- 1Department of Fisheries and Marine Science, Noakhali Science and Technology University, Sonapur, Bangladesh
- 2School of Engineering and Built Environment, Griffith University, Brisbane, QLD, Australia
- 3Analytical Chemistry Laboratory, Chemistry Division, Atomic Energy Centre Dhaka, Bangladesh Atomic Energy Commission, Dhaka, Bangladesh
- 4Materials Science Division, Atomic Energy Centre Dhaka, Bangladesh Atomic Energy Commission, Dhaka, Bangladesh
- 5State Key Laboratory of Biogeology and Environmental Geology, China University of Geosciences, Wuhan, China
Kuakata beach, known as Daughter of Sea in Bangladesh, has drawn a growing number of tourists from all over the world, leading to the higher use of single plastic products. This study was a first attempt to describe the occurrence, spatial distribution, and ecological risk of microplastics (MPs) in Kuakata beach sediments. A total of 24 surface sediment samples were collected from the intertidal zone of the beach, and MPs were extracted using the density separation method and a stereomicroscope. Fourier transform infrared (FTIR) spectroscopy was used for qualitative and quantitative identification. The results revealed that the average MPs in the beach sediment were 232 ± 52 items kg–1 dry weight, which was much higher than many other sandy beaches throughout the world. Analyses of variance showed a significant (p < 0.01) difference among the mean abundance of MPs in sampling points. Fibers were dominated in every sampling point with an average of 123 ± 27 item kg–1. Most of the MPs observed were colored (60%), and the rest were transparent (40%). It was found that the size range of 1–5 mm MPs constituted over half (55%) of total MPs covering an average value of 127 ± 34 items kg–1. Three polymer types were identified in the sediment samples through FTIR analysis which followed the decreasing order of polyethylene terephthalate > polyethylene > polypropylene. Correlation analysis showed a positive relationship between the abundance of MPs and the finer grain size of sediment (p = 0.055; r = 0.7), indicating grain size-controlled the density of MPs. The pollution load index was assessed to estimate the ecological risk and found that the beach sediment of Kuakata belonged to the risk category I of the pollution index. This investigation provided preliminary information on MPs pollution in the marine ecosystem that the policymakers can use to take appropriate management approaches.
Introduction
Microplastics (MPs) (<5 mm in size) were first reported in 1972 as an aquatic pollutant in marine environments, and now it has become a global concern for potential harmful effects not only on ecosystems but also on human health (Carpenter and Smith, 1972; Crew et al., 2020). Most of the MPs float on the ocean surface except high-density polymers, such as polyvinylchloride (PVC), polycarbonate (PC), and polyethylene terephthalate (PET). These floated MPs remain in the marine environment for longer periods due to their longevity, ubiquity, and impact resistance properties and cannot be easily removed (Geyer et al., 2017). Almost 367 million tons of plastic products were produced worldwide in 2020, from which 2–5% ended up in the oceans (Plastics Europe Market Research Group [PEMRG], 2021). If proper action is not taken to reduce the use of plastic products, the amount of plastic entering the ocean each year will be increased to 16 million tons by 2030 and approximately 32 million tons by 2050. It was estimated that in terms of weight, there will be more plastics in the ocean than fish by 2050 (Neufeld et al., 2016).
Plastic materials that have been widely used as virgin pellets, pharmaceuticals, cosmetics, or exfoliating scrubs (glitters and microbeads) in the plastic industry and cleaning products are generally referred to as primary MPs (Hahladakis et al., 2018; Camacho et al., 2019). The larger amount of plastic items are degraded into small, microscopic elements (<5 mm) due to mechanical abrasion caused by wave action, photochemical oxidation generated by UV-B radiations as well as biological processes (Corcoran et al., 2015), which are commonly known as secondary MPs. Plastics and their degraded products enter into the oceans from some point and nonpoint sources, such as incorrect disposal, sewage systems, loss during maritime activity, tourists activity, industrial effluents, roadside dust (vehicles tires, grease, etc.), beach adjacent hotels, motels, and restaurants, which are driven by riverine output, atmospheric outfall, and stormwater activity (Corcoran et al., 2015; Li et al., 2020). The key sources of synthetic fibers in aquatic ecosystems are from the washing process of synthetic textiles, which contribute about 35% to the worldwide release of MPs to the oceans (Boucher and Friot, 2017).
Microplastics are bioaccumulated into marine organisms and then infiltrated into the human food web via direct or indirect ingestion (Van Cauwenberghe and Janssen, 2014). Though some toxic pollutants and coexposure of MPs have health hazards, many ecotoxicological studies suggested that the physiological activities of marine organisms may not be significantly affected by a representative number of MPs (Rist et al., 2016; Canniff and Hoang, 2018). Furthermore, MPs alone has no effects on biochemical biomarkers in mussel. Still, the combined effects of MPs and triclosan, an antimicrobial agent, have enhanced the superoxide dismutase activity as well as lipid peroxidation and caused oxidative stress (Webb et al., 2020). Usually, some of the persistent organic pollutants, such as polycyclic aromatic hydrocarbons, polychlorinated biphenyls, pesticides, and medicinal agents, as well as heavy metals (e.g., Cu, Pb, Hg, Cd, Cr, etc.), are carried by MPs biofilms which can cause adverse health issues on aquatic organisms and humans (Wang and Wang, 2018; Camacho et al., 2019; Duan et al., 2020; Mahfooz et al., 2020). However, these toxicants are sorbed onto MPs following several mechanisms driven by the physicochemical properties of the MPs, toxicants, and the intermediate substances where the sorption takes place (Yu et al., 2019; Fred-Ahmadu et al., 2020). Plastic products, such as plastic bags, bottles, and other wrapping substances release polybrominated diphenyl ether (PBDE), nonylphenol, and bisphenol A, which are responsible for cardiovascular disease, reproductive disorders, and breast cancer in humans (Glausiusz, 2014; Weidemann et al., 2016; Ortiz-Villanueva et al., 2018). Hence, it is indispensable to estimate the ecological and environmental risks posed by MPs due to the versatility of numerous physiochemical features. The ecological risks which may usually drive-by MP particles can be illustrated by different initial assessment methods (Li et al., 2020; Wang et al., 2021). For instance, to calculate an index of MPs polymer types Xu et al. (2018) merged the hazard scores of plastic polymers and the pollution load index (PLI) which are discovered by Tomlinson et al. (1980) and Lithner et al. (2011).
However, the occurrence, distribution, and impacts of MPs had already been studied and detected in marine habitats from different parts of the world (Lo et al., 2018; Rodrigues et al., 2018; Anela et al., 2019; Botterell et al., 2019; Wieczorek et al., 2019; Li et al., 2020; Selvam et al., 2020; Wang Q. et al., 2020; Wong et al., 2020; Bhowmick et al., 2021; Gardon et al., 2021; Nithin et al., 2021; Sivagami et al., 2021; Taha et al., 2021; Wu et al., 2021; Yaranal et al., 2021). The presence of MPs was also documented in Bangladesh from sea salt (Parvin et al., 2022), fish species (Hossain et al., 2019, 2020; Ghosh et al., 2021; Parvin et al., 2021), and the beach sediment of Cox’s Bazar (Hossain et al., 2021). Those studies, however, were only a brief description of MPs occurrence, and none of them focused on the hazards connected with MPs.
Kuakata sea beach, also known as Shagor Kannya (Daughter of Sea), is the second-largest sandy beach along the Bay of Bengal coastline of Bangladesh. About 115,000 tourists from home and abroad visit this beach in the peak tourist season (November–March) (Rahman et al., 2015). However, beachside hotels, restaurants, and tourists’ activities produce many plastic wastes, often disposed of on the beach. The three main rivers in Bangladesh (The Padma, Meghna, and Jamuna) could enable that transport, although currents could also transport MPs from other parts of the Bay of Bengal into the coastline and beaches. Therefore, important to know the occurrence of MPs and understand the potential impacts of MPs on the environment and the health risk to humans and organisms therein. So far, no scientific study on MP contamination has been carried out along the entire coastal water and beach sediment in Kuakata beach. Therefore, this study was the foremost step toward elucidating the occurrence, spatial distribution of MPs particles in beach sediments from Kuakata, Bangladesh. Furthermore, ecological risk assessment of MPs and a relationship between sediment grain size and MPs distribution in Kuakata beach was studied for the first time in Bangladesh.
Materials and Methods
Study Site
Kuakata sea beach (90° 7′ 0.0012″ E and 21° 49′ 0.0012″ N) of Patuakhali district is one of the attractive tourist places on the southernmost tip of Bangladesh (Figure 1). This beach area is about 65 km away from Patuakhali town and is situated in the middle of the Galachipa river and Andharmanik river estuary. It has an unbroken natural sandy beach (approximately 18 km long and 3 km wide) along the Bay of Bengal. The straight coastline of Kuakata lies 7 m above the mean sea level. The yearly mean temperature and rainfall are 25.9°C (78.70°F) and 2,590 mm where the average sea surface temperature (SST) is 27.30°C (81.14°F), respectively. Moreover, the average UV index is recorded at 7–12 (Bangladesh Meteorological Department [BMD], 2016) and such climate is categorized as tropical monsoon (Am) climate based on the Köppen–Geiger system. The current arrangement of the Bay of Bengal is recorded as clockwise from January to July whereas counterclockwise from August to December with a mean wind speed of 8.2 miles/h. The semidiurnal tide with two high and two lows has been noticed daily. The study area is generally flat and smooth with an average elevation of 65 cm AMSL and lies on the mid-southern coast of the old Ganges delta, covered with recent tidal deposits. In addition, most of the area is blanketed by tidal flats and these tidal flats are broad and nearly horizontal, which is detected by numerous tidal creeks and channels. The formation of beach ridge is a continuous linear mound of relatively coarser sediment close to the high-water mark, and a well-developed dune is present in Cower Char which is about 400 m away from the shoreline. One of the major factors is wave energy for controlling the beach development and changes across the Kuakata shoreline. There are well-generated longshore and rip currents in Kuakata beach, which develop within the surf zone by wave action. The maximum velocity of longshore and rip currents may exceed 1 ms–1. This sandy beach with a gentle slope indicates that it has formed by faulting and down wrapping. The slope of Kuakata is 1–2° at Gangamatir char, 1–1.5° at Labur char, and 3–5° at Cower char which are the parts of Kuakata beach toward the Bay of Bengal (Rashid and Mahmood, 2011). Tourists usually enjoy the scenic beauty of Kuakata beach through scenic drives, boat tours, and bike tours. However, two forms of anthropogenic activities adversely affect the beach environment in this area: (1) the industrial and domestic waste discharge into Galachipa river in the East and Andharmanik river in the West and (2) exploration of tourists and the waste thrown by them.
Sample Collection
A total of 24 sediment samples were collected from eight sampling points, each having triplicates, in the pretourist season from September to October (postmonsoon) in 2019. These locations were S1 (90° 5′ 6″ E, 21° 50′ 34.8″ N), S2 (90° 7′ 12″ E, 21° 49′ 4.8″ N), S3 (90° 9′ 0″ E, 21° 48′ 28.8″ N), S4 (90° 11′ 9.6″ E, 21° 48′ 7.2″ N), S5 (90° 12′ 50.4″ E, 21° 48′ 49.68″ N), S6 (90° 13′ 55.2″ E, 21° 49′ 30″ N), S7 (90° 15′ 21.6″ E, 21° 50′ 56.4″ N), and S8 (90° 15′ 54″ E, 21° 52′ 8.4″ N) (Figure 1). All the samples were collected from strandline when there was ebb tide into the Bay of Bengal. Surface sand samples (top 5 cm) were collected using a metal quadrate (30 cm × 30 cm) following the methods of de Carvalho and Neto (2016) and Li et al. (2020). All the sands within the quadrate were carefully collected using the metal shovel and transferred into an aluminum foil bag. The sample bags were then carefully packed and shipped back to the laboratory for further processing.
Extraction Procedure of Microplastics
The extraction procedure of MPs in this study was conducted following the methodologies illustrated by Masura et al. (2015) and Hossain et al. (2020) with some modifications. First, wet sand samples of 400 g each were weighed and dried at 90°C using a hot air oven until their dryness. The dried samples were subsequently taken into an 800 ml glass beaker with 300 ml of ZnCl2 (1.8 g cm–3) salt solution (Coppock et al., 2017) and stirred with a spatula for a few minutes. Next, all solutions, e.g., H2O2 (Scharlab, Spain), ZnCl2, FeSO4, and NaCl (Loba Chemie, India), were filtered through a cellulose nitrate filter paper of 5.0 μm to remove indigenous MPs from them. After that, all the floating solids were sieved with a 0.3 mm sieve and moved into a 500 ml beaker. Then the beaker with the sample was dried at 90°C for 24 h. Finally, to eliminate organic matters from the dried sample, Fenton’s reagent (30% H2O2 + FeSO4) associated with 3 ml H2SO4 was added, and again heated to 75°C temperature on a hotplate for 30 min. A total of 6 g of salt (∼5 M NaCl) was added afterward per 20 ml of sample to intensify the density of the wet peroxide oxidation (WPO) solution and transferred to a density separator (Coppock et al., 2017) and kept overnight. After that, the floating solids from the separator were collected into a 500 ml beaker and filtered through a 5.0 μm of cellulose nitrate filter paper (Minipore, India) with 47 mm diameter (Bonello et al., 2018).
Visual Identification of Microplastics
A stereomicroscope (Leica EZ4E, Germany) with 8 to 35X magnification was used to quantify and identify the MPs from the filter paper with the method as in Hidalgo-Ruz et al. (2012), Cheung et al. (2016), and Catarino et al. (2018). For these, the filter paper was divided into four quarts pointing to the top clearly, and MPs were counted one by one quart from the filter paper (Lots et al., 2017). The images of MPs were taken with a high-resolution camera (DP-software) attached with the microscope, and measurements were done using ImageJ software (ver. 2.0.0) (Laglbauer et al., 2014). Besides, a hot needle test was conducted for suspicious plastic pieces (De Witte et al., 2014). The morphometric characteristics of MP particles were categorized into different types (microbeads, sheets, foams, films, fibers, and fragments) and shapes (irregular, elongated, rectangular, and cylindrical) (Hidalgo-Ruz et al., 2012; Lusher A. et al., 2017; Lusher A. L. et al., 2017; Frias and Nash, 2019), colors (Möller et al., 2020), and sizes (Zhang et al., 2016).
Polymer Type Identification
Comparatively larger particles were collected to Petri dish from filter papers to identify the polymer types of MPs. An FTIR 8400S manufactured by Shimadzu Corporation, Japan (wavenumber range of 4,000–400 cm–1) and potassium bromide (KBr) pellet technique were used for the polymer characterization. Nearly 200 mg of KBr powder was mixed with around 1–3 mg of finely ground sample. The mixture was then pressed for 1 min in a pellet maker with a continuous pressure of 10 tons to form a transparent pellet using a Shimadzu (IR Prestige-21) hydraulic press. During pellet preparation, the system was kept under evacuation. The pellet was analyzed immediately using an FTIR spectrometer with resolution 2 cm–1 in 30 no. of a scan. The identification process is performed through an automated contrast with the extensive spectral libraries. However, depending only on automated libraries may lead to false identification. Therefore, the FTIR spectra have also been contrasted to absorption bands of polymers reported in the previous studies (Noda et al., 2007; Jung et al., 2018).
Sand Grain Size Analysis
Wet sand samples of 250 g were collected from each sampling point (S1–S8) at Kuakata beach and dried at 105°C in a hot air oven until sample dryness (Urban-Malinga et al., 2020). The average grain size was assessed by sieving 40 g of dry sediment through a sorted sequence of sieves (2, 1, 0.5, 0.25, 0.125, 0.063, and 0.002 mm) with a sieve shaker (Biobase BK-TS 200, China), and shake for 15 min (Wu et al., 2021). After that, all sediments on each sieve were collected and weighed for analysis. The grain sizes were ascertained based on the Wentworth scale (Wentworth, 1922).
Risk Assessment
The PLI is generally used to evaluate the ecological risk in terrestrial and aquatic environments (Tomlinson et al., 1980). In this study, the concentration of MPs was considered the pollutant to estimate the ecological risk in the beach sediment of Kuakata Beach. The PLI was evaluated using the following equations (Xu et al., 2018; Wang et al., 2021).
where CFi is the quotient (contamination factor) of the MP concentration at each sampling site, Ci is the MP concentration at each sample site, and Coi is the background value of MP concentration in sediments before expanding the plastics industry. Nevertheless, there was no scientific study regarding MPs pollution in Kuakata to acquire the background values. Besides, there is no existing standard method to assess the risk of MPs. Coi has been suggested to be denoted by the minimum MP concentration (153 items kg–1) to assess the PLI (Isobe et al., 2014; Xu et al., 2018; Li et al., 2020). However, this approach has the potential that an unusually scattered minimum value can distort the PLI values. The MP concentration (154 items kg–1) at 5% cumulative probability was used to avoid this. The advantage is that this value is determined by the entire probability distribution and hence not significantly affected by a single point value. The PLI value of MPs pollution was categorized according to Wang et al. (2021). It provides a clear extent of MP risk and raises concerns about managing MP contamination (Xu et al., 2018).
Control of Contamination
In this study, all the cautious steps were taken to avoid possible contamination. While working with a toxic hydrogen peroxide mixed solution, special care was taken, and all the reaction was carried out under a fume hood. Precautions were also taken to prevent cross-contamination, predominantly with aerial contaminants and synthetic fibers from clothes. All equipment was washed with distilled deionized water before and after use. Moreover, working surfaces were continuously wiped with distilled H2O and 70% alcohol. Samples were kept retained, wrapping with aluminum foil. Two blank-control samples were run using the above procedure: one with pure sand and concentrated ZnCl2 solution and the other with concentrated ZnCl2 solution (Yu et al., 2016). The blank samples contained no plastics. As we sieved the sand salt (ZnCl2) solution through 0.3 mm mesh, the size range of particles used in this study was between 0.3 and 5 mm.
Statistical Analysis
Significant variations in the mean abundance of MPs among the sites were analyzed using one-way ANOVA followed by pairwise comparisons using Tukey’s HSD test. In all the cases, homogeneity of variances was tested with Levene’s test, and the data were transformed using square root or logarithm when needed. The granulometric data were analyzed on normalized data using Ward’s method. Statistical analyses were conducted using the computer package, PAST (PAleontological STatistics), Version 4.03.
Results and Discussion
Occurrence and Spatial Distribution of Microplastics
Microplastics were recognized in every sampling point of Kuakata Beach sediment with an overall mean value of 232 ± 52 items kg–1 dry sediment. In our investigation, the highest mean value of MPs was observed in the estuarine S8 sampling point (311 ± 11 items kg–1), followed by S7 (279 ± 8 items kg–1), S1 (270 ± 9 items kg–1), S4 (246 ± 8 items kg–1), S5 (221 ± 9 items kg–1), S3 (191 ± 11 items kg–1), S6 (182 ± 6 items kg–1), and lastly in the lowest interrupted zone S2 (157 ± 5 items kg–1) (Figure 2). One-way ANOVA showed that the abundance of MPs significantly varied (F = 123.8, df = 7, p < < 0.001) among the eight stations. Tukey’s pairwise comparisons showed significant differences between almost all sites pair, except for S1 and S4 (p = 0.12), S1 and S7 (p = 0.94), S3 and S6 (p = 0.75). In addition, the cumulative probabilistic distributions of all the sample values were calculated (Figure 3). The 5% and 95% values from the distributions were 154 and 318 items kg–1, respectively.
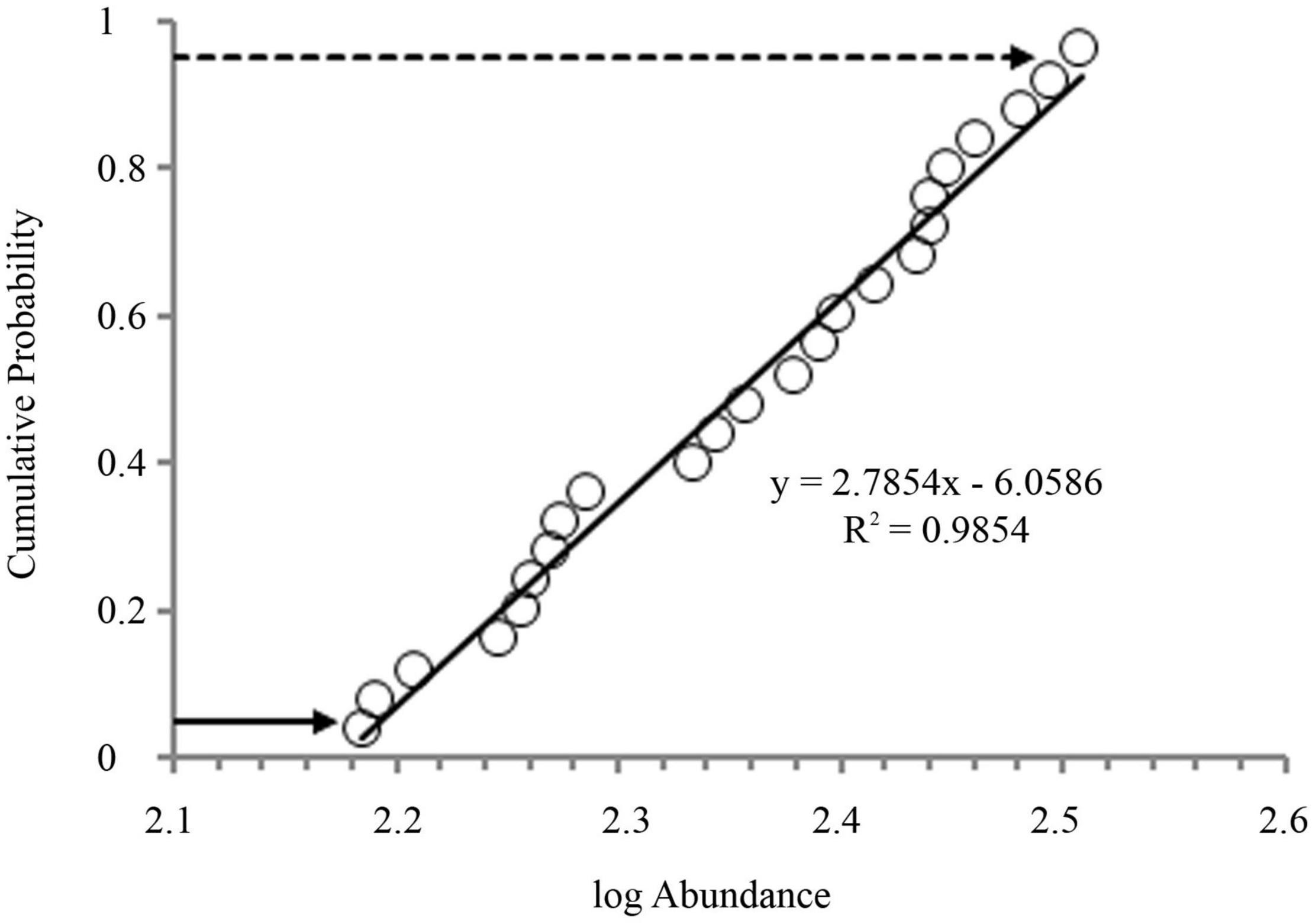
Figure 3. Cumulative probabilistic distributions of all the sample values indicating 5 and 95% values.
The higher abundance in S1, S7, and S8 sampling points indicated that MPs load in the estuarine beach might be attributed to extensive river discharge along with tourism activities (Zhang et al., 2019). Tourist activity would yield an incredible amount of plastic waste, leading to MP pollution on the beach. It was proposed that wind-driven oceanic circulation could also influence MP accumulation at beaches with higher concentrations (Vianello et al., 2013). On the other hand, the S2 sampling point was a remote area for the tourists, and therefore the abundance of MPs was recorded lowest at S2. Previous studies indicated that MP contamination was significantly positively correlated with population density, river discharge, and the spread of industrial zones (Fetner and Miller, 2021; Zhu et al., 2021). However, present outcomes were compared with other countries that used almost similar extraction and detection methods, as well as the quantification unit (Table 1). The mean abundance of MPs in this study site was found to be higher than those in Small Island, Fuji (Ferreira et al., 2020), Brest Bay, France (Frère et al., 2017), and some other beaches (Graca et al., 2017). Our results depicted the MPs pollution in the surface sediments of the Kuakata beach was lower than the sediments from Chennai, India (Sathish et al., 2019), Lido di Dante, Italy (Lots et al., 2017), Bohai sea, China (Zhu et al., 2021), and Da Nang, Vietnam (Nguyen et al., 2020).
Characteristics of Microplastics
The investigation on characteristics of MPs in this experiment exposed the types and shapes, color, and size of MPs presented in the Kuakata beach sediment. A total of six different types of MPs were noticed in the examined sediment, namely microbeads, sheets, foams, films, fibers, and fragments (Figure 4A). Among these, fibers were predominant in every sampling point. They contributed about 55% of total MPs with an average of 123 ± 27 items kg–1, followed by 15% fragments (35 ± 20 items kg–1), 14% films (30 ± 11 items kg–1), 10% microbeads (28 ± 30 items kg–1), 5% sheets (12 ± 9 items kg–1), and 2% foams (4 ± 6 items kg–1), respectively. Fibers, fragments, films, and microbeads were documented at all sampling points, and most of the fragments and films (98%) were irregular in shape. In addition, a little elongated, rectangular, and cylindrical-shaped particle were also visualized. While comparing with other studies conducted worldwide, fibers were found to contribute a significant portion of most beach sediment (Graca et al., 2017; Sathish et al., 2019; Nguyen et al., 2020), showing a similar pattern to our present findings (Table 1). Among the MPs types, fibers were also observed to be dominant in the surface sediment from Belgium, Singapore, Slovenia, and South Africa (Nor and Obbard, 2014; Nel and Froneman, 2015). However, a larger amount of fiber found in the beach sediment of Kuakata, Bangladesh, may be originated from clothing materials and industrial fabrics, domestic laundry effluents through river discharge, fishing activity using nets and ropes in the Bay of Bengal. Besides, food and beverage packaging plastics disposed of by the tourists and locals might have caused the presence of MPs in the study area.
Most of the MPs were found colored (60%) in the sediment of Kuakata, whereas 40% were transparent. The highest number of MPs were purple (27%) in color with an average of 62 ± 24 items kg–1, followed by red (12%; 29 ± 14 items kg–1), blue (10%; 23 ± 13 items kg–1), brown (6%; 15 ± 11 items kg–1), and others (5%; 12 ± 11 items kg–1). The mean abundance of transparent MPs was 92 ± 25 items kg–1. Colorful MPs were distributed on every sampling point of Kuakata Beach and highest (68%) in site S4 (Figure 4B), which might be degraded mainly from plastic food and beverage package. MPs’ color may be attributed to their various sources (Patterson et al., 2019). However, the finding of our study was consistent with some other studies conducted worldwide (Peng et al., 2017; Robin et al., 2019; Yuan et al., 2019), who reported that most MPs were colored particles in the sediment. As bleaching processes happen in the marine ecosystem, it is difficult to comment on MPs’ color (Stolte et al., 2015). Nevertheless, the color of the MPs is a significant factor as the marine organisms prey on colored MPs for resembling their prey, and the results have already been documented in many parts of the world (Ory et al., 2018; Botterell et al., 2019; Hossain et al., 2019, 2020; Hoellein et al., 2021; Liu et al., 2021; Muller, 2021; Wootton et al., 2021).
Microplastics ranging from 0.3 to 5 mm were the primary concern in this investigation. The observed MPs were thus categorized into three distinct groups among which size range between 1 and 5 mm comprised almost half (55%) of total MPs with an average of 127 ± 34 items kg–1, followed by 0.5 − 1 mm (31%; 72 ± 19 items kg–1), and <0.5 mm (14%; 34 ± 15 items kg–1) (Figure 4C). Most fibers were predominantly documented in a size range of 1–5 mm. Though our findings were similar to some authors (Zhang et al., 2016; Sagawa et al., 2018), many others disagree with these (Nor and Obbard, 2014; Klein et al., 2015; Lots et al., 2017; Peng et al., 2017; Urban-Malinga et al., 2020; Wang S. et al., 2020) who reported that MPs < 1 mm were more abundant. It was proven that MPs in sediment are accredited from large plastic particles and domestic laundry wash effluent (Yu et al., 2018). Approximately 6,000,000 microfibers ranging from 20 to 2,000 mm can be released from each 5 kg of polyester fabrics washing effluent (De Falco et al., 2017), which degrade to MPs (<5 mm) due to water turbulence, wave action, and high UV from sunlight (Auta et al., 2017).
Correlation With Grain Size Distribution
Beach sediments of Kuakata were primarily composed of very fine sands (39%) and mud (35%), followed by fine sand (24%), medium sand (1.5%), and coarse sand [0.5 (Figure 5)]. However, a positive correlation was found between the MPs abundance and the finer grain size distributions from each sampling point (p = 0.055; r = 0.7). McLachlan and Brown (2006) stated that beach sediments work as pollution traps that nonspecifically adsorb particles carried by tides and currents. It was proven that the finer the sediment is more effective to trap the particles. Besides, fine-grained sediments are typical for accumulation regions, therefore, may be susceptible to pollution. In this primary investigation, we have noticed higher MPs incidence in finer sediment grain size, which reinforced our assumption that finer sediments act as pollution traps for MPs on beaches. Nevertheless, the absence of the relationship between MPs occurrence and finer sediment grain size was previously observed from tidal beaches (Browne et al., 2013; Mathalon and Hill, 2014; Urban-Malinga et al., 2020) as well as shallow coastal sediment (Alomar et al., 2016). Such findings in our study might be due to the position of beaches in the river estuary (Galachiap river and Andharmanik river), where deposition happens with substantial domestic and industrial input.
Composition and Sources of Microplastics
A total of 12 representative samples of a size range between 1 and 5 mm were extracted for FTIR analysis, and 11 were found as plastic polymer, while 1 remained unidentified. Our investigation elucidated three types of polymers, namely, PET, polyethylene (PE), and polypropylene (PP), in the sediment samples of Kuakata, Bangladesh. PET was found to be the most abundant polymer type, contributing 45.5% of the total samples identified through FTIR analysis, whereas PP was found to be least (18.2%). The FTIR spectra of these polymers are shown in Figure 6. Due to the aging, natural weathering, and degradation of MPs, some identical peaks of those plastic polymers were not found in the FTIR spectrum (Wang et al., 2017; Sathish et al., 2019). However, these polymers were common MPs in the coastal ecosystems (Sathish et al., 2019; Godoy et al., 2020; Selvam et al., 2020; Wu et al., 2021; Zhu et al., 2021).
Recognized polymer type through FTIR analysis did not provide enough evidence to identify the exact sources of MPs origin (Claessens et al., 2011). We can only assume their potential sources based on the extensive discretion of our findings (Wang et al., 2019). The main source of MPs in the studied beach might be river discharge, surface runoff, and plastics deposited by tourists and locals. However, most of the fibers found in this study were PET and fragments were either PE or PP. The possible sources of PET and PP could be clothes and textile products as these polymers are extensively used industrially. PP is also used for packaging food, beverage, plastic containers, carpets, and pipes. PE is another type of polymer found in the studied area which is used widely as food-packaging film and containers for oil, shampoos, soap, etc. (Hossain et al., 2020; Wu et al., 2021).
Ecological Risk Assessment of Microplastics With Pollution Load Index
The PLI value of each sampling site (S1–S8) was calculated, and the findings were illustrated in Figure 7. The highest value of PLI was evaluated in the S8 sampling point (1.43 ± 0.02), followed by S7 (1.36 ± 0.02), S1 (1.33 ± 0.02), S4 (1.27 ± 0.01), S5 (1.20 ± 0.02), S3 (1.11 ± 0.02), S6 (1.09 ± 0.02), and S2 (1.01 ± 0.01). The PLI specified that the beach sediment of Kuakata belonged to the risk category I of the pollution index, indicating slightly polluted by the MPs. However, the results of one-way ANOVA showed that the values of PLI significantly varied (F = 211.7, p < < 0.001) among the eight stations.
The estuarine beach (i.e., S8, S7, and S1) possesses a higher PLI value due to massive river discharge from two main estuaries along with tourism activities (Zhang et al., 2019). Moreover, we mentioned earlier that the estuarine sediments of Kuakata mainly consist of fine sand, which acts as a more effective pollutant trapping agent. Tourist activity and beachside development programs would produce a significant number of plastic wastes which may dispose of on the beach. On the contrary, the S2 sampling point had a lower PLI value which might be due to the lower touristic and beach developmental activities. However, according to these findings, it can be concluded that the PLI value can assess the degree of MP contamination in an area, but it is not possible to calculate the precise MP concentration by the PLI values (Wang et al., 2021). The toxic effect of MPs is primarily associated with the hazard scores; therefore, the value of PLI is not a practical endpoint of health risk assessment. Besides, the evaluation of health risks allied with MPs exposure is quite deficient. MPs might be ingested through drink or food and inhaled (Rist et al., 2018; Cox et al., 2019; Vianello et al., 2019). Hence, advanced investigation regarding possible exposure pathways of MPs and their menace to humans is needed.
Conclusion
This investigation aimed to assess the occurrence and characteristics of MPs, for the first time, in beach sediments from Kuakata, Northern Bay of Bengal, Bangladesh. The results confirmed the presence of various types of MPs (fibers, microbeads, fragments, etc.) and polymer forms (PET, PE, and PP) in beach sediment samples with the highest density detected at sampling points near the estuary, which could be attributed to colossal river discharges along with tourism activities. The higher incidence of MPs was found in finer sediment grain sizes, which supports the assumption that finer sediments act on beaches as pollution traps for MPs. The abundance and nature of MPs indicate that these MPs are derived from land-based sources. PLI analyses showed the beach sediments of Kuakata were in category I of pollution index is slightly polluted. This finding can help inform improved management of local and regional plastic debris. More long-term and systematic studies on the impacts of MPs on marine life, habitats, and eventually on human health are recommended.
Data Availability Statement
The original contributions presented in the study are included in the article/supplementary material, further inquiries can be directed to the corresponding authors.
Author Contributions
PB contributed to sample collection, laboratory analysis, and draft writing. MH and JS contributed to conceptualization, design, writing, and supervision. A-AN contributed to data analysis and draft writing. TC and SL contributed to FTIR sample processing and analysis. JY contributed to writing and editing. MN contributed to formatting, writing, and editing. All authors contributed to the article and approved the submitted version.
Funding
This study was primarily supported financially by the National Key Research and Development Project of China (2019YFC1407800) and the National Natural Science Foundation of China (41876134) to JS.
Conflict of Interest
The authors declare that the research was conducted in the absence of any commercial or financial relationships that could be construed as a potential conflict of interest.
Publisher’s Note
All claims expressed in this article are solely those of the authors and do not necessarily represent those of their affiliated organizations, or those of the publisher, the editors and the reviewers. Any product that may be evaluated in this article, or claim that may be made by its manufacturer, is not guaranteed or endorsed by the publisher.
References
Alomar, C., Estarellas, F., and Deudero, S. (2016). Microplastics in the Mediterranean Sea: deposition in coastal shallow sediments, spatial variation and preferential grain size. Mar. Environ. Res. 115, 1–10. doi: 10.1016/j.marenvres.2016.01.005
Anela, C. C., Robison, B. H., Gagne, T. O., Erwin, B., Evan, F., Halden, R. U., et al. (2019). The vertical distribution and biological transport of marine microplastics across the epipelagic and mesopelagic water column. Sci. Rep. 9:7843. doi: 10.1038/s41598-019-44117-2
Auta, H. S., Emenike, C. U., and Fauziah, S. H. (2017). Distribution and importance of microplastics in the marine environment: a review of the sources, fate, effects, and potential solutions. Environ. Int. 102, 165–176. doi: 10.1016/j.envint.2017.02.013
Bangladesh Meteorological Department [BMD] (2016). Monthly Maximum and Minimum Temperature, Normal monthly rainfall. Dhaka, Bangladesh: Bangladesh Meteorological Department.
Bhowmick, G. D., Sarmah, A. K., and Dubey, B. (2021). Microplastics in the NZ environment: current status and future directions. Case Stud. Chem. Environ. Eng. 3:100076.
Bonello, G., Varrella, P., and Pane, L. (2018). First evaluation of microplastic content in benthic filter-feeders of the Gulf of La Spezia (Ligurian Sea). J. Aquat. Food Prod. Technol. 27, 284–291. doi: 10.1080/10498850.2018.1427820
Botterell, Z. L. R., Beaumont, N., Dorrington, T., Steinke, M., Thompson, R. C., and Lindeque, P. K. (2019). Bioavailability and effects of microplastics on marine zooplankton: a review. Environ. Pollut. 245, 98–110. doi: 10.1016/j.envpol.2018.10.065
Boucher, J., and Friot, D. (2017). Primary microplastics in the oceans: a global evaluation of sources Gland. Switzerland: IUCN.
Browne, M. A., Niven, S. J., Galloway, T. S., Rowland, S. J., and Thompson, R. C. (2013). Microplastic moves pollutants and additives to worms, reducing functions linked to health and biodiversity. Curr. Biol. 23, 2388–2392. doi: 10.1016/j.cub.2013.10.012
Camacho, M., Herrera, A., Gómez, M., Acosta-Dacal, A., Martínez, I., Henríquez-Hernández, L. A., et al. (2019). Organic pollutants in marine plastic debris from Canary Islands beaches. Sci. Total Environ. 662, 22–31. doi: 10.1016/j.scitotenv.2018.12.422
Canniff, P. M., and Hoang, T. C. (2018). Microplastic ingestion by Daphnia magna and its enhancement on algal growth. Sci. Total Environ. 633, 500–507. doi: 10.1016/j.scitotenv.2018.03.176
Carpenter, E. J., and Smith, K. L. (1972). Plastics on the Sargasso Sea surface. Science 175, 1240–1241. doi: 10.1126/science.175.4027.1240
Catarino, A. I., Macchia, V., Sanderson, W. G., Thompson, R. C., and Henry, T. B. (2018). Low levels of microplastics (MP) in wild mussels indicate that MP ingestion by humans is minimal compared to exposure via household fibres fallout during a meal. Environ. Pollut. 237, 675–684. doi: 10.1016/j.envpol.2018.02.069
Cheung, P. K., Cheung, L. T. O., and Fok, L. (2016). Seasonal variation in the abundance of marine plastic debris in the estuary of a subtropical macro-scale drainage basin in South China. Sci. Total Environ. 562, 658–665. doi: 10.1016/j.scitotenv.2016.04.048
Claessens, M., De Meester, S., Van Landuyt, L., De Clerck, K., and Janssen, C. R. (2011). Occurrence and distribution of microplastics in marine sediments along the Belgian coast. Mar. Pollut. Bull. 62, 2199–2204. doi: 10.1016/j.marpolbul.2011.06.030
Coppock, R. L., Cole, M., Lindeque, P. K., Queirós, A. M., and Galloway, T. S. (2017). A small-scale, portable method for extracting microplastics from marine sediments. Environ. Pollut. 230, 829–837. doi: 10.1016/j.envpol.2017.07.017
Corcoran, P. L., Norris, T., Ceccanese, T., Walzak, M. J., Helm, P. A., and Marvin, C. H. (2015). Hidden plastics of Lake Ontario, Canada and their potential preservation in the sediment record. Environ. Pollut. 204, 17–25. doi: 10.1016/j.envpol.2015.04.009
Cox, K. D., Covernton, G. A., Davies, H. L., Dower, J. F., Juanes, F., and Dudas, S. E. (2019). Human consumption of microplastics. Environ. Sci. Technol. 53, 7068–7074.
Crew, A., Gregory-Eaves, I., and Ricciardi, A. (2020). Distribution, abundance, and diversity of microplastics in the upper St. Lawrence River. Environ. Pollut. 260:113994. doi: 10.1016/j.envpol.2020.113994
de Carvalho, D. G., and Neto, J. A. B. (2016). Microplastic pollution of the beaches of Guanabara Bay, Southeast Brazil. Ocean Coast. Manage. 128, 10–17. doi: 10.1016/j.ocecoaman.2016.04.009
De Falco, F., Gullo, M. P., Gentile, G., Di Pace, E., Cocca, M., Gelabert, L., et al. (2017). Evaluation of microplastic release caused by textile washing processes of synthetic fabrics. Environ. Pollut. 236, 916–925. doi: 10.1016/j.envpol.2017.10.057
De Witte, B., Devriese, L., Bekaert, K., Hoffman, S., Vandermeersch, G., Cooreman, K., et al. (2014). Quality assessment of the blue mussel (Mytilus edulis): comparison between commercial and wild types. Mar. Pollut. Bull. 85, 146–155. doi: 10.1016/j.marpolbul.2014.06.006
Duan, H., Yu, L., Tian, F., Zhai, Q., Fan, L., and Chen, W. (2020). Gut microbiota: a target for heavy metal toxicity and a probiotic protective strategy. Sci. Total Environ. 742:140429. doi: 10.1016/j.scitotenv.2020.140429
Ferreira, M., Thompson, J., Paris, A., Rohindra, D., and Rico, C. (2020). Presence of microplastics in water, sediments and fish species in an urban coastal environment of Fiji, a Pacific small island developing state. Mar. Pollut. Bull. 153:110991. doi: 10.1016/j.marpolbul.2020.110991
Fetner, H., and Miller, S. A. (2021). Environmental payback periods of reusable alternatives to single-use plastic kitchenware products. Int. J. Life Cycle Asses. 26, 1521–1537.
Fred-Ahmadu, O. H., Bhagwat, G., Oluyoye, I., Benson, N. U., Ayejuyo, O. O., and Palanisami, T. (2020). Interaction of chemical contaminants with microplastics: principles and perspectives. Sci. Total Environ. 706:135978. doi: 10.1016/j.scitotenv.2019.135978
Frère, L., Paul-Pont, I., Rinnert, E., Petton, S., Jaffré, J., Bihannic, I., et al. (2017). Influence of environmental and anthropogenic factors on the composition, concentration and spatial distribution of microplastics: a case study of the Bay of Brest (Brittany, France). Environ. Pollut. 225, 211–222. doi: 10.1016/j.envpol.2017.03.023
Frias, J. P. G. L., and Nash, R. (2019). Microplastics: finding a consensus on the definition. Mar. Pollut. Bull. 138, 145–147. doi: 10.1016/j.marpolbul.2018.11.022
Gardon, T., El Rakwe, M., Paul-Pont, I., Le Luyer, J., Thomas, L., Prado, E., et al. (2021). Microplastics contamination in pearl-farming lagoons of French Polynesia. J. Hazard. Mater. 419:126396. doi: 10.1016/j.jhazmat.2021.126396
Geyer, R., Jambeck, J. R., and Law, K. L. (2017). Production, use, and fate of all plastics ever made. Sci. Adv. 3:e1700782. doi: 10.1126/sciadv.1700782
Ghosh, G. C., Akter, S. M., Islam, R. M., Habib, A., Chakraborty, T. K., Zaman, S., et al. (2021). Microplastics contamination in commercial marine fish from the Bay of Bengal. Reg. Stud. Mar. Sci. 44:101728. doi: 10.1016/j.rsma.2021.101728
Godoy, V., Prata, J. C., Blázquez, G., Almendros, A. I., Duarte, A. C., Rocha-Santos, T., et al. (2020). Effects of distance to the sea and geomorphological characteristics on the quantity and distribution of microplastics in beach sediments of Granada (Spain). Sci. Total Environ. 746:142023. doi: 10.1016/j.scitotenv.2020.142023
Graca, B., Szewc, K., Zakrzewska, D., Dołęga, A., and Szczerbowska-Boruchowska, M. (2017). Sources and fate of microplastics in marine and beach sediments of the Southern Baltic Sea—a preliminary study. Environ. Sci. Pollut. Res. 24, 7650–7661. doi: 10.1007/s11356-017-8419-5
Hahladakis, J. N., Velis, C. A., Weber, R., Iacovidou, E., and Purnell, P. (2018). An overview of chemical additives present in plastics: migration, release, fate and environmental impact during their use, disposal and recycling. J. Hazard. Mater. 344, 179–199. doi: 10.1016/j.jhazmat.2017.10.014
Hidalgo-Ruz, V., Gutow, L., Thompson, R. C., and Thiel, M. (2012). Microplastics in the marine environment: a review of the methods used for identification and quantification. Environ. Sci. Technol. 46, 3060–3075. doi: 10.1021/es2031505
Hoellein, T., Rovegno, C., Uhrin, A. V., Johnson, E., and Herring, C. (2021). Microplastics in invasive freshwater mussels (Dreissena sp.): spatiotemporal variation and occurrence with chemical contaminants. Front. Mar. Sci. 8:690401. doi: 10.3389/fmars.2021.690401
Hossain, M. B., Banik, P., Nur, A. A. U., and Rahman, T. (2021). Abundance and characteristics of microplastics in sediments from the world’s longest natural beach, Cox’s Bazar, Bangladesh. Mar. Pollut. Bull. 163:111956. doi: 10.1016/j.marpolbul.2020.111956
Hossain, M. S., Rahman, M. S., Uddin, M. N., Sharifuzzaman, S. M., Chowdhury, S. R., Sarker, S., et al. (2020). Microplastic contamination in Penaeid shrimp from the Northern Bay of Bengal. Chemosphere 238:124688. doi: 10.1016/j.chemosphere.2019.124688
Hossain, M. S., Sobhan, F., Uddin, M. N., Sharifuzzaman, S. M., Chowdhury, S. R., Sarker, S., et al. (2019). Microplastics in fishes from the Northern Bay of Bengal. Sci. Total Environ. 690, 821–830. doi: 10.1016/j.scitotenv.2019.07.065
Isobe, A., Kubo, K., Tamura, Y., Nakashima, E., and Fujii, N. (2014). Selective transport of microplastics and mesoplastics by drifting in coastal waters. Mar. Pollut. Bull. 89, 324–330. doi: 10.1016/j.marpolbul.2014.09.041
Jung, M. R., Horgen, F. D., Orski, S. V., Rodriguez, C. V., Beers, K. L., Balazs, G. H., et al. (2018). Validation of ATR FT-IR to identify polymers of plastic marine debris, including those ingested by marine organisms. Mar. Pollut. Bull. 127, 704–716. doi: 10.1016/j.marpolbul.2017.12.061
Klein, S., Worch, E., and Knepper, T. P. (2015). Occurrence and spatial distribution of microplastics in sediment of the Rhine-Main Area in Germany. Environ. Sci. Technol. 49, 6070–6076. doi: 10.1021/acs.est.5b00492
Laglbauer, B. J., Franco-Santos, R. M., Andreu-Cazenave, M., Brunelli, L., Papadatou, M., Palatinus, A., et al. (2014). Macrodebris and microplastics from beaches in Slovenia. Mar. Pollut. Bull. 89, 356–366. doi: 10.1016/j.marpolbul.2014.09.036
Li, R., Yu, L., Chai, M., Wu, H., and Zhu, X. (2020). The distribution, characteristics and ecological risks of microplastics in the mangroves of Southern China. Sci. Total Environ. 708:135025. doi: 10.1016/j.scitotenv.2019.135025
Lithner, D., Larsson, Å, and Dave, G. (2011). Environmental and health hazard ranking and assessment of plastic polymers based on chemical composition. Sci. Total Environ. 409, 3309–3324. doi: 10.1016/j.scitotenv.2011.04.038
Liu, S., Shi, J., Wang, J., Dai, Y., Li, H., Li, J., et al. (2021). Interactions between microplastics and heavy metals in aquatic environments: a review. Front. Microbiol. 12:652520. doi: 10.3389/fmicb.2021.652520
Lo, H. S., Xu, X., Wong, C. Y., and Cheung, S. G. (2018). Comparisons of microplastic pollution between mudflats and sandy beaches in Hong Kong. Environ. Pollut. 236, 208–217. doi: 10.1016/j.envpol.2018.01.031
Lots, F. A., Behrens, P., Vijver, M. G., Horton, A. A., and Bosker, T. (2017). A large-scale investigation of microplastic contamination: abundance and characteristics of microplastics in European beach sediment. Mar. Pollut. Bull. 123, 219–226. doi: 10.1016/j.marpolbul.2017.08.057
Lusher, A., Hollman, P., and Mendoza-Hill, J. (2017). Microplastics in fisheries and aquaculture: status of knowledge on their occurrence and implications for aquatic organisms and food safety. FAO Fisheries and Aquaculture Technical Paper. No. 615. Rome, Italy: FAO, 147.
Lusher, A. L., Welden, N. A., Sobral, P., and Cole, M. (2017). Sampling, isolating and identifying microplastics ingested by fish and invertebrates. Anal. Methods 9, 1346–1360. doi: 10.1039/C6AY02415G
Mahfooz, Y., Yasar, A., Guijian, L., Islam, Q. U., Akhtar, A. B. T., Rasheed, R., et al. (2020). Critical risk analysis of metals toxicity in wastewater irrigated soil and crops: a study of a semi-arid developing region. Sci. Rep. 10:12845. doi: 10.1038/s41598-020-69815-0
Masura, J., Baker, J. E., Foster, G. D., Arthur, C., and Herring, C. (2015). Laboratory methods for the analysis of microplastics in the marine environment: recommendations for quantifying synthetic particles in waters and sediments. NOAA Technical Memorandum NOS-OR&R-48. Washington, DC: NOAA.
Mathalon, A., and Hill, P. (2014). Microplastic fibers in the intertidal ecosystem surrounding Halifax Harbor, Nova Scotia. Mar. Pollut. Bull. 81, 69–79. doi: 10.1016/j.marpolbul.2014.02.018
McLachlan, A., and Brown, A. C. (2006). The Ecology of Sandy Shores, 2nd edition. Cambridge, Massachusetts: Academic Press, Elsevier.
Möller, J. N., Löder, M. G., and Laforsch, C. (2020). Finding microplastics in soils: a review of analytical methods. Environ. Sci. Technol. 54, 2078–2090. doi: 10.1021/acs.est.9b04618
Muller, C. (2021). Not as bad as it seems? A literature review on the case of microplastic uptake in fish. Front. Mar. Sci. 8:672768. doi: 10.3389/fmars.2021.672768
Nel, H. A., and Froneman, P. W. (2015). A quantitative analysis of microplastic pollution along the south-eastern coastline of South Africa. Mar. Pollut. Bull. 101, 274–279. doi: 10.1016/j.marpolbul.2015.09.043
Neufeld, L., Stassen, F., Sheppard, R., and Gilman, T. (2016). The new plastics economy: rethinking the future of plastics. Cologny: World Economic Forum.
Nguyen, Q. A. T., Nguyen, H. N. Y., Strady, E., Nguyen, Q. T., and Trinh-Dang, M. (2020). Characteristics of microplastics in shoreline sediments from a tropical and urbanized beach (Da Nang, Vietnam). Mar. Pollut. Bull. 161:111768. doi: 10.1016/j.marpolbul.2020.111768
Nithin, A., Sundaramanickam, A., Surya, P., Sathish, M., Soundharapandiyan, B., and Balachandar, K. (2021). Microplastic contamination in salt pans and commercial salts–A baseline study on the salt pans of Marakkanam and Parangipettai, Tamil Nadu, India. Mar. Pollut. Bull. 165:112101. doi: 10.1016/j.marpolbul.2021.112101
Noda, I., Dowrey, A. E., Haynes, J. L., and Marcott, C. (2007). “Group frequency assignments for major infrared bands observed in common synthetic polymers,” in Physical Properties of Polymers Handbook, ed. J. E. Mark (New York: Springer), 395–406. doi: 10.1007/978-0-387-69002-5_22
Nor, N. H. M., and Obbard, J. P. (2014). Microplastics in Singapore’s coastal mangrove ecosystems. Mar. Pollut. Bull. 79, 278–283. doi: 10.1016/j.marpolbul.2013.11.025
Ortiz-Villanueva, E., Jaumot, J., Martínez, R., Navarro-Martín, L., Piña, B., and Tauler, R. (2018). Assessment of endocrine disruptors effects on zebrafish (Danio rerio) embryos by untargeted LC-HRMS metabolomics analysis. Sci. Total Environ. 635, 156–166. doi: 10.1016/j.scitotenv.2018.03.369
Ory, N., Chagnon, C., Felix, F., Fernández, C., Ferreira, J. L., Gallardo, C., et al. (2018). Low prevalence of microplastic contamination in planktivorous fish species from the Southeast Pacific Ocean. Mar. Pollut. Bull. 127, 211–216. doi: 10.1016/j.marpolbul.2017.12.016
Parvin, F., Jannat, S., and Tareq, S. M. (2021). Abundance, characteristics and variation of microplastics in different freshwater fish species from Bangladesh. Sci. Total Environ. 784:147137. doi: 10.1016/j.scitotenv.2021.147137
Parvin, F., Nath, J., Hannan, T., and Tareq, S. M. (2022). Proliferation of microplastics in commercial sea salts from the world longest sea beach of Bangladesh. Environ. Adv. 7:100173.
Patterson, J., Jeyasanta, K. I., Sathish, N., Booth, A. M., and Edward, J. K. P. (2019). Profiling microplastics in the Indian edible oyster, Magallana bilineata collected from the Tuticorin coast, Gulf of Mannar, Southeastern India. Sci. Total Environ. 691, 727–735. doi: 10.1016/j.scitotenv.2019.07.063
Peng, G., Zhu, B., Yang, D., Su, L., Shi, H., and Li, D. (2017). Microplastics in sediments of the Changjiang Estuary, China. Environ. Pollut. 225, 283–290. doi: 10.1016/j.envpol.2016.12.064
Plastics Europe Market Research Group [PEMRG] (2021). Plastics–the Facts 2020: an analysis of European plastics production, demand and recovery for 2020. Brussels: PEMRG
Rahman, M. M., Rahman, M. A., and Nahar, A. (2015). Status of “Kuakata” a neglected natural scenic resource of Bangladesh. Eur. Sci. J. 11, 87–98.
Rashid, M. B., and Mahmood, A. (2011). Longshore currents and its effect on Kuakata beach, Bangladesh, national seminar on Bangladesh coast: geology hazards and resources, 29–30 July, 2011. People’s Republic of Bangladesh. Dhaka, Bangladesh: Geological Survey of Bangladesh.
Rist, S., Carney Almoroth, B., Hartmann, N. B., and Karlsson, T. M. (2018). A critical perspective on early communications concerning human health aspects of microplastics. Sci. Total Environ. 626, 720–726. doi: 10.1016/j.scitotenv.2018.01.092
Rist, S. E., Assidqi, K., Zamani, N. P., Appel, D., Perschke, M., Huhn, M., et al. (2016). Suspended micro-sized PVC particles impair the performance and decrease survival in the Asian green mussel Perna viridis. Mar. Pollut. Bull. 111, 213–220. doi: 10.1016/j.marpolbul.2016.07.006
Robin, R. S., Karthik, R., Purvaja, R., Ganguly, D., Anandavelu, I., Mugilarasan, M., et al. (2019). Holistic assessment of microplastics in various coastal environmental matrices, southwest coast of India. Sci. Total Environ. 703:134947. doi: 10.1016/j.scitotenv.2019.134947
Rodrigues, M. O., Abrantes, N., Gonçalves, F. J. M., Nogueira, H., Marques, J. C., and Gonçalves, A. M. M. (2018). Spatial and temporal distribution of microplastics in water and sediments of a freshwater system (Antuã River, Portugal). Sci. Total Environ. 633, 1549–1559. doi: 10.1016/j.scitotenv.2018.03.233
Sagawa, N., Kawaai, K., and Hinata, H. (2018). Abundance and size of microplastics in a coastal sea: comparison among bottom sediment, beach sediment, and surface water. Mar. Pollut. Bull. 133, 532–542. doi: 10.1016/j.marpolbul.2018.05.036
Sathish, N., Jeyasanta, K. I., and Patterson, J. (2019). Abundance, characteristics and surface degradation features of microplastics in beach sediments of five coastal areas in Tamil Nadu, India. Mar. Pollut. Bull. 142, 112–118. doi: 10.1016/j.marpolbul.2019.03.037
Selvam, S., Manisha, A., Venkatramanan, S., Chung, S. Y., and Paramasivam, C. R. (2020). Microplastic presence in commercial marine sea salts: a baseline study along Tuticorin Coastal salt pan stations, Gulf of Mannar, South India. Mar. Pollut. Bull. 150:110675. doi: 10.1016/j.marpolbul.2019.110675
Sivagami, M., Selvambigai, M., Devan, U., Velangani, A. A. J., Karmegam, N., Biruntha, M., et al. (2021). Extraction of microplastics from commonly used sea salts in India and their toxicological evaluation. Chemosphere 263:128181. doi: 10.1016/j.chemosphere.2020.128181
Stolte, A., Forster, S., Gerdts, G., and Schubert, H. (2015). Microplastic concentrations in beach sediments along the German Baltic coast. Mar. Pollut. Bull. 99, 216–229. doi: 10.1016/j.marpolbul.2015.07.022
Taha, Z. D., Amin, R. M., Anuar, S. T., Nasser, A. A. A., and Sohaimi, E. S. (2021). Microplastics in seawater and zooplankton: a case study from Terengganu estuary and offshore waters, Malaysia. Sci. Total Environ. 786:147466. doi: 10.1016/j.scitotenv.2021.147466
Tomlinson, D. L., Wilson, J. G., Harris, C. R., and Jeffrey, D. W. (1980). Problems in the assessment of heavy-metal levels in estuaries and the formation of a pollution index. Helgol. Mar. Res. 33, 566–575.
Urban-Malinga, B., Zalewski, M., Jakubowska, A., Wodzinowski, T., Malinga, M., Pałys, B., et al. (2020). Microplastics on sandy beaches of the southern Baltic Sea. Mar. Pollut. Bull. 155:111170. doi: 10.1016/j.marpolbul.2020.111170
Van Cauwenberghe, L., and Janssen, C. R. (2014). Microplastics in bivalves cultured for human consumption. Environ. Pollut. 193, 65–70. doi: 10.1016/j.envpol.2014.06.010
Vianello, A., Boldrin, A., Guerriero, P., Moschino, V., Rella, R., Sturaro, A., et al. (2013). Microplastic particles in sediments of Lagoon of Venice, Italy: first observations on occurrence, spatial patterns and identification. Estuar. Coast. Shelf Sci. 130, 54–61. doi: 10.1016/j.ecss.2013.03.022
Vianello, A., Jensen, R. L., Liu, L., and Vollertsen, J. (2019). Simulating human exposure to indoor airborne microplastics using a breathing thermal manikin. Sci. Rep. 9:8670. doi: 10.1038/s41598-019-45054-w
Wang, G., Lu, J., Li, W., Ning, J., Zhou, L., Tong, Y., et al. (2021). Seasonal variation and risk assessment of microplastics in surface water of the Manas River Basin, China. Ecotoxicol. Environ. Saf. 208:111477. doi: 10.1016/j.ecoenv.2020.111477
Wang, Q., Shan, E., Zhang, B., Teng, J., Wu, D., Yang, X., et al. (2020). Microplastic pollution in intertidal sediments along the coastline of China. Environ. Pollut. 263:114428. doi: 10.1016/j.envpol.2020.114428
Wang, S., Chen, H., Zhou, X., Tian, Y., Lin, C., Wang, W., et al. (2020). Microplastic abundance, distribution and composition in the mid-west Pacific Ocean. Environ. Pollut. 264:114125. doi: 10.1016/j.envpol.2020.114125
Wang, T., Zou, X., Li, B., Yao, Y., Zang, Z., Li, Y., et al. (2019). Preliminary study of the source apportionment and diversity of microplastics: taking floating microplastics in the South China Sea as an example. Environ. Pollut. 245, 965–974. doi: 10.1016/j.envpol.2018.10.110
Wang, W., Ndungu, A. W., Li, Z., and Wang, J. (2017). Microplastics pollution in inland freshwaters of China: a case study in urban surface waters of Wuhan, China. Sci. Total Environ. 575, 1369–1374. doi: 10.1016/j.scitotenv.2016.09.213
Wang, W., and Wang, J. (2018). Different partition of polycyclic aromatic hydrocarbon on environmental particulates in freshwater: microplastics in comparison to natural sediment. Ecotoxicol. Environ. Saf. 147, 648–655. doi: 10.1016/j.ecoenv.2017.09.029
Webb, S., Gaw, S., Marsden, I. D., and McRae, N. K. (2020). Biomarker responses in New Zealand green-lipped mussels Perna canaliculus exposed to microplastics and triclosan. Ecotoxicol. Environ. Saf. 201:110871. doi: 10.1016/j.ecoenv.2020.110871
Weidemann, E., Andersson, P. L., Bidleman, T., Boman, C., Carlin, D. J., Collina, E., et al. (2016). 14Th congress of combustion by-products and their health effectsdorigin, fate, and health effects of combustion-related air pollutants in the coming era of bio-based energy sources. Environ. Sci. Pollut. Res. 23, 8141–8159. doi: 10.1007/s11356-016-6308-y
Wentworth, C. K. (1922). A scale of grade and class terms for clastic sediments. J. Geol. 30, 377–392.
Wieczorek, A. M., Croot, P. L., Lombard, F., Sheahan, J. N., and Doyle, T. K. (2019). Microplastic ingestion by gelatinous zooplankton may lower efficiency of the biological pump. Environ. Sci. Technol. 53, 5387–5395. doi: 10.1021/acs.est.8b07174
Wong, G., Löwemark, L., and Kunz, A. (2020). Microplastic pollution of the Tamsui River and its tributaries in northern Taiwan: spatial heterogeneity and correlation with precipitation. Environ. Pollut. 260:113935. doi: 10.1016/j.envpol.2020.113935
Wootton, N., Ferreira, M., Reis-Santos, P., and Gillanders, B. M. (2021). A comparison of microplastic in fish from Australia and Fiji. Front. Mar. Sci. 8:690991. doi: 10.3389/fmars.2021.690991
Wu, X., Zhong, C., Wang, T., Zou, X., Zang, Z., Li, Q., et al. (2021). Occurrence and distribution of microplastics on recreational beaches of Haichow Bay, China. Environ. Sci. Pollut. Res. Int. 28, 6132–6145. doi: 10.1007/s11356-020-10987-7
Xu, P., Peng, G., Su, L., Gao, Y., Gao, L., and Li, D. (2018). Microplastic risk assessment in surface waters: a case study in the Changjiang Estuary, China. Mar. Pollut. Bull. 133, 647–654. doi: 10.1016/j.marpolbul.2018.06.020
Yaranal, N. A., Subbiah, S., and Mohanty, K. (2021). Identification, extraction of microplastics from edible salts and its removal from contaminated seawater. Environ. Technol. Innov. 21:101253.
Yu, F., Yang, C., Zhu, Z., Bai, X., and Ma, J. (2019). Adsorption behavior of organic pollutants and metals on micro/nanoplastics in the aquatic environment. Sci. Total Environ. 694:133643. doi: 10.1016/j.scitotenv.2019.133643
Yu, X., Ladewig, S., Bao, S., Toline, C. A., Whitmire, S., and Chow, A. T. (2018). Occurrence and distribution of microplastics at selected coastal sites along the southeastern United States. Sci. Total Environ. 61, 298–305. doi: 10.1016/j.scitotenv.2017.09.100
Yu, X., Peng, J., Wang, J., Wang, K., and Bao, S. (2016). Occurrence of microplastics in the beach sand of the Chinese inner sea: the Bohai Sea. Environ. Pollut. 214, 722–730. doi: 10.1016/j.envpol.2016.04.080
Yuan, W., Liu, X., Wang, W., Di, M., and Wang, J. (2019). Microplastic abundance, distribution and composition in water, sediments, and wild fish from Poyang Lake, China. Ecotoxicol. Environ. Saf. 170, 180–187. doi: 10.1016/j.ecoenv.2018.11.126
Zhang, B., Wu, D., Yang, X., Teng, J., Liu, Y., Zhang, C., et al. (2019). Microplastic pollution in the surface sediments collected from Sishili Bay, North Yellow Sea, China. Mar. Pollut. Bull. 141, 9–15. doi: 10.1016/j.marpolbul.2019.02.021
Zhang, K., Su, J., Xiong, X., Wu, X., Wu, C., and Liu, J. (2016). Microplastic pollution of lakeshore sediments from remote lakes in Tibet plateau, China. Environ. Pollut. 219, 450–455. doi: 10.1016/j.envpol.2016.05.048
Keywords: microplastic pollution, beach sediment, sand grain size, ecological risk assessment, Bangladesh
Citation: Banik P, Hossain MB, Nur A-AU, Choudhury TR, Liba SI, Yu J, Noman MA and Sun J (2022) Microplastics in Sediment of Kuakata Beach, Bangladesh: Occurrence, Spatial Distribution, and Risk Assessment. Front. Mar. Sci. 9:860989. doi: 10.3389/fmars.2022.860989
Received: 24 January 2022; Accepted: 23 February 2022;
Published: 13 April 2022.
Edited by:
Tanveer Adyel, Deakin University, AustraliaReviewed by:
Edward J. K. Patterson, Suganthi Devadason Marine Research Institute (SDMRI), IndiaGabriel Enrique De-la-Torre, Saint Ignatius of Loyola University, Peru
Copyright © 2022 Banik, Hossain, Nur, Choudhury, Liba, Yu, Noman and Sun. This is an open-access article distributed under the terms of the Creative Commons Attribution License (CC BY). The use, distribution or reproduction in other forums is permitted, provided the original author(s) and the copyright owner(s) are credited and that the original publication in this journal is cited, in accordance with accepted academic practice. No use, distribution or reproduction is permitted which does not comply with these terms.
*Correspondence: M. Belal Hossain, bWJobnN0dUBnbWFpbC5jb20=; Jun Sun, cGh5dG9wbGFua3RvbkAxNjMuY29t
†These authors have contributed equally to this work