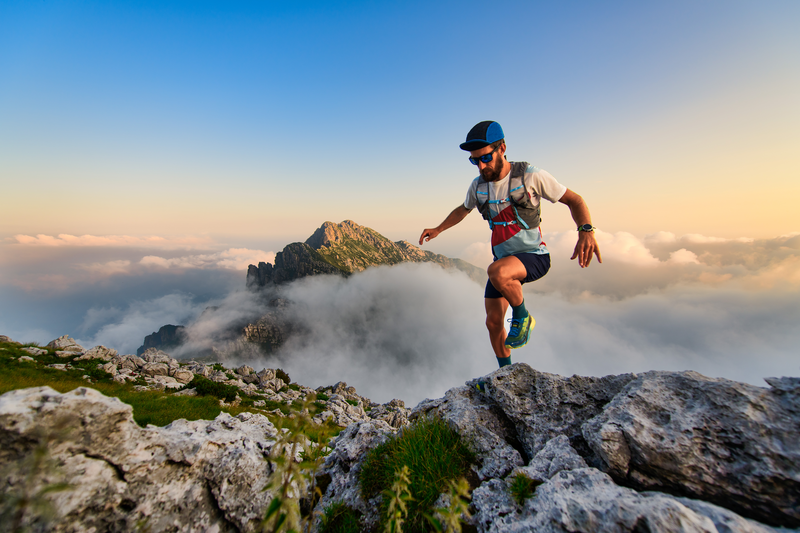
94% of researchers rate our articles as excellent or good
Learn more about the work of our research integrity team to safeguard the quality of each article we publish.
Find out more
ORIGINAL RESEARCH article
Front. Mar. Sci. , 10 May 2022
Sec. Marine Molecular Biology and Ecology
Volume 9 - 2022 | https://doi.org/10.3389/fmars.2022.862929
This article is part of the Research Topic Marine Biological Materials: Functional Mechanisms and Environmental Impacts from the Molecular to the Macro-Scale View all 11 articles
Molluscs produce rigid shells to protect their soft bodies from predators and physiochemical violations. The soft tissues attach to shells via the myostracum layer (also called adductor muscle scar, AMS) which bears tremendous contract force and is of vital importance to the survival of the molluscs. Considering the prevalence of tissue-shell attachment in molluscs, we speculate that certain homology may be shared among varied species. To test this speculation, scanning electron microscopy and Raman spectrum were applied to analyze the microstructure and calcium carbonate polymorphs of the myostracum in most of the molluscan classes. It was found that all the tested molluscan classes and genera contain similar columnar prisms which aligned vertically and were composed of aragonite. Moreover, this structure was found in ammonoid fossils dating back to the Permian period. Such peculiar mineral structure may contribute to the loading contract force, thus being evolutionally conservative among varied species and for hundreds of millions of years. Our study underscores the vital impact of physiological functions on the evolution of the shell structure.
Biominerals formed by living organisms exhibit excellent mechanical properties such as strength and stiffness (Raut et al., 2020). These organic-inorganic composites play a role in body supporting, protection, feeding, and directionality (Weaver et al., 2010; Addadi and Weiner, 2014; Guzman-Lastra et al., 2016; Deng et al., 2020). Characterizing biominerals with specific functions is fundamental for understanding how organisms make use of inorganic materials to orchestrate exquisite structures, and in return one can be inspired to build artificial analogous materials.
Molluscs can produce myriad shells with extraordinary morphology and microstructures. For example, one of the most adopted microstructures in Gastropoda is crossed-lamellar structure, and similar structure has been reprinted in plywood to improve its fracture resistance (Pramreiter et al., 2020). Nacre, also called mother of pearl, has been found in pearl oyster, mussel, abalone, and nautilus genera (Jackson et al., 1988; Sun and Bhushan, 2012). The salient feature of nacre is the mortar-and-brick arrangement consisting of stacking sheets of aragonite tablets separated by organic matrix, which leads to the dissipation of stress and results in extremely high toughness (Jackson et al., 1988; Marin et al., 2008; Huang et al., 2019; Deng et al., 2020). Therefore, intensive efforts have been made to reproduce nacre-like biomaterials to gain strength (Mao et al., 2016; Raut et al., 2020). Other microstructures, such as prismatic layer and homogeneous fine grains have been found in various molluscan species (Bayerlein et al., 2014; Checa et al., 2016; Zhu et al., 2016).
Most molluscan shells form exoskeletons except that some Cephalopoda genera have evolved endoskeletons inside the soft tissue (Mao et al., 2021). For the exoskeleton, the soft tissue is attached to the mineral surface via the myostracum layer which is also called the adductor muscle scar. The adductor muscle is responsible for shell closure in the Bivalvia and retraction of the soft body (such as in the Gastropoda) (Gilman, 2007; Castro-Claros et al., 2021). Therefore, the myostracum layer mainly bears internal stress compared with the outer shell layers which withstand external damages. The microstructure of the myostracum has been reported in several bivalves (Nakahara and Bevelander, 1970; John and Taylor, 1973; Zhu et al., 2016) and Gastropoda (Suzuki et al., 2010). In previous studies, myostracum layers were usually described as “prismatic aragonite” (Weiner, 1989); however, exceptions were reported (Zhu et al., 2016). In addition, it remains unclear whether all molluscs share similar features in this particular shell layer.
Since all the molluscs with exoskeleton have myostracum layers of similar function, we speculate that some similarities may be shared among various species. In this study, shell samples from five classes (Polyplacophora, Bivalvia, Gastropoda, Cephalopoda, and Scaphopoda) were collected and examined using scanning electronic microscopy and Raman spectroscopy. It was found that myostracum layers in these classes share a striking similarity in both microstructure and mineralogy, indicating that these shell structures are evolutionarily conserved or resulted from convergent evolution for similar functions.
Abalone Haliotis discus, Arcoida Tegillarca granosa, mussels Mytilus edulis and Pinna rudis, scallop Chlamys farreri, Pacific oyster Crassostrea gigas, Unionoida Cristaria plicata, clams Ruditapes philippinarum, Sinonovacula constricta (Lamarck), Dosinorbis japonica and Panopea abrupta were purchased from a seafood market in Beijing City, China. Limpets Cellana toreuma were collected from the intertidal zone of Zhoushan Island in Zhejiang Province, China. Unionoida Hyriopsis cumingii was provided by Shanghai Ocean University in Shanghai City, China. Pearl oyster Pinctada fucata were purchased from Guangdong Ocean University in Guangdong Province, China. Mud snail Bullacta exarata (Philippi, 1848), chiton Ischnochiton comptus (Gould) and tusk shell Pictodentalium vernerdi were collected from the Eastern China Sea. Fresh water snails Bradybaena ravida (Benson) were collected in Zhuhai City of Guangdong Province, China. Ammonoidea sp. was collected in Pearl River Delta of Guangdong Province, China. All samples were living animals except the P. vernerdi and Ammonoidea sp. which were shell specimens. The soft tissues were removed by scalpel, the shells were thoroughly washed with deionized water, and air-dried.
The shell samples were cut into small pieces with scissors and a diamond cutter. The resultant shell samples were coated with gold in a vacuum sputtering apparatus and examined with a scanning electronic microscope (FEI Quanta 200) with an accelerating voltage of 30 kV in a high vacuum mode.
Laser Raman Spectroscopy (Evolution, 111 HORIBA, France) was performed to determine the calcium carbonate polymorph with the detected wavenumber ranging from 100 cm-1 to 1200 cm-1. For each shell sample tested, more than three sites were randomly selected in the myostracum layers or the shell layers underlying myostracum layers.
Living molluscs contain seven classes, namely Aplacophora, Polyplacophora, Monoplacophora, Gastropoda, Scaphopoda, Cephalopoda, and Bivalvia. Since most of the bivalves have adductor muscle for the shell closure, we first examined the myostracum layer in this class. Interestingly, all the studied species contained a similar microstructure at the muscle-shell attachment (Figure 1 and Supplementary Figure 1). Though there exist some slight variations, the myostracum layers in bivalves consisted of simple regular prisms. Raman spectra showed that the mineral counterparts were all aragonite (Table 1 and Supplementary Figures 3, 7). Most of the species have a monolayer myostracum (Figures 1A–T). However, multilayered myostracum was observed in the Myoida P. abrupta (Figures 1U, V), thus forming regular alternate microstructure of prisms and fine granules. Usually, the height of the prismatic myostracum is 10-20 µm, except those in the P. abrupta and Arcoida T. granosa could reach more than 50 µm or even 200 µm in the latter (Figures 1W, X). Moreover, the myostracum in T. granosa differed from others in that the columns were much larger. The columns could reach 10 µm in diameter, while those in other species were only around 1 µm (e.g., in the C. gigas and P. fucata).
Figure 1 Microstructure of the myostracum layer in bivalve molluscs. (A, B) pen shell Pinna rudis; (C, D) mussel Mytilus edulis; (E, F) Pacific oyster Crassostrea gigas; (G, H) scallop Chlamys farreri; (I, J) pearl oyster Pinctada fucata; (K, L) Dosiniinae Dosinorbis japonica; (M, N) solen Sinonovacula constricta (Lamarck); (O, P) clam Ruditapes philippinarum; (Q, R) freshwater mussel Cristaria plicata; (S, T) freshwater mussel Hyriopsis cumingii; (U, V) geoduck Panopea abrupta; (W, X) ark shell Tegillarca granosa. In each sample, the latter image is the magnification of the former (black boxes), except that in (U–X), different positions of myostracum layers are shown. The myostracum layer is indicated by a purple star, and the boundary between the myostracum and the underlying shell layers is indicated by the yellow dashed line. The scale bar is 50 µm in the former image of each sample and 10 µm in the latter (magnification) as shown in (A, B), except (U–X) which are marked in the images.
The marvelous similarity of the myostracum layers among various bivalves suggest that such microstructure might have evolved in the ancient bivalve ancestor and remained conservative once that occurred. When considering the vast diversity of the shell layers underlying the myostracum, such a scheme was more convincing. In mussels, pearl oyster, and Unionoida, the shell layer underlying the myostracum adopt a sheet nacreous microstructure, and in scallop and Pacific oyster, foliated sheets could be seen, while both fine-grained homogeneous structure and crossed lamellar were found in clams (Table 1). Moreover, the polymorphs of these shell structures could be either aragonite or calcite (Supplementary Figure 4). For example, the nacreous layers in P. fucata were aragonite while foliated layers C. farreri were calcite, although both species belong to Pterioida. Therefore, the bulk shell structures in bivalves underwent divergent evolution which leads to the myriad microstructures. Oppositely, the myostracum layer might be under enormous evolutionary pressure and remain conservative in bivalves.
Then we wonder whether other molluscs have similar myostracum arrangement and when such shell structure evolved. Strikingly, prism columns were present in the innermost shell layer of the tested genera (Figure 2 and Supplementary Figure 2), and the mineral counterparts were all aragonite (Table 2 and Supplementary Figure 3). It should be noted that the fine structures were varied among different classes. In chiton, which belongs to Polyplacophora, the myostracum layer did not showcase regular prisms, instead, composite prisms were vertically aligned. The Gastropoda and Scaphopoda have more regular aligned prisms in the myostracum layer (Figures 2C–J) except that in the mud snail B. exarata, the prisms were irregular to some extent. Strikingly, a simple regular prismatic structure was found in the fossil specimen of ammonoid. Previous studies have proved that ammonoid fossils in south China dated back to the Permian period around 250 million years ago (Shen et al., 2019). The location was in the inner shell surface (Supplementary Figures 2, 8), and this microstructure was supposed to be the adductor muscle scar according to previous studies (Kazushige Tanabe and Mapes, 1998; Zhu et al., 2016). Unfortunately, it was technically difficult to determine the polymorph of this myostracum layer. Nevertheless, the absence of magnesium implied that the aragonite was more likely the case (Supplementary Figure 8) because calcite in molluscan shell usually contained a certain amount of magnesium (Cuif et al., 2012). We also examined the Brachiopod L. anatine whose shells are similar to those of molluscs but consist of calcium phosphate. It was shown that, although they contain multilayers, the myostracum layer with columnar aragonitic prisms was absent in the L. anatine shells (Figures 2O, P and Supplementary Figures 5, 6).
Figure 2 Microstructure of the myostracum layer in some molluscan classes. (A, B) chiton Ischnochiton comptus (Gould); (C, D) abalone Haliotis discus; (E, F) Limpets Cellana toreuma; (G, H) mud snail Bullacta exarata (Philippi, 1848); (I, J) fresh water snail Bradybaena ravida (Benson); (K, L) Scaphopoda Pictodentalium vernerdi; (M, N) Ammonoidea sp.; (O, P) Brachiopod Lingula anatine, serving as a comparison. In each sample examined, the latter image is the magnification of the black box in the former image. The myostracum layer was indicated by a purple star, and the boundary between the myostracum and the underlying shell layers was indicated by the yellow dashed line. The white dashed line in (N) shows the boundary between the myostracum and the filled impurities in the shell lumen.
Similar to Bivalvia, shell structures underlying the myostracum layer in the Polyplacophora, Gastropoda, Scaphopoda, and Cephalopoda exhibited a diverse display. Columnar nacreous were present in both Gastropoda and Cephalopoda, while composite prismatic, crossed lamellar, and simple lamellar were also found as the bulk microstructure of tested shells. Moreover, both calcite and aragonite were found to be the composition of the main part of the shell layers.
Molluscs have elaborate rigid shells to protect the soft bodies, and when encountered with danger, the shells are closed or the soft bodies retract into the shell tube. Therefore, it would be of significant importance to control the shell movement and maintain the firm attachment of soft tissues and mineral organs. Our study showed that the myostracum layer in most molluscs adopt a conservative microstructure and crystal polymorphism, namely columnar prisms (or composite prisms in some individual cases) of aragonitic calcium carbonate. The result was consistent with previous studies in mussel, oyster, and scallop (Lee et al., 2011; Song et al., 2013; Zhu et al., 2016). Although myostracum is considered as prismatic aragonite in general in some archives (John and Taylor, 1973; Weiner, 1989; Zhu et al., 2016), most are focused on bivalves, and our present study extended this conception to most molluscs.
Zhu et al. (2016) reported that no distinguishable myostracum layer could be found in the clam R. philippinarum, at odds with our study (Figures 1O, P). Such an inconsistency might be due to the different sampling methods. Because in Zhu’s work, they examined the fracture surface perpendicular to the shell growth direction, which might lead to their missing the myostracum. Indeed, the myostracum in R. philippinarum was only 5 µm in height (Figures 1O, P), which was quite difficult to figure out. It would be much easier to image this structure in the fracture surface along the shell growth direction, as we did in the present study.
In all the selected living species, the myostracum layers adopted aragonite polymorphs, coincident with previous studies (Lee et al., 2011; Wang et al., 2014; Zhu et al., 2016), except the scallop. Reported data showed that myostracum in scallop C. farreri contained both aragonite and calcite (Zhu et al., 2016). The authors used FTIR to analyze the shell powder collected in myostracum, which might contain contaminated foliated layer (calcite) underlying the object. Because it was near impossible to completely separate the myostracum layer and the foliated layer, we applied in situ measurement (Raman spectrum) to analyze the mineral composition, and only aragonite was present.
The highly conserved myostracum in most molluscan classes suggested that such shell structure may be a primitive signature of their ancient ancestors. Indeed, evidence from this study (Figure 2 and Supplementary Figure 8) and archive [Figure 4Ain Reference (Kazushige Tanabe and Mapes, 1998)] revealed that prismatic myostracum structures already existed in molluscan shells dating back to the Permian and Jurassic periods. An interesting finding reported by Porter showed that calcite and aragonite seas have a strong influence on the mineralogy of carbonate skeletons at the time they first evolve (Porter, 2010). In that case, skeletons evolved in calcite sea would be composed of calcite, and skeletons evolved in aragonite sea would be composed of aragonite. Because there were two aragonite seas before the Permian period (Porter, 2010), unique myostracum structure might have evolved in early Cambrian or Carboniferous periods or both.
The prismatic microstructures of the myostracum seemed to be more advanced in Bivalvia class compared with other classes (Figures 1, 2). Most of the bivalve genera have myostracum of simple regular prisms. In some particular samples, the myostracum layers could grow more than 200 µm in height (Figures 1W, X). This phenomenon may be due to the evolutionary pressure on closure of the two shell valves. As a result, the tissue-shell attachment in Bivalvia was one of the strongest ligaments in invertebrates (Castro-Claros et al., 2021). Nevertheless, Aplacophora completely lost their calcified shell, and some Gastropoda and Cephalopoda evolve endogenous skeletons or lose the shell. For example, slug Agriolimax agrestis (Linnaeus) is a terrestrial molluscs without a shell (Jiang et al., 2021). Some cuttlefish and squids have internal shells serving as buoyancy regulatory organs (Yang et al., 2020; Liu et al., 2021). We surmise that adductor muscle degenerated in these genera, although more studies are needed for verification.
In this study, we examined most of the molluscan genera to reveal the shell microstructure of the myostracum layers where the adductor muscle (soft tissue)-mineral attachment is located. The results showed that myostracum layers are composed of vertically aligned aragonitic prisms, and such microstructure is conserved among most molluscan classes and genera. Such evolution conservation implies pivotal functions of the unique myostracum layer with similar microstructure and mineralogy. How such shell structures are correlated with their physiological function remains elusive and deserves more in-depth studies.
The original contributions presented in the study are included in the article/Supplementary Material. Further inquiries can be directed to the corresponding author.
The animal study was reviewed and approved by the Animal Ethics Committee of Tsinghua University, Beijing, China.
WD carried out the lab work. JH conceived the project, participated in lab work and drafted the manuscript. CL helped with data analysis. HW and GZ revised the manuscript. LX and RZ provided financial supports and revised the manuscript. All authors gave final approval for publication.
This work was supported by National Natural Science Foundation of China Grants 31872543 and 32072951.
The authors declare that the research was conducted in the absence of any commercial or financial relationships that could be construed as a potential conflict of interest.
All claims expressed in this article are solely those of the authors and do not necessarily represent those of their affiliated organizations, or those of the publisher, the editors and the reviewers. Any product that may be evaluated in this article, or claim that may be made by its manufacturer, is not guaranteed or endorsed by the publisher.
The Supplementary Material for this article can be found online at: https://www.frontiersin.org/articles/10.3389/fmars.2022.862929/full#supplementary-material
Addadi L., Weiner S. (2014). Biomineralization: Mineral Formation by Organisms. Phys. Scripta 89 (9). doi: 10.1088/0031-8949/89/9/098003
Bayerlein B., Zaslansky P., Dauphin Y., Rack A., Fratzl P., Zlotnikov I. (2014). Self-Similar Mesostructure Evolution of the Growing Mollusc Shell Reminiscent of Thermodynamically Driven Grain Growth. Nat. Mater. 13 (12), 1102–1107. doi: 10.1038/nmat4110
Castro-Claros J. D., Checa A., Lucena C., Pearson J. R., Salas C. (2021). Shell-Adductor Muscle Attachment and Ca2+ Transport in the Bivalves Ostrea Stentina and Anomia Ephippium. Acta Biomaterialia. 120, 249–262. doi: 10.1016/j.actbio.2020.09.053
Checa A. G., Macias-Sanchez E., Harper E. M., Cartwright J. H. (2016). Organic Membranes Determine the Pattern of the Columnar Prismatic Layer of Mollusc Shells. Proc. Biol. Sci. 283 (1830). doi: 10.1098/rspb.2016.0032
Cuif J. P., Dauphin Y., Nehrke G., Nouet J., Perez-Huerta A. (2012). Layered Growth and Crystallization in Calcareous Biominerals: Impact of Structural and Chemical Evidence on Two Major Concepts in Invertebrate Biomineralization Studies. Minerals-Basel 2 (1), 11–39. doi: 10.3390/min2010011
Deng Z., Chen H., Yang T., Jia Z., Weaver J. C., Shevchenko P. D., et al. (2020). Strategies for Simultaneous Strengthening and Toughening via Nanoscopic Intracrystalline Defects in a Biogenic Ceramic. Nat. Commun. 11 (1), 5678. doi: 10.1038/s41467-020-19416-2
Gilman S. E. (2007). Shell Microstructure of the Patellid Gastropod Collisella Scabra (Gould): Ecological and Phylogenetic Implications. Veliger 48 (4), 235–242.
Guzman-Lastra F., Kaiser A., Lowen H. (2016). Fission and Fusion Scenarios for Magnetic Microswimmer Clusters. Nat. Commun. 7, 13519. doi: 10.1038/ncomms13519
Huang W., Restrepo D., Jung J. Y., Su F. Y., Liu Z. Q., Ritchie R. O., et al. (2019). Multiscale Toughening Mechanisms in Biological Materials and Bioinspired Designs. Adv. Mater. 31 (43). doi: 10.1002/adma.201901561
Jackson A. P., Vincent J. F. V., Turner R. M. (1988). The Mechanical Design of Nacre. Proc. R. Soc. London Ser. B Biol. Sci. 234 (1277), 415–440. doi: 10.1098/rspb.1988.0056
Jiang L., Zhao R. N., Tian H., Wu X. S., Guo F., Chen W. L. (2021). Functional Response and Predation Potential of Carabus Elysii Adults Against the Terrestrial Slug Agriolimax Agrestis. Insects 12 (12). doi: 10.3390/insects12121135
John D., Taylor W. J. K. (1973). The Shell Structure and Mineralogy of the Bivalvia II. Lucinacea-Clavagellacea, Conclusions: Bulletin of the British Museum (Natural History) 253–294. doi: 10.5962/p.314199
Kazushige Tanabe N. H. L., Mapes R. H. (1998). Muscle Attachment Scars in a Carboniferous Goniatite. Paleontol. Res. 2 (2), 130–136. doi: 10.2517/prpsj.2.130
Landman N. H., Davis R. A., Mapes R. H. (2007). Cephalopods Present and Past: New Insights and Fresh Perspectives (The Nitherlands: Springer Press.).
Lee S.-W., Jang Y.-N., Kim J.-C. (2011). Characteristics of the Aragonitic Layer in Adult Oyster Shells,Crassostrea Gigas: Structural Study of Myostracum Including the Adductor Muscle Scar. Evidence-Based Complement. Altern. Med. 2011, 1–10. doi: 10.1155/2011/742963
Liu C., Ji X., Huang J., Wang Z., Liu Y., Hincke M. T. (2021). Proteomics of Shell Matrix Proteins From the Cuttlefish Bone Reveals Unique Evolution for Cephalopod Biomineralization. ACS Biomater. Sci. Eng. doi: 10.1021/acsbiomaterials.1c00693
Mao L. B., Gao H. L., Yao H. B., Liu L., Colfen H., Liu G., et al. (2016). Synthetic Nacre by Predesigned Matrix-Directed Mineralization. Science 354 (6308), 107–110. doi: 10.1126/science.aaf8991
Mao A. R., Zhao N. F., Liang Y. H., Bai H. (2021). Mechanically Efficient Cellular Materials Inspired by Cuttlebone. Adv. Mater. 33 (15). doi: 10.1002/adma.202007348
Marin F., Luquet G., Marie B., Medakovic D. (2008). Molluscan Shell Proteins: Primary Structure, Origin, and Evolution. Curr. Top. Dev. Biol. 80, 209–276. doi: 10.1016/S0070-2153(07)80006-8
Nakahara H., Bevelander G. (1970). An Electron Microscope Study of the Muscle Attachment in the Mollusc Pinctada Radiata. Tex. Rep. Biol. Med. 28 (3), 279–286. doi: 10.1080/01459740.1984.9965887
Porter S. M. (2010). Calcite and Aragonite Seas and the De Novo Acquisition of Carbonate Skeletons. Geobiology 8 (4), 256–277. doi: 10.1111/j.1472-4669.2010.00246.x
Pramreiter M., Rohner M., Kumpenza C., Ungerer B., Stadlmann A., Keckes J., et al. (2020). Energy-Absorbing Wood Composite for Improved Damage Tolerance Inspired by Mollusc Shells. Mater. Res. Express 7 (9). doi: 10.1088/2053-1591/abb1f3
Raut H. K., Schwartzman A. F., Das R., Liu F., Wang L., Ross C. A., et al. (2020). Tough and Strong: Cross-Lamella Design Imparts Multifunctionality to Biomimetic Nacre. ACS Nano. 14 (8), 9771–9779. doi: 10.1021/acsnano.0c01511
Shen S. Z., Zhang H., Zhang Y. C., Yuan D. X., Chen B., He W. H., et al. (2019). Permian Integrative Stratigraphy and Timescale of China. Sci. China Earth Sci. 62 (1), 154–188. doi: 10.1007/s11430-017-9228-4
Song Y., Lu Y., Ding H., Lv H., Gao G., Sun C. (2013). Structural Characteristics at the Adductor Muscle and Shell Interface in Mussel. Appl. Biochem. Biotechnol. 171 (5), 1203–1211. doi: 10.1007/s12010-013-0194-2
Sun J. Y., Bhushan B. (2012). Hierarchical Structure and Mechanical Properties of Nacre: A Review. Rsc Adv. 2 (20), 7617–7632. doi: 10.1039/c2ra20218b
Suzuki M., Kameda J., Sasaki T., Saruwatari K., Nagasawa H., Kogure T. (2010). Characterization of the Multilayered Shell of a Limpet, Lottia Kogamogai (Mollusca: Patellogastropoda), Using SEM-EBSD and FIB-TEM Techniques. J. Struct. Biol. 171 (2), 223–230. doi: 10.1016/j.jsb.2010.04.008
Wang X. T., Li L., Zhu Y. B., Song X. R., Fang X. D., Huang R. L., et al. (2014). Aragonite Shells are More Ancient Than Calcite Ones in Bivalves: New Evidence Based on Omics. Mol. Biol. Rep. 41 (11), 7067–7071. doi: 10.1007/s11033-014-3620-9
Weaver J. C., Wang Q. Q., Miserez A., Tantuccio A., Stromberg R., Bozhilov K. N., et al. (2010). Analysis of an Ultra Hard Magnetic Biomineral in Chiton Radular Teeth. Mater. Today 13 (1-2), 42–52. doi: 10.1016/S1369-7021(10)70016-X
Yang T., Jia Z., Chen H. S., Deng Z. F., Liu W. K., Chen L. N., et al. (2020). Mechanical Design of the Highly Porous Cuttlebone: A Bioceramic Hard Buoyancy Tank for Cuttlefish. P Natl. Acad. Sci. U.S.A. 117 (38), 23450–23459. doi: 10.1073/pnas.2009531117
Keywords: molluscs, AMS, myostracum layer, microstructure, aragonite
Citation: Dong W, Huang J, Liu C, Wang H, Zhang G, Xie L and Zhang R (2022) Characterization of the Myostracum Layers in Molluscs Reveals a Conservative Shell Structure. Front. Mar. Sci. 9:862929. doi: 10.3389/fmars.2022.862929
Received: 26 January 2022; Accepted: 25 March 2022;
Published: 10 May 2022.
Edited by:
Shiguo Li, Research Center for Eco-environmental Sciences (CAS), ChinaCopyright © 2022 Dong, Huang, Liu, Wang, Zhang, Xie and Zhang. This is an open-access article distributed under the terms of the Creative Commons Attribution License (CC BY). The use, distribution or reproduction in other forums is permitted, provided the original author(s) and the copyright owner(s) are credited and that the original publication in this journal is cited, in accordance with accepted academic practice. No use, distribution or reproduction is permitted which does not comply with these terms.
*Correspondence: Rongqing Zhang, cnF6aGFuZ0BtYWlsLnRzaW5naHVhLmVkdS5jbg==
†These authors have contributed equally to this work
Disclaimer: All claims expressed in this article are solely those of the authors and do not necessarily represent those of their affiliated organizations, or those of the publisher, the editors and the reviewers. Any product that may be evaluated in this article or claim that may be made by its manufacturer is not guaranteed or endorsed by the publisher.
Research integrity at Frontiers
Learn more about the work of our research integrity team to safeguard the quality of each article we publish.