- 1Laboratory of Aquatic Animal Nutrition and Feed, Fisheries College, Guangdong Ocean University, Zhanjiang, China
- 2Aquatic Animals Precision Nutrition and High Efficiency Feed Engineering Research Center of Guangdong Province, Zhanjiang, China
- 3Key Laboratory of Aquatic, Livestock and Poultry Feed Science and Technology in South China, Ministry of Agriculture, Zhanjiang, China
- 4Guangdong Provincial Key Lab of Pathogenic Biology and Epidemiology for Aquatic Economic Animals, Zhanjiang, China
Little is known about the association of bile acids (BAs) with lipid deposition and lipid metabolism of fish in vitro. In the present study, we established the model of fatty-degenerated hepatocytes in pearl gentian grouper (Epinephelus fuscoguttatus♂ × Epinephelus lanceolatus♀), and explored the effects and mechanism of BAs regulating on lipid metabolism in this model. The results showed that fatty-degenerated hepatocytes induced by lipid emulsion accumulated the intracellular triglyceride (TG), the enhanced expression of sterol responsive element binding protein 1 (SREBP1) protein, and the repressed expression of peroxisome proliferator-activated receptor alpha (PPARA), and phosphorylated PPARA (P-PPARA) proteins. BAs co-incubation reduced the content of TG, while increasing the expression of PPARA, farnesoid X receptor (FXR), and G protein-coupled bile acid receptor 1 (TGR5) proteins. Activation of FXR by INT-747 reduced the content of TG, while down-regulating the expression of SREBP1 and PPARA, and up-regulating the expression of P-PPARA, FXR, small heterodimer partner (SHP), and TGR5. Inhibition of FXR by guggulsterone increased the level of TG, while decreasing the expression of TGR5, increasing the expression of SHP. Activation of TGR5 by INT-777 reduced the content of TG, while down-regulating the expression of SREBP1 and SHP, up-regulated the expression of TGR5. Inhibition of TGR5 by SBI-115 elevated the level of TG, while reducing the expression of P-PPARA and TGR5. In conclusion, the FXR and/or TGR5 signaling pathways which were inhibited in fatty-degenerated hepatocytes from pearl gentian grouper, were activated after BAs co-incubation, then altering the lipid metabolism by repressing lipogenesis and enhancing lipolysis, and regulating transcriptional factors, thus reduces the lipid accumulation in vitro.
Introduction
Groupers are the important mariculture species in China, with a total output of 192,000 tons in 2020, an increase of 4.87% over 2019 (Food Organization, 2020; China Agriculture Press, 2021). Among them, the hybrid grouper, Epinephelus fuscoguttatus♂ × Epinephelus lanceolatus♀, also known as the pearl gentian grouper, is a popular farmed marine fish in Southeast Asia and China, and is intensively cultured along the coastlines of these areas (Xu et al., 2021). High-lipid diets (>13%) are generally used in this fish species, but it will lead to abnormal lipid metabolism and fat accumulation, which results in the susceptibility of fatty liver, then impair the health of liver, and ultimately damage to the growth of fish (Shapawi et al., 2018; Zou et al., 2019). Therefore, it is of great significance to study the mechanism of lipid deposition in fish, and it is necessary to find effective ways to improve the adverse effects induced by high-fat intake.
Bile acids (BAs) are endocrine molecules with a number of basic functions, such as cholesterol catabolism, intestinal fat emulsification, lipid metabolism, and intestinal microbiota homeostasis (Xie et al., 2021b; Xu et al., 2022a). In fact, BAs have broader biological effects, as they are recognized as regulators of farnesoid X receptor (FXR) and G protein-coupled bile acid receptor 1 (TGR5), and therefore, hold important promise for altering lipid content in the treatment of metabolic diseases (Wang et al., 2016; Kumari et al., 2020). Specifically, FXR is a ligand-activated transcription factor that belongs to the nuclear receptor superfamily and plays a vital role in regulating genes network related to the maintenance of lipid homeostasis (Kumari et al., 2020). Many in vitro and in vivo studies have used mouse models to clarify the positive association of FXR with fatty liver disease and its regulatory role on lipid metabolism (Yang et al., 2010; Kumari et al., 2020). In addition, TGR5 is coupled to a stimulatory G-protein and is responsive to various BAs, such as taurolithocholic acid, lithocholic acid, deoxycholic acid, chenodeoxycholic acid, and cholic acid (Reich et al., 2016). In humans, the activation of TGR5 shows promise for the treatment of various metabolic diseases such as type 2 diabetes and obesity (Guo et al., 2016). In mice, TGR5 can prevent oxidative stress and lipid accumulation in kidney (Wang et al., 2016), and the development of steatosis in liver (Pols et al., 2011). Several studies explored the effects of dietary supplementation of BAs on fat metabolism in fish, such as largemouth bass (Micropterus salmoides) (Yu et al., 2019; Yin et al., 2021), tiger puffer (Takifugu rubripes) (Liao et al., 2020), Nile tilapia (Oreochromis niloticus) (Jiang et al., 2018; El-Shenawy et al., 2020), large yellow croaker (Larimichthys crocea) (Ding et al., 2020), grass carp (Ctenopharyngodon idella) (Zhou et al., 2018), and turbot (Scophthalmus maximus) (Huang et al., 2015); however, it is unclear whether the underlying mechanism is through the FXR and TGR5 signaling pathways.
Compared to in vivo experiments, in vitro experiments can be used as auxiliary models for whole-animal assays in vivo to assess the thorough effects of exogenous substances (Zhu et al., 2014). As an excellent in vitro platform, fish cell lines have been widely used in virology, immunology, toxicology, and nutritional analysis (Collet et al., 2018; Li et al., 2022). The liver is a critical organ in metabolic processes and plays a key role in the metabolism of nutrients (Zou et al., 2019). Therefore, fish liver cell lines from various species are commonly used for studying lipid metabolism, such as grass carp (Lu et al., 2012), pearl gentian grouper (Zou et al., 2018, 2019), yellow catfish (Pelteobagrus fulvidraco) (Chen et al., 2014; Song et al., 2015b), large yellow croaker (Lai et al., 2021), and zebrafish (Danio rerio) (Wang et al., 2019). However, so far, no research has focused on the functions of BAs in fish cells and the mechanism behind.
Therefore, the purpose of this study was to evaluate the role of BAs in the regulation of lipid deposition in primary hepatocytes from pearl gentian grouper. First, we explored the effects of BAs on lipid metabolism in a fatty-degenerated hepatocytes model. Then, we separately studied the effects of FXR and TGR5 signaling pathways on lipid metabolism in vitro. This study was firstly investigated the roles of BAs in the fat metabolism of hepatocytes in fish. The findings of the present study would be helpful in developing the new feed additives to improve the lipid deposition in fish.
Materials and Methods
Reagents and Animals
The taurocholic acid sodium (TCA, CAS: 345909-26-4, T4009) was purchased from Sigma Chemical Co. (Burlington, MA, United States). INT-747 (FXR agonist, CAS: 459789-99-2, HY-12222), guggulsterone (FXR antagonist, CAS: 95975-55-6, HY-107738), SBI-115 (TGR5 antagonist, CAS: 882366-16-7, HY-111534), and INT-777 (TGR5 agonist, CAS: 1199796-29-6, HY-15677) were purchased from the MedChemExpress Co. (Monmouth Junction, United States). Lipid emulsion solution (LE, 20% soybean oil, 1.2% lecithin, and 78.8% glycerinum) was purchased from Sichuan Kelun Pharmaceutical Co., Ltd (China). MEM medium was purchased from Procell Co. (Wuhan, China). Fetal bovine serum (FBS) was obtained from Gibco Invitrogen (UK). Penicillin and streptomycin were obtained from Sigma-Aldrich (United States). The kit of Cell Counting Kit-8 (CCK-8) was purchased from Glpbio Technology Co. (California, United States). The kits of triglyceride (TG, A110-1-1) and total protein (TP, A045-4) were purchased from Nanjing Jian Cheng Bioengineering Institute (Nanjing, China). The primary antibodies against sterol responsive element binding protein 1 (SREBP1, ab28481) and phosphor-peroxisome proliferator-activated receptor alpha (P-PPARA, S12, ab3484) were purchased from Abcam Co. (Cambridge, UK); PPARA (66836-1-Ig) from Proteintech Co. (Chicago, United States); FXR (bs-12867R) and small heterodimer partner (SHP, bs-4311R) from Bioss Co. (China); TGR5 (NBP2-23669SS) from Novus Co. (China); GAPDH (2118S) from Cell Signaling Technology (MA, United States).
All animals were handled and experimental procedures were conducted in accordance with the Care and Use of Laboratory Animals in China, Animal Ethical and Welfare Committee of China Experimental Animal Society. The study was approved by the Animal Ethical and Welfare Committee of Guangdong Ocean University. The permit number for conducting animal experiments is GDOU-AEWC-20180063.
Cell Culture and Treatments
The pearl gentian grouper (Epinephelus fuscoguttatus♀ × E. lanceolatus♂) was obtained and domesticated according to the method of our study (Xu et al., 2022b). Primary hepatocytes were isolated from pearl gentian grouper liver as described in previous study (Zou et al., 2019). Briefly, the fish was cleared of blood, disinfected with alcohol. The liver was carefully excised, rinsed twice with phosphate buffer saline (PBS) supplemented with streptomycin (200 μg/ml) and penicillin (200 IU/ml). Next, liver was aseptically minced, digested by sterile trypsin, neutralized with MEM medium containing FBS. Then, cell suspension was filtered, collected in centrifuge tubes, centrifuged twice at low speed (100 × g, 5 min), and once at a lower speed (50 × g, 5 min). Finally, the purified hepatocytes were resuspended with MEM medium containing 15% FBS, penicillin (100 IU/ml), and streptomycin (100 μg/ml), and counted by hemocytometer. When the viability of cells was > 92%, the cells suspension was plated onto 75 cm2 flasks at 106 cells/ml. The hepatocytes were cultured at 27°C with 5% (v/v) CO2, and the medium was replaced every 2–3 days. Upon reaching 80–90% confluency, cells were harvested by trypsin, and transferred into two new flasks.
The hepatocytes was treated as follows: (1) V-CN group (MEM medium without any treatment); (2) V-HL (0.5 ml/L LE); (3) V-TCA (0.5 ml/L LE + 100 μM TCA); (4) V-T747 (0.5 ml/L LE + 100 μM TCA + 5 μM obeticholic acid); (5) V-TGU (0.5 ml/L LE + 100 μM TCA + 10 μM guggulsterone); (6) V-T777 (0.5 ml/L LE + 100 μM TCA + 10 μM INT-777); (7) V-TSBI (0.5 ml/L LE + 100 μM TCA + 1 μM SBI-115). Each treatment was performed in triplicate, and three independent experiments were carried out. In the latter four groups, the activators or inhibitors were added at 48 h after adding the LE. The 2 h later (at 50 h), in the latter five groups, the TCA was added after adding the LE. Sampling was conducted after 96 h of incubation in all groups. The concentrations and periods of LE, TCA incubation, as well as specific inhibitors and activators, were selected according to our preliminary experiment and previous studies (Zou et al., 2019).
Liver Staining, Cell Viability, and Biochemical Assays
The Bodipy 493/503 staining for liver or hepatocytes was conducted according to the established protocol (Zhao et al., 2020), and hepatocytes were observed with a laser scanning confocal microscope (Leica Microsystems, Wetzlar, Germany) to visualize the intensity of fluorescence (the green dots were defined as lipid droplet) under 400 × magnification. Cell viability was determined using the CCK-8 assay (Zou et al., 2019). The contents of TG and TP were measured using commercial kits (Nanjing Jian Cheng Bioengineering Institute, Nanjing, China) (Zou et al., 2019).
qPCR Analyses
The details of total RNA extraction, cDNA synthesis, and qPCR assays of hepatocytes sample were described in a previous study (Xu et al., 2022b). Based on transcriptome sequences obtained by our team from pearl gentian grouper (Zhang et al., 2021), the present study chose genes such as (Table 1): 6pgd, 6-phosphogluconate dehydrogenase; acbp, acyl-CoA binding protein; acc, acetyl-CoA carboxylase; atgl, adipose triglyceride lipase; cpt1, carnitine palmitoyltransferase 1; dgat, acyl CoA diacylglycerol acyltransferase 2; dgka, diacylglycerol kinase alpha; fabp, fatty acid-binding protein; fas, fatty acid synthase; fxr, farnesoid X receptor; g6pd, glucose 6-phosphate dehydrogenase; hl, hepatic lipase; hsl, hormone-sensitive lipase; lxr, liver X receptor alpha; me, malic enzyme; ppara; pparr, peroxisome proliferator-activated receptor-gamma; srebp1; tgr5. The references genes 18s (F: AGCAACTTTAGTATACGCTATTG; R: CCTGAGAAACGGCTACCACATC) and β-actin (F: TACGAGC TGCCTGACGGACA; R: GGCTGTGATCTCCTTCTGC) were selected as the reference gene on the basis of its expression stability (Xu et al., 2022b). We calculated the relative expression of genes using the 2–ΔΔCT method (Xu et al., 2022a).
Western Blot Analyses
According to our previous methods (Xu et al., 2022b), phosphorylase inhibitors, protease inhibitors, cell lysate, PBS, and phenylmethanesulfonyl fluoride (PMSF) were added to tissues for fragmentation. After the concentration of protein was determined, loading buffer and PBS were added to make the final concentration at 4 mg/ml, then degeneration. Each gel hole was added 32 mg total protein in SDS-PAGE separation: electrophoresis running with 90 V for 30 min; transferring to PVDF membrane (Billerica, MA, United States); electrophoresis running with 110 V for 80 min. The membrane incubated with primary antibody overnight at 4°C, washed three times with TBST, and incubated with secondary antibody for 1 h. After visualizing with ECL reagents (Billerica, MA, United States), the Western bands were quantified using Image J (version 1.42, National Institutes of Health). The following antibodies were performed: SREBP1 (1:800), PPARA (1:1000), P-PPARA (1:800), FXR (1:500), SHP (1:800), TGR5 (1:500), and GAPDH (1:1000).
Statistical Analysis
The data were tested for normality and homogeneity of variances using the Shapiro–Wilk and Levene’s tests, and evaluated by one-way ANOVA and further analyzed by the Duncan’s multiple range tests. For the comparison between two groups, Student’s t-tests were used (unpaired, two-tailed). The analyses were performed with SPSS 23.0 (IBM, Armonk, NY, United States). Results were presented as means ± SD and statistically significant was set as p < 0.05.
Results
Fatty-Degenerated Hepatocytes Increased, While Bile Acids Co-incubation Reduced the Lipid Accumulation of Pearl Gentian Grouper in vitro
The CCK-8 assay showed that LE concentrations of 0.1–0.5 ml/L had no significant influence on the viability of hepatocytes (Figure 1). Thus, we chose the dose of 0.5 ml/L LE for the 48 h incubation to build the fatty degenerated hepatocytes model (FDH). The FDH was then incubated with LE for another 48 h (V-HL group). Same as the features of fatty degeneration in grass carp hepatocytes (Lu et al., 2012), the V-HL group increased the lipid accumulation (the area of green dots in Bodipy 493/503 staining and the level of TG) of hepatocytes compared to the V-CN group (hepatocytes without any treatment) (Figures 2, 3A). The V-HL group significantly up-regulated the expression of SREBP1 protein, significantly down-regulated the expression of PPARA and P-PPARA proteins (Figure 3). In addition, compared to V-CN treatment, the V-HL treatment significantly increased the expression of lipogenesis genes (6pgd, acc, fas, g6pd, and me) and their transcriptional factors genes (lxr and srebp1), whereas significantly decreased the expression of lipolysis genes (atgl, cpt1, dgat, dgka, and hl), fatty acid uptake genes (acbp and fabp), fxr, and tgr5 (Figure 4).
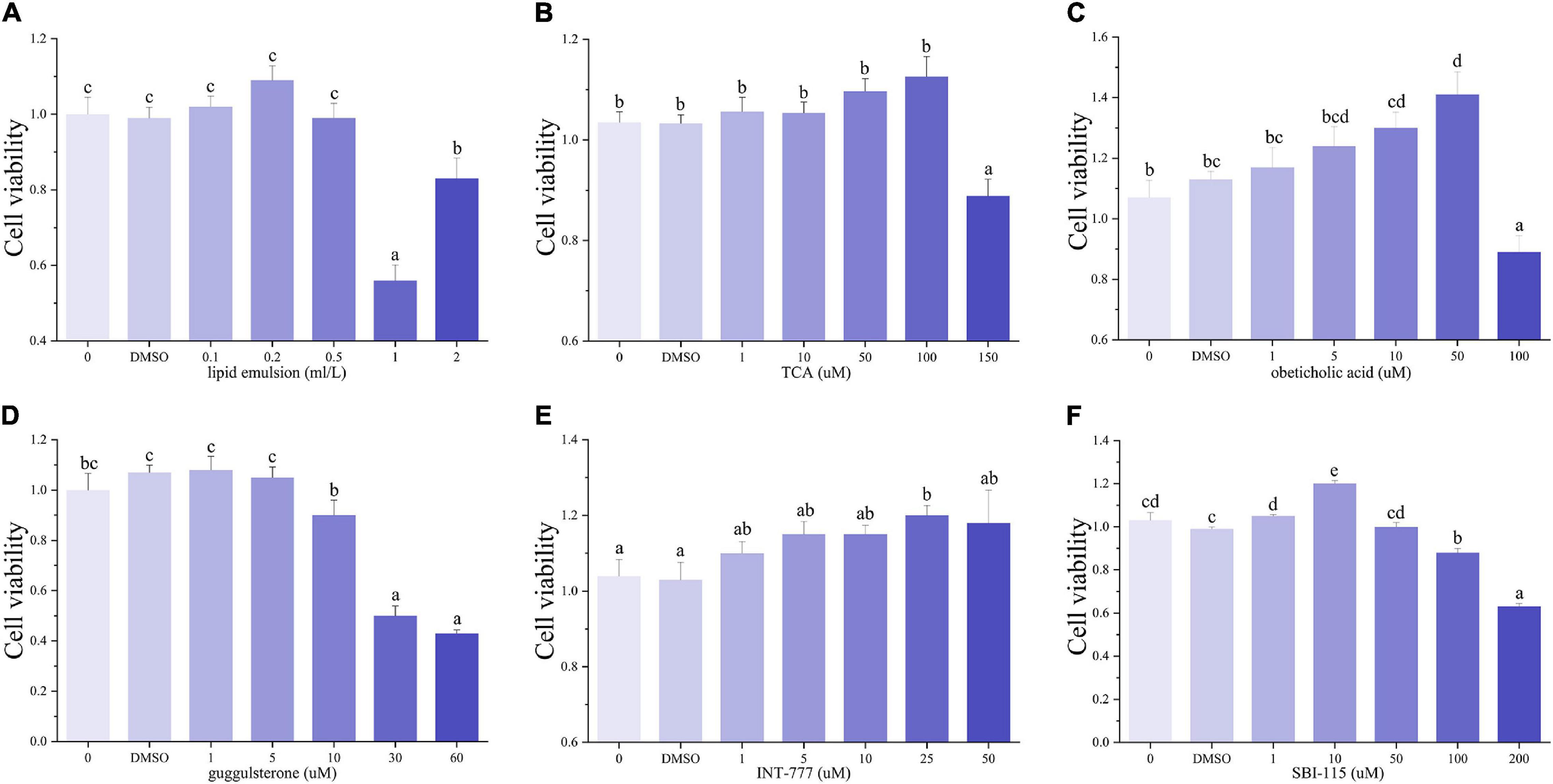
Figure 1. The cell viability of hepatocytes incubated with lipid emulsion (A), TCA (B), obeticholic acid (C), guggulsterone (D), SBI-115 (E), and INT-777 (F) at different concentrations (n = 9). TCA: taurocholic acid sodium; obeticholic acid: INT-747, FXR agonist; guggulsterone: FXR antagonist; SBI-115: TGR5 antagonist; INT-777: TGR5 agonist.
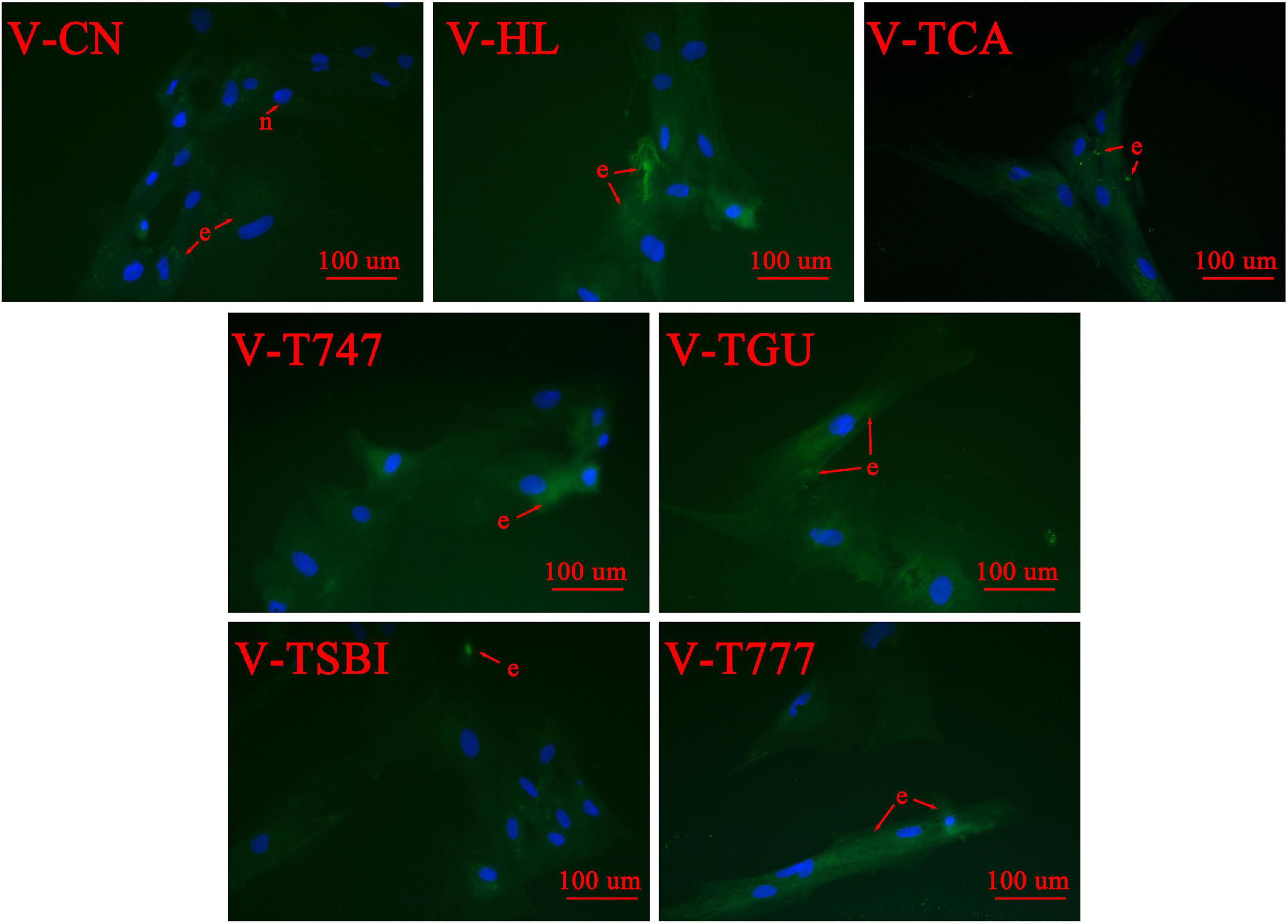
Figure 2. The Bodipy 493/503 staining of different treatments in 400 × magnification (n = 9). e, lipid droplets; n, nuclei. The lipid droplets are stained green and the nuclei are stained blue.
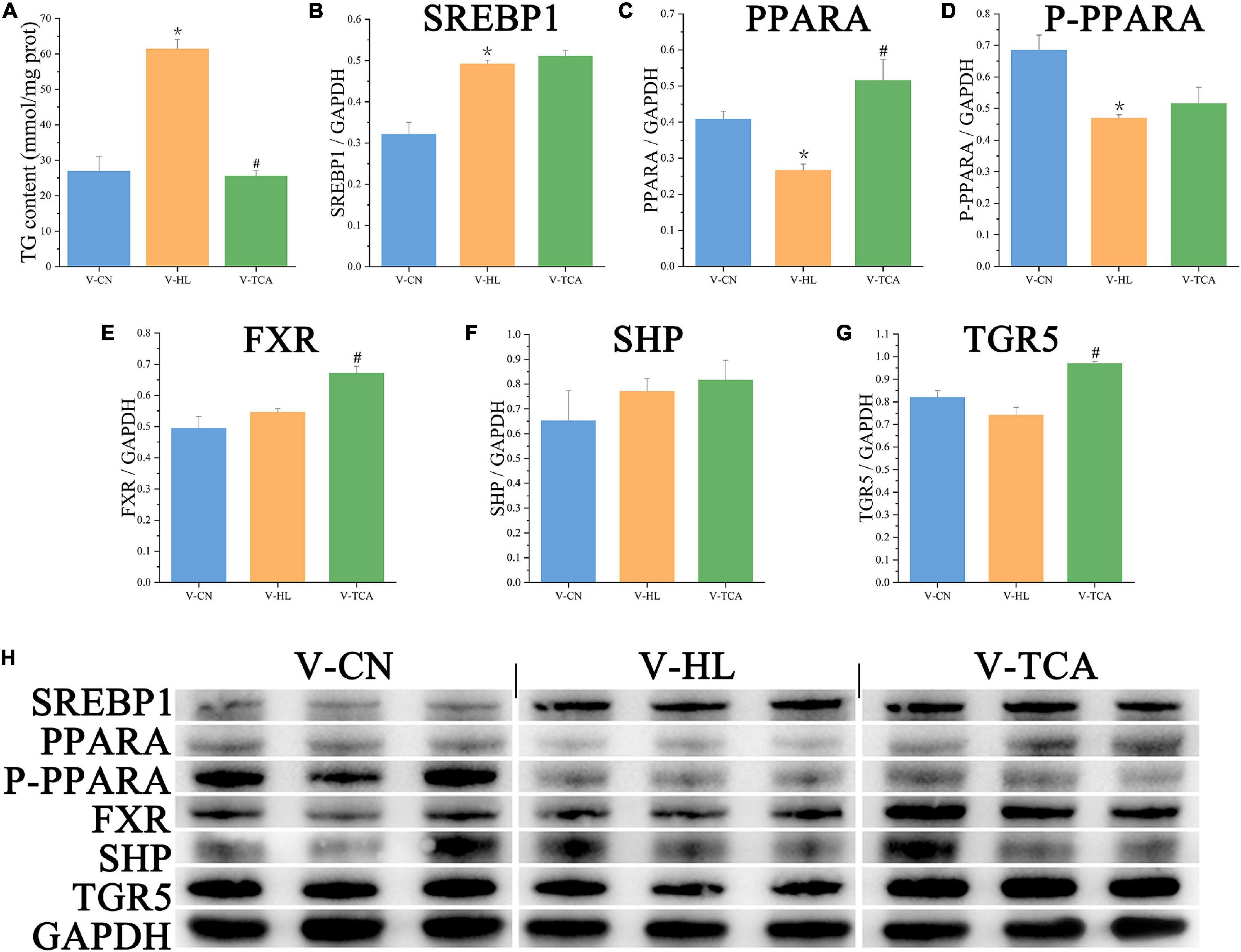
Figure 3. The lipid accumulation and metabolism of hepatocytes in lipid emulsion and BAs incubation. (A) The content of intracellular TG (n = 9). (B–G) The relative quantification of protein levels of SREBP1, PPARA, P-PPARA, FXR, SHP, and TGR5 normalized to GAPDH in hepatocytes (n = 3); (H) the Western blot band of hepatocyte samples (n = 3). Values are presented as means with SD, where “*” indicates significant (p < 0.05) differences in the V-HL group (compared to the V-CN group), and “#” indicates significant (p < 0.05) differences in the V-TCA group (compared to the V-HL group).
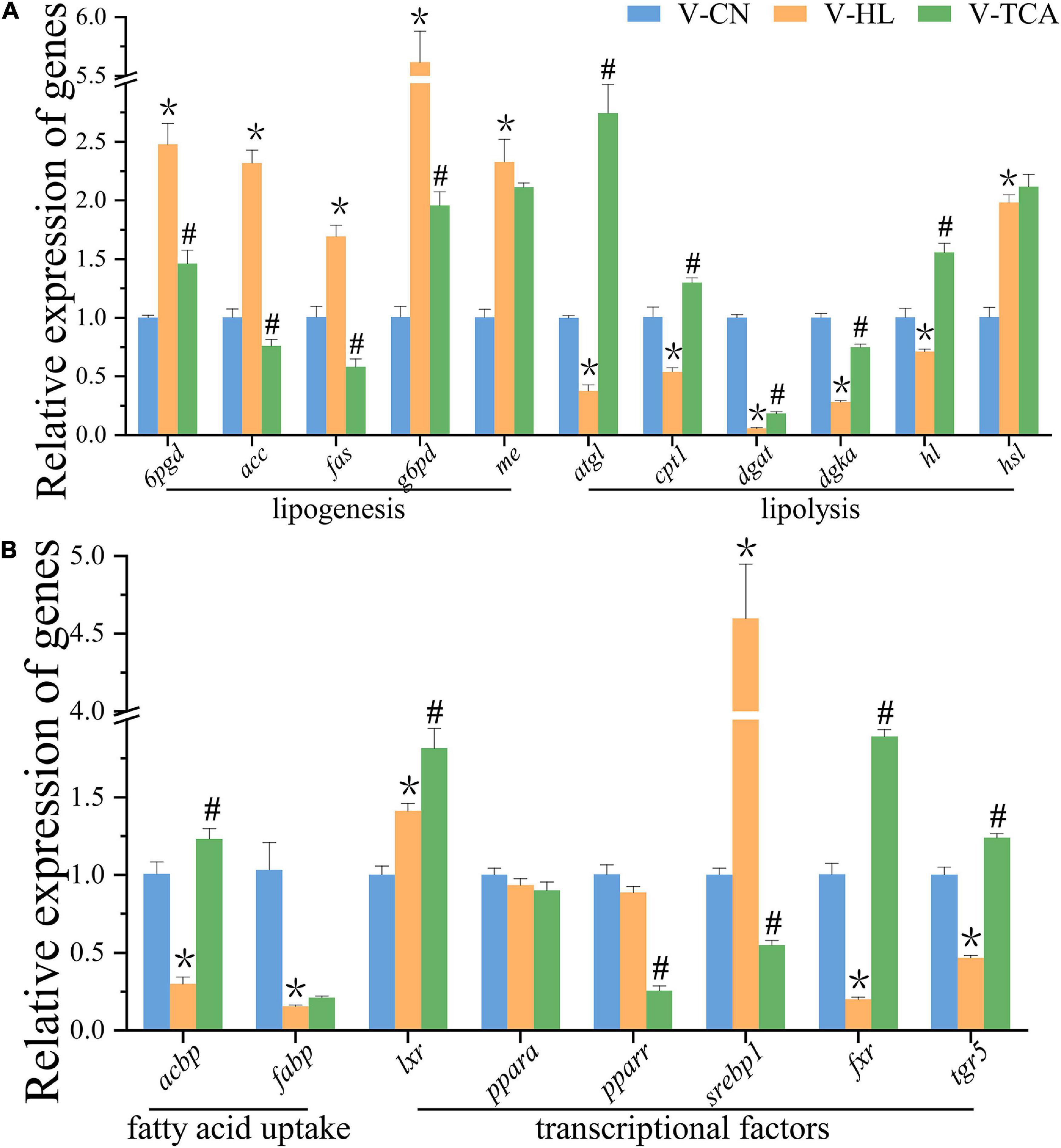
Figure 4. The relative expression of genes associated with lipid metabolism of hepatocytes normalized to 18s and β-actin in lipid emulsion and BAs incubation (A,B) (n = 9). Values are presented as means with SD, where “*” indicates significant (p < 0.05) differences in the V-HL group (compared to the V-CN group), and “#” indicates significant (p < 0.05) differences in the V-TCA group (compared to the V-HL group).
According to the results of CCK-8 assay, the FDH were co-incubated with 100 μM TCA for another 48 h (V-TCA group) (Figure 1). The TG concentration of the V-TCA group was significantly decreased, compared to the V-HL group (Figure 3A). The TCA incubation significantly elevated the expression of PPARA, FXR, and TGR5 proteins. Meanwhile, in V-TCA group, the expression of lipogenesis genes (6pgd, acc, fas, and g6pd) and their transcriptional factors genes (pparr and srebp1) were significantly decreased, whereas the expression of lipolysis genes (atgl, cpt1, dgat, dgka, and hl), as well as fxr and tgr5, significantly increased (Figure 4). These results showed that fatty-degenerated hepatocytes induced by LE increased the lipid accumulation via up-regulating the lipogenesis and down-regulating the lipolysis, also repressed the expression of fxr and tgr5; BAs co-incubation improved the lipid metabolism and reduced the fat accumulation of fatty-degenerated hepatocytes, while activated the FXR and TGR5 signaling pathways.
Regulation of Bile Acids on Lipid Deposition of Hepatocyte Was Dependent on the Activation of Farnesoid X Receptor Signaling in vitro
According to the CCK-8 assay, the 5 μM obeticholic acid and 10 μM guggulsterone had no significant influence on the viability of hepatocytes (Figure 1). Prior to the TCA incubation, the FDH pre-treated with obeticholic acid for 2 h (V-T747 group) or guggulsterone (V-TGU group) enhanced or alleviated the TCA-induced down-regulation of lipid accumulation, respectively (Figures 2, 5A). Compared to the V-TCA group, the V-T747 group exhibited a significantly decreased expression of SREBP1 and PPARA proteins, whereas a significantly increased expression of P-PPARA, FXR, SHP, and TGR5 proteins (Figure 5). Meanwhile, the V-T747 treatment significantly down-regulated the expression of lipogenesis genes (6pgd, acc, fas, and me) and their transcriptional factors genes (lxr, pparr, and srebp1), whereas significantly up-regulated the expression of fxr, fatty acid uptake gene (fabp), lipolysis genes (cpt1, dgat, dgka, hl, and hsl), and their transcriptional factors gene (ppara) (Figure 6).
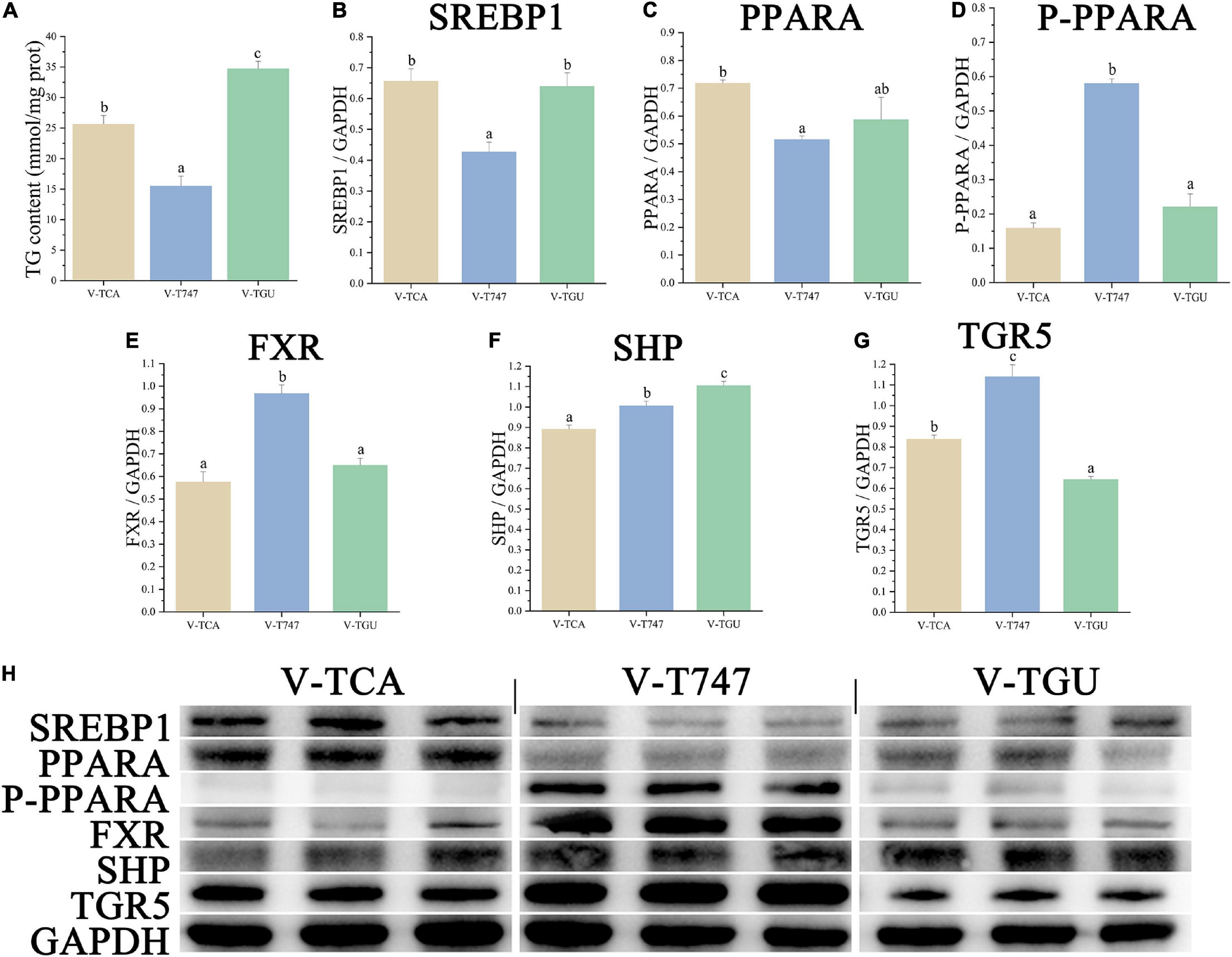
Figure 5. The lipid accumulation and metabolism of hepatocytes in FXR agonist or antagonist incubation. (A) The content of intracellular TG (n = 9). (B–G) The relative quantification of protein levels normalized to GAPDH in hepatocytes (n = 3). (H) the Western blot band of hepatocyte samples (n = 3). Values are presented as means with SD, where significant (p < 0.05) differences between groups are indicated by different letters.
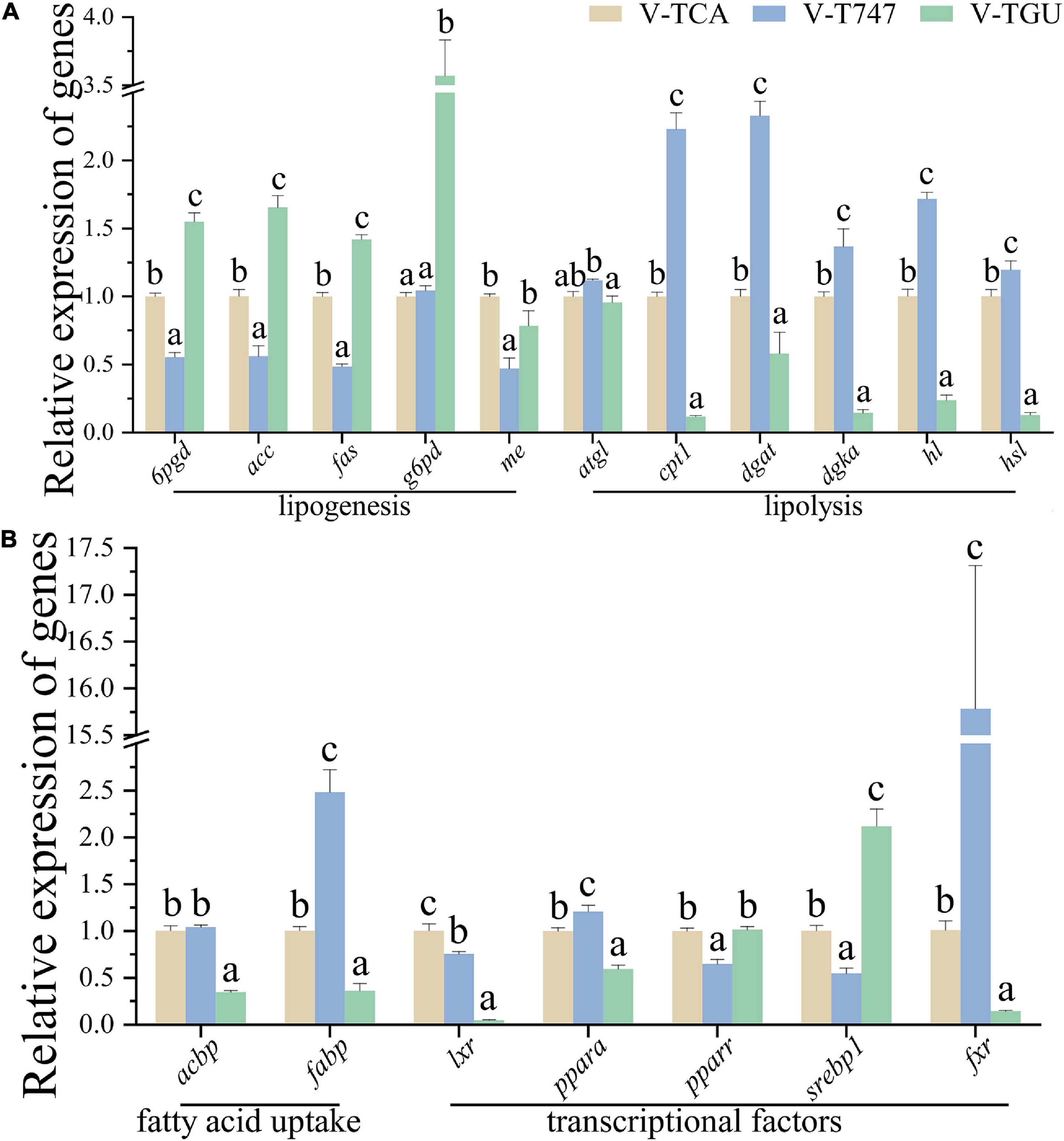
Figure 6. The relative expression of genes associated with lipid metabolism of hepatocytes normalized to 18s and β-actin in FXR agonist or antagonist incubation (A,B) (n = 9). Values are presented as means with SD, where significant (p < 0.05) differences between groups are indicated by different letters.
Compared to the V-TCA group, the V-TGU group significantly increased and decreased the expressions of SHP and TGR5 proteins, respectively. The V-TGU treatment significantly enhanced the expression of lipogenesis genes (6pgd, acc, fas, and g6pd) and their transcriptional factors gene (srebp1), significantly repressed the expression of fxr, fatty acid uptake genes (acbp and fabp), lipolysis genes (cpt1, dgat, dgka, hl, and hsl), and their transcriptional factors gene (ppara). These results showed that the lipid deposition of hepatocytes was decreased by the agonist of FXR, and increased by the antagonist of FXR, indicating that the effects of the BAs on lipid accumulation may be mediated through the FXR signaling pathway.
Regulation of Bile Acids on Lipid Deposition of Hepatocyte Was Dependent on the Activation of G Protein-Coupled Bile Acid Receptor 1 Signaling in vitro
According to the results of CCK-8 assay, the 1 μM SBI-115 and 10 μM INT-777 had no significant influence on the viability of hepatocytes (Figure 1). Before the TCA incubation, the FDH pre-treated with SBI-115 for 2 h (V-TSBI group) or INT-777 (V-T777 group) alleviated and enhanced the TCA-induced down-regulation of lipid accumulation (Figures 2, 7A), respectively. Compared to V-TCA treatment, the V-T777 treatment significantly increased the expression of TGR5, whereas significantly reduced the expression of SREBP1 and SHP proteins (Figure 7). In addition, the V-T777 group showed a significantly increased expression of tgr5 and lipolysis genes (atgl, cpt1, dgat, dgka, hl, and hsl), whereas a significantly decreased expression of lipogenesis genes (6pgd, acc, fas, g6pd, and me) (Figure 8).
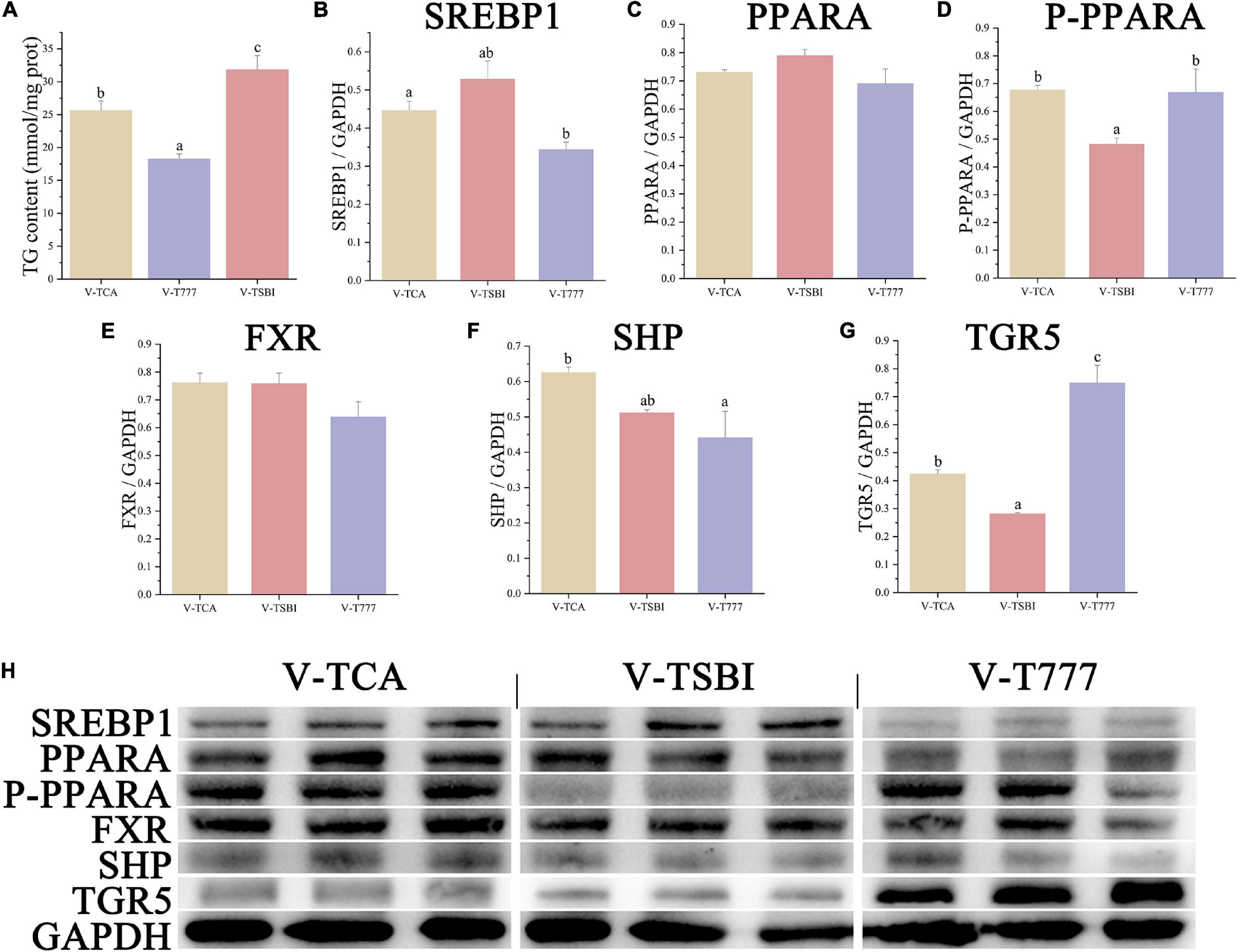
Figure 7. The lipid accumulation and metabolism of hepatocytes in TGR5 agonist or antagonist incubation. (A) The content of intracellular TG (n = 9). (B–G) The relative quantification of protein levels normalized to GAPDH in hepatocytes (n = 3). (H) The Western blot band of hepatocyte samples (n = 3). Values are presented as means with SD, where significant (p < 0.05) differences between groups are indicated by different letters.
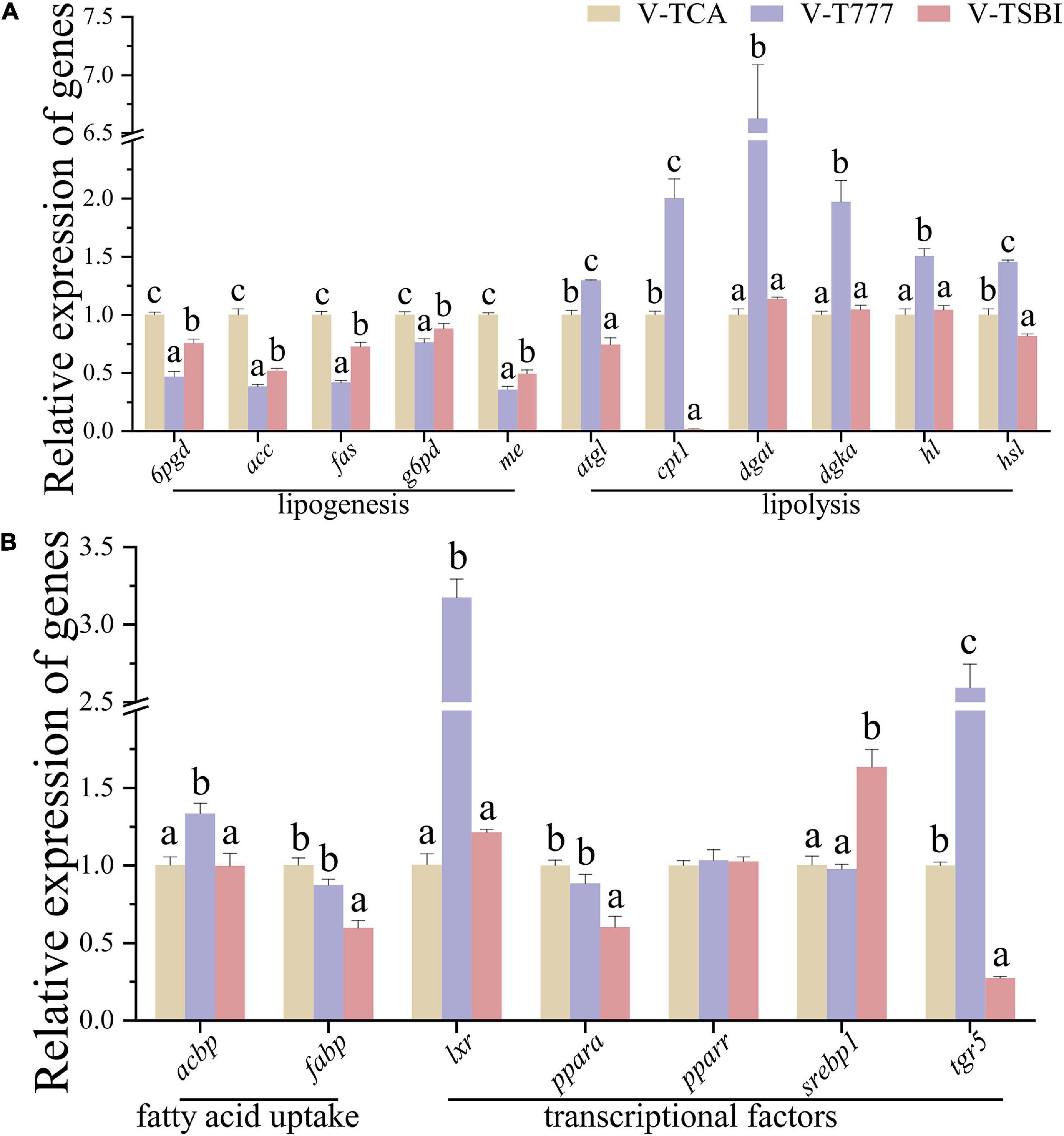
Figure 8. The relative expression of genes associated with lipid metabolism of hepatocytes normalized to 18s and β-actin in TGR5 agonist or antagonist incubation (A,B) (n = 9). Values are presented as means with SD, where significant (p < 0.05) differences between groups are indicated by different letters.
Compared to V-TCA group, the V-TSBI treatment significantly repressed the expression of P-PPARA and TGR5 proteins (Figure 7). Besides, the V-TSBI treatment significantly repressed the expression of tgr5, fatty acid uptake gene (fabp), lipolysis genes (atgl, cpt1, and hsl), and their transcriptional factors gene (ppara), whereas significantly enhanced the expression of lipogenesis genes (6pgd, acc, fas, g6pd, and me) and their transcriptional factors gene (srebp1) (Figure 8). These results showed the lipid deposition of hepatocytes was reduced by the agonist of TGR5, and elevated by the antagonist of TGR5, indicating that the effects of the BAs on lipid accumulation may be mediated through the TGR5 signaling pathway.
Discussion
Firstly, we established the model of fatty-degenerated hepatocytes in pearl gentian grouper. In grass carp, the hepatocytes of fatty degeneration were induced with media containing LE (Lu et al., 2012), which showed a significant accumulation of intracellular TG. Similarly, after exposing to LE in hybrid grouper liver cells, lipid droplets in Oil Red O staining and TG content were increased significantly (Zou et al., 2019). These observations were consistent with our results, that is, BODIPY 493/503 staining showed a large number of lipid droplets accumulated in hepatocytes in V-HL group, which can be proved by the increase of intracellular TG content. Generally, fat accumulation is caused by the imbalance of fatty acids synthesis and fat catabolism, and many key enzymes are involved in these processes (Song et al., 2015a). As providing NADPH essential compounds for fatty acids synthesis in the pentose phosphate pathway, G6PD and 6PGD act important roles in lipogenesis (Song et al., 2015b). As catalyzing the carboxylation of acetyl-CoA to malonyl-CoA, ACC act a rate-limiting enzyme for the synthesis of long-chain fatty acids (Zhu et al., 2014). In this study, the V-HL treatment up-regulated the mRNA level of these genes, as well as fas and me, indicating that the lipogenesis was enhanced in a fatty-degenerated hepatocytes model. Our results are similar to previous study in which LE induced the expression of adipogenesis genes (g6pd, me, and fas) in pearl gentian grouper hepatocytes (Zou et al., 2019). As catalyzing the conversion of fatty acids-CoAs into fatty acids-carnitine, CPT1 is regarded as a main regulatory enzyme in fatty acid oxidation (Xie et al., 2021a; Xu et al., 2022b). The reduced the mRNA level of cpt1 in V-HL group, as well as atgl, dgat, dgka, and hl, suggesting that lipolysis was suppressed in fatty-degenerated hepatocytes model. Similarly, LE co-incubation decreased the expression of lipolytic genes (atgl and lpl) in another reports in pearl gentian grouper hepatocytes (Zou et al., 2019). Furthermore, ppara is a ligand-dependent transcriptional activation of genes related to fatty acids oxidation pathway (Ribet et al., 2010; Zhu et al., 2014). While pparr and srebp1 are the major regulators of fatty acids/lipids biosynthesis genes, both of which promote lipid storage (Minghetti et al., 2011; Zhu et al., 2014). Same LE treatment decreased the expression of ppara (Zou et al., 2019), in the present study, the V-HL treatment significantly enhanced the expression of SREBP1 gene and protein, and repressed the expression of PPARA and P-PPARA proteins. Overall, compared to normal cell, fatty-degenerated hepatocytes increased lipid accumulation, and impaired lipid metabolism by enhancing lipogenesis, repressing lipolysis, and regulating transcriptional factors in pearl gentian grouper.
Then, we explored the effects of BAs on lipid deposition in a model of fatty-degenerated hepatocytes. Unlike 200 μM TCA did not affect the viability of hepatocytes in mice (Allen et al., 2011), the CCK-8 assay showed the TCA concentrations below 100 μM had no significant influence on the viability of hepatocytes in this study. This discrepancy might be due to the different tolerance of animals to BAs (Jia et al., 2018; Kumari et al., 2020). In vivo, exogenous BAs could decrease fat deposition caused by a high-lipid diet and improve the hepatic lipid metabolism in large yellow croaker and largemouth bass (Ding et al., 2020; Yin et al., 2021). Similarly, the V-TCA treatment reduced the lipid deposition in fatty degeneration liver cells. Furthermore, in present study, the increased expression of lipolysis genes, and decreased lipogenesis genes, and associated transcriptional factors, suggesting that TCA co-incubation could improve lipid metabolism by repressing lipogenesis and enhancing lipolysis. Notably, the low expression of fxr and tgr5 genes in the V-HL group, but high expression of both genes and proteins in the V-TCA group, might indicate that the fatty-degenerated hepatocytes inhibited, but TCA co-incubation activated, the FXR and TGR5 signaling pathways, thus altering the lipid metabolism and lipid accumulation in vitro.
Next, we explored whether the effects of BAs on lipid deposition were dependent on the activation of FXR signaling. The INT-747 (10 μM) is a classical FXR agonist that can significantly increase the luciferase activity of FXR reporter gene in HepG2 cells (Gao et al., 2018). Similarly, we also found the INT-747 (5 μM) co-incubation up-regulated the expression of FXR gene and protein in hybrid grouper hepatocytes, accompanied by a reduction in lipid accumulation. Our results are consistent with other studies in which INT-747 (1 μM) treatment significantly reduced TG level in mouse liver (AML12 cells) and human liver (Fa2N-4 cells) (Miyazaki et al., 2018). In human intestinal cells, the activation of FXR inhibited the expression of lxr and srebp1 genes, thereby decreasing the expression of fas gene and lipid synthesis via the SHP pathway (Watanabe et al., 2004; Yang et al., 2010). In addition, the activation of FXR stimulated the fatty acid β-oxidation by inducing the expression of PPARA (Alfaro-Viquez et al., 2018; Kumari et al., 2020). In accordance with this, we also found that the activation of FXR promoted the lipolysis and inhibited the lipogenesis. Same as the present study, the guggulsterone (20 μM) treatment significantly increased the content of TG in primary hepatocytes from yellow catfish (Wu et al., 2020). Thus, antagonizing FXR by guggulsterone, eliminated the lipid-lowering effects of TCA, suggesting that the activation of FXR might be the key step toward TCA-induced lipid-lowering outcome.
Besides, we explored whether the effects of BAs on lipid deposition was dependent on the activation of TGR5 signaling. The INT-777 had been discovered as a specific agonist for TGR5 (Guo et al., 2016). As with the human podocytes (Wang et al., 2016), the results of CCK-8 assay also showed 10 μM INT-777 had no significant effects on the viability of hepatocytes in this study. Since INT-777 treatment enhanced the expression of TGR5 and decreased the level of plasma TG in mice (Bianco et al., 1988; Wang et al., 2016), we also found the activation of TGR5 by INT-777 reduced the lipid deposition in hybrid grouper hepatocytes. In human podocytes cultured with high glucose, INT-777 co-incubation suppressed mRNA level of srebp1, induced the mRNA level of ppara and cpt1, leading to an increase in fatty acid β-oxidation (Wang et al., 2016). Partially agreeing with these, we observed that INT-777 treatment increased the expression P-PPARA protein and cpt1 gene in this study. At the same time, the inhibition of TGR5 by SBI-115 promoted lipid accumulation and impaired lipid metabolism of hybrid grouper hepatocytes. Our results were consistent with the results of another in vivo experiment, the ppara and cpt1 mRNA level were decreased in TGR5–/– mice (Wakil and Abu-Elheiga, 2009). Since TCA could active TGR5 in various cells (HEK293, Kupffer, and Sinusoidal endothelial cells) (Keitel et al., 2007, 2008), and TGR5 negatively regulated lipid deposition, we inferred that the activation of TGR5 might be a key step toward TCA-induced lipid-lowering outcome.
Conclusion
In pearl gentian grouper, we revealed: (1) a model of fatty degenerated hepatocytes induced by LE is characterized by increasing lipid accumulation and impairing lipid metabolism; (2) BAs co-incubation could improve lipid deposition and metabolism in fatty degenerated hepatocytes model; (3) the inhibition of FXR or TGR5 promoted lipogenesis and inhibited lipolysis in vitro; (4) the activation of FXR and/or TGR5 might be the key step toward the lipid-lowering effects of BAs. Understanding the functions of BAs may help to develop of management strategies to improve lipid metabolism and deposition in the liver of hybrid grouper.
Data Availability Statement
The original contributions presented in the study are included in the article/Supplementary Material, further inquiries can be directed to the corresponding authors.
Ethics Statement
The animal study was reviewed and approved by Animal Ethical and Welfare Committee of Guangdong Ocean University. The permit number for conducting animal experiments is GDOU-AEWC-20180063.
Author Contributions
JX: conceptualization, methodology, formal analysis, investigation, data curation, writing – original draft, and writing – review and editing. XY: data curation, investigation, and writing – original draft. XL: investigation, data curation, and visualization. SX: conceptualization, resources, and visualization. SC: methodology and conceptualization. SZ: conceptualization and funding acquisition. JC: methodology, conceptualization, and funding acquisition. BT: methodology, conceptualization, writing – review and editing, and funding acquisition. All authors read and approved the final manuscript.
Funding
This work was supported by the National Key R&D Program of China [2019YFD0900200], China’s Agricultural Research System of MOF and MARA [CARS-47], Science and Technology Project of Zhanjiang [2020A05003], National Natural Science Foundation of China [No. 31772864], and Natural Science Foundation of Guangdong Province [2018A030313154 & 2020A1515011129].
Conflict of Interest
The authors declare that the research was conducted in the absence of any commercial or financial relationships that could be construed as a potential conflict of interest.
Publisher’s Note
All claims expressed in this article are solely those of the authors and do not necessarily represent those of their affiliated organizations, or those of the publisher, the editors and the reviewers. Any product that may be evaluated in this article, or claim that may be made by its manufacturer, is not guaranteed or endorsed by the publisher.
Supplementary Material
The Supplementary Material for this article can be found online at: https://www.frontiersin.org/articles/10.3389/fmars.2022.861117/full#supplementary-material
References
Alfaro-Viquez, E., Roling, B. F., Krueger, C. G., Rainey, C. J., Reed, J. D., and Ricketts, M. L. (2018). An extract from date palm fruit (Phoenix dactylifera) acts as a co-agonist ligand for the nuclear receptor FXR and differentially modulates FXR target-gene expression in vitro. PLoS One 13:e0190210. doi: 10.1371/journal.pone.0190210
Allen, K., Jaeschke, H., and Copple, B. L. (2011). Bile acids induce inflammatory genes in hepatocytes: a novel mechanism of inflammation during obstructive cholestasis. Am. J. Pathol. 178, 175–186. doi: 10.1016/j.ajpath.2010.11.026
Bianco, A. C., Sheng, X., and Silva, J. E. (1988). Triiodothyronine amplifies norepinephrine stimulation of uncoupling protein gene transcription by a mechanism not requiring protein synthesis. J. Biol. Chem. 263, 18168–18175.
Chen, Q. L., Luo, Z., Song, Y. F., Wu, K., Huang, C., Pan, Y. X., et al. (2014). Hormone-sensitive lipase in yellow catfish Pelteobagrus fulvidraco: molecular characterization, mRNA tissue expression and transcriptional regulation by leptin in vivo and in vitro. Gen. Comp. Endocrinol. 206, 130–138. doi: 10.1016/j.ygcen.2014.06.031
China Agriculture Press. (2021). Fisheries and Fishery Administration Bureau of The Ministry of Agriculture and Rural Affairs, National Fishery Technology Promotion Station, China Society of Fisheries. China Fishery Statistics Yearbook 2020. Beijing: China Agriculture Press.
Collet, B., Collins, C., and Lester, K. (2018). Engineered cell lines for fish health research. Dev. Comp. Immunol. 80, 34–40. doi: 10.1016/j.dci.2017.01.013
Ding, T., Xu, N., Liu, Y., Du, J., Xiang, X., Xu, D., et al. (2020). Effect of dietary bile acid (BA) on the growth performance, body composition, antioxidant responses and expression of lipid metabolism-related genes of juvenile large yellow croaker (Larimichthys crocea) fed high-lipid diets. Aquaculture 518:734768. doi: 10.1016/j.aquaculture.2019.734768
El-Shenawy, A. M., Abeer, E.-K. M., Alsokary, E. T., Gad, D. M. J. I. J. O. F., and Studies, A. (2020). Impact of carbohydrate to lipid ratio and bile salts supplementation on performance, body gain and body composition of Nile tilapia fish. Int. J. Fish. Aquat. Stud. 8, 88–97.
Food Organization (2020). Sustainability in action. State of World Fisheries and Aquaculture. Rome: Food Organization. 200.
Gao, X., Fu, T., Wang, C., Ning, C., Liu, K., Liu, Z., et al. (2018). Yangonin protects against cholestasis and hepatotoxity via activation of farnesoid X receptor in vivo and in vitro. Toxicol. Appl. Pharmacol. 348, 105–116. doi: 10.1016/j.taap.2018.04.015
Guo, C., Chen, W. D., and Wang, Y. D. (2016). TGR5, Not Only a Metabolic Regulator. Front. Physiol. 7:646. doi: 10.3389/fphys.2016.00646
Huang, B., Baoshan, L. I., Zhang, L., Huadong, L. I., Sun, Y., Wang, S., et al. (2015). Effects of dietary bile acid on growth performance, fatty enzymatic activities and biochemical indices of turbot Scophthalmus maximus L. junveniles. J. Shanghai Ocean University. 24, 737–744.
Jia, W., Xie, G., and Jia, W. (2018). Bile acid-microbiota crosstalk in gastrointestinal inflammation and carcinogenesis. Nat. Rev. Gastroenterol. Hepatol. 15, 111–128. doi: 10.1038/nrgastro.2017.119
Jiang, M., Wen, H., Gou, G. W., Liu, T. L., Lu, X., and Deng, D. F. (2018). Preliminary study to evaluate the effects of dietary bile acids on growth performance and lipid metabolism of juvenile genetically improved farmed tilapia (Oreochromis niloticus) fed plant ingredient-based diets. Aquacult. Nutr. 24, 1175–1183. doi: 10.1111/anu.12656
Keitel, V., Donner, M., Winandy, S., Kubitz, R., and Haussinger, D. (2008). Expression and function of the bile acid receptor TGR5 in Kupffer cells. Biochem. Biophys. Res. Commun. 372, 78–84. doi: 10.1016/j.bbrc.2008.04.171
Keitel, V., Reinehr, R., Gatsios, P., Rupprecht, C., Gorg, B., Selbach, O., et al. (2007). The G-protein coupled bile salt receptor TGR5 is expressed in liver sinusoidal endothelial cells. Hepatology 45, 695–704. doi: 10.1002/hep.21458
Kumari, A., Pal Pathak, D., and Asthana, S. (2020). Bile acids mediated potential functional interaction between FXR and FATP5 in the regulation of Lipid Metabolism. Int. J. Biol. Sci. 16, 2308–2322. doi: 10.7150/ijbs.44774
Lai, W., Xu, D., Li, J., Wang, Z., Ding, Y., Wang, X., et al. (2021). Dietary polystyrene nanoplastics exposure alters liver lipid metabolism and muscle nutritional quality in carnivorous marine fish large yellow croaker (Larimichthys crocea). J. Hazard Mater. 419:126454. doi: 10.1016/j.jhazmat.2021.126454
Li, Y., Jia, P., Yu, F., Li, W., Mao, C., Yi, M., et al. (2022). Establishment and characterization of a liver cell line, ALL, derived from yellowfin sea bream, Acanthopagrus latus, and its application to fish virology. J. Fish. Dis. 45, 141–151. doi: 10.1111/jfd.13543
Liao, Z., Sun, B., Zhang, Q., Jia, L., Wei, Y., Liang, M., et al. (2020). Dietary bile acids regulate the hepatic lipid homeostasis in tiger puffer fed normal or high-lipid diets. Aquaculture 519:734935. doi: 10.1016/j.aquaculture.2020.734935
Lu, R. H., Liang, X. F., Wang, M., Zhou, Y., Bai, X. L., and He, Y. (2012). The role of leptin in lipid metabolism in fatty degenerated hepatocytes of the grass carp Ctenopharyngodon idellus. Fish. Physiol. Biochem. 38, 1759–1774. doi: 10.1007/s10695-012-9673-6
Minghetti, M., Leaver, M. J., and Tocher, D. R. (2011). Transcriptional control mechanisms of genes of lipid and fatty acid metabolism in the Atlantic salmon (Salmo salar L.) established cell line. SHK-1. Biochim. Biophys. Acta 1811, 194–202. doi: 10.1016/j.bbalip.2010.12.008
Miyazaki, T., Honda, A., Ikegami, T., Iida, T., and Matsuzaki, Y. (2018). Human-specific dual regulations of FXR-activation for reduction of fatty liver using in vitro cell culture model. J. Clin. Biochem. Nutr. 64,, 112-123. doi: 10.3164/jcbn.18-80
Pols, T. W., Nomura, M., Harach, T., Lo Sasso, G., Oosterveer, M. H., Thomas, C., et al. (2011). TGR5 activation inhibits atherosclerosis by reducing macrophage inflammation and lipid loading. Cell. Metab. 14, 747–757. doi: 10.1016/j.cmet.2011.11.006
Reich, M., Deutschmann, K., Sommerfeld, A., Klindt, C., Kluge, S., Kubitz, R., et al. (2016). TGR5 is essential for bile acid-dependent cholangiocyte proliferation in vivo and in vitro. Gut 65, 487–501. doi: 10.1136/gutjnl-2015-309458
Ribet, C., Montastier, E., Valle, C., Bezaire, V., Mazzucotelli, A., Mairal, A., et al. (2010). Peroxisome proliferator-activated receptor-α control of lipid and glucose metabolism in human white adipocytes. Endocrinology 151, 123–133. doi: 10.1210/en.2009-0726
Shapawi, R., Abdullah, F. C., Senoo, S., and Mustafa, S. (2018). Nutrition, growth and resilience of tiger grouper (Epinephelus fuscoguttatus) × giant Grouper (Epinephelus lanceolatus) hybrid− a review. Rev. Aquacult. 11, 1285–1296. doi: 10.1111/raq.12292
Song, Y. F., Luo, Z., Huang, C., Chen, Q. L., Pan, Y. X., and Xu, Y. H. (2015a). Endoplasmic Reticulum Stress-Related Genes in Yellow Catfish Pelteobagrus fulvidraco: Molecular Characterization. Tissue Expression, and Expression Responses to Dietary Copper Deficiency and Excess. G3 5, 2091–2104. doi: 10.1534/g3.115.019950
Song, Y. F., Wu, K., Tan, X. Y., Zhang, L. H., Zhuo, M. Q., Pan, Y. X., et al. (2015b). Effects of recombinant human leptin administration on hepatic lipid metabolism in yellow catfish Pelteobagrus fulvidraco: in vivo and in vitro studies. Gen. Comp. Endocrinol. 212, 92–99. doi: 10.1016/j.ygcen.2015.01.022
Wakil, S. J., and Abu-Elheiga, L. A. (2009). Fatty acid metabolism: target for metabolic syndrome. J. Lipid. Res. 50(Suppl.), S138–S143. doi: 10.1194/jlr.R800079-JLR200
Wang, J., Han, S. L., Lu, D. L., Li, L. Y., Limbu, S. M., Li, D. L., et al. (2019). Inhibited Lipophagy Suppresses Lipid Metabolism in Zebrafish Liver Cells. Front. Physiol. 10:1077. doi: 10.3389/fphys.2019.01077
Wang, X. X., Edelstein, M. H., Gafter, U., Qiu, L., Luo, Y., Dobrinskikh, E., et al. (2016). G Protein-Coupled Bile Acid Receptor TGR5 Activation Inhibits Kidney Disease in Obesity and Diabetes. J. Am. Soc. Nephrol. 27, 1362–1378. doi: 10.1681/ASN.2014121271
Watanabe, M., Houten, S. M., Wang, L., Moschetta, A., Mangelsdorf, D. J., Heyman, R. A., et al. (2004). Bile acids lower triglyceride levels via a pathway involving FXR. SHP, and SREBP-1c. J. Clin. Invest. 113, 1408–1418. doi: 10.1172/JCI21025
Wu, K., Zhao, T., Hogstrand, C., Xu, Y. C., Ling, S. C., Chen, G. H., et al. (2020). FXR-mediated inhibition of autophagy contributes to FA-induced TG accumulation and accordingly reduces FA-induced lipotoxicity. Cell. Commun. Signal. 18:47. doi: 10.1186/s12964-020-0525-1
Xie, S., Lin, Y., Wu, T., Tian, L., Liang, J., and Tan, B. (2021a). Dietary lipid levels affected growth performance, lipid accumulation, inflammatory response and apoptosis of japanese seabass (lateolabrax japonicus). Aquac. Nutr. 27, 807–816. doi: 10.1111/anu.13225
Xie, S., Wei, D., Tian, L., and Liu, Y. (2021b). Dietary supplementation of chenodeoxycholic acid improved the growth performance, immune response and intestinal health of juvenile Penaeus monodon fed a low fish-meal diet. Aquac. Rep. 20:100773.
Xu, J., He, G., Chen, L., Xie, S., Chi, S., Zhang, S., et al. (2022a). Farnesoid X receptor (FXR) and G protein-coupled bile acid receptor 1 (TGR5) signaling pathways improved the hepatic lipid metabolism in hybrid grouper. Aquac. Rep. 22:100997.
Xu, J., Xie, S., Chi, S., Zhang, S., Cao, J., and Tan, B. (2022b). Short-term dietary antibiotics altered the intestinal microbiota and improved the lipid metabolism in hybrid grouper fed medium and high-lipid diets. Aquaculture 547:737453. doi: 10.1016/j.aquaculture.2021.737453
Xu, X., Song, Z., Li, Z., Liu, X., Feng, Y., Wang, W., et al. (2021). Establishment and characterization of a gill cell line from pearl gentian grouper (Epinephelus lanceolatusmale symbolxEpinephelus fuscoguttatusfemale symbol) and its application in cadmium toxicology. Ecotoxicol. Environ. Saf. 208:111614. doi: 10.1016/j.ecoenv.2020.111614
Yang, Z. X., Shen, W., and Sun, H. (2010). Effects of nuclear receptor FXR on the regulation of liver lipid metabolism in patients with non-alcoholic fatty liver disease. Hepatol. Int. 4, 741–748. doi: 10.1007/s12072-010-9202-6
Yin, P., Xie, S., Zhuang, Z., He, X., Tang, X., Tian, L., et al. (2021). Dietary supplementation of bile acid attenuate adverse effects of high-fat diet on growth performance, antioxidant ability, lipid accumulation and intestinal health in juvenile largemouth bass (Micropterus salmoides). Aquaculture 531:735864. doi: 10.1016/j.aquaculture.2020.735864
Yu, H., Zhang, L., Chen, P., Liang, X., Cao, A., Han, J., et al. (2019). Dietary Bile Acids Enhance Growth, and Alleviate Hepatic Fibrosis Induced by a High Starch Diet via AKT/FOXO1 and cAMP/AMPK/SREBP1 Pathway in Micropterus salmoides. Front. Physiol. 10:1430. doi: 10.3389/fphys.2019.01430
Zhang, W., Tan, B., Deng, J., and Haitao, Z. (2021). Multiomics analysis of soybean meal induced marine fish enteritis in juvenile pearl gentian grouper. Epinephelus fuscoguttatus♂ × Epinephelus lanceolatus♀. Sci. Rep. 11:23319. doi: 10.1038/s41598-021-02278-z
Zhao, T., Wu, K., Hogstrand, C., Xu, Y. H., Chen, G. H., Wei, C. C., et al. (2020). Lipophagy mediated carbohydrate-induced changes of lipid metabolism via oxidative stress, endoplasmic reticulum (ER) stress and ChREBP/PPARgamma pathways. Cell. Mol. Life Sci. 77, 1987–2003. doi: 10.1007/s00018-019-03263-6
Zhou, J. S., Chen, H. J., Ji, H., Shi, X. C., Li, X. X., Chen, L. Q., et al. (2018). Effect of dietary bile acids on growth, body composition, lipid metabolism and microbiota in grass carp (Ctenopharyngodon idella). Aquac. Nutr. 24, 802–813. doi: 10.1111/anu.12609
Zhu, Q. L., Luo, Z., Zhuo, M. Q., Tan, X. Y., Sun, L. D., Zheng, J. L., et al. (2014). In vitro exposure to copper influences lipid metabolism in hepatocytes from grass carp (Ctenopharyngodon idellus). Fish. Physiol. Biochem. 40, 595–605. doi: 10.1007/s10695-013-9869-4
Zou, C., Su, N., Wu, J., Xu, M., Sun, Z., Liu, Q., et al. (2019). Dietary Radix Bupleuri extracts improves hepatic lipid accumulation and immune response of hybrid grouper (Epinephelus lanceolatusmale symbol x Epinephelus fuscoguttatusfemale symbol). Fish. Shellf. Immunol. 88, 496–507. doi: 10.1016/j.fsi.2019.02.052
Zou, C., Tan, X., Ye, H., Sun, Z., Chen, S., Liu, Q., et al. (2018). The hepatoprotective effects of Radix Bupleuri extracts against D-galactosamine/lipopolysaccharide induced liver injury in hybrid grouper (Epinephelus lanceolatus♂ × Epinephelus fuscoguttatus♀). Fish Shellf. Immunol. 83, 8–17. doi: 10.1016/j.fsi.2018.08.047
Keywords: FXR, tgr5, lipid deposition, fatty-degenerated hepatocytes, in vitro
Citation: Xu J, Yao X, Li X, Xie S, Chi S, Zhang S, Cao J and Tan B (2022) Bile Acids Reduced the Lipid Deposition in Fatty Degenerated Hepatocytes of Pearl Gentian Grouper (Epinephelus fuscoguttatus♂ × Epinephelus lanceolatus♀) in vitro. Front. Mar. Sci. 9:861117. doi: 10.3389/fmars.2022.861117
Received: 24 January 2022; Accepted: 17 February 2022;
Published: 12 April 2022.
Edited by:
Wei Huang, Second Institute of Oceanography, Ministry of Natural Resources, ChinaReviewed by:
Min Xue, Feed Research Institute (CAAS), ChinaXiaodan Wang, East China Normal University, China
Copyright © 2022 Xu, Yao, Li, Xie, Chi, Zhang, Cao and Tan. This is an open-access article distributed under the terms of the Creative Commons Attribution License (CC BY). The use, distribution or reproduction in other forums is permitted, provided the original author(s) and the copyright owner(s) are credited and that the original publication in this journal is cited, in accordance with accepted academic practice. No use, distribution or reproduction is permitted which does not comply with these terms.
*Correspondence: Shiwei Xie, eHN3enNkeEAxNjMuY29t; Beiping Tan, YnB0YW5AMTI2LmNvbQ==