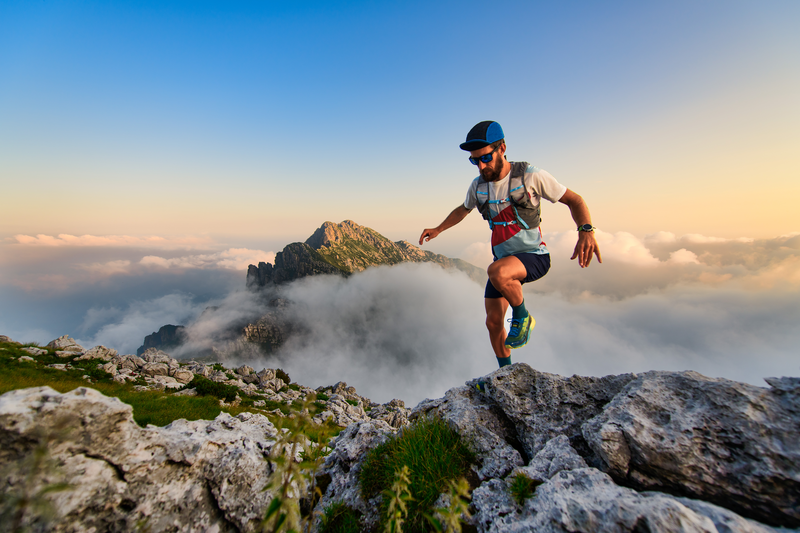
94% of researchers rate our articles as excellent or good
Learn more about the work of our research integrity team to safeguard the quality of each article we publish.
Find out more
ORIGINAL RESEARCH article
Front. Mar. Sci. , 23 May 2022
Sec. Marine Fisheries, Aquaculture and Living Resources
Volume 9 - 2022 | https://doi.org/10.3389/fmars.2022.862548
This article is part of the Research Topic The Ecological Function of Mariculture View all 14 articles
Oyster reefs are structurally complex habitats which are increasingly recognized for their importance in estuarine systems. With over 85% of oyster reefs lost to human activities globally, there is increasing interest in aquaculture to not only meet the growing need for food worldwide, but also enhance ecological functions and services. Prime among these services is the provision of habitat for marine biodiversity. We assessed the biodiversity associated with an abandoned benthic oyster farm to investigate the potential ecological benefits of benthic farming techniques used in traditional oyster farms. In addition, we made note of any invasive species which may use these abandoned structures as habitat. The macrobenthic assemblage in the oyster habitat formed by the farm was different from the surrounding mudflats, containing 61 and 26 species, respectively. Density of invertebrates was similar in both habitats (~ 5,777 ± 981 indiv·m−2), but biomass in the oyster farm over ten times greater than the mudflat substrate, which had a fauna dominated by small polychaetes (<5 mm long). Molluscs and crustaceans were especially abundant in the oyster habitat and contributed to 90% of the enhanced biomass associated with oyster habitat (excluding oyster biomass). Only one invasive species was documented, the mussel Xenostrobus secures, but at densities lower than nearby artificial substrates (e.g., concrete shorelines). Oyster development on this abandoned farm appears to be self-sustaining, provide habitat and trophic support for associated benthic macrofauna. In addition to supporting higher biodiversity and biomass of associated species, traditional oyster aquaculture could improve the restoration potential of oyster reefs by supporting broodstock of native oysters, thus maintaining larval supply.
Coastal environments have suffered from centuries of overexploitation, habitat degradation and pollution, with estuaries being the most degraded of marine ecosystems (Jackson et al., 2001). Despite declines in ecosystem functioning and biodiversity across marine environments globally, different habitats often receive strikingly disproportionate media attention and research effort due to the human propensity to focus on charismatic ecosystems such as coral reefs (Duarte et al., 2008). As a result, the loss of less charismatic but ecologically important coastal habitats continues. Oyster reefs were historically common and extensive in estuarine habitats globally but have experienced an 85% loss over recent centuries (Beck et al., 2011; Zu Ermgassen et al., 2012). Natural oyster reefs form complex three-dimensional structures and create habitats that maintain secondary production, trophic transfer, and coastal biodiversity (Grabowski and Powers, 2004; Tolley and Volety, 2005; Walles et al., 2016). The ecosystem goods and services provided by oyster reefs underpin the health and sustainability of subtropical and temperate estuarine waters (reviewed by Smaal et al., 2019). Therefore, loss at such a global scale significantly impacts not only the function of estuarine ecosystems but also the quality of services provided by oyster reefs to human communities. In addition to food provisioning through fisheries, oyster reefs perform a wide range of supporting, regulating, and cultural ecosystem services, including water filtration, nutrient cycling (Kellogg et al., 2014), shoreline protection, (Ysebaert et al., 2019) fish production (Zu Ermgassen et al., 2016) and tourism (Rusher, 2004). These services are primarily delivered in the nearshore and estuarine areas most impacted by human activities.
Increasing efforts in oyster reef conservation and restoration has meant that attention has largely focused on the role of mature oyster reefs in coastal systems. In contrast, the potential role of aquaculture in providing ecosystem functions and services within an integrated seascape has received relatively little attention (Theuerkauf et al., 2019; Gentry et al., 2020). Notably, benthic oyster aquaculture may provide some of the benefits of oyster reefs to coastal ecosystems (e.g., Coen et al., 2007; Kellogg et al., 2014; Humphries et al., 2016). Yet substantial knowledge gaps on the ecological benefits provided by aquaculture have hampered the development of restorative aquaculture in Asian coastal cities. For example, China is the largest global producer of oysters, which produced 5.14 million tonnes of oysters and contributed about 86% of global oyster aquaculture by weight in 2018 (FAO, 2021), yet there is no assessment of the ecological value of this oyster aquaculture. Given that oyster production in China has continued to increase at ~5% per annum from 1950 to 2016, compared to ~2% per annum for the rest of the world (Botta et al., 2020), there is huge incentive to understand how these oyster farms interact with natural systems, and provide guidance to direct future production growth to not only meet our consumption demand, but also to enhance ecosystem services in the most practical and sustainable way possible.
Hong Kong is a coastal megacity located at the lower basin of Pearl River Delta (PRD) in southern China and has a subtropical monsoonal climate which creates estuarine conditions that are particularly suitable for natural oyster reefs and aquaculture. The full historical extent of natural oyster reefs in Hong Kong is unknown, but archaeological records provide evidence of a natural community of oysters supporting the once-flourishing shell-based lime industry with nearly 30 kilns spread across the territory during the Tang Dynasty (618 to 907 AD) (Meacham 1979). It is evident that historically extensive harvesting and recent, rapid coastal development in southern China (Fang et al., 2007) have caused extensive loss of oyster reefs, leaving only sparse, degraded natural oyster beds in Hong Kong (Lau et al., 2020). Changes in land use practices, including extensive deforestation and coastal reclamation for agriculture, have dramatically increased sediment transport and deposition into the PRD and Greater Bay Area over past millennium (Weng, 2000; Weng, 2007), with increased sedimentation reducing the extent and persistence of sessile hard structured habitats such as oyster reefs (Colden and Lipcius, 2015). Oyster cultivation was first practiced in the PRD about 1,000 years ago during the Song Dynasty (Cheung, 2019) and has a continuous record of cultivation in the region even as harvest of natural oyster reefs declined.
The Hong Kong oyster Magallana hongkongensis has been cultivated on the mudflats of Deep Bay, northwest Hong Kong in the past 150–700 years for their meat and the extraction of lime from the shells (Morton and Wong, 1975). The traditional method of farming involved placing hard substrate such as rocks, concrete tiles, and posts on the soft mud to promote settlement of oyster larvae, a practice that is synonymous with the use of artificial substrate in oyster reef restoration. Farms are then managed until harvest, for example, young oysters could be lifted from a breeding ground and transferred to a more suitable growing or fattening grounds or concrete posts could be relocated to avoid sedimentation. One of the ancillary benefits of this method of benthic farming is that it provides hard substrate among soft sediment mudflats, allowing the aggregation of sessile and mobile benthic organisms. The only assessment of the fauna associated with oyster farms in Deep Bay found over 30 species inhabiting the traditional concrete posts (Morton and Morton, 1983). However, over the last few decades there has been a transition of oyster aquaculture in the region from benthic culture techniques to rope-suspended raft culture techniques introduced from Japan to enhance production (Botta et al., 2020). As a result, many traditional oyster farms are now left fallow and have been allowed to develop into more extensive habitats over several years. These fallow benthic farms are inherently different from natural oyster reefs which accumulate generations of oysters that settle, grow and form three-dimensional structures. The design and layout of substrate adopted in traditional oyster farms intentionally discourages the establishment of such complex structure. When abandoned or left fallow, however, these farms can form complex habitats as continuous recruitment and the formation of small oyster clusters suggests they could provide substrate and shelter for other species which enhance biodiversity of intertidal mudflats, even if not to the extent of natural oyster reefs. With the slow expansion of oyster reef restoration in the region, and as yet uncertain outcomes based on heavy harvest pressure (Lau et al., 2020), benthic oyster farms which are allowed to become fallow, but are still protected from recreational harvest, may provide some of the ecosystem benefits of oyster reefs which have previously been overlooked.
Most studies that have investigated fauna associated with aquaculture have focused on assessing mobile species that gather around fish farms (Gentry et al., 2020) meaning that there is limited information on the biodiversity benefits provided by bivalve aquaculture (e.g., D’Amours et al., 2008), especially oyster aquaculture which is closely associated with benthic habitats (e.g., soft-sediment shores). Yet, fallow benthic oyster farms may provide ecosystem functions and services akin to oyster reefs. Therefore, we investigated the macrobenthic biodiversity on an abandoned traditional oyster farm in Hong Kong. As oyster reefs are known to support greater density, biomass and diversity of resident macrobenthic invertebrates than unstructured mudflats (Shervette and Gelwick, 2008; Stunz et al., 2010; Humphries et al., 2011; Quan et al., 2013), we hypothesized that the resident benthic macroinvertebrate communities in the abandoned oyster farm would be more diverse than, and distinct from, the adjacent mudflat habitats. As a result, we aim to provide the first insights into the biodiversity value of the structured habitat of benthic aquaculture production, expand the discussion around the benefits of traditional aquaculture and its role in producing ecosystem services, and inform the potential to enhance the ecosystem services associated with traditional aquaculture practices.
Biodiversity surveys were conducted at an abandoned oyster farm (“oyster habitat”) and adjacent mudflats (“control”) at Pak Nai (Figure 1, 22° 26’ N 113° 56’ E), Deep Bay, Hong Kong. Deep Bay is subjected to a wide range of seasonal salinity (10.5 - 28.7‰) and temperature (17.8 - 31.1°C) (EPD, 2018). Contrary to its name, Deep Bay is shallow, with an average water depth of 2.9 m and a mean tidal range of 1.4 m (Young and Melville, 1993). As a result, extensive mudflat habitats are exposed during low tide, including the study site.
Figure 1 (A) A map of Hong Kong showing the location of Pak Nai in Deep Bay, north-western Hong Kong. (B) Close-up map showing the location of the study site in Pak Nai. Black lines represent major streams in the study area.
The site is composed of multiple patchy oyster habitats, formed by the remnant oyster populations from the abandoned farm, with mudflats interspersed in between. The oyster habitat is created by an abandoned benthic oyster farm which is characterized by lines of concrete posts with oysters attached, each spaced 30 – 50 cm apart (Figure 2 Traditionally, the short concrete posts (5 cm × 5 cm × 60 cm) were embedded in the sediment prior to the initial settlement peak (April in Deep Bay; Lau et al., 2020), leaving ~ 20 cm above the sediment to act as substrate for the natural settlement of oyster spat which were then allowed to grow for 1 – 2 years before harvest. This benthic method of oyster cultivation creates a unique habitat that is absent in modern suspended (e.g., line, basket or raft) oyster aquaculture. At Pak Nai, the farm areas have been left fallow – they have effectively abandoned but are protected from harvest by being within an aquaculture lease area. Without regular maintenance or harvesting, multiple generations of oysters have overgrown the concrete posts and become a habitat that share some resemblance to dispersed restored oyster beds. While farm abandonment was between 5–7 years ago, the oyster habitats in this study have yet to fuse together and form the extensive three-dimensional structures found in natural reefs. However, this site remains as potentially the best indication of the diversity of macrobenthic communities associated with historically natural oyster reef in Hong Kong due to the protection from recreational harvesting; even though the aquaculture lease is no longer managed or harvested, it still has legal protection and the absence of intervention over several years has allowed these oyster habitats to establish.
Figure 2 (Left) Regular spatial arrangement of the concrete posts used in traditional oyster culture in the Pearl River Delta and southern China, and (right) oysters growing on concrete posts forming a unique habitat at the abandoned farm of the study site. Note the more modern and intensively managed rafts now used for oyster aquaculture in the background of the left panel.
Biodiversity surveys took place in November 2018 and May 2019 during daylight low-tide period (<1.0 m Chart Datum) to sample both wet (summer) and dry (winter) months. To fully assess macrofauna associated with the oyster farm, both quadrat sampling and sediment core sampling were used to collect epifauna and infauna, respectively.
A total of six samples (n = 6) were haphazardly located across the abandoned farm using 0.25 m2 quadrats (0.5 m × 0.5 m) and adjacent, interspersed mudflat (controls). This size of quadrat was determined appropriate for moderate oyster densities of 100 to 500 oyster m2 (Baggett et al., 2014; Baggett et al., 2015). First, all oysters within the sampled quadrat were collected and recorded for their abundance and shell length to obtain size-frequency distributions. Shell length (the distance from the umbo to the distal margin of the shell) were measured to the nearest 0.1 mm using dial callipers.
All fauna were then removed from the live and dead oyster shells within each quadrat and were collected for later identification and enumeration in the laboratory. Sediment core samples were then taken to a depth of 10 cm below the substrate surface using a 10 cm diameter PVC pipe core sampler. The same sampling protocol was used for nearby mudflats as control (n = 6), but as there were no oysters or structured habitat in any of these samples, no epifauna were collected from the quadrats (i.e. all fauna were in the sediment cores). All samples were washed and sieved through a 500 μm stainless steel mesh sieves on the day of collection and preserved in 70% ethanol until processed in the laboratory. All samples were further sorted in the laboratory under dissecting microscope for macrobenthos before final enumeration and identification to the lowest possible taxonomic level. Samples with substantial volume of detritus and/or sediment were subsampled at fixed fraction (50%) prior to sorting and identification of organisms, yielding cost‐effective estimate of species richness and abundance (Barbour and Gerritsen, 1996; Cox et al., 2017).
Data produced from the samples were abundance (number of individuals), biomass, density (number of individuals per unit surface area), species richness (number of species), Shannon diversity index (Shannon, 1948) and J-evenness.
The effect of habitat type and season on macrobenthic assemblages (community composition) was examined with permutational multivariate analysis of variance (PERMANOVA) using Bray–Curtis dissimilarity. Non-parametric multidimensional scaling (nMDS) was then employed to visualize dissimilarities between habitats based on their corresponding benthic assemblages. Abundance data were square-root transformed prior to analysis to reduce the influence of the numerically dominant species (Clarke and Warwick, 2001).
Following nMDS, the means and standard deviation of species density, richness, Shannon diversity index and evenness were determined for each habitat type and season. All parameters were tested by two-way ANOVA with season (wet vs. dry) and habitat type (oyster vs. mudflat control) and their interactions as treatment variables for potential seasonal and spatial differences. The effect of season on oyster density and shell lengths were tested using one-way ANOVA because there were no oysters in control mudflats. Data sets with significant difference between habitat types which did not show homoscedasticity were tested additionally by non-parametric Kruskal–Wallis test by rank. Data on overall density for higher taxonomic groups of interest were also tested for differences using the same statistical methods (above), however the data are presented as pooled values only showing the effect of habitat for precise and comparative purposes.
All statistics were performed using R 3.6.3 (R Core Team, 2020), using vegan (v2.5-6; Oksanen et al., 2019) and BiodiversityR (v2.11-3; Kindt et al., 2005) packages via the R-Commander. All tests were assessed at the α = 0.05 significance level.
The abandoned farm allowed multiple generations of oysters to create relatively new but complex habitat. Oysters were young overall, with a mean length of 31.3 ± 0.8 mm and 26.8 ± 0.6 mm (mean ± SE) in the dry and wet seasons, respectively. Oyster shell length was greater in dry season than that of wet season (Figure 3, Kruskal–Wallis, H1 = 19.89, p < 0.001), probably representing the older juveniles from the previous spawning season. There were very few individuals > 100 mm in length, with none > 150 mm, meaning that the population is mostly comprised of individuals less than ~3 years old. In contrast to size, density of oysters was similar between seasons (ANOVA, F1,10 = 0.002, p > 0.05), with the mean density being 329 ± 103 ind·m−2 and 336 ± 138 ind·m−2 (mean ± SE) in the dry and wet seasons, respectively.
Figure 3 Percentage frequency distributions of the shell length (SL) of oysters growing on the abandoned oyster farm in Deep Bay, Hong Kong, in both dry and wet season. (n = number of individuals sampled, mean ± SE).
Habitat-forming oysters (Magallana spp.) were excluded from the analysis of the associated microbenthic fauna as they formed the habitat and they were not present in the control mudflat plots. There was a distinct macrobenthic assemblage present in oyster habitat created by the abandoned oyster farm compared to mudflat (Figure 4). Habitat type had a large effect on community assemblages. Differences in the benthic community likely exist during wet and dry seasons but would require long-term monitoring to confirm (Table 1; p = 0.09). The separation of macrobenthic assemblages between habitat types is clear based on density data.
Figure 4 Nonparametric multidimensional scaling (nMDS) ordination plot of dissimilarities between sampling sites based on Bray–Curtis distance metric of square‐root transformed abundance of 70 invertebrate taxa in a total of 24 sampling units (n = 6 from two intertidal sites during two sampling times) in Pak Nai. Symbols represent the placement of the faunal communities (labelled as ‘control’ and ‘oyster’) and the individual species which comprise those communities (red crosses) within the multidimensional space.
Table 1 Results of a two-factor PERMANOVA testing for the effects of Habitat (oyster farm vs. mudflat control) and Season (wet vs. dry) on macrobenthic assemblages (70 Taxa) from Pak Nai, based on square-root transformed relative abundances and Bray–Curtis dissimilarities.
Pak Nai harbours a diverse macrobenthic community. A total of 6,112 individuals were recorded in the biodiversity surveys across both oyster habitats and mudflats, representing 70 taxa, in which 52 (74%) were uniquely found in the oyster habitats surveyed in this study (Table S1). The mean density of macrobenthic invertebrates in oyster habitat (5,777 ± 981 indiv·m−2) and in mudflat (5,694 ± 1173 indiv·m−2) were similar and are comparable to the density of invertebrates reported from both natural and restored oyster reefs in China, the USA, and France (Table 2).
Table 2 Comparisons of species richness, biomass, and density of macrobenthos inhabiting various natural or restored oyster reefs and the abandoned benthic oyster farm in this study.
Species richness (Figure 5A) and diversity (Shannon diversity index) were greater in oyster habitat than mudflats (Table 3). The maximum value of Shannon diversity index (2.73) was found in the oyster habitat during wet season, with the mean values being 1.85 and 1.35 in oyster habitat and mudflats, respectively. Biomass of fauna was up to 10 times greater on oyster habitats than mudflats (Figure 5B, Kruskal–Wallis, H1 = 10.83, p < 0.01). Overall, season did not affect species richness or diversity in either habitat type (Table 3), though there was a trend towards greater faunal density in the dry season (Figure 5C; Table 3, p = 0.069). Polychaetes were the most numerically dominant taxa (up to 96%) and obscured abundance patterns in other taxa. When polychaetes are excluded, density of other taxa was greater in oyster habitat than on mudflats (Figure 5D, Kruskal–Wallis, H1 = 11.34, p < 0.001). Differences in species evenness across seasons may be present but undetectable due to low sample sizes (Table 3, p = 0.16). In general, both oyster habitat and mudflat exhibited a moderately even community with mean values ranging from 0.61 to 0.75, respectively.
Figure 5 Biodiversity survey for resident macrobenthic invertebrates conducted on oyster habitats and control mudflats in 2018 and 2019 in Pak Nai, Hong Kong. (A) Species richness; (B) Biomass (g·m−2); (C) Species density (indiv·m−2); and (D) Species density without polychaetes (indiv·m−2) of the sampling sites among habitat types across wet and dry seasons. All values are means ± SE.
Table 3 Summary of two-way ANOVAs testing the effects of habitats and seasons on six benthic assemblage parameters.
Three taxonomic groups accounted for the majority of the fauna found in oyster habitats; polychaetes (57%; 3,302 ± 556 ind·m−2), molluscs (23% excluding oysters; 1324 ± 352 ind·m−2) and crustaceans (19%; 1,098 ± 365 ind·m−2). The remaining 1% were numerically rare taxa such as Sipuncula (Phascolosoma agassizii) and barnacles. The same three taxonomic groups were present for the mudflat controls, but polychaetes accounted for ~96% of individuals in the mudflat. All species encountered in the surveys are known to either be native to Hong Kong, or occur within their natural Indo-Pacific range, except for the invasive mussel Xenostrobus securis which is native to Australia and was first recorded in the eastern waters of Hong Kong in 2010, from where it spread across Hong Kong (Morton and Leung, 2015). It is also worth noting that some fish (Blennidae) were occasionally observed among the oysters during surveys but were not encountered in the quadrats.
The density of the different taxonomic groups of interest varied between habitats and possibly differ among seasons (Figure 6; Table 4). The seasonal effects were on the margins of significance (Table 4, p = 0.07 to 0.1) potentially due to low sample sizes. Oyster habitats contained densities of molluscs and crustaceans one to two orders of magnitude greater than mudflats (Figure 6; Table 4). In contrast, the Sedentaria, a taxonomic group typified by sediment burrowing or tube-dwelling polychaete worms, were approximately twice as abundant in mudflats than oyster habitat (Figure 6, F1,20 = 5.15, p < 0.05). But, at higher taxonomic rank, the density of Polychaeta was considered equally abundant in both habitats. The small invasive mussel Xenostrobus securis (mean shell length 7.8 ± 0.3 mm) only occurred in oyster habitat (882 ± 253 indiv·m−2) and comprised approximately two-thirds of the total molluscan abundance, excluding oysters (Kruskal–Wallis, H1 = 12.8, p < 0.001). This density is two orders of magnitude less than on concrete structures (e.g. protective seawalls) on which X. securis dominates in other parts of Hong Kong (20,900 - 76,000 indiv·m−2; Morton and Leung, 2015) and an order of magnitude less than found on more modern suspended rope (raft) oyster aquaculture which is actively managed (up to 3,500 indiv·m−2; Lau et al., 2018).
Figure 6 The mean density of different taxonomic groups sampled on oyster habitats and control mudflats pooled across seasons. All values are means ± SE.
Table 4 Summary of two-way ANOVAs testing for the effects of habitats and seasons on the density of individuals (m-2) in different taxonomic groups.
Oyster reefs are well known to provide structured habitat for diverse assemblages of fauna (Meyer and Townsend, 2000; Tolley and Volety, 2005; Boudreaux et al., 2006; Shervette and Gelwick, 2008; Stunz et al., 2010). Here, we show that abandoned benthic oyster farms can provide habitat for a rich diversity of fauna and enhance the biodiversity of intertidal mudflats from which oyster reefs have been lost through anthropogenic activities. The macrobenthic assemblages in oyster habitats were distinct from that of the surrounding mudflat habitats. For instance, species richness of the benthic assemblages found in oyster habitat was substantially greater than unstructured mudflats, in particular for groups of epifauna such as crustaceans and molluscs (6 and 18 times greater, respectively). While both mudflat control and the oyster habitat had similar density of macroinvertebrates, the biomass of macrofauna on the mudflats was 9 - 10 times less than that of oyster habitats, given that 96% of individuals in the mudflat were small (2 – 5 mm) polychaetes found as infauna in the sediment matrix. These polychaetes were also present within the oyster habitat, while the other taxa were much more abundant, thus oyster habitats substantially increased biodiversity and biomass of organisms on the intertidal shore.
The only other biodiversity assessment of oyster farms within the region documented roughly half the number of species found in our study (Morton and Morton, 1983). That farm was, however, actively managed meaning that the benthic poles were cleaned of organisms other than large oysters at least annually and lacked the extra time that our abandoned farm had to develop greater structural complexity and the associated faunal community. Furthermore, the abundance of benthic species in the abandoned farm habitat is comparable to the established macrofaunal populations on remnant natural oyster reefs in the national marine park in Jiangsu Province, China, though a different species composition because they are in a different biogeographic region (Quan et al., 2012b). Accretion of oyster shells over longer periods is the single most unique component of natural oyster reefs, which are formed by high-density shell aggregations that introduce high complexity into benthic ecosystems akin to coral reefs (Gutiérrez et al., 2003). Therefore, while abandoned benthic oyster farms do not provide the same level of complexity as natural oyster reefs, they illustrate the potential for regenerative aquaculture in enhancing species diversity, biomass and abundance of both oyster reef restoration and by using the habitat provided by an abandoned oyster farm.
The presence of structurally complex habitats has a positive relationship with increased macrobenthic density and species richness (Hosack et al., 2006; Humphries et al., 2011). Increased diversity associated with biogenic habitats is well known; the structure and complexity created by seagrass beds (Wells et al., 1985; Hosack et al., 2006; McSkimming et al., 2016), algal mats (Dean and Connell, 1987), kelp forests (Fowler-Walker and Connell, 2002), and empty bivalve shells (Tolley and Volety, 2005) are known to be inhabited by greater densities and diversity of marine invertebrates compared to unstructured habitats. Therefore, the presence of oyster reef structure per se is likely a dominant factor determining nekton assemblages (Humphries et al., 2011). While the density and configuration of habitat can determine species diversity (Goodsell and Connell, 2002; Russell et al., 2005), the provision of structure on previously structureless mudflats will cause a rapid increase in diversity and abundance of organisms. Therefore, although the abandoned oyster farm habitat in this study lacks the vertical relief and consolidated structures found in natural oyster reefs, basic structures such as shell valves and clusters formed by several generations of oysters have already increased habitat complexity, surface area and interstitial spaces, which differentiates this oyster habitat from structurally simple habitats like mudflats.
Not only do structurally complex habitats provide structure per se, but they also provide enhanced resources and trophic flows thus increasing the number of species that utilize a habitat (Hiwatari et al., 2002; Grabowski and Peterson, 2007). In addition to offering refugia for macrofauna, the presence of dense epibenthic invertebrates may indicate that the oyster habitats, formed by the remnant oyster populations from the abandoned farm, has the capability to provide trophic support as a valuable foraging resource for intermediate predators (Dame, 1979; Meyer and Townsend, 2000; Grabowski et al., 2005; Humphries et al., 2011; Hanke et al., 2017). For example, high densities of crustaceans, resident crabs and polychaetes can provide important sources of prey for fishes, gulls and terns, including the vulnerable Saunders’s Gull (Chroicocephalus saundersi; BirdLife International, 2018) and the endangered Black-faced spoonbill (Platalea minor; BirdLife International, 2017), both of which are regularly recorded using Deep Bay as their wintering site. Indeed, wildlife taking advantage of open-system aquaculture operations is well-documented (Barrett et al., 2019), generally due to increased food availability either by direct feeding on the cultured species or indirect trophic subsidies from pelagic-benthic coupling due to filter feeding, transferring energy into the benthic environment in the form of faeces and undigested material (pseudofaeces). This deposition subsequently drives a series of trophic resources as the abundant benthic detritivores attracts higher order consumers, contributing to the higher biodiversity and biomass associated with oyster habitats (Rodney and Paynter, 2006; Grabowski and Peterson, 2007; Hancock and zu Ermgassen, 2019). Therefore, the structural complexity created by oysters not only increases abundance and biomass of resident fauna, but it also increases the functional roles of oyster habitats (and therefore abandoned oyster farms) as refugia and feeding areas for macrobenthic organisms.
Habitat-forming species can perform ecosystem functions differently depending on whether they are native to the community or not, and whether they are in natural configurations (Kochmann et al., 2008). When habitat-forming species, such as oysters, are associated with other hard substrate (e.g., concrete) or not allowed to form naturally (e.g., managed aquaculture farms) the resulting habitat can affect the relative abundances of associated organisms (Kochmann et al., 2008). Therefore, in soft sediment estuaries where hard substrate is a limiting resource, oyster aquaculture selects for organisms that utilize hard substrate, thus potentially providing habitat for invasive species (Heiman et al., 2008). In our surveys, we documented an invasive mussel, Xenostrobus securis, which was recently introduced to Hong Kong (Morton and Leung, 2015), in what may initially seem to be relatively high density (882 indiv·m−2) in the abandoned oyster farm. While the presence of the abandoned farm clearly allowed for the settlement of this mussel, because they weren’t recorded on the mudflat, the density in the farm is two orders of magnitude less than documented on manmade concrete structures (e.g., concrete foreshore; 20,900 - 76,000 indiv·m−2; Morton and Leung, 2015) and an order of magnitude less than on more modern, and actively managed, suspended rope (raft) oyster aquaculture (up to 3,500 indiv·m−2; Lau et al., 2018) in Hong Kong. Oyster reefs were historically extensive along the coastline in this region (Meacham 1979) and while some invasive species may inhabit restored reefs where they cannot occupy soft-sediment, restored reefs enhance local biodiversity and ecosystem services above what mudflats provide. Therefore, while there is potential for abandoned oyster farms to provide habitat for invasive species, the relatively low density of Xenostrobus securis suggests that the high density of native oysters (Magallana spp.) which form the basis of the habitat inhibits mussel populations (Gestoso et al., 2014), although further study is required to validate such a link.
Oyster reefs were historically an important estuarine habitat providing multiple ecosystem functions and services (Grabowski and Peterson, 2007; Gregalis et al., 2009; Grabowski et al., 2012). Native oyster reefs have been overharvested globally and are functionally extinct along many coastlines of the world (Beck et al., 2011). As a result, the restoration of oyster reefs is increasing globally (Fitzsimons et al., 2020). Restoring marine habitats can be expensive, requires both social and political will, and has had varying success rates in many parts of the world (Bayraktarov et al., 2016). Within southern China, oyster restoration is in its infancy, progressing slowly, and outcomes that are uncertain because of high levels of recreational harvest (Lau et al., 2020). In contrast, there is a history of benthic oyster aquaculture in the Pearl River Delta (which includes Hong Kong) stretching back over 1,000 years. This continuous aquaculture practice means that not only native oyster populations been prevented from functional extinction in the region, but these habitats may also have provided the key to cost-effective local reef restoration. Natural recruitment is extremely high in the western waters of Hong Kong (up to 197,550 indiv·m−2) despite natural oyster beds in the region being degraded and having sparse densities of individuals (2.3 indiv·m−2), suggesting an additional source of larval supply from local oyster farms (Lau et al., 2020). In contrast, the collapse of the oyster fisheries on the east coast of the USA since the 1800s has led to recruitment limitation in many areas, meaning that restoration needs to begin by re-establishing a breeding population to provide the larvae for recruitment. Therefore, even though Hong Kong is one of the most intensively developed coastal cities in Asia, providing multiple challenges for marine ecosystems such as habitat loss, eutrophication and coastal acidification (Williams et al., 2016), benthic oyster aquaculture has likely maintained breeding populations of native oysters and helped sustain reef-associated biodiversity over recent centuries even as oyster reefs have been in decline. Although implemented with seafood production in mind, the potential role of traditional oyster aquaculture in facilitating and sustaining native biodiversity and future ecosystem services should be considered. As inshore shellfish aquaculture continues to transition into offshore operations around the world, the potential for transforming abandoned intertidal oyster farms into protected reef habitat may provide opportunities in the future. These traditional farms could be revitalized to complement restoration and showcase an example of ‘restorative aquaculture’, which describes the intentional design of aquaculture to deliver direct ecological benefits to the surrounding area, in addition to the providing seafood or other commercial products and livelihoods through economic opportunities (Theuerkauf et al., 2019). When abandoned or left unmanaged, they might also provide an opportunity to consolidate material and biogenic structure to speed restoration.
Capturing this concept, ecologically conscious aquaculture techniques and infrastructure could effectively be integrated into restoration efforts and enhance the rebuilding of native oyster populations (Powers et al., 2009). Developing good relationships and the support of industry stakeholders is essential in this process, since local knowledge and collaborations must be involved in aligning farming practices with conservation objectives in a specific local context (Froehlich et al., 2017). Importantly, both environmental (e.g., anthropogenic pollution, heavy sedimentation) and economic (e.g. low return on investment) challenges will need to be addressed to create an opportunity for ongoing traditional oyster farming in Hong Kong if it is to be effective in providing support to restoration activities.
The raw data supporting the conclusions of this article will be made available by the authors.
SC: Formal analysis, Investigation, Writing - Original Draft. HTW: Methodology, Investigation, Writing - Review & Editing. MT: Conceptualization, Methodology, Investigation, Writing - Review & Editing. HA: Conceptualization, Writing - Review & Editing. BH: Conceptualization, Methodology, Investigation, Writing - Review & Editing. BR: Conceptualization, Methodology, Investigation, Writing - Original Draft, Review & Editing, Funding acquisition. All authors contributed to the article and approved the submitted version.
This project was funded by a grant from The Nature Conservancy Hong Kong and a Faculty of Science (HKU) Rising Star Fund to BR.
The authors declare that the research was conducted in the absence of any commercial or financial relationships that could be construed as a potential conflict of interest.
All claims expressed in this article are solely those of the authors and do not necessarily represent those of their affiliated organizations, or those of the publisher, the editors and the reviewers. Any product that may be evaluated in this article, or claim that may be made by its manufacturer, is not guaranteed or endorsed by the publisher.
We thank members of the Marine Futures Laboratory and the many volunteers who have been invaluable help in both field and lab work, particularly Fong Sing Yuen, Chu Pui Ching, Dwi Wai Shan Jaimie, Jessica Cheng, Michael Lau, Stan Shea, Kathleen Ho, and Justin Donohugh.
The Supplementary Material for this article can be found online at: https://www.frontiersin.org/articles/10.3389/fmars.2022.862548/full#supplementary-material
Baggett L. P., Powers S. P., Brumbaugh R. D., Coen L. D., DeAngelis B., Greene J., et al. (2015). Guidelines for Evaluating Performance of Oyster Habitat Restoration. Restor. Ecol. 23, 737–745. doi: 10.1111/rec.12262
Baggett L. P., Powers S. P., Brumbaugh R., Coen L. D., DeAngelis B., Green J., et al. (2014). Oyster Habitat Restoration Monitoring and Assessment Handbook (Arlington, VA: The Nature Conservancy).
Barbour M. T., Gerritsen J. (1996). Subsampling of Benthic Samples: A Defense of the Fixed-Count Method. J. North Am. Benthol. Soc. 15, 386–391. doi: 10.2307/1467285
Barrett L. T., Swearer S. E., Dempster T. (2019). Impacts of Marine and Freshwater Aquaculture on Wildlife: A Global Meta-Analysis. Rev. Aquacult. 11 (4), 1022–1044. doi: 10.1111/raq.12277
Bayraktarov E., Saunders M. I., Abdullah S., Mills M., Beher J., Possingham H. P., et al. (2016). The Cost and Feasibility of Marine Coastal Restoration. Ecol. Appl. 26, 1055–1074. doi: 10.1890/15-1077
Beck M. W., Brumbaugh R. D., Airoldi L., Carranza A., Coen L. D., Crawford C., et al. (2011). Oyster Reefs at Risk and Recommendations for Conservation, Restoration, and Management. BioScience 61, 107–116. doi: 10.1525/bio.2011.61.2.5
BirdLife International (2017) Platalea Minor (The IUCN Red List of Threatened Species 2017) (Accessed 8 February 2021).
BirdLife International (2018) Saundersilarus Saundersi (The IUCN Red List of Threatened Species 2018) (Accessed 13 May 2020).
Botta R., Asche F., Borsum J. S., Camp E. V. (2020). A Review of Global Oyster Aquaculture Production and Consumption. Mar. Policy 117, 103952. doi: 10.1016/j.marpol.2020.103952
Boudreaux M. L., Stiner J. L., Walters L. J. (2006). Biodiversity of Sessile and Motile Macrofauna on Intertidal Oyster Reefs in Mosquito Lagoon, Florida. J. Shellfish Res. 25, 1079–1089. doi: 10.2983/0730-8000(2006)25[1079:BOSAMM]2.0.CO;2
Cheung S. C. H. (2019). Floating Mountain in Pearl River: A Study of Oyster Cultivation and Food Heritage in Hong Kong. Asian Edu. Dev. Stud. 8, 433–442. doi: 10.1108/AEDS-02-2018-0048
Clarke K. R., Warwick R. M. (2001). Change in Marine Communities: An Approach to Statistical Analysis and Interpretation. 2nd Edition (Plymouth: PRIMER-E, Ltd., Plymouth Marine Laboratory).
Coen L. D., Brumbaugh R. D., Bushek D., Grizzle R., Luckenbach M. W., Posey M. H., et al. (2007). Ecosystem Services Related to Oyster Restoration. Mar. Ecol. Prog. Ser. 341, 303–307. doi: 10.3354/meps341303
Colden A. M., Lipcius R. (2015). Lethal and Sublethal Effects of Sediment Burial on the Eastern Oyster Crassostrea Virginica. Mar. Ecol. Prog. Ser. 527, 105–117. doi: 10.3354/meps11244
Cox K. D., Black M. J., Filip N., Miller M. R., Mohns K., Mortimor J., et al. (2017). Community Assessment Techniques and the Implications for Rarefaction and Extrapolation With Hill Numbers. Ecol. Evol. 7, 11213–11226. doi: 10.1002/ece3.3580
Dame R. F. (1979). The Abundance, Diversity and Biomass of Macrobenthos on North Inlet, South Carolina, Intertidal Oyster Reefs. In.: Proc. Natl. Shellfish Assoc. 69, 6–10.
D’Amours O., Archambault P., McKindsey C. W., Johnson L. E. (2008). Local Enhancement of Epibenthic Macrofauna by Aquaculture Activities. Mar. Ecol. Prog. Ser. 371, 73–84. doi: 10.3354/meps07672
Dean R. L., Connell J. H. (1987). Marine Invertebrates in an Algal Succession. III. Mechanisms Linking Habitat Complexity With Diversity. J. Exp. Mar. Biol. Ecol. 109, 249–273. doi: 10.1016/0022-0981(87)90057-8
Duarte C. M., Dennison W. C., Orth R. J. W., Carruthers T. J. B. (2008). The Charisma of Coastal Ecosystems: Addressing the Imbalance. Estuar. Coast. 31, 233–238. doi: 10.1007/s12237-008-9038-7
Environmental Protection Department, Hong Kong (2018) Marine Water Quality in Hong Kong in 2018. Available at: https://www.epd.gov.hk/epd/sites/default/files/epd/english/environmentinhk/water/hkwqrc/files/waterquality/annual-report/marinereport2018.pdf (Accessed 13 May 2020).
Fang E., Li W., Yu J. (2007). Sustainable Use of Live Oyster Reef in Bohai Gulf. Modern. Fish. Inf. 22, 12–14. doi: 10.3969/j.issn.1004-8340.2007.11.003
FAO (2021)Fishery and Aquaculture Statistics. Global Production Statistics 1950-2019 (Query Panel). In: FAO Fisheries and Aquaculture Department (Rome). Available at: www.fao.org/fishery/statistics/globalproduction/query/en (Accessed 8 February 2021).
Fitzsimons J. A., Branigan S., Gillies C. L., Brumbaugh R. D., Cheng J., DeAngelis B. M., et al. (2020). Restoring Shellfish Reefs: Global Guidelines for Practitioners and Scientists. Conserv. Sci. Pract. 2, e198. doi: 10.1111/csp2.198
Fowler-Walker M. J., Connell S. D. (2002). Opposing States of Subtidal Habitat Across Temperate Australia: Consistency and Predictability in Kelp Canopy-Benthic Associations. Mar. Ecol. Prog. Ser. 240, 49–56. doi: 10.3354/meps240049
Froehlich H. E., Gentry R. R., Halpern B. S. (2017). Conservation Aquaculture: Shifting the Narrative and Paradigm of Aquaculture’s Role in Resource Management. Biol. Conserv. 215, 162–168. doi: 10.1016/j.biocon.2017.09.012
Gentry R. R., Alleway H. K., Bishop M. J., Gillies C. L., Waters T., Jones R. (2020). Exploring the Potential for Marine Aquaculture to Contribute to Ecosystem Services. Rev. Aquac. 12 (2), 499–512. doi: 10.1111/raq.12328
Gestoso I., Arenas F., Olabarria C. (2014). Biotic Resistance and Facilitation of a non-Indigenous Mussel Vary With Environmental Context. Mar. Ecol. Prog. Ser. 506, 163–173. doi: 10.3354/meps10828
Goodsell P. J., Connell S. D. (2002). Can Habitat Loss be Treated Independently of Habitat Configuration? Implications for Rare and Common Taxa in Fragmented Landscapes. Mar. Ecol. Prog. Ser. 239, 37–44. doi: 10.3354/meps239037
Grabowski J. H., Brumbaugh R. D., Conrad R. F., Keeler A. G., Opaluch J. J., Peterson C. H., et al. (2012). Economic Valuation of Ecosystem Services Provided by Oyster Reefs. Bioscience 62 (10), 900–909. doi: 10.1525/bio.2012.62.10.10
Grabowski J. H., Hughes A. R., Kimbro D. L., Dolan M. A. (2005). How Habitat Setting Influences Restored Oyster Reef Communities. Ecology 86, 1926–1935. doi: 10.1890/04-0690
Grabowski J. H., Peterson C. H. (2007). “Restoring Oyster Reefs to Recover Ecosystem Services,” in Ecosystem Engineers: Plants to Protists. Eds. Cuddington Byers K.J. E., WG Hastings A. (Amsterdam: Academic Press), p 281–298.
Grabowski J., Powers S. (2004). Habitat Complexity Mitigates Trophic Transfer on Oyster Reefs. Mar. Ecol. Prog. Ser. 277, 291–295. doi: 10.3354/meps277291
Gregalis K. C., Johnson M. W., Powers S. P. (2009). Restored Oyster Reef Location and Design Affect Responses of Resident and Transient Fish, Crab, and Shellfish Species in Mobile Bay, Alabama. T. Am. Fish. So. 138, 314–327. doi: 10.1577/T08-041.1
Gutiérrez J. L., Jones C. G., Strayer D. L., Iribarne O. O. (2003). Molluscs as Ecosystem Engineers: The Role of Shell Production in Aquatic Habitats. Oikos 101, 79–90. doi: 10.1034/j.1600-0706.2003.12322.x
Hancock B., zu Ermgassen P. (2019). “Enhanced Production of Finfish and Large Crustaceans by Bivalve Reefs,” in Goods and Services of Marine Bivalves (Cham: Springer International Publishing), 295–312.
Hanke M. H., Posey M. H., Alphin T. D. (2017). The Influence of Habitat Characteristics on Intertidal Oyster Crassostrea Virginica Populations. Mar. Ecol. Prog. Ser. 571, 121–138. doi: 10.3354/meps12094
Heiman K., Vidargas N., Micheli F. (2008). Non-Native Habitat as Home for non-Native Species: Comparison of Communities Associated With Invasive Tubeworm and Native Oyster Reefs. Aquat. Biol. 2, 47–56. doi: 10.3354/ab00034
Hiwatari T., Kohata K., Iijima A. (2002). Nitrogen Budget of the Bivalve Mactra Veneriformis, and its Significance in Benthic-Pelagic Systems in the Sanbanse Area of Tokyo Bay. Estuar. Coast. Shelf. Sci. 55 (2), 299–308. doi: 10.1006/ecss.2001.0905
Hosack G. R., Dumbauld B. R., Ruesink J. L., Armstrong D. A. (2006). Habitat Associations of Estuarine Species: Comparisons of Intertidal Mudflat, Seagrass (Zostera Marina), and Oyster (Crassostrea Gigas) Habitats. Estuar. Coast. 29, 1150–1160. doi: 10.1007/BF02781816
Humphries A. T., Ayvazian S. G., Carey J. C., Hancock B. T., Grabbert S., Cobb D., et al. (2016). Directly Measured Denitrification Reveals Oyster Aquaculture and Restored Oyster Reefs Remove Nitrogen at Comparable High Rates. Front. Mar. Sci. 3. doi: 10.3389/fmars.2016.00074
Humphries A. T., La Peyre M. K., Kimball M. E., Rozas L. P. (2011). Testing the Effect of Habitat Structure and Complexity on Nekton Assemblages Using Experimental Oyster Reefs. J. Exp. Mar. Biol. Ecol. 409, 172–179. doi: 10.1016/j.jembe.2011.08.017
Jackson J. B. C., Kirby M. X., Berger W. H., Bjorndal K. A., Botsford L. W., Bourque B. J., et al. (2001). Historical Overfishing and the Recent Collapse of Coastal Ecosystems. Science 293, 629–637. doi: 10.1126/science.1059199
Kellogg M. L., Smyth A. R., Luckenbach M. W., Carmichael R. H., Brown B. L., Cornwell J. C., et al. (2014). Use of Oysters to Mitigate Eutrophication in Coastal Waters. Estuar. Coast. Shelf. Sci. 151, 156–168. doi: 10.1007/s10499-015-9953-0
Kindt R., Coe R., World Agroforestry Centre (ICRAF) (2005). Tree Diversity Analysis. A Manual and Software for Common Statistical Methods for Ecological and Biodiversity Studies (Nairobi: World Agroforestry Centre)
Kochmann J., Buschbaum C., Volkenborn N., Reise K. (2008). Shift From Native Mussels to Alien Oysters: Differential Effects of Ecosystem Engineers. J. Exp. Mar. Biol. Ecol. 364 (1), 1—10. doi: 10.1016/j.jembe.2008.05.015
Lau S. C. Y., Brettell D. L. D. F., Astudillo J. C. (2018). Rapid Assessment of the Invasive Xenostrobus Securis on Cultured Oysters in Hong Kong. Reg. Stud. Mar. Sci. 17, 11–16. doi: 10.1016/j.rsma.2017.11.008
Lau S. C. Y., Thomas M., Hancock B., Russell B. D. (2020). Restoration Potential of Asian Oysters on Heavily Developed Coastlines. Restor. Ecol. 28, 1643–1653. doi: 10.1111/rec.13267
Lejart M., Hily C. (2011). Differential Response of Benthic Macrofauna to the Formation of Novel Oyster Reefs (Crassostrea Gigas, Thunberg) on Soft and Rocky Substrate in the Intertidal of the Bay of Brest, France. J. Sea Res. 65 (1), 84–93. doi: 10.1016/j.seares.2010.07.004
McSkimming C., Connell S. D., Russell B. D., Tanner J. E. (2016). Habitat Restoration: Early Signs and Extent of Faunal Recovery Relative to Seagrass Recovery. Estuar. Coast. Shelf. Sci. 171, 51–57. doi: 10.1016/j.ecss.2016.01.028
Meacham W., The Hong Kong Archaeological Society (1979). A “Missing Link” in Hong Kong Archaeology Vol. Vol. VII (Hong Kong: Journal of the Hong Kong Archaeological Society), 110–117.
Meyer D. L., Townsend E. C. (2000). Faunal Utilization of Created Intertidal Eastern Oyster (Crassostrea Virginica) Reefs in the Southeastern United States. Estuaries 23, 34. doi: 10.2307/1353223
Morton B., Leung K. F. (2015). Introduction of the Alien Xenostrobus Securis (Bivalvia: Mytilidae) Into Hong Kong, China: Interactions With and Impacts Upon Native Species and the Earlier Introduced Mytilopsis Sallei (Bivalvia: Dreissenidae). Mar. Pollut. Bull. 92, 134–142. doi: 10.1016/j.marpolbul.2014.12.046
Morton B., Wong P. S. (1975). The Pacific Oyster Industry in Hong Kong (香港的蠔業). J. R. Asiatic. Soc. Hong. Kong. 15, 139–149.
Oksanen J., Blanchet F. G., Friendly M., Kindt R., Legendre P., McGlinn D., et al. (2019) Vegan: Community Ecology Package. R Package Version 2.5-6. Available at: https://CRAN.R-project.org/package=vegan.
Powers S. P., Peterson C. H., Grabowski J. H., Lenihan H. S. (2009). Success of Constructed Oyster Reefs in No-Harvest Sanctuaries: Implications for Restoration. Mar. Ecol. Prog. Ser. 389, 159–170. doi: 10.3354/meps08164
Quan W., An C., Ma C., Huang H., Cheng W., Wang Y., et al. (2012b). Biodiversity and Community Structure of Benthic Macroinvertebrates on the Xiaomiaohong Oyster Reef in Jiangsu Province, China. Oceanol. Limnol. Sin. 43, 992–999.
Quan W., Humphries A. T., Shen X., Chen Y. (2012a). Oyster and Associated Benthic Macrofaunal Development on a Created Intertidal Oyster (Crassostrea Ariakensis) Reef in the Yangtze River Estuary, China. J. Shellfish Res. 31, 599–610. doi: 10.2983/035.031.0302
Quan W., Zheng L., Li B., An C. (2013). Habitat Values for Artificial Oyster (Crassostrea Ariakensis) Reefs Compared With Natural Shallow-Water Habitats in Changjiang River Estuary. Chin. J. Ocean. Limnol. 31, 957–969. doi: 10.1007/s00343-013-2319-4
R Core Team (2020). R: A Language and Environment for Statistical Computing. R Foundation for Statistical Computing (Vienna, Austria: R Foundation for Statistical Computing). Available at: https://www.R-project.org/.
Rodney W. S., Paynter K. T. (2006). Comparisons of Macrofaunal Assemblages on Restored and non-Restored Oyster Reefs in Mesohaline Regions of Chesapeake Bay in Maryland. J. Exp. Mar. Biol. Ecol. 335 (1), 39–51. doi: 10.1016/j.jembe.2006.02.017
Rusher K. (2004). “The Bluff Oyster Festival and Regional Economic Development: Festivals as Culture Commodified,” in Food Tourism Around the World. Eds. Hall C. M., Sharpless L., Mitchell R., Macionis N., Cambourne B. (Jordan Hill: Taylor & Francis Group), 192–205.
Russell B. D., Gillanders B. M., Connell S. D. (2005). Proximity and Size of Neighbouring Habitat Affects Invertebrate Diversity. Mar. Ecol. Prog. Ser. 296, 30–38. doi: 10.3354/meps296031
Shannon C. E. (1948). A Mathematical Theory of Communication. Bell. system. Tech. J. 27, 379–423. doi: 10.1002/j.1538-7305.1948.tb01338.x
Shervette V. R., Gelwick F. (2008). Seasonal and Spatial Variations in Fish and Macroinvertebrate Communities of Oyster and Adjacent Habitats in a Mississippi Estuary. Estuar. Coast. 31, 584–596. doi: 10.1007/s12237-008-9049-4
Sigovini M., Keppel E., Tagliapietra D. (2016). Open Nomenclature in the Biodiversity Era. Methods Ecol. Evol. 7, 1217–1225. doi: 10.1111/2041-210X.12594
Smaal A. C., Ferreira J. G., Grant J., Petersen J. K., Strand Ø. (Eds.) (2019). Goods and Services of Marine Bivalves (Cham, Springer International Publishing).
Stunz G. W., Minello T. J., Rozas L. P. (2010). Relative Value of Oyster Reef as Habitat for Estuarine Nekton in Galveston Bay, Texas. Mar. Ecol. Prog. Ser. 406, 147–159. doi: 10.3354/MEPS08556
Theuerkauf S. J., Morris J. A. Jr., Waters T. J., Wickliffe L. C., Alleway H. K., Jones R. C. (2019). A Global Spatial Analysis Reveals Where Marine Aquaculture can Benefit Nature and People. PloS One 14 (10), e0222282. doi: 10.1371/journal.pone.0222282
Tolley S. G., Volety A. K. (2005). The Role of Oysters in Habitat Use of Oyster Reefs By Resident Fishes And Decapod Crustaceans. J. Shellfish Res. 24, 1007–1012. doi: 10.2983/0730-8000(2005)24[1007:TROOIH]2.0.CO;2
Walles B., Troost K., van den Ende D., Nieuwhof S., Smaal A. C., Ysebaert T. (2016). From Artificial Structures to Self-Sustaining Oyster Reefs. J. Sea. Res. 108, 1–9. doi: 10.1016/j.seares.2015.11.007
Wells F. E., Rose R. A., Lang S. (1985). An Analysis of Benthic Marine Invertebrate Communities in Subtidal Seagrass and Sand Habitats in Shark Bay, Western Australia. Records. Western. Aust. Museum. 12, 47–56.
Weng Q. (2000). Human-Environment Interactions in Agricultural Land Use in a South China's Wetland Region: A Study on the Zhujiang Delta in the Holocene. GeoJournal 51, 191–202. doi: 10.1023/A:1012021520392
Weng Q. (2007). A Historical Perspective of River Basin Management in the Pearl River Delta of China. J. Environ. Manage. 85, 1048–1062. doi: 10.1016/j.jenvman.2006.11.008
Williams G. A., Helmuth B., Russell B. D., Dong Y., Thyiagarajan T. (2016). Meeting the Climate Change Challenge: Pressing Issues in Southern China and SE Asian Coastal Ecosystems. Reg. Stud. Mar. Sci. 8, 373–381. doi: 10.1016/j.rsma.2016.07.002
Young L., Melville D. S. (1993). “Conservation of the Deep Bay Environment,” in The Marine Biology of the South China Sea, Proc 1st Inter Con Mar Biol of the South China Sea, Hong Kong. Ed. Morton B. (University Press, Hong Kong), 211–231.
Ysebaert T., Walles B., Haner J., Hancock B. (2019). “Habitat Modification and Coastal Protection by Ecosystem-Engineering Reef-Building Bivalves,” in Goods and Services of Marine Bivalves. Eds. Smaal A., Ferreira J., Grant J., Petersen J., Strand O. (Cham: Springer International Publishing)
Zu Ermgassen P. S., Grabowski J. H., Gair J. R., Powers S. P. (2016). Quantifying Fish and Mobile Invertebrate Production From a Threatened Nursery Habitat. J. Appl. Ecol. 53 (2), 596–606. doi: 10.1111/1365-2664.12576
Keywords: shellfish aquaculture, biodiversity enhancement, habitat provisioning, oyster reef restoration, restorative aquaculture
Citation: Chan SSW, Wong HT, Thomas M, Alleway HK, Hancock B and Russell BD (2022) Increased Biodiversity Associated With Abandoned Benthic Oyster Farms Highlight Ecosystem Benefits of Both Oyster Reefs and Traditional Aquaculture. Front. Mar. Sci. 9:862548. doi: 10.3389/fmars.2022.862548
Received: 26 January 2022; Accepted: 14 April 2022;
Published: 23 May 2022.
Edited by:
Dapeng Liu, Georgia Institute of Technology, United StatesReviewed by:
Christian Buschbaum, Alfred Wegener, Institute Helmholtz Centre for Polar and Marine Research (AWI), GermanyCopyright © 2022 Chan, Wong, Thomas, Alleway, Hancock and Russell. This is an open-access article distributed under the terms of the Creative Commons Attribution License (CC BY). The use, distribution or reproduction in other forums is permitted, provided the original author(s) and the copyright owner(s) are credited and that the original publication in this journal is cited, in accordance with accepted academic practice. No use, distribution or reproduction is permitted which does not comply with these terms.
*Correspondence: Bayden D. Russell, YnJ1c3NlbGxAaGt1Lmhr
Disclaimer: All claims expressed in this article are solely those of the authors and do not necessarily represent those of their affiliated organizations, or those of the publisher, the editors and the reviewers. Any product that may be evaluated in this article or claim that may be made by its manufacturer is not guaranteed or endorsed by the publisher.
Research integrity at Frontiers
Learn more about the work of our research integrity team to safeguard the quality of each article we publish.