- 1Marine Research Institute, Klaipėda University, Klaipeda, Lithuania
- 2Estonian Marine Institute, University of Tartu, Tallinn, Estonia
Submerged macrophytes are essential elements of sublittoral ecosystems to maintain a diverse fish fauna. The aim of this study was to assess juvenile fish associated with macrophyte habitats in the Curonian Lagoon by means of modified pop net method. We focused on two dominant littoral habitats with different structure (pondweed and charophyte stands). The pop nets sampling was carried out on two study sites located in the freshwater and oligohaline part of the lagoon in July and September 2019-2020. Our upgraded pop nets reduced the risk of under sampling and are recommended for monitoring fishes since this method has relatively low disturbance on fish behavior and on the habitat itself. The most efficient time of sampling with pop nets in shallow macrophyte habitats is at night, where at least 4 samples collected are sufficient to represent the biggest part (> 70%) of the fish assemblage, but the sampling covering different times of day is recommended. In total, 14 juvenile species were recorded, where perch and European roach dominated (respectively 48% and 24%). Half of the species (8) were associated with macrophyte stands, although water salinity and temperature were the most important factors explaining the variance of fish abundance followed by the sampling time and the biomass of macrophytes. Based on the abundance and the duration of species within these habitats, European roach, nine-spined and three-spined stickleback were mainly associated with the pondweed biomass and stands, while spined loach, round goby, perch, ruffe and gudgeon were mainly associated with the charophyte biomass and stands. The increase of charophyte stands since 2005 affected the fish assemblage where it should have enhanced the abundance of perch, ruffe, spined loach and partly round goby.
Introduction
Submerged vegetation is one of the essential elements of aquatic ecosystems to maintain a diverse fish fauna (Beck et al., 2001; Kraufvelin et al., 2018). Macrophytes are used directly as food, as a spawning substrate, as material for construction of nests, refuge from predators and as nurseries for larval and juvenile life stages (Persson and Eklov, 1995; Boström et al., 2006; Whitfield, 2017). In that sense, the patchiness of macrophyte stands may increase heterogeneity of habitat and structural complexity supporting fish abundance and diversity (Gray et al., 1998; Weaver et al., 2011). Connection of littoral vegetated habitats with pelagic feeding areas, determines the patterns of diel feeding migrations, feeding efficiency and survival of juveniles (Eklöv and VanKooten, 2001; Lv et al., 2016). Co-dominance of different macrophyte species creates increase in multifunctionality of the coastal communities, promotes recruitment of large predatory fish, grazer biomass, inverted ‘nuisance’ algal biomass, and increase in water clarity (Austin et al., 2021). The investigation methods of associated fauna are diverse and frequently non-systematic, providing scale and time dependent results.
Relations among the coverage and species composition of macrophytes and the temporal and spatial density and occurrence of fish have been the focus of many researchers (Beck et al., 2001; Boström et al., 2006; Whitfield, 2017; Kraufvelin et al., 2018). However, it’s not always clear how temporal changes in macrophyte biomass and canopy development influence shelter and food availability for juvenile fishes in these habitats; whether different fish species have specific preferences for plants as a shelter and if diel dynamic patterns in particular fish species abundance are associated with submerged macrophyte beds (Kapusta and Bogacka, 2006). If the fish-plant association is well expressed, the long-term changes in macrophyte communities, as disappearance of species or changed species composition induced by anthropogenic or natural factors, are important in terms of juvenile fish nursery and rearing habitat quality and availability (Beck et al., 2001). Therefore, the understanding of fish response to a change in habitat is necessary to provide stronger insight into how fish populations and ecosystem structure may be impacted and what conservation and management measures are most appropriate (Kraufvelin et al., 2018).
Curonian Lagoon is one of the most productive water bodies in the Baltic Sea, important for resident freshwater, semi-migratory and migratory fish populations and local fishery (Gasiūnaitė et al., 2008). In this transitional and hydrodynamically active water body, littoral vegetation is basically the only habitat structure, suitable and crucially important for juveniles as the main shelter from predators (fish and birds) and currents. Several last decades, the lagoon has been experiencing an increased exposure to brackish waters, nutrient loads and occurrence of the cyanobacteria blooms (Gasiūnaitė et al., 2008; Vaičiūtė et al., 2021). On the other hand, there has been a significant spread of charophyte habitats in the estuarine part of the lagoon during the recent decade (especially Chara contraria) changing the submerged vegetation community dominated by pondweeds in the past (Sinkevičienė et al., 2017; Stragauskaitė et al., 2021).
Therefore, one the objective of this study was to assess the fish community and spatial-temporal changes in the upper littoral part of the Curonian Lagoon. We focused on pondweed and charophyte stands which are dominant and represent habitats with different structure: pondweed stands form macro 3D structure from the bottom till surface while charophyte stands form complex 3D structure only near the bottom up to 0.4 m (Bučas et al., 2016). We expected that different fish communities could be supported by these habitats, therefore, the importance of macrophyte stands for fish species was determined by three approaches: (1) the fish occurrence, diel movement patterns in fish abundance (duration) within each macrophyte stands, (2) the correspondence of fish abundance with the biomass of charophytes and pondweeds taking into account other important environmental factors (salinity, temperature etc.), and (3) the long-term changes of fish occurrence associated with increase of charophyte stands over the last decade in the littoral zones.
We hypothesised that fish species associated with charophyte stands, which expanded during the last decade, should significantly differ from the fish assemblage recorded in 2005, when mainly pondweeds were dominant. This information is essential for understanding how habitat-animal relationships impact the ecology of an ecosystem undergoing broad-scale habitat change and may be useful to managers in a decision support process.
Methods
Study Area
The sampling was performed in the Curonian Lagoon, which is coastal water body connected to the South-Eastern Baltic Sea via a narrow channel through the Port of Klaipeda (Gasiūnaitė et al., 2008). The lagoon area covers approximately 1600 km2, with the mean depth of 3.8 m and it can be divided into two sub-basins (Ferrarin et al., 2010): estuarine and lacustrine. The estuarine basin is located in the northern part of the lagoon (from the Nemunas River Delta to the entry to the Baltic Sea) and is classified as transitional waters according to the Water Framework Directive, which are influenced by both the freshwater flow and the lagoon–sea exchange (where salinity fluctuates between 0.1 and 7). Due to river discharge, this part of the lagoon is influenced by a large amount of nutrient inflow (Zilius et al., 2018), which results in intensive eutrophication processes including cyanobacteria blooms, especially in the central part of the lagoon (Vaičiūtė et al., 2021). The lacustrine basin is mainly located in the Russian part of the lagoon, where hydrodynamics is mostly influenced by the wind.
Fish sampling was performed in 2019 and 2020 on two study sites on the eastern shore of the estuarine basin. The Northern site represents oligohaline water conditions, while the Southern one is more influenced by riverine waters (Figure 1). The littoral slope is gentle and very shallow (1–2 m), mainly composed of fine sand, which is suitable for extensive stands of macrophytes dominated by Potamogeton perfoliatus, P. rutilus, Stuckenia pectinata, Chara contraria, C. aspera (Bučas et al., 2019). One of the sites was located near the entry to the Baltic Sea (henceforth referred to as the Northern site), where salinity fluctuates from 0 to 7 and the annual mean is 2.5 (Umgiesser et al., 2016). The other site was selected closer to the Nemunas Delta (henceforth referred to as the Southern site), where the mean annual water salinity is ca. 0.5.
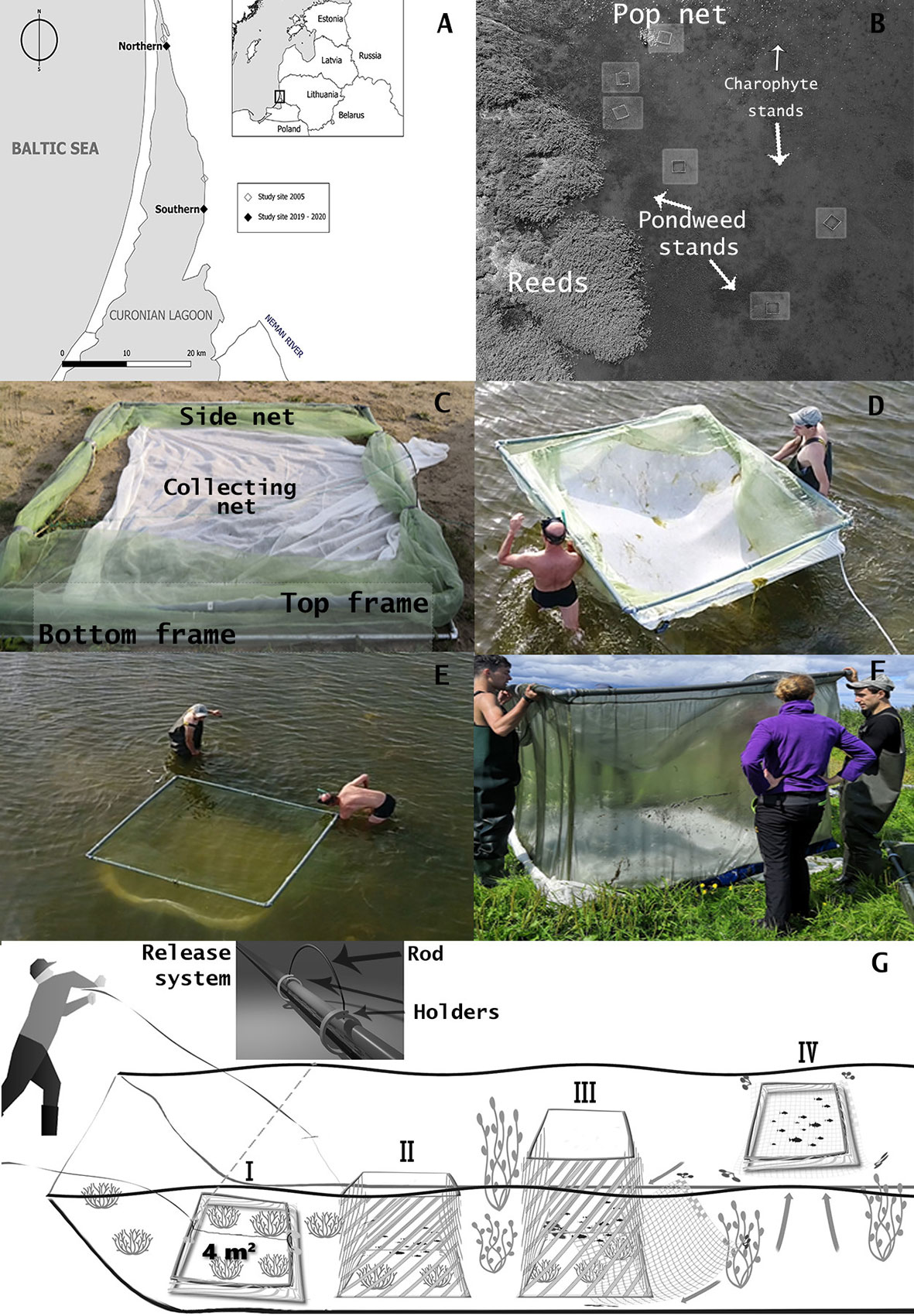
Figure 1 Study area and the two study sites (indicated by rhombus) in the estuarine part of the Curonian Lagoon (A) the distribution of pop nets in the littoral zone of the Southern site from a drone image in July, 2019 (B) the procedure with and schematic representation of procedure (G) with a pop net (C) (I) a 2x2m pop net is laid on the bottom in water (D) with trigger mechanism ready and left for at least 1 hour for fish to recolonise an area, (II) top buoyant frame is de-attaching from a steel bottom frame after remote (from a shore) release of pin-key (rods) attached to a rope, (III) after a pop net release, a separate collecting net is pulled under a steel frame to close an open bottom part of a pop net (E) by minimizing the risk of fish escape, (IV) a steel frame together with bottom net is lifted to the surface of water and carried to the shore for fish measurements (F).
There are 57 fish species found in the lagoon (Repečka, 2003), but the most common species are roach (Rutilus rutilus), perch (Perca fluviatilis) and common bream (Abramis brama) (Repečka et al., 2012). Migratory fish includes Atlantic salmon (Salmo salar), sea trout (Salmo trutta), smelt (Osmerus operlanus), twaite shad (Alosa fallax), European whitefish (Coregonus lavaretus), vimba (Vimba vimba), European eel (Anguilla anguilla) and river lamprey (Lampetra fluviatilis) (Gasiūnaitė et al., 2008).
Upgrade of Pop Net and Fish Sampling
The pop nets were made according to Dewey et al. (1989), but with three main modifications: (1) the shape of a pop net frame was square (2 x 2 m) in order to normalize side net effect; (2) the mechanical release system was attached to the bottom frame and modified to allow the release of a pop net from longer (up to 300 m) distance; (3) an open bottom of a pop net was closed with a separate net covering almost a whole frame of a pop net in order to reduce the risk of gaps between a bottom frame and a bottom net and the underestimation of fish.
In this study, a pop net consisted of top and bottom frames, which were respectively made of 20.0 mm diameter galvanized steel pipes and 40.0 mm diameter PVC pipes. The side walls of a pop net were composed of a fine mesh netting (1.0 mm diameter), which bottom edge was sewn around the perimeter of a bottom frame, whereas the top edge of the mesh was sewn around the perimeter of a top frame. The top and bottom of a pop net were open (no netting). Two parallel pipes of a top frame were sealed at the ends for buoyancy, which elevated the frame to a maximum height of 1.2 m. Before placing a net, top and bottom frames were pressed together and fixed by two release systems at opposite sides of a net (Figure 1C–I). A release system consisted of two split ring holders (the top and bottom frames) fixed to a metal plate in 0.7-0.8 m distance from each other. Holders were made from PVC pipe of 110 mm diameter, which was cut into rings of 40 mm wide and they were split on the upper part of a ring by transversely cut, where two holes of 15 mm were drilled on both sides of a holder in order to place a locking rod through the overlapped holes (which were adjusted by pushing ring sides of holders). Release systems were placed below a bottom frame, while a top frame with side nets were pressed between holders, which were locked by two plastic rods of 1.0 m length and 7.0 mm diameter. Both rods were connected by a green rope of 2 m length and 2.0 mm diameter, and the same rope of 300 m length was attached to one of the rods in order remotely (from the shore or reed belt) to pull out synchronously both rods from holders and release a pop net. After a pop net was set it was placed among macrophyte stands and left from 1 to 7 h (usually < 2 h) to deposit disturbed sediments and to return fish. After the recolonization period, a rope with rods was pulled out to release a buoyant top frame (Figure 1C–II). The top of a net surfaced within 2 seconds after release in 1 m deep. A separate semi-solid-framed collecting net (3 x 4 m) of the same mesh size was used to close the bottom of a pop net by pulling a collecting net under a bottom frame (Figure 1C–III). Captured fishes were retrieved by raising both a collecting net and a pop net to a surface and brought to a shore (Figure 1C–IV). We tested the effect of different recolonization times (i.e. time between set up and release of pop nets) on fish communities. The results did not show any significant differences in fish communities, supporting that release of pop nets can be performed at least after 1 h or even sooner. This agreed with the results of similar analyses in lakes (Slade et al., 2005), where the variability in soak time did not significantly influence fish abundance or fish species richness.
To account for seasonal (i.e. intensive and post vegetation period) and temporal variation, samples were collected twice a year (in July and September), and several times per day (approximately every 6 hours): morning (from sunrise till noon), afternoon (from 12:01 pm till 18:00), evening (from 18:01 till sunset) and night (from sunset till sunrise). The pop nets were placed within two common macrophyte stands in the upper littoral zone (0.5-1 m depth): (1) pondweeds (P. rutilus and Stuckenia pectinata on the Northern study site, Potamogeton perfoliatus and S. pectinata on the Southern study site) and (2) charophytes (Chara contraria and C. aspera). Due to the first trials of sampling by pop nets in 2019, the number of replicates considering all spatio-temporal aspects (study site, habitat, month and time) was in a random manner and resulted in 31 total number of samples (Table 1). A more systematic approach of replicates was used in 2020 and in total 72 samples were collected.
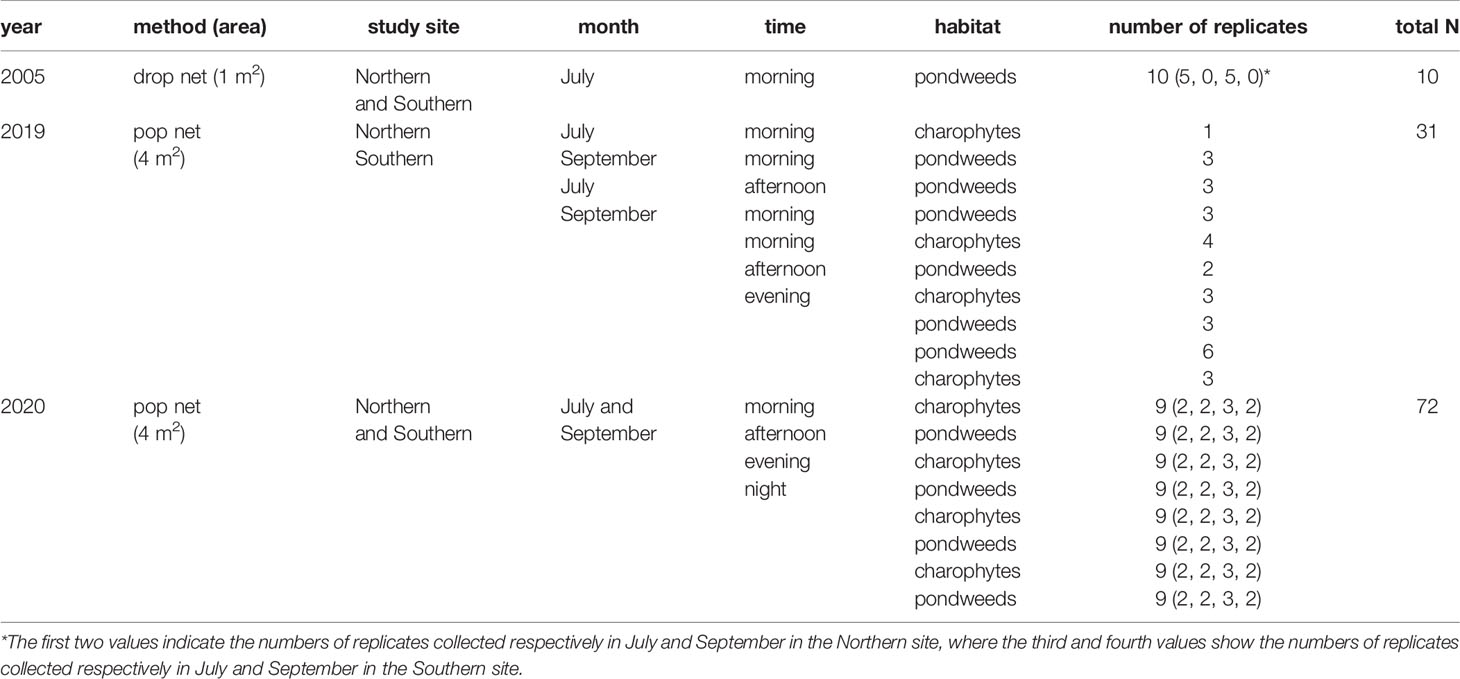
Table 1 The sampling design according to the spatio-temporal aspects such as study site, month, time and habitat (i.e. stands of pondweeds or charophytes), number of replicates and total number of samples (total N) using the drop and pop nets in the Curonian Lagoon in 2005, 2019 and 2020.
During the netting, water temperature and salinity were measured approximately every 6 hours by multimeter (YSI Pro1030 or FE30-Basic FiveEasy). Water turbidity was measured similarly but mainly in 2020 using a handheld spectrofluorometer (AlgaeTorch 10, bbe Moldaenke GmbH). The above ground biomass of macrophytes was collected at the end of the sampling using a hand-net (the area of opening 700 cm2) which was dredged near the bottom for 1 m distance in the nets or in their vicinity. Macrophytes were classified into three groups (charophytes, pondweeds and epiphytes), which were oven-dried at 60°C to constant weight for dry mass determination.
Fish caught during the work were assigned to juveniles or adults by measuring their total length in relation to the theoretical length at first maturation (Virbickas, 2000; Froese and Pauly, 2022). Most juvenile fish or small fish species were identified and measured at a sampling site and released, others were preserved in a 4% formaldehyde solution and later identified according to Virbickas (2000) under stereo microscope in a laboratory. The total length of individuals was measured to the nearest millimetre.
The sampling activities were stopped when the wind speed increased more than 10 m s-1 since generated waves and strong currents scattered about the side nets and lifted the buoyant frame before its release or after its release the upper frame was pushed under the surface increasing the risk of fish loss. Sometimes after the release of pop nets, one corner or side of a net has not fully surfaced due to entanglement of a net between its frames or dense vegetation. The fish communities between properly and improperly collected samples did not significantly differed (most likely due to the prevalence of fishes near the bottom or within the stands of macrophytes), therefore we used all samples in the analysis.
Historical Data by Drop Net Sampling
Unpublished historical data of fish abundance in the littoral of the Curonian Lagoon was based on fish surveys performed on two study sites (Figure 1) during morning (from 9:00 am to 12:00 am) in July, 2005. Fish were caught using a square enclosure net (1 mm mesh size), which was fastened to a 4 m pole and carried by two people. The net dropped down with sudden movement enclosing a vegetated area of 1 m2 in the upper littoral part (max sampling depth 0.9 m). The hand net (1 mm mesh size) was used to collect fish fry 0+ and juvenile fish from the enclosed area.
In each survey, 5 replicates of drop net samples were taken. All collected fish were fixed in 4% formaldehyde solution for further analyses in the laboratory. For the comparison of the fish community between 2005 and 2019–2020, the species occurrence data were used while quantitative estimates may significantly differ due to the size of nets and sampling procedure. On the Southern site in 2005, typical vegetation consisted of charophytes and P. perfoliatus which coverage ranged to 50%, while denser (80–100%) vegetation of P. perfoliatus, S. pectinata and charophytes covered the littoral on the Northern site. Considering water salinity in both surveys (1.1–1.8 on the Southern site and 5.4–6.7 on the Northern site) and sampling time in 2005, the final selected dataset consisted of 5 samples in each study site in 2005 (Table 1), and respectively 7 and 6 samples on the Northern and Southern sites in 2019–2020.
Data Analysis
In order to test any difference in fish assemblages (based on the species abundance data) between different recolonization times (1, 1.1, 1.5, 2, 4, 5 and 7 h) after the placing of the pop nets and releasing them, the multilevel pairwise comparison test based on permutational multivariate analysis of variance using the Bray-Curtis dissimilarity index (pairwise ADONIS) was performed using the “pairwiseAdonis” package (Martinez, 2017) in R 4.0.4 (Core Team, 2013). If pairwise ADONIS revealed a statistically significant result, the relative contribution of each species to group dissimilarities was quantified using a similarity percentage analysis (simper function), with a cut-off criterion of 90% dissimilarity index, to identify subsets of the most important species (Clarke, 1993). The same method was used to assess the differences in juvenile assemblages between the nets that popped up well and the nets where one corner or side did not properly surface.
For each study site, the differences in the species composition of juvenile assemblages (based on the species occurrence data) between the habitats (stands of charophytes and pondweeds), the sampling season (July and September) and the sampling time (morning, afternoon, evening and night) were tested by the pairwise ADONIS using the Jaccard dissimilarity index. The same approach was applied for the comparison of juvenile assemblages between 2019–2020 and 2005 on both study sites.
For the assessment of an optimal number of pop net samples required to properly represent the fish assemblages, the species abundance data were randomly sampled (with replacement) for different sample sizes (from 2 to 30) using the sample function in R. The species abundance was averaged for each group of sample size and the mean Bray-Curtis similarity was estimated between the mean abundance of all samples and each group of sample size using the “vegan” package (Oksanen, 2020) in R. This was performed for each study site, sampling season and time. For each sample size, 95% confidence intervals were estimated by repeating 99 times the sampling and calculation of the mean Bray-Curtis similarity.
Variation in the diel patterns of common fish abundance were analysed by the generalized additive models with a mixed effects structure (Pedersen et al., 2019) using explanatory variables such as the sampling time and the factor of interaction between the sampling site, season and habitat (SMH), and the interaction between the sampling time and SMH. The negative binomial error distribution model was selected for the species abundance. The penalized cyclic cubic smoothing term was selected for the sampling time with 4–5 degrees of freedom. Generalized additive models were performed with the “mgcv” package (Wood, 2017) in R. Additionally, the differences in the mean abundance of species were compared between months, sites or habitats using the Brunner–Munzel test in the “brunnermunzel” package (Ara, 2020).
Empirical relationships among fish assemblage (based on the abundance data) and environmental factors (water temperature, salinity, tubidity, sampling time, biomass of charophytes, pondweed and epiphytes) were assessed by the direct gradient analysis (Zuur et al., 2007). According to Lepš and Šmilauer (2003), we inspected how the fish abundances change along an ordination axis of detrended correspondence analysis (DCA). The length of the first DCA axis was 3.6 standard deviations; therefore, we carried out canonical correspondence analysis (CCA). The Spearman rank correlation and the variance inflation factors (VIF) in CCA between explanatory variables (environmental factors) were assessed, where the highest and statistically significant correlations were determined between water salinity and turbidity (rS = 0.52, p < 0.01; VIF > 3) and the biomass of epiphytes and pondweeds (rS = 0.42, p < 0.01; VIF > 7). For the assessment of which of two correlated variables had statistical significance on the fish assemblage, two CCA models were tested, where each variable was respectively partialed out. In the CCA model, statistical significance of canonical axes and marginal statistical significance of explanatory variables (environmental factors) were evaluated using permutation tests (n = 999). The explained variance with all explanatory variables was estimated and it was subtracted with the variance obtained after conditioning each variable in order to assess the explained variance of each explanatory variable. The CCA results were displayed in a three-dimensional ordination biplot using the “vegan3d” package (Oksanen et al., 2017) in R. Based on the coordinates of response and explanatory variables for the three CCA axes, the cosine similarities (CS) were estimated between the vectors in order to quantify the correspondence between each species and environmental factors. All the multivariate analysis was performed using the “vegan” package (Oksanen, 2020) in R.
Results
Species Composition of Fish Assemblages
A total of 1279 individuals of 14 fish species were caught in the 2019–2020 period (Figure 2). Roach (Rutilus rutilus), perch (Perca fluviatilis), rudd (Scardinius erythrophthalmus) pike-perch (Sander lucioperca), round goby (Neogobius melanostomus), bleak (Alburnus alburnus), Prussian carp (Carassius gibelio), asp (Aspius aspius) and gudgeon (Gobio gobio) consisted exclusively of juveniles (predominantly 0+ age group-99%). For other fish species, mature fish were also present in the samples. Three-spined stickleback (Gasterosteus aculeatus), nine-spined stickleback (Pungitius pungitius), spined loach (Cobitis taenia) and ruffe (Gymnocephalus cernuus), the juveniles accounted for 99% of the caught three-spined stickleback (1.3-4 cm length group), 58% of the nine-spined stickleback (0.9-3 cm length group), 19% of the spined loach (1-6 cm length group) and 68% of the ruffe (2.4-5 cm length group).
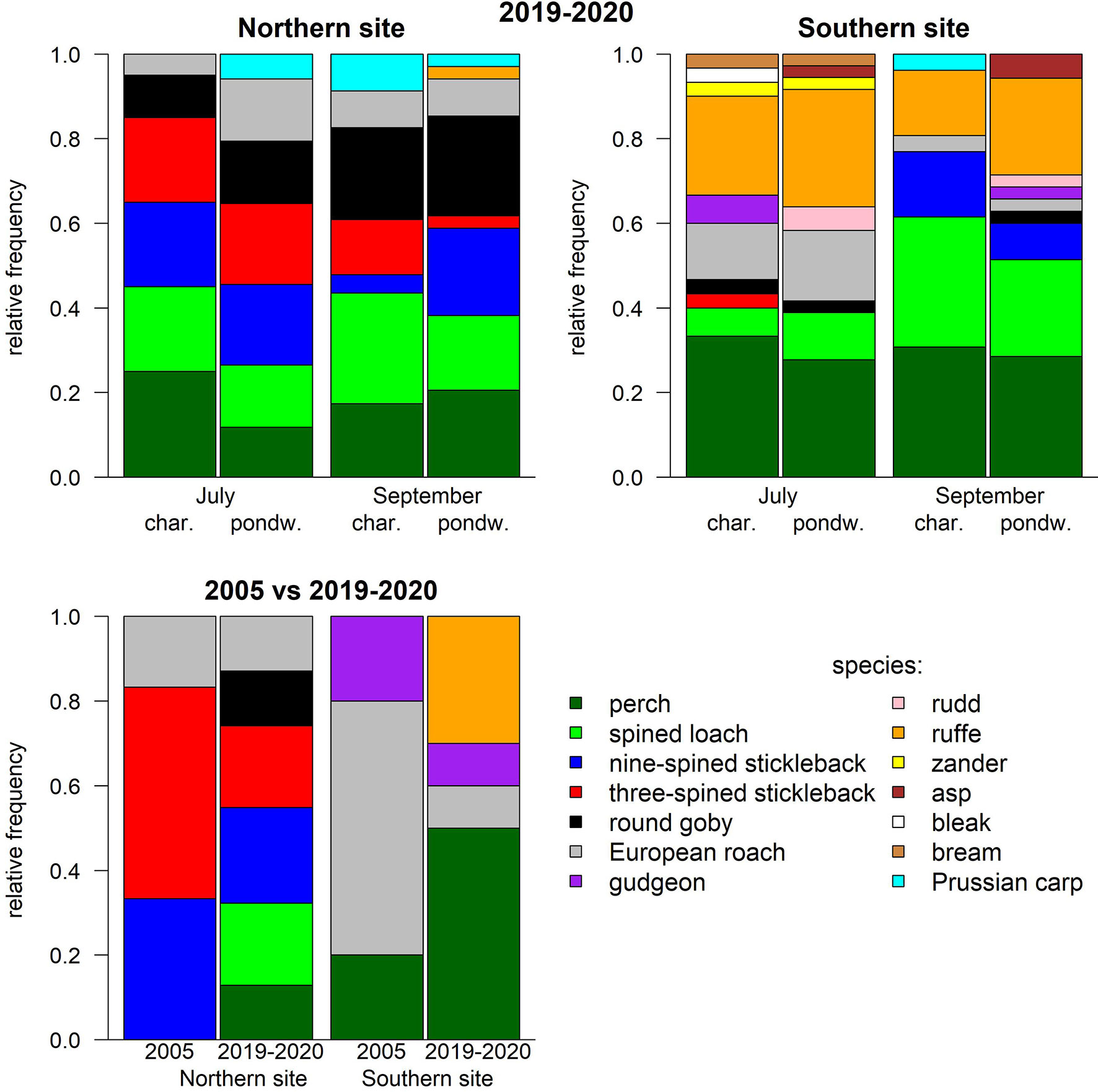
Figure 2 Fish community composition (relative species frequency) on the Northern and Southern study sites in 2019–2020 (upper) within different months (July and September) and habitats: charophyte stands (char.) and pondweed stands (pondw.). Comparison of juvenile community composition during the morning in July between 2005 and 2019–2020 on both study sites. In these years, the salinity ranged between 1.1 and 1.8 on the Southern site, while its range of 5.4–6.6 was on the Northern site.
in situ The species composition statistically significantly differed between the study sites and the sampling season (ADONIS test, p = 0.01), therefore the habitat effect was analysed separately for each site and month. Most species rich fish assemblage (12) was recorded within both pondweed and charophyte stands on the Southern site (Figure 2). In the pondweed stands, 300 individuals were collected, where four species were dominant: perch (40%), ruffe (24%), European roach (18%) and spined loach (12%). In the charophyte stands, 211 individuals were collected with similar proportions of the dominant species (pairwise ADONIS test, p = 0.68): perch (38%), ruffe (35%), European roach (12%) and spined loach (9%). The species composition significantly differed between the years (pairwise ADONIS test, p < 0.01) and did not significantly differ between the sampling season (pairwise ADONIS test, p = 0.06) and the time (pairwise ADONIS test, p > 0.13). Perch, European roach and spined loach occurred more frequently in 2019 than in 2020, while ruffe was found only in 2020.
On the Northern site, 768 individuals were collected, comprising 8 species (Figure 2). Within the pondweed stands, 655 individuals were collected, where three species were dominant: three-spined stickleback (38%), roach (30%) and nine-spined stickleback (15%). In the charophyte stands, 113 individuals (comprising 7 species) were captured, dominated by spined loach (30%), three-spined stickleback (20%), perch (20%) and round goby (17%). There were no significant differences in the species composition between the years (pairwise ADONIS test, p = 0.44), the sampling season (pairwise ADONIS test, p > 0.47) and habitats (pairwise ADONIS test, p = 0.17). Fish assemblage significantly differed between the habitats in the summer (pairwise ADONIS test, p < 0.05). European roach and round goby were more frequent in the pondweed stands, while perch and spined loach occurred more within the charophyte stands.
The juvenile assemblage (occurrence) significantly (pairwise ADONIS, p < 0.01) differed between 2005 and 2019–2020 on both the Northern and the Southern sites (Figure 2). On the Northern site, European roach and nine-spined stickleback were more frequently found in 2019–2020 (respectively 57% and 100%) than in 2005 (respectively 33% and 67%), while spined loach, perch and round goby were recorded only in 2019–2020 (respectively 86%, 57% and 57%). On the Southern site, the occurrence of European roach was more than 5 times higher in 2005 (100%) than in 2019–2020 (17%), while perch was more frequently found in 2019–2020 (83%) than in 2005 (33%) and ruffe was only recorded in 2019–2020 (50%).
The optimal number of samples required for the maximum number of species depended on the sampling time, month and study site (Figure 3). In general, the mean Bray-Curtis similarity between randomly sampled and in situ measured fish community was higher (over 80%) on the Southern site than on the Northern site. On the Northern site in July, at least 3 samples in the morning were needed to reach over 70% of the mean Bray-Curtis similarity, whereas 8 samples were necessary in the evening or night. In September, the samples collected in the morning never reached more than 70% of the mean Bray-Curtis similarity as the samples collected in the afternoon in July and September. On the Southern site in July, at least 2 samples in the evening and night and 3 samples in the afternoon were sufficient to reach over 70% of the mean Bray-Curtis similarity, whereas 4 samples in the afternoon, 3 samples in the evening and 2 samples in the night were needed in September. The samples collected in the morning never reached more than 50 and 60% of the mean Bray-Curtis similarity respectively in July and September.
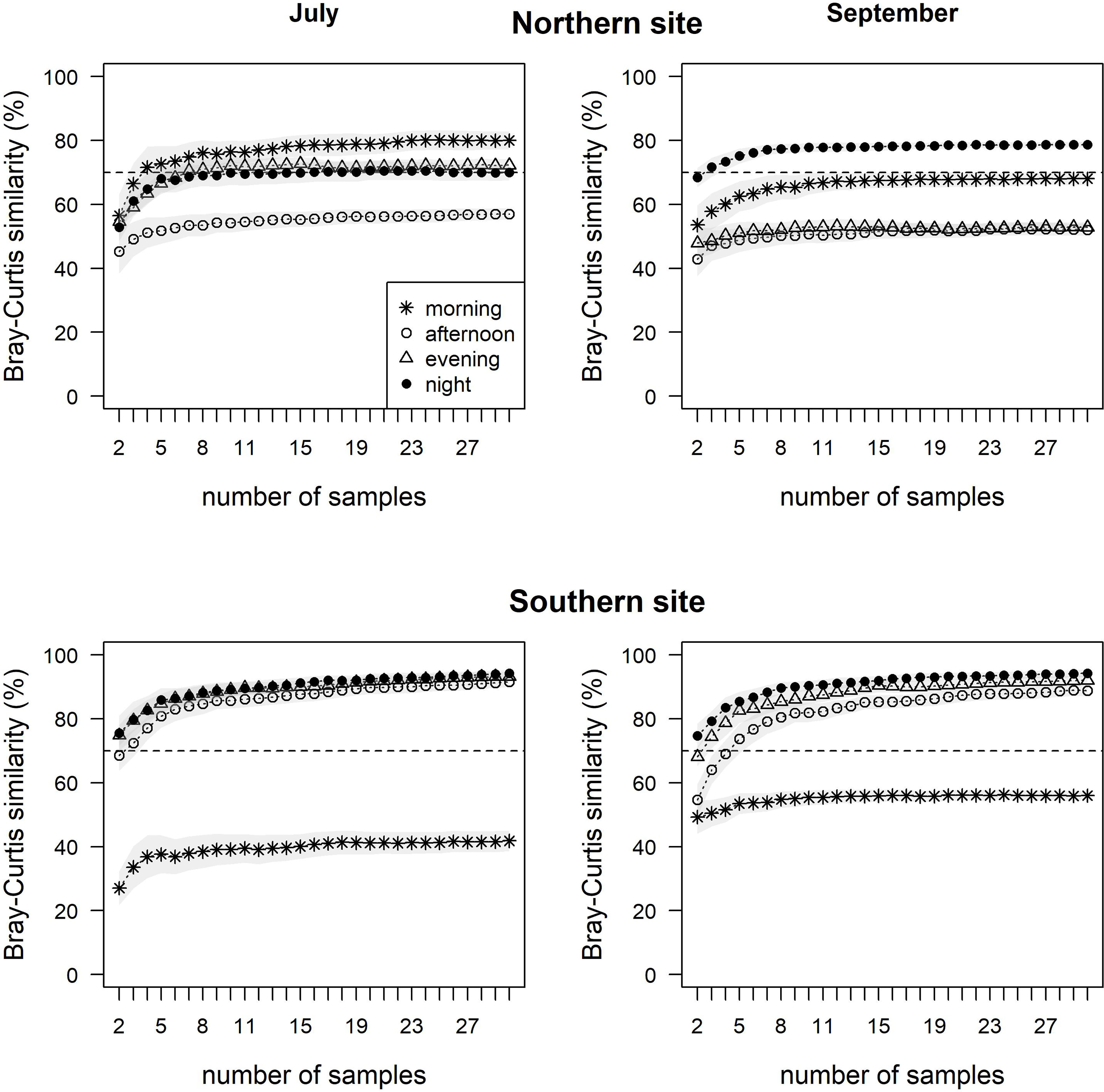
Figure 3 Optimal number of samples required for the fish community assessment by the pop nets at a different sampling time in July and September on the Northern and Southern study sites. Relationships between the Bray-Curtis similarity (between mean abundance of species from all samples and randomly selected from 2 to 30 samples) and the number of samples show how a randomly sampled community is similar to in situ measured fish community. The grey areas indicate 95% confidence intervals.
Diel Changes in Common Fish Habitat Use
The diel dynamics in the mean abundance of common juvenile fishes depended on the study site, sampling season and habitat (Figure 4). For example, three-spined stickleback and European roach were constantly recorded on the Northern site in July, where the first species was found in both habitats and the second only within the pondweed stands. In pondweed stands, the mean abundance of three-spined stickleback was x-fold higher in the night and morning (5–7 a.m., respectively, > 35 and > 55 indiv. m-2) than in the afternoon and evening, and was high in the late evening (> 40 indiv. m-2) again. The time effect was statistically significant (Chi2 = 11.85, p < 0.01) only for European roach. This species was found in low mean abundance (< 15 indiv. m-2) on the Southern site in July only in the late evening, whereas in September, it was recorded only in the night and morning. In September, both species were in low abundance (> 5 indiv. m-2) on the Northern site. The mean abundance of perch did not exceed 20 indiv. im-2 and during the day the species was constantly found within both habitats in all months and sites. In the Southern site, the mean abundance of perch significantly differed in the pondweed stands (Chi2 = 25.72, p < 0.01) and charophyte stands (Chi2 = 16.12, p < 0.01) in July. Within the pondweed stands, the mean abundance of perch increased (> 15 indiv. m-2) in the evening and night, while within the charophyte stands, it increased in the night.
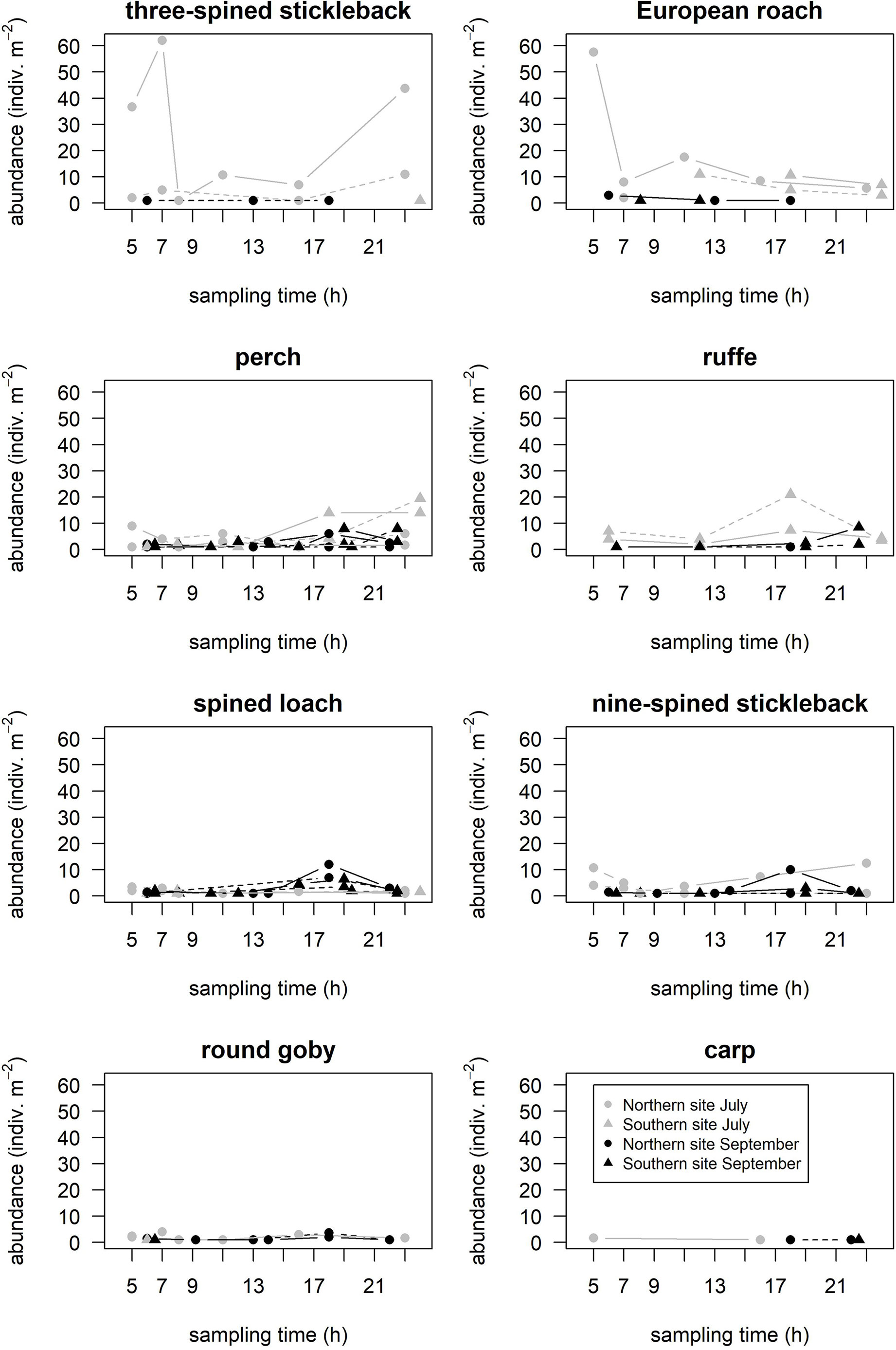
Figure 4 Diel changes in the mean abundance of six common species in July and September (indicated respectively by grey and black colour) on the Southern and Northern study sites (indicated respectively by dot and triangle) within the stands of charophytes and pondweeds (indicated respectively by dashed and solid lines).
The mean abundance of ruffe did not exceed 25 indiv. m-2 and during the day the species was constantly found on the Southern site within both habitats and months. The mean abundance of ruffe significantly increased (Chi2 = 6.51, p = 0.01) in the afternoon. In general, the species mean abundance was almost three times higher in the charophyte stands (21.00 ± 24.04 indiv. m-2) than in the pondweed stands (7.33 ± 2.89 indiv. m-2); however, it was not a significant difference (t = -0.20, df = 1.08, p = 0.87).
The mean abundance of spined loach did not exceed 15 indiv. m-2 and during the day the species was constantly found in all months and sites within the pondweed stands. The mean abundance of spined loach significantly increased (Chi2 = 2.13, p < 0.01) in the afternoon on both study sites in September. The mean species abundance was higher in the pondweed stands (8.33 ± 5.51 indiv. m-2) than in the charophyte stands (5.00 ± 4.15 indiv. m-2); however, the difference was not significant (t = 1.13, df = 2.48, p = 0.36).
The similar pattern was observed for nine-spined stickleback (Chi2 = 5.49, p < 0.03) on the Northern site, while the high mean abundance (> 10 indiv. m-2) was from the late evening till early morning in July. Nine-spined stickleback was absent within both habitats on the Southern site in July and only once in charophyte stands on the Northern site in September.
The mean abundance of round goby did not exceed 10 indiv. m-2 and during the day the species was constantly found only within the pondweed stands in both months on the Northern site. Round goby could be caught only occasionally in the morning, only once at each study site. However, its abundance increased in the evening on the charophyte habitat in the Northern site.
Prussian carp were occasionally found during the day with a relatively low mean abundance (< 5 indiv. m-2). On the Southern site, the species was recorded only once in the late evening in September within the charophyte stands. On the Northern site, the species was found only in the pondweed stands at night and in the afternoon in July, while in September, Prussian carp occurred only in the evening in both habitats.
Fish Community Relationships With Environmental Factors
The CCA models with partialled out correlated variables showed that the water turbidity and the biomass of epiphytes were not statistically significant factors (respectively F = 1.73, p = 0.10 and F = 1.02, p = 0.37). These variables were removed from the final CCA model. The first two CCA axes were statistically significant (p = 0.001), whereas the third one was marginal (p = 0.096). All explanatory variables with three axes explained 35% of the variance in the abundance of juveniles in 2019–2020 (Figure 5). Water salinity was the most statistically significant explanatory variable (F = 20.24, p < 0.01; explained 22% of the variance after conditioning the variable), followed by water temperature (F = 9.29, p < 0.01; explained 6% of the variance), sampling time (F = 4.30, p < 0.01; explained 4% of the variance), the biomass of pondweeds (F = 3.31, p < 0.01; explained 0.4% of the variance) and charophytes (F = 2.43, p=0.02; explained 5% of the variance). The high abundance of ruffe, gudgeon and perch strongly corresponded to freshwater conditions (CS > -0.75), whereas the high abundance of three-spined and nine-spined sticklebacks strongly corresponded to brackish water conditions (CS > 0.71).
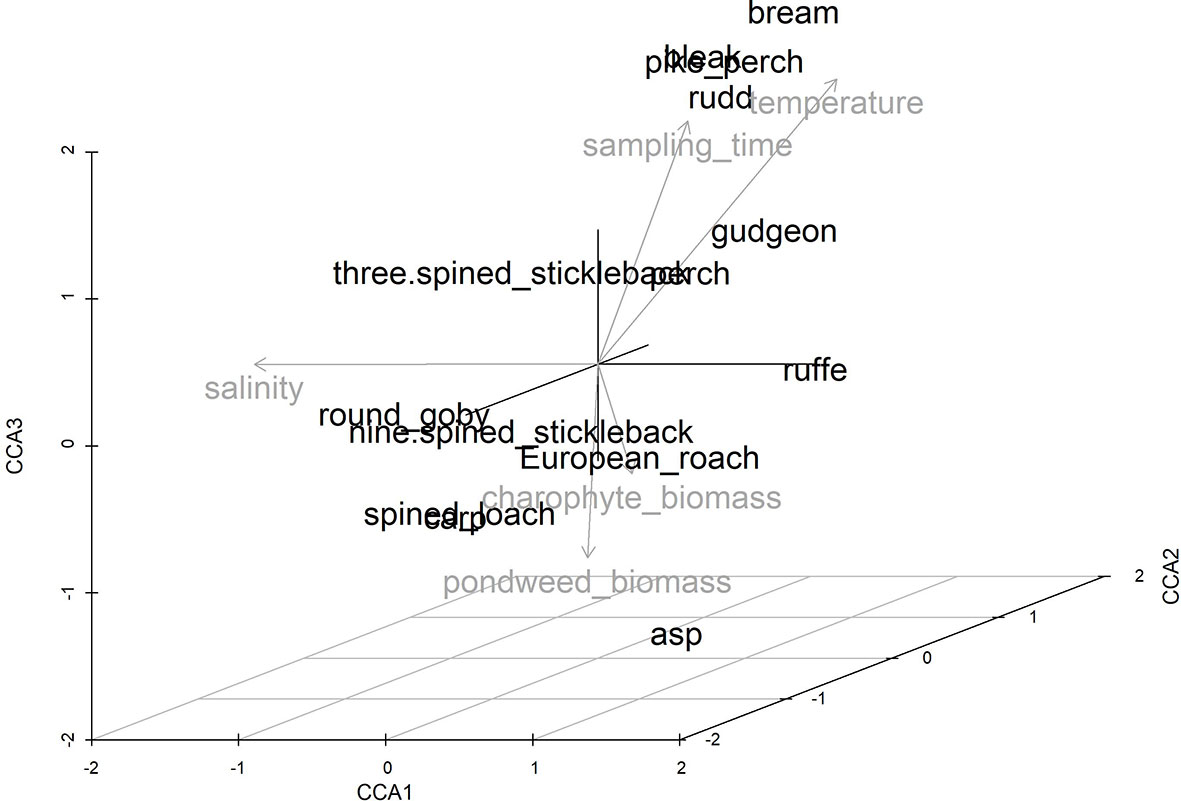
Figure 5 Three-dimensional CCA biplot of juvenile community based on the abundance data in 2019–2020 and relationships with explanatory variables (indicated by vectors and in grey).
The high abundance of rudd, pike-perch (Sander lucioperca), asp, bleak and bream moderately corresponded to freshwaters (CS > -0.45). The high abundance of Prussian carp, round goby and spined loach strongly corresponded to low water temperature (CS > -0.79), while a moderate correspondence was for high abundance of asp (Leuciscus aspius) and nine-spined stickleback (CS > -0.58). The high abundance of bream, gudgeon, rudd, three-spined stickleback, pike-perch, perch, ruffe and bleak weakly or moderately corresponded to high water temperature (CS > 0.34). The high abundance of bleak, pike-perch, rudd, bream, perch and gudgeon strongly corresponded to late sampling time (CS > 0.87), whereas it was the opposite for nine-spined stickleback and European roach (CS > -0.88), and a low correspondence was for high abundance of Prussian carp and asp (CS > -0.39). The high abundance of Europine roach, asp and nine-spined stickleback strongly corresponded to the high biomass of pondweeds (CS > 0.73), whereas it was the opposite for bream, rudd, pike-perch, bleak and perch (CS > -0.70). A moderate and low correspondence to the high biomass of pondweeds were for the high abundance of Prussian carp (CS = 0.63) and spined loach (CS = 0.36), while a moderate correspondence to the low biomass of pondweeds was for the high abundance of gudgeon and three-spined stickleback (CS > -0.57). The high abundance of spined loach and asp strongly corresponded to the high biomass of charophytes (CS ≥ 0.8), whereas it was the opposite for three-spined stickleback (CS > -0.82). A moderate correspondence to the high biomass of charophytes was for the high abundance of Prussian carp and round goby (CS > 0.36), while a low correspondence was for perch and ruffe (CS > 0.28).
Discussion
Sampling Aspects of Juvenile Fish Assemblages in Vegetated Habitats
To our knowledge this study is the first to use the pop net approach for the assessment of juvenile fish assemblages within the macrophyte stands in the Baltic Sea. We tested the performance of pop nets in two study sites (Southern and Northern), seasons (July and September) and different sampling hours (morning, afternoon, evening and night) to fill the knowledge gap about the performance of this method in the coastal waters. Based on the highest mean similarities of the fish assemblage between randomly sampled and all data, the results suggest that the most efficient time of sampling with pop nets in shallow macrophyte habitats is at night. This was in line with the results obtained from the Gulf of Finland (Taal et al., 2017), since most species are active at night and therefore susceptible to passive fishing gears due to low visibility (Henseler et al., 2019 and reference therein). Differently from such gears, a pop net approach should not significantly affect the avoidance of fish as the pop nets were laid within the macrophyte stands making the nets hardly visible or different from the surrounding. Thus, we strongly believe that obtained patterns of fish species with the time of day were not biased. These patterns depended on the sampling site and season, where at least 4 samples (pooled from charophyte and pondweed stands) used in this study were sufficient to represent the biggest part (> 70%) of the fish assemblage at night. The sampling only in the morning or in the afternoon should be avoided respectively on the Southern and the Northern sites due to the decrease of abundance of most common species within vegetated habitats (Figure 4). On the later site in September, the sampling only in the evening and partly in the morning should not be performed too as the species such as perch, three-spined stickleback, European roach and ruffe could be missed. We agree with (Taal et al., 2017) that for highly representative assessment of the fish assemblage, the sampling should cover all different times of day (dawn, noon, dusk, and midnight).
We compared the species composition determined by the pop nets with other techniques used in the Curonian and Vistula lagoons. In July 2019–2020, 14 fish species were recorded in total, while 13 fish species were recorded in catches by beach seines and multi-mesh gillnets close to our study sites in 2012 (Repečka et al., 2012). Similarly, to 2019–2020, perch and roach dominated respectively 48% and 24% in 2012. However, we did not catch rare or less common species such as ide (Leuciscus idus), smelt, flounder (Platichthys flesus trachurus), vimba, pike-perch and belica (Leucaspius delineatus). Ide is relatively rare in the study sites and is more abundant on the western shore of the lagoon (Repečka et al., 2012). Most of the smelt juveniles migrate to the Baltic Sea in the beginning of July (Žiliukienė and Žiliukas, 2000) which could explain the absence of species in the pop nets. Moreover, the location of the previous study site was closer to the Klaipeda channel which is more affected by brackish waters and therefore some brackish water species were recorded there. On the other hand, bream, rudd, ruffe and nine-spined stickleback were not caught in 2012 on the Southern site and rudd, gudgeon, spined loach were absent on the Northern site. These differences could be due to sampling methods since beach seine and multi-mesh gillnets emphasized the open habitat fish assemblage, while pop net method represented real vegetation-associated fish assemblages.
Our approach of closing the bottom of pop-nets with a separate net was time consuming and physical power demanding. It took at least 1 h for at least two persons to cover this net below four pop nets and to transport them to the shore and set them back (up to 0.5 km for one net). We tried to minimise the transportation effort by using an electrofishing removal method onsite to collect fish inside the pop net; however, most likely due to size-dependent inferior performance of electric field on larval and juvenile fish, substantial depth of sampled water column and/or dense vegetation fish could not be removed effectively by this method. This was in accordance with the results obtained by Dewey (1992), where the catches were significantly less with the electrofishing frame of the pop net than without it in vegetated and turbid water of the Lawrence Lake.
Although, our approach of using pop nets was time-consuming, previous studies have shown that density and biomass of small fishes estimated by using this type of nets were significantly higher than those using other methods (Dewey et al., 1989; Dewey, 1992). Species composition and abundance of fish caught in this study suggest that a pop net might be more suitable for demersal small fish with low swimming activity (e.g. spined loach and round goby) than for epipelagic fishes with high swimming activity (e.g. rudd and bleak). Despite this, a pop net compared with other methods is able to sample small fishes in a fixed area with little disturbance to their natural distribution and activity. If performed with caution, this approach does little damage to vegetation structure, which enables repetition of sampling from the same site. Considering all the sampling aspects, we support that a pop netting is irreplaceable in studies of heavily vegetated habitats where seining or electroshocking is difficult (Dewey et al., 1989).
In complex habitats, drop nets are also considered a relevant (Beesley and Gilmour, 2008). However, we strongly believe that pop nets superior drop nets in densely vegetated habitats since the bottom frame of a drop net after release may not evenly seal the bottom due to patches of macrophyte stands. While, a frame of a pop net can be carefully placed on the bottom between macrophyte stands. The limitation of drop nets to some extent could be reduced by increased weights attached on the bottom of nets, but this will simultaneously increase the size of boyant part of a frame, which may consequently affect fishes.
Fish collection from pop or drop nets after their release is the most critical, where mainly two approaches are used. One of them is rather destructive, where macrophytes within a net are usually removed and fish are collected with a small dip net (Connolly, 1994) or seine (Dewey, 1992). The passes of dip net or hauls of seine are made until several consecutive passes or hauls fail to find fish. Although this collection method can be performed without removing vegetation, the risk of underestimation increases as fishes may hide in the dense stands of macrophytes. Another approach is less destructive, since fishes within a frame of pop or drop net are enclosed either by pushing bottom nets from the sides of lower frame over vegetation stands (Dewey et al., 1989). Despite the advantages of the second approach, especially regarding the destruction of habitat, some gaps can be formed while lifting a pop net along the sides of the bottom frame without attached bottom nets resulting in loss of some fish. In our study this limitation was reduced by covering a whole bottom frame with a separate bottom net. This allowed the more reliably assessment of a whole-community dynamics and relationship with environmental factors, for instance how different fish species in their juvenile stages choose habitats.
Importance of Environmental Factors for Spatial-Temporal Variation in Juvenile Communities
Water salinity
The results of this study (CCA) showed that brackish water conditions and seasonality (water temperature or sampling month) are primary factors determining the fish community composition and abundance in the littoral of the Curonian Lagoon. The southern littoral area, less affected by brackish water intrusions, hosted a higher number of species (13) than on the Northern site (8). In the latter site, brackish water species, such as three-spined stickleback and round goby were more abundant than on the Southern site. The invasive round goby has spread within the lagoon since 2007 occurring at higher abundances in the north compared to the central inner part of the water body as the main habitat and population is in the coastal Baltic Sea (Rakauskas et al., 2013). Similar is with the sticklebacks, which are well adapted to brackish conditions and abundant in the Baltic Sea coastal waters, thus the local population could persist and potentially be supplemented with the individuals migrating from the sea to the transitional northern part of the lagoon.
Last decade in Lithuania it has been speculated that increasing inflow of brackish water into the Curonian Lagoon may significantly affect fish stocks and reduce fisheries productivity of the northern part of the lagoon. However, the negative effects of brackish salinity on freshwater fish species are highly questionable (Parry, 1966; Varsamos et al., 2005; Dainys et al., 2019). Cyprinids, percids and most other fishes of the lagoon, can easily bear low Baltic Sea salinity up to 7 (Parry, 1966; Jäger et al., 1981; Bøeuf and Payan, 2001; Varsamos et al., 2005; Dainys et al., 2019), and normally it should not cause any significant physiological effect and avoidance behaviour. Experimental study with Curonian Lagoon perch showed that young-of-the-year fish prefer brackish conditions when given a choice between 6 psu and fresh water (Dainys et al., 2019). From the other hand, typical freshwater fish in early ontogenetic stages due to incomplete osmoregulatory capacity may be suppressed, therefore avoid marine conditions, at least in the first months or year (Jäger et al., 1981; Varsamos et al., 2005; Härmä et al., 2008);. Brackish water had a negative effect on roach spawned egg development and early life stages (Härmä et al., 2008; Malakpour Kolbadinezhad et al., 2012), but have little to no effect later for grown individuals. Therefore, frequent and intensifying intrusions of brackish cold water in the summer (Dabuleviciene et al., 2020) may have a potentially negative effect on fish spawning and fry abundance on the Northern sampling site, forcing more sensitive species to retreat from this area to freshwater areas. This could explain the results of 2005 survey data (unpublished data), when roach and perch diminished, while the abundance of sticklebacks remained stable or even increased.
Beside osmotic properties and impact on fish through osmoregulation mechanisms, the effect of intruding brackish water on the northern part of the lagoon could be more complex and indirect. Due to high productivity of the Curonian Lagoon, the water in summer-autumn period is highly eutrophicated, therefore turbid, and warm. Wind or upwelling driven intrusions mostly bring cooler and more transparent sea water to the northern part. Experimental studies with perch (Dainys et al., 2019) showed that young-of-the-year fish avoided cooler water (12 °C versus 18 °C) in both salinity conditions (0 and 6), therefore, likely the temperature rather than the salinity of inflowing brackish water from the Baltic Sea are more important driver of short-term changes in fish distribution in the Curonian Lagoon. Additionally, the inflowing brackish water normally is much more transparent (Galkus, 2003), and may substantially increase underwater visibility in littoral area of the northern part of the lagoon. This may trigger juvenile fish predator avoidance behaviour, like lower activity and mobility or relocation to areas with more turbid water. The importance of water turbidity as predator avoidance factor, could be reflected in the lack of distinctive predation risk-sensitive diel patterns: higher activity and higher pop up net catches during night, and sheltering, low activity and consequently, lower catches during sampling in light hours.
Water temperature
Seasonal changes in the abundance of juvenile fish are determined by complex factors (Taal et al., 2017). In our study, the abundance of juveniles in the summer was significantly higher on both study sites compared with the autumn. The decreased juvenile abundance in the fall can be explained by usually high natural and predation mortality in the first year, also by seasonal shift in habitats of larger individuals, which usually move to deeper open waters (Perry et al., 2018).
Macrophyte habitats
We assessed the importance of macrophyte stands for fish species by three approaches: (1) the correspondence of fish abundance with the biomass of charophytes and pondweeds in CCA, (2) the fish occurrence and abundance within each macrophyte stand and (3) the long-term changes of fish occurrence associated with changes in macrophyte communities over the last decade (see in the chapter 3). According to the CCA results, perch, roach, both species of sticklebacks, Prussian carp, spined loach and round goby were associated with the macrophyte biomass.
Fish movement among the vegetated and open unvegetated areas is a common risk-sensitive foraging strategy, an important species-specific adaptation to tackle trade-off between predation risk and food availability (Perry et al., 2018). It was found that juvenile perch is a more efficient feeder on zooplankton within vegetation (Diehl, 1988; Burks et al., 2001). Previous study using trapping method (Lesutienė et al., 2005) showed that perch was more frequently caught in pondweed (P. perfoliatus) stands, compared to unvegetated habitat in the Curonian Lagoon. In this study, perch was constantly found with similar abundance within pondweed and charophyte stands, which indicates that macrophyte type has no major influence on fish behaviour.
European roach was mainly associated with pondweed biomass and was constantly found in high abundance (especially in the night) within their stands on the Northern site in July. According to Lesutienė et al. (2005), European roach preferred open habitat, but this preference was not significant, most likely due to high species movement between the habitats. It was shown experimentally, that roach individuals possess better predator avoidance and escaping abilities, therefore they are able to forage in open areas or close to vegetation and shift frequently between the habitats in the presence of predators (Eklöv and VanKooten, 2001). Therefore, vegetated-unvegetated areas in the littoral have key importance for young fish survival and coexistence with other species using macrophyte habitat to hide and forage.
In this study, both species of sticklebacks were more associated with densely vegetated habitat (i.e. high biomass of pondweeds and epiphytes) and they were constantly found with higher abundance within pondweed stands than within charophyte stands. The preference of pondweed habitat could probably be due to its better suitability for spawning and feeding (Burks et al., 2006). Three-spined sticklebacks also use such vegetation for nest-building during spawning (Bakker et al., 2000), which could be to some extent limited on charophyte covered bottom.
We found that a high abundance of spined loach and round goby was mainly associated with charophyte biomass, which could be explained by the species bottom-dwelling habit that minimises the risk of predation in more open habitats. From the diel dynamics data (see more in the chapter 2.4), the species were more constantly abundant within the pondweed stands than the charophyte stands, which agrees with other studies where these species abundance or habitat selection were usually correlated with macrophyte density (Kim et al., 2011; Kornis et al., 2012). Emergent fry of gobies was collected in charophyte beds where spawning presumably occurred (Jude et al., 1995). According to Bohlen (2003), the spined loach requires dense vegetation as suitable spawning habitats too, which occur naturally in shallow and calm areas. Although spined loach has the least concern (IUCN) status in the Baltic Sea (“HELCOM Red List Species Information Sheets (SIS) Fish,” 2013), the preferences for spawning in macrophyte habitat should be considered for conservation of this scarce species.
Diel dynamics
Most documented diel rhythm shifts have been associated with fish ontogenesis and transition through its life cycle (Reebs, 2002). Environmental factors may also play a role in the diel rhythm of fish. As described by Granqvist and Mattila (2004), light intensity and temperature affect behaviour patterns in different seasons, when daylight duration is changing. In the Curonian Lagoon case, a high variation in species abundance during the day was found, which depended more on the sampling site (proxy of salinity) and sampling season than on the habitat. The diel differences of fish assemblage composition associated with seasonal changes were also found in the coastal surf-zone area in the Gulf of Finland using seine (Taal et al., 2017). Similarly, to our results, the mean abundance of three-spined stickleback increased in the night and morning in July. However, the results differed in September, when it was in very low abundance during all day in the Curonian Lagoon, most likely due to its migration to the sea after spawning (Žiliukas, 2003). In July and September, the diel changes in the mean abundance of nine-spined stickleback in the Gulf of Finland (Taal et al., 2017) correspond to the patterns recorded in our study, where their number increased in the evening and especially in the pondweed stands. The preference of this habitat could be associated with favourable prey (chironomids and gammarids), which were determined in the Bothnian Sea (Thorman and Wiederholm, 1986) or Schlei fjord (Antholz et al., 1991), since the mean abundance of these invertebrates was lower in the charophyte stands than in the pondweed stands in the Curonian Lagoon (unpublished data).
In our study, more common fish (such as perch, European roach and ruffe) and a higher number of species were caught in the evening or night even though the sampling efforts were relatively lower compared with other sampling times of the day. This agreed with the results obtained in the coastal waters of the Bothnian Sea (Thorman and Wiederholm, 1986) and the Gulf of Finland (Taal et al., 2017), where perch and European roach were mainly caught at night. Helfman (1981) found that on sunny days small prey fish prefer to stay in shade to avoid visual predators, but our results do not support similar assumptions. Any type structure in the shallow bottom shore may inhabit higher abundances of invertebrates and other food objects (Nicolle et al., 2010). However, better feeding conditions attract more fish and increase competition, which, in contrast, may deplete the density of their prioritised food item (Hülsmann et al., 1999). Lewin et al. (2004) suggested that occurrence of juvenile fish in structured habitats in some cases can be considered as a trade-off between safety and food availability. Therefore, diel migration can be observed between the structured habitat during daytime in the littoral zone, towards the more dangerous open water habitat at night (Jacobsen and Berg, 1998; Hölker et al., 2002). The reasoning why sampling efficiency and community composition was different during the day could most probably be explained by food preference fish movement between adjacent habitats (Mustamäki et al., 2015; Perry et al., 2018). However, it is still underestimated due to the lack of intensive simultaneous sampling effort needed across different habitats (seascapes) and other environmental gradients (such as depth, salinity and temperature).
In the Curonian Lagoon, spined loach did not show any particular pattern in July within the charophyte stands, while its abundance in September increased in the afternoon within the pondweed stands. As this species lives buried in the bed (Robotham, 1982) most likely is less vulnerable to predation during the peak of vegetation period in more open habitats such as charophyte stands than in the autumn, when stands of charophyte are usually uprooted and washed out to shallow habitats such as reeds or pondweed stands (M. Bucas pers. obs.). No significant temporal patterns were observed for round goby too, which is also a bottom-dwelling species and prefers to hide between overgrowths of macrophytes or other substrate crevices (Jude and DeBoe, 1996).
How a Macrophyte Community Change may Impact Associated Fish Assemblage?
Earlier fish studies performed in the Curonian Lagoon (Žiliukas, 2003 and Lesutienė et al., 2005) show significant perch prevalence of vegetated vs. open littoral habitat: proportions of perch and European roach were respectively 80% and 20% in vegetated habitats compared to ~50% and 50% in open littoral areas. During the recent decade in the littoral, the open spaces between pondweed stands have overgrown with charophytes (Sinkevičienė et al., 2017). Based on the correspondence of fish species with the macrophyte biomass and the fish duration (diel dynamics) within both vegetated habitats, the increase of charophytes should have enhanced the abundance of perch, ruffe, spined loach and round goby. This agreed with the long-term changes in the fish community between 2005 and 2019-2020 (Figure 3), where the occurrence of these species significantly increased in 2019-2020. This agreement supports non-random relationships determined between the fish abundance and the macrophyte habitats, which allows empirically based predictions under different ecological scenarios and a better management of littoral habitats. In general, the increase of charophyte stands did not significantly reduce the area of open habitats (i.e. free of vegetation) resulting in the increase of diversity and occurrence of bottom-dwelling species. The charophytes have also spread within the pondweed stands (especially for P. perfoliatus) with coverage usually less than 50%; however, these stands occupy the entire water column of more shallow sites and at high densities may decrease to some extent the free movement of fish. Assuming that patchiness of pondweed and charophyte stands will increase due to natural succession processes in the shallow littoral, we speculate that it may reduce the abundance of European roach and three-spined stickleback as their decrease in occurrence was the most pronounced from all the species.
The potential macrophyte importance in ecological restoration and biodiversity conservation has been widely investigated providing insights about non-linear relationship between macrophyte coverage extent and community functional and diversity parameters (Warfe and Barmuta, 2006; Thomaz et al., 2010; He et al., 2021). Macrophytes serve as spawning grounds for many fish; therefore, the increase of vegetated habitats in the lagoon may enhance suitable substrate for spawning and shelter, especially for ruffe, which prefers the charophyte stands (de Nie and European Inland Fisheries Advisory Commission, 1987). Moreover, the changes in physical structure of habitats may affect water physical-chemical properties (e.g. light, pH, temperature, oxygen and nutrient concentrations), associated invertebrates and zooplankton too. These multi and synergetic effects in turn will have impact on fish communities, which is challenging to assess and predict and there are limited studies from natural systems (Austin et al., 2021). Thus, the results obtained in our study contribute to a better understanding of how the abundance fish populations may change due to alternations in macrophyte habitats and what are possible effects on a food web and ecosystem services. Such knowledge is important for ecosystem-based management aimed at maintaining both fisheries and healthy habitats. Our upgraded pop netting approach can be used to collect a time series of discrete, quantitative samples where seining would cause unacceptable disturbances within macrophyte stands (Dewey et al., 1989).
Conclusions
Our upgraded pop nets reduce the risk of under sampling and are highly recommended for monitoring juvenile fish changes in densely vegetated habitats since this method has relatively low disturbance on fish behaviour and on the habitat itself, especially if surveys are performed in protected and sensitive macrophyte stands. At least 4 samples (pooled from charophyte and pondweed stands) collected at night are sufficient to represent the biggest part (> 70%) of the fish assemblage, but the sampling covering different times of day is recommended.
In total 14 species were recorded, where perch and roach dominated (respectively 48% and 24%) in juvenile fish assemblage. Half of the species were associated with macrophyte stands, which indicates ecological significance of these habitats in the shallow littoral in one of the most productive lagoons in the Baltic Sea. A high variation in the species abundance during the day was found, which depended more on the sampling site (proxy of salinity) and sampling season (proxy of temperature) than on the habitat. Although, the pondweed and charophyte stands supported similar fish juvenile assemblages, based on their abundance and the duration of species within these habitats, European roach, nine-spined and three-spined stickleback were mainly associated with the pondweed biomass and stands, while spined loach, round goby, perch, ruffe and gudgeon were mainly associated with the charophyte biomass and stands.
Our results allow empirically based predictions under different ecological scenarios along with the improving water quality and further spread of charophytes in the lagoon.
Data Availability Statement
The raw data supporting the conclusions of this article will be made available by the authors, without undue reservation.
Ethics Statement
The studies involving animals were reviewed and approved by Environmental Protection Agency under the Ministry of Environment (Permission nr. 027).
Author Contributions
MB, AS, EI, GS, ET, JG, JL, AŠ and GM performed the field works. MB, AS, EI, ET and JG analyzed the data. MB, AS, EI and ET drafted the manuscript. MB, AS, EI, ET and JG designed the study. All authors discussed the results, edited the manuscript, and approved the submitted version.
Funding
This research was funded by the Research Council of Lithuania (no. S-MIP-19-29).
Conflict of Interest
The authors declare that the research was conducted in the absence of any commercial or financial relationships that could be construed as a potential conflict of interest.
Publisher’s Note
All claims expressed in this article are solely those of the authors and do not necessarily represent those of their affiliated organizations, or those of the publisher, the editors and the reviewers. Any product that may be evaluated in this article, or claim that may be made by its manufacturer, is not guaranteed or endorsed by the publisher.
Acknowledgments
We would like to thank Vilius Zeigis for engineering pop nets and turning their parts. We are very grateful to Jonas Lelys, Akvilė Jaugaitė, Vilius Klimavičius, Irma Saudargaite, Paola Forni, Tobia Politi and Reza Pashaei for assistance in pop netting. This study is partly dedicated in memory of Saulius Stakėnas.
References
Antholz B., Meyer-Antholz W., Zander C. D. (1991). Feeding Activities of Two Euryhaline Small-Sized Fish in a Western Baltic Brackish Fjord. Helgoländer. Meeresuntersuchungen. 45, 287–300. doi: 10.1007/BF02365521
Ara T. (2020). Brunnermunzel: (Permuted) Brunner-Munzel Test. R package version 1.4.1. https://CRAN.R-project.org/package=brunnermunzel
Austin Å., Hansen J. P., Donadi S., Bergström U., Eriksson B. K., Sundblad G., et al. (2021). Synergistic Effects of Rooted Aquatic Vegetation and Drift Wrack on Ecosystem Multifunctionality. Ecosystems 24, 1670–1686. doi: 10.1007/s10021-021-00609-9
Bøeuf G., Payan P. (2001). How Should Salinity Influence Fish Growth? Comparative Biochemistry and Physiology Part C. Toxicol. Pharmacol. 130, 411–423. doi: 10.1016/S1532-0456(01)00268-X
Bakker T., Kraak S., Hočevar S. (2000). Stickleback Males, Especially Large and Red Ones, are More Likely to Nest Concealed in Macrophytes. Behaviour 137, 907–919. doi: 10.1163/156853900502529
Beck M. W., Heck K. L., Able K. W., Childers D. L., Eggleston D. B., Gillanders B. M., et al. (2001). The Identification, Conservation, and Management of Estuarine and Marine Nurseries for Fish and Invertebrates: A Better Understanding of the Habitats That Serve as Nurseries for Marine Species and the Factors That Create Site-Specific Variability in Nursery Quality Will Improve Conservation and Management of These Areas. Bioscience 51, 633–641. doi: 10.1641/0006-3568(2001)051[0633:TICAMO]2.0.CO;2
Beesley L. S., Gilmour J. P. (2008). A Modified Drop Net for Sampling Fish Communities in Complex Habitats: A Description and Comparison With Other Techniques. North Am. J. Fisheries. Manage. 28, 1214–1222. doi: 10.1577/M07-050.1
Bohlen J. (2003). Spawning Habitat in the Spined Loach, Cobitis Taenia (Cypriniformes: Cobitidae). Ichthyol. Res. 50, 0098–0101. doi: 10.1007/s102280300016
Boström C., Jackson E. L., Simenstad C. A. (2006). Seagrass Landscapes and Their Effects on Associated Fauna: A Review. Estuarine. Coastal. Shelf. Sci. 68, 383–403. doi: 10.1016/j.ecss.2006.01.026
Bučas M., Šaškov A., Šiaulys A., Sinkevičienė Z. (2016). Assessment of a Simple Hydroacoustic System for the Mapping of Macrophytes in Extremely Shallow and Turbid Lagoon. Aquat. Bot. 134, 39–46. doi: 10.1016/j.aquabot.2016.06.009
Bučas M., Sinkevičienė Z., Kataržytė M., Vaičiūtė D., Petkuvienė J., Stragauskaitė V., et al. (2019). How Much can the Occurrence and Coverage of Charophytes in an Estuarine Lagoon (Curonian Lagoon) Be Explained by Environmental Factors? Estuarine. Coastal. Shelf. Sci. 216, 128–138. doi: 10.1016/j.ecss.2018.01.017
Burks R. L., Jeppesen E., Lodge D. M. (2001). Littoral Zone Structures as Daphnia Refugia Against Fish Predators. Limnol. Oceanography. 46, 230–237. doi: 10.4319/lo.2001.46.2.0230
Burks R. L., Mulderij G., Gross E., Jones I., Jacobsen L., Jeppesen E., et al. (2006). Center Stage: The Crucial Role of Macrophytes in Regulating Trophic Interactions in Shallow Lake Wetlands. Ecological Studies, 37–59. doi: 10.1007/978-3-540-33189-6_3
Clarke K. R. (1993). Non-Parametric Multivariate Analyses of Changes in Community Structure. Aust. J. Ecol. 18, 117–143. doi: 10.1111/j.1442-9993.1993.tb00438.x
Connolly R. M. (1994). Comparison of Fish Catches From a Buoyant Pop Net and a Beach Seine Net in a Shallow Seagrass Habitat. Marine. Ecology-Progress. Ser. 109, 305–305. doi: 10.3354/meps109305
Core Team, R (2013). R: A Language and Environment for Statistical Computing. R Foundation for Statistical Computing (Vienna). https://www.R-project.org/
Dabuleviciene T., Vaiciute D., Kozlov I. E. (2020). Chlorophyll-A Variability During Upwelling Events in the South-Eastern Baltic Sea and in the Curonian Lagoon From Satellite Observations. Remote Sens. 12, 3661. doi: 10.3390/rs12213661
Dainys J., Jakubavičiūtė E., Gorfine H., Pūtys Ž., Virbickas T., Jakimavičius D., et al. (2019). Predicted Climate Change Effects on European Perch (Perca Fluviatilis L.) - A Case Study From the Curonian Lagoon, South-Eastern Baltic. Estuarine. Coastal. Shelf. Sci. 221, 83–89. doi: 10.1016/j.ecss.2019.03.020
de Nie H. W. (1987). The Decrease in Aquatic Vegetation in Europe and its Consequences for Fish Populations. EIFAC Occasional Paper 19. Rome: FAO, 88.
Dewey M. R. (1992). Effectiveness of a Drop Net, a Pop Net, and an Electrofishing Frame for Collecting Quantitative Samples of Juvenile Fishes in Vegetation. North Am. J. Fisheries. Manage. 12, 808–813. doi: 10.1577/1548-8675(1992)012<0808:EOADNA>2.3.CO;2
Dewey M. R., Holland-Bartels L. E., Zigler S. J. (1989). Comparison of Fish Catches With Buoyant Pop Nets and Seines in Vegetated and Nonvegetated Habitats. North Am. J. Fisheries. Manage. 9, 249–253. doi: 10.1577/1548-8675(1989)009<0249:COFCWB>2.3.CO;2
Diehl S. (1988). Foraging Efficiency of Three Freshwater Fishes: Effects of Structural Complexity and Light. Oikos 53 (2), 207–214. doi: 10.2307/3566064
Eklöv P., VanKooten T. (2001). Facilitation Among Piscivorous Predators: Effects of Prey Habitat Use. Ecology 82, 2486–2494. doi: 10.1890/0012-9658(2001)082[2486:FAPPEO]2.0.CO;2
Ferrarin C., Razinkovas-Baziukas A., Gulbinskas S., Umgiesser G., Bliūdžiutė L. (2010). Erratum to: Hydraulic Regime-Based Zonation Scheme of the Curonian Lagoon. Hydrobiologia 652, 397–397. doi: 10.1007/s10750-010-0339-z
Froese R., Pauly D. (2022). FishBase (World Wide Web electronic publication). Available at: www.fishbase.org.
Galkus A. (2003). Summer Water Circulation and Spatial Turbidity Dynamics in the Lithuanian Waters of Curonian Lagoon and Baltic Sea. Vilnius. Chronics. Geography. 36 (2), 48–60.
Gasiūnaitė Z. R., Daunys D., Olenin S., Razinkovas A. (2008). “The Curonian Lagoon,” in Ecology of Baltic Coastal Waters, Ecological Studies. Ed. Schiewer U. (Berlin, Heidelberg: Springer), 197–215. doi: 10.1007/978-3-540-73524-3_9
Granqvist M., Mattila J. (2004). The Effects of Turbidity and Light Intensity on the Consumption of Mysids by Juvenile Perch (Perca Fluviatilis L.). Hydrobiologia 514, 93–101. doi: 10.1023/B:hydr.0000018210.66762.3b
Gray C. A., Chick R. C., McElligott D. J. (1998). Diel Changes in Assemblages of Fishes Associated With Shallow Seagrass and Bare Sand. Estuarine. Coastal. Shelf. Sci. 46, 849–859. doi: 10.1006/ecss.1997.0321
Härmä M. H., Lappalainen A. L., Urho L. U. (2008). Reproduction Areas of Roach (Rutilus Rutilus) in the Northern Baltic Sea: Potential Effects of Climate Change. Canadian Journal of Fisheries and Aquatic Sciences 65 (12), 2678–88. doi: 10.1139/F08-167
HELCOM Red List Species Information Sheets (SIS) Fish (2013). Available at: https://www.helcom.fi/wp-content/uploads/2019/08/HELCOM-RedList-All-SIS_Fish.pdf (Accessed 1.20.22).
Helfman G. S. (1981). The Advantage to Fishes of Hovering in Shade. Copeia 1981, 392–400. doi: 10.2307/1444228
Henseler C., Nordström M. C., Törnroos A., Snickars M., Pecuchet L., Lindegren M., et al. (2019). Coastal Habitats and Their Importance for the Diversity of Benthic Communities: A Species- and Trait-Based Approach. Estuarine. Coastal. Shelf. Sci. 226, 106272. doi: 10.1016/j.ecss.2019.106272
He Y., Zhao K., Zhang H., He L., Niu Y., Zhang M., et al. (2021). Linking Macrophyte Community Structure With Food Chain Length: A Case Study in the Largest Freshwater Lake in China and Ecological Restoration Implications. Ecol. Indic. 123, 107363. doi: 10.1016/j.ecolind.2021.107363
Hölker F., Haertel S. S., Steiner S., Mehner T. (2002). Effects of Piscivore-Mediated Habitat Use on Growth, Diet and Zooplankton Consumption of Roach: An Individual-Based Modelling Approach. Freshwater. Biol. 47, 2345–2358. doi: 10.1046/j.1365-2427.2002.01002.x
Hülsmann S., Mehner T., Worischka S., Plewa M. (1999). “Is the Difference in Population Dynamics of Daphnia Galeata in Littoral and Pelagic Areas of a Long-Term Biomanipulated Reservoir Affected by Age-0 Fish Predation?,” in Shallow Lakes ‘98: Trophic Interactions in Shallow Freshwater and Brackish Waterbodies, Developments in Hydrobiology. Eds. Walz N., Nixdorf B. (Dordrecht: Springer Netherlands), 57–63. doi: 10.1007/978-94-017-2986-4_6
Jacobsen L., Berg S. (1998). Diel Variation in Habitat Use by Planktivores in Field Enclosure Experiments: The Effect of Submerged Macrophytes and Predation. J. Fish. Biol. 53, 1207–1219. doi: 10.1111/j.1095-8649.1998.tb00242.x
Jäger T., Nellen W., Schöfer W., Shodjal F. (1981). Influence of Salinity and Temperature on Early Life Stages of Coregonus Albula, C. Lavaretus, R. Rutilus and L. lota 178, 345–348.
Jude D. J., DeBoe S. F. (1996). Possible Impact of Gobies and Other Introduced Species on Habitat Restoration Efforts. Can. J. Fisheries. Aquat. Sci 53(S1), 136–141. doi: 10.1139/f96-001
Jude D. J., Janssen J., Crawford G. (1995). Ecology, Distribution, and Impact of the Newly Introduced Round and Tubenose Gobies on the Biota of the St. Clair and Detroit Rivers. Lake. Huron. Ecosyst.: Ecol. Fisheries. Manage. Munawar M., Edsall T., Leach J.(Amsterdam: SPB Academic Publishing) 447–460. doi: 10.14321/j.ctt1pwtcm0.25
Kapusta A., Bogacka E. (2006). Diel Variability in the Occurrence of Juvenile Fish in the Shallow Littoral Zone: Do Macrophytes Impact the Structure of Fish Assemblages? Fisheries. Aquat. Life 14, 301–308.
Kim E.-J., Nakajima J., Onikura N. (2011). Habitat Selection During Spawning Season of the Spined Loach, Cobitis Sp. ‘yamato’. complex. Kyushu Island. Japan. fozo. 60, 315–321. doi: 10.25225/fozo.v60.i4.a8.2011
Kornis M. S., Mercado-Silva N., Vander Zanden M. J. (2012). Twenty Years of Invasion: A Review of Round Goby Neogobius Melanostomus Biology, Spread and Ecological Implications. J. Fish. Biol. 80, 235–285. doi: 10.1111/j.1095-8649.2011.03157.x
Kraufvelin P., Pekcan-Hekim Z., Bergström U., Florin A.-B., Lehikoinen A., Mattila J., et al. (2018). Essential Coastal Habitats for Fish in the Baltic Sea. Estuarine. Coastal. Shelf. Sci. 204, 14–30. doi: 10.1016/j.ecss.2018.02.014
Lepš J., Šmilauer P. (2003). Multivariate Analysis of Ecological Data Using CANOCO (University of South Bohemia, Czech Republic, Cambridge University Press). https://doi.org/10.1017/CBO9780511615146
Lesutienė J., Gasiūnaitė Z. R., Grinienė E. (2005). Habitat-Induced Heterogeneity in the Curonan Lagoon Littoral Assemblages: Mysids, Juvenile Fish and Plankton Crustaceans. Acta Zool. Lituanica. 15, 312–323. doi: 10.1080/13921657.2005.10512696
Lewin W.-C., Okun N., Mehner T. (2004). Determinants of the Distribution of Juvenile Fish in the Littoral Area of a Shallow Lake. Freshwater. Biol. 49, 410–424. doi: 10.1111/j.1365-2427.2004.01193.x
Lv D., Fan M., Kang Y., Blanco K. (2016). Modeling Refuge Effect of Submerged Macrophytes in Lake System. Bull. Math. Biol. 78, 662–694. doi: 10.1007/s11538-016-0154-4
Malakpour Kolbadinezhad S., Hajimoradloo A., Ghorbani R., Joshaghani H., Wilson J. M. (2012). Effects of Gradual Salinity Increase on Osmoregulation in Caspian Roach Rutilus Caspicus. J. Fish. Biol. 81, 125–134. doi: 10.1111/j.1095-8649.2012.03317.x
Martinez A. P. (2017). Pairwiseadonis: Pairwise Multilevel Comparison Using Adonis. R Package Version. 1.
Mustamäki N., Jokinen H., Scheinin M., Bonsdorff E., Mattila J. (2015). Seasonal Small-Scale Variation in Distribution Among Depth Zones in a Coastal Baltic Sea Fish Assemblage. ICES. J. Marine. Sci.: J. Du. Conseil. 72 (8), 2374–2384. doi: 10.1093/icesjms/fsv068
Nicolle A., Hansson L.-A., Brönmark C. (2010). Habitat Structure and Juvenile Fish Ontogeny Shape Zooplankton Spring Dynamics. Hydrobiologia 652, 119–125. doi: 10.1007/s10750-010-0323-7
Oksanen J. (2020) Vegan: Community Ecology Package. R Package Version 2. Available at: http://cran.r-project.org/package=vega.
Oksanen J., Kindt R., Simpson G. L. (2017). Vegan3d: Static and Dynamic 3D Plots for the ‘Vegan’package. R Package Version 1.
Parry G. (1966). Osmotic Adaptation in Fishes. Biol. Rev. 41, 392–444. doi: 10.1111/j.1469-185X.1966.tb01499.x
Pedersen E. J., Miller D. L., Simpson G. L., Ross N. (2019). Hierarchical Generalized Additive Models in Ecology: An Introduction With Mgcv. PeerJ 7, e6876. doi: 10.7717/peerj.6876
Perry D., Staveley T. A. B., Gullström M. (2018). Habitat Connectivity of Fish in Temperate Shallow-Water Seascapes. Front. Marine. Sci. 4. doi: 10.3389/fmars.2017.00440
Persson L., Eklov P. (1995). Prey Refuges Affecting Interactions Between Piscivorous Perch and Juvenile Perch and Roach. Ecology 76, 70–81. doi: 10.2307/1940632
Rakauskas V., Pūtis Ž., Dainys J., Lesutienė J., Ložys L., Arbačiauskas K. (2013). Increasing Population of the Invader Round Goby, Neogobius Melanostomus (Actinopterygii: Perciformes: Gobiidae), and its Trophic Role in the Curonian Lagoon, SE Baltic Sea. Acta Ichthyol. Piscatoria. 43, 95–108. doi: 10.3750/AIP2013.43.2.02
Reebs S. G. (2002). Plasticity of Diel and Circadian Activity Rhythms in Fishes. Rev. Fish. Biol. Fisheries. 12, 349–371. doi: 10.1023/A:1025371804611
Repečka R. (2003). The Species Composition of the Ichthyofauna in the Lithuanian Economic Zone of the Baltic Sea and the Curonian Lagoon and Its Changes in Recent Years. Acta Zool. Lituanica. 13, 149–157. doi: 10.1080/13921657.2003.10512558
Repečka R., Ložys L., Žiliukas V., Levickienė D., Jasiukonytė L. (2012). Research on the Rational Use of Fish Resources in the Curonian Lagoon. Rep. Vilnius., 64 p.
Robotham P. W. J. (1982). An Analysis of a Specialized Feeding Mechanism of the Spined Loach, Cobitis Taenia (L.), and a Description of the Related Structures. J. Fish. Biol. 20, 173–181. doi: 10.1111/j.1095-8649.1982.tb03918.x
Sinkevičienė Z., Bučas M., Ilginė R., Vaičiūtė D., Kataržytė M., Petkuvienė J. (2017). Charophytes in the Estuarine Curonian Lagoon: Have the Changes in Diversity, Abundance and Distribution Occurred Since the Late 1940s? Oceanol. Hydrobiol. Stud. 46, 186–198. doi: 10.1515/ohs-2017-0019
Slade J. G., Dibble E. D., Smiley P. C. (2005). Relationships Between Littoral Zone Macrophytes and the Fish Community in Four Urban Minnesota Lakes. J. Freshwater. Ecol. 20, 635–640. doi: 10.1080/02705060.2005.9664785
Stragauskaitė V., Bučas M., Martin G. (2021). Distribution of Charophyte Oospores in the Curonian Lagoon and Their Relationship to Environmental Forcing. Water 13, 117. doi: 10.3390/w13020117
Taal I., Saks L., Rohtla M., Jürgens K., Svirgsden R., Kesler M., et al. (2017). Diel Changes in the Fish Assemblage in a Coastal Surf-Zone Area in the Eastern Baltic Sea. Boreal. Environ. Res. 22, 83–96.
Thomaz S. M., Cunha E. R., Cunha E. (2010). The Role of Macrophytes in Habitat Structuring in Aquatic Ecosystems: Methods of Measurement, Causes and Consequences on Animal Assemblages’ Composition and Biodiversity. Acta Limnol. Brasiliensia. 22, 218–236. doi: 10.4322/actalb.02202011
Thorman S., Wiederholm A.-M. (1986). Food, Habitat and Time Niches in a Coastal Fish Species Assemblage in a Brackish Water Bay in the Bothnian Sea, Sweden. J. Exp. Marine. Biol. Ecol. 95, 67–86. doi: 10.1016/0022-0981(86)90088-2
Umgiesser G., Zemlys P., Erturk A., Razinkova-Baziukas A., Mėžinė J., Ferrarin C. (2016). Seasonal Renewal Time Variability in the Curonian Lagoon Caused by Atmospheric and Hydrographical Forcing. Ocean. Sci. 12, 391–402. doi: 10.5194/os-12-391-2016
Vaičiūtė D., Bučas M., Bresciani M., Dabulevičienė T., Gintauskas J., Mėžinė J., et al. (2021). Hot Moments and Hotspots of Cyanobacteria Hyperblooms in the Curonian Lagoon (SE Baltic Sea) Revealed via Remote Sensing-Based Retrospective Analysis. Sci. Total. Environ. 769, 145053. doi: 10.1016/j.scitotenv.2021.145053
Varsamos S., Nebel C., Charmantier G. (2005). Ontogeny of Osmoregulation in Postembryonic Fish: A Review. Comp. Biochem. Physiol. Part A. 141, 401–429. doi: 10.1016/j.cbpb.2005.01.013
Warfe D. M., Barmuta L. A. (2006). Habitat Structural Complexity Mediates Food Web Dynamics in a Freshwater Macrophyte Community. Oecologia 150, 141–154. doi: 10.1007/s00442-006-0505-1
Weaver M. J., Magnuson J. J., Clayton M. K. (1997). “Distribution of Littoral Fishes in Structurally Complex Macrophytes,” Canadian Journal of Fisheries and Aquatic Sciences 54 (10), 2277–2289. doi: 10.1139/f97-130
Whitfield A. K. (2017). The Role of Seagrass Meadows, Mangrove Forests, Salt Marshes and Reed Beds as Nursery Areas and Food Sources for Fishes in Estuaries. Rev. Fish. Biol. Fisheries. 27, 75–110. doi: 10.1007/s11160-016-9454-x
Wood S. N. (2017). Generalized Additive Models: An Introduction With R. 2nd ed. (New York: Chapman and Hall/CRC), 496.
Žiliukas V. (2003). Assessment of the Dynamics of Main Ecological Parameters of the Fish Fry Community in the Coastal Zone of the Curonian Lagoon Near Ventė Cape. Acta Zool. Lituanica. 13, 167–175. doi: 10.1080/13921657.2003.10512560
Žiliukienė V., Žiliukas V. (2000). Ecological Characteristics of the Ichthyoplankton of the Curonian Lagoon. Acta Zool. Lituanica. 10, 32–55. doi: 10.1080/13921657.2000.10512345
Zilius M., Vybernaite-Lubiene I., Vaiciute D., Petkuviene J., Zemlys P., Liskow I., et al. (2018). The Influence of Cyanobacteria Blooms on the Attenuation of Nitrogen Throughputs in a Baltic Coastal Lagoon. Biogeochemistry 141, 143–165. doi: 10.1007/s10533-018-0508-0
Keywords: diel dynamics, long-term changes, submerged macrophyte stands, pop net sampling, littoral fish assemblage
Citation: Bučas M, Lesutienė J, Nika N, Skersonas A, Ivanauskas E, Srėbalienė G, Tiškus E, Gintauskas J, Šaškov A and Martin G (2022) Juvenile Fish Associated With Pondweed and Charophyte Habitat in the Curonian Lagoon. Front. Mar. Sci. 9:862925. doi: 10.3389/fmars.2022.862925
Received: 26 January 2022; Accepted: 18 May 2022;
Published: 13 June 2022.
Edited by:
Alberto Basset, University of Salento, ItalyReviewed by:
Alan Whitfield, South African Institute for Aquatic Biodiversity, South AfricaHUGO SAULINO, Federal University of São Carlos, Brazil
Copyright © 2022 Bučas, Lesutienė, Nika, Skersonas, Ivanauskas, Srėbalienė, Tiškus, Gintauskas, Šaškov and Martin. This is an open-access article distributed under the terms of the Creative Commons Attribution License (CC BY). The use, distribution or reproduction in other forums is permitted, provided the original author(s) and the copyright owner(s) are credited and that the original publication in this journal is cited, in accordance with accepted academic practice. No use, distribution or reproduction is permitted which does not comply with these terms.
*Correspondence: Martynas Bučas, bWFydHluYXMuYnVjYXNAa3UubHQ=
†These authors have contributed equally to this work and share senior authorship