- 1Tropical Aquaculture Research and Development Center, South China Sea Fisheries Research Institute, Chinese Academy of Fishery Sciences, Sanya, China
- 2Key Laboratory of South China Sea Fishery Resources Exploitation and Utilization, Ministry of Agriculture and Rural Affairs, Guangzhou, China
- 3Sanya Tropical Fisheries Research Institute, Sanya, China
- 4State Key Laboratory of Marine Environmental Science, College of Ocean and Earth Sciences, Xiamen University, Xiamen, China
In order to understand the effects of dietary curcumin on the growth and digestive performance of Seriola dumerili, this study was conducted under laboratory conditions. The control group was added with 0% curcumin, and two treatment groups were added with 0.01% and 0.02% curcumin, respectively. Fish were fed with experimental diet for 8 weeks. The growth data, growth genes, intestinal digestive enzymes, tissue parameters, and blood lipid-related indicators were measured, and the correlation between digestive indicators, growth gene indicators, and growth characteristic indicators was analyzed. The results showed that the survival rate, visceral ratio, hepatosomatic ratio, and condition factor of Seriola dumerili increased first and then decreased with the increase of curcumin content in feed. The feed conversion ratio of the control group was significantly higher than that of the treatment group. With the increase of curcumin content in feed, lipase and trypsin increased first and then decreased with the increase of curcumin content. Compared with the control group, curcumin significantly increased the muscle thickness and the number of goblet cells of Seriola dumerili. High and low contents of curcumin can reduce the content of serum total cholesterol and triglyceride, and a low dose (0.01%) of curcumin has the strongest lipid-lowering effect. The relative gene expression of IGF1 and IGF2 reached the maximum in the 0.02% treatment group. The results showed that the addition of curcumin in feed could theoretically improve the survival rate, feed efficiency, and other growth characteristics of Seriola dumerili, improve intestinal digestion and structure, promote intestinal health, and reduce blood lipid levels. The best effect was achieved in the 0.01% treatment group. The results of this study provide a theoretical reference for the feasibility of adding curcumin to Seriola dumerili feed in production and realize the optimal proportion of feed additives for this species, which is beneficial to the sustainable development of aquaculture.
Introduction
Curcumin is a polyphenolic compound extracted from rhizomes of Zingiberaceae and Araceae (Indira Priyadarsini, 2013). It has multiple functions including antioxidant, anti-inflammatory, and antibacterial effects and has the advantages of being green pollution-free and natural and having no residue (Zhang and Xiao, 2021). A previous study has shown that when using 120 mg/kg dietary level of curcumin, the lipid peroxidation of juvenile tilapia (Oreochromis niloticus) can be significantly reduced, and the liver can be protected from injury, while the antioxidant function of the fish body is improved (Zhang et al., 2018). The addition of curcumin in feed could reduce the serum transaminase activity and liver inflammatory factor level and improve the body color of juvenile American eel (Anguilla rostrata) to a certain extent (Zhu et al., 2020). A dietary supplementation of 0.06% curcumin significantly increased the serum antioxidant capacity of juvenile turbot (Scophthalmus maximus) (Wang et al., 2016). Giri et al. (2019) found that the addition of curcumin in diets could not only increase the expression of anti-inflammatory factors in intestine and liver of common carp (Cyprinus carpio), reducing the expression of pro-inflammatory factors, but also promote its growth. Mahmoud et al. (2017) proposed that dietary curcumin levels of 50–100 mg/kg could improve the growth index and feed conversion rate of Oreochromis niloticus, reduce the number of intestinal Escherichia coli and Aeromonas, and improve serum immunity and liver antioxidant.
Recent advances in curcumin and its other different forms being adopted as dietary supplements have broad application prospects in fish nutrition (Mahmoud et al., 2020). Curcumin could promote the growth of fish and improve the intestinal flora and tissue structure, thereby improving the antioxidant capacity of the intestinal tract and improving its own immunity. A study has shown that adding 1 or 5 g curcumin per kilogram of feed can improve digestive enzyme activities such as lipase and protease, antioxidant capacity, and digestibility of feed in crucian carp (Carassius auratus), thereby promoting growth (Jiang et al., 2016). Khorshidi et al. (2018) also found that the use of curcumin increased the relative abundance of lactic acid bacteria in the intestine of common carp Cyprinus carpio. In addition, curcumin was added to the diet in the related application experiments of rainbow trout Oncorhynchus mykiss (Yonar et al., 2019), large yellow croaker Pseudosciaena crocea (Ji et al., 2021), yellow catfish Pelteobagrus fulvidraco (Zhang et al., 2017), tilapia Oreochromis mossambicus (Sruthi et al., 2018), Anguilla marmorata (Xie, 2017), and Juvenile American Eel (Anguilla rostrata) (Zhu, 2021).
The greater amberjack Seriola dumerili, belonging to Carangidae Seriola, is a warm-water oceanodromous migration fish (Chen, 2004). Due to its high flesh quality and fast growth, the greater amberjack has become a good candidate for aquaculture (Thakur et al., 2009). With the increase in consumption demand and the continuous development of the market, the commercial culturing scale of Seriola dumerili has expanded considerably. However, as the consequences of large-scale intensive culturing, disease outbreaks in this species have been frequently reported and have caused considerable economic losses (Wu and Pan, 2000). In this study, a physical response of Seriola dumerili to different dietary curcumin levels was evaluated. Growth characteristics, intestinal digestion, and growth gene expression were used as the evaluation criteria. Results from the present study will improve our understanding on the usage of dietary curcumin in Seriola dumerili.
Materials and methods
Experimental Fish and Feeding Management
The experimental fish Seriola dumerili was cultivated by the Tropical Aquaculture Research and Development Center, South China Sea Fishery Research Institute, Chinese Academy of Fishery Sciences. A total of 135 healthy fish were randomly divided into 3 groups. Dietary curcumin supplemental levels of 0% (control), 0.01%, and 0.02% with three replicates were tested in this study. A total of nine tanks were used in this study, and 15 fish were tested in each tank. After transferring to the experimental tank, fish were acclimated in the experimental system for 1 week before the feeding experiment was started. The feeding experiment was carried out in a RAS system, and the tank volume was 5,000 l. The initial mean body weights of each group were 149.02 ± 12.59, 153.51 ± 4.09, and 151.78 ± 3.84g, respectively. During the experimental period, water quality was maintained at temperatures 27°C–31.5°C, salinity at 35‰, and pH at 7.5–8, and ammonia nitrogen was maintained at <0.1 mg/l, nitrite at <0.02 mg/l, and dissolved oxygen >7.0 mg/l. Seriola dumerili were fed twice a day at 8:00 a.m. and 16:00 p.m. The residual feeds and feces were collected 1 h after feeding. The experimental period was 8 weeks.
Preparation of Experimental Feed
In this study, 0%, 0.01%, and 0.02% curcumin were added into the feed to prepare three kinds of isonitrogenous and isolipid experimental feeds. Then, the feed feeding experiment was carried out on Seriola dumerili. The experimental feed was self-designed and produced by the Lingshui Tropical Aquatic Research and Development Center. Curcumin (purity >95%) was provided by Xi’an Feida Biotechnology Co., Ltd. (Xi’an, China). All ingredients were crushed and screened (0.2 mm mesh), mixed through a commercial food mixer (Guangdong Li Feng Co., Ltd., Guangzhou, China), then mixed with oil, produced through a granulator (Shandong Hengfeng Co., Ltd., Dongying, China) to produce particles with a diameter of 4 mm, and air-dried at room temperature (25°C). The prepared feed was sealed in plastic bags and stored in a -20°C refrigerator for later use (Li et al., 2020). Experimental feed formula information is shown in Table 1.
According to each parallel 15,000-g feed, the weight of each component of the feed is shown in the table. The control group, the 0.01% treatment group, and the 0.02% treatment group represented the experimental groups fed with 0 (control), 100 mg/kg, and 200 mg/kg curcumin, respectively; that is, the amount of curcumin in each 15,000-g feed was 0, 1.5, and 3g, respectively.
Serum Biochemical Parameters
Feeding was stopped the day before the end of the trial, and each group was weighed separately. Using zinc oxide eugenol cement as anesthetics (Changshu Shang Dental Materials Co., Ltd., Shanghai, China), all groups were anesthetized and sampled; each parallel took 3 tails. The surface water was wiped with paper towels, and a 1-ml sterile syringe was used to extract blood from the tail veins of Seriola dumerili. After standing at room temperature for 4 h, blood samples were centrifuged for 10 min at 3,500 ×g, 4°C, and then the supernatants were collected. The collected supernatant was stored at −80°C for further measurement and analysis. Serum biochemical parameters were determined according to the instructions of the commercial kits (Nanjing Jiancheng Biological Co., Ltd., Nanjing, China), i.e., total cholesterol (TC) (Item No. A111-1-1); cholesterol oxidase–peroxidase aminoantipyrine method; and triglycerides (TG) (Item No. A110-1-1): phosphate oxidase–peroxidase aminoantipyrine method. All serum biochemical parameters were performed in triplicates.
Tissue Sample Collection and Growth Performance Measurement
Fish were then dissected with sterilized scalpel, tweezers, and so on, the intestines quickly separated out, and the whole operation process performed on the ice. The intestine samples were put into the 2-ml centrifuge tubes with labels. Part of them were frozen in liquid nitrogen and then transferred to a -80°C refrigerator for the determination of enzyme activity. The other part was used for making slices. Biochemical and gene expression samples were frozen in liquid nitrogen and stored at -80°C. In addition, 9 fish were randomly selected from each group, and the body length and weight were measured respectively. The liver and viscera were quickly separated and weighed to calculate the specific growth rate, weight gain rate, feed conversion ratio and condition factor, visceral ratio, and hepatosomatic ratio. The calculation formulas are as follows:
In the formula, the unit of the body length is cm, the unit of the final average weight and initial average weight is g, and the unit of breeding test days is d.
Digestive Enzymes and Intestinal Structure Determination
Intestine samples from each parallel were mixed and weighed. Under the condition of ice bath, 0.9% normal saline or sample homogenate medium was added to the tissue according to the weight volume ratio of 1:9 to make 10% homogenate. According to the requirements of the corresponding kits (Nanjing Jiancheng Biological Co., Ltd., Nanjing, China), the homogenates were centrifuged and the supernatants were extracted, and then the relevant indicators were measured. Intestinal digestion indicators included amylase (AMS) (Item No. C016-1-1), lipase (LPS) (Item No. A054-2-1), trypsin (TRYP) (Item No. A080-2-2), and total protein (TP) (Item No. A045-4-2). The starch-iodine colorimetric method, methyl halal substrate method (microplate method), colorimetric method, and BCA microplate method were used for determination, respectively.
The intestines of the three groups were fixed with 4% paraformaldehyde for 24 h, dehydrated with graded ethanol concentration, and embedded in paraffin. A Leica RM 2016 rotary microtome (Shanghai Leica Instrument Co., Ltd., Shanghai, China) was used to cut into sections of 4-μm thickness. Hematoxylin–eosin (HE) staining was used and finally permanently fixed with resinene. A Pannoramic 250/MIDI scanner and CaseViewer 2.0 (3DHISTECH Co., Ltd., Budapest, Hungary) were used to scan slices, collect images, and measure data. The height and width of intestinal folds, the height of the intestinal villus, the thickness of the muscular layer, and the number of goblet cells were quantified.
Growth Gene Expression
Genes selected for qPCR analysis were found based on the NCBI database (https://www.ncbi.nlm.nih.gov/). Primers of EF1α (internal reference gene), IGF1, and IGF2 genes were designed by the Primer Premier 5 program (Table 2). The method for proving the specificity and availability of synthetic primers was referred to Yang et al. (2020). RNA was extracted according to the method of Fu et al. (2019). The ND 5000 spectrophotometer (BioTeke Corporation, Beijing, China) was used to measure the absorbance at 260 and 280 nm to determine the purity and concentration of RNA extracted. Finally, agarose gel electrophoresis (1%) was used to detect the integrity of RNA. RNA was immediately used to synthesize cDNA. TransScript-Uni One-Step gDNA Removal and cDNA Synthesis SuperMix (TransGen Biotech Co., Ltd., Beijing, China) were used for reverse transcription of 1 μg total RNA. The synthesized cDNA samples were stored at -20°C for further use (Yang et al., 2020). SYBR Green (Tiangen Biotech Co., Ltd., Beijing, China) was used for real-time quantitative PCR analysis (Hangzhou LongGene Scientific Instrument Co., Ltd., Hangzhou, China) for three times for each sample (Fu et al., 2019).
Statistical Analysis
The relative mRNA expression level of the target gene was determined by the 2-ΔΔCt method. The standard deviation (SD) of the test data was determined by using the EF1α gene as an internal reference gene. Statistical analysis was performed using SPSS Statistics 26 software. One-way analysis of variance and Duncan’s test were used for comparison between groups. The significance level was set to p < 0.05 (Yang et al., 2020). Finally, Origin 2019 was used for mapping.
Results
Effect of Curcumin on the Growth Performance of Seriola dumerili
Indicators related to the growth performance after 8 weeks of feeding experiment are shown in Table 3. It can be found that the survival rate and condition factor of Seriola dumerili increased first and then decreased with the increase of curcumin content in feed (p < 0.05). Moreover, they reached the highest level in the 0.01% treatment group (p < 0.05), while there was no significant difference between the control group and the 0.02% treatment group (p > 0.05). The feed coefficient of Seriola dumerili in the control group was significantly higher than that in the treatment group (p < 0.05), and there was no significant difference in feed coefficient between 0.01% and 0.02% treatment groups (p > 0.05). Different contents of curcumin diet had no significant effects on final average weight, weight gain rate, specific growth rate, visceral ratio, and hepatosomatic ratio of Seriola dumerili (p > 0.05).
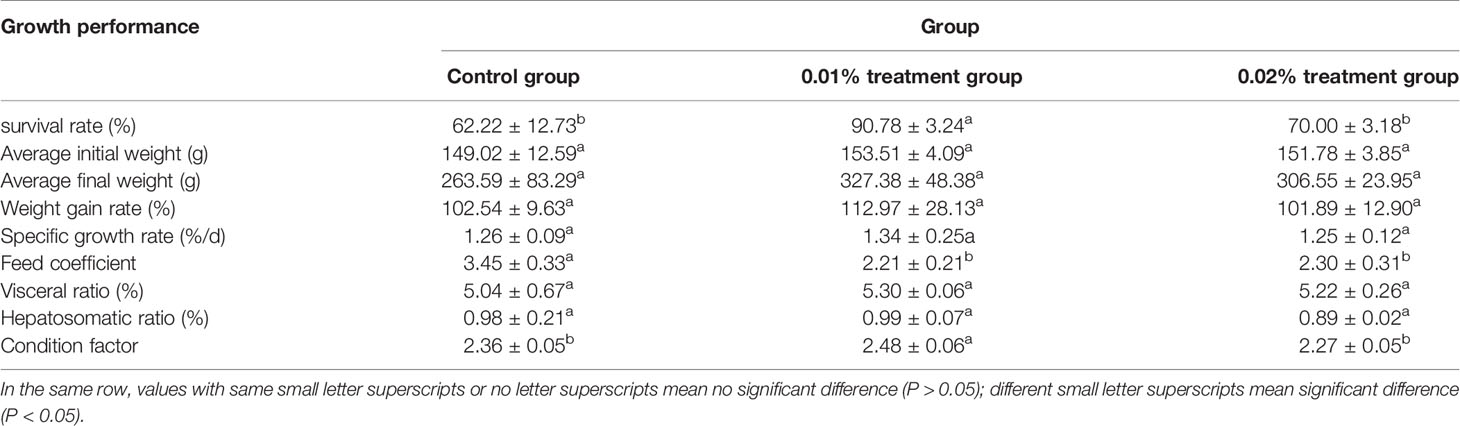
Table 3 Effects of different levels of curcumin in feed on the growth performance of Seriola dumerili.
Data are presented as mean ± SD. In the same row, the same lowercase letters on the right side of the data indicated no significant difference (p > 0.05). Different lowercase letters indicated significant difference (p < 0.05).
Effects of Curcumin on Digestive Enzymes and Intestinal Tissue of Seriola dumerili
After feeding with different levels of curcumin for 8 weeks, the intestinal digestive enzyme (lipase, trypsin, amylase) activity is as shown in Figure 1. The results showed that the content of curcumin in feed had a significant effect on the digestive enzyme of Seriola dumerili (p < 0.05). Among them, the intestinal amylase activity of Seriola dumerili decreased firstly and then increased with the increase of curcumin content (p < 0.05) and reached the maximum value in the control group (Figure 1: I). With the increase of curcumin content in diet, the activities of lipase and trypsin in the intestine of fish were firstly increased and then decreased (p < 0.05), and the activities of these two digestive enzymes reached the minimum values in the control group and 0.02% treatment group, respectively (Figure 1: II and III).
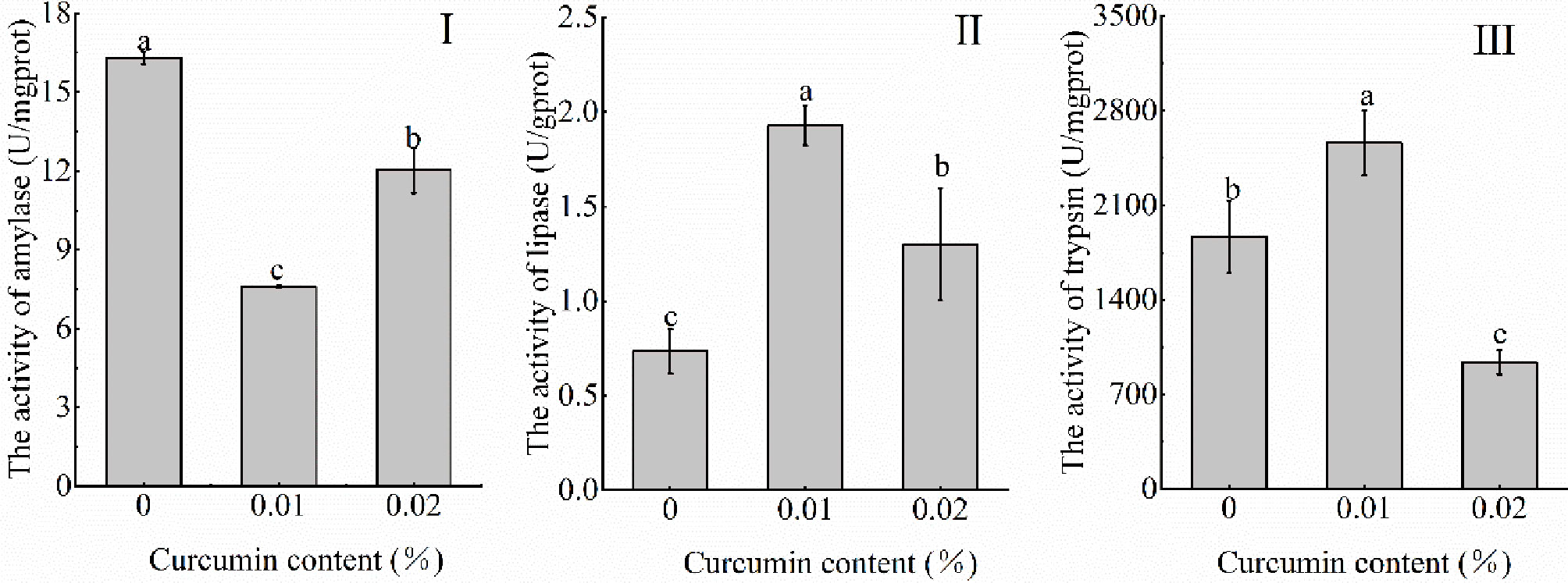
Figure 1 Effects of different levels of curcumin on the activities of intestinal digestive enzymes (I, amylase activity; II, lipase activity; III, trypsin activity). Different superscript letters indicate significant differences among treatments (P < 0.05).
As shown in Figure 2, compared with the control group, dietary curcumin significantly increased the thickness of the muscular layer and the number of goblet cells (p < 0.05) and had no significant effect on the height of the intestinal villus (p > 0.05). The intestinal fold width reached the maximum value in the 0.02% treatment group and the minimum value in the control group (p < 0.05), while there was no significant difference between the 0.01% treatment group and the control group and the 0.02% treatment group (p > 0.05). The intestinal fold height reached the minimum in the 0.02% group (p < 0.05), and there was no significant difference between the control group and the 0.01% group (p > 0.05).
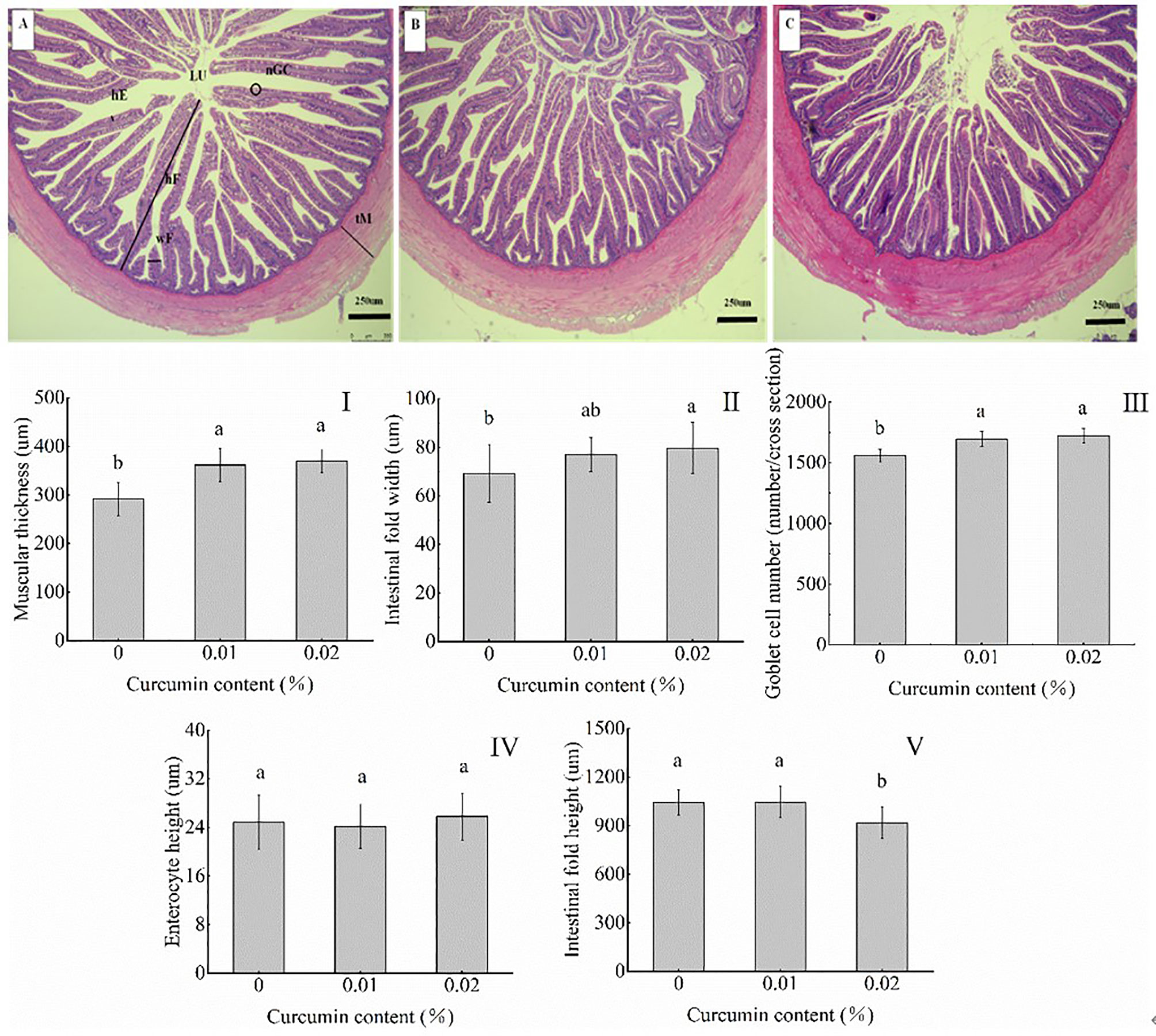
Figure 2 The intestinal tract of Seriola dumerili was affected by diets with different curcumin contents. Representative sections of intestinal tissue (50 magnification) are shown at the top [(A) control group; (B) 0.01% treatment group; (C) 0.02% treatment group]. tM represents muscle layer thickness; hF represents the height of intestinal fold; wF represents the intestinal fold width; hE stands for intestinal epithelial cell height; nGC represents the number of goblet cells; LU represents lumen. (I, muscle layer thickness; II, intestinal fold width; III, number of goblet cells; IV, intestinal epithelial cell height; III, intestinal fold height). Different superscript letters indicate significant differences between different treatments (p < 0.05).
Effects of Curcumin Content on Serum Biochemical Parameters
The results of serum triglyceride and cholesterol contents of Seriola dumerili are observed in Figure 3. It can be seen from the figure that the curcumin content in feed had a significant effect on the serum triglyceride content of Seriola dumerili (p < 0.05). With the increase of curcumin content in feed, the serum triglyceride content of Seriola dumerili firstly decreased and then increased (p < 0.05) and reached the highest value in the control group. The total cholesterol content reached the maximum value in the control group and the minimum value in the 0.01% treatment group (p < 0.05), and there was no significant difference between the 0.02% treatment group and the control group and the 0.01% treatment group (p > 0.05).
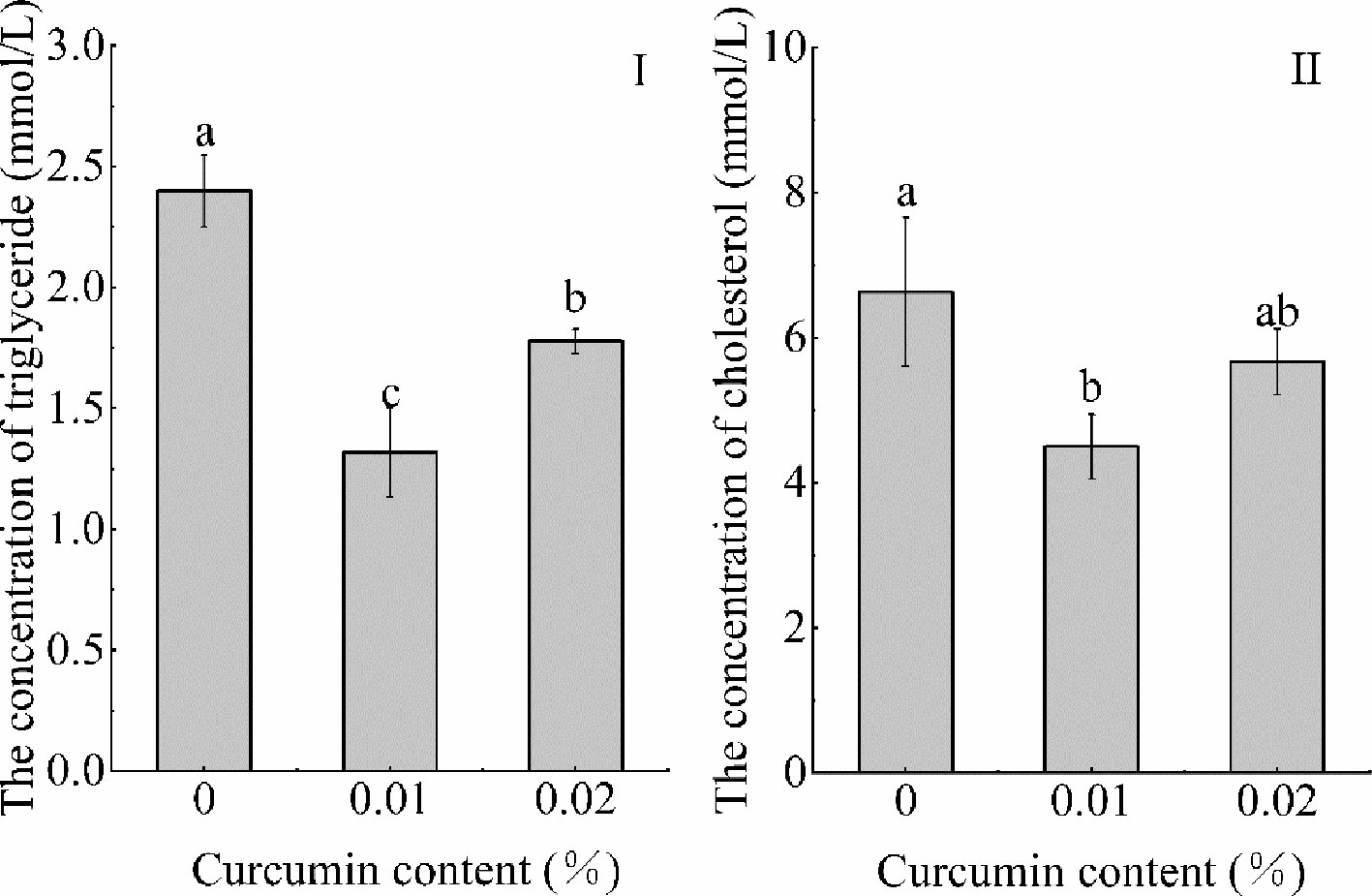
Figure 3 Effects of different levels of curcumin on serum triglyceride and total cholesterol content of Seriola dumerili (I, triglyceride content; II, total cholesterol content). Different superscript letters indicate significant differences among treatments (P < 0.05).
Effects of Curcumin on the Expression of Liver Growth Genes in Seriola dumerili
After feeding 0%, 0.01%, and 0.02% curcumin diets for 8 weeks, the relative gene expression levels of IGF1 and IGF2 in the liver of Seriola dumerili are shown in Figure 4. The relative gene expression levels of IGF1 and IGF2 reached the maximum in the 0.02% treatment group (p < 0.05), while there was no significant difference between the control group and 0.01% treatment group (p > 0.05).
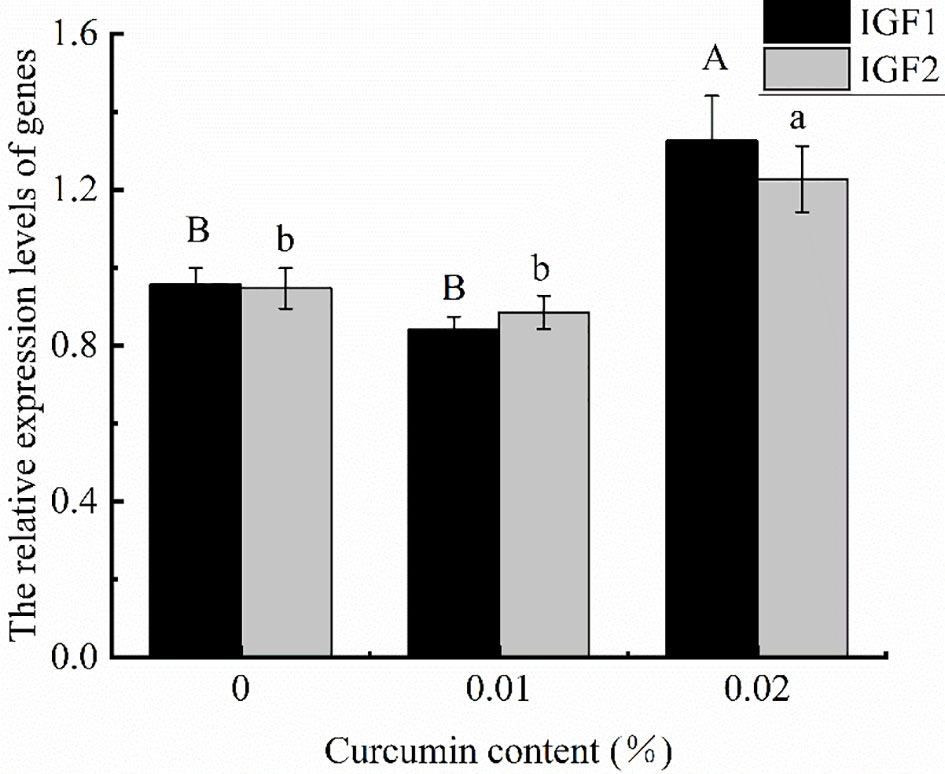
Figure 4 Effects of different levels of curcumin on the quantitative expression of IGF1 and IGF2 genes in the liver of Seriola dumerili. Different uppercase or lowercase letters indicate significant differences between treatments (p < 0.05).
Discussion
Curcumin has been widely used in traditional medicine for a long time because of its anti-inflammatory and antioxidant effects (Oetari et al., 1996; Nishikawa et al., 2013). In addition, recent studies have also reported that curcumin, as a popular immunostimulator, can promote the growth of aquatic animals and enhance disease resistance (Zhang et al., 2014; Guan et al., 2015; Jiang et al., 2016; Wang et al., 2016; Yonar et al., 2019; Ashry et al., 2021). Until now, a number of studies have confirmed that the addition of curcumin in diet can show great growth performance on some species of fish, including large yellow croaker (Pseudosciaena crocea) (Wang and Wu, 2007), grass carps (Ctenopharyngodon idella) (Zhongze et al., 2003), crucian carp (Carassius auratus) (Jiang et al., 2016), Asian see bass (Lates calcarifer) (Abdelwahab and El-Bahr, 2012), and Nile tilapia (Oreochromis niloticus) (Mahmoud et al., 2017). However, the growth of juvenile turbot (Scophthalmus maximus) was not significantly affected by dietary curcumin content, which may be related to various factors such as fish species, size, and culture environment (Wang et al., 2016). This study found that although there was no significant difference in the final average weight, weight gain rate, specific growth rate, visceral ratio, and hepatosomatic ratio between the experimental groups, they were higher in the 0.01% treatment group than in the control group. Moreover, the 0.01% treatment also significantly increased the survival rate and condition factor and reduced the feed coefficient. However, the 0.02% treatment group had a trend to increase the survival rate, final average weight, and visceral ratio and significantly reduced the feed coefficient of Seriola dumerili. The results showed that the addition of curcumin in the feed had a certain promoting effect on the growth of Seriola dumerili and promoted the feed conversion rate, while 0.01% curcumin could better improve the growth performance of Seriola dumerili.
Improvement of growth performance has been reported to be related to the role of curcumin in enhancing intestinal digestive enzyme activity (Jiang et al., 2016; Li et al., 2020; Zhang and Xiao, 2021). Digestive enzymes play a key role in the digestion of nutrients, and their activities directly reflect the digestive ability of fish and affect the growth rate (Ling et al., 2010; Jiang et al., 2016). Moreover, because of its important role in digestive tract digestion and absorption, the intestinal tract of fish has an important impact on the growth and nutrition of fish (El-Bakary, 2010). This study showed that the 0.01% curcumin diet significantly increased the activities of lipase and trypsin in the intestine of Seriola dumerili. That was consistent with the findings on grass carp (Ctenopharyngodon idella) (Li et al., 2020), crucian carp (Carassius auratus) (Jiang et al., 2016), and tilapia (Oreochromis mossambicus) (Midhun et al., 2016). However, this study was contrary to the results of previous studies on amylase. In general, there is a strong relationship between dietary preferences and digestive ability, and high proteolytic (trypsin and pepsin), carbohydrase (cellulase and amylase), and lipase activities reflect high protein, cellulose/starch, and fat diet habits, respectively (Johnston, 2003; Johnston and Freeman, 2005; Qi-Cun et al., 2007). The results of amylase in Seriola dumerili may be related to the fact that it is a carnivorous fish with less starch intake and amylase fluctuates within a low activity range. Carnivorous fish usually eat fat-rich foods that stimulate lipase activity in their digestive tract (Fu et al., 2021a). Compared with the control group, the significant increase of lipase in the treatment group may be related to the decrease of cholesterol level and the stimulation of fat digestion by dietary curcumin (Guan et al., 2015; Zhang and Xiao, 2021). In brief, this study showed that the appropriate amount of curcumin in the feed could enhance the digestive ability of the whole intestine (indicated by the activities of trypsin and lipase), and the 0.01% curcumin content in the feed was the best ratio to enhance the digestion of Seriola dumerili. The above analysis on growth traits confirmed this point well, indicating that curcumin may have the function as a digestive promoter, thereby improving growth performance. The attractive flavor of curcumin can also increase the palatability and intake of feed, while making it of more effective use as feed for fish, thereby improving feed utilization, which can be reflected in the feed conversion ratio. As the fermentation substrate and selective growth factor of beneficial gastrointestinal bacteria, plant chemicals and their metabolites can provide health benefits for organisms. At the same time, as a selective inhibitor of harmful intestinal bacteria, it can also play a prebiotic-like role in fish (Zheng et al., 2009; Harikrishnan et al., 2011). Curcumin contains a large number of bioactive compounds such as reducing sugars, triterpenoids, and alkaloids. These compounds may act as prebiotics which has immunomodulatory properties (Kurhekar, 2013). Therefore, curcumin may also be considered as a prebiotic that enhances the balance of the positive and negative flora in the intestine, increases intestinal absorption and digestion, and ultimately promotes fish growth and health (Midhun et al., 2016).
It has been reported that food composition strongly affects the activities of digestive enzymes and intestinal morphology of aquatic animals (Picos-Garcıía et al., 2000; Garcia-Esquivel and Felbeck, 2006; Wang et al., 2017). Intestinal tissue is the main component of the digestive system to absorb nutrients, and its morphological changes can be used as an important indicator of the health status of the organism (Wang et al., 2017; Amoah et al., 2019). According to previous studies, higher or wider intestinal folds, microvilli, and intestinal villus indicate higher nutrient intake and better health condition of aquatic animals (Li et al., 2020). In this experiment, the 0.02% treatment group significantly increased the intestinal fold width, indicating that curcumin can enhance digestive ability within a certain range. Muscle thickness, intestinal fold height, and goblet cell number are also important intestinal structural parameters, which can reflect the degree of intestinal health (Ma et al., 2018; Sun et al., 2018). Goblet cells have the function of secreting mucus and are the main source of mucin secretion; mucus can lubricate intestinal contents for easy passage, and it protects the intestinal surface from pathogens (Fu et al., 2021a). Reduced levels of mucin production and goblet cell response often occur when the intestine is impaired, such as intestinal infection, and sufficient goblet cells are required to have a possible positive impact on the intestinal host defense system (Kim and Khan, 2013). The number of goblet cells in the control group was significantly lower than in the other two treatment groups, which indicated that curcumin plays an active role in intestinal health and has a positive effect on fish intestinal morphology (Xie, 2017; Fu et al., 2021a) (Heidarieh et al., 2012). The higher feed efficiency in the treatment group may be due to the larger villi area. Curcumin can improve the intestinal digestive enzyme activities, which may be related to the increase in the number of intestinal mature cells. Studies have shown that intestinal digestive enzyme activity is positively correlated with the number of mature cells; that is, the increase in the number of mature cells leads to an increase in intestinal digestive enzyme activity (Zhu, 2021), which was consistent with the results of this study. Strikingly, the response of goblet cells was not consistent with increasing levels of turmeric supplementation and this warrants further research. At higher supplementation, minor effects observed might be due to the fact that high levels of turmeric could reduce feed intake and increased turmeric could also act as a phytoestrogen (Kaur et al., 2020). Muscle thickness is a common indicator to evaluate the body’s ability to absorb and digest nutrients. The thicker the muscle is, the stronger the digestive ability is (Miao et al., 2019). Compared with the control group, curcumin significantly increased the thickness of the muscle layer and goblet cell number of Seriola dumerili in this study, which was similar to the results of previous studies on Anguilla marmorata (Xie, 2017), tilapia (Zhang et al., 2014), and Pseudosciaena crocea (Yu, 2016). It indicated that proper addition of curcumin could improve the morphological structure of intestinal tissue, which may also be one of the reasons for the growth-promoting effect of curcumin.
It has been reported that curcumin can also reduce serum triglyceride and total cholesterol content and promote fat metabolism (Guan et al., 2015). Total cholesterol and triglyceride are collectively referred to as blood lipid levels. Although they only account for a very small part of total fish lipid levels, they are transported between tissues and are very active in metabolism, reflecting lipid metabolism in fish (Deng et al., 2001). Previous studies have shown that the addition of curcumin reduces the contents of triglyceride (TG) and total cholesterol (TC) in serum of grass carp and tilapia, showing a favorable regulatory effect on lipid metabolism (Guan et al., 2015). That was consistent with the results of this study. In vertebrate like fish, lipids are transported as lipoproteins to deliver endogenous and dietary lipids to peripheral tissues, where lipoprotein lipase (LPL) hydrolyzes the triglycerides (TGs) of very low-density lipoprotein (VLDL) or TG-rich lipoprotein and released fatty acids (FAs) are then taken up by the tissues for oxidation or storage with reduced plasma TG level (Salmerón, 2018). However, excessive fat deposits in fish can degrade health and product quality (Salmerón, 2018). In the study of Labeo rohita fingerlings (Chowdhury et al., 2021), the reduced serum TG level in the fish of phyto-additive fed groups compared to non-supplemented control might be mediated through bioactive compounds of dietary phyto-additives that probably enhanced the LPL activity for lipolysis of serum TGs and utilization of released FAs for energy at the cellular level with reduced lipid storage in adipocytes as we could observe significantly reduced body lipid in the fish of phyto-additive groups. Moreover, curcumin reduced fat deposition in the abdominal area of broiler chicks (Rajput et al., 2012) and fat content in liver of tilapia (Guan et al., 2015), respectively. The results are slightly different from those in this paper; this may be due to the different effects of curcumin on fat deposition in different tissues of different age species. Or it may be that the negative feedback effect of excessive fat deposition starts to lead to the reduction of blood lipids, so as to control the excessive accumulation of fat in fish culture. The blood lipid index measured in this study confirmed that both high and low curcumin can reduce the contents of serum total cholesterol and triglyceride, and the low-dose (0.01%) curcumin has the strongest effect on lowering blood lipid.
Insulin-like growth factors (IGFs) are mitogenic peptides, whose expression is generally regulated by exogenous factors (photoperiod and temperature) and endogenous factors (humoral factors and nutritional status) (Fu et al., 2021b). Reinecke et al. (2005) showed that blood levels of IGF-1 or tissue levels of its mRNA were positively proportional to protein content, dietary ration, and systemic growth rates in some fish. In addition, feeding methods also affect the gene expression of IGF-1 and IGF-2 (Gabillard et al., 2006). Studies have shown that polyphenol phytochemicals can increase the growth of fish by stimulating the production of IGF-1 and growth hormone and promoting the synthesis of protein, RNA, DNA, and other synthetic effects. IGFs have been proved to play an important role in fish growth, development, and nutritional metabolism and can be determined by the expression of liver mRNA (Rolland et al., 2015). For example, in the study of golden pompano (Trachinotus ovatus) (Tan et al., 2017), channel catfish (Ictalurus punctatus) (Peterson et al., 2005), clownfish (Amphiprion ocellaris) (Avella et al., 2010), and Barramundi (Lates calcarifer) (Fu et al., 2021b), it was found that IGF-1 and IGF-2 gene expression was positively correlated with fish growth performance. In this study, the expression of IGF-1 and IGF-2 in the 0.02% treatment group was significantly upregulated, and the gene expression was consistent with the growth performance (final average weight) of Seriola dumerili. The growth performance seems to be related to the visceral ratio and feed conversion rate. This association can also be explained by the upregulation of IGF-1, because IGF-1 can promote the proliferation and differentiation of adipose tissue (Chang et al., 2016). In the study of tilapia (Oreochromis mossambicus), curcumin significantly increased the real-time quantitative expression of IGF-1 and IGF-2 genes (Midhun et al., 2016), which was consistent with the results of the 0.02% treatment group in this study. Therefore, the improvement of Seriola dumerili growth performance may be related to the higher content of curcumin promoting IGF secretion. In addition, curcumin enhances nutrient availability, stimulates growth hormone expression, and subsequently increases the gene expression of IGF-1 and IGF-2, suggesting physiological mechanisms of growth.
Conclusion
In summary, the effects of different levels of curcumin in feed on growth performance, intestinal digestive enzyme activity and structural parameters, blood lipid levels, and growth genes of Seriola dumerili were studied. It showed that curcumin had a certain promoting effect on the growth of Seriola dumerili, promoted the feed conversion rate, and had no negative effect on the growth of Seriola dumerili. These increases may be attributed to the digestive promotion characteristics of curcumin and the potential of regulating intestinal structure and enhancing nutritional absorption and production performance. Based on these results, curcumin can promote the growth of Seriola dumerili. Therefore, curcumin can be safely used for fish feed, and it provides a theoretical reference for its application in Seriola dumerili.
Data Availability Statement
The original contributions presented in the study are included in the article/supplementary material, further inquiries can be directed to the corresponding author.
Ethics Statement
This animal study was reviewed and approved by the Animal Welfare Committee of Chinese Academy of Fishery Sciences.
Author Contributions
JY conceived the idea and wrote the manuscript. JH and ZF helped in the field experimental work. JH assisted in the data analysis. ZM supervised the manuscript. All authors contributed to the article and approved the submitted version.
Funding
This work was supported by the Central Public-Interest Scientific Institution Basal Research Fund, CAFS (no. 2020TD55) and Guangxi Innovation Driven Development Special Fund Project [Guike AA18242031].
Conflict of Interest
The authors declare that the research was conducted in the absence of any commercial or financial relationships that could be construed as a potential conflict of interest.
Publisher’s Note
All claims expressed in this article are solely those of the authors and do not necessarily represent those of their affiliated organizations, or those of the publisher, the editors and the reviewers. Any product that may be evaluated in this article, or claim that may be made by its manufacturer, is not guaranteed or endorsed by the publisher.
References
Abdelwahab A., El-Bahr S. (2012). Influence of Black Cumin Seeds (Nigella Sativa) and Turmeric (Curcuma Longa Linn.) Mixture on Performance and Serum Biochemistry of Asian Sea Bass, Lates Calcarifer. World J. Fish Marine Sci. 4 (5), 496–503. doi: 10.5829/idosi.wjfms.2012.04.05.6478
Amoah K., Huang Q.-C., Tan B.-P., Zhang S., Chi S.-Y., Yang Q.-H., et al. (2019). Dietary Supplementation of Probiotic Bacillus Coagulans ATCC 7050, Improves the Growth Performance, Intestinal Morphology, Microflora, Immune Response, and Disease Confrontation of Pacific White Shrimp, Litopenaeus Vannamei. Fish Shellfish Immunol. 87, 796–808. doi: 10.1016/j.fsi.2019.02.029
Ashry A. M., Hassan A. M., Habiba M. M., El-Zayat A., El-Sharnouby M. E., Sewilam H., et al. (2021). The Impact of Dietary Curcumin on the Growth Performance, Intestinal Antibacterial Capacity, and Haemato-Biochemical Parameters of Gilthead Seabream (Sparus Aurata). Animals 11 (6), 1779. doi: 10.3390/ani11061779
Avella M. A., Olivotto I., Silvi S., Place A. R., Carnevali O. (2010). Effect of Dietary Probiotics on Clownfish: A Molecular Approach to Define How Lactic Acid Bacteria Modulate Development in a Marine Fish. Am. J. Physiology-Regulatory Integr. Comp. Physiol. 298 (2), R359–R371. doi: 10.1152/ajpregu.00300.2009
Chang H. R., Kim H. J., Xu X., Ferrante A. W. Jr. (2016). Macrophage and Adipocyte IGF 1 Maintain Adipose Tissue Homeostasis During Metabolic Stresses. Obesity 24 (1), 172–183. doi: 10.1002/oby.21354
Chen C. (2004). “Study on Artificial Propagation, Seedling and Breeding Techniques of Seriola Dumerili,” in The Ichthyology Branch of Oceanology and Limnology Society of China, and the Ichthyology Branch of Zoology Society of China, vol. 1. Beibei, Chongqing, China: Zoology Society of China.
Chowdhury D. K., Sahu N. P., Sardar P., Deo A. D., Bedekar M. K., Singha K. P., et al. (2021). Feeding Turmeric in Combination With Ginger or Garlic Enhances the Digestive Enzyme Activities, Growth and Immunity in Labeo Rohita Fingerlings. Anim. Feed Sci. Technol. 277, 114964. doi: 10.1016/j.anifeedsci.2021.114964
Deng D.-F., Refstie S., Hung S. S. O. (2001). Glycemic and Glycosuric Responses in White Sturgeon (Acipenser Transmontanus) After Oral Administration of Simple and Complex Carbohydrates. Aquaculture 199 (1), 107–117. doi: 10.1016/S0044-8486(01)00515-4
El-Bakary N. (2010). Comparative Histological, Histochemical and Ultrastructural Studies on the Proximal Intestine of Flathead Gray Mullet (Mugil Cephalus) and Sea Bream (Sparus Aurata). World Appl. Sci. J. 8, 477–485.
Fu Z., Yang R., Chen X., Qin J. G., Gu Z., Ma Z. (2019). Dietary non-Protein Energy Source Regulates Antioxidant Status and Immune Response of Barramundi (Lates Calcarifer). Fish Shellfish Immunol. 95, 697–704. doi: 10.1016/j.fsi.2019.11.018
Fu Z., Yang R., Ma Z., Han M., Wang Y. (2021a). Dietary Non-Protein Energy Sources Regulate Growth and Digestion of Barramundi Juvenile (Lates Calcarifer). Pakistan J. Zool. 53, 2279–2287. doi: 10.17582/journal.pjz/20200425050411
Fu Z., Zhou S., Yu G., Yang R., Han M., Ma Z (2021b). Influence of non-Protein Diets on Hepatic Metabolism and Endocrine in Barramundi (Lates Calcarifer). Front. Marine Science 8. doi: 10.3389/fmars.2021.649747
Gabillard J.-C., Kamangar B. B., Montserrat N. (2006). Coordinated Regulation of the GH/IGF System Genes During Refeeding in Rainbow Trout (Oncorhynchus Mykiss). J. Endocrinol. 191 (1), 15–24. doi: 10.1677/joe.1.06869
Garcia-Esquivel Z., Felbeck H. (2006). Activity of Digestive Enzymes Along the Gut of Juvenile Red Abalone, Haliotis Rufescens, Fed Natural and Balanced Diets. Aquaculture 261 (2), 615–625. doi: 10.1016/j.aquaculture.2006.08.022
Giri S. S., Sukumaran V., Park S. C. (2019). Effects of Bioactive Substance From Turmeric on Growth, Skin Mucosal Immunity and Antioxidant Factors in Common Carp, Cyprinus Carpio. Fish Shellfish Immunol. 92, 612–620. doi: 10.1016/j.fsi.2019.06.053
Guan Y., Xie W., Ai C. (2015). The Physiological Function of Curcumin and its Application in Aquatic Feed. China Feed 16), 11–15. doi: 10.15906/j.cnki.cn11-2975/s.20151603
Harikrishnan R., Balasundaram C., Heo M.-S. (2011). Diet Enriched With Mushroom Phellinus Linteus Extract Enhances the Growth, Innate Immune Response, and Disease Resistance of Kelp Grouper, Epinephelus Bruneus Against Vibriosis. Fish Shellfish Immunol. 30 (1), 128–134. doi: 10.1016/j.fsi.2010.09.013
Heidarieh M., Mirvaghefi A. R., Akbari M., Farahmand H., Sheikhzadeh N., Shahbazfar A. A., et al. (2012). Effect of Dietary Ergosan on Growth Performance, Digestive Enzymes, Intestinal Histology, Hematological Parameters and Body Composition of Rainbow Trout (Oncorhynchus Mykiss). Fish Physiol. Biochem. 38 (4), 1169–1174. doi: 10.1007/s10695-012-9602-8
Indira Priyadarsini K. (2013). Chemical and Structural Features Influencing the Biological Activity of Curcumin. Curr. Pharm. design 19 (11), 2093–2100. doi: 10.2174/138161213805289228
Jiang J., Wu X.-Y., Zhou X.-Q., Feng L., Liu Y., Jiang W.-D., et al. (2016). Effects of Dietary Curcumin Supplementation on Growth Performance, Intestinal Digestive Enzyme Activities and Antioxidant Capacity of Crucian Carp Carassius Auratus. Aquaculture 463, 174–180. doi: 10.1016/j.aquaculture.2016.05.040
Ji R., Xiang X., Li X., Mai K., Ai Q. (2021). Effects of Dietary Curcumin on Growth, Antioxidant Capacity, Fatty Acid Composition and Expression of Lipid Metabolism-Related Genes of Large Yellow Croaker Fed a High-Fat Diet. Br. J. Nutr. 126 (3), 345–354. doi: 10.1017/S0007114520004171
Johnston D. (2003). Ontogenetic Changes in Digestive Enzyme Activity of the Spiny Lobster, Jasus Edwardsii (Decapoda; Palinuridae). Marine Biol. 143 (6), 1071–1082. doi: 10.1007/s00227-003-1154-0
Johnston D., Freeman J. (2005). Dietary Preference and Digestive Enzyme Activities as Indicators of Trophic Resource Utilization by Six Species of Crab. Biol. Bull. 208 (1), 36–46. doi: 10.2307/3593099
Kaur A., Shanthanagouda A. H., Kaur V. I., Bansal N., Billekallu Thammegowda N. K. (2020). Biochemical and Histomorphological Associated In Vivo Responses of Turmeric Supplemented Diets in Rohu, Labeo Rohita (Linn.). Aquaculture Res. 51 (9), 3915–3923. doi: 10.1111/are.14740
Khorshidi Z., Sarvi Moghanlou K., Imani A., Behrouzi S. (2018). The Interactive Effect of Dietary Curcumin and Silver Nanoparticles on Gut Microbiota of Common Carp (Cyprinus Carpio). Iranian J. Sci. Technol Trans. A: Sci. 42 (2), 379–387. doi: 10.1007/s40995-016-0130-8
Kim J. J., Khan W. I. (2013). Goblet Cells and Mucins: Role in Innate Defense in Enteric Infections. pathogens 2 (1), 55–70. doi: 10.3390/pathogens2010055
Kurhekar J. V. (2013). Curcuma Longa and Allium Sativum as Prebiotics. Bionano Front. 6 (2), 327–329.
Li C., Liu E., Li T., Wang A., Liu C., Gu Z. (2020). Suitability of Three Seaweeds as Feed in Culturing Strawberry Conch Strombus Luhuanus. Aquaculture 519, 734761. doi: 10.1016/j.aquaculture.2019.734761
Ling J., Feng L., Liu Y., Jiang J., Jiang W.-D., Hu K., et al. (2010). Effect of Dietary Iron Levels on Growth, Body Composition and Intestinal Enzyme Activities of Juvenile Jian Carp (Cyprinus Carpio Var. Jian) 16 (6), 616–624. doi: 10.1111/j.1365-2095.2009.00699.x
Li G., Zhou X., Jiang W., Wu P., Liu Y., Jiang J., et al. (2020). Dietary Curcumin Supplementation Enhanced Growth Performance, Intestinal Digestion, and Absorption and Amino Acid Transportation Abilities in on-Growing Grass Carp (Ctenopharyngodon Idella). Aquaculture Res. 51 (12), 4863–4873. doi: 10.1111/are.14777
Ma Z., Hassan M. M., Allais L., He T., Leterme S., Ellis A. V., et al. (2018). Replacement of Fishmeal With Commercial Soybean Meal and EnzoMeal in Juvenile Barramundi Lates Calcarifer. Aquaculture Res. 49 (10), 3258–3269. doi: 10.1111/are.13790
Mahmoud H. K., Al-Sagheer A. A., Reda F. M., Mahgoub S. A., Ayyat M. S. (2017). Dietary Curcumin Supplement Influence on Growth, Immunity, Antioxidant Status, and Resistance to Aeromonas Hydrophila in Oreochromis Niloticus. Aquaculture 475, 16–23. doi: 10.1016/j.aquaculture.2017.03.043
Mahmoud A., R F. M., A A. S., A.O D. M., S E. S., Kuldeep D. (2020). Curcumin and its Different Forms: A Review on Fish Nutrition. Aquaculture 532 (prepublish). 736030. doi: 10.1016/j.aquaculture.2020.736030
Miao S., Han B., Hu J., Zhu J., Zhang X., Dong X., et al. (2019). Effects of Dietary Different Concentrations of Tetracycline on Growth Performance, Intestinal Microbiota Composition and Morphology of Channa Argus. Chin. J. Anim. Nutr. 31 (12), 5813–5822.
Midhun S. J., Arun D., Edatt L., Sruthi M., Thushara V., Oommen O. V., et al. (2016). Modulation of Digestive Enzymes, GH, IGF-1 and IGF-2 Genes in the Teleost, Tilapia (Oreochromis Mossambicus) by Dietary Curcumin. Aquaculture Int. 24 (5), 1277–1286. doi: 10.1007/s10499-016-9984-1
Nishikawa H., Tsutsumi J., Kitani S. (2013). Anti-Inflammatory and Anti-Oxidative Effect of Curcumin in Connective Tissue Type Mast Cell. J. Funct. Foods 5 (2), 763–772. doi: 10.1016/j.jff.2013.01.022
Oetari S., Sudibyo M., Commandeur J. N. M., Samhoedi R., Vermeulen N. P. E. (1996). Effects of Curcumin on Cytochrome P450 and Glutathione S-Transferase Activities in Rat Liver. Biochem. Pharmacol. 51 (1), 39–45. doi: 10.1016/0006-2952(95)02113-2
Peterson B., Waldbieser G., Bilodeau L. (2005). Effects of Recombinant Bovine Somatotropin on Growth and Abundance of mRNA for IGF-I and IGF-II in Channel Catfish (Ictalurus Punctatus). J. Anim. Sci. 83 (4), 816–824. doi: 10.2527/2005.834816x
Picos-Garcıía C., Garcıía-Carreño F. L., Serviere-Zaragoza E. (2000). Digestive Proteases in Juvenile Mexican Green Abalone, Haliotis Fulgens. Aquaculture 181 (1), 157–170. doi: 10.1016/S0044-8486(99)00223-9
Qi-Cun Z., Zhou J.-B., Chi S.-Y., Yang Q.-H., Liu C.-W. (2007). Effect of Dietary Lipid Level on Growth Performance, Feed Utilization and Digestive Enzyme of Juvenile Ivory Shell, Babylonia Areolate. Aquaculture 272 (1-4), 535–540. doi: 10.1016/j.aquaculture.2007.07.236
Rajput N., Muhammah N., Yan R., Zhong X., Wang T. (2012). Effect of Dietary Supplementation of Curcumin on Growth Performance, Intestinal Morphology and Nutrients Utilization of Broiler Chicks. J. Poult Sci. 50 (1), 44–52. doi: 10.2141/jpsa.0120065
Reinecke M., Björnsson B. T., Dickhoff W. W., McCormick S. D., Navarro I., Power D. M., et al. (2005). Growth Hormone and Insulin-Like Growth Factors in Fish: Where We are and Where to Go. Gen. Comp. Endocrinol. 142 (1-2), 20–24. doi: 10.1016/j.ygcen.2005.01.016
Rolland M., Dalsgaard J., Holm J., Gómez-Requeni P., Skov P. V. (2015). Dietary Methionine Level Affects Growth Performance and Hepatic Gene Expression of GH–IGF System and Protein Turnover Regulators in Rainbow Trout (Oncorhynchus Mykiss) Fed Plant Protein-Based Diets. Comp. Biochem. Physiol. Part B: Biochem. Mol. Biol. 181, 33–41. doi: 10.1016/j.cbpb.2014.11.009
Salmerón C. (2018). Adipogenesis in Fish. J. Exp. Biol. 221 (Suppl_1), jeb161588. doi: 10.1242/jeb.161588
Sruthi M., Nair A. B., Arun D., Thushara V., Sheeja C., Vijayasree A. S., et al. (2018). Dietary Curcumin Influences Leptin, Growth Hormone and Hepatic Growth Factors in Tilapia (Oreochromis Mossambicus). Aquaculture 496, 105–111. doi: 10.1016/j.aquaculture.2018.06.083
Sun Z., Tan X., Ye H., Zou C., Ye C., Wang A. (2018). Effects of Dietary Panax Notoginseng Extract on Growth Performance, Fish Composition, Immune Responses, Intestinal Histology and Immune Related Genes Expression of Hybrid Grouper (Epinephelus Lanceolatus♂× Epinephelus Fuscoguttatus♀) Fed High Lipid Diets. Fish Shellfish Immunol. 73, 234–244. doi: 10.1016/j.fsi.2017.11.007
Tan X., Sun Z., Huang Z., Zhou C., Lin H., Tan L., et al. (2017). Effects of Dietary Hawthorn Extract on Growth Performance, Immune Responses, Growth-and Immune-Related Genes Expression of Juvenile Golden Pompano (Trachinotus Ovatus) and its Susceptibility to Vibrio Harveyi Infection. Fish Shellfish Immunol. 70, 656–664. doi: 10.1016/j.fsi.2017.09.041
Thakur D. P., Morioka K., Itoh N., Wada M., Itoh Y. (2009). Muscle Biochemical Constituents of Cultured Amberjack Seriola Dumerili and Their Influence on Raw Meat Texture. Fish Sci. 75 (6), 1489–1498. doi: 10.1007/s12562-009-0173-2
Wang Y., Wang Y., Mai K., Xu W., Zhang Y., Zhou H., et al. (2016). Effects of Dietary Curcumin on Growth Performance, Body Composition and Serum Antioxidant Enzyme Activity in Juvenile Turbot (Scophthalmus Maximus). J. Fish China 40 (9), 1299–1308.
Wang Y.-r., Wang L., Zhang C.-x., Song K. (2017). Effects of Substituting Fishmeal With Soybean Meal on Growth Performance and Intestinal Morphology in Orange-Spotted Grouper (Epinephelus Coioides). Aquaculture Rep. 5, 52–57. doi: 10.1016/j.aqrep.2016.12.005
Wang J., Wu T. (2007). Effect of Curcumin on the Feed in Large Yellow Croaker (Pseudosciaene Crocea). Reserv Fish 6, 105–106.
Wu H., Pan J. (2000). Experimental Studies On Antibiotic Treatment Of Vibriosis Of Marinecage-Cultured Seriola Dumerili. J. Trop. Oceanogr 01), 85–89.
Xie W. (2017). Effects of Four Functional Feed Additives on Growth Performance, Lipid Metabolism, Non-Specific Immunity and Health of Liver and Intestine of Anguilla Marmorata (Xiamen, Fujian, China: Xiamen University).
Yang R., Han M., Fu Z., Wang Y., Zhao W., Yu G., et al. (2020). Immune Responses of Asian Seabass Lates Calcarifer to Dietary Glycyrrhiza Uralensis. Animals 10 (9), 1629. doi: 10.3390/ani10091629
Yonar M. E., Yonar S. M., İspir Ü., Ural M.Ş. (2019). Effects of Curcumin on Haematological Values, Immunity, Antioxidant Status and Resistance of Rainbow Trout (Oncorhynchus Mykiss) Against Aeromonas Salmonicida Subsp. Achromogenes. Fish Shellfish Immunol. 89, 83–90. doi: 10.1016/j.fsi.2019.03.038
Yu J. (2016). Effects of Dietary Curcumin on Growth and Non-Specific Immunity in Pseudosciaena Crocea (Shanghai, China: Shanghai Ocean University).
Zhang T., Chen Q., Zhang B., Lin C., Zhu G., Fang Z., et al. (2017). The Effects of Curcumin on the Growth, Digestion and Antioxidant Ability of Yellow Catfish (Pelteobagrus Fulvidraco). Prog. Fishery Sci. 38 (06), 56–63.
Zhang Y., Song L., Hu B., Mao S., Xu P. (2018). Effect of Curcumin on Growth Performance and Protective Effect of Liver Injury Induced by Carbon Tetrachloride in Oreochromis Niloticus. J. Fishery Sci. China 25 (06), 1271–1280. doi: 10.3724/SP.J.1118.2018.17437
Zhang H., Xiao D. (2021). The Physiological Function of Curcumin and its Application in Aquaculture Industry. Feed Res. 44 (05), 133–136. doi: 10.13557/j.cnki.issn1002-2813.2021.05.032
Zhang B., Zhang B., Xiao P. (2014). Effects of Curcumin on Growth Performance, Serum Biochemical Parameters and Intestinal Morphology of Tilapia. China Feed 02), 34–37. doi: 10.15906/j.cnki.cn11-2975/s.2014.02.008
Zheng Z., Tan J. Y., Liu H., Zhou X., Xiang X., Wang K. (2009). Evaluation of Oregano Essential Oil (Origanum Heracleoticum L.) on Growth, Antioxidant Effect and Resistance Against Aeromonas Hydrophila in Channel Catfish (Ictalurus Punctatus). Aquaculture 292 (3-4), 214–218. doi: 10.1016/j.aquaculture.2009.04.025
Zhongze H., Jiufeng Y., Zhijing T. (2003). Effect of Curcumin on the Growth and Activity of Digestive Enzyme in Grass Carps (Ctenopharyngodon Idells). Cereal Feed Ind. 2003 (11), 29–30.
Zhu H. (2021). Effects of Dietary Curcumin Supplementation on Growth, Intestinal and Liver Health Ofjuvenile American Eel (Anguilla Rostrata) (Xiamen, Fujian, China: Jimei university).
Keywords: Seriola dumerili, curcumin, growth performance, digestion, growth gene
Citation: Yang J, Hong J, Fu Z and Ma Z (2022) Effects of Dietary Curcumin on Growth and Digestive Physiology of Seriola dumerili. Front. Mar. Sci. 9:862379. doi: 10.3389/fmars.2022.862379
Received: 25 January 2022; Accepted: 09 March 2022;
Published: 05 April 2022.
Edited by:
Jin Niu, Sun Yat-sen University, ChinaReviewed by:
Qingchao Wang, Huazhong Agricultural University, ChinaYongjiang Xu, Chinese Academy of Fishery Sciences (CAFS), China
Copyright © 2022 Yang, Hong, Fu and Ma. This is an open-access article distributed under the terms of the Creative Commons Attribution License (CC BY). The use, distribution or reproduction in other forums is permitted, provided the original author(s) and the copyright owner(s) are credited and that the original publication in this journal is cited, in accordance with accepted academic practice. No use, distribution or reproduction is permitted which does not comply with these terms.
*Correspondence: Zhenhua Ma, emhlbmh1YS5tYUBob3RtYWlsLmNvbQ==
†These authors have contributed equally to this work