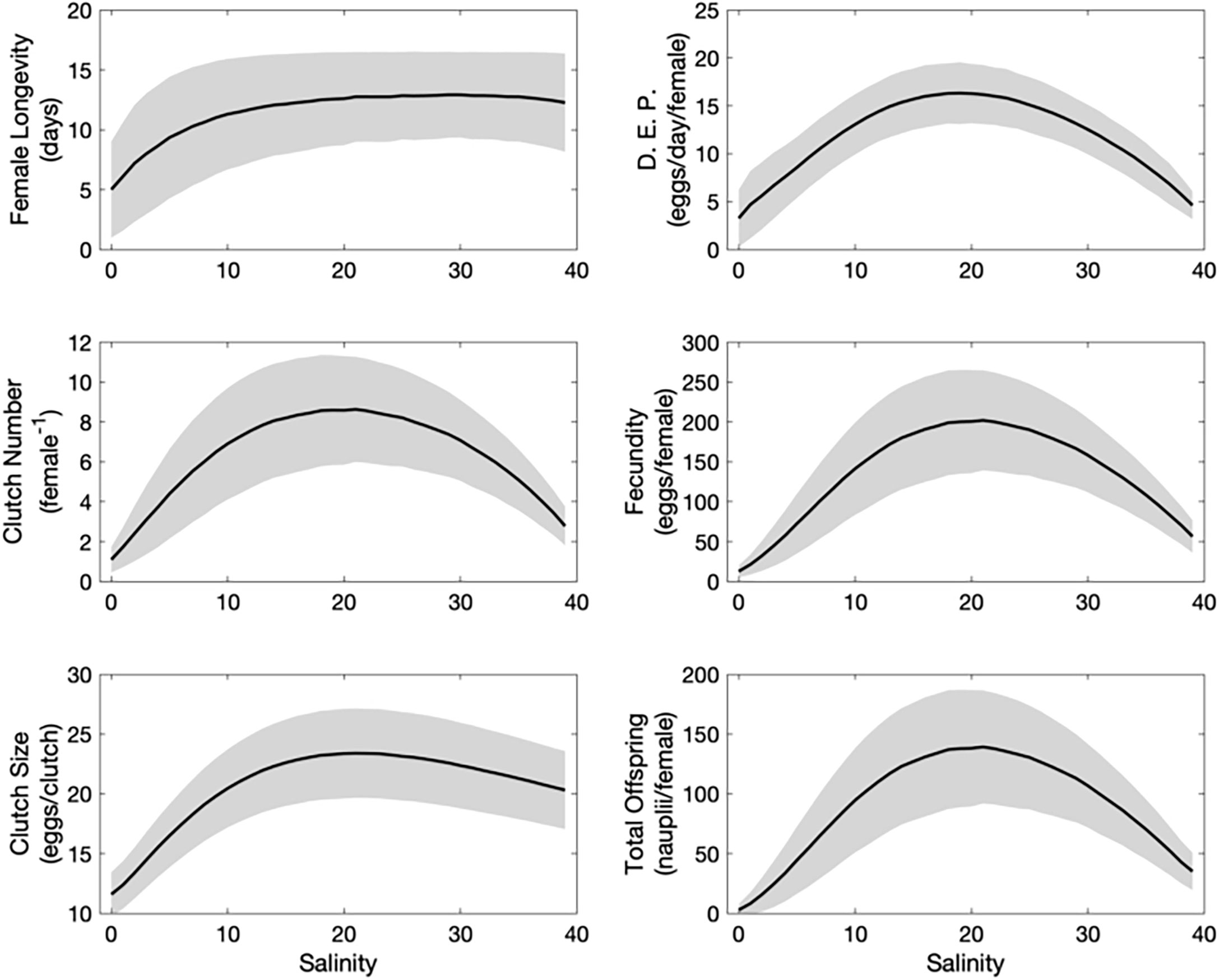
Figure 5 Average (black line) and standard deviation (gray area) of different reproductive traits (Left: female longevity, clutch number, clutch size) and the resulting individual production (right: daily egg production, total egg production, total offspring production) for 15,000 females (15 runs of 1000) exposed to different salinities.
Analysis of the distribution of parameter values “confirmed” the importance of female longevity (Figure 6). For all parameters besides clutch size, the distribution shifted toward zero as the salinity tends to 0. The span of the distribution of clutch number expanded when salinity is between 8 and 32. Conversely, the span of the clutch size distribution stayed constant for salinities higher than 8. The fecundity and total offspring distributions center slid slightly toward the higher class, with a tail toward the lower classes as salinity increased from 0 to 20 and exhibited a reverse pattern for salinities higher than 24.
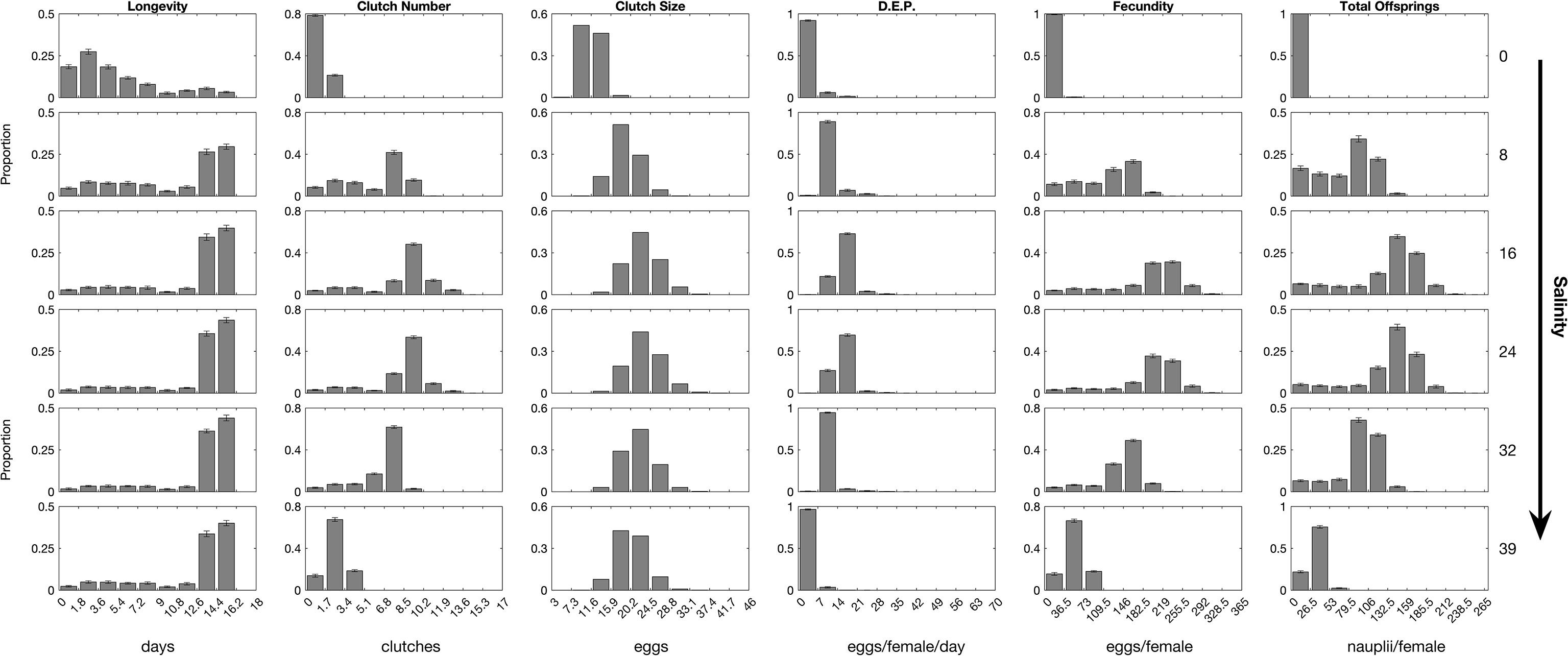
Figure 6 Probability density function of the individual endpoints for 1000 females exposed to different salinities. The histogram represents the average proportion per class over the 15 replicate simulations and the error bars represent the standard deviation.
Salinity Effect on Cohort Endpoints
At the cohort level, the model results showed the importance of the interclutch duration on the production (Figure 7). At low and high salinities, the time between the burst of production is quite long resulting in few eggs and nauplii production. Females can only produce two and three clutches at salinity 0 and 39, respectively. At salinities 3 and 39, the cumulated production of both eggs and nauplii is quite similar, but the dynamics are different. At salinity 3, two peaks of production appeared within the first 6 days of simulation, and they are followed by relatively low but constant production. As salinity increased, so did the regularity of production, leading to quasi-linear increases in the total production for salinities between 7 to 31. The dynamics of the egg and nauplii production were quite similar for salinities between 15 to 23.
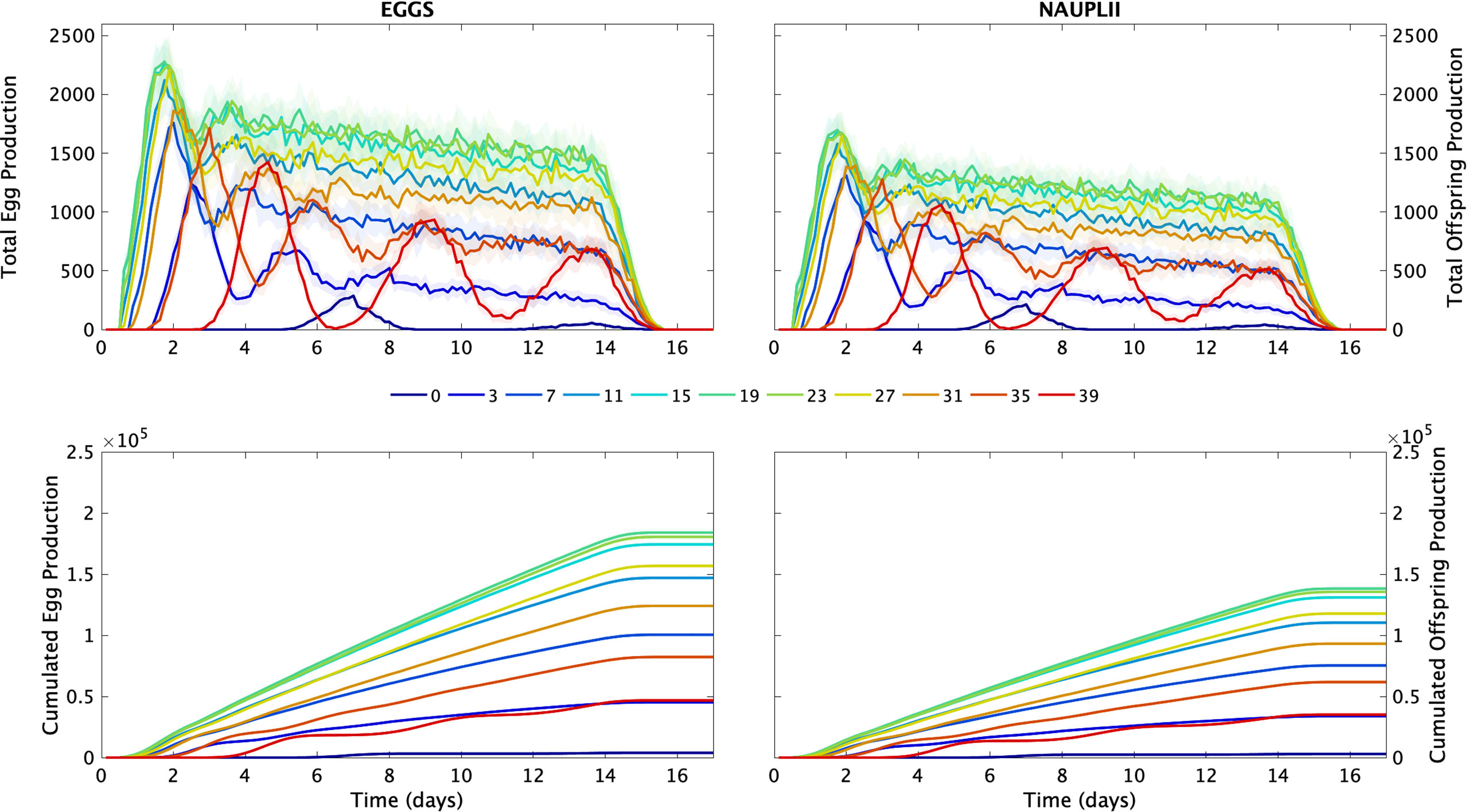
Figure 7 Total production (Top) and cumulative production (bottom) of eggs (left) and offspring (right) by 1000 females over 20 days at different salinity conditions (PSU). All individuals died before the end of the simulation. The x-axis was therefore limited to 17 days. Lines represent the average over the 15 replicate simulations. The shaded area is the standard deviation.
At the end of the 15 days of simulation, the highest total egg and nauplii productions were obtained at 21 PSU (1.85×105eggs,1.39×105 nauplii) and the lowest at 0 PSU (1.11×104 eggs, 8.23×103 nauplii) (Figure 8). The total egg and nauplii production decreased the further it goes out of the optimal range, which is related to the individual behavior (Figure 5). Between 19 and 22 PSU, the egg cumulated production exceeded 1.8x105. And for salinities between 18 and 23, nauplii cumulated production exceeded 1.35x105. Between 7 and 31 PSU, the egg and nauplii production is growing steadily, but outside this range, it is unstable and not increasing uniformly (Figure 7 bottom graphs). The difference between egg production and nauplii production is related to the constant hatching success (74.5%, data from Pan et al., 2016).
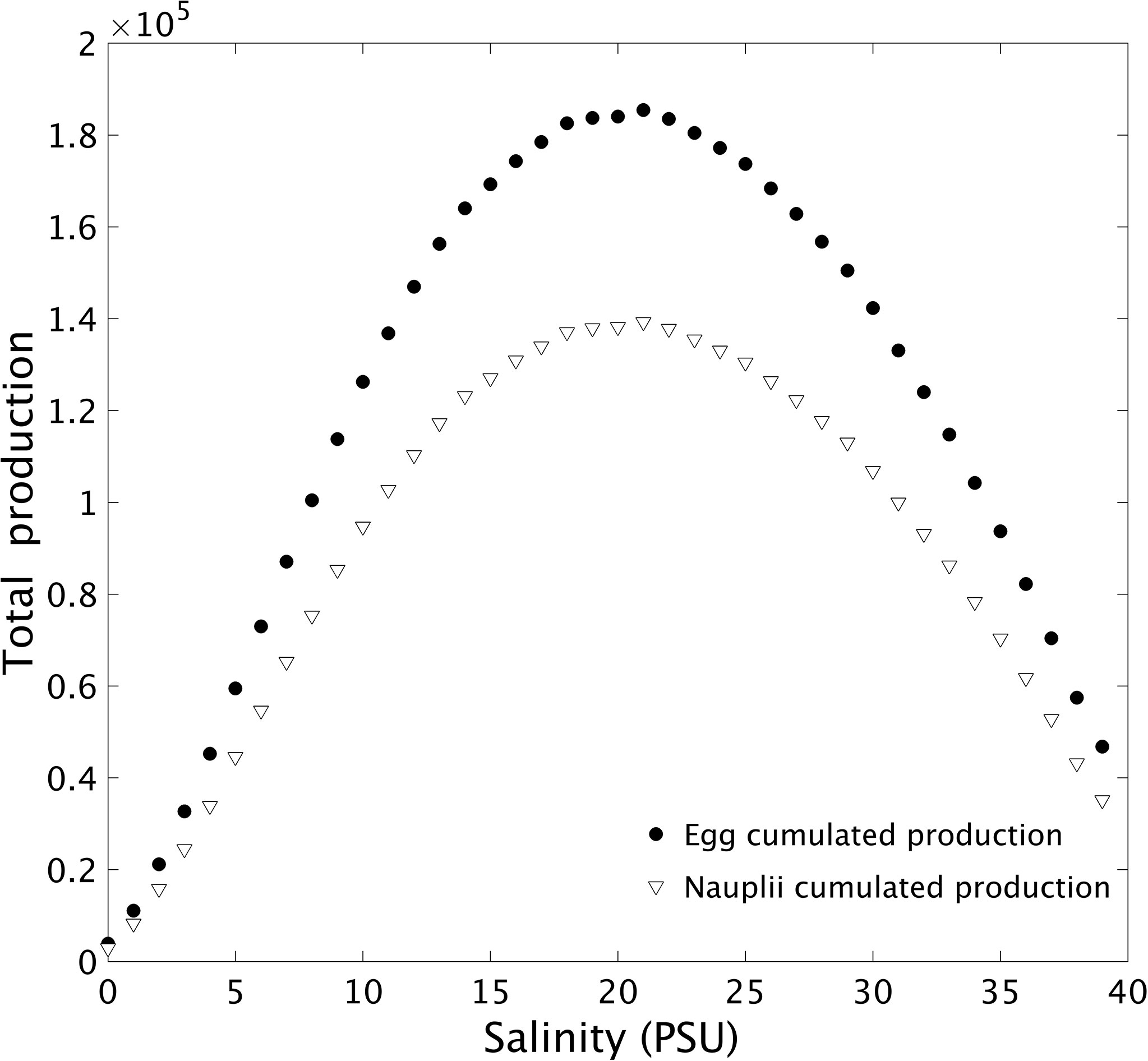
Figure 8 Relation between the cumulated mean production of eggs and nauplii by 1000 females after 20 days and the salinity condition (PSU).
Discussion
Copepod is a great live feed for aquaculture (Shields et al., 1999; Støttrup, 2000; Payne et al., 2001; Rajkumar and Kumaraguru Vasagam, 2006; van der Meeren et al., 2008; Piccinetti et al., 2014), but its application has yet to reach the practicality and cost-effectiveness required for the global aquaculture hatcheries due to unstable productivity. The present study developed a modeling tool to identify the risk of salinity variations on the mass culture of the cyclopoid copepod Apocyclops royi. Here, we focused on the effects on reproduction by first defining dose-response relationships based on the results of laboratory experiments on females. We then implemented those relationships in an IBM representing the reproductive cycle of egg-carrying copepods (Dur et al., 2009). The model represents a new approach to define the optimal salinity to induce maximal fecundity of A. royi and provides a mechanistic understanding of the effect of salinity on production.
Production of A. royi
Salinity influences the ecological and biological response of copepod and has been investigated extensively during the past decades. Chen et al. (2006) determined the effect of salinity of Pseudodiaptomus pelagicus, for 10 days with individual adult pairs to define the optimal salinity culture. In terms of total nauplii production, under 15g/L observed the highest value (131.2 ± 67.6). For Acartia species, egg production patterns were more irregular, but some species produced fewer eggs at the lowest salinity tested (Castro-Longoria, 2003). Castro-Longoria (2003) demonstrated that hatching success increased with increasing salinity and was maximal at 25 PSU of Acartia tonsa (Copepoda: Calanoida). In this study, the model output (Figure 7) suggested an optimal salinity for A. royi reproduction at 21 PSU and 0 PSU as the adverse salinity condition. Pan et al. (2016) conducted a population experiment to find the optimal salinity with eight different salinity conditions (0, 5, 10, 15, 20, 25, 30, and 35) with the same species. In their result, the highest mean number of the total populations was observed at 20 PSU and low at extreme salinity treatments (0,5,30, and 35). In terms of optimal and suboptimal conditions, these two results matched. However, the mean number of total populations cultivated at salinities higher than 30 was low in the experiment compared to our model results in terms of offspring production. This discrepancy may be due to the female mortality rate implemented in the model. The mortality data were obtained from Van Someren Gréve et al. (2020). Before testing the 24hour mortality of adult female copepod A. royi, Van Someren Gréve et al. (2020) acclimated the copepods to 32 PSU for at least 111 days. Because we used data of copepods that were adapted to high salinity conditions, the model results did not show a strong decrease in productivity at high salinity.
The negative effect of high salinity on female longevity and the clutch size is not as strong as for lower salinity conditions (Figure 4). The decrease in total production at salinities higher than 23 is however similar to that at lower salinities. Under high salinity conditions, the decrease in production is mainly due to extended interclutch duration. High salinity negatively affects LT and EDT (Figure 3). The lengthened LT and EDT lead to fewer spawning events, and a subsequent decrease in production. These results support the claim that, for egg-carrying copepods, it is important to take into consideration the effect of environmental factors on the time component to avoid misleading egg production rate estimation (Lloyd, 2006; Dur et al., 2009).
In order to achieve the goal of producing a large number of eggs, we documented that the salinity should be carefully maintained in a given range of salinities. A high production of ~18.104 eggs was predicted with the model for salinities between 18 and 23. Except in the rare case of equipment failure, salinity can be easily maintained in batch culture within indoor facilities. But outdoor cultures can exhibit significant salinity fluctuations (i.e., 8-15PSU, Blanda et al., 2015) and possibly affect considerably the production of the culture species. According to our model, a deviation of 7 PSU from the optimal range of salinity would lead to a loss of 22 to 25% in A. royi. This finding provides implications for risk control in copepod aquaculture, where the water exchange or management should be necessarily performed to avoid production loss.
Model Potential and Current Limitations
This version of the model allows already some valuable applications for aquaculture. In the case of outdoor ponds, the model can help to estimate the production in case of sudden rainfall and improve harvest management. In the case of indoor culture, the model can be used as a tool for salinity management to increase production. Nevertheless, several limitations of the current version should be highlighted.
Although several studies have investigated the effect of salinity on the reproductive capacity of copepods, none has provided functional relationship between environmental salinity and embryonic development time or latency time. The effect of salinity on the survival rate of the calanoid copepod Pseudodiaptomus annandalei was expressed by a two-order polynomial fit (Chen et al., 2006). Contrarily to P. annandalei, A. royi did not exhibit a decrease in survival at salinity 0. We therefore tested different equations to fit our data. There is no clear consensus as to what is the “best’ type of function to apply to salinity response data. Here, we tested temperature-dependent equations to fit our data as they presented similar types of response, i.e. faster development and lower mortality at optimal condition and longer development and higher mortality when the environmental condition goes further than this optimal. All equations commonly used to represent temperature-dependent relationship applied well to our study by fitting the relationship between salinity and the reproductive traits.
Euryhaline copepod has the ability to adapt relatively quickly to change in salinity after some acclimatation period (Hansen et al., 2022). This ability might result in changes in shape and position of the functional relationship with salinity after acclimation. In the present model, we did not consider any acclimation period and only focussed on the productivity of females at constant salinity. We therefore did not implement any adaptative process in the model. However, with appropriate data such implementation should be conducted for future version of the model.
Individual variability is important for population persistence and stability under stressful conditions (Morozov et al., 2013; Bi and Liu, 2017). In the present model, the individual variability in female longevity and interclutch variability in LT and EDT are represented by normal distributions, the parameters of which were constant. However, previous observations revealed an increase of the individual variability in the developmental duration of copepod in relation to non-optimal temperature (Souissi et al., 1997; Jiménez-Melero et al., 2005). The calibration of gamma distributions to represent individual variability is possible (Dur et al., 2009; Dur et al., 2013) but requires an important number of observations at the individual scale (Devreker et al., 2012; Liu et al., 2015).
Several studies showed that salinity affects the HS brackish copepods whether they carry their eggs (Nguyen et al., 2020) or release them (Holste and Peck, 2006; Choi et al., 2021). However, this relation between salinity and hatching success seems to be species-specific. For instance, for the egg-bearing copepod Eurytemora affinis, there was no effect of salinity on the hatching success which is always above 90% (Devreker et al., 2012). In the present model, we considered a constant HS of 74.5% based on the observation in the laboratory (data from Pan et al., 2016) that showed no effect of salinity on HS. While the eggs are tolerant to salinity changes, the nauplius stages could respond differently to salinity stressors. When exposed to adverse salinities, the nauplii suffer from retarded development, molting failure and even decreasing survival rate (Devreker et al., 2004). Besides the effect of salinity, nauplii might also be subject to cannibalism. Drillet and Lombard (2015) build a simple development model based on the well-known life-history traits of Acartia tonsa to define the optimal density condition with the effect of cannibalism in production. In the present study, cannibalism in A. royi is not considered as the model focuses only on reproduction. Considering the importance of the naupliar stages for population maintenance (Nagaraj, 1988), the effect of salinity and other factors, such as cannibalism, on nauplii life traits should be considered in the future development of the model which will simulate the entire life cycle of A. royi.
In the present model, some individuals died before their eggs hatched (i.e., they spawn but died before the EDT was completed). Other egg-bearing copepods, such as Eurytemora carolleeae could drop the egg sacs and allow the nauplii to be born independently (Nowakowski and Sługocki, 2021). In this study, the model is based on the hypothesis that A. royi’s egg sac does not detach and cannot survive if the female dies. However, if A. royi exhibit abilities similar to that of E. carolleeae, the productive capacity of A. royi would be even more than the present estimation.
Our model simulated the case of 1000 females, which if it is considered as 1000 ind/L can represent a high density. The present model did not consider any crowding effect. There are some contradictory results concerning the effect of high density on copepods. In Acartia tonsa, high-density can have a negative effect on adult survival, and fecundity (Drillet et al., 2014; Franco et al., 2017). However, the same species did not show any stress in terms of behavior, respiration, and gene expression when exposed to high culture densities (Nilsson et al., 2017). Similarly, the copepod Acartia steueri mortality and fecundity are independent of density due to a reallocation of its energy from the metabolic to the reproductive process (Takayama et al., 2020). Further research about the response of A. royi to high densities is necessary to decide the need for implementing any effect of high-density on its reproductive traits.
In outdoor culture, the temperature is another important parameter that exhibits some variation (Blanda et al., 2017) and influences considerably the production of egg-carrying copepods (Dur et al., 2009; Nguyen et al., 2020). The present model does consider only the effect of temperature variation on longevity. In the present model, the effect of temperature on LT, EDT and CS is not considered despite its relevance because of a lack of data. However, with proper laboratory data, this effect can easily be implemented in the model (Dur et al., 2009). Additionally, in practice, the model can investigate the effect of temperature change on production. For example, we can consider the hypothetical case where the salinity is maintained at 21, which is theoretically the optimal condition (Figure 7), and estimate the production at the minimum and maximum temperature observed in outdoor aquaculture pond in Taiwan (26.1, and 32°C, Blanda et al., 2017). The total egg production increased by 4.27% at 26.1°C and decreased by 10.1% at 32°C compared to 28°C. In the case of total nauplii production, the model predicted an increase of 9.97% at 26.1°C and a decrease of 10.1% at 32°C (Figure 9). One should note that this result does not consider the effect of temperature on other reproductive processes, such as clutch size and interclutch variability. The next improvement of the current model must focus on this aspect, considering the increase in the appearance of extreme temperatures due to global warming. It will be interesting to integer both the negative effect of high temperature on fecundity and nauplii production (Nguyen et al., 2020) and the positive effect of cold temperature on productivity (Pan et al., 2017).
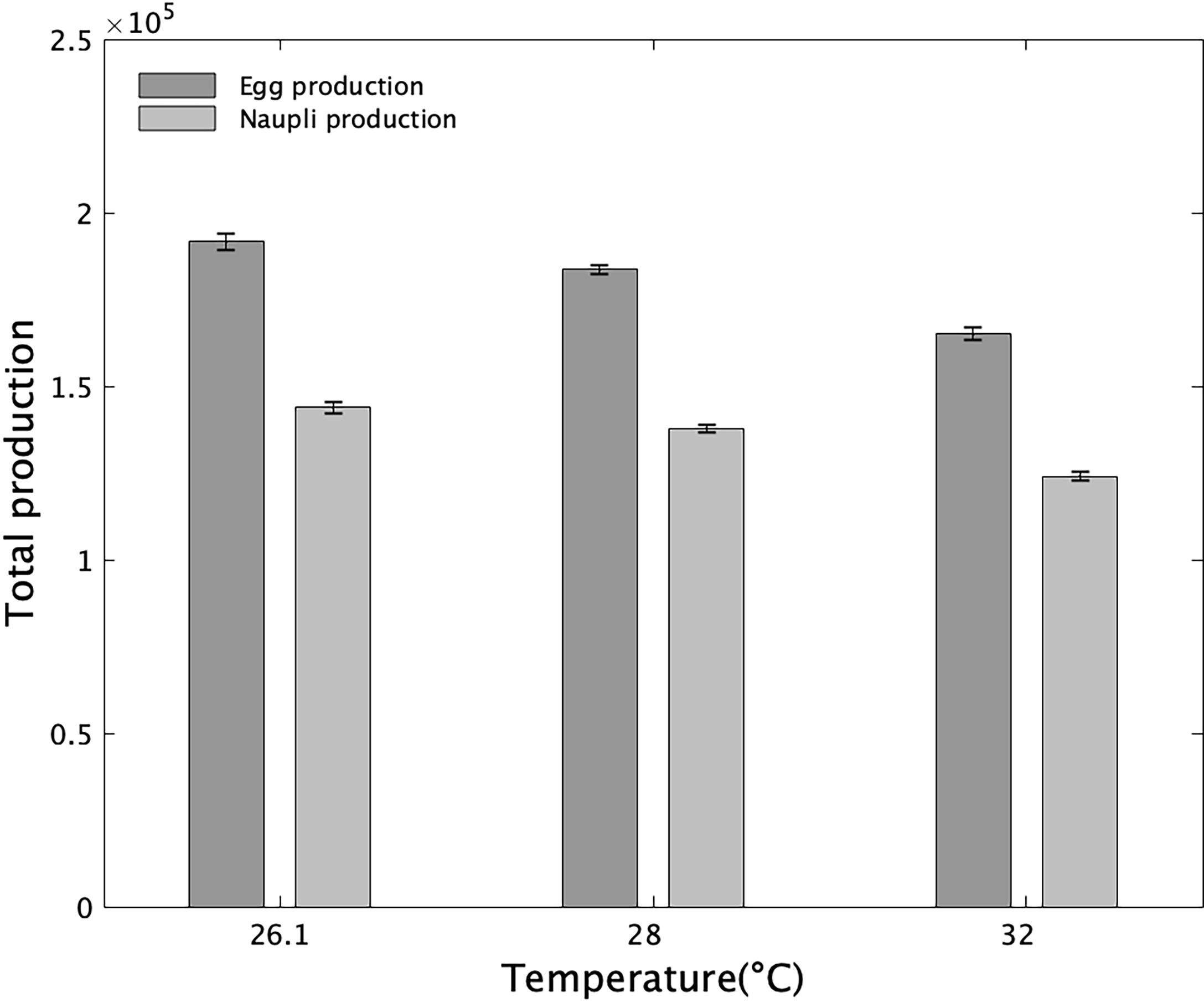
Figure 9 Cumulated total egg and nauplii production of 1000 females over a period of 20 days at three constant temperatures. Salinity was fixed at 21 PSU. Vertical bars represent the mean values over fifteen replicates and the error bars represent the standard error.
Although the Mobidyc platform was developed two decades ago (Ginot et al., 2002), it contributed to promoting and democratizing multi-agent systems to con-computer experts. Its development has been recently accelerated with the new RE : Mobidyc project (Oda et al., 2021). The recent development in collaboration with computer scientists will lead to a future version accessible to a wide range of users including the aquaculture field.
Conclusion
Compared to experimental investigation, our model presents the ability to integrate the effect of different environmental parameters on all reproductive traits as well as individual variability. On top of that, the model has the potential to aggregate fine-scale knowledge and investigate coarser-scale phenomena. Based on the present version of the model, other environmental factors such as temperature, food quality, and quantity could potentially be incorporated pending the availability of data.
In this study, we only focused on the reproductive traits of adult females of copepod. The goal of this model is to determine the optimal condition for reproduction and estimate the loss and gain of change in salinity. Ultimately, we are aiming to create a model that represents the entire life cycle of A. royi. The reproductive process constitutes a necessary step in the development of a complete population dynamics model (Prestidge, 1995; Souissi et al., 2005). In addition to the previously proposed improvements, the perspective of the model is to be included within an already developed population dynamics model integrating all development stages (Dur et al., 2013). This population dynamics model will be an important tool for establishing and maintaining copepod mass culture through the determination of optimal culture conditions for growth and reproduction, estimation of the production, and defining appropriate harvest time.
Data Availability Statement
The raw data supporting the conclusions of this article will be made available by the authors, without undue reservation.
Author Contributions
MY conducted the model calibration, run the model and the output analyses, and draft the manuscript. Y-JP conceived and run the complementary experiments, commented on the manuscript. GD conceived the model and the complementary experiments, helped in writing the manuscript. SS contributed to writing the manuscript, commented earlier version of the manuscript. All authors contributed to the article and approve the submitted version.
Funding
This work was supported by the research grant from the Faculty of Science of Shizuoka University to GD. This work is a contribution to the research projects supported by Taiwanese Ministry of Science and Technology; Council of Agriculture, Executive Yuan; and Ministry of Education (109-2636-M-019-001 and 110-2636-M-019-001; 110AS-1.3.2-ST-aO and 111AS-1.3.2-ST-aU; Higher Education Sprout Project to the CEO, NTOU) to Y-JP.
Conflict of Interest
The authors declare that the research was conducted in the absence of any commercial or financial relationships that could be construed as a potential conflict of interest.
Publisher’s Note
All claims expressed in this article are solely those of the authors and do not necessarily represent those of their affiliated organizations, or those of the publisher, the editors and the reviewers. Any product that may be evaluated in this article, or claim that may be made by its manufacturer, is not guaranteed or endorsed by the publisher.
Acknowledgments
This paper is a contribution to the International Associated Laboratory between Université de Lille and the National Taiwan Ocean University (IAL MULTIFAQUA). The Mobidyc platform (version V2.22), including codes and tutorials, is freely available in French and English for non-commercial use at https://mobidyc.cnrs.fr/index.php?title=English_summary. We express our gratitude to Dr. Guillaume Drillet for his comments on a previous version of the manuscript. This study is dedicated to the memory of V. Ginot for his exceptional work in creating and promoting the Mobidyc project.
Supplementary Material
The Supplementary Material for this article can be found online at: https://www.frontiersin.org/articles/10.3389/fmars.2022.863244/full#supplementary-material
References
Aganesova L. O. (2021). The Reproduction and Development of Brackish-Water Copepods That Were Fed Microalgae of Different Species. Russ. J. Mar. Biol. 47, 114–120. doi: 10.1134/S1063074021020024
Ajiboye O. O., Yakubu A. F., Adams T. E., Olaji E. D., Nwogu N. A. (2011). A Review of the Use of Copepods in Marine Fish Larviculture. Rev. Fish. Biol. Fish. 21, 225–246. doi: 10.1007/s11160-010-9169-3
Alajmi F., Zeng C. (2014). The Effects of Stocking Density on Key Biological Parameters Influencing Culture Productivity of the Calanoid Copepod, Parvocalanus Crassirostris. Aquaculture 434, 201–207. doi: 10.1016/j.aquaculture.2014.08.029
Anzeer F. M., Aneesh K. S., Abraham M. V., Darsana S., Santhosh B., Anil M. K., et al. (2019). Breeding, Early Development and Larval Rearing of Cloudy Damsel, Dascyllus Carneus Fische. Aquaculture 505, 374–385. doi: 10.1016/j.aquaculture.2019.03.001
Beaudouin R., Goussen B., Piccini B., Augustine S., Devillers J., Brion F., et al. (2015). An Individual-Based Model of Zebrafish Population Dynamics Accounting for Energy Dynamics. PloS One 10, e0125841. doi: 10.1371/journal.pone.0125841
Bell J. G., McEvoy L. A., Estevez A., Shields R. J., Sargent J. R. (2003). Optimising Lipid Nutrition in First-Feeding Flatfish Larvae. Aquaculture 227, 211–220. doi: 10.1016/S0044-8486(03)00504-0
Bi R., Liu H. (2017). Effects of Variability Among Individuals on Zooplankton Population Dynamics Under Environmental Conditions. Mar. Ecol. Prog. Ser. 564, 9–28. doi: 10.3354/meps11967
Blanda E., Drillet G., Huang C.-C., Hwang J.-S., Højgaard J. K., Jakobsen H. H., et al. (2017). An Analysis of How to Improve Production of Copepods as Live Feed From Tropical Taiwanese Outdoor Aquaculture Ponds. Aquaculture 479, 432–441. doi: 10.1016/j.aquaculture.2017.06.018
Blanda E., Drillet G., Huang C.-C., Hwang J.-S., Jakobsen H. H., Rayner T. A., et al. (2015). Trophic Interactions and Productivity of Copepods as Live Feed From Tropical Taiwanese Outdoor Aquaculture Ponds. Aquaculture 445, 11–21. doi: 10.1016/j.aquaculture.2015.04.003
Burgess A. I., Callan C. K., Touse R., Delos Santos M. (2020). Increasing Survival and Growth in Larval Leopard Coral Grouper (Plectropomus Leopardus) Using Intensively Cultured Parvocalanus Crassirostris Nauplii. J. World Aquacult. Soc. 51, 171–182. doi: 10.1111/jwas.12635
Buskey E. J. (2005). ““Behavioral Characteristics of Copepods That Affect Their Suitability as Food for Larval Fishes,”,” in Copepods in Aquaculture. Eds. Lee C.-S., O’Bryen P. J., Marcus N. H. (Ames, Iowa, USA: Blackwell Publishing Professional), 91–106. doi: 10.1002/9780470277522.ch8
Camus T., Rolla L., Jiang J., Zeng C. (2021). Effects of Microalgal Food Quantity on Several Productivity-Related Parameters of the Calanoid Copepod Bestiolina Similis (Calanoida: Paracalanidae). Front. Mar. Sci. 8. doi: 10.3389/fmars.2021.812240
Camus T., Zeng C. (2008). Effects of Photoperiod on Egg Production and Hatching Success, Naupliar and Copepodite Development, Adult Sex Ratio and Life Expectancy of the Tropical Calanoid Copepod Acartia Sinjiensis. Aquaculture 280, 220–226. doi: 10.1016/j.aquaculture.2008.05.008
Castro-Longoria E. (2003). Egg Production and Hatching Success of Four Acartia Species Under Different Temperature and Salinity Regimes. J. Crustac. Biol. 23 (2), 11.
Chen Q., Sheng J., Lin Q., Gao Y., Lv J. (2006). Effect of Salinity on Reproduction and Survival of the Copepod Pseudodiaptomus Annandalei Sewell 1919. Aquaculture 258, 575–582. doi: 10.1016/j.aquaculture.2006.04.032
Choi S. Y., Lee E. H., Soh H. Y., Jang M.-C. (2021). Effects of Temperature and Salinity on Egg Production, Hatching, and Mortality Rates in Acartia Ohtsukai (Copepoda, Calanoida). Front. Mar. Sci. 8. doi: 10.3389/fmars.2021.704479
Conceição L. E. C., Yúfera M., Makridis P., Morais S., Dinis M. T. (2010). Live Feeds for Early Stages of Fish Rearing. Aquacult. Res. 41, 613–640. doi: 10.1111/j.1365-2109.2009.02242.x
Costello C., Cao L., Gelcich S., Cisneros-Mata M.Á., Free C. M., Froehlich H. E., et al. (2020). The Future of Food From the Sea. Nature 588, 95–100. doi: 10.1038/s41586-020-2616-y
Das P., Mandal S. C., Bhagabati S. K., Akhtar M. S., Singh S. K. (2012). “Important Live Food Organisms and Their Role in Aquaculture,” in Frontiers in Aquaculture, New Delhi: Narendra Publishing House 69–86.
Dayras P., Bialais C., Lee J., Souissi S. (2020). Effects of Microalgal Diet on the Population Growth and Fecundity of the Cyclopoid Copepod Paracyclopina Nana. J. World Aquacult. Soc. 51, 1386–1401. doi: 10.1111/jwas.12685
Dayras P., Bialais C., Sadovskaya I., Lee M.-C., Lee J.-S., Souissi S. (2021). Microalgal Diet Influences the Nutritive Quality and Reproductive Investment of the Cyclopoid Copepod Paracyclopina Nana. Front. Mar. Sci. 8. doi: 10.3389/fmars.2021.697561
Devreker D., Pierson J. J., Souissi S., Kimmel D. G., Roman M. R. (2012). An Experimental Approach to Estimate Egg Production and Development Rate of the Calanoid Copepod Eurytemora Affinis in Chesapeake Bay, USA. J. Exp. Mar. Biol. Ecol., 416–417, 72–83. doi: 10.1016/j.jembe.2012.02.010
Devreker D., Souissi S., Seuront L. (2004). Development and Mortality of the First Naupliar Stages of Eurytemora Affinis (Copepoda, Calanoida) Under Different Conditions of Salinity and Temperature. J. Exp. Mar. Biol. Ecol. 303, 31–46. doi: 10.1016/j.jembe.2003.11.002
Devreker D., Souissi S., Winkler G., Forget-Leray J., Leboulenger F. (2009). Effects of Salinity, Temperature and Individual Variability on the Reproduction of Eurytemora Affinis (Copepoda; Calanoida) From the Seine Estuary: A Laboratory Study. J. Exp. Mar. Biol. Ecol. 368, 113–123. doi: 10.1016/j.jembe.2008.10.015
Dorman J. G., Sydeman W. J., Bograd S. J., Powell T. M. (2015). An Individual-Based Model of the Krill Euphausia Pacifica in the California Current. Prog. Oceanogr. 138, 504–520. doi: 10.1016/j.pocean.2015.02.006
Drillet G., Frouël S., Sichlau M. H., Jepsen P. M., Højgaard J. K., Joarder A. K., et al. (2011). Status and Recommendations on Marine Copepod Cultivation for Use as Live Feed. Aquaculture 315, 155–166. doi: 10.1016/j.aquaculture.2011.02.027
Drillet G., Lombard F. (2015). A First Step Towards Improving Copepod Cultivation Using Modelling: The Effects of Density, Crowding, Cannibalism, Tank Design and Strain Selection on Copepod Egg Production Yields. Aquac. Res. 46, 1638–1647. doi: 10.1111/are.12317
Drillet G., Maguet R., Mahjoub M.-S., Roullier F., Fielding M. J. (2014). Egg Cannibalism in Acartia Tonsa: Effects of Stocking Density, Algal Concentration, and Egg Availability. Aquacult. Int. 22, 1295–1306. doi: 10.1007/s10499-013-9747-1
Dur G., Jiménez-Melero R., Beyrend-Dur D., Hwang J.-S., Souissi S. (2013). Individual-Based Model of the Phenology of Egg-Bearing Copepods: Application to Eurytemora Affinis From the Seine Estuary, France. Ecol. Modell. 269, 21–36. doi: 10.1016/j.ecolmodel.2013.08.006
Dur G., Souissi S., Devreker D., Ginot V., Schmitt F. G., Hwang J.-S. (2009). An Individual-Based Model to Study the Reproduction of Egg Bearing Copepods: Application to Eurytemora Affinis (Copepoda Calanoida) From the Seine Estuary, France. Ecol. Modell. 220, 1073–1089. doi: 10.1016/j.ecolmodel.2008.12.013
FAO (2020) Global Proportion of Fish Stocks Within Biologically Sustainable Levels, (1974-2017). Available at: http://www.fao.org/sustainable-development-goals/indicators/1441/en/.
Franco S. C., Augustin C. B., Geffen A. J., Dinis M. T. (2017). Growth, Egg Production and Hatching Success of Acartia Tonsa Cultured at High Densities. Aquaculture 468, 569–578. doi: 10.1016/j.aquaculture.2016.10.044
Ginot V., Le Page C., Souissi S. (2002). A Multi-Agents Architecture to Enhance End-User Individual-Based Modelling. Ecol. Modell. 157, 23–41. doi: 10.1016/S0304-3800(02)00211-9
Grønning J., Doan N. X., Dinh N. T., Dinh K. V., Nielsen T. G. (2019). Ecology of Pseudodiaptomus Annandalei in Tropical Aquaculture Ponds With Emphasis on the Limitation of Production. J. Plankt. Res. 41, 741–758. doi: 10.1093/plankt/fbz053
Grimm V., Berger U., Bastiansen F., Eliassen S., Ginot V., Giske J., et al. (2006). A Standard Protocol for Describing Individual-Based and Agent-Based Models. Ecol. Modell. 198, 115–126. doi: 10.1016/j.ecolmodel.2006.04.023
Grimm V., Berger U., DeAngelis D. L., Polhill J. G., Giske J., Railsback S. F. (2010). The ODD Protocol: A Review and First Update. Ecol. Modell. 221, 2760–2768. doi: 10.1016/j.ecolmodel.2010.08.019
Grimm V., Railsback S. F., Vincenot C. E., Berger U., Gallagher C., DeAngelis D. L., et al. (2020). The ODD Protocol for Describing Agent-Based and Other Simulation Models: A Second Update to Improve Clarity, Replication, and Structural Realism. JASSS 23, 7. doi: 10.18564/jasss.4259
Hansen B. W., Ciappini G., Malmendal A., Rayner T. A. (2022). Can We Adjust a Marine Cyclopoid Copepod to Freshwater? First Step Towards a ‘Universal’ Live Feed Product for Fish and Shrimp Larvae. Aquacult. Res. 53, 178–190. doi: 10.1111/are.15563
Holste L., Peck M. A. (2006). The Effects of Temperature and Salinity on Egg Production and Hatching Success of Baltic Acartia Tonsa (Copepoda: Calanoida): A Laboratory Investigation. Mar. Biol. 148, 1061–1070. doi: 10.1007/s00227-005-0132-0
Jepsen P. M., Gréve H., Jørgensen K. N., Kjær K. G. W., Hansen B. W. (2021). Evaluation of High-Density Tank Cultivation of the Live-Feed Cyclopoid Copepod Apocyclops Royi (Lindberg 1940). Aquaculture 736125. doi: 10.1111/are.14970
Jiménez-Melero R., Santer B., Guerrero F. (2005). Embryonic and Naupliar Development of Eudiaptomus Gracilis and Eudiaptomus Graciloides at Different Temperatures: Comments on Individual Variability. J. Plankt. Res. 27, 1175–1187. doi: 10.1093/plankt/fbi083
Lee C.-H., Dahms H.-U., Cheng S.-H., Souissi S., Schmitt F. G., Kumar R., et al. (2010). Predation of Pseudodiaptomus Annandalei (Copepoda: Calanoida) by the Grouper Fish Fry Epinephelus Coioides Under Different Hydrodynamic Conditions. J. Exp. Mar. Biol. Ecol. 393, 17–22. doi: 10.1016/j.jembe.2010.06.005
Lee K.-W., Dahms H.-U., Park H. G., Kang J.-H. (2013). Population Growth and Productivity of the Cyclopoid Copepods Paracyclopina Nana, Apocyclops Royi and the Harpacticoid Copepod Tigriopus Japonicus in Mono and Polyculture Conditions: A Laboratory Study. Aquac. Res. 44, 836–840. doi: 10.1111/j.1365-2109.2011.03071.x
Lee K.-W., Kwon O.-N., Park H.-G. (2005). Effect of Temperature, Salinity and Diet on the Productivity of the Cyclopoid Copepod Apocyclops Royi. J. Aquacult. 18, 52–59. doi: 10.1002/9780470277522
Lee S.-H., Lee M.-C., Puthumana J., Park J. C., Kang S., Hwang D.-S., et al. (2017). Effects of Salinity on Growth, Fatty Acid Synthesis, and Expression of Stress Response Genes in the Cyclopoid Copepod Paracyclopina Nana. Aquaculture 470, 182–189. doi: 10.1016/j.aquaculture.2016.12.037
Lee K.-W., Rhee J.-S., Han J., Park H. G., Lee J.-S. (2012). Effect of Culture Density and Antioxidants on Naupliar Production and Gene Expression of the Cyclopoid Copepod, Paracyclopina Nana. Comp. Biochem. Physiol. Part A.: Mol. Integr. Physiol. 161, 145–152. doi: 10.1016/j.cbpa.2011.10.019
Liu X., Beyrend-Dur D., Dur G., Ban S. (2015). Combined Effects of Temperature and Food Concentration on Growth and Reproduction of Eodiaptomus Japonicus (Copepoda: Calanoida) From Lake Biwa (Japan). Freshw. Biol. 60 (10), 2003–2018. doi: 10.1111/fwb.12626
Liu J.-P., Zhao W., Wang S., Wang L., Xing Y.-N. (2007). The Embryonic and Larval Development of Cyclop Apocyclops Royi. J. Dalian. Fish. Univ. 20 (4), 239–245. doi: 10.3969/j.issn.1000-9957.2007.04.001
Lloyd S. S. (2006). Zooplankton Ecology in the Chesapeake Bay Estuarine Turbidity Maximum, With Emphasis on the Calanoid Copepod Eurytemora Affinis. Horn. Point. Lab. Univ. Maryl. Cent. Environ. Sci. p, 198.
Magouz F. I., Essa M. A., Matter M., Tageldein Mansour A., Alkafafy M., Ashour M. (2021). Population Dynamics, Fecundity and Fatty Acid Composition of Oithona Nana (Cyclopoida, Copepoda), Fed on Different Diets. Animals 11, 1188–899. doi: 10.3390/ani11051188
Mahjoub M.-S., Souissi S., Michalec F.-G., Schmitt F. G., Hwang J.-S. (2011). Swimming Kinematics of Eurytemora Affinis (Copepoda, Calanoida) Reproductive Stages and Differential Vulnerability to Predation of Larval Dicentrarchus Labrax (Teleostei, Perciformes). J. Plankt. Res. 33, 1095–1103. doi: 10.1093/plankt/fbr013
Marten G. G., Reid J. W. (2007). Cyclopoid Copepods. J. Am. Mosquit. Contr. Assoc. 23, 65–92. doi: 10.2987/8756-971X(2007)23[65:CC]2.0.CO;2
McKinnon A. D., Duggan S., Nichols P. D., Rimmer M. A., Semmens G., Robino B. (2003). The Potential of Tropical Paracalanid Copepods as Live Feeds in Aquaculture. Aquaculture 223, 89–106. doi: 10.1016/S0044-8486(03)00161-3
Mejri S. C., Tremblay R., Audet C., Wills P. S., Riche M. (2021). Essential Fatty Acid Requirements in Tropical and Cold-Water Marine Fish Larvae and Juveniles. Front. Mar. Sci. 8. doi: 10.3389/fmars.2021.680003
Mitsuzawa A., Miyamoto H., Ueda H. (2017). Feeding Selectivity of Early-Stage Fish Larvae on the Nauplii and Eggs of Different Copepod Species. Plankt. Benthos. Res. 12, 115–122. doi: 10.3800/pbr.12.115
Monroig Ó., Shu-Chien A. C., Kabeya N., Tocher D. R., Castro L. F. C. (2022). Desaturases and Elongases Involved in Long-Chain Polyunsaturated Fatty Acid Biosynthesis in Aquatic Animals: From Genes to Functions. Prog. Lipid Res. 86, 101157. doi: 10.1016/j.plipres.2022.101157
Morozov A., Pasternak A. F., Arashkevich E. G. (2013). Revisiting the Role of Individual Variability in Population Persistence and Stability. PloS One 8, e70576. doi: 10.1371/journal.pone.0070576
Nagaraj M. (1988). Combined Effects of Temperature and Salinity on the Complete Development of Eurytemora Velox (Crustacea: Calanoidea). Mar. Biol. 99, 353–358. doi: 10.1007/BF02112127
Nguyen T. T., Le M., Doan N. X., Nguyen S. T., Truong T. S. H., Vu M. T. T., et al. (2020). Salinity and Temperature Effects on Productivity of a Tropical Calanoid Copepod Pseudodiaptomus Incisus. Aquac. Res. 51, 3768–3779. doi: 10.1111/are.14727
Nielsen B. L. H., Gréve H. V. S., Hansen B. W. (2021). Cultivation Success and Fatty Acid Composition of the Tropical Copepods Apocyclops Royi and Pseudodiaptomus Annandalei Fed on Monospecific Diets With Varying PUFA Profiles. Aquac. Res. 52, 1127–1138. doi: 10.1111/are.14970
Nielsen B. L. H., Gréve H. V. S., Rayner T. A., Hansen B. W. (2020). Biochemical Adaptation by the Tropical Copepods Apocyclops Royi and Pseudodiaptomus Annandalei to a PUFA-Poor Brackish Water Habitat. Mar. Ecol. Prog. Ser. 655, 77–89. doi: 10.3354/meps13536
Nilsson B., Jakobsen H. H., Stief P., Drillet G., Hansen B. W. (2017). Copepod Swimming Behavior, Respiration, and Expression of Stress-Related Genes in Response to High Stocking Densities. Aquacult. Rep. 6, 35–42. doi: 10.1016/j.aqrep.2017.03.001
Nowakowski K., Sługocki Ł. (2021). Short-Term Heat Shock Perturbation Affects Populations of Daphnia Magna and Eurytemora Carolleeae: A Warning to the Water Thermal Pollution. Sci. Rep. 11, 16909. doi: 10.1038/s41598-021-96464-8
Nunes J. P., Ferreira J. G., Gazeau F., Lencart-Silva J., Zhang X. L., Zhu M. Y., et al. (2003). A Model for Sustainable Management of Shellfish Polyculture in Coastal Bays. Aquaculture 219, 257–277. doi: 10.1016/S0044-8486(02)00398-8
Oda T., Dur G., Ducasse S., Souissi S. (2021). “Re:Mobidyc – Reconstructing Modeling Based on Individual for the Dynamics of Community. Advance in Practical Applications of Agents and Multi-Agent Systems, and Social Good,” in The PAAMS collection. 19th International Conference, PAAMS21, Salamanca, Spain, October 6-8, 2021. 367–371, ISBN: 978-3-030-85739-4.
Pan Y.-J., Souissi A., Sadovskaya I., Hansen B. W., Hwang J.-S., Souissi S. (2017). Effects of Cold Selective Breeding on the Body Length, Fatty Acid Content, and Productivity of the Tropical Copepod Apocyclops Royi (Cyclopoida, Copepoda). J. Plankt. Res. 39, 994–1003. doi: 10.1093/plankt/fbx041
Pan Y.-J., Souissi A., Souissi S., Hwang J.-S. (2016). Effects of Salinity on the Reproductive Performance of Apocyclops Royi (Copepoda, Cyclopoida). J. Exp. Mar. Biol. Ecol. 475, 108–113. doi: 10.1016/j.jembe.2015.11.011
Pan Y.-J., Souissi S., Souissi A., Wu C.-H., Cheng S.-H., Hwang J.-S. (2014). Dietary Effects on Egg Production, Egg-Hatching Rate and Female Life Span of the Tropical Calanoid Copepod Acartia Bilobata. Aquac. Res. 45, 1659–1671. doi: 10.1111/are.12113
Pan Y.-J., Wang W.-L., Hwang J.-S., Souissi S. (2021). Effects of Epibiotic Diatoms on the Productivity of the Calanoid Copepod Acartia Tonsa (Dana) in Intensive Aquaculture Systems. Front. Mar. Sci. 8. doi: 10.3389/fmars.2021.728779
Parker R. A. (1974). Empirical Functions Relating Metabolic Processes in Aquatic Systems to Environmental Variables J. Fish. Res. Board. Can. 31, 1550–1552. doi: 10.1139/f74-192
Payne M. F., Rippingale R. J., Cleary J. J. (2001). Cultured Copepods as Food for West Australian Dhufish (Glaucosoma Hebraicum) and Pink Snapper (Pagrus Auratus) Larvae. Aquaculture 194 (1-2), 137–150. doi: 10.1016/S0044-8486(00)00513-5
Piccinetti C. C., Tulli F., Tokle N. E., Cardinaletti G., Olivotto I. (2014). The Use of Preserved Copepods in Sea Bream Small-Scale Culture: Biometric, Biochemical and Molecular Implications. Aquacult. Nutr. 20, 90–100. doi: 10.1111/anu.12055
Prestidge M. (1995). A Modelling Investigation of Copepod Egg Production in the Irish Sea. ICES J. Mar. Sci. 52, 693–703. doi: 10.1016/1054-3139(95)80082-4
Quinn B. K. (2017). A Critical Review of the Use and Performance of Different Function Types for Modeling Temperature-Dependent Development of Arthropod Larvae. J. Therm. Biol. 63, 65–77. doi: 10.1016/j.jtherbio.2016.11.013
Rajkumar M., Kumaraguru Vasagam K. P. (2006). Suitability of the Copepod, Acartia Clausi as a Live Feed for Seabass Larvae (Lates Calcarifer Bloch): Compared to Traditional Live-Food Organisms With Special Emphasis on the Nutritional Value. Aquaculture 261, 649–658. doi: 10.1016/j.aquaculture.2006.08.043
Rasdi N. W., Qin J. G. (2016). Improvement of Copepod Nutritional Quality as Live Food for Aquaculture: A Review. Aquac. Res. 47, 1–20. doi: 10.1111/are.12471
Rasdi N. W., Qin J. G. (2018). Impact of Food Type on Growth, Survival and Reproduction of the Cyclopoid Copepod Cyclopina Kasignete as a Potential Live Food in Aquaculture. Aquacult. Int. 26, 1281–1295. doi: 10.1007/s10499-018-0283-x
Shields R. J., Bell J. G., Luizi F. S., Gara B., Bromage N. R., Sargent J. R. (1999). Natural Copepods Are Superior to Enriched Artemia Nauplii as Feed for Halibut Larvae (Hippoglossus Hippoglossus) in Terms of Survival, Pigmentation and Retinal Morphology: Relation to Dietary Essential Fatty Acids. J. Nutr. 129, 1186–1194. doi: 10.1093/jn/129.6.1186
Souissi S. (2006). RôLe Des Strateígies De Vie Des Copeípodes Planctoniques Dans Le Fonctionnement Des Eícosystèmes Aquatiques: Approche Individu-Centreí Et Multi-Eíchelle. Habilit. à Diriger. Des. Recherches. Station Mar. Wimereux. Universiteí Des. Sci. Technol. Lille., 141.
Souissi S., Carlotti F., Nival P. (1997). Food- and Temperature-Dependent Function of Moulting Rate in Copepods: An Example of Parameterization for Population Dynamics Models. J. Plankt. Res. 19, 1331–1346. doi: 10.1093/plankt/19.9.1331
Souissi S., Seuront L., Schmitt F. G., Ginot V. (2005). Describing Space-Time Patterns in Aquatic Ecology Using IBMs and Scaling and Multi-Scaling Approaches. Nonlinear. Anal.: Real. World Appl. 6, 705–730. doi: 10.1016/j.nonrwa.2004.12.013
Støttrup J. G. (2000). The Elusive Copepods: Their Production and Suitability in Marine Aquaculture. Aquacult. Res. 31 (8-9), 703–711. doi: 10.1046/j.1365-2109.2000.318488.x
Støttrup J. G. (2003). ““Production and Nutritional Value of Copepods,”,” in Live Feeds in Marine Aquaculture. Eds. Støttrup J. G., McEvoy L. A. (Oxford, UK: Blackwell Science Ltd), 145–205. doi: 10.1002/9780470995143.ch5
Su H.-M., Cheng S.-H., Chen T.-I., Su M.-S. (2005). ““Culture of Copepods and Applications to Marine Finfish Larval Rearing in Taiwan,”,” in Copepods in Aquaculture. Eds. Lee C.-S., O’Bryen P. J., Marcus N. H. (Ames, Iowa, USA: Blackwell Publishing Professional), 183–194. doi: 10.1002/9780470277522.ch14
Takayama Y., Hirahara M., Liu X., Ban S., Toda T. (2020). Are Egg Production and Respiration of the Marine Pelagic Copepod Acartia Steueri Influenced by Crowding? Aquac. Res. 51, 3741–3750. doi: 10.1111/are.14723
Tordesillas D. T., Paredes P. M. F., Villaruel K. P. E., Queneri C. A. A. M., Rico J. L., Ban S., et al. (2018). Effects of Food Concentration on the Reproductive Capacity of the Invasive Freshwater Calanoid Copepod Arctodiaptomus Dorsalis (Mars) in the Philippines. J. Crustac. Biol. 38, 101–106. doi: 10.1093/jcbiol/rux089
van der Meeren T., Karlsen Ø., Liebig P. L., Mangor-Jensen A. (2014). Copepod Production in a Saltwater Pond System: A Reliable Method for Achievement of Natural Prey in Start-Feeding of Marine Fish Larvae. Aquacult. Eng. 62, 17–27. doi: 10.1016/j.aquaeng.2014.07.003
van der Meeren T., Olsen R. E., Hamre K., Fyhn H. J. (2008). Biochemical Composition of Copepods for Evaluation of Feed Quality in Production of Juvenile Marine Fish. Aquaculture 274, 375–397. doi: 10.1016/j.aquaculture.2007.11.041
Van Someren Gréve H., Jepsen P. M., Hansen B. W. (2020). Does Resource Availability Influence the Vital Rates of the Tropical Copepod Apocyclops Royi (Lindberg 1940) Under Changing Salinities? J. Plankt. Res. 42, 467–478. doi: 10.1093/plankt/fbaa031
Yamakawa T., Matsuda H. (1997). Improve BĕLehraídek’s Equation for Comprehensive Description of the Relationship Between Environmental Factors and Metabolic Rates Fisheries Sci 63, 725–730. doi: 10.2331/fishsci.63.725
Keywords: copepod culture, production estimation, reproduction, individual-based model (IBM), salinity
Citation: Yoshino M, Pan Y-J, Souissi S and Dur G (2022) An Individual-Based Model to Quantify the Effect of Salinity on the Production of Apocyclops royi (Cyclopoida, Copepoda). Front. Mar. Sci. 9:863244. doi: 10.3389/fmars.2022.863244
Received: 27 January 2022; Accepted: 11 May 2022;
Published: 13 June 2022.
Edited by:
Morten Omholt Alver, Norwegian University of Science and Technology, NorwayReviewed by:
Chaoshu Zeng, James Cook University, AustraliaBenni Winding Hansen, Roskilde University, Denmark
Copyright © 2022 Yoshino, Pan, Souissi and Dur. This is an open-access article distributed under the terms of the Creative Commons Attribution License (CC BY). The use, distribution or reproduction in other forums is permitted, provided the original author(s) and the copyright owner(s) are credited and that the original publication in this journal is cited, in accordance with accepted academic practice. No use, distribution or reproduction is permitted which does not comply with these terms.
*Correspondence: Gaël Dur, ZHVyLmdhZWxAc2hpenVva2EuYWMuanA=